- Collaborative Innovation Center of Recovery and Reconstruction of Degraded Ecosystem in Wanjiang Basin Co-founded by Anhui Province and Ministry of Education, School of Ecology and Environment, Anhui Normal University, Wuhu, China
Low-head dams are ubiquitous human disturbances that degrade aquatic ecosystem function worldwide. The localized effects of low-head dams have been relatively well documented; however, most previous studies have ignored the concealed process caused by native-invasive species. Based on fish assemblage data from the first-order streams of four basins in the Wannan Mountains, we used a quantitative approach to assess the effects of low-head dams on fish assemblages by distinguishing between native and native-invasive species using occurrence- and abundance-based data, respectively. Low-head dams significantly decreased native fish alpha diversity while favoring native-invasive fish. The opposite pattern between the two fish types partly masked changes in the whole fish assemblage. Meanwhile, the establishment of widespread native-invasive species and the loss of native species driven by low-head dams influenced the interaction network structure. The degree to which local fish assemblages were altered by low-head dams, i.e., beta diversity (β-diversity) was significantly higher for abundance-based approaches than for occurrence-based ones, suggesting that the latter underestimated the effects of low-head dams. Furthermore, the species contribution to β-diversity of native species was significantly higher than that of native-invasive species in both impoundments and free-flowing segments for abundance-based data. In communities or regions where native fish species are predominant, our results suggest that understanding which species contribute to β-diversity will offer new insights into the development of effective conservation strategies by taking the identities of native and native-invasive species into account.
Introduction
Habitat alteration and fragmentation resulting from anthropogenic disturbances greatly contribute to global freshwater biodiversity loss (Erős and Grant, 2015; Barbarossa et al., 2020; He et al., 2021). In a river network, headwaters have unique features and are extremely vulnerable to anthropogenic disturbances (Vannote et al., 1980; Meyer et al., 2007). Headwater streams not only export quantities of organic matter and nutrients to downstream ecosystems (Vannote et al., 1980; Eggert et al., 2012), but also provide heterogeneous habitats for a wide range of endemic species, which collectively contribute to the biodiversity of river networks (Meyer et al., 2007).
Low-head dams are ubiquitous human-made disturbances in mountainous headwater streams that are used for water supply, agricultural irrigation, and recreation (Yan et al., 2013; Jumani et al., 2020; Li et al., 2021). Dams can affect stream fish assemblages via multiple pathways, including blocking species dispersal between localities (Porto et al., 1999; Barbarossa et al., 2020) and changing the habitat and physicochemical conditions in streams (Smith et al., 2017; Hitchman et al., 2018; Turgeon et al., 2019). Specifically, the transformation from lotic to lentic conditions in impoundments can provide suitable conditions for potential invasive species to displace native species (Johnson et al., 2008; Liew et al., 2016; Turgeon et al., 2019). Further, habitat simplification can decrease resource and niche availability (Ruhí et al., 2016; Turgeon et al., 2019).
The localized effects of individual low-head dams have been relatively well documented, but the effects and conclusions differ among studies (see Fencl et al., 2017; Turgeon et al., 2019; Li et al., 2021). A potential reason for this inconsistency is that non-native or generalist native species can compensate for the difference in total local diversity in impoundments (Slawski et al., 2008; Liew et al., 2016; Turgeon et al., 2019). Invasions of non-native species caused by dams have a profound impact on global freshwater biodiversity and have drawn increasing attention from the public (Liew et al., 2016; Turgeon et al., 2019; Zhang et al., 2019). More importantly, in regions where native fish species are completely dominant, generalist native species that commonly occur in large streams would be potential invaders for upland streams and are usually termed as “native-invasive species” (Scott and Helfman, 2001; Dala-Corte et al., 2019). However, most previous studies on the ecological effects of low-head dams have ignored the invasion patterns of native-invasive species, as increased native diversity is generally viewed as a positive effect on ecosystem function.
Changes in species occurrences (i.e., local extinctions and colonizations) not only alter community α-diversity, but also affect community β-diversity and dynamics (Socolar et al., 2016; Erős et al., 2020), resulting in the formation of “novel communities” (Hobbs et al., 2006). A serious effect of native-invasive species invasion on native species is a change in trophic ecology through predation, competition, and the indirect effects in the context of environmental changes (Busst and Britton, 2017). Although the effects of low-head dams on habitat conditions and biodiversity have long been examined (Fencl et al., 2015, 2017; Turgeon et al., 2019), the responses in species interaction networks remain understudied. Recently, Momal et al. (2020) proposed a more robust method for inferring species interaction networks by incorporating environmental conditions (i.e., abiotic factors). Furthermore, native-invasive species typically have broad niches and environmental tolerances in headwater streams (Scott and Helfman, 2001). These native-invasive species with broad niches may contribute less to beta diversity than species with narrow or intermediate niches, because the latter species may occur in environmentally restricted conditions and contribute more to beta diversity (Heino and Grönroos, 2017). Therefore, exploring the contribution of each species to beta diversity may provide a platform for understanding the formation of community diversity patterns (Heino and Grönroos, 2017; Gavioli et al., 2019).
The Wannan Mountains of China, characterized by low-head dams becoming ubiquitous features of the landscape (Li et al., 2021), offer an excellent opportunity to evaluate the effects of low-head dams on fish assemblages. Empirical evidence has shown that spatial patterns in the ecological effects of low-head dams can be quantified effectively by selecting free-flowing reference segments (Fencl et al., 2017; Hitchman et al., 2018; Li et al., 2021). Based on fish assemblage data collected in the impoundments created by low-head dams (i.e., comparison sites) and free-flowing segments (i.e., reference sites) in the first-order streams of the Wannan Mountains, we used a quantitative approach to assess the patterns in low-head dams affecting fish assemblages by considering the distinction between native and native-invasive species. We aimed to: (1) determine the effects of low-head dams on within-community (i.e., α-diversity) and between-community (i.e., β-diversity) diversities, (2) quantify the contributions of each species to the β-diversity for each habitat type, and (3) assess the difference in species interaction networks between the habitat types.
Materials and Methods
Study Area
The Wannan mountainous region is located in the southern part of Anhui Province, China, and is composed of the Jiuhua, Huangshan, and Tainmu Mountains, with elevations of 1,342, 1,841, and 1,787 m, respectively. The study region is influenced by a subtropical monsoon climate, where the average annual temperature is approximately 17.8°C and the average annual rainfall is approximately 2,000 mm, with precipitation occurring mostly from May to September. Streams are abundant and extremely complex in this region, with major examples including the Qingyi, Qiupu, Chang, and Xin’an streams (Li et al., 2021).
Our study included 61 low-head dams in the first-order headwater streams (stream order, Strahler, 1957) of the Wannan Mountains, of which 16, 9, 9, and 27 low-head dams were located in the Qingyi, Qiupu, Chang, and Xin’an streams, respectively, (Figure 1). To properly identify and understand the effects of low-head dams, two types of sampling sites were set for each dam surveyed, comprising the impoundments created by low-head dams (i.e., comparison sites) and free-flowing segments (i.e., reference sites) far away from the dams. In this study, our criteria for selecting reference sites were as follows: (1) all sites should be wadable and accessible (i.e., first-order stream); (2) since the geomorphological footprints of low-head dams are less than 2 km, the free-flowing reference segments were ensured to be at least 2 km from each dam (Fencl et al., 2015); and (3) all the reference sites were located below dams to avoid confusion; references selected above the dams would ignore the blocking effects of the dam (Li et al., 2021).
