- 1AG Integrative Sensory Physiology, Institute for Animal Physiology, Justus-Liebig-Universität Gießen, Gießen, Germany
- 2Center for Mind, Brain and Behavior (CMBB), University of Marburg and Justus Liebig University Gießen, Gießen, Germany
- 3Zoological Research Museum Alexander Koenig, Leibniz Institute for Animal Biodiversity, Section Myriapoda, Bonn, Germany
- 4Institute of Evolutionary Biology and Animal Ecology, University of Bonn, Bonn, Germany
Leg chordotonal organs in insects show different adaptations to detect body movements, substrate vibrations, or airborne sound. In the proximal tibia of stick insects occur two chordotonal organs: the subgenual organ, a highly sensitive vibration receptor organ, and the distal organ, of which the function is yet unknown. The distal organ consists of a linear set of scolopidial sensilla extending in the tibia in distal direction toward the tarsus. Similar organs occur in the elaborate hearing organs in crickets and bushcrickets, where the auditory sensilla are closely associated with thin tympanal membranes and auditory trachea in the leg. Here, we document the position and attachment points for the distal organ in three species of stick insects without auditory adaptations (Ramulus artemis, Sipyloidea sipylus, and Carausius morosus). The distal organ is located in the dorsal hemolymph channel and attaches at the proximal end to the dorsal and posterior leg cuticle by tissue strands. The central part of the distal organ is placed closer to the dorsal cuticle and is suspended by fine tissue strands. The anterior part is clearly separated from the tracheae, while the distal part of the organ is placed over the anterior trachea. The distal organ is not connected to a tendon or muscle, which would indicate a proprioceptive function. The sensilla in the distal organ have dendrites oriented in distal direction in the leg. This morphology does not reveal obvious auditory adaptations as in tympanal organs, while the position in the hemolymph channel and the direction of dendrites indicate responses to forces in longitudinal direction of the leg, likely vibrational stimuli transmitted in the leg’s hemolymph. The evolutionary convergence of complex chordotonal organs with linear sensilla sets between tympanal hearing organs and atympanate organs in stick insects is emphasized by the different functional morphologies and sensory specializations.
Introduction
Structural differentiation in sensory organs commonly correlates to functional specializations (Steinbrecht, 1999; Ridgel et al., 2001; Homberg and Paech, 2002; Land and Nilsson, 2012; Scherberich et al., 2017; Strauß, 2017; Zhao and McBride, 2020). This is also found for chordotonal organs, which are internal mechanoreceptors occurring over the insect body plan (Howse, 1968; Field and Matheson, 1998; Kavlie and Albert, 2013). With scolopidial sensilla as sensory units, the chordotonal organs are versatile for adaptations to different mechanical stimuli acting on the sensilla by stretching or tilting of the dendrite. In one organ like the antennal Johnston’s organ, sensilla can be functionally specialized in different subgroups which are not anatomically separated (eg., Kamikouchi et al., 2009; Matsuo and Kamikouchi, 2013). In other cases, sub-groups of sensilla or several organs occur in close proximity (Field and Matheson, 1998). For the latter, the subgenual organ complex in orthopteroid insects provides an example of 2–4 chordotonal organs located in the leg (Strauß et al., 2014). This is a notable expansion of sensory structures, as the subgenual organ in several insect lineages is the sole chordotonal organ in the proximal tibia and may consist of only few sensilla which are sufficient for detection of vibration signals (Michel et al., 1982; Nishino et al., 2016; Čokl et al., 2019). The subgenual organ is the most sensitive receptor organ for substrate vibrations and it occurs in most insects (Čokl and Virant-Doberlet, 2009; Lakes-Harlan and Strauß, 2014).
Distinct types of chordotonal organs are recognized based on their functional morphologies, including connective chordotonal organs, or tympanal (auditory) organs. The different attachments to surrounding structures like joints, cuticle, or trachea, couple the sensory organs to the sites of stimulus transfer on the insect body. Depending on the coupling/attachment, chordotonal organs can thus adaptively function as proprioceptors or exteroceptors. The mechanical coupling structurally allows to transfer mechanical force to the sensory organ as a group of sensilla, and ultimately to the dendritic membrane of the sensilla (French, 1988).
