- 1Departamento de Ecología Humana, Centro de Investigación y de Estudios Avanzados del IPN (Cinvestav) Unidad Mérida, Mérida, Mexico
- 2Laboratorio de Inmunología, Centro de Investigaciones Regionales “Dr. Hideyo Noguchi”, Mérida, Mexico
- 3Instituto de Investigación Sobre la Salud Pública, Universidad de la Sierra Sur, Oaxaca, Mexico
- 4Instituto de Ecología, Universidad Nacional Autónoma de México, Ciudad de México, Mexico
Environmental change (i.e., urbanization) impacts species in contrasting ways, with some species experiencing benefits given their way of life (i.e., blood-sucking insects). How these species respond to such change is not well understood and for species involved in human diseases, this “how” question is particularly important. Most Triatominae bug species inhabit tropical and subtropical forests where their vertebrate hosts’ temporal abundance depends on climate seasonality. However, in human encroached landscapes, triatomines can benefit from resource stability which may lead to adaptive phenotypic change to track novel hosts. We tested for an association between different landscapes and morpho-functional traits linked to sensory, motion, and feeding functions in Triatoma dimidiata and compared fecundity (i.e., number of eggs) in each landscape as a proxy of fitness. Using geometric and traditional morphometric tools, we predicted a morphological simplification in bugs inhabiting urbanized areas. While wing morphology or proboscis were not influenced by landscape class, the opposite occurred for thorax morphology and number of sensilla. Wing and thorax morphology did not covary under modified landscape scenarios, yet we detected a morpho-functional convergence for thorax size and antennal phenotype in both sexes, with a simplification trend, from nature to urban settings. Given no fecundity differences across landscapes, there is no potential reproductive costs. Moreover, the convergence of thorax size and antennal phenotype suggests differences in flight/locomotion performance and host/environment perception, as a possible adaptive response to relaxed selective pressures of the bug’s native habitat. These results imply that T. dimidiata could be adapting to urbanized areas.
Introduction
Urbanization is an important selective driver that has given rise to phenotypes finely co-adapted to novel environments (Miles et al., 2021). Urbanized species have taken advantage of human-provided resources and some examples include arthropod vectors of diseases which have successfully “tracked” human evolution by either making use of their phenotypic plasticity (i.e., the ability of a single genotype to give rise to different phenotypes after being exposed to distinct environments) and/or evolving new traits (Beaty et al., 2016; Fouet et al., 2018; Suesdek, 2019). An example of such plasticity is the overwintering ability of Aedes albopictus which has allowed it to colonize northern (and, thus, colder) European areas in less than three decades (Wilke et al., 2020). However, we are unaware of how much phenotypic plasticity (i.e., the capacity of species to respond to environmental variation by producing different phenotypes) and evolution (i.e., change in the genetic composition of a population over successive generations) of new traits have contributed to explain such arthropod vector success in urban contexts. In any case, since most of this knowledge comes from work with mosquitoes (e.g., Beaty et al., 2016; Fouet et al., 2018), it is unclear whether some other vector taxa have gone through similar evolutionary or microevolutionary (i.e., evolution within and among populations) responses. Gathering vector-wide information is key as zoonotic diseases are on the rise (Allen et al., 2017; Magouras et al., 2020), and tracking the evolution of underlying vectors may be just one more angle to think about the spread of such diseases.
Most species of triatomine (Hemiptera: Reduviidae: Triatominae) bugs are specialized in hematophagy which involves multiple adaptations associated with host localization and blood suction (Weirauch, 2021). These “kissing bugs” are vectors of the parasite Trypanosoma cruzi, the etiological agent of Chagas disease (CD) (Noireau et al., 2009). Tropical and subtropical forest are the primary vectors’ habitat, yet the continued change of these landscapes by anthropogenic activities (Ellis et al., 2017) have promoted triatomine transient and seasonal invasion to human rural dwellings (Dumonteil et al., 2002, 2007; Nouvellet et al., 2011). Rural infestation by triatomines is the leading cause of CD epidemiology, which causes more than 15,000 human deaths and 40,000 new infections each year (Rassi et al., 2010). However, in the last two decades, more information is emerging that shows an “urbanization” scenario for CD for several Latin American countries (Moncayo and Silveira, 2009; Schmunis and Yadon, 2010; Sosa, 2010). Related to this, several studies have shown that at least 14 species of kissing bugs have invaded and colonized urban and peri-urban dwellings, increasing the frequency of vectorial infections in humans (Levy et al., 2006; Briceño-León, 2009; Provecho et al., 2014). Furthermore, records of urban infestation date back to 50 years for several of these countries, suggesting that it is not an emerging problem but an underestimated one (Gaspe et al., 2020).
Triatomines’ sources of blood have changed along with landscape modifications: a reduction of many blood-source vertebrates’ species from original forests (Cavada et al., 2019) and an increase of domestic hosts all-year-round from anthropic landscapes, including rural and urban settings (Ordóñez-Krasnowski et al., 2020). A shift in hosts would select for distinct functional and performance traits related to dispersal, foraging, and colonization in triatomines. Despite the shortage of studies on the effect of land-use change and urbanization on triatomine function and performance, these anthropogenic phenomena are repeatedly claimed as selective forces propelling the evolution of intraspecific morphological variation in triatomines (Bustamante et al., 2004; Dorn et al., 2007; Aldana et al., 2011).
The ability to detect and feed on vertebrate hosts by triatomines is mediated by their morphological traits (Dujardin et al., 2009). One first set of traits related to host detection is flight as this is largely involved in the infestation or reinfestation patterns in Triatominae (Ceballos et al., 2005). Some bionomic markers of this function are wing size, shape, and thorax (Dujardin et al., 2007; Gaspe et al., 2012; Hernández et al., 2015). Antennal sensilla are one second set of traits also under current selection (Dujardin et al., 1999; Schofield et al., 1999; Carbajal de la Fuente and Catalá, 2002), as they are related to dispersion and invasion ability to new habitats, as a signature of biotope-related selection (Catalá et al., 2005; Arroyo et al., 2007; Hernández et al., 2011). Finally, proboscis length is another functional trait that seems to have evolved in response to a wild-urban environmental gradient (Eggenberger et al., 2019). These flight- and feeding-related traits have led triatomine researchers to speculate about their covariation with habitat types (Dujardin et al., 1999; Schofield et al., 1999). Furthermore, wing morphology, antennal phenotypes, or the combination of both trait groups have been used to provide evidence related to “domiciliation” in triatomine bugs (Borges et al., 2005; Schachter-Broide et al., 2009; Hernández et al., 2011; Gaspe et al., 2012; Villacís et al., 2014). However, no studies have been carried out to understand how these and other functional traits have responded simultaneously to different landscapes, which has remained as one major question in the study of evolution of triatomine domiciliation (Flores-Ferrer et al., 2018). Given this, we have asked whether there is an association between the morpho-functional traits that underlie flight- and feeding-related traits and landscape type in triatomines. Having Triatoma dimidiata s. l as a study species and using geometric and traditional morphometric approaches, we hypothesized that morpho-functional traits associated with dispersal capacity and foraging behavior would reflect evolutionary responses to different landscapes in a nature-urban gradient. Furthermore, if such phenotypic change has an adaptative basis, we predicted similar fitness outcomes in different landscapes. According to this, we predict that: (a) the morphology related to flight and feeding will show patterns of simplification in urban habitats compared to non-urban habitats; and, (b) equal fitness payoffs across different nature-urban settings.
Materials and Methods
Study Area
The study was conducted in the Yucatan state, located at the north of the Yucatan Peninsula (Figure 1). T. dimidiata were collected from eight sites corresponding to the northeast (Mérida city) and southwest of Yucatan State (Figure 1 and Table 1). All sites are located at an elevation of <96 m. The collection sites were grouped into four landscape types: Urban, Homegarden in rural communities (“Solares Mayas”), continuous tropical forest, and secondary forest. The Urban (U) class is represented by Merida City, a large commercial hub for southern and southeastern Mexico (Biles and Lemberg, 2020). Until 2010, the city had grown to occupy an area from 15,944 to 27,027 ha (Secretaría de Desarrollo Urbano y Medio Ambiente [SEDUMA], 2018) and currently concentrates 43% of the Yucatan state’s population (García-Gil et al., 2010). It is estimated that between 1980 and 2015, population increased from 400.142 to 832.651 (Biles and Lemberg, 2020). T. dimidiata is the only Triatominae species in this region, with values of city infestation (percentage of houses with vector presence) and infection with T. cruzi of 38 and 48%, respectively (Guzmán-Tapia et al., 2007). According to data from the Secretaria de Salud de Yucatan (SSY, period 2012–2105), the presence of T. dimidiata nymphs has been documented in human housing all over the state, including Mérida city (González-Martínez, 2018). Mérida is organized in eight districts, and our collections were carried out in four of them: west, northwest, east, and south. The last one is a zone with active and significant circulation of T. cruzi, with evidence of seroprevalence in people, domestic dogs (Jiménez-Coello et al., 2010, 2015), marsupials and synanthropic rodents (Panti-May et al., 2017; Ucan-Euan et al., 2019).
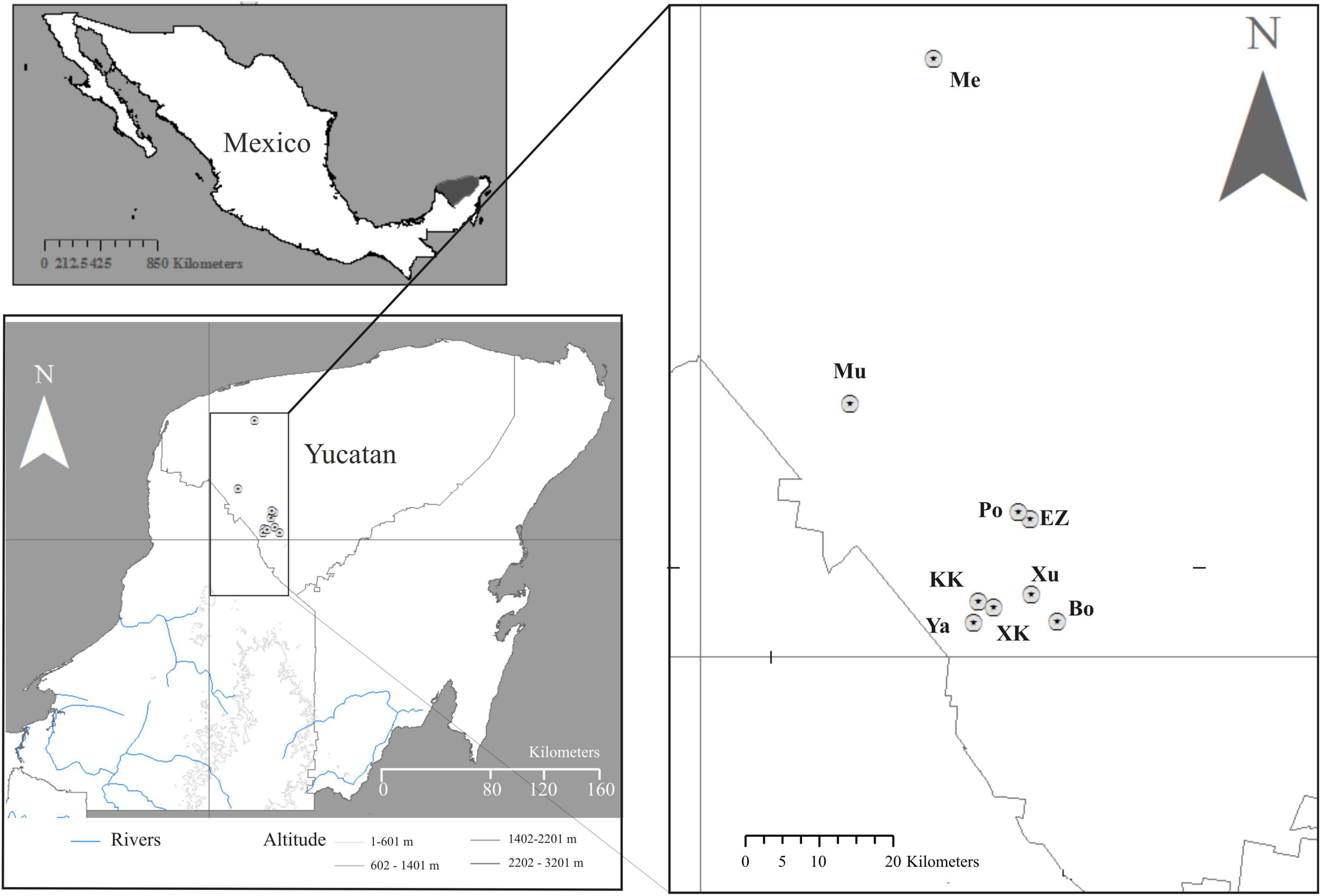
Figure 1. Study area in Mexico and Yucatan State Collection sites are indicated: Urban, Mérida City (Me); Homegarden (“Solares Mayas”: Emiliano Zapata (EZ) and Xul (Xu); Median subdeciduous tropical forest: Kaxil Kiuic (KK) and Yaxhachén (Ya); Secondary vegetation: X’kobenhaltun (XK), Bombahaltún (Bo), and Potoit (Po).
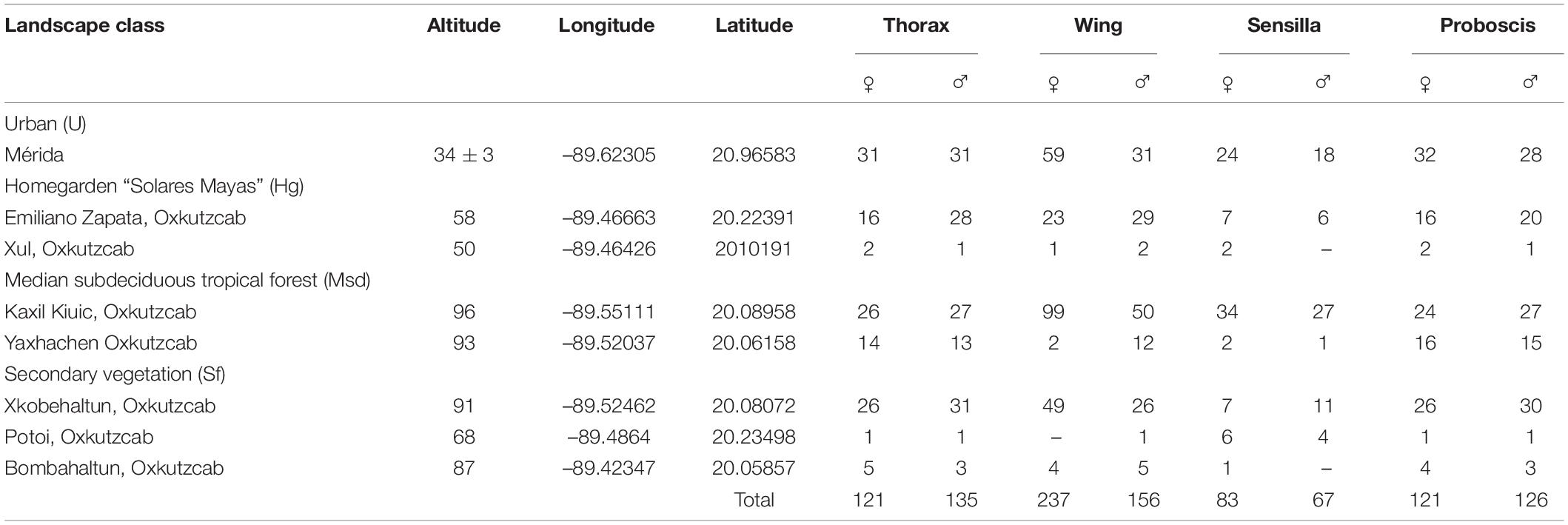
Table 1. Number of specimens of Triatoma dimidiata according to measured traits per landscape class.