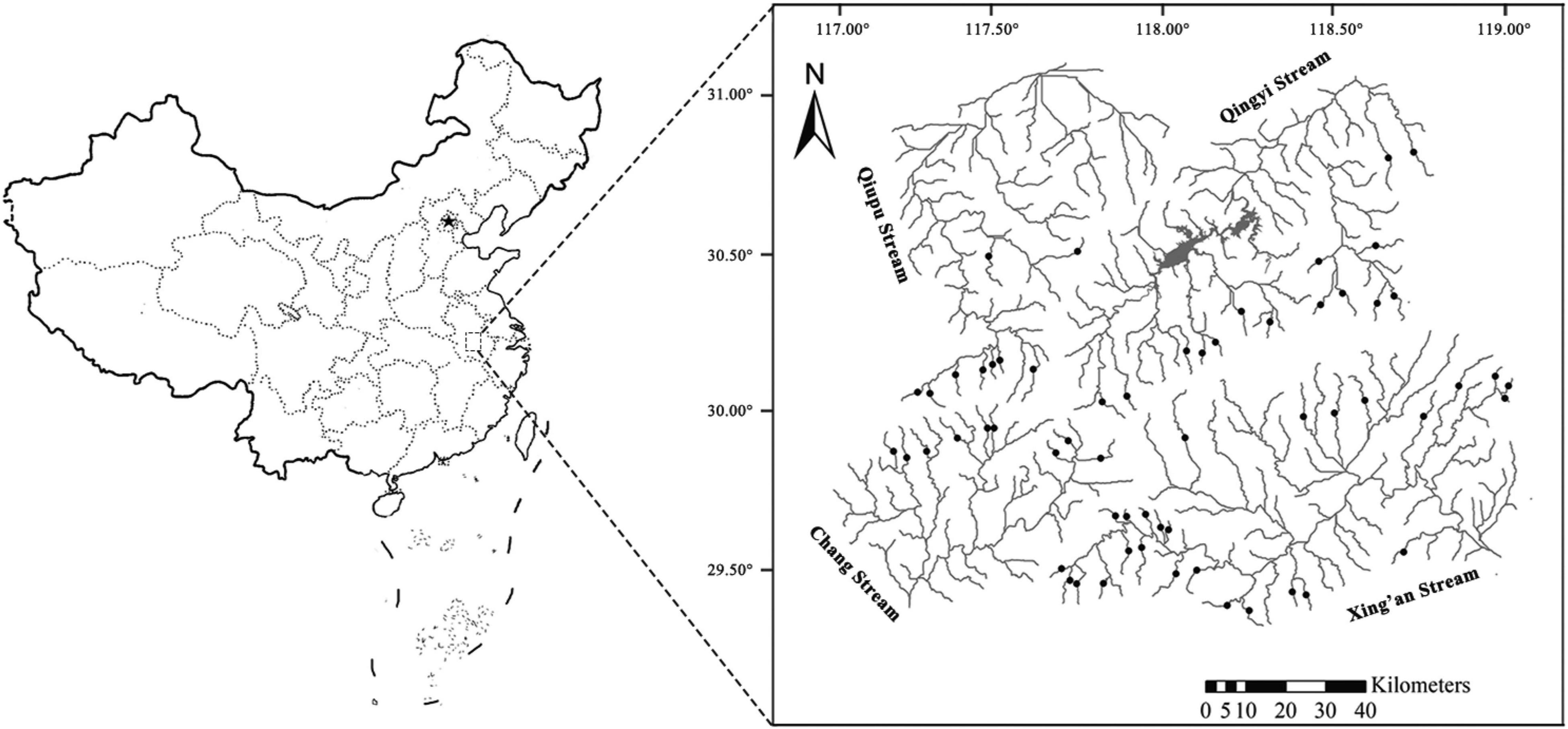
Figure 1. Map showing the survey of low-head dams distributed among four basins in the Wannan Mountains, China. One impoundment area and one free-flowing segment were sampled for each dam.
Field Survey
Fish assemblages were collected from each site using a backpack electrofishing unit (CWB-2000 P, China; 12V import, and 250V export) by wading in two passes during August 2015. For more details on the fish sampling methods and sampling effects, see Li et al. (2021). The fish were identified to the species level using Nelson’s (2006) classification method, counted, and released. Species were categorized as native and native-invasive species according to Scott and Helfman (2001), Chu et al. (2015); and Li et al. (2021).
The following local habitat conditions were measured at each site according to the methodology described by Li et al. (2021): (1) wetted width, (2) water depth, (3) current velocity, (4) dissolved oxygen, (5) substrate coarseness, and (6) heterogeneity. In addition, two dam metrics were used to quantify the size of each low-head dam: the dam height was estimated as the vertical height of the dam from the natural streambed to its lowest point on the dam crest, and the dam length was measured as the horizontal distance across the channel at the dam crest.
Data Statistics
The frequency of occurrence and relative abundance (RA) of each species were calculated for each habitat type. Species richness and abundance were regarded as the α-diversity. We used beta diversity to quantify the degree to which fish assemblages were altered by low-head dams. We calculated the temporal beta diversity (TBI) between the impoundment and free-flowing segment for each low-head dam for both occurrence and abundance data using the Sørensen dissimilarity index and its quantitative version, the Bray–Curtis dissimilarity index, respectively. The Sørensen indices, use occurrence-based data, which focus on species identities, i.e., abundant and rare species are treated equally (Koleff et al., 2003). However, abundance-based approaches give less weight to rare species, which means that they depend less on the sample size than occurrence-based approaches (Barwell et al., 2015).
For occurrence and abundance data, we obtained the fish community data (n site × p species) for two different habitat types, impoundments (Im) and free-flowing segments (Fr). We calculated the TBI between the impoundment and free-flowing reference segment for each dam. Specifically, we calculated the Sørensen (βSør) and Bray–Curtis dissimilarities (βBC) for both occurrence- and abundance-based data (Figure 2). The TBI (i.e., beta diversity) was further partitioned into gain and loss components, based on the method of Legendre (2019). In addition, we first obtained the dissimilarity matrix (n × n site) for each habitat type separately using the Hellinger-transformed community data (n site × p species). Based on the dissimilarity matrix, we calculated the species contribution to beta diversity (SCBD) of individual species for each habitat type separately (Figure 2; Legendre and De Cáceres, 2013). All of the above diversity indices were calculated through the “TBI” function (Legendre, 2015) and “adespatial” package with the function “beta.div” (Legendre and De Cáceres, 2013) in R 4.0.2.
