- 1Botany, School of Natural Sciences, Trinity College Dublin, Dublin, Ireland
- 2Department of Community Ecology, Helmholtz Centre for Environmental Research - UFZ, Theodor-Lieser- Strasse, Halle, Germany
- 3German Centre for Integrative Biodiversity Research (iDiv) Halle-Jena-Leipzig, Leipzig, Germany
- 4Agroecology and Environment, Agroscope, Reckenholzstrasse, Zurich, Switzerland
- 5Federation of Irish Beekeepers' Associations, Tullamore, Ireland
- 6Centre for Ecology, Evolution, and Behaviour, Department of Biological Sciences, Royal Holloway University of London, London, United Kingdom
- 7Centre for Agri-Environmental Research, School of Agriculture, Policy and Development, Reading University, Reading, United Kingdom
- 8CREA Research Centre for Agriculture and Environment, Council for Agricultural Research and Economics (CREA), Bologna, Italy
- 9Department of Zoology and Physical Anthropology, Faculty of Veterinary, University of Murcia, Murcia, Spain
- 10Department of Ecology, Swedish University of Agricultural Sciences, Uppsala, Sweden
- 11IPSP-CNR, Institute for Sustainable Plant Protection, The Italian National Research Council, Piazzale Enrico Fermi, Portici, Naples, Italy
- 12Research Centre in México, The National Institute of Forestry, Agriculture and Livestock Research (INIFAP), Texcoco, Mexico
- 13Federation of Swedish Farmers (LRF), Stockholm, Sweden
- 14Nature Conservation and Landscape Ecology, University of Freiburg, Freiburg, Germany
- 15Institute of Agricultural and Environmental Sciences, Estonian University of Life Sciences, Tartu, Estonia
- 16Department of Evolution, Ecology and Behaviour, Institute of Infection, Veterinary and Ecological Sciences, University of Liverpool, Liverpool, United Kingdom
- 17Department of Biology, Lund University, Lund, Sweden
- 18Department of Soil, Plant and Food Sciences (DiSSPA – Entomology and Zoology), University of Bari, Bari, Italy
- 19Departamento de Biología Animal, Facultad de Biología, Universidad de Salamanca, Salamanca, Spain
Introduction: Heterogeneity in composition and spatial configuration of landscape elements support diversity and abundance of flower-visiting insects, but this is likely dependent on taxonomic group, spatial scale, weather and climatic conditions, and is particularly impacted by agricultural intensification. Here, we analyzed the impacts of both aspects of landscape heterogeneity and the role of climatic and weather conditions on pollinating insect communities in two economically important mass-flowering crops across Europe.
Methods: Using a standardized approach, we collected data on the abundance of five insect groups (honey bees, bumble bees, other bees, hover flies and butterflies) in eight oilseed rape and eight apple orchard sites (in crops and adjacent crop margins), across eight European countries (128 sites in total) encompassing four biogeographic regions, and quantified habitat heterogeneity by calculating relevant landscape metrics for composition (proportion and diversity of land-use types) and configuration (the aggregation and isolation of land-use patches).
Results: We found that flower-visiting insects responded to landscape and climate parameters in taxon- and crop-specific ways. For example, landscape diversity was positively correlated with honey bee and solitary bee abundance in oilseed rape fields, and hover fly abundance in apple orchards. In apple sites, the total abundance of all pollinators, and particularly bumble bees and solitary bees, decreased with an increasing proportion of orchards in the surrounding landscape. In oilseed rape sites, less-intensively managed habitats (i.e., woodland, grassland, meadows, and hedgerows) positively influenced all pollinators, particularly bumble bees and butterflies. Additionally, our data showed that daily and annual temperature, as well as annual precipitation and precipitation seasonality, affects the abundance of flower-visiting insects, although, again, these impacts appeared to be taxon- or crop-specific.
Discussion: Thus, in the context of global change, our findings emphasize the importance of understanding the role of taxon-specific responses to both changes in land use and climate, to ensure continued delivery of pollination services to pollinator-dependent crops.
Introduction
Flower-visiting pollinating insects provide a vital ecological service, contributing to the pollination of both wild and cultivated plants (Ollerton et al., 2011). However, in recent decades, a decrease in the abundance and diversity of insect pollinator taxa has been recorded across different regions of the world (IPBES, 2016). Across Europe, between 37 and 65% of wild bee species are considered of conservation concern (Patiny et al., 2009; Nieto et al., 2014; Bretagnolle and Gaba, 2015), and a decline in bumble bee species richness has been documented for the last 60 years (Goulson et al., 2008). According to the European Red List of Butterflies, up to 10% of the butterfly species are considered threatened or nearly threatened (van Swaay et al., 2010, 2011; Warren et al., 2021), and over one-third of the European hover fly species are threatened as well (IUCN– International Union for Conservation of Nature, 2022). Scenarios of global change project further loss of butterflies (Settele et al., 2008), hover flies (Miličić et al., 2018), and bumble bees (Rasmont et al., 2015), supported by observed responses to historic climate change (Kerr et al., 2015).
These declines of pollinators are caused by multiple stressors (e.g., pesticides, climate change related factors, pathogens, invasive and alien species), with anthropogenic land use often considered as the main threat to flower-visiting insects (Goulson et al., 2008; Winfree et al., 2009; Potts et al., 2010; Scheper et al., 2013; Proesmans et al., 2021). Two main aspects of land use have been shown to affect biodiversity: compositional and configurational heterogeneity (Fahrig et al., 2011). The composition of the landscape describes the amount and the diversity of habitats or land use types that constitute the landscape; whereas the configuration of the landscape defines its spatial arrangement, e.g., the number and distribution of patches and their shapes, or their connectivity (Seppelt et al., 2016).
Landscapes characterized by high compositional heterogeneity are more diverse, and might offer additional foraging and breeding resources, and thus may support higher numbers of species (Dunning et al., 1992; Flick et al., 2012). In addition, high configurational heterogeneity enhances landscape connectivity, providing crucial structural elements for the movement of species and their orientation within the landscape, with positive consequences for population dynamics (population genetic structure and demography) and community interactions (Steffan-Dewenter and Tscharntke, 1999; Becher et al., 2016; Dominik et al., 2018; Hass et al., 2018). Thus, landscapes that are heterogeneous in both their composition and configuration are expected to support higher biodiversity, e.g., flower-visiting insects communities, by facilitating their dispersal providing extra nesting sites, and positively affecting the temporal and spatial distribution of floral resources (Steffan-Dewenter et al., 2002; Kremen et al., 2007; Fahrig et al., 2011; Cole et al., 2017; Senapathi et al., 2017; Hass et al., 2018). Conversely, habitats with low composition and configuration heterogeneity are usually associated with phenomena like fragmentation, habitat loss and degradation, which can result in the decrease of resource availability (Senapathi et al., 2017) and have negative impacts on flower-visiting insect movement, diet, reproduction, survival, and interaction with plants (Day, 1991; O'Toole, 1994; Steffan-Dewenter and Tscharntke, 1999; Gathmann and Tscharntke, 2002; Hadley and Betts, 2012).
Although the conversion of semi-natural land to intensive agriculture leads to habitat loss with adverse consequences for flower-visiting insects (Senapathi et al., 2017), the landscape surrounding cultivated crops may still support insect communities, depending on its composition and configuration (Steffan-Dewenter et al., 2002; Rundlöf et al., 2008a; Cranmer et al., 2012; Kennedy et al., 2013; Bourke et al., 2014). Semi-natural landscape features, hedgerows and field margins in particular, can promote insect diversity by providing additional food or nesting resources, and facilitating the movement of individuals between patches (Marshall and Moonen, 2002; Fahrig, 2003; Bengtsson et al., 2005; Hole et al., 2005; Jonason et al., 2011). Mass-flowering crops can also be attractive to flower-visiting insects, by offering food resources with short-term beneficial effects for pollinators (Westphal et al., 2003; Jauker et al., 2012; Holzschuh et al., 2016), while floral strips, hedges, bushes and field margins can fill nutritional gaps outside the blooming periods of these crops (Timberlake et al., 2019; Bottero et al., 2021).
Despite overall negative impacts of agricultural intensification on insect communities, different taxa may respond differently to landscape heterogeneity and land-use intensity due to the disparities in their diet, behavior, floral resource preferences and, nesting, and overwintering sites (Gathmann and Tscharntke, 2002; Fenster et al., 2004; Cane et al., 2006; Klein et al., 2012). A recent meta-analysis analyzing the combined effects of edge density length and percentage of semi-natural habitat on the abundance of different arthropod taxa across Europe, showed that the responses were highly context dependent (Martin et al., 2019). In large-scale studies covering multiple biogeographic regions, contrasting responses of landscape heterogeneity on insect communities may also be a result of varying weather conditions (daily temperature, wind, rain) and climate (annual temperature and precipitation, precipitation seasonality). Weather and climatic conditions can either have direct effects, by affecting the survival and fitness of individuals, or indirect effects, by impacting the availability of foraging resources and the phenology of both insects and plants (Vicens and Bosch, 2000; Brittain et al., 2013; Lawson and Rands, 2019; Martinet et al., 2021), with consequent cascading impacts on plant-pollinator interactions (Hegland et al., 2009; Vasiliev and Greenwood, 2021). While the responses of insects to these effects are mostly taxon-specific, they also differ according to the temporal (daily, seasonal, between years) and spatial scale, as landscape structure can buffer climate impacts (Papanikolaou et al., 2017; Herrera, 2019; Zoller et al., 2020; Ganuza et al., 2022). However, studies investigating the complex suite of landscape and environmental factors that influence flower-visiting insect communities over a larger (e.g., European) biogeographic scale are still scarce.
In this study, we investigated the impact of both the composition and configuration of the landscape on the abundance of several broad taxonomic groups of flower-visiting insects, in 128 crop dominated sites across Europe. At the landscape scale, we hypothesized that more heterogeneous landscapes, with a larger proportion of less-intensively managed habitat (i.e., non-crop and non-urban), and less isolated habitats sustained a greater abundance of flower-visiting insects. At the field scale, we hypothesized that mass-flowering crops and orchards adjacent to the sites could supplement flower-visiting insect abundance, by providing them with additional resources at the beginning of the spring season. In the face of climate change, we also investigated the effects of weather and climate on the abundance of different pollinator insect groups, distributed across multiple biogeographic regions.
Methods
Experimental design
Eight countries were selected within the PoshBee site network (https://poshbee.eu/; Figure 1), representing four of the main European biogeographic regions – Switzerland (CHE) and Germany (GER) for the Continental zone; Italy (ITA) and Spain (ESP) for the Mediterranean zone; Britain (GBR) and Ireland (IRE) for the Atlantic zone; and Estonia (EST) and Sweden (SWE) for the Boreal zone. In each country, we selected 16 sites to encompass a gradient of land use intensity: eight sites containing annual crops – winter-sown oilseed rape (OSR; Brassica napus) – and eight sites with perennial crops – apple orchards (APP), for a total of 128 sites (Figure 1; Hodge et al., 2022). The land use intensity gradient was defined by the proportion of cropland and orchards within a 1 km radius of the center of the sites (Hodge et al., 2022). We ensured a minimum distance of 3 km between the sites to avoid overlapping landscape buffers and violation of spatial independence for subsequent analyses (Hodge et al., 2022). Because of the large geographic range, and differences in cultivation patterns across this range, field sizes varied considerably: apple orchards varied between 0.32 and 45 hectares, while oilseed rape crops varied between 0.5 and 135 hectares (Hodge et al., 2022). A significant confounding factor in these studies was the presence of three honey bee (Apis mellifera) colonies and three bumble bee (B. terrestris) colonies in each site, and three Osmia bicornis trap nests in some of the sites, at the time of sampling – as sentinel colonies for other studies conducted at the same time in the PoshBee site network (Hodge et al., 2022). These sentinel colonies were introduced to the landscape immediately prior to blooming and removed immediately afterwards (Hodge et al., 2022), and thus will not have had long-term effects in the local pollinator community composition. However, they might have contribute to the insect survey data for these three pollinator groups. The statistical section describes how these biases were addressed during the analyses.
