- Department of Evolutionary Neuroethology, Max-Planck Institute for Chemical Ecology, Jena, Germany
For the nocturnal hawkmoth Manduca sexta, olfactory and gustatory cues are essential for finding partners, food, and oviposition sites. Three chemosensory receptor families, odorant receptors (ORs), ionotropic receptors (IRs), and gustatory receptors (GRs) are involved in the detection of these stimuli. While many chemosensory receptor genes have been identified, knowledge of their expression profile in potentially chemoreceptive organs is incomplete. Here, we studied the expression patterns of chemosensory receptors in different tissues including the antennae, labial palps, proboscis, legs, wings and ovipositor. We compared the receptors’ expression in female and male moths both before and after mating by using the NanoString platform. This tool allowed us to measure expression levels of chemosensory receptor genes in a single reaction using probes designed against 71 OR, 29 IR and 49 GR transcripts. In all tissues investigated, we detected expression of genes from all three receptor families. The highest number of receptors was detected in the antennae (92), followed by the ovipositor (59), while the least number was detected in the hindlegs (21). The highest number of OR genes were expressed in the antennae (63), of which 24 were specific to this main olfactory organ. The highest number of IRs were also expressed in the antennae (16), followed by the ovipositor (15). Likewise, antennae and ovipositor expressed the highest number of GRs (13 and 14). Expression of the OR co-receptor MsexORCo, presumably a prerequisite for OR function, was found in the antennae, labial palps, forelegs and ovipositor. IR co-receptors MsexIR25a and MsexIR76b were expressed across all tested tissues, while expression of the IR co-receptor MsexIR8a was restricted to antennae and ovipositor. Comparing the levels of all 149 transcripts across the nine tested tissues allowed us to identify sex-biased gene expression in the antennae and the legs, two appendages that are also morphologically different between the sexes. However, none of the chemosensory receptors was differentially expressed based on the moths’ mating state. The observed gene expression patterns form a strong base for the functional characterization of chemosensory receptors and the understanding of olfaction and gustation at the molecular level in M. sexta.
Introduction
Sensory systems help an organism to receive and respond to cues present in its environment and perform essential behaviors such as search for and selection of food, mate and shelter. For insects, the chemosensory system, which detects chemical signals via olfactory and gustatory means, is of great importance (Hansson and Stensmyr, 2011; Depetris-Chauvin et al., 2015; Haverkamp et al., 2018). These chemical signals can be volatile substances (odors) emitted by plants, microorganisms and animals or non-volatile substances (tastants) that remain on the surface of their sources, for example, on leaves. Insects use multiple peripheral organs to detect these signals, such as the antennae and mouthparts on the head, legs and wings attached to the thorax, and male or female genitalia at the tip of the abdomen (Dunipace et al., 2001; Depetris-Chauvin et al., 2015; Xu, 2020; Koutroumpa et al., 2021). These chemosensory organs have on their surfaces several hair-like structures, called sensilla, housing olfactory or gustatory sensory neurons. Different populations of sensory neurons detect different subsets of the chemical space surrounding the organism due to chemosensory receptor proteins expressed in their dendritic membranes (Dahanukar et al., 2005; Joseph and Carlson, 2015; Agnihotri et al., 2016). Three major chemosensory receptor gene families, the odorant receptors (ORs), the ionotropic receptors (IRs), and the gustatory receptors (GRs), encode these receptor proteins. ORs generally detect long-chain pheromone molecules (Zhang and Löfstedt, 2015) and plant-related volatiles of diverse chemical classes (Hallem and Carlson, 2006; de Fouchier et al., 2017). The molecular function of ORs relies on forming heteromeric complexes with the obligate co-receptor ORCo (Sato et al., 2008). IRs can detect both odors as well as tastants (Wicher and Miazzi, 2021). The olfactory function of IRs also requires co-receptors and is usually restricted to detecting acids, aldehydes or amines (Vulpe and Menuz, 2021). The co-receptor IR8a is involved in acid-sensing, while IR25a and IR76b are co-receptors for amine-sensing (Abuin et al., 2011; Vulpe and Menuz, 2021). GRs are mostly known for gustatory functions but are also involved in detecting CO2 (Jones et al., 2007; Ning et al., 2016) and a few other odorants such as acids (Kumar et al., 2020).
Initially, these chemosensory receptor genes were identified in the genome of Drosophila melanogaster (Clyne et al., 1999, 2000; Gao and Chess, 1999; Vosshall et al., 1999; Benton et al., 2009). Then, advancements in genome and transcriptome sequencing methods, and the development of optimized bioinformatic tools to identify homologous genes led to the identification of chemosensory receptor repertoires in many other insect species (Wanner et al., 2007; Grosse-Wilde et al., 2011; Koenig et al., 2015; Walker et al., 2016, 2019; Koutroumpa et al., 2021). Like all gene families, chemosensory receptors evolve by duplication, functional diversification and pseudogenization of their genes. Across insect orders, we find a considerable variation in the total number of receptors, high divergence in amino acid sequences and occurrence of clade-specific receptor subfamilies (Gouin et al., 2017; Pearce et al., 2017; Brand et al., 2018; Xu, 2020; Yin et al., 2021). These findings reveal that chemosensory receptors are under high selection pressure and evolve continuously in a birth and death process. Therefore, functional characterization of these genes in different species is necessary to understand the impact of chemical ecology on insect evolution.
The nocturnal hawkmoth Manduca sexta has served for decades as an important Lepidopteran model for research in chemosensory modalities providing deep insight into the physiological, neuroanatomical and behavioral aspects of olfaction and gustation. Recent work has generated a highly curated annotation of OR, IR and GR genes for M. sexta (Koenig et al., 2015). This paved the way for the application of CRISPR-Cas9 mediated targeted knock-out experiments revealing the function of the obligate co-receptors MsexORCo for ORs, and MsexIR8a and MsexIR25a for IRs in M. sexta (Fandino et al., 2019; Zhang J. et al., 2019). In addition, one sex pheromone receptor and two ORs detecting plant volatiles have been de-orphanized to date (Wicher et al., 2017; Guo et al., 2021; Zhang et al., 2022), whereas the functions of all other chemosensory receptors of M. sexta remain elusive to the best of our knowledge. A critical step in understanding any gene’s function is knowing when and where it is expressed. There is limited knowledge about the expression of some of these receptors in the antennae (Koenig et al., 2015), the proboscis (Haverkamp et al., 2016), and the female ovipositor (Klinner et al., 2016) in M. sexta. Few studies in other moth species have also investigated the expression of chemosensory genes in different tissues (Jacquin-Joly et al., 2012; Guo et al., 2018; Walker et al., 2019; Koutroumpa et al., 2021).
While input from the antennae can produce odor-directed flight responses in M. sexta moths (Schneiderman et al., 1986; Kalberer et al., 2010), a concerted role of information detected at multiple chemosensory tissues is likely for optimal behavioral output, especially regarding feeding and oviposition behaviors. Odor plumes originating from flowers guide moths toward rewarding nectar sources (Riffell et al., 2008). During such foraging, the proboscis which can both smell and taste (Reiter et al., 2015; Haverkamp et al., 2016) is extended, a behavior that is disrupted in knock-out MsexORCo mutants (Fandino et al., 2019). In case of oviposition, plant-released volatiles alone can attract gravid M. sexta females toward host-plants (Kariyat et al., 2013; Spaethe et al., 2013). When moths approach the host plant, they curl their abdomen even when contact with the plants is prevented (Mechaber et al., 2002). Results from Klinner et al. (2016) suggest that this behavior serves to sample odors with the odor-receptive ovipositor. After landing and before laying eggs, the female moth assesses the chemical profiles of leaves with its forelegs and midlegs, suggesting a gustatory function of these appendages (Yamamoto et al., 1969; Mechaber et al., 2002). Accordingly, putative chemosensory sensilla on the legs of M. sexta have been described (Kent and Griffin, 1990). Moreover, CO2 is an important cue for foraging (Guerenstein et al., 2004b; Thom et al., 2004), where CO2-detection has been shown to occur in specialized sensilla present on the labial palps (Kent et al., 1986; Guerenstein et al., 2004a). The chemosensory potential of wings has not been reported so far in M. sexta; however, it has been observed in other insects like mosquitoes (Yang et al., 2020) and vinegar flies (Raad et al., 2016; He et al., 2019; Yanagawa et al., 2019).
Males and female moths need to extract different information from their environment to perform their sex-specific tasks. A well-known example of sex-specificity in moths is their pheromone communication system. Male moths have specialized antennae to detect female-produced sex pheromones from afar and thereby find mates (Tumlinson et al., 1989). In M. sexta, the receptor for the main pheromone component bombykal, MsexOR1 (Wicher et al., 2017) has a male-specific expression (Grosse-Wilde et al., 2010) and mutant males lacking functional MsexORCo are unable to mate (Fandino et al., 2019). Likewise, searching for hostplants for oviposition is a female-specific task, which might require adaptations of the female chemosensory system and expression of female-specific receptors. Apart from behaviors related to reproduction, male and female hawkmoths exhibit different foraging preferences when given a choice between flowers from two plant species (Alarcón et al., 2010).
Moreover, physiological states such as age, nutrition and mating state can modify insect behavior (Gadenne et al., 2016). Mated female M. sexta, for example, shows an increased flight and abdomen curling response compared to virgin females when exposed to hostplant volatiles (Mechaber et al., 2002). Mating can also cause changes in physiological responses toward vegetative and floral plant odors in female M. sexta and Spodoptera littoralis (Saveer et al., 2012; Bisch-Knaden et al., 2022). For male hawkmoths, feeding after each mating is advantageous for repetitive mating success (Levin et al., 2016), and females benefit from post-mating feeding with increased longevity and more mature eggs than starved females (Sasaki and Riddiford, 1984; von Arx et al., 2013). These mating state-dependent differences might be linked to modulations of chemosensory receptor levels. Mating-dependent up- or downregulation of several ORs was seen in flies, Drosophila suzukii (Crava et al., 2019), Bactrocera dorsalis (Jin et al., 2017) and the moth Dendrolimus punctatus (Zhang et al., 2017).
In the present study, we investigated expression of genes from all three chemosensory receptor families in different chemosensory organs and compared the expression between males and females, pre- and post-mating. We used the NanoString digital platform for gene expression (Geiss et al., 2008; Kulkarni, 2011) to count individual mRNA transcripts using complementary nucleotide probes designed for each gene of interest. The NanoString technology was shown to be highly sensitive and comparable to qPCR and RNA-sequencing techniques (Geiss et al., 2008; Veldman-Jones et al., 2015; Bondar et al., 2020) and has been used to study expression of olfactory receptors in mice (Khan et al., 2011, 2013). Associating gene expression patterns with the known or suspected chemosensory function of the tested tissues enables us to identify potentially important receptors whose function could be revealed in future experiments.
Materials and methods
Insect rearing
Manduca sexta moths were either reared in our lab or pupae were kindly provided by Monika Stengl (University of Kassel). For rearing, we used an artificial diet (156.25 g soy flour, 144.38 g rye flour, 144.38 g wheat flour, 85 g agar-agar, 4.5 ml rapeseed oil, 1.45 g calcium carbonate, 1.45 g sodium chloride, 4.8 g methyl paraben, 4.8 g sorbic acid, 14.5 g ascorbic acid, 38.5 g milk powder, 32 mg nicotinic acid, 16 mg riboflavin, 7.5 mg thiamine, 7.5 mg pyridoxine, 7.5 mg folic acid, 0.64 mg biotin). We kept the larvae in a climate chamber with a 14 h:10 h light:dark cycle, a temperature of 26°C (light cycle) or 24°C (dark cycle), and at 60% relative humidity. Male and female pupae were sorted and moved to separate climate chambers with a 16 h:8 h light:dark cycle, a temperature of 25°C, and at 60% relative humidity (light cycle) or 70% relative humidity (dark cycle). Eclosing adults were collected daily and held in individual brown paper bags (17 cm × 26 cm) in the pupal climate chambers.
Tissue collection and RNA extraction
For virgin samples, males and females remained in separate chambers until tissue collection. For mated samples, males and females were paired 2 days after eclosion during early scotophase and observed every 2 h for successful mating. Tissues from virgin and mated moths (Supplementary Table 1) were collected 3 days after eclosion during the scotophase. Moths were first cooled down at 4°C for about 15 min to decrease movements, then tissues were dissected with microscissors, collected in 2 ml microcentrifuge tubes or 15 ml falcon tubes (forewings), immediately immersed in liquid N2, and stored at −80°C until further processing. The tissues (except forewings) were lysed and disrupted in RLT buffer (Qiagen, Germany1) using Tissue Lyser LT (Qiagen, Germany) with a steel bead (diameter: 5 mm) at 50 oscillations per second for 15 min. The forewings, due to their large size, were ground to a fine powder in liquid N2 using a mortar and a pestle. Total RNA was extracted from the lysed tissues using RNeasy Mini Kit (Qiagen, Germany) and RNase-free DNase Set (Qiagen, Germany) was used for the DNase digestion step. The concentration and purity of the extracted total RNA were checked first on a NanoDrop One (Thermo Fisher Scientific2). Three samples for each experimental group, leading to six ovipositor samples (3 virgin female, 3 mated female), and 12 samples for each of the remaining tissues (3 virgin female, 3 mated female, 3 virgin males, 3 mated males) were then run on 2,100 Bioanalyzer (Agilent Technologies, Inc.3) to confirm RNA integrity. Based on initial standardization assays, 200 ng total RNA from each tissue was used for the NanoString gene expression assay.
NanoString gene expression assay
We used the nCounter XT CodeSet gene expression assay (NanoString Technologies, Inc., United States4). The custom CodeSet (Zhang et al., 2022) contained 268 probes targeting 71 ORs, 29 IRs, 49 GRs, 47 odorant binding proteins, 5 pickpocket, 3 sensory neuron membrane proteins and 62 candidate reference gene transcripts (Supplementary Table 2). The probes were designed using the reference coding sequences of 74 ORs, 21 IRs, and 45 GRs (Koenig et al., 2015) that were submitted to M. sexta OGS2.0 (Kanost et al., 2016). Four isoforms of MsexGR11, six isoforms of MsexGR15 and 8 IRs that were identified later (MsexIR1.1, 7d.2, 7d.4, 85a, 100a, 100d, 100f, and 100h) (Liu et al., 2018) were included. Some of the receptor sequences had high homology with sequences of their duplicates, prohibiting the design of unique probes. Therefore, these receptors had to be excluded (MsexOR23, MsexGR15D, 25, 26, 27, and 36). For all final probes, we performed NCBI BLAST search against M. sexta reference mRNA. Except for a few cases with duplicate receptors for which the probes could cross-target, our probes did not have off-targets. The recent de novo M. sexta genome assembly (Gershman et al., 2021) indicated that MsexOR84 and MsexOR89 might be the same gene, and similarly MsexOR42 and MsexOR66 might be the same gene. Therefore, we provisionally named these receptors MsexOR84/89 and MsexOR42/66.
We followed the standard protocol from nCounter XT Gene Expression Assay User Manual (MAN-10023-11, page 165). All reagents and buffers were purchased from NanoString Technologies, Inc., United States. An attenuation mix (Eurofins Genomics, Germany6) to suppress counts of MsexABPx, MsexOBP1, MsexOBP5 and MsexOBP6 was used as these genes had very high expression levels causing high binding densities for the antennal samples. Briefly, a master-mix of 42 μl Reporter CodeSet, 28 μl Reporter-Plus reagent and 70 μl nCounter SPRINT hybridization buffer was created. For each hybridization reaction we combined 10 μl master-mix, 5 μl total RNA (200 ng), 1 μl attenuation-mix and 3 μl mixture of Capture ProbeSet and Capture-Plus reagent. Hybridization was done at 65°C for 22 h, after which 16 μl Merck water was added to the sample. The entire volume was loaded on nCounter SPRINT Cartridge (NanoString, United States) and processed on nCounter SPRINT Profiler (NanoString, United States).
