- 1Department of Urology, Sun Yat-sen University Cancer Center, State Key Laboratory of Oncology in South China, Collaborative Innovation Center of Cancer Medicine, Guangzhou, China
- 2Reproductive Center of Medicine, The First Affiliated Hospital of University of South China, Hengyang, China
Circular RNAs (circRNAs) are a panel of non-coding RNAs that mediate the regulation of gene expression, as well as pathological responses. Nonetheless, the function and expression pattern of circRNAs in urinary bladder cancer (UBC) remain unclear. Herein, we examined the function of circCA12 in UBC development. qRT-PCR results demonstrated remarkable circCA12 upregulation in UBC cell lines, as well as tissues. CCK-8, colony formation, and xenograft assays were employed to determine the effect of circCA12 on UBC. Our data illustrated silencing circCA12 repressed the proliferation along with the colony-formation capability of UBC cells. The migration and metastasis potential of UBC cells were remarkably abated in vivo, as well as in vitro after transfection with si-cirCA12 or sh-circCA12. Moreover, luciferase reporter and RIP assays indicated that circCA12 binds to miRNA-1184 through sponging miRNA, thereby up-regulating the expression of RAS family genes (NRAS, KRAS, and HRAS). In conclusion, the circCA12/miRNA-1184/RAS family was identified as a regulatory axis in UBC progression.
Introduction
Urinary bladder cancer (UBC) is responsible for about 549,000 new cases, as well as 200,000 deaths every year. It is the sixth most prevalent cancer in men and is ranked ninth among the main causes of cancer-linked deaths (Bray et al., 2018). Reports indicate that it is the most frequent malignant tumor in the urinary system (Antoni et al., 2017). Tobacco use, along with occupational exposure to carcinogens, are the primary predisposing factors of UBC occurrence (Cumberbatch et al., 2018). UBC is classified into different subtypes as per the extension of tumor invasion, namely NMIBC (non-muscle-invasive bladder cancer) and MIBC (muscle-invasive bladder cancer) (Sanli et al., 2017). Individuals with MIBC account for about 20–30% of the total number of UBC cases (Selth et al., 2017). The 5-year survival rate still remains around 60% despite the development of a wide range of treatment approaches including surgical resection and reconstruction, chemotherapy, and radiation therapy (Racioppi et al., 2012). Mounting research evidence opines that a group of molecular and clinicopathological heterogeneous factors regulate the development of UBC (Inamura, 2018). Identification of these factors can aid in the development of several new approaches for diagnosing, classifying, and treating UBC.
Several investigations have documented that circular RNAs (circRNAs) modulate UBC pathogenesis. CircRNAs constitute non-coding RNAs with a covalently closed loop structure, which exist widely in eukaryotic cells (Sanger et al., 1976). Recently, numerous investigations have documented diverse circRNAs in multiple cancer cell lines and tissues (Zhang et al., 2013; Mirzaei and Hamblin, 2020; Nahand et al., 2020; Zou et al., 2020), where they participate in the onset and progress of numerous forms of cancers, e.g., breast cancer (Sang et al., 2019; Zou et al., 2019b; Liu et al., 2020), Esophageal squamous cell cancer (Fan et al., 2019), gastric cancer (Zhang et al., 2017), ovarian cancer (Shabaninejad et al., 2019; Razavi et al., 2021), thyroid cancer (Borran et al., 2020), and gallbladder cancer (Wang et al., 2019). CircRNAs also have potential in regulating the tumor microenvironment (Zou et al., 2019a; Kristensen et al., 2020). Some investigations have postulated that circRNAs act as competing-endogenous RNAs (ceRNAs) through competing with other RNAs to dock miRNAs, a large class of sncRNAs associated with cancer proliferation, differentiation, metastasis, and carcinogenesis. Consequently, they suppress the function of targeted miRNAs (Romero-Cordoba et al., 2014; Li et al., 2015).
Given the promising role of circRNAs in the development of malignant tumor, we designed this study to determine whether endogenous circular RNA can facilitate progression of UBC. It was speculated that circCA12 might modulate the progress of UBC via influencing RAS oncogene expression in a miRNA-mediated approach. Hence, we conducted in vitro along with in vivo biological and molecular assays to elucidate the responsible mechanism. We established that circCA12 enhances UBC and regulates the carcinogenesis process through the circCA12/miRNA-1184/RAS axis.
Materials and Methods
Fresh UBC samples and matched non-malignant tissues which met the ethical standards were collected at Sun Yat-sen University Cancer Centre (SYSUCC). Tissues were immediately kept in RNAlater (Ambion, Austin, Texas) after resection. The Helsinki Declaration was strictly adhered to in this study. In addition, the Ethics Committee of SYSUCC approved the study. All participants signed informed consent before the study begun. Furthermore, animal experiments were approved by the Institutional Animal Care and Use Committee of SYSUCC.
Cell Culture and Treatment
The svhuc1, 5637, RT-112, and BIU-87 cell lines were supplied by the American Type Culture Collection (ATCC, United States). The cell lines were sub-cultured in our laboratory for less than 6 months and maintained as described by the manufacturer. Before use, DNA fingerprinting was used to verify mycoplasma infection and authenticity.
Colony Formation Assay and CCK-8 Assay
RT-112 and BIU-87 cell lines were planted onto petri dishes and incubated for 14 days. Next, cellular colonies were fixed with 4% PFA and dyed with crystal violet. Transfected cells were then planted into culture plates and inoculated with 10 μl of CCK-8 reagent, followed by incubation in accordance with the protocols described in the CCK-8 assay kit (Dojindo Japan). The absorbance was determined at 450 nm.
Transfection and qRT-PCR
RNA was isolated using Trizol (Invitrogen, United States). Afterward, M-MLV RT kit (Invitrogen, United States) was employed to synthesize cDNA as described in the manufacturer’s manual. SYBR Premix Ex Taq (Takara) was employed to conduct qRT-PCR assays in a 10 μl total volume including 2 μl cDNA, 5 μl 2 × Master Mix, 0.5 μl Forward Primer (10 μM), 0.5 μl Reverse Primer (10 μM), and 2 μl ddH2O. The amplification protocol involved heating the mixture at 95°C for 10 min, then at 95°C (10 s), and then at 60°C (60 s) for 40 cycles. Results were harvested in three independent wells.
