- 1Laboratorio de Enfermedades Neuromusculares, División de Neurociencias Clínicas, Instituto Nacional de Rehabilitación Luis Guillermo Ibarra Ibarra, Mexico City, Mexico
- 2Laboratorio de Aminoácidos Excitadores/Laboratorio de Neurofarmacología Molecular y Nanotecnología, Instituto Nacional de Neurología y Neurocirugía, Mexico City, Mexico
- 3Department of Molecular Pharmacology, Albert Einstein College of Medicine, Bronx, NY, United States
- 4Yaroslavl State University, Medical University (Sechenov University), Moscow, Russia
- 5Instituto de Investigaciones Biomédicas Maimonides de Córdoba, Departamento de Bioquímica Y Biología Molecular, Facultad de Medicina Y Enfermería, Red Española de Excelencia en Estimulación Cerebral (REDESTIM), Universidad de, Córdoba, Spain
- 6Departamento de Neuroquímica, Instituto Nacional de Neurología y Neurocirugía, Mexico City, Mexico
Thallium (Tl) is a toxic heavy metal responsible for noxious effects in living organisms. As a pollutant, Tl can be found in the environment at high concentrations, especially in industrial areas. Systemic toxicity induced by this toxic metal can affect cell metabolism, including redox alterations, mitochondrial dysfunction, and activation of apoptotic signaling pathways. Recent focus on Tl toxicity has been devoted to the characterization of its effects at the nuclear level, with emphasis on DNA, which, in turn, may be responsible for cytogenetic damage, mutations, and epigenetic changes. In this work, we review and discuss past and recent evidence on the toxic effects of Tl at the systemic level and its effects on DNA. We also address Tl’s role in cancer and its control.
1 Introduction
1.1 Thallium generalities
Thallium (Tl) is a ubiquitous natural trace metal discovered in 1861, considered one of the most toxic heavy metals to humans and animals. Since its discovery, Tl has been used in industrial applications as rodenticide, cement, insecticides, alloys, electronic devices, special glass, and optical lenses, in the industries of photography, semiconductors, and superconductivity (Taylor and McKillop, 1970). In addition, it has been used as a constituent of the growth media used for culture of some species of Mycoplasma (Hwang et al., 2010; Puschner et al., 2012). It is well documented that some anthropogenic activities such as the pyrite mining, smelting and related steel-making industry are also important source for Tl and other toxic metalloids pollution in the environment with the consequences in increasing the associated health risks (Wang et al., 2021). Unfortunately, Tl is still used as a rodenticide in many developing countries and in other novel technologies, raising concern about exposure risk to animals and humans. In the past, Tl has been employed for treating heavy persistent night sweats or ringworm (Galván-Arzate and Santamaría, 1998) as well as tuberculosis, sexually transmitted diseases such as syphilis and gonorrhea, for the production of depilatory products, as well as for homicidal purposes (Smith and Carson, 1977). Several decades ago, Tl isotopes have been used as radiotracers for cardiovascular and tumor imaging (National Minerals Information Center, 2022).
Thallium contributes to several health risk concerns (Liu et al., 2017) through accidental industrial occupational exposures such as coal burning and smelting (Smith and Carson, 1977; Brockhaus et al., 1981), environmental contamination, and therapeutic poisoning. Thallium is found in two oxidation states, thallous (Tl+) and thallic (Tl3+) salts. Because of its similarity to potassium (K+) ions, plants and animals readily absorb Tl+ through the skin and digestive and respiratory systems. When Tl is exposed to air and moisture, Tl oxidizes to form Tl(I) oxide (Tl2O) with chemical properties analogous to alkali metals, and when in its oxide form, at high temperatures it forms Tl(III) oxide (Tl2O3), behaving analogous to aluminum. Both of these species are highly toxic to living organisms (Rickwood et al., 2015), but Tl(I) is the predominant species. Thallium is found at low concentrations in the environment, and it is a trace compound for many minerals such as copper, gold, potassium, rubidium, and zinc. Some of the minerals with higher Tl concentrations (up to 16%–60% of Tl) are rookesite (found in Sweden and Brazil), lorandite (found in Greece and the United States), hutchinsonite (Switzerland), orabite (in Brazil and the United States), and berzelianite (in Germany) (Léonard and Gerber, 1997). There are few areas in the world with natural occurrence of high Tl concentrations, such as the southwestern Guizhou Province in China (Xiao et al., 2003), the Allchar area in Kavadarci, Macedonia (Percival and Radtke, 1994), and Lengenbach in Switzerland (Hofmann and Knill, 1996).
Thallium is released into the biosphere from both natural (volcanic activity and mineral ores) and anthropogenic (such as dust, vapors, or liquids from district heating, exhaust emissions, and coal combustion) sources, resulting in air pollution, thus allowing for its atmospheric spread and entry into terrestrial environment niches, binding to soil matrix (Savariar, 2014). Thallium is readily soluble in water and relatively more toxic than mercury, cadmium, lead, copper, or zinc (Cvjetko et al., 2010). The Tl-based salts are highly soluble, without color, odor, or taste, readily contaminating aquatic environments and accumulating in aquatic organisms. In addition, Tl compounds can leak into underground water streams or shallow soil and be taken up by roots leading to Tl accumulation in the plant biomass; therefore, once Tl has entered the food chain, it reaches living organisms with potentially toxic consequences (Karbowska, 2016; Liu et al., 2019). It has been demonstrated that some rivers and lakes downstream from coal mines and coal-fired power stations in Canada contain elevated Tl concentrations (Cheam et al., 2000; Cheam, 2001). Similarly, in North America and Europe, coal burning was determined as the main source of Tl, cadmium, and lead atmospheric pollution since the early 1900s, and the risk for increased levels of these elements is higher in the coal-driven economies (McConnell and Edwards, 2008).
Regarding some cases of intoxication, a recent study quantified the levels of Tl in urine from opium consumers developing symptoms similar to Tl poisoning. The study was carried out in the city of Kashan, in Iran, with 200 volunteers. Noteworthy, in the group of opium consumers, Tl concentrations reached an average of 38.93 μg/l, being significantly different from the control group (Eghtesadi et al., 2021). It was also reported in Malaysia that some baby diaper powders were contaminated with Tl+ and other heavy metals (Karwowski et al., 2017). In addition, unfortunately, Tl has also been used for war purposes as reported in Syria in 2015, where Tl-containing-candies were distributed among the population and were the cause of massive poisoning (Almassri and Sekkarie, 2018). Noteworthy, it has been determined that concentrations up to 0.43 μg/g of Tl+ have been found in tatoo inks obtained from some countries, hence constituting a health risk for the population who practice tattooing (Karbowska et al., 2020). Altogether, these and other cases demonstrate that more severe restrictions regarding the thallium and other metals pollution are needed (López Segura et al., 2013).
1.2 Systemic thallium toxicity
Since a biological function has yet to be demonstrated for Tl, it does not appear to be an essential element for humans or other living organisms (Cvjetko et al., 2010). One report in mice using field desorption mass spectrometry revealed that stomach was the organ showing the maximal levels of Tl accumulation, followed by kidney, heart, liver and brain during the first hours after exposure. Later, at 24 h after exposure, all organs (including the brain) were reported with higher accumulation (Achenbach et al., 1980). In rats, Tl was shown to accumulate in a higher concentration in kidney, followed by testis, spleen, lung, heart, liver and brain (Ríos et al., 1989). In human beings, Tl can be accumulated in several organs, and the resulting symptomatology is non-specific due to the multi-organ involvement, though the highest concentration has been reported in kidney, followed, in decreasing order, by bones, stomach, intestin e, spleen, liver, muscle, lung, and brain (Léonard and Gerber, 1997); the severity of intoxicat ion and t issue damage depends on the amount of Tl incorporated, age, the immune response, and each person’s tolerance to this poisoning element (Misra et al., 2003).
Regarding Tl toxic mechanisms, it has been suggested that thallium toxicity is related to the interference with relevant potassium-dependent processes, such as the (Na+/K+)- ATPase pump, since Tl+ and K+ are both univalent ions with similar ionic radii (173 pm and 165 pm respectively). In this regard, Tl+ ions are capable to mimics K+, therefore modifying the potassium cellular uptake and intracellular accumulation in mammals (Britten and Blank, 1968; Mulkey and Oehme, 1993; Galván-Arzate and Santamaría, 1998). Chen et al. (2000) demonstrated that Tl+ and K+ ions behave as analogous ions using murine-cultured rat cells. Authors reported that the previous treatment with extracellular K+ significantly evoked a reduction of thallium-201 ion influx in these cells, whereas the treatment with ouabain, (an inhibitor of sodium-potassium ATPase pump), diminished the uptake of thallium-201 ion in these cells via active transport.
