- 1Ecology, Evolution, and Marine Biology, University of California, Santa Barbara, Santa Barbara, CA, United States
- 2Cheadle Center for Biodiversity and Ecological Restoration, University of California, Santa Barbara, Santa Barbara, CA, United States
Habitat loss is a primary driver of global biodiversity decline, negatively impacting many species, including native bees. One approach to counteract the consequences of habitat loss is through restoration, which includes the transformation of degraded or damaged habitats to increase biodiversity. In this review, we survey bee habitat restoration literature over the last 14 years to provide insights into how best to promote bee diversity and abundance through the restoration of natural landscapes in North America. We highlight relevant questions and concepts to consider throughout the various stages of habitat restoration projects, categorizing them into pre-, during-, and post-restoration stages. We emphasize the importance of planning species- and site-specific strategies to support bees, including providing floral and non-floral resources and increasing nest site availability. Lastly, we underscore the significance of conducting evaluations and long-term monitoring following restoration efforts. By identifying effective restoration methods, success indicators, and areas for future research, our review presents a comprehensive framework that can guide land managers during this urgent time for bee habitat restoration.
1 Introduction
Ecological restoration, or habitat restoration, is the process of aiding the recovery of an ecosystem that has suffered degradation, damage, or destruction (Society for Ecological Restoration, 2004) to re-establish native plants and animals. In restoration, it is a common practice to focus on planting native plants, with the assumption that this is sufficient to restore the community and ecosystem (Kimball et al., 2015; Miller et al., 2017; Hamilton et al., 2022), as well as to provide habitat for targeted species in conservation (Hamilton et al., 2022). Generally, it has been shown that there is a positive effect of habitat restoration on bee population abundance and diversity, even if bees are not specifically included in the restoration plan (Heneberg, 2012; Tonietto and Larkin, 2018; Esque et al., 2021). However, by directly targeting the needs of local native bee species, we can ensure that the habitat requirements and floral resources are available for the highest bee diversity possible, including local at-risk species such as specialist bees (bees that forage pollen from one family, genera, or species of plant) (Griffin et al., 2017; Tonietto and Larkin, 2018; Griffin et al., 2021; Bullock et al., 2022). We propose that bee-centric restoration can further enhance bee abundance and diversity, thus increasing plant-pollinator interactions, and supporting the long-term sustainability of both diverse plant and bee species within an ecosystem (Griffin et al., 2017; Tonietto et al., 2017; de Araújo et al., 2018; Fantinato et al., 2018; Tonietto and Larkin, 2018; Cariveau et al., 2020; Griffin et al., 2021; Purvis et al., 2021; Meldrum et al., 2023).
To assemble the literature on bee habitat restoration, we conducted a topical search on Web of Science using the following three keywords: bee, habitat, and restoration. This search captured publications that included all three words in the title, abstract, or list of keywords. We followed this with two additional combinatorial searches, the first using the terms “bee” + “nesting” + “restoration” and the second using the terms “bee” + “floral resource” + “restoration.” We then restricted our literature search to the years from 2010-2024, following the publication of “The Conservation and Restoration of Wild Bees” by Winfree (2010), which addressed the restoration of bee communities. Together, these searches yielded 391 distinct articles. We restricted our review to 125 articles by focusing on North America, as well as by excluding most studies related to agricultural and urban environments, and non-native bees (i.e., honey bees). A few studies were included outside of these criteria (i.e., neonicotinoid exposure to bees) if they were critical to our recommendations for effective restoration practices. Our review does not attempt to prescribe universal solutions for habitat restoration because factors such as the size of the site being restored, the geographical location, and the type of habitat present can significantly influence the execution and objectives of a restoration project. Habitat restoration can range in size from thousands of acres to small-scale projects of less than one acre. While recommendations from this review can be integrated into restoration efforts at any scale, we aim to provide insights that are especially applicable to smaller-scale restoration projects.
The articles we reviewed revealed a number of biases. Out of the 125 articles reviewed, only 22% (28 articles) targeted specific taxonomic or functional groups of bees (e.g., eusocial, solitary). Of these 28 articles, 75% (21) were focused on bumble bees. While the risk of population decline faced by bumble bees is high (Colla et al., 2006, Colla et al., 2012; Mola et al., 2021b), it is important to note that solitary bee species represent 85% of bee diversity globally (Batra, 1984). There was a notable lack of research targeting solitary bees, which was the focus of only 9 of the 28 articles (32%). The remaining articles focused solely on enhancing overall bee diversity and discussed general conservation or restoration strategies applicable to bees and other pollinators. Furthermore, only three of the 125 articles examined were focused on specialist bees. While some studies on specialists have been conducted in Europe (Exeler et al., 2010; Sydenham et al., 2014; Heneberg et al., 2019), there is a distinct lack of research focusing on North American specialists. Finally, the majority of the 125 studies that focused on a particular habitat or region were conducted in grasslands, prairies, and forests, with few studies conducted in desert, alpine, and scrubland environments.
Nearly 90% of angiosperm species rely on insects, especially bees, for pollination (Ollerton et al., 2011; Koh et al., 2016; Almeida et al., 2023). However, continued development, expansion of agricultural monocultures, the spread of invasive plant species, and pollution all pose risks to bee species diversity and abundance (Winfree, 2010; Lázaro and Tur, 2018; LeBuhn and Vargas Luna, 2021; Mola et al., 2021b). Native bee species and their associated host plants are experiencing local extinction and population decline due to human activities (Winfree, 2010; Goulson et al., 2015; Koh et al., 2016; Sánchez-Bayo and Wyckhuys, 2019; Raiol et al., 2021; Lima et al., 2022). Anthropogenic threats (summarized in Table 1) can reduce the quantity and quality of floral resources and suitable nesting habitats, exacerbating the stressors faced by native bees (Goulson et al., 2015; Goulson and Nicholls, 2016; Kline and Joshi, 2020; Olynyk et al., 2021) and highlighting the need for conservation and habitat restoration efforts to protect these species (Winfree, 2010; Drossart and Gérard, 2020; Hanberry et al., 2021).
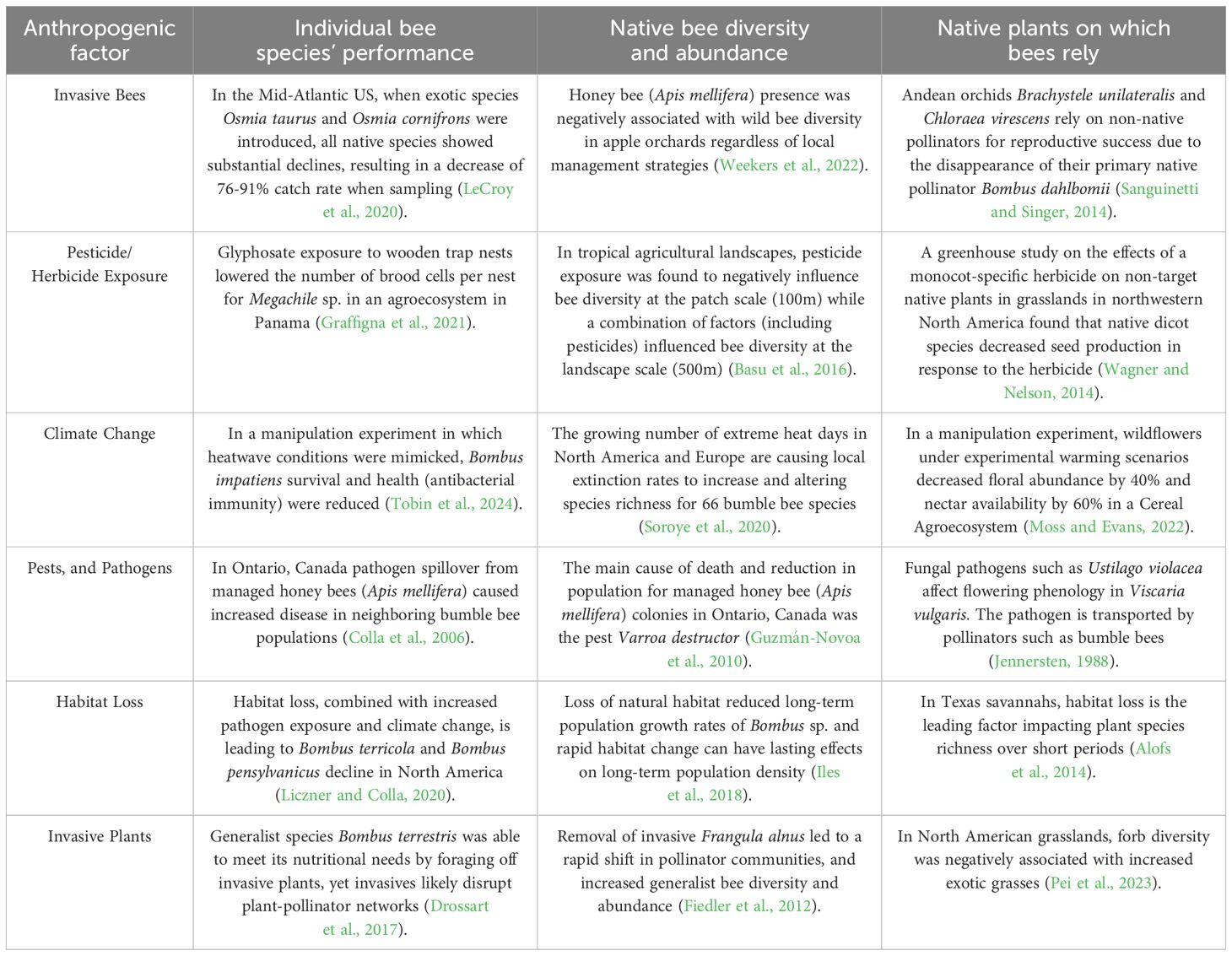
Table 1 The effects of different anthropogenic factors on individual bee performance, bee diversity and abundance, and the plants on which bees rely.
The majority of bee species in North America are solitary bees, which are non-eusocial and typically build their nests in the ground (Danforth et al., 2019; Antoine and Forrest, 2020). The life history traits of solitary bees differentiate them from eusocial bees; solitary bees are usually smaller and produce fewer offspring per female than eusocial bees (Danforth et al., 2019; Antoine and Forrest, 2020; Lima et al., 2022). Focusing conservation and restoration efforts specifically on solitary bees is especially important as their needs may differ from the needs of eusocial species (Danforth et al., 2019). Currently, conservation initiatives focusing on solitary bees are limited due to a lack of data on their abundance, diversity, and extinction rates (Danforth et al., 2019; Kline and Joshi, 2020; Lehmann and Camp, 2021). Despite a recent increase of studies on solitary bees in restoration (Sydenham et al., 2014; Sexton et al., 2021), continued research is needed to determine the best practices to support these bees in a variety of habitats. Due to the preferential number of studies on bumble bees and limited information for the majority of bee species, implementing and consolidating precise, targeted restoration protocols for most bee species can pose significant challenges.
Our goal is to identify important steps for successful bee habitat restoration (Figure 1) and to demonstrate how the needs of bees can be considered and integrated at every stage. Our recommendations were developed by reviewing the literature using a rubric to identify effective restoration strategies, taking into account the specific habitat type and the focal bee species, including their unique biological traits such as nesting and social behaviors. We aim to promote interest in bee habitat restoration by targeting an interdisciplinary audience. Bee habitat restoration is a relatively new field and there is currently limited research on how to apply what is known about bee biology to ecological restoration efforts. In addition, we hope to offer insights that may be useful to land managers and to highlight future research directions in bee biology and ecology that can be integrated into ecological restoration practices.
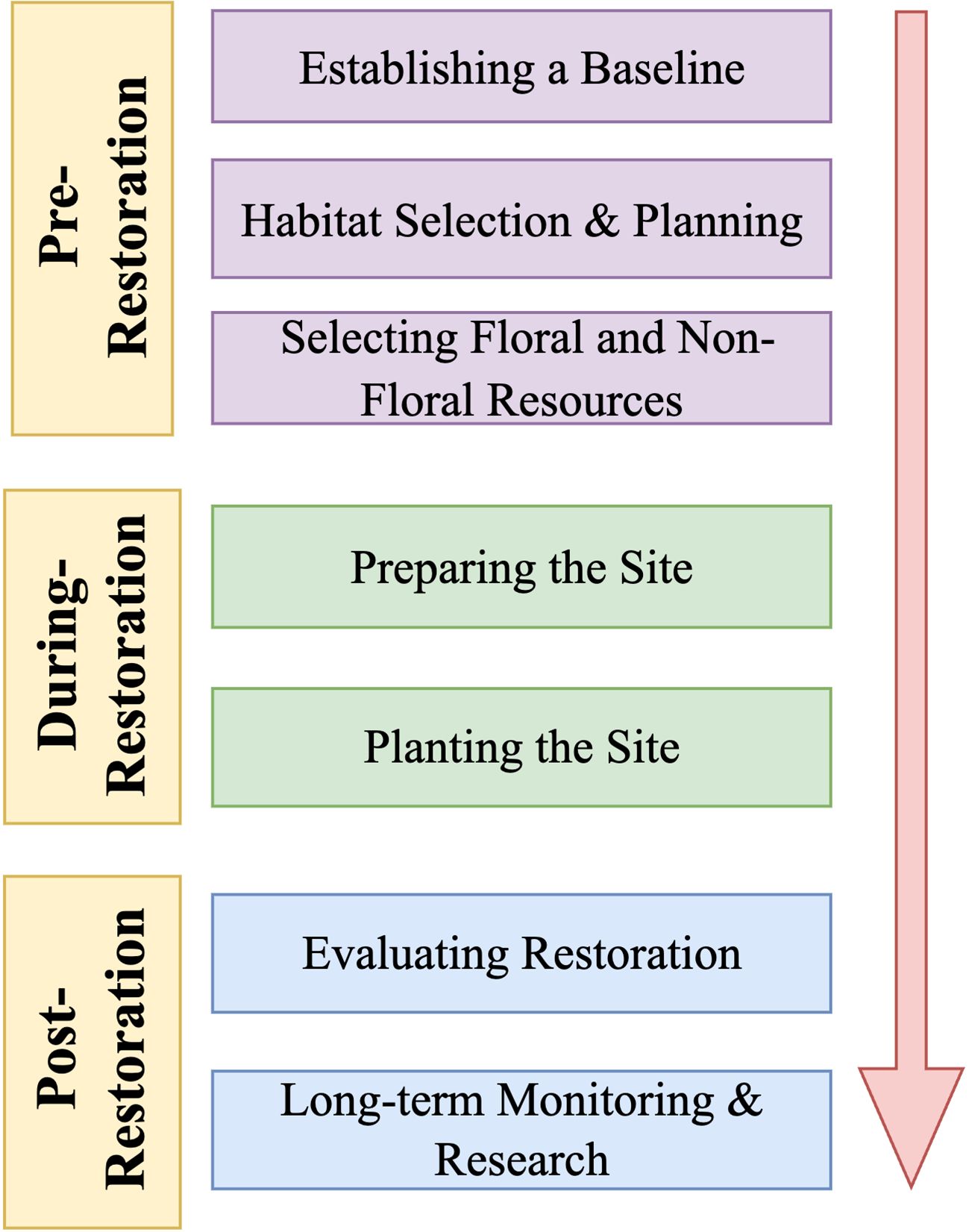
Figure 1 This paper provides a framework for integrating considerations of bees into each step of ecological restoration. While we chose this framework for clarity, it is important to note that the process is often non-linear. For example, results from post-restoration evaluations can prompt practitioners to revisit earlier stages of the restoration process such as planning, establishing new baselines, or continuing site maintenance.
2 Pre-restoration: planning and initial assessment
2.1 Establishing a baseline
Establishing ecological benchmarks using baseline data can be useful for assessing the impacts of habitat restoration by providing a reference point from which to measure change over time and setting realistic project goals (Hawkins et al., 2010; Downs et al., 2011). Baseline measures of pollinator diversity can be obtained through field sampling as well as by examining historical data from natural history collections (Lister, 2011; Breeze et al., 2021). Land managers can use this information to establish species-specific needs, prioritize the creation of habitat for targeted bee species by planting associated host-plant species, and provide suitable nesting habitat (Winfree, 2010; Danforth et al., 2019; Antoine and Forrest, 2020; Requier and Leonhardt, 2020).
During times of the year when bees are flying and plants are flowering, land managers can directly sample sites to determine species presence. Sampling relatively undisturbed areas nearby can aid in establishing realistic and site-specific goals for restoration projects (Curran et al., 2022), especially if these projects target habitat restoration towards species that are already occurring or nesting at nearby, undisturbed sites. Surveying sites before restoration is necessary to assess current bee diversity, identify existing nests for targeted conservation, evaluate available floral and non-floral resources, and devise strategies for managing invasive species (Ritchie and Berrill, 2020).
Historical specimen data from natural history collections, including those obtained from sources such as the Global Biodiversity Information Facility (GBIF, https://www.gbif.org/), are valuable for estimating local bee diversity, species distributions, species occurrence dates, and the floral resources visited by given bee species. When utilizing natural history collections, expanding searches to include specimen occurrences from adjacent sites can aid in identifying species that may be recruited from nearby regions, subsequently enabling their inclusion in targeted restoration efforts. In addition, natural history collections can be a critical tool for assessing species-specific flowering phenology and bee flight times (Ogilvie and Forrest, 2017), which may be used to select species of plants that are likely to form mutualistic relationships with bees in a given region or locality. For most species of plants and bees, specimens have not been collected equally across their ranges (Chesshire et al., 2023); in places where historical records do not exist, species distribution models may help to predict whether a location is suitable for a given plant or bee species. Researchers have used ecological niche modeling based on bee specimen records to estimate current and future species distributions (Carvalho and Del Lama, 2015; Beckham and Atkinson, 2017).
Determining targeted bee species nesting requirements is important when assessing the nesting conditions available at a given site. This can enable practitioners to find and protect bee nests before restoration or preserve nesting features (such as bare ground or woody debris; see Section 2.3.2 Nest Site Availability) that are already available on the landscape. However, for many bee species, these nesting requirements are unknown (Antoine and Forrest, 2020). Documentation of the nest site preferences (such as soil type or soil moisture) of different bee species in distinct environments is valuable so land managers can provide species and site-specific resources for nesting (Harmon-Threatt, 2020; Orr et al., 2022).
Recent community science efforts have been established to document the nesting habits of ground-nesting bees, such as when and where they nest (Liczner and Colla, 2019; Maher et al., 2019; Ground Nesting Bees, 2023). In the absence of species-specific nesting information, the nesting preferences of closely related congeners may be useful (Danforth et al., 2019). Contributions to shared databases can help correlate specific nesting conditions with bee observations, providing information for future bee conservation (Chesshire et al., 2023) and targeted bee habitat restoration.
2.2 Habitat selection and planning
Once ecological baseline data has been established, habitat restoration should create a detailed plan (Nilsson et al., 2016) which can include considerations of the needs of native bees. One approach is to begin by establishing baseline estimates that assess bee diversity, as well as the availability of floral and non-floral resources (such as materials for foraging and nest building) at or around the site. Based on information gathered from baseline surveys, specific plans can be developed for the bee species present or nearby. Consideration of floral and non-floral resources and nesting conditions for native bees can be included in these habitat restoration plans (Figure 2). Localized restoration initiatives, including small-scale habitat restoration projects, can provide floral resources and nesting habitats that support bee diversity and abundance (de Araújo et al., 2018; Phillips et al., 2019; Monasterolo et al., 2020; Phillips et al., 2020; Donkersley et al., 2023).
