- Institute for Plant Protection in Fruit Crops and Viticulture, Julius Kühn-Institut, Federal Research Institute for Cultivated Plants, Dossenheim, Germany
Over the past two decades, several headspace collection techniques have been used to detect and identify volatile organic compounds (VOCs) emitted by plants, pests, air, and soil. Volatiles emitted by pests and infected plants are usually found at low concentrations. The important challenge is to be able to capture an exact collection of relative quantities of the relevant VOCs. Here we present an innovative headspace collecting device (HSCD) for sampling VOCs, which ensures an exact regulation of mass flow velocity and collected total gas volume allowing besides qualitative analysis of collected VOCs, an exact comparison of their relative quantities in plant or insect headspace samples. The HSCD possess six parallel odor collection systems each consisting of a digital mass flow detector and controller connected to a vacuum pump, which are mounted in a trolley suitcase, connected by tubes, and wired electrically. The programming of the channels, can be done by a digital control unit. Using the HSCD, VOCs emitted by the brown marmorated stink bug (BMSB), Halyomorpha halys, from its different developmental stages including egg, nymphal stages, nymphal exuvia, and adults were collected on thermal desorption tubes and analyzed using an automated thermal desorber connected to a gas chromatography-mass spectrometry system. Forty-five VOCs were identified in total, which mainly consisted of tridecane (4.36-69.33%), E-2-decenal (4.24-26.48%), 2-undecenal (0.63-50.03%), and E-4-oxo-2-hexenal (1.22-20.58%). The random forest analysis revealed three different chemical patterns among BMSB life stages samples. In conclusion, these results indicate that the new developed HSCD, a mobile, easy to use, and reliable instrument, has a high potential to sample relevant VOCs of a specific life stage of an insect both qualitatively and quantitatively. It was demonstrated that this information can be used for identifying specific chemical signatures, which fit to specific life stages of the BMSB, and may be used as biomarker for on-site detection of this pest e.g. in shipping containers or agricultural areas.
1 Introduction
Volatile organic compounds (VOCs) are a large group of chemical compounds released by living organisms such as plants and insects for chemical communication and/or use as chemical defenses in respond to abiotic and biotic stressors (Vivaldo et al., 2017). In nature, VOCs play an important role in the adaptation of plants and their protection against various environmental stresses such as drought and heat (Karimi and Meiners, 2021), flooding and herbivory (Ngumbi and Ugarte, 2021), as well as in the reproductive behavior of many insects (Yew and Chung, 2015). Chemical interaction between plants and pests can provide species-specific signals to herbivorous insects that can attract or deter pests from feeding or ovipositing on their host plants (Helms et al., 2013).
Volatiles emitted by insect pests or infected plants are usually present in low concentrations. Thus, the major challenge is to collect the VOCs relevant for the identification of insect pests and plant pathogens in the field (Wong et al., 2019). Numerous collection techniques for VOCs have been developed in recent decades, such as headspace sampling, solid-phase microextraction (SPME), solid-phase extraction (SPE), stir-bar sorptive extraction (SBSE), and direct-contact sorptive extraction (Kfoury et al., 2017; Gross et al., 2019; Rering et al., 2020; Alborn et al., 2021; Tholl et al., 2021). All these different techniques have their limitations (Diez-Simon et al., 2020). The amounts of solid phase extraction depend strongly on the interactions of the solid phase with the volatiles to be collected. Due to its specific characteristics, SPME is suitable for sampling VOCs for qualitative rather than quantitative analysis (Weintraub and Gross, 2013). Furthermore, the flow in dynamic headspace collection systems is influenced by some internal and external factors: temperature, relative humidity and direct sunlight affect the amount of VOCs emitted and collected. Therefore, especially in field experiments, it is important to measure comparable samples at the same time to avoid distortions due to temperature fluctuations during the day or changing intensity of solar radiation due to e.g. clouds between the VOC collection of different samples. Finally, the thermal desorption tubes used for collection also differ often in their flow characteristics, which makes quantitative comparisons difficult. The attempt to collect relevant VOCs in qualitatively and, in particular, quantitatively comparable ratios has led us to develop an innovative dynamic headspace collection device (HSCD). It is a derivative of two prototypes we described earlier (Rid et al., 2016; Gross et al., 2019) and consists of six parallel odor collection systems. The technical features of the device are presented in more detail in this paper and tested using the VOCs emitted by Halyomorpha halys, the brown marmorated stink bug (BMSB).
The BMSB is an invasive insect pest that originated in East Asia, and has become established in many countries including many European countries, Russia, the United States, Canada, and South American countries such as Chile. The BMSB is a polyphagous and highly mobile pest that causes significant economic damage by infesting a wide range of horticultural crops such as vegetables, fruit, and ornamental plants (Wiman et al., 2015; Zobel et al., 2016; Leskey and Nielsen, 2018). The BMSB goes into diapause from early October to spring emergence, and during this time can be carried in new regions inside transportation containers or vehicles. Nixon et al. (2019) reported that container conditions and temperature changes did not significantly affect the mobility and mortality of diapausing H. halys, which may cause biosecurity issues at borders and increase the risk of H. halys invasion in other countries. In addition, the diapausing behavior of H. halys can be disrupted by unstable shipping conditions, affecting the efficacy of pheromone-baited monitoring traps (Morrison et al., 2017).
In the USA and Italy, the BMSB has been controlled mainly by pesticide applications, which strongly increased the amount of pesticide applied in many crops such as fruit and vegetables. Furthermore, the efficacy of several common synthetic insecticides was poor in controlling H. halys (Bergmann and Raupp, 2014). Interestingly, BMSB releases defensive VOCs in response to stress conditions. For example, tridecane, E-2-decenal, 5-ethyl-2(5H)-furanone, dodecane, and E-4-oxo-2-hexenal have been identified as the most abundant defensive compounds of H. halys (Solomon et al., 2013; Nixon et al., 2018; Nixon et al., 2022). These chemical compounds can be used as species-specific signature to detect the BMSB in containers or to monitor fields where the bugs are present. Hence, it is possible to develop a method such as electronic nose sensors (Fundurulic et al., 2023) based on the volatiles of H. halys, which can open new avenues for monitoring and detecting of this invasive pest in the fields where it occurs and in international freight shipments, preventing its arrival and establishment across the border (Nixon et al., 2019).
Therefore, the aims of this study are: (a) to develop a HSCD enabling to capture an exact collection of relative quantities of VOCs and (b) to evaluate the potential of this HSCD as well as to identify specific VOCs of the BMSB life stages, representing their chemical signature that could be used for their detection in shipping containers or in agricultural areas.
2 Materials and methods
2.1 Headspace collecting device
The Headspace Collecting Device (Figures 1A, B) was developed by Jürgen Gross [Julius Kühn-Institut (JKI), Dossenheim, Germany] together with Ralf Kunath (FLUSYS GmbH, Offenbach, Germany). The new device was developed on the basis of two prototypes that we have previously described (Rid et al., 2016; Gross et al., 2019) and that have been widely tested in scientific studies (Rid et al., 2019; Gallinger et al., 2020, 2023; Kais et al., 2023). It was produced as a small series as part of the PurPest project (European Commission, 2023).
