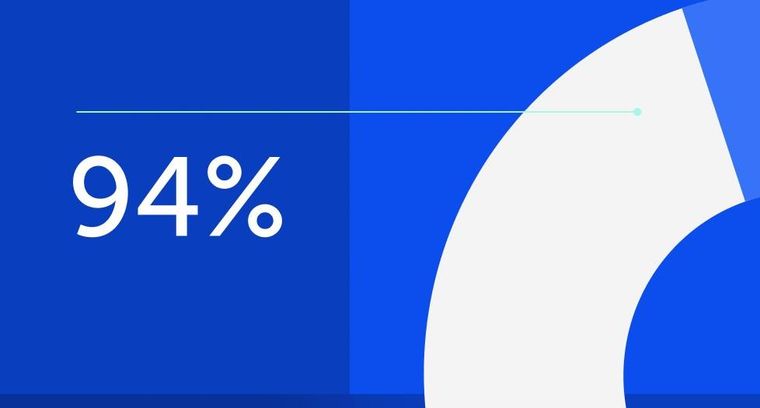
94% of researchers rate our articles as excellent or good
Learn more about the work of our research integrity team to safeguard the quality of each article we publish.
Find out more
ORIGINAL RESEARCH article
Front. Hortic., 03 October 2023
Sec. Sustainable Pest and Disease Management
Volume 2 - 2023 | https://doi.org/10.3389/fhort.2023.1175168
This article is part of the Research TopicEpidemiology and Management of Pome Fruit DiseasesView all 9 articles
Within integrated apple scab control there is a strong focus on reduction of Venturia inaequalis primary inoculum. The hypothesis that leaf shredding as an orchard sanitation practice would reduce the effective population size of the fungus (resulting in lower genetic variation due to reduction in sexual offspring) was tested. Assuming the allele causing fungicide resistance is already present in the population, it will be widely distributed at the end of the season, since selection occurs when the demethylation inhibitor (DMI) fungicide was applied. For short-term disease management a reduction of inoculum size, (i.e. potential ascospore dose) is most important. In the long-term resistant isolates/genotypes would be less likely to survive the winter and/or to infect in the spring, if that inoculum (i.e. in fallen leaves) has been removed. To sustain the use of highly effective synthetic fungicides, such as the DMIs, fungicide resistance management practices have to be evaluated. Fungicide resistance, which negatively affects pathogen fitness, is hypothetically reversible, if the selection pressure by the fungicide is removed. This study quantified the effect of leaf shredding on changes in the pathogen’s flusilazole sensitivity and population genetic structure using SSR markers. Venturia inaequalis populations in orchard trials, where sanitation practices had been applied, were tested for flusilazole sensitivity in planta and in vitro. Significant shifts towards flusilazole resistance were identified in orchards with a history of DMI application without sanitation treatment, with a mean sensitivity of EC50= 0.208 ug/ml (n=49) compared to an unexposed V. inaequalis population (EC50= 0.104 ug/ml, n=55). However, the isolates from the same sanitation trial orchards, from leaf shredding treatment in combination with a fungicide spray programme, had a mean EC50 of 0.110 ug/ml (n=41), similar to an unexposed V. inaequalis population. Furthermore, V. inaequalis offspring after sanitation treatment, showed shifts in microsatellite allele frequency distribution patterns used as an indicator of sexual reproduction. This study concludes that sanitation treatments, i.e. leaf shredding, impact on fungicide sensitivity and therefore effectively contributes to fungicide resistance management.
Apple scab caused by the ascomycete fungus Venturia inaequalis (Cooke) G. Wint. 1875 is responsible for large crop losses around the world, including South Africa, and is controlled by fungicide applications throughout the growing season (MacHardy, 1996). Despite the application of extensive spray programmes and the utilisation of multiple classes of contact and systemic fungicides, shifts towards fungicide resistance have been reported for V. inaequalis (Smith et al., 1991). This is also evident in South Africa (Schwabe et al., 1984; Schwabe et al., 1997). The development of demethylation inhibitor (DMI) fungicide resistance is a gradual process as multiple mutations have to be acquired (in multiple genes) for practical resistance to occur; hence, it is termed quantitative or directional resistance (Köller and Scheinpflug, 1987; Shirane et al., 1996; Köller et al., 1997; Schnabel and Jones, 2001; Schnabel and Jones, 2001; Cowen and Lindquist, 2005; Hoffmeister et al., 2021). For purposes of fungicide resistance management, it has therefore been recommended that fungicide sensitivity should be monitored. Furthermore, it is recommended to use fungicides, which are at risk of resistance development in the pathogen population, in conjunction with disease management practices to reduce inoculum pressure (Kunz et al., 1997; Holb and Kunz, 2016). Urea application has been used in Europe as standard orchard hygiene practice to reduce overwintering inoculum (Burchill, 1968). In South Africa, urea application was not found to have any effect on apple scab incidence (Schwabe and Matthee, 1972; Von Diest et al., 2016). Similarly, Mac an tSaoir et al. (2010) reported urea application to be ineffective in inhibiting V. inaequalis pseudothecia formation in Ireland, indicating that coastal regions with mild winters and extended leaf drop periods are unsuitable for this management strategy. Urea application to reduce primary V. inaequalis inoculum has therefore not been adopted in South Africa but should be further investigated in combination with defoliants (Burchill, 1968; Von Diest et al., 2016).
As part of an integrated management approach, cultural orchard practices, such as leaf shredding or the removal of fallen leaves for inoculum reduction, were found to have an impact on infection levels due to the increase in microbial activity, enabling leaf degradation and therefore preventing pseudothecia formation during winter. (Sutton et al., 2000; Holb, 2008; Von Diest et al., 2016). Dormant copper sprays and lower demethylation inhibitor (DMI) application frequencies have also been shown to cause reduced shifts towards DMI resistance (Pfeufer and Ngugi, 2012). Nevertheless, the use of copper sprays is not recommended due to the environmental and health risks associated with the use of this chemical (Holb and Kunz, 2016).
Despite studies that have shown the occurrence of DMI-resistant genotypes, their persistence in orchard environments has not been fully established yet. Other studies indicated that once resistant isolates can be detected in the orchard environment, this indicates that DMI resistance is present as a stable inherited trait with a fitness equal to the sensitive strains (Stanis and Jones, 1986). Fungicide resistance to benzimidazoles or strobilurins, for example, is known to persist in pathogen populations from orchards, while dicarboximide resistance was shown to be a less stable trait, which is lost once the fungicide use has been discontinued in the affected orchards (Shabi and Kaian, 1980; Northover, 1988; Fiaccadori, 2018).
Growth and sporulation of resistant isolates in artificial media has previously been regarded as a fitness measure in studies on the fungicide resistance of V. inaequalis (Stanis and Jones, 1986). Other factors mentioned in the study include abortion factors, which are associated with aborted spores after sexual crosses of isolates have been made, but these have not been reported for DMI-resistant isolates.
The prevention of sexual crosses through orchard sanitation treatments (such as the removal of fallen leaves) would have a similar effect as it reduces the chance of mating in V. inaequalis and results in fewer ascospores being dispersed as aerial primary inoculum (Sutton et al., 2000; Meitz-Hopkins et al., 2014). Until now, the effect of sanitation treatments on improving fungicide sensitivity levels or reversing resistance build-up has not been quantified.
The DMIs, such as the triazole group of fungicides, target a 14-α-demethylase utilized by the fungus during ergosterol biosynthesis (Joseph-Horne and Hollomon, 1997). Mechanisms previously reported to be associated with shifts towards DMI resistance due to the gradual predominance of resistant populations, include overexpression of the 14-α-demethylase gene (due to multiple promoter insertions at the CYP51A1 locus), modified efflux through ATP-binding cassette (ABC)-transporters, alternative modes of sterol biosynthesis, or changes in membrane composition (Nakaune et al., 1998; Schnabel and Jones, 2001; Cowen et al., 2002; Corkley et al., 2022). Van den Bosch et al. (2011) reviewed the dose-dependent development of DMI fungicide resistance. Models calculating the risk of fungicide resistance indicated that different resistance management strategies would have to be applied depending on the stage of resistance development (emergence, selection, or adjustment). After the emergence phase, the mutation responsible for fungicide resistance increases in a population through selection (if a fungicide of the same mode of action is applied) or genetic drift and eventually leads to a large fraction of the population carrying the mutations conferring fungicide resistance. Strategies such as alternating or mixing fungicide actives of different mode-of-action groups only defer the shifts towards resistance in a population and do not effectively prevent resistance from developing and therefore are not considered an effective anti-resistance strategy. Van den Bosch et al. (2011) concluded that most studies reported practical resistance towards DMI fungicides emerges when higher doses of fungicides were applied. Nevertheless, the authors said that more work was needed to assess partial fungicide resistance development in fungal populations.
Koopman et al. (2022) reported a highly variable ABC2 gene fragment in the South African apple scab population, although its effects on DMI resistance were not evaluated. Triazole resistance has also been found to be closely coupled with the ability of the fungus to accumulate the molecular chaperone HSP90, which in turn stabilises stress-mitigating signal transducers and membrane components (Cowen and Lindquist, 2005; Vincent et al., 2013). These multiple mechanisms contributing to resistance development result in a stepwise adaptation, which may explain the quantitative shift towards DMI resistance, i.e., the selection of multiple insertions in the CYP51A1 promoter (14-α-demethylase gene) in combination with variants capable of HSP90 overexpression and, possibly, stabilising modified ABC efflux (Joseph-Horne and Hollomon, 1997).