Thallium intoxication begins after this metal is introduced into the body by inhalation, ingestion of contaminated food or through the skin. Water-soluble Tl+ salts are able to enter in organisms and are widely distributed in organs and tissues, including the brain, heart, kidney, skeletal muscle and testis (Mulkey and Oehme, 1993; Galván-Arzate and Santamaría, 1998). Once Tl enters the body through the potassium uptake mechanisms, it is distributed throughout the organism, and accumulates intracellularly, where it may affect the functionality of various tissues and systems (Heim et al., 2002; Amiri et al., 2007; Fatemi et al., 2007; Osorio-Rico et al., 2017; Campanella et al., 2019).
Though the precise mechanisms of Tl toxicity remain unknown, its damaging effects have been related to: a) binding to blood proteins (Xiao et al., 2004; Peter and Viraraghavan, 2005); b) increase in the levels of reactive oxygen species (ROS) -which represents an important riskfactor for tissue injury and dysfunction (Radic et al., 2009)-; c) interference with the function of membrane channels and transporters associated with potassium (K+) (Kilic and Kutlu, 2010), induction of mitochondrial dysfunction -which interferes with vital potassium-dependent processes, disturbing the levels and expression of the Na+-K+-ATP enzymes inside cells (Zhao et al., 2008)-; d) triggering metabolic disturbances such as the inhibition of enzymes, coenzymes and structural proteins - which in turn could explain the structural changes observed in the affected cells that may correlate with physical, psychiatric, and neurological abnormalities present in these patients (Galván-Arzate and Santamarıa, 1998; Bragadin et al., 2003; Korotkov and Lapin, 2003)-; and e) inhibition of cellular respiration and disruption of calcium homeostasis when Tl forms complexes with proteins containing sulfhydryl groups (Kilic and Kutlu, 2010).
The estimated lethal dose for oral administration is between 10 and 15 mg/kg (Galván-Arzate and Santamaría, 1998; Conerly and Blain, 2008; Lennartson, 2015), sufficient to cause fever and gastrointestinal problems which appear within the few hours of acute poisoning, as well as seizures, memory impairment, mental disturbances, coma, and delirium (Saddique and Peterson, 1983). Reed et al. (1963) performed a 7-year study demonstrating that more than seventy children who ingested thallium-containing pesticides suffered neurological, gastrointestinal dysfunctions, and cardiovascular abnormalities. They estimated that renal excretion of thallium sulfate was slow, and this salt could be detected as late as 2 months after its ingestion. The biological half-time of Tl permanence in humans has been estimated between 10 and 30 days (World Health Organization WHO, 1996), with a mortality rate of 6%–15% (Ratti et al., 2018); therefore, it is important to determine an early diagnosis as to establish the most efficacious treatment.
Thallium (80%–100%) is readily absorbed through the skin and mucous membranes. The most common routes of exposure are via the gastrointestinal or pulmonary mucous by consuming contaminated food and drinking water or being exposed to air-borne contamination (Liu et al., 2020). During acute exposure, gastrointestinal symptoms such as severe abdominal pain, nausea, vomiting, and bloody diarrhea, appear within the first few hours of poisoning; whereas in chronic exposure the main symptoms are chest tightness, confusion, coma, and dyspnea, resulting in poor prognosis (Xu et al., 2010). These patients can also present non-specific skin abnormalities such as scaling of the palms and soles, acneiform lesions of the face, numbness, burning pain, hypertension, tachycardia, and hyperalgesia (Zhao et al., 2008). During chronic exposure, the main neurological symptoms include sensory and motor dysfunctions (Saddique and Peterson, 1983).
Clinical manifestations depend on the level of Tl intoxication, severity (acute, chronic, or sub-chronic), time of exposure, age and the general health status. Unfortunately, the lack of non-specific symptoms at the beginning of intoxication delays adequate diagnosis and decisions on appropriate therapeutic measures (Lech and Sadlik, 2007); nonetheless, the main symptom of Tl poisoning is alopecia, which appears on day 10–14, sometimes accompanied by loss of eyebrows and eyelashes, forcing the poisoned persons to contact a physician (Saddique and Peterson, 1983; Misra et al., 2003). The high affinity of Tl for sulfhydryl groups in proteins and other biomolecules may explain alopecia as Tl prevents keratinization of hair proteins by binding with cysteine residues, thus inducing atrophic and necrotic changes, which in turn, generate parakeratosis, necrotic sebaceous materials, dilated hair follicles filled with keratin, vascular degeneration of basal layer, and mild epidermal atrophy (Saha et al., 2004; Kuo et al., 2005; Jha et al., 2006; Lu et al., 2007). Approximately 1 month after Tl poisoning, the characteristic Mee’s lines appear in the nail plate of exposed people; these lesions manifest as transverse white lines presented as a result of complete erosion of the proximal parts of nails (Zhao et al., 2008).
Thallium can readily cross the blood-brain barrier leading to neurodegeneration, demyelination, and accumulation of end products of lipid oxidation in the brain of animal models (Galván-Arzate et al., 2000). Periodically, Tl poisoning is misdiagnosed as Guillain-Barré syndrome as some symptoms of neuropathy with muscle weakness, paresthesia, and hyporeflexia are detected (Jha et al., 2006). Also, some peripheral neuropathies with severe pain have been reported, accompanied by paresthesia of hands and lower limbs, distal motor weakness, and sensory impairment (Kuo et al., 2005). Thallium can also readily cross the placental barrier, and excreted in breast milk (Hoffman, 2000). During pregnancy, mothers exposed to Tl intoxication develop classic signs of thallium poisoning; however, their newborns appear normal and apparently, the only consistent reported effect is prematurity and low birth weight, despite the potential for severe maternal toxicity (Hoffman, 2000). Unfortunately, there are still insufficient data to evaluate prognostic markers of fetal outcome and the benefits or risks of treatment in Tl-poisoned pregnant patients.
Thallium is released slowly from the organism through sweat, saliva, tears, urine, and feces, and it is accumulated in the hair and nails. Elevated Tl levels in urine, saliva, and blood are better indicators of poisoning than its presence in hair, as Tl in hair could be explained by exogenous deposition (Tl-containing dust) (Frattini, 2009).
The misdiagnosis or delayed diagnosis leads patients to develop unfavorable outcomes (Hoffman, 2003; Jha et al., 2006; Lin et al., 2019) or severe or moderate sequelae of poisoning in sensory nerve fibers of the distal lower extremity and evokes problems in intelligence, depression, and memory. When hospital admission is delayed, it may lead to fatalities (Sun et al., 2012; Li et al., 2014).
1.3 Animal models
Tl-induced mechanisms of toxicity have been poorly studied and understood in living organisms due to their extreme toxicity. Therefore, only limited mutagenicity, genotoxicity, and epidemiological data to classify Tl carcinogenic potential have been reported (Aresini et al., 1993). Most of these reports have been focused on studying the efficacy of treatments for experimental thallium poisoning. Given that liver is the preferred site of Tl storage, it has been reported that Tl administration in a rat experimental liver injury model induced mitochondrial membrane potential collapse in isolated rat hepatocytes (Eskandari et al., 2015), which was evidenced by the impairment of mitochondrial fatty acid metabolism (Li et al., 2022). Using an in vitro model for studying the mitochondrial effects of Tl exposure, Eskandari and others showed a marked increase in oxidative stress characterized by elevated mitochondrial ROS generation, ATP depletion, oxidation of reduced glutathione (GSH), and mitochondrial membrane potential collapse, accompanied by mitochondrial outer membrane rupture and swelling and disruption of the mitochondrial respiratory chain. Combined, these effects triggered hepatocellular death secondary to the opening of the mitochondrial permeability transition pore (Eskandari et al., 2015).
2 Cellular effects of thallium exposure
Because of its similarity to potassium ions, Tl cannot only be absorbed through the skin, digestive, and respiratory systems, but also cross the placental, hematoencephalic, and gonadal barriers. Once the thallium ion (Tl+) reaches intracellular compartments, it can accumulate and interfere with the metabolism of potassium and other metal cations, mimicking or inhibiting their action. The toxicity of Tl compounds is predominantly related to their affinity for amino-, imino-, and sulfhydryl groups at catalytic enzymatic sites, similar to mercury, which possesses an affinity for selenol and sulfhydryl groups of bioligands (Rodríguez-Mercado and Altamirano-Lozano, 2013; Ajsuvakova et al., 2020).
