- The Department of Molecular and Cellular Physiology, Albany Medical College, Albany, NY, United States
The resolution of inflammation is a tissue protective program that is governed by several factors including specialized pro-resolving mediators (SPMs), proteins, gasses and nucleotides. Pro-resolving mediators activate counterregulatory programs to quell inflammation and promote tissue repair in a manner that does not compromise host defense. Phagocytes like neutrophils and macrophages play key roles in the resolution of inflammation because of their ability to remove debris, microbes and dead cells through processes including phagocytosis and efferocytosis. Emerging evidence suggests that failed resolution of inflammation and defective phagocytosis or efferocytosis underpins several prevalent human diseases. Therefore, understanding factors and mechanisms associated with enhancing these processes is a critical need. SPMs enhance phagocytosis and efferocytosis and this review will highlight mechanisms associated with their actions.
Introduction
Elie Metchnikoff uncovered the significance of phagocytosis nearly 100 years ago (1). Since then, phagocytosis has been recognized as a critical cellular program for innate and adaptive immune responses to foreign material. Moreover, we now appreciate that engulfment and neutralization of invading organisms is key to maintain health. Phagocytes like PMN and macrophages promote microbial removal and wound debridement. Mechanisms associated with phagocytosis continue to be uncovered which has aided in our understanding of inflammation and disease. There are now numerous endogenous factors like lipid mediators (LMs), proteins, metabolites and gasses, that can promote phagocytosis. For example, LMs like specialized pro-resolving mediators (SPMs) are biosynthesized from arachidonate (AA), eicosapentaenoic acid (EPA), docosahexaenoic acid (DHA), or n-3 docosapentaenoic acid (DPA) (2, 3). SPMs are named lipoxins, resolvins, protectins, their aspirin-triggered isomers, maresins, cysteinyl-conjugated SPMs (CTRs) and 13-series resolvins (RvTs) (2, 4–9). Each of the SPMs has a distinct chemical structure (2) and several of the SPMs bind and signal through distinct G-protein coupled receptors (GPCRs) (10–14). SPMs in general exert a tissue protective action in as much as they can temper pro-inflammatory factors and promote the clearance of harmful stimuli and dead cells (i.e. phagocytosis). Pro-phagocytic ligands are not limited to SPMs and indeed the repertoire of these factors are growing and is summarized in Table 1.
Non-resolving inflammation is an underpinning of several prevalent diseases, including cardiovascular and neurodegenerative diseases, cancer, arthritis, asthma etc. Phagocytes play a major role in the resolution of inflammation because of their ability to neutralize and contain harmful stimuli and clear dead cells and debris, which is a feed-forward process that helps repair tissue injury. Not surprisingly, phagocyte functions in several diseases mentioned above have been shown to be dysregulated and are nicely reviewed in the following reference (79). Instead, we appreciate that aging is a major risk factor for several diseases and is a scourge of modern medicine. Therefore, this mini review will highlight some of the pro-phagocytic bioactions of SPMs, with a focus on aging. Lastly, we will offer some suggestions for future studies in this arena.
Pro-Resolving Ligands Enhance the Clearance of Microbes and Debris
We now appreciate that phagocytosis and thus the engulfment and neutralization of invading organisms is key to maintain health. There are several manners in which phagocytes like neutrophils or macrophages ingest pathogens, which is nicely reviewed by Flannagan RS et al. (80). Briefly, the removal of pathogens is a highly orchestrated event that involves cell surface receptors and specific signaling pathways to initiate recognition, engulfment and degradation. SPMs have been shown to increase phagocytosis (Table 1) of pathogens and some of the initial findings are described below. One of the earliest observations involved the SPM called Resolvin E1 (RvE1). RvE1 was shown to enhance the clearance of Candida albicans by human neutrophils and in a mouse model of candidiasis (17). In this same paper, RvE1 was also shown to be biosynthesized by C. albicans. Other pathogens like Pseudomonas aeruginosa (81), T. gondii (82) and T. cruzi (83) were also shown to biosynthesize SPMs. Collectively, these studies demonstrate an intimate link between host-derived SPMs and pathogens. Some questions remain, like why would microbes make SPMs? From the viewpoint of pathogens like Candida, RvE1 could potentially limit the number of recruited polymorphonuclear lymphocytes (PMN), allowing for its persistence. From the perspective of the host, Candida also increases IL-8 (which is a neutrophil chemoattractant) and the presence of RvE1 can enhance local phagocytes to clear Candida (17). From an evolutionary perspective, it is possible that mammalian hosts hijacked SPMs as important armament to protect against foreign invaders. Therefore it is possible that evolutionary pressures on the host are what drove SPMs to possess the ability to enhance phagocytosis. Along these lines, several SPMs such as RvD5, PD1, RvD1 and RvD2 each enhanced the clearance and neutralization of E. coli by neutrophils and macrophages (23, 42). An important finding was that these SPMs do not directly kill E. coli, but rather act on phagocytes to enhance killing in a contained manner. Table 1 summarizes key papers associated with SPM’s ability to enhance phagocytosis in numerous contexts. SPMs also promote phagocytosis of pathogens found in the lungs (34, 84, 85), which may be important for a return to homeostasis post airway infections. Along these lines, RvD1 and Mar1 contain and limit Mycobacterium tuberculosis intracellular growth in macrophages (86). RvD1 increased antimicrobial peptides including bactericidal/permeability-increasing protein (BPI) and LL-37 and Mar1 only increased BPI. The control and containment of Tuberculosis relies heavily on the balance between pro-inflammatory and pro-resolving factors (87). Moreover, RvD1 and Mar1 each stimulated NF-κB nuclear translocation but only Mar1 promoted Nrf2 localization. These results suggest that while RvD1 and Mar1 have overlapping functions, they also exert distinct actions and thus highlight the utter importance of having several distinct SPM species available to promote optimal function. A deeper understanding of the common and distinct actions of SPMs on phagocytosis are of interest.
Along these lines, uncovering mechanisms associated with SPM clearance of microbes and debris is an emerging area of research. As an example, aspirin-triggered lipoxin A4 (or AT-LXA4) was shown to enhance phagocytosis in a mannose scavenger receptor dependent manner (61) because inhibition of the mannose receptor by treatment with mannin completely abrogated AT-LXA4’s ability to enhance the clearance of E. coli (61). In this same study, the authors also found that AT-LXA4 required PI3K and p110γ to enhance phagocytosis (61). AT-LXA4 binds and signals through a GPCR called ALX/FPR2 and so these results suggest that SPMs engage signaling pathways via their cell surface GPCRs. Accordingly, RvD2 binds and signals through a GPCR called GPR18 and knockdown of GPR18 abrogated RvD2’s ability to enhance phagocytosis (43). Moreover, RvD2 enhanced the expression of key phagocytic recognition receptors like CD206 and CD163 (43). In a subsequent study, RvD2 was also found to increase phagocytosis through mechanisms involving cAMP, PKA and STAT3 (44). Other SPMs, like 13S,14S-epoxy-DHA (or eMaR) and RvD1 also increased surface levels of CD163 and CD206 on human macrophages (88). Also, n-3 docosapentaenoic acid–derived resolvin D5 (RvD5n-3 DPA) enhanced phagocytosis through mechanisms involving a GPCR called GPR101 (14). Collectively, these are only a few examples of how SPMs enhance phagocytosis and further understanding of the intricate signaling pathways associated with SPM-initiated phagocytosis are of immense interest. SPMs have also been reported to enhance the clearance of debris, including fibrin clots (35), which is an important process to maintain vascular homeostasis. Overall, SPMs enhance the clearance of microbes and debris. There are several remaining questions: Do particular subsets of macrophages or neutrophils respond optimally to SPMs, and if so which species of SPM? How are SPM GPCRs regulated during phagocytosis? How do SPMs impact macrophage or PMN metabolism when ingesting and neutralizing pathogens? Addressing these questions will likely yield important information as to how cells integrate SPM signals and may help inform the development of targeted therapies for particular infections. Another critical aspect to the maintenance of homeostasis is the clearance of dead cells. The next sections will focus on how SPMs enhance dead cell removal by phagocytes.
Pro-Resolving Ligands Enhance the Clearance of Dead Cells
Billions of cells die daily in adult lives and so the efficient clearance of dead cells is utterly critical for homeostasis (89). Moreover, a large number of cells also die during the resolution of a pathological outcome, such as infection or tissue damage (89). The engulfment of dead cells by professional phagocytes like macrophages is called efferocytosis, which is a highly intricate process that ultimately allows for the recycling of cellular products and tissue repair (79, 90). Failure to clear dead cells can lead to accumulation of necrotic debris, which is associated with several prevalent human diseases, including atherosclerosis (91). Therefore, a major topic of interest in recent years has been the exploration of factors that increase efferocytosis to quell persistent inflammation, limit tissue necrosis and promote repair.
SPMs are of immense interest because of their ability to enhance efferocytosis. Lipoxin A4 (LXA4) was among the first SPMs that was published to enhance the clearance of apoptotic cells in vitro (62, 92). Mechanistically, the increase in efferocytosis is in part through LXA4’s ability to dephosphorylate MYH9, a protein involved with cytoskeletal rearrangement (92). This dephosphorylation results in the activation of components of a signaling cascade leading to cell polarization and increased phagocytosis. In addition to MYH9, LXA4 also activates CDC42, which promotes engulfment (92).
