- Pest, Pathology, and Ecology Department, NIAB East Malling, Kent, United Kingdom
Codling moth (CM), Cydia pomonella, is a significant pest of apple (Malus domestica) and other orchard crops worldwide, posing challenges due to the decrease in registered insecticides, rising resistance, and a changing climate. The pest exhibits a strong resistance capacity to both synthetic and natural insecticides, while shifting seasonal temperatures disrupt the reliability of phenology-temperature models for predicting targeted control strategies. Alternative control strategies are necessary to future-proof control of this pest. Current control methods primarily rely on chemical insecticide sprays or granulosis virus applications during egg hatching. This review focuses explicitly on semiochemical-based manipulation of CM adults and larvae for control in orchards. Topics covered include the role of semiochemicals in integrated pest management, area-wide control, mating disruption, female attractants, larval kairomones, and incorporation into monitoring and control strategies. The potential of CM repellents in a push–pull strategy is also discussed. Primary sources for identifying relevant literature included GoogleScholar and ResearchGate, with a focus on papers published since 2013 but also include relevant papers from 2003. Nine review papers and 119 papers were reviewed. The review emphasizes that effective control necessitates an area-wide approach targeting all life stages (eggs, larvae, pupae, and adults). Comprehensive monitoring is crucial for identifying CM “hot-spots” and enhancing targeted interventions. Growers must consider landscape context when designing control programs. Lastly, recommendations are provided for future research and CM management strategies. There are opportunities to explore and exploit female kairomone attractants and repellents in control strategies and modify monitoring traps to be more attractive and autonomous.
1 Introduction
In the last decade, tree fruit crops and consumer demands have seen a shift from the use of broad-spectrum insecticides to fewer, more selective products combined with biopesticides, augmented and conservation biocontrol, cultural practices, and novel semiochemical manipulation of insect pest populations to reduce the damage caused by pests and disease. However, the removal of broad-spectrum insecticides, in combination with a warmer and more unpredictable climate, has resulted in higher pest populations and unpredictable outbreaks of familiar native and less familiar non-native (invasive) species (Velásquez et al., 2018; Hulme, 2017).
This review was prompted because in the UK, tree fruit growers have recently lost the approval of several key pesticides, including those for codling moth (CM), Cydia pomonella (L.) (Lepidoptera: Tortricidae) control. The reduced range of products inevitably results in the same products being applied to crops sequentially, although restricted by approved maximum numbers of applications. Hence, other control measures with different modes of action are needed, which can be interspersed with the remaining conventional products to reduce resistance. For example, in the UK, chlorantraniliprole, used to control CM, is under registration changes in both number of uses and maximum field doses; similarly, indoxacarb will not be available for use from 2026. This, coupled with recently reported continuous flight of adult moths through the summer months (pers onal comm unication, UK agronomists), makes targeting hatching larvae more difficult and less reliable for short-term fruit damage control.
Currently available conventional and bio-protectant products need to be integrated with non-chemical controls to achieve consistent CM suppression within and between seasons. One of the future challenges for researchers and the industry is to ensure that legislation is practicable and flexible to ensure new pest control measures are accepted under future approval systems, enabling tree fruit growers to gain satisfactory control with measures that are less environmentally damaging with fewer residues on the fruit.
CM is still the main pest issue in apple orchards (Furmanczyk et al., 2022) and is globally distributed, having been introduced to most regions where apple (Malus) is grown but also damages pear, walnut, quince, and some stone fruits causing economic losses in fruit production (Ciglar, 1998). It is estimated that crop losses can reach 30% –50% without control measures, and in certain years, it can be as high as 80% (Pajač et al., 2012). Commercial fruit production rarely tolerates more than 1% CM damaged fruits and losses are generally less than 0.5%, utilizing various methods of fruit protection (Kovačević 1952; Ciglar, 1998). Levels of 7.5% damaged fruits are not unusual in organic orchards (Mangan et al., 2018).
Recent reviews have been published on CM morphology, biology, and genetics (e.g., Pajač Živković et al., 2011; Chen et al., 2017), integrated pest management (IPM) (e.g., Damos et al., 2015; Husain et al., 2018; Knight et al., 2019d; Shaw et al., 2021; Hatteland et al., 2023), and insecticide resistance (e.g., Pajač Živković et al., 2011; Balaško et al., 2020; Ju et al., 2021). Reviews on CM pheromones include Witzgall et al. (2008) and Witzgall et al. (2010). However, there have been no recent reviews focusing on kairomones and their application for CM control.
The aim of this review was to summarize the current state of semiochemical research and strategies for CM. The review gathered and summarized the latest published and peer-reviewed global studies on CM since 2003, with more emphasis on papers since 2013, through a Google Scholar search of the first three web pages of publications and ResearchGate searches on key terms. Search terms for each section in this review included the terms “pheromone”, “sterile insect”, “semiochemical”, “kairomone”, “attract”, “repel”, “pest ester” “monitoring”, “trap”, “mating disruption”, “monitoring”, and “management”, all preceded with “cydia pomonella” or “codling moth”, and, in some instances, “larva”. No papers were excluded based on their findings, but where relevant, the authors included critiques of methodology or interpretation of results. The authors worked individually on sub-section then peer reviewed each other’s sections.
The review finishes with a summary of the main conclusion of studies from the last 20 years and a list of recommendations for CM semiochemical control and future research directions for apples and pears.
2 Role of semiochemicals in integrated pest management
IPM is a sustainable approach to managing pests usually combining biological, cultural, physical, and chemical tools in a way that minimizes economic, health, and environmental risks. Semiochemicals are chemical substances produced by organisms such as plants and animals that elicit a physiological or behavioral response in individuals of the same or another species. Semiochemicals are classified into pheromones and allelochemicals. Pheromones are intraspecies semiochemicals, whereas allelochemicals are interspecies semiochemicals. For CM control, there are many options to exploit these interactions, for example, mass trapping or mating disruption (MD) using female-produced sex pheromones, or repellents and female attractants using allelochemicals. Strategies vary in efficacy; for example, mass trapping used alone is unreliable for economic thresholds (Mansour, 2010). While pheromone-based control and monitoring options are widely used, there are currently few CM control or monitoring options that utilize allelochemicals.
In the past, CM control has extensively relied upon insecticide applications leading to resistance and a reduction in natural biocontrols in orchards; indeed, this pest has developed resistance to many classes of insecticide, via both metabolic and target-site changes (Malone et al., 2017; Balaško et al., 2020; Ju et al., 2021). For CM, population monitoring using pheromone-baited traps, area-wide management including MD, and practices that harness natural biocontrols play a role in IPM and can be incorporated with other methods such as crop hygiene, granulosis virus, and entomopathogenic nematodes to kill overwintering larvae (Adolphi and Oeser, 2023). The strategy is also compatible with targeted habitat management (Warlop and Kienzle, 2022). Judd and Gardiner (2005) reviewed pheromone-based MD in British Columbia, Canada in the 1990s with the sterile insect technique (SIT) and tree banding in organic orchards. By 1997, no damaged fruit were found in any orchard, and by 1999, no wild moths, nor overwintering larvae were captured in any pheromone-supplemented orchard. However, wild moths and overwintering larvae were detectable in 15%–20% of insecticide-supplemented orchards in 1999. Catches of wild moths and overwintering larvae declined fastest in SIT orchards supplemented with pheromone and banding, further pointing to the need to target multiple life stages.
2.1 Area-wide management
MD is also an important part of area-wide IPM focusing on the preventive management of pest populations throughout the ecosystem. It seeks to treat all habitats of the pest population in space and time so that none produces migrants to re-establish significant infestations in areas of concern (Klassen and Vreysen, 2021), compared to conventional strategies (as used in the UK for CM control), which focus narrowly on defending the crop. Area-wide CM management requires multi-year planning and commitment, coordination among stakeholders, and an organization dedicated exclusively to its implementation, and integrates advanced technologies (Klassen and Vreysen, 2021).
Klassen and Vreysen (2021) defined area-wide IPM as “applied against an entire pest population within a delimited geographic area, with a minimum size large enough or protected by a buffer zone so that natural dispersal of the population occurs only within this area”.
Area-wide management is particularly important for pheromone-based MD strategies (Calkins et al., 2000; Coop et al., 1999), mass trapping and removal of host plants (Kovaleski and Mumford, 2007; Severin and Iosob, 2023), and SIT strategies (Dyck et al., 2005, in Klassen and Vreysen, 2021) where a given proportion of males and females may arrive from long distances. For CM, area-wide management “buffer zones” of 200 m of orchards are treated as this is predicted to be twice the estimated maximal movement distance of most wild mated females (Thistlewood and Judd, 2019). Using a fight mill, and depending on sex, mated status, and age, Schumacher et al. (1997) predicted that some CM individuals can disperse up to 11 km in the field. Ricci et al. (2009) examined characteristics of orchards and their surrounding landscape including orchard density and hedgerow network attributes and orchard buffer widths (50 to 500 m) and concluded that CM management should be organized over a minimum area of ~16 ha (Ricci et al., 2009).
Growers need to consider how their orchards fit into the wider landscape before deciding on control programs that utilize area-wide management approaches. For example, a grower wanting to utilize MD should consider factors such as proximity to other growers’ orchards, control methods used in those neighboring orchards, and whether there is potential for working together with neighbors within the same landscape. In addition, residential and unfarmed areas should be considered due to the risk from garden and wild apple trees providing a reservoir of mating CM, which circumnavigates the MD area affect.
2.2 Mating disruption
MD employs synthetic sex pheromones that interfere with the ability of males in finding female moths. Roelofs et al. (1971) identified the main pheromone components for CM attraction —codlemone (i.e., E8,E10-dodecadienol). Three main hypotheses explain how MD delays or prevents males mating with females (Miller et al., 2010; Miller and Gut, 2015):
1. “Competitive attraction,” where males follow the false plumes (false trail following), at the expense of finding a mate.
2. Exposure to high quantities of sex pheromones overwhelming male olfactory receptors rendering them non-functional, preventing the further detection of sex pheromones, synthetic or natural. The males’ ability to find females is blocked by the pheromone-saturated air, and they are unable to follow any plume (Murray and Alston, 2020).
3. Synthetic CM pheromone can also “ camouflage” wild female pheromone release, diverting male moths away from females and thus preventing mating (McGhee et al., 2014).
MD can be highly effective at controlling relatively low population levels of CM, but influxes of gravid females from neighboring farms or residential areas can result in control failure; hence, chance encounters and mating are known to occur even under MD where CM pressure is high (Murray and Alston, 2020). MD is globally used for control; however, preventing mating with female CM is difficult. A relatively high proportion (>60%) of female CM captured in traps placed in blocks treated with MD were mated (Knight et al., 2022). In older studies, 60%–85% of females were mated within MD orchards compared with 84%–88% in untreated orchards (Knight, 2000; Knight and Light, 2005; Knight, 2008).
Mathematical models suggest that 85% MD efficacy is not enough for export production in NZ (one larva in 1 million apples maximum) and control was highly susceptible to immigration of virgin or mated female moths. MD of 98% is needed for a reduction of CM to an extremely low population density, even in the presence of virgin female immigration up to ∼30 females/ha (Wearing, 2022).
