- 1Floral and Nursery Plants Research Unit, Agricultural Research Service, U.S. Department of Agriculture, McMinnville, TN, United States
- 2Tropical Research and Education Center, Department of Environmental Horticulture, University of Florida, Homestead, FL, United States
- 3Grapes Genetics Research Unit, Agricultural Research Service, U.S. Department of Agriculture, Geneva, NY, United States
Osmanthus fragrans Lour. is prized for its fragrant, cool-season blooms, smooth leaves, and compact tree form; however, the species is limited in production and use to USDA Hardiness Zones 7 and higher. Increasing the total amount of genetic variability available for evaluation, selection, and breeding is foundational to the development of novel Osmanthus cultivars with increased cold-hardiness and improved ornamental characteristics such as high rooting rates, faster growth, fragrant flowers, and an evergreen or semi-evergreen habit. Hybridization among Osmanthus species should generate novel phenotypes and allow trait introgression among populations and breeding lines. The objectives of this study were to produce, verify, and evaluate Osmanthus hybrids by making controlled and open-pollinated crosses among Osmanthus species, using SSR markers to determine parentage of cross progeny, and comparing flowering, leaf shape, and form of hybrids offspring with parents. Fifty-eight of 66 progenies (88%) were assigned paternity at the 95% confidence level or above and 47 progenies (71%) were assigned paternity at the 99% confidence level. Novel Osmanthus hybrids were produced including O. armatus × O. fortunei, O. armatus × O. heterophyllus, and O. armatus × O. fragrans. Open-pollinated families increased in height an average of 204% and 159% per year in the first and second growing seasons, respectively. Male flower type was significantly associated with progeny flower type (χ2 = 17.5, p < 0.0001) while leaf margin was not associated with cross family (χ2 = 0.83, p = 0.84). Evaluation of more Osmanthus accessions and subsequent hybridizations will be necessary to introgress favorable genes and generate the variation necessary to expand the genus into new markets.
1 Introduction
The genus Osmanthus (Family: Oleaceae Tribe: Oleinae) consists of about 35 species of evergreen trees and shrubs primarily distributed across mesic and temperate forests of China, Japan, and the Middle East (Green, 1963; Guo et al., 2011). All Osmanthus are diploid with a chromosome number of 2n = 46 and a base chromosome number of 23 (Li et al., 2004; Xiang and Liu, 2008). While classical morphological analysis divided the genus into five Sections (Leiolea [Spach] P. S. Green, Siphosmanthus Franch., Linocieroides P. S. Green, Notosmanthus P. S. Green, and Osmanthus), molecular analyses have shown Osmanthus to be a polyphyletic group containing three or four distinct lineages (Green, 1963; Wallander and Albert, 2000; Lu et al., 2010; Guo et al., 2011; Duan et al., 2013). The largest lineage consists of all species in former sections Osmanthus, Siphosomanthus, and Linocieroides. This lineage contains the majority of cultivated Osmanthus, including O. fragrans, O. heterophyllus, and O. armatus (Guo et al., 2011). An evaluation of genome size and cross-compatibility within this lineage showed that genome sizes among species are similar, ranging between 2.9 and 3.1 pg, and pollen tubes reached the ovaries in all attempted interspecific and intraspecific crosses. Germination of pollen on the stigma of self-pollinations with subsequent termination of pollen tube growth in the style provides support for a sporophytic self-incompatibility system in Osmanthus (Saumitou-Laprade et al., 2017; Alexander, 2023).
Osmanthus fragrans (Thunb.) Lour., commonly known as sweet osmanthus or fragrant tea-olive, is the most popular Osmanthus species with at least 166 named cultivars (Xiang and Liu, 2008). Four groups of O. fragrans (Albus, Asiaticus, Aurantiacus, and Luteus, a.k.a Thunbergii) are defined by morphological and phenological traits including flower color, peduncle length, and flowering time (Xiang and Liu, 2008). Osmanthus fragrans Lour. is prized in China for its fragrant, cool-season blooms, smooth leaves, compact tree form, and leaf extracts used in traditional medicine (Xiang and Liu, 2008; Cai et al., 2014). However, the species is limited in production and use to USDA Hardiness Zones 7 and higher (Dirr, 2009). Other Osmanthus species including O. heterophyllus, O. armatus, and O. fortunei (an O. fragrans × O. heterophyllus hybrid) have moderately fragrant flowers and a variety of forms but generally lack the small tree form and smooth leaves of O. fragrans. Two exceptions are the O. heterophyllus cultivars ‘Rotundifolius’ and ‘Rotundifolius Noveau’ which have smooth leaf margins.
Cold hardiness of Osmanthus varies between and within species. An evaluation of 16 taxa in a USDA Hardiness Zone 6/7 transition area showed that O. armatus and O. heterophyllus both had cultivars that grew and flowered in winter-exposed conditions (Alexander, 2019). O. fortunei had a cold-hardiness intermediate to that of its parents, suggesting that hybrid breeding can be an avenue for cold-hardiness improvement in Osmanthus. The least cold-hardy species was O. fragrans, though variation in cold-hardiness among O. fragrans cultivars has been reported (Yu et al., 2010). Low spring temperatures rather than low winter temperatures appear to be the chief factor limiting the northward cultivation of the species (Yu et al., 2010).
Hybrid breeding in Osmanthus is limited by diversity in breeding systems, disjunct flowering times, and low seed germination. Osmanthus is androdioecious, a rare breeding system where populations consist of both male and hermaphrodite individuals (Xiang and Liu, 2008; Xu et al., 2014). Clonally produced Osmanthus cultivars produce either male or perfect flowers. A study of 27 Osmanthus accessions showed that all O. armatus produced perfect flowers, all O. fortunei and O. fragrans produced male-only flowers, and 85% of O. heterophyllus cultivars produced perfect flowers while 15% produced male-only flowers (Alexander, 2023). Osmanthus fragrans has a 1:1 (perfect: male-only) sex ratio in natural populations across its native range in China (Xu et al., 2014), however, all of the O. fragrans cultivars commonly available on the U.S. market appear to be male-only. Additionally, some O. fragrans cultivars bloom in fall, some in spring, and some bloom year-round in favorable conditions. When compatible genotypes do overlap in flowering time and form a viable seed, germination is often low and inconsistent (Tang et al., 2013).
Co-dominant, highly informative SSR (simple sequence repeat) markers developed for O. fragrans were shown to amplify reliably across Osmanthus species (Zhang et al., 2011; Duan et al., 2013; Alexander et al., 2018). Osmanthus armatus is relatively cold hardy, has perfect flowers, and a long flowering time, making it suitable as a female parent. The flowers are fragrant but small, white, and non-descript. The objectives of this study were to produce, verify, and evaluate novel Osmanthus hybrids by making controlled and open-pollinated crosses among Osmanthus species, using SSR markers to determine parentage of cross progeny, and comparing flowering, leaf shape, and form of hybrids offspring with parents.
