- Warwick Crop Centre, School of Life Sciences, University of Warwick, Wellesbourne Campus, Wellesbourne, Warwick, United Kingdom
Vegetables are a key component of a healthy diet, but they are also exposed to a relatively high amount of pesticide usage in conventional growing systems. A generally agreed aim of Integrated Pest Management (IPM) is to reduce reliance on ‘chemical control’ using synthetic pesticides, usually placed at the top of the IPM pyramid. This review focuses on the approaches at the bottom of the IPM pyramid in relation to the pest insects of root, bulb and leafy vegetable crops in northern Europe. These concern establishment of the crop before any additional treatments are applied; summarised as 1) what to plant (crop and cultivar(s)), 2) where to plant it (considering a range of spatial scales) and 3) what to plant it with (companion species, wildflowers etc.). Were it possible to identify sources of resistance or partial resistance to all key pests and pathogens of vegetables and breed these into commercial cultivars, this would undoubtedly be an effective and ‘reliable’ way forward. Increased use of rotation and the isolation of ‘new’ crops from sources of infestation could also be a reliable approach to the management of certain pests, especially those with a limited capacity to disperse. For some growers, there would be a need to alter the ‘spatial arrangement’ of their cropping system to maximise the benefits and this might involve more cooperation with neighbours. Finally, although there appears to be much potential, the benefits of increasing the diversity of plant species within and around crops are currently hard to quantify with regard to pest management and thus might be considered to be less ‘reliable’ approaches at present. It is vital, therefore, that there is a greater understanding of the mechanisms involved and of how it may be possible to achieve a more consistent effect from the range of approaches available.
1 Introduction
Nearly 15 years ago, the European Parliament and Council established a framework to achieve sustainable use of Plant Protection Products (PPPs) by promoting integrated pest management (IPM) (2009/128/EC). IPM is an important part of the EU’s plant protection policy and became mandatory in 2014 (Article 14). However, progress has been slow. For example, whilst PPP sales do not directly correlate with the adverse effects associated with their use, overall sales of the active substances used in the EU have remained relatively stable in recent years and an audit undertaken in 2019 indicated that conditions were not optimal for stimulating the uptake of IPM (ECA, 2020). Through the European Green Deal the pressure is now on to reduce pesticide usage. Within the ‘Farm to Fork strategy’, which is one of the central pillars of the European Green Deal, the European Commission is setting more challenging targets for sustainable pesticide use. One of the targets is that by 2030, the use of, and risks attached to, chemical/more hazardous pesticides should be reduced by 50% (EU, 2023a). In parallel with the focus on pesticide reduction is an ambition to improve the diets of the global population as a whole (FAO and WHO, 2019). It is suggested that a major shift to healthy diets by 2050 will include a greater than 100% increase in consumption of certain foods, including vegetables (Willett et al., 2019). Obviously, the changes needed will differ in each region.
Vegetables are a key component of a healthy diet, but they are also exposed to a relatively high amount of pesticide usage in conventional growing systems. This is partly because of the stringent quality requirements, especially ‘cosmetic appearance’ standards, of retailers such as the large supermarkets (Porter et al., 2018). Pimentel et al. (1977) estimated that on average, fruits and vegetables are exposed to 10 - 20% more insecticide treatments to reduce the incidence of insects and/or to meet the standards set with regard to ‘cosmetic appearance’. In Europe, vegetable production has a small footprint compared with broad acre crops such as wheat, barley, maize and oil seed rape but IPM is equally important for vegetable crops, partly because much of the produce is consumed directly with minimal processing, but also, being minor crops, the pesticide armoury is dependent on the market shaped by the broad acre crops and the options for vegetable crops are often limited (Lamichhane et al., 2015). This article considers the potential for IPM in vegetable crops grown outdoors in northern Europe, with a focus on root, bulb and leafy crops and on pest insects. In 2017, fresh vegetables were produced on around 2.2 million hectares of land in the EU, which is about 1.2% of the land used for agriculture (EU, 2019). A considerable proportion of this area is crops grown in Spain, Italy and Portugal and of these some will be fruiting crops such as tomato, pepper, cucumber and aubergine.
1.1 Conceptual framework
The concept of IPM is not a new one and many definitions and diagrams have been produced (Stenberg, 2017; Deguine et al., 2021). In a review considering IPM, its origins and future, Kogan (1998) synthesized what seemed to be the consensus view from definitions generated over the previous 35 years: ‘IPM is a decision support system for the selection and use of pest control tactics, singly or harmoniously coordinated into a management strategy, based on cost/benefit analyses that take into account the interests of and impacts on producers, society, and the environment.’ There have also been critical publications, describing lack of progress, supporting the conclusions of the EU audit (ECA, 2020); a key indicator being that, overall, pesticide use remains as high as ever (Hokkanen, 2015; Deguine et al., 2021). Whilst it is undoubtedly true that a systems approach is required to implement effective IPM strategies, a linear/hirearchical description is probably more effective for explaining the concept, which is why the IPM pyramid has been adopted by a number of authors (Naranjo, 2011; Hokkanen, 2015; Stenberg, 2017; Deguine et al., 2021), projects (Figure 1) and organisations (e.g. International Biocontrol Manufacturers Association, the European Union), although the detail, and position within the pyramid of certain approaches (e.g. host plant resistance), varies. To quote Deguine et al. (2021) ‘the “IPM pyramid” concept provides a reminder that IPM is not only about “integrating pest management technologies” but that there should equally be a hierarchy or prioritization of practices (in which pesticides are listed as a measure of last resort.’… ‘In the absence of this prioritization, one can never achieve sustainable pest management and leave everything to chance while causing unacceptable environmental externalities.’
One of the main, generally agreed, aims of IPM is to reduce reliance on ‘chemical control’ using synthetic pesticides, shown at the top of the IPM pyramid. The shape of the pyramid also implies that the least focus should be on chemical pesticides and that they should be a ‘last resort’ and the greatest focus should be on what are broadly cultural approaches to prevent the development of infestations; the approaches at the bottom of the pyramid. If the use of synthetic pesticides is to be reduced in future, it is important that much more attention is paid to the bottom of the pyramid, as it is acknowledged that most of the control methods in the middle of the pyramid are not ‘silver bullets’ (Collier et al., 2020), and that they are likely only to be effective through integration with other approaches. This review will focus on the approaches at the bottom of the pyramid in relation to root, bulb and leafy vegetable crops, and pest insects, in northern Europe. To the author, these concern establishment of the crop before any additional treatments are applied, summarised as 1) what to plant (crop and cultivar(s)), 2) where to plant it (considering a range of spatial scales) and 3) what to plant it with (companion species, wildflowers etc.).
2 Approaches at the bottom of the IPM pyramid
2.1 What to plant
2.1.1 Resistant cultivars
Host plant resistance can be a very valuable tool for the management of pest insects, although usually most cultivars target just one species. For vegetable crops grown outdoors in northern Europe the focus has been principally on aphids and root-feeding fly pests.
The potential for resistance in lettuce to Pemphigus bursarius Linnaeus was identified many years ago (Dunn, 1974) and this was complete resistance. The original cultivars are not grown commercially nowadays but cultivars with resistance to P. bursarius are available. It is probable that sources of resistance are readily available, should new cultivars with resistance to P. bursarius be required (Ellis et al., 2002).
The development of cultivars resistant to the currant-lettuce aphid (Nasonovia ribisnigri Mosley) is more recent. Resistance was found in accessions of Lactuca virosa Linnaeus, providing almost complete control, and the resistance was due to a single (incompletely) dominant gene (Nr) (Eenink and Dieleman, 1983). The resistance was transferred to cultivated lettuce Lactuca sativa Linnaeus (Eenink et al., 1982) and over time, a wide variety of resistant cultivars came onto the market and they were grown widely. Unfortunately, aphids that were able to survive and reproduce on resistant plants became prevalent and the resistance broke down in some parts of Europe (e.g. Sauer-Kesper et al., 2011). Research is now being undertaken to identify new sources of resistance to N. ribisnigri (e.g. Walley et al., 2017). Partial resistance in lettuce to Myzus persicae Sulzer and Macrosiphum euphorbiae Thomas has been identified (Reinink et al., 1989) but has not been promoted as a trait in commercial cultivars.
