- 1Department of Biology, Boston University, Boston, MA, United States
- 2Villum Centre for Biodiversity Genomics, Section for Ecology and Evolution, Department of Biology, University of Copenhagen, Copenhagen, Denmark
Ants have remarkably diverse diets and extraordinary species richness, making them an excellent model system to study the evolution of taste. In this entirely eusocial clade, food choice and the mechanisms that regulate feeding have both individual and social dimensions. How taste receptors and sensory processing drive food preferences to generate dietary breadth in ants is poorly understood. It is additionally unclear how elements of colony organization such as division of labor and social food flow impact the mechanistic basis and evolution of taste. Previous work on dipteran, lepidopteran, and hymenopteran gustatory systems, while foundational, provide limited insights into ant dietary specialization. Here we synthesize and analyze research on ant gustation to identify mechanisms, sociobiological correlates, and phylogenetic patterns. We discuss the current state of genomic analyses of taste and future research. We propose that strikingly polymorphic species of Pheidole, Cephalotes, Camponotus, and leafcutter ants (Atta and Acromyrmex) offer compelling social systems to explore adaptive variation in gustation because of their pronounced division of labor in which morphologically, behaviorally, and neurally differentiated workers vary in feeding behavior. Research on ant gustation within and among species will advance our understanding of sensory systems and provide insight into the impact of taste on the evolution of species diversity and how social organization influences gustation.
Introduction
Sensory systems are interfaces between an animal and its environment, enabling adaptive response to variation in environmental stimuli. Because fitness depends on identifying and extracting nutrient and energy resources for metabolism, chemoreception is an important target of selection required for dietary optimization and fitness. Chemoreception includes olfaction (smell: detect volatile chemicals) and gustation (taste: detect soluble chemicals). Gustation coevolves with diet and nutritional ecology as the mechanistic basis of food preference and a driver of species diversification (Li and Zhang, 2013; Baldwin et al., 2014; Tu et al., 2018; Vizueta et al., 2019; Toda et al., 2021; Frank et al., 2022).
The exceptional dietary diversity of ants makes them excellent models to integrate foraging ecology, taste, nutrition, and sociobiology in phylogenetic context. An estimated 20 quadrillion ants inhabit the Earth (Schultheiss et al., 2022); there are 14,000 identified species in 16 extant subfamilies within the eusocial family Formicidae (Bolton, 2023). Feeding in ants has both individual and colony-level components that involve colony nutrition and growth, caste evolution, and division of labor. Ant foraging ecology has shaped community and ecosystem structure as well as biodiversity (Davidson et al., 2004; Parker and Kronauer, 2021). The evolutionary and ecological importance of ants can be attributed to their sociality and roles as scavengers, predators, herbivores, granivores, and plant mutualists. Gustation is a core determinant of ant feeding ecology.
Despite the importance of taste in ant foraging ecology, gustation is poorly understood. Indeed, in Hölldobler and Wilson’s (1990) seminal tome The Ants, the terms diet, feeding, food, and taste are not indexed. Open questions include: How does gustation vary intra- and interspecifically? How did molecular mechanisms of gustation evolve with changes in diet? How has sociality impacted taste? How has taste influenced species diversity? Here we address these questions and present a prospectus for future research.
Ant gustation
Diet preferences
In the well-studied fruit fly Drosophila melanogaster, sugars, amino acids (AAs), and water are attractive, whereas deterrent (“bitter”) compounds are aversive. Salts and acids are attractive at low concentrations but aversive at high concentrations (Ahn et al., 2017; Jaeger et al., 2018). D. melanogaster can also detect water, polyamines, lipopolysaccharides, ammonia, calcium, and H2O2 (Chen and Dahanukar, 2020). Honey bees (Apis mellifera) notably lack a bitter receptor or typical behavioral and electrophysiological responses to bitter chemicals, which instead inhibit sugar receptors (de Brito Sanchez et al., 2005, 2014).
Among ants, there are generalist and specialist predators, omnivorous scavengers, and consumers of extrafloral nectar, fungi, fruits, seeds, and honeydew. Army ants are voracious mass-raiding predators that rely on chemical senses (Kronauer, 2020; McKenzie et al., 2021). Specialist predators hunt spider eggs, springtails, centipedes, or termites (Azorsa et al., 2022). Nectarivores tend gardens to obtain extrafloral nectar (Mayer et al., 2014) or consume honeydew excretions of hemipteran mutualists (Cicconardi et al., 2020). Fungus-growing ants mulch their fungal garden with freshly cut leaves (Hölldobler and Wilson, 2010). This remarkably wide dietary variation is likely reflected in gustatory profiles.
Behavioral studies are foundational to understanding gustation, but research that comprehensively compares sugar preferences is limited to 30 species from four subfamilies. Comparisons are constrained by methodological inconsistencies (Ricks and Vinson, 1970; Shetty, 1982; Vander Meer et al., 1995; Cornelius et al., 1996; Cannon, 1998; Völkl et al., 1999; Tinti and Nofre, 2001; Barbani, 2002; Boevé and Wäckers, 2003; Blüthgen and Fiedler, 2004b; Detrain et al., 2010; Horta-Vega et al., 2010; Detrain and Prieur, 2014; Zhou et al., 2015a,b; Madsen et al., 2017; Madsen and Offenberg, 2020; Jaleel et al., 2021; Renyard et al., 2021). Studies suggest that ants discriminate among sugars and that preference for sucrose, a major component of nectar and honeydew (González-Teuber and Heil, 2009; Shaaban et al., 2020), is conserved. Sucrose attraction increases with concentration (Horta-Vega et al., 2010; Detrain and Prieur, 2014). Species, body size, and sucking pump activity affect sucrose uptake, which is regulated by serotonin (Davidson et al., 2004; Falibene et al., 2009; Josens et al., 2018, 2021). Melezitose (produced exclusively by hemipterans), glucose, fructose, raffinose, and maltose appear to be preferred to other sugars. In comparison, honey bees prefer sucrose, glucose, fructose, maltose, melezitose, and trehalose to other sugars (Von Frisch, 1934). While attractive sugars vary among ant species, preferences also sometimes differ with concentration or methodology (Horta-Vega et al., 2010).
There is limited research on taste in respect to other nutrients. Many ants prefer sugar to AAs and select a combination of sugar and AAs over sugar alone (Lanza, 1991; Blüthgen and Fiedler, 2004b). There is variability in AA preferences among species, as in lepidopterans (Lanza and Krauss, 1984; Blüthgen and Fiedler, 2004b; Agnihotri et al., 2016), but most studies have not comprehensively tested AA preference. Granivores prefer seeds with higher concentrations of AAs and fatty acids (Reifenrath et al., 2012). Leafcutter ants adjust plant substrates for their fungus based on protein-carbohydrate ratio, likely requiring sugar and AA detection (Shik et al., 2021). Electrolyte studies are limited, but Solenopsis richteri workers prefer zinc, magnesium, and ammonium, whereas sodium preference varies (Vinson, 1970). Ants exhibit a geographical gradient in sodium consumption correlating with distance from the ocean, and higher sodium consumption in non-predatory than predatory species (Kaspari et al., 2008, 2020). Bitter taste in ants has not been comprehensively evaluated, although quinine is aversive to Lasius niger (Wenig et al., 2021), high concentrations of caffeine in sucrose reduced feeding in Oecophylla smaragdina (Madsen and Offenberg, 2019), and alkaloids reduced feeding in Ectatomma ruidum (Bolton et al., 2017). Leafcutter ants avoid leaves with anti-fungal terpenoids (Howard et al., 1989), but anti-fungal tannins do not affect cutting (Crumière et al., 2022). Bitter chemical detection may be especially important for evaluating foods with high levels of toxins.
Cellular mechanisms
The cellular mechanisms underlying ant taste are unstudied. In insects, soluble chemicals are detected through activation of taste receptors on the dendrites of sensory neurons within gustatory sensilla, small hairs with a single lymph-filled pore (Clyne et al., 2000; Dunipace et al., 2001; Chen and Dahanukar, 2020). Putative gustatory sensilla have been identified on the antennae, mouthparts, and foreleg tarsi (Barsagade et al., 2013, 2017; Jaleel et al., 2021; Masram and Barsagade, 2021), mirroring the distribution in honey bees (Bestea et al., 2021). Gustatory receptor neurons in insects project primarily to the subesophageal zone of the brain in a pattern categorized by taste modality and sensilla location (Wang et al., 2004). Gustatory receptors (GRs)—a large family containing most arthropod taste receptors—detect sugars, AAs, and deterrent compounds. Some ionotropic receptors (IRs), a family that also functions in olfaction, detect organic acids, fatty acids, AAs, and salts (Benton et al., 2009; Croset et al., 2010; Ganguly et al., 2017; Rimal et al., 2019; Brown et al., 2021). Certain pickpocket channels (PPKs), insect orthologs of the degenerin/epithelial sodium channel (DEG/ENaC) superfamily, and transient receptor potential (TRP) channels are also taste receptors in invertebrates (Chen et al., 2010; Kang et al., 2010). More work is needed to determine broad preference patterns and cellular mechanisms and relate these to dietary variation in ants.