2 Materials and methods
2.1 Plant material
Twenty-two Osmanthus cultivars and six unnamed accessions representing five species (n = 28 genotypes) were obtained from the U.S. National Arboretum or commercial nurseries and grown in containers at the Tennessee State University Nursery Research Center in McMinnville, TN, USA (Table 1). Plants were maintained in 26.5 L pots under full sun and micro-irrigated using spray stakes. Growing media consisted of pine bark amended with 6.6 kg·m-3 19N-2.1P-7.4K Osmocote Pro fertilizer (Scotts-Sierra Horticultural Products Co., Maryville, Ohio), 0.6 kg·m-3 Micromax (Scotts-Sierra Horticultural Products Co.), 0.6 kg·m-3 iron sulfate, and 0.2 kg·m-3 Epsom salts. Pots were moved into a plastic covered poly-house heated to 6°C from November through May of each year.
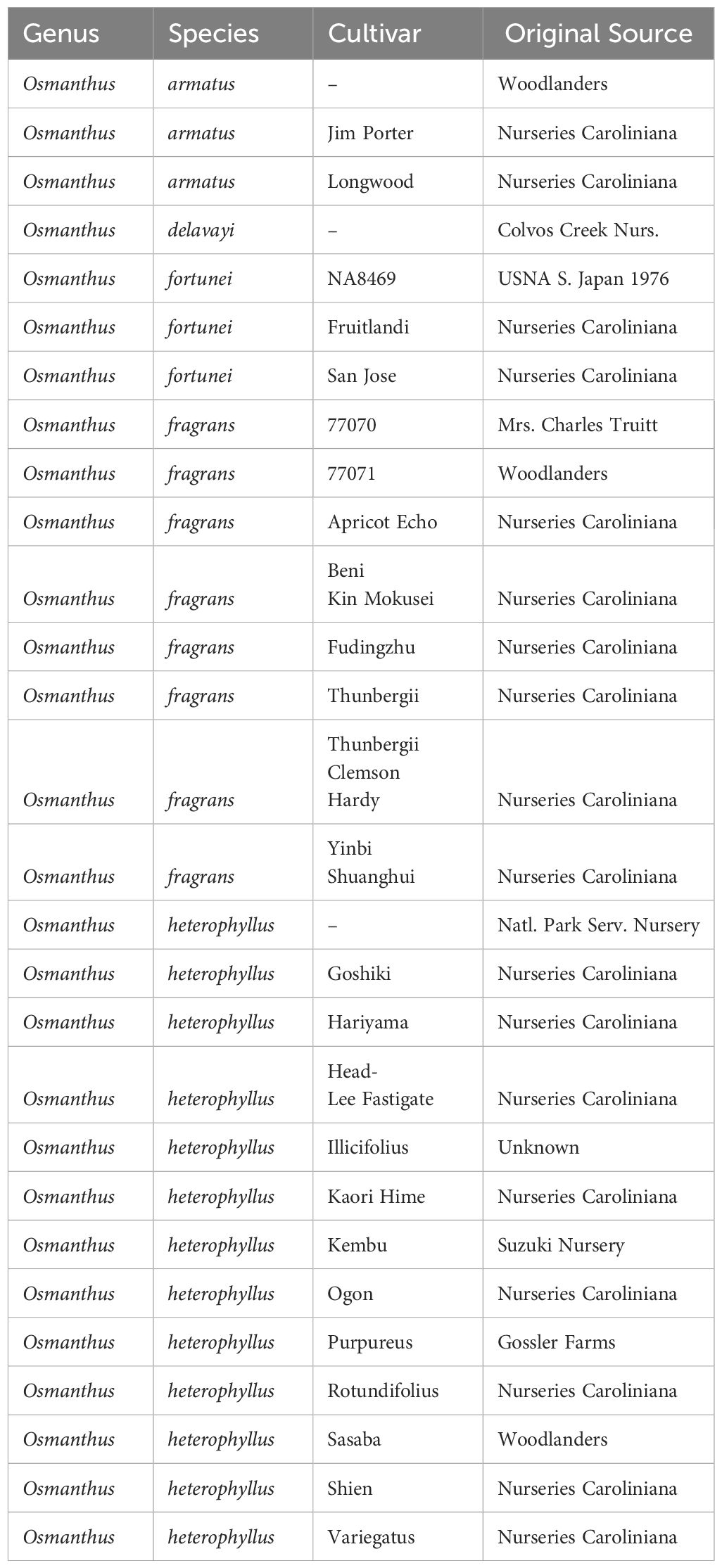
Table 1 Candidate male parents for SSR analysis of Osmanthus armatus and O. heterophyllus progenies.
2.2 Hybrid breeding and seed collection
Controlled crosses of Osmanthus armatus ‘Longwood’ × O. heterophyllus ‘Kaori Hime’ were made 30 December 2016. Fifty flowers spread over multiple branches of ‘Longwood’ were emasculated and fresh donor pollen was collected on a brush and placed on the stigma of the female flower the same day. A single ‘Kaori Hime’ plant was used as the pollen donor. Flowers were bagged after pollination and the bags were removed after 10 days. Osmanthus armatus ‘Jim Porter’, O. armatus ‘Longwood’, and O. heterophyllus ‘Rotundifolius’ were allowed to open-pollinate during their flowering times between September and December 2016.
Seed was collected May 2017 when the fruit turned greenish-purple and began to wrinkle. Seed was stratified in moist peat for 12 weeks at room temperature followed by 12 weeks at 4.5°C. Seeds were removed from stratification, rinsed with deionized water, and placed in 5 cm pots with pine bark media. Seeds germinated September–December 2017 at room temperature. After germination, seedlings were maintained in a greenhouse with overhead watering for 1 min twice per day.
2.3 SSR genotyping
DNA was isolated from leaf tissue of putative hybrids and candidate parents in June 2018 using the Qiagen DNeasy Plant Mini-kit (Qiagen, Carlsbad, California, USA) according to manufacturer’s instructions with a starting weight of 100 mg fresh leaf tissue. A panel of eight SSR loci previously shown to have a high probability of identity in Osmanthus genotypes (Alexander et al., 2018; Supplementary Table S1) was amplified using the Terra PCR Direct Polymerase Mix Kit (Takara Bio USA, San Jose, California, USA) according to manufacturer’s instructions with final reaction concentrations as follows: 4 µM of each primer, 1/2 volume of 2X Terra PCR Direct Buffer, 2.5U of Terra PCR direct Polymerase Mix and 10 ng template DNA. PCR conditions consisted of: 98°C for 3 min; 12 cycles of 98°C for 15 s, 63°C for 20 s, and 72°C for 30 s, decreasing 0.5°C annealing temp each cycle; followed by 20 cycles of 98°C for 15 s, 57°C for 20 s, and 72°C for 30 s; followed by a final extension at 72°C for 5 min. PCR products were analyzed on an ABI 3730xl DNA Analyzer (Applied Biosystems, Foster City, CA) using 0.02 µL of PCR product, 9 µL of formamide (Applied Biosystems), and 0.03 µL of GeneScan 500 ROX Size Standard (Applied Biosystems). Allele sizes and number of alleles per locus were determined with GeneMarker version 3.0.1 (SoftGenetics, State College, PA). Non-amplified loci were scored as missing data. Markers with irreconcilable stutter peaks were not included in downstream analysis.