Connective chordotonal organs are attached to a strand of connective tissue to a tendon or body parts (Howse, 1968; Wright, 1976; Field and Matheson, 1998). They can function as proprioceptors, responding to body movements if they are located at or linked to joints by a receptor apodeme (Bässler, 1965, 1977; Field and Pflüger, 1989), tendons (Godden, 1972), or occur at pleural membranes (Hustert, 1974). Here, they can respond to movements like leg extension and flexion (Bässler, 1993; Tuthill and Azim, 2018) and motion of abdominal segments (Hustert, 1974). Other chordotonal organs can function as exteroceptors, detecting environmental stimuli. In these cases, they are usually coupled e.g., to structures resonating to airborne sound, like tympanal membranes and associated tracheal spaces in hearing organs. In these cases, both the tympana of very thin cuticle, as well as enlarged trachea, can provide entrance for sound energy (Stumpner and Nowotny, 2014; Montealegre-Z and Robert, 2015; Römer and Schmidt, 2016; Windmill and Jackson, 2016). Another modality detected by chordotonal organs are substrate-borne vibrations transmitted over the legs and the body. Receptor organs adapted to substrate vibrations are often located in the legs, like the subgenual organ. The subgenual organ spans the hemolymph channel in the proximal tibia and is excited by substrate vibrations transferred over the leg and the hemolymph system (Lakes-Harlan and Strauß, 2014). However, vibration stimuli are also detected by connective chordotonal organs like the femoral chordotonal organ, which usually has the highest sensitivity at relatively low frequencies (locust: Field and Pflüger, 1989; green lacewing: Devetak and Amon, 1997; stick insect: Stein and Sauer, 1999; stink bug: Čokl et al., 2006; cerambycid beetle: Takanashi et al., 2016).
For insect chordotonal organs, which repeatedly evolved a complex structure or a high number of sensory neurons (Field and Matheson, 1998; Yack, 2004), the functional morphology can indicate their physiological role by identifying how sensilla are stimulated by mechanical energy from their coupling to different structures like cuticle or tracheae. Elaborate chordotonal organs in the legs with linearly arranged sensilla occur in Ensifera, the long-horned grasshoppers (Nishino and Field, 2003; Strauß and Lakes-Harlan, 2009; Strauß et al., 2017). Especially the tympanal hearing organs in crickets and tettigoniids are well studied, with the linear sensilla forming the basis for frequency analysis (Stumpner and Nowotny, 2014; Montealegre-Z and Robert, 2015; Hummel et al., 2017; Nishino et al., 2019). Notably, these hearing organs in Ensifera are located next to other chordotonal organs which are sensitive to substrate vibrations. The differentiation of distinct organs can allow functional specialization by divergent tuning of individual organs, or by multimodal stimulus detection (Lin et al., 1993; Kalmring et al., 1994). A sensory organ similar in neuroanatomy to these tympanal organs is the distal organ (DO) of stick insects (Phasmatodea), located also in the proximal tibia, which is not associated with tympanal membranes (Strauß and Lakes-Harlan, 2013; Strauß, 2020a). This neuroanatomical organization evolved in convergence in these two taxa, and in stick insects independent to the evolution of tympanal membranes (Strauß and Lakes-Harlan, 2013). The DO in stick insects contains ∼20 sensilla in a linear array with decreasing cell sizes from proximal to distal (Strauß and Lakes-Harlan, 2013; Strauß, 2020b). This neuroanatomical complexity strongly suggests an important physiological role of the stick insect DO, as well as a mechanosensory adaptation different to the subgenual organ. The physiological function of the DO has not been specifically studied, but it is possibly vibrosensitive (Strauß and Lakes-Harlan, 2017). A more detailed understanding of the DO functional anatomy and its attachments will also show the similarities and differences to the auditory organs of Ensifera, and likely give insights into the different adaptations in diverse sensory organs.
Here, we investigate the functional morphology and neuroanatomy of the DO in stick insects. Previously, the attachments of the organ in the tibia were not studied in detail (Strauß and Lakes-Harlan, 2013). We investigate the DO in three species of stick insects to provide information for the functional morphology and their similarity in different genera. We included Carausius morosus (Lonchodinae) as model species for neurophysiology (eg., Bässler, 1983; Bässler and Büschges, 1998; Mantziaris et al., 2020), Sipyloidea sipylus (Necrosciinae) as this species has been studied for the neuroanatomy and physiology of the subgenual organ complex (Strauß and Lakes-Harlan, 2013, 2017), and Ramulus artemis (Clitumninae) to sample a further species with leg sizes accessible for vital stainings. The aim here is to document the structure of the DO within the tibia (the hemoplymph channel), to identify the points of suspension or attachment for the DO, and thereby to indicate the possible mechanical coupling of the DO to other leg structures. For this, the organ is investigated for possible connections to tendons, connective tissue, trachea, and the leg’s cuticle. The connections to these surrounding structures can indicate possible mechanical input pathways and the sensory activation (eg., Shaw, 1994b; Strauß et al., 2017; Stritih-Peljhan et al., 2019). Understanding the functional morphology of the DO of stick insects in more detail will also give insights into the extent of evolutionary convergence in the DO to the auditory sensilla in crickets and tettigoniids.
Materials and Methods
Insects
This study investigated adult female Ramulus artemis (Westwood, 1859), Carausius morosus (Sinéty, 1901), and Sipyloidea sipylus (Westwood, 1859) for their sensory organs in the proximal tibia. R. artemis was included in the study to gain data on the subgenual organ complex for a previously unstudied species of stick insects [see Strauß and Lakes-Harlan (2013)]. The larger body size made preparations of the legs for vital stainings more feasible (see below). For all species, parthenogenetically reproducing females were reared in a laboratory culture at the Institute for Animal Physiology, Justus-Liebig-Universität Gießen. They were reared at 21–23°C, and under a 12:12 h light-dark cycle. The insects were provided with leaves of Rosaceae ad libitum and sprayed daily with water.
