- 1Pest and Pathogen Ecology, National Institute of Agricultural Botany (NIAB), West Malling, United Kingdom
- 2School of Agriculture, Policy and Development, University of Reading, Reading, United Kingdom
Cherry brown rot, primarily caused by Monilinia laxa, poses a significant threat to cherry production in the UK. Traditional control methods are labour-intensive or environmentally damaging, favouring alternative solutions such as using biological control agents (BCAs). This study investigated the survival and efficacy of two BCAs, Bacillus subtilis (B91) and Aureobasidium pullulans (Y126), in reducing M. laxa populations on mummified fruits and cherry blossoms. Field experiments were conducted to assess the survival of B91 and Y126 on mummified fruits over winter and their effect on M. laxa sporulation in spring. Additionally, the efficacy of the two BCAs when applied to cherry blossoms was evaluated, at different times of application relative to M. laxa inoculation. Y126 could persist on blossoms and throughout the growing season. B91 population level declined rapidly on blossoms but recovered once fruits appeared. Neither BCA usefully reduced the concentration of M. laxa. Y126 was marginally more effective at long-term reduction. On mummified fruits, Y126 population size declined rapidly within the first two weeks after inoculation before stabilising. B91 survived slightly longer. Longer interaction times between M. laxa and the BCAs in the late winter resulted in lower spore counts of M. laxa from mummified fruits. While both BCAs reduced M. laxa populations when applied close to M. laxa inoculation, BCA populations were not maintained at effective levels throughout the growing season. These results suggest that multiple applications targeting critical periods (blossom and pre-harvest) are necessary for effective control of M. laxa by either of these BCAs.
1 Introduction
Monilinia spp. are the main causal agent of brown rot, a serious disease in stone fruits worldwide (Rungjindamai et al., 2014a). The main species prevalent in the UK is Monilinia laxa (Mari et al., 2012). It can infect blossoms, twigs, and wounded and intact fruits causing blossom blights, cankers and fruit rots (Xu et al., 2007). Infected fruits that are left in the orchard over winter become mummified (Casals et al., 2015). Most M. laxa infections are by conidia (asexual spores) which are spread by wind, water splash and some vectors (Byrde and Willetts, 1977). The optimum temperature for conidium production for M. laxa is 10°C (Tamm and Fluckiger, 1993). However, it can grow at even lower temperatures as both M. laxa conidial germination and mycelial growth were observed at -4°C (Tian and Bertolini, 1999). This enables the pathogen to build up inoculum over winter on infected fruits that have mummified in orchards. These mummies are the primary source of inoculum causing blossom blight infections the following spring and a consequent increase in post-harvest brown rot (Villarino et al., 2010). The first symptom of the disease is blossom blight seen in spring (Holb, 2008). These early infections and blighted blossoms then lead to post-harvest rots causing crop losses in the field and in storage (Luo et al., 2005). Therefore, control of infection at the blossom stage, thereby reducing blossom wilt, is an important management tool for managing M. laxa on cherry.
Blossom blight is mainly controlled with one to three applications of fungicides during the bloom period (Holb and Kunz, 2013). However, legislative restrictions and an increase in environmental concerns have led to a demand for other control methods. Among non-chemical control methods, the removal of mummified fruits from the orchard is effective (Casals et al., 2015) but very labour-intensive. Biological control agents (BCAs) could be an alternative method to reduce inoculum arising from mummified fruits, reducing the disease pressure in subsequent years (Holb, 2008). There is one commercial biopesticide – BoniProtect (a formulated product of Aureobasidium pullulans) available, specifically targeting Monilinia spp. on stone fruit (Bio, 2008; Weisshaupt et al., 2011); This product has been recommended for pre-harvest use in order to reduce post-harvest brown rot. Another biopesticide - Serenade (formulated Bacillus subtilis QST713) - was also shown to suppress inoculation production on mummified plum fruit with brown rot (Rungjindamai et al., 2014b). Nevertheless, current brown rot management on cherry in the UK still relies on the scheduled application of fungicides.
Preliminary lab or small-scaled controlled field experiments suggested that the efficacy of BoniProtect and Serenade against M. laxa were not as good as two corresponding native strains from the same species: Bacillus subtilis (B91) and Aureobasidium pullulans (Y126) (Rungjindamai, 2013), which were isolated from the indigenous population of cherries within an orchard in the UK (Rungjindamai et al., 2013). Both microbes not only can suppress the conidium formation of M. laxa on mummified fruits but can survive a range of temperatures under lab conditions (Rungjindamai et al., 2013, 2014b). Y126 competes with the pathogen for nutrients and space and B91 produces antagonist compounds (Rungjindamai et al., 2013). We expect that an increased interaction time of a BCA with a pathogen would lead to increased biocontrol efficacy (Ruano-Rosa et al., 2016).
To effectively reduce the primary inoculum these BCAs would need to be able to persist on mummified fruits in the winter. Maintaining an optimum concentration level in the field is vital, as regular applications would become costly and time-consuming for growers. A good understanding of the interaction between the BCA and the brown rot pathogen over time is also needed. The study reported here looked at survival of the two new biocontrol microbes (B. subtilis - B91 and A. pullulans - Y126 in the winter and spring. Preliminary data suggested that Y126 may not survive below 0°C and B91 may not survive below 10°C (Rungjindamai et al., 2013). Therefore, we hypothesise a rapid decline in the viable populations of either organism (particularly for B91), when applied to mummified fruits over winter and to blossoms. This would potentially affect the biocontrol efficacy.
We extended previous research on the potential of the two strains (B91 and Y126) to suppress M. laxa development on cherry in the UK (Rungjindamai et al., 2013, 2014b). Specifically, following their application in the late autumn we studied the survival of B91 and Y126 over winter on mummified fruits. Next, we studied the effects of the number of application times during winter on M. laxa sporulation in the spring on mummified fruits. Then, we assessed the viable population sizes of B91, Y126 and M. laxa during the growing season following application onto blossoms in the spring. Finally, we determined the biocontrol potential of applying B91 and Y126 to cherry blossom before or after inoculation of blossom with M. laxa.