Taste evolution
Evolutionary history and genomics
Genomic analysis is needed to elucidate the mechanistic basis and evolution of taste in ants. GRs with gustatory functions likely evolved in the arthropod ancestor from a reduced number of GR-like genes which predated the ancestor of eukaryotes (Robertson, 2019; Benton et al., 2020; Vizueta et al., 2020). The GR family subsequently evolved across arthropods under a dynamic gene birth-and-death model influenced by episodic bursts of gene duplication yielding lineage-specific expansions (Robertson, 2019). Ionotropic receptors (IRs) and pickpocket channels (PPKs) also have ancient origins, but when they were historically co-opted for gustation remains unknown (Croset et al., 2010; Vizueta et al., 2020; Latorre-Estivalis et al., 2021). Drosophila have 60 GR, 66 IR, and 31 PPK genes (Croset et al., 2010; Joseph and Carlson, 2015; Latorre-Estivalis et al., 2021), but many have non-gustatory or unknown functions. The honey bee genome contains only 11 GRs, four of which have known functions, as well as yet-uncharacterized IRs and PPKs (Robertson and Wanner, 2006; Bestea et al., 2021).
Ancestral hymenopterans were once thought to have few chemosensory genes (Zhou et al., 2015c; McKenzie et al., 2016), but recent research suggests a parasitoid hymenopteran genome with many odorant receptor (OR) genes (Oeyen et al., 2020; Obiero et al., 2021). The early branching Symphyta (sawflies) similarly have more chemosensory genes than previously thought, including 30 GRs. Gene losses may explain lower numbers in some extant species like honey bees (Oeyen et al., 2020). Compared to ORs, hymenopterans other than ants have few GRs (10 to ∼40), excepting lineage-specific expansions in two wasps (Oeyen et al., 2020).
Based on 24 sequenced genomes with annotated GRs, GR number appears variable, ranging from 17 to 219 (Table 1), although these estimations may be inaccurate due to difficulty producing high-quality gene annotations for GRs. There are minimally 13 GR subfamilies in ants, inferred from GR phylogenies of four species (Zhou et al., 2012); their functions are largely unknown. Phylogenetic comparisons with other insects reveal candidate sugar, fructose, and AA receptors in Linepithema humile, Harpegnathos saltator, and Solenopsis invicta (Bestea et al., 2021; Obiero et al., 2021). IR numbers are less variable (18–32 genes) and comparable to most other hymenopterans (Zhou et al., 2012; Obiero et al., 2021; Table 1). Phylogenetic analyzes have also revealed 13 ant subfamilies of “divergent IRs” (Zhou et al., 2012), as well as orthologs of taste implicated IR76b and IR23a from Drosophila (Obiero et al., 2021). PPKs (6–8) from four subfamilies have been identified in two ant species (Latorre-Estivalis et al., 2021), and TRP channels (13–27) from six subfamilies have been identified in seven ant species (Peng et al., 2015), but their functions in gustation are unknown (Table 1). Recent research has elucidated the evolutionary origins of hymenopteran chemosensory receptors, but much remains unknown about their current identities and functions in ants.
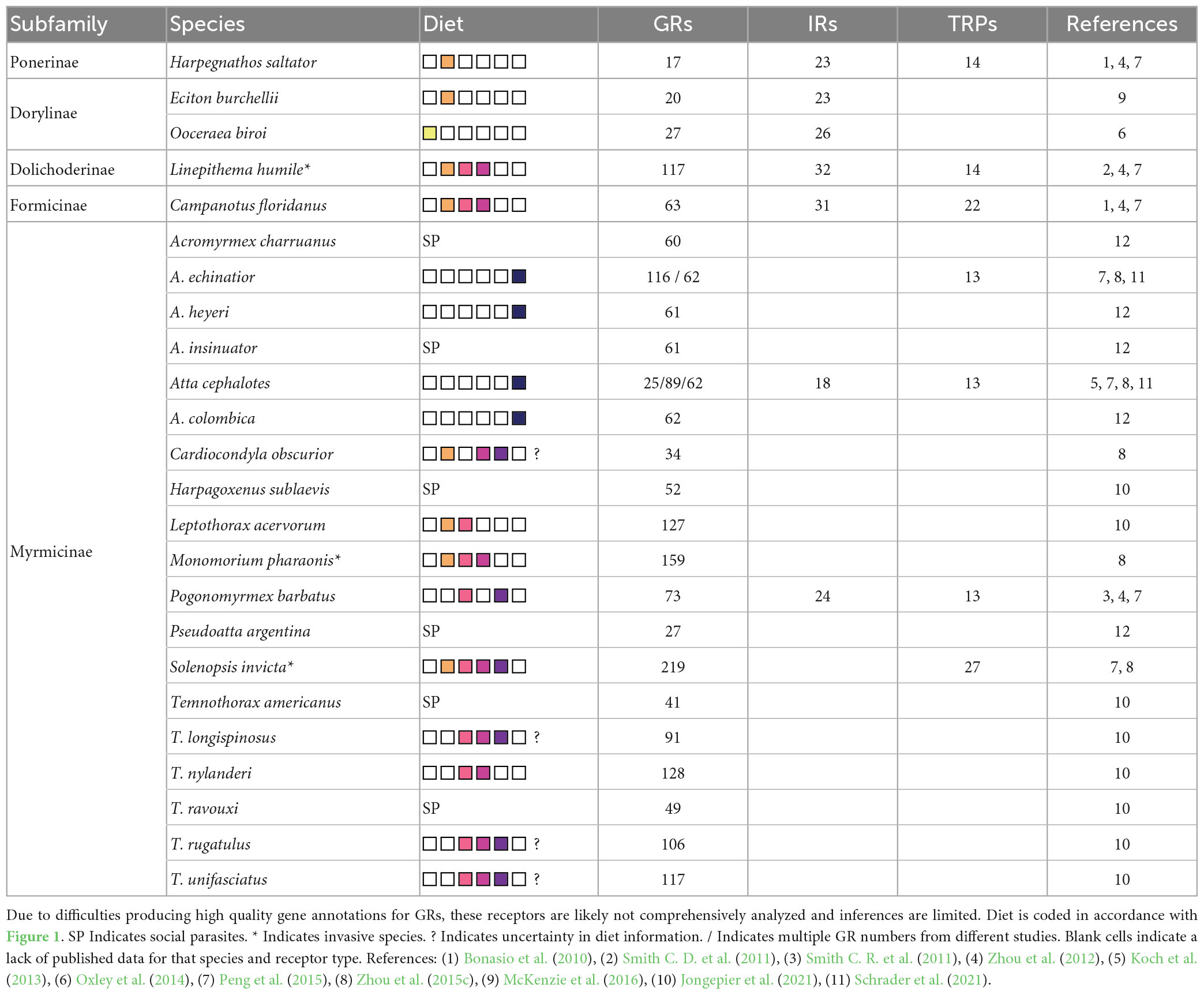
Table 1. Reported GR number, IR number, TRP channel number, and diet information of species with annotated genomes.
Phylogenetic patterns
Hummingbird umami receptors were co-opted to detect sugars (Baldwin et al., 2014; Toda et al., 2021), vertebrate bitter receptor numbers correlate with the degree of herbivory (Li and Zhang, 2013) and many carnivores lost sugar receptors through pseudogenization (Li et al., 2005; Zhao et al., 2010; Jiang et al., 2012). Dietary change in arthropods is also reflected in receptor evolution, with fewer GRs present in parasites than in generalists (Robertson, 2019). Within the Lepidoptera, divergent evolution resulted in GR expansion, including for lineage-specific receptors that detect bitter compounds (Wanner and Robertson, 2008; Engsontia et al., 2014). Lepidopterans with narrow diets are more sensitive to bitter compounds than species with broader diets (Zhang et al., 2013), suggesting that their dietary specialization is correlated with specific GR expansion (Agnihotri et al., 2016). In contrast, low GR numbers and lack of bitter taste in honey bees are also thought to result from diet specialization (Robertson and Wanner, 2006). Given the similarities and differences in gustatory coding and organization across phyla (Yarmolinsky et al., 2009), interspecific comparisons may reveal broad patterns in the evolution and mechanisms of gustation in ants.
