- 1Department of Forest Sciences, University of Helsinki, Helsinki, Finland
- 2Latvasilmu osk, Hankasalmi, Finland
Introduction: Urban areas are challenging environments for wetland species with complex habitat requirements and life cycles. However, even semiaquatic species, representing such complex wetland groups, can be provided with adequate conditions through thorough understanding of their habitat requirements coupled with comprehensive wetland management.
Methods: We studied the occurrence and abundance of the smooth newt (Lissotriton vulgaris), a widely distributed amphibian, in an urban landscape in metropolitan Helsinki, Finland. We classified 50 randomly selected urban wetlands based on their connectivity by applying isolation scores counted using principal component analysis (PCA) and measured the occurrence and abundance of smooth newts in these locations.
Results: Our analyses showed well-connected wetland sites to differ significantly in smooth newt occurrence from both isolated and partially connected sites. Additionally, smooth newt abundance in well-connected wetlands differed from isolated sites. A PCA model with residential buildings (negative effect) and aquatic vegetation (positive effect) best explained smooth newt occurrence and total and male abundance, and female newt occurrence was best explained by a model also incorporating forest and natural open areas. Predation pressure by fish negatively influenced both smooth newt occurrence and abundance.
Discussion: Tighter networks of constructed wetlands and stricter management guidelines for urban wetland planning and fish community management could increase the suitable habitat for smooth newts in urban landscapes. Managing such areas for the common smooth newt offers potential broad benefits to the conservation of other semiaquatic amphibians and reptiles in urban wetlands and to urban wetland biodiversity in general. Our results show that smooth newt presence may indicate sustained wetland connectivity in an urban landscape and could even be used to signal successful urban planning.
1 Introduction
Freshwater wetlands are biodiverse and economically paramount ecosystems worldwide (Emerton and Bos, 2004; Dudgeon et al., 2006; Russi et al., 2013) but have concurrently become some of the most endangered and deteriorated habitats (Darwall et al., 2009; Davidson, 2014). Kingsford et al. (2016) estimated over 70% of the world’s wetlands to have been destroyed or impaired, and two-thirds of European wetlands have experienced serious degradation or destruction (Amezaga et al., 2002). Urbanization is a significant driver of wetland degradation, inducing losses to connectivity and changes in water chemistry and temperature (e.g., Brabec et al., 2002). Anthropogenic disturbance is recognized as a major cause of habitat fragmentation, especially in freshwater ecosystems (Brauer and Beheregaray, 2020), leading to great reductions in wetland biodiversity and increasing species endangerment (Clark et al., 2014; Kingsford et al., 2016). The importance of connectivity is emphasized in urban environments, where dispersal barriers and anthropogenic habitat alteration are more profound.
Semiaquatic amphibians and reptiles require both terrestrial and aquatic habitats (Semlitsch and Bodie, 2003; Cosentino and Schooley, 2018). Amphibians are globally affected by habitat loss and fragmentation, often resulting from urbanization (Stuart et al., 2004; Cushman, 2006; Hamer and McDonnell, 2008). The need for aquatic habitat, along with low mobility and a seasonal migration between breeding ponds and hibernation sites cause amphibians to be particularly vulnerable to habitat fragmentation (deMaynadier and Hunter, 2000; Bowne and Bowers, 2004; Cushman, 2006), which leads to a loss in habitat functional connectivity (Joly et al., 2001; Baguette and Mennechez, 2004). Anthropogenic factors, such as roads and traffic, act as barriers to dispersal (Marsh et al., 2005) and gene flow (Garcia-Gonzalez et al., 2012; Pittman et al., 2014) and may impact amphibians through direct mortality (Beebee, 2013; Hamer et al., 2021; reviewed by Fahrig and Rytwinski, 2009). Buildings and altered light regimes caused by artificial lighting may also affect the breeding behavior of amphibians (Dias et al., 2019) and impede or even prevent their migration and distribution (Perry et al., 2008). Despite numerous threats, healthy amphibian and reptile populations can survive in urban environments (e.g., Perry et al., 2008; Brand and Snodgrass, 2010; Garcia-Gonzalez and Garcia-Vazquez, 2012; Guzy et al., 2013; Scheffers and Paszkowski, 2013; Konowalik et al., 2020).
The smooth newt (Lissotriton vulgaris) is a rather common and widely distributed European semiaquatic salamander, yet its populations are declining (IUCN Red List of Threatened Species 2018). Habitat fragmentation and isolation possibly play exceedingly large roles in altering its population dynamics (Denoël and Ficetola, 2007). Smooth newts inhabit both artificial and natural wetlands (Mulkeen et al., 2017; Mulkeen, 2018; Buono et al., 2019; Rannap et al., 2020), which allows breeding even in urban environments. They utilize a wide variety of waterbodies (Mulkeen et al., 2017) for reproduction, although they prefer still and shallow ponds (e.g., Kinne, 2004) and exhibit less strict aquatic habitat requirements when compared to other pond-reproducing newts (e.g., Skei et al., 2006; Gledhill et al., 2008; Vuorio, 2016). As smooth newts show extensive terrestrial stages in their life cycle and juveniles remain on land for several years until reaching reproductive readiness (e.g., Bell, 1977; Heiss et al., 2015), they require suitable terrestrial areas for hibernation, migration, and dispersal (Mulkeen et al., 2017).
Smooth newts and other European Lissotriton/Triturus newts show site fidelity by returning to the same breeding ponds (Joly and Miaud, 1993) and terrestrial refugia (Jehle, 2000; Müllner, 2001; Malmgren, 2002; Denoël et al., 2018) and by utilizing the same migration routes (Vuorio et al., 2015). However, habitat degradation and edge effect create challenges for their dispersal and migration to spawning areas even at these short distances (Semlitsch, 2000; Houlahan and Findlay, 2003). Smooth newts have a rather low annual survival rate, with ~50% yearly mortality according to Bell (1977). In addition to anthropogenic-related mortality, fish and invasive alien crayfish are known to affect smooth newt populations through predation (Stenson and Aronsson, 1995; Falaschi et al., 2022).
Because of its characteristics, the smooth newt is a potential species to aid in recognizing amphibian- and reptile-friendly urban environments. The species’ requirement for connected landscapes may make it particularly vulnerable to urban land use changes and therefore an indicator of well-connected urban wetlands (for non-urban areas see Malmgren, 2002). To understand the key mechanisms driving smooth newt occurrence in urban areas, we examined the presence and abundance of smooth newts in 50 wetlands in metropolitan Helsinki, Finland. Our aim was to investigate whether buildings, other constructed elements, environmental variables, and fish presence affect urban smooth newts. We first hypothesize that smooth newt occurrence and abundance will be greatest in well-connected sites and second that smooth newt occurrence and abundance will be greater in urban waterbodies with forest or meadow environments nearby. Third, we assume that smooth newt presence and abundance are greater in fishless waterbodies compared with aquatic habitats with predatory fish. Fourth, we hypothesize that road networks will negatively affect smooth newts in urban settings. To our best knowledge, this study is the first to investigate how urban infrastructure affects smooth newt occurrence and abundance.
2 Materials and methods
2.1 Study sites
Our study was conducted in metropolitan Helsinki (60°13’N, 24°51’E), located in southern Finland. The study area comprised three cities: Espoo, Helsinki, and Vantaa (Fig.1), with a total land area of 669 km2 and approximately 1.16 million residents. Metropolitan Helsinki is the most populous urban area in Finland. The area belongs to the southern boreal vegetation zone (Ahti et al., 1968), with a forest cover of >30%. Forests are predominantly coniferous, with interspersing deciduous patches. Metropolitan Helsinki is located by the Baltic Sea, with more than 100 km of coastline. Soils are low in nutrients, with glacial and sandy tills being the dominant soil types. Annual average precipitation reaches approximately 700 mm, and the thermal growing season is 175–185 days (Finnish Meteorological Institute, n.d.), although the growing season of 2018 was exceptionally long (214 days; Finnish Meteorological Institute, 2018). Four native amphibian species occur in the area: smooth newt, common frog (Rana temporaria), moor frog (Rana arvalis), and common toad (Bufo bufo).