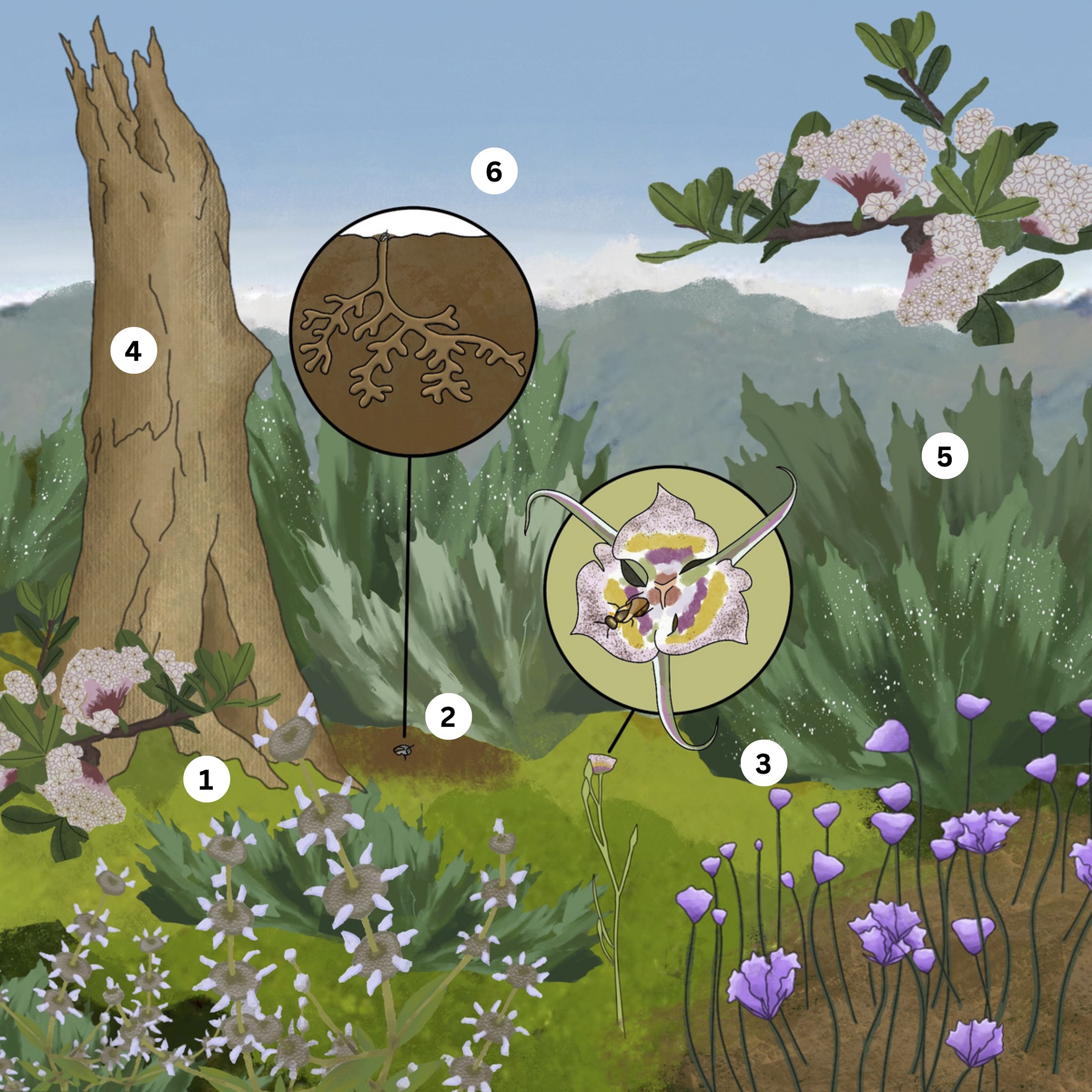
Figure 2 Key considerations for native bee habitat restoration planning in natural environments. (1) Plant native species that are nutritionally and phenologically diverse; (2) Implement restoration clearing and planting techniques that are bee-friendly, including the provision or protection of viable nesting sites; (3) Use empirical tools for optimizing pollinator species richness, including providing necessary host-plant species; (4) Provide non-floral resources for bee foraging and nesting; (5) Remove invasive plant species and replace with natives; (6) Increase bare ground and woody debris to enhance the availability of nesting habitat.
Unlike plant-centric restoration, where plants are introduced and established through seeds, cuttings, or outplanting entire individuals, the establishment of bee communities depends heavily on the natural recruitment of bee species from surrounding regions (M’Gonigle et al., 2015; Öckinger et al., 2018). The creation of suitable habitats and connectivity between habitats (corridors) can facilitate the movement and persistence of bee populations (Hanula et al., 2016; Keilsohn et al., 2018; Mola et al., 2021a), alter pollination services provided by bees (Mitchell et al., 2013), and affect the genetic diversity of founding populations (Bruns et al., 2024). For a review from 2013 on the relationship between landscape connectivity and ecosystem services, see Mitchell et al. (2013).
By identifying corridors between habitats in heterogeneous landscapes, restoration practitioners can better design projects that recruit diverse bee species to restored sites (Öckinger et al., 2018). Winsa et al. (2017) determined that trait composition (a trait-based approach for assessing bee diversity based on morphological, phenological, and behavioral traits) was positively correlated with connectivity to intact grassland habitat in restored pastures. Cusser and Goodell (2013) found as the distance to remnant habitat patches (areas from which bees would populate a restoration site) increased, bee diversity declined. However, they also observed that increasing floral richness promoted pollinator network stability, even at the sites furthest from remnant patches. Thus, Cusser and Goodell (2013) recommended prioritizing providing bee habitats that are diverse in floral resources far from remnant patches to increase pollinator network stability in new locations.
Proximity of restored landscapes to ecological threats can also impact bee communities. For example, numerous restoration projects are situated near roadways, raising the likelihood of bee fatalities resulting from traffic collisions (Keilsohn et al., 2018) and hurting more individual bees than they help (Keilsohn et al., 2018). Determining the optimal distance from roadways for bee habitat restoration sites (Keilsohn et al., 2018) and identifying the threshold of roadway activity that negatively affects bees are important goals for future research.
2.3 Selecting supplemental floral and non-floral resources
Consideration of floral and non-floral resources is important for bee habitat restoration (Requier and Leonhardt, 2020). Planting species representing a variety of growth forms (annual and perennial forbs and grasses, as well as shrubs and trees) can provide both floral and non-floral resources for native bees (Requier and Leonhardt, 2020), while also providing ecosystem functions such as shade and erosion control during restoration (Mitchell et al., 2022).
2.3.1 Supplemental floral resources
Enhancing flowering plant species richness at restoration sites can increase bee diversity and abundance (Fischer et al., 2016; Hanula et al., 2016; Purvis et al., 2020; Lane et al., 2022; Rubio et al., 2022; Beneduci et al., 2023) and bee visitation rates (Denning and Foster, 2018). A meta-analysis of observational studies by Kral-O’Brien et al. (2021) found that plant species richness was the strongest predictor of bee species richness. Other studies have reported comparable findings, indicating that vegetation type may significantly influence bee community assembly (Brooks, 2020; Novotny and Goodell, 2020). In addition, including high densities of flowering species through the implementation of seed mixes has also been found to increase the chances of pollination and reproductive success for some outcrossing plants (Cane et al., 2012), creating positive feedback loops between associated plant and bee species.
Nevertheless, the reintroduction or supplementation of appropriate combinations of native plant species at restoration sites may be difficult for several reasons. Seed mixes that represent local combinations of sympatric species are often unavailable, due either to their high cost in creating them, the difficulty of sourcing locally adapted genotypes, or challenges in producing seed mixes quickly enough (Nevill et al., 2018; Erickson and Halford, 2020). Nevertheless, carefully designed seed mixes that include seeds sourced from established “seed zones” (seeds from regions with similar environments; these seeds are considered the same in the context of locally adapted seed mixes; Erickson and Halford, 2020) can enhance bee diversity (Harmon-Threatt and Hendrix, 2015; Galea et al., 2016; Lybbert et al., 2022). Despite many benefits, seed zones are not defined for numerous important species in restoration (Johnson et al., 2023). Some research indicates that admixture seed sourcing (sourcing seeds from many different locations) can alter plant-arthropod interactions when flowering species richness is low (Hulting et al., 2024). However, there have been no studies examining the impact of admixture seed sourcing on pollination success or bee diversity.
Empirical tools can be beneficial for selecting plant species for bee habitat restoration (M’Gonigle et al., 2017; Esque et al., 2021; Purvis et al., 2021). M’Gonigle’s genetic algorithm, which uses phylogenetic relatedness, bee visitation rates, and bee diversity, is an effective tool for designing seed mixes (M’Gonigle et al., 2017) and has been empirically tested and used in multiple restoration efforts (Williams and Lonsdorf, 2018; Campbell et al., 2019; Bruninga-Socolar et al., 2023). Continued testing of empirical tools designed to facilitate the selection of plants that support generalist and specialist bee communities in different environments is needed.
2.3.1.1 Bee nutrition
The central focus of most bee-centric restoration efforts is to provide bees with ample floral resources to meet their nutritional needs (Winfree, 2010; Scheper et al., 2013; Image et al., 2022). The quality of these resources may be as important as their abundance (Vaudo et al., 2014). When foraging choices are insufficient, bee health and survival decline (Filipiak et al., 2022). High plant species diversity does not always guarantee nutritionally adequate pollen and nectar (Filipiak et al., 2022), which should be considered when designing bee conservation and restoration efforts (Vaudo et al., 2015, Vaudo et al., 2020; Crone et al., 2022; Filipiak et al., 2022). Moreover, bee microbiota is affected by the plants that bees forage, which can directly impact bee health (Nguyen and Rehan, 2023). Crone et al. (2022) recently published an extensive review of bee nutritional ecology, emphasizing the need to evaluate the diet preferences of all focal bee species. They also highlight the potential of emerging technologies (i.e., automated monitoring systems, DNA metabarcoding) to enhance bee habitat restoration for species of special concern in the future (see Section 4.2 Long-Term Monitoring & Research). Existing knowledge gaps include understanding the significance of macro and micronutrients for various bee species and discerning the nutritional requirements of specialist bees.
2.3.1.2 Plant and bee phenology
Phenology, or the biological timing of life events such as bee emergence or flowering time, is important for pollination success and bee survival. Plant reproduction and the availability of food for bee larvae largely depend on synchrony between plants and their associated pollinators (Kudo, 2014; Ogilvie and Forrest, 2017; Slominski and Burkle, 2021). A mismatch of just a few days can decrease bee fitness through increased mortality and decreased fecundity (Buckley and Nabhan, 2016; CaraDonna et al., 2018; Schenk et al., 2018). When selecting plant species for habitat restoration, seed mixes and propagules composed of species with overlapping and long bloom periods can benefit pollinator populations by decreasing the risk of a phenological mismatch and providing a long foraging season (Tilley et al., 2013; Havens and Vitt, 2016; Gross, 2017; Simanonok et al., 2022).
In restoration planning, practitioners should evaluate the distribution and diversity of floral resources throughout the flowering season, and ensure that both early and late-season floral resources are available (Curran et al., 2023). Gonzalez et al. (2013) found that bumble bee foraging areas shifted to different habitats throughout spring and summer based on the availability of floral resources in bunchgrass prairie habitats in the Pacific Northwest. Bees were supported by grasslands early in the flowering season and aspen stands in late summer. Providing variation in the flowering time of floral resources can establish alternate food sources for generalist bees during periods of scarce floral resources (Ogilvie and Forrest, 2017; Dibble et al., 2020).
2.3.1.3 Floral resources for specialist bees
Numerous studies have documented the advantages of providing supplemental floral resources for generalist bee species (Russo et al., 2013; Woodcock et al., 2014; Kremen and M’Gonigle, 2015; Eeraerts et al., 2019; Frankie et al., 2019; McCormick et al., 2019; Fuccillo Battle et al., 2021; Walston et al., 2023). However, there is a lack of research on specialist bees (Kremen and M’Gonigle, 2015; Fowler, 2016). Success in promoting specialized bee abundance and diversity in restoration efforts requires the inclusion of host plant species on which the local specialists rely (Frankie et al., 2009; Fowler, 2016; Brooks and Poulos, 2023). Additionally, many host plant species may depend on specialized pollinators for reproductive success (Page et al., 2019). Fowler (2016) emphasize the importance of host plants in habitat conservation for specialist bees in the Northeastern U.S., noting that approximately 15% of native bee species in this region specialize in pollen collection from a particular plant family or genus.
Similar research on specialist bees in other regions is needed, and focused restoration efforts could promote their conservation (Fowler, 2016). Sampling pollen loads carried by specialist bees can aid in identifying the plant species on which these bees rely (Kelly and Elle, 2021). Additionally, bee specimen data can provide insight into the floral resources historically associated with specialist bee species (Fowler, 2016). Recently available databases (Seltmann and Community, 2022; Wood et al., 2023) provide lists of plant-bee species interactions that may be used to improve bee-centric restoration efforts, while also facilitating data sharing and continued monitoring for a better understanding of the dietary requirements of these important pollinators.
2.3.2 Nest site availability
Although most bee-centric conservation plans focus on floral resources, the availability of nesting habitats should not be overlooked (Öckinger et al., 2018; Requier and Leonhardt, 2020). Bee nesting biology has been recently reviewed by Orr et al. (2022), and anthropogenic threats to bee nesting in wild bee communities have been reviewed by Harmon-Threatt (2020).
The majority of bee species, including both eusocial and solitary species, nest underground (Danforth et al., 2019; Liczner and Colla, 2019); for a review of ground-nesting bee biology, see Antoine and Forrest (2020). Different species create distinct nest architectures and prefer different microhabitat conditions (Danforth et al., 2019; Antoine and Forrest, 2020). In a study by Buckles and Harmon-Threatt (2019) in tall grass prairies, bee nesting was positively influenced by increasing floral resource abundance as well as increasing the availability of bare ground, low soil moisture, and warmer soil temperature (Purvis et al., 2020). However, some bee species (e.g., bumble bees) prefer increased litter over bare ground (Williams et al., 2019; Smith DiCarlo et al., 2020).
Studies of ground-nesting bees have assessed the effect of ground cover, temperature, texture, space, slope, soil compaction, and soil moisture on nest site selection (Cane, 1991; Xie et al., 2013; Sardiñas et al., 2016; Tsiolis et al., 2022). A multitude of studies have revealed that landscapes undergoing early successional stages, such as habitat restoration efforts, often provide nesting habitats that support diverse and specialized bee species (Rutgers-Kelly and Richards, 2013; Řehounková et al., 2016; Banaszak and Twerd, 2018; Seitz et al., 2019; Mola et al., 2020; Simanonok and Burkle, 2020; van der Heyde et al., 2022). Biotic factors, including plants, pathogens, parasites, predators, and conspecifics, can also influence the nesting density and nesting location at which bees choose their nesting sites (Potts and Willmer, 1997; Michener, 2000; Requier and Leonhardt, 2020). For example, in Hawaii, the nesting sites of Hylaeus anthracinus Smith, 1853 experience lower reproductive success due to invasive ants (Plentovich et al., 2021). Limited research has explored the biotic factors influencing bee nesting, such as soil microbial diversity.
Another group of bees is comprised of native cavity-nesting bee species, which require live or dead biotic material in which to nest. Studies have shown that areas with simplified vegetative structures have low cavity-nesting rates (Flores et al., 2018; de Araújo et al., 2019, de Araújo et al., 2021; Felderhoff et al., 2022). Many bee species are opportunistic nesters, choosing to nest in existing holes, stems, or downed woody debris (Galbraith et al., 2019; Davis et al., 2020; Foote et al., 2020; Glenny et al., 2023; Rappa et al., 2023). In addition, specific habitat types may be preferred as overwintering sites, such as forest habitats for many Bombus sp (Mola et al., 2021a). In sum, whether or not there is sufficient availability of nesting sites for the bees at a given locality will depend on the type of biotic material available, the complexity of the vegetation structure, the presence of existing holes and debris, and the prevailing habitat type, depending on the bee species’ preferences.
2.3.2.1 Nest building materials
Including supplemental non-floral resources at a restoration site is important for providing bees with the materials they need to construct their nests (Requier and Leonhardt, 2020). Both eusocial and solitary bee species use leaves, bark, trichomes, or resin for nest building and to protect their brood cells (Shanahan and Spivak, 2021). Some native bees (Apidae, Meliponini, Centridini, Euglossini, Apini, some Xylocopinae, and some Bombini) use herbaceous material or coarse woody debris to build their nests (Michener, 2000; Danforth et al., 2019; Requier and Leonhardt, 2020). An example is the genus Ceratina, which creates nests in the stems of dried herbaceous material or woody branches (Danforth et al., 2019). Furthermore, the plant species that bees rely upon for nest building may not be their floral host plant species. For example, many Anthidium spp. depend on the trichomes of hairy plant species for lining their brood cells, while they collect pollen most frequently from other, glabrous, species (e.g., Larrea spp.; Vitale et al., 2017). Thus, providing floral resources alone would not be sufficient to support the genus Anthidium. Resin, another non-floral resource foraged from plants that some bee species rely on, can function like concrete, solidifying nesting structures and preventing bacteria from contaminating brood cells (Chui et al., 2022).
2.3.2.2 Non-floral resources for food
The importance of non-floral resources for solitary bees has only recently been recognized (Chui et al., 2022). Non-floral sugars, such as honeydew produced by scale insects, provide additional carbohydrates for some bee species. Meiners et al. (2017) observed 42 wild bee species, including many solitary and native species, visiting Adenostoma fasciculatum Hook. & Arn. to obtain honeydew, which may serve as a supplemental food source outside the flowering season. Preserving scale insects, which produce honeydew, could help to extend the seasonal duration of bee foraging, mitigating the negative effects of potential phenological mismatches (Gérard et al., 2020). Additionally, other symbionts can be important for bees. A study of the generalist solitary species Osmia lignaria found that bacterial and fungal symbionts increased larval developmental success (Westreich et al., 2023). Documenting and sharing these interactions can be useful for restoration managers. Continued research identifying symbionts associated with native bee species and their impact on bee health and determining the most effective strategies for incorporating these non-floral resources into bee habitat restoration efforts is needed.
2.3.3 Non-native plants
The role of non-native plants in bee conservation is highly debated. Parra-Tabla and Arceo-Gómez (2021) provide an extensive review of the influence of invasive plants on plant-pollinator networks, although bees were not a focus. While native plants are recognized for supporting a wide array of bee species (Discua and Longing, 2022), several studies have found that non-native plants can also promote bee abundance and bolster pollination networks (Severns and Moldenke, 2010; Gaiarsa and Bascompte, 2022; Kovács-Hostyánszki et al., 2022). However, most studies of non-native plants and bees focus on the floral resources that non-natives provide (typically in urban environments) and do not account for competition in bee visitation rates between non-native and native plant species (Aizen and Morales, 2020) or disruptions in ecosystem function (Parra-Tabla and Arceo-Gómez, 2021; Tallamy et al., 2021). Hanula et al. (2016) note that when non-native plants outcompete native plant species, this typically negatively impacts pollinator communities, including bees. Additionally, Mathiasson and Rehan (2020) observed that the decline of native bees (particularly specialists) was associated with the proliferation of non-native plant taxa in northern New England due to the loss of their associated host plants. Moreover, non-native plants may affect other aspects of bee biology and ecology, including reproductive success (Hanula et al., 2016), the availability of ground-nesting sites, the abundance of native plant species (Barron and Beston, 2022), as well as floral visitor communities (Denning and Foster, 2017). In some habitats, the removal of non-native plants during restoration increased bee abundance and species richness (Fiedler et al., 2012; Tonietto and Larkin, 2018; Ulyshen et al., 2020). Forb diversity is often negatively associated with non-native grass abundance (Drobney et al., 2020; Molinari and D’Antonio, 2020), high levels of which may cause declines in bee abundance, the simplification of bee communities (Pei et al., 2023), and the alteration of entire insect communities (Luong et al., 2019). Furthermore, non-native grasses create dense litter layers that may block nesting sites for ground-nesting bees (Pei et al., 2023). For example, Pei et al. (2023) observed a decrease in ground-nesting bee abundance at sites occupied by increased leaf litter and high densities of non-native grass Poa pratensis L. in the Northern Great Plains. Other studies have hypothesized that non-native grasses are responsible for a decline in both forb and pollinator diversity and abundance (Lybbert et al., 2022).
