- Department of Urban Horticulture and Plant Use, Hochschule Geisenheim University, Geisenheim, Germany
Introduction: Urban green can be complemented by Living walls (LW). Knowledge of LW, including technique or their cooling effects, is well discussed, but little published data on plant diversity, design and development in LWs exists. The plants themselves determine whether LWs achieve their intended benefits. However, LW plants are exposed to extreme conditions such as temperature or drought stress.
Material and methods: Therefore, we observed plant development in a textile-based LW (mat) over a three-year experiment under a temperate oceanic climate in the south west of Germany. The aim was to establish higher plant diversity for use in LWs. We chose 34 perennials (shrubs, ferns, grasses, and geophytes) that require high soil moisture. The mat was soil-free and had to be overwatered with a nutrient solution. The perennials were grouped in the plant module “cascade” (tall, hanging plants) and “ground cover” (low-spreading plants). We created four experimental walls exposed to the south, north, west, and east and adapted the plant modules to the lighting conditions of the experiment wall. The modules were pre-cultivated and attached to the experimental walls in a randomised design and repetition of four. We determined the visual attractiveness and additional parameters of individual plants.
Results: We observed that Tellima grandiflora ‘Rubra’, Waldsteinia ternata, Pachysandra terminalis, and Heuchera Hybride ‘Purple Petticoats’ were the most attractive all year. Ajuga tenorii ‘Mauro’, Alchemilla caucasica, Hosta sieboldii ‘Harry van Trier’, Glechoma hederacea, and Geum coccineum ‘Carlskaer’ showed a nice ornamental effect and flowering during summer. The ferns and Waldsteinia ternata showed delayed growth but nice leaf texture gradually.
Discussion and conclusion: We saw differences in winter survival rates depending on minimum temperature in winter. The tested plants developed a special vertical growth form and height. We observed various results at different expositions but found suitable species for each exposition. With these results, we derived a table of plants along with their suitability for LWs and determining conditions.
1 Introduction
Plants are a fundamental part of living walls (LWs). So far, little attention has been paid to the role of plants in LWs. LWs are a type of urban green infrastructure possessing the potential to deal with the issues of urbanisation, such as the heat island effect (LW classification according to Tzoulas et al., 2007; Rouse et al., 2013; Radić et al., 2019). Compared to horizontal green infrastructure, LWs are installed on unused façade surfaces and do not conflict with roads and buildings (Ottelé et al., 2010; Böhm et al., 2016). LWs also provide benefits for the environment and humans (Perini et al., 2011; Perini and Magliocco, 2012; Perini et al., 2013; Pfoser et al., 2013; Manso and Castro-Gomes, 2015; Riley, 2017; Radić et al., 2019). LWs have positive effects on biodiversity (Francis and Lorimer, 2011), wellbeing, and social interaction (Tzoulas et al., 2007; Kowarik et al., 2017; Radić et al., 2019). LWs help manage high temperatures (Wong et al., 2010; Hunter et al., 2014), building insulation (Mazzali et al., 2013; Pérez et al., 2014), stormwater (Rouse et al., 2013; Pfoser, 2016), noise (Irvine et al., 2009; Pfoser et al., 2013), and air pollution (Ottelé et al., 2010; Wack, 2015).
The construction, technology, costs, and types of LWs have already been described (Manso and Castro-Gomes, 2015; Riley, 2017; Radić et al., 2019). Furthermore, green facades are well known too (Pfoser, 2016; Radić et al., 2019). The difference between both systems is that green facades need a connection to the soil and are limited to climbing plants. As LWs provide plant nutrients directly at the façade, it is possible to use many different perennials (Radić et al., 2019; Charoenkit and Yiemwattana, 2021).
Despite this advantage, only a few species are used currently. In the temperate oceanic climate (Germany), the cold winter (frost) is a specific challenge for plant choice. Presumably, robust species proven to withstand the conditions are used. Finally, plant failure can cause high costs (Riley, 2017). Bundesverband Gebäude Grün (BuGG) (German Green Building Association) has compiled the knowledge on greening practices (Brandhorst et al., 2015). Besides this, there are only a few scientific studies on the suitability of perennials for LWs (Mårtensson et al., 2016) and few studies on plants in LWs under climates with periods of frost (e.g. Germany: Roth-Kleyer and Gunkel, 2014; Günther et al., 2016 and Sweden: Mårtensson et al., 2014; Mårtensson et al., 2016). The development of roots in LWs under greenhouse conditions has been investigated in several studies (Jørgensen et al., 2014a; Jørgensen et al., 2014b; Jørgensen et al., 2018). Other studies have described plants for LWs in other climate zones (Charoenkit and Yiemwattana, 2021; Dvorak et al., 2021).
The general findings are that perennials are suitable for LW (Mårtensson et al., 2014). Perennials can survive extreme (hot summer, cold winter) and Mediterranean climate conditions (Devecchi et al., 2013; Mårtensson et al., 2014). Different plant species exhibited various development and other growth forms (Günther et al., 2016; Charoenkit and Yiemwattana, 2021). Depending on the substrate used and thus a variation in water content, differences in plant and root growth were observed (Jørgensen et al., 2014a).
Eppel (2015) compared LW systems of different manufacturers and showed that plant selection is often made without considering the location conditions. It is assumed that proven species are used for plant selection. Plant losses are caused by plant pests, drought stress, or the technique of the systems (Roth-Kleyer and Gunkel, 2014; Eppel, 2015).
From these results, first instructions for plant design of LWs can be developed: an attractive planting picture can be created with a well-considered plant selection. This should achieve a high degree of cover and comprise evergreen and perennial species (Jim, 2015). Plant selection for LWs are varied and should be done with different selection criteria than those for common horizontal use (Günther et al., 2016; Charoenkit and Yiemwattana, 2021). Higher plant diversity is recommended to optimise the effects and functions of LWs (Charoenkit and Yiemwattana, 2021; Dvorak et al., 2021) tested the suitability of native plants in Mexico for LWs and recommended it for further projects.
LW studies: (Cameron et al., 2014; Hunter et al., 2014; Günther et al., 2016; Riley, 2017; Radić et al., 2019; Charoenkit and Yiemwattana, 2021) call for more research on plants in LW.
In this regard, a key aspect of LWs is the plants themselves. Plants determine whether a LW is attractive and how much maintenance is necessary and whether the desired effects, such as cooling effect or biodiversity promotion, materialise (Charoenkit and Yiemwattana, 2021). On the other hand, LW plants are exposed to extreme conditions (Mårtensson et al., 2014). Therefore, their characteristics must be considered before selecting adapted species (Jørgensen et al., 2014a). Plants in LWs have to deal with special conditions. We have described three factors that influence plant vigor: (1) The environmental conditions and climate surrounding the plant and its growing space. (2) The root space, which describes the conditions in the substrate or area where the plant is rooted and where the plant is supplied with water and nutrients. And (3) the plant composition, i.e., the competition among the different plants within a LW system.
(1) Plants have to cope with environmental conditions and the surrounding climate: variations in the climate due to seasons and the vertical level (Riley, 2017); extreme microclimatic conditions near the plant occur due to variations in temperature, humidity, heat, wind, and radiation to extreme values (e.g. high radiation and frost simultaneously) (Mårtensson et al., 2014; Riley, 2017); dynamic environmental conditions depending on the exposition (e.g. shadow at a north exposition) and prevail on a façade surface (e.g. higher radiation at the top of the façade compared to the bottom) (Riley, 2017).
(2) The LW systems require growth area for the plants: the shoot and root space. For a vital plant especially, the root space and the characteristic of the substrate are of decisive importance (Jørgensen et al., 2014a). When there is limited root space as in LW, the requirements of the plants must be carefully met (Jørgensen et al., 2014a). The water availability and prevention of drought stress or water logging are important (Mårtensson et al., 2014; Jørgensen et al., 2014a; Riley, 2017). Furthermore, nutrient availability with fertilisation and control of pH in the substrate or nutrient solution is essential (Riley, 2017). Root zone parameters are also influenced by the choice of substrate type. Moreover, the different plants in one LW are in competition for root space (Jørgensen et al., 2014a).
(3) For the plant composition in one LW, different plant species are selected which have varied requirements and influence each other. Plant species has different requirements (e.g. water, light) (Jørgensen et al., 2014a). It is difficult to meet different demands through LW systems. Therefore, species that have the same habitat requirements must be selected. Moreover, the plants are in competition above ground and for root space (Jørgensen et al., 2014a). The different growth forms, different planting methods, and competition for light, water, nutrients and space should be noted. The plants are at a different, unnatural level and are exposed to a new type of interaction. So, they must cope with shading from other overlapping plants. Since this is not a natural site, the habitat has to be redefined.
In the early days of LWs, it was assumed that plants would simply grow in the systems. Plants exude natural simplicity, so their demands were underestimated (Riley, 2017). Some projects failed because plants were seen as the secondary and not the main factor of LWs. This was also because LWs emerged from disciplines that had little knowledge of plants. The influence of plant sciences (e.g. horticulture, botany) and the cooperation between disciplines was missing (Hunter et al., 2014). In summary, successful LWs are very complex and depend on many factors (Riley, 2017).
2 Hypotheses for the study
This study aimed to test a higher variety of plants for their suitability in LWs. The project was set up as interdisciplinary research. It integrated a scientific experimental design with the demands of common plant design in landscape architecture and knowledge from horticulture. We created a field experiment with differently exposed walls, a plant selection adapted to the conditions, and repetitions of plant modules. The plant modules were tested continuously for three years under all seasons. The following research questions were explored:
• What does the plant look like during different seasons and are there differences in plant aesthetics during the experiment years?
• How growth form, spread, and competition behavior changed in vertical planting? Is there different development depending on plant position? And what are the learnings from the different planting variants (cascade and groundcover)?
• How susceptible are the plantings to pests or pathogens?
• Is there a difference in development depending on the exposure of the experimental walls?
• Which methodology works best for describing the suitability of a plant?
3 Materials and methods
3.1 Materials and experimental conditions
For our research, we set up a larger field experiment with LWs for three years (2017–2019). The location was Hochschule Geisenheim University, Geisenheim, Germany (49° 59′ 11.161″ N 7° 58′ 0.099″ E). We tested two different plant modules, a seeding variant and a control, without planting on a textile-based LW (mat). The two plant modules were cascade and ground cover. This paper attempts to show whether plants in these modules are suitable for LWs. Parts of this research project have already been published. Two articles with practical advice were published in German practitioner journals (Stollberg et al., 2021; Hietel et al., 2022). We tested different planting variants: Among others seeding and we published the results in: Stollberg et al., 2022. In Stollberg and von Birgelen, 2022 we give an overview about the visual impression and development of all plants on the mat. In addition to our previous studies we describe in this article the results of all single plants and give detailed information about their characteristics.
3.2 Climatic conditions
The research location is classified under winter hardiness zone 8a and temperate oceanic climate (mean annual temperature (MAT): 12.7°C; mean annual precipitation (MAP): 531 mm/annum – over at least a 30-year period: 1989–2019). Between 2017 and 2019, the local annual temperatures were as follows: maximum – 35.0°C, minimum – -9.9°C, mean – 11.3°C (2017); maximum – 36.6°C, minimum – -8.6°C, mean – 12.4°C (2018); and maximum – 39.2°C, minimum – -9.2°C, mean – 11.8°C (2019) (data from weather station nearby). The number of hot days was 17, 25, and 30, respectively (hot days = maximum daytime temperature >30°C). The annual precipitation sums were 589.8 mm (2017), 480.2 mm (2018), and 489.7 mm (2019). A striking feature was a frost period (minimum temperatures: -8.6°C to -2.7°C and maximum temperatures: -2.5°C to 1.6°C) from 25 February to 3 March 2018 (see previous study: Stollberg et al., 2022; Stollberg and von Birgelen, 2022).
3.3 Experiment run and setup
The experiment was started in April 2017 with a two-month pre-cultivation on flood tables. The mats were planted with different plant modules and randomly placed (Figure 1). In June 2017, the plants were rooted in the mats, and we installed them outside on the experimental walls in a randomised design. The four freestanding experimental walls’ expositions were north, east, west, and south (Figure 1 and see (Hietel et al., 2022; Stollberg et al., 2022). The mats remained on the walls until the end of the experiment (September 2019). Necessary interventions were maintenance and replanting of failed plants after winter.
