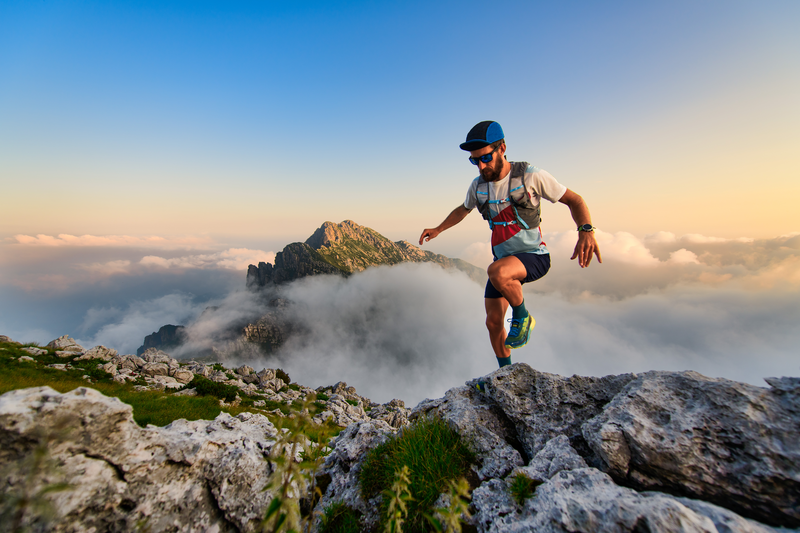
94% of researchers rate our articles as excellent or good
Learn more about the work of our research integrity team to safeguard the quality of each article we publish.
Find out more
REVIEW article
Front. Immunol. , 17 November 2022
Sec. Molecular Innate Immunity
Volume 13 - 2022 | https://doi.org/10.3389/fimmu.2022.1059376
This article is part of the Research Topic Immunomodulatory Role of Metalloproteases in Chronic Inflammatory Diseases View all 13 articles
ADAM17 is a member of the a disintegrin and metalloproteinase (ADAM) family of transmembrane proteases involved in the shedding of some cell membrane proteins and regulating various signaling pathways. More than 90 substrates are regulated by ADAM17, some of which are closely relevant to tumor formation and development. Besides, ADAM17 is also responsible for immune regulation and its substrate-mediated signal transduction. Recently, ADAM17 has been considered as a major target for the treatment of tumors and yet its immunomodulatory roles and mechanisms remain unclear. In this paper, we summarized the recent understanding of structure and several regulatory roles of ADAM17. Importantly, we highlighted the immunomodulatory roles of ADAM17 in tumor development, as well as small molecule inhibitors and monoclonal antibodies targeting ADAM17.
Transmembrane proteolysis is a post-translational modification that plays an important role in cellular biological processes, such as signal transduction and immune responses (1–3). Many transmembrane proteins need to be cleaved from the cell surface and released in a soluble form to initiate cellular or intercellular signal transduction (4–6). ADAM17, also known as tumor necrosis factor (TNF)-α converting enzyme (TACE), CD156B, NISBD1, and snake venom-like protease (cSVP), is a member of the disintegrin and metalloprotease family. ADAM17 exists in two forms: precursor and activated ADAM17. Activation of ADAM17 is required for the cleavage of its prodomain and exposure of the active site. In response to the inflammatory stimuli, activated ADAM17 prompts multiple receptor-mediated signal transduction by cleaving ectodomains of membrane proteins, including inflammatory cytokines, growth factors, receptors, and adhesion factors (7). The expression of ADAM17 in mouse articular cartilage is positively correlated with the development of arthritis, and its deletion attenuates articular cartilage degeneration (8). Moreover, ADAM17 is associated with glomerular inflammation and fibrosis (9). In diabetic mice, ADAM17 deletion in the proximal tubules improves glucose tolerance, prevents podocyte loss, and inhibits the accumulation of glomerular macrophages and collagen (9). More importantly, ADAM17 is contributory to the occurrence and development of cancers, including lung carcinoma (10), ovarian carcinoma (11), breast carcinoma (12–14), gastric carcinoma (15), and cervical carcinoma (16). Interestingly, it regulates some immune signaling pathways through the shedding activity, which may facilitate the inflammatory response in tumor development (17–19). However, the study of the relationship between the abnormal expression of this metalloproteinase in tumors and its immune regulation is still not well studied. Herein, we summarized and updated multiple regulatory roles of ADAM17 as well as the development of ADAM17 inhibitors with a focus on the immunomodulatory role of ADAM17 in tumor development, which may provide reasonable insights for the prevention and treatment of cancer diseases.
ADAM17 is a widely distributed transmembrane protein that is involved in different physiological processes such as inflammation, cell proliferation and apoptosis by its hydrolysis of various precursor membrane proteins, such as TNF-α, TNFRII, HB-EGF, IL-1R1, etc. It is localized in the membranes and cytoplasm of normal and tumor tissues and expressed in human lung, bronchus, nasopharynx, placenta, and lymphoid tissues (20, 21). In lung or respiratory tissues, activation of ADAM17 may contribute to the shedding of the collectrin-like part of ACE2, leading to the formation of soluble ACE2 (sACE2) (22, 23) and the development of inflammatory response (24). Furthermore, in distinct cells from the lung, ADAM17 expression is relatively high in pneumocytes and endothelial cells (20), suggesting that ADAM17 may be participating in the cleavage and shedding of key proteins in lung tissues. Activation of ADAM17 promotes the release of soluble fms-like tyrosine kinase 1 (sFlt1) in the placenta and induces preeclampsia (25). ADAM17 also induces T-cell activation in lymphoid tissues through the promotion of L-selectin hydrolysis and shedding (26). ADAM17 is lowly expressed in NK cells and its activation by IL-15 obstructs the proliferation of NK cells (19). Among multiple immune cells, ADAM17 is relatively high expressed in granulocytes and monocytes (20). ADAM17 mediates IL-6R shedding from neutrophils and induces apoptosis (27), which may be associated with a pro-inflammatory response mediated by the sIL-6R/IL-6 trans-signaling pathway (28).
ADAM17 is a member of the adamalysins subfamily of metzincin metalloproteinases consisting of 824 amino acids with zinc-dependent catalytic activities (29). The human ADAM17 protein sequence contains an N-terminal signal sequence (SS), a prodomain (PD), a catalytic metalloprotease domain (MD), a disintegrin domain (DD), a membrane-proximal protein domain (MPD), a conserved ADAM17 interaction sequence (CANDIS), a transmembrane domain (TM), and a C-terminal cytoplasmic domain (CD), which are located at amino acid residues 1-17, 18-216, 217-474, 480-559, 581-642, 643-666, 672-694, and 695-824, respectively (7, 30) (Figure 1A). Among them, the first five protein sequences that make up its extracellular domain may be involved in regulating multiple biological functions, including angiogenesis, cell migration, cell proliferation, inflammation, and immune responses. SS transfers the newly synthesized ADAM17 protein (110 kDa) to endoplasmic reticulum and Golgi apparatus (32). The PD obstructs the catalytic activity of metalloproteinases based on the cysteine-switch mechanism (33) (Figure 1B). During activation, furin, PC7 and PC5B pro-protein convertases are able to remove the prodomain of ADAM17 and induce production of the matured protein (80 kDa) (34). The cysteine-switch mechanism is not essential for the maintenance of inactivated ADAM17, which may be due to the presence of subdomains in the amino-terminal region of the prodomain (35). The MD serves as the main catalytic region of ADAM17 that contains a zinc-dependent HexGH-XXGXXHD motif (36). Amino acid residues His405, His409 and His415 located in this motif bind to zinc ions and determine the activity of the ADAM17 enzyme (31). The curved “Met turn” structure consisting of amino acid residues Tyr433, Val434, Met435, Tyr436, also known as 1,4-β-turn, is prone to ADAM17 cleavage and its mutations (37, 38). The DD can impair multiple functions of integrins, thereby affecting cell-cell/extracellular matrix interactions (20). In contrast to other members of ADAMs family, ADAM17 shows disulfide bonds in the MD, but its DD lacks typical calcium binding sites (39, 40). ADAM17 MPD plays crucial roles in substrate recognition and protein shedding. Due to the dimerization of ADAM17 and its substrate specificity, cysteine-rich and epidermal growth factor (EGF)-like domains are considered important components of MPD (41, 42). The hypervariable region of the former contributes to substrate recognition and shedding of extra-substrate domains and the latter affects the protein regulation of ADAM17 activity (43, 44). However, the existence of EGF-like domain remains controversial (45). In addition, the positively charged motif (Arg625-Lys628) in MPD binds to phosphatidylserine in the outer membrane, affects the conformation of ADAM17, and induces its activation (46). TM and CD mainly regulate the response of exocytodomain signaling molecule-related events (7, 38, 47), which may be attributed to the functional assembly of the Src SH3-binding motif (20). The CANDIS domain lies between MPD and TM, consisting of amino acid residues 643-666 (48), which binds to the type I transmembrane protein IL-6R but not the type II transmembrane protein TNF-α (49). As shown in Figure 1C, the visualized crystal structure of the catalytic domain of ADAM17 has five α-helices and five highly distorted β-sheet structures. The N-terminus binds to β1 and β3 sites, and the C-terminus binds to the α5 sites (31, 50). ADAM17 has shallower hydrophobic S1’ and very deep hydrophobic S3’ pockets linked by water channels, which facilitate the binding of the hydroxamic acid-based inhibitor TAPI-1 (also called an ADAM17 inhibitor) to the isobutyl side chain S1’ pocket and its other long chain to the S3’ pocket (31, 45). The structure and function of MD and MPD catalyzed by ADAM17 have been studied extensively, but the crystal structure and exact function of the remaining domains are still unclear.
Figure 1 Molecular structure of the ADAM17 protein. (A) Sequence and structure of ADAM17. ADAM17 protein mainly comprises five extracellular domains, a transmembrane domain, and a cytoplasmic domain. (B) The classic cysteine-switch mechanism. The conserved cysteine switch is located in the prodomain. It coordinates with Zn2+ at the catalytic site of the metalloproteinase domain to produce an inactivated enzyme (ADAM17 precursor). Once its prodomain is cleaved, the adjacent furin site (RVKR sequence) is responsible for catalyzing the separation of zinc from cysteine, ultimately leading to ADAM17 activation. (C) The 3D catalytic structure of ADAM17 (PDB CODE: 1BKC) (31) with a hydroxamic acid-based inhibitor INN and Zn2+ shows N-terminal domains, α-helix (blue), β-sheet (green), and C-terminal domains. INN stands for N-{(2R)-2-[2-(hydroxyamino)-2-oxoethyl]-4-methylpentanoyl}-3-methyl-L-valyl-N-(2-aminoethyl)-L-alaninamide with the chemical structure of C19H37N5O5. INN, also known as TAPI-2, is an analogue of TAPI-1. This 3D image was made with the SWISS-MODEL Expasy.
Post-translational modification of precursor proteins includes proteolysis, phosphorylation, glycosylation, methylation and acetylation (51). It can regulate the hydrolysis and cleavage of proteins, affect their activities, localization and interaction with other cellular molecules. As an irreversible post-translational modification, proteolysis/cleavage of transmembrane proteins is responsible for activating multiple cytokine-mediated signal transduction pathways. ADAM17 was first identified as the TNF-α converting enzyme, and its transmembrane proteolysis is related to inflammation (52) and immune regulation (26). TNF consists of TNF-α and TNF-β, to be secreted by macrophages and/or T lymphocytes (53, 54). TNF-α interacts with its receptors TNFR1 and TNFR2. TNFR1 is widely expressed in various human cells and is involved in cell survival and cellular damage (55, 56). The death domain of TNFR1 is occupied by the silencer of death domains (SODD) which blocks the binding of TRADD to TNFR1 and suppresses the TNFR1 signaling pathway (57). The binding of TNF-α and TNFR1 enables the shedding of SODD from the death domain of TNFR1 and leads to the formation of the TNFR1-TRADD-RIP1-TRAF2 complex, thus promoting cell survival (57). In addition, TNFR1 is also internalized by the clathrin protein, which subsequently triggers the assembly of intracellular death-inducing signaling complex and activation of caspase8, leading to apoptosis or necrosis (57, 58). TNFR2 is mainly distributed in immune cells and plays a role in regulating the function of the immune system (59). Numerous studies have shown that furin endopeptidase close to the prodomain can remove the NH2-terminus of ADAM17 by proteolysis/protein cleavage (60), thereby activating it and inducing shedding of pro-TNF-α, TNFR1, and TNFR2 and subsequent pro-inflammatory response. Besides, ADAM17 triggers the hydrolysis and release of more than 90 substrate proteins. These have been further discussed in “ADAM17 Mediates Substrate Shedding Activity” section. Phosphorylation of ADAM17’s cytoplasmic tail is another post-translational modification. ADAM17 is often hyperphosphorylated in patients with emphysema (61). As shown in Figure 2, ADAM17 can be phosphorylated by various protein kinases, such as PKC (3), PKL2 (3), PTK2 (18), MAPKs (3, 62), Akt/GSK (63), and Smad2/3 (64). Recent studies have shown that the extracellular domain of ADAM17 with a homodimer structure can bind tightly to selective ADAM17 inhibitors, while serine 819 (Ser819) and threonine 735 (Thr735) in the cytoplasmic tail release selective ADAM17 inhibitors, which activate ADAM17 by inhibiting its phosphorylation-induced dimerization (65, 66). In addition, ADAM17 phosphorylation further promotes the shedding of TNF-α and two TNF receptors (32). Short term pro-TNF-α shedding by ADAM17 substrate does not depend on rapid phosphorylation of pro-TNF-α or the cytoplasmic tail of ADAM17 and it is mainly regulated by serine/threonine kinases (67). It has been reported that serine phosphorylation of ADAM17 substrate NRG1-ICD can restrain its cleavage of these post-translational modified substrates to some extent (68). Glycosylation of ADAM17 plays an important role in regulating enzyme activity or binding to substrates (69). ADAM17 glycosylation is significantly different between mammalian and insect cells (69). The glycosylation of ADAM17 cannot be detected in CRIB-1 cells (70). ADAM17-mediated TNF-α shedding is associated with O-glycosylation in the extracellular proximal membrane region (71). O-glycosylation at Ser41, however, prevented ADAM17-dependent cleavage of β1-AR (72). Glycosylation not only alters protein folding and conformation and affects ADAM17 activity, but also regulates receptor-mediated signal transduction (73, 74) and facilitates drug interventions targeting non-zinc-binding exosome sites of ADAM17 (69). Chen et al. found that zidovudine-based treatment inhibited the glycosylation of ADAM17 and the lysis of monocyte CD163 (75), indicating the important role of glycosylation in ADAM17 activity and disease progression.
Figure 2 Multiple regulatory roles of ADAM17. ADAM17 activity is affected by transcriptional regulation, and post-transcriptional and post-translational modification. ADAM17 activity is also associated with substrate shedding. iRhoms affect the shedding of ADAM17 and regulation of its downstream signaling pathways.
In addition to post-translational modifications, ADAM17 also affects post-transcriptional regulation. ADAM17 is highly expressed or upregulated in cancer (76, 77) and other inflammation-related diseases, including kidney disease (78), sepsis (79), cicatrization (80), diabetic retinopathy (81), myocardial fibrosis (82), aortic dissection (83), arthritis (84) and atherosclerosis (7). The guanine-cytosine (G-C) sequences in the promoter region of ADAM17 are capable of binding specifically to many transcription factors (85–87). The gain- or loss-of-function of ADAM17 is attributed to the regulation of the following transcription factors, such as NF-κB (77, 88, 89), AP-1 (77, 88), SP1 (85), HIF-1α (82, 83), C/EBP-β (76), EGR1 (79), Sim1 (90), RUNX2 (91). For instance, inflammatory induction of inactive rhomboid protein 2 (iRhom2) stimulated by TNF and IFN-γ drives the activation and upregulation of ADAM17 expression and subsequent shedding of cell-surface molecules (77, 88, 89), which is blocked by NF-κB and AP-1 (77, 88, 89). However, ADAM17 can negatively regulate miR-449b-3p expression and its promoter activity via activating NF-κB transcription. MiR-449b-3p is a downstream target of ADAM17 and has a binding site of NF-κB in its promoter (77). He et al. found that EGR1 is bound to the ADAM17-172A>G (rs12692386) promoter region with affinity, leading to upregulation of ADAM17 promoter activity and transcription (79). However, the loss of EGR1 function prevents ADAM17 expression and induces a pro-inflammatory response. HIF-1α is an upstream target of ADAM17, and the transcriptional activation of HIF-1α promotes the upregulation of ADAM17 expression (82, 83). The latter regulates AMPK metabolism-related diseases through the adrenergic receptor (ADRA1A) (82). In addition, miR-145 downregulates ADAM17 expression by binding to the 3’-UTR of ADAM17, which leads to activation of the ADAM17-EGFR-Akt-C/EBP-β feedback loop and induction of tumor invasion (76). Epigenetic regulation of histone post-transcriptional modifications also plays a pivotal role in the post-transcriptional regulation of ADAM17. Recruitment/deletion of histone H3K4me3/H3K27me3 at the ADAM17 gene promoter downregulates ADAM17 expression (92), suggesting that dynamic chromatin modifications at this site lead to inflammatory responses.