First, we located all the wetlands in our study area using maps and satellite images. We found 152 urban wetlands and randomly chose 50 of these for our study (Figure 1; detailed information in Vehkaoja et al., 2020). Randomization was performed in the R program with the ‘randomizeR’ package. Our wetlands included both temporary (N = 10) and permanent (N = 40) waterbodies. Ten of the 40 permanent wetlands were classified as stormwater wetlands while the remaining 30 were natural.
2.2 Sampling
The smooth newt breeding season in the boreal zone begins in April at the earliest, and in Finland occurs after ice-melt, i.e., at the turn of April and May (Vuorio et al., 2015). Juveniles begin their post-metamorphic dispersal in search of suitable terrestrial habitats for overwintering around August and September in the boreal zone (Malmgren, 2002), including Finland (Koskela, 1984). Adult smooth newts leave the aquatic habitat after egg-laying (in May) and spend the rest of the year on land (Vuorio et al., 2015).
We collected the smooth newt data between April 30 and May 19, 2018. We standardized the weather conditions of the sampling days as much as possible by avoiding heavy wind (over 5 m/s), rain, and cold (below +5°C) conditions. We tested our capture success against the following weather variables (we calculated the daily means of all weather variables): air temperature, cloud cover, air pressure, rainfall, air humidity, and wind velocity. The weather variables did not significantly differ between the sampling days, nor did they have an effect on the number of captured individuals.
We selected spring (post-snowmelt) as the sampling season because smooth newts reproduce and are active in water at this time and are therefore more likely to be captured. We set 10 activity traps at even spacing in the waterbody of each site. We used 1-L glass jars and transparent plastic funnels with 120-mm openings at the wide end and 20-mm openings at the narrow end (see, e.g., Elmberg et al., 1992; Elmberg et al., 1993). The activity traps were submerged at an approximate depth of 1 m. Each wetland site was sampled for a total of 48 h, with traps emptied every 12 h to avoid premature death of the animals. With this method, the capture effort was the same for each site and the number of captured individuals does not indicate the actual size of the population. We then calculated and sexed all captured individuals in the field. The trapped individuals were afterwards released back into the wetland, which might have resulted in some individuals being recaptured.
2.3 Environmental and land cover variables
We measured riparian canopy cover and submerged and emergent vegetation coverage as environmental variables for all study sites (see more details for canopy cover and emergent vegetation measurements in Vehkaoja et al., 2020). Submerged vegetation coverage was calculated using four randomly chosen 1-m2 vegetation squares from each study site.
We calculated the land cover and road variables (length) from a 500-m radius circle around the study sites. This scale was selected to match the smooth newt’s average maximum dispersal distance from the breeding waterbody, as defined by Kovar et al. (2009). We processed the landscape and road data using ArcMap 10.3.1 (ESRI, 2015).
Land cover data were extracted from the Finnish national version of the CORINE Land Cover 2012 database, where land use in Finland is presented with a pixel size of 20 m × 20 m (Finnish Environment Institute, 2014). To reduce the number of land cover variables in our models, we reclassified CORINE data by combining ecologically comparable habitat subclasses such as various coniferous forest or wetland types. Reclassification was performed using ArcMap version 10.3.1 (ESRI, 2015). Our final land cover variables were the coverage of 1) residential buildings, 2) industrial and service buildings, 3) other buildings, 4) recreation area, 5) farming area, 6) deciduous forests, 7) coniferous forests, 8) mixed forests, 9) other open natural areas, 10) wetlands, and 11) water systems (mainly sea area).
The road data were extracted from Digiroad, the national database of the Finnish road and street network (Finnish Traffic Agency, 2018). We used the following road classes 1) main roads, 2) connecting roads, 3) streets, 4) walkways, and 5) all road and street types together.
2.4 Fish occurrence
We assessed fish occurrence using fish traps (wire mesh traps), activity traps, and visual sightings. One fish trap was submerged into each waterbody at an approximate depth of 1 m. The fish traps remained in the waterbody for the duration of the newt trapping, and each fish trap was emptied every 12 h concurrently with the newt traps. All fish were released back into the waterbody afterwards.
2.5 Principal component analysis
Initially, we analyzed the 18 environmental and land cover variables using principal component analysis (PCA; see, e.g., Pimental, 1979; Gauch, 1982) to investigate the main environmental factors defining the study sites. We chose PCA because it enables statistically categorizing the study sites into appropriate isolation gradients.
The first and second PCA components explained 29.1% and 17.6% of the total variation in the habitat data, so combined these two components explained 46.7% of the variation. The score values of the first component organized the wetlands on an isolation gradient: habitats with forest and recreation areas nearby, rich emergent vegetation, and location next to other wetlands were situated at the positive end of the gradient, while habitats with roads and various building types were at the negative end. All 50 wetlands were categorized according to their isolation scores received from the PCA. Sites with an isolation score between −3 and −0.5 were categorized as isolated. Wetlands that scored between −0.5 and 0.5 were categorized as partially connected, and wetlands with a score >0.5 were categorized as well connected.
2.6 Statistical analyses and model selection
The occurrence and abundance of smooth newts, and the number of females and males were calculated for each site (N = 50). We analyzed smooth newt occurrence by comparing the presence–absence data with the environmental and land cover variables, in addition to fish presence and the wetland type from the PCA. Smooth newt abundance was a count datum with a Poisson distribution (log). The abundance data were overdispersed due to the many zeros (no smooth newts on a site). Thus, we used negative binomial modeling with the glm.nb function, which is suitable for overdispersed data. We used the packages ‘mgcv’ (Wood, 2006) and ‘MASS’ (Venables and Ripley, 2002) from the software package R (R Development Core Team, 2013). Wetland type (received from the PCA: well connected, partially connected, and isolated) and fish presence were used as categorical parameters, and the other explanatory parameters were continuous parameters.
Smooth newt occurrence and abundance were tested against wetland type. These were tested separately with the glm.nb function. Next, we used model selection to determine the effect of fish and the 18 environmental and land cover variables against smooth newt occurrence, abundance, and the number of females and males. Model selections for presence–absence (i.e., occurrence) and for female, male, and total smooth newt abundance data were made by dropping out explanatory variables one at a time until all remaining variables had at least a 95% significance level (Zuur et al., 2009). With these model selections, we wanted to explore which variables are important for smooth newts.
3 Results
We captured a total of 447 smooth newts, which were recorded from 18 of our 50 study wetlands. Smooth newts were detected from both temporary (4/10) and permanent (14/40) waterbodies, including one permanent stormwater wetland. Of all newts caught in the whole study area, 72% were males and 28% were females. However, the sex ratio in the most populous site was approximately 50:50, with 41 males and 40 females. The sex ratio did not differ between wetland types (Partially connected t value: −1.320, SE: 0.128, p value: 0.207; Isolated t value: −0.088, p value: 0.931) or with fish presence (t value: −0.035, SE: 0.173, pvalue: 0.843).
Over 70% of the well-connected wetlands had smooth newts, whereas the species only occupied 22% and 29% of the partially connected and isolated wetlands, respectively (Table 1). The wetland isolation level significantly influenced smooth newt occurrence (Figure 2). Well-connected sites also had a significantly higher abundance of smooth newts when compared with isolated wetlands and a non-significantly higher abundance when compared with partially connected wetlands (Table 2).
