- 1Division of Paleontology (Invertebrates), American Museum of Natural History, New York, NY, United States
- 2Department of Earth and Atmospheric Sciences, University of Nebraska–Lincoln, Lincoln, NE, United States
- 3School of Biological Sciences, University of Nebraska–Lincoln, Lincoln, NE, United States
Some taxa occupy our imaginations as “living fossils” because they were known from the fossil record before being discovered alive today. Other taxa are considered “living fossils” because modern relatives bear a strong morphological resemblance to fossil relatives, or because they occupy a contracted geographic range or have less diversity now than in the past, or because they represent phylogenetic diversity that requires conservation. A characterizing feature of living fossils–and thus an implicit assumption of all criteria–is that the “living fossil” of interest is extant. However, the general research questions that “living fossils” inspire–Why do rates of evolution vary across organisms, across traits, and across time? Why do some clades decline in diversity over extended periods?–may be applied to any clade, including completely extinct clades. We propose that there is nothing special about “now” when it comes to pursuing these questions and that it is unnecessarily limiting to restrict research programs to clades for which an extant member meets some conception of the “living fossil” moniker. To this end, we investigate the extent to which Permian trilobites might resemble “living fossils,” albeit from the perspective of 253 million years ago, when the last trilobites were still alive. We do so by comparing the taxonomic diversity, geographic range, and morphological disparity of trilobites living in the Permian to earlier time periods. We find that Permian trilobites meet most definitions of living fossils, although our assessment of morphological change and character retention depend on taxonomic scale.
Introduction
Despite ongoing debate over the usefulness of the “living fossils” concept, there has been an increase over the last forty years in the number of biological entities identified as living fossils as well as an increase in the number of publications where the term is used (Lidgard and Love, 2021). Part of the appeal may lie in our imaginations: when we see a living fossil (e.g., horseshoe crab), we can see in front of us what ancient environments (e.g., beaches) might have been like (Turner, 2019). Another part of the appeal may be the breadth of definitions that exist for living fossils: although this draws much of the debate, it also reflects the rich and expansive research questions that they inspire. Such definitions include the following (modified from Lidgard and Love, 2018; Turner, 2019):
• Prolonged geologic duration or persistence of a lineage.
• Low taxonomic richness today compared to the past.
• Small geographic range today compared to the past.
• Low relative abundance today compared to the past.
• Genealogical divergence in the very distant past (“phylogenetically distinct”/high contribution to phylogenetic diversity).
• Slow rate of evolutionary change (or in the limiting case, stasis).
• Morphological similarity to fossils from the distant past.
• Presence of characters that are or seem plesiomorphic.
These definitions are not mutually exclusive. For example, stalked crinoids have been referred to as living fossils because they were more speciose and abundant in shallow marine environments in the Paleozoic and are now largely restricted to the deep sea, but still look like their Paleozoic ancestors and in some cases have been referred to the same genera (Meyer and Macurda, 1977; Bourseau et al., 1991). [Interestingly, there is evidence that some modern stalked crinoids may have evolved from stalk-less ones (Rouse et al., 2013), so this lifestyle and the associated anatomy could also be the product of convergent evolution, not retainment of plesiomorphic characters!].
These definitions also require a comparison to be made between ancestral and descendent individuals or to other “non-living-fossil” groups, with the implicit assumption that the comparison is between extinct (fossil) ancestors and still-living organisms. Indeed the characterization that “living fossil” groups contain extant members is codified in the term itself. Yet the pursuit of the research questions implied by “living fossils” concepts, such as “why do some but not all constellations of characters exhibit apparent stasis over long periods of time in the same lineage?” and “how are perceived declines in living fossil groups related to patterns in phylogenetic sister groups, and to origination and extinction dynamics?” (Lidgard and Love, 2018, p. 766) does not necessarily depend on the presence of living clade members in the study system. To demonstrate this, we turn to a completely extinct fossil clade, the Trilobita, and assess the extent to which its youngest members would have met the definition(s) of a living fossil at the time they were alive.
Did trilobites have a prolonged geologic duration?
Trilobites are crown arthropods (Daley et al., 2018) with an extensive fossil record thanks to their biomineralized exoskeleton and penchant for living along continental shelves where sedimentation rates are high (Hopkins, 2017). Over 22,000 species are known (Paterson, 2020) across a 270-million-year evolutionary history which comprised most of the Paleozoic; they went extinct during the Permo-Triassic mass extinction (252 million years ago). Although 270 million years is considerably shorter than the geologic duration of one of the quintessential living fossils, xiphosurid horseshoe crabs (480 million years, Bicknell and Pates, 2020), the dominant trilobite family during the last geologic period of their existence (the Permian) was the Proetidae, whose geologic duration spanned over 230 million years, rivaling that of Limulidae, the horseshoe crab family comprising living species (250 million years, Bicknell et al., 2022).
Were Permian trilobites “relicts” of the past?
Living fossils may be thought of as relicts in three different capacities: having reduced taxonomic richness, smaller geographic range, and/or low relative abundance.
Low taxonomic richness
The first extensive tabulation of the number of trilobite species (and genera) over time showed a peak in taxonomic diversity in the middle to late Cambrian followed by an ongoing decline in taxonomic richness through the rest of the Paleozoic. By the Permian, species diversity was only 2.2% of the maximum (1.8% maximum generic richness) (Foote, 1993). Compilations based on the Sepkoski dataset (Sepkoski, 2002; Supplementary Figure 1A), the Paleobiology Database (Alroy, 2010; Bault et al., 2022; Supplementary Figure 1B), the Geobiodiversity Database (Fan et al., 2020), and others (e.g., Owens and Hahn, 1993; Lerosey-Aubril and Feist, 2012) show similarly low levels of taxonomic diversity from the latest Carboniferous through the Permian. Only two genera, Acropyge and Pseudophillipsia, are known from the Changhsingian (254–252 million years ago) (Fortey and Owens, 1997; Supplementary Figure 1B).
