- 1School of Biological Sciences, The University of Western Australia, Perth, WA, Australia
- 2School of Biological Sciences, University of Southampton, Southampton, United Kingdom
- 3Faculty of Forestry, Universiti Putra Malaysia, Serdang, Malaysia
- 4Conservation Science Group, Department of Zoology, University of Cambridge, Cambridge, United Kingdom
Tropical montane forests (TMFs) are major centers of evolutionary change and harbor many endemic species with small geographic ranges. In this systematic map, we focus on the impacts of anthropogenic habitat degradation on TMFs globally. We first determine how TMF research is distributed across geographic regions, degradation type (i.e., deforestation, land-use conversion, habitat fragmentation, ecological level (i.e., ecosystem, community, population, genetic) and taxonomic group. Secondly, we summarize the impacts of habitat degradation on biodiversity and ecosystem services, and identify deficiencies in current knowledge. We show that habitat degradation in TMFs impacts biodiversity at all ecological levels and will be compounded by climate change. However, despite montane species being perceived as more extinction-prone due to their restricted geographic ranges, there are some indications of biotic resilience if the impacts to TMFs are less severe. Species richness and key species interactions can be maintained in mildly degraded sites, and gene flow can persist between TMF fragments. As such, minimally degraded areas such as secondary forests and restored areas could play a crucial role in maintaining the meta-community and ecosystem services of TMFs—either via resource provision or by linking patches of pristine forest. Research deficiencies highlighted include poor research representation in Asian and African TMFs, few assessments of population and genetic-level responses to fragmentation, and little assessment of the impacts of habitat fragmentation at all ecological levels. To address these concerns, we present a list of the top research priorities to urgently address the growing threat of habitat degradation in TMF.
Introduction
In addition to harboring rich biodiversity and endemism (Kessler and Kluge, 2008), tropical montane forests (TMF) provide crucial ecosystem services that include the production of water, carbon sequestration and storage, and prevention of erosion (Martinez et al., 2009; Spracklen and Righelato, 2014; Brenning et al., 2015). The occurrence of TMFs depends on the prevailing climate rather than a defined elevation, and they have lower temperatures and higher humidity relative to tropical lowland forests (Bruijnzeel et al., 1993). As such, TMFs can occur at elevations as low as 500 m above sea level (a.s.l.) on small islands, to 1,200 m a.s.l. on coastal or isolated mountain ridges, and as high as 2,000–3,000 m a.s.l. on large inland mountains (Scatena et al., 2010). Forest structure is similarly variable, with lower-elevation TMF characterized by emergent trees up to 35 m tall, an absence of large buttress roots, two layers of canopy, and many non-vascular epiphytes (Whitmore, 1998; Scatena et al., 2010). Upper TMFs feature shorter trees with high moss cover (Frahm and Gradstein, 1991; Scatena et al., 2010), while sub-alpine TMFs have small gnarled trees and a near absence of epiphytes (Kitayama, 1995; Scatena et al., 2010). About 7% of the 3.2 million square kilometers of TMF is tropical montane cloud forest (TMCF) which lies within the upper and lower bounds of cloud formation (Scatena et al., 2010).
Numerous species endemic to montane regions are threatened with extinction due to deforestation (Long, 1995; Brooks et al., 1999; Renner et al., 2006; Hall et al., 2009). From 2000 to 2010 more than 7 million ha of tropical forest (including forest types beyond TMFs) were lost each year, with a corresponding annual gain in agricultural land of 6 million ha (FAO, 2016). Temperatures in lowland tropical regions have increased by 0.25°C per decade (Malhi and Wright, 2004) and are projected to rise by 2.6–4.8°C (mean 3.7°C) over the twenty first century under the RCP8.5 greenhouse gas concentration trajectory (Cubasch et al., 2013), leading both to species' ranges contracting to higher elevations, and an increased risk of extirpation (Peters and Darling, 1985; La Sorte and Jetz, 2010; McCain and Colwell, 2011). Conversion of lowland regions to agriculture promotes drier conditions in adjacent TMFs due to reduced cloud formation; consequently, forests dependent on humid conditions become restricted to higher elevations, exacerbating habitat loss and fragmentation (Lawton et al., 2001).
Prior efforts to comprehend the collective impacts of habitat degradation on TMFs were focused either on TMCFs or TMFs in the neotropics. The first synthesis of research on TMCFs in 1987 concluded that deforestation, agricultural expansion, roads and human encroachment were major threats (Stadtmüller, 1987). Later, growing interest in the preservation of TMCFs led to the publication of symposium proceedings on TMCF in 1993 and 2004 (Hamilton et al., 1995; Bruijnzeel et al., 2010). Another symposium was conducted in 1993, with a regional focus on neotropical TMFs (Churchill et al., 1995). In Asia, a case-study in Malaysia highlighted the current threats to the local TMCF and proposed multi-sectoral strategies for their conservation (Peh et al., 2011).
Despite these contributions, clear research directions that could support the sustainable use of the world's TMFs are lacking. Research questions for the conservation of TMCF were consolidated in Bruijnzeel et al. (2010), based on a list of one hundred questions of importance to the conservation of global biodiversity by Sutherland et al. (2009). While the questions are valid and well-represented for ecosystem services and species-focused research, none addressed conservation concerns at the community, species-interaction and genetic levels. The Malaysian case-study by Peh et al. (2011) provided a holistic approach to mitigate the threats posed to TMCF; however, the strategies were aimed at tackling inadequacies in various commercial and governmental sectors (i.e., agriculture, forestry, infrastructure, tourism, and policy and legislation) without regard to current research gaps and priorities. To address these shortfalls, we systematically mapped all research on the effects of habitat degradation in TMFs globally to identify deficiencies in current knowledge, and to guide future research prioritization. Specifically, we asked how TMF research is distributed by geographic region, country, degradation type (i.e., deforestation, land-use conversion, habitat fragmentation, and edge effects), ecological level (i.e., ecosystem, community, population, genetic) and taxonomic group. We summarized the impacts of habitat degradation on biodiversity and ecosystem services and examined how degradation of TMFs has affected species richness and composition—two of the most commonly used response metrics for communities. Lastly, we list the top research priorities which, if addressed, would advance the goals of biodiversity conservation and sustainable use of resources in TMF.
Methods
We performed a search scoping exercise in Web of Science (WoS) to generate a suitable search string to gather relevant articles for a systematic map (Collaboration for Environmental Evidence, 2013) (see Figure 1 for flow diagram of protocol). Systematic maps or reviews differ primarily from traditional reviews in that the methods for the former show greater transparency (Collaboration for Environmental Evidence, 2013; Berger-Tal et al., 2018). We tested various combinations of keywords that returned a manageable number of hits (<5,000), while not being overly restrictive. Keywords were combined with appropriate Boolean operators (e.g., AND, OR), wildcards (*) to account for alternate word endings and parentheses for nested searches. The eventual search we selected was (trop* monta* forest* OR cloud forest* OR trop* high* elevation* forest* OR trop* mid* elevation* forest*) AND (degrad* OR deforest* OR fragment* OR disturb* OR land convers* OR land change*) which returned 2,495 hits on 23 January 2018. The keywords in the first set of parentheses represented the ecosystem of interest, which broadly covered tropical montane forests, and the keywords in the second set of parentheses were terms related to habitat degradation. Additionally, we also gathered articles from CAB Abstracts using the same search string as the search function allowed for nested searches as in WoS. CAB Abstracts returned 458 hits on 5 March 2018. In both cases, the forms of articles accepted included peer-reviewed journal papers, book chapters and conference papers. We also entered the same keywords with wildcard operators in Google Search to find relevant gray-literature that was possibly missed in WoS or CAB Abstracts (but without parentheses) as our original nested search performed poorly with a return of only five hits. Google Search returned 16,600 hits on 24 April 2019, so we assessed the first 100 hits as they were sorted in order of decreasing relevance.
For data extraction, we used four criteria to determine if an article was suited to the purposes of our systematic map. First, at least two study sites were required, where a control (e.g., primary forest) was compared to a site or sites that had undergone some form of anthropogenic impact (e.g., selective logging). Second, a biotic or abiotic (i.e., ecosystem function) response to the impact needed to have been measured. Third, we broadly considered all tropical forests under frequent cloud cover or mist between 1,000 and 3,000 m a.s.l. to be TMFs, in line with other studies (Brooks et al., 1999; La Sorte and Jetz, 2010; Spracklen and Righelato, 2014; Li et al., 2016; Dietrich et al., 2017). Last, study sites needed to occur within tropical latitudes (i.e., between 23°26′13.5″ N and S). However, we made a few exceptions to include studies where TMFs occurred lower than 1,000 m (e.g., montane forests on small islands where cloud base is lower than 1,000 m). We avoided the inclusion of research on forests 3,000 m a.s.l. or higher, as these biomes are regarded as sub-alpine cloud forests and have different floristic structure and lower biodiversity (Scatena et al., 2010). In total, 362 peer-reviewed articles were considered relevant, of which 344 were obtained from our first search using WoS, 16 were added from using CAB Abstracts and two more were included after using Google Search (Table S1).
This literature was systematically assessed by reading the title, abstract, and study site descriptions in the body of each article. We rejected studies that did not yield empirical data, such as reviews or studies that performed simulations or meta-analyses. Data extracted from relevant articles included the year of publication, journal/book/conference title, geographic information (i.e., continent and country where the research is conducted; the name, latitude, longitude and elevational range of the study sites), the duration of the study, the ecological level of the response variable (i.e., ecosystem, community, species-interactions, population, genetic), the taxonomic group or ecosystem service (i.e., water regulation (including erosion control) and purification, maintenance of soil fertility, carbon storage and sequestration, and nutrient cycling), the response and explanatory variables measured, and a summary of the key outcomes. We made the distinction between community and species interaction studies by assessing if the study examined symbiotic relationships (i.e., close relationships between species that include predation, competition, mutualism, parasitism and commensalism). For example, research that studied the effect of deforestation on species richness was considered a community study, while a study of the effect deforestation on nest predation was considered a species-interaction study. We collated the conservation status of all studied species, as listed in the International Union for the Conservation of Nature (IUCN) Red List on the 3 August 2018 (IUCN, 2018).
We classified the type of habitat degradation into four categories: deforestation (i.e., any habitat that has lost pristine forest due to anthropogenic causes such as selectively-logged forest, regenerating abandoned clear-cuts, and secondary forests), land-use conversion (i.e., any habitat that has been cleared and converted to pasture or plantations), and habitat fragmentation and edge effects. Species interactions were broadly classified as competition, predation, mutualism, commensalism or parasitism.
As there were several research deficiencies highlighted in our systematic map, it was useful to consider their relative importance, which we did via consensus among the authors. Research priorities in rank order were: (1) ecological level, (2) impact type, (3) geographic region, and (4) focal taxa. At each level, we highlighted sub-topics that deserved greater attention based on the current extent of our knowledge, and on the urgency for conservation action.
Results
The geographic region with the most number of articles was North America (127 publications, 35% of the global total), and the region with the fewest articles was Southeast Asia (15 publications, 4% of the global total; Figure 2A). The number of articles published on TMFs in the Americas more than doubled the number in Africa, and was at least an order of magnitude higher than the number in Asia (Table 1). Articles published on Mexican TMFs were the most numerous (121), and constituted 95% of all articles from North America (Figure 3). Articles on Columbian and Ecuadorian studies were the next most numerous (43 and 39 articles, respectively). Collectively, these results indicate a very strong research contribution from Latin America, with Mexico, Columbia and Ecuador representing more than 55% of articles considered in this mapping exercise. Among other geographic regions, Costa Rican TMFs were the focus of most articles from Central America (27), Kenyan TMFs were the focus of most articles from Africa (29), and Chinese TMFs were the focus of most articles from Asia (8).
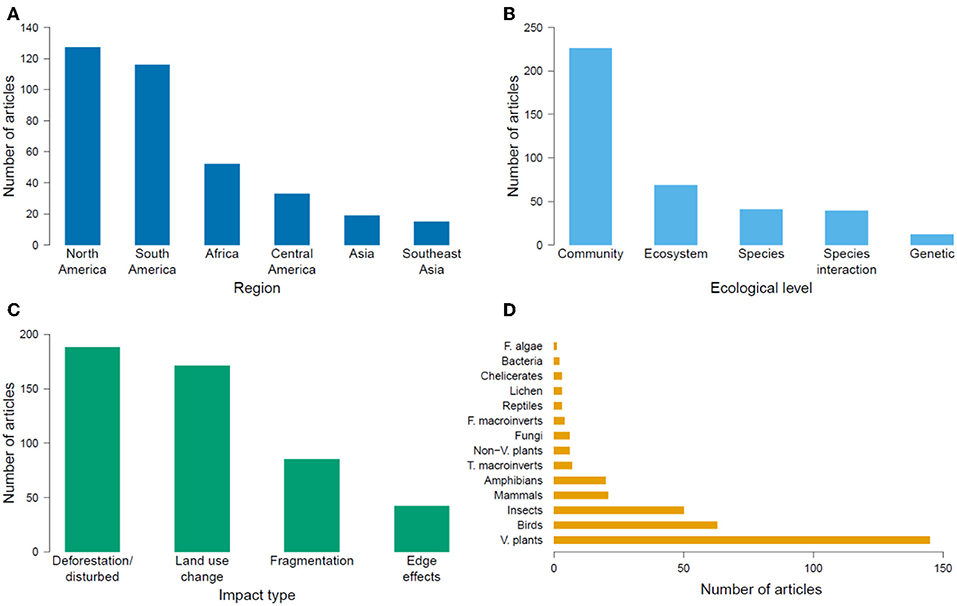
Figure 2. Number of articles published globally from 1991 to 2018 on the impacts of habitat degradation on tropical montane biodiversity in different (A) geographical regions, (B) ecological levels, (C) impact types, and (D) taxonomic groups. F, T, and V refer to freshwater, terrestrial, and vascular respectively.