The experiments documented here comply with the principles of animal care of the Justus-Liebig-Universität Gießen, Germany, and with the current law of the Federal Republic of Germany.
External Leg Morphology
The external leg cuticle was documented for isolated legs with a Leica 9Si dissection microscope and an in-build digital camera (1,024 × 768 pixels) via the Leica Application Suite version 4.12 (Leica Microsystems CMS GmbH, Wetzlar, Germany). The tibia was photographed from the anterior and the posterior side. Series of photographs of each leg were combined using the freeware program CombineZP1.
Neuroanatomy and Axonal Tracing
For neuroanatomical experiments, all insects were checked for intact legs and tarsi to avoid possible influences of regeneration after leg autotomy in postembryogenic development. The sensory organs of the subgenual organ complex and their neuronal innervation were stained intracellularly by axonal tracing using cobalt solution (5% CoCl2 × 6 H2O; Merck, Darmstadt, Germany, dissolved in Aqua dest.). The procedure for the dissection and tracing of the nervus cruris followed Strauß (2020a): legs were fixed with insect pins in a glass dish that was covered with Sylgard (Sylgard 184, Suter Kunststoffe AG, Fraubrunnen, Switzerland) with the ventral side facing upward. The ventral cuticle was removed with a piece of a blade (Feather FA-10, 0.1 mm, Feather, Osaka, Japan). The nerve dissection took place while covering the legs with Carausius saline [see Bässler (1977); 177.96 mmol NaC1, 17.4 mmol KC1, 25.1 mmol MgC12 × 6 H2O, Roth, Karlsruhe, Germany; 7.48 mmol CaC12 × 2 H2O, Merck, Darmstadt, Germany; 1.98 mmol Tris, from Sigma-Aldrich, St. Louis, MO, United States; dissolved in Aqua dest., adjusted to pH = 7.4]. The nervus cruris was cut with iridectomy scissors, and the ending of the nerve was placed in a glass capillary filled with a 5% cobalt solution. The preparations were incubated at 4°C for 48 hr. The neuronal staining was achieved by precipitating the cobalt with ammonium sulfide (Alpha Aesar, Karlsruhe, Germany) in a 1% solution in Carausius saline. Prior to the incubation, the tarsi and distal tibia was cut off. Legs were placed in the ammonium sulfide solution for 15 min, rinsed in Carausius saline, and fixed in paraformaldehyde (4%; Sigma-Aldrich, St. Louis, MO, United States) for 60 min. Following dehydration in a graded ethanol series (Carl Roth, Karlsruhe, Germany), the legs were cleared and stored in methyl salicylate (Merck, Darmstadt, Germany).
Light Microscopy and Documentation
Before microscopy of the tracing preparations, the posterior cuticle in the tibia of tracing preparations was removed with a piece of a blade. The presence of the posterior subgenual organ and its neuronal innervation (see section “Results”) were checked to ensure that the complete sensory organs were present after opening the leg. The preparations were mounted on a microscopy slide and were in most cases viewed from the posterior side. Occasionally, legs were also documented from dorsal direction.
The legs were viewed on a microscopy slide under methyl salicylate (Merck, Darmstadt, Germany) with an Olympus BH-2 microscope (Olympus, Shinjuku, Japan). Digital photographs were acquired with a Leica DFC 7000 T camera (1,920 × 1,440 pixel) attached to the microscope via the Leica Application Software V4.9 (Leica Microsystems CMS GmbH, Wetzlar, Germany). Series of photographs were combined using CombineZP. Photographs were assembled into panels, adjusted for contrast and brightness, and labeled using CorelDraw 11 (Corel, Ottawa, Canada).
The innervation pattern of the tibia was drawn using a Leitz microscope combined with a drawing attachment (Leitz, Wetzlar, Germany), and digitally redrawn and labeled using CorelDraw 11.
μCT Analysis of Sensory Organs
For the comparative micro-computed tomography (μCT) analysis, at least one tibia each of the foreleg and midleg of R. artemis, C. morosus, and S. sipylus were fixed for 24 h in Bouin’s solution and then stored in 70% ethanol. To increase soft tissue contrast in the μCT scans, all samples were subsequently stained in a solution of 0.3% phosphotungstic acid (PTA; Sigma-Aldrich, St. Louis, MO, United States) in 70% ethanol (Metscher, 2009) for 21 days, and subsequently washed and scanned in 70% ethanol. The tomography imaging was performed with a commercial μCT desktop system (Skyscan 1272, Bruker microCT, Kontich, Belgium) at the Zoological Research Museum A. Koenig (ZFMK). Scan settings are summarized in Table 1.
Thermal drift correction and digital section reconstruction was performed in NRecon 1.7 (Bruker microCT). The resulting image stacks were analyzed in DataViewer 1.5 (Bruker microCT).
Gray level-based three-dimensional volume renderings of the relevant organs were created in Drishti (Limaye, 2012) making use of the “crop” function and various “Clip” planes.