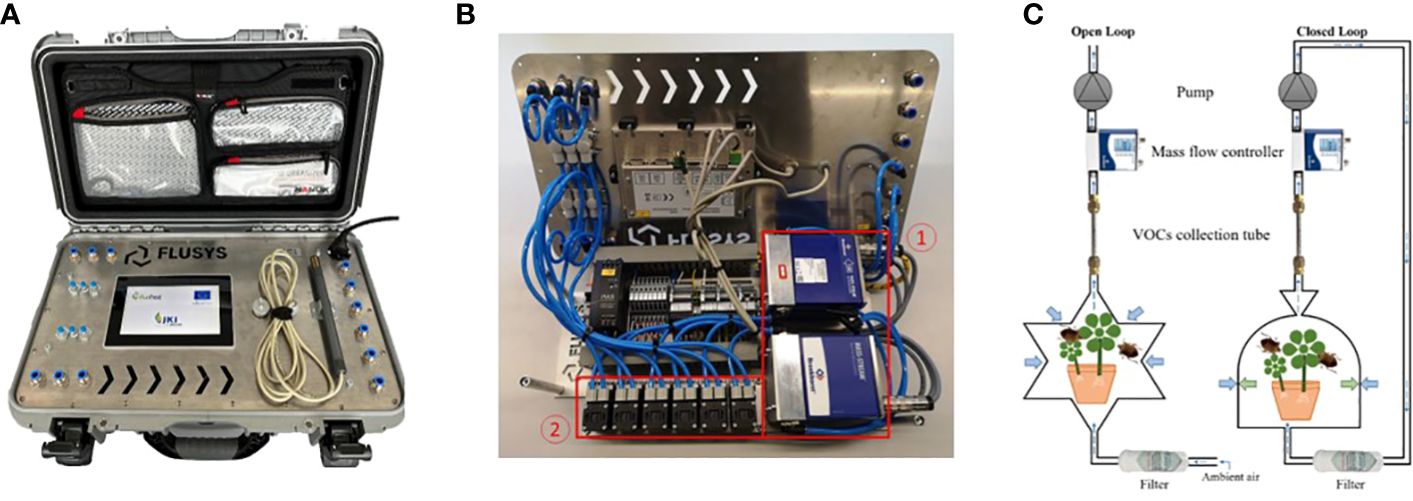
Figure 1 Portable digital 6-channel headspace collecting device (HSCD) for collecting VOCs in standardized manner. In the center is the touch screen, each left and right are six mechanical interfaces (blue). To the right of the screen are the sensors for temperature and rH on an external probe, which are connected to the device with a 2 m cable (A). Device opened to show the mass flow meters (1), suction pumps (2), tubing and wiring (B). The circuit diagrams of open loop system (OLS) and closed loop system (CLS) for sampling (C). Further information can be found in the text. Reproduced with permission.
The HSCD consists of six fragrance collection systems (“channels”) mounted in parallel, each consisting of a suction pump (KNF Neuberger GmbH, Freiburg, Germany) coupled to a digital mass flow meter (detector and controller; Bronkhorst High Tech B.V., AK Ruurlo, The Netherlands) with a measuring range of 0.1 to 1 ln/min air. To prevent cross contamination, there are no connections between single channels. The programming of both flow rate and total collected air volume of each single channel can be done by a digital control unit with a LCD touch screen allowing to switch on or off each channel individually. Flow rate [ln/min], volume [ln], volatile load [%], temperature [°C], humidity [%], date, and time of sampling, together with all status indicator are displayed in real time. The HSCD is adjusted to collect VOCs in a wide range of temperature (5 to 40°C). The final air volume can be set between 0.1 to 1.0 ln/min. Needle valves are interposed in each channel to adjust the suction power of the pumps. Furthermore, the user can select between two sampling methods, the open loop sampling (OLS) and the closed loop sampling (CLS) mode (Figure 1C). Using the 12 mechanical interfaces in the front plate of the device, tubes can just connect to the six input (suction) interfaces (OLS) or additionally to the output interfaces (CLS).
In addition, the ambient temperature and relative humidity are monitored by the HSCD during the sampling of VOCs, which are saved together with exact time and date as a DAT file on an USB storage medium (e.g. an USB stick). The temperature and humidity sensors are mounted on an external probe connected to the device via a 2 m cable. For temperature measurements in the field, the sensor should be hung close to the collected plants, but without being exposed to direct sunlight. All components are mounted in a trolley suitcase for mobility of the system, connected by tubes, and wired electrically. The HSCD can be operated from a 240 V socket or, e.g. for outdoor use, easily by using a portable power station.
For odor collection, each channel of the HSCD must be connected by mechanical interfaces to an air filter cartridge using Teflon tubing to remove VOCs from ambient air, and the stream of the new purified air is then passed over a suitable vessel containing the insect or plant sample and from there to a sample tube on which the VOCs are collected. To avoid negative pressure from the suction that can occur in OLS mode, the outlet of the sample tube can be connected to the output port of the HSCD by mechanical interfaces so that air is simultaneously pumped back into the system (CLS mode). Only ever connect the same input and output numbers to each other! In this way, the HSCD ensures that the air flow is pumped over the insect or plant at exactly the same speed until the final volume is reached. Each channel then switches off automatically and immediately.
2.2 Insect
Adults of Halyomorpha halys (BMSB) were collected at the JKI experimental orchard in Dossenheim, Germany. Rearing was accomplished in insect rearing cages (25 × 25 × 25 cm) in controlled climate chambers (Rütgers GmbH & Co KG), which were maintained at 25°C, 60% relative humidity, and 16L:8D photoperiod. The BMSB were fed on a diet of tomatoes, green beans, and sunflower seeds, and water was provided with soaked cotton wool in small containers. Inside each cage was covered by paper towels as oviposition substrate. Eggs were collected twice a week and transferred to plastic Petri dishes. After hatching, the nymphs were transferred to the rearing cages. To investigate VOCs of H. halys, volatiles released by egg masses (n = 5; approx. 50 eggs per replicate), first and second instar nymphs and their exuvia (n = 3-5; 10 nymphs/exuvia per replicate), and adult (n = 5; 3 males + 2 females per replicate) were sampled. Before sampling adult BMSB, VOCs from males and females were sampled separately. Comparison of the VOCs chromatograms from male and female BMSB showed very similar chromatograms and released VOCs. Hence, the mixed male and female adults were considered to be sampled for this study. To reduce mechanical agitation, the BMSB adults and nymphs were placed smoothly in glass bottles, and let them adapt to the sampling condition for 30 min, then collecting VOCs was carried out. Furthermore, eggs were separated from the paper towels and then their VOCs were collected.
2.3 Headspace sampling
To detect and identify plant-pest specific VOCs, single branches of plants or individual pests are wrapped in oven plastic bags or glass bottles as described by Rid et al. (2016). The chemical patterns among different life stages of H. halys were investigated by using the HSCD in CLS mode. To collect volatiles, the eggs, nymphs, exuvia, and adults of H. halys were placed in glass bottles [GL 45 DURAN®, 250 ml, PPS caps (250°C)]. Using clean air filter cartridges (ICAF 2X6, Sigma Scientific, Micanopy, USA), the stream of ambient air was purified and pumped, controlled by the HSCD, through each bottle until it reached the final volume of 30 L. Volatile organic compounds from headspace sampling were trapped on stainless steel, prepacked sample tubes with Tenax TA35/60 sorbent (Markes, Neu-Isenburg, Germany). Moreover, there were control glass bottles in each round of VOCs collection to avoid identifying unexpected VOCs from plastic, paper towels, and glass bottles in H. halys samples.