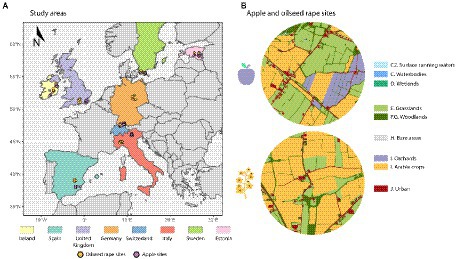
Figure 1. (A) Location of the 128 sites – oilseed rape sites (orange dots) and apple sites (purple dots) across the eight countries. (B) Examples of mapping land cover features within 1-km radius buffers around apple and oilseed rape sites.
Insect surveys
We recorded the presence of five groups of obligate flower-visiting insects – honey bees (Apis mellifera), bumble bees (Bombus spp.), bees other than honey bees and bumble bees – here called solitary bees (despite the fact that some of them might be primitively eusocial, communal or kleptoparasitic species; Hymenoptera, Apoidea, Anthophila), hover flies (Diptera, Syrphidae), and day-flying butterflies (Lepidoptera).
Insects belonging to the five groups were recorded along four transects per site. Two transect were placed in the center of the focal crop field or orchard and two along the respective margins. The two transects in the center of the crops were at least 30 m apart, at least 30 m from the edges of the field and as close as possible to the center of the field. The two transects on the margins were performed on the actual field borders (e.g., strips along the side of the crop, hedgerows, ditches, stonewalls, etc.). We surveyed the field borders rather than the edges of the crop itself, because our aim was to focus on landscape-level features, rather than to analyze variation within the crop field. Each transect was 50 m long and 2 m wide and walked for 5 min on three occasions during the main crop flowering period – at the beginning, peak and towards the end of flowering, resulting in a maximum of 12 transect walks per site. Transect walks were conducted from the 1st of April 2019 (oilseed rape in Ireland) to the 7th of June 2019 (oilseed rape in Germany; see Supplementary Table S1). Insect surveys were only performed during suitable weather conditions, and between 10.00 am and 4.00 pm (see Mahon and Hodge, 2022). Due to unfavorable weather conditions or the difficulties accessing the center of the crops at specific growing stages, 1,295 transect walks (84%) were completed (out of a possible total of 1,536). Transect walks were performed in a non-destructive manner (Hodge et al., 2022), which prevented a species-level identification, but allowed for the assessment of taxon-specific abundances.
Landscape heterogeneity
At the field scale, we identified the habitat type surrounding the focal sites, based on categories defined by the EUNIS habitat classification system (Davies et al., 2004), and recorded the number of the site borders with adjacent mass-flowering crops and orchards (such as apple orchards, oilseed rape crops, horticulture other than apples, and other types of crops, e.g., peas) in situ.
At the landscape scale, we quantified different aspects of landscape heterogeneity by calculating multiple metrics that best describe habitat composition and configuration. First, all landscape features were manually digitalized at a 1:2500 scale within a 1 km radius around the sampling sites (Figure 1), using a combination of head-up digitizing remote sensing data provided by World Imagery (ESRI) and GIS tools (ArcGIS Pro 2.4.1, ESRI). Following the EUNIS habitat classification reference, we classified all land cover categories into nine final categories: Surface Running Waters, Waterbodies, Wetlands, Grasslands (including both managed grassland for livestock, and semi-natural grassland), Woodlands (including also hedgerows, shrub plantations, lines of trees and gardens), Bare Areas, Orchards, Cropland, and Urban Areas (including different types of sealed areas such as roads and cities), see below and Figure 1. Although, the EUNIS reference offers a detailed classification of each land-cover that best defines ecological habitats, we harmonized and reclassified the land cover categories in accordance with the habitat requirements of flower-visiting insects. Therefore, woodlands and hedgerows were combined into the same land-use cover class, under the assumption that they both positively benefit flower-visiting insects, by providing potential additional nectar, pollen or nesting resources (Marshall and Moonen, 2002; Marini et al., 2012a; Alison et al., 2022). In contrast, sealed areas (urban areas of different intensity gradient) were grouped within the same land use type, as they may be an impediment to the survival of flower-visiting insects.
As a measure of compositional landscape heterogeneity, we measured the proportion of cropland, orchards, urban areas and less-intensively managed habitats (aggregation of wetland, woodlands and grasslands habitat types; Supplementary Table S2). Given the resolution of the habitat classification in our study, it was not possible to distinguish between highly managed grasslands (including pastures and silage fields) and semi-natural meadows, and between commercial forestry and woodlands, thus these land-uses were aggregated into less-intensively managed habitats. In addition of the proportion of cropland, orchards, urban areas and less-intensively managed habitats, we calculated a measure of landscape diversity (Shannon diversity index, SHDI) using all nine final land-cover categories (see Supplementary Table S2). Landscape diversity is generally perceived as a critical aspect of landscape heterogeneity, as many arthropods may be associated with a single land use category (e.g., pollinators respond positively to semi-natural habitats).
As a measure of configurational landscape heterogeneity, we used the number of patches (NP) for orchards and cropland, as a proxy for the fragmentation of those habitats (Supplementary Table S2). In addition, we calculated the Interspersion and Juxtaposition Index (IJI), which describes how the different land use types are mixed together in the landscape; and habitat isolation (using the coefficient of variation of Euclidean Nearest-Neighbor distance – ENN), which calculates the distance between near patches belonging to the same land use type – calculated separately for cropland, orchard and less-intensively managed patches. We did not include edge density in our analyses, despite the established use of this measure for the assessment of configuration heterogeneity of the landscape, as it was strongly correlated with the proportion of less-intensively managed habitat. Configurational and compositional landscape metrics were calculated with the R package “landscapemetrics” (Hesselbarth et al., 2019).
Weather and climate parameters
Temperature was measured in the field during each sampling, at 1 m above the ground level in the shade, using a thermometer (Supplementary Table S2). For each site, long-term climate parameters (30 years averages from 1970 to 2000, spatial resolution approximately 1 km2), related to multi-annual temperature and precipitation variables (such as the annual mean temperature and precipitation, or the precipitation seasonality which expresses the variation in monthly precipitation over the year), were extracted from the WorldClim database (v2.1; Fick and Hijmans, 2017; Supplementary Table S2).
Statistical analyses
The impacts of landscape structure, weather and climatic conditions on the abundance of the different insect groups were assessed using generalized linear mixed effects models (GLMM) with a Poisson distribution and a logit link, using country as a random factor (Supplementary Table S3). Because of the disparity in the insect surveys performed between countries (Supplementary Table S1), we pooled insect count data per site and used the number of visits to each site as an offset in the GLMMs.
Due to the co-occurrence of other experiments at the moment of sampling, notably the presence in each site of three honey bee hives, three Bombus terrestris colonies and three Osmia bicornis trap nests (Hodge et al., 2022), we did not directly compare the different groups of insects in an overall model. Instead, we used separate models for each of our response variables: total number of insects (excluding A. mellifera), A. mellifera, Bombus spp., solitary bees, hover flies, butterflies, and for the two types of crops (oilseed rape and apple). We removed A. mellifera counts from the “total Insects” group because of their high abundance in the samples (72%; Figure 2), boosted by three sentinel honey bee colonies nearby and the pervasive presence of beekeeping in many of the landscapes. The remaining 28% of the Total Insects record was split more-or-less evenly between hover flies, butterflies, bumble bees and solitary bees, including possible contributions by the two other experimentally placed sentinel bee species (B. terrestris and O. bicornis). We tested for multicollinearity between our initial set of explanatory variables, by using the variance inflation factor (VIF). A total of 18 variables were included as explanatory variables in the initial models (Supplementary Table S3). Because of the potentially strong impact of climate on the phenology of crop plants (Hegland et al., 2009), we considered potential interactions between annual temperature and the number of mass-flowering crops and orchards in the area adjacent to the sites, and landscape diversity, as well as an interaction between annual precipitation and mass-flowering crops and orchards (Supplementary Table S2).
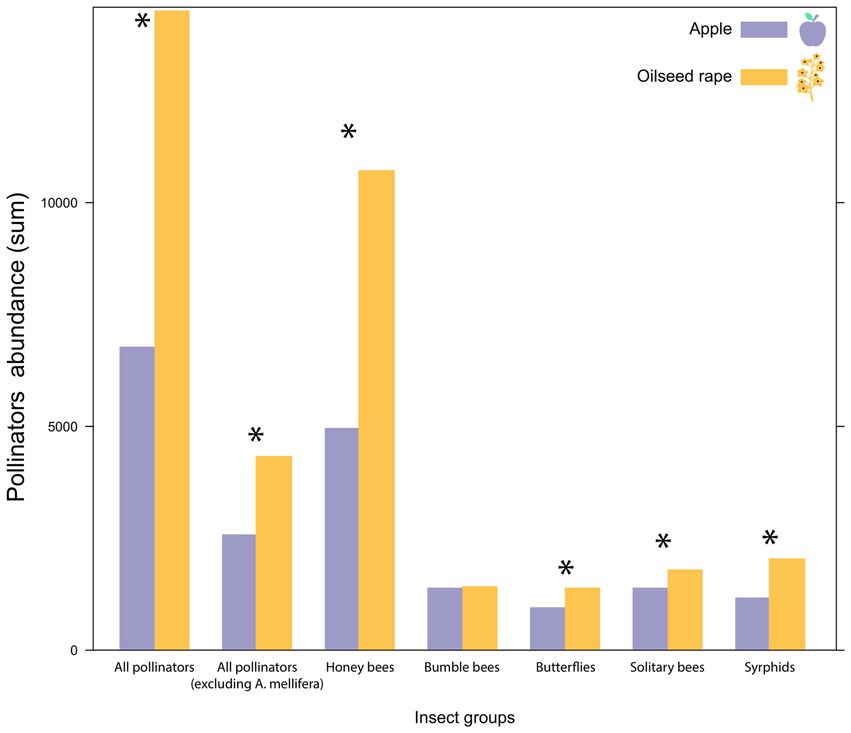
Figure 2. The total number of flower-visiting insects recorded across all sites (All pollinators), excluding honey bees (All pollinators (excluding A. mellifera)) and each insect group (Apis mellifera, Bumble bees, Butterflies, Solitary bees and Hover flies), according to crop type (purple = apple orchard sites, orange = oilseed rape sites). Asterisks show significant (p < 0.05) differences between crop types.
In case of overdispersion, we added an observer term to the random structure (Harrison, 2014). To avoid overfitting, we limited the maximum number of terms to 6 (ca. 10% of data points). If the model failed to converge and the variance of “country” as random factor was negligible, we removed” country” as a random factor from the model. For each analysis, the final selection of the best model was conducted following a multimodel inference approach (Burnham and Anderson, 2002); dredge function of the MuMIn R package (Multimodel inference approach). Finally, we ran model diagnostics to test if all statistical requirements were met for each model and checked for spatial autocorrelation (“check_model” and “testSpatialAutocorrelation” functions in “performance” and “DHARMa” packages (Lüdecke et al., 2021).
To test for differences in total insect abundances between the two crop types (oilseed rape and apple), we used generalized linear mixed effects models (GLMM) with a Poisson distribution and a logit link, for each insect taxon, where crop was the independent variable and the country as a random intercept.
All analyses were performed using R software Version 1.3.1093 (R Core Team, 2020). We used the libraries “ggplot2” and “effects” for the construction of the graphs (Wickham, 2016; Fox and Weisberg, 2019), and the libraries “vegan” and “RcolorBrewer” for building the PCA plot (Oksanen et al., 2022). The library “hclust” was used to check for collinearity among variables. The models were built under the library “lme4” (Bates et al., 2015).