Population monitoring using sex pheromone traps is less effective when MD is being used, resulting in growers being unaware when additional control measures are needed. Despite the sustainable farming benefits that MD offers, the use of CM and Adoxophyes orana (summer fruit tortrix) sex pheromones has declined in the UK over recent years; 53 ha in 2020, compared to 217 ha in 2018 out of a total of 2,736 ha surveyed (Ridley et al., 2021). In Switzerland, MD is employed in 50% of apple orchards and has resulted in two-thirds less synthetic pesticide use (Onstad and Knolhoff, 2023). In Italy, MD was used on 22,000 ha in 2016 because of reduced efficacy of chemical insecticides due to resistance and conflict with the public around spraying, resulting in a reduction of CM and insecticide applications (Ioriatti and Lucchi, 2016). A high level of grower education, public engagement, and collaboration is needed for MD success (Ioriatti and Lucchi, 2016).
Barić and Pajač Živković (2017) demonstrated 67.7% and 73.5% efficacy of MD when combined with the application of two insecticide treatments. The method was not deemed economically viable at ~150 €/ha given the level of first-class fruit losses (Barić and Pajač Živković, 2017). In Turkey, MD decreased the number of sprays required by up to 56.6%. The cost of insecticide sprays, application of pheromone dispensers, labor, machinery, fuel, and other pheromone-based expenses, such as pest monitoring, increased labor costs compared with conventional treatments. The cost of MD was $193.70 higher than the conventional treatment in cv. “ Gala” in 2013 (Kovanci, 2017). This author concluded that the price of pheromone dispensers would need to decrease by 22.2%–70.4%, depending on CM pressure (Kovanci, 2017). In 14 Hawke’s Bay, NZ apple orchards, seasonal pheromone trap catch was reduced from 40.1 to 11.7 CM/trap over the subsequent five seasons. Insecticide use was reduced from 5.9 applications/season in 2006–2007 to 2.3 in 2007–2008 and 3.7 since 2008–2009 where larvae in fruit are now only 0.01% in MD orchards (Walker et al., 2013). In 1 year of the study, CM increased, resulting in a greater reliance on granulosis virus (Walker et al., 2013).
When employing MD, much depends on the starting population, integration with other controls, dose of pheromone, and shape, size, isolation, and local environment of orchards (Balaško et al., 2020). Up to 100 g of pheromone/ha effectively controlled CM populations over an entire growing season (Witzgall et al., 2008), even though females are not affected (Yan et al., 1999). In a single study, comparing female CM that were exposed to MD, or not, there was a significant difference in sex pheromone composition for two of the minor components. The intraspecific variation was postulated to show a potential for a shift in female sexual signal when selection pressure is high (Duménil et al., 2014).
For many lepidopteran species (including CM), the emitters of sex pheromone also detect sex pheromone and change their behavior in response (Holdcraft et al., 2016), such as increasing calling frequency and intensity (Weissling and Knight, 1996). Pre-exposure of females to conspecific pheromone can alter their antennal sensitivity to the pheromone and, after transferring to an odor-free, clean area with naïve males, reduce copulation success; however, this effect was not seen in CM (Kuhns et al., 2012). The effects and implications of autodetection require further investigations, particularly due to the potential for changing the effectiveness of pheromone-based control strategies.
Usually, the synthetic female sex pheromone is deployed either as a device impregnated with the pheromone (passive) (Reinke et al., 2012), as a sprayable formulation (Kovanci, 2015), or via a timed-release aerosol (“puffer”) (see Shaw et al., 2021).
Passive dispensers are normally manually deployed at high densities, typically 200–3000 units/ha (Benelli et al., 2019). Pear ester (PE) can be combined with the pheromone for improved MD (Kovanci, 2015).
Recent studies in Albania, where there are two complete CM generations per year, have tested ISOMATE CTT duplicate plastic threads (E,E)-8, 10-dodecadien-1-ol (codlemone), on four blocks of approximately 2.66 ha at 500 dispensaries/ha. A single dispenser covered ~20 m2. A 200-m perimeter around the blocks was also treated and fruit damage was 0.3%–0.5%, with the treatment cost estimated to be 30% higher than using conventional insecticides (Isufi 2023).
Kutinkova et al. (2022) compared microencapsulated liquid sprayable pheromones (CIDETRAK® CM MEC) with dispensers (80/ha) (CIDETRAK® CMDA COMBO™ MESO™). CM flight was monitored using traps baited with standard PHEROCON® CM L2 lures and PHEROCON® CMDA COMBO™ - P + AA lures (Trécé Inc.) designed for use with MD strategies. Fruit damage was below the economical threshold (0.1% to 0.2%) in both years of the study using MD + insecticides compared to insecticides only (Kutinkova et al., 2022). This publication is a little unclear, but they likely applied CIDETRAK® CMDA COMBO™ MESO™ dispensers at 80/ha and CIDETRAK® CM MEC, microencapsulated CM pheromone, in the tank mix with insecticides, further reducing CM damage to apples (Kutinkova et al., 2022).
Aerosol dispensers [e.g., Checkmate Puffer (Suterra) and Isomate CM Mist (Pacific Biocontrol)] are typically deployed 2–5/ha, with plumes reaching up to 300 m downwind, and pheromone was released at specific times to coincide with the female pheromone release, which, for CM, occurs at dusk (Casado et al., 2014; McGhee et al., 2014; Benelli et al., 2019). Aerosol dispensers formulated with 50% less codlemone (3.5 mg/emission) provided orientation disruption equal to the standard commercial formulation (7 mg/emission). Decreased periods of dispenser operation (3 and 6 h) and frequency of pheromone emission (30 and 60 min) provided a level of orientational disruption equivalent to releasing pheromone over a 12-h period on a 15-min cycle, respectively. Combined with increasing dispenser densities to 5–7 units/ha (McGhee et al., 2014), this reduced the amount of pheromone needed and achieved higher levels of disruption (McGhee et al., 2016).
MD is most effective when used on an area-wide basis (McGhee et al., 2011), e.g., the 5-year area-wide management program “Codling moth Areawide Management Program” (CAMP) in the USA (Brunner et al., 2002; Knight, 2008; Balaško et al., 2020) and before males appear in the orchards. It is also compatible with other biological and insecticide programs (Brunner et al., 2002). MD can also be combined with SIT for further CM population suppression (Horner et al., 2020). An especially useful and grower-friendly factsheet produced by the Extension Service of the Utah State University recommends that >4 contiguous ha need to be treated for MD to be effective (Murray and Alston, 2020).
MD needs to be complemented with insect growth regulators (IGRs), mineral oils, granulosis virus, generalist predators (e.g., earwigs), orchard sanitation, and entomopathogenic nematodes for fully integrated control. Male and female moths mate more or less immediately after emergence; thus, the chance of a sterile male successfully finding and mating is decreased, and fruit damage can continue where there are “hot-spots”, even when a population is at a very low level (Proverbs et al., 1967). In orchards with high CM population pressure, supplementing MD with insecticides is required, but with long-term CM, populations are reduced (Murray and Alston, 2020). It is essential that monitoring continues in MD orchards, especially females, by using a lure such as the CM DA-Combo lure. However, action thresholds for CM insecticide sprays change in MD strategies, and this should be considered in orchards.
Causes of CM MD failure are listed by Murray and Alston (2020) and include (1) the use of MD in a small area (less than 4 ha) or use in an orchard with a high ratio of border to interior trees; (2) not increasing MD dispenser rates in hot-spots such as along borders with external moth sources (e.g., garden trees) or along upwind borders of strong prevailing winds; (3) not applying supplemental insecticides when necessary; (4) not monitoring and detecting increased moth populations and fruit injury; (5) applying MD dispensers after the first flight is detected; (6) not applying MD dispensers according to label recommendations; and (7) not maintaining sanitation practices (e.g., removing waste apples and bins).
A future approach to consider alongside MD of male moths would be the removal of females [female removal (FR)] (Knight et al., 2022). Although MD-FR studies in Italy significantly reduced pear injury by only 25% (Preti et al., 2021b), in a US study, Knight et al. (2022) reported lower proportions of CM fruit injury by MD-FR vs. MD (0.016 vs. 0.055; 0.014 vs. 0.040, and 0.015 vs. 0.071, in 2018, 2019 and 2020, respectively).
Unquestionably, for effective and economically viable MD strategies against CM, the incorporation of MD for other tortricids is practical; otherwise, these need to be treated separately, adding to costs including labor, thinning, and targeted insecticides (Lo et al., 2013). For example, the product 4-Play™^ (now 4-playMAX), used in NZ, consists of a range of pheromone components used for CM, light brown apple moth (Epiphyas postvittana), green-headed leafroller (Planotortrix octo), and brown-headed leafroller (Ctenopseustis obliquana) (Suckling et al., 2016) with approval for similar straight-chain lepidopteran pheromones all covered under one regulation. Encouragingly, the use of MD may promote the occurrence in orchards of non-target invertebrates in the long-term, benefiting pest suppression, decomposition, and ecosystem services (Malone et al., 2017).
2.3 Female attractants
Odors from host plants are also a key component of mate finding alongside the female-produced sex pheromone, which, in isolation, is significantly less attractive to males compared to codlemone combined with tree/fruit odors (Erdei et al., 2023). Although the sex pheromone has been used successfully in MD and monitoring male CM populations, the major limitation for crop protection is that it only attracts males, yet crop damage is the result of female oviposition and larval feeding. Consequently, removal of females has a greater impact on fruit damage and population reduction than removal of males (Gregg et al., 2016). Put another way, the catch efficiency of female attractants can be lower than for male attractants but still be comparatively effective at protecting crops. In the past 10 years, research has been directed towards developing attractants for female CM, building on the historic research that identified compounds such as pear ester (PE) (Light et al., 2001), acetic acid (AA) (Landolt et al., 2007), and (E)-4,8-dimethyl-1,3,7-nonatriene (DMNT) (Knight et al., 2011) (Table 1). Female attractants can be used to manipulate female moth behavior and control damage to the crop by reducing the female population and oviposition.
Research developments have occurred in several areas, including identifying and testing individual and blends of volatile organic compounds (VOCs), combining female and dual-sex attractants with other IPM control strategies, and improving population monitoring lures.
2.3.1 Identifying and testing VOCs for female CM attraction
PE was identified as an attractant for female CM in 2001 by Light et al. (2001), and when combined with AA, the duo-lure was significantly more attractive than PE alone (Landolt et al., 2007). In traps baited with PE or DMNT combined with AA, the proportion of females attracted was 60%–75% higher (Knight et al., 2011).
Traps baited with lures containing PE, DMNT, LOX, and AA (MegaLure 4K by Trécé Inc.), caught significantly more CM (> 2-fold), of which 55% were females compared to traps baited with sex pheromone, PE, and AA (30% females) (Knight et al., 2019b; Knight et al., 2022). Hence, the addition of LOX and DMNT greatly increases trap catch and the proportion of females. The 4-K blend has been tested in several geographic locations and proven to be a highly effective female and male CM attractant, attracting high proportions (>50%) of females (Knight et al., 2019a) and, in some instances, outperforming pheromone lures in total moth captures (Preti et al., 2021a). However, in trials in Italy, female captures were proportionally lower than males, perhaps suggesting that different CM populations may respond differently to these kairomones (Preti et al., 2021a).
