- 1College of Chemical and Environmental Engineering, Pingdingshan University, Pingdingshan, China
- 2Henan Key Laboratory of Germplasm Innovation and Utilization of Eco-economic Woody Plant (Pingdingshan University), Pingdingshan, China
The low hilly area is a major landform in the west of Henan province, China, and it is suffering soil and water loss because of human activities. The silvopastoral system that combines trees and grasses has been widely used to restore this fragile area. We conducted in situ field experiments in 2011 in the low hilly area of Henan province involving pure forests of Populus simonii (PS; Salicaceae), Platycladus orientalis (PO; Cupressaceae), Quercusvariabilis (QV; Fagaceae), and Robinia pseudoacacia (RP; Fabaceae), and also with each forest tree species being combined with Medicago sativa (MS; Fabaceae) as silvopastoral systems, i.e., PS-MS, PO-MS, QV-MS, and RP-MS, respectively. We recorded tree diameter at breast height (DBH) and tree height (TH) in the years 2014–2016, 2018, 2020, and 2022 for all the different vegetation types. Tree biomass load (TBL, i.e., tree biomass per unit area) was estimated based on DBH and TH using allometric equations for each tree species. Generally, the results showed that the DBH, TH, and TBL were promoted in the silvopastoral systems PS-MS, PO-MS, and QV-MS. Specially, the DBH and TBL of PS-MS and PO-MS tended to be higher and were significantly higher than the pure forests of PS and MS, respectively, in 2014–2016; the silvopastoral systems were not significantly different from the pure forests for DBH in 2018 and 2022, and for TBL in 2018 and 2020. TH was lower in PS-MS and PO-MS than that in PS and PO in 2014–2016, while there was no difference of TH between PS-MS and PS in 2018 and 2022, and TH was higher in PO-MS than that in PO in 2018, 2020 and 2022. The DBH, TH, and TBL were all higher in QV-MS than QV. The TBL in RP-MS was non-significant or lower compared to that in RP. Moreover, TBL tended to be lower in upslope positions than downslope in the pure forests, while there was no difference in most silvopastoral systems. In summary, PS and PO may be suggested as the tree species selection in vegetation restoration processes for about five years, QV may be a better alternative for a long term, while RP is not recommended.
1 Introduction
Low hilly areas cover about 28% of China with an elevation of 200–500 m (Li et al., 2023; Xiao et al., 2023). This landform is characterized as a species reservoir of plants and invertebrates (Chen and Cao, 2014), with the main ecological functions of conserving water resources and preventing soil erosion (Chen et al., 2007, 2019). However, these areas are experiencing water and soil loss because of human activities (i.e., overgrazing, firewood mining, stone mining, and tree over-cutting; Zhang et al., 2018; Minea et al., 2022), resulting in the soil becoming barren with stones. The serious soil erosion of the low hilly areas has side effects on ecosystem health and ecosystem services, e.g., soil quality degradation, biodiversity decreases, and carbon release (Kihara et al., 2020).
Pure forests and silvopastoral systems (i.e., forest and grass composite systems, see Nair, 1985) have been widely used to restore these ecologically fragile areas (Grewal et al., 1994; Chebli et al., 2021; Olaya-Montes et al., 2021). Compared to pure forests, silvopastoral systems can often increase soil fertility (Amatya et al., 2002; He et al., 2013; Fuchs et al., 2022) and increase herbaceous plant/animal biodiversity (Wyss, 1996; Kong et al., 2001; Santos et al., 2019). However, most studies are confined to the effect of silvopastoral systems on soil quality, and the responses of tree growth to silvopastoral systems are not well examined in low hilly areas.
Silvopastoral systems can increase the utilization rate of light energy and promote the forest tree growth, improving its economic yield. For example, some studies have shown that planting herbage, especially leguminous species in young woodland or open woodland, can significantly promote the forest tree growth, shorten the closure years, and improve woodland productivity (Clason, 1998). The tree-trunk circumference of the apple–alfalfa composite system increased compared with the clearing areas (Fan et al., 2004), and there was a higher tree height in Populus sp. (Salicaceae) × Melilotus alba (Fabaceae) than in the pure forest (Yin et al., 1994). Nevertheless, other studies have shown that the intercropping of forest and grass has negative effects on tree growth. For example, alfalfa may affect the diameter growth and branch length of Juglans regia (Juglandaceae) due to the competition for water and nutrients (Paris et al., 1995). These results collectively show that tree growth may respond in complex ways to different planting systems. However, the response of tree growth to silvopastoral systems is not well examined under long-term monitoring. Considering that the reciprocal/competition relationship between the tree and the herbaceous plants (Muthuri et al., 2005), the responses of tree growth to silvopastoral systems in the long term in the context of climate change and anthropogenic disturbance may provide guidance for the orderly restoration in low hilly areas.
We restored the vegetation of silvopastoral and pure forest systems on a slope of a low hilly area of Henan province over a long-term scale of about ten years. We assumed that (1) the diameter breast height (DBH), tree height (TH), and tree biomass load (TBL) may be higher in the silvopastoral systems than the pure forests, and (2) the growth rate in the silvopastoral systems may be higher than the pure forests. Moreover, given that, compared to pure forests, the higher plant cover of the silvopastoral systems can decrease surface runoff, thus retaining water and soil on the low hilly slopes (Olsson et al., 2009; Sepúlveda and Carrillo, 2015; Mackay-Smith et al., 2022), we assumed that (3) the tree biomass would be higher in the downslope areas than the upslope areas in the pure forests, while there may be no difference of tree biomass among slopes in the silvopastoral systems.
2 Materials and methods
2.1 Study sites
The study was conducted in the low hilly region of western Henan Province (E112°40′, N33°45′). The altitude is ca. 220 m with an annual rainfall of 800–950 mm and an average annual temperature of 13–14°C. The dominant tree species are Albizzia kalkora (Fabaceae), Platycarya strobilacea (Juglandaceae), Platycladus orientali (Juglandaceae), Populus simonii (Salicaceae), Quercus aliena (Fagaceae), Quercus glandulifera (Fagaceae), Quercus variabilis (Fagaceae), and Robinia pseudoacacia (Fabaceae). The dominant herbaceous species are Carex lanceolata (Cyperaceae), Carex rigescens (Cyperaceae), Cyperus rotundus (Cyperaceae), Dendranthema indicum (Asteraceae), Melica scabrosa (Poaceae), and Phlomis umbrosa (Lamiaceae). The common planted trees are Q. variabilis, P. orientali, P. simonii, and R. pseudoacacia (Liu et al., 2014).
2.2 Experimental design
The study site was built on a homogeneous wasteland with a slope of 22° that had not been used for more than 20 years. The wasteland is covered by loam soil with a volume proportion of 15–20% of gravels in soil depth 0–50 cm, with almost no trees and grass. The few saplings and grass were removed. Common trees were selected, including the P. simonii, P. orientalis, Q. variabilis, and R. pseudoacacia. Q. variabilis and R. pseudoacacia are endemic, and P. simonii and P. orientalis are exotic. Previous studies have proved that nitrogen-fixing plants (e.g., Medicago sativa) can serve as pioneer species in barren hills in vegetation restoration (Yang et al., 2020) because they can increase the soil quality (e.g., soil nutrients and moisture; Li and Liber, 2018). In the study site, 10 plots were designed horizontally along the contour line, including: one M. sativa (MS); four silvopastoral systems: P. simonii × M. sativa (PS-MS), P. orientalis × M. sativa (PO-MS), Q. variabilis × M. sativa (QV-MS), and R. pseudoacacia × M. sativa (RP-MS); four pure forest areas: PS, PO, QV, and RP; and one plot as a control (CK). Each plot had an area of 300 m2 (20 m length × 15 m width) with 3 m isolation zones from each other. Every plot was divided into a total of 12 subsections, each with an area of 25 m2 (5 m length × 5 m width; see Supplementary Figure S1). The slopes along the contour line were further divided into four plots, i.e., S1, S2, S3, and S4, with each plot being 75 m2 (5 m length × 15 m width).