2.4 Parentage analysis
The number of alleles per locus (k), observed (Ho) and expected (He) heterozygosity, total number of null alleles (Fnull), polymorphic information content (PIC), and the deviation from the Hardy–Weinberg equilibrium (HW) were calculated using CERVUS 3.0.7 software (Marshall et al., 1998; Kalinowski et al., 2007). Paternity inference analysis was performed with CERVUS 3.0.7 software using a likelihood-based approach where paternity is assigned to a particular male if the likelihood ratio (also known as a LOD score) is large relative to the likelihood ratios of alternative males (Marshall et al., 1998). The option to include known maternal genotypes was selected and the proportion of candidate fathers sampled was set to 0.99. Internal simulations were run (set to 10,000) to determine the significance of LOD scores using the Delta method (Greenberg and Berger, 1994: Marshall et al., 1998). Relaxed and strict confidence levels were set to 95 and to 99% respectively, and the proportion of loci mistyped was set to 0.01 (Supplementary Table S2).
2.5 Cluster analysis
A genetic distance matrix was generated based on Bruvo’s estimation (Bruvo et al., 2004) taking into account mutational distance between SSR alleles implemented in the ‘poppr’ package (v. 2.9.3 in R (v.4.2.2; Kamvar et al., 2015). Genotypes were then clustered using the UPGMA algorithm using bootstrap support based on 20,000 replications and plotted in a dendrogram using the ‘ape’ package (v.5.6-2; Paradis and Schliep, 2019).
2.6 Progeny phenotyping
Progenies were transplanted from 5 cm pots to 3.8 L pots in May of 2019. Height (cm), widest width (cm), and perpendicular width (cm) were measured 10 May 2019, 9 May 2020, 28 May 2021, and 20 May 2022. Plants were observed for flowers and fruit every two weeks from 21 Oct. 2021 to 15 Feb. 2023. Presence of flowers (Y/N), presence of fruit (Y/N), and flower type (male or perfect) was recorded. Leaf margins (smooth or toothed) were observed 15 February 2023. Progeny were transplanted to 11 L pots 22 May 2021 and remained for the duration of the test. Plants were not pruned during this evaluation. Average width was calculated from width measurements as: (widest width + perpendicular width) ÷ 2. Yearly height and width increases were converted to percentages as follows: Height increase (%) = [(Height Year 2 – Height Year 1) ÷ Height 1] × 100%.
Data analysis was performed using SAS® software, Version 9.4 of the SAS system for Microsoft (Copyright © 2013, SAS Institute Inc., Cary, NC, USA). The general linear model procedure (PROC GLM) was used to partition variance in height, average width, height increase, and average width increase to sources attributable to cross and error. Constancy of residual variance was checked using the Brown–Forsythe test. Means for each cross were compared using Fisher’s Least Significant Difference with an α = 0.05 significance level. Associations between paternal and progeny flower type and between family and leaf margin were tested for significance using a chi-square test for independence.
3 Results
3.1 Hybrid breeding and seed collection
Open-pollinated seed was collected from O. armatus ‘Longwood’ (n=22) and ‘Jim Porter’ (n = 89) and O. heterophyllus ‘Rotundifolius (n = 10). Twenty-two of 50 controlled pollinations between O. armatus ‘Longwood’ and O. heterophyllus ‘Kaori Hime’ produced seed. Of these 143 seeds, 74 germinated successfully (57.1%) and were available for genotyping and phenotyping [(O. armatus ‘Longwood’ × OP (n = 15), O. armatus ‘Longwood’ × O. heterophyllus ‘Kaori Hime’ (n = 14), O. heterophyllus ‘Rotundifolius’ × OP (n = 5), and O. armatus ‘Jim Porter’ × OP (n = 40)].
3.2 SSR Genotyping
High-quality DNA was isolated from 66 of 74 hybrid progeny. A total of 90 individuals (66 putative hybrids and 24 candidate parents) were genotyped at eight SSR loci. One locus was removed from analysis due to low amplification across individuals. For the remaining seven SSR loci, mean number of alleles per locus was 11 with a mean of 98.1% of loci successfully genotyped (Table 2). Mean polymorphic information content of loci was moderate at 0.71. The combined non-exclusion probability; that is, the probability of not excluding an unrelated male, was 0.0000303. Taken together, these metrics indicate that the seven loci used in this study were sufficient to resolve paternity of the seedlings with high confidence.
3.3 Parentage
Fifty-eight of 66 progenies (88%) were assigned paternity at the 95% confidence level or above and 47 progenies (71%) were assigned paternity at the 99% confidence level (Table 3). All seedlings of O. armatus ‘Longwood’ × O. heterophyllus ‘Kaori Hime’ were confirmed to be the desired cross (Table 4). This means that methods of bagging female parents and emasculating and pollinating on the same day are useful for obtaining desired crosses. Male parents of open-pollinated O. armatus ‘Jim Porter’ seedlings included eight cultivars from four species. Male parents of open-pollinated O. armatus ‘Longwood’ seedlings included nine cultivars from three species, and O. heterophyllus ‘Rotundifolius’ was pollinated by two cultivars from two species (Table 4).
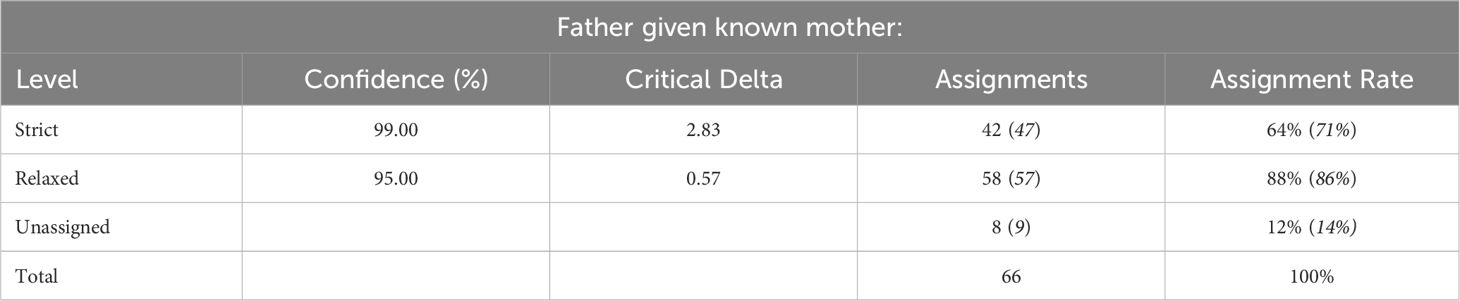
Table 3 Observed and expected (in italics) paternity assignment rates for 66 Osmanthus seedlings of known maternal origin using seven SSR markers.
