- 1Department of Botany, Bahona College, Jorhat, Assam, India
- 2Department of Botany, Pandit Deendayal Upadhyaya Adarsha Mahavidyalaya (PDUAM), Eraligool, Karimganj, Assam, India
- 3Botany Section, Women’s College, Aligarh Muslim University, Aligarh, India
Introduction: French bean (Phaseolus vulgaris L.) holds global significance as one of the most consumed legumes, with commercial value surpassing that of all other legume crops combined. In India, the consumption of French beans has grown steadily, especially in the North Eastern region, driven by heightened consumer interest in its nutritional benefits. Considering these factors, we initiated an induced mutagenesis program to enhance the genetic diversity of locally grown French bean genotypes, traditionally cultivated for their superior adaptability.
Methods: To achieve this, we initiated an induced mutagenesis program. Seeds from the village seed stock were subjected to treatments with varying doses of ethyl methane sulfonate (EMS) ranging from 0.1% to 0.4% and sodium azide (SA) from 0.1% to 0.4%. The objective was to increase yield potential and enhance genetic diversity.
Results: The treatment with EMS and SA led to a non-specific, dosage-independent reduction in biophysiological characteristics in French bean mutants. Notably, the 0.4% SA treatment significantly inhibited germination and fertility, causing a decrease in chlorophyll (10.02 mg. g-1 FW) and carotenoid (1.57 mg. g-1 FW) levels. This suggests a disruption in genes associated with chlorophyll and carotenoid synthesis. However, in the M2 generation, the mutagenic treatments substantially improved yield and associated traits. The highest pod yield per plant was recorded at 79.50 gm for the 0.2% EMS treatment. A character association study revealed strong correlations (0.217 to 0.995) between pod yield and other agronomic traits.
Discussion: The results indicate that selecting mutants based on these traits in populations treated with EMS and SA can significantly increase crop yield. The 0.2% SA and 0.2% EMS M2 mutant populations exhibited the highest induced variability, making them ideal for selecting higher-yielding mutant lines for further breeding generations. The increased yields in these mutant lines, derived from a local cultivar, show promise for meeting the growing demand for French bean production through their widespread cultivation.
Introduction
Phaseolus vulgaris (L.), commonly known as the French bean, holds a crucial position among protein-rich vegetables cultivated in developing nations. Nevertheless, its cultivation faces formidable obstacles due to low yields resulting from a multitude of biotic and abiotic stress factors (Bhawna et al., 2016). It is a self-pollinating member of the Fabaceae family, featuring a compact 588 MB sized genome and characterized by a diploid genetic constitution (2n =2x =22) (Arumuganthan and Earle, 1991). This versatile crop is commonly referred to by a variety of appellations such as French bean, common bean, snap bean, kidney bean, and garden bean. The genus Phaseolus encompasses a considerable diversity of around 80 cultivated and wild species, with Phaseolus vulgaris being the predominantly cultivated one among them (Porch et al., 2013). While Phaseolus vulgaris shares numerous characteristics with its botanical family, two distinctive features set the entire Phaseolus genus apart from other members of the family. Firstly, the keel of the blossom culminates in a spiral, which can exhibit between one and two rotations (Gentry, 1969). Secondly, the plant’s vegetative and reproductive components bear uncinate hairs (Freytag and Debouck, 2002). French bean holds particular importance as food crop and has its roots originating in Central and South America (Swiader et al., 1992). Cultivated varieties of the French bean display extensive diversity in various characteristics such as growth habit, flower color, as well as the configuration, dimensions, and hues of pods and seeds. The diversity in immature pod phenotypes includes variations in length and curvature (straight or curved), cross-sectional features (diameter, flat, round, sieve size), and color (yellow, green, purple) before seed development begins (García-Fernández et al., 2021). Certain bean genotypes have pods that are harvested and consumed as fresh green beans. These green beans are picked when the pods have attained their peak length, while the seeds are yet in the formative stages. On the other hand, parched legumes are ingested as completely developed seeds following soaking and culinary preparation. (García-Fernández et al., 2021). In 2021, India harvested beans (dry) from an area of 14.65 million hectares, producing approximately 6.12 million metric tons, while globally, the world harvested beans from 35.92 million hectares, resulting in a total production of approximately 27.72 million metric tons (FAOSTAT, 2023). India’s bean production accounted for about 22.1% of the global production, indicating a substantial contribution to worldwide output. The cultivation of French beans is widespread throughout India, including north-eastern states with small and medium-size farms being the primary producers. The beans are an integral part of crop rotations and intercropping systems in the region. Although, French bean can be grown on all types of soil, sandy loams and clay loams are suitable for a better yield of this crop. Except for the winter season in hilly region, it can be grown all year round. On the other hand, it can be grown in plains during winter.
The French bean plays a pivotal role in the diets of people across various continents, serving as a valuable source of essential minerals and proteins (Gepts et al., 2008). In India, there exists a deep-rooted historical custom of utilizing dried and vegetable legumes, and this practice has been on a consistent rise owing to the increasing desire of consumers and the acknowledgment of legumes as an essential nutritious dietary choice. French bean holds the position of being the most extensively ingested legume globally and is of utmost significance as a legume crop intended for immediate human consumption. Its economic worth surpasses that of all other leguminous crops collectively worldwide (Broughton et al., 2003; Porch et al., 2013). In the North Eastern region of India, the French bean stands out as one of the most vital vegetable crops among leguminous crop plants. It is cultivated for various purposes, such as shelled green beans, dry beans (rajmah), and most notably as tender vegetables, owing to its rich content of proteins, vitamins, and minerals. In north- east regions, it is cultivated during rabi. Furthermore, when irrigation facilities are available during dry periods, there exists the possibility of year-round cultivation. Notably, beans offer a plethora of health benefits, being rich in fiber, protein, folate, other B-vitamins, minerals, and antioxidants (Mullins and Arjmandi, 2021).
French bean encounters a wide array of yield constraints throughout its growth cycle. Among these, diseases and environmental strains such as aridity, soil compression, diminished soil nutrient content, and elevated temperatures play a pivotal role in limiting bean production, resulting in a significant gap between the actual and potential yields (Bhawna et al., 2016). This shortfall in yield leads to substantial financial losses for farmers annually. The combination of low yields, abrupt climate fluctuations, and a myriad of stressors continue to plague production, falling short of meeting the substantial demand for this crop. Consequently, there is a growing need to develop tolerant varieties of the crop which is not only environmentally friendly but also cost-effective and enhances the quality of the produce.
Genetic variability plays a crucial function in the progression of crop enhancement initiatives (Blixt and Gottschalk, 1975). In cases where the gene pool lacks sufficient diversity, hybridization and mutations are the sole viable means to augment allelic variations. As early as the 1940s, X-ray mutagenesis was employed to create a bush-type architecture in Mesoamerican white-seeded beans, specifically in the ‘Michelite’ variety at Michigan State University. This breakthrough paved the way for the development of several renowned cultivars like ‘Sanilac’ and ‘Seafarer’ throughout the 1950s and 1960s (Nichterlein, 1999). A diverse genetic pool is essential to begin a successful breeding program, and the level of induced mutation within genepool of the crop directly impacts the breeding outcomes. Nonetheless, the cultivated gene pool of french bean has already been extensively leveraged to maximize productivity. Consequently, to facilitate further progress, there is a pressing need to induce additional variations through mutations. French bean possesses a considerable amount of inherent alterations (Rukmanski, 2005). Globally induced mutagenesis has been employed as an approach to generate fresh genetic variations and develop novel strains or varieties Shu (2009). Among various mutagenesis agents, ethyl methanesulfonate (EMS) has shown remarkable success in inducing desired breeding traits (Ramandeep et al., 2018; Chen et al., 2023). EMS primarily alkylates guanine (Boysen et al., 2009), producing O6-ethylguanine and causing mispairing with thymine instead of cytosine. These EMS-induced mutations offer valuable opportunities for obtaining genotypes with higher yield potential, altered plant architecture suitable for mechanical harvesting and resistant to lodging, early ripening, increased protein and beta-carotene content, as well as resistance to diseases, pests, and drought, or exhibiting ecological plasticity (Tomlekova et al., 2014a; Tomlekova et al., 2014b). Sodium azide is highly mutagenic in various organisms including crop species, with its mutagenicity linked to the production of an organic metabolite and dependent on acidic pH, resulting in a relatively low frequency of chromosome breakage (Gruszka et al., 2012). In general, local French bean cultivars are cultivated by traditional farmers in challenging agricultural settings, including arid hilly regions, waterlogged lowlands, and other marginal lands. The preference for these traditional cultivars lies in their inherent adaptability and resilience to stresses, coupled with their favorable taste, as recognized by the local populace. Given these attributes, there is a significant opportunity to enhance their economic viability for farmers by harnessing their yield potential through induced mutagenesis. The effective utilization of induced mutation breeding has been demonstrated as a successful approach for enhancing locally adapted cultivars of sorghum (Wanga et al., 2018). Therefore, the objective of the present study was to generate high-yielding French bean mutants with broadened genetic diversity from a local, non-descript Phaseolus vulgaris L. cultivar through EMS and SA mutagenesis for breeding purposes.