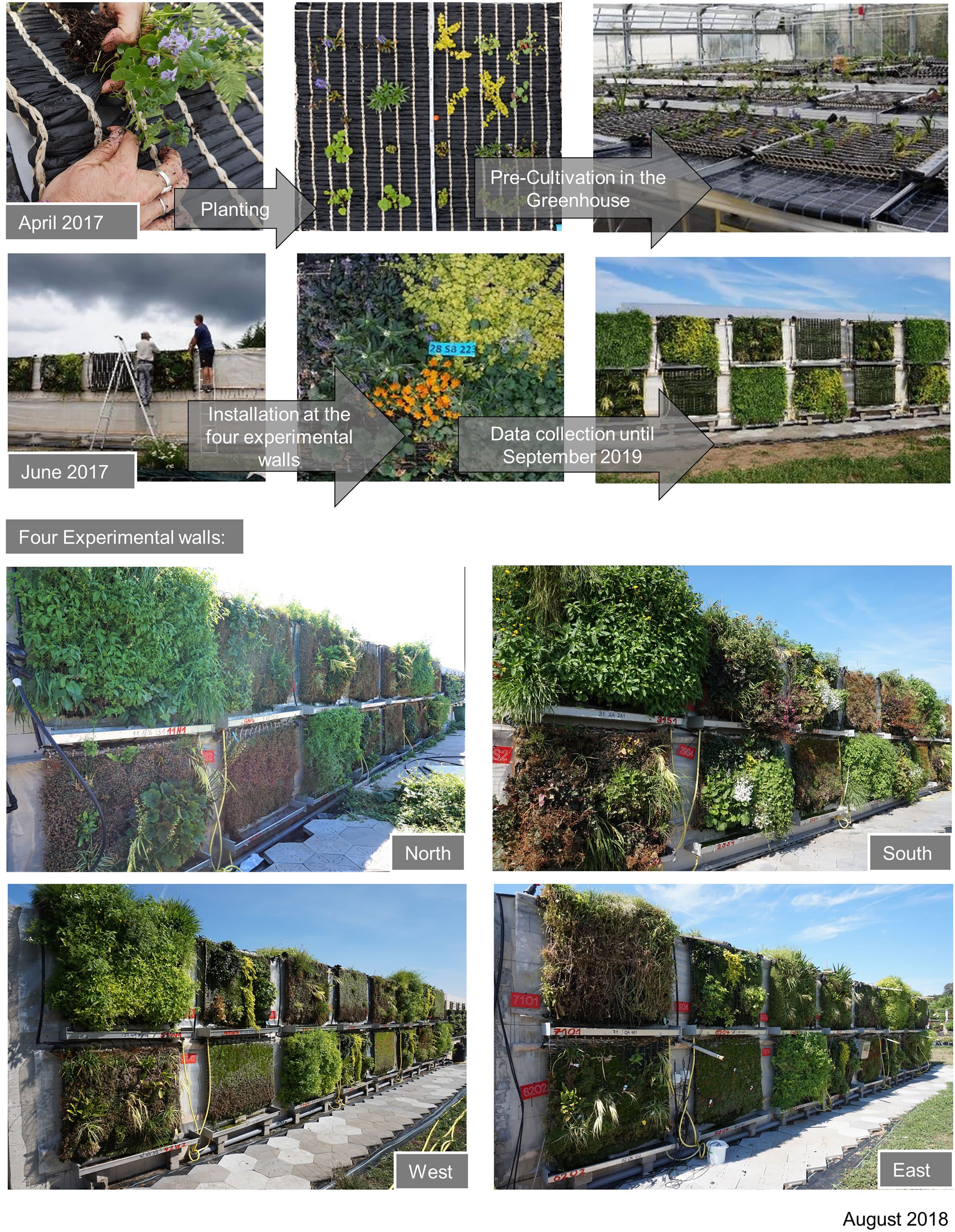
Figure 1 Experimental set up: Steps during the experiment run and the four experimental walls in August 2018.
The highest maintenance effort required was the irrigation and fertilisation of the mats (see (Hietel et al., 2022; Stollberg et al., 2022). The irrigation ran automatically at frequent intervalls, so that the mats were humid all the time.
The LW system was fertilized with a nutrient solution (35 mg N L-1): “Ferty 6” (6% N, 14% P2O5, 37% K2O, 2.4% MgO, 0,3% Mineral elements (Planta Düngemittel GmbH; 93,128 Regenstauf) as a basis which was supplemented by a nitrogen component (pH balancing).
Other maintenance steps were the cutting of a few plant species, removal of weeds, and maintenance after winter. We collected the plant material from the cutting and determined the dry mass. After winter, we removed the dead plant material. Over spring, we observed whether a plant had fallen out completely and replanted it in May at the latest. From this, we calculated the winter survival rate.
3.4 Textile vegetation layer (mat)
In this project, we tested a textile-based vegetation layer (mat, 1 x 1.20 m) (Table 1). We worked with Sächsisches Textilforschungsinstitut e.V., Chemnitz, Germany. They developed and produced the mat and also carried out research on it (Maehlmann, 2021; Stollberg et al., 2021). As mentioned in the “Maintenance” sub-section, the mat retained less water because it contained no soil. Instead of the substrate, the plants take root in a polyester-spun bounded fabric that framed an irrigation fleece (non-woven) (Table 1). The planting position of the plants is vertical (90°) to the experimental wall due to the flat system (mat).
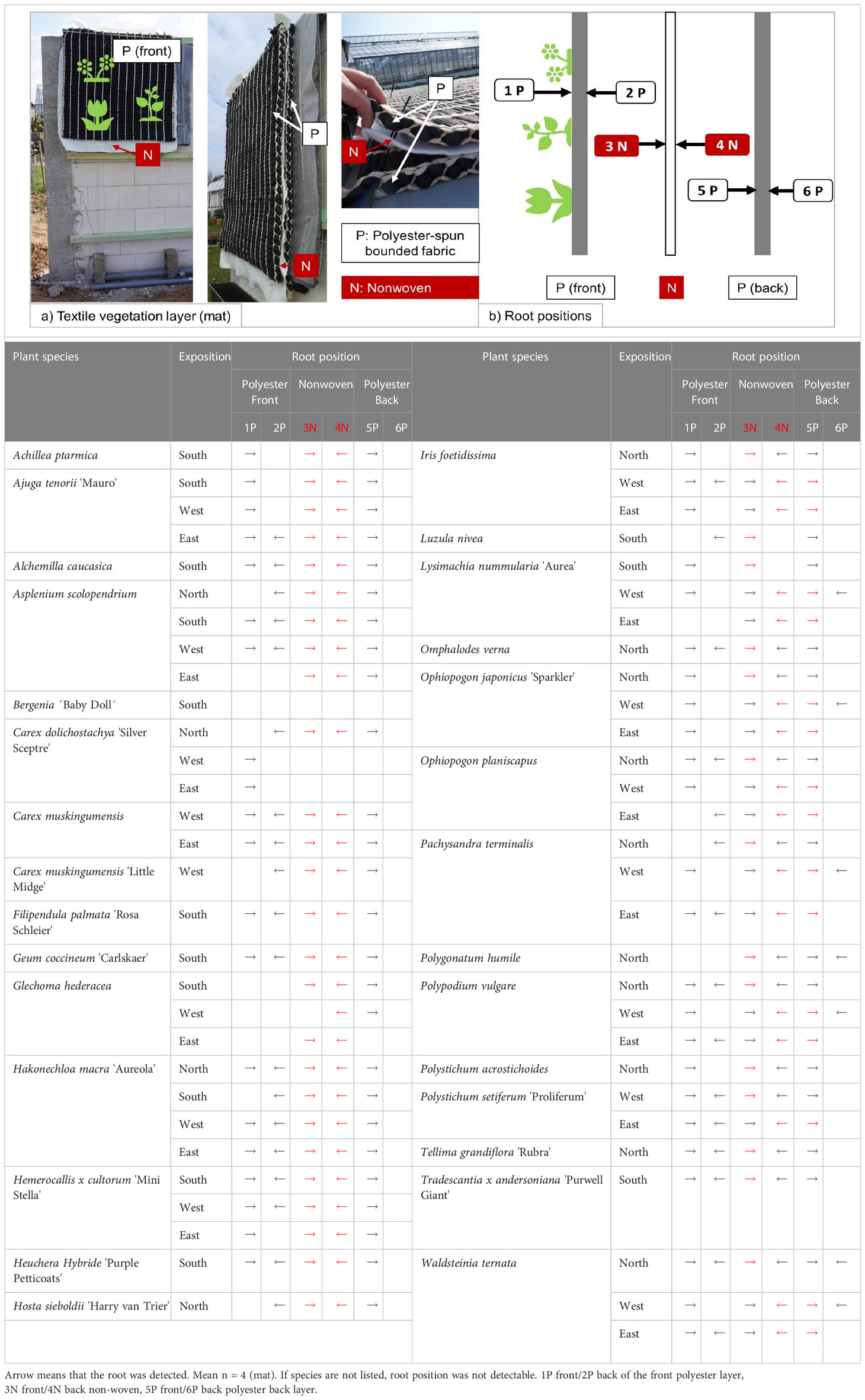
Table 1 Construction of the mat and schematic illustration of the root positions and root position documented at the final evaluation.
3.5 Plant choice and modules
In total, we tested 34 perennials as well as geophytes (e.g. different Narcissus species, Muscari armeniacum, Convallaria). Notably, we found that geophytes are not suitable for the mat; thus, they have not been discussed here.
We ordered the perennials as young plants or in a 9-pot (tb9x9). It was easier to plant the young ones. However, it was not possible to get all species young. After winter, we had to replace some of the plants. Over spring, it was possible to see which plants have survived winter. In 2018, we ordered the plants after we detected the missing plants. Therefore, we planted at the end of May 2018. For 2019, all the plants that had a high potential of failure were ordered in Autumn 2018. In this case, we planted earlier (April 2019).
Furthermore, we had to water frequently to create a moist/fresh habitat. For the plant choice, we considered light conditions of the respective experimental walls. For the north exposure, we chose shade tolerant plants, for the east and west semi-shade and -sun tolerant species, and for the south sun loving plants. As a basis for planning, we use the living areas for gardens according to Hansen (2016). We wanted to test two different plant compositions: cascade and ground cover. We chose tall species (up to 50 cm) for the cascade and hypothesised that these plants would grow so high that they would fall. The cascade should look like a waterfall. The ground cover is distinguished by species that expand over a wide area and have a lower growth height (maximum 30 cm). Subsequently, we named the variant by the exposition and plant module. All tested species and plant modules are shown in Figure 2. We tested species that had already proven themselves in projects by adapting to the conditions, and we risked the use of species that did not necessarily seem suitable (Figures 2, 3).
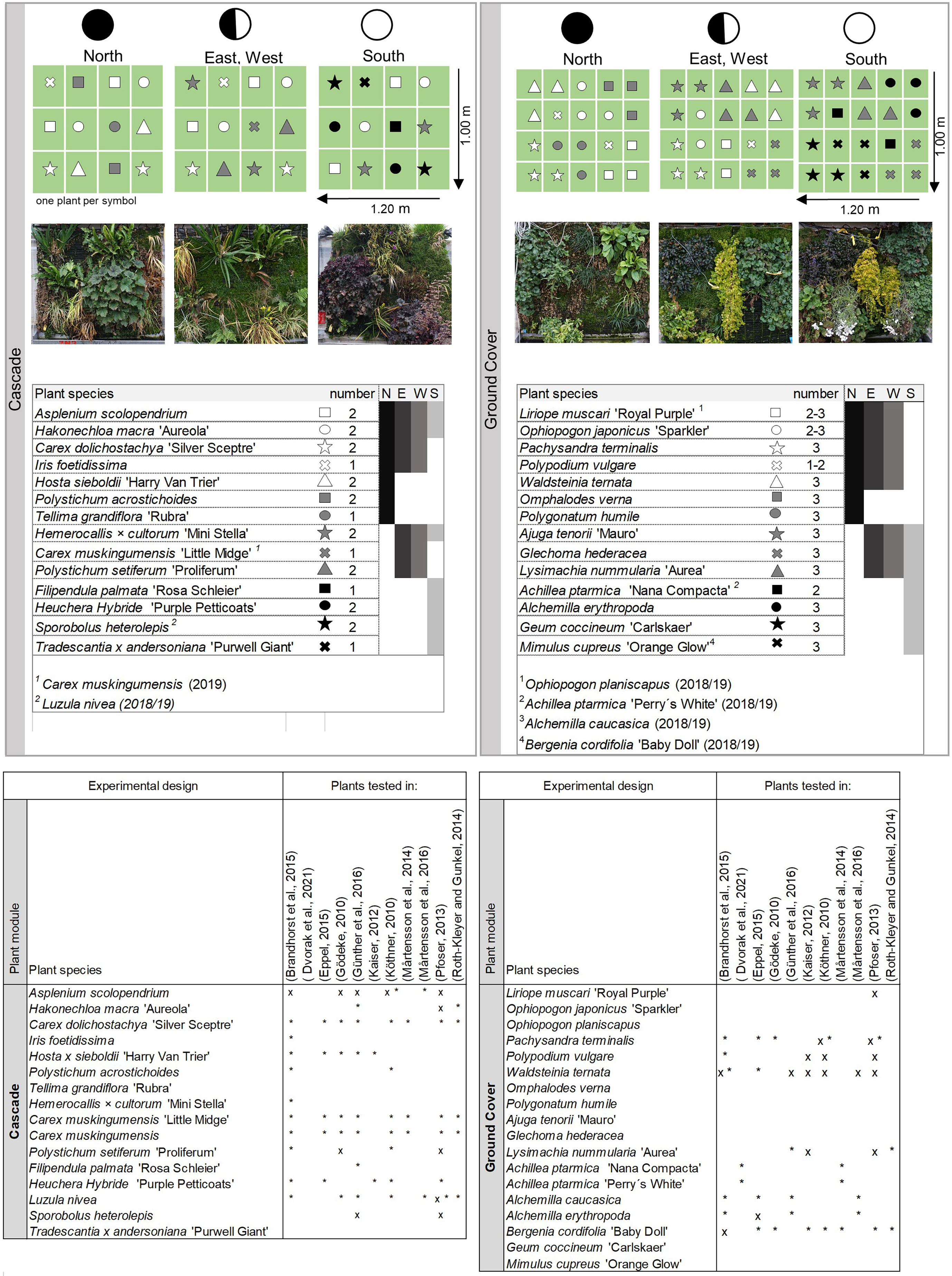
Figure 2 The plant modules “Cascade” and “Ground cover” at different expositions with species and position of the species in the mat (one symbol per plant). And list of studies that imply the species (Köthner, 2010; Kaiser, 2012; Mårtensson et al., 2014; Roth-Kleyer & Gunkel, 2014; Brandhorst et al., 2015; Eppel, 2015; Günther et al., 2016; Mårtensson et al., 2016; Dvorak et al., 2021).
