- 1Department of Biology, University of Texas Rio Grande Valley, Brownsville, TX, United States
- 2School of Earth, Environmental and Marine Sciences, University of Texas Rio Grande Valley, Brownsville, TX, United States
- 3Department of Environmental Affairs, Texas Department of Transportation, Austin, TX, United States
Movement is a key component of survival and reproduction, often causing wildlife to cross heavily trafficked highways, resulting in road mortalities by oncoming vehicles. Fencing and crossing structures are commonly regarded as effective mitigation structures to reduce these mortalities. In south Texas, ten wildlife exits (WE) were installed along State Highway 100 in conjunction with existing mitigation structures to provide the US endangered ocelot (Leopardus pardalis), a medium-sized spotted wild cat, a safe option to escape the right of way (ROW). The objectives of this study were to determine the effectiveness and species usage and to estimate the percentage of wildlife that crossed back into the habitat via a WE. Camera traps were used for monitoring with one on the roadside and one on the habitat side of each WE and ten at adjacent right-of-way (ROW) sites. Entry and exit rates through WE were calculated to determine where wildlife was entering and exiting the roadway. The total number of individuals for each target species was counted for all entries (H-R) and exits (R-H) at any mitigation structure within 200 m of an exit and was compared to those using a WE. Results showed that ten species – jackrabbit (Lepus californicus), bobcat (Lynx rufus), coyote (Canis latrans), domestic cat (Felis catus), cottontail (Sylvilagus floridanus), skunk (Mephitis mephitis), raccoon (Procyon lotor), opossum (Didelphis virginiana), armadillo (Dasypus novemcinctus), and weasel (Mustela frenata) – used a WE to return to the habitat. Coyote and bobcat usage at WE increased over time, with bobcats first exhibiting usage within 30 days while coyotes first used WE at 180 days. PERMANOVA showed significantly different assemblages of nine target species between the habitat side and all other groups along the roadside. The species assemblage using WE to escape the roadway was also significantly different from those using the WE to enter the roadway. Approximately 43% of bobcats, a surrogate species for the ocelot, used a WE to escape the ROW. Information on the effectiveness of these novel structures will be useful in the development of future WE to optimize placement and design.
1 Introduction
Roads and highways have significant impacts on wildlife. As humans expand and develop wild areas, interactions with wildlife are becoming more frequent, inhibiting wildlife viability along busy highways (Kindall and Manen, 2007; Grilo et al., 2008; Soulsbury and White, 2015). Roadways comprise an estimated network of 10.3 million km stretched across the United States (U.S. Department of Transportation, 2018), presenting a variety of threats to wildlife populations through habitat fragmentation and a loss of suitable habitat.
Roads and highways also influence wildlife negatively in a variety of ways, often causing habitat fragmentation, noise disruptions, loss of suitable habitat, and wildlife road mortalities (Forman and Alexander, 1998; Cain et al., 2003). Vegetation maintenance that is associated with many of these road structures can create barriers that may affect species differently (D'Amico et al., 2015; Andis et al., 2017). This combination of interrupted habitat and wildlife dispersal across roadways often results in road mortalities from wildlife–vehicle collisions (WVCs) (Grilo et al., 2008; Sawaya et al., 2014). Although wildlife–vehicle mortalities do not affect the population viability for more abundant species, they can greatly impact threatened or endangered species with small population sizes and low reproductive rates (Glista et al., 2008; Gilhooly et al., 2019), such as the US endangered ocelot (Leopardis pardalis). The consequences of a lack of landscape connectivity in south Texas have led to decreases in ocelot population size, a loss of genetic diversity via inbreeding (Janečka et al., 2007), and an increase in road mortalities (Haines et al., 2005a; Ascensão et al., 2013).
Over the past few decades, studies have shown that fencing, wildlife crossing structures (WCS), and other mitigation structures along highways are potential solutions to improving habitat connectivity and acting as a filter to reduce road permeability that typically results in WVCs (Cain et al., 2003), with varying levels of success. Wildlife crossing structures are meant to provide wildlife that approach a highway with a form of safe passage across it, limiting the overall number of WVCs that occur (Andis et al., 2017). Simpson et al. (2016) investigated WCS effectiveness for ungulates and found that underpasses and overpasses served as a successful means of connectivity for deer. The use of WCS by carnivores has also been documented where Florida panthers, bobcats, and black bears used WCS to travel across highways that separated their home ranges (Foster and Humphrey, 1995).
Other forms of mitigation structures such as wildlife guards (WG), fencing, and wildlife exits (WE) such as jump-outs modify the movements of wildlife of varying sizes and with different patterns of behavior. Wildlife guards, fencing, and jump-outs have all demonstrated success in reducing wildlife road mortalities and providing habitat connectivity for wildlife (Clevenger and Waltho, 2000; McCollister and Van Manen, 2010; Gagnon et al., 2011). For example, fencing in Europe was found to be effective at mitigating the effects of roads on stone martens (Ascensão et al., 2013), suggesting that even partial fencing may aid in the reduction in road mortalities. For larger mammals such as deer and elk, WE have been constructed to aid wildlife in leaving the area between the road and continuous fencing that separates the road from the habitat called the right of way (ROW). This mitigation exists in the form of earthen jump-outs (Bissonette and Hammer, 2000), escape ramps, and other one-way gates to create areas where wildlife can escape the ROW (Jackson and Griffin, 2000). VerCauteren et al. (2009) found that WE such as guards and bump gates for white-tailed deer prevented entry into fenced areas with mixed results. While bump gates demonstrated some success, deer-resistant guards were less effective and were not expected to provide long-term deterrence of deer (VerCauteren et al., 2009). Specifications are needed for these types of WE for roadside use, as gates and escapes do not take ungulate behavior into consideration (Bissonette and Hammer, 2000). Deer are prey animals that are typically hesitant to pass through narrow or constricting structures that could make them vulnerable to predators. Consequently, these studies on these types of WE have produced a mixture of results that need continued testing to measure their effectiveness.
Various factors can influence wildlife activity along roadways including canopy cover, amount of traffic, distances between crossing points, and seasonality. Consideration of structural and landscape attributes is important when determining the placement of mitigation structures (Clevenger and Waltho, 2000) and the target species being studied. Clevenger and Waltho (2000) investigated factors that influence the success of wildlife underpasses in Banff National Park in Alberta, Canada, revealing that carnivores responded differently than ungulates and exhibited greater impacts by the landscape as opposed to structural differences. For some carnivores, structural characteristics can play an important role in the species’ presence at WCS. A study by Clevenger and Waltho (2005) found that constricted structures that were narrower and longer in length had higher black bear and cougar crossing rates. Notably, the design and dimensions of mitigation structures should reflect the travel routes, behavior, and biology of all target species, especially regarding predators, in order to construct an effective structure (Foster and Humphrey, 1995). Additionally, there is evidence that habitat corridors are actively used by predators and their prey. Gloyne and Clevenger (2001) investigated the use of WCS by cougars along 45 km of the Trans-Canada Highway in Alberta, Canada. They detected a positive correlation between mule deer and cougars utilizing these corridors and found that cougars appeared to be making the most passage through areas with the highest habitat quality (Gloyne and Clevenger, 2001). Similar studies have found an overlap of predators and their prey (Dickson et al., 2005; Ford and Clevenger, 2010), but do not suggest that predator behavior at WCS is affected by prey movement. These findings support the idea that WCS are serving their intended purpose for predators, lending an optimistic view to the implementation of similar structures along other highways.
A common method of determining the effectiveness of a mitigation structure is to examine the number of crossing events for species that are observed using these structures (Grilo et al., 2008; Andis et al., 2017). Crossing events allow the behavior of an individual to be recorded and categorized to better understand the performance of a mitigation structure. Some of the ways of measuring effectiveness include road mortality surveys, monitoring telemetry movements, and camera trapping. Monitoring wildlife activity via cameras is a common method of documenting species interactions and passage rates given that camera trapping is effective for wildlife that is elusive, largely nocturnal, or maintains low population densities in a given area (Heilbrun et al., 2006). Camera monitoring is effective for various types of mitigation structures including fence ends (Huijser et al., 2016), WCS (Grilo et al., 2008; Huijser et al., 2016; Simpson et al., 2016), and WG (Allen et al., 2013).
Many mammals require expansive habitats that are commonly bisected by roads. As a result, these species would likely benefit from effective mitigation, such as properly tailored crossing structures (Ford and Clevenger, 2010). An ideal candidate to study is the ocelot (Leopardus pardalis) because this species is endangered in the United States and would allow the consequences of roadways to be viewed through a conservation lens by estimating effective population sizes of highly fragmented populations (Janečka et al., 2007). Although the ocelot’s range previously extended all the way to Arkansas, the loss of its preferred thornscrub habitat has caused populations to dwindle, limiting the ocelot’s range in the United States to southern Texas (Janečka et al., 2007). In south Texas, mitigation efforts are underway to conserve the two remaining US populations of the ocelot, a medium-sized carnivore that has been federally listed as endangered throughout its range in the United States since 1982 (U.S. Fish and Wildlife Service (USFWS), 1982). Currently, these ocelot populations are known from Willacy and Cameron Counties in south Texas (Janečka et al., 2007; Horne et al., 2009; Lombardi et al., 2020), which are separated from the larger Mexico population by roads and highways and areas of urban and agricultural development (Lehnen et al., 2021). Biologically, ocelots are highly sensitive to changes in their preferred habitat, which can amplify through the implementation of linear infrastructure and human development. As a result, one of the causes of ocelot mortality is collisions with vehicles along roads and highways that intersect areas of optimal habitat in which ocelots are known to occur (Haines et al., 2005a; Wilkins et al., 2019). Due to the rarity of ocelots and their similarities in ecology, bobcats (Lynx rufus) have often been used as a surrogate species for ocelots when considering responses to mitigation structures (Grigione and Mrykalo, 2004; Schmidt et al., 2020). This is largely due to their similarities in habitat, diet, body size, behavior, and overlapping home ranges (Cain et al., 2003; Horne et al., 2009; Booth-Binczik et al., 2013).
Various forms of mitigation structures for ocelots and other mesocarnivores in this area are being studied with the aim of reducing the number of highway entries that often result in road mortalities. Presently, an estimated 80 individual ocelots comprise the two isolated populations that inhabit areas of south Texas (Haines et al., 2005a; Horne et al., 2009). Cain et al. (2003) examined the effect that mitigation has had on bobcat mortalities and suggested that maintaining preferred habitats near highways may increase mortalities. Although favorable habitat is ideal for the persistence of ocelots, the presence of prime habitat bordering the highway could prove to have negative impacts on individuals. A new design of wildlife exit (WE) designed for ocelot use has the potential to reduce road mortality, and their effectiveness on State Highway 100 was considered by looking at bobcat activity. While offering jump-outs to enable wildlife to leave the roadway is a recommended practice at WCS (Bissonette and Hammer, 2000; Huijser et al., 2009), these are typically designed for large mammals, and to date, no wildlife exits have been installed in conjunction with structures along the roads of Texas.
In this study, 12 target species including the ocelot were expected to use the WEs due to their body size and occurrence in the area (Table 1). Two main objectives were assessed in this study. The first objective was to characterize the wildlife community occurring around and using the WE and adjacent ROW locations to determine differences in species composition. On roads and highways with wildlife mitigation structures present, wildlife communities occurring along the road can differ in species presence and abundance from adjacent wildlife communities that exist on the habitat side of the fencing. The following hypotheses were tested: (1) target species communities observed at WE will be significantly different in terms of their occurrence and movement category (exiting the roadway, entering the roadway, walking parallel to the fence on either side) regardless of canopy cover, side of the highway, or season (wet or dry months); (2) since the WE should serve as an escape from the roadway, the target species community will not significantly differ between wildlife observed traveling around WE and wildlife detected on cameras placed in the ROW away from the WE cameras; and (3) the average number of target species (S) and overall relative abundance (N) will not significantly differ between wildlife movement categories detected at WE and wildlife detected on cameras placed in the ROW.
The second objective of this study examined the effectiveness of WE by identifying the mitigation structures where wildlife enter and exit the roadway, calculating the percentage of wildlife remaining on the road that correctly used a WE to escape the ROW, and estimating the number of wildlife remaining on the road. The hypotheses for this objective were the following: (4) all target species will use a WE to correctly travel from road to habitat and will not use a WE incorrectly to access the roadway from the habitat side; (5) since the WE were designed for ocelots, more bobcats will use WE than coyotes to move from road to habitat because of size differences; and (6) WE exits with high prey species occurrence (cottontail and rodent) will be correlated with mesocarnivore occurrence with significant overlap in activity patterns.
2 Materials and methods
2.1 Study area
This study occurred along an 11.9-km stretch of State Highway (SH) 100 between Los Fresnos and Laguna Vista in Cameron County, Texas (Figure 1). The ROW on SH 100 has been fenced with continuous geomesh polypropylene fiber (GEO 55) chain-link fencing with 10 WE. Bordering the Laguna Atascosa National Wildlife Refuge, the habitat in this area primarily consists of dense Taumaulipan thornscrub, grassland communities, and Gulf coastal prairie, and has a flat topography with elevations ranging from 0 m to 10 m (Haines et al., 2005b; Horne et al., 2009; Watson et al., 2019). Vegetation along SH 100 is variable, from dense thornscrub with high canopy cover in some areas to wide stretches of open grassland. The climate in this area is subtropical and semi-arid (Jahrsdoerfer and Leslie, 1988; Harveson et al., 2004); annual rainfall throughout the region is 65 cm, with summer temperatures climbing to 35°C and winter temperatures averaging as low as 12°C (NOAA, 2021). Due to the dry and warm climate of south Texas, seasonality is more accurately assessed by specific climate factors such as precipitation or temperature.
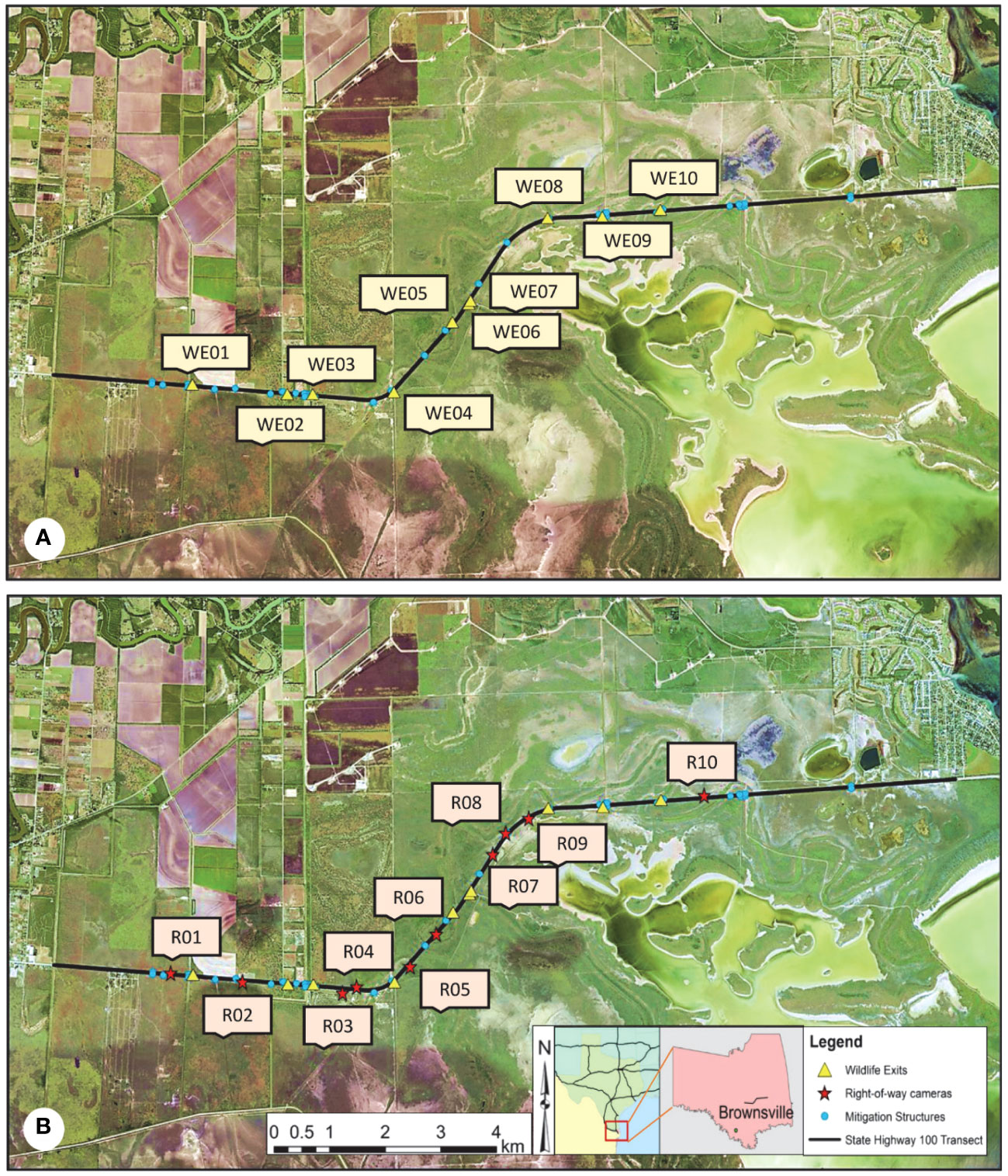
Figure 1 Map of the study area showing the locations of the ten wildlife exits (WE) (A) and the ten right-of-way (ROW) sites (B) along State Highway (SH) 100 in Cameron County, TX. The mitigation area covers an 11.9-km transect of SH 100 that stretches from Los Fresnos, TX to Laguna Vista, TX. Wildlife exits were installed on 13 February 2019, by the Texas Department of Transportation to give wildlife that entered the ROW using a wildlife guard an option to escape into the habitat, aiming to reduce the number of wildlife road mortalities that occur on SH 100. The ROW cameras were installed in December 2019 to capture wildlife activity occurring in the ROW that was not being recorded on cameras next to a monitored mitigation structure.