Luciferase Reporter Assay
3′UTR of HRAS/KRAS/NRAS and circCA12 sequence harboring wide-type (WT) or mutant-type (Mut) docking sites for miR-1184 were created in the pGL3 luciferase vector (Promega, Madison, WI, United States) to obtain circCA12-WT, circCA12-Mut, HRAS/KRAS/NRAS -3′UTR-WT (WT), and HRAS/KRAS/NRAS-3′UTR-Mut (Mut). The predicted miR-1184 binding sites of circCA12, 3′-UTR of HRAS/KRAS/NRAS, was mutated. miR-1184 with locked-nucleic acid (miR-1184-LNA), as well as miR-1184 mimics were inserted into UBC cells accompanied by the reporter plasmid (pRL-TK vector, Promega) via transfection. The activity of luciferase enzyme was then determined using DLR (dual-luciferase enzyme Reporter) assay system kit (Promega) 48 h post transfection, as described by the manufacturer.
RNA Immunoprecipitation (RIP)
RT-112 UBC cells were co-transfected with MS2bs-circCA12, MS2bs-miRNA-1184, MS2bs-KRAS, MS2bs-NRAS, and MS2bs-HRAS. The Magna RIP RNA-Binding Protein Immunoprecipitation Kit (Millipore) was employed to perform RIP and miR-1184 quantification was done after purification. Anti-Ago2 antibody (Cat No, Millipore) was employed to conduct the RIP assay for Ago2 and the abundance of circCA12, miRNA-1184, KRAS, NRAS, and HRAS was determined after purification.
Immunohistochemical Analysis (IHC)
Bladder tissues were sectioned into 4-μm-thick slices from embedded blocks. The slices were deparaffinized, rehydrated, and treated with a 90% methanol/3% Hydrogen peroxide solution for 15 min at 37°C. Next, the sections were inoculated in sodium citrate buffer (10 mM sodium citrate; 0.05% Tween 20, pH 6.0) for 5 min at 96°C. Afterward, the sections were blocked with BSA, followed by overnight inoculation with anti-circCA12 antibody (Santa Cruz Biotechnology, Europe; 1:100) at 4°C. Subsequently, they were inoculated at 37°C with a biotinylated secondary antibody for 10 min, then finally with Horseradish Peroxidase (HRP)-streptavidin for 10 min. Results were identified as positive or negative after DAB staining.
Mice Xenograft Model
Nude mice (28 days old) were provided by Sun Yat-sen University Laboratory Animal Center (Guangzhou, China). 5 × 106 BIU-87 cells were administered into the right flanks of nude mice to establish xenograft models. The injected cells had been stably transfected with shRNA for Control and shRNA for circCA12. Tumor sizes were then determined with a Vernier caliper, and micro-metastasis nodules were counted.
Statistical Analysis
All data are given as mean ± standard deviation (SD) and analyses were implemented in GraphPad Prism Software (V.8, La Jolla, CA, United States). One-way analysis of variance (ANOVA) along with Student’s t-test were implemented to compare the data. P < 0.05 signified statistical significance.
Results
Differential Expression and Circular Characterization of CircCA12 in Bladder Cancer
We explored circCA12 expression level using four bladder cell lines. Results demonstrated upregulation of expression of circCA12 in UBC cell lines including 5637, RT112, and BIU-87 in contrast with svhuc1 cells (Figure 1A). We also assessed circCA12 levels in 20 pairs of UBC tissues along with their neighboring non-malignant tissue. Among them, 70% (14/20) of the samples showed increased circCA12 contents (Figure 1B; P = 0.0017), illustrating elevated relative expression of circCA12 in UBC samples. Resistance to the digestive effect of RNase R exonuclease confirmed the circularization of circCA12 (Figure 1C). In addition, Actinomycin D data illustrated that the half-life of circCA12 mRNA was more than 24 h, implying that the stability of the isoform is higher relative to the linear CA12 mRNA in BIU87 cells (Figure 1D).
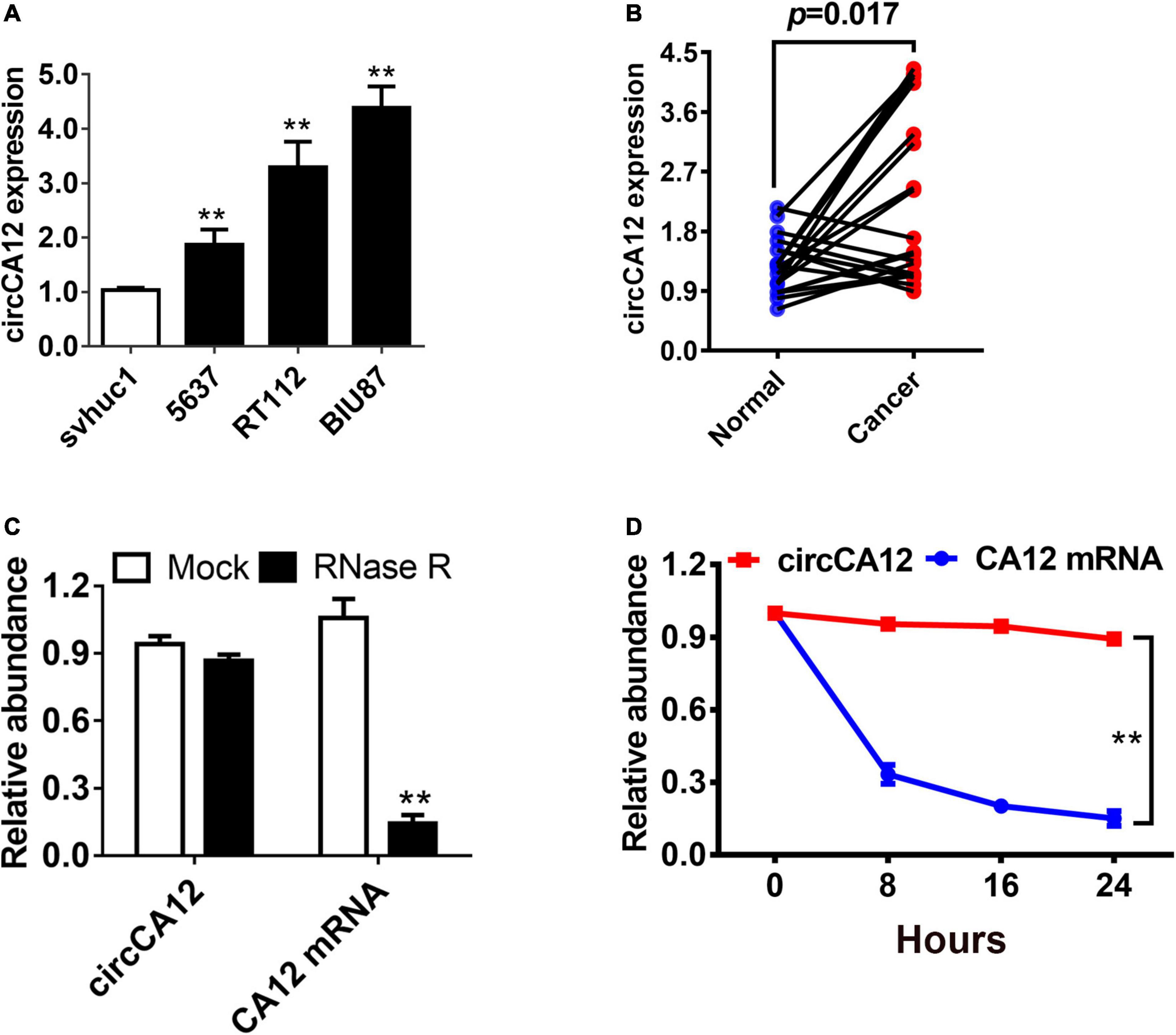
Figure 1. Differential expression and circular characterization of circCA12 in bladder cancer. (A) circCA12 relative expression in non-malignant bladder epithelial cells and bladder cancer cell lines. (B) circCA12 differential expression was verified in 20 bladder cancer tissues and paired non-malignant tissues. (C) qRT-PCR assessment of circCA12 and CA12 mRNA in BIU87 cells after RNase R treatment. (D) qRT-PCR assays of circCA12 and CA12 mRNA in BIU87 cells after Actinomycin D treatment. **P < 0.01.