3.6 Statistical analysis and calculations
Statistical analyses were performed with IBM SPSS Statistic 25. Since the vitality and ornamental effect data are ordinally scaled, the median is shown. For the growth height, length, and drymass, we calculated the mean with standard deviation. The data were not normally distributed. The data were tested for significant differences using a non-parametric Kruskal–Wallis test (multiple comparisons: all in pairs) and the Dunn–Bonferroni test as a post hoc test. We measured once a month and formed the mean or median from four repetition. In order to summarize the data, we formed averages for the vegetation periods (= three month with four repetition per month). We tested if data were significant different for ornamental effect between exposition in a vegetation period, for winter hardiness, for growth height between expositions and vegetation periods, for shoot-drymass between exposition and plant position at the final evaluation.
3.7 Methods
3.7.1 Growth height
We measured the growth height once a month for every species and, if possible, every single plant. Some plants spread out so it was not possible to differentiate single plants. Here, we measured all plants of one species together. We defined growth height as the vertical distance from the mat to the top of the plants. We measured the growth height with a plastic plate (25x25 cm²) and a scale and measured when 90% of the plant touched the plastic plate (Stollberg et al., 2022).
3.7.2 Rating
We visually assessed the individual plants once a month over the entire experiment using the rating method of the AK Pflanzenverwendung of the Bund deutscher Staudengärtner, 2013 (Association of German Perennial Gardeners) (Table 2). We rated the visual appearance according to parameters and a scale (Table 2). We did not assess dead plants or those that were dormant. The evaluation ranged from 1 (very poor) to 3, 5, 7, and 9 (very good). The parameters were vitality, hardiness, coexistence, flower-ornamental effect, leaf-ornamental effect, coverage, and overall impression. The AK Pflanzenverwendung also weighted the sum of all the parameters. It must be noted that individual parameters influence each other. Therefore, independent consideration of the parameters does not make sense.
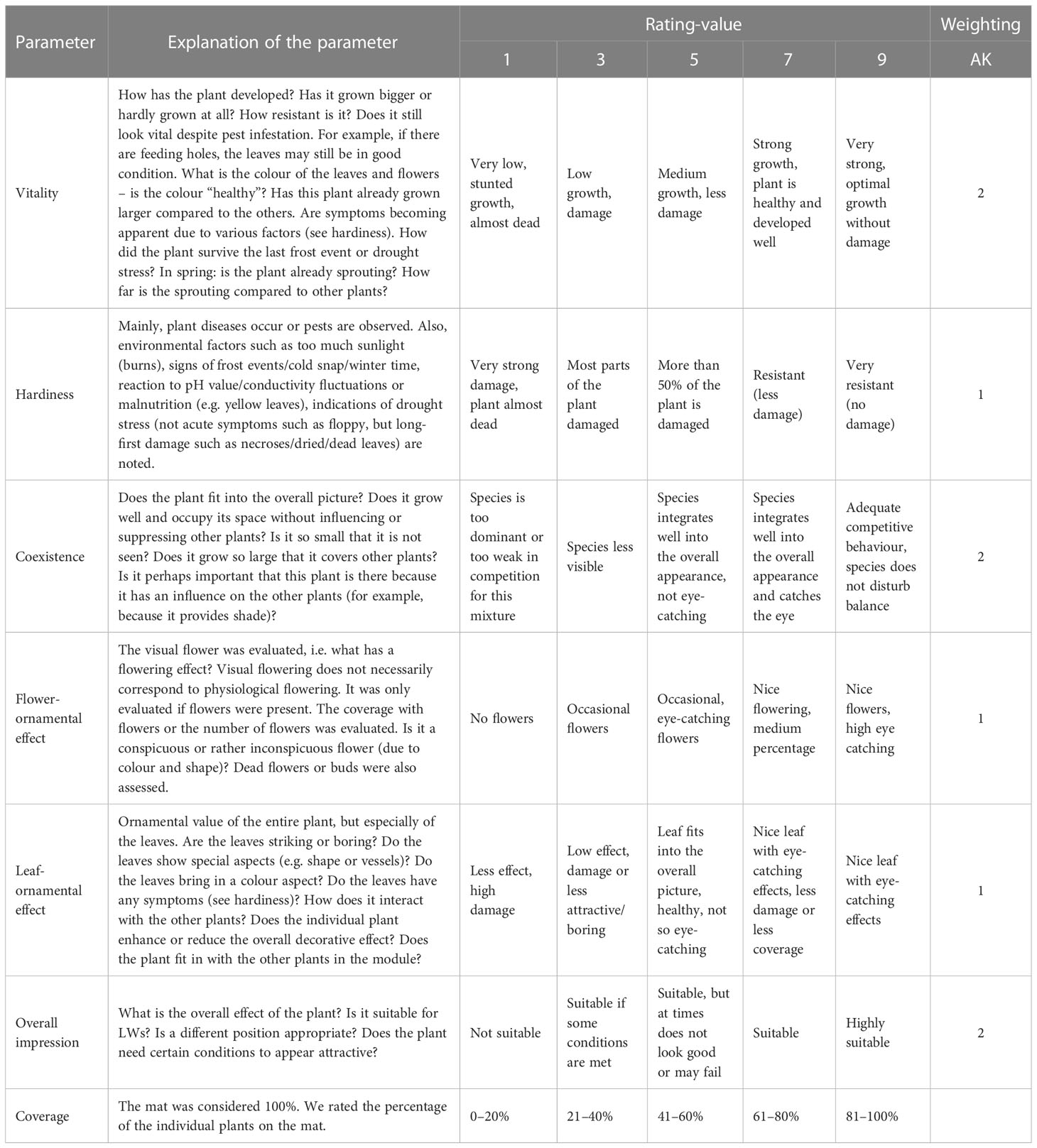
Table 2 Explanation of the rating parameters and the rating scale with the weighting of1 AK Pflanzenverwendung.
To determine the suitability of the individual plant species, we have calculated the high score values in different periods and qualities in with the single rating parameters (see Table 1 in 01_Supplementary Material). With the high score values, we determined whether individual plant species are suitable for LWs.
To calculate values for suitability, we first take the number of months of the whole year, summer, or winter. Please note that some plants were replaced and therefore not tested during the entire experiment run. For these species, we calculated the corresponding values for suitability (see 2. in 02_Supplementary Data). We multiplied the number of months with the rating value: 3 (low), 5 (medium), 9 (maximum), and 7 (nice) for the leaf ornamental effect. The calculated values were the basis for the evaluation of the rating data. These values were compared with the rating data from individual species. We determined the median for every month for every plant species and exposure.
We summed up the median for the whole year, summer, and winter (cumulative rating). We compared these cumulative ratings with the calculated rating values and determined the suitability using different rating parameters. We summed up the suitability value for each species, which occurred a minimum of four times. In the case of an equal number of votes, we chose the lower value. The high leaf-ornamental effect was determined according to the period.
3.7.3 Growth form
We documented the growth form of the different species by drawing and controlling them. From the drawings, we grouped the species in 9 categories. The categories lean on the elaborated growth form of (Günther et al., 2016).
3.7.4 Winter survival rate
We documented the number of plants that failed after the winter and listed the proportion of surviving plants [%] related to the target number. The failures between May and October were also documented.
3.7.5 Final evaluation
We conducted a final evaluation of all plants at the end of the experiment run in September 2019. Here, we determined the growth height (folding rule), plant height, fresh mass (leaves, stem, rhizome), and dry mass after drying the plant material at 60°C in the dryer for 2–3 days (see Figures 1, 2 in 01_Supplementary Material). We counted the number of leaves and determined the leaf area (randomly or of the total plant with LAI-meter – LI-3100 C Area Meter, LI-COR, Inc. Lincoin, Nebraska USA). We also attempted to determine the root mass and root height. These were very densely rooted in the material, so the values did not reflect the total root weight. We cut the mat apart and took photos from the different layers of the mat. This gives an impression of the root spreading. We also determined where the plants were rooted in the mat by taking them apart and determining whether the roots were growing in each part. The positions were named from 1P to 6P (Table 1). For example, if the roots grew in the irrigation mat (non-woven) and we observed this on the front of the non-woven mat, then the position would be 3N.
3.7.6 Final suitability table
From all the results, we calculated a parameter that provides an overview of the plants’ development and suitability for LWs. The single parameters are explained in the results and summarised as final results.
4 Results
4.1 Rating
4.1.1 Rating all parameters
During the experimental run, the individual plant species showed different characteristics (Figures 4, 5 and see Figures 3, 8 in 01_Supplementary Material). From spring to the first frost, Ajuga tenorii ‘Mauro’, Alchemilla caucasica, Hosta sieboldii ‘Harry van Trier’, Lysimachia nummularia ‘Aurea’ and Waldsteinia ternata showed an interesting and attractive leaf texture with a long distance effect (Figures 4, 5A). Moreover, these species (except Lysimachia nummularia ‘Aurea’), Glechoma hederacea and Geum coccineum ‘Carlskaer’ showed occasional flowering, and Hakonechloa macra ‘Aureola’ exhibited an eye-catching leaf colour.
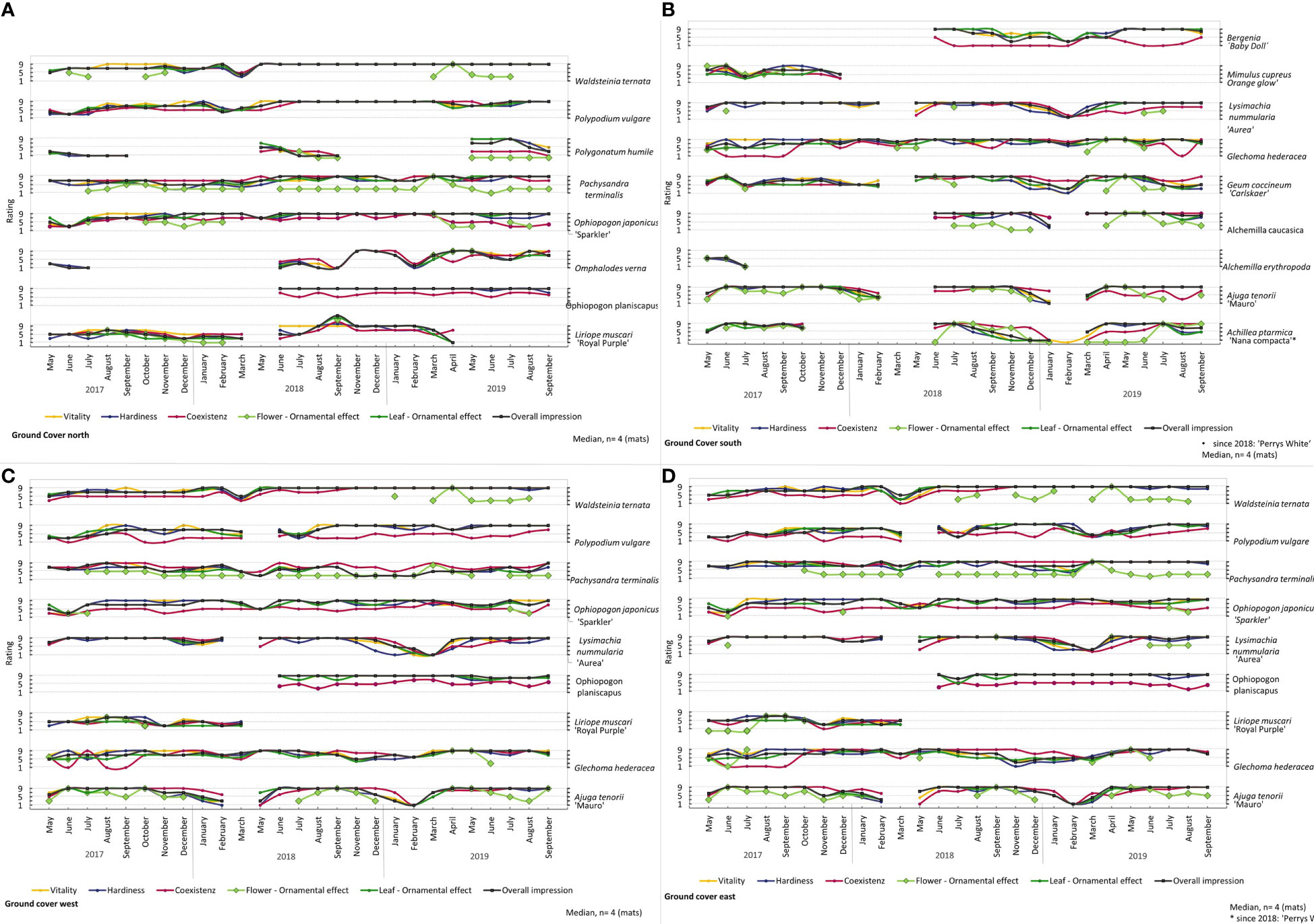
Figure 4 Rating of all plants in the Ground cover (A) north, (B) south, (C) west and (D) east during the experiment run. Median, n = 4 (mats).