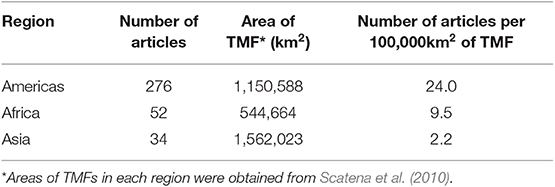
Table 1. Number of published articles on the impact of habitat degradation of tropical montane forests (TMF) in three major regions per unit area of forest.
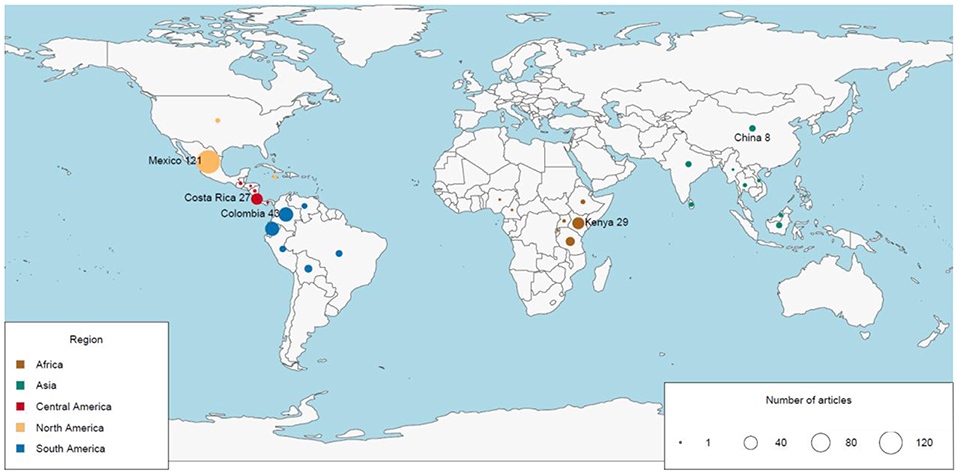
Figure 3. Map of the number of articles published from 1991 to 2018 on the impacts of habitat degradation on tropical montane biodiversity in different countries. The diameter of the circles is directly proportional to the number of published articles. Country names represent the highest number of articles published in the region.
At the ecological level, most research focused on community ecology, followed by research on ecosystem functioning, species interactions, population ecology and population genetics (Figure 2B). Most articles studied the effects of deforestation, followed by land-use change, fragmentation and edge effects (Figure 2C). Plants were the most studied taxon, followed by arthropods and birds (Figure 2D). When information regarding the duration of a study was available (82% of articles), short-term studies (<4 years) constituted 68% of this subset, while long-term studies (≥4 years) comprised 12%. However, 3% of articles examined long-term effects using historical data collected more than 4 years ago, or included study sites that had been deforested or restored more than 4 years earlier. Many articles yielded no information on the duration of the study (18%).
There were 227 articles focused on community ecology, with most articles from Latin America and the fewest from Southeast Asia. The best studied taxa were vascular plants followed by insects and birds (Table 2). Most studies used species richness and species composition (beta diversity) to measure the impacts of environmental change, with only a few measuring functional diversity (Figure 4A). Although the impact of deforestation or land conversion of TMF generally resulted in lower species richness (53% of 75 articles), many studies reported no change (29%) or higher species richness (14%) (Figure 4B). A few studies (4%) showed peak species richness occurred at an intermediate level of degradation (e.g., the highest number of species was in secondary forest, relative to primary forest and farmland). Species composition showed a more definitive pattern; in 87% of 55 papers, species composition changed in response to deforestation or land-use change (Figure 4C).
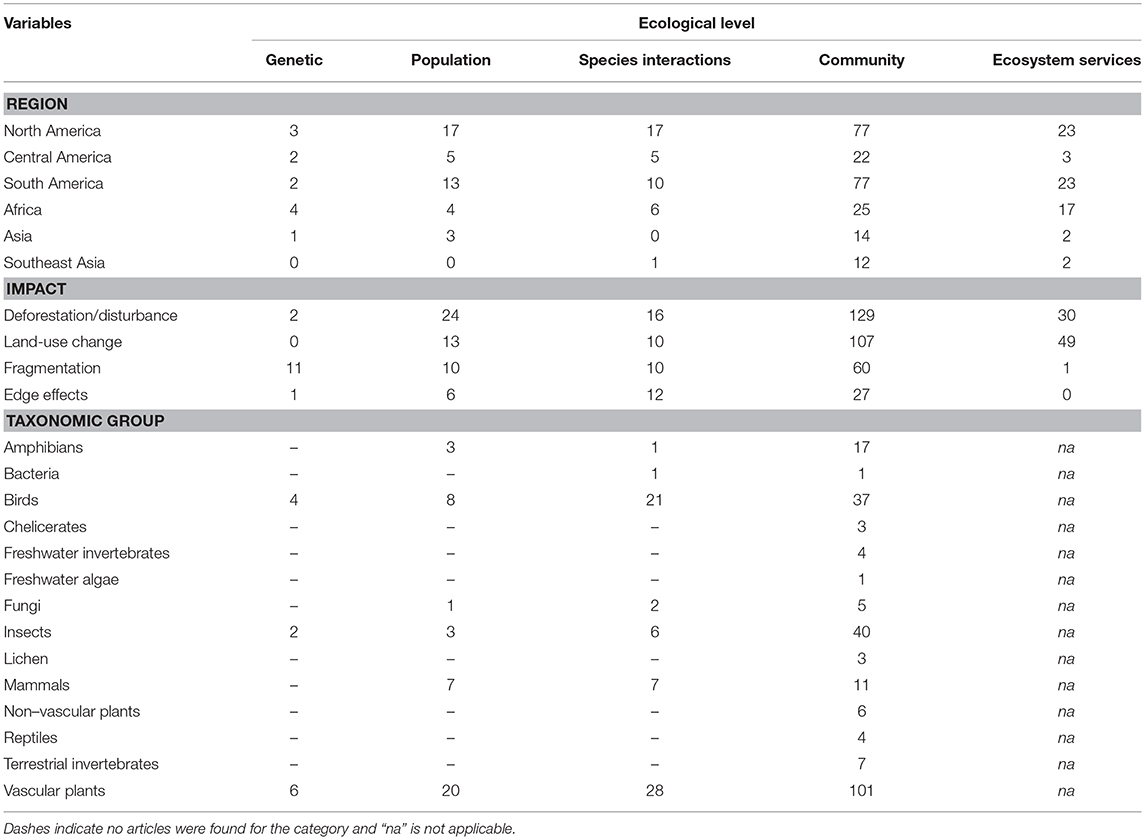
Table 2. Number of articles on the impact of habitat degradation of tropical montane forests at various ecological levels distributed across regions, impact types and taxonomic groups.
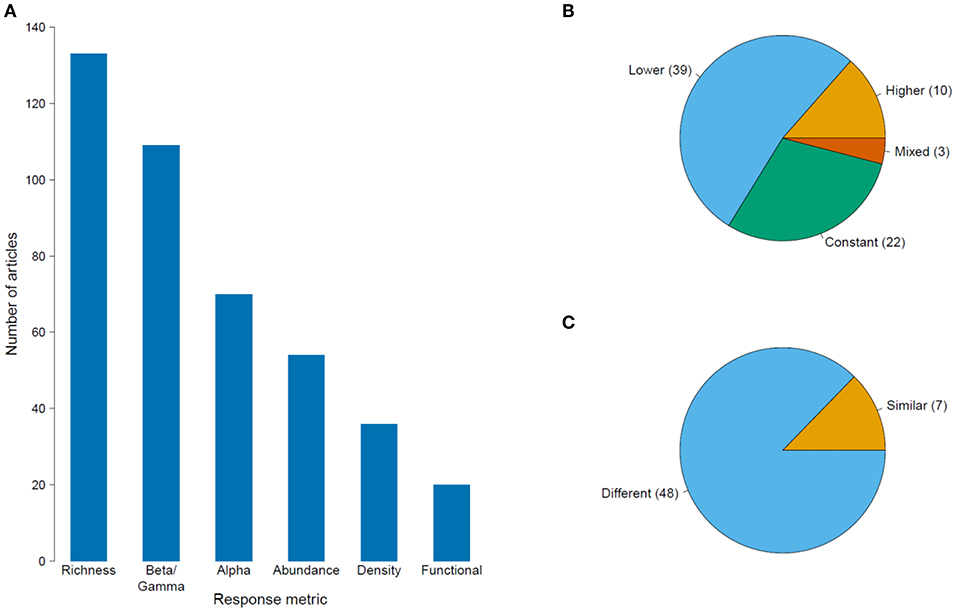
Figure 4. (A) Number of published articles on the impacts of habitat degradation on tropical montane biodiversity using different community response metrics. The proportion of articles showing the community responses to deforestation and habitat disturbance measured using (B) species richness; and (C) species composition. The numbers in parentheses refer to the number of articles for each type of response. For (B), labels “Lower,” “Higher,” and “Constant” refer to a decline, increase and no change in species richness in disturbed areas in comparison to pristine sites while “mixed” response refers to a unimodal peak along a disturbance gradient. For (C), labels “Similar” and “Difference” describe if the species composition is the same or different between disturbed and pristine sites.
Of 69 articles on ecosystem services, most were from North America (largely from Mexico; Table 2). Most articles (68%) focused on the impacts of land conversion on ecosystem services, while one article focused on fragmentation (Table 2). Ecosystem services were broadly classified as water regulation (including erosion control) and purification, maintenance of soil fertility, carbon storage and sequestration, and nutrient cycling. Each category was well-represented, with a slight bias toward articles exploring hydrological impacts and soil fertility (Figure 5A).
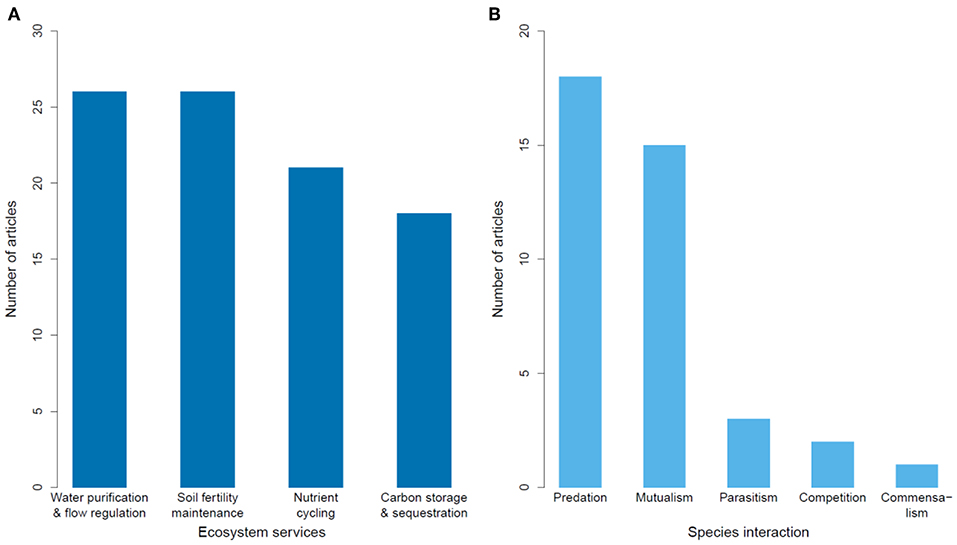
Figure 5. Number of articles published on the impacts of habitat degradation on tropical montane biodiversity studying different types of (A) ecosystem services, and (B) species interactions.
Species interactions were most commonly studied in Latin America (80% of 39 articles), with seven articles from Africa and one from Asia (Table 2). The impacts measured were almost equally represented, with edge effects slightly more studied than deforestation, land conversion or fragmentation (Table 2). The most studied species interaction was predation, followed by mutualism, which collectively accounted for 85% of articles (Figure 5B). Studies on predation often lacked identification of the predators, due to the rarity of documenting such events.
Population ecology (i.e., species-level processes) was the focus of 41 articles, with (80%) being from Latin America, largely studying vascular plants (Table 2). Vertebrates were also well-represented (19% birds, 17% mammals, and 7% for amphibians). Most articles investigated impacts of deforestation and land-use change (73%), with fewer (27%) measuring fragmentation and edge effects (Table 2). The demographic parameters measured varied, with population size, density and fecundity being most frequently recorded (61%) (Figure 6A). Survival rates and fitness-related traits (e.g., body condition and growth) were also often assessed (30%). To assess the conservation statuses of species in the population studies, we omitted two articles that measured demographic parameters but with a greater focus on community level responses (Hitimana et al., 2004; Cuervo and Restrepo, 2007). Thus, of the 41 remaining papers, 64 species were represented, of which 40% have not had their conservation status assessed by the IUCN, and 30% were considered threatened (Critically Endangered, Endangered or Vulnerable; Figure 6B).