Results
A total of 19,632 insects were recorded in our study across the two crops (6,122 in apple sites and 13,510 in oilseed rape sites; Figure 2). Honey bees (A. mellifera) were by far most abundant, accounting for the 72.44% of all individuals recorded (4,270 in the apple sites and 9,951 in the oilseed rape sites; Figure 2). Hover flies were the second most abundant group in our record, accounting for 8.68% of the individuals (428 in apple sites and 1,276 in oilseed rape sites; Figure 2), solitary bees contributed to 8.30% of the individuals (612 in apple sites and 1,017 in oilseed rape sites), while bumble bees and butterflies, respectively, comprised 6.57 and 4.02% of the samples (631 bumble bees in apple sites and 658 in oilseed rape sites; 181 butterflies in apple sites and 608 in oilseed rape sites; Figure 2). Total insect abundances and abundances of all taxonomic groups were significantly higher in oilseed rape sites than in the apple sites (all p < 0.001; Figure 2), except for bumble bees (p = 0.6; Figure 2). The extent to which the survey records were augmented by the sentinel honey bee colonies and O. bicornis trap nests at the center of the sites is not known. Wild and feral colonies of the bee species are also very common throughout the biogeographic range covered by the sites (hence their choice as sentinel species) and indistinguishable from sentinel-derived bees. However, since the number of bees in these sentinel colonies was rigorously standardized across sites prior to placement (Hodge et al., 2022), the numerical basis for their presence in the survey records is essentially identical for the sites. The actual presence of sentinel bees in the survey records is therefore primarily subjected to the same landscape and climate factors as their wild conspecifics, for the purpose of statistical analysis. Of course, any beekeeping around each of the 128 sites may have biased the number of non-sentinel honey bees at the sites, as function of the number of colonies within range, augmented by social recruitment to the site’s focal crop in competition with other floral resources: factors that are neither known nor can be modelled reliably. For this reason honey bees are analyzed separately throughout the study.
Landscape composition
At the field scale, the responses of insects to the number of mass-flowering crops adjacent to the site were taxon-specific (Figure 3). Bumble bee abundance was positively correlated with the extent of mass-flowering crops and orchards surrounding the apple sites (p < 0.001; Tables 1, 2; Figure 3). However, both honey bees and hover flies abundance was negatively correlated with mass-flowering crops and orchards surrounding the oilseed rape sites (respectively p =0.01 and p < 0.001; Tables 1, 2; Figure 3). Butterfly abundance in the apple sites showed a moderate decline with the increase of mass-flowering crops and orchards in the surrounding landscape (p = 0.07; Tables 1, 2).
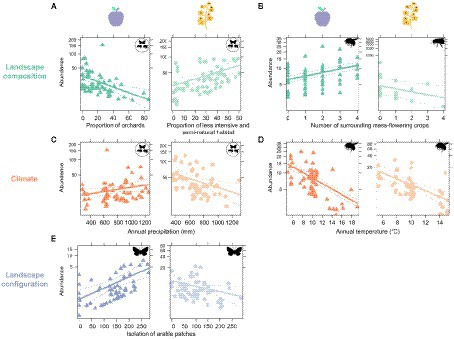
Figure 3. GLMM representing relationships found in both apple and oilseed rape sites between (A) the proportion of orchards or less intensive and semi-natural habitat, and abundance of all pollinators, (B) number of surrounding mass-flowering crops and abundance of bumble bees and honey bees, (C) annual precipitation and abundance of all pollinators, (D) annual temperature and abundance of bumble bees, and (E) isolation of arable patches and abundance of butterflies.
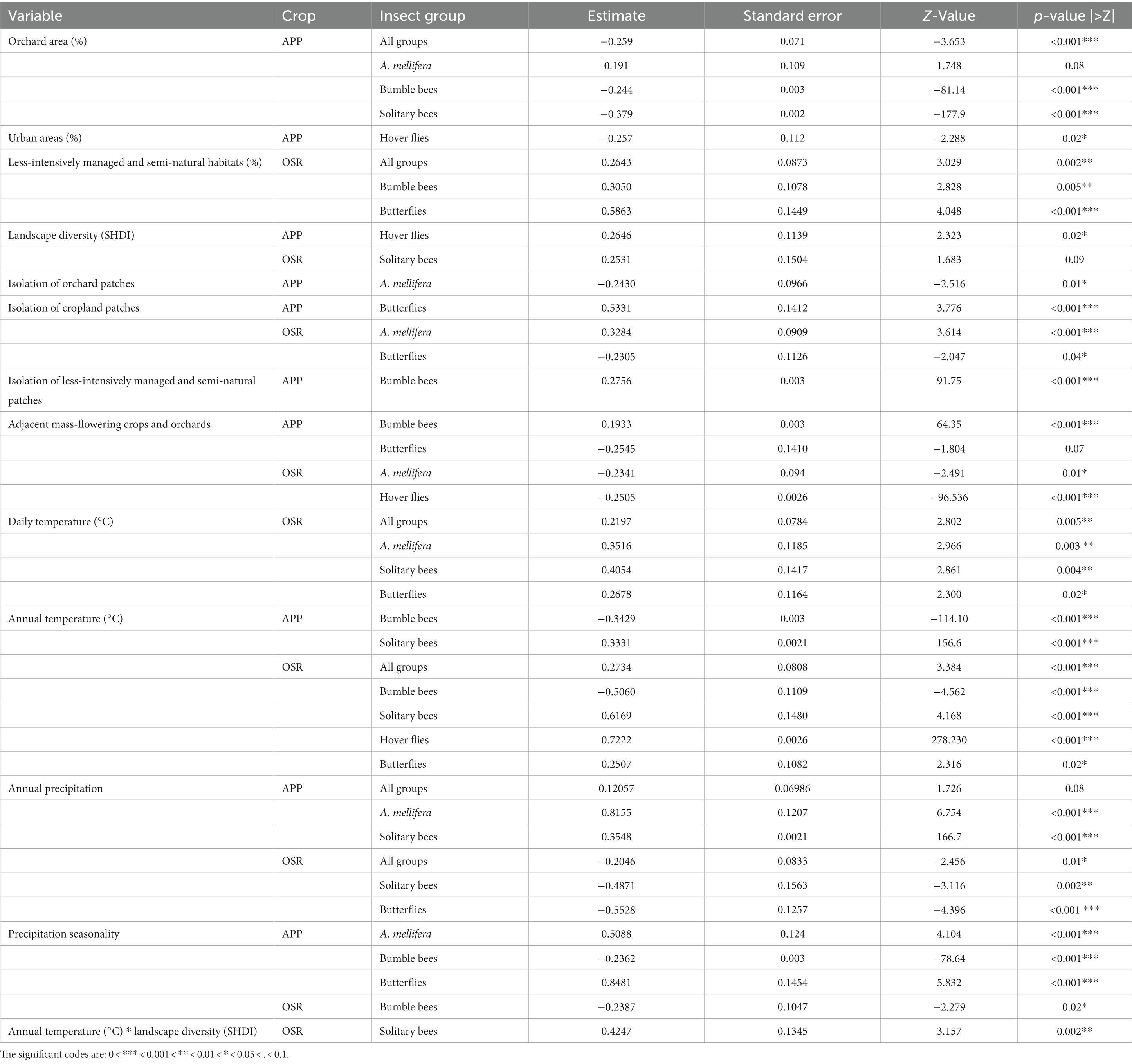
Table 1. The results from the mixed effect models (GLMM models testing the relationship between landscape and climatic variables and insect abundance in each crop – apple (APP) and oilseed rape (OSR).
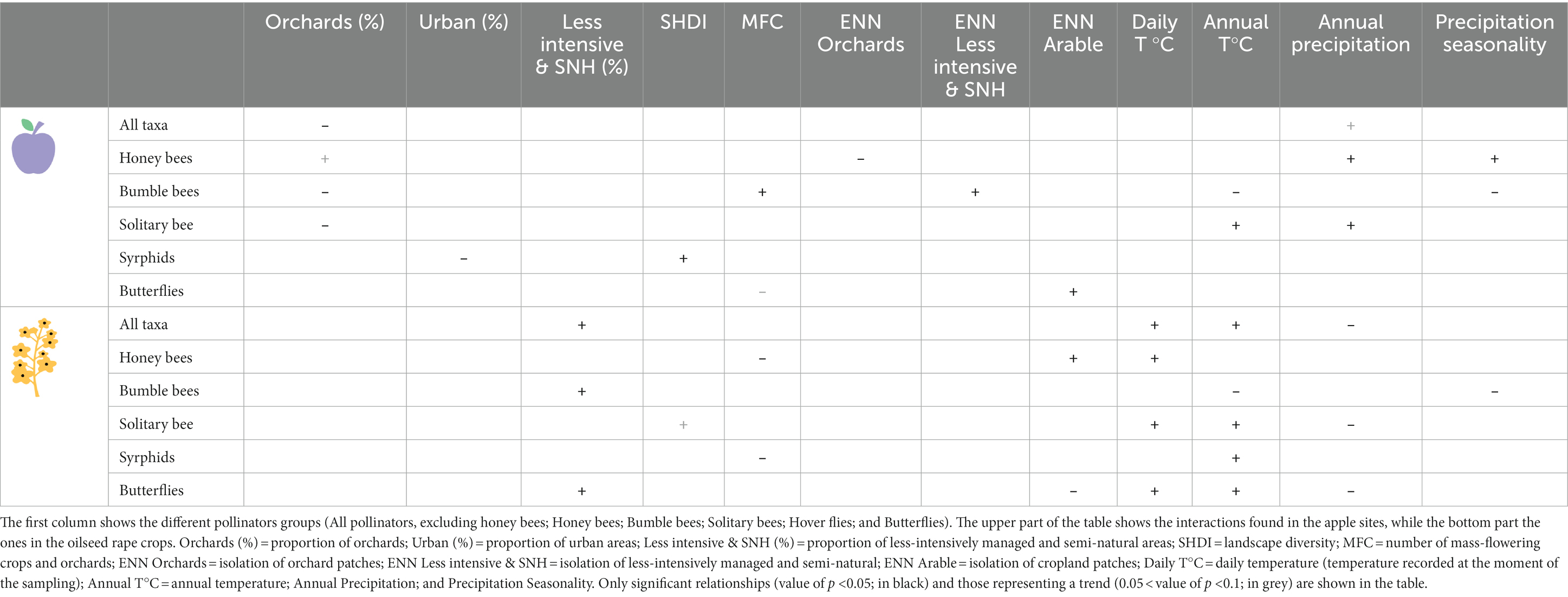
Table 2. Summary of the positive (“+”) and negative (“–”) effects of landscape and climate variables on the abundance of the six groups of pollinators.
A high proportion of orchards in the landscape surrounding the apple sites was negatively correlated with total insect abundance (excluding honey bees; p < 0.001; Tables 1, 2; Figure 3), bumble bees (p < 0.001; Tables 1, 2) and solitary bees (p < 0.001; Tables 1, 2). Honey bees were slightly more abundant in sites surrounded by a higher proportion of orchards, although this result was not statistically significant (p = 0.08; Tables 1, 2). Beekeeping is popular near fruit orchards, both for hobby and for pollination services, and could well have generated this marginal effect. The proportion of urban area negatively influenced hover flies in the apple sites (p = 0.02; Tables 1, 2). As expected, a positive relationship between the proportion of less-intensively managed areas and number of flower-visiting insects was found, though the effect was only observed in oilseed rape sites. The total number of insects (excluding honey bees) increased with the increasing proportion of less-intensively managed areas (p = 0.002; Tables 1, 2; Figure 3). Similar patterns were observed between the proportion of less-intensively managed areas and the abundance of bumble bees and butterflies (p = 0.004 and p < 0.001 respectively; Tables 1, 2).
High landscape diversity in the surrounding landscape increased the abundance of hover flies (p = 0.02; Tables 1, 2). Solitary bees were also positively influenced by landscape diversity, although this was only found in oilseed rape sites (p = 0.09; Tables 1, 2).
Landscape configuration
None of the selected explanatory variables describing landscape configuration explained insect abundance, except for habitat isolation (ENN). Contrary to our hypothesis, isolation of less-intensively managed habitat patches was positively correlated with abundance of bumble bees in apple sites (p < 0.001; Tables 1, 2). On the other hand, isolation of orchard patches was negatively correlated with the abundance of honey bees in apple sites (p = 0.01 Tables 1, 2). Honey bees in oilseed rape sites showed a positive correlation with the isolation of cropland patches (p < 0.001; Tables 1, 2). A positive relationship between the isolation of cropland and butterflies was also found in apple sites (p < 0.001; Tables 1, 2; Figure 3). However, the opposite was found for butterflies in oilseed rape sites, where abundance declined with increasing isolation of cropland patches (p = 0.04; Tables 1, 2; Figure 3).