The “4- K” blend is also attractive to Grapholita molesta (Tortricidae), the oriental fruit moth (OFM), particularly if benzaldehyde is added (Giri, 2022), and the same blend may also attract other species that inhabit fruit orchards. However, other researchers have suggested that benzaldehyde and butyl acetate are repellent to mated adult female CM (Vallat and Dorn, 2005).
Certain VOCs found in host plant odor profiles may be attractive to gravid females specifically and encourage oviposition. These include (E)-β-ocimene, DMNT, (Z)-3-hexenyl acetate, nonanal, β-caryophyllene, germacrene D, (E,E)-α-farnesene, and methyl salicylate (Witzgall et al., 2005). (E,E)-α-farnesene may be a key compound for virgin and gravid female CM, stimulating calling behavior and oviposition (Yan et al., 2003). Moreover, volatiles from fermenting yeasts of the genus Metschnikowia are attractive to gravid females and stimulate them to oviposit significantly more compared to apple wounds with an absence of the yeasts. Seventeen compounds were identified from the fermenting yeast odor profile, including relatively large proportions of isoamyl acetate, 3-methyl-3-butenol, nonanal, 2-phenylethanol, 2-heptanone, and 2-nonanone (Witzgall et al., 2012). These compounds offer the possibility of creating a lure with enhanced attraction of gravid CM, thus directly reducing the number of eggs laid in the crop.
Landolt et al. (2014) and Jaffe and Landolt (2018) conducted a series of field trials assessing the attractiveness of N-butyl sulfide (NBS) for CM. Their trials concluded that NBS was attractive on its own and enhanced the attractiveness of a combination of AA and PE. NBS is not known to be naturally produced and it is unknown why it is attractive to CM but it may be a chemical analog to other natural compounds.
2.4 Larval kairomone attractants
Larvae looking for pupation sites are attracted to the other conspecifics (Jumean et al., 2008). Jumean et al. (2004, 2005, 2007) identified an aggregation pheromone produced by cocoon-spinning fifth instar larvae preparing to pupate. The components of the odor blend include (E)-2-octenal, (E)-2-nonenal, sulcatone, and geranylacetone, in combination with either 3-carene and/or three saturated aldehydes (octanal, nonanal, and decanal), and these compounds elicit a behavioral response from conspecific larvae and from the prepupal parasitoid, Mastrus ridibundus.
Neonate larvae are also attracted to PE (Knight and Light, 2001) and (E,E)-α-farnesene (Hughes et al., 2003). When PE was formulated as a microencapsulated liquid and applied to trees, its release rate was sufficient to cause larval arrestment over a 2-week period in the field (Light and Beck, 2012). This offers potential for enhancing the effectiveness of control products that specifically target the larval stages as they locate their host (Knight et al., 2019d).
2.5 Combining attractants with other IPM control strategies
The combination of MD with female attractant lures was compared in extensive field studies in Washington, USA (Knight et al., 2022). Traps containing female attractant were deployed at various densities, e.g., 37, 62, and 97 traps/ha. The trials compared areas of orchards treated with either MD only or MD + FR using traps baited with 4-K. The authors use the term “female removal”, which is synonymous to mass trapping targeting female CM. The combination of MD + FR resulted in significant reductions in fruit damage from CM compared to using MD only. In all three years, the proportion of damaged fruit was at least halved for each moth generation with the addition of FR, from approximately 2% to 7% fruit injured with MD only to 1%–2% damaged with MD + FR. There is a clear synergy using MD combined with mass trapping. Knight et al. (2022) demonstrated success in combining MD with FR (via female attractants); however, the authors discuss the economics of using these methods and state that further research is required to understand whether these technologies are economically viable. More research is needed to determine the optimal trap density and arrangement for mass trapping females combined with MD, with the objective of lowering the number of lures and traps required. It is possible that traps with female attractants could be deployed at higher density around the edge of orchards to capture gravid females as they enter an orchard and at lower density within the orchard. This could capture those females that have mated outside the MD area before they enter the orchard to oviposit.
CM attractants could enhance the effectiveness of using granulovirus to control larvae Knight et al. (2019d). For example, in laboratory bioassays, Madex (granulovirus of CM) combined with microencapsulated PE doubled the mortality compared to Madex alone after 30 min (18.8% versus 41.9% mortality) (Schmidt et al., 2008). Unfortunately, the enhanced mortality rate did not translate to a decrease in fruit damage in field trials. It was posited that this was due to the high application rate of Madex used so the granulovirus was working at its maximum effectiveness for all treatments regardless of the addition of PE (10%–16% fruit damage for all treatments containing Madex). The authors proposed further trials testing lower rates of Madex combined with PE to assess whether the PE feeding attractant would allow for less granulovirus to be used with the same level of control. Other larval attractants [e.g., (E,E)-α-farnesene] could be assessed to identify other compounds that enhance the effectiveness of granulovirus.
Another major advantage of dual-sex attractants that capture a high proportion of females is that information such as percentage of mated females, egg density, and timing of hatching can be determined (Barros-Parada et al., 2015; Balaško et al., 2020; Knight et al., 2019c). These are important factors to inform IPM controls, e.g., MD, or granulovirus. MD is most effective when initiated while the CM population is low (Fernández et al., 2010 and references therein); thus, a reliable population monitoring system is essential. Including monitoring that measures the presence and status of females in the area allows better informed management decisions to be taken. Knowledge on female mated status and timing of egg hatch will allow for better timing of application of biocontrols, specifically targeting eggs and larvae (e.g., granulovirus or Beauveria bassiana), which are susceptible to degradation in the environment (Barros-Parada et al., 2015).
Female attractants also offer the potential for attract and kill strategies, either using mass traps or with baited sprays (sprays with an insecticide and attractant combined). There are no recent published research papers developing or assessing an attract- and-kill control strategy for CM. This method was tested over 20 years ago, but with the 4-K lure or other attractants, it would be sensible to revisit this form of control.
2.6 Repellents and deterrents
Repellents or deterrents can act against CM mobile life stages, deterring them from treated areas. Repellent compounds are usually VOCs (odors), either synthetic or naturally derived, while deterrents may be volatile, low-volatile, or non-volatile compounds that inhibit feeding. Compared to repellents, which do not need to be applied directly to the crop because the odor plume will spread over the orchard area, larval deterrents may be less suited to controlling pests on freshly consumed products like apples and pears due to flavor-taint or other residues. Some repellent compounds, such as plant defense compounds, act as both a pest repellent and a predator attractant.
Studies have investigated the use of naturally derived products or intercropping with aromatic plants to repel CM. Pszczolkowski (2023) reviewed several published papers for their methodology and the effectiveness of 23 botanical extracts and essential oils for repelling or deterring CM neonate larvae. While several of the tested compounds had a high potency (e.g., >95% neonates repelled/deterred) in repelling or deterring the larvae away from apples or from feeding in laboratory bioassays, very few large field studies have been published. This is perhaps in part due to the challenges of acquiring sufficient volumes of botanical extracts. Botanical extracts or essential oils from garlic Allium sativum (L.) (Amaryllidaceae), absinthe wormwood Artemisia absinthium (L.) (Asteraceae), tansy (Tanacetum vulgare) (L.) (Asteraceae), false hellebore Veratrum californicum (Durand) (Liliaceae), ginger Zingiber officinale (Roscoe) (Zingiberaceae), patchouli Pogostemon cablin (Blanco) (Lamiaceae), Ginkgo biloba (L.) (Ginkgoaceae), and rue Ruta graveolens (L.) (Rutaceae) repelled or deterred CM neonates from feeding (Pszczolkowski, 2023, and the references therein).
When crude extracts from several artemisia species were tested as a feeding deterrent on CM neonates, Artemisia arborescens (L.) (Asteraceae) (Creed et al., 2015) and Artemisia annua (L.) (Asteraceae) (Durden et al., 2011) were significant deterrents. In terms of identified and isolated compounds, ginkgolic acid had a strong deterrent effect on neonates, and α-thujone, artemisinin, 1,8-cineole, bilobalide, and ginkgolide B had weak deterrent activity (Durden et al., 2011; Pszczolkowski et al., 2011; Creed et al., 2015; Pszczolkowski, 2023). Future research could focus on synthesizing and formulating compounds, or the molecular targets involved in these repellent or deterrent interactions for field testing.
Formulation of repellent or deterrent compounds by microencapsulation can provide benefits such as enhancing activity, lowering the quantity of the active substance required, and increasing longevity in the field. Two microencapsulation formulations containing either mostly 1,8-cineole or a mixture of 1,8-cineole and α-terpinyl acetate compounds were extracted from cardamom, Elettaria cardamomum (Zingiberaceae), and oleoresin and tested in laboratory bioassays and field trials (Kovanci, 2016). The microencapsulation formulation containing both compounds showed high levels of feeding deterrent (>90%) and oviposition repellence (>80%). In field trials, significant reductions in the number of CM and fruit damage were observed in treated areas, and further research is needed to confirm these findings.
A series of field trials measuring the number of male and female CM adults caught in traps baited with previously identified attractive kairomone blends (3-K and 4-K) tested the addition of individual VOCs in the blends. Several of the added VOCs reduced the catches of female CM and may be repellent. These potential repellent compounds were (Z)-3-hexenol, (E)-2-hexanal, (Z)-3-hexenyl acetate, hexyl butanoate, and linalool (Preti et al., 2021b).
Intercropping with aromatic plants offers the potential for repelling the pest without the application of any products onto the crop. Apple trees intercropped with French marigolds (Tagetes patula) had not only slightly lower numbers of CM larvae compared to control plots, but also lower predatory and parasitoid insects, whereas apple trees intercropped with basil, Ocimum basilicum, had higher rates of parasitism compared to control trees or trees intercropped with T. patula (Laffon et al., 2022). The underlying mechanisms of repellence of the pest or attraction of parasitoids were not identified.
In conclusion, published research on repellents and deterrents for CM is somewhat limited, and there are very few recently published papers reporting field trials. However, several plant extracts and compounds have been identified that are strong repellents to adults or deterrents to larvae. Of particular interest are 1,8-cineole (eucalyptol) and α-terpinyl acetate, which proved effective in significantly reducing fruit damage in orchards when microencapsulated and applied by conventional spraying methods. Other repellents could be formulated by microencapsulation into sprayable liquids and tested in field trials. Deployment of sachets or other means of dispersing VOCs into orchards can be prohibitively expensive; thus, the development of sprayable formulations may be an affordable and practical solution. In addition, with the recent advances in attractants for CM, a combined “push– pull” approach is possible (Pyke et al., 1987; Cook et al., 2007).
2.7 Improving population monitoring lures
Precise knowledge of female population dynamics, and potentially their mated status, would greatly improve timing and efficacy of crop protection interventions (Barros-Parada et al., 2015). The development of female attractants offers substantial potential for improving the effectiveness of other control strategies.
Using quince cultivars, the impact of CM larvae infestation on the odor profile of the fruit was assessed. (E,E)-α-farnesene was the main component in healthy fruits, and the proportion of this volatile increased nearly 20% when fruit was infested by CM. PE was the second most common VOC but did not increase post-infestation (López et al., 2022). Low levels of (E,E)-α-farnesene stimulate oviposition and are attractive to neonate larvae (Hern and Dorn, 1999); VOCs from apple, pear, or walnut trees, which included (E,E)-α-farnesene, (E)-β-ocimene, DMNT, (Z)-3-hexenyl acetate, nonanal, β-caryophyllene, germacrene D, and methyl salicylate, all increased oviposition of wild CM, but not from laboratory-reared CM (Witzgall et al., 2005). It is postulated by López et al. (2022) that the ratio of (E,E)-α-farnesene and esters (e.g., PE) modulates female moth host finding to assist gravid females locate optimum oviposition sites in terms of fruit maturity and competition from conspecifics. Therefore, (E,E)-α-farnesene, or other compounds identified by Witzgall et al. (2005), and PE could be important VOCs to consider as a female attractant, particularly for attractants used in oviposition lures.