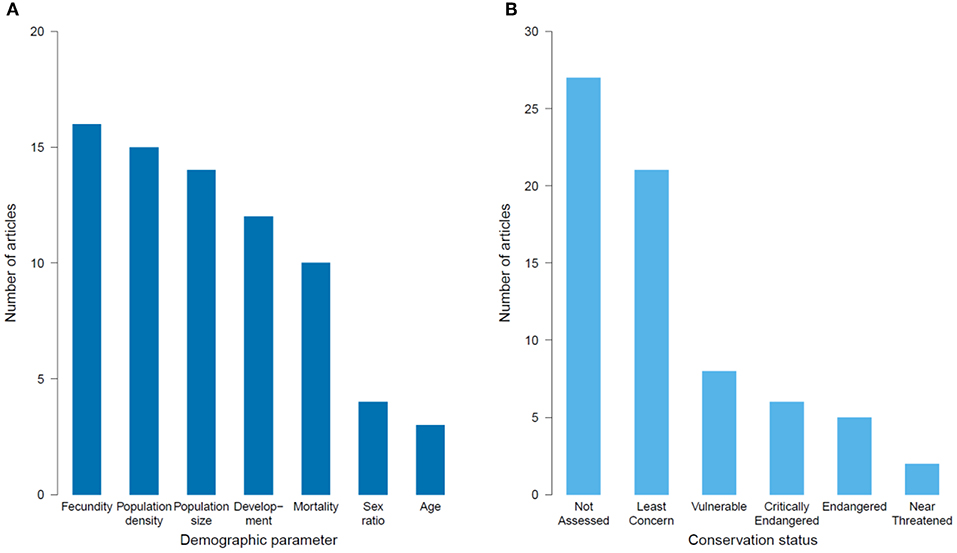
Figure 6. Number of population ecology articles published on the impacts of habitat degradation on tropical montane biodiversity that: (A) measured different demographic parameters, and (B) studied species listed in the various categories of the IUCN Red List.
Only 12 articles described genetic studies (Table 2). The earliest study from 2005 explored the impacts of habitat degradation (logging) on the genetics of an endemic species of oak in China (Zheng et al., 2005). Other genetic studies were published from 2011 to 2015, with six from Latin America, four from Africa and one from Asia (Table 2). Most explored the impacts of habitat degradation on a single focal species, but two drew comparisons between two species (Winkler et al., 2011; Quevedo et al., 2013), and another compared seven species (Callens et al., 2011). Most articles examined the impact of fragmentation or edge effects on genetic diversity or gene flow, while a few studied the effects of deforestation or land-use change (Table 2). In most cases (75%), deforestation and fragmentation adversely affected genetic variability and gene flow.
Effects of Habitat Degradation on TMF Biodiversity
A myriad of habitat degradation effects on biodiversity and ecosystem services in TMFs were reported (Figure 7). Equivocal or inverse responses in species richness may be due to sampling in habitats with intermediate levels of degradation, which often show higher species richness than pristine environments (e.g., Deloya et al., 2007; Vazquez et al., 2011; Mendez-Castro and Rao, 2014; Abella-Medrano et al., 2015; Badillo-Saldana et al., 2016; Gomez-Diaz et al., 2017). The main cause cited was higher resource availability (e.g., food abundance, breeding habitat or light availability) that attract resource-generalist species (Vazquez et al., 2011; Mendez-Castro and Rao, 2014; Abella-Medrano et al., 2015; Badillo-Saldana et al., 2016). Changes in species composition across a disturbance gradient were often reported, with resilient species more likely to be generalists (e.g., Pattanavibool and Dearden, 2002; Gove et al., 2013; Diaz-Garcia et al., 2017; Gomez-Diaz et al., 2017), not threatened (Basham et al., 2016), have broad elevational distributions (Escobar and de Ulloa, 2000), introduced (Gomez-Diaz et al., 2017), and adaptable to climatic change (Ariyanti et al., 2008).
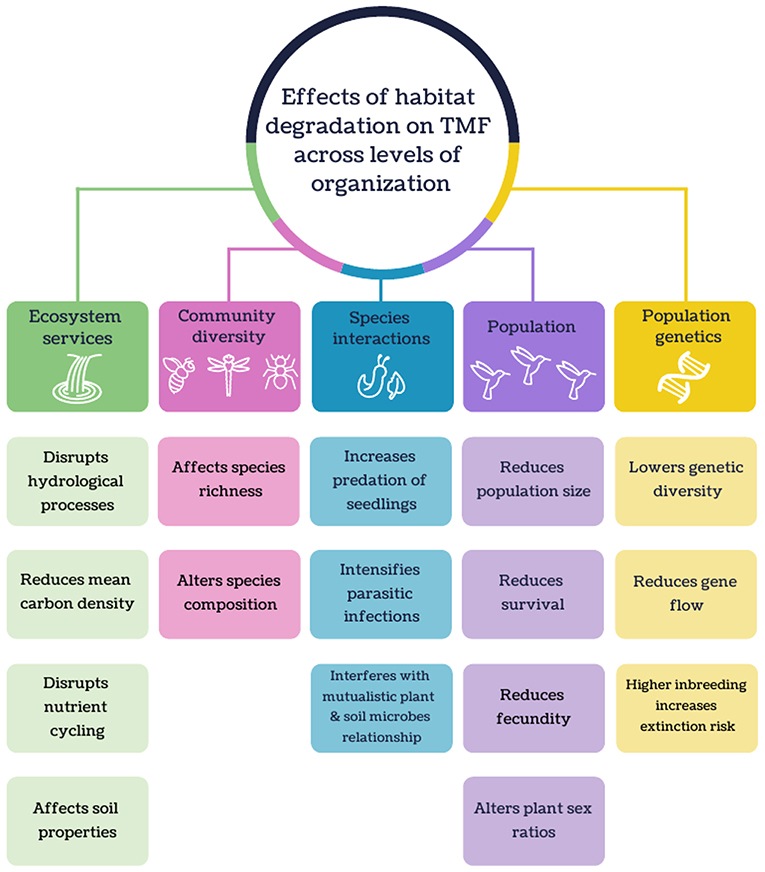
Figure 7. Schematic of the major effects of habitat degradation on tropical montane forest across levels of organization.
The degradation of TMF can be detrimental to some species interactions such as predator-prey (e.g., Goldsmith et al., 2007; Kumar and O'Donnell, 2007; Anthelme et al., 2014) and mutualistic (e.g., Maldonado et al., 2000; Lehouck et al., 2009; Pizano et al., 2017) relationships. Increased predation of seedlings was observed in deforested areas due to a lack of concealment from predators (Anthelme et al., 2014). Habitat degradation also interfered with mutualistic relationships between plant and soil microbes. For example, plant growth showed a positive response to soil filtrate from TMF due to the presence of beneficial soil microbes, but was negatively affected by soil filtrate from pastures (Pizano et al., 2017). Parasitic infections generally intensified with increased habitat disturbance. Amphibian chytrid fungus (Batrachochytrium dendrobatidis) was most prevalent in agroforests (Murrieta-Galindo et al., 2014), and mistletoe (Psittacanthus schiedeanus) infection of host trees was more common at the forest edge than the interior (Lopez de Buen et al., 2002).
There were a small number of TMF articles that have investigated how populations respond to habitat degradation. From these studies, habitat fragmentation was shown to reduce the population size of birds and foxes (Husemann et al., 2015; Gallina et al., 2016). For example, the effective of population sizes of the mountain white-eye (Zosterops poliogaster) in East Africa were higher in larger and interconnected forest patches (Husemann et al., 2015). Habitat fragmentation can also decrease fecundity in plants (Somanathan and Borges, 2000; Franceschinelli et al., 2015), which may be caused by a decrease in pollinator visits and an increase in seed predation (Franceschinelli et al., 2015). Fragmentation also resulted in reduced plant survival which could be attributed to higher desiccation and seedling predation (Alvarez-Aquino et al., 2004). Trees in isolated patches were also found to be to have altered plant sex ratios apart from natural populations due to a lack of pollinator visits to female trees (Somanathan and Borges, 2000).
While genetic studies conducted in TMFs were rare, most revealed that populations in isolated forests had lower genetic diversity due to inbreeding and reduced gene flow (Cascante-Marin et al., 2014; Habel et al., 2014; Husemann et al., 2015). For example, a lack of genetic variation in epiphytic bromeliad (Guzmania monostachia) populations in Costa Rican forest patches was attributed primarily to anthropogenic barriers to gene flow but could also be influenced by life history traits such as its selective breeding system and limited seed dispersal ability (Cascante-Marin et al., 2014). Higher inbreeding and genetic bottlenecks within a small TMF fragment can also increase the extinction risk of a population (Juárez et al., 2011).
Effects of Habitat Degradation on Ecosystem Services Provided by TMFs
Habitat degradation in TMFs has been shown to disrupt several hydrological processes like affecting water conduction in soils, with reduced hydraulic conductivity in secondary forests and plantations (Marin-Castro et al., 2016, 2017). In turn, this likely contributed to increased surface runoff in cultivated land (Lorup and Hansen, 1997; Munoz-Villers and McDonnell, 2013; Suescun et al., 2017). With increasing surface water runoff, streamflow in degraded landscapes can be higher following rainfall (Munoz-Villers et al., 2012; Ramirez et al., 2017) but in the dry season, stream flow from cultivated catchments can be drastically reduced (Castillo et al., 2012). Water storage was also lower in agricultural areas (Guardiola-Claramonte et al., 2010; Schrumpf et al., 2011), and may subsequently lead to a reduction in watershed discharge (Caballero et al., 2013).
Land conversion in TMFs can lead to declines in mean carbon densities due to biomass loss (De Jong et al., 1999; Restrepo et al., 2012). Impacts of land conversion on soil organic carbon (SOC) are less conclusive; most studies reported lower SOC in cultivated land relative to montane forest (e.g., Rhoades et al., 2000; Mendoza-Vega et al., 2003; Omoro et al., 2013; Tesfaye et al., 2016), but two showed weak or no effects (De Jong et al., 1999; Chacon et al., 2015) and another showed no clear trend (Twongyirwe et al., 2013). Such conflicting results may be attributed to variation in soil properties, age since disturbance, the type of cultivated land, and altitude (Twongyirwe et al., 2013; Chacon et al., 2015).
Habitat degradation in TMF can lead to marked changes in N storage and conversion rates. Conversion of TMFs to cultivated land led to a reduction in soil N (Rhoades and Coleman, 1999; Tesfaye et al., 2016) and decreased net N mineralization rates (Campos et al., 2014). Total dissolved nitrogen was higher in plantations than in TMF catchments, probably due to more leaching (Jacobs et al., 2017). Additionally, the rate of N decay from leaf litter in plantations, or in streams within pastures, was slower compared to natural TMFs (Encalada et al., 2010; Loaiza-Usuga et al., 2013; Ramirez et al., 2014).
Land use change alters the properties of montane soils, such as decreasing soil moisture (Schrumpf et al., 2011; Ramirez et al., 2017) and macroporosity (Pinto et al., 2016). Soil microbial biomass generally declines as land disturbance intensifies (Campos et al., 2007, 2014; Mganga et al., 2015, 2016). Macroinvertebrate diversity in soils is lower in deforested sites (Yankelevich et al., 2006; Negrete-Yankelevich et al., 2007) but can peak in secondary forests (De la Rosa and Negrete-Yankelevich, 2012).
Discussion
Potential Biases and Limitations of Our Systematic Map
Our method of gauging research effort per country, by aggregating the number of studies stemming from the country of interest, is likely biased by the extent of TMFs available. Estimating the number of studies per area unit of TMF in each country will provide better resolution. Another caveat to note is that community responses were tabulated without accounting for the intensity of disturbance, beyond broad classifications of habitat types. Also, our classifications did not consider spatial differences among studies, and the impacts of habitat degradation at a site, country or regional scale will vary. Last, our assessment of articles covering ecosystem services mainly focused on those that provided supporting and regulation services, with less emphasis on provisioning and cultural services (Alcamo and Bennett, 2003). A separate systematic review on the impacts of habitat degradation on the ecosystem services provided by TMFs is recommended by using additional search terms (e.g., “ecotourism” and “education”) to obtain relevant articles.
Regional Comparisons
Given that more than half the world's remaining TMFs occur in Asia (Scatena et al., 2010), the scant research in this region highlighted in this systematic map is of concern. Our results corroborate an earlier review that found only 5% of published biodiversity studies in Southeast Asia from 1990 to 2010 were conducted in montane forests (Peh et al., 2011). Average annual deforestation rates from 2000 to 2010 for montane forests in Southeast Asia ranged from 0.2 to 0.4% compared to 1.2% for lowland and 2.2% for peat swamp forests (Miettinen et al., 2011), but deforestation rates in the highlands are increasing due to recent expansion of roads (Peh et al., 2011; Margono et al., 2014). While roads are essential for economic development, they are a major threat to biodiversity (Laurance et al., 2009). In Peninsular Malaysia, the construction of the second East-West highway, completed in 2010, has led to rampant deforestation in the Lojing Highlands despite regulations that restrict logging above 1,000 m (Singh, 2013). Much of the cleared land has been converted to agricultural farms (Omar and Hamzah, 2010). Alarmingly, nearly half of montane primary forest loss in Indonesia has occurred within protected areas (Margono et al., 2014). To tackle illegal logging, Malaysian and Indonesian governments have implemented schemes that award certification to producers that promote sustainable logging practices (e.g., Malaysian Timber Certification Council and the Timber Legality Assurance System in Indonesia) (Peh et al., 2011; Margono et al., 2014). However, such initiatives have not stopped deforestation of protected areas (Peh et al., 2011; Chitra and Cetera, 2018). Imposing sanctions on non-compliant timber producers, and stricter assessments to gain certifications are needed to secure the remaining TMFs in Asia (Chitra and Cetera, 2018).