Although part of the land that encompasses the study area is managed by the US Fish and Wildlife Service, some areas are privately owned and used for agriculture or ranchlands. The area is home to many species that are unique to south Texas, including the state-threatened Texas tortoise (Gopherus berlandieri), and endangered species like the Aplomado falcon (Falco femoralis) and the ocelot. Numerous species in this area face threats of population decline, primarily due to a loss of habitat. Future development is expected to continue throughout the Lower Rio Grande Valley, likely further fragmenting the remaining habitat in this area.
Although designed with the endangered ocelot in mind, the dimensions of the WE are suitable for use across a wide range of species (Figures 2A, B). Six medium-duty PVC-coated T-posts measuring 4 ft high were driven 30 cm into the ground and secured to each exit with T-post clips to provide support. The material used for the exit fencing was 5 cm × 7.6 cm mesh 16-gauge black PVC-coated welded wire, from which two side panels were cut to dimensions of 0.60 m × 1.37 m long and secured using hog rings along the roadside of the existing chain-link fence. The height of the exits was 60 cm tall with the opening measured at 46 cm wide. The WE were funnel shaped and narrowed to a final width of approximately 13 cm across, extending to a depth of 84 cm when measured from the chain-link fence (Figures 2C, D).
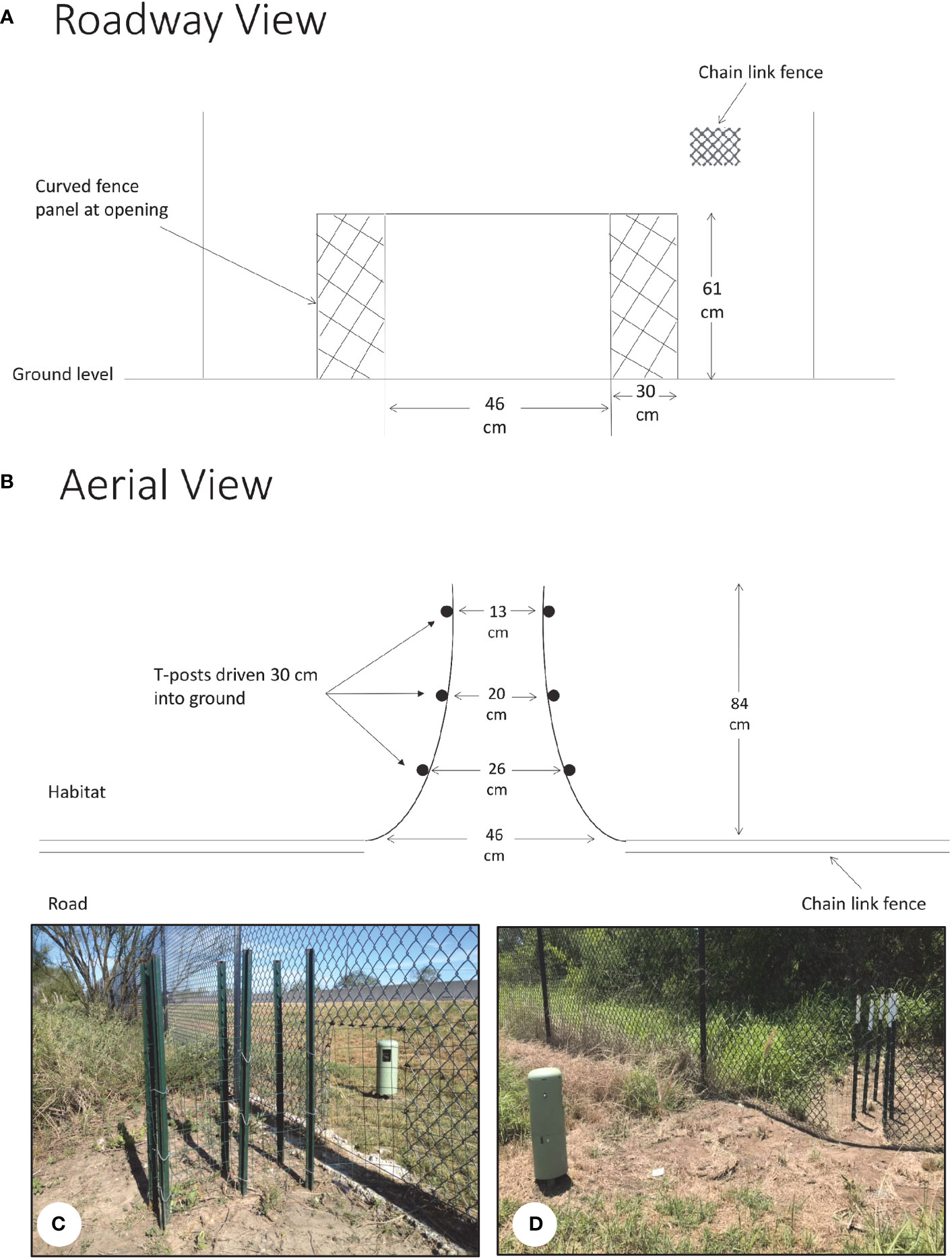
Figure 2 Wildlife exits (WE) installed on State Highway 100 to allow wildlife that entered the right-of-way via a wildlife guard an option to escape into the habitat. The dimensions of the WE were suitable for use across a wide range of species. (A) The exits were 53 cm tall with an opening of 46 cm wide. (B) As an exit funneled into the habitat, it narrowed to a final width of approximately 13 cm across, extending to a depth of 84 cm when measured from the chain-link fence. (C, D) Actual pictures of wildlife exits as viewed from the habitat side of the chain-link fence (C) and from the roadside (D).
2.2 Data collection
Camera monitoring of WE sites was carried out year-round from February 2019 to November 2020. A total of 20 Reconyx HyperFire 2™ series cameras (Reconyx, Holmen, WI, USA) were set up across the ten WE. At each WE, two cameras were installed 2–3 m from the exits, one on the roadside of the continuous fencing and one on the habitat side, each facing perpendicular to the exit (Figure 2).
All cameras were checked monthly at which point memory cards were replaced and batteries were changed as needed. Cameras were programmed to take a set of three pictures at 1-s intervals each time the camera was triggered by motion. On field days when pictures were collected, the surrounding vegetation was cleared out to an approximate distance of 3 m around each exit to reduce excessive camera triggers and to clearly view species interactions in each photo. The distance of this arc was chosen to discourage unwanted human interest in the cameras and to limit the visual alterations made to the habitat’s natural appearance that could repel wildlife entry from the roadside (White and Gehrt, 2009). In addition, a densitometer was used to measure the amount of canopy cover at each WE. A WE was closed once either a bobcat or coyote was observed using it to incorrectly access the roadway, due to the concern that an ocelot may do the same.
In addition to the cameras that were set up at each WE, 10 cameras were placed in the ROW on both the north and south sides of SH 100 to capture wildlife walking outside of the fencing along the highway from December 2019 through December 2020 (Figure 1). Using ArcMap 10.6.1, ROW locations were selected by identifying unmonitored gaps of chain-link fencing exceeding 500 m to the nearest mitigation structure. From these gaps, cameras were placed on the roadside of the fencing, approximately 3 m away and facing perpendicular to the fence (Figure 3A). The primary goal was to capture animal activity occurring along the road that was not being recorded on cameras next to a monitored opening in the fence. The surrounding vegetation was cleared 2–3 m around each ROW camera.
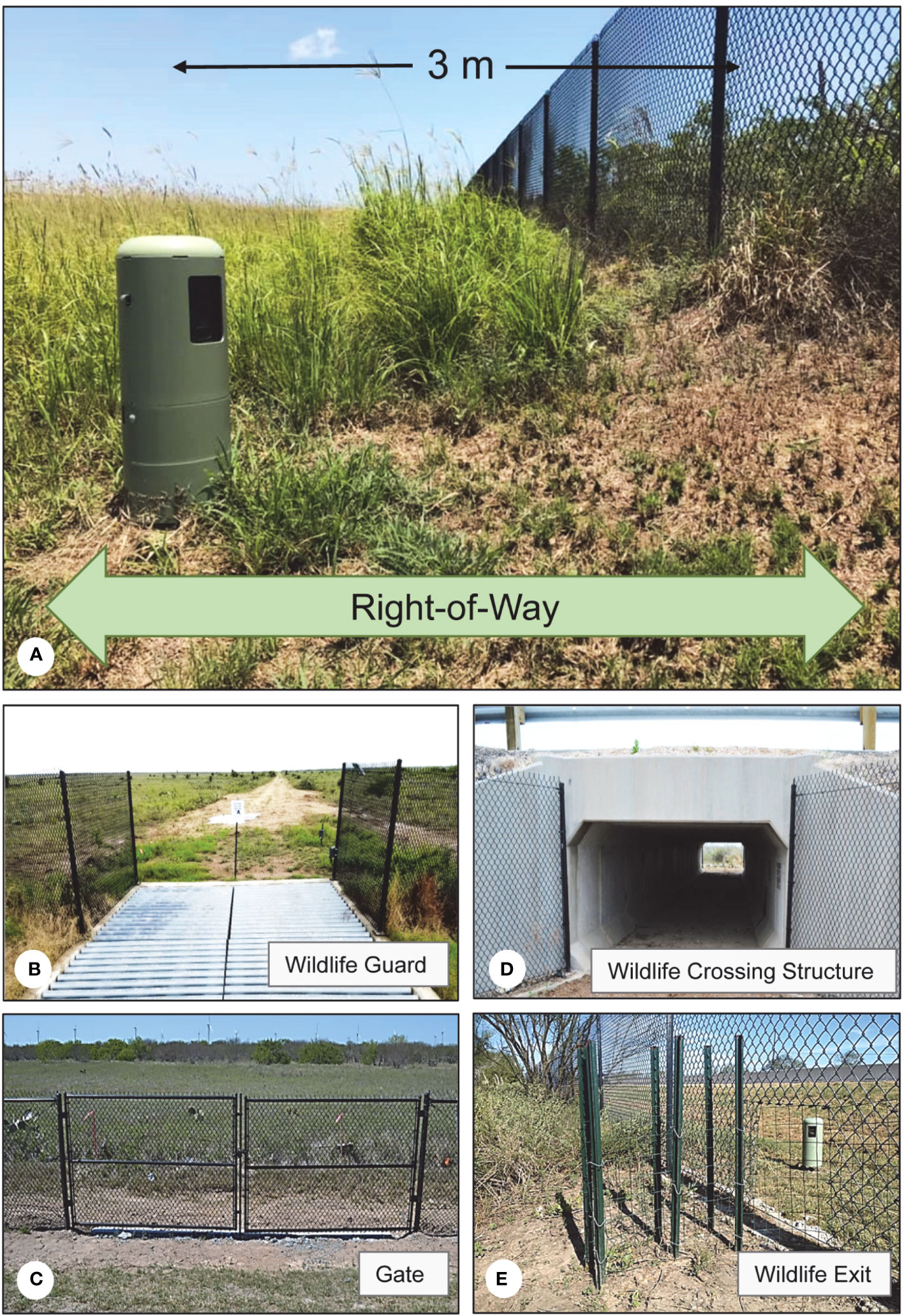
Figure 3 Monitoring of mitigation structures along State Highway 100. (A) A total of 10 right-of-way (ROW) cameras were placed on State Highway (SH) 100 in Cameron County, TX facing the chain-link fencing to capture unmonitored wildlife activity occurring along the roadside of the fence. The ROW here is defined as the area between the road and the continuous chain-link fencing that separates the road from the habitat, measuring approximately 5–10 m. Right-of-way cameras were placed approximately 3 m from the chain-link fence. All cameras were checked monthly at which point surrounding vegetation was cleared around each ROW camera. (B–E) In addition to wildlife exits (E), current monitoring of mitigation structures along State Highway to exists in the form of 16 wildlife guards (B), 5 wildlife crossing structures (D), and 16 gates (C).
Along with the WE and ROW, the SH 100 mitigation project monitored 5 WCS, 16 WG, 4 fence ends (FE), 16 gates, and 3 wing walls (WW), all of which were constructed to prevent wildlife road access in order to reduce wildlife road mortalities along with SH 100 (Figures 3B–E). These mitigation structures were also checked every two weeks during which memory cards were replaced and batteries were changed as needed. Total entries onto the road and exits into the habitat at every monitored WCS, WG, gate, and WW were counted for each of the target species from February 2019 to November 2020 to determine the number of individuals remaining on the roadway.
As a measure of seasonality, monthly average precipitation data were downloaded from the nearest weather station, University of Utah’s MesoWest Station ATRT2 at Laguna Atascosa National Wildlife Refuge. Monthly total precipitation data were converted into “Wet” (n = 10) and “Dry” (n = 14) months by binning them into above- and below-average annual monthly precipitation total of 5.0 cm.
2.3 Data management
Each WE and ROW camera was checked once per month, at which point pictures were downloaded, visually analyzed, and sorted into corresponding species folders. All pictures were renamed according to the date and time that the photo was captured using the software programs Renamer and Special Renamer (Sanderson and Harris, 2013). Additionally, DataOrganize was used to catalog pictures and highlight any organization errors that were missed during the sorting process.
All recorded species were identified to the lowest taxonomic level. For the purposes of this study, only mammals larger than rodents were assigned an interaction. Interactions were not assigned to humans, rodents, birds, and most herpetofauna except for species of concern, including the Texas tortoise and the Texas indigo snake (Drymarchon melanurus erebennus), and primarily ground-dwelling birds such as the northern bobwhite (Colinus virginianus) greater roadrunner (Geococcyx californianus). However, given the low numbers of these species and their ability to permeate the continuous chain-link fencing along SH 100, they were excluded from analysis. Due to their size, larger animals such as white-tailed deer, nilgai, and javelina were unable to use WE and were also excluded from analysis. Although eastern cottontails occasionally traveled directly through the WE fencing, this species was still included as a target species in the analysis due to their availability as a prey item for mesopredators (Booth-Binczik et al., 2013). Rodents in this area were small enough to permeate both the chain-link and WE fencing and were consequently only analyzed in relation to mesocarnivore presence by counting independent events. Additionally, all domestic animals except for domestic cats were excluded from analysis. Long-tailed weasels due to their low numbers were also excluded from further analyses. The purpose of these constraints was to encompass only the species that were expected to benefit the most from the installation of the WE.
Once classified by species, a second reviewer further sorted the pictures by the number of individuals observed in each picture. Finally, individual interaction categories were assigned depending on how the individual interacted with the WE, where “A” designated a successful crossing from one side of the fence to the other, “B” was an entry and exit on the same side of the fence, and “P” was assigned to interactions where an individual moved parallel to an exit and did not attempt to use it (Figure 4). Potential directions included “H,” which designated the habitat side of the fence, “R” indicated a direction on the road, and “F” was a wildlife crossing made via a hole or gap in the exit’s mesh fencing. Successful passages at WE from the roadside to the habitat side were labeled “R-H,” and passages from the habitat to the roadside were labeled “H-R” (Figure 5). Similarly, pictures from all other mitigation structures were sorted by species and number of individuals, and categorized by interaction type (Figure 5).