Trials assessing PE, AA, and NBS investigated the mated status of female CM caught in traps (Landolt et al., 2014) and confirmed the findings of previous studies, where female CM caught in kairomone-baited traps are usually gravid, and therefore their removal from the crop area has a high potential for reduction in eggs laid and larval damage to fruit.
Dual-sex attractants can be used to monitor the effectiveness of MD. Within apple and pear orchards treated with MD, trials demonstrated that traps baited with codlemone and PE in combination caught significantly more CM than traps baited with codlemone (high and low loading) or PE (Fernández et al., 2010), but the numbers were insufficient to use for population monitoring. However, in other orchards treated with MD, PE, and AA, substantial numbers of CM (both males and females but >50% females) were captured compared to high-loading pheromone-baited traps (Tóth et al., 2014). Over the season, the PE + AA-baited traps were effective at monitoring the population generations, whereas high-loading pheromone traps caught negligible numbers of male CM. In field trials assessing traps baited with the '4-K' odor blend in orchards treated with MD, the 4-K baited traps caught significantly more female and total moths than traps baited with pheromone+PE+AA, sufficient for population monitoring in MD orchards (Knight et al., 2019c). In addition, when pheromone was added to the 4-K blend, the number of male CM caught significantly increased.
Female attractants are also used to monitor the success of SIT. During release periods of sterilized males to inhibit successful mating of the local wild populations of females, traps baited with female attractants can be deployed to capture females and assess their mated status (Knight, 2022).
In conclusion, research has identified blends of VOCs that achieve similar levels of attraction for females as pheromones do for male CM. This development opens many possibilities for improving CM control, either from direct control of females and reduction of oviposition, or indirectly by enhancing monitoring systems to improve knowledge of the population dynamics within the area and subsequently increasing the effectiveness of IPM strategies such as MD, SIT, and bio-insecticides.
Following this, two potential directions to explore are (i) direct control strategies, e.g., attract and kill, baited spraying, or MD + FR, and (ii) indirect control, e.g., enhanced population monitoring and phenological modeling to improve timing of applications or monitoring populations during MD or SIT to maximize their effectiveness.
2.7.1 Trapping and monitoring
Trapping CM is an essential component of crop protection; the moth counts are used to determine when it first arrives in the crop area, the population dynamics, and when it is active (Witzgall et al., 2010). This information allows improved selection and timing of control measures (Barros-Parada et al., 2015). Consequently, pheromone-baited traps for monitoring CM have become commonplace in orchards.
Standard pheromone-baited “ Delta” traps or funnel traps are used to monitor CM and establish biofix (an identifiable point in the pest’s life cycle from which degree-day accumulation can be started or pest control actions can be taken). Traps utilize a pheromone lure to attract male CM and either a sticky base or funnel and bucket to capture them for counting. Although these traps work well when used correctly and are relatively cheap, they have disadvantages. Accurate timing of application for controlling CM requires precise temporal information on their population; however, for manually checked traps, the information on the time of arrival of CM males is dependent on how regularly the traps are checked. Even though species-specific pheromone lures are used, there are often additional species (“bycatch”) in the traps that must be discounted, and identifying the target species caught on sticky bases can be difficult due to damage and poor presentation of the insect. Thus, it takes time and an experienced practitioner to accurately count trapped CM. Using non-sticky traps may be quicker to check as the moths are less likely to be damaged and there is no sticky base to replace, but bucket/funnel traps are not as effective at capturing CM as traps with sticky bases (Knodel and Agnello, 1990).
Regularly traveling around a farm to check multiple traps is time-consuming. Because of the need for regular access for manual checking, the traps are often placed in easily accessible locations, which may not be optimal in terms of maximizing captures, identifying hot-spots, or ensuring all incoming flight directions around the farm are monitored. For example, to maximize trap captures, traps should be placed 2.0 –3.5 m from the ground and within the tree canopy (Howell et al., 1990), which can make manually checking the traps difficult. Because of these limitations, and the benefits that automation and digitization offers, various types of automated trap have been developed and commercialized to date (Table 2).
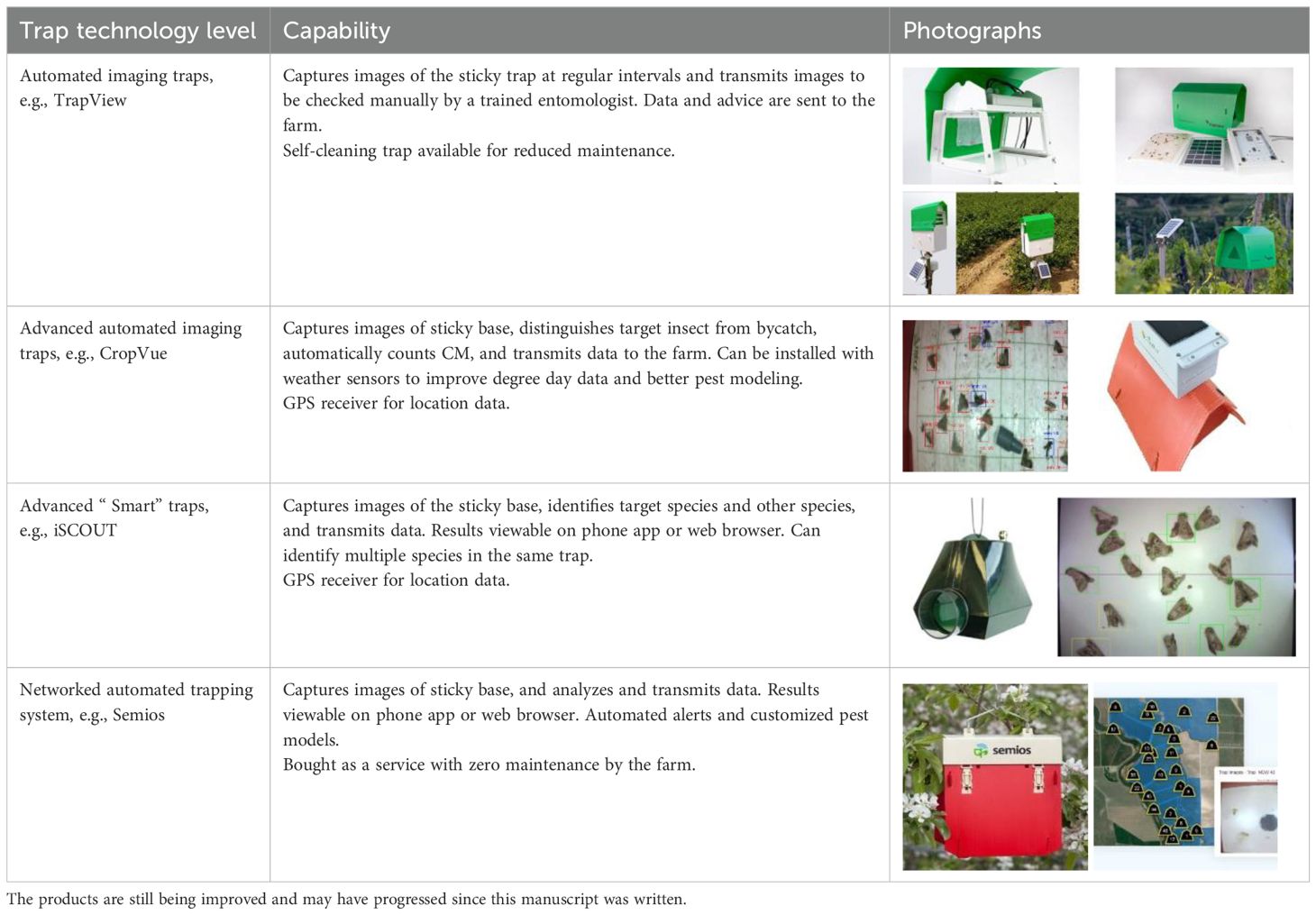
Table 2. Examples of commercial automated traps, their technology level, and capabilities (from Suto, 2022; Guyer and Whitfield, 2023).
In recent years, the main advancement in trapping hardware for CM has been the development of semi-automated and automated traps for population monitoring (Guarnieri et al., 2011; Ding and Taylor, 2016; Suto, 2022; Čirjak et al., 2022; 2023). These usually comprise of a sticky trap baited with a lure. Automated traps can have different levels of ability and complexity (Guyer and Whitfield, 2023). Basic automated traps take images of the sticky base at periodic intervals and then transmit the images to a data store for processing, either via a wireless network or by an operator downloading the images from the trap. The images are then manually reviewed by a trained scout or entomologist.
In more advanced traps, the images are processed automatically in a data server, with machine vision algorithms used to detect, identify, and count the target and other species. These semi- or fully automated traps are battery powered so that they can be deployed remotely in orchards or surrounding areas, and some fully automated traps include self-renewing sticky bases that require minimal maintenance lasting months without a human operator. Despite the additional hardware attached to automated traps and their different designs, automated traps catch equivalent numbers of CM compared to the standard white Delta traps and Pomotraps, and larger openings may further increase capture rates (Guarnieri et al., 2011; Frewin et al., 2022).
There are multiple benefits to using automated pest monitoring systems, including the following: (1) reduced labor traveling to and inspecting traps; (2) higher accuracy population data, due to more regular (e.g., daily) moth counts, better positioning of traps as they require less human intervention, and integration of local weather data for improved modeling; (3) digitization of the data, so that they can easily be integrated into farm management software, triggering pest alerts or other events; (4) enabling faster decision-making, combined with better temporal accuracy, will improve the timing of control applications; (5) networking/sharing of data across multiple farms or wider areas could improve knowledge of the wider population; and (6) potential for multi-species monitoring using kairomone-baited traps.
The main disadvantages of deploying automated traps are the cost and complexity of set up and maintenance. In the USA, commercial service models are available in which the farm pays a subscription to a pest monitoring service but does not have to buy or maintain the trap hardware.
Smart traps baited with kairomones (e.g., the 4-K lure) would be ideal for CM population monitoring and accessing performance of control strategies in orchards treated with SIT or MD. However, because kairomones are less species-specific compared to sex- pheromone-baited traps, they capture a species assemblage including other similar Lepidoptera, creating a more challenging problem for machine-based detection and identification. Trials with a commercially available automatic trapping system (TrapView) baited with 4-K + pheromone lures had low CM count accuracy (66%–87% count accuracy compared to manual counts) due to false-positive counts from high numbers of non-target species with similar morphology to CM (e.g., apple clearwing moth Synanthedon myopaeformis, OFM G. molesta, and carnation tortrix Cacoecimorpha pronubana; Preti et al., 2021a). The ability to distinguish male and female CM in sticky traps would be beneficial for monitoring and targeting sex-appropriate controls. Russell IPM in the UK have developed this for Drosophila suzukii. The technology uses smart phone photographic technology and could potentially be developed for CM.
