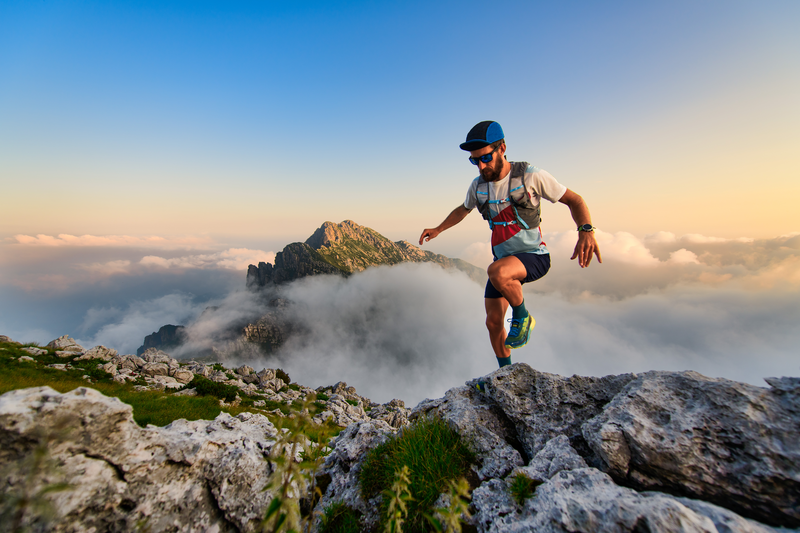
94% of researchers rate our articles as excellent or good
Learn more about the work of our research integrity team to safeguard the quality of each article we publish.
Find out more
ORIGINAL RESEARCH article
Front. For. Glob. Change , 21 February 2025
Sec. Tropical Forests
Volume 8 - 2025 | https://doi.org/10.3389/ffgc.2025.1412130
Tropical forests, known for their biodiversity and carbon (C) richness, face significant threats from biological invasions that disrupt structural and functional processes. Lantana camara (Family: Verbenaceae) is an invasive shrub that has spread across several Indian landscapes. The present study aimed to assess the changes in tree species richness and total ecosystem carbon (TEC) storage in Lantana camara-invaded (LI) and uninvaded (UI) sites in the tropical dry deciduous forests of Madhya Pradesh, India. Significantly lower species richness (SR), C storage of juveniles, total trees, and total biomass C were observed in LI sites than in UI sites. However, significantly higher C storage of shrubs + herbs (understorey), litter, and soil organic carbon (SOC) were found in LI sites than in UI sites. The percent allocation of C in tree juveniles, adults, understorey, detritus, and SOC to the TEC pool was 2.6, 39.1, 1.4, 5.5, and 51.3 in LI sites and 3.8, 49.7, 0.2, 4.0 and 42.3 in UI sites, respectively. The C stocks of tree juveniles, adults, and herbs were lower by 23.3, 15.7 and 20.3%, respectively, in LI sites than in UI sites, whereas shrub, detritus, and SOC stocks were higher by 95.1, 9.1 and 7.9%, respectively, in LI sites than in UI sites. A significant negative relationship was observed between L. camara density and SR, tree juvenile C, herb C, understorey C, and total ecosystem C storage, while the same had a significant positive relationship with shrub C, litter C, and SOC. The present findings revealed that the plant diversity and total C pools were altered by shrub invasion and have important implications for their quantification in these tropical forests.
Plant invasions alter the structural and functional diversity of ecosystems and are the major drivers of global change ecology (Ehrenfeld, 2010; Lone et al., 2019, 2022) when present in high numbers (Vilà et al., 2011). Invasive exotic species have affected the composition and structural diversity of several native ecosystems worldwide (Vitousek et al., 1996; Hughes et al., 2017). They differ from the native biota in terms of both resource acquisition and resource consumption, which, in turn, alter ecosystem properties, disturbance regimes, and elemental cycling (Asner et al., 2010; Mandal and Joshi, 2015a). The impacts of invasion are often long-lasting, persisting in native ecosystems, even after the removal of native species (Ramaswami and Sukumar, 2016). Plant invasion is a major driver of global change, but studies on its impacts on diversity and ecosystem-level C storage in forest ecosystems are limited and fragmented (Vitousek et al., 1997; Lone et al., 2022). Some studies have suggested that plant invasion enhances ecosystem-level C storage (Stock et al., 1995; Hibbard et al., 2001), whereas few others have reported that invasion leads to a decline in diversity, ecosystem C storage, and changes in soil physio-chemical properties (Jackson et al., 2002; Gooden et al., 2009a; Kumar et al., 2020). These contrasting outcomes may result from differences in the eco-physiological traits of invasive species and the characteristics of the ecosystems they invade (Liao et al., 2007). In terrestrial ecosystems, there are predominantly six major C pools: aboveground and belowground biomass of trees, understorey vegetation, deadwood, forest floor litter, and soil (Pearson et al., 2005). Understanding the influence of plant invasion on biodiversity and each of the C pools is essential to accurately assess its impacts on C cycling (Yang et al., 2013). Invasive species affect diversity, soils, and C dynamics in several ways. They usually possess greater reproductive potential and higher competitive ability, which help to displace native species (Gooden et al., 2009a; Hughes et al., 2017). Alteration of plant communities by invasion alters the primary productivity and C sequestration potential in forests (Ehrenfeld, 2010). The C pool sizes of forests are altered by plant invasions due to variations in above- and belowground processes, which reflect on C fluxes such as rates of photosynthesis, soil respiration, etc. (Nie et al., 2017), and ultimately ecosystem-level C.
Tropical forests are biodiverse and carbon (C)-rich ecosystems and play a crucial part in the global C cycle (Dixon et al., 1994; Sullivan et al., 2017). Overall, 7–10% of the earth’s area is covered by tropical forests, but they account for 34% of terrestrial primary productivity (Beer et al., 2010). These are huge C repositories, storing about 55% of the world’s forest C (471 ± 93 Pg C), and contain the highest C stocks (Pan et al., 2011). Among the different tropical forest types, dry forests are the most threatened and understudied ecosystems, and they are modulated by seasonal ecological processes. Seasonally dry forests cover 42% of tropical ecosystems and receive 250–2,100 mm of rainfall annually (Murphy and Lugo, 1986).
As per the India State of Forest Report (2019), tropical dry deciduous forests cover 40.9% of India’s total forest cover. Madhya Pradesh has the largest forest cover of tropical dry forests, comprising roughly 84% of the state’s forest cover. Lantana camara is a highly invasive plant with 650 varieties and occurs in over 60 countries (Global Invasive Species Database, 2020).
L. camara is an aggressive, fast-growing, perennial shrub of the Verbenaceae plant family. Native to tropical Central and South America, it exhibits significant genetic diversity and phenotypic plasticity, enabling it to survive under diverse climatic conditions (Adhikari et al., 2024). Initially introduced to India for ornamental purposes, it has since spread across diverse landscapes, including tropical rainforests, semi-arid regions, and mangrove ecosystems (Mungi et al., 2018). Its expansion is particularly pronounced in habitats that have experienced disturbances such as fires or floods, where native plant species are under stress. With increased canopy openings allowing light penetration and availability of soil resources, L. camara quickly colonizes the disturbed habitats. Seed dispersal by birds and the release of chemicals that inhibit other plants (allelopathy) give L. camara a competitive advantage over native species (Mungi et al., 2020). Despite long-term intensive control efforts in India, L. camara has only continued an upward trajectory of spread and invasion, and the spread has been projected to expand under climate change (Bhagwat et al., 2012; Adhikari et al., 2024). It covers over 13 million hectares, threatening 44% of India’s forest cover by invading almost all dry deciduous forests (Sharma et al., 2005; Sharma and Raghubanshi, 2006; Goyal et al., 2018; Mungi et al., 2020). L. camara has also extensively invaded Central India’s forest ecosystems (Love et al., 2009; Bhagwat et al., 2012; Wagh and Jain, 2018). The main goal of this research was to understand the effects of shrub (L. camara) invasion on plant species richness and C pools {trees, understorey, detritus (deadwood + forest floor litter), and soil organic carbon} of Central Indian tropical dry deciduous forests. Our specific objectives were: (1) to assess the impacts of L. camara invasion on plant diversity and ecosystem-level C pools and (2) quantify changes in these parameters due to shrub invasion.