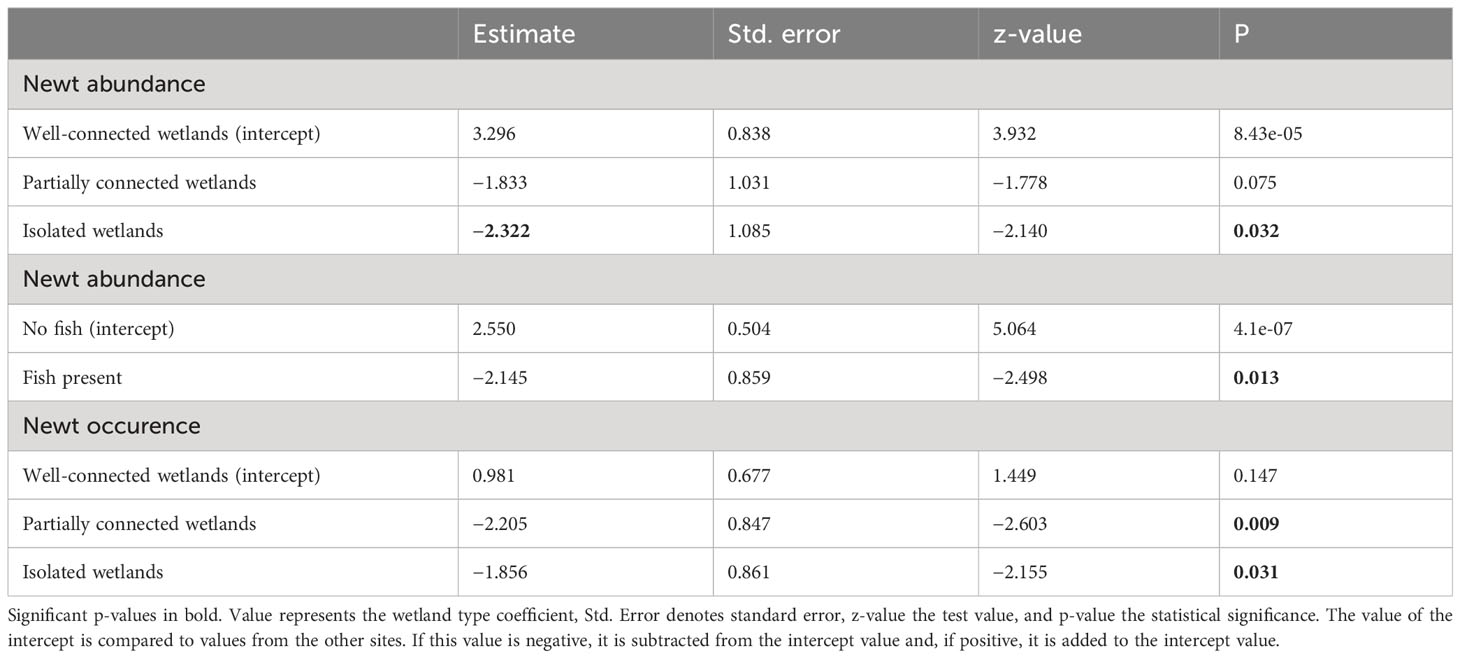
Table 2 Differences between well-connected, partially connected, and isolated wetlands in terms of smooth newt abundance and occurrence, in addition to the effect of fish presence on newts.
The model with fish, open natural areas, residential buildings, and aquatic vegetation was the best model at explaining smooth newt total abundance (males and females together) and male smooth newt abundance, when considering every environmental and infrastructural variable (Table 3). Residential buildings and fish had a significant negative effect on total abundance, i.e., the higher the share of residential buildings located near a smooth newt breeding site or the larger the number of fish, the fewer smooth newts were observed. On the other hand, aquatic vegetation cover and open natural areas had a significant positive effect on total abundance. Female smooth newt abundance, however, was best explained by the model that incorporated forests and natural open areas in addition to fish presence, residential buildings, and aquatic vegetation. Each of these five variables had a significant effect on female smooth newt abundance, with residential buildings and fish showing a significant negative effect and the remaining variables showing a significant positive effect (Table 3). The model with fish, residential buildings, and aquatic vegetation was the best model at explaining smooth newt occurrence. Fish and residential buildings had a negative effect on occurrence, whereas the effect of aquatic vegetation cover was positive.
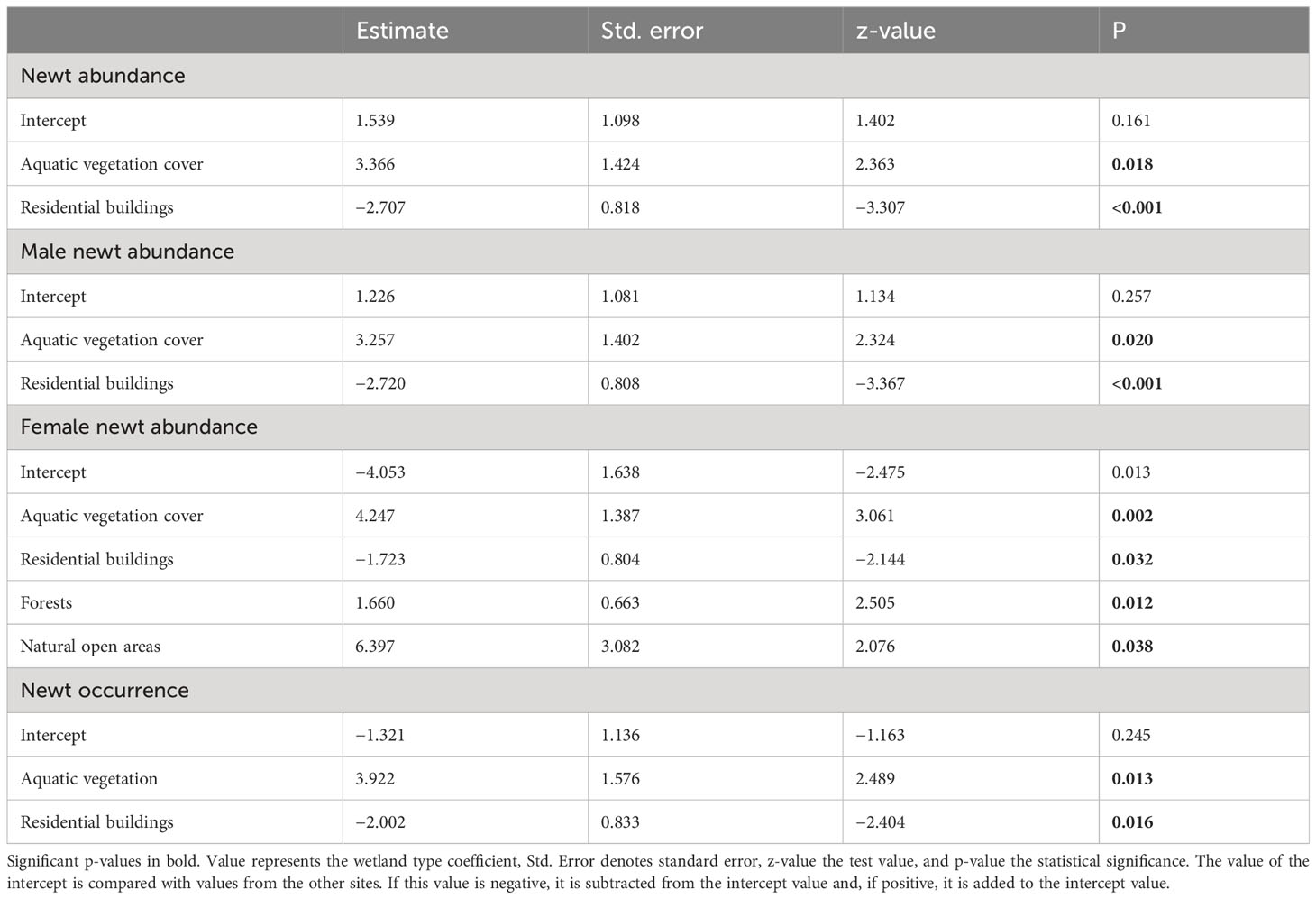
Table 3 Environmental factors affecting smooth newt abundance. The best models explaining newt abundance in urban wetlands.
We recoded several fish species in our study wetlands, e.g., perch (Perca fluviatilis), roach (Rutilus rutilus), pike (Esox lucious), and Crucian carp (Carassius carassius). All these species are predators of newts or newt eggs. We found more smooth newts from fishless wetlands, as 89% of the newt-inhabited wetlands (16/18) were fishless, and fish presence (18/50 wetlands) significantly influenced both smooth newt abundance and occurrence in our study (Table 3).
4 Discussion
Our results show that smooth newts can utilize all three of our wetland types (temporary and permanent, including permanent stormwater wetlands). As predicted, wetland isolation levels strongly affected smooth newt occurrences in our study. We observed a significant difference in smooth newt occurrence between well-connected (smooth newts recorded on 70% of sites) and partially connected and isolated sites (<33%). This is not surprising, as many studies have shown habitat fragmentation to strongly influence amphibian populations due to impaired movement caused by poor vagility ability, high traffic mortality (Cushman, 2006), patch isolation (Vuorio et al., 2015), and broken migration routes (Joly, 2019). Additionally, Gledhill et al. (2008) observed strong positive correlation between aquatic plant and invertebrate species richness and pond density. Well-connected sites therefore potentially increase the smooth newt’s chances of finding food resources, breeding sites with abundant aquatic vegetation for egg-laying and for juveniles, and suitable overwintering refugia. Despite less stringent habitat requirements, habitat diversity has been shown to drive the movement patterns of the species (Pittman et al., 2014; Vuorio et al., 2015).