Multiple applications of one fungicide class in an orchard during the same growing season are thought to act as a selection pressure on the pathogen population, leaving insensitive isolates to multiply in the next asexual cycle, while a lower fungicide application frequency might prolong the lifespan of the fungicide (Van den Berg et al., 2016). This was particularly problematic if DMI fungicides were applied curatively to save cost (Beckerman et al., 2014).
The effect of leaf shredding on the reduction in inoculum size of South African V. inaequalis populations has been clearly established (Von Diest et al., 2016). In this study, the objectives were a. to measure the effect of inoculum reduction on the fungal population in terms of sensitivities to DMI fungicides and b. to assess genetic variation using microsatellite genotype analysis. The in vitro DMI sensitivity shifts of the isolates from standard spray programmes were compared with the isolates from integrated programmes, including leaf shredding or removal, and fungicide efficacy in these different orchard populations was also quantified in inoculation trials in the glasshouse (in planta).
Apple scab samples were collected from sanitation trial orchards located more than 100 km apart at Elgin and the Koue Bokkeveld in the Western Cape. The trial was part of a parallel study to test the effect of leaf shredding or removal on apple scab incidence and severity in a randomised block design, which was replicated within each of the two orchards (Von Diest et al., 2016). Orchard treatments comprised two non-treated orchard sections (N), a leaf shredding treatment (LS), leaf shredding plus (mancozeb) fungicide application (LS+S), and (mancozeb) fungicide application alone (S). Apple and leaf lesions were collected from the Koue Bokkeveld (cultivar ‘Early Red’) and Elgin orchards (cultivar ‘Braeburn’) in spring (November 2011, 2012, and 2013) during the first appearance of apple scab symptoms on 2-cm-long fruitlets from the middle row of five in a high-density orchard (1 m distance between trees, 3 m between rows). The orchards had a history of high apple scab incidence, despite extensive fungicide programmes, including mancozeb and DMIs among other fungicide groups. At the Elgin site, the spray programme that was applied during the season before the start of the trial included one application of DMI fungicide (difenoconazole). Furthermore, the eradicative application of flusilazole in the orchard after harvest to curb leaf infections was common practice (Schwabe, 2015). At the Koue Bokkeveld site, one DMI application (active ingredient difenoconazole) was part of the standard spray programme before the start of the sanitation trial. During the trial, flusilazole and difenoconazole were applied once in season 2011/2012, and flusilazole alone was applied three times in season 2011/2012, and in the following season, both flusilazole and difenoconazole were applied three times each in the blocks with the standard spray programme (control treatment).
Venturia inaequalis conidiospores (from 30 leaves per orchard section) were multiplied on MM109 trees in the glasshouse through spray inoculation and stored as infected leaf samples at –80°C for further use in glasshouse inoculations (in planta trials). Spore concentrations were standardised to 1.5 × 105 spores/ml before inoculation. Orchard sections with leaf shredding treatment (either alone [LS] or in combination with fungicide spray application [LS + S]) were sampled in all seasons. The leaf shredding sanitation treatment was conducted as described by Von Diest et al. (2016). Briefly, leaf shredding involved manually raking leaves from under the trees to the middle of the tractor lane at 100% leaf drop (no leaves remaining on the tree), followed by shredding to a fine mulch using a Nobili RM 155 triturator mulcher (Southtrade, Cape Town South Africa). In the 2011 season, an additional treatment section (leaf removal, LR) was sampled. Leaf removal also involved raking leaves from under the trees to the middle of the tractor lanes followed by physical removal of the leaves from the orchard to be buried underground, away from the orchard. The LR treatment was not repeated in the following seasons due to grower concern about the removal of this source of organic matter from the orchard. Orchard sections treated with standard fungicide spray programmes (S) were used as a positive control treatment.
A V. inaequalis population from an orchard in Vyeboom (VB) with DMI resistance (field resistance) from a previous fungicide trial was used as an additional positive control (courtesy of Dr. Wolf Schwabe). Spray programmes applied in the Elgin and Koue Bokkeveld orchard sections during the trial included mancozeb only, at Elgin, while in the Koue Bokkeveld orchard, applications of the DMI difenoconazole, an anilinopyrimidine (cyprodinil), the anthraquinone dithianon, an adenosin deaminase inhibitor (bupirimate), and the dithiocarbamate mancozeb were used as a standard spray programme. Isolates from an abandoned orchard near Greyton (G) in the Western Cape (n=55) were analysed as the reference baseline population in vitro. This orchard had been out of commercial production for over 8 years.
Single sporing of apple scab samples was conducted in preparation for fungicide sensitivity tests in Petri dishes and DNA extractions for genotyping by plating out spore suspensions on 2% water agar. Samples (n=306) were collected from apple and leaf lesions from orchards in the Western Cape representing V. inaequalis isolates from sanitation trial orchard populations from the cultivars ‘Fuji’ and ‘Top Red’ in two climatically different geographic regions (Elgin and Koue Bokkeveld) and different seasons (2011, 2012, and 2013) (Supplementary Table 1).
Monoconidial isolates (n=306) were grown for 6 weeks on potato dextrose agar including streptomycin (50 mg/l; PDA+). Agar plugs (4 mm in diameter) were then transferred in triplicate onto PDA (including streptomycin at 50 mg/l, tetracycline at 50 mg/l, and chloramphenicol at 50 mg/l). Technical grade flusilazole (#45753, Sigma-Aldrich) was dissolved in acetone and acetone levels in all fungicide-amended and control media were adjusted to 0.01%. To establish relative growth percentages compared with the unamended negative control of each isolate, mycelial plugs were plated on fungicide-amended media at five concentrations, namely 0.00, 0.01, 0.10, 1.00, and 2.00 mg/l (flusilazole). Additionally, some isolates from the orchard sections where standard spray programmes or sanitation treatments were applied were also tested at lower doses of 0.001, 0.002, 0.005, and 0.05 mg/l of flusilazole to test for hormetic effects. To establish baseline sensitivity, the V. inaequalis population from the abandoned orchard at the Greyton site was tested using the same five fungicide concentrations. Mycelial growth diameter was measured at perpendicular angles using a calliper after 3 weeks of incubation at 20°C. The average of six measurements (two measurements per agar plug) was used to calculate the relative growth (% RG) for each isolate in comparison with the unamended control (Smith et al., 1991).
DNA was extracted from 60 mg of mycelial samples (from single-spored isolates on PDA+) using buffer that contained polyvinylpyrrolidone (Sigma, PVP40) at 2%, 50 mM EDTA (pH 8.0), 100 mM Tris-HCl (pH 8.0), 500 mM NaCl, 0.25% sodium dodecyl sulphate, and 0.7% β-mercaptoethanol and included a phenol-chloroform purification step as described by Meitz-Hopkins et al. (2014). DNA samples were resuspended in 100 ul of TE0.1 (10 mM Tris-HCl and 0.1 mM EDTA, pH 8.0) and stored at −20°C.
Microsatellite markers (simple sequence repeats, SSR) were used for genotyping single-spored fungal isolates. Venturia inaequalis isolates (n=390) were collected from orchard isolates exposed to specific sanitation practices or fungicide treatment over two seasons to evaluate differences in population diversity. Primers for six previously characterised polymorphic loci (Table 1; Tenzer et al., 1999; Guérin et al., 2004; Celton et al., 2010; Koopman et al., 2017) were synthesised and forward primers were labelled with fluorescent dyes by Applied Biosystems (UK). The six loci were amplified in two multiplex PCR reactions (MP1 and MP2; Table 1) and tested for polymorphisms in two regional V. inaequalis populations (Elgin and Koue Bokkeveld). A touchdown PCR program with annealing temperatures decreasing from 55°C to 45°C by −0.5°C per cycle, as previously described, was used (Koopman et al., 2017). Each PCR reaction (25 μl) contained 1× Kapa buffer (Kapa Biosystems (Pty) Ltd., Cape Town, South Africa), 0.08 mM dNTPs, 1.5 mM MgCl2, and 0.625 U of Kapa HotStart Taq polymerase (Kapa Biosystems, DKAPKK5513), with primers for MP1 (0.04 µM 1tctg, 0.032 µM EmVi10, and 0.16 µM Vitc2/D) or MP2 (0.08 µM 1tc1a, 0.04 µM Vitg9/129, and 0.04 µM Vitg11/70). As described by Koopman et al. (2017), following PCR, 1 μl of diluted product was mixed with a LIZ 500 internal size standard/formamide mix (1/14) (Applied Biosystems). Sizing was performed on an ABI 3100 genetic analyser (Applied Biosystems), and alleles were estimated using Gene Mapper 3.0 (ABI Prism) and confirmed manually.
Table 1 Primers used in microsatellite analysis of six loci and the size range found in two South African V. inaequalis populations.