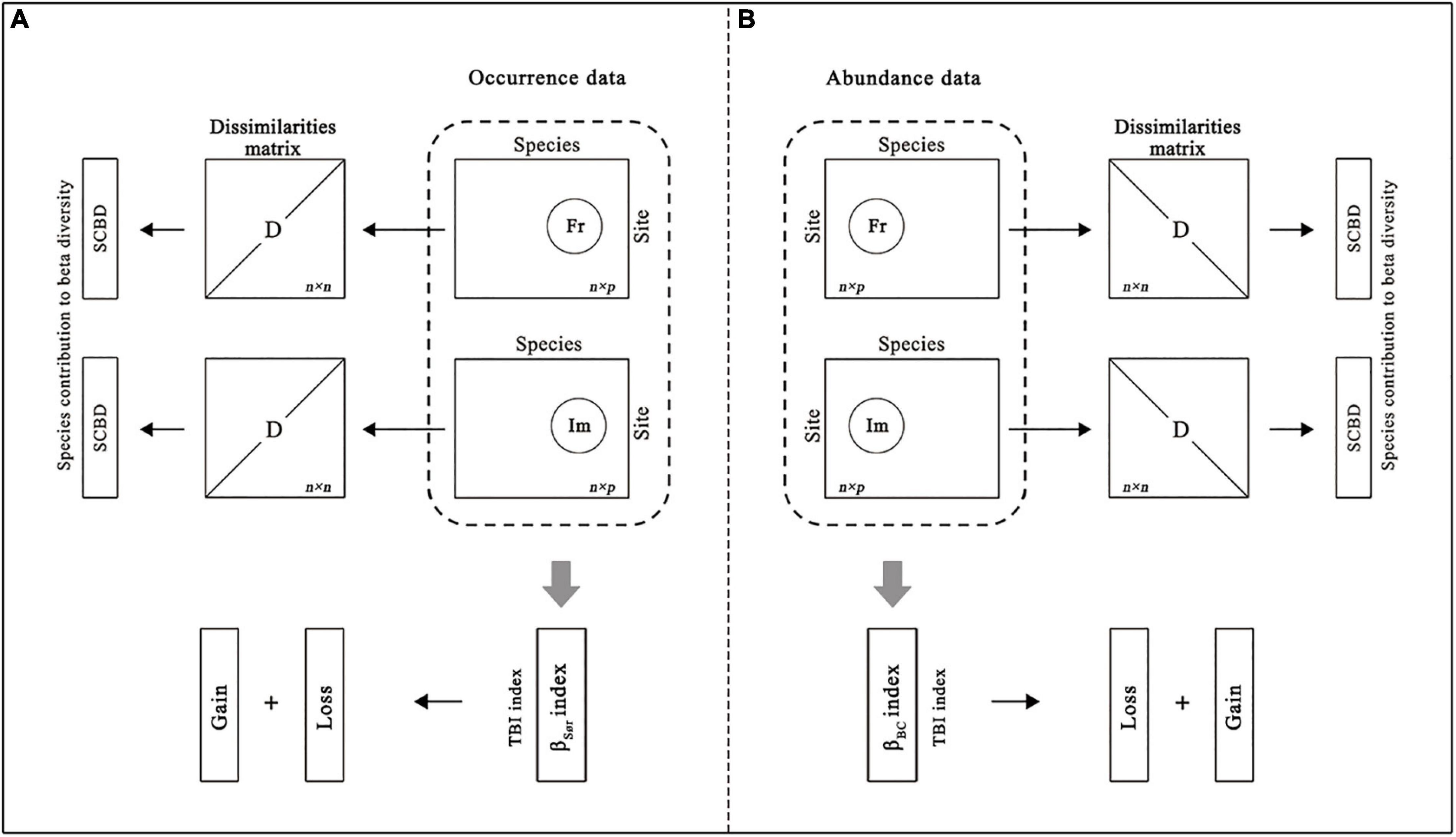
Figure 2. Schematic diagram of the comparison of community data (n site × p species) for two different habitat types, impoundments (Im) and free-flowing segments (Fr). We calculated the temporal beta diversity (TBI) using the Sørensen dissimilarity index (βSør) for (A) occurrence data and the Bray-Curtis dissimilarity index (βBC) for (B) abundance data. The TBI was further partitioned into gain and loss components, based on the method of Legendre (2019). In addition, we first obtained the dissimilarity matrix (n × n site) for each habitat type, and then we calculated the species contribution to beta diversity (SCBD) of individual species for each habitat type separately (adapted from Kuczynski et al., 2018).
Using the standardized environmental variables, we employed principal component analysis (PCA) and permutational multivariate analysis of variance (PERMANOVA) to test the differences in habitat conditions between impoundments and free-flowing segments. The paired sample t-test was used to test the differences in species richness and abundance between the impoundments and free-flowing reference segments. Given that changes in the local diversities of whole species may not mean any change for native species (Turgeon et al., 2019), the paired samples t-test was conducted by distinguishing between native and native-invasive species. To determine whether the ecological effects of low-head dams depended on the data type, we used the paired samples t-test to test the differences in beta diversity for each dam between occurrence- and abundance-based measures. In addition, changes in local diversity associated with anthropogenic disturbances often depend on the relative number between the species lost and gained. As a complement to local diversity changes, we also used the paired samples t-test to test the differences between gain and loss components for each data type.
To quantify the contributions of native and native-invasive species to the β-diversity for each habitat type, we first obtained the SCBD of individual species that reflected the relative importance of species for a region (Legendre and De Cáceres, 2013; Heino and Grönroos, 2017). Analysis of variance was used to test the difference in SCBD values between the native and native-invasive species in impoundments and free-flowing segments. Statistical analyses were performed for occurrence- and abundance-based data separately.
Poisson log-normal (PLN) models are joint species distribution models that can be used to infer species interactions while controlling for environmental factors and sampling efforts (Chiquet et al., 2019). Following Chiquet et al. (2019), we constructed a suite of PLN models to infer the species interaction network of fish assemblages in each habitat type using environmental factors and species abundance data. Here, we built 13 PLN models that included different combinations for the free-flowing reference segments, and 17 PLN models were built for the impoundments by considering the dam height and length (Supplementary Appendix 1). Additionally, the sampling effort (i.e., the total number of fish caught at each site) was included in our models to control for differences in fish abundance at different sites (Chiquet et al., 2019; Brimacombe et al., 2021). The PLN models were evaluated to determine the best model using non-traditional Bayesian information criterion (BIC) and cumulative root-mean-square error. Generally, higher BIC scores indicate better-fitting models. If the PLN models showed the same BIC scores, the model with the lowest RMSE score was selected as the best model. Furthermore, we inferred the fish interaction networks for each habitat type using the EMtree method proposed by Momal et al. (2020). The EMtree method, which represents a single undirected connection between species nodes, can infer both potential direct and indirect interactions between species. A criterion for assessing the reliability of species connections is to use a higher threshold such that at least one node (i.e., species) has at least one connection to build the network (Bassett et al., 2006). We created networks with the highest threshold that remained connected. PLN models were performed using the “PLN models” package (Chiquet et al., 2019), and EMtree (EMtree version 1.1.0) was accomplished using the “EMtree” package (Momal et al., 2020).
Results
Habitat Conditions
The first and second axes of PCA explained 32.43 and 19.99% of the habitat variance, respectively. Sampling sites were scattered at the right section of the ordination plot for the impoundments and at the left section for the free-flowing segments (Figure 3). PERMANOVA revealed that habitat conditions significantly differed between impoundments and free-flowing segments (R2 = 0.15, P < 0.001). Compared with free-flowing segments, conductivity, water width, and water depth were higher, and current velocity and substrate coarseness were lower in impoundments (Figure 3).
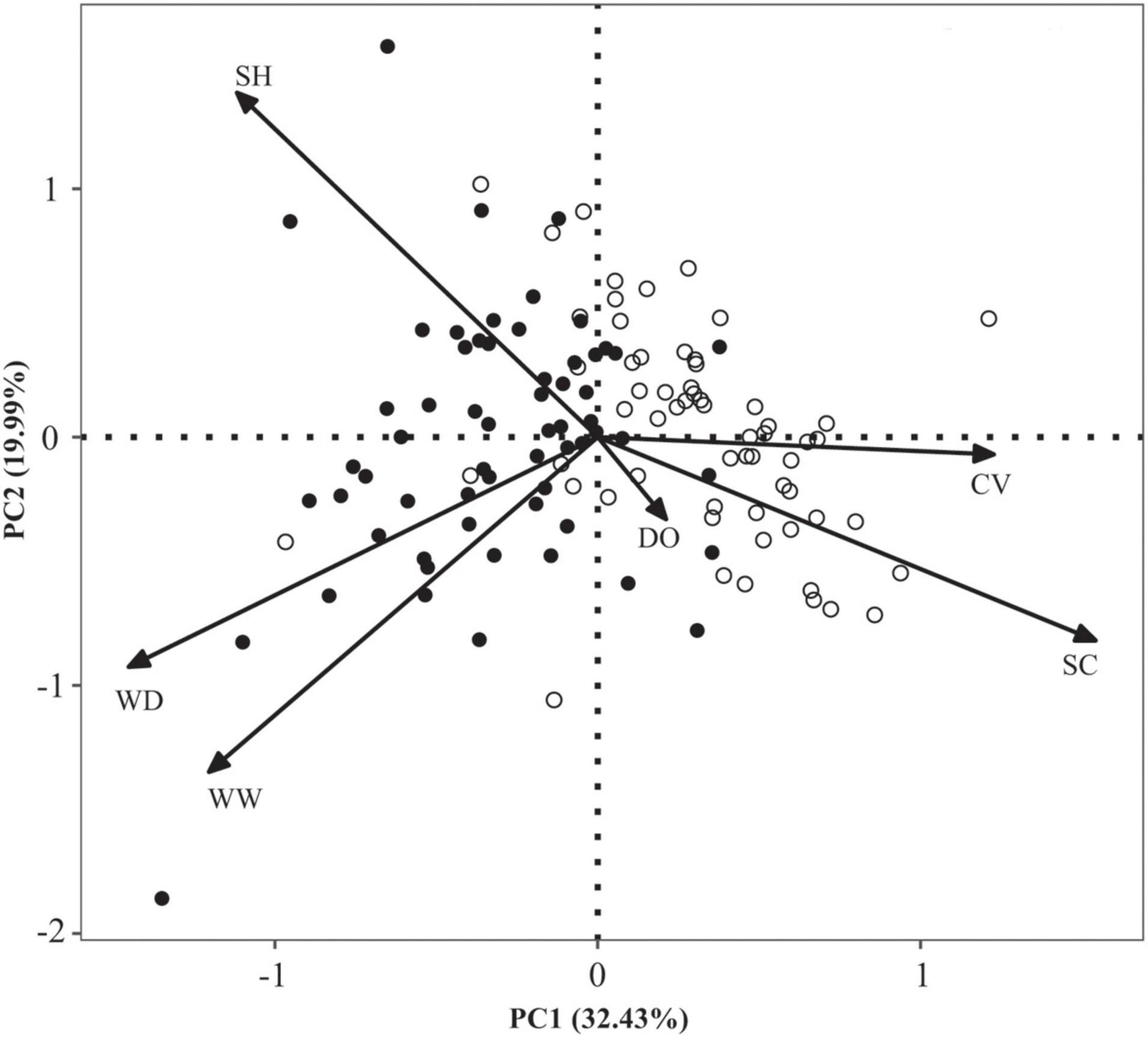
Figure 3. Ordination plots of the principal component analysis (PCA) between impoundments and reference free-flowing segments. Solid and hollow circles represent the impoundments and free-flowing segments, respectively. WW, wetted width; WD, water depth; SH, substrate heterogeneity; SC, substrate coarseness; DO, dissolved oxygen; and CV, current velocity.