Due to the shedding activity, ectodomains of many transmembrane proteins are hydrolyzed and released by ADAMs metalloproteinases. Studies over the past five years revealed that ADAM17 has more than 90 substrates (7, 32) with distinct functions (Table 1), which are involved in various cellular processes, including cell adhesion, migration, development, inflammation, immune response, tumorigenesis, signal transduction. The cleavage and release of substrates (inflammatory cytokines, growth factors, receptors, adhesion molecules, and others) for ADAM17 may result in different functions of substrate proteins. Some substrate proteins, such as glycocalyx (104), TNFR (173, 178), and JAM-A/FIIR (156), are shed by ADAM17 in the form of active molecules. Glycocalyx is a polysaccharide protein complex that covers the aperture membrane surface of vascular endothelial cells and regulates the homeostasis of the cytoplasmic membrane through proteoglycan-glycoprotein attachment to endothelial cells. Recent studies have shown that activation of S100B/RAGE signaling by traumatic brain injury contributes significantly to ADAM17-mediated endothelial calyx shedding, which aggravates blood-brain barrier dysfunction and increased vascular permeability (104). The sheddase activity of ADAM17 drives scramblase-dependent phosphatidylserine (PS) exposure to the membrane surface, allowing the substrate to be cleaved and shed at the membrane surface (178, 179). The inability of ADAM17 to interact directly with PS may be due to the ability of the ortho-phosphorylserine form of PS to competitively inhibit the shedding of ADAM17 substrates (179). ANO6 facilitates the regulation of phosphatidylserine on the plasma membrane due to its scramblase activity. Veit M et al. found that downregulation of ANO6 expression by RNA interference significantly reduced the cleavage and release of TNFR1 by ADAM17 in HUVECs (178) and that free TNFR1 promotes TNF-induced cell necrosis (173). ADAM17-mediated JAM-A/FIIR shedding is responsible for aging-related abnormal endothelial remodeling (156). However, other substrates, like EGFR ligands (17, 97, 180), E-cadherin (124), VLDLR (4), IL-11R (5), CD137 (94), P75 (11), GPIBα (6), HPP1 (119), and NRG1 (10) are precursor proteins or fusion proteins that can yield active components or soluble active receptors only after cleavage and release by ADAM17 (Figure 2). Evidence suggests that ADAM17 promotes tumor-associated macrophage polarization and angiotensin II-mediated pro-growth and pro-migration signals by shedding EGFR ligands, including heparin-binding EGF-like growth factor (HB-EGF) and AREG (members of the EGF family), from the cell membrane (17, 32). E-cadherin is a key substrate for ADAM17, which is conducive to epigenetic regulation, endocytosis and efflux of cells by cleaving and shedding E-cadherin. Once ADAM17 binds to CD82, ADAM17 metalloproteinase activity is inhibited, leading to a reduction in E-cadherin cleavage products (124). IL-11 is a member of the IL-6 family that binds to IL-11R and forms a complex with CP130 to mediate anti-inflammatory signal transduction. On the other hand, IL-11R is hydrolyzed to soluble IL-11R (sIL-11R) via ADAM17 overexpression, mediating IL-11 trans-signaling pathway (5), which confers pro-inflammatory cytokine activity. Similarly, the bidirectional regulation of CD137/CD137L-mediated cellular responses has been implicated in the development of tumors and autoimmunity. The shedding protease ADAM17 triggers the production of soluble CD137 (sCD137), a spliceosome of CD137, which subsequently enhances T cell proliferation, whereas inhibition of ADAM17 activity intercepts the sCD137 production (94). VLDLR, an apolipoprotein receptor, plays an important role in foam cell formation, plasma triglyceride metabolism and inflammation. Its soluble ectodomain-mediated anti-inflammatory effect is related to the activation of the Wnt signaling pathway. ADAM17 induces the release of soluble VLDLR (sVLDLR), which inhibits the Wnt pathway and leads to macular degeneration in eye tissue, whereas the shedding of sVLDLR is blocked by selective ADAM17 inhibitors (4). Carrido et al. revealed that tumor formation mechanisms were probably caused by ADAM17-mediated cleavage of the P75 ectodomain (11). In addition, the increased ectodomain cleavage of other ADAM17 substrates (GPIBα and HPP1) may be required for immune platelet clearance and tumor suppression (119, 181). However, in another study related to oncogenic KRAS, KRAS mutations triggered enhanced ADAM17-mediated NPG1 shedding of the SLC3A2-NPG1 fusion protein, which in turn promoted tumor cell growth (10). Collectively, the pro-inflammatory and anti-inflammatory effects induced by ADAM17 substrate shedding may be related to distinct regulatory effects and functions of the substrates.
ADAM17 regulates signal transduction in many pathophysiological processes, including inflammation, immunity and tumor. The upregulation of ADAM17 expression leads to increased EGFR ligand release and polarization of the EGFR signaling, which is responsible for cell proliferation, invasion, and migration (182, 183). However, downregulation of the ADAM17 expression urges the opposite effect by suppressing the EGFR/ERK, EGFR/Akt/C/EBP-β or EGFR/ErbB signaling pathways (76, 184). ADAM17-mediated EGFR signaling increases the levels of TGF-β and accumulates extracellular matrix (185), implying the role of TGF-β in the regulation of multiple immune cells under pro-inflammatory conditions. Emerging evidence suggest that blocking ADAM17 expression effectively alleviates inflammatory responses, which may be relevant to the regulation of pro-inflammatory cytokines IL-1β, IL-6 and TNF-α (186, 187). However, the loss of ADAM17 function with gene mutations triggers the development of inflammatory diseases (48, 188). Based on the aforementioned discussion, we suggest that ADAM17’s critical role in various signaling pathways ensures its activity is strictly regulated.
iRhoms, lacking the catalytic motif GxS, are members of the rhomboid protein family with important biological functions (189). Recently, iRhoms have been identified as key regulators of ADAM17 activation. In different tissues, iRhoms appear to form proteolytic complexes with ADAM17 sheddase, but not other ADAMs (190), thus mediating ADAM17 cell membrane surface transport. iRhoms contribute to the activation of ADAM17-dependent shedding events and substrate recognition, while deletion of iRhoms hinders ADAM17 activation, suggesting that iRhoms are required for ADAM17 maturation (190). iRhoms contain two inactive homologs, iRhom1 and iRhom2, also known as RHBDF1 and RHBDF2, respectively. iRhom1 is barely expressed in inflammatory/immune cells and yet iRhom2 is highly expressed in these cells and is responsible for ADAM17 activation (89). iRhom2 deficiency inhibits ADAM17-dependent substrate release, including bidirectional regulators and TNFs (191, 192). In iRhom2-mutated macrophages, ADAM17 remains in endoplasmic reticulum (ER), and cannot be activated by lysis of its prodomain (193). The cytoplasmic domain of iRhom2 participates in the regulation of ADAM17-dependent shedding events (189). Shed ADAM17 triggers phosphorylation of the N-terminus of iRhom2 and promotes the separation of ADAM17 from the iRhom2/ADAM17 complex by recruiting 14-3-3 protein (194). Despite the loss of protease activity, iRhom1 and iRhom2 maintain critical non-protease activities in regulating EGF and TNF-α signaling pathways (41, 195). Upregulated expression of iRhom1 in ER may enhance proteasome activity via the PAC1/2 pathway rather than via EGF signaling. Mice with iRhom2 deficiency had severe immunodeficiency and could neither produce the main inflammatory cytokine, TNF, nor could they respond to lipopolysaccharide-induced inflammation and immune responses. Therefore, iRhoms play an integral role in ADAM17-mediated downstream signal regulation. Hence, targeting iRhoms and ADAM17 may provide new strategies for anti-inflammatory treatment.
Due to the shedding activity, ADAM17 is closely related to the formation and development of distinct cancer types, including lung cancer, ovarian cancer, breast cancer, stomach cancer, colorectal cancer, bladder cancer, melanoma, cervical cancer, pancreatic cancer, etc.
Lung cancer has the highest incidence and mortality rate in the world. ADAM17 is usually an oncogene and its upregulation is associated with the progression of lung cancer. In LUAD, KRAS mutation contributes to the phosphorylation of ADAM17 threonine via p38 MAPK, thereby driving ADAM17 to selectively promote its substrate IL-6R shedding and subsequent ERK1/2 MAPK-IL-6-mediated trans-signal transduction, leading to malignant progression of the cancer (152). Enhanced ADAM17 activity mediated by KRAS mutation also facilitates the shedding of S-N (SLC3A2-NRG1) fusion protein NRG1 and the release of soluble NRG1 (sNRG1), which contributes to the increase in ERBB2-ERBB3 heterocomplex receptors and the activation of the downstream PI3K-AKT-mTOR pathway, leading to the growth of lung cancer cells (10). In addition, iRhom2, as a key binding protein for ADAM17, further promotes KRAS-induced tumor cell growth by modulating the release of ERBB ligands (196). However, the efficacy of radiotherapy for non-small cell lung cancer was enhanced when blockade of ADAM17 function with the neutralizing antibody (197). These findings suggest that ADAM17 is a cancer-promoting gene and a potential target for anti-lung cancer therapies.
Fabbi et al. found that ADAM17 is significantly upregulated in ovarian cancer, and its high-expression is associated with poor clinical prognosis in ovarian cancer patients (198). High levels of ADAM17 in serum and ascites fluid of patients with ovarian cancer may be used as a hematologic tumor marker for the detection of ovarian cancer (199). ADAM17 promotes the malignant progression of ovarian cancer and causes chemo-resistance by mediating ADAM17-dependent shedding of AREG, HB-EGF, IL-6Rα, TNF, TNFR1-α, TGFα and activating the EGFR signaling pathway (198, 200). Deletion of ADAM17 or treatment with selective ADAM17 inhibitor GW280264X is capable of declining substrate cleavage/release and promoting chemo-sensitization (198, 201). ADAM17-induced P75 cleavage may also be responsible for ovarian cancer-promoting activities (11).
ADAM17 functions as one of the highly expressed genes in breast cancer that plays an important role in the development of breast cancer. ADAM17 promotes cleavage of PD-L1 on the surface of breast cancer cells (12), regulates the interaction between PD-L1 and PD-1 (12), and may contribute to immune escape of triple-negative breast cancer cells (12, 202). ADAM17 can mediate the release of sTNFR1 and sTNFR2, which inhibit the secretion of metastasis-promoting chemokines (CXCL8, CCL5, CXCL) and induce anti-metastasis effects in triple-negative breast cancer cells (203). An earlier study indicated that breast cancer-associated fibroblasts stimulated breast cancer cell proliferation through ADAM17-mediated cleavage of TGF-α (204). Interestingly, ADAM17 is also present in platelets and is involved in tumor immune escape. It was found that downregulation of ADAM17 in activated platelets from breast cancer patients was associated with tumor metastasis and clinical stage of breast cancer (14). D8P1C1, an anti-ADAM17 monoclonal antibody, remarkably inhibited tumor growth in triple-negative breast cancer mouse models (205). Similar results were reported in another published paper (13). In summary, the critical role of ADAM17 in breast cancer makes it a potential target for breast cancer therapy.
ADAM17 is probably associated with aggressive metastasis and poor prognosis of gastric cancer. A meta-analysis associated with gastric cancer indicated that ADAM17 might be a tumor marker for poor prognosis in gastric cancer, and high expression of ADAM17 is associated with lymph node metastasis and clinical staging of lymph node metastasis in gastric cancer (15). ADAM17 promotes gastric cancer cell metastasis by activating the Notch-Wnt signaling pathway (206). Epithelial-mesenchymal transition (EMT) is a transformation of cell morphology that occurs in the development of tumors, including gastric cancer. It was reported that ADAM17 promotes EMT in gastric cancer cells (33, 64). The mechanism of ADAM17 in gastric cancer may be through TGF-β/p-Smad2/3-mediated EMT activation (207, 208).
ADAM17 is also highly expressed in cervical cancer, liver cancer, colorectal cancer and bladder cancer. ADAM17-modified bone marrow mesenchymal stem cells may stimulate the malignant growth of drug-resistant cervical cancer cells by activating the EGFR/PI3K/Akt pathway (16). ADAM17 is thought to cleave the Notch receptor and inactivate Notch signaling, thereby impeding the GPR50/ADAM17/Notch axis-mediated development of liver cancer (85). ADAM17 can interact with cellular integrin α5β1 to promote the binding and uptake of exosomes derived from colorectal cancer (209). Newly formed exosomes are associated with the malignant phenotype of tumors. In addition, ADAM17 also promotes STAT3 activation by induction of EGFR/IL-6 transduction signaling pathways, which ultimately lead to tumor progression; inhibition of the ADAM17/IL-6/STAT3 signaling axis significantly attenuated the growth of colon cancer cells (210). The ADAM17/EGFR/AKT/GSK3β axis plays a key role in regulating melanoma cell proliferation, migration, and cell sensitivity to chemotherapeutic drugs (211). ADAM17 is also involved in immune-related autocrine and paracrine regulation (40). However, knockdown of ADAM17 or treatment with anti-ADAM17 antibody MEDI3622 resulted in regression of pancreatic tumors, accompanied by down-regulated EGFR/STAT3 signaling, increased cytotoxic T cells, and decreased granulocyte-like medullary inhibitory cells in mouse models of pancreatic cancer (212).
Tumor microenvironment (TME) refers to a complex environment closely related to tumorigenesis, tumor growth and its metastasis, which is composed of a variety of cells (including macrophages, fibroblasts, lymphocytes, endothelial cells, etc.), extracellular matrix, and multiple signaling molecules (cytokines, growth factors, chemokines, hormones, etc.) (213). Autocrine and paracrine are conducive to the activation of multiple signaling pathways in tumor cells and non-tumor cells (e.g., macrophages, lymphocytes, endothelial cells) (214–216). In this way, the dynamic interaction between tumor cells and their surrounding matrix triggers tumor cell proliferation, immune evasion, distal metastasis, and drug resistance, and angiogenesis as well (217, 218).
Tumor-associated macrophage (TAM) is derived from mature monocyte in peripheral blood. Monocytes are recruited to TME through chemokines and cytokines secreted by tumor cells and become TAMs. TAMs are the most abundant immune cells in the TME and are closely relevant to tumor growth, invasion and metastasis (219). For one thing, macrophages serves as an important component of tumor stromal cells that are able to gather around blood vessels and promote angiogenesis, tumor invasion and metastasis (220, 221). For another thing, it also has the ability to remove tumor cells and reshape the TME (222). Due to the influence of cytokines in the TME, TAMs can be divided into two distinct polarized forms, M1 and M2 macrophages. The former is responsible for killing tumor cells; the latter is able to promote tumor growth (223). Macrophage M1/M2 polarization is adjustable and reversible. Increased M2-polarized TAMs are often associated with cytokines and growth factors, e.g., IL-4 (224), IL-10 (225), CSF-1 (226), TGF-β (227) secreted by tumor cells or Th2 cells in the TME, indicating a poor prognosis for tumor patients.
Metalloproteinase ADAM17 can shed distinct signaling proteins on the cell surface, making it a mediator for intercellular signal transduction (7, 20). Our previous study showed that the expression of ADAM17 was associated with infiltration of multiple immune cells, including macrophages (20), in TCGA pan-cancer samples and yet the specific regulatory mechanism of ADAM17 is unknown. Recently, Gnosa et al. have revealed the positive roles of ADAM17 in regulating the polarization of TAMs (17). By using bioinformatics analysis based on the TCGA dataset and immunohistochemical analysis from triple-negative breast cancer cohort, the authors first confirmed that highly expressed ADAM17 in tumors is positively correlated with tumorigenic macrophage markers CD163 or CD206. Deletion of ADAM17 gene inhibited tumor growth, increased the survival in tumor-bearing mouse models, and resulted in a significant decrease in CD163+ cell population. In a co-cultured mouse bone marrow-derived macrophages with ADAM17-WT or ADAM17-KO tumor cells, knockdown of ADAM17 significantly diminished the expression of CD163 or CD206, IL-6, IL-10, and CCR7 in bone marrow-derived polarized macrophages, suggesting an important role of ADAM17 in tumorigenic macrophages. Furthermore, the authors used cellular co-culture and zebrafish embryo propagation models to demonstrate that tumor cells, in an ADAM17-dependent manner, drive macrophage polarization into a tumor-promoting phenotype and accelerate tumor cell invasion. Based on the sheddase activity of ADAM17 (38), this macrophage polarization is regulated by ADAM17-mediated shedding of EGFR ligands (HB-EGF, AREG). Actually, the mechanism of macrophage polarization driven by tumor cells has been reported in many previous works. For instance, the EGFR/PI3K/AKT/mTOR axis plays an important role in promoting TAM M2 polarization by secreting EGF from colon cancer cells (228). Pancreatic cancer triggers the polarization of TAM M2 by secreting REG4 through the EGFR/AKT/CREB pathway (229). These findings further indicate that EGFR ligand shedding mediated by ADAM17 may be beneficial to activating the EGFR signaling pathway and inducing the polarization of tumor-promoting TAMs. Finally, they further demonstrated the promoting effect of macrophage-derived CXCL1 secretion on tumor cell invasion by RNA-seq analysis of transcriptome data from co-cultured macrophages. Taken together, these findings suggest a critical role of the ADAM17-EGFR (HB-EGF/AREG) axis in the polarization of TAMs (Figure 3), which also provides a new strategy for the anti-tumor immunotherapy.