Numerous SPMs, including the E-series, D-series, DPA-derived resolvins, protectins and maresins enhance the clearance of dead cells (93). This redundancy again suggests that SPMs and efferocytosis are utterly critical for tissue repair mechanisms and our survival. The mechanisms by which SPMs enhance efferocytosis are under investigation. Because SPMs have distinct structures, the first step in our understanding toward mechanisms is to investigate the receptors to which they bind. Indeed, several SPM receptors have been discovered and are highlighted in further detail by Chiang et al. (2). Removal of key receptors for SPMs demonstrate that SPMs initiate pro-efferocytotic programs through their cell surface receptors (14, 36, 43, 47).
Intracellular signaling associated with SPM mechanisms are also of immense interest. Resolvin D1 for example binds and signals through a GPCR called ALX/FPR2 (36). RvD1 initiates a cAMP-PKA signaling event and also limits the phosphorylation of p47, a crucial molecule involved in NADPH oxidase (NOX) activation (37). Limiting the activation of NOX aids in quelling inflammation by reducing the amount of ROS which trigger oxidative stress induced cellular damage (37). To this end, it is appreciated that certain pro-inflammatory cytokines, like TNF-α can limit efferocytosis through increased ROS in macrophages (94). RvD1 also limits LPS-induced TNF-α expression to rescue defective efferocytosis by controlling the classical NF-kB1 pathway and activating an atypical pathway that suppresses the secretion of TNF-α and IL-1β (28). Moreover, through C-terminal cleavage of NF-kB1, RvD1 initiates formation of a p50/p50 homodimer which competes for DNA binding with the classical heterodimer (28). Therefore, RvD1’s actions in limiting the release of pro-inflammatory factors (28, 37, 95–97) may also aid in its ability to enhance efferocytosis. Another interesting angle is that oral administration of RvD1 was recently shown to control key transcriptional profiles in ingesting macrophages in vivo (98). For example, RvD1 reduced transcript levels of coactivator‐associated arginine methyltransferase 1 (CARM1), histone aminotransferase 1 (HAT1), histone deacetylase 5 and 7 (HDAC5 and HDAC7), protein arginine N‐methyltransferase 2 (PMRT2), and ribosomal protein S6 kinase, polypeptide 5 (RPS6KA5) in macrophages from inflammatory loci ex vivo (98). With regard to the regulation of CARM1, adoptive transfer of siCARM1‐transfected human macrophages to zymosan‐challenged mice resulted in a significant increase in PMN clearance, which suggests an important counter regulatory role for CARM1 and efferocytosis (98). RvD1 also decreased HDAC5 and HDAC7, which suggests that RvD1 may regulate epigenetic mechanisms (98). How RvD1-initated epigenetic control of macrophages impacts its efferocytic function is of interest. Along these lines, RvD1 also regulated a panel of miRNAs that might contribute to phagocytosis, efferocytosis and resolution of inflammation (99) and a deeper exploration of roles and mechanisms of pro-resolving and pro-phagocytic miRNAs are also of interest.
Another emerging area of interest is the macrophage’s ability to carry out continual efferocytosis, which is the ability of individual macrophages to engulf multiple apoptotic cells consecutively. Early work in this arena demonstrated that CD11b levels were associated with a macrophage’s ability to eat “a little” or “a lot” of apoptotic cells (100). We now appreciate that macrophages within tissues are very diverse with regard to function and phenotype and newer research suggests that macrophage metabolism is an important player in the ability of a macrophage to eat apoptotic cells (101–105). Apoptotic cell engulfment and the eventual breakdown of the corpse provides the fuel for metabolic programs like fatty acid oxidation (FAO) and oxidative phosphorylation (OXPHOS) (101). Macrophages also engage aerobic glycolysis to ingest apoptotic cells (105). Recent work by Yurdagul A et al. demonstrates a critical role for amino acid metabolism and continual efferocytosis (102, 103). Briefly, they found that arginine can be metabolized to ornithine by Arginase-1 in pro-resolving macrophages. Moreover they also found that pro-resolving macrophages converted ornithine into putrescine via ornithine decarboxylase (ODC) for continual efferocytosis. To further this mechanism they then found that ODC-dependent putrescine synthesis drives IL-10 production and inflammation-resolution in vivo. Moreover, putrescine promoted MerTK levels through sH3K9 di/trimethylation mechanisms. Together, these results uncover key players in our understanding of the link between macrophage metabolism, efferocytosis and inflammation resolution programs.
Efferocytosis Promotes SPM Biosynthesis
Another fascinating finding is that the process of efferocytosis itself leads to the biosynthesis of more SPMs (15, 106, 107). This feed-forward circuit was first demonstrated in murine systems (15). Human macrophages that had ingested apoptotic PMN also had increased biosynthesis of SPMs, including RvD1, RvD2 and LXB4 (106). A potential mechanism for the increase in SPMs during efferocytosis is transcellular biosynthesis (106). From a mechanistic perspective and to determine whether released SPMs enhance efferocytosis in a feed forward manner, Chiang N et al. knocked down a key SPM biosynthetic enzyme called 15-lipoxygenase (15-LOX) in human macrophages. They found the 15-LOX silenced macrophages had significantly impaired efferocytosis, which suggests a critical role for SPM synthesis and efficient clearance (76). Another mechanism that promotes SPMs during efferocytosis is through MerTK signaling (107). Cai B. et al. demonstrated that MerTK signaling led to increased SPMs and that silencing of MerTK resulted in less SPMs (107). Mechanisms through which MerTK increases SPMs may be through non-nuclear subcellular localization of a key SPM biosynthetic enzyme called 5-lipoxyeganse (5-LOX) (95, 107). Collectively, these papers point to a critical feed-forward mechanism in which efferocytosis stimulates SPMs and SPMs act locally to further enhance efferocytosis.
Released SPMs as Important“Good-Bye” Tissue Messengers
SPMs and other lipid mediators were also shown to be released by apoptotic neutrophils (106). Interestingly some SPMs enhance the migration of monocytes and macrophages and so released SPMs by apoptotic cells may act as a signal for phagocytes to find these cells for swift clearance. Moreover, these findings suggest that apoptotic cells themselves are active participants in their own clearance. Newer work suggests that metabolites such as putrescine released by apoptotic cells also participate in their own clearance (108) and so released products from apoptotic cells may play critical roles in their swift clearance. More work regarding apoptotic cell secretomes and how released factors impact their clearance are of interest. In fact, Medina et al. profiled the metabolite secretome of apoptotic lymphocytes and macrophages and showed specific metabolites released by apoptotic cells act as “good-bye” messengers to modulate tissue functions. These metabolites reprogram the genes of neighboring healthy cells to facilitate an anti-inflammatory phenotype and promote tissue homeostasis (108).
Moreover, we know there are many modes of cell death beyond apoptosis. Lytic cell death like necroptosis, impacts phagocyte function given the pro-inflammatory nature of their death (109). In addition to the release of DAMPs, cytokines and chemokines, recent work from our lab suggests that necroptotic cells release prostanoids (26). Advanced atherosclerotic plaques from hypercholesterolemic Mlkl-/- mice had significantly more prostanoids like PGE2, PGD2, PGF2α, and thromboxane (TX) compared with wild type (Wt) controls (26). In vitro studies revealed that necroptotic macrophages and endothelial cells also released prostanoids, including TX and PGE2 (26). Receptor antagonists for DP (i.e. PGD2 receptor), EP2 (i.e. PGE2 receptor) and TP (i.e. TX receptor) rescued defective efferocytosis caused by necroptotic cell releasate, suggesting that a prostanoid storm negatively impacts phagocyte behavior (26). Moreover, macrophages stimulated with a TP agonist called U46619 impaired the clearance of both apoptotic and necroptotic cells and so TX may be a novel “avoid me” signal (26). As mentioned above, certain pro-inflammatory cytokines also limit efferocytosis and so released factors from necroptotic cells (or cells that undergo a pro-inflammatory mode of death) may play a large role in negatively impacting phagocyte behavior in tissues.