In our study, well-connected sites also harbored larger newt abundances, which shows the importance of well-connected habitats in upholding smooth newt populations. Our results on urban smooth newts are widely applicable in urban wetland biodiversity conservation, as they are in line with other studies that have explored the role of connectivity for semiaquatic amphibians and reptiles. Terrestrial connectivity and proximity to and size of green spaces benefit amphibians (Semlitsch and Bodie, 2003; Simon et al., 2009; Guderyahn et al., 2016) and turtles (Hamer and Parris, 2011; Guzy et al., 2013; Hamer et al., 2018; Santoro et al., 2020). Concurrently, aquatic connectivity increases amphibian and reptile occurrences and abundances in urban settings (e.g., Roe et al., 2003; Attum et al., 2006; Hamer et al., 2012; Fyson and Blouin-Demers, 2021). Hamer et al. (2018) showed that without adequate proximal terrestrial habitats, urban wetland conservation and management do not increase the populations of freshwater turtles in Australia, while Trovillion et al. (2023) found that adding ponds to connected greenspaces can help mitigate the adverse effects of urbanization on semiaquatic species richness in the United States. Associations across taxa have also been found, for example positive bird–amphibian co-occurrence patterns in Sweden, indicating that conservation efforts of one taxon may facilitate another (Kačergytė et al., 2023). The importance of connectivity is emphasized in built-up areas, where dispersal barriers, such as roads, other infrastructure, and habitat alteration are more profound (Bierwagen, 2007). The role of connectivity may become pronounced at the species’ range margin, such as in Finland, as suggested by Rannap et al. (2012). Both range margins and urban environments emphasize the challenges faced by smooth newts and possibly other amphibian species as well. Our study combines both aspects, and our conservation suggestions can be used as guidelines in urban management planning and potentially even in rural range margin areas.
The model with residential buildings and aquatic vegetation was best at explaining smooth newt presence (46.7% of variation explained) and male and total abundances. Residential buildings negatively associated with smooth newt populations in our urban environment to a significant degree. Vehkaoja et al. (2020) previously found that residential buildings and roads also had a negative effect on aquatic invertebrate family richness and abundances in the same study area, and these lower invertebrate levels may be reflected in the results of this study. Other building types (e.g., industrial buildings) did not show a significant effect on smooth newts in our study sites. Both residential and industrial buildings require heavy infrastructure (roads, asphalt etc.) and maintenance. This leaves little favorable terrestrial habitat for newt dispersal or overwintering and hinders dispersal by decreasing connectivity. However, the lots on which industrial buildings are located may incorporate less built-up environment compared with residential areas, and may be managed less, to where such lots may contain more fallow, higher terrestrial vegetation, and other refugia (stones, dead wood) required by newts (Schabetberger et al., 2004; Denoël et al., 2013; Vuorio et al., 2015; Denoël et al., 2018) and may even be less affected by pesticides used to manage residential building lots.
Smooth newts are crepuscular (Griffiths, 1985; Paterson, 2018), suggesting individuals prefer intermediate to low light conditions (Hill et al., 2018). Evidence also indicates their activity levels may be influenced by the lunar cycle and cloud cover (Deeming, 2008; Warren and Büttner, 2008; Phillips, 2020; but see also Paterson, 2018). Residential buildings or areas may more strongly influence local light conditions than industrial buildings, which are well lit during the day compared with residential buildings, where lighting is brightest in the mornings and evenings. Light pollution, resulting from this high-intensity lighting of residential buildings and street lighting in the mornings and evenings, may interfere with how smooth newts perceive natural illumination regimes (including the lunar cycle), thus hindering smooth newt activity in adjacent waterbodies and terrestrial patches (Feuka, 2016).
Likewise to the Rannap et al. (2012) review, our study shows a significant positive association between aquatic vegetation and smooth newts. Newt eggs are laid on the leaves of aquatic vegetation. The larval stage prefers dense vegetation for foraging, and both adult and larval smooth newts utilize aquatic vegetation as refuges against predatory fish. Invertebrate assemblages are most diverse in ponds with richest aquatic plant diversity (Gledhill et al., 2008), potentially leading to more food sources for newts. This effect was heightened at broader landscape scales, i.e., assemblage diversities were larger when looking at pond networks compared with individual ponds.
The best model to explain female newt abundance also included forests and natural open areas, both of which associated positively with female numbers. Why these two variables were important only for females remains an open question. Previous studies (e.g., Malmgren, 2002; Vuorio et al., 2015) show canopy cover and various forested and open natural areas to play roles, but there is no distinguishing difference between newt sexes. Male lekking behavior may lead to female-biased dispersal in newts (Matos et al., 2017; Cayuela et al., 2020). In our study, this phenomenon may be exacerbated by both predator avoidance and avoidance of density-dependent male harassment. These factors may push female smooth newts towards more frequent and longer-distanced dispersal. In turn, this may lead to the exaggerated importance of terrestrial habitat factors (forest and natural open areas) for females as opposed to male smooth newts. The females’ larger size may also either be an attribute of female-biased dispersal, where larger size aids in migratory survival dispersal (Matos et al., 2017; Cayuela et al., 2020) or it may cause females to need more sheltered terrestrial habitat to guard against predators.
Somewhat surprisingly, we found no significant effect of road networks on smooth newt occurrence or abundance, despite this factor having previously been identified as playing a negative role (e.g., Fahrig et al., 1995; Glista et al., 2007). It is possible that the scale chosen for our study fails to show this effect; 75% of dispersing newts have been found to stay within ~300 meters of a pond (Kovar et al., 2009). On the other hand, our study is also located in an area with Finland’s densest road network crisscrossing the entire study area, which may cause subtle impacts to go unnoticed.
Fish presence negatively influenced smooth newt occurrence in our study. Our observation was in line with previous studies, which have found that smooth newts can coexist with fish (Dolmen, 1988; Skei et al., 2006; Rannap et al., 2012; Kačergytė et al., 2021), but their populations may be limited in waterbodies with fish (Denoël et al., 2013).
Negative responses by numerous organism groups, including invertebrates, amphibians, and birds, have previously been reported for fish-related predator–prey interactions, competition, and trophic cascading (e.g., Carpenter et al., 1985; Stenson and Aronsson, 1995; Reshetnikov, 2003; Eaton et al., 2005; Väänänen et al., 2012; Milardi et al., 2016). In amphibians, predator avoidance may determine breeding site selection more than competition avoidance (Indermaur et al., 2010). Nomadic behavior is typical for smooth newts, not only when migrating between overwintering and reproductive areas, but also when choosing sites according to other habitat characteristics such as predator prevalence (Malmgren, 2002; Denoël et al., 2013; Denoël et al., 2018). High waterbody connectivity leads to increased movement opportunities between individual ponds during the breeding season. Newts may aggregate into few suitable predator-free waterbodies, thereby exhibiting predator avoidance over competition avoidance.
For example, Winandy et al., 2017 showed alpine newts (Ichthyosaura alpestris) to exhibit clear predator avoidance by leaving/aggregating in ponds with fish presence/absence. While predator avoidance may be an effective short-term strategy, such circumstances may lead to density-dependence problems, e.g., harassment in dense populations, food resource shortages, or reproductive disruptions (Winandy et al., 2017). An example of this was shown in Winandy et al. (2017), where predator avoidance indirectly led to increased female aggregation in terrestrial habitats, with female newts using the terrestrial habitats seven times more frequently than males during the breeding season. Urbanization may further exacerbate the fish effect because urban green and blue spaces incorporate numerous disturbances and hazards affecting newts. However, a Norwegian study (Skei et al., 2006) did not detect any significant vulnerability of smooth newts to fish, and a review by Rannap et al. (2012) observed that the fish effect was not geographically unified, as smooth newts appeared to tolerate fish presence to a larger degree in more northerly regions of Europe.
