- 1Key Laboratory of Wetland Ecology and Environment, Northeast Institute of Geography and Agroecology, Chinese Academy of Sciences, Changchun, China
- 2University of Chinese Academy of Sciences, Beijing, China
- 3School of Environment, Northeast Normal University, Changchun, China
- 4Shandong Key Laboratory of Eco-Environmental Science for the Yellow River Delta, Binzhou University, Binzhou, China
- 5Shijiazhuang University, Shijiazhuang, China
- 6The Institute for Advanced Study of Coastal Ecology, Key Laboratory of Ecological Restoration and Conservation of Coastal Wetlands in Universities of Shandong, Ludong University, Yantai, China
Melatonin priming has been widely reported to positively affect seed germination under abiotic stresses. However, there is still a gap in knowledge on how melatonin priming impacts the seed germination and physiological change of wetland plant species. We assessed the effects of different melatonin concentrations on germination characteristics, antioxidant defense, and reserve mobilization of Suaeda corniculata seeds. Priming of S. corniculata seeds with 50 μM melatonin significantly improved the germination rate, germination speed, germination index, superoxide dismutase and peroxidase activity, and soluble sugar content as compared with the control, and effectively reduced the malondialdehyde content, promoted starch, soluble protein, and fat mobilization. However, the stress tolerance ability of S. corniculata seeds was reduced by high melatonin concentration. The structural equation model indicated that the melatonin priming directly affects the seed germination, while also indirectly regulating the antioxidant defense system and reserve mobilization. In conclusion, melatonin priming affects the S. corniculata seed germination under salinization stress in a concentration-dependent manner via both direct and indirect regulatory pathways. Insights into these aspects will advance our understanding of how melatonin priming affects S. corniculata seed germination and provide invaluable information and technical support for the restoration of salinized wetlands in the Momoge National Nature Reserve.
Introduction
Salinization causes major losses in the earth’s ecosystem, with nearly 831 million hectares of land undergoing salinization globally (Ma et al., 2015; Zhao et al., 2021a). Salinization is becoming more widespread due to the interactions between the global climate change and anthropogenic hydrological modifications, especially in the wetland ecosystems in arid and semi-arid regions (An et al., 2019; Feghhenabi et al., 2020; Wang et al., 2021). Salinization not only changes the fundamental physicochemical properties of soil, but also negatively affects the seed germination and plant growth by disturbing the physio-biochemical processes including ionic imbalance, oxidative stress, and osmotic stress, thereby reducing the effective wetlands area, thereby resulting in gradual changes or even disappearance of wetland ecosystem services (Tahjib et al., 2018; Wang et al., 2019; Zhang et al., 2019). Therefore, the quick and effective restoration of salinity-degraded wetlands has become an urgent global concern.
Seeds are pivotal propagative organs which carry the genetic information and support the multifunctional evolution in plants (Kettenring and Tarsa, 2020). Employing strategic seed-based approaches in wetland restoration is key to faster and complete recovery of the targeted underlying vegetation structure and composition (James and Carrick, 2016; Zhang M. Y. et al., 2021). Besides being the beginning of the life cycle of higher plants, seed germination is also the most environmentally stress-sensitive stage of the plant’s life history (Chen et al., 2021). Previous studies had identified that salinization weakens the seeds’ ability to absorb water from the soil as well as inhibits the growth of the seed and the embryo (Li et al., 2019). Moreover, salinization disturbs the delicate balance between the intracellular ROS production and scavenging, and also reduces the ability of reserve mobilization in seeds (Ibrahim, 2016). The seed’s stress-amelioration techniques, which can address limiting environmental constraints, are crucial for improving outcomes in degraded salinized wetlands (Kettenring and Tarsa, 2020). In recent decades, various strategies have been adopted to enhance the abiotic stress tolerance of seeds, such as genetic approach, genetic engineering, and plant breeding. However, these methods are complex, time-consuming, expensive, and have biosafety issues (Cao et al., 2019; Johnson and Puthur, 2021). Therefore, the development of a simple, effective, and economical strategy will be significant in improving the salinization stress tolerance of seeds.
Priming is a pretreatment technology which hydrates the seed in specific solutions for initiating specific metabolic processes before germination (Masondo et al., 2018). It is proven to be an effective and safe strategy for addressing the current and future issues of seed germination under abiotic stress conditions (Forti et al., 2021). The priming treatment enhances the inherent tolerance potential of seeds through many mediums, which, based on hormone, have been widely applied in the pretreatment of commercial seeds (Li et al., 2020; Zhang Y. et al., 2021). Melatonin (N-acetyl-5-methoxtryptamine), an endogenous indoleamine, is a highly evolutionarily conserved multifunctional molecule, which is proven to be an abiotic anti-stress hormone in seeds and is recently being considered as a research hotspot in the priming domain (Li et al., 2016; Arnao and Hernandez-Ruiz, 2019; Zhang T. G. et al., 2022). Recent studies have demonstrated that melatonin priming boosted the activities and transcript levels of antioxidant enzymes in seeds for effectively scavenging salinization stress-induced ROS, and consequently improving their salinization stress tolerance ability during seed germination (Yan et al., 2020). Additionally, melatonin priming also promotes seed germination under salinization stress by regulating carbohydrate metabolism and enhancing reserve mobilization (Zhao et al., 2015; Seneviratne et al., 2019). However, due to the differences in seed parameters, the most optimum effect of melatonin priming needs to be quantified according to the target seed. Additionally, most studies have focused only on how melatonin priming affects crops and fodder grasses, but little is known about their effect on wetland species under salinization stress.
The Ramsar-listed Momoge National Nature Reserve (MNNR), a vital stopover habitat on the migration route of East Asian-Australasian migratory birds, serves as an ecological barrier in preventing the soil salinization and desertification in the Songnen plain (Tang et al., 2021; Zhang M. Y. et al., 2022). Over the past 50 years, soil salinization due to climate change and anthropogenic activities has resulted in a loss of wetland area and severely limited the ecological service functions in the MNNR (Li X. Y. et al., 2017). As an indigenous species, Suaeda corniculata is widely distributed in the lake, river, and saline wetlands in MNNR. It was selected as the pioneer species for vegetation restoration in this region due to their ability to absorb soluble salt from soil and consequently increase their organic matter content (Zhao et al., 2003; Yang et al., 2017; Wang D. W. et al., 2020). Recently, the MNNR plans to not only implement the seed-based wetland restoration project, but also enhance the salinization stress tolerance ability of S. corniculata to improve their impact on wetland restoration. Therefore, studying the effect of melatonin priming on S. corniculata seed germination, antioxidant defense, and reserve mobilization is of great importance in achieving wetland restoration.
Due to the primary aim of restore degraded salinization wetlands, current studies have deepened our comprehension of sexual propagation-based restoration strategies. However, little is known about the positive effects of improve stress tolerance of germinating seeds by priming treatment (Wang X. Y. et al., 2020; Zhao et al., 2021b). In this study, laboratory experiment was carried out to investigate the effects of melatonin priming on S. corniculata seed germination, antioxidant enzyme activity, and reserve mobilization. The aims of this study are: (1) to examine the response of S. corniculata seed germination to melatonin priming; (2) to identify the effect of melatonin priming on S. corniculata seed’s antioxidant enzyme activity and reserve mobilization; and (3) to reveal the tolerance regulatory pathways induced by melatonin priming during S. corniculata seed germination under salinization stress conditions. We hypothesized that the accelerating effect of exogenous melatonin happens in a concentration-dependent manner during S. corniculata seed germination under salinization stress. This study will assist facilitate the understanding of the protective effects of seed priming under abiotic stress conditions, and also provide technique guidelines for salinized wetland restoration in the MNNR.
Materials and Methods
Seed Materials
Matures seeds were collected in late September 2020 from the wild S. corniculata populations in the Ertou wetlands in the MNNR (45°53′ – 45°55′ N, 123°36′ – 123°41′ E). The collected seeds were air-dried indoors under dark conditions and then stored in cloth bags at 4°C until the start of the experiment in January 2022. Healthy S. corniculata seeds were surface-sterilized using 75% ethanol for 10 min and then rinsed 9 times with distilled water, and the water was wiped off the seeds surface with sterile filter paper in the super clean bench before being used in the experiments.
