- 1Department of Chemical Sciences, University of Padova, Padova, Italy
- 2Department of Molecular Biology and Radiobiology, Faculty of AgriSciences, Mendel University in Brno, Brno, Czechia
- 3Division of Biotechnology and Plant Health, Norwegian Institute of Biotechnology Research, As, Norway
- 4Faculty of Science, Institute for Biology, University of Neuchâtel, Neuchatel, Switzerland
- 5INIAV, I.P., National Institute for Agrarian and Veterinary Research, Oeiras, Portugal
- 6GREEN-IT Bioresources for Sustainability, Instituto de Tecnologia Química e Biológica, Universidade Nova de Lisboa, Oeiras, Portugal
- 7Department of Forest Protection and Wildlife Management, Mendel University in Brno, Brno, Czechia
- 8R&D Department, Chromatotec, Saint-André de Cubzac, France
- 9Department of Agronomy, Food, Natural Resources, Animals, Environment (DAFNAE), University of Padova, Padova, Italy
- 10Faculty of Agricultural, Environmental and Food Sciences, Free University of Bolzano, Bolzano, Italy
Invasive pests and plant pathogens pose a significant threat to ecosystems and economies worldwide, prompting the need of anticipatory strategies. Preventing their introduction by detection at the ports of entry has been proven extremely difficult. This review explores the potential of biogenic volatile detection as a reliable preventive solution. It underscores the importance of early detection and rapid response as integral components of effective invasive pest management, and it discusses the limitations of current control measures and the increasing globalization that facilitates the spread of pests and pathogens. Through a synthesis of existing literature, this review analyzes the Volatile Organic Compound (VOC) emissions in five invasive model species: three insects, Halyomorpha halys, Spodoptera frugiperda, Helicoverpa armigera, a nematode, Bursaphelenchus xylophilus, and an oomycete, Phytophthora ramorum. The review focuses on the specific volatiles, released by both the invasive organisms and the infested host plants. If available, the volatiles emitted from similar species were considered for comparison. Ultimately, this review highlights specific pest volatile and shared Herbivore Induced Plant Volatiles (HIPVs) as a reliable and innovative solution in pest detection. If possible, candidate compounds are provided, whilst the lack of some emphasizes the urge of expanding the information available.
1 Introduction
In the global context of agriculture, the continuous threat posed by quarantine and severe pest species has become a pressing concern for researchers, policymakers, and farmers. The expansion of international trade and travel has facilitated the inadvertent spread of invasive pests, resulting in substantial economic losses and ecological imbalances worldwide. Management of these pests can be achieved through several approaches, but the most used ones are harmful chemicals, such as insecticides and fungicides in plant protection. The reduction by 50% of pesticide use is among the proposals adopted by the European Commission, in line with the EU’s Farm to Fork and Biodiversity strategies (EC, 2020b). To achieve this goal, it is important to control new pest invasions and already established pests. The detection of invasive pests is a fundamental aspect of contemporary agricultural practices and ecological conservation. Swift and accurate detection allows for the implementation of timely control measures, preventing the establishment and spread of invasive species, minimizing economic and ecological impacts (MacDougall et al., 2022). One innovative approach to enhance the detection of invasive pests involves exploiting the volatile organic compounds (VOCs) released by the target pests or induced in attacked plants (Cui et al., 2018). Insects and pathogens produce VOCs as a mean of communication (semiochemicals in insects) or as metabolic derivatives (Bos et al., 2013; Gullan and Cranston, 2014; Fennine et al., 2024). Plants use VOCs to interact with other plants and insects, by luring pollinators, recruiting an herbivore’s adversaries, camouflaging other plants, spotting invading plants, alerting other plants to impending danger, and exhibiting allelopathy (Baldwin, 2010; Heil, 2014; Karban et al., 2014; Turlings and Erb, 2018; MacDougall et al., 2022; Schuman, 2023). Once attacked by an enemy (either animals, fungi, bacteria, virus or nematodes), plants change their volatile profile induced volatiles (Dicke et al., 2009). They are a plant defense mechanism released by plants attacked by herbivores as a signal for higher trophic levels or other plants (Paré and Tumlinson, 1999; Gebreziher, 2018; Turlings and Erb, 2018; War et al., 2011).
The unique chemical signatures of these VOCs serve as indirect early warning signals, enabling to identify the presence of invasive pests before establishment and significant damage occur (Cui et al., 2018). These volatile signals can be used as valuable cues for monitoring and identifying pest infestations, enabling timely interventions, reducing potential crop damage and optimizing pest management strategies (MacDougall et al., 2022). Nixon et al. (2018), proposed to use the compounds released by Halyomorpha halys to detect the diapausing insects in shipments and selected possible target VOCs after a Gas Chromatography - Mass Spectrometry (GC-MS) analysis. Similarly, pest VOCs were taken into consideration to detect the presence of bed bugs (Akhoundi et al., 2023), a serious human health-related issue. Forty-nine compounds emitted by Cimex lectuarius L. and C. hemipterus Fabricius through their life stages were considered as valuable indicators of the bug presence. The same approach has also been proposed for the detection of a pine fungal pathogen, Fusarium circinatum Nirenberg & O'Donnell (Nordström et al., 2022), where through VOCs collection, GC-MS and automated data analysis, they managed to correctly distinguish infested and healthy seedlings of Pinus radiata Don and P. sylvestris L.
Volatiles can be collected in various ways (Brezolin et al., 2018; Tholl et al., 2021). Pre-concentration of VOCs on solid adsorbents followed by thermal desorption has become one of the standard methods for both field and laboratory studies. The type of adsorbent must be carefully selected to match the physicochemical characteristics of the target compounds. Additionally, the time from sampling to analysis must be minimized to prevent sample degradation due to reactive gases like ozone. However, most commercially available adsorbents work well, and they can be stored for a long time in a freezer without severely affect the outcomes of the analysis (Chu et al., 2016; Ho et al., 2018, authors personal observation). Among the different analytical techniques available for VOC analysis, including Proton Transfer Reaction Mass Spectrometry (PTR-MS) or electronic e-nose, Gas chromatography-Mass Spectrometry (GC-MS) has proven to efficiently separate and identify trace levels of VOCs in complex mixtures. However, both PTR-MS and GC-MS requires the use of large, expensive laboratory equipment that is unsuitable for use in the field. Moreover, the collection, processing, and analysis of the samples is time consuming and requires trained people. Therefore, for timely detection of pest, rapid and accurate diagnostic techniques that can be applied in the field are required. Efforts to achieve this goal have focused on the employment of so-called electronic noses to identify plant diseases and pests. An electronic nose uses a variety of gas sensors in conjunction with techniques for feature extraction and pattern recognition to identify and differentiate between distinct odors. The work of Fundurulic et al. (2023) provides an overview of the most recent developments in the field and emphasizes the application of cutting-edge methods for the prompt, non-destructive identification and control of harmful plant pests. Still, deploying e-nose for accurate and reliable characterization of specific VOCs in the field requires addressing challenges like sensor stability, specificity and reproducibility.
This review provides a context on the biology and, importantly, summarizes the current knowledge on the signature VOCs of five invasive pest model species: the brown marmorated stink bug (Halyomorpha halys), the fall armyworm (Spodoptera frugiperda), the cotton bollworm (Helicoverpa armigera), the pinewood nematode (Bursaphelenchus xylophilus) and Phytophthora ramorum. They are three insects, a nematode and an oomycete, and they were chosen because of their relevance to the EU pest surveillance programs. Induced volatiles released by attacked plants are also reported. The purpose of this review goes beyond the evaluation of the scientific soundness of the reference works, as they span a time of decades and a wide array of VOC collection techniques. It aims instead at presenting the current state of available information on VOCs of some relevant key pests, defining, when possible, a list of candidate VOCs for the pest identification to be used in pest surveillance and monitoring. The candidate VOCs were selected following the criteria of specificity and consistency. Specificity refers to the quality of clearly define or identify the target pest, whilst reducing unrelated false positive signals. Consistency on the other hand is crucial for scientific reproducibility and defines uniformity and stability of measurements over time and across different conditions or experiment. Therefore, findings that were confirmed by multiple studies were accounted as reliable. Each pest is presented in a dedicated section, reporting the biology, the management and its related VOCs.
2 Volatile organic compounds produced and induced by Halyomorpha halys
2.1 The brown marmorated stink bug: distribution, biology and management
Halyomorpha halys (Stål) (Hemiptera: Pentatomidae), known as the Brown Marmorated Stink Bug (BMSB) (Figures 1A, B), is an insect native to eastern Asia, and is now considered one of the most harmful invasive pests in North America and Europe (Zobel et al., 2016).
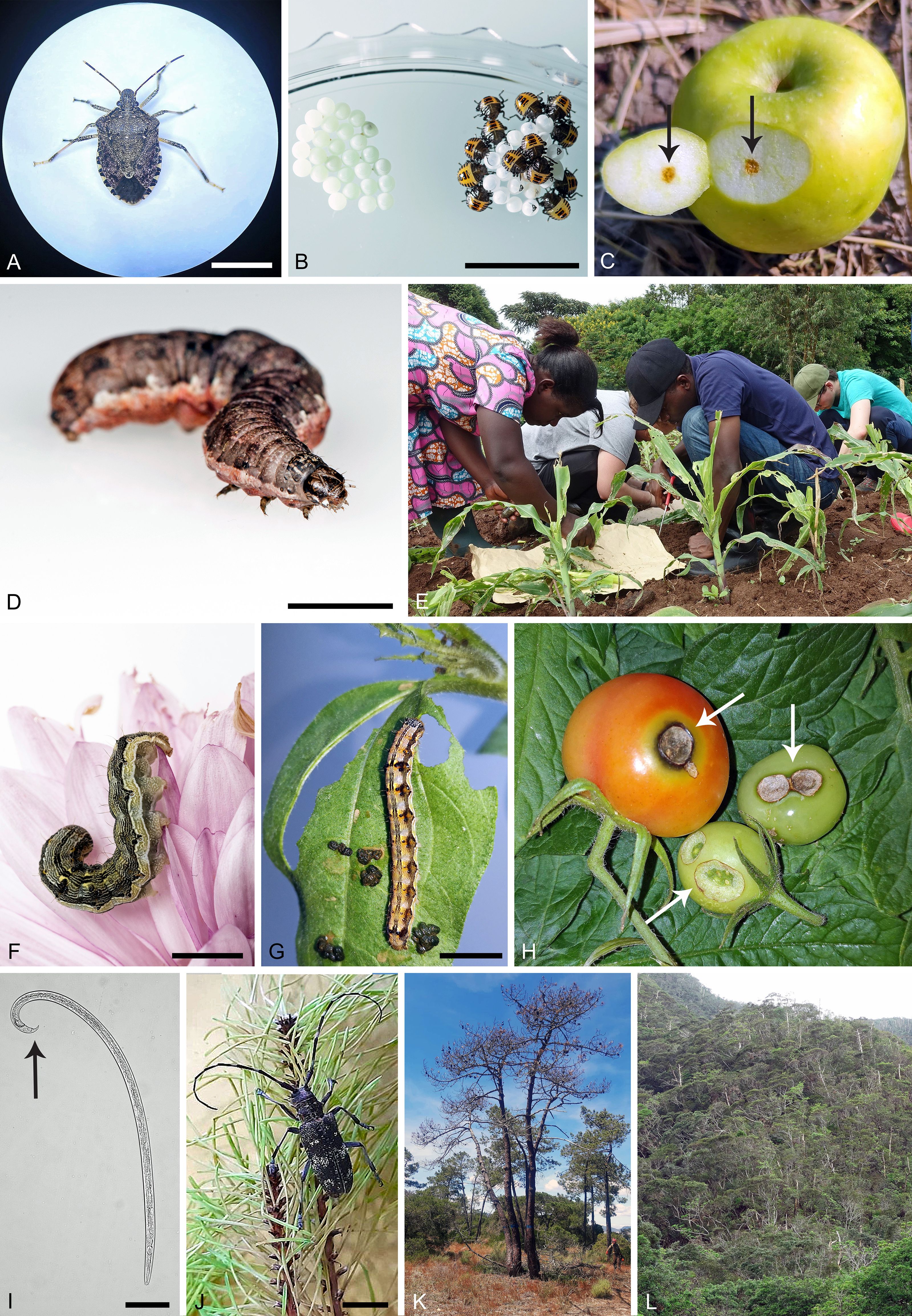
Figure 1. Pests and representative disease symptoms. (A–C) Brown marmorated stink bug (Halyomorpha halys). Scale bar = 1 cm. (A) Adult bug (photo courtesy: Diana La Forgia, Agroscope, Switzerland). (B) Eggs (left) and newly hatched nymphs (right) (photo courtesy: Carole Paroli, Agroscope, Switzerland). (C) Apple with sucking damage (arrows) (photo courtesy: Veronica Carnio, Free University of Bolzano, Italy). (D, E) Fall armyworm (Spodoptera frugiperda). (D) Mature caterpillar with distinctive inverted Y suture on the forehead (photo courtesy: Neil Villard, University of Neuchâtel, Switzerland). Scale bar = 1 cm. (E) Fall armyworm frass and feeding damage in a maize field in Rwanda (photo courtesy: Stefan Toepfer, CABI, Switzerland). (F–H) Cotton bollworm (Helicoverpa armigera). Scale bar = 1 cm. (F) Mature caterpillar on Chrysanthemum flower (photo courtesy: Erling Fløistad, NIBIO, Norway). (G) Mature caterpillar with frass and feeding damage on a sunflower leaf (photo: Gunda Thöming). (H) Damage caused by caterpillar frass on tomato fruits (arrows) (photo: Gunda Thöming). (I) Male Pinewood Nematode (PWN; Bursaphelenchus xylophilus) with characteristic pointed tale. Scale bar = 100 nm (photo courtesy: INIAV, Portugal). (J) Monochamus galloprovincialis, main vector of the PWN in Europe. Scale bar = 1 cm (photo courtesy: INIAV, Portugal). (K) Mature Pinus pinaster tree in Portugal showing acute wilting and mortality caused by the PWN (photo courtesy: INIAV, Portugal). (L) Natural Pinus densiflora forest on Amami Island, Japan with high mortality due to PWN damage (photo: Thomas Jung).
Halyomorpha halys consumes plant juices for nutrition feeding on the green parts of the plant, such as leaves and stems, but fruits are typically preferred (Figure 1C). The most significant crop damage comes from piercing fruiting structure on pome, stone and other fruit crops as well as seeds in legume pods such as soybeans (Leskey and Nielsen, 2018). The fruits are Highly deformed in cases of severe infestations, and there may be significant financial losses (Zobel et al., 2016). Fruit attacked by H. halys showed also increase in damage by fruit pathogens (Rice et al., 2014; Moore et al., 2019). The economic implications in the agricultural production are considerable, reaching billions of euros in crops worldwide (Rice et al., 2014). From a detailed estimation conducted in Northern Italy, it has emerged that it causes damages in the production of pears, apples, peaches, and kiwis for an estimated economic loss of 740 million euros (Fornasiero et al., 2023). In the eastern USA, the effects on apple and peach orchards reached up to total loss in 2010 (Leskey et al., 2012).
Due to its extreme polyphagia, H. halys has roughly 40 hosts among domesticated plants and much more (around 300) wild hosts (EPPO Global Database, 2023). Halyomorpha halys has one or two generations per year in the USA and Europe, but there have been reports up to 5–6 generations per year in the species’ native range (Lee et al., 2013; Haye et al., 2014; Costi et al., 2017). In its adult stage, the stink bug spends the winter in natural shelters or anthropogenic structures. On the underside of the leaves, in clusters of 20–30 eggs, females lay 50–150 eggs, but they can also lay up to 400 eggs per female. There are five nymphal stages before reaching the adult stage (Lee et al., 2013) (Figure 1B). The control of H. halys relies on insecticides (Leskey et al., 2012). Recently, adventive populations of Asian egg parasitoid Trissolcus spp. (Hymenoptera: Scelionidae), in particular Trissolcus japonicus Ashmead and Trissolcus mitsukurii Ashmead were discovered in America and Europe (Talamas et al., 2019; Abram et al., 2019; Stahl et al., 2019; Sabbatini Peverieri et al., 2018; Scaccini et al., 2020) and may serve as viable antagonists. Other management options for the control of H. halys are staking, trap crops, perimeter reshaping in orchards, push-pull, exclusion nets, and behavioral manipulation. These had the potential to drastically minimize fruit loss (e.g., Blaauw et al., 2015; Candian et al., 2020; Falagiarda et al., 2023; Fornasiero et al., 2023; Carnio et al., 2024).
The use of semiochemicals plays an important role in the management of this pest. The discovery of the pest aggregation pheromone (Khrimian et al., 2014) opened for further pest control strategies. It is solely produced by adult males, and it attracts both adult males, adult females, and nymphs (Weber, 2015). For this reason, the pheromone lures are employed in traps for monitoring, early detection (Vandervoet et al., 2019) and for pest management decision making.
2.2 Summary of literature on Halyomorpha halys VOCs
Numerous studies have identified and characterized the volatile compounds emitted by H. halys (Table 1). These volatiles primarily consist of a diverse array of aldehydes, alcohols, esters, terpenes, and sulphur-containing compounds. Some of the most notable compounds include the aldehydes (E)-2-decenal, (E)-2-octenal, (E)-2-hexenal, sesquiterpenes and alkanes. The composition and ratios of these volatiles can vary depending on factors such as developmental stage, sex, feeding status, and environmental conditions.
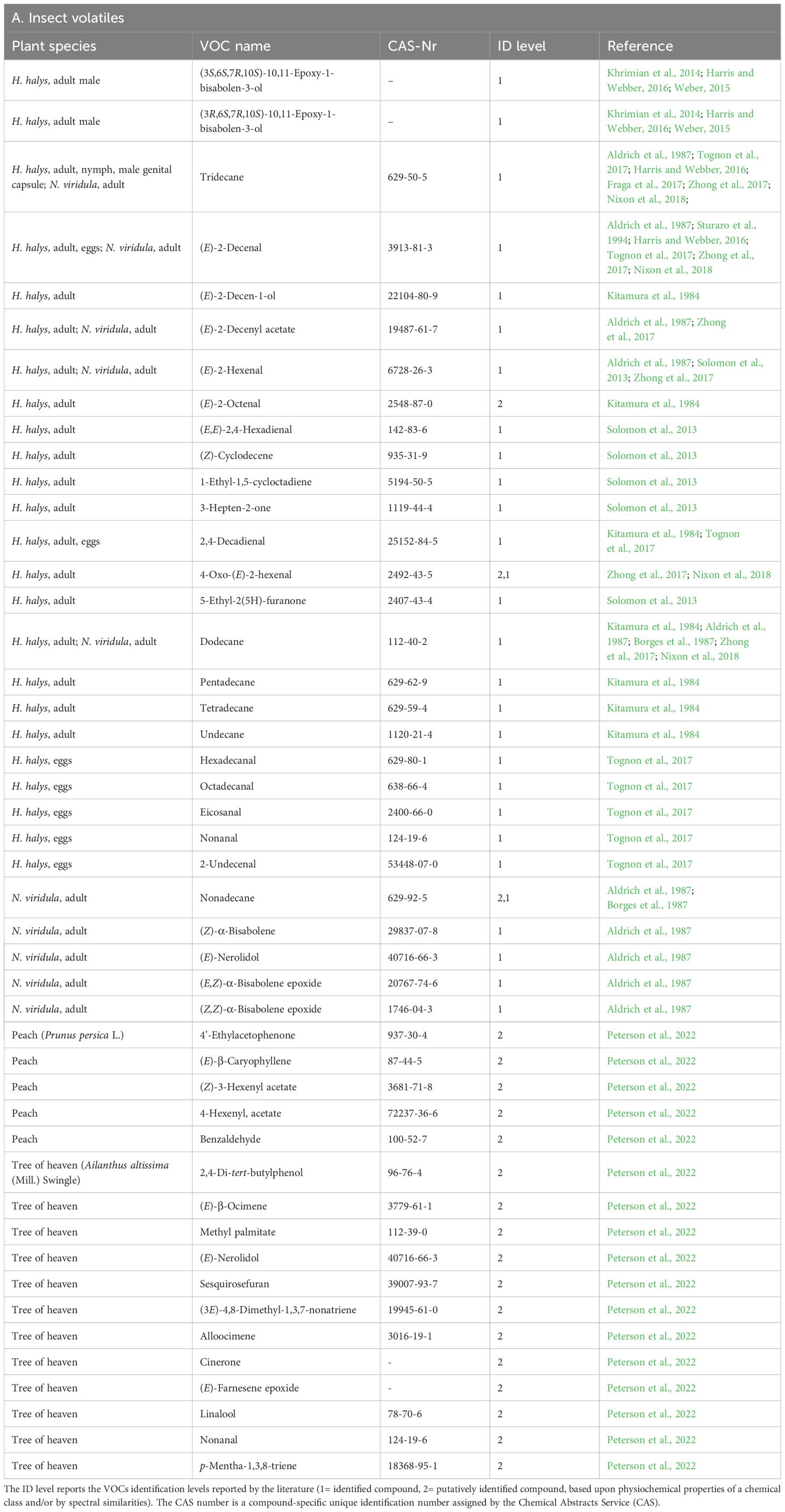
Table 1. List of Volatile Organic Compounds (VOCs) released by Halyomorpha halys and Nezara viridula (A), and list of Herbivore Induced Plant Volatiles (HIPVs) released by H. halys-infested plants (B) described in the literature.
