- 1Co-Innovation Center for Sustainable Forestry in Southern China, Bamboo Research Institute, College of Forestry and Grassland, Nanjing Forestry University, Nanjing, China
- 2Department of Geosciences and Natural Resource Management, The University of Copenhagen, Frederiksberg C, Denmark
- 3Department of Biosciences Engineering, University of Antwerp, Antwerp, Belgium
Editorial on the Research Topic
The adaptation, plasticity and extinction of forest plants to climate change: mechanisms behind the morphological, physiological, phenological and ecological traits
Climate change is one of the greatest threats to humankind’s current and future survival. Forests are one of the most essential solutions to addressing the effects of climate change by absorbing huge amounts of carbon dioxide (Pugh et al., 2019; Jiang et al., 2020; Wang et al., 2020). Furthermore, forest loss and degradation are both a cause and an effect of our changing climate (Sasaki and Putz, 2009; Griscom et al., 2017). The expected future climate with increasing drought episodes, seasonally warm temperatures, and severe storms, poses a challenge for forest management since it will affect the growth, mortality, species composition, and distribution of future forests (Figure 1; Huang et al., 2017; Shi et al., 2020). However, how the growth of different species responds differently to long-term drought and high temperatures has been poorly understood. Climate-growth relationships are an important tool for investigating tree growth responses under changing climate and thus provide a scientific basis for future forest management (Huang et al., 2022; Leifsson et al., 2024).
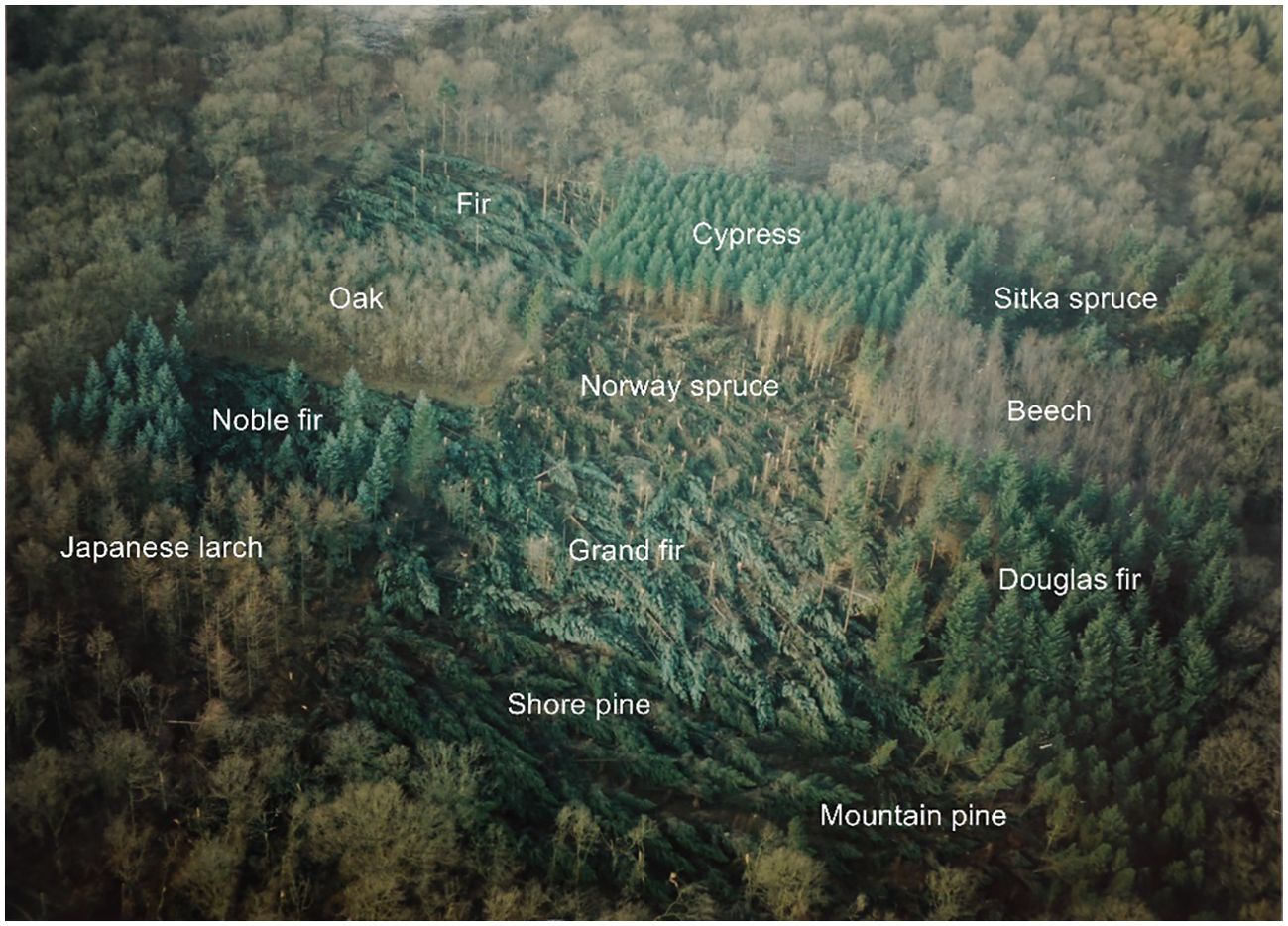
Figure 1. The tree species trial in Lovrup Forest in Denmark (NST Vadehavet) after the storm on December 3, 1999. The trial provides an example of the effect of storms on individual tree species and their potential to withstand increasing and more powerful storms. This picture was obtained from Huang et al. (2018; Foto: Feb. 2000, B. Bilde Jørgensen).
The papers collected in this Research Topic cover some important topics associated with climate change, including phenology, morphology, tree-ring growth, and wood anatomical traits of trees’ response to long-term climate change in Australia’s cool temperate rainforest, Amazon flooding forest, and Canada’s temperate forest, and also includes drought and heavy metal control experiments, as well as an article investigating the mathematical intricacies of bamboo internode elongation.