Varietal resistance might also provide an option for protecting carrots from aphids/transmission of virus. Some varieties appear to be less affected by virus than cv. Nairobi which is grown widely in the UK (H. Hinds, personal communication). However, such varieties may be less attractive to growers compared with Nairobi, which is high-yielding when not affected by virus, and is resilient to damage during harvesting and packing (H. Hinds, personal communication).
Also for carrot, following a 15 year programme in the UK, plant material with partial resistance to carrot fly (Psila rosae Fabricius) was transferred to seed companies for the development of new cultivars (Ellis, 1999). Resistance had been identified originally in a cultivar called ‘Sytan’ and greater levels of resistance were found in wild species of Daucus. Two partially resistant cultivars were released subsequently (Flyaway and Resistafly) and are used by gardeners in the UK, but these have not been taken up by the industry because they lack some of the other traits that are considered to be more important, and at present, P. rosae is generally controlled effectively using other approaches. However, it is possible that more cultivars with resistance to P. rosae will appear on the market, based on the original material and on resistant material identified from other sources. There have also been attempts, so far with little success, to identify resistance to cabbage root fly (Delia radicum Linnaeus) in the Brassicaceae (e.g. Crisp et al., 1977).
2.2 Where to plant it
2.2.1 Crop rotation
Careful consideration of crop rotation is recommended for all outdoor crop production systems and this should consider pest, disease and weed management (e.g. Chongtham et al., 2017). With regard to many pest insects, crop species from different plant families should be grown in sequence to minimise the carry-over of pests, particularly those with limited dispersal ability and which will overwinter in the vicinity. Crop rotation is particularly important for organic systems, because of the restrictions on the application of soluble mineral fertilisers and synthetic pesticides (Chongtham et al., 2017).
Most pest insects have some capacity to disperse, which means that the rotation and situation of crops should ideally be considered at a landscape scale. It has long been known that populations of P. rosae can be managed to a great extent by the spatial separation of ‘new’ crops from ‘old’ ones and the minimum distance of separation is likely to be in the order of 1 km (Finch and Collier, 2004). This may be easily achievable for one business, but in areas where more than one business is growing a host crop, there may need to be an element of cooperation between them. Ironically, many small-scale organic growers have a single block of land, so that the insects can move easily from one crop to another, even if rotation in time is pursued rigorously on each piece of land. A recent small scoping study on swede midge (Contarinia nasturtii Kieffer) in the UK, to determine the extent of what seems to be an increasing problem, showed that the most heavily infested crops were indeed grown organically and were rotated around a relatively small block of land (unpublished data). Current strategies in north America, where this pest became a problem relatively recently, following its accidental introduction (Chen et al., 2011), include long (temporal) and wide (geographically distant) crop rotations and planting fewer Brassicaceous vegetables, as well as the use of insect-proof netting, biological control measures and insecticides (Hodgdon et al., 2022).
Another example highlighting the need to consider the spatial and temporal aspects of rotation is the production of radish (Raphanus sativus Linnaeus) outdoors in the UK. Changes in the availability and acceptability of the insecticides to manage D. radicum meant that one of the largest growers in the UK decided to re-appraise their approach to managing this pest (Witkowska et al., 2018). After considering all the possible options, they opted to cover their crops in fine mesh netting to exclude the adult flies and prevent oviposition on emerging radish plants. However, although the nets should have been effective, there was damage to some of the crops. The company’s practice had been to grow, within a year, several radish crops in succession on one piece of land, since the time from sowing to harvest is relatively short. Further investigation revealed that once the first crop had been uncovered and harvested, female flies moved in to lay eggs on the ‘trash’. These eggs/larvae survived the cultivation activities associated with sowing the following crop, so that once this new crop was covered with fine mesh netting, they were trapped inside, and able to move to, and feed on, the new radish plants. Thus, the company had to adapt its cropping cycle by allowing more time between radish crops grown on the same piece of land; essentially to allow the generation of D. radicum laid as eggs on the fresh trash to emerge as adults before a new crop is sown. Interestingly, this re-appraisal of their cropping system had a number of other benefits to the company (Witkowska et al., 2018).
2.2.2 Impact of proximity to certain arable crops
As mentioned above, horticultural crops are minor crops and occupy a small footprint. It is worth considering how their proximity to certain arable crops may impact on pest colonisation. More than 30 years ago, British cauliflower (Brassica oleracea var. botrytis Linnaeus) crops were infested by large numbers of bronzed-blossom (pollen) beetles – Meligethes aeneus Fabricius and M. viridescens Fabricius (Finch et al., 1990). It became clear that these beetles were adults of the ‘new’ summer generation, which would subsequently overwinter and lay eggs in the following spring and that they were using the developing cauliflower curds as a food source. This population had clearly completed the first part of its life cycle (oviposition, larval and pupal development) on oil seed rape (Brassica napus subsp. napus Linnaeus), which is far more extensive in cultivation in many areas than cauliflower. Whilst an outbreak on such a scale has not occurred subsequently, the initial outbreak led to the development of a weather-based forecasting system to predict the timing of the emergence of the new generation of adults and their subsequent dispersal to find food (Phelps et al., 1993).
Oil seed rape is undoubtedly a ‘source’ of pests (and pathogens) that infest horticultural brassica crops (Wheatley and Finch, 1984). It is also likely that changes in the management of oil seed rape that impact on the abundance and/or insecticide resistance status of brassica pests will impact on horticultural crops. Brassica pests whose resistance status has changed include M. persicae (Bass et al., 2014) (this species uses a number of plant families as hosts – crops including potato and oil seed rape), M. aeneus (Slater et al., 2011), and the cabbage stem flea beetle (Psylliodes chrysocephala Linnaeus) (Højland et al., 2015). Recent changes in insecticidal management of oil seed rape pests, specifically the withdrawal of neonicotinoid seed treatments, coupled with selection for resistance to pyrethroid insecticides, have increased the abundance of P. chrysocephala (Ortega-Ramos et al., 2022), with possible impacts on horticultural brassicas. It seems likely that this same change in the management of oil seed rape may be one reason why C. nasturtii is becoming an increasing problem in horticultural brassica crops in the UK, although this is impossible to prove. However, it might be difficult to persuade neighbouring arable farmers to adapt their cropping cycle to make life ‘easier’ for vegetable growers.
For some species it is simply not known what impact the local/regional cropping system has on pest abundance in any particular crop and that applies, for example, to adult D. radicum which can disperse over distances of 2-3 km (Finch and Skinner, 1975). It is likely that although individual oil seed rape plants are less suitable hosts than most horticultural brassicas (Finch and Ackley, 1977), the high density of plants, combined with the area of crop grown, will have an impact on the population of D. radicum within a region. For many years, when oil seed rape was grown close to Warwick Crop Centre at Wellesbourne, the second generation of D. radicum was the largest (unpublished data), suggesting that large numbers of individuals completed the first generation on oil seed rape and when that matured the emerging flies dispersed to alternative hosts. Recent research in the UK has focused on managing bean seed flies (Delia platura Meigen/D. florilega Zetterstedt). These species have a range of host plants and whilst alliums and legumes are generally the crops most affected in Europe, it is impossible to know where the majority of the population ‘resides’ and so what the risk factors of planting crops in any area will be. There is also concern that the increasing use of cover crops may exacerbate the problems caused by these two species – a concern shared with regard to the impact of D. platura on soybean crops (Hammond and Cooper, 1993).
Finally, there are species that travel large distances and some species of aphid may be included amongst these (Fereres et al., 2017). Of particular interest for production of vegetable and salad crops in northern Europe have been two species of ‘migrant’ moth, Plutella xylostella Linnaeus and Autographa gamma Linnaeus. Neither of these species overwinter successfully in the UK at present, and this is likely to apply to a number of other countries in northern Europe, but this may alter in the future with climate change. At present, they tend to arrive in northern Europe as migrants, travelling from further south and/or east (Chapman et al., 2002; Chapman et al., 2012; Wainwright et al., 2020). There is currently no understanding of the factors that lead to outbreak years in northern Europe, the last significant one for P. xylostella being in 2016, but whatever they are, it is the development of the moths in the locations in which the migrating population is produced that is important, together with wind direction when the moths are ready to migrate.