Natural killer (NK) cells are important lymphocytes to fight against tumor escape or immune evasion. A large number of studies have shown that the activity and function of NK cells in peripheral blood of some cancer patients are significantly reduced (230), which may be conducive to the development of malignant tumors. In non-small cell lung cancer, the function of NK cells has been shown to be significantly impaired. Therefore, immunotherapy targeting NK cells has become a therapeutic concept for this type of cancer (231). One of the reasons for the anti-tumor immune activity of NK cells is attributed to the binding of its surface-activated receptor natural killer cell group 2D (NKG2D) to MHC class I chain-related protein A/B (MICA/B), an NKG2D ligand on the surface of tumor cells, thus activating NK cell function and enables NK cells to kill tumor cells (232). Studies have shown that inhibition of the ADAM9 activity significantly blocked MICA shedding and affected the immune killing effect of NK cells on tumor cells (233). ADAM17 is also a member of the metalloproteinase family that may have a similar function. Recently it was found that ADAM17 has the ability to hydrolyze MICA/B on the surface of tumor cells to generate soluble MICA/B (sMICA/B) (52), the latter of which alters the conformation of NKG2D on the surface of NK cells (234) and affects the recognition and binding of membranous MICA with NKG2D, thereby inhibiting NK activation signals and reducing the killing sensitivity of NK cells to tumor cells (235). Knockdown of ADAM17 prohibits MICA shedding and boosts MICA expression on the surface of hepatocellular carcinoma cells (131). In addition, hypoxia-induced shedding of MICA on the surface of pancreatic cancer cells enables tumor cells to evade NK cell immune killing (235). The function of MICA/B monoclonal antibodies is to inhibit MICA/B shedding by binding antibodies at key epitopes in the MICA/M proximal membrane domain, and its antitumor immunity activity is associated with NKG2D and CD16 Fc receptor activation (236). Inhibition of MICA/B shedding with monoclonal antibodies drives NK cell-mediated antitumor immunity (237), suggesting that the sMICA levels may be correlated with decreased NK cell function. Therefore, blocking ADAM17-mediated hydrolytic activity to inhibit MICA shedding may be one of the ways to improve NK cell killing of tumor cells (Figure 3).
The antitumor immune activity of NK cells is also related to the antibody-dependent cell-mediated cytotoxicity (ADCC) induced by CD16 Fc receptor (Figure 3). ADCC is a key cytolytic mechanism of NK cells. NK cells, on the one hand, interact with the Fc region of antibodies that recognize proteins on the surface of tumor cells through their IgG Fc receptors to target tumor antigens and produce cytotoxic effects. On the other hand, it also mediates adaptive immune responses. In human beings, IgG’s FcR family consists of six receptors, including FcγRI (CD64), FcγRIIa (CD32a), FcγRIIb (CD32b), FcγRIIc (CD32c), FcγRIIIa (CD16a), and FcγRIIIb (CD16b), of which CD16a is primarily responsible for triggering NK cell-mediated ADCC. Therefore, exploring the mechanism of CD16a contributes to the development of anti-tumor immunotherapy drugs that enhance ADCC activity. The metalloprotease ADAM17 has been reported to shed CD16a (238), leading to decreased ADCC activity and reduced IFN-γ production (239). However, Blocking CD16 shedding or avoiding cleavage prompted a stronger tumor cell killing by NK cells (240, 241) and increased IFN-γ production (242). Paradoxically, treatment with an ADAM17 inhibitor did not increase IFN-γ levels induced by stimulated NK cells (242). CD16a is a hot topic discussed in recent NK cell anti-tumor immunity, and more information about the role of ADAM17 in the regulation of CD16a in NK cells can be seen in some recent studies (238, 243).
In addition, IL-15, an immunomodulatory factor, also plays a key role in the development, homeostasis, activation and proliferation of NK cells (244). IL-15 can differentiate hematopoietic progenitor cells into CD56+ NK cells to induce pro-proliferative responses. In NOG-IL-15 Tg mice expressing transgenic human IL-15, there is a significant increase in transplanted NK cells from healthy subjects’ peripheral blood (19, 245). In different tumor-bearing animal models, IL-15 treatment contributes to tumor regression, reduction of tumor metastasis, and improvement of animal survival. Currently, the developed IL-15 mutant (IL-15N72D) or its stable soluble complex, ALT-803, has been shown to have similar functions as IL-15 and significantly improved the antitumor activity of anti-CD20 monoclonal antibody in NK cells and the immunotherapeutic efficacy of PD-1/PD/L1 monoclonal antibody (232). ADAM17 is present in various immune cells, including NK cells (20), which mediates lysis and shedding of cell surface receptors. CD62L/L-selectin is an immune cell homing receptor that regulates the migration of white blood cells to sites of inflammation. It was found that CD62L expression is increased in IL-15-stimulated NK cells (19). Expression of ADAM17 on NK cells promotes the downregulation of CD62L expression (242). Mishra et al. first indicated that ADAM17 reduced IL-15-stimulated NK cell proliferation with the participation of CD62L (19). The blockade of ADAM17 reversed this event. Overall, IL-15-mediated NK cell proliferation promotes an increase in CD62L levels, while prolonged activation of ADAM17 leads to CD62L shedding and impaired NK cell proliferation stimulated by IL-15 (Figure 3).
Metastasis is a form of tumor progression. 90% of tumor-related deaths are caused by metastasis of tumor cells. The process includes: 1) the shedding of tumor cells from the primary tumor; 2) intravasation; 3) survival in the blood circulation; 4) extravasation of blood vessels and metastases. The interaction between endothelial cells and tumor cells is an important step in tumor metastasis. Tumor cell-endothelial cell tight contacts promote tumor cell adhesion to the vascular wall through justacrine or paracrine signaling (246). As shown in Figure 3, endothelial cells secrete a series of adherent molecules, such as E-selectin, VCAM-1, etc., to increase the adhesion of tumor cells with endothelial cells, and further promote tumor metastasis. The mechanism of tumor metastasis may be related to EMT, angiogenesis, tumor stem cell characteristics, and the increase of circulating tumor cells. ADAM17 is widely present in endothelial cells and is positively correlated with immune infiltration levels of endothelial cells in multiple cancer species (20, 88, 173). It is speculated that endothelial ADAM17 may help tumor metastasis. Recent emerging evidence supports this speculation (173). Julia et al. also confirmed that endothelial ADAM17 is required for endothelial necrosis, tumor cell extravasation and metastasis (247). ADAM17-dependent death receptor TNFR1 ectodomain shedding promotes endothelial cell necrosis and tumor cell extravasation (173, 247). In addition, CCL2 secreted by tumor cells and macrophages promotes PKCβ activation by binding to endothelial CCR2, which further leads to ADAM17 activation (247). ADAM17 appears to be closely associated with pathological angiogenesis (138). In ADAM17flox/flox/Tie2-Cre mice, loss of endothelial ADAM17 inhibits chord formation and impedes ectopic injected tumor growth (138). In endothelial cells, soluble Robo4 (sRobo4) is shed and released by ADAM1, which subsequently inhibits SLIT3-induced angiogenesis (248). Meanwhile, SLIT3 obstructs Robo4 shedding and enhances its signal transduction (248). ADAM17 may disrupt the barrier effect of vascular endothelial cells by affecting their attachment and tight junctions (249). Beyond vascular endothelial cells, ADAM17 is also important in lymphatic endothelial cell-induced tumor migration and metastasis. Sun et al. indicated that ADAM17 activation by MAPK14/T180 promoted the secretion of soluble CX3CL1, which further led to malignant metastasis of liver cancer cells (18). In addition, Macrophage M2 polarization is also associated with ADAM17-dependent CX3CL1 secretion (18). As a critical binding protein for ADAM17, iRhom1 has been found to promote independent regulation of ADAM17 under physiological shear stress (88). However, there is no report yet on the regulation of ADAM17 by iRhom1 in endothelial cells and its effect on tumor malignant progression, which may be an interesting topic.
ADAM17 has over 90 substrates, some of which are mediators of cancer diseases, which implies that substrate based ADAM17 inhibitors have the potential to be used for the treatment of malignant tumors. In this section, we outline recent advances in potent and selective ADAM17 inhibitors containing hydroxamate and non-hydroxamate moieties, as well as anti-ADAM17 monoclonal antibodies (Figure 4, Table 2).
The metalloproteinase domain of ADAM17 has a catalytic site containing a sequence of zinc-dependent amino acid residues that can bind to zinc ions to interfere with ADAM17 enzyme activity. The hydroxamate moiety is a common zinc-binding motif, and hydroxamate-based small-molecule inhibitors targeting the catalytic site may be an effective strategy against tumors. Marimastat and apratastst are the earliest synthesized hydroxamate-based inhibitors with limited selectivity. Marimastat inhibits the cleavage of TNF-α and CD44 and reduces the invasion of tumor cells with an IC50 of 4.75 μM (102). Shu et al. found that apratastst significantly inhibited TNF-α cleavage with IC50 of 81.7 ng/mL ex vivo and 144 ng/mL in vitro, respectively (250). INCB7839 is not ideal as a single agent, but it enhances the efficacy of trastuzumab in metastatic HER2-positive breast cancer. INCB7839 suppresses ADAM10/17-dependent EGFR ligand shedding and potentiates the antitumor effects of the recombinant peptidase PEPDG278D (251). Since January 2009, INCB7839 has been used in Phase I/II clinical trials alone or in combination with rituximab/trastuzumab + vinorelbine/trastuzumab + docetaxel for the treatment of diffuse large B cells non-hodgkin lymphoma gliomas, breast cancer or solid tumors (Table 3). In a subset of subjects, INCB7839 at a dose of 300 mg b.i.d. (Phase II) in combination with rituximab resulted in a range of serious side effects, including thromboembolism, pain, and infections (NCT02141451). However, other anticancer clinical trials associated with INCB7839 were terminated for some reason or were not conducted or not yet reported (NCT01254136; NCT00864175; NCT0429575; NCT00820560). INCB3619, an early hydroxamate-based inhibitor with the IC50 value of 14 nmol/L, significantly inhibits tumor cell survival by blocking the shedding of ErbB ligands (252). INCB3619 also enhances the sensitivity of gefitinib (264), cisplatin (252), and lapatinib (265), and acts synergistically with CD16 × 33 bispecific killer cell conjugates against acute myelogenous leukemia (266). KP457 increases the production of platelets derived from functional human induced pluripotent stem cells by inhibiting the exodomain shedding of platelet glycoprotein Iba (GPIba), with an IC50 value of 10.6 nmol/L for KP457 (253). GW280264X facilitates the anti-ovarian cancer effect of cisplatin (201) and restrains the development of lung adenocarcinoma cells (254). The IC50 value of PF-5480090/TMI-002 in MDA-MB-468 cells is approximately 1696.6 RFU/mg, which reduces the release of TGF-α and increases the cytotoxic effects of anti-EGFR/HER drugs (255). GI254023X is a selective inhibitor of ADAM10 and ADAM17, but its selectivity for ADAM10 is 100 times higher than that of ADAM17, with IC50 values of 5.3 μM and 541 μM for ADAM10 and ADAM17, respectively (256). The hydroxamate derivative batimastat inhibits ADAM17 shedding (267) and has prevented the progression of multiple tumors in clinical trials, particularly the formation of peritoneal carcinomas (268). TAPI-1 with IC50 value of 8.09 μM is capable of inhibiting matrix metalloproteinase and blocking the shedding of cytokine receptors (107). Recent studies have shown that TAPI-1 appreciably restrains ADAM17 activation during pseudomonas aeruginosa infection (269). Additionally, we previously retrieved four novel hydroxamate-based small molecule compounds 11-14 targeting ADAM17 from the DrugBank database, but no in vitro and in vivo experimental data were reported (20). BMS-561392 reduced ADAM17 activity with an IC50 of 0.2 nM. Overall, most hydroxamate-based inhibitors exhibit potent ADAM17 shedding activity and resist tumor progression. Compounds with the hydroxamate group, however, are usually poorly bioavailable and produce toxic hydroxylamine through metabolism, which somewhat limits the clinical use of these compounds (270).
To avoid side effects and toxicity caused by the hydroxamate group and to improve bioavailability, research on new ADAM17 inhibitors has been directed toward non-hydroxamate-based small-molecule compounds (46). By searching the literature published in the last five years, we have selected the following four new compounds for description. With computerized virtual screening, Lu et al. identified a non-hydroxamate-based inhibitor, called thioxodihydro pyrimidindione ZLDI-8, which reversed taxol resistance, displayed an IC50 value equal to 6.85 μM against ADAM17 (259), and inhibited metastasis of hepatocellular carcinoma (271). It also enhanced the antitumor effects of sorafenib and 5-fluorouracil (272, 273). Another non-hydroxamate-based inhibitor, SN-4 specifically impedes ADAM17-mediated cleavage of TNF-α and CD44 with a higher activity than malistamate and an IC50 value of 3.22 μM (102). SN-4(Nps)2, a prodrug of SN-4, can markedly enhance its bioavailability. A thiadiazolone derivative JTP-96193 showed 1800 times more selectivity toward ADAM17 over other matrix metalloproteinases with an IC50 value of 5.4 μM (258). Compounds 20-23 are novel non-hydroxamate-based small molecules targeting ADAM17 from the DrugBank database, whereas their ADAM17 inhibitory activity and potential mechanism remain to be further explored (20).
The development of anti-ADAM17 monoclonal antibodies has accelerated the progress of innovative ADAM17 inhibitors. Anti-ADAM17 monoclonal antibodies include A300E, A9 (B8), D1 (A12), MEDI3622, etc. A300E is rapidly internalized by ADAM17-expressing cells (274), and its IC50 against ADAM17 is approximately 0.7 μg/mL (260). Trad et al. suggested that A300E plays a role in cancer cells by transporting a conjugated toxin to target cells (260). A9 (B8) cross-reacts with both human and mouse ADAM17, whereas D1 (A12) binds only to human ADAM17. D1 (A12) is bound to both the catalytic and non-catalytic domains of ADAM17. Yang et al. found that A9 (B8) conferred EGFR-TKI-mediated antitumor effects in NSCLC cells with IC50 values of 0.22 nM and 0.25 nM against human and murine ADAM17, respectively (261). Ye et al. revealed that A9 (B8) inhibited the shedding of ADAM17 substrate and contributed to the growth inhibition of pancreatic ductal adenocarcinoma in vivo and in vitro (275). D1 (A12) at 4.7 nM inhibits 50% TNF-α shedding and induces anti-ovarian cancer effects (262). Besides, D1 (A12) restrains the progression of head and neck squamous cell carcinoma by reducing HERS-transactivation induced by retarded hormone and even has therapeutic prospects for EGFR TKI-resistant head and neck squamous cell carcinoma (276). Another anti-ADAM17 monoclonal antibody, MEDI3622, inhibits tumor-dependent EGFR activity with IC50 values of 39 pmol/L and 132 pmol/L against human and murine ADAM17, respectively (263). In esophageal and colorectal tumors, the antitumor effect of MEDI3622 was superior to that of the EGFR/HER pathway inhibitor, suggesting that MEDI3622 inhibits tumor growth by partially modulating non-EGFR-mediated pathways (263). In addition, MEDI3622 enhances the release of antibody-bound tumor cells binding IFN-γ in NK cells by blocking CD16A shedding (239).
To date, there are no clinically available ADAM17 inhibitors. The high toxicity and low selectivity of existing ADAM17 inhibitors and the high structural homology between the catalytic domain of ADAM17 and other metalloproteases (e.g., ADAM10) have limited the development of selective ADAM17 inhibitors. However, the advent of small molecule compounds and anti-ADAM17 monoclonal antibodies targeting the non-catalytic domain of ADAM17 or the catalytic and non-catalytic domains of ADAM17 (44, 46, 277–279) further overcome these problems and improve bioavailability, which may provide a new strategy for the development of the highly effective low-toxicity ADAM17 inhibitors. In addition, as iRhom2 is a specific binding protein of ADAM17, targeting iRhom2 to inhibit ADAM17 activity is also a trend in the development of ADAM17 inhibitors.