2 Materials and methods
2.1 Inoculum production and inoculation
For all experiments, single colonies of B91 and Y126 were grown in liquid media - B91 in LB broth (ThermoFisher) and Y126 in potato dextrose broth - for 24 hours on a shaking incubator (180 rpm, 25°C). The concentration was measured using a spectrophotometer and adjusted to OD600 0.2 (B91) and 0.01 (Y126), equivalent to 1x107 CFU/ml for inoculation. This concentration was determined from a preliminary experiment where B91 and Y126 were applied at four doses (108, 107, 106, 105 CFU/ml) onto cherries that were inoculated with M. laxa 24 h later. The biocontrol suspensions, along with fungicide or the sterile distilled water control, were applied to mummified fruit or blossoms using handheld sprayers. To produce M. laxa inoculum for inoculum, a M. laxa isolate from the East Malling collection was first inoculated onto wounded ripe plums and then spores were collected by washing the sporulating fruit 2 weeks after. A haemocytometer was used to adjust the M. laxa concentration to 1x105 conidia/ml; M. laxa inoculum was applied similarly to the biocontrol suspensions via a hand-held sprayer.
2.2 Quantification of viable population sizes of biocontrol microbes and M. laxa
2.2.1 Propidium monoazide treatment and DNA extraction
Samples of mummified fruits, blossoms or fruit were washed in Maximum Recovery (Sigma-Aldrich) for one hour on a shaking incubator at 180 rpm. The wash was then spun down and the pellet was resuspended in 500 µl of sterile distilled water. The suspension was then treated with PMAxx™ (Propidium monoazide) at a concentration of 25 μM for B91, 50 μM for Y126 and 60 μm for M. laxa (Tut et al., 2021). The samples were then incubated at room temperature, in the dark, for 15 minutes on a rocker at 35 rpm. This was followed by a light exposure stage of 20 minutes to bind PMAxx™ to DNA of degraded cells. Samples were kept on ice on a rocker at 35 rpm to ensure full light exposure and reduce heating from the light source. DNA was then extracted from the samples using TRI Reagent® (Sigma-Aldrich) following the manufacturer’s instructions with an additional step of ethanol (70%) precipitation to remove any residual salts.
2.2.2 Quantitative real-time PCR
To quantify B91, Y126 and M. laxa genomic DNA a qPCR (Bio-Rad CFX96) with SYBR green (Bioline) were used. Primers were selected from published literature: M. laxa, Mlx368-F CCAAGGGCTCCGTAGGTAA and Mlx368-R TCCACACCGTCGAACAATAA [annealing temperature, 55°C] (Guinet et al., 2016); B91, Bs_dnaK1154F, ACACGACGATCCCAACAAGC and Bs_dnaK1254R, AGACATTGGGCGCTCACCT [60°C] (Tut et al., 2021); and Y126, ELO2-F CACTCTTGACCGTCCCTTCGG, and ELO2-R GCGGTGATGTACTTCTTCCACCAG [62°C] (Zalar et al., 2008). All reactions were performed in triplicate with the final volume of 20 µl containing 10 µl of extracted DNA, 8 µl of SensiFAST™ SYBR® no-rox kit and 1 µl of each forward and reverse primer. The cycle conditions for B91, Y126 and M. laxa were 94°C for 3 min followed by 40 cycles of 15 s at 94°C, 30 s at annealing temperature and 30 s at 72°C. Annealing temperatures for each microbe were B91: 60°C, Y126: 62°C, M. laxa: 55°C. Within each plate there were standards made from a ten-fold serial dilution of genomic DNA from an initial concentration of 108 CFU, and a negative control containing 10 µl of DNAse-free water instead of DNA sample. The standards were used to generate a standard curve. The CFU were calculated using the standard curve and adjusted to CFU per blossom or per fruit.
2.3 Single application of biocontrol microbes or fungicide onto mummified fruit in late autumn
In 2017, mummified damsons from Damson plums of an unknown cultivar from the University of Reading were used, whereas in 2018 mummies of the cherry variety “Kordia” were collected from a non-commercial orchard at East Malling, Kent, England. The fruits were kept in a cold store at 4°C until needed. Single colonies of B91 and Y126 were grown in liquid media for 24 hours on a shaking incubator (180 rpm, 25°C). A commercial fungicide, Luna® Sensation (250 g/L fluopyram + 250 g/L trifloxystrobin), was prepared according to the manufacturer’s instructions. Mummified fruits were sprayed until runoff with a handheld sprayer, within polythene bags to avoid cross-contamination between treatments. For biocontrol experiments, each mummified fruit was estimated to have received ca. 4 x 107 CFU for the biocontrol microbe. In addition, a control treatment was included where mummified fruits were sprayed with distilled water.
Treated fruits were placed in green mesh (ca. 0.5 cm) bags (10 fruits per bag) and placed in an orchard at East Malling. For each treatment, treated fruits were sampled at six time points (Table 1) to quantify viable population sizes of B91, Y126 and M. laxa. Four cherry trees in a non-commercial orchard were used, each tree as an experimental block; mesh bags of different treatments were hung on branches at similar heights. On each tree, there were 24 mesh bags (six bags for each treatment, one for each sampling time point). Immediately after removal from the orchard, all fruit from a single bag was washed before PMA treatment and DNA extraction as described above.
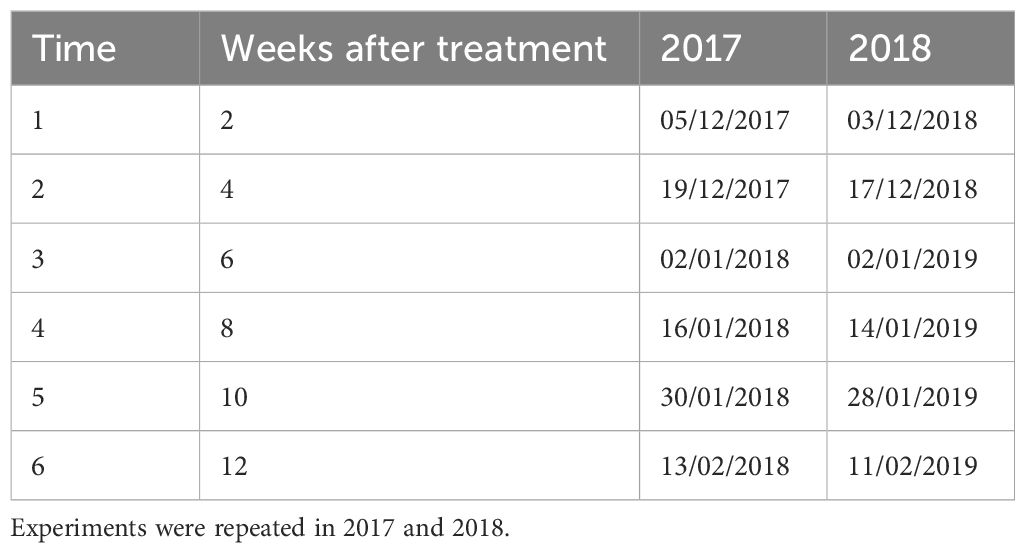
Table 1. The sampling times after mummified fruits were treated with biocontrol microbes or a fungicide to assess the treatment effects on M. laxa.
