- 1Department of Plant and Environmental Sciences, New Mexico State University, Las Cruces, NM, United States
- 2Department of Tropical Plant and Soil Sciences, University of Hawaii at Manoa, Honolulu, HI, United States
- 3Hawaii Agriculture Research Center, Honolulu, HI, United States
- 4Department of Horticulture, University of Georgia (UGA), Tifton, GA, United States
Climate change creates a leverage point in plant breeding. We must rethink the way we employ plant breeding, utilize genetic diversity, and prioritize edible plant production across the landscape. A long-term strategy to ‘redirect’ the process of crop domestication that prioritizes the generation of abiotic and biotic stress-tolerant cultivars is needed. A proposed neo-domestication strategy is presented as a case study. It entails the re-domestication of chile by retro-crossing of modern plant cultivars with landraces and a desert-adapted wild relative. An organized, collaborative effort could reintroduce stress tolerance genes that will render new cultivars more tolerant to challenges imposed by climate change. Purposeful populations generated using a broad spectrum of genetic diversity could also provide the basis for an evolutionary–participatory plant breeding process. The process must recognize the need for a paradigm shift in our resource allocation and breeding strategies. To succeed, a shared vision for neo-domestication of chile must recognize the importance of human cultural values and the need for sustained cooperation among stakeholders.
Introduction
Climate change is an impetus for neo-domestication of modern crop cultivars
Climate change has caused economic losses to agricultural production in many geographical regions (Mora et al., 2018; Brás et al., 2021). Sustainable crop production has been imperiled by climate change-induced weather events (Lobell et al., 2008; Bradshaw et al., 2021). The impacts of temperature and rainfall extremes range from seasonal reductions in crop yields or quality to long-term issues such as altered crop adaptation (McCoy et al., 2022; Gao et al., 2023).
The rate of average genetic improvements in crops must increase by an estimated 38% per year to keep up with growing food demand (Tester and Langridge, 2010). There seems to be an inherent assumption that increased yield potential of crops in our dominant food systems will be achieved by multinational seed and biotechnology corporations employing proprietary technologies. Improvements in major genetically modified organism (GMO) crops produced in developed nations will not address the caloric and nutritional needs of food-insecure regions of the world suffering marked impacts of climate change (climate risk ‘hot spots’) (Lobell et al., 2008).
The rising challenge of climate change compels the need for more stress-tolerant varieties (Zhang et al., 2017). The primary source of genetic diversity to achieve genetic gain through selection in modern crop cultivars would come preferentially from other elite cultivars whenever possible, with ‘vintage’ varieties and landraces a second-choice. Wild species would be a reluctant last preference when desired traits are not present in modern cultivars or cannot be made available through a novel gene technology (Smith et al., 2022).
Genetic diversity is reduced during the process of crop domestication and improvement (Figure 1). Populations growing in marginal environments may also experience a loss of genetic diversity depending on the severity of stress imposed on them. Both the limited genetic diversity and the lack of adaptation to stressful environments in elite cultivars restrict our ability to confront the growing challenge. During crop domestication, many genes that conferred fitness of crop wild relatives (CWR) in their natural environment were lost (Byrne et al., 2020; Krug et al., 2023). In addition to the loss of adaptive alleles through drift, it is unlikely that there was adequate sampling of stress tolerance alleles during the early, spatially limited phases of crop domestication. Natural populations inhabiting more stressful or marginal environments were less conducive to sustained human selection for agronomically desirable traits. Understandably, a modern selection achieved higher crop responsiveness to inputs in favorable environments rather than the resilience necessary for meeting the challenges of climate change (Snowdon et al., 2021). Considering the events related to domestication, artificial selection, and the current status of breeding practices, we are led to ask the question:
