- 1Department of General Surgery, Peking Union Medical College Hospital, Chinese Academy of Medical Sciences, Peking Union Medical College, Beijing, China
- 2Department of Immunology, School of Basic Medical Sciences, Peking University, Beijing, China
Traditionally, immunoglobulin (Ig) was believed to be produced by only B-lineage cells. However, increasing evidence has revealed a high level of Ig expression in cancer cells, and this Ig is named cancer-derived Ig. Further studies have shown that cancer-derived Ig shares identical basic structures with B cell-derived Ig but exhibits several distinct characteristics, including restricted variable region sequences and aberrant glycosylation. In contrast to B cell-derived Ig, which functions as an antibody in the humoral immune response, cancer-derived Ig exerts profound protumorigenic effects via multiple mechanisms, including promoting the malignant behaviors of cancer cells, mediating tumor immune escape, inducing inflammation, and activating the aggregation of platelets. Importantly, cancer-derived Ig shows promising potential for application as a diagnostic and therapeutic target in cancer patients. In this review, we summarize progress in the research area of cancer-derived Ig and discuss the perspectives of applying this novel target for the management of cancer patients.
Introduction
Immunoglobulin (Ig) molecules comprise two immunoglobulin heavy (IgH) chains and two immunoglobulin light (IgL) chains, which are linked by disulfide bridges to form a structure with twofold symmetry. IgH chains are classified into five isotypes, namely, Igμ, Igγ, Igα, Igδ and Igϵ, four subclasses of Igγ (Igγ1, Igγ2, Igγ3 and Igγ4) and two subclasses of Igα (Igα1 and Igα2). IgL chains have the following two isotypes: Igκ and Igλ. The N-terminal regions of the IgH and IgL chains, which are known as variable (V) regions, are highly variable in their sequences and are responsible for antigen recognition. In contrast, the C-terminal regions of the IgH and IgL chains are constant in their sequences and, thus, are called constant (C) regions. The generation of V region diversity is essential for functional Ig expression and the ability of Ig molecules to recognize various antigens. Multiple molecular mechanisms participate in this process, including V(D)J recombination, somatic hypermutation (SHM) and class-switch recombination (CSR) (1, 2). Ig molecules are important components of humoral immune responses. These molecules function as antibodies in immune defense by neutralizing antigens and mediating antibody-dependent cellular cytotoxicity (ADCC), complement-dependent cytotoxicity (CDC) and antibody-dependent cellular phagocytosis (ADCP) (3, 4).
In patients with cancer, elevated levels of Ig molecules and monoclonal gammopathy were identified decades ago (5). These Ig molecules were considered tumor-reactive antibodies but seemed to be indicative of malignancy progression rather than defense functions. Since Ig was believed to be solely expressed by B lymphocytes, these phenomena were paradoxically interpreted as the “rebel” roles of B lymphocytes in antitumor immunity.
In 1996, the existence of an “Ig-like” protein was unexpectedly identified using anti-human IgG antibody and Protein A in several types of cancer cells (6). Due to the classic theory that Ig is produced by only B lymphocytes, it was difficult to understand at that time whether this “Ig-like” protein was an Ig molecule or another protein sharing a common epitope with Ig. Subsequently, several research groups have started to explore this molecule. First, the transcripts of the IgH variable region and constant region were detected in several epithelial cancer cell lines (7). Then, the expression of IgG in human epithelial cancer cells was comprehensively confirmed at both the transcriptional and protein levels (8). To avoid contamination with B lymphocytes, laser capture microdissection and flow cytometry were used to purify cancer cells from cancer tissues, and the expression of IgG was further validated in long-term-cultured cancer cell lines. During almost the same period, other isotypes of Ig, such as Igκ (9), IgA (10), and IgM (11), were also found to be expressed in cancer cells. Thus, clearly, Ig can be expressed by cancer cells, and this Ig is named cancer-derived Ig. In this review, we summarize current research concerning cancer-derived Ig and its critical roles in tumorigenesis.
Wide Expression of Ig in Cancer Cells
Expression Profile of Ig in Cancers
Generally, cancer-derived Ig is mainly detected in epithelial cancer cells, including those of breast cancer (12–15), colon cancer (16, 17), cervical cancer (16, 18), lung cancer (18–20), laryngeal cancer (21), nasopharyngeal cancer (22), pancreatic cancer (23–25), liver cancer (16, 18), prostate cancer (26–28), oral cancer (29, 30), thyroid cancer (31), parathyroid cancer (32), esophageal cancer (33), gastric cancer (34), renal cancer (35), and bladder cancer (36). At the subcellular level, cancer-derived Ig is mainly located in the cytoplasm and on the cell membrane. Cancer-derived Ig can also be detected in secreted forms in the supernatant of cultured cancer cells (8, 10). Moreover, cancer-derived Ig is detected in a wide variety of soft tissue tumors including malignant fibrous histiocytomas, fibrosarcomas, and leiomyosarcomas (37). The expression of cancer-derived Ig in sarcomas was confirmed in the sarcoma cell lines A673, U-2 OS and HT1080 (38). In addition, cancer-derived Ig is frequently expressed in acute myeloid leukemia cells (39–42). Thus, cancer-derived Ig is validated to be widely expressed in different cancer lineages, including epithelial cancers, soft tissue tumors, and hematopoietic neoplasms. The IGHG1, IGHG2, IGHG3, and IGHG4 mRNA levels in tumor and peritumoral tissues from The Cancer Genome Atlas (TCGA) database and Genotype-Tissue Expression (GTEx) database are shown according to GEPIA (http://gepia.cancer-pku.cn/) (43) (Supplementary Figure 1). The detailed information of cancer-derived Ig expression in cancers is summarized in Table 1.
Characteristics of the Variable Region of Cancer-Derived Ig
V(D)J rearrangement is a process required for Ig expression and is initiated by recombination activating 1 (RAG1) and recombination activating 2 (RAG2) proteins. These two essential enzymes cooperate to generate double-strand breaks at recombination signal sites (RSSs), and the cleaved ends are joined through processes involving the end joining DNA repair system (59, 60). Although RAG1 and RAG2 were previously considered lymphoid-specific proteins, similar to cancer-derived Ig, these proteins have been detected in cancer cells (8, 18). Zheng et al. (61) sequenced 89 VHDJH transcripts from cancer cells purified by laser capture microdissection from eight different types of epithelial cancers and cells from two cancer cell lines (HT-29 and HeLa). The results suggested that the VHDJH transcripts of cancer-derived Ig share some features with Ig derived from B lymphocytes, such as V(D)J recombination, N-region insertion, and a high mutation rate. However, the VHDJH transcripts of cancer-derived Ig also show several characteristics distinct from those of B cell-derived Ig. First, in contrast to the highly variable sequences of classical Ig, the VHDJH transcripts of cancer-derived Ig present restricted VHDJH sequences. The VHDJH transcripts of cancer cells from one tumor sample or even different tumor samples possess identical VHDJH recombination patterns, junctions, and V region mutations. For example, VH5-51/D3-9/JH4 was repeatedly detected in half of the evaluated cases (19/38) of breast cancer, colon cancer, lung cancer, and oral cancer. In addition, cancer-derived IgG production in cancer cells might not follow CSR mechanisms. No identical patterns of VHγDγJHγ and VHμDμJHμ were detected in the study, indicating that IgM-producing cancer cells might not be the precursors of IgG producers. Subsequently, several unique VHDJH recombination patterns, such as VH3-33/D6-19/JH5 in oral cancer (29), VH3-48/D4-7/JH4 in acute myeloid leukemia (39), VH3-30/D6-6/JH4 in bladder cancer (57), VH3-7/D3-22/JH5 in colon cancer (48), and VH5-51/D3-16/JH4 in pancreatic cancer (25), have been identified.