Data processing
The raw data from the gene expression assay (see Data Availability Statement) was processed using nSolver4.0 (NanoString, United States). Quality control for mRNA data was done on each sample using default parameters for nCounter SPRINT Profiler according to NanoString Gene Expression Data Analysis Guidelines (MAN-C0011-047). The parameters were Imaging QC: 75; Binding Density QC: 0.1 – 1.8; Positive Control Linearity QC: 0.95; Positive Control Limit of Detection QC: 2 standard deviations. Due to high expression levels of chemosensory genes in the antennae, those samples had a slightly higher binding density than the default threshold of 1.8. We visually inspected the raw data from antennae following instructions from the user manual and confirmed that the data was robust and usable. However, the raw counts of positive spike-in controls in antennae were lower than those of the other tissues (Supplementary Figure 1). To avoid normalization flags, we normalized the antennal samples separate from the samples of the other tissues. For antennae, background subtraction was done first using the raw counts of the 8 negative control probes (mean + 2 standard deviations). After that, two normalization steps were performed, first using the geometric mean counts of the 6 external positive control probes, and second, using the geometric mean counts of three endogenous reference genes that were selected based on their coefficient of variation (CV < 30%). The endogenous reference genes for antennal samples were melastatin, smoothened, and dunce. For the remaining tissue samples, background subtraction and positive control normalization was done similar to the antennae. The endogenous reference genes used for the second normalization were melastatin, smoothened and two G protein genes (Gbeta13F and concertina) with a CV < 25%. After these two normalization steps, the minimum normalized count for each sample was defined as “background,” and any chemosensory receptor gene with counts above this background in at least 2 of the 3 samples was considered to be expressed in that experimental group. The normalized data are available online (see section “Data availability statement”).
Data analysis
The double-normalized counts (referred to as “counts” in the Results and Discussion) were log2 transformed and used for making heatmaps and for statistical analyses using R (version 4.1.2)8 and Rstudio.9 The heatmaps were generated using the libraries heatmaply and RColorBrewer and later edited on Adobe Illustrator CS5. For identifying sex and mating state dependent gene expression in a given tissue (except ovipositor), we performed a nested ANOVA for each gene with sex as the main factor and mating state as the nested factor and a significance level of 0.05. The test was done separately for each tissue only for those genes that were expressed in at least one of the four experimental groups for that tissue. We then calculated the false discovery rate (FDR) using the Benjamini-Yekutieli procedure with the sets of p-values for the two factors for each tissue. Finally, a gene was considered to be significantly differentially expressed between sexes or mating states if FDR < 0.05 and log2 fold-change ≥ 2. Ovipositor samples from virgin and mated females were compared with an unpaired t-test. For a p-value < 0.05 and a log2 fold-change = 2 between both groups, a gene was considered to be differently expressed.
Results and discussion
NanoString gene expression assay for Manduca sexta chemosensory receptors
We measured the expression of chemosensory receptors in different tissues (antennae, labial palps, proboscis, forelegs, midlegs, hindlegs, forewings, hindwings) of female and male M. sexta moths, both before and after mating. In addition, we examined the expression of chemosensory genes in the ovipositor of virgin and mated females. The probe set targeted 71 OR transcripts including the co-receptor MsexORCo, 29 IR transcripts including the co-receptors MsexIR8a, MsexIR25a, MsexIR76b, and 49 GR transcripts (see section “Materials and methods” for details). Out of these 149 chemosensory receptors, transcripts for two ORs, seven IRs and 25 GRs were not detected in any of the investigated tissues, that is, counts of these transcripts were in the range of background counts (see section “Materials and methods” for details, Table 1 and Supplementary Table 3). On the other hand, three ORs, three IRs and two GRs were detected in each of the tissues tested.
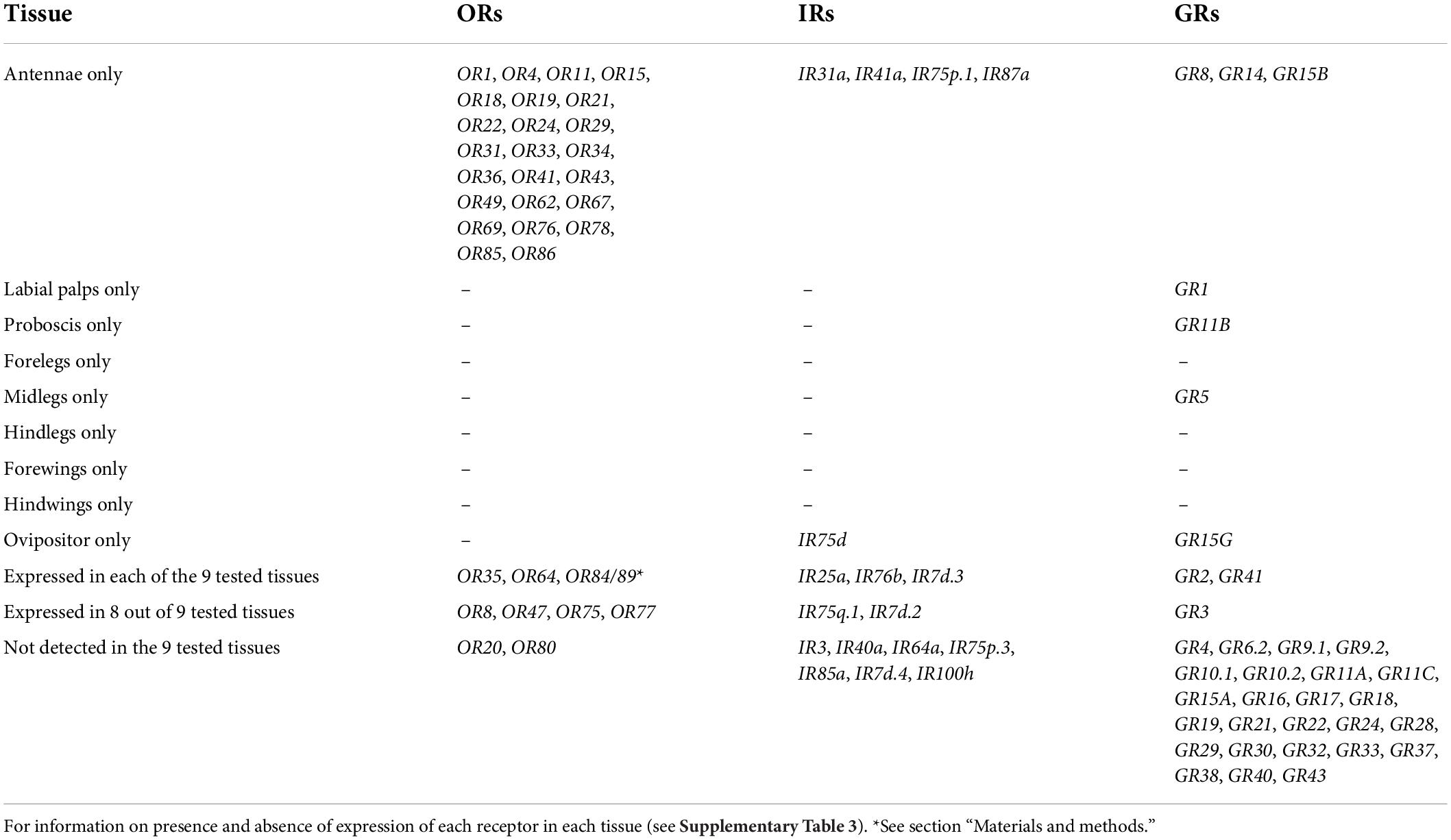
Table 1. Chemosensory receptor gene transcripts that were tissue-specific, broadly expressed or not detected.
We identified several genes with tissue-specific expression (Table 1). We also found sex-biased expression of several ORs and one GR (FDR corrected p < 0.05, nested ANOVA), whereas the expression of IRs seemed to be independent of sex in the investigated tissues (FDR corrected p > 0.05, nested ANOVA, Table 2). In contrast, the mating status of female or male moths had no statistically significant effect on the expression level of any chemosensory receptor in any tissue (FDR corrected p > 0.05, nested ANOVA).
Antennae
In total, in the male and female antennae, we detected transcripts of 63 out of the 71 ORs (89%, Figure 1A). Twelve of these antennal ORs had a sex-biased gene expression (Table 2). Sixteen out of the 29 IRs (55%) and 13 out of the 49 GR transcripts (27%) were also expressed in the antennae (Figures 1B,C).
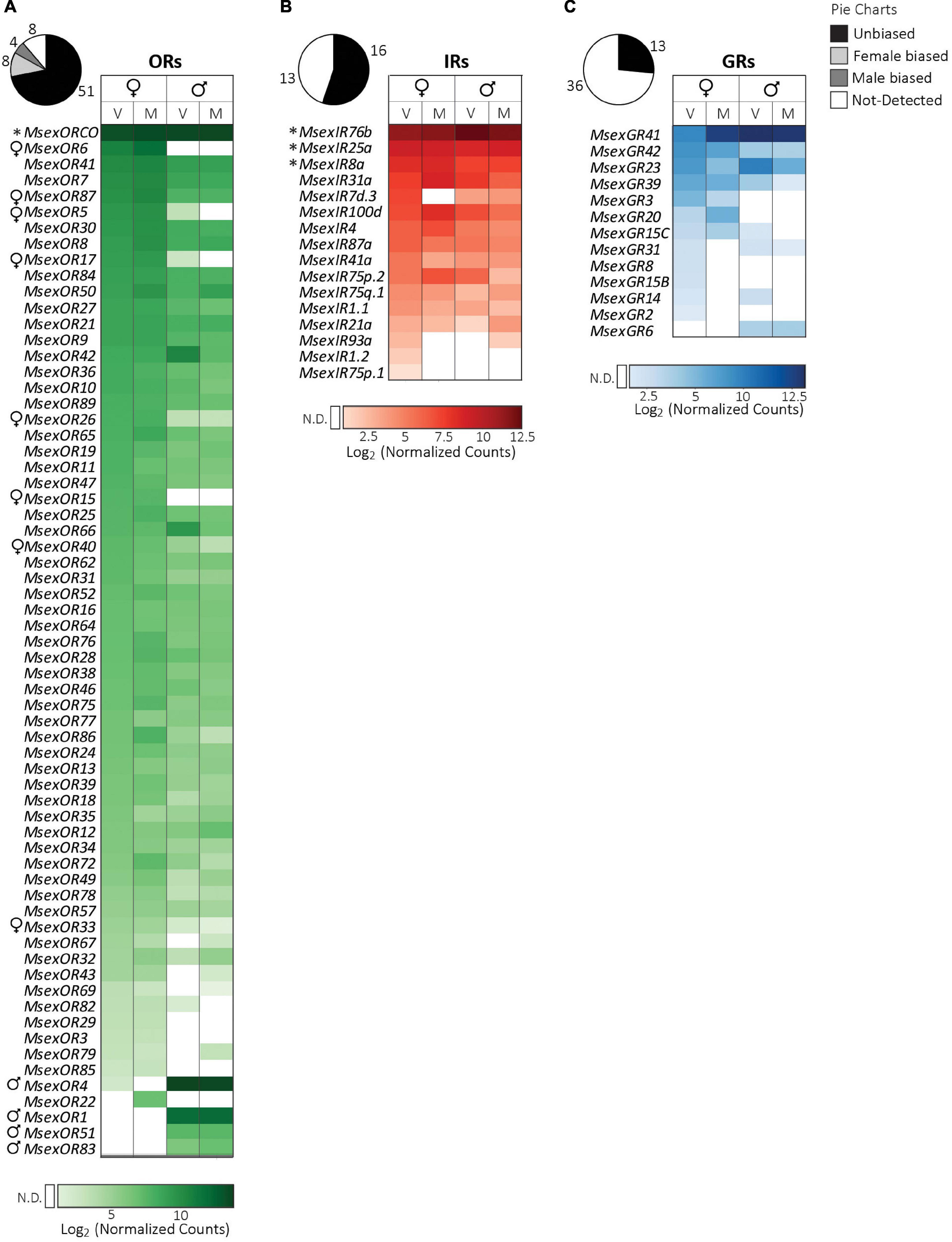
Figure 1. Expression of chemosensory receptor genes in the antennae of adult M. sexta. (A) Odorant receptors, (B) ionotropic receptors, (C) gustatory receptors. Pie charts depict the percentage of expressed genes (absolute numbers next to the charts) of each receptor family (see legend on top right). Heatmaps were created separately for each receptor family using the log2 of the geometric mean (n = 3 biological replicates) of normalized counts obtained from the NanoString assay (see section “Materials and methods”). Light to dark shades indicate low to high counts (see color bar at bottom of each heatmap); white cells indicate no detection (N.D.) of transcripts (for a definition see section “Materials and methods”). Columns represent female and male moths of different mating states (V: virgin, M: mated). Rows represent receptors that were expressed in the antennae of at least one of the four groups, and are sorted first according to values in virgin females, followed by mated females, virgin males and mated males. Symbols next to receptor gene names: *, OR or IR co-receptors; ♀, female-biased receptors; ♂, male-biased receptors (FDR corrected p < 0.05, nested ANOVA). Mating states of males and females did not have a significant effect on receptor gene expression (FDR corrected p > 0.05, nested ANOVA).
ORs. The OR co-receptor MsexORCo had high counts for the antennal samples (Figure 1A). A high expression of MsexORCo was expected as its co-expression in all OR expressing neurons is considered necessary for OR-mediated odor detection (Sato et al., 2008) and knocking out MsexORCo reduces or completely abolishes antennal, antennal lobe and behavioral responses toward plant odors (Fandino et al., 2019).
We could confirm previous findings that MsexOR1, MsexOR4, MsexOR51, and MsexOR83 had a male-biased expression in the antennae (Grosse-Wilde et al., 2010; Koenig et al., 2015). MsexOR1 detects the major female sex-pheromone bombykal (Wicher et al., 2017), which, together with MsexOR4 and MsexOR51, belongs to the expanded pheromone receptor clade (Koenig et al., 2015). Likewise, the fourth male-biased receptor MsexOR83 belongs to a newly discovered lineage of pheromone receptors (Bastin-Héline et al., 2019). Hence, all three orphan, male-biased receptors might be involved in detecting minor components of the female-produced pheromone blend, containing in total 12 components (Kaissling et al., 1989; Kalinova et al., 2001). MsexOR1, 51, and 83 had a male-specific antennal expression, whereas MsexOR4 transcripts were also detected in female antennae. Such expression of a pheromone receptor in females is seen in other moth species (Bengtsson et al., 2012; Walker et al., 2016), suggesting that these females can detect their own pheromone (Holdcraft et al., 2016). M. sexta female antenna is known to only respond to the minor pheromone component Z11-hexadecanal (Kalinova et al., 2001). However, in male M. sexta, MsexOR4-expressing olfactory sensory neurons are housed in the most abundant type of sensilla in the male antenna, the long trichoid sensilla (Grosse-Wilde et al., 2010), and neurons in these sensilla do not respond to Z-11-hexadecanal (Kalinova et al., 2001). Therefore, it seems unlikely that MsexOR4 is the receptor for this minor pheromone component. One OR from the pheromone receptor clade, MsexOR15, is female-specific, a rare feature for this subfamily (Bastin-Héline et al., 2019). Its absence of expression in male antennae negates the possibility of MsexOR15 being the Z-11-hexadecanal receptor, as males can detect this component with neurons housed in short trichoid sensilla (Kalinova et al., 2001). MsexOR38, which belongs to the same group as MsexOR83 (Koenig et al., 2015) has an unbiased expression in males and females, and might therefore be the putative receptor for Z-11-hexadecanal. These ORs of the pheromone receptor clade may also detect pheromones of sympatric hawkmoths, such as M. quinquemaculata and M. rustica, thus ensuring reproductive isolation (Daimon et al., 2012). However, in the codling moth, C. pomonella, an OR belonging to the pheromone clade detects a plant volatile (Bengtsson et al., 2014), opening the possibility that some of the uncharacterized pheromone clade members of M. sexta might have a non-pheromonal function.