Material and method
Plant material
Induced mutagenesis was employed in a farmer’s cultivar of French bean using different concentrations of Ethyl methanesulfonate (EMS) and Sodium azide (SA). The seeds of the cultivar were collected from farmers of village in Bahona, Jorhat and are widely adapted to this agroclimatic region of North East India.
Methodology
The cultivar’s robust and viable seeds, with a moisture content of 11.0%, were utilized in the mutagenic treatment. Moisture content percentage was determined in accordance with the guidelines of the International Seed Testing Association, which relies on the distinction between the seeds’ fresh and dry weights. For chemical treatments, seeds pre-soaked for 6 hours were exposed to various concentrations (v/v) of EMS and SA, specifically 0.1%, 0.2%, 0.3%, and 0.4%, at a room temperature of 25 ± 2°C for 9 hours. Each treatment involved 90 seeds, and they were cultivated at the Bahona College’s experimental house in Jorhat between mid-November 2020 and March 2021. This was done using a randomized complete block design (RCBD), with each treatment consisting of 90 seeds distributed across 3 replications, each replication comprises 30 seeds, organized into three rows of ten seeds each. Each block consist of one replication from each of the 9 treatments, all of which were subjected to uniform agronomic practices throughout the study. Each self-pollinated and fertile M1 plant was harvested and stored separately according to treatment after being sun-dried to achieve a moisture content level of 10-12% over a period of 10-12 days. To propagate the M2 generation, five viable seeds from each healthy M2 plant were planted in rows according to their plant lineage. We gathered quantitative data on the physical characteristics of the top 30 plant lineages in each experimental group, which displayed remarkable survival rates and trait expression. The average measurements of 10 quantitative traits namely the days to flowering, days to maturity, plant height (cm), no. of branches per plant, no. of pods per plant, pod length (cm), single pod weight (gm), pod yield per plant (gm), seed per pod and 100 seed weight (gm) were used in a statistical analysis to evaluate genetic diversity within the mutant population and determine the extent of divergence caused by the mutagenic treatments in relation to their corresponding control plants.
The spectrophotometric method was employed to measure the chlorophyll and carotenoid contents. The green beans were crushed using a mortar, and aqueous acetone (80%) chlorophyll extract was prepared. Afterward, the extract was filtered, and the resulting filtrate was transferred into a cuvet for subsequent measure of the total chlorophyll and carotenoid contents. the levels of chlorophyll and carotenoid were determined based on fresh weight (mg.g-1FW) using Arnon’s (1949) prescribed formula.
Where, OD645, OD663, OD480, OD510 = Optical densities at OD645, OD663, OD480 and OD510, respectively V = Volume of an extract W = Mass of leaf tissues d = Length of light path (d= 1.4 cm).
Statistical analysis of quantitative traits
Estimation of seed germination (%), fertile plants %, chlorophyll (mg g−1 FW) and carotenoid (mg g−1 FW) were done to analyze the biophysiological damage created by the mutagen doses in M1 generation. The information concerning 10 quantitative phenotypic characteristics, specifically the days to flowering, days to maturity, plant height (cm), no. of branches per plant, no. of pods per plant, pod length (cm), single pod weight (gm), pod yield per plant (gm), seed per pod and 100 seed weight (gm) were recorded throughout M2 generation. The pods were harvested while they are young and tender in 2-3 weeks after first flowering for pod yield estimate. The crop was harvested three times per week over a four-week period, with the focus on removing pods that had reached horticultural maturity during each harvest. The pods harvested from each tagged plant were counted separately at each harvest, and the recorded numbers were later used to calculate the average number of pods per plant.
Mean values for various parameters investigated in the current study across all mutagen concentrations within the M2 generation were computed through descriptive statistics. To ascertain whether the doses of mutagens employed had any impact on the recorded observations, a One-Way ANOVA was conducted between treatments. Additionally, after identifying statistically significant differences in the ANOVA, the Duncan Multiple Range Test (DMRT) was employed to assess the nature of distinctions between the control group and various treatment means. To assess trait linkage, Pearson’s correlation coefficient (r) was employed in character association investigations. The International Business Machines Corporation Statistical Package for the Social Sciences (IBM SPSS 20.0) was utilized for quantitative data analysis.
Results and discussion
Genetic variation in traits
The findings unveiled an arbitrary and dosage-agnostic decrease in the biophysiological characteristics within the subjected groups of EMS and SA (Table 1). Biophysiological study outcome showed mutagen dose strength dependent increase in inhibitions, with maximum in higher treatment (0.4%). The mutagenic doses considerably inhibited the germination and fertility in the mutant population of French bean. The maximum inhibition was observed in 0.4% SA treatment followed by 0.4% EMS treatment. A significantly higher chlorophyll (10.02 mg.g-1FW), and carotenoid contents (1.57 mg.g-1FW) were recorded in the green pod of control population of the local cultivar of French bean. The administration of mutagenic treatments led to a significant decline in biophysiological characteristics. Chlorophyll assumes a pivotal function in the process of photosynthesis and exerts a substantial influence on numerous mechanisms that regulate the growth and maturation of plants (Li et al., 2018). The process of synthesizing chlorophyll is a complex one, involving numerous enzymes and steps, such as the incorporation of Mg2+ into protoporphyrin IX, which directly impacts the production of chlorophyll as described by Tanaka and Tanaka (2007). If any of the enzymatic reactions or steps are obstructed, it can result in a decrease in chlorophyll content, as reported by Willows and Hansson (2003). In our research, we observed a reduction in the average levels of chlorophyll in mutagenized populations, which corroborated the findings of Borzouei et al. (2010) for Triticum aestivum, Verma et al. (2010) for Euryale ferox, and Raina and Khan (2023) for Vigna unguiculata. This decline in chlorophyll content can likely be attributed to the disruption of genes involved in chlorophyll synthesis caused by mutagenesis. Additionally, the decrease in chlorophyll levels may also be due to dephytolization and pheophytinization caused by EMS and SA, which ultimately impairs chlorophyll synthesis. This is supported by the findings of Saha et al. (2010), who reported similar mutagenic effects caused by gamma radiation. Carotenoids play a vital role as secondary pigments in the light-harvesting process by mitigating excess light energy, scavenging free radicals, and establishing a plant’s defense system, as elucidated by Fukuzawa et al. (1998); Ramel et al. (2012), and Hashimoto et al. (2016). Our experimental results demonstrated a significant reduction in carotenoid levels in treated populations of both EMS and SA. These findings align with the observations made by Amin et al. (2019) regarding black cumin populations treated with mutagens, suggesting that the decrease in carotenoid content can likely be credited to the mutagen-induced impairment of genes responsible for carotenoid synthesis. Consequently, the lowered carotenoid levels in the treated seeds may result in decreased light-harvesting and photosynthetic capabilities.
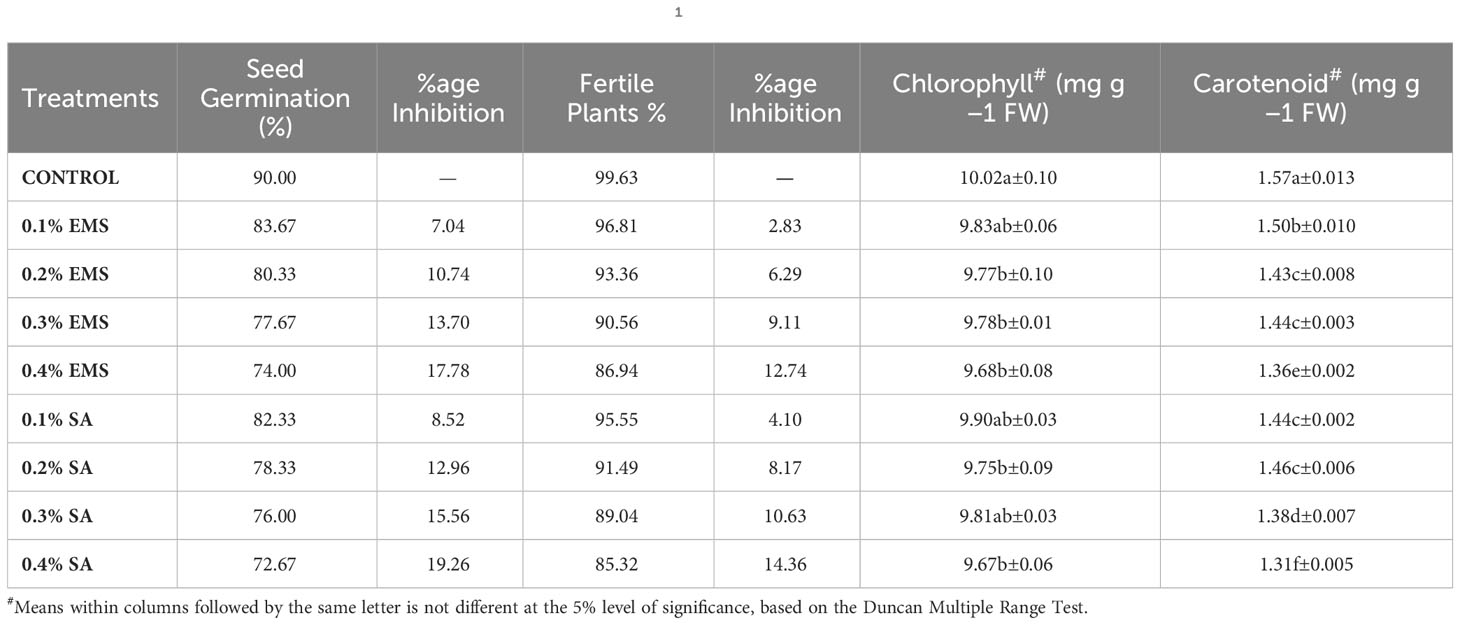
Table 1 Estimates of mutagenic effect on biophysiological traitsin M1 generation of French bean (Phaseolus vulgaris L.).