2.4 BCA application to mummified fruit at different times in spring
For this study, plums of variety “Victoria” were purchased in the early winter of 2021, surface sterilised. Each fruit was then inoculated with an M. laxa mycelial plug placed into a freshly created wound, and then placed on plastic trays in a glasshouse compartment to mummify for 6 weeks.
As for the single application in autumn, there were four product treatments: B91, Y126, fungicide and control (distilled water). Each product was applied on the following three dates: 17/01/2022, 24/01/2022 and 01/02/2022; any given fruit only received one application. Thus, there were in total 12 treatments: four products each applied on three different dates. All experimental fruit, irrespective of application timing, were placed into mesh bags and hung on branches of cherry trees in a netted orchard on 14/01/2022, three days before the first application. All fruit were taken back to the laboratory on 07/02/2022 and incubated under 100% moisture conditions for one week before estimation of M. laxa conidia density with a haemocytometer. Treated mummified fruits were washed in 200 ml of Maximum Recovery (Sigma-Aldrich) for one hour on a shaking incubator at 180 rpm. The wash was then filtered through a sterile muslin. A serial dilution was then performed before a haemocytometer was used to estimate the number of M. laxa spores in each aliquot.
2.5 Survival of biocontrol microbes on blossoms and their effects on M. laxa
In spring 2019, an orchard trial was conducted to assess the survival of B91 and Y126 on blossoms of cherry variety “Kordia” from late spring through to the summer/fruit harvest. The experiment included five treatments: B91, Y126, a fungicide (Luna Sensation), Serenade (a commercially formulated B. subtilis QTS713 strain), and a sterile distilled water control. These products were applied to all blossoms/fruits in selected and marked branches of cherry trees in an orchard at East Malling just a few days after full bloom. Following application, blossoms/fruit treated with B91, Y126 and distilled water were taken at six time points (1 [blossom], 8 [blossom], 34 [green fruit], 45 [green fruit], 79 [ripening fruit] and 92 [ripe fruit] days after application) to assess the viable population size of B91, Y126 and M. laxa. For comparison, fruits or blossoms of Serenade and Luna Sensation treatments were only sampled at the first and last time points since our focus was on the dynamics of M. laxa in relation to B91 and Y126.
A randomised block design was used for the experiment. There were three blocks; within each block, there were four trees - a single tree was randomly assigned to the B91, Y126, or water control treatment, and the remaining tree was used for both Luna Sensation and Serenade. Thus, there were a total of 12 trees. On each tree assigned to the B91, Y126, or water control treatment, one of six branches was randomly assigned to one of the six sampling time points. On each tree allocated to Luna Sensation and Serenade, two branches were first randomly allocated to one product and then a single branch was assigned to either the first or last sampling time point.
At each sampling time point, all treated fruits/blossoms on each branch allocated to the time point were taken back to the lab. For the ripening and ripe fruit samples, 10 similar-sized fruits were selected from each branch; for all the other four sampling points, 20 similar-sized flowers/green fruits were taken from each branch. These selected flowers/fruits were immediately washed in Maximum Recovery (Sigma-Aldrich) for one hour on a shaking incubator at 180 rpm. The washes were collected, spun down and the pellet resuspended in 500 µl of sterile distilled water. Washes were treated with PMA before subsequent DNA extraction and qPCR analysis. Estimated viable CFU were expressed as number per fruit/blossom.
2.6 Applying biocontrol microbes on blossoms before or after M. laxa inoculation
In 2020, an inoculation experiment was conducted on potted trees of two cherry cultivars (“Kordia” and “Sweetheart”) in a polythene tunnel at East Malling to study BCA application timing relative to inoculation with M. laxa. The three products (B91, Y126 and distilled water as control) were applied on the same day but blossoms were inoculated with M. laxa at two different time points: 4 days before and 1 day after product application. To assess the background level of M. laxa, fruits or blossoms were not inoculated with M. laxa on one randomly selected branch on each tree, namely the control treatment.
A random block design with four blocks was used for this experiment. Within each block, there were three potted trees for each of the two cultivars; a single tree of a given cultivar was randomly allocated to one of the three product treatments. On each tree, three branches were selected, two of which randomly assign to the two M. laxa inoculation times (one for each inoculation time); the remaining branch was used as the control to assess the background level of M. laxa. Branches were prepared by removing old and new (un-opened) blossoms, so all inoculated and treated blooms were open and of similar age. M. laxa spore suspension was adjusted to 1x104 spores ml-1 with a haemocytometer for inoculation. Both pathogen inoculation and microbial applications were via a hand-held sprayer sprayed until runoff. On each tree, all experimental blossoms were sampled 5 days after the application of treatment products, and PMA-qPCR used to estimate viable population sizes of B91, Y126 and M. laxa as for the field experiment.
2.7 Data analysis
In all analyses, all CFU data were log10 transformed before statistical analysis. In many cases where PMA-qCPR failed to generate an estimate, we used the detection/quantification limit instead (which was equal to 3.63 on the log10 scale).
2.7.1 Single autumn application to suppress sporulation
The two season data from the single application onto mummified fruit in autumn were combined together and subjected to a linear mixed model analysis in which there were two random effect factors (replication [combining the year and blocks within a year] and individual treatments within a block [a subject for repeated assessment]). The assessment time and treatments were treated as fixed effect factors. Three separate analyses were made: (1) comparing the viable B91 population size in the B91 treatment with the Control; (2) comparing the viable Y126 population size in the Y126 treatment with the Control; and (3) assessing the effects of all four treatments on the viable M. laxa population size.
2.7.2 Single spring application to suppress sporulation
ANOVA was applied to the data; in addition to the block factor, there were two study factors in ANOVA: product treatments and application timing.
2.7.3 Microbial dynamics on flowers/fruits
A similar linear mixed model analysis was applied to the temporal data on the blossom or fruit data except that both Senerade and fungicide treatments were excluded since they were sampled at two time points instead of six. As for the inoculum suppression experiments, the same three specific comparisons were made.
2.7.4 Inoculation of flowers with M. laxa and biocontrol microbes
ANOVA was applied to the data with two study factors in addition to the block factor: product treatments and application timing (before or after M. laxa inoculation). Since the randomisation unit for products was the ‘tree’ whereas application timing was the ‘branch’, a split-plot ANOVA was used.