Apart from MsexOR15, we found seven more female-biased ORs. These include those previously identified as female-biased (MsexOR5, 6, 15, and 87; Koenig et al., 2015), and four newly identified female-biased ORs (MsexOR17, 26, 33, and 40). MsexOR6, like MsexOR15, was not only female-biased but also female-specific for the antennae. MsexOR5 and 6 are encoded by two gene duplicates orthologous to the female-specific Bombyx mori linalool receptor BmorOR19 (Anderson et al., 2009; Koenig et al., 2015). Therefore, one of these ORs might be responsible for the enantioselective response to (+)-linalool of the lateral large female glomerulus (latLFG) in the female M. sexta antennal lobe (Reisenman et al., 2004) and for the female moth’s oviposition preference for (+)-linalool (Reisenman et al., 2010).
It is interesting to note that we have two times more female-biased than male-biased ORs. All male-biased ORs belong to pheromone receptor lineages; however, seven of the eight female-biased ORs are from different clades of potential plant volatile receptors (Guo et al., 2021). These ORs might be important in the context of the female-specific behavior of searching an appropriate oviposition site directed by host-plant volatiles. According to Bastin-Héline et al. (2019), another possible function of these polyphyletic, female-biased receptors might be the detection of male-produced pheromones, such as those presumably emitted from male hairpencils in M. sexta (Grant and Eaton, 1973; Birch et al., 1990).
The MsexORCo-associated glomeruli on the dorsal surface of the M. sexta antennal lobe (Fandino et al., 2019) respond to volatile headspace collections of native, nectar-rich flowers, and host and non-host plant foliage (Bisch-Knaden et al., 2022). This suggests that ORs might be responsible for the antennal detection of a large variety of floral and vegetative odors in M. sexta (Fraser et al., 2003; Spaethe et al., 2013). Ligands can be predicted based on homology to other de-orphanized Lepidopteran ORs for highly conserved OR clades. Such an approach has led to identification of the floral odor phenyl acetaldehyde as a ligand for MsexOR67 (Guo et al., 2021). The second identified plant volatile-detecting OR, MsexOR35, detects α-copaene, a volatile abundant in the headspace of the beetle-infested host-plant Datura wrightii that attracts ovipositing M. sexta (Zhang et al., 2022).
Overall, our results for the antennal expression of ORs largely agree with a previously performed RNA sequencing analysis, especially for the sex-biased ORs (Koenig et al., 2015), providing support for using the NanoString assay as a valid method for the study of insect chemosensory gene expression (Supplementary Figure 2).
IRs. Several IRs of the conserved antennal IR group (Croset et al., 2010; Yin et al., 2021) were expressed in the M. sexta antennae. These included the IR co-receptor genes MsexIR8a, 25a, 76b which had the highest counts among all IRs expressed in the antennae (Figure 1B). MsexIR8a and MsexIR25a are required for native responses of antennal coeloconic sensilla to carboxylic acids and amines, respectively (Zhang J. et al., 2019). For other antennal IRs, functions can be predicted based on D. melanogaster orthologs. MsexIR76b is ortholog to DmelIR76b, which is involved in both smell and taste of polyamines (Hussain et al., 2016) and also mediates gustatory detection of low salt concentrations and sour substances (Zhang et al., 2013; Chen and Amrein, 2017). Similarly, MsexIR31a, together with the co-receptor IR8a, is likely involved in acid detection, while MsexIR41a, when co-expressed with the co-receptor MsexIR25a or MsexIR76b, might be involved in detecting amines (Silbering et al., 2011; Hussain et al., 2016). IRs can also have non-chemosensory roles. Based on homology to Drosophila IRs, MsexIR21a, MsexIR93a and MsexIR25a could have cool-temperature-sensing functions (Knecht et al., 2016; Ni et al., 2016). Alternatively, MsexIR93a and MsexIR25a might be involved in hygrosensation (Knecht et al., 2016) by neurons present in the styliform sensilla of the antenna (Dahake et al., 2022).
We also found expression of nine Lepidoptera-specific IRs in the antennae (Koenig et al., 2015; Yin et al., 2021). Seven of these, MsexIR1.1, MsexIR1.2, MsexIR4, MsexIR75p.1, MsexIR75p.2, MsexIR75q.1, and MsexIR87a putatively encode IRs nested within the antennal IRs and are considered to have an olfactory function (Yin et al., 2021). All except MsexIR87a belong to a monophyletic group of putative acid-detectors (Hou et al., 2022). This group includes two acid-sensing IRs of Agrotis segetum, AsegIR75q.1, which is tuned specifically to octanoic acid and AsegIR75p.1, which has a broader tuning and detects acids, aldehydes and alcohols. Two Lepidoptera-specific IRs, MsexIR7d.3 and MsexIR100d, lie among the divergent IRs (Yin et al., 2021; Hou et al., 2022), which are defined as IRs that are not expressed in the antennae but in other tissues (Croset et al., 2010; Yin et al., 2021). However, the expression pattern of MsexIR7d.3 resembles that of its A. segetum ortholog AsegIR7d.3, which is highly expressed in the antennae (Hou et al., 2022). The function of receptors in the IR7d subfamily is unknown; however, the phylogenetically related DmelIR7a is expressed in neurons of the gustatory organs of larval and adult D. melanogaster (Croset et al., 2010), suggesting a gustatory function for these receptors.
Sex-dependent IR expression has been observed in other moth species. For instance, both female- and male-biased IRs have been identified in B. mori (Yin et al., 2021). In contrast, we did not find any significant effect of sex on IR expression in the antennae of M. sexta, although MsexIR8a-mediated detection of acids from larval feces is crucial for oviposition deterrence behavior, which prevents larval crowding (Zhang J. et al., 2019). This female-specific behavior seems to be independent of differential expression of the IRs investigated in our study.
GRs. Consistent with a previous report, we detected antennal expression of genes of two putative CO2-receptors MsexGR2 and MsexGR3, sugar receptor MsexGR6 and bitter receptors MsexGR41 and MsexGR42 (Koenig et al., 2015). However, we could not confirm the antennal expression of the fructose receptor genes MsexGR9.1, 9.2 and 10.2. In addition, we found transcripts of seven more bitter receptors and another putative sugar receptor MsexGR8, whose ortholog BmorGR8 detects the sugar alcohol inositol, which is ubiquitously found in plants (Zhang et al., 2011).
MsexGR41 had the highest counts among all GRs expressed in the antennae (Figure 1C) and encodes a highly conserved and unduplicated putative bitter GR in the Lepidopteran order. The ortholog of MsexGR41 in the silkmoth (BmorGR63) is broadly expressed in different chemosensory tissues of adults and larvae (Guo et al., 2017). Similarly, MsexGR41 transcripts were detected not only in the adult and larval antennae but also in non-chemosensory tissues such as gut, fat bodies, brain and gonads (Koenig et al., 2015), suggesting additional roles for this receptor. Functional studies of bitter receptors in Lepidopteran species are sparse (reviewed in Xu, 2020). Moreover, predictions based on sequence similarity might be difficult since bitter receptors, like ORs, are highly divergent and often go through lineage-specific expansions within Lepidoptera (Koenig et al., 2015).
Although putative gustatory sensilla chaetica are present on the antennae of M. sexta (Lee and Strausfeld, 1990), the functional roles of antennal gustatory neurons are unknown. In the moths Heliothis virescens and Agrotis ipsilon, sugar and bitter detecting gustatory neurons in the antennae influence the proboscis extension behavior (Jørgensen et al., 2007; Hostachy et al., 2019). Similarly, antennal GRs might also influence proboscis extension in M. sexta, a behavior which is dependent on both olfactory and visual cues (Raguso and Willis, 2002) and MsexORCo function (Fandino et al., 2019).
In Drosophila, co-expression of the two CO2 receptors, DmelGR21a and DmelGR63a, in the fly antennae is required for CO2 detection (Jones et al., 2007). Similarly, expression of two putative CO2 receptor genes, MsexGR2 and MsexGR3, suggests that the M. sexta antennae could also detect CO2; a function that is not reported so far. In moths, however, CO2 detection is generally assigned to labial palps. In fact, the CO2 sensitive glomerulus in the antennal lobe in M. sexta is targeted by sensory neurons in the labial pit organ of the labial palp (Guerenstein et al., 2004a). In contrast to Drosophila, Lepidopteran species have three GRs (GR1, GR2 and GR3) in the CO2 receptor subfamily (Robertson and Kent, 2009). Functional analysis in Helicoverpa armigera revealed that co-expression of HarmGR1 and HarmGR3 is necessary for CO2 reception (Ning et al., 2016). Assuming that the functions of these GRs are conserved, the absence of expression of MsexGR1, the ortholog of HarmGR1, might actually indicate the inability of M. sexta antennae to detect CO2. MsexGR2 and MsexGR3 might be involved in olfaction as GRs of the CO2 receptor family were shown to be activated or inhibited by various odorants in D. melanogaster and Aedes aegypti (MacWilliam et al., 2018; Kumar et al., 2020).
Mouthparts
In contrast to the antennae, transcripts of only 17 ORs each were detected in labial palps and proboscis (Figure 2A). The labial palps in addition expressed six IRs and seven GRs, whereas in the proboscis 11 IRs and nine GRs were expressed (Figures 2B,C).
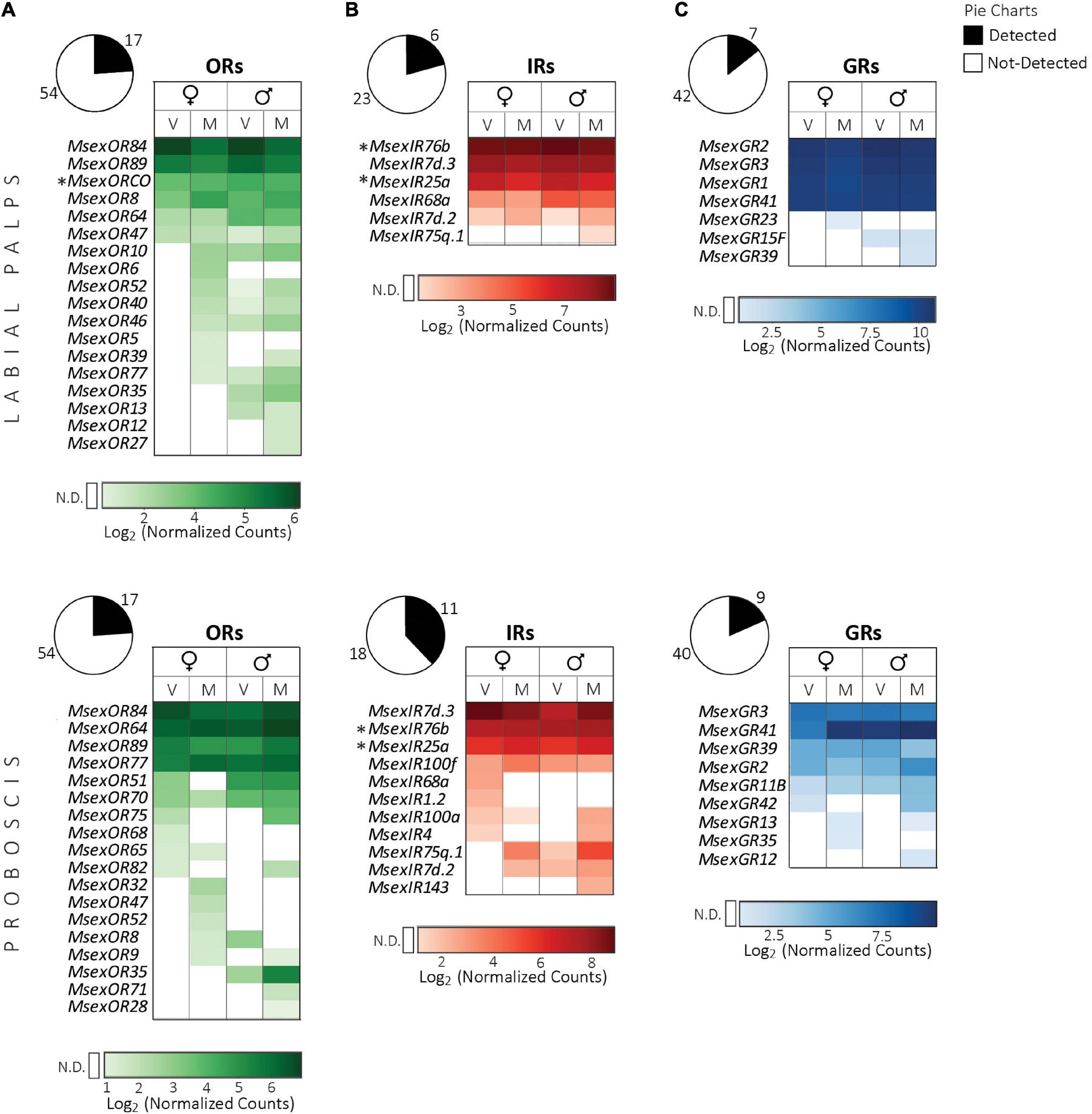
Figure 2. Expression of chemosensory receptor genes in the mouthparts (top panel, labial palps; bottom panel, proboscis) of adult M. sexta. (A) Odorant receptors, (B) ionotropic receptors, (C) gustatory receptors. Pie charts depict the percentage of expressed genes (absolute numbers next to the charts) of each receptor family (see legend on top right). Heatmaps were created separately for each receptor family using the log2 of the geometric mean (n = 3 biological replicates) of normalized counts obtained from the NanoString assay (see section “Materials and methods”). Light to dark shades indicate low to high counts (see color bar at bottom of each heatmap); white cells indicate no detection (N.D.) of transcripts (for a definition see section “Materials and methods”). Columns represent female and male moths of different mating states (V: virgin, M: mated). Rows represent receptors that were expressed in the mouthparts of at least one of the four groups, and are sorted first according to values in virgin females, followed by mated females, virgin males and mated males. Symbols next to receptor gene names: *, OR or IR co-receptors. Sex and mating states of males and females did not have a significant effect on receptor gene expression (FDR corrected p > 0.05, nested ANOVA).
ORs. Of the 17 ORs expressed in each mouthpart, only seven ORs were common between both tissues, indicating that labial palps and proboscis have different chemosensory functions (Figure 2A). MsexORCo expression was consistently detected in male and female labial palp samples at high levels, whereas in the proboscis, it seemed absent. In M. sexta, like in several other moth species, the labial palps are so far known only to detect CO2 (Guerenstein et al., 2004a; Ning et al., 2016). The expression of several ORs, like the potential linalool-receptors MsexOR5 and MsexOR6 (Grosse-Wilde et al., 2010), and more importantly, MsexORCo, strongly indicates that the labial palps of M. sexta might have an olfactory function beyond the detection of CO2. We found expression of 13 ORs in labial palps of mated females compared to only five ORs in virgins. Although this difference was not significant according to the nested ANOVA we applied, it might reflect a slightly increased olfactory sensitivity of the palps to foraging- or oviposition-associated volatiles in females following mating. For the proboscis, expression of MsexORCo in male M. sexta has been demonstrated previously using RT-PCR and immunohistochemistry methods (Haverkamp et al., 2016). In our study, however, we detected MsexORCo transcripts only in one out of three samples each of virgin and mated males, thus not fulfilling our criterion for gene expression (see section “Materials and methods” for details). MsexORCo is expressed only in a single neuronal cell in a single sensillum at the tip of the proboscis (Haverkamp et al., 2016). As we extracted RNA from the entire length of the proboscis and not only from the tip, such cell-specific expression might have gone undetected. This sensillum responds strongly to benzyl acetone (the major component of N. attenuata flowers) but in addition detects other typical floral odors such as cis-jasmone, linalool, geraniol, nerol and benzyl alcohol (Haverkamp et al., 2016; Bisch-Knaden et al., 2022). MsexOR84/89, MsexOR64 and MsexOR77 were highly expressed in the proboscis and might be candidate receptors involved in the detection of these floral odors. Another intriguing feature was expression of one of the male-specific antennal pheromone receptors, MsexOR51, in the proboscis of female virgin M. sexta. This suggests that females might have multiple mechanisms of detecting their own pheromone, and that MsexOR51 might not really be “male-specific.”