All collections of Triatomine from southwest of Yucatan state were carried out in a region with a long history of ancient Mayan settlements (McAnany, 2016). This region is the central agricultural zone of the state after transformation from tropical dry forests (Klepeis and Vance, 2003; Manson and Evans, 2007; Hernández-Stefanoni et al., 2014).
The “Solares” (S) are essential in the cultural traditions and family economy of contemporary Mayan populations. They are environmental units that includes the house-room and spaces destined for agricultural production to subsistence. Blood sources in the Solares are humans, domestic vertebrates (pigs, cow, turkey, chicken, and dogs), and opportunistic vertebrates such as mice, marsupials, and birds that benefit from the presence of fruit trees [e.g., Mangifera indica, Manilkara zapota (L.) P. Royen, Guabana, Anona, Saramuyo], trees for local use [Brosimum alicastrum Swartz, Bursera simaruba (L.) Sarg, Ceiba pentandra (L.) Gaertn.] Hylocereus undatus [(Haw.) Britton and Rose] and other plants (Ordóñez-Díaz, 2018). Therefore, these systems offer good conditions for the infestation by T. dimidiata. We included two sites within this category: Emiliano Zapata and Xul, which are separated by 13.55 km (Figure 1 and Table 1).
Kaxil Kiuic biocultural reserve (KKBR) and forest fragments around Yaxhachén (separated by 4.03 km) are the two localities with continuous forest and fragments of Median sub deciduous tropical forest (Msb), respectively (Figure 1 and Table 1). KKBR has an area of 1,642 ha of Msd that has existed for more than 100 years (Essens and Hernández-Stefanoni, 2013). Trees reach a height of 13–20 m (Dupuy et al., 2012) in both localities, dominating the arboreal and shrubby elements, with scarce climbing plants and epiphytes. Vertebrate communities of potential vector hosts at KKBR are composed by birds (151 species), mammals (40 species), and reptiles (36 species) (Callaghan and Pasos, 2010). Several mammal species present in this region have been implied in the in enzootic cycle of T. cruzi (Dumonteil et al., 2018; Moo-Millan et al., 2019; Torres-Castro et al., 2021).
Finally, secondary vegetation (Sv) is represented by three sites (X’kobehaltun, Potoi, and Bombahaltún) with different ages of abandonment after use of traditional agriculture with the method of roza-tumba-quema (Table 1). Among the most abundant tree species are Neomillspaughia emarginata (Grooss) Blake, Gymnopodium floribundum Rolfe, Bursera simaruba (L.) Sarg, and Piscidia piscipula (L.) Sarg (Rico-Gray and García-Franco, 1992). Vertebrate community and potential reservoirs of T. cruzi at these sites are species that tolerate agricultural land-use such as Peromyscus yucatanicus, Oryzomys couesi, Sigmodon hispidus rodents, Artibeus jamaicensis, Desmodus rotundus bats, and Dasypus spp., Didelphis virginiana, and Nasua narica mammals (Panti-May et al., 2021).
Insect Collections
Triatomine bug adults were collected from April 2014 to June 2019. We conducted sampling for 3 h (20:00–23:00 h) using 3 × 3 m, white blankets and led lamps (Light traps) with standardized light intensity (2000 lumens). We also searched for insects (e.g., under fallen leaves, rocks, trunks, houses) at a radius of five meters from the light traps. Collected bugs were preserved in ethanol (90%). Insects from the city were reported and delivered alive at inhabitants’ homes the same or following day of report.
Measurement of Fitness Proxy and Feeding Information
Both males and females were dissected to extract the intestine, visually register the feeding status (i.e., blood presence), and calculate the proportion of feed insects. We quantified egg production as a fitness proxy registering the number of eggs per female in each landscape and year. For dissections, bugs were placed in a ventral view in Petri dishes sterilized with 96% alcohol. For each specimen, we used sterilized scalpels and made two lateral cuts in the ventral region of the abdomen from the anterior part to the anal section. Both dissections made it possible to remove the ventral area of the abdomen as a lid. The digestive tract was removed using sterilized entomological forceps. Dissections and egg number counting were performed by a single person to minimize sources of error.
Flight Morphology Data
The right wing and thorax for females (wings n = 248 and thorax n = 131) and males (wings n = 162 and thorax n = 134) were used to characterize flight morphology among the four landscape classes (Table 1). We obtained wing and thorax images with an integrated photo-macroscope model Leica Z16APOA (Leica Microsystems AG, Wetzlar, Germany). Images were processed in MakeFan8 (Sheets, 2003) to draw templates that allowed us to register the contours. For wings, we outlined three templates by registering 37 semi-landmarks. For thorax images, we removed four templates by registering 19 semi-landmarks (black dots, Figures 2A,B).
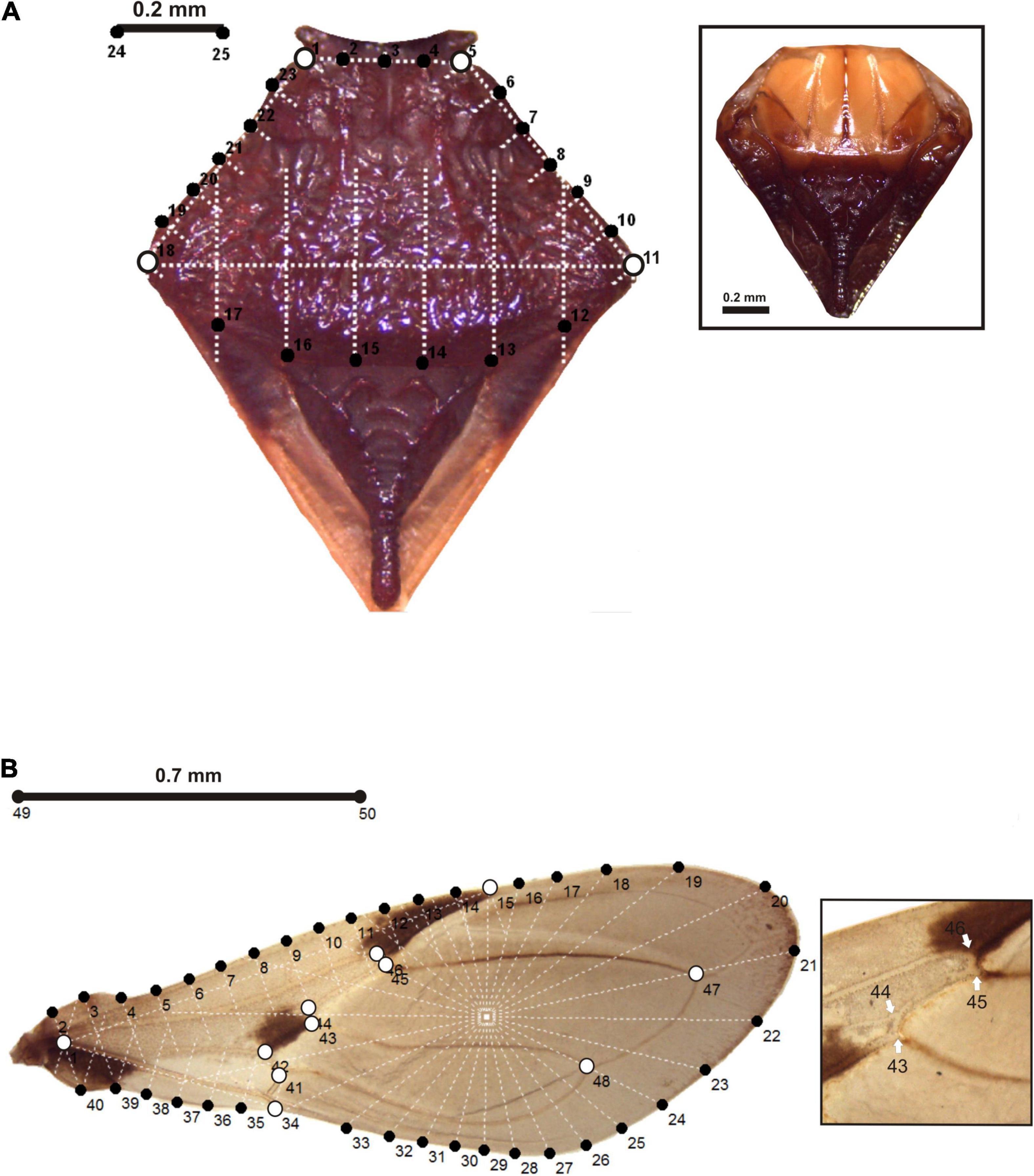
Figure 2. Landmarks used to capture the configuration of T. dimidiata shape: (A) thorax (Landmarks, LM = 4 and Semilandmarks, SML = 19; right, muscle mass in thorax); and, (B) wing (Landmarks, LM = 11 and Semilandmarks, SML = 37). White dots are LM, and black dots are SLM.
Landmarks type I were also recorded for wing venation origins and intersections (white dots, Figure 2), while for the thorax, we selected four landmarks type II (Bookstein, 1991). We employed TPSDig (Rohlf, 2007) to register the Cartesian coordinates of the 48 and 23 points for wings and thorax, respectively. Landmark configurations were adjusted with CoordGen8 (Sheets, 2005) using a Generalized Procrustes analysis based on the sum of the least-squares at each point. This adjustment allows to minimize the variation associated with the effects of positioning, orientation, and scale (Bookstein, 1991; Zelditch et al., 2004). A second adjustment was applied to eliminate variation by sliding the semi-landmarks based on the alignment distance criteria of the Semiland8 (Sheets, 2002). The superimposed and aligned Procrustes coordinates were used to calculate shape variables (Partial Warps, PW) and centroid size (Cs, as a measure of size) for each anatomical structure.
The wing aspect ratio (WAR) was calculated for each individual as wing span2/wing area (Females n = 248 and Males n = 162). Measurements were carried out in ImageJ v. 1.52p (image processing and analysis in Java, free access1, Rasband, 1997–2019).
Antennal Phenotype Data
We examined the antennal phenotype (defined by the number of sensilla) of 150 adult bugs (n = 83 females and n = 67 males) (Table 1). Previously, the antenna was dissected and processed with sodium hydroxide 4% for 6 h at 60°C, and then neutralized with glacial acetic acid (5%) for 2 min. Subsequently, each antenna was preserved and mounted temporally with glycerin for identification and counting of sensilla under a Leica DM300 microscope (Leica Microsystems AG, Wetzlar, Germany) at 400x. We followed the nomenclature proposed by Catalá and Schofield (1994) for olfactory sensillum identification. We identified and counted the followed sensilla for the ventral region of Pedicel (P), Flagellomere I (FI), and Flagellomere II (FII): Basiconic (Ba), bristles (Br), thin-walled trichoid (TH), and thick-walled trichoid (TK).
Feeding Morphology Data
We measured the proboscis length of 246 adult bugs (n = 121 females and n = 126 males, Table 1) from its basis to distal region. Measurement (in mm) was taken with a squared sheet (1 × 1 mm) under a Leica Z4 stereoscope (Leica Microsystems AG, Wetzlar, Germany).
The person who took all measurements was unaware of the bug’s collection site to avoid any observer’s bias.
Statistical Analyses
Feeding Status and Fitness Proxy Differences
We carried out a generalized linear model setting a negative binomial distribution and Tukey post hoc tests to evaluate differences in feeding status among landscapes. Also, to evaluate differences between the number of eggs by females among landscapes we performed a Kruskal–Wallis test and post hoc test with Holm correction. The analysis was performed in R v. 3.1.0; MASS and emmeans packages were used for construction of generalized linear model and post hoc tests, respectively.
Geometric Morphometric Analysis
Given size-related gender differences in our study species (Lehmann et al., 2005), we first analyzed shape and size differences between sexes (dimorphism) in thorax and wings. Sexual dimorphism in size was tested through two-way analysis of variance (ANOVA), where shape dimorphism was determined through a permutation test using Goodall’s F test implemented in TwoGroup8 with 3,600 bootstrap permutations (Sheets and Zeldich, 2001). Given the significant differences in both structures (see section “Results”), the subsequent analyses were performed by sex (Supplementary Table S1).
Because the two principal structures related to flight dispersion in Triatominae bugs are the thorax and wings (Hernández et al., 2015), we analyzed the possible covariation between these two structures, and also whether such covariation was affected by landscape type, thus producing different selective pressures and promote patterns, for example, in favor of individuals or populations with increased flight performance or dispersion. Therefore, we performed a partial least square analysis (PLS) to assess the covariance between the aligned morpho-anatomical structures (wing and thorax). Covariation analysis was performed using the function “two.b. pls” as implemented in the geomorph package v. 3.1.0 in R (Adams et al., 2020).
For each structure, we explored patterns of generalized shape variance among all individuals using the function “gm.prcomp” on Procrustes shape as implemented in geomorph package v. 3.1.0 (Adams et al., 2020). We used the function “mshape” to generate the consensus shape and the function “shape.predictor” to estimate the predicted shapes along the principal component axes. These inferred configurations were employed to visualize the shape differences among individuals along the principal component axes. Both functions are implemented in the “geomorph” package.
We conducted a linear mixed model (LMM) to evaluate the landscape effect on WAR, shape variables, and centroid size of both structures (wing and thorax). The year of the collection (period 2014–2018) was considered a random effect while body size and the interaction among variables were considered in the model. Additionally, for LMM where wing and thorax shape were entered as a response variable, we tested the relation with the WAR to detected patterns of covariation among these variables related to flight/dispersion. Because the detection of an allometric effect could influence shape inferences, we also tested if a significant association between shape and size (as Log transformed) existed. The LMM was performed according to a residual randomization permutation procedures (RRPP) approach with the “rpp.lm” function (with 2,500 permutations) as implemented in the RRPP package in R v.3.5.0 (Collyer and Adams, 2020). To assume that landscape type effects are independent of geographic location, we included a geographical covariance matrix in the “rpp.lm” function with the argument “cov”. This allowed driving the non-independence of the error by geographic location in the estimation of the coefficients of the landscape effect via GLS (Generalized least square). We computed geographic covariance matrix with the “cov.sp” function in SpatialTool package v.1.0.4 (French, 2008).
Antennal Data Analysis
We carried out univariate and multivariate analyses to assess differences in the number of antennal sensilla among the four landscape classes. The univariate analysis allowed us to describe and identify the type of sensilla by segment that exhibited differences between the triatomines. Sensilla that did not show significant differences among landscapes classes were discarded in subsequent analyses. As recommend in antennal phenotypic analysis of Triatominae bugs (May-Concha et al., 2016), we performed the univariate analysis at three levels: sensillum (Ba, Br, TH, TK), segment (P, FI, FII expressed as Total), and antenna (defined as TOTAL). All univariate analyses were performed by sex. At the sensillum level, the mean and standard deviation for each type of sensillum was calculated by each segment, and differences among landscape type were evaluated. The mean and standard deviation of the total abundance by segment were estimated at the segment level, and differences between landscape classes were calculated. Finally, we assessed differences in the total abundance (number) of sensilla considering all segments (antenna level). Significant differences were evaluated following generalized linear mixed models (GLMM), considering collection year as a random effect (period 2015–2019). GLMM was performed using a Poisson distribution model, and multiple comparisons were estimated using Tukey’s contrasts. The analysis was performed in R v3.5.0. “lmer4” and “multcomp” packages which were used to construct all GLMMs and multiple comparisons, respectively.