Weather and climate parameters
Daily temperature, annual temperature, annual precipitation, and precipitation seasonality played a role in shaping insect abundance. Although the positive effect of daily temperature only emerged in oilseed rape sites, annual temperature, annual precipitation and the precipitation seasonality affected insect abundance in both crop types, albeit the effect was positive or negative depending on the insect group and crop type (Tables 1, 2; Figure 3).
The total number of insects in oilseed rape sites was positively correlated with both daily and annual temperatures (both p < 0.001; Tables 1, 2), but was negatively correlated with annual precipitation (p = 0.01; Tables 1, 2; Figure 3). In contrast, annual precipitation had a positive relationship with insect abundance in apple sites, albeit non-significantly (p = 0.08; Tables 1, 2; Figure 3).
By analyzing the responses of different insect groups to weather and climatic conditions, we found that daily temperature was positively correlated with the number of honey bees, solitary bees and butterflies (respectively p = 0.003, p = 0.004, p = 0.02; Tables 1, 2), while the responses of insects to annual temperature were mostly taxon-specific. The abundance of solitary bees in apple and oilseed rape sites, and hover flies and butterflies in oilseed rape sites were positively correlated with annual temperature (p < 0.001, p < 0.001, p < 0.001 and p = 0.02; Tables 1, 2), whereas the abundance of bumble bees was negatively correlated with annual temperature in both crop types (p < 0.001; Tables 1, 2; Figure 3). Moreover, a positive interaction of annual temperature and landscape diversity on the abundance of solitary bees in oilseed rape sites was found (p = 0.002; Table 1), i.e., that positive effects of landscape diversity were even stronger under warmer climates and vice versa.
The effect of annual precipitation on insect abundance varied across crop type and insect group (Tables 1, 2). The abundance of honey bees and solitary bees in apple sites were positively correlated with increasing annual precipitation (p < 0.001; Tables 1, 2). On the other hand, solitary bee and butterfly abundances in oilseed rape sites responded negatively to annual precipitation (respectively p = 0.002 and p < 0.001; Tables 1, 2). Finally, precipitation seasonality was negatively correlated with the abundance of bumble bees in both apple and oilseed rape sites (p < 0.001 and p = 0.02; Tables 1, 2), although it was positively associated with the abundance of honey bees and butterflies in apple sites (p < 0.001; Tables 1, 2).
Discussion
Impact of landscape composition and configuration on insect abundance
Our results demonstrate that both the composition and configuration of the landscape, such as landscape diversity, the presence of less-intensively managed land, but also the complexity and connectivity of the landscape, were major drivers of flower-visiting insect abundances in agriculturally dominated landscapes. As expected, a higher proportion of less-intensively managed habitats was found to support higher numbers of flower-visiting insects. However, we found these effects to be highly context dependent, in regard to both taxon and crop-type. Because we used a non-destructive sampling method (Hodge et al., 2022), our analyses only considered insect abundance in very broad taxonomic categories. While previous studies found hover flies responses to surrounding habitat structure can vary within these broad groups, probably due to scale-dependent ecological requirements of species, particularly within the species rich groups of hover flies and solitary bees (Stanley et al., 2013), we were not able to investigate species-level patterns, except for honey bees.
In addition, differences related to crop-type emerged in relation to insect abundance, with more individuals recorded in oilseed rape crops, compared to apple orchards – with the exception of bumble bees. Oilseed rape crops are known to be highly attractive to bees, and the pollen diet of some species (e.g., honey bees and red mason bees) consists predominantly of mass flowering crops when available (Holzschuh et al., 2013; Stanley and Stout, 2013). However, bumble bees also include other plant species in their diet, even when mass-flowering crops are abundant (Kovács-Hostyánszki et al., 2013). Similarly, apples are pollinator-dependent, attracting a wide diversity of insects (Russo et al., 2015; Burns and Staney, 2022; Gamonal Gomez et al., 2023). However, their nectar production is lower compared to oilseed rape flowers, and some groups of insects (i.e., honey bees) have been shown to be attracted away from apple orchards, when oilseed rape crops were co-occurring in the vicinity (Quinet et al., 2016; Carruthers et al., 2017; Osterman et al., 2021). On the other hand, oilseed rape crops can attract some insects species from the surrounding landscape, given their abundant floral resources and high flower density (Hoyle et al., 2007; Rollin et al., 2013; Vrdoljak et al., 2016; Woodcock et al., 2016; Magrach et al., 2017; Van Reeth et al., 2018). Another explanation for the different number of insects recorded in the two crops could be related to management. Previous studies showed that apple orchards were associated with high level of pesticides (with fungicide contributing to over the 98% of the total pesticides residues in pollen collected by bees; Šlachta et al., 2020). Although generally not toxic to bees (Rondeau and Raine, 2022), some fungicides can negatively impact the behavior and fitness of honey bees [European Commission Implementing Regulation EU, 2018, (2018/1865 of 28 November 2018); Liao et al., 2019]. Moreover, some pollinator groups might have been attracted to the target crops by other species of plant growing within and along the margins of the fields, despite the pollen and nectar resources offered by the target crops during sampling. The composition and abundance of these non-crop plants may also differ between sites, as a result of fundamental differences in cultivation between our two focal crops; oilseed rape being an annual field crop and apples a woody orchard crop. In fact, previous studies show that some insect groups are more abundant along the margins of the cultivation rather than in the center of the crop itself (e.g., butterflies and hover flies; Bottero et al., 2021). Similarly, the larvae of butterflies and some hover fly species feed on plant tissues (particularly the larvae of Pieris butterfly species that favour Brassicacea), whilst other hover fly larvae are saprophagous or predatory (Speight et al., 2010). Thus butterfly and hover fly abundance in crops may be determined by factors other than the availability of floral resources.
Less-intensively managed habitats
Our results show that the abundance of different groups of pollinators increased with the proportion of less-intensively managed habitats and with habitat diversity, confirming that heterogeneous habitats can support beneficial insects in agricultural landscapes, likely by offering a greater diversity of food and resources (Rundlöf et al., 2008b; Marini et al., 2012b; Nayak et al., 2015; Raderschall et al., 2021; Martínez-Núñez et al., 2022). Different pollinator taxa have different ecological and physiological requirements, and even individuals of the same species might benefit from diets based on a diversity of plant species (Cane and Sipes, 2007; Eckhardt et al., 2014; Bertrand et al., 2019), and during different stages of their life cycle (Erhardt, 1985; Erhardt and Mevi-Schütz, 2009; Meyer et al., 2009). Therefore, less-intensively managed and semi-natural habitats might fill nutritional gaps at specific times of the year, such as at the end of the abundant, yet temporally constrained flowering period of mass-flowering crops (Timberlake et al., 2019; Bottero et al., 2021). The less-intensively managed habitats in the landscape surrounding the fields, may also play an important role in promoting pollinators. For instance, Maurer et al. (2022) reported that different types of semi-natural features (meadows, floral strips in the cultivated crops, hedgerows) have a different impact on the richness and the abundance of different flower-visiting insects, depending on the insects’ needs and the time of year they are active. Similarly, the presence of floral strips in cultivated crops promoted bumble bees across seasons (Bommarco et al., 2021), while hover flies and butterflies were shown to favour the crops’ flowering margins (Bottero et al., 2021). Similarly, the diversity and growth stages of the plants present in the floral strips can support pollinator communities in cultivated crops, ultimately promoting pollination services in agricultural landscapes (Albrecht et al., 2020). In addition, Raderschall et al. (2021) showed that higher crop diversity (and semi-natural habitats) may support bumble bee density in agricultural landscape.
Highly managed crop and urban habitats
Urban areas and highly managed crops such as orchards decreased the abundance of different groups of flower-visiting insects. The negative impact of anthropogenic habitats on insect communities is likely to be related to habitat disturbance and/or management intensity (McKinney, 2008; Vanbergen and Initiative, 2013). An increase in the proportion of both cropland and orchards adds to the overall intensification burden throughout the landscape, through agrochemical inputs and reduced nesting opportunities, not only in the actual cropland fields and orchards, but also outside of these, due to crop rotation and the persistence and dispersal of agrochemicals through soil and groundwater to areas beyond their initial application.
Apple orchards are usually subjected to intensive application of plant protection products to maximize crop value (Damos et al., 2015). As a result, they may directly lead to declines in pollinator abundance, or precipitate their departure from target crop sites to the surrounding areas – the latter may be particularly true for honey bees and bumble bees, which are known for their long foraging distances (Beekman and Ratnieks, 2000; Knight et al., 2005; Carvell et al., 2012). Many of the adjacent patches in our apple sites were mass-flowering crops, including oilseed rape and other orchards, which may have caused a dilution of flower-visiting pollinators for high floral rewards in the vicinity, especially when these mass-flowering crops bloom at the same time (Stanley and Stout, 2013; Riedinger et al., 2015; Holzschuh et al., 2016; Grab et al., 2017; Bänsch et al., 2021; Osterman et al., 2021). However, in contrast to our results, Osterman et al. (2021) did not observe a shift in bumble bee abundance when apple sites were surrounded by oilseed rape, but found more solitary bees in apple sites. These differences could be explained by the different types of crops surrounding our sites, though we do not have the necessary information regarding the cultivar nor the intensity of inputs used in the surrounding crops.
Mass-flowering crops and orchards adjacent to the sites
Competition for better floral rewards between different crop types may also explain the negative relationships found between the presence of mass-flowering crops and orchards in the adjacent patches and the abundance of both honey bees and hover flies at the focal site. The proximity of competing mass-flowering crops and orchards in the vicinity of a site could lead to the dilution of pollinators (Robinson et al., 2022). Alternatively, low plant richness in mass-flowering monocultures may explain the low abundance of pollinators found in mass-flowering dominated landscapes. Indeed, butterflies, bees and hover flies require different plants to properly complete their life cycle (Erhardt, 1985; Erhardt and Mevi-Schütz, 2009), and thus can strongly benefit from the presence of semi-natural habitats that offer a greater diversity of floral and nesting resources (Steffan-Dewenter et al., 2002; Rundlöf et al., 2008a, 2008b; Nayak et al., 2015). On the other hand, bumble bees seemed to profit from the presence of mass-flowering crops in the vicinity, likely due to their longer foraging ranges combined with the highly attractive nature of these crops, which corroborates the findings of previous studies (Holzschuh et al., 2013; Stanley and Stout, 2013).
Habitat configuration
Although the spatial arrangement of crop fields and other habitats has been shown to promote insects in agroecosystems (Martin et al., 2019), habitat isolation was the only configurational landscape metric that influenced the abundance of flower-visiting insects. The effects of habitat isolation on pollinators in our study sites appeared to be highly context dependent. In oilseed rape fields, more honey bees were found when the nearest croplands were further away, as opposed to butterflies which were more abundant when croplands were close by. We found the opposite trend in apple sites, where more honey bees were found when the nearest orchard was close, and more butterflies when the nearest cropland was further away. Contrary to our expectations (Fahrig, 2013; Perović et al., 2015), we found more bumble bees in apple sites when the less-intensively managed habitat patches were further away.
The opposing trends observed for honey bees and butterflies may be due to the differences in their foraging behavior and ranges, the composition of the landscape surrounding the sites, and the crop’s attractiveness in regards to flower rewards. Honey bees can forage over large distances, and are known to be central-place foragers that recruit individuals to more rewarding patches (Seeley, 1995; Dyer, 2002). The placement of honey bee hives is usually managed by beekeepers to optimize both access and proximity to a diversity of high-yielding floral resources, especially in highly managed crops such as apple orchards. Thus, the high number of honey bees found in our apple sites may be explained by the presence of numerous honey bee hives managed by beekeepers, especially since many other orchards were found in the vicinity, and may have influenced the abundance of other insect groups. Butterflies on the other hand are part of a much more diverse group that is influenced by a number of factors mostly related to foraging behavior, mating opportunities and oviposition resources at the patch and landscape level (Dover and Settele, 2009). Butterflies generally benefited from the isolation of the cropland in apple sites, suggesting that cropland offered poor rewards to butterflies, as opposed to less-intensively managed habitats. Similarly, we did not assess overall crop diversity and thus lack the information about specific crops in the vicinity of oilseed rape and apple sites. Although honey bees seem to favour oilseed rape sites that are further away from croplands, we can only presume that our oilseed rape sites were in landscape dominated by less attractive crops for honey bees, e.g., cereal fields. In contrast, oilseed rape fields may need to be less-isolated to attract butterflies, suggesting that the temporary boost of early floral resource pulse provided by mass-flowering crops are not sufficient to support butterflies in more intensive landscapes.