IRs. All IRs expressed in the labial palps were also detected in the proboscis (Figure 2B). In both mouthparts, the IRs with the highest counts were those encoding co-receptors, MsexIR25a and MsexIR76b, and the Lepidoptera-specific IR MsexIR7d.3, which were expressed in each of the tested tissues (Table 1). The IR-co-receptor MsexIR8a, on the other hand, was not detected in the mouthparts. This is contrary to a previous report where both MsexIR8a and MsexIR25a were shown to be expressed in the proboscis by using RT-PCR (Haverkamp et al., 2016). We detected MsexIR8a transcripts in only one sample of a virgin female, indicating that MsexIR8a is expressed in very low levels in the proboscis, and PCR amplification would be a better technique for detecting this expression. The proboscis expression of genes of three IRs from the “expanded acid-sensing cluster” (MsexIR1.2, MsexIR4 and MsexIR75q.1) suggests that the proboscis could detect volatile carboxylic acids (Hou et al., 2022); however, based on the functional characterization of IRs from this cluster in the turnip moth A. segetum, such acid-sensing would require expression of the co-receptor IR8a.
Several IRs that were not detected in the antennae, were expressed in the mouthparts. These are MsexIR7d.2, a duplicate of MsexIR7d.3 expressed in the antenna, and MsexIR68a, whose Drosophila ortholog is involved in hygrosensation (Knecht et al., 2017). Additionally, the proboscis expressed three more Lepidoptera-specific IRs (MsexIR100a, MsexIR100f, and MsexIR143; Liu et al., 2018) with unknown functions.
GRs. Four GRs were commonly expressed between labial palps and proboscis (Figure 2C). High counts of the ubiquitously expressed MsexGR41 (Table 1) were found in both mouthparts. A distinct and high expression of all three putative CO2 receptor genes, MsexGR1, 2 and 3, was observed only in the labial palps, the organ known for CO2 detection in M. sexta (Guerenstein et al., 2004a). In the noctuid moth H. armigera, a similar high expression of the orthologous GRs, HarmGR1, 2, and 3, was reported for the labial palps (Guo et al., 2018). In the proboscis, like in the antennae, transcripts of MsexGR2 and MsexGR3, but not of MsexGR1 were detected. Therefore, CO2-detection in M. sexta seems to be restricted to the labial palps.
All other GRs expressed in the mouthparts belong to the clade of bitter receptors (Koenig et al., 2015) and, surprisingly, no sugar or fructose receptors were detected. However, as the proboscis is considered the major gustatory organ, we expected to find expression of sugar or fructose receptors. In H. armigera, for example, sugar receptors are expressed in the proboscis (Guo et al., 2018). Reiter et al. (2015) showed that gustatory neurons in the proboscis of M. sexta can discriminate various tastants including sucrose and other sugars, strongly suggesting the presence of sugar receptors in the proboscis. However, gustatory neurons are mainly found in the distal one-third of the proboscis (Reiter et al., 2015) and the expression level of these sugar receptors might be very low.
Legs
We detected 19, 18, and 9 OR transcripts in fore-, mid- and hindlegs respectively (Figure 3). In addition, 11, 10, and 7 IRs, and 9, 10, and 5 GRs were expressed in fore-, mid- and hindlegs, respectively.
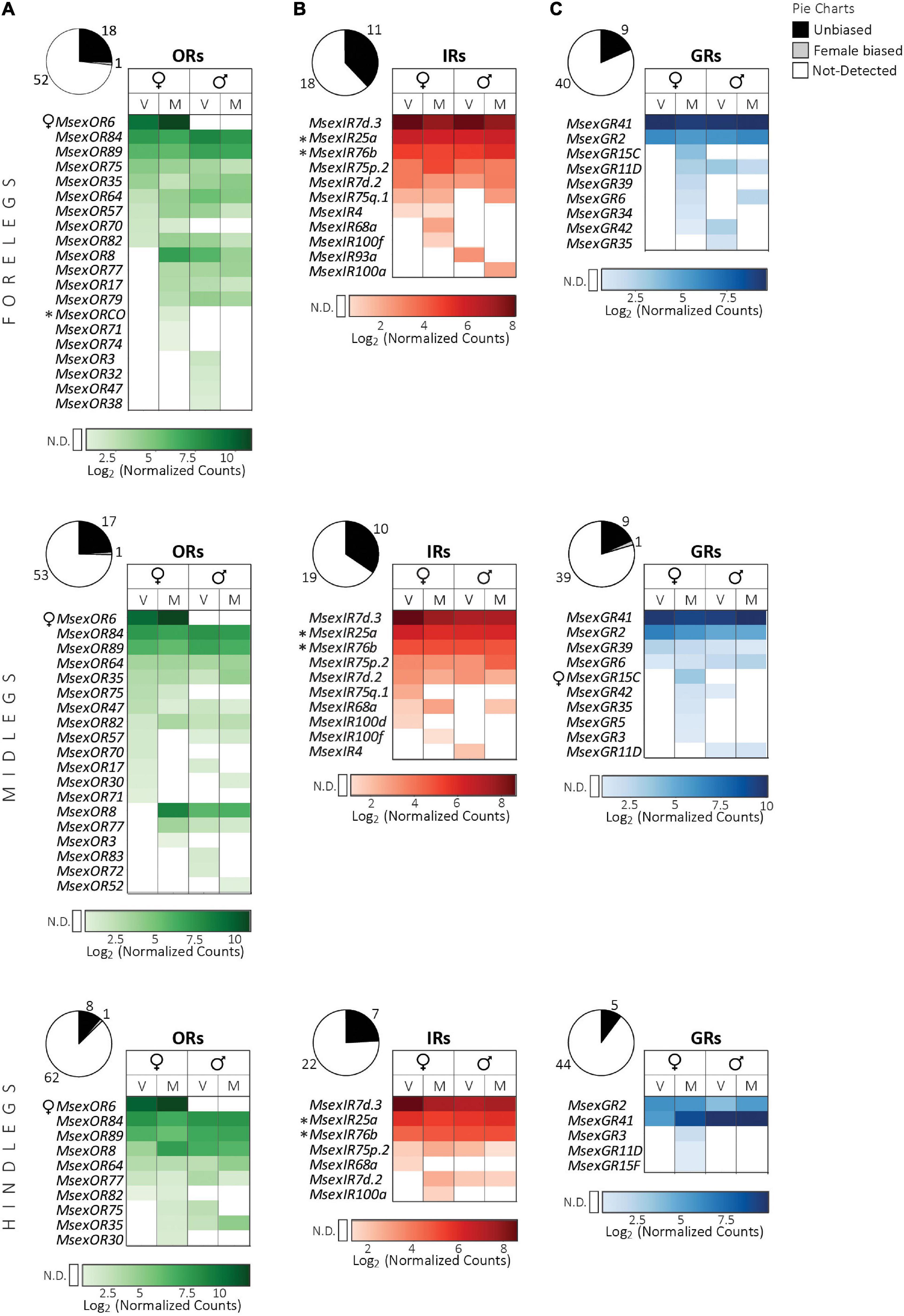
Figure 3. Expression of chemosensory receptor genes in the legs of adult M. sexta (top panel, forelegs; middle panel, midlegs; bottom panel, hindlegs). (A) Odorant receptors, (B) ionotropic receptors, (C) gustatory receptors. Pie charts depict the percentage of expressed genes (absolute numbers next to the charts) of each receptor family (see legend on top right). Heatmaps were created separately for each receptor family using the log2 of the geometric mean (n = 3 biological replicates) of normalized counts obtained from the NanoString assay (see section “Materials and methods”). Light to dark shades indicate low to high counts (see color bar at bottom of each heatmap); white cells indicate no detection (N.D.) of transcripts (for a definition see section “Materials and methods”). Columns represent female and male moths of different mating states (V: virgin, M: mated). Rows represent receptors that were expressed in the legs of at least one of the four groups and are sorted first according to values in virgin females, followed by mated females, virgin males and mated males. Symbols next to receptor gene names: *, OR or IR co-receptors; ♀, female-biased receptors (FDR corrected p < 0.05, nested ANOVA). Mating states of males and females did not have a significant effect on receptor gene expression (FDR corrected p > 0.05, nested ANOVA).
ORs. Nine ORs were common among all three leg pairs (Figure 3A). The ORs with the highest counts in all leg pairs were MsexOR6 and the ubiquitous MsexOR84/89 (Table 1). The putative linalool receptor gene MsexOR6, which was highly expressed and female-specific in the antennae, was also highly expressed and female-specific in all three leg pairs. Transcripts of its paralog MsexOR5, however, was not detected in the legs. Based on our criteria (see section “Materials and methods”), the obligatory co-receptor MsexORCo was only expressed in forelegs of mated females. MsexORCo was, however, detected in one foreleg sample each of virgin females and mated males. We also note that there are 15 ORs expressed in the mated female versus only eight in virgin females (see discussion of a similar trend in female labial palps). It could be possible that expression of ORs including MsexORCo is upregulated in female forelegs after mating and that the forelegs might be involved in the assessment of oviposition substrates via olfaction. Transcripts of putative pheromone receptor gene MsexOR83, which is male-specific in the antennae, were expressed in the virgin male midlegs. This suggests that in addition to the antennae, legs may also detect pheromones.
Our results indicate a potential olfactory function of the legs of M. sexta. A class of chemosensory sensilla found on the M. sexta legs, the scattered blunt sensilla, has been hypothesized to have olfactory function due to the presence of wall pores (Kent and Griffin, 1990). Both male and female legs possess an equal number of these sensilla; however, their olfactory function still needs to be tested.
IRs. Six IRs were common among all leg pairs (Figure 3B). The transcripts of IR co-receptor genes MsexIR25a, MsexIR76b were detected in all leg pairs, whereas expression of acid-sensing co-receptor MsexIR8a seemed to be absent in leg tissues. However, we detected expression of IRs that potentially code for IRs tuned to acids based on their homolog’s functions: MsexIR4, MsexIR75p.2, and Msex75q.1 (Ai et al., 2010; Rytz et al., 2013; Hou et al., 2022). This suggests that like in the mouthparts, MsexIR8a expression might be very low in the legs. Alternatively, these receptors might have a different function in the legs of M. sexta. In fact, some A. segetum homologs AsegIR75p.2 and AsegIR75q.2 did not respond to any acid, aldehyde or alcohol stimulation when co-expressed with AsegIR8a in Xenopus oocytes (Hou et al., 2022), either indicating that their volatile ligands were not tested, or that they have a non-olfactory function. Like in the labial palps and proboscis, the Lepidoptera-specific MsexIR7d.2 and the putative gene for humidity sensor MsexIR68a are expressed in all leg pairs (Knecht et al., 2017). MsexIR93a, which could have both hygro- and thermosensory roles, was detected only in the forelegs (Knecht et al., 2016).
GRs. Among the three leg pairs, four GRs were commonly expressed (Figure 3C). Once again, MsexGR41 had the highest counts in each of them. Due to the crucial gustatory function of a moth’s legs, especially for oviposition, we expected high expression of several GRs. However, we detected transcripts of fewer GRs in the legs than in the antennae. A similar, unexpectedly low GR expression was also seen in the legs of S. littoralis (Koutroumpa et al., 2021). Interestingly, we found expression of more GRs in the legs of mated females, than in virgin females or in males. Expression of a bitter receptor transcript, MsexGR15C, was detected only in the fore- and midlegs of mated females, and in the midlegs we found a statistically significant, female-biased expression of this GR. This is interesting as there is evidence of sexual dimorphism in the chemosensory sensilla on the legs of M. sexta. A class of putative contact chemosensilla, the “spine-associated clustered sensilla”, is more abundant on the females’ fore-, mid- and hindlegs than on the males’ legs (Kent and Griffin, 1990). The female might have subclasses of these sensilla that express female specific receptors. Transcripts of sugar receptors MsexGR5 and MsexGR6 (Koenig et al., 2015) were detected in the fore- and midlegs of both sexes but not in the hindleg samples.
As in the antennae and proboscis, GRs from the CO2 receptor group, MsexGR2 and MsexGR3, were expressed. While MsexGR2 had a consistent and high expression in males and females, MsexGR3 was only detected in mated female mid- and hindlegs. As these receptors are known for detecting odorants rather than tastants, they could be associated with the scattered blunt sensilla on the legs.
Overall, fore- and midlegs expressed about two times more GRs than the hindlegs. After arriving at a potential hostplant via multisensory cues such as olfaction, vision, CO2, humidity, and temperature, a gravid female M. sexta touches the leaves with its fore- and midlegs while still hovering in front of the plant. Such direct contact is important for the stimulation of egg laying behavior in hawkmoths (Yamamoto et al., 1969; Mechaber et al., 2002) as well as in butterflies (Staedler et al., 1995), and allows the assessment of primary and secondary plant metabolites to decide whether the plant is suitable for oviposition. Both the expressed sugar and bitter receptors might be involved in this behavior. The spines on the moth’s legs may help them to pierce through the leaf surface to allow these metabolites to come in contact with the gustatory sensilla, such as the spine-associated clustered sensilla (Kent and Griffin, 1990). In another Lepidopteran species, Cydia pomonella, primary metabolites, like plant surface sugars can influence egg laying decisions (Lombarkia and Derridj, 2008).
Wings
We detected expression of 16 ORs, eight IRs and five GRs in forewings and 16 ORs, nine IRs and four GRs in hindwings (Figure 4).
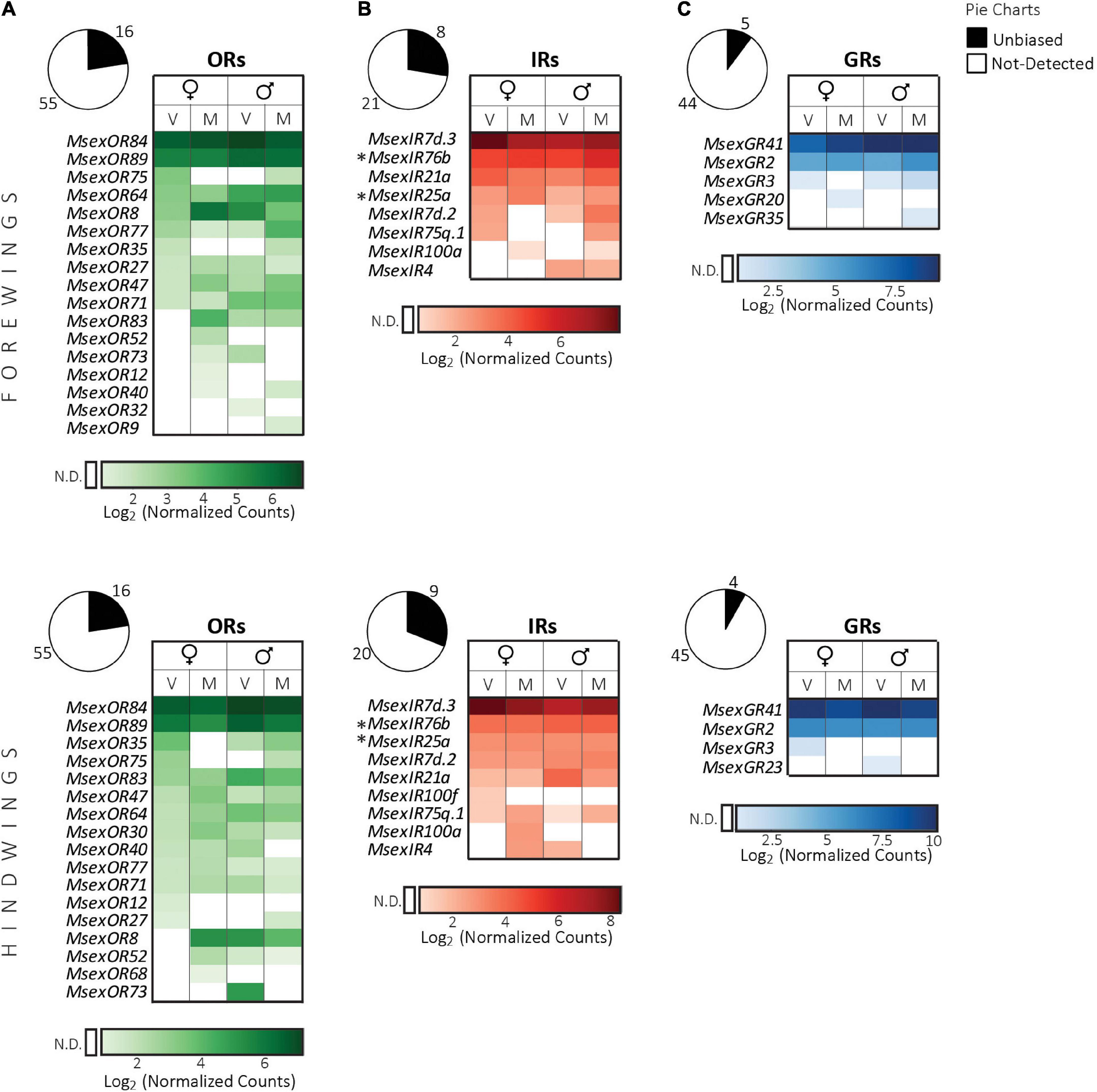
Figure 4. Expression of chemosensory receptor genes in the wings of adult M. sexta (top panel, forewings; bottom panel, hindwings). (A) Odorant receptors, (B) ionotropic receptors, (C) gustatory receptors. Pie charts depict the percentage of expressed genes (absolute numbers next to the charts) of each receptor family (see legend on top right). Heatmaps were created separately for each receptor family using the log2 of the geometric mean (n = 3 biological replicates) of normalized counts obtained from the NanoString assay (see section “Materials and methods”). Light to dark shades indicate low to high counts (see color bar at bottom of each heatmap); white cells indicate no detection (N.D.) of transcripts (for a definition see section “Materials and methods”). Columns represent female and male moths of different mating states (V: virgin, M: mated). Rows represent receptors that were expressed in the wings of at least one of the four groups, and are sorted first according to values in virgin females, followed by mated females, virgin males and mated males. Symbols next to receptor gene names: *, OR or IR co-receptors. Sex or mating states of males and females did not have a significant effect on receptor gene expression (FDR corrected p > 0.05, nested ANOVA).