Stink bugs, including H. halys, possess specialized scent glands located on their thorax and abdomen that release volatiles when disturbed or threatened (Kitamura et al., 1984). The emission of volatiles is primarily a passive process, relying on the release of pressure built up within the scent gland reservoir. These defense compounds are shared among many species and (E)-2-decenal, (E)-2-octenal, (E)-2-hexenal, (E)-2-decenyl acetate are reported in not only H. halys (Harris and Webber, 2016; Zhong et al., 2017; Nixon et al., 2018, Nixon et al., 2019, 2021; Karimi and Gross, 2024), but also in a cosmopolitan species, the green stink bug Nezara viridula L (Aldrich et al., 1987).These volatiles were collected from either full insect after immersion in solvent (Zhong et al., 2017) or from headspace by using different adsorption materials: Super-Q (Khrimian et al., 2014; Harris and Webber, 2016; Nixon et al., 2018, 2019, 2021), Tenax (Karimi and Gross, 2024), activated charcoal (Aldrich et al., 1987).
Generally, these (E)-2-aldehydes are reported as defensive compounds inducing avoidance by predators (Noge et al., 2012), and likely connected to the disturbance level the insects are exposed (Nixon et al., 2021). It was showed that they function also as alarm pheromones (Harris and Webber, 2016), acting as warning signal to conspecifics, indicating the presence of threats, and as dispersal signals, causing other stink bugs to disperse quickly, reducing the likelihood of multiple individuals being preyed upon simultaneously (Nixon et al., 2018, 2021). The implication of these VOCs in an intraspecific context is supported by the data reported in Nixon et al. (2021), in which the proportion of samples that released these defensive odors was null in singularly agitated insects, whilst increased proportionally to the number of insects in the group. Khrimian et al (2014) characterized the male-produced aggregation pheromone of H. halys as a 3.5:1 mixture of two stereoisomers, (3S,6S,7R,10S)-10,11-epoxy-1-bisabolen-3-ol and (3S,6S,7R,10S)-10,11-epoxy-1-bisabolen-3-ol. According to Harris and Webber (2016), mature males started producing pheromone at a mean age of 13 days. Males who were housed alone produced a mean of 843 ng of pheromone per day, in daily volatile collections in levels that ranged fivefold. Males in groups emitted <10% pheromone per bug per day than lone males due to a strong negative reaction to male density. The pheromone is mainly emitted during the day and it is effective to both adult sexes and nymphs (Weber, 2015).
An array of linear hydrocarbons has been detected in H. halys: undecane, dodecane, tridecane and pentadecane (Kitamura et al., 1984; Baldwin, 2010; Harris and Webber, 2016; Zhong et al., 2017). Among them, tridecane was the most frequently found. Linear hydrocarbons are also reported among the emissions of N. viridula: dodecane, tridecane and nonadecane (Borges et al., 1987). It is unclear whether these volatiles might play a role as bioactive compounds (Weber, 2015). A likely explanation proposed that such hydrocarbons serve as solvents or carriers (Calam and Youdeowei, 1968), as they would facilitate the efficient evaporation of active substances such as aldehydes on the scent gland system in a variety of pentatomid species (Kment and Vilimova, 2010). However, behavioral studies showed that the exposure of adult H. halys to the insect most abundant alkane, tridecane, led to an increased speed (Lockwood and Story 1985, 1987; Nixon et al., 2018), total distance and mean angular velocity (Nixon et al., 2021), and it also significantly reduced the emission of pheromones in adult males (Harris and Webber, 2016). This evidence might reveal a possible biological function of tridecane, but its mechanisms have yet to be understood. As addressed by Weber (2015), the data proposed by Harris and Webber (2016) lack statistical significance and should be considered carefully. On the other hand, the more recent work from Nixon et al. (2021) shows statistically sound evidence of an actual tridecane effect on the stink bugs behavior. It is worth considering however, that tridecane has always been found in all samples of H. halys VOCs studies, despite a more occasional presence of the E-2-aldehydes (Aldrich et al., 1987; Tognon et al., 2017; Harris and Webber, 2016; Zhong et al., 2017; Nixon et al., 2018), opening up a question on the biological relevance of an ubiquitous compound.
2.3 Summary of literature on Halyomorpha halys - induced plant VOCs
Despite the relevance of H. halys on crops worldwide, only one study has so far explored the HIPVs released after infestation. Peterson et al. (2022), analyzed direct and systemic emissions of potted peach (Prunus persica L.) and tree of heaven (Ailanthus altissima (Mill.) Swingle) plants following insect feeding and oviposition. The VOCs were collected from the headspace of a bag-enclosed branch for 24 hours by using a Hayesep adsorbent (Sigma-Aldrich, St. Louis, MO) and later eluted in dichloromethane and analyzed in GC-MS. The study showed a species-specific response in the VOCs released. Nerolidol was released at a greater rate by tree of heaven branches that were directly exposed to H. halys oviposition and feeding than by branches exposed to only feeding and control trees. In comparison to plants exposed to oviposition and feeding or control trees, tree of heaven leaves treated to H. halys feeding alone emitted greater rates of (E)-4,8-dimethyl-1,3,7-nonatriene. On the other hand, when peaches were subjected to H. halys oviposition, there was a reduction in (Z)-3-hexenyl acetate in both the directly and systemically exposed branches. Other compounds in peach plants varied between treatments: 4’-ethylacetophenone, (E)-β-caryophyllene, 4-hexenyl acetate and benzaldehyde. Similarly in tree of heaven plants, differences appeared between treatments: 2,4-di-tert-butylphenol, (E)-β-ocimene, methyl palmitate, sesquirosefuran, alloocimene, cinerone, (E)-farnesene epoxide, linalool, nonanal, p-mentha-1,3,8-triene.
2.4 Candidate VOCs for Halyomorpha halys detection
So far, the most unique compounds are the two stereoisomers (3S,6S,7R,10S)-10,11-epoxy-1-bisabolen-3-ol and (3R,6S,7R,10S)-10,11-epoxy-1-bisabolen-3-ol, identified as the main components of the aggregation pheromone released by adult males. All the other VOCs (Table 2) are generic of stink bugs or other organisms. It is however worth considering the aldehydes (E)-2-decenal, (E)-2-octenal, (E)-2-hexenal and the ester (E)-2-decenyl acetate, the unpleasant odors released by stink bugs when disturbed (alarm/defense pheromones). These VOCs, even if very generic, can at least indicate presence of stink bugs. Tridecane, the most frequently compound detected from H. halys and other stink bugs, occurs commonly in the environment as it is largely emitted by biotic and abiotic sources. The candidate VOCs here selected should answer to a specificity requirement, and therefore tridecane, due to its ubiquitous nature, has not been considered. The question on whether plants may respond in a similar way after an insect attack is crucial to increase the number of candidate HIPVs. Induced compounds that would be shared among a considerable number of host plant species following H. halys infestation would be extremely valuable as candidates for detection. However, the scarce literature available does not provide enough confidence to select any HIPVs, and more plant species need to be tested.
3 Volatile organic compounds produced and induced by Spodoptera frugiperda
3.1 The fall armyworm: distribution, biology and management
The Fall Armyworm (FAW), Spodoptera frugiperda (J. E. Smith) (Lepidoptera: Noctuidae), is native to the Americas (Todd and Poole, 1980) and has been reported to attack a wide range of host plants and causes serious damage to many economical plants (Kenis et al., 2022). Although the larvae (Figure 1D) are known to feed on many host plants, they exhibit a preference for grasses and cereal crops like maize (Figure 1E), rice, sorghum, and wheat (Sparks, 1979; Pitre and Hogg, 1983). The high invasiveness potential of S. frugiperda is attributed to the exceptional capacity of the adult moths to migrate (Johnson, 1987; Westbrook et al., 2019). Spodoptera frugiperda has invaded all of sub-Saharan Africa after it was first observed in Nigeria in 2016 (Cock et al., 2017; Day et al., 2017) and also made its way from Africa to Asia (Sharanabasappa et al., 2018; Liu et al., 2020). More recently it was found in Oceania (Day et al., 2017; Lamsal et al., 2020) and entered continental Europe in 2024 (https://www.fao.org/fall-armyworm/monitoring-tools/faw-map/en/). It is now one of the biggest threats to food security on multiple continents (FAO, 2020), causing tremendous yield losses, especially in maize (Day et al., 2017; Baudron et al., 2019; Hruska and Gould, 1997; Rwomushana et al., 2018; Wan et al., 2021), threatening the livelihoods of millions of farmers and the food security of over 65 million people in Africa alone (Day et al., 2017; Rwomushana et al., 2018; Babendreier et al., 2020) (Figure 1E). According to estimates, it causes in maize up to 73% of global economic losses (Guo et al., 2018). In Africa, S. frugiperda alone results in annual yield losses of 9.4 billion US dollars (Eschen et al., 2021). Between 2017 and 2019, the pest reduced Ethiopia’s grain yield by 0.225 million tons, with an average yearly loss of 36% in maize production (Abro et al., 2021). According to De Groote et al. (2020), the pest results in losses of around one-third of Kenya’s yearly maize crop, or one million tons, with significant regional variations.
As a consequence of the S. frugiperda invasion, the use of pesticides has dramatically increased (Tambo et al., 2020; Yang et al., 2021), potentially causing health problems, harming the environment, and threatening biodiversity. The FAO considers S. frugiperda one of the most important threats to food security in these regions (http://www.fao.org/fall-armyworm/en/). Management of S. frugiperda involves, however, not only chemical insecticides but a wide array of integrated pest management strategies (Kenis et al., 2022). Many pathogens (bacteria, fungi, viruses), parasitoids (Diptera and Hymenoptera), and predators (Coleoptera, Dermaptera, Hemiptera, Hymenoptera) attack it throughout its natural habitat. Of all the natural enemies, egg parasitoids are the simplest to raise in high quantities for augmentative releases, and several studies are testing its viability (Vieira et al., 2017; Firake and Behere, 2020). Experiments on host plant choice have been carried out throughout the pest invasive range, mostly to look at the viability of intercropping and push-pull control techniques (Tay et al., 2023). Biopesticides involving entomopathogenic fungi, baculoviruses, entomopathogenic bacteria and nematodes have also been extensively explored (Kenis et al., 2022).
Research on the chemical ecology of S. frugiperda has focused on two aspects: the pheromone produced by the female moths to attract males, and the caterpillar-induced plant volatiles that attract natural enemies of the caterpillars. The sex pheromone of S. frugiperda is a blend of several volatile acetates, dominated by (Z)-9-tetradecenyl acetate (Z9-14:Ac). A combination of Z9-14:Ac with (Z)-7-dodecenyl acetate (Z7-12:Ac) is highly attractive to males in the field (Tumlinson et al., 1986), also to invasive populations in Japan (Wakamura et al., 2021). In a study in China a pheromone lure was optimized by still adding (Z)-11-hexadecenyl acetate (Z11-16:Ac) to the blend that was initially identified. Indeed, the S. frugiperda pheromone blend has been shown to be different for different geographic regions (Batista-Pereira et al., 2006; Groot et al., 2008). Spodoptera. frugiperda was one of the first insects studied in the context of herbivore-induced plant volatiles (HIPVs). Maize plants in particular are very responsive to caterpillar attacks and have been shown to emitted large amounts of mainly terpenoids, but also indole in response to such attacks (Tumlinson et al., 1990, Turlings et al., 1993). The emissions of the truly inducible compounds are systemic and not just limited to the damaged site (Turlings and Tumlinson, 1992), enhancing their detectability. The fatty acid-amino acid conjugate volicitin (N-[17-hydroxylinolenoyl]-L glutamine) present in the caterpillar oral secretions was found to be the main elicitor that triggers this response (Alborn et al., 1997; Turlings et al., 2000). Spodoptera frugiperda also emits such volatiles (Turlings et al., 1993), but to a lesser extent, possibly because it is able to somewhat suppress the emissions (De Lange et al., 2020). There is tremendous variation among maize genotypes in the amounts of volatiles that they release upon caterpillar attack (Degen et al., 2004), yet the overall volatile profile shows clear consistencies in their caterpillar-induced emissions (Hoballah et al., 2002; Gouinguené and Turlings, 2002; Gouinguené et al., 2003). Studying the S. frugiperda-maize model is therefore has not only a great economic importance, but also an ideal model to demonstrate the potential of odor-based detection technologies (Turlings and Erb, 2018; Turlings and Degen, 2022).
3.2 Summary of literature on Spodoptera frugiperda - related VOCs
The literature on volatiles directly emitted by S. frugiperda is limited to publications on the identification of the sex pheromone blend emitted by female moths. The first identification was done by extracting the pheromone directly from the female moth glands (Sekul and Sparks, 1967), which composition is different from the pheromone released by the moths (Tumlinson et al., 1986). The female moths were found to release (Z)-7-dodecenyl acetate (Z7-12:Ac), dodecanyl acetate (12:Ac), 11-dodecenyl acetate (11-12:Ac), (Z)-9-tetradecenyl acetate (Z9-14:Ac), and (Z)-11-hexadecen-yl acetate (Z11-16:Ac). To the best of our knowledge, this latter publication is the only one that used the dynamic headspace technique to collect and identify the sex pheromone of Spodoptera, including S. frugiperda. The composition of the sexual pheromone of two closely related species, S. exigua and S. frugiperda, can be distinguished by the exclusive presence of the 12:Ac, Z7-12:Ac and 11-12:Ac in the S. frugiperda sex pheromone blend. Tests on the biological function of Z7-12:Ac have shown that it is the main compound responsible for the attraction of males (Tumlinson et al., 1986; Andrade et al., 2000; Cruz-Esteban et al., 2018). Their concentration is not very accurate, and composition and ratios of volatiles can vary depending on factors such as developmental stage, sex, feeding status, and environmental conditions. In subsequent studies it was shown that Z9-14:Ac and Z7-12:Ac are universal pheromone components of S. frugiperda, but other compounds, such as Z9-12:Ac, Z11-16:Ac and E7-12:Ac were also found to be released in different geographic populations (Tumlinson et al., 1986; Descoins et al., 1988; Fleischer et al., 2005; Batista-Pereira et al., 2006; Groot et al., 2008; Lima and McNeil, 2009; Jiang et al., 2022). In addition to the pheromone work, considerable information is available on plant volatiles induced by the caterpillars of S. frugiperda and other Spodoptera species have been extensively studied (Turlings and Erb, 2018; Turlings and Degen, 2022). In Table 3 we list the most relevant papers on inducible volatiles emitted by maize plants. It is important to note that different varieties of maize were used in different studies and they were also conducted under varying conditions ranging for the laboratory to the field. It can be concluded that different induction techniques and Spodoptera species induce similar volatile profiles. S. frugiperda does not appear to differ from other species in the volatile profile that they induce in maize plants, but there are quantitative differences, resulting in differences in ratios among VOCs that may facilitate the detection of specific pest species. For maize plants attacked by S. frugiperda and other Spodoptera species about 25 different volatiles have been reported. These include a diverse array of green leaf volatiles (GLV’s), monoterpenes, sesquiterpenes and aromatic compounds. Some of the most notable compounds include (Z)-3-hexenyl acetate, linalool, indole, (3E)-4,8-dimethyl-1,3,7-nonatriene (DMNT), (3E, 7E)-4,8,12-trimethyl-1,3,7,11-tridecatetraene (TMTT) and (E)-β-farnesene. The release of the GLVs is induced within seconds, whereas the others are released after 4 to 6 hours after S. frugiperda attack (terpenoids and indole) small maize plants typically release these compounds at rates of 50-200 ng per hour.
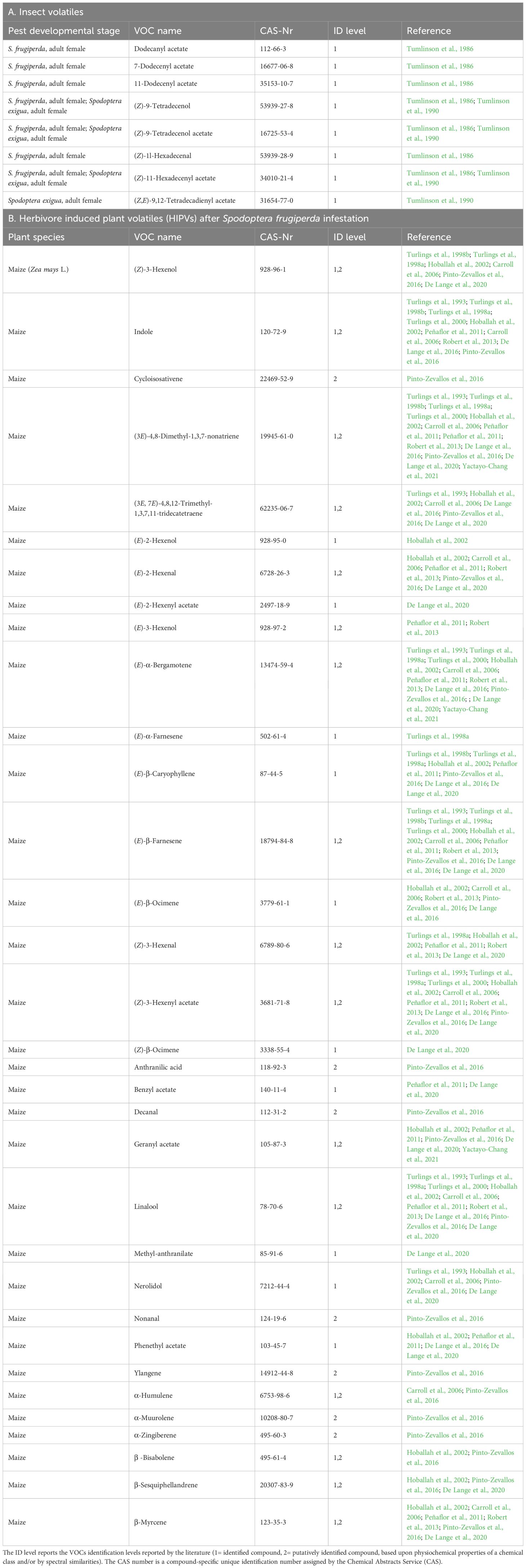
Table 3. List of Volatile Organic Compounds (VOCs) released by Spodoptera frugiperda and S. exigua adult females (A), and list of Herbivore Induced Plant Volatiles (HIPVs) released by damaged plants (B) described in the literature.