The present study was undertaken in three forest study sites in Sagar, Madhya Pradesh, Central India (Figure 1; Table 1). The study area is located in north-central Madhya Pradesh and is surrounded by the Vindhyachal mountain range. The forest type in the study region belongs to group 4b as per Champion and Seth’s (1968) classification. A subtropical climate with a hot summer (March to mid-June), followed by a rainy season (mid-June to September) and a cool winter (October to February), prevails in the study region. The mean annual minimum temperature is 11.6°C, and the mean maximum temperature is 40.7°C, whereas the mean annual rainfall is 1197.6 mm in the study area (WorldClim, 2024). The southwestern region of the district receives the heaviest rainfall, and the intensity decreases toward the north and east. The soil type is clayey to sandy loam in all the study sites. All three study sites are comprised of open forests, with grazing, firewood, and non-timber forest produce collected by local people being observed. Butea monosperma, Tectona grandis, Lagerstroemia parviflora, and Diospyros melanoxylon are the most prevalent tree species in this forest, which has now been invaded by L. camara (Dar et al., 2019).
Table 1. Study site characteristics of uninvaded (UI) and Lantana-invaded (LI) sites of tropical dry deciduous forests in Madhya Pradesh, Central India.
Fieldwork was carried out in 2017 and 2018, and three study sites were chosen after an extensive reconnaissance survey (Supplementary Figure 2). The study sites were chosen based on the differences in altitude, structure, and composition (Table 1; Supplementary Tables 1–3). Each site was divided into two sub-sites based on the presence or absence of the shrub, L. camara – they were classified as uninvaded (UI; L. camara cover absent) and L. camara-invaded (LI; presence of L. camara cover >50%) following Gooden et al. (2009a). In each sub-site, 10 plots of 2,500 m2 (50 m × 50 m) were laid randomly in the study site (a total of 60 plots – 30 in UI and 30 in LI). The study plots were randomized based on a rarefaction curve analysis to ensure representative sampling (Figure 2).
Figure 2. Rarefaction curves with a 95% confidence interval of estimated species richness to sample plots.
Phytosociological attributes such as tree richness, frequency, density, basal area, and size class distribution were enumerated in all the plots. Each 2,500 m2 plot was further partitioned into 25 (100 m2) quadrats for easy sampling (Misra, 1968). The stems were considered as trees: >3–9.9 cm DBH (juveniles) and ≥ 10 cm DBH (adults) (Chaturvedi et al., 2012a; Chaturvedi et al., 2012b; Baboo et al., 2017). Tree and liana stem with a diameter greater than 3 cm at breast height (DBH) were measured (Knight, 1963). The girths of the multi-stemmed trees were measured separately. The above- and belowground (AGB and BGB, respectively) C storage was estimated following the standard biomass equations using tree DBH, height, and wood density (Zanne et al., 2009). The height of all trees was measured using a digital Haglof clinometer.
Allometric equations used:
The tree AGB was estimated following Chave et al. (2014):
The AGB of tree juveniles was calculated following Chaturvedi et al. (2012a), Chaturvedi et al. (2012b):
The woody climber biomass was estimated following Schnitzer et al. (2006):
Tree BGB was calculated following Cairns et al. (1997):
The BGB of tree juveniles and climbers was estimated following Ravindranath and Ostwald (2008):
To sample the understorey, ten 25 m2 (5 m × 5 m) quadrats were laid in each plot to record shrubs (including L. camara) and climbers. An additional ten 1 m2 (1 m × 1 m) quadrats were used for herbs. The understorey vegetation was clipped at the base during the peak growing period. The clipped biomass was weighed fresh on-site with a digital balance in the field, and five replicate samples were transferred to the lab. These samples were dried in a hot-air oven at 105 ± 5°C for 72 h and were weighed again for dry mass. The detritus biomass (deadwood + litter) was calculated following Ravindranath and Ostwald (2008). The estimated biomass was converted to C by following the conversion factor (0.5) as per the Intergovernmental Panel on Climate Change (IPCC, 2006).
The soil samples in the LI and UI subsites were collected in all the plots. Five random points were chosen in each plot, and standing crop litter was cleared before collecting the soil samples. A soil core sampler with an internal diameter of 6 cm and a total of 60 soil samples (at a depth of 0–50 cm) were collected in labeled polyethylene bags before being transported to the laboratory. The samples were air-dried, sieved, and ground to a fine powder in the laboratory.
Walkley and Black’s (1934) method was used to estimate soil organic carbon (SOC). For bulk density (BD; g/cm3) estimation, three replicates of undisturbed soil cores (0–50 cm) were collected in each plot. The soil samples were taken to the laboratory, oven-dried at 105 ± 5°C for 72 h, and then re-weighed. The coarse rocky fragments were removed using a 2 mm sieve and re-weighed. Soil BD was calculated following Pearson et al. (2005):
The SOC of soil samples was calculated following Pearson et al. (2005).
Soil moisture (M%) was measured using the gravimetric method, while soil pH (1:2.5 ratio of soil to water) was determined using a digital pH meter. Climatic variables, such as minimum and maximum temperatures, mean annual temperature (MAT), and mean annual precipitation (MAP), were obtained for all plots from the WorldClim dataset (WorldClim, 2024) using the sp. and raster packages in R 3.6.2. Additionally, topographic variables, such as slope and aspect, were derived using R 3.6.2.
Ecosystem-level carbon storage was calculated by summing carbon stored in trees, the understorey, detritus, and soil organic carbon (SOC) (Ravindranath and Ostwald, 2008; Kothandaraman et al., 2020). A one-way analysis of variance (ANOVA) was used for statistical analysis. Tukey’s HSD test tested the differences in mean ± standard error of diversity, vegetation C stocks, and SOC (p < 0.05). All the statistical analyses were conducted using SPSS 22.0 (SPSS Inc, 2013). Linear correlations were conducted to assess the relationships of L. camara density with other descriptor variables (Supplementary Table 5).
Significantly lower (p < 0.05) species richness (SR, 98 species) was observed in LI sites than in UI sites (132 species). The mean SR of trees was significantly (p < 0.05) lower in LI sites (saplings - 13, juveniles - 30, and adult trees - 29) than in the UI sites (saplings - 21, juveniles - 33, and adult trees - 40). For saplings, juveniles, and adults, the total SR was 55, 72, and 54 in LI, and 71, 98, and 76 in UI sites, respectively (Figure 3; Supplementary Table 1; Supplementary Figures 5–9).
Figure 3. Species richness (SR; No. of species) of tree saplings, juveniles, herbs, and total plants (trees, herbs, and shrubs) in uninvaded (UI) and Lantana-invaded (LI) sub-sites. Different letters show (p < 0.05) different means significantly between them by Tukey’s HSD post hoc test.
The mean aboveground total tree C stock (juvenile carbon + adult carbon) was significantly (p = 0.032) lower in LI sites (48.1 ± 3.6 Mg C ha−1) than in UI sites (66.7 ± 4.8 Mg C ha−1; Figure 4). The total tree aboveground C ranged from 43.1 to 50.6 Mg C ha−1 in LI sites and 59.4 to 72.2 Mg C ha−1 in UI sites. The belowground C stock also showed the same trend as the latter, which has been calculated from the former. Significantly (p < 0.001), higher mean shrub C stock was observed in LI sites (1.87 ± 0.13 Mg C ha−1) than in UI sites (0.008 ± 0.004 Mg C ha−1) sites. Overall, significantly (p < 0.001) higher total understorey C stock (shrub C + herb C) was observed in LI sites than in UI sites. The total understorey C ranged from 1.7 to 2.4 Mg C ha−1 in LI sites and from 0.08 to 0.41 Mg C ha−1 in UI sites.
