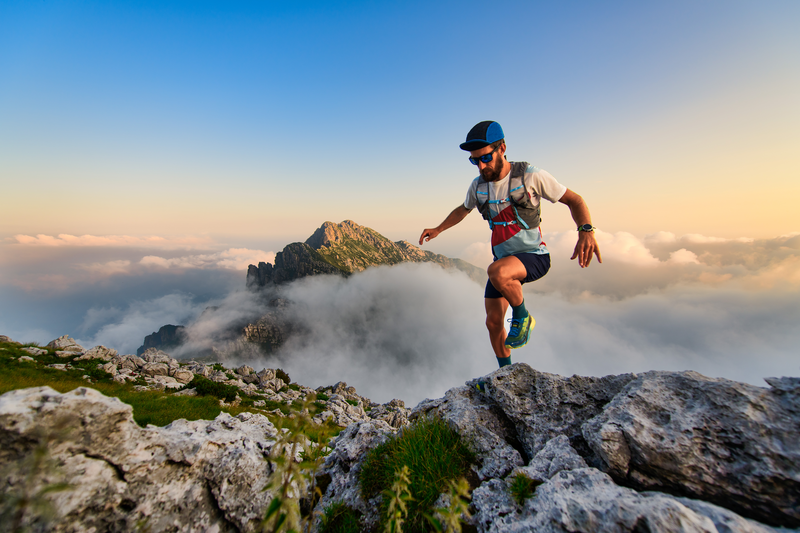
94% of researchers rate our articles as excellent or good
Learn more about the work of our research integrity team to safeguard the quality of each article we publish.
Find out more
OPINION article
Front. Ecol. Evol. , 06 May 2022
Sec. Behavioral and Evolutionary Ecology
Volume 10 - 2022 | https://doi.org/10.3389/fevo.2022.873557
At its core, life history reflects the outcome of energy allocation toward maintenance, growth, and reproduction over an individual's lifetime (van Noordwijk and de Jong, 1986; Stearns, 1992). This allocation of energy can be estimated via life history traits, such as the age at weaning or first reproduction and the rate of reproduction (Lande, 1982). In primates and other mammals, life history traits are negatively allometrically related to adult female body mass (Harvey et al., 1987; Martin et al., 2005). However, gorillas seem to defy this principle as previous estimates suggested the mountain gorilla (Gorilla beringei) to be heavier and to have a faster life history (Table 1) than the lighter western gorilla (Gorilla gorilla) (Stoinski et al., 2013). We will argue in the following that some of the body mass data used in the past were flawed. Recent data (see below) confirm that females in both species are of similar mass and consequently their life histories should be similar, all else being equal.
Table 1. Examples of phenotypic plasticity in primate life history (mean values in months, except when noted).
Apart from the question of body mass, variation in gorilla life history has mainly been interpreted in light of two ecological hypotheses, namely “ecological risk aversion” and the importance of “folivory” (Janson and van Schaik, 1993; Leigh, 1994). Both hypotheses predict slower life histories in more frugivorous primates. This is relevant because the diet of western gorillas—with the slower life history (Stoinski et al., 2013)—contains much more fruits compared to that of mountain gorillas (e.g., Lodwick and Salmi, 2019). On the one hand, more frugivorous primates are assumed to face higher food uncertainty given the seasonally restricted availability of fruits, in combination with extended periods of low fruit availability (Knott and Harwell, 2020). Frugivores should thus have a generally slower life history (late weaning, late onset of reproduction, and a slow reproductive rate) to avoid starvation (ecological risk aversion, Janson and van Schaik, 1993). On the other hand, the mainly folivorous diet of the faster mountain gorilla, is much less seasonal (Wright et al., 2015). The year-round consumption of protein contained in high-quality leaves is assumed to promote growth and thus to accelerate their life history (Janson and van Schaik, 1993; Leigh, 1994).
The above concept of ecological risk aversion implies that the life histories of gorillas living in different environments are the consequence of adaptation to the respective food availability. However, we will argue here that it is more likely that the variation in gorilla growth and reproductive rates (i.e., life history) is simply a direct and flexible phenotypic response to the actual nutrient availability (phenotypic plasticity below). In addition, we challenge, based on earlier and recent data, the assumed importance of protein intake for growth and reproduction (advantages of folivory below).