ORs. Of the 16 expressed ORs, 14 were common in fore- and hindwings (Figure 4A). In both wing pairs, MsexOR84/89 transcripts had the highest counts. However, MsexORCo transcripts were not detected in any of the samples. Similar expression of ORs but not ORCo was reported for the wings of the geometrid moth Ectropis obliqua (Ma et al., 2016). Such expression of ORs without that of ORCo in the wings, suggests that ORs may have more functions than the detection of odor molecules. Alternatively, some ORs might function without ORCo as in the case of basal insect species that lack the ORCo gene (del Mármol et al., 2021). MsexOR83, whose transcripts were specifically detected in antennae and midlegs of males, was in addition expressed in wings of both males and females. Therefore, moth wings might detect female pheromones. For females, this information might be useful to regulate pheromone production or release. In moths, pheromone perception with wings has not been reported to the best of our knowledge. However, an IR in gustatory sensilla on the wings of Drosophila is required for normal mating behavior in both males and females and is thought to detect pheromonal cuticular hydrocarbons (He et al., 2019).
IRs. Except for one additional IR (MsexIR100f) in the hindwings, we detected expression of the same set of IR transcripts in both wings (Figure 4B). MsexIR7d.3 transcripts had the highest counts in the wings like in other tissues. In both pairs of wings, two IR co-receptor genes, MsexIR25a and MsexIR76b, were expressed, but the third one MsexIR8a was not. The expression of MsexIR25a and MsexIR76b might indicate olfactory sensing of amines, or in case of MsexIR76b, gustatory detection of salts, sour acids and amino acids (Zhang et al., 2013; Hussain et al., 2016; Knecht et al., 2016, 2017; Chen and Amrein, 2017). Of special interest is the expression of MsexIR21a in fore- and hindwings of both sexes. The only other tissue where this gene was expressed was the antennae. MsexIR21a is potentially involved in thermosensation (cool sensing) as reported for its ortholog DmelIR21a (Knecht et al., 2016; Ni et al., 2016).
GRs. Three GRs that were detected in almost all tissues described so far, MsexGR2 and MsexGR3 of the CO2 subfamily, and MsexGR41, were expressed in both wing pairs, with MsexGR41 having the highest counts (Figure 4C). Three more bitter receptor transcripts were detected. No putative sugar or fructose receptors (Koenig et al., 2015) were found in the wings. So far, no chemosensory sensilla have been reported on the wings of M. sexta. GR expression was also found in the wings of E. obliqua including a putative sugar receptor gene (Ma et al., 2016). In Drosophila, functional gustatory sensilla on the wings are important for sugar and bitter detection eliciting food exploration behavior and grooming (Raad et al., 2016; Yanagawa et al., 2019).
Ovipositor
We detected 30 OR, 15 IR and 14 GR transcripts in the ovipositor (Figure 5). This is a higher number of receptor genes than was found to be expressed previously (Klinner et al., 2016). This difference might be due to different sample collection methods applied in both studies. As opposed to the previous study, where the last four abdominal segments were taken, our sample consisted only of the anal papillae at the last abdominal segment that bears the sensilla. Hence, it is possible that we were able to detect more genes expressed in chemosensory neurons housed in these sensilla because potential receptor transcripts were more concentrated in our samples.
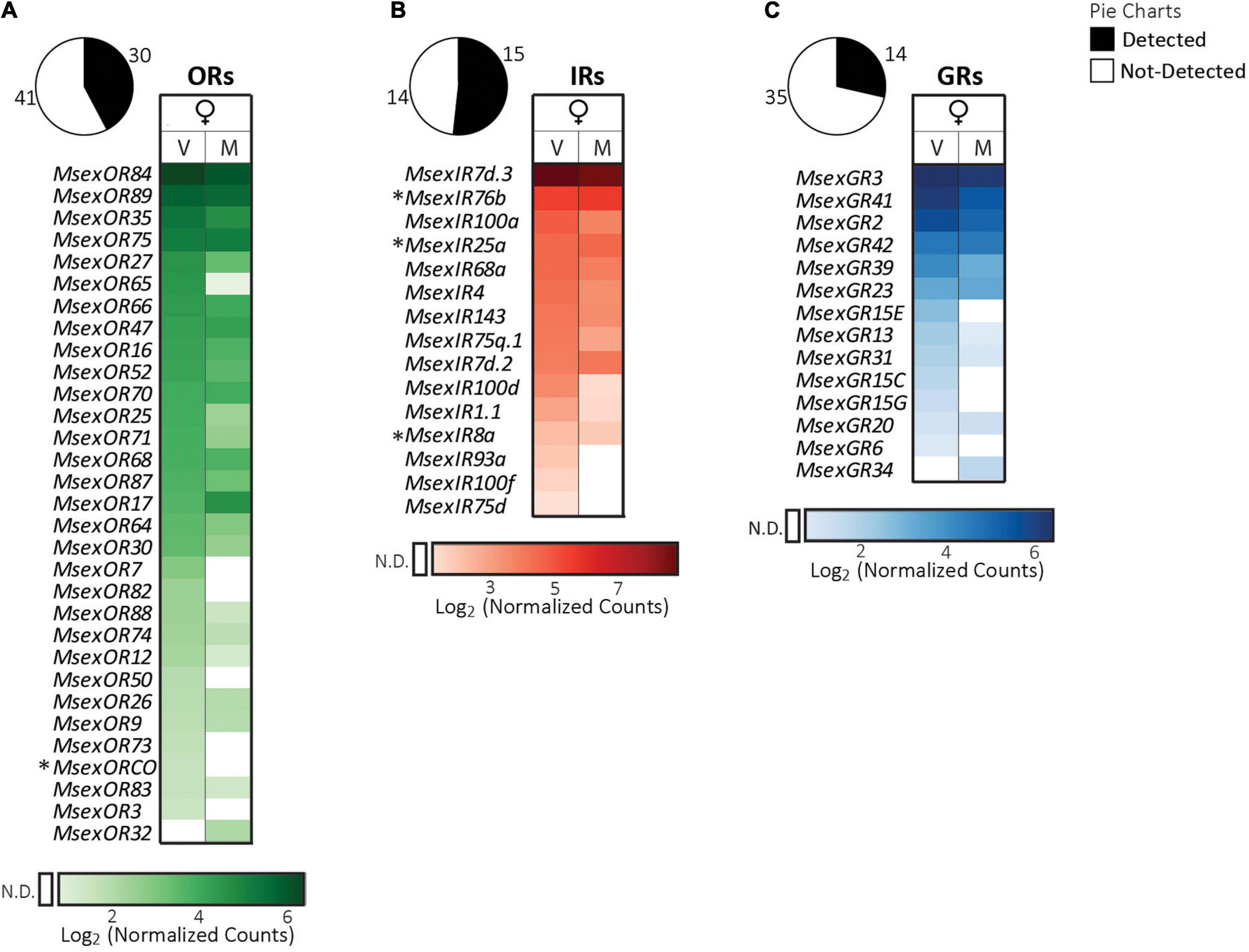
Figure 5. Expression of chemosensory receptor genes in the ovipositor of female adult M. sexta. (A) Odorant receptors, (B) ionotropic receptors, (C) gustatory receptors. Pie charts depict the percentage of expressed genes (absolute numbers next to the charts) of each receptor family (see legend on top right). Heatmaps were created separately for each receptor family using the log2 of the geometric mean (n = 3 biological replicates) of normalized counts obtained from the NanoString assay (see section “Materials and methods”). Light to dark shades indicate low to high counts (see color bar at bottom of each heatmap); white cells indicate no detection (N.D.) of transcripts (for a definition see section “Materials and methods”). Columns represent female moths of different mating states (V: virgin, M: mated). Rows represent receptors that were expressed in the ovipositor of at least one of the two groups, and are sorted first according to values in virgin females, followed by mated females. Symbols next to receptor gene names: *, OR or IR co-receptors. Mating state of females did not have a significant effect on receptor gene expression (FDR corrected p > 0.05, nested ANOVA).
ORs. The highest counts were found for MsexOR84/89 transcripts, followed by MsexOR35 and MsexOR75 (Figure 5A). Our results furthermore, included the three ORs that were previously reported to be expressed in the ovipositor using RNA-sequencing, MsexOR9, MsexOR26, and MsexORCo (Klinner et al., 2016). The expression of MsexORCo in our NanoString assay was detected only in the virgin female ovipositor as it was the case for the RNA sequencing data in our previous study (Klinner et al., 2016). However, MsexORCo expression was confirmed using RT-PCR and western blot analysis for both mating states (Klinner et al., 2016). Hence, MsexORCo might be expressed in both mating states, but the expression level is most probably lower in mated than in virgin females. This indicates that OR-mediated olfactory function of the ovipositor might be involved in courtship rather than in oviposition behavior. MsexOR17, MsexOR26, and MsexOR87 that were female-biased on the antennae, were expressed in the ovipositor as well and might be involved in detection of mating or oviposition related cues.
Our result of low expression of ORCo and higher expression of several ORs is similar to reports from other moth species. Transcriptome sequencing together of pheromone gland and ovipositor of Spodoptera frugiperda revealed the expression of SfruORCo and 11 SfruORs, of which the transcript level of SfruORCo was the lowest (Sun et al., 2022). Furthermore, in the ovipositor of Helicoverpa assulta, the expression level of a tuning OR gene, HassOR31, is higher than that of HassORCo (Li et al., 2020). HassOR31 is co-expressed with HassORCo in some, but not all sensilla on the ovipositor. Heterologous expression in Xenopus oocyte show that HassORCo is necessary for olfactory detection of host-plant volatiles by HassOR31, therefore cells where HassORCo is not co-expressed with HassOR31 might use this OR in a non-olfactory function. One such example is CpomOR1, the pheromone receptor of C. pomonella, whose expression in the ovipositor has been suggested to play a role in the process of egg production and maturation (Garczynski et al., 2017). In H. virescens, HvirOR13, the receptor for its major pheromone component (Z)-11-hexadecenal was detected in the ovipositor, along with the pheromone binding protein PBP2, which is needed for the function of the pheromone receptor. Pheromone receptor and binding protein were proposed to be involved in a potential feedback mechanism (Widmayer et al., 2009). As the putative pheromone receptor gene MsexOR83 was expressed in the ovipositor of M. sexta, it might accordingly be involved in the regulation of pheromone emission or in other non-chemosensory cellular functions.
IRs. Similar to the proboscis, legs and wings, among the expressed IRs, MsexIR7d.3 transcripts again had the highest counts (Figure 5B). Expression of all known IR co-receptors (MsexIR8a, 25a, and 76b) were detected in the ovipositor, and also of most previously reported IRs (MsexIR4, 7d, 68a, and 75d; Klinner et al., 2016). In fact, MsexIR75d expression was found exclusively in the ovipositor in our study. Based on homology with Drosophila, we predict that MsexIR75d might be involved in the detection of pyrrolidine or other amines (Silbering et al., 2011; Rytz et al., 2013), in line with the strong activation of sensilla on the ovipositor upon stimulation with pyrrolidine (Klinner et al., 2016). MsexIR8a is involved in acid detection (Zhang J. et al., 2019) and sensilla at the ovipositor of M. sexta also detect acids, especially hexanoic acid (Klinner et al., 2016). Acids play a key role in oviposition deterrence of M. sexta (Zhang J. et al., 2019). As the ovipositor is the only tissue apart from the antennae where we find MsexIR8a expression, the role of the ovipositor in acid-induced oviposition deterrence would be worth investigating. We also found expression of other IR8a-associated putatively acid-sensing IR genes: MsexIR1.1, MsexIR4, and MsexIR75q.1 (Hou et al., 2022). Non-chemosensory IRs such as MsexIR68a and MsexIR93a were also expressed in the ovipositor. We found a very clear expression of a Lepidoptera-specific IR, MsexIR143, in the ovipositor. The only other tissue where this IR was expressed is the male proboscis.
GRs. MsexGR3 and MsexGR41 transcripts had the highest counts among all GRs in the ovipositor (Figure 5C). In agreement with a previous report, the putative genes for CO2 receptors MsexGR2 and MsexGR3 were expressed in the ovipositor (Klinner et al., 2016). The gene for sugar receptor MsexGR6 and several bitter receptors (Koenig et al., 2015) were expressed in addition, and might be involved in detecting sugars and other plant metabolites that can be used for evaluating leaf quality for oviposition.
Of the chemosensory tissues we examined, the ovipositor expressed the highest number of GRs. At the anal papillae of the M. sexta ovipositor, there are blunt tipped sensilla, which have both a terminal pore and wall pores, indicating olfactory and gustatory functions of the neurons residing in them (Klinner et al., 2016). Single sensillum recordings (Klinner et al., 2016) have demonstrated only an olfactory function so far. In the moth S. littoralis, however, gustatory responses of ovipositor sensilla to salts, sugar and bitter compounds have been shown (Seada et al., 2016). It is possible, that gravid female moths, after landing on a potential host plant, use the spines on their legs to scratch the leaf surface and expose essential sugars and bitter secondary plant metabolites. Sensing these substances with sensilla on both the leg and the ovipositor might help the females to make an oviposition choice. As more GRs are present in the ovipositor than in the legs (Figure 6), gustation by the ovipositor might have the most important contribution to this decision-making in M. sexta.