We employed the number of sensilla by segment (by each individual) to perform a principal component analysis (PCA) to explore patterns of variance among all individuals. We used the results of corresponding PC’s to reduce the number of independent variables in the multivariate analyses. We discarded the PC axes whose contribution to the cumulative variance was not significant. Then, we conducted a multivariate analysis of variance with a canonical variate analysis (CVA) using Wilk’s lambda test. CVA’s was performed with all specimens for the four landscape class groups. The scores of each canonical variate were used for the univariate ANOVA according to the Scheffé test to determine the landscape class discriminated by CVA axes. The correct classification and CVA performance were evaluated considering the posterior probabilities of assignation. The multivariate analysis was implemented in the “MASS” and “Candisc” package in R v3.5.0.
Feeding Morphology Analyses
We carried out an LMM to evaluate differences in the proboscis length among landscape classes. The model was constructed considering sex and body size as independent variables, and entering collection year (period 2014–2018) as a random effect. LMM was performed with 4,000 permutations in the RRPP package (Collyer and Adams, 2020) in R v3.5.0.
Morphological Disparity Analysis
The general concept of disparity refers to the relative quantity of variability scaled in a sample of measures. The disparity is measured from the quantitative description of the morphological variation and the empirical distribution of samples in some morpho-space (i.e., PCA, Foote, 1991). We considered that relative low values of disparities are equivalent to decreased variance in the phenotype. Our interest was to investigate whether the levels of morphological disparity in the four morphological traits were different between landscapes.
For proboscis length we calculated the coefficient of variation, which is the metric of variability in the univariate case (Van Valen, 2005). We evaluated differences in coefficient of variation with the “asymptotic test” function implemented in the “cvequality” package in R (Marwick and Krishnamoorthy, 2019). In the multivariate case, disparities in wing and thorax were evaluated using the Procrustes pairwise distances between specimens and the sample mean as metric of the morpho-space occupied by all specimens in a landscape class. We estimated the values of disparities of wing and thorax with the “morphological.disparities” function implemented in geomorph package in R. We used a permutation test (2,500 iterations) to assess statistical significance differences (Adams et al., 2020). Morphological disparities in the antennal phenotypes were estimated through principal components scores using the “dispRity” package in R (Guillerme, 2018). We used a bootstrapping procedure (2,500 iterations) to assess 95% confidence intervals and a t-test to evaluate significant differences between values of morphological disparity after Bonferroni correction.
Pattern of Similarities in Morphometric and Morphological Data
We calculated a Mahalanobis distance matrix for each morphometric and morphological data by sex. These distance matrices were employed to construct a pattern of hierarchical similarities among landscape classes that were visualized in a cluster analysis based on the UPGMA (Unweighted Pair Group Method using Arithmetic averages) algorithm performed in R v3.5.0. We compared the topology and correlation between clusters to detect patterns of similarity/concordance between dendrograms generated by each morphological and morphometric data. Due to the uneven number of samples between the morphometric and morphological data set, dendrogram comparisons were carried out with the dendextend package in R v.3.5.0 (Galili, 2015). We calculated the quality of the alignment among clusters (topology) with the “entanglement” function, where values range from 0 to 1 where a lower entanglement coefficient corresponds to a good alignment (values close to 0). Also, we calculated a correlation coefficient through de Goodman and Kruskal’s gamma index (γ). The values of this index vary from 0 (absence of agreement) to 1 (100% positive association or perfect agreement).
Results
Abundance, Fitness Proxy and Feeding Information
Eight hundred and seven bugs were collected during 2014–2019 (55% Msd, 18% Sv, and 15% U; Supplementary Table S2). Overall, 67% of the triatomines showed blood ingestion, of which 74% were females and 57% were males. Seventy nine percent and 70% of triatomines that were captured in Secondary vegetation (Sv) and forest (Msd) respectively were fed, while 65% of the urban bugs were fed. These frequencies were significantly different (Chi-squared = 18.938, df = 3, p-value = 0.00028; Table 2). A post hoc comparison showed further differences between forest (Msd) and Urban (U) (p = 0.018) and Secondary vegetation (Sv) and Urban landscape class (p = 0.015, Table 2). Only 18% of females were gravid (n = 84). Most gravid females were observed in 2015, 2016, and 2018. Two hundred and forty-two females were recorded in Msd, and 26% of them were gravid, while of the 100 females in Sv, 38% were gravid. In Homegardens (n = 52) and Urban (n = 79), only 21 and 19% of the females were gravid, respectively. The number of eggs per female between landscapes was barely significantly different (Kruskal–Wallis chi-squared = 8.4297, df = 3, p-value = 0.04; Supplementary Table S2), However, post hoc comparisons showed only one marginally significant difference between urban (U) and forest (Msd) landscape class (p = 0.049), with the former having fewer eggs.
Flight Morphology Variation
Thorax
The LMM detected significant differences in thorax size among landscape classes (Table 2). The same variation pattern was detected in both sexes. A statistically significant smaller thorax size was found in Urban (U) individuals, while conserved and rural landscapes had a significantly larger thorax size (Figure 3 and Table 3).
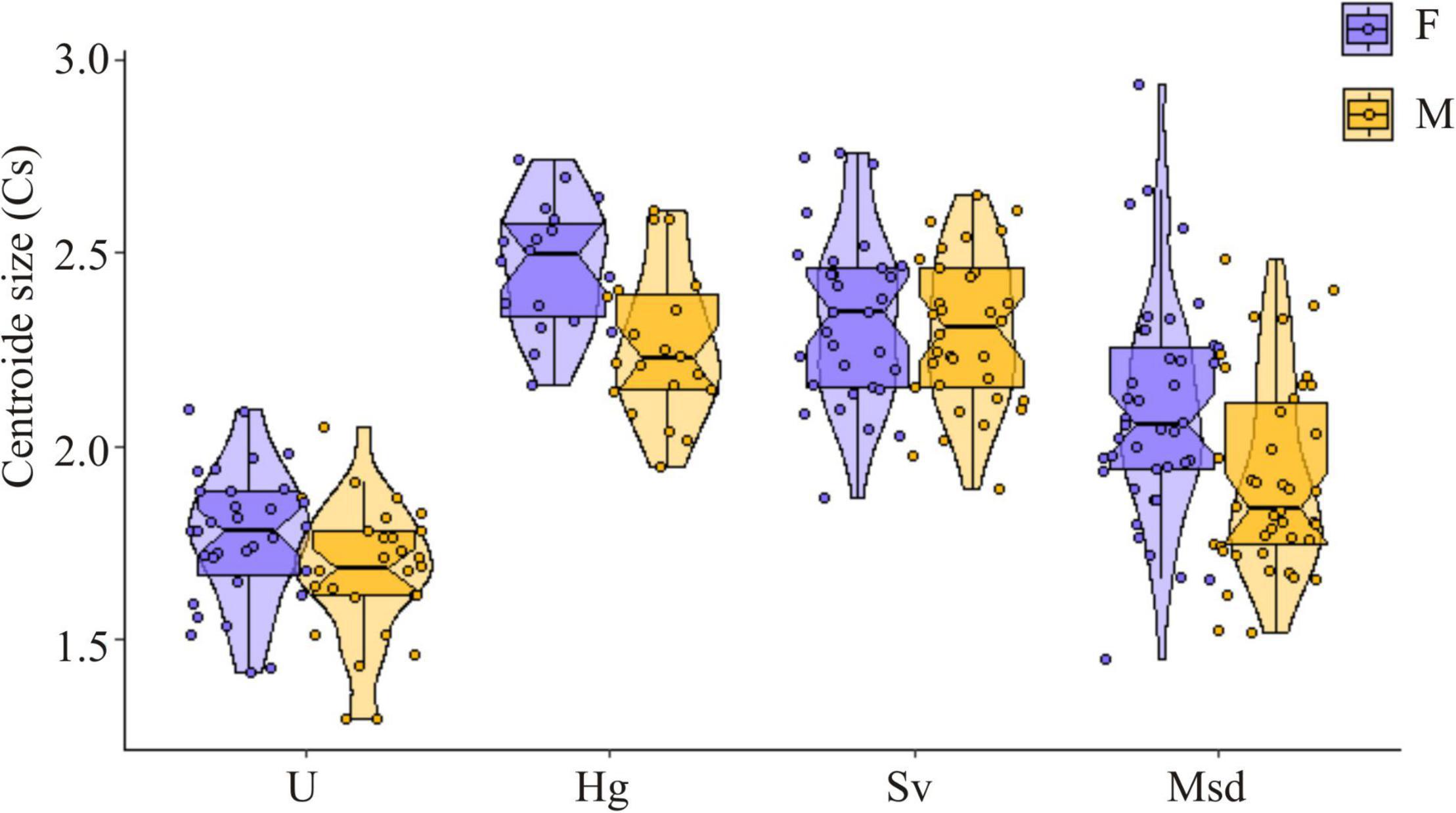
Figure 3. Thorax size variation among landscapes for T. dimidiata female (F) and male (M). Landscape: Urban (U), Homegarden (Hg), Median subdeciduous tropical forest (Msd), and Secondary vegetation (Sv).
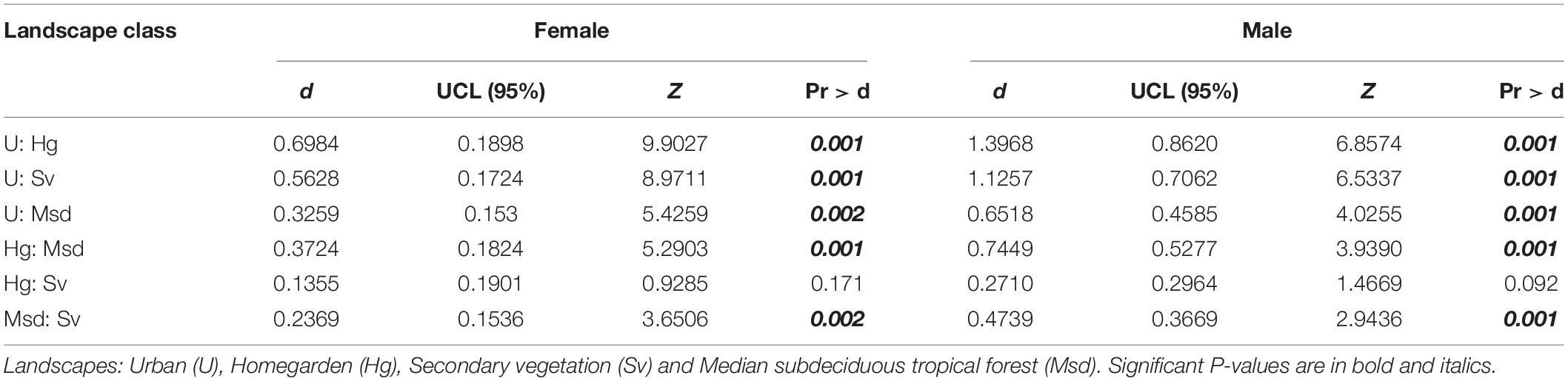
Table 3. Results of pairwise comparison of thorax size of T. dimidiata among landscapes Z and P are based on 2,500 random permutations.
A large thorax size was observed in individuals from Hg and Sv (Figure 3). For both sexes, the PCA based on thorax shape indicates a small proportion of variance explained by the first two components (females: PC1 35%, PC2 18%; males: PC1 38%, PC2: 15%) (Figures 4A,B). According to the LMM, no significant differences in thorax shape among landscapes were detected (Supplementary Tables S3, S4).
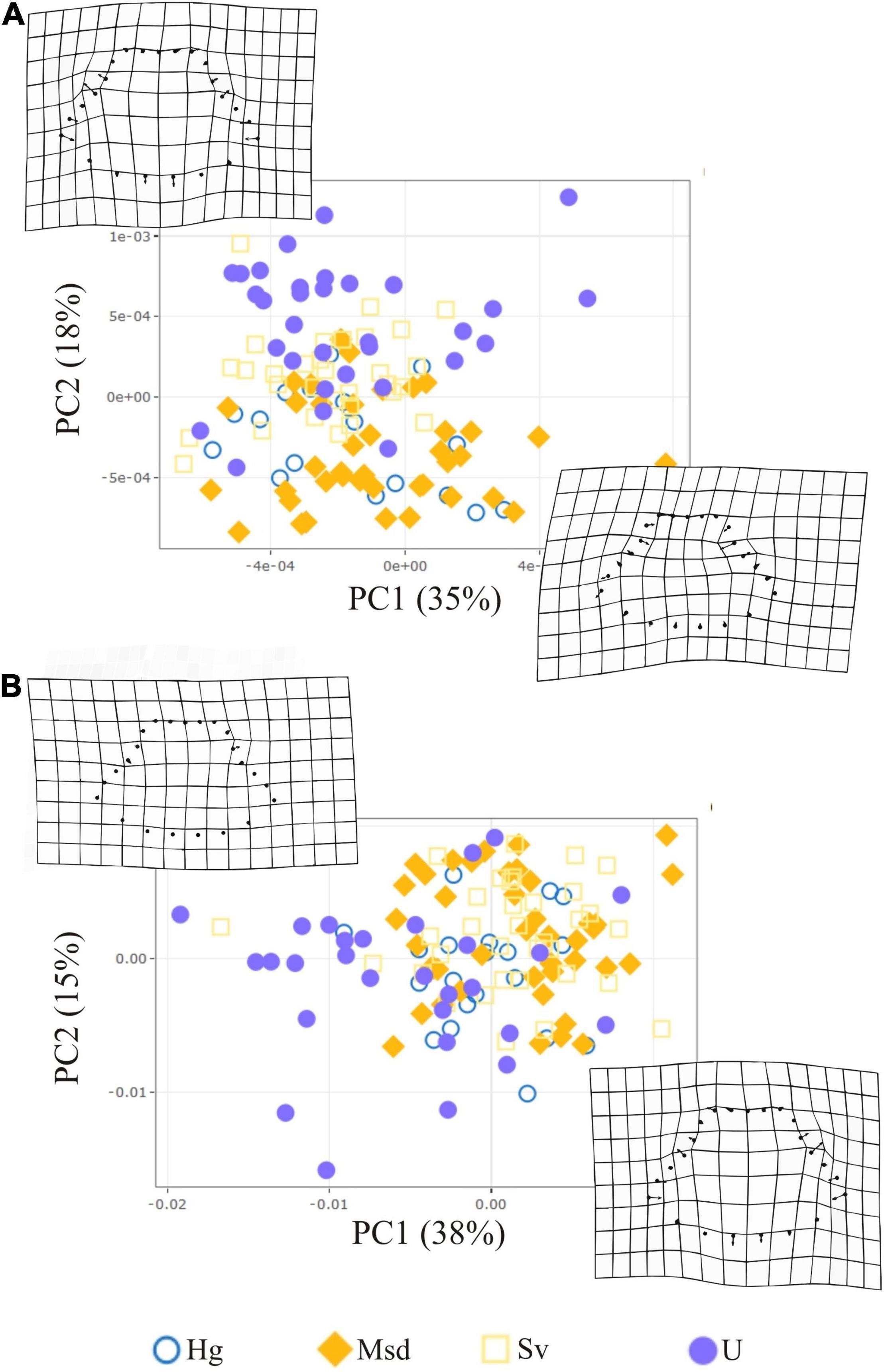
Figure 4. PCA of thorax shape variation for T. dimidiata females (A) and males (B). Landscape: Urban (U), Homegarden (Hg), Secondary vegetation (Sv), and Median subdeciduous tropical forest (Msd).
