- 1Department of Microbial Ecology, Netherlands Institute of Ecology (NIOO-KNAW), Wageningen, Netherlands
- 2Institute of Biology, Leiden University, Leiden, Netherlands
- 3Soil Biology Group, Wageningen University and Research, Wageningen, Netherlands
- 4Department of Plant Science & Huck Institutes of the Life Sciences, The Pennsylvania State University, University Park, PA, United States
Introduction
Microbial volatile compounds (mVCs) can provide numerous functional benefits to the producing organism, for instance, by contributing to the invasion of previously unexploited niches and/or inhibiting competitors, by promoting beneficial interactions or regulating abiotic stress tolerance (Tyc et al., 2016; Sharifi and Ryu, 2020). Over time, the benefits provided by mVCs can translate to increased fitness of the volatile-producing microbe, leading to the selection and evolution of specific mVC-associated traits. Simultaneously, interacting (micro)organisms can evolve mechanisms for mVC-perception and tolerance to attain fitness gains. The balancing of fitness gains in producing and perceiving microbial populations can be considered components of eco-evolutionary dynamics, broadly defined as interactions between ecological and evolutionary processes affecting organismal fitness (Hendry, 2017). More specifically, these dynamics occur when ecological changes result in evolutionary changes (e.g., adaptation to new environments) or when evolutionary changes lead to ecological changes (e.g., trait change altering ecosystem function). When these processes reciprocally influence each other, positively or negatively, these dynamics are considered eco-evolutionary feedbacks. To date, however, reliable assessments of the strength of selection acting on mVC-associated traits are still lacking. This occurs primarily because most research focused on mVCs has been dedicated to their ecological functions (e.g., communication, plant growth promotion, ecological competition), relatively with less attention given to their evolution in producing and perceiving microbes. In this opinion, we discuss examples of yet-underexplored evolutionary consequences of mVCs acting as mediators of ecological intra- and interspecific interactions, ranging from microbe-microbe communication to cross-domain interactions. In particular, we discuss whether selection acting on mVC-associated traits can lead to eco-evolutionary dynamics and feedbacks in both producing and perceiving (micro)organisms across varying phylogenetic distances (Figure 1).
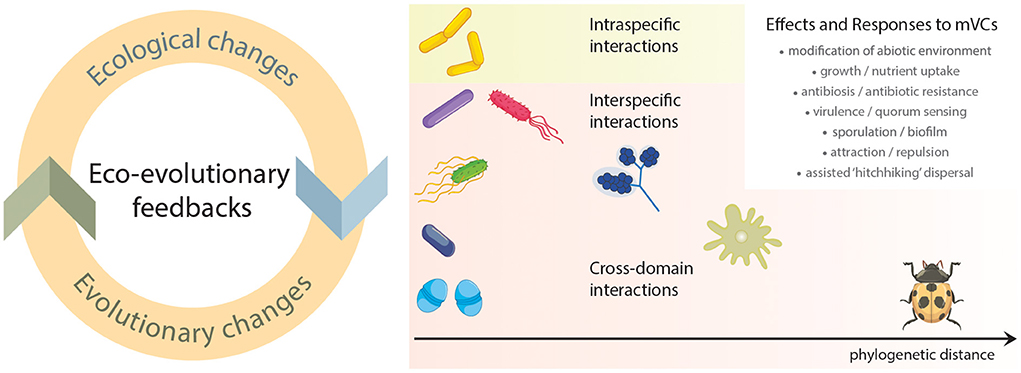
Figure 1. Schematic representation of mVCs mediating eco-evolutionary dynamics. The left panel displays a simplified diagram of an eco-evolutionary feedback. In brief, these occur when ecological changes sequentially impact evolutionary trajectories of interacting organisms, which in turn lead to changes in their ecological interactions. The right panel depicts (micro)organisms ecological interactions at increasing phylogenetic distances, i.e., form intraspecific (same species) to cross-domain interactions.
