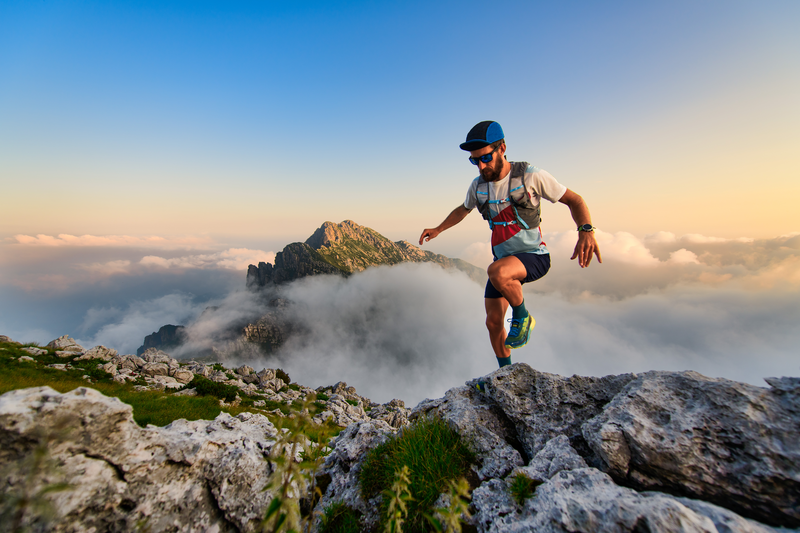
95% of researchers rate our articles as excellent or good
Learn more about the work of our research integrity team to safeguard the quality of each article we publish.
Find out more
ORIGINAL RESEARCH article
Front. Ecol. Evol. , 22 February 2023
Sec. Models in Ecology and Evolution
Volume 11 - 2023 | https://doi.org/10.3389/fevo.2023.1113474
Introduction: Invasive alien plants (IAPs) are major hazards to biodiversity, human health, and the agricultural economy. As one of the most aggressive species of IAPs, the distribution area of Centaurea solstitialis L. has increased exponentially in the past two years since its invasion into Xinjiang, China, in July 2014. Predicting the potential geographic distributions (PGDs) of C. solstitialis in China can provide theoretical support for preventing the continued spread of this weed.
Methods: In this study, based on 5,969 valid occurrence records of C. solstitialis and 33 environmental variables, we constructed an ensemble model to predict suitable habitats for C. solstitialis under climate change scenarios.
Results: Our results showed that the mean true skill statistic (TSS) values, area under the receiver operating characteristic (ROC) curve (AUC), and Cohen’s Kappa (KAPPA) for the ensemble model were 0.954, 0.996, and 0.943, respectively. The ensemble model yielded more precise predictions than those of the single model. Temperature seasonality (Bio4), minimum temperature of the coldest month (Bio6), precipitation of the driest month (Bio14), and human influence index (HII) have significantly disrupted the PGDs of C. solstitialis in China. The total (high) suitability habitat area of C. solstitialis in China was 275.91 × 104 (67.78 × 104) km2, accounting for 71.26 (7.06)% of China. The PGDs of C. solstitialis in China under the current climate were mainly in East China (Shandong, Jiangsu, Shanghai, Zhejiang, and Anhui), Central China (Henan, southwestern Shanxi, southern Shaanxi, southern Gansu, Hubei, Hunan, Jiangxi, Chongqing, and Guizhou), and South China (southern Tibet, eastern Sichuan, Yunnan, Guangxi, Guangdong, Fujian, and Taiwan). Under future climate scenarios, the total suitability habitat area for C. solstitialis will expand, whereas the high suitability habitat area will decrease.
Discussion: The main manifestation is that the shift of southeast China into a moderate suitability habitat, and the total suitability habitats will be extended to northwest China. More focus needs to be placed on preventing further spread of C. solstitialis in northwest China.
Invasive alien plants (IAPs) profoundly influence productivity, biomass, and carbon fixation in natural ecosystems (Early et al., 2016; Sitzia et al., 2018; Skubel et al., 2020). It is subject to the effects of global environmental changes, particularly climate warming (Ju et al., 2015; Ferrarini et al., 2016). Climate warming makes IAPs more prone to encroach on unoccupied ecological niches, thereby increasing the risk of plant invasion (Moraira et al., 2020; Turbelin and Catford, 2021). It has been documented that there are above 13,000 naturalized plant species worldwide at present (Majewska, 2018; Thapa et al., 2018; Bartz and Kowarik, 2019). In Europe alone, there are 12,000 species, 15% of which are known to negatively impact ecosystem biodiversity (Kleunen et al., 2015). To date, more than 268 IAPs have been discovered in China. For instance, native to North America, Ambrosia artemisiifolia L. invaded several coastal provinces of China in the early 1930s, resulting in the disruption of native ecosystems (Csontos et al., 2010). Mikania micrantha Kunth, which is native to South America and was discovered in Hong Kong in 1919, has encroached on nearly 20% of the native ecological niche as a weed (Banerjee et al., 2019). Among IAPs, Asteraceae accounts for nearly 26% (49 species in total) in China, and Asteraceae are not only a threat to human health but also an ecological hazard as a result of their toxic pollen, seeds, and spiny hairs (Huang et al., 2018). Due to its rapid growth and high biomass, the invasive Solidago canadensis L. in Shanghai and Tithonia diversifolia L. in Yunnan were able to form new communities promptly in the second year, thus dissipating local biodiversity (Zhou et al., 2004). The damage of Eupatorium odoratum L. has resulted in more than 20% annual crop failure in most of southern Yunnan (Fang et al., 2021), causing significant economic losses. Moreover, rapid climate warming and increased anthropogenic activities exacerbate the current situation of Asteraceae invasion (Teller et al., 2016; Fitchett et al., 2017). Therefore, it is essential to elucidate the patterns of Asteraceae under warming conditions in order to develop early warning, prevention, and control strategies in China.