3 Results
Open field weather conditions (temperature) during the experimental period are shown in Figure 1 for all years. There were many days with minimum temperature < 0°C during the winter period in all three years (Figures 1A, B, D). During the fruiting period in 2019, weather conditions were cold during April and May with daily average temperature hovering around 10°C (Figure 1C).
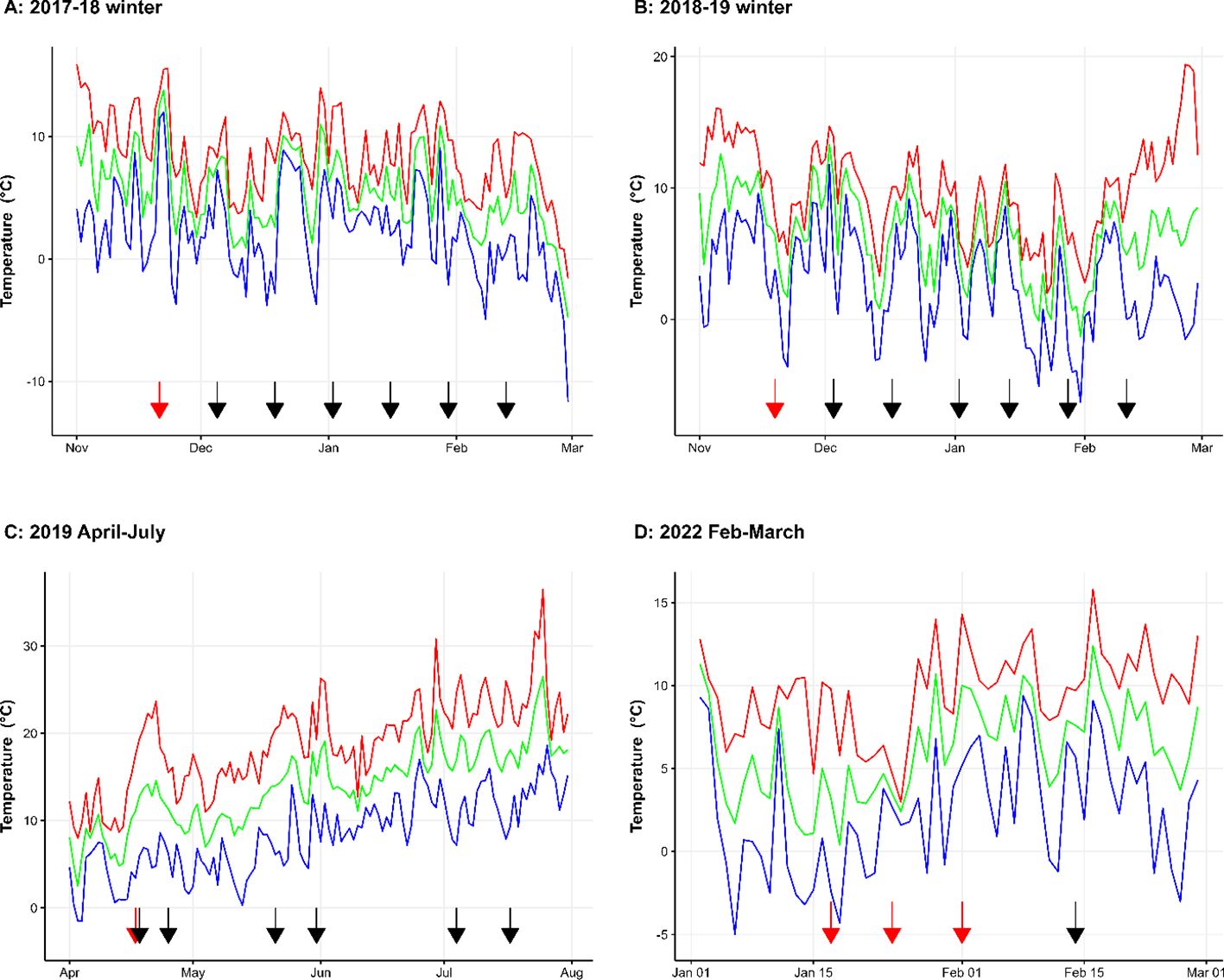
Figure 1. Maximum (red line), average (green line) and minimum (blue line) temperature during the experimental periods in the four years when biocontrol suppression of M. laxa production in mummified cherry fruit (2017-18 (A), 2018-19 (B) and 2022 (D)) and biocontrol application on cherry blossoms (2019 (C)) were studied in a cherry orchard at NIAB East Malling, England. Red arrows indicate the dates of application of biocontrol microbes; the black arrows indicate the dates of assessment/sampling.
3.1 Single application of biocontrol microbes or fungicide onto mummified fruit in autumn
Applying B91 resulted in a large and highly significant (P < 0.001) increase in the viable B91 population size at the first sampling. Overall, the application of B91 in the autumn led to an increase in the viable population size of B91 over the winter when compared to the control: 5.95 (± 0.174) vs. 4.69 (± 0.136). Although the overall B91 population size did not change much over time (from 4.97 ± 0.191 to 5.52 ± 0.237), there were significant interactions between treatments and time (P < 0.001, Figure 2A). Specifically, there were no differences between the two treatments on the 3rd and last sampling points, but applying B91 led to a much higher B91 population size for the other four time points.