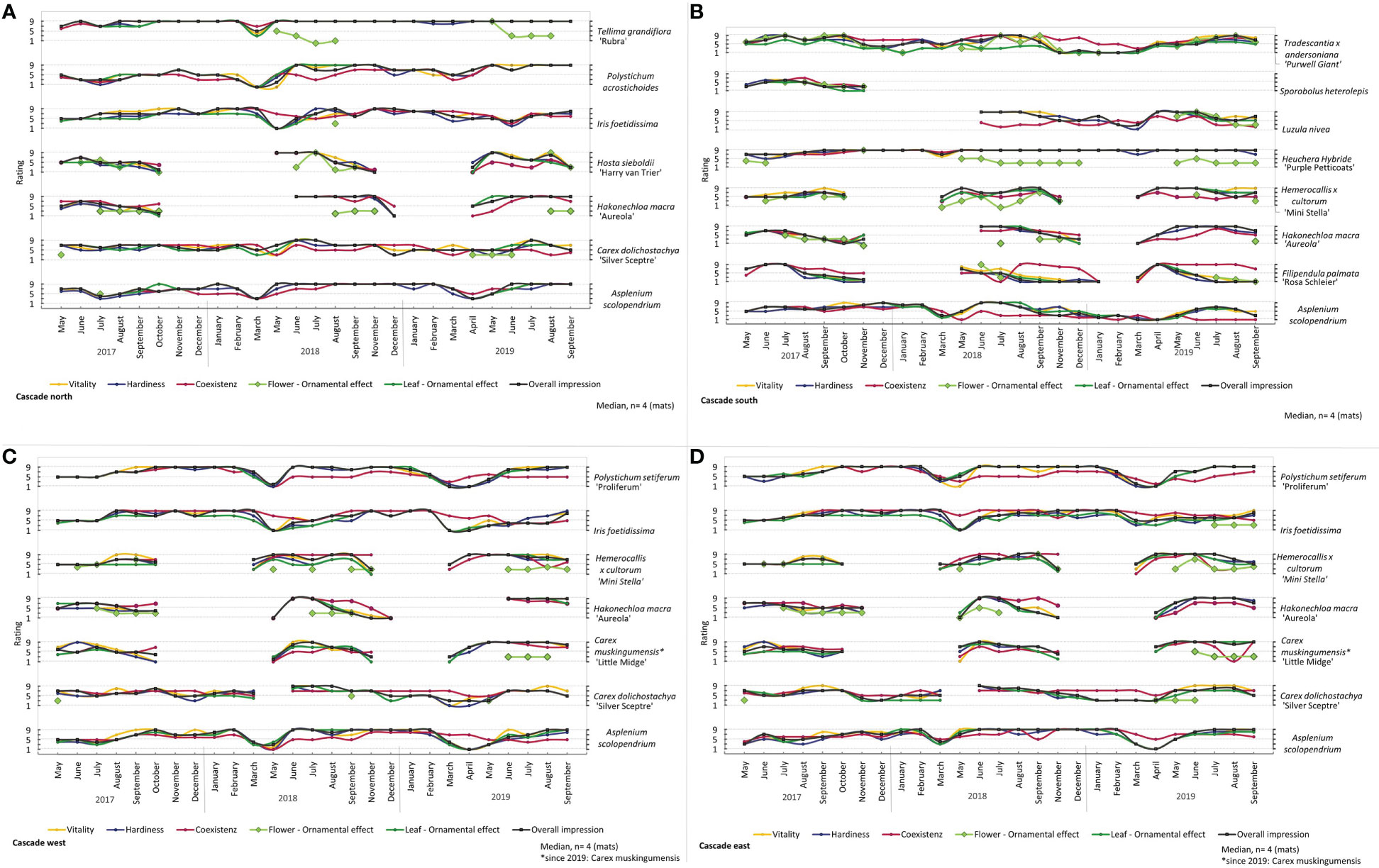
Figure 5 Rating of all plants in the Cascade (A) north, (B) south, (C) west and (D) east during the experiment run. Median, n = 4 (mats).
Other plant species did not have a conspicuous leaf texture, but spread throughout the mat with green leaves and ensured a closed canopy. We observed this for Hemerocallis x cultorum ‘Mini Stella’, Iris foetidissima, Geum coccineum ‘Carlskaer’, Glechoma hederacea, and Carex muskingumensis.
It is considered good if a few species in the plantation provide closed cover. However, Glechoma hederacea spread so much that it overran other plants, so we had to cut it back occasionally. We planted Carex muskingumensis in 2019 to replace Carex muskingumensis ‘Little Midge’. but the species was far too tall for vertical planting and covered other plants.
The ferns (Asplenium scolopendrium, Polypodium vulgare, Polystichum acrostichoides, and Polystichum setiferum ‘Proliferum’) and Waldsteinia ternata in particular showed delayed growth. Over time, we saw good development and a nice leaf texture (Figures 4A, C, D, 5A, C, D).
Tellima grandiflora ‘Rubra’, Waldsteinia ternata, Pachysandra terminalis, and Heuchera Hybride ‘Purple Petticoats’ showed a good impression throughout the experiment and also during winter periods (Figures 4A, C, D, 5B). Other plants died or went into dormancy in winter. Lysimachia nummularia ‘Aurea’, Glechoma hederaceae, and Ajuga tenorii ‘Mauro’ had nice leaf texture until frost (Figures 4B, D). After the frost period, most aboveground biomass was dead. Between the winter of 2017 and 2018, most plants died because of a late and long frost period (Figure 6). Glechoma hederacea displayed a fast recovery from frost (Figures 4B, D). Dead plants were replaced. For replanting in 2018, Sporobolus heterolepsis, Mimilus cupreus, and Liriope muscari were not available and therefore not tested further (Figures 4, 5A). While most plant species displayed frost damage in March 2018 and indicated that they had to be replaced, Iris foetidissima showed consequences of frost later in May 2018, which was too late for replacement (Figures 5A, C, D). We evaluated Iris foetidissima in summer 2017, with low values for vitality. Other vulnerable plants were Alchemilla erythropoda, which failed immediately after the experiment started and did not grow after replanting (Figure 4B). Therefore, this species was replaced with Alchemilla caucasica in the following year. Furthermore, Polygonatum humile and Sporobolus heterolepsis did not grow well (Figures 4A, 5A). Carex dolichostachya “Silver Sceptre” showed brown leaf tips. We recognised problems with pest and pathogens for Lysimachia nummularia ‘Aurea’ with mealy root aphids, and we rarely saw mildew on Ajuga tenorii ‘Mauro’ and frequently for Filipendula palmata ‘Rosa Schleier’.
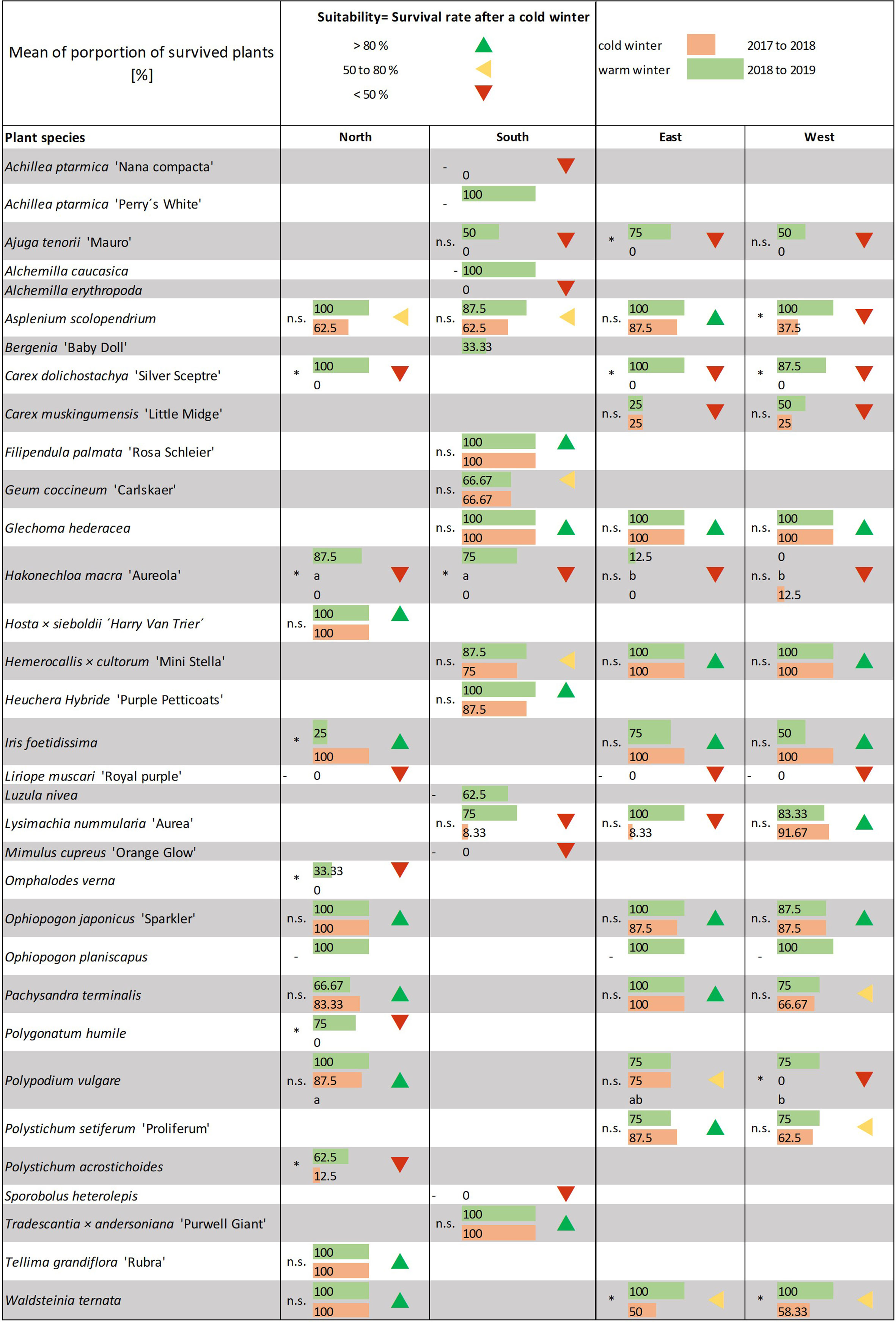
Figure 6 Determination of suitability depending on the winter survival rate of the individual plants. Mean of proportion of surviving plants [%]. Data not normally distributed as per a Kruskal–Wallis test with post hoc test (all pairwise). Significant differences between winter 2017–2018 and 2018–2019 are given with*, - means statistic was unable to compute. Letters indicate significant differences between the expositions. Suitability based on survival rate after the cold winter of 2018.
Some plants showed dynamic development. In this regard, the rating parameters differed, and evaluation values changed from one month to the next. We also recognised that plant development varied in between the repetition in one variant. We made these observations for Omphalodes verna, Polystichum setiferum ‘Proliferum’, Hemerocallis x cultorum ‘Mini Stella’ and Tradescantia x andersoniana ‘Purwell Giant’ (Figure 5A). Especially for Tradescantia x andersoniana ‘Purwell Giant’ we saw a big difference between the mat which hung at the upper part of the experimental wall to the mat which hung in the lower part of the wall.
Bergenia’ Baby Doll’, Luzula nivea, Ophiopogon japonicus ‘Sparkler’ and Ophiopogon planiscapus presented an attractive picture but were too small, with too few individuals represented, and therefore not visible (Figure 4).
The arrangement of the plants was in a specific plant plan (Figure 2). We saw that some plants were covered and crowd out by other plants, performed poorly in competition and did not grow that much: Polypodium vulgare (east and west, Figures 4C, D) and Asplenium scolopendrium (south, Figure 4B). For some plants, we saw differences in the development based on the exposition (see Figures 5, 8 in 01_Supplementary Material). Asplenium scolopendrium showed the best performance in the north. In the east and west, it developed dynamically. In the south, it was highly vulnerable and survived only because of a bigger plant that provided shade. Moreover, for Hakonechloa macra “Aureola”, we saw the best development in the north and the worst in the south and west.
Polypodium vulgare were smaller and hidden in the west and east, while it performed really well in the north. Pachysandra terminalis made for an attractive picture in the north and east and was less impressive in the west.
4.1.2 Weighted rating and determination of LW suitability with rating
With the calculated weighted rating, we saw that some species developed well. Other species showed problems at certain times or over the whole experiment time (see Table 2 in 01_Supplementary Material). The weighted rating provides an overview of the plants’ development in the entire experiment. Consequently, we realised that the determination of suitability based on only one rating value did not make sense. Therefore, we extended the determination to include other parameters. We calculated the suitability depending on different periods – whole year, summer, and winter – and for the ornamental effect of leaves and flowers. In the following table, we also present other parameters that we considered for the suitability of plants. In the “list of plants LW suitability” a final summary of the suitability determination is given (Table 3 and see additional data: 03_List of plants LW suitability). The detailed table with all parameters and suitability determination is presented in the additional data: 2. in 02_Supplementary Data).
4.2 Growth form
Furthering Günther et al. (2016) work, we described the different growth forms of the plant species (Figure 7). We differentiated the “growing stage” and the “established plant”. We saw that some plants showed another growth form shortly after planting at a juvenile stage (“growing stage”). We observed this growth for plants that were resprouting, replanting, or poorly growing. We visualised a “growing stage” form if the adult form differed from this juvenile growth. For this “growing stage”, we categorised three different forms: (a) standout, (b) upright/spreading, and (c) direct upright. Standout means that the plants have less connection to the mat and reach out in all directions. Upright/spreading plants are attached to the mat, spreading upwards or in all directions. Direct upright plants face the sun and do not have a connection to the mat or grow in another direction. There were also plants that stayed in the juvenile growth form while growing poorly. For these species, we did not observe an adult form and could not visualise it. These species were Polygonatum humile, Bergenia “Baby Doll”, Liriope muscari, and Sporobolus heterolepis.