Centaurea solstitialis L. (Asteraceae; common name: yellow starthistle), an aggressive IAP worldwide, is native to southern Europe and western Eurasia (Mediterranean countries) and is widely distributed in Europe, Oceania, Africa, and Asia (Tomaso, 2005). It is a winter annual plant that is propagated from seeds germinating in the Autumn or Spring. The bracts are numerous and end with hard yellow spines up to 1.2-2.5 cm long, with one or two pairs of very short spines at the base (CABI, 2022). Seeds of C. solstitialis can be spread naturally by media such as wind or water (Pitcairn et al., 2021), accidental dispersal by pollinators and wood products (Eagle et al., 2007), or by trade, such as agricultural products intended for export (Maddox, 1981). Centaurea solstitialis L. first invaded California, USA (North America) in 1869, and was carried by contaminated seeds of M. Sativa (Tomaso, 2005; Pitcairn et al., 2006). After the 21st century, one case of C. solstitialis has been recorded in China (Xinyuan County, Yili Kazakh Autonomous Prefecture, Xinjiang), which was attributed to its strong natural dispersal capacity (Yang et al., 2017), where it dispersed along roadsides, grasslands, water banks, abandoned fields, and garbage dumps in Xinyuan County (Fornasari et al., 1991). According to the previous study, the long spines of C. solstitialis have grown up to 2 cm, forming closed colonies that make it difficult for plants to survive and livestock to feed, which hinders the development of agriculture, forestry, and livestock in Xinjiang (Yang et al., 2017). As such, it has been classified as a priority management IAP in China. Moreover, no studies have yet gathered on the geographical distribution of C. solstitialis in China. Thus, elucidating the patterns of C. solstitialis under climate change conditions can provide an accurate measurement of the dynamics of its potential geographical distribution (PGDs) and guide prevention strategies in China.
Multi-species distribution models (SDMs), including the maximum entropy model (Maxent), CLIMEX, generalized linear model (GLM), genetic algorithm for rule-set prediction (GARP), multiple adaptive regression spline (MARS) model, surface range envelope (SRE), flexible discriminant analysis (FDA), categorical regression tree analysis (CTA), gradient boosting model (GBM), random forest (RF), and artificial neural network (ANN), have substantial practical applications in identifying PGDs for IAPs under both current and future climate change conditions (Merow et al., 2017; Fusco et al., 2019; McMahon et al., 2021). Previous studies have usually used a single model, such as Maxent or GARP, to predict the PGDs of IAPs (Tu et al., 2021). However, in subsequent studies, the biomod platform was generated in 2003 to bring the SDMs prediction results closer to real-life conditions (Thuiller et al., 2003). Numerous studies have used single SDMs or ensemble models (EM) in the biomod2 platform to predict the PGDs of IAPs, and studies have shown that EM is gaining increasing attention for predicting the PGDs of IAPs because it provides more reliable results than single models (Wagner et al., 2017; Gong et al., 2020; Fang et al., 2021).
In this study, an optimal EM consisting of a combination of eight single models was used to predict the PGDs of C. solstitialis under current and future climate change conditions. We propose the hypothesis that PGDs of C. solstitialis in China will shift to higher latitudes (mainly toward the northwest) in the future. Thus, we (1) selected four optimal SDMs to combine with an EM model that showed high accuracy after simulation trials; (2) analyzed key environmental variables influencing PGDs of C. solstitialis; (3) predicted PGDs of C. solstitialis under current climate and climate change conditions in the 2030s and 2050s using an optimal EM model; and (4) analyzed centroid shifts of C. solstitialis under current climate conditions versus climate change conditions in the 2030s and 2050s. Our findings can help better formulate strategies related to the prevention of C. solstitialis invasion in China.
The 5,712 occurrence records of C. solstitialis were obtained from the Global Biodiversity Information Facility (GBIF),1 one occurrence record of C. solstitialis was obtained from the Chinese Virtual Herbarium (CVH),2 and 925 occurrence records of C. solstitialis were obtained from the National Specimen Information Infrastructure (NSII)3. Finally, 6,638 occurrence records of C. solstitialis worldwide were obtained from databases. Thereafter, we removed occurrence data that did not contain detailed geographic information. ENMTools (Warren et al., 2010) was used to screen the occurrence data such that only one distribution point was retained within each 5 km × 5 km raster. Finally, we obtained 5,969 occurrence records of C. solstitialis for modeling PGDs in China using SDMs (Figure 1).
We obtained the current climate data (19 bioclimatic variables) from the PaleoClim database4 with a resolution of 2.5 for 1979–2013 (Brown et al., 2018). Future climate data, as well as altitude, originated from the WorldClim database version 1.45 (Fick and Hijmans, 2017). Two representative concentration pathways, RCP2.6, and RCP8.5, from future climate data with the Community Climate System Model (CCSM) 4.0, for the 2030s and 2050s, were used. In addition, several soil variables were downloaded from the Harmonized World Soil Database6, and the Human Influence Index (HII) was obtained from the Global Human Influence Index (Geographic)7. When the correlation coefficient (Pearson’s) of any two environmental variables is greater than 0.8 (|r| > 0.8), the more meaningful one retains. Ultimately, 11 environmental variables were effectively modeled: mean diurnal range (bio2), temperature seasonality (bio4), minimum temperature of the coldest month (bio6), precipitation of the driest month (bio14), precipitation seasonality (bio15), precipitation of the warmest quarter (bio18), precipitation of the coldest quarter (bio19), Altitude, T_CaCO3, T_ph (H2O), and HII.
In our work, to construct an optimal EM, we modeled PGDs of C. solstitialis using eight different algorithms (GLM, GBM, CTA, ANN, SRE, FDA, RF, and MaxEnt) with the biomod2 package (Thuiller et al., 2016) in R Studio version 4.2.2 based on occurrence records and environmental variables. In each process, 75% of the occurrence records were randomly selected as the training dataset, and the opposite 25% were selected as the testing dataset. The random processing for the two types of data was repeated five times. In addition, 1,000 pseudoabsence points were randomly selected and repeated three times. Ultimately, 120 models were available. To evaluate the performance of the eight models, four individual models with high accuracy were selected to construct an EM. Moreover, the EM was used to predict the PGDs of C. solstitialis under different climate conditions.
The true skill statistic (TSS) (Allouche et al., 2006), area under the receiver operating characteristic (ROC) curve (AUC), and Cohen’s kappa (KAPPA) values were used to assess the accuracy of the models (Fielding and Bell, 1997; Bolliger et al., 2000). The ROC curve is a sensitivity curve in which the horizontal and vertical coordinates indicate the false-positive rate (1-specificity) and true-positive rate (1-omission rate), respectively (Peterson et al., 2008). KAPPA indicates random prediction accuracy, which is influenced by incidence data and thresholds (Monserud and Leemans, 1992). As a rule, the accuracy of the SDMs improves with an increase in these three values.