Microsatellite analysis was conducted on samples from the four orchard sections of two sanitation trial sites (2012/2013, Elgin [n=26] and Koue Bokkeveld [n=23]) using six highly polymorphic dinucleotide repeat SSR markers described above. As described by Koopman et al. (2017), alleles that appeared to have 1-bp differences in product size at one locus were sequenced to check for distinctiveness. Three additional markers (EmVi1, EmVi3, and Vitc1/2) that did not amplify well, possibly due to null alleles, were not used further for analysis; therefore, a reduced set of isolates were used for subsequent analyses for the 2013 dataset (Supplementary Table 2), while the full dataset was used for the multilocus genotype and principal coordinate analysis (PCoA).
In the initial analysis, only 2012/2013 data from sanitation trial orchard sections were used as these represented the most complete set from the leaf shredding and combined LS_+S sanitation treatments. For structure analysis, data from the previous and the following seasons (mainly orchard blocks with a standard spray programme) were also analysed (2011/2012, Elgin [n=17] and KB [n=29]; 2013/2014, Elgin [n=144] and KB [n=158]) and a small set of isolates from the orchard with practical resistance (2006/2007; VB: n=7) were also included. All data were clone-corrected, and loci were tested for neutrality using the Ewen Watterson test in POPGENE version 3.2 (Manly, 1985; Yeh and Boyle, 1997).
GenAlex (v.6.501; Peakall and Smouse, 2012) was used to calculate Shannon diversity indices, number of alleles, and number of private alleles and to conduct the PCoA using total genetic differentiation between regional populations and isolates from different sanitation treatments, as described by Li et al. (2016). Furthermore, the amount and distribution of genetic variation within and among populations (pairwise population differentiation, FST) were determined using analysis of molecular variance (AMOVA, 999 permutations), which tested the null hypothesis of no genetic difference among the populations (ΦPT=0). A genotype accumulation curve was generated for the V. inaequalis populations from Elgin and Koue Bokkeveld to visualise the proportion of multilocus genotypes based on the number of loci sampled, as described by Kamvar et al. (2015).
The program bottleneck was used to estimate whether the population had undergone a recent severe reduction in effective population size under the stepwise mutation model (SMM; Cornuet and Luikart, 1996; Luikart et al., 1998; Piry et al., 1999). The standardised index of association (IA; Brown et al., 1980) was estimated for each regional population split into sanitation treatment subpopulations in LIAN vs.3.7 using the Monte Carlo simulation with 100,000 iterations and 20 replicates (Haubold and Hudson, 2000).
Multilocus genotype analysis was conducted using STRUCTURE vs. 2.3.4 to infer clusters according to the populations present using the six SSR markers analysed from clone corrected data (n=379) (Pritchard et al., 2000; Hubisz et al., 2009). Structure output (Q-matrix) was analysed as the mean of the estimated Ln probability of data divided by the standard deviation of the 20 replicates to estimate the appropriate number of populations (K) (Evanno et al., 2005; reviewed by Porras-Hurtado et al., 2013). A STRUCTURE bar plot was drawn using Distruct v.1.1 (Rosenberg, 2004; reviewed by Porras-Hurtado et al., 2013), assigning three regional populations (Elgin, Koue Bokkeveld, and Vyeboom) sampled over three seasons from varying orchard treatments [I. sanitation including leaf removal, or leaf shredding alone (SANI), compared with II. leaf shredding plus a spray programme (LS+S), III. a standard spray programme (S), and IV. non-treated (N)].
A subset of 20 V. inaequalis isolates from each sanitation trial orchard (Elgin and Koue Bokkeveld), representing different multilocus genotypes, was tested for promoter insertions, as described by Schnabel and Jones (2001), using a polymerase chain reaction with primers that specifically amplified the promoter insertion of the CYP51A1 gene (AJ455, 5′-CGACGGCGATGTTGTTTACGAGATGTA-3′; and AJ414, 5′-CTGCCAAGAAGAATGAAGG-TAAA-3′) in DMI resistant isolates. Schnabel and Jones (2001) reported that the CYP51A1 promoter region may contain insertions of 500 bp or 553 bp that contain additional promoter elements. The study also found that the 553 bp insertion has been associated with increased DMI resistance. Reaction mixtures (40 µl) contained 2 µl of DNA, 0.5 µM each primer, 200 µM dNTPs (#BIO-03925, Bioline, UK), 1.25 units of BioTaq DNA polymerase (#BIO-21040; Bioline; UK), 150 µM MgCl2, 1 mg/ml of BSA, 4 µl of 10× PCR Buffer, and PCR water (to make up to 40 µl). The PCR amplification was conducted in an ABI mini cycler PCR machine (Model GeneAmp PCR System 2700, Applied Biosystems, USA) and consisted of 94°C for 2 min followed by 35 cycles of 94°C for 30 s, 50°C for 1 min, and 72°C for 1 min. The PCR product was analysed using electrophoresis with 2% agarose gels in 1× Tris-acetate-EDTA (TAE) buffer (40 mM Tris base, 2 mM acetic acid, 2 mM EDTA, pH 8.0). The PCR products were scored as genotypes containing either a 500 bp insertion or a 553 bp insertion according to the product size identified on a 2% agarose gel stained with ethidium bromide (0.001% in the gel) and visualised under UV light.
MM109 trees were stored at 0–5°C until used in the glasshouse trial as described by Schwabe et al. (1984). Per experiment, 50 trees were grown for 4 weeks at 26°C (18 h day) and then inoculated with each V. inaequalis population sample (from the different orchard treatments described below) using a conidiospore suspension of 2×105 spores/ml on six tree replicates per fungicide treatment or non-treated control. The fungal populations used were collected at the following locations: positive control (VB) from a DMI field-resistant population from Vyeboom, standard spray programme (S) from Elgin and Koue Bokkeveld, sanitation treatment leaf removal (LR) from Koue Bokkeveld, and negative controls (N) from non-treated populations from Elgin and Koue Bokkeveld. Trees were left in an inoculation chamber while humidity was maintained at a high level by spraying water mist for 20 s every 30 min at 18°C for 48 h. Seventy-two hours after inoculation, the commercial fungicide formulation (active ingredient flusilazole) was applied at concentrations ranging from 1–40 mg/l a.i., until runoff, and ten trees were ‘mock-sprayed’ with water as a negative control. Plants were left to dry and placed in the glasshouse between 25°C and 28°C. Scab incidence and severity were evaluated after 3 weeks incubation in the glasshouse, as described by Kremer and Unterstenhöfer (1967). All leaves of the tree were evaluated (not just the susceptible three youngest ones) after 21 days in the glasshouse. Scoring of apple scab leaf infection severity was carried out according to a scale from 1 to 5 and calculated using the equation described by Kremer and Unterstenhöfer (1967).
Results from in planta and in vitro fungicide sensitivity tests were analysed by the Centre for Statistical Consultation at Stellenbosch University, using Statistica version 13.1 (Dell Inc., USA). To analyse in planta data, a linear mixed model analysis of variance (ANOVA) was used to compare the reduction in scab incidence and severity at four fungicide concentrations with the water-sprayed control trees. Tree replicates were included as a random effect. Treatments and regions were treated as fixed effects.
For in vitro data, the mean concentration to inhibit 50% mycelial growth (EC50) was determined for isolates from each treatment category. Log-logistic models were fitted using the R package drc (R Development Core Team 2005; Ritz and Streibig, 2006). Low concentrations caused a spike of growth in most isolates, which, for analysis, was capped at 100%. The mean EC50 was calculated per region or year of sampling or orchard sanitation treatment. The mean EC50 dataset of each orchard treatment was correlated against the apple scab incidence on leaves and fruit of week 44 of the 2012 season (Von Diest et al., 2016) in Statistica (Spearman, 1904), with Spearman correlations being reported as r and p values. Isolates were further categorised as sensitive or resistant to flusilazole on the basis of their relative growth (RG) on PDA amended with 1 μg/ml or 2 μg/ml of flusilazole. Isolates showing >20% RG on 1 μg/ml of flusilazole were classed as resistant. Mycelial growth of the two groups of isolates was compared on unamended medium to identify potential differences in the fitness of resistant phenotypes. The statistical significance of results from ANOVA of weighted EC50 means (due to varying sample size) using orchard treatments (sample origin) as fixed factors were further evaluated using a Games–Howell means post-hoc test.
Isolates that had been exposed to orchard sanitation treatments in` combination with a standard spray programme had a significantly (P= 0.07) lower flusilazole EC50 in the mycelial growth test. Data also indicated that in the Koue Bokkeveld orchard the spray programme was successful and eradicated resistant isolates, resulting in flusilazole sensitivity levels similar to the SA baseline from Greyton (mean EC50 = 0.104 μg/ml; Figure 1).
Figure 1 (A) Average EC50 (mg/ml) in box and whiskers plots of isolates from all treatments of the Elgin (E) orchard or Koue Bokkeveld (KB) or Greyton (G) orchards (LS, leaf shredding; LS+S, leaf shredding plus spray programme; N, non-treated negative control; S, standard spray programme) compared with NE organic orchard (baseline population from Greyton). (B) Relative growth differences at individual doses indicating hormesis (induced growth at low doses) visualised as crossing of the 100% relative growth (dashed) line; Sani, sanitation treatments such as leaf removal and leaf shredding (combined).