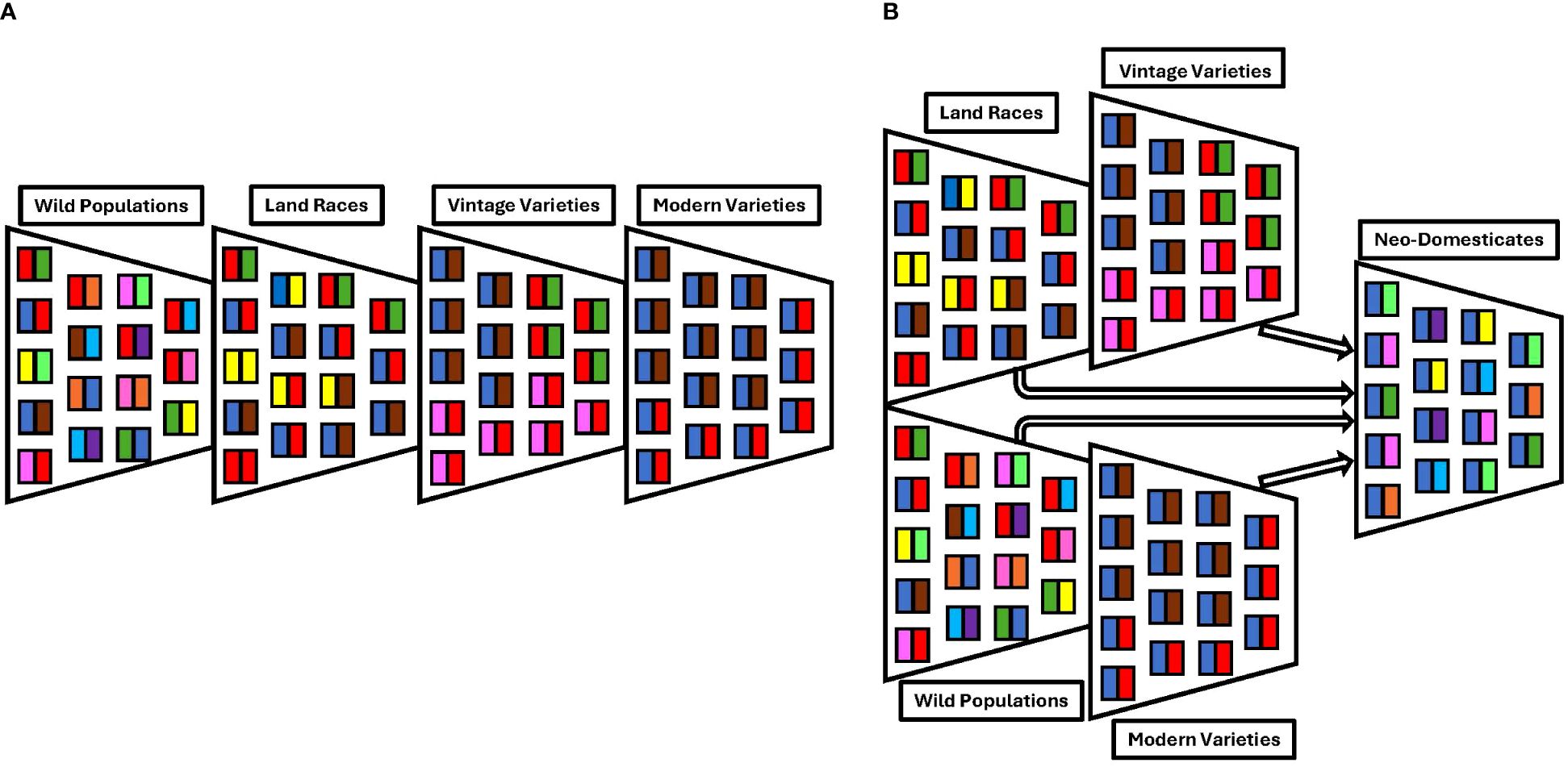
Figure 1. Here, we present two models of the loss of genetic diversity during domestication. In these representations, each colored box represents an allele and each funnel represents a population or demographic effect. (A) Classic domestication bottleneck where there is a reduction in genetic diversity driven both by demography and selection. Here, the loss of genetic diversity during the domestication of modern elite cultivars due to bottleneck events is illustrated. (B) Potential way to reintroduce alleles by using a different population structure showing the anticipated retention of genetic diversity achieved during the neo-domestication of more stress-tolerant chile varieties.
“Where will we find suitable genetic diversity for stress-tolerance traits, and how can we ensure the development of more stress-tolerant cultivars?”
The answer is that suitable diversity would probably not be identified in gene pools comprised of elite cultivars; however, it may likely be found in the broader germplasm pool that comprises landraces and CWR (secondary gene pool) (Byrne et al., 2020). Some breeding programs rely on landraces to develop breeding material, at least in the pre-breeding stages, but the use of ancestral or related species is limited (Pérez-Martínez et al., 2022). In this paper, we propose a new conceptual model for producing resilient chile varieties capable of addressing the growing challenges of climate change. We posit that through a process of neo-domestication, we will be able to accomplish this, and retain chile’s iconic status as a part of human culture.
The case for neo-domestication of pepper
Pepper (Capsicum sp.) is economically and culturally important across the globe, with ~36,972,494 tons harvested on ~2,020,816 ha in 2022 (FAOSTAT, 2022). While most Capsicum peppers were domesticated in Mesoamerica (Kraft et al., 2014), they have become a global staple across the globe (Tripodi et al., 2021). The species range has changed considerably over the course of their domestication history with wild and landraces inhabiting a large number of novel environments (Martínez-Ainsworth et al., 2023). Modern chile breeding has resulted in the offering of diverse hybrids selected to meet the needs of growers and processors who seek yield, synchronized maturity, field holding capacity, flavor, and processing line adaptability (Anderson, 2021). Pepper is an ideal test case crop for neo-domestication due to the known histories and the large amount of available germplasm resources.
Available germplasm resources in Capsicum annuum L. var. annuum Capsicum annuum landraces
Here, we adopt the definition of a landrace provided by Khoury et al. (2022):
“A crop variety or population managed by farmers through cultivation, selection and diffusion, which is typically adapted to a local area and to traditional farming systems, has a recognizable identity and geographic origin, and is often genetically heterogeneous.”
Landraces are still actively grown in some regions while in others, many have disappeared, perhaps pushed out of production by new hybrid cultivars. The decline of locally adapted landraces may result not only in the loss of genetic diversity, but also in income, culinary quality, and a part of the local cultural heritage (Casañas et al., 2017). Fortunately, many landraces are preserved in seed banks. A recent large-scale characterization of the global core collection of Capsicum annuum in response to climate change, showed there was substantial variation across the collection, often housed in landraces (Fumia et al., 2023). Similarly, core collections established at the regional level, such as the Balkan Capsicum collection, have also displayed significant variation for phenotypic and biochemical traits among landraces (Nankar et al., 2020). As our appreciation of landraces has grown, so too has our realization of the need for enhanced collection, characterization, and utilization of CWR (Barchenger and Bosland, 2019).