2.3 Tree biomass
The tree saplings for each species were uniform in thickness during the initial period of experimental plot construction in 2011. Each of the plots was planted with eight lines of trees for a total of 80 trees, and each slope included two lines of trees. The M. sativa in each silvopastoral system was sown with a sowing rate of 22.49–37.48 kg/ha along the contour (see Supplementary Figure S1).
In order to address the responses of tree growth, in the year of 2014–2016, 2018, 2020, and 2022, the diameter at breast height (cm; DBH) was recorded using a diameter-at-breast-height ruler (HYSTIC gnjz-680, Beijing) with an accuracy of 0.1 cm. Tree height (m; TH) was estimated using a Blume-Leiss Model BL6 ALTIMeter. The tree biomasses of PS, PO, QV, and RP were estimated based on DBH and TH, incorporating the allometric equations of previous studies (Liu et al., 1998; Li et al., 2007; Wang et al., 2009; Niu et al., 2017), and the whole individual tree biomass (WT) for each tree species including parts of the trunk, roots, and leaves was obtained (see Supplementary Table S1). Due to data loss of the downslopes of the pure forest of P. simonii in 2020, we only calculated the biomass of PO, QV, and RP in this year. To compare the tree biomass between the silvopastoral systems and pure forests, the tree biomass load (TBL) was defined as the biomass per individual tree per unit (g m−2):
where k is the number of individuals of trees on a slope and WT is one individual tree biomass.
2.4 Alfalfa biomass
In order to measure the alfalfa biomass, we randomly placed twelve 1 m × 1 m quadrats in each plot, each of which were divided into 10 × 10 cm grids to facilitate estimates of plant abundance in October 2015 and 2016. We then recorded species presence and abundance. Moreover, we harvested aboveground plant organs by clipping using scissors. Then, all the aboveground plant organs were divided into individual plant species by hand-sorting. The aboveground plant biomass for each plant species was weighed after drying for 72 h at 65°C.
2.5 Soil nutrients
A total of 96 soil cores were collected from the 0–20 cm soil layer using a soil auger of 100 cm3 in each subsection of silvopastoral systems and pure forests in these years. The soil nutrient contents of soil total nitrogen were examined for the years 2014, 2016, 2018, 2020, and 2022, and soil total carbon only for 2018, 2020, and 2022. The soil samplings were firstly crushed, and then the gravels and other impurities were removed. Then the soil was naturally dried in the shade and passed through a 100 mesh screen (0.149 mm). A CHNOS Elemental Analyzer (Vario Macro Cube; specific instructions on www.elementar.de) was used to determine the soil total carbon and nitrogen.
2.6 Data analysis
To approach normality, the DBH, TH, TBL, and soil nutrients were log (x + 1)-transformed. The t-test was used to determine the differences in DBH, TH, and TBL between PS-MS and PS, PO-MS and PO, QV-MS and QV, and RP-MS and RP in different years. In order to compare the tree growth rate between the silvopastoral systems and corresponding pure forests, the allometric relationships between TBL and years (the years 2014–2016, 2018, 2020, and 2022 were defined as 1–6) in different vegetation types were established. The slopes were assessed by the function “diffslope” in the package “simba” in the R platform (Jurasinski and Retzer, 2015). In addition, a linear regression equation was used to determine whether the soil total carbon and total nitrogen could significantly account for the nutrient retention capacity in the silvopastoral systems.
The changes in relative alfalfa biomass were calculated using the formula: RAlf = (SilAlf – PurAlf)/ SilAlf, where SilAlf and PurAlf are the alfalfa biomass in the silvopastoral systems and pure forests, respectively. The changes in relative tree growth of DBH, TH, and TBL were calculated using RDBH = (SilDBH – PurDBH)/ SilDBH, RTH = (SilTH – PurTH)/ SilTH, and RTBL = (SilTBL – PurTBL)/ SilTBL, where SilDBH, SilTH, and SilTBL are the diameter at breast height, tree height, and tree biomass load in the silvopastoral systems, respectively, and PurDBH, PurTH, and PurTBL are those in pure forests, respectively. RAlf, RDBH, RTH, and RTBL were all standardized to mean = 0 and variance = 1 (z-score transformation) using the function “decostand” in the package “vegan” (Oksanen et al., 2019). In order to test whether the alfalfa biomass affected tree growth, a linear regression equation was used to determine the relationships between RAlf and RDBH, RTH, RTBL for the years 2015 and 2016.
All the statistical analyses were conducted in R v4.0.0 (R Core Team, 2020).
3 Results
The DBH and TBL of PS-MS and PO-MS were significantly higher than the PS and PO in 2015 and 2016, whereas there were no differences in 2018 and 2022 (except DBH and TBL between PO-MS and PO in 2022). From 2014 to 2016, the TH in the silvopastoral systems of PS-MS and PO-MS were (almost all significantly) lower than those in the pure forests of PS and PO, whereas there was a trend in 2018 and 2022 that the TH in PS-MS and PO-MS was not significant with PS and PO or higher than PS and PO, indicating that the TH showed a higher growth rate in silvopastoral systems. The DBH, TH, and TBL in QV-MS were all higher in QV-PS than those in QV. From 2014 to 2016, TH tended to be higher in RP-MS than in RP, whereas the DBH was higher in RP than in RP-MS, and there was no difference for the TBL between RP-MS and RP. In 2018, 2020, and 2022, the TH was not significantly different between RP-MS and RP, the DBH tended to be lower in RP-MS than in RP, and the TBL was non-significantly different between RP-MS and RP (except in 2020 when it was lower in RP-MS than in RP; see Figure 1).