Australian cold temperate moist forests are known to have the highest aboveground biomass carbon stocks of all forest types (Keith et al., 2009). However, little is known about the effects of Australia’s cool temperate rainforest climate on long-term phenology. Vogado et al. provided 20 years of defoliation data from cool-temperature Nothofagus rainforests in New South Wales, Australia. They found that defoliation at the community level was mainly affected by Nothofagus moorei, driven by temperature and wind speed, and Ceratopetalum apetalum, driven by temperature, rainfall, and solar radiation. In addition, the average dates of community defoliation were increased by advanced solar radiation. All species presented seasonality in phenological behaviors, but seasonality peaked in different months and is influenced by different climate variables.
The Amazon floodplain forest is one of the largest flood-pulsed environments in the world (Junk et al., 2011), whose phenology and diameter increment are mainly triggered by flood pulses. de Sá et al. found that the tree growth and xylem anatomical characteristics of Hydrochorea corymbosa in várzea flooded forest in Central Amazon responded diversely to flooding and non-flooding periods. High flood levels during the end of the flood negatively affected vessel diameter in June and positively influenced parenchyma quantity in September and October. During the non-flooded period in December, the annual tree growth negatively correlated with the vapor pressure deficit. The vessel diameter was negatively affected by the September maximum temperature. The authors suggest that intensification of the hydrological regime and the severe droughts during the non-flooded periods can be a risk for H. corymbosa in the Central Amazonian floodplains.
Different tree species growing under the same conditions showed different plasticity and adaptation to climate change (Huang et al., 2017). Populations of a species descending from different origins may also exhibit different climatic adaptations. Zhou et al. observed that Acer saccharum seedlings originating from inland areas showed higher plasticity of bud burst than those from coastal areas at the beginning of leaf development in Quebec, Canada. Trees experiencing a wider climatic fluctuation may exhibit higher plasticity.
