- 1Department of Immunology, School of Basic Medical Sciences, Peking University, NHC Key Laboratory of Medical Immunology (Peking University), Beijing, China
- 2Department of Clinical Medicine, Mudanjiang Medical University, Mudanjiang, China
Proallergic type 2 helper T (Th2A) cells are a subset of memory Th2 cells confined to atopic individuals, and they include all the allergen-specific Th2 cells. Recently, many studies have shown that Th2A cells characterized by CD3+ CD4+ HPGDS+ CRTH2+ CD161high ST2high CD49dhigh CD27low play a crucial role in allergic diseases, such as atopic dermatitis (AD), food allergy (FA), allergic rhinitis (AR), asthma, and eosinophilic esophagitis (EoE). In this review, we summarize the discovery, biomarkers, and biological properties of Th2A cells to gain new insights into the pathogenesis of allergic diseases.
Introduction
Allergic diseases are the greatest prevalent chronic immunological diseases, including AD, asthma, and AR, which are estimated to affect more than 230 million, 330 million, and 400 million people worldwide, respectively (1–3). The prevalence of allergic diseases is increasing in both industrial and developing countries, making it a global epidemic (4). Notably, people with allergic diseases are more likely to have a family disposition known as atopy, defined by Coca and Cooke in 1923 (5). Many studies show that the progression of allergic diseases occurs in a predictable time sequence and is widely distributed to various organs, which is referred to as the atopic march (6). It is known that AD and FA in infancy gradually develop into asthma and AR in childhood, even EoE (7). However, the mechanism of the atopic march remains unclear.
It is known that allergic diseases are mainly driven by type 2 inflammation, mediated by Th2 cells (8). When atopic individuals are exposed to allergens, their epithelial cells start to secrete IL-25, IL-33, and TSLP, leading to the activation of dendritic cells, which promote the differentiation of allergen-specific Th2 cells (9, 10). The activated allergen-specific Th2 cells can not only favor B cells to produce allergen-specific IgE, but also recruit and activate basophils, mast cells, and eosinophils by secreting IL-4, IL-5, and IL-13 (11, 12). Thus, allergen-specific Th2 cells play a crucial role in the allergic response. Recently, pMHCII tetramer staining was widely used to study the allergen-specific Th2 cells (10, 13–17). However, the allergens in most allergic diseases are unclear. It is noted that Erik Wambre et al. defined a subset of memory Th2 cells confined to atopic individuals that include all allergen-specific Th2 cells as Th2A cells, which are characterized by CD4+ CRTH2+ CD161high HPGDS+ CD27low CD49dhigh ST2high, providing a novel approach to study the allergen-specific Th2 cells (18). Here, we review the discovery, biomarkers, and biological properties of Th2A cells to gain new insights into the pathogenesis of allergic diseases.
Discovery of Th2A cells
In type 2 inflammation, Th2 cells mainly trigger an inflammatory response, in which a subset of pathogenic effector Th2 cells (peTh2) are characterized by secreting high-level IL-5 cytokines (19, 20). After antigen elimination, most peTh2 cells are induced to undergo cell apoptosis, and part of them differentiate into memory-type Th2 cells (21). The memory-type Th2 cells in allergic diseases are activated by exposure to various allergens, which contribute to the recurrence of diseases. For instance, Kurokawa et al. demonstrate that the expression of ST2 in T cells is responsible for the pathogenesis of asthma (22). In addition, Calman Prussin et al. found that IL-5+ Th2 cells are highly differentiated Th2 cells that demand repetitive allergen exposure to generate, demonstrating that Th2-dominant diseases characterized by recurrent allergen stimulation possibly promote the generation of IL-5 Th2 cells, leading to the occurrence of eosinophilic inflammation (23). Moreover, Mitson et al. found that the CRTH2+ CD161high HPGDS+ memory Th2 cells play a central role in AD and eosinophilic gastrointestinal disease (EGID) (20). Numerous studies demonstrate that the CD45ROhigh CD69high ST2high IL17RBhigh memory Th2 cells are the primary pathogenic subset in eosinophilic chronic rhinosinusitis (24–26). Meanwhile, Stephen Till et al. proposed that IL-17RB+ CD4+ Th2 cells may represent a subset that co-expresses ST2 during activation and may be pathogenic for chronic rhinosinusitis with nasal polyposis via the IL-25/IL-33 axis (27).
In 2012, Wambre et al. found the pathogenicity of allergen-specific CD4+ T cells to be associated with the CRTH2+ CD27low phenotype (13). Wambre et al. proposed the Th2A cells, which can evaluate the severity of allergic diseases and the therapeutic effect of the allergen-specific immunotherapy (AIT) (28). In 2015, Wambre et al. clarified the importance of Th2A cells for allergic diseases and the possibility of Th2A cells as a target in AIT (29). Importantly, in 2017, Wambre et al. defined a subset of memory Th2 cells confined to atopic individuals that include all allergen-specific Th2 cells as Th2A cells, characterized by the expression of CD4+ CRTH2+ CD161high HPGDS+ CD27low CD49dhigh ST2high (18). Wambre et al. discovered that expression of a cardinal Th2 cytokine by peanut-reactive T cells was mainly restricted to allergic individuals and the Th2A cell subset and that a decrease in Th2A cells is also associated with peanut desensitization, confirming that Th2A cells are involved in the pathogenesis of FA (18, 30–32). Chiang et al. found the heterogeneity of effector Th2 subsets in food allergies (33). On the other hand, Renand et al. show that a decrease in grass pollen–specific CD4+ T cell frequency most closely paralleled the transient clinical outcome of allergen immunotherapy via either the subcutaneous or sublingual route (34). In 2020, Luce et al. found a decrease in Th2A cells after a period of food oral immunotherapy (OIT), but the frequency of DCs and ILCs remained, confirming the possibility of Th2A frequency as a new marker for OIT (35). In 2021, Bangert et al. found that Th2A cells were persistently maintained in the tissues of AD patients, suggesting that Th2A cells may be responsible for AD recurrence (36). Meanwhile, Luce et al. observed the frequency of CD38+ Th2A cells to be only significantly decreased in patients who received the treatment of HDM tablets, demonstrating the potential value of CD38+ Th2A cells as the new biomarker of asthma (37). In the meantime, Morgan et al. found that Th2A cells are enriched in esophageal tissue from EoE patients and associated with the recruitment of eosinophils to the esophageal tissue, indicating that Th2A cells are the pathogenic cells for EoE (38). A recent study by Vandamme et al. using TCR and transcriptomic analysis of dog allergen–specific T cell responses in allergic subjects revealed a close relationship between Th2-like, Th2, and Th2A cells (39).
Biomarkers of Th2A cells
HPGDS
Unlike conventional Th2 cells, Th2A cells have a high expression of hematopoietic prostaglandin D2 synthase (HPGDS). HPGDS is a glutathione transferase expressed mainly in mast cells and antigen-presenting cells (40). Upon the stimulation of allergens, arachidonic acid is liberated from phospholipids under the mediation of phospholipase A2 (PLA2), and some arachidonic acid can be transformed into PGH2 by the action of COX (41). HPGDS then catalyzes the conversion of arachidonic acid–derivative PGH2 to PGD2 with the assistance of GSH and Mg2+ (42). PGD2 acts on the G-protein coupled receptor DP1 and receptor DP2/CRTH2 expressed in Th2 cells to promote inflammation by the secretion of IL-4, IL-5, and IL-13 cytokines (43) (Figure 1). Thus, the high-level expression of HPGDS in Th2A cells helps to promote the type 2 inflammatory response. The study shows that the HPGDS inhibitor improved AD symptoms, possibly due to a reduction in PGD2 production, indicating that HPGDS might be a potential therapeutic target for allergic diseases (44, 45).