Most of our sites showed male preponderance, corresponding with previous studies (e.g., Arntzen, 2002; Grayson et al., 2012). Our result may be due to intense mate competition by males. A female within a trap can lure several reproductive males into the trap, resulting in trapping favoring male newts. Newt males often exhibit coercive behavior during the mating season. The arrival time of male and female smooth newts into the breeding ponds may be another explanation for our skewed sex ratios. Previous studies (e.g., Griffiths, 1984; Arntzen, 2002) have investigated whether males enter breeding ponds earlier after ice-melt than females, with varying results. However, in our study area, ice-melt occurred in the second half of April in 2018 (Finnish Environment Institute TARKKA portal). It is therefore likely that both females and males were present in the breeding ponds during our study period (30 April–19 May 2018). Also, only males (no females) were trapped from two of our study wetlands and only females (no males) were caught from one site. A third possibility is that females in our sample may be showing stronger predator avoidance to fish presence, leading females to enter the breeding ponds less frequently.
Given increasing global urbanization along with the continued decrease in smooth newt numbers, understanding how to conserve and construct suitable aquatic and terrestrial habitats for newts is important for their future. Smooth newts can utilize artificial wetlands, which is a positive aspect for their conservation. However, several factors should be ensured. Urban wetland management and conservation should ensure adequate pond densities and adjacent terrestrial habitats, maintain sufficient landscape connectivity between breeding sites, uphold sufficient distances between urban waterbodies and residential buildings, and keep fish removal and aquatic vegetation assemblages in mind. Introducing artificial overwintering hibernacula or newt hotels and migration tunnels and fences (Matos et al., 2017; Helldin and Petrovan, 2019; Matos et al., 2019) should also be considered. However, tunnels and fences should be studied more to ensure newts truly utilize the structures rather than them being obstructive to dispersal (Matos et al., 2017; Helldin and Petrovan, 2019; Jarvis et al., 2019; Matos et al., 2019). They must also lead to suitable breeding and/or overwintering habitats to be worthwhile.
Our study contains certain limitations. We excluded artificial lighting as a study variable and were thus unable to examine its role in newt habitat selection. Future studies should investigate the topic in addition to how artificial wetland types affect smooth newts at various developmental stages. We also did not examine the effect of any physicochemical elements, such as pollutants or calcium levels, which may directly affect smooth newt occurrence/abundance (e.g., Skei et al., 2006) and which concurrently may be greatly altered in urban wetlands. Understanding how such mechanisms affect newts in an urban setting is an important step for their conservation. The 20 × 20-m CORINE data may also be slightly coarse, leading to very fine-scale results being lost in the analyses. However, the data are equal in all habitat types, causing no systematic bias in the results, and data of this scale are widely used in habitat studies.
5 Conclusions
Urban wetlands are potentially suitable habitats for several semiaquatic amphibian and reptile species. Amphibians indicate terrestrial and aquatic conditions. Smooth newts exhibit less stringent habitat requirements than many other newt species, meaning they may be able to tolerate human presence and modification to a greater degree. Nonetheless, our study shows that wetland connectivity, aquatic vegetation, terrestrial habitat, and residential buildings play important roles in determining smooth newt population occurrence and abundance in urban areas. Smooth newts in urban areas may experience a bottleneck caused by their terrestrial habitat preferences. Their less strict aquatic requirements allow them to inhabit and breed in urban or artificial waterbodies, whereas occurrence in adjacent terrestrial habitats may prove detrimental or impossible if these habitats are unsuitable or if habitat isolation prevents the migration and dispersal of various age groups and between aquatic and terrestrial life stages. Fish presence also influenced newt occurrence and abundance. Fish impede numerous organism groups through predator–prey interactions, competition, and trophic cascading, in both natural waterbodies and man-made ponds. None of the fish species we detected are endangered in Finland but rather are common and abundant throughout the country. On the contrary, smooth newts and several other amphibian species have declined widely in Europe. Controlling fish populations is therefore an important step in upholding biodiversity in systems with naturally fishless waterbodies, such as our study area. As smooth newts in urban areas favor high connectivity, the species can be used by urban planning and management to identify well-connected wetland areas. Also, managing such areas for smooth newts offers potential broad benefits to wetland biodiversity conservation in general. Protecting any semiaquatic species requires treating aquatic and terrestrial areas jointly. Failing to uphold the connectivity and condition of both habitats leads to weakened biodiversity of urban wetlands. Identifying not only potential smooth newt breeding ponds but essential terrestrial corridors could help urban planners prioritize and conserve important amphibian and reptile environments, construct artificial wetlands if needed, and thus maintain urban wetland biodiversity.
Data availability statement
The datasets presented in this study can be found in online repositories. The names of the repository/repositories and accession number(s) can be found below: https://doi.org/10.5281/zenodo.7598682.
Ethics statement
The animal study was approved by the Centre for Economic Development, Transport, and the Environment (issued on 14 March 2018). The study was conducted in accordance with the local legislation and institutional requirements.
Author contributions
MV, MN, ST, and V-MV contributed to the conception and design of the study. MV performed the data collection, fieldwork, and statistical analyses. MN performed the GIS analyses. ST wrote the first draft of the manuscript, and all authors contributed to editing and revising it. All authors have read and approved the submitted version.
Funding
This project was funded by the Maj and Tor Nessling Foundation (201800264). The foundation did not have a role in the formulation of the study design, or in the collection, analyses, or interpretation of the data. They additionally did not influence the writing of the report or the decision to submit our paper for publication.
Conflict of interest
Author MN was employed by the company Latvasilmu osk.
The remaining authors declare that the research was conducted in the absence of any commercial or financial relationships that could be construed as a potential conflict of interest.
Publisher’s note
All claims expressed in this article are solely those of the authors and do not necessarily represent those of their affiliated organizations, or those of the publisher, the editors and the reviewers. Any product that may be evaluated in this article, or claim that may be made by its manufacturer, is not guaranteed or endorsed by the publisher.
References
Ahti T., Hämet-Ahti L., Jalas J. (1968). Vegetation zones and their sections in north-western Europe. Ann. Bot. Fenn 5, 169–211.
Amezaga J. M., Santamaria L., Green A. J. (2002). Biotic wetland connectivity — supporting a new approach for wetland policy. Acta Oecologica 23, 213–222. doi: 10.1016/S1146-609X(02)01152-9
Arntzen J. W. (2002). Seasonal variation in sex ratio and asynchronous presence at ponds of male and Triturus newts. J. Herpetol. 36 (1), 30–35. doi: 10.1670/0022-1511(2002)036[0030:SVISRA]2.0.CO;2
Attum O., Lee Y. M., Roe J. H., Kingsbury B. A. (2006). Upland–wetland linkages: relationship of upland and wetland characteristics with watersnake abundance. J. Zool. 271, 134–139. doi: 10.1111/j.1469-7998.2006.00178.x
Baguette M., Mennechez G. (2004). Resource and habitat patches, landscape ecology and metapopulation biology: A consensual viewpoint. Oikos 106 (2), 399–403. doi: 10.1111/j.0030-1299.2004.13120.x
Beebee T. J. (2013). Effects of road mortality and mitigation measures on amphibian populations. Conserv. Biol. 27 (4), 657–668. doi: 10.1111/cobi.12063
Bell G. (1977). The life of the smooth newt (Triturus vulgaris) after metamorphosis. Ecol. Monogr. 47, 279–299. doi: 10.2307/1942518
Bierwagen B. G. (2007). Connectivity in urbanizing landscapes: The importance of habitat configuration, urban area size, and dispersal. Urban Ecosyst. 10, 29–42. doi: 10.1007/s11252-006-0011-6
Bowne D. R., Bowers M. A. (2004). Interpatch movements in spatially structured populations: a literature review. Landsc. Ecol. 19 (1), 1–20. doi: 10.1023/B:LAND.0000018357.45262.b9
Brabec E., Schulte S., Richards P. L. (2002). Impervious surfaces and water quality: a review of current literature and its implications for watershed planning. J Plan Lit 16(4), 499–514. doi: 10.1177/088541202400903563
Brand A. B., Snodgrass J. W. (2010). Value of artificial habitats for amphibian reproduction in altered landscapes. Conserv. Biol. 24, 295–301. doi: 10.1111/j.1523-1739.2009.01301.x
Brauer C. J., Beheregaray L. B. (2020). Recent and rapid anthropogenic habitat fragmentation increases extinction risk for freshwater biodiversity. Evol. Appl. 13 (10), 2857–2869. doi: 10.1111/eva.13128
Buono V., Galliani G., Bissattini A. M., Vignoli L. (2019). Can a cow save a newt? The role of cattle drinking troughs in amphibian conservation. Aquat. Conserv.: Mar. Freshw. Ecosyst. 29 (6), 964–975. doi: 10.1002/aqc.3126
Carpenter S. R., Kitchell J. F., Hodgson J. R. (1985). Cascading trophic interactions and lake productivity: Fish predation and herbivory can regulate lake ecosystems. BioScience 35 (10), 634–639. doi: 10.2307/1309989
Cayuela H., Valenzuela-Sanchez A., Teulier L., Martínez-Solano Í., Léna J.-P., Merilä J., et al. (2020). Determinants and consequences of dispersal in vertebrates with complex life cycles: a review of pond-breeding amphibians. Quart. Rev. Biol. 95 (1), 1–36. doi: 10.1086/707862
Clark N. E., Lovell R., Wheeler B. R., Higgins S. L., Depledge M. H., Norris K. (2014). Biodiversity, cultural pathways, and human health: a framework. Trends Ecol. Evol. 29 (4), 198–204. doi: 10.1016/j.tree.2014.01.009
Cosentino B. J., Schooley R. L. (2018). “Dispersal and wetland fragmentation,” in The Wetland Book. Ed. Finlayson C. M., Everard M., Irvine K., McInnes R. J., Middleton B. A., van Dam A. A. (Dordrecht: Springer), 105–111. doi: 10.1007/978-90-481-9659-3_56
Cushman S. A. (2006). Effects of habitat loss and fragmentation on amphibians: A review and prospectus. Biol. Conserv. 128, 231–240. doi: 10.1016/j.biocon.2005.09.031
Darwall W. R. T., Smith K. G., Allen D., Seddon M. B., McGregor, Reid G., Clausnitzer V., et al. (2009). “Freshwater biodiversity: a hidden resource under threat,” in Wildlife in a Changing World. Eds. Vié J.-C., Hilton-Taylor C., Stuart S. N. (Gland: IUCN), 43–54.