Research in African TMFs was also poorly represented globally, yet much of Africa's TMF is threatened from overexploitation through illegal logging and poaching, and habitat loss via land conversion to agriculture and charcoal burning (Cronin et al., 2014; Jacobs et al., 2017). Although there are designated protected areas in Africa, their coverage is inadequate and many protected sites are poorly managed (Cronin et al., 2014; Jacobs et al., 2017). Further, the heavy reliance of fertilizers in farms increases nutrient loads in streams that lead to a deterioration in water quality and eutrophication (Jacobs et al., 2017). Political unrest in countries such as Sudan also affect the state of natural resources, such as those in the Imatong Mountains and surrounds which are part of the Eastern Afro-montane ecosystem—considered to be one of Africa's biodiversity hot spots (Uma, 2016). Two decades of civil war have decimated large swathes of forest, particularly on Mount Dongotomea, with two-thirds of the forest lost since 1986 (Gorsevski, 2012; African Wildlife Foundation, 2014). A lack of livelihoods for returning refugees and strong dependence on natural resources has led to increased poaching for bushmeat, illegal logging and fires set deliberately for shifting agriculture (Gorsevski, 2012; African Wildlife Foundation, 2014).
Country Comparisons
Articles describing research conducted in Mexico comprised a third of all relevant papers in this mapping exercise. Although TMFs in Mexico occupy only 1% of the country's land area, they have exceptionally high biodiversity owing to the diversity of habitats and the contribution of biota from Holarctic and Neotropical origins (González-Espinosa et al., 2011; Ponce-Reyes et al., 2012). More than half of the TMFs in Mexico have been converted to crops (Toledo-Aceves et al., 2011) and consequently 70% of forest trees in this ecosystem are threatened (González-Espinosa et al., 2011). Deforestation rates in the highlands of Mexico have also intensified sharply (Cayuela et al., 2006a; Gómez-Mendoza et al., 2006). In the oak and mountain cloud forests of Sierra Norte of Oaxaca, the annual deforestation rates from 1980 to 2000 was 1–2% (Gómez-Mendoza et al., 2006). In the Chiapas highlands, the annual rate increased from 1.3% during 1975 to 1990, to 4.8% during 1990 to 2000 (Cayuela et al., 2006a). More recent estimates of TMF deforestation rates are lacking in Mexican TMF, and considering that the last reported deforestation rates were rising nearly two decades ago, an updated estimate is crucial to assess the current extent of forest loss and re-valuate its impact to montane biota. Additionally, only 12% of Mexico's cloud forests are protected (Ponce-Reyes et al., 2012), and if unprotected forests are cleared, the combined effects of habitat loss and climate change could lead to the extinction of up to 70% of Mexico's endemic vertebrates (Ponce-Reyes et al., 2012).
Integrating the Impacts of Climate Change
Several studies in our systematic map explored the synergistic effects of climatic/microclimatic change and habitat degradation on biotic and abiotic responses. These included changes in species distribution and population sizes, germination and seedling development, community structure, food webs and nutrient availability. For example, the altitudinal distribution of several dung beetle species was higher in deforested areas (where it was hotter and drier) than in intact landscapes (Larsen, 2012). When projected changes in both climate and forested areas were combined, declines in range and population sizes of montane birds were amplified, resulting in 19–42 and 19–62% reductions, respectively (Harris et al., 2014). Harsher microclimates in altered habitats negatively affected germination rates, seedling development and recruitment, which in turn hampered recolonization rates (Werner and Gradstein, 2008; Anthelme et al., 2011; Hietz et al., 2012). Epiphyte species richness declined due to warmer and drier microclimates in disturbed forests (Barthlott et al., 2001) but recovered in restored sites if microclimatic conditions simulated those in primary forests (Diaz-Garcia et al., 2017). The interaction of habitat degradation and climate can also affect nutrient and pollutant deposition (Ponette-González et al., 2010) and is more pronounced in drier seasons, affecting processes such as plant transpiration (Ramirez et al., 2017). Collectively, these studies indicate that the effects of habitat degradation on biodiversity and ecosystem functioning are compounded when climatic/microclimatic change is also considered.
Research Priorities for Habitat Degradation in TMF
Clearly, the most urgent research priority with regards the impacts of habitat degradation on TMF is to understand its effects on population genetics (Figure 8). Aside from there being so few genetic studies of species occurring in this forest type, the preservation of genetic diversity is fundamental in maintaining viable populations that have adaptive potential. We suggest applying next-generation sequencing (NGS) in future genetic research, as inferences from NGS are drawn genome-wide (Angeloni et al., 2011). This is unlike the studies identified via our systematic map, where traditional methods like Sanger sequencing or microsatellites targeted only a few genes.
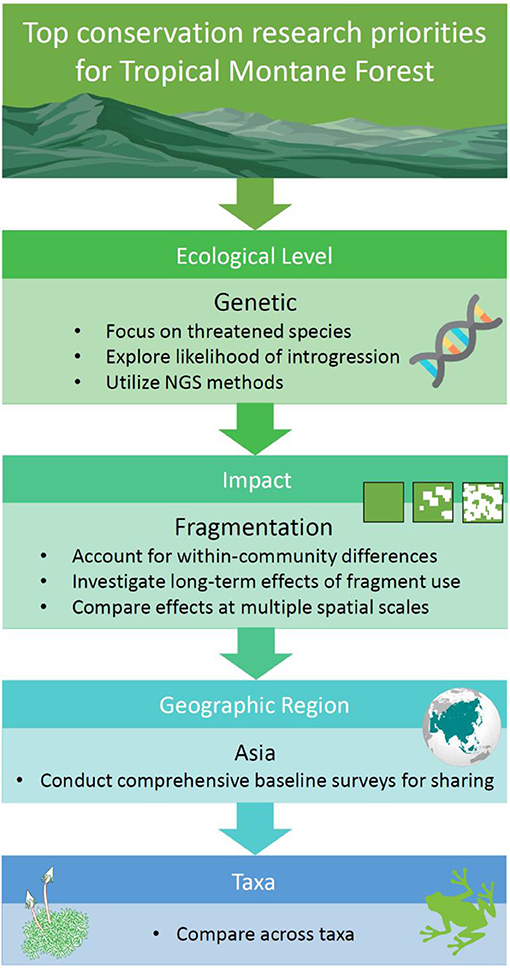
Figure 8. Research priorities for conserving the world's tropical montane forest ecosystems, indicated in order of importance.
A logical group for thorough evaluation of their genetic structure are threatened or endemic species, many of which are likely to exist as small populations that are vulnerable to the effects of genetic drift. The findings from the limited number of studies conducted in TMF have shown expected results: (1) habitat fragmentation can impede gene flow and lead to a loss of genetic variation, and (2), improving fragment connectivity can help reserve this trend. Where possible, drawing inferences on gene flow and genetic diversity from multiple species within an ecosystem is ideal, as species responses to fragmentation can differ in concert with variation in species traits. For instance, a study of montane forest birds in Kenya revealed tighter genetic clustering among sedentary species compared to more mobile species (Callens et al., 2011). Further, generalist species are often more robust to the impacts of habitat fragmentation (Janecka et al., 2016).
Not yet documented in our mapping exercise are studies that examine the role played by habitat degradation (and likely interactions with climate change) on introgression in TMF biota. Introgression is the hybridization of closely related species accompanied by repeated back-crossing of the hybrid with a parent species (Anderson, 1949). It is pervasive in natural populations and can accelerate the loss in genetic diversity (Harrison and Larson, 2014). A study in the Ethiopian highlands found that the wild gene pool for Coffea arabica had admixed with cultivars grown in close proximity to natural populations (Aerts et al., 2013). This example illustrates the threat posed by non-native species, and it is not inconceivable that increasing habitat loss and/or climate change in TMFs could broaden hybrid zones and increase the likelihood of introgression between related lowland and montane species. If so, montane endemics may experience genetic swamping (i.e., replacement of local genotypes by hybrids) and increased risk of extinction (Ellstrand and Rieseberg, 2016).
Research that investigates the impacts of habitat fragmentation and edge effects on montane biota should also be prioritized, as the results will have profound implications for sustainable land-use planning (e.g., implementing green corridors and evaluating land-sparing vs. land-sharing options) (Figure 8). Although fragmentation is well-known for reducing gene flow, it has far-reaching consequences at all ecological levels, including ecosystem services. In general, fragmentation has a negative impact on communities; resulting in a decline in species richness (e.g., Borgella et al., 2001; Kumar and O'Donnell, 2007; Hundera et al., 2013; Mohandass et al., 2014; Rovero et al., 2014), and changes in species composition in response to fragmentation has also been consistently reported (e.g., Larrea and Werner, 2010; Mohandass et al., 2014; Rueda-Hernandez et al., 2015; García-Martínez et al., 2017; Herrera-Paniagua et al., 2017). However, some studies have highlighted that certain spatial characteristics such as fragment area and isolation have no effect on abundance, density or diversity (Muriel and Kattan, 2009; Ulrich et al., 2016). While the extent of degradation may lead to conflicting results, deeper examination of species functional traits, which are indicators of habitat use, reveal that some groups within a community are more affected than others. For instance, two avian studies independently concluded that understory insectivores and canopy frugivores were more sensitive to fragmentation than other functional groups (Kattan et al., 1994; Rueda-Hernandez et al., 2015). Thus, within-community differences should be accounted for in future fragmentation research in TMF.
Our current understanding of the long-term effects of fragmentation is also limited by the scarcity of relevant historical data. In the short term, diversity may not be adversely affected by fragmentation and may even increase (Rey-Benayas et al., 2007). However, the effects are generally negative over the long term, with studies in TMFs showing a decline in plant diversity (>20 years) (Rey-Benayas et al., 2007; Koester et al., 2009), loss of plant biomass and change in species composition (>40 years) (Zahawi et al., 2017), and local extinction of birds (31% loss in over 80 years) (Kattan et al., 1994). Elucidating the environmental factors driving colonization and extinction patterns will allow better comprehension of community dynamics in a fragmented landscape. As such, a multi-season or dynamic occupancy models (DCMs), which are variants of more widely applied multi-species occupancy models, are well-suited for the task (Fernández-Chacón et al., 2014). Both are hierarchical models, but the dynamic model accounts for changes in occupancy over time by including sub-models of colonization and persistence that affect the previous occurrence state. Crucially, DCM models do not assume perfect detection among species, which can lead to misconstrued interpretations of occupancy dynamics.
A key recommendation from our mapping exercise is that effects of fragmentation should be examined at multiple spatial scales, as scaling dependencies in fragmented landscapes are vital for conservation planning (Cushman and McGarigal, 2004). While broad spatial effects have been documented to affect biotic responses (Chiavacci et al., 2018; Froehly et al., 2019) fine scale features such as vegetation heterogeneity were found to be better predictors in other studies (Cayuela et al., 2006b; Banks and Gagic, 2016; Michael et al., 2017). For example, research on golden-cheek warblers, Setophaga chrysoparia, showed that landscape composition best predicted species density, but vegetation characteristics was the best predictor of nesting success (Reidy et al., 2017).
Region-wise, research in Asian TMF is under-represented at all ecological levels, impact type and taxa (Figure 8). The future discovery of new species is particularly likely in Southeast Asia, given the regions' unique biogeographical history (Holloway and Hall, 1998). In the last 5 years, several new species were discovered in TMFs in Southeast Asia, including amphibians and reptiles from Vietnam (Grismer et al., 2013, 2015; Rowley et al., 2013, 2014, 2015). In these regions, attaining baseline ecological data such as a country-by-country species inventory represents a crucial first step in efficient data-sharing, and accumulation of large databases that facilitate multidisciplinary ecological research. Although we encourage further research of poorly studied taxa, available data for better represented groups such as vascular plants and birds are already useful for comparative studies, and to gain perspective on the overall response of the biome to habitat degradation (Figure 8). Comparisons between the responses of endotherms and ectotherms will be of interest, as the latter are more likely to be adversely affected by degradation due to their sensitivity to microclimatic change and generally lower dispersal abilities.
Suggestions for Future Research
Greater attention should be given to how functional diversity, which characterizes the range of ecological roles played by species in a community, may be affected by anthropogenic change in TMFs (Petchey and Gaston, 2006). Species richness estimates and related indices assume all species perform the same roles, but high species richness does not necessarily beget high functional diversity (Stuart-Smith et al., 2013). For example, while bird species richness and density in medium-sized fragments were higher than larger fragments in Mexican TMFs, larger fragments had a distinct functional composition, with a greater proportion of understory insectivore species and canopy frugivores (Rueda-Hernandez et al., 2015). Furthermore, functional diversity may be a better predictor of ecosystem function than species richness or abundance indices (Gagic et al., 2015).