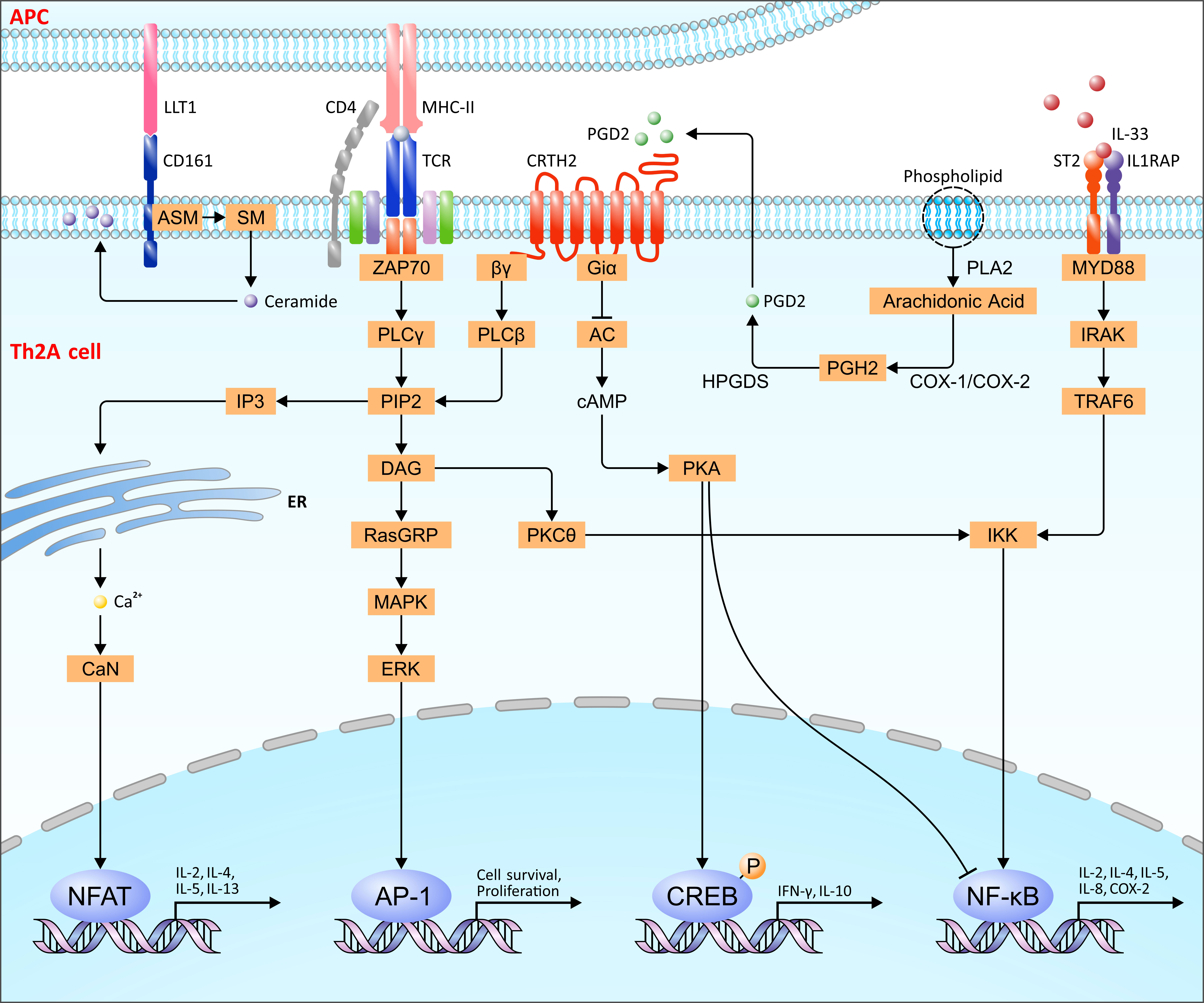
Figure 1 The signaling pathway of Th2A cells. The interaction of ST2 with IL-33 recruits MYD88 to activate TRAF6 by IRAK, leading to the activation of NF-kB released from the IKK complex. After CD161 combination with LLT1, aSMase is recruited to catalyze SM into ceramide, resulting in the aggregation in the membrane and inside the cells to mediate distinct functions. CRTH2 activated by PGD2 reduces cAMP production, thereby inhibiting NF-κB activity and phosphorylates CREB protein. Meanwhile, the same as TCR, CRTH2 also activates PKCθ, ERK/MAPK, and Ca2+ calcineurin signaling pathways to mediate cell survival, proliferation, and cytokine production through the transcription factor NF-κB, AP-1, and NFAT. Arachidonic acid derived from phospholipids is converted to PGH2 by COX-1/2, which can then be catalyzed into PGD2 by HPGDS. IKK, IκB kinase; ZAP70, ζ-chain associated protein kinase of 70 kDa; PLCγ, phospholipase Cγ; PIP2, phosphatidylinositol bisphosphate; DAG, diacylglycerol; PKCθ, protein kinase Cθ; RASGRP, RAS guanyl nucleotide–releasing protein; MAPK, mitogen-activated protein kinase; ERK, extracellular signal-regulated kinase; IP3, inositol trisphosphate; cAMP, cyclic adenosine 3, 5′-monophosphate; PKA, protein kinase A; COX-1/2, cyclooxygenases I and II; ASM, acid sphingomyelinase; CaN, calcineurin.
CRTH2
The chemoattractant receptor homologous molecule expressed on T-helper-type-2 cells (CRTH2) is a g-protein–coupled receptor of prostaglandin (PG) D2, mainly expressed in Th2 cells, eosinophils, basophils, and ILC2 (46–48). Many studies show that CRTH2 is one of the biomarkers of Th2A cells (18, 20). The combination of CRTH2 with PGD2 can induce the chemotaxis of Th2 cells, basophils, and eosinophils, recruiting circulating inflammatory cells from blood vessels to the site of inflammation (49–51). CRTH2 activated by PGD2 restrains adenylate cyclase (AC) activity through the Giα protein subunit, reducing the intracellular cAMP level, which can reduce the transcriptional activity of NF-κB and induce the phosphorylation of CREB protein to inhibit inflammation (52, 53). CRTH2 also activates the Gβγ complex to provoke the formation of PIP2 hydrolyzed by phospholipase Cβ, leading to the production of DAG and IP3 (52, 54). DAG can activate PKCθ and the MAPK/ERK pathway, activating the transcription factors NF-κB and AP-1, respectively (55). IP3 can induce the release of Ca2+ from the endoplasmic reticulum to activate transcription factor NFAT by the phosphatase calcineurin (52, 55) (Figure 1). As is known, CRTH2 is a significant marker of ILC2, which promotes the effector function in ILC2 (48). More importantly, the activation of CRTH2 stimulates Th2 cells to produce IL-4, IL-5, and IL-13 (56, 57). Moreover, CRTH2+ Th2 cells express more CD200R, which strongly correlates with Th2A pathology (58, 59).
CD161
CD161, namely, NKR-P1, is a C-type lectin-like receptor mainly expressed in ILC2, NK cells, TH17 cells, and circulating memory T cells (48, 60). As the most significant biomarker in Th2A cells, CD161 is commonly regarded as the co-signaling receptor in T cells, the expression of which is related to memory phenotype (61). The binding of CD161 to LLT1 may recruit aSMase, which can upregulate Bcl-XL expression by mediating IL-2 secretion, resulting in the reduction of susceptibility to apoptosis, leading to the prolonged survival of effector memory T cells (62, 63). More importantly, aSMase catalyzes sphingomyelin (SM) to generate ceramide (64) (Figure 1). Ceramide aggregates into a platform, which leads to the permeability alternation of the membrane (65–68). Intracellular ceramide affects the multifarious cellular processes and protein kinase activation (Ras/PKC), mediating the Akt-mTOR and MAPK cellular pathways downstream of CD28 and CD3 to regulate cell apoptosis, proliferation, and differentiation (62, 69, 70). Similar to Th2A, CD161 and CRTH2 co-expression are characteristic of ILC2 as well (48).