Although rarely emphasized in the literature, the negative allometric relationship between adult female body mass and life history did not seem to apply to species within the genus Gorilla, as previous estimates suggested wild mountain gorillas to be heavier [97.5 kg (Smith and Jungers, 1997)] and to have a faster life history (e.g., Stoinski et al., 2013) than the lighter wild western gorillas [71.5 kg, (Smith and Jungers, 1997)]. This may relate to the fact that the mass value for mountain gorillas was based on a single female specimen of undisclosed origin. Recently, new body mass data for mountain gorillas reversed this relationship. Rather than being much heavier, adult female mountain gorillas at Karisoke turned out to be slightly lighter [66.3 kg (Burgess et al., 2018)] than western gorillas. This much smaller difference (−5.2 kg vs. formerly +26.0 kg) should only marginally affect life history, if at all.
In addition, genetic data suggest a late split between western and mountain gorillas with gene flow occurring up until about 80–500 kya (Thalmann et al., 2007; Scally et al., 2012). This recent divergence is consistent with their similar body mass and with craniometric data demonstrating a much higher phenotypic variation within (80%) rather than between species (20%) (Leigh et al., 2003). Similarly, gestation length, the only life history trait that is tightly linked to phylogeny (Martin and MacLarnon, 1985; Lee, 2012), is identical in the different gorilla species (Smith et al., 1999; Doran-Sheehy et al., 2009; Habumuremyi et al., 2016). This all adds to the notion of small genetic distance such that strong similarities in life history are to be expected for all gorillas although genetic differences in gorilla life history cannot be ruled out.
The different gorilla populations vary greatly in the proportion of leaves and herbs in their diet (Rogers et al., 2004; Rothman et al., 2007). Consequently, the higher protein intake in the more folivorous mountain gorillas (Plumptre, 1995) was thought to contribute to their faster growth and reproduction (Janson and van Schaik, 1993).
However, the effect of protein on the body is dependent on intake, as was for example shown in deprivation experiments on capuchins (Cebus spp.), where signs of protein deficiency emerged only in animals with <3% protein intake but not in those with 6.7% intake (Fleagle et al., 1975; Elias and Samonds, 1977). An intake of 6.4–8.0% protein has been established as sufficient for several primate species (National Research Council US, 2003). In comparison, for the three gorilla populations for which protein intake has been estimated, it averaged at least 12% [i.e., mountain gorillas: 18% at Karisoke, 17% at Bwindi, (Rothman et al., 2007); western gorillas: 12% at Mondika (Lodwick and Salmi, 2019, with 8.4% as the lowest monthly protein intake)]. From a growth perspective, the protein intake is, thus, always sufficient across sites. Furthermore, studies of nutrient intake (Rothman et al., 2011) suggested that folivorous primates usually did not select for protein [this generally only happened in primates when protein availability is very low (Ganzhorn et al., 2017)] but instead prioritized non-protein energy (Rothman et al., 2011). Especially in tropical forests, nitrogen, the building block of proteins, is not a limiting factor for folivores, and thus differences in protein intake are unlikely to explain the variation in gorilla life history. If more protein is consumed than can be assimilated, it is metabolized and may render the energy balance more positive (see also below), but it cannot be stored as protein or nitrogen (Pesta and Samuel, 2014). Thus, a protein heavy diet per se will not accelerate growth.
As has been demonstrated in several species, individuals respond to local ecological conditions (Ricklefs and Wikelski, 2002; Lee and Kappeler, 2003; Wells and Stock, 2011), a phenomenon known as phenotypic plasticity (Scheiner, 1993). Within the inherited limits, the same genotype can produce different phenotypes in different environments or changing environments, leading to changes in phenotype over time (Fusco and Minelli, 2010). While the range of possible reactions to environmental influences is genetically determined, gene expression and cellular processes are regulated by hormones, thus implementing flexible responses to the current environmental conditions (Emery Thompson, 2017).