Experimental Design
The experiment was conducted in the Key Laboratory of Wetland Ecology and Environment of the Northeast Institute of Geography and Agroecology, Chinese Academy of Sciences. We selected six priming treatments: MT10 (10 μM melatonin), MT20 (20 μM melatonin), MT50 (50 μM melatonin), MT100 (100 μM melatonin), MT200 (200 μM melatonin), and CK (distilled water). Seeds were incubated in each priming solution for 12 h. For each treatment, 25 seeds were evenly placed in Petri dishes (diameter 9 cm) containing double Whatman No. 1 filter paper soaked with 2 ml of 30 mM NaHCO3 solution (the salt solution concentration data comes from the MNNR), and sealed with Parafilm® to prevent moisture evaporation. The seeds were then germinated in an artificial climate box (RDN-300B-4, China) under an alternating cycle of 12/12 h light and dark at an average temperature of 22.5/8.6°C (the climate data represents field conditions in May in the MNNR). For each germination treatment, five independent replicates were used (three petri dishes were used to test the germination rate of S. corniculata seeds, while two petri dishes were used to cultivate testable seed materials). Germinated seeds were recorded daily, and germination was considered having occurred when the radicle ≥ 2 mm. The entire experiment lasted for 7 days (from 6th to 12th January, 2022) and partial seeds were harvested on days 1, 3, 5, and 7, and immediately frozen in liquid nitrogen and stored at −80°C until further analysis of antioxidant enzymes and reserve substances.
Determination of Germination
In order to describe seed germination changes, the germination rate (GR), germination speed (GS), and germination index (GI), were measured according to following Biju et al. (2017):
where n is the number of germinated seeds, N is the total number of the tested seeds, Nt is the percentage of germinated seeds at the tth days, Gt is the number of germinated seeds in t days, and Dt is the corresponding germination days.
Determination of Antioxidant Enzyme Activity and Reserve Content
The activities of superoxide (SOD), peroxidase (POD), and the malondialdehyde (MDA) content were determined via WST-8, visible-spectrophotometry and TBA-spectrophotometry by using detection kits (M0102B, M0105B, M0106B, Michy Biomedical Technology Co., Ltd., Suzhou, China), respectively. The contents of starch (ST), soluble sugar (SS), and soluble protein (SP) were determined by detection kits (M1101B, M1503B, M1806B, Michy Biomedical Technology Co., Ltd., Suzhou, China) which are based on anthrone-sulfuric acid colorimetry, anthrone colorimetry, and Lowry’s method, respectively. The fat content was determined by the supercritical fluid-Soxhlet extractor using petroleum ether as the extraction buffer (Soriano et al., 2011). All the samples were conducted in three independent biological replicates.
Statistical Analysis
Statistical analysis was conducted using SPSS 22.0 (SPSS, Chicago, IL, United States) and Origin Pro 2022 (OriginLab, Northampton, MA, United States). The data normality and homogeneity were checked before further analyses; seed GR, SOD, and ST were log-transformed and square root transformed to meet the assumptions of homoscedasticity. Effects of melatonin priming on S. corniculata seeds under salinization stress were evaluated using one-way analysis of variance (ANOVA). Duncan’s multiple comparison test was used to determine the significant differences at the 0.05 significance level. Pearson’s correlation analysis was performed to identify the correlational relationship between antioxidant enzymes, reserve contents, as well as seed germination. Structural equation model (SEM) analysis was performed to evaluate the direct and indirect regulatory pathways of melatonin priming on S. corniculata seed germination in SPSSAU1.
Results
Seed Germination Characteristics
Among the different priming treatments, we observed significant differences in the S. corniculata seed GR (F = 20.64, p < 0.05), ranging from 51.33 to 87.33%, with the maximum and minimum being recorded in the M50 and M200 treatments, respectively (Figure 1A). Priming treatment significantly affected the GS (F = 13.20, p < 0.05), which peaked in M50 (7.47) and was 1.80 times greater than in the M200 treatment (Figure 1B). Additionally, the GI was significantly affected by the priming treatments (F = 19.75, p < 0.05). The GI values recorded in the M50 treatment (27.41 ± 0.89) were 61.05 and 92.28% higher than those in CK and M200 treatment, respectively (Figure 1B).
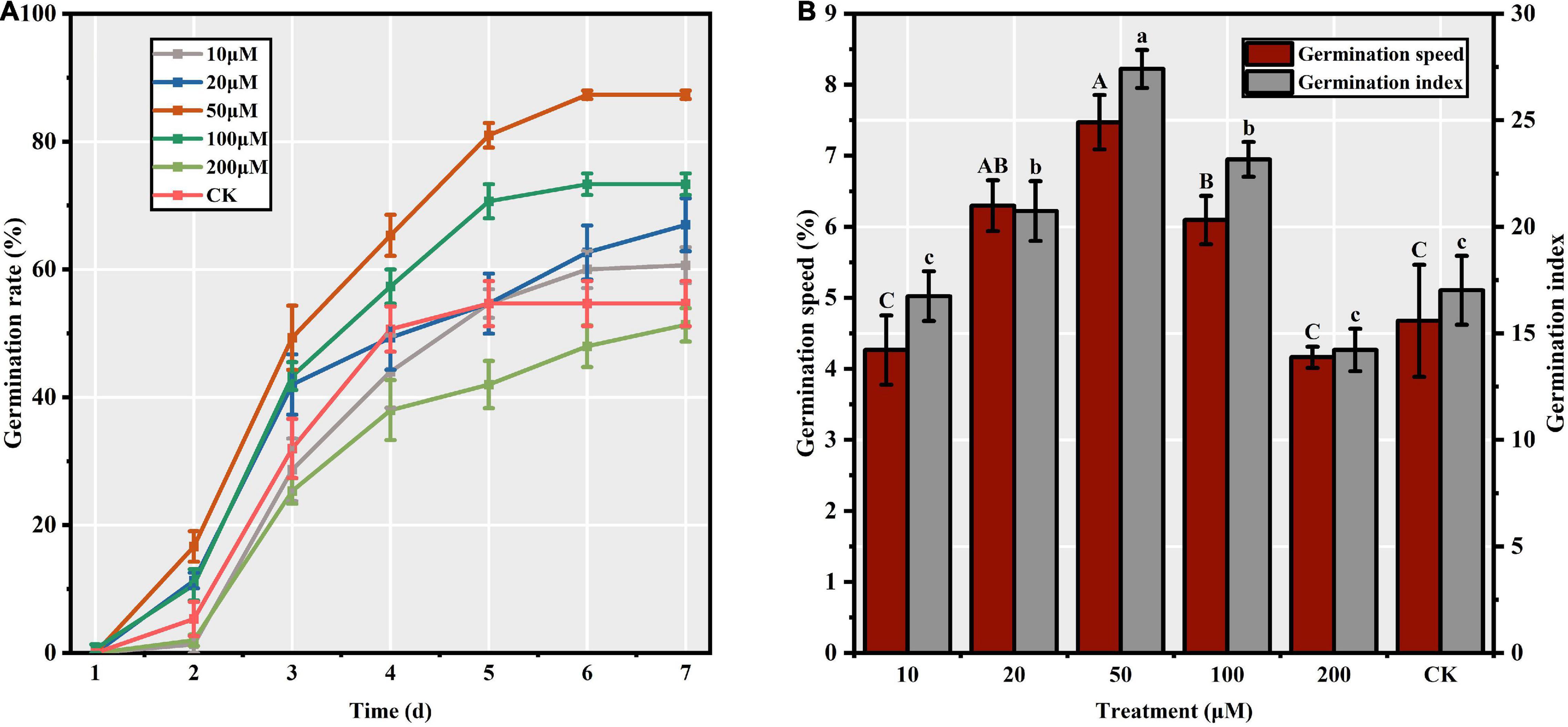
Figure 1. Effect of melatonin priming on Suaeda corniculata seed germination (means ± standard errors, n = 3). (A) Germination rate of Suaeda corniculata seeds. (B) Germination speed and Germination index of Suaeda corniculata seeds. Different capital letters indicate the significant differences in GS at the 0.05 significance level. Different small letters indicate the significant differences in GI at the 0.05 significance level.
Antioxidant Enzymes Activity and Malondialdehyde Content
We identified significant differences in the activities of antioxidant enzymes of S. corniculata seeds at each stage under different melatonin priming treatments (Table 1 and Figure 2). The antioxidant enzymes activities showed a gradually increasing trend during germination stage, with both SOD and POD activities peaking in the M50 treatment on the 7th day, and which were 2.59 and 3.67 times greater than the M200 treatment during the same period (Figures 2D,H). The degree of membrane lipid peroxidation in S. corniculata seeds was significantly affected by the melatonin priming treatments (Figure 2). The MDA content gradually decreased with germination time, with its lowest value appearing in the M50 treatment on the 7th day, and it was 27.33% lower than that on the 1st day (Figures 2I,L).