ST2
Serum stimulation-2 (ST2), known as IL1RL1, is an orphan receptor mainly expressed in Th2 cells, Treg cells, Th9 cells, and ILC2s (71, 72). ST2 expression on peripheral allergen-specific CD41 T cells is confined to allergy individuals and restricted to Th2A cells and, on circulating CD4+ T cells, represents a transient phenotype associated with Th2A cell activation, allowing these cells to sense locally elicited tissue cytokines (73, 74). ST2 exists in two ways, which are different in signal transduction: a membrane-bound form and a soluble form (sST2) (71). After ST2 binds to its only ligand IL-33, the myeloid differentiation factor 88 (MyD88) is recruited to the intracellular domain, activating TRAF6 signaling via IL-1R-associated kinase (IRAK) (71, 75). Downstream MAPK kinases and the IKK complex activated by TRAF6 regulate the activation of AP-1 and the release of NF-κB from the complex, respectively (72) (Figure 1). However, sST2 inhibits IL-33/ST2 signaling by isolating free IL-33 (71). IL-33 selectively amplifies pathogenic Th2 cell effector functions, suggesting a tissue checkpoint that may regulate adaptive allergic immunity (73). Many studies show that ST2 in Th2A cells presents a transient phenotype related to cell activation, leading to the expression of IL-5 and IL-13 (71, 73, 76).
PPAR
Peroxisome proliferator–activated receptor (PPAR) is a member of the nuclear receptor superfamily that is mainly expressed on macrophages and Th2A cells (77, 78). Activated PPAR forms a heterodimer with the retinoid X receptor (RXR), which can combine with the upstream PPAR response element to regulate the transcription of the target genes included in adipogenesis, lipid metabolism, inflammation, and metabolic homeostasis (79). PPARG can directly regulate the generation of IL-5 and the expression of ST2 both in vitro and in vivo (80). As mentioned, the IL-33/ST2 axis can strongly accelerate the Th2 cell function, indicating that PPARG might be a potential target for the treatment of allergic diseases (73, 80).
Biological properties of Th2A cells
In the type 2 inflammatory response, epithelial cells and keratinocytes secrete IL-25, IL-33, and TSLP to activate ILC2, APCs, and Th2A cells, which induce the Th2 response to secrete IL-4, IL-5, IL-9, IL-10, and IL-13 in response to allergen stimulation (10, 11, 48). It is known that Th2A cells play a significant role in allergic diseases, but the mechanism remains unclear (18). We believe that, after exposure of atopic individuals to allergens, APCs present allergens to the tissue-resident Th2A cells, inducing cell activation (81). The activated Th2A cells may produce IL-5 and IL-9 to favor the recruitment and activation of eosinophils, which is supported by the fact that the Th2A subset presents a significant positive correlation with the number of eosinophils in the peripheral blood of atopic individuals (19, 20, 82). Meanwhile, Th2A cells may secrete IL-4 and IL-13, stimulating B cells to produce allergen-specific IgE antibodies that sensitize mast cells and basophils (11). In addition, Th2A cells may produce IL-4, IL-9, and IL-10 to activate the sensitized mast cells and basophils (11) (Figure 2). PGD2 secreted by the activated mast cells, in turn, acts on CRTH2 in Th2A cells, further promoting the activation of Th2A cells (83). Besides this, Th2A cells can survive in the tissues and blood for years, reserving the ability of allergen memory (73). Notably, ILC2s can induce tissue repair and result in the recruitment and activation of eosinophils and mast cells, which are functionally like Th2A cells and also essential to triggering the type 2 response but differ in that ILC2s have no TCR and allergen specificity (19, 84).
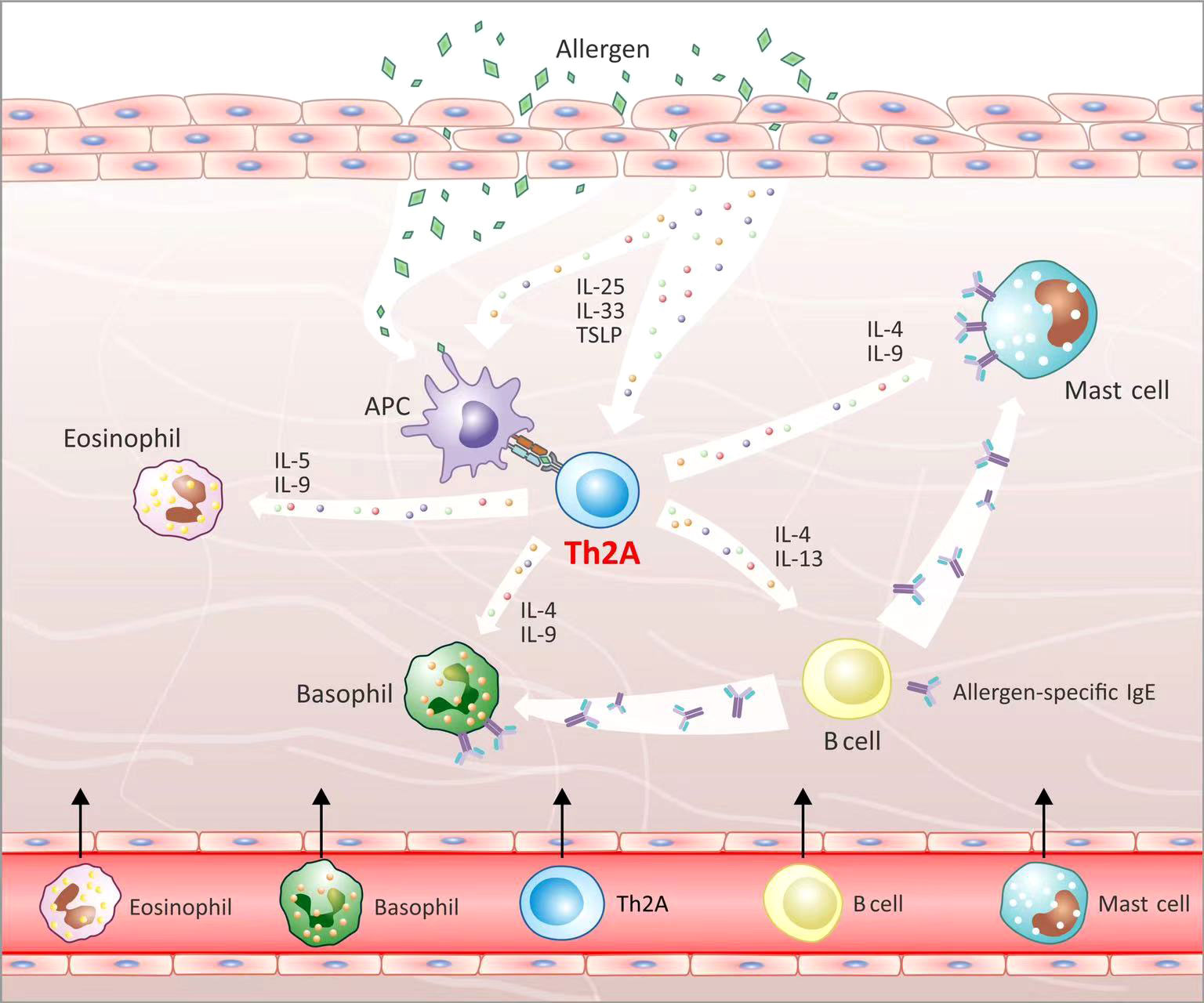
Figure 2 Th2A cells’ biological function. After allergen stimulation, epithelial cells secrete IL-25, IL-33, and TSLP to activate APCs and Th2A cells. APCs present allergens to activate tissue-resident Th2A cells. Th2A cells that have been activated secrete IL-5 and IL-9, which aid in the recruitment and activation of eosinophils. Th2A cells produce IL-4 and IL-13, stimulating B cells to produce allergen-specific IgE antibodies. Meanwhile, Th2A cells secrete IL-4, IL-9, and IL-10 to activate mast cells and basophils.
Th2A cells in Allergic Diseases
Th2A cells in AD
AD is the most common chronic inflammatory skin disease characterized by severe pruritus, recurrent eczema, and fluctuating course, which would gradually develop into FA, asthma, and even EoE (1). In 2021, Bangert et al. analyzed samples from the antecubital fossa of AD patients who had received the treatment of IL-4Rα blockers for 16 weeks and a year with single-cell RNA sequencing (scRNA-seq). Notably, a cluster of cells with IL17RB+ CRTH2+ CD161+ CD27low phenotype most in accord with Th2A cells was found in the tissues of AD patients. Moreover, it is shown that even after a year of treatment, Th2A cells persistently existed in the patient tissues but were not present in healthy skin, indicating that Th2A cells are the main pathogenic subset for AD, possibly resulting in recurrent AD (36).