Davidson N. (2014). How much wetland has the world lost? Long-term and recent trends in global wetland area. Mar. Freshw. Res. 65, 934–942. doi: 10.1071/MF14173
Deeming D. C. (2008). Capture of smooth newts (Lissotriton vulgaris) and great crested newts (Triturus cristatus) correlates with the lunar cycle. Herpetol. J. 18, 171–174.
deMaynadier P. G., Hunter M. L. Jr. (2000). Road effects on amphibian movements in a forested landscape. Nat. Areas J. 20, 56–65.
Denoël M., Dalleur S., Langrand E., Besnard A., Cayuela H. (2018). Dispersal and alternative breeding site fidelity strategies in an amphibian. Ecography 41 (9), 1543–1555. doi: 10.1111/ecog.03296
Denoël M., Ficetola G. F. (2007). Landscape-level thresholds and newt conservation. Ecol. Appl. 17 (1), 302–309. doi: 10.1890/1051-0761(2007)017[0302:LTANC]2.0.CO;2
Denoël M., Perez A., Cornet Y., Ficetola G. F. (2013). Similar local and landscape processes affect both a common and a rare newt species. PloS One 8 (5), e62727. doi: 10.1371/journal.pone.0062727
Dias K. S., Dosso E. S., Hall A. S., Schuch A. P., Tozetti A. M. (2019). Ecological light pollution affects anuran calling season, daily calling period, and sensitivity to light in natural Brazilian wetlands. Sci. Nat. 106 (46), 1–9. doi: 10.1007/s00114-019-1640-y
Dolmen D. (1988). Coexistence and niche segregation in the newts Triturus vulgaris (L.) and T. cristatus (Laurenti). Amphibia–Reptilia 9, 365–374. doi: 10.1163/156853888X00044
Dudgeon D., Arthington A. H., Gessner M. O., Kawabata Z.-I., Knowler D. J., Lévêque C. (2006). Freshwater biodiversity: importance, threats, status and conservation challenges. Biol. Rev. 81, 163–182. doi: 10.1017/S1464793105006950
Eaton B. R., Tonn W. M., Paszkowski C. A., Danylchuk A. J., Boss S. M. (2005). Indirect effects of fish winterkills on amphibian populations in boreal lakes. Can. J. Zool. 83 (12), 1532–1539. doi: 10.1139/z05-151
Elmberg J., Nummi P., Pöysä H., Sjöberg K. (1992). Do intruding predators and trap position affect the reliability of catches in activity traps? Hydrobiologia 239, 187–193. doi: 10.1007/BF00007676
Elmberg J., Nummi P., Pöysä H., Sjöberg K. (1993). Factors affecting species numbers and density of dabbling duck guilds in North Europe. Ecography 16 (3), 251–260. doi: 10.1111/j.1600-0587.1993.tb00214.x
Emerton L., Bos E. (2004). Value. Counting ecosystems as an economic part of water infrastructure (Report) (Gland, Switzerland and Cambridge, UK: IUCN), 88 pp. Available at: cmsdata.iucn.org/downloads/value_en.pdf.
Environmental Systems Research Institute Inc. (ESRI) (2015). ArcGIS Desktop: Release 10.3.1 (Redlands, CA).
Fahrig L., Pedlar J. H., Pope S. E., Taylor P. D., Wegner J. F. (1995). Effect of road traffic on amphibian density. Biol. Conserv. 73, 177–182. doi: 10.1016/0006-3207(94)00102-V
Fahrig L., Rytwinski T. (2009). Effects of roads on animal abundance: an empirical review and synthesis. Ecol. Soc. 14 (1). doi: 10.5751/ES-02815-140121
Falaschi M., Muraro M., Gibertini C., Monache D. D., Parrino E. L., Faraci F., et al. (2022). Explaining declines of newt abundance in northern Italy. Freshw. Biol. 67, 1174–1187. doi: 10.1111/fwb.13909
Feuka A. B. (2016). Effects of light pollution on habitat selection in post-metamorphic wood frogs and unisexual blue-spotted salamanders. Honors College, University of Maine. 379. https://digitalcommons.library.umaine.edu/honors/379.
Finnish Environment Institute SYKE TARKKA portal. Lake Ice Extent for Northern Europe (Helsinki: Finnish Environment Institute). Available at: https://ckan.ymparisto.fi/dataset/%7B236BE975-A61C-42CF-987C-FD9714F3E2D0%7D (Accessed 15.8.2021).
Finnish Environment Institute SYKE (2014). Corine Land Cover 2012 Finland (Helsinki: Finnish Environment Institute). Available at: https://ckan.ymparisto.fi/dataset/corine-maanpeite-2012.
Finnish Meteorological Institute Terminen kasvukausi. Available at: https://www.ilmatieteenlaitos.fi/terminen-kasvukausi (Accessed December 21, 2022). [in Finnish].
Finnish Meteorological Institute (2018) Finnish Meteorological Institute Weather Radar Data. Available at: https://registry.opendata.aws/fmi-radar (Accessed 10.8.2023).
Fyson V. K., Blouin-Demers G. (2021). Effects of landscape composition on wetland occupancy by Blanding’s Turtles (Emydoidea blandingii) as determined by environmental DNA and visual surveys. Can. J. Zool. 99, 672–680. doi: 10.1139/cjz-2021-0004
Garcia-Gonzalez C., Campoa D., Polaa I. G., Garcia-Vazquez E. (2012). Rural road networks as barriers to gene flow for amphibians: Species-dependent mitigation by traffic calming. Landsc. Urb. Plan 104, 171–180. doi: 10.1016/j.landurbplan.2011.10.012
Garcia-Gonzalez C., Garcia-Vazquez E. (2012). Urban ponds, neglected Noah’s Ark for amphibians. J. Herpetol. 46 (4), 507–514. doi: 10.1670/10-227
Gauch H. G. Jr. (1982). Multivariate analysis in community ecology (Cambridge: Cambridge University Press).
Gledhill D. G., James P., Davies D. H. (2008). Pond density as a determinant of aquatic species richness in an urban landscape. Landsc. Ecol. 23, 1219–1230. doi: 10.1007/s10980-008-9292-x
Glista D. J., DeVault T. L., DeWoody J. A. (2007). Vertebrate road mortality predominantly impacts amphibians. Herpetol. Conserv. Biol. 3 (1), 77–87.
