- 1Institute of Plant Breeding and Genetic Resources, Hellenic Agricultural Organization-DIMITRA (ELGO-DIMITRA), Thessaloniki, Greece
- 2Department of Horticulture, School of Agriculture, Aristotle University of Thessaloniki, Thessaloniki, Greece
- 3Laboratory of Animal Physiology, Department of Zoology, School of Biology, Aristotle University of Thessaloniki, Thessaloniki, Greece
- 4Laboratory of Ichthyology and Fisheries, Department of Animal Production, School of Agriculture, Faculty of Agriculture, Forestry and Natural Environment, Aristotle University of Thessaloniki, Thessaloniki, Greece
Introduction: Aquaponics is an integrated food production system that links recirculating aquaculture with hydroponics, leading to higher water use efficiency than conventional food production systems while also saving on fertilizers. In the present study, baby lettuce and baby rocket plants cultivated hydroponically in a deep water culture system using wastewater from rainbow trout were evaluated as part of a vertical decoupled aquaponic system.
Materials and methods: More specifically, three different nutrient solutions were supplied: a) using fish wastewater only (designated as Fish); b) fish wastewater enriched with synthetic fertilizers (Mix); and c) a typical nutrient solution (Hoagland) as the control. Both lettuce and rocket plants were monitored in these nutrient solutions and in two different substrates, peat and perlite, as an organic and an inert substrate, respectively. The purpose of this study was to assess a vertical decoupled aquaponic system with regard to the resource use efficiency, such as water, land, and energy, while evaluating plant cultivation in the different treatments in terms of yield, growth, nitrate concentration on the leaf tissue, and foliar analysis. The photosynthetic rate and leaf color indices were also considered in the plant evaluation.
Results: The Mix–Peat treatment was the most efficient growing combination in terms of land and water use efficiency, with approximately 7% better land use efficiency while using 38% less water and 10% less fertilizer than Hoagland–Peat. Moreover, lettuce plants had the highest yield in the Mix–Peat treatment, at 2,497 g m−2, which was approximately 6% higher than that of Hoagland–Peat while not being inferior in the quality measures. On the other hand, the yield of rocket was significantly higher in the Hoagland–Peat treatment, being 18% higher than that of Mix–Peat and 30% higher than that of Fish–Peat. Overall, the study confirmed that aquaponic systems could lead to higher water use efficiency and savings in fertilizers without undermining the yield and quality of lettuce, while the vertical arrangement developed within the scope of this study can increase the land useefficiency of the system.
1 Introduction
Intensive crop farming, as a means of addressing the food requirements of the expanding population, entails significant detrimental effects on the environment, principally resulting in soil degradation and water pollution (Mózner et al., 2012). Hydroponics, being a soilless production system in controlled environments, eradicates many of the impacts of intensive crop farming. Moreover, it leads to higher yields and better quality products as a result of year-round cultivation, higher crop densities, and fewer applications of plant protection products (Tomasi et al., 2015), while deep water culture (DWC), in particular, offers the advantage of low installation costs and maximum greenhouse land use (Karnoutsos et al., 2021). On the other hand, the substantial requirement for nutrients, along with the large water volume needed, can lead to increased disposal challenges in hydroponic systems (Blidariu and Grozea, 2011).
Aquaponics, the combined cultivation of fish (aquaculture) and plants in a soilless system (hydroponics) is a developing method for sustainable food production, offering a potential solution to the adverse environmental effects of intensive aquaculture and crop farming (Rakocy et al., 2003). Aquaculture, the practice of farming commercial fishes under controlled environments in enclosed tanks or ponds (Blidariu and Grozea, 2011), plays a significant role in meeting the increasing global demand for fish while also alleviating pressure on wild fish populations (Subasinghe et al., 2009). In terms of sustainability and as a response to environmental regulations, recirculating aquaculture systems (RAS), in which water can be reused, have been developed mainly for freshwater species (Martins et al., 2010). These systems are designed to cultivate significant amounts of fish within confined water volumes by processing the water to eliminate toxic waste products before recycling it (Rakocy et al., 2003). The ammonia accumulated from uneaten fish feed, feces, and urea could reach toxic concentrations in RAS. The nitrification process, which usually takes place in properly designed biofilters, can contribute to lowering the ammonia concentrations. During nitrification, specific autotrophic bacteria (Nitrosomonas) oxidize ammonia into nitrite, while others (Nitrobacter) oxidize nitrite into nitrate, which is less toxic to fish (Rakocy et al., 2003; Tyson et al., 2004). However, nitrates in higher concentrations can also be toxic to fish; thus, excess nutrients should occasionally be removed from the system (Blidariu and Grozea, 2011). Aquaponics, as a combined technique, utilizes the nitrate-rich fish water from the RAS, which needs to be replenished, as a nutrient solution for hydroponic cultivation (Rakocy et al., 2003).
Warm-water fish species, particularly the Nile tilapia (Oreochromis niloticus), have been mainly reared in aquaponic systems (Lennard and Leonard, 2006; Pantanella et al., 2010; Nicoletto et al., 2018; Stathopoulou et al., 2021), but there are fewer reports on cold-water species, such as the rainbow trout (Oncorhynchus mykiss) (Adler et al., 2000; Buzby and Lin, 2014). Nevertheless, O. mykiss is the main freshwater fish species bred in Europe, which has significant market value (Martsikalis et al., 2014). With regard to the plant species cultivated in aquaponic systems, several vegetables and herbs have been assessed. Lettuce, tomato, and basil are more widely used, while there are very few reports on rockets (Adler et al., 2000; Suhl et al., 2016; Alcarraz et al., 2018; Nicoletto et al., 2018; Velichkova et al., 2019; Stathopoulou et al., 2021).
Aquaponics contributes to conserving freshwater, achieving a higher water use efficiency (WUE) compared with conventional agriculture while also saving on nutrients (Ibrahim et al., 2023). A typical aquaponic system consists of a fish tank, a mechanical filter to remove any solid particles such as fish feed or feces, a biological filter where the nitrification process takes place, a hydroponic unit, and a sump, where water is collected if intended for use in the aquaculture (Rakocy et al., 2003). To simplify the procedure, the hydroponic unit may also serve as a biofilter (Birolo et al., 2020), while flow-through systems have also been evaluated (Johnson et al., 2016). Therefore, aquaponic systems can either be coupled or decoupled. A coupled aquaponic system integrates hydroponics into the RAS. This might be the most common system, although the simultaneous symbiosis of fish, plants, and bacteria makes managing the system more difficult (Delaide et al., 2019). On the contrary, decoupled aquaponics allows for optimum fish and plant cultivation, as the two systems are independent and the fish wastewater from the RAS does not return to the aquaculture after passing through the hydroponic unit. This double recirculating aquaponic system is more challenging to install, but provides the opportunity to easily control both the RAS and the hydroponic system and to supplement the fish wastewater with synthetic fertilizers, with the aim of maximizing plant yield (Suhl et al., 2016).
Decoupled aquaponic systems with supplemented nutrients have been previously studied in the cultivation of basil (Rodgers et al., 2022), lettuce (Tsoumalakou et al., 2022), and tomato (Suhl et al., 2016; Delaide et al., 2019). However, to our knowledge, although the symbiosis of fish and plants in a single-level cultivation system is less complicated to install, aquaculture occupies valuable cultivable land. Furthermore, cold-water species might have difficulty adjusting when reared inside a greenhouse due to the higher temperatures. In the present study, the combined cultivation of rainbow trout and leafy vegetables is examined in a developed vertical arrangement. The system was implemented in a two-level greenhouse, with the RAS of rainbow trout established belowground and the plants cultivated in the upper greenhouse space. The main objective was to assess a vertical aquaponic system, focusing on the optimization of the WUE and land use efficiency (LUE) while maintaining the optimum yield and quality of the produced vegetables. With this aim, baby lettuce and baby rocket plants were cultivated in a DWC system, both in supplemented and in plain fish wastewater, using both an organic and an inert substrate.
2 Materials and methods
2.1 Experimental layout
The experiment was carried out in July 2022 in a two-level pilot greenhouse in the premises of the Sustainable Agricultural Structures and Renewable Energy Resources Lab (SASRER Lab) IPBGR, Hellenic Agricultural Organization-Dimitra, Thessaloniki, Greece (40°32′16.98″ N, 22°59′57.3684″ E). The aboveground and underground spaces of the greenhouse were utilized for plant cultivation following a DWC system and a fish rearing system, respectively. The two levels were combined to create a vertical decoupled aquaponic system (Figure 1).
The greenhouse, which has a total surface area of 106.4 m2 (11.2 m × 9.6 m), was of a pitched-roof type, with a galvanized iron frame and is covered with 8-mm-thick hard polycarbonate sheets, both sideways and on the roof. Natural ventilation and cooling were accomplished through roof openings and with a fan and a pad cooling system, respectively. Both roof vents and the cooling system were automatically set to function via a PLC system (PR-18DC-DAI-R-N, Rievtech Electronic Co., Nanjing, China), which was based on the air temperature recorded inside the greenhouse. Relative humidity (RH) was not controlled in any way; for photosynthetically active radiation (PAR) shading, curtains were operated when needed. The air temperature, RH, and PAR inside the greenhouse, as well as the external meteorological data, were always monitored through the PLC and recorded at an interval of 10 min (GP2 Data Logger, Delta-T Devices Ltd., Cambridge, UK).
The gutters set around the perimeter of the greenhouse ensured the collection of rainwater in a tank, which has a capacity of 14 m3 and placed outside the greenhouse. Inside the greenhouse, six tanks made of galvanized steel, with dimensions of 2.50 m × 1.00 m × 0.25 m (L × W × H) were used, and each one was filled with 0.50 m3 of the nutrient solution of different concentrations. Each tank was equipped with an autonomous water recirculation pump, as well as an air pump, to ensure even distribution of the nutrient solution and sufficient oxygen supply.
The rainwater collected in the outside tank was filtered to remove any solid particles and stored in a collection tank (1 m3) inside the belowground fish rearing system space, ready to use whenever needed in aquaculture. The fish rearing system in the underground space of the greenhouse (surface area of 52.6 m2) consisted of: 1) two fish tanks (1.5 m3 each); 2) a buffer tank (0.5 m3) that collects water from both fish tanks; 3) a mechanical/biological filter (ProfiDrum Combi Bio 15, ProfiDrum UK, Rockley, Retford, Nottinghamshire, UK) that removes solid particles from the remaining fish feed or feces, which also does part of the nitrification process; 4) an extra biological filter (0.5 m3) filled with ceramic rings, where the main nitrification process takes place; and 5) two storage tanks (2 m3 each), hereafter referred to as sumps. Sumps, as a part of the recirculation system, were used to increase the total water volume of the RAS, reaching up to 8 m3 in total. Each tank was equipped with an air pump to ensure enough oxygen supply. Fish water was recirculated in the rearing system through pipes and pumps using a UV lamp to ensure water disinfection. The above- and underground spaces of the greenhouse were also connected through pipes and a pump so that the fish water discarded from the RAS could be supplied into the cultivation tanks of the greenhouse, not continuously but based on the cultivation needs (Figure 2).