Wing
The LMM revealed no significant differences in wing size and WAR among sexes between landscapes (Table 2 and Supplementary Table S3). For males and females, the PCA based on wing shape indicated that the first two components explained a small proportion of variance (female, PC1 30%, PC214%; male, PC1 35%, PC2 15%) (Figures 5A,B). Also, the LMM did not detect significant differences in wing shape among landscapes (Supplementary Table S4).
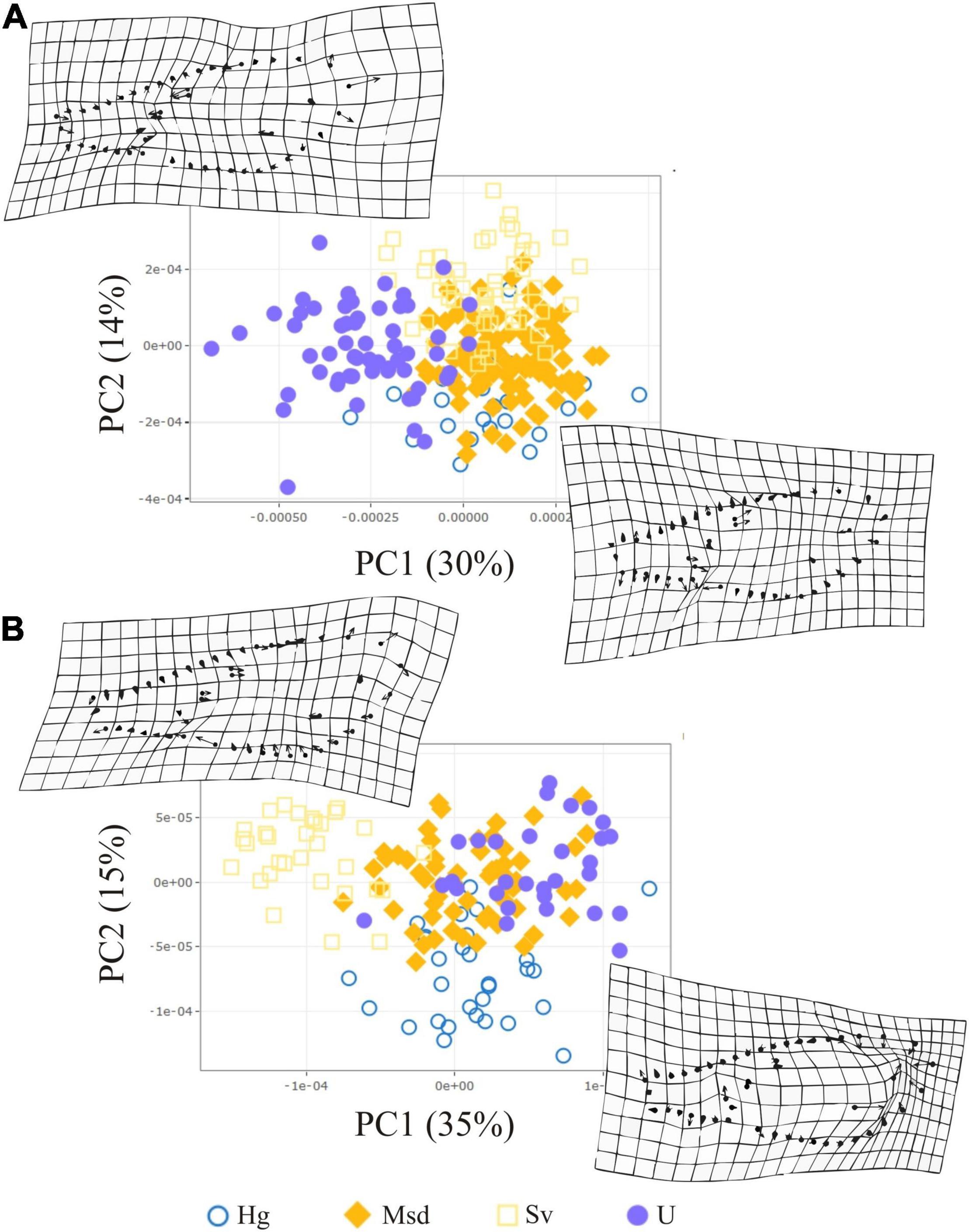
Figure 5. PCA of wing shape variation for T. dimidiata females (A) and males (B). Urban (U), Homegarden (Hg), Secondary vegetation (Sv), and Median subdeciduous tropical forest (Msd).
The PLS showed that wing and thorax morphology did not covariate (female, r = 0.359, P = 0.094; male, r = 0.302, P = 0.061).
Antennal Phenotype Variation
The univariate analysis rendered similar patterns in sensillum abundance in both sexes. Our results indicated significant differences between landscapes at the sensillum level, determined by the number of sensillum types TK and TH. Among landscapes, differences in TK and TH were observed in the three segments (Supplementary Figures S1, S2 and Supplementary Table S5). In all segments, differences in the number of sensilla type Ba among landscapes were identified only in males.
At the segment level, in both sexes, the number of sensilla from Pedicel and Flagellomere I (FI) were greater in Msd and Sv than in Urban landscape (Supplementary Figures S3, S4 and Supplementary Table S5). In Flagellomere II, differences in sensillum total abundance were only observed in males but not in females. Also, in FII, we found fewer sensory receptors for Hg and U, yet a high abundance for Msd and Sv (Supplementary Table S5).
The CVA detected significant differences in sensory phenotypes among landscapes in both sexes (Supplementary Table S6). For each sex, only one canonical ax allowed discrimination among landscapes (female, Wilks’ λ1 = 0.5157, χ2 = 2.5957, d.f = 21, p < 0.001; male, Wilks’ λ1 = 0.4422, χ2 = 2.2066, d.f = 24, P = 0.0019). In both sexes, the most different groups were the anthropized landscapes (U) and conserved ones (Msd) (Figures 6A–D). The proportion of correct assignment of specimens based on posterior probabilities obtained from the CVA scores supported the differences among U and Msd landscapes. In females, the main difference between the landscapes was observed in TH of the FII (0.088), while in males, it was observed in Ba of the FI (0.066) (Supplementary Tables S6, S7).
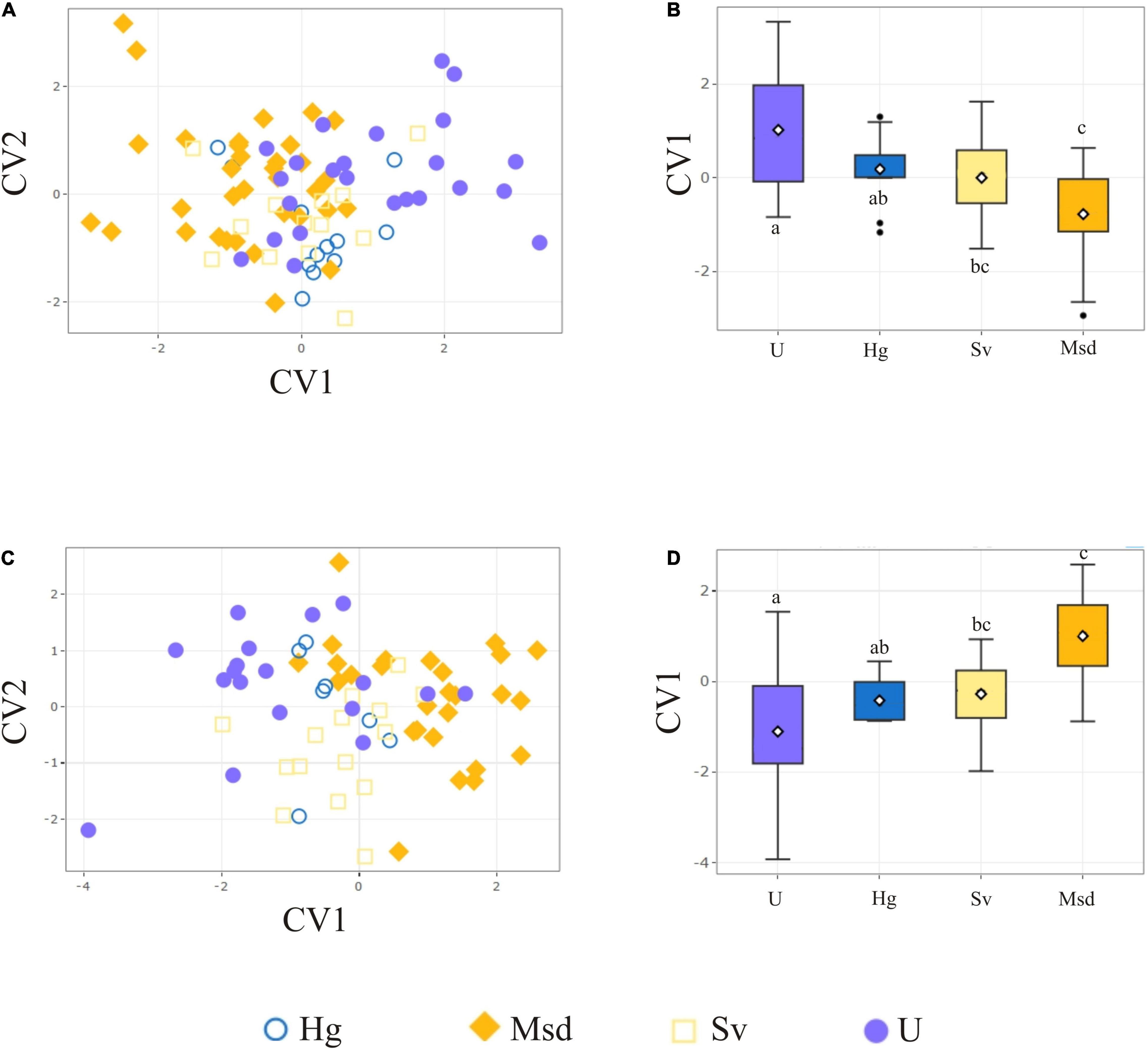
Figure 6. Antennal phenotype variation of T dimidiata among landscapes by sex (A,C). Distribution of individuals by landscape class along the first two canonical axes (B,D). Female (A,B) and Male (C,D). Groups with the same letter did not present significant difference (p < 0.05) according to the Scheffé test. Urban (U), Homegarden (Hg), Secondary vegetation (Sv), and Median subdeciduous tropical forest (Msd).
Feeding Morphology Variation
No significant differences were detected among landscape types for the length of the proboscis (F3,245 = 2521, P = 0.069, R2 = 0.027, Supplementary Table S8). Also, the results showed that the length of the proboscis was not influenced by sex or body size (Supplementary Table S8).
Morphological Disparities
The disparity values of the morpho-functional traits revealed differences between landscapes, but not all the disparity values follow the urban and non-urban gradient (Table 4). For example, for females the results showed that the Procrustes variance in thorax size and AP decreases in urban landscapes, while the disparity values of the thorax shape, wing shape and proboscis length decrease in Msd landacape. The highest values of disparity in AP and proboscis length were observed in Hg and Sv, which exhibit about three times greater variance than Urban or Msd (Table 4).
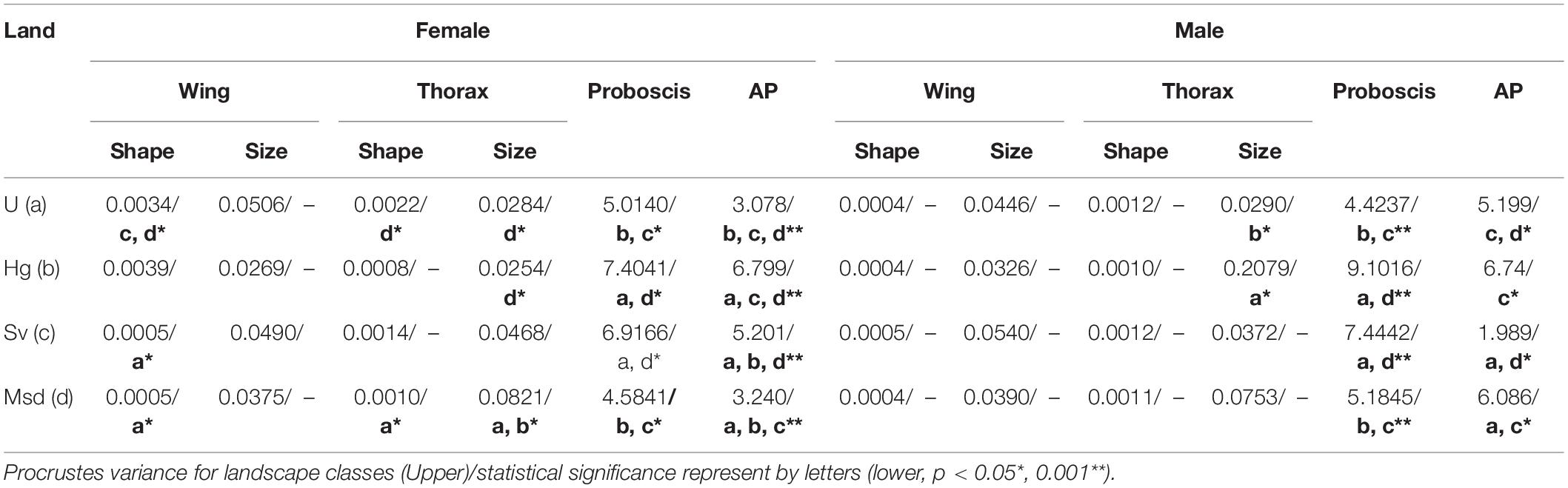
Table 4. Disparity values of four morpho-functional traits of T. dimidiata s. l. along a nature-urban gradient.
In males, the pairwise disparity comparisons of the wing morphology (shape and size) and thorax shape did not reveal significant differences among landscapes, while the Procrustes variance of thorax size and proboscis length was significantly lower in Urban and Msd classes, respectively. On the contrary, disparity values in AP showed significant differences between Urban and Msd, but the lower value was observed in Sv class (Table 4).
Patterns of Hierarchical Similarities
Thorax size and antennal phenotype similarities among landscapes were visualized in a cluster analysis based on the UPGMA algorithm. Thorax size and phenotype dendrograms showed entanglement values of 0.14 and 0.16 for females and males, respectively. For both sexes, the correlation coefficients for both dendrogram pairs were 0.85, suggesting high association and agreement. All dendrograms showed two groups, being the Urban the most distinct class. The second group is integrated by heterogeneous but conserved sites (Msd, Sv, and Hg) (Figure 7).