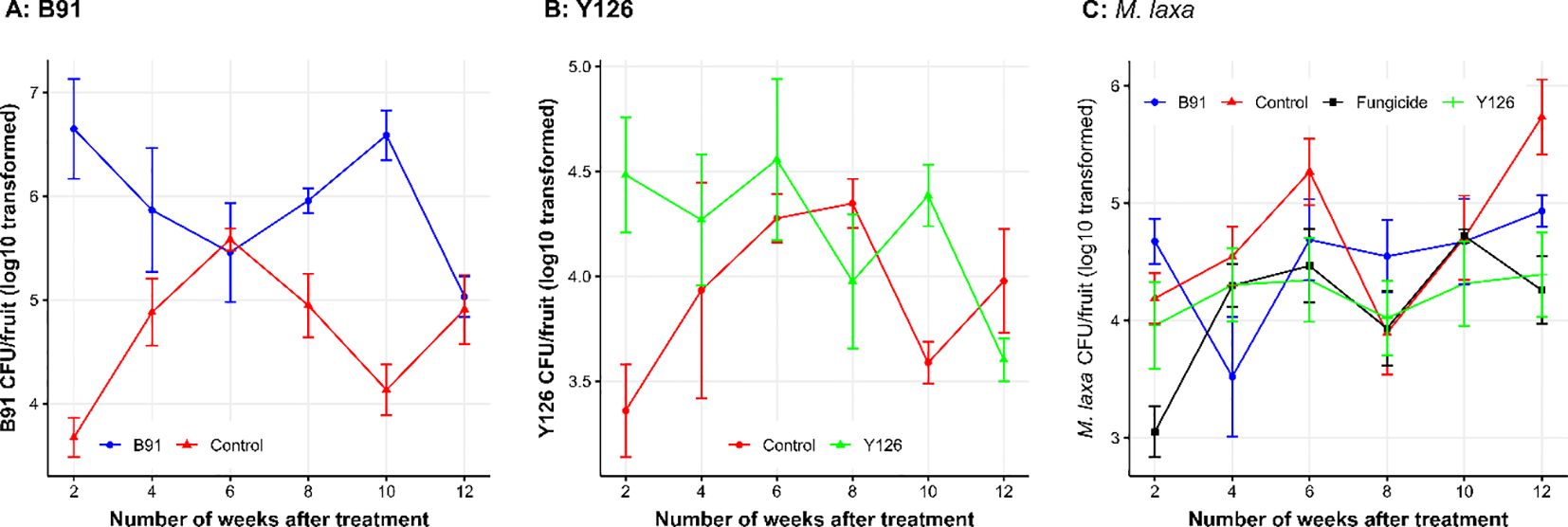
Figure 2. The log10 transformed viable population size of B91 (A), Y126 (B) and M. laxa (C) over time; biocontrol microbes (B91 and Y126), fungicide and distilled water (Control) were hand-sprayed onto mummified fruit in the autumn 2017 and 2018, and fruits sampled during the winter for estimation of viable population sizes of B91, Y126 and M. laxa via the PMA-based qPCR method. The error bars represent one standard error of individual observed mean.
Overall, applying Y126 did not consistently alter the Y126 population size over the following winter (ranging from 3.79 ± 0.138 to 4.42 ± 0.0.198; P = 0.097), although initially it led to a large increase in Y126 population (Figure 2B). Although the mean Y126 population size did not vary much over time, there were significant interactions between treatments and time (P < 0.05, Figure 2B).
The average effect of treatment on the population size of M. laxa was affected by treatments (P < 0.05) but the interpretation of this was complicated by the significant interactions between treatment and assessment time (P < 0.01, Figure 2C) – relative performance of treatments varied greatly with time. The average number of M. laxa CFU was, in decreasing order: control 4.72 (± 0.148); B91 4.51 (± 0.144); Y126 4.22 (± 0.136) and fungicide 4.14 (± 0.121). For individual samples there was no significant correlation between M. laxa CFU and either of the B91 and Y126 CFU counts.
3.2 Single application of biocontrol microbes or fungicide onto mummified fruit at different times in spring
Increasing the interval between applying products and assessment led to reduced (P < 0.001) M. laxa sporulation on mummified fruit. The average M. laxa spore counts were 4.16 (± 0.061), 5.27 (± 0.042) and 6.51 (± 0.069) for fruits receiving treatments on 17/01/2022, 24/01/2022 and 01/02/2024, respectively. Products also differed (P < 0.001) in their effect on M. laxa sporulation. The average spore counts of M. laxa were 5.23 (± 0.026), 5.55 (± 0.030), 5.38 (± 0.032) and 5.10 (± 0.028) for the B91, Control, Fungicide and Y126 treatment, respectively. There was also a significant interaction between treatments and the application timing (P < 0.001) (Figure 3).
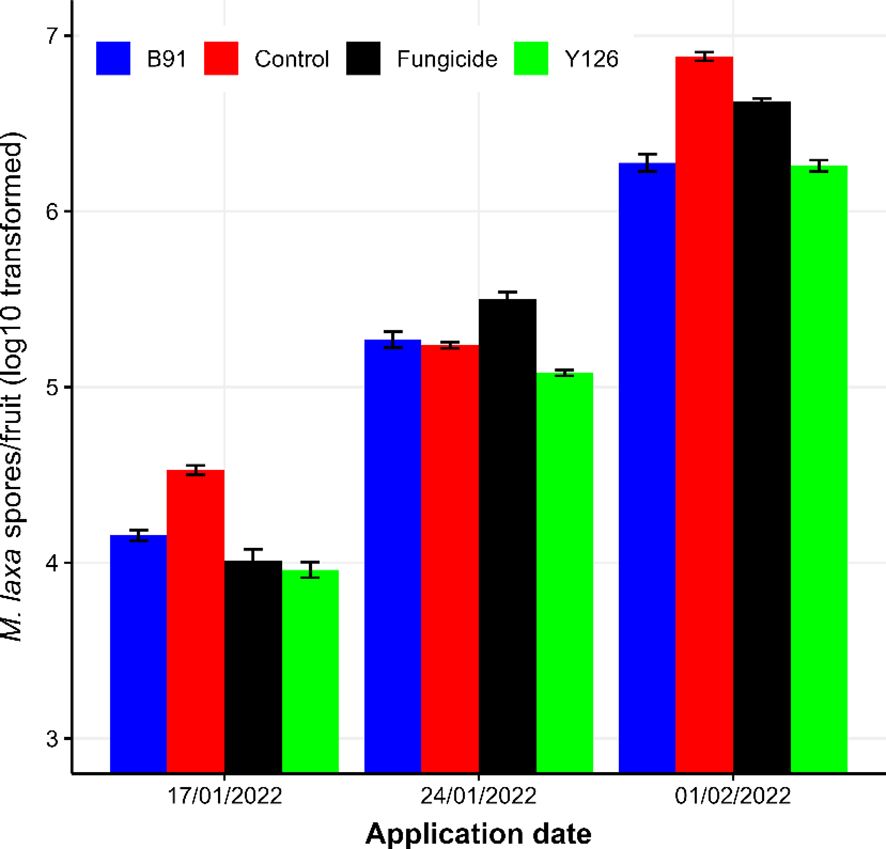
Figure 3. The log10 transformed spore counts of M. laxa per fruit for the four products when they were applied on three different dates during the late winter period. For the control treatment, distilled water was used. Treated mummified fruits were taken back to the lab on 07/02/2022 and incubated under moist conditions for one week before assessment. The error bars represent one standard error of individual observed mean.
3.3 Survival of biocontrol microbes on blossoms and their effects on M. laxa
B91 was detected in several samples at the first two sampling points. The viable population size was similar to the water control at all time points. Applying B91 to cherry blossoms in an orchard did not result in appreciable differences in the viable population size of B91 (Figure 4A) over time. The viable B91 population size (P < 0.001) rose asymptotically between the six time points, primarily because of the higher level from the green fruit onwards (Figure 4A). For the Serenade treatment, B. subtilis was not detected on the first sampling date and was 9.59 (± 0.008) on the last date, compared to 9.59 (± 0.440) and 7.48 (± 1.930) for the B91 and control treatment, respectively.