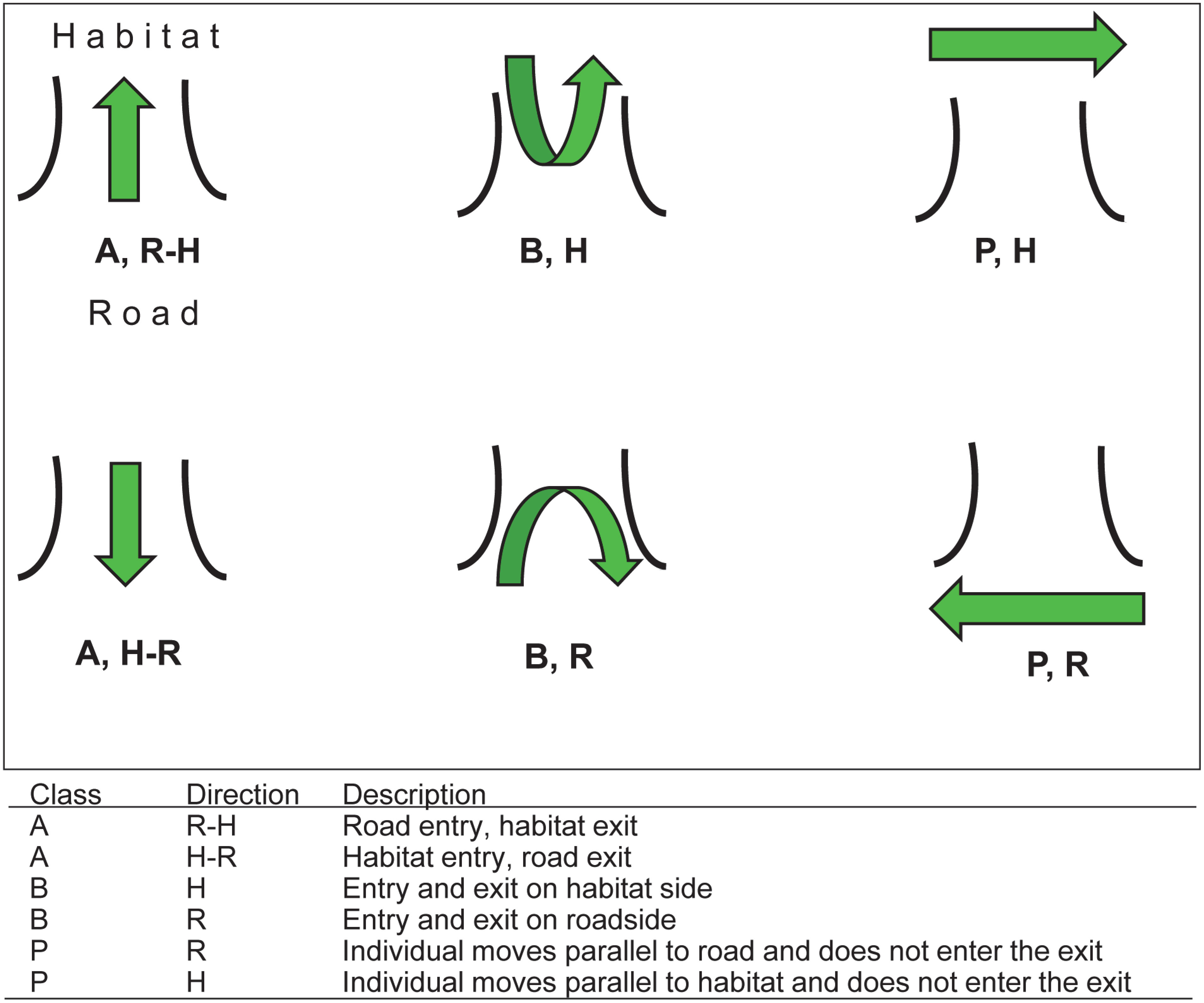
Figure 4 Potential interactions and categorizations of individual wildlife activity at wildlife exits on State Highway 100 in Cameron County, TX. Interaction classes include successful crossings from one side of the chain-link fence to the other (A), entries/exits on the same side of the chain-link fence (B), and parallel interactions in which an individual walked along the chain-link fence and did not attempt to use the exit (P). Directions for species travel included habitat (H) and roadway (R).
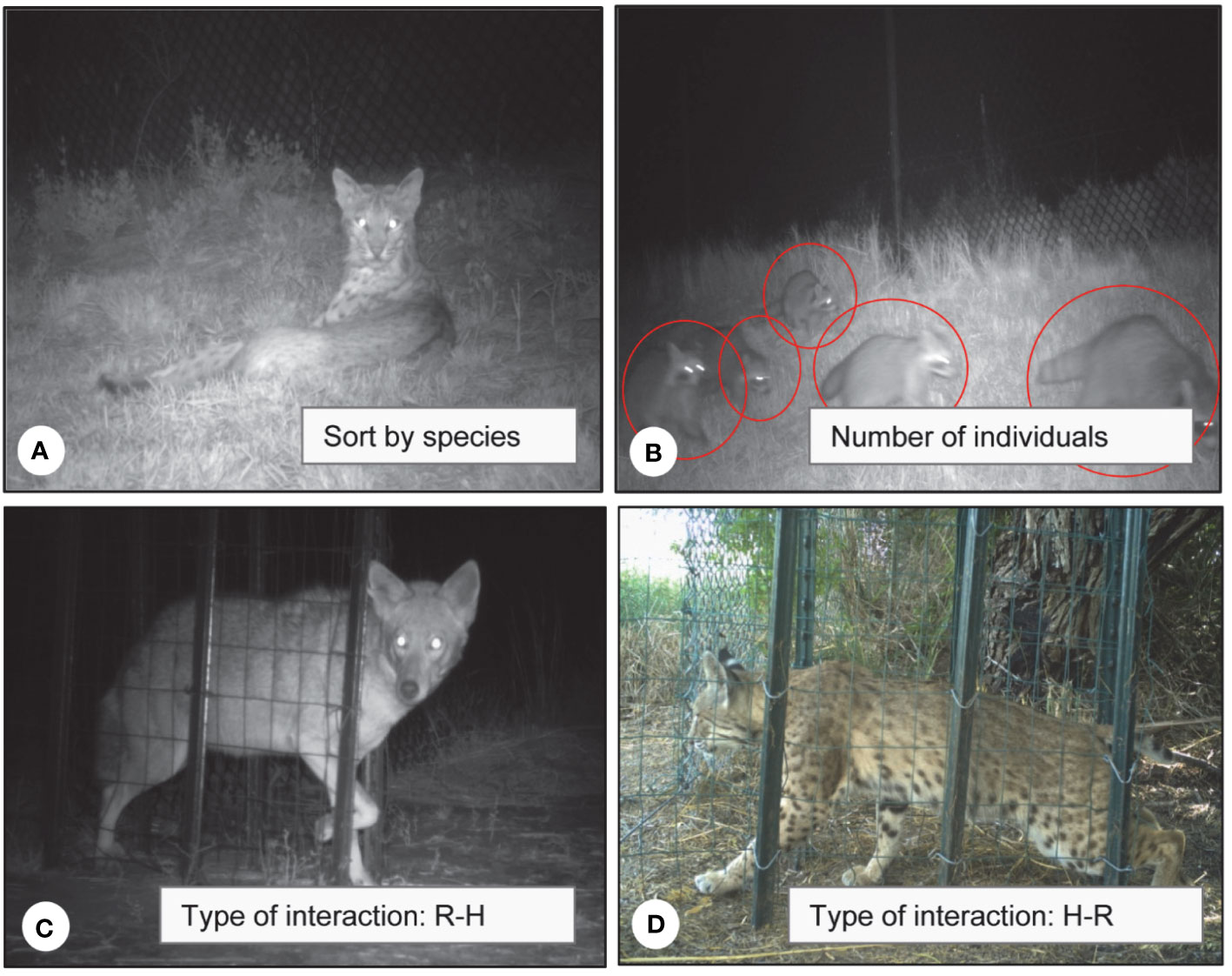
Figure 5 After wildlife exit pictures were collected from the field, they were sorted first by species captured in photo (A), then by the number of individuals in that photo (B), and finally by the type of interaction that an individual had with the wildlife exit (C, D). Interactions depicted include a successful road-to-habitat (R-H) crossing of a coyote (C) and an incorrect habitat-to-road (H-R) crossing of a bobcat (D).
To avoid counting the same animal as multiple individuals, a time frame of 30 min was established to determine independent interaction events (O'Brien et al., 2003). If multiple interactions of an individual of the same species occurred at a WE under this time frame, it was considered the same individual and counted as a single event. For example, if an animal was documented crossing onto the road and then captured within 30 min using a mitigation structure to travel back into the habitat, it was considered the same individual unless overwhelmingly evident (by means of markings, injuries, size, etc.) that there were two distinct individuals.
2.4 Data analysis
Recording, compilation, and visualization of the data was performed in Excel. To address hypotheses 1 and 2, multivariate analyses of the target species community were performed in PRIMER-e v7 with PERMANOVA+ (PRIMER-E, Albany, Auckland, NZ). To address hypothesis 3, generalized linear models (GLMs) were conducted on the number of species and relative abundance counts at each category in SPSS v25 (IBM Corp). To address hypothesis 4, target species counts and percentages were calculated in Microsoft Excel. Due to low counts and a single percentage calculated of each species, these were analyzed qualitatively. To address hypothesis 5, bobcat and coyote counts were calculated in Excel and analyzed qualitatively. For hypothesis 6, comparing predator and prey occurrence, Spearman correlations were conducted with SPSS v25, and activity analyses were performed in R (R Core Team, 2022).
To determine the level of transformation needed in PERMANOVA, visual examinations of shade plots were conducted (Clarke et al., 2013). All factors included in the PERMANOVA models were fixed. PERMANOVAs were run with 9,999 permutations. Post-hoc pair-wise PERMANOVAs were used to further test combinations of each level within a factor to determine the source of the differences. For multiple comparisons, the Benjamini–Hochberg method (Benjamini and Hochberg, 1995) was used to control for the false discovery rate. Similarity percentages (SIMPER) analyses set at a cutoff of 95% were used to identify species that were responsible for any patterns of variation observed between species communities. A bootstrapped metric multidimensional scaling plots (mMDS) with 100 bootstraps per group was created to visualize the differences in the wildlife communities.
Error distribution models considered for the GLMs were linear, gamma with log link, Poisson loglinear, and negative binomial with log link. To determine the best error distribution model for the GLMs, comparisons of the Akaike’s Information Criterion with small-sample correction (AICc) values and the results of the Omnibus test were used. Where significant effects were detected, post-hoc pair-wise Tukey tests were used to determine differences between groups.
2.4.1 Characterization of the wildlife community at wildlife exits and right-of-way locations
To test if target species assemblages observed at WE were significantly different in terms of their occurrence and movement category, a PERMANOVA with four factors was used to compare movement categories of wildlife communities at WE over the entire period from February 2019 to November 2020. The four factors were direction of travel, canopy cover, side of the highway, and season (“wet” or “dry” based on monthly precipitation). A Bray–Curtis similarity matrix was applied to the Log(x+1) transformed data with a dummy variable of one. A post-hoc pairwise PERMANOVA was used to further test combinations of each level within a factor to determine the source of the differences.
To test if the target species community was different between wildlife observed traveling around WE and wildlife detected on cameras placed in the ROW away from the WE cameras, a PERMANOVA was used to compare the wildlife communities over the period December 2019 through November 2020 when ROW and WE monitoring overlapped. A Bray–Curtis similarity matrix was applied to the Log(x+1) transformed data with a dummy variable of one. A post-hoc pairwise PERMANOVA was used to further test combinations of each level within a factor to determine the source of the differences. A similarity percentages (SIMPER) analysis set at a cutoff of 95% was used to identify species that were responsible for any patterns of variation observed.
Additionally, generalized linear models (GLMs) were used on the December 2019 through November 2020 dataset to compare the total number of species recorded (S) and total number of individuals observed (N) for the five movement categories. Post-hoc pair-wise Tukey tests were used to determine any differences between groups.
2.4.2 Use of wildlife exits
To further understand how wildlife were using the WE, entry and escape rates were calculated to determine where wildlife were entering and exiting the roadway outside of WE. The total number of individuals for each target species was counted for all entries (H-R) and exits (R-H) at any WG, gate, or WW within 200 m of an exit. This distance was chosen given the average walking speed per hour of a bobcat is approximately 400 m per hour (Elizalde-Arellano et al., 2012) and the 30-min photo capture interval for individual identification events. Using ArcMap 10.6.1, a 200-m buffer around each WE was created to identify mitigation structures that were included within this range. Wildlife guard crossing counts were drawn from eight WG that were adjacent to the 10 WE. In this analysis, additional species including cottontails, armadillos, and raccoons were excluded due to their ability to permeate the continuous chain-link fencing along SH 100 by burrowing or climbing directly over the fence.
To calculate the number of individuals that remained on the roadway on SH 100 for each species, four categories of individual interactions were created: (1) “Mit H-R,” which encompassed all entries onto the roadway made via a WG, gate, or WW; (2) “Mit R-H,” included all exits from the road into the habitat made via a WG, gate, or WW; (3) “WE R-H,” which considered all road to habitat crossings through a WE; and (4) “WE H-R,” indicated habitat to road access through a WE. Total entries onto the road were determined by adding all Mit H-R and all WE H-R movements:
The number of individuals remaining on the road after all Mit R-H escapes was calculated by taking the difference of all Mit R-H from the total entries:
Percentages of individuals that left via a WE were calculated for each of the target species using the following formula:
To determine the number of individuals of each species that were not recorded leaving the highway via a monitored mitigation structure within the 200-m buffer around each WE (the total unaccounted for), all “WE R-H” were removed from the “remaining on-road” count:
Data were examined to determine the bobcat and coyote use in the road to habitat direction over the study period. The total R-H events for each of these species across all 10 WE were counted per 30-day period from February 2019 to November 2020 to identify when each species initially began to use the WE. Patterns in mesocarnivore usage of WE and the total number of WE accessed were also determined. Due to the relatively low number of bobcat and coyote observations, these data are presented as a qualitative analysis.
2.4.3 Mesopredator-prey species associations at WE
To test if wildlife exits with high prey species occurrence would be correlated with mesopredator occurrence, activity patterns at WE as a function of time of day were reported for all bobcat, coyote, cottontail, and rodent interactions between February 2019 and November 2020. Peak activity times and activity pattern overlap were assessed. In order to assess patterns in presence at the wildlife exits, kernel density distributions were created using “fitact” function in the R package “activity,” and the “compareCkern” function, also in the R package “activity,” was used to identify any significant differences between the density distributions of coyotes, bobcats, cottontails, and rodents (Rowcliffe and Rowcliffe, 2016).
To test if wildlife exits with high cottontail and rodent usage exhibited high mesocarnivore activity due to the presence of prey, Spearman correlations were conducted on counts of rodents by bobcats, rodents by coyotes, cottontails by bobcats, and cottontails by coyotes.
3 Results
3.1 Characterization of the wildlife community at wildlife exits and right-of-way locations
Of the 12 species that were expected to use WE to travel from the road to the habitat, 10 of them used WE in the R-H direction (Table 2) from February 2019 through the time of the first WE closure on 13 April 2020. At the end of the data collection period in November 2020, four of the ten WEs had been closed at the direction of USFWS due to some bobcats and coyotes using them in the unintended direction of habitat to road.