2.2 Plant material and growing conditions
Romaine lettuce (Lactuca sativa var. Parris Island Cos) and rocket (Eruca sativa Mill.) seeds (AGRIS, Kleidi, Imathia, Greece) were sown, in two types of substrates, directly in polystyrene double trays (33 cm × 67 cm) (DTS-T300, INA PLASTICS S.A., Aspropyrgos, Athens, Greece) with a planting density of 1,200 plants/m2. Peat (Klasmann TS2, Klasmann-Deilmann GmbH, Geeste, Germany) was used as an organic substrate; however, given its inherent nutrient enrichment, which supplies plants with nutrients, an inert substrate was also employed to isolate the effects of the nutrient solutions without substrate interference. Coarse-grained agricultural perlite (1–5 mm) (Perterra, Nordia SA, Thessaloniki, Greece) was selected as the inert substrate. Both substrates were used across all nutrient solution treatments. A total of 30 trays were sown with lettuce seeds, 15 in peat and 15 in perlite, which was similarly done for the rocket seeds as well. After seeding, all trays were placed in a closed chamber of constant temperature and humidity, set at 20 ± 2°C and 70% ± 10%, respectively, and with the absence of light until sprouting. The trays were watered daily with simple tap water until the formation of the first true leaf, after which they were placed in the cultivation tanks without transplanting.
Three different treatments were evaluated in terms of the nutrient solutions (Hoagland, Mix, and Fish) in the experiment, and each nutrient solution was examined for both substrates. Thus, a total of six treatments, one in each tank, were evaluated for each plant species, with each tank containing five trays of each species. More specifically, the nutrient solutions were as follows: a) Hoagland treatment (which served as the control) consisting of rainwater derived from the tank outside the greenhouse, which was filtered to remove any solids and enriched with regular synthetic fertilizers at the desired concentrations; b) Mix treatment consisting of fish wastewater, which was analyzed and enriched with synthetic fertilizers up until reaching Hoagland’s concentration; and c) Fish treatment consisting of fish wastewater, without any additions. pH was not adjusted in any of the treatments so that the added acid would not interfere with the composition of the nutrient solution. Nonetheless, pH, as well as the electrical conductivity (EC) and water temperature of the nutrient solution in all cultivation tanks, was monitored daily throughout the experiment using a portable measuring instrument (HI-98129, Hanna Instruments, Inc., Woonsocket, RI, USA). Dissolved oxygen (DO) was assessed regularly using a portable oxygen meter (HI-9142, Hanna Instruments, Inc., Woonsocket, RI, USA) to ensure that the oxygen in the root system was within the acceptable levels for cultivation.
Both the fish wastewater and rainwater used were analyzed to determine their nutrient contents and then adjusted according to Hoagland and Arnon (1950). The water samples collected from the fish rearing system and the rainwater tank were infiltrated and analyzed an inductively coupled plasma–optical emission spectrometry (ICP-OES) instrument (Avio 220 Max ICP-OES Scott/Cross-Flow Configuration, PerkinElmer, Shelton, CT, USA) to determine all micro- and macronutrients, except for those related to the nitrogen concentration. Nitrogen was only determined when in the form of nitrate, as in measurements conducted in fish wastewater prior to the cultivation setup. Ammonium ions were detected in quite low concentrations; therefore, no adjustments were performed in the nutrient solution with regard to ammonium. Nitrates were determined with a spectrophotometer through the absorbance in two different wavelengths (210 and 275 nm) in a solution of 50 mL of the water sample with 1 mL of hydrochloric acid (HCl).
2.3 Fish species and growing conditions
Rainbow trout (O. mykiss) was chosen for the purpose of the experiment. Approximately a thousand juveniles of the same size were distributed evenly in the two fish tanks 3 months before the plant setup. Fish were fed daily on a quantity corresponding to 2%–3% of their total weight. The water temperature (17.60 ± 0.71°C), pH (7.62 ± 0.39), EC (0.337 ± 0.139 mS cm−1), and the DO (6.90 ± 0.78 mg L−1) of the rearing system were monitored constantly through a PLC system (PR-18DC-DAI-R-N, Rievtech Electronic Co., Nanjing, China) and kept at the desired levels. Nitrogen compounds (i.e., total ammonia nitrogen, nitrite, and nitrate) were also recorded twice daily using commercial test kits (Tetra, Melle, Germany) and were kept at safe levels. The air temperature was monitored and controlled through an air conditioning unit, so that the air temperature and the water temperature, consequently, would not exceed 19.00°C. By the time plant cultivation was established in the greenhouse, fish had a mean initial weight of 7.35 ± 0.28 g and a mean length of 8.32 ± 0.41 cm.
2.4 Crop evaluation
Cultivations were primarily evaluated based on the produced fresh weight per square meter (g FW m−2). The nitrate concentration on FW and foliar analysis on the plant dry weight (DW) are also crucial for evaluating the differences in the nutrient solutions and substrates. Furthermore, plant growth per treatment was assessed, while photosynthesis and leaf color were considered.
2.4.1 Yield and growth
Harvesting was carried out at the baby leaf stage (The European Commission, 2014) and at different periods between treatments, with length as the main criterion at approximately 13 ± 2 cm for lettuce and 8 ± 1 cm for rocket plants, with four to six leaves depending on the plant species. Each tray, per treatment, was considered as a different replication. Thus, the harvested leaves were weighed separately for each tray. FW, measured in grams per square meter, was later calculated for each treatment. After the 15th day of cultivation and up until harvesting, measurements of the plants’ phenotypic characteristics were taken daily. Three plants per tray were randomly chosen at the beginning of the experiment so that the growth differences between treatments could be assessed. The leaf number, the length, and the width of the biggest leaf per chosen plant were measured. For growth evaluation, a comparison was carried out between the measurements taken on the same dates for each treatment. The initial measurement was at 15 days after seeding (DAS), while the latest was at 21 DAS, when peat treatments were harvested. For each of the examined parameters, growth was evaluated as the percentage of the change between two measurements (ΔT) at different time points using the following equation:
where T2 corresponds to the measurement taken at the final time point and T1 the measurement at the initial time point.
2.4.2 Nitrate concentration
The leaf samples randomly harvested from all trays of each treatment were pooled and split to create three different replicates. The nitrate concentration was defined in a spectrophotometer, as described by Cataldo et al. (1975). Samples (2.5 g of plant tissue) were extracted into 25.0 mL of distilled water. Of the aqueous plant extract, 0.2 mL was added into two test tubes along with 0.8 mL H2SO4 and 0.8 mL H2SO4 with 5% salicylic acid, respectively, followed by the addition of 19.0 mL 2 N NaOH. The absorbance of the colored product was measured at 410 nm and the results expressed as milligrams per kilogram FW.
2.4.3 Foliar analysis
Three samples from each treatment were randomly collected on harvest day. The samples were washed both with tap and distilled water, dried at 68°C for 48 h, and ground to a fine powder. A quantity of 1 g of the ground sample was ash-dried in a muffle furnace at 515°C for 5 h. The remaining ash, which contains all inorganic substances, was dissolved with 5 mL of 6 N HCl and diluted with distilled water up to 50 mL. For the determination of macronutrients, 2 mL of the solution was further diluted with 50 mL of distilled water. The concentrations of P, K, Ca, Mg, Fe, Mn, Zn, and Cu were determined using ICP-OES (Avio 220 Max ICP-OES Scott/Cross-Flow Configuration, PerkinElmer, Shelton, CT, USA). Total nitrogen was determined using the Kjeldahl method. The macronutrient concentrations were expressed as the percentage of DW, while those of the micronutrients were expressed in parts per million (or milligrams per kilogram).
2.4.4 Leaf color
On harvest day of each treatment, three random leaves from each tray were used to assess leaf color. Measurements were taken on the leaf tip immediately after harvesting, without prior processing of the leaves, using a digital colorimeter (CR-400 Chroma Meter, Konica Minolta Inc., Tokyo, Japan). The results were given in three coordinates, interpreted as lightness (L*), ranging from black = 0 to white = 100, a* (red–green), b* (yellow–blue), hue angle (b*/a*), and chroma (a*2 + b*2) according to Mcguire (1992).
2.4.5 Photosynthetic parameters
The day before the harvesting of each treatment, measurements of the photosynthetic rate (in micromoles of CO2 per square meter per second) and the transpiration rate (in millimoles of H2O per square meter per second) were taken. Three plants per treatment were randomly chosen and measured around noon, in full sunlight, using an infrared gas analyzer (LCi-SD Portable Photosynthesis System, ADC BioScientific Ltd., Hoddesdon, UK).
2.5 Evaluation of fish performance in the aquaculture tanks
Fish behavior was observed both remotely, through cameras, and directly four times a day during manual feeding. The relative health and condition of fish was estimated using Fulton’s condition factor:
where W is the weight (in grams) of fish and L is the total length (in centimeters).
The efficiency of the fish species to convert feed into body mass was measured through the feed conversion ratio (FCR), as follows:
where W1 and W2 are the initial and final weights (in grams) of fish, respectively. A lower FCR indicates better efficiency, as less feed is required to produce a unit of fish.
2.6 Resource use efficiency
For the calculation of the resource use efficiency (RUE), the land area and the water and energy consumptions were used. RUE was calculated as follows:
The output was expressed as the sum of the FWs of the vegetables per cultivation tank and the increase in the weight of fish (in the Mix and Fish treatments). The values in Table 1 for each indicator were used as the input. Differences in the energy use in the Mix and Fish treatments were explained using the different durations of each treatment cultivation. The water input was calculated based on the nutrient solution consumed throughout the cultivation period, as measured by the reduction in the total water volume with the use of a flow meter. Energy input was recorded through kilowatt-hour meters established in the fish rearing system space and the greenhouse. Such devices, along with the PLC systems, were used to monitor and control the aquaponic system. Furthermore, they ensured the accuracy of the measurements, which help to better understand what the hotspots regarding each particular input are, thus more effectively moving toward the sustainability of the aquaponic system. The LUE, WUE, and energy use efficiency (EUE) were calculated for the six treatments for all vegetables produced in an area of 2.2 m2, occupied by 10 cultivation trays.
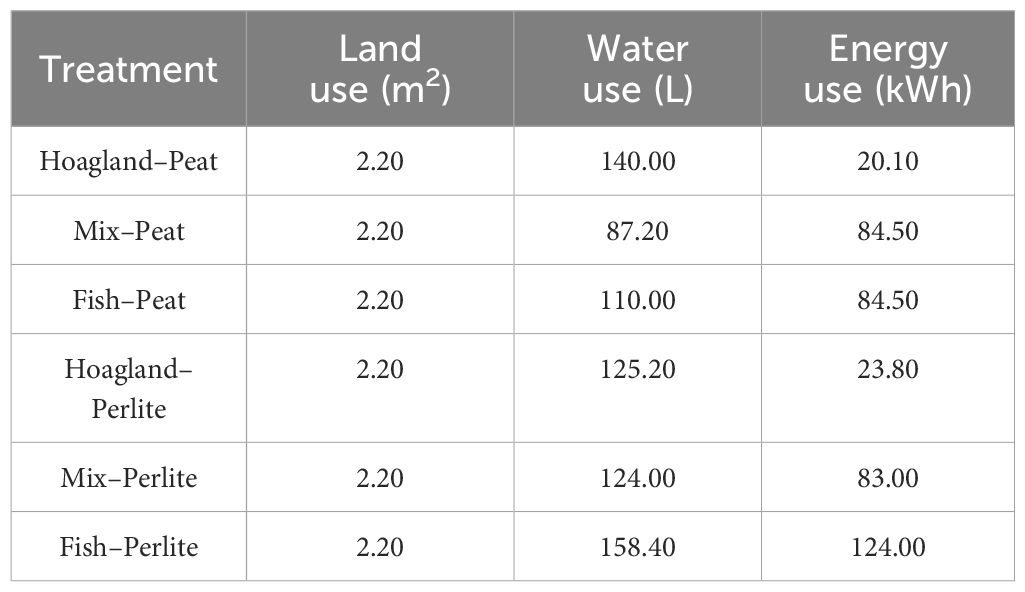
Table 1 System inputs for each examined indicator—land, water, and energy—in terms of the resource use efficiency of the treatments.