So far, it has not been possible to distinguish between male and female CM from images and is challenging because of the similarity between the sexes (Preti et al., 2021a). However, new developments could enable sex differentiation, for example, using spectral imaging techniques combined with machine learning models, as demonstrated for differentiating male and female silkworm larvae (Bombyx mori) (Tao et al., 2019). Counting male and female moths individually in kairomone-baited traps would benefit growers by providing precise knowledge of when females arrive/emerge in the crop. Within a generation, males outnumber females for the first 30–35 days; after that, females outnumber males (Higbee et al., 2001 and references therein).
It may also be possible to use machine vision systems and smart traps to detect resistant phenotypes in CM populations. CM phenotypes that are resistant to insecticides have different forewing morphologies, and these differences could be used as biomarkers to detect the presence of resistance (Pajač Živković et al., 2019; Balaško et al., 2022).
Smart trapping technology will continue to advance as new knowledge and capabilities are developed. While some current commercial devices utilize artificial intelligence (AI) to detect and identify CM and bycatch, this is done by transmitting the images to a server where they are processed. An alternative, and potentially more energy-efficient method (Čirjak et al., 2023), is to use Edge computers and advanced deep neural networks (DNNs) that can process the images within the trap and only transmit count data. These DNN systems will also have higher levels of detection and identification accuracy, such as the system developed for CM using an EfficientDet-4 DNN-based model with a 99% accuracy (Čirjak et al., 2023).
A key piece of information needed from trap catches is the number of CM in the area. This can be accurately estimated by determining (i) the moth’s temporal and spatial distribution, (ii) the movement patterns before and after encountering the pheromone plume, (iii) the maximum distance from a trap that a moth will locate and enter the trap, and (iv) the average probability of capture in the trapping area of known size (Miller et al., 2015; Adams et al., 2017). These elements were explored and determined from field studies conducted in Michigan, USA. Although, the plume reach of a standard pheromone trap was calculated to be <5 m, males from 240 to 275 m distance were captured, meaning a single trap could potentially cover approximately 18–24 ha; however, the authors also suggest that deploying multiple traps per hectare would provide better accuracy on CM abundance (Adams et al., 2017). The probability of capture varied depending on the distance from the trap and population density with a range of 0.01–0.025, and an effective probability of capture in commercial Michigan orchards was determined to be 0.02. Therefore, trap captures of 1, 3, 10, and 30 equate to 50, 150, 500, and 1,500 males per 21 ha (the average of the trapping range). As CM has a 1:1 sex ratio, the same numbers can be used for female CM (Adams et al., 2017).
The potential for using new types of female or dual sex attractants, developed in recent decades, in mass-trapping strategies has been assessed in field trials. The dual-sex lure comprising PE, NBS, and AA was tested by Jaffe and Landolt (2018) in Washington, USA. The plots treated with the mass-trapping kairomone-baited traps had significantly lower post-treatment fruit damage (4.5%) compared to the control plots (10.4%). The number of CM removed from mass-trapped 1. 6-ha (4-acre) plots ranged from 336 to 8,598. None of the plots received insecticides and the kairomone-baited traps were placed at 50 traps per 0.4 ha (1 acre) (equivalent to 125 traps/ha) with 8 –12 m space between them. Further studies using different kairomone blends including the 4-K blend, and “ Combo” blend (PE, AA, and codlemone), combined with low-powered LED lights were assessed as a new tool for mass trapping CM and other orchard pests (Figure 1) (Knight et al., 2023). Traps baited with either the 4-K or Combo lures and UV-A-LEDs caught significantly more CM than with other LED colors, and combining UV-A lights with 4-K lures more than doubled the captures of CM compared to traps without the lights.
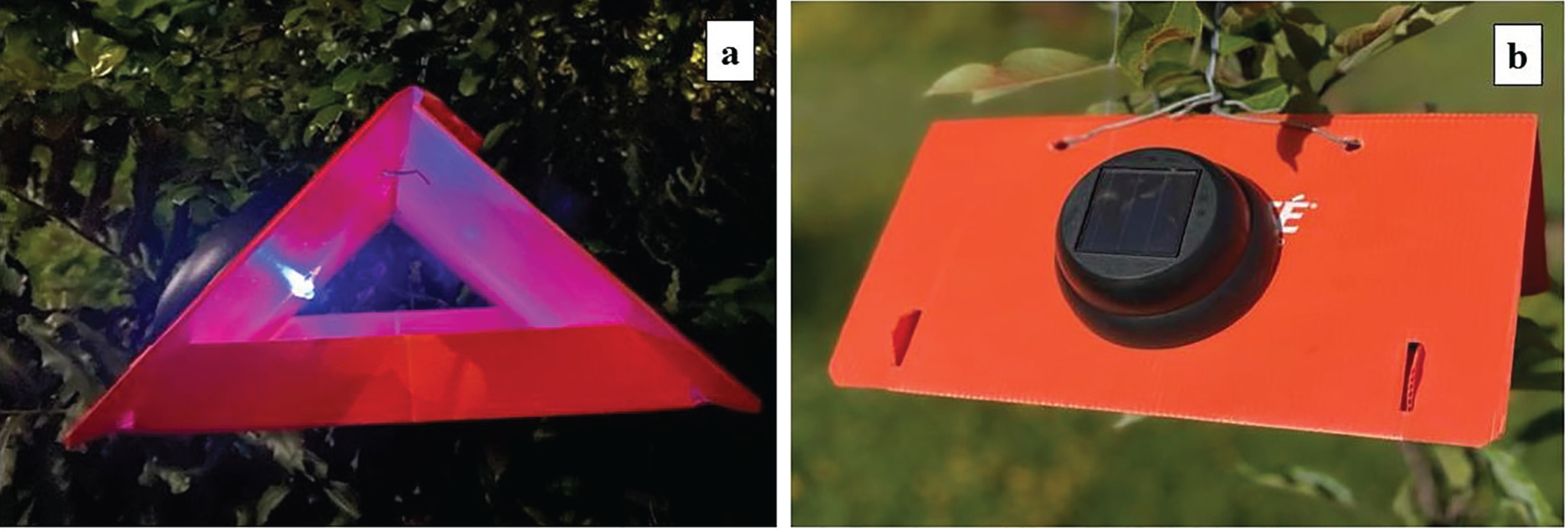
Figure 1. (A) Orange Delta trap equipped with a low-powered UV-A and blue light (spectral range 380 –500 nm, peak at 398 nm) and (B) solar panel (from Knight et al., 2023).
Another development in trap technology is a newly designed light trap, which was assessed as a mass-trapping device in field trials in Turkey. Light traps were placed above the canopy (~4 m from the ground), 25–30 m apart on the outer rows of the plot, powered by solar panels and batteries (Erler and Tosun, 2023). Light trap-treated plots were not sprayed with insecticides to control CM, and control plots were treated with the farm’s standard insecticide program. Pheromone monitoring and fruit damage assessments were used to determine the traps’ effectiveness. Both the number of adults and damage to the fruit was significantly lower in the mass-trapping plots compared to the conventionally treated plots and below 10%. The light traps also caught other crop pests.
These studies confirm mass trapping by kairomone or in conjunction with light as a viable alternative to pesticide application and could form part of an IPM strategy. Future research directions could include the comparison and assessment of automated traps against manual counts in regional orchards, including the software services, population models, and reliability of the smart traps; investigating the potential for developing a system to distinguish male and female CM (Figure 2); or if kairomone dual-sex attractants can be enhanced by addition of UV-A lights.
3 Conclusions
Codling moth is becoming increasingly difficult to control globally since the withdrawal of many chemical insecticides, development of resistance, and changes in climate. Control is best achieved on an area-wide basis, which combines multiple control tactics including semiochemicals, biological control, and selective insecticides that target each life stage (e.g., Schmidt et al., 2008; Klassen and Vreysen, 2021; Adolphi and Oeser, 2023). For example, the use of CM control programs, less reliant on chemical insecticides, would improve the survival and therefore efficacy of biological control agents such as parasitoid wasps and generalist predators. Area-wide management (minimum of 16 ha with 200 m buffer zones) is essential due to the long flight that a small proportion of the population can achieve. Area-wide management is part of an IPM approach and can include MD and SIT. These measures are also preventative rather than reactive. Approvals for MD should include the pheromones of other leaf rolling tortricids for cost-effectiveness.
The 5-year area-wide management program CAMP in the USA managed to reduce broad-spectrum insecticide use up to 80% (Knight, 2008; Balaško et al., 2020). The success of this program was attributed to (i) operational factors, i.e. the availability of several effective and selective tactics for both the key and secondary pests backed by technical support, and (ii) organizational factors which were the well-funded coordinated programs directly involving growers, researchers, industry leaders, community, and governmental administrators (Knight, 2008). The program included growers, outreach, government, researchers, and farm practitioners in a coordinated approach that was closely monitored and employed the required skill set.
To properly monitor progress with area-wide management, innovative and remote CM monitoring is needed so that hot-spots of CM can be identified and more easily targeted —preventing spread to less infested areas of orchards. Suggested monitoring trap densities to achieve this are debated (between 2.5 traps/ha and 1 trap per 21 ha). Perhaps, 0.25 traps/ha would be viable, but more research would be needed to determine this for a UK situation. Certainly, higher trapping densities are more likely to identify sources of CM invasion.
Most monitoring is based on the detection of male CM flight. However, there are already products available to monitor female CM flight. MD requires at least four contiguous hectares of orchard to be treated with buffer zones of 200 m or more. It is essential that monitoring continues in MD orchards, especially assessing the mated status of females, by using a lure such as CM DA-Combo or 4-K lure. These latter products might even be used to trap-out female CM in the future. The intensive trapping densities needed for adequate monitoring could be resolved with remote camera trap and moth identification and count technology. Action thresholds for CM insecticide sprays change in MD strategies and should be investigated in UK orchards.
Globally, there is evidence that in some areas, CM already has an extra generation due to changing climate, and perhaps changes to its phenology caused by insecticide pressure. Changes to seasonal average temperatures are expanding the flight periods and phenology- temperature-based prediction models may also need revising.
Future recommendations on furthering semiochemical control could include the following: (1) exploit existing female attractants to mass trap female CM alone or in combination with MD for male CM; (2) consider incorporating other VOCs including (E)-β-ocimene, DMNT, (Z)-3-hexenyl acetate, nonanal, β-caryophyllene, germacrene D, (E,E)-α-farnesene, and methyl salicylate; (3) determine action thresholds for CM insecticide sprays under MD strategies; (4) investigate the potential of repellent spray formulations at peak egg laying (e.g., benzaldehyde and butyl acetate are repellent to mated adult female CM); (5) develop microencapsulated repellent [e.g., 1,8-cineole (eucalyptol) and α-terpinyl acetate] as sprayable products to reduce the quantity of active ingredient needed; (6) combine pear ester as feeding attractant with granulovirus to test if this enhances control; (7) combine female attractants and repellents in a “push– pull” approach; and (8) kairomone dual-sex attractants can be enhanced by the addition of UV-A lights, and may be suitable for using as a mass-trapping control strategy.