CircCA12 Promotes Cellular Growth and Metastasis in Bladder Cancer
To study the role of circCA12 in UBC, we knocked down circCA12 using si-RNA assay and verified its efficiency in two cell lines as indicated in Figure 2A. CCK-8 assay results indicated that silencing circCA12 inhibited UBC cell proliferation (RT-112 as well as BIU-87) as indicated in Figure 2B. Suppressing circCA12 significantly curtailed UBC cell colony formation as demonstrated in Figure 2C. Moreover, Transwell assay data indicated that suppression of circCA12 decreased UBC cell’s infiltration, as illustrated in Figure 2D.
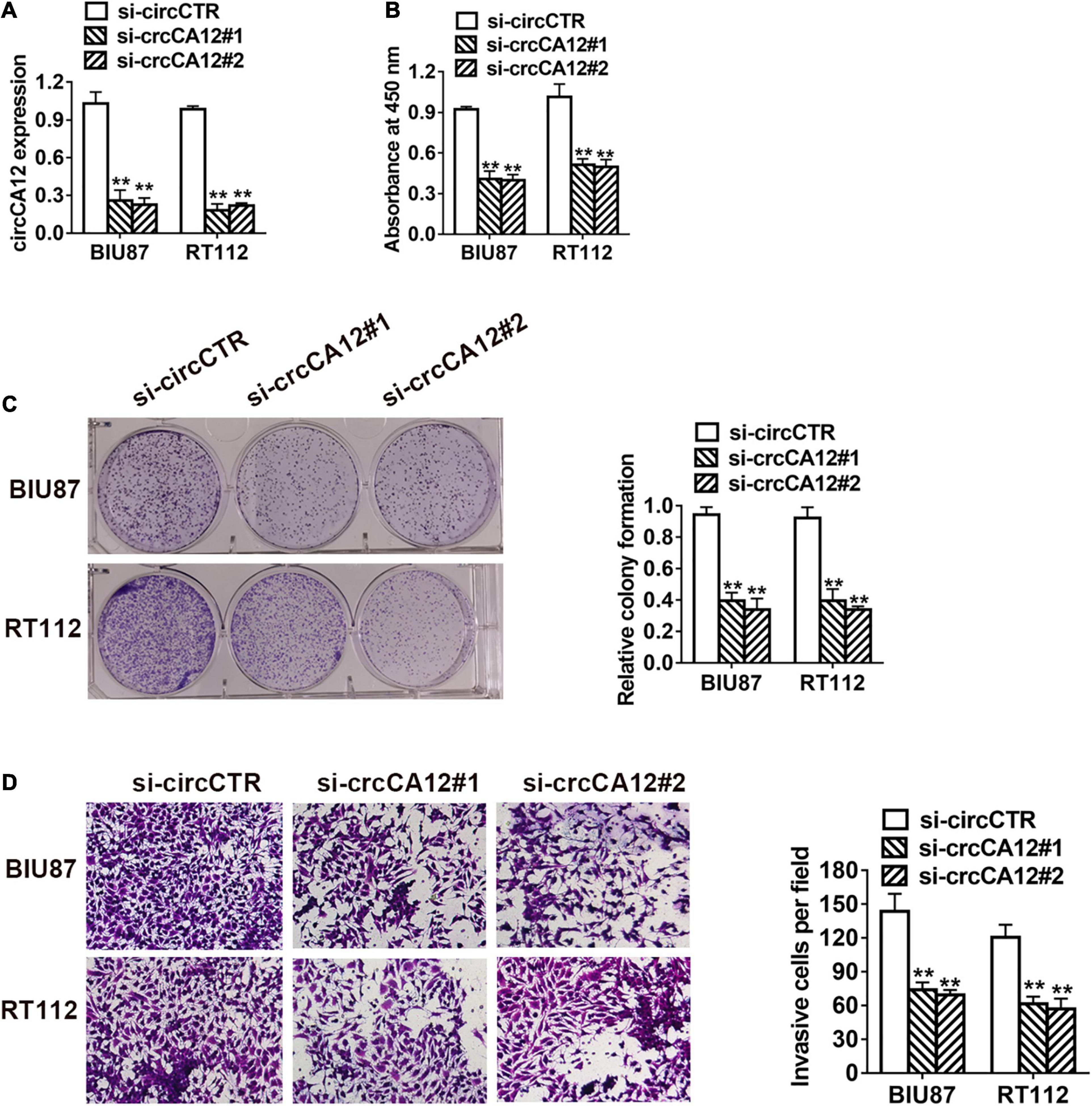
Figure 2. CircCA12 promotes cellular growth along with metastasis in bladder cancer. (A) The knockdown of circCA12 determined using qRT-PCR. (B) CCK-8 assay of cell proliferation. (C) Colony formation assays. (D) Suppression of circKIF4A decreased the infiltration potential in the Transwell assay. **P < 0.01.
CircCA12 Knockdown Suppresses Growth and Metastasis of Bladder Cancer in vivo
We established mice xenograft models for further evaluation of the roles of circCA12 in UBC. Results revealed that the tumor weight of circCA12 knockdown group was remarkably reduced in contrast with the control group, as indicated in Figure 3A. Next, we prepared lung metastasis sections (4 μm) and used them to determine the impact of circCA12 on cancerous metastasis. Repression of circCA12 remarkably diminished the number of lung metastatic nodules (Figure 3B).