Grayson K. L., De Lisle S. P., Jackson J. E., Black S. J., Crespi E. J. (2012). Behavioral and physiological female responses to male sex ratio bias in a pond-breeding amphibian. Front. Zool. 9 (1), 1–10. doi: 10.1186/1742-9994-9-24
Griffiths R. A. (1984). Seasonal behaviour and intrahabitat movements in an urban population of Smooth newts, Triturus vulgaris, (Amphibia: Salamandridae). J. Zool. Lond. 203, 241–251. doi: 10.1111/j.1469-7998.1984.tb02330.x
Griffiths R. A. (1985). Diel profile of behaviour in the smooth newt, Triturus vulgaris (L.): an analysis of environmental cues and endogenous timing. Anim. Behav. 33, 573–582. doi: 10.1016/S0003-3472(85)80081-6
Guderyahn L. B., Smithers A. P., Mims M. C. (2016). Assessing habitat requirements of pond-breeding amphibians in a highly urbanized landscape: implications for management. Urb. Ecosys. 19, 1801–1821. doi: 10.1007/s11252-016-0569-6
Guzy J. C., Price S. J., Dorcas M. E. (2013). The spatial configuration of greenspace affects semi-aquatic turtle occupancy and species richness in a suburban landscape. Landsc. Urb. Plan 117, 46–56. doi: 10.1016/j.landurbplan.2013.04.011
Hamer A. J., Barta B., Bohus A., Gál B., Schmera D. (2021). Roads reduce amphibian abundance in ponds across a fragmented landscape. Glob. Ecol. Conserv. 28, 1–16. doi: 10.1016/j.gecco.2021.e01663
Hamer A. J., Harrison L. J., Stokeld D. (2018). Terrestrial habitat and individual fitness increase survival of a freshwater turtle in an urban landscape. Urb. Ecosyst. 21, 71–83. doi: 10.1007/s11252-017-0708-8
Hamer A. J., McDonnell M. J. (2008). Amphibian ecology and conservation in the urbanising world: A review. Biol. Conserv. 141 (10), 2432–2449. doi: 10.1016/j.biocon.2008.07.020
Hamer A. J., Parris K. M. (2011). Local and landscape determinants of amphibian communities in urban ponds. Ecol. Appl. 21 (2), 378–390. doi: 10.1890/10-0390.1
Hamer A. J., Smith P. J., McDonnell M. J. (2012). The importance of habitat design and aquatic connectivity in amphibian use of urban stormwater retention ponds. Urb. Ecosys. 15, 451–471. doi: 10.1007/s11252-011-0212-5
Heiss E., Aerts P., Van Wassenbergh S. (2015). Flexibility is everything: prey capture throughout the seasonal habitat switches in the smooth newt Lissotriton vulgaris. Org. Divers. Evol. 15, 127–142. doi: 10.1007/s13127-014-0187-1
Helldin J. O., Petrovan S. O. (2019). Effectiveness of small road tunnels and fences in reducing amphibian roadkill and barrier effects at retrofitted roads in Sweden. PeerJ 7, e7518. doi: 10.7717/peerj.7518
Hill I. D. C., Rossi C. A., Petrovan S. O., Hartup M., Clark F., Downie J. R. (2018). Mitigating the effects of a road on amphibian migrations: a Scottish case study of road tunnels. Glasgow Naturalist 27, 1–12. doi: 10.37208/tgn27s06
Houlahan J. E., Findlay C. S. (2003). The effects of adjacent land use on wetland amphibian species richness and community composition. Can. J. Fish Aquat. Sci. 60 (9), 1078–1094. doi: 10.1139/f03-095
Indermaur L., Schaub M., Jokela J., Tockner K., Schmidt B. R. (2010). Differential response to abiotic conditions and predation risk rather than competition avoidance determine breeding site selection by anurans. Ecography 33 (5), 887–895. doi: 10.1111/j.1600-0587.2010.06150.x
Jarvis L. E., Hartup M., Petrovan S. O. (2019). Road mitigation using tunnels and fences promotes site connectivity and population expansion for a protected amphibian. Eur. J. Wildl. Res. 65 (2), 1–11. doi: 10.1007/s10344-019-1263-9
Jehle R. (2000). The terrestrial summer habitat of radio-tracked great crested newts (Triturus cristatus) and marbled newts (T. marmoratus). Herpetol. J. 10, 137–142.
Joly P. (2019). Behavior in changing landscape: using movement ecology to inform the conservation of pond-breeding amphibians. Front. Ecol. Evol. 155. doi: 10.3389/fevo.2019.00155
Joly P., Miaud C. (1993). How does a newt find its pond? The role of chemical cues in migrating newts (Triturus alpestris). Ethol. Ecol. Evol. 5 (4), 447–455.
Joly P., Miaud C., Lehmann A., Grolet O. (2001). Habitat matrix effects on pond occupancy in newts. Conserv. Biol. 15 (1), 239–248. doi: 10.1111/j.1523-1739.2001.99200.x
Kačergytė I., Petersson E., Arlt D., Hellström M., Knape J., Spens J., et al. (2021). Environmental DNA metabarcoding elucidates patterns of fish colonisation and co-occurrences with amphibians in temperate wetlands created for biodiversity. Freshw. Biol. 66, 1915–1929. doi: 10.1111/fwb.13800
Kačergytė I., Knape J., Żmihorski M., Arlt D., Pärt T. (2023). Community associations of birds with amphibians and fish in wetlands created for biodiversity. Biol. Conserv 282, 1–9. doi: 10.1016/j.biocon.2023.110031
Kingsford R. T., Basset A., Jackson L. (2016). Wetlands: conservation’s poor cousins. Aquat. Conserv.: Mar. Freshw. Ecosyst. 26 (5), 892–916. doi: 10.1002/aqc.2709
Kinne O. (2004). Successful re-introduction of the newts Triturus cristatus and T. vulgaris. Endanger Species Res. 1, 25–40. doi: 10.3354/esr001025
Konowalik A., Najbar A., Konowalik K., Dylewski Ł., Frydlewicz M., Kisiel P., et al. (2020). Amphibians in an urban environment: a case study from a central European city (Wrocław, Poland). Urban Ecosys. 23, 235–243. doi: 10.1007/s11252-019-00912-3
Koskela P. (1984). Vesilisko In: Suomen eläimet: Kalat, sammakkoeläimet ja matelijat. Ed. Koli L. (Helsinki, Finland: Weilin+Göös). [In Finnish].
Kovar R., Brabec M., Vita R., Bocek R. (2009). Spring migration distances of some Central European amphibian species. Amphibia–Reptilia 30 (3), 367–378. doi: 10.1163/156853809788795236
Malmgren J. C. (2002). How does a newt find its way from a pond? Migration patterns after breeding and metamorphosis in great crested newts (Triturus cristatus) and smooth newts (T. vulgaris). Herpetol. J. 12 (1), 29–35.
Marsh D. M., Milam G. S., Gorham N. P., Beckman N. G. (2005). Forest roads as partial barriers to terrestrial salamander movement. Conserv. Biol. 19 (6), 2004–2008. doi: 10.1111/j.1523-1739.2005.00238.x
Matos C., Petrovan S., Ward A. I., Wheeler P. (2017). Facilitating permeability of landscapes impacted by roads for protected amphibians: patterns of movement for the great crested newt. PeerJ 5, e2922. doi: 10.7717/peerj.2922
Matos C., Petrovan S., Wheeler P. M., Ward A. I. (2019). Landscape connectivity and spatial prioritization in an urbanising world: A network analysis approach for a threatened amphibian. Biol. Conserv. 237, 238–247. doi: 10.1016/j.biocon.2019.06.035
Milardi M., Käkelä R., Weckström J., Kahilainen K. (2016). Terrestrial prey fuels the fish population of a small, high-latitude lake. Aquat. Sci. 78, 695–706. doi: 10.1007/s00027-015-0460-1
Mulkeen C. J. (2018). Biodiversity and performance of constructed wetlands; a comparison with natural wetlands. [dissertation] (Galway: National University of Ireland).