Metalloproteinase ADAM17 holds a vital role in post-translational protein modification, gene transcription and post-transcriptional regulation, and is closely associated with tumors and inflammation. ADAM17 regulates cell membrane protein shedding and subsequent signal transduction. It can also be impacted by the interacting proteins and thus participate in the regulation of its downstream signaling pathways. ADAM17 has been implicated in immune regulation of tumor development. However, its immunomodulatory functions and mechanisms in cancer diseases are not well studied, and therefore more studies are needed to further determine the role of ADAM17 in tumor development. In this article, we summarized the structure and multiple regulatory roles of ADAM17, the latest immune regulation of ADAM17 in tumor formation and development, as well as the progress in the development of ADAM17 inhibitors. On the one hand, although the regulatory effect of ADAM17 on macrophages, NK cells, and endothelial cells has been confirmed in tumor, more key proteins or genes related to ADAM17 need to be identified, and the immune response involved in TME needs to be further explored. In addition, the role of ADAM17 in post-translational modifications, such as proteolysis, phosphorylation, glycosylation, and post-transcriptional regulation in cancer progression remains unclear. On the other hand, due to the structural homology of ADAM17’s catalytic domain with other metalloproteinases, more three-dimensional crystal structures associated with ADAM17 need to be uncovered to make them more conducive to highly selective and toxic drug design for ADAM17 inhibitors. How to reduce or avoid the toxic side effects of ADAM17 is also a potential research direction. Therefore, studying the key role and immunomodulatory mechanisms of ADAM17 in tumor development will provide new strategies for the prevention, diagnosis and treatment of cancer diseases.
KW and HW conceived and designed this work. KW drafted the manuscript. KW and ZX edited and revised the manuscript. CY, XL, and MZ provided constructive suggestions for the manuscript and literature. All authors contributed to the article and approved the submitted version.
This work was financially supported by the Research Funding of Luzhou Science and Technology Bureau (2021-JXJ-55; 41/00140011) (KW) and Support Funding of Southwest Medical University (41/00190026) (KW), Start-Up Research Funding of Southwest Medical University (41/00040179) (KW), and Research Funding of Southwest Medical University (41/00160216; 2021ZKQN108) (KW).
We thank all the participants for their careful revision and intense discussion of the manuscript, and especially thank Prof. Asaduzzaman Khan and Prof. Yongxue Liu for their critical review for the manuscript.
The authors declare that the research was conducted in the absence of any commercial or financial relationships that could be construed as a potential conflict of interest.
All claims expressed in this article are solely those of the authors and do not necessarily represent those of their affiliated organizations, or those of the publisher, the editors and the reviewers. Any product that may be evaluated in this article, or claim that may be made by its manufacturer, is not guaranteed or endorsed by the publisher.
1. Lokau J, Kespohl B, Kirschke S, Garbers C. The role of proteolysis in interleukin-11 signaling. Biochim Biophys Acta Mol Cell Res (2022) 1869(1):119135. doi: 10.1016/j.bbamcr.2021.119135
2. Hossain MG, Tang YD, Akter S, Zheng C. Roles of the polybasic furin cleavage site of spike protein in sars-Cov-2 replication, pathogenesis, and host immune responses and vaccination. J Med Virol (2022) 94(5):1815–20. doi: 10.1002/jmv.27539
3. Gonzalez SM, Siddik AB, Su RC. Regulated intramembrane proteolysis of Ace2: A potential mechanism contributing to covid-19 pathogenesis? Front Immunol (2021) 12:612807. doi: 10.3389/fimmu.2021.612807
4. Ma X, Takahashi Y, Wu W, Liang W, Chen J, Chakraborty D, et al. Adam17 mediates ectodomain shedding of the soluble vldl receptor fragment in the retinal epithelium. J Biol Chem (2021) 297(4):101185. doi: 10.1016/j.jbc.2021.101185
5. Sammel M, Peters F, Lokau J, Scharfenberg F, Werny L, Linder S, et al. Differences in shedding of the interleukin-11 receptor by the proteases Adam9, Adam10, Adam17, meprin alpha, meprin beta and Mt1-mmp. Int J Mol Sci (2019) 20(15):3677. doi: 10.3390/ijms20153677
6. Wei G, Luo Q, Wang X, Wu X, Xu M, Ding N, et al. Increased gpibalpha shedding from platelets treated with immune thrombocytopenia plasma. Int Immunopharmacol (2019) 66:91–8. doi: 10.1016/j.intimp.2018.11.011
7. Tang BY, Ge J, Wu Y, Wen J, Tang XH. The role of Adam17 in inflammation-related atherosclerosis. J Cardiovasc Trans Res (2022). doi: 10.1007/s12265-022-10275-4
8. Kaneko T, Horiuchi K, Chijimatsu R, Mori D, Nagata K, Omata Y, et al. Regulation of osteoarthritis development by Adam17/Tace in articular cartilage. J Bone mineral Metab (2022) 40(2):196–207. doi: 10.1007/s00774-021-01278-3
9. Palau V, Villanueva S, Jarrin J, Benito D, Marquez E, Rodriguez E, et al. Redefining the role of Adam17 in renal proximal tubular cells and its implications in an obese mouse model of pre-diabetes. Int J Mol Sci (2021) 22(23):13093. doi: 10.3390/ijms222313093
10. Shin DH, Kim SH, Choi M, Bae YK, Han C, Choi BK, et al. Oncogenic kras promotes growth of lung cancer cells expressing Slc3a2-Nrg1 fusion Via Adam17-mediated shedding of Nrg1. Oncogene (2022) 41(2):280–92. doi: 10.1038/s41388-021-02097-6
11. Garrido MP, Vallejos C, Girardi S, Gabler F, Selman A, Lopez F, et al. Ngf/Trka promotes Adam17-dependent cleavage of P75 in ovarian cells: Elucidating a pro-tumoral mechanism. Int J Mol Sci (2022) 23(4):2124. doi: 10.3390/ijms23042124
12. Romero Y, Wise R, Zolkiewska A. Proteolytic processing of pd-L1 by Adam proteases in breast cancer cells. Cancer Immunol Immunother: CII (2020) 69(1):43–55. doi: 10.1007/s00262-019-02437-2
13. Lofgren KA, Sreekumar S, Jenkins EC Jr., Ernzen KJ, Kenny PA. Anti-tumor efficacy of an mmae-conjugated antibody targeting cell surface Tace/Adam17-cleaved amphiregulin in breast cancer. Antibody Ther (2021) 4(4):252–61. doi: 10.1093/abt/tbab026
14. Zhou Y, Heitmann JS, Kropp KN, Hinterleitner M, Koch A, Hartkopf AD, et al. Regulation of platelet-derived Adam17: A biomarker approach for breast cancer? Diagnostics (2021) 11(7):1188. doi: 10.3390/diagnostics11071188
15. Ni P, Yu M, Zhang R, He M, Wang H, Chen S, et al. Prognostic significance of Adam17 for gastric cancer survival: A meta-analysis. Medicina (2020) 56(7):322. doi: 10.3390/medicina56070322
16. Zhou C, Chen F, Li L. A disintegrin and metalloprotease 17 (Adam17)-modified bone marrow mesenchymal stem cells (Bmscs) enhance drug-resistant cervical cancer development. J Biomaterials Tissue Eng (2022) 12(7):1302–8(7). doi: 10.1166/jbt.2022.3057
17. Gnosa SP, Puig Blasco L, Piotrowski KB, Freiberg ML, Savickas S, Madsen DH, et al. Adam17-mediated egfr ligand shedding directs macrophage-promoted cancer cell invasion. JCI Insight (2022) 7(18):e155296. doi: 10.1172/jci.insight.155296
18. Sun C, Hu A, Wang S, Tian B, Jiang L, Liang Y, et al. Adam17-regulated Cx3cl1 expression produced by bone marrow endothelial cells promotes spinal metastasis from hepatocellular carcinoma. Int J Oncol (2020) 57(1):249–63. doi: 10.3892/ijo.2020.5045
19. Mishra HK, Dixon KJ, Pore N, Felices M, Miller JS, Walcheck B. Activation of Adam17 by il-15 limits human nk cell proliferation. Front Immunol (2021) 12:711621. doi: 10.3389/fimmu.2021.711621
20. Wang K, Deng H, Song B, He J, Liu S, Fu J, et al. The correlation between immune invasion and sars-Cov-2 entry protein Adam17 in cancer patients by bioinformatic analysis. Front Immunol (2022) 13:923516. doi: 10.3389/fimmu.2022.923516
21. Blanchot-Jossic F, Jarry A, Masson D, Bach-Ngohou K, Paineau J, Denis MG, et al. Up-regulated expression of Adam17 in human colon carcinoma: Co-expression with egfr in neoplastic and endothelial cells. J Pathol (2005) 207(2):156–63. doi: 10.1002/path.1814
22. Healy EF, Lilic M. A model for covid-19-Induced dysregulation of Ace2 shedding by Adam17. Biochem Biophys Res Commun (2021) 573:158–63. doi: 10.1016/j.bbrc.2021.08.040
23. Healy EF. How tetraspanin-mediated cell entry of sars-Cov-2 can dysregulate the shedding of the Ace2 receptor by Adam17. Biochem Biophys Res Commun (2022) 593:52–6. doi: 10.1016/j.bbrc.2022.01.038
24. Zipeto D, Palmeira JDF, Arganaraz GA, Arganaraz ER. Ace2/Adam17/Tmprss2 interplay may be the main risk factor for covid-19. Front Immunol (2020) 11:576745. doi: 10.3389/fimmu.2020.576745
25. Wang G, Huang Y, Hu T, Zhang B, Tang Z, Yao R, et al. Contribution of placental 11beta-Hsd2 to the pathogenesis of preeclampsia. FASEB journal: Off Publ Fed Am Societies Exp Biol (2020) 34(11):15379–99. doi: 10.1096/fj.202001003RR
26. Mohammed RN, Wehenkel SC, Galkina EV, Yates EK, Preece G, Newman A, et al. Adam17-dependent proteolysis of l-selectin promotes early clonal expansion of cytotoxic T cells. Sci Rep (2019) 9(1):5487. doi: 10.1038/s41598-019-41811-z
27. Wang Y, Robertson JD, Walcheck B. Different signaling pathways stimulate a disintegrin and metalloprotease-17 (Adam17) in neutrophils during apoptosis and activation. J Biol Chem (2011) 286(45):38980–8. doi: 10.1074/jbc.M111.277087
28. Du P, Geng J, Wang F, Chen X, Huang Z, Wang Y. Role of il-6 inhibitor in treatment of covid-19-Related cytokine release syndrome. Int J Med Sci (2021) 18(6):1356–62. doi: 10.7150/ijms.53564
29. Sen P, Kandasamy T, Ghosh SS. In-silico evidence of Adam metalloproteinase pathology in cancer signaling networks. J biomolecular structure dynamics (2021), 1–16. doi: 10.1080/07391102.2021.1964602
30. Meharenna YT, Doukov T, Li H, Soltis SM, Poulos TL. Crystallographic and single-crystal spectral analysis of the peroxidase ferryl intermediate. Biochemistry (2010) 49(14):2984–6. doi: 10.1021/bi100238r
31. Maskos K, Fernandez-Catalan C, Huber R, Bourenkov GP, Bartunik H, Ellestad GA, et al. Crystal structure of the catalytic domain of human tumor necrosis factor-Alpha-Converting enzyme. Proc Natl Acad Sci USA (1998) 95(7):3408–12. doi: 10.1073/pnas.95.7.3408
32. Kawai T, Elliott KJ, Scalia R, Eguchi S. Contribution of Adam17 and related adams in cardiovascular diseases. Cell Mol Life Sci: CMLS (2021) 78(9):4161–87. doi: 10.1007/s00018-021-03779-w
33. Sisto M, Ribatti D, Lisi S. Adam 17 and epithelial-to-Mesenchymal transition: The evolving story and its link to fibrosis and cancer. J Clin Med (2021) 10(15):3373. doi: 10.3390/jcm10153373
34. Lopes PH, van den Berg CW, Tambourgi DV. Sphingomyelinases d from loxosceles spider venoms and cell membranes: Action on lipid rafts and activation of endogenous metalloproteinases. Front Pharmacol (2020) 11:636. doi: 10.3389/fphar.2020.00636
35. Buckley CA, Rouhani FN, Kaler M, Adamik B, Hawari FI, Levine SJ. Amino-terminal tace prodomain attenuates Tnfr2 cleavage independently of the cysteine switch. Am J Physiol Lung Cell Mol Physiol (2005) 288(6):L1132–8. doi: 10.1152/ajplung.00429.2004
36. Qian M, Shen X, Wang H. The distinct role of Adam17 in app proteolysis and microglial activation related to alzheimer’s disease. Cell Mol Neurobiol (2016) 36(4):471–82. doi: 10.1007/s10571-015-0232-4
37. Dobert JP, Cabron AS, Arnold P, Pavlenko E, Rose-John S, Zunke F. Functional characterization of colon-Cancer-Associated variants in Adam17 affecting the catalytic domain. Biomedicines (2020) 8(11):463. doi: 10.3390/biomedicines8110463
38. Rossello A, Nuti E, Ferrini S, Fabbi M. Targeting Adam17 sheddase activity in cancer. Curr Drug Targets (2016) 17(16):1908–27. doi: 10.2174/1389450117666160727143618
39. Adu-Amankwaah J, Adzika GK, Adekunle AO, Ndzie Noah ML, Mprah R, Bushi A, et al. Adam17, a key player of cardiac inflammation and fibrosis in heart failure development during chronic catecholamine stress. Front Cell Dev Biol (2021) 9:732952. doi: 10.3389/fcell.2021.732952
40. Stolarczyk M, Scholte BJ. The egfr-Adam17 axis in chronic obstructive pulmonary disease and cystic fibrosis lung pathology. Med Inflamm (2018) 2018:1067134. doi: 10.1155/2018/1067134
41. Schmidt-Arras D, Rose-John S. Regulation of fibrotic processes in the liver by Adam proteases. Cells (2019) 8(10):1226. doi: 10.3390/cells8101226
42. Dusterhoft S, Babendreyer A, Giese AA, Flasshove C, Ludwig A. Status update on irhom and Adam17: It’s still complicated. Biochim Biophys Acta Mol Cell Res (2019) 1866(10):1567–83. doi: 10.1016/j.bbamcr.2019.06.017
43. Yamashita Y, Shimada M. The release of egf domain from egf-like factors by a specific cleavage enzyme activates the egfr-Mapk3/1 pathway in both granulosa cells and cumulus cells during the ovulation process. J Reprod Dev (2012) 58(5):510–4. doi: 10.1262/jrd.2012-056
44. Reed SG, Ager A. Immune responses to iav infection and the roles of l-selectin and Adam17 in lymphocyte homing. Pathogens (2022) 11(2):150. doi: 10.3390/pathogens11020150
45. Bienstein M, Minond D, Schwaneberg U, Davari MD, Yildiz D. In silico and experimental Adam17 kinetic modeling as basis for future screening system for modulators. Int J Mol Sci (2022) 23(3):1368. doi: 10.3390/ijms23031368
46. Calligaris M, Cuffaro D, Bonelli S, Spano DP, Rossello A, Nuti E, et al. Strategies to target Adam17 in disease: From its discovery to the irhom revolution. Molecules (2021) 26(4):944. doi: 10.3390/molecules26040944
47. Lora J, Weskamp G, Li TM, Maretzky T, Shola DTN, Monette S, et al. Targeted truncation of the Adam17 cytoplasmic domain in mice results in protein destabilization and a hypomorphic phenotype. J Biol Chem (2021) 296:100733. doi: 10.1016/j.jbc.2021.100733
48. Imoto I, Saito M, Suga K, Kohmoto T, Otsu M, Horiuchi K, et al. Functionally confirmed compound heterozygous Adam17 missense loss-of-Function variants cause neonatal inflammatory skin and bowel disease 1. Sci Rep (2021) 11(1):9552. doi: 10.1038/s41598-021-89063-0
49. Dusterhoft S, Hobel K, Oldefest M, Lokau J, Waetzig GH, Chalaris A, et al. A disintegrin and metalloprotease 17 dynamic interaction sequence, the sweet tooth for the human interleukin 6 receptor. J Biol Chem (2014) 289(23):16336–48. doi: 10.1074/jbc.M114.557322
50. Niu X, Umland S, Ingram R, Beyer BM, Liu YH, Sun J, et al. Ik682, a tight binding inhibitor of tace. Arch Biochem biophysics (2006) 451(1):43–50. doi: 10.1016/j.abb.2006.03.034
51. Li W, Li F, Zhang X, Lin HK, Xu C. Insights into the post-translational modification and its emerging role in shaping the tumor microenvironment. Signal transduction targeted Ther (2021) 6(1):422. doi: 10.1038/s41392-021-00825-8
52. Dusterhoft S, Lokau J, Garbers C. The metalloprotease Adam17 in inflammation and cancer. Pathol Res Pract (2019) 215(6):152410. doi: 10.1016/j.prp.2019.04.002
53. Jang DI, Lee AH, Shin HY, Song HR, Park JH, Kang TB, et al. The role of tumor necrosis factor alpha (Tnf-alpha) in autoimmune disease and current tnf-alpha inhibitors in therapeutics. Int J Mol Sci (2021) 22(5):2719. doi: 10.3390/ijms22052719
54. Ramesh P, Shivde R, Jaishankar D, Saleiro D, Le Poole IC. A palette of cytokines to measure anti-tumor efficacy of T cell-based therapeutics. Cancers (2021) 13(4):821. doi: 10.3390/cancers13040821
55. Wang Y, Guo L, Yin X, McCarthy EC, Cheng MI, Hoang AT, et al. Pathogenic tnf-alpha drives peripheral nerve inflammation in an aire-deficient model of autoimmunity. Proc Natl Acad Sci USA (2022) 119(4):e2114406119. doi: 10.1073/pnas.2114406119