Th2A cells in FA
FA is an adverse immune response induced by food allergens, including peanuts, milk, soy, and fish et al. (85, 86). Studies indicate that FA is caused by allergen-specific TH2 cells through the mediation of IgE switching and expansion of lymphocytes (87). Wambre et al. completed a longitudinal study on patients with peanut allergies who underwent characterized oral desensitization immunotherapy in 2017 (CODIT). In this randomized, double-blind, placebo-controlled experiment, most of the CD4+ T cells responding to peanuts were verified to be Th2A cells by a CD154 upregulation assay, which can observe the quantity of peanut-specific T cells in vitro. Furthermore, it is shown that a decrease in Th2A cell frequency is positively correlated with peanut desensitization, demonstrating the association of Th2A cells with the pathogenesis of FA (18). In 2020, Luce et al. recruited 60 desirable patients to be treated with omalizumab at weeks 1 to 16 and multi-OIT that included two to five kinds of allergens at weeks 8 to 30, and the patients received a food challenge at week 30. Fluorescent antibody labeling revealed a decrease in Th2A cell frequency at week 30 compared with the baseline. Inversely, no changes in ILC and DC frequency were observed through flow cytometry, indicating the value of Th2A frequency as a new marker for OIT (35). Meanwhile, Monian et al. subdivided the observed cells into six subsets through single-cell RNA-Seq and paired T cell receptor α/β sequencing, where inhibition of the Th2 signature in the Th2A subpopulation correlates with clinical outcomes of OIT (32). Moreover, Chiang et al. discovered that Tregs activated by IL-2 could partially inhibit the Th2 response, but the heterogeneous Th2 response was not significantly inhibited (33). Then, Lozano-Ojalvo found that IL-2 produced by allergen-specific cells can induce the activation of Treg cells with suppressive properties during an oral food challenge (88). In 2022, Bajzik et al. demonstrated that, during an experiment, the Th2A cell level observed in the peripheral blood of peanut-allergic patients is associated with not only T-cell reactivity to peanuts but also serum peanut-specific IgE and IgG4 levels (89).
Th2A cells in Asthma
Asthma is a chronic allergic inflammatory disease characterized by multiple respiratory symptoms, airflow limitation, and reversible airway obstruction (2). A study was performed in 2021 by Luce et al. on 182 patients with allergies to house dust mites (HDM) to indicate the role of Th2A cells in allergic diseases. During the randomized, double-blind, placebo-controlled trial, these patients were treated with daily either 300 IR (n = 58), 500 IR (n = 63) HDM, or placebo (n = 61) tablets for 12 months. Flow cytometry was used to examine the changes in Th2A cell frequency between baseline and the end of AIT. Because both the active and placebo groups showed a decrease in Th2A cells, the same analysis was performed with CD38, which is upregulated in Th2A cells. The results suggest that a significant reduction in CD38+ Th2A was observed only in all active groups, confirming the potential value of CD38+ Th2A cells as the new clinical biomarker of asthma (37). Meanwhile, Blinova, EA et al. observed that, after treatment, the proportion of TH2A/Th2 cells decreased in the peripheral blood of asthma patients (90). Interestingly, in the ovalbumin-induced asthma mouse model, the expression of pathogenic Th2 cells in ST2-/- and IL33-/- mice is reduced significantly compared with ST2+/+ and IL33+/+ mice, resulting in observable improvement of pulmonary fibrosis, collagen deposition, and the prominent decrease in fibrosis-related gene expression, which indicates that ST2hi memory pathogenic Th2 cells are involved in the establishment of airway fibrosis (91).
Th2A cells in EoE
EoE is a chronic esophageal inflammatory disease characterized by pathological eosinophil infiltration, leading to dysphagia, food impaction, and impaired esophagus function (92, 93). In 2019, Rothenberg et al. utilized scRNA-seq to discover the specific enrichment of HPGDS+ CRTH2+ IL-17RB+ FFAR3+ CD4+ Th2 cell in EoE (94). In 2021, Morgan et al. collected biopsy tissue from the esophagus and duodenum of 10 patients with EoE (n = 6 active disease, n = 4 remission disease) and enzymatically hydrolyzed the patient tissues into a single-cell suspension for scRNA-seq. Th2A cells were prominently higher in the patients with active diseases due to the calculation of the two-sided Wilcoxon rank-sum test and enriched in the esophageal tissue of patients with EoE. In addition, the result of ligand-receptor pathway analysis shows that receptors selectively expressed on eosinophils are matched with the ligands on Th2A cells, indicating that Th2A cells may be related to the recruitment of eosinophils to the esophageal tissue (38).
Immunotherapy targeting Th2A cells
It is known that, with insight into the immune mechanism, many targeted drugs are now applied to treat moderate and severe allergic diseases. As Th2A cells are pathogenic for allergic diseases, relative monoclonal antibodies are widely used, such as IL-4 monoclonal antibody (Pitrakinra, Dupilumab) (95–97), IL-5 monoclonal antibody (Mepolizumab, Reslizumab, Benralizumab) (98), IL-9 monoclonal antibody (MEDI-528) (99), IL-13 monoclonal antibody (Lebrikizumab, Tralokinumab) (100, 101), IL-33 monoclonal antibody (Etokimab, AMG 282) (102, 103), TSLP monoclonal antibody (Tezepelumab) (104), and IgE monoclonal antibody (Omalizumab, Ligelizumab) (105, 106). Importantly, JAK can regulate the full activation of Th2A cells, thus, JAK inhibitors also play an indispensable role in allergic disease treatment (107–114) (Table 1).
Moreover, Th2A cells have the possibility to be deleted selectively. Studies demonstrate that a persistent high dose of allergen cumulation in AIT might lead to the selective functional deletion of proallergic Th2 cells, which permits the occurrence of other T cell responses (29). Moreover, this chronic stimulation of high-dose allergens may induce autocrine IL-10 generation, which is the salient mediator of T cell exhaustion and the methylation of the GATA-3 promoter related to the decrease in Th2 cell activity (115, 116).
Conclusion
Th2A cells are a subset of memory Th2 cells confined to atopic individuals, including all the allergen-specific Th2 cells. Notably, Th2A cells are characterized by the expression of CD4+ CRTH2+ CD161high HPGDS+ CD27low CD49dhigh ST2high, which exhibit multiple biological properties leading to cell survival, activation, and cytokine secretion. After allergen exposure, APCs involve in allergen presentation to activate Th2A cells which can secrete IL-4, IL-5, IL-9, IL-10, and IL-13, leading to the activation of mast cells, basophils, and eosinophils. It is known that Th2A cells are demonstrated to be the central pathogenic cells in various allergic diseases, including AD, FA, asthma, and EoE. The frequency of Th2A cells can be used to assess the efficacy of allergic disease treatment, and selective Th2A cell deletion may result in long-term clinical benefits. Subsequently, Th2A cells are expected to be the focal point of research into the pathogenesis of allergic diseases and a critical target for disease treatment.
Author contributions
ZH wrote most of the review. ZH and MC contributed to writing and correcting the manuscript. XC, ZW, LJ, and YM participated in critically correcting the manuscript. MC and YW contributed to the manuscript structure and supervised the work.
Funding
Peking University Medicine Seed Fund for Interdisciplinary Research supported by “the Fundamental Research Funds for the Central Universities” (No. BMU2021MX021, No. BMU2022MX017). This work was supported by the National Natural Science Foundation of China (81603119) and the Natural Science Foundation of Beijing Municipality (7174316).
Conflict of interest
The authors declare that the research was conducted in the absence of any commercial or financial relationships that could be construed as a potential conflict of interest.
Publisher’s note
All claims expressed in this article are solely those of the authors and do not necessarily represent those of their affiliated organizations, or those of the publisher, the editors and the reviewers. Any product that may be evaluated in this article, or claim that may be made by its manufacturer, is not guaranteed or endorsed by the publisher.