The cropped but otherwise unchanged μCT-scans of the tibiae are available as Supplementary Material at Zenodo2.
Vital Staining of Sensory Organs
Vital staining of the subgenual organ complex was achieved with Janus Green B solution [Sigma-Aldrich, St. Louis, Missouri; dissolved at a concentration of 0.02% in Carausius saline; see Yack (1993)]. Isolated legs were fixed with insect pins in a glass dish with the leg’s dorsal side facing upward. The cuticle was cut open dorsally with a piece of a blade and covered with Carausius saline. After removal of the saline, Janus Green B solution was applied for up to 60 s, and the legs then rinsed repeatedly with Carausius saline. The sensory organs were viewed with a dissection microscope (Leica), and digital photographs were taken with a Leica DFC 7000 T camera (1,920 × 1,440 pixel) mounted on the dissection microscope.
Terminology of Nerves and Nerve Branches
The tibia is innervated from the main leg of the nerve, termed nervus cruris. The terminology of nerve branches from the nervus cruris in the tibia follows that established for the more proximal leg segments (Bässler, 1983) by numbering the branches consecutively from proximal to distal. The smaller nerve branches originating from these first-order branches were numbered accordingly [see Strauß (2020a) for the subgenual organ complex in S. sipylus]. The terminology for the sections of the tibia along the different leg axis follows Ball and Field (1981).
Statistical Analysis
Statistical analyses were performed in GraphPad Prism 4 (GraphPad, San Diego, CA, United States) to test for differences in the number of sensilla among the legs pairs in Ramulus artemis with ANOVA, omnibus normality test, and Tukey’s Multiple Comparison Test.
Results
Neuroanatomy of the Subgenual Organ Complex
Ramulus artemis is an apterous stick insect with the body form characteristic for many Phasmatodea with thin legs (Figure 1). All legs are slender and elongated, with shorter middle legs (Figure 1 and Supplementary Figure 1). The leg’s cuticle at the position of the subgenual organ complex next to the femur-tibia joint was solid and showed no thinner cuticle compared to adjacent lateral leg areas. There were no differences between the anterior and posterior sides (Supplementary Figure 1).
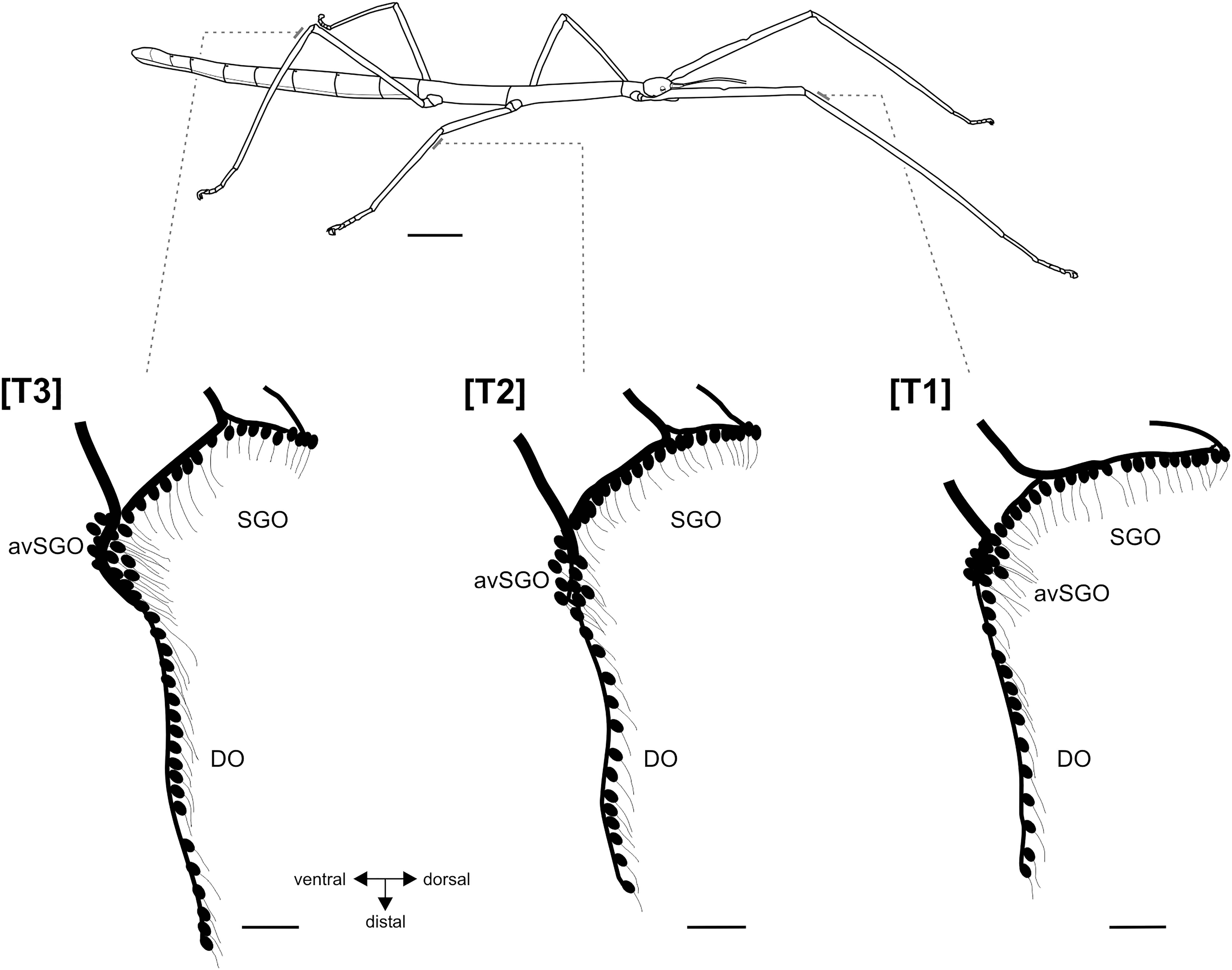
Figure 1. Habitus of female R. artemis (top) and the serial organization of the subgenual organ complex (below). Bars at the legs indicate the position of the sensory organs in the proximal tibia. Schematics of the sensory neurons in the subgenual organ complex, redrawn from tracing preparations. Scales: habitus = 1 cm, sensory organs = 100 μm. avSGO, anterior-ventral subgenual organ; DO, distal organs; SGO, subgenual organ; [T1], foreleg; [T2], midleg; [T3], hindleg.