Community Composition
Thirty-two species belonging to 11 families and 5 orders were collected. Thirty and 29 species were collected in impoundments and free-flowing segments, respectively, of which the two habitat types shared 27 species, including 14 native and 13 native-invasive species. Additionally, two native-invasive species (Micropercops swinhonis and Pelteobagrus fulvidraco) were only collected in impoundments, whereas two native (Leptobotia guilinensis and Parasinilabeo assimilis) and one native-invasive (Silurus asotus) species only occurred in free-flowing segments (Table 1).
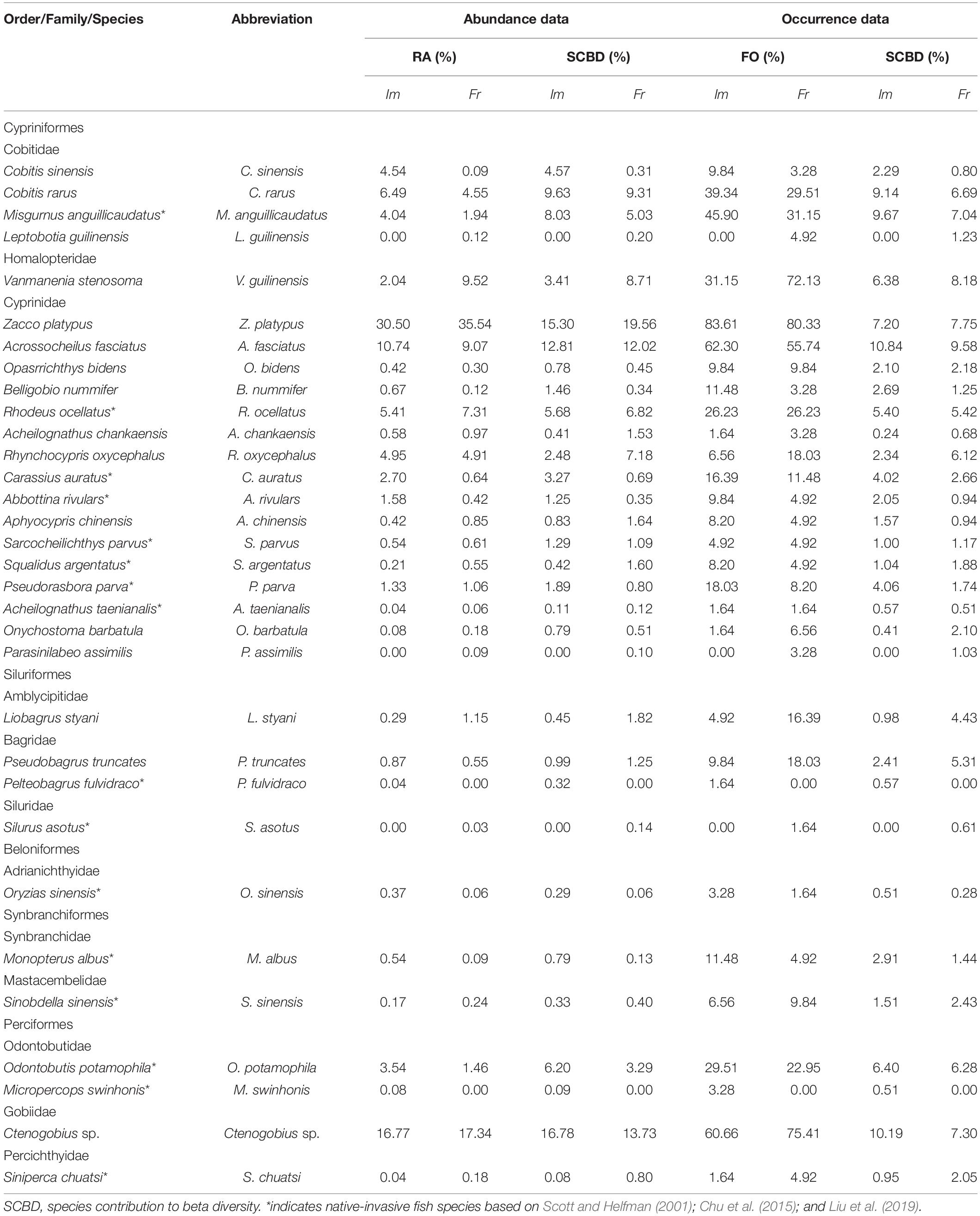
Table 1. Species compositions, occurrence of frequency (FO), and relative abundance (RA) of fishes collected in impoundments (Im) and free-flowing segments (Fr).
Changes in α- and β-Diversity
Regarding the whole assemblages, the paired samples t-test showed that the mean species abundance significantly decreased in impoundments compared to that in free-flowing segments (P < 0.05), whereas the species richness did not significantly vary between the two habitat types (Table 1). After distinguishing the two categories of fish assemblages, the species richness and abundance of native assemblages significantly varied between the two habitat types (P < 0.05), both of which were significantly lower in impoundments than in free-flowing segments. In addition, when only native-invasive assemblages were considered, the species richness was significantly higher in impoundments than in free-flowing segments (P < 0.05), whereas no significant difference was observed for species abundance (Table 2).
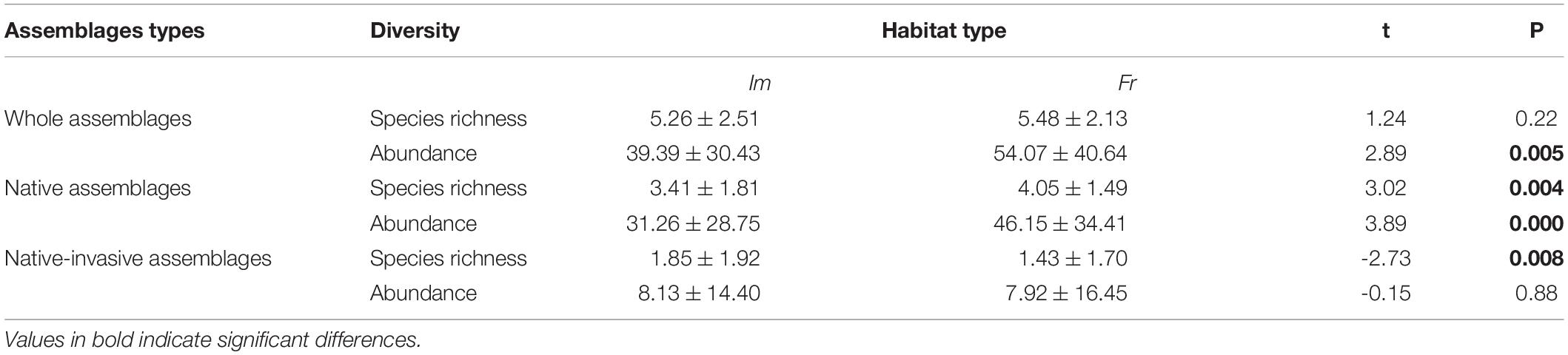
Table 2. Spatial variations in species richness and fish abundance (mean ± standard deviation) between the impoundments (Im) and free-flowing segments (Fr) for whole, native, and native-invasive assemblages based on the paired samples t-test.