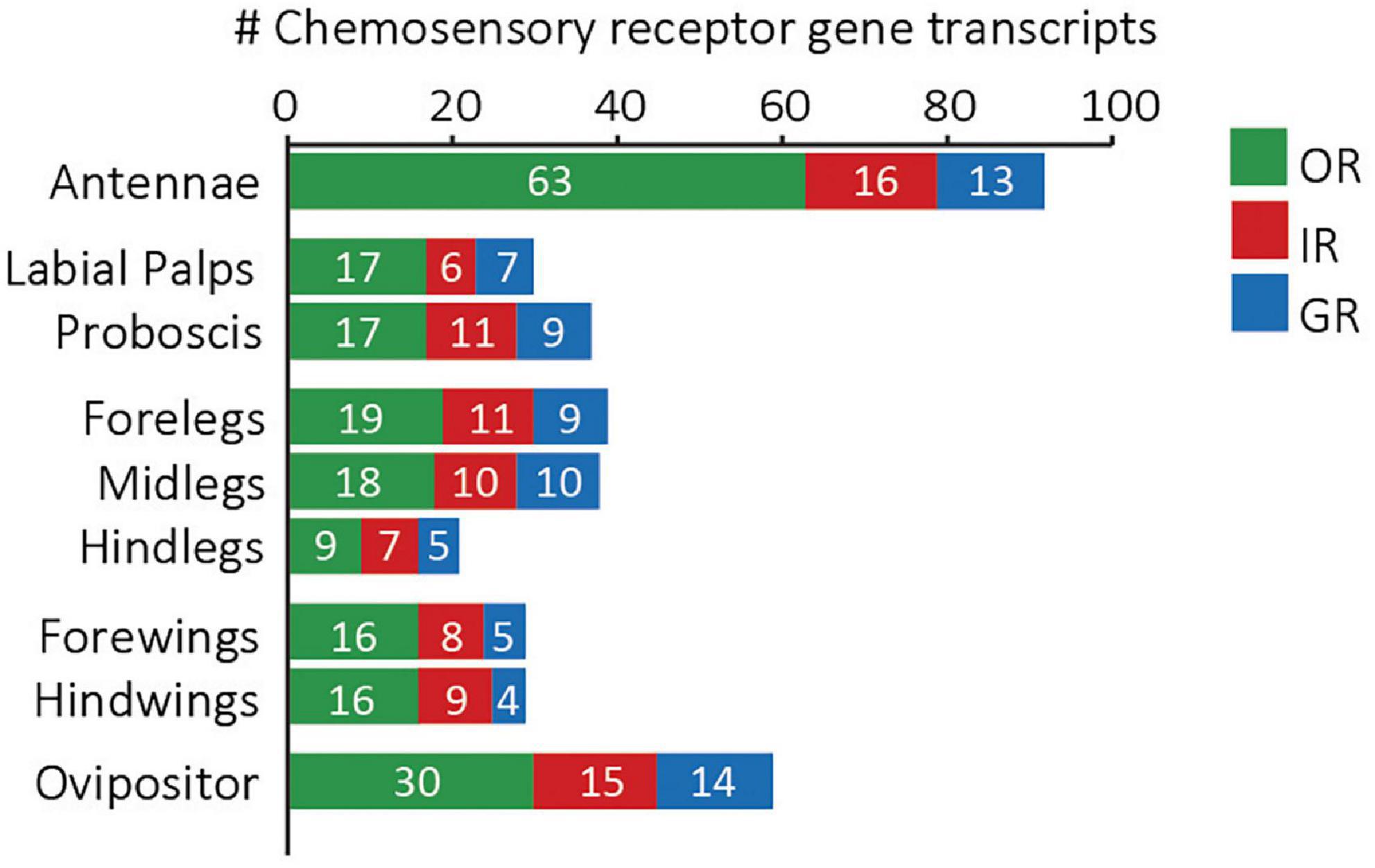
Figure 6. Number of chemosensory receptor gene transcripts expressed in different tissues of adult M. sexta.
Comparison among different tissues
In our results, the antennae and the ovipositor of M. sexta expressed every co-receptor known so far: MsexORCo, MsexIR8a, MsexIR25a, and MsexIR76b (Table 3), indicating that all pathways of odor detection take place in these organs. The labial palps and the forelegs express all co-receptors except MsexIR8a. The striking expression of MsexORCo in the labial palps suggests that these might be the second most important olfactory organs in Manduca, analogous to the maxillary palps in Drosophila. In the noctuid moth S. frugiperda labial palps detect other volatiles in addition to CO2 (Chen et al., 2021), although the response profile indicates IR-mediated olfaction. Transcripts of two IR co-receptor (MsexIR25a and MsexIR76b) were detected in all nine tissues, similar to the expression patterns observed in other insects and even mollusks. These receptors are expressed in neurons of gustatory organs all over the body (Croset et al., 2010; van Schooten et al., 2016; Liu et al., 2018; Zhu et al., 2018). However, the expression of the MsexIR25a and MsexIR76b in peripheral tissues may not only indicate chemosensory activity as these genes could be involved in hygro- and thermosensation.
Apart from these two IR-coreceptors, 13 more receptors were expressed ubiquitously in all or almost all tissues (Table 1). The expression of three of these receptors (MsexOR64, MsexOR75, and MsexOR77) would require further validation due to possible cross-hybridization of the probes with duplicate receptor transcripts. For the remaining 12 receptors our probes did not have any off-targets. MsexOR84/89 was broadly expressed even in tissues where no ORCo was detected, suggesting a possible non-olfactory function of this gene. The ortholog of this gene in the silkmoth, BmorOR23, is expressed only in the larvae, but its function remains unknown (Tanaka et al., 2009; Koenig et al., 2015). However, expression of some ORs in several tissues including non-chemosensory ones was also detected in other moth species like Spodoptera littoralis (Walker et al., 2019). MsexIR7d.2 and MsexIR7d.3 belong to the Lepidoptera-specific IR7d group and homologs of their genes in other moth species have a broad expression in several tissues in adult and larval stages (Liu et al., 2018; Zhu et al., 2018). However, their paralog MsexIR7d.4 was not detected in any of the tissues investigated here. MsexGR41, similar to its ortholog BmorGR63, is expressed in different chemosensory tissues (Guo et al., 2017).
In contrast to the broad expression of several genes, expression of 25 GRs were not detected in any of the tissues under investigation. We hypothesize that the low expression levels of GRs underlay the underrepresentation of this gene family in our expression data which has also hampered the detection of these genes in transcriptomes and RNA in situ hybridizations (Clyne et al., 2000; Koutroumpa et al., 2021). Another aspect is the spatially restricted distribution of cells expressing different receptors, for example, the segregation of MsexORCo and MsexIR8a expression in the proboscis (Haverkamp et al., 2016). Extracting RNA from smaller segments of each organ and increasing the amount of input RNA could also aid in detection of lowly expressed genes. Alternatively, these genes could be expressed in internal organs such as the brain or gut, where they might be involved in cell signaling acting as transmembrane receptors for various molecules. In addition, many of these not expressed GRs in adults could be larvae-specific, as larval feeding behaviors are strongly influenced by gustation (Glendinning et al., 2000; Zhang Z. J. et al., 2019).
Among all the tissues investigated, we found the highest number of expressed chemosensory receptors in the antennae (92), followed by the ovipositor (59, Figure 6). The hindlegs, in contrast, showed the lowest number of chemosensory receptor genes, particularly the least number of ORs compared to all other tissues, indicating that hindlegs might have a minor role in chemoreception, a phenomenon also seen in mosquitoes (Yang et al., 2020). In female Ae. aegypti, electrophysiological responses from different tissues (antennae, mouthparts, wings and tarsal segments of legs) to seven insect repellents and attractants showed that all these tissues can detect odors with different capacities. The hindlegs were the least chemoreceptive as they responded only to one odor, triethylamine. This amine response is likely due to an IR25a or IR76b pathway, as these receptors are known to be broadly expressed and amine-detecting in many insects.
Furthermore, the antennae had the highest number of exclusively expressed genes, with 24 ORs, 4 IRs and 3 GRs (Table 1). One antennal GR is a putative inositol receptor gene, MsexGR8 (Zhang et al., 2011). In M. sexta caterpillars inositol, an alcohol sugar that occurs in host plants (Nelson and Bernays, 1998), promotes feeding and helps overcome the inhibitory effects of bitter compounds (Glendinning et al., 2000), whereas, in adults, this GR may help identify host plants. Remarkably, MsexGR1 showed specific expression in the labial palps, which differs from the broad expression patterns of the other two candidate CO2 receptor genes MsexGR2 and MsexGR3. If MsexGR1 is necessary for CO2 perception, then no tissue other than the labial palps might be able to detect it.
Sex-dependent expression
We found sex-dependent expression of chemosensory receptor genes only in the antennae (12 ORs) and legs (1 OR and 1 GR), the two organs for which sexual dimorphism has been described. In M. sexta, similar to other moth species, the antenna is sexually dimorphic with respect to its shape as well as the size, number and type of its sensilla (Sanes and Hildebrand, 1976; Keil, 1989; Shields and Hildebrand, 1999). For all three pairs of legs, females have more spine-associated sensilla than males (Kent and Griffin, 1990). No sexual dimorphism is observed in the palps (Kent et al., 1986), and none has been reported for proboscis or wings.
However, sex-biased receptors in one tissue may have a sex-independent expression in other tissues. Examples are the expression of the “male-specific” putative pheromone receptor gene MsexOR51 in the proboscis of virgin females and the expression of the “female-biased” MsexOR17 in the legs of males. A receptor could thus have multiple functions, being involved in certain sex-specific roles when expressed in one tissue and a role common for both sexes when expressed in another tissue.
Mating-status dependent expression
Few studies have shown plasticity in insect chemosensory receptor gene expression dependent on mating state. In female D. suzukii, for example, several ORs are upregulated after mating (Crava et al., 2019). In the moth Dendrolimus punctatus, both up- and down-regulation have been reported for ORs and other chemosensory genes in both males and females (Zhang et al., 2017). In H. armigera, two antennal IRs are downregulated after mating in females (Liu et al., 2018). Such differential expression of chemosensory receptors can modulate sensitivity to essential cues and lead to changes in behavior and physiology.
For M. sexta, post-mating changes of odor-evoked behavioral and physiological responses have been described. Host plant volatiles induce more often a directed upwind flight and abdomen curling in mated than in virgin female M. sexta (Mechaber et al., 2002). In addition, some plant odor-evoked activation patterns in the antennal lobe of female M. sexta change after mating (Bisch-Knaden et al., 2022). However, we did not find significant differences between virgin and mated moths in the expression levels of ORs in the antennae or other tissues, suggesting that other mechanisms are responsible for the observed state-dependent changes in physiology and behavior.
Conclusion
We provide an extensive data set of the expression pattern of 149 chemosensory receptor genes across nine peripheral chemosensory tissues and both sexes in different mating states in adults of the hawkmoth M. sexta. Our data suggest that all peripheral tissues under investigation are potentially multimodal, having both olfactory and gustatory functions. Based on this information, we can predict functions or discover potential new functions for the repertoire of chemosensory receptors in different tissues of this insect species.
Data availability statement
The datasets presented in this study can be found in online repositories. The name of the repository and accession number can be found below: Edmond, https://edmond.mpdl.mpg.de/, doi: 10.17617/3.AEVVZV.
Author contributions
SB-K and BH: study conception and editing the manuscript. MT, LC, SB-K, and BH: study design. MT and SB: sample and data collection. MT, LC, and SB-K: data analysis. MT: writing of first draft. All authors revised the manuscript and approved the submitted version.
Funding
This study was supported by the Max-Planck Society.
Acknowledgments
We thank Sabine Brase for help with RNA extraction; Richard Fandino for introducing us to the NanoString technique; Klaus Gase and Chidambareswaren Mahadevan for their help and advice; and Monika Stengl for providing us with additional moths.
Conflict of interest
The authors declare that the research was conducted in the absence of any commercial or financial relationships that could be construed as a potential conflict of interest.
Publisher’s note
All claims expressed in this article are solely those of the authors and do not necessarily represent those of their affiliated organizations, or those of the publisher, the editors and the reviewers. Any product that may be evaluated in this article, or claim that may be made by its manufacturer, is not guaranteed or endorsed by the publisher.
Supplementary material
The Supplementary Material for this article can be found online at: https://www.frontiersin.org/articles/10.3389/fevo.2022.976521/full#supplementary-material
Footnotes
- ^ https://www.qiagen.com/de
- ^ https://www.thermofisher.com/de/de/home.html
- ^ https://www.agilent.com/
- ^ https://nanostring.com/
- ^ https://nanostring.com/wp-content/uploads/MAN-10023-11_nCounter_XT_Assay_User_Manual.pdf
- ^ https://eurofinsgenomics.eu/
- ^ https://nanostring.com/wp-content/uploads/Gene_Expression_Data_Analysis_Guidelines.pdf
- ^ https://www.r-project.org/
- ^ https://www.rstudio.com
References
Abuin, L., Bargeton, B., Ulbrich, M. H., Isacoff, E. Y., Kellenberger, S., and Benton, R. (2011). Functional architecture of olfactory ionotropic glutamate receptors. Neuron 69, 44–60.
Agnihotri, A. R., Roy, A. A., and Joshi, R. S. (2016). Gustatory receptors in Lepidoptera: chemosensation and beyond. Insect. Mol. Biol. 25, 519–529. doi: 10.1111/imb.12246
Ai, M., Min, S., Grosjean, Y., Leblanc, C., Bell, R., Benton, R., et al. (2010). Acid sensing by the Drosophila olfactory system. Nature 468, 691–695.
Alarcón, R., Riffell, J. A., Davidowitz, G., Hildebrand, J. G., and Bronstein, J. L. (2010). Sex-dependent variation in the floral preferences of the hawkmoth Manduca sexta. Animal Behav. 80, 289–296.
Anderson, A. R., Wanner, K. W., Trowell, S. C., Warr, C. G., Jaquin-Joly, E., Zagatti, P., et al. (2009). Molecular basis of female-specific odorant responses in Bombyx mori. Insect Biochem. Mol. Biol. 39, 189–197. doi: 10.1016/j.ibmb.2008.11.002
Bastin-Héline, L., de Fouchier, A., Cao, S., Koutroumpa, F., Caballero-Vidal, G., Robakiewicz, S., et al. (2019). A novel lineage of candidate pheromone receptors for sex communication in moths. eLife 8:e49826.
Bengtsson, J. M., Gonzalez, F., Cattaneo, A. M., Montagné, N., Walker, W. B., Bengtsson, M., et al. (2014). A predicted sex pheromone receptor of codling moth Cydia pomonella detects the plant volatile pear ester. Front. Ecol. Evol. 2:33. doi: 10.3389/fevo.2014.00033
Bengtsson, J. M., Trona, F., Montagné, N., Anfora, G., Ignell, R., Witzgall, P., et al. (2012). Putative chemosensory receptors of the codling moth, Cydia pomonella, identified by antennal transcriptome analysis. PLoS One 7:e31620. doi: 10.1371/journal.pone.0031620
Benton, R., Vannice, K. S., Gomez-Diaz, C., and Vosshall, L. B. (2009). Variant ionotropic glutamate receptors as chemosensory receptors in drosophila. Cell 136, 149–162.
Birch, M. C., Poppy, G. M., and Baker, T. C. (1990). Scents and eversible scent structures of male moths. Ann. Rev. Entomol. 35, 25–58. doi: 10.1007/BF00376363
Bisch-Knaden, S., Rafter, M. A., Knaden, M., and Hansson, B. S. (2022). Unique neural coding of crucial versus irrelevant plant odors in a hawkmoth. eLife 11:e77429. doi: 10.7554/eLife.77429
Bondar, G., Xu, W., Elashoff, D., Li, X., Faure-Kumar, E., Bao, T. M., et al. (2020). Comparing NGS and NanoString platforms in peripheral blood mononuclear cell transcriptome profiling for advanced heart failure biomarker development. J. Biol. Methods 7:e123. doi: 10.14440/jbm.2020.300
Brand, P., Robertson, H. M., Lin, W., Pothula, R., Klingeman, W. E., Jurat-Fuentes, J. L., et al. (2018). The origin of the odorant receptor gene family in insects. eLife 7:e38340.
Chen, Q., Liu, X., Cao, S., Ma, B., Guo, M., Shen, J., et al. (2021). Fine structure and olfactory reception of the labial palps of Spodoptera frugiperda. Front. Physiol. 12:680697. doi: 10.3389/fphys.2021.680697
Chen, Y., and Amrein, H. (2017). Ionotropic receptors mediate drosophila oviposition preference through sour gustatory receptor neurons. Curr. Biol. 27, 2741-2750.e4. doi: 10.1016/j.cub.2017.08.003
Clyne, P. J., Warr, C. G., and Carlson, J. R. (2000). Candidate taste receptors in Drosophila. Science 287, 1830–1834.