SPMs Promote the Clearance of Necroptotic Cells
Necroptosis is a pro-inflammatory form of cell death (110, 111) and so the accumulation of necroptotic cells can be damaging to tissues (112). Therefore, uncovering mechanisms associated with necroptotic cell clearance is of immense interest. Earlier work demonstrated that necroptotic cells were cleared by macrophages in a distinct and less efficient manner than apoptotic cells (113, 114). However, detailed molecular mechanisms, quantification methods, and factors that augment the clearance of necroptotic cells were not known. We recently found that necroptotic macrophages express high levels of a “don’t eat me” signal called CD47 (27). Other work suggests that the exuberant expression of CD47 results from pro-inflammatory cytokines, like TNF-α (115), and so the pro-inflammatory nature of this cell death likely drives the increase of CD47. Moreover, because necroptotic cells have regions of disrupted membrane (109), we also found that elevated levels of CD47 were present in clusters on the surface of the necroptotic cell (27). The elevated levels and clustering of CD47 led to an inefficient “nibbling” of necroptotic cells via a RhoA-pMLC signaling event (27). RvD1 when given in vitro and in vivo was able to enhance the clearance of necroptotic cells by promoting whole cell engulfment (26, 27). RvD1-stimulated whole cell engulfment of necroptotic cells by macrophages was non-phlogistic and RvD1 acted by limiting RhoA-pMLC signaling and promoting CDC42 (27). Additionally, RvD1-stimulated macrophages swiftly recognized necroptotic cells for their engulfment and how RvD1 overcame the “don’t eat me” recognition was of interest. ER-mediated phagocytosis is a process in which macrophages release calreticulin onto their target. In other contexts, ER-mediated phagocytosis has been described and is thought to be important for eating large cargo (116, 117). Our work suggests that RvD1 promotes the release of calreticulin from macrophages (117) and may be a mechanism through which RvD1 can swiftly recognize these cells for whole-cell clearance. This work highlights the intricate set of signals/signaling that a macrophage needs to decode for efficient engulfment and clearance of dead cells.
From a metabolic perspective RvD1 stimulates p-AMPK, fatty acid oxidation (FAO) and oxidative phosphorylation (OXHPOS) mechanisms in macrophages to allow for enhanced clearance of necroptotic cells (26). We found OXPHOS is not as readily activated in vehicle-treated macrophages that were ingesting necroptotic cells (26), which suggests that the cargo load within the macrophage may be important for initiating these programs. As mentioned above, apoptotic cell uptake and its eventual breakdown provides the fuel for FAO and OXPHOS in macrophages (101) and so these data suggest that RvD1 maintains this protective metabolic phenotype.
Pro-Resolving Ligands Rescue Age-Related Defects in Efferocytosis
The population is rapidly aging, health care costs are already insurmountable and therapeutics to manage several age-related diseases are limited. Therefore aging and age-related diseases are the scourges of modern medicine. Aging is a complex process that involves genetic, environmental and biological factors. Therefore understanding a common mechanism that may link all of these diseases will inform the development of therapeutics that can promote health span. Persistent, non-resolving inflammation, or inflammaging, largely contributes to a panoply of age-related diseases including periodontal disease, neurodegenerative diseases, macular degeneration, and atherosclerotic cardiovascular disease (118). Defective efferocytosis in aging is well appreciated (119–123). Linehan et al. observed that peritoneal macrophages from old mice had diminished efferocytosis compared with peritoneal macrophages from young mice (121). Interestingly, they transferred peritoneal macrophages from young mice into the peritoneum of old mice, and found that the young macrophages exhibited defective efferocytosis similar to that of macrophages from old mice (121), which suggests that the aging milieu drives defective efferocytosis (120, 121). Lung macrophages from old mice also exhibited defective efferocytosis, which may account for the accumulation of leukocytes and failed tissue repair in lungs from influenza-infected aged mice (124, 125).
Accumulation of senescent cells is also associated with inflammaging (126). Senescent cells acquire a senescence-associated secretory phenotype (SASP) which exacerbates inflammation (126) and contributes to inefficient clearance of dead cells (38). Briefly, we found that released factors from senescent cells promote the cleavage of a critical efferocytosis receptor on macrophages called MerTK to limit efferocytosis (38). RvD1 rescued senescent cell-induced defective efferocytosis in vitro. RvD1 treatment to old mice also increased in situ efferocytosis in lungs post hind-limb ischemia-reperfusion injury (38). MerTK is an interesting efferocytosis receptor because of its ability to stimulate a pro-resolution feed-forward circuit. In this regard, MerTK cleavage is associated with delayed temporal resolution, impaired SPM synthesis over pro-inflammatory lipid mediators and has been shown to promote atherosclerosis (107, 127). Also, with regard to other TAM receptors and aging, Frisch et al. found that Gas6, which is a ligand for MerTK (and other TAM receptors), was down regulated in the bone marrow from aged mice (123) which may account for defective efferocytosis in the aging bone marrow milieu. Moreover, they demonstrated loss-of-function of another efferocytic TAM receptor, Axl in bone marrow macrophages from aged mice which further leads to a significant increase in pro-inflammatory IL-1β signaling. Together, these results suggest that efferocytosis receptors and thus a feed-forward pro-resolution circuit may be dysfunctional in aging.
Along these lines, imbalances in the SPM to pro-inflammatory lipid mediator ratios have been observed in mice and humans in the context of inflammaging (122, 128). In elderly humans, urinary lipoxins (LXs) were decreased resulting in a profound imbalance between pro-resolving LXs and leukotrienes (LTs) (128). Other relevant age-related diseases like atherosclerosis, peripheral vascular disease, periodontal disease and Alzheimer’s disease are also associated with imbalances in this critical ratio and restoration of defective SPMs to these pre-clinical models of disease result in protection (129–132). Nevertheless, how these imbalances arise in aging is of interest but work suggests that one mechanism (of many) may be through MerTK signaling (38). MerTK signaling in human macrophages decreased activity of the mitogen-activated protein kinase (MAPK) p38 and the kinase MK2, resulting in the increased non-phosphorylated, cytoplasmic form of 5-LOX and enhanced SPM biosynthesis (133). Therefore, downstream signaling events from efferocytic responses may drive SPM synthesis of pro-inflammatory mediators. Of note, RvD1 was shown to limit p38 activation which promotes a pro-resolution circuit in macrophages (95). Therefore, continued activation of p38 may not only drive defective efferocytosis but also impair resolution in aging (Figure 1).
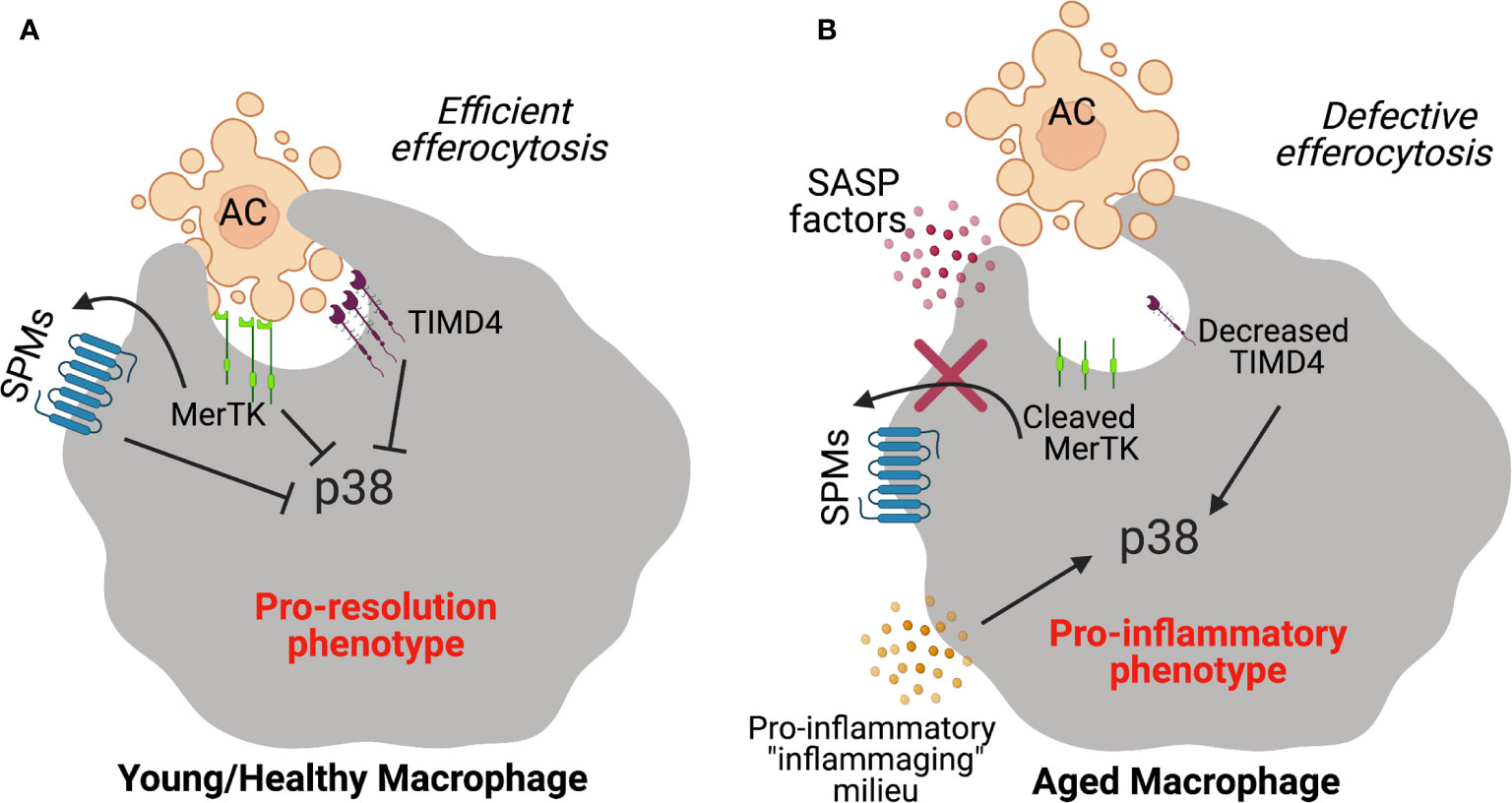
Figure 1 Schematic diagram depicting failed efferocytosis mechanisms during aging. (A) Efferocytic receptors like MerTK or TIMD4 on young healthy macrophages interact with phosphatidyl serine on apoptotic cells to promote efficient clearance. SPMs promote efferocytosis and MerTK signaling in a feed forward manner which stimulates the synthesis of SPMs. In response to apoptotic cell ingestion, the pro-inflammatory p38 MAPK pathway is inhibited and macrophages prevent the production of pro-inflammatory programs. (B) In the context of aging, MerTK is cleaved by released factors from senescent cells (i.e. factors from the senescence-associated secretory phenotype or SASP) which limits apoptotic cell uptake and the feed-forward pro-resolution circuit. TIMD4 expression is also significantly decreased on macrophages from aging humans which drives the activation of p38 to propagate inflammation.