2.4 Analysis of VOCs
The chemical analysis of the sampled VOCs was performed using an automated thermal desorption system (TurboMatrix™ ATD 650, PerkinElmer, Rodgau, Germany) connected to a gas chromatograph coupled with a mass spectrometer (TD-GC-MS). The GC was equipped with a 30 m × 0.25 mm i.d., 0.25 μm film thickness, nonpolar Elite-5MS column as described previously (Gallinger et al., 2023). In brief, the carrier gas was Helium. The oven temperature was programmed at 40°C for 1 min, heating from 50 to 180°C at 5 K min-1, and increased to the final temperature of 280°C by a rate of 20 K min-1, and held at 280 °C for 6 min. The GC inlet line temperature and the ion source temperature were set at 250°C and 180°C, respectively. The quadrupole mass detector was operated at 70 eV ionization energy.
Using “Automated Mass spectral Deconvolution and Identification System” (AMDIS, V. 2.71; National Institute of Standards and Technology NIST, Boulder, CO, USA) the chromatograms of analyzed samples were evaluated as described by Gross et al. (2019). In brief, mass spectra, retention times and retention indices of detected compounds were compared with the standard compounds according to previous studies to identify volatiles (Gross et al., 2019; Czarnobai De Jorge et al., 2022). The following identification criteria were set to identify VOCs: match factor, ≥ 80% and the relative retention index deviation, ≤ 5% from reference value. For quantification, the deconvolution settings was set as follows: component width, 32; adjacent peak subtraction, one; resolution, low; sensitivity, medium; shape requirements, low; maximum penalty, 20; and signal to noise ratio, > 50. Ethyl ester dodecanoic acid (10 ng/µl) was used as internal standard and was injected into the Tenax tubes before the analysis. The relative percentage composition of individual VOCs was computed from the GC-MS peak areas obtained without using correction factors, comparing for each chromatogram the peak area of individual VOCs with that of the internal standard.
2.5 Statistical analysis
Statistical analyses were conducted using R statistical software (Version 4.3.0). The normality of data was tested using the Shapiro-Wilk test. Unsupervised random forest classification was carried out using the function randomForest from the package “randomForest” (version 4.3.1) to visualize the overall differences in the BMSB volatile profiles as well as selection of the most discriminant volatiles between the BMSB samples. Principal component analysis (PCA) was performed using concentrations of the most discriminant VOCs to exhibit differences in VOCs released by the BMSB sample. The data were mean-centered and PCA was carried out using the function fviz_pca from the package “factoextra” (version 1.0.7.999). Random forest classification and PCA were performed based on the abundance of VOCs peak area. Furthermore, one-way analysis of variance (ANOVA) using the function anova from the package “stats” (version 4.3.0) was performed to investigate whether there were differences in the main VOCs levels among the BMSB samples. Tukey’s Honest Significant Difference (HSD) test (p ≤ 0.05) was used to compare the mean values of VOCs. For this purpose, the relative percentage of main VOCs were used.
3 Results
3.1 Volatiles released by H. halys
According to the ATD-GC-MS analyses, 45 VOCs released by H. halys samples were identified (Table 1). Figure 2 shows the differences in GC-MS chromatograms of VOCs from two H. halys life stages (egg mass and adult; see Supplementary Figure S1 for nymphs and their exuvia). The differences were mostly related to the detected VOCs quantitatively. A comparison of chromatographic profiles from H. halys life stages revealed that VOCs released mainly consisted of tridecane, E-2-decenal, E-4-oxo-2-hexenal, and 2-undecenal. The BMSB adults released higher relative content of tridecane (69.33%) whilst lower relative amount of this compound was measured in the exuvia from first instar nymphs (4.36%) and the egg masses (7.72%). High relative content of E-2-decenal (26.48%) was measured in first instar nymphs, whereas the egg masses (4.24%) contained lower relative amount of this compound. In addition, E-4-oxo-2-hexenal (20.58%) and 2-undecenal (50.03%) were found in high amounts in the exuvia of first instar nymphs. Four VOCs including E-2-decenyl acetate (2.02%), 1-pentadecene (0.32%), Z-2-decenal (0.25%), and E-4-decenal (0.11%) were detected only in adults. Moreover, other VOCs such as undecane, nonanal, dodecane, decanal, and 2,6,10-trimethyltridecane were found in the BMSB samples.
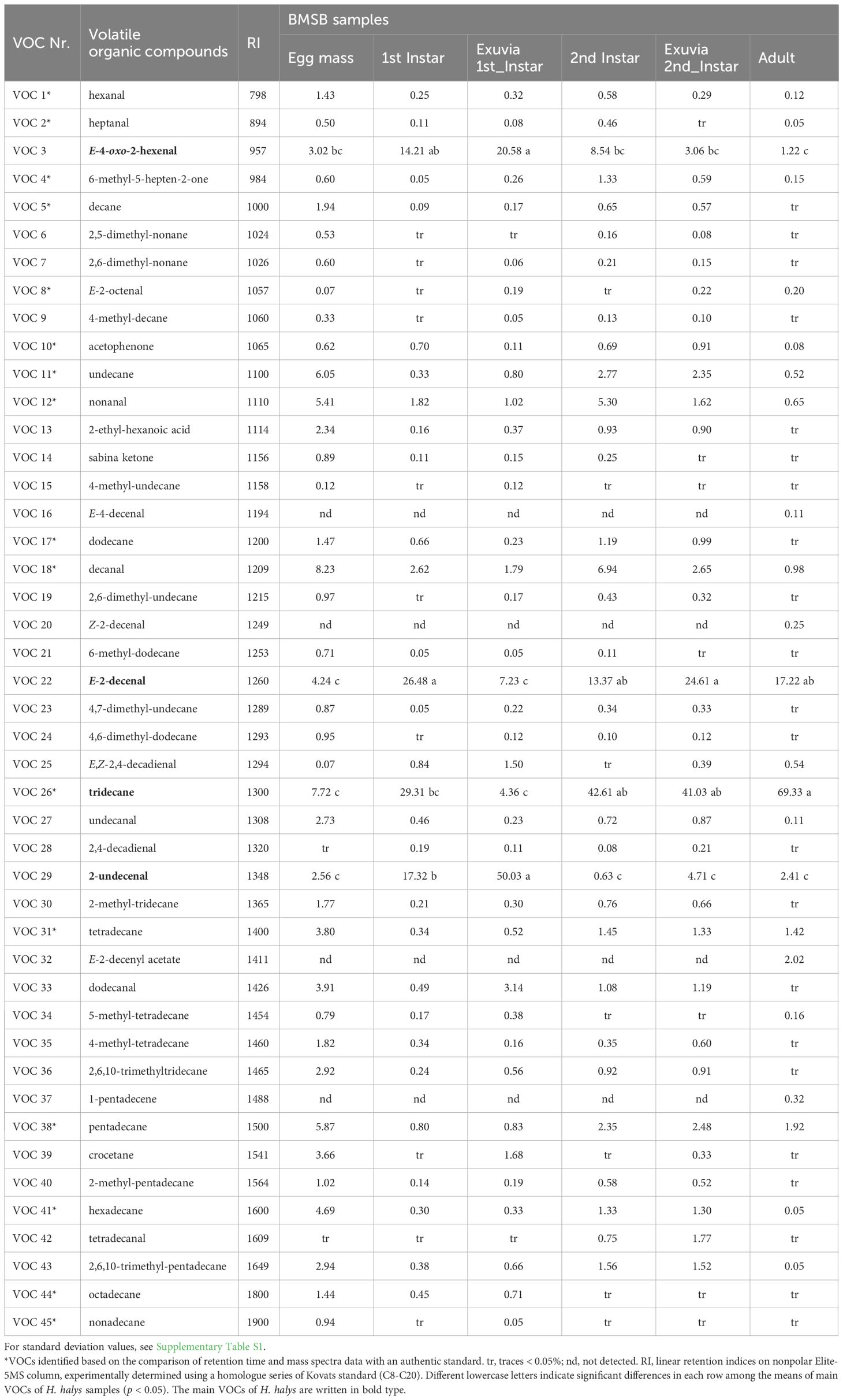
Table 1 Mean relative percentage of VOCs [(%), in comparison with that of the internal standard] identified in the headspace samples of H. halys.