Ancestral wild species
Chiltepin (Capsicum annuum var. glabriusculum (Dunal) Heiser & Pickersgill) is considered the ‘mother’ of modern chiles. Its center of origin is in Mexico and it is widely distributed from northern South America to southern North America. It grows in an arid region of the Sonoran Desert in the north of Mexico and the southwestern USA. A perennial, it regularly survives heat and drought but is susceptible to winter freezes. Commercial gathering of the highly desired fruit has endangered these populations. In response to that threat, a protected area was created. The Wild Chile Botanical Area consists of 1,012 ha where the northernmost outlier population grows (Votava et al., 2002). It is the first botanical area set aside to protect CWR of domesticated crops in the USA (Riordan and Nabhan, 2019).
‘Retro-crossing’ of Capsicum annuum with landraces and wild chile to create highly diverse breeding populations
The breadth of genetic diversity available in landraces makes them a potential resource for abiotic and biotic stress-tolerance traits (McCoy et al., 2022). Casañas et al. (2017) proposed crossing of preserved (no longer cultivated seed bank accessions) with landraces still in cultivation. That strategy would preserve the agricultural utility of both the ‘active’ and ‘preserved’ landraces with beneficial traits. Landrace populations would also lend themselves to participatory plant breeding programs (Ceccarelli and Grando, 2022). Varieties developed from such a revitalized evolutionary diversity may not achieve the exceptional yield potential of elite varieties, but they could be bred to achieve enhanced production stability when subjected to environmental stressors from year to year. This fuller utilization of genetic diversity would be in contrast to the traditional use of landraces as an occasional resource for the improvement of modern elite cultivars, usually through backcrossing of mono- or oligogenic insect and disease resistance traits (Hajjar and Hodgkin, 2007; Maxted and Kell, 2009; Brumlop et al., 2013; Dempewolf et al., 2017).
The superior adaptation of wild chile populations to stress-prone environments (relative to domesticates) makes them an appealing source of abiotic stress tolerance (Bernau et al., 2023; Martínez-Ainsworth et al., 2023). Ideally, collections of CWR would represent a diversity of source habitats and “encompass the breadth of segregating genetic variation and adaptations characteristic of the target species” (Warschefsky et al., 2014). Sadly, there are only a handful of chiltepin accessions from arid environments such as the Sonoran Desert. Three accessions from southern Arizona are available in the USDA seed bank (GRIN search).
In relation to other solanaceous crop species, trait introgression using CWR has been relatively underutilized in Capsicum (Barchenger and Bosland, 2019) and limited to few traits, mostly those for disease resistance (Suwor et al., 2017; Jo et al., 2023). Tanksley and McCouch (1997) suggested that systematic introgressions (into the domesticate genome) would enable quick recovery of both stress tolerance from CWR and cultivated agronomic traits through advance generation backcrosses. Gur and Zamir (2004) have demonstrated progress in breeding tomato (Solanum lycopersicum) for increased yield in both wet and dry field conditions using genotypes carrying three independent yield-promoting genomic regions introduced from the drought-tolerant wild relative Solanum pennellii.
We would like to consider not backcrossing to elite cultivars, but instead ‘crossing back’ or ‘retro-crossing’ to more stress-tolerant landraces and/or CWR. An incredible amount of genetic diversity would be ‘unleashed’ by effectively manifesting the breadth of diversity generated during the course of domestication. This approach would also provide a platform for a new domestication pathway, or neo-domestication. This domestication ‘do-over’ would incorporate new selection criteria and technologies (Valle-Echevarria et al., 2021), and be performed in present day environments strongly affected by climate change.
Of course, we would assume that it would take a long time, perhaps hundreds of years, to recover competitive varieties. Anderson and Stebbins (1954) posited that at times, there have been
“…bursts of creative evolution (that) may well have been due to hybridization. At such times diverse faunas and floras were brought together in the presence of new or greatly disturbed habitats where some hybrid derivates would have been at a selective advantage.”
Perhaps, using modern breeding and population genetics theory, combined with genomic and phenomic tools (Zhang et al., 2017), integrated with a global evolutionary–participatory breeding program (Murphy et al., 2005), we could create such an evolutionary burst capable of providing neo-domesticates with superior adaptation to our changing climate.
Reorienting crop improvement strategies to accomplish neo-domestication in chile
Neo-domestication refers to re-domestication of a species whereas de novo domestication seeks to create a new crop species (Fernie and Yan, 2019). It initially took humans thousands of years to domesticate CWA, but leveraging modern breeding techniques in a strategic framework could perhaps enable the process to occur on a multi-decadal time scale (Zsögön et al., 2018; Valle-Echevarria et al., 2021). Neo-domestication programs would typically target ‘domestication syndrome’ traits and market-specific traits. We propose that retro-crossing of modern cultivars to landraces and CWA would not only allow us to ‘re-access’ diversity that was present throughout the initial domestication pathway, but also re-introduce genes that were previously lost during bottlenecks. This expanded scope of diversity would allow us to chart a new pathway for domestication. Coupled with selected modern technology and participatory breeding in diverse global environments, a new pathway without bottlenecks could unfold more rapidly. The participatory aspect would also help ensure the retention of cultural traits relevant to society, indirectly maintaining genetic diversity driven by societal components.
Crop improvement, genetic diversity, and cultural heritage
Such a democratized version of crop improvement is consistent with our emerging worldview of holistic cropping systems. The concept of genotype by environment interaction (G × E) could be said to be the ‘lens’ through which plant breeders have viewed varietal performance. We have also recognized that human management has a key, interactive role with both genotype and environment as portrayed (Beres et al., 2020). Considering the interest of society in the culinary and cultural aspects of crop improvement, it seems that larger society and stakeholders should be involved in the goal-setting of breeding programs (Seabrook and Avison, 2010). These terms may not be in the lexicon of farmers, but we are reminded that crop domestication was accomplished initially by ‘smallholder’ farmers who imposed their individual and cultural values during the selection process by unconsciously using this model.