Recent work demonstrated that the efferocytosis receptor, TIM-4 was decreased on macrophages from elderly humans in self-limited inflammatory loci (134). Reduced levels of TIM4 in elderly humans was attributed to increased p38 activation (134) and blockade of elevated p38 restored efferocytosis in the elderly. This work provides strong rationale for therapeutic strategies that target p38 to promote efferocytosis in aging (135). Together, phagocyte function in aging is impaired, which may be one of several factors that contributes to limited tissue repair in aging. A deeper exploration of efferocytosis mechanisms in aging may help inform the development of new tissue-reparative therapies.
Closing Remarks: Therapeutic Opportunity
SPMs and other pro-resolving ligands offer tremendous opportunities for therapeutic use. Currently, treating inflammation is difficult because we evolved inflammatory reactions to fight infection and repair wounds. Therefore anti-inflammatories possess a risk in which critical host defense mechanisms are weakened. Anti-inflammatories may halt the progression of ongoing inflammation, but do very little to repair the already damaged tissue. Ultimately what is lacking is a therapeutic strategy that can repair tissue damage once it has occurred. SPMs are protective in numerous pre-clinical models of disease and most recently have been shown to reduce PMN infiltration during acute sterile inflammation in humans (136). SPMs stimulate phagocytes to clear, and neutralize pathogens or accelerate the removal of unwanted dead cells or debris, all while dampening inflammatory mediators and stimulating necessary pro-repair factors (58). Thus, SPMs offer an entirely new way to control inflammation which includes enhancing the removal of harmful stimuli and promoting tissue repair.
Author Contributions
CD, SS and GF wrote the manuscript. All authors contributed to the article and approved the submitted version.
Funding
This work was supported by NIH grants HL141127 (GF), HL153019 (GF).
Conflict of Interest
The authors declare that the research was conducted in the absence of any commercial or financial relationships that could be construed as a potential conflict of interest.
References
1. Gordon S. Phagocytosis: The Legacy of Metchnikoff. Cell (2016) 166(5):1065–8. doi: 10.1016/j.cell.2016.08.017
2. Chiang N, Serhan CN. Specialized Pro-Resolving Mediator Network: An Update on Production and Actions. Essays Biochem (2020) 64(3):443–62. doi: 10.1042/EBC20200018
3. Serhan CN. Pro-Resolving Lipid Mediators Are Leads for Resolution Physiology. Nature (2014) 510(7503):92–101. doi: 10.1038/nature13479
4. Serhan CN, Hamberg M, Samuelsson B. Lipoxins: Novel Series of Biologically Active Compounds Formed From Arachidonic Acid in Human Leukocytes. Proc Natl Acad Sci USA (1984) 81(17):5335–9. doi: 10.1073/pnas.81.17.5335
5. Serhan CN, Clish CB, Brannon J, Colgan SP, Chiang N, Gronert K. Novel Functional Sets of Lipid-Derived Mediators With Antiinflammatory Actions Generated From Omega-3 Fatty Acids Via Cyclooxygenase 2-Nonsteroidal Antiinflammatory Drugs and Transcellular Processing. J Exp Med (2000) 192(8):1197–204. doi: 10.1084/jem.192.8.1197
6. Serhan CN, Hong S, Gronert K, Colgan SP, Devchand PR, Mirick G, et al. Resolvins: A Family of Bioactive Products of Omega-3 Fatty Acid Transformation Circuits Initiated by Aspirin Treatment That Counter Proinflammation Signals. J Exp Med (2002) 196(8):1025–37. doi: 10.1084/jem.20020760
7. Hong S, Gronert K, Devchand PR, Moussignac RL, Serhan CN. Novel Docosatrienes and 17S-Resolvins Generated From Docosahexaenoic Acid in Murine Brain, Human Blood, and Glial Cells. Autacoids in Anti-Inflammation. J Biol Chem (2003) 278(17):14677–87. doi: 10.1074/jbc.M300218200
8. Dalli J, Chiang N, Serhan CN. Identification of 14-Series Sulfido-Conjugated Mediators That Promote Resolution of Infection and Organ Protection. Proc Natl Acad Sci USA (2014) 111(44):E4753–61. doi: 10.1073/pnas.1415006111
9. Dalli J, Chiang N, Serhan CN. Elucidation of Novel 13-Series Resolvins That Increase With Atorvastatin and Clear Infections. Nat Med (2015) 21(9):1071–5. doi: 10.1038/nm.3911
10. Fiore S, Maddox JF, Perez HD, Serhan CN. Identification of a Human cDNA Encoding a Functional High Affinity Lipoxin A4 Receptor. J Exp Med (1994) 180(1):253–60. doi: 10.1084/jem.180.1.253
11. Arita M, Bianchini F, Aliberti J, Sher A, Chiang N, Hong S, et al. Stereochemical Assignment, Antiinflammatory Properties, and Receptor for the Omega-3 Lipid Mediator Resolvin E1. J Exp Med (2005) 201(5):713–22. doi: 10.1084/jem.20042031
12. Krishnamoorthy S, Recchiuti A, Chiang N, Fredman G, Serhan CN. Resolvin D1 Receptor Stereoselectivity and Regulation of Inflammation and Proresolving Micrornas. Am J Pathol (2012) 180(5):2018–27. doi: 10.1016/j.ajpath.2012.01.028
13. Chiang N, Serhan CN. Structural Elucidation and Physiologic Functions of Specialized Pro-Resolving Mediators and Their Receptors. Mol Aspects Med (2017) 58:114–29. doi: 10.1016/j.mam.2017.03.005
14. Flak MB, Koenis DS, Sobrino A, Smith J, Pistorius K, Palmas F, et al. GPR101 Mediates the Pro-Resolving Actions of RvD5n-3 DPA in Arthritis and Infections. J Clin Invest (2020) 130(1):359–73. doi: 10.1172/JCI131609
15. Schwab JM, Chiang N, Arita M, Serhan CN. Resolvin E1 and Protectin D1 Activate Inflammation-Resolution Programmes. Nature (2007) 447(7146):869–74. doi: 10.1038/nature05877
16. Ohira T, Arita M, Omori K, Recchiuti A, Van Dyke TE, Serhan CN. Resolvin E1 Receptor Activation Signals Phosphorylation and Phagocytosis. J Biol Chem (2010) 285(5):3451–61. doi: 10.1074/jbc.M109.044131
17. Haas-Stapleton EJ, Lu Y, Hong S, Arita M, Favoreto S, Nigam S, et al. Candida Albicans Modulates Host Defense by Biosynthesizing the Pro-Resolving Mediator Resolvin E1. PloS One (2007) 2(12):e1316. doi: 10.1371/journal.pone.0001316
18. Oh SF, Pillai PS, Recchiuti A, Yang R, Serhan CN. Pro-Resolving Actions and Stereoselective Biosynthesis of 18S E-series Resolvins in Human Leukocytes and Murine Inflammation. J Clin Invest (2011) 121(2):569–81. doi: 10.1172/JCI42545
19. Fredman G, Oh SF, Ayilavarapu S, Hasturk H, Serhan CN, Van Dyke TE. Impaired Phagocytosis in Localized Aggressive Periodontitis: Rescue by Resolvin E1. PloS One (2011) 6(9):e24422. doi: 10.1371/journal.pone.0024422
20. Oh SF, Dona M, Fredman G, Krishnamoorthy S, Irimia D, Serhan CN. Resolvin E2 Formation and Impact in Inflammation Resolution. J Immunol (2012) 188(9):4527–34. doi: 10.4049/jimmunol.1103652
21. Libreros S, Shay AE, Nshimiyimana R, Fichtner D, Martin MJ, Wourms N, et al. A New E-Series Resolvin: Rve4 Stereochemistry and Function in Efferocytosis of Inflammation-Resolution. Front Immunol (2020) 11:631319. doi: 10.3389/fimmu.2020.631319