In addition, the mean β-diversity using occurrence-based approaches (βsør) was significantly lower than that using abundance-based approaches (βBC; P < 0.05). Meanwhile, the abundance-based approaches detected a higher loss component (0.34 ± 0.22) than the gain component (0.20 ± 0.19) for all assemblages (P < 0.05), but no significant difference was observed for occurrence-based approaches (Table 3).

Table 3. Variation in beta diversity (β-diversity) between the data types and the difference between losses and gains components for each data type based on paired samples t-test.
Species Contribution to β-Diversity for Native and Native-Invasive Species
When using occurrence data, there was no significant difference in SCBD values between native and native-invasive species in either the impoundments or free-flowing segments (P > 0.05, Figure 4A). However, abundance data showed significant differences in SCBD values between native and native-invasive species in both habitat types (P < 0.05, Figure 4B). Specifically, the SCBD values were significantly higher for native fish species than for native-invasive ones in both the impoundments and free-flowing segments (Figure 4B).
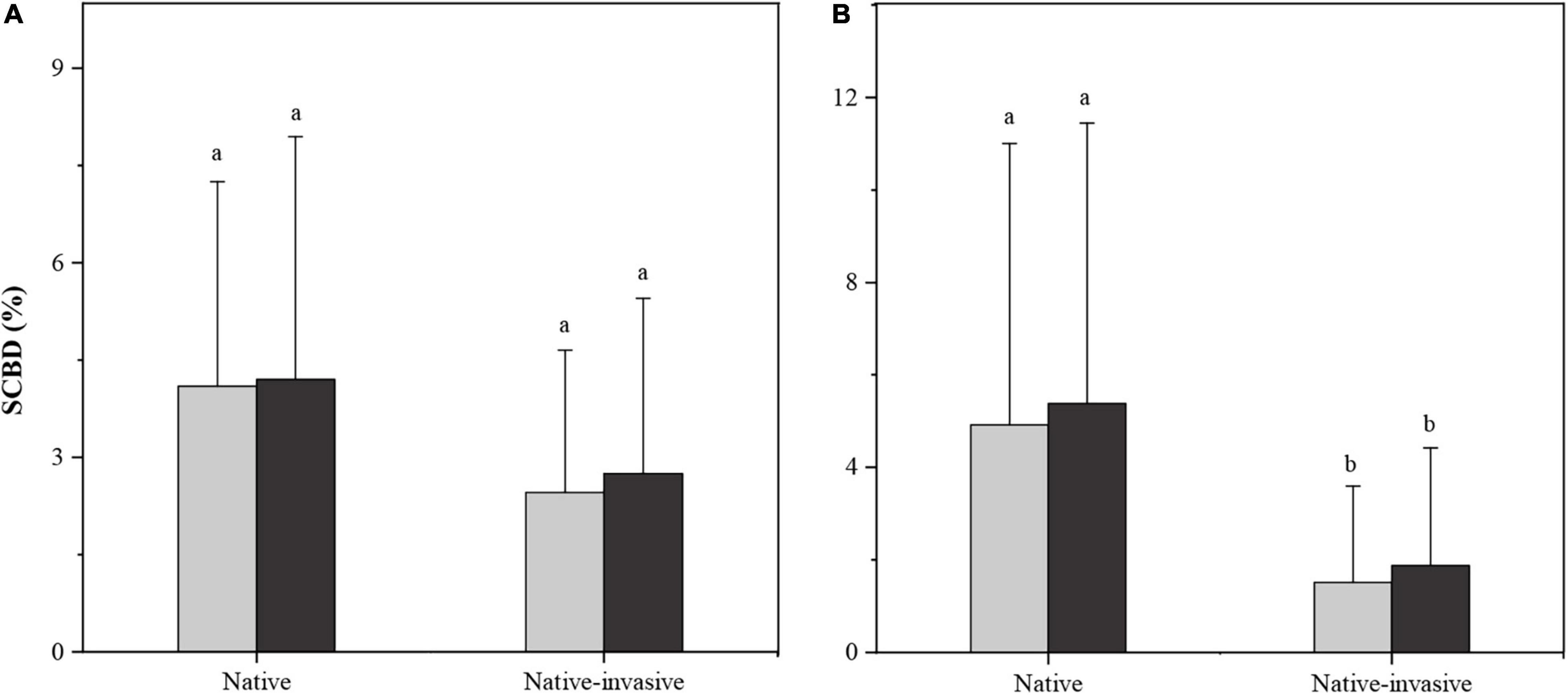
Figure 4. Bar plots showing the mean ± SD of the species contribution to β-diversity (SCBD) of native species and native-invasive species in impoundments (black) and free-flowing segments (gray). Different letters represent significant differences (P < 0.05). (A) occurrence-based SCBD, (B) abundance-based SCBD.
Inferred Species Interaction Networks From EMtree
Within each habitat type, the best-fit PLN model was selected to infer the species interaction networks. Specifically, the PLN model with “Site name” was chosen for both habitat types, which had the highest BIC scores (free-flowing segments: -2,503.34; impoundments: -2,315.86; Supplementary Appendix 1). Meanwhile, native species, such as Zacco platypus and Cobitis sinensis, showed the highest betweenness centrality values (larger nodes in the figures) in the free-flowing segments (Figure 5A). However, native-invasive species, such as Sarcocheilichthys parvus and Acheilognathus taenianalis, achieved the highest betweenness centrality values in the impoundments (Figure 5B).
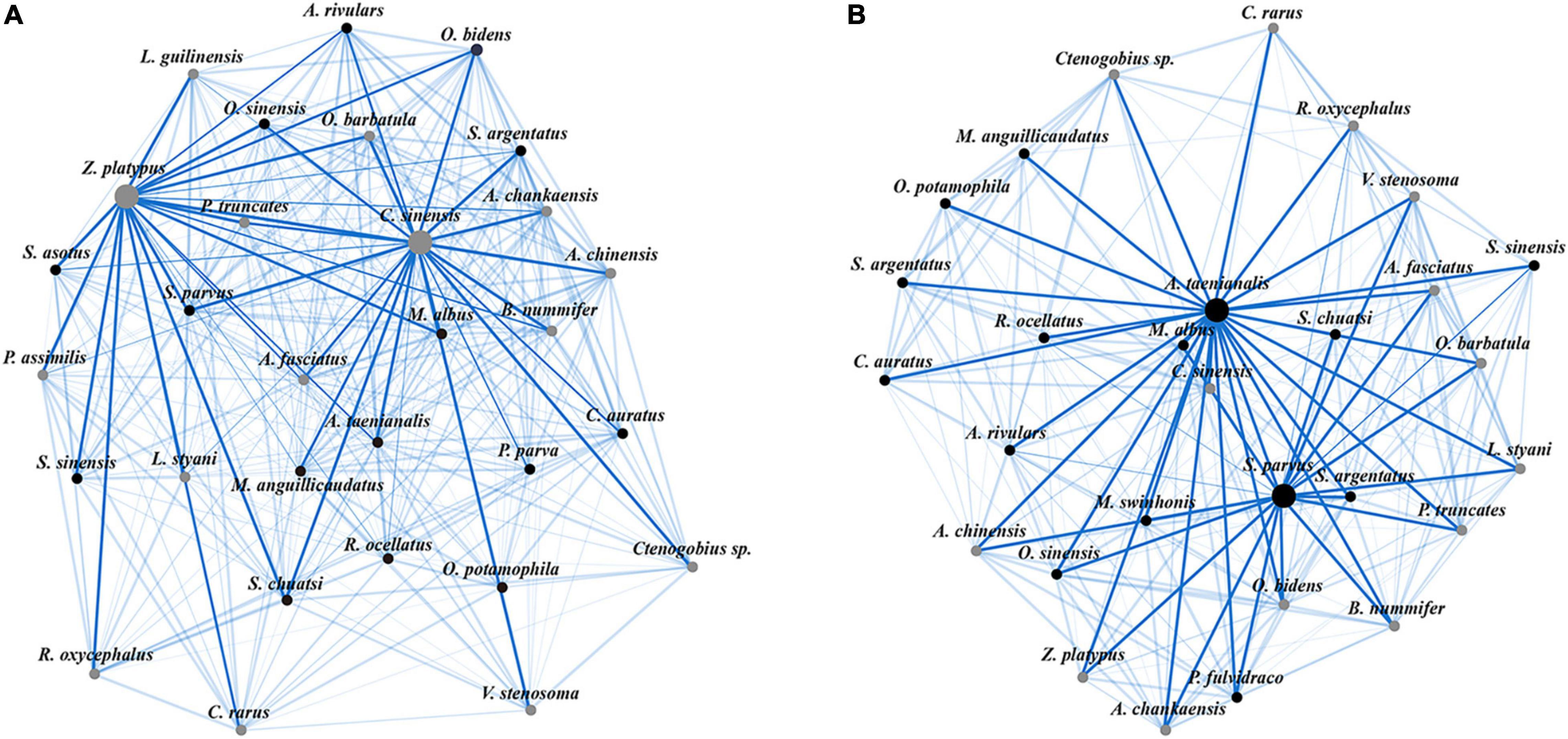
Figure 5. Species interaction networks of stream fish assemblages in the free-flowing segments (A) and impoundments (B). Each species is represented by a node, and each edge width is proportional to its conditional probability. The native (gray) and native-invasive species (black) are marked differently. For more details about species abbreviations, see Table 1.