Impact of weather and climate variables on flower-visiting insects
In the context of general concern about the impact that heat waves, droughts, and changes in temporal dynamics (including precipitation seasonality) can have on flower-visiting insects, our study collected important information about the responses of different groups of pollinators at a European level, albeit the relationships were highly context dependent.
As expected, the abundance of several taxa of flower-visiting insects decreased with increasing annual precipitation and precipitation seasonality. On the other hand, only bumble bees responded negatively to annual temperature.
Daily and annual temperature
Our results showed that both daily and annual temperatures positively influenced the abundance of most of the studied insect groups, as could be expected given that most insects are ectotherms and more active during warmer day periods. Fewer bumble bees were found when annual temperatures were higher though; as temperate species, they are generally more suited to northern latitudes in Europe and lower temperatures (Rasmont and Iserbyt, 2010-2014). Changes in temperature, especially when rising above specific levels and during the developmental stages of the species, can negatively affect flower-visiting insects by impacting foraging activities, fertility, morphology (wing and tongue length and body size), colony productivity and development time, and survival (Tepedino and Parker, 1986; Weidenmüller et al., 2002; Radmacher and Strohm, 2010; O’Neill et al., 2011; Holland and Bourke, 2015; Miller-Struttmann et al., 2015; Gerard et al., 2018a,b; Martinet et al., 2021). Moreover, higher temperatures are linked to earlier emergence of flower-visiting insects, which can have repercussions on plant-pollinators interactions (Hegland et al., 2009). Furthermore, higher temperatures are often related to drought, extreme weather phenomena, and to changes in seasonality with possible adverse consequences on plant communities and the resulting cascading effects on food resources (Lawson and Rands, 2019; Höfer et al., 2021). In the face of climate change, a better understanding of the relationships between pollinator abundance and temperature is crucial, given the risk that higher temperatures may result in more homogeneous pollinator communities, likely associated with higher dispersion rates, with a consequent decrease of the species pool (Ganuza et al., 2022).
Annual precipitation and precipitation seasonality
Precipitation can directly affect insects, e.g., their flight mechanism and sensory signals, but also indirectly affect their food resources (Lawson and Rands, 2019). It is also responsible for nectar dilution and pollen damage in some plant species, but the corolla shape and the position of nectaries, nectar spurs and anthers can facilitate the protection of pollen and nectar from rain or drought (Lawson and Rands, 2019). Although both apple and oilseed rape flowers are characterized by an open corolla, we found contrasting responses of insects to annual precipitation in both crop types, suggesting that the differences in landscape composition and configuration, rather than direct impacts of precipitation on food resources, are more important in shaping pollinator communities. Apple sites were surrounded by a higher proportion of both diverse and less-intensively managed habitats, and associated with a lower isolation between semi-natural patches. Such landscape structures might facilitate access to different flower resources, e.g., when pollen was damaged, or when the nectar of the mass-flowering crops was diluted. The contrasting effects of precipitation on different taxonomic groups might be explained by morphological differences in body size and wing structure of the different taxa (Lawson and Rands, 2019), or indirectly mediated through forage resources. Flowers pollinated by butterflies usually have more dilute nectar, while bee-pollinated ones show higher sugar concentrations (Pyke and Waser, 1981; Baker and Baker, 1983; Lawson and Rands, 2019), suggesting that the different responses to the precipitation seasonality on butterflies might be related to taxa preferences for nectar resources. However, changes in floral communities related to different climate event may also be responsible for a shift in flower-visiting insect community.
Conclusion
The adoption of a standardized insect sampling protocol across 128 structurally different sites characterized by different climatic and weather conditions, and the decision to focus on multiple groups of insects, allowed us to properly account for context dependency when disentangling the effects of landscape heterogeneity and climate on pollinator communities at a European level. Despite being constrained to a single flowering season, and due to logistical constraints in conducting the study at the European scale, our study offers important insights on the combined effects that climate and landscape structure have on flower-visiting insect communities. Overall, our results indicate that heterogeneous landscapes, characterized by diverse and less-intensively managed habitats, with low levels of patch isolation, can have a positive impact on the communities of flower-visiting insects, even when the landscape is dominated by intensive agricultural land use. Conversely, structurally simple landscapes will likely be associated with a loss of flower-visiting insects (Senapathi et al., 2017).
Moreover, our study offers new evidence about the importance of both weather and climate parameters on shaping flower-visiting insect communities across Europe. This is particularly relevant in the context of climate change, which will have direct or indirect repercussions on insects and plants communities in the next few decades. Furthermore, due to the strong impact of climate on pollinators shown in this study, we recommend including weather and climate parameters in studies investigating pollinator communities, notably in regard to different biogeographic ranges and fluctuating weather patterns. Additionally, future studies that aim to generate a better understanding of the impact of landscape configuration on insect population dynamics should also focus on the natural structural elements present in the landscape, which have previously been shown to play a major role in influencing insects, especially in an intensive agricultural context (Dover and Sparks, 2000; Marshall and Moonen, 2002; Cranmer et al., 2012).
Broadly, our take-home message is that despite some taxonomic variation, landscape simplification negatively affects some important pollinating insect taxa. In addition, our results show a negative impact of high temperature on bumble bee abundance. Taken with other studies, which have reported similar findings for other taxonomic groups, there may be widespread implications of landscape simplification and climate change on multifunctionality and the delivery of multiple ecosystem services (Mooney et al., 2009; Dainese et al., 2017; Martin et al., 2019; Le Provost et al., 2021). Together, these findings support the implementation of land-use plans and policies to preserve heterogeneity and semi-natural features at a field and landscape level in Europe, to sustain the communities of beneficial insects in agricultural landscapes. For example, increasing the amount of less-intensively managed and semi-natural habitats in landscapes characterized by oilseed rape cultivation, could promote pollinator abundance in oilseed rape crops. On the other hand, in habitats dominated by apple orchards, decreasing the total orchard area, and/or increasing crop diversity and the number of types of mass-flowering crop, could have a positive impact on pollinating insect communities. As well as helping to reverse decline and restore pollinator populations, which are key global and European biodiversity targets, this could have knock-on benefits for other taxa and the restoration of biodiversity more broadly in agriculturally-dominated landscapes across Europe.
Data availability statement
The raw data supporting the conclusions of this article will be made available by the authors, without undue reservation.
Author contributions
MA, MB, PR, CD, SH, KI, A-MK, MM, VM-L, SP, MR, OS, and JSt: study design. MB, PR, A-MK, MM, OS, CC, JM, MA, MR, SP, and JSt: funding and resources. MA, EA, IB, MB, CC, PR, JM, GP, SH, AK, VM-L, SP, PM, RR, MR, OS, and JSt: protocol development. MA, IB, EC, CC, PR, GP, CD, DDU, SH, A-MK, AK, VM-L, PM, HP-P, MR, JSc, OS, DS, GT, and ET: data collection. IB, CD, OS, and JSt: analysis and interpretation of results. IB, CD, and JSt: draft manuscript preparation. All authors contributed to the article and approved the submitted version.
Funding
This project received funding from the European Horizon 2020 research and innovation program under grant agreement no. 773921. VM-L was funded by a postdoctoral fellowship (21260/PD/19), Fundación Séneca, Región de Murcia (Spain).
Acknowledgments
A special acknowledgement is expressed to the farmers who made us feel welcomed, and allowed us access to their crops. We would like to thank our excellent field crews for help with running the transects and identifying the insects: Adam Bates, Glenn Svensson, Karin Hill, Karen Johnson, Sachin Anand, Christine Sandberg, Vera Kauna, Sigmar Naudi, Margret Jürison, Madis Järvis, Kaarel Pent, Ronalds Krams, Aile Rummel, Egle Liiskman, Norbertas Noreika, Tiit Hallikma, Jonas Borth, Simon Lotz, Manuel Hügelmann, Muireann Cotter, Amy Turner, Ciarán Ó Cuív, Melissa Else, Ilaria Cardaio, Irene Guerra, Vittorio Capano, Sara Danielli, Micaela Sánchez Domingo, Irene Muñoz, Bettina Schaer, Jenny Panziera, Laura Junk, Sarina Kivelitz, Dominik Ganser, Stephan Bosshart, Ross George, and Louise Hutchinson.
Conflict of interest
The authors declare that the research was conducted in the absence of any commercial or financial relationships that could be construed as a potential conflict of interest.
Publisher’s note
All claims expressed in this article are solely those of the authors and do not necessarily represent those of their affiliated organizations, or those of the publisher, the editors and the reviewers. Any product that may be evaluated in this article, or claim that may be made by its manufacturer, is not guaranteed or endorsed by the publisher.
Supplementary material
The Supplementary material for this article can be found online at: https://www.frontiersin.org/articles/10.3389/fevo.2023.1128228/full#supplementary-material
References
Albrecht, M., Kleijn, D., Williams, N. M., Tschumi, M., Blaauw, B. R., Bommarco, R., et al. (2020). The effectiveness of flower strips and hedgerows on pest control, pollination services and crop yield: a quantitative synthesis. Ecol. Lett. 23, 1488–1498. doi: 10.1111/ele.13576
Alison, J., Botham, M., Maskell, L. C., Garbutt, A., Seaton, F. M., Skates, J., et al. (2022). Woodland, cropland and hedgerows promote pollinator abundance in intensive grassland landscapes, with saturating benefits of flower cover. J. Appl. Ecol. 59, 342–354. doi: 10.1111/1365-2664.14058
Baker, H. G., and Baker, I. (1983). “Floral nectar sugar constituents in relation to pollinator type” in Handbook of experimental pollination biology. eds. C. E. Jones and R. J. Little (New York: Van Nostrand Reinhold), 117–141.