In addition to field experiments, control experiments are a method to study the effects of climate on trees by precisely controlling a single factor or multiple factors to examine the impacts of factor changes on tree growth. Xiao et al. set a pot experiment to investigate the effects of droughts and re-watering on the dynamics of non-structural carbohydrates (NSCs) in the different organs of two-year-old Pinus yunnanensis seedlings. Under drought, when the carbohydrates produced by photosynthesis could not satisfy the energy required for respiration, plants began to consume stored NSC. “Carbon starvation” occurs when stored NSCs cannot meet the energy required for cellular metabolism (McDowell et al., 2008, 2011). The author found that during the early stage of drought, the drought resistance of P. yunnanensis seedlings was enhanced by increasing soluble sugar concentration; in the later stages of drought, stored starch in organs, stems, and coarse roots were consumed. Growth under moderate drought was promoted after re-watering, suggesting that moderate drought stress can enhance drought tolerance and compensatory growth. Different drought treatments may lead to discrepancies in results. Zhao et al. found a diminishing relationship between trait patterns versus soil water content (SWC) from N-fixing non-legumes, N-fixing legumes to non-N-fixing plants. Whereas, several other studies have shown that drought intensification is more detrimental to non-N-fixing plants compared to N-fixing plants (Hofer et al., 2016, 2017). Climate change may increase runoff and accelerate leaching, thereby increasing heavy metal concentrations in soils. Alotaibi found that the increasing concentration of Pb and Cd delayed the Calligonum comosum seeds’ germination rate and speed. The authors suggest that the enhanced redox proteins and proteins involved in ATP synthesis may be a possible mechanism for seed tolerance to heavy metals.
Bamboo is one of the fastest-growing plants on earth, which can grow almost a meter in a single day and grow to its full height of 2 m to above 30 m within a few months (Shi et al., 2017), and sequester large amounts of atmospheric carbon to mitigate climate change. However, the relationship between internode length and serial number changes with culm height growth is not well understood. Tan et al. investigated the mathematical intricacies of the internode elongation pattern of Phyllostachys edulis, Phyllostachys iridescens, and Pseudosasa amabilis involved in the rapid culm growth.
In conclusion, the papers published in this Research Topic expand the current understanding of the adaptation and plasticity of different species growing in diverse continents with diverse climate conditions to climate change, as well as the mechanisms behind the morphological, physiological, phenological, and ecological traits.
Author contributions
WH: Writing – original draft, Writing – review & editing. JG: Writing – review & editing. PS: Writing – review & editing.
Funding
The author(s) declare that financial support was received for the research, authorship, and/or publication of this article. WH was supported by the National Natural Science Foundation of China (No. 32271973) and the Natural Science Foundation of Jiangsu Province, China (No. BK20220413).
Acknowledgments
We extend our gratitude to the authors, reviewers, and editors who played significant roles in publishing this Research Topic. Their dedication and expertise have been instrumental in bringing this Research Topic to fruition.
Conflict of interest
The authors declare that the research was conducted in the absence of any commercial or financial relationships that could be construed as a potential conflict of interest.
Publisher’s note
All claims expressed in this article are solely those of the authors and do not necessarily represent those of their affiliated organizations, or those of the publisher, the editors and the reviewers. Any product that may be evaluated in this article, or claim that may be made by its manufacturer, is not guaranteed or endorsed by the publisher.
References
Griscom B. W., Adams J., Ellis P. W., Houghton R. A., Lomax G., Miteva D. A., et al. (2017). Natural climate solutions. Proc. Natl. Acad. Sci. U.S.A. 114, 11645–11650. doi: 10.1073/pnas.1710465114
Hofer D., Suter M., Buchmann N., Luscher A. (2017). Nitrogen status of functionally different forage species explains resistance to severe drought and post-drought overcompensation. Agric. Ecosyst. Environ. 236, 312–322. doi: 10.1016/j.agee.2016.11.022
Hofer D., Suter M., Haughey E., Finn J. A., Hoekstra N. J., Buchmann N., et al. (2016). Yield of temperate forage grassland species is either largely resistant or resilient to experimental summer drought. J. Appl. Ecol. 53, 1023–1034. doi: 10.1111/1365-2664.12694
Huang W., Fonti P., Larsen J. B., Ræbild A., Callesen I., Pedersen N. B., et al (2017). Projecting tree-growth responses into future climate: A study case from a Danish-wide common garden. Agr. For. Meteorol 247, 240–251. doi: 10.1016/j.agrformet.2017.07.016
Huang W., Fonti P., Lundqvist S.-O., Larsen J. B., Hansen J. K., Thygesen L. G. (2022). Differences in xylem response to drought provide hints to future species selection. New For. 53, 759–777. doi: 10.1007/s11056-021-09885-8
Huang W., Hansen J. K., Ræbild A., Thygesen L. G., Jørgensen B. B., Larsen J. B. (2018). Hvordan påvirkes skovtræarternes vækst af klimæt [How is the growth of forest tree species affected by climate. Skoven 10, 385–387.