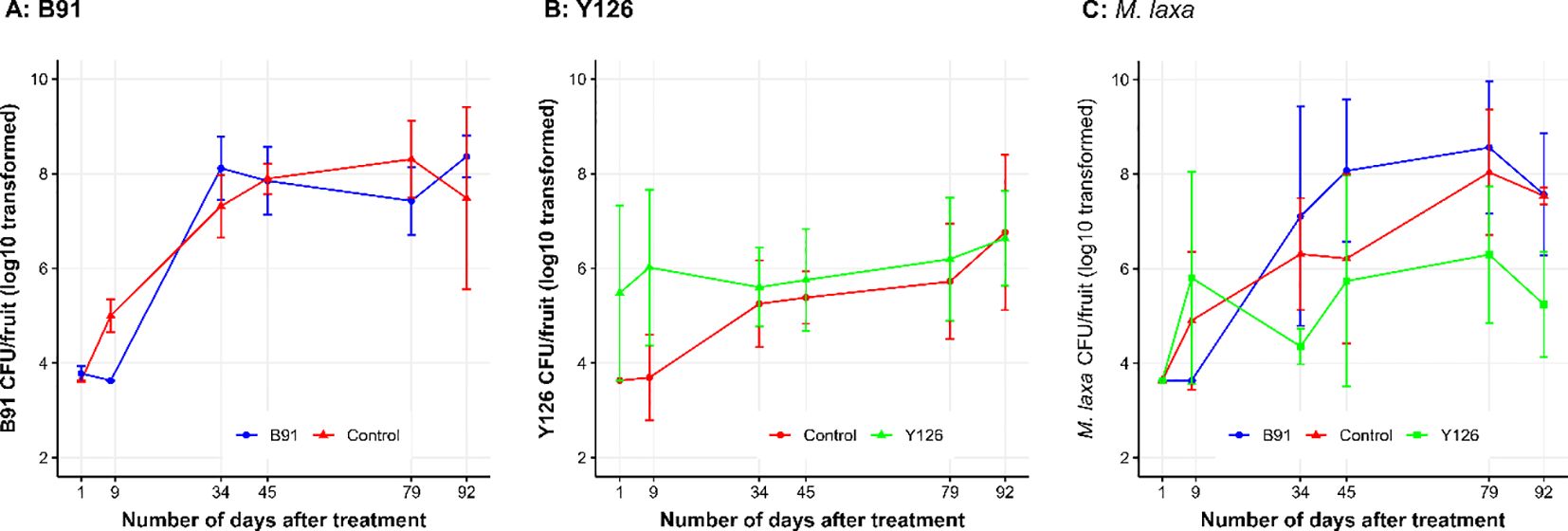
Figure 4. The log10 transformed viable population size of B91 (A), Y126 (B) and M. laxa (C) over time; biocontrol microbes (B91 and Y126), fungicide and distilled water (Control) were hand-sprayed onto blossoms in the spring 2019, and blossoms/fruits were sampled on six occasions for estimation of viable population sizes of B91, Y126 and M. laxa via the PMA-based qPCR method. On the last sampling day (92 days after application), fruits were ripe and ready for harvest. The error bars represent one standard error of individual observed mean.
Y126 was not detected in several samples at the first and second time points, particularly for the control treatment, and hence set to the detection limit. Applying Y126 to cherry blossoms appeared to increase the viable population size of Y126 initially (Figure 4B) when compared to the control treatment. Unlike B91, the differences in the viable Y126 population size between the six time points were not significant (P = 0.089). There were no significant interactions between treatment and assessment time.
M. laxa was not detected in any sample at the first time point, and in few samples at the second time point. Although Y126 appeared to reduce M. laxa CFU (Figure 4C), differences between treatments were not statistically significant because of the large variation between replicate samples. The average M. laxa CFU was 6.43 (± 0.681), 6.11 (± 0.547) and 5.18 (± 0.559 for the B91, Control, and Y126 treatment, respectively. Overall, M. laxa CFU increased with time (P < 0.01, Figure 4C). On the final sampling date, the average M. laxa CFU was 7.57 (± 1.290), 7.54 (± 0.181), 9.88 (± 1.370), 8.38 (± 2.100) and 5.24 (± 1.111) for the B91, Control, Fungicide, Serenade and Y126 treatment, respectively.
3.4 Applying biocontrol microbes on blossoms before or after M. laxa inoculation
M. laxa was only detected in six inoculated samples, all from the control treatment (namely not treated with biocontrol microbes; later inoculation with M. laxa led to higher M. laxa CFU values than earlier inoculation although this difference was statistically not significant (P = 0.21). As M. laxa was not detected in treated samples, no statistical analysis could be carried out. In all 16 samples taken from fruits treated with B91, B91 was above the detection level, ranging from 1.45x105 CFU/fruit to 7.88x107 CFU/fruit. Y126 was above the detection level in 14 of the 16 samples treated with Y126.
4 Discussion
We extended previous research on the potential of two microbial strains (B91 and Y126) to suppress M. laxa development on cherry in the UK (Rungjindamai et al., 2013, 2014b). The two strains both showed some potential in suppressing sporulation of overwintered mummified fruit in the spring. Furthermore, the long interaction time between biocontrol microbes and M. laxa on mummified fruits also increased biocontrol efficacy in suppressing sporulation. When the two strains were applied soon before or after M. laxa inoculation they can effectively prevent the fungus from infecting blossoms. However, when applied to blossoms, neither microbe was able to maintain a sufficiently high density until the fruiting period to prevent fruit infection.
Applying B91 and Y126 to mummified fruits in the winter led to increased viable population sizes of both B91 and Y126 on mummified fruit for the first 4 weeks after application. Thereafter, the viable population sizes of both microbes were similar to the background levels. This suggests multiple applications of these microbial products throughout the winter would be necessary to reduce the overwintering M. laxa inoculum to harmless levels. Although yeasts are generally more tolerant of changing environmental conditions, such as humidity, than bacteria (Ippolito and Nigro, 2000), this was not observed in the present study. Although B91 suffered more from temperatures below 10°C than Y126 (Rungjindamai, 2013), B91 in the present study was able to survive at least at a level similar to Y126. There was a large year-to-year variability on the sporulation suppression achieved by the two biocontrol microbes. One possible reason is the difference in temperature between the two seasons, particularly in the period between late December and early January (ca. six weeks after product application). A complication factor is whether the extent of temperature fluctuation over time also affects the survival of the two biocontrol strains. Another possible explanation for the observed year-to-year variability is that plum fruits were used in the 2017-18 winter, but cherry fruits were used in the 2018-19 winter. Further studies are necessary to understand whether the extent of temperature fluctuations and pathogen substrate affect biocontrol suppression of M. laxa sporulation on mummified fruit.