Figure 4. Variation in carbon stocks (total tree C, shrub+herb+understorey C, litter+deadwood+detritus C, SOC) in uninvaded (UI) and Lantana-invaded (LI) sub-sites. Different letters show (p < 0.05) different means significantly between them by Tukey’s HSD post hoc test.
The mean litter C stock was significantly (p < 0.0001) higher in LI sites (1.8 ± 0.1 Mg C ha−1) than in UI sites (1.5 ± 0.1 Mg C ha−1). Overall, the mean detritus C stock (litter carbon + deadwood carbon) was higher in LI sites (8.1 ± 1.3 Mg C ha−1) than in UI sites (6.2 ± 0.7 Mg C ha−1), although non-significant. The mean total biomass C stock of all the pools (tree C + understorey C + detritus C) was found to be significantly (p < 0.05) lower (69.2 ± 3.4 Mg C ha−1) in LI sites than in UI sites (88.5 ± 5.9 Mg C ha−1; Figure 4; Supplementary Table 4).
SOC stocks were significantly (F = 10.67, p < 0.001) higher in LI sites than in UI sites. In LI sites, SOC stocks ranged from 64.6 to 84.1 Mg C ha−1, with a mean of 73.1 ± 2.7 Mg C ha−1, whereas, in UI sites, the SOC stocks ranged from 55.7 to 76.1 Mg C ha−1, with the mean of 65.2 ± 2.8 Mg C ha−1 for a depth of 0–50 cm (Supplementary Table 4). L. camara density was related to changes in soil properties. The values of BD (g cm−3) and pH were significantly (F = 12.96, p < 0.05) lower in LI sites than in UI sites, whereas moisture (M%) was significantly (F = 68.55, p < 0.001) higher in LI sites than in UI sites. The SOC and soil total nitrogen (STN) stocks were significantly (F = 48.3, p < 0.001; F = 51.7, p < 0.001) higher in LI sites than in UI sites (Figure 5).
Figure 5. Soil organic carbon (SOC; Mg C ha−1), soil total nitrogen (STN; Mg C ha−1), moisture (M; %), pH, and bulk density (BD; g cm−3) in uninvaded (UI) and Lantana-invaded (LI) sites. Data are presented as mean values. Different letters show significant differences at a p-value of <0.05.
The total ecosystem C (TEC) storage, comprising tree juveniles, adults, understorey (shrubs + herbs), detritus (litter + deadwood), and SOC, varied significantly (p < 0.001) among LI sites and UI sites (Supplementary Table 4). Significantly (p < 0.05) lower TEC pool was found in LI sites (mean 142.4 Mg C ha−1) than in UI sites (mean 153.7 Mg C ha−1).
The C allocation pattern among the different ecosystem components was markedly different in LI sites from those of UI sites. Overall, tree juveniles, adults, understorey, detritus, and SOC contribute 3.7, 55.5, 2.0, 8.0, and 73.1 Mg C ha−1, and 5.9, 76.1, 0.21, 6.27, and 65.3 Mg C ha−1 in LI sites and UI sites, respectively (Supplementary Table 4). The percent allocation of tree juveniles, adults, understorey, detritus, and SOC to the total ecosystem C pool was 2.6, 39.1, 1.4, 5.5 and 51.3% in LI sites and 3.8, 49.7, 0.2, 4.0 and 42.3% in UI sites, respectively (Figure 6). In LI sites, carbon stocks of tree juveniles, adults, and herbs were reduced by 23.3, 15.7, and 20.3%, respectively, compared to UI sites. Conversely, shrub, detritus, and SOC stocks increased by 95.1, 9.1, and 7.9%, respectively (Supplementary Figures 1,4).
Figure 6. Percent allocation of different ecosystem components in uninvaded (UI) and Lantana-invaded (LI) sites of tropical dry deciduous forests in Madhya Pradesh, Central India.
In all three LI sites, the 20.1–30 cm diameter class held the highest carbon stock, followed by the 30.1–40 cm diameter class (Figure 7A). The C stocks were low by 35, 23.7, 23, and 21.8% in the 60.1–70, >80.1, 0–10, and 30.1–4-40 cm diameter classes, respectively, in LI sites than UI sites (Figure 7B).
Figure 7. (A) Diameter class-wise distribution of saplings, juveniles, and adult trees in terms of stem density, and (B) Percent (%) change in C stocks in different DBH classes in uninvaded (UI) and Lantana-invaded (LI) sites of tropical dry deciduous forests in Madhya Pradesh, Central India.
A significantly negative relationship has been found between L. camara density and plant diversity (r = −0.459), tree juvenile C (r = −0.475), herb C (r = −0.384), understorey C (r = −0.686), and total ecosystem C storage (r = −0.208). On the other hand, a significant positive relationship was observed with shrub C (r = 0.645), litter C (r = 0.425), and SOC (r = 0.89; Table 2). The SOC, STN, and M% showed significant positive correlations, whereas soil pH correlated significantly negatively with L. camara density. Soil bulk density had a non-significant negative relationship with L. camara density.
The Principal Components Analysis (PCA) assessed the relationships among diversity, structural attributes, and environmental factors with L. camara density (Supplementary Figure 3). Eigenvalues of the dominant axis were 6.25, 4.92, 3.93, 2.03, and 1.04, respectively, and the corresponding percentage variances were 28.4, 22.4, 17.9, 9.2, and 5.8. These values reflect variations in elevation, mean annual precipitation, slope, moisture, and pH, which accounted for 83.7% of the variation in L. camara density.
Biological invaders represent a major change element in forest ecosystems (Vitousek et al., 1996). They are known for their impacts on the community structure, species composition, and diversity of both above- and belowground communities, with significant changes in functioning and processes (Gaertner et al., 2009; Ehrenfeld, 2010; Vilà et al., 2011; Richardson et al., 2011; Gioria et al., 2014). The C stocks of different pools are greatly impacted by shrub invasion by changing their structure, above- and belowground biomass, litter production, and SOC (Tilman et al., 2001; Liao et al., 2008a, b; Fornara and Tilman, 2008). L. camara is a widely distributed invasive weed and has engulfed the maximum area of tropical dry forests in the Central Indian landscape (Mungi et al., 2020). Thus, the invasion could drastically alter the structural and functional processes. Any such change by invasion would significantly influence regional and global C cycling, exacerbating the effects of climate change.
The total carbon stocks of adult trees (above- and belowground) were 15.4% lower in LI than in UI sites, though this difference was not statistically significant (p = 0.060). This insignificance in total adult tree biomass C could be attributed to the predominance of Tectona grandis trees with almost similar densities, basal areas, and representation in higher diameter classes (≥ 60 cm DBH). Similar results were obtained by Litton et al. (2006), who reported insignificantly higher aboveground tree biomass stocks between native and Pennisetum setaceum-invaded tropical dry deciduous forests in Hawaii, and Gaudel et al. (2016), who reported lower aboveground tree biomass and C stocks in areas that are highly invaded by Mikania micrantha compared to medium- and lowly invaded areas in Parsa Wildlife Reserve, Nepal.
In the present study, biomass C stocks of juvenile trees were significantly (p < 0.0001) lower by 22.9% in LI sites than in UI sites (Supplementary Table 4). Our results confirm with Litton et al. (2006) and Gaudel et al. (2016), who reported significantly lower biomass C stocks in tree seedlings in areas invaded by Pennisetum setaceum and Mikania micrantha, respectively. This significant difference in juvenile tree biomass and C stock may be due to the presence of greater L. camara density/cover, which was above the threshold limit (> 70% cover) in these sites (Gooden et al., 2009a), which could have impacted the growth of tree saplings and juveniles, thereby reducing their density and basal area. Plant invasion can result in changes in the structure, composition, diversity, evenness, and regeneration potential of forests (Saxena and Singh, 1985; Saxena, 1991; Ambika et al., 2003; Taylor et al., 2012; Kumar et al., 2020). The higher density of L. camara alters the structure, species composition, diversity, and biomass of native plants. The impacts of invasion by woody plants on native tree juvenile and adult biomass C stocks are reported to be greater than those of non-woody and non-nitrogen-fixing plants (Liao et al., 2008b).