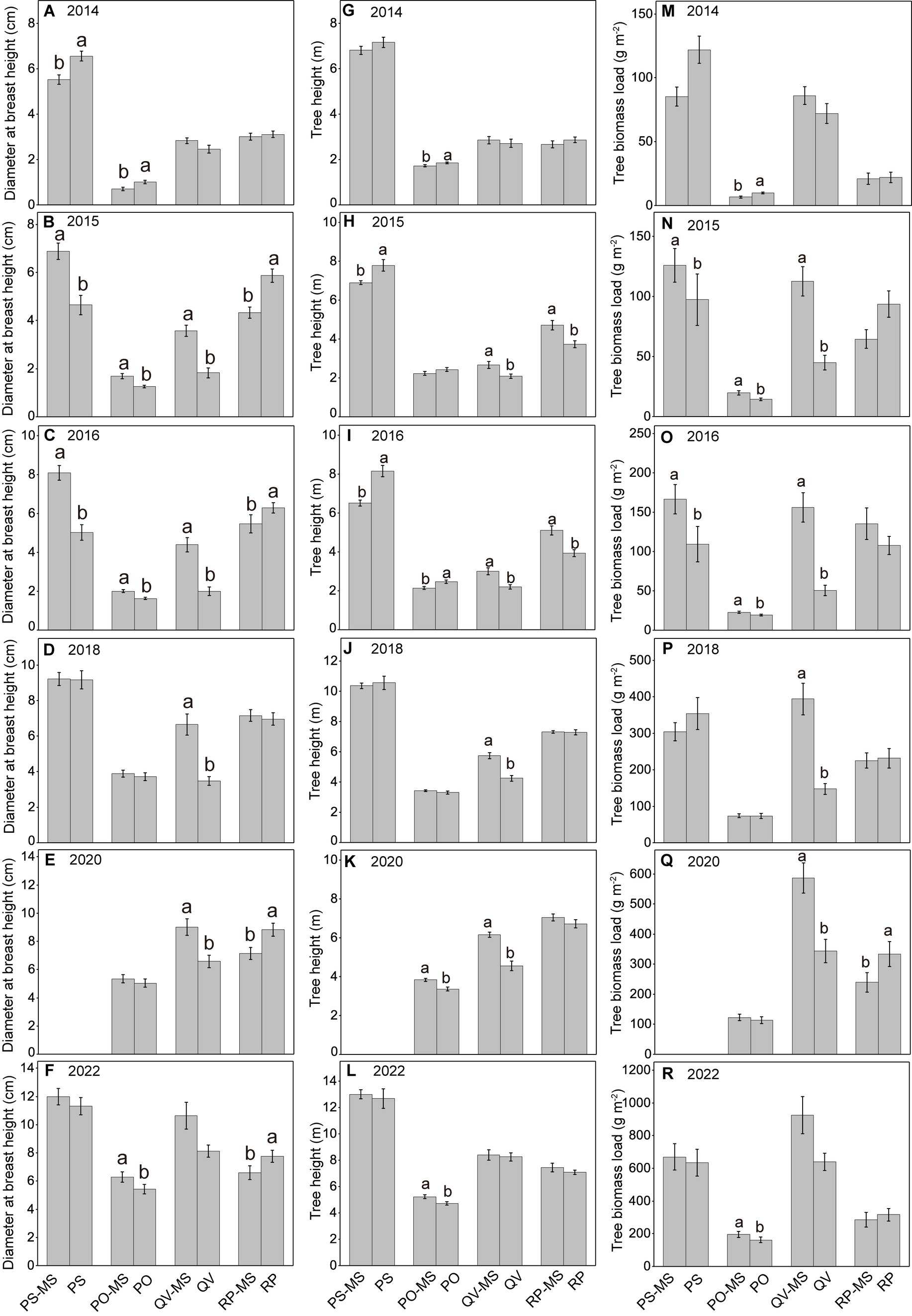
Figure 1. Variations in diameter breast height (A–F), tree height (G–L), and tree biomass load (M–R) with increasing years. Different lowercase letters above the error bars denote statistically significant differences between PS-MS (Populus simonii × Medicago sativa) and PS, between PO-MS (Platycladus orientalis× Medicago sativa) and PO, between QV-MS (Quercus variabilis× Medicago sativa) and QV, and between RP-MS (Robinia pseudoacacia × Medicago sativa) and RP. The differences between means are non-significant if no letters are provided in each panel.
The TBLs were all significantly positive with the increasing planting years (2014–2016, 2018, 2020, and 2022) in both the silvopastoral systems (Figures 2A,C,E,G) and pure forests (Figures 2B,D,F,H). In all the years, the growth rate of TBL was significantly higher in the PS-MS and PO-MS than in the PS and PO (p = 0.028 and 0.01, respectively; Figures 2A–F), while it was significantly lower in QV-MS than in QV (p = 0.038; Figures 2E,F), and the RP-MS was non-significantly different from RP (p = 0.209; Figures 2G,H). Moreover, the pattern of growth rate between the silvopastoral systems and pure forests from 2014 to 2016 was similar to that in the whole experimental period (PS-MS vs. PS: p = 0.001; PO-MS vs. PO: p = 0.001; QV-MS vs. QV: p = 0.001; RP-MS vs. RP: p = 0.392).
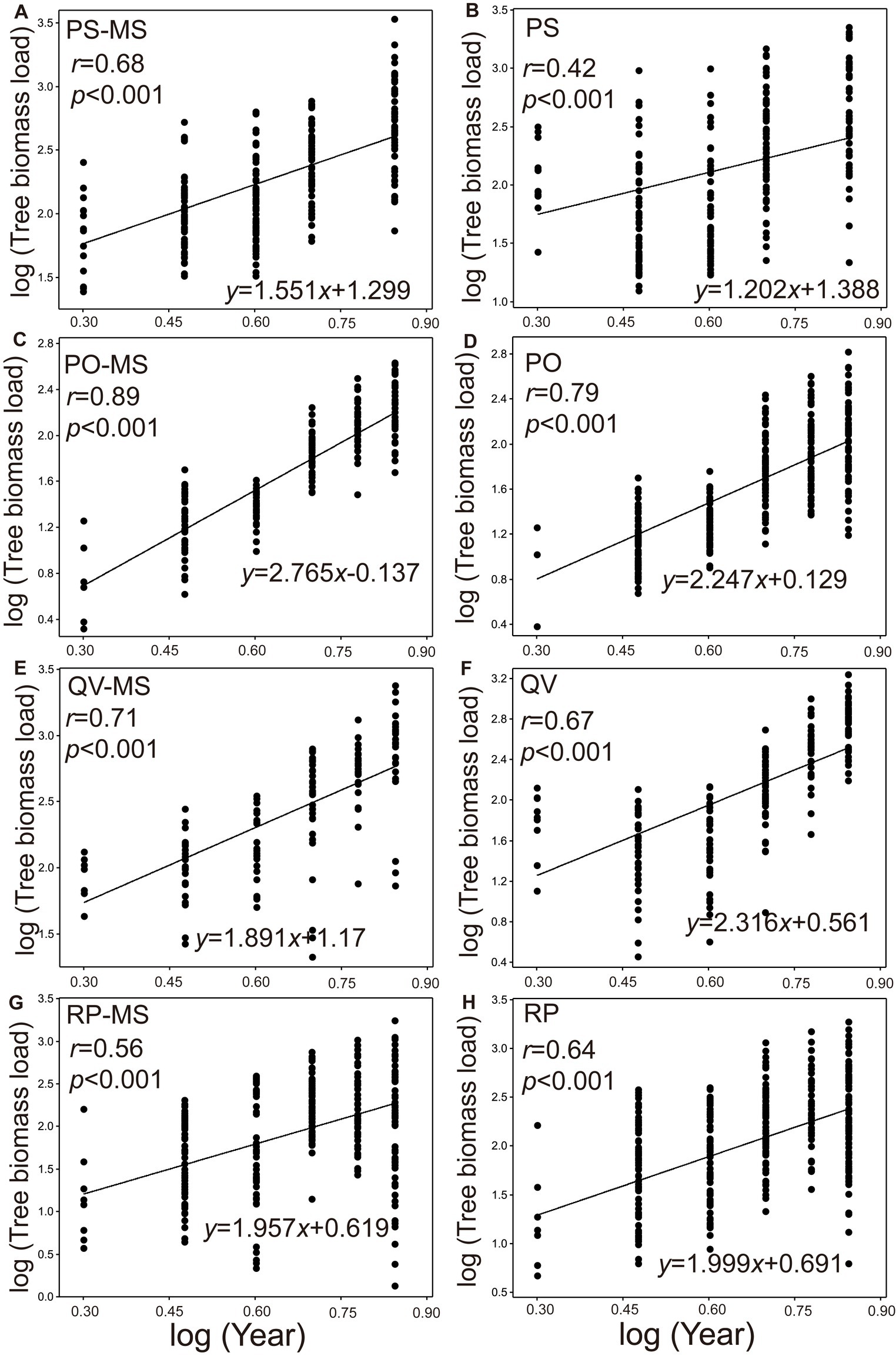
Figure 2. Log–log bivariate plots for the relationships between the year and tree biomass load of PS-MS [Populus simonii × Medicago sativa] (A), PS (B), PO-MS [Platycladus orientalis × Medicago sativa] (C), PO (D), QV-MS [Quercus variabilis × Medicago sativa] (E), QV (F), RP-MS [Robinia pseudoacacia × Medicago sativa] (G) and RP (H) (original units for year and tree biomass load are ind. and mg m−2, respectively). Each point represents the biomass per individual tree per unit for all the relationships.