Applying an ecological network is a useful approach to test the effects of degradation on species interactions at the community-level. An ecological network is a collection of nodes represented by species that are joined by links that either represent antagonistic or mutualistic interactions (Pascual and Dunne, 2006). The resilience of ecological networks can be compared along a disturbance gradient (Harvey et al., 2017; Tylianakis and Morris, 2017), and networks that are more resilient to environmental perturbations are generally characterized by having a greater number of nodes (e.g., species or functional diversity) and links (interactions) (Pascual and Dunne, 2006). In one study, seed-dispersal networks of birds in the montane forest interior and edge were compared, revealing that functional and interaction diversity were higher at forest edges (Saavedra et al., 2014). In contrast, parasitoid–host interaction networks were more homogenized in agricultural sites than in lowland forests (Laliberté and Tylianakis, 2010). Such conflicting responses to habitat degradation may relate to different levels of disturbance in these networks (i.e., edge vs. forest interior compared to open agriculture vs. forested habitats). As montane species are highly sensitive to disturbance (Long, 1995; Brooks et al., 1999; Soh et al., 2006), exploring the sensitivity of ecological networks in TMFs across varying disturbance intensities, while factoring the extent of disturbance and time passed since degradation began, will deepen our understanding of how species assemblages change with continued habitat modification.
The lack of population studies of TMF species in Southeast Asia is troubling given that the number of montane species threatened with extinction in this region may be underestimated (Brooks et al., 1999). Further, many species studied in TMFs have not been assessed against IUCN Red List criteria, which may indicate how little is known of their population dynamics. Unsurprisingly, most species without Red List assessments (“Not Evaluated,” NE) were vascular plants (>95%, n = 40), but included one insect and one fungus. While “NE” are often species new to science, they can potentially be under serious threat, particularly if their habitats are rapidly being lost or are unprotected (Tapley et al., 2018). Indeed, some NE species may be included in national threatened species lists, however, evaluation against IUCN Red List criteria is crucial for formulating policy and prioritizing management of threatened species at multiple scales (e.g., from site-specific environmental impact assessments, to national plans for conservation, to global initiatives like the Convention on International Trade in Endangered Species of Wild Fauna and Flora, Rodrigues et al., 2006).
Disappointingly, only a few articles investigated the effects of habitat fragmentation or edge effects on ecosystem services in tropical montane environments (Table 2). The potential impact of habitat fragmentation cannot be underestimated given that 70% of forest remaining globally is within 1 km of the forest's edge (Haddad et al., 2015). Factors such as fragment size and isolation can influence ecosystem services; for example, regulation of crop pests is more effective next to a forest, but crop production is greatest at intermediate distances from a forest (Mitchell et al., 2014). Careful consideration of the ecosystem services affected by fragmentation is crucial, as trade-offs between different services can be expected. For instance, in a study of montane heathlands, decreasing fragment size reduced biodiversity and recreational value, but increased carbon storage and timber value (Cordingley et al., 2015). Lastly, most studies investigating fragmentation effects on ecosystem services consider “supply” aspects (the potential of a natural resource to provide a benefit to people), without regard for their realization. This realization is affected by the “demand” (the desire for the service) and the “flow”, which represents the delivery of the service to the people. The framework provided by Mitchell et al. (2015) considers all three aspects, and will be useful in testing hypotheses on the realization of ecosystem services in forest fragments.
Conclusion
Our systematic map has shown that habitat degradation in TMFs has had discernible impacts on biodiversity and ecosystem services. While the impacts of this degradation are fairly well-studied at the community level, and adequate data may be available for meta-analysis, the impacts on genetic diversity and gene flow are less well-understood. Thus, population genetic studies should be prioritized for endemic species that are extinction-prone. We also advocate studying the long-term impacts of habitat degradation (particularly habitat fragmentation) on TMF at multiple spatial and taxonomic scales, and greater support for research based in Asia and Africa.
Data Availability Statement
The dataset generated for this study are included in the article/Supplementary Material.
Author Contributions
MS and KP conceived the systematic mapping. MS and CB performed the literature search and data extraction. MS wrote the manuscript and all co-authors reviewed the manuscript.
Funding
We thank the Institute of Life Sciences, University of Southampton, grant no. 511206105; for funding this work, along with the School of Biological Science at The University of Western Australia. MS was supported by the Australian Government Research Training Program Scholarship.
Conflict of Interest
The authors declare that the research was conducted in the absence of any commercial or financial relationships that could be construed as a potential conflict of interest.
Supplementary Material
The Supplementary Material for this article can be found online at: https://www.frontiersin.org/articles/10.3389/ffgc.2019.00083/full#supplementary-material
References
Abella-Medrano, C. A., Ibáñez-Bernal, S., MacGregor-Fors, I., and Santiago-Alarcon, D. (2015). Spatiotemporal variation of mosquito diversity (Diptera: Culicidae) at places with different land-use types within a neotropical montane cloud forest matrix. Parasit. Vectors 8:487. doi: 10.1186/s13071-015-1086-9
Aerts, R., Berecha, G., Gijbels, P., Hundera, K., Glabeke, S., Vandepitte, K., et al. (2013). Genetic variation and risks of introgression in the wild Coffea arabica gene pool in south-western Ethiopian montane rainforests. Evol. Appl. 6, 243–252. doi: 10.1111/j.1752-4571.2012.00285.x
African Wildlife Foundation (2014). Imatong Forest Water Consultancy Report. Institute of Natural Resources.
Alcamo, J., and Bennett, E. (2003). Ecosystems and Human Well-Being: A Framework for Assessment. Washington, DC: Island Press.
Alvarez-Aquino, C., Williams-Linera, G., and Newton, A. C. (2004). Experimental native tree seedling establishment for the restoration of a Mexican cloud forest. Restor. Ecol. 12, 412–418. doi: 10.1111/j.1061-2971.2004.00398.x
Angeloni, F., Wagemaker, N., Vergeer, P., and Ouborg, J. (2011). Genomic toolboxes for conservation biologists. Evol. Appl. 5, 130–143. doi: 10.1111/j.1752-4571.2011.00217.x
Anthelme, F., Gomez-Aparicio, L., and Montufar, R. (2014). Nurse-based restoration of degraded tropical forests with tussock grasses: experimental support from the Andean cloud forest. J. Appl. Ecol. 51, 1534–1543. doi: 10.1111/1365-2664.12311
Anthelme, F., Lincango, J., Gully, C., Duarte, N., and Montufar, R. (2011). How anthropogenic disturbances affect the resilience of a keystone palm tree in the threatened Andean cloud forest? Biol. Conserv. 144, 1059–1067. doi: 10.1016/j.biocon.2010.12.025
Ariyanti, N. S., Bos, M. M., Kartawinata, K., Tjitrosoedirdjo, S. S., Guhardja, E., and Gradstein, S. R. (2008). Bryophytes on tree trunks in natural forests, selectively logged forests and cacao agroforests in Central Sulawesi, Indonesia. Biol. Conserv. 141, 2516–2527. doi: 10.1016/j.biocon.2008.07.012
Badillo-Saldana, L. M., Ramirez-Bautista, A., and Wilson, L. D. (2016). Effects of establishment of grazing areas on diversity of amphibian communities in tropical evergreen forests and mountain cloud forests of the Sierra Madre Oriental. Rev. Mex. Biodivers. 87, 133–139. doi: 10.1016/j.rmb.2015.09.019
Banks, J. E., and Gagic, V. (2016). Aphid parasitoids respond to vegetation heterogeneity but not to fragmentation scale: an experimental field study. Basic Appl. Ecol. 17, 438–446. doi: 10.1016/j.baae.2016.01.007
Barthlott, W., Schmit-Neuerburg, V., Nieder, J., and Engwald, S. (2001). Diversity and abundance of vascular epiphytes: a comparison of secondary vegetation and primary montane rain forest in the Venezuelan Andes. Plant Ecol. 152, 145–156. doi: 10.1023/A:1011483901452
Basham, E. W., del Pliego, P. G., Acosta-Galvis, A. R., Woodcock, P., Medina, C. A., Haugaasen, T., et al. (2016). Quantifying carbon and amphibian co-benefits from secondary forest regeneration in the Tropical Andes. Anim. Conserv. 19, 548–560. doi: 10.1111/acv.12276
Berger-Tal, O., Greggor, A. L., Macura, B., Adams, C. A., Blumenthal, A., Bouskila, A., et al. (2018). Systematic reviews and maps as tools for applying behavioral ecology to management and policy. Behav. Ecol. 130, 1–8. doi: 10.1093/beheco/ary130
Borgella, R., Snow, A. A., and Gavin, T. A. (2001). Species richness and pollen loads of hummingbirds using forest fragments in southern Costa Rica. Biotropica 33, 90–109. doi: 10.1111/j.1744-7429.2001.tb00160.x
Brenning, A., Schwinn, M., Ruiz-Paez, A. P., and Muenchow, J. (2015). Landslide susceptibility near highways is increased by 1 order of magnitude in the Andes of southern Ecuador, Loja province. Nat. Hazards Earth Syst. Sci. 15, 45–57. doi: 10.5194/nhess-15-45-2015
Brooks, T. M., Pimm, S. L., Kapos, V., and Ravilious, C. (1999). Threat from deforestation to montane and lowland birds and mammals in insular South-east Asia. J. Anim. Ecol. 68, 1061–1078. doi: 10.1046/j.1365-2656.1999.00353.x
Bruijnzeel, L. A., Bruijnzeel, L. A., Scatena, F. N., and Hamilton, L. S. (2010). Tropical Montane Cloud Forests Science for Conservation and Management. International Hydrology Series. Cambridge; New York, NY: Cambridge University Press.
Bruijnzeel, L. A., Waterloo, M. J., Proctor, J., Kuiters, A. T., and Kotterink, B. (1993). Hydrological observations in montane rain-forests on Gunung Silam, Sabah, Malaysia, with special reference to the Massenerhebung effect. J. Ecol. 81, 145–167. doi: 10.2307/2261231
Caballero, L. A., Easton, Z. M., Richards, B. K., and Steenhuis, T. S. (2013). Evaluating the bio-hydrological impact of a cloud forest in Central America using a semi-distributed water balance model. J. Hydrol. Hydromech. 61, 9–96. doi: 10.2478/jhh-2013-0003
Callens, T., Galbusera, P., Matthysen, E., Durand, E. Y., Githiru, M., Huyghe, J. R., et al. (2011). Genetic signature of population fragmentation varies with mobility in seven bird species of a fragmented Kenyan cloud forest. Mol. Ecol. 20, 1829–1844. doi: 10.1111/j.1365-294X.2011.05028.x
Campos, A. C., Etchevers, J. B., Oleschko, K. L., and Hidalgo, C. M. (2014). Soil microbial biomass and nitrogen mineralization rates along an altitudinal gradient on the Cofre de Perote Volcano (Mexico): the importance of landscape position and land use. Land Degrad. Dev. 25, 581–593. doi: 10.1002/ldr.2185
Campos, A. C., Klaudia, O. L., Jorge, E. B., and Claudia, H. M. (2007). Exploring the effect of changes in land use on soil quality on the eastern slope of the Cofre de Perote Volcano (Mexico). For. Ecol. Manage. 248, 174–182. doi: 10.1016/j.foreco.2007.05.004
Cascante-Marin, A., Oostermeijer, G., Wolf, J., and Fuchs, E. J. (2014). Genetic diversity and spatial genetic structure of an epiphytic bromeliad in costa rican montane secondary forest patches. Biotropica 46, 425–432. doi: 10.1111/btp.12119
Castillo, M. M., Morales, H., Valencia, E., Morales, J. J., and Cruz-Motta, J. J. (2012). The effects of human land use on flow regime and water chemistry of headwater streams in the highlands of Chiapas. Knowledge Manage. Aquat. Ecosyst. 407:9. doi: 10.1051/kmae/2013035
Cayuela, L., Benayas, J. M. R., and Echeverria, C. (2006a). Clearance and fragmentation of tropical montane forests in the Highlands of Chiapas, Mexico (1975-2000). For. Ecol. Manage. 226, 208–218. doi: 10.1016/j.foreco.2006.01.047
Cayuela, L., Golicher, D. J., Benayas, J. M. R., Gonzalez-Espinosa, M., and Ramirez-Marcial, N. (2006b). Fragmentation, disturbance and tree diversity conservation in tropical montane forests. J. Appl. Ecol. 43, 1172–1181. doi: 10.1111/j.1365-2664.2006.01217.x
Chacon, P., Lorenz, K., Lal, R., Calhoun, F. G., and Fausey, N. (2015). Soil organic carbon in some land uses of Costa Rica. Acta Agricult. Scand. B Soil Plant Sci. 65, 310–320. doi: 10.1080/09064710.2015.1008563
Chiavacci, S. J., Benson, T. J., and Ward, M. P. (2018). Linking landscape composition to predator-specific nest predation requires examining multiple landscape scales. J. Appl. Ecol. 55, 2082–2092. doi: 10.1111/1365-2664.13090
Chitra, J., and Cetera, K. (2018). Indonesia Has a Carrot to End Illegal Logging; Now it Needs a Stick. World Resources Institute. Available online at: http://www.wri.org/print/57351 (accessed May 18, 2019).
Churchill, S. P., Balslev, H., Forero, E., and Luteyn, J. L. (1995). Biodiversity and Conservation of Neotropical Montane Forests. New York, NY: The New York Botanical Garden.