Clyne, P. J., Warr, C. G., Freeman, M. R., Lessing, D., Kim, J., and Carlson, J. R. (1999). A novel family of divergent seven-transmembrane proteins: candidate odorant receptors in Drosophila. Neuron 22, 327–338. doi: 10.1016/s0896-6273(00)81093-4
Crava, C. M., Sassù, F., Tait, G., Becher, P. G., and Anfora, G. (2019). Functional transcriptome analyses of Drosophila suzukii antennae reveal mating-dependent olfaction plasticity in females. Insect Biochem. Mol. Biol. 105, 51–59. doi: 10.1016/j.ibmb.2018.12.012
Croset, V., Rytz, R., Cummins, S. F., Budd, A., Brawand, D., Kaessmann, H., et al. (2010). Ancient protostome origin of chemosensory ionotropic glutamate receptors and the evolution of insect taste and olfaction. PLoS Genet. 6:e1001064. doi: 10.1371/journal.pgen.1001064
Dahake, A., Jain, P., Vogt, C., Kandalaft, W., Stroock, A., and Raguso, R. A. (2022). Floral humidity as a signal – not a cue – in a nocturnal pollination system. bioRxiv [Preprint] doi: 10.1101/2022.04.27.489805v1
Dahanukar, A., Hallem, E. A., and Carlson, J. R. (2005). Insect chemoreception. Curr. Opin. Neurobiol. 15, 423–430.
Daimon, T., Fujii, T., Fujii, T., Yokoyama, T., Katsuma, S., Shinoda, T., et al. (2012). Reinvestigation of the sex pheromone of the wild silkmoth Bombyx mandarina: The effects of Bombykal and Bombykyl Acetate. J. Chem. Ecol. 38, 1031–1035. doi: 10.1007/s10886-012-0164-0
de Fouchier, A., Walker, W. B. III, Montagne, N., Steiner, C., Binyameen, M., Schlyter, F., et al. (2017). Functional evolution of Lepidoptera olfactory receptors revealed by deorphanization of a moth repertoire. Nat. Commun. 8:15709. doi: 10.1038/ncomms15709
del Mármol, J., Yedlin, M. A., and Ruta, V. (2021). The structural basis of odorant recognition in insect olfactory receptors. Nature 597, 126–131.
Depetris-Chauvin, A., Galagovsky, D., and Grosjean, Y. (2015). Chemicals and chemoreceptors: ecologically relevant signals driving behavior in Drosophila. Front. Ecol. Evol. 3:41. doi: 10.3389/fevo.2015.00041
Dunipace, L., Meister, S., McNealy, C., and Amrein, H. (2001). Spatially restricted expression of candidate taste receptors in the Drosophila gustatory system. Curr. Biol. 11, 822–835. doi: 10.1016/s0960-9822(01)00258-5
Fandino, R. A., Haverkamp, A., Bisch-Knaden, S., Zhang, J., Bucks, S., Nguyen, T. A. T., et al. (2019). Mutagenesis of odorant coreceptor ⁢em>Orco⁢/em> fully disrupts foraging but not oviposition behaviors in the hawkmoth ⁢em>Manduca sexta⁢/em>. Proc. Natl. Acad. Sci. 116:15677.
Fraser, A. M., Mechaber, W. L., and Hildebrand, J. G. (2003). Electroantennographic and behavioral responses of the sphinx moth Manduca sexta to host plant headspace volatiles. J. Chem. Ecol. 29, 1813–1833. doi: 10.1023/a:1024898127549
Gadenne, C., Barrozo, R. B., and Anton, S. (2016). Plasticity in insect olfaction: To smell or not to smell? Annu. Rev. Entomol. 61, 317–333.
Gao, Q., and Chess, A. (1999). Identification of candidate Drosophila olfactory receptors from genomic DNA sequence. Genomics 60, 31–39.
Garczynski, S. F., Martin, J. A., Griset, M., Willett, L. S., Cooper, W. R., Swisher, K. D., et al. (2017). CRISPR/Cas9 editing of the codling moth (Lepidoptera: Tortricidae) CpomOR1 gene affects egg production and viability. J. Econ. Entomol. 110, 1847–1855. doi: 10.1093/jee/tox166
Geiss, G. K., Bumgarner, R. E., Birditt, B., Dahl, T., Dowidar, N., Dunaway, D. L., et al. (2008). Direct multiplexed measurement of gene expression with color-coded probe pairs. Nat. Biotechnol. 26, 317–325.
Gershman, A., Romer, T. G., Fan, Y., Razaghi, R., Smith, W. A., and Timp, W. (2021). De novo genome assembly of the tobacco hornworm moth (Manduca sexta). G3 11:jkaa047. doi: 10.1093/g3journal/jkaa047
Glendinning, J. I., Nelson, N. M., and Bernays, E. A. (2000). How do inositol and glucose modulate feeding in Manduca sexta caterpillars? J. Exp. Biol. 203, 1299–1315. doi: 10.1242/jeb.203.8.1299
Gouin, A., Bretaudeau, A., Nam, K., Gimenez, S., Aury, J.-M., Duvic, B., et al. (2017). Two genomes of highly polyphagous lepidopteran pests (Spodoptera frugiperda Noctuidae) with different host-plant ranges. Sc. Rep. 7:11816. doi: 10.1038/s41598-017-10461-4
Grant, G. G., and Eaton, J. L. (1973). Scent brushes of the male tobacco hornworm Manduca sexta (Lepidoptera: Sphingidae)1. Ann. Entomol. Soc. Am. 66, 901–904.
Grosse-Wilde, E., Kuebler, L. S., Bucks, S., Vogel, H., Wicher, D., and Hansson, B. S. (2011). Antennal transcriptome of Manduca sexta. Proc. Natl. Acad. Sci. 108:7449.
Grosse-Wilde, E., Stieber, R., Forstner, M., Krieger, J., Wicher, D., and Hansson, B. (2010). Sex-specific odorant receptors of the tobacco hornworm Manduca Sexta. Front. Cell. Neurosci. 4:22. doi: 10.3389/fncel.2010.00022
Guerenstein, P. G., Christensen, T. A., and Hildebrand, J. G. (2004a). Sensory processing of ambient CO2 information in the brain of the moth Manduca sexta. J. Comp. Physiol. A 190, 707–725. doi: 10.1007/s00359-004-0529-0
Guerenstein, P. G., Yepez, E. A., van Haren, J., Williams, D. G., and Hildebrand, J. G. (2004b). Floral CO2 emission may indicate food abundance to nectar-feeding moths. Naturwissenschaften 91, 329–333. doi: 10.1007/s00114-004-0532-x
Guo, H., Cheng, T., Chen, Z., Jiang, L., Guo, Y., Liu, J., et al. (2017). Expression map of a complete set of gustatory receptor genes in chemosensory organs of Bombyx mori. Insect Biochem. Mol. Biol. 82, 74–82. doi: 10.1016/j.ibmb.2017.02.001
Guo, M., Chen, Q., Liu, Y., Wang, G., and Han, Z. (2018). Chemoreception of Mouthparts: Sensilla Morphology and Discovery of Chemosensory Genes in Proboscis and Labial Palps of Adult Helicoverpa armigera (Lepidoptera: Noctuidae). Front. Physiol. 9:97. doi: 10.3389/fphys.2018.0097
Guo, M., Du, L., Chen, Q., Feng, Y., Zhang, J., Zhang, X., et al. (2021). Odorant receptors for detecting flowering plant cues are functionally conserved across moths and butterflies. Mol. Biol. Evol. 38, 1413–1427. doi: 10.1093/molbev/msaa300
Hallem, E. A., and Carlson, J. R. (2006). Coding of odors by a receptor repertoire. Cell 125, 143–160.
Haverkamp, A., Hansson, B. S., and Knaden, M. (2018). Combinatorial codes and labeled lines: How insects use olfactory cues to find and judge food, mates, and Oviposition sites in complex environments. Front. Physiol. 9:49. doi: 10.3389/fphys.2018.00049
Haverkamp, A., Yon, F., Keesey, I. W., Mißbach, C., Koenig, C., Hansson, B. S., et al. (2016). Hawkmoths evaluate scenting flowers with the tip of their proboscis. eLife 5:e15039. doi: 10.7554/eLife.15039
He, Z., Luo, Y., Shang, X., Sun, J. S., and Carlson, J. R. (2019). Chemosensory sensilla of the Drosophila wing express a candidate ionotropic pheromone receptor. PLoS Biol. 17:e2006619. doi: 10.1371/journal.pbio.2006619
Holdcraft, R., Rodriguez-Saona, C., and Stelinski, L. L. (2016). Pheromone Autodetection: Evidence and implications. Insects 7:17. doi: 10.3390/insects7020017
Hostachy, C., Couzi, P., Hanafi-Portier, M., Portemer, G., Halleguen, A., Murmu, M., et al. (2019). Responsiveness to Sugar Solutions in the Moth Agrotis ipsilon: Parameters affecting proboscis extension. Front. Physiol. 10:1423. doi: 10.3389/fphys.2019.01423
Hou, X. Q., Zhang, D. D., Powell, D., Wang, H. L., Andersson, M. N., and Lofstedt, C. (2022). Ionotropic receptors in the turnip moth Agrotis segetum respond to repellent medium-chain fatty acids. BMC Biol. 20:34. doi: 10.1186/s12915-022-01235-0
Hussain, A., Üçpunar, H. K., Zhang, M., Loschek, L. F., and Grunwald Kadow, I. C. (2016). Neuropeptides modulate female chemosensory processing upon mating in Drosophila. PLoS Biol. 14:e1002455. doi: 10.1371/journal.pbio.1002455
Jacquin-Joly, E., Legeai, F., Montagné, N., Monsempes, C., François, M.-C., Poulain, J., et al. (2012). Candidate chemosensory genes in female antennae of the noctuid moth Spodoptera littoralis. Int. J. Biol. Sci. 8, 1036–1050. doi: 10.7150/ijbs.4469
Jin, S., Zhou, X., Gu, F., Zhong, G., and Yi, X. (2017). Olfactory plasticity: Variation in the expression of chemosensory receptors in Bactrocera dorsalis in different physiological states. Front. Physiol. 8:672. doi: 10.3389/fphys.2017.00672
Jones, W. D., Cayirlioglu, P., Kadow, I. G., and Vosshall, L. B. (2007). Two chemosensory receptors together mediate carbon dioxide detection in Drosophila. Nature 445, 86–90. doi: 10.1038/nature05466
Jørgensen, K., Almaas, T. J., Marion-Poll, F., and Mustaparta, H. (2007). Electrophysiological characterization of responses from gustatory receptor neurons of sensilla chaetica in the Moth Heliothis virescens. Chem. Senses 32, 863–879. doi: 10.1093/chemse/bjm057
Joseph, R. M., and Carlson, J. R. (2015). Drosophila Chemoreceptors: A molecular interface between the chemical world and the brain. Trends Genet. 31, 683–695. doi: 10.1016/j.tig.2015.09.005
Kaissling, K.-E., Hildebrand, J. G., and Tumlinson, J. H. (1989). Pheromone receptor cells in the male moth Manduca sexta. Arch. Insect Biochem. Physiol. 10, 273–279.
Kalberer, N. M., Reisenman, C. E., and Hildebrand, J. G. (2010). Male moths bearing transplanted female antennae express characteristically female behaviour and central neural activity. J. Exp. Biol. 213, 1272–1280. doi: 10.1242/jeb.033167
Kalinova, B., Hoskovec, M., Liblikas, I., Unelius, C. R., and Hansson, B. S. (2001). Detection of sex pheromone components in Manduca sexta (L.). Chem. Senses 26, 1175–1186. doi: 10.1093/chemse/26.9.1175
Kanost, M. R., Arrese, E. L., Cao, X., Chen, Y. R., Chellapilla, S., Goldsmith, M. R., et al. (2016). Multifaceted biological insights from a draft genome sequence of the tobacco hornworm moth Manduca sexta. Insect Biochem. Mol. Biol. 76, 118–147. doi: 10.1016/j.ibmb.2016.07.005
Kariyat, R. R., Mauck, K. E., Balogh, C. M., Stephenson, A. G., Mescher, M. C., and De Moraes, C. M. (2013). Inbreeding in horsenettle (Solanum carolinense) alters night-time volatile emissions that guide oviposition by Manduca sexta moths. Proc. Biol. Sci. 280:20130020. doi: 10.1098/rspb.2013.0020
Keil, T. A. (1989). Fine structure of the pheromone-sensitive sensilla on the antenna of the hawkmoth Manduca sexta. Tissue Cell 21, 139–151. doi: 10.1016/0040-8166(89)90028-1
Kent, K. S., and Griffin, L. M. (1990). Sensory organs of the thoracic legs of the moth Manduca sexta. Cell Tissue Res. 259, 209–223. doi: 10.1007/BF00318442
Kent, K. S., Harrow, I. D., Quartararo, P., and Hildebrand, J. G. (1986). An accessory olfactory pathway in Lepidoptera: the labial pit organ and its central projections in Manduca sexta and certain other sphinx moths and silk moths. Cell Tissue Res. 245, 237–245. doi: 10.1007/BF00213927
Khan, M., Vaes, E., and Mombaerts, P. (2011). Regulation of the probability of mouse odorant receptor gene choice. Cell 147, 907–921.
Khan, M., Vaes, E., and Mombaerts, P. (2013). Temporal patterns of odorant receptor gene expression in adult and aged mice. Mol. Cell Neurosci. 57, 120–129. doi: 10.1016/j.mcn.2013.08.001
Klinner, C. F., König, C., Missbach, C., Werckenthin, A., Daly, K. C., Bisch-Knaden, S., et al. (2016). Functional olfactory sensory neurons housed in olfactory sensilla on the ovipositor of the Hawkmoth Manduca sexta. Front. Ecol. Evol. 4:130. doi: 10.3389/fevo.2016.00130
Knecht, Z. A., Silbering, A. F., Cruz, J., Yang, L., Croset, V., Benton, R., et al. (2017). Ionotropic Receptor-dependent moist and dry cells control hygrosensation in Drosophila. eLife 6:e26654. doi: 10.7554/eLife.26654
Knecht, Z. A., Silbering, A. F., Ni, L., Klein, M., Budelli, G., Bell, R., et al. (2016). Distinct combinations of variant ionotropic glutamate receptors mediate thermosensation and hygrosensation in Drosophila. eLife 5:e17879. doi: 10.7554/eLife.17879
Koenig, C., Hirsh, A., Bucks, S., Klinner, C., Vogel, H., Shukla, A., et al. (2015). A reference gene set for chemosensory receptor genes of Manduca sexta. Insect Biochem. Mol. Biol. 66, 51–63. doi: 10.1016/j.ibmb.2015.09.007
Koutroumpa, F. A., Monsempes, C., François, M.-C., Severac, D., Montagné, N., Meslin, C., et al. (2021). Description of chemosensory genes in unexplored tissues of the moth Spodoptera littoralis. Front. Ecol. Evol. 9:678277. doi: 10.3389/fevo.2021.678277
Kulkarni, M. M. (2011). Digital multiplexed gene expression analysis using the NanoString nCounter system. Curr. Protoc. Mol. Biol. Chapter 25:Unit25B10.
Kumar, A., Tauxe, G. M., Perry, S., Scott, C. A., Dahanukar, A., and Ray, A. (2020). Contributions of the conserved insect carbon dioxide receptor subunits to odor detection. Cell Rep. 31:107510. doi: 10.1016/j.celrep.2020.03.074
Lee, J. K., and Strausfeld, N. J. (1990). Structure, distribution and number of surface sensilla and their receptor cells on the olfactory appendage of the male moth Manduca sexta. J. Neurocytol. 19, 519–538. doi: 10.1007/BF01257241
Levin, E., Mitra, C., and Davidowitz, G. (2016). Fed males increase oviposition in female hawkmoths via non-nutritive direct benefits. Animal Behav. 112, 111–118.