Cancer-Derived Ig Exhibits Aberrant Glycosylation
In carcinogenesis, glycans play important roles in cellular communication, cancer cell migration and invasion, cell-matrix interactions, metastasis formation and immune modulation (62). Several glycoconjugates, such as CA19-9, CEA, and CA125, have been applied clinically as tumor biomarkers (63). Glycosylation can profoundly regulate the physiochemical and biological properties of Ig molecules (64). For example, N-glycosylation at asparagine 297 (Asn297) is a consensus glycosylation event responsible for maintaining the effector functions of IgG (65). RP215 is a monoclonal antibody developed to recognize a glycan-associated epitope specifically expressed in cancer cells (66). Studies have shown that the antigen recognized by RP215 is an IgG molecule with aberrant glycosylation expressed by cancer cells (67). The RP215-recognized glycosylation of cancer-derived IgG was further revealed to be N-glycosylation at asparagine 162 (Asn162), located in the CH1 domain of the IgG heavy chain and carrying a sialic acid modification (44, 68). In subsequent studies, RP215 showed more specificity than commercial anti-IgG antibodies in discriminating cancer-derived IgG from B cell-derived IgG due to its capacity to recognize the specific sialylation site of cancer-derived Ig (25, 69).
Regulatory Mechanisms of Ig Expression in Cancer Cells
The transcription of the Ig gene depends on the activation of Ig promoters and enhancers, which requires the participation of multiple transcription factors such as E2A, EBF, Pax5 and Oct-2 (70–72). Since Ig was previously thought to be selectively expressed in B lymphocytes, how Ig transcription is regulated in cancer cells is unknown. The Ig transcription factors E2A and Oct-1 were first found to be widely expressed in cancer cell lines (73). Using a dual-luciferase reporter assay, the 5’-flanking sequence of VH4-59 was revealed to present promoter activity in cancer cell lines. An enhancer-like element and a copromoter-like element were identified, and the octamer element (ATGCAAAT) was shown to be crucial for Ig gene transcription in cancer cells. In contrast to B cells, which utilize the Ig transcription factor Oct-2, cancer cells tend to utilize Oct-1. Similarly, the 1,200-bp fragment upstream of VH6-1 was also identified to exhibit promoter activity in cancer cells (74). Additionally, the IgIα1 promoter was found to be activated by the transcription factor Ets-1 in cancer cells (75). Other Ig transcription factors, including EBF and Pax5, have also been detected in cancer cells (76). The above studies initially describe how cancer-derived Ig is regulated at the transcriptional level.
In addition to internal Ig transcription factors, several external factors, such as pathogen infection or cytokine stimulation, can regulate Ig expression in cancer cells. Epstein-Barr virus (EBV)-encoded latent membrane protein 1 (LMP1) is an EBV latent gene that plays important roles in carcinogenesis. Previous researchers found that the expression of Igκ in LMP1-positive nasopharyngeal cancer cells was significantly higher than that in LMP1-negative cells (77). Further investigations revealed that the Igκ transcription factors NF-κB (p52/p65) and AP-1 (c-Jun/c-Fos) could be stimulated by LMP1 and then bind the Igκ gene enhancer iEκ, resulting in the upregulation of Igκ expression (78). Merkel cell carcinoma (MCC) is a type of skin cancer that can be divided into the following two types: Merkel cell polyomavirus (MCPyV)-positive MCC and MCPyV-negative MCC. Ig is expressed in 70% of MCPyV-positive MCCs, while no MCPyV-negative MCCs have been shown to express Ig, suggesting that Ig expression in MCC may be induced by MCPyV infection (79). Furthermore, Toll-like receptor 9 (TLR9) agonist stimulation, which mimics bacterial infection, can increase the secretion of IgM via the TLR9-MyD88 pathway (16). The above phenomena imply that pathogen infection may participate in regulating the expression of cancer-derived Ig. In addition, several crucial cytokines, including TGF-β and TNF-α, have been proven to regulate Ig expression in cancer cells in a dose-dependent manner (75, 80). A comparison of the characteristics of cancer-derived Ig and B cell-derived Ig is provided in Table 2.
Cancer-Derived Ig Performs Critical Roles in Cancer Progression
The functions of cancer-derived Ig are distinct from those of B cell-derived Ig, which exerts antibody functions in humoral immunity. Studies have shown that cancer-derived Ig acts as a crucial protumorigenic molecule that promotes carcinogenesis via several different mechanisms.
Cancer-Derived Ig Promotes Malignant Behaviors in Cancer Cells
Cancer-derived Ig acts as a growth factor-like molecule that directly promotes the malignant behaviors of cancer cells including proliferation, migration, invasion, and apoptosis resistance. The protumorigenic effect of cancer-derived Ig on cancer cells has been confirmed in multiple different types of cancers (20, 25, 39, 54). The blockade of cancer-derived IgG by antisense DNA or a specific antibody profoundly suppresses the growth of cancer cells (8, 68). Furthermore, cancer-derived IgG is highly expressed in basal-like cancer cells and cancer cells at the tumor boundary. After sorting different populations of cancer cells based on the IgG expression level, cancer cells with high IgG expression display cancer stem cell-like properties, such as the coexpression of CD44v6, a high sphere-forming capability and resistance to chemotherapy (69). To elucidate the molecular mechanisms of action involving cancer-derived Ig, the major downstream pathways that cancer-derived Ig may regulate have been further studied. Twenty-seven potential IgG-interacting proteins were previously identified by a coimmunoprecipitation assay in cancer cells (81). Among the identified proteins, RACK1, RAN, and PRDX1, which are closely associated with cell growth and oxidative stress, were confirmed to interact with IgG. The induction of intracellular reactive oxygen species (ROS) was further revealed to be an important pathway regulated by cancer-derived IgG. Cancer-derived IgG also interacts with many membrane proteins involved in cell-cell adhesion junctions, focal adhesion and hemidesmosomes (69). For example, cancer-derived IgG can be secreted in autocrine manners by cancer cells, specifically interact with the integrin α6β4 complex and subsequently activate the FAK-Src pathway, which is a critical downstream pathway of integrins (68, 82). The protumorigenic roles of secreted cancer-derived IgG can be blocked by its specific monoclonal antibody RP215. In another study, the MEK/ERK/c-Myc pathway was demonstrated to be another downstream pathway of cancer-derived IgG regulating cell growth and the cell cycle (83). Moreover, Igκ and Igλ have been shown to be responsible for maintaining high expression of the antiapoptotic molecule Bcl-xL and thus perform roles in resisting apoptosis (84).