Discussion
As a common anthropogenic disturbance in headwater streams, low-head dams may modify the local habitat in stream, including deeper water, slower flow, smaller substrate in impoundments upstream, and faster flow and larger substrate in the plunging areas downstream (Tiemann et al., 2004; Yan et al., 2013; Fencl et al., 2015). Due to the backwater effect of dams, the wetted width and water depth are greater in impoundments than that in free-flowing segments (Fencl et al., 2015). As the water depth increases in impoundments, current velocity is reduced and its sediment transport ability in the water column increases, generally resulting in an increase of sediment deposition (Tiemann et al., 2004; Yan et al., 2013). In this study, we found that low-head dams significantly decreased substrate coarseness, current velocity, and increased water depth, wetted width, and substrate heterogeneity in impoundments, which is consistent with the findings of other studies (Yan et al., 2013; Fencl et al., 2015).
Habitat modifications associated with anthropogenic activities not only cause the native species population to decline and even cause local extinctions, but also facilitate the invasion of alien/native-invasive species into upland streams (Slawski et al., 2008; Dala-Corte et al., 2019; Turgeon et al., 2019). A serious consequence associated with low-head dams is the addition of native-invasive species and exclusion of native species (Liew et al., 2016; Smith et al., 2017; Liu et al., 2019). Our study clearly documented that the species richness of native species significantly decreased in impoundments, while that of native-invasive species increased (Table 2). Changes in local diversities associated with anthropogenic activities often depend on the relative number of lost and gained species and their abundances (Turgeon et al., 2019). Unsurprisingly, increases in native-invasive species richness can largely compensate for the loss of native species, which could explain this pattern of changes in the total species richness in impoundments; this effect masked changes in the whole fish assemblage. When the loss of native species (abundance change: 14.89) outnumbers the gain of native-invasive species (abundance change: 0.21), changes in the species abundance patterns of whole assemblages may be driven by native species (Table 2). Overall, low-head dams favor native-invasive species, while negatively affecting native species with high sensitivity to environmental change (Slawski et al., 2008; Liu et al., 2019).
The variation in both the identity and abundance of species between different habitats can also affect the degree to which local fish community compositions differ (Barwell et al., 2015; Li et al., 2021). More importantly, our results showed that the local community changes in fish assemblages (i.e., the total β-diversity) associated with low-head dams were significantly higher for abundance-based approaches than for occurrence-based approaches (Table 3), suggesting that the latter could underestimate the localized effects of individual low-head dams. As low-head dams are less likely to result in local-scale species extinctions than large dams, most of the remaining species often experience changes in abundance (decreased, increased, or unchanged; Yan et al., 2013; Fencl et al., 2017). Previous studies have shown that changes in fish diversity patterns may be primarily driven by changes in the abundance of native species (Tiemann et al., 2004; Liu et al., 2019) in communities or regions where native-invasive species are still not predominant. If the number of shared species between communities does not change, but the abundance does, the dissimilarity index based on abundance approaches will show changes that depend on the magnitude of shifts in abundance (Cassey et al., 2008; Baselga, 2013; Legendre, 2014). In this study, 29 of the 32 species were found in both impoundments and free-flowing segments. However, besides the variations in the abundances of dominant native species, we also found that some native species (e.g., Vanmanenia stenosoma and Aphyocypris chinensis) drastically decreased and other native-invasive species (e.g., Carassius auratus and Odontobutis potamophila) markedly increased in abundance in the impoundments compared to in the free-flowing segments (Table 1). Therefore, the results of our study are not surprising when formerly rare species become dominant or vice versa, given that species abundances can change drastically enough to produce large differences relative to the changes in species composition from one community to another.
For occurrence- and abundance-based approaches, we also found that native species had higher SCBD values than native-invasive species in both habitat types, but significant variation was observed only in abundance-based approaches (Figure 4). Headwater streams with highly heterogeneous habitats nourish unique species assemblages (Meyer et al., 2007), where native species with small niche breadths occurring under environmentally restricted conditions may contribute more to β-diversity (Heino and Grönroos, 2017). As is generally observed in natural ecosystems, communities often exhibit heterogeneous species–abundant distributions (Magurran et al., 2011). In this study, the RA of most native species across sites was higher than that of native-invasive species, especially in regions where native fish species were completely predominant. Previous studies have shown that species with high total abundance across sites contribute most to the abundance-based β-diversity (Heino and Grönroos, 2017; Gavioli et al., 2019). When the identities of native and native-invasive species are considered, the high SCBD values can reveal which native-invasive species are the most abundant and widespread, whereas the low SCBD values can identify rare native species. Although some rare native species have a relatively lower contribution to β-diversity, such species may still need conservation actions owing to their local extinction risk (Gavioli et al., 2019). Meanwhile, the native-invasive species with high abundance should be strictly controlled to mitigate the ecological consequences of low-head dams.
Complex ecological networks of interacting species are crucial for maintaining biodiversity in local ecosystems and the effects of species interactions can differ either spatially or temporally (Tylianakis and Morris, 2017). Normally, a fraction of native species may primarily contribute to local community dynamics (Meyer et al., 2007), especially in headwater streams where native-invasive fish species are still not predominant. However, changes in species diversity and basal food resources resulting from habitat modifications can affect the species interaction networks (Brimacombe et al., 2021; Danet et al., 2021). Previous studies have shown that impoundments created by dams can act as powerful environmental filters that may select sets of species that are better adapted to particular habitat conditions (Liew et al., 2016). In this study, we found that the betweenness centrality of native-invasive species (i.e., omnivorous fish) was the highest in the impoundments (Figure 5), which reflected the importance of species for the interaction network structure. Indeed, betweenness centrality measures the ability of nodes (i.e., species) to act as a bridge in the interaction network, which can efficiently identify the “keystone species” in ecosystems (González et al., 2010; Momal et al., 2020). High betweenness centrality values of species represent high network connectance among species, which can strengthen the importance of species interaction networks (Thébault and Fontaine, 2010). In addition, the transformation of natural lotic habitats into artificial lentic habitats not only facilitate the establishment of native-invasive species (Chu et al., 2015; Liew et al., 2016), but also greatly change basal food resources (Ruhí et al., 2016). Feeding habits can play a crucial role in maintaining the stability of species interactions, and tropical streams are typically dominated by omnivorous mechanisms (Ruhí et al., 2016; Brimacombe et al., 2021). Therefore, native-invasive species (omnivorous fish) can take full advantage of niches specific to lentic habitats in impoundments, which are essential for maintaining the stability of interaction networks.