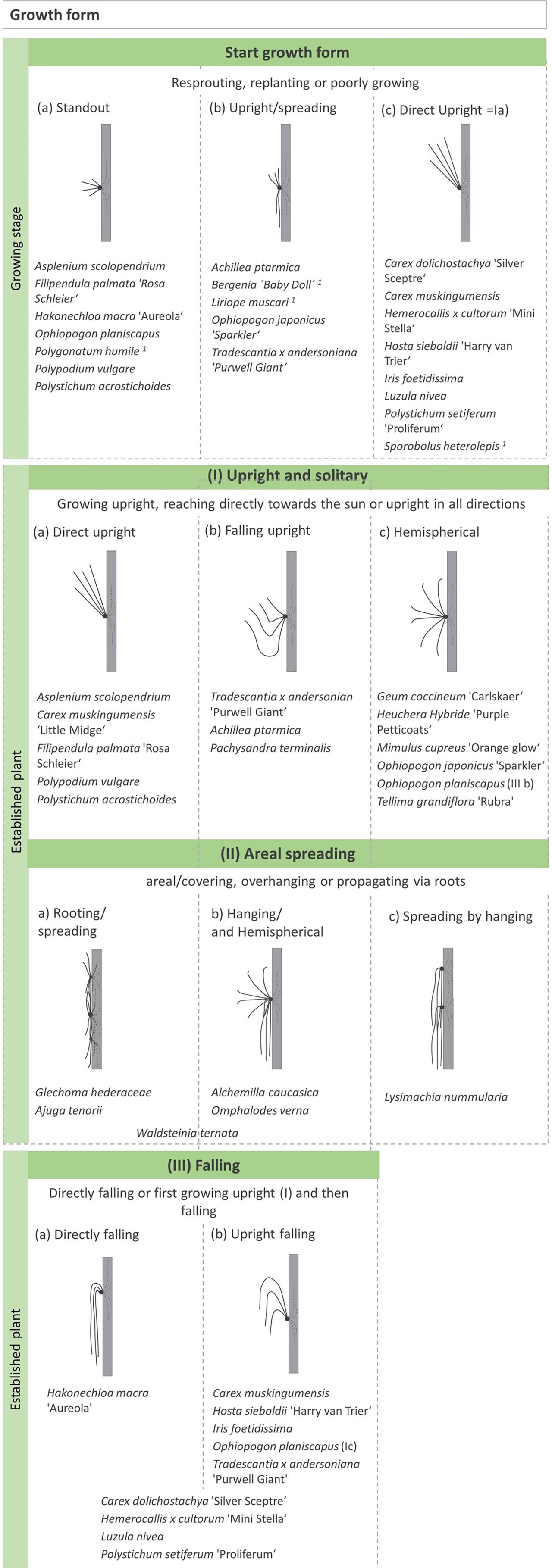
Figure 7 Growth forms: “Growing stage”. Growth form of juvenile plants that were resprouting, replanting, or poorly growing. The start growth form was determined if the adult form differed. One poorly growing plant, no adult form. No determination of Alchemilla erythropoda. “Adult growth form”. If several growth forms were observed, this is marked in brackets, or the plants are classified in two categories. The * indicates plants that form a hemispherical form and spread areally. The growth forms are based on of Günther et al. (2016).
The “established plant” was categorised in three groups with subdivisions. The three groups are called (I) upright and solitary, (II) areal spreading, and (III) falling (Figure 7). First, the form upright and solitary describes plants that did not cover the mat but stood out and showed a unique feature. This could be (a) directly upright, (b) first falling and then upright, or (c) hemispherical in all directions. The form (Ia) corresponds to the juvenile form (c).
Second, the form areal spreading sums up all growth forms that cover the mat and create a closed canopy. We observed this for species from the groundcover variant. We also used differing categorisations: (a) Plants spread out in all directions and rotted at “new” positions. (b) Plants grew a lot, had hemispherical growth, and developed long shoots which then hung low and covered large parts of the mat. In total, it was difficult to differentiate the plants in this category. (c) Another observation was Lysimachia nummularia, which spread out by rooting and, at another repletion or time, remained hanging.
Especially for grasses and ferns, we observed the third growth form falling. We classified plants that fall directly (a) but have no contact with the mat, which differentiates it from (IIb). (b) Then, we divided plants that first grew upright and then fell. We observed that some plants showed both growth form (a) and (b).
4.3 Growth height
Most species showed an increasing growth height from the experiment’s start in 2017 (see Table 3 and Figures 9–27 in 01_Supplementary Material and 3. in 02_Supplementary Data). These species were Asplenium scolopendrium (north and east), Geum coccineum ‘Carlskaer’, Glechoma hederacea, Heuchera Hybride ‘Purple Petticoats’, Hosta sieboldii ‘Harry van Trier’’, Hakonechloa macra ‘Aureola’, Ophiopogon planiscapus, Luzula nivea, Alchemilla caucasica, Tellima grandiflora ‘Rubra’, Pachysandra terminalis (east), and Polypodium vulgare (north). Moreover, there were species that showed a clearly higher growth height but not until 2019: Omphalodes verna, Ophiopogon japonicus ‘Sparkler’, Polystichum acrostichoides, and Waldsteinia ternata. The species planted first in 2018 showed an increasing growth height: Achillea ptarmica, Alchemilla caucasica, and Bergenia’ Baby Doll’. We observed a dynamic development of the growth height during the seasons for Achillea ptarmica, Ajuga tenorii ‘Mauro’, Geum coccineum ‘Carlskaer’, Lysimachia nummularia ‘Aurea’, Hakonechloa macra ‘Aureola’, Hemerocallis x cultorum ‘Mini Stella’ and Hosta sieboldii ‘Harry van Trier’. The other plants showed a more or less constant growth height (see Figures 15 – 27 in 01_Supplementary Material). The exceptions were that after the frost-intense winter of 2017–2018, Ajuga tenorii ‘Mauro’, Lysimachia nummularia ‘Aurea’, and Carex muskingumensis ‘Little Midge’ showed a lower growth height between April and June compared to 2017 and 2019. Iris foetidissima showed growth height the other way around. The growth height of Iris foetidissima was higher in 2017 compared to 2019 due to a changing growth form from falling to more upright. Moreover, Iris foetidissima showed frost consequences much later after the 2017–2018 winter. Therefore, we did not replant Iris foetidissima, as it did not recover well. Also, Polygonatum humile showed a decreasing growth height.
Most species showed a decreasing growth height or no growth towards winter. This phenomenon was not observed for Asplenium scolopendrium and Pachysandra terminalis. Carex dolichostachya ‘Silver Sceptre’ showed a delay in decreasing growth height. Glechoma hederacea had a lower growth height in the winter months but recovered faster and had a relatively higher growth height in spring compared to other species. Filipendula palmata ‘Rosa Schleier’showed a special growth height development. The growth height varied strongly between the vegetation phases since Filipendula palmata ‘Rosa Schleier’ was vulnerable to mildew and had to be cut a few times.
Differences between the expositions were observed for the whole year for Pachysandra terminalis, which were higher in the north and east, and Polypodium vulgare, which had a higher growth height in the north (see Figures 9 – 14 in 01_Supplementary Material). For Asplenium scolopendrium, we mostly documented a higher growth height in the north and east. One exception we found was the vegetation phase from October to December in both experiment winters, where only at the West Asplenium scolopendrium showed a lower growth height.
We also saw differences depending on the expositions in certain vegetation phases for the following: Hakonechloa macra ‘Aureola’ had higher growth height in the north between July and September; Waldsteinia ternata in the north from July to September; and Ajuga tenorii ‘Mauro’ and Hemerocallis x cultorum ‘Mini Stella ‘in the south between October and March.
In the final suitability , we calculated the mean, min, and max growth height of the individual species (Table 3). From these results, we decided to visualise the maximum growth height above 20 cm with two arrows and below 20 cm with one arrow.
4.4 Position of the plant on the vegetation mat and the wall
We designed a practice-oriented plant plan for one vegetation mat. The plant design as a group should be tested as closely to practice as possible. Thus, we did not want to test single plants or a randomised arrangement of plants, as this would not correspond to real plant design.
In this plant plan, the individual plants were planted at different positions. We compared the development of the plants at the different positions and observed similar trends. For the parameter “overall impression”, we kept deviations of the maximum rating value. It must be mentioned that we did not place the individual plant species in all possible positions on the vegetation mat. Therefore, we cannot exclude the possibility that another position of the plant would have presented a different result.
In our experiment, we combined planting design methods with a scientific setup. Therefore, we repeated the same planting plan four times. The vegetation mats were attached to the top and bottom of the wall in duplicate. We analysed the data of growth height and overall impression, based on the wall placement. No differences can be found here except for Tradescantia x andersoniana ‘Purwell Giant’. We found that these plants on the upper mats grew higher (about 10 cm) compared to those on the lower mats. During the final evaluation we saw less differences between the positions, only for Heuchera Hybride ‘Purple Petticoats’ we found significant higher biomass for the individual which were planted in the middle (see Figures 33 – 41 in 01_Supplementary Material and 5.2 in 02_Supplementary Data).
4.5 Plant number and winter survival rate
The plant number was documented over the year too. During spring, summer, and autumn, we saw only a few plants die. Alchemilla erythropoda did not grow and fell out shortly after planting. In some cases, Polygonatum humile and Bergenia ‘Baby Doll’ did not grow properly. Very rarely, we recorded failures (one to two individuals) of Carex dolichostachya ‘Silver Sceptre’, Hakonechloa macra’ Aureola’, Liriope muscari, Luzula nivea, and Pachysandra terminalis.
We observed most plant losses after the winter (Figure 6 and see 4. in 02_Supplementary Data). The survival rates after winter 2017–2018 (2018) and after 2018–2019 (2019) were very different owing to the significantly colder temperatures in winter 2017–2018. We found only six species that 100% survived the winter of 2018. These were Filipendula palmata ‘Rosa Schleier’, Glechoma hederacea (south, west, and east), Hosta sieboldii ‘Harry van Trier’, Tradescantia x andersoniana ‘Purwell Giant’ and Tellima grandiflora ‘Rubra’. These species can be used in LWs without the risk of loss through frost. Moreover, for Iris foetidissima, we saw a 100% survival rate during the data collection. However, it only seemed to have survived the winter and showed frost stress symptoms later (May to June 2018) and failed after all. We saw a lower survival rate in 2019. In total, 11 species failed completely in 2018.
In 2019, a 100% survival rate was observed for 14 species. The other species showed a clearly (partly significant) higher survival rate compared to 2018. Only Hakonechloa macra ‘Aureola’ failed completely in the west.
In both years, we saw differences between the survival rates at different expositions. A higher survival rate was found for the north exposition. Only Pachysandra terminalis showed a lower survival rate in the north (and west) compared to east in both years. We found that, in the south, Hemerocallis x cultorum ‘Mini Stella’ had a lower survival rate than east and west in both years. The same phenomena repeated for Lysimachia nummularia ‘Aurea’, which had a lower survival rate in the east in 2018 and west in 2019. For Ajuga tenorii ‘Mauro’, we documented a higher survival rate in the east in 2019. A higher failure was mostly found in the west and east compared to north. Asplenium scolopendrium, Ophiopogon japonicus ‘Sparkler’, and Waldsteinia ternata had higher survival rates in the north. In both years, we found a low survival rate for Polypodium vulgare in the east and west, which was significant for the west in 2018 (Figure 6 and see 4. in 02_Supplementary Data). Hakonechloa macra ‘Aureola’ had a high survival in the north and showed a significantly low survival rate in the east and west in 2019 and west in 2018.
From the survival rate after Winter 2018, we calculated the suitability of the plants. The winter of 2018 was frigid with a long frost period, and therefore, a high failure of plants was observed. Plants in LWs have less root space and roots are exposed to extreme conditions even in mild winters. Thus, we started from the worst case when calculating the winter survival suitability. We recommend species that showed a winter survival rate above 80% without restrictions. Species in which we observed a survival rate between 50% and 80% can be used in LWs, but the conditions and the risk of losing these species to frost should be considered. If a species survived the winter at a proportion less than 50%, the high risk of failure and therefore high probability of replanting in the next spring must be remembered.
4.6 Final evaluation
In the final evaluation, we studied the different parameters that indicate plant biomass and the leaf area of the individual plants. The first impression of the root development determined where on the vegetation mat the root was found.
We derived a canopy parameter for the final suitability list from biomass and leaf area (Table 3 and see Table 4 in 01_Supplementary Material). For biomass, we formed three groups. The first group (less biomass) included species that showed a biomass (drymass of the shoot and stem) up to 50 g, and the second group (medium biomass) was up to 150 g (Figure 8A). Third, we summed up plants with a high biomass (150–400 g) (Figure 8B). Plants with high biomass were Carex muskingumensis, Glechoma hederacea, Heuchera Hybride ‘Purple Petticoats’, Tradescantia x andersoniana ‘Purwell Giant’, Tellima grandiflora ‘Rubra’ and Waldsteinia ternata.