Smaller geographic range
Despite the low taxonomic diversity, late Permian trilobites occupied a range of environments. While the majority of Lopingian (260–242 million years ago) genera occupied shallow tropical carbonate settings (Bault et al., 2022), trilobite fragments have been recovered from sediments deposited in polar settings (Hyden et al., 1982; paleo-coordinates based on Paleobiology Database, see collection 98,149) and from deeper water clastic environments (Shen and He, 1994). Broad geographic ranges are also seen at low taxonomic scale, for example Pseudophillipsia remained widespread even in the latest Permian (Owens and Hahn, 1993).
Lower abundance
Trilobites started becoming a less dominant component of marine ecosystems during the Ordovician radiation (Westrop and Adrain, 1998; Peters, 2004), but even in later periods, they could make up a high percentage of particular faunas (e.g., Brett, 1974), occasionally occurring in overwhelming numbers (Speyer and Brett, 1985, 1986). By the Permian, however, trilobites were “limited in diversity and abundance worldwide” (Hanger, 1998, p. 12). In a summary of Permian fossil assemblages from southeast Asia, Fontaine (2002, p. 583) describes the timing for extinction of major marine invertebrate groups, noting that “Trilobites are not in abundance at many Permian outcrops; [so the timing of] their disappearance is not immediately obvious.” Grant (1966, p. 64) noted that while the late Permian Productus Limestone of the Salt Range, Pakistan, is important for its unusual abundance and excellent preservation of marine invertebrates, only a very few trilobites specimens had been found and “their extreme rarity makes it unlikely that additional trilobite specimens will be available soon.” A more recent quantitative study of relative abundances across the Permo-Triassic boundary at sections on the Yangtze Platform noted the rare presence of trilobites, including them with three other classes in an “other” category comprising only 9% of the sample (Gong et al., 2022).
In summary, although Permian trilobites continued to occupy a range of environments, their diversity and local abundance were much lower than that of contemporaneous marine invertebrate groups and much lower than that of trilobites earlier in their evolutionary history.
Were Permian trilobites phylogenetically distinct?
Although there is ongoing debate about whether trilobites and other artiopods were more closely related to mandibulates or chelicerates, they are currently estimated to have diverged from other arthropod groups in the earliest Cambrian (539–521 million years ago) before the appearance of the first trilobite fossils (Paterson et al., 2019; Edgecombe, 2020). With minimally 270 million years of divergence having occurred before their extinction, an argument could be made that by the Permian, they were phylogenetically distinct from other arthropods as well as other animals more generally.
Proponents of this definition for living fossils note that relative phylogenetic distinctness could be useful for directing conservation efforts (e.g., Turner, 2019). This is a modern concern which is more meaningful if we restrict ourselves to discussing extant groups. However, if there were an entity living 253 million years ago interested in conservation of rare and phylogenetically distinct groups, trilobites would have qualified for consideration.
Were Permian trilobites morphologically “boring?”
This question attempts to address three definitions– slow rates of evolution, morphological similarity to ancestors, and retention of plesiomorphic characters – that are different characterizations of the same essential observation: that modern “living fossils” look at least superficially like their very ancient ancestors.
In spite of Proetidae being the longest lived, most diverse trilobite family (1,927 species, Adrain, 2011), there is less debate about familial affinity for proetid trilobites than for many other groups (Paterson, 2020; Hopkins and To, 2022). In fact, “a proetid is a proetid is a proetid” has become a mantra among the three of us (MH, PW, KJ), a view which implies that the family had relatively low morphological disparity. Similarly, proetid trilobites have previously been referred to as “garden variety” (Lieberman and Karim, 2010), implying few novelties over the clade’s evolutionary history. More importantly, these sentiments imply that Permian trilobites may be similar enough to their earliest ancestors that they could qualify as living fossils from a morphological perspective.
Testing this perception, however, is non-trivial, requiring comparisons within Proetidae, between Proetidae and other trilobites, and between trilobites and other arthropod groups, ideally within the same framework. In lieu of this, we approach this question from two different angles.
Morphological variation in the shape of the head shield
The most taxonomically comprehensive morphological datasets for trilobites use morphometrics to capture some aspect of shape in the head shield (cranidium, Foote, 1993; cephalon, Suárez and Esteve, 2021; see Supplementary Figure 2 for description of basic trilobite anatomy). Despite differences in how the datasets sample trilobite taxonomic diversity (Supplementary Information), both datasets show low levels of morphological diversity overall in the Carboniferous and Permian and there is no statistically significant shape change across these time intervals (Figure 1 and Supplementary Table 1). These patterns are similar to those recently reported for shape variation in xiphosurid horseshoe crabs: a decrease in morphological variation and subsequent lack in statistically significant shape change from the Middle Triassic to the Recent (Bicknell et al., 2022). In these later intervals of evolutionary history, both groups are also dominated by a single family (Proetidae and Limulidae, respectively).