Total tree biomass and carbon stocks were significantly lower (by 17.5%; p < 0.05) in LI sites compared to UI sites. Invasion could exert positive, negative, or neutral impacts on different C pools, depending on the type of invader and its characteristics and the type of community undergoing invasion (Hughes et al., 2017). In the present study, the lower total tree biomass and C stocks in LI sites than UI sites are due to the lower tree densities and basal areas in LI sites. The invasion of L. camara has altered the structural diversity, changing the size of saplings and juveniles and their relative dominance (Ens and French, 2008; Fisher et al., 2009). Invasive plants impact native plants by changing their structural composition (Mason and French, 2008). Hejda et al. (2009) reported a reduction in structural diversity in invaded plots compared to the uninvaded plots. High L. camara invasion suppresses the growth and recruitment of vegetation by changing the microenvironment and inhibits germination by the release of allelochemicals in soil (Gentle and Duggin, 1997; Lewis et al., 2004). L. camara thickets impede the recruitment of smaller individuals by outcompeting them for essential resources, including light and space (Gooden et al., 2009b; Ramaswami and Sukumar, 2011, 2016; Sundaram and Hiremath, 2012). This reduces basal area, which is an indicator of biomass C (Litton et al., 2006; Adomako et al., 2019; Raha et al., 2020).
The shrub C stock was significantly more at 95.1% in LI sites than in UI sites, whereas the herbaceous C stock was significantly less at 23.2% in LI sites than in UI sites. The significantly (p < 0.0001) higher C stocks of shrubs in LI sites are due to the presence of higher density, basal area, and biomass of L. camara in these sites than in the UI sites. However, Lantana density did not significantly correlate with shrub biomass, excluding L. camara (R2 = 0.10). Our values of shrub biomass and C stocks are in concurrence with the findings reported by Mandal and Joshi (2015b) in the subtropical deciduous forests of western Himalaya, India (3.82 Mg C ha−1), Pande (2005) from tropical dry deciduous teak forests (0.69–3.77 Mg ha−1), Oraon (2012) in tropical dry deciduous forests, Chhattisgarh (1.15 and 6.79 Mg ha−1), Jhariya et al. (2014) in the tropics of Chhattisgarh, India (2.48–5.88 Mg ha−1), but lower than the values reported by Jhariya (2017) from Bhoramdeo Wildlife Sanctuary, Chhattisgarh, India (6.82–15.71 and 2.93–6.76 Mg ha−1 biomass and C, respectively), Singh and Singh (1991) from tropical dry forest of Vindhyan region (7.1 and 20.98 Mg ha−1) and Swamy et al. (2010) from tropical evergreen forests of Western Ghats (38.1–86.3 Mg ha−1). The significantly (p < 0.0001) lower C stock of herbs in LI sites could be due to the lower herb density, basal cover, and evenness. L. camara canopies are known to intercept the incoming sunlight, causing shade (Asner et al., 2008, 2010), inhibiting the recruitment of understorey herbaceous vegetation by displacing them through competition for resources (water, nutrients, light, space, etc.), allelopathy and by altering soil properties (Walck et al., 1999; Vila and Weiner, 2004; Yurkonis et al., 2005; Bjerknes et al., 2007; Pejchar and Mooney, 2009; Timsina et al., 2011). Similarly, Hughes et al. (2006) have also reported a decline in herbaceous C pool due to Prosopis glandulosa encroachment in the Southern Great Plains of the United States.
Significantly (p < 0.001), higher litter and deadwood C stocks (non-significant) were recorded in LI sites than in UI sites. Litter and deadwood C were higher by 9.1 and 14.5%, respectively, in LI sites. The higher litter C stocks in LI sites are due to a large accumulation of L. camara litter and non-extraction of deadwood timber by the local people and the forest department. L. camara thickets also hinder entering the forest for timber extraction. High L. camara density might be the reason for higher detritus biomass and C stocks in LI sites than in UI sites because the latter are relatively open and have less surface litter.
More SOC stocks in LI sites than in the UI sites could have been due to significantly higher litter inputs with varying litter composition, higher nitrogen concentration, higher moisture content, and lower lignin and cellulose content that might have enabled rapid decomposition of L. camara litter (Ehrenfeld, 2003; Liao et al., 2008a; Li et al., 2016). This implies that the litter quantity and quality may alter soil nutrients.
The TEC stocks and allocation patterns showed significant changes between LI sites and UI sites. Invasion by woody plants tends to have considerable impacts on C stocks and profoundly alters the allocation patterns (Liao et al., 2008a). In this study, the greater allocation of C stocks in shrubs, detritus, and soil is due to higher L. camara density, non-extraction of deadwood, and greater litter inputs, which may have resulted in higher allocation in these components. On the other hand, lower allocation of C stocks in tree juveniles, adults, and herbs could be because high L. camara density could have inhibited their growth and recruitment by intercepting light, releasing allelochemicals, resource competition, and so on, as suggested by Asner et al. (2010).
In the present study, SOC showed significant positive correlations with L. camara density, basal area, biomass, and litter. Similarly, Sharma and Raghubanshi (2006, 2007) have found a positive relationship between L. camara cover and SOC in tropical dry deciduous forests in India. Mandal and Joshi (2014, 2015b) have found a strong relationship between L. camara biomass and SOC in the central Himalayas, India. Gómez-Rey et al. (2013) have also found a positive relationship between shrub density and soil organic matter in Mediterranean oak woodland.
The distribution of different size classes indicates the C storage and regeneration potential of any forest landscape. Small, medium-sized, and large trees are important in C storage in any forest ecosystem (Brown et al., 1997; Baishya et al., 2009; Borah et al., 2013). In the present study, C stocks were reduced in most of the diameter classes in LI sites. This could be due to lower density and basal area in the invaded sites. L. camara impedes the recruitment of small-sized tree individuals into larger diameter classes and the growth of large DBH classes, causing a reduction in density and basal area (Alemu and Terefe, 2015). The conversion of these forests to shrublands will reduce the tree C pools (Jaramillo et al., 2003; Bonino, 2006).
Mitigating the spread of L. camara using fire and biological control has been recorded as early as 1921 in India (Troupe, 1921; Bhagwat et al., 2012). However, fire is known to facilitate further expansion of this invasive shrub (Hiremath and Sundaram, 2005). However, chemical control followed by planting fast-growing native species has yielded positive results in Punjab, India (Luna et al., 2009). There have also been efforts to contain L. camara spread while boosting economic benefits by using it as a green compost and using the stems to make baskets (Nanjappa et al., 2005; Sharma et al., 2005). Love et al. (2009) developed a new strategy to manage L. camara invasion in Indian forest ecosystems, which is to first remove the L. camara plants by cutting rootstock method followed by weeding of its saplings under the trees (to prevent further seed dispersal) and ecological restoration of grasslands/forests as per the stakeholder needs. Cost–benefit analyses of different management options need to be considered while devising the appropriate control plan (Negi et al., 2019). Successful management of L. camara invasion required an integrated approach involving all stakeholders, including state governments, national networks, and, where necessary, global coordination (Adhikari et al., 2024).