The above model also reminds us that the selection of landraces occurred not just through selection by individuals, but under the influence of broader human culture (Casañas et al., 2017). An examination of the importance of culture (not just management) is well-portrayed in traditional potato cultivation in the Andes Mountains of South America. Brush et al. (1981) described the attributes of potato cultivation:
“Native agriculture associated with this diverse genetic material has 3 consequences: (a) the maintenance of numerous genotypes over space and time, (b) the wide distribution of particular genotypes, and (c) the generation or amplification of new genotypes. In order to understand these consequences, major cultural patterns (emphases added) of traditional potato agriculture in the Andes must be examined in relation to the genetic diversity of that crop. … Another significant feature of native agriculture relevant to genetic diversity of potatoes is the presence of wild and weedy potatoes in and around fields (emphasis added). This promotes the introgression of germplasm from those sources into cultivated stocks (Ugent, 1968).”
A more recent, systematic examination of genetic diversity in the Andean Center of Crop Domestication revealed the potato crop population structure was impacted by adoption of modern varieties, and an emphasis on fewer native varieties, but diversity within lower yielding plots of mixed native varieties still had a major influence of the diversity of the overall population (Brush et al., 1995). Traditional cropping systems such as these retain agrobiodiversity, which enables both the preservation of genetic diversity and its creation. Investment and retention of ‘metapopulations’, the “interconnected groups of species and subspecies,” ensures an inherent capacity, or ‘ecological versatility’, in the cropping system for dealing with environmental changes (Zimmerer, 1998).
Participatory plant breeding with purposeful populations
We have proposed a new paradigm for the breeding of more climate-resilient chile varieties (Figure 2). Such a schema would be most effective with a new breeding agenda—with breeding populations produced and distributed to many public cooperators. This would allow the populations to be exposed to more environments than those experienced during the initial domestication. Selection priorities are multi-genic stress-tolerance traits, not modern market requirements. This philosophical reorientation might be controversial because it places paramount importance on the resilience of crops, not their market appeal, or on a smaller local market rather than a potential global market.
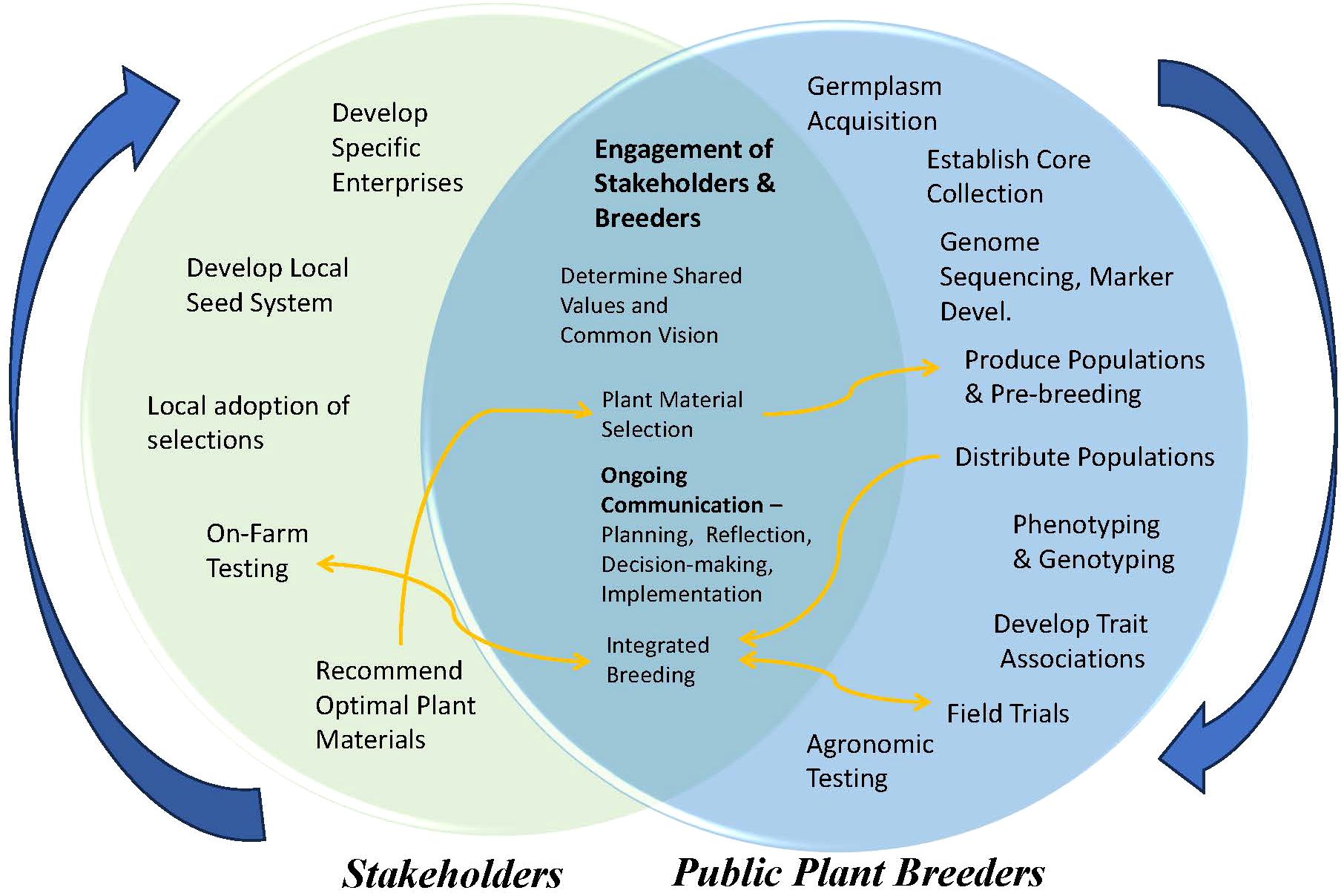
Figure 2. Cooperative pathways that could be used to create a seed system for localized varieties developed through neo-domestication.