Both the change in relative DBH and change in relative TBL manifested a significant positive correlation with the change in relative alfalfa biomass (p < 0.001, Figure 3A; p = 0.022, Figure 3C, respectively), but the change in tree height was not significantly associated with the change in relative alfalfa biomass (p = 0.234, Figure 3B). The alfalfa species was not found since the year 2018.
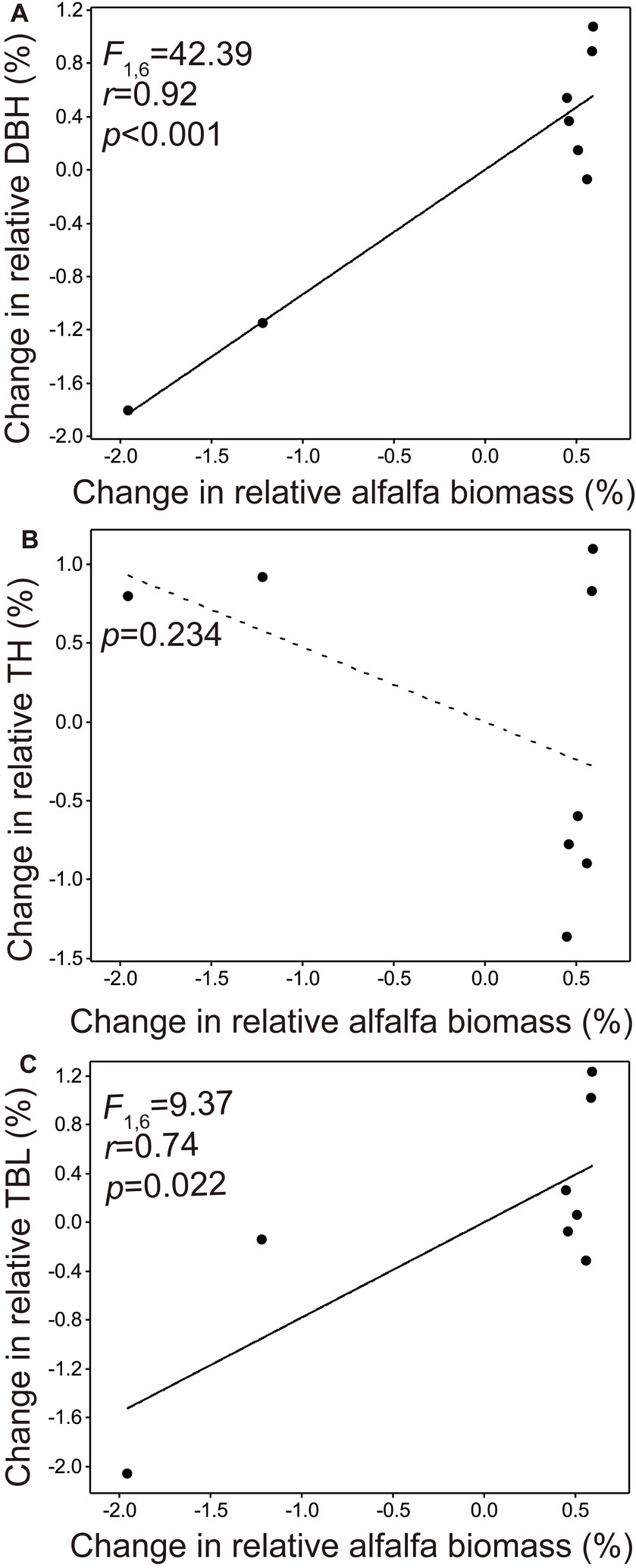
Figure 3. Relationships of the change in relative diameter at breast height (A), change in relative tree height (B), and change in relative tree biomass load (C) against the change in relative alfalfa biomass.
Almost all the TBLs of PS-MS and PS were higher in the downslopes (or S3 + S4) than the upslopes (or S1 + S2) in all years. In the PO-MS, QV-MS, and RP-MS, most of the TBLs in the slopes showed no differences in all the years, whereas the TBLs were higher on downslopes (or S3 + S4) than on upslopes (S1 + S2) in the PO, QV, and RP (Figure 4).
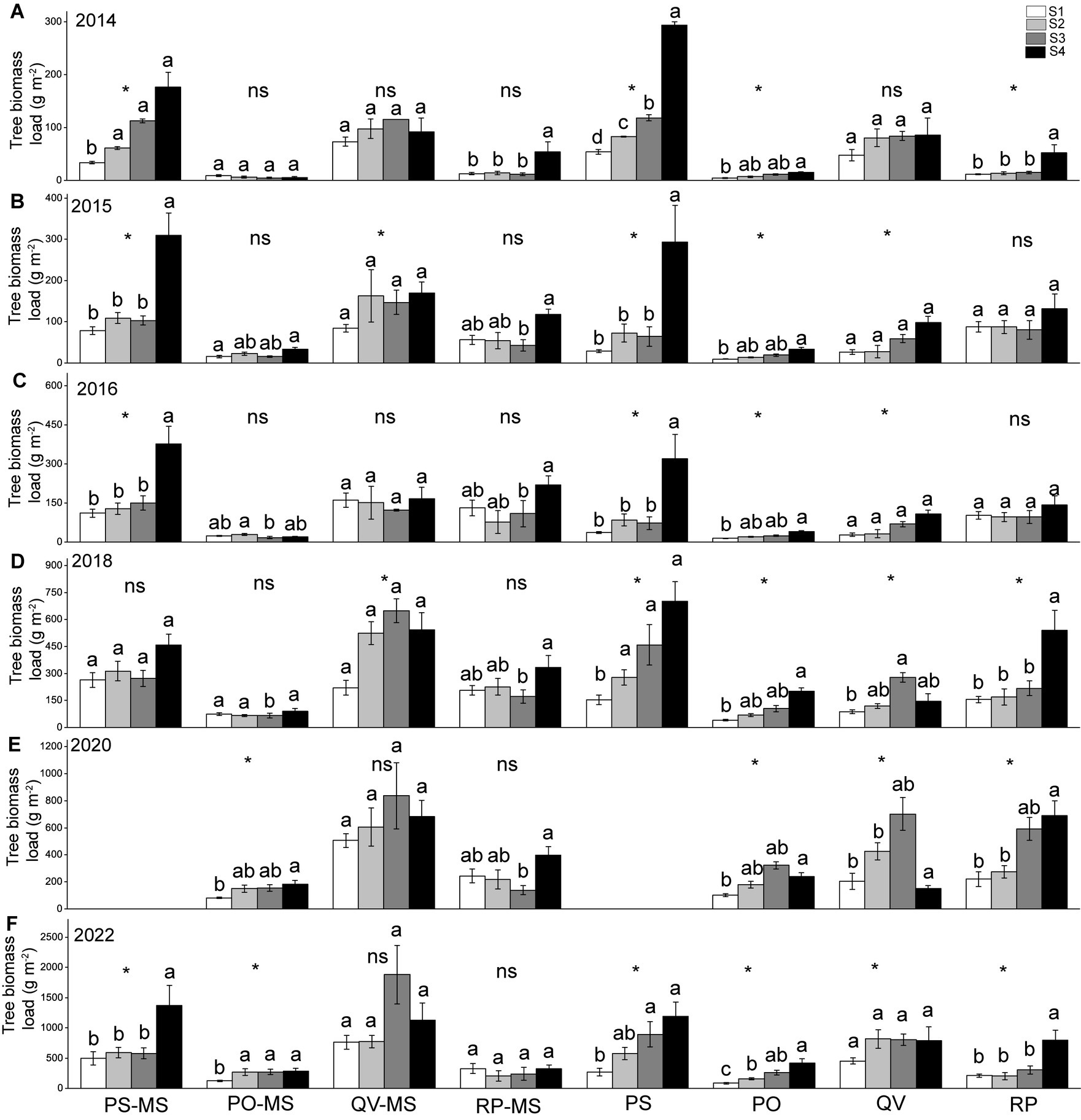
Figure 4. Variations in tree biomass load of slope positions of different vegetation types in different years of 2014 (A), 2015 (B), 2016 (C), 2018 (D), 2020 (E), 2022 (F). The different lowercase letters within a plant type indicate a significant difference among slope positions at 0.05 level; the * indicates a significant difference between S1 + S2 and S3 + S4, and ns indicates a non-significant difference between S1 + S2 and S3 + S4.