2.2.3 Other factors to consider in the landscape
Apart from arable crops, other features in the landscape may have an impact on the abundance of pests. Over time, wild hosts have been of particular interest and in some cases, concern, with regard to pest infestation and also transmission of plant viruses. Many years ago, the lettuce root aphid (P. bursarius) was considered to be a threat to lettuce crops in the UK (Dunn, 1959) and at least one UK grower took steps to remove its primary host, poplar (Populus nigra, Linnaeus) trees, from the area where lettuce was grown (J. Shropshire, personal communication). For some years more recently, P. bursarius has not been considered to be a priority pest, due to the use of neonicotinoid seed treatments, but more consideration may need to be given in the future now that these seed treatments have been withdrawn.
There has been, in the past, interest in the role of wild hosts in supporting populations of P. rosae (Hardman and Ellis, 1982; Hardman et al., 1990). The presence of the wild host plant, Conium maculatum Linnaeus, in the boundaries of carrot fields in eastern England, was considered to be an important factor influencing the numbers of P. rosae present. Hardman and Ellis (1982) suggested that if each plant produced two adult P. rosae, as they observed in an experiment, the fly population produced might reach 260,000/ha. This is considerably lower than the numbers that might emerge from a carrot crop (up to 5 million/ha). However, it still represents a substantial reservoir of insects that could infest new crops. More recently, questions have been raised about the role of wild hosts in the transmission pathways of viruses of carrot (North et al., 2004; Defra, 2013) and this is the subject of current research (A. Fox, personal communication). The willow-carrot aphid (Cavariella aegopodii Scopoli) is likely to be an important vector.
There are also questions about the role of wild hosts in the life cycle of currant-lettuce aphids (N. ribisnigri) infesting lettuce, this includes consideration of the role of wild herbaceous hosts in overwintering of anholocyclic N. ribisnigri, which is feasible when weather conditions are relatively mild (Hough, 2013). Obviously outdoor crops of lettuce are not viable in northern Europe and so provide no opportunities for overwintering aphids. Recently, Garrett (2022) has considered the role of wild hosts during the spring and summer period and his findings suggest that N. ribisnigri is using an alternative ‘intermediate’ secondary host plant prior to migrating onto outdoor lettuce later in the summer. Finally, the mirid bug Orthops campestris Linnaeus has become a problem in crops of celery grown in organic systems in the UK and this species spends a good proportion of its life cycle on wild apiaceous hosts that can be found in the field margins (AHDB, 2017; Garrett, 2022). With all of the pest species described above, there is a trade-off between the importance of the presence of these various wild hosts for biodiversity, especially for pollinators and natural enemies of pests, and their role as alternative hosts for pests, sometimes providing a ‘green bridge’. This can provide a dilemma for growers who are actively promoting biodiversity within their cropping systems.
Over the last 20 years there has been increased consideration of the influence of the structure of the surrounding landscape on populations of pests and their natural enemies. Overall, reviews of the literature suggest that crops within diversified landscapes which have a greater proportion of semi-natural areas/non-crop habitats have lower numbers of pests and/or a higher abundance of natural enemies than 'simple' large-scale landscapes with a lack of non-crop habitat (Bianchi et al., 2006; Veres et al., 2013). The reviews are based on published studies covering a range of crop/pest combinations. There are a few examples related to vegetables in Europe, specifically the Netherlands. In one study, batches of cabbage moth (Mamestra brassicae Linnaeus) eggs presented on cards were placed in crops of Brussels sprout grown organically. After 2 days, Bianchi et al. (2005) found that rates of predation and parasitism (which were 7.2 and 12.4%, respectively) were related to landscape variables at scales up to 10 km. Predation rates were positively associated with the presence of woods and parasitism rates were positively associated with the area of pasture and/or associated negatively with the area of horticultural crops. A later study on P. xylostella suggested that forests and road verges might be important for supporting parasitoids (Bianchi et al., 2008). Data collected by Den Belder et al. (2002) indicated that onion thrips (Thrips tabaci Lindeman) were less abundant in leek fields in landscapes with a larger total area of woodland. None of the specific mechanisms have been elucidated for these associations.
2.3 What to plant it with
Over several decades, there has been consideration of, and discussion about, the impact of monocultures on the development of pest infestations (Andow, 1983; Gurr et al., 2003; Crews et al., 2018), and to quote Matson et al. (1997) ‘The low planned diversity of monocultural agricultural systems typically results in greater crop losses from an insect pest complex that is less diverse but more abundant’. Not only do fields or groups of fields generally contain only one crop species (and often just one cultivar) but there is also an emphasis on complete removal of weeds. Whilst the presence of weeds is very challenging for the production of many horticultural crops, there are opportunities to manage weeds or introduced plant species in a structured way. The introduced species might be other crops (intercropping/trap crops), other cultivated species e.g., clover (companion planting/undersowing), wild species or even trees (agroforestry), often collectively termed ‘polyculture’.
2.3.1 Polyculture
There has been a good amount of research in Europe (and elsewhere) with regard to undersowing and companion planting in vegetable crops, although the uptake of these approaches in practice has been minimal to date. The main topics of the studies have been pests of brassica crops, particularly D. radicum (Finch and Kienegger, 1997; Finch and Collier, 2000; Finch et al., 2003; Morley et al., 2005; Parsons et al., 2007; George et al., 2013), onion (Uvah and Coaker, 1984 (T. tabaci); Finch et al., 2003 (Delia antiqua Meigen)), carrot (Uvah and Coaker, 1984; Varis, 1991; Rämert, 1996 (P. rosae) and leek (Theunissen and Schelling, 1998 (T. tabaci)). Intercropping has not been considered very seriously as an approach commercially, mainly because of the problems of managing two crops/species in the field. However, it may be practiced on a small scale by some growers where much of the work is done by hand. The considerable advances in technology in recent years, including GPS and robotics, make the options for managing more than one plant species in a field much more feasible in the future.
The approaches to, and applications of, using trap crops were reviewed by Shelton and Badenes-Perez, 2006 At that time, they considered that were only 10 examples of successful use of trap cropping in agricultural and forest systems, approximately half of which were outdoor vegetable systems. In an earlier review, Hokkanen (1991) identified a similar number of systems where trap crops were successfully applied in agricultural practice and went into some detail about the use of trap crops to manage Meligethes spp. in cauliflower in Finland (Hokkanen et al., 1986). Here a system using several species of trap plant (Chinese cabbage, oilseed and turnip rape, sunflower, and marigold), often in a mixture, was used to deter adult beetles from moving to the cauliflower plants. In a more recent review Sarkar et al. (2018) stated that ‘Only a few successful cases of trap crop application have been conducted at the commercial level targeting mainly Coleoptera, Hemiptera and Lepidoptera species. These cases involved insects that directed their movement and tended to aggregate on a highly attractive trap crop’, implying that the application of this approach commercially is still limited. There are a range of reasons for this, including the need to devote productive land to the trap crop, the complexities of managing the trap crop so that it is maximally attractive at the time that the pests arrive, and of ensuring that insects do not ‘spill over’ into the cash crop. The ideal situation with regard to the risk of overspill would be a dead-end trap crop (Shelton and Nault, 2004) where the trap crop is attractive to the adult pest insect for oviposition but does not support larval development.
2.3.2 Flower strips and margins
Of increasing interest is the introduction of non-crop plant species as strips into fields or around field margins, incorporating resources for insects – which could include pollinators and species that will assist in the regulation of pest numbers. In many cases these are being deployed to deliver a range of ecosystem services, rather than pest control alone (Marshall and Moonen, 2002; Haaland et al., 2011). Much of the emphasis has understandably been on arable crops (e.g. Woodcock et al., 2016; Serée et al., 2023) and the focus to a great extent has been on monitoring the impacts of such approaches on beneficial species. Bischoff et al. (2016) state that ‘in spite of the great number of studies demonstrating a positive effect of semi-natural landscape structures on important predator and parasitoid groups, evidence for a corresponding negative effect on crop herbivores and their damage is still poor’. They suggest that reasons for this are the smaller number of studies, and that these have produced inconsistent results.