The invasion of L. camara in tropical dry deciduous forests significantly alters diversity, biomass, and C pools, as well as their allocation patterns, in Central Indian forests. However, it is important to note that this study is based on a one-time survey, meaning that the invaded sites may have had lower diversity and carbon levels even before the invasion. However, efforts were made to ensure that the study design adequately captured the differences between uninvaded and invaded sites. For example, the UI plots were captured at distances of 50–100 m from LI plots under similar site conditions. These findings emphasize the critical need for long-term monitoring and effective management of L. camara to prevent its further spread and mitigate future ecological and biodiversity-related challenges.
In the present study, LI sites had lower diversity and biomass C stocks in tree juveniles and herbs but higher SOC and litter C compared to UI sites. The density of L. camara was negatively correlated with plant diversity, tree juvenile C, herb C, and total ecosystem C storage, while it was positively correlated with litter C and SOC. These findings reveal that plant species richness and C pools tend to be altered by invasion, which has important implications for C cycling. As L. camara reduces tree regeneration, it could gradually shift diversity patterns across the landscape. Furthermore, although shrub biomass may increase due to L. camara invasion, the reduction in tree regeneration is likely to disrupt the overall balance of ecosystem-level C pool partitioning in the long run. This study improves our understanding of how shrub invasion impacts diversity and ecosystem-level carbon storage, highlighting the need for long-term monitoring, conservation, and restoration efforts to mitigate these effects.
To effectively manage the spread of this invasive shrub, an integrated approach should be adopted, involving a thorough analysis of the cost–benefit dynamics and the ecological and socioeconomic consequences. Additionally, more research is needed to understand periodic changes in plant diversity and C pool partitioning following L. removal, which could inform more effective management and restoration strategies.
The original contributions presented in the study are included in the article/Supplementary materials, further inquiries can be directed to the corresponding author/s.
PL: Data curation, Formal analysis, Investigation, Project administration, Software, Writing – original draft, Writing – review & editing. SK: Conceptualization, Data curation, Formal analysis, Investigation, Methodology, Resources, Software, Supervision, Validation, Visualization, Writing – original draft, Writing – review & editing. JD: Data curation, Formal analysis, Investigation, Project administration, Software, Writing – original draft, Writing – review & editing, Conceptualization, Funding acquisition, Methodology, Resources, Supervision, Validation, Visualization. KH: Formal analysis, Writing – review & editing. MK: Conceptualization, Data curation, Formal analysis, Funding acquisition, Investigation, Methodology, Project administration, Resources, Software, Supervision, Validation, Visualization, Writing – original draft, Writing – review & editing.
The author(s) declare that financial support was received for the research, authorship, and/or publication of this article. JD would like to thank the Science & Engineering Research Board, Department of Science & Technology (DST-SERB), Government of India, for funding (Reference No.: SRG/2022/002286), SRM University-AP for the Seed Grant (SRMAP/URG/E&PP/2022–23/012), and SK acknowledges the Science and Engineering Research Board, Department of Science and Technology (DST-SERB) for the National Postdoctoral Fellowship (PDF/2021/003742/LS). MK thankfully acknowledges the Department of Biotechnology (DBT), Government of India (No. BT/PR12899/NDB/39/506/2015 dt. 20/06/2017) for partial funding.
We are thankful to the Madhya Pradesh State Forest Department for permission and for providing the necessary facilities and staff support during the fieldwork.
The authors declare that the research was conducted in the absence of any commercial or financial relationships that could be construed as a potential conflict of interest.
The author(s) declared that they were an editorial board member of Frontiers, at the time of submission. This had no impact on the peer review process and the final decision.
All claims expressed in this article are solely those of the authors and do not necessarily represent those of their affiliated organizations, or those of the publisher, the editors and the reviewers. Any product that may be evaluated in this article, or claim that may be made by its manufacturer, is not guaranteed or endorsed by the publisher.
The Supplementary material for this article can be found online at: https://www.frontiersin.org/articles/10.3389/ffgc.2025.1412130/full#supplementary-material
Adhikari, P., Lee, Y. H., Adhikari, P., Poudel, A., Choi, S. H., Yun, J. Y., et al. (2024). Global invasion risk assessment of Lantana camara, a highly invasive weed, under future environmental change. Global Ecol. Conserv. 55:e03212. doi: 10.1016/j.gecco.2024.e03212
Adomako, M. O., Ning, L., Tang, M., Du, D. L., van Kleunen, M., and Yu, F. H. (2019). Diversity-and density-mediated allelopathic effects of resident plant communities on invasion by an exotic plant. Plant Soil 440, 581–592. doi: 10.1007/s11104-019-04123-9
Alemu, S. C., and Terefe, A. A. (2015). Impact of invasion: a case study on the ecological and socioeconomic impact of lantana Camara (L.) in abay millennium park (AMP), Bahir Dar, Ethiopia. J Ecol Nat Environ 7, 132–145. doi: 10.5897/JENE2015.0514
Ambika, S. R., Poornima, S., Palaniraj, R., Sati, S. C., and Narwal, S. S. (2003). Allelopathic plants. 10. Lantana camara L. Allelopath. J. 12, 147–161.
Asner, G. P., Flint, R., Varga, T. A., Knapp, D. E., and Kennedy-Bowdoin, T. (2008). Environmental and biotic controls over aboveground biomass throughout a tropical rain forest. Ecosystems 12, 261–278. doi: 10.1007/s10021-008-9221-5
Asner, G. P., Martin, R. E., Knapp, D. E., and Kennedy-Bowdoin, T. (2010). Effects of Morella faya tree invasion on aboveground carbon storage in Hawaii. Biol. Invasions 12, 477–494. doi: 10.1007/s10530-009-9452-1
Baboo, B., Sagar, R., Bargali, S. S., and Verma, H. (2017). Tree species composition, regeneration and diversity of an Indian dry tropical forest protected area. Trop. Ecol. 58, 409–423.
Baishya, R., Barik, S. K., and Upadhaya, K. (2009). Distribution pattern of aboveground biomass in natural and plantation forests of humid tropics in northeast India. Trop. Ecol. 50, 295–304.
Beer, C., Reichstein, M., Tomelleri, E., Ciais, P., Jung, M., Carvalhais, N., et al. (2010). Terrestrial gross carbon dioxide uptake: global distribution and covariation with climate. Science 329, 834–838. doi: 10.1126/science.1184984
Bhagwat, S. A., Breman, E., Thekaekara, T., Thornton, T. F., and Willis, K. J. (2012). A battle lost? Report on two centuries of invasion and management of Lantana camara L. in Australia, India and South Africa. PLoS One 7:e32407. doi: 10.1371/journal.pone.0032407
Bjerknes, A. L., Totland, Ø., Hegland, S. J., and Nielsen, A. (2007). Do alien plant invasions really affect pollination success in native plant species? Biol. Conserv. 138, 1–12. doi: 10.1016/j.biocon.2007.04.015
Bonino, E. E. (2006). Changes in carbon pools associated with a land-use gradient in the Dry Chaco, Argentina. For. Ecol. Manag. 223:183. doi: 10.1016/j.foreco.2005.10.069
Borah, N., Nath, A. J., and Das, A. K. (2013). Aboveground biomass and carbon stocks of tree species in tropical forests of Cachar District, Assam, Northeast India. Int. J. Ecol. Environ. Sci. 39, 97–106.
Brown, S., Schroeder, P., and Birdsey, R. (1997). Aboveground biomass distribution of US eastern hardwood forests and the use of large trees as an indicator of forest development. For. Ecol. Manag. 96, 37–47. doi: 10.1016/S0378-1127(97)00044-3
Cairns, M. A., Brown, S., Helmer, E. H., and Baumgardner, G. A. (1997). Root biomass allocation in the world's upland forests. Oecologia 111, 1–11.
Champion, H. G., and Seth, S. K. (1968). A Revised Survey of the Forest Types of India. Delhi, India: The Manager of Publications.