Collaboration for Environmental Evidence (2013). Guidelines for Systematic Review and Evidence Synthesis in Environmental Management. Version 4.2. Environmental Evidence. Available online at: www.environmentalevidence.org/Documents/Guidelines/Guidelines4.2.pdf (accessed May 18, 2019).
Cordingley, J. E., Newton, A. C., Rose, R. J., Clarke, R. T., and Bullock, J. M. (2015). Habitat fragmentation intensifies trade-offs between biodiversity and ecosystem services in a heathland ecosystem in Southern England. PLoS ONE 10:e0130004. doi: 10.1371/journal.pone.0130004
Cronin, D. T., Libalah, M. B., Bergl, R. A., and Hearn, G. W. (2014). Biodiversity and conservation of tropical montane ecosystems in the Gulf of Guinea, West Africa. Arctic Antarctic Alpine Res. 46, 891–904. doi: 10.1657/1938-4246-46.4.891
Cubasch, U., Wuebbles, D., Chen, D., Facchini, M. C., Frame, D., Mahowald, N., et al. (2013). “Introduction,” in Climate Change 2013: The Physical Science Basis. Contribution of Working Group I to the Fifth Assessment Report of the Intergovernmental Panel on Climate Change, eds T. F. Stocker, D. Qin, G.-K. Plattner, M. Tignor, S. K. Allen, J. Boschung, A. Nauels, Y. Xia, V. Bex, and P. M. Midgley (Cambridge; New York, NY: Cambridge University Press).
Cuervo, A. M., and Restrepo, C. (2007). Assemblage and population-level consequences of forest fragmentation on bilateral asymmetry in tropical montane birds. Biol. J. Linnean Soc. 92, 119–133. doi: 10.1111/j.1095-8312.2007.00884.x
Cushman, S. A., and McGarigal, K. (2004). Patterns in the species-environment relationship depend on both scale and choice of response variables. Oikos 105, 117–124. doi: 10.1111/j.0030-1299.2004.12524.x
De Jong, B. H. J., Cairns, M. A., Haggerty, P. K., Ramírez-Marcial, N., Ochoa-Gaona, S., Mendoza-Vega, J., et al. (1999). Land-use change and carbon flux between 1970s and 1990s in central highlands of Chiapas, Mexico. Environ. Manage. 23, 373–385. doi: 10.1007/s002679900193
De la Rosa, I. N., and Negrete-Yankelevich, S. (2012). Spatial distribution of soil macrofauna in cloud forest, secondary forest and grassland in La Cortadura reserve, Coatepec, Veracruz, Mexico. Rev. Mex. Biodivers. 83, 201–215. doi: 10.22201/ib.20078706e.2012.1.788
Deloya, C., Parra-Tabla, V., and Delfín-González, H. (2007). Fauna of Scarabaeidae-laparosticti and Trogidae (Coleoptera: Scarabaeoidea) associated with cloud forest, shaded coffee plantations and transformed communities in Central Veracruz, Mexico. Neotrop. Entomol. 36, 5–21. doi: 10.1590/S1519-566X2007000100002
Diaz-Garcia, J. M., Pineda, E., Lopez-Barrera, F., and Moreno, C. E. (2017). Amphibian species and functional diversity as indicators of restoration success in tropical montane forest. Biodivers. Conserv. 26, 2569–2589. doi: 10.1007/s10531-017-1372-2
Dietrich, K., Spohn, M., Villamagua, M., and Oelmann, Y. (2017). Nutrient addition affects net and gross mineralization of phosphorus in the organic layer of a tropical montane forest. Biogeochemistry 136, 223–236. doi: 10.1007/s10533-017-0392-z
Ellstrand, N. C., and Rieseberg, L. H. (2016). When gene flow really matters: gene flow in applied evolutionary biology. Evol. Appl. 9, 833–836. doi: 10.1111/eva.12402
Encalada, A. C., Calles, J., Ferreira, V., Canhoto, C. M., and Graca, M. A. S. (2010). Riparian land use and the relationship between the benthos and litter decomposition in tropical montane streams. Freshw. Biol. 55, 1719–1733. doi: 10.1111/j.1365-2427.2010.02406.x
Escobar, F., and de Ulloa, P. C. (2000). Space and time distribution along a succesional gradient of coprofagous coleopterans (Scarabaeinae, Aphodiinae) in a tropical montane forest, Narino Colombia. Rev. Biol. Trop. 48, 961–975.
FAO (2016). “State of the World's Forests, 2016,” in Forests and Agriculture: Land-Use Challenges and Opportunities (Rome: FAO), 1–107. Available online at: http://www.fao.org/3/a-i5588e.pdf (accessed May 18, 2019).
Fernández-Chacón, A., Stefanescu, C., Genovart, M., Nichols, J. D., Hines, J. E., Páramo, F., et al. (2014). Determinants of extinction-colonization dynamics in Mediterranean butterflies: the role of landscape, climate and local habitat features. J. Anim. Ecol. 83, 276–285. doi: 10.1111/1365-2656.12118
Frahm, J. P., and Gradstein, S. R. (1991). An altitudinal zonation of tropical rain-forests using byrophytes. J. Biogeogr. 18, 669–678. doi: 10.2307/2845548
Franceschinelli, E. V., do Carmo, R. M., Neto CdMe, S., Goncalves, B. B., and Bergamini, L. L. (2015). Reproductive success of Cabralea canjerana (Meliaceae) in Atlantic forest fragments, Brazil. Rev. Biol. Trop. 63, 515–524. doi: 10.15517/rbt.v63i2.14160
Froehly, J. L., Tegeler, A. K., Jachowski, C. M. B., and Jachowski, D. S. (2019). Effects of scale and land cover on loggerhead shrike occupancy. J. Wildl. Manage. 83, 426–434. doi: 10.1002/jwmg.21587
Gagic, V., Bartomeus, I., Jonsson, T., Taylor, A., Winqvist, C., Fischer, C., et al. (2015). Functional identity and diversity of animals predict ecosystem functioning better than species-based indices. Proc. R. Soc. B Biol. Sci. 282, 1–8. doi: 10.1098/rspb.2014.2620
Gallina, S., López Colunga, P., Valdespino, C., and Farías, V. (2016). Relative abundance of the gray fox Urocyon cinereoargenteus (Carnivora: Canidae) in Veracruz central area, Mexico. Rev. Biol. Trop. 64, 221–233. doi: 10.15517/rbt.v64i1.18237
García-Martínez, M. Á., Valenzuela-González, J. E., Escobar-Sarria, F., López-Barrera, F., and Castaño-Meneses, G. (2017). The surrounding landscape influences the diversity of leaf-litter ants in riparian cloud forest remnants. PLoS ONE 12:e0172464. doi: 10.1371/journal.pone.0172464
Goldsmith, S., Gillespie, A., and Weatherby, C. (2007). Restoration of Hawaiian montane wet forest: endemic longhorned beetles (Cerambycidae: Plagithmysus) in koa (Fabaceae: Acacia koa) plantations and in intact forest. Southwestern Nat. 52, 356–363. doi: 10.1894/0038-4909(2007)52[356:ROHMWF]2.0.CO;2
Gomez-Diaz, J. A., Kromer, T., Carvajal-Hernandez, C. I., Gerold, G., and Heitkamp, F. (2017). Richness and distribution of herbaceous angiosperms along gradients of elevation and forest disturbance in central Veracruz, Mexico. Bot. Sci. 95, 307–328. doi: 10.17129/botsci.859
Gómez-Mendoza, L., Vega-Pena, E., Isabel Ramirez, M., Palacio-Prieto, J. L., and Galicia, L. (2006). Projecting land-use change processes in the Sierra Norte of Oaxaca, Mexico. Appl. Geogr. 26, 276–290. doi: 10.1016/j.apgeog.2006.09.003
González-Espinosa, M., Meave, J. A., Lorea-Hernández, F. G., Ibarra-Manríquez, G., and Newton, A. C. (2011). The Red List of Mexican Cloud Forest Trees. Cambridge: Fauna and Flora International.
Gorsevski, V. B. (2012). Impacts of conflict on land use and land cover in the Imatong mountain region of south Sudan and northern Uganda (Unpublished doctor of philosophy). The University of Maryland, College Park, MD, United States, 157.
Gove, A. D., Hylander, K., Nemomissa, S., Shimelis, A., and Enkossa, W. (2013). Structurally complex farms support high avian functional diversity in tropical montane Ethiopia. J. Trop. Ecol. 29, 87–97. doi: 10.1017/S0266467413000023
Grismer, L. L., Wood, P. L., Anuar, S., Muin, M. A., Quah, E. S. H., McGuire, J. A., et al. (2013). Integrative taxonomy uncovers high levels of cryptic species diversity in Hemiphyllodactylus Bleeker, 1860 (Squamata: Gekkonidae) and the description of a new species from Peninsular Malaysia. Zool. J. Linn. Soc. 169, 849–880. doi: 10.1111/zoj.12064
Grismer, L. L., Wood, P. L., Lee, C. H., Quah, E. S., Anuar, S., Ngadi, E., et al. (2015). An integrative taxonomic review of the agamid genus Bronchocela (Kuhl, 1820) from Peninsular Malaysia with descriptions of new montane and insular endemics. Zootaxa 3948, 1–23. doi: 10.11646/zootaxa.3948.1.1
Guardiola-Claramonte, M., Troch, P. A., Ziegler, A. D., Giambelluca, T. W., Durcik, M., Vogler, J. B., et al. (2010). Hydrologic effects of the expansion of rubber (Hevea brasiliensis) in a tropical catchment. Ecohydrology 3, 306–314. doi: 10.1002/eco.110
Habel, J. C., Mulwa, R. K., Gassert, F., Rödder, D., Ulrich, W., Borghesio, L., et al. (2014). Population signatures of large-scale, long-term disjunction and small-scale, short-term habitat fragmentation in an Afromontane forest bird. Heredity 113, 205–214. doi: 10.1038/hdy.2014.15
Haddad, N. M., Brudvig, L. A., Clobert, J., Davies, K. F., Gonzalez, A., Holt, R. D., et al. (2015). Habitat fragmentation and its lasting impact on Earth's ecosystems. Sci. Adv. 1, 1–9. doi: 10.1126/sciadv.1500052
Hall, J., Burgess, N. D., Lovett, J., Mbilinyi, B., and Gereau, R. E. (2009). Conservation implications of deforestation across an elevational gradient in the Eastern Arc Mountains, Tanzania. Biol. Conserv. 142, 2510–2521. doi: 10.1016/j.biocon.2009.05.028
Hamilton, L. S., Juvik, J. O., and Scatena, F. N. (1995). “The Puerto Rico tropical montane cloud forest symposium: introduction and workshop synthesis,” in Tropical Montane Cloud Forests, eds L. S. Hamilton, J. O. Juvik, and F. N. Scatena (New York, NY: Springer-Verlag), 1–16.
Harris, J. B. C., Putra, D. D., Gregory, S. D., Brook, B. W., Prawiradilaga, D. M., Sodhi, N. S., et al. (2014). Rapid deforestation threatens mid-elevational endemic birds but climate change is most important at higher elevations. Divers. Distribut. 20, 773–785. doi: 10.1111/ddi.12180
Harrison, R. G., and Larson, E. L. (2014). Hybridization, introgression, and the nature of species boundaries. J. Heredity 105, 795–809. doi: 10.1093/jhered/esu033
Harvey, E., Gounand, I., Ward, C. L., and Altermatt, F. (2017). Bridging ecology and conservation: from ecological networks to ecosystem function. J. Appl. Ecol. 54, 371–379. doi: 10.1111/1365-2664.12769
Herrera-Paniagua, P., Martinez, M., Hernandez-Sandoval, L., and Garcia-Franco, J. (2017). Epiphytic mosses in the Humid Mountain Forests of the Sierra Madre Oriental, Mexico - species richness, rarity and composition. Cryptogamie Bryol. 38, 171–190. doi: 10.7872/cryb/v38.iss2.2017.171
Hietz, P., Winkler, M., Scheffknecht, S., and Huelber, K. (2012). Germination of epiphytic bromeliads in forests and coffee plantations: microclimate and substrate effects. Biotropica 44, 197–204. doi: 10.1111/j.1744-7429.2011.00791.x
Hitimana, J., Kiyiapi, J. L., and Njunge, J. T. (2004). Forest structure characteristics in disturbed and undisturbed sites of Mt. Elgon moist lower montane forest, western Kenya. For. Ecol. Manage. 194, 269–291. doi: 10.1016/j.foreco.2004.02.025
Holloway, J. D., and Hall, R. (1998). “SE Asian geology and biogeography: an introduction,” in Biogeography and Geological Evolution of SE Asia, eds R. Hall and J. D. Holloway (Lieden: Backhuys Publishers).
Hundera, K., Aerts, R., De Beenhouwer, M., Van Overtveld, K., Helsen, K., Muys, B., et al. (2013). Both forest fragmentation and coffee cultivation negatively affect epiphytic orchid diversity in Ethiopian moist evergreen Afromontane forests. Biol. Conserv. 159, 285–291. doi: 10.1016/j.biocon.2012.10.029
Husemann, M., Cousseau, L., Borghesio, L., Lens, L., and Habel, J. C. (2015). Effects of population size and isolation on the genetic structure of the East African mountain white-eye Zosterops poliogaster (Aves). Biol. J. Linnean Soc. 114, 828–836. doi: 10.1111/bij.12468
IUCN (2018). The IUCN Red List of Threatened Species. Version 2017-3. Available online at: https://www.iucnredlist.org (accessed May 18, 2019).