We used the Cutoff value of the EM as the threshold between PGDs and non-PGDs in this study (Supplementary Table 2). The ASCII raster layer, which ranged from 0 to 1,000 of the EM, indicated the occurrence probability (p) of C. solstitialis. Finally, we divided the PGDs of C. solstitialis into four segments using ArcGIS: high-suitability habitat (500 ≤ P ≤ 1000), moderate-suitability habitat (400 ≤ P < 600), low-suitability habitat (200 ≤ P < 400), and unsuitable habitat (0 ≤ P < 200). The centroids shift of PGDs in IAPs can be captured scientifically and visually using the statistical tools of ArcGIS software (Bates et al., 2020).
In the current climate conditions, the evaluation accuracies were calculated for several SDMs, including GLM, GBM, CTA, ANN, SRE, FDA, RF, and MaxEnt. In our results, GLM, GBM, FDA, and RF were more accurate than CTA, ANN, SRE, and MaxEnt (Figure 2). The mean TSS values for GLM, GBM, FDA, and RF were 0.872, 0.886, 0.833, and 0.877, respectively. The mean AUC values for GLM, GBM, FDA, and RF were 0.968, 0.981, 0.943, and 0.973, respectively. The mean KAPPA values for GLM, GBM, FDA, and RF were 0.833, 0.833, 0.795, and 0.849, respectively. Therefore, the EM was constructed using these four models. The mean TSS, AUC, and KAPPA values for the EM were 0.954, 0.996, and 0.943, respectively. The results revealed that the EM improved the fitting accuracy and reduced the fitting uncertainty. These results demonstrate that the PGDs of C. solstitialis predicted using the EM were more reliable than those obtained using any of the single models.
Figure 2. True skill statistics (TSS), area under the receiver operating characteristic curve (AUC), and Cohen’s Kappa (KAPPA) values of eight species distribution models.
We estimated and analyzed the contribution values among environmental variables based on GBM, GLM, RF, and FDA. Our results indicated that the top three environmental variables, which significantly influenced the PGDs of C. solstitialis with the highest mean contribution values, were temperature seasonality (Bio4, 0.049), precipitation of the coldest quarter (Bio19, 0.200), and human influence index (HII, 0.136) in the GBM, Figure 3A; temperature seasonality (Bio4, 0.217), precipitation of the driest month (Bio14, 0.582), and precipitation of the warmest quarter (Bio18, 0.203) in the GLM, Figure 3B; temperature seasonality (Bio4, 0.124), minimum temperature of the coldest month (Bio6, 0.281), and precipitation of the coldest quarter (Bio19, 0.089) in the RF, Figure 3C; and minimum temperature of the coldest month (Bio6, 0.561), precipitation of the driest month (Bio14, 0.250), and human influence index (HII, 0.200) in the FDA, Figure 3D. Overall, the prominent environmental variables influencing the PGDs of C. solstitialis were one temperature, two precipitation, and one human influence variable: temperature seasonality (Bio4), minimum temperature of the coldest month (Bio6), precipitation of the driest month (Bio14), and human influence index (HII).
Figure 3. Contribution values of the environmental variables in (A) (GBM), (B) (GLM), (C) (RF), and (D) (FDA).
In the Mediterranean regions, C. solstitialis was first discovered in 2014 in Xinjiang (83.25°E, 43.42°N), with a unique recorded occurrence in China at present, and it has not been successfully colonized elsewhere. We predicted the PGDs of C. solstitialis in China under the current climate conditions using the EM in biomod2. As shown in Figure 4, the PGDs of C. solstitialis under the current climate conditions were mainly in East China (Shandong, Jiangsu, Shanghai, Zhejiang, and Anhui), Central China (Henan, southwestern Shanxi, southern Shaanxi, southern Gansu, Hubei, Hunan, Jiangxi, Chongqing, and Guizhou), and South China (southern Tibet, eastern Sichuan, Yunnan, Guangxi, Guangdong, Fujian, and Taiwan). Specifically, the high-suitability habitat area was 67.78 × 104km2, accounting for 7.06% of China, including Jiangsu, Zhejiang, southern Hubei, southern Hunan, Jiangxi, and Fujian, mainly distributed in East and Central China south of the Yangtze River basin. The moderate suitability habitat area was 101.76 × 104 km2, accounting for 10.6% of China, including Anhui, southern Henan, northern Hubei, northern Hunan, northern Yunnan, Guizhou, and Chongqing. The low suitability habitat area was 106.37 × 104 km2, accounting for 11.08% of China, including Shandong, southern Hebei, northern Henan, southwestern Shanxi, Shaanxi, a small part of southeastern Gansu, southeastern Sichuan, central Yunnan, central Guangxi, central Guangdong, central Taiwan, and a small part of Xinjiang and Tibet. The moderate suitability and low suitability habitat zones were distributed in the northwest direction in turn, where the dividing line between them was nearly consistent with the 800 mm isoprecipitation line.
The PGDs of C. solstitialis under RCP2.6 and RCP8.5, in the 2030s and the 2050s, are shown in Figure 5. The changes in PGDs under climate change are shown in Figure 6. Under future climate change scenarios, PGDs of C. solstitialis were also distributed in East, Central, and South China. The significant change was that the range of high suitability habitats for C. solstitialis decreased and became sparse compared to the current climate. Under future climate change, parts of the high suitability habitats in Central China have changed to moderate suitability habitats.
Figure 6. Changes in PGDs of Centaurea solstitialis in China under future climate conditions based on the EM.
The low suitability habitat areas of C. solstitialis for the 2030s and 2050s were 123.07 × 104 and 125.67 × 104 km2, respectively, under RCP2.6 (accounting for 12.82 and 13.09% of the Chinese total area, respectively), and 145.88 × 104 and 169.58 × 104 km2, respectively, under RCP8.5 (accounting for 15.2 and 17.66% of the Chinese total area, respectively). The low-suitability habitat areas showed an increasing trend from the current to the 2030s and the 2050s, and increased significantly under RCP8.5, in the 2050s (Figure 7).
Figure 7. Area of Suitable habitats of Centaurea solstitialis for current and future climate conditions.