The TBL was all significantly positive with soil total nitrogen in pure forests in 2018, 2020, and 2022 (Figures 5C,E,G) and with soil total carbon in 2018, 2020, and 2022 (Figures 5D,F,H), while TBL was not significantly associated with soil total nitrogen in the silvopastoral systems in 2014, 2016, 2018, 2020, and 2022 (Figures 5A–C,E,G) and with soil total carbon in 2018, 2020, and 2022 (Figures 5D,F,H).
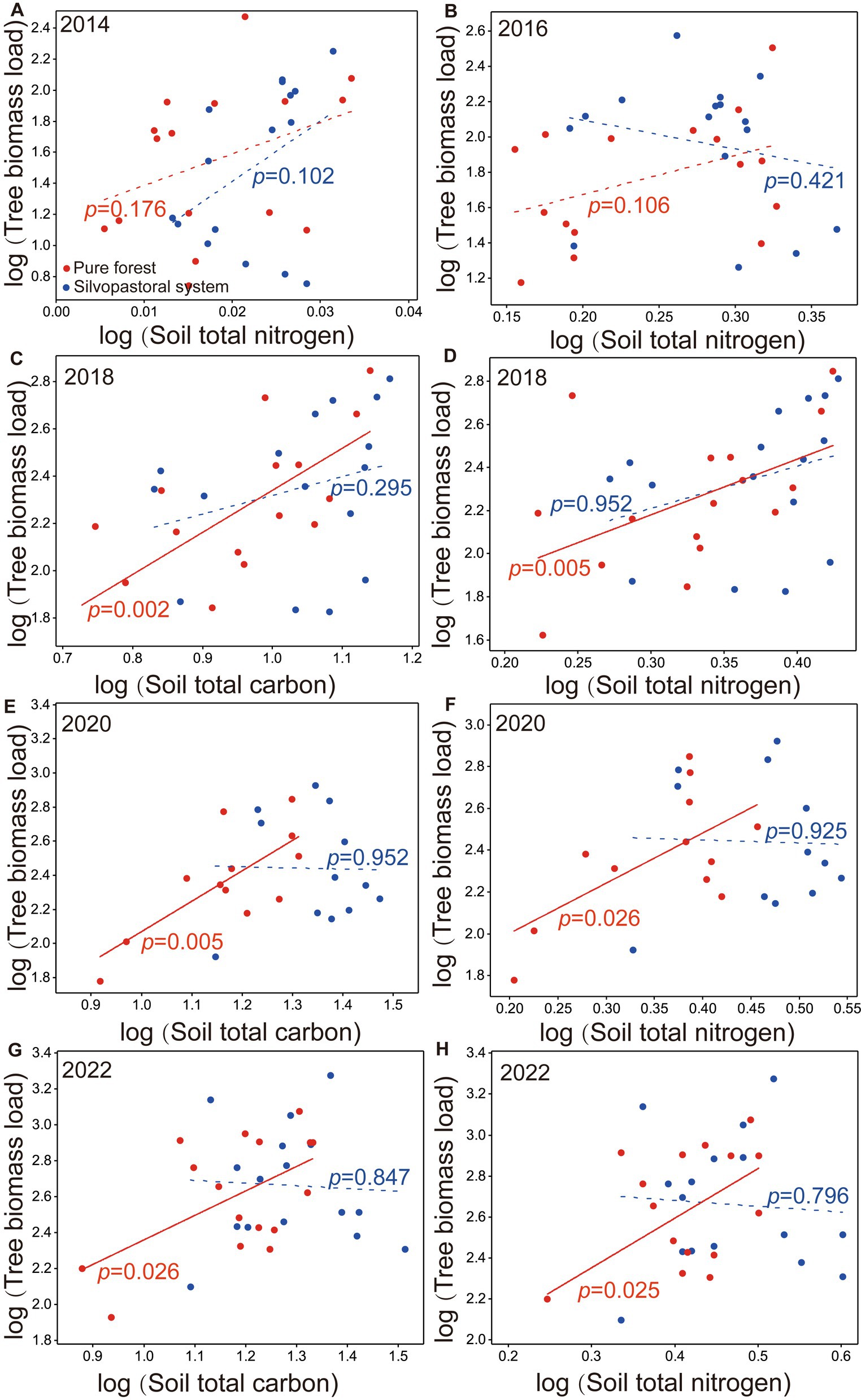
Figure 5. Log–log bivariate plots for the relationships between soil total nitrogen and tree biomass load in years of 2014 (A), 2016 (B), 2018 (D), 2020 (F), 2022 (H), and between soil total carbon and tree biomass load in years of 2018 (C), 2020 (E), 2022 (G) in silvopastoral systems and pure forests. Red points indicate the slopes of pure forests and blue points indicate the slopes of silvopastoral systems.
4 Discussion
Silvopastoral systems have been regarded as sustainable restoration ways to improve soil quality (Polanía-Hincapié et al., 2021), species diversity (Barros et al., 2018), and tree growth (Ares et al., 2003). This study is one of the first studies to explore the tree growth of silvopastoral systems compared to pure forest systems over a long-term period. Consistent with our hypothesis, the tree growth has been generally improved in the silvopastoral systems, although the effect varies with time duration (i.e., PS-MS and PO-MS systems performed well in the first five years, and the QV-MS always performed well during the entire experimental period). Through monitoring of the tree growth in different restored vegetations, this study provides experimental evidence on tree species choice.
Our results showed that the DBH and TBL were higher in the silvopastoral systems of PS-MS and PO-MS than in the pure forests of PS and PO in the years 2014–2016, consistent with previous studies (Meng et al., 1994). This may be due to the fact that M. sativa may generally form nodules attacked by rhizobia with increasing years which can increase the nitrogen fixation rate (Manchanda and Garg, 2007). A higher N content in silvopastoral systems promotes the growth of DBH significantly, and the effect on the DBH growth of poplars is parabolic (Yang et al., 2018), resulting in no difference of PS-MS vs. PS and PO-MS vs. PO at the later stage (e.g., 2018 and 2022).
TH may respond slowly to the effects of the silvopastoral systems of PS-MS and PO-MS; this is indicated by a lower TH in PS-MS and PO-MS than in PS and PO in 2014–2016, while there was no difference between them in 2018 and 2022. This indicated that the effects on tree height mainly occurred in the late stages of the silvopastoral systems. It is likely that the rapid growth duration of tree height occurred later than that of DBH, and the application of M. sativa is conducive to the cultivation of large diameter wood (Shi et al., 2013).
Generally, the tree biomass was not significantly different or lower in the RP-MS silvopastoral system compared with RP. This is because the DBH tended to be lower in RP-MS than in RP, while the TH of RP was higher or non-significant in RP-MS compared with RP. The most likely reason may be that the canopy closure may be promoted in an early year in the silvopastoral system (Clason, 1998), and the canopy closure affected the diameter at breast height more than the tree height (Guo et al., 2010). Therefore, thinning is necessary to reduce the black locust tree canopy closure in tree planting.