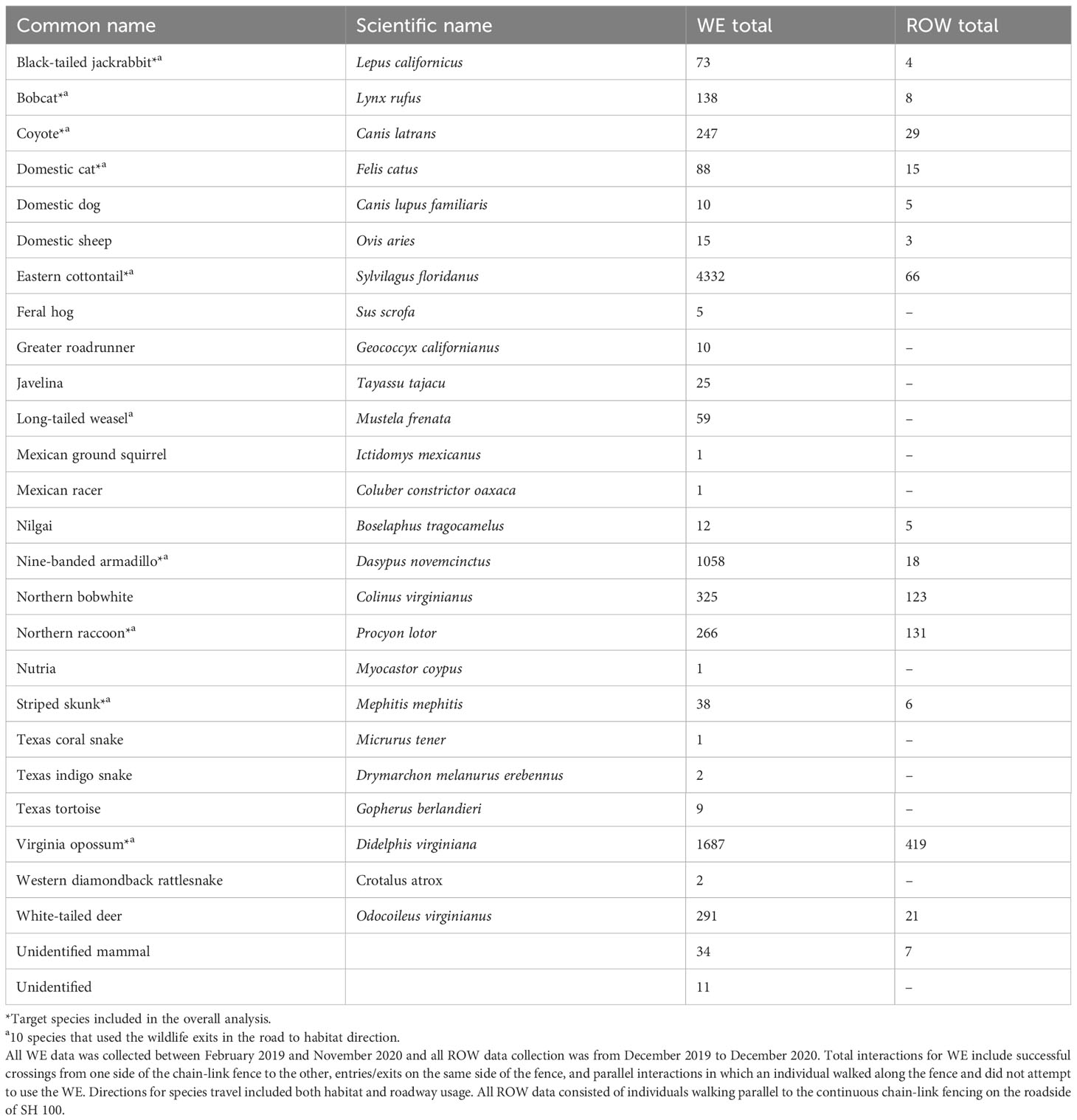
Table 2 All species observed interacting with wildlife exits (WE) and passing by right-of-way (ROW) sites, as well as their respective total individual counts recorded on State Highway (SH) 100 in Cameron County, Texas.
3.1.1 Target species community at wildlife exits
Results of the PERMANOVA on the WE target species communities showed that all four factors were significant in predicting the target species communities that were tested in the PERMANOVA including movement category (pseudo-F = 9.075, p = 0.0001), canopy cover (pseudo-F = 8.184, p = 0.0001), side of highway (pseudo-F = 4.842, p = 0.0029), and precipitation (pseudo-F = 5.528, p = 0.0004), and the interaction term canopy cover X side of highway (pseudo-F = 7.948, p = 0.0001). All other interaction terms of the model were not significant (p > 0.10). Since there were no significant interactions between movement category and any other factor in the model, movement category was analyzed separately in subsequent analyses.
Post-hoc pair-wise comparisons between P-H and all three other movement categories were significant: P-H and R-H (t = 3.66, p = 0.0002), P-H and H-R (t = 4.155, p = 0.0002), and P-H and P-R (t = 3.892, p = 0.0002). In addition, the communities for movement categories H-R and R-H were significantly different (t = 1.803, p = 0.02055). However, the communities found going parallel to the roadway (P-R) were not significant (p > 0.08) from those using wildlife exits in either direction (H-R or R-H).
Results from the SIMPER analysis showed that the primary species responsible for the average dissimilarity between P-H and all other groups was the nine-banded armadillo (≤ 21.3%). Eastern cottontails (≤ 20.7%) and Virginia opossums (≤ 12.9%) followed armadillos in the overall percentage of species contributions to the dissimilarities observed. Furthermore, cottontails were the primary species driving differences between R-H and H-R, contributing approximately 36.6% to the dissimilarity between this group. Coyotes and bobcats showed their highest contribution to the average dissimilarity between R-H and H-R, with bobcats contributing 7.8% and coyotes contributing 5.5% and to the average dissimilarity in this group. The primary species responsible for the average dissimilarity for all ROW sites grouped with R-H, H-R, and P-R were eastern cottontails (≤ 24.9%).
3.1.2 Target species communities at wildlife exits and right-of-ways
The PERMANOVA results testing differences in target species communities between ROW and WE sites showed that movement category had a significant effect (pseudo-F = 3.463, p = 0.0001; Figure 6). Post-hoc pair-wise comparisons between ROW and all of the movement categories were significantly different: ROW and R-H (t = 1.97, p = 0.00987), ROW and H-R (t = 2.64, p = 0.0012), ROW and P-R (t = 1.68, p = 0.040), and ROW and P-H (t = 2.23, p = 0.0028).
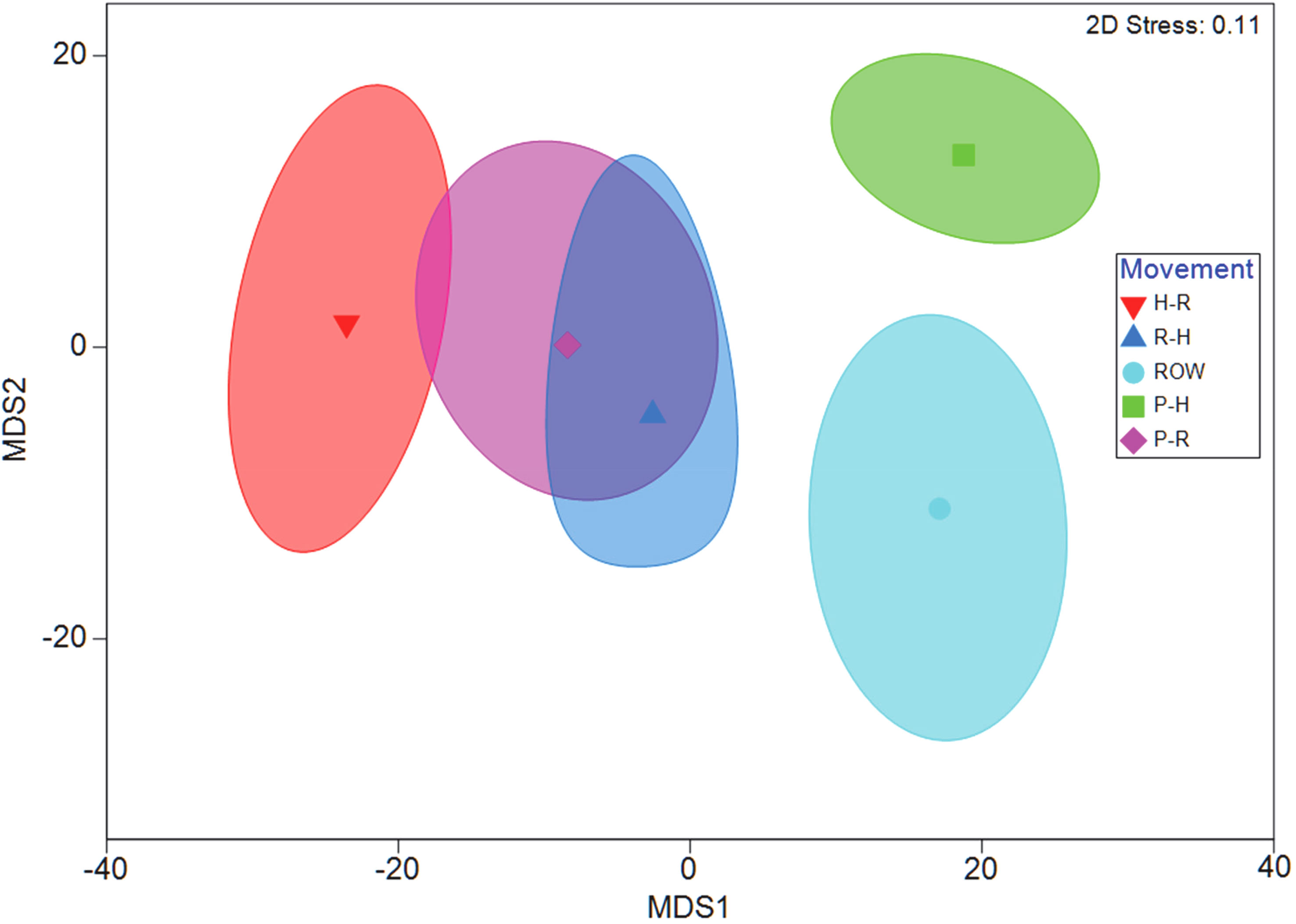
Figure 6 Bootstrapped metric multidimensional scaling (mMDS) plot produced with a 95% confidence region of Log(x+1) transformed data on a Bray–Curtis similarity matrix provided a visual representation of the differences between interaction types observed at all 10 wildlife exits and 10 ROW sites on State Highway. PERMANOVA results showed that movement type was a significant factor (p = 0.0001). Pair-wise tests revealed that the species assemblage making movements Parallel to the Habitat (P-H) side of the chain-link fencing were significantly different than the species assemblages of the other three interaction groups Habitat to Road (H-R), Road to Habitat (R-H), and Parallel to Road (P-R) (all p-values < 0.0002). Additionally, wildlife activity for (R-H) and (H-R) pair-wise comparisons were significantly different (p = 0.021). All pair-wise tests for interactions were significantly different from Right-of-Way (ROW) locations away from the wildlife exits (all p-values < 0.025).
The SIMPER analysis showed that the primary species responsible for the average dissimilarity between ROW and P-H was the nine-banded armadillo (≤21.9%). Among mesocarnivores, bobcats contributed the most to the dissimilarities seen between ROW and P-H, comprising approximately 9.0%. In contrast, coyotes had the highest contribution to dissimilarities between ROW and H-R, providing approximately 11.1% to the average dissimilarity in this group.
3.1.3 Richness and relative abundance at wildlife exits and right-of-ways
A GLM with gamma with a linear error distribution provided the best fit (likelihood ratio chi-square = 38.38, p < 0.001) and further highlighted significant differences in the number of target species observed between R-H, H-R, P-H, P-R, and ROW movement categories (intercept: Wald chi-square = 590.6, df = 1, p = 0.000; movement category: Wald chi-square = 57.7, df = 4, p < 0.001; Figure 7A). The post-hoc Tukey test showed significantly higher average number species in parallel to habitat (6.7 ± 0.6) movements than all the other four movement categories R-H (4.0 ± 0.1) H-R (2.4 ± 0.4), P-R (4.3 ± 0.6), and ROW (4.7 ± 0.3) (all p < 0.015). There were more species observed on ROW than H-R (p = 0.004) and more species in P-R than H-R movements (p = 0.024). All other comparisons were not significantly different (p > 0.08). Although the average relative abundance for all target species was considerably higher for the P-H movement category (234.1 ± 59.8) as compared to R-H (132.9 ± 58.4), H-R (2.4 ± 0.4), P-R (84.3 ± 25.8), and ROW (4.7 ± 0.3), the GLM with negative binomial with log link error distribution for relative abundance observed between movement categories provided the best fit (likelihood ratio chi-square = 8.316, p = 0.081), but was not significant (intercept: Wald chi-square = 1133.9, df = 1, p = 0.000; movement category: Wald chi-square = 7.88, df = 4, p = 0.096; Figure 7B).
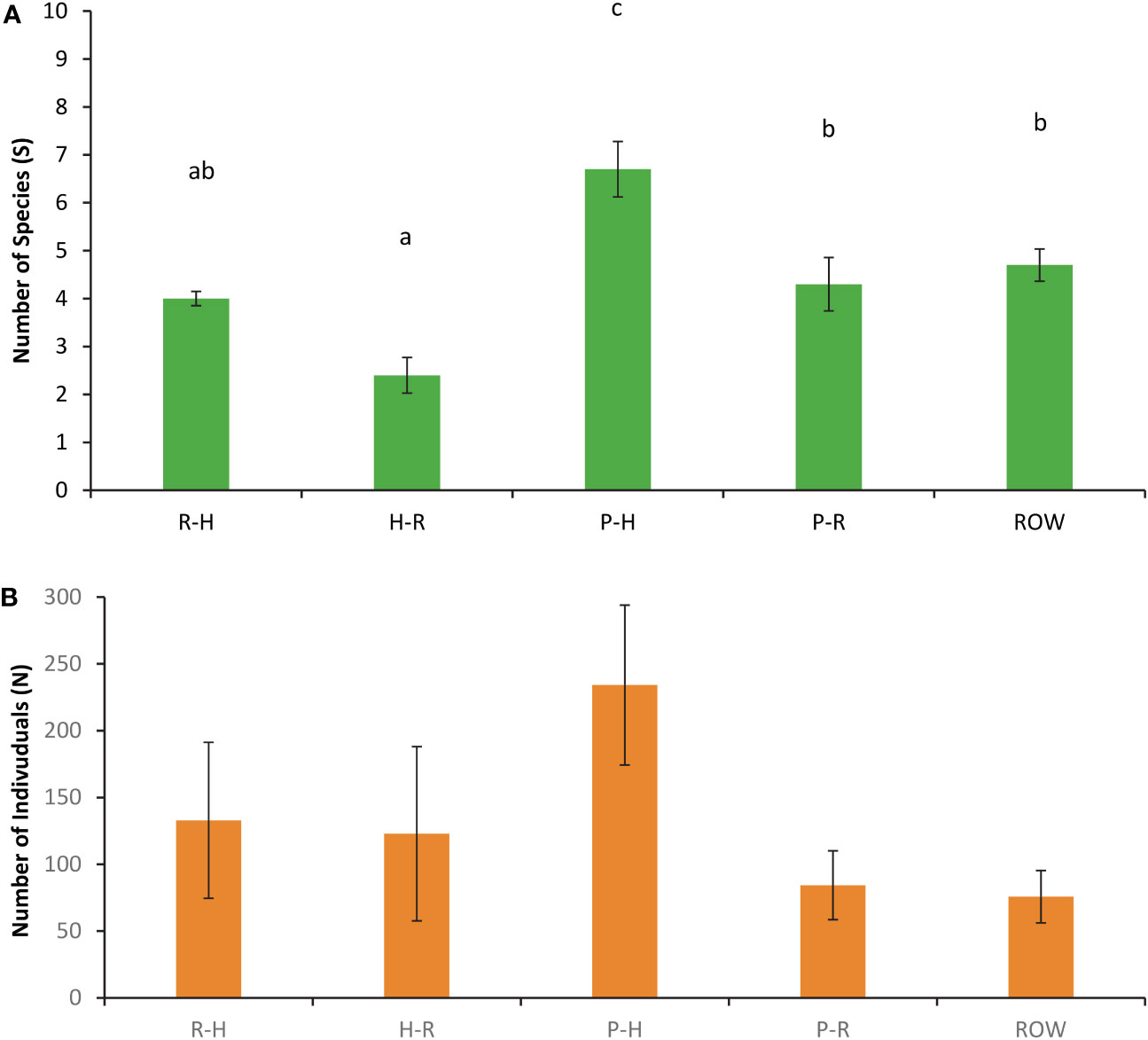
Figure 7 (A) Number of species (S) and (B) number of individuals observed (N) for all five movement types observed at wildlife exits and right-of-way cameras on State Highway 100 in Cameron County, TX, consisting of road-to-habitat (R-H) crossings, habitat-to-road (H-R) crossings, parallel events on the habitat (P-H) side of the chain-link fencing, parallel events on the roadside (P-R), and individuals traveling parallel on the road next to right-of-way locations (ROW). Data are from December 2019 to November 2020. Significant pairwise differences (p < 0.05) are indicated with letters.