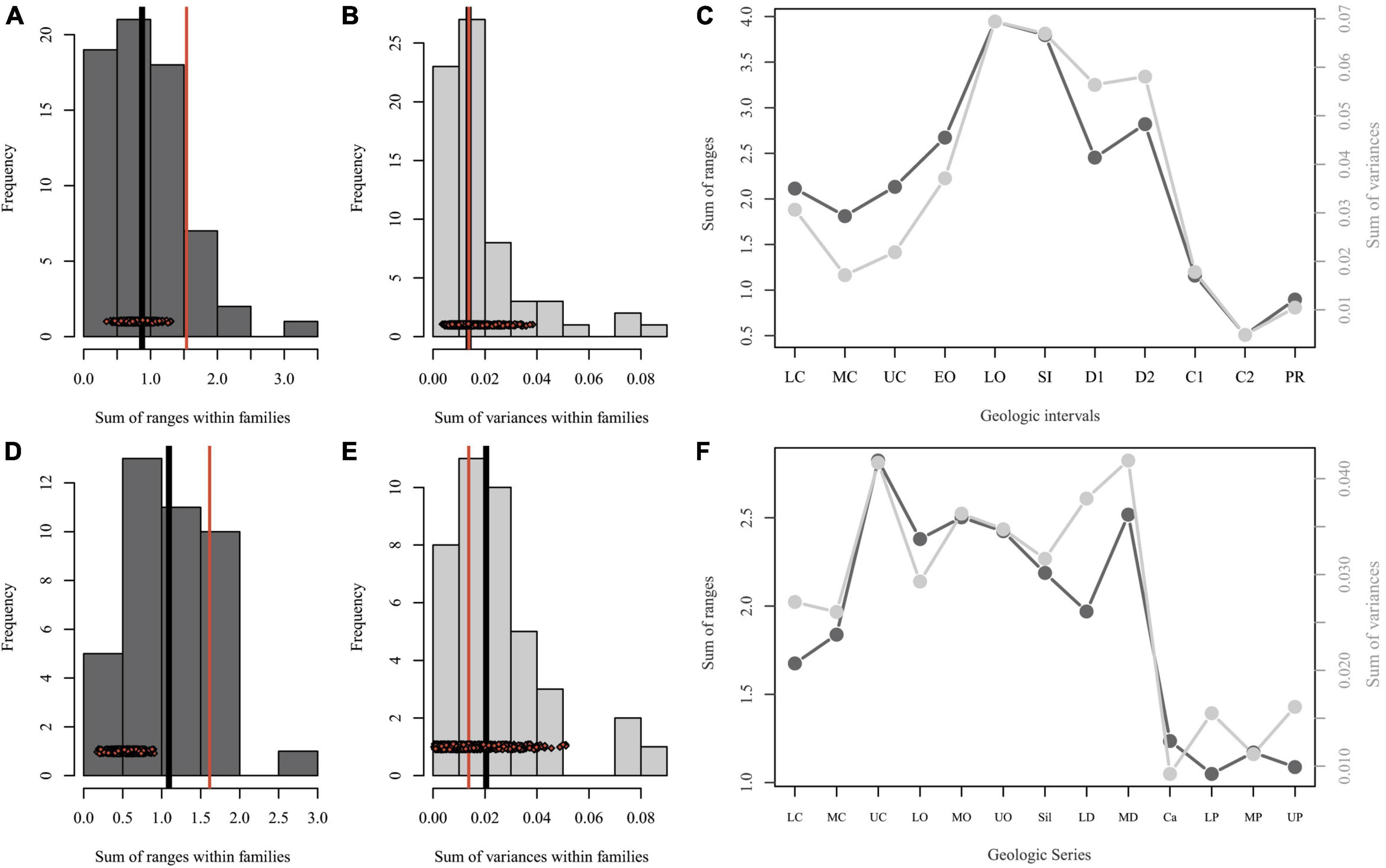
Figure 1. Disparity within trilobite head shape, represented either by Fourier analysis of the outline of the cranidium (medial sclerite of the head shield) (A–C) or by Procrustes superimposition of semi-landmark data describing the cephalon (entire head shield). (D–F) Disparity is estimated using sum of ranges, which describes overall breadth of morphospace occupation, and sum of variances, which describes the density of points within the space occupied (Hopkins and Gerber, 2017; Guillerme et al., 2020). (A,D) Disparity (sum of ranges) within families; black line shows median and red line shows disparity for Proetidae. Red diamonds show disparity estimates for Proetidae when subsampled to median sample size within families. (B,E) Disparity (sum of variances) within families; black line shows median and red line shows disparity for Proetidae. Red diamonds show disparity estimates for Proetidae when subsampled to median sample size within families. (C,F) Disparity (sum of ranges in dark gray; sum of variances in light gray) through time. Cranidial dataset (A–C) modified from Foote (1993); cephalic dataset (D–F) modified from Suárez and Esteve (2021); see Supplementary materials for details. C1 = early Carboniferous; C2 = late Carboniferous; Ca = Carboniferous; D1 = early Devonian; D2 = later Devonian; EO = early Ordovician; LC = lower Cambrian; LO = late Ordovician; LP = lower Permian; MC = middle Cambrian; MO = middle Ordovician; MP = middle Permian; PR = Permian; SL = Sil = Silurian; UC = upper Cambrian; UO = upper Ordovician; UP = upper Permian.