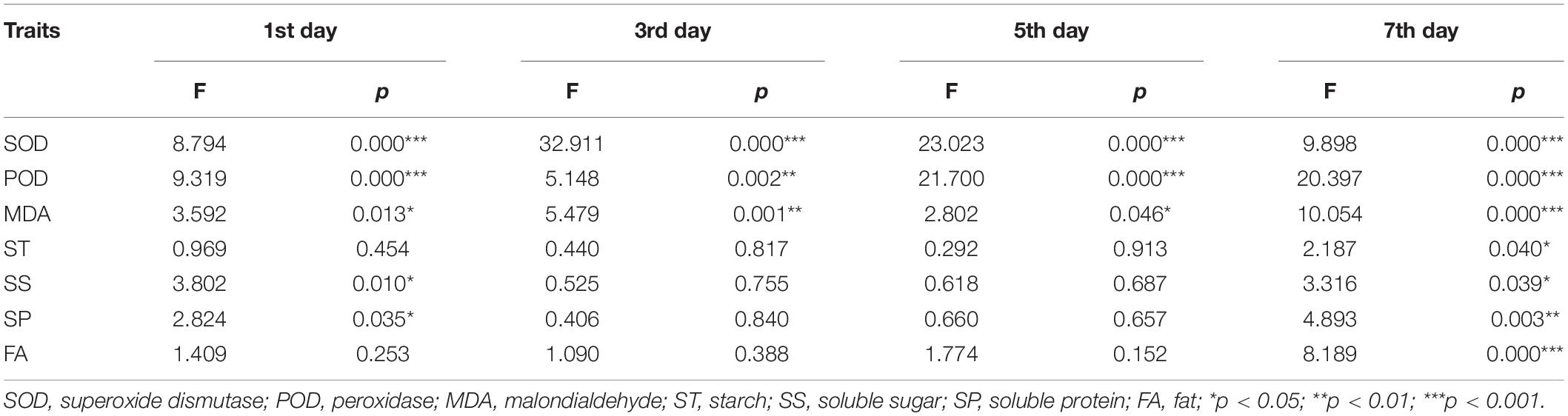
Table 1. Result (F and p-values) of one-way ANOVAs about the priming with melatonin effects on Suaeda corniculata seed antioxidant enzymes activity and reserve traits.
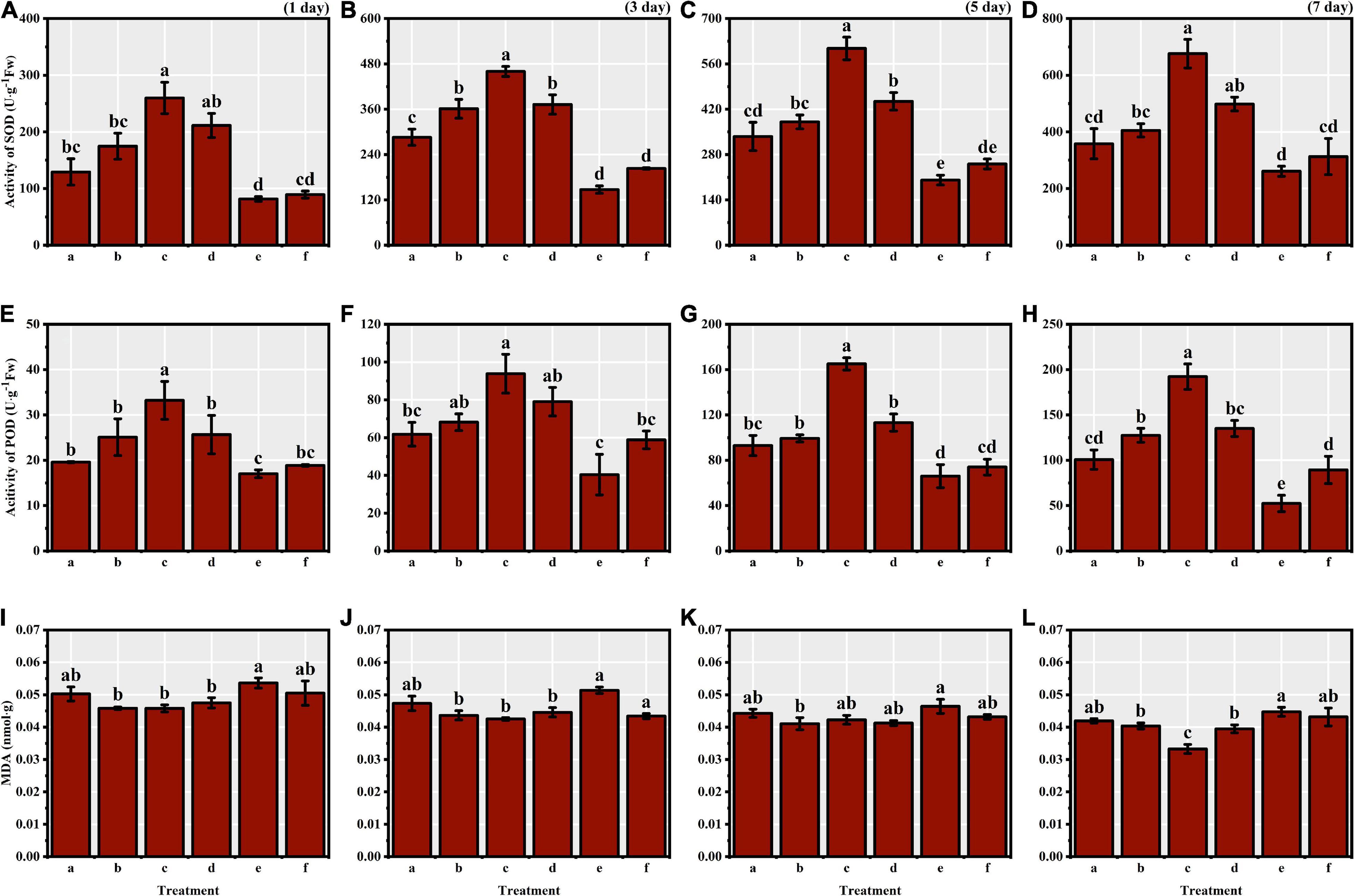
Figure 2. The antioxidant enzymes’ activity and MDA content of Suaeda corniculata seeds under melatonin priming during the entire germination period (means ± standard errors, n = 3). Different letters indicate the significant differences at the 0.05 significance level. SOD, superoxide dismutase; POD, peroxidase; MDA, malondialdehyde; (a) M10; (b) M20; (c) M50; (d) M100; (e) M200; (f) CK. (A) SOD of 1st day; (B) SOD of 3th day; (C) SOD of 5th day; (D) SOD of 7th day; (E) POD of 1st day; (F) POD of 3th day; (G) POD of 5th day; (H) POD of 7th day; (I) MDA of 1st day; (J) MDA of 3th day; (K) MDA of 5th day; (L) MDA of 7th day.
Reserve Mobilization
Melatonin priming significantly affected the reserve mobilization process in S. corniculata seeds (Table 1). The trend of changes in the starch and fat contents was basically uniform across each stage, i.e., they decreased significantly with the increase in germination days, where the seed starch and fat contents under M50 treatment decreasing up to 40.36 and 8.87%, respectively (Figures 3A,D). The soluble sugar content continuously increased with the germination stage, with the increase ranging from 13.90 to 56.11% under each melatonin treatment (Figure 3B). Additionally, the soluble protein content initially increased before decreasing during the process of S. corniculata seed germination (Figure 3C). By the 7th day, the soluble protein content of the M200 treatment was the highest, thus indicating that its mobilization level was significantly lower than in the other treatments.