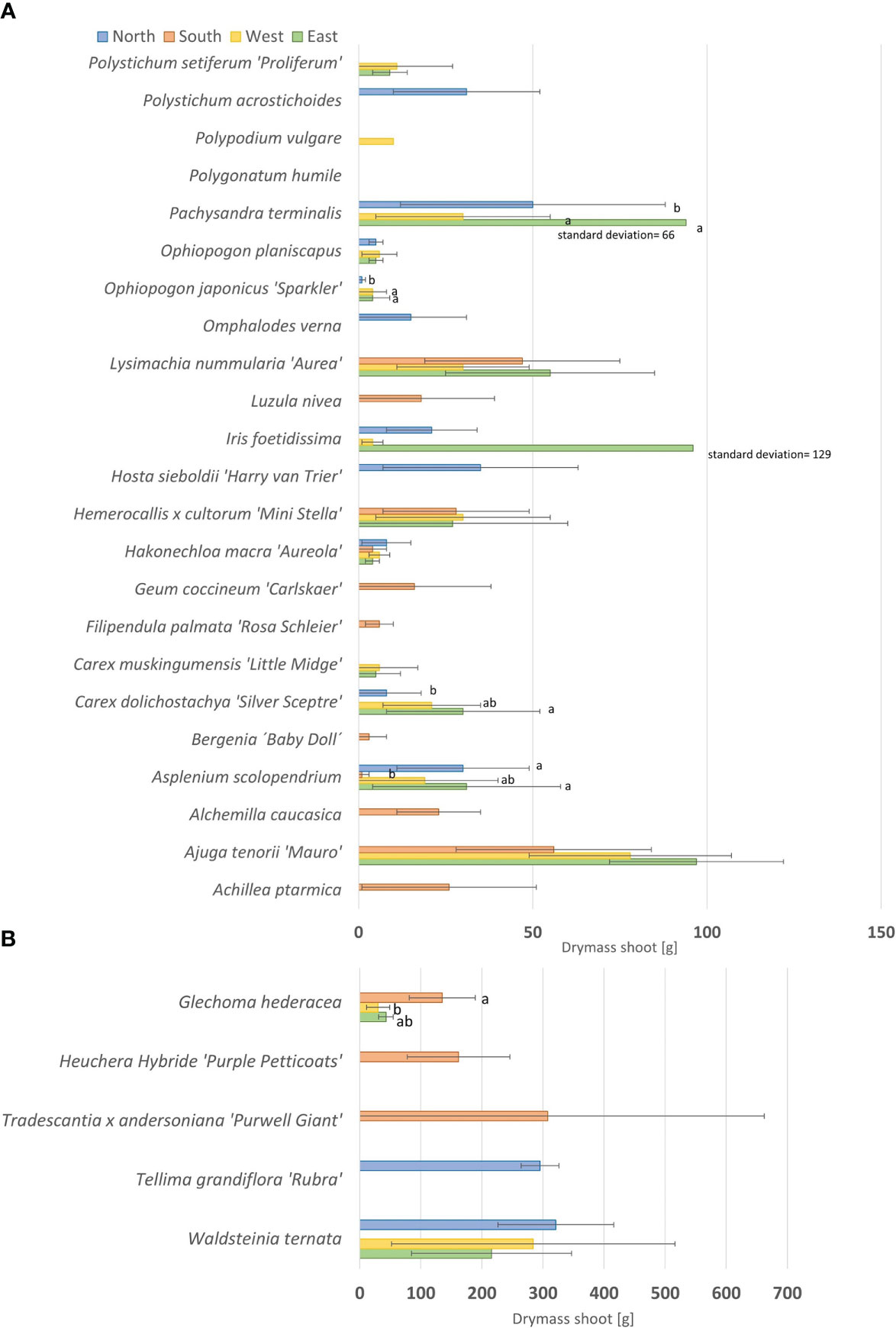
Figure 8 Final biomass evaluation for smaller plants (A) and bigger plants (B). Mean of drymass of the shoot [g], +/- 1 standard deviation. Determination at final evaluation in October 2019. Data not normally distributed as per a Kruskal–Wallis test with post hoc test (all pairwise). Significant differences are shown with letters. If not indicated, there was no significant difference.
We found differences in biomass based on the exposition (see Figures 29 – 32 in 01_Supplementary Material). The biomass of Carex dolichostachya ‘Silver Sceptre’ and Ophiopogon japonicus ‘Sparkler’ in the north and Ajuga tenorii ‘Mauro’ and Asplenium scolopendrium in the south was low. We observed higher biomass for Glechoma hederacea in the south, for Iris foetidissima, Pachysandra terminalis, and Asplenium scolopendrium in the east, and for Asplenium scolopendrium, Polypodium vulgare, and Waldsteinia ternata in the north.
We differentiated the entire above biomass into leaves and stems if the plant had both (see Figure 28 in 01_Supplementary Material). We found that stems and leaves mostly had the same proportion of biomass. For Ajuga tenorii ‘Mauro’, there was a higher stem biomass. A higher leave biomass was found for Lysimachia nummularia ‘Aurea’ and Tellima grandiflora ‘Rubra’. Waldsteinia ternata was a special case because it had rhizomes as well. We observed that there was less rhizome mass in the east compared to north and west.
For leaf area, we found the maximum values around 40,000 cm² for Ajuga tenorii ‘Mauro’ (east), Waldsteinia ternata (north and east) and Glechoma hederacea (south) (Figure 9). Moreover, their mean was above 35,000 cm² much like Waldsteinia ternata (west), Tellima grandiflora ‘Rubra’, and Lysimachia nummularia ‘Aurea’ (south). We categorised these species under “high leaf area”. In total, seven plants were grouped into “medium leaf area”, as they showed values between 5,000 and 20,000 cm². There were 35 plants that had a leaf area below 5,000 cm² (less leaf area).
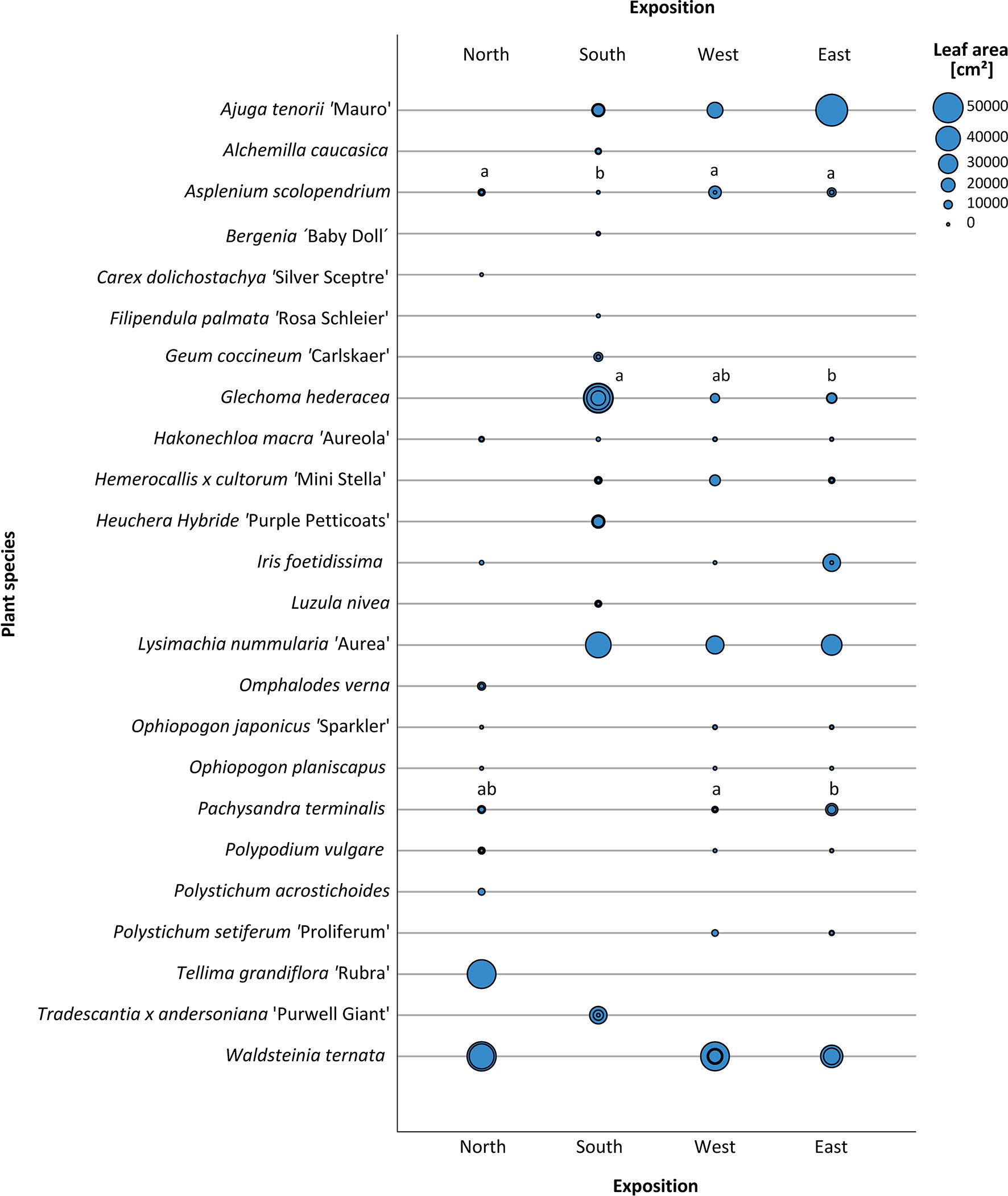
Figure 9 Leaf area [cm²]. Cumulative leaf area of individual plants at different expositions. Determination at final evaluation in October 2019. One circle shows the individual leaf area of the plant species per repetition. Data not normally distributed as per a Kruskal–Wallis test with post hoc test (all pairwise). Significant differences are shown with letters. If not indicated, there was no significant difference. (Data from Carex dolichostachya “Silver Sceptre” for west and east were not documented).
The leaf area also differed depending on exposition. We found a higher leaf area in the east for Ajuga tenorii ‘Mauro’, Iris foetidissima, and Pachysandra terminalis. In the south, we found less leaf area for Asplenium scolopendrium and a higher leaf area for Lysimachia nummularia ‘Aurea’, and Glechoma hederacea. In the north, there was a higher leaf area for Hakonechloa macra ‘Aureola’ and Polypodium vulgare. In the west, we saw higher leaf area values for Hemerocallis x cultorum ‘Mini Stella’.
For the final determination of the “canopy” as a parameter for the plants’ suitability for LWs, we summed up the biomass and leaf area (Figures 8, 9 and see Table 8 in 01_Supplementary Material). For both, we categorised the results and signed the data with symbols. The canopy depends on the highest category of biomass or leaf area. Thus, we categorised all species in comparison to each other in three canopy groups: high, medium, and less. In total, eight species had a high canopy, seven a medium canopy, and the other tested species had a less canopy. Since this parameter was only recorded once, it should not be overvalued.
During the final evaluation, we also determined the root position and root biomass (Table 3, see Photo 1 - 3 in 01_Supplementary Material and 5.3 in 02_Supplementary Data). Since it was not possible to remove all root biomass from the mat, this data is not represented.
We analysed the root position (Tables 1, 3), finding most roots in the non-woven layer on both sides (3N and 4N), except for Glechoma hederacea and Carex dolichostachya ‘Silver Sceptre’ (west and east). These species did not grow in the front part of the back polyester layer (5P), while all other plants were rooted there. Less roots were found on the back side of the back polyester layer (6P). We documented 26 plants rooted in the front polyester layer on both sides (1P and 2P). Other plants were rooted either in the front of the front polyester layer 1P or the back of the front polyester layer 2P. For some species, we documented less than 3 root positions. These were Asplenium scolopendrium (east), Carex dolichostachya ‘Silver Sceptre’ (west and east), Glechoma hederacea, Lysimachia nummularia ‘Aurea’ (south and east), and Luzula nivea. Since our LW system is a special construction, no general parameter for suitability can be derived from the root position.
For the additional parameters of the final evaluation see 5.4. in 02_Supplementary Data.
4.7 Final list of plants’ suitability for LWs
All of the parameters described above are included as results in the list of suitable plants for LWs (Tab.3). Furthermore, this list is available as an Excel sheet that can be sorted according to certain conditions (e.g. light) (see additional data: 03_List of plants LW suitability). The list enables better planning because the desired parameters, such as a high ornamental effect or a high probability of surviving the winter, can be filtered.
5 Discussion
Theoretically, with our understanding of plants, it is possible to grow plants in extreme locations, for example: vertically (Ghazalli et al., 2019). The current opinion from previous studies is that only a few perennials are suitable for LWs and, that they may not look attractive (Mårtensson et al., 2014; Ghazalli et al., 2019; Lausen et al., 2020). At the same time, there are only a few studies on plants in LW (Ghazalli et al., 2019; Lausen et al., 2020). And Ghazalli et al. (2019) criticized the planner’s choose plants because of their visual effects and note that previous studies analyzed plant’s suitability for the vertical level and the substrate, but they did not consider the natural habitat. Research on plant species for vertical greenery systems was done mainly in Europe and Asia (Ghazalli et al., 2019).