3.2 Use of wildlife exits
3.2.1 R-H and H-R movements
Nine target species were observed using the WE to travel from R-H and H-R from February 2019 through the end of the data collection period in November 2020 (Table 3). All H-R events at WG, gates, and WW combined resulted in 123 bobcat and 685 coyote events (Table 4). After all of the R-H events were made via a WG, gate, or WW, 38 bobcats and 229 coyotes were observed remaining in the ROW. Percentages of successful WE R-H events for all species remaining on the road after all exits via a mitigation structure other than a WE were as follows: bobcats = 43%, jackrabbits = 38%, skunks = 38%, opossums = 37%, domestic cats = 15%, and coyotes = 6% (Figure 8). Of the initial 123 bobcats that entered the roadway over the course of this study, 29 of those successfully found and used a WE to cross back into the habitat. In contrast, only 15 of the remaining 229 coyotes on the roadway were recorded traveling from R-H. Jackrabbits and skunks followed bobcats in the overall percentage of successful exits from the ROW via a WE, both of which had 38% of their respective species exiting from the road. Opossums and domestic cats were the next two species that used a WE to escape the ROW.
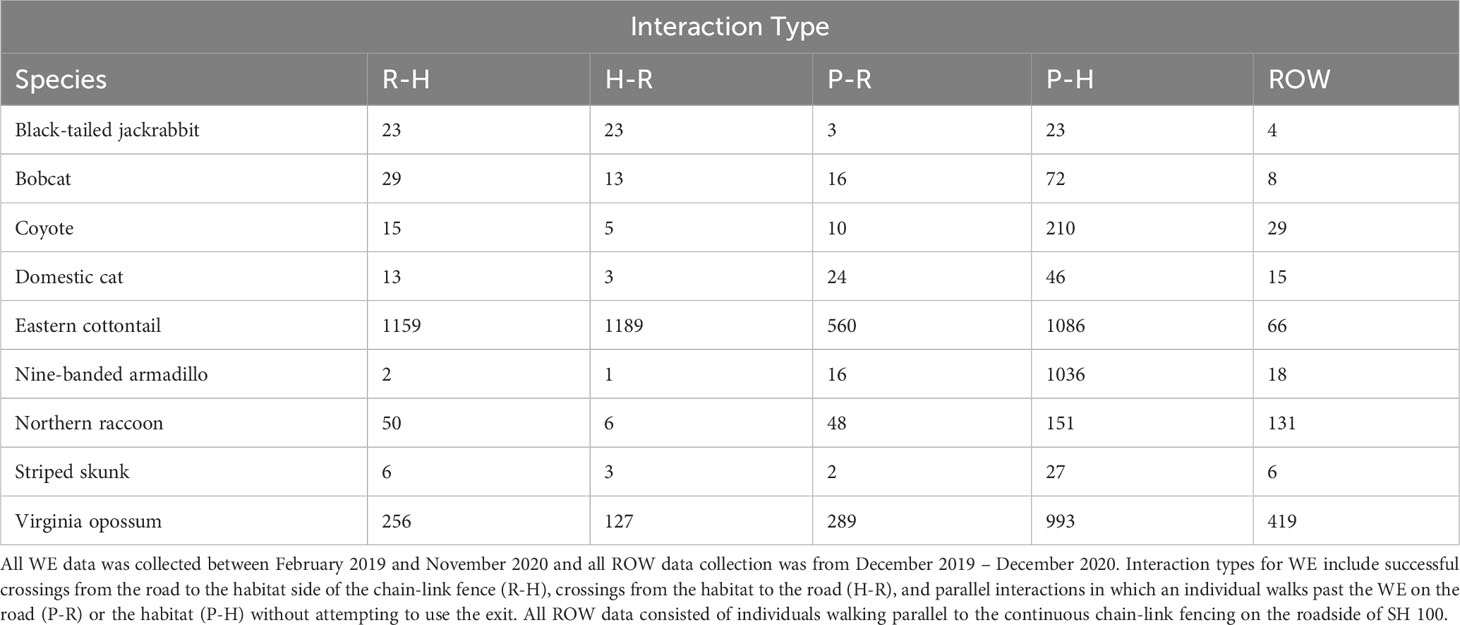
Table 3 Total number of interactions at all ten wildlife exits (WE) and ten right-of-way (ROW) locations for all target species recorded on State Highway (SH) 100 in Cameron County, Texas.
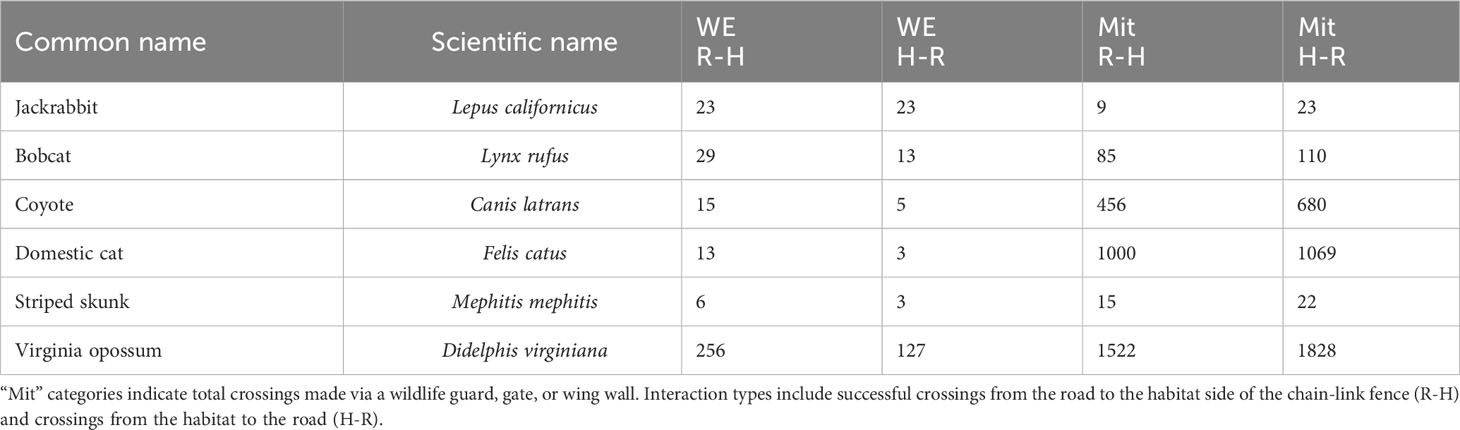
Table 4 Total entries and exits of target species at wildlife exits (WE) and all mitigation structures within 200 m of a WE on State Highway 100 in Cameron County, Texas between February 2019 and November 2020.
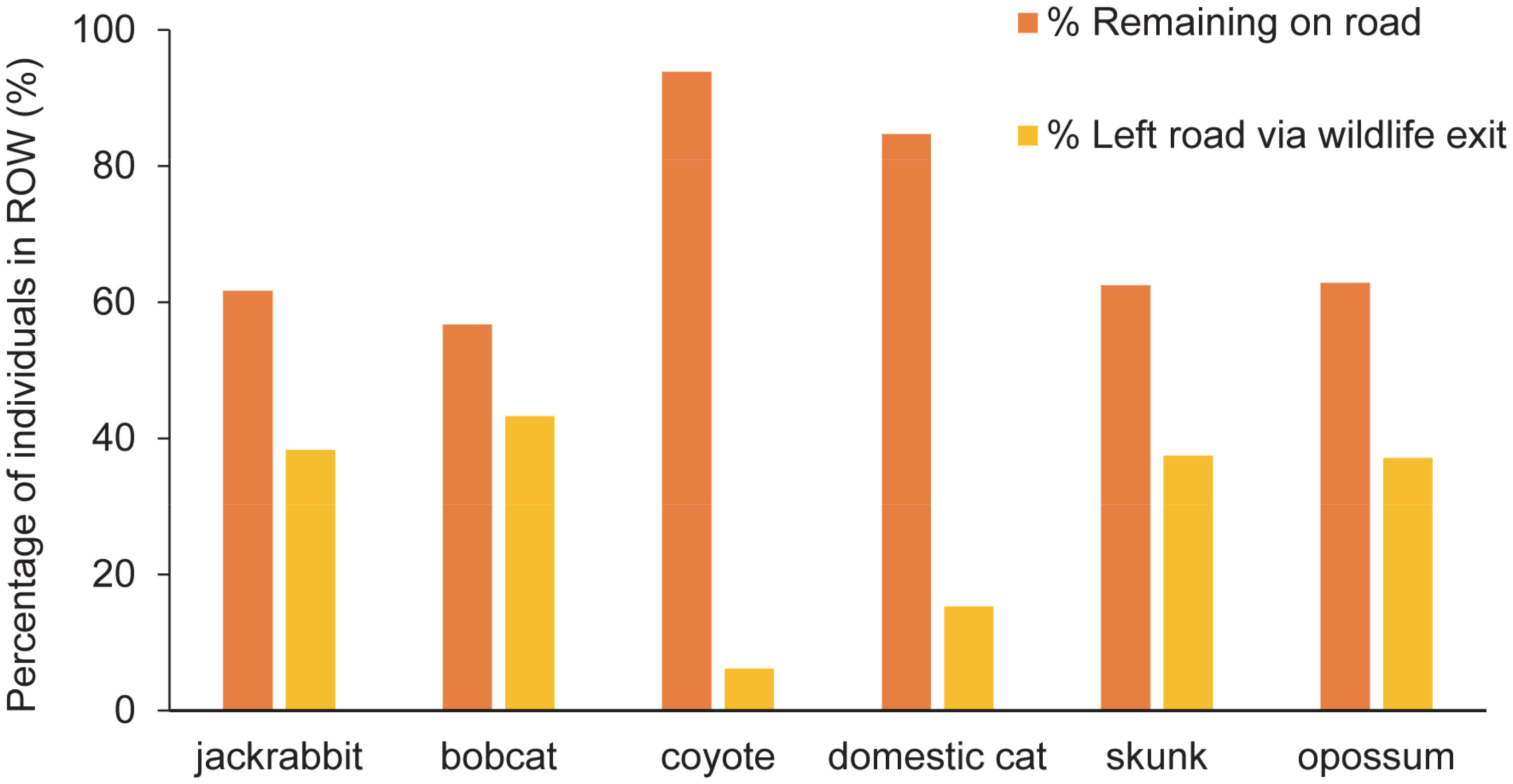
Figure 8 Percentage of wildlife that left the highway using a wildlife exit (WE) along State Highway 100 in Cameron County, TX, compared to the number of individuals remaining on the road after all road to habitat (R-H) exits via a mitigation structure. The time period for all entries and exits used in the analysis was from February 2019 to November 2020. Of the bobcats remaining on the road, approximately 43% of them found and used a WE to escape into the habitat. Approximately 6% of coyotes left on the road successfully used a WE to return to the habitat.
Species density maps for all target species showed that nearly 90% of all wildlife traffic in the R-H direction occurred in the section of SH 100 between WE02 and WE07 (Figure 9A). Wildlife exits that represented the least R-H activity were WE08, WE09, and WE10, contributing < 5% of the total traffic. Approximately 83% of wildlife traffic in the H-R direction occurred between WE04 and WE07, with all other WE sites exhibiting low activity levels in this direction.
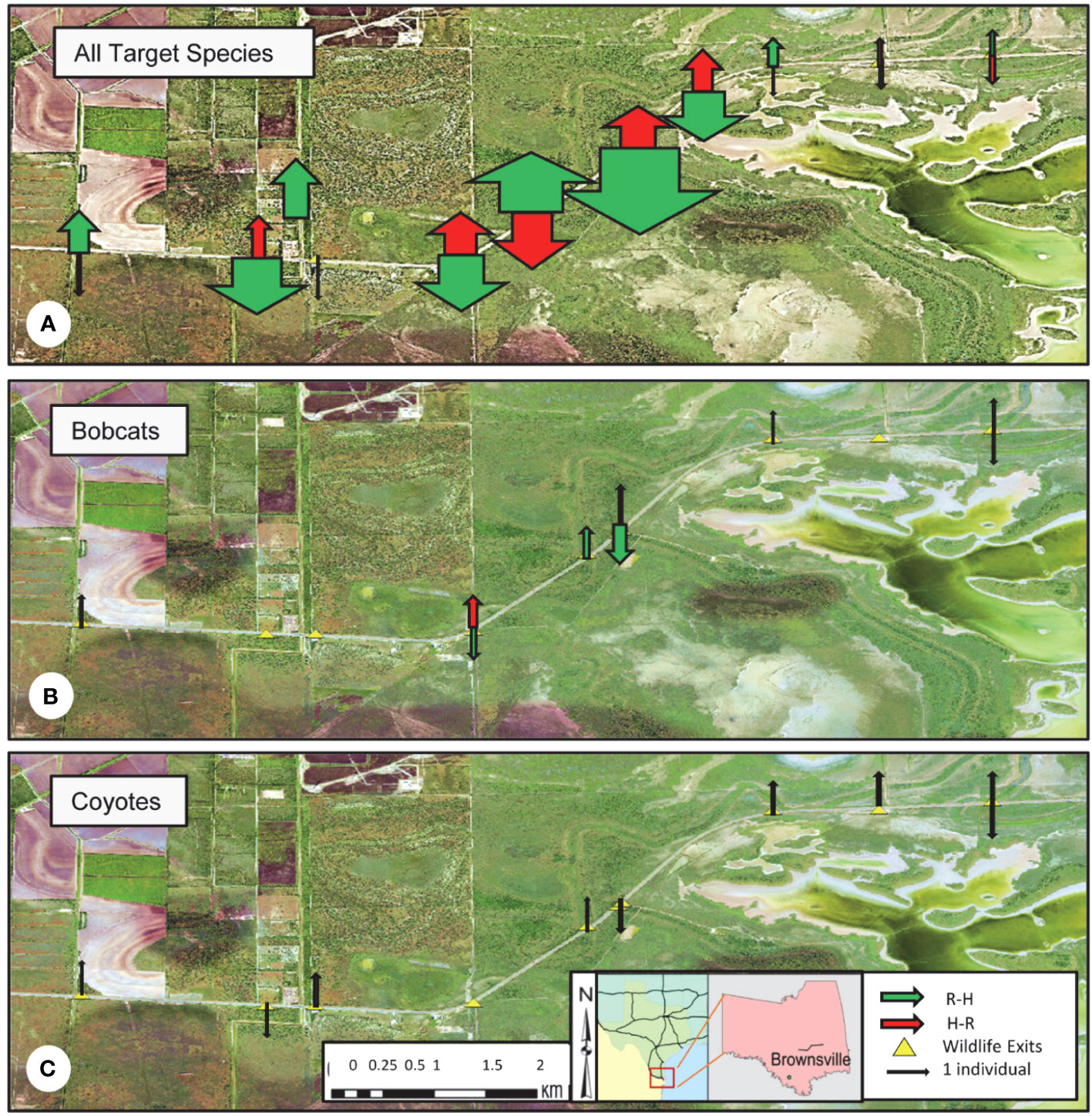
Figure 9 Species density maps indicating the proportion of total wildlife traffic in the intended direction of road-to-habitat (R-H, green arrow), and unintended direction of habitat-to-road (H-R, red arrow) along State Highway 100 in Cameron County, TX, from February 2019 to November 2020. (A) Species included were black-tailed jackrabbits, bobcats, coyotes, domestic cats, striped skunks, and Virginia opossums. (B) Bobcat density map illustrating the proportion of bobcat traffic in the R-H and H-R directions. Bobcats successfully used 6 of the 10 wildlife exits to travel from the road to the habitat during the data collection period. (C) Coyote density map illustrating the proportion of coyote traffic in the R-H and H-R directions. Coyotes successfully used 8 of the 10 wildlife exits to travel from the road to the habitat during the data collection period. All road to habitat (green) arrows designate the side of the highway where the wildlife exit is present.