56. Hwang HS, Park YY, Shin SJ, Go H, Park JM, Yoon SY, et al. Involvement of the tnf-alpha pathway in tki resistance and suggestion of Tnfr1 as a predictive biomarker for tki responsiveness in clear cell renal cell carcinoma. J Korean Med Sci (2020) 35(5):e31. doi: 10.3346/jkms.2020.35.e31
57. Kondylis V. Ripk1 and allies in the battle against hepatocyte apoptosis and liver cancer. Trans Cancer Res (2017) 6(Suppl 3):S569–S77. doi: 10.21037/tcr.2017.04.16
58. Fritsch J, Frankenheim J, Marischen L, Vadasz T, Troeger A, Rose-John S, et al. Roles for Adam17 in tnf-R1 mediated cell death and survival in human U937 and jurkat cells. Cells (2021) 10(11):3100. doi: 10.3390/cells10113100
59. Chen Y, Jia M, Wang S, Xu S, He N. Antagonistic antibody targeting Tnfr2 inhibits regulatory T cell function to promote anti-tumor activity. Front Immunol (2022) 13:835690. doi: 10.3389/fimmu.2022.835690
60. Jann J, Gascon S, Roux S, Faucheux N. Influence of the tgf-beta superfamily on Osteoclasts/Osteoblasts balance in physiological and pathological bone conditions. Int J Mol Sci (2020) 21(20):7597. doi: 10.3390/ijms21207597
61. Saad MI, McLeod L, Hodges C, Vlahos R, Rose-John S, Ruwanpura S, et al. Adam17 deficiency protects against pulmonary emphysema. Am J Respir Cell Mol Biol (2021) 64(2):183–95. doi: 10.1165/rcmb.2020-0214OC
62. Jaiswal AK, Makhija S, Stahr N, Sandey M, Suryawanshi A, Saxena A, et al. Dendritic cell-restricted progenitors contribute to obesity-associated airway inflammation Via Adam17-P38 mapk-dependent pathway. Front Immunol (2020) 11:363. doi: 10.3389/fimmu.2020.00363
63. Sharma AK, Thanikachalam PV, Bhatia S. The signaling interplay of gsk-3beta in myocardial disorders. Drug Discovery Today (2020) 25(4):633–41. doi: 10.1016/j.drudis.2020.01.017
64. Xu M, Zhou H, Zhang C, He J, Wei H, Zhou M, et al. Adam17 promotes epithelial-mesenchymal transition Via tgf-Beta/Smad pathway in gastric carcinoma cells. Int J Oncol (2016) 49(6):2520–8. doi: 10.3892/ijo.2016.3744
65. Zhong S, Khalil RA. A disintegrin and metalloproteinase (Adam) and Adam with thrombospondin motifs (Adamts) family in vascular biology and disease. Biochem Pharmacol (2019) 164:188–204. doi: 10.1016/j.bcp.2019.03.033
66. Sharma D, Singh NK. The biochemistry and physiology of a disintegrin and metalloproteinases (Adams and Adam-tss) in human pathologies. Rev physiology Biochem Pharmacol (2022). doi: 10.1007/112_2021_67
67. Schwarz J, Broder C, Helmstetter A, Schmidt S, Yan I, Muller M, et al. Short-term tnfalpha shedding is independent of cytoplasmic phosphorylation or furin cleavage of Adam17. Biochim Biophys Acta (2013) 1833(12):3355–67. doi: 10.1016/j.bbamcr.2013.10.005
68. Parra LM, Hartmann M, Schubach S, Li Y, Herrlich P, Herrlich A. Distinct intracellular domain substrate modifications selectively regulate ectodomain cleavage of Nrg1 or Cd44. Mol Cell Biol (2015) 35(19):3381–95. doi: 10.1128/MCB.00500-15
69. Chavaroche A, Cudic M, Giulianotti M, Houghten RA, Fields GB, Minond D. Glycosylation of a disintegrin and metalloprotease 17 affects its activity and inhibition. Analytical Biochem (2014) 449:68–75. doi: 10.1016/j.ab.2013.12.018
70. Zaruba M, Chen HW, Pietsch OF, Szakmary-Braendle K, Auer A, Motz M, et al. Adam17 is an essential factor for the infection of bovine cells with pestiviruses. Viruses (2022) 14(2):381. doi: 10.3390/v14020381
71. Goth CK, Halim A, Khetarpal SA, Rader DJ, Clausen H, Schjoldager KT. A systematic study of modulation of Adam-mediated ectodomain shedding by site-specific O-glycosylation. Proc Natl Acad Sci USA (2015) 112(47):14623–8. doi: 10.1073/pnas.1511175112
72. Zhu J, Steinberg SF. Beta1-adrenergic receptor n-terminal cleavage by Adam17; the mechanism for redox-dependent downregulation of cardiomyocyte Beta1-adrenergic receptors. J Mol Cell Cardiol (2021) 154:70–9. doi: 10.1016/j.yjmcc.2021.01.012
73. Yang G, Cui M, Jiang W, Sheng J, Yang Y, Zhang X. Molecular switch in human diseases-disintegrin and metalloproteinases, Adam17. Aging (2021) 13(12):16859–72. doi: 10.18632/aging.203200
74. Dusterhoft S, Kahveci-Turkoz S, Wozniak J, Seifert A, Kasparek P, Ohm H, et al. The irhom homology domain is indispensable for Adam17-mediated tnfalpha and egf receptor ligand release. Cell Mol Life Sci (2021) 78(11):5015–40. doi: 10.1007/s00018-021-03845-3
75. Chen S, Wang X, Zhu H, Tang Q, Du W, Cao H, et al. Zidovudine-based treatments inhibit the glycosylation of Adam17 and reduce Cd163 shedding from monocytes. J acquired Immune Deficiency Syndromes (2018) 79(1):126–34. doi: 10.1097/QAI.0000000000001769
76. Guo Y, He X, Zhang M, Qu Y, Gu C, Ren M, et al. Reciprocal control of Adam17/Egfr/Akt signaling and mir-145 drives gbm invasiveness. J Neuro-Oncol (2020) 147(2):327–37. doi: 10.1007/s11060-020-03453-4
77. Fei Q, Du MY, Qian LX, Chen HB, Chen J, Zhu HM, et al. Feedback loop in mir-449b-3p/Adam17/Nf-Kappab promotes metastasis in nasopharyngeal carcinoma. Cancer Med (2019) 8(13):6049–63. doi: 10.1002/cam4.2469
78. Palau V, Pascual J, Soler MJ, Riera M. Role of Adam17 in kidney disease. Am J Physiol Renal Physiol (2019) 317(2):F333–F42. doi: 10.1152/ajprenal.00625.2018
79. He J, Zhao T, Liu L, Liao S, Yang S, Lu F, et al. The -172 a-to-G variation in Adam17 gene promoter region affects Egr1/Adam17 pathway and confers susceptibility to septic mortality with sepsis-3.0 criteria. Int Immunopharmacol (2022) 102:108385. doi: 10.1016/j.intimp.2021.108385
80. Le X, Fan YF. Adam17 regulates the proliferation and extracellular matrix of keloid fibroblasts by mediating the Egfr/Erk signaling pathway. J Plast Surg Handb Surg (2022), 1–8. doi: 10.1080/2000656X.2021.2017944
81. Abu El-Asrar AM, Nawaz MI, Ahmad A, De Zutter A, Siddiquei MM, Blanter M, et al. Evaluation of proteoforms of the transmembrane chemokines Cxcl16 and Cx3cl1, their receptors, and their processing metalloproteinases Adam10 and Adam17 in proliferative diabetic retinopathy. Front Immunol (2020) 11:601639. doi: 10.3389/fimmu.2020.601639
82. Xue F, Cheng J, Liu Y, Cheng C, Zhang M, Sui W, et al. Cardiomyocyte-specific knockout of Adam17 ameliorates left ventricular remodeling and function in diabetic cardiomyopathy of mice. Signal Transduction Targeted Ther (2022) 7(1):259. doi: 10.1038/s41392-022-01054-3
83. Lian G, Li X, Zhang L, Zhang Y, Sun L, Zhang X, et al. Macrophage metabolic reprogramming aggravates aortic dissection through the Hif1alpha-Adam17 pathway(). EBioMedicine (2019) 49:291–304. doi: 10.1016/j.ebiom.2019.09.041
84. Ishii S, Isozaki T, Furuya H, Takeuchi H, Tsubokura Y, Inagaki K, et al. Adam-17 is expressed on rheumatoid arthritis fibroblast-like synoviocytes and regulates proinflammatory mediator expression and monocyte adhesion. Arthritis Res Ther (2018) 20(1):159. doi: 10.1186/s13075-018-1657-1
85. Saha SK, Choi HY, Yang GM, Biswas PK, Kim K, Kang GH, et al. Gpr50 promotes hepatocellular carcinoma progression Via the notch signaling pathway through direct interaction with Adam17. Mol Ther oncolytics (2020) 17:332–49. doi: 10.1016/j.omto.2020.04.002
86. Mizui Y, Yamazaki K, Sagane K, Tanaka I. Cdna cloning of mouse tumor necrosis factor-alpha converting enzyme (Tace) and partial analysis of its promoter. Gene (1999) 233(1-2):67–74. doi: 10.1016/s0378-1119(99)00155-9
87. Szalad A, Katakowski M, Zheng X, Jiang F, Chopp M. Transcription factor Sp1 induces Adam17 and contributes to tumor cell invasiveness under hypoxia. J Exp Clin Cancer research: CR (2009) 28:129. doi: 10.1186/1756-9966-28-129
88. Babendreyer A, Rojas-Gonzalez DM, Giese AA, Fellendorf S, Dusterhoft S, Mela P, et al. Differential induction of the Adam17 regulators Irhom1 and 2 in endothelial cells. Front Cardiovasc Med (2020) 7:610344. doi: 10.3389/fcvm.2020.610344
89. Giese AA, Babendreyer A, Krappen P, Gross A, Strnad P, Dusterhoft S, et al. Inflammatory activation of surface molecule shedding by upregulation of the pseudoprotease Irhom2 in colon epithelial cells. Sci Rep (2021) 11(1):24230. doi: 10.1038/s41598-021-03522-2
90. Mukerjee S, Gao H, Xu J, Sato R, Zsombok A, Lazartigues E. Ace2 and Adam17 interaction regulates the activity of presympathetic neurons. Hypertension (2019) 74(5):1181–91. doi: 10.1161/HYPERTENSIONAHA.119.13133
91. Araya HF, Sepulveda H, Lizama CO, Vega OA, Jerez S, Briceno PF, et al. Expression of the ectodomain-releasing protease Adam17 is directly regulated by the osteosarcoma and bone-related transcription factor Runx2. J Cell Biochem (2018) 119(10):8204–19. doi: 10.1002/jcb.26832
92. Katakia YT, Thakkar NP, Thakar S, Sakhuja A, Goyal R, Sharma H, et al. Dynamic alterations of H3k4me3 and H3k27me3 at Adam17 and jagged-1 gene promoters cause an inflammatory switch of endothelial cells. J Cell Physiol (2022) 237(1):992–1012. doi: 10.1002/jcp.30579
93. Tang J, Frey JM, Wilson CL, Moncada-Pazos A, Levet C, Freeman M, et al. Neutrophil and macrophage cell surface colony-stimulating factor 1 shed by Adam17 drives mouse macrophage proliferation in acute and chronic inflammation. Mol Cell Biol (2018) 38(17):e00103–18. doi: 10.1128/MCB.00103-18
94. Seidel J, Leitzke S, Ahrens B, Sperrhacke M, Bhakdi S, Reiss K. Role of Adam10 and Adam17 in regulating Cd137 function. Int J Mol Sci (2021) 22(5):2730. doi: 10.3390/ijms22052730
95. Bech-Serra JJ, Santiago-Josefat B, Esselens C, Saftig P, Baselga J, Arribas J, et al. Proteomic identification of desmoglein 2 and activated leukocyte cell adhesion molecule as substrates of Adam17 and Adam10 by difference gel electrophoresis. Mol Cell Biol (2006) 26(13):5086–95. doi: 10.1128/MCB.02380-05
96. Iwagishi R, Tanaka R, Seto M, Takagi T, Norioka N, Ueyama T, et al. Negatively charged amino acids in the stalk region of membrane proteins reduce ectodomain shedding. J Biol Chem (2020) 295(35):12343–52. doi: 10.1074/jbc.RA120.013758
97. Hosur V, Farley ML, Burzenski LM, Shultz LD, Wiles MV. Adam17 is essential for ectodomain shedding of the egf-receptor ligand amphiregulin. FEBS Open Bio (2018) 8(4):702–10. doi: 10.1002/2211-5463.12407
98. Garton KJ, Gough PJ, Blobel CP, Murphy G, Greaves DR, Dempsey PJ, et al. Tumor necrosis factor-Alpha-Converting enzyme (Adam17) mediates the cleavage and shedding of fractalkine (Cx3cl1). J Biol Chem (2001) 276(41):37993–8001. doi: 10.1074/jbc.M106434200
99. Sahin U, Blobel CP. Ectodomain shedding of the egf-receptor ligand epigen is mediated by Adam17. FEBS Lett (2007) 581(1):41–4. doi: 10.1016/j.febslet.2006.11.074
100. Parekh RU, Sriramula S. Activation of kinin B1r upregulates Adam17 and results in Ace2 shedding in neurons. Int J Mol Sci (2020) 22(1):145. doi: 10.3390/ijms22010145
101. Lartey NL, Valle-Reyes S, Vargas-Robles H, Jimenez-Camacho KE, Guerrero-Fonseca IM, Castellanos-Martinez R, et al. Adam17/Mmp inhibition prevents neutrophilia and lung injury in a mouse model of covid-19. J leukocyte Biol (2022) 111(6):1147–58. doi: 10.1002/JLB.3COVA0421-195RR
102. Tateishi H, Tateishi M, Radwan MO, Masunaga T, Kawatashiro K, Oba Y, et al. A new inhibitor of Adam17 composed of a zinc-binding dithiol moiety and a specificity pocket-binding appendage. Chem Pharm Bull (2021) 69(11):1123–30. doi: 10.1248/cpb.c21-00701
103. Takamune Y, Ikebe T, Nagano O, Nakayama H, Ota K, Obayashi T, et al. Adam-17 associated with Cd44 cleavage and metastasis in oral squamous cell carcinoma. Virchows Archiv: an Int J Pathol (2007) 450(2):169–77. doi: 10.1007/s00428-006-0350-y
104. Zou Z, Li L, Li Q, Zhao P, Zhang K, Liu C, et al. The role of S100b/Rage-enhanced Adam17 activation in endothelial glycocalyx shedding after traumatic brain injury. J Neuroinflamm (2022) 19(1):46. doi: 10.1186/s12974-022-02412-2
105. Horiuchi K, Morioka H, Takaishi H, Akiyama H, Blobel CP, Toyama Y. Ectodomain shedding of Flt3 ligand is mediated by tnf-alpha converting enzyme. J Immunol (2009) 182(12):7408–14. doi: 10.4049/jimmunol.0801931
106. Sahin U, Weskamp G, Kelly K, Zhou HM, Higashiyama S, Peschon J, et al. Distinct roles for Adam10 and Adam17 in ectodomain shedding of six egfr ligands. J Cell Biol (2004) 164(5):769–79. doi: 10.1083/jcb.200307137
107. Slack BE, Ma LK, Seah CC. Constitutive shedding of the amyloid precursor protein ectodomain is up-regulated by tumour necrosis factor-alpha converting enzyme. Biochem J (2001) 357(Pt 3):787–94. doi: 10.1042/0264-6021:3570787
108. Li Y, Brazzell J, Herrera A, Walcheck B. Adam17 deficiency by mature neutrophils has differential effects on l-selectin shedding. Blood (2006) 108(7):2275–9. doi: 10.1182/blood-2006-02-005827
109. Chen CD, Podvin S, Gillespie E, Leeman SE, Abraham CR. Insulin stimulates the cleavage and release of the extracellular domain of klotho by Adam10 and Adam17. Proc Natl Acad Sci USA (2007) 104(50):19796–801. doi: 10.1073/pnas.0709805104
110. Kanzaki H, Shinohara F, Suzuki M, Wada S, Miyamoto Y, Yamaguchi Y, et al. A-disintegrin and metalloproteinase (Adam) 17 enzymatically degrades interferon-gamma. Sci Rep (2016) 6:32259. doi: 10.1038/srep32259
111. Murthy A, Defamie V, Smookler DS, Di Grappa MA, Horiuchi K, Federici M, et al. Ectodomain shedding of egfr ligands and Tnfr1 dictates hepatocyte apoptosis during fulminant hepatitis in mice. J Clin Invest (2010) 120(8):2731–44. doi: 10.1172/JCI42686
112. Vidlickova I, Dequiedt F, Jelenska L, Sedlakova O, Pastorek M, Stuchlik S, et al. Apoptosis-induced ectodomain shedding of hypoxia-regulated carbonic anhydrase ix from tumor cells: A double-edged response to chemotherapy. BMC Cancer (2016) 16:239. doi: 10.1186/s12885-016-2267-4
113. Nanba D, Toki F, Asakawa K, Matsumura H, Shiraishi K, Sayama K, et al. Egfr-mediated epidermal stem cell motility drives skin regeneration through Col17a1 proteolysis. J Cell Biol (2021) 220(11):e202012073. doi: 10.1083/jcb.202012073
114. Franzke CW, Bruckner-Tuderman L, Blobel CP. Shedding of collagen Xvii/Bp180 in skin depends on both Adam10 and Adam9. J Biol Chem (2009) 284(35):23386–96. doi: 10.1074/jbc.M109.034090
115. Franzke CW, Tasanen K, Borradori L, Huotari V, Bruckner-Tuderman L. Shedding of collagen Xvii/Bp180: Structural motifs influence cleavage from cell surface. J Biol Chem (2004) 279(23):24521–9. doi: 10.1074/jbc.M308835200
116. Rhee M, Kim JW, Lee MW, Yoon KH, Lee SH. Preadipocyte factor 1 regulates adipose tissue browning Via tnf-Alpha-Converting enzyme-mediated cleavage. Metabolism: Clin Exp (2019) 101:153977. doi: 10.1016/j.metabol.2019.153977
117. Wang Y, Sul HS. Ectodomain shedding of preadipocyte factor 1 (Pref-1) by tumor necrosis factor alpha converting enzyme (Tace) and inhibition of adipocyte differentiation. Mol Cell Biol (2006) 26(14):5421–35. doi: 10.1128/MCB.02437-05
118. Coglievina M, Guarnaccia C, Zlatev V, Pongor S, Pintar A. Jagged-1 juxtamembrane region: Biochemical characterization and cleavage by Adam17 (Tace) catalytic domain. Biochem Biophys Res Commun (2013) 432(4):666–71. doi: 10.1016/j.bbrc.2013.02.022
119. Elahi A, Ajidahun A, Hendrick L, Getun I, Humphries LA, Hernandez J, et al. Hpp1 ectodomain shedding is mediated by Adam17 and is necessary for tumor suppression in colon cancer. J Surg Res (2020) 254:183–90. doi: 10.1016/j.jss.2020.04.010
120. Trino LD, Granato DC, Neves LX, Hansen HP, Paes Leme AF. Chapter 9 - Proteinase imbalance in oral cancer and other diseases. In: Zelanis A editor. Proteolytic signaling in health and disease Cambridge, Massachusetts: Academic Press (2022), p.165–208.