Conclusion
In this study, we used a quantitative approach to assess the patterns of low-head dams affecting stream fish assemblages by distinguishing between native and native-invasive species. Our results suggest that low-head dams affect stream fish diversity in two ways: by adding native-invasive species and by excluding native species typical of headwaters. However, changes in species richness and abundance not only alter the local community composition (Barwell et al., 2015; Li et al., 2021), but also affect the community stability (Brimacombe et al., 2021). Although species interactions were not directly observed in our system, we provide initial evidence that low-head dams influence the interaction network structure. Additionally, we found that occurrence-based approaches could underestimate the localized effects of low-head dams when fish assemblages differ primarily in abundance rather than species composition. SCBD values can determine which species contribute the most to β-diversity (Legendre and De Cáceres, 2013). We found that the abundance-based SCBD values were significantly higher for native fish species than for native-invasive ones in both habitat types. In communities or regions where native fish species are predominant, our results suggest that understanding which species contribute to β-diversity will offer new insights into the development of effective conservation strategies, by taking the identities of native and native-invasive species into account.
Data Availability Statement
The original contributions presented in this study are included in the article/Supplementary Material, further inquiries can be directed to the corresponding authors.
Ethics Statement
The animal study was reviewed and approved by the Animal Ethics Committee of the Anhui Normal University.
Author Contributions
YY and QL designed the study. QL, XL, KT, CZ, and YY conducted the field and/or laboratory work. QL, XL, and CZ analyzed data. LC, HF, and YG contributed materials, reagents, and analytical tools. QL drafted the manuscript with the assistance of CZ and YY. All authors contributed to the article and approved the submitted version.
Funding
Funding was provided by the National Natural Science Foundation of China (Grant Numbers: 31372227, 31500452, and 31872251) and the Special Fund for Anhui Agriculture Research System [Anhui Agricultural Science letter No. (2021) 711].
Conflict of Interest
The authors declare that the research was conducted in the absence of any commercial or financial relationships that could be construed as a potential conflict of interest.
Publisher’s Note
All claims expressed in this article are solely those of the authors and do not necessarily represent those of their affiliated organizations, or those of the publisher, the editors and the reviewers. Any product that may be evaluated in this article, or claim that may be made by its manufacturer, is not guaranteed or endorsed by the publisher.
Acknowledgments
We would like to express our thank to all of our colleagues for their assistance in field surveys and fish identification. This study was approved by the Animal Ethics Committee of the Anhui Normal University. We would also like to express our gratitude to Chuanbo Guo and two reviewers for their comments, which significantly improved the manuscript.
Supplementary Material
The Supplementary Material for this article can be found online at: https://www.frontiersin.org/articles/10.3389/fevo.2022.916873/full#supplementary-material
References
Barbarossa, V., Schmitt, R., Huijbregts, M., Zarfl, C., and Schipper, A. M. (2020). Impacts of current and future large dams on the geographic range connectivity of freshwater fish worldwide. Proc. Natl. Acad. Sci. U.S.A. 117, 3648–3655. doi: 10.1073/pnas.1912776117
Barwell, L. J., Isaac, N. J. B., and Kunin, W. E. (2015). Measuring β-diversity with species abundance data. J. Anim. Ecol. 84, 1112–1122. doi: 10.1111/1365-2656.12362
Baselga, A. (2013). Separating the two components of abundance-based dissimilarity: balanced changes in abundance vs. abundance gradients. Methods Ecol. Evol. 4, 552–557. doi: 10.1111/2041-210X.12029
Bassett, D. S., Meyer-Lindenberg, A., Achard, S., Duke, T., and Bullmore, E. (2006). Adaptive reconfiguration of fractal small-world human brain functional networks. Proc. Natl. Acad. Sci. U.S.A. 103, 19518–19523. doi: 10.1073/pnas.0606005103
Brimacombe, C., Bodner, K., and Fortin, M. J. (2021). Inferred seasonal interaction rewiring of a freshwater stream fish network. Ecography 44, 219–230. doi: 10.1111/ecog.05452
Busst, G. M. A., and Britton, J. R. (2017). Comparative trophic impacts of two globally invasive cyprinid fishes reveal species-specific invasion consequences for a threatened native fish. Freshw. Biol. 62, 1587–1595. doi: 10.1111/fwb.12970
Cassey, P., Lockwood, J. L., Olden, J. D., and Blackburn, T. M. (2008). The varying role of population abundance in structuring indices of biotic homogenization. J. Biogeogr. 35, 884–892. doi: 10.1111/j.1365-2699.2007.01827.x
Chiquet, J., Mariadassou, M., and Robin, S. (2019). Variational inference of sparse network from count data. Proc. Mach. Learn. Res. 97, 1162–1171. doi: 10.1186/s12859-018-2125-2
Chu, L., Wang, W. Z., Zhu, R., Yan, Y. Z., Chen, Y. F., and Wang, L. Z. (2015). Variation in fish assemblages across impoundments of low-head dams in headwater streams of the Qingyi River, China: effects of abiotic factors and native invaders. Environ. Biol. Fish. 98, 101–112. doi: 10.1007/s10641-014-0239-6
Dala-Corte, R. B., Melo, A. S., Becker, F. G., and Teresa, F. (2019). Testing the native invasion hypothesis to explain anthropogenic influence on stream fish assemblages. Aquat. Sci. 81:66. doi: 10.1007/s00027-019-0663-y
Danet, A., Mouchet, M. A., Bonnaffé, W., Thébault, E., and Fontaine, C. (2021). Species richness and food-web structure jointly drive community biomass and its temporal stability in fish communities. Ecol. Lett. 24, 2364–2377. doi: 10.1111/ele.13857
Eggert, S. L., Wallace, J. B., Meyer, J. L., and Webster, J. R. (2012). Storage and export of organic matter in a headwater stream: responses to long-term detrital manipulations. Ecosphere 3:75. doi: 10.1890/ES12-00061.1
Erős, T., Czeglédi, I., Tóth, R., and Schmera, D. (2020). Multiple stressor effects on alpha, beta and zeta diversity of riverine fish. Sci. Total. Environ. 748:141407. doi: 10.1016/j.scitotenv.2020.141407
Erős, T., and Grant, E. H. C. (2015). Unifying research on the fragmentation of terrestrial and aquatic habitats: patches, connectivity and the matrix in riverscapes. Freshw. Biol. 60, 1487–1501. doi: 10.1111/fwb.12596
Fencl, J. S., Mather, M. E., Costigan, K. H., and Daniels, M. D. (2015). How big of an effect do small dams have? Using geomorphological footprints to quantify spatial impact of low-head dams and identify patterns of across-dam variation. PLoS One 10:e0141210. doi: 10.1371/journal.pone.0141210
Fencl, J. S., Mather, M. E., Smith, J. M., and Hitchman, S. M. (2017). The blind men meet the elephant at the dam: alternative spatial and taxonomic components reveal different insights about how low-head dams impact fish biodiversity. Ecosphere 8:e01973. doi: 10.1002/ecs2.1973
Gavioli, A., Milardi, M., Castaldelli, G., Fano, E. A., and Soininen, J. (2019). Diversity patterns of native and exotic fish species suggest homogenization processes, but partly fail to highlight extinction threats. Divers. Distrib. 25, 983–994. doi: 10.1111/ddi.12904
González, A. M. M., Dalsgaard, B., and Olesen, J. M. (2010). Centrality measures and the importance of generalist species in pollination networks. Ecol. Complex. 7, 36–43. doi: 10.1016/j.ecocom.2009.03.008
He, F., Thieme, M., Zarfl, C., Grill, G., Lehner, B., Hogan, Z., et al. (2021). Impacts of loss of free-flowing rivers on global freshwater megafauna. Biol. Conserv. 263:109335. doi: 10.1016/j.biocon.2021.109335
Heino, J., and Grönroos, M. (2017). Exploring species and site contributions to beta diversity in stream insect assemblages. Oecologia 183, 151–160. doi: 10.1007/s00442-016-3754-7
Hitchman, S. M., Mather, M. E., Smith, J. M., and Fencl, J. S. (2018). Habitat mosaics and path analysis can improve biological conservation of aquatic biodiversity in ecosystems with low-head dams. Sci. Total. Environ. 619–620, 221–231. doi: 10.1016/j.scitotenv.2017.10.272
Hobbs, R. J., Arico, S., Aronson, J., Baron, J. S., Bridgewater, P., Cramer, V. A., et al. (2006). Novel ecosystems: theoretical and management aspects of the new ecological world order. Glob. Ecol. Biogeogr. 15, 1–7. doi: 10.1111/j.1466-822X.2006.00212.x
Johnson, P. T., Olden, J. D., and Vander Zanden, M. J. (2008). Dam invaders: impoundments facilitate biological invasions into freshwaters. Front. Ecol. Environ. 6, 357–363. doi: 10.1890/070156
Jumani, S., Deitch, M. J., Kaplan, D., Anderson, E. P., Krishnaswamy, J., Lecours, V., et al. (2020). River fragmentation and flow alteration metrics: a review of methods and directions for future research. Environ. Res. Lett. 15:123009. doi: 10.1088/1748-9326/abcb37
Koleff, P., Gaston, K. J., and Lennon, J. J. (2003). Measuring beta diversity for presence–absence data. J. Anim. Ecol. 72, 367–382.