Impact of mutagenic treatments on quantitative traits
This section presents the results of the M2 generation, where we assessed the genetic diversity in yield and related characteristics of a locally adapted French bean cultivar induced by EMS and SA doses. Descriptive statistics revealed that lower and moderate mutagenic treatments were linked to an increase in the means of quantitative trait phenotypes, while higher doses led to the maximum inhibition of trait expression in comparison to their corresponding control. To determine the statistical significance of the mutagenic effects, we conducted an independent ANOVA between treatment groups, which showed a significant effect (p<0.05) for all quantitative phenotypic attributes, encompassing green pod yield per plant, with the exception of days to flowering and seeds per pod, where the treatment impact was relatively reduced (Table 2). These results indicate that the null hypothesis of no distinctions among the means of different mutagenized populations was rejected, suggesting that the mutagen treatments successfully created an array of genetic variability by random mutations. The estimates of coefficient of variation (CV%) provided insights into the variability and stability of traits within the individual treatment population. In the context of genetic gain, the CV% helps estimate the potential improvement that can be achieved by selecting individuals with superior trait performance within a specific mutagenized population. Traits with higher CV% offer more room for improvement through selection. Furthermore, the Duncan Multiple Range Test (DMRT) provided valuable insights regarding treatment groups significantly deviating from their corresponding controls, indicating a significant alteration in the average values of quantitative phenotypic traits caused by the mutagen dosages. These findings demonstrate the potential of induced mutagenesis in generating diverse genetic variations, which could pave the way for further improvements in French bean cultivation, particularly in yield and related traits. The results of the quantitative traits showing significant mean shift and genetic variability (Tables 3, 4) are elaborated here. In M2 generation, the estimation of days to flowering showed negative shift in mean values in all the treated populations. It was reduced from 37.20 days in control to 34.20 days in 0.4% SA. In days to maturity estimation, the mean shifted in negative directions in the treated population. The maximum reduction was observed in 0.1% SA (57.97 days) compared to the control (62.07 days). The decrease in flowering caused by mutagen aligns with the earlier report by Laskar et al. (2017). We believe that this reduction in flowering could be linked to changes in the expression of genes related to flowering, specifically MADS-box genes related to flowering time and development (Colquhoun et al., 2011). It was reported that the overexpression of genes belonging to the MADS-box gene family caused early flowering in transgenic plants like chrysanthemums (Shinoyama et al., 2012) and dendrobium orchids (Sawettalake et al., 2017). In the specific plant variety under consideration, each experimental treatment that induced mutations led to a decrease in plant height compared to the average values observed in the control group during the M2 generation, as indicated by the recorded data. In all the populations subjected to mutagen treatment, there was a notable decrease in the average plant height when compared to their corresponding control groups. Maximum reduction of 4.90 was observed with the treatment 0.4% SA compared to control 35.79 cm. In the 0.1% EMS treatment, the highest CV% was 3.79%. This confirms that this treatment caused the highest dispersion and produced the mutant population with the highest variation. The findings demonstrated a notable decline in plant height at elevated mutagen doses. The results align with the observations made by Raina et al. (2020), who emphasized a significant reduction in plant stature within mutant lineages. The variability in plant height could be attributed to the influences of SA and MMS on the regulation of cell divisions, as reported by Rao (1988). Mutagens have the capacity to accelerate or halt mitotic divisions, contingent upon the location and nature of the mutation. In the M2 generation, there was a noticeable shift towards the positive side in the average number of fertile branches for a majority of the mutagen treatments in both EMS and SA populations. The most significant increase in the average, which reached 11.47, was observed in the 0.2% SA treatment, when compared to the control mean of 9.73. The CV% experienced a substantial increase across various treatments, with the highest recorded at 11.40% in the 0.1% SA treatment. Mutagenesis resulted in a notable rise in the number of fertile branches per plant, aligning with the earlier observations by Khursheed et al. (2019) in faba bean mutant lines that were subjected to gamma rays and EMS treatment. This augmentation in plant branches can be attributed to the prevalence of favorable mutations. When estimating the number of pods per plant, the average generally moved in a favorable direction, exhibiting negative variations in the most extreme treatments. Notable enhancements in the average pod count per plant were noted in the case of lower and moderate doses of mutagens. The mean figures displayed the greatest increase in the 0.1% SA treatment (14.03), closely trailed by the 0.2% EMS treatment (14.00), in contrast to the control group’s mean of 9.67. The analysis of the CV% revealed significant variability in the number of pods per plant within both mutagenic populations. In the M2 generation, we observed reductions in mean values for pod length (measured in centimeters) across all the treated populations, when compared to the control group. The mean pod length (cm) of increased from 9.37 cm in control to 11.93 in 0.2% SA and 11.12 in 0.2% EMS. Overall, substantial variations induced in all the mutant populations as estimated with the CV%. Pod length is a crucial characteristic that plays a key role in enhancing yield, as a greater pod length enables the accommodation of a higher number of seeds. The substantial rise in pod length caused by mutagenesis aligns well with the observations made by Raina et al. (2020). It was believed that the expansion of pod length within a population subjected to mutagenesis can be attributed to the interplay between mutagens and the genes responsible for regulating pod length. Significant alterations in mean values compared to the control group were noted for single pod weight (in grams) in both the mutagenic treatments. The mean single pod weight (g) of the cultivar increased from control (5.60 gm) to the maximum in 0.2% EMS (5.68 gm). The heightened pod count per plant resulting from mutagen exposure could potentially be linked to modified genes associated with the flowering process, given that pod development in any plant is influenced by the quantity of flowers present. As a consequence, the amplified pod count per plant within mutagen-altered populations might be ascribed to an escalated presence of floral buds. Notably, a significant rise in the number of seeds per pod was documented in populations treated with SA and EMS. These findings closely aligned with the outcomes reported by Laskar et al. (2017). The augmentation in seed quantity per pod among mutagenized populations could potentially be attributed to the interplay between mutagens and subsequent changes in gene expression that oversee the regulation of seed quantity per pod. The M2 population that underwent mutagenic treatments to produce the M3 generation displayed a noticeable rise in the average pod yield per plant for both EMS and SA treatments. These treated groups showed a substantial disparity in mean values when compared to the control group. Looking at it from a productivity angle, the number of pods per plant is a crucial characteristic, and a higher count of pods per plant is directly linked to an enhanced yield. Horn et al. (2016) and Kumar et al. (2021) reported a significant increase in the number of pods per plant due to the mutagenic impact. The mean pod yield was found to be highest at 0.2% EMS (79.50 gm) and 0.2% SA (79.05 gm) in the treated populations as compared to the control population (54.14 gm). The highest CV% (25.36%) was observed at 0.2% EMS. At maturity, data on mean seed per pod showed significant deviations from the mean. It was found to be highest in 0.1% EMS (6.73) compared to the control (6.47). The findings indicated a meaningful and statistically significant rise in green pod yield within mutagen-altered populations. These outcomes closely aligned with the reports by Mahamune and Kothekar (2012); Sood and Pathania (2014); Kumar et al. (2021) regarding French bean populations subjected to mutagenesis. Among the altered populations, the most substantial enhancement in seed yield was observed in those with lower mutagen exposure.