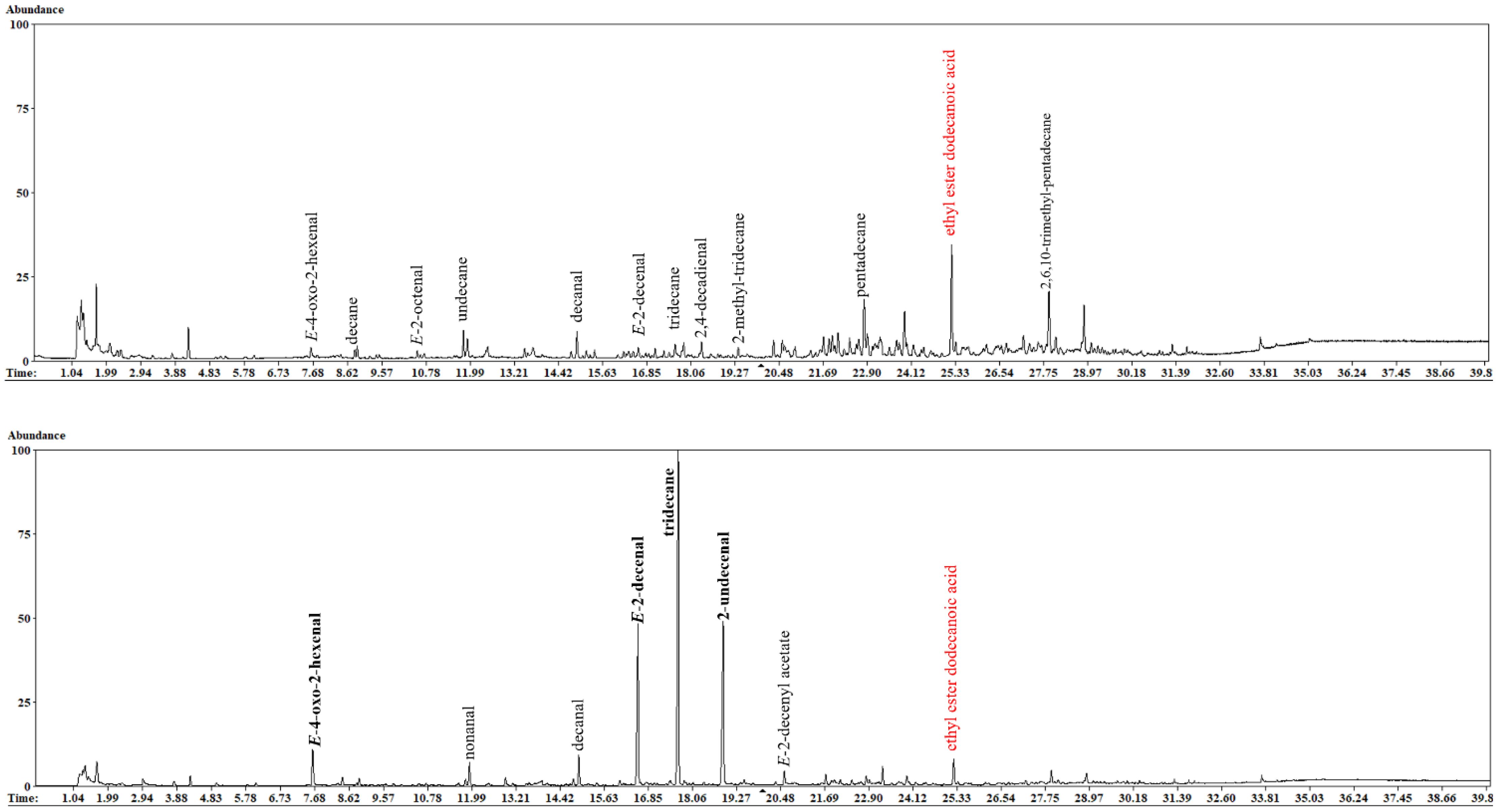
Figure 2 Representative chromatograms obtained from an egg sample (upper panel) and adult (lower panel) of H. halys. For nymphs and their exuvia, see Supplementary Figure S1.
To evaluate differences in VOCs levels among the BMSB samples, the detected VOCs including tridecane, E-2-decenal, E-4-oxo-2-hexenal, and 2-undecenal were analyzed by one way ANOVA followed by Tukey HSD (Table 1). Based on the results, there were significant differences in VOCs levels among the BMSB life stages. As shown in Table 1 and Supplementary Figure S2, a significant difference (p < 0.05) was found, as tridecane and E-2-decenal levels differed between the life stages, where the BMSB adults and first instar nymphs contained highest relative amounts of these VOCs. Furthermore, the BMSB adults and second instar nymphs contained significantly less relative amounts of E-4-oxo-2-hexenal and 2-undecenal comparing to first instar nymphs and their exuvia. Moreover, a small relative amount of these VOCs were found in the egg samples, too.
3.2 Semiochemical variation of H. halys
To estimate the semiochemical variation among H. halys samples, unsupervised random forest (RF) classification was performed (Figure 3). The RF analysis prioritized the twenty top VOCs as discriminating between the BMSB samples (Supplementary Figure S3). Tridecane, the main compound of the BMSB, was highlighted as one of the top compounds which discriminated the BMSB samples. The RF classification distinguished three chemical patterns among BMSB life stages samples based on all detected VOCs. The first pattern, characterized by high relative content of tridecane and E-2-decenal, contained only adults of BMSB. The second pattern consisted of first instar nymphs including their exuvia, characterized by high relative content of E-4-oxo-2-hexenal and 2-undecenal. The third pattern consisted of second instar nymphs and their exuvia characterized by moderate relative content of tridecane and E-2-decenal. Moreover, egg samples were scattered in both second and third pattern (Figure 3).