Improvements to monitoring using semiochemicals include (1) implementation and demonstration of a fully integrated automated monitoring system on a farm to identify and target treatments towards CM hot-spots; (2) assessing the reliability of the latest female or dual-sex attractants for capturing females, to improve the knowledge of female flight, oviposition, and egg hatch; (3) incorporation of new “dual- sex” attractant semiochemicals into monitoring traps to assess if control strategies are working; (4) comparing and assessing automated traps against manual counts in orchards, including comparison of the software services, population models, and reliability of the smart traps; and (5) investigating the potential for developing a system to distinguish male and female CM.
Author contributions
EW: Conceptualization, Data curation, Formal analysis, Writing – original draft, Writing – review & editing. MF: Conceptualization, Data curation, Funding acquisition, Investigation, Writing – original draft, Writing – review & editing.
Funding
The author(s) declare financial support was received for the research, authorship, and/or publication of this article. The authors declare that this study received funding from British Apples and Pears Ltd., through the project titled Opportunities for Integrated Pest and Disease Management in Apples and Pears. The funder was not involved in the study design, collection, analysis, interpretation of data, the writing of this article or the decision to submit it for publication.
Acknowledgments
We are grateful to the East Malling Trust for their support and to Rebecca Griffiths (NIAB) for her help organizing the references.
Conflict of interest
The authors declare that the research was conducted in the absence of any commercial or financial relationships that could be construed as a potential conflict of interest.
Publisher’s note
All claims expressed in this article are solely those of the authors and do not necessarily represent those of their affiliated organizations, or those of the publisher, the editors and the reviewers. Any product that may be evaluated in this article, or claim that may be made by its manufacturer, is not guaranteed or endorsed by the publisher.
References
Adams C. G., Schenker J. H., McGhee P. S., Gut L. J., Brunner J. F., Miller J. R. (2017). Maximizing information yield from pheromone-baited monitoring traps: estimating plume reach, trapping radius, and absolute density of Cydia pomonella (Lepidoptera: Tortricidae) in Michigan apple. J. Economic Entomology 110, 305–318. doi: 10.1093/jee/tow258
Adolphi C., Oeser N. (2023). Beneficial nematodes against codling moth in organic apple production. BIOFRUITNET Pract. Abstract.
Balaško M. K., Bažok R., Mikac K. M., Benítez H. A., Suazo M. J., Viana J. P. G., et al. (2022). Population genetic structure and geometric morphology of codling moth populations from different management systems. Agronomy 12, 1278. doi: 10.3390/agronomy12061278
Balaško M. K., Bažok R., Mikac K. M., Lemic D., Pajač Živković I. (2020). Pest management challenges and control practices in codling moth: A review. Insects 11, 38. doi: 10.3390/insects11010038
Barić B., Pajač Živković I. (2017). The efficacy of mating disruption in the control of codling moth in Croatia, with special reference to the costs. Pomologia Croatica: Glasilo Hrvatskog Agronomskog Društva 21, 125–132. doi: 10.33128/pc.21.3-4.1
Barros-Parada W., Knight A. L., Fuentes-Contreras E. (2015). Modelling codling moth (Lepidoptera: Tortricidae) phenology and predicting egg hatch in apple orchards of the Maule Region, Chile. Chilean J. Agric. Res. 75, 57–62. doi: 10.4067/S0718-58392015000100008
Benelli G., Lucchi A., Thomson D., Ioriatti C. (2019). Sex pheromone aerosol devices for mating disruption: Challenges for a brighter future. Insects 10, 308. doi: 10.3390/insects10100308
Brunner J., Welter S., Calkins C., Hilton R., Beers E., Dunley J., et al. (2002). Mating disruption of codling moth: a perspective from the Western United States. IOBC WPRS Bull. 25, 11–20.
Calkins C. O., Knight A. L., Richardson G., Bloem K. A. (2000). “Areawide population suppression of codling moth,” in Area-wide Control of Fruit Flies and Other Insect Pests. Ed. Tan K. H. (Penerbit Universiti Sains Malaysia, Pulau Pinang, Malaysia), 215–219. International Conference on Area-Wide Control of Insect Pests, and the 5th International Symposium on Fruit Flies of Economic Importance, 28 May–5 June 1998, Penang, Malaysia.
Casado D., Cave F., Welter S. (2014). “Puffer®-CM Dispensers for mating disruption of codling moth: area of influence and impacts on trap finding success by males,” in Eds. Tasin M., Kovanci O. B.. Proceedings of the IOBC/WPRS Pheromones and other Semiochemicals in integrated production, 1-5 October 2012. (Bursa, Turkey: International Organization for Biological and Integrated Control), Vol. 99. 25–31, IOBC/WPRS Bulletin 2014.
Chen M., Duan X., Li Y., Men Q., Wan F. (2017). “Codling moth Cydia pomonella (L.),” in Biological Invasions and its Management in China. (Germany: Springer Nature), vol. 2, 285–298.
Čirjak D., Aleksi I., Lemic D., Pajač Živković I. (2023). Efficientdet-4 deep neural network-based remote monitoring of codling moth population for early damage detection in apple orchard. Agriculture 13, 961. doi: 10.3390/agriculture13050961
Čirjak D., Miklečić I., Lemić D., Kos T., Pajač Živković I. (2022). Automatic pest monitoring systems in apple production under changing climatic conditions. Horticulturae 8, 520. doi: 10.3390/horticulturae8060520
Cook S., Khan Z., Pickett J. (2007). The use of push-pull strategies in integrated pest management. Annu. Rev. Entomology 52, 375–400. doi: 10.1146/annurev.ento.52.110405.091407
Coop L. B., Kogan M., Bajwa W., Center I. P. P. (1999). Areawide codling moth: extending the principles and lessons learned outside the project and to other commodities. Washington Hortic. Assoc. Proc. 95, 176–183. Available at: https://pnwpest.org/awcm/wsha99.html.
Creed C., Mollhagen A., Mollhagen N., Pszczolkowski M. A. (2015). Artemisia arborescens “Powis Castle“ extracts and α-thujone prevent fruit infestation by codling moth neonates. Pharm. Biol. 53, 1458–1464. doi: 10.3109/13880209.2014.985796
Damos P., Colomar L.-A. E., Ioriatti C. (2015). Integrated fruit production and pest management in Europe: the apple case study and how far we are from the original concept? Insects 6, 626–657. doi: 10.3390/insects6030626
Ding W., Taylor G. (2016). Automatic moth detection from trap images for pest management. Comput. Electron. Agric. 123, 17–28. doi: 10.1016/j.compag.2016.02.003
Duménil C., Judd G. J., Bosch D., Baldessari M., Gemeno C., Groot A. T. (2014). Intraspecific variation in female sex pheromone of the codling moth Cydia pomonella. Insects 5, 705–721. doi: 10.3390/insects5040705
Durden K., Sellars S., Cowell B., Brown J. J., Pszczolkowski M. A. (2011). Artemisia annua extracts, artemisinin and 1, 8-cineole, prevent fruit infestation by a major, cosmopolitan pest of apples. Pharm. Biol. 49, 563–568. doi: 10.3109/13880209.2010.528433
Dyck V. A., Hendrichs J., Robinson A. S. (2005). Sterile Insect Technique: Principles and Practice in Area-Wide Integrated Pest Management. Dordrecht, The Netherlands: Springer.
Erdei A., Preti M., Bengtsson M., Witzgall P. (2023). Host plant odour and sex pheromone are integral to mate finding in codling moth. bioRxiv, 2023–2010. doi: 10.1101/2023.10.02.560528
Erler F., Tosun H. S. (2023). Mass-trapping the codling moth, Cydia pomonella (L.)(Lepidoptera: Tortricidae), using newly designed light trap reduces fruit damage in apple orchards. J. Plant Dis. Prot. 130, 795–807. doi: 10.1007/s41348-023-00735-7
Fernández D. E., Cichon L., Garrido S., Ribes-Dasi M., Avilla J. (2010). Comparison of lures loaded with codlemone and pear ester for capturing codling moths, Cydia pomonella, in apple and pear orchards using mating disruption. J. Insect Sci. 10, 139. doi: 10.1673/031.010.13901
Frewin A.J., Adams C., Judd G., Hazell J. (2022). Wind Tunnel and Field Evaluation of Trapping Efficiency of Semiochemical Baited Camera-Traps for Capturing Codling Moth (Lepidoptera: Tortricidae). J Econ Entomol. 115 (6), 2004–2012. doi: 10.1093/jee/toac132
Furmanczyk E. M., Parveaud C. E., Jacquot M., Warlop F., Kienzle J., Kelderer M., et al. (2022). An overview of pest and disease occurrence in organic pome fruit orchards in Europe and on the implementation of practices for their control. Agriculture 12, 2136. doi: 10.3390/agriculture12122136
Giri A. P. (2022). Evaluation of semiochemicals for attractiveness to multiple tortricid (Lepidoptera) pests in apple orchards. (Massachusetts, USA: University of Massachusetts Amherst, Plant & Soil Sciences department), 1239.
Gregg P. C., Del Socorro A. P., Hawes A. J., Binns M. R. (2016). Developing bisexual attract-and-kill for polyphagous insects: ecological rationale versus pragmatics. J. Chem. Ecol. 42, 666–675. doi: 10.1007/s10886-016-0725-8
Guarnieri A., Maini S., Molari G., Rondelli V. (2011). Automatic trap for moth detection in integrated pest management. Bull. Insectol. 64, 247–251.
Guyer D., Whitfield C. (2023). “Advances in image-based identification and analysis of crop insect pests,” in Advances in Monitoring of Native and Invasive Insect Pests of Crops, 1st ed. Eds. Pope T., Fountain M. T. (Burleigh Dodds Science Publishing, Cambridge, UK).
Hatteland B. A., Andrade Moral R. D., Jaastad G., Myren G., Bjotveit E., Sekse I. L., et al. (2023). Pesticide use, arthropod fauna and fruit damage in apple orchards in a Nordic climate. Pest Manage. Sci. 79, 5292–5303. doi: 10.1002/ps.v79.12
Hern A., Dorn S. (1999). Sexual dimorphism in the olfactory orientation of adult Cydia pomonella in response to α-farnesene. Entomologia Experimentalis Applicata 92, 63–72. doi: 10.1046/j.1570-7458.1999.00525.x
Higbee B. S., Calkins C. O., Temple C. A. (2001). Overwintering of codling moth (Lepidoptera: Tortricidae) larvae in apple harvest bins and subsequent moth emergence. J. Economic Entomology 94, 1511–1517. doi: 10.1603/0022-0493-94.6.1511
Holdcraft R., Rodriguez-Saona C., Stelinski L. L. (2016). Pheromone autodetection: evidence and implications. Insects 7, 17. doi: 10.3390/insects7020017
Horner R. M., Lo P. L., Rogers D. J., Walker J. T., Suckling D. M. (2020). Combined effects of mating disruption, insecticides, and the sterile insect technique on Cydia pomonella in New Zealand. Insects 11, 837. doi: 10.3390/insects11120837
Howell J. F., Schmidt R. S., Horton D. R., Khattak S. U. K., White L. D. (1990). Codling moth: male moth activity in response to pheromone lures and pheromone-baited traps at different elevations within and between trees. Environ. Entomology 19, 573–577. doi: 10.1093/ee/19.3.573
Hughes W. O., Gailey D., Knapp J. J. (2003). Host location by adult and larval codling moth and the potential for its disruption by the application of kairomones. Entomologia Experimentalis Applicata 106, 147–153. doi: 10.1046/j.1570-7458.2003.00020.x
Hulme P. E. (2017). Climate change and biological invasions: evidence, expectations, and response options. Biol. Rev. 92, 1297–1313. doi: 10.1111/brv.2017.92.issue-3
Husain M., Rathore J., Sharma A., Raja A., Qadri I., Wani A. B. W. (2018). Description and management strategies of important pests of pear: A review. J. Entomology Zoology Stud. 6, 677–683.