Figure 1 illustrates subfamily variation in ant diets and their hypothesized origins, indicating a predator ancestor. The greatest dietary diversity is found in formicoid ants, which include the species-rich formicines and myrmicines. Correlations between diet diversity and speciation are yet unknown and may be confounded by other ecological factors and life history traits. Subfamily-specific patterns in mouthpart anatomy differ with diet (Paul and Roces, 2019) and receptors may similarly vary. Because ants can discriminate among sugars and have species-specific preferences, they may, like other insects, have multiple GRs tuned to different sugars (Chen and Dahanukar, 2020; Bestea et al., 2021). Receptors share a common insect ancestor, with evolution influenced by episodic bursts of gene duplication yielding lineage-specific expansions (Kent and Robertson, 2009). Variability in AA preferences suggests that AA receptors are similarly lineage-specific, as in other insects (Agnihotri et al., 2016).
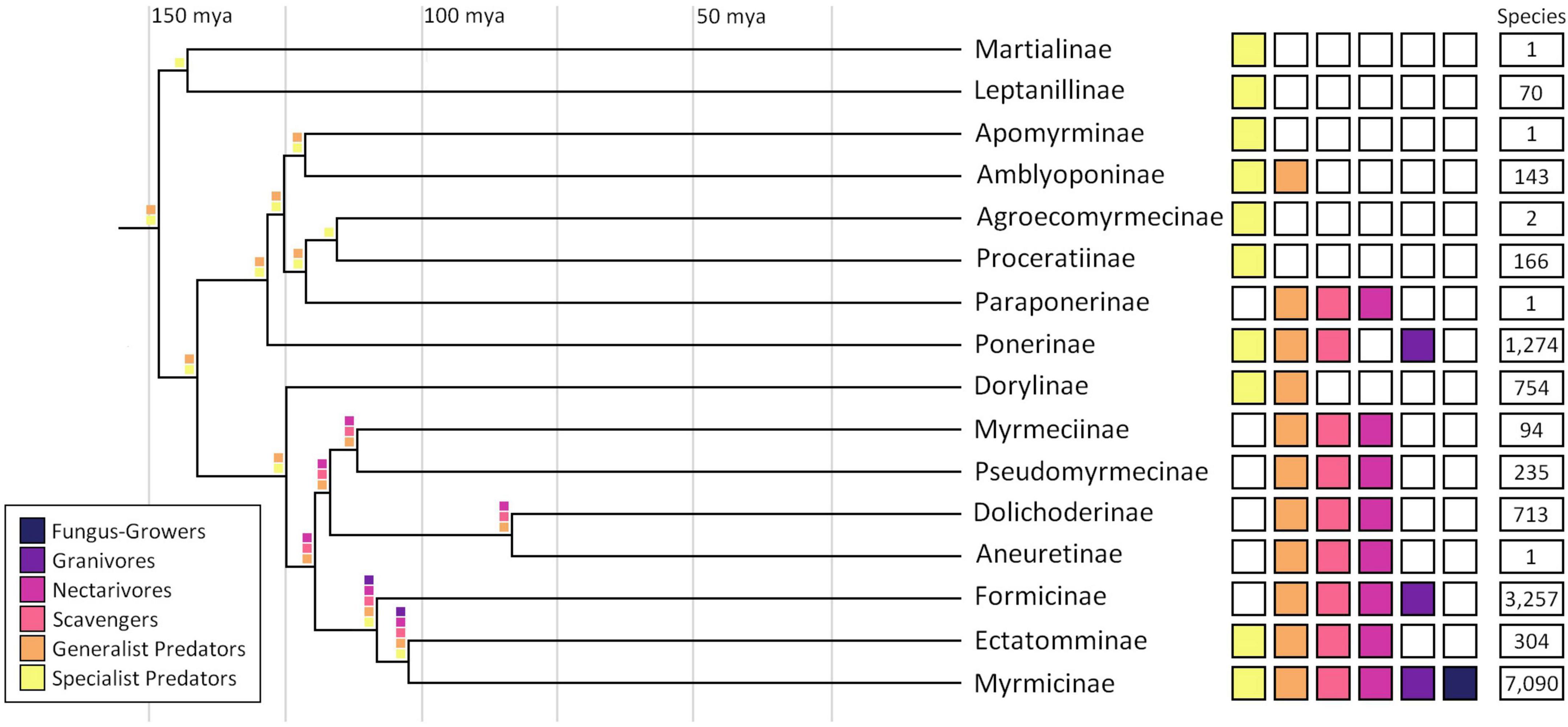
Figure 1. Ant phylogeny and dietary breadth. Phylogeny based on Romiguier et al. (2022). Diet descriptions from Jayasuriya and Traniello (1985), Lachaud (1990), Cook and Davidson (2006), Heterick (2009), Lenhart et al. (2013), and Ward (2014). Species richness from Bolton (2023).
Omnivorous generalists may require more receptors to evaluate diverse foods. Many invasive ant species are generalist scavengers that “overeat” imbalanced foods to acquire limiting nutrients (Csata et al., 2020; Shik and Dussutour, 2020). The ability to detect diverse foods by gene duplication or combinatorial pattern may partially underpin the success of these species as invasives. The high number of GRs in the invasive generalists L. humile (117), Monomorium pharaonis (159), and S. invicta (219) supports this hypothesis (Table 1). In contrast, primarily predatory ants may have comparatively few GRs and detect a narrower range of foods. These species do not appear to have the opportunity to sample their food before capture. Trap-jaw ants (e.g., Odontomachus sp.) use “spring-loaded” mandibles that rapidly close on prey in response to activation of mechanosensory hairs (Gronenberg et al., 1993) and may instead rely on olfaction to detect prey repellency (Traniello, 1981; Manubay and Powell, 2020) or vision to identify prey (Azorsa et al., 2022). This hypothesis is supported by the fewer GRs in the generalist predators Eciton burchellii (20) and H. saltator (17) (Table 1).
Dietary specialists may vary in receptor profiles. Dulotic ants, which rely on workers of their host species obtained in raids to forage and tend brood, have half as many GRs as their hosts (Jongepier et al., 2021). Similar patterns are observed across other social parasites (Schrader et al., 2021). Other species have stronger attraction to macronutrients provided by their plant or hemipteran mutualists (Völkl et al., 1999; Blüthgen and Fiedler, 2004a; González-Teuber and Heil, 2009). Such patterns may be underpinned by positive adaptive selection in receptors that detect mutualist-provided chemicals, or relaxed selection on the remaining genes (Wang et al., 2019). In contrast, fungus-growing ants specialize on their fungal mutualist, but workers adjust foraging based on the nutritional needs of their cultivar (Shik et al., 2021), a complex adaptation likely requiring a wide range of receptors. Annotated genomes also support this hypothesis (Table 1). Further research is required to understand the relationship between diet and GR expression.
Social dimensions of taste
Foraging ecology and dietary evolution in ants involves social behavior, and colony nutritional state regulates worker response to food (Traniello, 1977; Dussutour and Simpson, 2009; Greenwald et al., 2018). Hunger is communicated through solicitations between workers for regurgitated food, while satiation is regulated by speed of food offloading by returning foragers (Csata and Dussutour, 2019). Foragers deposit trail pheromone to share food quality and location (Czaczkes et al., 2015) and social and environmental cues regulate food acceptance or rejection during foraging (Arenas and Roces, 2017, 2018; Csata and Dussutour, 2019). Trophallaxis, the sharing of stored liquids by regurgitation, is a social mechanism that informs foraging decisions (Farina et al., 2005; Csata and Dussutour, 2019) and may connect individual- and colony-level nutrition by influencing taste preferences. Gustation may thus transmit information that impacts foraging and nutrition and play a key role in ant social organization.
Because some GRs and PPKs detect pheromones (Montell, 2009; Liu et al., 2020), the number and combinatorial pattern of GRs and PPKs may also correlate with social complexity of colony organization, including queen number, colony size, worker polymorphism, foraging activity, and social communication (Anderson and McShea, 2001; Zhou et al., 2012). Colony size may also correlate with diet, as many large colonies are generalist omnivores or leafcutter ants, whereas many species that form small colonies are predatory (Dornhaus et al., 2012; Romiguier et al., 2022).