22. Norris PC, Libreros S, Serhan CN. Resolution Metabolomes Activated by Hypoxic Environment. Sci Adv (2019) 5(10):eaax4895. doi: 10.1126/sciadv.aax4895
23. Chiang N, Fredman G, Backhed F, Oh SF, Vickery T, Schmidt BA, et al. Infection Regulates Pro-Resolving Mediators That Lower Antibiotic Requirements. Nature (2012) 484(7395):524–8. doi: 10.1038/nature11042
24. Rogerio AP, Haworth O, Croze R, Oh SF, Uddin M, Carlo T, et al. Resolvin D1 and Aspirin-Triggered Resolvin D1 Promote Resolution of Allergic Airways Responses. J Immunol (2012) 189(4):1983–91. doi: 10.4049/jimmunol.1101665
25. Winkler JW, Orr SK, Dalli J, Cheng CY, Sanger JM, Chiang N, et al. Resolvin D4 Stereoassignment and its Novel Actions in Host Protection and Bacterial Clearance. Sci Rep (2016) 6:18972. doi: 10.1038/srep18972
26. Hosseini Z, Marinello M, Decker C, Sansbury BE, Sadhu S, Gerlach BD, et al. Resolvin D1 Enhances Necroptotic Cell Clearance Through Promoting Macrophage Fatty Acid Oxidation and Oxidative Phosphorylation. Arterioscler Thromb Vasc Biol (2021) 2021:ATVBAHA120315758. doi: 10.1161/ATVBAHA.120.315758
27. Gerlach BD, Marinello M, Heinz J, Rymut N, Sansbury BE, Riley CO, et al. Resolvin D1 Promotes the Targeting and Clearance of Necroptotic Cells. Cell Death Differ (2019) 27:525–39. doi: 10.1038/s41418-019-0370-1
28. Lee HN, Kundu JK, Cha YN, Surh YJ. Resolvin D1 Stimulates Efferocytosis Through p50/p50-Mediated Suppression of Tumor Necrosis Factor-Alpha Expression. J Cell Sci (2013) 126(Pt 17):4037–47. doi: 10.1242/jcs.131003
29. McCauley LK, Dalli J, Koh AJ, Chiang N, Serhan CN. Cutting Edge: Parathyroid Hormone Facilitates Macrophage Efferocytosis in Bone Marrow Via Proresolving Mediators Resolvin D1 and Resolvin D2. J Immunol (2014) 193(1):26–9. doi: 10.4049/jimmunol.1301945
30. Mizwicki MT, Liu G, Fiala M, Magpantay L, Sayre J, Siani A, et al. 1alpha,25-Dihydroxyvitamin D3 and Resolvin D1 Retune the Balance Between Amyloid-Beta Phagocytosis and Inflammation in Alzheimer’s Disease Patients. J Alzheimers Dis (2013) 34(1):155–70. doi: 10.3233/JAD-121735
31. Croasdell A, Lacy SH, Thatcher TH, Sime PJ, Phipps RP. Resolvin D1 Dampens Pulmonary Inflammation and Promotes Clearance of Nontypeable Haemophilus Influenzae. J Immunol (2016) 196(6):2742–52. doi: 10.4049/jimmunol.1502331
32. Abdulnour RE, Sham HP, Douda DN, Colas RA, Dalli J, Bai Y, et al. Aspirin-Triggered Resolvin D1 Is Produced During Self-Resolving Gram-Negative Bacterial Pneumonia and Regulates Host Immune Responses for the Resolution of Lung Inflammation. Mucosal Immunol (2016) 9(5):1278–87. doi: 10.1038/mi.2015.129
33. Matte A, Recchiuti A, Federti E, Koehl B, Mintz T, El Nemer W, et al. Resolution of Sickle Cell Disease-Associated Inflammation and Tissue Damage With 17R-Resolvin D1. Blood (2019) 133(3):252–65. doi: 10.1182/blood-2018-07-865378
34. Codagnone M, Cianci E, Lamolinara A, Mari VC, Nespoli A, Isopi E, et al. Resolvin D1 Enhances the Resolution of Lung Inflammation Caused by Long-Term Pseudomonas Aeruginosa Infection. Mucosal Immunol (2018) 11(1):35–49. doi: 10.1038/mi.2017.36
35. Elajami TK, Colas RA, Dalli J, Chiang N, Serhan CN, Welty FK. Specialized Proresolving Lipid Mediators in Patients With Coronary Artery Disease and Their Potential for Clot Remodeling. FASEB J (2016) 30(8):2792–801. doi: 10.1096/fj.201500155R
36. Krishnamoorthy S, Recchiuti A, Chiang N, Yacoubian S, Lee CH, Yang R, et al. Resolvin D1 Binds Human Phagocytes With Evidence for Proresolving Receptors. Proc Natl Acad Sci USA (2010) 107(4):1660–5. doi: 10.1073/pnas.0907342107
37. Lee HN, Surh YJ. Resolvin D1-Mediated NOX2 Inactivation Rescues Macrophages Undertaking Efferocytosis From Oxidative Stress-Induced Apoptosis. Biochem Pharmacol (2013) 86(6):759–69. doi: 10.1016/j.bcp.2013.07.002
38. Rymut N, Heinz J, Sadhu S, Hosseini Z, Riley CO, Marinello M, et al. Resolvin D1 Promotes Efferocytosis in Aging by Limiting Senescent Cell-Induced MerTK Cleavage. FASEB J (2020) 34(1):597–609. doi: 10.1096/fj.201902126R
39. Luo B, Han F, Xu K, Wang J, Liu Z, Shen Z, et al. Resolvin D1 Programs Inflammation Resolution by Increasing TGF-Beta Expression Induced by Dying Cell Clearance in Experimental Autoimmune Neuritis. J Neurosci (2016) 36(37):9590–603. doi: 10.1523/JNEUROSCI.0020-16.2016
40. Gilligan MM, Gartung A, Sulciner ML, Norris PC, Sukhatme VP, Bielenberg DR, et al. Aspirin-Triggered Proresolving Mediators Stimulate Resolution in Cancer. Proc Natl Acad Sci USA (2019) 116(13):6292–7. doi: 10.1073/pnas.1804000116
41. Merched AJ, Ko K, Gotlinger KH, Serhan CN, Chan L. Atherosclerosis: Evidence for Impairment of Resolution of Vascular Inflammation Governed by Specific Lipid Mediators. FASEB J (2008) 22(10):3595–606. doi: 10.1096/fj.08-112201
42. Spite M, Norling LV, Summers L, Yang R, Cooper D, Petasis NA, et al. Resolvin D2 Is a Potent Regulator of Leukocytes and Controls Microbial Sepsis. Nature (2009) 461(7268):1287–91. doi: 10.1038/nature08541
43. Chiang N, Dalli J, Colas RA, Serhan CN. Identification of Resolvin D2 Receptor Mediating Resolution of Infections and Organ Protection. J Exp Med (2015) 212(8):1203–17. doi: 10.1084/jem.20150225
44. Chiang N, de la Rosa X, Libreros S, Serhan CN. Novel Resolvin D2 Receptor Axis in Infectious Inflammation. J Immunol (2017) 198(2):842–51. doi: 10.4049/jimmunol.1601650
45. Dalli J, Winkler JW, Colas RA, Arnardottir H, Cheng CY, Chiang N, et al. Resolvin D3 and Aspirin-Triggered Resolvin D3 Are Potent Immunoresolvents. Chem Biol (2013) 20(2):188–201. doi: 10.1016/j.chembiol.2012.11.010
46. Winkler JW, Libreros S, De La Rosa X, Sansbury BE, Norris PC, Chiang N, et al. Structural Insights Into Resolvin D4 Actions and Further Metabolites Via a New Total Organic Synthesis and Validation. J Leukoc Biol (2018) 103:995–1010. doi: 10.1002/JLB.3MI0617-254R
47. Chiang N, Libreros S, Norris PC, de la Rosa X, Serhan CN. Maresin 1 Activates LGR6 Receptor Promoting Phagocyte Immunoresolvent Functions. J Clin Invest (2019) 129(12):5294–311. doi: 10.1172/JCI129448
48. Serhan CN, Dalli J, Karamnov S, Choi A, Park CK, Xu ZZ, et al. Macrophage Proresolving Mediator Maresin 1 Stimulates Tissue Regeneration and Controls Pain. FASEB J (2012) 26(4):1755–65. doi: 10.1096/fj.11-201442
49. Deng B, Wang CW, Arnardottir HH, Li Y, Cheng CY, Dalli J, et al. Maresin Biosynthesis and Identification of Maresin 2, A New Anti-Inflammatory and Pro-Resolving Mediator From Human Macrophages. PloS One (2014) 9(7):e102362. doi: 10.1371/journal.pone.0102362
50. Serhan CN, Yang R, Martinod K, Kasuga K, Pillai PS, Porter TF, et al. Maresins: Novel Macrophage Mediators With Potent Antiinflammatory and Proresolving Actions. J Exp Med (2009) 206(1):15–23. doi: 10.1084/jem.20081880