Such a cooperative venture could allow for neo-domestication to occur in a far faster timeframe than the initial domestication. This pathway would require sustained institutional and stakeholder investment in comprehensive, long-term strategies. Plant breeders would also be required to act not only as occasional technical consultants, but as co-facilitators of a democratic, evolutionary–participatory breeding program. To further speed up the process, specific population structures could be used, for example, possible inclusion of synthetics, MAGIC, or RIL populations crossed with CWR representing arid environments (Warschefsky et al., 2014). The inclusion of smallholder farmers and active ‘seed savers’ would provide a base of stakeholders to work with. Such decentralized testing would expose breeding populations to a landscape of highly variable physical edaphic and climatic environments, and to producers and breeders who would select from diverse cultural, technical, and scientific perspectives (Runck et al., 2014). Examples include the Oregon Naked Barely Blend (Hayes et al., 2024), the ‘RePioneer’ maize population (Buffalo Seed Co., 2024), and the ‘Ultracross’ okra population (Experimental Farm Network, 2024).
Purposeful use of plant breeding infrastructure
From the experience of wide hybridization breeding programs, it will be important to maintain large population sizes. Phenotyping precision will be less important as its effects are typically large, and due to these large effects the importance of genomic technologies may be limited unless causative mutations are known (Fumia, 2023). Within a neo-domestication program, larger effective population sizes will be beneficial regardless of selection scheme, mating types, and cropping systems. The larger population sizes will also allow for an opportunity to identify and select transgressive segregates (Rieseberg et al., 1999). Cost-effective maintenance and sustainable gain for a complex trait such as stress tolerance will be achieved using a large number of parents, mid-level number of progeny per cross, low narrow-sense heritability, and mate allocation methods with low index weighting (Fumia, 2023).
Plant breeders will not be able to completely reorient their programs. A minimal commitment might entail planting one extra row of ‘purposeful’ populations in a nursery border, or as a guard row in performance trials. Some producers may even wish to plant the populations in a ‘biodiversity row’ including pollinator plant species to encourage outcrossing. If sufficient support could be garnered, some breeders would be able to devote a large portion of their program to neo-domestication. If many cooperators across diverse environments were to participate in such a scheme, the odds of selecting beneficial germplasm would be magnified.
Discussion
A new era of crop domestication
Examples of introgressed polygenic traits from CWR into modern cultivars remain in the minority. Genetic diversity held in seed banks has most often been utilized as a source of mono- and oligogenic insect and disease resistance traits for incorporation into elite cultivars (Hajjar and Hodgkin, 2007; Maxted and Kell, 2009; Brumlop et al., 2013; Dempewolf et al., 2017). Our proposed schema for incorporating (polygenic) stress tolerance into modern cultivars via neo-domestication is in line with the proposed next era of plant breeding presented by Krug et al. (2023). Those authors’ vision for the next era of plant breeding would broaden the palette of crop diversity by (re)engaging the three elements of domestication: crops, ecosystems, and humans. We posit that when there are no constraining traits (e.g., market ideotypes), the gain through selection for complex traits will be more rapid. With concomitant societal selection for cultural traits, new phenotypes may be drawn directly into local production, where breeding for the improvement of the harvestable organ and cultivar release could be conducted concurrently with production.
Unraveling the negative aspects of ‘domestication syndrome’ (loss of genetic diversity, accumulation of deleterious mutations, altered metabolomic profiles, and decreased nutritional content) (Krug et al., 2023) will also undo its positive aspects that may be preferred by consumers or producers. Thus, the neo-domestication process will require a scale of resources and cooperation that would be best afforded by a comprehensive reshaping of how we approach public plant breeding. It would likely also require reprioritization of funding allocation and germplasm exchange policies. Bold new approaches and long-term investment would be required to achieve a new set of goals for our food systems, and those systems should be defined by different communities. There are some promising approaches that include ‘open-source’ breeding (Luby et al., 2016; Lozada et al., 2022) and evolutionary–participatory methods (Murphy et al., 2005). Making use of the Global Capsicum Conservation Strategy (Barchenger and Khoury, 2022).
Conservation Strategy, and the over 3-dozen species it is focused on will also require rethinking how we conduct wide hybridization and domestication. The neo-domestication approach we propose should bolster genetic, agroecosystem, and food system diversity.
The neo-domestication pathway contrasts with the consideration of market pull typically given at the outset of variety development programs. Conceivably, advanced selections entering local markets due to novel traits or specific adaptation, would be unlikely to enter into broader markets until competitive advantage over elite cultivars is demonstrated, either through new nutritional complexes, ecosystem services, or other benefit to farmers, consumers, and society. Chile is unique in that chiltepin is already grown in home gardens (McKittrick, 2009), and landraces are still cultivated in many regions. We are optimistic that it should be feasible to channel that interest into opportunities that foster evolutionary–participatory plant breeding approaches to the neo-domestication of chile and other suitable crops (Ceccarelli, 2006).