(Ghazalli et al. (2019) reviewed vertical greenery systems and listed plants tested for these systems. They listed all tested species and categorized perennials, climbing plants, grasses, and the region. In Europe, in total, 72 shrubs species are tested. Not all of them are suitable for the climate in Germany. Perennials that had the same genre compared to our study were: Achillea millefolia, Asplenium nidus, Bergenia ssp., Bergenia cordifolia, Carex brunnea, Carey morrowi, Geranium sanguineum, Iris sibirica, Polystichum rigens Tagawa, Tradescantia spathacea and Tradescantia zebrina. Also, ornamental grasses could be suitable for LW because they are visually attractive and need low maintenance (Suárez-Cáceres et al., 2022). At the beginning of our research, we hypothesised that we would find a higher variety of suitable plants for LWs. We described plant development and made conclusions about the suitability of the tested species. Therefore, we used different methods and parameters.
First, we wanted to know what the plants looked like during the different seasons. We used different perennials and assumed that it is possible to create attractive plant plans for LW because of the plants ornamental effects: sizes, texture, or colour (Suárez-Cáceres et al., 2022).
Our research project took three years, and we saw differences in plant aesthetics between the experiment years. Shortly after the experiment started, plants grew slowly and there was no closed coverage, especially in the cascade variant in the north. During the time, the plants became bigger, and the gaps were filled with them or wild species.
The plants mostly had the same growth height and rating during the three experiment years. Nevertheless, we saw a varying, dynamic, but always attractive, plant picture. We observed differences in plant development depending on the climate and light conditions. Plants in LW do not grow so high, which could be due to less space for roots and the shoot (Suárez-Cáceres et al., 2022). The main difference between the experiment years was the hard winter between 2017 and 2018, where we observed that the plants needed more time to recover after the hard winter. More research is needed to analyze: the plant’s hardiness against extreme conditions (Ghazalli et al., 2019).
Our main aim was to determine how an attractive plant picture can be created in different years and seasons. Using the results of our research project, we created an LW-suitable plant list. If planning a LW, many things should be considered. First, the appropriate climate and light conditions should be created. We suggest using robust species that would survive the winter. If you want to use vulnerable species, you should budget for possible plant failures. Next, it should be clear what kind of goal the LW must achieve. If it is only for “greenery”, then an easy plant composition should be sufficient; less species variation and species that spread widely, fill the gaps, and need less maintenance can be used. If a really attractive plant picture is desired, then plants that have a high leaf ornamental effect and even better developed flowers should be chosen. Intense maintenance management must also be set up. Furthermore, it is also possible to consider what kind of ecosystem functions you want to achieve. Biodiversity can certainly be promoted using animal-aided design, water supply, and the right plant choice. Thorpert et al. (2022) pointed out that choosing plants for living walls with the color theory is possible and that the plants could promote biodiversity simultaneously. Moreover, the creation of LWs, which provides a great temperature-lowering effect, is an appropriate consideration in the context of climate change and heat stress in urban areas. A great temperature-lowering effect can be attained using a high canopy with its water consumption and evaporation. Therefore, we also added the canopy parameter to the suitability list. We observed an attractive plant picture for almost the whole year. The tested plants could be categorised as plants that looked well the whole year and only showed an attractive appearance from spring to summer. In general, it is important to include plants that appeared to have a nice ornamental effect in winter too. A crucial factor for the visual attractiveness of the LWs is winter and the survival rate after winter. Plants in LWs do not have much root space and roots are exposed to extreme conditions even in milder winters (Jørgensen et al., 2014a; Jørgensen et al., 2014b; Jørgensen et al., 2018). We recommend that, for the plant choice of LWs, a higher failure should be assumed because of the increased frost compared to a normal horizontal design. Additionally, robust/winter-hardy species should be considered for use. Perhaps, native or adapted species can survive winter with low temperatures, as seen in a study in another climate (Dvorak et al., 2021).
Shortly after the frost period, we observed an unattractive plant picture for a limited period. Furthermore, plants that survived winter died above ground and left only necromass behind. Even if plants recovered quickly, it took at least a month before an attractive plant picture was created again. This remains a problem for future LW projects in regions with frosty winters. Compared to horizontal plantings, vertical greening is much more obvious and is therefore viewed much more critically. There are different ways to deal with this problem. One possibility is to use only plants that have high winter hardiness. However, even these species can die above ground and look unattractive after winters with low temperatures.
Another option is intense maintenance management. With this, the necromass can be removed directly after the winter, and the plant picture will appear more beautiful. If a plant fails, it can also be replanted quickly. Moreover, the plant image can be enhanced with geophytes. Overall, a more attractive plant image can be created more quickly with higher maintenance.
Rather unusual approaches are conceivable as well. LWs should look as nice as possible and be visually attractive, even without plants. Alternatively, LWs can be covered with something over the “after frost period”, e.g. an advertising poster. It is also imaginable to remove the LW over the winter period and replace it with another element.
A rather technically demanding approach is to equip the LW with a heating system. Higher temperatures prevent plants from dying in winters. However, irrigation management must also be considered in that case.
We observed that the plants had different growth forms in the LW during the experiment. There were species that showed similar and different growth forms, as is the case in horizontal planting as well. The plants in our system were planted vertically, which means they grew parallel to the normal ground (Suárez-Cáceres et al., 2022). This 90° rotation led to an adapted growth direction of some species. Some of them grew straight and directly towards the sun; other species first grew facing the sun and then fell due to gravity. We also observed that the plants changed their growth strategies over time.
We observed different strategies of different plants in terms of competition and spreading behaviour. Most of the plants spread roughly around their planting positions and grew upwards or in all directions away from the mat. Only a few of the species we spread over the entire mat, some filled the gaps between species, as originally planned. Especially in the cascade variant, many gaps remained between the plants. These gaps were filled by self-established moss and Sagina. Thus, we also recommend considering wild species in the planting concept (Hietel et al., 2022).
Besides competition and spreading behaviour, the position of the plant is also crucial. We observed that bigger plants hung over and thus covered plants below them. Hence, smaller species grew poorly and were not visible. For further projects, we recommend putting smaller plants in the upper part of LWs to give them enough space. Perhaps, temporary plants can be added to cover empty spaces until the intended plant establishes itself. In general, we chose the wrong position for Asplenium scolopendrium (south), Bergenia’ Baby Doll’ (south), Luzula nivea (south), and Polypodium vulgare (west and east).
Also Suárez-Cáceres et al. (2022) make clear that for the designing process of LW, knowing about the growth type, competition, and development in different LW systems is necessary. For the composition of the plant plan, the designer must consider the “right” place for the species: Plants should not overlay each other and should all get enough light (intra and interspecific interactions). In addition, to reach a closed coverage, the growth form or dimension (“up” and “down”), the “volume”, and also the “horizontal space” should be considered (Suárez-Cáceres et al., 2022). Another factor to consider is plant combination. For our experiment, we classified the 34 perennials into two variants and tested this at the different expositions. We wanted to know what are the learnings from the different planting variants (cascade and groundcover)? The idea behind the different variants was to create different plant pictures. The cascade contained species that grew higher, and we hypothesised that the species would fall like a waterfall. Most plants in the cascade variant developed a growth form that was exposed to the sun. We saw the “falling down effect” for several species in the cascade. The cascade contained solitary plants that did not spread around the mat and left gaps. For future LW projects, we recommend using these solitary plants together with species that spread to fill the gaps. Analyzing and describing different growth forms is essential for an attractive design (Suárez-Cáceres et al., 2022).
The vegetation mats were attached to the top and bottom of the wall in duplicate. We did not find a difference depending on the wall position for most plants, except for Tradescantia x andersoniana ‘Purwell Giant’, which grew higher in the upper mats. This could be due to the higher radiation at the top or the possibility of growing more naturally from a horizontal starting point. We observed that Tradescantia x andersoniana ‘Purwell Giant’ grew from a different layer in the upper part of the mat. Therefore, it could be that the irrigation gutter above the mat at the bottom was a limiting factor. Maybe it could be due to the mat’s nutrient and water distribution which could differ (Ghazalli et al., 2019).
We did not analyse the water consumption as we overwatered the plants. From Prodanovic et al. (2019) it is knowing that there is a high need for water, especially in summer, which plants used for transpiration. In winter, water consumption is a result of evaporation. Plants showed different development depending on the LW conditions, e.g., the substrate (Prodanovic et al., 2019; Lausen et al., 2020). A plant species provision of water is needed, and planners should choose the plant placement because of the plant’s water requirement (Prodanovic et al., 2019).
Another limitation for plant growth is vulnerability to pests or pathogens. We observed fewer problems due to this. We did not apply pest management because this would not be feasible in urban areas. If we faced problems with pests and pathogens, the infected parts were removed. In general, we recommend choosing more robust species. Pruning due to pests and pathogens should be considered in maintenance planning.
We observed differences due to the different light conditions of our experimental walls. As mentioned, the plants in the north grew slowly, which could be due to limited light. On the other hand, we documented a more attractive plant picture in the winter month there compared to the south. For some plants, we observed a better performance in the north compared to other expositions. Hence, it is possible to design an attractive LW at a façade with low light intensity.
For the summer months, we saw greater plant growth in the south, east, and west compared to north. More flowers and a more colourful leaf ornamental effect were also detected in these expositions.
Regarding the winter survival rate (after 2017–2018), we found differences between expositions. We observed the highest failure of Lysimachia nummularia ‘Aurea’ in the east. This species had high water consumption. In the morning, during winter months, the LW and roots were frozen. Meanwhile, the sun shone (extremely in the east), so the plant got dehydrated, and as it did not get water, its dried up (frost drought). This was not observed for all species in the east. In contrast, we found higher survival of Pachysandra terminalis and Asplenium scolopendrium in the east compared to the west, which can be explained by the incredibly high radiation in the west for these species.
For further planning, we recommend considering the light conditions. The façade is an extreme habitat, and if it is exposed in the south, the radiation and temperature are different and higher than the ground. Therefore, the common light information of the perennial should be considered and, if possible, overvalued.
Finally, in this list, we provide a table of plants and the conditions needed for good development in a vertical system, but further research is needed. The next question is as follows: which methodology is best for describing the suitability of the plant?
We documented the plants’ appearance with ratings, which entailed a subjective evaluation but provided a good overview of their development and were a good basis for the calculation of the general suitability of the plants for LWs. Moreover, the continuity and description of the rating parameters resulted in reproducibility. We took different rating values and derived various parameters (leaf/flower and high ornamental effect). Other studies showed that it is not effective to use only the coverage and one rating parameter (quality) to derive plants’ LW suitability (Mårtensson et al., 2014). The parameter coverage during the experimental run was missing due to methodology problems. Instead, we documented the biomass and the leaf area of the individual plants at the final evaluation. The derived canopy parameter provided information about plant biomass and leaf area only from one time point in this case. For further research, the canopy and biomass should be documented at different points to correlate it with the coverage. These parameter can also be interesting in the context of temperature-lowering or air-purifying effects. For e.g. the amount of biomass is crucial for the amount of transpiration. (Lausen et al., 2020).
Furthermore, a factor for plant LW suitability is winter hardiness, as this depends on the extreme conditions underlying LW systems, and at the façade, it is difficult to derive this information from common knowledge on perennials. For this factor, longer term research in various climate zones is needed.
To plan an attractive LW design, the growth form and height should also be considered. These parameters are not crucial for the survival of the plants in LWs but should be documented. Only attractive LWs are accepted by people in cities. We took our results from a special textile LW system without a substrate. It is not clear to what extent these results can be transferred to other LWs or even other vertical greenery systems. There are many different constructed LW systems which resulted in various conditions for plants and make plant selection more complicated (Suárez-Cáceres et al., 2022). The same must be mentioned for the climate zone where we conducted our research.
6 Conclusions
Our main aim was to test a large variety of plants, describe their development, and determine their suitability for LWs. The challenge of this research was the interdisciplinary approach, especially the combination of a scientific experimental design with the demands of a practice-orientated concept and knowledge from horticulture. Finally, this interdisciplinary approach resulted in success and produced a list of the tested plant species that can be used for future designs of LWs. Notably, this list is not complete and is limited to our studied climate zone and the system we used. There are many different constructed LW systems which resulted in various conditions for plants and make plant selection more complicated. Therefore, research on LW plants in different climate zones should be conducted with an interdisciplinary approach. Consider what kind of goal the LW should achieve (e.g. promoting biodiversity) to choose the optimal plant composition.
For further LW projects, we recommend considering the following:
• Choose robust species (related to pests, pathogens, and winter hardiness); be prepared for higher failure because of frost and calculate the budget
• Competition, spreading behaviour, water needs, substrate, the construction type of the LW and light preference of the plants
• Maintenance: intensity and frequency (e.g. pruning due to pest and pathogens)
• Considering growth type, competition, dimension, volume and development and using these solitary plants together with species that spread to fill the gaps. Position of the individual plant in the plant plan and in the façade area (give small plants enough space and do not position bigger plants directly above them)
• Vertical growth form and height of plants
• Plants that have a nice ornamental effect even in winter and considering wild species in the planting concept
• Unusual approaches to creating an attractive LW (overlap without plants or heating system)
Data availability statement
The original contributions presented in the study are included in the article/Supplementary Material. Further inquiries can be directed to the corresponding author.
Author contributions
Both authors designed the experimental set up, methodology, and conceptualization of the manuscript. AB raised the project fund, conceived and supervised the project. MS served as project administrator, performed the field trial, and recorded the data. MS analyzed and visualized the data, conducted the literature review, and wrote the manuscript. AB edited the manuscript. All authors contributed to the article and approved the submitted version.
Funding
We acknowledge the support received from the Open Access Publishing Fund of Geisenheim University, GFHG Gesellschaft zur Förderung der Hochschule Geisenheim e.V. and the financier ZIM for the project “Begrünte Fassadenkacheln” (FKZ 4013810HF6).