Microbial volatiles mediating intraspecific interactions
The ability of mVCs to steer evolutionary outcomes within species is linked to their capacity to change the chemical properties of the niche (e.g., medium alkalinization), to interfere with chemical communication between taxa (e.g., disruption of quorum sensing), and regulate virulence (e.g., toxin production). For example, a recent study revealed that the bacterium Streptomyces venezuelae synthesizes trimethylamine during its exploratory behavior. This volatile compound acts by increasing the local pH, which in turn reduces local iron availability (Jones et al., 2019) in the system – as similarly shown by the production of the alkaline mVC ammonia. In doing so, the producing strain gains a competitive advantage by reducing the fitness (i.e., the efficiency of iron acquisition) of competing species. It is tempting to speculate, as it remains to be tested, whether this trait in S. venezuelae might have co-evolved with siderophore biosynthesis and uptake, thus providing an additional fitness gain to the mVC-producing bacterium (Jones et al., 2019). Besides, there are also examples of intraspecific modulation of chemical communication and physiology by mVCs. For example, the blend of mVCs produced by Pseudomonas chlororaphis – composed of 1-undecene, methyl thiolacetate and dimethyl disulfide – was shown to quench quorum-sensing signals required for phenazine biosynthesis, and suppress the transcription of the biosynthetic genes for N-acyl-homoserine lactones (Chernin et al., 2011). In another example, the volatile compound leudiazen was shown to regulate the production of mangotoxin in Pseudomonas syringae pv. syringae (Sieber et al., 2021). This volatile – produced by the non-ribosomal peptide synthetase MgoA – triggers its own mangotoxin production that not only causes disease symptoms in plants but also acts as an antimicrobial molecule, facilitating colonization of the phyllosphere by P. syringae pv. syringae.
Microbial volatiles mediating interspecific interactions
The broad diversity of mVCs mediates complex and yet-underexplored interactions between different microbial taxa. These occur via diverse mechanisms and can be associated with eco-evolutionary dynamics, and in some cases, with the establishment of eco-evolutionary feedbacks. First, mVCs can reduce the metabolic cost associated with the biosynthesis of other active metabolites. For example, some Streptomyces strains produce ammonia (a “low cost” molecule) at high enough concentrations to kill other bacteria at a distance and to potentiate the activity of soluble antibiotics (e.g., polyketides, non-ribosomal peptides, β-lactams – “high cost” molecules) produced locally by other Streptomyces species (Avalos et al., 2020). In another example, a yet-unidentified mVOC produced by Xanthomonas perforans (a non-motile organism) was shown to trigger surface motility in Paenibacillus vortex (Hagai et al., 2014). The same study showed that X. perforans was able to “hitchhike” with the swarming colony of P. vortex to reach new sites for infection on tomato leaves. As such, it is plausible the plant pathogenic Xanthomonads are benefiting from the volatile-mediated interspecific interaction by reducing the cost of an energetically costly trait, in this case, motility. Whether this type of ecological interaction can be characterized as mutualism or commensalism is still unclear. For different bacterial taxa, however, the “hitchhiking” bacteria provide functional benefits to their interactive motile partners. These benefits can occur via local degradation of antibiotics (Finkelshtein et al., 2015) or by breaking down complex carbon sources leading to co-metabolism (Wang et al., 2021). Hence, given the mutual fitness benefits to both interacting partners described in these studies, it is more likely that these interactions are in most cases mutualistic.
Perhaps one of the most known examples of how microbial volatiles mediate eco-evolutionary feedbacks is illustrated by the interaction between the taxonomically distant organisms Streptomyces and the soil arthropod Folsomia candida. This example originated from studies addressing the longstanding question about the possible biological function(s) of the ubiquitous soil volatile geosmin (Becher et al., 2020). Several studies have shown that the production of geosmin and 2-methylisoborneol by Streptomyces attracts F. candida. This arthropod assists in the dispersal of bacterial spores through feeding and their attachment to the arthropod's cuticle, whereas the arthropod benefits from this attraction by feeding on the bacteria, directly increasing its reproductive success (i.e., higher arthropod molting and egg laying). Interestingly, the biosynthesis of these mVCs by Streptomyces is restricted to the sporulating life stage of the bacterium, which is tightly controlled by the transcription factors BldM and WhiH. Moreover, the hydrophobic cuticle of this arthropod favors the attachment of hydrophobic Streptomyces spores. Taken together, the functional interplay between these traits possesses signatures of an eco-evolutionary feedback between distantly related organisms across domains of life. Another example is provided by the interaction between the bacteria Listeria monocytogenes and the protozoan Euglena gracilis in soil. Listeria monocytogenes can produce an array of mVCs (including decanal, 2-ethylhexyl acetate, 3,5-dimethylbenzaldehyde, and ethyl acetate) that attract E. gracilis (Gaines et al., 2019). This protozoan feeds on specific bacterial taxa, imposing a strong selective pressure by favoring the persistence and evolution of adaptive traits to resist predation (e.g., secondary metabolites, motility, cell surface, and multicellular behaviors, such as biofilm formation) (Matz and Kjelleberg, 2005).