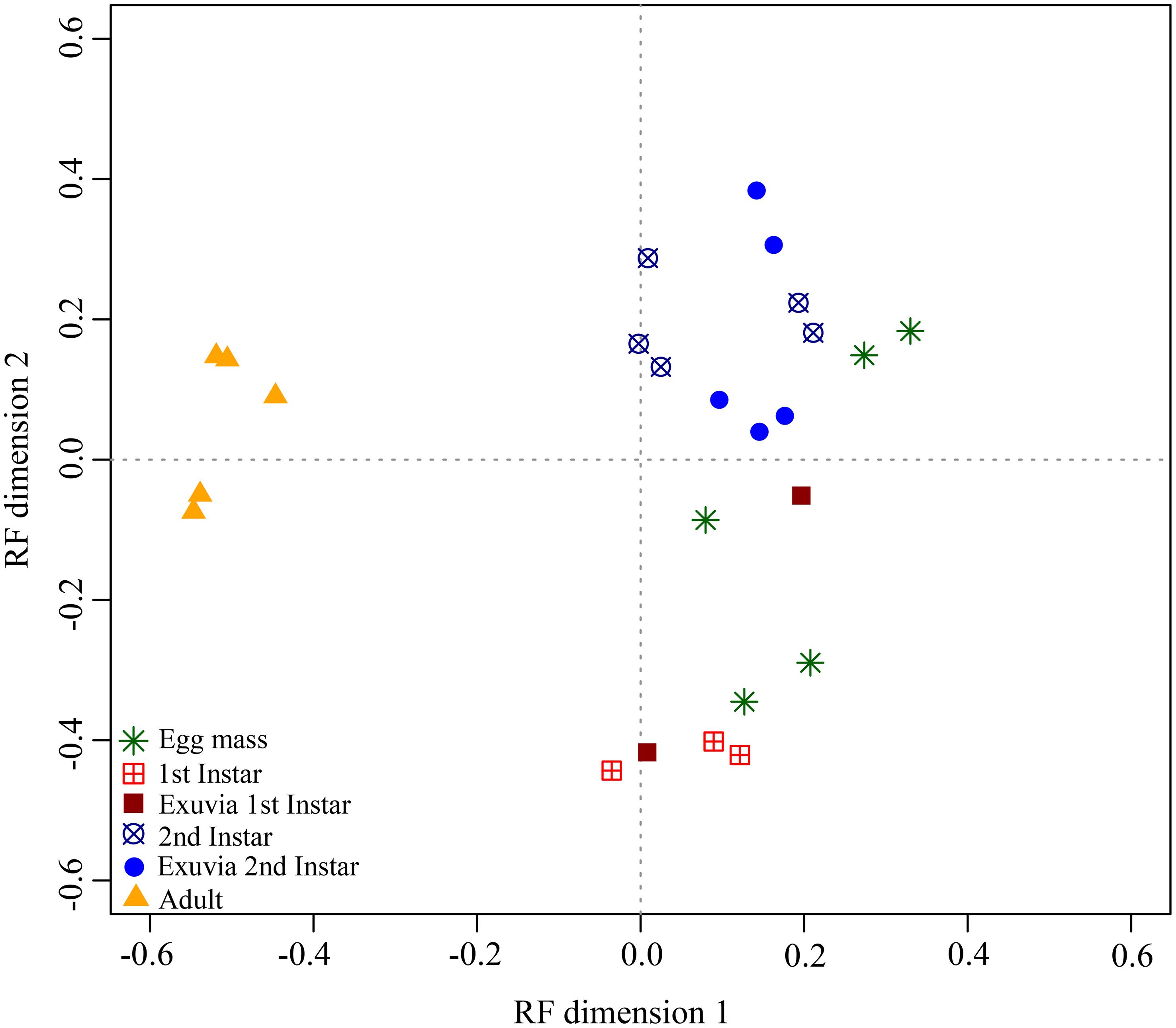
Figure 3 Random forest (RF) classification of volatile profiles obtained from different life stages of H. halys.
Furthermore, PCA analysis clustered the BMSB samples into distinguished chemical patterns and displayed the correlation between the discriminant VOCs and the BMSB samples (Figure 4). The first two PCs represented 90.9% of the total variation in the data set. However, tridecane (0.93), 6-methyl-5-hepten-2-one (0.92), E-2-decenyl acetate (0.92), decane (-0.97), 2,6-dimethyl-nonane (-0.92), 2,6,10-trimethyltridecane (-0.90), and 2-methyl-tridecane (-0.88), contributed more than the other compounds to the PC1 whereas nonanal (0.90), E-4-oxo-2-hexenal (0.81), E,Z-2,4-decadienal (0.76), and 2-undecenal (0.75) were the compounds that influence the PC2 value (Table 2).
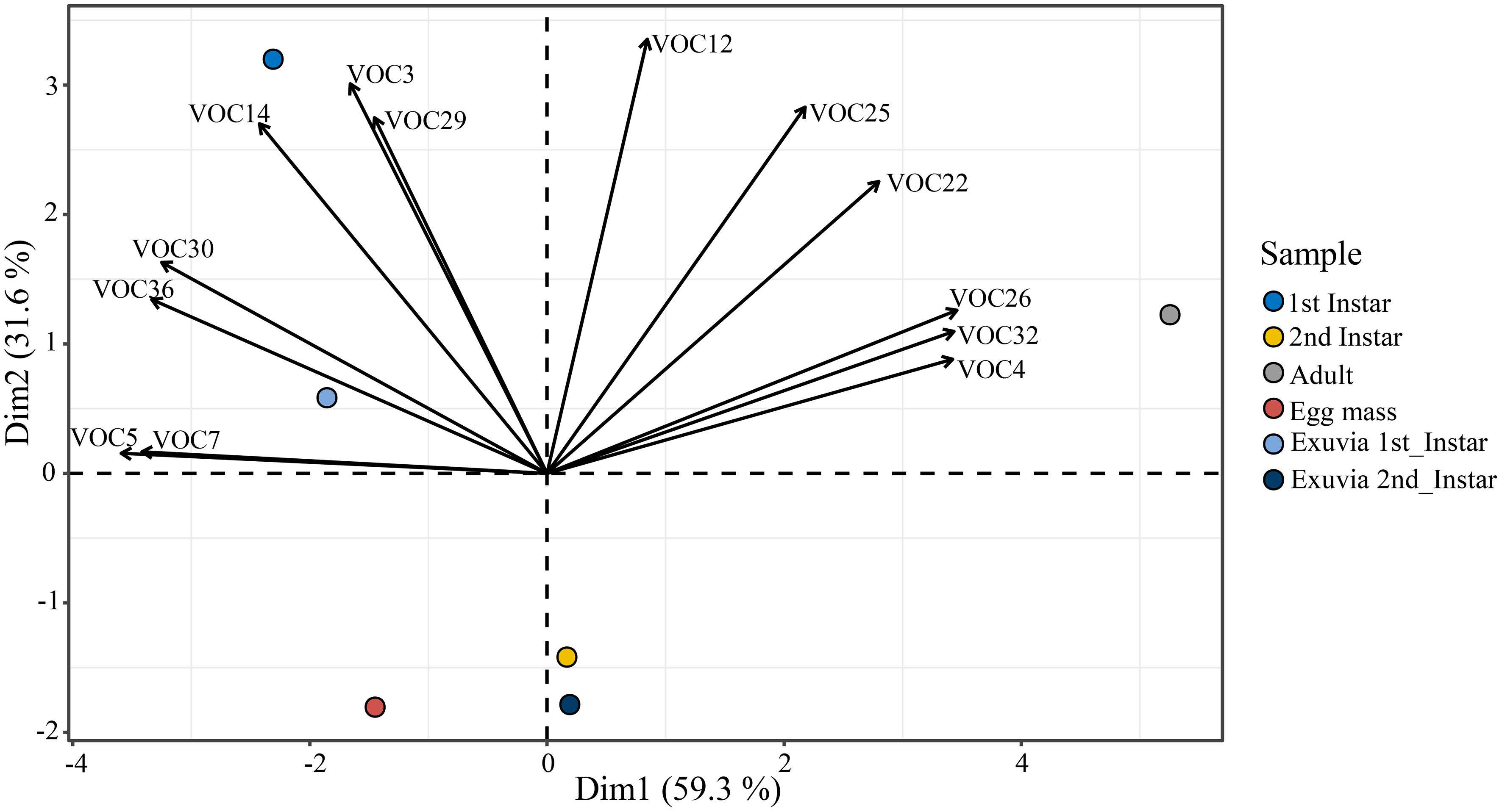
Figure 4 Principal Component Analysis (PCA) biplot showing the correlation of the most discriminant volatiles in the BMSB samples (see Table 2 for the identifiers legend).