Cancer-Derived Ig Promotes Tumor Immune Escape
Ig is well known for mediating a wide range of effector functions that modulate several aspects of innate and adaptive immunity (85). Modified by given glycosylation, the functions of Ig can dramatically switch from immunoreactive roles to immunosuppressive roles (86). For example, it has been reported that the anti-inflammatory effect of intravenous immunoglobulin (IVIG) depends on a small fraction of sialylated IgG (87). These sialylated IgG molecules can inhibit the functions of dendritic cells (DCs) and CD4+ T cells by binding the sialic acid receptor DC-SIGN on DCs. Considering that cancer-derived Ig is not only distributed in the cytoplasm but is also located on the cell membrane and secreted, it could be significant to explore whether cancer-derived Ig molecules can modulate other cell types, such as immune cells. Wang et al. (44) purified cancer-derived IgG from the tumor microenvironment and identified a large fraction of sialylated cancer-derived IgG (SIA-CIgG). Using in vitro and in vivo models, these authors demonstrated that SIA-CIgG could significantly inhibit T cell proliferation and reduce effector T cell frequencies in a dose-dependent manner. As SIA-CIgG was purified from the general tumor microenvironment, the immunosuppressive effect of SIA-CIgG may attribute to both membrane-bound and secreted cancer-derived Ig. Further experiments are needed to elucidate whether membrane-bound and secreted cancer-derived Ig share the same function. Furthermore, the inhibitory effect of SIA-CIgG on effector T cells was revealed to rely on its binding with sialic acid-binding immunoglobulin-type lectins (Siglecs). The immunosuppressive function of SIA-CIgG significantly depends on its sialyation modification at the Ans162 site. Siglecs are found on most immune cells and have a common N-terminal domain that recognizes sialic acid-containing glycans (88). Recent studies have shown that Siglecs are highly expressed in tumor infiltrating immune cells and serve as immune checkpoints regulating anti-cancer immunity (89, 90). However, the ligands of Siglecs in tumor immunity are largely unknown. Thus, SIA-CIgG is considered a ligand of Siglec, and combined, they may serve as a pair of potential immune checkpoint molecules mediating tumor immune escape. Targeting SIA-CIgG/Siglecs with specific antibodies could be a promising approach to reversing immune suppression in cancer. In addition, cancer-derived Ig can downregulate the cytotoxic activity of natural killer (NK) cells via the inhibition of ADCC function (23, 45). The presence of cancer-derived Ig specifically reduces the ADCC effect induced by anti-human epithelial growth factor receptor (EGFR) antibodies in a dose-dependent manner.
Other Mechanisms of Cancer-Derived Ig Involved in Promoting Carcinogenesis
Cancer-derived Ig also plays important roles in the regulation of protumorigenic inflammation. After stimulation with lipopolysaccharide (LPS), cancer-derived IgG expression is significantly upregulated. A reduction in the IgG level can directly downregulate Toll-like receptor 4 (TLR4) expression, resulting in the suppressed phosphorylation of NF-κB and MAPK and attenuated production of LPS-induced proinflammatory cytokines (53). In addition, cancer-derived IgG is identified as a functional component of pancreatic cancer cell debris that can induce inflammation by stimulating IL-1β release from tumor-associated macrophages via the TLR4/TRIF/NF-κB pathway (50). Furthermore, free Ig light chain (FLC) exerts an essential promotive effect on colitis-associated colon carcinogenesis by activating the inflammasome (91). The FLC blocker F991 can significantly impede the process of colon carcinogenesis by inhibiting the activation of the inflammasome and reducing the levels of cleaved caspase-1, IL-1β and IL-18. Additionally, cancer-derived IgG has the ability to activate platelets and, thus, participates in tumor-associated thrombosis (92). This effect is mediated by the interaction between cancer-derived IgG and platelet FcγRIIa, which upregulates the expression of CD62P and PAC-1, platelet aggregation, and ATP release. The above molecular mechanisms of action of cancer-derived IgG are summarized in Figure 1.
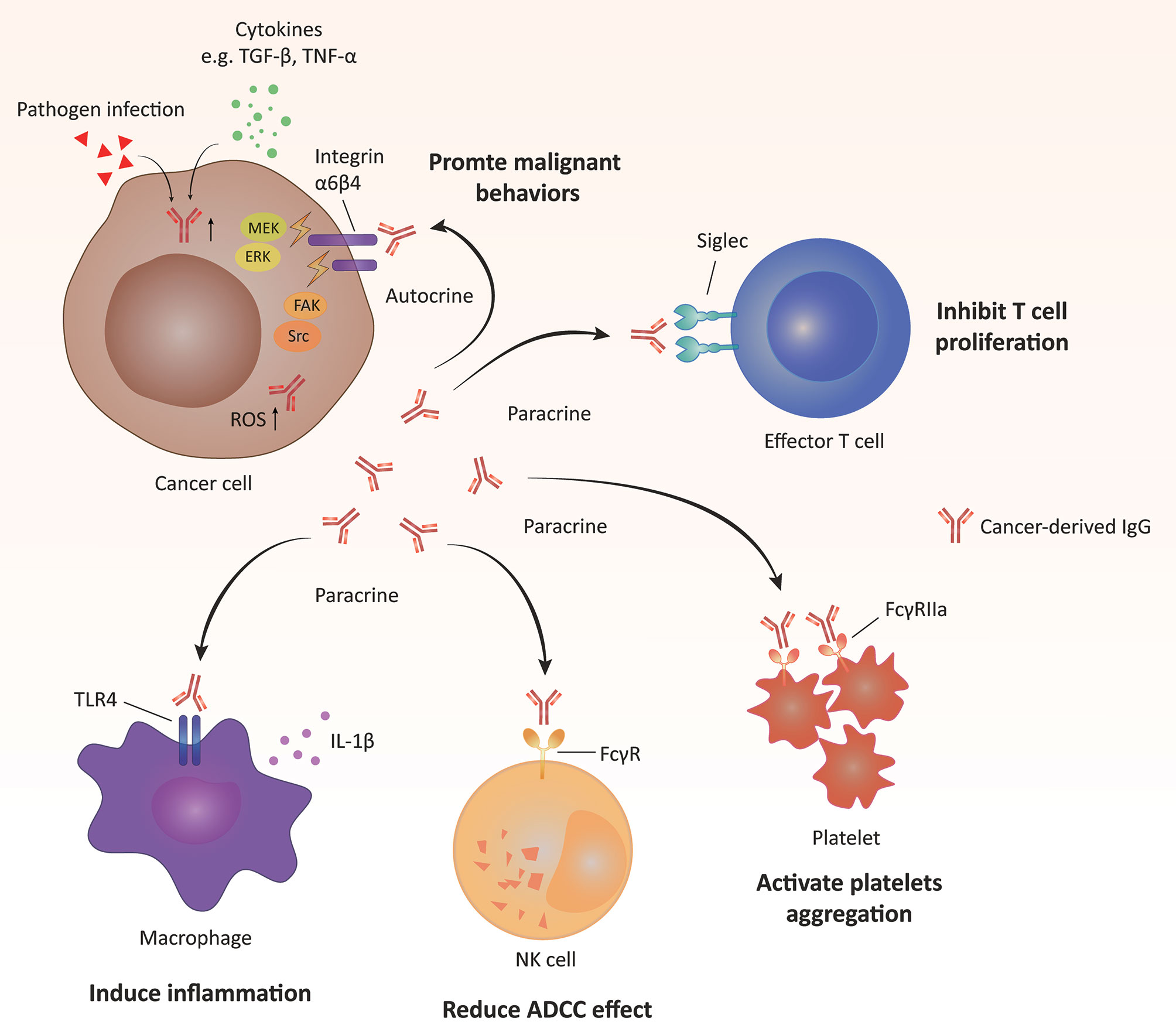
Figure 1 Molecular mechanisms of action of cancer-derived IgG. Cancer-derived IgG promotes tumorigenesis in autocrine and paracrine manners.(i) Cancer-derived IgG interacts with integrins located on the tumor cell membrane and then activates downstream pathways, including FAK/Src, MEK/ERK/c-Myc and reactive oxygen species (ROS) pathways. (ii) Cancer-derived IgG interacts with different membrane receptors of other types of cells, including Siglec of T cells, Toll-like receptor (TLR) of macrophages and FcγR of natural killer (NK) cells and platelets, and further regulates the functions of these cell types. ADCC, antibody-dependent cellular cytotoxicity.