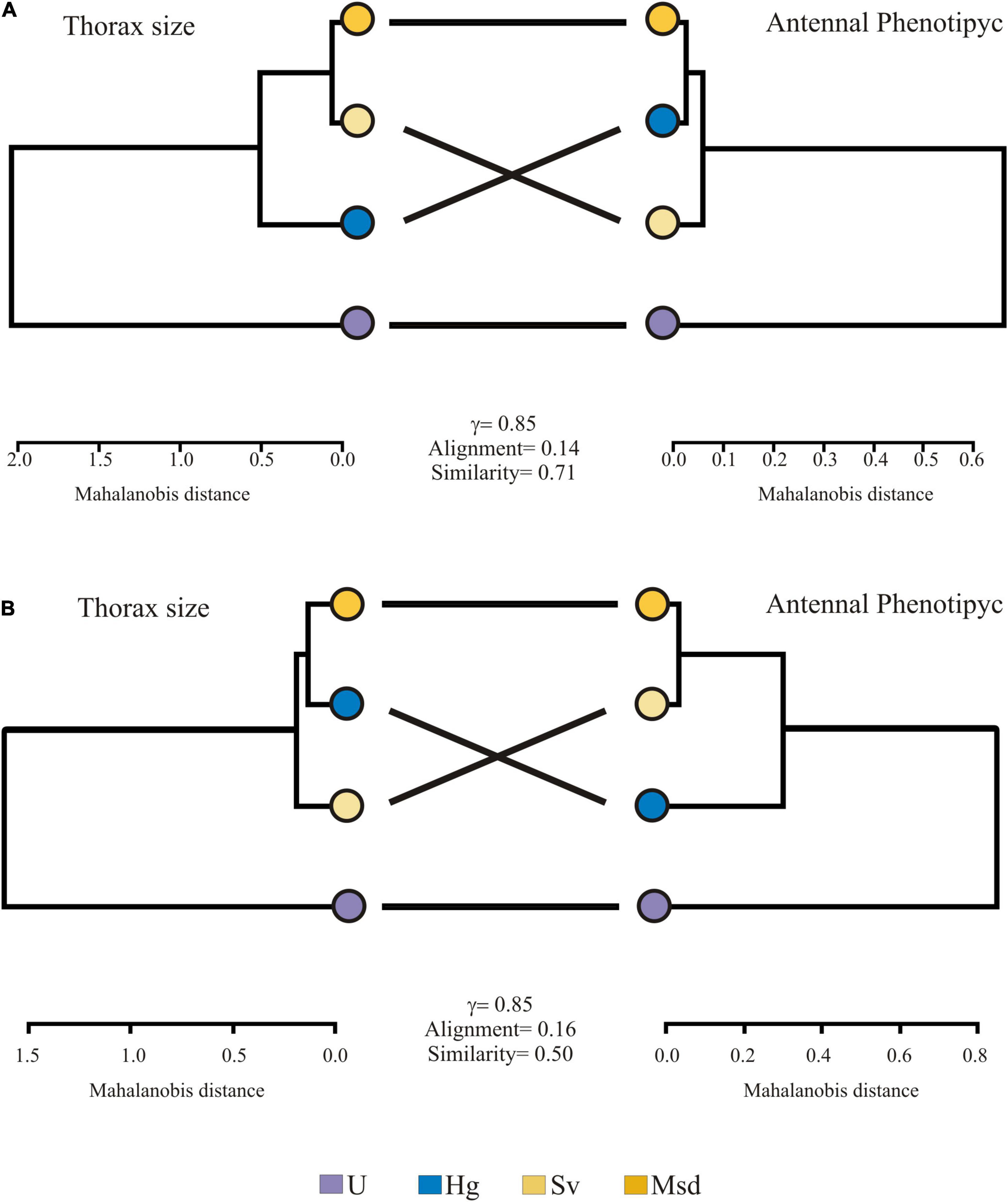
Figure 7. Patterns of hierarchical similarities in thorax size (left) and antennal phenotypic (right) by landscape for T. dimidiata (A) female and (B) male. Landscape: Urban (U), Homegarden (Hg), Secondary vegetation (Sv), Median subdeciduous tropical forest (Msd) Goodman-Kruskal-gamma index (γ); Alignment quality (Alignment).
Differences in all dendrograms were related to the position of Hg and Sv. For females, the thorax size of Msd was most similar to Sv, but not so when considering the antennal phenotype. On the contrary, for males, the thorax size in Msd was similar to Hg but with an antennal phenotype similar to Sv (Figure 7).
Discussion
Our general assessment of how morpho-functional traits of T. dimidiata have responded to a non-urban and urban gradient provides new insights of likely drivers underlying adaptive processes for triatomines. In what follows, we discuss our results in terms of how microevolution is presumably acting in our study species at the population level. First, there were similar fitness payoffs among landscapes. Although only urbanized animals showed some reduction in egg number, gravid females were similarly common in urbanized and non-urbanized areas. Second, our results suggest that changes in wing morphology are not associated with landscape modification (e.g., Urban, Rural) in contrast to thorax size. Third, thorax size and antennal phenotype reveal a morpho-functional convergence as both show a similar trend in phenotypic simplification. For both sexes, the phenotypic pattern follows a simplification in the non-urban and urban gradient. And fourth, convergence of thorax size and antennal phenotype suggests that T. dimidiata s. l. exhibits differences in flight/locomotion performance and dispersion as an adaptive response to host availability. We discuss these results at length below.
There was a decrease in thorax (pronotum) size and sensillum antennal density in urban individuals relative to their sylvatic and rural counterparts. Previous studies had documented intra and interspecific variation in flight capacity in triatomine bugs associated to a lack of development of flight muscle or thorax size (e.g., T. sherlocki) (Almeida et al., 2012; Hernández et al., 2015; Nattero et al., 2017). Given that flight initiation and dispersion in triatomine bugs is modulated by starvation or inanition (Lehane and Schofield, 1982; Lehane et al., 1992; McEwen and Lehane, 1993; Galvão et al., 2001), a large thoracic area would grant bugs an enhanced ability to fly and, thus, disperse to track diverse and scattered host burrows. This could explain why in sylvatic and regenerating landscapes (i.e., abandoned fields) and rural ecotopes, triatomines exhibit a large thoracic size and disparity values. However, it is also possible that the similarities in thorax size between Msd, Sv, and Hp are explained by the fact that the great majority of individuals within these categories (Sv and Hp) have a sylvatic origin, which coincides with the infestation/movement process documented in rural landscapes in this region (Dumonteil et al., 2002, 2007; Nouvellet et al., 2011). Our findings suggest that occupying stable landscapes (i.e., urban landscape class), where hosts do not have seasonal changes in abundance and are thus predictable throughout the year, would allow a reduction in triatomine investment in structures of reduced use.
The morpho-functional pattern documented in thorax size is similar to that of antennal sensilla, showing a clear morphological simplification in T. dimidiata s. l. in the urban landscape. The antennal phenotype of this species is characterized by a high diversity of chemoreceptor sensilla in the pedicel (Catalá et al., 2005; Arroyo et al., 2007; May-Concha et al., 2016, Supplementary Table S9), which coincides with the complexity of the niche it occupies throughout its geographical distribution (Ibarra-Cerdeña et al., 2014; Villalobos et al., 2019). Given that TK and TH chemoreceptors are sensitive markers of habitat influence in our study species (Catalá et al., 2005; Arroyo et al., 2007; May-Concha et al., 2016, 2018), our findings indicate that these odor-detecting traits may be responding to selection via a reduction in complexity in urbanized environments. Interestingly, abundance of TK flagellum in T. infestans (Catalá and Dujardin, 2001) and T. dimidiata (Catalá et al., 2005) was higher in urbanized areas in South America. Notwithstanding, we found that the highest abundance of TK sensilla was observed in individuals from Msd and Sv and not in stable landscapes as represented by U. Also, we found a complex relationship between antennal phenotype and sylvatic habitats (Msd and Sv), as evidenced by the density of TK and TH sensilla and the total abundance of sensilla per segment. In this regard, sensillum density in the pedicel and FI was higher in Msd and Sv than in Urban and Hg for both sexes. Additionally, unlike females, males showed a nature-urban gradient in the total abundance of sensilla in FII and significant differences in Ba abundance between landscapes. These sexual differences may be related to mate searching activities by males because Ba is related to the perception of sex-pheromone components (Bohman et al., 2018). A functional explanation for the decrease in the density of sensilla in urban environments is that a high density of such structures is not needed in stable landscapes as blood sources can be, as indicated above, less diverse and more predictable. This idea would be supported mainly by the sensillum abundance at the pedicel in urban triatomines as this trait has been described in domiciled species that either occupy stable habitats (e.g., T. infestans, Catalá, 1997; Catalá et al., 2005) or use humans and other domestic animals (canids, chickens, cats) as the main hosts (Ceballos et al., 2005; Rabinovich et al., 2011; Alvedro et al., 2021).
One remarkable aspect of our findings is that these are compatible with Schofield’s domiciliation hypothesis which proposes a pattern of phenotypic/genotypic simplification in triatomines from urbanized areas (Schofield et al., 1999; Flores-Ferrer et al., 2018). Although the methodological approach is different, our detected simplification pattern matches with that documented for Panstrongylus geniculatus in Caracas, Venezuela (Aldana et al., 2011). Because both sexes are strictly blood-sucking, host-feeding traits in urban landscapes will show convergence, which is the case of thorax size and antennal phenotype. Moreover, our results are also in agreement with those of other insects, where morpho-functional structures are smaller in urban individuals than their rural counterparts and that such phenotypic variation would be related to the respective foraging landscape (Eggenberger et al., 2019). The phenotypic simplification in T. dimidiata may imply a reduced ability during flight/locomotion and dispersion, related to a less diverse yet more predictable hosts in urban landscapes. This logic has a sound background: it has been shown that while bugs make use of a larger range of hosts in rural environments, bugs that infest and colonize urban dwellings make use of humans and domestic animals (Alvedro et al., 2021). In this sense, the phenotypic simplification in urban bugs, could be supported by the blood-feeding profiles so far documented in bugs from the city (Guzmán-Tapia et al., 2007), which contrasts with the full range of host types identified in sylvatic and rural individuals (López-Cancino et al., 2015; Dumonteil et al., 2018; Moo-Millan et al., 2019).
Opposite to our predictions, we did not find variation in proboscis length and wing morphology related to landscape, possibly showing that these structures are equally economic and functional among the different landscape classes. These results reflect the generalist and opportunistic nature that characterizes this species (López-Cancino et al., 2015; Dumonteil et al., 2018; Moo-Millan et al., 2019). On the contrary, specialization has been the main driver of the differentiation of proboscis length under a rural-urban gradient in other insects (Eggenberger et al., 2019). As for wing morphology, our findings agree with previous studies in the YP (Dumonteil et al., 2007; Nouvellet et al., 2011). Notice that wing shape is a genetically determined character (Birdsall et al., 2000; Zimmerman et al., 2000) so that the absence of differentiation documented in our study match with the low genetic structure detected (Dumonteil et al., 2007; Nouvellet et al., 2011) and the fact that there is mainly a single haplogroup (Hg1) circulating in Yucatan state (Pech-May et al., 2019). Our interpretation of the morphological stability between landscapes and the lack of functional integration with thorax is that the variation in wing morphology does not affect the flight performance and dispersion in T. dimidiata s. l. Actually, walking is another important dispersal mechanism in triatomines (Vazquez-Prokopec et al., 2004, 2005; Abrahan et al., 2011) and the muscle involved with such locomotion is also concentrated in the thorax (Davis and Holden, 2015; Hernández et al., 2015). The evidence suggests that through this dispersal mechanism (walking), triatomine bugs could disperse more actively, regardless of their nutritional status or even if they are not fed, which favors the invasion and colonization of new habitats (Lobbia et al., 2019). Thus, possibly a small thorax is related to a lower capacity for locomotion performance in urban bugs.
Nutritional status (as a body weight/body length ratio or feeding status) is associated with population structure and dispersal in triatomine bugs (Lehane and Schofield, 1982; Lehane et al., 1992). Although this study was carried out in different periods of time during certain months, the nutritional status would be an immediate indicator of the individual’s physiological state, while the morpho-functional variables quantified in this study would reflect the conditions (environmental, nutritional, etc.) that prevailed during development of nymphal stages (Hernández et al., 2011). The former would also explain the significant differences in the frequency of blood feeding documented in this work. This is, it is a momentary indicator of the physiological state and blood-source availability. Although the frequency of blood feeding between urban versus rural and sylvatic individuals were significantly different, our results show that bugs from all landscapes are fairly successful in gaining access to blood (higher than 65%). For example. this value is higher than that reported for peridomicile bugs of Rhodnius pallescens in Panama (less than 40%; Gottdenker et al., 2011), and shows a less contrast between sylvatic and urban/rural bugs (a difference of 14% in T. dimidiata in our study vs. 40% difference in Rhodnius pallescens).
Several aspects of the morpho-functional simplification in T. dimidiata in urban areas are unknown and need to be addressed in future studies. First, would this simplification generate a trade-off with fitness-related traits? For example, could feeding on an urban host generate nutritional stress that affects their development, reproduction, and/or survival? Related to this, developmental instability (Dujardin et al., 1999; Nattero et al., 2015a,b) and reduced size and survival (Gutiérrez-Cabrera et al., 2021) has been documented in triatomines that feed on specific hosts that occupy predictable habitats. However, we are not aware of any fitness-related difference when triatomines use urban vs. non-urban hosts. On the other hand, despite providing data on egg number as a fitness proxy, there is not any apparent benefit of occupying urban landscapes where the availability of resources would be stable. Future studies should include several fitness measures (e.g., number of eggs laid, hatching nymphs and/or survival) to unravel the costs/benefits of occupying urban niches for T. dimidiata. Second, because the intraspecific morphological variation in Triatominae does not necessarily have a linear relationship with the genetic structure (Dujardin et al., 1999, 2009), the microevolutionary potential that urban environments could exert on this species remains unknown. T. dimidiata is a species sub-complex integrated by at least four evolutionary lineages (Pech-May et al., 2019) with records in several cities of Mexico (Ramsey et al., 2015) and other Central and South America countries (Zeledon and Rabinovich, 1981; Zeledón et al., 2005; Dorn et al., 2007). In this sense, because the ecological pressures in cities would be similar (Santangelo et al., 2018), it is expected that urban traits will be highly canalized, independently of genetically distinct lineages, as has been documented in the south American triatomine, R. ecuadoriensis (Abad-Franch et al., 2021). Third, related to the wide distribution of T. dimidiata, the question remains as for whether the morphological changes are explained by phenotypic plasticity or are evolutionary responses to selection at the population level. One key feature of vectors that are expanding their distribution range is their phenotypic plasticity (Lefèvre et al., 2009). Future research should answer this for triatomines. This can be done with selection experiments whereby phenotypic plasticity is examined via fitness payoffs in different environments. And fourth, although we have provided a general discussion on how the phenotypic traits we measured are related to urbanization, a more intimate link may occur at the level of microhabitat structure. For example, the antennal traits can be used to detect places with particular humidity and temperature ranges where bugs can hide (e.g., Triatoma infestans Vazquez-Prokopec et al., 2002). Thus, one further step would be to link such traits with microhabitat structure.