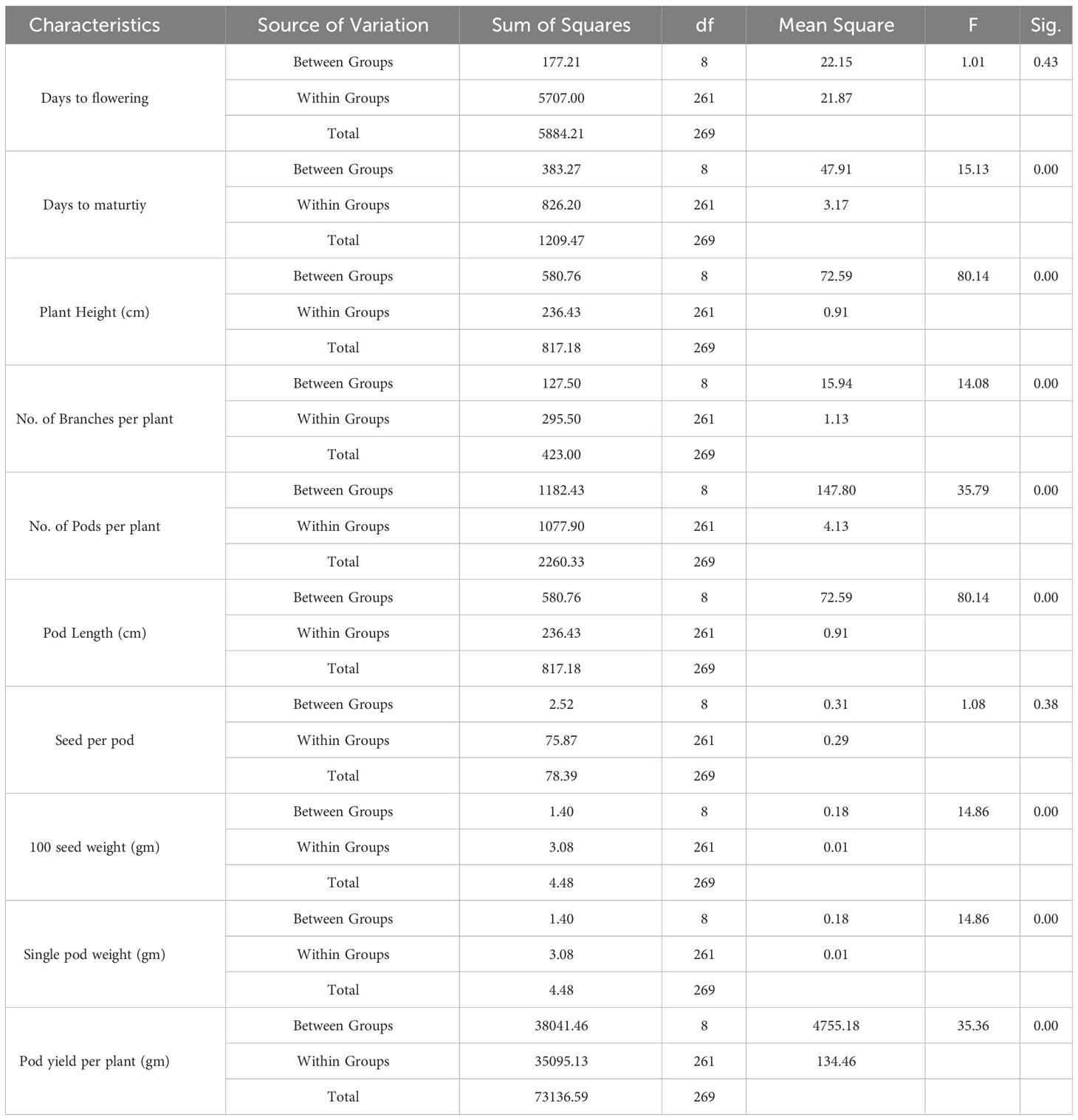
Table 2 Significance in terms of probability (p) of F-test for the analysis of variance (ANOVA) for each of the ten traits of French bean local cultivar evaluated in M2 generation.
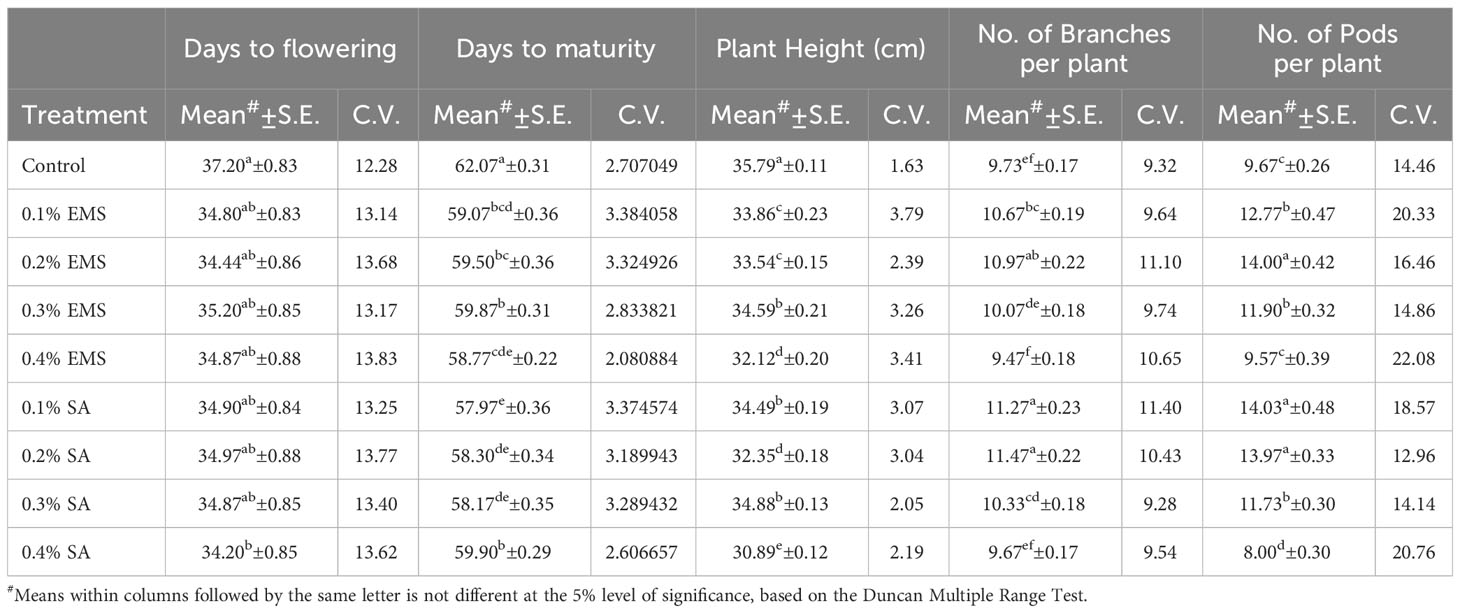
Table 3 Estimates of Mean and Coefficient of Variation (C.V. %) for different quantitative traits in M2 generation of French bean (Phaseolus vulgaris L.).
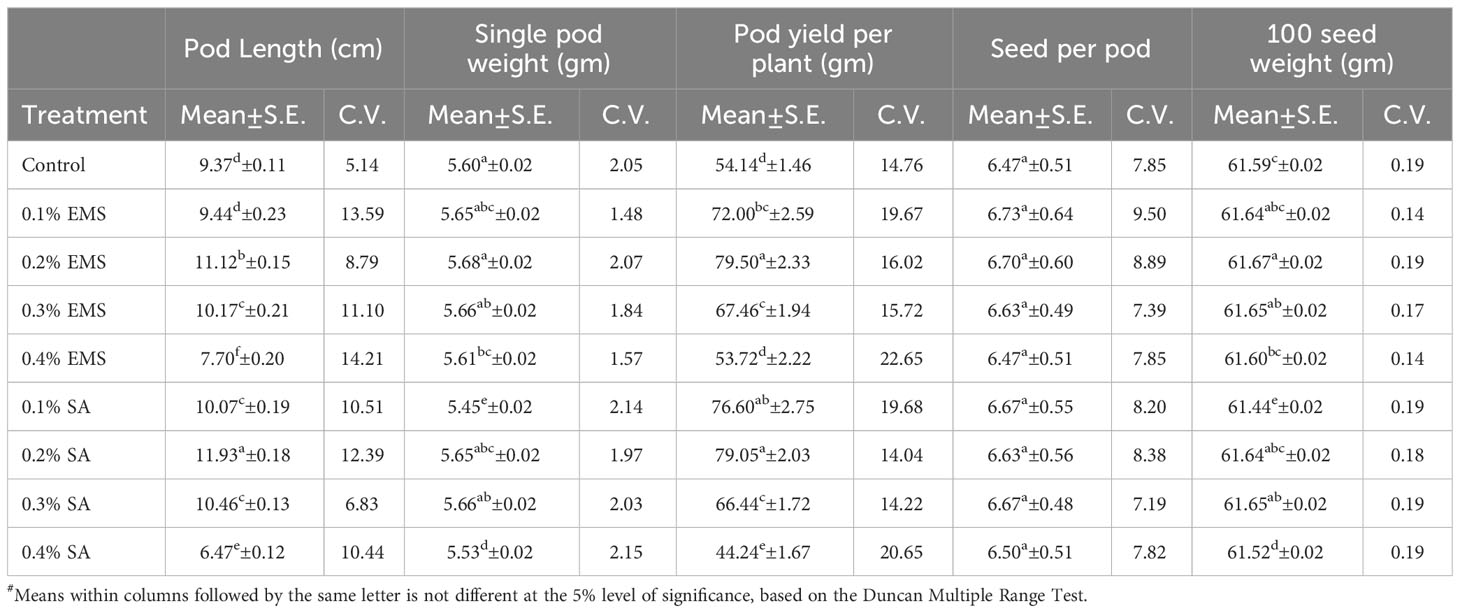
Table 4 Estimates of Mean and Coefficient of Variation (C.V. %) for different quantitative traits in M2 generation of French bean (Phaseolus vulgaris L.).