Kuczynski, L., Legendre, P., and Grenouillet, G. (2018). Concomitant impacts of climate change, fragmentation and non-native species have led to reorganization of fish communities since the 1980s. Glob. Ecol. Biogeogr. 27, 213–222.
Legendre, P. (2014). Interpreting the replacement and richness difference components of beta diversity. Glob. Ecol. Biogeogr. 23, 1324–1334. doi: 10.1111/j.1365-2656.2010.01771.x
Legendre, P. (2015). R function TBI: Difference Between Multivariate Observations at T1 and T2. Available online at: http://adn.biol.umontreal.ca/~numericalecology/Rcode/
Legendre, P. (2019). A temporal beta-diversity index to identify sites that have changed in exceptional ways in space-time surveys. Ecol. Evol. 9, 3500–3514. doi: 10.1002/ece3.4984
Legendre, P., and De Cáceres, M. (2013). Beta diversity as the variance of community data: dissimilarity coefficients and partitioning. Ecol. Lett. 16, 951–963. doi: 10.1111/ele.12141
Li, Q., Li, Y. R., Jiang, M. D., Wang, Y. X., Xu, D. P., Chu, L., et al. (2021). Effects of low-head dams on fish assemblages in subtropical streams: context dependence on local habitat and landscape conditions. Ecol. Indic. 121:107190.
Liew, J. H., Tan, H. H., and Yeo, D. C. J. (2016). Dammed rivers: impoundments facilitate fish invasions. Freshw. Biol. 61, 1421–1429.
Liu, Y., Wang, Y. R., Zhu, Q., Li, Y. R., Kang, B., Chu, L., et al. (2019). Effects of low-head dams on fish assemblages in subtropical streams: context dependence on species category and data type. River Res. Appl. 35, 396–404.
Magurran, A. E., Khachonpisitsak, S., and Ahmad, A. B. (2011). Biological diversity of fish communities: pattern and process. J. Fish. Biol. 79, 1393–1412.
Meyer, J. L., Strayer, D. L., Wallace, J. B., Eggert, S. L., Helfman, G. S., and Leonard, N. E. (2007). The contribution of headwater streams to biodiversity in river networks. J. Am. Water. Resour. Assoc. 43, 86–103.
Momal, R., Robin, S., and Ambroise, C. (2020). Tree-based inference of species interaction networks from abundance. Methods Ecol. Evol. 11, 621–632.
Porto, L. M., Mclaughlin, R. L., and Noakes, D. L. G. (1999). Low-head barrier dams restrict the movements of fishes in two lake Ontario streams. N. Am. J. Fish. Manage. 19, 1028–1036.
Ruhí, A., Muñoz, I., Tornés, E., Batalla, R. J., Vericat, D., Ponsatí, L., et al. (2016). Flow regulation increases food-chain length through omnivory mechanisms in a Mediterranean river network. Freshw. Biol. 61, 1536–1549.
Scott, M. C., and Helfman, G. S. (2001). Native invasions, homogenization, and the mismeasure of integrity of fish assemblages. Fisheries 26, 6–15.
Slawski, T. M., Veraldi, F. M., Pescitelli, S. M., and Pauers, M. J. (2008). Effects of tributary spatial position, urbanization, and multiple low-head dams on warmwater fish community structure in a midwestern stream. N. Am. J. Fish. Manage. 28, 1020–1035.
Smith, S. C. F., Meiners, S. J., Hastings, R. P., Thomas, T., and Colombo, R. E. (2017). Low-head dam impacts on habitat and the functional composition of fish communities. River Res. Appl. 33, 680–689.
Socolar, J. B., Gilroy, J. J., Kunin, W. E., and Edwards, D. P. (2016). How should beta-diversity inform biodiversity conservation? Trends. Ecol. Evol. 30, 67–80.
Strahler, A. N. (1957). Quantitative analysis of watershed geomorphology. Trans. Am. Geophys. Union 38, 913–920.
Thébault, E., and Fontaine, C. (2010). Stability of ecological communities and the 566 architecture of mutualistic and trophic networks. Science 329, 853–856. doi: 10.1126/science.1188321
Tiemann, J. S., Gillette, D. P., Wildhaber, M. L., and Edds, D. R. (2004). Effects of lowhead dams on riffle-dwelling fishes and macroinvertebrates in a midwestern river. Trans. Am. Fish. Soc. 133, 705–717.
Turgeon, K., Turpin, C., and Gregory-Eaves, I. (2019). Dams have varying impacts on fish communities across latitudes: a quantitative synthesis. Ecol. Lett. 22, 1501–1516. doi: 10.1111/ele.13283
Tylianakis, J. M., and Morris, R. J. (2017). Ecological metworks across environmental gradients. Annu. Rev. Ecol. Evol. Syst. 48, 25–48.
Vannote, R. L., Minshall, G. W., Cummins, K. W., Sedell, J. R., and Cushing, C. E. (1980). The river continuum concept. Can. J. Fish. Aquat. Sci. 37, 130–137.
Yan, Y. Z., Wang, H., Zhu, R., Chu, L., and Chen, Y. F. (2013). Influences of low-head dams on the fish assemblages in the headwater streams of the Qingyi watershed, China. Environ. Biol. Fish. 96, 495–506.
Keywords: low-head dams, stream fish, diversity patterns, interaction networks, native-invasive species
Citation: Li Q, Li X, Fu H, Tan K, Ge Y, Chu L, Zhang C and Yan Y (2022) Role of Impoundments Created by Low-Head Dams in Affecting Fish Assemblages in Subtropical Headwater Streams in China. Front. Ecol. Evol. 10:916873. doi: 10.3389/fevo.2022.916873
Received: 10 April 2022; Accepted: 17 June 2022;
Published: 04 July 2022.
Edited by:
Chuanbo Guo, Institute of Hydrobiology (CAS), ChinaReviewed by:
Chengzhi Ding, Yunnan University, ChinaKatrine Turgeon, University of Quebec in Outaouais, Canada
Copyright © 2022 Li, Li, Fu, Tan, Ge, Chu, Zhang and Yan. This is an open-access article distributed under the terms of the Creative Commons Attribution License (CC BY). The use, distribution or reproduction in other forums is permitted, provided the original author(s) and the copyright owner(s) are credited and that the original publication in this journal is cited, in accordance with accepted academic practice. No use, distribution or reproduction is permitted which does not comply with these terms.
*Correspondence: Yunzhi Yan, eWFueXVuemhpQGFobnUuZWR1LmNu; Chen Zhang, Y3poYW5naGVuQGFobnUuZWR1LmNu