51. Bang S, Xie YK, Zhang ZJ, Wang Z, Xu ZZ, Ji RR. GPR37 Regulates Macrophage Phagocytosis and Resolution of Inflammatory Pain. J Clin Invest (2018) 128(8):3568–82. doi: 10.1172/JCI99888
52. Mukherjee PK, Marcheselli VL, de Rivero Vaccari JC, Gordon WC, Jackson FE, Bazan NG. Photoreceptor Outer Segment Phagocytosis Attenuates Oxidative Stress-Induced Apoptosis With Concomitant Neuroprotectin D1 Synthesis. Proc Natl Acad Sci USA (2007) 104(32):13158–63. doi: 10.1073/pnas.0705963104
53. Serhan CN, Gotlinger K, Hong S, Lu Y, Siegelman J, Baer T, et al. Anti-Inflammatory Actions of Neuroprotectin D1/protectin D1 and its Natural Stereoisomers: Assignments of Dihydroxy-Containing Docosatrienes. J Immunol (2006) 176(3):1848–59. doi: 10.4049/jimmunol.176.3.1848
54. Serhan CN, Fredman G, Yang R, Karamnov S, Belayev LS, Bazan NG, et al. Novel Proresolving Aspirin-Triggered DHA Pathway. Chem Biol (2011) 18(8):976–87. doi: 10.1016/j.chembiol.2011.06.008
55. Ramon S, Dalli J, Sanger JM, Winkler JW, Aursnes M, Tungen JE, et al. The Protectin Pctr1 Is Produced by Human M2 Macrophages and Enhances Resolution of Infectious Inflammation. Am J Pathol (2016) 186(4):962–73. doi: 10.1016/j.ajpath.2015.12.012
56. Dalli J, Sanger JM, Rodriguez AR, Chiang N, Spur BW, Serhan CN. Identification and Actions of a Novel Third Maresin Conjugate in Tissue Regeneration: Mctr3. PloS One (2016) 11(2):e0149319. doi: 10.1371/journal.pone.0149319
57. Chiang N, Riley IR, Dalli J, Rodriguez AR, Spur BW, Serhan CN. New Maresin Conjugates in Tissue Regeneration Pathway Counters Leukotriene D4-stimulated Vascular Responses. FASEB J (2018) 32(7):4043–52. doi: 10.1096/fj.201701493R
58. Dalli J, Ramon S, Norris PC, Colas RA, Serhan CN. Novel Proresolving and Tissue-Regenerative Resolvin and Protectin Sulfido-Conjugated Pathways. FASEB J (2015) 29(5):2120–36. doi: 10.1096/fj.14-268441
59. de la Rosa X, Norris PC, Chiang N, Rodriguez AR, Spur BW, Serhan CN. Identification and Complete Stereochemical Assignments of the New Resolvin Conjugates in Tissue Regeneration in Human Tissues That Stimulate Proresolving Phagocyte Functions and Tissue Regeneration. Am J Pathol (2018) 188(4):950–66. doi: 10.1016/j.ajpath.2018.01.004
60. Dalli J, Colas RA, Serhan CN. Novel N-3 Immunoresolvents: Structures and Actions. Sci Rep (2013) 3:1940. doi: 10.1038/srep01940
61. Prescott D, McKay DM. Aspirin-Triggered Lipoxin Enhances Macrophage Phagocytosis of Bacteria While Inhibiting Inflammatory Cytokine Production. Am J Physiol Gastrointest Liver Physiol (2011) 301(3):G487–97. doi: 10.1152/ajpgi.00042.2011
62. Godson C, Mitchell S, Harvey K, Petasis NA, Hogg N, Brady HR. Cutting Edge: Lipoxins Rapidly Stimulate Nonphlogistic Phagocytosis of Apoptotic Neutrophils by Monocyte-Derived Macrophages. J Immunol (2000) 164(4):1663–7. doi: 10.4049/jimmunol.164.4.1663
63. Mitchell S, Thomas G, Harvey K, Cottell D, Reville K, Berlasconi G, et al. Lipoxins, Aspirin-Triggered Epi-Lipoxins, Lipoxin Stable Analogues, and the Resolution of Inflammation: Stimulation of Macrophage Phagocytosis of Apoptotic Neutrophils In Vivo. J Am Soc Nephrol (2002) 13(10):2497–507. doi: 10.1097/01.ASN.0000032417.73640.72
64. Norris PC, Libreros S, Chiang N. And Serhan Cn. A Cluster of Immunoresolvents Links Coagulation to Innate Host Defense in Human Blood. Sci Signal (2017) 10(490):eaan1471. doi: 10.1126/scisignal.aan1471
65. Maderna P, Yona S, Perretti M, Godson C. Modulation of Phagocytosis of Apoptotic Neutrophils by Supernatant From Dexamethasone-Treated Macrophages and Annexin-Derived Peptide Ac(2-26). J Immunol (2005) 174(6):3727–33. doi: 10.4049/jimmunol.174.6.3727
66. Kourtzelis I, Li X, Mitroulis I, Grosser D, Kajikawa T, Wang B, et al. DEL-1 Promotes Macrophage Efferocytosis and Clearance of Inflammation. Nat Immunol (2019) 20(1):40–9. doi: 10.1038/s41590-018-0249-1
67. Chiang N, de la Rosa X, Libreros S, Pan H, Dreyfuss JM, Serhan CN. Cysteinyl-Specialized Proresolving Mediators Link Resolution of Infectious Inflammation and Tissue Regeneration Via TRAF3 Activation. Proc Natl Acad Sci USA (2021) 118(10):e2013374118. doi: 10.1073/pnas.2013374118
68. Kumaran Satyanarayanan S, El Kebir D, Soboh S, Butenko S, Sekheri M, Saadi J, et al. IFN-Beta is a Macrophage-Derived Effector Cytokine Facilitating the Resolution of Bacterial Inflammation. Nat Commun (2019) 10(1):3471. doi: 10.1038/s41467-019-10903-9
69. Rinaldi M, Thomas L, Mathieu P, Carabias P, Troncoso MF, Pasquini JM, et al. Galectin-1 Circumvents Lysolecithin-Induced Demyelination Through the Modulation of Microglial Polarization/Phagocytosis and Oligodendroglial Differentiation. Neurobiol Dis (2016) 96:127–43. doi: 10.1016/j.nbd.2016.09.003
70. Yaseen H, Butenko S, Polishuk-Zotkin I, Schif-Zuck S, Perez-Saez JM, Rabinovich GA, et al. Galectin-1 Facilitates Macrophage Reprogramming and Resolution of Inflammation Through IFN-Beta. Front Pharmacol (2020) 11:901. doi: 10.3389/fphar.2020.00901
71. Wright RD, Souza PR, Flak MB, Thedchanamoorthy P, Norling LV, Cooper D. Galectin-3-null Mice Display Defective Neutrophil Clearance During Acute Inflammation. J Leukoc Biol (2017) 101(3):717–26. doi: 10.1189/jlb.3A0116-026RR
72. Fernandez GC, Ilarregui JM, Rubel CJ, Toscano MA, Gomez SA, Beigier Bompadre M, et al. Galectin-3 and Soluble Fibrinogen Act in Concert to Modulate Neutrophil Activation and Survival: Involvement of Alternative MAPK Pathways. Glycobiology (2005) 15(5):519–27. doi: 10.1093/glycob/cwi026
73. Montero-Melendez T, Patel HB, Seed M, Nielsen S, Jonassen TE, Perretti M. The Melanocortin Agonist AP214 Exerts Anti-Inflammatory and Proresolving Properties. Am J Pathol (2011) 179(1):259–69. doi: 10.1016/j.ajpath.2011.03.042
74. Dalli J, Norling LV, Montero-Melendez T, Federici Canova D, Lashin H, Pavlov AM, et al. Microparticle alpha-2-macroglobulin Enhances Pro-Resolving Responses and Promotes Survival in Sepsis. EMBO Mol Med (2014) 6(1):27–42. doi: 10.1002/emmm.201303503
75. Dufton N, Natividad J, Verdu EF, Wallace JL. Hydrogen Sulfide and Resolution of Acute Inflammation: A Comparative Study Utilizing a Novel Fluorescent Probe. Sci Rep (2012) 2:499. doi: 10.1038/srep00499
76. Chiang N, Shinohara M, Dalli J, Mirakaj V, Kibi M, Choi AM, et al. Inhaled Carbon Monoxide Accelerates Resolution of Inflammation Via Unique Proresolving Mediator-Heme Oxygenase-1 Circuits. J Immunol (2013) 190(12):6378–88. doi: 10.4049/jimmunol.1202969
77. Salmon JE, Cronstein BN. Fc Gamma Receptor-Mediated Functions in Neutrophils are Modulated by Adenosine Receptor Occupancy. A1 Receptors are Stimulatory and A2 Receptors Are Inhibitory. J Immunol (1990) 145(7):2235–40.