References
1. Langan SM, Irvine AD, Weidinger S. Atopic dermatitis. Lancet (2020) 396(10247):345–60. doi: 10.1016/S0140-6736(20)31286-1
2. Papi A, Brightling C, Pedersen SE, Reddel HK. Asthma. Lancet (2018) 391(10122):783–800. doi: 10.1016/S0140-6736(17)33311-1
3. Greiner AN, Hellings PW, Rotiroti G, Scadding GK. Allergic rhinitis. Lancet (2011) 378(9809):2112–22. doi: 10.1016/S0140-6736(11)60130-X
4. Rutkowski K, Sowa P, Rutkowska-Talipska J, Sulkowski S, Rutkowski R. Allergic diseases: the price of civilisational progress. Adv Dermatol Allergology/Postȩpy Dermatologii i Alergologii (2014) 31(2):77. doi: 10.5114/pdia.2014.40936
5. Ring J. Terminology of allergic phenomena. Chem Immunol Allergy (2014) 100:46–52. doi: 10.1159/000358500
6. Yang L, Fu J, Zhou YF. Research progress in atopic march. Front Immunol (2020) 11:1907. doi: 10.3389/fimmu.2020.01907
7. Aw M, Penn J, Gauvreau GM, Lima H, Sehmi R. Atopic march: collegium internationale allergologicum update 2020. Int Arch Allergy Immunol (2020) 181(1):1–0. doi: 10.1159/000502958
8. Gandhi NA, Bennett BL, Graham NM, Pirozzi G, Stahl N, Yancopoulos GD. Targeting key proximal drivers of type 2 inflammation in disease. Nat Rev Drug Discovery (2016) 15(1):35–50. doi: 10.1038/nrd4624
9. Saenz SA, Taylor BC, Artis D. Welcome to the neighborhood: epithelial cell-derived cytokines license innate and adaptive immune responses at mucosal sites. Immunol Rev (2008) 226:172–90. doi: 10.1111/j.1600-065X.2008.00713.x
10. Wambre E, James EA, Kwok WW. Characterization of CD4+ T cell subsets in allergy. Curr Opin Immunol (2012) 24(6):700–6. doi: 10.1016/j.coi.2012.07.009
11. Romagnani S. The role of lymphocytes in allergic disease. J Allergy Clin Immunol (2000) 105(3):399–408. doi: 10.1067/mai.2000.104575
12. Teran LM. Chemokines and IL-5: major players of eosinophil recruitment in asthma. Clin Exp Allergy: J Br Soc Allergy Clin Immunol (1999) 29(3):287–90. doi: 10.1046/j.1365-2222.1999.00522.x
13. Wambre E, DeLong JH, James EA, LaFond RE, Robinson D, Kwok WW. Differentiation stage determines pathologic and protective allergen-specific CD4+ T-cell outcomes during specific immunotherapy. J Allergy Clin Immunol (2012) 129:544–51. doi: 10.1016/j.jaci.2011.08.034
14. Macaubas C, Wahlstrom J, da Silva APG, Forsthuber TG, Sønderstrup G, Kwok WW, et al. Allergen-specific MHC class II tetramer+ cells are detectable in allergic, but not in nonallergic, individuals. J Immuno (2006) 176(8):5069–77. doi: 10.4049/jimmunol.176.8.5069
15. Wambre E, DeLong JH, James EA, Torres-Chinn N, Pfützner W, Möbs C, et al. Specific immunotherapy modifies allergen-specific CD4+ T-cell responses in an epitope-dependent manner. J Allergy Clin Immunol (2014) 133(3):872–9.e7. doi: 10.1016/j.jaci.2013.10.054
16. Archila L, DeLong J, Wambre E, James E, Robinson D, Kwok W. Grass-specific CD 4+ T-cells exhibit varying degrees of cross-reactivity, implications for allergen-specific immunotherapy. Clin Exp Allergy (2014) 44(7):986–98. doi: 10.1111/cea.12324
17. Van Overtvelt L, Wambre E, Maillère B, von Hofe E, Louise A, Balazuc AM, et al. Assessment of bet v 1-specific CD4+ T cell responses in allergic and nonallergic individuals using MHC class II peptide tetramers. J Immuno (2008) 180(7):4514–22. doi: 10.4049/jimmunol.180.7.4514
18. Wambre E, Bajzik V, DeLong JH, O'Brien K, Nguyen QA, Speake C, et al. A phenotypically and functionally distinct human TH2 cell subpopulation is associated with allergic disorders. Sci Transl Med (2017) 9(401):eaam9171. doi: 10.1126/scitranslmed.aam9171
19. Licona-Limón P, Kim LK, Palm NW, Flavell RA. TH2, allergy and group 2 innate lymphoid cells. Nat Immunol (2013) 14(6):536–42. doi: 10.1038/ni.2617
20. Mitson-Salazar A, Yin Y, Wansley DL, Young M, Bolan H, Arceo S, et al. Hematopoietic prostaglandin d synthase defines a proeosinophilic pathogenic effector human TH2 cell subpopulation with enhanced function. J Allergy Clin Immunol (2016) 137(3):907–18. doi: 10.1016/j.jaci.2015.08.007
21. Nakayama T, Yamashita M. Initiation and maintenance of Th2 cell identity. Curr Opin Immunol (2008) 20(3):265–71. doi: 10.1016/j.coi.2008.03.011
22. Kurokawa M, Matsukura S, Kawaguchi M, Ieki K, Suzuki S, Odaka M, et al. Expression and effects of IL-33 and ST2 in allergic bronchial asthma: IL-33 induces eotaxin production in lung fibroblasts. Int Arch Allergy Immunol (2011) 155(Suppl. 1):12–20. doi: 10.1159/000327259
23. Upadhyaya B, Yin Y, Hill BJ, Douek DC, Prussin C. Hierarchical IL-5 expression defines a subpopulation of highly differentiated human Th2 cells. J Immunol (2011) 187(6):3111–20. doi: 10.4049/jimmunol.1101283
24. Endo Y, Hirahara K, Iinuma T, Shinoda K, Tumes DJ, Asou HK, et al. The interleukin-33-p38 kinase axis confers memory T helper 2 cell pathogenicity in the airway. Immunity (2015) 42(2):294–308. doi: 10.1016/j.immuni.2015.01.016
25. Shinoda K, Hirahara K, Iinuma T, Ichikawa T, Suzuki AS, Sugaya K, et al. Thy1+ IL-7+ lymphatic endothelial cells in iBALT provide a survival niche for memory T-helper cells in allergic airway inflammation. Proc Natl Acad Sci (2016) 113(20):E2842–51. doi: 10.1073/pnas.1512600113
26. Iinuma T, Okamoto Y, Yamamoto H, Inamine-Sasaki A, Ohki Y, Sakurai T, et al. Interleukin-25 and mucosal T cells in noneosinophilic and eosinophilic chronic rhinosinusitis. Ann Allergy Asthma Immunol (2015) 114(4):289–98. doi: 10.1016/j.anai.2015.01.013
27. Lam EP, Kariyawasam HH, Rana BM, Durham SR, McKenzie AN, Powell N, et al. IL-25/IL-33–responsive TH2 cells characterize nasal polyps with a default TH17 signature in nasal mucosa. J Allergy Clin Immunol (2016) 137(5):1514–24. doi: 10.1016/j.jaci.2015.10.019
28. Wambre ER, DeLong JH, James EA, Robinson D, Kwok WW. TH2A cells as a unique TH2 cell subset in allergic individuals: Steps toward a T cell biomarker for allergy. J Allergy Clin Immunol (2012) 129(2):AB129. doi: 10.1016/j.jaci.2011.12.429
29. Wambre E. Effect of allergen-specific immunotherapy on CD4+ T cells. Curr Opin Immunol (2015) 15(6):581. doi: 10.1097/ACI.0000000000000216
30. Bajzik V, DeBerg HA, Garabatos N, Rust BJ, Obrien KK, Nguyen QA, et al. Oral desensitization therapy for peanut allergy induces dynamic changes in peanut-specific immune responses. Allergy (2022) 00:1–15. doi: 10.1111/all.15276
31. Ruiter B, Smith NP, Monian B, Tu AA, Fleming E, Virkud YV, et al. Expansion of the CD4+ effector T-cell repertoire characterizes peanut-allergic patients with heightened clinical sensitivity. J Allergy Clin Immunol (2020) 145(1):270–82. doi: 10.1016/j.jaci.2019.09.033