The moderate suitability habitat areas of C. solstitialis for the 2030s and 2050s were 112.61 × 104 and 104.34 × 104 km2, respectively, under RCP2.6 (accounting for 11.73 and 10.87% of the Chinese total area, respectively), and 116.64 × 104 and 107.67 × 104 km2, respectively, under RCP8.5 (accounting for 12.15 and 11.22% of the Chinese total area, respectively). Moderate suitability habitat areas showed an increasing trend, followed by a decrease from the current to the 2030s and the 2050s, and increased significantly under RCP8.5, in the 2030s (Figure 7).
The high suitability habitats of C. solstitialis for the 2030s and 2050s were 49.82 × 104 and 51.59 × 104 km2, respectively, under RCP2.6 (accounting for 5.19 and 5.37% of the Chinese total area, respectively), and 35.67 × 104 and 25.22 × 104 km2, respectively, under RCP8.5 (accounting for 3.71 and 2.63% of the Chinese total area, respectively). The high suitability habitats showed a decreasing trend from the present to the 2030s and 2050s and decreased significantly under RCP8.5 in the 2030s (Figure 7).
The total suitability habitats of C. solstitialis for the 2030s and 2050s were 285.51 × 104 and 281.64 × 104 km2, respectively, under RCP2.6 (accounting for 29.74 and 29.33% of the Chinese total area, respectively), and 298.28 × 104 and 302.55 × 104 km2, respectively, under RCP8.5 (accounting for 31.06 and 31.51% of the Chinese total area, respectively). Compared to the current climate conditions, the total suitability habitats in the 2030s under RCP2.6 and RCP8.5, and the 2050s under RCP2.6, showed a generally increasing trend and increased significantly in the 2030s under RCP8.5.
The centroids of C. solstitialis suitable habitats are shown in Figure 8. In the current climate conditions, the centroid was located at the point (110.34°E, 29.23°N). Under RCP2.6, the centroid of the suitable habitats shifted from the present to the point (110.05°E, 29.7°N) in the 2030s and then to the point (109.56°E, 29.68°N) in the 2050s; it shifted 0.29°E and 0.47°N from the current state to the 2030s and 0.49°E and 0.02°N from the 2030s to 2050s. Under RCP8.5, from the present to the 2030s, the centroid of suitable habitats shifted to the point (109.4°E, 30.01°N) and then to the next point (108.94°E, 30.46°N) in the 2050s. It shifted 0.94°E and 0.78°N from the current state to the 2030s and shifted 0.46°E and 0.45°N from the 2030s to 2050s (Figure 8).
In summary, under both climate conditions, the centroid of C. solstitialis suitable habitats showed a northwestward shift in the 2030s and 2050s. The centroid of suitable habitats under RCP8.5 moved the furthest distance, approximately 1.68 km. Therefore, the centroid of C. solstitialis suitable habitats tended to shift to the northwest, lower longitudes, and higher latitudes with future climate change conditions (Figure 8).
Centaurea solstitialis L. is considered an important invasive plant worldwide, and its invasion in China has disturbed local biodiversity and led to livestock mortality as well as crop yield reduction; however, the current concerns are not obvious. Existing studies have mainly focused on the description of its morphological characteristics and speculation of its hazard and transmission sources (Yang et al., 2017). Only a few studies have focused on the PGDs in China. Therefore, this study is the first attempt to predict the PGDs of C. solstitialis in China using an EM and provides some theoretical guidance for early warning signs and further control of C. solstitialis in China.
Our study reveals that the PGDs of C. solstitialis in China are mainly in East, Central, and South China, which are primarily located in subtropical monsoon climate regions and part of temperate monsoon climate regions. For C. solstitialis, the optimum annual average temperature is 4.3–18°C and the average annual precipitation is between 250–1,500 mm (Widmer and Guermache, 2006). In our study, temperature variables, including temperature seasonality and minimum temperature of the coldest month, were the most significant variables influencing the PGDs of C. solstitialis in China; this is followed by precipitation (precipitation of the driest month) and the human influence index. The successful invasion of C. solstitialis was primarily attributed to the synergistic effects of the temperature, precipitation, and human influence index variables. In successfully invaded regions, suitable temperature and precipitation conditions have enhanced the existence and expansion of C. solstitialis, allowing individuals of invasive C. solstitialis populations to have greater body size, stem diameter, and foliage width than those of other native populations (Dukes et al., 2011). In addition, epigenetic modifications can contribute to the spread of C. solstitialis to cooler regions of China (Barker et al., 2017). For example, invasive populations of C. solstitialis prefer lower temperatures in their new environments than in their native environments. Previous studies have illustrated that winter temperatures and the minimum temperature of the coldest month slow down the flowering time of C. solstitialis in western USA rangelands (Roché et al., 1997). In general, C. solstitialis prefers to grow in a warm atmosphere, such as between 10 and 25°C (Gutierrez et al., 2005). Several studies have confirmed that experimental warming improves biomass and canopy productivity, thereby reducing mortality of the Asteraceae (Wan et al., 2002; Cao et al., 2018). Compared to native environments, epigenetic modifications facilitate the spread of C. solstitialis to cooler regions after its invasion into new environments (Montesinos et al., 2019). Therefore, climate warming increases the environmental tolerance of C. solstitialis, thereby increasing its invasive range. The results of these studies support and validate our findings. Our results also show that precipitation during the driest month influence the PGDs of C. solstitialis. Variations in climate have altered precipitation distribution patterns worldwide (Pendergrass et al., 2017), while disrupting the invasion of IAPs by making the population more invasive and robust (Guan et al., 2019). Some studies have confirmed that the invasion of IAPs is advanced by genetic changes and rapid evolution due to changes in precipitation patterns caused by climate change; an example, two annual grass species, Avena barbata and Bromus madritensis in reduced precipitation habitats in the United States (Puritty et al., 2018).
Anthropogenic influences have also partially determined the invasion of C. solstitialis. plants, which spread diffusely in woodlands away from rivers, have been found to have weaker growth (Yang et al., 2017). In contrast, the distribution was preferred in areas disturbed by anthropogenic activities, including cultivated land, plantations, grazing grasslands, and roadsides. Meanwhile, anthropogenic influences have made it possible for C. solstitialis to spread in more ways than before; for example, it can follow the multi-directional dispersal of vehicles such as mobile people and goods in trade (Montesinos et al., 2019). Anthropogenic influences have accelerated spreading. In this study, the important environmental variables that influence the PGDs of C. solstitialis in China do not include any soil variables, because C. solstitialis is highly adaptable to soil and has low soil condition requirements (Morghan and Rice, 2005). Therefore, with the appropriate temperature, precipitation, and a certain level of anthropogenic activity, they will flourish.