Mulkeen C. J., Gibson-Brabazon S., Carlin C., Williams C. D., Healy M. G., Mackey P. (2017). Habitat suitability assessment of constructed wetlands for the smooth newt (Lissotriton vulgaris [Linnaeus 1758]): A comparison with natural wetlands. Ecol. Eng. 106, 532–540. doi: 10.1016/j.ecoleng.2017.06.005
Müllner A. (2001). Spatial patterns of migrating Great Crested Newts and Smooth Newts: The importance of the terrestrial habitat surrounding the breeding pond. Rana 4, 279–293.
Paterson E. (2018). Nocturnal variation in population size estimate counts of male palmate and smooth newts (Lissotriton helveticus and L. vulgaris). Herpetol. Bull. 145, 1–7.
Perry G., Buchanan B. W., Fisher R. N., Salmon M., Wise S. E. (2008). Effects of artificial night lighting on amphibians and reptiles in urban environments. Herpetol. Conserv. 3, 239–256.
Phillips J. (2020). Over the moon: the effect of the lunar cycle on three species of newt in the UK. [dissertation] (United Kingdom: University of Kent).
Pimental R. A. (1979). Morphometrics. The multivariate analysis of biological data. Dubuque (Dubuque, Iowa, United States of America: Kendall/Hunt Publishing Company), 276.
Pittman S. E., Osbourn M. S., Semlitsch R. D. (2014). Movement ecology of amphibians: A missing component for understanding population declines. Biol. Conserv. 169, 44–53. doi: 10.1016/j.biocon.2013.10.020
Rannap R., Kaart M. M., Kaart T., Kill K., Uuemaa E., Mander Ü., et al. (2020). Constructed wetlands as potential breeding sites for amphibians in agricultural landscapes: A case study. Ecol. Eng. 158, 1–9. doi: 10.1016/j.ecoleng.2020.106077
Rannap R., Lõhmus A., Linnamägi M. (2012). Geographic variation in habitat requirements of two coexisting newt species in Europe. Acta Zool. Acad. Sci. Hungaricae 58 (1), 69–86.
R Development Core Team (2013). R: A Language and Environment for Statistical Computing. R Foundation for Statistical Computing (Vienna Austria: The R Foundation for Statistical Computing). Available at: http://www.R-project.org/.
Reshetnikov A. N. (2003). The introduced fish, rotan (Perccottus glenii), depresses populations of aquatic animals (macroinvertebrates, amphibians, and a fish). Hydrobiologia 510 (1), 83–90. doi: 10.1023/B:HYDR.0000008634.92659.b4
Roe J. H., Kingsbury B. A., Herbert N. R. (2003). Wetland and upland use patterns in semi-aquatic snakes: Implications for wetland conservation. Wetlands 23 (4), 1003–1014. doi: 10.1672/0277-5212(2003)023[1003:WAUUPI]2.0.CO;2
Russi D., ten Brink P., Farmer A., Badura T., Coates D., Förster J., et al. (2013). The Economics of Ecosystems and Biodiversity for Water and Wetlands (London and Brussels; Ramsar Secretariat, Gland: IEEP).
Santoro A., Chambers J. M., Robson B. J., Beatty S. J. (2020). Land use surrounding wetlands influences urban populations of a freshwater turtle. Aquat. Conserv.: Mar. Freshw. Ecosyst. 30, 1050–1060. doi: 10.2307/3802732
Schabetberger R., Jehle R., Maletzky A., Pesta J., Sztatecsny M. (2004). Delineation of terrestrial reserves for amphibians: post-breeding migrations of Italian crested newts (Triturus c. carnifex) at high altitude. Biol. Conserv. 117 (1), 95–104. doi: 10.1016/S0006-3207(03)00268-4
Scheffers B. R., Paszkowski C. A. (2013). Amphibian use of urban stormwater wetlands: The role of natural habitat features. Landsc. Urb. Plan 113, 139–149. doi: 10.1016/j.landurbplan.2013.01.001
Semlitsch R. D. (2000). Principles for management of aquatic-breeding amphibians. J Wildl Manag. 64, 615–631. doi: 10.2307/3802732
Semlitsch R. D., Bodie J. D. (2003). Biological criteria for buffer zones around wetlands and Riparian habitats for amphibians and reptiles. Conserv. Biol. 17 (5), 1219–1228. doi: 10.1046/j.1523-1739.2003.02177.x
Simon J. A., Snodgrass J. W., Casey R. E., Sparling D. W. (2009). Spatial correlates of amphibian use of constructed wetlands in an urban landscape. Lands Ecol. 24, 361–373. doi: 10.1007/s10980-008-9311-y
Skei J. K., Dolmen D., Ronning L., Ringsby T. H. (2006). Habitat use during the aquatic phase of the newts Triturus vulgaris (L.) and T. cristatus (Laurenti) in central Norway: proposition for a conservation and monitoring area. Amphib. Reptil. 27, 309–324. doi: 10.1163/156853806778189972
Stenson J. A. E., Aronsson S. (1995). Newt-fish interactions in a small forest lake. Amphibia–Reptilia 16 (2), 177–184. doi: 10.1163/156853895X00352
Stuart S. N., Chanson J. S., Cox N. A., Young B. E., Rodrigues A. S. L., Fischman D. L., et al. (2004). Status and trends of amphibian declines and extinctions worldwide. Science 306 (5702), 1783–1786. doi: 10.1126/science.1103538
Trovillion D. C., Sauer E. L., Shay G., Crone E. R., Preston D. L. (2023). Habitat complexity, connectivity, and introduced fish drive pond community structure along an urban to rural gradient. Ecol. Appl. 33, 1–13. doi: 10.1002/eap.2828
Väänänen V.-M., Nummi P., Pöysä H., Rask M., Nyberg K. (2012). Fish-duck interactions in boreal lakes in Finland as reflected by abundance correlations. Hydrobiologia 697, 85–93. doi: 10.1007/s10750-012-1172-3
Vehkaoja M., Niemi M., Väänänen V.-M. (2020). Effects of urban infrastructure on aquatic invertebrate diversity. Urban Ecosyst. 23, 831–840. doi: 10.1007/s11252-020-00947-x
Venables W. N., Ripley B. D. (2002). Modern Applied Statistics with S, 4th edn (New York: Springer).
Vuorio V. (2016). Conservation biology of the great crested newt in managed boreal forests in Finland. Dissertationes Forestales (Turku, Finland: Finnish Society of Forest Science; Faculty of Agriculture and Forestry, University of Helsinki; School of Forest Sciences of the University of Eastern Finland) Vol. 214. 45 p. doi: 10.14214/df.214
Vuorio V., Tikkanen O.-P., Mehtätalo L., Kouki J. (2015). The effects of forest management on terrestrial habitats of a rare and a common newt species. Eur. J. For Res. 134, 377–388. doi: 10.1007/s10342-014-0858-7
Warren S. D., Büttner R. (2008). Relationship of endangered amphibians to landscape disturbance. J. Wildl. Manag. 72 (3), 738–744. doi: 10.2193/2007-160
Winandy L., Legrand P., Denoël M. (2017). Habitat selection and reproduction of newts in networks of fish and fishless aquatic patches. Anim. Behav. 123, 107–115. doi: 10.1016/j.anbehav.2016.10.027
Keywords: amphibians, connectivity, infrastructure, newts, urban landscape, urban planning, urban wetlands, wetland conservation
Citation: Vehkaoja M, Thompson SMA, Niemi M and Väänänen V-M (2023) Connectivity, land use, and fish presence influence smooth newt (Lissotriton vulgaris) occurrence and abundance in an urban landscape. Front. Ecol. Evol. 11:1157297. doi: 10.3389/fevo.2023.1157297
Received: 02 February 2023; Accepted: 06 October 2023;
Published: 20 October 2023.
Edited by:
Olivia Sanllorente, University of Granada, SpainReviewed by:
Maider Iglesias, Spanish National Research Council (CSIC), SpainSteven Price, University of Kentucky, United States
Copyright © 2023 Vehkaoja, Thompson, Niemi and Väänänen. This is an open-access article distributed under the terms of the Creative Commons Attribution License (CC BY). The use, distribution or reproduction in other forums is permitted, provided the original author(s) and the copyright owner(s) are credited and that the original publication in this journal is cited, in accordance with accepted academic practice. No use, distribution or reproduction is permitted which does not comply with these terms.
*Correspondence: Mia Vehkaoja, mia.vehkaoja@helsinki.fi