32. Monian B, Tu AA, Ruiter B, Morgan DM, Petrossian PM, Smith NP, et al. Peanut oral immunotherapy differentially suppresses clonally distinct subsets of T helper cells. J Clin Invest (2022) 132(2):e150634. doi: 10.1172/JCI150634
33. Chiang D, Chen X, Jones SM, Wood RA, Sicherer SH, Burks AW, et al. Single-cell profiling of peanut-responsive T cells in patients with peanut allergy reveals heterogeneous effector TH2 subsets. J Allergy Clin Immuno (2018) 141(6):2107–20. doi: 10.1016/j.jaci.2017.11.060
34. Renand A, Shamji MH, Harris KM, Qin T, Wambre E, Scadding GW, et al. Synchronous immune alterations mirror clinical response during allergen immunotherapy. J Allergy Clin Immuno (2018) 141(5):1750–60.e1. doi: 10.1016/j.jaci.2017.09.041
35. Luce S, Chinthrajah S, Lyu SC, Nadeau K, Mascarell L. Th2A and Th17 cell frequencies and regulatory markers as follow-up biomarker candidates for successful multifood oral immunotherapy. Allergy (2020) 75(6):1513. doi: 10.1111/all.14180
36. Bangert C, Rindler K, Krausgruber T, Alkon N, Thaler FM, Kurz H, et al. Persistence of mature dendritic cells, TH2A, and Tc2 cells characterize clinically resolved atopic dermatitis under IL-4Rα blockade. Sci Immunol (2021) 6(55):eabe2749. doi: 10.1126/sciimmunol.abe2749
37. Luce S, Batard T, Bordas-Le Floch V, Le Gall M, Mascarell L. Decrease in CD38+ TH2A cell frequencies following immunotherapy with house dust mite tablet correlates with humoral responses. Clin Exp Allergy (2021) 51(8):1057–68. doi: 10.1111/cea.13891
38. Morgan DM, Ruiter B, Smith NP, Tu AA, Monian B, Stone BE, et al. Clonally expanded, GPR15-expressing pathogenic effector TH2 cells are associated with eosinophilic esophagitis. Sci Immunol (2021) 6(62):eabi5586. doi: 10.1126/sciimmunol.abi5586
39. Vandamme C, Rytkönen-Nissinen M, Lönnberg T, Randell J, Harvima RJ, Kinnunen T, et al. Single-cell characterization of dog allergen–specific T cells reveals TH2 heterogeneity in allergic individuals. J Allergy Clin Immunol (2022) 149(5):1732–43.e15. doi: 10.1016/j.jaci.2021.11.018
40. Kanaoka Y, Urade Y. Hematopoietic prostaglandin d synthase. Prostaglandins Leukotrienes Essential Fatty Acids (2003) 69(2-3):163–7. doi: 10.1016/s0952-3278(03)00077-2
41. Urade Y. Biochemical and structural characteristics, gene regulation, physiological, pathological and clinical features of lipocalin-type prostaglandin D2 synthase as a multifunctional lipocalin. Front Physiol (2021) 12:. doi: 10.3389/fphys.2021.718002
42. Inoue T, Irikura D, Okazaki N, Kinugasa S, Matsumura H, Uodome N, et al. Mechanism of metal activation of human hematopoietic prostaglandin d synthase. Nat Struct Bio (2003) 10(4):291–6. doi: 10.1038/nsb907
43. Pettipher R. The roles of the prostaglandin D(2) receptors DP(1) and CRTH2 in promoting allergic responses. Br J Pharmacol (2008) 153 Suppl 1(Suppl 1):S191–9. doi: 10.1038/sj.bjp.0707488
44. Rittchen S, Heinemann A. Therapeutic potential of hematopoietic prostaglandin D2 synthase in allergic inflammation. Cells (2019) 8(6):619. doi: 10.3390/cells8060619
45. Hau CS, Kanda N, Watanabe S. Suppressive effects of antimycotics on thymic stromal lymphopoietin production in human keratinocytes. J Dermatol Sci (2013) 71(3):174–83. doi: 10.1016/j.jdermsci.2013.04.023
46. Hirai H, Tanaka K, Yoshie O, Ogawa K, Kenmotsu K, Takamori Y, et al. Prostaglandin D2 selectively induces chemotaxis in T helper type 2 cells, eosinophils, and basophils via seven-transmembrane receptor CRTH2. J Exp Med (2001) 193(2):255–62. doi: 10.1084/jem.193.2.255
47. Nagata K, Hirai H, Tanaka K, Ogawa K, Aso T, Sugamura K, et al. CRTH2, an orphan receptor of T-helper-2-cells, is expressed on basophils and eosinophils and responds to mast cell-derived factor (s). FEBS Letters (1999) 459(2):195–9. doi: 10.1016/s0014-5793(99)01251-x
48. Hazenberg MD, Spits H. Human innate lymphoid cells. Blood J Am Soc Hematol (2014) 124(5):700–9. doi: 10.1182/blood-2013-11-427781
49. Gyles SL, Xue L, Townsend ER, Wettey F, Pettipher R. A dominant role for chemoattractant receptor-homologous molecule expressed on T helper type 2 (Th2) cells (CRTH2) in mediating chemotaxis of CRTH2+ CD4+ Th2 lymphocytes in response to mast cell supernatants. Immunology (2006) 119(3):362–8. doi: 10.1111/j.1365-2567.2006.02440.x
50. Nagata K, Hirai H. The second PGD2 receptor CRTH2: structure, properties, and functions in leukocytes. Prostaglandins Leukotrienes Essential Fatty Acids (2003) 69(2-3):169–77. doi: 10.1016/s0952-3278(03)00078-4
51. Marone G, Galdiero MR, Pecoraro A, Pucino V, Criscuolo G, Triassi M, et al. Prostaglandin D2 receptor antagonists in allergic disorders: safety, efficacy, and future perspectives. Expert Opin Investigational Drugs (2019) 28(1):73–84. doi: 10.1080/13543784.2019.1555237
52. Kostenis E, Ulven T. Emerging roles of DP and CRTH2 in allergic inflammation. Trends Mol Med (2006) 12:148–58. doi: 10.1016/j.molmed.2006.02.005
53. Tavares LP, Negreiros-Lima GL, Lima KM, Silva PM, Pinho V, Teixeira MM, et al. Blame the signaling: Role of cAMP for the resolution of inflammation. Pharmacol Res (2020) 159:105030. doi: 10.1016/j.phrs.2020.105030
54. Xue L, Gyles SL, Wettey FR, Gazi L, Townsend E, Hunter MG, et al. Prostaglandin D2 causes preferential induction of proinflammatory Th2 cytokine production through an action on chemoattractant receptor-like molecule expressed on Th2 cells. J Immunol (2005) 175(10):6531–6. doi: 10.4049/jimmunol.175.10.6531
55. Gaud G, Lesourne R, Love PE. Regulatory mechanisms in T cell receptor signalling. Nat Rev Immunol (2018) 18(8):485–97. doi: 10.1038/s41577-018-0020-8
56. Kupczyk M, Kuna P. Targeting the PGD2/CRTH2/DP1 signaling pathway in asthma and allergic disease: current status and future perspectives. Drugs (2017) 77(12):1281–94. doi: 10.1007/s40265-017-0777-2
57. Xue L, Barrow A, Pettipher R. Interaction between prostaglandin D2 and chemoattractant receptor-homologous molecule expressed on Th2 cells mediates cytokine production by Th2 lymphocytes in response to activated mast cells. Clin Exp Immunol (2009) 156(1):126–33. doi: 10.1111/j.1365-2249.2008.03871.x
58. Blom LH, Martel BC, Larsen LF, Hansen CV, Christensen MP, Juel-Berg N, et al. The immunoglobulin superfamily member CD 200R identifies cells involved in type 2 immune responses. Allergy (2017) 72(7):1081–90. doi: 10.1111/all.13129
59. Wambre ER, Farrington M, Bajzik V, DeBerg HA, Ruddy M, DeVeaux M, et al. Clinical and immunological evaluation of cat-allergic asthmatics living with or without a cat. Clin Exp Allergy (2021) 51(12):1624–33. doi: 10.1111/cea.14024
60. Wyrożemski Ł, Qiao SW. Immunobiology and conflicting roles of the human CD161 receptor in T cells. Scand J Immunol (2021) 94(3):e13090. doi: 10.1111/sji.13090