Clinical Significance of Cancer-Derived Ig in Cancer
With the identification of the critical roles of cancer-derived Ig in tumorigenesis, studies have begun to explore whether cancer-derived Ig can serve as a promising target for clinical applications. The expression of cancer-derived IgG is found to be closely associated with tumor differentiation in colon cancer (17), lung cancer (20), renal cancer (35), and pancreatic cancer (25, 93). The expression of cancer-derived Ig is also related to cancer metastasis. The potential of a high expression of cancer-derived IgG to act as a predictor of lymph node metastasis has been indicated in breast cancer (15), lung cancer (46), colon cancer (17), and thyroid cancer (31). Furthermore, cancer-derived Ig can serve as a promising prognostic factor predicting worse outcomes in patients with lung cancer (20), pancreatic cancer (25), gastric cancer (34), renal cancer (34), salivary gland cancer (94), and parathyroid cancer (32). The above studies indicate that cancer-derived Ig could be a diagnostic and prognostic marker in clinical applications in cancer patients. More importantly, cancer-derived Ig is specifically expressed in cancer cells, rendering it a potentially anti-cancer therapeutic target. Specific antibodies targeting cancer-derived IgG have shown promising anti-cancer therapeutic effects in preclinical models (44, 68). Considering its localization on cancer cell membranes, a new generation of chimeric antigen (CAR)-T cell targeting cancer-derived IgG is also worth exploring. Future translational studies and clinical trials are needed to evaluate whether cancer-derived Ig could serve as a novel target or joint target for cancer therapy. Moreover, cancer-derived Ig can be detected by the RP215 antibody in the serum of cancer patients and shows considerable value in monitoring cancer progression (95). Recently, serum IgG glycosylation has been identified as having great significance in differential diagnosis, disease progression monitoring, and therapeutic efficacy prediction in cancer patients (96–100). Similarly, autoantibodies have also been considered next generation biomarkers of cancers (101–104). It will be interesting to perform more studies to elucidate whether these serum aberrant glycosylated Ig or autoantibodies are secreted by cancer cells and evaluate whether secreted cancer-derived Ig can serve as a valuable circulating biomarker in cancer patients.
Conclusions and Perspectives
Ig is a well-known molecule essential for the humoral immune response, but its roles in tumorigenesis are far from clear. Some studies have elucidated the effects of Ig on B cell infiltration in the tumor microenvironment, but many phenomena are still difficult to understand from the perspective of B cell-derived Ig (105–107).
The identification of cancer-derived Ig is undoubtedly a significant finding in the current research area of Ig and largely improves the understanding of Ig in tumorigenesis. Ig is highly expressed in various types of malignancies and is produced by cancer cells. Cancer-derived Ig is located in the cytoplasm and on the cell membrane of cancer cells and can be secreted extracellularly. Compared to B cell-derived Ig, cancer-derived Ig presents distinct characteristics, such as restricted variable region sequences and aberrant glycosylation. Functionally, cancer-derived Ig performs protumorigenic roles via several different mechanisms. Moreover, preliminary studies have shown that cancer-derived Ig has the potential to serve as a novel diagnostic and therapeutic target in clinical applications.
Although the current results are promising, more work is needed to understand the roles of cancer-derived Ig in tumorigenesis. First, the variable region of Ig is essential for recognizing diverse antigens based on its specific sequence. Several molecules have been identified to interact with cancer-derived Ig (24, 89). Considering the restricted patterns of the variable region of cancer-derived Ig, it could be critical to identify the types of molecules that interact with cancer-derived Ig and the specific functions of the variable regions of cancer-derived Ig. Second, cancer-derived Ig has shown pivotal modulatory effects on immune cells, including NK cells and T cells, supporting its function as an immune checkpoint molecule. More studies are needed to elucidate the roles of cancer-derived Ig in antitumor immunity. Finally, it could be important to explore the significance of cancer-derived Ig in clinical applications. Previous studies have demonstrated that cancer-derived Ig can serve as a promising diagnostic and prognostic biomarker in cancer patients. Therapies targeting cancer-derived Ig have shown promising effects in preclinical studies. Future studies are expected to evaluate whether it is feasible to apply cancer-derived Ig as a therapeutic target in cancer patients.
Author Contributions
MC and JH drafted the manuscript. SZ and QFL collected related literature. XQ and QL directed the work and revised the manuscript. All authors contributed to the article and approved the submitted version.
Funding
This work was supported by grants from the National Natural Science Foundation of China (81872501 and 81673023), the Key Project of the National Natural Science Foundation of China (82030044), the Key Support Projects of the National Natural Science Foundation’s Major Research Program (91642206), and the Non-profit Central Research Institute Fund of the Chinese Academy of Medical Sciences (2018PT32014).
Conflict of Interest
The authors declare that the research was conducted in the absence of any commercial or financial relationships that could be construed as a potential conflict of interest.
Supplementary Material
The Supplementary Material for this article can be found online at: https://www.frontiersin.org/articles/10.3389/fimmu.2021.613530/full#supplementary-material
References
1. Tonegawa S. Somatic generation of antibody diversity. Nature (1983) 302:575–81. doi: 10.1038/302575a0
2. Chi X, Li Y, Qiu X. V(D)J recombination, somatic hypermutation and class switch recombination of immunoglobulins: mechanism and regulation. Immunology (2020) 160:233–47. doi: 10.1111/imm.13176
3. Bournazos S, Ravetch JV. Diversification of IgG effector functions. Int Immunol (2017) 29:303–10. doi: 10.1093/intimm/dxx025
4. Vidarsson G, Dekkers G, Rispens T. IgG subclasses and allotypes: from structure to effector functions. Front Immunol (2014) 5:520. doi: 10.3389/fimmu.2014.00520
5. Gerçel-Taylor Ç, Bazzett LB, Taylor DD. Presence of aberrant tumor-reactive immunoglobulins in the circulation of patients with ovarian cancer. Gynecol Oncol (2001) 81:71–6. doi: 10.1006/gyno.2000.6102
6. Qiu X, Yang G. Existance of Ig-like protein in malignant tumor cells. J Norman Bethune Univ Med Sci (1996) 22:575–7.
7. Kimoto Y. Expression of heavy-chain constant region of immunoglobulin and T-cell receptor gene transcripts in human non-hematopoietic tumor cell lines. Genes Chromosomes Cancer (1998) 22:83–6. doi: 10.1002/(SICI)1098-2264(1998)22:1<83::AID-GCC12>3.0.CO;2-O
8. Qiu X, Zhu X, Zhang L, Mao Y, Zhang J, Hao P, et al. Human epithelial cancers secrete immunoglobulin g with unidentified specificity to promote growth and survival of tumor cells. Cancer Res (2003) 63:6488–95.