Hantson et al. (1997) reported some preliminary observations showing that Tl can induce cytogenetic damage in vivo when analyzing samples of peripheral blood lymphocytes from a patient who self-ingested a dose of 200 mg of thallium sulfate. These authors reported drastic increases in binucleated cells presenting micronuclei, suggesting that Tl2SO4 interferes more with chromosome distribution than with the structural chromosome integrity or the sister chromatid exchange process.
Rodríguez-Mercado et al. (2013) determined in vitro that increased concentrations of thallium(I) acetate induced a concentration-dependent reduction in the mitotic and replicative indices in human peripheral blood cells when assayed for structural chromosomal aberrations and sister chromatid exchanges. Additionally, increased structural chromosomal aberrations were observed in the Tl treated group compared with the control group.
Hanzel et al. (2012) demonstrated that Tl triggered apoptosis. Here, the authors treated PC12 cells for 3 h with 100 μM Tl(III) and observed marked endosomal acidification content characterized by altered profile of acridine orange uptake. This was associated with increased levels of Cathepsins B and D activities and decreased the co-localization of these enzymes with the lysosomal marker Lamp-1. The authors hypothesized that Tl may be taken up via iron uptake mechanisms, in turn, leading to lysosomal functions. Notably, this group observed that Cathepsin D activated the pro-apoptotic protein BID, which is involved in the intrinsic pathway of apoptosis. When PC12 cells were preincubated with pepstatin A, a cathepsin D inhibitor, further treatment with Tl(III) did not activate caspase 3 and therefore, apoptosis progression was blocked. These results support the concept that Tl(III) is incorporated by the acidic cell compartments and the role played in the early steps of Tl-mediated apoptosis in PC12 cells.
Rodríguez-Mercado and Altamirano-Lozano (2013) also reported that Tl induced cytotoxic and mutagenic effects, as well as DNA breaks, reduced cell viability, interferes with the cell cycle progression, induced apoptosis, inhibited DNA synthesis, and blocked cell proliferation in a variety of mammalian cells such as embryo fibroblasts, osteoblasts, hepatocytes, glioma cells, ovarian cells, and lymphocytes from mice, rats, Chinese hamsters, and human cells. In an elegant review, these authors discussed several cell lines and animal models such as C57B1/6 strain, CBA strain, 3T3 cells, MC3T3-E1 cells, L929 cells, rat and human primary hepatocytes, C6 glioma cells, Chinese hamster’s ovary cells, lymphocyte cultures, HepG2 cells, Jurkat cells, and Chinese hamster’s bone marrow, all of which have been used to elucidate the mechanism of Tl-induced cellular damage.
Recently, it was shown that human peripheral lymphocytes treated with increased concentrations (0.5–100 μg/mL) of thallium (I) acetate, thallium (I) sulfate, and thallium (III) chloride had decreased viability secondary to accelerated apoptosis and necrosis in a concentration and time-dependent manner. When the cells were treated with these 3 Tl compounds in the G0 phase, the mitotic index was diminished in a concentration-dependent manner, suggesting that cell membrane is the main target of Tl. In addition, it was observed that these Tl salts decreased the cellular proliferative rate, characterized by dysfunction in cellular energetics, and suggesting that mitochondria are the most sensitive organelles to Tl (Rodríguez-Mercado et al., 2019).
3 Mutations induced by thallium
Although the effects of Tl on genetic material have yet to be fully explored, it is known that Tl+ is molecularly more stable than Thallic cation (Tl3+), which has a strong oxidizing capacity (Harris and Messori, 2002). Under experimental UV irradiation and the presence of molecular oxygen, Tl+ can be oxidized to Tl3+, which in turn, can oxidize guanine residues on DNA to 8-oxoguanine to induce mutagenic effects. Thus, Tl compounds likely exert changes in cell-cycle progression (Nowicka, et al., 2013; Rodríguez-Mercado et al., 2019).
Zasukhina et al. (1983) demonstrated with hydroxyapatite chromatography assays that increased doses of thallium carbonate added for 24 h to rodent embryonic fibroblast cells induced single-stranded DNA breaks. In addition, the mutagenic potential of Tl was tested using the dominant lethal test, which consists of chronically Tl exposed males crossed with unexposed females. The effects were characterized by an increase in the total number of embryonic deaths. However, these results must be confirmed using a better sample size. Additionally, these authors explored the Vaccinia virus (Lister) strain upon exposure to Tl carbonate, and the induced lethal mutations on DNA virus were estimated by comparing the virus titer between the control and thallium-treated group, showing that thallium carbonate reduced virus survival and suggesting that a significant rate of mutability resulting in non-viable viruses was induced by this genotoxic agent.
Several groups have demonstrated that thallium sulfate administered to chick embryos reduced the growth of the long bones and induced areas of necrotic chondrocytes containing low levels of acid mucopolysaccharide. It is likely that Tl sulfate can induce mutations in an enzyme essential for acid mucopolysaccharide synthesis, or in constitutive membrane proteins of chondrocytes, thus altering the release of the acid mucopolysaccharide. These mutations might be related to the fact that the resulting progenies of the Tl-treated embryos had short-limbed dwarfism even when the parents were healthy (Léonard and Gerber, 1997).
4 Epigenetic changes
Exogenous DNA damage occurs when environmental, physical, and chemical agents affect the DNA integrity, as it occurs with exposure to UV and ionizing radiation, alkylating agents, external DNA-binding molecules, and crosslinking agents (Chatterjee and Walker, 2017). Nowicka et al. (2013) reported that Tl can interact with selected oligonucleotide gene sequences such as those present in the human gene OGG1 responsible for repairing DNA damage. This gene encodes for the enzyme responsible for the excision of 8- oxoguanine, a mutagenic base byproduct that is generated by exposure to reactive oxygen species. Using cyclic voltammetry assays, these authors showed that under basal conditions, nitrogen N-7 in guanine structure can be oxidized. The binding of this residue to Tl(I) leads to the loss of this electroactivity. The resulting binding failed to induce changes in DNA conformation, but increased the probability of a concurrent introduction of an incorrect base and propagation in subsequent rounds of DNA replications. Authors also demonstrated that the addition of guanine-enriched telomeric sequences in one of the strands of the DNA helix limited the interaction of Tl with dsDNA. Despite these results, authors concluded that the presence of monovalent thallium cations did not alter the DNA double helix nor induced its oxidization, though the possibility of introducing and preserving mutations on DNA sequence remained.
Although this study concluded that Tl did not alter the DNA conformation substantially, this element should be considered a DNA-damaging agent due to its ability to form numerous bonds within the major groove of DNA, to methylate guanine profile, to elicit 8-oxo-guanine lesions, and to favor single and double-strand breaks, as well as its interstrand guanine crosslinking effect. These abnormalities trigger specific DNA repairing pathways such as repairing replication mismatch errors, base-excision enzyme machinery, repairing stranded breaks in the DNA backbone, and nucleotide excision to eliminate intra-strand crosslinks (Chatterjee and Walker, 2017). The interaction of Tl, especially with the guanine residues on DNA, strongly suggests that Tl might be involved in the modulation of methylation of CpG islands present in promoter regions of several genes subjected to epigenetic control to regulate their transcription as it is known to occur during developmental programming of fetal growth to adult organisms, preventing or allowing the access of transcription factors or protein complexes to DNA. Alternatively, it has been reported that epigenetic control is responsible for silencing tumor suppressor genes associated with carcinogenic processes (John and Rougeulle, 2018; Nishiyama and Nakanishi, 2021), though it is necessary to perform more studies to confirm these hypotheses.
Mitochondrial DNA is susceptible to oxidative damage when protective histones and effective DNA repair mechanisms are absent. Histones modulate the cellular accessibility of nuclear factors to DNA. An excess in histone proteins supply has been invoked in increased DNA damage susceptibility and blocking the DNA repair process by interfering with the homologous recombination-mediated DNA repair mechanisms (Kumar et al., 2020). The mitochondrial DNA copy number is altered during physiological malfunctions or adverse environmental conditions. This parameter is considered a cellular marker that indicates deficits in the energy production balance required to maintain optimal cellular and physiological functions. Wu et al. (2019) analyzed the urine of pregnant women exposed to environmental thallium. These investigators demonstrated a significant decrease in the amounts of mitochondrial DNA copy numbers, mainly during the first trimester. In addition, this group determined the neonatal relative telomere length by quantitative real-time polymerase chain reaction assays in cord blood leukocytes. The relative telomere length is a hallmark of cell aging and it has been involved in preserving genomic stability and chromosomal integrity (Wu et al., 2021). These results suggest a close relationship between prenatal exposure to Tl and decreased mitochondrial DNA copy number and shortened neonatal telomere length due to epigenetic changes in histone proteins, such as H3 and H4. Table 1 summarizes the main effects of Tl on DNA damage and epigenetic modifications.