Predicting PGDs is an essential part of IAP risk assessments. Our results show that, under current climatic conditions, the C. solstitialis PGDs were mainly in East, Central, and South China. However, it will spread to northwest China under climate change conditions. Currently, the mild climate and abundant moisture in East, Central, and South China provide suitable conditions for the invasion and colonization of C. solstitialis (Widmer and Guermache, 2006). Already, various studies agree that changes in climate have enlarged or narrowed the PGDs of the IAPs. For instance, the PGDs of six IAPs may expand in Nepal under climate change conditions (Shrestha et al., 2018), whereas in Brazil, the PGDs are predicted to decrease in 67.5% of IAPs (Fulgêncio-Lima et al., 2021). In our study, the suitability and invasion risk in southeastern China declined under climate change conditions, which appears to be due to climate warming, thus making temperatures in southeastern China unsuitable C. solstitialis (Shi et al., 2010; Dlugosch et al., 2015). We found that under future climate change conditions (in the 2030s and 2050s), the total suitable habitats for C. solstitialis in China are projected to increase. In contrast, the high suitability habitats for C. solstitialis decreased under climate change conditions. A previous study indicated that the distribution of C. solstitialis is currently in 209 counties and 23 states and that PGDs may expand in western America in the future (Maddox et al., 2019). Our results were generally consistent with these findings.
Previous studies have suggested that 11 IAPs of the Compositae, Basellaceae, Verbenaceae, Chenopodiaceae, and Convolvulaceae families will shift to higher latitudes (northward) in China under warming conditions (Guan et al., 2019). Recently, Galinsoga quadriradiata (Asteraceae) has also been recognized to shift its ecological niche to northern China (Zhang et al., 2022). The results of our study suggest that under climate change conditions, the PGDs of C. solstitialis are at risk of shifting to northwestern China. Our findings for C. solstitialis were consistent with this pattern. Hence, PGDs of C. solstitialis in China should be injected given attention to prevent its further spread in northwest China.
Currently C. solstitialis is still spreading in China, and there is still a large potential hazard to agriculture, forestry, and animal husbandry. Therefore, we propose the following management recommendations based on our findings: (1) From the perspective of introduction, the main routes of long-distance spread of C. solstitialis are natural (dispersal by water and wind), global trade, and accidental (Caughlin et al., 2014). Available evidence suggests that snowmelt-type flooding has occurred in Xinyuan County in the Ili River Valley since March 2014 (Huang et al., 2020). We speculate that the floods facilitated the introduction of C. solstitialis into China. The routes of short-distance spread mainly refer to pollinators, plants, crops, and soil carriages (CABI, 2022). For instance, previous studies have shown that C. solstitialis can spread via native pollinators, including bees and flies (Swope and Parker, 2013). Given that, to prevent the introduction of C. solstitialis in PGDs, the customs offices of Jinan, Zhengzhou, Xi’an, Chengdu, Nanjing, Hefei, Wuhan, Chongqing, Guiyang, Changsha, Nanchang, Hangzhou, Fuzhou, Guangzhou, Nanning, Kunming, and Taiwan should strengthen the quarantine of imported containers and wood products, especially for the Mediterranean countries, Oceania and Africa. (2) From the perspective of colonization, the above customs should be better managed, as there are no records of C. solstitialis invasion in these areas. Other municipalities should refer to their suitable habitats and modes of short-distance spread, conduct distribution surveys, and periodically determine their distribution in China. (3) From the perspective of prevention and control, if a wild population of C. solstitialis is found in China, cultural, mechanical, chemical, and biological control measures should be implemented immediately to eradicate it (CABI, 2022). Cultural control mainly refers to replanting the optimum combination of species to prevent soil invasion by C. solstitialis; one way is to deploy dense stands of perennial wheat, oats, and legumes (Adams et al., 2020). Mechanical control measures consisted of uprooting or mechanical tillage before C. solstitialis plants produce seeds (Huang et al., 2018). Chemical control measures included repeated application of herbicides, such as picloram, clopyralid, and 2,4-D (Widmer and Guermache, 2006) after the emergence of C. solstitialis. In addition, three weevils (Bangasternus orientalis, Eustenopus villosus, and Larinus curtus) and three flies (Urophora sirunaseva, Chaetorellia australis, and Chaetorellia succinea) can be used as natural enemies as biological control measures for C. solstitialis to increase seedling mortality (Swope, 2014). Finally, comprehensive management measures for the early introduction, colonization, and control of C. solstitialis invasion were established. bitat (0 ≤ P < 200).
The EM, constructed using the best single-species distribution models, has relatively high accuracy in predicting the PGDs of C. solstitialis. Our results suggest that under current climatic conditions, C. solstitialis PGDs were mainly in East, Central, and South China. However, it will spread to northwest China under climate change conditions. Those are the results of synergistic effects on natural and anthropogenic factors. Specifically, the temperature and precipitation variables play a key role in invasion and expansion in China. Consequently, more focus needs to be placed on preventing the further spread of C. solstitialis in the northwest China. In future studies, we plan to combine species distribution models with information on genetic variation and local adaptation to make the results more desirable and scientific.
The raw data supporting the conclusions of this article will be made available by the authors, without undue reservation.
TJ, YQ, HZ, WY, and W-XL: conception and design of the research. TJ, YQ, WY, and W-XL: acquisition of data, analysis and interpretation of data, statistical analysis, and drafting the manuscript. HZ, XX, JL, and HH: manuscript revision. All authors contributed to the article and approved the submitted version.
This research was funded by the National Key R&D Program of China (Grant Nos. 2021YFC2600400 and 2022YFC2601100) and Technology Innovation Program of Chinese Academy of Agricultural Sciences (Grant No. caascx-2022-2025-IAS).
The authors declare that the research was conducted in the absence of any commercial or financial relationships that could be construed as a potential conflict of interest.
All claims expressed in this article are solely those of the authors and do not necessarily represent those of their affiliated organizations, or those of the publisher, the editors and the reviewers. Any product that may be evaluated in this article, or claim that may be made by its manufacturer, is not guaranteed or endorsed by the publisher.