It is important to recognize that not all non-native plants are invasive, and some non-native species can provide floral resources to support bee abundance (Carson et al., 2016; Frankie et al., 2019; Gibson et al., 2019; Niemuth et al., 2021; Ulyshen et al., 2022). This is especially the case at the beginning and end of the flowering season, when non-natives may be less likely to disrupt native plant-pollinator networks (Staab et al., 2020). During restoration efforts, non-native plants may serve as temporary food sources for bees while native plants become established (Lybbert et al., 2022; Thapa-Magar et al., 2023). While non-native plant species may support generalist bee abundance in some habitats, prioritizing native plants is recommended as they provide habitat for a broader range of native insects and contribute to ecosystem function (Tallamy et al., 2021).
3 During-restoration: implementation
3.1 Preparing the site
Before undertaking habitat restoration, land managers are frequently required to remove debris, infrastructure, pollutants, or invasive species (Elmqvist et al., 2013). Different methods of vegetation removal have advantages and disadvantages for native bees (Table 2). For the effective execution of these strategies, they frequently require multiple iterations during and following the restoration process (Kimball et al., 2015; Oliveira et al., 2021; Keeley et al., 2023).
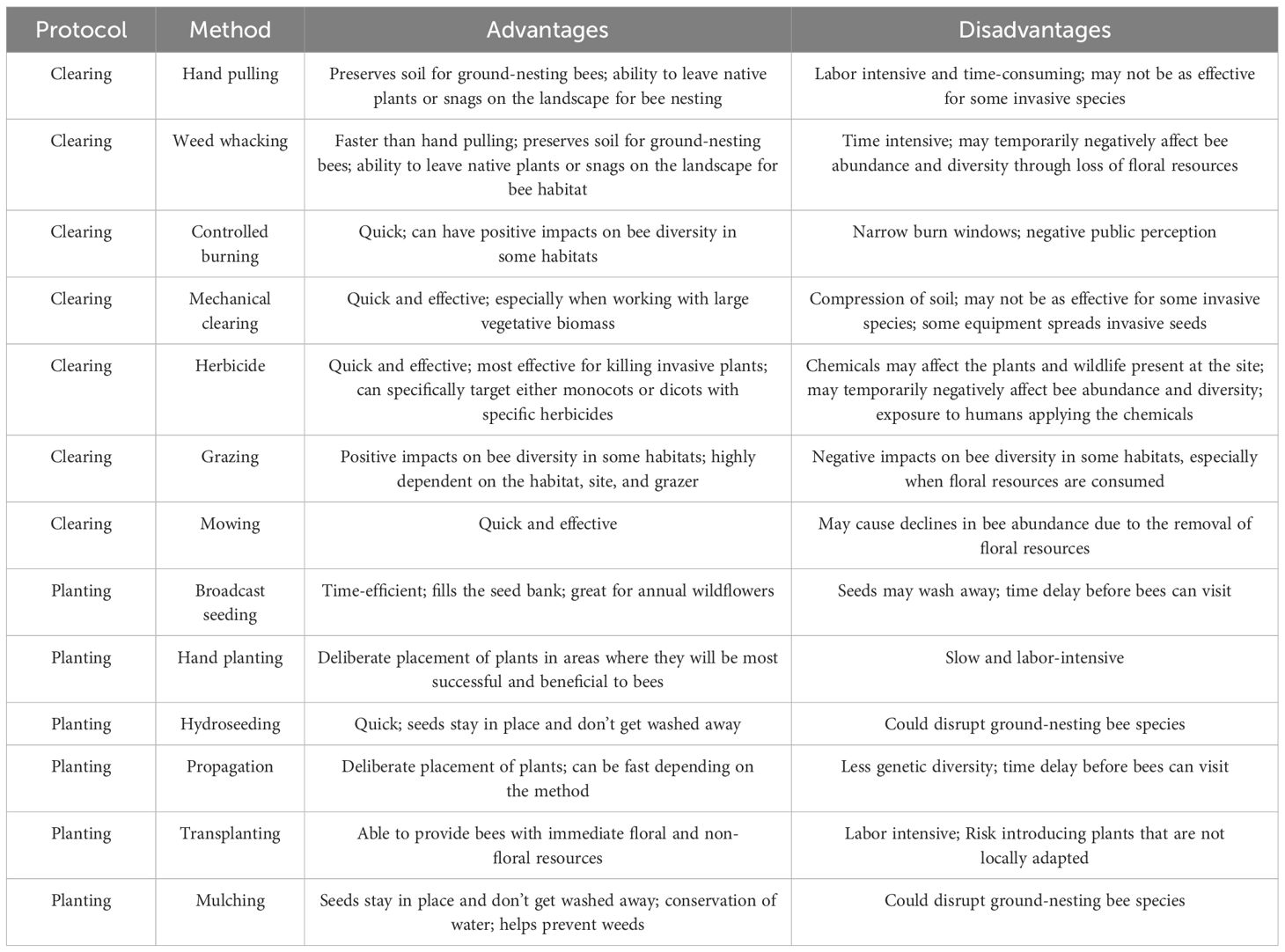
Table 2 Advantages and disadvantages of different methods used to clear restoration sites and to introduce plants during bee-centric habitat restoration. Continuous implementation of these methods may be necessary to maintain resources and achieve restoration objectives.
3.1.1 Mechanical and hand thinning
Heavy machinery used to move soil, water, or vegetation during restoration can affect soil structure (Schäffer et al., 2007; Nawaz et al., 2013). Many species of ground-nesting bees require specific soil characteristics to build their nests (Antoine and Forrest, 2020). Christmann et al. (2022) suggest that heavy machinery could threaten existing nesting sites for ground-nesting bees; however, no studies to date have looked directly at the effect of soil movement or compaction from heavy machinery on bee nesting success.
Mechanical thinning has been found to have several effects on bee communities. When Ealy et al. (2023) compared pollinator communities in old-growth forests with logged early seral forests, they detected negative long-term effects on the bee communities in clear-cut sites, including a decrease in specialist bees. However, when comparing clear-cut vs. young forests with dense understories, clear-cut forests have higher bee diversity, likely due to decreased canopy cover (Ealy et al., 2023). Odanaka et al. (2020) conducted an experiment measuring the effects of mechanical thinning on bee diversity and abundance in longleaf pine savannas compared to untouched remnant plots. They found that bee diversity and abundance were positively correlated with thinning and negatively correlated with canopy cover. Lettow et al. (2018) found thinning coupled with controlled burns significantly increased pollinator richness and abundance in oak savannas relative to unmanaged controls. Other studies observed similar results (Hanula et al., 2015, Hanula et al., 2016; Abella et al., 2017; Milam et al., 2018; Rivers et al., 2018; Glenny et al., 2022; Davies et al., 2023). These findings highlight that mechanical thinning can increase bee abundance and diversity in certain vegetation types such as forests.
Hand thinning offers the advantage of leaving woody debris on the landscape which can support cavity, stem, and opportunistic nesting bees (Rappa et al., 2023). The maintenance of structural heterogeneity to provide nesting sites for diverse bee species should be supported during and following bee habitat restoration (Antoine and Forrest, 2020; Image et al., 2022).
3.1.2 Prescribed fire
Some plant and animal communities in North America have adaptations that enable them to thrive when exposed to periodic wildfires (Simmons and Bossart, 2020). Prescribed fire (designed to mimic conditions of periodic low-intensity wildfires) can result in bare ground which provides nesting habitat for ground-nesting bees (Hanula et al., 2016; Sitters et al., 2016; Decker and Harmon-Threatt, 2019; Bruninga-Socolar et al., 2022; Brokaw et al., 2023). Ulyshen et al. (2021) examined the effects of frequent prescribed fires on bee abundance and species richness in southeastern U.S. forests. They found that bee abundances significantly increased in burned plots compared to unburned plots, although bee species differed in their tolerance to burn frequencies. Similar results were obtained following controlled burns of tallgrass prairies (Harmon-Threatt and Chin, 2016) and mixed conifer forests.
However, not all species benefit from controlled burns, particularly cavity and stem nesters such as Bombus, Ceratina, and Osmia (Galbraith et al., 2019). Bruninga-Socolar et al. (2022) determined that ground-nesting bee abundance and diversity responded positively to fire, while cavity-nesting bee abundance and diversity increased in the absence of fire, highlighting the importance of heterogeneity in fire regimes. Moreover, plant-pollinator interactions can be disrupted in certain habitats after fire due to the elimination of floral host plants (Love and Cane, 2019). Despite this, Cole et al. (2019) found that burn scars, which contribute to environmental heterogeneity in riparian environments, were positively correlated with bee diversity. Other studies have observed similar results in different habitats (Gelles et al., 2022). In addition, controlled burns have been found to reduce non-native grasses (Ditomaso et al., 2006; Weidlich et al., 2020) and to increase annual wildflower diversity (Peterson and Reich, 2008; Davies and Sheley, 2011; Decker and Harmon-Threatt, 2019; Lybbert et al., 2022; Gelles et al., 2023), which may lead to increases in bee abundance and diversity (Smith DiCarlo et al., 2019). In general, pyrodiversity (the variability in burn size, frequency, duration, and severity across a landscape), including some exposure to high-severity wildfires, has been found to increase bee species richness in fire-adapted regions (Galbraith et al., 2019). Creating a mosaic containing different burn histories will likely provide habitat and resources for the greatest diversity of bee species (Ponisio et al., 2016; Rodríguez and Kouki, 2017; Galbraith et al., 2019).
3.1.3 Mowing and grazing
Mowing is often used to manage weed and grass growth in restored habitats, especially during spring. Mowing has been found to promote forb diversity (Lybbert et al., 2022) but can have the opposite effect if done too frequently (Smith et al., 2018). Additionally, increased mowing frequency has been found to be negatively associated with bee species richness and abundance (Audet et al., 2021; Serret et al., 2022). The “No Mow May’’ movement has spread, in which residents are urged to reduce mowing during peak pollinator flight time (Andrews, 2023). This practice has been found to promote bee abundance on the US East Coast and elsewhere (Lerman et al., 2018), but it may require alterations when applied to other major geographic regions such as the western US, where peak pollinator foraging and flight times occur later in the season. Another method, such as the reintroduction of grazing animals such as wild horses, has been found to enhance forb diversity and boost bee abundance in habitats that historically evolved under herbivory from large ungulates (Garrido et al., 2019). Similarly, Bruninga-Socolar et al. (2022) found that the heterogeneity in vegetation cover caused by cattle grazing and controlled burns benefited ground-nesting bees by providing more bare ground, but implementation of specific grazing regimes is necessary to minimize soil compaction as well as providing habitat for stem and hole-nesting bees. In contrast, Stein et al. (2020) detected that grazing in grassland communities in the upper Midwestern United States led to a reduction in native flowering plant species abundance. In this study, body mass and lipid stores were also measured to assess nutritional health indicators in three sweat bees (Agapostemon spp.). It was found that in ungrazed sites, Agapostemon virescens (Fabricius, 1775) showed greater body mass compared to individuals sampled in grazed areas. Beckett et al (Beckett et al., 2022). determined that deer presence in British Columbia negatively affected bumble bee abundance indirectly by depleting floral resources, indicating a potential decline in colony success. For a review of the known roles of mowing and grazing in restoration as of 2016, see Tälle et al. (2016).
3.1.4 Herbicides and insecticides
The impacts of pesticide use on native bee health are poorly understood. Experimental studies on honey bees are frequently used to infer the effects of pesticides on all bee species (Franklin and Raine, 2019; Lehmann and Camp, 2021). The U.S. Environmental Protection Agency’s Policy Mitigating Acute Risk to Bees from Pesticide Products states that protecting managed bees will “also protect native solitary and eusocial bees that are also in and around treatment areas” (EPA, 2015). However, honey bee sensitivity to pesticides may differ from the responses of native bees (Chan et al., 2019; Franklin and Raine, 2019). Because some solitary bee species are more vulnerable than honey bees to pesticide exposure, it is crucial to avoid relying solely on honey bees as the risk assessment model when observing the toxic effects of pesticides (Franklin and Raine, 2019). This increased susceptibility can be attributed to solitary bee consumption of fresher pollen and nectar, as well as increased exposure to pesticides through their nesting sites (Goulson, 2013; Chan et al., 2019; Franklin and Raine, 2019; Lehmann and Camp, 2021). In addition, solitary bees have a smaller body size than honey bees (Chole et al., 2019); thus, a dosage calibrated to honey bees could pose a significant risk to most wild bee species. This is particularly concerning as body size is one of the primary predictors of bee species’ vulnerability to pesticides (Schmolke et al., 2021). Furthermore, honey bees are eusocial and thrive in large colonies, whereas native bees are typically solitary and relatively scarce across the landscape. This trait makes them particularly vulnerable to population declines if negatively impacted by pesticides (Straub et al., 2015; Sgolastra et al., 2019).
Herbicides can be useful for removing invasive plants during restoration, but their costs and benefits should be considered before implementation (Bennion et al., 2020). Whenever feasible, employing biological controls can be highly effective and bypass the hazards associated with herbicides (Auld, 1998; Peterson et al., 2020). However, biological control agents are not available for all plant species (Singh et al., 2020). In a review of 372 published articles, Weidlich et al. (2020) reported that 42.3% of the restoration projects used chemicals to eradicate invasive plants. Of these, 40% used glyphosate, an active ingredient in most herbicides (Weidlich et al., 2020). Glyphosate, marketed as Roundup™, can be harmful and sometimes lethal to non-target pollinators including honey bees, bumble bees, and solitary bee species (Abraham et al., 2018; Battisti et al., 2021; Straw et al., 2021). When cavity nests were sprayed with glyphosate, solitary bee reproductive success declined due to reduced brood cell production (Graffigna et al., 2021). In an acute exposure experiment conducted under realistic field conditions, glyphosate exposure impaired fine-scale color recognition and long-term memory in bumble bees, which may disrupt their foraging behavior and lead to overall declines in colony success (Helander et al., 2023). Glyphosate use is restricted or banned in several European countries due to human and environmental concerns, including its negative effect on bee development, behavior, and survival (Kudsk and Mathiassen, 2020; Battisti et al., 2021). Further research is required to investigate the sublethal effects of glyphosate on native bee species observed in field settings, as noted by Battisti et al. (2021). While the effects of herbicides on bee health are considered in agricultural practices, these impacts have not been evaluated in habitat restoration efforts.
Although not commonly used in restoration, insecticides can occasionally be used to protect rare plants that are vulnerable to insect herbivores (Bevill et al., 1999; Flower et al., 2018). However, more commonly insecticides leach into native landscapes from neighboring agricultural fields or watersheds. Neonicotinoids, a class of widely used systemic neuro-acting insecticides absorbed by plants and spread throughout their tissues, are extremely harmful to bees (Alkassab and Kirchner, 2017). Transferred through pollen and nectar consumption, neonicotinoids cause bee mortality or have sub-lethal effects by altering bee communication, foraging behavior, or navigation (Fischer et al., 2014; Alkassab and Kirchner, 2017). Only two studies have investigated neonicotinoid exposure through soil contamination for ground-nesting bees, employing differing experimental designs and yielding conflicting results (Willis Chan et al., 2019; Tetlie and Harmon-Threatt, 2024).
While research on the effects of insecticides on native bee species has increased in recent years, more research is needed, especially on the sub-lethal effects of these chemicals (Dirilgen et al., 2023; Tetlie and Harmon-Threatt, 2024). Further research is needed to assess the impacts of pesticides on bee-centric restoration and to identify or discover practices that minimize negative outcomes. For instance, it has been shown that nighttime spraying is effective in reducing exposure to honey bees (Decourtye et al., 2023). Such studies will provide insights for practitioners to develop more informed, bee-friendly conservation and restoration strategies.
3.2 Planting the site
Various planting methods have been devised for bee habitat restoration (Leverkus et al., 2021), the pros and cons of which in relation to bee habitat restoration are summarized in Table 2. One planting technique that promotes annual plant species over time is continuous reseeding (Applestein et al., 2018). Ongoing research indicates that regular reseeding can boost wildflower populations (Barr et al., 2017; Applestein et al., 2018), and annual wildflowers may be replaced by a few perennial species over time without strategic, planned disturbance regimes (i.e. burning, mowing, or grazing) (Lybbert et al., 2022). Questions remain regarding the optimal frequency and density of reseeding to support bee species. Barr et al. (2017) highlighted that if land managers have to choose between prioritizing reseeding rates and plant species diversity when sowing seed mixes, prioritizing plant species diversity is best for improving restoration success in grassland habitats.
In addition to implementing bee-conscious planting techniques, the density and size of floral patches are important considerations in bee habitat restoration. Some research suggests that including corridors or gaps in vegetation for bees to fly through can provide bee-friendly habitat, especially in areas of dense woods or shrubs (Jackson et al., 2014; Hanula et al., 2016). Other research has found that distributing bee seed mixes at low densities increases nectar production per plant, providing higher-quality floral resources for bees (Neece et al., 2023). These results suggest that planting at lower densities could be strategic for bee habitat restoration.
Floral resources are often unequally distributed across a landscape, and patch size may influence bee foraging behavior, especially in fragmented habitats. Harmon-Threatt and Anderson (2023) found that bees in a naturally patchy Ozark Mountain glade ecosystem rarely traveled between patches, demonstrating the importance of nearby floral resources. Bumble bees and solitary bees respond to both patch size and isolation when foraging for resources (Fragoso et al., 2021; Fragoso and Brunet, 2023), and bumble bee foraging is considered particularly sensitive to habitat fragmentation (Osborne et al., 2008; Goulson et al., 2011). Fragoso and Brunet (2023) reported that Bombus impatiens Cresson, 1863 preferred larger, more closely spaced patches, while Megachile rotundata Fabricius, 1787 preferred patches located nearby their nests regardless of the patch size. Although bumble bees may prefer closely spaced patches, they can forage over greater distances than solitary bees. For example, an average-sized eusocial bee (intertegular distance = 2.5mm for a female foraging bee) has a foraging range of ~3,300 meters whereas a similarly sized solitary bee has a foraging distance of ~1,200 meters (Grüter and Hayes, 2022). Fragoso and Brunet (2023) determined that both solitary and eusocial bees use complex learning to determine which patches to visit. The composition of flowering patches may be expected to influence bees’ foraging preferences. To our knowledge, however, no studies have investigated how a patch’s plant diversity or the relative abundances of different species influence bee foraging distance or behavior in restored landscapes.
When establishing patches of floral resources, the provenance of seeds or plants can influence plant-pollinator interactions (Thomas et al., 2014; Bucharova et al., 2022; Höfner et al., 2022). For example, due to local adaptation, wild populations of plants differ with respect to flowering phenology, which in turn can affect bee foraging. If seeds or propagules are relocated for restoration, the flowering window of each plant species’ population at the restoration site may differ from the window of the flight times of sympatric bee populations (Buisson et al., 2017). This could lead to a phenological mismatch between the flowering phenology of a restored site vs. its neighboring landscapes (Buisson et al., 2017; MacTavish and Anderson, 2022) increasing the risk of mismatches between plant species and their associated bees (see Section 2.3.1.2 Plant and Bee Phenology). Utilization of locally sourced seeds could potentially avoid this problem, but locally sourced seeds may not be physiologically adapted to changing climatic conditions (Bucharova et al., 2022). Managers should consider planting floral resources that are better suited for future climatic conditions (Oliver et al., 2016). Stephenson et al. (2020) found that in emergent wetlands, sites that were passively managed (allowing the establishment of native perennials through natural succession) after active restoration was completed had similar bee diversity and species richness compared to actively restored sites. No other studies within our review compared active and passive restoration methods.
4 Post-restoration: assessment & monitoring
Post-restoration refers to the assessment and monitoring that occurs after the initial steps of a project, but it does not necessarily signify the project’s completion. Ecological restoration is an iterative process that requires continuous upkeep and evaluation to ensure that specific goals are achieved. Assessments and monitoring are beneficial at any stage of a project; however, they are particularly important for post-restoration evaluation.