The fungal isolates were tested at a discriminatory dose of 1.00 µg/ml of flusilazole, resulting in two categories of fungicide sensitivity (either sensitive or shifted towards resistance). When a subset of the growth of 104 isolates was compared, there was no significant difference between sensitive and resistant isolates on unamended medium (no fungicide), with an average of 10.66 mm (±0.62 SD) in isolates categorised as sensitive vs. 10.87 mm (± 1.33) in isolates categorised as resistant. We concluded that sensitive isolates did not have a growth advantage over resistant isolates in the absence of the selection for resistance. At the discriminatory dose (1.0 μg/ml), flusilazole-sensitive isolates showed a mean growth of 0.14 mm (±0.10), while resistant isolates had a mean growth of 3.14 mm (±0.63). The mean growth of 84 isolates categorised as sensitive on medium amended with one-tenth flusilazole at 0.1 μg/ml had a mean diameter of 5.04 mm (±0.45) compared with the growth of 20 resistant isolates with a mean diameter of 6.98 mm (±0.65).
In a total of 306 isolates, 60 isolates (19.6%) from the four sampled locations in the Western Cape were resistant to flusilazole. Flusilazole sensitivity tests showed the highest incidence of isolates categorised as resistant in samples from the orchard at Vyeboom, where field resistance to DMIs had been reported (67%; n= 12), followed by the leaf removal treatment alone (36%, n= 22), the negative control (16%, n= 80), and the leaf shredding treatment alone (15%, n= 59). The lowest incidence of resistant isolates was found in the combined treatment of leaf shredding plus mancozeb (a non-DMI fungicide) application (7%, n=41) and in the baseline population from the abandoned orchard at Greyton (4%, n=55).
A significantly improved flusilazole sensitivity, as tested by EC50 estimation, could be seen in isolates from the LS+S treatment-orchard sections, where leaf shredding and fungicides had been applied (Table 2). The high level of fungicide sensitivity from isolates of the Koue Bokkeveld and Elgin orchards (pooled data) of the combined treatment of leaf shredding plus spray programme (LS+S) was not significantly different from the EC50 of the baseline population at the abandoned Greyton (G) orchard (EC50(LS+S)= 0.110 μg/ml vs. EC50(G)= 0.104 μg/ml). This EC50(LS+S) value of 0.110 μg/ml was also significantly lower than the EC50 values of isolates from the other treatments. Surprisingly, in season 2012/13, after 3 years of DMI omission, isolates from the KB orchard section, where only the standard spray programme (mancozeb) had been applied, also had a high DMI sensitivity (mean EC50 = 0.104 μg/ml), which was not significantly different from the abandoned orchard. In the KB orchard, an overall trend towards lower numbers of resistant isolates could be detected throughout the study (from season to season), while the numbers of resistant isolates dramatically increased in the Elgin orchard (Table 2).
Table 2 Flusilazole sensitivity (mycelial growth) of V. inaequalis isolates collected from Western Cape orchards at Vyeboom (VB), Elgin (E), Koue Bokkeveld (KB), and Greyton (G) (2007–2014)
A correlation could be seen between leaf scab incidence and leaf scab severity [r(leaf incidence) = 0.81, r(leaf severity)=0.79], but less significantly between fruit scab incidence (r=0.49, p=0.14) and severity (r=0.62, p=0.04), early in the apple growing season (week 44) in the 2012 season, when data from both orchards (Elgin and Koue Bokkeveld) were compared (Figures 2A, B).
Figure 2 (A, B) Correlation between leaf scab incidence (A) and leaf scab severity (B) and EC50 in orchard sections of the sanitation trial (combined data for leaf shredding, leaf removal, standard spray programme, and non-treated plots) from two Western Cape apple growing regions (Elgin and Koue Bokkeveld).
Seventeen out of 40 tested samples contained the 500 bp insertion in the promoter region, while only four contained the 553 bp insertion, previously associated with DMI resistance. No trend to a lower number of isolates containing such a resistance-conferring promoter insertion was observed in the population from the LS treatments; five out of 22 isolates in the sanitation treatment vs. one isolate out of nine from the (non-sanitation) control treatment orchard sections contained the 553 bp insert, while seven contained the 500 bp insertion, and one did not contain either insertion (subset of 15 genotyped samples in Supplementary Table 1).
Microsatellite markers were used to detect population differences in the isolates from orchard blocks receiving either the fungicide spray programme (S) or sanitation treatments (LR, LS, and LS + S) or no treatment (N). Six out of the nine tested microsatellite markers were found to be highly variable in the South African V. inaequalis populations, with gene diversity ranging from h=0.30 (low diversity) to h=0.84 (high diversity) and up to a total of 17 alleles per locus between the two regions (marker Vitc2/D; Table 3; Supplementary Figure 1). Clone correction identified 21 isolates (Table 4). Some of the identified clones represented a lack of data as more loci would further differentiate between isolates (Supplementary Figure 2). The samples were collected from primary ascospore lesions (spore traps were used to monitor ascospore discharge), which explains the low frequency of clones. Analysis of molecular variance (AMOVA) showed a moderate ΦPT value of 0.19 (p=0.004) in 2012, and 0.05 (p=0.09) in the 2013 datasets between the Elgin and Koue Bokkeveld regions. The two geographic populations were highly differentiated over all seasons. Analysis of isolates from the sanitation trial orchards resulted in a total of two and 18 private alleles in seasons 2012 and 2013, respectively, which were unique to Elgin isolates, as well as seven and 15 private alleles in the Koue Bokkeveld populations (Table 2; Figure 3). This indicated significant differences in allele frequencies at simple sequence repeat (SSR) loci as determined by Shannon analysis (P < 0.01 for six loci). The number of private alleles was slightly higher in the subpopulation from the non-treated (negative) control than from the sanitation treatment (LS) section in the Elgin population, with two vs. zero private alleles found in three isolates in 2012 and five vs. three private alleles in seven and four isolates, respectively, in 2013. Although the number of private alleles did not differ significantly for isolates between treatments in the Koue Bokkeveld population, a trend towards a lower number of private alleles (indicated within brackets next to Na) in the LS treatment was observed (Table 3). A trend towards lower allelic diversity could also be observed (h values) in the 2013 dataset (Table 4; Figure 4A). Multilocus analysis of the orchard sections where leaves were shredded or removed, showed an over-representation of certain genotypes compared with the population from the negative control treatment (mean index of association IA(LS)=−0.06 vs. IA(N)=0.07). The effect was more significant in the Koue Bokkeveld population (IA(LS)=−0.065, P=1.0 and IA(N)=0.115, P=0.06). Using the single stepwise model (SSM) model, bottlenecks were identified in populations from sanitation treatments by testing for heterozygosity excess and numbers of alleles (30). An unusual allele distribution was observed in the 2013 season at Elgin, with three alleles appearing at the most common frequency of 25% each for locus Vitg11/70 (Table 3; Supplementary Figure 1). Shannon partition analysis over loci for pairwise population combinations showed significant differences between the two Elgin subpopulations (sHua= 0.19; P=0.09). The total number of alleles from the orchard sections where sanitation in the form of leaf shredding was applied was lower than the number of alleles in V. inaequalis isolates from the non (sanitation)-treated orchard sections (54 vs. 64 alleles) over six loci. A bottleneck was detected in the V. inaequalis population from the Koue Bokkeveld, where leaf shredding had been applied, using the SMM model (P=0.04).
Table 3 Genetic diversity (observed vs. expected) in South African V. inaequalis isolates from Elgin (n=46) and Koue Bokkeveld (n=43) within six SSR loci.
Table 4 Allelic patterns across sanitation trial V. inaequalis populations (regions Elgin [E] [N=173] and Koue Bokkeveld [KB] [N=202] combined) of six SSR loci and multilocus genotypes per region.
Figure 3 Principal coordinate analyses showing genetic distance among populations of V. inaequalis isolates from different apple growing regions (A) Elgin and (B) Koue Bokkeveld), indicating the different origins of isolates from sanitation trial orchard sections of the following treatments: sani, leaf removal or leaf shredding (LS) treatment; LSPS, leaf shredding plus spray programme; N, non-treated; S, standard spray programme; and (C), combined populations from Elgin and Koue Bokkeveld.
Figure 4 (A) Allelic patterns across sanitation trial V. inaequalis populations (2013 data) of six SSR loci (NE, negative control Elgin; NK, negative control Koue Bokkeveld; SaniE, leaf shredding treatment Elgin; SaniK, leaf shredding treatment Koue Bokkeveld). Na, total number of alleles; Ne, effective population size; No. L, Common alleles, number of less common alleles (occurring ≤50%); h, diversity. (B) STRUCTURE bar plot assigning three populations (Clusters I and III, Elgin [E]; I and II, Koue Bokkeveld [KB]; and I, Vyeboom [VB]) sampled over three seasons from varying orchard treatments (Ia, sanitation treatment (Sani) including leaf removal, leaf shredding, or leaf shredding plus spray programme (Elgin) or non-treated (from Elgin and Koue Bokkeveld) and ancestral sprayed population with practical resistance [VB]; and Ib, Sani from Koue Bokkeveld; IV, Standard spray programme, Elgin and KB). Data were analysed either treating null alleles as missing data (B) or including null alleles as valid alleles (C). Division by sampling year (2006, 2011, 2023, 2013, and 2014) and orchard treatment (LS, leaf shredding; LS+S, leaf shredding plus spray programme; N, non-treated; S/Spray, spray programme).