78. Loiola RA, Wickstead ES, Solito E, McArthur S. Estrogen Promotes Pro-Resolving Microglial Behavior and Phagocytic Cell Clearance Through the Actions of Annexin A1. Front Endocrinol (Lausanne) (2019) 10:420. doi: 10.3389/fendo.2019.00420
79. Doran AC, Yurdagul A Jr., Tabas I. Efferocytosis in Health and Disease. Nat Rev Immunol (2020) 20(4):254–67. doi: 10.1038/s41577-019-0240-6
80. Flannagan RS, Jaumouille V, Grinstein S. The Cell Biology of Phagocytosis. Annu Rev Pathol (2012) 7:61–98. doi: 10.1146/annurev-pathol-011811-132445
81. Vance RE, Hong S, Gronert K, Serhan CN, Mekalanos JJ. The Opportunistic Pathogen Pseudomonas Aeruginosa Carries a Secretable Arachidonate 15-Lipoxygenase. Proc Natl Acad Sci USA (2004) 101(7):2135–9. doi: 10.1073/pnas.0307308101
82. Bannenberg GL, Aliberti J, Hong S, Sher A, Serhan C. Exogenous Pathogen and Plant 15-Lipoxygenase Initiate Endogenous Lipoxin A4 Biosynthesis. J Exp Med (2004) 199(4):515–23. doi: 10.1084/jem.20031325
83. Colas RA, Ashton AW, Mukherjee S, Dalli J, Akide-Ndunge OB, Huang H, et al. Trypanosoma Cruzi Produces the Specialized Proresolving Mediators Resolvin D1, Resolvin D5, and Resolvin E2. Infect Immun (2018) 86(4):e00688–17. doi: 10.1128/IAI.00688-17
84. Sham HP, Walker KH, Abdulnour RE, Krishnamoorthy N, Douda DN, Norris PC, et al. 15-Epi-Lipoxin A4, Resolvin D2, and Resolvin D3 Induce NF-Kappab Regulators in Bacterial Pneumonia. J Immunol (2018) 200(8):2757–66. doi: 10.4049/jimmunol.1602090
85. Wang H, Anthony D, Yatmaz S, Wijburg O, Satzke C, Levy B, et al. Aspirin-Triggered Resolvin D1 Reduces Pneumococcal Lung Infection and Inflammation in a Viral and Bacterial Coinfection Pneumonia Model. Clin Sci (Lond) (2017) 131(18):2347–62. doi: 10.1042/CS20171006
86. Ruiz A, Sarabia C, Torres M, Juarez E. Resolvin D1 (RvD1) and Maresin 1 (Mar1) Contribute to Human Macrophage Control of M. Tuberculosis Infection While Resolving Inflammation. Int Immunopharmacol (2019) 74:105694. doi: 10.1016/j.intimp.2019.105694
87. Tobin DM, Ramakrishnan L. TB: The Yin and Yang of Lipid Mediators. Curr Opin Pharmacol (2013) 13(4):641–5. doi: 10.1016/j.coph.2013.06.007
88. Dalli J, Zhu M, Vlasenko NA, Deng B, Haeggstrom JZ, Petasis NA, et al. The Novel 13S,14S-Epoxy-Maresin is Converted by Human Macrophages to Maresin 1 (MaR1), Inhibits Leukotriene A4 Hydrolase (LTA4H), and Shifts Macrophage Phenotype. FASEB J (2013) 27(7):2573–83. doi: 10.1096/fj.13-227728
89. Boada-Romero E, Martinez J, Heckmann BL, Green DR. The Clearance of Dead Cells by Efferocytosis. Nat Rev Mol Cell Biol (2020) 21(7):398–414. doi: 10.1038/s41580-020-0232-1
90. Arandjelovic S, Ravichandran KS. Phagocytosis of Apoptotic Cells in Homeostasis. Nat Immunol (2015) 16(9):907–17. doi: 10.1038/ni.3253
91. Tabas I. Macrophage Death and Defective Inflammation Resolution in Atherosclerosis. Nat Rev Immunol (2010) 10(1):36–46. doi: 10.1038/nri2675
92. Reville K, Crean JK, Vivers S, Dransfield I, Godson C. Lipoxin A4 Redistributes Myosin IIA and Cdc42 in Macrophages: Implications for Phagocytosis of Apoptotic Leukocytes. J Immunol (2006) 176(3):1878–88. doi: 10.4049/jimmunol.176.3.1878
93. Dalli J, Serhan CN. Identification and Structure Elucidation of the Pro-Resolving Mediators Provides Novel Leads for Resolution Pharmacology. Br J Pharmacol (2019) 176(8):1024–37. doi: 10.1111/bph.14336
94. McPhillips K, Janssen WJ, Ghosh M, Byrne A, Gardai S, Remigio L, et al. TNF-Alpha Inhibits Macrophage Clearance of Apoptotic Cells Via Cytosolic Phospholipase A2 and Oxidant-Dependent Mechanisms. J Immunol (2007) 178(12):8117–26. doi: 10.4049/jimmunol.178.12.8117
95. Fredman G, Ozcan L, Spolitu S, Hellmann J, Spite M, Backs J, et al. Resolvin D1 Limits 5-Lipoxygenase Nuclear Localization and Leukotriene B4 Synthesis by Inhibiting a Calcium-Activated Kinase Pathway. Proc Natl Acad Sci USA (2014) 111(40):14530–5. doi: 10.1073/pnas.1410851111
96. Schmid M, Gemperle C, Rimann N, Hersberger M. Resolvin D1 Polarizes Primary Human Macrophages Toward a Proresolution Phenotype Through GPR32. J Immunol (2016) 196(8):3429–37. doi: 10.4049/jimmunol.1501701
97. Gu Z, Lamont GJ, Lamont RJ, Uriarte SM, Wang H, Scott DA. Resolvin D1, Resolvin D2 and Maresin 1 Activate the GSK3beta Anti-Inflammatory Axis in TLR4-Engaged Human Monocytes. Innate Immun (2016) 22(3):186–95. doi: 10.1177/1753425916628618
98. Recchiuti A, Codagnone M, Pierdomenico AM, Rossi C, Mari VC, Cianci E, et al. Immunoresolving Actions of Oral Resolvin D1 Include Selective Regulation of the Transcription Machinery in Resolution-Phase Mouse Macrophages. FASEB J (2014) 28(7):3090–102. doi: 10.1096/fj.13-248393
99. Recchiuti A, Krishnamoorthy S, Fredman G, Chiang N, Serhan CN. MicroRNAs in Resolution of Acute Inflammation: Identification of Novel Resolvin D1-miRNA Circuits. FASEB J (2010) 25(2):544–60. doi: 10.1096/fj.10-169599
100. Schif-Zuck S, Gross N, Assi S, Rostoker R, Serhan CN, Ariel A. Saturated-Efferocytosis Generates Pro-Resolving CD11b Low Macrophages: Modulation by Resolvins and Glucocorticoids. Eur J Immunol (2011) 41(2):366–79. doi: 10.1002/eji.201040801
101. Zhang S, Weinberg S, DeBerge M, Gainullina A, Schipma M, Kinchen JM, et al. Efferocytosis Fuels Requirements of Fatty Acid Oxidation and the Electron Transport Chain to Polarize Macrophages for Tissue Repair. Cell Metab (2019) 29(2):443–56 e5. doi: 10.1016/j.cmet.2018.12.004
102. Yurdagul A Jr., Subramanian M, Wang X, Crown SB, Ilkayeva OR, Darville L, et al. Macrophage Metabolism of Apoptotic Cell-Derived Arginine Promotes Continual Efferocytosis and Resolution of Injury. Cell Metab (2020) 31(3):518–33 e10. doi: 10.1016/j.cmet.2020.01.001
103. Yurdagul A Jr., Kong N, Gerlach BD, Wang X, Ampomah P, Kuriakose G, et al. Odc (Ornithine Decarboxylase)-Dependent Putrescine Synthesis Maintains MerTK (Mer Tyrosine-Protein Kinase) Expression to Drive Resolution. Arterioscler Thromb Vasc Biol (2021) 2021:ATVBAHA120315622. doi: 10.1161/ATVBAHA.120.315622
104. Butenko S, Satyanarayanan SK, Assi S, Schif-Zuck S, Sher N, Ariel A. Transcriptomic Analysis of Monocyte-Derived Non-Phagocytic Macrophages Favors a Role in Limiting Tissue Repair and Fibrosis. Front Immunol (2020) 11:405. doi: 10.3389/fimmu.2020.01003
105. Morioka S, Perry JSA, Raymond MH, Medina CB, Zhu Y, Zhao L, et al. Efferocytosis Induces a Novel SLC Program to Promote Glucose Uptake and Lactate Release. Nature (2018) 563(7733):714–8. doi: 10.1038/s41586-018-0735-5
106. Dalli J, Serhan CN. Specific Lipid Mediator Signatures of Human Phagocytes: Microparticles Stimulate Macrophage Efferocytosis and Pro-Resolving Mediators. Blood (2012) 120(15):e60–72. doi: 10.1182/blood-2012-04-423525
107. Cai B, Thorp EB, Doran AC, Subramanian M, Sansbury BE, Lin CS, et al. MerTK Cleavage Limits Proresolving Mediator Biosynthesis and Exacerbates Tissue Inflammation. Proc Natl Acad Sci USA (2016) 113(23):6526–31. doi: 10.1073/pnas.1524292113
108. Medina CB, Mehrotra P, Arandjelovic S, Perry JSA, Guo Y, Morioka S, et al. Metabolites Released From Apoptotic Cells Act as Tissue Messengers. Nature (2020) 580(7801):130–5. doi: 10.1038/s41586-020-2121-3