The observation that perceiving microorganisms can develop resistance mechanisms to mVCs, i.e., becoming “deaf” to mVCs as chemical signals, may affect eco-evolutionary dynamics and feedbacks. Examples of such mechanisms include the reduced transcription of the ompR porin master regulator in Escherichia coli, preventing the influx of ammonia into the cells, thereby enabling this bacterium to grow at high ammonia concentrations (Avalos et al., 2020). However, when E. coli was exposed to sub-inhibitory concentrations of ammonia, this volatile served as an intracellular signal enhancing the production of polyamines. This, in turn, resulted in a reduction of cell membrane permeability and enhanced resistance to antibiotics (Bernier et al., 2011). When mVCs exert specific regulatory roles inside cells, it is likely that developing such resistance mechanisms will have consequences on the perception and functioning of these compounds in the perceiving organisms. This is also exemplified by the toxic inorganic volatile hydrogen cyanide (HCN). In this case, perceiving microorganisms have evolved strategies to resist its toxicity via cyanide-resistant respiratory pathways or via the breakdown of HCN into the less toxic formamide (Duffy et al., 2003). Volatiles can also have broader impacts on the evolution of interactive microbial species by specifically targeting signaling cascades. This was demonstrated in the case of the mVCs 2,3-butanedione and glyoxylic acid produced by Bacillus subtilis. In brief, these molecules were shown to negatively regulate the expression of the gene ypdB in E. coli and the respective downstream transcription factors (soxS, rpoS and yhjU) (Kim et al., 2013) involved in cell motility and antibiotic resistance.
The evolutionary role of mVCs can be further inferred from the emergence of congruent patterns when assessing microbial phylogeny from metabolomics (Elmassry et al., 2020), or “chemotyping.” For example, a phylogenetic signal for the volatile profile was observed in Actinobacteria (Choudoir et al., 2019), with metabolomics leading to more refined phylogenies in a collection of Streptomyces species as compared to classical taxonomic markers (i.e., 16S rRNA, atpD and recA genes) (Cordovez et al., 2015). On the other hand, when analyzing biosynthetic genes of the 10 most abundant terpene classes in Streptomyces, the overall phylogenomic and phylogenetic analyses were incongruent (Martín-Sánchez et al., 2019). This points to the fact that the evolution of mVCs genes may differ in terms of their vertical and horizontal gene transfer, where the latter may lead to a more rapid and phylogenomic-disconnected evolution of specific mVCs, in this case, terpenes. However, some terpene synthases were shown to be restricted to one phylogenomic (sub)group, such as epi-isozizaene, cyclooctat-9-en-7-ol, and isoafricanol synthases, suggesting functional specialization and highlighting the ecological relevance of these terpenoid compounds for specific phylogenetically related Streptomyces species.
Perspectives and concluding remarks
Here, we postulate that mVCs offer several opportunities for the creation, mediation, and manipulation of intra- and inter-specific eco-evolutionary feedbacks. Nevertheless, challenges remain in quantifying and determining how specific mVCs contribute to the fitness of particular microbial species (both, producer and perceiver). This is primarily due to the complexity of properly identifying and quantifying eco-evolutionary dynamics across multiple potential biological interactions. Besides, this challenge can be exacerbated by the chemical nature of volatile molecules, which due to their high diffusibility, can serve as “common goods” in the system (Netzker et al., 2020). This means that locally produced mVCs can be accessed by a diverse set of (micro)organisms in a non-specific and concentration-dependent manner, further confounding the identification of their role as signals modulating eco-evolutionary dynamics. The local microbial community composition can also affect the production and functioning of mVCs, adding another layer of complexity. This can occur, for example, when bacteria are interacting at a distance and changes in interacting partners elicit changes in emitted mVCs (Tyc et al., 2015), or by non-enzymatic reactions between compounds produced by two distinct bacterial populations leading to the formation of novel compounds (Kai et al., 2015). The environmental conditions and nutrient availability also play key roles in defining the chemical diversity and concentration of mVCs produced by microbial populations (Choudoir et al., 2019). Since most of the current data derives from in vitro studies, significant constraints in our interpretation of the true eco-evolutionary consequences of mVCs in natural settings still exist. Nevertheless, we consider that in vitro studies offer optimal conditions, currently not fully leveraged in literature, to test whether specific mVCs are the key molecules driving eco-evolutionary dynamics and feedbacks. This can be based on the design of prospective experimental evolution assays aiming at unraveling the mechanisms of species interactions mediated by mVCs, thus discerning between correlation (based on indirect experimental evidence) and causation (biological mechanistic evidence). Last, embracing the complexity of mVC-mediated communication from an eco-evolutionary perspective is a necessary step toward advancing our understanding of the mechanisms driving the emergence and evolution of interactions between producing and perceiving microbial populations across ecosystems.