Ample evidence has shown that the biotic and abiotic characteristics in urbanized environments are responsible for the nearly 1600 phenotypic changes that have been documented in plant and animal species worldwide (Alberti et al., 2017). Cities are fragmented landscapes with a high degree of human disturbance, characterized by pollution, noise, light intensity, where the habitat structure is shaped by artificial buildings that tend to increase the temperature (urban heat island effect) and thus affect important biophysical and physiological processes (Liker, 2020). The availability of food and nutrients in the urban landscape is also another factor that influences inter/intraspecific competition, predation and diversity (Biard et al., 2017; Liker, 2020). All of these ecological conditions can generate strong selection that leads to the evolution of specific urban phenotypes. In this regard, while we know that the triatomine phenotype is modulated by ecological factors in the sylvatic-rural gradient within a context of an anthropogenic drive (domiciliation), the role of cities as new adaptive filters has been little investigated. While in many cases, urban evolution is explained by non-adaptive processes (i.e., genetic drift), there is evidence that suggests that genetic diversity and gene-flow among populations of T. dimidiata are not associated to anthropic gradients in Yucatan state (Dumonteil et al., 2002; Pech-May et al., 2019). This gives support to the hypothesis that differentiation in thorax size, density of sensilla, and their morphological convergence, cannot be explained by genetic drift. The feeding characteristics of T. dimidiata (i.e., opportunistic and host generalist status), and assisted movement of bugs by rural human migration to Mérida (Chi-Méndez, 2016) could help sylvatic insects to move into the urban landscape, thereby increasing genetic diversity and reducing the genetic structuration (e.g., “Urban facilitation models,” Miles et al., 2019). Since infestation and transmission of CD in urban settings has increased elsewhere (Vallvé et al., 1996; Levy et al., 2006; Medrano-Mercado et al., 2008; Carvalho et al., 2014; Parente et al., 2017; Alvedro et al., 2021), there is a potential signal for urban evolution in triatomines, due to the presence of a suitable niche where susceptible hosts are abundant and not intermittent (Bradley and Altizer, 2007). What factors could be driving such niche search? Food resource availability in cities appear to be a leading factor driving phenotypic adaptation in several species (Serruys and Van Dyck, 2014; Alberti et al., 2017; Biard et al., 2017; Eggenberger et al., 2019; Liker, 2020) including, possibly, our study species. We do not rule out that other evolutionary mechanisms (e.g., orthogenesis) or phenomena (e.g., epigenetics/pleiotropy) could be related to morphological simplification in urban individuals of T. dimidiata. However, our findings should foster research linking genetic bases of this morpho-functional structuring and possible fitness benefits in the urban landscape. Also key is to answer if the availability of food resources (as host diversity, nutritional stress, etc.) is one driver underlying these covariations and phenotypic structuring (Donihue and Lambert, 2015; Santangelo et al., 2018; Lambert et al., 2020).
From an epidemiological point of view, the morpho-functional simplification of T. dimidiata in the natural-urban gradient could suggest a “domiciliation” event which implies that this species maintains resident urban populations. This actually challenges the view that T. dimidiata occurrence is restricted to the warmer months (dry season: March to June) uniformly for the Yucatan peninsula (Dumonteil et al., 2007; Waleckx et al., 2015). Thus, a more realistic estimation of Chagas disease risk should take into consideration the temporal patterns of abundance in the appropriate landscape (Ribeiro et al., 2021). In the region, the potential of T. dimidiata to establish colonies has not yet been clearly determined (Ibarra-Cerdeña et al., 2020). For instance, some studies have shown that T. dimidiata persists in the city throughout the year (albeit with low densities) with infection rates even higher than those documented in rural communities (48%, Guzmán-Tapia et al., 2007; 90%, Ibarra-Cerdeña et al., in preparation) where presence of abandoned backyards are associated with the house infestation by providing temporary shelter, where synanthropic and domestic animals are available as a source of blood (Guzmán-Tapia et al., 2007). This could be the result of two non-exclusive processes, the urban expansion, and the passive transport of bugs due to rural human migration from the countryside to the city (Chi-Méndez, 2016). Since urban expansion and interstate migration in this region continues to increase (Goujon et al., 2000; Colditz et al., 2017), it is likely that bug infestation will also augment. In any case, our approach can be used to track the process of bug domiciliation.
Conclusion
Our study reveals site-specific, simplified morpho-functional architecture that renders similar fitness pay offs in urban environments for an insect disease vector. We admit that selective forces, other than urbanization, may be acting and thus explaining our results. However, urbanization properties are so pervasive that it is hard to think about such other forces. It is necessary to promote and increase research on the “evolvability” of other morpho-functional and “plastic” traits of T. dimidiata in urban landscapes. Given a functional specialization in urban areas, there are implications for triatomine control. One example would be that urban populations of this species perceive different wavelengths than those that inhabit rural environments. Related to this, it has been documented an intense attraction of triatomines to incandescent light whose use is typical of many Mexican and Latin American villages (Pacheco-Tucuch et al., 2012). One may learn whether light sensitivity to such light sources is part of the novel adaptive repertoire for urbanized triatomines and, if it is the case, how we can use such information for bug attraction and control.
Data Availability Statement
The raw data supporting the conclusions of this article will be made available by the authors, without undue reservation.
Author Contributions
CI-C and ACMO-A contributed to conceptualization, methodology, and wrote original draft. RC-G, AG-M, PI-L, and SS-Á performed the methodology and data collection. ACMO-A performed the analysis. CI-C, ACMO-A, and AC-A reviewed and edited the manuscript. All authors approved the final manuscript for submission.
Funding
CI-C received financial support from Sep-Cinvestav (Project: FIDSC2018/160) and from Secretaría de Educación Pública-Programa para el Desarrollo Profesional Docente (Project: CA27). ACMO-A thanks Conacyt for a postdoctoral fellowship (414043). RC-G and PI-L thank Conacyt for scholarships to conduct graduate studies at the Human Ecology Graduate Program of Cinvestav.
Conflict of Interest
The authors declare that the research was conducted in the absence of any commercial or financial relationships that could be construed as a potential conflict of interest.
Publisher’s Note
All claims expressed in this article are solely those of the authors and do not necessarily represent those of their affiliated organizations, or those of the publisher, the editors and the reviewers. Any product that may be evaluated in this article, or claim that may be made by its manufacturer, is not guaranteed or endorsed by the publisher.
Acknowledgments
We appreciate the support of Miguel Munguía-Rosas and Daniela Martínez for allowing us using the Ecología Terrestre Lab at Cinvestav Mérida. We are grateful to James Callaghan and staff from the Reserva Biocultural Kaxil Kiuic and inhabitants of the rural towns and Mérida for their help in bug collection. We also thank Irving May-Concha for suggestions to characterize the antennal phenotype.
Supplementary Material
The Supplementary Material for this article can be found online at: https://www.frontiersin.org/articles/10.3389/fevo.2022.805040/full#supplementary-material
Footnote
References
Abad-Franch, F., Monteiro, F. A., Pavan, M. G., Patterson, J. S., Bargues, M. D., Zuriaga, M. A., et al. (2021). Under pressure: phenotypic divergence and convergence associated with microhabitat adaptations in Triatominae. Parasit Vectors 14:195. doi: 10.1186/s13071-021-04647-z
Abrahan, L. B., Gorla, D. E., and Catalá, S. S. (2011). Dispersal of Triatoma infestans and other Triatominae species in the arid Chaco of Argentina: flying, walking or passive carriage? the importance of walking females. Mem. Inst. Oswaldo Cruz 106, 232–239. doi: 10.1590/S0074-02762011000200019
Adams, D., Collyer, M., and Kaliontzopoulou, A. (2020). Geomorph: Software for Geometric Morphometric Analyses. R package version 3.2.1. Available online at: https://cran.r-project.org/package=geomorph. (Accessed 20, 2020)
Alberti, M., Correa, C., Marzluff, J. M., Hendry, A. P., Palkovacs, E. P., Gotanda, K. M., et al. (2017). Global urban signatures of phenotypic change in animal and plant populations. Proc. Natl. Acad. Sci. U S A. 114, 8951–8956. doi: 10.1073/pnas.1606034114
Aldana, E., Heredia-Coronado, E., Avendaño-Rangel, F., Lizano, E., Concepción, J. L., Bonfante-Cabarcas, R., et al. (2011). Análisis morfométrico de Panstrongylus geniculatus de Caracas. Venezuela. Biomédica 31, 108–117. doi: 10.7705/biomedica.v31i1.341
Allen, T., Murray, K. A., Zambrana-Torrelio, C., Morse, S. S., Rondinini, C., Di Marco, M., et al. (2017). Global hotspots and correlates of emerging zoonotic diseases. Nat. Commun. 8:1124. doi: 10.1038/s41467-017-00923-928
Almeida, C. E., Oliveira, H. L., Correia, N., Dornak, L. L., Gumiel, M., Neiva, V. L., et al. (2012). Dispersion capacity of Triatoma sherlocki, Triatoma juazeirensis and laboratory-bred hybrids. Acta Trop. 122, 71–79. doi: 10.1016/j.actatropica.2011.12.001
Alvedro, A., Gaspe, M. S., Milbourn, H., Macchiaverna, N. P., Laiño, M. A., Enriquez, G. F., et al. (2021). Trypanosoma cruzi infection in Triatoma infestans and high levels of human-vector contact across a rural-to-urban gradient in the Argentine Chaco. Parasites Vectors 14, 1–13. doi: 10.1186/s13071-020-04534-z
Arroyo, C. M., Esteban, L., Catalá, S. S., and Angulo, V. M. (2007). Variación del fenotipo antenal de poblaciones del domicilio, peridomicilio y silvestres de Triatoma dimidiata (Hemiptera: Reduviidae) en Santander. Colombia. Biomédica 27, 92–100.
Beaty, B. J., Black, W. C., Eisen, L., Flores, A. E., García-Rejón, J. E., Loroño-Pino, M., et al. (2016). “The intensifying storm: domestication of Aedes aegypti, urbanization of arboviruses, and emerging insecticide resistance,” in Proceedings of the Global Health Impacts of Vector-Borne Diseases: Workshop Summary, (Washington, D. C: National Academies Press).
Biard, C., Brischoux, F., Meillère, A., Michaud, B., Nivière, M., Ruault, S., et al. (2017). Growing in cities: an urban penalty for wild birds? a study of phenotypic differences between urban and rural great tit chicks (Parus major). Front. Ecol. Evol. 5:79. doi: 10.3389/fevo.2017.00079
Biles, J. J., and Lemberg, D. S. (2020). A multi-scale analysis of urban warming in residential areas of a Latin American City: the case of mérida, Mexico. J. Plan Educ. Res. doi: 10.1177/0739456X20923002 [Epub ahead of print].
Birdsall, K., Zimmerman, E., Teeter, K., and Gibson, G. (2000). Genetic variation for the positioning of wing veins in Drosophila melanogaster. Evol. Dev. 2, 16–24. doi: 10.1046/j.1525-142x.2000.00034.x
Bohman, B., Weinstein, A. M., Unelius, C. R., and Lorenzo, M. G. (2018). Attraction of Rhodnius prolixus males to a synthetic female-pheromone blend. Parasit Vectors 11:418. doi: 10.1186/s13071-018-2997-z
Bookstein, F. L. (1991). Morphometric Tools for Landmark Data: Geometry and Biology. New York, NY: Cambridge University Press.
Borges, ÉC., Dujardin, J. P., Schofield, C. J., Romanha, A. J., and Diotaiuti, L. (2005). Dynamics between sylvatic, peridomestic and domestic populations of Triatoma brasiliensis (Hemiptera: Reduviidae) in Ceará State, Northeastern Brazil. Acta Trop. 93, 119–126. doi: 10.1016/j.actatropica.2004.10.002
Bradley, C. A., and Altizer, S. (2007). Urbanization and the ecology of wildlife diseases. Trends Ecol. Evol. 22, 95–102. doi: 10.1016/j.tree.2006.11.001
Briceño-León, R. (2009). La enfermedad de Chagas en las Américas: una perspectiva de ecosalud. Cad Saúde Pública 25, (Suppl. 1), 71–82. doi: 10.1590/S0102-311X2009001300007
Bustamante, D. M., Monroy, C., Menes, M., Rodas, A., Salazar-Schettino, P. M., Rojas, G., et al. (2004). Metric variation among geographic populations of the Chagas vector Triatoma dimidiata (Hemiptera: Reduviidae: Triatominae) and related species. J. Med. Entomol. 41, 296–301. doi: 10.1603/0022-2585-41.3.296
Callaghan, J., and Pasos, R. (2010). “Reserva biocultural kaxil kiuic,” in Biodiversidad y Desarrollo Humano en Yucatán, eds R. Durán and M. Méndez (Mexico: Cicy, Ppdfmam, Conabio, Seduma).
Carbajal de la Fuente, A. L., and Catalá, S. (2002). Relationship between antennal sensilla pattern and habitat in six species of Triatominae. Mem. Inst. Oswaldo Cruz 97, 1121–1125. doi: 10.1590/S0074-02762002000800010
Carvalho, D. B., Almeida, C. E., Rocha, C. S., Gardim, S., Mendonca, V. J., Ribeiro, A. R., et al. (2014). A novel association between Rhodnius neglectus and the Livistona australis palm tree in an urban center foreshadowing the risk of Chagas disease transmission by vectorial invasions in Monte Alto City. São Paulo. Brazil Acta Trop. 130, 35–38. doi: 10.1016/j.actatropica.2013.10.009
Catalá, S. S. (1997). Antennal sensilla of Triatominae (Hemiptera, Reduviidae): a comparative study of five genera. Int. J. Insect Morphol. Embryol. 26, 67–73. doi: 10.1016/s0020-7322(97)00014-7
Catalá, S., and Dujardin, J. P. (2001). Antennal sensilla patterns indicate geographic and ecotopic variability among Triatoma infestans (Hemiptera: Reduviidae) populations. J. Med. Entomol. 38, 423–428. doi: 10.1093/jmedent/38.3.423
Catalá, S., and Schofield, C. (1994). Antennal sensilla of Rhodnius. J. Morphol. 219, 193–203. doi: 10.1002/jmor.1052190208
Catalá, S., Sachetto, C., Moreno, M., Rosales, R., Salazar-Schettino, P. M., Gorla, D., et al. (2005). Antennal phenotype of Triatoma dimidiata populations and its relationship with species of Phyllosoma and Protracta complexes. J. Med. Entomol. 42, 719–725. doi: 10.1093/jmedent/42.5.719
Cavada, N., Worsøe-Havmøller, R., Scharff, N., and Rovero, F. (2019). A landscape-scale assessment of tropical mammals reveals the effects of habitat and anthropogenic disturbance on community occupancy. PLoS One 14:e0215682. doi: 10.1371/journal.pone.0215682
Ceballos, L. A., Vazquez-Prokopec, G. M., Cecere, M. C., and Gürtler, R. E. (2005). Feeding rates, nutritional status and flight dispersal potential of peridomestic populations of Triatoma infestans in rural north-western Argentina. Acta Trop. 95, 149–159. doi: 10.1016/j.actatropica.2005.05.010
Chi-Méndez, C. G. (2016). Efectos Potenciales de la Migración Rural y el Ambiente Construido Sobre la Infestación y Colonización de Vectores de la Enfermedad de Chagas en Mérida, Yucatán. Tesis de Doctorado, Mexico: Centro de Investigaciones y de Estudios Avanzados del Instituto Politécnico Nacional.
Colditz, R. R., López, M. I. C., Martínez, A. G. A., Rosas, J. M. D., and Ressl, R. A. (2017). “Urbanization in Latin America with a particular emphasis on Mexico,” in Urban Expansion, Land Cover and Soil Ecosystem Services, ed. C. Gardi (Milton Park: Routledge), 238–257. doi: 10.4324/9781315715674-15
Collyer, M. L., and Adams, D. C. (2020). RRPP: Linear Model Evaluation with Randomized Residuals in a Permutation Procedure. R package version 052 Available online at: https://github.com/mlcollyer/RRPP (accessed July 13, 2020).