Skirvin et al. (2011) compared the numbers of pest aphids in plots of lettuce grown next to wildflower strips with those in plots grown without wildflowers. A decrease in the numbers of aphids on lettuce plants was associated with the presence of wildflower strips during June-July, but an association was less evident in August-September. The decrease was greatest close to the wildflower strips and the effect declined with increasing distance from them, up to a distance of 10 metres, where there was little effect. The main species of natural enemy in the crop were able to disperse aerially; which is consistent with research findings from cereal crops. Analysis of natural enemy data was difficult due to low numbers. However, the numbers of natural enemies seemed to follow changes in aphid numbers rather than being related to the presence of wildflower strips. When the wildflower strips were cut to remove the flowers, this had no impact on the reduction in aphid numbers that occurred during June-July, but the ‘effect’ of the flower-less wildflower strips was decreased during August-September.
In a recent study, McGrath (2021) investigated whether the inclusion of annual wildflower strips in conventional carrot crops would attract sufficient numbers of beneficial insects to reduce aphid abundance and thereby transmission of virus. To quote her conclusions ‘Invertebrate sampling in trials across the plot, field and commercial-scale revealed that seed mix composition manipulated insect community composition but cannot be linked with direct evidence for pest control delivery as assessed by pest aphid numbers, sentinel prey predation, and insect-damaged carrots’. A practical problem with such an approach is in establishing sufficient flowering plants in time for the May migration of C. aegopodii, the most important pest aphid (H. Hinds, personal communication).
Finally, a well-documented approach that is used in commercial production of organic lettuce in California is the deployment of alyssum (Lobularia maritima Linnaeus) to provide resources for hoverflies, which subsequently lay their eggs on the lettuce plants; the larvae that hatch consume aphids (Brennan, 2013). Although well-known, it has not been taken up on a wide basis in northern Europe. One area to investigate is whether hoverflies would be as abundant, or present over as long a period of time, in northern Europe as those in California. Additionally, it is important to point out that the harvested lettuce may not be completely free of aphids nor of hoverfly larvae; ‘We don’t generally worry about hoverfly larvae in the lettuce. I can find them but most consumers are not able to. They generally wash out when the lettuce is washed’ (E. Brennan, personal Communication).
3 Integration
According to Stenberg (2017), the science of IPM should elucidate interactions between different actions and formulate strategies to optimize the synergy between them. His paper focused on 7 interactions between combinations of 1) intrinsic heritable plant resistance, 2) plant vaccination, 3) inter- and intra-specific botanical diversity, 4) biorational synthetic volatiles and 5) biological control. Whilst a synergistic effect is undoubtedly a very desirable outcome for IPM, additive effects are also important when dealing with actions that on their own would not achieve adequate reductions in pest numbers.
Two recent examples of experimental work investigating interactions, as described by Stenberg, concern management of D. radicum (Lamy et al., 2018) and Brevicoryne brassicae Linnaeus (Gladman, 2022), both important pests of brassica crops in northern Europe. As part of a European project on root-feeding fly pests of vegetables (FlyIPM), Lamy et al. tested a strategy for managing D. radicum in a cash crop of broccoli by combining, in a “push-pull” approach, dimethyl disulfide as an oviposition deterrent, and a trap crop of Chinese cabbage grown in strips and treated with Z-3-hexenyl-acetate as an attractant. The dimethyl disulfide appeared to reduce oviposition on broccoli by almost 30%, and application of Z-3-hexenylacetate to Chinese cabbage plants increased oviposition on them by 40%. Overall, the numbers of pupae were 40% higher in ‘control’ Chinese cabbage compared to ‘control’ broccoli, and parasitism by the wasp, Trybliographa rapae Westwood, was four times higher in pupae recovered from the trap plants. Lamy et al. (2020) further investigated the preference of D. radicum for cultivars of Chinese cabbage subsp. Pekinensis, and within the small range of cultivars tested, they observed ten-fold differences in oviposition, suggesting that varietal selection is important not only for host plant resistance but also for approaches such as trap cropping. In the second study, Gladman (2022) combined accessions of brassicas with partial resistance to aphids with the application of entomopathogenic fungi (EPF), using B. brassicae as the target pest species. The study showed that the partial host plant resistance had a positive effect on the virulence of the entomopathogenic fungi, depending on the structure of the aphid population with regard to age, and on the fungal isolate. Thus, in principle, it would be possible to breed new cultivars that improve the control of aphids with entomopathogenic fungi. This is an example of potentiation, where the partial host plant resistance is augmenting the activity of the entomopathogen.
4 The future
It is evident that something has to change with regard to approaches to pest and disease management in northern Europe – and that this change must be wider than vegetable production. However, as emphasised above, vegetable producers have particular challenges through growing minor crops and with the high standards for the ‘cosmetic appearance’ of marketed produce. They will also be greatly affected by what happens on the much larger area of arable production with regard to pressure from pests and pathogens.
Recently, the withdrawal of neonicotinoid seed treatments in Europe has led to new challenges for those who grow several important crops, both arable crops and vegetables. With oil seed rape and sugar beet this has contributed to a re-think about pest management approaches for P. chrysocephala and M. persicae (transmission of virus yellows; Dewar and Qi, 2021) respectively. It is interesting and encouraging that, for P. chrysocephala, research effort in the UK is now focused on an integrated approach to management in oil seed rape, including host plant resistance, agronomic approaches and biopesticides. Some components can be utilised immediately (e.g. trap crops and changes in sowing date) while others require further research to determine their effectiveness (e.g. resistant cultivars and winter defoliation of crops) (AHDB, 2020). Similarly, the British Beet Research Organisation (BBRO) are investigating approaches to manage virus yellows in sugar beet, which include: assessing new varieties for resistance/tolerance to the virus, use of undersown cover crops to reduce aphid colonization, flower mixtures to increase the numbers of beneficial insects and brassica companion plants to ‘pull’ aphids away from the sugar beet (M. Stevens, personal communication).
Table 1 summarises the approaches ‘at the bottom of the IPM pyramid’ evaluated for the pest insects named in this article. Those that, in the author’s opinion, have been clearly demonstrated to be used in a commercial situation are highlighted. They are few in number and three are groups of cultivars, each with resistance to a single pest species. Were it possible to identify sources of resistance or partial resistance to all key pests and pathogens of vegetables and breed these into commercial cultivars, this would undoubtedly be an effective and ‘reliable’ way forward. Seed companies are working on several pest and disease resistance traits and in the UK the importance of crop improvement has been recognised by the Department for the Environment, Food and Rural Affairs through their support for a UK Vegetable Genetic Improvement Network (VeGIN). VeGIN is a network of researchers and members of the industry, who work together to promote the development and delivery of improved vegetable cultivars and has, for example, supported work on resistance to N. ribisnigri (Walley et al., 2017). There is a strong argument that partial resistance, which could then be integrated with other approaches (e.g. entomopathogenic fungi; Gladman, 2022), would be more durable and not as easily ‘broken’ as was the complete resistance in lettuce to N. ribisnigri. Additionally, there is a case for managing the deployment of any particular resistance trait more carefully, as has been attempted to avoid the development of resistance to synthetic pesticides e.g. through the Insecticide Resistance Action Committee (IRAC). Finally, there is an opportunity to breed companion and trap crops that fit in better with certain vegetable production systems and which may be more effective in terms of manipulating pest behaviour (e.g Lamy et al., 2020).