The subgenual organ complex in R. artemis consisted of the subgenual organ (SGO) and the distal organ (DO) recognized by distinct anatomies and innervation (Figures 1, 2a). The sensory organs were present in all leg pairs with no obvious differences in the overall organization (Figure 1). Axonal tracing showed that the SGO was oriented perpendicularly to the leg’s main axis with dendrites pointing distally (Figures 2a,b). The most anterior sensilla of the SGO formed a dense group, termed the anterior-ventral subgenual organ (avSGO; Strauß and Lakes-Harlan, 2013) with ∼15 sensilla in all leg pairs (Figures 2a,e). The avSGO sensilla were placed next to the remaining subgenual sensilla (Figure 2a), and their dendrites were oriented in dorso-distal direction. The DO had a linear organization of sensilla (Figure 2c), while gaps in the line of cell bodies were occasionally seen (Figure 2d; in five out of 30 leg preparations). The DO dendrites were oriented in distal direction (Figures 2c,d). The overall neuroanatomy of the subgenual organ complex in R. artemis resembled that of the other stick insect species (S. sipylus, C. morosus) included in this study.
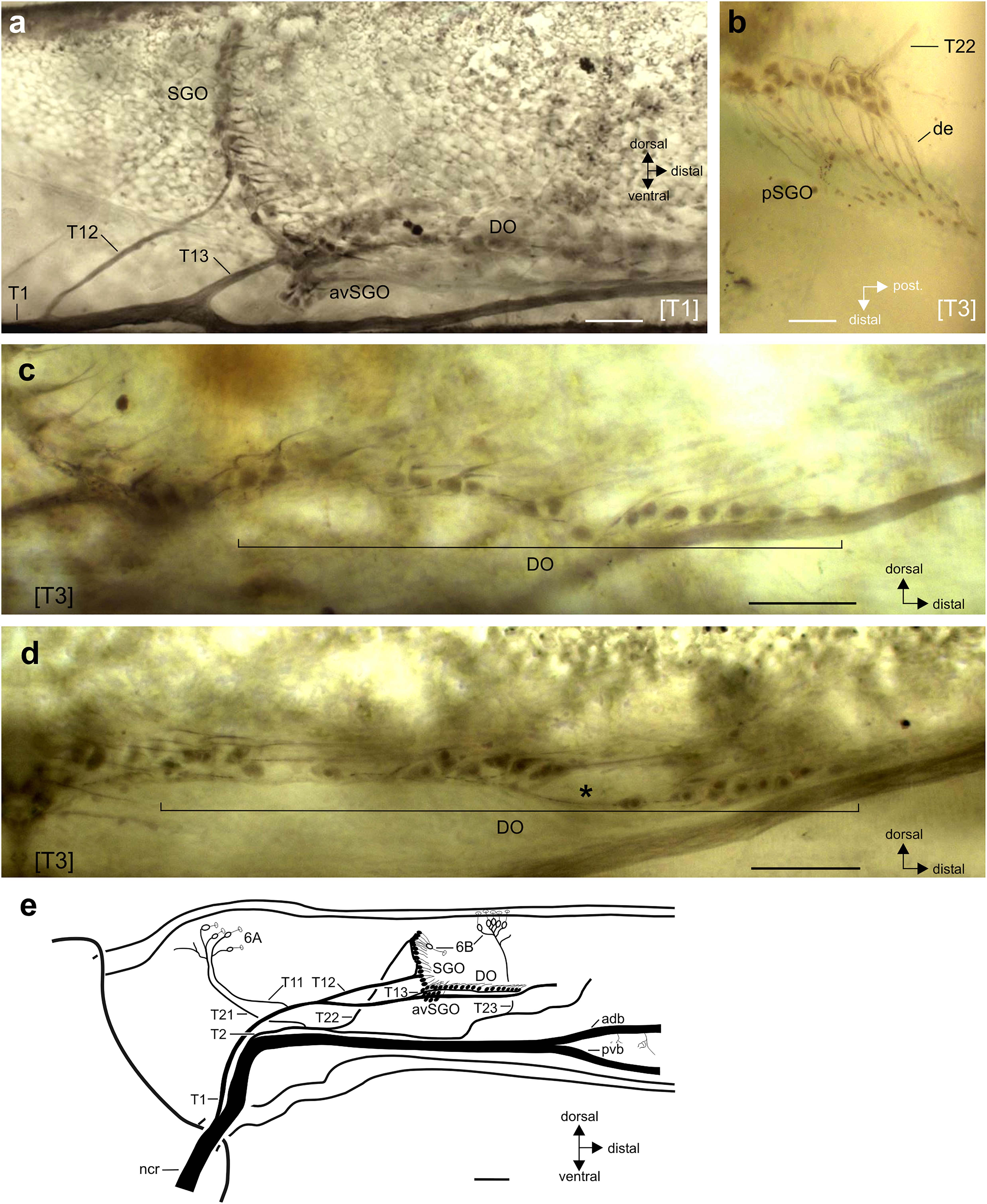
Figure 2. Neuroanatomy of the subgenual organ complex in R. artemis. Perspective is from lateral unless stated otherwise. (a) Wholemount staining of the subgenual organ complex with the subgenual organ (SGO), anterior-ventral subgenual organ (avSGO) and distal organ (DO). (b) The sensilla in the posterior subgenual organ (pSGO) are innervated by a distinct nerve branch, T22. The sensilla’s dendrites (de) point in posterior-distal direction. Viewed from dorsal. (c,d) Sensilla in the distal organ are arranged linearly in proximo-distal direction. They are usually continuously organized (c) but can show gaps between the somata (d; indicated by asterisk). (e) Schematic of the innervation pattern of the subgenual organ complex and campaniform sensilla in R. artemis, viewed in lateral perspective from the anterior side. The cell bodies of scolopidial sensilla are shown in black, the cell bodies of campaniform sensilla (groups 6A, 6B) in white. Scales: (a,c,d) = 100 μm; (b) = 50 μm; (e) = 200 μm. adb, anterior dorsal branch of nervus cruris; avSGO, anterior-ventral subgenual organ; de, dendrite; DO, distal organ; ncr, nervus cruris; pvb, posterior-ventral branch of nervus cruris; SGO, subgenual organ; [T1], foreleg; [T3], hindleg.