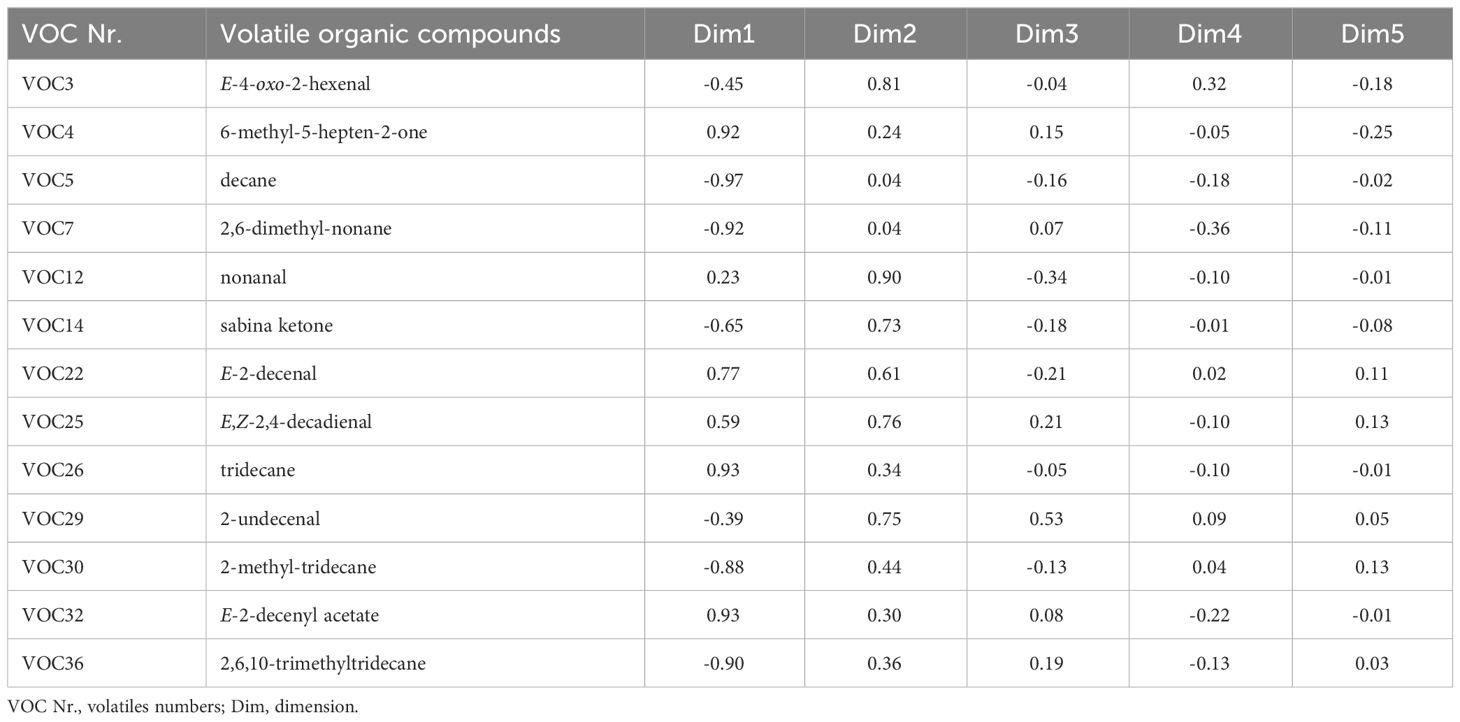
Table 2 Eigenvalues for five factors obtained from principal component analysis (PCA) based on VOCs of BMSB samples.
4 Discussion
The headspace collection technique is a simple method that can be used to sample a variety of VOCs from different materials such as plants, insect pests, air and soil (Deasy et al., 2016). Volatile matter collection techniques have been used for decades, however, these techniques have their limitations, especially when sampling VOCs in field-based experiments. Furthermore, extraction methods have a major impact on the metabolites detected. For example, different trapping techniques such as SPME and dynamic headspace sampling yielded significantly different profiles for volatile compounds in food (Diez-Simon et al., 2020). In this study, we aimed to develop a headspace sampling device that enables the collection of a wide range of VOCs from plants and insects as well as other organisms, not only in laboratory experiments but also in field trials under different environmental conditions. Compared to our previous device (Rid et al., 2016; Gross et al., 2019), the newly developed HSCD is a mobile, easy-to-use, and reliable instrument. The HSCD was designed to sample VOCs in both OLS and CLS mode (Figure 1), which was not possible with the former prototypes. When the VOCs are collected by wrapping plant in polyethylene terephthalate oven bags, which are commonly used for VOCs collection (Rid et al., 2019; Gallinger et al., 2023), the bags may due to negative shrink pressure, causing stress to plants or pests, while in CLS mode the oven bags do not shrink. In contrast, OLS mode is more suitable for sampling VOCs in glass bottles. The channels can be programmed via a 255 digital control unit, whereas our previous prototypes were controlled manually (Gross et al., 2019). The digital control unit can ensure precise control of the mass flow rate and the total volume of gas collected. As the HSCD permanently monitors the actual flow rate and readjusts in case of deviations from the set values, distortions due to differently filled sample tubes or clogging by aspirated leaves are avoided. This allows an accurate comparison of the relative amounts of collected VOCs between the six headspace samples collected in parallel and simultaneously, as was the case in the previous prototypes. In this new HSCD, all settings are stored in an Excel file, along with information on ambient temperature, relative humidity, date and time of sampling and other information such as plant and pest species.
Using the HSCD, 45 VOCs were identified in the BMSB samples, which mainly consisted of tridecane, E-2-decenal, E-4-oxo-2-hexenal, and 2-undecenal. The volatile compounds found in our study are according to previous reports (Solomon et al., 2013; Baldwin et al., 2014; Tognon et al., 2016). However, we also detected VOCs that have not been previously identified in the BMSB samples, for instance, 2,6,10-trimethyltridecane which ranked as most important VOCs in discriminating between the BMSB samples. Figure 3 presents the appropriate RF classification showing the separation of the BMSB samples into three chemical patterns. In compliance with our results, tridecane, E-2-decenal, and E-4-oxo-2-hexenal have been identified as the most abundant VOCs of the BMSB adults and nymphs in previous studies (Harris et al., 2015; Nixon et al., 2018), suggesting that these compounds are chemical cues of this species. However, the presence of tridecane, E-2-decenal, and E-4-oxo-2-hexenal, together with small amount of dodecane, were linked with defensive odors of diapausing and diapause-disrupted adult H. halys after mechanical agitation (Nixon et al., 2018). Although, tridecane, E-2-decenal, and E-4-oxo-2-hexenal were found in the exuvia from first and second instar nymphs, high relative amounts of these VOCs mainly tridecane in BMSB adults might relate to the mechanical agitation and/or the presence of males and females together which are known to elicit a defensive odor response. Harris et al. (2015) reported that the BMSB nymphs released 100-fold more E-2-decenal than adults. Moreover, the BMSB adults produced significantly lower content of tridecane than nymphs (Fraga et al., 2017). Based on our results, the first instar nymphs produced more E-2-decenal, E-4-oxo-2-hexenal and 2-undecenal, whereas the BMSB adults released high amount of tridecane. In addition, the BMSB egg masses released 10-fold smaller amount of tridecane compared to adults. In conclusion we can show that the BMSB releases different VOCs during different life stages.