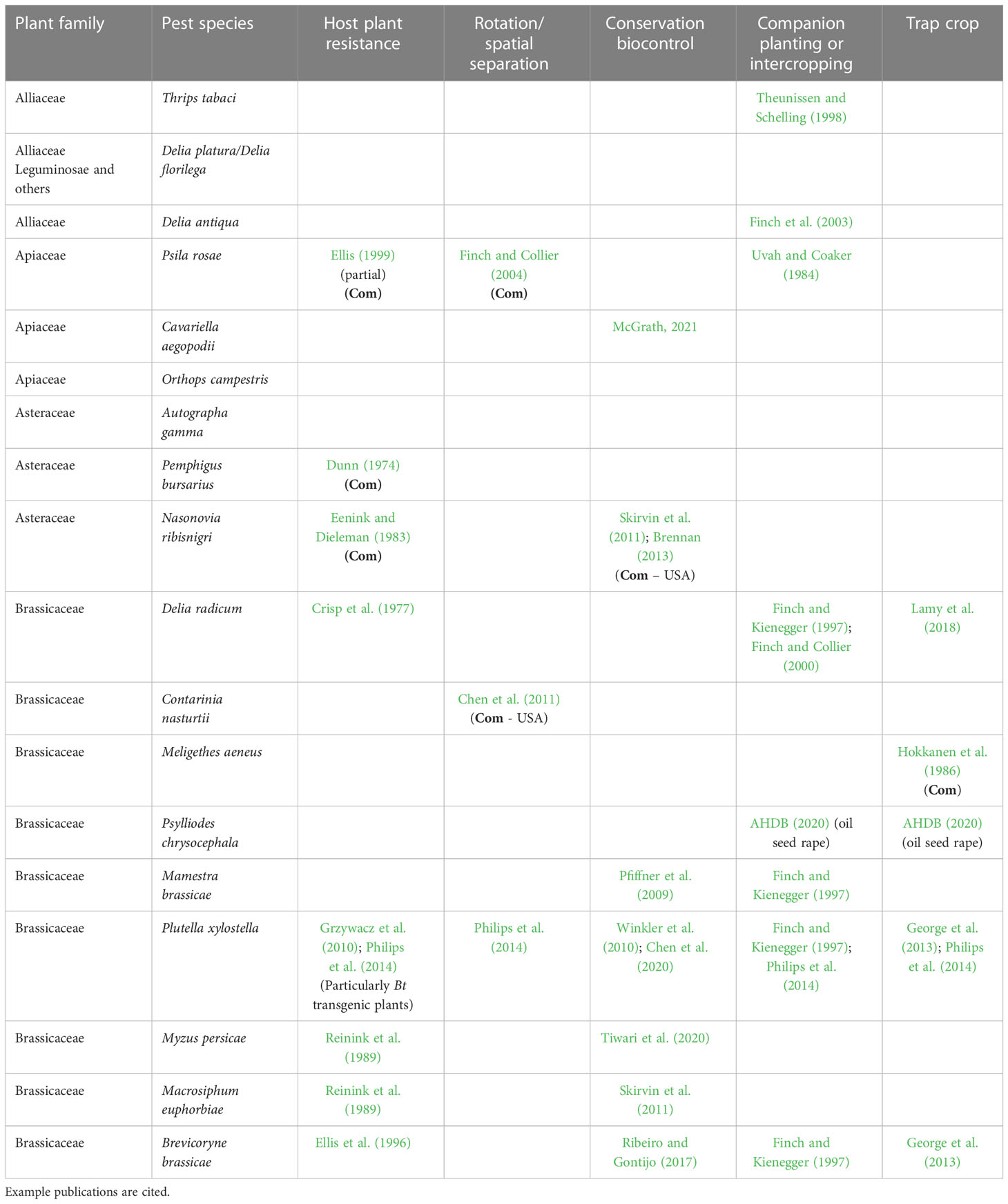
Table 1 Summary of approaches ‘at the bottom of the IPM pyramid’ that have been investigated for management of the pest insects of vegetables named in this article (Com - known by the author to have been used in commercial production/available commercially).
Increased use of rotation and the isolation of ‘new’ crops from sources of infestation could also be a reliable approach to the management of certain pests, especially those with a limited capacity to disperse. For some growers, there would be a need to alter the ‘spatial arrangement’ of their cropping system to maximise the benefits and this might involve more cooperation with neighbours. Indeed, some British vegetable growers grow a proportion of their crops on land rented from arable farms, which can benefit both parties with regard to rotations. However, this approach does limit the ‘control’ that vegetable growers have over other aspects of land management and, for example, mean that there may be insufficient time to establish flower margins/strips if this was considered a desirable option.
Although there appears to be much potential, the benefits of increasing the diversity of plant species within and around crops are currently hard to quantify with regard to pest management and thus might be considered to be less ‘reliable’ approaches at present. It is vital that there is a greater understanding of the mechanisms involved and of how it may be possible to achieve a more consistent ‘effect’ from the range of approaches available. There is an urgent need to halt the loss of biodiversity globally, of which reduced pesticide use is a part, and there are global (Sustainable Development Goal 15), European (EU, 2023b) and national (Gov.UK, 2023) initiatives. The best outcome would be that actions to address biodiversity loss, and specifically to reduce pesticide use, are complementary.
As monoculture and crop specialisation, together with an overall loss of natural habitat, appear to exacerbate pest infestations, a re-think of the overall structure of the cropping system, including pest management, might be the eventual ‘solution’. A return to mixed farming systems could be one approach (Oomen et al., 1998). Deguine et al. (2021) propose the concept of Agroecological Crop Protection which describes ‘an interdisciplinary approach that comprises an orderly strategy (and clear prioritization) of practices at the field, farm, and agricultural landscape level and a dimension of social and organizational ecology’. This would require ‘the redesign of entire farming systems using a “cropping system-oriented” approach’. Similarly, Van Der Werf and Bianchi (2022) consider that a greater use of ‘nature-based solutions’ will depend on a paradigm shift where farmers are rewarded not only for food production but also for ‘being stewards of the environment’.
5 Conclusion
This article has focused on the actions at the bottom of at least one version of the IPM pyramid (Figure 1), summarised as: 1) what to plant, 2) where to plant it and 3) what to plant it with. It has also focused on pest insects, thus ignoring truly ‘integrated’ management of pests, pathogens and weeds and the complex interactions and trade-offs this might entail. It has also not given much detailed consideration to the other tools/approaches summarised in Figure 1 that might be integrated subsequently to achieve an acceptable level of pest control. However, if the actions at the bottom of pyramid are used to provide the soundest basis possible this gives more scope for effective integration with other solutions, such as biopesticides, that may not be ‘silver bullets’ but can contribute to incremental improvement of pest control (Chandler, 2017). This could certainly include the careful and very targeted use of synthetic pesticides in situations where available and where justified.
Author contributions
The author confirms being the sole contributor of this work and has approved it for publication.
Funding
At the time of writing, the author was working on projects funded by the European Union’s Horizon 2020 research and innovation programme under grant agreement No. 862563. (SmartProtect Thematic Network), the UK Department for the Environment, Food and Rural Affairs (Vegetable Genetic Improvement Network) and by the UK Agriculture and Horticulture Development Board (AHDB).
Acknowledgments
The author is grateful for discussions with colleagues including David Chandler, Howard Hinds, Andrew Jukes and members of the SmartProtect Thematic Network Consortium.
Conflict of interest
The author declares that the research was conducted in the absence of any commercial or financial relationships that could be construed as a potential conflict of interest.
Publisher’s note
All claims expressed in this article are solely those of the authors and do not necessarily represent those of their affiliated organizations, or those of the publisher, the editors and the reviewers. Any product that may be evaluated in this article, or claim that may be made by its manufacturer, is not guaranteed or endorsed by the publisher.
References
AHDB. (2017). Celery: Investigation of strategies to control mirid bugs in outdoor crops Final report project FV 441.
AHDB. (2020). Integrated pest management of cabbage stem flea beetle in oilseed rape. Report No.: 623.