Structure analysis of multilocus genotypes (MLGT) from six loci on the extended dataset including orchard sections under standard fungicide treatment (treating null alleles as missing data) revealed a clear clustering by year (2006–2011, cluster I) and/or region (Elgin vs. Koue Bokkeveld). The data was estimated to contain K=3 populations (Supplementary Figure 3). When null alleles were treated as valid data, the isolates from DMI fungicide-sprayed (S) orchard sections (2012–2014) predominately clustered together, either in MLGT cluster II or III. Cluster I was found predominantly in the sanitation treatments (Sani) and non-treated (N) orchard sections and comprised the 2006 population collected from a third (DMI sprayed) sampling region (Vyeboom; Figure 4; Supplementary Table 2). Principal coordinate analysis of individual isolates from regional sanitation trial orchard sections revealed that two main components accounted for 81.6% (Elgin) or 79.8% (Koue Bokkeveld) of the total variability (Figures 3A, B) but did not clearly distinguish isolates from orchard sanitation treatments. A combined analysis of populations comprising sanitation treatments from both regions (Elgin and Koue Bokkeveld) showed a higher total variability (96.5%) of the two main components, clearly distinguishing the population from the orchard sections under standard fungicide treatment, even though they originated from two geographically distinct regions (Figure 3C).
The results of curative fungicide application confirmed flusilazole sensitivity shifts towards resistance from the V. inaequalis samples from the Vyeboom (VB) orchard, where field resistance had been reported. The mean infection incidence (i.e., the proportion of leaves with visual sporulating scab lesions) for VB (over all flusilazole concentrations) was 12%, which corresponds to a 33% reduction in the mean infection rate of the water-sprayed control. The lowest mean infection rate was found in the sample from the leaf removal treatment with 6% (equates to 53% mean inhibition). This indicates the orchard sanitation (LR) treatment improved the action of the fungicide by 20%. Samples from the sanitation treatment (LR) indicated a significantly increased fungicide sensitivity; the leaf removal treatment showed a trend towards lower infection incidence compared with the sprayed orchard section (analysis of variance effect F=2.9, P= 0.031).
Glasshouse tests further identified significant infection levels above the discriminatory flusilazole dose of 20 mg/l. A sporulation incidence of approximately 7% was observed at a flusilazole concentration of 40 mg/l, but no sporulation was observed at doses recommended by the manufacturer (110 mg/l). In samples from orchard sections at Elgin receiving mancozeb application compared with orchard sections at Elgin and at Koue Bokkeveld treated with the standard spray programme (S)), a scab infection incidences of up to 17% at the 20 mg/l dose were found, corresponding to 9% inhibition for relative infection compared with the (mock treated) water control. Furthermore, a significantly lower scab severity was found for samples from the leaf removal treatment than for samples from other sources, where either no orchard sanitation treatment or spray programmes had been applied (F(severity)=4.2; P= 0.006; Figure 5).
Figure 5 Mean percentage incidence of leaves with scab lesions (infection) and scab severity (averaged over all leaves) on MM109 trees with curative flusilazole application within 72 h of inoculation. Bulk conidiospore inoculum from Koue Bokkeveld orchard sections with leaf removal treatment [LR(KB)], non-treated (negative) control [N(KB)], mancozeb spray programme [S(KB)]; the Elgin orchard with flusilazole and mancozeb application [S(E)]; and the Vyeboom orchard [S(VB), positive control for practical DMI resistance]. Bars marked with the same letter had mean percentages (either infection or severity) that were not significantly different (P < 0.05).
In planta studies are regarded as the most reliable measure for shifts in fungicide sensitivity (Kunz et al., 1997). When compared with baseline EC50 values from the literature, the calculated resistance factor was comparable to data for resistant populations with a mean EC50 of 12.5 mg/l, but did not cross the factor of the 40 times increase in resistance threshold suggested by Kunz et al., (1997) for a significant shift in sensitivity. Whereas glasshouse studies with flusilazole had shown a good response of V. inaequalis isolates sensitive to DMIs, such as flusilazole at 25 mg/l (Kunz et al., 1997), in this study, there was no total loss of control but rather a shift towards resistance (no sporulation at the recommended dose). When the abandoned SA orchard at Greyton was tested for baseline flusilazole sensitivity, only two isolates (3.6%) grouped in the resistant category, with a mean EC50 of 0.71 μg/ml. No fungicides had been applied in this orchard for >8 years.
Primary fungal inoculum reduction through orchard sanitation practices in the form of leaf removal or leaf shredding has been shown to reduce disease pressure and the incidence of apple scab in South Africa (Von Diest et al., 2016). The current study was conducted to ascertain whether the reduction in primary sexual inoculum would influence the effective population size, causing a genetic bottleneck, which was previously suggested as a possible fungicide resistance management practice (McDonald and Linde, 2002). As fungicide sensitivity often has a beneficial effect on pathogen fitness, a genetic bottleneck could favour the isolates with higher fungicide sensitivity due to their increased fitness, which would result in a reversal of shifts in fungicide resistance, provided that no postharvest DMI applications are applied (see later). However, such an effect has so far not been quantified. Here, we show the reduced effective population size of V. inaequalis after leaf shredding and its increased fungicide sensitivity in two populations, which have shifted towards demethylation inhibitor fungicide resistance. Other potential explanations of the results include the long dispersal of ascospores from non-treated control plots and sampling error due to small sample size.
A reduced incidence and severity of apple scab was reported in the sanitation trial section of the two orchards, where leaf shredding was applied (Von Diest et al., 2016). However, the treatment was more successfully implemented in the colder apple growing region of the Koue Bokkeveld, possibly due to larger amounts of viable asexual overwintering inoculum in the warmer apple growing region of Elgin. This had also been reflected in the lower incidence of apple scab on developing fruit before fruit thinning in the Koue Bokkeveld (−49%) than in the Elgin orchard (−20%; Von Diest et al., 2016). Another possible explanation for the higher DMI resistance levels observed in the Elgin orchard is the common practice of orchard treatment with DMIs after harvest. This practice is likely to select resistant isolates in the leaf scab infections, which are present late in the season. According to the Fungicide Resistance Action Committee (www.frac.info; Goertz, 2023), repeated application of DMIs alone in one season should be avoided under high disease pressure, and preventative applications should be favoured over curative applications, as they are more effective at controlling the pathogen (Beckerman et al., 2014). In South Africa, these recommendations are even more relevant due to the high risk of overwintering asexual inoculum in mild winter climates (Von Diest et al., 2015a). The selected resistant isolates would be available to form mating structures in the fallen leaves and sexual inoculum for the next season.
Furthermore, other FRAC recommendations appear relevant when differences in fungicide sensitivity were analysed between the populations from orchards of varying spray programmes, such as the use of more than one active ingredient of a systemic fungicide (of different mode-of-action groups) within one growing season, either as a mixture or in alternation to delay a selection of resistant isolates (Van den Bosch et al., 2011). This might explain the significant increase in fungicide sensitivity from treatments in the Koue Bokkeveld orchard trial, where multiple active ingredients were used in the spray programme. By contrast, only a trend towards a lower resistance level could be observed (Figure 2) in the Elgin trial, where only mancozeb and difenoconazole were applied.
It has also been shown that copper application during tree dormancy effectively reduces fungicide resistance (Pfeufer and Ngugi, 2012). This could be the result of the reduction in asexual overwintering inoculum caused by copper sprays (Passey et al., 2017). The conidiospores that remained from the previous season’s infections would have been exposed to multiple DMI applications and through this selection process would have increased the proportion of resistant isolates present in the population. As copper products are being phased out due to the high toxicity and environmental concerns, lime sulphur products present an appropriate alternative as they have similar properties that eradicate overwintering asexual inoculum when applied at the green tip (Gadoury et al., 1994; Holb et al., 2005). The use of leaf shredding, on the other hand, to improve fungicide sensitivity could also be applicable to other apple pathogens, the primary inoculum source of which stems from leaf material, such as Alternaria mali and other Alternaria spp., the causal agents of Alternaria leaf blotch and fruit spot of apple (Harteveld et al., 2014).
Pfeufer and Ngugi (2012) further hypothesised that increased cultivar variability and a lower number of fungicide applications have an effect on lowering DMI fungicide resistance levels. The study confirmed the common notion of using integrated control strategies such as alternation of a variety of protectively applied systemic fungicides and multisite inhibitors with mixed plantings of plant host cultivars within an orchard. This would aid in lowering inoculum pressure as an active fungicide resistance management strategy. In this study, the higher incidence of resistant isolates in the Elgin region might be due to an increased relative importance of asexual overwintering inoculum and a history of postharvest “eradication” application of DMI fungicides in the orchard (Von Diest et al., 2015a; Schwabe, 2015). Furthermore, the observed clonality of samples from a parallel study (Koopman et al., 2017) indicated asexual inoculum could have overwintered, as previously suggested, in the form of conidiospores in buds or on pygmy apples. To have a greater impact on apple scab control and lower the occurrence of resistant isolates overwintering, it would therefore be advisable to apply a fungicide spray on dormant trees and remove pygmy apples (Gadoury et al., 1994; Pfeufer and Ngugi, 2012).