Davis, A. K., and Holden, M. T. (2015). Measuring intraspecific variation in flight-related morphology of monarch butterflies (Danaus plexippus): which sex has the best flying Gear? J. Insects 2015:591705. doi: 10.1155/2015/591705
Donihue, C. M., and Lambert, M. R. (2015). Adaptive evolution in urban ecosystems. ambio 44, 194–203. doi: 10.1007/s13280-014-0547-542
Dorn, P. L., Monroy, C., and Curtis, A. (2007). Triatoma dimidiata (Latreille, 1811): a review of its diversity across its geographic range and the relationship among populations. Infect. Genet. Evol. 7, 343–352. doi: 10.1016/j.meegid.2006.10.001
Dujardin, J. P., Beard, C. B., and Ryckman, R. (2007). The relevance of wing geometry in entomological surveillance of Triatominae, vectors of Chagas disease. Infect. Genet. Evol. 7, 161–167. doi: 10.1016/j.meegid.2006.07.005
Dujardin, J. P., Costa, J., Bustamante, D., Jaramillo, N., and Catalá, S. (2009). Deciphering morphology in Triatominae: the evolutionary signals. Acta Trop. 110, 101–111. doi: 10.1016/j.actatropica.2008.09.026
Dujardin, J. P., Panzera, P., and Schofield, C. J. (1999). Triatominae as a model of morphological plasticity under ecological pressure. Mem. Inst. Oswaldo Cruz 94, 223–228. doi: 10.1590/S0074-02761999000700036
Dumonteil, E., Gourbière, S., Barrera-Pérez, M., Rodriguez-Félix, E., Ruiz-Piña, H., Baños-Lopez, O., et al. (2002). Geographic distribution of Triatoma dimidiata and transmission dynamics of Trypanosoma cruzi in the Yucatan peninsula of Mexico. Am. J. Trop. Med. Hyg. 67, 176–183. doi: 10.4269/ajtmh.2002.67.176
Dumonteil, E., Ramirez-Sierra, M. J., Pérez-Carrillo, S., Teh-Poot, C., Herrera, C., Gourbière, S., et al. (2018). Detailed ecological associations of triatomines revealed by metabarcoding and next-generation sequencing: implications for triatomine behavior and Trypanosoma cruzi transmission cycles. Sci. Rep. 8:4140. doi: 10.1038/s41598-018-22455-x
Dumonteil, E., Tripet, F., Ramirez-Sierra, M. J., Payet, V., Lanzaro, G., and Menu, F. (2007). Assessment of Triatoma dimidiata dispersal in the Yucatan Peninsula of Mexico by morphometry and microsatellite markers. Am. J. Trop. Med. Hyg. 76, 930–937.
Dupuy, J. M., Hernández-Stefanoni, J. L., Hernández-Juárez, R. A., Tetetla-Rangel, E., López-Martínez, J. O., Leyequién-Abarca, E., et al. (2012). Patterns and correlates of tropical dry forest structure and composition in a highly replicated chronosequence in Yucatan, Mexico. Biotropica 44, 151–162. doi: 10.1111/j.1744-7429.2011.00783.x
Eggenberger, H., Frey, D., and Pellissier, L. (2019). Urban bumblebees are smaller and more phenotypically diverse than their rural counterparts. J. Animal Ecol. 88, 1522–1533. doi: 10.1111/1365-2656.13051
Ellis, E. A., Gómez, U. H., and Romero-Montero, J. A. (2017). Los procesos y causas del cambio en la cobertura forestal de la Península Yucatán. México. Rev. Ecos. 26, 101–111. doi: 10.7818/ECOS.2017.26-1.16
Essens, T., and Hernández-Stefanoni, J. L. (2013). Mapping Lepidoptera and plant alpha-diversity across a heterogeneous tropical dry forest using field and remotely sensed data with spatial interpolation. J. Insect Conserv. 17, 725–773. doi: 10.1007/s10841-013-9556-x
Flores-Ferrer, A., Marcou, O., Waleckx, E., Dumonteil, E., and Gourbière, S. (2018). Evolutionary ecology of Chagas disease; what do we know and what do we need? Evol. Appl. 11, 470–487. doi: 10.1111/eva.12582
Foote, M. (1991). “Analysis of morphological data,” in Analytical Paleobiology. Short Courses in Paleontology, eds N. L. Gilinsky and Signor, P. W. (Knoxville, TN: Paleontological Society), 59–86.
Fouet, C., Atkinson, P., and Kamdem, C. (2018). Human interventions: driving forces of mosquito evolution. Trends Parasitol. 34, 127–139. doi: 10.1016/j.pt.2017.10.012
French, J. (2008). SpatialTools: Tools for Spatial Data Analysis. Available online at: https://r-forge.r-project.org/R/?group_id=1492 (accessed October 28, 2018).
Galili, T. (2015). endextend: an R package for visualizing, adjusting, and comparing trees of hierarchical clustering. Bioinformatics 31, 3718–3720. doi: 10.1093/bioinformatics/btv428
Galvão, C., Rocha, D. D. S., Jurberg, J., and Carcavallo, R. (2001). Início da atividade de vôo em Triatoma infestans (Klug, 1834) e T melanosoma Martínez, Olmedo & Carcavallo, 1987 (Hemiptera, Reduviidae). Mem. Inst. Oswaldo Cruz 96, 137–140. doi: 10.1590/s0074-02762001000100017
García-Gil, G., Oliva-Peña, Y., and Ortiz-Pech, R. (2010). Distribution especial de la marginación urbana en la Ciudad de Mérida, Yucatán, México. Bol. Inst. Geo. 77, 89–106.
Gaspe, M. S., del Pilar, Fernández, M., Cardinal, M. V., Enriquez, G. F., Rodríguez-Planes, L. I., et al. (2020). Urbanisation, risk stratification and house infestation with a major vector of Chagas disease in an endemic municipality of the Argentine Chaco. Parasit Vectors 13:316. doi: 10.1186/s13071-020-04182-4183
Gaspe, M. S., Schachter-Broide, J., Gurevitz, J. M., Kitron, U., Gürtler, R. E., and Dujardin, J. P. (2012). Microgeographic spatial structuring of Triatoma infestans (Hemiptera: Reduviidae) populations using wing geometric morphometry in the Argentine Chaco. J. Med. Entomol. 49, 504–514. doi: 10.1603/ME11176
González-Martínez, A. (2018). Prevalencia de la Enfermedad de Chagas Asociada al Género y su Ámbito de Ocupación, en el Estado de Yucatán. Unpublished Doctoral Dissertation, México: Universidad Autónoma de Nuevo León.
Gottdenker, N. L., Calzada, J. E., Saldaña, A., and Carroll, C. R. (2011). Association of anthropogenic land use change and increased abundance of the Chagas disease vector Rhodnius pallescens in a rural landscape of Panama. Am. J. Trop. Med. Hyg. 84, 70–77. doi: 10.4269/ajtmh.2011.10-0041
Goujon, A., Kohler, I., and Lutz, W. (2000). Future population and education trends: scenarios to 2030 by socioecological region. Res. Rep. Int. Inst. Appl. Systems Anal. 14, 141–172.
Guillerme, T. (2018). dispRity: a modular R package for measuring disparity. Methods Ecol. Evol. 9, 1755–1763. doi: 10.1111/2041-210X.13022
Gutiérrez-Cabrera, A. E., Bello-Bedoy, R., Patiño-Uriostegui, N. M., Lecona-Valera, A. N., and Córdoba-Aguilar, A. (2021). Effects of food source and feeding frequency on Chagasic bug (Triatoma pallidipennis) fitness. Entomol. Gen. 43, 143–150. doi: 10.1127/entomologia/2021/1169
Guzmán-Tapia, Y., Ramírez-Sierra, M. J., and Dumonteil, E. (2007). Urban infestation by Triatoma dimidiata in the city of Mérida, Yucatan, Mexico. J. Vector Borne Dis. 7, 597–606. doi: 10.1089/vbz.2007.0133
Hernández, M. L., Abrahan, L. B., Dujardin, J. P., Gorla, D. E., and Catalá, S. (2011). Phenotypic variability and population structure of peridomestic Triatoma infestans in rural areas of the arid Chaco (Western Argentina): spatial influence of macro-and microhabitats. J. Vector Borne Dis. 11, 503–513. doi: 10.1089/vbz.2009.0253
Hernández, M. L., Dujardin, J. P., Gorla, D. E., and Catalá, S. S. (2015). Can body traits, other than wings, reflect the flight ability of Triatominae bugs? Rev. Soc. Bras Med. Trop. 48, 682–691. doi: 10.1590/0037-8682-0249-2015
Hernández-Stefanoni, J. L., Dupuy, J. M., Johnson, K. D., Birdsey, R., Dzul, F. T., Peduzzi, A., et al. (2014). Improving species diversity and biomass estimates of tropical dry forest using airborne LiDAR. Remote Sensing 6, 4741–4763. doi: 10.3390/rs6064741
Ibarra-Cerdeña, C. N. González-Martínez, A. Valdez-Tah, A. R. Chi-Méndez, C. G. Castillo-Burguete, M. T., and Ramsey, J. M. (2020). “Tackling exposure to Chagas disease in the Yucatan from a human ecology perspective,” in Culture, Environment and Health in the Yucatan Peninsula, eds H. Azcorra and Dickinson, F. (Cham: Springer). doi: 10.1007/978-3-030-27001-8_16
Ibarra-Cerdeña, C. N., Zaldívar-Riverón, A., Peterson, A. T., Sánchez-Cordero, V., and Ramsey, J. M. (2014). Phylogeny and niche conservatism in North and Central American triatomine bugs (Hemiptera: Reduviidae: Triatominae), vectors of Chagas’ disease. PLoS Negl. Trop. Dis. 8:e3266. doi: 10.1371/journal.pntd.0003266
Jiménez-Coello, M., Acosta-Viana, K., Guzmán-Marín, E., Bárcenas-Irabién, A., and Ortega-Pacheco, A. (2015). American trypanosomiasis and associated risk factors in owned dogs from the major city of Yucatan. J. Venomous Animals Toxins Including Trop. Dis. 21:37. doi: 10.1186/s40409-015-0039-32
Jiménez-Coello, M., Guzmán-Marín, E., Ortega-Pacheco, A., and Acosta-Viana, K. Y. (2010). Serological survey of American trypanosomiasis in dogs and their owners from an urban area of Merida Yucatan, Mexico. Transbound Emerg. Dis. 57, 33–36. doi: 10.1111/j.1865-1682.2010.01130.x
Klepeis, P., and Vance, C. (2003). Neoliberal policy and deforestation in southeastern Mexico: an assessment of the PROCAMPO program. Econ. Geogr. 79, 221–240.
Lambert, M. R., Brans, K. I., Des Roches, S., Donihue, C. M., and Diamond, S. E. (2020). Adaptive evolution in cities: progress and misconceptions. Trends Ecol. Evol. 36, 239–257. doi: 10.1016/j.tree.2020.11.002
Lefèvre, T., Gouagna, L. C., Dabiré, K. R., Elguero, E., Fontenille, D., Renaud, F., et al. (2009). Beyond nature and nurture: phenotypic plasticity in blood-feeding behavior of Anopheles gambiae when humans are not readily accessible. Am. J. Trop. Med. Hyg. 81, 1023–1029. doi: 10.4269/ajtmh.2009.09-0124
Lehane, M. J., and Schofield, C. J. (1982). Flight initiation in Triatoma infestans (Klug) (Hemiptera: Reduviidae). Bull. Entomol. Res. 72, 497–510. doi: 10.1017/S0007485300013687
Lehane, M. J., McEwen, P. K., Whitaker, C. J., and Schofield, C. J. (1992). The role of temperature and nutritional status in flight initiation by Triatoma infestans. Acta Trop. 52, 27–38. doi: 10.1016/0001-706X(92)90004-H
Lehmann, P., Ordoñez, R., Ojeda-Baranda, R., Lira, J., Hidalgo-Sosa, L., Monroy, C., et al. (2005). Morphometric analysis of Triatoma dimidiata populations (Reduviidae: Triatominae) from Mexico and northern Guatemala. Mem. Inst. Oswaldo Cruz 100, 477–486. doi: 10.1590/S0074-02762005000500006
Levy, M. Z., Bowman, N. M., Kawai, V., Waller, L. A., Cornejo, del Carpio, J. G., et al. (2006). Periurban Trypanosoma cruzi-infected Triatoma infestans, Arequipa. Peru. Emerg. Infect. Dis. 12, 1345–1352. doi: 10.3201/eid1209.051662
Liker, A. (2020). Biologia Futura: adaptive changes in urban populations. Biol. Futura 71, 1–8. doi: 10.1007/s42977-020-00005-9
Lobbia, P. A. Rodríguez, C. Mougabure-Cueto, G. (2019). Effect of nutritional state and dispersal on the reproductive efficiency in Triatoma infestans (Klug, 1834) (Hemiptera: Reduviidae: Triatominae) susceptible and resistant to deltamethrin. Acta Trop. 191, 228–238. doi: 10.1016/j.actatropica.2019.01.012
López-Cancino, S. A., Tun-Ku, E., De la Cruz-Felix, H. K., Ibarra-Cerdeña, C. N., Izeta-Alberdi, A., et al. (2015). Landscape ecology of Trypanosoma cruzi in the southern Yucatan Peninsula. Acta Trop. 151, 58–72. doi: 10.1016/j.actatropica.2015.07.021
Magouras, I., Brookes, V. J., Jori, F., Martin, A., Pfeiffer, D. U., and Dürr, S. (2020). Emerging zoonotic diseases: should we rethink the animal-human interface? Front. Vet. Sci. 7:582743. doi: 10.3389/fvets.2020.582743
Manson, S. M., and Evans, T. (2007). Agent-based modeling of deforestation in southern Yucatan, Mexico, and reforestation in the Midwest United States. Proc. Natl. Acad. Sci. U S A. 104, 20678–20683. doi: 10.1073/pnas.0705802104
Marwick, B., and Krishnamoorthy, K. (2019). cvequality: Tests for the Equality of Coefficients of Variation from Multiple Groups. R software package version 0.1.3. Available online at: https://github.com/benmarwick/cvequality. (accessed December 15, 2019).
May-Concha, I. J., Guerenstein, P. G., Malo, E. A., Catalá, S., and Rojas, J. C. (2018). Electroantennogram responses of the Triatoma dimidiata complex to volatiles produced by its exocrine glands. Acta Trop. 185, 336–343. doi: 10.1016/j.actatropica.2018.06.018
May-Concha, I., Guerenstein, P. G., Ramsey, J. M., Rojas, J. C., and Catalá, S. (2016). Antennal phenotype of Mexican haplogroups of the Triatoma dimidiata complex, vectors of Chagas disease. Infect. Genet. Evol. 40, 73–79. doi: 10.1016/j.meegid.2016.02.027
McAnany, P. A. (2016). Maya Cultural Heritage: how Archaeologists and Indigenous Communities Engage the Past. Lanham, MD: Rowman & Littlefield.