2.7 Statistical analysis
Five trays per treatment were used for each plant species, with each one considered as a different replication. Each tray was harvested separately. Samples were acquired evenly from each replication, pooled, and split to create three different samples for determining the nitrate concentration and for foliar analysis. For the LCI and color measurements, three leaves per treatment and per tray, respectively, were randomly chosen. The average measurements of all acquired data per treatment were analyzed with one-way analysis of variance (ANOVA). Comparisons of the means were performed using Tukey’s post-hoc test at the 0.05 level. The IBM SPSS Statistics (version 28.0; IBM Corp, Armonk, NY, USA) was used for all statistical analyses conducted during the experiment.
3 Results and discussion
The results of the evaluation of baby lettuce and baby rocket plants in a decoupled aquaponic system, as well as the RUE of the system, are presented below. Both plant species cultivations were evaluated primarily based on the yield and growth of plants. The nitrate concentration on the leaf tissue, the foliar analysis, and the photosynthetic rate were also taken into account to evaluate the adaptability of the plants in the applied nutrient solutions in two different substrates [peat (organic) and perlite (inert)]. Fish performance was assessed according to Fulton’s condition factor and the FCR.
3.1 Nutrient solution and growing conditions
The fish wastewater analyzed at the beginning of the experiment showed a nitrate (NO3−) concentration of 90.00 mg L−1. The target value for a typical hydroponic cultivation was set, according to Hoagland and Arnon (1950), at 745.00 mg L−1, meaning that approximately 12.00% of the desired nitrate concentration was of a biofertilizer. The calcium (Ca2+) and magnesium (Mg2+) in the fish wastewater were detected at 19.90% and 47.70% of the target concentrations, respectively, while phosphorus (P2O5) was at 5.50%. The nutrient solution finally used in the Mix treatment ended up using 12.50% less millimoles per liter of NO3− and 21.40% less millimoles per liter of Ca2+. To balance the nutrient solution, only 28.50% less millimoles per liter of Mg2+ was used. Phosphorus did not undergo any adjustment as its concentration in the fish wastewater was inadequate. Ammonium cations, as measured before the cultivation setup, were detected at approximately 0.08 mg L−1, probably due to the conversion of ammonia to nitrites and then nitrates (Birolo et al., 2020). Likewise, the micronutrient concentrations were below the detectable values in fish wastewater. Thus, the ammonium and micronutrients were not quantified anew, and the nutrient solution used in the Mix treatment was not adjusted for either of the two. Overall, in total, approximately 10% less fertilizer were used in the Mix treatments in comparison to the control.
The reported concentrations of NO3− in rainbow trout cultivation systems varied from 0.3 mg L−1 in a flow-through aquaponic system (Johnson et al., 2016) to 417.0 mg L−1 in a high-density recirculating aquaponic system (Birolo et al., 2020). The concentration detected in the present experiment was closer to that reported by Adler et al. (2000), which was at 110.8 mg L−1. The phosphorus concentrations were higher than those of the latter; however, overall, the concentrations of potassium, calcium, and magnesium in the effluent were lower. The differences found can be explained both by the fish density in aquaculture (Birolo et al., 2020) and the quantity and the quality of their feed (Buzby and Lin, 2014).
Hoagland treatment (control) was not adjusted beyond what was described in the original instructions of Hoagland and Arnon (1950) for the nutrient solution. Rainwater, which supplied the control, was analyzed, but the macro- and micronutrient concentrations were extremely low to adjust the original recipe accordingly.
The target concentrations for each nutrient in the nutrient solution, as well as the concentrations detected in the fish wastewater and rainwater tanks, as analyzed before cultivation was set, are presented in Table 2. The final amounts of fertilizers used in the Hoagland and Mix treatments for both macro- and micronutrients (in milligrams per liter) are presented in Table 3.
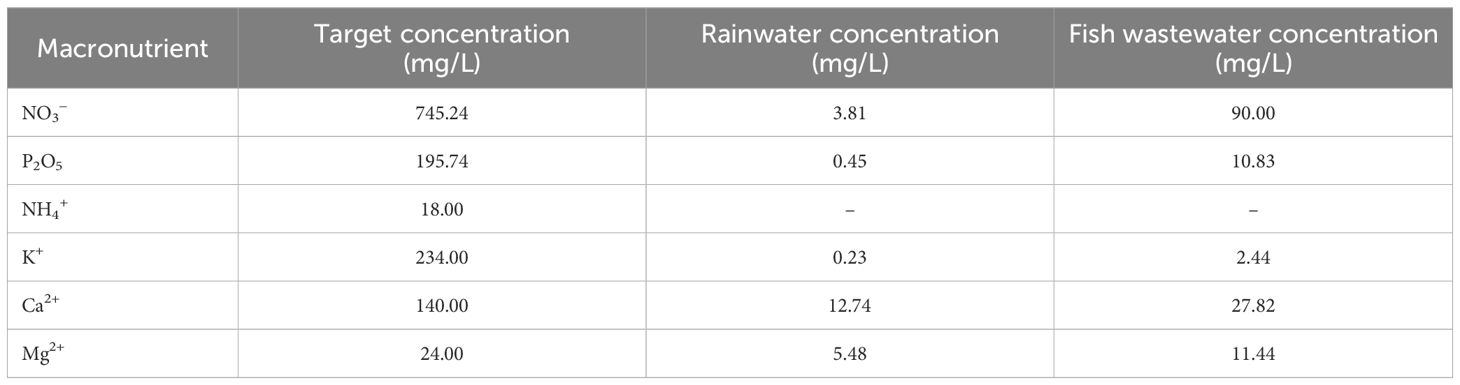
Table 2 Macronutrient concentrations of the target nutrient solution, the analyzed rainwater, and the fish wastewater presented per chemical compound and element.
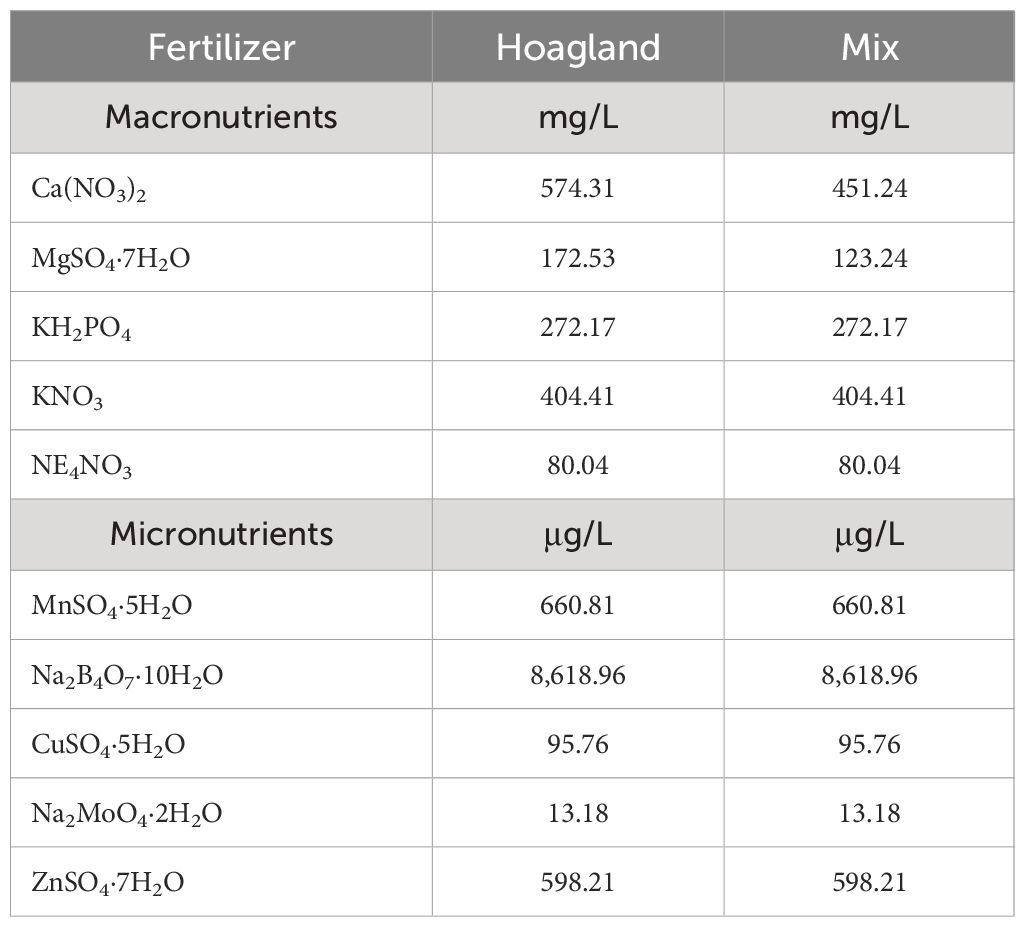
Table 3 Fertilizers, macronutrients, and micronutrients used per treatment of nutrient solution in both substrates.
The different sources of water used, as well as the different substrates, resulted in differences in both pH and EC (Table 4). The treatments with peat as the substrate resulted in slightly lower pH and higher EC than the corresponding treatments with perlite as the substrate, as peat has a pH of 6, acidifying water, and is enriched with nutrients of its own, leading to a higher EC. On the other hand, perlite, being an inert substrate, did not affect the pH or EC of the water. All treatments except for Fish–Perlite resulted in pH values lower than 7, which are acceptable for most hydroponic cultivations (Jones, 2014).
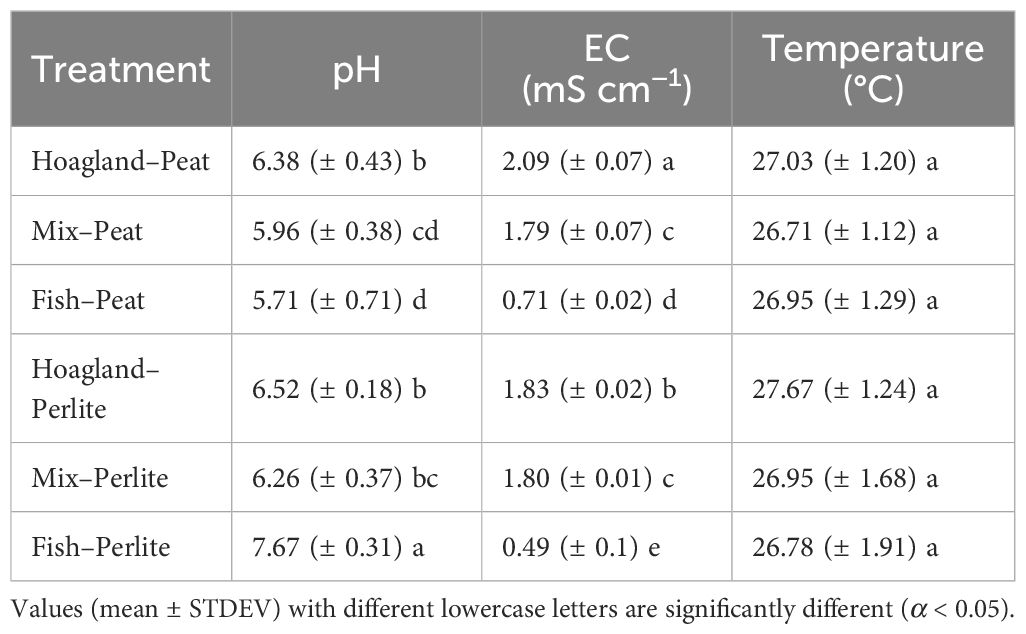
Table 4 Average pH, electrical conductivity (EC), and temperature of the daily record throughout the experiment in all treatments.