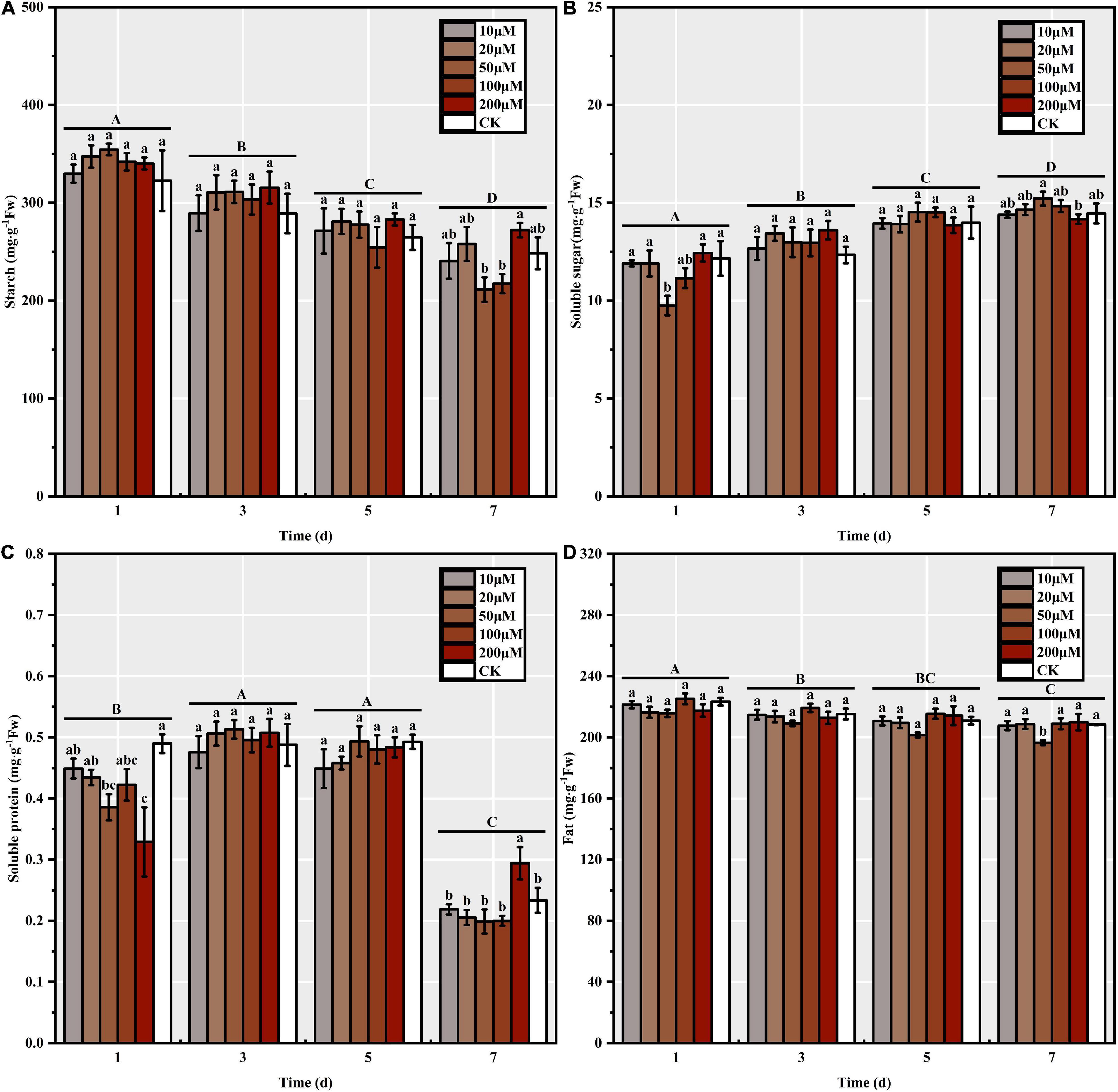
Figure 3. The reserve mobilization of Suaeda corniculata seeds under melatonin priming during the entire germination period (means ± standard errors, n = 3). (A) Starch, (B) Soluble sugar, (C) Soluble protein, and (D) Fat of Suaeda corniculata seeds. Different capital letters indicate significant differences in the germination stages at the 0.05 significance level; different small letters indicate significant differences in the treatments at the 0.05 significance level.
The Coupling Relationships of Seed Germination, Antioxidant Enzyme Activity, and Reserve Mobilization Under Melatonin Priming Treatments
We observed significant positive relationships between SOD, POD, and soluble sugar, while there were significant negative relationships with MDA, starch, soluble protein, and fat (Figure 4). Additionally, the GR was significantly correlated with antioxidant enzymes’ activity and reserve mobilization. The SEM analysis showed that the differences in melatonin concentration priming were directly related to the antioxidant enzymes’ activity, reserve mobilization, and seed germination (Figure 5). Meanwhile, melatonin priming also indirectly affected S. corniculata seed germination by affecting their antioxidant enzymes’ activity and reserve mobilization. Additionally, the degree of reserve mobilization directly affected the level of antioxidant enzymes’ activity.
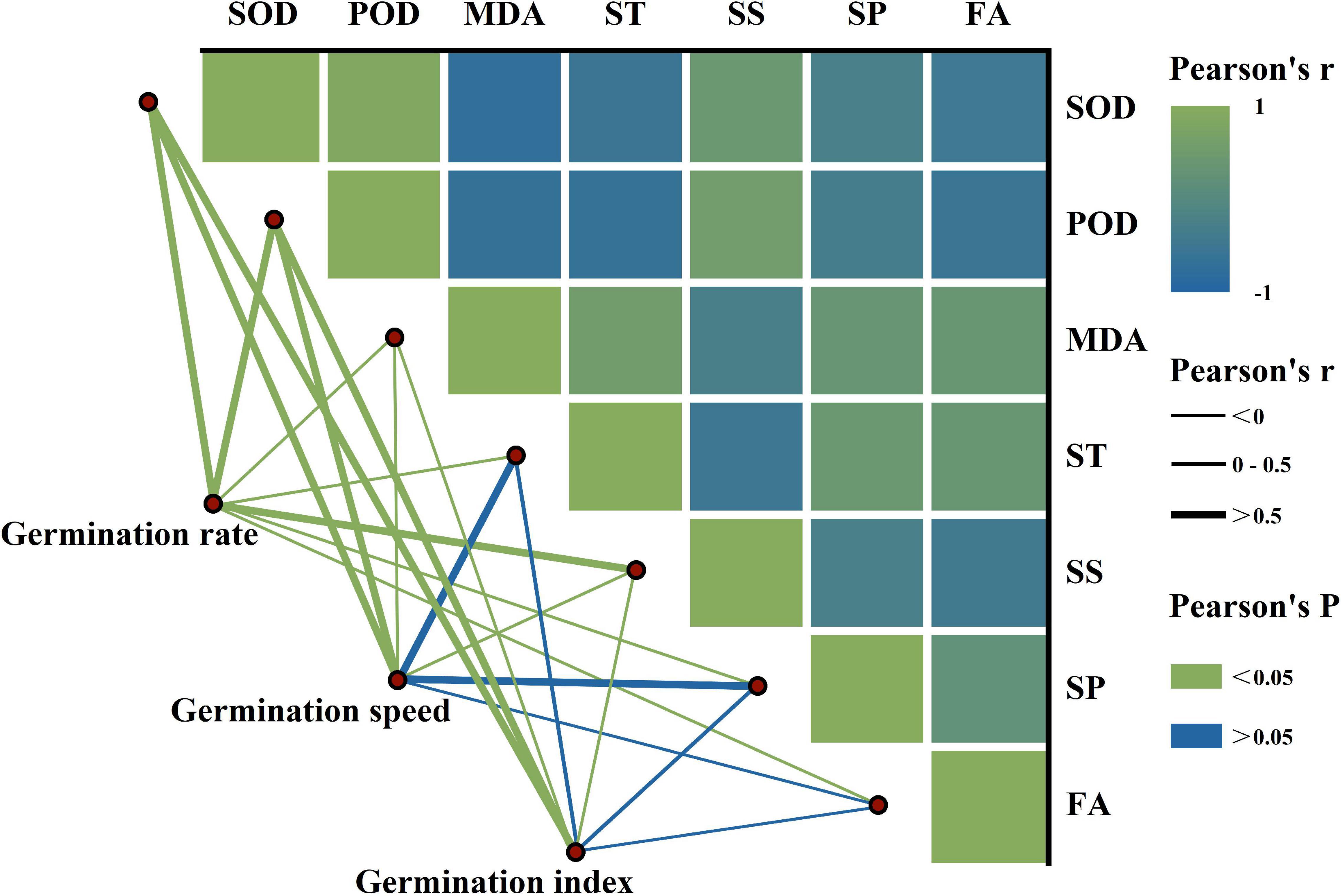
Figure 4. The relationship between seed germination, antioxidant enzymes’ activity, and reserve mobilization of Suaeda corniculata. The color gradient denotes the Pearson’s correlation coefficient. The line width denotes the Pearson’s r value. The line color denotes the statistical significance level. SOD, superoxide dismutase; POD, peroxidase; MDA, malondialdehyde; ST, starch; SP, soluble protein; SS, soluble sugar; FA, fat.