Li, R.-T., Huang, L.-Q., Dong, J.-F., and Wang, C.-Z. (2020). A moth odorant receptor highly expressed in the ovipositor is involved in detecting host-plant volatiles. eLife 9:e53706. doi: 10.7554/eLife.53706
Liu, N.-Y., Xu, W., Dong, S.-L., Zhu, J.-Y., Xu, Y.-X., and Anderson, A. (2018). Genome-wide analysis of ionotropic receptor gene repertoire in Lepidoptera with an emphasis on its functions of Helicoverpa armigera. Insect Biochem. Mol. Biol. 99, 37–53. doi: 10.1016/j.ibmb.2018.05.005
Lombarkia, N., and Derridj, S. (2008). Resistance of apple trees to Cydia pomonella egg-laying due to leaf surface metabolites. Entomol. Exp. Appl. 128, 57–65.
Ma, L., Li, Z.-Q., Bian, L., Cai, X.-M., Luo, Z.-X., Zhang, Y.-J., et al. (2016). Identification and comparative study of chemosensory genes related to host selection by legs transcriptome analysis in the tea geometrid Ectropis obliqua. PLoS One 11:e0149591. doi: 10.1371/journal.pone.0149591
MacWilliam, D., Kowalewski, J., Kumar, A., Pontrello, C., and Ray, A. (2018). Signaling Mode of the Broad-Spectrum Conserved CO2 receptor is one of the important determinants of odor valence in drosophila. Neuron, 97, 1153-1167.e4. doi: 10.1016/j.neuron.2018.01.028
Mechaber, W. L., Capaldo, C. T., and Hildebrand, J. G. (2002). Behavioral responses of adult female tobacco hornworms, Manduca sexta, to hostplant volatiles change with age and mating status. J. Insect Sci. 2:5.
Nelson, N., and Bernays, E. (1998). Inositol in two host plants of Manduca sexta. Entomol. Exp. Appl. 88, 189–193.
Ni, L., Klein, M., Svec, K. V., Budelli, G., Chang, E. C., Ferrer, A. J., et al. (2016). The Ionotropic Receptors IR21a and IR25a mediate cool sensing in Drosophila. eLife 5:e13254.
Ning, C., Yang, K., Xu, M., Huang, L. Q., and Wang, C. Z. (2016). Functional validation of the carbon dioxide receptor in labial palps of Helicoverpa armigera moths. Insect Biochem. Mol. Biol. 73, 12–19. doi: 10.1016/j.ibmb.2016.04.002
Pearce, S. L., Clarke, D. F., East, P. D., Elfekih, S., Gordon, K. H. J., Jermiin, L. S., et al. (2017). Genomic innovations, transcriptional plasticity and gene loss underlying the evolution and divergence of two highly polyphagous and invasive Helicoverpa pest species. BMC Biol. 15:63. doi: 10.1186/s12915-017-0402-6
Raad, H., Ferveur, J. F., Ledger, N., Capovilla, M., and Robichon, A. (2016). Functional gustatory role of chemoreceptors in drosophila wings. Cell Rep. 15, 1442–1454.
Raguso, R. A., and Willis, M. A. (2002). Synergy between visual and olfactory cues in nectar feeding by naïve hawkmoths Manduca sexta. Animal Behav. 64, 685–695.
Reisenman, C. E., Christensen, T. A., Francke, W., and Hildebrand, J. G. (2004). Enantioselectivity of projection neurons innervating identified olfactory glomeruli. J. Neurosci. 24, 2602–2611. doi: 10.1523/JNEUROSCI.5192-03.2004
Reisenman, C. E., Riffell, J. A., Bernays, E. A., and Hildebrand, J. G. (2010). Antagonistic effects of floral scent in an insect-plant interaction. Proc. Biol. Sci. 277, 2371–2379. doi: 10.1098/rspb.2010.0163
Reiter, S., Campillo Rodriguez, C., Sun, K., and Stopfer, M. (2015). Spatiotemporal coding of individual chemicals by the gustatory system. J. Neurosci. 35, 12309–12321. doi: 10.1523/JNEUROSCI.3802-14.2015
Riffell, J. A., Alarcón, R., Abrell, L., Davidowitz, G., Bronstein, J. L., and Hildebrand, J. G. (2008). Behavioral consequences of innate preferences and olfactory learning in hawkmoth–flower interactions. Proc. Natl. Acad. Sci. U.S.A. 105, 3404–3409. doi: 10.1073/pnas.0709811105
Robertson, H. M., and Kent, L. B. (2009). Evolution of the gene lineage encoding the carbon dioxide receptor in insects. J. Insect. Sci. 9:19.
Rytz, R., Croset, V., and Benton, R. (2013). Ionotropic Receptors (IRs): Chemosensory ionotropic glutamate receptors in Drosophila and beyond. Insect Biochem. Mol. Biol. 43, 888–897. doi: 10.1016/j.ibmb.2013.02.007
Sanes, J. R., and Hildebrand, J. G. (1976). Structure and development of antennae in a moth Manduca sexta. Dev. Biol. 51, 280–299.
Sasaki, M., and Riddiford, L. M. (1984). Regulation of reproductive-behavior and egg maturation in the tobacco hawk moth Manduca-Sexta. Physiol. Entomol. 9, 315–327.
Sato, K., Pellegrino, M., Nakagawa, T., Nakagawa, T., Vosshall, L. B., and Touhara, K. (2008). Insect olfactory receptors are heteromeric ligand-gated ion channels. Nature 452, 1002–1006.
Saveer, A. M., Kromann, S. H., Birgersson, G., Bengtsson, M., Lindblom, T., Balkenius, A., et al. (2012). Floral to green: mating switches moth olfactory coding and preference. Proc. R. Soc. B Biol. Sci. 279, 2314–2322. doi: 10.1098/rspb.2011.2710
Schneiderman, A. M., Hildebrand, J. G., Brennan, M. M., and Tumlinson, J. H. (1986). Trans-sexually grafted antennae alter pheromone-directed behaviour in a moth. Nature 323, 801–803. doi: 10.1038/323801a0
Seada, M. A., Ignell, R., and Anderson, P. (2016). Morphology and distribution of ovipositor sensilla of female cotton leaf worm Spodoptera littoralis (Lepidoptera: Noctuidae), and evidence for gustatory function. Entomol. Sci. 19, 9–19.
Shields, V. D. C., and Hildebrand, J. G. (1999). Fine structure of antennal sensilla of the female sphinx moth, Manduca sexta (Lepidoptera: Sphingidae). II. Auriculate, coeloconic, and styliform complex sensilla. Can. J. Zool. 77, 302–313.
Silbering, A. F., Rytz, R., Grosjean, Y., Abuin, L., Ramdya, P., Jefferis, G. S. X. E., et al. (2011). Complementary function and integrated wiring of the evolutionarily distinct Drosophila factory subsystems. J. Neurosci. 31, 13357–13375. doi: 10.1523/JNEUROSCI.2360-11.2011
Spaethe, A., Reinecke, A., Olsson, S. B., Kesavan, S., Knaden, M., and Hansson, B. S. (2013). Plant species- and status-specific odorant blends guide oviposition choice in the moth Manduca sexta. Chem. Senses 38, 147–159. doi: 10.1093/chemse/bjs089
Staedler, E., Renwick, J. A. A., Radke, C. D., and Sachdev-Gupta, K. (1995). Tarsal contact chemoreceptor response to glucosinolates and cardenolides mediating oviposition in Pieris rape. Physiol. Entomol. 20, 175–187.
Sun, Y.-L., Dong, J.-F., Yang, H.-B., Li, D.-X., and Tian, C.-H. (2022). Identification and Characterization of Chemosensory Receptors in the Pheromone Gland-Ovipositor of Spodoptera frugiperda (J. E. Smith). Insects 13:481. doi: 10.3390/insects13050481
Tanaka, K., Uda, Y., Ono, Y., Nakagawa, T., Suwa, M., Yamaoka, R., et al. (2009). Highly selective tuning of a silkworm olfactory receptor to a key mulberry leaf volatile. Curr. Biol. 19, 881–890. doi: 10.1016/j.cub.2009.04.035
Thom, C., Guerenstein, P. G., Mechaber, W. L., and Hildebrand, J. G. (2004). Floral CO2 reveals flower profitability to moths. J. Chem. Ecol. 30, 1285–1288. doi: 10.1023/b:joec.0000030298.77377.7d
Tumlinson, J. H., Brennan, M. M., Doolittle, R. E., Mitchell, E. R., Brabham, A., Mazomenos, B. E., et al. (1989). Identification of a pheromone blend attractive to Manduca-sexta (L) males in a wind-tunnel. Arch. Insect Biochem. Physiol. 10, 255–271.
van Schooten, B., Jiggins, C. D., Briscoe, A. D., and Papa, R. (2016). Genome-wide analysis of ionotropic receptors provides insight into their evolution in Heliconius butterflies. BMC Genomics 17:254. doi: 10.1186/s12864-016-2572-y
Veldman-Jones, M. H., Brant, R., Rooney, C., Geh, C., Emery, H., Harbron, C. G., et al. (2015). Evaluating robustness and sensitivity of the nanostring technologies nCounter platform to enable multiplexed gene expression analysis of clinical samples. Cancer Res. 75, 2587–2593. doi: 10.1158/0008-5472.CAN-15-0262
von Arx, M., Sullivan, K. A., and Raguso, R. A. (2013). Dual fitness benefits of post-mating sugar meals for female hawkmoths (Hyles lineata). J. Insect Physiol. 59, 458–465. doi: 10.1016/j.jinsphys.2013.01.006
Vosshall, L. B., Amrein, H., Morozov, P. S., Rzhetsky, A., and Axel, R. (1999). A spatial map of olfactory receptor expression in the Drosophila antenna. Cell 96, 725–736. doi: 10.1016/s0092-8674(00)80582-6
Vulpe, A., and Menuz, K. (2021). Ir76b is a co-receptor for amine responses in drosophila olfactory neurons. Front. Cell. Neurosci. 15:759238. doi: 10.3389/fncel.2021.759238
Walker, W. B. III, Gonzalez, F., Garczynski, S. F., and Witzgall, P. (2016). The chemosensory receptors of codling moth Cydia pomonella-expression in larvae and adults. Sci. Rep. 6:23518. doi: 10.1038/srep23518
Walker, W. B. III, Roy, A., Anderson, P., Schlyter, F., Hansson, B. S., and Larsson, M. C. (2019). Transcriptome analysis of gene families involved in chemosensory function in Spodoptera littoralis (Lepidoptera: Noctuidae). BMC Genomics 20:428. doi: 10.1186/s12864-019-5815-x
Wanner, K. W., Anderson, A. R., Trowell, S. C., Theilmann, D. A., Robertson, H. M., and Newcomb, R. D. (2007). Female-biased expression of odourant receptor genes in the adult antennae of the silkworm Bombyx mori. Insect Mol. Biol. 16, 107–119. doi: 10.1111/j.1365-2583.2007.00708.x
Wicher, D., and Miazzi, F. (2021). Functional properties of insect olfactory receptors: ionotropic receptors and odorant receptors. Cell Tissue Res. 383, 7–19.
Wicher, D., Morinaga, S., Halty-deLeon, L., Funk, N., Hansson, B., Touhara, K., et al. (2017). Identification and characterization of the bombykal receptor in the hawkmoth Manduca sexta. J. Exp. Biol. 220(Pt 10), 1781–1786. doi: 10.1242/jeb.154260
Widmayer, P., Heifetz, Y., and Breer, H. (2009). Expression of a pheromone receptor in ovipositor sensilla of the female moth (Heliothis virescens). Insect Mol. Biol. 18, 541–547. doi: 10.1111/j.1365-2583.2009.00894.x
Xu, W. (2020). How do moth and butterfly taste?—Molecular basis of gustatory receptors in Lepidoptera. Insect Sci. 27, 1148–1157. doi: 10.1111/1744-7917.12718
Yamamoto, R. T., Jenkins, R. Y., and McClusky, R. K. (1969). Factors determining the selection of plants for oviposition by the tobacco hornworm Manduca sexta. Entomol. Exp. Appl. 12, 504–508.
Yanagawa, A., Couto, A., Sandoz, J. C., Hata, T., Mitra, A., Ali Agha, M., et al. (2019). LPS perception through taste-induced reflex in Drosophila melanogaster. J. Insect. Physiol. 112, 39–47. doi: 10.1016/j.jinsphys.2018.12.001
Yang, L., Agramonte, N., Linthicum, K. J., and Bloomquist, J. R. (2020). A survey of chemoreceptive responses on different mosquito appendages. J. Med. Entomol. 58, 475–479. doi: 10.1093/jme/tjaa154
Yin, N. N., Nuo, S. M., Xiao, H. Y., Zhao, Y. J., Zhu, J. Y., and Liu, N. Y. (2021). The ionotropic receptor gene family in Lepidoptera and Trichoptera: Annotation, evolutionary and functional perspectives. Genomics 113(1 Pt 2), 601–612. doi: 10.1016/j.ygeno.2020.09.056
Zhang, D.-D., and Löfstedt, C. (2015). Moth pheromone receptors: gene sequences, function, and evolution. Front. Ecol. Evol. 3:105. doi: 10.3389/fevo.2015.00105
Zhang, H.-J., Anderson, A. R., Trowell, S. C., Luo, A. R., Xiang, Z.-H., and Xia, Q.-Y. (2011). Topological and functional characterization of an insect gustatory receptor. PLoS One 6:e24111. doi: 10.1371/journal.pone.0024111
Zhang, J., Bisch-Knaden, S., Fandino, R. A., Yan, S. W., Obiero, G. F., Grosse-Wilde, E., et al. (2019). The olfactory coreceptor IR8a governs larval feces-mediated competition avoidance in a hawkmoth. Proc. Natl. Acad. Sci. 116:21828. doi: 10.1073/pnas.1913485116
Zhang, Z.-J., Zhang, S.-S., Niu, B.-L., Ji, D.-F., Liu, X.-J., Li, M.-W., et al. (2019). A determining factor for insect feeding preference in the silkworm Bombyx mori. PLoS Biol. 17:e3000162. doi: 10.1371/journal.pbio.3000162
Zhang, J., Komail Raza, S. A., Wei, Z., Keesey, I. W., Parker, A. L., Feistel, F., et al. (2022). Competing beetles attract egg laying in a hawkmoth. Curr. Biol. 32, 861-869.e8. doi: 10.1016/j.cub.2021.12.021
Zhang, S. F., Zhang, Z., Kong, X. B., Wang, H. B., and Liu, F. (2017). Dynamic changes in chemosensory gene expression during the Dendrolimus punctatus mating process. Front. Physiol. 8:1127. doi: 10.3389/fphys.2017.01127
Zhang, Y. V., Ni, J., and Montell, C. (2013). The molecular basis for attractive salt-taste coding in Drosophila. Science 340, 1334–1338. doi: 10.1126/science.1234133
Keywords: hawkmoth, sex-specific, mating status, chemosensory organs, NanoString, chemosensory receptor, Manduca sexta
Citation: Tom MT, Cortés Llorca L, Bucks S, Bisch-Knaden S and Hansson BS (2022) Sex- and tissue-specific expression of chemosensory receptor genes in a hawkmoth. Front. Ecol. Evol. 10:976521. doi: 10.3389/fevo.2022.976521
Received: 23 June 2022; Accepted: 03 August 2022;
Published: 22 August 2022.
Edited by:
William Benjamin Walker III, Temperate Tree Fruit and Vegetable Research Unit, Agricultural Research Service, United States Department of Agriculture (USDA-ARS), United StatesReviewed by:
Nicolas Montagné, Sorbonne Universités, FranceXin-Cheng Zhao, Henan Agricultural University, China
Copyright © 2022 Tom, Cortés Llorca, Bucks, Bisch-Knaden and Hansson. This is an open-access article distributed under the terms of the Creative Commons Attribution License (CC BY). The use, distribution or reproduction in other forums is permitted, provided the original author(s) and the copyright owner(s) are credited and that the original publication in this journal is cited, in accordance with accepted academic practice. No use, distribution or reproduction is permitted which does not comply with these terms.
*Correspondence: Sonja Bisch-Knaden, sbisch-knaden@ice.mpg.de
†These authors share senior authorship