The nutrient solution analyzed after harvest presented an uneven fluctuation of the nutrients in each applied treatment (Table 5). It is notable that, in most of the treatments using fish wastewater, the phosphorus concentrations increased by the end of the experiment. The uneaten fish feed and feces that accumulated, which were transferred into the cultivation tanks and decomposed later during the cultivation, could possibly explain the irregularity between the initial and final concentrations of the fertilizers (Johnson et al., 2016). Similarly, peat, as an organic, nutrient-enriched substrate also appeared to increase the final nutrient solution concentrations, especially in comparison to the perlite treatments where a more obvious reduction was observed.
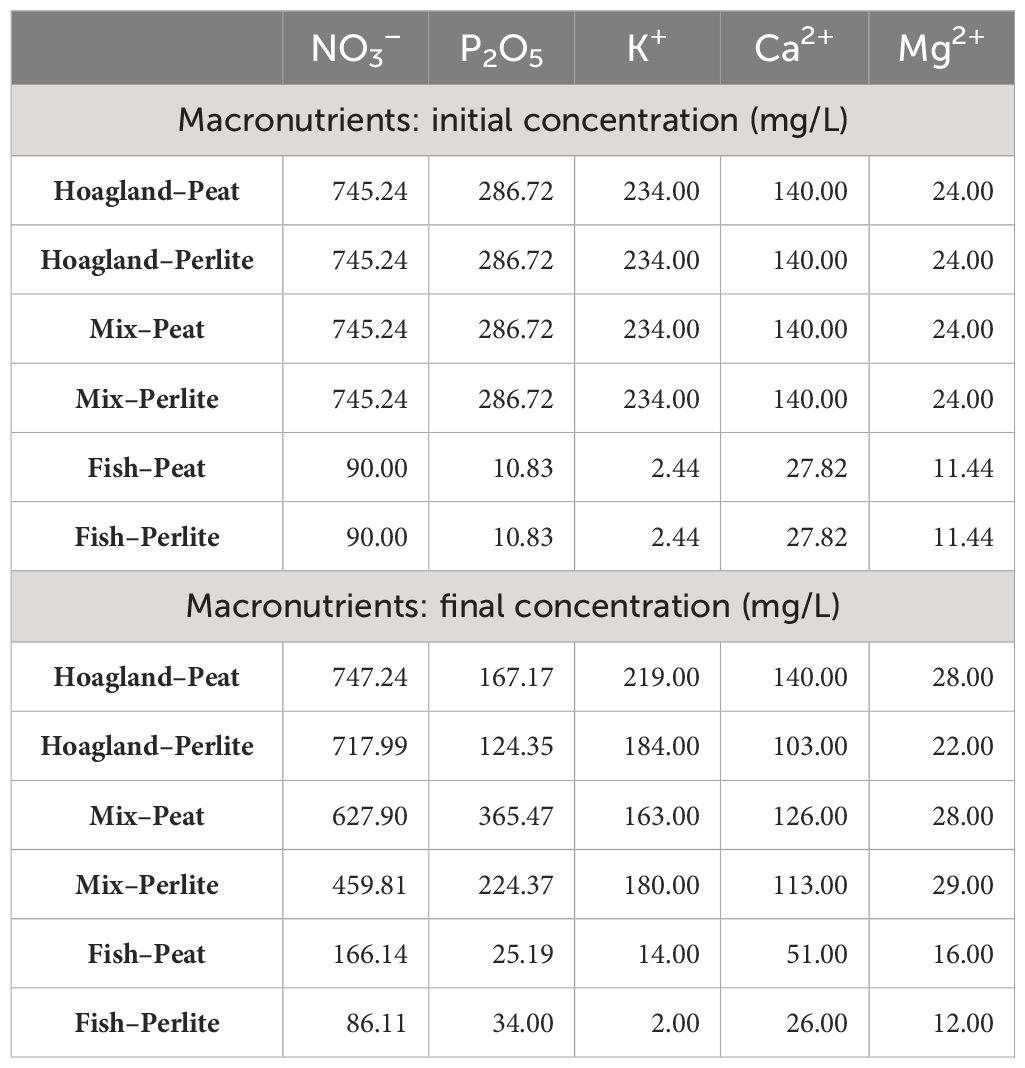
Table 5 Macronutrient initial concentrations of the nutrient solutions, as applied in the corresponding treatments, of both substrates used, in comparison to macronutrients’ final concentrations (mg L−1), separately for each nutrient solution and substrate treatment.
For the climate conditions, the air temperature during the experiment was controlled and kept, on average, at 25.86 ± 1.36°C, while the average daily minimum and maximum temperatures were at 22.78 ± 1.43°C and 31.19 ± 2.88°C, respectively. The optimum air temperatures for lettuce ranged from 17°C to 28°C in the daytime (Wurr et al., 1992), while these were lower for the rocket plants, which were set between 10°C and 25°C (Doležalová et al., 2013). Although the average temperatures were higher than the optimum values for lettuce and rocket growth, neither tip burn nor bolting occurred.
3.2 Yield and plant growth evaluation
The plants sown in perlite sprouted later than those in peat, exiting the germination chamber 3 days later. Phenotypic measurements taken throughout the experiment indicated a slight delay in the growth of the plants in perlite, which was particularly noticeable until approximately the first week of the experiment (12 DAS). However, this discrepancy gradually balanced out, leading to a higher growth from the 15th day onward. Regarding leaf length, which was the main criterion for harvest, all perlite-treated lettuce plants had a higher growth change than the peat-treated plants of the corresponding nutrient solution. Comparing Mix–Perlite to Mix–Peat, Hoagland–Perlite to Hoagland–Peat, and Fish–Perlite to Fish–Peat, it was found that the plants in perlite had 72%, 69%, and 53% higher growth percentage change, respectively, for each comparison. Differences were not as evident when comparing the leaf length percentage change of rocket plants, which led to 27%, 35%, and 73% higher growth change between the perlite and peat treatments in the Mix-, Hoagland-, and Fish-treated plants, respectively. Overall, the plants grown in peat exhibited a smoother growth throughout the experiment, whereas those in perlite tended to accelerate their growth later on. Nevertheless, despite the higher growth change of the plants in perlite during the final week, this was not adequate to keep up with the plants in peat, resulting in the harvest of peat and perlite plants occurring at different times. Comparison of the differences in the growth change percentage between the nutrient solutions of the same substrate showed that lettuce had the highest growth change percentage in Mix, both with peat and with perlite. On the other hand, the growth change of rocket in peat showed no statistically significant differences between the three nutrient solutions, while in perlite, Fish had the highest growth change in comparison to Hoagland and Mix; however, the final leaf growth was not sufficient according to the criteria set for harvesting. Finally, although the purpose of the experiment was not to compare lettuce and rocket directly, it is worth mentioning that the lettuce plants were better adjusted in the perlite substrate compared with the rocket plants.
All treatments with peat as a substrate were harvested at 21 DAS, while the perlite treatments for Hoagland and Mix were harvested 2 days later (23 DAS), both in lettuce and in rocket plants. For the Fish–Perlite treatment, due to the lack of sufficient growth, cultivation was extended at 32 days; nevertheless, the length was insufficient according to the set criteria in both cultivations, only reaching approximately 6 cm in lettuce and 2.5 cm in rocket plants.
The yield per treatment in lettuce varied from 1,070 to 2,497 g m−2 in the Fish–Perlite and Mix–Peat treatments, respectively. The Hoagland and Mix treatments in both substrates showed no statistically significant differences between them; however, they all differed significantly in both Fish treatments (Figure 3A). The results reported above indicate a significantly lower yield of the aquaponic treatment (Fish) in comparison to that of the other treatments. These results differed to a great extent from those reported by Alcarraz et al. (2018), who also cultivated baby lettuce in rainbow trout aquaponic effluent, but reported a higher yield in the aquaponic treatment in comparison to the hydroponic one. Nonetheless, the limited information provided on the composition of both the aquaponic and hydroponic nutrient solutions used in this study did not permit a safe comparison of the final yield or a meaningful interpretation of the observed differences. More studies have been conducted regarding the aquaponic cultivation of lettuce, either with rainbow trout or other fish species. However, these have primarily referred to head lettuce cultivation (Lennard and Leonard, 2006; Pantanella et al., 2010; Johnson et al., 2016; Velichkova et al., 2019; Birolo et al., 2020); therefore, the results on yield cannot be directly compared with those of the present findings.
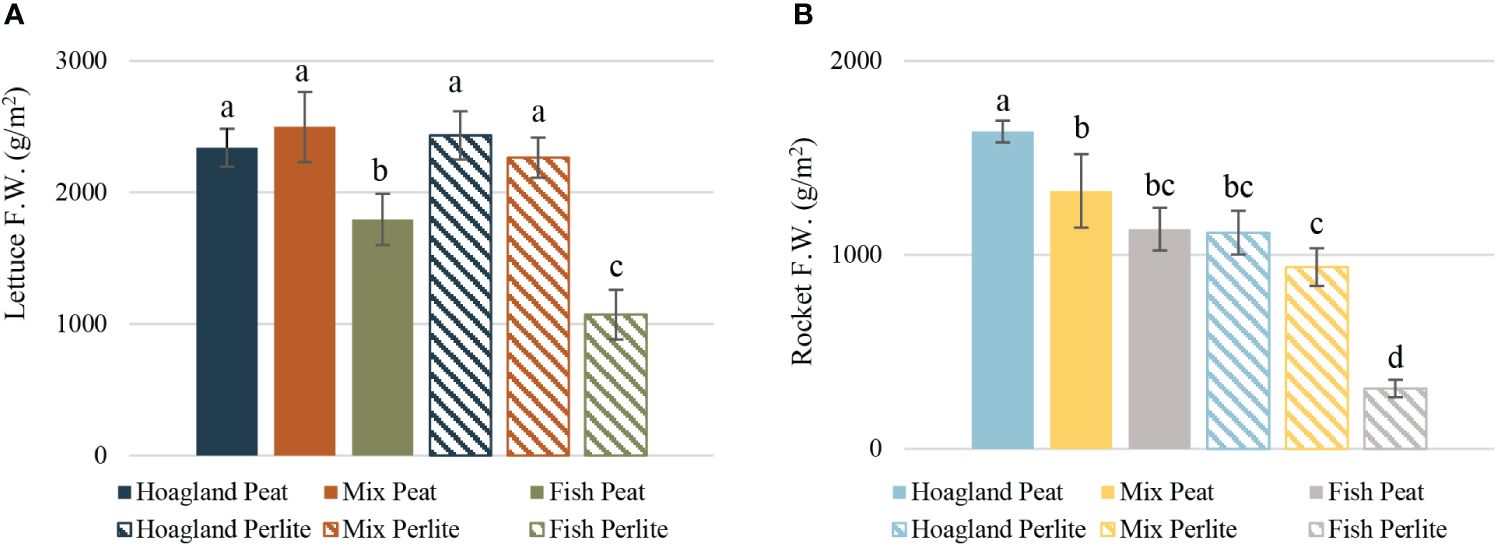
Figure 3 Yield of baby lettuce (A) and baby rocket (B) plants cultivated under different nutrient solutions of aquaponic water, mixed with fertilizers (Mix), or plain (Fish) and two different substrates (peat and perlite). Columns (FW g/m2 ± STDEV) with different letters are significantly different (α < 0.05).