One of the strongest phenotypic effects on life history traits likely results from differences in energy balance. Energy balance is the net amount of energy available to the body for maintenance, growth, and reproduction (Emery Thompson, 2017). If food is superabundant and of high quality, energy intake of the individuals can exceed their energy expenditure, thus rendering energy balance positive (Ellison, 2017). This surplus energy can be stored as fat, which is, however, rare in wild primates (Altmann et al., 1993). Extra energy is mainly allocated for growth and reproduction leading to faster growth, younger maturation ages, and higher reproductive rates (Emery Thompson, 2013).
Phenotypic plasticity is a well-established phenomenon that has been documented in various animal orders (Sadleir, 1969; Gilmore and Cook, 1981; Stearns and Koella, 1986), including primates (Table 1). At Amboseli, Kenya, one of the yellow baboon groups (Papio cynocephalus) frequently fed at a dumpster, which led to high energy intake and a particularly low energy expenditure. The individuals in this group grew, matured, and reproduced significantly faster than those in groups lacking access to energy-dense foods (Altmann and Alberts, 2003, 2005). We documented similar, significant effects in an intrageneric comparison of gray langurs (Semnopithecus spp.) from a provisioned (India) and an unprovisioned (Nepal) population (Borries and Koenig, 2000; Borries et al., 2001). In these two comparisons, the surplus energy was provided by humans, but natural habitats can also differ markedly in quality, as in the Kibale forest, Uganda (Potts et al., 2015). Here, the chimpanzees (Pan troglodytes) inhabiting the area with high fruit availability at Ngogo, had a mean interbirth interval that was 19 months shorter compared to the neighboring community at Kanyawara (Emery Thompson et al., 2007; Watts, 2012).
Phenotypic plasticity becomes even more apparent when changes in energy balance occur within the course of an individuals' lifetime (Table 1). For example, one group of the Amboseli baboons eventually relocated to a more productive habitat, which shortened interbirth intervals (Altmann and Alberts, 2003). Similar changes, although in the opposite direction, occurred in free-ranging Japanese macaques (Macaca fuscata) from Mt. Ryozen, Japan, after regular provisioning was discontinued (Sugiyama and Ohsawa, 1982). The same individuals, with identical genetic makeup, exhibited different speeds of life history, contingent on energy availability. More broadly, all of the above examples illustrate that excess energy can lead to predictable and non-trivial accelerations of primate life history.
Currently, it remains difficult to apply this concept to gorillas because very few comparative data on energy intake and expenditure, and thus on energy balance, are available. However, mountain gorillas at the long-term site, Karisoke, have a very short daily path length and a high energy intake from mainly terrestrial herbaceous vegetation (Supplementary Table S1). A recent analysis of C-peptide concentrations in feces, a proxy for energy intake, suggests that there were no seasonal, energetic bottlenecks (Grueter et al., 2014). It seems therefore reasonable to assume that gorillas at Karisoke live year-round under nearly ideal energetic conditions similar to those experienced in captivity (Wright et al., 2015). This could be the reason why previous comparisons found gorilla life history to be unaffected by the improved nutritional conditions in captivity (Harcourt et al., 1980; Tutin, 1994). The conclusion was based on data for wild mountain gorillas from Karisoke and captive western gorillas. Only more recently has it become clear that wild western gorillas have a much slower life history compared to their captive counterparts (Breuer et al., 2009; Stoinski et al., 2013; Manguette et al., 2019; cf. Table 1). The latter do not experience seasonal, nutritional restrictions and have a low energy expenditure.
We hope to have shown that variation in gorilla life history is unlikely to be an adaptive strategy with a generally reduced speed of life history in habitats where negative energy balances are likely to occur regularly (ecological risk aversion, Janson and van Schaik, 1993). Rather, growth and reproduction could simply fluctuate locally in relation to how positive the current energy balance is and remains over time. Furthermore, we now know that the gorilla species are very similar in adult female body mass and that excess protein intake per se does not accelerate life history. It seems therefore most parsimonious to predict that the overall availability of nutrients and seasonal fluctuations in energy balance should directly and flexibly affect the speed of gorilla life history.