Because the Proetidae is the longest-lived and most diverse trilobite family, we may expect that they would also have a large amount of disparity overall. In terms of shape of the cranidium or cephalon, the Proetidae shows a median or smaller amount of disparity (sum of variances) across a larger-than-median area of morphospace (sum of ranges) relative to other families. However, Proetidae is also the most heavily sampled family in both datasets and subsampling at the median sample size shows a wide range of smaller estimates (Figure 1). Furthermore, there is no statistically significant shape change across the entire sampled history of Proetidae in either dataset (Supplementary Table 1), which implies relatively slow rates of evolution across the family. Nor is there statistically significant shape difference between the oldest sampled specimens and the youngest sampled specimens (Supplementary Table 1), which implies retention of ancestral morphology, at least in terms of the shape of the cranidium/cephalon.
Morphological diversification across the exoskeleton
Variation in some aspect of the head shield shape underlies our understanding of trilobite morphological diversification at many taxonomic levels. However, the outline shape of the cranidium or cephalon (as analyzed above) comprises a small part of the morphological variation across the entire exoskeleton. Many researchers turn to semi-quantitative character datasets to describe variation across the organism or at higher taxonomic levels (e.g., Wagner and Erwin, 2006). Although considerable phylogenetic work has been done for trilobites at lower taxonomic scales, including proetids (e.g., Lieberman, 1994; Lamsdell and Selden, 2015), there is currently no suitable character matrix available that could be co-opted for this study (for example the most extensive published character matrix is limited to Cambrian trilobites, Paterson et al., 2019; see also Lieberman and Karim, 2010; Paterson, 2020).
Instead, we designed a taxon sampling and coding protocol for the specific purpose of characterizing the basic or typical trilobite body form. We developed a list of 37 notable or unusual exoskeletal traits. We then queried the Paleobiology Database (PBDB) for the most common genera within orders within each geologic period. For each of these genera, we then recorded if any observable congenerics expressed any of those 37 traits (see Supplementary material for details).
Across orders, there is a strong association between the average proportion of unusual exoskeletal traits within genera and the average pairwise distance among genera within each order (Figure 2A). This suggests that orders that have more genera with unusual traits are also more diverse in terms of the combination of unusual characters expressed across those genera. Exceptions to this rule include the orders Odontopleurida and Redlichiida, both of which show high proportions of unusual traits but more consistency among the combinations of traits which are unusual. Compared to other orders, Proetida has the lowest values. Amongst families for which more than five genera were sampled, Proetidae comprise genera with the lowest proportions of unusual traits and smaller pairwise distances among genera (Figure 2A).
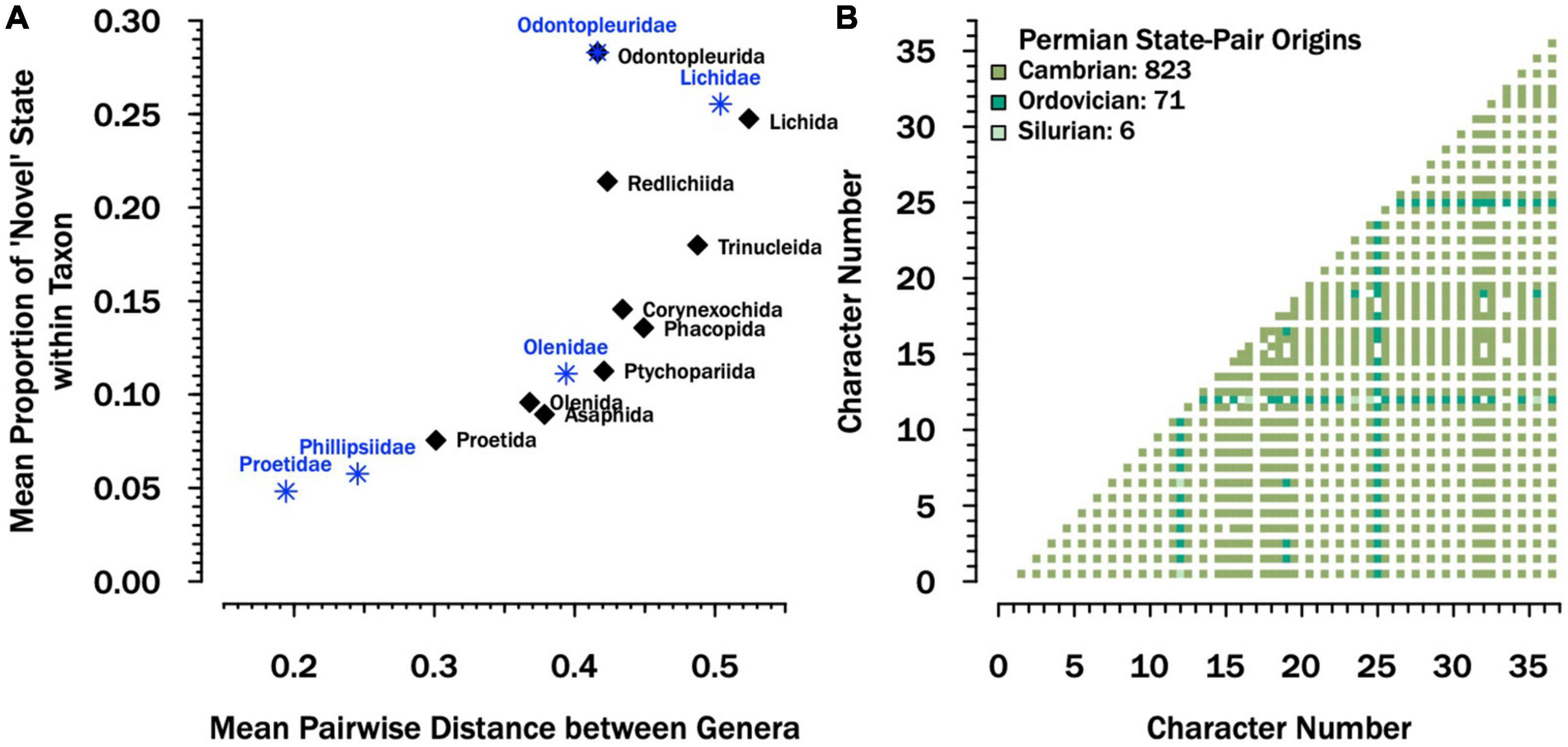
Figure 2. Disparity across the exoskeleton. (A) Scatterplot showing the average proportion of unusual traits (those coded as “1” in the matrix) compared to the average pairwise distance within orders (black dots) and families with more than 5 sampled genera (blue asterisks). Here “Proetidae” consists of non-phillipsiid taxa. The taxonomic status of “Phillipsidae” is debated, but it is currently thought to be a subclade within the Proetidae (Vanderlaan and Ebach, 2015). Together, they comprise 67% of the genera assigned to order Proetida in the character matrix. See Supplementary material for details about sampling, coding, and summary statistics presented here. (B) Combinations of paired states from the character matrix that are observed in Permian taxa (lower triangle), color-coded by the previous period in which the pair is observed in other trilobite taxa. The majority (80%) are first observed in the Cambrian; the rest are first observed in either the Ordovician or Silurian and none of them are novel to the Permian.