4.1 Evaluating restoration for bees
To gauge the success of a restoration project, land managers must have specific, measurable outcomes and goals (Hallett et al., 2013). This may consist of setting targets that include specific biodiversity metrics, such as species richness, species diversity, or the presence of endangered species, which may be based on historical baselines (Michener, 1997). These metrics are possible to measure for small-scale bee habitat restoration initiatives; however, for larger projects that may take a rewilding approach (which involves allowing nature to reclaim a site rather than actively restoring it), other metrics may be more appropriate. Rewilding-focused strategies (Perino et al., 2019; Carver et al., 2021) emphasize the need to evaluate ecological complexity, which can be gauged by examining pollinator networks and redundancy (Elle et al., 2012; Bullock et al., 2022; Gawecka and Bascompte, 2023) as well as through the delivery of ecosystem services (Perino et al., 2019), which may be estimated by floral visitation rates, pollen transfer by bees (Plentovich et al., 2021), or the reproductive success of plants.
Assessing the success of restoration efforts should involve evaluating multiple ecological indicators (Prach et al., 2019); however, to date, bee diversity has not been commonly included in such assessments due to the difficulty and expense of monitoring (Bruninga-Socolar et al., 2023). Animals, particularly pollinators, can serve as excellent indicators of environmental health because of their interdependence with native plants (Buisson et al., 2017; Montoya-Pfeiffer et al., 2020) and their sensitivity to environmental toxins. Honey bee colony growth and performance have served as a useful bio-monitor for contaminants, pesticides, pathogens, and climate change (Quigley et al., 2019) and therefore may serve as useful indicators for assessing ecosystem health (CaraDonna et al., 2018; Herrera et al., 2023; Schenk et al., 2018; Willis Chan et al., 2019). Solitary bees are considered more sensitive to climate change and other anthropogenic factors than honey bees (Cunningham et al., 2022). Thus, solitary bees may be an even better proxy for ecosystem health, although no studies to date have tested this.
By utilizing multiple bee-capturing methods, sampling efforts can encompass bee species with different life histories (Begosh et al., 2020; Prendergast et al., 2020). For example, Sardiñas and Kremen (2014) employed emergence traps to estimate ground-nesting bee diversity, which differed from the composition of bee taxa estimated using aerial nets and pan trapping. Other, more indirect indicators of bee population health can be used to assess the long-term success of restoration projects. For example, native parasites (particularly brood parasites, found in bees’ nests) indicate healthy populations that are able to sustain native parasitic species (Hudson et al., 2006; Dougherty et al., 2016; Araujo et al., 2018). Additionally, sex ratios can be used as an indicator of bee population health. In many species, including Osmia rufa Linnaeus, 1758, Megachile apicalis Spinola, 1808, and Bombus sp., bee sex ratios can be sensitive to resource availability and parasitism rates, both of which influence larvae provisioning (Bourke, 1997; Kim, 1999; Seidelmann et al., 2010). When larvae receive less food, there is a decrease in female offspring (Kim, 1999; Seidelmann et al., 2010). Female bees are primarily responsible for nest building and provisioning brood cells (Danforth et al., 2019); thus, when populations are female-limited, nest density and birth rates decrease, negatively affecting population size.
4.2 Long-term monitoring & research
Long-term bee monitoring at current restoration sites may help to improve future bee habitat restoration if used to identify practices that sustain native bee populations (see Section 2.2 Establishing a Baseline; Woodard et al., 2020; Droege et al., 2023). Sampling native bee species richness and estimating population abundances are useful metrics for evaluating restoration success (Williams, 2011; Tonietto and Larkin, 2018). Long-term monitoring of restored habitats is necessary to detect habitat and community changes over time, as short-term assessments (one to five years following the termination of a project) can provide incomplete or misleading indicators of a project’s overall success (Herrick et al., 2006; Griffin et al., 2017; Onuferko et al., 2018; Sexton and Emery, 2020; Tang et al., 2023). For example, Abella et al. (2020) observed floristic quality (an index where plants are ranked by the commonality of a plant at a site) throughout 20 years, rather than just sampling at the beginning and end of monitoring. They found the difference observed across years better accounted for temporal fluctuations in vegetation growth and plant diversity. Thus, it may be meaningful to continuously assess the accumulation of restoration benefits considering the impacts of the restored landscape over time.
Long-term monitoring of bee populations and communities at a given location is challenging because observations can be sensitive to sampling methods (Portman et al., 2020; Bruninga-Socolar et al., 2023) and the costs associated with identifying bees and processing bee specimens can be high (Bruninga-Socolar et al., 2023). Surveillance monitoring, or broadly sampling bee communities to determine species presence, may provide measures of bee diversity. However, increased bee diversity does not guarantee that local populations of all bee species are sustainable; some populations may be thriving while others are not (Kammerer et al., 2021). Monitoring needs to occur across years; increased bee species occurrences across a season do not necessarily indicate an increase in population size (Portman et al., 2020; Woodard et al., 2020).
Alternatively, targeted monitoring is an emerging method for assessing bee populations. It is based on specifically monitoring certain bees or ecosystem functions that are the focal points of a given restoration project (Portman et al., 2020; Woodard et al., 2020). Tepedino and Portman (2021) contend that targeted monitoring is more effective than surveillance monitoring methods. Moreover, targeted monitoring is hypothesis-driven, which may facilitate the discovery of species-specific restoration practices rather than just observing broad trends (Tepedino and Portman, 2021). For example, targeted monitoring of rare plant reproductive success can benefit specialist bees because of their unique association with specialist pollinators (Motta et al., 2022). In addition, innovative techniques, such as using camera traps with deep learning technologies are emerging (Barlow and O’Neill, 2020; Spiesman et al., 2021; Bjerge et al., 2023). These approaches, which offer cost-effective and non-invasive methods for monitoring bee diversity, are expected to continue to improve in the near future (Bjerge et al., 2023).
Community science approaches can also provide cost-effective long-term monitoring strategies (Huddart et al., 2016; Edwards et al., 2018; MacPhail et al., 2020). Developing standardized protocols for community science efforts allows high-quality data to be obtained while educating the public about local environmental concerns (MacPhail et al., 2020). To assess bee abundance or diversity, community efforts could include catching and photographing specimens for identification, locating and counting nests, or quantifying floral resources and their phenology (Vilen et al., 2023). Moreover, new methods such as passive crowdsourcing can be a valuable screening tool for determining potential plant candidates for bee habitat restoration (Bahlai and Landis, 2016). Utilizing public resources such as iNaturalist and BugGuide for species identification can contribute to the growth of databases and more accurate distribution records (Orr et al., 2023). However, it is important to understand the strengths and limitations of community science data (Kosmala et al., 2016) and account for this when designing studies and analyzing data recorded by members of the public.
5 Discussion
Throughout this review, we provide insights for bee-centric habitat restoration through our pre-, during-, and post-restoration framework. We also emphasize promising directions for future research. Table 3 summarizes the most promising research areas needed to advance bee-centric restoration. Despite the limited knowledge of many aspects of bee habitat restoration, prioritizing the research gaps identified here can guide the application of restoration practices based on empirical evidence. Ultimately, bee habitat restoration aims to enhance native bee diversity and abundance, contributing to the persistence of bee populations, bee communities, and plant-pollinator interactions (Winfree, 2010; Tonietto and Larkin, 2018).
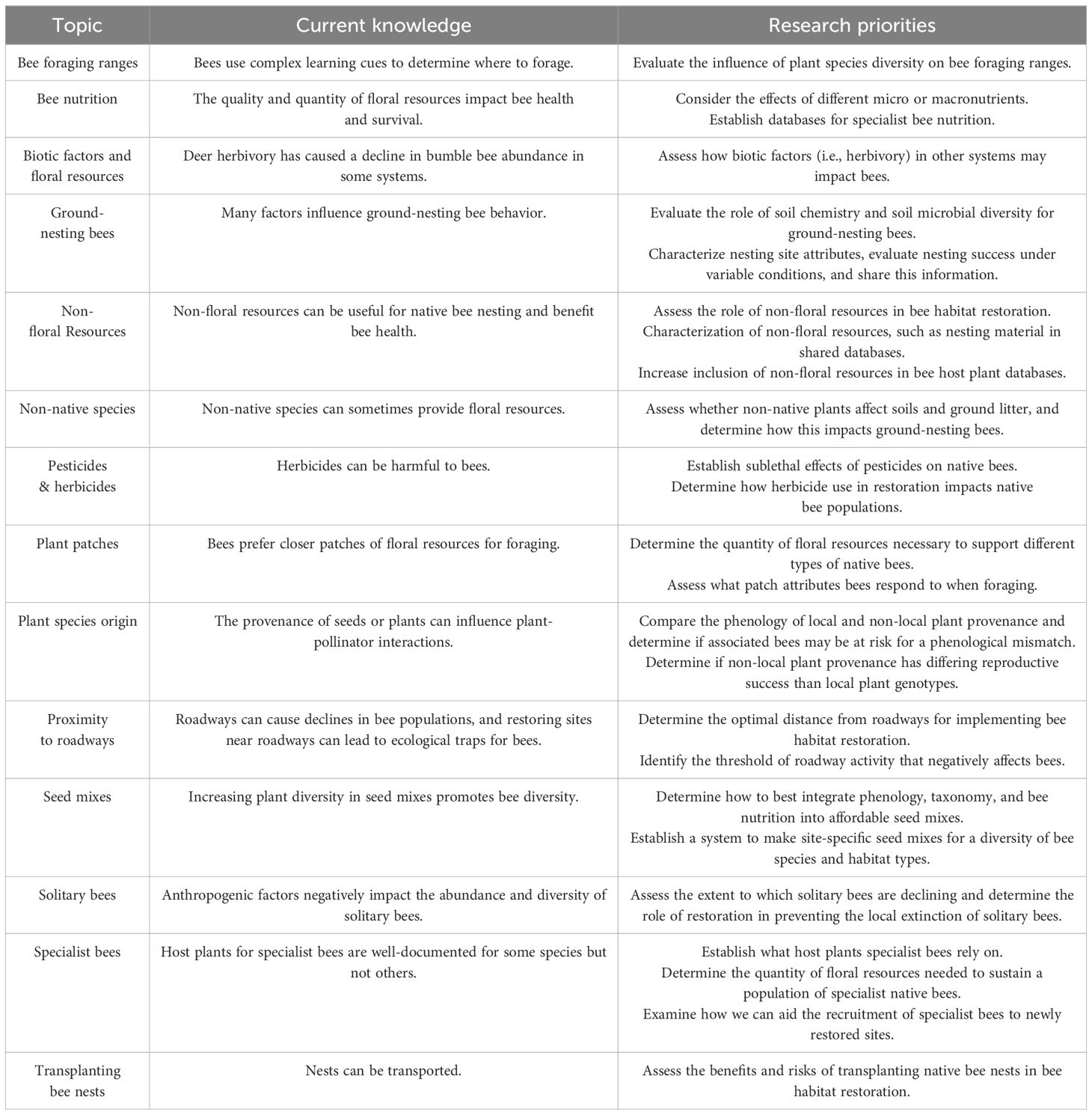
Table 3 Research priorities that are critical for advancing the fields of bee conservation and habitat restoration.
The effects of habitat restoration on native bee species diversity and abundance are currently data-limited but can be expanded through the open sharing of restoration plans and monitoring outcomes (Woodard et al., 2020). Throughout this review, we found the majority of studies reported an increase in bee abundance and diversity following restoration; however, we found only 21 studies that focused on species-specific responses of bees to restoration. Including species-specific responses in future studies can provide detailed information that can be used when restoring habitat for targeted bee species.
In the absence of species-specific data for most wild bee species, the best approach is to use strategies that will likely benefit a wide range of bee species. Implementing empirical tools such as M’Gonigle’s Genetic Algorithm can aid in the selection of plant species to be used when restoring bee habitat (M’Gonigle et al., 2017). Despite significant gaps in our understanding of the specific nutritional requirements of native bees (Crone et al., 2022; Filipiak et al., 2022), a prudent approach would include planting phenologically overlapping floral resources (in which multiple related host plant species flower simultaneously), and augmenting floral resources both early and late in the flowering season in order to increase flowering duration at the community level. These approaches should consider plant nutritional variability when possible, increasing the chances that the nutritional requirements of most bee species will be met (Rowe et al., 2018). Additionally, including native keystone plant species that support a wide array of generalist bees and other insects should be a priority (James et al., 2014, 2016; Fantinato et al., 2018).
It is important to recognize that plant species that are important for bees may not always be bee-pollinated. For example, many bee species rely on willow (a wind-pollinated species) for pollen in riparian habitats (Mitchell et al., 2022). Moreover, not all sites may require the addition of supplemental floral resources. Many restoration projects can improve the habitat for native bees through the removal of non-native species and allowing the natural recruitment of native plants from nearby areas and from the existing seed bank (Hanula and Horn, 2011).
Nesting habitat can be provided by leaving dead plant debris at restoration sites and reducing the use of mulch to provide some bare ground for ground-nesting bees (Vaughan and Black, 2008; Eckerter et al., 2021; Rappa et al., 2023). If cavity nests are present during pre-site surveys, restoration efforts should be timed for spring to minimize net loss (when many cavity nesters are less likely to be overwintering). When applicable, care should be taken to reconstitute the original vegetation structure of the site using native plants. In addition, retaining dead piles of shrubs can increase nest site availability for bumble bees (Liczner and Colla, 2020). By providing nesting sites that attract bees and lead to high-quality nests, as well as by protecting existing nests, restoration efforts can contribute to the preservation of bee populations (Harmon-Threatt, 2020). Conserving existing nesting sites, however, is not the only option; occupied nests can be transplanted (Davison and Field, 2018), although the risks associated with this practice are unknown. Although no direct studies compare planting techniques for bee habitat restoration, implementing a mixture of techniques (e.g., transplanting, seed spreading, and propagation) to provide heterogeneity in habitat structure and plant diversity would likely best support bee habitat restoration.
Habitat loss and degradation are major factors driving insect declines (Wagner et al., 2021), and refocusing restoration practices on bees may help conserve native bee diversity and abundance. Developing metrics for use as part of a rapid assessment protocol for bees is necessary to ensure clear quantitative and standardized outcomes of bee-centric restoration work across projects. If the establishment of metrics is widely adopted, this can provide specific information on the causes of successful or unsuccessful bee-centric restoration projects. Rapid assessment protocols are already employed in various restoration contexts (Obrist and Duelli, 2010; Collins and Stein, 2018). Similar protocols are currently being adapted to gauge the success of bee habitat restoration across ecological scales, from individual species to ecosystem functionality (Woodard et al., 2020). These protocols play a crucial role in providing standardized methodologies for evaluating the effectiveness of restoration efforts, facilitating the advancement of research, and promoting the implementation of bee-centric habitat restoration practices.
The restoration of bee habitats contributes to the overarching objectives of ecological restoration by increasing plant diversity and enhancing pollination services (Menz et al., 2011; Wratten et al., 2012; Wojcik et al., 2018; Cole et al., 2019). For example, restoring native plant communities to support bee populations can provide habitat and nutritional resources for a range of other species, including birds, mammals, and other invertebrates (Tallamy, 2020). Moreover, strategies that target the conservation of specialist bees can lead to the preservation of rare and endemic plant species, further contributing to the conservation of unique ecosystems (Motta et al., 2022). Restoring bee habitats within the framework of general restoration efforts can enhance pollination networks (Kaiser-Bunbury et al., 2017), promote ecosystem services, and improve plant and bee reproductive success (Albrecht et al., 2012; Danforth et al., 2019).
Author contributions
HP: Conceptualization, Investigation, Methodology, Project administration, Resources, Validation, Visualization, Writing – original draft, Writing – review & editing. SM: Conceptualization, Supervision, Validation, Writing – review & editing. KS: Conceptualization, Funding acquisition, Investigation, Methodology, Project administration, Resources, Supervision, Validation, Visualization, Writing – original draft, Writing – review & editing.
Funding
The author(s) declare financial support was received for the research, authorship, and/or publication of this article. This work was supported by two National Science Foundation (NSF) awards: (DBI-2102006) to KS and (DEB-1655727) to SM.
Acknowledgments
We thank Dr. Lisa Stratton and Dr. Adam Lambert (Cheadle Center for Biodiversity and Ecological Restoration, UC Santa Barbara) for their insightful feedback as restoration practitioners who include pollinators in their projects. We also appreciate Rachel Payne for creating Figure 2.
Conflict of interest
The authors declare that the research was conducted in the absence of any commercial or financial relationships that could be construed as a potential conflict of interest.
Publisher’s note
All claims expressed in this article are solely those of the authors and do not necessarily represent those of their affiliated organizations, or those of the publisher, the editors and the reviewers. Any product that may be evaluated in this article, or claim that may be made by its manufacturer, is not guaranteed or endorsed by the publisher.
References
Abella S. R., Menard K. S., Schetter T. A., Sprow L. A., Jaeger J. F. (2020). Rapid and transient changes during 20 years of restoration management in savanna-woodland-prairie habitats threatened by woody plant encroachment. Plant Ecol. 221, 1201–1217. doi: 10.1007/s11258-020-01075-4
Abella S. R., Schetter T. A., Walters T. L. (2017). Restoring and conserving rare native ecosystems: A 14-year plantation removal experiment. Biol. Conserv. 212, 265–273. doi: 10.1016/j.biocon.2017.06.034
Abraham J., Benhotons G. S., Krampah I., Tagba J., Amissah C., Abraham J. D. (2018). Commercially formulated glyphosate can kill non-target pollinator bees under laboratory conditions. Entomol. Exp. Appl. 166, 695–702. doi: 10.1111/eea.12694
Aizen M. A., Morales C. L. (2020). Impacts of non-native plants on plant-pollinator interactions. Plant Invasions Role Biot. Interact., 241–255. doi: 10.1079/9781789242171.0013
Albrecht M., Schmid B., Hautier Y., Müller C. B. (2012). Diverse pollinator communities enhance plant reproductive success. Proc. R. Soc B Biol. Sci. 279, 4845–4852. doi: 10.1098/rspb.2012.1621
Alkassab A. T., Kirchner W. H. (2017). Sublethal exposure to neonicotinoids and related side effects on insect pollinators: honeybees, bumblebees, and solitary bees. J. Plant Dis. Prot. 124, 1–30. doi: 10.1007/s41348-016-0041-0
Almeida E. A. B., Bossert S., Danforth B. N., Porto D. S., Freitas F. V., Davis C. C., et al. (2023). The evolutionary history of bees in time and space. Curr. Biol. CB 33, 3409–3422.e6. doi: 10.1016/j.cub.2023.07.005
Alofs K. M., González A. V., Fowler N. L. (2014). Local native plant diversity responds to habitat loss and fragmentation over different time spans and spatial scales. Plant Ecol. 215, 1139–1151. doi: 10.1007/s11258-014-0372-5
Andrews H. (2023). What is “No Mow May”? It’s a way to save the bees. In: Fox Weather. Available online at: https://www.foxweather.com/lifestyle/no-mow-may-not-lazy-saving-the-bees (Accessed September 19, 2023).
Antoine C. M., Forrest J. R. K. (2020). Nesting habitat of ground-nesting bees: a review. Ecol. Entomol. 46, 143–159. doi: 10.1111/een.12986
Applestein C., Bakker J. D., Delvin E. G., Hamman S. T. (2018). Evaluating seeding methods and rates for prairie restoration. Nat. Areas J. 38, 347–355. doi: 10.3375/043.038.0504
Araujo G. J., Fagundes R., Antonini Y. (2018). Trap-nesting hymenoptera and their network with parasites in recovered Riparian forests Brazil. Neotrop. Entomol. 47, 26–36. doi: 10.1007/s13744-017-0504-4
Audet T., Romero N., Richards M. (2021). Negative effects of early spring mowing on a bee community: a case study in the Niagara Region. J. Entomol. Soc Ont. 152, 39–55.