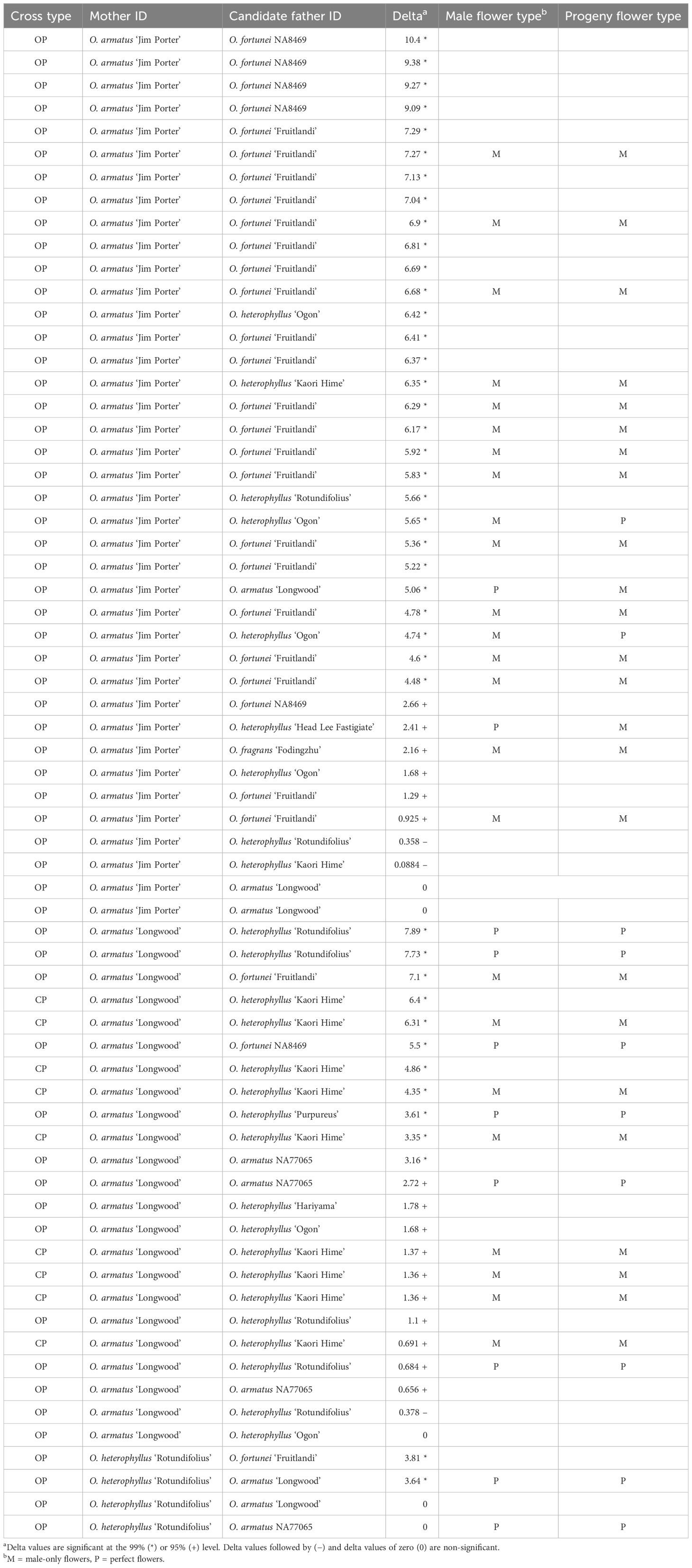
Table 4 Osmanthus hybrid parentage and flower type as determined with Cervus 3.0.7 software using seven SSR markers.
Clustering analysis revealed two major groups of cultivars and hybrids (Figure 1). Cluster A includes O. fragrans (Subgroup 1) and O. fortunei and O. armatus × O. fortunei hybrids (Subgroup 2). Cluster B includes O. heterophyllus (Subgroup 1) and O. armatus, and O. heterophyllus × O. armatus hybrids (Subgroups 2 and 3; Figure 1).
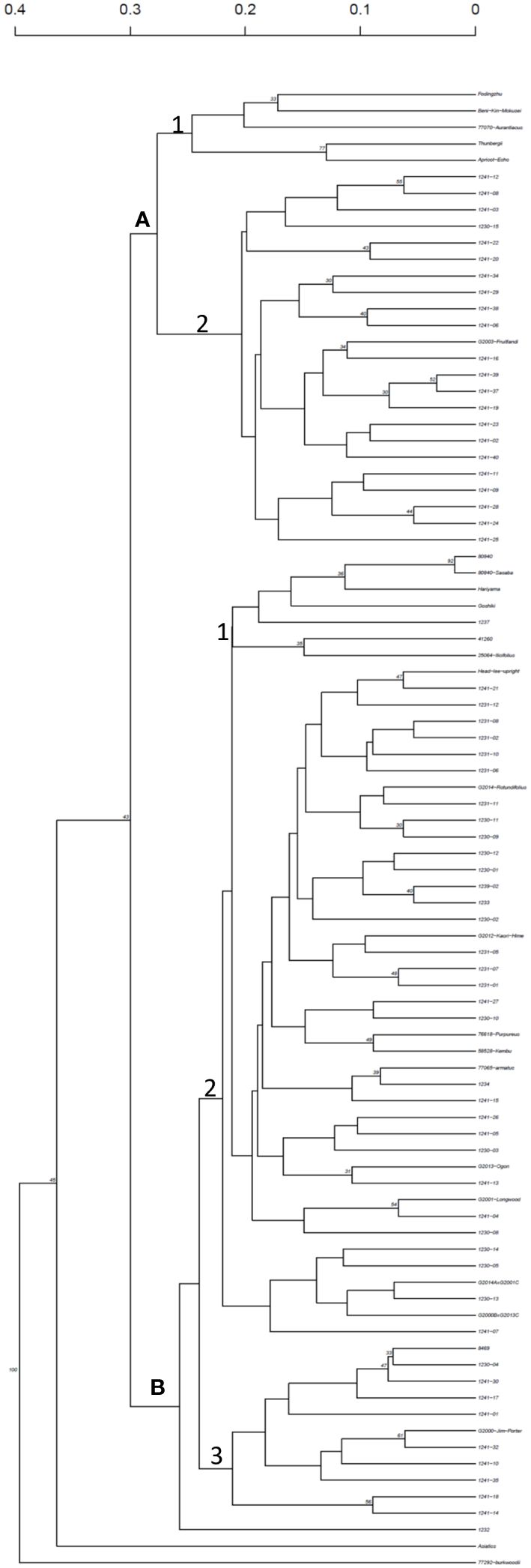
Figure 1 UPGMA cluster analysis of Osmanthus cultivars and hybrid progenies based on Bruvo’s distance calculated using seven SSR markers. Nodes are ordered by increasing genetic distance.
3.4 Phenotypic analysis
Seventy-four hybrid progenies were evaluated for growth rate, flower type, and leaf margin (smooth or toothed). Progenies were evaluated by the cross: O. armatus ‘Longwood’ × OP (n = 15 progenies), O. armatus ‘Longwood’ × O. heterophyllus ‘Kaori Hime’ (n = 14 progenies), O. heterophyllus ‘Rotundifolius’ × OP (n = 5 progenies), and O. armatus ‘Jim Porter’ × OP (n = 40 progenies). At the start of the test, average heights ranged between 9.9 and 24.5 cm; after three growing seasons, average heights ranged between 105 and 138 cm (Figure 2). O. armatus ‘Jim Porter’ × OP and O. armatus ‘Longwood’ × OP had significantly tallest progeny at all time points except the initial measurement. Starting widths ranged between 12 and 22 cm; after three growing seasons, average widths ranged between 59.9 and 76.2 cm. O. armatus ‘Jim Porter’ × OP and O. heterophyllus ‘Rotundifolius’ × OP had the widest progenies at all time points (Figure 3). By the end of the evaluation, O. armatus ‘Longwood’ × OP was both the tallest and narrowest family (Figures 2, 3). Height growth among families ranged between 158% and 238% in year 1 (2019–2020), between 124% and 221% in year 2 (2020–2021), and between 39% and 64.7% in year 3 (2021–2022). Osmanthus heterophyllus ‘Rotundifolius’ × OP had the largest height increase in year one, while O. armatus ‘Longwood’ × OP had the largest height increase in the following two years (Table 5). Width ranges increased between 54.5 and 121% in year 1, between 44.1 and 118% in year 2, and between 39.0 and 64.7% in year 3 for all cross progeny. O. heterophyllus ‘Rotundifolius’ × OP had the largest width increase in years 1 and 2, and O. armatus ‘Longwood’ × OP had the largest width increase in year 3 (Table 5). For all four cross families, growth rates were highest in years 1 and 2 and slowed significantly in year 3 (Table 5).