The early Cambrian was dominated by genera from the orders Redlichiida, Corynexochida, and Ptychopariida. These groups have moderate to high proportions of unusual traits (Figure 2A), some of which may arguably be considered ancestral despite this. Nonetheless, there were genera in the Cambrian and Ordovician that also expressed very few unusual traits (Supplementary Figure 3). More importantly, there were no pairs of characters expressed by Permian taxa that were not already present in early Paleozoic taxa; in fact, 80% were expressed in taxa as early as the Cambrian (Figure 2B and Supplementary Figure 4). Notably, the writing might have been on the wall as early as the Devonian. Although trilobites were still fairly diverse anatomically and taxonomically, of the three dominant trilobite orders (Proetida, Phacopida, and Lichida), only the Lichida show new character combinations in the Devonian; the youngest proetide combinations are Silurian and the youngest phacopide character combinations are Ordovician (Supplementary Figure 5).
Discussion
The concept of living fossils was originally used to describe the persistence of “ancient” morphologies observed in fossil taxa and extant taxa separated in time (Darwin, 1859). More recently, it has been proposed that the potential of the living fossils concept to further current research in evolutionary biology is realized through the specific questions they inspire (Lidgard and Love, 2018). This shift in perspective motivated us to look beyond what happens to be alive today in search of extinct organisms that could be considered “living fossils” at a particular time in their evolutionary history with the idea that such an exercise would expand the potential systems to which we can apply pertinent questions. Further, there may even be a benefit to working on extinct clades if knowing the timing of extinction matters for a particular question about relict clades or clade decline. In this study, we focus on one such putative group, trilobites, and assessed the extent to which the living fossil moniker could be applied to Permian trilobites. According to the criteria presented above, Permian trilobites belonged to a subclade with a relatively long geologic duration and lineage persistence and did exhibit low taxonomic richness and abundance in the Permian compared to previous levels, just as do modern horseshoe crabs relative to their ancestors. However, Permian trilobites were not relicts in all ways and continued to occupy broad geographic and environmental ranges not unlike their past ranges.
Similarly, our morphological analyses support the living fossil moniker to different degrees. The family to which Permian trilobites belonged, the Proetidae, were more morphologically conservative in comparison with other lineages (Figure 2A), and included members with few “unusual” traits, especially in comparison to common members of some “ostentaceous” trilobite groups like odontopleurides, lichides, and trinucleides (Figure 2A). Furthermore, Permian proetids did not express novel combinations of characters, they had all been realized by Cambrian and Ordovician trilobites (Figure 2B). There are also aspects of proetid morphology, like the shape of the cephalon and cranidium, that not only showed historically low disparity in the Permian (Figure 1), but did not change significantly from the Ordovician to the Permian (Supplementary Table 1). These results imply some degree of morphological stasis within proetids relative to other trilobite groups. However, Proetidae also expressed few of the “unusual” traits observed in common members of some of the oldest trilobite groups, such as the Redlichiida and Ptychopariida. If we consider these basal groups to represent ancestral trilobites (Paterson et al., 2019), then a case could be made that despite low disparity and a paucity of novel characters, Permian trilobites did not retain some plesiomorphic characters. One caveat to our results concerning the retention of primitive character combinations is that our null expectation is that primitive combinations should be the most diverse throughout clade history (Raup and Gould, 1974). Moreover, in some cases, “average” morphologies have longer lifespans in the fossil record (Liow, 2006, 2007). However, the more general pattern is for “old” character state combinations to be replaced by “younger” ones, and although Cambrian trilobites are the sole known exception to this, post-Cambrian trilobites are not (Wagner and Estabrook, 2014).