Auld B. A. (1998). On the social value of biological control of weeds. Int. J. Soc Econ. 25, 1199–1206. doi: 10.1108/03068299810212685
Bahlai C. A., Landis D. A. (2016). Predicting plant attractiveness to pollinators with passive crowdsourcing. R. Soc Open Sci. 3, 150677. doi: 10.1098/rsos.150677
Banaszak J., Twerd L. (2018). Importance of thermophilous habitats for protection of wild bees (Apiformes). Community Ecol. 19, 239–247. doi: 10.1556/168.2018.19.3.5
Barlow S. E., O’Neill M. A. (2020). Technological advances in field studies of pollinator ecology and the future of e-ecology. Curr. Opin. Insect Sci. 38, 15–25. doi: 10.1016/j.cois.2020.01.008
Barr S., Jonas J. L., Paschke M. W. (2017). Optimizing seed mixture diversity and seeding rates for grassland restoration. Restor. Ecol. 25, 396–404. doi: 10.1111/rec.12445
Barron J., Beston J. (2022). Pollinator abundance and diversity under differing wet prairie management. Nat. Areas J. 42, 313–318. doi: 10.3375/22-13
Basu P., Parui A. K., Chatterjee S., Dutta A., Chakraborty P., Roberts S., et al. (2016). Scale dependent drivers of wild bee diversity in tropical heterogeneous agricultural landscapes. Ecol. Evol. 6, 6983–6992. doi: 10.1002/ece3.2360
Batra S. W. T. (1984). Solitary bees. Sci. Am. 250, 120–127. doi: 10.1038/scientificamerican0284-120
Battisti L., Potrich M., Sampaio A. R., de Castilhos Ghisi N., Costa-Maia F. M., Abati R., et al. (2021). Is glyphosate toxic to bees? A meta-analytical review. Sci. Total Environ. 767, 145397. doi: 10.1016/j.scitotenv.2021.145397
Beckett K., Elle E., Kremen C., Sherwood A., McComb S., Martin T. G. (2022). Hyperabundant black-tailed deer impact endangered Garry oak ecosystem floral and bumblebee communities. Glob. Ecol. Conserv. 38, e02237. doi: 10.1016/j.gecco.2022.e02237
Beckham J. L., Atkinson S. (2017). An updated understanding of Texas bumble bee (Hymenoptera: Apidae) species presence and potential distributions in Texas, USA. PeerJ 5, e3612. doi: 10.7717/peerj.3612
Begosh A., Smith L. M., Park C. N., Mcmurry S. T., Lagrange T. G. (2020). Effects of wetland presence and upland land use on wild hymenopteran and dipteran pollinators in the rainwater basin of Nebraska, USA. Wetlands 40, 1017–1031. doi: 10.1007/s13157-019-01244-w
Beneduci Z. J., Scott D. A., Byrd S. M., Swab R. M. (2023). We built it; did they come? Pollinator diversity and community structure in a post-mining prairie restoration project. Ecol. Restor. 41, 180–188. doi: 10.3368/er.41.4.180
Bennion L. D., Ferguson J. A., New L. F., Schultz C. B. (2020). Community-level effects of herbicide-based restoration treatments: structural benefits but at what cost? Restor. Ecol. 28, 553–563. doi: 10.1111/rec.13118
Bevill R. L., Louda S. M., Stanforth L. M. (1999). Protection from natural enemies in managing rare plant species. Conserv. Biol. 13, 1323–1331. doi: 10.1046/j.1523-1739.1999.98450.x
Bjerge K., Alison J., Dyrmann M., Frigaard C. E., Mann H. M. R., Høye T. T. (2023). Accurate detection and identification of insects from camera trap images with deep learning. PloS Sustain. Transform. 2, e0000051. doi: 10.1371/journal.pstr.0000051
Bourke A. F. G. (1997). Sex ratios in bumble bees. Philos. Trans. R. Soc Lond. B. Biol. Sci. 352, 1921–1933. doi: 10.1098/rstb.1997.0179
Breeze T. D., Bailey A. P., Balcombe K. G., Brereton T., Comont R., Edwards M., et al. (2021). Pollinator monitoring more than pays for itself. J. Appl. Ecol. 58, 44–57. doi: 10.1111/1365-2664.13755
Brokaw J., Portman Z. M., Bruninga-Socolar B., Cariveau D. (2023). Prescribed fire increases the number of ground-nesting bee nests in tall grass prairie remnants. Insect Conserv. Divers. 16, 355–367. doi: 10.1111/icad.12628
Brooks M. G. (2020). Plant-pollinator interactions across California grassland and coastal scrub vegetation types on San Bruno Mountain, San Mateo County (Middletown, CT: Wesleyan University). doi: 10.14418/wes01.1.2282
Brooks M. G., Poulos H. M. (2023). Plant–pollinator interactions in a northern california coastal habitat, San Bruno Mountain, San Mateo County, California, USA. Nat. Areas J. 43, 212–224. doi: 10.3375/0885-8608-43.4.212
Bruninga-Socolar B., Griffin S. R., Portman Z. M., Gibbs J. (2022). Variation in prescribed fire and bison grazing supports multiple bee nesting groups in tallgrass prairie. Restor. Ecol. 30, e13507. doi: 10.1111/rec.13507
Bruninga-Socolar B., Lonsdorf E. V., Lane I. G., Portman Z. M., Cariveau D. P. (2023). Making plant–pollinator data collection cheaper for restoration and monitoring. J. Appl. Ecol. 60, 2031–2039. doi: 10.1111/1365-2664.14472
Bruns C. E., Demastes J. W., Berendzen P. B., Wen A. (2024). The genetic structure of founding bumblebee populations in reconstructed prairie habitat 3 years after planting. Restor. Ecol. n/a, e14176. doi: 10.1111/rec.14176
Bucharova A., Lampei C., Conrady M., May E., Matheja J., Meyer M., et al. (2022). Plant provenance affects pollinator network: Implications for ecological restoration. J. Appl. Ecol. 59, 373–383. doi: 10.1111/1365-2664.13866
Buckles B. J., Harmon-Threatt A. N. (2019). Bee diversity in tallgrass prairies affected by management and its effects on above- and below-ground resources. J. Appl. Ecol. 56, 2443–2453. doi: 10.1111/1365-2664.13479
Buckley S., Nabhan G. P. (2016). Food chain restoration for pollinators: regional habitat recovery strategies involving protected areas of the southwest. Nat. Areas J. 36, 489–497. doi: 10.3375/043.036.0414
Buisson E., Alvarado S. T., Le Stradic S., Morellato L. P. C. (2017). Plant phenological research enhances ecological restoration. Restor. Ecol. 25, 164–171. doi: 10.1111/rec.12471
Bullock J. M., Fuentes-Montemayor E., McCarthy B., Park K., Hails R. S., Woodcock B. A., et al. (2022). Future restoration should enhance ecological complexity and emergent properties at multiple scales. Ecography 2022, e05780. doi: 10.1111/ecog.05780
Campbell A. J., Gigante Carvalheiro L., Gastauer M., Almeida-Neto M., Giannini T. C. (2019). Pollinator restoration in Brazilian ecosystems relies on a small but phylogenetically-diverse set of plant families. Sci. Rep. 9, 17383. doi: 10.1038/s41598-019-53829-4
Cane J. H. (1991). Soils of ground-nesting bees (Hymenoptera: apoidea): texture, moisture, cell depth and climate. J. Kans. Entomol. Soc 64, 406–413.
Cane J. H., Weber M., Miller S. (2012). Breeding biologies, pollinators, and seed beetles of two prairie-clovers, Dalea ornata and Dalea searlsiae (Fabaceae: Amorpheae), from the Intermountain West, USA. West. North Am. Nat. 721, 16–20. doi: 10.3398/064.072.0102
CaraDonna P. J., Cunningham J. L., Iler A. M. (2018). Experimental warming in the field delays phenology and reduces body mass, fat content and survival: Implications for the persistence of a pollinator under climate change. Funct. Ecol. 32, 2345–2356. doi: 10.1111/1365-2435.13151
Cariveau D. P., Bruninga-Socolar B., Pardee G. L. (2020). A review of the challenges and opportunities for restoring animal-mediated pollination of native plants. Emerg. Top. Life Sci. 4, 99–109. doi: 10.1042/ETLS20190073
Carson B. D., Bahlai C. A., Gibbs J., Landis D. A. (2016). Flowering phenology influences bee community dynamics in old fields dominated by the invasive plant Centaurea stoebe. Basic Appl. Ecol. 17, 497–507. doi: 10.1016/j.baae.2016.04.004
Carvalho A. F., Del Lama M. A. (2015). Predicting priority areas for conservation from historical climate modelling: stingless bees from Atlantic Forest hotspot as a case study. J. Insect Conserv. 19, 581–587. doi: 10.1007/s10841-015-9780-7
Carver S., Convery I., Hawkins S., Beyers R., Eagle A., Kun Z., et al. (2021). Guiding principles for rewilding. Conserv. Biol. 35, 1882–1893. doi: 10.1111/cobi.13730
Chan D. S. W., Prosser R. S., Rodríguez-Gil J. L., Raine N. E. (2019). Assessment of risk to hoary squash bees (Peponapis pruinosa) and other ground-nesting bees from systemic insecticides in agricultural soil. Sci. Rep. 14, 11870. doi: 10.1101/434498
Chesshire P. R., Fischer E. E., Dowdy N. J., Griswold T. L., Hughes A. C., Orr M. C., et al. (2023). Completeness analysis for over 3000 United States bee species identifies persistent data gap. Ecography 2023, e06584. doi: 10.1111/ecog.06584
Chole H., Woodard S. H., Bloch G. (2019). Body size variation in bees: regulation, mechanisms, and relationship to social organization. Curr. Opin. Insect Sci. 35, 77–87. doi: 10.1016/j.cois.2019.07.006
Christmann S. (2022). Regard and protect ground-nesting pollinators as part of soil biodiversity. Ecol. Appl. 32, e2564. doi: 10.1002/eap.2564
Chui S. X., Keller A., Leonhardt S. D. (2022). Functional resin use in solitary bees. Ecol. Entomol. 47, 115–136. doi: 10.1111/een.13103
Cole J. S., Siegel R. B., Loffland H. L., Tingley M. W., Elsey E. A., Johnson M. (2019). Explaining the birds and the bees: deriving habitat restoration targets from multi-species occupancy models. Ecosphere 10, e02718. doi: 10.1002/ecs2.2718
Colla S. R., Gadallah F., Richardson L., Wagner D., Gall L. (2012). Assessing declines of North American bumble bees (Bombus spp.) using museum specimens. Biodivers. Conserv. 21, 3585–3595. doi: 10.1007/s10531-012-0383-2
Colla S. R., Otterstatter M. C., Gegear R. J., Thomson J. D. (2006). Plight of the bumble bee: Pathogen spillover from commercial to wild populations. Biol. Conserv. 129, 461–467. doi: 10.1016/j.biocon.2005.11.013
Collins J., Stein E. D. (2018). “Chapter 4.3.3 - California rapid assessment method for wetlands and riparian areas (CRAM),” in Wetland and Stream Rapid Assessments. eds. Dorney J., Savage R., Tiner R. W., Adamus P. (Cambridge, MA: Academic Press), 353–361. doi: 10.1016/B978-0-12-805091-0.00044-X
Crone M. K., Biddinger D. J., Grozinger C. M. (2022). Wild bee nutritional ecology: integrative strategies to assess foraging preferences and nutritional requirements. Front. Sustain. Food Syst. 6. doi: 10.3389/fsufs.2022.847003
Cunningham M. M., Tran L., McKee C. G., Ortega Polo R., Newman T., Lansing L., et al. (2022). Honey bees as biomonitors of environmental contaminants, pathogens, and climate change. Ecol. Indic. 134, 108457. doi: 10.1016/j.ecolind.2021.108457
Curran M. F., Robinson T. J., Guernsey P., Sorenson J., Crow T. M., Smith D. I., et al. (2022). Insect abundance and diversity respond favorably to vegetation communities on interim reclamation sites in a semi-arid natural gas field. Land 11, 527. doi: 10.3390/land11040527
Curran M. F., Sorenson J. R., Craft Z. A., Crow T. M., Robinson T. J., Stahl P. D. (2023). Ecological Restoration Practices within a Semi-arid Natural Gas Field Improve Insect Abundance and Diversity during Early and Late Growing Season. Animals 13, 134. doi: 10.3390/ani13010134
Cusser S., Goodell K. (2013). Diversity and distribution of floral resources influence the restoration of plant–pollinator networks on a reclaimed strip mine. Restor. Ecol. 21, 713–721. doi: 10.1111/rec.12003
Danforth B. N., Minckley R. L., Neff J. L., Fawcett F. (2019). “The solitary bee life cycle,” in The Solitary Bees (New Jersey: Princeton University Press), 37–69. doi: 10.2307/j.ctvd1c929.6
Davies C. B., Davis T. S., Griswold T. (2023). Forest restoration treatments indirectly diversify pollination networks via floral- and temperature-mediated effects. Ecol. Appl. n/a, e2927. doi: 10.1002/eap.2927
Davies K. W., Sheley R. L. (2011). Promoting native vegetation and diversity in exotic annual grass infestations. Restor. Ecol. 19, 159–165. doi: 10.1111/j.1526-100X.2009.00548.x
Davis T. S., Rhoades P. R., Mann A. J., Griswold T. (2020). Bark beetle outbreak enhances biodiversity and foraging habitat of native bees in alpine landscapes of the southern Rocky Mountains. Sci. Rep. 10, 16400. doi: 10.1038/s41598-020-73273-z
Davison P. J., Field J. (2018). Environmental barriers to sociality in an obligate eusocial sweat bee. Insectes Sociaux 65, 549–559. doi: 10.1007/s00040-018-0642-7
de Araújo G. J., Izzo T. J., Storck-Tonon D., Paolucci L. N., Didham R. K. (2021). Re-establishment of cavity-nesting bee and wasp communities along a reforestation gradient in southern Amazonia. Oecologia 196, 275–288. doi: 10.1007/s00442-021-04920-z
de Araújo G. J., Monteiro G. F., Messias M. C. T. B., Antonini Y. (2018). Restore it, and they will come: trap-nesting bee and wasp communities (Hymenoptera: Aculeata) are recovered by restoration of riparian forests. J. Insect Conserv. 22, 245–256. doi: 10.1007/s10841-018-0058-8
de Araújo G. J., Storck-Tonon D., Izzo T. J. (2019). Is planting trees enough? The effect of different types of reforestation on the offspring of trypoxylon (Trypargilum) lactitarse (Hymenoptera: crabronidae) in the Southern Amazon. Neotrop. Entomol. 48, 572–582. doi: 10.1007/s13744-019-00682-9
Decker B. L., Harmon-Threatt A. N. (2019). Growing or dormant season burns: the effects of burn season on bee and plant communities. Biodivers. Conserv. 28, 3621–3631. doi: 10.1007/s10531-019-01840-6
Decourtye A., Rollin O., Requier F., Fabrice A., Rüger C., Vidau C., et al. (2023). Decision-making criteria for pesticide spraying considering the bees’ presence on crops to reduce their exposure risk. Front. Ecol. Evol. 11, 1062441. doi: 10.3389/fevo.2023.1062441
Denning K., Foster B. (2017). Flower visitor communities are similar on remnant and reconstructed tallgrass prairies despite forb community differences. Restor. Ecol. 26, 751–759. doi: 10.1111/rec.12615
Denning K. R., Foster B. L. (2018). Taxon-specific associations of tallgrass prairie flower visitors with site-scale forb communities and landscape composition and configuration. Biol. Conserv. 227, 74–81. doi: 10.1016/j.biocon.2018.08.023
Dibble A. C., Drummond F. A., Stack L. B. (2020). Bee visitation on flowers in Maine, United States, reveals the relative attractiveness of plants through space and time: part I. Environ. Entomol. 49, 726–737. doi: 10.1093/ee/nvaa028
Dirilgen T., Herbertsson L., O’Reilly A. D., Mahon N., Stanley D. A. (2023). Moving past neonicotinoids and honeybees: A systematic review of existing research on other insecticides and bees. Environ. Res. 235, 116612. doi: 10.1016/j.envres.2023.116612
Discua S. A., Longing S. D. (2022). Attractiveness of drought-tolerant plants to insect pollinators in the southern high plains region. J. Kans. Entomol. Soc 94, 167–182. doi: 10.2317/0022-8567-94.3.167
Ditomaso J. M., Brooks M. L., Allen E. B., Minnich R., Rice P. M., Kyser G. B. (2006). Control of invasive weeds with prescribed burning. Weed Technol. 20, 535–548. doi: 10.1614/WT-05-086R1.1
Donkersley P., Witchalls S., Bloom E. H., Crowder D. W. (2023). A little does a lot: Can small-scale planting for pollinators make a difference? Agric. Ecosyst. Environ. 343, 108254. doi: 10.1016/j.agee.2022.108254
Dougherty E. R., Carlson C. J., Bueno V. M., Burgio K. R., Cizauskas C. A., Clements C. F., et al. (2016). Paradigms for parasite conservation. Conserv. Biol. 30, 724–733. doi: 10.1111/cobi.12634
Downs P. W., Singer M. S., Orr B. K., Diggory Z. E., Church T. C. (2011). Restoring ecological integrity in highly regulated rivers: the role of baseline data and analytical references. Environ. Manage. 48, 847–864. doi: 10.1007/s00267-011-9736-y
Drobney P., Larson D. L., Larson J. L., Viste-Sparkman K. (2020). Toward improving pollinator habitat: reconstructing prairies with high forb diversity. Nat. Areas J. 40, 252–261. doi: 10.3375/043.040.0322
Droege S., Irwin E., Malpass J., Mawdsley J. (2023). The bee lab. U.S. Geological Survey. doi: 10.3133/fs20233023
Drossart M., Gérard M. (2020). Beyond the decline of wild bees: optimizing conservation measures and bringing together the actors. Insects 11, 649. doi: 10.3390/insects11090649
Drossart M., Michez D., Vanderplanck M. (2017). Invasive plants as potential food resource for native pollinators: A case study with two invasive species and a generalist bumble bee. Sci. Rep. 7, 16242. doi: 10.1038/s41598-017-16054-5
Ealy N., Pawelek J., Hazlehurst J. (2023). Effects of forest management on native bee biodiversity under the tallest trees in the world. Ecol. Evol. 13, e10286. doi: 10.1002/ece3.10286
Eckerter T., Buse J., Bauhus J., Förschler M. I., Klein A. M. (2021). Wild bees benefit from structural complexity enhancement in a forest restoration experiment. For. Ecol. Manage. 496, 119412. doi: 10.1016/j.foreco.2021.119412
Edwards P. M., Shaloum G., Bedell D. (2018). A unique role for citizen science in ecological restoration: a case study in streams. Restor. Ecol. 26, 29–35. doi: 10.1111/rec.12622
Eeraerts M., Smagghe G., Meeus I. (2019). Pollinator diversity, floral resources and semi-natural habitat, instead of honey bees and intensive agriculture, enhance pollination service to sweet cherry. Agric. Ecosyst. Environ. 284, 106586. doi: 10.1016/j.agee.2019.106586
Elle E., Elwell S. L., Gielens G. A. (2012). The use of pollination networks in conservation1This article is part of a Special Issue entitled “Pollination biology research in Canada: Perspectives on a mutualism at different scales. Botany 90, 525–534. doi: 10.1139/b11-111
Elmqvist T., Fragkias M., Goodness J., Güneralp B., Marcotullio P. J., McDonald R. I., et al. eds. (2013). Urbanization, Biodiversity and Ecosystem Services: Challenges and Opportunities (Dordrecht: Springer Netherlands). doi: 10.1007/978-94-007-7088-1
EPA (2015). Policy mitigating acute risk to bees from pesticide products. U.S. Environmental Protection Agency. Available at: https://www.epa.gov/pollinator-protection/policy-mitigating-acute-risk-bees-pesticide-products#:~:text=EPA's%20Policy%20Mitigating%20Acute%20Risk,plans%20and%20best%20management%20practices. (accessed 31 July 2024).