A potential impact on the fitness of the flusilazole resistant isolates compared with the sensitive group was inferred by comparing the average growth of isolates on medium unamended with flusilazole. Isolates categorised as flusilazole sensitive did not produce significantly larger mycelial colonies than the average resistant isolate. However, a possible effect on growth could be evaluated as a time course experiment instead of just the endpoint reading after 21 days to confirm results, as described by Pfeufer and Ngugi (2012). Von Diest et al. (2015b) observed that the population from Elgin, where a higher rate of flusilazole-resistant isolates was found, produced fewer ascospores, suggesting reduced reproduction fitness. Nevertheless, growth studies of DMI-resistant and -sensitive isolates in planta and an evaluation of other parameters indicating the fitness of isolates, such as sporulation and the ability to invade the host, should be tested in future studies to confirm or refute the findings. A more appropriate measure for the fitness of the resistant isolates would be to assess their spore production, i.e., by plating them on cellophane medium (Koopman et al., 2022). Furthermore, glasshouse inoculation experiments could be evaluated for sporulating lesions (severity), and re-isolations of these fungicide resistant isolates from sporulating lesions should be quantified for spore production in planta, i.e., using qPCR.
This study indicated that the apple scab population from the orchard section, where leaf shredding had been applied, had lower genetic diversity and a lower chance of resistant phenotypes occurring. Polymorphic microsatellite loci were used to genotype V. inaequalis isolates from seasons 2011/2012 and 2012/2013 (h=0.30-0.81). The index of association indicates that the Elgin population has experienced less sexual recombination; in this region, a milder winter climate enables more asexual overwintering inoculum (Koopman et al., 2017; Von Diest et al., 2015a.). Additionally, this could be reflected in the over-representation of some of the multilocus genotypes in specific orchard sections when sanitation treatments were applied in comparison with the more diverse orchard sections under standard spray programmes. A subset of isolates collected from orchards at Elgin, Koue Bokkeveld, and Vyeboom clustered as one population despite their regional source differences of over 100 km, while the isolates from the orchard sections under a standard spray programme showed genotypes clustering by region. In future studies, a minimum of 30 fungal isolates should be collected from each sanitation trial orchard section to enable analysis of a more robust dataset. Bottlenecks observed in the population where leaf shredding had been applied indicated that this treatment could reduce the effective population size. Thus, the appearance of DMI-resistant genotypes would be delayed as recombination could cause the accumulation of resistance-inducing polymorphisms after selection pressure had been applied, as previously hypothesised (Zhan et al., 2015). It is possible that the plasticity in the ascomycete genome related to DMI sensitivity is due to transposable elements combined with a repeat-induced point mutation inserted into the promoter region of the fungicide target’s gene, where its overexpression can be switched on when the fungicide is present (Grandaubert et al., 2014; Yang et al., 2020). A specific characteristic of these so-called AT-isochores is their increased AT content (Galagan and Selker, 2004). The effect of gene duplication (or deletion) occurred during the recombination phase in the sexual stages of ascomycete fungi. Further analysis of the structure and function of the transposable elements is required to identify their mechanism in resistance development in V. inaequalis.
Population genetic tools enable the evolutionary potential of plant pathogens and the effect of control strategies on pathogen populations to be assessed (McDonald and Linde, 2002; Mikaberidze and McDonald, 2015). Events such as migration, mutation, and recombination typically lead to an increased variation in the sexually outcrossing V. inaequalis population. In agro-ecosystems, fungal pathogens are generally under high selection pressure due to agricultural practices, which reduces diversity in the populations (Stefansson et al., 2014). Additionally, it has been hypothesised that an effectively smaller population size due to primary inoculum reduction could, in the long term, reduce the chances of resistant isolates occurring in that population (Zhan et al., 2015). On the other hand, bottlenecks followed by genetic drift associated with a small population size have a neutral effect on the trait under consideration (i.e., fungicide resistance), which leads to an increase in the variability in allele frequency due to large sampling errors in small populations. The amount and distribution of genetic variation within and among populations can provide insight into the genetic structure of the pathogen population, the evolutionary processes that have shaped the population, and the potential of the population to overcome fungicide control measures (McDonald and Linde, 2002). As a large effective population size results in a higher number of mutants in that population, this can lead to a higher evolutionary potential to develop practical fungicide resistance. A disease management programme, such as leaf shredding for primary inoculum reduction, could lower the fungicide resistance risk in the long term as a reduced population size will also reduce the absolute number of new mutations in the population, especially if the resistance development is due to multiple mutations. This so-called quantitative or stepwise resistance development has been well documented for the DMI group of fungicides (Schnabel and Jones, 2001).
Polymorphisms in the CYP51A1 promoter region associated with DMI resistance (553 bp insert) were found in 10% of the total number of isolates tested, which indicates a shift towards DMI resistance. The fact that many isolates (43%) contained the 500-bp promoter insert indicates that a complete loss of DMI sensitivity is a real threat for the tested populations as these isolates are just one recombination event away from a total shift towards resistance. Owing to the small sample number, no difference could be observed between samples from different orchard treatments. Further analysis should be carried out to identify the motifs inserted into the promoter region and associated mechanisms of expression due to transposable elements (as reviewed by Wells and Feschotte, 2020). Furthermore, a more detailed study of growth induction at lower doses of fungicide is required to determine whether hormesis (increased growth at sublethal doses) is an indication of the presence of fungicide resistance in V. inaequalis (Flores and Garzon, 2012; Pradhan et al. 2019). In other pathogens, such as Scerotinia spp., it was shown that the preconditioning of fungal isolates containing sublethal doses of fungicide resulted in an increased incidence of fungicide resistance (Amaradasa and Everhardt, 2016). Although highly sensitive isolates did not show a hermetic effect, the fungicide-resistant isolates showed growth stimulation of up to 20% compared with the untreated control. Thus, hormesis could be an indication of fungicide resistance in a population.
We conclude that leaf shredding in combination with fungicide application was effective in managing shifts towards DMI resistance in the V. inaequalis population. The fungicide sensitivity levels increased to what would be found in an unexposed population. Therefore, the use of leaf shredding combined with protectively applied spray programmes is highly recommended for the management of DMI fungicide resistance.
The original contributions presented in the study are publicly available. This data can be found here: https://figshare.com/s/f269dbbf6949c6bfaa5c.
JM-H conceptualised the study, and carried out fungicide sensitivity testing in vitro and in vivo as well as molecular analysis and preparation of the manuscript. SD carried out the orchard sanitation study and participated in sample collection. TK acquired funding, carried out sample collection, fungicide sensitivity testing in planta as well as molecular analysis and editing of the manuscript. KT was involved in supervising the study, funding acquisition and editing the manuscript. XX developed the molecular markers and edited the manuscript. CL supervised the study and acquired funding. All authors contributed to the article and approved the submitted version.
This study was supported by Hortgro Science for project and MSc bursary funding, the Claude Leon Foundation for postdoc bursary funding, and the South African National Research Foundation (NRF) for incentive and THRIP funding.
We would like to thank the South African fruit growers for their participation in this study and Michell Leibrandt and Elveresha Davids for technical assistance. Many thanks also to Prof. Jasper Rees and his research group for providing V. inaequalis genome information and Prof. Douglas E. Rawlings for editing the manuscript.
The authors declare that the research was conducted in the absence of any commercial or financial relationships that could be construed as a potential conflict of interest.
The authors JM-H, XX, and CL declared that they were an editorial board member of frontiers, at the time of submission. This had no impact on the peer review process and the final decision.
All claims expressed in this article are solely those of the authors and do not necessarily represent those of their affiliated organizations, or those of the publisher, the editors and the reviewers. Any product that may be evaluated in this article, or claim that may be made by its manufacturer, is not guaranteed or endorsed by the publisher.
The Supplementary Material for this article can be found online at: https://www.frontiersin.org/articles/10.3389/fhort.2023.1175168/full#supplementary-material
Supplementary Figure 1 | SSR allele distribution for individual loci comparing the two regions, Grabouw and Koue Bokkeveld, from isolates collected from a sanitation trial orchard.
Supplementary Figure 2 | Genotype accumulation curve for V. inaequalis populations from Elgin (E) and Koue Bokkeveld (KB) based on six SSR markers.
Supplementary Figure 3 | Population clustering based on K clustering.
Supplementary Table 1 | Genotyping data of six microsatellite loci and CYP51A1 promoter of South African Venturia inaequalis isolates.
Supplementary Table 2 | List of multilocus genotype data.