Moving forward, quantifying individual female energy balance (intake and expenditure) over time in a standardized manner at different gorilla study sites is required to determine whether phenotypic plasticity is indeed the main explanation for variation in gorilla life history. Such a study will need to also account for differences e.g., in the digestibility of leaves and in the amount of energy invested in thermoregulation (Wright et al., 2015), as well as in arboreal locomotion. Non-energetic factors, such as the density of large food competitors (impacting food availability) and genetic differences between gorilla populations, resulting in different metabolic or other physiological adaptations should also be examined.
CB collated the core arguments and wrote the first draft. The ideas were refined and tailored to gorillas by all authors who also commented on and edited all versions of the manuscript. All authors contributed to the article and approved the submitted version.
The open access publication fees were provided by the Dean of the College of Arts and Sciences.
The authors declare that the research was conducted in the absence of any commercial or financial relationships that could be construed as a potential conflict of interest.
All claims expressed in this article are solely those of the authors and do not necessarily represent those of their affiliated organizations, or those of the publisher, the editors and the reviewers. Any product that may be evaluated in this article, or claim that may be made by its manufacturer, is not guaranteed or endorsed by the publisher.
We thank two reviewers for their comments, Diane Doran-Sheehy for countless discussions of all things gorilla, and Angela Meder for pointing out references on captive gorillas.
The Supplementary Material for this article can be found online at: https://www.frontiersin.org/articles/10.3389/fevo.2022.873557/full#supplementary-material
Altmann, J., and Alberts, S. C. (2003). “Intraspecific variability in fertility and offspring survival in a nonhuman primate: behavioral control of ecological and social sources”, in Offspring: Human Fertility Behavior in Biodemographic Perspective, Wachter, K. W., and Bulatao, R. A., editors. Washington DC: National Academies Press. p 140–169.
Altmann, J., and Alberts, S. C. (2005). Growth rates in a wild primate population: ecological influences and maternal effects. Behav. Ecol. Sociobiol. 57, 490–501. doi: 10.1007/s00265-004-0870-x
Altmann, J., Schoeller, D., Altmann, S. A., Muruthi, P., and Sapolsky, R. M. (1993). Body size and fatness of free-living baboons reflect food availability and activity levels. Am. J. Primatol. 30, 149–161. doi: 10.1002/ajp.1350300207
Borries, C., and Koenig, A. (2000). “Infanticide in Hanuman langurs: social organization, male migration, and weaning age” in, Infanticide by Males and its Implications, van Schaik, C. P., and Janson, C. H., editors. Cambridge: Cambridge University Press. p. 99–122. doi: 10.1017/CBO9780511542312.007
Borries, C., Koenig, A., and Winkler, P. (2001). Variation of life history traits and mating patterns in female langur monkeys (Semnopithecus entellus). Behav. Ecol. Sociobiol. 50, 391–402. doi: 10.1007/s002650100391
Breuer, T., Breuer-Ndoundou, H. M., Olejniczak, C., Parnell, R. J., and Stokes, E. J. (2009). Physical maturation, life-history classes and age estimates of free-ranging western gorillas - insights from Mbeli Bai, Republic of Congo. Am. J. Primatol. 71, 106–119. doi: 10.1002/ajp.20628
Burgess, M. L., McFarlin, S. C., Mudakikwa, A., Cranfield, M. R., and Ruff, C. B. (2018). Body mass estimation in hominoids: age and locomotor effects. J. Hum. Evol. 115, 36–46. doi: 10.1016/j.jhevol.2017.07.004
Doran-Sheehy, D. M., Fernandez, D., and Borries, C. (2009). The strategic use of sex in wild female western gorillas. Am. J. Primatol. 71:1011–1020. doi: 10.1002/ajp.20743