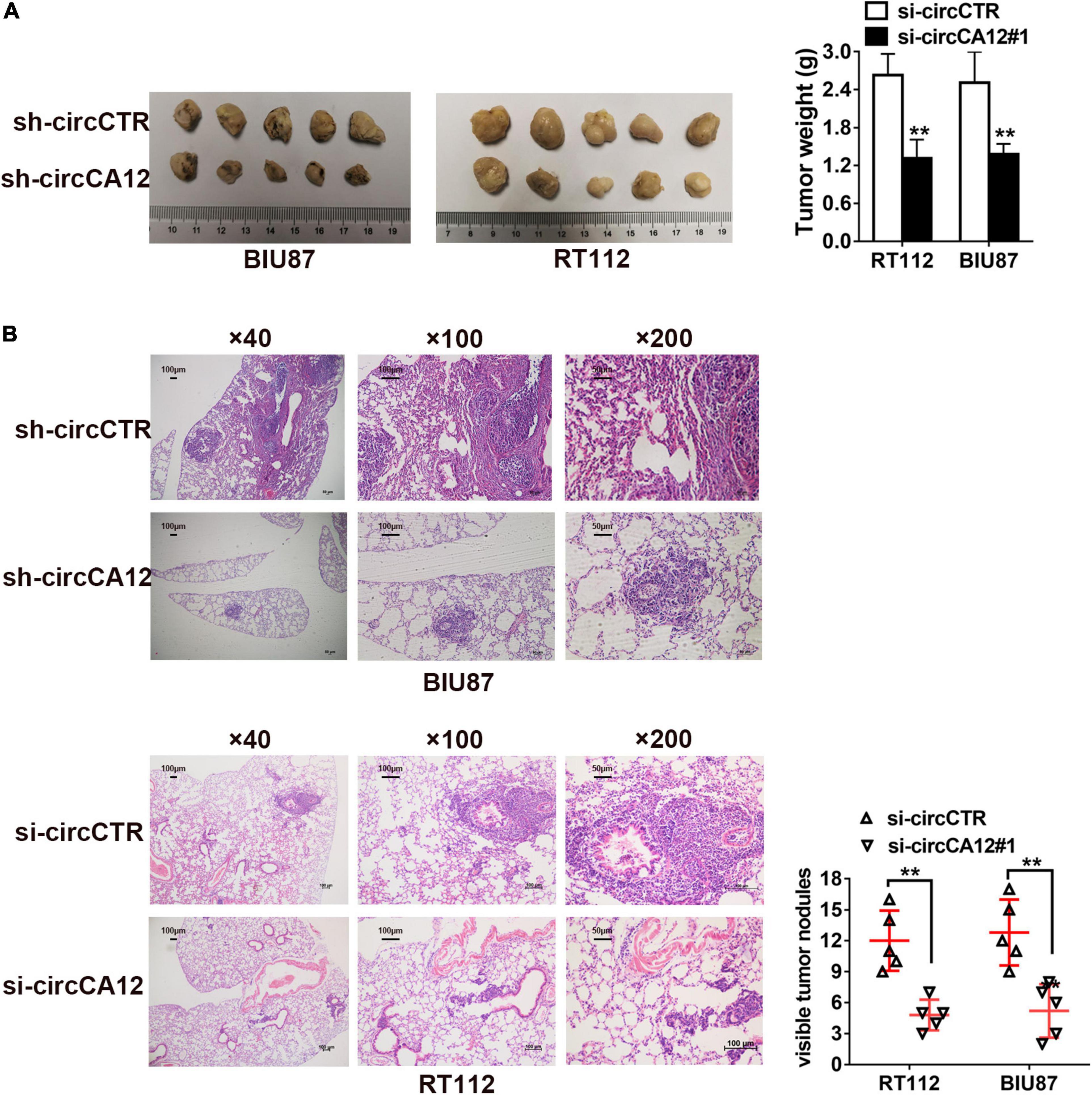
Figure 3. Knockdown of circCA12 represses the proliferation along with metastasis of bladder cancer in vivo. (A) Mice xenograft models were constructed using RT-112 and BIU-87 cell lines. The weight of tumors was measured after 28 days. (B) Lung metastases HE staining images illustrating metastatic nodule numbers in the lung. **P < 0.01.
CircCA12 Sponges miR-1184
We employed the Circular RNA Interactome web resource to identify possible circRNA and miRNA cross talks. From our results, we selected miR-1184 for further research (Figure 4B). CircCA12 was mainly localized in the cytoplasm (Figure 4A). Dual luciferase enzyme reporter assay demonstrated that relative luciferase enzyme activity was abated after transfection with wild-type circCA12, as well as miR-1184 mimics. The predicted miR-1184 binding sites of circCA12 was mutated (Figure 4C). Moreover, RNA immunoprecipitation (RIP) assay results revealed that miR-1184 were abundant in the non-mutant MS2bs-circCA12 group (Figure 4D).
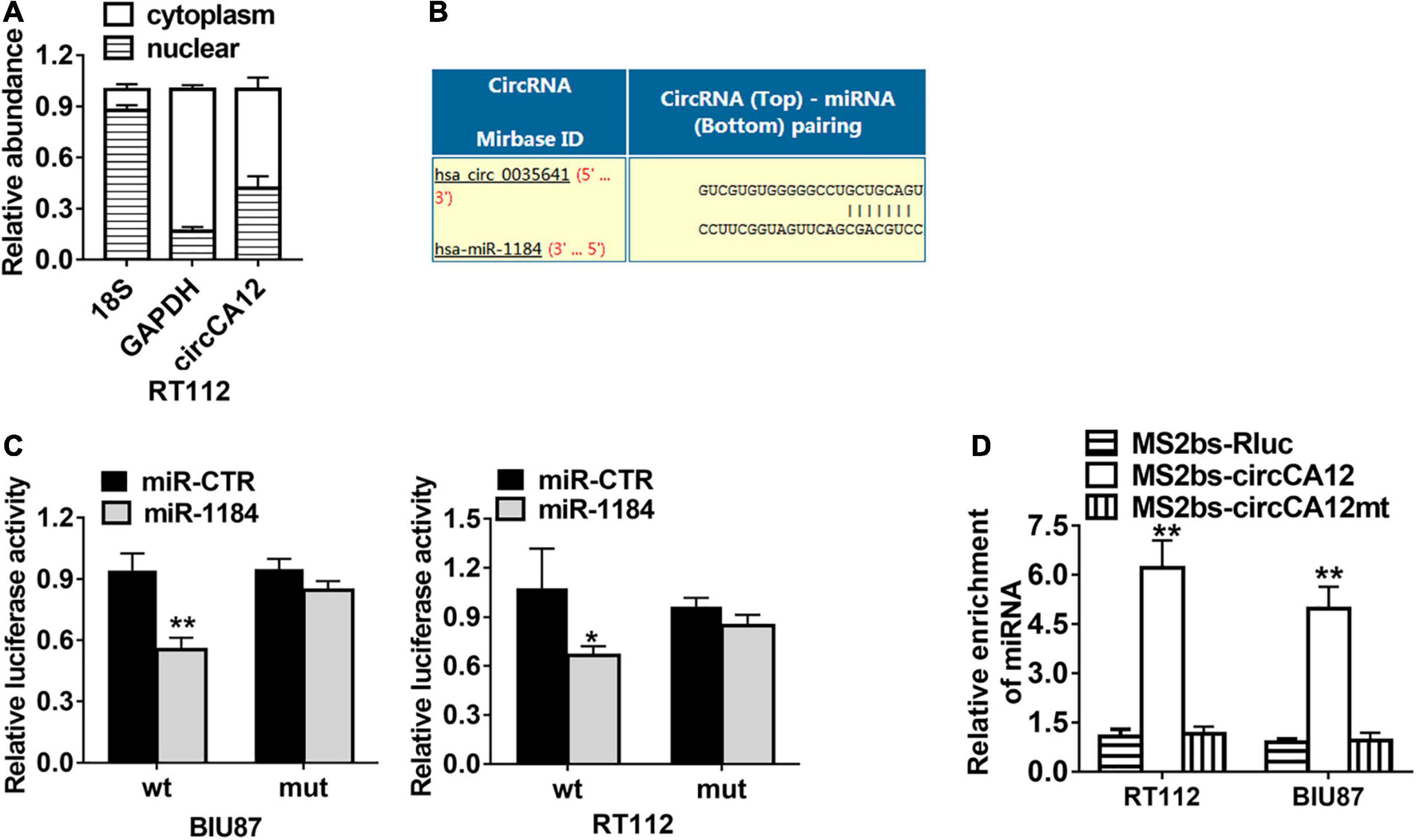
Figure 4. CircCA12 serves as a sponge for miR-1184. (A) 18S, GAPDH, and circCA12 expressions levels in the cytoplasmic and nuclear fractions. (B) Prediction of the docking sites of miR-1184 in the circCA12 sequence (https://circinteractome.nia.nih.gov). (C) Luciferase reporter assay results. RT-112 and BIU-87 cells were transfected with miR-1184 mimics, accompanied by wild-type or mutant-type circCA12 luciferase reporter assay. The predicted miR-1184 binding sites of circCA12 was mutated. (D) MS2-based RIP assay in RT-112 and BIU-87 cells inserted with MS2bs-circCA12, MS2bs-circCA12-mt, or MS2bs-Rluc via transfection. *P < 0.05, **P < 0.01.