The inferences we make about modes and rates of morphological evolution depend critically on the aspect of morphology that is under investigation (e.g., Hopkins and Lidgard, 2012; Hunt et al., 2015). How this impacts our view of living fossils is exemplified by studies showing that the internal anatomy of Cambrian lingulid brachiopods is in fact notably different from descendants alive today, despite remarkable stasis in the shape of the shell (Zhang et al., 2005). Trilobites are also a potentially interesting system in this context. For example, trilobites exhibit morphological diversity in the exoskeleton, but trilobite appendages appear to have changed relatively little in comparison (Supplementary Information). Why did some trilobite morphological traits exhibit apparently slower rates of evolution than other traits? Why were the last rare trilobites relatively “boring” morphologically, and how did they retain such broad environmental distributions despite this? Why and how were some ancestral traits retained over hundreds of millions of years while others disappeared? Why was trilobite diversification so constrained following the late Devonian mass extinctions and possibly even earlier? These evolutionary questions are independent of whether trilobites were “living fossils” in the Permian, and yet emerged from our inquiry into the question.
Data availability statement
The datasets presented in this study can be found in online repositories. The names of the repository/repositories and accession number(s) can be found in this article/ Supplementary material.
Author contributions
MH and PW performed the data analysis. MH wrote the first draft of the manuscript. All authors contributed to the conception and design of the study, data collection, manuscript revision, and approved the submitted version.
Acknowledgments
We thank SL and Alan Love for inviting us to contribute to this special issue on living fossils and to BL and KV for their helpful comments. We thank past and current contributors to the Paleobiology Database, particularly W. Kiessling, A. Miller, B. Kröger, M. Foote, and M. Patzkowsky. We also thank all those whose primary work forms the basis for the data entered into the Paleobiology Database. For this study, the relevant occurrence data reflect over 1,700 publications, the most prominent of which include: Hintze (1953), Palmer (1954, 1965), Harrington and Leanza (1957), Ergaliev (1980), Chlupáč (1983), Stitt and Perfetta (2000), Chatterton and Ludvigsen (2004), Peng et al. (2004), and Loch (2007), with extensive updating of the generic assignments of species based on more recent published opinions. This is Paleobiology Database publication 452.
Conflict of interest
The authors declare that the research was conducted in the absence of any commercial or financial relationships that could be construed as a potential conflict of interest.
Publisher’s note
All claims expressed in this article are solely those of the authors and do not necessarily represent those of their affiliated organizations, or those of the publisher, the editors and the reviewers. Any product that may be evaluated in this article, or claim that may be made by its manufacturer, is not guaranteed or endorsed by the publisher.
Supplementary material
The Supplementary Material for this article can be found online at: https://www.frontiersin.org/articles/10.3389/fevo.2023.1166126/full#supplementary-material
References
Adrain, J. M. (2011). Animal biodiversity: An outline of higher-level classification and survey of taxonomic richness. Zootaxa 3148, 1–237. doi: 10.11646/zootaxa.3148.1.1
Alroy, J. (2010). The shifting balance of diversity among major marine animal groups. Science 329, 1191–1194. doi: 10.1126/science.1189910
Bault, V., Balseiro, D., Monnet, C., and Crônier, C. (2022). Post-Ordovician trilobite diversity and evolutionary faunas. Earth Sci Rev. 230:104035. doi: 10.1016/j.earscirev.2022.104035
Bicknell, R. D., Kimmig, J., Budd, G. E., Legg, D. A., Bader, K. S., Haug, C., et al. (2022). Habitat and developmental constraints drove 330 million years of horseshoe crab evolution. Biol. J. Linn. Soc. 136, 155–172. doi: 10.1093/biolinnean/blab173
Bicknell, R. D. C., and Pates, S. (2020). Pictorial atlas of fossil and extant horseshoe crabs, with focus on Xiphosurida. Front. Earth Sci. 8:98. doi: 10.3389/feart.2020.00098
Bourseau, J.-P., Améziane-Cominardi, N., Avocat, R., and Roux, M. (1991). Echinodermata: Les crinoïdes pédonculés de Nouvelle-Calédonie. Résultats des Campagnes Musorstom 8, 229–333.
Brett, C. E. (1974). “Biostratigraphy and paleoecology of the Windom Shale Member (Moscow Formation) in Erie County, New York,” in New York State Geological Association 46th annual meeting guidebook, ed. D. N. Peterson (New York, NY: State University College at Fredonia), G1–G11.
Chatterton, B. D. E., and Ludvigsen, R. (2004). Early Silurian trilobites of Anticosti Island, Québec, Canada. Palaeontogr. Can. 22, 1–264.
Chlupáč, I. (1983). Trilobite assemblages in the Devonian of the Barrandian area and their relations to palaeoenvironments. Geol. Palaentol. 17, 45–73.
Daley, A. C., Antcliffe, J. B., Drage, H. B., and Pates, S. (2018). Early fossil record of Euarthropoda and the Cambrian Explosion. Proc. Natl. Acad. Sci. U.S.A. 115, 5323–5331. doi: 10.1073/pnas.1719962115
Edgecombe, G. D. (2020). Arthropod origins: Integrating paleontological and molecular evidence. Annu. Rev. Ecol. Evol. Syst. 51, 1–25. doi: 10.1146/annurev-ecolsys-011720-124437
Ergaliev, G. K. (1980). Cambrian malyy Karatau range, Kazakhstan. Alma-Ata: Akademiya Nauk Kazakhskoy SSR.