5 Thallium and cancer
The negative effects exerted by Tl on severThe negative effects exerted by Tl on several cell functions, such as cell survival, the introduction of mutations, disruption of DNA methylation control mechanisms, and inducible DNA damages, have been well documented. These abnormalities might trigger several diseases, including cancer. Previously, Tl was shown to exert missense mutations, insertions, translocations on DNA, changes into the DNA backbone, histone profile modifications, and chromatin architecture, to name a few, which can modulate gene expression while reprograming the development and progression of tumor cells (Casalino and Verde, 2020). These studies highlight the ability of Tl to induce some changes in the DNA methylation patterns, especially the methylation of promoter CpG islands and transcriptional silencing of tumor suppressors genes, thus modifying genomic stability and gene expression of key oncogenic genes (Jones and Baylin, 2007; Casalino and Verde, 2020; Nishiyama and Nakanishi, 2021).
Nowicka et al. (2013) reported that Tl(I) can decrease the activity of doxorubicin (DOX), a popular anticancer drug directed to DNA that targets a group of three guanines. These results suggest that Tl(I) interferes with the metabolism of certain antineoplastic agents, modifying or inhibiting their anti-carcinogenic action and therefore allowing the progression of tumorigenesis (Léonard and Gerber, 1997). In addition, it has been demonstrated that acute in vivo administration of Tl induces dysfunctions in the assembly of ribosomes due to structural changes observed in the endoplasmic reticulum of hepatocytes, decreasing the activity of several drug-metabolizing enzymes dependent on cytochrome P-450. Consequently, the Tl-induced effects might confer carcinogenic susceptibility to certain cell populations (Fowler et al., 1993).
Further studies are warranted using these two oxidation states of thallium, as thallium (I) sulfate and thallium (III) chloride showed different effects in diminishing the mitotic index in human peripheral lymphocytes at all tested concentrations (Rodríguez-Mercado et al., 2017). These studies might contribute to a better understanding as to whether the intercalating property of Tl to DNA is more potent in affecting the survival and proliferation rate of neoplastic cells.
On the other hand, Tl has been used for tumor imaging due to its similarity to alkali metals such as cesium (Nadel, 1993; Kumar et al., 2017). However, the exact mechanism of Tl specificity to tumor cells remains unclear. Noteworthy, other studies have demonstrated that Tl might show anti-tumor activity. Hart and Adamson (1971) demonstrated that Tl trichloride (2–5 mg/kg) increased more than 700% the survival of rats implanted with the ascites form of Walker 256 carcinosarcoma compared to the control group.
Recently, 3 Tl(III) synthetic complexes were obtained showing selective cytotoxic effects against the cancer cell lines A375 (derived from human melanoma) and HT29 (derived from human colon adenocarcinoma), though these inhibitory effects were weaker on the normal fibroblast cell line HFF (isolated from human foreskin). The cytotoxic activity of these Tl derivatives was determined by MTT assays reporting a significant reduction of cell viability in these cell lines after treatment with thallium complexes, particularly by C1 and C3 compounds. The cellular uptake of Tl compounds was analyzed revealing that the cellular concentration of Tl was lower in normal cells than in cancer cells. When the generation of reactive oxygen species (ROS) was analyzed in A375 cells, all three complexes increased the ROS levels, which contrasted with much lower levels in HFF cells. ROS generation was accompanied by the reduction of mitochondrial membrane potential in A375 cells, whereas in Tl -treated HFF cells no significant changes in this parameter were observed. By flow cytometry assays on A375 cells, it was shown that Tl complexes induced arrest in the G2-M cell cycle phase with the consequent induction of apoptosis. The cell cycle did not show significant changes in HFF cells. The type of cell death induced in this cell line depicted increases in the late apoptosis pattern compared to Tl-untreated A375 cells. The suggested apoptotic pathway activated in this cell line was confirmed by western blotting assays which showed an increase in p53 protein, Bax upregulation, Bcl-2 down-regu ation, cytochrome c release, and augmented expression of procaspase-9, and 3 (Abdolmaleki et al., 2021).
Our group recently demonstrated that Tl exerted cytotoxic effects in two glioblastoma cell lines (C6 and U373) exposed to increased concentrations of thallium (I) acetate. By means of atomic absorption assays, it was shown to accumulate in the cytoplasm of C6 and U373 cells, supporting an efficient uptake mechanism. The cytotoxic effect of Tl in these cells was spectrophotometrically determined by the incorporation of the neutral red dye, showing that cell viability decreased in a concentration-dependent manner in both cell lines compared to primary cell cultures. The loss of confluence and morphological changes in cancer cells was determined by light microscopy. The type of cell death observed in these neoplastic cells was assayed by the incorporation of ethidium bromide and the enzymatic metabolism of the acridine orange. Epifluorescence assays demonstrated chromatin condensation and nuclear fragmentation, as well as the formation of apoptotic bodies compared to the control group and brain primary cultures. Propidium iodide staining assays revealed that Tl exposure evoked changes in the cell cycle in C6 cells, where a prominent G1 phase was induced, while U373 cells were arrested in the subG1 phase. This study established that Tl was able to exert toxic effects preferentially in tumor cells compared to primary brain cultures (Rangel-López et al., 2022).
Altogether, the above-described evidence establishes a dual scenario where, in some instances, Tl might be responsible for mutagenesis and alterations possibly leading to tumorigenesis, while opposite to these changes, Tl might exert direct toxic effects on tumor cells due to its affinity for these cells. Studies clarifying the effects of this metal on tumor phenotypes are warranted, searching for the conditions by which Tl may act as a tumorigenic or antitumor element.
6 Concluding remarks
Although cytotoxic, mutagenic, and genotoxic effects have been ascribed to Tl decades ago, it was not considered a carcinogenic element due to the lack of more relevant human and animal studies (IARC, 1989). Here, we noted several studies which suggest that Tl has damaging properties like those observed by other metals, such as beryllium (Gordon and Bowser, 2003), cadmium (Waisberg et al., 2003), lead (García- Lestón et al., 2010) and mercury (Aschner and Aschner, 1990; Ajsuvakova et al., 2020). It has been documented that the adverse effects of Tl in living organisms are a consequence of chronic exposure, and these elevated concentrations may be a consequence of anthropogenic activities, such as mining, releasing this metal into the environment. Once inside cells, it has been suggested that mitochondria are the main target for Tl toxicity, affecting cell viability by activating ERK and caspase-3 proteins involved in the apoptotic pathway, as it has been reported for mercury (Aschner and Carvalho, 2019). Combined, these harmful features evoke DNA damage that cells try to offset through base excision repair, nucleotide excision repair, mismatch repair, homologous recombination, and non-homologous end joining, which are active throughout different stages of the cell cycle. These repairing processes and DNA tolerance pathways are essential to preserving the genetic stability in cells (Chatterjee and Walker, 2017). This scenario represents a relevant challenge for researchers and mandates additional studies to further characterize the adverse effects of Tl.
In addition, it is important to consider that Tl not only exerts negative effects on human health but its chemical resemblance as a potassium analog and its ability to be taken up and accumulate in cancer cells at higher rates than normal cells have allowed for its use as the radioisotope 201TI in imaging as an accurate radiotracer to detect and delimit tumors (Jo et al., 2020; Poudyal et al., 2022).
Regarding the antineoplastic effects induced by Tl compounds, it seems that the ROS levels generated by the administration of this metal induce more cytotoxic effects in neoplastic cells than in normal cells, suggesting that the cellular levels of ROS play a crucial role in inducing cancer cell death. Finally, it has been demonstrated that Tl has a special affinity for cancer cells likely due to the mimicry between Tl to potassium (Abdolmaleki et al., 2021; Rangel-López et al., 2022). Considering its biochemical and physical properties, its effects on DNA damage, and the energetic requirements of neoplastic cells future studies should focus on strategies to reduce the toxic effects of Tl and to design novel thallium-based drugs to inhibit the development and progression of several cancer types.