3.3 Candidate VOCs for Spodoptera frugiperda detection
There are three compounds that adult females of S. frugiperda emit (dodecanyl acetate; (Z)-7-dodecenyl acetate and (Z)-11-dodecenyl acetate) and are found only in this species compared to other species of Spodoptera. They have biological relevance for male attraction in the field. Plant-produced VOCs induced by S. frugiperda caterpillars are released in considerably larger amounts and easier to detect. As mentioned in the previous section, there are no unique compounds emitted by maize plants under S. frugiperda attack in comparison to attacks by other Spodoptera species, but ratios differences can be used to determine which species is attacking a plant. The most relevant compounds that are consistently emitted and have an ecological relevance are (Z)-3-hexenyl acetate, linalool, indole, (3E)-4,8-dimethyl-1,3,7-nonatriene (DMNT), (3E,7E)-4,8,12-trimethyl-1,3,7,11-tridecatetraene (TMTT) and (E)-β-farnesene (Table 4).
4 Volatile organic compounds produced and induced by Helicoverpa armigera
4.1 The cotton bollworm: distribution, biology and management
The Cotton Bollworm (CBW) Helicoverpa armigera Hübner (Lepidoptera: Noctuidae) (Figures 1F, G) is considered as one of the major pests in tropical and warm-temperate regions worldwide (Jones et al., 2019). Global economic losses caused by this species are estimated at over 3 billion US dollars per year (Haile et al., 2021; Riaz et al., 2021). Helicoverpa armigera is widely distributed throughout Asia, Oceania, Africa, and southern Europe, and has recently invaded South America (Tay et al., 2013; Jones et al., 2019).
Helicoverpa armigera is a highly polyphagous pest infesting more than 200 host plant species of diverse plant families. Many crops of high economic importance are included in its host range, such as cotton, maize, tomato (Figure 1H), sunflower (Figure 1G), soybean, and several legumes (Cunningham et al., 1999; Cunningham and Zalucki, 2014). The adults of H. armigera are excellent flyers and can migrate over long distances up to 2000 km (Behere et al., 2013; Jones et al., 2015). The species has a high fecundity and rapid reproduction rates, resulting in average in 4-6 generations per year and up to 10-11 generations per year in tropical regions (Riaz et al., 2021). The larvae are highly destructive plant feeders and very polyphagous, not only regarding plant species but also concerning plant parts (Figures 1F–H). The species has the ability to adapt its diapause depending on environmental conditions, in order to optimize survival. All these characteristics in their biology – its polyphagy, high mobility and reproduction rates and its facultative diapause - make H. armigera a serious pest, quickly invading new areas.
A blind trust of synthetic pesticides as main control measure for H. armigera has led to resistance development to all major classes of synthetic insecticides across many regions of the world (Downes et al., 2016; Jones et al., 2019; Riaz et al., 2021). As an alternative pest control measure have genetically modified crops, such as Bt (with a toxin from Bacillus thuringiensis) cotton, shown a good control effect of H. armigera over a period. But, as for synthetic pesticides, resistant populations have developed also for Bt crops, making well deliberated resistance management strategies necessary (Jin et al., 2015; Downes et al., 2016; Tabashnik and Carrière, 2017). Today, IPM strategies based on forecast, monitoring and decision support systems combined with biological, chemical, and physical control measures must be developed and used for successful control of H. armigera (Downes et al., 2016; Jones et al., 2019; Riaz et al., 2021).
4.2 Summary of literature on Helicoverpa armigera VOCs
We focus here on studies on H. armigera-related VOCs, which have shown that the pest itself (4.2) or plants infested by H. armigera (4.3) can release herbivore-specific signals which can be measured by chemical analyzes and behavioral and/or electrophysiological bioassays. The huge number of studies regarding other issues of the chemical ecology of H. armigera are not included here.
Research on H. armigera VOCs has started in the 1970s with the identification of sex pheromones in H. armigera, namely (Z)-9-hexadecenal, (Z)-11-hexedecenal, hexadecanol, (Z)-11-hexadecenol and (Z)-9-tetradecenal (Piccardi et al., 1977; Nesbitt et al., 1980; Wu et al., 1997; Zhang et al., 2012). We want to highlight here for our purpose the most promising pheromones, the oviposition marking pheromones (OMPs) or oviposition deterring pheromones (ODPs) (Table 5). ODPs have been identified for H. armigera around the turn of the millennium (Guoqing et al., 2001; Xu et al., 2006; Liu et al., 2008). ODPs are deposited by many parasitic and phytophagous insects associated with egg-laying, aiming for modification of the oviposition behavior of conspecifics such that subsequent eggs are not deposited into an already utilized resource. After behavioral observations on H. armigera have indicated the existence of oviposition-deterrent compounds, the three compounds 4-hydroxy-4-methyl-2-pentanone, hexadecanoic acid (palmitic acid) and (Z)-9-octadecenoic acid (oleic acid) have been identified from the tarsi of female H. armigera as oviposition-deterrent compounds (Guoqing et al., 2001). In further studies on ODPs in larval frass of H. armigera, a blend of fatty acid and corresponding methyl esters was found in the larval frass. Some compounds were found independent of the diet of the larvae, while others seem to be dependent on the food source. All compounds elicited responses in H. armigera moth antennae using electroantennography (EAG) analyzes (Xu et al., 2006). Moreover, it was found that laid eggs resulted in similar EAG responses. Compounds identified from the laid eggs were the 4-oviposition deterring fatty acids myristic, palmitic, stearic, and oleic acid and their corresponding methyl esters (Liu et al., 2008).
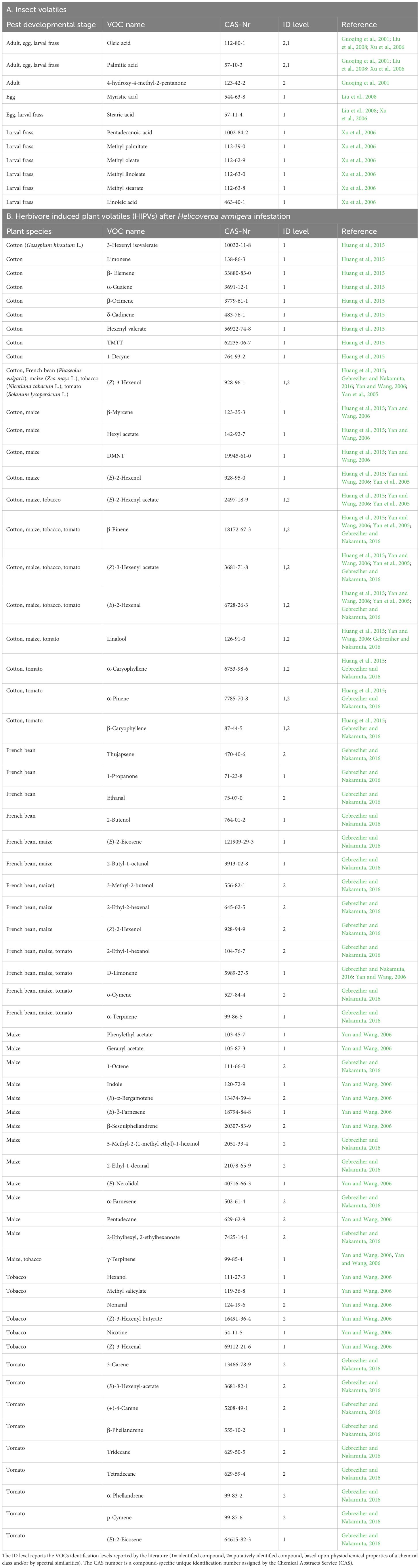
Table 5. List of Volatile Organic Compounds (VOCs) released by Helicoverpa armigera (A), and list of Herbivore Induced Plant Volatiles (HIPVs) released by damaged plants (B) described in the literature.
4.3 Summary of literature on Helicoverpa armigera - induced plant VOCs
Another important type of H. armigera related VOCs is herbivore induced plant volatiles (HIPVs) (Table 5). The HIPVs emission of tobacco plants induced by larvae feeding of the sibling species H. armigera and H. assulata were studied, and the corresponding behavioral response (wind tunnel bioassay) of a main parasitoid of both species, Campoletis chlorideae Uchida (Hymenoptera: Ichneumonidae), towards the different HIPV blends were recorded. GC/MS analyzes showed that β-pinene was specifically measured after feeding of H. armigera larvae, whereas (Z)-3-hexenal was particularly measured after infestation of both species, and hexyl acetate by mechanical damage (Yan et al., 2005). In another study, the HIPVs emission of maize plants induced by feeding of larvae of H.armigera and Pseudaletia separata Walker (Lepidoptera: Noctuidae) and the behavioral response of C. chlorideae in a wind tunnel were investigated. After infestation of H. armigera particularly the four terpenoids β-pinene, β-myrcene, D-limonene, and (E)-nerolidol were measured. All these compounds were not measured after attack of P. separata or mechanical damage (Yan and Wang, 2006). Also the volatile characteristics of cotton plants after larvae infestation of H. armigera have been investigated. GC/MS analyzes showed that several green leaf volatiles and terpenoids were measured after H. armigera infestation of cotton plants, whereas other compounds were found in both, infested and non-infested plants (Huang et al., 2015). Further studies compared by chemical analyzes the HIPV emission of tomato, French bean, and maize plants after infestation of H. armigera larvae, and by Y-tube olfactometer bioassays the behavioral response of the predator Orius strigicollis Poppius (Heteroptera: Anthocoridae). In all three plant species, a higher number and larger amounts of VOCs were found on H. armigera infested plants than undamaged or mechanically damaged plants (Gebreziher and Nakamuta, 2016). In some of these studies the odor profile of H. armigera infested plants have been compared with mechanical damaged plants, as both types of damage, biotic and abiotic, are stresses for the plants and induce specific VOC emission. However, to our knowledge no study compares the volatile profiles from H. armigera-infested plants with those of plants stressed by other abiotic factors such as water logging, drought, darkness, or extreme temperatures, or even volatile profiles of plants stressed by both, H. armigera infestation and abiotic factors, at the same time.
4.4 Candidate VOCs for Helicoverpa armigera detection
As ODPs are species-specific VOCs, detectable also in absence of the adult pest insect and identified for H. armigera, these compounds might be potential candidates for detection of H. armigera. In all three studies, we found the specific fatty acids myristic, palmitic, stearic, and oleic acid and their corresponding methyl esters have been identified as ODPs of H. armigera (Guoqing et al., 2001; Xu et al., 2006; Liu et al., 2008). Particularly, palmitic and oleic acid have been extracted from female moths (tarsi), larval frass and laid eggs of H. armigera, which might render them as robust and reliable candidates for detection purpose (Table 6).
With a view to detecting an herbivore-specific volatile blend measured from plants in response to H. armigera larvae feeding, we compared the volatile profiles from H. armigera infested plants with those from non-infested plants of different plant species found in literature. Compounds reported to be measured after H. armigera larval infestation of different plant species, but not or in very small amounts only from non-infested, mechanically damaged or plants infested of another pest species, might be possible candidates for detection of H. armigera. The terpenoids β-pinene, β-myrcene, D-limonene, and (E)-nerolidol were found to be species-specific for H. armigera larval infestation of maize plants (Yan and Wang, 2006) (Table 6). The compound β-myrcene was found in maize and cotton particularly after H. armigera larval infestation (Yan and Wang, 2006; Huang et al., 2015). D-limonene was species-specific measured after H. armigera larval infestation on maize, French bean and tomato (Gebreziher and Nakamuta, 2016; Yan and Wang, 2006). A compound noticed in four different studies as species-specific volatile measured from a plant in response to H. armigera larvae feeding on maize, cotton, tobacco, and tomato is β-pinene (Huang et al., 2015; Yan and Wang, 2006; Yan et al., 2005; Gebreziher and Nakamuta, 2016).
5 Volatile organic compounds produced and induced by Bursaphelenchus xylophilus
5.1 The pinewood nematode: distribution, biology and management
The Pinewood Nematode (PWN), Bursaphelenchus xylophilus (Steiner & Buhrer 1934) (Rhabditida: Aphelenchoididae), is a migratory plant parasitic nematode (Figure 1I) responsible for pine wilt disease (PWD), a serious forest disease that has devastated vast pine stands in Asia and Europe (Figures 1K, L) causing substantial ecological, economic, and cultural impacts (Back et al., 2024). Originally from North America, where the incidence of B. xylophilus is very low due to a co-evolution between the nematode and native pine species (Sutherland, 2008), the nematode was first noted in Japan in the early 20th century Futai, 2008. Bursaphelenchus xylophilus spread to Taiwan, China (in 1982), and Korea (in 1988), and was detected in Portugal, within the European Union, in 1999 (Mota et al., 1999). By 2008, mainland Portugal was declared a quarantine zone, and wood export restrictions extended nationwide (Rodrigues, 2008). Bursaphelenchus xylophilus was found in Madeira Island in 2010 (Fonseca et al., 2012) and in Spain in 2011 (Abelleira et al., 2011), even attacking different species of Pinus trees (Inácio et al., 2015; Zamora et al., 2015).
The European Plant Protection Organization (EPPO) classifies B. xylophilus as an A2 type quarantine pest in the EU, given its extreme pathogenicity and the abundance of susceptible pines in Europe (e.g., Pinus pinaster, P. sylvestris L., and P. nigra Arnold) (EPPO, 2023). Although currently limited to Portugal and Spain, future climate conditions in northern Europe might create a highly susceptible environment for B. xylophilus, threatening northern pine forests (Hirata et al., 2017; De la Fuente et al., 2018).
The infection mechanism of PWD involves the host pine tree, an insect vector (mainly Monochamus sp. beetles) (Figure 1J), B. xylophilus, and associated microbiota (Zhao et al., 2014; Vicente et al., 2012). During beetle maturation feeding, B. xylophilus enters healthy pines through beetle wounds, causing severe damage by invading resin canals, attacking epithelial cells, and disrupting water and mineral transport (Mamiya, 1983). This leads to pine wilting within three weeks, resulting in tree collapse within 40 to 60 days post-infection, with millions of nematodes infecting the trunk and branches (Kuroda, 2008) (Figures 1K, L). The decaying pine becomes attractive to the adult Monochamus beetles and, consequently, a source for new infections spread by the insect-vector (Jones et al., 2008; Futai, 2013).
Control strategies for PWD include breeding resistant pine species (Nose and Shiraishi, 2008; Carrasquinho et al., 2018; Menéndez-Gutiérrez et al., 2018) eradicating infected trees, treating wood (Kamata, 2008; Rodrigues, 2008; Xu, 2008) and controlling beetle populations. Infected wood can be treated chemically or thermally before its use for exportation or industrial purposes. Chemical insecticides prevent beetle spread, though they may harm beneficial organisms and accumulate in ecosystems (Kamata, 2008; Bi et al., 2015). Alternative controls include pheromone traps for controlling the spread of insect-vector populations and biological control using beetle predators or parasites (Nakamura, 2008; Shimazu, 2008; Kim et al., 2016). Trunk injection of nematicides is also effective, despite the toxicity risks associated with chemicals (Kamata, 2008). Integrated management strategies combining various methods are crucial for effective B. xylophilus containment.
5.2 Summary of literature on Bursaphelenchus xylophilus - related VOCs
Literature on the volatiles emitted by B. xylophilus -infected plant material is very scarce and only six volatiles were reportedly influenced by B. xylophilus infection, in field and greenhouse grown infected pines (Table 7). Although no induced volatiles were reported, some constitutive pine compounds were reportedly emitted in greater proportions by the affected trees. In a study using 30-year-old Pinus thunbergii Parlatore trees, the proportions of sativene, carvacrol methyl ether and camphor were seen to increase, however the number of samples was low (7 infected individuals) and this response was detected on a single tree alone (Takeuchi et al., 2006). For volatiles capture, TENAX-TA absorbent filled glass tubes were used to sample 6 L of air surrounding the stem (ca. 2h at 50 mL/min). In a different study using 2-year-old P. thunbergii seedlings, slightly higher proportions of borneol were signaled as a result of B. xylophilus inoculation in a susceptible variety (Wang et al., 2022). However, sampling was performed using SPME (65 µm PDMS/DVB) adapted to a headspace vial with 1 cm sections of 500 mg pine needles, for 30 min. For P. densiflora Siebold & Zuccarini and P. koraiensis Siebold & Zuccarini 5-year-old trees, the emission of the monoterpene hydrocarbon 3-carene was 9.7 and 54.7 times higher than in control trees, when analyzed by HS-SPME/GC-MS, by using plastic wrapped plants and analyzing with DVB/CAR/PDMS fibers (df 50/30 µm) for 1 h, at room temperature (Hwang et al., 2021). For P. pinaster, limonene emission was seen to increase in B. xylophilus -infected trees, however, this was only detected for two out of four tested trees (Gaspar et al., 2020). Sampling was performed with 1.0 g of sample/100 mL of air, using a 65 µm PDMS/DVB coated fiber with 5 min exposure time at 35°C.
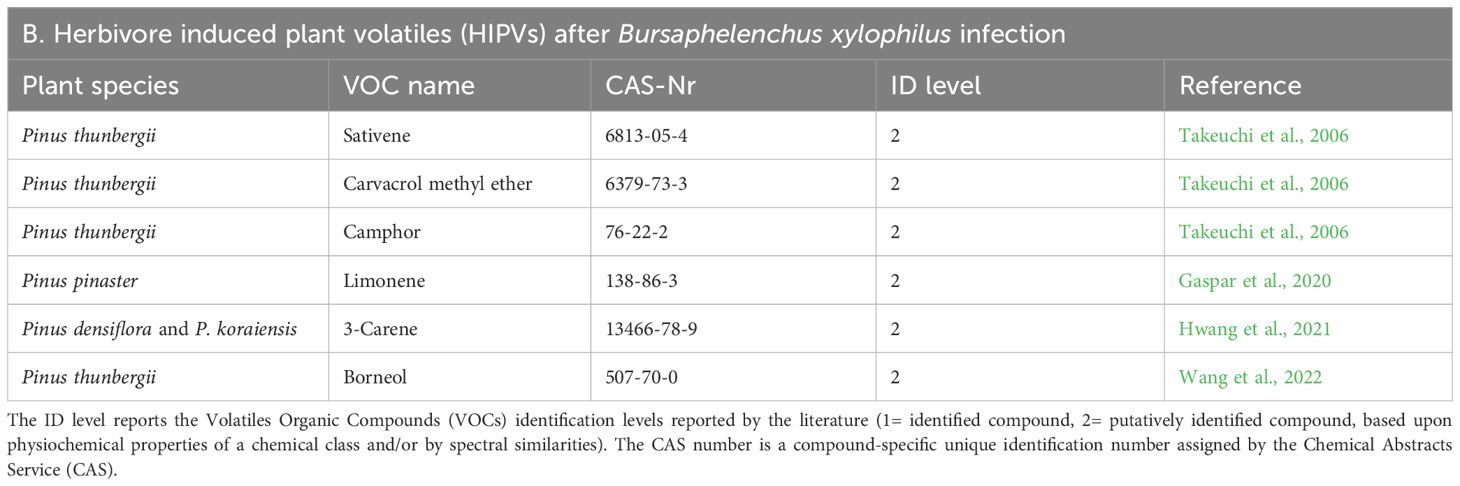
Table 7. List of Herbivore Induced Plant Volatiles (HIPVs) released by Bursaphelenchus xylophilus infected plants (B) described in the literature.
5.3 Candidate VOCs for Bursaphelenchus xylophilus detection
The available literature lacks an acceptable sample size or repeatability in results as well as variability in the conditions of sampling to conclude on suitable VOC candidates for detection of B. xylophilus.
6 Volatile organic compounds produced and induced by Phytophthora
6.1 Phytophthora ramorum and other important Phytophthora species: distribution, biology, diseases and management
The oomycete genus Phytophthora de Bary 1876 (Peronosporales: Peronosporaceae) currently includes eight obligate biotrophic unculturable species and ca 260 hemibiotrophic or necrotrophic culturable species and is widely distributed on all continents except Antarctica. Approximately half of the known species have been spread from their native areas to other continents where they became invasive causing severe diseases on non-coevolved host plants in horticultural, forest and natural ecosystems (Erwin and Ribeiro, 1996; Yang et al., 2017; Jung et al., 2018a, Jung et al., 2022, Jung et al., 2024; Brasier et al., 2022; Chen et al., 2022; Abad et al., 2023). Since the 1960s, the global number of epidemic diseases of forests and natural ecosystems caused by invasive Phytophthora species has increased exponentially from 5 to currently 41 (Brasier et al., 2022).