Jacobs, S. R., Breuer, L., Butterbach-Bahl, K., Pelster, D. E., and Rufino, M. C. (2017). Land use affects total dissolved nitrogen and nitrate concentrations in tropical montane streams in Kenya. Sci. Tot. Environ. 603, 519–532. doi: 10.1016/j.scitotenv.2017.06.100
Janecka, J. E., Tewes, M. E., Davis, I. A., Haines, A. M., Caso, A., Blankenship, T. L., et al. (2016). Genetic differences in the response to landscape fragmentation by a habitat generalist, the bobcat, and a habitat specialist, the ocelot. Conserv. Genet. 17, 1093–1108. doi: 10.1007/s10592-016-0846-1
Juárez, L., Montana, C., and Ferrer, M. M. (2011). Genetic structure at patch level of the terrestrial orchid Cyclopogon luteoalbus (Orchidaceae) in a fragmented cloud forest. Plant Syst. Evol. 297, 237–251. doi: 10.1007/s00606-011-0511-6
Kattan, G. H., Alvarezlopez, H., and Giraldo, M. (1994). Forest fragmentation and bird extinctions - San-Antonio 80 years later. Conserv. Biol. 8, 138–146. doi: 10.1046/j.1523-1739.1994.08010138.x
Kessler, M., and Kluge, J. (2008). “The tropical mountain forest: Patterns and processes in a biodiversity hotspot,” in Biodiversity and Ecology Series, eds S. R. Gradstein, J. Homeier, and D. Gansert (Göttingen: Gottingen Centre for Biodiversity and Ecology), 35–50.
Kitayama, K. (1995). Biophysical conditions of the montane cloud forests of Mount Kinabalu, Sabah, Malaysia. Trop. Montane Cloud For. 110, 183–197. doi: 10.1007/978-1-4612-2500-3_12
Koester, N., Friedrich, K., Nieder, J., and Barthlott, W. (2009). Conservation of epiphyte diversity in an andean landscape transformed by human land use. Conserv. Biol. 23, 911–919. doi: 10.1111/j.1523-1739.2008.01164.x
Kumar, A., and O'Donnell, S. (2007). Fragmentation and elevation effects on bird-army ant interactions in neotropical montane forest of Costa Rica. J. Trop. Ecol. 23, 581–590. doi: 10.1017/S0266467407004270
La Sorte, F. A., and Jetz, W. (2010). Projected range contractions of montane biodiversity under global warming. Proc. R. Soc. B Biol. Sci. 277, 3401–3410. doi: 10.1098/rspb.2010.0612
Laliberté, E., and Tylianakis, J. M. (2010). Deforestation homogenizes tropical parasitoid-host networks. Ecology 91, 1740–1747. doi: 10.1890/09-1328.1
Larrea, M. L., and Werner, F. A. (2010). Response of vascular epiphyte diversity to different land-use intensities in a neotropical montane wet forest. For. Ecol. Manage. 260, 1950–1955. doi: 10.1016/j.foreco.2010.08.029
Larsen, T. H. (2012). Upslope range shifts of Andean dung beetles in response to deforestation: compounding and confounding effects of microclimatic change. Biotropica 44, 82–89. doi: 10.1111/j.1744-7429.2011.00768.x
Laurance, W. F., Goosem, M., and Laurance, S. G. (2009). Impacts of roads and linear clearings on tropical forests. Trends Ecol. Evol. 24, 659–669. doi: 10.1016/j.tree.2009.06.009
Lawton, R. O., Nair, U. S., Pielke, R. A., and Welch, R. M. (2001). Climatic impact of tropical lowland deforestation on nearby montane cloud forests. Science 294, 584–587. doi: 10.1126/science.1062459
Lehouck, V., Spanhove, T., Colson, L., Adringa-Davis, A., Cordeiro, N. J., and Lens, L. (2009). Habitat disturbance reduces seed dispersal of a forest interior tree in a fragmented African cloud forest. Oikos 118, 1023–1034. doi: 10.1111/j.1600-0706.2009.17300.x
Li, B. V., Hughes, A. C., Jenkins, C. N., Ocampo-Peñuela, N., and Pimm, S. L. (2016). Remotely sensed data informs red list evaluations and conservation priorities in Southeast Asia. PLoS ONE 11:e0160566. doi: 10.1371/journal.pone.0160566
Loaiza-Usuga, C. J., Leon-Pelaez, J. D., Ramirez-Correa, J., Gonzalez-Hernandez, M. I., Gallardo-Lancho, F. J., Osorio-Vega, W., et al. (2013). Alterations in litter decomposition patterns in tropical montane forests of Colombia: a comparison of oak forests and coniferous plantations (vol 43, pg 528, 2013). Can. J. For. Res. 43:776. doi: 10.1139/cjfr-2013-0237
Long, A. J. (1995). “The importance of tropical montane cloud forests for endemic and threatened birds,” in: Ecological Studies; Tropical montane cloud forests, eds L. S. Hamilton, J. O. Juvik, and F. N. Scantena (New York, NY: Springer-Verlag Inc.), 79–106.
Lopez de Buen, L., Ornelas, J. F., and Guadalupe Garcia-Franco, J. (2002). Mistletoe infection of trees located at fragmented forest edges in the cloud forests of Central Veracruz, Mexico. For. Ecol. Manage. 164, 293–302. doi: 10.1016/S0378-1127(01)00624-7
Lorup, J. K., and Hansen, E. (1997). “Effect of land use on the streamflow in the southwestern highlands of Tanzania,” in Sustainability of Water Resources under Increasing Uncertainty, eds D. Rosbjerg, N.-E. Boutayeb, A. Gustard, Z. W. Kundzewicz, and P. F. Rasmussen (Great Yarmouth: IAHS Press), 227–236.
Maldonado, J. D., Tainter, F. H., Skipper, H. D., and Lacher, T. E. (2000). Arbuscular mycorrhiza inoculum potential in natural and managed tropical montane soils in Costa Rica. Trop. Agric. 77, 27–32.
Malhi, Y., and Wright, J. (2004). Spatial patterns and recent trends in the climate of tropical rainforest regions. Philos. Trans. R. Soc. Lond. Ser. B Biol. Sci. 359, 311–329. doi: 10.1098/rstb.2003.1433
Margono, B. A., Potapov, P. V., Turubanova, S., Stolle, F., and Hansen, M. C. (2014). Primary forest cover loss in Indonesia over 2000-2012. Nat. Clim. Chang. 4, 730–735. doi: 10.1038/nclimate2277
Marin-Castro, B. E., Geissert, D., Negrete-Yankelevich, S., and Chavez, A. G. T. (2016). Spatial distribution of hydraulic conductivity in soils of secondary tropical montane cloud forests and shade coffee agroecosystems. Geoderma 283, 57–67. doi: 10.1016/j.geoderma.2016.08.002
Marin-Castro, B. E., Negrete-Yankelevich, S., and Geissert, D. (2017). Litter thickness, but not root biomass, explains the average and spatial structure of soil hydraulic conductivity in secondary forests and coffee agroecosystems in Veracruz, Mexico. Sci. Tot. Environ. 607, 1357–1366. doi: 10.1016/j.scitotenv.2017.07.064
Martinez, M. L., Perez-Maqueo, O., Vazquez, G., Castillo-Campos, G., García-Franco, J., Mehltreter, K., et al. (2009). Effects of land use change on biodiversity and ecosystem services in tropical montane cloud forests of Mexico. For. Ecol. Manage. 258, 1856–1863. doi: 10.1016/j.foreco.2009.02.023
McCain, C. M., and Colwell, R. K. (2011). Assessing the threat to montane biodiversity from discordant shifts in temperature and precipitation in a changing climate. Ecol. Lett. 14, 1236–1245. doi: 10.1111/j.1461-0248.2011.01695.x
Mendez-Castro, E. F., and Rao, D. (2014). Spider diversity in epiphytes: can shade coffee plantations promote the conservation of cloud forest assemblages? Biodivers. Conserv. 23, 2561–2577. doi: 10.1007/s10531-014-0739-x
Mendoza-Vega, J., Karltun, E., and Olsson, M. (2003). Estimations of amounts of soil organic carbon and fine root carbon in land use and land cover classes, and soil types of Chiapas highlands, Mexico. For. Ecol. Manage. 177, 191–206. doi: 10.1016/S0378-1127(02)00439-5
Mganga, K. Z., Razavi, B. S., and Kuzyakov, Y. (2015). Microbial and enzymes response to nutrient additions in soils of Mt. Kilimanjaro region depending on land use. Eur. J. Soil Biol. 69, 33–40. doi: 10.1016/j.ejsobi.2015.05.001
Mganga, K. Z., Razavi, B. S., and Kuzyakov, Y. (2016). Land use affects soil biochemical properties in Mt. Kilimanjaro region. Catena 141, 22–29. doi: 10.1016/j.catena.2016.02.013
Michael, D. R., Ikin, K., Crane, M., Okada, S., and Lindenmayer, D. B. (2017). Scale-dependent occupancy patterns in reptiles across topographically different landscapes. Ecography 40, 415–424. doi: 10.1111/ecog.02199
Miettinen, J., Shi, C. H., and Liew, S. C. (2011). Deforestation rates in insular Southeast Asia between 2000 and 2010. Glob. Chang. Biol. 17, 2261–2270. doi: 10.1111/j.1365-2486.2011.02398.x
Mitchell, M. G., Suarez-Castro, A. F., Martinez-Harms, M., Maron, M., McAlpine, C., Gaston, K. J., et al. (2015). Reframing landscape fragmentation's effects on ecosystem services. Trends Ecol. Evol. 30, 190–198. doi: 10.1016/j.tree.2015.01.011
Mitchell, M. G. E., Bennett, E. M., and Gonzalez, A. (2014). Forest fragments modulate the provision of multiple ecosystem services. J. Appl. Ecol. 51, 909–918. doi: 10.1111/1365-2664.12241
Mohandass, D., Hughes, A. C., Campbell, M., and Davidar, P. (2014). Effects of patch size on liana diversity and distributions in the tropical montane evergreen forests of the Nilgiri Mountains, southern India. J. Trop. Ecol. 30, 579–590. doi: 10.1017/S0266467414000455
Munoz-Villers, L. E., Holwerda, F., Gomez-Cardenas, M., Equihuaa, V., Asbjornsend, H., Bruijnzee, L. A., et al. (2012). Water balances of old-growth and regenerating montane cloud forests in central Veracruz, Mexico. J. Hydrol. 462, 53–66. doi: 10.1016/j.jhydrol.2011.01.062
Munoz-Villers, L. E., and McDonnell, J. J. (2013). Land use change effects on runoff generation in a humid tropical montane cloud forest region. Hydrol. Earth System Sci. 17, 3543–3560. doi: 10.5194/hess-17-3543-2013
Muriel, S. B., and Kattan, G. H. (2009). Effects of patch size and type of coffee matrix on Ithomiine butterfly diversity and dispersal in cloud-forest fragments. Conserv. Biol. 23, 948–956. doi: 10.1111/j.1523-1739.2009.01213.x
Murrieta-Galindo, R., Parra-Olea, G., Gonzalez-Romero, A., Lopez-Barrera, F., and Vredenburg, V. T. (2014). Detection of Batrachochytrium dendrobatidis in amphibians inhabiting cloud forests and coffee agroecosystems in central Veracruz, Mexico. Eur. J. Wildl. Res. 60, 431–439. doi: 10.1007/s10344-014-0800-9
Negrete-Yankelevich, S., Fragoso, C., Newton, A. C., and Heal, O. W. (2007). Successional changes in soil, litter and macroinvertebrate parameters following selective logging in a Mexican Cloud Forest. Appl. Soil Ecol. 35, 340–355. doi: 10.1016/j.apsoil.2006.07.006
Omar, I. C., and Hamzah, Z. (2010). Conserving Lojing Highlands for Sustainable Development. Kota Bharu: Ubay Solution.
Omoro, L. M. A., Starr, M., and Pellikka, P. K. E. (2013). Tree biomass and soil carbon stocks in indigenous forests in comparison to plantations of exotic species in the Taita Hills of Kenya. Silva Fennica 47, 255–264. doi: 10.14214/sf.935
Pascual, M., and Dunne, J. A. (2006). Ecological Networks: Linking Structure to Dynamics in Food Webs. New York, NY: Oxford University Press.