Amaradasa B. S., Everhardt S. E. (2016). Effects of sublethal fungicides on mutation rates and genomic variation in fungal plant pathogen, Sclerotinia sclerotiorum. PLOSone 11, e0168079. doi: 10.1371/journal.pone.0168079
Beckerman J. L., Sundin G. W., Rosenberger D. A. (2014). Do some IPM concepts contribute to the development of fungicide resistance? Lessons learned from the apple scab pathosystem in the United States. Pest Manage. Sci. 71, 331–342. doi: 10.1002/ps.3715
Brown A. H. D., Feldman M. W., Nevo E. (1980). Multilocus structure of natural populations of Hordeum spontanum. Genetics 96, 523–536. doi: 10.1093/genetics/96.2.523
Burchill R. T. (1968). Field and laboratory studies on the effect of urea on ascospore production of Venturia inaequalis (Cke.) Wint. Ann. Appl. Biol. 62, 297–307. doi: 10.1111/j.1744-7348.1968.tb02825.x
Celton J.-M., Christoffels A., Sargent D. J., Xu X., Rees D. J. (2010). Genome-wide SNP identification by high-throughput sequencing and selective mapping allows sequence assembly positioning using a framework genetic linkage map. BMC Biol. 8, 155. doi: 10.1186/1741-7007-8-155
Corkley I., Fraaije B., Hawkins N. (2022). Fungicide resistance management: Maximising the effective life of plant protection products. Plant Pathol. 71, 150–169. doi: 10.1111/ppa.13467
Cornuet J. M., Luikart G. (1996). Description and power analysis of two tests for detecting recent population bottlenecks from allele frequency data. Genetics 144, 2001–2014. doi: 10.1093/genetics/144.4.2001
Cowen L. E., Anderson J. B., Kohn L. M. (2002). Evolution of drug resistance in. Candida albicans. Ann. Rev. Microbiol. 56, 139–165. doi: 10.1146/annurev.micro.56.012302.160907
Cowen L. E., Lindquist S. (2005). Hsp90 potentiates the rapid evolution of new traits: drug resistance in diverse fungi. Science 309, 2185–2189. doi: 10.1126/science.1118370
Evanno G., Regnaut S., Goudet J. (2005). Detecting the number of clusters of individuals using the software STRUCTURE: a simulation study. Mol. Ecol. 14, 2611–2620. doi: 10.1111/j.1365-294X.2005.02553.x
Fiaccadori R. (2018). Persistence of Venturia inaequalis populations resistant to strobilurins in the field and in the glasshouse. Am. J. Plant Sci. 9, 552–560. doi: 10.4236/ajps.2018.94042
Flores F., Garzon C. D. (2012). Detection and assessment of chemical hormesis on the radial growth in vitro of oomycetes and fungal plant pathogens. Dose Response 11, 361–373. doi: 10.2203/dose-response.12-026.Garzon
Gadoury D. M., Pearson R. C., Riegel D. G., Seem R. C. (1994). Reduction of powdery mildew and other diseases by over-the-trellis applications of lime sulfur to dormant grapevines. Plant Dis. 78, 83–87. doi: 10.1094/PD-78-0083
Galagan J. E., Selker E. U. (2004). RIP: the evolutionary cost of genome defense. Trends Genet. 20, 417–423. doi: 10.1016/j.tig.2004.07.007
Goertz A. (2023) Fungicide resistance action committee (FRAC) recommendations for SBI fungicides. Available at: www.frac.info.
Grandaubert J., Lowe R. G. T., Soyer J. L., Schoch C. L., van den Wouw A. P., Fudal I., et al. (2014). Transposable element-assisted evolution and adaptation to host plant within the Leptosphaeria maculans-Leptosphaeria biglobosa species complex of fungal pathogens. BMC Genomics 15, 891. doi: 10.1186/1471-2164-15-891
Guérin F., Franck P., Loiseau A., Devaux M., LeCam B. (2004). Isolation of 21 new polymorphic microsatellite loci in the phytopathogenic fungus Venturia inaequalis. Mol. Ecol. Notes 4, 268–270. doi: 10.1111/j.1471-8286.2004.00637.x
Harteveld D. O. C., Akinsanmi O. A., Dullahide S., Drenth A. (2014). Sources and seasonal dynamics of Alternaria inoculum associated with leaf blotch and fruit spot of apples. Crop Protect. 59, 35–42. doi: 10.1016/j.cropro.2014.01.011
Haubold H., Hudson R. R. (2000). LIAN 3.0: Detecting linkage disequilibrium in multilocus data. Bioinformatics 16, 847–848. doi: 10.1093/bioinformatics/16.9.847
Hoffmeister M., Zito R., Böhm J., Stammler G. (2021). Mutations in Cyp51 of Venturia inaequalis and their effects on DMI sensitivity. J. Plant Dis. Protect. 128, 1467–1478. doi: 10.1007/s41348-021-00516-0
Holb I. J. (2008). Timing of first and final sprays against apple scab combined with leaf removal and pruning in organic apple production. Crop Protect. 27, 814–822. doi: 10.1016/j.cropro.2007.11.009
Holb I. J., Heijne B., Jeger M. J. (2005). The widespread occurrence of overwintered conidial inoculum of Venturia inaequalis on shoots and buds in organic and integrated apple orchards across the Netherlands. Eur. J. Plant Pathol. 111, 157–168. doi: 10.1007/s10658-004-1883-z
Holb I. J., Kunz S. (2016). Integrated control of apple scab and powdery mildew in an organic apple orchard by combining potassium carbonates with wettable sulfur, pruning, and cultivar susceptibility. Plant Dis. 100, 1894–1905. doi: 10.1094/PDIS-12-15-1416-RE
Hubisz M. J., Falush D., Stephens M., Pritchard J. K. (2009). Inferring weak population structure with the assistance of sample group information. Mol. Ecol. Res. 9, 1322–1332/. doi: 10.1111/j.1755-0998.2009.02591.x
Joseph-Horne T., Hollomon D. W. (1997). Molecular mechanisms of azole resistance in fungi. FEMS Microbiol. Lett. 149, 141–149. doi: 10.1111/j.1574-6968.1997.tb10321.x
Kamvar Z. N., Brooks J. C., Grünwald N. J. (2015). Novel R tools for analysis of genome wide population genetic data with emphasis on clonality. Front. Genet. 6. doi: 10.3389/fgene.2015.00208
Köller W., Scheinpflug H. (1987). Fungal resistance to sterol biosynthesis inhibitors: A new challenge. Plant Dis. 71, 1066–1074. doi: 10.1094/PD-71-1066
Köller W., Wilcox W. F., Barnard J., Jones A. L., Braun P. G. (1997). Detection and quantification of resistance of V. inaequalis populations to sterol demethylation inhibitors. Phytopathology 87, 184–190. doi: 10.1094/PHYTO.1997.87.2.184
Koopman T. A., Meitz-Hopkins J. C., Bester-van der Merwe A. E., Tobutt K. R., Bester C., Lennox C. L. (2017). Genetic diversity and gene flow of four South African Venturia inaequalis (apple scab) populations. Phytopathology 107, 455–462. doi: 10.1094/PHYTO-07-16-0279-R
Koopman T. A., Meitz-Hopkins J. C., Tobutt K. R., Bester C., Lennox C. L. (2022). Pathogenicity and virulence of South African isolates of Venturia inaequalis. Eur. J. Plant Pathol. 164, 45–58. doi: 10.1007/s10658-022-02537-3
Kremer W., Unterstenhöfer G. (1967). Computation of results of crop protection experiments by the method of Townsend and Heuberger. Pflanzenschutz-Nachrichten 20, 625–628.
Kunz S., Deising H., Mendgen K. (1997). Acquisition of resistance to sterol demethylation inhibitors by populations of Venturia inaequalis. Phytopathology 87, 1272–1277. doi: 10.1094/PHYTO.1997.87.12.1272
Li H., Zhou G.-Y., Liu J.-A., Xu J. (2016). Population genetic analysis of the fungal pathogen Colletotrichum fructicola on tea-oil trees in China. PloS One 11 (6), e0156841. doi: 10.1371/journal.pone.0156841
Luikart G., Allendorf F. W., Cornuet J. M., Sherwin W. B. (1998). Distortion of allele frequency distributions provides a test for recent population bottlenecks. J. Hered. 89, 238–247. doi: 10.1093/jhered/89.3.238
Mac an tSaoir S., Cooke L. R., McCracken A. R. (2010). The effects of leaf litter treatments, post-harvest urea and omission of early fungicide sprays on the overwintering of apple scab on Bramley’s Seedling grown in a maritime environment. Irish J. Agric. Food Res. 49, 55–66. doi: 10.2307/20788565
MacHardy W. E. (1996). Apple scab: biology, epidemiology, and management (St. Paul, Minnesota USA: American Phytopathological Society, APS Press).
Manly B. F. J. (1985). The statistics of natural selection on animal populations (London, UK: Chapman and Hall).