61. Fergusson JR, Fleming VM, Klenerman P. CD161-expressing human T cells. Front Immunol (2011) 2:36. doi: 10.3389/fimmu.2011.00036
62. Adam D, Heinrich M, Kabelitz D, Schütze S. Ceramide: does it matter for T cells? Trends Immunol (2002) 23(1):1–4. doi: 10.1016/s1471-4906(01)02091-9
63. Tischner D, Theiss J, Karabinskaya A, van den Brandt J, Reichardt SD, Karow U, et al. Acid sphingomyelinase is required for protection of effector memory T cells against glucocorticoid-induced cell death. J Immunol (2011) 187(9):4509–16. doi: 10.4049/jimmunol.1100911
64. Zeidan YH, Hannun YA. The acid sphingomyelinase/ceramide pathway: biomedical significance and mechanisms of regulation. Curr Mol Med (2010) 10(5):454–66. doi: 10.2174/156652410791608225
65. Bollinger CR, Teichgräber V, Gulbins E. Ceramide-enriched membrane domains. Biochim Biophys Acta (BBA)-Molecular Cell Res (2005) 1746(3):284–94. doi: 10.1016/j.bbamcr.2005.09.001
66. Grassmé H, Jekle A, Riehle A, Schwarz H, Berger J, Sandhoff K, et al. CD95 signaling via ceramide-rich membrane rafts. J Biol Chem (2001) 276(23):20589–96. doi: 10.1074/jbc.M101207200
67. Gulbins E. Regulation of death receptor signaling and apoptosis by ceramide. Pharmacol Res (2003) 47(5):393–9. doi: 10.1016/s1043-6618(03)00052-5
68. Gulbins E, Kolesnick R. Raft ceramide in molecular medicine. Oncogene (2003) 22(45):7070–7. doi: 10.1038/sj.onc.1207146
69. Pozo D, Valés-Gómez M, Mavaddat N, Williamson SC, Chisholm SE, Reyburn H. CD161 (human NKR-P1A) signaling in NK cells involves the activation of acid sphingomyelinase. J Immunol (2006) 176(4):2397–406. doi: 10.4049/jimmunol.176.4.2397
70. Bai A, Guo Y. Acid sphingomyelinase mediates human CD4+ T-cell signaling: Potential roles in T-cell responses and diseases. Cell Death Disease (2017) 8(7):e2963. doi: 10.1038/cddis.2017.360
71. Griesenauer B, Paczesny S. The ST2/IL-33 axis in immune cells during inflammatory diseases. Front Immunol (2017) 8:475. doi: 10.3389/fimmu.2017.00475
72. Baumann C, Bonilla WV, Fröhlich A, Helmstetter C, Peine M, Hegazy AN, et al. T-Bet- and STAT4-dependent IL-33 receptor expression directly promotes antiviral Th1 cell responses. Proc Natl Acad Sci USA (2015) 112(13):4056–61. doi: 10.1073/pnas.1418549112
73. Calise J, Garabatos N, Bajzik V, Farrington M, Robinson D, Jeong D, et al. Optimal human pathogenic TH2 cell effector function requires local epithelial cytokine signaling. J Allergy Clin Immunol (2021) 148(3):867–75. doi: 10.1016/j.jaci.2021.02.019
74. Van Dyken SJ, Nussbaum JC, Lee J, Molofsky AB, Liang H-E, Pollack JL, et al. A tissue checkpoint regulates type 2 immunity. Nat Immunol (2016) 17(12):1381–7. doi: 10.1038/ni.3582
75. Kakkar R, Lee RT. The IL-33/ST2 pathway: therapeutic target and novel biomarker. Nat Rev Drug Discov (2008) 7(10):827–40. doi: 10.1038/nrd2660
76. Liew FY, Girard JP, Turnquist HR. Interleukin-33 in health and disease. Nat Rev Immunol (2016) 16(11):676–89. doi: 10.1038/nri.2016.95
77. Takano H, Hasegawa H, Zou Y, Komuro I. Pleiotropic actions of PPARg activators thiazolidinediones in cardiovascular diseases. Curr Pharm Design (2004) 10(22):2779–86. doi: 10.2174/1381612043383719
78. Bonvalet M, Moussu H, Wambre E, Ricarte C, Horiot S, Rimaniol AC, et al. Allergen-specific CD 4+ T cell responses in peripheral blood do not predict the early onset of clinical efficacy during grass pollen sublingual immunotherapy. Clin Exp Allergy (2012) 42(12):1745–55. doi: 10.1111/cea.12015
79. Tontonoz P, Spiegelman BM. Fat and beyond: the diverse biology of PPARγ. Annu Rev Biochem (2008) 77:289–312. doi: 10.1146/annurev.biochem.77.061307.091829
80. Nobs SP, Natali S, Pohlmeier L, Okreglicka K, Schneider C, Kurrer M, et al. PPARγ in dendritic cells and T cells drives pathogenic type-2 effector responses in lung inflammation. J Exp Med (2017) 214(10):3015–35. doi: 10.1084/jem.20162069
81. Holt PG, Stumbles PA. Regulation of immunologic homeostasis in peripheral tissues by dendritic cells: the respiratory tract as a paradigm. J Allergy Clin Immunol (2000) 105(3):421–9. doi: 10.1067/mai.2000.105010
82. Yang D, Chen Q, Su SB, Zhang P, Kurosaka K, Caspi RR, et al. Eosinophil-derived neurotoxin acts as an alarmin to activate the TLR2–MyD88 signal pathway in dendritic cells and enhances Th2 immune responses. J Exp Med (2008) 205(1):79–90. doi: 10.1084/jem.20062027
83. Bischoff SC. Role of mast cells in allergic and non-allergic immune responses: comparison of human and murine data. Nat Rev Immunol (2007) 7(2):93–104. doi: 10.1038/nri2018
84. Halim TY. Group 2 innate lymphoid cells in disease. Int Immunol (2016) 28(1):13–22. doi: 10.1093/intimm/dxv050
85. Bruijnzeel-Koomen CA, Ortolani C, Aas K, Bindslev-Jensen C, Björksten B, Wüthrich B. Adverse reactions to food: Position paper of the European academy of allergy and clinical immunology. Allergy (1995) 50:623–35. doi: 10.1111/j.1398-9995.1995.tb02579.x
86. Sicherer SH, Sampson HA. Food allergy. J Allergy Clin Immunol (2010) 125(2):S116–25. doi: 10.1016/j.jaci.2009.08.028
87. Tordesillas L, Berin MC, Sampson HA. Immunology of food allergy. Immunity (2017) 47(1):32–50. doi: 10.1016/j.immuni.2017.07.004
88. Lozano-Ojalvo D, Tyler SR, Aranda CJ, Wang J, Sicherer SH, Sampson HA, et al. Allergen recognition by specific effector Th2 cells enables IL-2-dependent activation of regulatory T cell responses in humans. medRxiv (2022). doi: 10.1101/2022.05.17.22275017
89. Yii A, Tay TR, Choo X, Koh M, Tee A, Wang DY. Precision medicine in united airways disease: a “treatable traits” approach. Allergy (2018) 73(10):1964–78. doi: 10.1111/all.13496
90. Blinova EA, Galdina VA, Pashkina EA, Makarova AE, Demina DV, Kozlov VA. Proportion of TH2A/Th2 cells in the peripheral blood of patients with asthma decreases after therapy. Allergy: European Journal of Allergy and Clinical Immunology Supplement (2021) 76(S110):133. doi: 10.1111/all.15095
91. Morimoto Y, Hirahara K, Kiuchi M, Wada T, Ichikawa T, Kanno T, et al. Amphiregulin-producing pathogenic memory T helper 2 cells instruct eosinophils to secrete osteopontin and facilitate airway fibrosis. Immunity (2018) 49(1):134–50. doi: 10.1016/j.immuni.2018.04.023
92. Furuta GT, Katzka DA. Eosinophilic esophagitis. N Engl J Med (2015) 373(17):1640–8. doi: 10.1056/NEJMra1502863
93. Davis BP, Rothenberg ME. Mechanisms of disease of eosinophilic esophagitis. Annu Rev Pathol: Mech Dis (2016) 11:365–93. doi: 10.1146/annurev-pathol-012615-044241
94. Wen T, Aronow BJ, Rochman Y, Rochman M, Kiran K, Dexheimer PJ, et al. Single-cell RNA sequencing identifies inflammatory tissue T cells in eosinophilic esophagitis. J Clin Invest (2019) 129(5):2014–28. doi: 10.1172/JCI125917