Worker size variation and colony demography may also affect the evolution of taste. The genera Pheidole, Cephalotes, Camponotus, Atta and Acromyrmex are characterized by morphologically distinct (polymorphic) worker subcastes with task specialization. The diversification of tasks by polymorphic workers may have favored the evolution of gustatory phenotypes that vary between subcastes. Foragers, for example, may require expression of more taste receptors than non-foraging defense-specialized soldiers, which may rely on other sensory modalities (Arganda et al., 2020). This hypothesis is preliminarily supported by differences in sensilla size and number among polymorphic workers (Renthal et al., 2003; Masram and Barsagade, 2021), although there are only two GRs differentially expressed between major and minor workers of Camponotus floridanus (Zhou et al., 2012). Subcastes of Camponotus mus differ in sucrose feeding threshold, which may promote specialization on different nectar resources (Josens et al., 2018). C. floridanus major and minor workers also differ in olfactory sensitivity (Ferguson et al., 2023). Leafcutter ants have worker subcastes that perform leaf-cutting, fungus care, brood care, and defense (Muratore et al., 2022). Remarkable morphological, neural, and behavioral differentiation among subcastes (Arganda et al., 2020; Muratore et al., 2022) suggest that gustatory polyphenisms may support taste-based tasks in leaf selection and fungal condition assessment. Through roles in regulating colony nutrition and informing division of labor, taste likely plays an important role in ant social behavior.
Discussion
Future research
To understand the evolution and mechanisms of gustation in ants, we need to assess feeding behavior and preferences, annotate taste receptors to study phylogenetic patterns and their role in speciation, and investigate the role of taste in sociality. Quantifying taste preference is foundational, but research is methodologically inconsistent, phylogenetically narrow, and primarily investigates responsiveness to sugars. Behavioral assays should be complemented by dietary data for species in their natural habitats and encompass basal clades (Leptanillinae, Martialinae), predatory ants (poneroids), and dietary specialists (granivores, fungus-growers, prey specialists) to reveal patterns of taste evolution.
Transcriptomic data from sensory tissues, whole genome sequencing, and high-quality gene annotations of chemoreceptors across dietary-diverse species are also needed to study gustatory gene evolution, receptor function, and the role of receptors in dietary change and species diversification. The Global Ant Genomics Alliance (GAGA) aims to sequence and compare diverse taxa (Boomsma et al., 2017). Such datasets will provide the basis for annotating ant GRs and studying their evolution and relationship with diet and sociality. GRs are often located in tandem duplication, which makes high-quality gene annotation difficult using common analysis pipelines. GRs thus require specific analyses or manual annotation to acquire high-quality datasets that capture the complete gene family (McKenzie et al., 2016; McKenzie and Kronauer, 2018) necessary to study the evolution of taste in ants.
Phylogenetic analysis of taste receptors could reveal conserved genes, pointing to potential functions of ant receptors through orthologs with known ligands in other species, although functional studies will be required to define their physiological role. Gene subfamilies that originated in ants can also be identified, as well as patterns associated with evolutionary convergence (e.g., in honeydew specialization). Birth and death rate estimations for gustatory gene families may additionally reveal evolutionary patterns associated with dietary adaptation and speciation. The impact of colony size and worker size frequency distributions on diet in phylogenetic context require further research.
Polymorphic species provide models for elucidating the role of gustation in division of labor. Preference, sensitivity, and receptor expression differences between polymorphic workers may correlate with task specialization. Given their strongly differentiated subcastes and multiple taste-reliant tasks, polymorphic leafcutter ants (Atta) can be models to study the coevolution of gustation and division of labor. In Pheidole pallidula, differential expression of the P. pallidula foraging gene (ppfor) regulates behavioral differences between the defensive major and forager minor workers, with ppfor activation increasing defensive behaviors in majors and lowering foraging behaviors in majors and minors (Lucas and Sokolowski, 2009). Similarly, in C. floridanus, pharmacological reduction of histone deacetylation increased foraging behavior of majors and minors (Simola et al., 2016). Taste likely varies across subcastes and these manipulations could provide inroads to explore the pathways that regulate differences in taste.
Finally, ant gustation may adaptively evolve with dietary shifts in rapidly changing environments. Pavement ants (Tetramorium sp. E) colonizing urbanized landscapes have a dietary reliance on processed human foods (Penick et al., 2015). The role of taste receptors in such urban dietary adaptation is not known and may provide opportunities to examine the evolution of gustation over relatively short time scales.
Conclusion
Gustation has evolved to meet nutritional needs as the mechanism underpinning food choice and dietary optimization. Nutritional decisions require taste perception; evolutionary changes in receptor alleles enable assessment of new food sources, driving selection for adaptive chemoreception. The remarkable dietary and species diversity of ants presents an outstanding opportunity to explore the evolution and mechanisms of gustation in eusocial species that make individual- and colony-level decisions based on taste.
Gustation influences species diversity through its role in dietary niche evolution. Dietary evolution in ants has broader significance due to their striking ecological and evolutionary impact on the broad range of organisms they consume or with which they have dietary mutualisms, thus significantly affecting biodiversity. Gustation is therefore critical to understanding the impact of ants on ecosystem health.
Author contributions
ES and JT conceived the review and prepared initial drafts of the manuscript. JV, MY, and SM developed and edited the manuscript. All authors read and approved the submitted version.
Funding
JT was supported by National Science Foundation grant IOS 1953393. MY was supported by funding from the Searle Scholars Program, the Esther A. and Joseph Klingenstein Fund, and the Simons Foundation.
Acknowledgments
We thank Zach Coto and Frank Azorsa for their insights on multiple drafts of the manuscript.
Conflict of interest
The authors declare that the research was conducted in the absence of any commercial or financial relationships that could be construed as a potential conflict of interest.
Publisher’s note
All claims expressed in this article are solely those of the authors and do not necessarily represent those of their affiliated organizations, or those of the publisher, the editors and the reviewers. Any product that may be evaluated in this article, or claim that may be made by its manufacturer, is not guaranteed or endorsed by the publisher.
References
Agnihotri, A. R., Roy, A. A., and Joshi, R. S. (2016). Gustatory receptors in Lepidoptera: Chemosensation and beyond. Insect Mol. Biol. 25, 519–529. doi: 10.1111/imb.12246
Ahn, J.-E., Chen, Y., and Amrein, H. (2017). Molecular basis of fatty acid taste in Drosophila. eLife 6:e30115. doi: 10.7554/eLife.30115
Anderson, C., and McShea, D. W. (2001). Individual versus social complexity, with particular reference to ant colonies. Biol. Rev. Camb. Philos. Soc. 76, 211–237. doi: 10.1017/s1464793101005656
Arenas, A., and Roces, F. (2017). Avoidance of plants unsuitable for the symbiotic fungus in leaf-cutting ants: Learning can take place entirely at the colony dump. PLoS One 12:e0171388. doi: 10.1371/journal.pone.0171388
Arenas, A., and Roces, F. (2018). Appetitive and aversive learning of plants odors inside different nest compartments by foraging leaf-cutting ants. J. Insect Physiol. 109, 85–92. doi: 10.1016/j.jinsphys.2018.07.001
Arganda, S., Hoadley, A. P., Razdan, E. S., Muratore, I. B., and Traniello, J. F. A. (2020). The neuroplasticity of division of labor: Worker polymorphism, compound eye structure and brain organization in the leafcutter ant Atta cephalotes. J. Comp. Physiol. A 206, 651–662. doi: 10.1007/s00359-020-01423-9
Azorsa, F., Muscedere, M. L., and Traniello, J. F. A. (2022). Socioecology and evolutionary neurobiology of predatory ants. Front. Ecol. Evol. 9:804200. doi: 10.3389/fevo.2021.804200
Baldwin, M. W., Toda, Y., Nakagita, T., O’Connell, M. J., Klasing, K. C., Misaka, T., et al. (2014). Evolution of sweet taste perception in hummingbirds by transformation of the ancestral umami receptor. Science 345, 929–933. doi: 10.1126/science.1255097
Barbani, L. E. (2002). Foraging activity and food preferences of the odorous house ant (Tapinoma sessile Say) (Hymenoptera: Formicidae). Ph.D. thesis. Blacksburg, VA: Virginia Tech.