McDonald B. A., Linde C. (2002). Pathogen population genetics, evolutionary potential, and durable resistance. Ann. Rev. Phytopathol. 40, 349–379. doi: 10.1146/annurev.phyto.40.120501.101443
Meitz-Hopkins J. C., von Diest S. G., Koopman T. A., Bahramisharif A., Lennox C. L. (2014). A method to monitor airborne Venturia inaequalis ascospores using volumetric spore traps and quantitative PCR. Eur. J. Plant Pathol. 140, 527–541. doi: 10.1007/s10658-014-0486-6
Mikaberidze A., McDonald B. A. (2015). “Fitness cost of resistance: Impact on Management,” in Fungicide Resistance in Plant Pathogens. Eds. Ishii H., Hollomon D. W. (Japan: Springer), 77. doi: 10.1007/978-4-431-55642-8_6
Nakaune R., Adachi K., Nawata O., Tomiyama M., Akutsu K., Hibi T. (1998). A novel ATP-binding cassette transporter involved in multidrug resistance in the phytopathogenic fungus Penicillium digitatum. Appl. Environ. Microbiol. 64, 3983–3988. doi: 10.1128/AEM.64.10.3983-3988.1998
Northover J. (1988). Persistence of dicarboximide-resistant Botrytis cinerea in Ontario vineyards. Can. J. Plant Pathol. 10, 123–132. doi: 10.1080/07060668809501744
Passey T. A. J., Robinson J. D., Shaw M. W., Xu X.-M. (2017). The relative importance of conidia and ascospores as primary inoculum of Venturia inaequalis in the United Kingdom. Plant Pathol. 66, 1445–1451. doi: 10.1111/ppa.12686
Peakall R., Smouse P. E. (2012). GenAlEx 6.5: genetic analysis in Excel. Population genetic software for teaching and research–an update. Bioinformatics 28, 2537–2539. doi: 10.1093/bioinformatics/bts460
Pfeufer E. E., Ngugi H. K. (2012). Orchard factors associated with resistance and cross resistance to sterol demethylation inhibitor fungicides in populations of Venturia inaequalis from Pennsylvania. Phytopathology 102, 272–282. doi: 10.1094/PHYTO-04-11-0117
Piry S., Luikart G., Cornuet J. M. (1999). BOTTLENECK: A computer program for detecting recent reductions in the effective population size using allele frequency data. J. Hered. 90, 502–503. doi: 10.1093/jhered/90.4.502
Porras-Hurtado L., Ruitz Y., Santos C., Phillips C., Carracedo A., Lareu M. (2013). An overview of STRUCTURE: applications, parameter settings, and support software. Front. Genet. 4. doi: 10.3389/fgene.2013.00098
Pradhan S., Miller L., Marcillo V., Koche A. R., Grachet N. G., Molineros J. E., et al. (2019). Hormetic effects of thiophanate-methyl in multiple isolates of Sclerotinia homeocarpa. Plant Dis. 103, 89–94. doi: 10.1094/PDIS-05-18-0872-RE
Pritchard J. K., Stephens M., Donnelly P. (2000). Inference of population structure using multilocus genotype data. Genetics 155, 945–959. doi: 10.1093/genetics/155.2.945
R Development Core Team (2005). R: A language and environment for statistical computing (Vienna, Austria: R foundation for statistical computing). Available at: http://www.R-project.org.
Ritz C., Streibig J. C. (2006). Bioassay analysis using R. J. Stat. Softw. 12, 1–17. doi: 10.18637/jss.v012.i05
Rosenberg N. A. (2004). Distruct: a program for the graphical display of population structure. Mol. Ecol. Notes 4, 137–138. doi: 10.1046/j.1471-8286.2003.00566.x
Schnabel G., Jones A. L. (2001). The 14 alpha-demethylase (CYP51A1) gene is overexpressed in Venturia inaequalis strains resistant to myclobutanil. Phytopathology 91, 102–110. doi: 10.1094/PHYTO.2001.91.1.102
Schwabe W. F. S. (2015). Effective post-harvest eradication of EBI tolerant Venturia inaequalis with EBI fungicides in South Africa. IOBC-WPRS Bull. 110, 229–240.
Schwabe W. F. S., Jones A. L., Jonker J. P. (1984). Greenhouse evaluation of curative and protective action of sterol-inhibiting fungicides against apple scab. Phytopathology 74, 249–252. doi: 10.1094/Phyto-74-249
Schwabe W. F. S., Matthee F. N. (1972). Fusicladium: Can urea eradicate the primary infection source?. Deciduous Fruit Grower 22, 179-181.
Schwabe W. F. S., van der Rust M., Van der Rijst M. (1997). Tolerance of Venturia inaequalis to ergosterol biosynthesis inhibiting fungicides in South African commercial apple orchards. Decid. Fruit Grow. 47, 303–307.
Shabi E., Kaian I. (1980). Fitness of Venturia pirina isolates resistant to benzimidazoles fungicides. Phytopathology 70, 1172–1174. doi: 10.1094/Phyto-70-1172
Shirane N., Takenaka H., Ueda K., Hashimoto Y., Katoh K., Ishii H. (1996). Sterol analysis of DMI resistant and -sensitive strains of Venturia inaequalis. Phytochemistry 41, 1301–1308. doi: 10.1016/0031-9422(95)00787-3
Smith F. D., Parker D. M., Köller W. (1991). Sensitivity distribution of Venturia inaequalis to the sterol demethylation inhibitor flusilazole - base-line sensitivity and implications for resistance monitoring. Phytopathology 81, 392–396. doi: 10.1094/Phyto-81-392
Spearman C. E. (1904). The proof and measurement of association between two things. Am. J. Psychol. 15, 72–101. doi: 10.2307/1412159
Stanis V. F., Jones A. L. (1986). Reduced sensitivity to sterol-inhibiting fungicides in field isolates of Venturia inaequalis. Phytopathology 75, 1098–1101. doi: 10.1094/Phyto-75-1098
Stefansson T. S., McDonald B. A., Willi Y. (2014). The influence of genetic drift and selection on quantitative traits in a plant pathogenic fungus. PloS One 9, e112523. doi: 10.1371/journal.pone.0112523
Sutton D. K., MacHardy W. E., Lord W. G. (2000). Effects of shredding or treating apple leaf litter with urea on ascospore dose of Venturia inaequalis and disease buildup. Plant Dis. 84, 1319–1326. doi: 10.1094/PDIS.2000.84.12.1319
Tenzer I., Degli Ivanissevich S., Morgante M., Gessler C. (1999). Identification of microsatellite markers and their application to population genetics of Venturia inaequalis. Phytopathology 89, 748–753. doi: 10.1094/PHYTO.1999.89.9.748
Van den Berg F., Paveley N. D., van den Bosch F. (2016). Dose and number of applications that maximize fungicide effective life exemplified by Zymoseptoria tritici on wheat – a model analysis. Plant Pathol. 65, 1380–1389. doi: 10.1111/ppa.12558
Van den Bosch F., Paveley N., Shaw M., Hobbelen P., Oliver R. (2011). The dose rate debate: does the risk of fungicide resistance increase or decrease with dose? Plant Pathol. 60, 597–606. doi: 10.1111/j.1365-3059.2011.02439.x
Vincent B. M., Lancaster A. K., Scherz-Shouval R., Whitesell L. (2013). Fitness trade-offs restrict the evolution of resistance to amphotericin B. PloS Biol. 11, e1001692. doi: 10.1371/journal.pbio.1001692
Von Diest S. G., Meitz J. C., MacHardy W. E., Lennox C. L. (2016). The effect of leaf shredding on apple scab in South African orchards. Plant Dis. 100, 2094–2098. doi: 10.1094/PDIS-03-15-0294-RE
Von Diest S. G., Meitz-Hopkins J. C., MacHardy W. E., Lennox C. L. (2015a). Overwintering of Venturia inaequalis conidiospores in South African apple orchards. IOBC-WPRS Bull. 110, 123–125.
Von Diest S. G., Rabie A., MacHardy W. E., Lennox C. L. (2015b). Venturia inaequalis pseudothecia development in relatively warm and cold winter regions. IOBC-WPRS Bull. 110, 119–122.
Wells J. N., Feschotte C. (2020). A field guide to Eukaryotic transposable elements. Annu. Rev. Genet. 54, 539–561. doi: 10.1146/annurev-genet-040620-022145
Yang Y., Marcroft S. J., Forsyth L. M., Zhao J., Li Z., Van de Wouw A. P., et al (2020). Sterol demethylation inhibitor fungicide resistance in Leptosphaeria maculans caused by modifications in the regulatory region of ERG11. Plant Dis. 104, 1280–1290.
Yeh F. C., Boyle T. J. B. (1997). Population genetic analysis of co-dominant and dominant markers and quantitative traits. Belg. J. Bot. 129, 157.
Keywords: fungicide resistance and management, flusilazole, Venturia inaequalis (Cke.) wint, SSR (microsatellite), orchard sanitation
Citation: Meitz-Hopkins JC, von Diest SG, Koopman TA, Tobutt KR, Xu X and Lennox CL (2023) Leaf shredding as an alternative strategy for managing apple scab resistance to demethylation inhibitor fungicides. Front. Hortic. 2:1175168. doi: 10.3389/fhort.2023.1175168
Received: 27 February 2023; Accepted: 30 August 2023;
Published: 03 October 2023.
Edited by:
Bhabesh Dutta, University of Georgia, United StatesReviewed by:
Sydney Everhart, University of Connecticut, United StatesCopyright © 2023 Meitz-Hopkins, von Diest, Koopman, Tobutt, Xu and Lennox. This is an open-access article distributed under the terms of the Creative Commons Attribution License (CC BY). The use, distribution or reproduction in other forums is permitted, provided the original author(s) and the copyright owner(s) are credited and that the original publication in this journal is cited, in accordance with accepted academic practice. No use, distribution or reproduction is permitted which does not comply with these terms.
*Correspondence: Julia C. Meitz-Hopkins, anVsaWFtQHN1bi5hYy56YQ==
Disclaimer: All claims expressed in this article are solely those of the authors and do not necessarily represent those of their affiliated organizations, or those of the publisher, the editors and the reviewers. Any product that may be evaluated in this article or claim that may be made by its manufacturer is not guaranteed or endorsed by the publisher.
Research integrity at Frontiers
Learn more about the work of our research integrity team to safeguard the quality of each article we publish.