All Phytophthora species produce sporangia (Figures 2A, B) which usually release biflagellate zoospores (Figure 2B) or germinate directly. Aerial Phytophthora species spread during periods of high humidity with caducous sporangia (Figure 2B) via rain splash, fog and wind whereas soilborne Phytophthoras spread during wet periods via zoospores in soil and surface water (Erwin and Ribeiro, 1996; Chen et al., 2022). Many Phytophthora species form chlamydospores as vegetative survival structures (Figure 2C). Most Phytophthora species are characterized by the production of sexually derived enduring oospores (Figure 2D) (Erwin and Ribeiro, 1996; Chen et al., 2022; Jung et al., 2022, Jung et al., 2024).
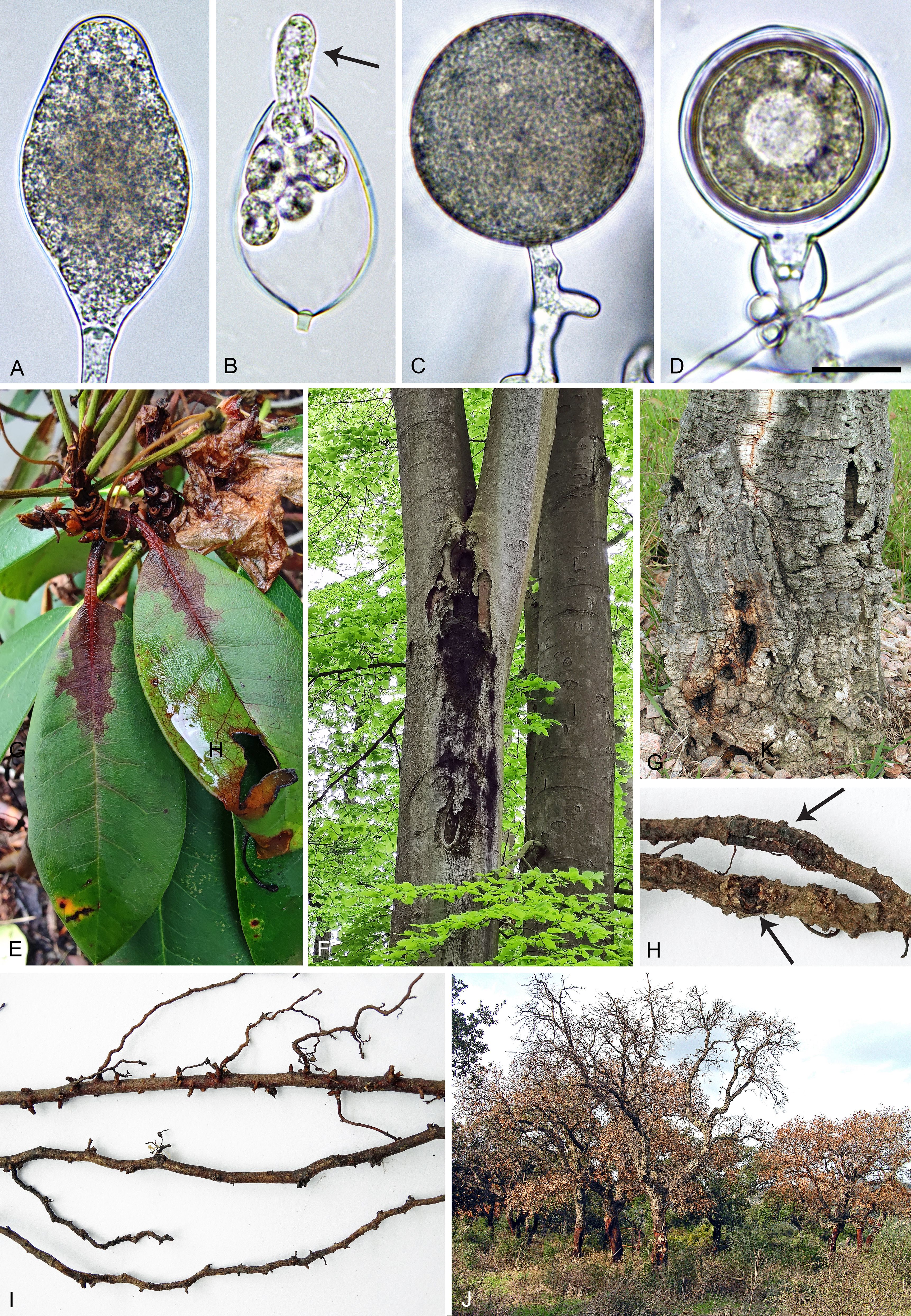
Figure 2. Phytophthora structures (on V8-juice agar) and typical disease symptoms (all photos: Thomas Jung). (A) Mature nonpapillate sporangium of the soilborne Phytophthora ×cambivora. (B) Caducous sporangium of the aerial Phytophthora ramorum releasing zoospores (arrow). (C) Chlamydospore of the soilborne Phytophthora cinnamomi. (D) Oogonium of Phytophthora cinnamomi with mature thick-walled oospore and amphigynous antheridium. Scale bar = 20 µm and applies to (A–D). (E) Shoot and leaf blight of a Rhododendron shrub caused by Phytophthora ramorum. (F) Stem of a mature beech (Fagus sylvatica) tree with an aerial bark canker with dark exudations caused by Phytophthora plurivora. (G) Stembase of a young cork oak (Quercus suber) tree with a bark canker with orange-brown exudations caused by Phytophthora cinnamomi. (H) Woody roots (diameters 0.5-1.0 cm) of a mature sessile oak (Quercus petraea) tree with bark cankers (arrows) caused by Phytophthora ×cambivora. (I) Woody roots (diameters <0.6 cm) of a mature sessile oak tree with severe losses of lateral roots and fine roots caused by Phytophthora plurivora and Phytophthora ×cambivora. (J) Acute mortality of mature cork oak trees due girdling bark cankers at the stembase and main roots caused by Phytophthora cinnamomi.
Generally, the management of Phytophthora diseases includes a wide range of measures including the prevention of pathogen introduction by using non-infested nursery stock, substrates and irrigation water, disinfesting of tools, cleaning of vehicles and boots from adhering soil particles, and phytosanitary controls using both visual inspections and high-throughput molecular detection tests; best-practice management in nurseries; avoiding of soil compaction and building of drainage systems to prevent waterlogging and flooding; application of potassium phosphite to stimulate defense reactions of roots (horticulture and forestry); fungicide applications (horticulture and agriculture); eradication via host removal and destruction; use of nanoparticle technologies; resistance screening programs and the use of resistant host genotypes or rootstocks (horticulture, agriculture and forestry); use of effectors and NLR resistance genes; and the development and use of general models to predict regions that might be most susceptible to epidemics by certain Phytophthora species (e.g. P. cinnamomi Rands, P. ramorum Werres, De Cock & Man in’ t Veld) or regional models to predict periods with environmental conditions conducive to disease development (e.g. P. infestans (Mont.) de Bary) (Harris, 1991; Erwin and Ribeiro, 1996; Colquhoun and Hardy, 2000; Hardy, 2001; Meentemeyer et al., 2004; Rizzo et al., 2005; Robin et al., 2006; Henderson et al., 2007; Stukely et al., 2007; Frankel, 2008; Garbelotto et al., 2009; Brasier and Webber, 2010; Filipe et al., 2012; Pérez-Sierra and Jung, 2013; Crane and Shearer, 2014; Santos et al., 2017; Peterson et al., 2015; Jung et al., 2016, Jung et al., 2018a; O’Hanlon et al., 2016, O’Hanlon et al., 2018; Lu et al., 2019; Sniezko et al., 2019; González et al., 2020; Solla et al., 2021; Santos et al., 2022; Brandano et al., 2023; Martínez et al., 2023).
Phytophthora ramorum (Figure 2B), an airborne pathogen, originates from the Laurisilva forests of East Asia (Jung et al., 2020, Jung et al., 2021). Since the early 1990s, each two lineages have been introduced to Europe (EU1 and EU2) and the Pacific Northwest (NA1 and NA2) where they became highly invasive causing leaf and shoot blights and bark cankers on a wide range of more than 100 host species, including Rhododendron (Figure 2E), Camelia and Viburnum spp., and the devastating epidemics “Sudden Oak Death” (California and Oregon) and “Sudden Larch Death” (UK and Republic of Ireland) which killed millions of oak, tanoak and larch trees (Werres et al., 2001; Rizzo et al., 2002; Brasier and Webber, 2010; Grünwald et al., 2012; Van Poucke et al., 2012; Harris and Webber, 2016; Jung et al., 2018a; Cobb et al., 2020). In the EU, all P. ramorum lineages not yet introduced (= all lineages except of EU1) are listed as A1 quarantine pests.
The panglobal soilborne pathogen Phytophthora cinnamomi (Figures 2C, D) is the most notorious and invasive member of the genus infecting and causing root rot, bark cankers (Figure 2G), dieback and mortality (Figure 2J) of more than 5000 woody plant species worldwide (Erwin and Ribeiro, 1996; Hardham and Blackman, 2018). A recent population genomic study showed that P. cinnamomi originates in Southeast Asia and that the global pandemic is driven by two clonal A2 mating type lineages (Shakya et al., 2021). Besides being a major pathogen of many horticultural crops and ornamentals, P. cinnamomi causes some of the most devastating epidemics of forest trees and natural ecosystems including decline and dieback of eucalypt forests across Australia; fynbos heathlands in South Africa; Valdivian rainforests and Araucaria forests in Chile; oak and chestnut forests in Southern Europe (Figures 2G, J) and the USA (Von Broembsen and Kruger, 1985; Shearer and Tippett, 1989; Brasier et al., 1993; Erwin and Ribeiro, 1996; Shearer et al., 2004; Vettraino et al., 2005; Dos Santos et al., 2011; Jung et al., 2016, Jung et al., 2018a, Jung et al., 2018b; McConnell and Balci, 2014; Sanfuentes et al., 2022).
Phytophthora cactorum (Leb. and Cohn) Schroeter is native to North America and has reached a panglobal distribution (Bourret et al., 2022). It causes both air- and soilborne diseases on a wide range of host plants including many ornamentals; forest trees like Fagus sylvatica L. (damping-off, root and collar rot, aerial bleeding cankers) and Betula pendula Roth (root and collar rot); horticultural crops like strawberries (collar rot and leather rot of fruits); and fruit trees, in particular apple trees (root, collar rot and fruit rot) (Harris, 1991; Erwin and Ribeiro, 1996; Jung et al., 2016, Jung et al., 2018a, Jung et al., 2019; Hantula et al., 2000; Jung, 2009; Corcobado et al., 2020).
Phytophthora plurivora Jung & Burgess originates from East Asia (Vettraino et al., 2011; Huai et al., 2013; Jung et al., 2017a, Jung et al., 2024). It is a soilborne introduced pathogen in both Europe and North America causing root and collar rot, aerial bleeding bark cankers (Figures 2F, I), and leaf and shoot blight on a wide range of woody host plants in natural ecosystems, nurseries and planting sites across; it is also one of the main drivers of current oak and beech declines across Europe (Jung, 2009; Jung and Burgess, 2009; Orlikowski et al., 2011; Reeser et al., 2011; Hansen et al., 2012; Jung et al., 2016, Jung et al., 2018a, Jung et al., 2019; Bienapfl and Balci, 2014; Brazee et al., 2016; Corcobado et al., 2020; Frankel et al., 2020).
Phytophthora ×cambivora (Petri) Buisman (Figure 2A) is a soilborne pathogen with a cosmopolitan distribution which originates from East Asia (Erwin and Ribeiro, 1996; Jung et al., 2017b; Mullett et al., 2023). It causes root (Figures 2H, I) and collar rot and infrequently aerial bleeding bark cankers on a wide range of woody host plants including many ornamentals, fruit trees and forest trees, and is one of the main drivers of the devastating Ink disease of sweet chestnut (Castanea sativa) in Europe and oak and beech declines across Europe (Mircetich and Matheron, 1976; Erwin and Ribeiro, 1996; Jung et al., 2000, Jung et al., 2018a, Jung et al., 2019; Vettraino et al., 2005; Jung, 2009; Corcobado et al., 2020).
6.2 Summary of literature on VOCs produced and induced by Phytophthora species
In this summary we focus on studies with Phytophthora-infection related VOCs from different Phytophthora-inoculated substrates i.e., chemical analyzes of VOCs directly from the pathogens or from the plants infected by Phytophthora species. A limited number of studies have identified and described VOCs emitted from substrates infected with Phytophthora species (and other oomycetes). For this work we included 12 studies, but only two studies are focused on or include VOCs from quarantine pathogen P. ramorum, the target pathogen in this review.
McCartney et al. (2018), used headspace sorptive extraction (HSSE), stir bar sorptive extraction (SBSE) and SPME combined with GC-MS to find specific VOCs and VOC profiles from P. ramorum-infected Rhododendron hybrid ‘Cunningham’s White’ plants. This is currently the only published study that investigates VOCs from P. ramorum-infected plants. For the HSSE method (in situ branch enclosure technique), 79 VOCs were detected. Three compounds were statistically different for P. ramorum-inoculated Rhododendron plants vs controls: linalool, (Z)-4-hexenol and (Z)-3-hexenyl pentanoate. For the SBSE liquid extraction method (leaf volatiles from a methanol extract), 115 VOCs were detected, and 31 compounds were statistically different for the inoculated Rhododendron plants (see Table 8). One compound, (Z)-3-hexenyl pentanoate, was produced in higher abundances in healthy plants (control) for both HSSE and SBSE. The SPME method (water runoff from the soil of potted healthy and inoculated plants), four compounds were only present in runoff water from soil infested with P. ramorum: (Z)-11-hexadecenoic acid, (Z)-9-hexadecenoic acid, cyclic octaatomic sulfur, oleic acid. These identified fatty acids have boiling points higher than 350°C and can be difficult to detect in ambient air except under specific experimental conditions. Loulier et al (2020), utilized SPME/GC-MS to investigate VOCs from cultures in potato dextrose agar (PDA) of P. ramorum, P. plurivora, P. cinnamomi, P. cactorum and a range of fungi. It was found that ethanol was shared between P. ramorum and P. cinnamomi (see Table 8). Other VOCs detected (but also present in various species of fungi) were 3-octanone, 1-octen-3-ol, 2-methylbutanol and isoamyl alcohol from P. ramorum. P. ramorum also emitted 2-phenylethanol. Loulier et al. (2020) further found that P. ramorum emitted higher amounts of compounds compared to the other Phytophthora species, and this was also confirmed in an analysis using an e-nose instrument developed in the same study. The e-nose could discriminate between VOCs emitted by P. ramorum, Fusarium poae (Peck) Wollenweber, Trichoderma asperellum Samuels, Lieckfeldt & Nirenberg and Rhizoctonia solani (Prillieux & Delacroix) Donk. Interestingly, P. plurivora emitted two monoterpenes α-pinene and 3-carene. Furthermore, Loulier et al. (2020) found that a major difference between the Phytophthora species and the fungi could be the amount of sesquiterpene produced, where the Phytophthora tested does not release these compounds/VOCs, but all tested fungal species did (except one). A recent study by Sherwood et al. (2024) used SPME/GC-MS to analyze the volatile organic compounds (VOCs) emitted by eight Phytophthora species cultivated on medium: P. cambivora, P. cinnamomi, P. citricola, P. gonapodyides, P. multivora, P. plurivora, P. polonica, and P. syringae. A total of 58 compounds were identified. However, identification from mass spectral libraries was not possible for some compounds, and these were excluded from Table 8. Surprisingly, there was very little overlap with the VOCs identified in the Loulier et al. (2020), sharing only three VOCs: 1-octen-3-ol, heptanol, and 4-ethyl guaiacol. Additionally, the sesquiterpene aristolochene was identified in P. cinnamomi, which contradicts Loulier et al. (2020)’s results. Some Phytophthora species may lack some genes for terpene biosynthesis (Chen et al., 2016), limiting their ability to produce a diverse range of terpenes compared to fungal organisms.

Table 8. List of Volatile Organic Compounds (VOCs) released by Phytophthora sp. (A), and list of pathogen-induced plant volatiles released by Phytophthora sp. - infected plants (B) described in the literature.
Qiu et al., 2014a; Qiu et al., 2014b optimized and used SPME to find specific VOCs from Phytophthora cinnamomi. After inoculation of different substrates [V8A, PDA, lupin seedlings (Lupinus angustifolius L. ‘Danja’), soil, and soil + lupin seedlings] with P. cinnamomi, this study identified 87 VOCs from infected and non-infected substrate. Four of these, 4-ethyl guaiacol (4-ethyl-2-methoxy phenol), 4-ethylphenol, butyrolactone, and phenylethanol, were significant and specific for P. cinnamomi-infections. This study shows that it is possible to detect differences between inoculated and non-inoculated plants and substrates. Borowik et al. (2021a) used SPME and found specific VOCs for P. plurivora and Pythium intermedium (de Bary) Uzuhashi, Tojo & Kakishima from in vitro infected germinated acorns of Quercus robur L. In total, four VOCs were detected on the inoculated acorns, which were not found in the control acorns. Three of them, neophytadiene isomer 2, neophytadiene isomer 3 and isoamyl alcohol were significant and specific for acorns infected with P. plurivora, whereas methylcarveol were specific for Pythium intermedium-infected acorns. Furthermore, Borowik et al. (2021b) also developed a low-cost electronic nose that applies six non-specific Figaro Inc. metal oxide sensors. A machine learning approach with this system was able to distinguish between P. plurivora and Pythium intermedium grown on Petri dishes with V8-Agar media (Borowik et al., 2021b) and using in vitro infected germinated acorns of Q. robur (Borowik et al., 2021a). Durkovic et al. (2021) used HS/GC-MS to analyze emissions from field-grown hybrid poplar infected with P. cactorum and P. plurivora. Their findings showed that the emissions of both sesquiterpenes, α-cubebene and germacrene D, were induced solely by the Phytophthora inoculations (both species).
De Lacy Costello et al. (2001) used improvised thermal desorption system (sorbent Tenax TA, Tenax GR and Carbosieve III) for P. infestans- and Fusarium coeruleum Libert ex Saccardo inoculated potato tubers (Solanum tuberosum L. cv. Maris Piper). The four most abundant and significant VOCs were common for both pathogens, but not present in the control: benzothiazole, 2-ethyl-1-hexanol, 3-hydroxy-2,2-dimethyl-1-(1-methylethyl)propyl isobutyrate and propanoic acid, 2-methyl-, 3-hydroxy-2,4,4-trimethylpentyl ester (see Table 8). Laothawornkitkul et al. (2010) detected three VOCs specific for P. infestans-infected potato leaves: 5-ethyl-2(5H)-furanone, (E)-2-hexenal, and phenylethanol (VOCs were trapped on volatile traps (Tenax sorbent) and then eluted with a solvent). Even though both studies on potato used similar technology the experimental conditions, potato growth stage and variety differed could explain the difference in the VOCs emitted from P. infestans-infected potato. Li et al. (2019), developed a smartphone-based VOC fingerprinting platform that could detect P. infestans in tomato (Solanum lycopersicum L.) both in vitro and in vivo. They suggest that (E)-2-hexenal is a major diagnostic VOC marker for P. infestans infection, which aligns with the findings of an earlier study by Laothawornkitkul et al. (2010). However, Xu et al. (2021) later demonstrated that (E)-2-hexenal is involved in Botrytis cinerea infection in tomato plants.