Bänsch, S., Tscharntke, T., Gabriel, D., and Westphal, C. (2021). Crop pollination services: complementary resource use by social vs solitary bees facing crops with contrasting flower supply. J. Appl. Ecol. 58, 476–485. doi: 10.1111/1365-2664.13777
Bates, D., Mächler, M., Bolker, B., and Walker, S. (2015). Fitting linear mixed-effects models using lme4. J. Stat. Softw. 67, 1–48. doi: 10.18637/jss.v067.i01
Becher, M. A., Grimm, V., Knapp, J., Horn, J., Twiston-Davies, G., and Osborne, J. L. (2016). BEESCOUT: a model of bee scouting behaviour and a software tool for characterizing nectar/pollen landscapes for BEEHAVE. Ecol. Model. 340, 126–133. doi: 10.1016/j.ecolmodel.2016.09.013
Beekman, M., and Ratnieks, F. L. W. (2000). Long-range foraging by the honey-bee, Apis mellifera L. Funct. Ecol. 14, 490–496. doi: 10.1046/j.1365-2435.2000.00443.x
Bengtsson, J., Ahnström, J., and Weibull, A.-C. (2005). The effects of organic agriculture on biodiversity and abundance: a meta-analysis. J. Appl. Ecol. 42, 261–269. doi: 10.1111/j.1365-2664.2005.01005.x
Bertrand, C., Eckerter, P. W., Ammann, L., Entling, M. H., Gobet, E., Herzog, F., et al. (2019). Seasonal shifts and complementary use of pollen sources by two bees, a lacewing and a ladybeetle species in European agricultural landscapes. J. Appl. Ecol. 56, 2431–2442. doi: 10.1111/1365-2664.13483
Bommarco, R., Lindström, S. A. M., Raderschall, C. A., Gagic, V., and Lundin, O. (2021). Flower strips enhance abundance of bumble bee queens and males in landscapes with few honey bee hives. Biol. Conserv. 263:109363. doi: 10.1016/j.biocon.2021.109363
Bottero, I., Hodge, S., and Stout, J. (2021). Taxon-specific temporal shifts in pollinating insects in mass-flowering crops and field margins in Ireland. J. Pollin. Ecol. 28, 90–107. doi: 10.26786/1920-7603(2021)628
Bourke, D., Stanley, D., O’Rourke, E., Thompson, R., Carnus, T., Dauber, J., et al. (2014). Response of farmland biodiversity to the introduction of bioenergy crops: effects of local factors and surrounding landscape context. GCB Bioenergy 6, 275–289. doi: 10.1111/gcbb.12089
Bretagnolle, V., and Gaba, S. (2015). Weeds for bees? A review. Agron. Sustain. Dev. 35, 891–909. doi: 10.1007/s13593-015-0302-5
Brittain, C., Kremen, C., and Klein, A.-M. (2013). Biodiversity buffers pollination from changes in environmental conditions. Glob. Chang. Biol. 19, 540–547. doi: 10.1111/gcb.12043
Burnham, K. P., and Anderson, D. R. (2002). Model selection and multimodel inference: A practical information-theoretic approach. 2nd ed. New York, Springer-Verlag
Burns, K. L. W., and Staney, D. (2022). The importance and value of insect pollination to apples: a regional case study of key cultivars. Agric. Ecosyst. Environ. 331:107911. doi: 10.1016/j.agee.2022.107911
Cane, J., Minckley, R., Kervin, L., Roulston, T., and Williams, N. (2006). Complex responses within a desert bee guild (Hymenoptera: Apiformes) to urban habitat fragmentation. Ecol. Appl. 16, 632–644. doi: 10.1890/1051-0761(2006)016[0632:CRWADB]2.0.CO;2
Cane, J., and Sipes, S. (2007). “Characterizing floral specialization by bees: analytical methods and a revised lexicon for oligolecty” in Plant-pollinator interactions: From specialization to generalization. eds. N. M. Waser and J. Ollerton (University of Chicago Press), 99–122.
Carruthers, J. M., Cook, S. M., Wright, G. A., Osborne, J. L., Clark, S. J., Swain, J. L., et al. (2017). Oilseed rape (Brassica napus) as a resource for farmland insect pollinators: quantifying floral traits in conventional varieties and breeding systems. GCB Bioenergy 9, 1370–1379. doi: 10.1111/gcbb.12438
Carvell, C., Jordan, W. C., Bourke, A. F. G., Pickles, R., Redhead, J. W., and Heard, M. S. (2012). Molecular and spatial analyses reveal links between colony-specific foraging distance and landscape-level resource availability in two bumblebee species. Oikos 121, 734–742. doi: 10.1111/j.1600-0706.2011.19832.x
Cole, L., Brocklehurst, S., Robertson, D., Harrison, W., and McCracken, D. (2017). Exploring the interactions between resource availability and the utilisation of semi-natural habitats by insect pollinators in an intensive agricultural landscape. Agric. Ecosyst. Environ. 246, 157–167. doi: 10.1016/j.agee.2017.05.007
Cranmer, L., McCollin, D., and Ollerton, J. (2012). Landscape structure influences pollinator movements and directly affects plant reproductive success. Oikos 121, 562–568. doi: 10.1111/j.1600-0706.2011.19704.x
Dainese, M., Isaac, N. J. B., Powney, G. D., Bommarco, R., Öckinger, E., Kuussaari, M., et al. (2017). Landscape simplification weakens the association between terrestrial producer and consumer diversity in Europe. Glob. Chang. Biol. 23, 3040–3051. doi: 10.1111/gcb.13601
Damos, P., Colomar, L.-A. E., and Ioriatti, C. (2015). Integrated fruit production and pest management in Europe: the apple case study and how far we are from the original concept? Insects 6, 626–657. doi: 10.3390/insects6030626
Davies, C. E., Moss, D., and Hill, M.O. (2004). EUNIS habitat classification revised 2004. European environment agency. European topic centre on nature protection and biodiversity.
Day, MC (1991). Towards the conservation of aculeate hymenoptera in Europe: an outline of the case for recognition of the high value of hymenoptera Aculeata as indicators of biotype integrity and diversity, with relevant examples and proposals for conservation actions. Nature and environment Series no. 45. Council of Europe, Strasbourg.
Dominik, C., Seppelt, R., Horgan, F. G., Settele, J., and Vaclavik, T. (2018). Landscape composition, configuration, and trophic interactions shape arthropod communities in rice agroecosystems. J. Appl. Ecol. 55, 2461–2472. doi: 10.1111/1365-2664.13226
Dover, J., and Settele, J. (2009). The influences of landscape structure on butterfly distribution and movement: a review. J. Insect Conserv. 13, 3–27. doi: 10.1007/s10841-008-9135-8
Dover, J., and Sparks, T. (2000). A review of the ecology of butterflies in British hedgerows. J. Environ. Manag. 60, 51–63. doi: 10.1006/jema.2000.0361
Dunning, J. B., Danielson, B. J., and Pulliam, H. R. (1992). Ecological processes that affect populations in complex landscapes. Oikos 65, 169–175. doi: 10.2307/3544901
Dyer, F. C. (2002). The biology of the dance language. Annu. Rev. Entomol. 47, 917–949. doi: 10.1146/annurev.ento.47.091201.145306
Eckhardt, M., Haider, M., Dorn, S., and Müller, A. (2014). Pollen mixing in pollen generalist solitary bees: a possible strategy to complement or mitigate unfavourable pollen properties? J. Anim. Ecol. 83, 588–597. doi: 10.1111/1365-2656.12168
Erhardt, A. (1985). Diurnal Lepidoptera: sensitive indicators of cultivated and abandoned grassland. J. Appl. Ecol. 22, 849–861. doi: 10.2307/2403234
Erhardt, A., and Mevi-Schütz, J. (2009). “Adult food resources in butterflies” in Ecology of butterflies in Europe (Scopus; Cambridge University Press), 9–16.
European Commission Implementing Regulation (EU) (2018). 2018/1865 of 28 November 2018 Concerning the non-renewal of approval of the active substance propiconazole, in accordance with regulation (EC) no 1107/2009 of the European Parliament and of the council concerning the placing of plant protection products on the market, and amending commission implementing regulation (EU) no 540/2011. Official journal of European Union (304), 6–9. Available at: https://eur-lex.europa.eu/legal-content/EN/TXT/?qid=1543505797502&uri=CELEX:32018R1865 [Accessed September 25, 2020].
Fahrig, L. (2003). Effects of habitat fragmentation on biodiversity. Annu. Rev. Ecol. Evol. Syst. 34, 487–515. doi: 10.1146/annurev.ecolsys.34.011802.132419
Fahrig, L. (2013). Rethinking patch size and isolation effects: the habitat amount hypothesis. J. Biogeogr. 40, 1649–1663. doi: 10.1111/jbi.12130
Fahrig, L., Baudry, J., Brotons, L., Burel, F. G., Crist, T. O., Fuller, R. J., et al. (2011). Functional landscape heterogeneity and animal biodiversity in agricultural landscapes. Ecol. Lett. 14, 101–112. doi: 10.1111/j.1461-0248.2010.01559.x
Fenster, C. B., Armbruster, W. S., Wilson, P., Dudash, M. R., and Thomson, J. D. (2004). Pollination syndromes and floral specialization. Annu. Rev. Ecol. Evol. Syst. 35, 375–403. doi: 10.1146/annurev.ecolsys.34.011802.132347
Fick, S. E., and Hijmans, R. J. (2017). WorldClim 2: new 1km spatial resolution climate surfaces for global land areas. Int. J. Climatol. 37, 4302–4315. doi: 10.1002/joc.5086
Flick, T., Feagan, S., and Fahrig, L. (2012). Effects of landscape structure on butterfly species richness and abundance in agricultural landscapes in eastern Ontario, Canada. Agric. Ecosyst. Environ. 156, 123–133. doi: 10.1016/j.agee.2012.05.006
Fox, J, and Weisberg, S (2019). An R companion to applied regression, 3rd edition. Sage, Thousand Oaks CA.
Gamonal Gomez, N., Sørensen, D. H., Chua, P. Y. S., and Sigsgaard, L. (2023). Assessing flower-visiting arthropod diversity in apple orchards through metabarcoding of environmental DNA from flowers and visual census. Environ. DNA 5, 117–131. doi: 10.1002/edn3.362
Ganuza, C., Redlich, S., Uhler, J., Tobisch, C., Rojas-Botero, S., Peters, M. K., et al. (2022). Interactive effects of climate and land use on pollinator diversity differ among taxa and scales. Science. Advances 8:eabm9359. doi: 10.1126/sciadv.abm9359
Gathmann, A., and Tscharntke, T. (2002). Foraging ranges of solitary bees. J. Anim. Ecol. 71, 757–764. doi: 10.1046/j.1365-2656.2002.00641.x
Gerard, M., Michez, D., Debat, V., Fullgrabe, L., Meeus, I., Piot, N., et al. (2018a). Stressful conditions reveal decrease in size, modification of shape but relatively stable asymmetry in bumblebee wings. Sci. Rep. 8:15169. doi: 10.1038/s41598-018-33429-4
Gerard, M., Vanderplanck, M., Franzen, M., Kuhlmann, M., Potts, S. G., Rasmont, P., et al. (2018b). Patterns of size variation in bees at a continental scale: does Bergmann’s rule apply? Oikos 127, 1095–1103. doi: 10.1111/oik.05260
Goulson, D., Lye, G. C., and Darvill, B. (2008). Decline and conservation of bumble bees. Annu. Rev. Entomol. 53, 191–208. doi: 10.1146/annurev.ento.53.103106.093454
Grab, H., Blitzer, E. J., Danforth, B., Loeb, G., and Poveda, K. (2017). Temporally dependent pollinator competition and facilitation with mass flowering crops affects yield in co-blooming crops. Sci. Rep. 7:45296. doi: 10.1038/srep45296
Hadley, A. S., and Betts, M. G. (2012). The effects of landscape fragmentation on pollination dynamics: absence of evidence not evidence of absence. Biol. Rev. 87, 526–544. doi: 10.1111/j.1469-185X.2011.00205.x
Harrison, X. A. (2014). Using observation-level random effects to model overdispersion in count data in ecology and evolution. PeerJ 2:e616. doi: 10.7717/peerj.616
Hass, A. L., Kormann, U. G., Tscharntke, T., Clough, Y., Baillod, A. B., Sirami, C., et al. (2018). Landscape configurational heterogeneity by small-scale agriculture, not crop diversity, maintains pollinators and plant reproduction in western Europe. Proc. R. Soc. B Biol. Sci. 285:20172242. doi: 10.1098/rspb.2017.2242
Hegland, S. J., Nielsen, A., Lázaro, A., Bjerknes, A.-L., and Totland, Ø. (2009). How does climate warming affect plant-pollinator interactions? Ecol. Lett. 12, 184–195. doi: 10.1111/j.1461-0248.2008.01269.x
Herrera, C. M. (2019). Complex long-term dynamics of pollinator abundance in undisturbed Mediterranean montane habitats over two decades. Ecol. Monogr. 89:e01338. doi: 10.1002/ecm.1338
Hesselbarth, M. H. K., Sciaini, M., With, K. A., Wiegand, K., and Nowosad, J. (2019). Landscapemetrics: an open-source R tool to calculate landscape metrics. Ecol. Softwares Notes 42, 1648–1657. doi: 10.1111/ecog.04617
Hodge, S., Schweiger, O., Klein, A.-M., Potts, S. G., Costa, C., Albrecht, M., et al. (2022). Design and planning of a transdisciplinary investigation into farmland pollinators: rationale, co-design, and lessons learned. Sustainability 14:10549. doi: 10.3390/su141710549
Höfer, R. J., Ayasse, M., and Kuppler, J. (2021). Bumblebee behavior on flowers, but not initial attraction, is altered by short-term drought stress. Front. Plant Sci. 11:564802. doi: 10.3389/fpls.2020.564802
Hole, D. G., Perkins, A. J., Wilson, J. D., Alexander, I. H., Grice, P. V., and Evans, A. D. (2005). Does organic farming benefit biodiversity? Biol. Conserv. 122, 113–130. doi: 10.1016/j.biocon.2004.07.018
Holland, J. G., and Bourke, A. F. G. (2015). Colony and individual life-history responses to temperature in a social insect pollinator. Funct. Ecol. 29, 1209–1217. doi: 10.1111/1365-2435.12480
Holzschuh, A., Dainese, M., González-Varo, J. P., Mudri-Stojnić, S., Riedinger, V., Rundlöf, M., et al. (2016). Mass-flowering crops dilute pollinator abundance in agricultural landscapes across Europe. Ecol. Lett. 19, 1228–1236. doi: 10.1111/ele.12657
Holzschuh, A., Dormann, C. F., Tscharntke, T., and Steffan-Dewenter, I. (2013). Mass-flowering crops enhance wild bee abundance. Oecologia 172, 477–484. doi: 10.1007/s00442-012-2515-5
Hoyle, M., Hayter, K., and Cresswell, J. E. (2007). Effect of pollinator abundance on self-fertilization and gene flow: application to GM canola. Ecol. Appl. 17, 2123–2135. doi: 10.1890/06-1972.1
IPBES (2016) in The assessment report of the intergovernmental science-policy platform on biodiversity and ecosystem services on pollinators, pollination and food production. eds. S. G. Potts, V. L. Imperatriz-Fonseca, and H. T. Ngo (Bonn, Germany: Secretariat of the Intergovernmental Science-Policy Platform on Biodiversity and Ecosystem Services), 1–36.