The Supplementary Material for this article can be found online at: https://www.frontiersin.org/articles/10.3389/fevo.2023.1113474/full#supplementary-material
Adams, S. N., Jennings, S., and Warnock, N. (2020). Plant invasion depresses native species richness, but control of invasive species does little to restore it. Plant. Ecol. Divers. 13, 257–266. doi: 10.1080/17550874.2020.1817998
Allouche, O., Tsoar, A., and Kadmon, R. (2006). Assessing the accuracy of species distribution models: prevalence, kappa and the true skill statistic (TSS). J. Appl. Ecol. 43, 1223–1232. doi: 10.1111/j.1365-2664.2006.01214.x
Banerjee, A. K., Mukherjee, A., Guo, W., Liu, Y., and Huang, Y. (2019). Spatio-temporal patterns of climatic niche dynamics of an invasive plant Mikania micrantha Kunth and its potential distribution under projected climate change. Front. Ecol. Evol. 7:291. doi: 10.3389/fevo.2019.00291
Barker, B. S., Andonian, K., Swope, S. M., Luster, D. G., and Dlugosch, K. M. (2017). Population genomic analyses reveal a history of range expansion and trait evolution across the native and invaded range of yellow starthistle (Centaurea solstitialis). Mol. Ecol. 26, 1131–1147. doi: 10.1111/mec.13998
Bartz, R., and Kowarik, I. (2019). Assessing the environmental impacts of invasive alien plants: a review of assessment approaches. Neobiota 43, 69–99. doi: 10.3897/neobiota.43.30122
Bates, O. K., Ollier, S., and Bertelsmeier, C. (2020). Smaller climatic niche shifts in invasive than non-invasive alien ant species. Nat. Commun. 11:5213. doi: 10.1038/s41467-020-19031-1
Bolliger, J., Kienest, F., and Bugmann, H. (2000). Comparing models for tree distribution: concept, structures and behavior. Ecol. Model. 134, 89–102. doi: 10.1016/S0304-3800(00)00338-0
Brown, J. L., Hill, D. J., Dolan, A. M., Carnaval, A. C., and Haywood, A. M. (2018). PaleoClim, high spatial resolution paleoclimate surfaces for global land areas. Sci. Data 5:180254. doi: 10.1038/sdata.2018.254
CABI (2022). Centaurea solstitialis (yellow starthistle), CABI Compendium. CABI International. doi: 10.1079/cabicompendium.12043
Cao, Y. S., Xiao, Y. A., Zhang, S. S., and Hu, W. H. (2018). Simulated warming enhances biological invasion of Solidago canadensis and Bidens frondosa by increasing reproductive investment and altering flowering phenology pattern. Sci. Rep. 8:16073. doi: 10.1038/s41598-018-34218-9
Caughlin, T. T., Ferguson, J. M., Lichstein, J. W., Bunyavejchewin, S., and Levey, D. J. (2014). The importance of long-distance seed dispersal for the demography and distribution of a canopy tree species. Ecology 95, 952–962. doi: 10.1890/13-0580.1
Csontos, P., Vitalos, M., Barina, Z., and Kiss, L. (2010). Early distribution and spread of Ambrosia artemisiifolia in Central and Eastern Europe. Bot. Helv. 120, 75–78. doi: 10.1007/s00035-010-0072-2
Dlugosch, K. M., Cang, F. A., Barker, B. S., Andonian, K., Swope, S. M., and Rieseberg, L. H. (2015). Evolution of invasiveness through increased resource use in a vacant niche. Nat. Plants 1:15066. doi: 10.1038/NPLANTS.2015.66
Dukes, J. S., Chiariello, N. R., Loarie, S. R., and Field, C. B. (2011). Strong response of an invasive plant species (Centaurea solstitialis L.) to global environmental changes. Ecol. Appl. 21, 1887–1894. doi: 10.1890/11-0111.1
Eagle, A. J., Eiswerth, M. E., Johnson, W. S., Schoenig, S., and Van, K. G. C. (2007). Costs and losses imposed on California ranchers by yellow starthistle. Rangel. Ecol. Manag. 60, 369–377.
Early, R., Bradley, B. A., Dukes, J. S., Lawler, J. J., Olden, J. D., and Blumenthal, D. M. (2016). Global threats from invasive alien species in the twenty-first century and national response capacities. Nat. Commun. 7:12485. doi: 10.1038/ncomms12485
Fang, Y. Q., Zhang, X. H., Wei, H. Y., Wang, D. J., Chen, R. D., and Wang, L. K. (2021). Predicting the invasive trend of exotic plants in China based on the ensemble model under climate change: a case for three invasive plants of Asteraceae. Sci. Total Environ. 756:143841. doi: 10.1016/j.scitotenv.2020.143841
Ferrarini, A., Selvaggi, A., Abeli, T., Alatalo, J. M., Orsenigo, S., and Gentili, R. (2016). Planning for assisted colonization of plants in a warming world. Sci. Rep. 6:28542. doi: 10.1038/srep28542
Fick, S. E., and Hijmans, R. J. (2017). WorldClim 2: new 1-km spatial resolution climate surfaces for global land areas. Int. J. Climatol. 37, 4302–4315. doi: 10.1002/joc.5086
Fielding, A. H., and Bell, J. F. (1997). A review of methods for the assessment of prediction errors in conservation presence/absence models. Environ. Conserv. 24, 38–49. doi: 10.1017/S0376892997000088
Fitchett, J. M., Bamford, M. K., Mackay, A. W., and Grab, S. W. (2017). Chrysocoma ciliata L. (Asteraceae) in the lesotho highlands: an anthropogenically introduced invasive or a niche colonizer? Biol. Invasions. 19, 2711–2728. doi: 10.1007/s10530-017-1478-1
Fornasari, L., Turner, C. E., and Andres, L. A. (1991). Eustenopus villosus (Coleoptera: Curculionidae) for biological control of yellow starthistle (Asteraceae: Cardueae) in North America. Environ. Entomol. 20, 1187–1194. doi: 10.1093/ee/20.4.1187
Fulgêncio-Lima, L. G., Andrade, A. F. A., Vilela, B., Lima-Júnior, D. P., Souza, R. A., and Sgarbi, L. F. (2021). Invasive plants in Brazil: climate change effects and detection of suitable areas within conservation units. Biol. Invasions. 23, 1577–1594. doi: 10.1007/s10530-021-02460-4
Fusco, E. J., Finn, J. T., Balch, J. K., and Bradley, B. A. (2019). Invasive grasses increase fire occurrence and frequency across US ecoregions. PNAS. 116, 23594–23599. doi: 10.1073/pnas.1908253116
Gong, X., Chen, Y. J., Wang, T., Jiang, X. F., Hu, X. K., and Feng, J. M. (2020). Double-edged effects of climate change on plant invasions: ecological niche modeling global distributions of two invasive alien plants. Sci. Total Environ. 740:139933. doi: 10.1016/j.scitotenv.2020.139933
Guan, B. C., Guo, H. J., Chen, S. S., Li, D. M., Liu, X., and Gong, X. (2019). Shifting ranges of eleven invasive alien plants in China in the face of climate change. Ecol. Inform. 55:101024. doi: 10.1016/j.ecoinf.2019.101024
Gutierrez, A. P., Pitcairn, M. J., Ellis, C. K., Carruthers, N., and Ghezelbash, R. (2005). Evaluating biological control of yellow starthistle (Centaurea solstitialis) in California: a GIS based supply–demand demographic model. Biol. Control. 34, 115–131.