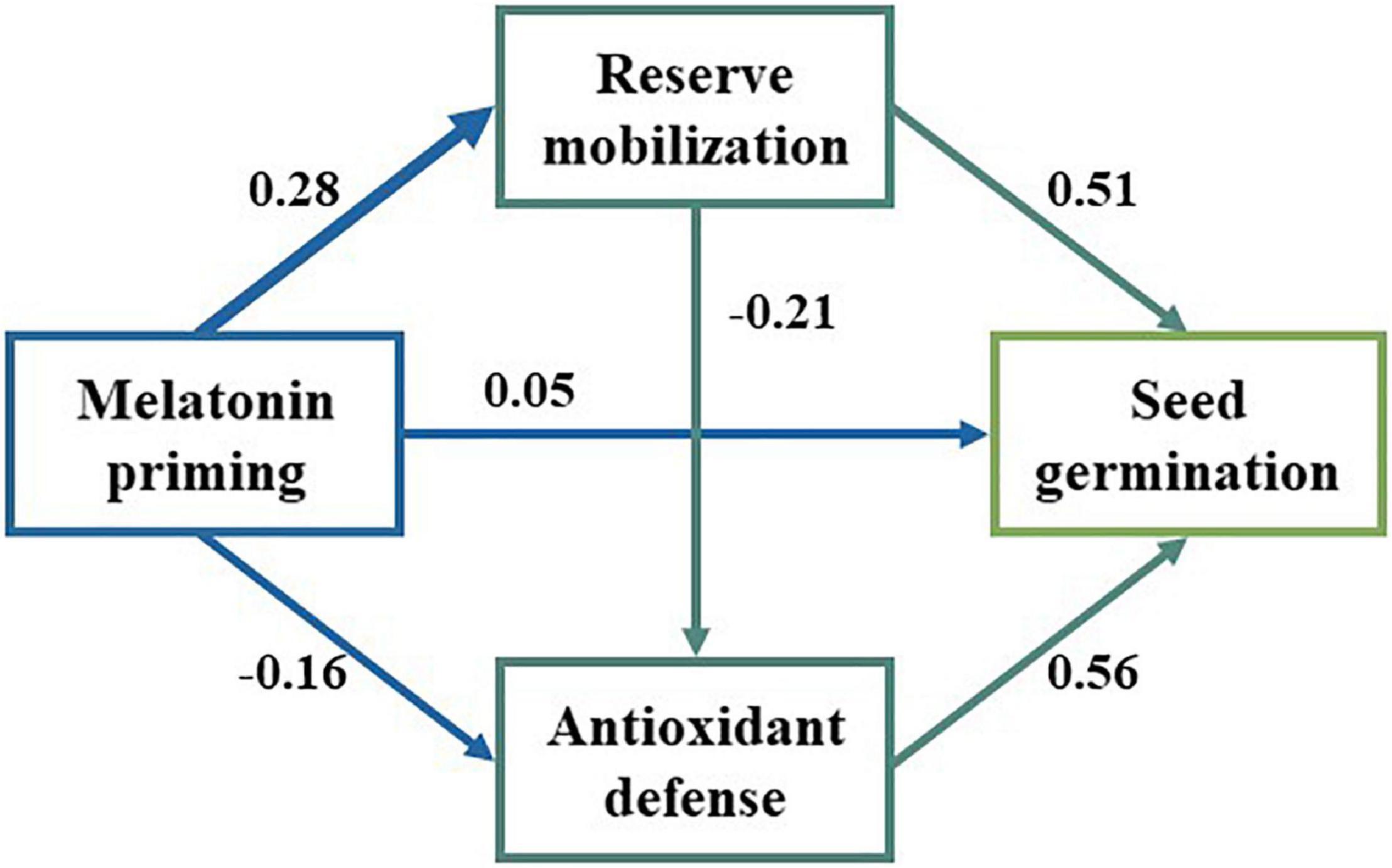
Figure 5. The structural equation model (SEM) analysis was performed to evaluate the effect of the influential pathways of melatonin priming on reserve mobilization, antioxidant activity (SOD and POD) and seed germination. The arrows indicate the assumed pathways. The arrow width is proportional to the strength of the pathways. The number on the arrow is the path coefficients (χ2 = 4.311, dfp = 2, p = 0.116, CFI = 0.995, RMSEA = 0.094).
Discussion
Since soil salinization had caused losses of ecological functions and also in wetland area in the MNNR, the process of effectively restoring degraded wetlands has attracted a lot of attention from the wetland manager and policymakers (Zhao et al., 2021a). Priming treatment, a key technique to alleviate the effects of abiotic stress on seed germination, had been attempted recently in wetland restoration engineering (Zhang M. Y. et al., 2021). It boosts the antioxidant and DNA repair systems, and also modulates the reserve mobilization for improving seed germination (Yan et al., 2020; Forti et al., 2021). Our results support the notion of effectively applying the priming treatment to wetland plant seed germination, and thus confirm the previously proposed hypothesis that melatonin priming had a concentration-dependent effect on S. corniculata seed germination during salinization stress. Priming with M50 had significantly positive effects on the seed germination, antioxidant defense, and reserve mobilization of S. corniculata.
Effect of Melatonin Priming on the Seed Germination of Suaeda corniculata
Seed propagation is preferred for maintaining plant genetic variation, and it will also play an important role in the process of near-naturalized wetland restoration (Kim, 2019). The seed germination process is usually affected by salinization stress, which leads to the decrease in germination quality and even results in an incomplete plant life cycle (Jia et al., 2018). Multiple studies have shown that melatonin is a major regulator not only involved in seed germination, but also in diverse physiological and biochemical processes like stress resistance-related gene expression, boosting the antioxidant defense system, and reserve mobilization (Cao et al., 2019; Chen et al., 2021; Zhang T. G. et al., 2022). Our results showed that melatonin priming positively affected seed germination of S. corniculata in a concentration-dependent manner, while significantly increasing the GR, GS, and GI of S. corniculata seed under the M50 treatment. This finding was consistent with Qin and Zeevaart (2002) and Ogawa et al. (2003), where they found that exogenous melatonin priming upregulates the expression of gibberellins (GA) biosynthesis genes (GA20ox and GA30ox), while downregulating the key abscisic acid (ABA) biosynthesis genes (LbNCED1 and LbNCED3), both of which affect seed germination. Additionally, the appropriate melatonin priming concentration activated the SOS pathway to improve the seed’s salinity tolerance at the germination stage by upregulating the expression of chloride channel proteins (CLC1 and CLC2) (Li X. J. et al., 2017). Meanwhile, priming with the appropriate melatonin concentration could up-regulate the ethylene, kinetin, and brassinolide biosynthetic gene expression and the plant hormone signal pathways in response to salinization stress (Zhang Y. X. et al., 2021). However, the mitigative effect of melatonin priming treatment on salinization stress could be counterproductive at high concentration. Our results suggested that the M200 treatment inhibited the S. corniculata seed germination as compared with the CK treatment. This may be because high melatonin concentration promoted the synthesis of endogenous ABA, which regulated seed germination via ABI3, ABI4, and ABI5 and promotes dormancy via the response factors like SCHNARCHZAPFEN (SNZ) and ABA overly sensitive 5 (ABO5) (Liu et al., 2010; Zou et al., 2013). This explanation has been supported by the fact that 1,000 μM melatonin treatment significantly inhibited the Arabidopsis seed germination as compared with the untreated control in Lv et al. (2021).
Effect of Melatonin Priming on Antioxidant Defense of Suaeda corniculata Seeds
The excessive accumulation of reactive oxygen species (ROS) not only has irreversible negative effects on seed germination, but also affects the future seedling growth (Goud and Kachole, 2011). Antioxidant system is one of the major physiological defense systems protecting against salinization-induced oxidative stress by improving the antioxidant enzyme activity and antioxidant contents (Ibrahim, 2016). In this sense, studying the antioxidant defense is crucial for a holistic understanding of priming treatments. Previous studies have demonstrated that melatonin get through the free radical scavenging cascade, extends the free radical scavenging capacity of antioxidants to its multi-stage metabolites. Especially N1-acetyl-N2-formyl-5-methoxykynuramine, a primary metabolite derived from melatonin, also has strong antioxidant capacity, thus effectively protecting organisms from oxidative stress (Balabusta et al., 2016). Simultaneously, melatonin helps scavenge free radicals through its receptors by modulating the antioxidant enzymes in the cells and tissues in a dose-dependent manner (Li et al., 2018; Cao et al., 2019). Our results showed that the SOD and POD activities of S. corniculata seeds continued increasing with the germination stage, which was significantly higher than others in the M50 treatment. Exogenous melatonin priming may have indirectly upregulated the expression of antioxidant enzyme and related genes, which then improves their efficiency as antioxidants (Arora and Bhatla, 2017; Wang et al., 2017). However, other studies have demonstrated that exogenous melatonin priming did not change the antioxidant enzymes’ activity of Lolium perenne (Zhang et al., 2016; Lu et al., 2022), and we suspect that melatonin possibly uses different physiological mechanisms to strengthen the antioxidant defense. MDA is the indicator of oxidative-stress induced membrane damage under salinization stress (Ibrahim, 2016). Our study results showed the MDA content in S. corniculata seeds continuously decreased during the experiment, and it decreased up to 27.33% in the M50 treatment. This result was in agreement with previous findings indicating that a negative correlation exists between the MDA content and the activity of antioxidant enzymes (Esfandiari et al., 2008; Younesi and Moradi, 2015). The possible explanation is that melatonin treatment increases the autophagy induction capacity and thereby reduces the accumulation of oxidized proteins, while also simultaneously controlling the ROS accumulation by upregulating the antioxidant enzymes activity and their transcript levels to mitigate membrane lipid peroxidation under salinization stress (Wang et al., 2015; Sun et al., 2020; Zhang T. G. et al., 2022). Additionally, it improves the activity of non-enzymatic antioxidants by regulating the activity of enzymes in the ASA-GSH cycle, thereby further improving the free radical scavenging capacity (Ni et al., 2018). Simultaneously, by up-regulating the genes related to glutathione metabolic pathway and activating the downstream signal transduction pathway, also may help explain how melatonin priming improves the antioxidant defense of seeds under salinization stress (Zhang Y. X. et al., 2021).