IUCN–International Union for Conservation of Nature (2022). The IUCN red list of threatened species.
Jauker, F., Peter, F., Wolters, V., and Diekötter, T. (2012). Early reproductive benefits of mass-flowering crops to the solitary bee Osmia rufa outbalance post-flowering disadvantages. Basic Appl. Ecol. 13, 268–276. doi: 10.1016/j.baae.2012.03.010
Jonason, D., Andersson, G. K. S., Öckinger, E., Rundlöf, M., Smith, H. G., and Bengtsson, J. (2011). Assessing the effect of the time since transition to organic farming on plants and butterflies. J. Appl. Ecol. 48, 543–550. doi: 10.1111/j.1365-2664.2011.01989.x
Kennedy, C. M., Lonsdorf, E., Neel, M. C., Williams, N. M., Ricketts, T. H., Winfree, R., et al. (2013). A global quantitative synthesis of local and landscape effects on wild bee pollinators in agroecosystems. Ecol. Lett. 16, 584–599. doi: 10.1111/ele.12082
Kerr, J. T., Pindar, A., Galpern, P., Packer, L., Potts, S. G., Roberts, S. M., et al. (2015). Climate change impacts on bumblebees converge across continents. Science 349, 177–180. doi: 10.1126/science.aaa7031
Klein, A.-M., Brittain, C., Hendrix, S. D., Thorp, R., Williams, N., and Kremen, C. (2012). Wild pollination services to California almond rely on semi-natural habitat. J. Appl. Ecol. 49, 723–732. doi: 10.1111/j.1365-2664.2012.02144.x
Knight, T. M., Steets, J. A., Vamosi, J. C., Mazer, S. J., Burd, M., Campbell, D. R., et al. (2005). Pollen limitation of plant reproduction: pattern and process. Annu. Rev. Ecol. Evol. Syst. 36, 467–497. doi: 10.1146/annurev.ecolsys.36.102403.115320
Kovács-Hostyánszki, A., Haenke, S., Batáry, P., Jauker, B., Báldi, A., Tscharntke, T., et al. (2013). Contrasting effects of mass-flowering crops on bee pollination of hedge plants at different spatial and temporal scales. Ecol. Appl. 23, 1938–1946. doi: 10.1890/12-2012.1
Kremen, C., Williams, N. M., Aizen, M. A., Gemmill-Herren, B., LeBuhn, G., Minckley, R., et al. (2007). Pollination and other ecosystem services produced by mobile organisms: a conceptual framework for the effects of land-use change. Ecol. Lett. 10, 299–314. doi: 10.1111/j.1461-0248.2007.01018.x
Lawson, D. A., and Rands, S. A. (2019). The effects of rainfall on plant–pollinator interactions. Arthropod Plant Interact. 13, 561–569. doi: 10.1007/s11829-019-09686-z
Le Provost, G., Thiele, J., Westphal, C., et al. (2021). Contrasting responses of above- and belowground diversity to multiple components of land-use intensity. Nat. Commun. 12:3918. doi: 10.1038/s41467-021-23931-1
Liao, L. H., Wu, W. Y., Dad, A., and Berenbaum, M. R. (2019). Fungicide suppression of flight performance in the honeybee (Apis mellifera) and its amelioration by quercetin. Proc. R. Soc. B: Biol. Sci. 286:20192041. doi: 10.1098/rspb.2019.2041
Lüdecke, D., Ben-Shachar, M., Patil, I., Waggoner, P., and Makowski, D. (2021). Performance: an R package for assessment, comparison and testing of statistical models. J. Open Source Software 6:3139. doi: 10.21105/joss.03139
Magrach, A., Holzschuh, A., Bartomeus, I., Riedinger, V., Roberts, S. P., Rundlöf, M., et al. (2017). Plant–pollinator networks in semi-natural grasslands are resistant to the loss of pollinators during blooming of mass-flowering crops. Ecography 41, 62–74. doi: 10.1111/ecog.02847
Mahon, N., and Hodge, S. (2022). High density floral patches attract more pollinators, but not as an ideal free distribution. Wētā 56, 51–58.
Marini, L., Bruun, H. H., Heikkinen, R. K., Helm, A., Honnay, O., Krauss, J., et al. (2012a). Traits related to species persistence and dispersal explain changes in plant communities subjected to habitat loss. Divers. Distrib. 18, 898–908. doi: 10.1111/j.1472-4642.2012.00893.x
Marini, L., Quaranta, M., Fontana, P., Biesmeijer, J. C., and Bommarco, R. (2012b). Landscape context and elevation affect pollinator communities in intensive apple orchards. Basic Appl. Ecol. 13, 681–689. doi: 10.1016/j.baae.2012.09.003
Marshall, E. J. P., and Moonen, A. C. (2002). Field margins in northern Europe: their functions and interactions with agriculture. Agric. Ecosyst. Environ. 89, 5–21. doi: 10.1016/S0167-8809(01)00315-2
Martin, E. A., Dainese, M., Clough, Y., Báldi, A., Bommarco, R., Gagic, V., et al. (2019). The interplay of landscape composition and configuration: new pathways to manage functional biodiversity and agroecosystem services across Europe. Ecol. Lett. 22, 1083–1094. doi: 10.1111/ele.13265
Martinet, B., Zambra, E., Przybyla, K., Lecocq, T., Anselmo, A., Nonclercq, D., et al. (2021). Mating under climate change: impact of simulated heatwaves on the reproduction of model pollinators. Funct. Ecol. 35, 739–752. doi: 10.1111/1365-2435.13738
Martínez-Núñez, C., Kleijn, D., Ganuza, C., Heupink, D., Raemakers, I., Vertommen, W., et al. (2022). Temporal and spatial heterogeneity of semi-natural habitat, but not crop diversity, is correlated with landscape pollinator richness. J. Appl. Ecol. 59, 1258–1267. doi: 10.1111/1365-2664.14137
Maurer, C., Sutter, L., Martínez-Núñez, C., Pellissier, L., and Albrecht, M. (2022). Different types of semi-natural habitat are required to sustain diverse wild bee communities across agricultural landscapes. J. Appl. Ecol. 59, 2604–2615. doi: 10.1111/1365-2664.14260
McKinney, M. L. (2008). Effects of urbanization on species richness: a review of plants and animals. Urban Ecosyst. 11, 161–176. doi: 10.1007/s11252-007-0045-4
Meyer, B., Jauker, F., and Steffan-Dewenter, I. (2009). Contrasting resource-dependent responses of hover fly richness and density to landscape structure. Basic Appl. Ecol. 10, 178–186. doi: 10.1016/j.baae.2008.01.001
Miličić, M., Vujić, A., and Cardoso, P. (2018). Effects of climate change on the distribution of hover fly species (Diptera: Syrphidae) in Southeast Europe. Biodivers. Conserv. 27, 1173–1187. doi: 10.1007/s10531-017-1486-6
Miller-Struttmann, N. E., Geib, J. C., Franklin, J. D., Kevan, P. G., Holdo, R. M., Ebert-May, D., et al. (2015). Functional mismatch in a bumble bee pollination mutualism under climate change. Science (New York, N.Y.) 349, 1541–1544. doi: 10.1126/science.aab0868
Mooney, H., Larigauderie, A., Cesario, M., Elmquist, T., Hoegh-Guldberg, O., Lavorel, S., et al. (2009). Biodiversity, climate change, and ecosystem services. Curr. Opin. Environ. Sustain. 1, 46–54. doi: 10.1016/j.cosust.2009.07.006
Nayak, G. K., Roberts, S. P. M., Garratt, M., Breeze, T. D., Tscheulin, T., Harrison-Cripps, J., et al. (2015). Interactive effect of floral abundance and semi-natural habitats on pollinators in field beans (Vicia faba). Agric. Ecosyst. Environ. 199, 58–66. doi: 10.1016/j.agee.2014.08.016
Nieto, A., Roberts, S. P.M., Kemp, J., Rasmontv, P., Kuhlmann, M., Criado, M. G., et al. (2014). European red list of bees.
O’Neill, K. M., O’Neill, R. P., Kemp, W. P., and Delphia, C. M. (2011). Effect of temperature on post-wintering development and total lipid content of alfalfa leafcutting bees. Environ. Entomol. 40, 917–930. doi: 10.1603/EN10320
Oksanen, J., Simpson, G., Blanchet, F., Kindt, R., Legendre, P., Minchin, P., et al. (2022). Vegan: Community ecology package_. R package version 2.6-4. Available at: https://CRAN.R-project.org/package=vegan (Accessed October 12, 2022).
Ollerton, J., Winfree, R., and Tarrant, S. (2011). How many flowering plants are pollinated by animals? Oikos 120, 321–326. doi: 10.1111/j.1600-0706.2010.18644.x
Osterman, J., Theodorou, P., Radzevičiūtė, R., Schnitker, P., and Paxton, R. J. (2021). Apple pollination is ensured by wild bees when honey bees are drawn away from orchards by a mass co-flowering crop, oilseed rape. Agric. Ecosyst. Environ. 315:107383. doi: 10.1016/j.agee.2021.107383
O'Toole, C. (1994). “Who cares for the solitary bees?” in Forage for bee in an agricultural landscape. ed. A. Matheson (Cardiff, UK: International Bee Research Association), 47–56.