McEwen, P. K., and Lehane, M. J. (1993). The effect of varying feed interval on nymph development, subsequent adult flight behaviour, and autogeny in the triatomine bug Triatoma infestans (Klug) (Hem, Reduviidae). J. Appl. Entomol. 115, 90–96. doi: 10.1111/j.1439-0418.1993.tb00368.x
Medrano-Mercado, N., Ugarte-Fernández, R., Butrón, V., Uber-Busek, S., Guerra, H. L., de Araújo-Jorge, T. C., et al. (2008). Urban transmission of Chagas desease in Cochabamba, Bolivia. Mem. Isnt Oswaldo Cruz. 103, 423–430. doi: 10.1590/S0074-02762008000500003
Miles, L. S., Carlen, E. J., Winchell, K. M., and Johnson, M. T. (2021). Urban evolution comes into its own: emerging themes and future directions of a burgeoning field. Evol. Appl. 14, 3–11. doi: 10.1111/eva.13165
Miles, L. S., Rivkin, L. R., Johnson, M. T., Munshi-South, J., and Verrelli, B. C. (2019). Gene flow and genetic drift in urban environments. Mol. Ecol. 28, 4138–4151. doi: 10.1111/mec.15221
Moncayo, A., and Silveira, A. C. (2009). Current epidemiological trends for Chagas disease in Latin America and future challenges in epidemiology, surveillance and health policy. Mem. Inst. Oswaldo Cruz 104, 17–30. doi: 10.1590/s0074-02762009000900005
Moo-Millan, J. I., Arnal, A., Pérez-Carrillo, S., Hernández-Andrade, A., Ramírez-Sierra, M. J., and Rosado-Vallado, M. (2019). Disentangling Trypanosoma cruzi transmission cycle dynamics through the identification of blood meal sources of natural populations of Triatoma dimidiata in Yucatán. Mexico. Parasites Vectors 12:572. doi: 10.1186/s13071-019-3819-3817
Nattero, J., Dujardin, J. P., del Pilar, Fernández, M., and Gürtler, R. E. (2015a). Host-feeding sources and habitats jointly affect wing developmental stability depending on sex in the major Chagas disease vector Triatoma infestans. Infect. Genet. Evol. 36, 539–546. doi: 10.1016/j.meegid.2015.08.032
Nattero, J., Leonhard, G., Gürtler, R. E., and Crocco, L. B. (2015b). Evidence of selection on phenotypic plasticity and cost of plasticity in response to host-feeding sources in the major Chagas disease vector Triatoma infestans. Acta Trop. 152, 237–244. doi: 10.1016/j.actatropica.2015.09.022
Nattero, J., Piccinali, R. V., Lopes, C. M., Hernández, M. L., Abrahan, L., Lobbia, P. A., et al. (2017). Morphometric variability among the species of the Sordida subcomplex (Hemiptera: Reduviidae: Triatominae): evidence for differentiation across the distribution range of Triatoma sordida. Parasit Vectors 10:412. doi: 10.1186/s13071-017-2350-y
Noireau, F., Diosque, P., and Jansen, A. M. (2009). Trypanosoma cruzi: adaptation to its vectors and its hosts. Vet Res. 40:26. doi: 10.1051/vetres/2009009
Nouvellet, P., Ramirez-Sierra, M. J., Dumonteil, E., and Gourbière, S. (2011). Effects of genetic factors and infection status on wing morphology of Triatoma dimidiata species complex in the Yucatan Peninsula. Mexico Infect Genet Evol. 11, 1243–1249. doi: 10.1016/j.meegid.2011.04.008
Ordóñez-Díaz, M. D. J. (2018). Atlas Biocultural de Huertos Familiares en México: Chiapas, Hidalgo, Oaxaca, Veracruz y Península de Yucatán. Mexico : Universidad Nacional Autónoma de México Press.
Ordóñez-Krasnowski, P. C., Lanati, L. A., Gaspe, M. S., Cardinal, M. V., Ceballos, L. A., and Gürtler, R. E. (2020). Domestic host availability modifies human-triatomine contact and host shifts of the Chagas disease vector Triatoma infestans in the humid Argentine Chaco. Med. Vet. Entomol. 34, 459–469. doi: 10.1111/mve.12463
Pacheco-Tucuch, F. S., Ramírez-Sierra, M. J., Gourbière, S., and Dumonteil, E. (2012). Public street lights increase house infestation by the Chagas disease vector Triatoma dimidiata. PLoS One 7:e36207. doi: 10.1371/journal.pone.0036207
Panti-May, J. A., De Andrade, R. R. C., Gurubel-González, Y., Palomo-Arjona, E., Sodá-Tamayo, L., Meza-Sulú, J., et al. (2017). A survey of zoonotic pathogens carried by house mouse and black rat populations in Yucatan. Mexico. Epidemiol. Infect. 145, 2287–2295. doi: 10.1017/S0950268817001352
Panti-May, J. A. Torres-Castro, M. A.Hernández-Betancourt, S. F. (2021). Parásitos zoonóticos y micromamíferos en la Península de Yucatán. México: Contribuciones del ccba-uady.
Parente, C. C., Bezerra, F. S., Parente, P. I., Dias-Neto, R. V., Xavier, S. C., and Ramos, A. N. Jr., et al. (2017). Community-based entomological surveillance reveals urban foci of Chagas disease vectors in Sobral, State of Ceará, Northeastern Brazil. PLoS One 12:e0170278. doi: 10.1371/journal.pone.0170278
Pech-May, A., Mazariegos-Hidalgo, C. J., Izeta-Alberdi, A., López-Cancino, S. A., Tun-Ku, E., De la Cruz-Félix, K., et al. (2019). Genetic variation and phylogeography of the Triatoma dimidiata complex evidence a potential center of origin and recent divergence of haplogroups having differential Trypanosoma cruzi and DTU infections. PLoS Negl. Trop. Dis. 13:e0007044. doi: 10.1371/journal.pntd.0007044
Provecho, Y. M., Gaspe, M. S., Fernández, M. P., Enriquez, G. F., Weinberg, D., and Gürtler, R. E. (2014). The peri-urban interface and house infestation with Triatoma infestans in the Argentine Chaco: an underreported process? Mem. Inst. Oswaldo Cruz 109, 923–934. doi: 10.1590/0074-0276140225
Rabinovich, J. E., Kitron, U. D., Obed, Y., Yoshioka, M., Gottdenker, N., and Chaves, L. F. (2011). Ecological patterns of blood-feeding by kissing-bugs (Hemiptera: Reduviidae: Triatominae). Mem. Inst. Oswaldo Cruz 106, 479–494. doi: 10.1590/S0074-02762011000400016
Ramsey, J. M., Peterson, T., Carmona-Castro, O., Moo-Llanes, D., Nakazawa, Y., Butrick, M., et al. (2015). Atlas of Mexican Triatominae (Reduviidae: Hemiptera) and vector transmission of Chagas disease. Mem. Inst. Oswaldo Cruz 110, 339–352. doi: 10.1590/0074-02760140404
Rassi, A. Jr., Rassi, A., and Marin-Neto, J. A. (2010). Chagas disease. Lancet 375, 1388–1402. doi: 10.1016/S0140-6736(10)60061-X
Rico-Gray, V., and García-Franco, J. G. (1992). Vegetation and soil seed bank of successional stages in tropical lowland deciduous forest. J. Veg Sci. 3, 617–624. doi: 10.2307/3235828
Rohlf, F. J. (2007). TpsDig, Program for Digitalizing Morphologic Landmark and Outlines for Geometric Morphometric Analyses, Version 204. New York, NY: Department of Ecology and Evolution, State University of New York at Stony Brook.
Ribeiro-Jr, G. Abad-Franch, F. de Sousa, O. M. Dos Santos, C. G., Fonseca, E. O. Dos Santos, R. F., et al. (2021). TriatoScore: an entomological-risk score for Chagas disease vector control-surveillance. Parasit. Vectors 14:492. doi: 10.1186/s13071-021-04954-5
Santangelo, J. S., Rivkin, L. R., and Johnson, M. T. J. (2018). The evolution of city life. Proc. Royal Soc. B. 285:20181529. doi: 10.1098/rspb.2018.1529
Schachter-Broide, J., Gürtler, R. E., Kitron, U., and Dujardin, J. P. (2009). Temporal variations of wing size and shape of Triatoma infestans (Hemiptera: Reduviidae) populations from northwestern Argentina using geometric morphometry. J. Med. Entomol. 46, 994–1000. doi: 10.1603/033.046.0504
Schmunis, G. A., and Yadon, Z. E. (2010). Chagas disease: a Latin American health problem becoming a world health problem. Acta Trop. 115, 14–21. doi: 10.1016/j.actatropica.2009.11.003
Schofield, C. J., Diotaiuti, L., and Dujardin, J. P. (1999). The process of domestication in Triatominae. Mem. Inst. Oswaldo Cruz 94, 375–378. doi: 10.1590/s0074-02761999000700073
Secretaría de Desarrollo Urbano y Medio Ambiente [SEDUMA] (2018). Crecimiento de la Mancha Urbana (1950-1978-1998-2010). Mexico: SEDUMA.
Serruys, M., and Van Dyck, H. (2014). Development, survival, and phenotypic plasticity in anthropogenic landscapes: trade-offs between offspring quantity and quality in the nettle-feeding peacock butterfly. Oecologia 176, 379–387. doi: 10.1007/s00442-014-3016-5
Sheets, H. D. (2002). Semiland A Tool for Processing Semi-landmarks Physics Department, Canisius College, Buffalo, New York. Available online at: https://www.animal-behaviour.de/imp/ (accessed September 28, 2018).
Sheets, H. D. (2003). MakeFan6. Available online at: http://www3canisiusedu/~sheets/. View (accessed September 28, 2018)
Sheets, H. D. (2005). CoordGen, Coordinate Generation Program for Calculating Shape Coordinates. Available online at: https://www.animal-behaviour.de/imp/ (accessed September 28, 2018).
Sheets, H. D., and Zeldich, M. L. (2001). Tmorphgen6traditional Morphometrics Variables Generation Utility Part of IMPIntegrated Morphometrics Package Physics Department, Canisius College, Buffalo, New York. Available online at: https://www.animal-behaviour.de/imp/ (accessed September 28, 2018).
Sosa, F. J. (2010). “Chagas: Más allá de la frontera del bosque. Desmonte, desocupación y migración en Tucumán,” in Chagas en el Siglo XXI, ed. R. Storino (Argentina: Buenos Aires).
Suesdek, L. (2019). Microevolution of medically important mosquitoes-a review. Acta Trop. 191, 162–171. doi: 10.1016/j.actatropica.2018.12.013
Torres-Castro, M., Cuevas-Koh, N., Hernández-Betancourt, S., Noh-Pech, H., Estrella, E., Herrera-Flores, B., et al. (2021). Natural infection with Trypanosoma cruzi in bats captured in Campeche and Yucatán, México. Bioméd 41, 131–140.
Ucan-Euan, F., Hernández-Betancourt, S., Arjona-Torres, M., Panti-May, A., and Torres-Castro, M. (2019). Estudio histopatológico de tejido cardiaco de roedores infectados con Trypanosoma cruzi capturados en barrios suburbanos de Mérida, México. Biomed 39, 32–43.
Vallvé, S. L., Rojo, H., and Wisnivesky-Colli, C. (1996). Urban ecology of Triatoma infestans in San Juan, Argentina. Mem Isnt Oswaldo Cruz. 91, 40–408. doi: 10.1590/s0074-02761996000400003
Van Valen, L. (2005). The Statistics of Variation. in: Variation: a Central Concept in Biology. Burlington: Elsevier.
Vazquez-Prokopec, G. M., Ceballos, L. A., Cecere, M. C., and Gürtler, R. E. (2002). Seasonal variations of microclimatic conditions in domestic and peridomestic habitats of Triatoma infestans in rural northwest Argentina. Acta Trop. 84, 229–238. doi: 10.1016/s0001-706x(02)00204-8
Vazquez-Prokopec, G. M., Ceballos, L. A., Kitron, U., and Gürtler, R. E. (2004). Active dispersal of natural populations of Triatoma infestans (Hemiptera: Reduviidae) in rural northwestern Argentina. J. Med. Entomol. 41, 614–621. doi: 10.1603/0022-2585-41.4.614
Vazquez-Prokopec, G. M., Cecere, M. C., Canale, D. M., Gürtler, R. E., and Kitron, U. (2005). Spatiotemporal patterns of reinfestation by Triatoma guasayana (Hemiptera: Reduviidae) in a rural community of northwestern Argentina. J. Med. Entomol. 42, 571–581. doi: 10.1093/jmedent/42.4.571
Villacís, A. G., Grijalva, M. J., and Catalá, S. S. (2014). Phenotypic variability of Rhodnius ecuadoriensis populations at the Ecuadorian central and southern Andean region. J. Med. Entomol. 47, 1034–1043. doi: 10.1603/ME10053
Villalobos, G., Nava-Bolaños, A., De Fuentes-Vicente, J. A., Téllez-Rendón, J. L., Huerta, H., Martínez-Hernández, F., et al. (2019). A reduction in ecological niche for Trypanosoma cruzi-infected triatomine bugs. Parasit Vectors 12:240. doi: 10.1186/s13071-019-3489-5
Waleckx, E. Gourbière, S., and Dumonteil, E. (2015). Intrusive versus domiciliated triatomines and the challenge of adapting vector control practices against Chagas disease. Mem. Inst. Oswaldo Cruz 110, 324–338. doi: 10.1590/0074-02760140409
Weirauch, C. (2021). Origin and Evolution of Triatominae: The Biology of Chagas Disease Vectors, Entomology in Focus. Cham: Springer.
Wilke, A. B., Benelli, G., and Beier, J. C. (2020). Beyond frontiers: on invasive alien mosquito species in America and Europe. PLoS Negl. Trop. Dis. 14:e0007864. doi: 10.1371/journal.pntd.0007864
Zelditch, M. L., Swiderski, D. L., and Sheets, H. D. (2004). Geometric Morphometrics for Biologists: A Primer Elsevier. London: Academic Press.
Zeledon, R., and Rabinovich, J. E. (1981). Chagas’ disease: an ecological appraisal with special emphasis on its insect vectors. Annu. Rev. Entomol. 26, 101–133. doi: 10.1146/annurev.en.26.010181.000533
Zeledón, R., Calvo, N., Montenegro, V. M., Lorosa, E. S., and Arévalo, C. (2005). A survey on Triatoma dimidiata in an urban area of the province of Heredia, Costa Rica. Memo Inst Oswaldo Cruz. 100, 507–512. doi: 10.1590/S0074-02762005000600002
Keywords: Triatoma dimidiata, adaptation, urbanization, domiciliation, phenotypic variation, traditional morphometric, geometric morphometric
Citation: Montes de Oca-Aguilar AC, González-Martínez A, Chan-González R, Ibarra-López P, Smith-Ávila S, Córdoba-Aguilar A and Ibarra-Cerdeña CN (2022) Signs of Urban Evolution? Morpho-Functional Traits Co-variation Along a Nature-Urban Gradient in a Chagas Disease Vector. Front. Ecol. Evol. 10:805040. doi: 10.3389/fevo.2022.805040
Received: 29 October 2021; Accepted: 25 February 2022;
Published: 24 March 2022.
Edited by:
Emily J. Flies, University of Tasmania, AustraliaReviewed by:
Irving May, Universidad Autónoma de Yucatán, MexicoFernando Otalora-Luna, University of Richmond, United States
Elis Aldana, Universidad de Los Andes (Venezuela), Venezuela, in collaboration with reviewer FO-L
Copyright © 2022 Montes de Oca-Aguilar, González-Martínez, Chan-González, Ibarra-López, Smith-Ávila, Córdoba-Aguilar and Ibarra-Cerdeña. This is an open-access article distributed under the terms of the Creative Commons Attribution License (CC BY). The use, distribution or reproduction in other forums is permitted, provided the original author(s) and the copyright owner(s) are credited and that the original publication in this journal is cited, in accordance with accepted academic practice. No use, distribution or reproduction is permitted which does not comply with these terms.
*Correspondence: Carlos N. Ibarra-Cerdeña, Y2liYXJyYUBjaW52ZXN0YXYubXg=