Finally, Figure 1 summarizes the most relevant effects induced by cellular uptake of Tl. Since the Tl ion resembles potassium due its ionic radii, the sodium-potassium pump takes thallium instead of potassium (Álvarez-Barrera et al., 2019). Once inside the cell, Tl binds to cellular proteins, enzymes and organelles involved in several physiological functions. The function of ribosomes is altered by binding of Tl to enriched-sulfhydryl groups in their structure, affecting the protein synthesis (Chou and Lo, 2019), and the resulting proteins show misfolding mistakes and/or mutated amino acid sequences. Similarly, Tl decreases glutathione synthesis and reduces the levels of superoxide dismutase (SOD), thus interfering with cellular antioxidant mechanisms and inducing the generation of reactive oxygen species (ROS) (Rodríguez-Mercado and Altamirano-Lozano, 2013). The increase in ROS levels elicits membrane damage by lipoperoxidation, which in turn, generates death signals that are transduced by membrane Death Receptors (DR’s). These receptors trigger the apoptotic pathway mediated by caspases 9 and 3 through the apoptosome complex. Similarly, Tl induces an increase in Cathepsine D levels which activates the protein BID, involved in the intrinsic pathway of apoptosis (Hanzel et al., 2012). In addition, secondary to increased ROS levels induced by intracellular Tl, damage to mitochondria ensues, including electron transport chain disruption. In turn, ATP levels decrease and Ca+ cytoplasmic levels increase, leading to the release of cytochrome c and activation of apoptotic protease activating factor-1 (Apaf- 1) (Fani et al., 2016; Abdolmaleki et al., 2021). At the nuclear level, Tl alters the expression of p21 which inhibits the cyclin/CDK complex, blocking the G1/S and G2/M transitions, thus disturbing the cell cycle (Chia et al., 2005), In addition, Tl induces telomere shortening and modifies epigenetic patterns due the structural modifications of histones H3 and H4 from nucleosomes and the sites susceptible of methylations (ME), thus eliciting chromosomal aberrations (Wu et al., 2021). At the DNA level, Tl elicits DNA breaks and favors dysfunctions on enzymatic DNA repairing mechanisms due to the conversion of guanine residues to the mutagenic 8-oxo guanine, affecting the expression of CpG enriched sequences (Nowicka et al., 2013). Combined, these malfunctions inhibit cell viability and cellular proliferation eliciting the apoptosis of the affected cells (Rodríguez-Mercado and Altamirano-Lozano, 2013).
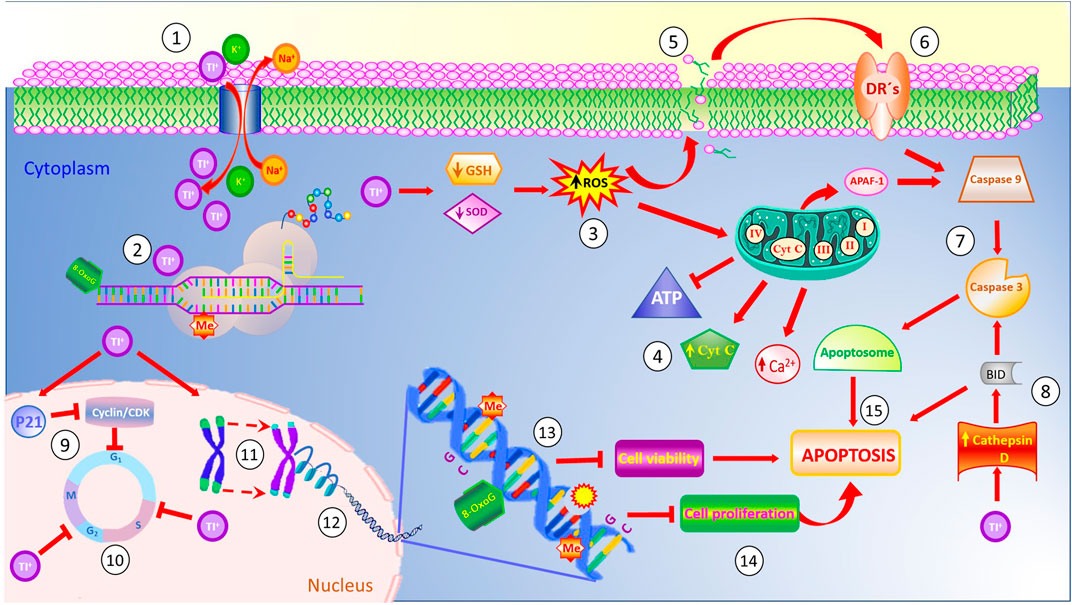
FIGURE 1. Thallium affects the function of the Na+/K+ pump ATPase due to its chemical similarity to potassium (1). Once inside the cell, Tl binds to sulfhydryl-enriched proteins affecting their normal functions, inhibiting the stability of ribosomes and protein synthesis (2). Also, cytoplasmic levels of Tl induce poor antioxidant responses, thus decreasing the levels of reduced glutathione (GSH) and superoxide dismutase (SOD), and generating high levels of reactive oxygen species (ROS) (3). These reactive species affect the mitochondrial membrane permeability, causing disruption in the electron transport chain, releasing Ca+ and cytochrome C to the cytoplasm, and inhibiting the generation of the energetic molecule of ATP (4). Similarly, high levels of ROS evoke membrane damage via increased lipoperoxidation (5), which, in turn, release factors activating the membrane death receptors (6) and triggering the caspases cascade (7). Cytoplasmic Tl increases the Cathepsin D levels which activate the BID protein involved in the apoptotic pathway (8). On the other hand, Tl also disturbs cell cycle progression (9,10), decreases telomere length (11), and affects the integrity of nucleosomes (12). Inside the DNA molecule, thallium induces the generation of the mutagenic 8-oxo guanine, disturbs the expression of CpG islands in promoter gene sequences, induces DNA breaks, and modifies the methylation pattern of the DNA (13). Combined, these effects decrease cell viability and cell proliferation (14), evoking cell death by apoptosis (15).
Author contributions
ER-L, SG-A, and AS conceived and designed the study. LS-C, SG-A, LO-R, and ER-L wrote the manuscript under the guidance of AS. MA, TK, AT, and IT added significant suggestions to improve the review. All authors have read, edited, commented on, and approved the final manuscript.
Funding
MA was supported in part by a grant from the National Institute of Environmental Health Sciences (NIEHS) R01ES07331. AAT was supported by the Russian Ministry of Science and Higher Education, Project No. FENZ-2023-0004.
Conflict of interest
The authors declare that the research was conducted in the absence of any commercial or financial relationships that could be construed as a potential conflict of interest.
Publisher’s note
All claims expressed in this article are solely those of the authors and do not necessarily represent those of their affiliated organizations, or those of the publisher, the editors and the reviewers. Any product that may be evaluated in this article, or claim that may be made by its manufacturer, is not guaranteed or endorsed by the publisher.
References
Abdolmaleki, S., Ghadermazi, M., and Aliabadi, A. (2021). Novel Tl(III) complexes containing pyridine-2,6-dicarboxylate derivatives with selective anticancer activity through inducing mitochondria-mediated apoptosis in A375 cells. Sci. Rep. 11, 15699. doi:10.1038/s41598-021-95278-y
Achenbach, C., Hauswirth, O., Heindrichs, C., Ziskoven, R., Köhler, F., and Bahr, U. (1980). Quantitative measurement of time-dependent thallium distribution in organs of mice by field desorption mass spectrometry. J. Toxicol. Environ. Health 6, 519–528. doi:10.1080/15287398009529870
Ajsuvakova, O. P., Tinkov, A. A., Aschner, M., Rocha, J. B. T., Michalke, B., Skalnaya, M. G., et al. (2020). Sulfhydryl groups as targets of mercury toxicity. Coord. Chem. Rev. 417, 213343. doi:10.1016/j.ccr.2020.213343
Almassri, I., and Sekkarie, M. (2018). Cases of thallium intoxication in Syria: A diagnostic and a therapeutic challenge. Avicenna J. Med. 8, 78–81. doi:10.4103/ajm.AJM_17_18
Álvarez-Barrera, L., Rodríguez-Mercado, J. J., Mateos-Nava, R. A., Vázquez-Martínez, Y., and Altamirano-Lozano, M. A. (2019). Effect on the offspring of pregnant females CD-1 mice treated with a single thallium(I) application. Rep. Toxicol. 90, 1–7. doi:10.1016/j.reprotox.2019.07.022
Amiri, A., Fatemi, S. J., and Fatemi, S. N. (2007). Removal of thallium by combining desferrioxamine and deferiprone chelators in rats. Biometals 20, 159–163. doi:10.1007/s10534-006-9023-1
Aresini, G., Draper, M. H., Duffus, J. H., and van der Venne, M. (1993). The toxicology of chemicals – series 1. Carcinogenicity. Vol IV - summary review of the scientific evidence. Luxembourg: Commission of the European Communities.