Effect of Melatonin Priming on Reserve Mobilization of Suaeda corniculata Seeds
It is well known that the seed reserve is an important factor which affects germination characteristics, and reserve mobilization plays an important role in the energy supply and maintains osmotic balance (Zhao et al., 2018; Lei et al., 2021). Recent experimental data suggested that the priming treatment regulates the carbohydrate, protein, and fat metabolism, thereby resulting in increased seed germination (Li et al., 2019; Chen et al., 2021). However, the effect of melatonin priming treatment on reserve mobilization under abiotic stress still needs to be studied according to the target species. Our results showed that there is a strong negative correlation between starch and soluble sugar content variation. A plausible explanation for this phenomenon could be that melatonin priming upregulated the α-amylase gene expression, to ensure that starch is broken down into smaller and simpler molecules which will be used as an energy source by the organism for seed germination (Cao et al., 2019; Yan et al., 2020). Previous studies also found that the priming treatment induced a phased effect on the change of seed soluble protein content under abiotic stress (Adawy et al., 2003). Priming treatment induced seeds to adopt the defensive strategy of accumulating soluble protein for improving the cell’s water holding capacity and protect its membrane (Li et al., 2010). During the later stage of germination, hydrolysis of storage proteins provides the energy for both the hypocotyl growth and new protein synthesis (Wahid and Bounoua, 2013). Meanwhile, the fat metabolism also pushed the seed’s developmental switch from dormancy to germination (Footitt et al., 2002), which was similar to our data.
Pathways of Melatonin Priming in Suaeda corniculata Seed Germination
The action pathway of priming treatment is the basis for revealing the driving mechanisms. The SEM analysis showed that there are both direct and indirect effect pathways of melatonin priming on the S. corniculata seed germination under salinization stress conditions (Figure 5). On the one hand, melatonin priming promoted seed germination by regulating the expression of stress tolerance, hormone synthesis, and metabolism-related genes (Zhang Y. et al., 2021). On the other hand, priming treatment also upregulated the antioxidant enzyme activities and accelerated the level of reserve mobilization, ultimately improving the seed germination (Arora and Bhatla, 2017; Cao et al., 2019; Lu et al., 2022). This study is the first to reveal the effect pathways of melatonin priming on the S. corniculata seed germination under salinization stress, and also proved the feasibility of melatonin priming in improving the germination of S. corniculata seeds. It is also worth noting that the positive effects of the melatonin priming we found were concentration-dependent, which reconfirms our hypothesis.
Conclusion
In summary, we comprehensively assessed the effect of melatonin priming on the S. corniculata seed germination under salinization stress. Our results showed that the stress-alleviating effect of melatonin priming is concentration-dependent. The melatonin priming concentration of 50 μM significantly improved the germination characteristics of S. corniculata seeds, increased the activity of antioxidant enzymes to alleviate the salinity stress-induced membrane lipid peroxidation, and promoted the reserve mobilization during germination. However, the treatment of 200 μM melatonin inhibited the above mentioned physiological activities. Melatonin priming directly influences the seed germination of S. corniculata, while also acting via indirect pathways to regulate the antioxidant defense and reserve mobilization. Our study is the first to explore the effects of exogenous melatonin on S. corniculata seed germination, antioxidant defense, and reserve mobilization under salinization stress, thereby providing invaluable information and technical support for the restoration of salinized wetlands. However, further studies are required to understand the synthetic effects of salinization stress intensity on the S. corniculata seed germination post-priming treatments, before a general conclusion can be drawn.
Data Availability Statement
The original contributions presented in this study are included in the article/Supplementary Material, further inquiries can be directed to the corresponding author.
Author Contributions
MZ, ST, and XW designed the study. MZ, SL, and YW collected the data. DZ, QQ, and YA analyzed the data. ST and XL lead the writing with all co-authors. All authors gave final approval for publication.
Funding
This research was supported by the National Natural Science Foundation of China (No. 41871101) and the Strategic Priority Research Program of the Chinese Academy of Sciences (No. XDA23060402).
Conflict of Interest
The authors declare that the research was conducted in the absence of any commercial or financial relationships that could be construed as a potential conflict of interest.
Publisher’s Note
All claims expressed in this article are solely those of the authors and do not necessarily represent those of their affiliated organizations, or those of the publisher, the editors and the reviewers. Any product that may be evaluated in this article, or claim that may be made by its manufacturer, is not guaranteed or endorsed by the publisher.
Acknowledgments
We would like to express their gratitude to EditSprings (https://www.editsprings.cn) for the expert linguistic services provided.
Supplementary Material
The Supplementary Material for this article can be found online at: https://www.frontiersin.org/articles/10.3389/fevo.2022.941032/full#supplementary-material
Footnotes
References
Adawy, T. A. E., Rahma, E. H., Bedawey, A. A. E., and Beltagy, A. E. E. (2003). Nutritional potential and functional properties of germinated mung bean, pea and lentil seeds. Plant Foods Hum. Nutr. 58, 1–13. doi: 10.1023/b:qual.0000040339.48521.75
An, Y., Gao, Y., Zhang, Y., Tong, S. Z., and Liu, X. H. (2019). Early establishment of Suaeda salsa population as affected by soil moisture and salinity: implications for pioneer species introduction in saline-sodic wetlands in Songnen Plain, China. Ecol. Indic. 107:105654. doi: 10.1016/j.ecolind.2019.105654
Arnao, M. B., and Hernandez-Ruiz, J. (2019). Melatonin: a new plant hormone and/or a plant master regulator? Trends Plant Sci. 24, 38–48. doi: 10.1016/j.tplants.2018.10.010
Arora, D., and Bhatla, S. C. (2017). Melatonin and nitric oxide regulate sunflower seedling growth under salt stress accompanying differential expression of Cu/Zn SOD and Mn SOD. Free Radic. Biol. Med. 106, 315–328. doi: 10.1016/j.freeradbiomed.2017.02.042
Balabusta, M., Szafranska, K., and Posmyk, M. M. (2016). Exogenous melatonin improves antioxidant defensein Cucumber seeds (Cucumis sativus L.) germinated under chilling stress. Front. Plant Sci. 7:575. doi: 10.3389/fpls.2016.00575
Biju, S., Fuentes, S., and Gupta, D. (2017). Silicon improves seed germination and alleviates drought stress lentil crops by regulating osmolytes, hydrolytic enzymes and antioxidant defense system. Plant Physiol. Biochem. 119, 250–264. doi: 10.1016/j.plaphy.2017.09.001
Cao, Q. J., Li, G., Cui, Z. G., Yang, F. T., Jiang, X. L., Diallo, L., et al. (2019). Seed priming with melatonin improves the seed germination of waxy maize under chilling stress via promoting the antioxidant system and starch metabolism. Sci. Rep. 9:15044. doi: 10.1038/s41598-019-51122-y
Chen, L., Lu, B., Liu, L. T., Duan, W. J., Jiang, D., Li, J., et al. (2021). Melatonin promotes seed germination under salt stress by regulating ABA and GA(3) in cotton (Gossypium hirsutum L.). Plant Physiol. Biochem. 162, 506–516. doi: 10.1016/j.plaphy.2021.03.029
Esfandiari, E., Shakiba, M. R., Mahboob, S. A., Alyari, H., and Shahabivand, S. (2008). The effect of water stress on the antioxidant content, protective enzyme activities, proline content and lipid peroxidation in wheat seedling. Pak. J. Biol. Sci. 11, 1916–1922. doi: 10.3923/pjbs.2008.1916.1922
Feghhenabi, F., Hadi, H., Khodaverdiloo, H., and van Genuchten, M. T. (2020). Seed priming alleviated salinity stress during germination and emergence of wheat (Triticum aestivum L.). Agric. Water Manage. 231:106022. doi: 10.1016/j.agwat.2020.106022
Footitt, S., Slocombe, S. P., Larner, V., Kurup, S., Wu, Y. S., Larson, T., et al. (2002). Control of germination and lipid mobilization by COMATOSE, the Arabidopsis homologue of human ALDP. EMBO J. 21, 2912–2922. doi: 10.1093/emboj/cdf300
Forti, C., Ottobrino, V., Doria, E., Bassolino, L., Toppino, L., Rotino, G. L., et al. (2021). Hydropriming applied on fast germinating Solanum villosum miller seeds: impact on pre-germinative metabolism. Front. Plant Sci. 12:639336. doi: 10.3389/fpls.2021.639336
Goud, P. B., and Kachole, M. S. (2011). Effect of exogenous hydrogen peroxide on peroxidase and polyphenol oxidase activities in Cajanus cajan (L.) Millsp, Detached leaves. Int. J. Curr. Res. 3, 61–65.