Chaturvedi, R. K., Raghubanshi, A. S., and Singh, J. S. (2012a). Biomass estimation of dry tropical woody species at juvenile stage. The Sci. World J 2012, 1–5. doi: 10.1100/2012/790219
Chaturvedi, R. K., Raghubanshi, A. S., and Singh, J. S. (2012b). Effect of grazing and harvesting on diversity, recruitment and carbon accumulation of juvenile trees in tropical dry forests. For. Ecol. Manag. 284, 152–162. doi: 10.1016/j.foreco.2012.07.053
Chave, J., Réjou-Méchain, M., Búrquez, A., Chidumayo, E., Colgan, M. S., Delitti, W. B. C., et al. (2014). Improved allometric models to estimate the aboveground biomass of tropical trees. Glob. Chang. Biol. 20, 3177–3190. doi: 10.1111/gcb.12629
Dar, J. A., Subashree, K., Sundarapandian, S., Saikia, P., Kumar, A., Khare, P. K., et al. (2019). “Invasive species and their impact on tropical forests of Central India: A Review” in Tropical Ecosystems: Structure, Functions and Challenges in the Face of Global Change (Singapore: Springer), 69–109.
Dixon, R. K., Solomon, A. M., Brown, S., Houghton, R. A., Trexier, M. C., and Wisniewski, J. (1994). Carbon pools and flux of global forest ecosystems. Science 263, 185–190. doi: 10.1126/science.263.5144.185
Ehrenfeld, J. G. (2003). Effects of exotic plant invasions on soil nutrient cycling processes. Ecosystems 6, 503–523. doi: 10.1007/s10021-002-0151-3
Ehrenfeld, J. G. (2010). Ecosystem consequences of biological invasions. Annu. Rev. Ecol. Evol. Syst. 41, 59–80. doi: 10.1146/annurev-ecolsys-102209-144650
Ens, E. J., and French, K. (2008). Exotic woody invader limits the recruitment of three indigenous plant species. Biol. Conserv. 141, 590–595. doi: 10.1016/j.biocon.2007.12.012
Fisher, J. L., Loneragan, W. A., Dixon, K., Delaney, J., and Veneklaas, E. J. (2009). Altered vegetation structure and composition linked to fire frequency and plant invasion in a biodiverse woodland. Biological Conservation 142, 2270–2281.
Fornara, D. A., and Tilman, D. (2008). Plant functional composition influences rates of soil carbon and nitrogen accumulation. J. Ecol. 96, 314–322. doi: 10.1111/j.1365-2745.2007.01345.x
Gaertner, M., Den Breeyen, A., Hui, C., and Richardson, D. M. (2009). Impacts of alien plant invasions on species richness in Mediterranean-type ecosystems: a meta-analysis. Progress in Physical Geography 33, 319–338.
Gaudel, G., Hui, Z. W., Hung, D. Q., Hien, L. T., and Xiao, L. (2016). Assessment of Invasive Alien Plant Species: Impact on Carbon Sequestration. Imp. J. Interdiscip. Res. 2, 51–65.
Gentle, C. B., and Duggin, J. A. (1997). Allelopathy as a competitive strategy in persistent thickets of Lantana camara L. in three Australian forest communities. Plant Ecol. 132, 85–95. doi: 10.1023/A:1009707404802
Gioria, M., Jarošík, V., and Pyšek, P. (2014). Impact of invasions by alien plants on soil seed bank communities: emerging patterns. Perspec. Plant Ecol. Evol. System. 16, 132–142.
Global Invasive Species Database (2020). Species profile: Lantana camara. Available at: https://www.iucngisd.org/gisd/species.php?sc=56 on 28-12-2020.
Gómez-Rey, M. X., Madeira, M., Gonzalez-Prieto, S. J., and Coutinho, J. (2013). Soil C and N dynamics in a Mediterranean oak woodland with shrub encroachment. Plant Soil 371, 339–354. doi: 10.1007/s11104-013-1695-z
Gooden, B., French, K., and Turner, P. J. (2009b). Invasion and management of a woody plant, Lantana camara L., alters vegetation diversity within wet sclerophyll forest in southeastern Australia. For. Ecol. Manag. 257, 960–967. doi: 10.1016/j.foreco.2008.10.040
Gooden, B., French, K., Turner, P. J., and Downey, P. O. (2009a). Impact threshold for an alien plant invader, Lantana camara L., on native plant communities. Biol. Conserv. 142, 2631–2641. doi: 10.1016/j.biocon.2009.06.012
Goyal, N., Esler, K. J., and Sharma, G. P. (2018). What drives performance potential of Lantana camara L. (sensu lato) in the invaded range? Tropic. Ecol. 59, 57–68.
Hejda, M., Pyšek, P., and Jarošík, V. (2009). Impact of invasive plants on the species richness, diversity and composition of invaded communities. J. Ecol. 97, 393–403. doi: 10.1111/j.1365-2745.2009.01480.x
Hibbard, K. A., Archer, S., Schimel, D. S., and Valentine, D. W. (2001). Biogeochemical changes accompanying woody plant encroachment in a subtropical savanna. Ecology 82, 1999–2011. doi: 10.1890/0012-9658(2001)082[1999:BCAWPE]2.0.CO;2
Hiremath, A. J., and Sundaram, B. (2005). The fire-lantana cycle hypothesis in Indian forests. Conserv. Soc. 3, 26–42.
Hughes, R. F., Archer, S. R., Asner, G. P., Wessman, C. A., McMurtry, C., Nelson, J., et al. (2006). Changes in aboveground primary production and carbon and nitrogen pools accompanying woody plant encroachment in a temperate savanna. Glob. Chang. Biol. 12, 1733–1747. doi: 10.1111/j.1365-2486.2006.01210.x
Hughes, R.F., Asner, G.P., Litton, C.M., Selmants, P.C., Hawbaker, T.J., Jacobi, J.D., et al., (2017). Influence of invasive species on carbon storage in Hawai‘i's ecosystems. Baseline and projected future carbon storage and carbon fluxes in ecosystems of Hawai'I (pp.43–55). US Geological Survey Professional Paper 1834 U.S. Geological Survey Publications Warehouse.
India State of Forest Report (2019) Forest Survey of India, Edition 16, Ministry of Environment, Forest & Climate Change, Government of India. Available at:https://www.fsi.nic.in/forest-report-2019.
IPCC Guidelines for national greenhouse gas inventories. Vol. 4. Hayama (Japan): Agriculture, Forestry and Other Land Use (AFLOLU), Institute for Global Environmental Strategies ; Task Force on National Greenhouse Gas Inventories. (2006)
Jackson, R. B., Banner, J. L., Jobbágy, E. G., Pockman, W. T., and Wall, D. H. (2002). Ecosystem carbon loss with woody plant invasion of grasslands. Nature 418, 623–626. doi: 10.1038/nature00910
Jaramillo, V. J., Kauffman, J. B., Rentería-Rodríguez, L., Cummings, D. L., and Ellingson, L. J. (2003). Biomass, carbon, and nitrogen pools in Mexican tropical dry forest landscapes. Ecosystems 6, 609–629. doi: 10.1007/s10021-002-0195-4
Jhariya, M. K. (2017). Vegetation ecology and carbon sequestration potential of shrubs in tropics of Chhattisgarh, India. Environ. Monit. Assess. 189, 1–15. doi: 10.1007/s10661-017-6246-2
Jhariya, M. K., Bargali, S. S., Swamy, S. L., Kittur, B., Kiran, B., and Pawar, G. V. (2014). Impact of forest fire on biomass and carbon storage pattern of tropical deciduous forests in Bhoramdeo Wildlife Sanctuary, Chhattisgarh. Int. J. Ecol. Environ. Sci. 40, 57–74.
Knight, D. H. (1963). A distance method for constructing forest profile diagrams and obtaining structural data. Trop. Ecol. 4, 89–94.