Huang, Q. Q., Fan, Z. W., Li, X. X., Wang, Y., Liu, Y., and Shen, Y. D. (2018). Effects of nutrient addition and clipping on biomass production of invasive and native annual Asteraceae plants. Weed Res. 58, 318–326. doi: 10.1111/wre.12308
Huang, X., Zhou, Y. S., and Liu, L. (2020). Occurrence and development of an extreme precipitation event in the ili valley, Xinjiang, China and analysis of gravity waves. Atmosphere-Basel 11:752. doi: 10.3390/atmos11070752
Ju, R. T., Zhu, H. Y., Gao, L., Zhou, X. H., and Li, B. (2015). Increases in both temperature means and extremes likely facilitate invasive herbivore outbreaks. Sci. Rep. 5:15715. doi: 10.1038/srep15715
Kleunen, M. V., Dawson, W., Essl, F., Pergl, J., Winter, M., and Weber, E. (2015). Global exchange and accumulation of non-native plants. Nature 525, 100–103. doi: 10.1038/nature14910
Maddox, D. M. (1981). Introduction, Phenology and Density of Yellow Starthistle in Coastal, Intercoastal and Central Valley Situations in California; USDA-ARS Publication W-20. Oakland, CA: USDA-ARS.
Maddox, D. M., Mayfield, A., and Poritz, N. H. (2019). Distribution of yellow starthistle (Centaurea solstitialis) and Russian Knapweed (Centaurea repens). Weed. Sci. 33, 315–327. doi: 10.1017/S0043174500082357
Majewska, M. L. (2018). Do the impacts of alien invasive plants differ from expansive native ones? an experimental study on arbuscular mycorrhizal fungi communities. Biol. Fertil. Soils 54, 631–643. doi: 10.1007/s00374-018-1283-8
McMahon, D. E., Urza, A. K., Brown, J. L., Phelan, C., and Chambers, J. C. (2021). Modelling species distributions and environmental suitability highlights risk of plant invasions in western United States. Divers. Distrib. 27, 710–728. doi: 10.1111/ddi.13232
Merow, C., Bois, S. T., Allen, J. M., and Silander, J. A. (2017). Climate change both facilitates and inhibits invasive plant ranges in New England. PNAS. 114, 3276–3284. doi: 10.1073/pnas.1609633114
Monserud, R. A., and Leemans, R. (1992). Comparing global vegetation maps with the Kappa statistic. Ecol. Model. 62, 275–293.
Montesinos, D., Graebner, R. C., and Callaway, R. M. (2019). Evidence for evolution of increased competitive ability for invasive Centaurea solstitialis, but not for naturalized C. calcitrapa. Biol. Invasions. 21, 99–110. doi: 10.1007/s10530-018-1807-z
Moraira, S. H., Vitales, D., Nualart, N., Bellver, C. G., Ibáñez, N., and Massó, S. (2020). Global distribution patterns and niche modelling of the invasive Kalancho houghtonii (Crassulaceae). Sci. Rep. 10:3143. doi: 10.1038/s41598-020-60079-2
Morghan, K. J. R., and Rice, K. J. (2005). Centaurea solstitialis invasion success is influenced by Nassella pulchra size. Restor Ecol. 13, 524–528. doi: 10.1111/j.1526-100X.2005.00065.x
Pendergrass, A. G., Knutti, R., Lehner, F., Deser, C., and Sanderson, B. M. (2017). Precipitation variability increases in a warmer climate. Sci. Rep. 7:17966. doi: 10.1038/s41598-017-17966-y
Peterson, A., Papes, M., and Soberón, J. (2008). Rethinking receiver operating characteristic analysis applications in ecological niche modeling. Ecol. Model. 213, 63–72. doi: 10.1016/j.ecolmodel.2007.11.008
Pitcairn, M. J., Schoenig, S., Yacoub, R., and Gendron, J. (2006). Yellow starthistle continues its spread in California. Calif. Agric. 60, 83–90. doi: 10.3733/ca.v060n02p83
Pitcairn, M. J., Woods, D. M., Joley, D. B., and Turner, C. E. (2021). Evaluation of the impact of Eustenopus villosus on Centaurea solstitialis seed production in California. Insects 12:606. doi: 10.3390/insects12070606
Puritty, C. E., Mayfield, M. M., Azcárate, F. M., and Cleland, E. E. (2018). Different traits predict competitive effect versus response by Bromus madritensis in its native and invaded ranges. Biol. Invasions. 20, 2553–2565. doi: 10.1007/s10530-018-1719-y
Roché, C. T., Thill, D. C., and Shafii, B. (1997). Reproductive phenology in yellow starthistle (Centaurea solstitialis). Weed. Sci. 45, 763–770. doi: 10.1017/s0043174500088949
Shi, J., Luo, Y. Q., Zhou, F., and He, P. (2010). The relationship between invasive alien species and main climatic zones. Biodivers. Conserv. 19, 2485–2500. doi: 10.1007/s10531-010-9855-4
Shrestha, U. B., Sharma, K. P., Devkota, A., Siwakoti, M., and Shrestha, B. B. (2018). Potential impact of climate change on the distribution of six invasive alien plants in Nepal. Ecol. Indic. 95, 99–107. doi: 10.1016/j.ecolind.2018.07.009
Sitzia, T., Campagnaro, T., Kotze, D. J., Nardi, S., and Ertani, A. (2018). The invasion of abandoned fields by a major alien tree filters understory plant traits in novel forest. Sci. Rep. 8:8410. doi: 10.1038/s41598-018-26493-3
Skubel, S. A., Su, X. Y., Alexander, P., Foxcroft, L. C., Dushenkov, V., and Raskin, I. (2020). Metabolomic differences between invasive alien plants from native and invaded habitats. Sci. Rep. 10:9749. doi: 10.1038/s41598-020-66477-w