Baby rocket plants presented higher variability in terms of yield among treatments. The Hoagland–Peat plants significantly had the highest yield at 1,636 g m−2, followed by the Mix–Peat, Fish–Peat, and Hoagland–Perlite plants, which showed no statistically significant differences between them. The yield of Fish–Perlite was the lowest (311 g m−2), being significantly different from the rest (Figure 3B). Baby leaf rocket production was also evaluated by Nicoletto et al. (2018) using the effluent of a pangasius fish aquaculture, which led to a yield of approximately 500 g m−2, varying between treatments. The differences in the yield between the latter and the present study could be justified by the dissimilarities in the nutrient solutions, as evident from the different ECs reported. Nonetheless, the same trend was followed in both experiments, where the Fish (aquaponic) treatment had the lowest yield in comparison to the Hoagland (hydroponic) treatment, which had the highest yield. Similar results have also been reported by Stathopoulou et al. (2021); however, plants of higher final size were evaluated in their study.
Overall, in baby lettuce, a clear pattern can be seen regarding the effect of the nutrient solution, with the substrate not appearing to affect the lettuce yield as much. On the other hand, the yield of rocket appeared to be significantly affected by the substrate used, and not only by the nutrient solution itself. In rocket, evaluation of the yield separately for each substrate showed that there was an obvious pattern where the yield of Hoagland was higher than that of Mix and the yield of Fish was the lowest, in both substrates. However, in total, there was significant dissimilarity between treatments in the same nutrient solution, but with the opposite substrate. The fact that the yield of Hoagland–Perlite was almost equal to that of Fish–Peat, for example, even though one was in full nutrient solution and the other was only grown on plain fish wastewater, strengthened the conclusion of the significant effect of the substrate used in rocket plants. For perlite, this might be justified by the absence of nutrients during the initial developmental stages of the plants and until trays were set in the cultivation tanks. This appeared to significantly affect the rocket plants, and this initial difference cannot be balanced later in the growth. On the contrary, lettuce plants can compensate for the difference during their growth, which was also confirmed by their higher difference in growth change in comparison to rocket. However, to our knowledge, there is no relevant study to compare our results with; therefore, further research is needed to elaborate better on these findings.
3.3 Nitrate concentrations in plant tissue
The nitrate concentrations in lettuce leaf tissue were the highest in Hoagland–Perlite (1,048 mg NO3− g−1 FW) and Mix–Perlite (1,018 mg NO3− g−1 FW), which were statistically significantly different from all other treatments. Furthermore, both had approximately 40% higher concentration than the corresponding nutrient solutions with the peat substrate. This, however, did not apply for Fish–Perlite in comparison to Fish–Peat, where the concentration was also higher in the perlite treatment, but not statistically significantly different from that of Fish–Peat (Figure 4A). Other aquaponic experiments on head lettuce reported concentrations varying from approximately 285 to 1,501 mg kg−1 FW (Pantanella et al., 2010) depending on the cultivation period and the fish density. In baby leaf lettuce, Alcarraz et al. (2018) reported 1,087 mg kg−1 FW in the aquaponic treatment, being significantly lower than that of the hydroponic one. This difference is in agreement with our findings when comparing Fish, regardless of the substrate, to the Hoagland and Mix–Perlite treatments; however, the nitrate concentration in the corresponding Fish treatment in the present study is almost half that reported by Alcarraz et al. (2018).
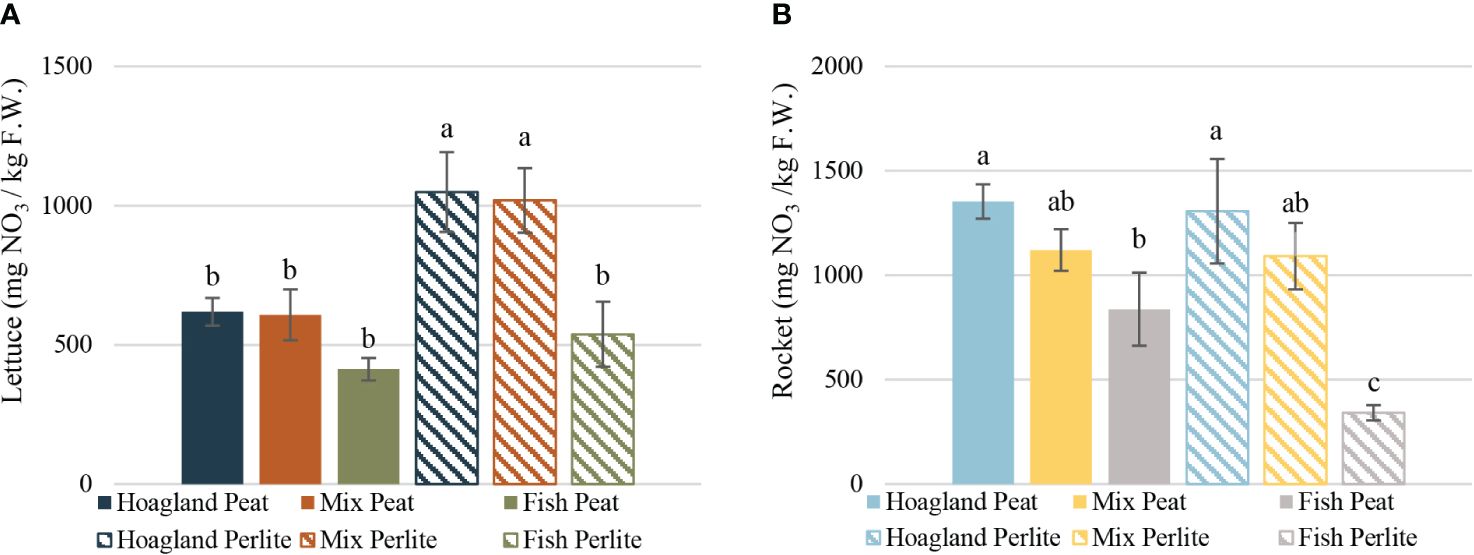
Figure 4 Nitrate content of baby lettuce (A) and baby rocket (B) plants cultivated under different nutrient solutions of aquaponic water, mixed with fertilizers (Mix), or plain (Fish) and two different substrates (peat and perlite). Columns (mean ± STDEV) with different letters are significantly different (α < 0.05).
In baby rocket plants, the concentration was the highest in Hoagland–Peat (1,352 mg NO3− g−1 FW), but was not statistically significantly different from that in Hoagland–Perlite or any of the Mix treatments. However, both Hoagland treatments differed significantly from both Fish treatments, while the Fish treatments also differed between them (Figure 4B). To the best of our knowledge, no study has been published yet regarding the nitrate concentration on the FW of rocket leaves in aquaponic cultivation.
The nitrate accumulation on leaf tissue could vary depending on the fertilization and environmental factors, mainly light intensity (Cantliffe, 1973). Despite any differences between treatments, as a result of varying nutrient solutions, no extreme value has been reported, while all the reported values were lower than those of the EU regulation limits (The European Commission, 2011).
3.4 Foliar analysis and color evaluation
Baby lettuce plants, despite the differences found between treatments regarding nutrient assimilation (Figure 5A), overall had little to no deficiencies according to the nutrient value ranges described in Neokleous (2014). Plants grown in the Hoagland and Mix treatments, regardless of the substrate used, showed no deficiency with regard to the macronutrient concentrations. The total nitrogen in these treatments ranged from 4.15% in Hoagland–Peat to 4.71% in Hoagland–Perlite, with no statistical differences with the Mix treatments. The total nitrogen in the Fish treatments ranged from 3.25% in perlite to 3.64% in peat, which were barely in range according to Neokleous (2014), while Fish–Perlite was the only treatment that was significantly lower in potassium concentration. Furthermore, the plants in Hoagland–Perlite and Mix–Perlite were barely below the limits set for Mn and were in range with regard to the other micronutrients. Plants with Fish–Peat treatment were inadequate only with regard to the boron concentrations (16.64 ppm), but this did not affect the overall plant image. On the other hand, Fish–Perlite plants showed deficiencies of most micronutrients, such as Fe (49.60 ppm), Mn (6.71 ppm), and boron (25.24 ppm), resulting in obvious chlorotic leaves that are unsuitable for consumption. Plants in both the Hoagland and Mix treatments, whether in peat or perlite, exhibited higher concentrations of all macronutrients, except for magnesium, compared with the hydroponic treatment in the study of Pantanella et al. (2010). The plants in the Fish treatments showed nutrient concentrations close to those of their respective aquaponic treatments, except for potassium in Fish–Perlite, which was significantly lower, and calcium and magnesium, which were notably higher.