Fan, J.-X., Shen, S.-Z., Erwin, D. H., Sadler, P. M., MacLeod, N., Cheng, Q.-M., et al. (2020). A high-resolution summary of Cambrian to early Triassic marine invertebrate biodiversity. Science 367, 272–277. doi: 10.1126/science.aax4953
Fontaine, H. (2002). Permian of Southeast Asia: An overview. J. Asian Earth Sci. 20, 567–588. doi: 10.1016/S1367-9120(01)00076-1
Foote, M. (1993). Discordance and concordance between morphological and taxonomic diversity. Paleobiology 19, 185–204. doi: 10.1017/S0094837300015864
Fortey, R. A., and Owens, R. M. (1997). “Evolutionary history,” in Treatise on invertebrate paleontology, part o, Arthropoda 1, Trilobita, Revised, ed. R. L. Kaesler (Boulder: Geological Society of America), 249–287. doi: 10.17161/dt.v0i0.5613
Gong, L., Gao, X., Stow, D., Qu, F., Zhang, G., and Liu, P. (2022). What continued after the mass extinction: Insights from carbonate microfacies and biological evolution around the Permian–Triassic boundary in the middle Upper Yangtze Platform, SW China. Geol. Mag. 160, 35–59. doi: 10.1017/S0016756822000632
Grant, R. E. (1966). Late Permian trilobites from the Salt Range, West Pakistan. Palaeontology 9, 64–73.
Guillerme, T., Cooper, N., Brusatte, S. L., Davis, K. E., Jackson, A. L., Gerber, S., et al. (2020). Disparities in the analysis of morphological disparity. Biol. Lett. 16:20200199. doi: 10.1098/rsbl.2020.0199
Hanger, R. A. (1998). Miscellaneous Permian taxa of western North America: Paleobiogeographic, paleogeographic, biostratigraphic and paleoecologic implications. Permophiles 31, 12–14.
Harrington, H. J., and Leanza, A. F. (1957). Ordovician trilobites of Argentina. Department of Geology, University of Kansas special publication. Lawrence: University of Kansas Press.
Hintze, L. F. (1953). Lower Ordovician trilobites from western Utah and eastern Nevada. Utah Geol. Mineral. Surv. Bull. 48, 1–249.
Hopkins, M. J. (2017). Development, trait evolution, and the evolution of development in trilobites. Integr. Comp. Biol. 57, 488–498. doi: 10.1093/icb/icx033
Hopkins, M. J., and Gerber, S. (2017). “Morphological disparity,” in Evolutionary developmental biology: A reference guide, eds L. Nuno de la Rosa and G. Müller (Cham: Springer International Publishing), 1–12. doi: 10.1007/978-3-319-33038-9_132-1
Hopkins, M. J., and Lidgard, S. (2012). Evolutionary mode routinely varies among morphological traits within fossil species lineages. Proc. Natl. Acad. Sci. U.S.A. 109, 20520–20525. doi: 10.1073/pnas.1209901109
Hopkins, M. J., and To, R. (2022). Long-term clade-wide shifts in trilobite segment number and allocation during the Palaeozoic. Proc. R. Soc. B Biol. Sci. 289:20221765. doi: 10.1098/rspb.2022.1765
Hunt, G., Hopkins, M. J., and Lidgard, S. (2015). Simple versus complex models of trait evolution, and stasis as a response to environmental change. Proc. Natl. Acad. Sci. U.S.A. 112, 4885–4890. doi: 10.1073/pnas.1403662111
Hyden, G., Begg, J., Campbell, H., and Campbell, J. (1982). Permian fossils from the Countess Formation, Mossburn, Southland. N. Z. J. Geol. Geophys. 25, 101–108. doi: 10.1080/00288306.1982.10422508
Lamsdell, J. C., and Selden, P. A. (2015). Phylogenetic support for the monophyly of proetide trilobites. Lethaia 48, 375–386. doi: 10.1111/let.12113
Lerosey-Aubril, R., and Feist, R. (2012). “Quantitative approach to diversity and decline in late Palaeozoic trilobites,” in Earth and Life, ed. J. A. Talent (Berlin: Springer), 535–555. doi: 10.1007/978-90-481-3428-1_16
Lidgard, S., and Love, A. C. (2018). Rethinking living fossils. BioScience 68, 760–770. doi: 10.1093/biosci/biy084
Lidgard, S., and Love, A. C. (2021). The living fossil concept: Reply to Turner. Biol. Philos. 36:13. doi: 10.1007/s10539-021-09789-z
Lieberman, B. S. (1994). Evolution of the trilobite subfamily Proetinae Salter, 1864, and the origin, diversification, evolutionary affinity, and extinction of the Middle Devonian proetid fauna of eastern North America. Bull. Am. Mus. Nat. Hist. 223:176.
Lieberman, B. S., and Karim, T. S. (2010). Tracing the trilobite tree from the root to the tips: A model marriage of fossils and phylogeny. Arthropod Struct. Dev. 39, 111–123. doi: 10.1016/j.asd.2009.10.004
Liow, L. H. (2006). Do deviants live longer? Morphology and longevity in trachyleberidid ostracodes. Paleobiology 32, 55–69. doi: 10.1666/05004.1
Liow, L. H. (2007). Lineages with long durations are old and morphologically average: An analysis using multiple datasets. Evolution 61, 885–901. doi: 10.1111/j.1558-5646.2007.00077.x
Loch, J. D. (2007). Trilobite biostratigraphy and correlation of the Kindblade Formation (Lower Ordovician) of Carter and Kiowa counties, Oklahoma. Oklahoma Geol. Surv. Bull. 149, 1–154.
Meyer, D. L., and Macurda, D. B. (1977). Adaptive radiation of the comatulid crinoids. Paleobiology 3, 74–82. doi: 10.1017/S0094837300005121
Owens, R. M., and Hahn, G. (1993). Biogeography of Carboniferous and Permian trilobites. Geol. Palaeontol. 27, 165–180.