Author contributions
RM and FD-A discussed and wrote the first draft. The concepts were refined and further discussed by all authors who also provided comments on and edited all versions of the manuscripts. All authors contributed and approved the submitted version.
Funding
This work was supported by the Bill & Melinda Gates Foundation, Seattle, WA, via grant OPP1082853: RSM Systems Biology for Sorghum.
Conflict of interest
The authors declare that the research was conducted in the absence of any commercial or financial relationships that could be construed as a potential conflict of interest.
The handling editor DL-B declared a past co-authorship with the author JR.
Publisher's note
All claims expressed in this article are solely those of the authors and do not necessarily represent those of their affiliated organizations, or those of the publisher, the editors and the reviewers. Any product that may be evaluated in this article, or claim that may be made by its manufacturer, is not guaranteed or endorsed by the publisher.
References
Avalos, M., Garbeva, P., Raaijmakers, J. M., and van Wezel, G. P. (2020). Production of ammonia as a low-cost and long-distance antibiotic strategy by Streptomyces species. ISME J. 14, 569–583. doi: 10.1038/s41396-019-0537-2
Becher, P. G., Verschut, V., Bibb, M. J., Bush, M. J., Molnár, B. P., Barane, E., et al. (2020). Developmentally regulated volatiles geosmin and 2-methylisoborneol attract a soil arthropod to Streptomyces bacteria promoting spore dispersal. Nat. Microbiol. 5, 821–829. doi: 10.1038/s41564-020-0697-x
Bernier, S. P., Létoff,é, S., Delepierre, M., and Ghigo, J.-M. (2011). Biogenic ammonia modifies antibiotic resistance at a distance in physically separated bacteria. Mol. Microbiol. 81, 705–716. doi: 10.1111/j.1365-2958.2011.07724.x
Chernin, L., Toklikishvili, N., Ovadis, M., Kim, S., Ben-Ari, J., Khmel, I., et al. (2011). Quorum-sensing quenching by rhizobacterial volatiles. Environ. Microbiol. Rep. 3, 698–704. doi: 10.1111/j.1758-2229.2011.00284.x
Choudoir, M., Rossabi, S., Gebert, M., Helmig, D., and Fierer, N. (2019). A phylogenetic and functional perspective on volatile organic production by Actinobacteria. mSystems. 4, e00295–e00218. doi: 10.1128/mSystems.00295-18
Cordovez, V., Carrion, V. J., Etalo, D. W., Mumm, R., Zhu, H., van Wezel, G. P., et al. (2015). Diversity and functions of volatile organic compounds produced by Streptomyces from a disease-suppressive soil. Front. Microbiol. 6, 1081. doi: 10.3389/fmicb.2015.01081
Duffy, B., Schouten, A., and Raaijmakers, J. M. (2003). Pathogen self-defense: mechanisms to counteract microbial antagonism. Annu. Rev. Phytopathol. 41, 501–538. doi: 10.1146/annurev.phyto.41.052002.095606
Elmassry, M. M., Farag, M. A., Preissner, R., Gohlke, B.-O., Piechulla, B., et al. (2020). Sixty-one volatiles have phylogenetic signals across bacterial domain and fungal kingdom. Front. Microbiol. 11, 557253. doi: 10.3389/fmicb.2020.557253
Finkelshtein, A., Roth, D., Eshel, B. J., and Ingham, C. J. (2015). Bacterial swarms recruit cargo bacteria to pave the way in toxic environments. mBio. 6, e00074–s15. doi: 10.1128/mBio.00074-15
Gaines, A., Ludovice, M., Xu, J., Zanghi, M., Meinersmann, R. J., Berrang, M., et al. (2019). The dialogue between protozoa and bacteria in a microfluidic device. PLoS ONE. 14, e0222484. doi: 10.1371/journal.pone.0222484
Hagai, E., Dvora, R., Havkin-Blank, T., Zelinger, E., Porat, Z., Schulz, S., et al. (2014). Surface-motility induction, attraction and hitchhiking between bacterial species promote spore dispersal on solid surfaces. ISME J. 8, 1147–1151. doi: 10.1038/ismej.2013.218