Significant changes in mean values compared to the control were observed in both mutagens for the 100-gram seed weight. The mean 100 seed weight (g) was highest in 0.2% EMS (61.67 gm) compared to the control (61.59 gm). The mutagenic influence resulted in a noteworthy rise in the average weight of 100 seeds, aligning closely with the prior observations of Laskar et al. (2018) in altered lentil lines exposed to mutagenesis. This enhancement in seed weight is likely attributed to the beneficial impacts of SA and EMS dosage. Kumar et al. (2021) reported a similar observation regarding induced mutagenesis in locally adapted landraces of French Bean (Phaseolus vulgaris L.), where these cultivars showed higher mutagenic sensitivity and mutability for quantitative improvement thus supporting the findings of the current study. Breeding trials are essential for evaluating these populations with the aim of releasing French bean mutant varieties that exhibit high yields. Moreover, these productive mutagenized populations can play a pivotal role as parental sources within crossbreeding initiatives, facilitating the attainment of targeted objectives.
Chemical mutagenesis has proven to be highly effective, reliable, and well-understood for common bean, yet there remains scope for protocol improvements, which are actively being pursued (Blair et al., 2007). Plant architecture and other morphological characteristics have captivated the attention of bean breeders from the inception of modern bean breeding. Among the various methods at our disposal for generating a new genetic mix in crop genomes, mutation induction technology has proven to be the most effective, enabling plant breeders to efficiently identify and choose desired combinations of economically important traits (Laskar and Khan, 2017). Analyzing induced micromutations and their impact on quantitative traits is crucial yet demanding, requiring extensive statistical tools to identify the most promising improved mutant genotype.
Trait correlations
Achieving yield improvement through direct selection proves challenging due to the influence of multiple genes with minor additive effects on yield (Rasik et al., 2022). Nonetheless, the effective indirect selection in plant breeding initiatives can be enhanced by focusing on attributes that wield a significant, direct impact on yield. Therefore, it is imperative to possess a comprehensive understanding of the relationship between yield and its various attributes in order to optimize selection gains (Khursheed et al., 2016). Utilizing correlation analysis becomes vital in evaluating the strength and nature of the connections between these traits, providing valuable insights into their roles in yield enhancement (Cruz et al., 2012). Table 5 displays the results of the character association, and the Pearson’s correlation coefficients obtained in M2 generations revealed strong correlations between certain traits and pod yield for the common bean cultivar viz. no. of pods per plant (r =0.995**), no. of branches per plant (r =0.410**), seed per pod (r =0.257**), plant height (r =0.230**), pod length (r =0.230**), 100 seed weight (r =0.217**), single pod weight (r =0.217**). These findings suggest that selecting mutants based on these traits within treated populations is a viable approach for identifying high-yield candidates. The significant character association were observed between different quantitative traits in the French bean local cultivar as depicted in Figure 1). In the study, pod length vs plant height, pod weight vs seed weight and yield per pod vs seed weight showed complete linkage with maximum significant correlation coefficients. Furthermore, Heatmap correlation analysis revealed that the positive variation of some economically important yield traits was significantly related to medium range (0.2% & 0.3%) doses of the mutagen EMS and SA (Figure 2). Fresh green pod yield was found positively associated with the 0.2% concentration of both EMS and SA, in comparison to other treatment concentrations. The number of pods per plant and pod yield are clustered together due their significantly higher correlation. Also, 100 seed weight and single pod weight showed significant trait association. Correlation analysis assumes a crucial role in establishing the connection between various yield-attributing characteristics (Ramesh, 2016). The strength of the linear correlation between two traits is influenced by selection, gene linkage, and pleiotropy (Sakai and Suzuki, 1964; Laskar and Khan, 2017). Earlier reports indicated that mutagens play a role in modifying correlations between traits in various cultivars (Toker and Cagirgan, 2004; Shin et al., 2011). Enhancing crop yield, which is a key objective in crop improvement initiatives, necessitates a comprehensive comprehension of the connection between yield and the contributing traits. In this study, yield was considered the outcome variable, while other traits were regarded as causal variables. A high positive and significant correlation value was found with yield. Out of the 9 component characters examined, 7 of them displayed significant positive correlations with plant yield. In the French bean cultivar, the strength of trait correlation with pod yield was ranked as follows: pods per plant > no. of branches per plant > seed per pod > plant height > pod length > 100 seed weight > single pod weight. These correlated traits likely influence the plant’s food production through processes like photosynthesis or other physiological activities, thus directly impacting the yield (Laskar and Khan, 2017). The findings suggest that opting for any of these correlated characteristics associated with crop yield could potentially result in an increase in grain production. Therefore, when conducting mutation breeding experiments on French beans, it is crucial to prioritize the selection of these traits alongside yield enhancement. In this present investigation, the assessment of various attributes unveiled a noteworthy degree of diversity among them, which was influenced by distinct treatments involving EMS and SA, administered individually. An analysis of the average values for different quantitative characteristics primarily revealed favorable shifts in traits contributing to yield, with only a few minor exceptions where mutagenic treatments led to decreased average values. Given the intricate nature of quantitative trait expression, which involves multiple genes, and the stochastic manner in which mutagens interact with the targeted genome, it is anticipated that variations in both directions from the control averages will occur.
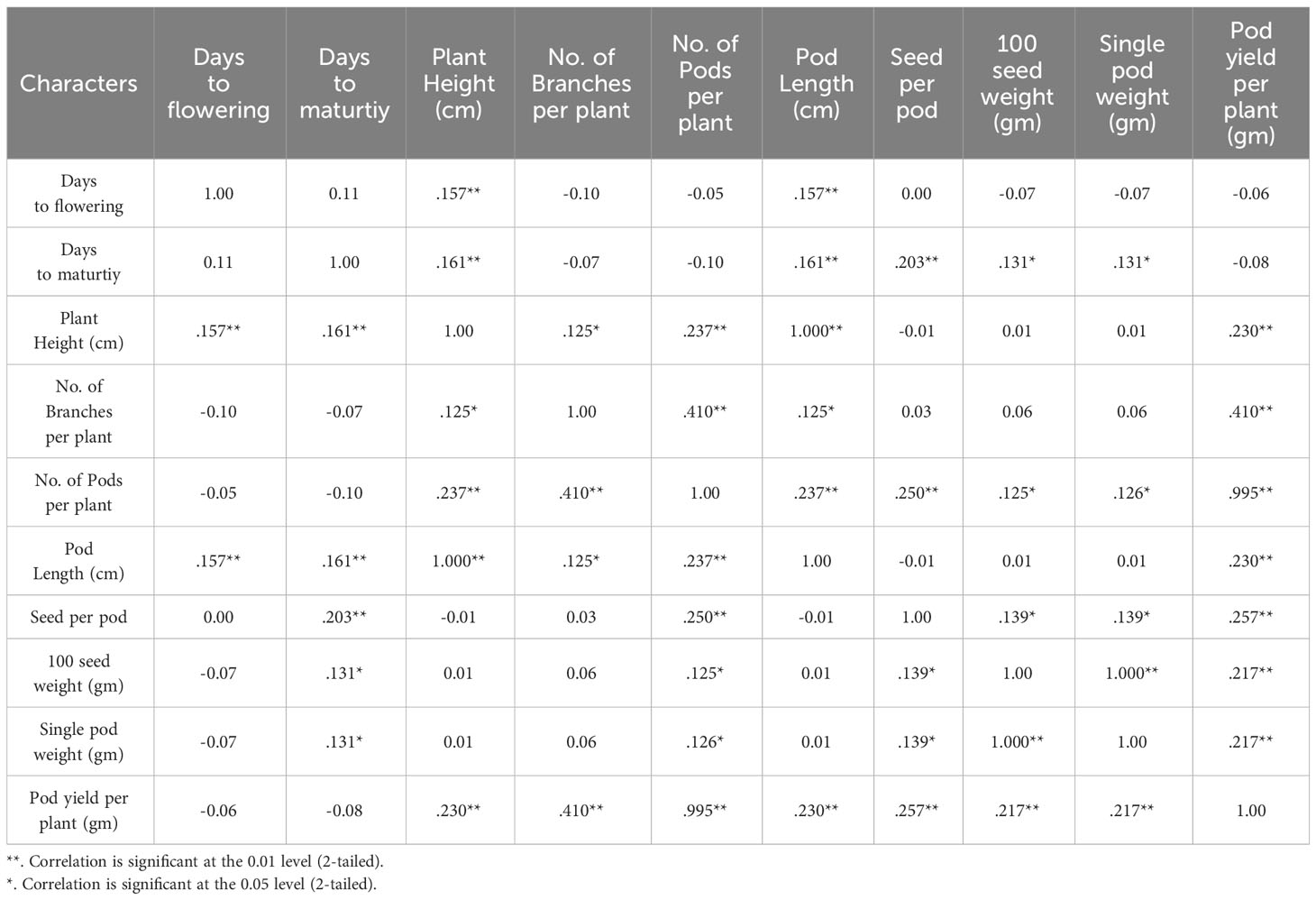
Table 5 Pearson's correlation coefficients of different quantitative traits in the French bean local cultivar.