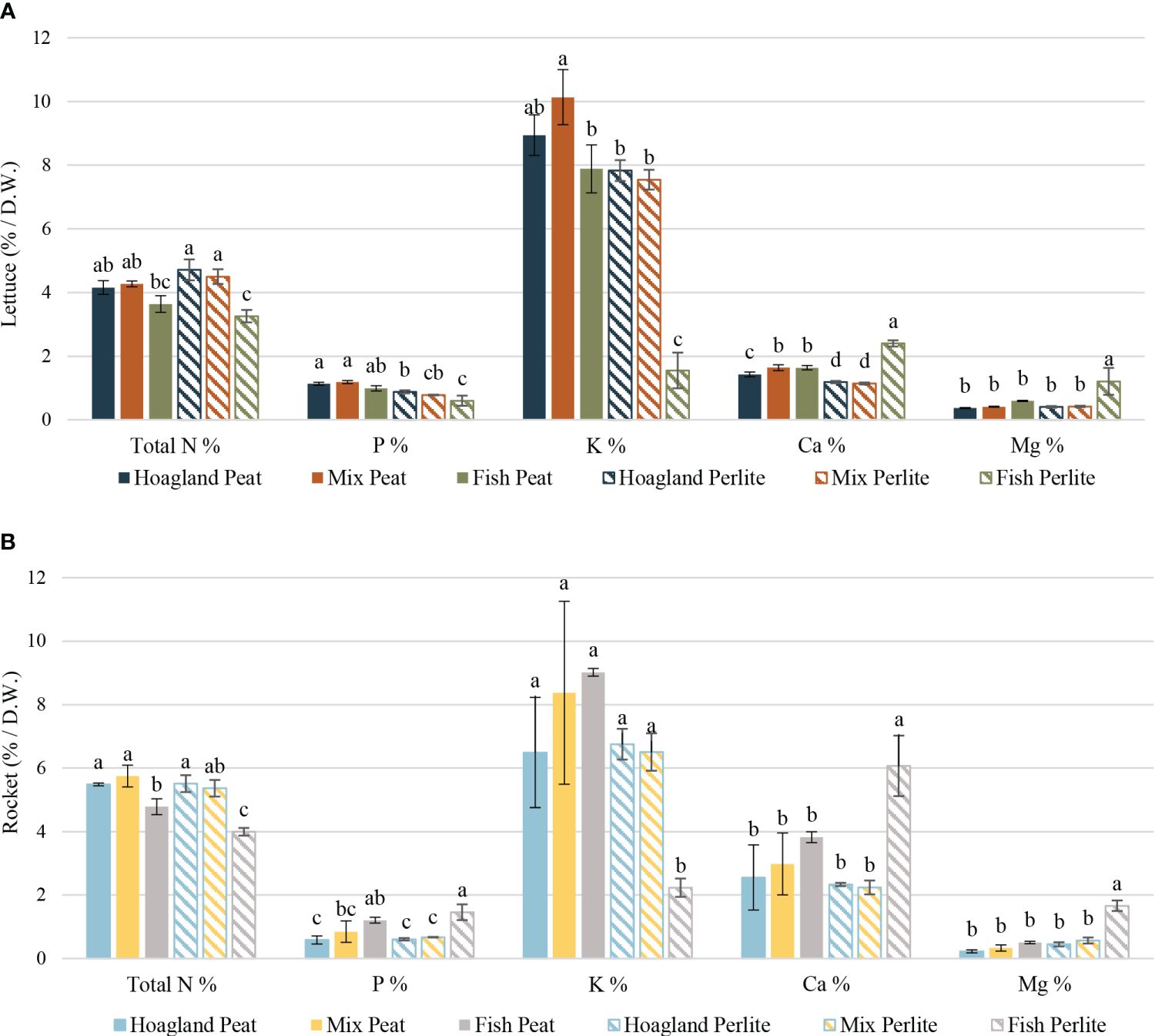
Figure 5 Plant nutrient concentrations of baby lettuce (A) and baby rocket (B) plants cultivated under different nutrient solutions of aquaponic water, mixed with fertilizers (Mix), or plain (Fish) and two different substrates (peat and perlite). Columns (mean ± STDEV) with different letters are significantly different (α < 0.05).
In baby rocket plants, important differences in the concentration of most macronutrients were mainly detected in the Fish–Perlite treatment in comparison to others (Figure 5B). Fish–Perlite plants exhibited significantly low concentrations of total nitrogen (4.00%) and potassium (2.23%), while conversely showing significantly high concentrations of calcium (6.07%) and magnesium (1.66%). In terms of phosphorus, both Fish treatments had higher concentrations compared with others, 1.20% and 1.46% for Peat and Perlite, respectively, particularly those utilizing the Hoagland nutrient solution. Similarly to lettuce plants, with respect to micronutrients, the Fish–Perlite plants demonstrated notably lower concentrations of boron (35.74 ppm), iron (35.87 ppm), and manganese (5.44 ppm) compared with other treatments. Nicoletto et al. (2018) also confirmed the effect of different aquaponic or hydroponic nutrient solutions on the plant nutrient concentrations, but specific data between the applied treatments have not been provided. In total, the results of the plant nutrient concentrations indicate that rocket plants grown in Fish–Perlite treatment are unsuitable for consumption due to the widely chlorotic leaves.
Overall, both species were influenced by the nutrient solution. In both plants grown in the Fish–Perlite treatment, similarities were observed in the particularly low potassium uptake and the higher calcium and magnesium uptake in comparison to the other treatments. The fish wastewater was characterized by a low EC (0.51 mS cm−1) and low potassium concentrations (2.44 mg L−1). On the other hand, perlite, which served as an inert substrate, did not contribute additional nutrients to the plants. The low EC and low K/Ca ratio, which was the case in the Fish–Perlite nutrient solution, have been reported to lead to lower potassium and increased magnesium concentrations on the fully expanded leaves of lettuce (Huett, 1994), justifying the findings of the present study for both lettuce and rocket plants.
Comparing both the lettuce and rocket plants to the corresponding cultivations in the DWC hydroponic system in Karnoutsos et al. (2021), not many differences were observed in the Hoagland and Mix treatments of either substrate. The potassium, calcium, and magnesium concentrations were similar to those in our study. Nitrogen was approximately 1% higher, and phosphorus also had certain differences depending on the substrate used.
The chlorosis observed in the Fish–Perlite plant leaves of both cultivations was also established by the leaf color measurements taken after harvest (Figures 6A, B). The hue angle (h°), being the main index for the color parameters (Mcguire, 1992), as well as the lightness (L*) data, confirmed that the Fish–Perlite plants had a smaller ratio of green and yellow and a higher lightness, meaning that they are more yellow than the rest of the treatments. Severe Fe and Mn deficiencies could be responsible for such a discoloration; however, it cannot be attributed solely to one nutrient. In lettuce, the Mix–Peat plant leaves were also statistically different from the rest, although were greener and darker. In rocket plants, the Hoagland–Perlite leaves had a deeper green color than the rest, while any other differences detected regarding the lightness of the leaves did not affect the overall color and the b*/a* ratio.
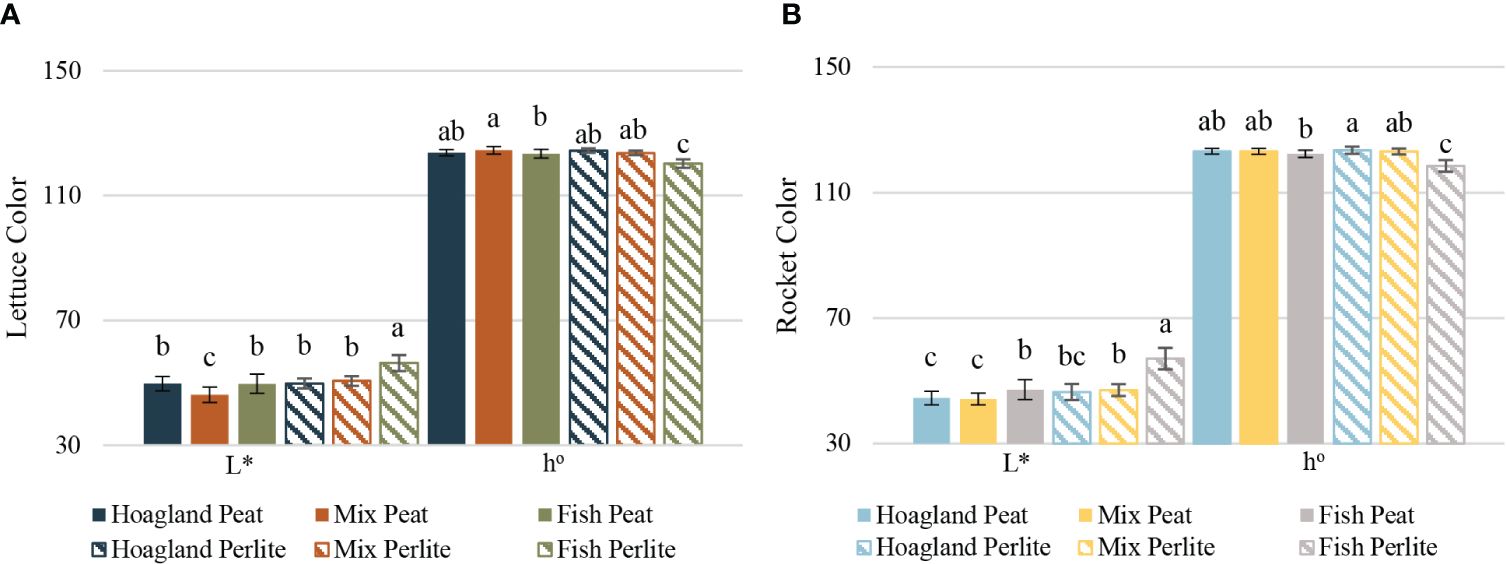
Figure 6 Color parameters of baby lettuce (A) and baby rocket (B) plants cultivated under different nutrient solutions of aquaponic water, mixed with fertilizers (Mix), or plain (Fish) and two different substrates (peat and perlite). The lightness (L*) and hue angle (b*/a*) are presented for each plant species. Columns (mean ± STDEV) with different letters are significantly different (α < 0.05).
3.5 Photosynthetic parameters
The photosynthetic rate, as measured the day before harvesting, showed no statistically significant differences between treatments in lettuce plants (Figure 7A); in rocket (Figure 7B), only the Fish–Perlite plants showed significantly lower photosynthetic rates than the rest. The values in lettuce varied from 9.30 μmol CO2 m−2 s−1 in the Fish treatments to 10.99 μmol CO2 m−2 s−1 in Hoagland–Perlite, similar to the lettuce in a Hoagland solution by Karnoutsos et al. (2021). Rocket, on the other hand, deviated largely from 7.23 μmol CO2 m−2 s−1 in Fish–Perlite to 20.96 μmol CO2 m−2 s−1 in the Mix–Perlite treatment, being higher overall than that reported by Karnoutsos et al. (2021) in a similar rocket cultivation. The differences observed in the photosynthesis in rocket plants between the two studies could be explained by the differences in the climatic conditions during the measurements. Although the results cannot be directly compared, they provide certain information on the general condition of our plants.
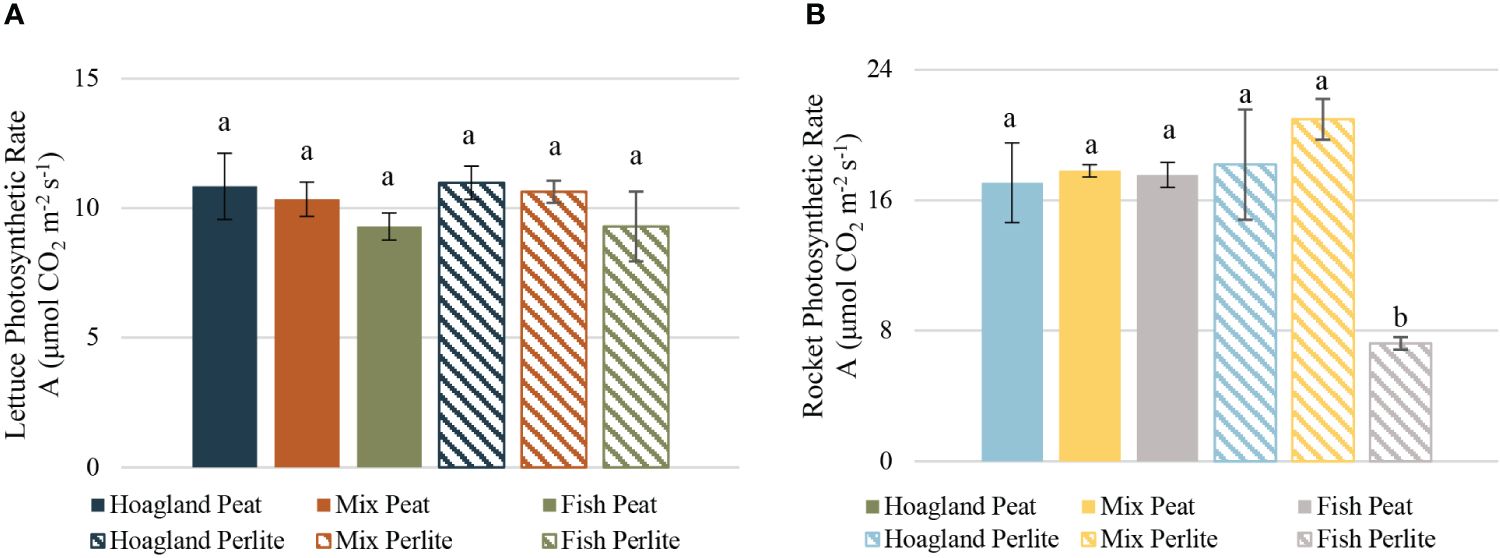
Figure 7 Photosynthetic rates of baby lettuce (A) and baby rocket (B) plants cultivated under different nutrient solutions of aquaponic water, mixed with fertilizers (Mix), or plain (Fish) and two different substrates (peat and perlite). Columns (mean ± STDEV) with different letters are significantly different (α < 0.05).