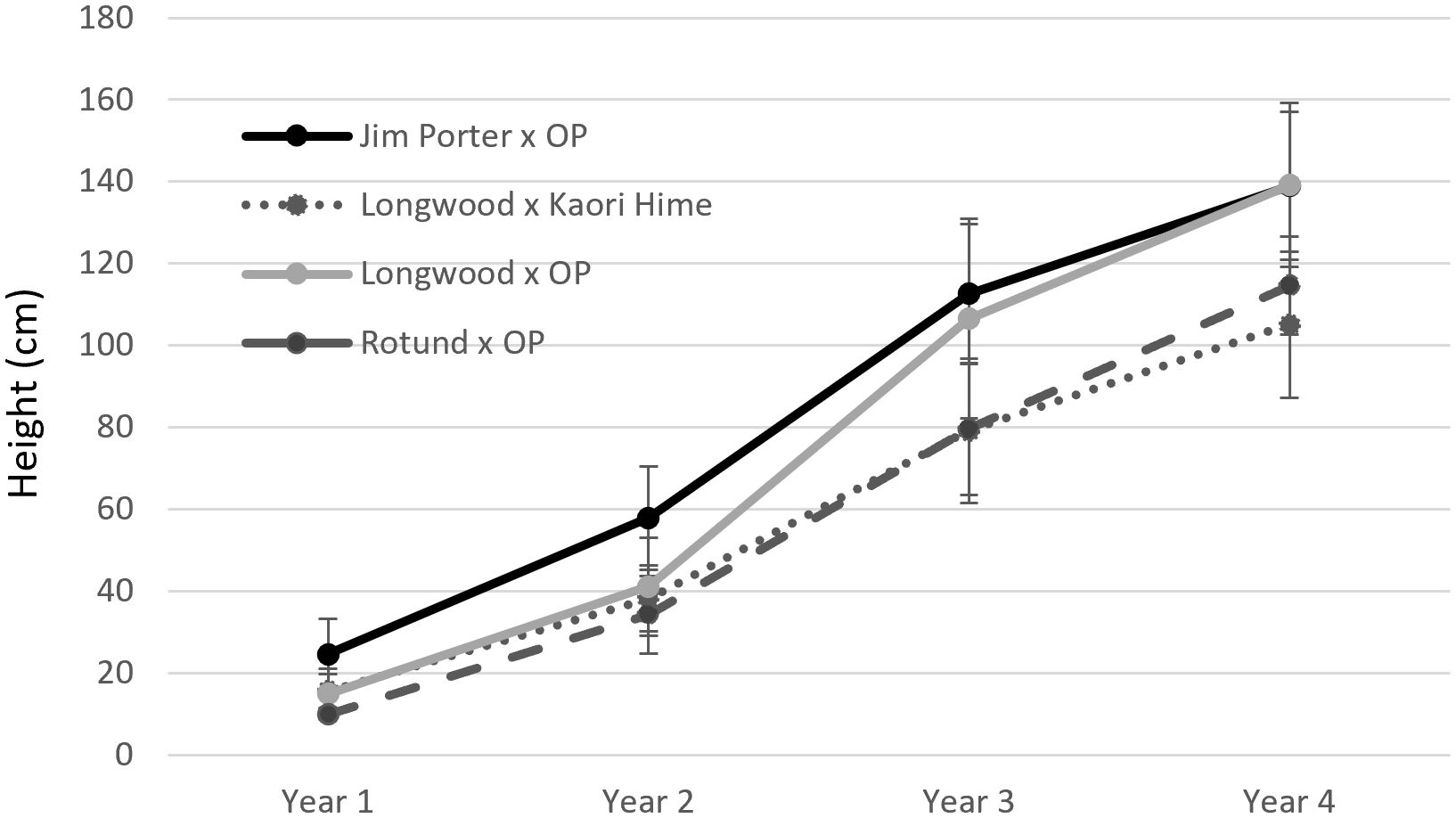
Figure 2 Mean height of four container-grown Osmanthus families measured between 2019 and 2022 in McMinnville, Tennessee, USA. Error bars represent standard deviation.
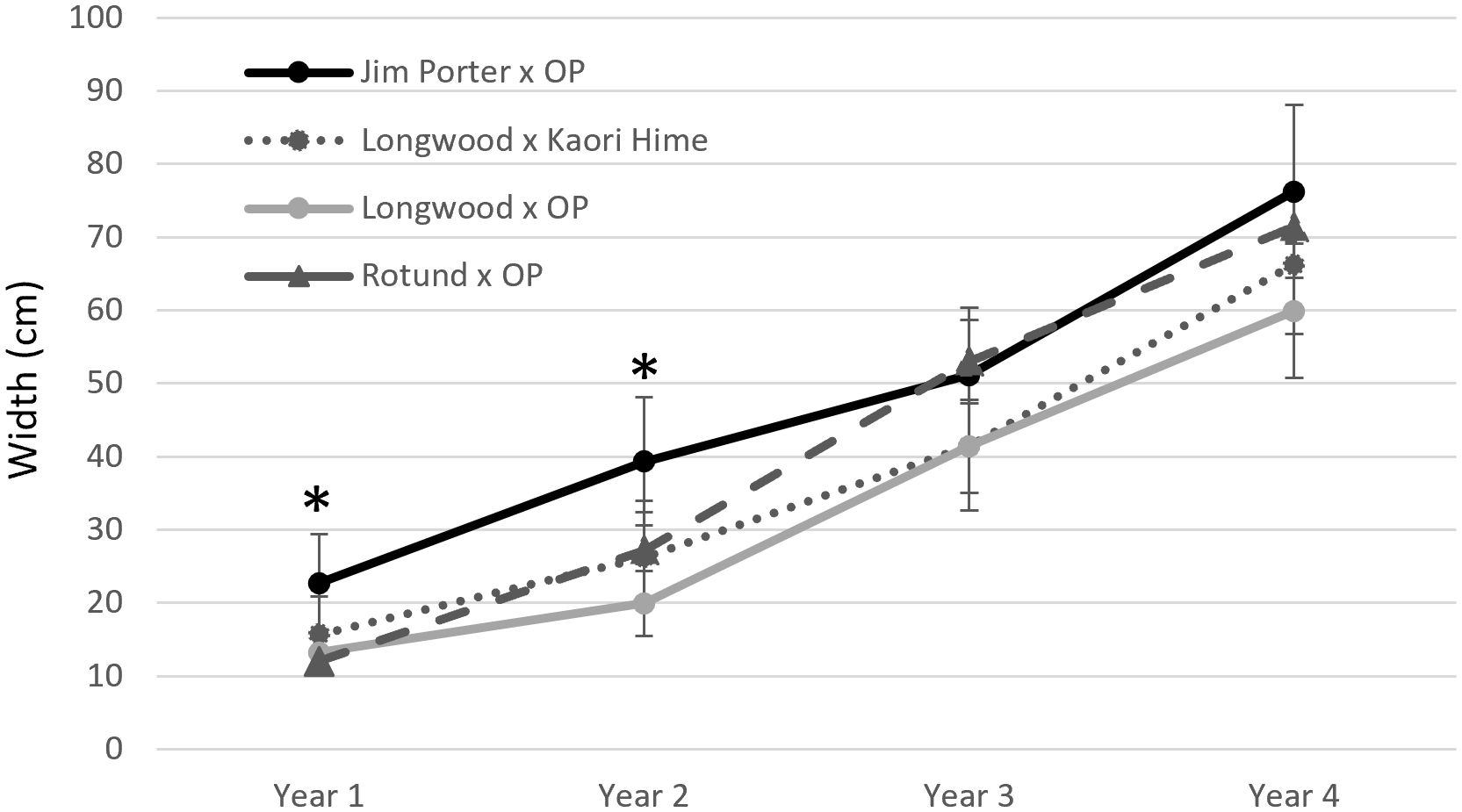
Figure 3 Mean width of four container-grown Osmanthus families measured between 2019 and 2022 in McMinnville, Tennessee, USA. Error bars represent standard deviation. Means significantly different at the α = 0.05 level are shown with an asterisk (*).