In nature, natural enemies such as egg parasitoids use chemicals associated with H. halys in host finding and recognition (Conti et al., 2020). For instance, egg parasitoid Trissolcus japonicus behaved differently in response to n-tridecane and E-2-decenal, two defensive volatiles emitted by the BMSB. Interestingly, T. japonicus females were attracted by n-tridecane, whereas strongly repelled by E-2-decenal (Zhong et al., 2017). A possible explanation for the smaller amount of tridecane in the BMSB egg masses is to prevent further attraction to egg parasitoids. Therefore, we assume that the different life stages of BMSB have each a specific chemical signature (VOC pattern), which may be influenced by environmental conditions, and the presence of natural enemies. However, further studies are needed to investigate whether these chemical signatures change in response to biotic and abiotic stresses. These chemical signatures might be used as specific biomarkers for the detection of BMSB in shipping containers or in an agricultural areas by specific electronic noses (Fundurulic et al., 2023).
Data availability statement
The raw data supporting the conclusions of this article will be made available by the authors, without undue reservation.
Author contributions
AK: Conceptualization, Data curation, Formal analysis, Investigation, Methodology, Validation, Writing – original draft, Writing – review & editing. JG: Conceptualization, Funding acquisition, Investigation, Methodology, Project administration, Supervision, Validation, Writing – original draft, Writing – review & editing.
Funding
The author(s) declare financial support was received for the research, authorship, and/or publication of this article. This work was funded by the European Union (Horizon 2020 Farm2Fork) under the PurPest project through grant agreement 101060634.
Acknowledgments
The authors thank Ralf Kunath and FLUSYS GmbH (Offenbach, Germany) for help in development, construction and production of a small series of HSCDs as well as their valuable support. In addition, we would like to thank Alicia Koßmann and Katharina Klostermann for excellent assistance in the lab and insect rearing.
Conflict of interest
The authors declare that the research was conducted in the absence of any commercial or financial relationships that could be construed as a potential conflict of interest.
The author(s) declared that they were an editorial board member of Frontiers, at the time of submission. This had no impact on the peer review process and the final decision.
Publisher’s note
All claims expressed in this article are solely those of the authors and do not necessarily represent those of their affiliated organizations, or those of the publisher, the editors and the reviewers. Any product that may be evaluated in this article, or claim that may be made by its manufacturer, is not guaranteed or endorsed by the publisher.
Supplementary material
The Supplementary Material for this article can be found online at: https://www.frontiersin.org/articles/10.3389/fhort.2024.1380008/full#supplementary-material
References
Alborn H. T., Bruton R. G., Beck J. J. (2021). Sampling of volatiles in closed systems: a controlled comparison of three solventless volatile collection methods. J. Chem. Ecol. 47, 930–940. doi: 10.1007/s10886-021-01306-6
Baldwin R., Zhang A., Fultz S. W., Abubeker S., Harris C., Connor E. E., et al. (2014). Hot topic: Brown marmorated stink bug odor compounds do not transfer into milk by feeding bug-contaminated corn silage to lactating dairy cattle. J. Dairy Sci. 97, 1877–1884. doi: 10.3168/jds.2013-7545
Bergmann E. J., Raupp M. J. (2014). Efficacies of common ready to use insecticides against Halyomorpha halys (Hemiptera: Pentatomidae). Fla. Entomol. 97, 791–800. doi: 10.1653/024.097.0262
Conti E., Avila G., Barratt B., Cingolani F., Colazza S., Guarino S., et al. (2020). Biological control of invasive stink bugs: Review of global state and future prospects. Entomol. Exp. Appl. 169, 28–51. doi: 10.1111/eea.12967
Czarnobai De Jorge B., Hummel H. E., Gross J. (2022). Repellent activity of clove essential oil volatiles and development of nanofiber-based dispensers against Pear psyllids (Hemiptera: Psyllidae). Insects 13, 743. doi: 10.3390/insects13080743
Deasy W., Shepherd T., Alexander C. J., Birch A. N. E., Evans K. A. (2016). Development and validation of a SPME-GC-MS method for in situ passive sampling of root volatiles from glasshouse-grown broccoli plants undergoing below-ground herbivory by larvae of cabbage root fly, Delia radicum L. Phytochem. Anal. 27, 375–393. doi: 10.1002/pca.v27.6
Diez-Simon C., Ammerlaan B., van den Berg M., van Duynhoven J., Jacobs D., Mumm R., et al. (2020). Comparison of volatile trapping techniques for the comprehensive analysis of food flavourings by Gas Chromatography-Mass Spectrometry. J. Chromatogr. A 1624, 461191. doi: 10.1016/j.chroma.2020.461191
European Commission (2023). Plant pest prevention through technology-guided monitoring and site-specific control. CORDIS, Publications Office of the European Union. doi: 10.3030/101060634
Fraga D. F., Parker J., Busoli A. C., Hamilton G. C., Nielsen A. L., Rodriguez-Saona C. (2017). Behavioral responses of predaceous minute pirate bugs to tridecane, a volatile emitted by the brown marmorated stink bug. J. Pest Sci. 90, 1107–1118. doi: 10.1007/s10340-016-0825-9
Fundurulic A., Faria J. M., Inácio M. L. (2023). Advances in electronic nose sensors for plant disease and pest detection. Eng. Proc. 48, 14. doi: 10.3390/CSAC2023-14890
Gallinger J., Jarausch B., Jarausch W., Gross J. (2020). Host plant preferences and detection of host plant volatiles of the migrating psyllid species Cacopsylla pruni, the vector of European Stone Fruit Yellows. J. Pest Sci. 93, 461–475. doi: 10.1007/s10340-019-01135-3
Gallinger J., Rid-Moneta M., Becker C., Reineke A., Gross J. (2023). Altered volatile emission of pear trees under elevated atmospheric CO2 levels has no relevance to pear psyllid host choice. Environ. Sci. pollut. Res. 30, 43740–43751. doi: 10.1007/s11356-023-25260-w
Gross J., Gallinger J., Rid M. (2019). Collection, identification, and statistical analysis of volatile organic compound patterns emitted by Phytoplasma infected plants. Phytoplasmas: Methods Protoc. 1875, 333–343. doi: 10.1007/978-1-4939-8837-2_25
Harris C., Abubeker S., Yu M., Leskey T., Zhang A. (2015). Semiochemical production and laboratory behavior response of the brown marmorated stink bug, Halyomorpha halys. PloS One 10, e0140876. doi: 10.1371/journal.pone.0140876
Helms A. M., De Moraes C. M., Tooker J. F., Mescher M. C. (2013). Exposure of Solidago altissima plants to volatile emissions of an insect antagonist (Eurosta solidaginis) deters subsequent herbivory. PNAS 110, 199–204. doi: 10.1073/pnas.1218606110
Kais B., Köhler J., Werner P., Gross J. (2023). Does the causative agent of Syndrome Basses Richesses (SBR), Candidatus Arsenophonus phytopathogenicus, alter sugar beet phloem composition or plant-emitted volatiles? IOBC-WPRS Bull. 166, 128–133.