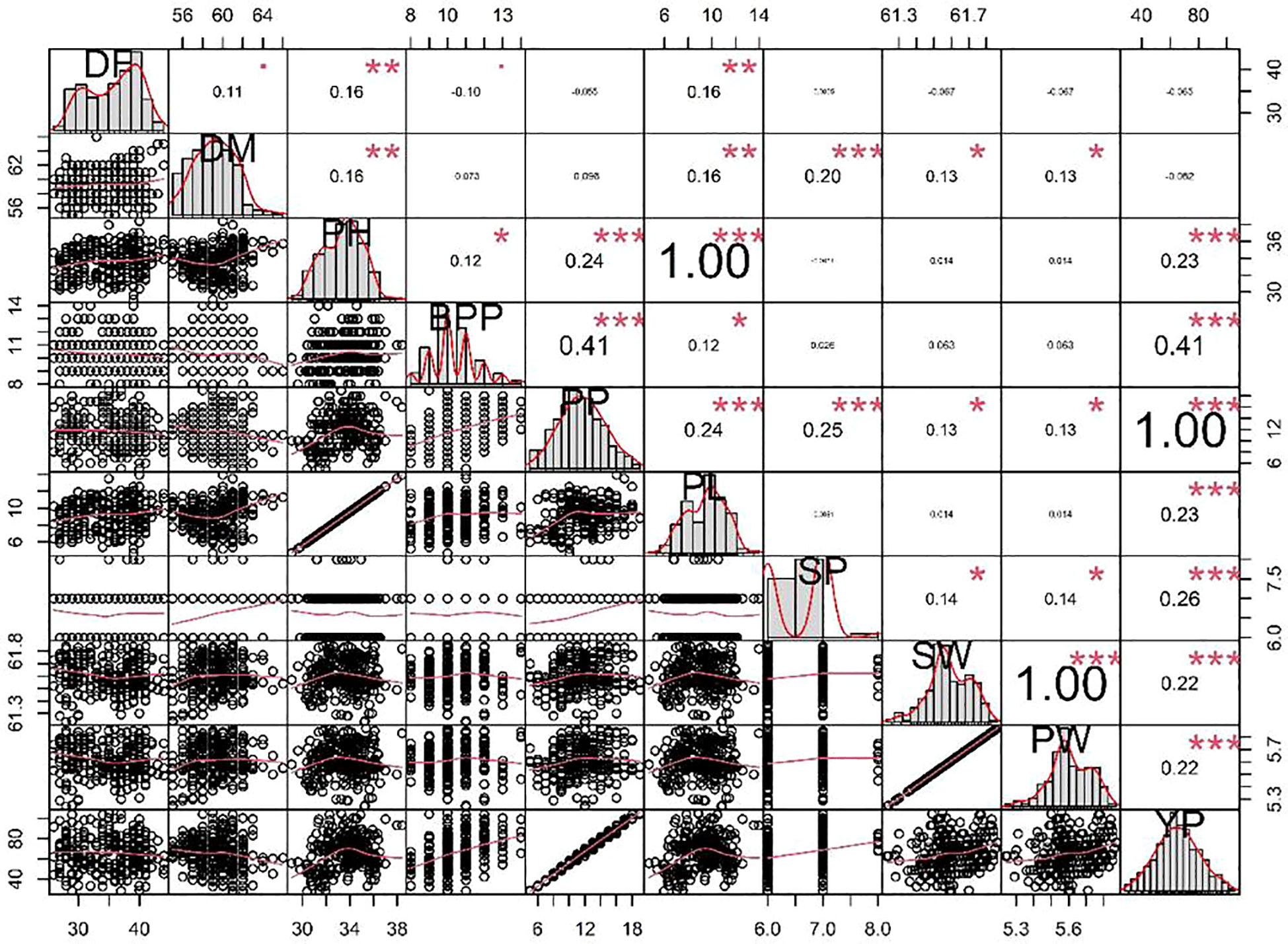
Figure 1 Character association analysis of different quantitative traits in the French bean local cultivar. *DF, Days to flowering; DM, Days to maturity; PH, plant height; BPP, branches per plant; PP, pods per plant; PL, pod length; SP, seed per pod; SW, seed weight; PW, single pod weight; YP, Yield per pod. “*”, “**” and “***” represent significance at p < 0.05, p < 0.01, and p <0.001, respectively.
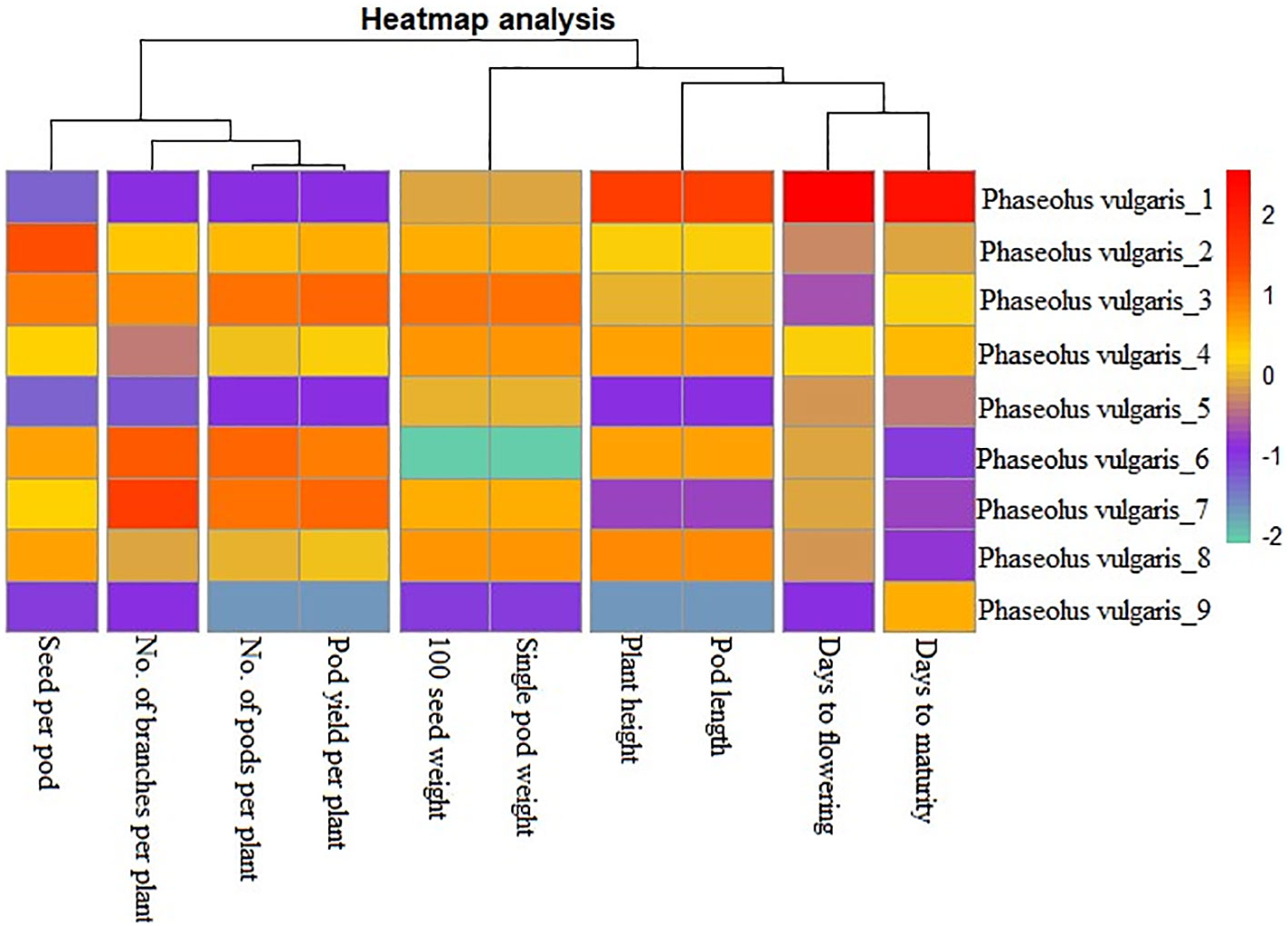
Figure 2 Heatmap analysis for the character association study on ten quantitative traits of French bean cultivar in M2 generation. Phaseolus vulgaris_1 : Control; Phaseolus vulgaris_2-5: EMS 0.1-04% and Phaseolus vulgaris_6-9: SA 0.1-0.4%.