CircCA12 Enhances Bladder Cancer Development Through the circCA12/miR-1184/RAS Family Pathway
We then employed the TargetScan web resource1 to identify the down-stream miR-1184 targets. Results identified RAS family genes, including KRAS, NRAS, and HRAS, as the potential targets of miR-1184 (Figure 5A). Luciferase enzyme reporter assays illustrated that the relative luciferase enzyme activity was diminished after transfection with miR-1184 mimics, as well as the non-mutant RAS family (Figure 5B). On the other hand, the relative luciferase activity was increased when the miR-1184 and wild-type 3′-UTR-RAS family combination was blocked (Figure 5C). Moreover, Ago2-related RIP assays revealed that low-expression of circCA12 significantly boosted the enrichment of Ago2 by the RAS family (Figure 5D).
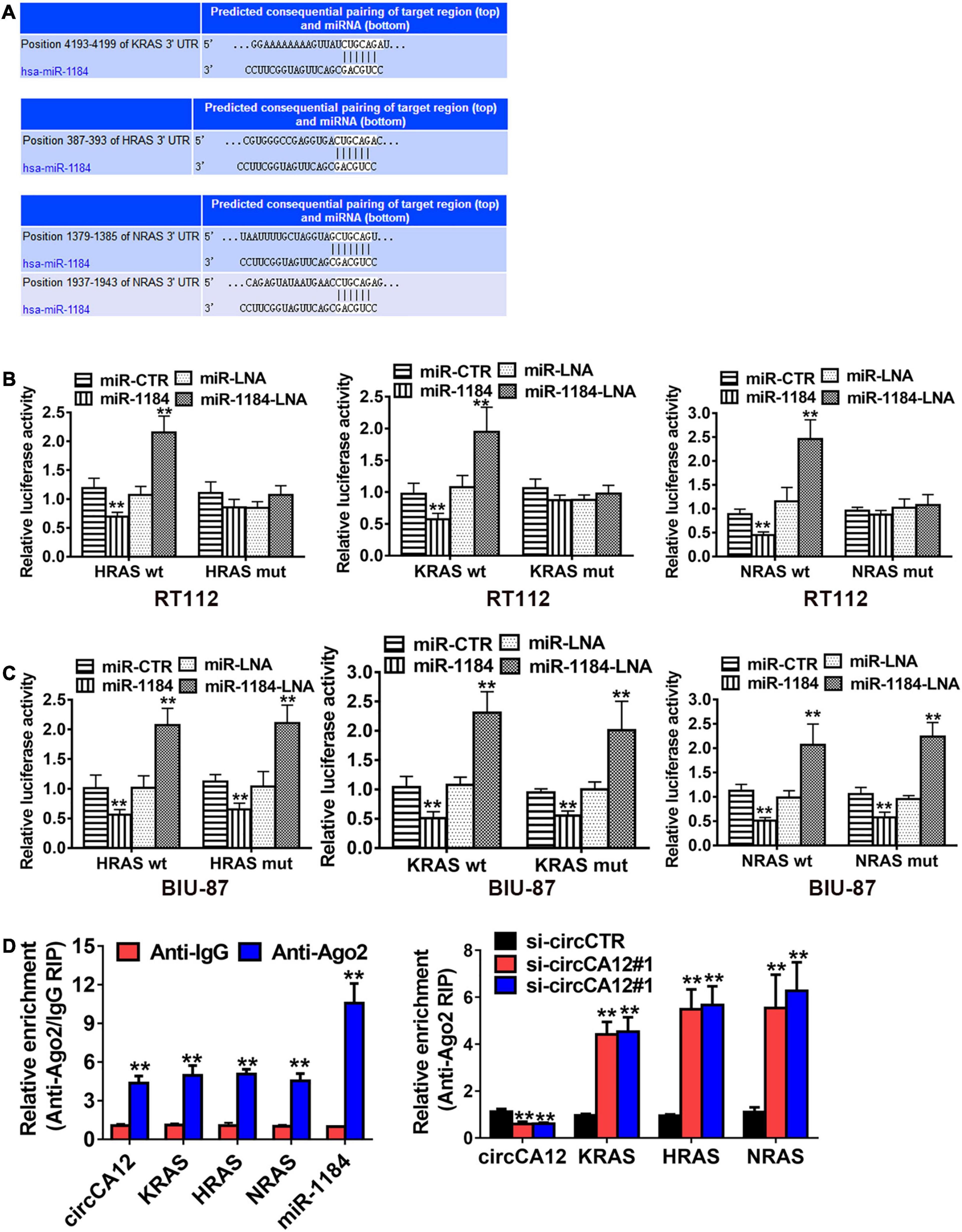
Figure 5. CircCA12 enhances bladder cancer development through the circCA12/mir-1184/RAS family pathway. (A) Docking sites of miR-1184 in the 3′-UTR of KRA/HRA/NRAS predicted by TargetScan web resource (http://www.targetscan.org). (B,C) Luciferase enzyme reporter analysis. We transfected miR-1184 mimics, locked nucleic acid (LNA), as well as control groups into circCA12 wild type or mutant luciferase reporter in RT-112 and BIU-87 cells. The predicted miR-1184 binding sites of 3′-UTR of HRAS/KRAS/NRAS were mutated. (D) RIP assay. CircCA12, miRNA-1184, and KRAS/HRAS/NRAS were abundant in the Ago2 complex in contrast with the lgG group, and enrichment of KRAS/HRAS/NRAS was elevated after silencing circCA12. CircCA12, miRNA-1184, and KRAS/HRAS/NRAS expression were measured by RT-qPCR analysis. **P < 0.01.