9. Li M, Feng DY, Ren W, Zheng L, Zheng H, Tang M, et al. Expression of immunoglobulin kappa light chain constant region in abnormal human cervical epithelial cells. Int J Biochem Cell Biol (2004) 36:2250–7. doi: 10.1016/j.biocel.2004.03.017
10. Zheng H, Li M, Ren W, Zeng L, Liu HD, Hu D, et al. Expression and secretion of immunoglobulin alpha heavy chain with diverse VDJ recombinations by human epithelial cancer cells. Mol Immunol (2007) 44:2221–7. doi: 10.1016/j.molimm.2006.11.010
11. Babbage G, Ottensmeier CH, Blaydes J, Stevenson FK, Sahota SS. Immunoglobulin heavy chain locus events and expression of activation-induced cytidine deaminase in epithelial breast cancer cell lines. Cancer Res (2006) 66:3996–4000. doi: 10.1158/0008-5472.CAN-05-3704
12. Kang S, Maeng H, Kim BG, Qing GM, Choi YP, Kim HY, et al. In situ identification and localization of IGHA2 in the breast tumor microenvironment by mass spectrometry. J Proteome Res (2012) 11:4567–74. doi: 10.1021/pr3003672
13. Ma C, Wang Y, Zhang G, Chen Z, Qiu Y, Li J, et al. Immunoglobulin G expression and its potential role in primary and metastatic breast cancers. Curr Mol Med (2013) 13:429–37. doi: 10.2174/156652413805076731
14. Welinder C, Baldetorp B, Blixt O, Grabau D, Jansson B. Primary breast cancer tumours contain high amounts of IgA1 immunoglobulin: an immunohistochemical analysis of a possible carrier of the tumour-associated Tn antigen. PLoS One (2013) 8:e61749. doi: 10.1371/journal.pone.0061749
15. Yang B, Ma C, Chen Z, Yi W, McNutt MA, Wang Y, et al. Correlation of immunoglobulin G expression and histological subtype and stage in breast cancer. PLoS One (2013) 8:e58706. doi: 10.1371/journal.pone.0058706
16. Hu F, Zhang L, Zheng J, Zhao L, Huang J, Shao W, et al. Spontaneous production of immunoglobulin M in human epithelial cancer cells. PLoS One (2012) 7:e51423. doi: 10.1371/journal.pone.0051423
17. Niu N, Zhang J, Huang T, Sun Y, Chen Z, Yi W, et al. IgG expression in human colorectal cancer and its relationship to cancer cell behaviors. PLoS One (2012) 7:e47362. doi: 10.1371/journal.pone.0047362
18. Chen Z, Gu J. Immunoglobulin G expression in carcinomas and cancer cell lines. FASEB J (2007) 21:2931–8. doi: 10.1096/fj.07-8073com
19. Xiao T, Ying W, Li L, Hu Z, Ma Y, Jiao L, et al. An approach to studying lung cancer-related proteins in human blood. Mol Cell Proteomics (2005) 4:1480–6. doi: 10.1074/mcp.M500055-MCP200
20. Liu Y, Liu D, Wang C, Liao Q, Huang J, Jiang D, et al. Binding of the monoclonal antibody RP215 to immunoglobulin G in metastatic lung adenocarcinomas is correlated with poor prognosis. Histopathology (2015) 67:645–53. doi: 10.1111/his.12686
21. Wang H, Cao X, Liu EC, He D, Ma Y, Zhang T, et al. Prognostic significance of immunoglobulin M overexpression in laryngeal squamous cell carcinoma. Acta Otolaryngol (2013) 133:1080–7. doi: 10.3109/00016489.2013.799776
22. Hu D, Duan Z, Li M, Jiang Y, Liu H, Zheng H, et al. Heterogeneity of aberrant immunoglobulin expression in cancer cells. Cell Mol Immunol (2011) 8:479–85. doi: 10.1038/cmi.2011.25
23. Li X, Ni R, Chen J, Liu Z, Xiao M, Jiang F, et al. The presence of IGHG1 in human pancreatic carcinomas is associated with immune evasion mechanisms. Pancreas (2011) 40:753–61. doi: 10.1097/MPA.0b013e318213d51b
24. Wan X, Lei Y, Li Z, Wang J, Chen Z, McNutt M, et al. Pancreatic expression of immunoglobulin G in human pancreatic cancer and associated diabetes. Pancreas (2015) 44:1304–13. doi: 10.1097/MPA.0000000000000544
25. Cui M, You L, Zheng B, Huang X, Liu Q, Huang J, et al. High expression of cancer-derived glycosylated immunoglobulin G predicts poor prognosis in pancreatic ductal adenocarcinoma. J Cancer (2020) 11:2213–21. doi: 10.7150/jca.39800
26. Liu Y, Chen Z, Niu N, Chang Q, Deng R, Korteweg C, et al. IgG gene expression and its possible significance in prostate cancers. Prostate (2011) 72:690–701. doi: 10.1002/pros.21476
27. Pan B, Zheng S, Liu C, Xu Y. Suppression of IGHG1 gene expression by siRNA leads to growth inhibition and apoptosis induction in human prostate cancer cell. Mol Biol Rep (2012) 40:27–33. doi: 10.1007/s11033-012-1944-x
28. Qin C, Sheng Z, Huang X, Tang J, Liu Y, Xu T, et al. Cancer-driven IgG promotes the development of prostate cancer though the SOX2-CIgG pathway. Prostate (2020) 80:1134–44. doi: 10.1002/pros.24042
29. Zhu X, Li C, Sun X, Mao Y, Li G, Liu X, et al. Immunoglobulin mRNA and protein expression in human oral epithelial tumor cells. Appl Immunohistochem Mol Morphol (2008) 16:232–8. doi: 10.1097/PAI.0b013e31814c915a
30. Wang LM, Gan YH. Cancer-derived IgG involved in cisplatin resistance through PTP-BAS/Src/PDK1/AKT signaling pathway. Oral Dis (2021) 27:464–74. doi: 10.1111/odi.13583
31. Qiu Y, Korteweg C, Chen Z, Li J, Luo J, Huang G, et al. Immunoglobulin G expression and its colocalization with complement proteins in papillary thyroid cancer. Mod Pathol (2012) 25:36–45. doi: 10.1038/modpathol.2011.139
32. Cui M, Hu Y, Zheng B, Zhang S, Zhang X, Wang M, et al. Cancer-derived immunoglobulin G: a novel marker for differential diagnosis and relapse prediction in parathyroid carcinoma. Clin Endocrinol (2020) 92:461–7. doi: 10.1111/cen.14158
33. Zhang L, Hu S, Korteweg C, Chen Z, Qiu Y, Su M, et al. Expression of immunoglobulin G in esophageal squamous cell carcinomas and its association with tumor grade and Ki67. Hum Pathol (2012) 43:423–34. doi: 10.1016/j.humpath.2011.05.020
34. Li L, Cheng X, Zheng Z, Xing X, Wang X, Zhang L, et al. Overexpression of cancer cell-derived immunoglobulin G correlates with poor prognosis in gastric cancer patients. Transl Cancer Res (2016) 5:285–93. doi: 10.21037/tcr.2016.06.06