Ioriatti C., Lucchi A. (2016). Semiochemical strategies for tortricid moth control in apple orchards and vineyards in Italy. J. Chem. Ecol. 42, 571–583. doi: 10.1007/s10886-016-0722-y
Isufi E. (2023). Protection of apple fruits, from codling moth (Cydia pomonella), using the mating disrupting pheromones, ISOMATE CTT. Unpublished manuscript. UDC: 634.11-278:632.951(496.5).
Jaffe B. D., Landolt P. J. (2018). Field experiment of a three-chemical controlled-release dispensers to attract codling moth (Cydia pomonella) (Lepidoptera: Tortricidae). J. Economic Entomology 111, 1268–1274. doi: 10.1093/jee/toy045
Ju D., Mota-Sanchez D., Fuentes-Contreras E., Zhang Y.-L., Wang X.-Q., Yang X.-Q. (2021). Insecticide resistance in the Cydia pomonella (L): Global status, mechanisms, and research directions. Pesticide Biochem. Physiol. 178, 104925. doi: 10.1016/j.pestbp.2021.104925
Judd G. J., Gardiner M. G. (2005). Towards eradication of codling moth in British Columbia by complimentary actions of mating disruption, tree banding and sterile insect technique: five-year study in organic orchards. Crop Prot. 24, 718–733. doi: 10.1016/j.cropro.2004.12.009
Jumean Z., Gries R., Unruh T., Rowland E., Gries G. (2005). Identification of the larval aggregation pheromone of codling moth, Cydia pomonella. J. Chem. Ecol. 31, 911–924. doi: 10.1007/s10886-005-3552-x
Jumean Z., Lafontaine J. P., Wood C., Judd G. J., Gries G. (2007). Pheromone-based trapping of larval codling moth, Cydia pomonella, in apple orchards. Entomologia Experimentalis Applicata 122, 87–91. doi: 10.1111/j.1570-7458.2006.00478.x
Jumean Z., Rowland E., Judd G. J., Gries G. (2004). Male and female Cydia pomonella (Lepidoptera: Olethreutidae) larvae produce and respond to aggregation pheromone. Can. Entomologist 136, 871–873. doi: 10.4039/n04-050
Jumean Z., Wood C., Gries G. (2008). Does larval aggregation pheromone of codling moth, Cydia pomonella, induce attraction or arrestment of receivers? Bull. Entomological Res. 98, 425–429. doi: 10.1017/S0007485308005646
Klassen W., Vreysen M. J. B. (2021). “Area-wide integrated pest management and the sterile insect technique,” in Sterile Insect Technique, Principles and Practice in Area-Wide Integrated Pest Management. (Florida, USA, and Oxfordshire UK: CRC Press Taylor and Francis Group), 75–112.
Knight A. L. (2000). Monitoring codling moth (Lepidoptera: Tortricidae) with passive interception traps in sex pheromone-treated apple orchards. J. Economic Entomology 93, 1744–1751. doi: 10.1603/0022-0493-93.6.1744
Knight A. L. (2008). “Codling moth areawide integrated pest management,” in Theory and Implementation, 1st ed. Eds. Koul O., Cuperus G., Elliott N. (CAB International, Oxfordshire, UK), 159–190.
Knight A. (2022). Two Decades of Research Yields Non-Pheromone Lure Composed of Plant Volatiles, [and] Acetic Acid (Good Fruit Grower) (Accessed 05/12/2023).
Knight A. L., Judd G. J., Gilligan T., Fuentes-Contreras E., Walker W. B. (2019d). “Integrated management of tortricid pests of tree fruit,” in Integrated management of diseases and insect pests of tree fruit, 1st ed. Eds. Xu X., Fountain M. T. (Burleigh Dodds Sciences Publishing, Cambridge, UK), 377–424.
Knight A. L., Light D. M. (2001). Attractants from Bartlett pear for codling moth, Cydia pomonella (L.), larvae. Naturwissenschaften 88, 339–342.
Knight A. L., Light D. M. (2005). Developing action thresholds for codling moth (Lepidoptera: Tortricidae) with pear ester-and codlemone-baited traps in apple orchards treated with sex pheromone mating disruption. Can. Entomologist 137, 739–747. doi: 10.4039/n05-040
Knight A. L., Light D. M., Trimble R. M. (2011). Identifying (E)-4, 8-dimethyl-1, 3, 7-nonatriene plus acetic acid as a new lure for male and female codling moth (Lepidoptera: Tortricidae). Environ. Entomology 40, 420–430. doi: 10.1603/EN10283
Knight A. L., Mujica V., Herrera S. L., Tasin M. (2019a). “A new bisexual kairomone lure for codling moth,” in Eds. Branco M., Franco J. C., Gross J., Ioriatti C.. Proceedings of the IOBC/WPRS Pheromones and other Semiochemicals in integrated production. International Organization for Biological and Integrated Control, vol. 146, 19–22.
Knight A. L., Mujica V., Herrera S. L., Tasin M. (2019b). Addition of terpenoids to pear ester plus acetic acid increases catches of codling moth (Lepidoptera: Tortricidae). J. Appl. Entomology 143, pp.813–pp.821. doi: 10.1111/jen.v143.8
Knight A. L., Mujica V., Larsson Herrera S., Tasin M. (2019c). Monitoring codling moth (Lepidoptera: Tortricidae) with a four-component volatile blend compared to a sex pheromone-based blend. J. Appl. Entomology 143, pp.942–pp.947. doi: 10.1111/jen.v143.9
Knight A. L., Preti M., Basoalto E., Fuentes-Contreras E. (2023). Increasing catches of adult moth pests (Lepidoptera: Tortricidae) in pome fruit with low-intensity LED lights added to sex pheromone/kairomone lure-baited traps. J. Appl. Entomology 147, 843–856. doi: 10.1111/jen.v147.9
Knight A. L., Preti M., Basoalto E., Mujica V. M., Favaro R., Angeli S. (2022). Combining female removal with mating disruption for management of Cydia pomonella in apple. Entomologia Generalis 42, 309–321. doi: 10.1127/entomologia/2021/1316
Knodel J. J., Agnello A. M. (1990). Field comparison of nonsticky and sticky pheromone traps for monitoring fruit pests in western New York. J. Economic Entomology 83, 197–204. doi: 10.1093/jee/83.1.197
Kovačević Zcaron;. (1952). Primijenjena entomologija. II. Knjiga Poljoprivredni štetnici. 479 stranica, II izdanje 1961. (Zagreb: Sveučilište Zagreb).
Kovaleski A., Mumford J. (2007). “Pulling out the evil by the root: The codling moth Cydia pomonella eradication programme in Brazil,” in Area-Wide Control of Insect Pests: From Research to Field Implementation (Springer Netherlands, Dordrecht), 581–590.
Kovanci O. B. (2015). Co-application of microencapsulated pear ester and codlemone for mating disruption of Cydia pomonella. J. Pest Sci. 88, 311–319. doi: 10.1007/s10340-014-0619-x
Kovanci O. B. (2016). Feeding and oviposition deterrent activities of microencapsulated cardamom oleoresin and eucalyptol against Cydia pomonella. Chilean J. Agric. Res. 76, 62–70. doi: 10.4067/S0718-58392016000100009
Kovanci O. B. (2017). Comparison of the costs of mating disruption with traditional insecticide applications for control of codling moth in apple orchards in Turkey. Sci. Papers. Ser. B. Horticulture 61, 455–560.
Kuhns E. H., Pelz-Stelinski K., Stelinski L. L. (2012). Reduced mating success of female tortricid moths following intense pheromone auto-exposure varies with sophistication of mating system. J. Chem. Ecol. 38, 168–175. doi: 10.1007/s10886-012-0076-z
Kutinkova H., Dzhuvinov V., Palagacheva N., Staneva I., Gandev S., Kornov G., et al. (2022). Sustainable control of codling moth, Cydia pomonella L. @ in some apple orchards of Bulgaria. J. Biopesticides 15, 15–19. doi: 10.57182/jbiopestic.15.2.103-109
Laffon L., Bischoff A., Gautier H., Gilles F., Gomez L., Lescourret F., et al. (2022). Conservation biological control of Codling Moth (Cydia pomonella): Effects of two aromatic plants, Basil (Ocimum basilicum) and French Marigolds (Tagetes patula). Insects 13, 908. doi: 10.3390/insects13100908
Landolt P. J., Ohler B., Lo P., Cha D., Davis T. S., Suckling D. M., et al. (2014). N-Butyl sulfide as an attractant and coattractant for male and female codling moth (Lepidoptera: Tortricidae). Environ. Entomology 43, 291–297. doi: 10.1603/EN13178
Landolt P. J., Suckling D. M., Judd G. J. R. (2007). Positive interaction of a feeding attractant and a host kairomone for trapping the codling moth, Cydia pomonella (L.). J. Chem. Ecol. 33, 2236–2244. doi: 10.1007/s10886-007-9391-1
Light D. M., Beck J. J. (2012). Behavior of codling moth (Lepidoptera: Tortricidae) neonate larvae on surfaces treated with microencapsulated pear ester. Environ. Entomology 41, 603–611. doi: 10.1603/EN11273
Light D. M., Knight A. L., Henrick C. A., Rajapaska D., Lingren B., Dickens J. C., et al. (2001). A pear-derived kairomone with pheromonal potency that attracts male and female codling moth, Cydia pomonella (L.). Naturwissenschaften 88, 333–338. doi: 10.1007/s001140100243
Lo P. L., Walker J. T. S., Horner R. M., Hedderley D. I. (2013). Development of multiple species mating disruption to control codling moth and leafrollers (Lepidoptera Tortricidae). New Z. Plant Prot. 66, 264–269. doi: 10.30843/nzpp.2013.66.5646
López M. L., Gomez M. P., Díaz A., Barud F. J., Camina J. L., Dambolena J. S. (2022). Changes in the volatile profile of four cultivars of quince (Cydonia oblonga) produced by codling moth (Cydia pomonella) infestation. Phytochem. Lett. 49, 187–191. doi: 10.1016/j.phytol.2022.03.019
Malone L. A., Burgess E. P., Barraclough E. I., Poulton J., Todd J. H. (2017). Comparison of invertebrate biodiversity in New Zealand apple orchards using integrated pest management, with or without codling moth mating disruption, or organic pest management. Agriculture Ecosyst. Environ. 247, 379–388. doi: 10.1016/j.agee.2017.06.046
Mangan A. M., Piaggio A. J., Hopken M. W., Werner S. J., Pejchar L. (2018). A molecular analysis to assess codling moth Cydia pomonella L.(Lepidoptera: Tortricidae) predation by orchard birds. Ecol. Indic. 93, 1222–1225. doi: 10.1016/j.ecolind.2018.06.025
Mansour M. (2010). Attract and kill for codling moth Cydia pomonella (Linnaeus)(Lepidoptera: Tortricidae) control in Syria. J. Appl. Entomology 134, 234–242. doi: 10.1111/j.1439-0418.2009.01393.x
McGhee P. S., Epstein D. L., Gut L. J. (2011). Quantifying the benefits of areawide pheromone mating disruption programs that target codling moth (Lepidoptera: Tortricidae). Am. Entomologist 57, 94–100. doi: 10.1093/ae/57.2.94
McGhee P. S., Gut L. J., Miller J. R. (2014). Aerosol emitters disrupt codling moth, Cydia pomonella, competitively. Pest Manage. Sci. 70, 1859–1862. doi: 10.1002/ps.2014.70.issue-12
McGhee P. S., Miller J. R., Thomson D. R., Gut L. J. (2016). Optimizing aerosol dispensers for mating disruption of codling moth, Cydia pomonella L. J. Chem. Ecol. 42, 612–616. doi: 10.1007/s10886-016-0724-9
Miller J. R., Adams C. G., Weston P. A., Schenker J. H. (2015). Trapping of small organisms moving randomly: principles and applications to pest monitoring and management. (Springer Cham Heidelberg New York Dordrecht London: Springer).