The intracellular staining allowed reconstructing the innervation pattern for the chordotonal organs and campaniform sensilla from the nervus cruris (Figure 2e). In general, the sensory neurons at the anterior and posterior side of the tibia were innervated by separate nerve branches on the anterior and posterior side: the sensilla of the subgenual organ were innervated by separate nerves on the anterior side (innervated by nerve branch T12) and posterior side (pSGO, innervated by nerve branch T22; see Figures 2a,b,e). The sensilla of the SGO occur continuous without gaps between the sensilla with different innervating nerve branches [Figure 2e; also Strauß (2020a) for S. sipylus]. The sensilla of the DO were innervated jointly with the anterior-ventral subgenual organ by nerve branch T13 (Figures 2a,e). The campaniform sensilla in the proximal tibia (group 6A) on either side were innervated by two nerve branches (anterior: T11, posterior: T12, Figure 2e).
The subgenual organ contained 37 ± 5 sensilla in the foreleg, 36 ± 4 sensilla in the midleg, and 39 ± 3 sensilla in the hindleg (n = 10 for each leg). The differences in the numbers of subgenual organ sensilla among the leg pairs were not statistically significant (ANOVA: p = 0.3912; F = 0.9720; df = 29; D’Agostino and Pearson omnibus normality test: p = 0.1745–0.6189). The distal organ contained 17 ± 2 sensilla in the foreleg, 16 ± 1 sensilla in the midleg, and 21 ± 3 sensilla in the hindleg (n = 10 for each leg). These differences in the distal organ sensilla among leg pairs were statistically significant (ANOVA: p < 0.0001; F = 17.54; df = 29; D’Agostino and Pearson omnibus normality test: p = 0.3336–0.4518), with sensillum numbers significantly higher in the distal organ of the hindleg compared to the fore- and midlegs (Tukey’s Multiple Comparison Test: p < 0.001).
Functional Morphology of the Subgenual Organ Complex
The two sensory organs of the subgenual organ complex and associated tissues were stained in situ with Janus Green B for R. artemis (Figure 3), and analyzed by μCT for R. artemis (Figures 3, 4, 6), C. morosus and S. sipylus (Figures 5, 6). The anatomy was highly similar for R. artemis (Figure 4), C. morosus (Figures 5d–f,h) and S. sipylus (Figures 5a–c,g).