35. Sheng Z, Liu Y, Qin C, Liu Z, Yuan Y, Hu F, et al. IgG is involved in the migration and invasion of clear cell renal cell carcinoma. J Clin Pathol (2016) 69:497–504. doi: 10.1136/jclinpath-2015-202881
36. Liang PY, Li HY, Zhou ZY, Jin YX, Wang SX, Peng XH, et al. Overexpression of immunoglobulin G prompts cell proliferation and inhibits cell apoptosis in human urothelial carcinoma. Tumour Biol (2013) 34:1783–91. doi: 10.1007/s13277-013-0717-z
37. Chen Z, Huang X, Ye J, Pan P, Cao Q, Yang B, et al. Immunoglobulin G is present in a wide variety of soft tissue tumors and correlates well with proliferation markers and tumor grades. Cancer (2010) 116:1953–63. doi: 10.1002/cncr.24892
38. Chen Z, Li J, Xiao Y, Zhang J, Zhao Y, Liu Y, et al. Immunoglobulin G locus events in soft tissue sarcoma cell lines. PLoS One (2011) 6:e21276. doi: 10.1371/journal.pone.0021276
39. Qiu X, Sun X, He Z, Huang J, Hu F, Chen L, et al. Immunoglobulin gamma heavy chain gene with somatic hypermutation is frequently expressed in acute myeloid leukemia. Leukemia (2013) 27:92–9. doi: 10.1038/leu.2012.184
40. Huang J, Sun X, Gong X, He Z, Chen L, Qiu X, et al. Rearrangement and expression of the immunoglobulin μ-chain gene in human myeloid cells. Cell Mol Immunol (2013) 11:94–104. doi: 10.1038/cmi.2013.45
41. Wang C, Xia M, Sun X, He Z, Hu F, Chen L, et al. IGK with conserved IGKV/IGKJ repertoire is expressed in acute myeloid leukemia and promotes leukemic cell migration. Oncotarget (2015) 6:39062–72. doi: 10.18632/oncotarget.5393
42. Wu L, Xia M, Sun X, Han X, Zu Y, Jabbour EJ, et al. High levels of immunoglobulin expression predict shorter overall survival in patients with acute myeloid leukemia. Eur J Haematol (2020) 105:449–59. doi: 10.1111/ejh.13466
43. Tang Z, Li C, Kang B, Gao G, Li C, Zhang Z. GEPIA: a web server for cancer and normal gene expression profiling and interactive analyses. Nucleic Acids Res (2017) 45:W98–102. doi: 10.1093/nar/gkx247
44. Wang Z, Geng Z, Shao W, Liu E, Zhang J, Tang J, et al. Cancer-derived sialylated IgG promotes tumor immune escape by binding to Siglecs on effector T cells. Cell Mol Immunol (2020) 17:1148–62. doi: 10.1038/s41423-019-0327-9
45. Li M, Zheng H, Duan Z, Liu H, Hu D, Bode A, et al. Promotion of cell proliferation and inhibition of ADCC by cancerous immunoglobulin expressed in cancer cell lines. Cell Mol Immunol (2012) 9:54–61. doi: 10.1038/cmi.2011.40
46. Lee G. Cancer cell-expressed immunoglobulins: CA215 as a pan cancer marker and its diagnostic applications12. Cancer Biomark (2009) 5:137–42. doi: 10.3233/CBM-2009-0610
47. Jiang C, Huang T, Wang Y, Huang G, Wan X, Gu J. Immunoglobulin G expression in lung cancer and its effects on metastasis. PLoS One (2014) 9:e97359. doi: 10.1371/journal.pone.0097359
48. Geng ZH, Ye CX, Huang Y, Jiang HP, Ye YJ, Wang S, et al. Human colorectal cancer cells frequently express IgG and display unique Ig repertoire. World J Gastrointest Oncol (2019) 11:195–207. doi: 10.4251/wjgo.v11.i3.195
49. Jiang H, Kang B, Huang X, Yan Y, Wang S, Ye Y, et al. Cancer IgG, a potential prognostic marker, promotes colorectal cancer progression. Chin J Cancer Res (2019) 31:499–510. doi: 10.21147/j.issn.1000-9604.2019.03.12
50. Chen Q, Wang J, Zhang Q, Zhang J, Lou Y, Yang J, et al. Tumour cell-derived debris and IgG synergistically promote metastasis of pancreatic cancer by inducing inflammation via tumour-associated macrophages. Br J Cancer (2019) 121:786–95. doi: 10.1038/s41416-019-0595-2
51. Lei Y, Huang T, Su M, Luo J, Korteweg C, Li J, et al. Expression and distribution of immunoglobulin G in the normal liver, hepatocarcinoma and postpartial hepatectomy liver. Lab Invest (2014) 94:1283–95. doi: 10.1038/labinvest.2014.114
52. Lee G, Ge B. Cancer cell expressions of immunoglobulin heavy chains with unique carbohydrate-associated biomarker. Cancer Biomark (2009) 5:177–88. doi: 10.3233/CBM-2009-0102
53. Wang J, Lin D, Peng H, Shao J, Gu J. Cancer-derived immunoglobulin G promotes LPS-induced proinflammatory cytokine production via binding to TLR4 in cervical cancer cells. Oncotarget (2014) 5:9727–43. doi: 10.18632/oncotarget.2359
54. Zheng H, Li M, Liu H, Ren W, Hu D, Shi Y, et al. Immunoglobulin alpha heavy chain derived from human epithelial cancer cells promotes the access of S phase and growth of cancer cells. Cell Biol Int (2007) 31:82–7. doi: 10.1016/j.cellbi.2006.09.009
55. Ji F, Chang X, Liu C, Meng L, Qu L, Wu J, et al. Prognostic value and characterization of the ovarian cancer-specific antigen CA166-9. Int J Oncol (2015) 47:1405–15. doi: 10.3892/ijo.2015.3115
56. Xu Y, Chen B, Zheng S, Wen Y, Xu A, Xu K, et al. IgG silencing induces apoptosis and suppresses proliferation, migration and invasion in LNCaP prostate cancer cells. Cell Mol Biol Lett (2016) 21:27. doi: 10.1186/s11658-016-0029-6
57. Sheng Z, Liu Y, Qin C, Liu Z, Yuan Y, Yin H, et al. Involvement of cancer-derived IgG in the proliferation, migration and invasion of bladder cancer cells. Oncol Lett (2016) 12:5113–21. doi: 10.3892/ol.2016.5350
58. Lv WQ, Peng J, Wang HC, Chen DP, Yang Y, Zhao Y, et al. Expression of cancer cell-derived IgG and extra domain A-containing fibronectin in salivary adenoid cystic carcinoma. Arch Oral Biol (2017) 81:15–20. doi: 10.1016/j.archoralbio.2017.04.010
59. Bassing CH, Swat W, Alt FW. The mechanism and regulation of chromosomal V(D)J recombination. Cell (2002) 109:S45–55. doi: 10.1016/S0092-8674(02)00675-X
60. Gellert M. V(D)J recombination: RAG proteins, repair factors, and regulation. Annu Rev Biochem (2002) 71:101–32. doi: 10.1146/annurev.biochem.71.090501.150203