Acknowledgments
We would like to thank the student assistants and our colleagues from the Institute for Urban Horticulture and Plant Use for the setup, supervision, and maintenance of the experiment. We would like to express our gratitude specially for the excellent support.
Conflict of interest
The authors declare that the research was conducted in the absence of any commercial or financial relationships that could be construed as a potential conflict of interest.
Publisher’s note
All claims expressed in this article are solely those of the authors and do not necessarily represent those of their affiliated organizations, or those of the publisher, the editors and the reviewers. Any product that may be evaluated in this article, or claim that may be made by its manufacturer, is not guaranteed or endorsed by the publisher.
Supplementary material
The Supplementary Material for this article can be found online at: https://www.frontiersin.org/articles/10.3389/fhort.2023.1091026/full#supplementary-material
References
Böhm J., Böhme C., Bunzel A., Kühnau C., Landua D., Reinke M. (2016). “Urbanes grün in der doppelten innenentwicklung: Abschlussbericht zum F+E-vorhaben,” in Entwicklung von naturschutzfachlichen zielen und orientierungswerten für die planerische umsetzung der doppelten innenentwicklung sowie als grundlage für ein entsprechendes flächenmanagement" (FKZ 3513 82 0500) (Bonn-Bad Godesberg: Bundesamt für Naturschutz).
Brandhorst S., Zorn G., Steenis N. v., Mann G. (2015) Pflanzen wandgebunden arten 2015. FBB pflanzliste. Available at: http://www.gebaeudegruen.info/fileadmin/website/gruen/Fassadenbegruenung/basiswissen/Pflanzenliste_wandgeb_arten.pdf (Accessed October 25, 2016).
Cameron R. W. F., Taylor J. E., Emmett M. R. (2014). What's ‘cool’in the world of green façades? how plant choice influences the cooling properties of green walls. Building Environ. 73, 198–207. doi: 10.1016/j.buildenv.2013.12.005
Charoenkit S., Yiemwattana S. (2021). The performance of outdoor plants in living walls under hot and humid conditions. Landscape Ecol. Eng. 14, 193. doi: 10.1007/s11355-020-00433-8
Devecchi M., Merlo F., Vigetti A., Larcher F. (2013). THE CULTIVATION OF MEDITERRANEAN AROMATIC PLANTS ON GREEN WALLS. Acta Hortic. 999, 243–247. doi: 10.17660/ActaHortic.2013.999.34
Dvorak B., Yang S., Menotti T., Pace Z., Mehta S., Ali A. K. (2021). Native plant establishment on a custom modular living wall system in a humid subtropical climate. Urban Forestry Urban Greening 63, 127234. doi: 10.1016/j.ufug.2021.127234
Eppel J. (2015). Hang over!? – vetrikales grün in nürnberg (Germany: Veichtshömer Berichte aus der Landespflege), 17–30.
Francis R. A., Lorimer J. (2011). Urban reconciliation ecology: the potential of living roofs and walls. J. Environ. Manage. 92, 1429–1437. doi: 10.1016/j.jenvman.2011.01.012
Ghazalli A. J., Brack C., Bai X., Said I. (2019). Physical and non-physical benefits of vertical greenery systems: A review. J. Urban Technol. 26 (4), 53–78. doi: 10.1080/10630732.2019.1637694
Günther H., von Birgelen A., Kühn N. (2016). Potenziale von stauden in der vertikalbegrünung: Ergebnisse eines forschungsberichtes zu grüner infrastruktur (Germany: Stadt+Grün), 43–48.
Hansen R. (2016) Die stauden und ihre lebensbereiche. Available at: https://ebookcentral.proquest.com/lib/kxp/detail.action?docID=6968113.
Hietel E., Stollberg M., von Birgelen A. (2022). Unkräuter in der dach- und fassadenbegrünung: Erfahrungsbericht aus der forschung in bingen und geisenheim (Germany: Stadt+Grün).
Hunter A. M., Williams N. S. G., Rayner J. P., Aye L., Hes D., Livesley S. J. (2014). Quantifying the thermal performance of green facades: A critical review. Ecol. Eng. 63, 102–113. doi: 10.1016/j.ecoleng.2013.12.021
Irvine K. N., Devine-Wright P., Payne S. R., Fuller R. A., Painter B., Gaston K. J. (2009). Green space, soundscape and urban sustainability: an interdisciplinary, empirical study. Local Environ. 14, 155–172. doi: 10.1080/13549830802522061
Jørgensen L., Dresbøll D. B., Thorup-Kristensen K. (2014a). Root growth of perennials in vertical growing media for use in green walls. Scientia Hortic. 166, 31–41. doi: 10.1016/j.scienta.2013.12.006
Jørgensen L., Dresbøll D. B., Thorup-Kristensen K. (2014b). Spatial root distribution of plants growing in vertical media for use in living walls. Plant Soil 380, 231–248. doi: 10.1007/s11104-014-2080-2
Jørgensen L., Thorup-Kristensen K., Dresbøll D. B. (2018). Against the wall–root growth and competition in four perennial winter hardy plant species grown in living walls. Urban Forestry Urban Greening 29, 293–302. doi: 10.1016/j.ufug.2017.12.012
Jim C. Y. (2015). Greenwall classification and critical design-management assessments. Ecol. Eng. 77, 348–362. doi: 10.1016/j.ecoleng.2015.01.021
Kaiser D. (2012). “Aktuelle forschungsergebnisse zur fassadenbegrünung: Vergleichende unterschung an verschiedenen FassadenbegrünungsSystemen: Ergebnisse aus dem ersten untersuchungsjahr zu lärmadsorption, bewässerung und vegetationsentwicklung,” in FBB-symposium fassadenbegrünung 2012: Vortragsreihe zu themen der fassadenbegrünung. (Germany: Fachvereinigung Bauwerksbegrünung e.V. (FBB)), 6–11.
Köthner K. B. (2010). Fassadenbegrünungspflanzen für pflanzkörbe. Available at: http://www.gebaeudegruen.info/fileadmin/website/gruen/Fassadenbegruenung/basiswissen/PflanzenFassadengebundeneBegruenung.pdf (Accessed October 25, 2016).
Kowarik I., Bartz R., Brenck M., Hansjürgens B. (2017). Ökosystemleistungen in der stadt: Gesundheit schützen und lebensqualität erhöhen : Kurzbericht für entscheidungträger (Leipzig: Naturkapital Deutschland: TEEB DE).
Lausen E. D., Emilsson T., Jensen M. B. (2020). “Water use and drought responses of eight native herbaceous perennials for living wall systems,” in Urban forestry & urban greening. (Denmark, Sweden: Elsevier Urban Forestry & Urban Greening), vol. 54. doi: 10.1016/j.ufug.2020.126772
Maehlmann J. (2021). Mit textilen begrünungslösungen senkrecht druchstarten: Kettengewirkte textile vegetationsträger für die fassadenbe (Germany: Kettenwirkpraxis), 24–28.
Manso M., Castro-Gomes J. (2015). Green wall systems: A review of their characteristics. Renewable Sustain. Energy Rev. 41, 863–871. doi: 10.1016/j.rser.2014.07.203
Mårtensson L.-M., Fransson A.-M., Emilsson T. (2016). Exploring the use of edible and evergreen perennials in living wall systems in the Scandinavian climate. Urban Forestry Urban Greening 15, 84–88. doi: 10.1016/j.ufug.2015.12.001
Mårtensson L.-M., Wuolo A. A., Fransson A.-M., Emilsson T. T. (2014). Plant performance in living wall systems in the Scandinavian climate. Ecol. Eng. 71, 610–614. doi: 10.1016/j.ecoleng.2014.07.027
Mazzali U., Peron F., Romagnoni P., Pulselli R. M., Bastianoni S. (2013). Experimental investigation on the energy performance of living walls in a temperate climate. Building Environ. 64, 57–66. doi: 10.1016/j.buildenv.2013.03.005
Ottelé M., van Bohemen H. D., Fraaij A. L. A. (2010). Quantifying the deposition of particulate matter on climber vegetation on living walls. Ecol. Eng. 36, 154–162. doi: 10.1016/j.ecoleng.2009.02.007
Pérez G., Coma J., Martorell I., Cabeza L. F. (2014). Vertical greenery systems (VGS) for energy saving in buildings: A review. Renewable Sustain. Energy Rev. 39, 139–165. doi: 10.1016/j.rser.2014.07.055
Perini K., Magliocco A. (2012). The integration of vegetation in architecture, vertical and horizontal greened surfaces. IJB 4, 79–91. doi: 10.5539/ijb.v4n2P79
Perini K., Ottelé M., Haas E. M., Raiteri R. (2011). Greening the building envelope, façade greening and living wall systems. Open J. Ecol. 1, 1. doi: 10.4236/oje.2011.11001
Perini K., Ottelé M., Haas E. M., Raiteri R. (2013). Vertical greening systems, a process tree for green façades and living walls. Urban Ecosyst. 16, 265–277. doi: 10.1007/s11252-012-0262-3
Pfoser N. (2016). Fassade und pflanze: Potenziale einer neuen fassadengestaltung (Germany: Technische Universität Darmstadt). Dissertation.
Pfoser N., Jenner N., Henrich J., Heusinger J., Weber S., Schreiner J., et al. (2013). Gebäude, begrünung und energie: Potenziale und wechselwirkungen, Interdisziplinärer Leitfaden als Planungshilfe zur Nutzung energetischer, klimatischer und gestalterischer Potenziale sowie zu den Wechselwirkungen von Gebäude, Bauwerksbegrünung und Gebäudeumfeld.
Prodanovic V., Wang A., Deletic A. (2019). Assessing water retention and correlation to climate conditions of five plant species in greywater treating green walls. Water Res. 167, 115092. doi: 10.1016/j.watres.2019.115092
Radić M., Brković Dodig M., Auer T. (2019). Green facades and living walls–a review establishing the classification of construction types and mapping the benefits. Sustainability 11, 4579. doi: 10.3390/su11174579
Riley B. (2017). The state of the art of living walls: Lessons learned. Building Environ. 114, 219–232. doi: 10.1016/j.buildenv.2016.12.016
Roth-Kleyer S., Gunkel S. (2014). Machbarkeitsstudie vertikale gärten (Palmengarten Frankfurt/Main: Neue Landschaft), 47–53.
Rouse D. C., AICP, Bunster-Ossa I. F. (2013). Green infrastructure: A landscape approach // green infrastructure: A landscape approach (Chicago: APA Planners Press).
Stollberg M., Moser G., Müller C., von Birgelen A. (2022). Meadow-living walls: Creating attractive vertical greenery with meadow seed mixtures – experiences and recommendations from a three year field trial. Ecol. Eng. 185, 106817. doi: 10.1016/j.ecoleng.2022.106817
Stollberg M., von Birgelen A. (2022). Plants and polyester: suitable plants for a textile-based living wall system. Acta Hortic. 100, 189–196. doi: 10.17660/ActaHortic.2022.1345.25
Stollberg M., von Birgelen A., Mählmann J. (2021). Begrünte fassadenkacheln - pflanzen für die wandbegrünung: Eignung von stauden in textilen vegetationsträgern. (Germany: Stadt und Grün).
Suárez-Cáceres G. P., Mejía-Sampedro D. K., Fernández-Cañero R., Loges V., Pérez-Urrestarazu L. (2022). Establishment and development of ornamental grasses on green roofs and living walls. Landscape Ecol. Eng. 45 (8), 1779. doi: 10.1007/s11355-022-00527-5
Thorpert P., Rayner J., Haaland C., Englund J.-E., Fransson A.-M. (2022). Exploring the integration between colour theory and biodiversity values in the design of living walls. Front. Ecol. Evol. 10, 804118. doi: 10.3389/fevo.2022.804118
Tzoulas K., Korpela K., Venn S., Yli-Pelkonen V., Kaźmierczak A., Niemela J., et al. (2007). Promoting ecosystem and human health in urban areas using green infrastructure: A literature review. Landscape Urban Plann. 81, 167–178. doi: 10.1016/j.landurbplan.2007.02.001
Wack H. (2015). Konzept und realisierung einer vertikalen begrünungsmethode mit dem ziel der feinstaubsorption im urbanen raum. ANLiegen Natur 37/2, 1–5.
Keywords: urban green infrastructure, vertical greenery systems, living wall, planting design, perennials, species development, maintenance
Citation: Stollberg M and von Birgelen A (2023) Vertical plants: Plant design of Living walls – evaluation of 34 perennials in a textile based Living wall over a three years experiment. Front. Hortic. 2:1091026. doi: 10.3389/fhort.2023.1091026
Received: 06 November 2022; Accepted: 24 February 2023;
Published: 30 March 2023.
Edited by:
Daniela Romano, University of Catania, ItalyReviewed by:
Michele Valquíria Reis, Universidade Federal de Lavras, BrazilAlessandra Francini, Sant’Anna School of Advanced Studies, Italy
Copyright © 2023 Stollberg and von Birgelen. This is an open-access article distributed under the terms of the Creative Commons Attribution License (CC BY). The use, distribution or reproduction in other forums is permitted, provided the original author(s) and the copyright owner(s) are credited and that the original publication in this journal is cited, in accordance with accepted academic practice. No use, distribution or reproduction is permitted which does not comply with these terms.
*Correspondence: Maren Stollberg, TWFyZW4uU3RvbGxiZXJnQGhzLWdtLmRl