Jeleń et al., 2005 used SPME/GC-MS to find specific VOCs for P. cactorum-infected strawberries (Fragaria × ananassa (Duchesne ex Weston) Duchesne ex Rozier). Of 160 VOCs, 17 compounds were specific for inoculated strawberries and were absent in non-inoculated strawberries. Of these VOCs, two were found to be causing the characteristic off-odor from P. cactorum-infected strawberries (using gas chromatography–olfactometry): 4-ethylphenol and 4-ethyl guaiacol.
Each of these studies found different VOCs and different VOC profiles obtained from the different Phytophthora species-infected substrates, indicating there are Phytophthora species-specific VOCs and VOC profiles. Hence, enabling the development of e-noses for aiding detection of these pathogens, especially those of quarantine status and high destructive potential. However, of the above referenced papers, only twelve species of oomycetes (P. cactorum, P. cinnamomi, P. infestans, P. ramorum, P. plurivora, P. cambivora, P. citricola, P. gonapodyides, P. multivora, P. polonica, P. syringae and Pythium intermedium) have been investigated so far and they have utilized several different infected substrates and various methods to collect VOCs from the pathogens themselves or the infected plants. The VOC information for Phytophthora is very scarce compared to other pests such as the fall armyworm Spodoptera frugiperda, the brown marmorated stink bug Halyomorpha halys or the cotton Boll worm Helicoverpa armigera. It is not yet possible for any Phytophthora species to find a VOC profile that is robustly produced in connection with the target organisms i.e., VOCs that are not only produced in one infected plant variety or under one certain temperature/light regime. Three VOCs were consistently identified in at least three independent studies of Phytophthora: 1-octen-3-ol, 4-ethylguaiacol, and phenylethanol (see Table 8). However, a limitation of these potential biomarkers is that they are also produced by several other organism.
Finding suitable VOCs for the early detection of Phytophthora in woody plants is challenging. The genus Phytophthora often lacks genes necessary for the biosynthesis of secondary metabolites which are commonly used for fungal detection. This could be potentially utilized. Research by Loulier et al. (2020) using electronic noses (E-noses) demonstrates that the detection of specific terpenes can potentially distinguish between fungal infections and Phytophthora infections. Another challenge is that a significant portion of the pathogen resides within the roots, unlike infections caused by organisms like P. infestans. For the latter, a simpler device for VOC collection was successfully developed (Li et al., 2019). To capture sufficient VOCs for future analysis from this root-dwelling pathogen, a more sensitive method of collection and analysis, such as TD-GC-MS, is recommended.
6.3 Candidate VOCs for Phytophthora ramorum detection
Since only two studies included VOCs from P. ramorum, either P. ramorum-infected plants (McCartney et al., 2018) or from the pathogen in culture (Loulier et al., 2020), it is not possible to select any robust candidate VOCs for this pathogen. Therefore, all current VOCs that do not appear in the controls in these studies are listed as P. ramorum candidate VOCs in Table 8. More VOC profiling of P. ramorum and other Phytophthora species and Phytophthora-infected plants are urgently needed to find functioning candidate VOCs for these pathogens.
7 Discussion
Exploiting VOCs released by the pests and infested plants has shown to be a promising approach to assess the presence of quarantine organisms (MacDougall et al., 2022). Our review, however, highlights how crucial it is to have clear target signature VOCs for prompt and accurate pest detection. Volatiles specific to the organism (in case of insect, the pheromones) and herbivore-induced plant volatiles clearly appear as reliable VOCs for pest detection and identification. In the three insects we considered, at least one candidate compound was found among aggregation, defense, alarm, sexual and oviposition deterrent pheromones. No specific volatile has yet been identified for the nematode Bursaphelenchus xylophilus and for the oomycete Phytophtora ramorum.
Among the five organisms we analyzed, the insect pheromones appeared to be the most reliable candidates. Although the specificity of insect pheromones is geared toward precise communication within a species, the generalization and quantities of HIPVs released by plants serves a broader ecological context, influencing a wide array of interactions within an ecosystem (Shivaramu et al., 2017). In the case of insect pheromones, the specificity is often a result of coevolution between the emitter and the receiver within the same species. The accuracy of the signal is crucial for reproductive success. On the other hand, the generalization of HIPVs in plants is likely to be a strategy to maximize the benefits of indirect defense against herbivores and to establish complex ecological relationships with various organisms in the environment. For a precise detection through VOCs to be effective and applicable, pest-specific signals independent of the plant species should be desirable, pheromones satisfy this requirement more than HIPVs. Pheromones present, however, limitations. Since pheromones primary function is to deliver precise information within the species only at specific time periods, they are not constantly emitted. For instance, H. halys does not release defensive odors if not disturbed (Nixon et al., 2018), and the aggregation pheromone is emitted by males only (Weber et al., 2017). In Spodoptera frugiperda only adult virgin females emit the sexual pheromone. Therefore, eggs and immature insect stages would not be identified based on the pheromones that we currently know about. In contrast, the oviposition deterrent pheromones in Helicoverpa armigera are not only produced by females after oviposition, but also by eggs and larval frass (Guoqing et al., 2001; Xu et al., 2006; Liu et al., 2008), making them a more reliable VOCs for pest identification. It is highly desirable that similar compounds would be found for other insect species.
The HIPVs described in this review may have limitations as candidates in the case of H. halys and S. frugiperda. In H. halys, the HIPVs are different depending on plant species, and no apparent common VOC was released following H. halys damage. In the case of maize plants infested with different species of the genus Spodoptera, the HIPV blends lack unique molecules that could identify the attacking species. However, there are consistent difference in ratios that could provide such information. Further research is needed to determine consistencies and specificities in host plant responses to determine reliable combinations of compounds as candidates of pest identification.
A more favorable situation emerged in the HIPVs of H. armigera. Four terpenoids were found to be specific to CBW infestation of maize plants, among which β-myrcene was found in cotton too, and D-limonene also in French bean and tomato. β-Pinene was common in maize, cotton, tobacco, and tomato. Specificity and consistency allowed to select these HIPVs as candidates for the CBW detection, also showing that the use of HIPVs as identification cues is possible.
The use of HIPVs as marker signals should be considered carefully, because of the complexity that emerges from real-life conditions. Pest surveillance usually applies to international trade, and plant might face long and stressful journeys. Abiotic stress factors, such as drought, temperature extremes, and nutrient deficiencies, can vary widely in intensity and duration. The plant volatilome responses to such stressors must be considered when targeting for specific VOCs. In an extensive review, Loreto and Schnitzler (2010) reported how abiotic stresses enhance biogenic VOC emission rates and patterns, and how these stressors can alter the constitutive VOCs, causing them to either increase or decrease in emission, or they can cause the synthesis of new VOCs and suppression of others. The capacity of discerning HIPVs from abiotic stress-related plant VOCs becomes crucial. More data are needed to get a full picture of the diversity of plant responses under different stress combinations.
In case of the nematode Bursaphelenchus xylophilus, the scarce literature available did not provide any robust data to allow the selection of candidate VOCs. The few compounds mentioned are common terpenoids that lack consistency between the pine species and are thus unreliable. A considerable effort is required to expand the information available on the B. xylophilus-induced plant volatiles. Similarly, the number of studies on Phytophthora ramorum did not provide enough evidence to confidently select signature VOCs. Only one research paper (Loulier et al., 2020) analyzed the volatiles released by a pure P. ramorum culture, and another one (McCartney et al., 2018) from infected hybrid Rhododendron plants. As for the nematode, the need to expand the literature on the subject is essential.
8 Conclusions
This review collected and analyzed the available literature concerning pest VOCs and pest-induced VOCs from five selected pests/pathogens relevant to the international pest surveillance programs. The aim was to select the pest signature volatiles that can be employed in specific volatile detection. The picture that appears shows that insects pheromones are reliable indicators of the pest presence, albeit with limitations. The use of induced plant VOCs is a viable solution but requires in depth exploration that takes into account the complexity of the plant response to abiotic and biotic factors. As the development of volatiles-based approaches are advancing, their use is increasingly seen as a viable solution for early pest detection. It is imperative to increase the number of studies and the quality of information available on the most crucial pest species. The research in this direction should be methodical, precise and rigorous, aiming at offering a broad database of volatiles signatures.
Author contributions
RF: Conceptualization, Writing – original draft, Writing – review & editing. MP: Writing – original draft, Writing – review & editing. GT: Writing – original draft, Writing – review & editing. CA: Writing – original draft, Writing – review & editing. MI: Writing – original draft, Writing – review & editing. TT: Writing – review & editing. JF: Writing – original draft, Writing – review & editing. TJ: Writing – original draft, Writing – review & editing. DB: Writing – review & editing. AP: Writing – review & editing. SA: Writing – review & editing. LC: Conceptualization, Writing – original draft, Writing – review & editing. MB: Writing – review & editing.
Funding
The author(s) declare financial support was received for the research, authorship, and/or publication of this article. This research was funded by the project PurPest, grant number 101060634 supported under HORIZON-CL6-2021-FARM2FORK-01-04-Tackling outbreaks of plant pests, funded under the HORIZON Research and Innovation Action (RIA) from the European Research Executive Agency (REA)-Green Europe REA.B.
Conflict of interest
The authors declare that the research was conducted in the absence of any commercial or financial relationships that could be construed as a potential conflict of interest.
Publisher’s note
All claims expressed in this article are solely those of the authors and do not necessarily represent those of their affiliated organizations, or those of the publisher, the editors and the reviewers. Any product that may be evaluated in this article, or claim that may be made by its manufacturer, is not guaranteed or endorsed by the publisher.
References
Abad Z. G., Burgess T. I., Bourret T., Bensch K., Cacciola S., Scanu B., et al. (2023). Phytophthora: taxonomic and phylogenetic revision of the genus. Stud. Mycology 106, 259–348. doi: 10.3114/sim.2023.106.05
Abelleira A., Picoaga A., Mansilla J. P., Aguin O. (2011). Detection of Bursaphelenchus Xylophilus, Causal Agent of Pine Wilt Disease on Pinus Pinaster in Northwestern Spain. Plant Dis. 95, 776–776. doi: 10.1094/PDIS-12-10-0902
Abram P. K., Talamas E. J., Acheampong S., Mason P. G., Gariepy T. D. (2019). First detection of the samurai wasp, Trissolcus japonicus (Ashmead) (Hymenoptera, Scelionidae), in Canada. J. Hymenoptera Res. 68, 29–36. doi: 10.3897/jhr.68.32203
Abro Z., Kimathi E., De Groote H., Tefera T., Sevgan S., Niassy S., et al. (2021). Socioeconomic and health impacts of fall armyworm in Ethiopia. PloS One 16, e0257736. doi: 10.1371/journal.pone.0257736
Akhoundi M., Chebbah D., Elissa N., Brun S., Jan J., Lacaze I., et al. (2023). Volatile organic compounds: A promising tool for bed bug detection. Int. J. Environ. Res. Public Health 20, 5214. doi: 10.3390/ijerph20065214
Alborn H. T., Turlings T. C. J., Jones T. H., Stenhagen G., Loughrin J. H., Tumlinson J. H. (1997). An elicitor of plant volatiles from beet armyworm oral secretion. Science 276, 945–949. doi: 10.1126/science.276.5314.945
Aldrich J. R., Oliver J. E., Lusby W. R., Kochansky J. P., Lockwood J. A. (1987). Pheromone strains of the cosmopolitan pest, Nezara viridula (Heteroptera: Pentatomidae). J. Exp. Zoology 244, 171–175. doi: 10.1002/jez.1402440121
Andrade R., Rodriguez C., Oechlschlager A. C. (2000). Optimization of a pheromone lure for Spodoptera frugiperda (Smith) in Central America. J. Braz. Chem. Soc. 11, 609–613. doi: 10.1590/S0103-50532000000600009
Babendreier D., Koku Agboyi L., Beseh P., Osae M., Nboyine J., Ofori S. E. K., et al. (2020). The efficacy of alternative, environmentally friendly plant protection measures for control of fall armyworm, Spodoptera frugiperda, in maize. Insects 11 240, 1–21. doi: 10.3390/insects11040240
Back M. A., Bonifácio L., Inácio M. L., Mota M., Boa E. (2024). Pine wilt disease: A global threat to forestry. Plant Pathol. 73, 1026–1041. doi: 10.1111/ppa.13875
Batista-Pereira L. G., Stein K., de Paula A. F., Moreira J. A., Cruz I., Figueiredo M. D. L. C., et al. (2006). Isolation, identification, synthesis, and field evaluation of the sex pheromone of the Brazilian population of Spodoptera frugiperda. J. Chem. Ecol. 32, 1085–1099. doi: 10.1007/s10886-006-9048-5
Baudron F., Zaman-Allah M. A., Chaipa I., Chari N., Chinwada P. (2019). Understanding the factors influencing fall armyworm (Spodoptera frugiperda J.E. Smith) damage in African smallholder maize fields and quantifying its impact on yield. A case study in Eastern Zimbabwe. Crop Prot. 120, 141–150. doi: 10.1016/j.cropro.2019.01.028
Behere G. T., Tay W. T., Russell D. A., Kranthi K. R., Batterham P. (2013). Population genetic structure of cotton bollworm Helicoverpa armigera (Hübner) (Lepitoptera: Noctuidae) in India as inferred from EPIC-PCR DNA markers. PloS One 8, e53448. doi: 10.1371/journal.pone.0053448
Bi Z., Gong Y., Huang X., Yu H., Bai L., Hu J. (2015). Efficacy of four nematicides against the reproduction and development of pinewood nematode, Bursaphelenchus xylophilus. J. Nematol. 47, 126–132.
Bienapfl J. C., Balci Y. (2014). Movement of Phytophthora spp. in Maryland’s nursery trade. Plant Dis. 98, 134–144. doi: 10.1094/PDIS-06-13-0662-RE
Blaauw B. R., Polk D., Nielsen A. L. (2015). IPM-CPR for peaches: incorporating behaviorally-based methods to manage Halyomorpha halys and key pests in peach. Pest Manage. Sci. 71, 1513–1522. doi: 10.1002/ps.2015.71.issue-11
Borges M., Jepson P. C., Howse P. E. (1987). Long-range mate location and close-range courtship behaviour of the green stink bug, Nezara viridula and its mediation by sex pheromones. Entomologia Experimentalis Applicata 44, 205–212. doi: 10.1111/j.1570-7458.1987.tb00546.x
Borowik P., Adamowicz L., Tarakowski R., Wacławik P., Oszako T., Ślusarski S., et al. (2021b). Application of a low-cost electronic nose for differentiation between pathogenic oomycetes Pythium intermedium and Phytophthora plurivora. Sensors 21, 1326. doi: 10.3390/s21041326
Borowik P., Adamowicz L., Tarakowski R., Wacławik P., Oszako T., Ślusarski S., et al. (2021a). Electronic nose differentiation between Quercus robur acorns infected by pathogenic oomycetes Phytophthora plurivora and Pythium intermedium. Molecules 26, 5272. doi: 10.3390/molecules26175272
Bos L. D., Sterk P. J., Schultz M. J. (2013). Volatile metabolites of pathogens: a systematic review. PloS Pathog. 9, e1003311. doi: 10.1371/journal.ppat.1003311
Bourret T. B., Fajardo S. N., Engert C. P., Rizzo D. M. (2022). A barcode-based phylogenetic characterization of Phytophthora cactorum identifies two cosmopolitan lineages with distinct host affinities and the first report of Phytophthora pseudotsugae in California. J. Fungi 8, 303. doi: 10.3390/jof8030303
Brandano A., Serra S., Hardy G. E. S. J., Scanu B. (2023). Potassium phosphonate induces resistance in sweet chestnut against ink disease caused by Phytophthora species. Pathogens 12, 365. doi: 10.3390/pathogens12030365
Brasier C. M., Robredo F., Ferraz J. F. P. (1993). Evidence for Phytophthora cinnamomi involvement in Iberian oak decline. Plant Pathol. 42, 140–145. doi: 10.1111/j.1365-3059.1993.tb01482.x
Brasier C., Scanu B., Cooke D., Jung T. (2022). Phytophthora: an ancient, historic, biologically and structurally cohesive and evolutionarily successful generic concept in need of preservation. IMA Fungus 13, 12. doi: 10.1186/s43008-022-00097-z
Brazee N. J., Wick R. L., Hulvey J. P. (2016). Phytophthora species recovered from the Connecticut River Valley in Massachusetts, USA. Mycologia, 108, 6–19doi: 10.3852/15-038
Brezolin A. N., Martinazzo J., Muenchen D. K., de Cezaro A. M., Rigo A. A., Steffens C., et al. (2018). Tools for detecting insect semiochemicals: a review. Analytical Bioanalytical Chem. 410, 4091–4108. doi: 10.1007/s00216-018-1118-3
Calam D. H., Youdeowei A. (1968). Identification and functions of secretion from the posterior scent gland of fifth instar larva of the bug Dysdercus intermedius. J. Insect Physiol. 14, 1147–1158. doi: 10.1016/0022-1910(68)90054-1
Candian V., Pansa M. G., Santoro K., Spadaro D., Tavella L., Tedeschi R. (2020). Photoselective exclusion netting in apple orchards: Effectiveness against pests and impact on beneficial arthropods, fungal diseases and fruit quality. Pest Manage. Sci. 76, 179–187. doi: 10.1002/ps.v76.1
Carnio V., Favaro R., Preti M., Angeli S. (2024). Impact of Aggregation Pheromone Traps on Spatial Distribution of Halyomorpha halys Damage in Apple Orchards. Insects 15, 791. doi: 10.3390/insects15100791
Carrasquinho I., Lisboa A., Inácio M. L., Gonçalves E. (2018). Genetic variation in susceptibility to pine wilt disease of maritime pine (Pinus Pinaster Aiton) half-sib families. Ann. For. Sci. 75, 85. doi: 10.1007/s13595-018-0759-x
Carroll M. J., Schmelz E. A., Meagher R. L., et al. (2006). Attraction of spodoptera frugiperda larvae to volatiles from herbivore-damaged maize seedlings. J. Chem. Ecol. 32, 1911–1924. doi: 10.1007/s10886-006-9117-9
Chen Q., Bakhshi M., Balci Y., Broders K. D., Cheewangkoon R., Chen S. F., et al. (2022). Genera of phytopathogenic fungi: GOPHY 4. Stud. Mycology 101, 417–564. doi: 10.3114/sim.2022.101.06
Chen X., Köllner T. G., Jia Q., Norris A., Santhanam B., Rabe P., et al. (2016). Terpene synthase genes in eukaryotes beyond plants and fungi: Occurrence in social amoebae. Proc. Natl. Acad. Sci. 113, 12132–12137. doi: 10.1073/pnas.1610379113
Chu L., Deng S., Zhao R., Deng J., Kang X. (2016). Comparison of adsorption/desorption of volatile organic compounds (VOCs) on electrospun nanofibers with tenax TA for potential application in sampling. PloS One 11, e0163388. doi: 10.1371/journal.pone.0163388
Cobb R. C., Haas S. E., Kruskamp N., Dillon W. W., Swiecki T. J., Rizzo D. M., et al. (2020). The magnitude of regional-scale tree mortality caused by the invasive pathogen Phytophthora ramorum. Earth’s Future 8, e2020EF001500. doi: 10.1029/2020EF001500
Cock M. J. W., Beseh P. K., Buddie A. G., Cafá G., Crozier J. (2017). Molecular methods to detect Spodoptera frugiperda in Ghana, and implications for monitoring the spread of invasive species in developing countries. Sci. Rep. 7, 4103. doi: 10.1038/s41598-017-04238-y
Colquhoun I. J., Hardy G. E. S. J. (2000). Managing the risks of Phytophthora root and collar rot during bauxite mining in the Eucalyptus marginata (Jarrah) forest of Western Australia. Plant Dis. 84, 116–127. doi: 10.1094/PDIS.2000.84.2.116
Corcobado T., Cech T. L., Brandstetter M., Daxer A., Hüttler C., Kudláček T., et al. (2020). Decline of European beech in Austria: involvement of Phytophthora spp. and contributing biotic and abiotic factors. Forests 11, 895. doi: 10.3390/f11080895
Costi E., Haye T., Maistrello L. (2017). Biological parameters of the invasive brown marmorated stink bug, Halyomorpha halys, in Southern Europe. J. Pest Sci. 90, 1059–1067. doi: 10.1007/s10340-017-0899-z
Crane C. E., Shearer B. L. (2014). Comparison of phosphite application methods for control of Phytophthora cinnamomi in threatened communities. Australas. Plant Pathol. 43, 143–149. doi: 10.1007/s13313-013-0260-9
Cruz-Esteban S., Rojas J. C., Sánchez-Guillén D., Cruz-López L., Malo E. (2018). Geographic variation in pheromone component ratio and antennal responses, but not in attraction, to sex pheromones among fall armyworm populations infesting corn in Mexico. J. Pest Sci. 91, 973–983. doi: 10.1007/s10340-018-0967-z
Cui S., Ling P., Zhu H., Keener H. M. (2018). Plant pest detection using an artificial nose system: A review. Sensors 18, 378. doi: 10.3390/s18020378
Cunningham J. P., Zalucki M. P. (2014). Understanding heliothine (Lepidoptera: Heliothinae) pests: What is a host plant? J. Economic Entomology 107, 881–896. doi: 10.1603/EC14036
Cunningham J. P., Zalucki M. P., West S. A. (1999). Learning in Helicoverpa armigera (Lepitoptera: Noctuidae): A new look at the behaviour and control of a polyphagous pest. Bull. Entomological Res. 89, 201–207. doi: 10.1017/S0007485399000310
Day R., Abrahams P., Bateman M., Beale T., Clottey V., Cock M., et al. (2017). Fall armyworm: Impacts and implications for Africa. Outlooks Pest Manage. 28, 196–201. doi: 10.1564/v28_oct_02
Degen T., Dillmann C., Marion-Poll F., Turlings T. C. J. (2004). Genetic variability in herbivore-induced volatile emission within a broad range of maize inbred lines. Plant Physiol. 135, 1928–1938. doi: 10.1104/pp.104.039891
De Groote H., Kimenju S. C., Munyua B., Palmas S., Kassie M., Bruce A. (2020). Spread and impact of fall armyworm (Spodoptera frugiperda JE Smith) in maize production areas of Kenya. Agriculture Ecosyst. Environ. 292, 106804. doi: 10.1016/j.agee.2019.106804
De Lacy Costello B. P. J., Evans P., Ewen R. J., Gunson H., Ratcliffe N., Spencer-Phillips P. (2001). GC-MS analyzes of volatile organic compounds from potato tubers inoculated with Phytophthora infestans or Fusarium coeruleum. Plant Pathol. 50, 489–496. doi: 10.1046/j.1365-3059.2001.00594.x
De la Fuente B., Saura S., Beck P. S. (2018). Predicting the spread of an invasive tree pest: the pine wood nematode in Southern Europe. J. Appl. Ecol. 55, 2374–2385. doi: 10.1111/jpe.2018.55.issue-5
De Lange E., Laplange D., Guo H., Xu W., Vlimant M., Erb M., et al. (2020). Spodoptera frugiperda caterpillars suppress herbivore-induced volatile emissions in maize. J. Chem. Ecol. 46, 344–360. doi: 10.1007/s10886-020-01153-x
de Lange E. S., Farnier K., Gaudillat B., et al. (2016). Comparing the attraction of two parasitoids to herbivore-induced volatiles of maize and its wild ancestors, the teosintes. Chemoecology 26, 33–44. doi: 10.1007/s00049-015-0205-6
Descoins C., Silvain J. F., Lalanne-Cassou B., Cheron H. (1988). Monitoring of crop pests by sexual trapping of males in Guadeloupe and Guyana. Agriculture Ecosyst. Environ. 21, 53–56. doi: 10.1016/0167-8809(88)90139-9
Dicke M., Van Loon J. J., Soler R. (2009). Chemical complexity of volatiles from plants induced by multiple attack. Nat. Chem. Biol. 5, 317–324. doi: 10.1038/nchembio.169
Dos Santos A., Tessmann D. J., Alves T. C. A., Vida J. B., Harakava R. (2011). Root and crown rot of Brazilian Pine (Araucaria angustifolia) caused by *Phytophthora cinnamomi*. J. Phytopathol. 159, 194–196. doi: 10.1111/j.1439-0434.2010.01741.x
Downes S., Kriticos D., Parry H., Paull C., Schellhorn N., Zalucki M. P. (2016). A perspective on management of Helicoverpa armigera: transgenic Bt cotton, IPM, and landscape. Pest Manage. Sci. 73, 485–492. doi: 10.1002/ps.4461
Durkovic J., Bubeníková T., Gužmerová A., Fleischer P., Kurjak D., Čaňová I., et al. (2021). Effects of Phytophthora inoculations on photosynthetic behaviour and induced defence responses of plant volatiles in field-grown hybrid poplar tolerant to bark canker disease. J. Fungi 7, 969. doi: 10.3390/jof7110969
EPPO (2023). EPPO standard on diagnostics - PM 7/44(4) bursaphelenchus xylophilus. EPPO Bull. 53, 156–183. doi: 10.1111/epp.12915
Erwin D. C., Ribeiro O. K. (1996). Phytophthora diseases worldwide (St. Paul, Minnesota: APS Press).