109. Gong YN, Guy C, Olauson H, Becker JU, Yang M, Fitzgerald P, et al. Escrt-Iii Acts Downstream of MLKL to Regulate Necroptotic Cell Death and Its Consequences. Cell (2017) 169(2):286–300.e16. doi: 10.1016/j.cell.2017.03.020
110. Wallach D, Kang TB, Dillon CP, Green DR. Programmed Necrosis in Inflammation: Toward Identification of the Effector Molecules. Science (2016) 352(6281):aaf2154. doi: 10.1126/science.aaf2154
111. Enyedi B, Jelcic M, Niethammer P. The Cell Nucleus Serves as a Mechanotransducer of Tissue Damage-Induced Inflammation. Cell (2016) 165(5):1160–70. doi: 10.1016/j.cell.2016.04.016
112. Rayner KJ. Cell Death in the Vessel Wall: The Good, the Bad, the Ugly. Arterioscler Thromb Vasc Biol (2017) 37(7):e75–81. doi: 10.1161/ATVBAHA.117.309229
113. Krysko DV, Denecker G, Festjens N, Gabriels S, Parthoens E, D’Herde K, et al. Macrophages Use Different Internalization Mechanisms to Clear Apoptotic and Necrotic Cells. Cell Death Differ (2006) 13(12):2011–22. doi: 10.1038/sj.cdd.4401900
114. Karunakaran D, Geoffrion M, Wei L, Gan W, Richards L, Shangari P, et al. Targeting Macrophage Necroptosis for Therapeutic and Diagnostic Interventions in Atherosclerosis. Sci Adv (2016) 2(7):e1600224. doi: 10.1126/sciadv.1600224
115. Kojima Y, Volkmer JP, McKenna K, Civelek M, Lusis AJ, Miller CL, et al. CD47-Blocking Antibodies Restore Phagocytosis and Prevent Atherosclerosis. Nature (2016) 536(7614):86–90. doi: 10.1038/nature18935
116. Gagnon E, Duclos S, Rondeau C, Chevet E, Cameron PH, Steele-Mortimer O, et al. Endoplasmic Reticulum-Mediated Phagocytosis Is a Mechanism of Entry Into Macrophages. Cell (2002) 110(1):119–31. doi: 10.1016/S0092-8674(02)00797-3
117. Becker T, Volchuk A, Rothman JE. Differential Use of Endoplasmic Reticulum Membrane for Phagocytosis in J774 Macrophages. Proc Natl Acad Sci USA (2005) 102(11):4022–6. doi: 10.1073/pnas.0409219102
118. Franceschi C, Campisi J. Chronic Inflammation (Inflammaging) and Its Potential Contribution to Age-Associated Diseases. J Gerontol A Biol Sci Med Sci (2014) 69(Suppl 1):S4–9. doi: 10.1093/gerona/glu057
119. Aprahamian T, Rifkin I, Bonegio R, Hugel B, Freyssinet JM, Sato K, et al. Impaired Clearance of Apoptotic Cells Promotes Synergy Between Atherogenesis and Autoimmune Disease. J Exp Med (2004) 199(8):1121–31. doi: 10.1084/jem.20031557
120. Aprahamian T, Takemura Y, Goukassian D, Walsh K. Ageing is Associated With Diminished Apoptotic Cell Clearance In Vivo. Clin Exp Immunol (2008) 152(3):448–55. doi: 10.1111/j.1365-2249.2008.03658.x
121. Linehan E, Dombrowski Y, Snoddy R, Fallon PG, Kissenpfennig A, Fitzgerald DC. Aging Impairs Peritoneal But Not Bone Marrow-Derived Macrophage Phagocytosis. Aging Cell (2014) 13(4):699–708. doi: 10.1111/acel.12223
122. Arnardottir HH, Dalli J, Colas RA, Shinohara M, Serhan CN. Aging Delays Resolution of Acute Inflammation in Mice: Reprogramming the Host Response With Novel Nano-Proresolving Medicines. J Immunol (2014) 193(8):4235–44. doi: 10.4049/jimmunol.1401313
123. Frisch BJ, Hoffman CM, Latchney SE, LaMere MW, Myers J, Ashton J, et al. Aged Marrow Macrophages Expand Platelet-Biased Hematopoietic Stem Cells Via Interleukin1B. JCI Insight (2019) 4(10):e124213. doi: 10.1172/jci.insight.124213
124. Kulkarni U, Zemans RL, Smith CA, Wood SC, Deng JC, Goldstein DR. Excessive Neutrophil Levels in the Lung Underlie the Age-Associated Increase in Influenza Mortality. Mucosal Immunol (2019) 12(2):545–54. doi: 10.1038/s41385-018-0115-3
125. Wong CK, Smith CA, Sakamoto K, Kaminski N, Koff JL, Goldstein DR. Aging Impairs Alveolar Macrophage Phagocytosis and Increases Influenza-Induced Mortality in Mice. J Immunol (2017) 199(3):1060–8. doi: 10.4049/jimmunol.1700397
126. Campisi J. Aging, Cellular Senescence, and Cancer. Annu Rev Physiol (2013) 75:685–705. doi: 10.1146/annurev-physiol-030212-183653
127. Cai B, Thorp EB, Doran AC, Sansbury BE, Daemen MJ, Dorweiler B, et al. MerTK Receptor Cleavage Promotes Plaque Necrosis and Defective Resolution in Atherosclerosis. J Clin Invest (2017) 127(2):564–8. doi: 10.1172/JCI90520
128. Gangemi S, Pescara L, D’Urbano E, Basile G, Nicita-Mauro V, Davi G, et al. Aging is Characterized by a Profound Reduction in Anti-Inflammatory Lipoxin A4 Levels. Exp Gerontol (2005) 40(7):612–4. doi: 10.1016/j.exger.2005.04.004
129. Fredman G, Hellmann J, Proto JD, Kuriakose G, Colas RA, Dorweiler B, et al. An Imbalance Between Specialized Pro-Resolving Lipid Mediators and Pro-Inflammatory Leukotrienes Promotes Instability of Atherosclerotic Plaques. Nat Commun (2016) 7:12859. doi: 10.1038/ncomms12859
130. Fredman G, Van Dyke TE, Serhan CN. Resolvin E1 Regulates Adenosine Diphosphate Activation of Human Platelets. Arterioscler Thromb Vasc Biol (2010) 30(10):2005–13. doi: 10.1161/ATVBAHA.110.209908
131. Whittington RA, Planel E, Terrando N. Impaired Resolution of Inflammation in Alzheimer’s Disease: A Review. Front Immunol (2017) 8:1464. doi: 10.3389/fimmu.2017.01464
132. Ho KJ, Spite M, Owens CD, Lancero H, Kroemer AH, Pande R, et al. Aspirin-Triggered Lipoxin and Resolvin E1 Modulate Vascular Smooth Muscle Phenotype and Correlate With Peripheral Atherosclerosis. Am J Pathol (2010) 177(4):2116–23. doi: 10.2353/ajpath.2010.091082
133. Cai B, Kasikara C, Doran AC, Ramakrishnan R, Birge RB, Tabas I. MerTK Signaling in Macrophages Promotes the Synthesis of Inflammation Resolution Mediators by Suppressing CaMKII Activity. Sci Signal (2018) 11(549):eaar3721. doi: 10.1126/scisignal.aar3721
134. De Maeyer RPH, van de Merwe RC, Louie R, Bracken OV, Devine OP, Goldstein DR, et al. Blocking Elevated P38 MAPK Restores Efferocytosis and Inflammatory Resolution in the Elderly. Nat Immunol (2020) 21(6):615–25. doi: 10.1038/s41590-020-0646-0
135. Gilroy DW, De Maeyer RPH, Tepper M, O’Brien A, Uddin M, Chen J, et al. Treating Exuberant, Non-Resolving Inflammation in the Lung; Implications for Acute Respiratory Distress Syndrome and COVID-19. Pharmacol Ther (2020) 221:107745. doi: 10.1016/j.pharmthera.2020.107745
Keywords: macrophage, phagocytosis, efferocytosis, resolvin, inflammation
Citation: Decker C, Sadhu S and Fredman G (2021) Pro-Resolving Ligands Orchestrate Phagocytosis. Front. Immunol. 12:660865. doi: 10.3389/fimmu.2021.660865
Received: 29 January 2021; Accepted: 06 April 2021;
Published: 10 June 2021.
Edited by:
Valentin Jaumouillé, Simon Fraser University, CanadaReviewed by:
Nan Chiang, Brigham and Women’s Hospital and Harvard Medical School, United StatesAmiram Ariel, University of Haifa, Israel
Jesmond Dalli, Queen Mary University of London, United Kingdom
Copyright © 2021 Decker, Sadhu and Fredman. This is an open-access article distributed under the terms of the Creative Commons Attribution License (CC BY). The use, distribution or reproduction in other forums is permitted, provided the original author(s) and the copyright owner(s) are credited and that the original publication in this journal is cited, in accordance with accepted academic practice. No use, distribution or reproduction is permitted which does not comply with these terms.
*Correspondence: Gabrielle Fredman, ZnJlZG1hZ0BhbWMuZWR1