Andow D. (1983). The extent of monoculture and its effects on insect pest populations with particular reference to wheat and cotton. Agriculture Ecosyst. Environ. 9 (1), 25–35. doi: 10.1016/0167-8809(83)90003-8
Bass C., Puinean A. M., Zimmer C. T., Denholm I., Field L. M., Foster S. P., et al. (2014). The evolution of insecticide resistance in the peach potato aphid, Myzus persicae. Insect Biochem. Mol. Biol. 51, 41–51. doi: 10.1016/j.ibmb.2014.05.003
Bianchi F. J., Booij C. J. H., Tscharntke T. (2006). Sustainable pest regulation in agricultural landscapes: A review on landscape composition, biodiversity and natural pest control. Proc. R. Soc. B: Biol. Sci. 273 (1595), 1715–1727. doi: 10.1098/rspb.2006.3530
Bianchi F. J. J. A., Goedhart P. W., Baveco J. M. (2008). Enhanced pest control in cabbage crops near forest in the Netherlands. Landscape Ecol. 23 (5), 595–602. doi: 10.1007/s10980-008-9219-6
Bianchi F. J. J. A., Van Wingerden W. K. R. E., Griffioen A. J., van der Veen M., van der Straten M. J. J., Wegman R. M. A., et al. (2005). Landscape factors affecting the control of Mamestra brassicae by natural enemies in Brussels sprout. Agriculture Ecosyst. Environ. 107 (2-3), 145–150. doi: 10.1016/j.agee.2004.11.007
Bischoff A., Pollier A., Lamarre E., Salvadori O., Cortesero A. M., Le Ralec A., et al. (2016). Effects of spontaneous field margin vegetation and surrounding landscape on Brassica oleracea crop herbivory. Agriculture Ecosyst. Environ. 223, 135–143. doi: 10.1016/j.agee.2016.02.029
Brennan E. B. (2013). Agronomic aspects of strip intercropping lettuce with alyssum for biological control of aphids. Biol. Control 65 (3), 302–311. doi: 10.1016/j.biocontrol.2013.03.017
Chandler D. (2017). “Basic and applied research on entomopathogenic fungi,” in Microbial agents of insect and mite pests (Amsterdam; Boston; Heidelberg; London; New York; Oxford; Paris San Diego; San Francisco; Singapore; Sydney; Tokyo: Academic Press), 42–60. doi: 10.1016/B978-0-12-803527-6.00005-6
Chapman J. W., Bell J. R., Burgin L. E., Reynolds D. R., Pettersson L. B., Hill J. K., et al. (2012). Seasonal migration to high latitudes results in major reproductive benefits in an insect. Proc. Natl. Acad. Sci. 109 (37), 14924–14929. doi: 10.1073/pnas.1207255109
Chapman J. W., Reynolds D. R., Smith A. D., Riley J. R., Pedgley D. E., Woiwod I. P. (2002). High-altitude migration of the diamondback moth Plutella xylostella to the UK: A study using radar, aerial netting, and ground trapping. Ecol. Entomol 27 (6), 641–650. doi: 10.1046/j.1365-2311.2002.00472.x
Chen Y., Mao J., Reynolds O. L., Chen W., He W., You M., et al. (2020). Alyssum (Lobularia maritima) selectively attracts and enhances the performance of Cotesia vestalis, a parasitoid of Plutella xylostella. Sci. Rep. 10 (1), 6447. doi: 10.1038/s41598-020-62021-y
Chen M., Shelton A. M., Hallett R. H., Hoepting C. A., Kikkert J. R., Wang P. (2011). Swede midge (Diptera: Cecidomyiidae), ten years of invasion of crucifer crops in north America. J. Economic Entomol 104 (3), 709–716. doi: 10.1603/EC10397
Chongtham I. R., Bergkvist G., Watson C. A., Sandström E., Bengtsson J., Öborn I. (2017). Factors influencing crop rotation strategies on organic farms with different time periods since conversion to organic production. Biol. Agric. Horticulture 33 (1), 14–27. doi: 10.1080/01448765.2016.1174884
Collier R., Mazzi D., Folkedal Schjøll A., Schorpp Q., Thöming G., Johansen T. J., et al. (2020). The potential for decision support tools to improve the management of root-feeding fly pests of vegetables in Western Europe. Insects 11 (6), p.369. doi: 10.3390/insects11060369
Crews T. E., Carton W., Olsson L. (2018). Is the future of agriculture perennial? Imperatives and opportunities to reinvent agriculture by shifting from annual monocultures to perennial polycultures. Global Sustainability 1, e11.1–18. doi: 10.1017/sus.2018.11
Crisp P., Johnson A. G., Ellis P. R., Hardman J. A. (1977). Genetical and environmental interactions affecting resistance in radish to cabbage root fly. Heredity 38 (2), 209–218. doi: 10.1038/hdy.1977.26
Defra. (2013). Plant pathogen populations in wild and agricultural hosts and interactions with plant genotype. final report project IF0188. science search. Available at: defra.gov.uk (Accessed 5 February 2023).
Deguine J. P., Aubertot J. N., Flor R. J., Lescourret F., Wyckhuys K. A., Ratnadass A. (2021). Integrated pest management: good intentions, hard realities. a review. Agron. Sustain. Dev. 41 (3), 38. doi: 10.1007/s13593-021-00689-w
Den Belder E., Elderson J., Van Den Brink W. J., Schelling G. (2002). Effect of woodlots on thrips density in leek fields: A landscape analysis. Agriculture Ecosyst. Environ. 91 (1-3), 139–145. doi: 10.1016/S0167-8809(01)00264-X
Dewar A. M., Qi A. (2021). The virus yellows epidemic in sugar beet in the UK in 2020 and the adverse effect of the EU ban on neonicotinoids on sugar beet production. Outlooks Pest Manage. 32 (2), 53–59. doi: 10.1564/v32_apr_02
Dunn J. A. (1959). The biology of lettuce root aphid. Ann. Appl. Biol. 47 (3), 475–491. doi: 10.1111/j.1744-7348.1959.tb07280.x
Dunn J. A. (1974). Study on inheritance of resistance to root aphid, Pemphigus bursarius, in lettuce. Ann. Appl. Biol. 76 (1), 9–18. doi: 10.1111/j.1744-7348.1974.tb01352.x
ECA. (2020). Special report: Sustainable use of plant protection products: limited progress in measuring and reducing risks EN 2020 05. European court of auditors. sustainable use of plant protection products (Publications Office of the EU) (Accessed 28 January 2023).
Eenink A. H., Dieleman F. L. (1983). Inheritance of resistance to the leaf aphid Nasonovia ribis-nigri in the wild lettuce species Lactuca virosa. Euphytica 32 (3), 691–695. doi: 10.1007/BF00042148
Eenink A. H., Groenwold R., Dieleman F. L. (1982). Resistance of lettuce (Lactuca) to the leaf aphid Nasonovia ribis nigri. L. transfer of resistance from L. virosa to L. sativa by interspecific crosses and selection of resistant breeding lines. Euphytica 31 (2), 291–299. doi: 10.1007/BF00021643
Ellis P. R. (1999). The identification and exploitation of resistance in carrots and wild umbelliferae to the carrot fly, Psila rosae (F.). Integrated Pest Manage. Rev. 4 (3), 259–268. doi: 10.1023/A:1009609414192
Ellis P. R., McClement S. J., Saw P. L., Phelps K., Vice W. E., Kift N. B., et al. (2002). Identification of sources of resistance in lettuce to the lettuce root aphid, Pemphigus bursarius. Euphytica 125 (3), 305–315. doi: 10.1023/A:1016050706560
Ellis P. R., Singh R., Pink D. A. C., Lynn J. R., Saw P. L. (1996). Resistance to Brevicoryne brassicae in horticultural brassicas. Euphytica 88, 85–96. doi: 10.1007/BF00032439
EU. (2019). The fruit and vegetable sector in the EU - a statistical overview. the fruit and vegetable sector in the EU - a statistical overview - statistics explained (europa.eu) (Accessed 28 January 2023).
EU. (2023a). Food safety – sustainable use of pesticides. sustainable use of pesticides (europa.eu) (Accessed 28 January 2023).
EU. (2023b). Biodiversity: how the EU protects nature biodiversity: how the EU protects nature - consilium (europa.eu) (Accessed 28 January 2023).
FAO, WHO. (2019). Sustainable healthy diets – guiding principles (Rome: Food and Agriculture Organization of the United Nations, World Health Organization), 37.
Fereres A., Irwin M. E., Kampmeier G. E. (2017). Aphid movement: process and consequences. aphids as crop pests, 2nd ed. Eds. van Emden H. F., Harrington R. (Wallingford, Oxford: CAB International), 196–224.
Finch S., Ackley C. M. (1977). Cultivated and wild host plants supporting populations of the cabbage root fly. Ann. Appl. Biol. 85 (1), 13–22. doi: 10.1111/j.1744-7348.1977.tb00626.x
Finch S., Billiald H., Collier R. H. (2003). Companion planting–do aromatic plants disrupt host-plant finding by the cabbage root fly and the onion fly more effectively than non-aromatic plants? Entomologia experimentalis applicata 109 (3), 183–195. doi: 10.1046/j.0013-8703.2003.00102.x
Finch S., Collier R. H. (2000). Host-plant selection by insects–a theory based on ‘appropriate/inappropriate landings’ by pest insects of cruciferous plants. Entomologia experimentalis applicata 96 (2), 91–102. doi: 10.1046/j.1570-7458.2000.00684.x
Finch S., Collier R. H. (2004). A simple method–based on the carrot fly–for studying the movement of pest insects. Entomologia experimentalis applicata 110 (3), 201–205. doi: 10.1111/j.0013-8703.2004.00136.x
Finch S., Collier R. H., Elliott M. S. (1990). “Seasonal variations in the timing of attacks of bronzed blossom beetles (Meligethes aeneus/Meligethes viridescens) on horticultural brassicas,” in Brighton Crop protection conference, pests and diseases-1990, vol. 1. (Thornton Heath, UK: British Crop Protection Council), 349–354.