Ibrahim, E. A. (2016). Seed priming to alleviate salinity stress in germinating seeds. J. Plant Physiol. 192, 38–46. doi: 10.1016/j.jplph.2015.12.011
James, J. J., and Carrick, P. J. (2016). Toward quantitative dryland restoration models. Restor. Ecol. 24, S85–S90. doi: 10.1111/rec.12393
Jia, J., Huang, C., Bai, J. H., Zhang, G. L., Zhao, Q. Q., and Wen, X. J. (2018). Effects of drought and salt stresses on growth characteristics of euhalophyte Suaeda salsa in cosatal wetlands. Phys. Chem. Earth 103, 68–74. doi: 10.1016/j.pce.2017.01.002
Johnson, R., and Puthur, J. T. (2021). Seed priming as a cost effective technique for developing plants with cross tolerance to salinity stress. Plant Physiol. Biochem. 162, 247–257. doi: 10.1016/j.plaphy.2021.02.034
Kettenring, K. M., and Tarsa, E. E. (2020). Need to seed? Ecological, genetic, and evolutionary keys to seed-based wetland restoration. Front. Environ. Sci. 8:109. doi: 10.3389/fenvs.2020.00109
Kim, D. H. (2019). Practical methods for rapid seed germination from seed coat-imposed dormancy of Prunus yedoensis. Sci. Hortic. 243, 451–456. doi: 10.1016/j.scienta.2018.08.039
Lei, K., Sun, S., Zhong, K., Li, S., Hu, H., Sun, C., et al. (2021). Seed soaking with melatonin promotes seed germination under chromium stress via enhancing reserve mobilization and antioxidant metabolism in wheat. Ecotox. Environ. Safe. 220:112241. doi: 10.1016/j.ecoenv.2021.112241
Li, D. X., Batchelor, W. D., Zhang, D., Miao, H. X., Li, H. Y., Song, S. J., et al. (2020). Analysis of melatonin regulation of germination and antioxidant metabolism in different wheat cultivars under polyethylene glycol stress. PLoS One 15:e0237536. doi: 10.1371/journal.pone.0237536
Li, J. P., Zhao, C., Zhang, M. J., Yuan, F., and Chen, M. (2019). Exogenous melatonin improves seed germination in Limonium bicolor under salt stress. Plant Signal. Behav. 14:1659705. doi: 10.1080/15592324.2019.1659705
Li, X. J., Yang, M. F., Chen, H., Qu, L. Q., Chen, F., and Shen, S. H. (2010). Abscisic acid pretreatment enhances salt tolerance of rice seedlings: proteomic evidence. Biochim. Biophys. Acta Proteins Proteom. 1804, 929–940. doi: 10.1016/j.bbapap.2010.01.004
Li, X. J., Yu, B. J., Cui, Y. Q., and Yin, Y. F. (2017). Melatonin application confers enhanced salt tolerance by regulating Na+ and Cl– accumulation in rice. Plant Growth Regul. 83, 441–454. doi: 10.1007/s10725-017-0310-3
Li, X. N., Brestic, M., Tan, D. X., Zivcak, M., Zhu, X. C., Liu, S. Q., et al. (2018). Melatonin alleviates low PS I-limited carbon assimilation under elevated CO2 and enhances the cold tolerance of offspring in chlorophyll -deficient mutant wheat. J. Pineal Res. 64:12453. doi: 10.1111/jpi.12453
Li, X. N., Tan, D. X., Jiang, D., and Liu, F. L. (2016). Melatonin enhances cold tolerance in drought-primed wild-type and abscisic acid -deficient mutant barley. J. Pineal Res. 61, 328–339. doi: 10.1111/jpi.12350
Li, X. Y., Wen, B. L., Yang, F., Hartley, A., and Li, X. J. (2017). Effects of alternate flooding-drought conditions on degenerated Phragmites australis salt marsh in northeast China. Restor. Ecol. 25, 810–819. doi: 10.1111/rec.12500
Liu, Y., He, J. N., Chen, Z. Z., Ren, X. Z., Hong, X. H., and Gong, Z. Z. (2010). ABA overly-sensitive 5 (ABO5), encoding a pentatricopeptide repeat protein required for cis-splicing of mitochondrial nad2 intron 3, is involved in the abscisic acid response in Arabidopsis. Plant J. 63, 749–765. doi: 10.1111/j.1365-313x.2010.04280.x
Lu, X. P., Min, W. F., Shi, Y. F., Tian, L., Li, P. F., Ma, T. L., et al. (2022). Exogenous melatonin alleviates alkaline stress by removing reactive oxygen species and promoting antioxidant defence in rice seedlings. Front. Plant Sci. 13:849553. doi: 10.3389/fpls.2022.849553
Lv, Y., Pan, J. J., Wang, H. P., Reiter, R. J., Li, X., Mou, Z. M., et al. (2021). Melatonin inhibits seed germination by crosstalk with abscisic acid, gibberellin, and auxin in Arabidopsis. J. Pineal Res. 70, e12736. doi: 10.1111/jpi.12736
Ma, H. Y., Yang, H. Y., Lu, X. T., Pan, Y. P., Wu, H. T., Liang, Z. W., et al. (2015). Does high pH give a reliable assessment of the effect of alkaline soil on seed germination? A case study with Leymus chinensis (Poaceae). Plant Soil 394, 35–43. doi: 10.1007/s11104-015-2487-4
Masondo, N. A., Kulkarni, M. G., Finnie, J. F., and Van Staden, J. (2018). Influence of biostimulants-seed-priming on Ceratotheca triloba germination and seedling growth under low temperatures, low osmotic potential and salinity stress. Ecotox. Environ. Safe. 147, 43–48. doi: 10.1016/j.ecoenv.2017.08.017
Ni, J., Wang, Q. J., Shah, F. A., Liu, W. B., Wang, D. D., Huang, S. W., et al. (2018). Exogenous melatonin confers cadmium tolerance by counterbalancing the hydrogen peroxide homeostasis in wheat seedlings. Molecules 23:799. doi: 10.3390/molecules23040799
Ogawa, M., Hanada, A., Yamauchi, Y., Kuwahara, A., Kamiya, Y., and Yamaguchi, S. (2003). Gibberellin biosynthesis and response during Arabidopsis seed germination. Plant Cell 15, 1591–1604. doi: 10.1105/tpc.011650
Qin, X. Q., and Zeevaart, J. A. D. (2002). Overexpression of a 9-cis-epoxycarotenoid dioxygenase gene in Nicotiana plumbaginifolia increases abscisic acid and phaseic acid levels and enhances drought tolerance. Plant Physiol. 128, 544–551. doi: 10.1104/pp.010663
Seneviratne, M., Rajakaruna, N., Rizwan, M., Madawala, H. M. S. P., Ok, Y. S., and Vithanage, M. (2019). Heavy metal-induced oxidative stress on seed germination and seedling development: a critical review. Environ. Geochem. Health 41, 1813–1831. doi: 10.1007/s10653-017-0005-8
Soriano, D., Orozco-Segovia, A., Marquez-Guzman, J., Kitajima, K., Gamboa-de Buen, A., and Huante, P. (2011). Seed reserve composition in 19 tree species of a tropical deciduous forest in Mexico and its relationship to seed germination and seedling growth. Ann. Bot. 107, 939–951. doi: 10.1093/aob/mcr041
Sun, C. L., Lv, T., Huang, L., Liu, X. X., Jin, C. W., and Lin, X. Y. (2020). Melatonin ameliorates aluminum toxicity through enhancing aluminum exclusion and reestablishing redox homeostasis in roots of wheat. J. Pineal Res. 68:e12642. doi: 10.1111/jpi.12642
Tahjib, U. A., Roy, P. R., Sohag, A. A. M., Afrin, S., Rady, M. M., and Hossain, M. A. (2018). Exogenous calcium supplementation improves salinity tolerance in BRRI Dhan28; a salt susceptible high-yielding Oryza Sativa cultivar. J. Crop Sci. Biotech. 21, 383–394. doi: 10.1007/s12892-018-0098-0
Tang, H. R., Bai, J. S., Chen, F. Y., Liu, Y., and Lou, Y. J. (2021). Effects of salinity and temperature on tuber sprouting and growth of Schoenoplectus nipponicus. Ecosphere 12:e03448. doi: 10.1002/ecs2.3448
Wahid, N., and Bounoua, L. (2013). The relationship between seed weight, germination and biochemical reserves of maritime pine (Pinus pinaster Ait.) in Morocco. New For. 44, 385–397. doi: 10.1007/s11056-012-9348-2