Pattanavibool, A., and Dearden, P. (2002). Fragmentation and wildlife in montane evergreen forests, northern Thailand. Biol. Conserv. 107, 155–164. doi: 10.1016/S0006-3207(02)00056-3
Peh, K. S. H., Soh, M. C. K., Sodhi, N. S., Laurance, W. F., Ong, D. J., and Clements, R. (2011). Up in the clouds: is sustainable use of tropical montane cloud forests possible in malaysia? BioScience 61, 27–38. doi: 10.1525/bio.2011.61.1.8
Petchey, O. L., and Gaston, K. J. (2006). Functional diversity: back to basics and looking forward. Ecol. Lett. 9, 741–758. doi: 10.1111/j.1461-0248.2006.00924.x
Peters, R. L., and Darling, J. D. S. (1985). The greenhouse-effect and nature reserves. BioScience 35, 707–717. doi: 10.2307/1310052
Pinto, L. C., de Mello, C. R., Owens, P. R., Norton, L. D., and Curi, N. (2016). Role of inceptisols in the hydrology of mountainous catchments in southeastern Brazil. J. Hydrol. Eng. 21, 05015017–105015017-10. doi: 10.1061/(ASCE)HE.1943-5584.0001275
Pizano, C., Mangan, S. A., Graham, J. H., and Kitajima, K. (2017). Host-specific effects of soil microbial filtrates prevail over those of arbuscular mycorrhizae in a fragmented landscape. Ecol. Appl. 27, 1946–1957. doi: 10.1002/eap.1579
Ponce-Reyes, R., Reynoso-Rosales, V. H., Watson, J. E. M., VanDerWal, J., Fuller, R. A., Pressey, R. L., et al. (2012). Vulnerability of cloud forest reserves in Mexico to climate change. Nat. Clim. Chang. 2, 448–452. doi: 10.1038/nclimate1453
Ponette-González, A. G., Weathers, K. C., and Curran, L. M. (2010). Tropical land-cover change alters biogeochemical inputs to ecosystems in a Mexican montane landscape. Ecol. Appl. 20, 1820–1837. doi: 10.1890/09-1125.1
Quevedo, A. A., Schleuning, M., Hensen, I., Saavedra, F., and Durka, W. (2013). Forest fragmentation and edge effects on the genetic structure of Clusia sphaerocarpa and C. lechleri (Clusiaceae) in tropical montane forests. J. Trop. Ecol. 29, 321–329. doi: 10.1017/S0266467413000345
Ramirez, B. H., van der Ploeg, M., Teuling, A. J., Ganzeveld, L., and Leemans, R. (2017). Tropical Montane Cloud Forests in the Orinoco river basin: The role of soil organic layers in water storage and release. Geoderma 298, 14–26. doi: 10.1016/j.geoderma.2017.03.007
Ramirez, J. A., Leon-Pelaez, J. D., Craven, D., Herrera, D. A., Zapata, C. M., González-Hernández, M. I., et al. (2014). Effects on nutrient cycling of conifer restoration in a degraded tropical montane forest. Plant Soil 378, 215–226. doi: 10.1007/s11104-014-2024-x
Reidy, J. L., Thompson, F. R., and O'Donnell, L. (2017). Density and nest survival of golden-cheeked warblers: Spatial scale matters. J. Wildl. Manage. 81, 678–689. doi: 10.1002/jwmg.21234
Renner, S. C., Waltert, M., and Muhlenberg, M. (2006). Comparison of bird communities in primary vs. young secondary tropical montane cloud forest in Guatemala. Biodivers. Conserv. 15, 1545–1575. doi: 10.1007/s10531-005-2930-6
Restrepo, H. I., Orrego, S. A., and Galeano, O. J. (2012). Estructura de bosques secundarios y rastrojos montano bajos del norte de Antioquia, Colombia. Colombia Forestal 15, 173–189. doi: 10.14483/udistrital.jour.colomb.for.2012.2.a03
Rey-Benayas, J. M., Cayuela, L., Gonzalez-Espinosa, M., Echeverría, C., Manson, R. H., Williams-Linera, G., et al. (2007). “Plant diversity in highly fragmented forest landscapes in Mexico and Chile: implications for conservation,” in Biodiversity Loss and Conservation in Fragmented Forest Landscapes: The Forests of Montane Mexico and Temperate South America, ed A. C. Newton (King's Lynn: Biddles Ltd, 43–68.
Rhoades, C. C., and Coleman, D. C. (1999). Nitrogen mineralization and nitrification following land conversion in montane Ecuador. Soil Biol. Biochem. 31, 1347–1354. doi: 10.1016/S0038-0717(99)00037-1
Rhoades, C. C., Eckert, G. E., and Coleman, D. C. (2000). Soil carbon differences among forest, agriculture, and secondary vegetation in lower montane Ecuador. Ecol. Appl. 10, 497–505. doi: 10.1890/1051-0761(2000)010[0497:SCDAFA]2.0.CO;2
Rodrigues, A. S., Pilgrim, J. D., Lamoreux, J. F., Hoffmann, M., and Brooks, T. M. (2006). The value of the IUCN Red List for conservation. Trends Ecol. Evol. 21, 71–76. doi: 10.1016/j.tree.2005.10.010
Rovero, F., Menegon, M., Fjeldsa, J., Collett, L., Doggart, N., Leonard, C., et al. (2014). Targeted vertebrate surveys enhance the faunal importance and improve explanatory models within the Eastern Arc Mountains of Kenya and Tanzania. Divers. Distribut. 20, 1438–1449. doi: 10.1111/ddi.12246
Rowley, J. J., Dau, V. Q., and Nguyen, T. T. (2013). A new species of Leptolalax (Anura: Megophryidae) from the highest mountain in Indochina. Zootaxa 3737, 415–428. doi: 10.11646/zootaxa.3737.4.5
Rowley, J. J., Le, D. T., Dau, V. Q., Hoang, H. D., and Cao, T. T. (2014). A striking new species of phytotelm-breeding tree frog (Anura: Rhacophoridae) from central Vietnam. Zootaxa 3785, 25–37. doi: 10.11646/zootaxa.3785.1.2
Rowley, J. J., Tran, D. T., Frankham, G. J., Dekker, A. H., Le, D. T., Nguyen, T. Q., et al. (2015). Undiagnosed cryptic diversity in small, microendemic frogs (Leptolalax) from the central highlands of Vietnam. PLoS ONE 10:e0128382. doi: 10.1371/journal.pone.0128382
Rueda-Hernandez, R., MacGregor-Fors, I., and Renton, K. (2015). Shifts in resident bird communities associated with cloud forest patch size in Central Veracruz, Mexico. Avian Conserv. Ecol. 10:2. doi: 10.5751/ACE-00751-100202
Saavedra, F., Hensen, I., Beck, S. G., Böhning-Gaese, K., Lippok, D., Töpfer, T., et al. (2014). Functional importance of avian seed dispersers changes in response to human-induced forest edges in tropical seed-dispersal networks. Oecologia 176, 837–848. doi: 10.1007/s00442-014-3056-x
Scatena, F. N., Bruijnzeel, L. A., Bubb, P., and Das, S. (2010). Setting the Stage in Tropical Montane Cloud Forests: Science for Conservation and Management. Cambridge: Cambridge University Press.
Schrumpf, M., Axmacher, J. C., Zech, W., and Lyaruu, H. V. M. (2011). Net precipitation and soil water dynamics in clearings, old secondary and old-growth forests in the montane rain forest belt of Mount Kilimanjaro, Tanzania. Hydrol. Process. 25, 418–428. doi: 10.1002/hyp.7798
Soh, M. C. K., Sodhi, N. S., and Lim, S. L. H. (2006). High sensitivity of montane bird communities to habitat disturbance in Peninsular Malaysia. Biol. Conserv. 129, 149–166. doi: 10.1016/j.biocon.2005.10.030
Somanathan, H., and Borges, R. M. (2000). Influence of exploitation on population structure, spatial distribution and reproductive success of dioecious species in a fragmented cloud forest in India. Biol. Conserv. 94, 243–256. doi: 10.1016/S0006-3207(99)00170-6
Spracklen, D. V., and Righelato, R. (2014). Tropical montane forests are a larger than expected global carbon store. Biogeosciences 11, 2741–2754. doi: 10.5194/bg-11-2741-2014
Stadtmüller, T. (1987). Cloud forests in the Humid Tropics: A Bibliographic Review. Tokyo; Turrialba: United Nations University, CATIE.
Stuart-Smith, R. D., Bates, A. E., Lefcheck, J. S., Duffy, J. E., Baker, S. C., Thomson, R. J., et al. (2013). Integrating abundance and functional traits reveals new global hotspots of fish diversity. Nature 501, 539–42. doi: 10.1038/nature12529
Suescun, D., Villegas, J., Leon, J. D., Florez, C. P., Garcia-Leoz, V., and Correa-Londono, G. A. (2017). Vegetation cover and rainfall seasonality impact nutrient loss via runoff and erosion in the Colombian Andes. Reg. Environ. Change 17, 827–839. doi: 10.1007/s10113-016-1071-7
Sutherland, W. J., Adams, W. M., Aronson, R. B., Aveling, R., Blackburn, T. M., Broad, S., et al. (2009). One Hundred Questions of Importance to the Conservation of Global Biological Diversity. Conserv. Biol. 23, 557–567. doi: 10.1111/j.1523-1739.2009.01212.x
Tapley, B., Michaels, C. J., Gumbs, R., Bohm, M., Luedtke, J., Pearce-Kelly, P., et al. (2018). The disparity between species description and conservation assessment: a case study in taxa with high rates of species discovery. Biol. Conserv. 220, 209–214. doi: 10.1016/j.biocon.2018.01.022
Tesfaye, M. A., Bravo, F., Ruiz-Peinado, R., Pando, V., and Bravo-Oviedo, A. (2016). Impact of changes in land use, species and elevation on soil organic carbon and total nitrogen in Ethiopian Central Highlands. Geoderma 261, 70–79. doi: 10.1016/j.geoderma.2015.06.022
Toledo-Aceves, T., Meave, J. A., González-Espinosa, M., and Ramírez-Marcial, N. (2011). Tropical montane cloud forests: current threats and opportunities for their conservation and sustainable management in Mexico. J. Environ. Manage. 92, 974–981. doi: 10.1016/j.jenvman.2010.11.007
Twongyirwe, R., Sheil, D., Majaliwa, J. G. M., Ebanyat, P., Tenywa, M. M., van Heist, M., et al. (2013). Variability of soil organic carbon stocks under different land uses: a study in an afro-montane landscape in southwestern Uganda. Geoderma 193, 282–289. doi: 10.1016/j.geoderma.2012.09.005
Tylianakis, J. M., and Morris, R. J. (2017). Ecological networks across environmental gradients. Ann. Rev. Ecol. Evol. Syst. 48, 25–48. doi: 10.1146/annurev-ecolsys-110316-022821
Ulrich, W., Lens, L., Tobias, J. A., and Habel, J. C. (2016). Contrasting patterns of species richness and functional diversity in bird communities of east African cloud forest fragments. PLoS ONE 11:e0163338. doi: 10.1371/journal.pone.0163338
Vazquez, G., Ake-Castillo, J. A., and Favila, M. E. (2011). Algal assemblages and their relationship with water quality in tropical Mexican streams with different land uses. Hydrobiologia 667, 173–189. doi: 10.1007/s10750-011-0633-4
Werner, F. A., and Gradstein, S. R. (2008). Seedling establishment of vascular epiphytes on isolated and enclosed forest trees in an Andean landscape, Ecuador. Biodivers. Conserv. 17, 3195–3207. doi: 10.1007/s10531-008-9421-5
Whitmore, T. C. (1998). An introduction to Tropical Rain Forests. Oxford; New York, NY: Oxford University Press.
Winkler, M., Koch, M., and Hietz, P. (2011). High gene flow in epiphytic ferns despite habitat loss and fragmentation. Conserv. Genet. 12, 1411–1420. doi: 10.1007/s10592-011-0239-4
Yankelevich, S. N., Fragoso, C., Newton, A. C., Russell, G., and Heal, O. W. (2006). Spatial patchiness of litter, nutrients and macroinvertebrates during secondary succession in a Tropical Montane Cloud Forest in Mexico. Plant Soil 286, 123–139. doi: 10.1007/s11104-006-9031-5
Zahawi, R. A., Oviedo-Brenes, F., and Peterson, C. J. (2017). A degradation debt? Large-scale shifts in community composition and loss of biomass in a tropical forest fragment after 40 years of isolation. PLoS ONE 12:e0183133. doi: 10.1371/journal.pone.0183133
Zheng, J.-W., An, S.-Q., Chen, L., Leng, X., Wang, Z.-S., and Xiang, H.-J. (2005). Effects of logging on the genetic diversity of Quercus tiaoloshanica Chun et Ko in a tropical montane forest of Hainan island, southern China. J. Integr. Plant Biol. 47, 1184–1192. doi: 10.1111/j.1744-7909.2005.00143.x
Keywords: tropical montane forest, cloud forest, habitat degradation, disturbance, deforestation, fragmentation, biodiversity, ecosystem service
Citation: Soh MCK, Mitchell NJ, Ridley AR, Butler CW, Puan CL and Peh KS-H (2019) Impacts of Habitat Degradation on Tropical Montane Biodiversity and Ecosystem Services: A Systematic Map for Identifying Future Research Priorities. Front. For. Glob. Change 2:83. doi: 10.3389/ffgc.2019.00083
Received: 19 May 2019; Accepted: 29 November 2019;
Published: 17 December 2019.
Edited by:
Mark Andrew Adams, Swinburne University of Technology, AustraliaReviewed by:
Giuliano Maselli Locosselli, University of São Paulo, BrazilDaniel J. Wieczynski, UCLA Department of Ecology and Evolutionary Biology, United States
Copyright © 2019 Soh, Mitchell, Ridley, Butler, Puan and Peh. This is an open-access article distributed under the terms of the Creative Commons Attribution License (CC BY). The use, distribution or reproduction in other forums is permitted, provided the original author(s) and the copyright owner(s) are credited and that the original publication in this journal is cited, in accordance with accepted academic practice. No use, distribution or reproduction is permitted which does not comply with these terms.
*Correspondence: Malcolm C. K. Soh, bWFsY29sbXNvaEBnbWFpbC5jb20=