Numerous studies have reported that higher nitrogen concentrations in the nutrient solution can lead to an increased photosynthetic rate and, consequently, to a higher FW of plants (Petropoulos et al., 2016). In the present study, the higher nitrogen concentration was associated, to some extent, with higher yields; however, this was not confirmed by the differences in the photosynthetic rates between treatments. In lettuce, the Fish treatments had lower photosynthetic rates, corresponding to lower yields, but were not statistically significant. In rocket plants, the individual differences in yield did not imply a difference in the rate of photosynthesis, except for the plants treated with Fish–Perlite, where the significantly lower photosynthetic rates corresponded to a significantly lower yield as well. An increased photosynthetic rate might be a requirement for higher yield, but does not necessarily lead to that, as the photosynthesis measured in single leaves does not always correlate with the total plant biomass (Dannehl et al., 2021).
The transpiration rate was also evaluated in the present study, giving results slightly lower than those reported by Karnoutsos et al. (2021). However, the transpiration rates, being directly related to the air temperature and RH, were not comparable either between the different studies or between all our treatments, as measurements were performed on different days based on the harvest date.
3.6 Fish performance
Fish generally coped very well throughout the cultivation period, and mortality was only 2.65%. Minor agonistic behavior was detected, but there were no signs of abnormal swimming. Following the first 2 days, the fish were generally highly active in terms of swimming and feeding behaviors which was reflected in the great condition factor, the satisfactory FCRs, and the minor mortalities throughout the rearing period. The initial Fulton’s condition factor was 1.05 ± 0.21, while the condition factor of the fish during the experiment was 1.42 ± 0.16. The mean FCR was 0.89 ± 0.08 in the two tanks, which is similar to that in Becke et al. (2017) or better (Fanizza et al., 2023) compared with other works on rainbow trout inside RAS. Overall, this result is excellent considering that trout growers are normally satisfied with FCR values of 1.20–1.40 (Uysal and Alpbaz, 2001).
3.7 Resources use efficiency
From the comparison of the LUE index values, it emerged that the best case of successful aquaponics was the Mix–Peat treatment, where an increased yield was observed in both baby leaf vegetables and fish in the same area unit (Table 6), resulting in 7% higher LUE than that of Hoagland–Peat. The same indicator was greatly increased especially in relation to the scenario where the fish farming system would be in an adjacent area and not under the greenhouse. In this case, the area unit would be double; therefore, the index would have been calculated at 1,079.8 g m−2.
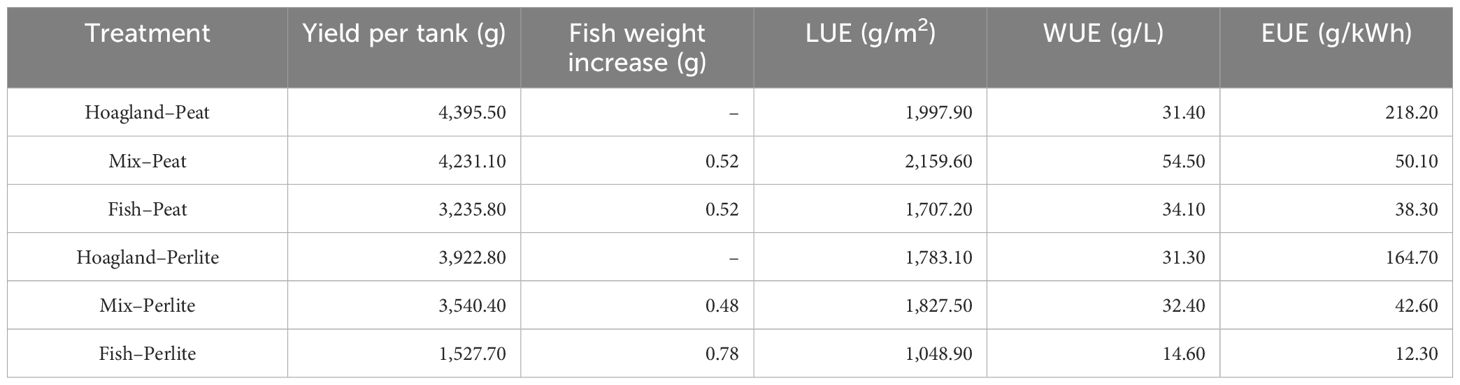
Table 6 Resource use efficiency—land use efficiency (LUE), water use efficiency (WUE), and energy use efficiency (EUE)—of the examined treatments considering the yield of both cultivations per tank and the weight increase in fish during the experiment.
Similar findings were observed for the WUE index, where the best case was still Mix–Peat, saving approximately 38% more water than Hoagland–Peat. The water used for the Mix and Fish treatments was a processed by-product from the RAS and was hence reused, in contrast to the water in the Hoagland treatments. Thus, the amount of water that would have to be used if the hydroponics and fish farming systems were autonomous would be double, confirming the importance of aquaponics in optimizing water use. Moreover, it has been mentioned that the WUE in aquaponics reaches 90% in relation to conventional agriculture (Ibrahim et al., 2023), which was also verified in the present work. The WU in aquaponics has been reported to be 2.7–3.0 m3 kg−1 of shrimp produced, while this value was calculated at 0.5–0.8 m3 kg−1 when both products were considered as outputs (Alarcón-Silvas et al., 2021).
Finally, for the EUE index, due to the high energy consumption required for the operation of the fish rearing system and the simultaneous small weight gain of fish due to their young age, its value was particularly low in the treatments where fish wastewater was used (Mix and Fish). This result shows the importance of using renewable energy sources, such as solar energy, for the operation of aquaponic systems. Nonetheless, in other studies, it has been reported that the EUE in the nutrient film technique (NFT) was calculated at 31.30 g kWh−1, while that in a DWC system was 24.53 g kWh−1 (Gillani et al., 2022), being, overall, close to our findings in Mix–Peat and Mix–Perlite.
4 Conclusions
Baby lettuce and baby rocket plants grown in a greenhouse, as part of a vertical decoupled aquaponic system, were evaluated in terms of yield and quality in three different nutrient solutions (Hoagland, Mix, and Fish) and two different substrates (peat and perlite). Each treatment was also evaluated based on its land, water, and energy use efficiency to assess the use of resources of the vertical decoupled aquaponic system developed in this study. In total, mixing fish wastewater with synthetic fertilizers (the Mix treatment) can save approximately 38% of water and 10% fertilizers in comparison to the control. The vertical layout offered optimal land use in comparison to the one-level combined cultivation, with approximately 7% better LUE. The lettuce cultivated in peat adjusted perfectly in the Mix treatment, showing the highest yield and not lagging in quality. The rocket plants, however, did not adjust as well, which showed optimum yield in the Hoagland–Peat (control) treatment. The present study, which used the wastewater of rainbow trout, confirmed that the combined cultivation of vegetables and fish benefits the simultaneous production of two products while also sustaining resources in terms of water, land, and, partially, fertilizers. Energy optimization using renewable energy sources would be interesting to investigate in future studies along with a techno-economic feasibility analysis.
Data availability statement
The raw data supporting the conclusions of this article will be made available by the authors, without undue reservation.
Ethics statement
The Ethics approval for the experimental use of the rainbow trout (Oncorhynchus mykiss) was granted by the University of Western Macedonia local ethics committee given the protocol code 101, whereas fish receipt was confirmed by the Department of Aquaculture Development, Greek Ministry of Rural Development and Food, Decision number 167/41275/02–06-2022.
Author contributions
IC: Data curation, Formal Analysis, Investigation, Methodology, Visualization, Writing – original draft. MR: Data curation, Investigation, Validation, Writing – original draft. DP: Data curation, Investigation, Validation, Writing – original draft. GK: Data curation, Writing – review & editing. IG: Writing – review & editing. AT: Project administration, Resources, Supervision, Writing – review & editing. AK: Resources, Writing – review & editing. GN: Conceptualization, Funding acquisition, Project administration, Resources, Supervision, Writing – review & editing.
Funding
The author(s) declare financial support was received for the research, authorship, and/or publication of this article. This research was co-financed by the European Regional Development Fund of the European Union and Greek national funds under the framework of the Action «Investment Plans of Innovation» of the Operational Program «Central Macedonia 2014 2020» (project code: KMR6–0279604).
Conflict of interest
The authors declare that the research was conducted in the absence of any commercial or financial relationships that could be construed as a potential conflict of interest.
Publisher’s note
All claims expressed in this article are solely those of the authors and do not necessarily represent those of their affiliated organizations, or those of the publisher, the editors and the reviewers. Any product that may be evaluated in this article, or claim that may be made by its manufacturer, is not guaranteed or endorsed by the publisher.
References
Adler P. R., Harper J. K., Takeda F., Wade E. M., Summerfelt S. T. (2000). Economic evaluation of hydroponics and other treatment options for phosphorus removal in aquaculture effluent. Am. Soc. Hortic. Sci. 35, 993–999. doi: 10.21273/HORTSCI.35.6.993
Alarcón-Silvas S. G., León-Cañedo J. A., Fierro-Sañudo J. F., Ramírez-Rochín J., Fregoso-López M. G., Frías-Espericueta M. G., et al. (2021). Water quality, water usage, nutrient use efficiency and growth of shrimp Litopenaeus vannamei in an integrated aquaponic system with basil Ocimum basilicum. Aquaculture 543. doi: 10.1016/j.aquaculture.2021.737023
Alcarraz E., Flores M., Tapia M. L., Bustamante A., Wacyk J., Escalona V. (2018). “Quality of lettuce (Lactuca sativa L.) grown in aquaponic and hydroponic systems,” in Acta Horticulturae (Leuven, Belgium: International Society for Horticultural Science), 31–38. doi: 10.17660/ActaHortic.2018.1194.6
Becke C., Steinhagen D., Schumann M., Brinker A. (2017). Physiological consequences for rainbow trout (Oncorhynchus mykiss) of short-term exposure to increased suspended solid load. Aquac Eng. 78, 63–74. doi: 10.1016/j.aquaeng.2016.11.001
Birolo M., Bordignon F., Trocino A., Fasolato L., Pascual A., Godoy S., et al. (2020). Effects of stocking density on the growth and flesh quality of rainbow trout (Oncorhynchus mykiss) reared in a low-tech aquaponic system. Aquaculture 529. doi: 10.1016/j.aquaculture.2020.735653
Blidariu F., Grozea A. (2011). Increasing the Economical Efficiency and Sustainability of Indoor Fish Farming by Means of Aquaponics-Review. Scientific Papers: Animal Science and Biotechnologies, 44.