Barsagade, D. D., Tembhare, D. B., and Kadu, S. G. (2013). Microscopic structure of antennal sensilla in the carpenter ant Camponotus compressus (Fabricius) (Formicidae: Hymenoptera). Asian Myrmecol. 5, 113–120. doi: 10.20362/am.005012
Barsagade, D., Thakre, M., Kirsan, J., Nagose, S., Nagarkar, D., and Bodele, A. M. (2017). Mouthparts sensilla of the worker ant Leptogenys dentilobis (Hymenoptera: Formicidae). Hisl. J. 10:2017. doi: 10.7324/JABB.2019.70201
Benton, R., Dessimoz, C., and Moi, D. (2020). A putative origin of the insect chemosensory receptor superfamily in the last common eukaryotic ancestor. eLife 9:e62507. doi: 10.7554/eLife.62507
Benton, R., Vannice, K. S., Gomez-Diaz, C., and Vosshall, L. B. (2009). Variant ionotropic glutamate receptors as chemosensory receptors in Drosophila. Cell 136, 149–162. doi: 10.1016/j.cell.2008.12.001
Bestea, L., Réjaud, A., Sandoz, J.-C., Carcaud, J., Giurfa, M., and de Brito Sanchez, M. G. (2021). Peripheral taste detection in honey bees: What do taste receptors respond to? Eur. J. Neurosci. 54, 4417–4444. doi: 10.1111/ejn.15265
Blüthgen, N., and Fiedler, K. (2004b). Preferences for sugars and amino acids and their conditionality in a diverse nectar-feeding ant community. J. Anim. Ecol. 73, 155–166. doi: 10.1111/j.1365-2656.2004.00789.x
Blüthgen, N., and Fiedler, K. (2004a). Competition for composition: Lessons from nectar-feeding ant communities. Ecology 85, 1479–1485. doi: 10.1890/03-0430
Boevé, J.-L., and Wäckers, F. L. (2003). Gustatory perception and metabolic utilization of sugars by Myrmica rubra ant workers. Oecologia 136, 508–514. doi: 10.1007/s00442-003-1249-9
Bolton, B. (2023). An online catalog of the ants of the world. Available online at: https://antcat.org (accessed January 27, 2023).
Bolton, S. K., Dickerson, K., and Saporito, R. A. (2017). Variable alkaloid defenses in the dendrobatid poison frog oophaga pumilio are perceived as differences in palatability to arthropods. J. Chem. Ecol. 43, 273–289. doi: 10.1007/s10886-017-0827-y
Bonasio, R., Zhang, G., Ye, C., Mutti, N. S., Fang, X., Qin, N., et al. (2010). Genomic comparison of the ants Camponotus floridanus and Harpegnathos saltator. Science 329, 1068–1071. doi: 10.1126/science.1192428
Boomsma, J. J., Brady, S., Dunn, R., Gadau, J., Heinze, J., Keller, L., et al. (2017). Forum The Global Ant Genomics Alliance (GAGA). Myrmecol. News 25, 61–66
Brown, E. B., Shah, K. D., Palermo, J., Dey, M., Dahanukar, A., and Keene, A. C. (2021). Ir56d-dependent fatty acid responses in Drosophila uncover taste discrimination between different classes of fatty acids. eLife 10:e67878. doi: 10.7554/eLife.67878
Cannon, C. A. (1998). Nutritional ecology of the carpenter ant Camponotus pennsylvanicus (De Geer): Macronutrient preference and particle consumption. Ph.D. thesis. Blacksburg, VA: Virginia Polytechnic Institute and State University.
Chen, Y. C., and Dahanukar, A. (2020). Recent advances in the genetic basis of taste detection in Drosophila. Cell. Mol. Life Sci. 77, 1087–1101. doi: 10.1007/s00018-019-03320-0
Chen, Z., Wang, Q., and Wang, Z. (2010). The amiloride-sensitive epithelial na+ channel ppk28 is essential for Drosophila gustatory water reception. J. Neurosci. 30, 6247–6252. doi: 10.1523/JNEUROSCI.0627-10.2010
Cicconardi, F., Krapf, P., D’Annessa, I., Gamisch, A., Wagner, H. C., Nguyen, A. D., et al. (2020). Genomic signature of shifts in selection in a subalpine ant and its physiological adaptations. Mol. Biol. Evol. 37, 2211–2227. doi: 10.1093/molbev/msaa076
Clyne, P. J., Warr, C. G., and Carlson, J. R. (2000). Candidate taste receptors in Drosophila. Science 287, 1830–1834. doi: 10.1126/science.287.5459.1830
Cook, S. C., and Davidson, D. W. (2006). Nutritional and functional biology of exudate-feeding ants. Entomol. Exp. Appl. 118, 1–10. doi: 10.1111/j.1570-7458.2006.00374.x
Cornelius, M. L., Grace, J. K., and Yates, J. R. (1996). Acceptability of different sugars and oils to three tropical ant species (Hymen., Formicidae). Anz. Schadlingskde Pflanzenschutz Umweltschutz 69, 41–43. doi: 10.1007/BF01907668
Croset, V., Rytz, R., Cummins, S. F., Budd, A., Brawand, D., Kaessmann, H., et al. (2010). Ancient protostome origin of chemosensory ionotropic glutamate receptors and the evolution of insect taste and olfaction. PLoS Genet. 6:e1001064. doi: 10.1371/journal.pgen.1001064
Crumière, A. J. J., Mallett, S., Michelsen, A., Rinnan, R., and Shik, J. Z. (2022). Nutritional challenges of feeding a mutualist: Testing for a nutrient–toxin tradeoff in fungus-farming leafcutter ants. Ecology 103:e3684. doi: 10.1002/ecy.3684
Csata, E., and Dussutour, A. (2019). Nutrient regulation in ants (Hymenoptera: Formicidae): A review. Myrmecol. News 29, 111–124. doi: 10.25849/MYRMECOL.NEWS_029:111
Csata, E., Gautrais, J., Bach, A., Blanchet, J., Ferrante, J., Fournier, F., et al. (2020). Ant foragers compensate for the nutritional deficiencies in the colony. Curr. Biol. 30, 135–142.e4. doi: 10.1016/j.cub.2019.11.019
Czaczkes, T. J., Grüter, C., and Ratnieks, F. L. W. (2015). Trail pheromones: An integrative view of their role in social insect colony organization. Annu. Rev. Entomol. 60, 581–599. doi: 10.1146/annurev-ento-010814-020627
Davidson, D. W., Cook, S. C., and Snelling, R. R. (2004). Liquid-feeding performances of ants (Formicidae): Ecological and evolutionary implications. Oecologia 139, 255–266. doi: 10.1007/s00442-004-1508-4
de Brito Sanchez, M. G., Giurfa, M., De Paula Mota, T. R., and Gauthier, M. (2005). Electrophysiological and behavioural characterization of gustatory responses to antennal ‘bitter’ taste in honeybees. Eur. J. Neurosci. 22, 3161–3170. doi: 10.1111/j.1460-9568.2005.04516.x
de Brito Sanchez, M. G., Lorenzo, E., Songkung, S., Liu, F., and Giurfa, M. (2014). The tarsal taste of honey bees: Behavioral and electrophysiological analyses. Front. Behav. Neurosci. 8:25. doi: 10.3389/fnbeh.2014.00025
Detrain, C., and Prieur, J. (2014). Sensitivity and feeding efficiency of the black garden ant Lasius niger to sugar resources. J. Insect Physiol. 64, 74–80. doi: 10.1016/j.jinsphys.2014.03.010
Detrain, C., Verheggen, F. J., Diez, L., Wathelet, B., and Haubruge, E. (2010). Aphid–ant mutualism: How honeydew sugars influence the behaviour of ant scouts. Physiol. Entomol. 35, 168–174. doi: 10.1111/j.1365-3032.2010.00730.x
Dornhaus, A., Powell, S., and Bengston, S. (2012). Group size and its effects on collective organization. Annu. Rev. Entomol. 57, 123–141. doi: 10.1146/annurev-ento-120710-100604
Dunipace, L., Meister, S., McNealy, C., and Amrein, H. (2001). Spatially restricted expression of candidate taste receptors in the Drosophila gustatory system. Curr. Biol. 11, 822–835. doi: 10.1016/s0960-9822(01)00258-5
Dussutour, A., and Simpson, S. J. (2009). Communal nutrition in ants. Curr. Biol. 19, 740–744. doi: 10.1016/j.cub.2009.03.015
Engsontia, P., Sangket, U., Chotigeat, W., and Satasook, C. (2014). Molecular evolution of the odorant and gustatory receptor genes in lepidopteran insects: Implications for their adaptation and speciation. J. Mol. Evol. 79, 21–39. doi: 10.1007/s00239-014-9633-0
Falibene, A., Gontijo, A., de, F., and Josens, R. (2009). Sucking pump activity in feeding behaviour regulation in carpenter ants. J. Insect Physiol. 55, 518–524. doi: 10.1016/j.jinsphys.2009.01.015
Farina, W. M., Grüter, C., and Díaz, P. C. (2005). Social learning of floral odours inside the honeybee hive. Proc. R. Soc. B Biol. Sci. 272, 1923–1928. doi: 10.1098/rspb.2005.3172
Ferguson, S. T., Bakis, I., Edwards, N. D., and Zwiebel, L. J. (2023). Olfactory sensitivity differentiates morphologically distinct worker castes in Camponotus floridanus. BMC Biol. 21:3. doi: 10.1186/s12915-022-01505-x
Frank, H. E. R., Amato, K., Trautwein, M., Maia, P., Liman, E. R., Nichols, L. M., et al. (2022). The evolution of sour taste. Proc. R. Soc. B Biol. Sci. 289:20211918. doi: 10.1098/rspb.2021.1918
Ganguly, A., Pang, L., Duong, V.-K., Lee, A., Schoniger, H., Varady, E., et al. (2017). A molecular and cellular context-dependent role for Ir76b in detection of amino acid taste. Cell Rep. 18, 737–750. doi: 10.1016/j.celrep.2016.12.071
González-Teuber, M., and Heil, M. (2009). The role of extrafloral nectar amino acids for the preferences of facultative and obligate ant mutualists. J. Chem. Ecol. 35, 459–468. doi: 10.1007/s10886-009-9618-4
Greenwald, E. E., Baltiansky, L., and Feinerman, O. (2018). Individual crop loads provide local control for collective food intake in ant colonies. eLife 7:e31730. doi: 10.7554/eLife.31730
Gronenberg, W., Tautz, J., and Hölldobler, B. (1993). Fast trap jaws and giant neurons in the ant Odontomachus. Science 262, 561–563. doi: 10.1126/science.262.5133.561
Heterick, B. E. (2009). A guide to the ants of South-western Australia, Records of the Western Australian Museum. Supplement. Perth, WA: Western Australian Museum.