Hendry, A. P. (2017). Eco-evolutionary Dynamics. Princenton, NJ: Princeton University Press. doi: 10.1515/9781400883080
Jones, S. E., Pham, C. A., Zambri, M. P., McKillip, J., Carlson, E. E., Elliot, M. A., et al. (2019). Streptomyces volatile compounds influence exploration and microbial community dynamics by altering iron availability. mBio. 10, e00171–e00119. doi: 10.1128/mBio.00171-19
Kai, M., Effmert, U., Lemfack, M. C., and Piechulla, B. (2015). Interspecific formation of the antimicrobial volatile schleiferon. Sci. Rep. 8, 16852. doi: 10.1038/s41598-018-35341-3
Kim, K.-S., Lee, S., and Ryu, C.-M. (2013). Interspecific bacterial sensing through airborne signals modulates locomotion and drug resistance. Nat. Commun. 4, 1809. doi: 10.1038/ncomms2789
Martín-Sánchez, L., Singh, K. S., Avalos, M., van Wezel, G. P., Dickschat, J. S., Garbeva, P., et al. (2019). Phylogenomic analyses and distribution of terpene synthases among Streptomyces. Beilstein J. Org. Chem. 15, 1181–1193. doi: 10.3762/bjoc.15.115
Matz, C., and Kjelleberg, S. (2005). Off the hook – how bacteria survive protozoan grazing. Trends Microbiol. 13, 302–327. doi: 10.1016/j.tim.2005.05.009
Netzker, T., Shepherdson, E. M. F., Zambri, M. P., and Elliot, M. A. (2020). Bacterial volatile compounds: functions in communication, cooperation, and competition. Annu. Rev. Microbiol. 74, 409–430. doi: 10.1146/annurev-micro-011320-015542
Sharifi, R., and Ryu, C.-M. (2020). “Contribution of bacterial volatiles to chemical ecology,” in Bacterial Volatile Compounds as Mediators of Airborne Interactions, eds C.-M. Ryu, L. Weisskopf, B. Piechulla (Singapore: Springer), 167–186. doi: 10.1007/978-981-15-7293-7_6
Sieber, S., Mathew, A., Jenul, C., Kohler, T., Bär, M., Carrión, V. J., et al. (2021). Mitigation of Pseudomonas syringae virulence by signal inactivation. Sci. Adv. 7:eabg2293. doi: 10.1126/sciadv.abg2293
Tyc, O., Song, C., Dickschat, J. S., Vos, M., and Garbeva, P. (2016). The ecological role of volatile and soluble secondary metabolites produced by soil bacteria. Trends Microbiol. 25, 280–292. doi: 10.1016/j.tim.2016.12.002
Tyc, O., Zweers, H., Boer, D. E., and Garbeva, W. P. (2015). Volatiles in inter-specific bacterial interactions. Front. Microbiol. 6, 1412. doi: 10.3389/fmicb.2015.01412
Keywords: eco-evo dynamics, microbial volatile compounds, interactions, evolution, chemical ecology
Citation: Masteling R, de Boer W, Raaijmakers JM, Garbeva P and Dini-Andreote F (2022) Microbial volatiles as mediators of eco-evolutionary dynamics. Front. Ecol. Evol. 10:960198. doi: 10.3389/fevo.2022.960198
Received: 02 June 2022; Accepted: 08 September 2022;
Published: 26 September 2022.
Edited by:
Dani Lucas-Barbosa, Research Institute of Organic Agriculture (FiBL), SwitzerlandReviewed by:
Rouhallah Sharifi, Razi University, IranCopyright © 2022 Masteling, de Boer, Raaijmakers, Garbeva and Dini-Andreote. This is an open-access article distributed under the terms of the Creative Commons Attribution License (CC BY). The use, distribution or reproduction in other forums is permitted, provided the original author(s) and the copyright owner(s) are credited and that the original publication in this journal is cited, in accordance with accepted academic practice. No use, distribution or reproduction is permitted which does not comply with these terms.
*Correspondence: Raul Masteling, ci5tYXN0ZWxpbmcmI3gwMDA0MDtuaW9vLmtuYXcubmw=