Erickson V. J., Halford A. (2020). Seed planning, sourcing, and procurement. Restor. Ecol. 28, S219–S227. doi: 10.1111/rec.13199
Esque T. C., DeFalco L. A., Tyree G. L., Drake K. K., Nussear K. E., Wilson J. S. (2021). Priority species lists to restore desert tortoise and pollinator habitats in Mojave Desert Shrublands. Nat. Areas J. 41, 145–158. doi: 10.3375/043.041.0209
Exeler N., Kratochwil A., Hochkirch A. (2010). Does recent habitat fragmentation affect the population genetics of a heathland specialist, Andrena fuscipes (Hymenoptera: Andrenidae)? Conserv. Genet. 11, 1679–1687. doi: 10.1007/s10592-010-0060-5
Fantinato E., Del Vecchio S., Silan G., Buffa G. (2018). Pollination networks along the sea-inland gradient reveal landscape patterns of keystone plant species. Sci. Rep. 8, 15221. doi: 10.1038/s41598-018-33652-z
Felderhoff J., Gathof A. K., Buchholz S., Egerer M. (2022). Vegetation complexity and nesting resource availability predict bee diversity and functional traits in community gardens. Ecol. Appl. 33, e2759. doi: 10.1002/eap.2759
Fiedler A. K., Landis D. A., Arduser M. (2012). Rapid shift in pollinator communities following invasive species removal. Restor. Ecol. 20, 593–602. doi: 10.1111/j.1526-100X.2011.00820.x
Filipiak Z. M., Denisow B., Stawiarz E., Filipiak M. (2022). Unravelling the dependence of a wild bee on floral diversity and composition using a feeding experiment. Sci. Total Environ. 820, 153326. doi: 10.1016/j.scitotenv.2022.153326
Fischer L. K., Eichfeld J., Kowarik I., Buchholz S. (2016). Disentangling urban habitat and matrix effects on wild bee species. PeerJ 4, e2729. doi: 10.7717/peerj.2729
Fischer J., Müller T., Spatz A.-K., Greggers U., Grünewald B., Menzel R. (2014). Neonicotinoids interfere with specific components of navigation in honeybees. PloS One 9, e91364. doi: 10.1371/journal.pone.0091364
Flores L. M. A., Zanette L. R. S., Araujo F. S. (2018). Effects of habitat simplification on assemblages of cavity nesting bees and wasps in a semiarid neotropical conservation area. Biodivers. Conserv. 27, 311–328. doi: 10.1007/s10531-017-1436-3
Flower C. E., Fant J. B., Hoban S., Knight K. S., Steger L., Aubihl E., et al. (2018). Optimizing conservation strategies for a threatened tree species: in situ conservation of white ash (Fraxinus americana L.) genetic diversity through insecticide treatment. Forests 9, 202. doi: 10.3390/f9040202
Foote G. G., Foote N. E., Runyon J. B., Ross D. W., Fettig C. J. (2020). Changes in the summer wild bee community following a bark beetle outbreak in a Douglas-fir forest. Environ. Entomol. 49, 1437–1448. doi: 10.1093/ee/nvaa119
Fowler J. (2016). Specialist bees of the northeast: host plants and habitat conservation. Northeast. Nat. 23, 305–320. doi: 10.1656/045.023.0210
Fragoso F. P., Brunet J. (2023). The decision-making process of leafcutting bees when selecting patches. Biol. Lett. 19, 20220411. doi: 10.1098/rsbl.2022.0411
Fragoso F. P., Jiang Q., Clayton M. K., Brunet J. (2021). Patch selection by bumble bees navigating discontinuous landscapes. Sci. Rep. 11, 8986. doi: 10.1038/s41598-021-88394-2
Frankie G., Feng I., Thorp R., Pawelek J., Chase M. H., Jadallah C. C., et al. (2019). Native and non-native plants attract diverse bees to urban gardens in California. J. Pollinat. Ecol. 25. doi: 10.26786/1920-7603(2019)505
Frankie G., Thorp R., Hernandez J., Rizzardi M., Ertter B., Pawelek J., et al. (2009). Native bees are a rich natural resource in urban California gardens. Calif. Agric. 63, 113–120. doi: 10.3733/ca.v063n03p113
Franklin E. L., Raine N. E. (2019). Moving beyond honeybee-centric pesticide risk assessments to protect all pollinators. Nat. Ecol. Evol. 3, 1373–1375. doi: 10.1038/s41559-019-0987-y
Fuccillo Battle K., de Rivera C. E., Cruzan M. B. (2021). The role of functional diversity and facilitation in small-scale pollinator habitat. Ecol. Appl. 31, e02355. doi: 10.1002/eap.2355
Gaiarsa M. P., Bascompte J. (2022). Hidden effects of habitat restoration on the persistence of pollination networks. Ecol. Lett. 25, 2132–2141. doi: 10.1111/ele.14081
Galbraith S. M., Cane J. H., Moldenke A. R., Rivers J. W. (2019). Wild bee diversity increases with local fire severity in a fire-prone landscape. Ecosphere 10, e02668. doi: 10.1002/ecs2.2668
Galea M. B., Wojcik V., Dunn C. (2016). Using pollinator seed mixes in landscape restoration boosts bee visitation and reproduction in the rare local endemic Santa Susana Tarweed, Deinandra minthornii. Nat. Areas J. 36, 512–522. doi: 10.3375/043.036.0416
Garrido P., Mårell A., Öckinger E., Skarin A., Jansson A., Thulin C.-G. (2019). Experimental rewilding enhances grassland functional composition and pollinator habitat use. J. Appl. Ecol. 56, 946–955. doi: 10.1111/1365-2664.13338
Gawecka K. A., Bascompte J. (2023). Habitat restoration and the recovery of metacommunities. J. Appl. Ecol. 60, 1622–1636. doi: 10.1111/1365-2664.14445
Gelles R. V., Davis T. S., Barrett K. J. (2023). Prescribed fire is associated with increased floral richness and promotes short-term increases in bee biodiversity in the ponderosa pine forest of the Southern Rocky Mountains. Agric. For. Entomol. 25, 435–448. doi: 10.1111/afe.12565
Gelles R. V., Davis T. S., Stevens-Rumann C. S. (2022). Wildfire and forest thinning shift floral resources and nesting substrates to impact native bee biodiversity in ponderosa pine forests of the Colorado Front Range. For. Ecol. Manage. 510, 120087. doi: 10.1016/j.foreco.2022.120087
Gérard M., Vanderplanck M., Wood T., Michez D. (2020). Global warming and plant–pollinator mismatches. Emerg. Top. Life Sci. 4, 77–86. doi: 10.1042/ETLS20190139
Gibson S. D., Liczner A. R., Colla S. R. (2019). Conservation Conundrum: At-risk Bumble Bees (Bombus spp.) Show Preference for Invasive Tufted Vetch (Vicia cracca) While Foraging in Protected Areas. J. Insect Sci. 19, 10. doi: 10.1093/jisesa/iez017
Glenny W., Runyon J. B., Burkle L. A. (2022). A review of management actions on insect pollinators on public lands in the United States. Biodivers. Conserv. 31, 1995–2016. doi: 10.1007/s10531-022-02399-5
Glenny W., Runyon J. B., Burkle L. A. (2023). Habitat characteristics structuring bee communities in a forest-shrubland ecotone. For. Ecol. Manage. 534, 120883. doi: 10.1016/j.foreco.2023.120883
Gonzalez N., DeBano S., Kimoto C., Taylor R., Tubbesing C., Strohm C. (2013). Native bees associated with isolated aspen stands in Pacific Northwest bunchgrass prairie. Nat. Areas J. 33, 374–383. doi: 10.3375/043.033.0415
Goulson D. (2013). REVIEW: An overview of the environmental risks posed by neonicotinoid insecticides. J. Appl. Ecol. 50, 977–987. doi: 10.1111/1365-2664.12111
Goulson D., Nicholls E. (2016). The canary in the coalmine; bee declines as an indicator of environmental health. Sci. Prog. 99, 312–326. doi: 10.3184/003685016X14685000479908
Goulson D., Nicholls E., Botías C., Rotheray E. L. (2015). Bee declines driven by combined stress from parasites, pesticides, and lack of flowers. Science 347, 1255957. doi: 10.1126/science.1255957
Goulson D., Rayner P., Dawson B., Darvill B. (2011). Translating research into action; bumblebee conservation as a case study. J. Appl. Ecol. 48, 3–8. doi: 10.1111/j.1365-2664.2010.01929.x
Graffigna S., Marrero H. J., Torretta J. P. (2021). Glyphosate commercial formulation negatively affects the reproductive success of solitary wild bees in a Pampean agroecosystem. Apidologie 52, 272–281. doi: 10.1007/s13592-020-00816-8
Griffin S. R., Bruninga-Socolar B., Gibbs J. (2021). Bee communities in restored prairies are structured by landscape and management, not local floral resources. Basic Appl. Ecol. 50, 144–154. doi: 10.1016/j.baae.2020.12.004
Griffin S. R., Bruninga-Socolar B., Kerr M. A., Gibbs J., Winfree R. (2017). Wild bee community change over a 26-year chronosequence of restored tallgrass prairie. Restor. Ecol. 25, 650–660. doi: 10.1111/rec.12481
Gross C. L. (2017). Improving vegetation quality for the restoration of pollinators – the relevance of co-flowering species in space and time. Rangel. J. 39, 499–522. doi: 10.1071/RJ17066
Ground Nesting Bees (2023). iNaturalist. Available online at: https://www.inaturalist.org/projects/ground-nesting-bees-3e6882c0-a112-4ddb-b043-1da25638ce96 (Accessed September 19, 2023).
Grüter C., Hayes L. (2022). Sociality is a key driver of foraging ranges in bees. Curr. Biol. 32, 5390–5397.e3. doi: 10.1016/j.cub.2022.10.064
Guzmán-Novoa E., Eccles L., Calvete Y., Mcgowan J., Kelly P. G., Correa-Benítez A. (2010). Varroa destructor is the main culprit for the death and reduced populations of overwintered honey bee (Apis mellifera) colonies in Ontario, Canada. Apidologie 41, 443–450. doi: 10.1051/apido/2009076
Hallett L. M., Diver S., Eitzel M. V., Olson J. J., Ramage B. S., Sardinas H., et al. (2013). Do we practice what we preach? Goal setting for ecological restoration. Restor. Ecol. 21, 312–319. doi: 10.1111/rec.12007
Hamilton H., Smyth R. L., Young B. E., Howard T. G., Tracey C., Breyer S., et al. (2022). Increasing taxonomic diversity and spatial resolution clarifies opportunities for protecting US imperiled species. Ecol. Appl. 32, e2534. doi: 10.1002/eap.2534
Hanberry B. B., DeBano S. J., Kaye T. N., Rowland M. M., Hartway C. R., Shorrock D. (2021). Pollinators of the great plains: disturbances, stressors, management, and research needs. Rangel. Ecol. Manage. 78, 220–234. doi: 10.1016/j.rama.2020.08.006
Hanula J. L., Horn S. (2011). Removing an invasive shrub (Chinese privet) increases native bee diversity and abundance in riparian forests of the southeastern United States. Insect Conserv. Divers. 4, 275–283. doi: 10.1111/icad.2011.4.issue-4
Hanula J. L., Horn S., O’Brien J. J. (2015). Have changing forests conditions contributed to pollinator decline in the southeastern United States? For. Ecol. Manage. 348 2015 142–152 348, 142–152. doi: 10.1016/j.foreco.2015.03.044
Hanula J. L., Ulyshen M. D., Horn S. (2016). Conserving pollinators in North American forests: A review. Nat. Areas J. 36, 427–439. doi: 10.3375/043.036.0409
Harmon-Threatt A. (2020). Influence of nesting characteristics on health of wild bee communities. Annu. Rev. Entomol. 65, 39–56. doi: 10.1146/annurev-ento-011019-024955
Harmon-Threatt A. N., Anderson N. L. (2023). Bee movement between natural fragments is rare despite differences in species, patch, and matrix variables. Landsc. Ecol. 38, 2519–2531. doi: 10.1007/s10980-023-01719-6
Harmon-Threatt A., Chin K. (2016). Common methods for tallgrass prairie restoration and their potential effects on bee diversity. Nat. Areas J. 36, 400–411. doi: 10.3375/043.036.0407
Harmon-Threatt A. N., Hendrix S. D. (2015). Prairie restorations and bees: The potential ability of seed mixes to foster native bee communities. Basic Appl. Ecol. 16, 64–72. doi: 10.1016/j.baae.2014.11.001
Havens K., Vitt P. (2016). The importance of phenological diversity in seed mixes for pollinator restoration. Nat. Areas J. 36, 531–537. doi: 10.3375/043.036.0418
Hawkins C. P., Olson J. R., Hill R. A. (2010). The reference condition: predicting benchmarks for ecological and water-quality assessments. J. North Am. Benthol. Soc. 29, 312–343. doi: 10.1899/09-092.1
Helander M., Lehtonen T. K., Saikkonen K., DeSpains L., Nyckees D., Antinoja A., et al. (2023). Field-realistic acute exposure to glyphosate-based herbicide impairs fine-color discrimination in bumblebees. Sci. Total Environ. 857, 159298. doi: 10.1016/j.scitotenv.2022.159298
Heneberg P. (2012). Flagship bird species habitat management supports the presence of ground-nesting aculeate hymenopterans. J. Insect Conserv. 16, 899–908. doi: 10.1007/s10841-012-9477-0
Heneberg P., Bogusch P., Řezáč M. (2019). Tiny fragments of acidophilous steppic grasslands serve as yet unknown habitats of endangered aeolian sand specialists among Aculeata (Hymenoptera). Biodivers. Conserv. 28, 183–195. doi: 10.1007/s10531-018-1646-3
Herrera C. M., Núñez A., Valverde J., Alonso C. (2023). Body mass decline in a Mediterranean community of solitary bees supports the size shrinking effect of climatic warming. Ecology 104, e4128. doi: 10.1002/ecy.4128
Herrick J. E., Schuman G. E., Rango A. (2006). Monitoring ecological processes for restoration projects. J. Nat. Conserv. 14, 161–171. doi: 10.1016/j.jnc.2006.05.001
Höfner J., Klein-Raufhake T., Lampei C., Mudrak O., Bucharova A., Durka W. (2022). Populations restored using regional seed are genetically diverse and similar to natural populations in the region. J. Appl. Ecol. 59, 2234–2244. doi: 10.1111/1365-2664.14067
Huddart J. E. A., Thompson M. S. A., Woodward G., Brooks S. J. (2016). Citizen science: from detecting pollution to evaluating ecological restoration. WIREs Water 3, 287–300. doi: 10.1002/wat2.1138
Hudson P. J., Dobson A. P., Lafferty K. D. (2006). Is a healthy ecosystem one that is rich in parasites? Trends Ecol. Evol. 21, 381–385. doi: 10.1016/j.tree.2006.04.007
Hulting K. A., Kemmerling L. R., Griffin S. R., Webb J., Brown A. K., Haddad N. M. (2024). Seed mix design and floral resources drive multitrophic interactions in prairie restoration. J. Appl. Ecol. 61, 859–868. doi: 10.1111/1365-2664.14605
Iles D. T., Williams N. M., Crone E. E. (2018). Source-sink dynamics of bumblebees in rapidly changing landscapes. J. Appl. Ecol. 55, 2802–2811. doi: 10.1111/1365-2664.13175
Image M., Gardner E., Clough Y., Smith H. G., Baldock K. C. R., Campbell A., et al. (2022). Does agri-environment scheme participation in England increase pollinator populations and crop pollination services? Agric. Ecosyst. Environ. 325, 107755. doi: 10.1016/j.agee.2021.107755
Jackson M. M., Turner M. G., Pearson S. M. (2014). Logging legacies affect insect pollinator communities in southern appalachian forests. Southeast. Nat. 13, 317–336. doi: 10.1656/058.013.0213
James D. G., Seymour L., Lauby G., Buckley K. (2014). Beneficial insects attracted to native flowering buckwheats (Eriogonum Michx) in central Washington. Environ. Entomol. 43, 942–948. doi: 10.1603/EN13342
James D. G., Seymour L., Lauby G., Buckley K. (2016). Beneficial Insect Attraction to Milkweeds (Asclepias speciosa, Asclepias fascicularis) in Washington State, USA. Insects 7, 30. doi: 10.3390/insects7030030
Jennersten O. (1988). Insect dispersal of fungal disease: effects of ustilago infection on pollinator attraction in Viscaria vulgaris. Oikos 51, 163–170. doi: 10.2307/3565638
Johnson R. C., Love S. L., Carver D., Irish B. M. (2023). Using climate-driven adaptive evolution to guide seed sourcing for restoration in a diverse North American herb-shrub species. Restor. Ecol. 31, e13856. doi: 10.1111/rec.13856
Kaiser-Bunbury C. N., Mougal J., Whittington A. E., Valentin T., Gabriel R., Olesen J. M., et al. (2017). Ecosystem restoration strengthens pollination network resilience and function. Nature 542, 223–227. doi: 10.1038/nature21071
Kammerer M., Goslee S. C., Douglas M. R., Tooker J. F., Grozinger C. M. (2021). Wild bees as winners and losers: Relative impacts of landscape composition, quality, and climate. Glob. Change Biol. 27, 1250–1265. doi: 10.1111/gcb.15485
Keeley J. E., Klinger R. C., Brennan T. J., Lawson D. M., La Grange J., Berg K. N. (2023). A decade-long study of repeated prescription burning in California native grassland restoration. Restor. Ecol. 31, e13939. doi: 10.1111/rec.13939
Keilsohn W., Narango D. L., Tallamy D. W. (2018). Roadside habitat impacts insect traffic mortality. J. Insect Conserv. 22, 183–188. doi: 10.1007/s10841-018-0051-2
Kelly T. T., Elle E. (2021). Investigating bee dietary preferences along a gradient of floral resources: how does resource use align with resource availability? Insect Sci. 28, 555–565. doi: 10.1111/1744-7917.12785
Kim J. (1999). Influence of resource level on maternal investment in a leaf-cutter bee (Hymenoptera: Megachilidae). Behav. Ecol. 10, 552–556. doi: 10.1093/beheco/10.5.552
Kimball S., Lulow M., Sorenson Q., Balazs K., Fang Y.-C., Davis S. J., et al. (2015). Cost-effective ecological restoration. Restor. Ecol. 23, 800–810. doi: 10.1111/rec.12261
Kline O., Joshi N. K. (2020). Mitigating the effects of habitat loss on solitary bees in agricultural ecosystems. Agriculture 10, 115. doi: 10.3390/agriculture10040115
Koh I., Lonsdorf E. V., Williams N. M., Brittain C., Isaacs R., Gibbs J., et al. (2016). Modeling the status, trends, and impacts of wild bee abundance in the United States. Proc. Natl. Acad. Sci. 113, 140–145. doi: 10.1073/pnas.1517685113
Kosmala M., Wiggins A., Swanson A., Simmons B. (2016). Assessing data quality in citizen science. Front. Ecol. Environ. 14, 551–560. doi: 10.1002/fee.1436
Kovács-Hostyánszki A., Piross I. S., Shebl M. A. (2022). Non-native plant species integrate well into plant-pollinator networks in a diverse man-made flowering plant community. Urban Ecosyst. 25, 1491–1502. doi: 10.1007/s11252-022-01242-7
Kral-O’Brien K. C., O’Brien P. L., Hovick T. J., Harmon J. P. (2021). Meta-analysis: higher plant richness supports higher pollinator richness across many land use types. Ann. Entomol. Soc Am. 114, 267–275. doi: 10.1093/aesa/saaa061
Kremen C., M’Gonigle L. K. (2015). EDITOR’S CHOICE: Small-scale restoration in intensive agricultural landscapes supports more specialized and less mobile pollinator species. J. Appl. Ecol. 52, 602–610. doi: 10.1111/1365-2664.12418