121. Motani K, Kosako H. Activation of stimulator of interferon genes (Sting) induces Adam17-mediated shedding of the immune semaphorin Sema4d. J Biol Chem (2018) 293(20):7717–26. doi: 10.1074/jbc.RA118.002175
122. Kawaguchi N, Horiuchi K, Becherer JD, Toyama Y, Besmer P, Blobel CP. Different adams have distinct influences on kit ligand processing: Phorbol-Ester-Stimulated ectodomain shedding of Kitl1 by Adam17 is reduced by Adam19. J Cell Sci (2007) 120(Pt 6):943–52. doi: 10.1242/jcs.03403
123. Hammood M, Craig AW, Leyton JV. Impact of endocytosis mechanisms for the receptors targeted by the currently approved antibody-drug conjugates (Adcs)-a necessity for future adc research and development. Pharmaceuticals (2021) 14(7):674. doi: 10.3390/ph14070674
124. Ma Z, Gao Y, Liu W, Zheng L, Jin B, Duan B, et al. Cd82 suppresses Adam17-dependent e-cadherin cleavage and cell migration in prostate cancer. Dis Markers (2020) 2020:8899924. doi: 10.1155/2020/8899924
125. Malapeira J, Esselens C, Bech-Serra JJ, Canals F, Arribas J. Adam17 (Tace) regulates tgfbeta signaling through the cleavage of vasorin. Oncogene (2011) 30(16):1912–22. doi: 10.1038/onc.2010.565
126. Li N, Wang Y, Forbes K, Vignali KM, Heale BS, Saftig P, et al. Metalloproteases regulate T-cell proliferation and effector function via lag-3. EMBO J (2007) 26(2):494–504. doi: 10.1038/sj.emboj.7601520
127. Peschon JJ, Slack JL, Reddy P, Stocking KL, Sunnarborg SW, Lee DC, et al. An essential role for ectodomain shedding in mammalian development. Science (1998) 282(5392):1281–4. doi: 10.1126/science.282.5392.1281
128. Yashiro M, Ohya M, Mima T, Nakashima Y, Kawakami K, Sonou T, et al. Excessive Adam17 activation occurs in uremic patients and may contribute to their immunocompromised status. Immunity Inflammation Dis (2020) 8(2):228–35. doi: 10.1002/iid3.298
129. Contin C, Pitard V, Itai T, Nagata S, Moreau JF, Dechanet-Merville J. Membrane-anchored Cd40 is processed by the tumor necrosis factor-Alpha-Converting enzyme. implications for Cd40 signaling. J Biol Chem (2003) 278(35):32801–9. doi: 10.1074/jbc.M209993200
130. Maetzel D, Denzel S, Mack B, Canis M, Went P, Benk M, et al. Nuclear signalling by tumour-associated antigen epcam. Nat Cell Biol (2009) 11(2):162–71. doi: 10.1038/ncb1824
131. Arai J, Goto K, Tanoue Y, Ito S, Muroyama R, Matsubara Y, et al. Enzymatic inhibition of mica sheddase Adam17 by lomofungin in hepatocellular carcinoma cells. Int J Cancer (2018) 143(10):2575–83. doi: 10.1002/ijc.31615
132. Peng M, Guo S, Yin N, Xue J, Shen L, Zhao Q, et al. Ectodomain shedding of fcalpha receptor is mediated by Adam10 and Adam17. Immunology (2010) 130(1):83–91. doi: 10.1111/j.1365-2567.2009.03215.x
133. Tsakadze NL, Sithu SD, Sen U, English WR, Murphy G, D’Souza SE. Tumor necrosis factor-Alpha-Converting enzyme (Tace/Adam-17) mediates the ectodomain cleavage of intercellular adhesion molecule-1 (Icam-1). J Biol Chem (2006) 281(6):3157–64. doi: 10.1074/jbc.M510797200
134. Boutet P, Aguera-Gonzalez S, Atkinson S, Pennington CJ, Edwards DR, Murphy G, et al. Cutting edge: The metalloproteinase Adam17/Tnf-Alpha-Converting enzyme regulates proteolytic shedding of the mhc class I-related chain b protein. J Immunol (2009) 182(1):49–53. doi: 10.4049/jimmunol.182.1.49
135. Yang Y, Wang Y, Zeng X, Ma XJ, Zhao Y, Qiao J, et al. Self-control of hgf regulation on human trophoblast cell invasion Via enhancing c-met receptor shedding by Adam10 and Adam17. J Clin Endocrinol Metab (2012) 97(8):E1390–401. doi: 10.1210/jc.2012-1150
136. Maretzky T, Schulte M, Ludwig A, Rose-John S, Blobel C, Hartmann D, et al. L1 is sequentially processed by two differently activated metalloproteases and Presenilin/Gamma-secretase and regulates neural cell adhesion, cell migration, and neurite outgrowth. Mol Cell Biol (2005) 25(20):9040–53. doi: 10.1128/MCB.25.20.9040-9053.2005
137. Kanzaki H, Makihira S, Suzuki M, Ishii T, Movila A, Hirschfeld J, et al. Soluble rankl cleaved from activated lymphocytes by tnf-Alpha-Converting enzyme contributes to osteoclastogenesis in periodontitis. J Immunol (2016) 197(10):3871–83. doi: 10.4049/jimmunol.1601114
138. Weskamp G, Mendelson K, Swendeman S, Le Gall S, Ma Y, Lyman S, et al. Pathological neovascularization is reduced by inactivation of Adam17 in endothelial cells but not in pericytes. Circ Res (2010) 106(5):932–40. doi: 10.1161/CIRCRESAHA.109.207415
139. Kalus I, Bormann U, Mzoughi M, Schachner M, Kleene R. Proteolytic cleavage of the neural cell adhesion molecule by Adam17/Tace is involved in neurite outgrowth. J neurochemistry (2006) 98(1):78–88. doi: 10.1111/j.1471-4159.2006.03847.x
140. Bell JH, Herrera AH, Li Y, Walcheck B. Role of Adam17 in the ectodomain shedding of tnf-alpha and its receptors by neutrophils and macrophages. J leukocyte Biol (2007) 82(1):173–6. doi: 10.1189/jlb.0307193
141. Lattenist L, Ochodnicky P, Ahdi M, Claessen N, Leemans JC, Satchell SC, et al. Renal endothelial protein c receptor expression and shedding during diabetic nephropathy. J Thromb Haemost. (2016) 14(6):1171–82. doi: 10.1111/jth.13315
142. Buchanan PC, Boylan KLM, Walcheck B, Heinze R, Geller MA, Argenta PA, et al. Ectodomain shedding of the cell adhesion molecule nectin-4 in ovarian cancer is mediated by Adam10 and Adam17. J Biol Chem (2017) 292(15):6339–51. doi: 10.1074/jbc.M116.746859
143. Young J, Yu X, Wolslegel K, Nguyen A, Kung C, Chiang E, et al. Lymphotoxin-alphabeta heterotrimers are cleaved by metalloproteinases and contribute to synovitis in rheumatoid arthritis. Cytokine (2010) 51(1):78–86. doi: 10.1016/j.cyto.2010.03.003
144. Ruhe JE, Streit S, Hart S, Ullrich A. Egfr signaling leads to downregulation of ptp-lar Via tace-mediated proteolytic processing. Cell Signalling (2006) 18(9):1515–27. doi: 10.1016/j.cellsig.2005.12.003
145. Schantl JA, Roza M, Van Kerkhof P, Strous GJ. The growth hormone receptor interacts with its sheddase, the tumour necrosis factor-Alpha-Converting enzyme (Tace). Biochem J (2004) 377(Pt 2):379–84. doi: 10.1042/BJ20031321
146. Garton KJ, Gough PJ, Philalay J, Wille PT, Blobel CP, Whitehead RH, et al. Stimulated shedding of vascular cell adhesion molecule 1 (Vcam-1) is mediated by tumor necrosis factor-Alpha-Converting enzyme (Adam 17). J Biol Chem (2003) 278(39):37459–64. doi: 10.1074/jbc.M305877200
147. Sun S, Qiao B, Han Y, Wang B, Wei S, Chen Y. Posttranslational modifications of platelet adhesion receptors. Pharmacol Res (2022) 183:106413. doi: 10.1016/j.phrs.2022.106413
148. Bender M, Hofmann S, Stegner D, Chalaris A, Bosl M, Braun A, et al. Differentially regulated gpvi ectodomain shedding by multiple platelet-expressed proteinases. Blood (2010) 116(17):3347–55. doi: 10.1182/blood-2010-06-289108
149. Leksa V, Loewe R, Binder B, Schiller HB, Eckerstorfer P, Forster F, et al. Soluble M6p/Igf2r released by tace controls angiogenesis Via blocking plasminogen activation. Circ Res (2011) 108(6):676–85. doi: 10.1161/CIRCRESAHA.110.234732
150. Muller M, Saunders C, Senftleben A, Heidbuechel JPW, Halwachs B, Bolik J, et al. Tetraspanin 8 subfamily members regulate substrate-specificity of a disintegrin and metalloprotease 17. Cells (2022) 11(17):2683. doi: 10.3390/cells11172683
151. Reddy P, Slack JL, Davis R, Cerretti DP, Kozlosky CJ, Blanton RA, et al. Functional analysis of the domain structure of tumor necrosis factor-alpha converting enzyme. J Biol Chem (2000) 275(19):14608–14. doi: 10.1074/jbc.275.19.14608
152. Saad MI, Alhayyani S, McLeod L, Yu L, Alanazi M, Deswaerte V, et al. Adam17 selectively activates the il-6 trans-Signaling/Erk mapk axis in kras-addicted lung cancer. EMBO Mol Med (2019) 11(4):e9976. doi: 10.15252/emmm.201809976
153. Althoff K, Reddy P, Voltz N, Rose-John S, Mullberg J. Shedding of interleukin-6 receptor and tumor necrosis factor alpha. contribution of the stalk sequence to the cleavage pattern of transmembrane proteins. Eur J Biochem (2000) 267(9):2624–31. doi: 10.1046/j.1432-1327.2000.01278.x
154. Li Y, Ren Z, Wang Y, Dang YZ, Meng BX, Wang GD, et al. Adam17 promotes cell migration and invasion through the integrin Beta1 pathway in hepatocellular carcinoma. Exp Cell Res (2018) 370(2):373–82. doi: 10.1016/j.yexcr.2018.06.039
155. Fan D, Takawale A, Shen M, Samokhvalov V, Basu R, Patel V, et al. A disintegrin and metalloprotease-17 regulates pressure overload-induced myocardial hypertrophy and dysfunction through proteolytic processing of integrin Beta1. Hypertension (2016) 68(4):937–48. doi: 10.1161/HYPERTENSIONAHA.116.07566
156. Tian Y, Fopiano KA, Buncha V, Lang L, Rudic RD, Filosa JA, et al. Aging-induced impaired endothelial wall shear stress mechanosensing causes arterial remodeling Via jam-a/F11r shedding by Adam17. GeroScience (2022) 44(1):349–69. doi: 10.1007/s11357-021-00476-1
157. Gandhi R, Yi J, Ha J, Shi H, Ismail O, Nathoo S, et al. Accelerated receptor shedding inhibits kidney injury molecule-1 (Kim-1)-Mediated efferocytosis. Am J Physiol Renal Physiol (2014) 307(2):F205–21. doi: 10.1152/ajprenal.00638.2013
158. Sommer C, Lee S, Gulseth HL, Jensen J, Drevon CA, Birkeland KI. Soluble leptin receptor predicts insulin sensitivity and correlates with upregulation of metabolic pathways in men. J Clin Endocrinol Metab (2018) 103(3):1024–32. doi: 10.1210/jc.2017-02126
159. Sagar D, Gaddipati R, Ongstad EL, Bhagroo N, An LL, Wang J, et al. Lox-1: A potential driver of cardiovascular risk in sle patients. PloS One (2020) 15(3):e0229184. doi: 10.1371/journal.pone.0229184
160. Zhao XQ, Zhang MW, Wang F, Zhao YX, Li JJ, Wang XP, et al. Crp enhances soluble lox-1 release from macrophages by activating tnf-alpha converting enzyme. J Lipid Res (2011) 52(5):923–33. doi: 10.1194/jlr.M015156
161. Liu Q, Zhang J, Tran H, Verbeek MM, Reiss K, Estus S, et al. Lrp1 shedding in human brain: Roles of Adam10 and Adam17. Mol neurodegeneration (2009) 4:17. doi: 10.1186/1750-1326-4-17
162. Tushaus J, Muller SA, Shrouder J, Arends M, Simons M, Plesnila N, et al. The pseudoprotease Irhom1 controls ectodomain shedding of membrane proteins in the nervous system. FASEB J (2021) 35(11):e21962. doi: 10.1096/fj.202100936R
163. Zhang Y, Wang Y, Zhou D, Zhang LS, Deng FX, Shu S, et al. Angiotensin ii deteriorates advanced atherosclerosis by promoting mertk cleavage and impairing efferocytosis through the At1r/Ros/P38 Mapk/Adam17 pathway. Am J Physiol Cell Physiol (2019) 317(4):C776–C87. doi: 10.1152/ajpcell.00145.2019
164. Brou C, Logeat F, Gupta N, Bessia C, LeBail O, Doedens JR, et al. A novel proteolytic cleavage involved in notch signaling: The role of the disintegrin-metalloprotease tace. Mol Cell (2000) 5(2):207–16. doi: 10.1016/s1097-2765(00)80417-7
165. Cho RW, Park JM, Wolff SB, Xu D, Hopf C, Kim JA, et al. Mglur1/5-dependent long-term depression requires the regulated ectodomain cleavage of neuronal pentraxin npr by tace. Neuron (2008) 57(6):858–71. doi: 10.1016/j.neuron.2008.01.010
166. Chow JP, Fujikawa A, Shimizu H, Suzuki R, Noda M. Metalloproteinase- and gamma-Secretase-Mediated cleavage of protein-tyrosine phosphatase receptor type z. J Biol Chem (2008) 283(45):30879–89. doi: 10.1074/jbc.M802976200
167. Kapp K, Siemens J, Haring HU, Lammers R. Proteolytic processing of the protein tyrosine phosphatase alpha extracellular domain is mediated by Adam17/Tace. Eur J Cell Biol (2012) 91(9):687–93. doi: 10.1016/j.ejcb.2012.04.003
168. Pruessmeyer J, Martin C, Hess FM, Schwarz N, Schmidt S, Kogel T, et al. A disintegrin and metalloproteinase 17 (Adam17) mediates inflammation-induced shedding of syndecan-1 and -4 by lung epithelial cells. J Biol Chem (2010) 285(1):555–64. doi: 10.1074/jbc.M109.059394
169. Ishimoto T, Miyake K, Nandi T, Yashiro M, Onishi N, Huang KK, et al. Activation of transforming growth factor beta 1 signaling in gastric cancer-associated fibroblasts increases their motility, via expression of rhomboid 5 homolog 2, and ability to induce invasiveness of gastric cancer cells. Gastroenterology (2017) 153(1):191–204 e16. doi: 10.1053/j.gastro.2017.03.046
170. Yang WS, Kim JJ, Lee MJ, Lee EK, Park SK. Adam17-mediated ectodomain shedding of toll-like receptor 4 as a negative feedback regulation in lipopolysaccharide-activated aortic endothelial cells. Cell Physiol Biochem (2018) 45(5):1851–62. doi: 10.1159/000487876
171. Banerjee H, Kane LP. Immune regulation by Tim-3. F1000Research (2018) 7:316. doi: 10.12688/f1000research.13446.1
172. Zhao D, Li C, Yang X, Yan W, Zhang Y. Elevated soluble Tim-3 correlates with disease activity of systemic lupus erythematosus. Autoimmunity (2021) 54(2):97–103. doi: 10.1080/08916934.2021.1891535
173. Bolik J, Krause F, Stevanovic M, Gandrass M, Thomsen I, Schacht SS, et al. Inhibition of Adam17 impairs endothelial cell necroptosis and blocks metastasis. J Exp Med (2022) 219(1):e20201039. doi: 10.1084/jem.20201039
174. Wanger TM, Dewitt S, Collins A, Maitland NJ, Poghosyan Z, Knauper V. Differential regulation of Trop2 release by pkc isoforms through vesicles and Adam17. Cell signalling (2015) 27(7):1325–35. doi: 10.1016/j.cellsig.2015.03.017