Elias, M. F., and Samonds, K. W. (1977). Protein and calorie malnutrition in infant cebus monkeys: growth and behavioral development during deprivation and rehabilitation. Am. J. Clin. Nutr. 30, 355–366. doi: 10.1093/ajcn/30.3.355
Ellison, P. T. (2017). Endocrinology, energetics, and human life history: a synthetic model. Horm. Behav. 91, 97–106. doi: 10.1016/j.yhbeh.2016.09.006
Emery Thompson, M. (2013). Comparative reproductive energetics of human and nonhuman primates. Annu. Rev. Anthropol. 42, 287–304. doi: 10.1146/annurev-anthro-092412-155530
Emery Thompson, M. (2017). Energetics of feeding, social behavior, and life history in non-human primates. Horm. Behav. 91, 84–96. doi: 10.1016/j.yhbeh.2016.08.009
Emery Thompson, M., Kahlenberg, S. M., Gilby, I. C., and Wrangham, R. W. (2007). Core area quality is associated with variance in reproductive success among female chimpanzees at Kibale National Park. Anim. Behav. 73, 501–512. doi: 10.1016/j.anbehav.2006.09.007
Fleagle, J. G., Samonds, K. W., and Hegsted, D. M. (1975). Physical growth of cebus monkeys, Cebus albifrons, during protein or calorie deficiency. Am. J. Clin. Nutr. 28, 246–253. doi: 10.1093/ajcn/28.3.246
Fusco, G., and Minelli, A. (2010). Phenotypic plasticity in development and evolution: facts and concepts. Phil. Trans. R. Soc. B. 365, 547–556. doi: 10.1098/rstb.2009.0267
Ganzhorn, J. U., Arrigo-Nelson, S., Carrai, V., Chalise, M. K., Donati, G., Droescher, I., et al. (2017). The importance of protein in leaf selection of folivorous primates. Am. J. Primatol. 79:e22550. doi: 10.1002/ajp.22550
Gilmore, D., and Cook, B. (1981). Environmental Factors in Mammal Reproduction. London: Macmillan Publishers. doi: 10.1007/978-1-349-03964-7
Grueter, C. C., Deschner, T., Behringer, V., Fawcett, K. A., and Robbins, M. M. (2014). Socioecological correlates of energy balance using urinary C-peptide measurements in wild female mountain gorillas. Physiol. Behav. 127, 13–19. doi: 10.1016/j.physbeh.2014.01.009
Habumuremyi, S., Stephens, C., Fawcett, K. A., Deschner, T., and Robbind, M. M. (2016). Endocrine assessment of ovarian cycle activity in wild female mountain gorillas (Gorilla beringei beringei). Physiol. Behav. 157, 185–195. doi: 10.1016/j.physbeh.2016.02.017
Harcourt, A. H., Fossey, D., Stewart, K. J., and Watts, D. P. (1980). Reproduction by wild gorillas and some comparisons with the chimpanzee. J. Reprod. Fertil. Suppl. 28, 59–70.
Harvey, P. H., Martin, R. D., and Clutton-Brock, T. H. (1987). “Life histories in comparative perspective” in, Primate Societies, Smuts, B. B., Cheney, D. L., Seyfarth, R. M., Wrangham, R. W., and Struhsaker, T. T., editors. Chicago: University of Chicago Press. p. 181–196.
Janson, C. H., and van Schaik, C. P. (1993). “Ecological risk aversion in juvenile primates: slow and steady wins the race”, in Juvenile primates: life history, development, and behavior. Pereira, M. E., and Fairbanks, L. A., editors. New York: Oxford University Press. p 57–74.
Knott, C. D., and Harwell, F. S. (2020). “Ecological risks and the evolution of great ape life histories”, in Chimpanzees in Context: a Comparative Perspective on Chimpanzee Behavior, Cognition, Conservation, and Welfare. Hopper, L. M., and Ross, S. R., editors. Chicago: University of Chicago Press. p 3–35. doi: 10.7208/chicago/9780226728032.003.0001
Lande, R. (1982). A quantitative genetic theory of life history evolution. Ecology. 63, 607–615. doi: 10.2307/1936778
Lee, P. C. (2012). Growth and investment in hominin life history evolution: patterns, processes, and outcomes. Int. J. Primatol. 33, 1309–1331. doi: 10.1007/s10764-011-9536-5
Lee, P. C., and Kappeler, P. M. (2003). “Socioecological correlates of phenotypic plasticity of primate life histories”, in Primate Life Histories and Socioecology, Kappeler, P. M., and Pereira, M. E., editors. Cambridge: Cambridge University Press. p. 41–65.