Author contributions
RP: Conceptualization, Writing – original draft, Writing – review & editing. MK: Conceptualization, Writing – review & editing. NF: Writing – review & editing. AN: Writing – review & editing.
Funding
The author(s) declare financial support was received for the research, authorship, and/or publication of this article. The authors gratefully acknowledge the Bulgarian National Academy of Sciences.
Conflict of interest
The authors declare that the research was conducted in the absence of any commercial or financial relationships that could be construed as a potential conflict of interest.
Publisher’s note
All claims expressed in this article are solely those of the authors and do not necessarily represent those of their affiliated organizations, or those of the publisher, the editors and the reviewers. Any product that may be evaluated in this article, or claim that may be made by its manufacturer, is not guaranteed or endorsed by the publisher.
References
Anderson B. B. (2021). “A market on fire,” in Seed World, Seed World USA, Grand Forks, North Dakota, 50–52.
Anderson E., Stebbins G. L. (1954). Hybridization as an evolutionary stimulus. Evolution 8, 378–388. doi: 10.2307/2405784
Barchenger D. W., Bosland P. W. (2019). “Wild Chile pepper (Capsicum L.) of North America,” in North American Crop Wild Relatives. Eds. Greene S., Williams K., Khoury C., Kantar M., Marek L. (Springer International Publishing, New York: Cham), 225–242.
Barchenger D. W., Khoury C. K. (2022). A global Strategy for the conservation and use of Capsicum genetic resources (Germany: Global Crop Diversity Trust. Bonn). doi: 10.5281/zenodo.8367264
Beres B. L., Hatfield J. L., Kirkegaard J. A., Eigenbrode S. D., Pan W. L., Lollato R. P. (2020). A Better understanding of Genotype × Environment × Management Interactions—A global wheat initiative agronomic research strategy. Front. Plant Sci. 11. doi: 10.3389/fpls.2020.00828
Bernau V., Kantar M., Jardon Barbolla L., McCoy J., Mercer K. L., McHale L. K. (2023). Genomic signatures of adaptation to abiotic stress from a geographically diverse collection of Chile peppers (Capsicum spp.) from Mexico. bioRxiv, 2023–2008. doi: 10.1101/2023.08.13.553093
Bradshaw C., Ehrlich J. A., Paul R., Beattie A., Ceballos G., Crist E., et al. (2021). Underestimating the challenges of avoiding a ghastly future. Front. Cons. Sci. 1. doi: 10.3389/fcosc.2020.615419
Brás T. A., Seixas J., Carvalhais N., Jägermeyr J. (2021). Severity of drought and heatwave crop losses tripled over the last five decades in Europe. Env. Res. Lett. 16, 065012. doi: 10.1088/1748-9326/abf004
Brumlop S., Reichenbecher W., Tappeser B., Finckh M. R. (2013). What is the SMARTest way to breed plants and increase agrobiodiversity? Euphytica 194, 53–66. doi: 10.1007/s10681-013-0960-9
Brush S. B., Carney H. J., Huamán Z. (1981). Dynamics of andean potato agriculture. Econ. Bot. 35, 70–88. doi: 10.1007/BF02859217
Brush S., Kesseli R., Ortega R., Cisneros P., Zimmerer K., Quiros C. (1995). Potato diversity in the andean center of crop domestication. Cons. Biol. 9, 1189–1198. doi: 10.1046/j.1523-1739.1995.9051176.x-i1
Buffalo Seed Company (2024). Maize (Dent): Re-Pioneer. Available online at: https://www.thebuffaloseedcompany.com/product/maize-dent-re-pioneer-50-or-100-seeds-/19 (Accessed 02/28/2024).
Byrne P., Richards C., Volk G. M. (2020).From wild species to landraces and cultivars. In: Crop wild relatives and their use in plant breeding (Fort Collins, Colorado: Colorado State University). Available online at: https://colostate.pressbooks.pub/cropwildrelatives/chapter/from-wild-species-to-landraces-and-cultivars/ (Accessed 03/21/2024).
Casañas F., Simó J., Casals J., Prohens J. (2017). Toward an evolved concept of landrace. Front. Plant Sci. 8. doi: 10.3389/fpls.2017.00145
Ceccarelli S. (2006). “Decentralized-participatory plant breeding: Lessons from the south - Perspectives in the north,” in Eco-PB Proceedings on Participatory Plant Breeding: Relevance for Organic Agriculture? Eds. Desclaux D., Hédont M. (La Besse, France, European Consortium for Organic Plant Breeding, Driebergen, The Netherlands), 8–15. Available at: http://www.eco-pb.org.
Ceccarelli S., Grando S. (2022). Return to agrobiodiversity: Participatory plant breeding. Diversity 14, 126. doi: 10.3390/d14020126
Dempewolf H., Baute G., Anderson J., Kilian B., Smith C., Guarino L. (2017). Past and future use of wild relatives in crop breeding. Crop Sci. 57, 1070–1082. doi: 10.2135/cropsci2016.10.0885
Experimental Farm Network (2024). Ultracross okra. Available online at: https://store.experimentalfarmnetwork.org/products/ultracross-okra (Accessed 02/28/2024).
FAOSTAT (2022). Crops: Chillies and peppers. Available online at: http://www.fao.org/faostat/en/#data.