61. Zheng J, Huang J, Mao Y, Liu S, Sun X, Zhu X, et al. Immunoglobulin gene transcripts have distinct VHDJH recombination characteristics in human epithelial cancer cells. J Biol Chem (2009) 284:13610–9. doi: 10.1074/jbc.M809524200
62. Rodrigues JG, Balmaña M, Macedo JA, Poças J, Fernandes Â, de-Freitas-Junior JCM, et al. Glycosylation in cancer: selected roles in tumour progression, immune modulation and metastasis. Cell Immunol (2018) 333:46–57. doi: 10.1016/j.cellimm.2018.03.007
63. Pinho SS, Reis CA. Glycosylation in cancer: mechanisms and clinical implications. Nat Rev Cancer (2015) 15:540–55. doi: 10.1038/nrc3982
64. Kiyoshi M, Tsumoto K, Ishii-Watabe A, Caaveiro JMM. Glycosylation of IgG-Fc: a molecular perspective. Int Immunol (2017) 29:311–7. doi: 10.1093/intimm/dxx038
65. Xue J, Zhu LP, Wei Q. IgG-Fc N-glycosylation at Asn297 and IgA O-glycosylation in the hinge region in health and disease. Glycoconj J (2013) 30:735–45. doi: 10.1007/s10719-013-9481-y
66. Lee G, Laflamme E, Chien CH, Ting HH. Molecular identity of a pan cancer marker, CA215. Cancer Biol Ther (2008) 7:2007–14. doi: 10.4161/cbt.7.12.6984
67. Lee G, Cheung AP, Li B, Ge B, Chow PM. Molecular and immuno-characteristics of immunoglobulin-like glycoproteins in cancer cell-expressed biomarker, CA215. Immunol Invest (2012) 41:429–46. doi: 10.3109/08820139.2012.661007
68. Tang J, Zhang J, Liu Y, Liao Q, Huang J, Geng Z, et al. Lung squamous cell carcinoma cells express non-canonically glycosylated IgG that activates integrin-FAK signaling. Cancer Lett (2018) 430:148–59. doi: 10.1016/j.canlet.2018.05.024
69. Liao Q, Liu W, Liu Y, Wang F, Wang C, Zhang J, et al. Aberrant high expression of immunoglobulin G in epithelial stem/progenitor-like cells contributes to tumor initiation and metastasis. Oncotarget (2015) 6:40081–94. doi: 10.18632/oncotarget.5542
70. Lin H, Grosschedl R. Failure of B-cell differentiation in mice lacking the transcription factor EBF. Nature (1995) 376:263–7. doi: 10.1038/376263a0
71. Yabuki M, Ordinario EC, Cummings WJ, Fujii MM, Maizels N. E2A acts in cis in G1 phase of cell cycle to promote Ig gene diversification. J Immunol (2008) 182:408–15. doi: 10.4049/jimmunol.182.1.408
72. Roy AL, Sen R, Roeder RG. Enhancer–promoter communication and transcriptional regulation of Igh. Trends Immunol (2011) 32:532–9. doi: 10.1016/j.it.2011.06.012
73. Zhu X, Wu L, Zhang L, Hao P, Zhang S, Huang J, et al. Distinct regulatory mechanism of immunoglobulin gene transcription in epithelial cancer cells. Cell Mol Immunol (2010) 7:279–86. doi: 10.1038/cmi.2010.13
74. Wu L, Liu Y, Zhu X, Zhang L, Chen J, Zhang H, et al. The immunoglobulin heavy chain VH6-1 promoter regulates Ig transcription in non-B cells. Cancer Cell Int (2014) 14:114. doi: 10.1186/s12935-014-0114-8
75. Duan Z, Zheng H, Xu S, Jiang Y, Liu H, Li M, et al. Activation of the Ig Iα1 promoter by the transcription factor Ets-1 triggers Ig Iα1–Cα1 germline transcription in epithelial cancer cells. Cell Mol Immunol (2014) 11:197–205. doi: 10.1038/cmi.2013.52
76. Geng LY, Shi ZZ, Dong Q, Cai XH, Zhang YM, Cao W, et al. Expression of SNC73, a transcript of the immunoglobulin alpha-1 gene, in human epithelial carcinomas. World J Gastroenterol (2007) 13:2305–11. doi: 10.3748/wjg.v13.i16.2305
77. Liu HD, Zheng H, Li M, Hu DS, Tang M, Cao Y. Upregulated expression of kappa light chain by Epstein–Barr virus encoded latent membrane protein 1 in nasopharyngeal carcinoma cells via NF-κB and AP-1 pathways. Cell Signal (2007) 19:419–27. doi: 10.1016/j.cellsig.2006.07.012
78. Liu H, Zheng H, Duan Z, Hu D, Li M, Liu S, et al. LMP1-augmented kappa intron enhancer activity contributes to upregulation expression of Ig kappa light chain via NF-kappaB and AP-1 pathways in nasopharyngeal carcinoma cells. Mol Cancer (2009) 8:92. doi: 10.1186/1476-4598-8-92
79. Murakami I, Takata K, Matsushita M, Nonaka D, Iwasaki T, Kuwamoto S, et al. Immunoglobulin expressions are only associated with MCPyV-positive Merkel cell carcinomas but not with MCPyV-negative ones. Am J Surg Pathol (2014) 38:1627–35. doi: 10.1097/PAS.0000000000000279
80. Duan Z, Zheng H, Liu H, Li M, Tang M, Weng X, et al. AID expression increased by TNF-α is associated with class switch recombination of Igα gene in cancers. Cell Mol Immunol (2015) 13:484–91. doi: 10.1038/cmi.2015.26
81. Wang J, Lin D, Peng H, Huang Y, Huang J, Gu J. Cancer-derived immunoglobulin G promotes tumor cell growth and proliferation through inducing production of reactive oxygen species. Cell Death Dis (2013) 4:e945. doi: 10.1038/cddis.2013.474
82. Mitra SK, Schlaepfer DD. Integrin-regulated FAK–Src signaling in normal and cancer cells. Curr Opin Cell Biol (2006) 18:516–23. doi: 10.1016/j.ceb.2006.08.011
83. Chu J, Li Y, Deng Z, Zhang Z, Xie Q, Zhang H, et al. IGHG1 regulates prostate cancer growth via the MEK/ERK/c-Myc pathway. BioMed Res Int (2019) 2019:1–10. doi: 10.1155/2019/7201562
84. Yang SB, Chen X, Wu BY, Wang MW, Cai CH, Cho DB, et al. Immunoglobulin kappa and immunoglobulin lambda are required for expression of the anti-apoptotic molecule Bcl-xL in human colorectal cancer tissue. Scand J Gastroenterol (2009) 44:1443–51. doi: 10.3109/00365520903369953
85. Lu LL, Suscovich TJ, Fortune SM, Alter G. Beyond binding: antibody effector functions in infectious diseases. Nat Rev Immunol (2017) 18:46–61. doi: 10.1038/nri.2017.106
86. Wang TT, Ravetch JV. Functional diversification of IgGs through Fc glycosylation. J Clin Invest (2019) 129:3492–8. doi: 10.1172/JCI130029