Leigh, S. R. (1994). Ontogenetic correlates of diet in anthropoid primates. Am. J. Phys. Anthropol. 94, 499–522. doi: 10.1002/ajpa.1330940406
Leigh, S. R., Relethford, J. H., Park, P. B., and Konigsberg, L. W. (2003). “Morphological differentiation of Gorilla subspecies”, in Gorilla Biology - a Multidisciplinary Perspective, Taylor, A. B., and Goldsmith, M. L., editors. Cambridge: Cambridge University Press. p. 104–131. doi: 10.1017/CBO9780511542558.007
Lodwick, J. L., and Salmi, R. (2019). Nutritional composition of the diet of the western gorilla (Gorilla gorilla): interspecific variation in diet quality. Am. J. Primatol. 81:e23044. doi: 10.1002/ajp.23044
Manguette, M. L., Robbin, A. M., Breuer, T., Stokes, E. J., Parnell, R. J., and Robbins, M. M. (2019). Intersexual conflict influences female reproductive success in a female-dispersing primate. Behav. Ecol. Sociobiol. 73:118. doi: 10.1007/s00265-019-2727-3
Martin, R. D., Genoud, M., and Hemelrijk, C. K. (2005). Problems of allometric scaling analysis: examples from mammalian reproductive biology. J. Exp. Biol. 208, 1731–1747. doi: 10.1242/jeb.01566
Martin, R. D., and MacLarnon, A. M. (1985). Gestation period, neonatal size and maternal investment in placental mammals. Nature. 313, 220–223. doi: 10.1038/313220a0
National Research Council US (2003). Nutrient Requirements of Nonhuman Primates. Washington, DC: National Academies Press.
Pesta, D. H., and Samuel, V. T. (2014). A high-protein diet for reducing body fat: mechanisms and possible caveats. Nutr Metab 11:53. doi: 10.1186/1743-7075-11-53
Plumptre, A. J. (1995). The chemical composition of montane plants and its influence on the diet of the large mammalian herbivores in the Parc National des Volcans, Rwanda. J Zool 235:323–337. doi: 10.1111/j.1469-7998.1995.tb05148.x
Potts, K. B., Baken, E., Ortmann, S., Watts, D. P., and Wrangham, R. W. (2015). Variability in population density is paralleled by large differences in foraging efficiency in chimpanzees (Pan troglodytes). Int. J. Primatol. 36, 1101–1119. doi: 10.1007/s10764-015-9880-y
Ricklefs, R. E., and Wikelski, M. C. (2002). The physiology/life-history nexus. Trends Ecol. Evol. 17, 462–468. doi: 10.1016/S0169-5347(02)02578-8
Rogers, M. E., Abernethy, K., Bermejo, M., Cipolletta, C., Doran, D. M., McFarland, K., et al. (2004). Western gorilla diet: a synthesis from six sites. Am J Primatol 64, 173–192. doi: 10.1002/ajp.20071
Rothman, J. M., Plumptre, A. J., Dierenfeld, E. S., and Pell, A. N. (2007). Nutritional composition of the diet of the gorilla (Gorilla beringei): a comparison between two montane habitats. J Trop Ecol. 23, 673–682. doi: 10.1017/S0266467407004555
Rothman, J. M., Raubenheimer, D., and Chapman, C. A. (2011). Nutritional geometry: gorillas prioritize non-protein energy while consuming surplus protein. Biol Lett. 7, 847–849. doi: 10.1098/rsbl.2011.0321
Sadleir, R. M. F. S. (1969). The role of nutrition in the reproduction of wild mammals. J Reprod Fertil Suppl. 6, 39–48.