Kudo G. (2014). Vulnerability of phenological synchrony between plants and pollinators in an alpine ecosystem. Ecol. Res. 29, 571–581. doi: 10.1007/s11284-013-1108-z
Kudsk P., Mathiassen S. K. (2020). Pesticide regulation in the European Union and the glyphosate controversy. Weed Sci. 68, 214–222. doi: 10.1017/wsc.2019.59
Lane I. G., Portman Z. M., Herron-Sweet C. H., Pardee G. L., Cariveau D. P. (2022). Differences in bee community composition between restored and remnant prairies are more strongly linked to forb community differences than landscape differences. J. Appl. Ecol. 59, 129–140. doi: 10.1111/1365-2664.14035
Lázaro A., Tur C. (2018). Los cambios de uso del suelo como responsables del declive de polinizadores. Ecosistemas 27, 23–33. doi: 10.7818/ECOS.1378
LeBuhn G., Vargas Luna J. (2021). Pollinator decline: what do we know about the drivers of solitary bee declines? Curr. Opin. Insect Sci. 46, 106–111. doi: 10.1016/j.cois.2021.05.004
LeCroy K. A., Savoy-Burke G., Carr D. E., Delaney D. A., Roulston T. H. (2020). Decline of six native mason bee species following the arrival of an exotic congener. Sci. Rep. 10, 18745. doi: 10.1038/s41598-020-75566-9
Lehmann D. M., Camp A. A. (2021). A systematic scoping review of the methodological approaches and effects of pesticide exposure on solitary bees. PloS One 16, e0251197. doi: 10.1371/journal.pone.0251197
Lerman S. B., Contosta A. R., Milam J., Bang C. (2018). To mow or to mow less: Lawn mowing frequency affects bee abundance and diversity in suburban yards. Biol. Conserv. 221, 160–174. doi: 10.1016/j.biocon.2018.01.025
Lettow M. C., Brudvig L. A., Bahlai C. A., Gibbs J., Jean R. P., Landis D. A. (2018). Bee community responses to a gradient of oak savanna restoration practices. Restor. Ecol. 26, 882–890. doi: 10.1111/rec.12655
Leverkus A. B., Lázaro González A., Andivia E., Castro J., Jiménez M. N., Navarro F. B. (2021). Seeding or planting to revegetate the world’s degraded land: systematic review and experimentation to address methodological issues. Restor. Ecol. 29, e13372. doi: 10.1111/rec.13372
Liczner A. R., Colla S. R. (2019). A systematic review of the nesting and overwintering habitat of bumble bees globally. J. Insect Conserv. 23, 787–801. doi: 10.1007/s10841-019-00173-7
Liczner A. R., Colla S. R. (2020). One-size does not fit all: at-risk bumble bee habitat management requires species-specific local and landscape considerations. Insect Conserv. Divers. 13, 558–570. doi: 10.1111/icad.12419
Lima M. A. P., Cutler G. C., Mazzeo G., Hrncir M. (2022). Editorial: The decline of wild bees: Causes and consequences. Front. Ecol. Evol. 10. doi: 10.3389/fevo.2022.1027169
Lister A. M. (2011). Natural history collections as sources of long-term datasets. Trends Ecol. Evol. 26, 153–154. doi: 10.1016/j.tree.2010.12.009
Love B. G., Cane J. H. (2019). Mortality and flowering of great basin perennial forbs after experimental burning: implications for wild bees. Rangel. Ecol. Manage. 72, 310–317. doi: 10.1016/j.rama.2018.11.001
Luong J. C., Turner P. L., Phillipson C. N., Seltmann K. C. (2019). Local grassland restoration affects insect communities. Ecol. Entomol. 44, 471–479. doi: 10.1111/een.12721
Lybbert A. H., Cusser S. J., Hung K.-L. J., Goodell K. (2022). Ten-year trends reveal declining quality of seeded pollinator habitat on reclaimed mines regardless of seed mix diversity. Ecol. Appl. 32, e02467. doi: 10.1002/eap.2467
M’Gonigle L. K., Ponisio L. C., Cutler K., Kremen C. (2015). Habitat restoration promotes pollinator persistence and colonization in intensively managed agriculture. Ecol. Appl. 25, 1557–1565. doi: 10.1890/14-1863.1
M’Gonigle L. K., Williams N. M., Lonsdorf E., Kremen C. (2017). A tool for selecting plants when restoring habitat for pollinators. Conserv. Lett. 10, 105–111. doi: 10.1111/conl.12261
MacPhail V. J., Gibson S. D., Colla S. R. (2020). Community science participants gain environmental awareness and contribute high quality data but improvements are needed: insights from Bumble Bee Watch. PeerJ 8, e9141. doi: 10.7717/peerj.9141
MacTavish R., Anderson J. T. (2022). Water and nutrient availability exert selection on reproductive phenology. Am. J. Bot. 109, 1702–1716. doi: 10.1002/ajb2.16057
Maher S., Manco F., Ings T. C. (2019). Using citizen science to examine the nesting ecology of ground-nesting bees. Ecosphere. 10, e02911. doi: 10.1002/ecs2.2911
Mathiasson M. E., Rehan S. M. (2020). Wild bee declines linked to plant-pollinator network changes and plant species introductions. Insect Conserv. Divers. 13, 595–605. doi: 10.1111/icad.12429
McCormick M. L., Aslan C. E., Chaudhry T. A., Potter K. A. (2019). Benefits and limitations of isolated floral patches in a pollinator restoration project in Arizona. Restor. Ecol. 27, 1282–1290. doi: 10.1111/rec.12995
Meiners J. M., Griswold T. L., Harris D. J., Ernest S. K. M. (2017). Bees without flowers: before peak bloom, diverse native bees find insect-produced honeydew sugars. Am. Nat. 190, 281–291. doi: 10.1086/692437
Meldrum J. R., Larson D. L., Hoelzle T. B., Hinck J. E. (2023). Considering pollinators’ ecosystem services in the remediation and restoration of contaminated lands: Overview of research and its gaps. Integr. Environ. Assess. Manag. 20, 322–336. doi: 10.1002/ieam.4808
Menz M. H. M., Phillips R. D., Winfree R., Kremen C., Aizen M. A., Johnson S. D., et al. (2011). Reconnecting plants and pollinators: challenges in the restoration of pollination mutualisms. Trends Plant Sci. 16, 4–12. doi: 10.1016/j.tplants.2010.09.006
Michener W. K. (1997). Quantitatively evaluating restoration experiments: research design, statistical analysis, and data management considerations. Restor. Ecol. 5, 324–337. doi: 10.1046/j.1526-100X.1997.00546.x
Milam J. C., Litvaitis J. A., Warren A., Keirstead D., King D. I. (2018). Bee assemblages in managed early-successional habitats in Southeastern New Hampshire. Northeast. Nat. 25, 437–459. doi: 10.1656/045.025.0309
Miller B. P., Sinclair E. A., Menz M. H. M., Elliott C. P., Bunn E., Commander L. E., et al. (2017). A framework for the practical science necessary to restore sustainable, resilient, and biodiverse ecosystems. Restor. Ecol. 25, 605–617. doi: 10.1111/rec.12475
Mitchell M. G. E., Bennett E. M., Gonzalez A. (2013). Linking landscape connectivity and ecosystem service provision: current knowledge and research gaps. Ecosystems 16, 894–908. doi: 10.1007/s10021-013-9647-2
Mitchell S. R., DeBano S. J., Rowland M. M., Burrows S. (2022). Feed the bees and shade the streams: riparian shrubs planted for restoration provide forage for native bees. Restor. Ecol. 30, e13525. doi: 10.1111/rec.13525
Mola J. M., Hemberger J., Kochanski J., Richardson L. L., Pearse I. S. (2021a). The importance of forests in bumble bee biology and conservation. BioScience 71, 1234–1248. doi: 10.1093/biosci/biab121
Mola J. M., Miller M. R., O’Rourke S. M., Williams N. M. (2020). Wildfire reveals transient changes to individual traits and population responses of a native bumble bee Bombus vosnesenskii. J. Anim. Ecol. 89, 1799–1810. doi: 10.1111/1365-2656.13244
Mola J. M., Richardson L. L., Spyreas G., Zaya D. N., Pearse I. S. (2021b). Long-term surveys support declines in early season forest plants used by bumblebees. J. Appl. Ecol. 58, 1431–1441. doi: 10.1111/1365-2664.13886
Molinari N. A., D’Antonio C. M. (2020). Where have all the wildflowers gone? The role of exotic grass thatch. Biol. Invasions 22, 957–968. doi: 10.1007/s10530-019-02135-1
Monasterolo M., Poggio S. L., Medan D., Devoto M. (2020). Wider road verges sustain higher plant species richness and pollinator abundance in intensively managed agroecosystems. Agric. Ecosyst. Environ. 302, 107084. doi: 10.1016/j.agee.2020.107084
Montoya-Pfeiffer P. M., Rodrigues R. R., Alves dos Santos I. (2020). Bee pollinator functional responses and functional effects in restored tropical forests. Ecol. Appl. 30, e02054. doi: 10.1002/eap.2054
Moss E. D., Evans D. M. (2022). Experimental climate warming reduces floral resources and alters insect visitation and wildflower seed set in a cereal agro-ecosystem. Front. Plant Sci. 13. doi: 10.3389/fpls.2022.826205
Motta C. I., Luong J. C., Seltmann K. C. (2022). Plant–arthropod interactions of an endangered California lupine. Ecol. Evol. 12, e8688. doi: 10.1002/ece3.8688
Nawaz M. F., Bourrié G., Trolard F. (2013). Soil compaction impact and modelling. A review. Agron. Sustain. Dev. 33, 291–309. doi: 10.1007/s13593-011-0071-8
Neece J., Brokaw J., Coker A., Bruninga-Socolar B. (2023). Seeding density of wildflower mixes affects nectar production in a focal plant species. Restor. Ecol. 31, e13912. doi: 10.1111/rec.13912
Nevill P. G., Cross A. T., Dixon K. W. (2018). Ethical seed sourcing is a key issue in meeting global restoration targets. Curr. Biol. 28, R1378–R1379. doi: 10.1016/j.cub.2018.11.015
Nguyen P. N., Rehan S. M. (2023). Environmental effects on bee microbiota. Microb. Ecol. 86, 1487–1498. doi: 10.1007/s00248-023-02226-6
Niemuth N. D., Wangler B., LeBrun J. J., Dewald D., Larson S., Schwagler T., et al. (2021). Conservation planning for pollinators in the U.S. Great Plains: considerations of context, treatments, and scale. Ecosphere 12, e03556. doi: 10.1002/ecs2.3556
Nilsson C., Aradottir A. L., Hagen D., Halldórsson G., Høegh K., Mitchell R. J., et al. (2016). Evaluating the process of ecological restoration. Ecol. Soc. 21, 41. doi: 10.5751/ES-08289-210141
Novotny J. L., Goodell K. (2020). Rapid recovery of plant–pollinator interactions on a chronosequence of grassland-reclaimed mines. J. Insect Conserv. 24, 977–991. doi: 10.1007/s10841-020-00268-6
Obrist M. K., Duelli P. (2010). Rapid biodiversity assessment of arthropods for monitoring average local species richness and related ecosystem services. Biodivers. Conserv. 19, 2201–2220. doi: 10.1007/s10531-010-9832-y
Öckinger E., Winsa M., Roberts S. P. M., Bommarco R. (2018). Mobility and resource use influence the occurrence of pollinating insects in restored seminatural grassland fragments. Restor. Ecol. 26, 873–881. doi: 10.1111/rec.12646
Odanaka K., Gibbs J., Turley N. E., Isaacs R., Brudvig L. A. (2020). Canopy thinning, not agricultural history, determines early responses of wild bees to longleaf pine savanna restoration. Restor. Ecol. 28, 138–146. doi: 10.1111/rec.13043
Ogilvie J. E., Forrest J. R. (2017). Interactions between bee foraging and floral resource phenology shape bee populations and communities. Curr. Opin. Insect Sci. 21, 75–82. doi: 10.1016/j.cois.2017.05.015
Oliveira C. D. C., de Durigan G., Putz F. E. (2021). Thinning temporarily stimulates tree regeneration in a restored tropical forest. Ecol. Eng. 171, 106390. doi: 10.1016/j.ecoleng.2021.106390
Oliver T. H., Smithers R. J., Beale C. M., Watts K. (2016). Are existing biodiversity conservation strategies appropriate in a changing climate? Biol. Conserv. 193, 17–26. doi: 10.1016/j.biocon.2015.10.024
Ollerton J., Winfree R., Tarrant S. (2011). How many flowering plants are pollinated by animals? Oikos 120, 321–326. doi: 10.1111/j.1600-0706.2010.18644.x
Olynyk M., Westwood A. R., Koper N. (2021). Effects of natural habitat loss and edge effects on wild bees and pollination services in remnant prairies. Environ. Entomol. 50, 732–743. doi: 10.1093/ee/nvaa186
Onuferko T. M., Skandalis D. A., León Cordero R., Richards M. H. (2018). Rapid initial recovery and long-term persistence of a bee community in a former landfill. Insect Conserv. Divers. 11, 88–99. doi: 10.1111/icad.12261
Orr M. C., Hung K.-L. J., Wilson-Rankin E. E., Simpson P. M., Yanega D., Kim A. Y., et al. (2023). Scientific note: First mainland records of an unusual island bee (Anthophora urbana clementina) highlight the value of community science for adventive species detection and monitoring. Apidologie 54, 46. doi: 10.1007/s13592-023-01025-9
Orr M. C., Jakob M., Harmon-Threatt A., Mupepele A.-C. (2022). A review of global trends in the study types used to investigate bee nesting biology. Basic Appl. Ecol. 62, 12–21. doi: 10.1016/j.baae.2022.03.012
Osborne J. L., Martin A. P., Carreck N. L., Swain J. L., Knight M. E., Goulson D., et al. (2008). Bumblebee flight distances in relation to the forage landscape. J. Anim. Ecol. 77, 406–415. doi: 10.1111/j.1365-2656.2007.01333.x
Page M. L., Ison J. L., Bewley A. L., Holsinger K. M., Kaul A. D., Koch K. E., et al. (2019). Pollinator effectiveness in a composite: a specialist bee pollinates more florets but does not move pollen farther than other visitors. Am. J. Bot. 106, 1487–1498. doi: 10.1002/ajb2.1383
Parra-Tabla V., Arceo-Gómez G. (2021). Impacts of plant invasions in native plant–pollinator networks. New Phytol. 230, 2117–2128. doi: 10.1111/nph.17339
Pei C. K., Hovick T. J., Limb R. F., Harmon J. P., Geaumont B. A. (2023). Invasive grass and litter accumulation constrain bee and plant diversity in altered grasslands. Glob. Ecol. Conserv. 41, e02352. doi: 10.1016/j.gecco.2022.e02352
Perino A., Pereira H. M., Navarro L. M., Fernández N., Bullock J. M., Ceauşu S., et al. (2019). Rewilding complex ecosystems. Science 364, eaav5570. doi: 10.1126/science.aav5570
Peterson P. G., Merrett M. F., Fowler S. V., Barrett D. P., Paynter Q. (2020). Comparing biocontrol and herbicide for managing an invasive non-native plant species: Efficacy, non-target effects and secondary invasion. J. Appl. Ecol. 57, 1876–1884. doi: 10.1111/1365-2664.13691
Peterson D. W., Reich P. B. (2008). Fire frequency and tree canopy structure influence plant species diversity in a forest-grassland ecotone. Plant Ecol. 194, 5–16. doi: 10.1007/s11258-007-9270-4
Phillips B. B., Gaston K. J., Bullock J. M., Osborne J. L. (2019). Road verges support pollinators in agricultural landscapes, but are diminished by heavy traffic and summer cutting. J. Appl. Ecol. 56, 2316–2327. doi: 10.1111/1365-2664.13470
Phillips B. B., Wallace C., Roberts B. R., Whitehouse A. T., Gaston K. J., Bullock J. M., et al. (2020). Enhancing road verges to aid pollinator conservation: A review. Biol. Conserv. 250, 108687. doi: 10.1016/j.biocon.2020.108687
Plentovich S., Graham J. R., Haines W. P., King C. B. A. (2021). Invasive ants reduce nesting success of an endangered Hawaiian yellow-faced bee, Hylaeus anthracinus. NeoBiota 64, 137–154. doi: 10.3897/neobiota.64.58670
Ponisio L. C., Wilkin K., M’Gonigle L. K., Kulhanek K., Cook L., Thorp R., et al. (2016). Pyrodiversity begets plant–pollinator community diversity. Glob. Change Biol. 22, 1794–1808. doi: 10.1111/gcb.13236
Portman Z. M., Bruninga-Socolar B., Cariveau D. P. (2020). The state of bee monitoring in the United States: A call to refocus away from bowl traps and towards more effective methods. Ann. Entomol. Soc Am. 113, 337–342. doi: 10.1093/aesa/saaa010
Potts S., Willmer P. (1997). Abiotic and biotic factors influencing nest-site selection by Halictus rubicundus, a ground-nesting halictine bee. Ecol. Entomol. 22, 319–328. doi: 10.1046/j.1365-2311.1997.00071.x
Prach K., Durigan G., Fennessy S., Overbeck G. E., Torezan J. M., Murphy S. D. (2019). A primer on choosing goals and indicators to evaluate ecological restoration success. Restor. Ecol. 27, 917–923. doi: 10.1111/rec.13011
Prendergast K. S., Menz M. H. M., Dixon K. W., Bateman P. W. (2020). The relative performance of sampling methods for native bees: an empirical test and review of the literature. Ecosphere 11, e03076. doi: 10.1002/ecs2.3076
Purvis E. E. N., Best L. R., Galpern P. (2021). Identifying key forage plants to support wild bee diversity and a species at risk in the Prairie Pothole Region. Insect Conserv. Divers. 14, 851–861. doi: 10.1111/icad.12524
Purvis E. E. N., Vickruck J. L., Best L. R., Devries J. H., Galpern P. (2020). Wild bee community recovery in restored grassland-wetland complexes of prairie North America. Biol. Conserv. 252, 108829. doi: 10.1016/j.biocon.2020.108829
Quigley T. P., Amdam G. V., Harwood G. H. (2019). Honey bees as bioindicators of changing global agricultural landscapes. Curr. Opin. Insect Sci. 35, 132–137. doi: 10.1016/j.cois.2019.08.012
Raiol R. L., Gastauer M., Campbell A. J., Borges R. C., Awade M., Giannini T. C. (2021). Specialist bee species are larger and less phylogenetically distinct than generalists in tropical plant–bee interaction networks. Front. Ecol. Evol. 9. doi: 10.3389/fevo.2021.699649
Rappa N. J., Staab M., Ruppert L.-S., Frey J., Bauhus J., Klein A.-M. (2023). Structural elements enhanced by retention forestry promote forest and non-forest specialist bees and wasps. For. Ecol. Manage. 529, 120709. doi: 10.1016/j.foreco.2022.120709
Řehounková K., Čížek L., Řehounek J., Šebelíková L., Tropek R., Lencová K., et al. (2016). Additional disturbances as a beneficial tool for restoration of post-mining sites: a multi-taxa approach. Environ. Sci. pollut. Res. 23, 13745–13753. doi: 10.1007/s11356-016-6585-5
Requier F., Leonhardt S. D. (2020). Beyond flowers: including non-floral resources in bee conservation schemes. J. Insect Conserv. 24, 5–16. doi: 10.1007/s10841-019-00206-1
Ritchie M., Berrill J.-P. (2020). “Precommercial thinning in California forests,” in Reforestation Practices for Conifers in California. University of California Agriculture and Natural Resources., 471–524.