Discussion
CircRNAs are comprised of recently identified non-coding RNAs that have become a hotspot in medical biotechnology. Previous investigations have documented that circRNAs’ expression is cell type-distinct, as well as tissue-distinct, and the differential expression of some circRNAs could function as potential biomarkers, thereby aiding in diagnosis and therapy of various kinds of cancer (Guo et al., 2014). Urinary bladder cancer (UBC) is the most frequently diagnosed cancer of the urinary system, as well as one of the most commonly diagnosed cancers globally (Miyazaki and Nishiyama, 2017). Several researches have documented that circRNAs can exert remarkable influence in the malignant procession of UBC. Herein, we discovered a potential circular RNA, circCA12, and determined its expression level in bladder cell lines and tissues. Our data illustrated that the relative expression of circCA12 was elevated in the cancerous part in contrast with non-malignant bladder epithelial cells and tissues, suggesting that over expression of circCA12 may be involved in the malignant cascade of UBC.
Firstly, we explored differential circCA12 levels in UBC cells and tissues. Results illustrated circCA12 upregulation in the UBC cells, as well as tissues, implying that circCA12 is potentially linked to UBC onset. Secondly, we silenced circCA12 expression in RT112, as well as BIU-87 UBC cell lines, and used them to conduct functional experiments. We found that down-regulation of circCA12 significantly repressed the growth, migration, and infiltration of UBC cells. Thereafter, animal assays were employed to further confirm our results in vivo. Mice xenograft models were established, followed by resection of the cancerous nodule. The weight of the tissue obtained from mice treated with silenced circCA12 expression was remarkably lower in contrast with the control group. Our in vitro along with the in vivo data demonstrated that circCA12 promotes the malignant process of bladder cancer.
Recent research evidence demonstrates that the localization of circRNAs in cytoplasm is closely associated with miRNA sponge (Hansen et al., 2013; Chen, 2016; Ye et al., 2019). The nuclear and cytoplasmic fraction assays conducted in this study verified that circACVR2A was distributed predominantly in the cytoplasm. Moreover, RIP and dual-luciferase reporter assays indicated that circCA12 could cross talk with miR-1184 in UBC cells. Similar results were obtained when circCA12 mutant-type or wild-type plasmids were transfected into UBC cells. Theoretically, miRNAs post-transcriptionally diminish the expression profiles of targeted genes by occupying the 3′UTR of the targeted mRNAs, thereby halting the translation procession or degrading the mRNA strand (Bartel, 2018; Chen et al., 2018). The TargetScan algorithm identified the RAS oncogene family as the potential downstream target of miR-1184. The human genome possesses three RAS genes (KRAS, HRAS, and NRAS), which code for the RAS proteins. RAS proteins, RAS superfamily members of small GTPases, are found at the center of a highly complex signaling network, which modulates numerous aspects of fundamental cellular processes consisting of cell survival, apoptosis, differentiation, and proliferation (Zhou et al., 2016). Moreover, RAS genes modulate human oncogenesis (Karnoub and Weinberg, 2008). In particular, functional activation of RAS genes by point mutations has been reported in approximately 30% of all human cancers (Pylayeva-Gupta et al., 2011). Recent bladder cancer studies have shown that genetic mutations in bladder epithelial cells frequently occur in FGFR3, PIK3CA, and RAS (all associated with RAS signaling networks) (Bockorny et al., 2018; Tsujino et al., 2019). In addition, a 2020 study demonstrated that the RAS mutation can be a favorable biomarker for diagnosing cancer patients (Santha et al., 2020). With regard to the relationship between RAS oncogene and microRNAs (miRNAs), accumulating evidence suggests that some miRNAs directly target RAS signaling pathways, thereby impeding RAS-driven tumorigenesis (Jinesh et al., 2018; Ding et al., 2020). Armed with the knowledge that circRNAs primarily serve as miRNA sponges to exert diverse biological effects, we hypothesized that the underlying mechanism through which RAS promotes UBC may be associated with ceRNA function (Thomson and Dinger, 2016). Thus, we conducted Ago2-related RIP and luciferase reporter assays in order to verify our hypothesis. Our results indicated that miR-1184 promotes tumor progression by directly targeting KRAS/NRAS/HRAS in UBC.
Conclusion
In conclusion, results obtained herein illustrated upregulation of circCA12 in bladder cancer and was closely linked to the malignant process. MicroRNA-1184 was identified as a special target of circCA12, and circCA12 sponge acts on miRNA-1184 to abate its biological functions. We also identified the RAS oncogene family (KRAS, NRAS, and HRAS) as a bladder cancer stimulus factor. This study has discovered that miRNA-1184 interacts with KRAS, NRAS, and HRAS coding genes, thereby regulating their effect in bladder cancer.
Data Availability Statement
The raw data supporting the conclusions of this article will be made available by the authors, without undue reservation.
Ethics Statement
The studies involving human participants were reviewed and approved by Ethics Committee of Sun Yat-sen University Cancer Centre (SYSUCC). The patients/participants provided their written informed consent to participate in this study. The animal study was reviewed and approved by Ethics Committee of Sun Yat-sen University Cancer Centre (SYSUCC).
Author Contributions
YL and XW designed the all experiments. LJ conducted the experiments. HT gathered specimens. XL, CH, ZL, and FZ analyzed the data. LJ, YL, and XW wrote and revised the manuscript. All authors read and approved the final manuscript.