Conclusion
In conclusion, our induced mutagenesis program aimed at broadening the genetic base of locally grown French bean genotypes has yielded valuable insights and promising outcomes. The exposure of seeds to varying doses of ethyl methane sulfonate (EMS) and sodium azide (SA) resulted in a notable increase in the average measurements of agronomic characteristics, signifying a potential for enhanced yield. The observed non-specific and dosage-independent decrease in biophysiological characteristics in French bean mutants, particularly the significant inhibition in germination and fertility at mutagenic doses, offers valuable information on the impact of mutagenic treatments. Additionally, the M2 generation showcased substantial improvements in both yield and associated traits within the French bean cultivar, registering the highest pod yield per plant under the 0.2% EMS treatment. The character association study uncovered robust correlations between pod yield and specific traits, providing a strategic approach to selecting mutants within treated populations influenced by EMS and SA, thus significantly enhancing crop yield. The M2 mutant populations treated with 0.2% SA and 0.2% EMS displayed the highest induced variability, positioning them as prime candidates for selecting higher-yielding mutant lines for advancement in subsequent breeding generations. This initiative holds great promise in meeting the increasing demand for French bean-based products, thereby contributing to the sustainable enhancement of French bean cultivation and addressing the nutritional needs of diverse communities. The comprehensive findings from this study provide a valuable foundation for further research and practical applications in the field of French bean improvement and productivity.
Data availability statement
The original contributions presented in the study are included in the article/supplementary material. Further inquiries can be directed to the corresponding authors.
Author contributions
RL: Conceptualization, Writing – original draft, Writing – review & editing. BD: Data curation, Visualization, Writing – review & editing. DT: Formal analysis, Writing – review & editing. SD: Writing – review & editing. PB: Writing – review & editing. AR: Methodology, Writing – review & editing.
Funding
The author(s) declare that no financial support was received for the research, authorship, and/or publication of this article.
Acknowledgments
The authors are thankful to the Dr. Prasanna Kumar Dutta, Principal, Bahona College, Jorhat, Assam, India for providing necessary facilities during this research work. The authors are also thankful to the Institutional Biotech-Hub (IBH), Bahona College for providing laboratory facility for the analysis.
Conflict of interest
The authors declare that the research was conducted in the absence of any commercial or financial relationships that could be construed as a potential conflict of interest.
The author(s) declared that they were an editorial board member of Frontiers, at the time of submission. This had no impact on the peer review process and the final decision.
Publisher’s note
All claims expressed in this article are solely those of the authors and do not necessarily represent those of their affiliated organizations, or those of the publisher, the editors and the reviewers. Any product that may be evaluated in this article, or claim that may be made by its manufacturer, is not guaranteed or endorsed by the publisher.
References
Amin R., Wani M. R., Raina A., Khursheed S., Khan S. (2019). Induced morphological and chromosomal diversity in the mutagenized population of black cumin (Nigella sativa L.) using single and combination treatments of gamma rays and ethyl methane sulfonate. Jordan J. Biol. Sci. 1, 12.
Arnon D. I. (1949). Copper enzymes in isolated chloroplast polyphenoloxidase in Beta vulgaris. Plant Physiol. 24, 1–15. doi: 10.1104/pp.24.1.1
Arumuganthan K., Earle E. (1991). Nuclear DNA content of some important plant species. Plant Mol. Biol. Rep. 9, 208–218. doi: 10.1007/BF02672069
Bhawna, Bonthala V. S., Gajula M. P. (2016). PvTFDB: a Phaseolus vulgaris transcription factors database for expediting functional genomics in legumes. Database. 2016, baw114. doi: 10.1093/database/baw114
Blair M. W., Porch T., Cichy K., Galeano C. H., Lariguet P., Pankhurst C., et al. (2007). Induced mutants in common bean (Phaseolus vulgaris), and their potential use in nutrition quality breeding and gene discovery. Israel J. Plant Sci. 55 (2), 191–200. doi: 10.1560/IJPS.55.2.191
Blixt S., Gottschalk W. (1975). Mutation breeding in leguminosae. Agri HortiqueGenetica 33 (1-4), 33–85.
Borzouei A., Kafi M., Khazaei H., Naseriyan B., Majdabadi A. (2010). Effects of gamma radiation on germination and physiological aspects of wheat (Triticum aestivum L.) seedlings. Pak. J. Bot. 42 (4), 2281–2290.
Boysen G., Pachkowski B., Nakamura J., Swenberg J. (2009). The formation and biological significance of N7-guanine adducts. Mutat. Res. 678, 76–94. doi: 10.1016/j.mrgentox.2009.05.006
Broughton W. J., Hernández G., Blair M. W., Beebe S. E., Gepts P., Vanderleyden J. (2003). “Beans (Phaseolus Spp.) – Model food legumes,” in Plant and soil. 252, 55–128. doi: 10.1023/a:1024146710611
Chen L., Duan L., Sun M., Yang Z., Li H., Hu K., et al. (2023). Current trends and insights on EMS mutagenesis application to studies on plant abiotic stress tolerance and development. Front. Plant Sci. 13. doi: 10.3389/fpls.2022.1052569
Colquhoun T. A., Kim J. Y., Wedde A. E., Levin L. A., Schmitt K. C., Schuurink R. C., et al. (2011). PhMYB4 fine-tunes the floral volatile signature of petunia × hybrida through PhC4H. J. Exp. Bot. 62, 1133–1143. doi: 10.1093/jxb/erq342
Cruz C. D., Regazzi A. J., Carneiro P. C. S. (2012). Modelos Biometricos Aplicados ao Melhoramento genetico (UFV, Vi¸cosa).
FAOSTAT (2023). Food and agriculture organization statistics database. Available at: https://www.fao.org/faostat/en/#data (Accessed 21/10/2023).
Freytag G. F., Debouck D. G. (2002). Taxonomy, distribution, and ecology of the genus phaseolus (Leguminosae-papilionoideae) in North America, Mexico and Central America. Botanical Research Institute of Texas (BRIT), Forth Worth, TX, USA. 298 p. (Sida, botanical miscellany no. 23). Available at: https://hdl.handle.net/10568/54291.
Fukuzawa K., Inokami Y., Terao J., Suzuki A. (1998). Rate constants for quenching singlet oxygen and activities for inhibiting lipid peroxidation of carotenoids and a-tocopherol in liposomes. Lipids 3, 751–756. doi: 10.1007/s11745-998-0266-y
García-Fernández C., Campa A., Soler Garzón A., Miklas P. N., Fernández J. J.F. (2021). GWAS of pod morphological and color characters in common bean. BMC Plant Biol. 21, 184. doi: 10.1186/s12870-021-02967-x
Gentry H. S. (1969). Origin of the common bean, Phaseolus vulgaris. Economic Bot. 23 (1), 55–69. doi: 10.1007/BF02862972
Gepts P., Moore P. H., Aragão F. J. L., Barros E., Blair M. W., Brondani R., et al. (2008). “Genomics of tropical crop plants. genomics of phaseolus beans, a major source of dietary protein and micronutrients in the tropics,” in Plant genetics and genomics: Crops and models. Eds. Moore P. H., Ming R. (New York: Springer), 113–143.
Gruszka D., Szarejko I., Maluszynski M. (2012). “Sodium azide as a mutagen,” in Plant mutation breeding and biotechnology. Eds. Shu Q. Y., Forster B. P., Nakagawa H. (Wallingford: CABI), 159–166.