Rivers J. W., Mathis C. L., Moldenke A. R., Betts M. G. (2018). Wild bee diversity is enhanced by experimental removal of timber harvest residue within intensively managed conifer forest. GCB Bioenergy 10, 766–781. doi: 10.1111/gcbb.12531
Rodríguez A., Kouki J. (2017). Disturbance-mediated heterogeneity drives pollinator diversity in boreal managed forest ecosystems. Ecol. Appl. 27, 589–602. doi: 10.1002/eap.1468
Rowe L., Gibson D., Landis D., Gibbs J., Isaacs R. (2018). A comparison of drought-tolerant prairie plants to support managed and wild bees in conservation programs. Environ. Entomol. 47, 1128–1142. doi: 10.1093/ee/nvy091
Rubio A., Wright K., Longing S. (2022). Bee and flowering plant communities in a riparian corridor of the lower Rio Grande River (Texas, USA). Environ. Entomol. 51, 229–239. doi: 10.1093/ee/nvab108
Russo L., DeBarros N., Yang S., Shea K., Mortensen D. (2013). Supporting crop pollinators with floral resources: network-based phenological matching. Ecol. Evol. 3, 3125–3140. doi: 10.1002/ece3.703
Rutgers-Kelly A. C., Richards M. H. (2013). Effect of meadow regeneration on bee (Hymenoptera: Apoidea) abundance and diversity in southern Ontario, Canada. Can. Entomol. 145, 655–667. doi: 10.4039/tce.2013.42
Sánchez-Bayo F., Wyckhuys K. A. G. (2019). Worldwide decline of the entomofauna: A review of its drivers. Biol. Conserv. 232, 8–27. doi: 10.1016/j.biocon.2019.01.020
Sanguinetti A., Singer R. B. (2014). Invasive bees promote high reproductive success in Andean orchids. Biol. Conserv. 175, 10–20. doi: 10.1016/j.biocon.2014.04.011
Sardiñas H. S., Kremen C. (2014). Evaluating nesting microhabitat for ground-nesting bees using emergence traps. Basic Appl. Ecol. 15, 161–168. doi: 10.1016/j.baae.2014.02.004
Sardiñas H. S., Ponisio L. C., Kremen C. (2016). Hedgerow presence does not enhance indicators of nest-site habitat quality or nesting rates of ground-nesting bees. Restor. Ecol. 24, 499–505. doi: 10.1111/rec.12338
Schäffer B., Stauber M., Müller R., Schulin R. (2007). Changes in the macro-pore structure of restored soil caused by compaction beneath heavy agricultural machinery: a morphometric study. Eur. J. Soil Sci. 58, 1062–1073. doi: 10.1111/j.1365-2389.2007.00886.x
Schenk M., Krauss J., Holzschuh A. (2018). Desynchronizations in bee-plant interactions cause severe fitness losses in solitary bees. J. Anim. Ecol. 87, 139–149. doi: 10.1111/1365-2656.12694
Scheper J., Holzschuh A., Kuussaari M., Potts S. G., Rundlöf M., Smith H. G., et al. (2013). Environmental factors driving the effectiveness of European agri-environmental measures in mitigating pollinator loss – a meta-analysis. Ecol. Lett. 16, 912–920. doi: 10.1111/ele.12128
Schmolke A., Galic N., Feken M., Thompson H., Sgolastra F., Pitts-Singer T., et al. (2021). Assessment of the vulnerability to pesticide exposures across bee species. Environ. Toxicol. Chem. 40, 2640–2651. doi: 10.1002/etc.5150
Seidelmann K., Ulbrich K., Mielenz N. (2010). Conditional sex allocation in the Red Mason bee, Osmia rufa. Behav. Ecol. Sociobiol. 64, 337–347. doi: 10.1007/s00265-009-0850-2
Seitz N., VanEngelsdorp D., Leonhardt S. (2019). Conserving bees in destroyed landscapes: The potentials of reclaimed sand mines. Glob. Ecol. Conserv. 19, e00642. doi: 10.1016/j.gecco.2019.e00642
Seltmann K. C., Global Biotic Interaction Community (2022). Data from: Global Bee Interaction Data. Zenodo. doi: 10.5281/zenodo.7315159
Serret H., Andersen D., Deguines N., Clauzel C., Park W.-H., Jang Y. (2022). Towards ecological management and sustainable urban planning in Seoul, South Korea: mapping wild pollinator habitat preferences and corridors using citizen science data. Animals 12, 1469. doi: 10.3390/ani12111469
Severns P. M., Moldenke A. R. (2010). Management tradeoffs between focal species and biodiversity: endemic plant conservation and solitary bee extinction. Biodivers. Conserv. 19, 3605–3609. doi: 10.1007/s10531-010-9897-7
Sexton A. N., Benton S., Browning A. C., Emery S. M. (2021). Reproductive patterns of solitary cavity-nesting bees responsive to both local and landscape factors. Urban Ecosyst. 24, 1271–1280. doi: 10.1007/s11252-021-01116-4
Sexton A. N., Emery S. M. (2020). Grassland restorations improve pollinator communities: a meta-analysis. J. Insect Conserv. 24, 719–726. doi: 10.1007/s10841-020-00247-x
Sgolastra F., Hinarejos S., Pitts-Singer T. L., Boyle N. K., Joseph T., Lūckmann J., et al. (2019). Pesticide exposure assessment paradigm for solitary bees. Environ. Entomol. 48, 22–35. doi: 10.1093/ee/nvy105
Shanahan M., Spivak M. (2021). Resin use by stingless bees: A review. Insects 12, 719. doi: 10.3390/insects12080719
Simanonok M. P., Burkle L. A. (2020). High-severity wildfire limits available floral pollen quality and bumble bee nutrition compared to mixed-severity burns. Oecologia 192, 489–499. doi: 10.1007/s00442-019-04577-9
Simanonok S. C., Otto C. R. V., Iovanna R. (2022). Forbs included in conservation seed mixes exhibit variable blooming detection rates and cost-effectiveness: implications for pollinator habitat design. Restor. Ecol. 30, e13657. doi: 10.1111/rec.13657
Simmons S. A., Bossart J. L. (2020). Apparent resilience to fire of native bee (Hymenoptera: apoidea) communities from upland longleaf pine forests in Louisiana and Mississippi. Southeast. Nat. 19, 567–581. doi: 10.1656/058.019.0316
Singh S., Kumar V., Dhanjal D. S., Singh J. (2020). “Biological control agents: diversity, ecological significances, and biotechnological applications,” in Natural Bioactive Products in Sustainable Agriculture. Eds. Singh J., Yadav A. N. (Springer, Singapore), 31–44. doi: 10.1007/978-981-15-3024-1_3
Sitters H., Di Stefano J., Christie F., Swan M., York A. (2016). Bird functional diversity decreases with time since disturbance: Does patchy prescribed fire enhance ecosystem function? Ecol. Appl. 26, 115–127. doi: 10.1890/14-1562
Slominski A. H., Burkle L. A. (2021). Asynchrony between solitary bee emergence and flower availability reduces flower visitation rate and may affect offspring size. Basic Appl. Ecol. 56, 345–357. doi: 10.1016/j.baae.2021.08.003
Smith A. L., Barrett R. L., Milner R. N. C. (2018). Annual mowing maintains plant diversity in threatened temperate grasslands. Appl. Veg. Sci. 21, 207–218. doi: 10.1111/avsc.12365
Smith DiCarlo L. A., DeBano S. J., Burrows S. (2019). Short-term response of two beneficial invertebrate groups to wildfire in an Arid Grassland System, United States. Rangel. Ecol. Manage. 72, 551–560. doi: 10.1016/j.rama.2018.11.011
Smith DiCarlo L. A., DeBano S. J., Burrows S. (2020). Arid grassland bee communities: associated environmental variables and responses to restoration. Restor. Ecol. 28, A54–A64. doi: 10.1111/rec.13074
Society for Ecological Restoration (2004). SER international primer on ecological restoration. Available online at: https://www.ser.org/resource/resmgr/custompages/publications/SER_Primer/ser_primer.pdf.
Soroye P., Newbold T., Kerr J. (2020). Climate change contributes to widespread declines among bumble bees across continents. Science 367, 685–688. doi: 10.1126/science.aax8591
Spiesman B. J., Gratton C., Hatfield R. G., Hsu W. H., Jepsen S., McCornack B., et al. (2021). Assessing the potential for deep learning and computer vision to identify bumble bee species from images. Sci. Rep. 11, 7580. doi: 10.1038/s41598-021-87210-1
Staab M., Pereira-Peixoto M. H., Klein A.-M. (2020). Exotic garden plants partly substitute for native plants as resources for pollinators when native plants become seasonally scarce. Oecologia 194, 465–480. doi: 10.1007/s00442-020-04785-8
Stein D. S., Debinski D. M., Pleasants J. M., Toth A. L. (2020). Evaluating native bee communities and nutrition in managed grasslands. Environ. Entomol. 49, 717–725. doi: 10.1093/ee/nvaa009
Stephenson P. L., Dowling A. P. G., Krementz D. G. (2020). Bee communities of emergent wetlands under restoration in the lower Mississippi Alluvial Valley of Arkansas. Southeast. Nat. 19, 472–490. doi: 10.1656/058.019.0303
Straub L., Williams G. R., Pettis J., Fries I., Neumann P. (2015). Superorganism resilience: eusociality and susceptibility of ecosystem service providing insects to stressors. Curr. Opin. Insect Sci. 12, 109–112. doi: 10.1016/j.cois.2015.10.010
Straw E. A., Carpentier E. N., Brown M. J. F. (2021). Roundup causes high levels of mortality following contact exposure in bumble bees. J. Appl. Ecol. 58, 1167–1176. doi: 10.1111/1365-2664.13867
Sydenham M. A. K., Eldegard K., Totland Ø. (2014). Spatio-temporal variation in species assemblages in field edges: seasonally distinct responses of solitary bees to local habitat characteristics and landscape conditions. Biodivers. Conserv. 23, 2393–2414. doi: 10.1007/s10531-014-0729-z
Tallamy D. W. (2020). Nature’s Best Hope: A New Approach to Conservation that Starts in Your Yard. (Oregon: Timber Press).
Tallamy D. W., Narango D. L., Mitchell A. B. (2021). Do non-native plants contribute to insect declines? Ecol. Entomol. 46, 729–742. doi: 10.1111/een.12973
Tälle M., Deák B., Poschlod P., Valkó O., Westerberg L., Milberg P. (2016). Grazing vs. mowing: A meta-analysis of biodiversity benefits for grassland management. Agric. Ecosyst. Environ. 222, 200–212. doi: 10.1016/j.agee.2016.02.008
Tang J., Nolan M., D’Antonio C., Cooper S. D., Stratton L. (2023). Reinvasion of restored California vernal pools reveals the importance of long-term restoration planning. Restor. Ecol. 31, e13991. doi: 10.1111/rec.13991
Tepedino V. J., Portman Z. M. (2021). Intensive monitoring for bees in North America: indispensable or improvident? Insect Conserv. Divers. 14, 535–542. doi: 10.1111/icad.12509
Tetlie J., Harmon-Threatt A. (2024). Neonicotinoid contamination in conservation areas affects bees more sharply than beetles. Front. Ecol. Evol. 12. doi: 10.3389/fevo.2024.1347526
Thapa-Magar K. B., Davis T. S., Galbraith S. M., Grant-Hoffman M. (2023). Effects of sage-grouse habitat restoration efforts on pollination networks in an arid ecosystem. Rangel. Ecol. Manage. 91, 95–104. doi: 10.1016/j.rama.2023.08.005
Thomas E., Jalonen R., Loo J., Boshier D., Gallo L., Cavers S., et al. (2014). Genetic considerations in ecosystem restoration using native tree species. For. Ecol. Manage. 333, 66–75. doi: 10.1016/j.foreco.2014.07.015
Tilley D., Taliga C., Burns C., John L. S. (2013). Plant materials for pollinators and other beneficial insects in eastern Utah and western Colorado. Tech. Note 2C. Boise, ID: U.S. Department of Agriculture, Natural Resources Conservation Service. 54 p.
Tobin K. B., Mandes R., Martinez A., Sadd B. M. (2024). A simulated natural heatwave perturbs bumblebee immunity and resistance to infection. J. Anim. Ecol. 93, 171–182. doi: 10.1111/1365-2656.14041
Tonietto R. K., Ascher J. S., Larkin D. J. (2017). Bee communities along a prairie restoration chronosequence: similar abundance and diversity, distinct composition. Ecol. Appl. 27, 705–717. doi: 10.1002/eap.1481
Tonietto R. K., Larkin D. J. (2018). Habitat restoration benefits wild bees: A meta-analysis. J. Appl. Ecol. 55, 582–590. doi: 10.1111/1365-2664.13012
Tsiolis K., Potts S., Garratt M., Tilston E., Burman J., Rintoul-Hynes N., et al. (2022). The importance of soil and vegetation characteristics for establishing ground-nesting bee aggregations. J. Pollinat. Ecol. 32, 186–200. doi: 10.26786/1920-7603(2022)682
Ulyshen M. D., Wilson A. C., Ohlson G. C., Pokswinksi S. M., Hiers J. K. (2021). Frequent prescribed fires favour ground‐nesting bees in southeastern U.S. forests. Insect Conserv. Divers. 14, 527–534. doi: 10.1111/icad.12484
Ulyshen M. D., Horn S., Hanula J. L. (2020). Effects of Chinese privet on bees and their vertical distribution in Riparian forests. For. Sci. 66, 416–423. doi: 10.1093/forsci/fxz088
Ulyshen M. D., Wilson A. C., Ohlson G. C., Pokswinksi S. M., Hiers J. K. (2021). Frequent prescribed fires favour ground-nesting bees in southeastern U.S. forests. Insect Conserv. Divers. doi: 10.1111/icad.12484
van der Heyde M., Bunce M., Dixon K. W., Fernandes K., Majer J., Wardell-Johnson G., et al. (2022). Evaluating restoration trajectories using DNA metabarcoding of ground-dwelling and airborne invertebrates and associated plant communities. Mol. Ecol. 31, 2172–2188. doi: 10.1111/mec.16375
Vaudo A. D., Patch H. M., Mortensen D. A., Grozinger C. M., Tooker J. F. (2014). Bumble bees exhibit daily behavioral patterns in pollen foraging. Arthropod-Plant Interact. 8, 273–283. doi: 10.1007/s11829-014-9312-5
Vaudo A. D., Tooker J. F., Grozinger C. M., Patch H. M. (2015). Bee nutrition and floral resource restoration. Curr. Opin. Insect Sci. 10, 133–141. doi: 10.1016/j.cois.2015.05.008
Vaudo A. D., Tooker J. F., Patch H. M., Biddinger D. J., Coccia M., Crone M. K., et al. (2020). Pollen protein: lipid macronutrient ratios may guide broad patterns of bee species floral preferences. Insects 11, 132. doi: 10.3390/insects11020132
Vaughan M., Black S. H. (2008). Native pollinators: how to protect and enhance habitat for native bees. Native Plants J. 9, 80–91. doi: 10.2979/NPJ.2008.9.2.80
Vilen L., Mason L., Viders S., Zarestky J. (2023). Community science online: building capacity for native bee monitoring. J. Hum. Sci. Ext. 11, 12. doi: 10.55533/2325-5226.1353
Vitale N., Gonzalez V. H., Vázquez D. P. (2017). Nesting ecology of sympatric species of wool carder bees (Hymenoptera: Megachilidae: Anthidium) in South America. J. Apic. Res. 56, 497–509. doi: 10.1080/00218839.2017.1370902
Wagner D. L., Grames E. M., Forister M. L., Berenbaum M. R., Stopak D. (2021). Insect decline in the Anthropocene: Death by a thousand cuts. Proc. Natl. Acad. Sci. 118, e2023989118. doi: 10.1073/pnas.2023989118
Wagner V., Nelson C. R. (2014). Herbicides can negatively affect seed performance in native plants. Restor. Ecol. 22, 288–291. doi: 10.1111/rec.12089
Walston L. J., Hartmann H. M., Fox L., Macknick J., McCall J., Janski J., et al. (2023). If you build it, will they come? Insect community responses to habitat establishment at solar energy facilities in Minnesota, USA. Environ. Res. Lett. 19, 014053. doi: 10.1088/1748-9326/ad0f72
Weekers T., Marshall L., Leclercq N., Wood T. J., Cejas D., Drepper B., et al. (2022). Dominance of honey bees is negatively associated with wild bee diversity in commercial apple orchards regardless of management practices. Agric. Ecosyst. Environ. 323, 107697. doi: 10.1016/j.agee.2021.107697
Weidlich E. W. A., Flórido F. G., Sorrini T. B., Brancalion P. H. S. (2020). Controlling invasive plant species in ecological restoration: A global review. J. Appl. Ecol. 57, 1806–1817. doi: 10.1111/1365-2664.13656
Westreich L. R., Westreich S. T., Tobin P. C. (2023). Bacterial and fungal symbionts in pollen provisions of a native solitary bee in urban and rural environments. Microb. Ecol. 86, 1416–1427. doi: 10.1007/s00248-022-02164-9
Williams N. M. (2011). Restoration of nontarget species: bee communities and pollination function in Riparian forests. Restor. Ecol. 19, 450–459. doi: 10.1111/j.1526-100X.2010.00707.x
Williams N. M., Lonsdorf E. V. (2018). Selecting cost-effective plant mixes to support pollinators. Biol. Conserv. 217, 195–202. doi: 10.1016/j.biocon.2017.10.032
Williams N. M., Mola J. M., Stuligross C., Harrison T., Page M. L., Brennan R. M., et al. (2019). Fantastic bees and where to find them: locating the cryptic overwintering queens of a western bumble bee. Ecosphere 10, e02949. doi: 10.1002/ecs2.2949
Willis Chan D. S., Prosser R. S., Rodríguez-Gil J. L., Raine N. E. (2019). Assessment of risk to hoary squash bees (Peponapis pruinosa) and other ground-nesting bees from systemic insecticides in agricultural soil. Sci. Rep. 9, 11870. doi: 10.1038/s41598-019-47805-1
Winfree R. (2010). The conservation and restoration of wild bees. Ann. N. Y. Acad. Sci. 1195, 169–197. doi: 10.1111/j.1749-6632.2010.05449.x
Winsa M., Öckinger E., Bommarco R., Lindborg R., Roberts S. P. M., Wärnsberg J., et al. (2017). Sustained functional composition of pollinators in restored pastures despite slow functional restoration of plants. Ecol. Evol. 7, 3836–3846. doi: 10.1002/ece3.2924
Wojcik V., Smith L., Carromero W., Adams L. D., Davis S., DeBano S. J., et al. (2018). New research and BMPs in natural areas: A synthesis of the pollinator management symposium from the 44th natural areas conference, October 2017. Nat. Areas J. 38, 334–346. doi: 10.3375/043.038.0503
Wood T. J., Müller A., Praz C., Michez D. (2023). Elevated rates of dietary generalization in eusocial lineages of the secondarily herbivorous bees. BMC Ecol. Evol. 23, 67. doi: 10.1186/s12862-023-02175-1
Woodard S. H., Federman S., James R. R., Danforth B. N., Griswold T. L., Inouye D., et al. (2020). Towards a U.S. national program for monitoring native bees. Biol. Conserv. 252, 108821. doi: 10.1016/j.biocon.2020.108821
Woodcock B. A., Savage J., Bullock J. M., Nowakowski M., Orr R., Tallowin J. R. B., et al. (2014). Enhancing floral resources for pollinators in productive agricultural grasslands. Biol. Conserv. 171, 44–51. doi: 10.1016/j.biocon.2014.01.023
Wratten S. D., Gillespie M., Decourtye A., Mader E., Desneux N. (2012). Pollinator habitat enhancement: Benefits to other ecosystem services. Agric. Ecosyst. Environ. 159, 112–122. doi: 10.1016/j.agee.2012.06.020
Keywords: bee habitat restoration, pollination services, ground-nesting bees, floral resource availability, native bee monitoring
Citation: Payne HE, Mazer SJ and Seltmann KC (2024) Native bee habitat restoration: key ecological considerations from recent North American literature. Front. Ecol. Evol. 12:1358621. doi: 10.3389/fevo.2024.1358621
Received: 20 December 2023; Accepted: 17 July 2024;
Published: 05 August 2024.
Edited by:
Michael D. Ulyshen, United States Department of Agriculture, United StatesReviewed by:
Will Glenny, German Centre for Integrative Biodiversity Research (iDiv), GermanyEmilee Poole, United States Department of Agriculture, United States
Copyright © 2024 Payne, Mazer and Seltmann. This is an open-access article distributed under the terms of the Creative Commons Attribution License (CC BY). The use, distribution or reproduction in other forums is permitted, provided the original author(s) and the copyright owner(s) are credited and that the original publication in this journal is cited, in accordance with accepted academic practice. No use, distribution or reproduction is permitted which does not comply with these terms.
*Correspondence: Helen E. Payne, helenpayne@ucsb.edu