Hölldobler, B., and Wilson, E. O. (2010). The Leafcutter Ants: Civilization by Instinct. New York, NY: W. W. Norton & Company.
Horta-Vega, J. V., Eguía-López, B., Ruíz-Cancino, E., and Brussolo-Ceballos, R. (2010). Recruitment response to six carbohydrates in the ant Pheidole dentigula Smith, 1927 (Hymenoptera: Formicidae). Entomol. News 121, 290–297. doi: 10.3157/021.121.0311
Howard, J. J., Green, T. P., and Wiemer, D. F. (1989). Comparative deterrency of two terpenoids to two genera of attine ants. J. Chem. Ecol. 15, 2279–2288. doi: 10.1007/BF01012081
Jaeger, A. H., Stanley, M., Weiss, Z. F., Musso, P.-Y., Chan, R. C., Zhang, H., et al. (2018). A complex peripheral code for salt taste in Drosophila. eLife 7:e37167. doi: 10.7554/eLife.37167
Jaleel, W., Li, Q., Shi, Q., and Lyu, L. (2021). Preference and effect of gustatory sense on sugar-feeding of fire ants. PeerJ 9:e11943. doi: 10.7717/peerj.11943
Jayasuriya, A. K., and Traniello, J. F. A. (1985). The biology of the primitive ant Aneuretus simoni (Emery). I: Distribution, abundance, colony structure, and foraging ecology. Insect. Soc. 32, 363–374.
Jiang, P., Josue, J., Li, X., Glaser, D., Li, W., Brand, J. G., et al. (2012). Major taste loss in carnivorous mammals. Proc. Natl. Acad. Sci. U.S.A. 109, 4956–4961. doi: 10.1073/pnas.1118360109
Jongepier, E., Séguret, A., Labutin, A., Feldmeyer, B., Gstöttl, C., Foitzik, S., et al. (2021). Convergent loss of chemoreceptors across independent origins of slave-making in ants. Mol. Biol. Evol. 39:1. doi: 10.1101/2021.05.11.443570
Josens, R., Giacometti, A., and Giurfa, M. (2021). Inhibition of serotonergic signaling induces higher consumption of both sucrose solution and toxic baits in carpenter ants. Sci. Rep. 11:19176. doi: 10.1038/s41598-021-98030-8
Josens, R., Lopez, M. A., Jofré, N., and Giurfa, M. (2018). Individual size as determinant of sugar responsiveness in ants. Behav. Ecol. Sociobiol. 72, 1–9.
Joseph, R. M., and Carlson, J. R. (2015). Drosophila Chemoreceptors: A molecular interface between the chemical world and the brain. Trends Genet. 31, 683–695. doi: 10.1016/j.tig.2015.09.005
Kang, K., Pulver, S. R., Panzano, V. C., Chang, E. C., Griffith, L. C., Theobald, D. L., et al. (2010). Analysis of Drosophila TRPA1 reveals an ancient origin for human chemical nociception. Nature 464, 597–600. doi: 10.1038/nature08848
Kaspari, M., Welti, E. A. R., and Beurs, K. M. (2020). The nutritional geography of ants: Gradients of sodium and sugar limitation across North American grasslands. J. Anim. Ecol. 89, 276–284. doi: 10.1111/1365-2656.13120
Kaspari, M., Yanoviak, S. P., and Dudley, R. (2008). On the biogeography of salt limitation: A study of ant communities. Proc. Natl. Acad. Sci. U.S.A. 105, 17848–17851. doi: 10.1073/pnas.0804528105
Kent, L. B., and Robertson, H. M. (2009). Evolution of the sugar receptors in insects. BMC Evol. Biol. 9:41. doi: 10.1186/1471-2148-9-41
Koch, S. I., Groh, K., Vogel, H., Hannson, B. S., Kleineidam, C. J., and Grosse-Wilde, E. (2013). Caste-specific expression patterns of immune response and chemosensory related genes in the leaf-cutting ant, Atta vollenweideri. PLoS One 8:e81518. doi: 10.1371/journal.pone.0081518
Kronauer, D. J. C. (2020). Army ants: Nature’s ultimate social hunters. Cambridge: Harvard University Press.
Lachaud, J.-P. (1990). Foraging activity and diet in some neotropical ponerine ants. I. Ectatomma ruidum roger (Hymenoptera, Formicidae). Folia Entomol. Mexic. 78, 241–256.