Hashimoto H., Uragami C., Cogdell R. J. (2016). Carotenoids and photosynthesis. Carotenoids Nat. 79, 111–139. doi: 10.1007/978-3-319-39126-7_4
Horn L. N., Ghebrehiwot H. M., Shimelis H. A. (2016). Selection of novel cowpea genotypes derived through gamma irradiation. Front. Plant Sci. 7, 262. doi: 10.3389/fpls.2016.00262
Khursheed S., Raina A., Parveen K., Khan S. (2016). Improvement of Yield and Mineral Content in Two Cultivars of Vicia faba L Through Physical and Chemical Mutagenesis and their Character Assoc. Anal. Arch. Curr. Res. Intl. 4 (1), 1–7. doi: 10.9734/ACRI/2016/24802
Khursheed S., Raina A., Parveen K., Khan S. (2019). Induced phenotypic diversity in the mutagenized populations of faba bean using physical and chemical mutagenesis. J. Saudi Soc Agric. Sci. 18, 113–119. doi: 10.1016/j.jssas.2017.03.001
Kumar S., Singh M., Malhotra N., Saha A. J., Jambhulkar S., Sharma J. P., et al. (2021). Induced Mutants in Locally Adapted Landraces of French Bean (Phaseolus vulgaris L.), their Mutagenic Sensitivity and Mutability for Crop Improvement. Acta Sci. Agric. 5 (12), 10–16.
Laskar R. A., Khan S. (2017). Assessment on induced genetic variability and divergence in the mutagenized lentil populations of microsperma and macrosperma cultivars developed using physical and chemical mutagenesis. PloS One 12 (9), e0184598. doi: 10.1371/journal.pone.0184598
Li Y., He N., Hou J., Xu L., Liu C., Zhang J., et al. (2018). Factors influencing leaf chlorophyll content in natural forests at the biome scale. Front. Ecol. Evol. 6. doi: 10.3389/fevo.2018.00064
Mahamune S. E., Kothekar V. S. (2012). Induced chemical and physical mutagenic studies in M1 generation of French bean (Phaseolus vulgaris L.). Curr. Bot. 3 (3), 17–21.
Mullins A. P., Arjmandi B. H. (2021). Health benefits of plant-based nutrition: focus on beans in cardiometabolic diseases. Nutrients 13, 519. doi: 10.3390/nu13020519
Nichterlein K. (1999). The role of induced mutations in the improvement of common beans (Phaseolus vulgaris L.) Mutat. Breed. Newsl. 44, 6–9.
Porch T., Beaver J. S., Debouck D. G., Jackson S. A., Kelly J. D., Dempewolf H. (2013). Use of wild relatives and closely related species to adapt common bean to climate change. Agronomy 3 (2), 433–461. doi: 10.3390/agronomy3020433
Raina A., Khan S. (2023). Field assessment of yield and its contributing traits in cowpea treated with lower, intermediate, and higher doses of gamma rays and sodium azide. Front. Plant Sci. 14. doi: 10.3389/fpls.2023.1188077
Raina A., Laskar R. A., Tantray Y. R., Khursheed S., Wani M. R., Khan S. (2020). Characterization of induced high yielding cowpea mutant lines using physiological, biochemical and molecular markers. Sci. Rep. 10(1). doi: 10.1038/s41598-020-60601-6
Ramandeep, Dhillon T. S., Dhall R. K., Gill B. S. (2018). Effect of mutagen-ethyl methane sulphonate on yield increasing parameters of french bean (Phaseolus vulgarisl.). Genetika 50, 1 (199–1 207). doi: 10.2298/GENSR1801199R
Ramel F., Birtic S., Ginies C., Soubigou-Taconnat L., Triantaphylidès C., Havaux M. (2012). Carotenoid oxidation products are stress signals that mediate gene responses to singlet oxygen in plants. Proc. Natl. Acad. Sci. 109 (14), 5535–5540. doi: 10.1073/pnas.1115982109
Ramesh M., Muniswamy S., Yamanura (2016). Correlation and path analysis in recombinant inbred lines (RILs) of wheat (Trticum aestivum l.). J. Appl. Natural Sci. 8 (2), 826–832. doi: 10.31018/jans.v8i2.880
Rasik S., Raina A., Laskar R. A., Wani M. R., Reshi Z. A., Khan S., et al. (2022). Lower doses of Sodium azide and Methyl methane sulphonate improved yield and pigment contents in vegetable cowpea [Vigna unguiculata (L.) Walp.]. South Afr. J. Botany 148, 727–736. doi: 10.1016/j.sajb.2022.04.034
Saha P., Raychaudhuri S. S., Chakraborty A., Sudarshan M. (2010). PIXE analysis of trace elements in relation to chlorophyll concentration in plantago ovata forsk. Appl. Radiat. Isotopes 68 (3), 444–449. doi: 10.1016/j.apradiso.2009.12.003
Sakai K. I., Suzuki A. (1964). Induced mutation and pleiotropy of genes responsible for quantitative characters in rice. Radiat. Bot. 4, 141–151. doi: 10.1016/S0033-7560(64)80065-X
Sawettalake N., Bunnag S., Wang Y., Shen L., Yu H. (2017). DOAP1 promotes flowering in the orchid Dendrobium Chao Praya Smile. Front. Plant Sci. 8, 400. doi: 10.3389/fpls.2017.00400
Shin J.-M., Kim B., Seo S., Jeon S. B., Kim J., Jun B., et al. (2011). Mutation breeding of sweet potato by gamma radiation. Afr. J. Agric. Res. 6 (6), 1447–1454.
Shinoyama H., Sano T., Saito M., Ezura H., Aida R., Nomura Y., et al. (2012). Induction of male sterility in transgenic chrysanthemums (Chrysanthemum morifolium Ramat.) by expression of a mutated ethylene receptor gene, cm-ETR1/H69A, and the stability of this sterility at varying growth temperatures. Mol. Breed 29, 285–295. doi: 10.1007/s11032-010-9546-6
Shu Q. Y. (2009). Induced plant mutations in the genomics era (Rome: Food and Agriculture Organization of the United Nations). Available at: https://www.fao.org/3/i0956e/i0956e00.html.
Sood M., Pathania N. K. (2014). Gene effects for pod yield and related traits in French bean (Phaseolus vulgaris L.) population developed through induced mutation. Am. Int. J. Res. Formal Appl. Natural Sci. 2 (6), 109–113.
Swiader J. M., Ware G. M., Mc colum J. P. (1992). Producing vegetable crops. 4th ed (Danville, Ilions, USA: Interstate publishers, Inc), 233–249.
Tanaka R., Tanaka A. (2007). Tetrapyrrole biosynthesis in higher plants. Annu. Rev. Plant Biol. 58, 321–346. doi: 10.1146/annurev.arplant.57.032905.105448
Toker C., Cagirgan M. I. (2004). The use of phenotypic correlations and factor analysis in determining characters for grain yield selection in chickpea (Cicer arietinum l.). Hereditas 140, 226–228. doi: 10.1111/j.1601-5223.2004.01781.x
Tomlekova N., Panayotov N., Kozgar M. I., Wani M. R., Serdaris P., Balacheva E. (2014a). Inducing and exploring new mutant donors of tomato. Mutagenesis: exploring genetic diversity of crops (The Netherlands: Wageningen Academic Publishers), 283–306. doi: 10.3920/9789086867967_016
Tomlekova N. B. (2014b). 21. Use of mutant genes for exploring and altering carotenoid biosynthetic pathway of sweet pepper: the Bulgarian viewpoint. Mutagenesis: exploring novel genes and pathways (The Netherlands: Wageningen Academic Publishers), 433–454. doi: 10.3920/978-90-8686-787-5_21
Verma A. K., Banerji B. K., Chakrabarty D., Datta S. K. (2010). Studies on makhana (Euryale ferox Salisbury). Curr. Sci. 99, 795–800.
Wanga M. A., Kumar A. A., Kangueehi G. N., Shimelis H., Horn L. N., Sarsu F., et al. (2018). Breeding sorghum using induced mutations: future prospect for Namibia. Am. J. Plant Sci. 9, 2696–2707. doi: 10.4236/ajps.2018.913196
Keywords: French bean (Phaseolus vulgaris L.), ethyl methane sulfonate (EMS), sodium azide (SA), yield improvement, local cultivar, induced mutagenesis, quantitative traits, character association
Citation: Laskar RA, Dowarah B, Tamang D, Das S, Borah P and Raina A (2024) Improving French bean yield potential through induced mutagenesis using EMS and SA. Front. Hortic. 2:1288720. doi: 10.3389/fhort.2023.1288720
Received: 04 September 2023; Accepted: 22 December 2023;
Published: 22 January 2024.
Edited by:
Samuel Kwame Offei, University of Ghana, GhanaReviewed by:
Mukesh Choudhary, ICAR-Indian Institute of Maize Research, IndiaHans Adu-Dapaah, Council for Scientific and Industrial Research (CSIR), Ghana
Copyright © 2024 Laskar, Dowarah, Tamang, Das, Borah and Raina. This is an open-access article distributed under the terms of the Creative Commons Attribution License (CC BY). The use, distribution or reproduction in other forums is permitted, provided the original author(s) and the copyright owner(s) are credited and that the original publication in this journal is cited, in accordance with accepted academic practice. No use, distribution or reproduction is permitted which does not comply with these terms.
*Correspondence: Rafiul Amin Laskar, cmFmaWhrZEBnbWFpbC5jb20=; Aamir Raina, YWFtaXI4NTRAZ21haWwuY29t