87. Galeotti C, Kaveri SV, Bayry J. IVIG-mediated effector functions in autoimmune and inflammatory diseases. Int Immunol (2017) 29:491–8. doi: 10.1093/intimm/dxx039
88. Duan S, Paulson JC. Siglecs as Immune Cell Checkpoints in Disease. Annu Rev Immunol (2020) 38:365–95. doi: 10.1146/annurev-immunol-102419-035900
89. Wang J, Sun J, Liu LN, Flies DB, Nie X, Toki M, et al. Siglec-15 as an immune suppressor and potential target for normalization cancer immunotherapy. Nat Med (2019) 25:656–66. doi: 10.1038/s41591-019-0374-x
90. Adams OJ, Stanczak MA, von Gunten S, Laubli H. Targeting sialic acid-Siglec interactions to reverse immune suppression in cancer. Glycobiology (2018) 28:640–7. doi: 10.1093/glycob/cwx108
91. Ma J, Jiang D, Gong X, Shao W, Zhu Z, Xu W, et al. Free immunoglobulin light chain (FLC) promotes murine colitis and colitis-associated colon carcinogenesis by activating the inflammasome. Sci Rep (2017) 7:5165. doi: 10.1038/s41598-017-05468-w
92. Miao S, Shu D, Zhu Y, Lu M, Zhang Q, Pei Y, et al. Cancer cell-derived immunoglobulin G activates platelets by binding to platelet FcγRIIa. Cell Death Dis (2019) 10:87. doi: 10.1038/s41419-019-1367-x
93. Cui M, Liao Q, Li J, Habib JR, Kinny-Köster B, Dong Y, et al. Expression of cancer-derived immunoglobulin G during malignant progression in intraductal papillary mucinous neoplasms. Pancreas (2020) 49:e51–4. doi: 10.1097/MPA.0000000000001572
94. Peng J, Wang HC, Liu Y, Jiang JH, Lv WQ, Yang Y, et al. Involvement of non-B cell-derived immunoglobulin G in the metastasis and prognosis of salivary adenoid cystic carcinoma. Oncol Lett (2017) 14:4491–8. doi: 10.3892/ol.2017.6782
95. Lee G, Ge B, Huang TK, Zheng G, Duan J, Wang IHY. Positive identification of CA215 pan cancer biomarker from serum specimens of cancer patients. Cancer Biomark (2010) 6:111–7. doi: 10.3233/CBM-2009-0134
96. Vuckovic F, Theodoratou E, Thaci K, Timofeeva M, Vojta A, Stambuk J, et al. IgG glycome in colorectal cancer. Clin Cancer Res (2016) 22:3078–86. doi: 10.1158/1078-0432.CCR-15-1867
97. Qin R, Yang Y, Qin W, Han J, Chen H, Zhao J, et al. The value of serum immunoglobulin G glycome in the preoperative discrimination of peritoneal metastasis from advanced gastric cancer. J Cancer (2019) 10:2811–21. doi: 10.7150/jca.31380
98. Mandili G, Follia L, Ferrero G, Katayama H, Hong W, Momin AA, et al. Immune-complexome analysis identifies immunoglobulin-bound biomarkers that predict the response to chemotherapy of pancreatic cancer patients. Cancers (2020) 12:746. doi: 10.3390/cancers12030746
99. Qin R, Yang Y, Chen H, Qin W, Han J, Gu Y, et al. Prediction of neoadjuvant chemotherapeutic efficacy in patients with locally advanced gastric cancer by serum IgG glycomics profiling. Clin Proteom (2020) 17:4. doi: 10.1186/s12014-020-9267-8
100. Zou Y, Hu J, Jie J, Lai J, Li M, Liu Z, et al. Comprehensive analysis of human IgG Fc N-glycopeptides and construction of a screening model for colorectal cancer. J Proteom (2020) 213:103616. doi: 10.1016/j.jprot.2019.103616
101. Fernandes E, Peixoto A, Neves M, Afonso LP, Santos LL, Ferreira JA. Humoral response against sialyl-Le(a) glycosylated protein species in esophageal cancer: Insights for immunoproteomic studies. Electrophoresis (2015) 36:2902–7. doi: 10.1002/elps.201500270
102. Zornig I, Halama N, Lorenzo BJ, Ziegelmeier C, Dickes E, Migdoll A, et al. Prognostic significance of spontaneous antibody responses against tumor-associated antigens in malignant melanoma patients. Int J Cancer (2015) 136:138–51. doi: 10.1002/ijc.28980
103. Tramentozzi E, Ruli E, Angriman I, Bardini R, Campora M, Guzzardo V, et al. Grp94 in complexes with IgG is a soluble diagnostic marker of gastrointestinal tumors and displays immune-stimulating activity on peripheral blood immune cells. Oncotarget (2016) 7:72923–40. doi: 10.18632/oncotarget.12141
104. Yu L, Lin X, Zhang L, Wu Q, Zhang S, Chen D, et al. The Combination of IgA and IgG autoantibodies against Transcriptional Intermediary Factor-1γ contributes to the early diagnosis of Lung Cancer. Int J Med Sci (2020) 17:1561–8. doi: 10.7150/ijms.47463
105. Affara NI, Ruffell B, Medler TR, Gunderson AJ, Johansson M, Bornstein S, et al. B cells regulate macrophage phenotype and response to chemotherapy in squamous carcinomas. Cancer Cell (2014) 25:809–21. doi: 10.1016/j.ccr.2014.04.026
106. Gu Y, Liu Y, Fu L, Zhai L, Zhu J, Han Y, et al. Tumor-educated B cells selectively promote breast cancer lymph node metastasis by HSPA4-targeting IgG. Nat Med (2019) 25:312–22. doi: 10.1038/s41591-018-0309-y
Keywords: immunoglobulin, cancer-derived Ig, IgG, glycosylation, immune escape, metastasis
Citation: Cui M, Huang J, Zhang S, Liu Q, Liao Q and Qiu X (2021) Immunoglobulin Expression in Cancer Cells and Its Critical Roles in Tumorigenesis. Front. Immunol. 12:613530. doi: 10.3389/fimmu.2021.613530
Received: 02 October 2020; Accepted: 08 March 2021;
Published: 24 March 2021.
Edited by:
Milan Raska, Palacký University, Olomouc, CzechiaReviewed by:
Andreia Ferreira Peixoto, Portuguese Oncology Institute, PortugalWei Chen, Stanford University, United States
Copyright © 2021 Cui, Huang, Zhang, Liu, Liao and Qiu. This is an open-access article distributed under the terms of the Creative Commons Attribution License (CC BY). The use, distribution or reproduction in other forums is permitted, provided the original author(s) and the copyright owner(s) are credited and that the original publication in this journal is cited, in accordance with accepted academic practice. No use, distribution or reproduction is permitted which does not comply with these terms.
*Correspondence: Quan Liao, lqpumc@126.com; Xiaoyan Qiu, qiuxy@bjmu.edu.cn