Finch S., Kienegger M. (1997). A behavioural study to help clarify how undersowing with clover affects host-plant selection by pest insects of brassica crops. Entomologia experimentalis applicata 84 (2), 165–172. doi: 10.1046/j.1570-7458.1997.00211.x
Finch S., Skinner G. (1975). Dispersal of the cabbage root fly. Ann. Appl. Biol. 81 (1), 1–19. doi: 10.1111/j.1744-7348.1975.tb00490.x
Garrett D. (2022). Utilising ecology and molecular techniques to identify alternative hosts, green bridges, and population structure of a serious aphid pest PhD Thesis. (Coventry, UK: University of Warwick).
George D. R., Collier R. H., Whitehouse D. M. (2013). Can imitation companion planting interfere with host selection by brassica pest insects? Agric. For. Entomol 15 (1), 106–109. doi: 10.1111/j.1461-9563.2012.00598.x
Gladman A. K. (2022). Understanding interactions between biopesticides and partial crop resistance for management of aphid pests of brassica crops. PhD Thesis. (Coventry, UK: University of Warwick).
Gov.UK. (2023). Nature recovery network. nature recovery network - GOV.UK. Available at: www.gov.uk (Accessed 28 January 2023).
Grzywacz D., Rossbach A., Rauf A., Russell D. A., Srinivasan R., Shelton A. M. (2010). Current control methods for diamondback moth and other brassica insect pests and the prospects for improved management with lepidopteran-resistant bt vegetable brassicas in Asia and Africa. Crop Prot. 29 (1), 68–79. doi: 10.1016/j.cropro.2009.08.009
Gurr G. M., Wratten S. D., Luna J. M. (2003). Multi-function agricultural biodiversity: pest management and other benefits. Basic Appl. Ecol. 4 (2), 107–116. doi: 10.1078/1439-1791-00122
Højland D. H., Nauen R., Foster S. P., Williamson M. S., Kristensen M. (2015). Incidence, spread and mechanisms of pyrethroid resistance in European populations of the cabbage stem flea beetle, Psylliodes chrysocephala L. (Coleoptera: Chrysomelidae). PloS One 10 (12), e0146045. doi: 10.1371/journal.pone.0146045
Haaland C., Naisbit R. E., Bersier L. F. (2011). Sown wildflower strips for insect conservation: A review. Insect Conserv. Diversity 4 (1), 60–80. doi: 10.1111/j.1752-4598.2010.00098.x
Hammond R. B., Cooper R. L. (1993). Interaction of planting times following the incorporation of a living, green cover crop and control measures on seedcorn maggot populations in soybean. Crop Prot. 12 (7), 539–543. doi: 10.1016/0261-2194(93)90096-2
Hardman J. A., Ellis P. R. (1982). An investigation of the host range of the carrot fly. Ann. Appl. Biol. 100 (1), 1–9. doi: 10.1111/j.1744-7348.1982.tb07186.x
Hardman J. A., Ellis P. R., Saw P. L. (1990). Further investigations of the host range of the carrot fly, Psila rosae (F.). Ann. Appl. Biol. 117 (3), 495–506. doi: 10.1111/j.1744-7348.1990.tb04816.x
Hodgdon E. A., Campbell A. E., Conner D. S., Hoepting C. A., Galimberti A. K., Chen Y. H. (2022). Farm-level losses and grower willingness to try management strategies for swede midge in vegetable crops. HortTechnology 32 (5), 471–478. doi: 10.21273/HORTTECH05063-22
Hokkanen H. M. (1991). Trap cropping in pest management. Annu. Rev. Entomol 36 (1), 119–138. doi: 10.1146/annurev.en.36.010191.001003
Hokkanen H. M. (2015). Integrated pest management at the crossroads: Science, politics, or business (as usual)? Arthropod-Plant Interact. 9 (6), 543–545. doi: 10.1007/s11829-015-9403-y
Hokkanen H., Granlund H., Husberg G. B., Markkula M. (1986). “Trap crops used successfully to control Meligethes aeneus (Col., nitidulidae), the rape blossom beetle,” in Annales entomologici fennici, vol. 52. (Helsinki, Finland: Entomological Society of Finland), 115–120.
Hough G. (2013). Biology and control of currant lettuce aphid Nasonovia ribisnigri. PhD thesis. (Coventry, UK: University of Warwick).
Kogan M. (1998). Integrated pest management: historical perspectives and contemporary developments. Annu. Rev. Entomol 43 (1), 243–270. doi: 10.1146/annurev.ento.43.1.243
Lamichhane J. R., Arendse W., Dachbrodt-Saaydeh S., Kudsk P., Roman J. C., van Bijsterveldt-Gels J. E., et al. (2015). Challenges and opportunities for integrated pest management in Europe: A telling example of minor uses. Crop Prot. 74, 42–47. doi: 10.1016/j.cropro.2015.04.005
Lamy F., Bellec L., Rusu-Stievenard A., Clin P., Ricono C., Olivier D., et al. (2020). Oviposition preference of the cabbage root fly towards some Chinese cabbage cultivars: A search for future trap crop candidates. Insects 11 (2), 127. doi: 10.3390/insects11020127
Lamy F., Dugravot S., Cortesero A. M., Chaminade V., Faloya V., Poinsot D. (2018). One more step toward a push-pull strategy combining both a trap crop and plant volatile organic compounds against the cabbage root fly Delia radicum. Environ. Sci. Pollut. Res. 25 (30), 29868–29879. doi: 10.1007/s11356-017-9483-6
Marshall E. J. P., Moonen A. C. (2002). Field margins in northern Europe: their functions and interactions with agriculture. Agriculture Ecosyst. Environ. 89 (1-2), 5–21. doi: 10.1016/S0167-8809(01)00315-2
Matson P. A., Parton W. J., Power A. G., Swift M. J. (1997). Agricultural intensification and ecosystem properties. Science 277 (5325), 504–509. doi: 10.1126/science.277.5325.504
McGrath H. (2021). Bespoke field margins delivering multiple benefits to fresh produce. PhD thesis. (Reading, UK: University of Reading).
Morley K., Finch S., Collier R. H. (2005). Companion planting–behaviour of the cabbage root fly on host plants and non-host plants. Entomologia Experimentalis Applicata 117 (1), 15–25. doi: 10.1111/j.1570-7458.2005.00325.x
Naranjo S. E. (2011). Impacts of Bt transgenic cotton on integrated pest management. J. Agric. Food Chem. 59 (11), 5842–5851. doi: 10.1021/jf102939c
North J., Morton A., Barbara D., Spence N., Morgan D., Boonham N. (2004). Development of TaqMan® assays towards the detection of parsnip yellow fleck virus and anthriscus yellows virus. Latvijas Entomologs 41, 87–92.