Funding
This work was funded by the National Natural Science Foundation of China (Nos. 81772726 and 81802553) and the Natural Science Foundation of Guangdong Province (No. 2018A030310235).
Conflict of Interest
The authors declare that the research was conducted in the absence of any commercial or financial relationships that could be construed as a potential conflict of interest.
The reviewers HT and YZ declared a shared affiliation, with no collaboration, with several of the authors LJ, YW, HT, XL, CH, ZL, FZ, and YL, to the handling editor at the time of review.
Footnotes
References
Antoni, S., Ferlay, J., Soerjomataram, I., Znaor, A., Jemal, A., and Bray, F. (2017). Bladder Cancer Incidence and Mortality: a Global Overview and Recent Trends. Eur. Urol. 71, 96–108. doi: 10.1016/j.eururo.2016.06.010
Bockorny, B., Rusan, M., Chen, W., Liao, R. G., Li, Y., Piccioni, F., et al. (2018). RAS-MAPK Reactivation Facilitates Acquired Resistance in FGFR1-Amplified Lung Cancer and Underlies a Rationale for Upfront FGFR-MEK Blockade. Mol. Cancer Ther. 17, 1526–1539. doi: 10.1158/1535-7163.MCT-17-0464
Borran, S., Ahmadi, G., Rezaei, S., Anari, M. M., Modabberi, M., Azarash, Z., et al. (2020). Circular RNAs: new players in thyroid cancer. Pathol. Res. Pract. 216:153217. doi: 10.1016/j.prp.2020.153217
Bray, F., Ferlay, J., Soerjomataram, I., Siegel, R. L., Torre, L. A., and Jemal, A. (2018). Global cancer statistics 2018: GLOBOCAN estimates of incidence and mortality worldwide for 36 cancers in 185 countries. CA Cancer J. Clin. 68, 394–424. doi: 10.3322/caac.21492
Chen, B., Wei, W., Huang, X., Xie, X., Kong, Y., Dai, D., et al. (2018). circEPSTI1 as a Prognostic Marker and Mediator of Triple-Negative Breast Cancer Progression. Theranostics 8, 4003–4015. doi: 10.7150/thno.24106
Chen, L. L. (2016). The biogenesis and emerging roles of circular RNAs. Nat. Rev. Mol. Cell Biol. 17, 205–211. doi: 10.1038/nrm.2015.32
Cumberbatch, M. G. K., Jubber, I., Black, P. C., Esperto, F., Figueroa, J. D., Kamat, A. M., et al. (2018). Epidemiology of Bladder Cancer: a Systematic Review and Contemporary Update of Risk Factors in 2018. Eur. Urol. 74, 784–795. doi: 10.1016/j.eururo.2018.09.001
Ding, C., Xi, G., Wang, G., Cui, D., Zhang, B., Wang, H., et al. (2020). Exosomal Circ-MEMO1 Promotes the Progression and Aerobic Glycolysis of Non-small Cell Lung Cancer Through Targeting MiR-101-3p/KRAS Axis. Front. Genet. 11:962. doi: 10.3389/fgene.2020.00962
Fan, L., Cao, Q., Liu, J., Zhang, J., and Li, B. (2019). Circular RNA profiling and its potential for esophageal squamous cell cancer diagnosis and prognosis. Mol. Cancer 18:16. doi: 10.1186/s12943-019-1050-y
Guo, J. U., Agarwal, V., Guo, H., and Bartel, D. P. (2014). Expanded identification and characterization of mammalian circular RNAs. Genome Biol. 15:409. doi: 10.1186/s13059-014-0409-z
Hansen, T. B., Jensen, T. I., Clausen, B. H., Bramsen, J. B., Finsen, B., Damgaard, C. K., et al. (2013). Natural RNA circles function as efficient microRNA sponges. Nature 495, 384–388. doi: 10.1038/nature11993
Inamura, K. (2018). Bladder Cancer: new Insights into Its Molecular Pathology. Cancers 10:100. doi: 10.3390/cancers10040100
Jinesh, G. G., Sambandam, V., Vijayaraghavan, S., Balaji, K., and Mukherjee, S. (2018). Molecular genetics and cellular events of K-Ras-driven tumorigenesis. Oncogene 37, 839–846. doi: 10.1038/onc.2017.377
Karnoub, A. E., and Weinberg, R. A. (2008). Ras oncogenes: split personalities. Nat. Rev. Mol. Cell Biol. 9, 517–531. doi: 10.1038/nrm2438
Kristensen, L. S., Ebbesen, K. K., Sokol, M., Jakobsen, T., Korsgaard, U., Eriksen, A. C., et al. (2020). Spatial expression analyses of the putative oncogene ciRS-7 in cancer reshape the microRNA sponge theory. Nat. Commun. 11:4551. doi: 10.1038/s41467-020-18355-2
Li, Y., Zheng, Q., Bao, C., Li, S., Guo, W., Zhao, J., et al. (2015). Circular RNA is enriched and stable in exosomes: a promising biomarker for cancer diagnosis. Cell Res. 25, 981–984. doi: 10.1038/cr.2015.82
Liu, P., Zou, Y., Li, X., Yang, A., Ye, F., Zhang, J., et al. (2020). circGNB1 Facilitates Triple-Negative Breast Cancer Progression by Regulating miR-141-5p-IGF1R Axis. Front. Genet. 11:193. doi: 10.3389/fgene.2020.00193
Mirzaei, H., and Hamblin, M. R. (2020). Regulation of Glycolysis by Non-coding RNAs in Cancer: switching on the Warburg Effect. Mol. Ther. Oncolytics 19, 218–239. doi: 10.1016/j.omto.2020.10.003
Miyazaki, J., and Nishiyama, H. (2017). Epidemiology of urothelial carcinoma. Int. J. Urol. 24, 730–734. doi: 10.1111/iju.13376
Nahand, J. S., Jamshidi, S., Hamblin, M. R., Mahjoubin-Tehran, M., Vosough, M., Jamali, M., et al. (2020). Circular RNAs: new Epigenetic Signatures in Viral Infections. Front. Microbiol. 11:1853. doi: 10.3389/fmicb.2020.01853
Pylayeva-Gupta, Y., Grabocka, E., and Bar-Sagi, D. (2011). RAS oncogenes: weaving a tumorigenic web. Nat. Rev. Cancer 11, 761–774. doi: 10.1038/nrc3106
Racioppi, M., D’Agostino, D., Totaro, A., Pinto, F., Sacco, E., D’Addessi, A., et al. (2012). Value of current chemotherapy and surgery in advanced and metastatic bladder cancer. Urol. Int. 88, 249–258. doi: 10.1159/000335556