M. sativa tends to have a positive effect in the first 5–6 years after restoration. This may be in relation to the growth of M. sativa. As a perennial forage, M. sativa has its highest yield in the 3–5 years after planting and gradually decreases after >6 years (Li and Shao, 2005). In the study, tree growth was associated with the alfalfa biomass, and this is indicated by a positive relationship between the change in relative alfalfa biomass and the change in relative diameter at breast height and tree biomass load. However, the alfalfa species was not found since 2018, indicating a rapid community succession in the ecologically vulnerable areas such as the low hilly areas. Moreover, the planting of M. sativa can significantly accelerate the natural succession process of vegetation with increasing years, and the invasion of herbaceous plants in the late growth stage of M. sativa will lead to the increase in plant species diversity. For example, M. sativa grassland can naturally succeed into a stable prairie community in 10 years (Stipa bungeana community) (Li et al., 2006). Considering that M. sativa may facilitate plant communities and soil conditions and interact with trees reciprocally or competitively (Muthuri et al., 2005), these factors may collectively have an effect on tree growth. Further studies should be conducted to explore the mechanisms of tree growth involving these factors.
In this study, DBH, TH, and TBL were all higher in QV-MS than in QV in all years. The main reason may be that the Chinese cork oak has obvious deeper roots compared to M. sativa, and the oak has a strong ecological adaptability, resulting in a better ability to retain water and cement soil (Gautam et al., 2002; Ma et al., 2013; Jia et al., 2022). Moreover, trees with allelopathic effects on herbaceous plants mostly belong to Quercus spp. and may inhibit seed germination and radicle growth of understory grassland, as well as their competitive ability (Lodhi, 1976; Facelli et al., 1991).
The growth rates were higher in PS-MS and PO-MS than in PS and PO, indicating that the silvopastoral systems play a role in growth rates during the long term; similar results were also found in other plants of Pinus radiata (Gautam et al., 2002). The main reason may be that large amounts of soil water and nutrients may be absorbed by the fast-growing poplars (Lukac et al., 2003; Randriamanana et al., 2014). However, the growth rate of QV in the pure forest system was higher than that in QV-MS, and this may be due to the fact that the tree growth rate in QV-MS may have a relatively smooth growth in the early stages. Previous studies have shown that pure forests have inconspicuous growth in the first few years but a higher growth rate than mingled forests after more than 5 years (He et al., 2021). It is noteworthy that the effect was more pronounced in the first few years, but gradually diminished in the last few years, and this is mostly likely because of the decline of M. sativa as noted above. Therefore, it is suggested that rational topdressing is key to the high yields of M. sativa, and fertilization can also properly delay the M. sativa decline time (Li, 2002; He et al., 2022), especially after planting for more than five years.
TBL tended to be higher in the downslope positions (S3, S4, or S3 + S4) than the upslope positions (S1, S2 or S1 + S2) in the pure forests, while there were no differences in TBL in most silvopastoral systems, indicating that M. sativa has a balanced effect on the tree biomass among the slope positions in the silvopastoral systems. This is presumably because the TBL was significantly positive with the soil total carbon and soil total nitrogen in the pure forests and was not significant in the silvopastoral systems, indicating that the silvopastoral systems have better nutrient retention capacities. Studies have shown that silvopastoral systems can reduce surface runoff through the interception of rainfall by herbaceous plants (Liu et al., 2008; Wang et al., 2012), and the effect may also be significantly enhanced with increasing planting years (Zhang et al., 2000; Xu et al., 2001). Thus, silvopastoral systems may contribute to an even tree growth, especially on the slope positions in low hilly areas.
5 Conclusion
In summary, tree growth could be an indicator for the effects of silvopastoral systems compared to pure forests. Specifically, the tree biomass load in the Populus simonii × Medicago sativa and Platycladus orientalis × Medicago sativa has a short-term growth effect, while Quercus variabilis × Medicago sativa may last for a longer term although the growth rate was lower in the silvopastoral system than the pure forest. As tree growth was not promoted in the pure forest of Robinia pseudoacacia, R. pseudoacacia may not be considered a suitable tree species for silvopastoral systems. Moreover, the tree biomass load showed no difference among slope positions in the silvopastoral systems, while it was higher on the downslope positions than the upslope positions in the pure forests, and this may be associated with a better nutrient retention capacity in the silvopastoral systems than the pure forests.
Data availability statement
The original contributions presented in this study are included in the article, further inquiries can be directed to the corresponding author.
Author contributions
PL conceived and designed the experiments. XW, ZL, and LK performed the experiments. LK analyzed the data. All authors contributed to the article and approved the submitted version.
Funding
This study was supported by supported by Henan Province Science and Technology Research Project (222102110336), the National Project Cultivation Fund of Pingdingshan University (PXY-PYJJ-202005), the Research Fund for High-Level Talents of Pingdingshan University (PXY-BSQD-2023041), and Henan Province Science and Technology Research Project (212102310840).
Acknowledgments
We are grateful to Jun Hu, Meng Li, Min Zhang and Yongzhen Li for field assistance, Xinqiang Xi, Xiaoli Hu, and Fei Chen (from Nanjing University) for their comments and data analysis, and the Key Laboratory of Ecological Restoration in Hilly Area for providing research facilities.
Conflict of interest
The authors declare that the research was conducted in the absence of any commercial or financial relationships that could be construed as a potential conflict of interest.
Publisher’s note
All claims expressed in this article are solely those of the authors and do not necessarily represent those of their affiliated organizations, or those of the publisher, the editors and the reviewers. Any product that may be evaluated in this article, or claim that may be made by its manufacturer, is not guaranteed or endorsed by the publisher.