Kothandaraman, S., Dar, J. A., Sundarapandian, S., Dayanandan, S., and Khan, M. L. (2020). Ecosystem-level carbon storage and its links to diversity, structural and environmental drivers in tropical forests of Western Ghats, India. Sci. Rep. 10:13444. doi: 10.1038/s41598-020-70313-6
Kumar, M., Verma, A. K., and Garkoti, S. C. (2020). Lantana camara and Ageratina adenophora invasion alter the understory species composition and diversity of chir pine forest in central Himalaya, India. Acta Oecol. 109:103642. doi: 10.1016/j.actao.2020.103642
Lewis, S. L., Phillips, O. L., Baker, T. R., Lloyd, J., Malhi, Y., Almeida, S., et al. (2004). Concerted changes in tropical forest structure and dynamics: evidence from 50 South American long-term plots. Philos. Trans. R. Soc. Lond. Ser. B Biol. Sci. 359, 421–436. doi: 10.1098/rstb.2003.1431
Li, H., Shen, H., Chen, L., Liu, T., Hu, H., Zhao, X., et al. (2016). Effects of shrub encroachment on soil organic carbon in global grasslands. Sci. Rep. 6, 1–9. doi: 10.1038/srep28974
Liao, C. Z., Luo, Y. Q., Fang, C. M., Chen, J. K., and Li, B. (2008b). Litter pool sizes, decomposition, and nitrogen dynamics in Spartina alterniflora-invaded and native coastal marshlands of the Yangtze Estuary. Oecologia 156, 589–600. doi: 10.1007/s00442-008-1007-0
Liao, C., Luo, Y., Jiang, L., Zhou, X., Wu, X., Fang, C., et al. (2007). Invasion of Spartina alterniflora enhanced ecosystem carbon and nitrogen stocks in the Yangtze Estuary, China. Ecosystems 10, 1351–1361. doi: 10.1007/s10021-007-9103-2
Liao, C. Z., Peng, R., Luo, Y., Zhou, X., Wu, X., Fang, C., et al. (2008a). Altered ecosystem carbon and nitrogen cycles by plant invasion: a meta-analysis. New Phytol. 177, 706–714. doi: 10.1111/j.1469-8137.2007.02290.x
Litton, C. M., Sandquist, D. R., and Cordell, S. (2006). Effects of non-native grass invasion on aboveground carbon pools and tree population structure in a tropical dry forest of Hawaii. For. Ecol. Manag. 231:105. doi: 10.1016/j.foreco.2006.05.008
Lone, P. A., Dar, J. A., Subashree, K., and Khan, M. L. (2022). Invasive shrub Lantana camara L. alters the flora and soils in tropical dry deciduous forests of Central India. Biotropica 54, 1412–1427. doi: 10.1111/btp.13112
Lone, P. A., Dar, J. A., Subashree, K., Raha, D., Pandey, P. K., Ray, T., et al. (2019). Impact of plant invasion on physical, chemical and biological aspects of ecosystems: A review. Trop. Plant Res. 6, 528–544. doi: 10.22271/tpr.2019.v6.i3.067
Love, A., Babu, S., and Babu, C. R. (2009). Management of Lantana, an invasive alien weed, in forest ecosystems of India. Curr. Sci., 97, 1421–1429.
Luna, R. K., Manhas, R. K., Banyal, S., and Kamboj, S. K. (2009). Effect of Lantana camara Linn. on biomass production and carrying capacity of forest areas of Shiwalik hills of Punjab. Indian Forester 135, 869–879.
Mandal, G., and Joshi, S. P. (2014). The role of habitat types and soil physicochemical properties in the spread of a non- native shrub Lantana camara in the Doon valley, Western Himalaya, India. J. Environ. Geog. 7, 31–42. doi: 10.2478/jengeo-2014-0010
Mandal, G., and Joshi, S. P. (2015a). Eco-physiology and habitat invasibility of an invasive, tropical shrub (Lantana camara) in western Himalayan forests of India. For. Sci. Tech. 11, 182–196. doi: 10.1080/21580103.2014.990062
Mandal, G., and Joshi, S. P. (2015b). Estimation of above-ground biomass and carbon stock of an invasive woody shrub in the subtropical deciduous forests of Doon Valley, western Himalaya, India. J. Fores. Res. 26, 291–305. doi: 10.1007/s11676-015-0038-8
Mason, T. J., and French, K. (2008). Impacts of a woody invader vary in different vegetation communities. Diversity and Distributions 14, 829–838.
Mungi, N. A., Coops, N. C., Ramesh, K., and Rawat, G. S. (2018). How global climate change and regional disturbance can expand the invasion risk? Case study of Lantana camara invasion in the Himalaya. Biol. Invasions 20, 1849–1863. doi: 10.1007/s10530-018-1666-7
Mungi, N. A., Qureshi, Q., and Jhala, Y. V. (2020). Expanding niche and degrading forests: Key to the successful global invasion of Lantana camara (sensu lato). Glob. Ecol. Conserv. 23:e01080. doi: 10.1016/j.gecco.2020.e01080
Murphy, P. G., and Lugo, A. E. (1986). Ecology of tropical dry forest. Annu. Rev. Ecol. Evol. Syst. 17, 67–88. doi: 10.1146/annurev.es.17.110186.000435
Nanjappa, H. V., Saravanane, P., and Ramachandrappa, B. K. (2005). Biology and management of Lantana camara L.–a review. Agric. Rev. 26, 272–280.
Negi, G. C., Sharma, S., Vishvakarma, S. C., Samant, S. S., Maikhuri, R. K., Prasad, R. C., et al. (2019). Ecology and use of Lantana camara in India. Bot. Rev. 85, 109–130. doi: 10.1007/s12229-019-09209-8
Nie, M., Shangc, L., Liao, C., and Li, B. (2017). “Changes in primary production and carbon sequestration after plant invasions” in Impact of biological invasions on ecosystem services (Cham: Springer), 17–31.
Oraon, P. R. (2012). Structure and dry matter dynamics along thedisturbance gradient of tropical dry deciduous forest in Bhoramdeo Wildlife Sanctuary, Chhattisgarh. [Ph.D. thesis], I.G.K.V., Raipur (C.G.).
Pan, Y., Birdsey, R. A., Fang, J., Houghton, R., Kauppi, P. E., Kurz, W. A., et al. (2011). A large and persistent carbon sink in the world’s forests. Science 333, 988–993. doi: 10.1126/science.1201609
Pande, P. K. (2005). Biomass and productivity in some disturbed tropical dry deciduous teak forests of Satpura plateau, Madhya Pradesh. Trop. Ecol. 46, 229–239.
Pearson, T., Walker, S., and Brown, S. (2005). Sourcebook for land use, land-use change and forestry. USA: Projects Winrock International, 35.
Pejchar, L., and Mooney, H. A. (2009). Invasive species, ecosystem services and human well-being. Trends Ecol. Evol. 24, 497–504. doi: 10.1016/j.tree.2009.03.016
Raha, D., Dar, J. A., Pandey, P. K., Lone, P. A., Verma, S., Khare, P. K., et al. (2020). Variation in tree biomass and carbon stocks in three tropical dry deciduous forest types of Madhya Pradesh, India. Carbon Manag. 11, 109–120. doi: 10.1080/17583004.2020.1712181
Ramaswami, G., and Sukumar, R. (2011). Woody plant seedling distribution under invasive Lantana camara thickets in a dry-forest plot in Mudumalai, southern India. J. Trop. Ecol. 27, 365–373. doi: 10.1017/S0266467411000137
Ramaswami, G., and Sukumar, R. (2016). Invasive plants in the tropics and the Case of Lantana camara. Tropical Conservation: Perspectives on Local and Global Priorities, 154.
Ravindranath, N. H., and Ostwald, M. (2008). Carbon inventory methods: handbook for greenhouse gas inventory, carbon mitigation and roundwood production projects : Springer Science & Business Media, Springer Dordrecht.
Richardson, D. M., Pysek, P., and Carlton, J. T. (2011). “A compendium of essential concepts and terminology in biological invasions” in Fifty years of invasion ecology: the legacy of Charles Elton. ed. D. M. Richardson (Oxford, UK: Wiley-Blackwell), 409–420.