Fernie A. R., Yan J. (2019). De novo domestication: An Alternative Route toward New Crops for the Future. Mol. Plant 12, 615–631. doi: 10.1016/j.molp.2019.03.016
Fumia N., Kantar M., Lin Y. P., Schafleitner R., Lefebvre V., Paran I., et al. (2023). Exploration of high-throughput data for heat tolerance selection in Capsicum annuum. Plant Phenome Jour. 6, e20071. doi: 10.1002/ppj2.20071
Fumia N. J. (2023). Breeding scheme development and optimization during neo-domestication and wide hybridization (Doctoral dissertation, University of Hawai'i at Manoa). 91 pp. Available online at: https://scholarspace.manoa.hawaii.edu/server/api/core/bitstreams/9cfa8926-09b0-4a74-b66f-1a80bd4f3535/content.
Gao L., Kantar M. B., Moxley D., Ortiz-Barrientos D., Rieseberg L. H. (2023). Crop adaptation to climate change: An evolutionary perspective. Mol. Plant 16, 1518–1546. doi: 10.1016/j.molp.2023.07.011
Gur A., Zamir D. (2004). Unused natural variation can lift yield barriers in plant breeding. PloS Biol. 2, e245. doi: 10.1371/journal.pbio.0020245
Hajjar R., Hodgkin T. (2007). The use of wild relatives in crop improvement: A survey of developments over the last 20 years. Euphytica 156, 1–13. doi: 10.1007/s10681-007-9363-0
Hayes P., Fisk S., Helgerson L., Meints B., Rocha G. (2024). The Oregon Naked Barley Blend - A germplasm release for education and collaborative research. Available online at: https://barleyworld.org/barley-info/osu-barley-project-varieties-and-germplasms (Accessed 03/21/24).
Jo J., Kim G. W., Back S., Jang S., Kim Y., Han K., et al. (2023). Exploring horticultural traits and disease resistance in Capsicum baccatum through segmental introgression lines. Theor. Appl. Genet. 136, 233. doi: 10.1007/s00122-023-04422-x
Khoury C. K., Brush S., Costich D. E., Curry H. A., De Haan S., Engels J. M., et al. (2022). Crop genetic erosion: understanding and responding to loss of crop diversity. New Phytol. 233, 84–118. doi: 10.1038/s41558-018-0315-6
Kraft K. H., Brown C. H., Nabhan G. P., Luedeling E., Luna Ruiz J. D. J., Coppens d’Eeckenbrugge G., et al. (2014). Multiple lines of evidence for the origin of domesticated chili pepper, Capsicum annuum, in Mexico. Proc. Natl. Acad. Sci. 111, 6165–6170. doi: 10.1073/pnas.1308933111
Krug A. S., Drummond E. B. M., Van Tassel D. L., Warschefsky E. J. (2023). The next era of crop domestication starts now. Proc. Natl. Acad. Sci. U.S.A. 120, e2205769120. doi: 10.1073/pnas.2205769120
Lobell D. B., Burke M. B., Tebaldi C., Mastrandrea M. D., Falcon W. P., Naylor R. L. (2008). Prioritizing climate change adaptation needs for food security in 2030. Science 319, 607–610. doi: 10.1002/9781119279723.ch6
Lozada D. N., Bosland P. W., Barchenger D. W., Haghshenas-Jaryani M., Sanogo S., Walker S., et al. (2022). Chile pepper (capsicum) breeding and improvement in the "multi-omics" era. Front Plant Sci. 13, 879182. doi: 10.3389/fpls.2022.879182
Luby C. H., Kloppenburg J., Goldman I. L. (2016). Open source plant breeding and the Open Source Seed Initiative. In Janick J. ed. Plant Breeding Reviews 4013, 271298. Hoboken, NY: Wiley-Blackwell.
Martínez-Ainsworth N. E., Scheppler H., Moreno-Letelier A., Bernau V., Kantar M. B., Mercer K. L., et al. (2023). Fluctuation of ecological niches and geographic range shifts along Chile pepper’s domestication gradient. Ecol. Evol. 13, e10731. doi: 10.1002/ece3.10731
Maxted N., Kell S. P. (2009). Establishment of a global network for the in situ conservation of crop wild relatives: Status and needs (Rome, Italy: FAO Commission on Genetic Resources for Food and Agriculture).
McCoy J. E., McHale L. K., Kantar M., Jardón-Barbolla L., Mercer K. L. (2022). Environment of origin and domestication affect morphological, physiological, and agronomic response to water deficit in Chile pepper (Capsicum sp.). PloS One 17, e0260684. doi: 10.1371/journal.pone.0260684
McKittrick L. (2009). Mother Chiltepin. In: Seedhead News. Available online at: https://cdn.shopify.com/s/files/1/0157/0808/files/SeedheadNews104-2009-Fall-Winter.pdf?5863920513817280179 (Accessed 03/22/2024).