Lanza, J. (1991). Response of fire ants (Formicidae: Solenopsis invicta and S.gerninata) to artificial nectars with amino acids. Ecol. Entomol. 16, 203–210. doi: 10.1111/j.1365-2311.1991.tb00210.x
Lanza, J., and Krauss, B. R. (1984). Detection of amino acids in artificial nectars by two tropical ants, Leptothorax and Monomorium. Oecologia 63, 423–425. doi: 10.1007/BF00390676
Latorre-Estivalis, J. M., Almeida, F. C., Pontes, G., Dopazo, H., Barrozo, R. B., and Lorenzo, M. G. (2021). Evolution of the Insect PPK Gene Family. Genome Biol. Evol. 13:evab185. doi: 10.1093/gbe/evab185
Lenhart, P., Dash, S. T., and Mackay, W. P. (2013). A revision of the giant Amazonian ants of the genus Dinoponera (Hymenoptera, Formicidae). J. Hymenopt. Res. 31, 119–164. doi: 10.3897/jhr.31.4335
Li, D., and Zhang, J. (2013). Diet shapes the evolution of the vertebrate bitter taste receptor gene repertoire. Mol. Biol. Evol. 31, 303–309. doi: 10.1093/molbev/mst219
Li, X., Li, W., Wang, H., Cao, J., Maehashi, K., Huang, L., et al. (2005). Pseudogenization of a sweet-receptor gene accounts for cats’ indifference toward sugar. PLoS Genet. 1:e3. doi: 10.1371/journal.pgen.0010003
Liu, T., Wang, Y., Tian, Y., Zhang, J., Zhao, J., and Guo, A. (2020). The receptor channel formed by ppk25, ppk29 and ppk23 can sense the Drosophila female pheromone 7,11-heptacosadiene. Genes Brain Behav. 19:e12529. doi: 10.1111/gbb.12529
Lucas, C., and Sokolowski, M. B. (2009). Molecular basis for changes in behavioral state in ant social behaviors. Proc. Natl. Acad. Sci. U.S.A. 106, 6351–6356. doi: 10.1073/pnas.0809463106
Madsen, N. E. L., and Offenberg, J. (2019). Effect of caffeine and γ-aminobutyric acid on preference for sugar solutions in two ant species. Asian Myrmecol. 11:e011003. doi: 10.20362/am.011003
Madsen, N. E. L., and Offenberg, J. (2020). Seasonal changes in sugar and amino acid preference in red wood ants of the Formica rufa group. Sociobiology 67, 144–152. doi: 10.13102/sociobiology.v67i2.3760
Madsen, N. E. L., Sørensen, P. B., and Offenberg, J. (2017). Sugar and amino acid preference in the black garden ant Lasius niger (L.). J. Insect Physiol. 100, 140–145. doi: 10.1016/j.jinsphys.2017.05.011
Manubay, J. A., and Powell, S. (2020). Detection of prey odours underpins dietary specialization in a Neotropical top-predator: How army ants find their ant prey. J. Anim. Ecol. 89, 1165–1174. doi: 10.1111/1365-2656.13188
Masram, P., and Barsagade, D. (2021). Morphological and surface ultra-structural studies of legs in polymorphs of weaver ant Oecophylla smaragdina Fabricius (Hymenoptera: Formicidae) with reference to sensilla. Int. J. Zool. Invest. 7, 26–39. doi: 10.33745/ijzi.2021.v07i02s.005
Mayer, V. E., Frederickson, M. E., McKey, D., and Blatrix, R. (2014). Current issues in the evolutionary ecology of ant–plant symbioses. New Phytol. 202, 749–764. doi: 10.1111/nph.12690
McKenzie, S. K., and Kronauer, D. J. C. (2018). The genomic architecture and molecular evolution of ant odorant receptors. Genome Res. 28, 1–9. doi: 10.1101/gr.237123.118
McKenzie, S. K., Fetter-Pruneda, I., Ruta, V., and Kronauer, D. J. C. (2016). Transcriptomics and neuroanatomy of the clonal raider ant implicate an expanded clade of odorant receptors in chemical communication. Proc. Natl. Acad. Sci. U.S.A. 113, 14091–14096. doi: 10.1073/pnas.1610800113
McKenzie, S. K., Winston, M. E., Grewe, F., Vargas Asensio, G., Rodríguez-Hernández, N., Rubin, B. E. R., et al. (2021). The genomic basis of army ant chemosensory adaptations. Mol. Ecol. 30, 6627–6641. doi: 10.1111/mec.16198
Montell, C. (2009). A taste of the Drosophila gustatory receptors. Curr. Opin. Neurobiol. Sens. Syst. 19, 345–353. doi: 10.1016/j.conb.2009.07.001
Muratore, I. B., Fandozzi, E. M., and Traniello, J. F. A. (2022). Behavioral performance and division of labor influence brain mosaicism in the leafcutter ant Atta cephalotes. J. Comp. Physiol. A Neuroethol. Sens. Neural. Behav. Physiol. 208, 325–344. doi: 10.1007/s00359-021-01539-6
Obiero, G. F., Pauli, T., Geuverink, E., Veenendaal, R., Niehuis, O., and Große-Wilde, E. (2021). Chemoreceptor diversity in apoid wasps and its reduction during the evolution of the pollen-collecting lifestyle of bees (Hymenoptera: Apoidea). Genome Biol. Evol. 13:evaa269. doi: 10.1093/gbe/evaa269
Oeyen, J. P., Baa-Puyoulet, P., Benoit, J. B., Beukeboom, L. W., Bornberg-Bauer, E., Buttstedt, A., et al. (2020). Sawfly genomes reveal evolutionary acquisitions that fostered the mega-radiation of parasitoid and eusocial Hymenoptera. Genome Biol. Evol. 12, 1099–1188. doi: 10.1093/gbe/evaa106
Oxley, P. R., Ji, L., Fetter-Pruneda, I., McKenzie, S. K., Li, C., Hu, H., et al. (2014). The genome of the clonal raider ant cerapachys biroi. Curr. Biol. 24, 451–458. doi: 10.1016/j.cub.2014.01.018
Parker, J., and Kronauer, D. J. C. (2021). How ants shape biodiversity. Curr. Biol. 31, R1208–R1214. doi: 10.1016/j.cub.2021.08.015
Paul, J., and Roces, F. (2019). “Comparative Functional Morphology of Ant Mouthparts and Significance for Liquid Food Intake,” in Insect mouthparts: Form, function, development and performance, zoological monographs, Vol. 5, ed. H. W. Krenn (Cham: Springer International Publishing), 335–359. doi: 10.1007/978-3-030-29654-4_10
Peng, G., Shi, X., and Kadowaki, T. (2015). Evolution of TRP channels inferred by their classification in diverse animal species. Mol. Phylogenet. Evol. 84, 145–157. doi: 10.1016/j.ympev.2014.06.016
Penick, C. A., Savage, A. M., and Dunn, R. R. (2015). Stable isotopes reveal links between human food inputs and urban ant diets. Proc. R. Soc. B Biol. Sci. 282:20142608. doi: 10.1098/rspb.2014.2608
Reifenrath, K., Becker, C., and Poethke, H. J. (2012). Diaspore trait preferences of dispersing ants. J. Chem. Ecol. 38, 1093–1104. doi: 10.1007/s10886-012-0174-y
Renthal, R., Velasquez, D., Olmos, D., Hampton, J., and Wergin, W. P. (2003). Structure and distribution of antennal sensilla of the red imported fire ant. Micron 34, 405–413. doi: 10.1016/S0968-4328(03)00050-7
Renyard, A., Gries, R., Lee, J., Chalissery, J. M., Damin, S., Britton, R., et al. (2021). All sugars ain’t sweet: Selection of particular mono-, di- and trisaccharides by western carpenter ants and European fire ants. R. Soc. Open Sci. 8:210804. doi: 10.1098/rsos.210804
Ricks, B. L., and Vinson, B. S. (1970). Feeding acceptability of certain insects and various water-soluble compounds to two varieties of the imported fire Ant12. J. Econ. Entomol. 63, 145–148. doi: 10.1093/jee/63.1.145
Rimal, S., Sang, J., Poudel, S., Thakur, D., Montell, C., and Lee, Y. (2019). Mechanism of acetic acid gustatory repulsion in Drosophila. Cell Rep. 26, 1432–1442.e4. doi: 10.1016/j.celrep.2019.01.042
Robertson, H. M. (2019). Molecular evolution of the major arthropod chemoreceptor gene families. Annu. Rev. Entomol. 64, 227–242. doi: 10.1146/annurev-ento-020117-043322
Robertson, H. M., and Wanner, K. W. (2006). The chemoreceptor superfamily in the honey bee, Apis mellifera: Expansion of the odorant, but not gustatory, receptor family. Genome Res. 16, 1395–1403. doi: 10.1101/gr.5057506
Romiguier, J., Borowiec, M. L., Weyna, A., Helleu, Q., Loire, E., Mendola, C. L., et al. (2022). Ant phylogenomics reveals a natural selection hotspot preceding the origin of complex eusociality. Curr. Biol. 32, 2942–2947. doi: 10.1016/j.cub.2022.05.001
Schrader, L., Pan, H., Bollazzi, M., Schiøtt, M., Larabee, F. J., Bi, X., et al. (2021). Relaxed selection underlies genome erosion in socially parasitic ant species. Nat. Commun. 12:2918. doi: 10.1038/s41467-021-23178-w
Schultheiss, P., Nooten, S. S., Wang, R., Wong, M. K. L., Brassard, F., and Guénard, B. (2022). The abundance, biomass, and distribution of ants on Earth. Proc. Natl. Acad. Sci. U.S.A. 119:e2201550119. doi: 10.1073/pnas.2201550119
Shaaban, B., Seeburger, V., Schroeder, A., and Lohaus, G. (2020). Sugar, amino acid and inorganic ion profiling of the honeydew from different hemipteran species feeding on Abies alba and Picea abies. PLoS One 15:e0228171. doi: 10.1371/journal.pone.0228171
Shetty, P. S. (1982). Gustatory preferences of ants (Camponotus compressus) for urea and sugars. Experientia 38, 259–260. doi: 10.1007/BF01945100
Shik, J. Z., and Dussutour, A. (2020). Nutritional dimensions of invasive success. Trends Ecol. Evol. 35, 691–703. doi: 10.1016/j.tree.2020.03.009
Shik, J. Z., Kooij, P. W., Donoso, D. A., Santos, J. C., Gomez, E. B., Franco, M., et al. (2021). Nutritional niches reveal fundamental domestication trade-offs in fungus-farming ants. Nat. Ecol. Evol. 5, 122–134. doi: 10.1038/s41559-020-01314-x
Simola, D. F., Graham, R. J., Brady, C. M., Enzmann, B. L., Desplan, C., Ray, A., et al. (2016). Epigenetic (re)programming of caste-specific behavior in the ant Camponotus floridanus. Science 351:aac6633. doi: 10.1126/science.aac6633
Smith, C. D., Zimin, A., Holt, C., Abouheif, E., Benton, R., Cash, E., et al. (2011). Draft genome of the globally widespread and invasive Argentine ant (Linepithema humile). Proc. Natl. Acad. Sci. U.S.A. 108, 5673–5678. doi: 10.1073/pnas.1008617108
Smith, C. R., Smith, C. D., Robertson, H. M., Helmkampf, M., Zimin, A., Yandell, M., et al. (2011). Draft genome of the red harvester ant Pogonomyrmex barbatus. Proc. Natl. Acad. Sci. U.S.A. 108, 5667–5672. doi: 10.1073/pnas.1007901108
Tinti, J.-M., and Nofre, C. (2001). Responses of the ant Lasius niger to various compounds perceived as sweet in humans: A structure–activity relationship study. Chem. Sens. 26, 231–237. doi: 10.1093/chemse/26.3.231
Toda, Y., Hayakawa, T., Itoigawa, A., Kurihara, Y., Nakagita, T., Hayashi, M., et al. (2021). Evolution of the primate glutamate taste sensor from a nucleotide sensor. Curr. Biol. 31, 4641–4649.e5. doi: 10.1016/j.cub.2021.08.002
Traniello, J. F. A. (1981). Enemy deterrence in the recruitment strategy of a termite: Soldier-organized foraging in Nasutitermes costalis. Proc. Natl. Acad. Sci. U.S.A. 78, 1976–1979. doi: 10.1073/pnas.78.3.1976
Traniello, J. F. A. (1977). Recruitment behavior, orientation, and the organization of foraging in the carpenter ant Camponotus pennsylvanicus DeGeer (Hymenoptera: Formicidae). Behav. Ecol. Sociobiol. 2, 61–79.