95. Hambly N, Nair P. Monoclonal antibodies for the treatment of refractory asthma. Curr Opin Pulm Med (2014) 20(1):87–94. doi: 10.1097/MCP.0000000000000007
96. Kraft M, Worm M. Dupilumab in the treatment of moderate-to-severe atopic dermatitis. Expert Rev Clin Immunol (2017) 13(4):301–10. doi: 10.1080/1744666X.2017.1292134
97. Wenzel S, Ford L, Pearlman D, Spector S, Sher L, Skobieranda F, et al. Dupilumab in persistent asthma with elevated eosinophil levels. N Engl J Med (2013) 368(26):2455–66. doi: 10.1056/NEJMoa1304048
98. Ridolo E, Pucciarini F, Nizi MC, Makri E, Kihlgren P, Panella L. Mabs for treating asthma: omalizumab, mepolizumab, reslizumab, benralizumab, dupilumab. J Hum Vaccin Immunother (2020) 16:2349–56. doi: 10.1080/21645515.2020.1753440
99. Parker J, Oh C, LaForce C, Miller S, Pearlman D, Le C, et al. MEDI-528 clinical trials group: Safety profile and clinical activity of multiple subcutaneous doses of MEDI-528, a humanized anti-interleukin-9 monoclonal antibody, in two randomized phase 2a studies in subjects with asthma. BMC Pulm Med (2011) 11:14. doi: 10.1186/1471-2466-11-14
100. Gandhi NA, Pirozzi G, Graham NM. Commonality of the IL-4/IL-13 pathway in atopic diseases. Expert Rev Clin Immunol (2017) 13(5):425–37. doi: 10.1080/1744666X.2017.1298443
101. Corren J, Lemanske Jr.RF, Hanania NA, Korenblat PE, Parsey MV, Arron JR, et al. Lebrikizumab treatment in adults with asthma. N Engl J Med (2011) 365(12):1088–98. doi: 10.1056/NEJMoa1106469
102. Chen YL, Gutowska-Owsiak D, Hardman CS, Westmoreland M, Mackenzie T, Cifuentes L, et al. Proof-of-concept clinical trial of etokimab shows a key role for IL-33 in atopic dermatitis pathogenesis. Sci Transl Med (2019) 11(515):eaax2945. doi: 10.1126/scitranslmed.aax2945
103. Chen Y, Wang W, Yuan H, Li Y, Lv Z, Cui Y, et al. Current state of monoclonal antibody therapy for allergic diseases. Engineering (2021) 7(11):1552–6. doi: 10.1016/j.eng.2020.06.029
104. Menzies-Gow A, Corren J, Bourdin A, Chupp G, Israel E, Wechsler ME, et al. Tezepelumab in adults and adolescents with severe, uncontrolled asthma. N Engl J Med (2021) 384(19):1800–9. doi: 10.1056/NEJMoa2034975
105. Holgate S, Djukanovic R, Casale T, Bousquet J. Anti-immunoglobulin e treatment with omalizumab in allergic diseases: an update on anti-inflammatory activity and clinical efficacy. Clin Exp Allergy (2005) 35(408):e16. doi: 10.1111/j.1365-2222.2005.02191.x
106. Gauvreau GM, Arm JP, Boulet L-P, Leigh R, Cockcroft DW, Davis BE, et al. Efficacy and safety of multiple doses of QGE031 (ligelizumab) versus omalizumab and placebo in inhibiting allergen-induced early asthmatic responses. J Allergy Clin Immunol (2016) 138(4):1051–9. doi: 10.1016/j.jaci.2016.02.027
107. Blauvelt A, Teixeira HD, Simpson EL, Costanzo A, De Bruin-Weller A, Barbarot S, et al. Efficacy and safety of upadacitinib vs dupilumab in adults with moderate-to-severe atopic dermatitis: a randomized clinical trial. JAMA Dermatology (2021) 157(9):1047–55. doi: 10.1001/jamadermatol.2021.3023
108. Gooderham MJ, Forman SB, Bissonnette R, Beebe JS, Zhang W, Banfield C, et al. Efficacy and safety of oral janus kinase 1 inhibitor abrocitinib for patients with atopic dermatitis: a phase 2 randomized clinical trial. JAMA Dermatol (2019) 155(12):1371–9. doi: 10.1001/jamadermatol.2019.2855
109. Cosgrove SB, Wren JA, Cleaver DM, Walsh KF, Follis SI, King VI, et al. A blinded, randomized, placebo-controlled trial of the efficacy and safety of the J anus kinase inhibitor oclacitinib (A poquel®) in client-owned dogs with atopic dermatitis. Vet Dermatol (2013) 24(6):587–e142. doi: 10.1111/vde.12088
110. Guttman-Yassky E, Silverberg JI, Nemoto O, Forman SB, Wilke A, Prescilla R, et al. Baricitinib in adult patients with moderate-to-severe atopic dermatitis: a phase 2 parallel, double-blinded, randomized placebo-controlled multiple-dose study. J Am Acad Dermatol (2019) 80(4):913–21.e9. doi: 10.1016/j.jaad.2018.01.018
111. Simpson E, Lacour JP, Spelman L, Galimberti R, Eichenfield L, Bissonnette R, et al. Baricitinib in patients with moderate-to-severe atopic dermatitis and inadequate response to topical corticosteroids: results from two randomized monotherapy phase III trials. Br J Dermatol (2020) 183(2):242–55. doi: 10.1111/bjd.18898
112. Szalus K, Trzeciak M, Nowicki RJ. JAK-STAT inhibitors in atopic dermatitis from pathogenesis to clinical trials results. Microorganisms (2020) 8(11):1743. doi: 10.3390/microorganisms8111743
113. Bissonnette R, Maari C, Forman S, Bhatia N, Lee M, Fowler J. The oral JAK/SYK inhibitor ASN002 demonstrates efficacy and improves associated systemic inflammation in patients with moderate-to-severe atopic dermatitis: results from a randomised, double-blind, placebo-controlled study. Br J Dermatol (2019) 181(4):733–42. doi: 10.1111/bjd.17932
114. Ross JA, Nagy ZS, Cheng H, Stepkowski SM, Kirken RA. Regulation of T cell homeostasis by JAKs and STATs. Arch Immunol Ther Exp (2007) 55(4):231–45. doi: 10.1007/s00005-007-0030-x
115. Saraiva M, Christensen JR, Veldhoen M, Murphy TL, Murphy KM, O'Garra A. Interleukin-10 production by Th1 cells requires interleukin-12-induced STAT4 transcription factor and ERK MAP kinase activation by high antigen dose. Immunity (2009) 31(2):209–19. doi: 10.1016/j.immuni.2009.05.012
Keywords: allergic disease, Th2A cells, allergen-specific Th2 cells, HPGDS, CRTH2, CD161, ST2
Citation: Huang Z, Chu M, Chen X, Wang Z, Jiang L, Ma Y and Wang Y (2022) Th2A cells: The pathogenic players in allergic diseases. Front. Immunol. 13:916778. doi: 10.3389/fimmu.2022.916778
Received: 10 April 2022; Accepted: 12 July 2022;
Published: 08 August 2022.
Edited by:
Graham Le Gros, Victoria University of Wellington, New ZealandReviewed by:
Takeshi Nabe, Setsunan University, JapanNahir Garabatos, Institut de Recerca Biomèdica August Pi i Sunyer (IDIBAPS), Spain
Eric Wambre, Benaroya Research Institute, United States
Copyright © 2022 Huang, Chu, Chen, Wang, Jiang, Ma and Wang. This is an open-access article distributed under the terms of the Creative Commons Attribution License (CC BY). The use, distribution or reproduction in other forums is permitted, provided the original author(s) and the copyright owner(s) are credited and that the original publication in this journal is cited, in accordance with accepted academic practice. No use, distribution or reproduction is permitted which does not comply with these terms.
*Correspondence: Ming Chu, ZmFtb3VzQGJqbXUuZWR1LmNu; Yuedan Wang, d2FuZ3l1ZWRhbkBiam11LmVkdS5jbg==