Flowers were first observed 3 December 2021, when plants were approximately four years old. By the end of the observation period (February 2023), 51 of 74 progenies had flowered. Male flower type was significantly associated with progeny flower type (χ2 = 17.5, p < 0.0001). Progeny with a male-flowered male parent had male flowers and progeny with a perfect-flowered male parent had perfect flowers in all but four comparisons (Table 4).
All 74 progenies had toothed leaf margins on immature growth. The newest growth on about half of the plants (n = 27) was transitioning from toothed to entire, while new growth on the remaining plants was as toothed as the immature growth. Leaf margin was not associated with cross family (χ2 = 0.83, p = 0.84).
4 Discussion
This is the first report of novel Osmanthus hybrids including O. armatus × O. fortunei, O. armatus × O. heterophyllus, and O. armatus × O. fragrans. Osmanthus armatus is a relatively cold-hardy species that can grow and flower into USDA Hardiness Zone 6 (Dirr, 2009). The hybrids developed herein are expected to have intermediate cold-hardiness relative to their parents based on observations of O. fortunei, an O. fragrans × O. heterophyllus hybrid (Alexander, 2019). Hybrids will be evaluated in the field for at least three growing seasons in USDA Plant Hardiness Zone 6b to determine relative cold-hardiness, growth rates, and plant architecture. Symptoms of cold-injury were observed in hybrids after the first winter in the field, including yellowed foliage, defoliation, and tip die-back (Figures 4, 5). The cultivars ‘Longwood’ and ‘Jim Porter’ used as female parents herein are relatively cold hardy, but very closely related (Alexander et al., 2018) with similar morphology including perfect flowers and a long flowering period; however, the toothed leaves, appressed flowers, and shrub-like habit are traits warranting improvement. Further crosses between O. fragrans and smooth-leafed O. heterophyllus species will generate variability for continued development of new Osmanthus cultivars.
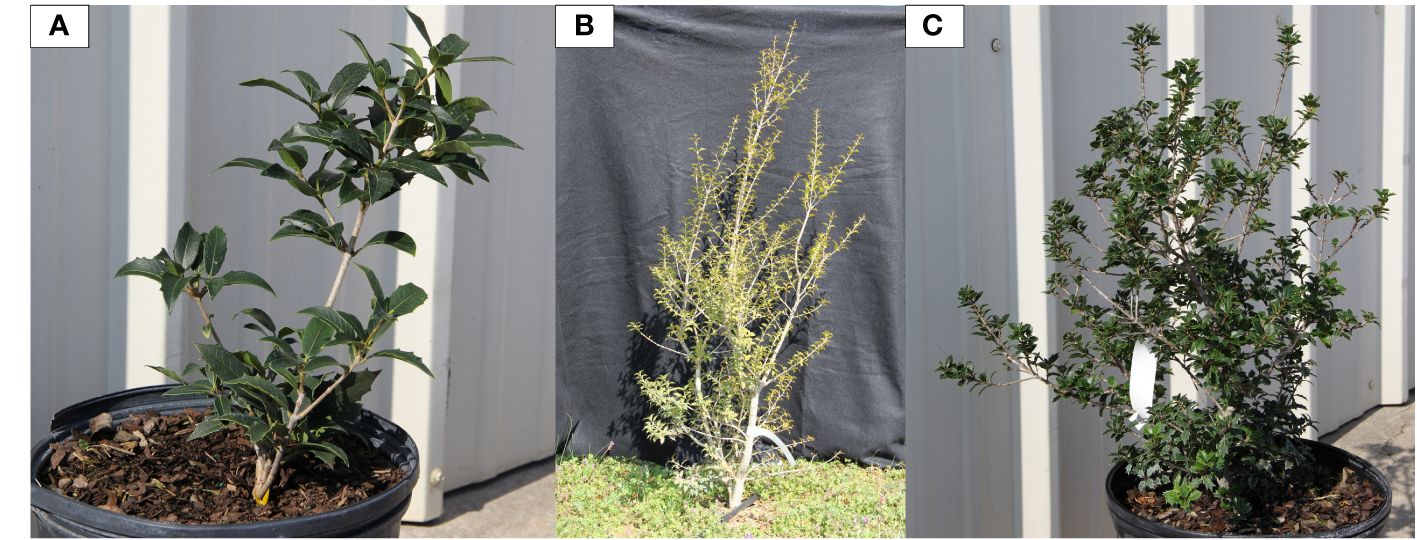
Figure 4 Osmanthus parents and hybrid. (A) O. armatus ‘Longwood’, (B) O. armatus ‘Longwood’ × O. heterophyllus ‘Kaori Hime’, (C) O. heterophyllus ‘Kaori Hime’. Photographed 13 March 2024, McMinnville, Tennessee, USA.