Tu, Y.-H., Cooper, A. J., Teng, B., Chang, R. B., Artiga, D. J., Turner, H. N., et al. (2018). An evolutionarily conserved gene family encodes proton-selective ion channels. Science 359, 1047–1050. doi: 10.1126/science.aao3264
Vander Meer, R. K., Lofgren, C. S., and Seawright, J. A. (1995). Specificity of the red imported fire ant (Hymenoptera: Formicidae) Phagostimulant response to carbohydrates. Fla. Entomol. 78, 144–154. doi: 10.2307/3495679
Vinson, S. B. (1970). Gustatory response by the imported fire ant to various electrolytes. Ann. Entomol. Soc. Am. 63, 932–935. doi: 10.1093/aesa/63.4.932
Vizueta, J., Escuer, P., Frías-López, C., Guirao-Rico, S., Hering, L., Mayer, G., et al. (2020). Evolutionary History of major chemosensory gene families across panarthropoda. Mol. Biol. Evol. 37, 3601–3615. doi: 10.1093/molbev/msaa197
Vizueta, J., Macías-Hernández, N., Arnedo, M. A., Rozas, J., and Sánchez-Gracia, A. (2019). Chance and predictability in evolution: The genomic basis of convergent dietary specializations in an adaptive radiation. Mol. Ecol. 28, 4028–4045. doi: 10.1111/mec.15199
Völkl, W., Woodring, J., Fischer, M., Lorenz, M. W., and Hoffmann, K. H. (1999). Ant-aphid mutualisms: The impact of honeydew production and honeydew sugar composition on ant preferences. Oecologia 118, 483–491. doi: 10.1007/s004420050751
Von Frisch, K. (1934). Über den Geschmackssinn der Biene. Z. F. Vergl. Physiol. 21, 1–156. doi: 10.1007/BF00338271
Wang, Y., Jiao, H., Jiang, P., and Zhao, H. (2019). Functional divergence of bitter taste receptors in a nectar-feeding bird. Biol. Lett. 15:20190461. doi: 10.1098/rsbl.2019.0461
Wang, Z., Singhvi, A., Kong, P., and Scott, K. (2004). Taste representations in the Drosophila brain. Cell 117, 981–991. doi: 10.1016/j.cell.2004.06.011
Wanner, K. W., and Robertson, H. M. (2008). The gustatory receptor family in the silkworm moth Bombyx mori is characterized by a large expansion of a single lineage of putative bitter receptors. Insect. Mol. Biol. 17, 621–629. doi: 10.1111/j.1365-2583.2008.00836.x
Ward, P. S. (2014). The phylogeny and evolution of ants. Annu. Rev. Ecol. Evol. Syst. 45, 23–43. doi: 10.1146/annurev-ecolsys-120213-091824
Wenig, K., Bach, R., and Czaczkes, T. J. (2021). Hard limits to cognitive flexibility: Ants can learn to ignore but not avoid pheromone trails. J. Exp. Biol. 224:jeb242454. doi: 10.1242/jeb.242454
Yarmolinsky, D. A., Zuker, C. S., and Ryba, N. J. P. (2009). Common sense about taste: From mammals to insects. Cell 139, 234–244. doi: 10.1016/j.cell.2009.10.001
Zhang, H.-J., Faucher, C. P., Anderson, A., Berna, A. Z., Trowell, S., Chen, Q.-M., et al. (2013). Comparisons of contact chemoreception and food acceptance by larvae of polyphagous Helicoverpa armigera and oligophagous Bombyx mori. J. Chem. Ecol. 39, 1070–1080. doi: 10.1007/s10886-013-0303-2
Zhao, H., Yang, J.-R., Xu, H., and Zhang, J. (2010). Pseudogenization of the umami taste receptor gene Tas1r1 in the giant panda coincided with its dietary switch to bamboo. Mol. Biol. Evol. 27, 2669–2673. doi: 10.1093/molbev/msq153
Zhou, A. M., Wu, D., Liang, G. W., Lu, Y. Y., and Xu, Y. J. (2015a). Effects Of Tending By Solenopsis Invicta (Hymenoptera: Formicidae) On The Sugar Composition And Concentration In The Honeydew Of An Invasive Mealybug, Phenacoccus Solenopsis (Hemiptera: Pseudococcidae). Ethology 121, 492–500. doi: 10.1111/eth.12363
Zhou, A. M., Kuang, B. Q., Gao, Y. R., and Liang, G. W. (2015b). Sucrose Triggers Honeydew Preference in the Ghost Ant, Tapinoma melanocephalum (Hymenoptera: Formicidae). Fla. Entomol. 98, 1217–1222. doi: 10.1653/024.098.0431
Zhou, X., Rokas, A., Berger, S. L., Liebig, J., Ray, A., and Zwiebel, L. J. (2015c). Chemoreceptor evolution in Hymenoptera and its implications for the evolution of eusociality. Genome Biol. Evol. 7, 2407–2416. doi: 10.1093/gbe/evv149
Keywords: diet, foraging ecology, Formicidae, genomics, gustatory receptors, sensory shift, taste perception, worker polymorphism
Citation: Smith EJ, Vizueta J, Younger MA, Mullen SP and Traniello JFA (2023) Dietary diversity, sociality, and the evolution of ant gustation. Front. Ecol. Evol. 11:1175719. doi: 10.3389/fevo.2023.1175719
Received: 28 February 2023; Accepted: 30 March 2023;
Published: 02 May 2023.
Edited by:
Alberto Maria Cattaneo, Swedish University of Agricultural Sciences, SwedenReviewed by:
Juergen Liebig, Arizona State University, United StatesClaude Desplan, New York University, United States
Copyright © 2023 Smith, Vizueta, Younger, Mullen and Traniello. This is an open-access article distributed under the terms of the Creative Commons Attribution License (CC BY). The use, distribution or reproduction in other forums is permitted, provided the original author(s) and the copyright owner(s) are credited and that the original publication in this journal is cited, in accordance with accepted academic practice. No use, distribution or reproduction is permitted which does not comply with these terms.
*Correspondence: E. Jordan Smith, smithej@bu.edu
†ORCID: Meg A. Younger, orcid.org/0000-0003-4967-7939