Razavi, Z. S., Tajiknia, V., Majidi, S., Ghandali, M., Mirzaei, H. R., Rahimian, N., et al. (2021). Gynecologic cancers and non-coding RNAs: epigenetic regulators with emerging roles. Crit. Rev. Oncol. Hematol. 157:103192. doi: 10.1016/j.critrevonc.2020.103192
Romero-Cordoba, S. L., Salido-Guadarrama, I., Rodriguez-Dorantes, M., and Hidalgo-Miranda, A. (2014). miRNA biogenesis: biological impact in the development of cancer. Cancer Biol. Ther. 15, 1444–1455. doi: 10.4161/15384047.2014.955442
Sang, Y., Chen, B., Song, X., Li, Y., Liang, Y., Han, D., et al. (2019). circRNA_0025202 Regulates Tamoxifen Sensitivity and Tumor Progression via Regulating the miR-182-5p/FOXO3a Axis in Breast Cancer. Mol. Ther. 27, 1638–1652. doi: 10.1016/j.ymthe.2019.05.011
Sanger, H. L., Klotz, G., Riesner, D., Gross, H. J., and Kleinschmidt, A. K. (1976). Viroids are single-stranded covalently closed circular RNA molecules existing as highly base-paired rod-like structures. Proc. Natl. Acad. Sci. U. S. A. 73, 3852–3856. doi: 10.1073/pnas.73.11.3852
Sanli, O., Dobruch, J., Knowles, M. A., Burger, M., Alemozaffar, M., Nielsen, M. E., et al. (2017). Bladder cancer. Nat. Rev. Dis. Primers 3:17022. doi: 10.1038/nrdp.2017.22
Santha, S., Ling, X., Aljahdali, I. A. M., Rasam, S. S., Wang, X., Liao, J., et al. (2020). Mutant Kras as a Biomarker Plays a Favorable Role in FL118-Induced Apoptosis, Reactive Oxygen Species (ROS) Production and Modulation of Survivin, Mcl-1 and XIAP in Human Bladder Cancer. Cancers 12:3413. doi: 10.3390/cancers12113413
Selth, L. A., Das, R., Townley, S. L., Coutinho, I., Hanson, A. R., Centenera, M. M., et al. (2017). A ZEB1-miR-375-YAP1 pathway regulates epithelial plasticity in prostate cancer. Oncogene 36, 24–34. doi: 10.1038/onc.2016.185
Shabaninejad, Z., Vafadar, A., Movahedpour, A., Ghasemi, Y., Namdar, A., Fathizadeh, H., et al. (2019). Circular RNAs in cancer: new insights into functions and implications in ovarian cancer. J. Ovarian Res. 12:84. doi: 10.1186/s13048-019-0558-5
Thomson, D. W., and Dinger, M. E. (2016). Endogenous microRNA sponges: evidence and controversy. Nat. Rev. Genet. 17, 272–283. doi: 10.1038/nrg.2016.20
Tsujino, T., Sugito, N., Taniguchi, K., Honda, R., Komura, K., Yoshikawa, Y., et al. (2019). MicroRNA-143/Musashi-2/KRAS cascade contributes positively to carcinogenesis in human bladder cancer. Cancer Sci. 110, 2189–2199. doi: 10.1111/cas.14035
Wang, S., Zhang, Y., Cai, Q., Ma, M., Jin, L. Y., Weng, M., et al. (2019). Circular RNA FOXP1 promotes tumor progression and Warburg effect in gallbladder cancer by regulating PKLR expression. Mol. Cancer 18:145. doi: 10.1186/s12943-019-1078-z
Ye, F., Gao, G., Zou, Y., Zheng, S., Zhang, L., Ou, X., et al. (2019). circFBXW7 Inhibits Malignant Progression by Sponging miR-197-3p and Encoding a 185-aa Protein in Triple-Negative Breast Cancer. Mol. Ther. Nucleic Acids 18, 88–98. doi: 10.1016/j.omtn.2019.07.023
Zhang, Y., Liu, H., Li, W., Yu, J., Li, J., Shen, Z., et al. (2017). CircRNA_100269 is downregulated in gastric cancer and suppresses tumor cell growth by targeting miR-630. Aging 9, 1585–1594. doi: 10.18632/aging.101254
Zhang, Y., Zhang, X. O., Chen, T., Xiang, J. F., Yin, Q. F., Xing, Y. H., et al. (2013). Circular intronic long noncoding RNAs. Mol. Cell 51, 792–806. doi: 10.1016/j.molcel.2013.08.017
Zhou, B., Der, C. J., and Cox, A. D. (2016). The role of wild type RAS isoforms in cancer. Semin. Cell Dev. Biol. 58, 60–69. doi: 10.1016/j.semcdb.2016.07.012
Zou, Y., Zheng, S., Deng, X., Yang, A., Kong, Y., Kohansal, M., et al. (2020). Diagnostic and prognostic value of circular RNA CDR1as/ciRS-7 for solid tumours: a systematic review and meta-analysis. J. Cell Mol Med. 24, 9507–9517. doi: 10.1111/jcmm.15619
Zou, Y., Zheng, S., Deng, X., Yang, A., Xie, X., Tang, H., et al. (2019a). The Role of Circular RNA CDR1as/ciRS-7 in Regulating Tumor Microenvironment: a Pan-Cancer Analysis. Biomolecules 9:429. doi: 10.3390/biom9090429
Keywords: circCA12, miR-1184, RAS, sponge, bladder cancer
Citation: Jiang L, Wang Y, Tang H, Li X, Huang C, Liu Z, Zhou F, Wang X and Li Y (2021) CircCA12 Promotes Malignant Process via Sponging miR-1184 and Upregulating RAS Family in Bladder Cancer. Front. Genet. 12:663982. doi: 10.3389/fgene.2021.663982
Received: 04 February 2021; Accepted: 26 April 2021;
Published: 21 June 2021.
Edited by:
Daniele Vergara, University of Salento, ItalyReviewed by:
Hailin Tang, Sun Yat-sen University Cancer Center (SYSUCC), ChinaYutian Zou, Sun Yat-sen University Cancer Center (SYSUCC), China
Hamed Mirzaei, Kashan University of Medical Sciences, Iran
Copyright © 2021 Jiang, Wang, Tang, Li, Huang, Liu, Zhou, Wang and Li. This is an open-access article distributed under the terms of the Creative Commons Attribution License (CC BY). The use, distribution or reproduction in other forums is permitted, provided the original author(s) and the copyright owner(s) are credited and that the original publication in this journal is cited, in accordance with accepted academic practice. No use, distribution or reproduction is permitted which does not comply with these terms.
*Correspondence: Yonghong Li, liyongh@sysucc.org.cn; Xiaolan Wang, lanlan7483@163.com
†These authors have contributed equally to this work