Karimi A., Meiners T. (2021). Antifungal activity of Zataria multiflora Boiss. essential oils and changes in volatile compound composition under abiotic stress conditions. Ind. Crops Prod. 171, 113888. doi: 10.1016/j.indcrop.2021.113888
Kfoury N., Scott E., Orians C., Robbat A. (2017). Direct contact sorptive extraction: a robust method for sampling plant volatiles in the field. J. Agric. Food. Chem. 65, 8501–8509. doi: 10.1021/acs.jafc.7b02847
Leskey T. C., Nielsen A. L. (2018). Impact of the invasive brown marmorated stink bug in North America and Europe: history, biology, ecology, and management. Annu. Rev. Entomol. 63, 599–618. doi: 10.1146/annurev-ento-020117-043226
Morrison W. R. III, Acebes-Doria A., Ogburn E., Kuhar T. P., Walgenbach J. F., Bergh J. C., et al. (2017). Behavioral response of the brown marmorated stink bug (Hemiptera: Pentatomidae) to semiochemicals deployed inside and outside anthropogenic structures during the overwintering period. J. Econ. Entomol. 110, 1002–1009. doi: 10.1093/jee/tox097
Ngumbi E. N., Ugarte C. M. (2021). Flooding and herbivory interact to alter volatile organic compound emissions in two maize hybrids. J. Chem. Ecol. 47, 707–718. doi: 10.1007/s10886-021-01286-7
Nixon L. J., Morrison W. R., Rice K. B., Brockerhoff E. G., Leskey T. C., Guzman F., et al. (2018). Identification of volatiles released by diapausing brown marmorated stink bug, Halyomorpha halys (Hemiptera: Pentatomidae). PloS One 13, e0191223. doi: 10.1371/journal.pone.0191223
Nixon L. J., Tabb A., Morrison W. R., Rice K. B., Brockerhoff E. G., Leskey T. C., et al. (2019). Volatile release, mobility, and mortality of diapausing Halyomorpha halys during simulated shipping movements and temperature changes. J. Pest Sci. 92, 633–641. doi: 10.1007/s10340-019-01084-x
Nixon L., Morrison III W. R., Rice K. B., Goldson S., Brockerhoff E. G., Khrimian A., et al. (2022). Behavioural responses of diapausing Halyomorpha halys (Hemiptera: Pentatomidae) to conspecific volatile organic compounds. J. Appl. Entomol. 146, 319–327. doi: 10.1111/jen.12955
Rering C. C., Gaffke A. M., Rudolph A. B., Beck J. J., Alborn H. T. (2020). A comparison of collection methods for microbial volatiles. Front. Sustain. Food Syst. 4. doi: 10.3389/fsufs.2020.598967
Rid M., Markheiser A., Stein S., Hoffmann C., Gross J. (2019). Volatiles of several grapevine cultivars emitted at different phenological stages linked to discriminatory ability of grapevine moths. J. Plant Dis. Prot. 126, 115–127. doi: 10.1007/s41348-019-00214-y
Rid M., Mesca C., Ayasse M., Gross J. (2016). Apple proliferation phytoplasma influences the pattern of plant volatiles emitted depending on pathogen virulence. Front. Ecol. Evol. 3. doi: 10.3389/fevo.2015.00152
Solomon D., Dutcher D., Raymond T. (2013). Characterization of Halyomorpha halys (brown marmorated stink bug) biogenic volatile organic compound emissions and their role in secondary organic aerosol formation. J. Air Waste Manage. Assoc. 63, 1264–1269. doi: 10.1080/10962247.2013.819047
Tholl D., Hossain O., Weinhold A., Röse U. S., Wei Q. (2021). Trends and applications in plant volatile sampling and analysis. Plant J. 106, 314–325. doi: 10.1111/tpj.15176
Tognon R., Sant’Ana J., Zhang Q. H., Millar J. G., Aldrich J. R., Zalom F. G. (2016). Volatiles mediating parasitism of Euschistus conspersus and Halyomorpha halys eggs by Telenomus podisi and Trissolcus erugatus. J. Chem. Ecol. 42, 1016–1027. doi: 10.1007/s10886-016-0754-3
Vivaldo G., Masi E., Taiti C., Caldarelli G., Mancuso S. (2017). The network of plants volatile organic compounds. Sci. Rep. 7, 11050. doi: 10.1038/s41598-017-10975-x
Weintraub P., Gross J. (2013). “Capturing insect vectors of phytoplasmas,” in Phytoplasma, Methods in molecular biology (methods and protocols), vol. 938 . Eds. Dickinson M., Hodgetts J. (Humana Press, Totwa, NJ), 61–72. doi: 10.1007/978-1-62703-089-2_6
Wiman N. G., Walton V. M., Shearer P. W., Rondon S. I., Lee J. C. (2015). Factors affecting flight capacity of brown marmorated stink bug, Halyomorpha halys (Hemiptera: Pentatomidae). J. Pest Sci. 88, 37–47. doi: 10.1007/s10340-014-0582-6
Wong Y. F., Yan D., Shellie R. A., Sciarrone D., Marriott P. J. (2019). Rapid plant volatiles screening using headspace SPME and person-portable gas chromatography–mass spectrometry. Chromatographia 82, 297–305. doi: 10.1007/s10337-018-3605-2
Yew J. Y., Chung H. (2015). Insect pheromones: An overview of function, form, and discovery. Prog. Lipid Res. 59, 88–105. doi: 10.1016/j.plipres.2015.06.001
Zhong Y. Z., Zhang J. P., Ren L. L., Tang R., Zhan H. X., Chen G. H., et al. (2017). Behavioral responses of the egg parasitoid Trissolcus japonicus to volatiles from adults of its stink bug host, Halyomorpha halys. J. Pest Sci. 90, 1097–1105. doi: 10.1007/s10340-017-0884-6
Keywords: brown marmorated stink bug, chemical signature, headspace sampling, pheromone, volatile organic compound, tridecane, (E)-2-decenal
Citation: Karimi A and Gross J (2024) Development and validation of an innovative headspace collection technique: volatile organic compound patterns emitted by different developmental stages of Halyomorpha halys. Front. Hortic. 3:1380008. doi: 10.3389/fhort.2024.1380008
Received: 31 January 2024; Accepted: 15 April 2024;
Published: 08 May 2024.
Edited by:
Peter Ojiambo, North Carolina State University, United StatesReviewed by:
Mohammad Shaef Ullah, Bangladesh Agricultural University, BangladeshChunhua Zhou, Yangzhou University, China
Sergio Angeli, Free University of Bozen-Bolzano, Italy
Copyright © 2024 Karimi and Gross. This is an open-access article distributed under the terms of the Creative Commons Attribution License (CC BY). The use, distribution or reproduction in other forums is permitted, provided the original author(s) and the copyright owner(s) are credited and that the original publication in this journal is cited, in accordance with accepted academic practice. No use, distribution or reproduction is permitted which does not comply with these terms.
*Correspondence: Jürgen Gross, anVlcmdlbi5ncm9zc0BqdWxpdXMta3VlaG4uZGU=