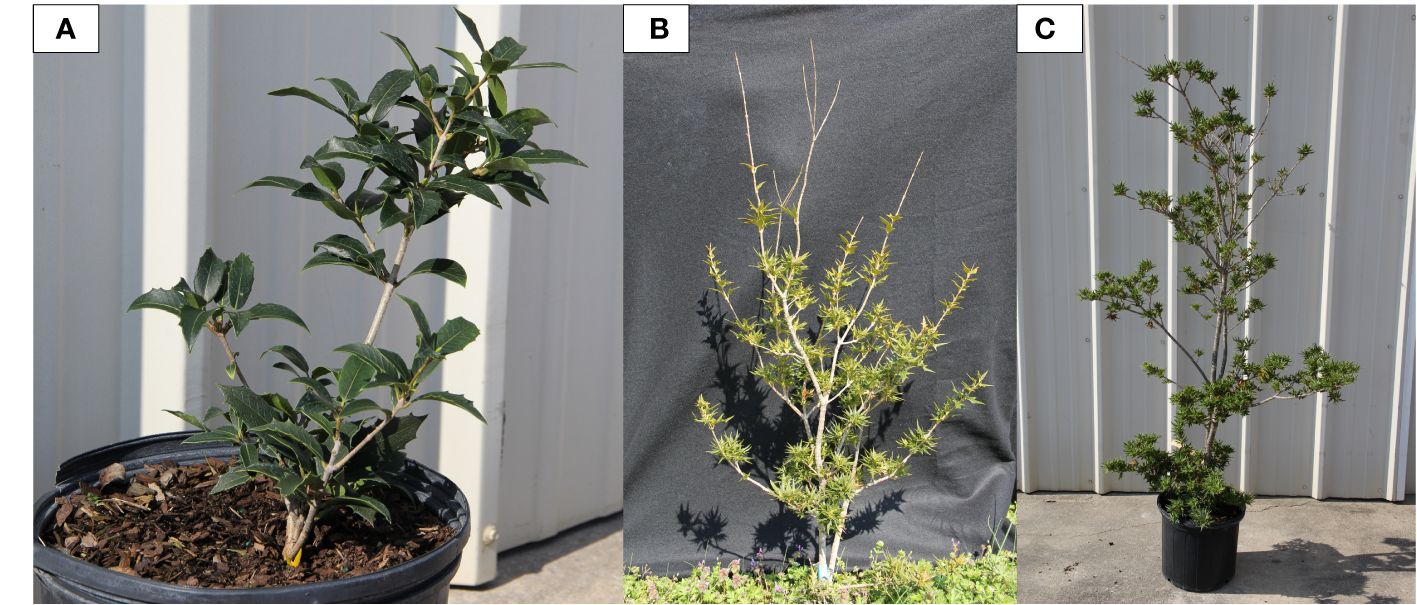
Figure 5 Osmanthus parents and hybrid. (A) O. armatus ‘Longwood’, (B) O. armatus ‘Longwood’ × O. heterophyllus ‘Hariyama’, (C) O. heterophyllus ‘Hariyama’. Photographed 13 March 2024, McMinnville, Tennessee, USA.
The complex breeding system of Osmanthus makes open-pollinated family reconstruction an attractive option for trait introgression, a strategy known as “breeding without breeding” (El-Kassaby and Lstibůrek, 2009). Controlled crosses among Osmanthus genera are often difficult to make due to the presence of androdioecy and diverse flowering times and may be only moderately successful; 22 of 50 controlled pollinations between O. armatus ‘Longwood’ and O. heterophyllus ‘Kaori Hime’ produced seed in this study. Osmanthus flowers each produce only one fruit, each fruit produces only one seed, and germination is difficult (Dirr, 2009; Tang et al., 2013). In this study, the average germination rate was 52%. More research is needed on germination of Osmanthus seed, where both the woody endocarp and endogenous dormancy within the embryo likely play key roles (Morales-Sillero et al., 2012; Alexander, 2023).
In this study, seven SSR markers provided unique fingerprints for 66 progenies, with most progenies assigned parentage at the 95% confidence level or above. Unassigned progeny was of two kinds – those with missing alleles and those with closely related parents as the two most likely parents. For example, three of the six unassigned parents were cases where O. armatus ‘Longwood’ and ‘Jim Porter’ were indistinguishable. Cluster analysis identified two major genetic groupings; an O. fragrans/fortunei group and an O. heterophyllus/armatus group. The difference between the subgroups reflects the genetic distance of O. fragrans from the other species (Lu et al., 2010; Duan et al., 2013). The utility of the markers used here was further validated by cluster analysis which placed hybrids, including O. fortunei (a hybrid of O. fragrans and O. heterophyllus) and the progenies from controlled crosses into subgroups with their known parents as expected.
Osmanthus is considered a slow-growing species which may hamper its adoption by industry (Dirr, 2009). In this study, progenies of O. armatus ‘Jim Porter’ had both the tallest and widest progenies, while the progenies of O. heterophyllus ‘Kaori Hime’ were smallest, as expected since ‘Kaori Hime’ is a small, highly branched cultivar. These results underscore the diversity and novel forms that can be obtained by hybridization among genera. Open-pollinated progenies grew an average of 204% and 159% per year in the first and second growing seasons, respectively, but only 44% in the third growing season. Considering this, it would be possible to finish a germinated seedling or rooted cutting in a single growing season.
Floral traits including fragrance, bright color, and long flowering time are highly valued in Osmanthus cultivars (Cai et al., 2014). Understanding flower inheritance among Osmanthus species and cultivars provides insight into the specific system of androdioecy and informs the expected flower sex ratios of progenies (Xu et al., 2014). In this study, male flower type was significantly associated with progeny flower type (χ2 = 17.5, p < 0.0001). Progeny with a male-flowered male parent had male flowers and progeny with a perfect-flowered male parent had perfect flowers in all but four cases. Two progenies of O. armatus ‘Jim Porter’ × O. heterophyllus ‘Ogon’ had perfect flowers while ‘Ogon’ produces male-only flowers. Two O. armatus ‘Jim Porter’ progenies have male-only flowers while their male-parents, O. armatus ‘Longwood’ and O. heterophyllus ‘Head-Lee Fastigiate’, produce perfect flowers. These four instances could be misassigned parentage; yet the LOD scores were highly significant. As O. fortunei is an O. heterophyllus × O. fragrans hybrid, it is possible that male-only flowers may be a dominant trait, or simply that genotypes with male flowers are more attractive and thus selected as cultivars. Larger sample sizes are needed to determine sex ratio in Osmanthus hybrid offspring. New sources of perfect flowers in O. fragrans are needed to develop novel Osmanthus breeding lines, increase cold hardiness of commercially available cultivars, and promote Osmanthus culture in expanded markets.
5 Conclusion
Novel Osmanthus hybrids including O. armatus × O. fortunei, O. armatus × O. heterophyllus, and O. armatus × O. fragrans were produced via controlled and open-pollination and verified using a suite of SSR markers. The hybrids developed herein are expected to have intermediate cold-hardiness relative to their parents and will be evaluated in the field in USDA Plant Hardiness Zone 6b for at least three growing seasons to determine relative cold-hardiness, growth rates, and plant architecture. The O. armatus cultivars ‘Longwood’ and ‘Jim Porter’ used as female parents herein are relatively cold hardy, but very closely related. Further crosses among Osmanthus species will generate variability for continued development of new Osmanthus cultivars. These Osmanthus hybrids may be obtained for certain uses under agreement with the U.S. Department of Agriculture by contacting the corresponding author.
Data availability statement
The raw data supporting the conclusions of this article will be made available by the authors, without undue reservation.
Author contributions
LA: Writing – review & editing, Writing – original draft, Visualization, Validation, Supervision, Software, Resources, Project administration, Methodology, Investigation, Funding acquisition, Formal Analysis, Data curation, Conceptualization. XW: Writing – review & editing, Methodology, Investigation. FG: Writing – review & editing, Visualization, Software, Methodology, Investigation, Data curation.
Funding
The author(s) declare financial support was received for the research, authorship, and/or publication of this article. This work was supported by the Floral and Nursery Research Initiative and USDA-ARS in-house project #8020-21000-086-000D.
Acknowledgments
Carrie Witcher, Benjamin Moore, and Joseph Davis maintained plants and provided field support. Mention of a trademark, proprietary product, or vendor does not constitute a guarantee or warranty of the product by the U.S. Dept. of Agriculture and does not imply its approval to the exclusion of other products or vendors that also may be suitable.
Conflict of interest
The authors declare that the research was conducted in the absence of any commercial or financial relationships that could be construed as a potential conflict of interest.