Eschen R., Beale T., Bonnin J. M., Constantine K. L., Duah S., Finch E. A., et al. (2021). Towards estimating the economic cost of invasive alien species to African crop and livestock production. CABI Agric. Bioscience 2, 1–18. doi: 10.1186/s43170-021-00038-7
European Commission (2020). “A farm to fork strategy for a fair, healthy and environmentally-friendly food system,” in COM 2020 381 final (European Commission, Brussels). Available at: https://eur-lex.europa.eu/legal-content/EN/TXT/?uri=CELEX%3A52020DC0381.
Falagiarda M., Carnio V., Chiesa S. G., Pignalosa A., Anfora G., Angeli G., et al. (2023). Factors influencing short-term parasitoid establishment and efficacy for the biological control of Halyomorpha halys with the samurai wasp Trissolcus japonicus. Pest Manage. Sci. 79 (7), 2397–2414. doi: 10.1002/ps.7423
FAO (2020). “The global action for fall armyworm control: Action framework 2020–2022,” in Working together to tame the global threat (FAO, Rome), 50.
Fennine C., Favaro R., Khomenko I., Biasioli F., Cappellin L., Angeli S. (2024). Diel rhythm of volatile emissions from males and females of the olive fruit fly Bactrocera oleae using PTR-ToF and GC–MS. J. Insect Physiol. 153, 104596. doi: 10.1016/j.jinsphys.2023.104596
Filipe J. A. N., Cobb R. C., Meentemeyer R. K., Lee C. A., Valachovic Y. S., Cook A. R., et al. (2012). Landscape epidemiology and control of pathogens with cryptic and long-distance dispersal: Sudden Oak Death in Northern Californian forests. PloS Comput. Biol. 8, e1002328. doi: 10.1371/journal.pcbi.1002328
Firake D. M., Behere G. T. (2020). Natural mortality of invasive fall armyworm, Spodoptera frugiperda (JE Smith) (Lepidoptera: Noctuidae) in maize agroecosystems of northeast India. Biol. control 148, 104303. doi: 10.1016/j.biocontrol.2020.104303
Fleischer S. J., Harding C. L., Blom P. F., White J., Grehan J. (2005). ). Spodoptera frugiperda pheromone lures to avoid nontarget captures of Leucania phragmatidicola. J. Economic Entomology 98, 66–71. doi: 10.1093/jee/98.1.66
Fonseca L., Cardoso J. M. S., Lopes A., Pestana M., Abreu F., Nunes N., et al. (2012). The pinewood nematode, Bursaphelenchus xylophilus, in Madeira Island. Helminthologia. doi: 10.2478/s11687-012-0020-3
Fornasiero D., Scaccini D., Lombardo V., Galli G., Pozzebon A. (2023). Effect of exclusion net timing of deployment and color on Halyomorpha halys (Hemiptera: Pentatomidae) infestation in pear and apple orchards. Crop Prot. 172, 106–331. doi: 10.1016/j.cropro.2023.106331
Fraga D. F., Parker J., Busoli A. C., Hamilton G. C., Nielsen A. L., Rodriguez-Saona C. (2017). Behavioral responses of predaceous minute pirate bugs to tridecane, a volatile emitted by the brown marmorated stink bug. J. Pest Sci. 90, 1107–1118. doi: 10.1007/s10340-016-0825-9
Frankel S. J. (2008). Sudden oak death and Phytophthora ramorum in the USA: a management challenge. Australas. Plant Pathol. 37, 19–25. doi: 10.1071/AP07088
Frankel S. J., Conforti C., Hillman J., Ingolia M., Benner D., Alexander J. M., et al. (2020). Phytophthora introductions in restoration areas: Responding to protect California native flora from human-assisted pathogen spread. Forests 11, 1291. doi: 10.3390/f11121291
Fundurulic A., Faria J. M., Inácio M. L. (2023). Advances in electronic nose sensors for plant disease and pest detection. Eng. Proc. 48, 14. doi: 10.3390/CSAC2023-14890
Futai K. (2008). “Pine wilt in Japan: From first incidence to the present,” in Pine wilt disease. Eds. Zhao B. G., Futai K., Sutherland J. R., Takeuchi Y. (Springer, Tokyo), 5–12.
Futai K. (2013). Pine wood nematode, Bursaphelenchus xylophilus. Annu. Rev. Phytopathol. 51, 61–83. doi: 10.1146/annurev-phyto-081211-172910
Garbelotto M., Harnik T. Y., Schmidt D. J. (2009). Efficacy of phosphonic acid, metalaxyl-M and copper hydroxide against Phytophthora ramorum in vitro and in planta. Plant Pathol. 58, 111–119. doi: 10.1111/j.1365-3059.2008.01894.x
Gaspar M. C., Agostinho B., Fonseca L., Abrantes I., de Sousa H. C., Braga M. E. M. (2020). Impact of the pinewood nematode on naturally-emitted volatiles and scCO2 extracts from Pinus pinaster branches: a comparison with P. pinea. J. Supercritical Fluids 159, 104784. doi: 10.1016/j.supflu.2020.104784
Gebreziher H. G. (2018). The role of herbivore-induced plant volatiles (HIPVs) as indirect plant defense mechanisms in a diverse plant and herbivore species; a review. Int. J. Agriculture Environ. Food Sci. 2, 139–147. doi: 10.31015/jaefs.18024
Gebreziher H. G., Nakamuta K. (2016). Responses of a predatory bug to a mixture of herbivore-induced plant volatiles from multiple plant species. Arthropod-Plant Interact. 10, 429–444. doi: 10.1007/s11829-016-9456-6
González M., Romero M.-A., Serrano M.-S., Sánchez M.-E. (2020). Fosetyl-aluminium injection controls root rot disease affecting Quercus suber in southern Spain. Eur. J. Plant Pathol. 156, 101–109. doi: 10.1007/s10658-019-01865-1
Gouinguené S., Alborn H., Turlings T. C. J. (2003). Induction of volatile emissions in maize by different larval instars of Spodoptera littoralis. J. Chem. Ecol. 29, 145–162. doi: 10.1023/A:1021984715420
Gouinguené S., Turlings T. C. J. (2002). The effects of abiotic factors on induced volatile emissions in corn plants. Plant Physiol. 129, 1296–1307. doi: 10.1104/pp.001941
Groot A. T., Marr M., Schöfl G., Lorenz S., Svatos A., Heckel D. G. (2008). Host strain specific sex pheromone variation in Spodoptera frugiperda. Front. Zoology 5, 20. doi: 10.1186/1742-9994-5-20
Grünwald N. J., Garbelotto M., Goss E. M., Heungens K., Prospero S. (2012). Emergence of the Sudden Oak Death pathogen Phytophthora ramorum. Trends Microbiol. 20, 131–138. doi: 10.1016/j.tim.2011.12.006
Gullan P. J., Cranston P. S. (2014). The insects: an outline of entomology (Chichester, UK: John Wiley & Sons).
Guo G. J., Zhao JianZhou Z. J., He KangLai H. K., Zhang Feng Z. F., Wang ZhenYing W. Z. (2018). Potential invasion of the crop-devastating insect pest fall armyworm Spodoptera frugiperda to China. Plant Prot. 44, 1–10. doi: 16688/j.zwbh.2018452
Guoqing L., Zhaojun H., Lili M., Xiaoran Q., Changkun C., Yinchang W. (2001). Natural oviposition-deterring chemicals in female cotton bollworm, Helicoverpa armigera (Hubner). J. Insect Phys. 47, 951–956.
Haile F., Nowatzki T., Storer N. (2021). Overview of pest status, potential risk, and management considerations of Helicoverpa armigera (Lepitoptera: Noctuidae) for U.S. Soybean production. J. Integrated Pest Manage. 12, 1. doi: 10.1093/jipm/pmaa030
Hansen E. M., Reeser P. W., Sutton W. (2012). Phytophthora beyond agriculture. Annu. Rev. Phytopathol. 50, 359–378. doi: 10.1146/annurev-phyto-081211-172946
Hantula J., Lilja A., Nuorteva H., Parikka P., Werres S. (2000). Pathogenicity, morphology and genetic variation of *Phytophthora cactorum* from strawberry, apple, rhododendron, and silver birch. Mycol. Res. 104, 1062–1068. doi: 10.1017/S0953756200002999
Hardham A. R., Blackman L. M. (2018). Phytophthora cinnamomi. Mol. Plant Pathol. 19, 260–285. doi: 10.1111/mpp.2018.19.issue-2
Hardy G., Barrett S., Shearer B. L. (2001). The future of phosphite as a fungicide to control the soilborne plant pathogen Phytophthora cinnamomi in natural ecosystems. Australas. Plant Pathol. 30, 133–139. doi: 10.1071/AP01012
Harris D. C. (1991). The Phytophthora diseases of apple. J. Hortic. Sci. 66, 513–544. doi: 10.1080/00221589.1991.11516181
Harris A. R., Webber J. (2016). Sporulation potential, symptom expression and detection of Phytophthora ramorum on larch needles and other foliar hosts. Plant Pathol. 65, 1441–1451. doi: 10.1111/ppa.2016.65.issue-9
Haye T., Abdallah S., Gariepy T., Wyniger. D. (2014). Phenology, life table analysis and temperature requirements of the invasive brown marmorated stink bug, Halyomorpha halys, in Europe. J. Pest Sci. 87, 407–418. doi: 10.1007/s10340-014-0560-z
Heil M. (2014). Herbivore-induced plant volatiles: targets, perception and unanswered questions. New Phytol. 204, 297–306. doi: 10.1111/nph.2014.204.issue-2
Henderson D., Williams C. J., Miller J. S. (2007). Forecasting late blight in potato crops of Southern Idaho using logistic regression analysis. Plant Dis. 91, 951–956. doi: 10.1094/PDIS-91-8-0951
Hirata A., Nakamura K., Nakao K., Kominami Y., Tanaka N., Ohashi H. (2017). Potential distribution of pine wilt disease under future climate change scenarios. PloS One 12, e0182837. doi: 10.1371/journal.pone.0182837
Ho S. S. H., Wang L., Chow J. C., Watson J. G., Xue Y., Huang Y., et al. (2018). Optimization and evaluation of multi-bed adsorbent tube method in collection of volatile organic compounds. Atmospheric Res. 202, 187–195. doi: 10.1016/j.atmosres.2017.11.026
Hoballah M. E. F., Tamò C., Turlings T. C. (2002). Differential attractiveness of induced odors emitted by eight maize varieties for the parasitoid Cotesia marginiventris: is quality or quantity important? J. Chem. Ecol. 28, 951–968. doi: 10.1023/A:1015253600083
Hruska A. J., Gould F. (1997). Fall armyworm (Lepidoptera: Noctuidae) and Diatraea lineolate (Lepidoptera: Pyralidae): impact of larval population level and temporal occurrence on maize yield in Nicaragua. J. Economic Entomology 90, 611–622. doi: 10.1093/jee/90.2.611
Huai W. X., Tian G., Hansen E. M., Zhao W.-X., Goheen E. M., Grünwald N. J., et al. (2013). Identification of Phytophthora species baited and isolated from forest soil and streams in northwestern Yunnan province, China. For. Pathol. 43, 87–103. doi: 10.1111/efp.2013.43.issue-2
Huang X.-Z., Chen J.-Y., Xiao H.-J., Xiao Y.-T., Wu J., Wu J.-X., et al. (2015). Dynamic transscriptome analysis and volatile profiling of Gossypium hirsutum in response to the cotton bollworm Helicovera armigera. Sci. Rep. 5, 11867. doi: 10.1038/srep11867
Hwang H. S., Han J. Y., Choi Y. E. (2021). Enhanced emission of Monoterpene 3-Carene in Pinus densiflora infected by pine wood nematode and characterization of 3-Carene Synthase. Forests 12, 514. doi: 10.3390/f12050514
Inácio M. L., Nóbrega F., Vieira P., Bonifácio L., Naves P., Sousa E., et al. (2015). First detection of Bursaphelenchus xylophilus associated with Pinus nigra in Portugal and in Europe. For. Pathol. 45, 235–238. doi: 10.1111/efp.2015.45.issue-3
Jeleń H. H., Krawczyk J., Larsen T. O., Jarosz A., Gołębniak B. (2005). Main compounds responsible for off-odour of strawberries infected by Phytophthora cactorum. Lett. Appl. Microbiol. 40, 255–259. doi: 10.1111/j.1472-765X.2005.01668.x
Jiang N. J., Mo B. T., Guo H., Yang J., Tang R., Wang C. Z. (2022). Revisiting the sex pheromone of the fall armyworm Spodoptera frugiperda, a new invasive pest in South China. Insect Sci. 29, 865–878. doi: 10.1111/1744-7917.12956
Jin L., Zhang H., Lu Y., Yang Y., Wu K., Tabashnik B. E., et al. (2015). Large-scale test of natural refuge strategy for delaying insect resistance to transgenic bt crops. Nat. Biotechnol. 33, 169–174. doi: 10.1038/nbt.3100
Johnson S. J. (1987). Migration and the life history strategy of the fall armyworm, Spodoptera frugiperda in the Western Hemisphere. Insect Sci. Appl. 8, 543–549. doi: 10.1017/S1742758400022591
Jones J. T., Moens M., Mota M., Li H., Kikuchi T. (2008). Bursaphelenchus xylophilus: opportunities in comparative genomics and molecular host–parasite interactions. Mol. Plant Pathol. 9, 357–368. doi: 10.1111/j.1364-3703.2007.00461.x
Jones C. M., Papanicolaou A., Mironidis G. K., Vontas J., Yang Y., Lim K., et al. (2015). Genomewide transcriptional signatures of migratory flight activity in a globally invasive insect pest. Mol. Ecol. 24, 4901–4911. doi: 10.1111/mec.2015.24.issue-19
Jones M. J., Parry H., Tay W. T., Reynolds D. R., Chapman J. W. (2019). Movement ecology of pest Helicoverpa: Implications for ongoing spread. Annu. Rev. Entomology 64, 277–295. doi: 10.1146/annurev-ento-011118-111959
Jung T. (2009). Beech decline in Central Europe driven by the interaction between Phytophthora infections and climatic extremes. For. Pathol. 39, 73–94. doi: 10.1111/j.1439-0329.2008.00566.x
Jung T., Blaschke H., Oßwald W. (2000). Involvement of soilborne Phytophthora species in Central European oak decline and the effect of site factors on the disease. Plant Pathol. 49, 706–718. doi: 10.1046/j.1365-3059.2000.00521.x
Jung T., Burgess T. I. (2009). Re-evaluation of Phytophthora citricola isolates from multiple woody hosts in Europe and North America reveals a new species. Phytophthora plurivora nov. Persoonia sp 22, 95–110. doi: 10.3767/003158509X442612
Jung T., Chang T.-T., Bakonyi J., Seress D., Pérez-Sierra A., Yang X., et al. (2017a). Diversity of Phytophthora species in natural ecosystems of Taiwan and association with disease symptoms. Plant Pathol. 66, 194–211. doi: 10.1111/ppa.2017.66.issue-2
Jung T., Durán A., von Stowasser E. S., Schena L., Mosca S., Fajardo S., et al. (2018b). Diversity of Phytophthora species in Valdivian rainforests and association with severe dieback symptoms. For. Pathol. 48, e12443. doi: 10.1111/efp.2018.48.issue-5
Jung T., Horta Jung M., Scanu B., Seress D., Kovács D. M., Maia C., et al. (2017b). Six new Phytophthora species from ITS Clade 7a including two sexually functional heterothallic hybrid species detected in natural ecosystems in Taiwan. Persoonia 38, 100–135. doi: 10.3767/003158517X693615
Jung T., Horta Jung M., Webber J. F., Kageyama K., Hieno A., Masuya H., et al. (2021). The destructive tree pathogen Phytophthora ramorum originates from the Laurosilva forests of East Asia. J. Fungi 7, 226. doi: 10.3390/jof7030226
Jung T., La Spada F., Pane A., Aloi F., Evoli M., Horta Jung M., et al. (2019). Diversity and distribution of Phytophthora species in protected natural areas in Sicily. Forests 10, 259. doi: 10.3390/f10030259
Jung T., Milenković I., Balci Y., Janoušek J., Kudláček T., Nagy Z.Á., et al. (2024). Worldwide forest surveys reveal forty-three new species in Phytophthora major Clade 2 with fundamental implications for the evolution and biogeography of the genus and global plant biosecurity. Stud. Mycology 107, 251–388. doi: 10.3114/sim.2024.107.04
Jung T., Milenković I., Corcobado T., Májek T., Janoušek J., Kudláček T., et al. (2022). Extensive morphological and behavioural diversity among fourteen new and seven described species in Phytophthora Clade 10 and its evolutionary implications. Persoonia 49, 1–57. doi: 10.3767/persoonia.2022.49.01
Jung T., Orlikowski L., Henricot B., Abad-Campos P., Aday A. G., Aguín Casal O., et al. (2016). Widespread Phytophthora infestations in European nurseries put forest, semi-natural and horticultural ecosystems at high risk of Phytophthora diseases. For. Pathol. 46, 134–163. doi: 10.1111/efp.2016.46.issue-2
Jung T., Pérez-Sierra A., Durán A., Horta Jung M., Balci Y., Scanu B. (2018a). Canker and decline diseases caused by soil- and airborne Phytophthora species in forests and woodlands. Persoonia 40, 182–220. doi: 10.3767/persoonia.2018.40.08
Jung T., Scanu B., Brasier C. M., Webber J., Milenković I., Corcobado T., et al. (2020). A survey in natural forest ecosystems of Vietnam reveals high diversity of both new and described Phytophthora taxa including P. ramorum. Forests 11, 93. doi: 10.3390/f11010093
Kamata N. (2008). “Integrated pest management of pine wilt disease in Japan: Tactics and strategies,” in Pine wilt disease (Springer Japan, Tokyo), 304–322.