3.3 Bobcat and coyote activity
3.3.1 Bobcats and coyotes use of WE over time
Bobcat and coyote use of WE increased over time for crossing events observed in the road to habitat direction. Two bobcats used WE within the first 30 days of the installation of the WE to move from R-H, with both species correctly using WE after approximately six months post-installation (Figure 10A).
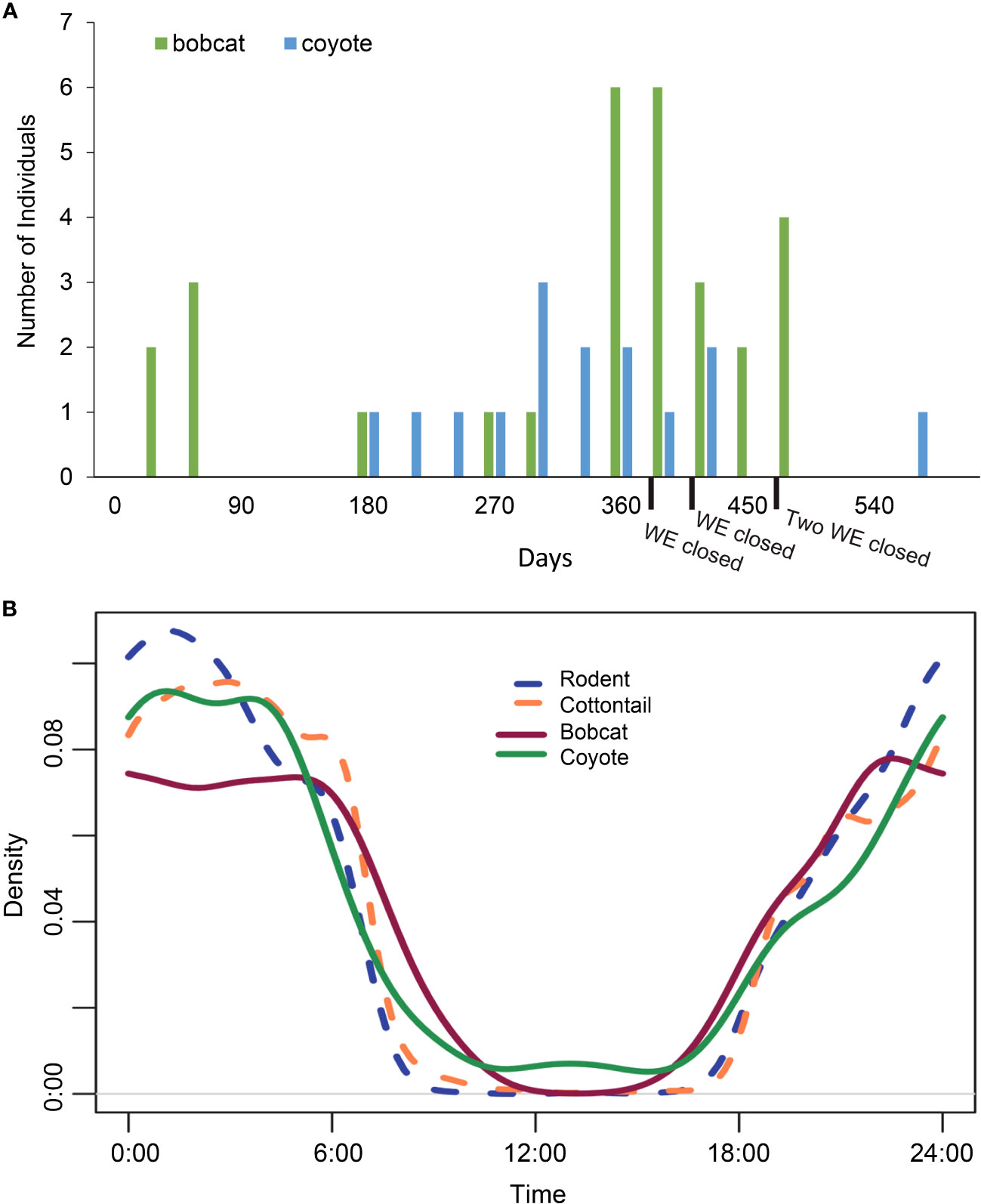
Figure 10 Bobcat and coyote activity. (A) Total number of bobcats and coyotes on State Highway 100 in Cameron County, TX, that learned to use the wildlife exits to travel from the road to the habitat from February 2019 to November 2020. At the end of this collection period, 4 of the 10 wildlife exits had been closed, which could explain the decrease in mesocarnivore R-H activity around a year and a half into the collection period. Bobcats used 6 of the 10 wildlife exits and coyotes used 8 of the 10 wildlife exits. (B) Bobcat, coyote, cottontail, and rodent activity as a function of the hour of the day across all interaction types captured on cameras at the wildlife exits (WE) on State Highway 100 in Cameron County, TX, between February 2019 and November 2020. The overlap in activity patterns was high and ranged from 87% to 92%.
3.3.2 Bobcat vs. coyote use of WE
Of all complete crossing events made across all 10 WE in either direction, 69% of bobcats and 75% of coyotes were observed using WE to correctly travel from R-H (Table 4). Bobcats used six of the ten WE and coyotes used eight of them. Furthermore, there was substantial overlap with coyotes using all WE that bobcats used, with the exception of WE04. Bobcat and coyote activity decreased nearly a year and a half into this study due to WE closure from unintended H-R use (Figure 10A).
Bobcat activity in the R-H direction showed that approximately 83% of successful crossings were concentrated around WE04, WE05, and WE06 (Figure 9B). For bobcats accessing the road via WE in the H-R direction, WE04 displayed the highest level of activity. When considering coyote activity, R-H crossing events were relatively evenly distributed across all ten WE sites (Figure 9C). Conversely, all H-R attempts for coyotes during this time period were restricted to two exits, WE09 and WE10.
The total number of coyote observations at WE was nearly double the total number of bobcat observations. For all WE combined, diel activity analysis showed that bobcats and coyotes had a high activity overlap that was not significant (87.3%; range 80.9–94.1, p = 0.050, Figure 10B). Bobcat activity reached its peak at 10 p.m. and then again during the hours 12 a.m.–5 a.m. Comparatively, coyote activity climbed at 10 p.m., increasing again from 12 a.m. to 4 a.m. Although there were no bobcat occurrences at exits between the hours of 10 a.m. and 3 p.m. throughout this time period, coyotes were recorded near a WE at every hour of the day except 11 a.m. and 3 p.m.
3.4 Mesocarnivore and prey activity
Wildlife exits were observed to have a high number of cottontails and rodents (Table 5). The average number of cottontails across all 10 sites was 433.2 ± 190.3, and the average number of rodents was 318.3 ± 75.42. Cottontail densities were highest at WE03, which also harbored the most coyote activity (58%) of all WE. This exit was followed by relatively equal relative abundances of cottontails found at WE01 and WE06. Approximately 70% of rodent activity was concentrated between WE05 and WE08, with WE03 contributing an additional 14% (Table 5). Rodent activity was highest at WE06, with nearly 25% of all activity occurring at this one site, followed closely by rodent activity at WE07 (19%). However, across all of the WE, none of the correlations were significant for: “rodents × bobcats” rs = 0.505, p (one-tailed = 0.068), “rodents × coyotes” rs = 0.177, p (one-tailed = 0.310), “cottontails × bobcats” rs = 0.340, p (one-tailed = 0.168), or “cottontails × coyotes” rs = 0.323, p (one-tailed = 0.181). For all WE combined, diel activity analysis showed that bobcats had significant activity overlaps with cottontails (89.2%; range, 85.7–97.0; p = 0.02) and rodents 87.1% (82.8–95.3; p = 0.003). Coyotes had higher significant activity overlaps with cottontails (92.0%, 90.2–97.2; p = 0.023) and rodents (90.1%, 87.6–95.7; p = 0.002, Figure 10B).
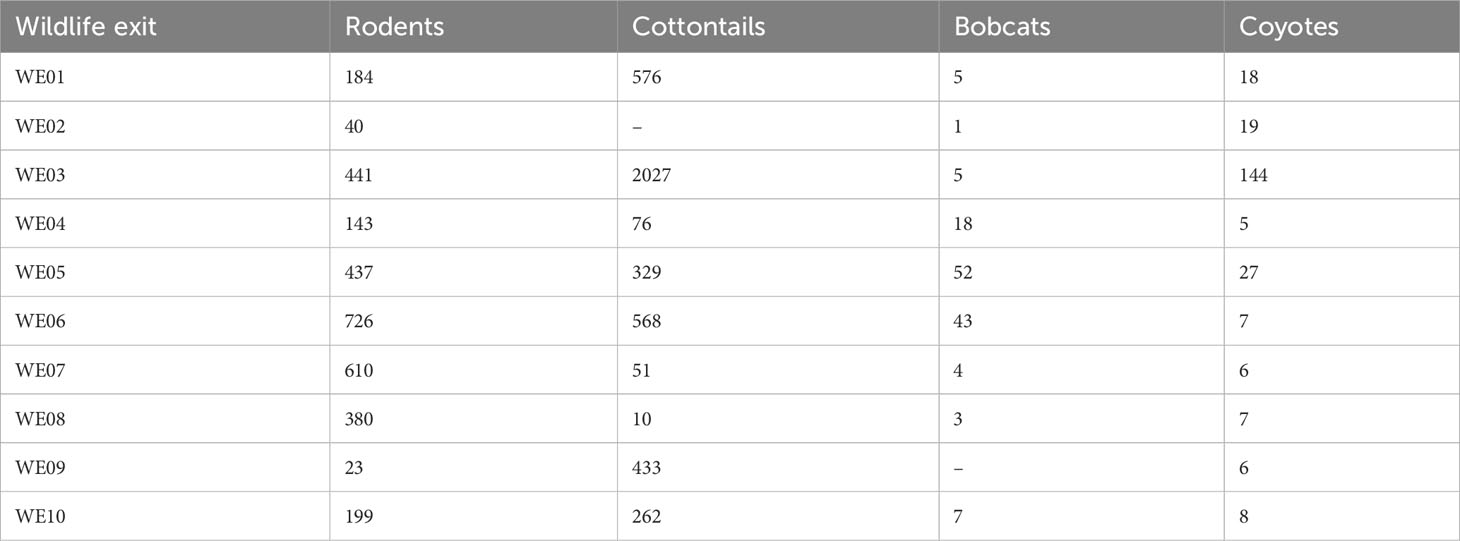
Table 5 Total number of rodents, cottontails, bobcats, and coyotes observed at each wildlife exit (WE) on State Highway 100 in Cameron County, Texas between February 2019 and November 2020.
4 Discussion
4.1 Characterization of the wildlife community at wildlife exits and right-of-way locations
The initial hypothesis in this study testing the differences in occurrence and behavior of target species communities occurring at WE along SH 100 was supported. Of the factors considered, movement category, particularly those animals using the WE to get off the road, showed significant distances. In addition, canopy cover, side of the highway, monthly precipitation, and movement category were all significant factors in describing the target species assemblages interacting with WE locations in the present study. Wildlife crossing structures have been documented to have variable wildlife activity depending on location (Rodriguez et al., 1996; Glista et al., 2009) and differences in species presence and abundance along a road or highway are often dependent on multiple factors (Dickson et al., 2005; Leblond et al., 2013; Cuyckens et al., 2016) including seasonality (Craveiro et al., 2019), suitable habitat (Grilo et al., 2008), availability of and proximity to water sources (Ng et al., 2004), and vehicle traffic (Chruszcz et al., 2003; Grilo et al., 2008). Finally, the species composition and relative abundance between WE and ROW locations were expected to be similar. This hypothesis was partially rejected, as the assemblages at ROW sites were significantly different from all other categories. However, the species richness and overall relative abundance were not significantly different between ROW and P-R.
Research carried out by Van der Grift and Van der Ree (2015) outlines guidelines for evaluating species’ use of WCS, stating that wildlife communities using WCS should eventually reflect actual species communities in the surrounding area. Further testing of the movement categories revealed significant dissimilarities between parallel events on the habitat side (P-H) when compared to the other three interaction types, including parallel movements on the road (P-R), the road to habitat (R-H) crossings, and habitat to the road (H-R) crossings. Potential explanations behind these results could be that there were more resources accessible to wildlife on the habitat side of the chain-link fence. Ng et al. (2004) found that sources of water are important factors that have been associated with raccoon presence near WCS that contained water. Additionally, a sense of security in the form of dens, burrows, and trees common in denser habitats does not exist in the ROW area along with SH 100. The grassy area is often mowed, leaving no form of cover for species that rely on bushes and canopy cover either to hunt for prey or escape a predator. McDonald and St Clair (2004) investigated the small mammal communities near crossing structures in Banff National Park in Alberta, Canada, and found that translocated rodents had a higher return success across crossing structures with greater vegetative cover. An abundant food source present in habitats with high canopy cover is likely to support more carnivores such as ocelots, bobcats, and coyotes (Harveson et al., 2004; Booth-Binczik et al., 2013; Lombardi et al., 2017). For the carnivores in this study area, a large population of prey such as rodents and the eastern cottontail is key to sustaining small populations of predators, including the endangered ocelot (Booth-Binczik et al., 2013).
Analysis between the R-H and H-R groups showed significant differences as well, suggesting that the present dimensional design of the WE could be influencing the type of wildlife activity observed. This association was found in a related study that established a relationship between the dimensions of a crossing structure and the size of an animal, demonstrating that larger animals tend to utilize larger structures (Mata et al., 2005). These wildlife exits were originally created to allow ocelots that entered the ROW an option to escape into the habitat via a WE and, as a result, were designed for wildlife the size of a small felid. However, many of the species included in the analysis were found to be small enough to travel in either direction in the current WE design. The smallest target species, cottontails, contributed the highest dissimilarity of all species observed between these two groups (36%). A potential reason for these differences, apart from their small size and ease of travel through the WE, could be that the ROW along SH 100 is typically covered in grass. Grasses are a primary source of food for cottontails (Dalke and Sime, 1941), which could explain why they access the ROW via a WE more frequently than other species.
An important finding of this study was that bobcats and coyotes were found on the lower end of species contributions for the groups that produced dissimilar results in relative abundance. However, coyotes and bobcats displayed the highest percentage of contribution between the R-H and H-R groups, reflecting their use of the WE structures. These observations suggest a potential for ocelot behavior for individuals that approach a WE from the road. Given their similarities in behavior and ecology to felids such as bobcats (Grigione and Mrykalo, 2004; Booth-Binczik et al., 2013), it is likely that they would respond the same and attempt to use a WE to escape the ROW. Although the WE was designed for felids, coyotes were able to use WE to access the habitat from the roadside. Nearly twice as many coyotes as bobcats were captured on cameras across all WE during this study; however, a higher percentage of bobcats successfully used the WE. Of the coyotes that approached the WE from the roadside, several of the attempts did not result in successful crossings. While reviewing pictures, it was apparent that many of the coyotes that managed to completely cross from R-H often struggled to fit through the narrowest section of the WE, whereas this did not appear to physically limit bobcat R-H crossings.
4.2 Right-of-way communities
In the present study, the composition of the wildlife community at ROW cameras was observed to be significantly different than the WE communities. Examination of the species contribution for each comparison showed that eastern cottontails were responsible for the highest dissimilarity seen between ROW sites when compared to R-H, H-R, and P-R. Cottontails were the most abundant species observed in this study overall and often crossed through the WE to access the preferred grass in the ROW many times in a single camera trap night. Moreover, foraging opportunities in the ROW in the form of forbs and grasses are known to draw wildlife such as deer and rabbits onto roadways and can result in mortalities (Feldhamer et al., 1986; Clevenger and Kociolek, 2013; Huijser et al., 2016). In contrast, nine-banded armadillos were the primary species responsible for dissimilarities between ROW and P-H. There were only three armadillos that made a successful crossing via a WE. Most armadillos were observed in the P-H category, with few in any other interaction category. The size of the armadillos in relation to the narrowest measurement of the WE (13 cm) may have discouraged WE usage in either direction. Armadillos typically prefer areas in dense habitats where they can burrow, rather than open areas with less cover that may increase predator detection (Platt et al., 2004).