Palmer, A. R. (1954). The faunas of the Riley Formation in central Texas. J. Paleontol. 28, 709–786.
Palmer, A. R. (1965). Trilobites of the late Cambrian pterocephaliid biomere in the Great Basin, United States. U. S. Geol. Surv. Prof. Paper 493, 1–105. doi: 10.3133/pp493
Paterson, J. R. (2020). The trouble with trilobites: Classification, phylogeny and the cryptogenesis problem. Geol. Mag. 157, 35–46. doi: 10.1017/S0016756819000426
Paterson, J. R., Edgecombe, G. D., and Lee, M. S. Y. (2019). Trilobite evolutionary rates constrain the duration of the Cambrian explosion. Proc. Natl. Acad. Sci. U.S.A. 116, 4394–4399. doi: 10.1073/pnas.1819366116
Peng, S. C., Babcock, L. E., and Lin, H. L. (2004). Polymerid trilobites from the Cambrian of northwestern Hunan, China; Volume 1 Corynexochida, Lichida, and Asaphida. Beijing: Science Press.
Peters, S. E. (2004). Evenness of Cambrian-Ordovician benthic marine communities in North America. Paleobiology 30, 325–346. doi: 10.1666/0094-8373(2004)030<0325:EOCBMC>2.0.CO;2
Raup, D. M., and Gould, S. J. (1974). Stochastic simulation and evolution of morphology–towards a nomothetic paleontology. Syst. Zool. 23, 305–322. doi: 10.2307/2412538
Rouse, G. W., Jermiin, L. S., Wilson, N. G., Eeckhaut, I., Lanterbecq, D., Oji, T., et al. (2013). Fixed, free, and fixed: The fickle phylogeny of extant Crinoidea (Echinodermata) and their Permian–Triassic origin. Mol. Phylogenet. Evol. 66, 161–181. doi: 10.1016/j.ympev.2012.09.018
Sepkoski, J. J. (2002). A compendium of fossil marine animal genera. Bull. Am. Paleontol. 363, 1–560.
Shen, S., and He, X. (1994). Changhsingian brachiopod fauna from Guiding Guizhou. Acta Palaeontol. Sin. 33, 440–454.
Speyer, S. E., and Brett, C. E. (1985). Clustered trilobite assemblages in the Middle Devonian Hamilton group. Lethaia 18, 85–103. doi: 10.1111/j.1502-3931.1985.tb00688.x
Speyer, S. E., and Brett, C. E. (1986). Trilobite taphonomy and Middle Devonian taphofacies. Palaios 1, 312–327. doi: 10.2307/3514694
Stitt, J. H., and Perfetta, P. J. (2000). Trilobites, biostratigraphy, and lithostratigraphy of the Crepicephalus and Aphelaspis zones, lower Deadwood Formation (Marjuman and Steptoean stages, Upper Cambrian), Black Hills, South Dakota. J. Paleontol. 74, 199–223. doi: 10.1666/0022-3360(2000)074<0199:TBALOT>2.0.CO;2
Suárez, M. G., and Esteve, J. (2021). Morphological diversity and disparity in trilobite cephala and the evolution of trilobite enrolment throughout the Palaeozoic. Lethaia 54, 752–761. doi: 10.1111/let.12437
Turner, D. D. (2019). In defense of living fossils. Biol. Philos. 34:23. doi: 10.1007/s10539-019-9678-y
Vanderlaan, T. A., and Ebach, M. C. (2015). A review of the Carboniferous and Permian trilobites of Australia. Zootaxa 3926, 1–56. doi: 10.11646/zootaxa.3926.1.1
Wagner, P. J., and Erwin, D. H. (2006). Patterns of convergence in general shell form among Paleozoic gastropods. Paleobiology 32, 316–337. doi: 10.1666/04092.1
Wagner, P. J., and Estabrook, G. F. (2014). Trait-based diversification shifts reflect differential extinction among fossil taxa. Proc. Natl. Acad. Sci. U.S. A. 111, 16419–16424. doi: 10.1073/pnas.1406304111
Westrop, S. R., and Adrain, J. M. (1998). Trilobite alpha diversity and the reorganization of Ordovician benthic marine communities. Paleobiology 24, 1–16. doi: 10.1017/S009483730001993X
Keywords: morphological stasis, rates of evolutionary change, morphometrics, arthropod, morphological diversity, Proetidae, relict taxa, diversity curve
Citation: Hopkins MJ, Wagner PJ and Jordan KJ (2023) Permian trilobites and the applicability of the “living fossil” concept to extinct clades. Front. Ecol. Evol. 11:1166126. doi: 10.3389/fevo.2023.1166126
Received: 14 February 2023; Accepted: 27 March 2023;
Published: 17 April 2023.
Edited by:
Scott Lidgard, Field Museum, United StatesReviewed by:
Kjetil Voje, University of Oslo, NorwayBruce S. Lieberman, University of Kansas, United States
Copyright © 2023 Hopkins, Wagner and Jordan. This is an open-access article distributed under the terms of the Creative Commons Attribution License (CC BY). The use, distribution or reproduction in other forums is permitted, provided the original author(s) and the copyright owner(s) are credited and that the original publication in this journal is cited, in accordance with accepted academic practice. No use, distribution or reproduction is permitted which does not comply with these terms.
*Correspondence: Melanie J. Hopkins, bWhvcGtpbnNAYW1uaC5vcmc=