Our initial overwintering study suggested that applying B91 and Y126 in the late autumn only led to increased levels of B91 and Y126 on mummified fruit for up to 4 weeks. We then examined whether the length of time for which the biocontrol agents and M. laxa interacted within the four-week window affected suppression of M. laxa sporulation. The results confirmed our expectation that the longer the BCA has interacted with the pathogen on the fruit surface the more effective it is. This increased efficacy on mummified fruit may be explained by the fact that neither fruit nor the pathogen is undergoing any noticeable growth. Recent research suggests that reduced biocontrol microbial density due to host expansion is often a key factor responsible for lack of biocontrol effects during active crop growing periods (Tut et al., 2023). This may also largely explain biocontrol successes under post-harvest storage conditions (Wisniewski et al., 2007; Zhou et al., 2008). Interestingly, applying distilled water also led to significant reductions in M. laxa sporulation which increased with increasing interaction time. We suggest two hypotheses which might explain this. Firstly, applying distilled water to fruit surfaces may lead to increased activity of other microbes that are better adapted to cold conditions. Secondly, applied water may lead to sporulation of M. laxa in the absence of any susceptible host tissues, thus depleting resources for M. laxa growth. Further research is needed to confirm the result and find its biological explanation.
When B91 was applied to blossoms its population rapidly dropped within the first day of application on blossoms, similar to the background level (which was close to the detection limit). This rapid decline on blossom is unlikely to be due to mortality since B91 can survive on mummified fruit for 2-4 weeks during the winter. We suggest two possible reasons for the decline. Firstly, some petals may have dropped on the sampling day; since petals accounted for a large proportion of flower surface area, this may have led to a substantial loss in microbial population. Secondly, B91 may not adhere well onto the surface of flower tissues. The second hypothesis is unlikely since the application of commercially formulated B. subtilis (Serenade) led to a similar level of B. subtilis on flowers as B91. In contrast, Y126 was able to sustain its population size for the first two weeks after application to blossoms.
The B91 variable population size increased several-fold between two and five weeks after blossom on both B91 treated and untreated fruits. This increase most probably resulted from the increase in the fruit surface area and/or B. subtilis density in the air. Similar to B91, from green fruit onwards there were no discernible differences in the viable Y126 population size between the Y126 treatment and background level, suggesting that naturally occurring Aureobasidium dominates the introduced inoculum during fruiting stage.
The viable M. laxa population size did not reach the detection limit on blossoms but then increased gradually but with large variabilities among replicate samples. However, no treatment (including fungicide) when applied at blossom significantly affected the viable M. laxa population size on the ripe fruit, suggesting products applied at blossoms failed to alter the pathogen on ripe fruit, the most susceptible stage to M. laxa infection (Xu et al., 2007). When both B91 and Y126 were applied to shortly before or after inoculation of cherry blossoms with M. laxa, they were able to reduce M. laxa population size below the detection level. As cherry fruits are most susceptible to infection by M. laxa within 2-3 weeks of fruit harvest (Xu et al., 2007), BCAs need thus to target this specific period of fruit development instead of only the blossom period to reduce fungal infection. Recently we demonstrated that applying B91 and Y126 close to harvest led to up to 60% reduction in post-harvest brown rot (Bellamy et al., 2022).
In summary, this study has highlighted the potential of using biocontrol microbes to suppress sporulation of M. laxa on overwintered mummified microbes provided the spray timing is correct. Although both microbes can effectively reduce M. laxa on blossoms when applied very close to M. laxa inoculation, neither strain can persist on the surface of developing fruits at a sufficiently high density to usefully reduce M. laxa rot. Thus, in addition to suppressing primary inoculum production in the spring, managing blossom wilt and fruit infection due to M. laxa needs to target the most susceptible periods (namely, blossom and 2-3 weeks before fruit harvest) with a couple of applications. Further commercial field studies are needed to demonstrate the effectiveness of this microbial-based approach for brown rot management.
Data availability statement
The raw data supporting the conclusions of this article will be made available by the authors, without undue reservation.
Author contributions
SB: Conceptualization, Data curation, Formal analysis, Investigation, Methodology, Project administration, Validation, Writing – original draft, Writing – review & editing. MS: Conceptualization, Funding acquisition, Methodology, Supervision, Visualization, Writing – review & editing. XX: Conceptualization, Formal analysis, Funding acquisition, Methodology, Project administration, Resources, Supervision, Visualization, Writing – review & editing.
Funding
The author(s) declare financial support was received for the research, authorship, and/or publication of this article. This work was funded as part of a Biotechnology and Biological Science Research Council (BBSRC) Collaborative Training Partnership for Fruit Crop Research (CTP-FCR, project number: BB/T509061/1).
Acknowledgments
The research was conducted as NIAB in East Malling and we thank the pathology team at East Malling who provided help in conducting the experiments.
Conflict of interest
The authors declare that the research was conducted in the absence of any commercial or financial relationships that could be construed as a potential conflict of interest.
The author(s) declared that they were an editorial board member of Frontiers, at the time of submission. This had no impact on the peer review process and the final decision.
Generative AI statement
The author(s) declare that no Generative AI was used in the creation of this manuscript.
Publisher’s note
All claims expressed in this article are solely those of the authors and do not necessarily represent those of their affiliated organizations, or those of the publisher, the editors and the reviewers. Any product that may be evaluated in this article, or claim that may be made by its manufacturer, is not guaranteed or endorsed by the publisher.
References
Bellamy S., Shaw M., Xu X.-M. (2022). Field application of Bacillus subtilis and Aureobasidium pullulans to reduce Monilinia laxa post-harvest rot on cherry. Eur. J. Plant Pathol. 163, 761–766. doi: 10.1007/s10658-022-02508-8
Byrde R. J. W., Willetts H. J. (1977). The brown rot fungi of fruit: their biology and control (Oxford, UK: Pergamon Press).