The purpose of installing ROW cameras was to characterize wildlife traveling along the roadway that could potentially use a WE. The ROW camera locations were largely covered in grass, with no canopy cover, which is necessary for many species that require dense habitat for shelter (McDonald and St Clair, 2004; Brehme et al., 2013; Lehnen et al., 2021). The average number of species and individuals was significantly lower at ROW than P-H sites, further confirming strong differences in wildlife observed walking along the road side of the fence and wildlife traveling parallel along the habitat near a WE. These results support barrier fencing as an effective method for preventing wildlife from accessing the road (Huijser et al., 2016). Although not significant, the average number of individuals was higher on the habitat side of the fence compared to the number captured on cameras placed in the ROW. The existing mitigation structures likely restrict permeability, limiting the number of individuals that could access the highway (Yanes et al., 1995; Mysłajek et al., 2020). Differences in species richness were apparent between H-R and ROW, where more species, on average, were detected walking parallel along the ROW than using a WE to cross from H-R. This finding provides additional evidence that restriction in the movement through WE may depend on the size, and shape of wildlife (Donaldson, 2007; Mysłajek et al., 2020). Comparisons for P-H and ROW were also significant, indicating strong differences in species communities walking parallel on the habitat near a WE and parallels in the ROW. With data collection spanning only one year, longer monitoring of the ROW would be needed to examine patterns of seasonality that coincide with landscape changes and species abundance.
4.3 Use of wildlife exits
One aim of this study was to determine if the target species would correctly use WE to travel from R-H after entering the roadway via a nearby mitigation structure. Although previous studies investigated wildlife exits such as jump-outs and one-way escapes for large mammals (Bissonette and Hammer, 2000; Huijser et al., 2016), these studies have reported limited success and, moreover, were not designed for mesopredators. A genuine concern after the installation of these exits was that they would potentially create additional points of entry leading to the roadway, which could result in ocelot road mortalities. Incorrect usage has also been observed at WG constructed along highways, which exhibit higher levels of effectiveness for ungulates than carnivores (Allen et al., 2013). Ideally, all individuals remaining in the ROW should successfully find and use a WE solely to travel into the habitat. However, while all of the target species in the present study used WE correctly to travel from R-H, some individuals crossed from H-R via a WE.
Although many forms of mitigation structures such as WG near access roads have been effective in acting as a barrier to wildlife that attempt to enter the roadway (Allen et al., 2013), they do not prevent all wildlife entries onto the road. Similarly, in the present study, WE did not remove all individuals trapped in the ROW. Of the target species that were analyzed, bobcats showed the highest percentage (43%) of successful R-H crossings through a WE. Interestingly, only 6% of coyotes that remained in the ROW after all other exits through other mitigation structures managed to correctly use a WE to return to the habitat. The larger number of coyotes remaining along the road could be attributed to a few different things. Coyotes are the largest species that were observed using WE during this study and visually struggled in their attempts to use them, since the narrow design of the exits was developed for felid use. Ford and Clevenger (2019) found that structural design in culverts can contribute to connectivity, suggesting that openings should be on the habitat side of the fencing due to size limitations for species such as coyotes that have difficulty in crossing fences. The size of an animal combined with its motivation to cross a mitigation structure is thought to contribute to the effectiveness of WG (VerCauteren et al., 2009; Allen et al., 2013). On SH 100, individuals were often seen having trouble using a WE to escape the roadway and would occasionally give up after several attempts at squeezing through the thinnest section. Another possibility could be that some of these coyotes were actually returning to the habitat via a WG and were not detected on the camera because the individual moved past the camera too quickly.
4.4 Mesocarnivore activity
Another goal of this study was to determine if WE would be effective for ocelots and bobcats in this area, and how many of the WE would be used. Previous findings suggest that there may be a strong learning curve for carnivores that use WCS over time, stressing the importance of long-term monitoring (Clevenger and Waltho, 2000; Clevenger and Waltho, 2005; Gagnon et al., 2011). This study found that bobcats and coyotes can learn to use WE over time to travel not only from R-H, but also from H-R. Other studies have speculated on the learning abilities of bears (Sawaya et al., 2014; Huijser et al., 2016), wolves, ungulates (Gagnon et al., 2011; Huijser et al., 2016), and other mammals (Jackson and Griffin, 2000) to use mitigation structures over time. On SH 100, bobcats learned within the first month to travel from R-H using a WE, while it took coyotes six times longer to learn to correctly use a WE. Overall, the majority of bobcats and coyotes that successfully crossed using a WE performed this crossing in the R-H direction. Since bobcat behavior has been used as a predictor for ocelots (Cain et al., 2003; Horne et al., 2009), these results provide hope that a future ocelot that is trapped in the ROW may use a WE as a means of escape.
Concentrations of activity in the R-H direction differed for bobcats and coyotes across the study area. Bobcats and coyotes used 60% and 80% of the WE, respectively, with twice as many bobcat R-H events as coyote R-H events. One potential reason for the high bobcat R-H usage may be due to size differences, which allow felids to walk through narrow openings with greater ease than their canid counterparts. Ruediger (2007) suggested that biological criteria may be useful when designing mitigation structures for carnivore use, noting that the size of the structure could influence usage by target species. Although Ruediger (2007) grouped bobcats and coyotes into the same size category, for structures as small as WE, dimensions appear to impact these two species differently. Another explanation may be found when considering habitat types. Bobcat activity was highest in areas near WE that contained some degree of canopy cover, whereas coyotes were detected across habitats with varying levels of canopy cover. Although bobcats can occur in different environments, they generally prefer dense habitats with high canopy cover that is useful for hunting prey (Cain et al., 2003; Horne et al., 2009), while coyotes have broader diet ranges that allow them to hunt or forage in areas that are more open (Andelt et al., 1987; Lombardi et al., 2020). From these bobcat results, inferences could be made for ocelots in this area. Ocelots are habitat specialists that show a preference for dense thornscrub (Horne et al., 2009; Satter et al., 2018; Lombardi et al., 2020; Schmidt et al., 2020) and would likely benefit from using WE installed in areas of denser habitat along SH 100.
Interestingly, no bobcats or coyotes used WE07 to cross in either direction. This exit was approximately 60 m away from WE06, which had 13 bobcat R-H crossings and was the most frequently accessed WE for bobcats. These two WEs are the closest in proximity to each other, yet they had opposite habitat types, with high canopy cover around WE06 and open habitat surrounding WE07. The type of habitat can be significant for the persistence of carnivore populations across their range (Horne et al., 2009; Booth-Binczik et al., 2013; Satter et al., 2018) and could explain why neither bobcats nor coyotes chose to use WE07. Horne et al. (2009) found significant differences in habitat selection for ocelots and bobcats, demonstrating that ocelots consistently selected areas of denser habitat than bobcats. Indeed, canopy cover was a significant factor in this study and bobcat activity occurred near sites with some of the densest habitats.
Although the bobcat–cottontail and coyote–cottontail correlations in this study were not statistically significant, significant activity pattern overlaps were observed with both species and could be an incentive for bobcat H-R movements through WE. Cottontails are one of the primary sources of prey for mesocarnivores in this area (Beasom and Moore, 1977; Andelt, 1985; Andelt et al., 1987; Booth-Binczik et al., 2013). Most of the cottontails were detected on cameras at three sites: WE03, WE01, and WE06. Comparatively, WE03 also had the largest coyote presence of all 10 WEs, a common predator of the eastern cottontail across its range (Andelt et al., 1987; Cepek, 2004). For bobcats, WE05 and WE06 had the highest occurrences of bobcat activity. While WE06 was a popular exit for both bobcats and cottontails, the two species did not compare when considering the overall percentages across all sites. Approximately 33% of bobcats visited this site, whereas only 14% of cottontails did. Bobcats tend to specialize in cottontails as a food source across their range. Tewes et al. (2002) reviewed 54 scientific sources for information on bobcat food habits and found that lagomorphs and rodents were dominant in bobcat diets across the United States.
Following the same pattern, the bobcat–rodent and coyote–rodent correlations were not statistically significant. However, significant activity pattern overlaps were observed with both species. Rodents also comprise a significant portion of the diet of ocelots, bobcats, and coyotes in south Texas (Haines et al., 2005a; Booth-Binczik et al., 2013). The majority of rodent activity on SH 100 was concentrated between WE05 and WE08, and WE03. The WE with the highest level of bobcat R-H activity was at WE06, which was also the site that had the most rodent activity (25%). Coyotes showed a moderate peak with rodent activity at WE03. This WE has extensive canopy cover that is likely to support an abundance of mesocarnivore prey. These findings align with previous studies that suggest that the placement of wildlife structures maybe even more important than the design (Rodriguez et al., 1996; Cain et al., 2003), especially if suitable habitat is present along distinct sections of roadway.
Studies suggest that wildlife can become used to mitigation structures once they learn of their presence (McCollister and Van Manen, 2010; Simpson et al., 2016), particularly resident populations that are likely to use structures more frequently than migratory populations. This could lead to the possibility of a reduction in the effectiveness of WE for wildlife that repeatedly approach from the habitat and attempt to cross onto the road. To mitigate this issue, once an H-R event for a bobcat or coyote was recorded, the WE it occurred at was manually closed in an effort to discourage future crossings made from the habitat side of the chain-link fence. In some instances, bobcats or coyotes were captured visiting the same WE in the days following the WE’s closure with clear interest or attempts to pass through the fencing. Wildlife can learn the locations of crossings and other mitigation structures, increasing the frequency of use (Clevenger and Waltho, 2005; Gagnon et al., 2011). In two cases, a bobcat approached a closed WE and jumped over the chain-link fence after realizing that it was unable to cross. These cases provide further support that carnivores such as the ocelot should be capable of learning the location of WE and will repeatedly return to these sites.
5 Conclusions and future research
Overall, WE were being used correctly by most target species to escape the roadway in this study. Although it was expected that wildlife would eventually learn to use these exits to travel from H-R, most used it correctly and traveled in the intended R-H direction. However, there was a learning component involved that was observed in bobcats and coyotes, and one year into this study, bobcats and coyotes began using WE to access the road more frequently. Considerations must be made for the future of ocelot conservation, especially for the two small US populations remaining in south Texas. Ocelots have been recorded in areas near SH 100 and have previously been hit by vehicles on this highway. If they learn to access the roadway via a WE, this offers concerns about the design of the exits and emphasizes the need for modifications. At this time, improvements to WE design should be investigated. Potential modifications could include unidirectional doors that may make it possible for animals to travel one way so that they cannot be opened from the habitat side. A reduction in the width of WE could make them more difficult to travel in the H-R direction. Narrowness may not be an issue for felids, as they typically utilize small or constricted spaces to avoid exposed habitats (Clevenger and Waltho, 2005). Although regular maintenance of the WE mesh has not been necessary, minor repairs or adjustments may be required in time to avoid problems with the integrity of the fencing (Bissonette and Hammer, 2000). Future work investigating factors that are expected to influence wildlife activity at WE is recommended to determine elements that may enhance WE usage. Although the purpose of mitigation structures such as WG, gates, and WW is to prevent wildlife from entering the road entirely, the activity at WE is expected to vary, as these structures are known to be circumvented by wildlife at times. Activity is expected to depend on the distance of each exit to its nearest mitigation structure. Close proximity to nearby mitigation structures will likely result in an increase in WE use, as animals that cross onto the road will have more opportunities to exit the ROW using a WE. Similarly, the type of mitigation structure could influence the amount of wildlife activity recorded at the exits and should be examined.
Data availability statement
The original contributions presented in the study are included in the article/supplementary material. Further inquiries can be directed to the corresponding author.
Ethics statement
Ethical approval was not required for the study involving animals in accordance with the local legislation and institutional requirements because the data collected in this study was observational camera trap data only.
Author contributions
Conceptualization: RK, KR, JY, and ZS; Methodology: RK, JL, and ZS; Visualization: ZS, JL, and KR; Software: RK and ZS; Validation and formal analysis: RK, ZS, JL, and CG; Supervision: RK, CG, and JY; Writing – original draft preparation: ZS, RK, and MR; Writing – review and editing: ZS, RK, MR, JL, and CG; Funding: RK. All authors contributed to the article and approved the submitted version.
Funding
This study was supported by funding from the Texas Department of Transportation (grant number 57-3XXIA002) to RK.
Acknowledgments
We thank the Texas Department of Transportation, Pharr District for background information about this project. We thank the US Fish and Wildlife Service for land access. Additionally, we thank individual members of the Kline lab for their assistance and support during my time on this project: T. Miles Hopkins, Thomas Yamashita, Anna Rivera Roy, Adam Sanjar, Bradley Beer, Victoria Rodriguez, and Alexa Campos for helping with fieldwork and sorting countless photos.
Conflict of interest
The authors declare that the research was conducted in the absence of any commercial or financial relationships that could be construed as a potential conflict of interest.
Publisher’s note
All claims expressed in this article are solely those of the authors and do not necessarily represent those of their affiliated organizations, or those of the publisher, the editors and the reviewers. Any product that may be evaluated in this article, or claim that may be made by its manufacturer, is not guaranteed or endorsed by the publisher.
Abbreviations
H-R, habitat to road; P-H, parallel events on the habitat; P-R, parallels events on the road; R-H, road to habitat; ROW, right-of-way; SH, state highway; WCSs, wildlife crossing structures; WE, wildlife exits; WG, wildlife guards, WVCs, wildlife–vehicle collisions; WW, wing walls.
References
Allen T. D., Huijser M. P., Willey D. W. (2013). Effectiveness of wildlife guards at access roads. Wildl. Soc Bull. 37 (2), 402–408. doi: 10.1002/wsb.253
Andelt W. F., Kie J. G., Knowlton F. F., Cardwell K. (1987). Variation in coyote diets associated with season and successional changes in vegetation. J. Wildl. Manage. 51 (2), 273–277. doi: 10.2307/3801002
Andis A. Z., Huijser M. P., Broberg L. (2017). Performance of arch-style road crossing structures from relative movement rates of large mammals. Front. Ecol. Evol. 5. doi: 10.3389/fevo.2017.00122
Ascensão F., Clevenger A., Santos-Reis M., Urbano P., Jackson N. (2013). Wildlife-vehicle collision mitigation: Is partial fencing the answer? An agent-based model approach. Ecol. Model. 257, 36–43. doi: 10.1016/j.ecolmodel.2013.02.026
Beasom S. L., Moore R. A. (1977). Bobcat food habit response to a change in prey abundance. Southwestern Nat. 21 (4), 451–457.
Benjamini Y., Hochberg Y. (1995). Controlling the false discovery rate: a practical and powerful approach to multiple testing. J. R. Stat. society: Ser. B (Methodological) 57 (1), 289–300. doi: 10.1111/j.2517-6161.1995.tb02031.x
Bissonette J. A., Hammer M. (2000). Effectiveness of earthen return ramps in reducing big game highway mortality in Utah. Utah Cooperative Fish and Wildlife Research Unit Report Series, 1–29. Available at: https://escholarship.org/uc/item/2f1080nm.
Booth-Binczik S. D., Bradley R. D., Thompson C. W., Bender L. C., Huntley J. W., Harvey J. A., et al. (2013). Food habits of ocelots and potential for competition with bobcats in southern Texas. Southwestern Nat. 58 (4), 403–410. doi: 10.1894/0038-4909-58.4.403
Brehme C. S., Tracey J. A., McClenaghan L. R., Fisher R. N. (2013). Permeability of roads to movement of scrubland lizards and small mammals. Conserv. Biol. 27 (4), 710–720. doi: 10.1111/cobi.12081
Cain A. T., Tuovila V. R., Hewitt D. G., Tewes M. E. (2003). Effects of a highway and mitigation projects on bobcats in Southern Texas. Biol. Conserv. 114, 189–197. doi: 10.1016/S0006-3207(03)00023-5
Cepek J. (2004). Diet composition of coyotes in the Cuyahoga Valley National Park, Ohio. Ohio J. Sci. 104 (3), 60–64.