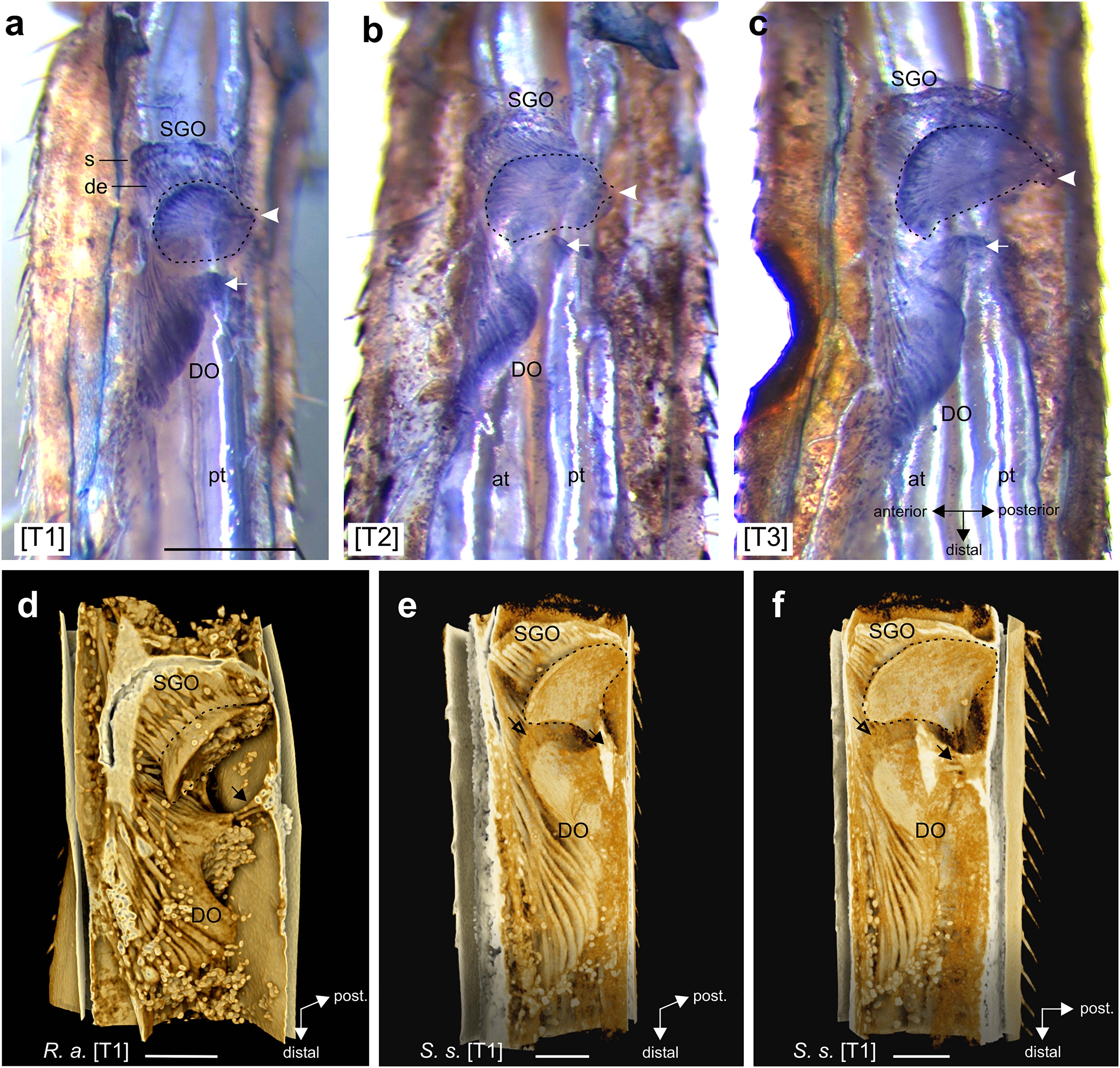
Figure 3. The subgenual organ complex in the legs. (a–c) Vital staining in R. artemis with Janus Green B in (a) foreleg, (b) midleg, and (c) hindleg. In the subgenual organ (SGO), the dendrites (de) point distally from the somata (s) into the SGO tissue (outlined by hatched line) that attaches at the dorsal cuticle (arrowhead). The distal organ (DO) has a triangular form and attaches at the dorsal cuticle (arrow). (d–f) 3D renderings of the subgenual organ complex from μCT scans in (d) R. artemis and (e,f) S. sipylus with the SGO and DO. The SGO tissue (hatched line) and the proximal DO attach at the dorsal cuticle (solid arrow). Empty arrow indicates the position of the membrane between SGO and DO. Note the globular fat at the sensory organs. Scales: (a) = 500 μm; (d–f) = 200 μm. at, anterior trachea; de, dendrites; DO, distal organ; pt, posterior trachea; s, somata; SGO, subgenual organ; [T1], foreleg; [T2], midleg; [T3], hindleg.