Karban R., Yang L. H., Edwards K. F. (2014). Volatile communication between plants that affects herbivory: a meta-analysis. Ecol. Lett. 17, 44–52. doi: 10.1111/ele.2013.17.issue-1
Karimi A., Gross J. (2024). Development and validation of an innovative headspace collection technique: volatile organic compound patterns emitted by different developmental stages of Halyomorpha halys. Front. Horticulture 3, 1380008. doi: 10.3389/fhort.2024.1380008
Kenis M., Benelli G., Biondi A., Calatayud P. A., Day R., Desneux N., et al. (2022). Invasiveness, biology, ecology, and management of the fall armyworm, Spodoptera frugiperda. Entomologia Generalis 43, 187–1241. doi: 10.1127/entomologia/2022/1659
Khrimian A., Zhang A., Weber D. C., Ho H.-Y., Aldrich J. R., Vermillion K. E., et al. (2014). Discovery of the aggregation pheromone of the brown marmorated stink bug (Halyomorpha halys) through the creation of stereoisomeric libraries of 1-bisabolen-3-ols. J. Natural Products 77, 1708–1717. doi: 10.1021/np5003753
Kim J., Lee S. M., Park C. G. (2016). Bursaphelenchus xylophilus is killed by homologues of 2-(1-undecyloxy)-1-ethanol. Sci. Rep. 6, 1–5. doi: 10.1038/srep29300
Kitamura C., Wakamura S., Takahashi S. (1984). Identification and functions of ventral glands secretion of some Heteroptera. Appl. Entomology Zoology 19, 33–41. doi: 10.1303/aez.19.33
Kment P., Vilimova J. (2010). Thoracic scent efferent system of Pentatomoidea (Hemiptera: Heteroptera): a review of terminology. Zootaxa 2706, 1–77. doi: 10.11646/zootaxa.2706.1.1
Kuroda K. (2008). “Physiological incidences related to symptom development and wilting mechanism,” in Pine wilt disease (Springer, Japan, Tokyo), 204–222.
Lamsal S., Sibi S., Yadav S. (2020). Fall armyworm in South Asia: Threats and management. Asian J. Adv. Agric. Res. 13, 21–34. doi: 10.9734/ajaar/2020/v13i330106
Laothawornkitkul J., Jansen R. M. C., Smid H. M., Bouwmeester H. J., Muller J., Van Bruggen A. H. C. (2010). Volatile organic compounds as a diagnostic marker of late blight infected potato plants: A pilot study. Crop Prot. 29, 872–878. doi: 10.1016/j.cropro.2010.03.003
Lee D. H., Short B. D., Joseph S. V., Bergh J. C., Leskey T. C. (2013). Review of the biology, ecology, and management of Halyomorpha halys (Hemiptera: Pentatomidae) in China, Japan, and the Republic of Korea. Environ. Entomology 42, 627–641. doi: 10.1603/EN13006
Leskey T. C., Nielsen A. L. (2018). Impact of the invasive brown marmorated stink bug in north america and europe: history, biology, ecology, and Management. Annu. Rev. Entomology 63, 599–618. doi: 10.1146/annurev-ento-020117-043226
Leskey T. C., Short B. D., Butler B. R., Wright S. E. (2012). Impact of the invasive brown marmorated stink bug, Halyomorpha halys (Stål), in mid-Atlantic tree fruit orchards in the United States: case studies of commercial management. Psyche 2012, 535062. doi: 10.1155/2012/535062
Li Z., Paul R., Ba Tis T., Saville A. C., Hansel J. C., Yu T., et al. (2019). Non-invasive plant disease diagnostics enabled by smartphone-based fingerprinting of leaf volatiles. Nat. Plants 5, 856–866. doi: 10.1038/s41477-019-0476-y
Lima E. R., McNeil J. N. (2009). Female sex pheromones in the host races and hybrids of the fall armyworm, Spodoptera frugiperda (Lepidoptera: Noctuidae). Chemoecology 19, 29–36. doi: 10.1007/s00049-009-0005-y
Liu T., Wang J., Hu X., Feng J. (2020). Land-use change drives present and future distributions of Fall armyworm, Spodoptera frugiperda (J.E. Smith) (Lepidoptera: Noctuidae). Sci. Total Environ. 706, 135872. doi: 10.1016/j.scitotenv.2019.135872
Liu M., Yu H., Li G. (2008). Oviposition deterrents from eggs of the cotton bollworm, Helicoverpa armigera (Lepidoptera: Noctuidae): chemical identification and analysis by electroantennogram. J. Insect Phys. 54, 656–662. doi: 10.1016/j.jinsphys.2008.01.002
Lockwood J. A., Story R. N. (1985). Bifunctional pheromone in the first instar of the southern green stink bug, nezara viridula (L.) (Hemiptera: Pentatornidae): its characterization and interaction with other stimuli. Ann. Entomological Soc. America 78 (4), 474–479.
Lockwood J. A., Story R. N. (1987). Defensive secretion of the southern green stink bug (Hemiptera: Pentatomidae) as an alarm pheromone. Ann. Entomological Soc. America 80 (5), 686–691.
Loreto F., Schnitzler J. P. (2010). Abiotic stresses and induced BVOCs. Trends Plant Sci. 15, 154–166. doi: 10.1016/j.tplants.2009.12.006
Loulier J., Lefort F., Stocki M., Asztemborska M., Szmigielski R., Siwek K., et al. (2020). Detection of fungi and oomycetes by volatiles using E-Nose and SPME-GC/MS platforms. Molecules 25, 5749. doi: 10.3390/molecules25235749
Lu X., Sun D., Rookes J. E., Kong L., Zhang X., Cahill D. M. (2019). Nanoapplication of a resistance inducer to reduce Phytophthora disease in pineapple (Ananas comosus L.). Front. Plant Sci. 10, 1238. doi: 10.3389/fpls.2019.01238
MacDougall S., Bayansal F., Ahmadi A. (2022). Emerging methods of monitoring volatile organic compounds for detection of plant pests and disease. Biosensors 12, 239. doi: 10.3390/bios12040239
Mamiya Y. (1983). Pathology of the pine wilt disease caused by Bursaphelenchus xylophilus. Annu. Rev. Phytopathol. 21, 201–220. doi: 10.1146/annurev.py.21.090183.001221
Martínez M. T., Cuenca B., Mosteiro F., Piñeiro P., Pérez F., Solla A., et al. (2023). Screening of cork oak for resistance to Phytophthora cinnamomi and micropropagation of tolerant seedlings. Horticulturae 9, 692. doi: 10.3390/horticulturae9060692
McCartney M. M., Roubtsova T. V., Yamaguchi M. S., Kasuga T., Ebeler S. E., Davis C. E., et al. (2018). Effects of Phytophthora ramorum on volatile organic compound emissions of Rhododendron using gas chromatography–mass spectrometry. Analytical Bioanalytical Chem. 410, 1475–1487. doi: 10.1007/s00216-017-0789-5
McConnell M., Balci Y. (2014). Phytophthora cinnamomi as a contributor to white oak decline in mid-Atlantic United States forests. Plant Dis. 98, 319–327. doi: 10.1094/PDIS-06-13-0649-RE
Meentemeyer R. K., Rizzo D., Mark W., Lotz E. (2004). Mapping the risk of establishment and spread of sudden oak death in California. For. Ecol. Manage. 200, 195–214. doi: 10.1016/j.foreco.2004.06.021
Menéndez-Gutiérrez M., Alonso M., Toval G., Díaz R. (2018). Testing of selected Pinus pinaster half-sib families for tolerance to pinewood nematode (Bursaphelenchus Xylophilus). Forestry 91, 38–48. doi: 10.1093/forestry/cpx030
Mircetich S. M., Matheron M. E. (1976). Phytophthora root and crown rot of cherry trees. Phytopathology 66, 549–558. doi: 10.1094/Phyto-66-549
Moore L. C., Tirello P., Scaccini D., Toews M. D., Duso C., Pozzebon A. (2019). Characterizing damage potential of the brown marmorated stink bug (Hemiptera: Pentatomidae) in cherry orchards. Entomologia Generalis 39, 271–283. doi: 10.1127/entomologia/2019/0799
Mota M. M., Braasch H., Bravo M. A., Penas A. C., Burgermeister W., Metge K., et al. (1999). First report of Bursaphelenchus xylophilus in Portugal and in Europe. Nematology 1, 727–734. doi: 10.1163/156854199508757
Mullett M. S., Van Poucke K., Haegeman A., Focquet F., Cauldron N. C., Knaus B. J., et al. (2023). Phylogeography and population structure of the global, wide host-range hybrid pathogen Phytophthora × cambivora. IMA Fungus 14, 4. doi: 10.1186/s43008-023-00109-6
Nakamura K. (2008). “Attraction trap for monitoring Monochamus alternatus adults - its usefulness and limitations,” in Pine wilt disease: A worldwide threat to forest ecosystems (Springer Netherlands, Dordrecht), 369–378.
Nesbitt B., Beevor P., Hall D., Lester R. (1980). (Z)-9-Hexadecenal: a minor component of the female sex pheromone of Heliothis armigera (Hübner) (Lepidoptera, Noctuidae). Entomologia Experimentalis Applicata 27, 306–308. doi: 10.1111/j.1570-7458.1980.tb02979.x
Nixon L. J., Ludwick D. C., Leskey T. C. (2021). Horizontal and vertical dispersal capacity and effects of fluorescent marking on Lycorma delicatula nymphs and adults. Entomologia Experimentalis et Applicata 169 (2), 219–226. doi: 10.1093/jee/toad038
Nixon L. J., Morrison W. R., Rice K. B., Brockerhoff E. G., Leskey T. C., Guzman F., et al. (2018). Identification of volatiles released by diapausing brown marmorated stink bug, Halyomorpha halys (Hemiptera: Pentatomidae). PloS One 13, e0191223. doi: 10.1371/journal.pone.0191223
Nixon L. J., Tabb A., Morrison W. R., Rice K. B., Brockerhoff E. G., Leskey T. C., et al. (2019). Volatile release, mobility, and mortality of diapausing halyomorpha halys during simulated shipping movements and temperature changes. J. Pest Sci. 92, 633–641.
Noge K., Prudic K. L., Becerra J. X. (2012). Defensive roles of (E)-2-Alkenals and related compounds in heteroptera. J. Chem. Ecol. 38, 1050–1056. doi: 10.1007/s10886-012-0166-y
Nordström I., Sherwood P., Bohman B., Woodward S., Peterson D. L., Niño-Sánchez J., et al. (2022). Utilizing volatile organic compounds for early detection of Fusarium circinatum. Sci. Rep. 12, 21661. doi: 10.1038/s41598-022-26078-1
Nose M., Shiraishi S. (2008). “Breeding for resistance to pine wilt disease,” in Pine wilt disease. Eds. B.G. Z., Futai K., Sutherland J. R., Takeuchi Y. (Springer, Tokyo), 334–350.
O’Hanlon R., Choiseul J., Brennan J. M., Grogan H. (2018). Assessment of the eradication treatments applied to Phytophthora ramorum in Irish Larix kaempferi forests. For. Pathol. 48, e12389. doi: 10.1111/efp.12389
O’Hanlon R., Choiseul J., Corrigan M., Catarame T., Destefanis M. (2016). Diversity and detections of Phytophthora species from trade and non-trade environments in Ireland. EPPO Bull. 46, 594–602. doi: 10.1111/epp.12331
Orlikowski L. B., Ptaszek M., Rodziewicz A., Nechwatal J., Thinggaard K., Jung T. (2011). Phytophthora root and collar rot of mature Fraxinus excelsior in forest stands in Poland and Denmark. For. Pathol. 41, 510–519. doi: 10.1111/j.1439-0329.2011.00714.x
Paré P. W., Tumlinson J. H. (1999). Plant volatiles as a defense against insect herbivores. Plant Physiol. 121, 325–331. doi: 10.1104/pp.121.2.325
Peñaflor M. F. G. V., Erb M., Robert C. A. M., et al. (2011). Oviposition by a moth suppresses constitutive and herbivore-induced plant volatiles in maize. Planta 234, 207–215. doi: 10.1007/s00425-011-1409-9
Pérez-Sierra A., Jung T. (2013). “Phytophthora in woody ornamental nurseries,” in Phytophthora: A global perspective. Ed. Lamour K. (CABI, Wallingford, UK), 166–177.
Peterson E. K., Hansen E. M., Kanaskie A. (2015). Temporal epidemiology of sudden oak death in oregon. Phytopathology 105, 937–946. doi: 10.1094/PHYTO-12-14-0348-FI
Peterson H. M., Ray S., Ali J. G., Krawczyk G. (2022). Feeding and oviposition by the brown marmorated stink bug, Halyomorpha halys (Stål) induce direct and systemic changes in volatile compound emissions from potted peach and tree of heaven. Arthropod-Plant Interact. 16, 227–247. doi: 10.1007/s11829-022-09893-1
Piccardi P., Capizzi A., Cassani G., Spinelli P., Arsura E., Massardo P. (1977). A sex pheromone component of the old world bollworm Heliothis armigera. J. Insect Physiol. 23, 1443–1445. doi: 10.1016/0022-1910(77)90170-6
Pinto-Zevallos D. M., Strapasson P., Zarbin P. H. G. (2016). Herbivore-induced volatile organic compounds emitted by maize: Electrophysiological responses in Spodoptera frugiperda females. Phytochem. Lett. 16, 70–74. doi: 10.1016/j.phytol.2016.03.005
Pitre H. N., Hogg D. B. (1983). Development of the fall armyworm on cotton, soybean and corn. J. Georgia Entomological Soc. 18, 187–194.
Qiu R., Qu D., Hardy G. E. S. J., Trengove R., Agarwal M., Ren Y. (2014a). Optimization of headspace solid-phase microextraction conditions for the identification of Phytophthora cinnamomi rands. Plant Dis. 98, 1088–1098. doi: 10.1094/PDIS-12-13-1258-RE
Qiu R., Qu D., Trengove R., Agarwal M., Hardy G. E. S. J., Ren Y. (2014b). Headspace solid-phase microextraction and gas chromatography-mass spectrometry for analysis of VOCs produced by Phytophthora cinnamomi. Plant Dis. 98, 1099–1105. doi: 10.1094/PDIS-01-14-0049-RE
Reeser P. W., Sutton W., Hansen E. M., Remigi P., Adams G. C. (2011). Phytophthora species in forest streams in Oregon and Alaska. Mycologia 103, 22–35. doi: 10.3852/10-013
Riaz S., Johnson J. B., Ahmad M., Fitt G. P., Naiker M. (2021). A review in biological interactions and management of the cotton bollworm, Helicoverpa armigera (Lepidoptera: Noctuidae). J. Appl. Entomology 145, 467–498. doi: 10.1111/jen.v145.6
Rice K. B., Bergh C. J., Bergmann E. J., Biddinger D. J., Dieckhoff C., Dively G., et al. (2014). Biology, ecology, and management of brown marmorated stink bug (Hemiptera: Pentatomidae). J. Integrated Pest Manage. 5, 1–13. doi: 10.1603/IPM14002
Rizzo D. M., Garbelotto M., Davidson J. M., Slaughter G. W., Koike S. T. (2002). Phytophthora ramorum as the cause of extensive mortality of Quercus spp. and Lithocarpus densiflorus in California. Plant Dis. 86, 205–214. doi: 10.1094/PDIS.2002.86.3.205
Rizzo D. M., Garbelotto M., Hansen E. M. (2005). Phytophthora ramorum: integrative research and management of an emerging pathogen in California and Oregon forests. Annu. Rev. Phytopathol. 43, 309–335. doi: 10.1146/annurev.phyto.42.040803.140418
Robert C. A., Erb M., Hiltpold I., Hibbard B. E., Gaillard M. D., Bilat J., et al. (2013). Genetically engineered maize plants reveal distinct costs and benefits of constitutive volatile emissions in the field. Plant Biotechnol. J. 11 (5), 628–639. doi: 10.1111/pbi.12053
Robin C., Morel O., Vettraino A. M., Perlerou C., Diamandis S., Vannini A. (2006). Genetic variation in susceptibility to Phytophthora cambivora in European chestnut (Castanea sativa). For. Ecol. Manage. 226, 199–207. doi: 10.1016/j.foreco.2006.01.035
Rodrigues J. M. (2008). “National eradication programme for the pinewood nematode,” in Pine wilt disease: A worldwide threat to forest ecosystems. Eds. Mota M. M., Vieira P. (Springer Netherlands, Dordrecht), 5–14.