175. Stoyanova T, Goldstein AS, Cai H, Drake JM, Huang J, Witte ON. Regulated proteolysis of Trop2 drives epithelial hyperplasia and stem cell self-renewal Via beta-catenin signaling. Genes Dev (2012) 26(20):2271–85. doi: 10.1101/gad.196451.112
176. Jin Y, Liu Y, Lin Q, Li J, Druso JE, Antonyak MA, et al. Deletion of Cdc42 enhances Adam17-mediated vascular endothelial growth factor receptor 2 shedding and impairs vascular endothelial cell survival and vasculogenesis. Mol Cell Biol (2013) 33(21):4181–97. doi: 10.1128/MCB.00650-13
177. Hermey G, Sjogaard SS, Petersen CM, Nykjaer A, Gliemann J. Tumour necrosis factor alpha-converting enzyme mediates ectodomain shedding of Vps10p-domain receptor family members. Biochem J (2006) 395(2):285–93. doi: 10.1042/BJ20051364
178. Veit M, Koyro KI, Ahrens B, Bleibaum F, Munz M, Rovekamp H, et al. Anoctamin-6 regulates Adam sheddase function. Biochim Biophys Acta Mol Cell Res (2018) 1865(11 Pt A):1598–610. doi: 10.1016/j.bbamcr.2018.08.011
179. Reiss K, Leitzke S, Seidel J, Sperrhacke M, Bhakdi S. Scramblases as regulators of proteolytic Adam function. Membranes (2022) 12(2):185. doi: 10.3390/membranes12020185
180. Tang B, Li X, Maretzky T, Perez-Aguilar JM, McIlwain D, Xie Y, et al. Substrate-selective protein ectodomain shedding by Adam17 and Irhom2 depends on their juxtamembrane and transmembrane domains. FASEB J (2020) 34(4):4956–69. doi: 10.1096/fj.201902649R
181. Wang Q, Wei J, Jia X, Feng X, Ji Z, Ji X, et al. Downregulation of Adam17 in pediatric immune thrombocytopenia impairs proplatelet formation. BMC Pediatr (2022) 22(1):164. doi: 10.1186/s12887-022-03237-x
182. Theret N, Bouezzedine F, Azar F, Diab-Assaf M, Legagneux V. Adam And adamts proteins, new players in the regulation of hepatocellular carcinoma microenvironment. Cancers (2021) 13(7):1563. doi: 10.3390/cancers13071563
183. Chun KH. Molecular targets and signaling pathways of microrna-122 in hepatocellular carcinoma. Pharmaceutics (2022) 14(7):1380. doi: 10.3390/pharmaceutics14071380
184. Liu J, Zhu G, Jia N, Wang W, Wang Y, Yin M, et al. Cd9 regulates keratinocyte migration by negatively modulating the sheddase activity of Adam17. Int J Biol Sci (2019) 15(2):493–506. doi: 10.7150/ijbs.29404
185. Chen JY, Cheng WH, Lee KY, Kuo HP, Chung KF, Chen CL, et al. Abnormal Adam17 expression causes airway fibrosis in chronic obstructive asthma. Biomedicine Pharmacother = Biomedecine Pharmacother (2021) 140:111701. doi: 10.1016/j.biopha.2021.111701
186. Schumacher N, Rose-John S. Adam17 orchestrates interleukin-6, tnfalpha and egf-r signaling in inflammation and cancer. Biochim Biophys Acta Mol Cell Res (2022) 1869(1):119141. doi: 10.1016/j.bbamcr.2021.119141
187. Matthews J, Villescas S, Herat L, Schlaich M, Matthews V. Implications of Adam17 activation for hyperglycaemia, obesity and type 2 diabetes. Bioscience Rep (2021) 41(5):BSR20210029. doi: 10.1042/BSR20210029
188. Hartl D, May P, Gu W, Mayhaus M, Pichler S, Spaniol C, et al. A rare loss-of-Function variant of Adam17 is associated with late-onset familial Alzheimer disease. Mol Psychiatry (2020) 25(3):629–39. doi: 10.1038/s41380-018-0091-8
189. Dulloo I, Muliyil S, Freeman M. The molecular, cellular and pathophysiological roles of irhom pseudoproteases. Open Biol (2019) 9(3):190003. doi: 10.1098/rsob.190003
190. Geesala R, Issuree PD, Maretzky T. Novel functions of inactive rhomboid proteins in immunity and disease. J Leukocyte Biol (2019) 106(4):823–35. doi: 10.1002/JLB.3VMR0219-069R
191. Skurski J, Penniman CM, Geesala R, Dixit G, Pulipati P, Bhardwaj G, et al. Loss of Irhom2 accelerates fat gain and insulin resistance in diet-induced obesity despite reduced adipose tissue inflammation. Metabolism (2020) 106:154194. doi: 10.1016/j.metabol.2020.154194
192. Sundaram B, Behnke K, Belancic A, Al-Salihi MA, Thabet Y, Polz R, et al. Irhom2 inhibits bile duct obstruction-induced liver fibrosis. Sci Signaling (2019) 12(605):eaax1194. doi: 10.1126/scisignal.aax1194
193. Brooke MA, Etheridge SL, Kaplan N, Simpson C, O’Toole EA, Ishida-Yamamoto A, et al. Irhom2-dependent regulation of Adam17 in cutaneous disease and epidermal barrier function. Hum Mol Genet (2014) 23(15):4064–76. doi: 10.1093/hmg/ddu120
194. Cavadas M, Oikonomidi I, Gaspar CJ, Burbridge E, Badenes M, Felix I, et al. Phosphorylation of Irhom2 controls stimulated proteolytic shedding by the metalloprotease Adam17/Tace. Cell Rep (2017) 21(3):745–57. doi: 10.1016/j.celrep.2017.09.074
195. Qing X, Chinenov Y, Redecha P, Madaio M, Roelofs JJ, Farber G, et al. Irhom2 promotes lupus nephritis through tnf-alpha and egfr signaling. J Clin Invest (2018) 128(4):1397–412. doi: 10.1172/JCI97650
196. Sieber B, Lu F, Stribbling SM, Grieve AG, Ryan AJ, Freeman M. Irhom2 regulates erbb signalling to promote kras-driven tumour growth of lung cancer cells. J Cell Sci (2022) 135(17):jcs259949. doi: 10.1242/jcs.259949
197. Tschanz F, Bender S, Telarovic I, Waller V, Speck RF, Pruschy M. The Adam17-directed inhibitory antibody Medi3622 antagonizes radiotherapy-induced vegf release and sensitizes non–small cell lung cancer for radiotherapy. Cancer Res Commun (2021) 1(3):164–77. doi: 10.1158/2767-9764.crc-21-0067
198. Fabbi M, Costa D, Russo D, Arenare L, Gaggero G, Signoriello S, et al. Analysis of a disintegrin and metalloprotease 17 (Adam17) expression as a prognostic marker in ovarian cancer patients undergoing first-line treatment plus bevacizumab. Diagnostics (2022) 12(9):2118. doi: 10.3390/diagnostics12092118
199. Rogmans C, Kuhlmann JD, Hugendieck G, Link T, Arnold N, Weimer JP, et al. Adam17-a potential blood-based biomarker for detection of early-stage ovarian cancer. Cancers (2021) 13(21):5563. doi: 10.3390/cancers13215563
200. Hedemann N, Rogmans C, Sebens S, Wesch D, Reichert M, Schmidt-Arras D, et al. Adam17 inhibition enhances platinum efficiency in ovarian cancer. Oncotarget (2018) 9(22):16043–58. doi: 10.18632/oncotarget.24682
201. Hedemann N, Herz A, Schiepanski JH, Dittrich J, Sebens S, Dempfle A, et al. Adam17 inhibition increases the impact of cisplatin treatment in ovarian cancer spheroids. Cancers (2021) 13(9):2039. doi: 10.3390/cancers13092039
202. Jiang X, Wang J, Deng X, Xiong F, Ge J, Xiang B, et al. Role of the tumor microenvironment in pd-L1/Pd-1-Mediated tumor immune escape. Mol Cancer (2019) 18(1):10. doi: 10.1186/s12943-018-0928-4
203. Baram T, Oren N, Erlichman N, Meshel T, Ben-Baruch A. Inflammation-driven regulation of pd-L1 and pd-L2, and their cross-interactions with protective soluble tnfalpha receptors in human triple-negative breast cancer. Cancers (2022) 14(14):3513. doi: 10.3390/cancers14143513
204. Gao MQ, Kim BG, Kang S, Choi YP, Yoon JH, Cho NH. Human breast cancer-associated fibroblasts enhance cancer cell proliferation through increased tgf-alpha cleavage by Adam17. Cancer Lett (2013) 336(1):240–6. doi: 10.1016/j.canlet.2013.05.011
205. Saha N, Xu K, Zhu Z, Robev D, Kalidindi T, Xu Y, et al. Inhibitory monoclonal antibody targeting Adam17 expressed on cancer cells. Trans Oncol (2022) 15(1):101265. doi: 10.1016/j.tranon.2021.101265
206. Li W, Wang D, Sun X, Zhang Y, Wang L, Suo J. Adam17 promotes lymph node metastasis in gastric cancer Via activation of the notch and wnt signaling pathways. Int J Mol Med (2019) 43(2):914–26. doi: 10.3892/ijmm.2018.4028
207. Lee CL, Lee KS, Chuang CK, Su CH, Chiu HC, Tu RY, et al. Otorhinolaryngological management in Taiwanese patients with mucopolysaccharidoses. Int J Med Sci (2021) 18(15):3373–9. doi: 10.7150/ijms.61827
208. Xu J, Qin N, Jiang W, Chen T. Amygdalin suppresses the proliferation, migration and emt of gastric cancer cells by inhibiting tgf-B/Smad signal pathway. Trop J Pharm Res (2022) 21(4):721–6. doi: 10.4314/tjpr.v21i4.6
209. Cardenes B, Clares I, Toribio V, Pascual L, Lopez-Martin S, Torres-Gomez A, et al. Cellular integrin Alpha5beta1 and exosomal Adam17 mediate the binding and uptake of exosomes produced by colorectal carcinoma cells. Int J Mol Sci (2021) 22(18):9938. doi: 10.3390/ijms22189938
210. Shi W, Men L, Pi X, Jiang T, Peng D, Huo S, et al. Shikonin suppresses colon cancer cell growth and exerts synergistic effects by regulating Adam17 and the Il6/Stat3 signaling pathway. Int J Oncol (2021) 59(6):99. doi: 10.3892/ijo.2021.5279
211. Huang L, Chen J, Quan J, Xiang D. Rosmarinic acid inhibits proliferation and migration, promotes apoptosis and enhances cisplatin sensitivity of melanoma cells through inhibiting Adam17/Egfr/Akt/Gsk3beta axis. Bioengineered (2021) 12(1):3065–76. doi: 10.1080/21655979.2021.1941699
212. Wen HJ, Crawford H. Disruption of cellular crosstalk by blockage of Adam17 inhibits tumor progression of pancreatic ductal adenocarcinoma. Cancer Res (2022) 82(Suppl 12):Abstract nr 341. doi: 10.1158/1538-7445.AM2022-341
214. Cotzomi-Ortega I, Rosas-Cruz A, Ramirez-Ramirez D, Reyes-Leyva J, Rodriguez-Sosa M, Aguilar-Alonso P, et al. Autophagy inhibition induces the secretion of macrophage migration inhibitory factor (Mif) with autocrine and paracrine effects on the promotion of malignancy in breast cancer. Biology (2020) 9(1):20. doi: 10.3390/biology9010020
215. Lu Y, Yang Y, Xiao L, Li S, Liao X, Liu H. Autocrine and paracrine effects of vascular endothelial cells promote cutaneous wound healing. BioMed Res Int (2021) 2021:6695663. doi: 10.1155/2021/6695663
216. Toumi R, Yuzefpolskiy Y, Vegaraju A, Xiao H, Smith KA, Sarkar S, et al. Autocrine and paracrine il-2 signals collaborate to regulate distinct phases of Cd8 T cell memory. Cell Rep (2022) 39(2):110632. doi: 10.1016/j.celrep.2022.110632
217. Khalaf K, Hana D, Chou JT, Singh C, Mackiewicz A, Kaczmarek M. Aspects of the tumor microenvironment involved in immune resistance and drug resistance. Front Immunol (2021) 12:656364. doi: 10.3389/fimmu.2021.656364
218. Liu H, Zhao H, Sun Y. Tumor microenvironment and cellular senescence: Understanding therapeutic resistance and harnessing strategies. Semin Cancer Biol (2021) 86(Pt 3):769–81. doi: 10.1016/j.semcancer.2021.11.004
219. Zhou K, Cheng T, Zhan J, Peng X, Zhang Y, Wen J, et al. Targeting tumor-associated macrophages in the tumor microenvironment. Oncol Lett (2020) 20(5):234. doi: 10.3892/ol.2020.12097
220. Bai R, Li Y, Jian L, Yang Y, Zhao L, Wei M. The hypoxia-driven crosstalk between tumor and tumor-associated macrophages: Mechanisms and clinical treatment strategies. Mol Cancer (2022) 21(1):177. doi: 10.1186/s12943-022-01645-2
221. Yang Y, Guo Z, Chen W, Wang X, Cao M, Han X, et al. M2 macrophage-derived exosomes promote angiogenesis and growth of pancreatic ductal adenocarcinoma by targeting E2f2. Mol therapy: J Am Soc Gene Ther (2021) 29(3):1226–38. doi: 10.1016/j.ymthe.2020.11.024
222. Mantovani A, Allavena P, Marchesi F, Garlanda C. Macrophages as tools and targets in cancer therapy. Nat Rev Drug Discov (2022) 21(11):799–820. doi: 10.1038/s41573-022-00520-5
223. Gao J, Liang Y, Wang L. Shaping polarization of tumor-associated macrophages in cancer immunotherapy. Front Immunol (2022) 13:888713. doi: 10.3389/fimmu.2022.888713
224. Gocheva V, Wang HW, Gadea BB, Shree T, Hunter KE, Garfall AL, et al. Il-4 induces cathepsin protease activity in tumor-associated macrophages to promote cancer growth and invasion. Genes Dev (2010) 24(3):241–55. doi: 10.1101/gad.1874010
225. Yang L, Dong Y, Li Y, Wang D, Liu S, Wang D, et al. Il-10 derived from M2 macrophage promotes cancer stemness Via Jak1/Stat1/Nf-Kappab/Notch1 pathway in non-small cell lung cancer. Int J Cancer (2019) 145(4):1099–110. doi: 10.1002/ijc.32151
226. Lin EY, Gouon-Evans V, Nguyen AV, Pollard JW. The macrophage growth factor csf-1 in mammary gland development and tumor progression. J mammary gland Biol neoplasia (2002) 7(2):147–62. doi: 10.1023/a:1020399802795
227. Bose D, Banerjee S, Chatterjee N, Das S, Saha M, Saha KD. Inhibition of tgf-beta induced lipid droplets switches M2 macrophages to M1 phenotype. Toxicol vitro: an Int J published Assoc BIBRA (2019) 58:207–14. doi: 10.1016/j.tiv.2019.03.037
228. Lian G, Chen S, Ouyang M, Li F, Chen L, Yang J. Colon cancer cell secretes egf to promote M2 polarization of Tam through Egfr/Pi3k/Akt/Mtor pathway. Technol Cancer Res Treat (2019) 18:1533033819849068. doi: 10.1177/1533033819849068
229. Ma X, Wu D, Zhou S, Wan F, Liu H, Xu X, et al. The pancreatic cancer secreted Reg4 promotes macrophage polarization to M2 through Egfr/Akt/Creb pathway. Oncol Rep (2016) 35(1):189–96. doi: 10.3892/or.2015.4357
230. Pockley AG, Vaupel P, Multhoff G. Nk cell-based therapeutics for lung cancer. Expert Opin Biol Ther (2020) 20(1):23–33. doi: 10.1080/14712598.2020.1688298
231. Russell E, Conroy MJ, Barr MP. Harnessing natural killer cells in non-small cell lung cancer. Cells (2022) 11(4):605. doi: 10.3390/cells11040605