Saxena, K. G. (1991). “Biological invasions in the Indian subcontinent: review on invasion by plants” in Ecology of Biological Invasion in the Tropics. ed. P. S. Ramakrishnan (New Delhi, India: International Scientific Publishers), 53–73.
Saxena, A. K., and Singh, J. S. (1985). Tree population structure of certain Himalayan forest associations and implications concerning their future composition. Vegetatio 58, 61–69. doi: 10.1007/BF00044928
Schnitzer, S. A., DeWalt, S. J., and Chave, J. (2006). Censusing and Measuring Lianas: A Quantitative Comparison of the Common Methods 1. Biotropica 38, 581–591. doi: 10.1111/j.1744-7429.2006.00187.x
Sharma, G. P., and Raghubanshi, A. S. (2006). Tree population structure, regeneration and expected future composition at different levels of Lantana camara L. invasion in the Vindhyan tropical dry deciduous forest of India. Lyonia 11, 27–39.
Sharma, G. P., and Raghubanshi, A. S. (2007). Effect of Lantana camara L. cover on local depletion of tree population in the Vindhyan tropical dry deciduous forest of India. Appl. Ecol. Environ. Res. 5, 109–121. doi: 10.15666/aeer/0501_109121
Sharma, G. P., Raghubanshi, A. S., and Singh, J. S. (2005). Lantana invasion: an overview. Weed Biol. Manag. 5, 157–165. doi: 10.1111/j.1445-6664.2005.00178.x
Singh, L., and Singh, J. S. (1991). Species structure, dry matter dynamics and carbon flux of a dry tropical forest in India. Ann. Bot. 68, 263–273. doi: 10.1093/oxfordjournals.aob.a088252
SPSS Inc (2013). Released 2013. IBM SPSS Statistics for Windows, Version 22.0. Armonk, NY: IBM Corp.
Stock, W. D., Wienand, K. T., and Baker, A. C. (1995). Impacts of invading N 2-fixing Acacia species on patterns of nutrient cycling in two Cape ecosystems: evidence from soil incubation studies and 15 N natural abundance values. Oecologia 101, 375–382. doi: 10.1007/BF00328825
Sullivan, M. J., Talbot, J., Lewis, S. L., Phillips, O. L., Qie, L., Begne, S. K., et al. (2017). Diversity and carbon storage across the tropical forest biome. Sci. Rep. 7:39102. doi: 10.1038/srep39102
Sundaram, B., and Hiremath, A. J. (2012). Lantana camara invasion in a heterogeneous landscape: patterns of spread and correlation with changes in native vegetation. Biol. Invasions 14, 1127–1141. doi: 10.1007/s10530-011-0144-2
Swamy, S. L., Dutt, C. B. S., Murthy, M. S. R., Mishra, A., and Bargali, S. S. (2010). Floristics and dry matter dynamics of tropical wet evergreen forests of Western Ghats, India. Curr. Sci. 99, 353–364.
Taylor, S., Kumar, L., Reid, N., and Kriticos, D. J. (2012). Climate change and the potential distribution of an invasive shrub, Lantana camara. PLoS One 7:e35565. doi: 10.1371/journal.pone.0035565
Tilman, D., Reich, P. B., Knops, J., Wedin, D., Mielke, T., and Lehman, C. (2001). Diversity and productivity in a long-term grassland experiment. Science 294, 843–845. doi: 10.1126/science.1060391
Timsina, B., Shrestha, B. B., Rokaya, M. B., and Münzbergová, Z. (2011). Impact of Parthenium hysterophorus L. invasion on plant species composition and soil properties of grassland communities in Nepal. Flora Morphol. Distrib. Funct. Ecol. Plants 206, 233–240. doi: 10.1016/j.flora.2010.09.004
Vilà, M., Espinar, J. L., Hejda, M., Hulme, P. E., Jarošík, V., and Maron, J. L. (2011). Ecological impacts of invasive alien plants: a meta-analysis of their effects on species, communities and ecosystems. Ecol. Lett. 14, 702–708. doi: 10.1111/j.1461-0248.2011.01628.x
Vila, M., and Weiner, J. (2004). Are invasive plant species better competitors than native plant species? Evidence from pairwise experiments. Oikos 105, 229–238. doi: 10.1111/j.0030-1299.2004.12682.x
Vitousek, P. M., D’Antonio, C. M., Loope, L. L., and Westbrooks, R. (1996). Biological invasions as global environmental change. Am. Sci. 84, 468–478.
Vitousek, P. M., D'antonio, C. M., Loope, L. L., Rejmanek, M., and Westbrooks, R. (1997). Introduced species: a significant component of human-caused global change. N. Z. J. Ecol. 21, 1–16.
Wagh, V. V., and Jain, A. K. (2018). Status of ethnobotanical invasive plants in western Madhya Pradesh, India. S. Afr. J. Bot. 114, 171–180. doi: 10.1016/j.sajb.2017.11.008
Walck, J. L., Baskin, J. M., and Baskin, C. C. (1999). Effects of competition from introduced plants on establishment, survival, growth and reproduction of the rare plant Solidago shortii (Asteraceae). Biol. Conserv. 88, 213–219. doi: 10.1016/S0006-3207(98)00097-4
Walkley, A., and Black, I. A. (1934). An examination of the Degtjareff method for determining soil organic matter, and a proposed modification of the chromic acid titration method. Soil Sci. 37, 29–38. doi: 10.1097/00010694-193401000-00003
WorldClim (2024). Available at:https://www.worldclim.org/
Yang, W., Zhao, H., Chen, X., Yin, S., Cheng, X., and An, S. (2013). Consequences of short-term C4 plant Spartina alterniflora invasions for soil organic carbon dynamics in a coastal wetland of Eastern China. Ecol. Eng. 61, 50–57. doi: 10.1016/j.ecoleng.2013.09.056
Yurkonis, K. A., Meiners, S. J., and Wachholder, B. E. (2005). Invasion impacts diversity through altered community dynamics. J. Ecol. 93, 1053–1061. doi: 10.1111/j.1365-2745.2005.01029.x
Zanne, A.E., Lopez-Gonzalez, G., Coomes, D.A., Ilic, J., Jansen, S., Lewis, S.L., et al., (2009). Global wood density database. Dryad Identifier. Available at:http://hdl.handle.net/10255/dryad.235
Keywords: shrub invasion, tree diversity, carbon pools, tropical dry deciduous forests, Central India
Citation: Lone PA, Kothandaraman S, Dar JA, Hakeem KR and Khan ML (2025) Invasive shrub (Lantana camara L.) alters the tree diversity and ecosystem-level carbon pools in tropical forests of Central India. Front. For. Glob. Change. 8:1412130. doi: 10.3389/ffgc.2025.1412130
Received: 04 April 2024; Accepted: 23 January 2025;
Published: 21 February 2025.
Edited by:
Greg R. Guerin, University of Adelaide, AustraliaCopyright © 2025 Lone, Kothandaraman, Dar, Hakeem and Khan. This is an open-access article distributed under the terms of the Creative Commons Attribution License (CC BY). The use, distribution or reproduction in other forums is permitted, provided the original author(s) and the copyright owner(s) are credited and that the original publication in this journal is cited, in accordance with accepted academic practice. No use, distribution or reproduction is permitted which does not comply with these terms.
*Correspondence: Javid Ahmad Dar, amF2aWRhaG1hZC5kQHNybWFwLmVkdS5pbg==
Disclaimer: All claims expressed in this article are solely those of the authors and do not necessarily represent those of their affiliated organizations, or those of the publisher, the editors and the reviewers. Any product that may be evaluated in this article or claim that may be made by its manufacturer is not guaranteed or endorsed by the publisher.
Research integrity at Frontiers
Learn more about the work of our research integrity team to safeguard the quality of each article we publish.