Swope, S. M. (2014). Biocontrol attack increases pollen limitation under some circumstances in the invasive plant Centaurea solstitialis. Oecologia 174, 205–215. doi: 10.1007/s00442-013-2750-4
Swope, S. M., and Parker, I. M. (2013). Complex interactions among biocontrol agents, pollinators, and an invasive weed: a structural equation modeling approach. Ecol. Appl. 22, 2122–2134. doi: 10.1890/12-0131.1
Teller, B. J., Zhang, R., and Shea, K. (2016). Seed release in a changing climate: initiation of movement increases spread of an invasive species under simulated climate warming. Divers. Distrib. 22, 708–716. doi: 10.1111/ddi.12436
Thapa, S., Chitale, V., Rijal, S. J., Bisht, N., and Shrestha, B. (2018). Understanding the dynamics in distribution of invasive alien plant species under predicted climate change in Western Himalaya. PLoS. One 13:e0195752. doi: 10.1371/journal.pone.0195752
Thuiller, W., Araújo, M. B., and Lavorel, S. (2003). Generalized models vs. classification tree analysis: predicting spatial distributions of plant species at different scales. J. Veg. Sci. 14, 669–680. doi: 10.1111/j.1654-1103.2003.tb02199.x
Thuiller, W., Georges, D., Engler, R., and Breiner, F. (2016). Package “biomod2”. Ensemble Platform for Species Distribution Modeling Version 3.3-7. Available Online at: https://cran.rproject.org/web/packages/biomod2/biomod2.pdf. (accessed Nov 5, 2016).
Tomaso, D. (2005). “Yellow starthistle, Centaurea solstitialis L,” in Invasive Plants of Range and Wildlands and Their Environmental, Economic, and Societal Impacts, (Lawrence, KS: Weed Science Society of America), 36–50.
Tu, W. Q., Xiong, Q. L., Qiu, X. P., and Zhang, Y. M. (2021). Dynamics of invasive alien plant species in China under climate change scenarios. Ecol. Indic. 129:107919. doi: 10.1016/j.ecolind.2021.107919
Turbelin, A., and Catford, J. A. (2021). Invasive plants and climate change. Nat. Clim. Change 2021, 515–539. doi: 10.1016/B978-0-12-821575-3.00025-6
Wagner, V., Chytrý, M., Jiménez-Alfaro, B., Pergl, J., Hennekens, S., and Biurrun, I. (2017). Alien plant invasions in European woodlands. Divers. Distrib. 23, 969–981. doi: 10.1111/ddi.12592
Wan, S. Q., Yuan, T., Bowdish, S., Wallace, L., Russell, S. D., and Luo, Y. Q. (2002). Response of an allergenic species, Ambrosia psilostachya (Asteraceae), to experimental warming and clipping: implications for public health. Am. J. Bot. 9, 1843–1846. doi: 10.3732/ajb.89.11.1843
Warren, D. L., Glor, R. E., and Turelli, M. (2010). ENMTools: a toolbox for comparative studies of environmental niche models. Ecography 33, 607–611. doi: 10.1111/j.1600-0587.2009.06142.x
Widmer, T. L., and Guermache, F. (2006). Factors affecting infection of Yellow starthistle (Centaurea solstitialis) by synchytrium solstitiale, causal agent of false rust disease. Plant Dis. 90, 425–428. doi: 10.1094/PD-90-0425
Yang, Y. G., Wu, S. M., Chen, M., Cheng, X. T., Cheng, D. H., and Wu, Y. (2017). Dentification and transmission risk analysis of exotic plant Centaurea solstitialis L. Forest Pest Dis. 36, 36–37.
Zhang, W. G., Chen, X. Y., Liu, R. L., Song, X. J., Liu, G., and Zou, J. B. (2022). Realized niche shift associated with Galinsoga quadriradiata (Asteraceae) invasion in China. J. Plant. Ecol. 15, 538–548. doi: 10.1093/jpe/rtab086
Keywords: Centaurea solstitialis, climate change, ensemble model, invasive alien plants, potential geographical distribution
Citation: Jia T, Qi Y, Zhao H, Xian X, Li J, Huang H, Yu W and Liu W-x (2023) Estimation of climate-induced increased risk of Centaurea solstitialis L. invasion in China: An integrated study based on biomod2. Front. Ecol. Evol. 11:1113474. doi: 10.3389/fevo.2023.1113474
Received: 01 December 2022; Accepted: 06 February 2023;
Published: 22 February 2023.
Edited by:
Donald DeAngelis, United States Department of the Interior, United StatesReviewed by:
Shanshan Qi, Jiangsu University, ChinaCopyright © 2023 Jia, Qi, Zhao, Xian, Li, Huang, Yu and Liu. This is an open-access article distributed under the terms of the Creative Commons Attribution License (CC BY). The use, distribution or reproduction in other forums is permitted, provided the original author(s) and the copyright owner(s) are credited and that the original publication in this journal is cited, in accordance with accepted academic practice. No use, distribution or reproduction is permitted which does not comply with these terms.
*Correspondence: Wan-xue Liu, bGl1d2FueHVlQGNhYXMuY24=; Wentao Yu,
eXV3ZW50YW9AY3VzdG9tcy5nb3YuY24=
†These authors have contributed equally to this work
Disclaimer: All claims expressed in this article are solely those of the authors and do not necessarily represent those of their affiliated organizations, or those of the publisher, the editors and the reviewers. Any product that may be evaluated in this article or claim that may be made by its manufacturer is not guaranteed or endorsed by the publisher.
Research integrity at Frontiers
Learn more about the work of our research integrity team to safeguard the quality of each article we publish.