232. Niu YX, Xu ZX, Yu LF, Lu YP, Wang Y, Wu C, et al. Advances of research of fc-fusion protein that activate nk cells for tumor immunotherapy. Int Immunopharmacol (2022) 109:108783. doi: 10.1016/j.intimp.2022.108783
233. Oh S, Park Y, Lee HJ, Lee J, Lee SH, Baek YS, et al. A disintegrin and metalloproteinase 9 (Adam9) in advanced hepatocellular carcinoma and their role as a biomarker during hepatocellular carcinoma immunotherapy. Cancers (2020) 12(3):745. doi: 10.3390/cancers12030745
234. Schmidt D, Ebrahimabadi S, Gomes KRS, de Moura Aguiar G, Cariati Tirapelle M, Nacasaki Silvestre R, et al. Engineering car-nk cells: How to tune innate killer cells for cancer immunotherapy. Immunotherapy Adv (2022) 2(1):ltac003. doi: 10.1093/immadv/ltac003
235. Ou ZL, Luo Z, Wei W, Liang S, Gao TL, Lu YB. Hypoxia-induced shedding of mica and Hif1a-mediated immune escape of pancreatic cancer cells from nk cells: Role of Circ_0000977/Mir-153 axis. RNA Biol (2019) 16(11):1592–603. doi: 10.1080/15476286.2019.1649585
236. Ferrari de Andrade L, Tay RE, Pan D, Luoma AM, Ito Y, Badrinath S, et al. Antibody-mediated inhibition of mica and micb shedding promotes nk cell-driven tumor immunity. Science (2018) 359(6383):1537–42. doi: 10.1126/science.aao0505
237. Oliviero B, Varchetta S, Mele D, Pessino G, Maiello R, Falleni M, et al. Mica/B-targeted antibody promotes nk cell-driven tumor immunity in patients with intrahepatic cholangiocarcinoma. Oncoimmunology (2022) 11(1):2035919. doi: 10.1080/2162402X.2022.2035919
238. Wu J, Mishra HK, Walcheck B. Role of Adam17 as a regulatory checkpoint of Cd16a in nk cells and as a potential target for cancer immunotherapy. J leukocyte Biol (2019) 105(6):1297–303. doi: 10.1002/JLB.2MR1218-501R
239. Mishra HK, Pore N, Michelotti EF, Walcheck B. Anti-Adam17 monoclonal antibody Medi3622 increases ifngamma production by human nk cells in the presence of antibody-bound tumor cells. Cancer Immunol Immunother: CII (2018) 67(9):1407–16. doi: 10.1007/s00262-018-2193-1
240. Zhu H, Blum RH, Bjordahl R, Gaidarova S, Rogers P, Lee TT, et al. Pluripotent stem cell-derived nk cells with high-affinity noncleavable Cd16a mediate improved antitumor activity. Blood (2020) 135(6):399–410. doi: 10.1182/blood.2019000621
241. Dixon KJ, Wu J, Walcheck B. Engineering anti-tumor monoclonal antibodies and fc receptors to enhance adcc by human nk cells. Cancers (2021) 13(2):312. doi: 10.3390/cancers13020312
242. Romee R, Foley B, Lenvik T, Wang Y, Zhang B, Ankarlo D, et al. Nk cell Cd16 surface expression and function is regulated by a disintegrin and metalloprotease-17 (Adam17). Blood (2013) 121(18):3599–608. doi: 10.1182/blood-2012-04-425397
243. Coenon L, Villalba M. From Cd16a biology to antibody-dependent cell-mediated cytotoxicity improvement. Front Immunol (2022) 13:913215. doi: 10.3389/fimmu.2022.913215
244. Lorenzo-Anota HY, Martinez-Loria AB, Tamez-Guerra RS, Scott-Algara D, Martinez-Torres AC, Rodriguez-Padilla C. Changes in the natural killer cell repertoire and function induced by the cancer immune adjuvant candidate immunepotent-crp. Cell Immunol (2022) 374:104511. doi: 10.1016/j.cellimm.2022.104511
245. Katano I, Nishime C, Ito R, Kamisako T, Mizusawa T, Ka Y, et al. Long-term maintenance of peripheral blood derived human nk cells in a novel human il-15- transgenic nog mouse. Sci Rep (2017) 7(1):17230. doi: 10.1038/s41598-017-17442-7
246. Maishi N, Hida K. Tumor endothelial cells accelerate tumor metastasis. Cancer Sci (2017) 108(10):1921–6. doi: 10.1111/cas.13336
247. Bolik J. The role of the metalloprotease Adam17 in metastasis. der Christian, Germany: Kiel University Library, Albrechts-Universität (2019).
248. Xiao W, Pinilla-Baquero A, Faulkner J, Song X, Prabhakar P, Qiu H, et al. Robo4 is constitutively shed by adams from endothelial cells and the shed Robo4 functions to inhibit Slit3-induced angiogenesis. Sci Rep (2022) 12(1):4352. doi: 10.1038/s41598-022-08227-8
249. Shen M, Hu M, Fedak PWM, Oudit GY, Kassiri Z. Cell-specific functions of Adam17 regulate the progression of thoracic aortic aneurysm. Circ Res (2018) 123(3):372–88. doi: 10.1161/CIRCRESAHA.118.313181
250. Shu C, Zhou H, Afsharvand M, Duan L, Zhang H, Noveck R, et al. Pharmacokinetic-pharmacodynamic modeling of apratastat: A population-based approach. J Clin Pharmacol (2011) 51(4):472–81. doi: 10.1177/0091270010372389
251. Yang L, Bhattacharya A, Li Y, Sexton S, Ling X, Li F, et al. Depleting receptor tyrosine kinases egfr and Her2 overcomes resistance to egfr inhibitors in colorectal cancer. J Exp Clin Cancer Res:: CR (2022) 41(1):184. doi: 10.1186/s13046-022-02389-z
252. Fridman JS, Caulder E, Hansbury M, Liu X, Yang G, Wang Q, et al. Selective inhibition of Adam metalloproteases as a novel approach for modulating erbb pathways in cancer. Clin Cancer research: an Off J Am Assoc Cancer Res (2007) 13(6):1892–902. doi: 10.1158/1078-0432.CCR-06-2116
253. Hirata S, Murata T, Suzuki D, Nakamura S, Jono-Ohnishi R, Hirose H, et al. Selective inhibition of Adam17 efficiently mediates glycoprotein ibalpha retention during ex vivo generation of human induced pluripotent stem cell-derived platelets. Stem Cells Trans Med (2017) 6(3):720–30. doi: 10.5966/sctm.2016-0104
254. Saad MI, McLeod L, Yu L, Ebi H, Ruwanpura S, Sagi I, et al. The Adam17 protease promotes tobacco smoke carcinogen-induced lung tumorigenesis. Carcinogenesis (2020) 41(4):527–38. doi: 10.1093/carcin/bgz123
255. McGowan PM, Mullooly M, Caiazza F, Sukor S, Madden SF, Maguire AA, et al. Adam-17: A novel therapeutic target for triple negative breast cancer. Ann oncology: Off J Eur Soc Med Oncol (2013) 24(2):362–9. doi: 10.1093/annonc/mds279
256. Hoettecke N, Ludwig A, Foro S, Schmidt B. Improved synthesis of Adam10 inhibitor Gi254023x. Neuro-degenerative Dis (2010) 7(4):232–8. doi: 10.1159/000267865
257. Yahiaoui S, Voos K, Haupenthal J, Wichelhaus TA, Frank D, Weizel L, et al. N-aryl mercaptoacetamides as potential multi-target inhibitors of metallo-Beta-Lactamases (Mbls) and the virulence factor lasb from pseudomonas aeruginosa. RSC medicinal Chem (2021) 12(10):1698–708. doi: 10.1039/d1md00187f
258. Maekawa M, Tadaki H, Tomimoto D, Okuma C, Sano R, Ishii Y, et al. A novel tnf-alpha converting enzyme (Tace) selective inhibitor jtp-96193 prevents insulin resistance in kk-a(Y) type 2 diabetic mice and diabetic peripheral neuropathy in type 1 diabetic mice. Biol Pharm Bull (2019) 42(11):1906–12. doi: 10.1248/bpb.b19-00526
259. Lu HY, Zu YX, Jiang XW, Sun XT, Liu TY, Li RL, et al. Novel Adam-17 inhibitor zldi-8 inhibits the proliferation and metastasis of chemo-resistant non-Small-Cell lung cancer by reversing notch and epithelial mesenchymal transition in vitro and in vivo. Pharmacol Res (2019) 148:104406. doi: 10.1016/j.phrs.2019.104406
260. Trad A, Hansen HP, Shomali M, Peipp M, Klausz K, Hedemann N, et al. Adam17-overexpressing breast cancer cells selectively targeted by antibody-toxin conjugates. Cancer Immunol Immunother. (2013) 62(3):411–21. doi: 10.1007/s00262-012-1346-x
261. Yang Z, Chan KI, Kwok HF, Tam KY. Novel therapeutic anti-Adam17 antibody A9(B8) enhances egfr-Tki-Mediated anticancer activity in nsclc. Trans Oncol (2019) 12(11):1516–24. doi: 10.1016/j.tranon.2019.08.003
262. Richards FM, Tape CJ, Jodrell DI, Murphy G. Anti-tumour effects of a specific anti-Adam17 antibody in an ovarian cancer model in vivo. PloS One (2012) 7(7):e40597. doi: 10.1371/journal.pone.0040597
263. Rios-Doria J, Sabol D, Chesebrough J, Stewart D, Xu L, Tammali R, et al. A monoclonal antibody to Adam17 inhibits tumor growth by inhibiting egfr and non-Egfr-Mediated pathways. Mol Cancer Ther (2015) 14(7):1637–49. doi: 10.1158/1535-7163.MCT-14-1040
264. Zhou BB, Peyton M, He B, Liu C, Girard L, Caudler E, et al. Targeting Adam-mediated ligand cleavage to inhibit Her3 and egfr pathways in non-small cell lung cancer. Cancer Cell (2006) 10(1):39–50. doi: 10.1016/j.ccr.2006.05.024
265. Witters L, Scherle P, Friedman S, Fridman J, Caulder E, Newton R, et al. Synergistic inhibition with a dual epidermal growth factor Receptor/Her-2/Neu tyrosine kinase inhibitor and a disintegrin and metalloprotease inhibitor. Cancer Res (2008) 68(17):7083–9. doi: 10.1158/0008-5472.CAN-08-0739
266. Wiernik A, Foley B, Zhang B, Verneris MR, Warlick E, Gleason MK, et al. Targeting natural killer cells to acute myeloid leukemia in vitro with a Cd16 X 33 bispecific killer cell engager and Adam17 inhibition. Clin Cancer Res (2013) 19(14):3844–55. doi: 10.1158/1078-0432.CCR-13-0505
267. Scharfenberg F, Helbig A, Sammel M, Benzel J, Schlomann U, Peters F, et al. Degradome of soluble Adam10 and Adam17 metalloproteases. Cell Mol Life Sci (2020) 77(2):331–50. doi: 10.1007/s00018-019-03184-4
268. Koch J, Monch D, Maass A, Mangold A, Guzvic M, Murdter T, et al. Pharmacologic targeting of Mmp2/9 decreases peritoneal metastasis formation of colorectal cancer in a human ex vivo peritoneum culture model. Cancers (2022) 14(15):3760. doi: 10.3390/cancers14153760
269. Aljohmani A, Andres NN, Yildiz D. Pseudomonas aeruginosa alters critical lung epithelial cell functions through activation of Adam17. Cells (2022) 11(15):2303. doi: 10.3390/cells11152303
270. Murumkar PR, Giridhar R, Yadav MR. Novel methods and strategies in the discovery of tace inhibitors. Expert Opin Drug Discovery (2013) 8(2):157–81. doi: 10.1517/17460441.2013.744745
271. Lu HY, Chu HX, Tan YX, Qin XC, Liu MY, Li JD, et al. Novel Adam-17 inhibitor zldi-8 inhibits the metastasis of hepatocellular carcinoma by reversing epithelial-mesenchymal transition in vitro and in vivo. Life Sci (2020) 244:117343. doi: 10.1016/j.lfs.2020.117343
272. Zhang Y, Li D, Jiang Q, Cao S, Sun H, Chai Y, et al. Novel Adam-17 inhibitor zldi-8 enhances the in vitro and in vivo chemotherapeutic effects of sorafenib on hepatocellular carcinoma cells. Cell Death Dis (2018) 9(7):743. doi: 10.1038/s41419-018-0804-6
273. Li DD, Zhao CH, Ding HW, Wu Q, Ren TS, Wang J, et al. A novel inhibitor of Adam17 sensitizes colorectal cancer cells to 5-fluorouracil by reversing notch and epithelial-mesenchymal transition in vitro and in vivo. Cell proliferation (2018) 51(5):e12480. doi: 10.1111/cpr.12480
274. Yamamoto K, Trad A, Baumgart A, Huske L, Lorenzen I, Chalaris A, et al. A novel bispecific single-chain antibody for Adam17 and Cd3 induces T-Cell-Mediated lysis of prostate cancer cells. Biochem J (2012) 445(1):135–44. doi: 10.1042/BJ20120433
275. Ye J, Yuen SM, Murphy G, Xie R, Kwok HF. Anti-tumor effects of a ‘Human & mouse cross-reactive’ anti-Adam17 antibody in a pancreatic cancer model in vivo. Eur J Pharm Sci (2017) 110:62–9. doi: 10.1016/j.ejps.2017.05.057
276. Huang Y, Benaich N, Tape C, Kwok HF, Murphy G. Targeting the sheddase activity of Adam17 by an anti-Adam17 antibody D1(A12) inhibits head and neck squamous cell carcinoma cell proliferation and motility Via blockage of bradykinin induced hers transactivation. Int J Biol Sci (2014) 10(7):702–14. doi: 10.7150/ijbs.9326
277. Verhulst E, Garnier D, De Meester I, Bauvois B. Validating cell surface proteases as drug targets for cancer therapy: What do we know, and where do we go? Cancers (2022) 14(3):624. doi: 10.3390/cancers14030624
278. Camodeca C, Cuffaro D, Nuti E, Rossello A. Adam Metalloproteinases as potential drug targets. Curr medicinal Chem (2019) 26(15):2661–89. doi: 10.2174/0929867325666180326164104
Keywords: ADAM17, tumor microenvironment, shedding activity, immune response, inflammation
Citation: Wang K, Xuan Z, Liu X, Zheng M, Yang C and Wang H (2022) Immunomodulatory role of metalloproteinase ADAM17 in tumor development. Front. Immunol. 13:1059376. doi: 10.3389/fimmu.2022.1059376
Received: 01 October 2022; Accepted: 03 November 2022;
Published: 17 November 2022.
Edited by:
Yi Wu, Xi’an Jiaotong University, ChinaReviewed by:
Johannes Zeller, Baker Heart and Diabetes Institute, AustraliaCopyright © 2022 Wang, Xuan, Liu, Zheng, Yang and Wang. This is an open-access article distributed under the terms of the Creative Commons Attribution License (CC BY). The use, distribution or reproduction in other forums is permitted, provided the original author(s) and the copyright owner(s) are credited and that the original publication in this journal is cited, in accordance with accepted academic practice. No use, distribution or reproduction is permitted which does not comply with these terms.
*Correspondence: Haiyong Wang, d2FuZ2hhaXlvbmc2Njg4QDEyNi5jb20=; Chao Yang, eWM1MjAyOEBob3RtYWlsLmNvbQ==
†These authors have contributed equally to this work
Disclaimer: All claims expressed in this article are solely those of the authors and do not necessarily represent those of their affiliated organizations, or those of the publisher, the editors and the reviewers. Any product that may be evaluated in this article or claim that may be made by its manufacturer is not guaranteed or endorsed by the publisher.
Research integrity at Frontiers
Learn more about the work of our research integrity team to safeguard the quality of each article we publish.