Supplementary material
The Supplementary material for this article can be found online at: https://www.frontiersin.org/articles/10.3389/ffgc.2023.1244303/full#supplementary-material
References
Amatya, G., Chang, S. X., Beare, M. H., and Mead, D. J. (2002). Soil properties under a Pinus radiata – ryegrass silvopastoral system in New Zealand. Part II. C and N of soil microbial biomass, and soil N dynamics. Agrofor. Syst. 54, 149–160. doi: 10.1023/A:1015076607090
Ares, A., St Louis, D., and Brauer, D. (2003). Trends in tree growth and understory yield in silvopastoral practices with southern pines. Agrofor. Syst. 59, 27–33. doi: 10.1023/A:1026132918914
Barros, F., Fracetto, G., Fracetto, F., Júnior, J., Araújo, V., and Lira Junior, M. (2018). Silvopastoral systems drive the nitrogen-cycling bacterial community in soil. Ciência e Agrotecnologia 42, 281–290. doi: 10.1590/1413-70542018423031117
Chebli, Y., El Otmani, S., Fouad, E., Moula, N., Chentouf, M., Hornick, J. L., et al. (2021). Silvopastoral system in Morocco: focus on their importance, strategic functions, and recent changes in the mediterranean side. Sustainability 13, 1–17. doi: 10.3390/su131910744
Chen, Y., and Cao, Y. (2014). Response of tree regeneration and understory plant species diversity to stand density in mature Pinus tabulaeformis plantations in the hilly area of the loess plateau. China. Ecol. Eng. 73, 238–245. doi: 10.1016/j.ecoleng.2014.09.055
Chen, L., Huang, Z., Gong, J., Fu, B., and Huang, Y. (2007). The effect of land cover/vegetation on soil water dynamic in the hilly area of the loess plateau, China. Catena 70, 200–208. doi: 10.1016/j.catena.2006.08.007
Chen, J., Xiao, H., Li, Z., Liu, C., Wang, D., Wang, L., et al. (2019). Threshold effects of vegetation coverage on soil erosion control in small watersheds of the red soil hilly region in China. Ecol. Eng. 132, 109–114. doi: 10.1016/j.ecoleng.2019.04.010
Clason, T. R. (1998). Silvopastoral practices sustain timber and forage production in commercial loblolly pine plantations of Northwest Louisiana, USA. Agroforest. Syst. 44, 293–303. doi: 10.1023/a:1006267114962
Facelli, J. M., Pickett, S., and Facelli, J. M. (1991). Indirect effects of litter on woody seedlings subject to herb competition. Oikos 62, 129–138. doi: 10.2307/3545257
Fan, W., Kong, L., Yin, S., and Tian, Z. (2004). Effects of apple-alfalfa system on growth fruit yield and quality of apple tree in the drought hilly regions of Taihang mountain. J. Henan Agr. Univ. 38, 423–440. doi: 10.16445/j.cnki.1000-2340.2004.04.014
Fuchs, L. E., Orero, L., Ngoima, S., Kuyah, S., and Neufeldt, H. (2022). Asset-based adaptation project promotes tree and shrub diversity and above-ground carbon stocks in smallholder agroforestry systems in Western Kenya. Front. For. Global Change 4:773170. doi: 10.3389/ffgc.2021.773170
Gautam, M. K., Mead, D. J., Chang, S. X., and Clinton, P. W. (2002). Spatial variation and understorey competition effect of Pinus radiata fine roots in a silvopastoral system in New Zealand. Agrofor. Syst. 55, 89–98. doi: 10.1023/A:1020559624831
Grewal, S. S., Juneja, M. L., Singh, K., and Singh, S. (1994). A comparison of two agroforestry systems for soil, water and nutrient conservation on degraded land. Soil Technol. 7, 145–153. doi: 10.1016/0933-3630(94)90016-7
Guo, J., Zhao, Z., and Luo, H. (2010). Growth status of artificial black locust forest with different densities in the hilly and gully area of western Shanxi province. Bull. Soil Water Conserv. 30, 80–84. doi: 10.13961/j.cnki.stbctb.2010.01.043
He, W., Li, T., Zhang, W., Wu, S., Su, Y., Yan, W., et al. (2021). Research on species diversity of pinus radiata plantation under different afforestation models in dry-hot valley of the Minjiang river. J. Sichuan For. Sci. Tec. Tech. 42, 61–67. doi: 10.12172/202012010001
He, F., Wang, G., Wang, L., Li, Z., Tong, Z., Wang, Y., et al. (2022). Effects of organic base fertilizer and inorganic topdressing on alfalfa productivity and the soil bacterial community in saline soil of the Huanghe river delta in China. Agronomy 12:2811. doi: 10.3390/agronomy12112811
He, Y., Zhou, Y. G., Li, X. W., Miao, Y., Fan, C., and Chen, L. L. (2013). Seasonal dynamics of soil microbial biomass carbon in Alnus formosana forest-grass compound models. Sci. Silvae Sin. 49, 26–33. doi: 10.11707/j.1001-7488.20130704
Jia, G., Nehemy, M. F., Chen, L., Yu, X., and Liu, Z. (2022). Ephemeral connectivity between trees and groundwater in a temperate forest in China. J. Hydrol. 610:127887. doi: 10.1016/j.jhydrol.2022.127887
Jurasinski, G., and Retzer, V. (2015). R package “simba”: a collection of functions for similarity analysis of vegetation data. Version 0.3-5. Available at: https://cran.r-project.org/web/packages/simba/index.html
Kihara, J., Bolo, P., Kinyua, M., Nyawira, S. S., and Sommer, R. (2020). Soil health and ecosystem services: lessons from sub-Sahara Africa (SSA). Geoderma 370:114342. doi: 10.1016/j.geoderma.2020.114342
Kong, J., Wang, H., Zhao, B., Ren, Y., Liu, Y., Chen, H., et al. (2001). Study on ecological regulation system of the pest control in apple orchard. Acta Ecol. Sin. 21, 789–794. doi: 10.3321/j.issn:1000-0933.2001.05.015
Li, Y. (2002). Productivity dynamic of alfalfa and its effects on water eco-environment. Acta Pedologica Sin. 39, 404–411. doi: 10.11766/trxb200007270316
Li, J., Li, C., and Peng, S. (2007). Study on the biomass expansi on factor of poplar plantation. J. Nanjing For. Univ. (Nat. Sci. Ed.) 31, 37–40. doi: 10.3969/j.jssn.1000-2006.2007.04.008
Li, X., Li, R., and Sha, Z. (2023). Modeling carbon uptake by vegetation of grassland ecosystems and its associated factors in China based on remote sensing. Front. Earth Sci. 10:1077885. doi: 10.3389/feart.2022.1077885
Li, S., and Liber, K. (2018). Influence of different revegetation choices on plant community and soil development nine years after initial planting on a reclaimed coal gob pile in the Shanxi mining area. China. Sci. Total Environ. 618, 1314–1323. doi: 10.1016/j.scitotenv.2017.09.252
Li, Y., and Shao, M. (2005). Degradation process and plant diversity of alfalfa grassland in north loess plateau of China. Chin. J. Appl. Ecol. 16, 2321–2327. doi: 10.13287/j.1001-9332.2005.0343
Li, Y., Shao, M., Shangguan, Z. P., Fan, J., and Wang, L. (2006). Study on the degrading process and vegetation succession of Medicago sativa grassl and in north loess plateau, China. Acta Prataculturae Sin. 15, 85–92. doi: 10.3321/j.issn:1004-5759.2006.02.014
Liu, B., Luo, Q., Chang, W., and Xu, X. (2008). Relationship between percentage of vegetative cover and soil erosion. Sci. Soil Wat. Conserv. 6, 68–73. doi: 10.16843/j.sswc.2008.06.01
Liu, P., Wang, J., Chu, C., Jiang, Y., Guo, Q., and Wen, Z. (2014). Effect of different land use types on soil properties of steep slope in low hilly areas of western Henan. Agr. Res. Arid. 32, 208–212. doi: 10.7606/j.issn.1000-7601.2014.01.034
Liu, Y., Wu, M., Guo Zong, M., Jiang, Y., and Liu, S. (1998). Biomass and net productivity of Quercus variabilis forest in Baotianman natural reserve. Chin. J. Appl. Ecol. 15, 931–933. doi: 10.1088/0256-307X/15/12/025
Lodhi, M. (1976). Role of allelopathy as expressed by dominating trees in a lowland forest in controlling the productivity and pattern of herbaceous growth. Am. J. Bot. 63, 1–8. doi: 10.1002/j.1537-2197.1976.tb11781.x
Lukac, M., Calfapietra, C., and Godbold, D. L. (2003). Production, turnover and mycorrhizal colonization of root systems of three Populus species grown under elevated CO2 (POPFACE). Glob. Chang. Biol. 9, 838–848. doi: 10.1046/j.1365-2486.2003.00582.x
Ma, C., Zhang, W., Wu, M., Xue, Y., Ma, L., and Zhou, J. (2013). Effect of aboveground intervention on fine root mass, production, and turnover rate in a Chinese cork oak (Quercus variabilis Blume) forest. Plant Soil 368, 201–214. doi: 10.1007/s11104-012-1512-0
Mackay-Smith, T. H., Burkitt, L. L., López, I. F., and Reid, J. I. (2022). The impact of a kānuka silvopastoral system on surface runoff and sediment and nutrient losses in New Zealand hill country. Catena 213:106215. doi: 10.1016/j.catena.2022.106215
Manchanda, G., and Garg, N. (2007). Endomycorrhizal and rhizobial symbiosis: how much do they share? J. Plant Interact. 2, 79–88. doi: 10.1080/17429140701558000
Meng, Q., Wen, Z., and Hui, Y. (1994). A preliminary study on planting legumes in sparse woodlands in bashang plateau in Hebei province. Grassland China 4, 25–28.