Rwomushana I., Bateman M., Beale T., Beseh P., Cameron K., Chiluba M., et al. (2018). Fall armyworm: impacts and implications for Africa October 2018. Evidence note Update Outlooks Pest Manage. 28, 18–19. doi: 10.1564/v28_oct_02
Sabbatini Peverieri G., Talamas E., Bon M. C., Marianelli L., Bernardinelli I., Malossini G., et al. (2018). Two Asian egg parasitoids of Halyomorpha halys (Stål) (Hemiptera, Pentatomidae) emerge in northern Italy: Trissolcus mitsukurii (Ashmead) and Trissolcus japonicus (Ashmead) (Hymenoptera, Scelionidae). J. Hymenoptera Res. 67, 37–53. doi: 10.3897/jhr.67.30883
Sanfuentes E., González M., Castillo M., Rubilar R., Besoain X., Sanhueza C., et al. (2022). Detection of Phytophthora cinnamomi on declining Araucaria araucana forests. For. Pathol. 52, e12765. doi: 10.1111/efp.12765
Santos C., Nelson C. D., Zhebentyayeva T., MaChado H., Gomes-Laranjo J., Costa R. L. (2017). First interspecific genetic linkage map for Castanea sativa x Castanea crenata revealed QTLs for resistance to Phytophthora cinnamomi. PloS One 12, e0184381. doi: 10.1371/journal.pone.0184381
Santos M. D. ,. L., Resend M. L. V., Alves G. S. C., Huguet-Tapia J. C., Resende M. F. R. Jr., Brawner J. T. (2022). Genome-Wide Identification, characterization, and comparative analysis of NLR resistance genes in. Coffea Front. Plant Sci. spp 13, 868581. doi: 10.3389/fpls.2022.868581
Scaccini D., Falagiarda M., Tortorici F., Martinez-Sañudo I., Tirello P., Reyes-Domínguez Y., et al. (2020). An insight into the role of Trissolcus mitsukurii as biological control agent of Halyomorpha halys in Northeastern Italy. Insects 11, 1–16. doi: 10.3390/insects11050306
Schuman M. C. (2023). Where, when, and why do plant volatiles mediate ecological signaling? The answer is blowing in the wind. Annual Review of. Plant Biol. 74, 609–633. doi: 10.1146/annurev-arplant-040121-114908
Sekul A. A., Sparks A. N. (1967). Sex pheromone of the fall armyworm moth: Isolation, identification, and synthesis. J. Economic Entomology 60, 1270–1272. doi: 10.1093/jee/60.5.1270
Shakya S. K., Grünwald N. J., Fieland V. J., Knaus B. J., Weiland J. E., Maia C., et al. (2021). Phylogeography of the wide-host range panglobal plant pathogen Phytophthora cinnamomi. Mol. Ecol. 30, 5164–5178. doi: 10.1111/mec.v30.20
Sharanabasappa D., Kalleshwara Swamy C. M., Asokan R., Swamy H. M. M., Maruthi M. S., Pavithra H. B., et al. (2018). First report of the Fall armyworm, Spodoptera frugiperda (JE Smith) (Lepidoptera: Noctuidae), an alien invasive pest on maize in India. Pest Manage. Hortic. Ecosyst. 24, 23–29.
Shearer B. L., Crane C. E., Cochrane A. (2004). Quantification of the susceptibility of the native flora of the South-West Botanical Province, Western Australia, to Phytophthora cinnamomi. Aust. J. Bot. 52, 435–443. doi: 10.1071/BT03131
Shearer B. L., Tippett J. T. (1989). Jarrah Dieback: The dynamics and management of Phytophthora cinnamomi in the Jarrah Eucalyptus marginata forest of South-Western Australia Vol. 3 (Como, Western Australia: Department of Conservation and Land Management, Research Bulletin), 76.
Sherwood P., Nordström I., Woodward S., Bohman B., Cleary M. (2024). Detecting pathogenic Phytophthora species using volatile organic compounds. Molecules 29, 1749. doi: 10.3390/molecules29081749
Shimazu M. (2008). “Biological control of the Japanese pine sawyer beetle, monochamus alternatus,” in Pine wilt disease. Eds. Zhao B. G., Futai K., Sutherland J. R., Takeuchi Y. (Springer, Tokyo), 351–370.
Shivaramu S., Jayanthi P. D. K., Kempraj V., Anjinappa R., Nandagopal B., Chakravarty A. K. (2017). What signals do herbivore-induced plant volatiles provide conspecific herbivores? Arthropod-Plant Interact. 11, 815–823. doi: 10.1007/s11829-017-9536-2
Sniezko R. A., Johnson J. S., Reeser P., Kegley A., Hansen E. M., Sutton W., et al. (2019). Genetic resistance to Phytophthora lateralis in Port-Orford cedar (Chamaecyparis lawsoniana) – Basic building blocks for a resistance program. Plants People Planet 2, 69–83. doi: 10.1002/ppp3.10081
Solla A., Moreno G., Malewski T., Jung T., Klisz M., Tkaczyk M., et al. (2021). Phosphite spray for the control of oak decline induced by Phytophthora in Europe. For. Ecol. Manage. 485, 118938. doi: 10.1016/j.foreco.2021.118938
Solomon D., Dutcher D., Raymond T. (2013). Characterization of Halyomorpha halys (brown marmorated stink bug) biogenic volatile organic compound emissions and their role in secondary organic aerosol formation. J. Air Waste Manage. Assoc. 63, 1264–1269. doi: 10.1080/10962247.2013.819047
Sparks A. N. (1979). A review of the biology of the fall armyworm. Florida Entomologist 62, 82–87. doi: 10.2307/3494083
Stahl J., Tortorici F., Pontini M., Bon M. C., Hoelmer K., Marazzi C., et al. (2019). First discovery of adventive populations of Trissolcus japonicus in Europe. J. Pest Sci. 92, 371–379. doi: 10.1007/s10340-018-1061-2
Stukely M. J. C., Crane C. E., McComb J. A., Bennett I. J. (2007). Field survival and growth of clonal, micropropagated Eucalyptus marginata selected for resistance to Phytophthora cinnamomi. For. Ecol. Manage. 238, 330–334. doi: 10.1016/j.foreco.2006.10.028
Sturaro A., Parvoli G., Doretti L. (1994). A simple and fast sampling method for the characterization of volatile compounds released by nezara viridula. Chromatographia 39 (1), 103–106.
Sutherland J. R. (2008). “A brief overview of the pine wood nematode and pine wilt disease in Canada and the United States,” in Pine wilt disease. Eds. Zhao B. G., Futai K., Sutherland J. R., Takeuchi Y. (Springer, Tokyo), 13–17.
Tabashnik B. E., Carrière Y. (2017). Surge in insect resistance to transgenic crops and prospects for sustainability. Nat. Biotechnol. 31, 926–935. doi: 10.1038/nbt.3974
Takeuchi Y., Kanzaki N., Futai K. (2006). Volatile compounds in pine stands suffering from pine wilt disease: qualitative and quantitative evaluation. Nematology 8, 869–879. doi: 10.1163/156854106779799295
Talamas E. J., Herlihy M. V., Dieckhoff C., Hoelmer K. A., Buffington M., Bon M. C., et al. (2019). Trapping for early detection of the brown marmorated stink bug, Halyomorpha halys, in New Zealand. New Z. Plant Prot. 72, 36–43. doi: 10.30843/nzpp.2019.72.316
Tambo J. A., Day R. K., Lamontagne-Godwin J., Silvestri S., Beseh P. K., Oppong-Mensah B., et al. (2020). Tackling fall armyworm (Spodoptera frugiperda) outbreak in Africa: an analysis of farmers’ control actions. Int. J. Pest Manage. 66, 298–310. doi: 10.1080/09670874.2019.1646942
Tay W. T., Meagher R. L. Jr., Czepak C., Groot A. T. (2023). Spodoptera frugiperda: ecology, evolution, and management options of an invasive species. Annu. Rev. Entomology 68, 299–317. doi: 10.1146/annurev-ento-120220-102548
Tay W. T., Soria M. F., Walsh T., Thomazoni D., Silvie P., Behere G. T., et al. (2013). A brave new world for an old world pest Helicoverpa armigera (Lepitoptera: Noctuidae) in Brazil. PloS One 8, e80134. doi: 10.1371/journal.pone.0080134
Tholl D., Hossain O., Weinhold A., Röse U. S., Wei Q. (2021). Trends and applications in plant volatile sampling and analysis. Plant J. 106, 314–325. doi: 10.1111/tpj.v106.2
Todd E. L., Poole R. W. (1980). Keys and illustrations for the armyworm moths of the noctuid genus Spodoptera Guenée from the Western Hemisphere. Ann. Entomological Soc. America 73, 722–738. doi: 10.1093/aesa/73.6.722
Tognon R., Aldrich J. R., Buffington M. L., Talamas E. J., Sant’Ana J., Zalom F. G. (2017). Halyomorpha halys (Heteroptera: Pentatomidae) egg surface chemicals inhibit North American Telenomus and Trissolcus (Hymenoptera: Scelionidae) parasitism. Biol. Control 114, 39–44. doi: 10.1016/j.biocontrol.2017.07.014
Tumlinson J. H., Mitchell E. R., Teal P. E. A., Heath R. R., Mengelkoch L. J. (1986). Sex pheromone of fall armyworm, Spodoptera frugiperda (J. E. Smith), identification of components critical to attraction in the field. J. Chem. Ecol. 12, 1909–1926. doi: 10.1007/BF01041855
Tumlinson J. H., Mitchell E. R., Yu H. S. (1990). Analysis and field evaluation of volatile blend emitted by calling virgin females of beet armyworm moth,Spodoptera exigua (Hübner). J. Chem. Ecol. 16, 3411–3423. doi: 10.1007/BF00982107
Turlings T. C. J., Alborn H. T., Loughrin J. H., Tumlinson J. H. (2000). Volicitin, an elicitor of maize volatiles in the oral secretion of Spodoptera exigua: its isolation and bio-activity. J. Chem. Ecol. 26, 189–202. doi: 10.1023/A:1005449730052
Turlings T. C., Bernasconi M., Bertossa R., Bigler F., Caloz G., Dorn S. (1998a). The induction of volatile emissions in maize by three herbivore species with different feeding habits: possible consequences for their natural enemies. Biol. Control. 11 (2), 122–129. doi: 10.1006/bcon.1997.0591
Turlings T. C., Degen T. (2022). The role of herbivore-induced plant volatiles in trophic interactions: The Swiss Connection. Chimia 76, 900–900. doi: 10.2533/chimia.2022.900
Turlings T. C., Erb M. (2018). Tritrophic interactions mediated by herbivore-induced plant volatiles: mechanisms, ecological relevance, and application potential. Annu. Rev. Entomology 63, 433–452. doi: 10.1146/annurev-ento-020117-043507
Turlings T., Lengwiler U., Bernasconi M., et al. (1998b). Timing of induced volatile emissions in maize seedlings. Planta 207, 146–152. doi: 10.1007/s004250050466
Turlings T. C. J., McCall P. J., Alborn H. T., Tumlinson J. H. (1993). An elicitor in caterpillar oral secretions that induces corn seedlings to emit chemical signals attractive to parasitic wasps. J. Chem. Ecol. 19, 411–425. doi: 10.1007/BF00994314
Turlings T. C., Tumlinson J. H. (1992). Systemic release of chemical signals by herbivore-injured corn. Proc. Natl. Acad. Sci. 89, 8399–8402. doi: 10.1073/pnas.89.17.8399
Vandervoet T. F., Bellamy D. E., Anderson D., MacLellan R. (2019). Trapping for early detection of the brown marmorated stink bug, halyomorpha halys, in new zealand. New Z. Plant Prot. 72, 36–43. doi: 10.30843/nzpp.2019.72.316
Van Poucke K., Franceschini S., Webber J. F., Vercauteren A., Turner J. A., McCracken A. R., et al. (2012). Discovery of a fourth evolutionary lineage of Phytophthora ramorum: EU2. Fungal Biol. 116, 1178–1191. doi: 10.1016/j.funbio.2012.09.003
Vettraino A. M., Brasier C. M., Brown A. V., Vannini A. (2011). Phytophthora himalsilva sp. nov. an unusually phenotypically variable species from a remote forest in Nepal. Fungal Biol. 115, 275–287. doi: 10.1016/j.funbio.2010.12.013
Vettraino A. M., Morel O., Perlerou C., Robin C., Diamandis S., Vannini A. (2005). Occurrence and distribution of Phytophthora species associated with Ink Disease of chestnut in Europe. Eur. J. Plant Pathol. 111, 169–180. doi: 10.1007/s10658-004-1882-0
Vicente C., Espada M., Vieira P., Mota M. (2012). Pine wilt disease: a threat to European forestry. Eur. J. Plant Pathol. 133, 89–99. doi: 10.1007/s10658-011-9924-x
Vieira N. F., Pomari-Fernandes A., Lemes A. A., Vacari A. M., De Bortoli S. A., de Freitas Bueno A. (2017). Cost of production of Telenomus remus (Hymenoptera: Platygastridae) grown in natural and alternative hosts. J. Economic Entomology 110, 2724–2726. doi: 10.1093/jee/tox271
Von Broembsen S. L., Kruger F. J. (1985). Phytophthora cinnamomi associated with mortality of native vegetation in South Africa. Plant Dis. 69, 715–717. doi: 10.1094/PD-69-715
Wakamura S., Arakaki N., Yoshimatsu S. I. (2021). Sex pheromone of the fall armyworm Spodoptera frugiperda (Lepidoptera: Noctuidae) of a “Far East. population Okinawa Japan. Appl. Entomology Zoology 56, 19–25. doi: 10.1007/s13355-020-00703-9
Wan J., Huang C., Li C. Y., Zhou H. X., Ren Y. L., Li Z. Y., et al. (2021). Biology, invasion and management of the agricultural invader: Fall armyworm, Spodoptera frugiperda (Lepidoptera: Noctuidae). J. Integr. Agric. 20, 646–663. doi: 10.1016/S2095-3119(20)63367-6
Wang W. Y., Wu X. Q., Wen T. Y., Feng Y., Zhang Y. (2022). Terpene production varies in Pinus thunbergii Parl. with different levels of resistance, with potential effects on pinewood nematode behavior. Forests 13, 1140. doi: 10.3390/f13071140
War A. R., Sharma H. C., Paulraj M. G., War M. Y., Ignacimuthu S. (2011). Herbivore induced plant volatiles. Their role in plant defense for pest management. Plant Signaling Behav. 6, 1973–1978. doi: 10.4161/psb/6.12.18053
Weber D. C. (2015). Trissolcus japonicus (Ashmead) (Hymenoptera, Scelionidae) emerges in North America. J. Hymenoptera Res. 43, 119–128. doi: 10.3897/JHR.43.4661
Weber D. C., Morrison W. R., Khrimian A., Rice K. B., Leskey T. C., Rodriguez-Saona C., et al. (2017). Chemical ecology of halyomorpha halys: discoveries and applications. J. Pest Sci. 90, 989–1008. doi: 10.1007/s10340-017-0876-6
Werres S., Marwitz R., Man in ‘t Veld W. A., De Cock A. W. A. M., Bonants P. J. M., De Weerdt M., et al. (2001). Phytophthora ramorum sp. nov., a new pathogen on Rhododendron and Viburnum. Mycological Res. 105, 1155–1165. doi: 10.1016/S0953-7562(08)61986-3
Westbrook J., Fleischer S., Jairam S., Meagher R., Nagoshi R. (2019). Multigenerational migration of fall armyworm, a pest insect. Ecosphere 10, e02919. doi: 10.1002/ecs2.v10.11
Wu D., Yan Y., Cui J. (1997). Sex pheromone components of Helicoverpa armigera; chemical analysis and field tests. Entomologia Sin. 4, 350–356. doi: 10.1111/j.1744-7917.1997.tb00109.x
Xu F. (2008). “Recent advances in the integrated management of the Pine Wood Nematode in China,” in Pine wilt disease. Eds. Zhao B. G., Futai K., Sutherland J. R., Takeuchi Y. (Springer, Tokyo), 323–333.
Xu H., Li G., Liu M., Xing G. (2006). Oviposition deterrents in larval frass of the cotton bollworm, Helicoverpa armigera (Lepidoptera: Noctuidae): chemical identification and analysis by electroantennogram. J. Insect Phys. 52, 320–326. doi: 10.1016/j.jinsphys.2005.11.011
Xu Y., Tong Z., Zhang X., Zhang X., Luo Z., Shao W., et al. (2021). Plant volatile organic compound (E)-2-hexenal facilitates Botrytis cinerea infection of fruits by inducing sulfate assimilation. New Phytol. 231, 432–446. doi: 10.1111/nph.v231.1
Yactayo-Chang J. P., Mendoza J., Willms S. D., et al. (2021). Zea mays volatiles that influence oviposition and feeding behaviors of spodoptera frugiperda. J. Chem. Ecol. 47, 799–809. doi: 10.1007/s10886-021-01302-w
Yan Z. G., Wang C. Z. (2006). Similar attractiveness of maize volatiles induced by Helicoverpa armigera and Pseudaletia separata to the generalist parasitoid Campoletis chlorideae. Entomologia Experimentalis Applicata 118, 87–96. doi: 10.1111/j.1570-7458.2006.00368.x
Yan Z., Yan Y., Wang C. (2005). Attractiveness of tobacco volatiles induced by Helicoverpa armigera and Helicoverpa assulta to Campoletis chlorideae. Chin. Sci. Bull. 50, 1334–1341. doi: 10.1360/982005-388
Yang X., Tyler B. M., Hong C. (2017). An expanded phylogeny for the genus *Phytophthora*. IMA Fungus 8, 355–384. doi: 10.5598/imafungus.2017.08.02.09
Yang X., Wyckhuys K. A. G., Jia X., Nie F., Wu K. (2021). Fall armyworm invasion heightens pesticide expenditure among Chinese smallholder farmers. J. Environ. Manage. 282, 111949. doi: 10.1016/j.jenvman.2021.111949
Zamora P., Rodríguez V., Renedo F., Sanz A. V., Domínguez J. C., Pérez-Escolar G., et al. (2015). First report of Bursaphelenchus xylophilus causing pine wilt disease on Pinus radiata in Spain. Plant Dis. 99, 1449. doi: 10.1094/PDIS-03-15-0252-PDN
Zhang J. P., Salcedo C., Fang Y. L., Zhang R. J., Zhang Z. N. (2012). An overlooked component: (Z)-9-tetradecenal as a sex pheromone in Helicoverpa armigera. J. Insect Physiol. 58, 1209–1216. doi: 10.1016/j.jinsphys.2012.05.018
Zhao L., Mota M., Vieira P., Butcher R. A., Sun J. (2014). Interspecific communication between pinewood nematode, its insect vector, and associated microbes. Trends Parasitol. 30, 299–308. doi: 10.1016/j.pt.2014.04.007
Zhong Y. Z., Zhang J. P., Ren L. L., Tang R., Zhan H. X., Chen G. H., et al. (2017). Behavioral responses of the egg parasitoid Trissolcus japonicus to volatiles from adults of its stink bug host, Halyomorpha halys. J. Pest Sci. 90, 1097–1105. doi: 10.1007/s10340-017-0884-6
Keywords: headspace, VOC collection, detection, Halyomorpha halys, Spodoptera frugiperda, Helicoverpa armigera, Bursaphelenchus xylophilus, Phytophthora ramorum
Citation: Favaro R, Berka M, Pettersson M, Thöming G, Arce CCM, Inácio ML, Turlings TCJ, Faria JMS, Jung T, Bazin D, Pozzebon A, Angeli S and Cappellin L (2024) The use of volatile organic compounds in preventing and managing invasive plant pests and pathogens. Front. Hortic. 3:1379997. doi: 10.3389/fhort.2024.1379997
Received: 31 January 2024; Accepted: 29 October 2024;
Published: 28 November 2024.
Edited by:
Prateek Gupta, SRM University, IndiaCopyright © 2024 Favaro, Berka, Pettersson, Thöming, Arce, Inácio, Turlings, Faria, Jung, Bazin, Pozzebon, Angeli and Cappellin. This is an open-access article distributed under the terms of the Creative Commons Attribution License (CC BY). The use, distribution or reproduction in other forums is permitted, provided the original author(s) and the copyright owner(s) are credited and that the original publication in this journal is cited, in accordance with accepted academic practice. No use, distribution or reproduction is permitted which does not comply with these terms.
*Correspondence: Luca Cappellin, bHVjYS5jYXBwZWxsaW5AdW5pcGQuaXQ=