Aschner, M., and Aschner, J. L. (1990). Mercury neurotoxicity: Mechanisms of blood-brain barrier transport. Neurosci. Biobehav Rev. 14, 169–176. doi:10.1016/S0149-7634(05)80217-9
Aschner, M., and Carvalho, C. (2019). The biochemistry of mercury toxicity. Biochim. Biophys. Acta 1863, 129412. doi:10.1016/j.bbagen.2019.129412
Bragadin, M., Toninello, A., Bindoli, A., Rigobello, M. P., and Anton, M. (2003). Thallium induces apoptosis in Jurkat cells. Ann. N. Y. Acad. Sci. 1010, 283–291. doi:10.1196/annals.1299.049
Britten, J. S., and Blank, M. (1968). Thallium activation of the (Na+-K+)-activated ATPase of rabbit kidney. Biochim. Biophys. Acta 159, 160–166. doi:10.1016/0005-2744(68)90254-4
Brockhaus, A., Dolgner, R., Ewers, U., Krämer, U., Soddemann, H., and Wiegand, H. (1981). Intake and health effects of thallium among a population living in the vicinity of a cement plant emitting thallium containing dust. Int. Arch. Occup. Environ. Health 48, 375–389. doi:10.1007/BF00378686
Campanella, B., Colombaioni, L., Benedetti, E., Di Ciaula, A., Ghezzi, L., Onor, M., et al. (2019). Toxicity of thallium at low doses: A review. Int. J. Environ. Res. Public Health 16, 4732. doi:10.3390/ijerph16234732
Casalino, L., and Verde, P. (2020). Multifaceted roles of DNA methylation in neoplastic transformation, from tumor suppressors to EMT and metastasis. Genes (Basel). 11, 922. doi:10.3390/genes11080922
Chatterjee, N., and Walker, G. C. (2017). Mechanisms of DNA damage, repair, and mutagenesis. Environ. Mol. Mutagen 58, 235–263. doi:10.1002/em.22087
Cheam, V., Garbai, G., Lechner, J., and Rajkumar, J. (2000). Local impacts of coal mines and power plants across Canada: I. Thallium in waters and sediments. Water Qual. Res. J. Can. 35, 581–608. doi:10.2166/wqrj.2000.034
Cheam, V. (2001). Thallium contamination of water in Canada. Water Qual. Res. J. Can. 36, 851–877. doi:10.2166/wqrj.2001.046
Chen, Y. W., Yeh, J. L., Huang, Y. C., Chen, I. J., Huang, Y. F., and Liu, G. C. (2000). The interference of uptake of thallium-201 in cultured rat myocardial cells with existence of potassium related pharmaceuticals-a preliminary report. Kaohsiung J. Med. Sci. 16, 134–140. PMID: 10846349.
Chia, C. F., Chen, S. C., Chen, C. S., Shih, C. M., Lee, H. M., and Wu, C. H. (2005). Thallium acetate induces C6 glioma cell apoptosis. Ann. N. Y. Acad. Sci. 1042, 523–530. doi:10.1196/annals.1338.064
Chou, Y. T., and Lo, K. Y. (2019). Thallium(I) treatment induces nucleolar stress to stop protein synthesis and cell growth. Sci. Rep. 9, 6905. doi:10.1038/s41598-019-43413-1
Conerly, O., and Blain, R. B. (2008). “Toxicological review of thallium and compounds,” in Support of summary information on the integrated risk information system (IRIS) (Washington, DC: US Environmental Protection Agency).
Cvjetko, P., Cvjetko, I., and Pavlica, M. (2010). Thallium toxicity in humans. Arh. Za Hig. Rada I Toksikol. 61 (1), 111–119. doi:10.2478/10004-1254-61-2010-1976
Eghtesadi, R., Esmaili-Shahzade-Ali-Akbari, P., Shahmirzaei, F., Banafsheh, H., and Ghaderi, A. (2021). Evaluation of thallium levels in opioid substance users: A case–control study. Int. Arch. Health Sci. 8, 285. doi:10.4103/iahs.iahs_109_21
Eskandari, M. R., Mashayekhi, V., Aslani, M., and Hosseini, M. J. (2015). Toxicity of thallium on isolated rat liver mitochondria: The role of oxidative stress and MPT pore opening. Environ. Toxicol. 30, 232–241. doi:10.1002/tox.21900
Fani, S., Kamalidehghan, B., Lo, K. M., Nigjeh, S. E., Keong, Y. S., Dehghan, F., et al. (2016). Anticancer activity of a monobenzyltin complex C1 against MDA-MB-231 cells through induction of Apoptosis and inhibition of breast cancer stem cells. Sci. Rep. 6, 38992. doi:10.1038/srep38992
Fatemi, S. J., Amiri, A., Bazargan, M. H., Tubafard, S., and Fatemi, S. N. (2007). Clinical evaluation of desferrioxamine (DFO) for removal of thallium ions in rat. Int. J. Artif. Organs 30, 902–905. doi:10.1177/039139880703001007
Fowler, B. A., Yamauchi, H., Conner, E. A., and Akkerman, M. (1993). Cancer risks for humans from exposure to the semiconductor metals. Scand. J. Work Environ. Health 19 (1), 101–103. PMID: 8159952.
Frattini, P. (2009). Thallium properties and behavior - a literature study. Available at: http://arkisto.gtk.fi/s41/S41_0000_2005_2.pdf.
Galván-Arzate, S., Martínez, A., Medina, E., Santamaría, A., and Ríos, C. (2000). Subchronic administration of sublethal doses of thallium to rats: Effects on distribution and lipid peroxidation in brain regions. Toxicol. Lett. 116, 37–43. doi:10.1016/s0378-4274(00)00200-9
Galván-Arzate, S., and Santamaría, A. (1998). Thallium toxicity. Toxicol. Lett. 99, 1–13. doi:10.1016/s0378-4274(98)00126-x
García-Lestón, J., Méndez, J., Pásaro, E., and Laffon, B. (2010). Genotoxic effects of lead: An updated review. Environ. Int. 36, 623–636. doi:10.1016/j.envint.2010.04.011
Gordon, T., and Bowser, D. (2003). Beryllium: Genotoxicity and carcinogenicity. Mutat. Res. 533, 99–105. doi:10.1016/j.mrfmmm.2003.08.022
Hantson, P., Desoir, R., Léonard, E. D., Crutzen-Fayt, M. C., Léonard, A., and Mahieu, P. (1997). Cytogenetic observations following thallium poisoning. J. Toxicol. Environ. Health 50, 97–100. doi:10.1080/009841097160500
Hanzel, C. E., Almeira-Gubiani, M. F., and Verstraeten, S. V. (2012). Endosomes and lysosomes are involved in early steps of Tl(III)-mediated apoptosis in rat pheochromocytoma (PC12) cells. Arch. Toxicol. 86, 1667–1680. doi:10.1007/s00204-012-0878-3
Harris, W., and Messori, L. (2002). A comparative study of aluminum (III), gallium (III), indium (III), and thallium (III) binding to human serum transferrin. Coord. Chem. Rev. 228, 237–262. doi:10.1016/S0010-8545(02)00037-1
Hart, M. M., and Adamson, R. H. (1971). Antitumor activity and toxicity of salts of inorganic group 3a metals: Aluminum, gallium, indium, and thallium. Proc. Natl. Acad. Sci. U. S. A. 68, 1623–1626. doi:10.1073/pnas.68.7.1623
Heim, M., Wappelhorst, O., and Markert, B. (2002). Thallium in terrestrial environments – occurrence and effects. Ecotoxicology 11, 369–377. doi:10.1023/a:1020513523462
Hoffman, R. S. (2000). Thallium poisoning during pregnancy: A case report and comprehensive literature review. J. Toxicol. Clin. Toxicol. 38, 767–775. doi:10.1081/clt-100102390
Hoffman, R. S. (2003). Thallium toxicity and the role of Prussian blue in therapy. Toxicol. Rev. 22, 29–40. doi:10.2165/00139709-200322010-00004
Hofmann, B. A., and Knill, M. D. (1996). Geochemistry and Genesis of the Lengenbach Pb-Zn-as-Tl-Ba-mineralisation, binn valley, Switzerland. Binn Val. Switz. Min. Depos. 31, 319–339. doi:10.1007/BF02280795
Hwang, M. H., Damte, D., Cho, M. H., Kim, Y. H., and Park, S. C. (2010). Optimization of culture media of pathogenic Mycoplasma hyopneumoniae by a response surface methodology. J. Vet. Sci. 11, 327–332. doi:10.4142/jvs.2010.11.4.327
Jha, S., Kumar, R., and Kumar, R. (2006). Thallium poisoning presenting as paresthesias, paresis, psychosis and pain in abdomen. J. Assoc. Physicians India 54, 53–55. PMID: 16649741.