Oomen G. J. M., Lantinga E. A., Goewie E. A., van der Hoek K. W. (1998). Farming systems as a way towards a more efficient use of nitrogen in European union agriculture. Environ. Pollut. 102 (1), 697–704. doi: 10.1016/S0269-7491(98)80101-2
Ortega-Ramos P. A., Cook S. M., Mauchline A. L. (2022). How contradictory EU policies led to the development of a pest: The story of oilseed rape and the cabbage stem flea beetle. GCB Bioenergy 14 (3), 258–266. doi: 10.1111/gcbb.12922
Parsons C. K., Dixon P. L., Colbo M. (2007). Relay cropping cauliflower with lettuce as a means to manage first-generation cabbage maggot (Diptera: Anthomyiidae) and minimize cauliflower yield loss. J. economic Entomol 100 (3), 838–846. doi: 10.1093/jee/100.3.838
Pfiffner L., Luka H., Schlatter C., Juen A., Traugott M. (2009). Impact of wildflower strips on biological control of cabbage lepidopterans. Agriculture Ecosyst. Environ. 129 (1-3), 310–314. doi: 10.1016/j.agee.2008.10.003
Phelps K., Collier R. H., Reader R. J., Finch S. (1993). Monte Carlo Simulation method for forecasting the timing of pest insect attacks. Crop Prot. 12 (5), 335–342. doi: 10.1016/0261-2194(93)90075-T
Philips C. R., Fu Z., Kuhar T. P., Shelton A. M., Cordero R. J. (2014). Natural history, ecology, and management of diamondback moth (Lepidoptera: Plutellidae), with emphasis on the united states. J. Integrated Pest Manage. 5 (3), D1–D11. doi: 10.1603/IPM14012
Pimentel D., Terhune E. C., Dritschilo W., Gallahan D., Kinner N., Nafus D., et al. (1977). Pesticides, insects in foods, and cosmetic standards. BioScience 27 (3), 178–185. doi: 10.2307/1297579
Porter S. D., Reay D. S., Bomberg E., Higgins P. (2018). Avoidable food losses and associated production-phase greenhouse gas emissions arising from application of cosmetic standards to fresh fruit and vegetables in Europe and the UK. J. Cleaner Production 201, 869–878. doi: 10.1016/j.jclepro.2018.08.079
Rämert B. (1996). Intercropping as a strategy for reducing damage to carrots caused by the carrot fly, Psila rosae (F.). Biol. Agric. Horticulture 13 (4), 359–369. doi: 10.1080/01448765.1996.9754794
Reinink K., Dieleman F. L., Jansen J., Montenarie A. M. (1989). Interactions between plant and aphid genotypes in resistance of lettuce to Myzus persicae and Macrosiphum euphorbiae. Euphytica 43 (3), 215–222. doi: 10.1007/BF00023056
Ribeiro A. L., Gontijo L. M. (2017). Alyssum flowers promote biological control of collard pests. BioControl 62 (2), 185–196. doi: 10.1007/s10526-016-9783-7
Sarkar S. C., Wang E., Wu S., Lei Z. (2018). Application of trap cropping as companion plants for the management of agricultural pests: A review. Insects 9 (4), 128. doi: 10.3390/insects9040128
Sauer-Kesper C., Lucia N., Buser H., Vogler U. (2011). The new biotype nr: 1 of the currant lettuce aphid: its distribution and impact on Swiss lettuce production. Agrarforschung Schweiz 18 (10), 462–469.
Serée L., Barbottin A., Chiron F., Valantin-Morison M., Gardarin A. (2023). Within-field floral resources have the potential to increase parasitism rates in winter oilseed rape pests more than resources at field margins. Agriculture Ecosyst. Environ. 344, 108288. doi: 10.1016/j.agee.2022.108288
Shelton A. M., Badenes-Perez F. R. (2006). Concepts and applications of trap cropping in pest management. Annu. Rev. Entomol 51 (1), 285–308. doi: 10.1146/annurev.ento.51.110104.150959
Shelton A. M., Nault B. A. (2004). Dead-end trap cropping: A technique to improve management of the diamondback moth, Plutella xylostella (Lepidoptera: Plutellidae). Crop Prot. 23 (6), 497–503. doi: 10.1016/j.cropro.2003.10.005
Skirvin D. J., Kravar-Garde L., Reynolds K., Wright C., Mead A. (2011). The effect of within-crop habitat manipulations on the conservation biological control of aphids in field-grown lettuce. Bull. Entomological Res. 101 (6), 623–631. doi: 10.1017/S0007485310000659
Slater R., Ellis S., Genay J. P., Heimbach U., Huart G., Sarazin M., et al. (2011). Pyrethroid resistance monitoring in European populations of pollen beetle (Meligethes spp.): A coordinated approach through the insecticide resistance action committee (IRAC). Pest Manage. Sci. 67 (6), 633–638. doi: 10.1002/ps.2101
Stenberg J. A. (2017). A conceptual framework for integrated pest management. Trends Plant Sci. 22 (9), 759–769. doi: 10.1016/j.tplants.2017.06.010
Theunissen J., Schelling G. (1998). Infestation of leek by Thrips tabaci as related to spatial and temporal patterns of undersowing. BioControl 43 (1), 107–119. doi: 10.1023/A:1009986917903
Tiwari S., Sharma S., Wratten S. D. (2020). Flowering alyssum (Lobularia maritima) promote arthropod diversity and biological control of Myzus persicae. J. Asia-Pacific Entomol 23 (3), 634–640. doi: 10.1016/j.aspen.2020.05.002
Uvah I. I. I., Coaker T. H. (1984). Effect of mixed cropping on some insect pests of carrots and onions. Entomologia experimentalis applicata 36 (2), 159–167. doi: 10.1111/j.1570-7458.1984.tb03422.x
Van Der Werf W., Bianchi F. (2022). Options for diversifying agricultural systems to reduce pesticide use: Can we learn from nature? Outlook Agric. 51 (1), 105–113. doi: 10.1177/00307270221077442
Varis A. L. (1991). Effect of intercropping carrots and onions on damage caused by the carrot fly, Psila rosae (F.)(Dipt., Psilidae). Agric. Food Sci. 63 (5), 411–414. doi: 10.23986/afsci.72420
Veres A., Petit S., Conord C., Lavigne C. (2013). Does landscape composition affect pest abundance and their control by natural enemies? a review. Agriculture Ecosyst. Environ. 166, 110–117. doi: 10.1016/j.agee.2011.05.027
Wainwright C., Jenkins S., Wilson D., Elliott M., Jukes A., Collier R. (2020). Phenology of the diamondback moth (Plutella xylostella) in the UK and provision of decision support for brassica growers. Insects 11 (2), 118. doi: 10.3390/insects11020118
Walley P. G., Hough G., Moore J. D., Carder J., Elliott M., Mead A., et al. (2017). Towards new sources of resistance to the currant-lettuce aphid (Nasonovia ribisnigri). Mol. Breed. 37 (1), 1–18. doi: 10.1007/s11032-016-0606-4
Wheatley G. A., Finch S. (1984). “Effects of oilseed rape on the status of insect pests of vegetable brassicas,” in 1984 British crop protection conference. pests and diseases. proceedings of a conference held at Brighton metropole, England, November 19-22, 1984, vol. 2. (Croydon, UK: British Crop Protection Council), 807–814.
Willett W., Rockström J., Loken B., Springmann M., Lang T., Vermeulen S., et al. (2019). Food in the anthropocene: the EAT–lancet commission on healthy diets from sustainable food systems. Lancet 393 (10170), 447–492. doi: 10.1016/S0140-6736(18)31788-4
Winkler K., Wäckers F. L., Termorshuizen A. J., van Lenteren J. C. (2010). Assessing risks and benefits of floral supplements in conservation biological control. BioControl 55, 719–727. doi: 10.1007/s10526-010-9296-8
Witkowska E., Moorhouse E. R., Jukes A., Elliott M. S., Collier R. H. (2018). Implementing integrated pest management in commercial crops of radish (Raphanus sativus). Crop Prot. 114, 148–154. doi: 10.1016/j.cropro.2018.08.008
Keywords: IPM (integrated pest management), vegetables, EUR = Europe, IPM pyramid, pest insects
Citation: Collier R (2023) Pest insect management in vegetable crops grown outdoors in northern Europe – approaches at the bottom of the IPM pyramid. Front. Hortic. 2:1159375. doi: 10.3389/fhort.2023.1159375
Received: 05 February 2023; Accepted: 28 February 2023;
Published: 04 April 2023.
Edited by:
Anders Huseth, North Carolina State University, United StatesReviewed by:
Panagiotis Eliopoulos, University of Thessaly, GreeceMehmet Bora Kaydan, Çukurova University, Türkiye
Copyright © 2023 Collier. This is an open-access article distributed under the terms of the Creative Commons Attribution License (CC BY). The use, distribution or reproduction in other forums is permitted, provided the original author(s) and the copyright owner(s) are credited and that the original publication in this journal is cited, in accordance with accepted academic practice. No use, distribution or reproduction is permitted which does not comply with these terms.
*Correspondence: Rosemary Collier, cm9zZW1hcnkuY29sbGllckB3YXJ3aWNrLmFjLnVr