Publisher’s note
All claims expressed in this article are solely those of the authors and do not necessarily represent those of their affiliated organizations, or those of the publisher, the editors and the reviewers. Any product that may be evaluated in this article, or claim that may be made by its manufacturer, is not guaranteed or endorsed by the publisher.
Supplementary material
The Supplementary Material for this article can be found online at: https://www.frontiersin.org/articles/10.3389/fhort.2024.1382450/full#supplementary-material
References
Alexander L. (2019). Growth, cold-hardiness, and flowering of sweet olive, fortune’s osmanthus, fragrant tea olive, and holly tea olive in Tennessee. Horttechnology 29, 78–84. doi: 10.21273/HORTTECH04166-18
Alexander L. (2023). Genome size, flowering, and breeding compatibility in Osmanthus accessions. Horticulturae 9, 56. doi: 10.3390/horticulturae9010056
Alexander L. W., Thammina C. S., Kramer M. (2018). Cross-transferability of SSR markers in Osmanthus. Genet. Resour. Crop Evol. 65, 125–136. doi: 10.1007/s10722-017-0514-4
Bruvo R., Michiels N. K., D’Souza T. G., Schulenburg H. (2004). A simple method for the calculation of microsatellite genotype distances irrespective of ploidy level. Mol. Ecol. 13, 2101–2106. doi: 10.1111/j.1365-294X.2004.02209.x
Cai X., Mai R., Zou J., Zhang H., Zeng X., Zheng R., et al. (2014). Analysis of aroma-active compounds in three sweet osmanthus (Osmanthus fragrans) cultivars by GC-olfactometry and GC-MS. J. Zhejiang Univ. Sci. B. 15, 638–648. doi: 10.1631/jzus.B1400058
Dirr M. A. (2009). Manual of woody landscape plants: Their identification, ornamental characteristics, culture, propagation and uses. 6th ed (Champaign, IL: Stipes Publishing, L.L.C).
Duan Y., Wang X., Qibai X., Liang L., Li X., Liu Y., et al. (2013). Genetic diversity of androdioecious Osmanthus fragrans (Oleaceae) cultivars using microsatellite markers. Appl. Plant Sci. 7, 1. doi: 10.3732/apps.1200092
El-Kassaby Y. A., Lstibůrek M. (2009). Breeding without breeding. Genet. Res. 91, 111–120. doi: 10.1017/S001667230900007X
Green P. S. (1963). A revision of the new Caledonian species of Osmanthus. J. Arnold Arb. 44, 268–283. doi: 10.5962/p.185667
Greenberg D. A., Berger B. (1994). Using lod-score differences to determine mode of inheritance: a simple, robust method even in the presence of heterogeneity and reduced penetrance. Am. J. Hum. Genet. 55, 834–840.
Guo S., Xiong M., Ji C., Zhang Z., Li D., Zhang Z. (2011). Molecular phylogenetic reconstruction of Osmanthus Lour. (Oleaceae) and related genera based on three chloroplast intergenic spacers. Plant Syst. Evol. 294, 57–64. doi: 10.1007/s00606-011-0445-z
Kalinowski S. T., Taper M. L., Marshall T. C. (2007). Revising how the computer program CERVUS accommodates genotyping error increases success in paternity assignment. Mol. Ecol. 16, 1099–1106. doi: 10.1111/j.1365-294X.2007.03089.x
Kamvar Z. N., Brooks J. C., Grünwald N. J. (2015). Novel R tools for analysis of genome-wide population genetic data with emphasis on clonality. Front. Genet. 6. doi: 10.3389/fgene.2015.00208
Li Y., Yuan W., Dong M., Shang F. (2004). A study on the karyotype of Osmanthus fragrans Lour. J. Nanjing Forestry Univ. 28, 87–90. doi: 10.3969/j.issn.1000-2006.2004.z1.014
Lu Z., Duan H., Lu L., Sun X. (2010). Genetic relationships of Osmanthus based on ISSR-PCR. Biologia 65, 459–464. doi: 10.2478/s11756-010-0039-1
Marshall T. C., Slate J., Kruuk L. E., Pemberton J. M. (1998). Statistical confidence for likelihood-based paternity inference in natural populations. Mol. Ecol. 7, 639–655. doi: 10.1046/j.1365-294x.1998.00374.x
Morales-Sillero A., Suárez M. P., Jiménez M. R., Casanova L., Ordovás J., Rallo P. (2012). Olive seed germination and initial seedling vigor as influenced by stratification treatment and the female parent. HortScience 47, 1672–1678. doi: 10.21273/HORTSCI.47.12.1672
Paradis E., Schliep K. (2019). ape 5.0: an environment for modern phylogenetics and evolutionary analyses in R. Bioinformatics 35, 526–528. doi: 10.1093/bioinformatics/bty633
Saumitou-Laprade P., Vernet P., Vekemans X., Billiard S., Gallina S., Essalouh L., et al. (2017). Elucidation of the genetic architecture of self-incompatibility in olive: Evolutionary consequences and perspectives for orchard management. Evol. Appl. 10, 867–880. doi: 10.1111/eva.12457
Tang A., Zhao Z., Luo A. (2013). Deep physiological dormancy in desiccation-sensitive seeds of Osmanthus fragrans (Oleaceae). Seed Sci. Technol. 41, 336–344. doi: 10.15258/sst
Wallander E., Albert V. A. (2000). Phylogeny and classification of Oleaceae based on rps16 and trnL-F sequence data. Am. J. Bot. 87, 1827–1841. doi: 10.2307/2656836
Xiang Q. B., Liu Y. L. (2008). An illustrated monograph of the sweet osmanthus cultivars in China (Zhejiang Science and Technology Publishing: Hangzhou).
Xu Y. C., Zhou L., Hu S., Hao R., Huang C., Zhao H. (2014). The differentiation and development of pistils of hermaphrodites and pistillodes of males in androdioecious Osmanthus fragrans L. implications Evol. to androdioecy. Plant Syst. Evol. 300, 843–849. doi: 10.1007/s00606-013-0923-6
Yu D., Wang X., Zhang W., Shang F. (2010). A study on cold resistance of Osmanthus fragrans cultivars in Kaifeng, China. J. Life Sci. 4, 17–20.
Keywords: tea olive, hybrid breeding, microsatellite, SSR, paternity analysis, germplasm, cultivar diversity
Citation: Alexander L, Wu X and Gouker F (2024) Production and verification of novel Osmanthus hybrids. Front. Hortic. 3:1382450. doi: 10.3389/fhort.2024.1382450
Received: 05 February 2024; Accepted: 29 March 2024;
Published: 11 April 2024.
Edited by:
Vagner A. Benedito, West Virginia University, United StatesReviewed by:
Mukesh Choudhary, ICAR-Indian Institute of Maize Research, IndiaReeta Bhatia, Indian Council of Agricultural Research, India
Copyright © 2024 Alexander, Wu and Gouker. This is an open-access article distributed under the terms of the Creative Commons Attribution License (CC BY). The use, distribution or reproduction in other forums is permitted, provided the original author(s) and the copyright owner(s) are credited and that the original publication in this journal is cited, in accordance with accepted academic practice. No use, distribution or reproduction is permitted which does not comply with these terms.
*Correspondence: Lisa Alexander, bGlzYS5hbGV4YW5kZXJAdXNkYS5nb3Y=