Jo, O., Schlicht, S., Slavin, J., Di Bella, C., Pang, G., Powell, G., et al. (2020). The role of Thallium-201 scintigraphy and Tc-99m pentavalent dimercaptosuccinic acid in diagnosis and grading of chondrosarcoma. Eur. J. Radiol. 125, 108846. doi:10.1016/j.ejrad.2020.108846
John, R. M., and Rougeulle, C. (2018). Developmental epigenetics: Phenotype and the flexible epigenome. Front. Cell Dev. Biol. 6, 130. doi:10.3389/fcell.2018.00130
Jones, P. A., and Baylin, S. B. (2007). The epigenomics of cancer. Cell 128, 683–692. doi:10.1016/j.cell.2007.01.029
Karbowska, B. (2016). Presence of thallium in the environment: Sources of contaminations, distribution and monitoring methods. Environ. Monit. Assess. 188, 640. doi:10.1007/s10661-016-5647-y
Karbowska, B., Rębiś, T., Zembrzuska, J., and Nadolska, K. (2020). Thallium in color tattoo inks: Risk associated with tattooing. Med. P. R. 71, 405–411. doi:10.13075/mp.5893.00934
Karwowski, M. P., Morman, S. A., Plumlee, G. S., Law, T., Kellogg, M., and Woolf, A. D. (2017). Toxicants in folk remedies: Implications of elevated blood lead in an American-born infant due to imported diaper powder. Environ. Geochem Health 39, 1133–1143. doi:10.1007/s10653-016-9881-6
Kilic, G. A., and Kutlu, M. (2010). Effects of exogenous metallothionein against thallium-induced oxidative stress in rat liver. Food Chem. Toxicol. 48, 980–987. doi:10.1016/j.fct.2010.01.013
Korotkov, S. M., and Lapin, L. V. (2003). Thallium induces opening of the mitochondrial permeability transition pore in the inner membrane of rat liver mitochondria. Dokl. Biochem. Biophys. 392, 247–252. doi:10.1023/a:1026182511897
Kumar, A. V. S. A., Kumar, P. G., Sampath, S., and Suhag, V. (2017). To study the efficacy of thallium-201 as tumor seeking agent and to study its role in therapeutic response. Med. J. Armed Forces India 73, 242–249. doi:10.1016/j.mjafi.2017.01.001
Kumar, K., Moirangthem, R., and Kaur, R. (2020). Genome protection: Histone H4 and beyond. Curr. Gen. 66, 945–950. doi:10.1007/s00294-020-01088-6
Kuo, H. C., Huang, C. C., Tsai, Y. T., Chu, C. C., Hsieh, S. T., and Chu, N. S. (2005). Acute painful neuropathy in thallium poisoning. Neurology 65, 302–304. doi:10.1212/01.wnl.0000169021.26172.f8
Lech, T., and Sadlik, J. K. (2007). Thallium intoxication in humans. Toxicol. Lett. 172, S85–S240. doi:10.1016/j.toxlet.2007.05.239
Léonard, A., and Gerber, G. B. (1997). Mutagenicity, carcinogenicity and teratogenicity of thallium compounds. Mutat. Res. 387, 47–53. doi:10.1016/s1383-5742(97)00022-7
Li, D., Yao, H., Du, L., Zeng, X., and Xiao, Q. (2022). Thallium(I and III) exposure leads to liver damage and disorders of fatty acid metabolism in mice. Chemosphere 307, 135618. doi:10.1016/j.chemosphere.2022.135618
Li, J. M., Wang, W., Lei, S., Zhao, L. L., Zhou, D., and Xiong, H. (2014). Misdiagnosis and long-term outcome of 13 patients with acute thallium poisoning in China. Clin. Toxicol. 52, 181–186. doi:10.3109/15563650.2014.892123
Lin, G., Yuan, L., Peng, X., Long, J., Wang, C., Bai, L., et al. (2019). Clinical characteristics and treatment of thallium poisoning in patients with delayed admission in China. Medicine 98, e16471. doi:10.1097/MD.0000000000016471
Liu, J., Li, N., Zhang, W., Wei, X., Tsang, D. C. W., Sun, Y., et al. (2019). Thallium contamination in farmlands and common vegetables in a pyrite mining city and potential health risks. Environ. Pollut. 248, 906–915. doi:10.1016/j.envpol.2019.02.092
Liu, J., Wang, J., Chen, Y., Lippold, H., Xiao, T., Li, H., et al. (2017). Geochemical transfer and preliminary health risk assessment of thallium in a riverine system in the Pearl River Basin, South China. J. Geochem Explor 176, 64–75. doi:10.1016/j.gexplo.2016.01.011
Liu, J., Wei, X., Zhou, Y., Tsang, D. C. W., Bao, Z., Yin, M., et al. (2020). Thallium contamination, health risk assessment and source apportionment in common vegetables. Sci. Total Environ. 703, 135547. doi:10.1016/j.scitotenv.2019.135547
López Segura, N., Aznar Laín, G., Nolla Salas, J., and Mur Sierra, A. (2013). Thallium poisoning in an adolescent girl. Med. Clin. Barc. 891 141, 557–558. Spanish. doi:10.1016/j.medcli.2013.04.002
Lu, C. I., Huang, C. C., Chang, Y. C., Tsai, Y. T., Kuo, H. C., Chuang, Y. H., et al. (2007). Short-term thallium intoxication: Dermatological findings correlated with thallium concentration. Arch. Dermatol 143, 93–98. doi:10.1001/archderm.143.1.93
McConnell, J. R., and Edwards, R. (2008). Coal burning leaves toxic heavy metal legacy in the Arctic. PNAS 34, 12140–12144. doi:10.1073/pnas.0803564105
Misra, U. K., Kalita, J., Yadav, R. K., and Ranjan, P. (2003). Thallium poisoning: Emphasis on early diagnosis and response to haemodialysis. Postgrad. Med. J. 79, 103–105. doi:10.1136/pmj.79.928.103
Mulkey, J. P., and Oehme, F. W. (1993). A review of thallium toxicity. Vet. Hum. Toxicol. 35, 445–453.
Nadel, H. R. (1993). Thallium-201 for oncological imaging in children. Semin. Nucl. Med. 23, 243–254. doi:10.1016/s0001-2998(05)80105-9
National Minerals Information Center (2022). Thallium statistics and information. Available at: https://pubs.usgs.gov/periodicals/mcs2022/mcs2022-thallium.pdf [Displayed 913 January 15th 2023].
Nishiyama, A., and Nakanishi, M. (2021). Navigating the DNA methylation landscape of cancer. Trends Genet. 37, 1012–1027. doi:10.1016/j.tig.2021.05.002
Nowicka, A. M., Mackiewicz, M., Matysiak, E., Krasnodebska-Ostrega, B., and Stojek, Z. (2013). Voltammetric and electrochemical gravimetric selective detection of interactions between Tl(I) and guanine and the influence on activity of DNA drug-intercalators. Talanta 106, 85–91. doi:10.1016/j.talanta.2012.12.018
Osorio-Rico, L., Santamaria, A., and Galván-Arzate, S. (2017). Thallium toxicity: General issues, neurological symptoms, and neurotoxic mechanisms. Adv. Neurobiol. 18, 345–353. doi:10.1007/978-3-319-60189-2_17
Percival, T. J., and Radtke, A. S. (1994). Sedimentary-rock-hosted disseminated gold mineralization in the Alsar district, Macedonia. Can. Mineral. 32, 649–665.
Peter, A. K. J., and Viraraghavan, T. (2005). Thallium: A review of public health andenvironmental concerns. Environ. Int. 31, 493–501. doi:10.1016/j.envint.2004.09.003
Poudyal, B., Shrestha, P., and Chowdhury, Y. S. (2022). “Thallium-201,” in StatPearls (Tampa, Florida, United States: StatPearls Publishing).