Chruszcz B., Clevenger A. P., Gunson K. E., Gibeau M. L. (2003). Relationships among grizzly bears, highways, and habitat in the Banff-BOW Valley, Alberta, Canada. Can. J. Zool. 81 (8), 1378–1391. doi: 10.1139/z03-123
Clarke K. R., Tweedley J. R., Valesini F. J. (2013). Simple shade plots aid better long-term choices of data pre-treatment in multivariate assemblage studies. J. Mar. Biol. Assoc. UK 94 (1), 1–16. doi: 10.1017/s0025315413001227
Clevenger A. P., Kociolek A. V. (2013). Potential impacts of highway median barriers on wildlife: State of the practice and gap analysis. Environ. Manage. 52 (5), 1299–1312. doi: 10.1007/s00267-013-0155-0
Clevenger A. P., Waltho N. (2000). Factors influencing the effectiveness of wildlife underpasses in Banff National Park, Alberta, Canada. Cons. Biol. 14 (1), 47–56. doi: 10.1046/j.1523-1739.2000.00099-085.x
Clevenger A. P., Waltho N. (2005). Performance indices to identify attributes of highway crossing structures facilitating movement of large mammals. Biol. Conserv. 121 (3), 453–464. doi: 10.1016/j.biocon.2004.04.025
Craveiro J., Bernardino J., Mira A., Vaz P. G. (2019). Impact of culvert flooding on carnivore crossings. J. Environ. Manage. 231, 878–885. doi: 10.1016/j.jenvman.2018.10.108
Cuyckens G. A., Mochi L. S., Vallejos M., Perovic P. G., Biganzoli F. (2016). Patterns and composition of road-killed wildlife in Northwest Argentina. Environ. Manage. 58 (5), 810–820. doi: 10.1007/s00267-016-0755-6
D'Amico M., Périquet S., Román J., Revilla E. (2015). Road avoidance responses determine the impact of heterogeneous road networks at a regional scale. J. Appl. Ecol. 53 (1), 181–190. doi: 10.1111/1365-2664.12572
Dalke P. D., Sime P. R. (1941). Food habits of the eastern and New England cottontails. J. Wildl. Manage. 5 (2), 216. doi: 10.2307/3795589
Dickson B. G., Jenness J. S., Beier P. (2005). Influence of vegetation, topography, and roads on cougar movement in southern california. J. Wildl. Manage. 69 (1), 264–276. doi: 10.2193/0022-541x(2005)0692.0.co;2
Donaldson B. (2007). Use of highway underpasses by large mammals and other wildlife in Virginia. J. Transport. Res. Board 2011 (1), 157–164. doi: 10.3141/2011-17. Transportation Research Record.
Elizalde-Arellano C., Hernández L., López-Vidal J., Laundre J. W. (2012). Home range, size, and activity patterns of bobcats (Lynx rufus) in the southern part of their range in the Chihuahuan Desert, Mexico. Am. Midland Nat. 168 (2), 247–264. doi: 10.1674/0003-0031-168.2.247
Feldhamer G. A., Gates J. E., Harman D. M., Loranger A. J., Dixon K. R. (1986). Effects of interstate highway fencing on white-tailed deer activity. J. Wildl. Manage. 50 (3), 497. doi: 10.2307/3801112
Ford A. T., Clevenger A. P. (2010). Validity of the prey-trap hypothesis for carnivore-ungulate interactions at wildlife crossing structures. Conserv. Biol. 24 (6), 1679–1685. doi: 10.1111/j.1523-1739.2010.01564.x
Ford A. T., Clevenger A. P. (2019). Factors affecting the permeability of road mitigation measures to the movement of small mammals. Can. J. Zool. 97 (4), 379–384. doi: 10.1139/cjz-2018-0165
Forman R. T. T., Alexander L. E. (1998). Roads and their major ecological effects. Annu. Rev. Ecol. Systemat. 29, 207–231. doi: 10.1146/annurev.ecolsys.29.1.207
Foster M. L., Humphrey S. R. (1995). Use of highway underpasses by Florida panthers and other wildlife. Wildl. Soc Bull. 23 (1), 95–100.
Gagnon J. W., Dodd N. L., Ogren K. S., Schweinsburg R. E. (2011). Factors associated with use of wildlife underpasses and importance of long-term monitoring. J. Wildl. Manage. 75 (6), 1477–1487. doi: 10.1002/jwmg.160
Gilhooly P. S., Nielsen S. E., Whittington J., St. Clair C. C. (2019). Wildlife mortality on roads and railways following highway mitigation. Ecosphere 10 (2). doi: 10.1002/ecs2.2597
Glista D. J., DeVault T. L., DeWoody J. (2008). Vertebrate road mortality predominantly impacts amphibians. Herpetol. Conserv. Biol. 3 (1), 77–87.
Glista D. J., DeVault T. L., DeWoody J. A. (2009). A review of mitigation measures for reducing wildlife mortality on roadways. Landscape Urban Plann. 91 (1), 1–7. doi: 10.1016/j.landurbplan.2008.11.001
Gloyne C. C., Clevenger A. P. (2001). Cougar Puma concolor use of wildlife crossing structures on the Trans-Canada highway in Banff National Park, Alberta. Wildl. Biol. 7, 117–1124. doi: 10.2981/wlb.2001.009
Grigione M. M., Mrykalo R. (2004). Effects of artificial night lighting on endangered ocelots (Leopardus paradalis) and nocturnal prey along the United States-Mexico border: A literature review and hypotheses of potential impacts. Urban Ecosyst. 7 (1), 65–77. doi: 10.1023/b:ueco.0000020173.70355.ab
Grilo C., Bissonette J. A., Santos-Reis M. (2008). Response of carnivores to existing highway culverts and underpasses: Implications for road planning and mitigation. Biodiver. Conserv. 17 (7), 1685–1699. doi: 10.1007/s10531-008-9374-8
Haines A., Caso A., Tewes M., Redeker E. (2005b). “Using LANDSAT imagery to identify potential ocelot habitat in Tamaulipas, Mexico,” in Proceedings of the 20th Biennial Workshop on Aerial Photography, Videography, and High Resolution Digital Imagery for Resource Assessment. Vol. 71. Welasco, TX.
Haines A. M., Tewes M. E., Laack L. L. (2005a). Survival and sources of mortality in ocelots. J. Wildl. Manage. 69 (1), 255–263. doi: 10.2193/0022-541x(2005)0692.0.co;2
Harveson P. M., Tewes M. E., Anderson G. L., Laack L. L. (2004). Habitat use by ocelots in south Texas: Implications for restoration. Wildl. Soc Bull. 32 (3), 948–954. doi: 10.2193/0091-7648(2004)032[0948:hubois]2.0.co;2
Heilbrun R. D., Silvy N. J., Peterson M. J., Tewes M. E. (2006). Estimating bobcat abundance using automatically triggered cameras. Wildl. Soc. Bull. 34 (1), 69–73. doi: 10.2193/0091-7648(2006)34[69:EBAUAT]2.0.CO;2
Horne J. S., Haines A. M., Tewes M. E., Laack L. L. (2009). Habitat partitioning by sympatric ocelots and bobcats: Implications for recovery of ocelots in southern Texas. 54 119-126, 118. doi: 10.1894/PS-49.1
Huijser M. P., Duffield J. W., Clevenger A. P., Ament R. J., McGowen P. T. (2009). Cost-benefit analyses of mitigation measures aimed at reducing collisions with large ungulates in the United States and Canada: A decision support tool. Ecol. Soc 14 (2), 15. doi: 10.5751/es-03000-140215
Huijser M. P., Fairbank E. R., Camel-Means W., Graham J., Watson V., Basting P., et al. (2016). Effectiveness of short sections of wildlife fencing and crossing structures along highways in reducing wildlife-vehicle collisions and providing safe crossing opportunities for large mammals. Biol. Conserv. 197, 61–68. doi: 10.1016/j.biocon.2016.02.002
Jackson S. D., Griffin C. R. (2000). “A strategy for mitigating highway impacts on wildlife,” in Wildlife and highways: seeking solutions to an ecological and socio-economic dilemma. Eds. Messmer T. A., West B. (Bethesda, MD: The Wildlife Society), 143–159.
Jahrsdoerfer S. E., Leslie D. M. Jr. (1988). “Tamaulipan brushland of the Lower Rio Grande Valley of south Texas: Description, human impacts, and management options” in Biological report, Vol. 88 (36). (Washington, DC: U.S. Fish and Wildlife Service).
Janečka J., Tewes M., Laack L., Grassman L. I. Jr., Haines A., Honeycutt R. (2007). Small effective population sizes of two remnant ocelot populations (Leopardus pardalis albescens) in the United States. Conserv. Genet. 9, 869–878. doi: 10.1007/s10592-007-9412-1
Kindall J. L., Manen F. T. V. (2007). Identifying habitat linkages for American black bears in North Carolina, USA. J. Wildl. Manage. 71, 487–495. doi: 10.2193/2005-709
Leblond M., Dussault C., Ouellet J. (2013). Impacts of human disturbance on large prey species: Do behavioral reactions translate to fitness consequences? PloS One 8 (9). doi: 10.1371/journal.pone.0073695
Lehnen S. E., Sternberg M. A., Swarts H. M., Sesnie S. E. (2021). Evaluating population connectivity and targeting conservation action for an endangered cat. Ecosphere 12 (2). doi: 10.1002/ecs2.3367
Lombardi J. V., Comer C. E., Scognamillo D. G., Conway W. C. (2017). Coyote, fox, and bobcat response to anthropogenic and natural landscape features in a small urban area. Urban Ecosyst. 20 (6), 1239–1248. doi: 10.1007/s11252-017-0676-z
Lombardi J. V., MacKenzie D. I., Tewes M. E., Perotto-Baldivieso H. L., Mata J. M., Campbell T. A. (2020). Co-occurrence of bobcats, coyotes, and ocelots in Texas. Ecol. Evol. 10 (11), 4903–4917. doi: 10.1002/ece3.6242
Mata C., Hervás I., Herranz J., Suárez F., Malo J. (2005). Complementary use by vertebrates of crossing structures along a fenced Spanish motorway. Biol. Conserv. 124 (3), 397–405. doi: 10.1016/j.biocon.2005.01.044
McCollister M. F., Van Manen F. T. (2010). Effectiveness of wildlife underpasses and fencing to reduce wildlife-vehicle collisions. J. Wildl. Manage. 74 (8), 1722–1731. doi: 10.2193/2009-535
McDonald W., St Clair C. C. (2004). Elements that promote highway crossing structure use by small mammals in Banff National Park. J. Appl. Ecol. 41 (1), 82–93. doi: 10.1111/j.1365-2664.2004.00877.x
Mysłajek R. W., Olkowska E., Wronka-Tomulewicz M., Nowak S. (2020). Mammal use of wildlife crossing structures along a new motorway in an area recently recolonized by wolves. Euro. J. Wildl. Res. 66, 79. doi: 10.1007/s10344-020-01412-y
Ng S. J., Dole J. W., Sauvajot R. M., Riley S. P., Valone T. J. (2004). Use of highway undercrossings by wildlife in southern California. Biol. Conserv. 115 (3), 499–507. doi: 10.1016/s0006-3207(03)00166-6
NOAA (2021). National centers for environmental information, climate at a glance: county time series. Available at: https://www.ncdc.noaa.gov/cag/.
O'Brien T. G., Kinnaird M. F., Wibisono H. T. (2003). Crouching tigers, hidden prey: Sumatran tiger and prey populations in a tropical forest landscape. Anim. Conserv. 6 (2), 131–139. doi: 10.1017/s1367943003003172
Platt S., Rainwater T., Brewer S. (2004). Aspects of the burrowing ecology of nine-banded armadillos in northern Belize. Mammal. Biol. 69 (4), 217–224. doi: 10.1078/1616-5047-00138
R Core Team (2022). R: A language and environment for statistical computing (Vienna, Austria: R Foundation for Statistical Computing). Available at: https://www.R-project.org/.
Rodriguez A., Crema G., Delibes M. (1996). Use of non-wildlife passages across a high speed railway by terrestrial vertebrates. J. Appl. Ecol. 33 (6), 1527–1540. doi: 10.2307/2404791
Rowcliffe M., Rowcliffe M. M. (2016). “Package ‘activity’,” in Animal activity statistics R Package Version, 1. Available at: https://cran.irsn.fr/web/packages/activity/activity.pdf.
Ruediger W. C. (2007). “Management considerations for designing carnivore highway crossings,” in Proceedings of the 2007 International Conference on Ecology and Transportation Chapter 7. Eds. Irwin C. L., Nelson D., McDermott K. P.. (Raleigh, NC: Center for Transportation and the Environment, North Carolina State University), 546–555.
Sanderson J., Harris G. (2013). Automatic data organization, storage, and analysis of camera trap pictures. J. Indonesian Nat. Hist. 1 (1), 11–19.
Satter C. B., Augustine B. C., Harmsen B. J., Foster R. J., Sanchez E. E., Wultsch C., et al. (2018). Long-term monitoring of ocelot densities in Belize. J. Wildlife Manag 83 (2), 283–294. doi: 10.1002/jwmg.21598
Sawaya M. A., Kalinowski S. T., Clevenger A. P. (2014). Genetic connectivity for two bear species at wildlife crossing structures in Banff National Park. Proc. R. Soc B: Biol. Sci. 281, 20131705–20131705. doi: 10.1098/rspb.2013.1705
Schmidt G. M., Lewison R. L., Swarts H. M. (2020). Identifying landscape predictors of ocelot road mortality. Landscape Ecol. 35 (7), 1651–1666. doi: 10.1007/s10980-020-01042-4
Simpson N. O., Stewart K. M., Schroeder C., Cox M., Huebner K., Wasley T. (2016). Overpasses and underpasses: effectiveness of crossing structures for migratory ungulates. J. Wildl. Manag., 1370–1378. doi: 10.1002/jw.21132
Soulsbury C. D., White P. C. L. (2015). Human-wildlife interactions in urban areas: a review of conflicts, benefits and opportunities. Wildl. Res. 42, 541–553. doi: 10.1071/WR14229
Tewes M. E., Mock J. M., Young J. H. (2002). Bobcat predation on quail, birds, and mesomammals. Proc. Natl. Quail Symp. 5, 65–70.
U.S. Department of Transportation (2018). National transportation statistics (Washington D.C: Bureau of Transportation Statistics, U.S. Department of Transportation).
U.S. Fish and Wildlife Service (USFWS) (1982). Endangered and threatened wildlife and plants; endangered status for U.S. population of the ocelot. Federal Registar 47, 31670–31672.
Van der Grift E. A., Van der Ree R. (2015). “Guidelines for evaluating use of wildlife crossing structures,” in Handbook of road ecology. Chapter 15. Eds. Ree R., Smith D. J., Grilo C. (UK: John Wiley & Sons), 119–128. doi: 10.1002/9781118568170.ch15
VerCauteren K. C., Seward N., Lavelle M., Fischer J., Phillips G. (2009). Deer guards and bump gates for excluding white-tailed deer from fenced resources. Human-Wildl. Conflicts 3 (1), 145–153. doi: 10.1016/j.rama.2018.11.005
Watson P. A., Alexander H. D., Moczygemba J. D. (2019). Coastal prairie recovery in response to shrub removal method and degree of shrub encroachment. Rangel. Ecol. Manag. 72, 275–282. doi: 10.1016/j.rama.2018.11.005
White L. A., Gehrt S. D. (2009). Coyote attacks on humans in the United States and Canada. Hum. Dimensions Wildl. 14, 419–432. doi: 10.1080/10871200903055326
Wilkins D. C., Kockelman K. M., Jiang N. (2019). Animal-vehicle collisions in Texas: How to protect travelers and animals on roadways. Accident Anal. Preve. 131, 157–170. doi: 10.1016/j.aap.2019.05.030
Keywords: wildlife exits, highway, endangered species, mitigation, wildlife road mortalities, ocelots, mesocarnivores
Citation: Sheikh ZN, Langbein JE, Ryer K, Rahman MS, Gabler CA, Young JH Jr and Kline RJ (2023) Use and effectiveness of wildlife exits designed for ocelots and other mesocarnivores on a south Texas highway. Front. Ecol. Evol. 11:1235223. doi: 10.3389/fevo.2023.1235223
Received: 05 June 2023; Accepted: 01 September 2023;
Published: 13 October 2023.
Edited by:
Darryl Jones, Griffith University, AustraliaReviewed by:
Sean Murphy, University of Kentucky, United StatesGianpasquale Chiatante, University of Tuscia, Italy
Copyright © 2023 Sheikh, Langbein, Ryer, Rahman, Gabler, Young and Kline. This is an open-access article distributed under the terms of the Creative Commons Attribution License (CC BY). The use, distribution or reproduction in other forums is permitted, provided the original author(s) and the copyright owner(s) are credited and that the original publication in this journal is cited, in accordance with accepted academic practice. No use, distribution or reproduction is permitted which does not comply with these terms.
*Correspondence: Richard J. Kline, cmljaGFyZC5rbGluZUB1dHJndi5lZHU=