Jiang M., Medlyn B. E., Drake J. E., Duursma R. A., Anderson I. C., Barton C. V. M., et al. (2020). The fate of carbon in a mature forest under carbon dioxide enrichment. Nature 580, 227–231. doi: 10.1038/s41586-020-2128-9
Junk W. J., Piedade M. T. F., Schöngart J., Cohn-Haft M., Adeney J. M., Wittmann F. (2011). A classification of major naturally-occurring amazonian lowland wetlands. Wetlands 31, 623–640. doi: 10.1007/s13157-011-0190-7
Keith H., Mackey B. G., Lindenmayer D. B. (2009). Re-evaluation of forest biomass carbon stocks and lessons from the world’s most carbon-dense forests. Proc. Natl. Acad. Sci. 106, 11635–11640. doi: 10.1073/pnas.0901970106
Leifsson C., Buras A., Klesse S., Baittinger C., Bat-Enerel B., Battipaglia G., et al. (2024). Identifying drivers of non-stationary climate-growth relationships of European beech. Sci. Total Environ. 937, 173321. doi: 10.1016/j.scitotenv.2024.173321
McDowell N. G., Beerling D. J., Breshears D. D., Fisher R. A., Raffa K. F., Stitt M. (2011). The interdependence of mechanisms underlying climate-driven vegetation mortality. Trends Ecol. Evol. 26, 523–532. doi: 10.1016/j.tree.2011.06.003
McDowell N., Pockman W. T., Allen C. D., Breshears D. D., Cobb N., Kolb T., et al. (2008). Mechanisms of plant survival and mortality during drought: why do some plants survive while others succumb to drought? New Phytol. 178, 719–739. doi: 10.1111/j.1469-8137.2008.02436.x
Pugh T. A. M., Lindeskog M., Smith B., Poulter B., Arneth A., Haverd V., et al. (2019). Role of forest regrowth in global carbon sink dynamics. Proc. Natl. Acad. Sci. U.S.A. 116, 4382–4387. doi: 10.1073/pnas.1810512116
Sasaki N., Putz F. E. (2009). Critical need for new definitions of “forest” and “forest degradation” in global climate change agreements. Conserv. Lett. 2, 226–232. doi: 10.1111/j.1755-263X.2009.00067.x
Shi P., Fan M., Ratkowsky D. A., Huang J., Wu H., Chen L., et al. (2017). Comparison of two ontogenetic growth equations for animals and plants. Ecol. Model. 349, 1–10. doi: 10.1016/j.ecolmodel.2017.01.012
Shi P., Preisler H. K., Quinn B. K., Zhao J., Huang W., Röll A., et al. (2020). Precipitation is the most crucial factor determining the distribution of moso bamboo in Mainland China. Glob. Ecol. Conser 22, e00924. doi: 10.1016/j.gecco.2020.e00924
Keywords: climate change, forests, bamboo, dendroecology, wood anatomy, plant physiology, statistical modeling
Citation: Huang W, Gielis J and Shi P (2024) Editorial: The adaptation, plasticity and extinction of forest plants to climate change: mechanisms behind the morphological, physiological, phenological and ecological traits. Front. Ecol. Evol. 12:1488465. doi: 10.3389/fevo.2024.1488465
Received: 30 August 2024; Accepted: 08 October 2024;
Published: 22 October 2024.
Edited and Reviewed by:
Giovanna Battipaglia, University of Campania Luigi Vanvitelli, Caserta, ItalyCopyright © 2024 Huang, Gielis and Shi. This is an open-access article distributed under the terms of the Creative Commons Attribution License (CC BY). The use, distribution or reproduction in other forums is permitted, provided the original author(s) and the copyright owner(s) are credited and that the original publication in this journal is cited, in accordance with accepted academic practice. No use, distribution or reproduction is permitted which does not comply with these terms.
*Correspondence: Weiwei Huang, d2hAbmpmdS5lZHUuY24=