Minea, G., Ciobotaru, N., Ioana-Toroimac, G., Mititelu-Ionuș, O., Neculau, G., Gyasi-Agyei, Y., et al. (2022). Designing grazing susceptibility to land degradation index (GSLDI) in hilly areas. Sci. Rep. 12:9393. doi: 10.1038/s41598-022-13596-1
Muthuri, C. W., Ong, C. K., Black, C. R., Ngumi, V. W., and Mati, B. M. (2005). Tree and crop productivity in Grevillea, Alnus and Paulownia-based agroforestry systems in semi-arid Kenya. For. Ecol. Manag. 212, 23–39. doi: 10.1016/j.foreco.2005.02.059
Nair, P. K. R. (1985). Classification of agroforestry systems. Agrofor. Syst. 3, 97–128. doi: 10.1007/BF00122638
Niu, C., Guan, J., Cheng, R., Li, G., Wu, C., Cheng, J., et al. (2017). Ecosystem carbon density of Robinia pseudoacacia plantations and influencing factors in the middle and western loess plateau. Acta Ecol. Sin. 37, 5049–5058. doi: 10.5846/stxb201604290824
Oksanen, J., Blanchet, F. G., Friendly, M., Kindt, R., Legendre, P., McGlinn, D., et al. (2019). Package ‘vegan’: community ecology package. Available at: https://cran.r-project.org/web/packages/vegan/index.html
Olaya-Montes, A., Llanos-Cabrera, M. P., Cherubin, M. R., Herrera-Valencia, W., Ortiz-Morea, F. A., and Silva-Olaya, A. M. (2021). Restoring soil carbon and chemical properties through silvopastoral adoption in the Colombian Amazon region. Land Degrad. Dev. 32, 3720–3730. doi: 10.1002/ldr.3832
Olsson, M., Erlandsson, M., Lundin, L., Nilsson, T., Nilsson, Å., and Stendahl, J. (2009). Organic carbon stocks in swedish podzol soils in relation to soil hydrology and other site characteristics. Silva Fennica 43, 209–222. doi: 10.14214/sf.207
Paris, P., Cannata, F., and Olimpieri, G. (1995). Influence of alfalfa (Medicago sativa L.) intercropping and polyethylene mulching on early growth of walnut (Juglans spp.) in Central Italy. Agrofor. Syst. 31, 169–180. doi: 10.1007/BF00711724
Polanía-Hincapié, K. L., Olaya-Montes, A., Cherubin, M. R., Herrera-Valencia, W., Ortiz-Morea, F. A., and Silva-Olaya, A. M. (2021). Soil physical quality responses to silvopastoral implementation in Colombian Amazon. Geoderma 386:114900. doi: 10.1016/j.geoderma.2020.114900
R Core Team. (2020). R: A language and environment for statistical computing. R Foundation for Statistical Computing, Vienna Austria.
Randriamanana, T. R., Nybakken, L., Lavola, A., Aphalo, P. J., Nissinen, K., and Julkunen-Tiitto, R. (2014). Sex-related differences in growth and carbon allocation to defence in Populus tremula as explained by current plant defence theories. Tree Physiol. 34, 471–487. doi: 10.1093/treephys/tpu034
Santos, P. Z. F., Crouzeilles, R., and Sansevero, J. B. B. (2019). Can agroforestry systems enhance biodiversity and ecosystem service provision in agricultural landscapes? A meta-analysis for the Brazilian Atlantic Forest. For. Ecol. Manag. 433, 140–145. doi: 10.1016/j.foreco.2018.10.064
Sepúlveda, B. R., and Carrillo, A. A. (2015). Soil erosion and erosion thresholds in an agroforestry system of coffee (Coffea arabica) and mixed shade trees (Inga spp and Musa spp) in northern Nicaragua. Agric. Ecosyst. Environ. 210, 25–35. doi: 10.1016/j.agee.2015.04.032
Shi, X., Tang, C., and Zhou, F. (2013). Growth model and process of Populus nigra var. thevestina in the Junggar basin. Arid Zone Res. 30, 652–658. doi: 10.13866/j.azr.2013.04.009
Wang, H., Jiang, Y., Cao, J., Zeng, H., Liang, Y., Wu, H., et al. (2012). Study on benefits of soil and water conservationin eucalypt plantations inter-cropped with different crops. Sci. Soil Wat. Conserv. 10, 104–107. doi: 10.16843/j.sswc.2012.04.019
Wang, T., Yuan, Z., Ye, Y., and Zhang, J. (2009). Primary study on biomass of Platycladus orientalis plantation at different age stands in the Songshan mountains national forest park. Henan Sci. 27, 817–820. doi: 10.13537/j.issn.1004-3918.2009.07.024
Wyss, E. (1996). The effects of artificial weed strips on diversity and abundance of the arthropod fauna in a Swiss experimental apple orchard. Agric. Ecosyst. Environ. 60, 47–59. doi: 10.1016/S0167-8809(96)01060-2
Xiao, S., Chen, M., Cheng, X., and Lai, Z. (2023). Spatial differentiation and driving forces behind non-grain agricultural production in the low mountain and hilly areas of southern Jiangxi: a case study of Xunwu County. J. Agr. Res. Environ. In press. doi: 10.13254/j.jare.2023.0176
Xu, M., Wen, S., and Gao, J. (2001). Effects of different forage planting model on soil and water conservation and environments in red hilly regions. J. Soil Water Conserv. 15, 77–80. doi: 10.3321/j.issn:1009-2242.2001.01.021
Yang, Y., Tang, Y., Tang, J., Li, Y., Li, Z., and Wu, M. (2018). Formula fertilization by soil testing and its correlation with nutrient content of poplar plantation around Dongting lake region. J. Cent. S. Univ. For. Technol. 38, 103–107. doi: 10.14067/j.cnki.1673-923x.2018.12.014
Yang, T., Zhu, Y., Duan, Z. P., Lu, W. H., Zhang, F. F., Wan, S. M., et al. (2020). Root distribution and productivity in a poplar tree + alfalfa silvopastoral system in Northwest China’s Xinjiang Province. Agrofor. Syst. 94, 997–1010. doi: 10.1007/s10457-019-00466-5
Yin, J., Zhu, S., Yang, Z., and Wu, J. (1994). The effect of water and soil conservation of intercropping of forest and grass. For. Sci. Technol. 19, 17–18.
Zhang, J., Duan, S., Luo, S., and Li, H. (2000). Eco-environmental effect of fruit trees and different pasture inter-planting systems in yong orchard on slope land of latored soils (hapludox). Soil Environ. Sci. 9, 42–44. doi: 10.16258/j.cnki.1674-5906.2000.01.011
Keywords: silvopastoral systems, tree growth, slope position, vegetation restoration, low hilly areas
Citation: Liu P, Cheng F, Wang X, Liu Z, Cheng L, Tong W, Qi G and Kou L (2023) Tree growth as an effect indicator of silvopastoral systems in the low hilly area of western Henan province, China. Front. For. Glob. Change. 6:1244303. doi: 10.3389/ffgc.2023.1244303
Edited by:
Youssef Chebli, National Institute for Agricultural Research (Morocco), MoroccoReviewed by:
Rafael Arturo Torres Fajardo, Federal University of Maranhão, BrazilAbdelhafid Keli, Ecole Nationale d'Agriculture de Meknès, Morocco
El Otmani Samira, National Institute for Agricultural Research (Morocco), Morocco
Copyright © 2023 Liu, Cheng, Wang, Liu, Cheng, Tong, Qi and Kou. This is an open-access article distributed under the terms of the Creative Commons Attribution License (CC BY). The use, distribution or reproduction in other forums is permitted, provided the original author(s) and the copyright owner(s) are credited and that the original publication in this journal is cited, in accordance with accepted academic practice. No use, distribution or reproduction is permitted which does not comply with these terms.
*Correspondence: Lixuan Kou, a291bHgyMDE1QDE2My5jb20=