Casals C., Segarra J., De Cal A., Lamarca N., Usall J. (2015). Overwintering of Monilinia spp. on mummified stone fruit. J. Phytopathol. 163, 160–167. doi: 10.1111/jph.12298
Guinet C., Fourrier-Jeandel C., Cerf-Wendling I., Ioos R. (2016). One-step detection of Monilinia fructicola, M. fructigena, and M. laxa on Prunus and Malus by a multiplex real-time PCR assay. Plant Dis. 100, 2465–2474. doi: 10.1094/PDIS-05-16-0655-RE
Holb I. J. (2008). Brown rot blossom blight of pome and stone fruits: symptom, disease cycle, host resistance, and biological control. Int. J. Hortic. Sci. 14, 15–21. doi: 10.31421/ijhs/14/3/796
Holb I. J., Kunz S. (2013). Integrated control of brown rot blossom blight by combining approved chemical control options with Aureobasidium pullulans in organic cherry production. Crop Prot. 54, 114–120. doi: 10.1016/j.cropro.2013.07.003
Ippolito A., Nigro F. (2000). Impact of preharvest application of biological control agents on postharvest diseases of fresh fruits and vegetables. Crop Prot. 19, 715–723. doi: 10.1016/S0261-2194(00)00095-8
Luo Y., Michailides T. J., Morgan D. P., Krueger W. H., Buchner R. P. (2005). Inoculum dynamics, fruit infection and development of brown rot in prune orchards in California. Phytopathology 95, 1132–1136. doi: 10.1094/phyto-95-1132
Mari M., Martini C., Guidarelli M., Neri F. (2012). Postharvest biocontrol of Monilinia laxa, Monilinia fructicola and Monilinia fructigena on stone fruit by two Aureobasidium pullulans strains. Biol. Control 60, 132–140. doi: 10.1016/j.biocontrol.2011.10.013
Ruano-Rosa D., Prieto P., Rincón A. M., Gómez-Rodríguez M. V., Valderrama R., Barroso J. B., et al. (2016). Fate of Trichoderma harzianum in the olive rhizosphere: time course of the root colonization process and interaction with the fungal pathogen Verticillium dahliae. BioControl 61, 269–282. doi: 10.1007/s10526-015-9706-z
Rungjindamai N. (2013). Biological control of brown rot disease caused by Monilinia laxa in cherries and plums (Canterbury, UK: University of Kent).
Rungjindamai N., Jeffries P., Xu X.-M. (2014a). Epidemiology and management of brown rot on stone fruit caused by Monilinia laxa. Eur. J. Plant Pathol. 140, 1–17. doi: 10.1007/s10658-014-0452-3
Rungjindamai N., Jeffries P., Xu X.-M. (2014b). A novel strategy to reduce overwintering inoculum of Monilinia laxa. Eur. J. Plant Pathol. 140, 591–596. doi: 10.1007/s10658-014-0473-y
Rungjindamai N., Xu X.-M., Jeffries P. (2013). Identification and characterisation of new microbial antagonists for biocontrol of Monilinia laxa, the causal agent of brown rot on stone fruit. Agronomy 3, 685–703. doi: 10.3390/agronomy3040685
Tamm L., Fluckiger W. (1993). Influence of temperature and moisture on growth, spore production, and conidial germination of Monilinia laxa. Phytopathology 83, 1321–1326. doi: 10.1094/Phyto-83-1321
Tian S. P., Bertolini P. (1999). Effect of temperature during conidial formation of Monilinia laxa on conidial size, germination and infection of stored nectarines. J. Phytopathol. 147, 635–641. doi: 10.1046/j.1439-0434.1999.00440.x
Tut G., Magan N., Brain P., Xu X.-M. (2021). Molecular assay development to monitor the kinetics of viable populations of two biocontrol agents, Bacillus subtilis QST 713 and Gliocladium catenulatum J1446, in the phyllosphere of lettuce leaves. Biology 10, 224. doi: 10.3390/BIOLOGY10030224
Tut G., Magan N., Xu X.-M. (2023). Influence of abiotic factors on kinetics of viable populations of biocontrol agents in the phyllosphere of lettuce and strawberry leaves. Plant Pathol. 72, 1069–1080. doi: 10.1111/ppa.13719
Villarino M., Melgarejo P., Usall J., Segarra J., Cal D. A. (2010). Primary inoculum sources of Monilinia spp. in Spanish peach orchards and their relative importance in brown rot. Plant Dis. 94, 1048–1054. doi: 10.1094/PDIS-94-8-1048
Weisshaupt S., Hinze M., Weiss A., Ertl C., Kunz S. (2011) “Application of BoniProtectagainst posthavest diseases” in 9th international IOBC-WPRS workshop on pome fruit diseases. ed. Creemers P.. (Darmstadt, Germany: IOBC/WPRS) 143–149.
Wisniewski M. E., Wilson C. L., Droby S., Chalutz E., El Ghaouth A., Stevens C. (2007). “Post-harvest biocontrol: New concepts and applications,” in Biological control: A global perspective. Eds. Vincent C., Goettel M. S., Lazarovits G. (CAB International, Florida), 262–273.
Xu X.-M., Bertone C., Berrie A. (2007). Effects of wounding, fruit age and wetness duration on the development of cherry brown rot in the UK. Plant Pathol. 56, 114–119. doi: 10.1111/j.1365-3059.2006.01502.x
Zalar P., Gostincar C., De Hoog G. S., Ursic V., Sudhadham M., Gunde-Cimerman N. (2008). Redefinition of Aureobasidium pullulans and its varieties. Stud. Mycology 61, 21–38. doi: 10.3114/sim.2008.61.02
Keywords: Monilinia, biocontrol, brown rot, cherry, blossom, overwintering Monilinia laxa, mummified fruit, survival
Citation: Bellamy S, Shaw M and Xu X (2024) Using microbes to suppress overwintering inoculum of Monilinia laxa on mummified fruits and to protect cherry blossoms and fruit from infection. Front. Hortic. 3:1504505. doi: 10.3389/fhort.2024.1504505
Received: 30 September 2024; Accepted: 03 December 2024;
Published: 18 December 2024.
Edited by:
María Luisa Pérez-Bueno, University of Granada, SpainReviewed by:
Aditi Satpute, Texas A&M University Kingsville, United StatesHannah M. Rivedal, Agricultural Research Service (USDA), United States
Copyright © 2024 Bellamy, Shaw and Xu. This is an open-access article distributed under the terms of the Creative Commons Attribution License (CC BY). The use, distribution or reproduction in other forums is permitted, provided the original author(s) and the copyright owner(s) are credited and that the original publication in this journal is cited, in accordance with accepted academic practice. No use, distribution or reproduction is permitted which does not comply with these terms.
*Correspondence: Xiangming Xu, eGlhbmdtaW5nLnh1QG5pYWIuY29t