Papanikolaou, A. D., Kühn, I., Frenzel, M., and Schweiger, O. (2017). Semi-natural habitats mitigate the effects of temperature rise on wild bees. J. Appl. Ecol. 54, 527–536. doi: 10.1111/1365-2664.12763
Patiny, S., Rasmont, P., and Michez, D. (2009). A survey and review of the status of wild bees in the West-Palaearctic region. Apidologie 40, 313–331. doi: 10.1051/apido/2009028
Perović, D., Gámez-Virués, S., Börschig, C., Klein, A.-M., Krauss, J., Steckel, J., et al. (2015). Configurational landscape heterogeneity shapes functional community composition of grassland butterflies. J. Appl. Ecol. 52, 505–513. doi: 10.1111/1365-2664.12394
Potts, S. G., Biesmeijer, J. C., Kremen, C., Neumann, P., Schweiger, O., and Kunin, W. E. (2010). Global pollinator declines: trends, impacts and drivers. Trends Ecol. Evol. 25, 345–353. doi: 10.1016/j.tree.2010.01.007
Proesmans, W., Albrecht, M., Gajda, A., Neumann, P., Paxton, R. J., Pioz, M., et al. (2021). Pathways for novel epidemiology: plant-pollinator-pathogen networks and global change. Trends Ecol. Evol. 36, 623–636. doi: 10.1016/j.tree.2021.03.006
Pyke, G. H., and Waser, N. M. (1981). The production of dilute nectars by hummingbird and honeyeater flowers. Biotropica 13, 260–270. doi: 10.2307/2387804
Quinet, M., Warzée, M., Vanderplanck, M., Michez, D., Lognay, G., and Jacquemart, A.-L. (2016). Do floral resources influence pollination rates and subsequent fruit set in pear (Pyrus communis L.) and apple (Malus x domestica Borkh) cultivars? Eur. J. Agron. 77, 59–69. doi: 10.1016/j.eja.2016.04.001
R Core Team (2020). R: A language and environment for statistical computing. Vienna, Austria: R Foundation for Statistical Computing. Available at: http://www.r-project.org/index.html
Raderschall, C. A., Bommarco, R., Lindström, S. A. M., and Lundin, O. (2021). Landscape crop diversity and semi-natural habitat affect crop pollinators, pollination benefit and yield. Agric. Ecosyst. Environ. 306:107189. doi: 10.1016/j.agee.2020.107189
Radmacher, S., and Strohm, E. (2010). Factors affecting offspring body size in the solitary bee Osmia bicornis (Hymenoptera, Megachilidae). Apidologie 41, 169–177. doi: 10.1051/apido/2009064
Rasmont, P., Franzén, M., Lecocq, T., Harpke, A., Roberts, S., Biesmeijer, J. C., et al. (2015). Climatic risk and distribution atlas of European bumblebees. BioRisk 10, 1–236. doi: 10.3897/biorisk.10.4749
Rasmont, P., and Iserbyt, I. (2010-2014). Atlas of the European bees: Genus Bombus. 3d Edition. STEP Project, Atlas Hymenoptera, Mons, Gembloux.
Riedinger, V., Mitesser, O., Hovestadt, T., Steffan-Dewenter, I., and Holzschuh, A. (2015). Annual dynamics of wild bee densities: attractiveness and productivity effects of oilseed rape. Ecology 96, 1351–1360. doi: 10.1890/14-1124.1
Robinson, S. V. J., Hoover, S. E., Pernal, S. F., and Cartar, R. V. (2022). Optimal distributions of central-place foragers: honey bee foraging in a mass flowering crop. Behav. Ecol. 33, 386–397. doi: 10.1093/beheco/arab143
Rollin, O., Bretagnolle, V., Decourtye, A., Aptel, J., Michel, N., Vaissiere, B. E., et al. (2013). Differences of floral resource use between honey bees and wild bees in an intensive farming system. Agric. Ecosyst. Environ. 179, 78–86. doi: 10.1016/j.agee.2013.07.007
Rondeau, S., and Raine, N. E. (2022). Fungicides and bees: a review of exposure and risk. Environ. Int. 165:107311. doi: 10.1016/j.envint.2022.107311
Rundlöf, M., Bengtsson, J., and Smith, H. G. (2008a). Local and landscape effects of organic farming on butterfly species richness and abundance. J. Appl. Ecol. 45, 813–820. doi: 10.1111/j.1365-2664.2007.01448.x
Rundlöf, M., Nilsson, H., and Smith, H. G. (2008b). Interacting effects of farming practice and landscape context on bumble bees. Biol. Conserv. 141, 417–426. doi: 10.1016/j.biocon.2007.10.011
Russo, L., Park, M., Gibbs, J., and Danforth, B. (2015). The challenge of accurately documenting bee species richness in agroecosystems: bee diversity in eastern apple orchards. Ecol. Evol. 5, 3531–3540. doi: 10.1002/ece3.1582
Scheper, J., Holzschuh, A., Kuussaari, M., Potts, S. G., Rundlöf, M., Smith, H. G., et al. (2013). Environmental factors driving the effectiveness of European Agri-environmental measures in mitigating pollinator loss – a meta-analysis. Ecol. Lett. 16, 912–920. doi: 10.1111/ele.12128
Seeley, T.D. (1995). The wisdom of the hive: The social physiology of honey bee colonies. Harvard University Press, Cambridge
Senapathi, D., Goddard, M. A., Kunin, W. E., and Baldock, K. C. R. (2017). Landscape impacts on pollinator communities in temperate systems: evidence and knowledge gaps. Funct. Ecol. 31, 26–37. doi: 10.1111/1365-2435.12809
Seppelt, R., Beckmann, M., Ceauşu, S., Cord, A. F., Gerstner, K., Gurevitch, J., et al. (2016). Harmonizing biodiversity conservation and productivity in the context of increasing demands on landscapes. Bioscience 66, 890–896. doi: 10.1093/biosci/biw004
Settele, J., Kudrna, O., Harpke, A., Kühn, I., van Swaay, C., Verovnik, R., et al. (2008). Climatic risk atlas of European butterflies. BioRisk 1, 1–712. doi: 10.3897/biorisk.1
Šlachta, M., Erban, T., Votavová, A., Bešta, T., Skalský, M., Václavíková, M., et al. (2020). Domestic gardens mitigate risk of exposure of pollinators to pesticides—an urban-rural case study using a red Mason bee species for biomonitoring. Sustainability 12:9427. doi: 10.3390/su12229427
Speight, M. C. D., Monteil, C., Castella, E., and Sarthou, J.-P. (2010). “StN 2010” in Syrph the net on CD, Issue 7. The database of European Syrphidae. eds. M. C. D. Speight, E. Castella, J.-P. Sarthou, and C. Monteil (Dublin: Syrph the Net Publications)
Stanley, D. A., Knight, M. E., and Stout, J. C. (2013). Ecological variation in response to mass-flowering oilseed rape and surrounding landscape composition by members of a cryptic bumblebee complex. PLoS One 8:e65516. doi: 10.1371/journal.pone.0065516
Stanley, D. A., and Stout, J. C. (2013). Quantifying the impacts of bioenergy crops on pollinating insect abundance and diversity: a field-scale evaluation reveals taxon-specific responses. J. Appl. Ecol. 50, 335–344. doi: 10.1111/1365-2664.12060
Steffan-Dewenter, I., Münzenberg, U., Bürger, C., Thies, C., and Tscharntke, T. (2002). Scale-dependent effects of landscape context on three pollinator guilds. Ecology 83, 1421–1432. doi: 10.1890/0012-9658(2002)083[1421:SDEOLC]2.0.CO;2
Steffan-Dewenter, I., and Tscharntke, T. (1999). Effects of habitat isolation on pollinator communities and seed set. Oecologia 121, 432–440. doi: 10.1007/s004420050949
Tepedino, V., and Parker, F. (1986). Effect of rearing temperature on mortality, second-generation emergence, and size of adult in Megachile rotundata (Hymenoptera: Megachilidae). J. Econ. Entomol. 79, 974–977. doi: 10.1093/jee/79.4.974
Timberlake, T. P., Vaughan, I. P., and Memmott, J. (2019). Phenology of farmland floral resources reveals seasonal gaps in nectar availability for bumblebees. J. Appl. Ecol. 56, 1585–1596. doi: 10.1111/1365-2664.13403
Van Reeth, C., Michel, N., Bockstaller, C., and Caro, G. (2018). Current and previous spatial distributions of oilseed rape fields influence the abundance and the body size of a solitary wild bee, Andrena cineraria, in permanent grasslands. PLoS One 13:e0197684. doi: 10.1371/journal.pone.0197684
van Swaay, C. A. M., Cuttelod, Annabelle, Collins, Sue, Maes, Dirk, Munguira, Miguel López, Martina, Šašić, et al. (2010). European red list of butterflies Publications Office of the European Union, Luxembourg.
van Swaay, C., Maes, D., Collins, S., Munguira, M. L., Šašić, M., Settele, J., et al. (2011). Applying IUCN criteria to invertebrates: how red is the red list of European butterflies? Biol. Conserv. 144, 470–478. doi: 10.1016/j.biocon.2010.09.034
Vanbergen, A. J., and Initiative, I. P. (2013). Threats to an ecosystem service: pressures on pollinators. Front. Ecol. Environ. 11, 251–259. doi: 10.1890/120126
Vasiliev, D., and Greenwood, S. (2021). The role of climate change in pollinator decline across the Northern Hemisphere is underestimated. Sci. Total Environ. 775:145788. doi: 10.1016/j.scitotenv.2021.145788
Vicens, N., and Bosch, J. (2000). Weather-dependent pollinator activity in an apple orchard, with special reference to Osmia cornuta and Apis mellifera (Hymenoptera: Megachilidae and Apidae). Environ. Entomol. 29, 413–420. doi: 10.1603/0046-225X-29.3.413
Vrdoljak, S. M., Samways, M. J., and Simaika, J. P. (2016). Pollinator conservation at the local scale: flower density, diversity and community structure increase flower visiting insect activity to mixed floral stands. J. Insect Conserv. 20, 711–721. doi: 10.1007/s10841-016-9904-8
Warren, M. S., Maes, D., van Swaay, C. A. M., Goffart, P., Dyck, H. V., Bourn, N. A. D., et al. (2021). The decline of butterflies in Europe: problems, significance, and possible solutions. Proc. Natl. Acad. Sci. 118. doi: 10.1073/pnas.2002551117
Weidenmüller, A., Kleineidam, C., and Tautz, J. (2002). Collective control of nest climate parameters in bumblebee colonies. Anim. Behav. 63, 1065–1071. doi: 10.1006/anbe.2002.3020
Westphal, C., Steffan-Dewenter, I., and Tscharntke, T. (2003). Mass flowering crops enhance pollinator densities at a landscape scale. Ecol. Lett. 6, 961–965. doi: 10.1046/j.1461-0248.2003.00523.x
Winfree, R., Aguilar, R., Vázquez, D. P., LeBuhn, G., and Aizen, M. A. (2009). A meta-analysis of bees’ responses to anthropogenic disturbance. Ecology 90, 2068–2076. doi: 10.1890/08-1245.1
Woodcock, B. A., Isaac, N. J. B., Bullock, J. M., Roy, D. B., Garthwaite, D. G., Crowe, A., et al. (2016). Impacts of neonicotinoid use on long-term population changes in wild bees in England. Nat. Commun. 7:12459. doi: 10.1038/ncomms12459
Keywords: habitat heterogeneity, intensity gradient of land-use, pollinators, standardized approach, European biogeographic regions
Citation: Bottero I, Dominik C, Schweiger O, Albrecht M, Attridge E, Brown MJF, Cini E, Costa C, De la Rúa P, de Miranda JR, Di Prisco G, Dzul Uuh D, Hodge S, Ivarsson K, Knauer AC, Klein A-M, Mänd M, Martínez-López V, Medrzycki P, Pereira-Peixoto H, Potts S, Raimets R, Rundlöf M, Schwarz JM, Senapathi D, Tamburini G, Talaván ET and Stout JC (2023) Impact of landscape configuration and composition on pollinator communities across different European biogeographic regions. Front. Ecol. Evol. 11:1128228. doi: 10.3389/fevo.2023.1128228
Edited by:
Pierre Lau, United States Department of Agriculture (USDA), United StatesReviewed by:
Joshua Campbell, United States Department of Agriculture (USDA), United StatesHannah Levenson, North Carolina State University, United States
Copyright © 2023 Bottero, Dominik, Schweiger, Albrecht, Attridge, Brown, Cini, Costa, De la Rúa, de Miranda, Di Prisco, Dzul Uuh, Hodge, Ivarsson, Knauer, Klein, Mänd, Martínez-López, Medrzycki, Pereira-Peixoto, Potts, Raimets, Rundlöf, Schwarz, Senapathi, Tamburini, Talaván and Stout. This is an open-access article distributed under the terms of the Creative Commons Attribution License (CC BY). The use, distribution or reproduction in other forums is permitted, provided the original author(s) and the copyright owner(s) are credited and that the original publication in this journal is cited, in accordance with accepted academic practice. No use, distribution or reproduction is permitted which does not comply with these terms.
*Correspondence: Irene Bottero, Ym90dGVyb2lAdGNkLmll
†These authors have contributed equally to this work and share first authorship