Miller J. R., Gut L. J. (2015). Mating disruption for the 21st century: matching technology with mechanism. Environ. Entomology 44, 427–453. doi: 10.1093/ee/nvv052
Miller J. R., McGhee P. S., Siegert P. Y., Adams C. G., Huang J., Grieshop M. J., et al. (2010). General principles of attraction and competitive attraction as revealed by large-cage studies of moths responding to sex pheromone. Proc. Natl. Acad. Sci. 107, 22–27. doi: 10.1073/pnas.0908453107
Murray M., Alston D. G. (2023). Chapter one - Major issues in insect resistance management. In: Eds. Onstad D. W., Knolhoff L. M. Insect Resistance Management (Third Edition) (Academic Press), 1–29. doi: 10.1016/B978-0-12-823787-8.00008-8
Onstad D. W., Knolhoff L. M. (2023). “Major issues in insect resistance management,” in Insect Resistance Management (Academic Press), 1–29. doi: 10.1016/B978-0-12-823787-8.00008-8
Pajač I., Barić B., Mikac K. M., Pejić I. (2012). New insights into the biology and ecology of Cydia pomonella from apple orchards in Croatia. Bull. Insectol. 65, 185–193.
Pajač Živković I., Benitez H. A., Barić B., Drmić Z., Kadoić Balaško M., Lemic D., et al. (2019). Codling moth wing morphology changes due to insecticide resistance. Insects 10, 310. doi: 10.3390/insects10100310
Pajač Živković I., Pejic I., Barić B. (2011). Codling Moth, Cydia pomonella (Lepidoptera: Tortricidae) – Major Pest in Apple Production: an Overview of its Biology, Resistance, Genetic Structure and Control Strategies. Agriculturae Conspectus Scientificus 76, 87–92. Available at: https://acs.agr.hr/acs/index.php/acs/article/view/618.
Preti M., Knight A. L., Mujica M. V., Basoalto E., Favaro R., Angeli S. (2021b). Developing female removal for Cydia pomonella (Lepidoptera: Tortricidae) in organic pear in the USA and Italy. J. Appl. Entomology 145, 856–868. doi: 10.1111/jen.v145.9
Preti M., Knight A. L., Mujica M. V., Basoalto E., Larsson Herrera S., Tasin M., et al. (2021a). Development of multi-component non-sex pheromone blends to monitor both sexes of Cydia pomonella (Lepidoptera: Tortricidae). J. Appl. Entomology 145, 822–830. doi: 10.1111/jen.v145.8
Proverbs M. G., Newton J. R., Logan D. M. (1967). Autocidal control of the codling moth by release of males and females sterilized as adults by gamma radiation. J. Economic Entomology 60, 1302–1306. doi: 10.1093/jee/60.5.1302
Pszczolkowski M. A. (2023). Prospects of codling moth management on apples with botanical antifeedants and repellents. Agriculture 13, 311. doi: 10.3390/agriculture13020311
Pszczolkowski M. A., Durden K., Sellars S., Cowell B., Brown J. J. (2011). Effects of Ginkgo biloba constituents on fruit-infesting behavior of codling moth (Cydia pomonella) in apples. J. Agric. Food Chem. 59, 10879–10886. doi: 10.1021/jf202386c
Pyke B., Rice M., Sabine B., Zalucki M. P. (1987). The push-pull strategy—behavioural control of Heliothis. Aust. Cotton Grower 9 (1), 7–9.
Reinke M. D., Miller J. R., Gut L. J. (2012). Potential of high-density pheromone-releasing microtraps for control of codling moth Cydia pomonella and obliquebanded leafroller Choristoneura rosaceana. Physiol. Entomology 37, 53–59. doi: 10.1111/j.1365-3032.2011.00816.x
Ricci B., Franck P., Toubon J. F., Bouvier J. C., Sauphanor B., Lavigne C. (2009). The influence of landscape on insect pest dynamics: a case study in southeastern France. Landscape Ecol. 24, 337–349. doi: 10.1007/s10980-008-9308-6
Ridley L., Mace A., Stroda E., Parrish G., Rainford J., MacArthur R., et al. (2021). Pesticide Usage Survey Report 297: Orchards in the United Kingdom 2020 (York, UK: Fera).
Roelofs W., Comeau A., Hill A., Milicevic G. (1971). Sex attractant of the codling moth: characterization with electroantennogram technique. Science 174, 297–299. doi: 10.1126/science.174.4006.297
Schmidt S., Tomasi C., Pasqualini E., Ioriatti C. (2008). The biological efficacy of pear ester on the activity of granulosis virus for codling moth. J. Pest Sci. 81, 29–34. doi: 10.1007/s10340-007-0181-x
Schumacher P., Weyeneth A., Weber D. C., Dorn S. (1997). Long flights in Cydia pomonella L. (Lepidoptera: Tortricidae) measured by a flight mill: influence of sex, mated status and age. Physiol. Entomology 22, 149–160. doi: 10.1111/j.1365-3032.1997.tb01152.x
Severin D., Iosob G. A. (2023). “. Enhancing pest control through biological methods: A case study on Cydia pomonella L. using pheromonal traps,” in Scientific Studies & Research, vol. 32. (Vegetable Research and Development Station Bacau, Bacău, Romania.).
Shaw B., Nagy C., Fountain M. T. (2021). Organic control strategies for use in IPM of invertebrate pests in apple and pear orchards. Insects 12, 1106. doi: 10.3390/insects12121106
Suckling D. M., El-Sayed A. M., Walker J. T. (2016). Regulatory innovation, mating disruption and 4-PlayTM in New Zealand. J. Chem. Ecol. 42, 584–589. doi: 10.1007/s10886-016-0728-5
Suto J. (2022). Codling moth monitoring with camera-equipped automated traps: A review. Agriculture 12, 1721. doi: 10.3390/agriculture12101721
Tao D., Wang Z., Li G., Xie L. (2019). Sex determination of silkworm pupae using VIS-NIR hyperspectral imaging combined with chemometrics. Spectrochimica Acta Part A: Mol. Biomolecular Spectrosc. 208, 7–12. doi: 10.1016/j.saa.2018.09.049
Thistlewood H. M., Judd G. J. (2019). Twenty-five years of research experience with the sterile insect technique and area-wide management of codling moth, Cydia pomonella (L.), in Canada. Insects 10, 292. doi: 10.3390/insects10090292
Tóth M., Jósvai J., Hári K., Pénzes B., Vuity Z., Holb I., et al. (2014). Pear ester based lures for the codling moth Cydia pomonella L. - A summary of research efforts in Hungary. Acta Phytopathologica Entomologica Hungarica 49, 37–47. doi: 10.1556/APhyt.49.2014.1.4
Vallat A., Dorn S. (2005). Changes in volatile emissions from apple trees and associated response of adult female codling moths over the fruit-growing season. J. Agric. Food Chem. 53, 4083–4090. doi: 10.1021/jf048499u
Velásquez A. C., Castroverde C. D. M., He S. Y. (2018). Plant–pathogen warfare under changing climate conditions. Curr. Biol. 28, R619–R634. doi: 10.1016/j.cub.2018.03.054
Walker J. T. S., Lo P. L., Horner R. M., Park N. M., Hughes J. G., Fraser T. M. (2013). Codling moth (Cydia pomonella) mating disruption outcomes in apple orchards. New Z. Plant Prot. 66, 259–263. doi: 10.30843/nzpp.2013.66.5642
Warlop F., Kienzle J. (2022). Codling moth prevention: Preserve antagonists in organic apple and pear orchards. BIOFRUITNET Pract. Abstract.
Wearing C. H. (2022). Life table simulations of a univoltine codling moth, Cydia pomonella, population 3. Impact of immigration on the effectiveness of mating disruption. Biocontrol Sci. Technol. 32, 1156–1176. doi: 10.1080/09583157.2022.2091112
Weissling T. J., Knight A. L. (1996). Oviposition and calling behavior of codling moth (Lepidoptera: tortricidae) in the presence of codlemone. Ann. Entomological Soc. America 89, 142–147. doi: 10.1093/aesa/89.1.142
Witzgall P., Ansebo L., Yang Z., Angeli G., Sauphanor B., Bengtsson M. (2005). Plant volatiles affect oviposition by codling moths. Chemoecology 15, 77–83. doi: 10.1007/s00049-005-0295-7
Witzgall P., Kirsch P., Cork A. (2010). Sex pheromones and their impact on pest management. J. Chem. Ecol. 36, 80–100. doi: 10.1007/s10886-009-9737-y
Witzgall P., Proffit M., Rozpedowska E., Becher P. G., Andreadis S., Coracini M., et al. (2012). This is not an apple”–yeast mutualism in codling moth. J. Chem. Ecol. 38, 949–957. doi: 10.1007/s10886-012-0158-y
Witzgall P., Stelinski L., Gut L., Thomson D. (2008). Codling moth management and chemical ecology. Annu. Rev. Entomology 53, 503–522. doi: 10.1146/annurev.ento.53.103106.093323
Yan F., Bengtsson M., Makranczy G., Löfqvist J. (2003). Roles of α-farnesene in the behaviors of codling moth females. Z. für Naturforschung C 58, pp.113–pp.118. doi: 10.1515/znc-2003-1-220
Keywords: Codling moth, area wide, semiochemical, attractant, repellent, integrated pest management
Citation: Whitfield EC and Fountain MT (2024) Future semiochemical control of codling moth, Cydia pomonella. Front. Hortic. 3:1446806. doi: 10.3389/fhort.2024.1446806
Received: 10 June 2024; Accepted: 17 September 2024;
Published: 11 October 2024.
Edited by:
Isabel Gomez, National Autonomous University of Mexico, MexicoReviewed by:
Eduardo Fuentes-Contreras, Universidad de Talca, ChileMuhammad Zeeshan Ahmed, Bahauddin Zakariya University, Pakistan
Copyright © 2024 Whitfield and Fountain. This is an open-access article distributed under the terms of the Creative Commons Attribution License (CC BY). The use, distribution or reproduction in other forums is permitted, provided the original author(s) and the copyright owner(s) are credited and that the original publication in this journal is cited, in accordance with accepted academic practice. No use, distribution or reproduction is permitted which does not comply with these terms.
*Correspondence: E. Charles Whitfield, Q2hhcmxlcy53aGl0ZmllbGRAbmlhYi5jb20=