Buzby K. M., Lin L. S. (2014). Scaling aquaponic systems: Balancing plant uptake with fish output. Aquac Eng. 63, 39–44. doi: 10.1016/j.aquaeng.2014.09.002
Cantliffe D. J. (1973). Nitrate accumulation in table beets and spinach as affected by nitrogen, phosphorus, and potassium nutrition and light intensity 1. Agron. J. 65, 563–565. doi: 10.2134/agronj1973.00021962006500040012x
Cataldo D. A., Haroon M. H., Schrader L. E., Youngs V. L. (1975). Rapid colorimetric determination of nitrate in plant tissue by nitration of salicylic acid. Commun. Soil Sci. Plant Anal. 6, 71–80. doi: 10.1080/00103627509366547
Dannehl D., Schwend T., Veit D., Schmidt U. (2021). Increase of yield, lycopene, and lutein content in tomatoes grown under continuous PAR spectrum LED lighting. Front. Plant Sci. 12. doi: 10.3389/fpls.2021.611236
Delaide B., Teerlinck S., Decombel A., Bleyaert P. (2019). Effect of wastewater from a pikeperch (Sander lucioperca L.) recirculated aquaculture system on hydroponic tomato production and quality. Agric. Water Manag 226. doi: 10.1016/j.agwat.2019.105814
Doležalová I., Duchoslav M., Dušek K. (2013). Biology and Yield of Rocket (Eruca sativa Mill.) under Field Conditions of the Czech Republic (Central Europe). Not Bot. Horti Agrobo 41, 530–537. doi: 10.15835/nbha4129281
Fanizza C., Trocino A., Stejskal V., Prokešová M. D., Zare M., Tran H. Q., et al. (2023). Practical low-fishmeal diets for rainbow trout (Oncorhynchus mykiss) reared in RAS: Effects of protein meals on fish growth, nutrient digestibility, feed physical quality, and faecal particle size. Aquac Rep. 28. doi: 10.1016/j.aqrep.2022.101435
Gillani S. A., Abbasi R., Martinez P., Ahmad R. (2022). “Comparison of energy-use efficiency for lettuce plantation under nutrient film technique and deep-water culture hydroponic systems,” in Procedia Computer Science (Amsterdam, Netherlands: Elsevier B.V), 11–19. doi: 10.1016/j.procs.2022.12.197
Hoagland D. R., Arnon D. I. (1950). The water-culture method for growing plants without soil., ed. Arnon D. I.. Berkeley, California: College of Agriculture, University of California.
Huett D. O. (1994). “Growth, nutrient uptake and tipburn severity of hydroponic lettuce in response to electrical conductivity and K : Ca ratio in solution,” in Aust. J. Agric. Res. 45, 251–67. doi: 10.1071/AR9940251
Ibrahim L. A., Shaghaleh H., El-Kassar G. M., Abu-Hashim M., Elsadek E. A., Alhaj Hamoud Y. (2023). Aquaponics: A sustainable path to food sovereignty and enhanced water use efficiency. Water (Switzerland) 15. doi: 10.3390/w15244310
Johnson G. E., Buzby K. M., Semmens K. J., Waterland N. L. (2016). Year-round lettuce (Lactuca sativa L.) production in a flow-through aquaponic system. J. Agric. Sci. 9, 75. doi: 10.5539/jas.v9n1p75
Jones J. B. (2014). Complete Guide for Growing Plants Hydroponically. 1st Edn (Boca Raton, Florida, USA: CRC Press, Taylor and Francsis Group). doi: 10.1201/b16482
Karnoutsos P., Karagiovanidis M., Bantis F., Chatzistathis T., Koukounaras A., Ntinas G. K. (2021). Controlled root-zone temperature effect on baby leaf vegetables yield and quality in a floating system under mild and extreme weather conditions. J. Sci. Food Agric. 101, 3933–3941. doi: 10.1002/jsfa.11033
Lennard W. A., Leonard B. V. (2006). A comparison of three different hydroponic sub-systems (gravel bed, floating and nutrient film technique) in an Aquaponic test system. Aquaculture Int. 14, 539–550. doi: 10.1007/s10499-006-9053-2
Martins C. I. M., Eding E. H., Verdegem M. C. J., Heinsbroek L. T. N., Schneider O., Blancheton J. P., et al. (2010). New developments in recirculating aquaculture systems in Europe: A perspective on environmental sustainability. Aquac Eng. 43, 83–93. doi: 10.1016/j.aquaeng.2010.09.002
Martsikalis P., Gkafas G. A., Apostolidis A., Exadactylos A. (2014). Genetic structure profile of rainbow trout (Oncorhynchus mykiss) farmed strains in Greece. Turk J. Fish Aquat Sci. 14, 749–757. doi: 10.4194/1303-2712-v14_3_17
Mcguire R. G. (1992). Reporting of Objective Color Measurements. Am. Soc. Horticultural Sci. 27, 1254–1255. doi: 10.21273/HORTSCI.27.12.1254
Mózner Z., Tabi A., Csutora M. (2012). Modifying the yield factor based on more efficient use of fertilizer - The environmental impacts of intensive and extensive agricultural practices. Ecol. Indic 16, 58–66. doi: 10.1016/j.ecolind.2011.06.034
Neokleous D. (2014). Hydroponics Manual. (Nicosia, Cyprus: Ministry of Agriculture, Rural Development and the Environment).
Nicoletto C., Maucieri C., Mathis A., Schmautz Z., Komives T., Sambo P., et al. (2018). Extension of aquaponic water use for NFT baby-leaf production: mizuna and rocket salad. Agronomy 8. doi: 10.3390/agronomy8050075
Pantanella E., Cardarelli M., Colla G., Rea E., Marcucci A. (2010). Aquaponics vs. Hydroponics: Production and Quality of Lettuce Crop., in XXVIIIth IHC – IS on Greenhouse and Soilless Cultivation, ed. Castilla N. (in Leuven, Belgium: Acta Horticulturae), 887–893. doi: 10.17660/actahortic.2012.927
Petropoulos S. A., Chatzieustratiou E., Constantopoulou E., Kapotis G. (2016). Yield and quality of lettuce and rocket grown in floating culture system. Not Bot. Horti Agrobot Cluj Napoca 44, 603–612. doi: 10.15835/nbha44210611
Rakocy J. E., Masser M. P., Losordo T. M. (2003). Recirculating Aquaculture Tank Production Systems: Aquaponics-Integrating Fish and Plant Culture. Available online at: http://osufacts.okstate.edu.
Rodgers D., Won E., Timmons M. B., Mattson N. (2022). Complementary nutrients in decoupled aquaponics enhance basil performance. Horticulturae 8. doi: 10.3390/horticulturae8020111
Stathopoulou P., Tsoumalakou E., Levizou E., Vanikiotis T., Zaoutsos S., Berillis P. (2021). Iron and potassium fertilization improve rocket growth without affecting tilapia growth and histomorphology characteristics in aquaponics. Appl. Sci. (Switzerland) 11. doi: 10.3390/app11125681
Subasinghe R., Soto D., Jia J. (2009). Global aquaculture and its role in sustainable development. Rev. Aquac 1, 2–9. doi: 10.1111/j.1753-5131.2008.01002.x
Suhl J., Dannehl D., Kloas W., Baganz D., Jobs S., Scheibe G., et al. (2016). Advanced aquaponics: Evaluation of intensive tomato production in aquaponics vs. conventional hydroponics. Agric. Water Manag 178, 335–344. doi: 10.1016/j.agwat.2016.10.013
The European Commission (2011). COMMISSION REGULATION (EU) no 1258/2011. Off. J. Eur. Union. 54, 17–19. doi: 10.2903/j.efsa.2010.1935
The European Commission (2014). COMMISSION REGULATION (EU) No 752/2014 (Brussels). Available online at: https://eur-lex.europa.eu/legal-content/EN/TXT/?uri=celex%3A32014R0752 (Accessed February 8, 2024).
Tomasi N., Pinton R., Dalla Costa L., Cortella G., Terzano R., Mimmo T., et al. (2015). New “solutions” for floating cultivation system of ready-to-eat salad: A review. Trends Food Sci. Technol. 46, 267–276. doi: 10.1016/j.tifs.2015.08.004
Tsoumalakou E., Mente E., Kormas K. A., Katsoulas N., Vlahos N., Kapsis P., et al. (2022). Precise monitoring of lettuce functional responses to minimal nutrient supplementation identifies aquaponic system’s nutrient limitations and their time-course. Agric. (Switzerland) 12. doi: 10.3390/agriculture12081278
Tyson R. V., Simonne E. H., White J. M., Lamb E. M. (2004). Reconciling Water Quality Parameters Impacting Nitrification In Aquaponics: The Ph Levels, in 117th conference of Florida State Horticultural Society, (Florida, USA: Watten and Busch), 79–83.
Uysal I., Alpbaz A. (2001). Food Intake and Feed Conversion Ratios in Abant Trout (Salmo trutta abanticus T. 1954) and Rainbow Trout (Oncorhynchus mykiss W. 1792) in Pond Culture. Turkish J. Biol. 26, 83–88.
Velichkova K., Sirakov I., Stoyanova S., Staykov Y. (2019). Cultivation of lettuce (Lactuca sativa L.) and rainbow trout (oncorhynchus mykiss w.) in the aquaponic recirculation system. J. Cent. Eur. Agric. 20, 967–973. doi: 10.5513/JCEA01/20.3.2223
Keywords: decoupled aquaponics, recirculating aquaculture system, hydroponics, deep water culture, land use efficiency, water use efficiency
Citation: Chatzigeorgiou I, Ravani M, Papadopoulos DK, Kelesidis G, Giantsis IA, Tsaballa A, Koukounaras A and Ntinas GK (2024) Greenhouse production of baby leaf vegetables using rainbow trout wastewater in a high-tech vertical decoupled aquaponic system. Front. Hortic. 3:1418447. doi: 10.3389/fhort.2024.1418447
Received: 16 April 2024; Accepted: 10 June 2024;
Published: 01 July 2024.
Edited by:
Jung Eek Son, Seoul National University, Republic of KoreaReviewed by:
Jiangtao Hu, Chinese Academy of Agricultural Sciences, ChinaBeppe Benedetto Consentino, University of Palermo, Italy
Dongpil Kim, Rural Development Administration, Republic of Korea
Copyright © 2024 Chatzigeorgiou, Ravani, Papadopoulos, Kelesidis, Giantsis, Tsaballa, Koukounaras and Ntinas. This is an open-access article distributed under the terms of the Creative Commons Attribution License (CC BY). The use, distribution or reproduction in other forums is permitted, provided the original author(s) and the copyright owner(s) are credited and that the original publication in this journal is cited, in accordance with accepted academic practice. No use, distribution or reproduction is permitted which does not comply with these terms.
*Correspondence: Georgios K. Ntinas, Z250aW5hc0BlbGdvLmdy