Puschner, B., Basso, M. M., and Graham, T. W. (2012). Thallium toxicosis in a dog consequent to ingestion of Mycoplasma agar plates. J. Vet. Diagn Invest. 24, 227–230. doi:10.1177/1040638711425941
Radic, S., Cvjetko, P., Glavas, K., Roje, V., Pevalek-Kozlina, B., and Pavlica, M. (2009). Oxidative stress and DNA damage in broad bean (Vicia faba L.) seedlings induced by thallium. Environ. Toxicol. Chem. 28, 189–196. doi:10.1897/08-188.1
Rangel-López, E., Robles-Bañuelos, B., Guadiana-Ramírez, N., Alvarez-Garduño, V., Galván- Arzate, S., Zazueta, C., et al. (2022). Thallium induces antiproliferative and cytotoxic activity in glioblastoma C6 and U373 cell cultures via apoptosis and changes in cell cycle. Neurotox. Res. 40, 814–824. doi:10.1007/s12640-022-00514-6
Ratti, F., Facchini, A., Beck, E., Cazzaniga, S., Francesconi, S., Tedesco, C., et al. (2018). Familial venoms": A thallium intoxication cluster. Intensive Care Med. 44, 2298–2299. doi:10.1007/s00134-018-5403-6
Reed, D., Crawley, J., Faro, S. N., Pieper, S. J., and Kurland, L. T. (1963). Thallotoxicosis. Acute manifestations and sequelae. JAMA. 183, 516–522.
Rickwood, C. J., King, M., and Huntsman-Mapila, P. (2015). Assessing the fate and toxicity of Thallium I and Thallium III to three aquatic organisms. Ecotoxicol. Environ. Saf. 115, 300–308. doi:10.1016/j.ecoenv.2014.12.024
Ríos, C., Galván-Arzate, S., and Tapia, R. (1989). Brain regional thallium distribution in rats acutely intoxicated with Tl2SO4. Arch. Toxicol. 63, 34–37. doi:10.1007/BF00334631
Rodríguez-Mercado, J. J., and Altamirano-Lozano, M. A. (2013). Genetic toxicology of thallium: A review. Drug Chem. Toxicol. 36, 369–383. doi:10.3109/01480545.2012.710633
Rodríguez-Mercado, J. J., Altamirano-Lozano, M., and Mateos-Nava, R. (2019). Induction of cytotoxicity in human cells exposed to thallium(I) and thallium (III). Preprint. doi:10.13140/RG.2.2.21251.04641
Rodríguez-Mercado, J. J., Hernández-de la Cruz, H., Felipe-Reyes, M., Jaramillo- Cruz, E., and Altamirano-Lozano, M. A. (2013). Evaluation of cytogenetic and DNA damage caused by thallium(I) acetate in human blood cells. Environ. Toxicol. 30, 572–580. doi:10.1002/tox.21934
Rodríguez-Mercado, J. J., Mosqueda-Tapia, G., and Altamirano-Lozano, M. A. (2017). Genotoxicity assessment of human peripheral Lymphocytes induced by thallium(I) and thallium(III). Toxicol. Environ. Chem. 99, 987–998. doi:10.1080/02772248.2017.1307377
Saddique, A., and Peterson, C. D. (1983). Thallium poisoning: A review. Vet. Hum. Toxicol. 25 (1), 16–22. PMID: 6338655.
Saha, A., Sadhu, H. G., Karnik, A. B., Patel, T. S., Sinha, S. N., and Saiyed, H. N. (2004). Erosion of nails following thallium poisoning: A case report. Occup. Environ. Med. 61, 640–642. doi:10.1136/oem.2003.009464
Savariar, V. (2014). Environmental health monitoring: A pragmatic approach. Int. J. Waste Resour. 4, 164. doi:10.4172/2252-5211.1000164
Smith, I. C., and Carson, B. L. (1977). Trace metals in the environment, Thallium. Michigan: Ann Arbor Science Publishers Inc.
Sun, T. W., Xu, Q. Y., Zhang, X. J., Wu, Q., Liu, Z. S., Kan, Q. C., et al. (2012).Management of thallium poisoning in patients with delayed hospital admission. Clin. Toxicol. 50, 65–69. doi:10.3109/15563650.2011.638926
Taylor, E. C., and McKillop, A. (1970). Thallium in organic synthesis. Acc. Chem. Res. 3, 338–346. doi:10.1021/ar50034a003
Waisberg, M., Joseph, P., Hale, B., and Beyersmann, D. (2003). Molecular and cellular mechanisms of cadmium carcinogenesis. Toxicology 192, 95–117. doi:10.1016/s0300-483x(03)00305-6
Wang, J., Wang, L., Wang, Y., Tsang, D. C. W., Yang, X., Beiyuan, J., et al. (2021). Emerging risks of toxic metal(loid)s in soil-vegetables influenced by steel-making activities and isotopic source apportionment. Environ. Int. 146, 106207. doi:10.1016/j.envint.2020.106207
World Health Organization (WHO) (1996). International programme on chemical safety (IPCS). Thallium. Environmental health criteria. 182. Geneva: WHO.
Wu, M., Shu, Y., Song, L., Liu, B., Zhang, L., Wang, L., et al. (2019). Prenatal exposure to thallium is associated with decreased mitochondrial DNA copy number in newborns: Evidence from a birth cohort study. Environ. Int. 129, 470–477. doi:10.1016/j.envint.2019.05.053
Wu, M., Wang, L., Song, L., Liu, B., Liu, Y., Bi, J., et al. (2021). The association between prenatal exposure to thallium and shortened telomere length of newborns. Chemosphere 265, 129025. doi:10.1016/j.chemosphere.2020.129025
Xiao, T., Boyle, D., Guha, J., Rouleau, A., Hong, Y., and Zheng, B. (2003). Groundwater-related thallium transfer processes and their impacts on the ecosystem: Southwest Guizhou Province, China. Appl. Geochem 18, 675–691. doi:10.1016/s0883-2927(02)00154-3
Xiao, T., Guha, J., Boyle, D., Liu, C. Q., and Chen, J. (2004). Environmental concerns related to high thallium levels in soils and thallium uptake by plants in southwest Guizhou, China. China Sci. Total Environ. 318, 223–244. doi:10.1016/S0048-9697(03)00448-0
Xu, X., Zhang, Y., and Zhao, Z. (2010). Review of cases of acute thallium poisoning at home and abroad for nearly 40 years. Zhonghua Lao Dong Wei Sheng Zhi Ye Bing Za Zhi 28, 233–235. PMID: 20635708.
Zasukhina, G. D., Vasilyeva, I. M., Sdirkova, N. I., Krasovsky, G. N., Vasyukovich, L. Ya., Kenesariev, U. I., et al. (1983). Mutagenic effect of thallium and mercury salts on rodent cells with different repair activities. Mutat. Res. 124, 163–173. doi:10.1016/0165-1218(83)90176-3
Keywords: thallium and DNA damage, thallium systemic toxicity, DNA alterations, cytogenetic damage, mutations, epigenetic changes, cancer
Citation: Sánchez-Chapul L, Santamaría A, Aschner M, Ke T, Tinkov AA, Túnez I, Osorio-Rico L, Galván-Arzate S and Rangel-López E (2023) Thallium-induced DNA damage, genetic, and epigenetic alterations. Front. Genet. 14:1168713. doi: 10.3389/fgene.2023.1168713
Received: 18 February 2023; Accepted: 10 April 2023;
Published: 20 April 2023.
Edited by:
Douglas Mark Ruden, Wayne State University, United StatesReviewed by:
Sanjay Saini, Columbia University Irving Medical Center, United StatesJin Wang, Guangzhou University, China
Copyright © 2023 Sánchez-Chapul, Santamaría, Aschner, Ke, Tinkov, Túnez, Osorio-Rico, Galván-Arzate and Rangel-López. This is an open-access article distributed under the terms of the Creative Commons Attribution License (CC BY). The use, distribution or reproduction in other forums is permitted, provided the original author(s) and the copyright owner(s) are credited and that the original publication in this journal is cited, in accordance with accepted academic practice. No use, distribution or reproduction is permitted which does not comply with these terms.
*Correspondence: Edgar Rangel-López, cmFsZWRnQGhvdG1haWwuY29t; Sonia Galván-Arzate, c29uaWFfZ2FsdmFuQHlhaG9vLmNvbQ==