Wang, D. W., Bai, J. H., Wang, W., Ma, X., Guan, Y. N., Gu, C. H., et al. (2020). Micro-topography manipulations facilitate Suaeda Salsa Marsh restoration along the lateral gradient of a tidal creek. Wetlands 40, 1657–1666. doi: 10.1007/s13157-020-01308-2
Wang, L., Feng, C., Zheng, X. D., Guo, Y., Zhou, F. F., Shan, D. Q., et al. (2017). Plant mitochondria synthesize melatonin and enhance the tolerance of plants to drought stress. J. Pineal Res. 63:e12429. doi: 10.1111/jpi.12429
Wang, P., Sun, X., Wang, N., Tan, D. X., and Ma, F. (2015). Melatonin enhances the occurrence of autophagy induced by oxidative stress in Arabidopsis seedlings. J. Pineal Res. 58, 479–489. doi: 10.1111/jpi.12233
Wang, Q., Xie, T., Luo, M., Bai, J. H., Chen, C., Ning, Z. H., et al. (2021). How hydrological connectivity regulates the plant recovery process in salt marshes. J. Appl. Ecol. 58, 1314–1324. doi: 10.1111/1365-2664.13879
Wang, X. H., Zhang, D. J., Qi, Q., Tong, S. Z., An, Y., Lu, X. G., et al. (2019). The restoration feasibility of degraded Carex tussock in soda-salinization area in arid region. Ecol. Indic. 98, 131–136. doi: 10.1016/j.ecolind.2018.08.066
Wang, X. Y., Cheng, R., Zhu, H., Cheng, X. W., Shutes, B., and Yan, B. X. (2020). Seed germination and early seedling growth of six wetland plant species in saline-alkaline environment. Int. J. Phytoremediat. 22, 1185–1194. doi: 10.1080/15226514.2020.1748565
Yan, H. F., Jia, S. G., and Mao, P. S. (2020). Melatonin priming alleviates aging-induced germination inhibition by regulating β-oxidation, protein translation, and antioxidant metabolism in oat (Avena sativa L.) seeds. Int. J. Mol. Sci. 21:1898. doi: 10.3390/ijms21051898
Yang, F., Baskin, J. M., Baskin, C. C., Yang, X. J., Cao, D. C., and Huang, Z. (2017). Divergence in life history traits between two populations of a seed-dimorphic halophyte in response to soil salinity. Front. Plant Sci. 8:1028. doi: 10.3389/fpls.2017.01028
Younesi, O., and Moradi, A. (2015). Effect of priming of seeds of Medicago sativa ‘Bami’ with gibberellic acid on germination, seedlings growth and antioxidant enzymes activity under salinity stress. J. Hortic. Res. 22, 167–174. doi: 10.2478/johr-2014-0034
Zhang, D. J., Qi, Q., Tong, S. Z., Wang, X. H., An, Y., Zhang, M. Y., et al. (2019). Soil degradation effects on plant diversity and nutrient in tussock meadow wetlands. J. Soil Sci. Plant Nutr. 19, 535–544. doi: 10.1007/s42729-019-00052-9
Zhang, J., Li, H. B., Xu, B., Li, J., and Huang, B. R. (2016). Exogenous melatonin suppresses dark-induced leaf senescence by activating the superoxide dismutase-catalase antioxidant pathway and down-regulating chlorophyll degradation in excised leaves of perennial ryegrass (Lolium perenne L.). Front. Plant Sci. 7:1500. doi: 10.3389/fpls.2016.01500
Zhang, M. Y., Qi, Q., Zhang, D. J., Tong, S. Z., Wang, X. H., An, Y., et al. (2021). Effect of priming on Carex schmidtii seed germination and seedling growth: implications for tussock wetland restoration. Ecol. Eng. 171:106389. doi: 10.1016/j.ecoleng.2021.106389
Zhang, M. Y., Zhang, D. J., Qi, Q., Tong, S. Z., Wang, X. H., An, Y., et al. (2022). Flooding effects on population and growth characteristics of Bolboschoenus planiculmis in Momoge wetland, northeast China. Ecol. Indic. 137:108730. doi: 10.1016/j.ecolind.2022.108730
Zhang, T. G., Wang, J., Sun, Y. P., Zhang, L., and Zheng, S. (2022). Versatile roles of melatonin in growth and stress tolerance in plants. J. Plant Growth Regul. 41, 507–523. doi: 10.1007/s00344-021-10317-2
Zhang, Y., Zhou, X. J., Dong, Y. T., Zhang, F., He, Q. L., Chen, J. H., et al. (2021). Seed priming with melatonin improves salt tolerance in cotton through regulating photosynthesis, scavenging reactive oxygen species and coordinating with phytohormone signal pathways. Ind. Crop. Prod. 169:113671. doi: 10.1016/j.indcrop.2021.113671
Zhang, Y. X., Fan, Y. P., Rui, C., Zhang, H., Xu, N., Dai, M. H., et al. (2021). Melatonin improves cotton salt tolerance by regulating ROS scavenging system and Ca2+ signal transduction. Front. Plant Sci. 12:693690. doi: 10.3389/fpls.2021.693690
Zhao, H. B., Xu, L. F., Su, T., Jiang, Y., Hu, L. Y., and Ma, F. W. (2015). Melatonin regulates carbohydrate metabolism and defenses against Pseudomonas syringae pv. tomato DC3000 infection in Arabidopsis thaliana. J. Pineal Res. 59, 109–119. doi: 10.1111/jpi.12245
Zhao, K. F., Fan, H., Zhou, S., and Song, J. (2003). Study on the salt and drought tolerance of Suaeda salsa and Kalanchoe claigremontiana under iso-osmotic salt and water stress. Plant Sci. 165, 837–844. doi: 10.1016/s0168-9452(03)00282-6
Zhao, M., Zhang, H. X., Yan, H., Qiu, L., and Baskin, C. C. (2018). Mobilization and role of starch, protein, and fat reserves during seed germination of six wild grassland species. Front. Plant Sci. 9:234. doi: 10.3389/fpls.2018.00234
Zhao, Y. T., Wang, G. D., Zhao, M. L., Wang, M., and Jiang, M. (2021a). Direct and indirect effects of soil salinization on soil seed banks in salinizing wetlands in the Songnen Plain, China. Sci. Total Environ. 819:152035. doi: 10.1016/j.scitotenv.2021.152035
Zhao, Y. T., Wang, G. D., Zhao, M. L., Wang, M., Hu, N. L., Jiang, M., et al. (2021b). The potentials of wetland restoration after farming differ between community types due to their differences in seed limit and salt tolerances in the Songnen Plain. China. Ecol. Indic. 131:108145. doi: 10.1016/j.ecolind.2021.108145
Keywords: antioxidant defense, melatonin priming, reserve mobilization, salinization stress, seed germination, Suaeda corniculata, wetland restoration
Citation: Zhang M, Liu S, Tong S, Zhang D, Qi Q, Wang Y, Wang X, An Y and Lu X (2022) Effects of Melatonin Priming on Suaeda corniculata Seed Germination, Antioxidant Defense, and Reserve Mobilization: Implications for Salinized Wetland Restoration. Front. Ecol. Evol. 10:941032. doi: 10.3389/fevo.2022.941032
Received: 11 May 2022; Accepted: 06 June 2022;
Published: 04 July 2022.
Edited by:
He Yixin, Chengdu Institute of Biology (CAS), ChinaReviewed by:
Junhong Bai, Beijing Normal University, ChinaJun You, Oil Crops Research Institute (CAAS), China
Copyright © 2022 Zhang, Liu, Tong, Zhang, Qi, Wang, Wang, An and Lu. This is an open-access article distributed under the terms of the Creative Commons Attribution License (CC BY). The use, distribution or reproduction in other forums is permitted, provided the original author(s) and the copyright owner(s) are credited and that the original publication in this journal is cited, in accordance with accepted academic practice. No use, distribution or reproduction is permitted which does not comply with these terms.
*Correspondence: Shouzheng Tong, dG9uZ3Nob3V6aGVuZ0BpZ2EuYWMuY24=
†These authors have contributed equally to this work