Scally, A., Dutheil, J. Y., Hillier, L. W., Jordan, G. E., Goodhead, I., Herrero, J., et al. (2012). Insights into hominid evolution from the gorilla genome sequence. Nature. 483, 169–175. doi: 10.1038/nature10842
Scheiner, S. M. (1993). Genetics and evolution of phenotypic plasticity. Annu. Rev. Ecol. Syst. 24, 35–68. doi: 10.1146/annurev.es.24.110193.000343
Smith, R., Wickings, E. J., Bowman, M. E., Belleoud, A., Dubreuil, G., Davies, J. J., et al. (1999). Corticotropin-releasing hormone in chimpanzee and gorilla pregnancies. J. Clin. Endocr. Metab. 84, 2820–2825. doi: 10.1210/jcem.84.8.5906
Smith, R. J., and Jungers, W. L. (1997). Body mass in comparative primatology. J. Hum. Evol. 32, 523–559. doi: 10.1006/jhev.1996.0122
Stearns, S. C., and Koella, J. C. (1986). The evolution of phenotypic plasticity in life-history traits: predictions of reaction norms for age and size at maturity. Evolution. 40, 893–913. doi: 10.1111/j.1558-5646.1986.tb00560.x
Stoinski, T. S., Perdue, B. M., Breuer, T., and Hoff, M. P. (2013). Variability in the developmental life history of the genus Gorilla. Am J Phys Anthropol. 152, 165–172. doi: 10.1002/ajpa.22301
Sugiyama, Y., and Ohsawa, H. (1982). Population dynamics of Japanese monkeys with special reference to the effect of artificial feeding. Folia Primatol. 39, 238–263. doi: 10.1159/000156080
Thalmann, O., Fischer, A., Lankester, F., Paeaebo, S., and Vigilant, L. (2007). The complex evolutionary history of gorillas: insights from genomic data. Mol Biol Evol. 24, 146–158. doi: 10.1093/molbev/msl160
Tutin, C. E. G. (1994). “Reproductive success story: variability among chimpanzees and comparisons with gorillas”, in Chimpanzee cultures, Wrangham, R. W., McGrew, W. C., de Waal, F. B. M., and Heltne, P. G., editors. Cambridge: Harvard University Press. p 181–193.
van Noordwijk, A. J., and de Jong, G. (1986). Acquisition and allocation of resources: their influence on variation in life history tactics. Am Nat. 128:137–142. doi: 10.1086/284547
Watts, D. P. (2012). “Long-term research on chimpanzee behavioral ecology in Kibale National Park, Uganda”, in Long-Term Field Studies of Primates, Kappeler, P. M., and Watts, D. P., editors. Berlin: Springer. p. 313–338. doi: 10.1007/978-3-642-22514-7_14
Wells, J. C. K., and Stock, J. T. (2011). Re-examining heritability: genetics, life history and plasticity. Trends Endocrin. Met. 22:421–428. doi: 10.1016/j.tem.2011.05.006
Keywords: body mass, captivity, energy balance, growth rate, protein intake, reproductive rate
Citation: Borries C, Lodwick JL, Salmi R and Koenig A (2022) Phenotypic Plasticity Rather Than Ecological Risk Aversion or Folivory Can Explain Variation in Gorilla Life History. Front. Ecol. Evol. 10:873557. doi: 10.3389/fevo.2022.873557
Received: 10 February 2022; Accepted: 19 April 2022;
Published: 06 May 2022.
Edited by:
Patricia Izar, University of São Paulo, BrazilReviewed by:
Hjalmar Kühl, German Centre for Integrative Biodiversity Research (iDiv), GermanyCopyright © 2022 Borries, Lodwick, Salmi and Koenig. This is an open-access article distributed under the terms of the Creative Commons Attribution License (CC BY). The use, distribution or reproduction in other forums is permitted, provided the original author(s) and the copyright owner(s) are credited and that the original publication in this journal is cited, in accordance with accepted academic practice. No use, distribution or reproduction is permitted which does not comply with these terms.
*Correspondence: Carola Borries, Y2Fyb2xhLmJvcnJpZXNAc3Rvbnlicm9vay5lZHU=
Disclaimer: All claims expressed in this article are solely those of the authors and do not necessarily represent those of their affiliated organizations, or those of the publisher, the editors and the reviewers. Any product that may be evaluated in this article or claim that may be made by its manufacturer is not guaranteed or endorsed by the publisher.
Research integrity at Frontiers
Learn more about the work of our research integrity team to safeguard the quality of each article we publish.