Mora C., Spirandelli D., Franklin E. C., Lynham J., Kantar M. B., Miles W. (2018). Broad threat to humanity from cumulative climate hazards intensified by greenhouse gas emissions. Nature. Clim. Change 8, 1062–1107. doi: 10.1038/s41558-018-0315-6
Murphy K., Lammer D., Lyon S., Carter B., Jones S. S. (2005). Breeding for organic and low-input farming systems: An evolutionary–participatory breeding method for inbred cereal grains. Renewable Agric. Food Syst. 20, 48–55. doi: 10.1079/RAF200486
Nankar A. N., Todorova V., Tringovska I., Pasev G., Radeva-Ivanova V., Ivanova V., et al. (2020). A step towards Balkan Capsicum annuum L. core collection: Phenotypic and biochemical characterization of 180 accessions for agronomic, fruit quality, and virus resistance traits. PloS One 15, e0237741. doi: 10.1371/journal.pone.0237741
Pérez-Martínez A. L., Eguiarte L. E., Mercer K. L., Martínez-Ainsworth N. E., McHale L., van der Knaap E., et al. (2022). Genetic diversity, gene flow, and differentiation among wild, semiwild, and landrace Chile pepper (Capsicum annuum) populations in Oaxaca Mexico. Am. J. Bot. 109, 1157–1176. doi: 10.1002/ajb2.16019
Rieseberg L. H., Archer M. A., Wayne R. K. (1999). Transgressive segregation, adaptation and speciation. Heredity 83, 363–372. doi: 10.1038/sj.hdy.6886170
Riordan E. C., Nabhan G. P. (2019). Trans situ conservation of crop wild relatives. Crop Sci. 59, 2387–2403. doi: 10.2135/cropsci2019.06.0356
Runck B. C., Kantar M. B., Jordan N. R., Anderson J. A., Wyse D. L., Eckberg J. O., et al. (2014). The reflective plant breeding paradigm: A robust system of germplasm development to support strategic diversification of agroecosystems. Crop Sci. 54, 1939–1948. doi: 10.2135/cropsci2014.03.0195
Seabrook J. A., Avison W. R. (2010). Genotype-environment interaction and sociology: contributions and complexities. Soc. Sci. Med. 70, 1277–1284. doi: 10.1016/j.socscimed.2010.01.016
Smith J. S., Trevisan W., McCunn A., Huffman W. E. (2022). Global dependence on Corn Belt Dent maize germplasm: Challenges and opportunities. Crop Sci. 62, 2039–2066. doi: 10.1002/csc2.20802
Snowdon R. J., Wittkop B., Chen T. W., Stahl A. (2021). Crop adaptation to climate change as a consequence of long-term breeding. Theor Appl Genet 134, 1613–1623. doi: 10.1007/s00122-020-03729-3
Suwor P., Sanitchon J., Thummabenjapone P., Kumar S., Techawongstien S. (2017). Inheritance analysis of anthracnose resistance and marker-assisted selection in introgression populations of chili (Capsicum annuum L.). Scientia Hortic. 220, 20–26. doi: 10.1016/j.scienta.2017.03.032
Tanksley S. D., McCouch S. R. (1997). Seed banks and molecular maps: unlocking genetic potential from the wild. Science 277, 1063–1066. doi: 10.1126/science.277.5329.1063
Tester M., Langridge P. (2010). Breeding technologies to increase crop production in a changing world. Science 327, 818–822. doi: 10.1126/science.1183700
Tripodi P., Rabanus-Wallace M. T., Barchi L., Kale S., Esposito S., Acquadro A., et al. (2021). Global range expansion history of pepper (Capsicum spp.) revealed by over 10,000 genebank accessions. Procational Acad. Sci. 118, e2104315118. doi: 10.1073/pnas.2104315118
Ugent D. (1968). The potato in Mexico: Geography and primitive culture. Econ. Bot. 22, 108–123. doi: 10.1007/BF02860554
Valle-Echevarria A. D., Fumia N., Gore M. A., Kantar M. (2021). Accelerating crop domestication in the era of gene editing. Plant Breed. Rev. 45, 185–211. doi: 10.1002/9781119828235.ch4
Votava E. J., Nabhan G. P., Bosland P. W. (2002). Genetic diversity and similarity revealed via molecular analysis among and within an in situ population and ex situ accessions of chiltepin (Capsicum annuum var. glabriusculum). Cons. Genet. 3, 125–129. doi: 10.1073/pnas.2104315118
Warschefsky E., Penmetsa R. V., Cook D. R., Von Wettberg E. J. B. (2014). Back to the wilds: Tapping evolutionary adaptations for resilient crops through systematic hybridization with crop wild relatives. Am. Jour. Bot. 101, 1791–1800. doi: 10.3732/ajb.1400116
Zhang H., Mittal N., Leamy L. J., Barazani O., Song B.-H. (2017). Back into the wild—Apply untapped genetic diversity of wild relatives for crop improvement. Evol. Appl. 10, 5–24. doi: 10.1111/eva.12434
Zimmerer K. S. (1998). The ecogeography of Andean potatoes: Versatility in farm regions and fields can aid sustainable development. Bioscience 48, 45–54. doi: 10.2307/1313242
Keywords: ancestral crop relatives, biodiversity, climate change, germplasm utilization, neo-domestication, genetic diversity, participatory plant breeding
Citation: Pratt RC, Kantar MB, Fumia N and Nankar AN (2024) From wild to mild and back again: envisioning a new model of crop improvement strategies. Front. Hortic. 3:1409398. doi: 10.3389/fhort.2024.1409398
Received: 29 March 2024; Accepted: 19 July 2024;
Published: 23 August 2024.
Edited by:
Jaime Prohens, Universitat Politècnica de València, SpainReviewed by:
Valeria Negri, University of Perugia, ItalyCopyright © 2024 Pratt, Kantar, Fumia and Nankar. This is an open-access article distributed under the terms of the Creative Commons Attribution License (CC BY). The use, distribution or reproduction in other forums is permitted, provided the original author(s) and the copyright owner(s) are credited and that the original publication in this journal is cited, in accordance with accepted academic practice. No use, distribution or reproduction is permitted which does not comply with these terms.
*Correspondence: Richard C. Pratt, cmljcHJhdHRAbm1zdS5lZHU=