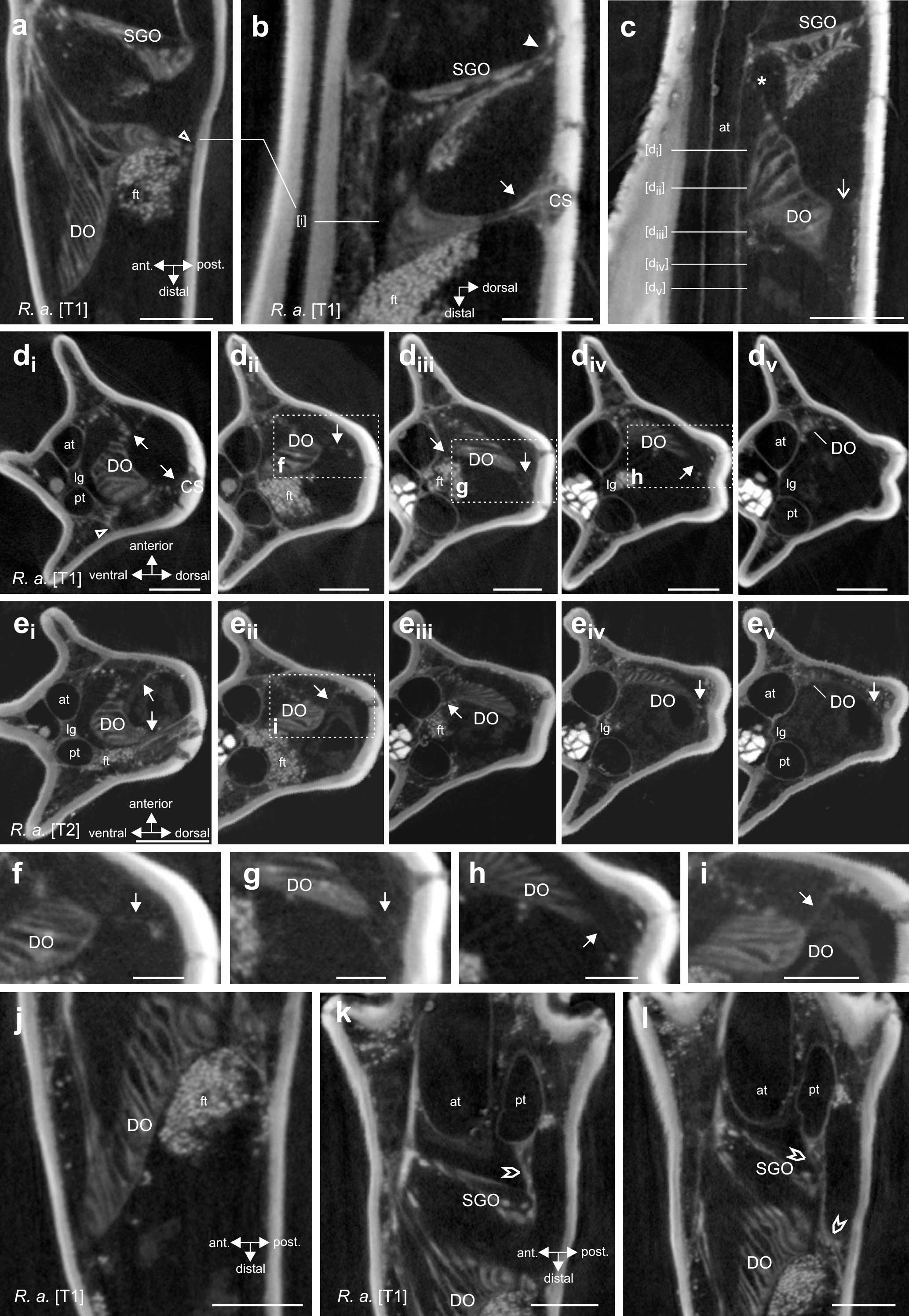
Figure 4. Morphology and attachment structures of the distal organ (DO) in R. artemis. (a) The DO is placed at the anterior cuticle of the tibia, with an extension to the posterior side (empty arrowhead) (foreleg, horizontal longitudinal section). (b) At the dorsal tibia, a strand of tissue extends from the DO to the cuticle (solid arrow) (foreleg, vertical longitudinal section). The subgenual organ (SGO) is located more proximally in the tibia with a separate connection at the dorsal cuticle (solid arrowhead). (c) The main part of the DO extends to the dorsal cuticle by a fine strand (open arrow), with diffuse tissue in the space between the DO and the dorsal cuticle (foreleg, vertical longitudinal section). A strand from the DOI extends to the ventral SGO (asterisk). (d) Transversal sections of the foreleg show the DO suspended by the two larger tissue strands [(di), solid arrows] and thin tissue strands [(dii–div), solid arrows] from the cuticle. Section levels are indicated in (c). The tissue strand to the posterior side originates from the posterior trachea (empty arrowhead). Note the distance of the DO to the anterior trachea in proximal sections. (ei–v) Transversal sections of the midleg DO at corresponding levels with identical strands. (f–i) Details of the boxed areas in (dii–iv,eii) with thin strands and tissue indicated by arrows. (j) The distal end of the DO is close to the anterior cuticle (foreleg, horizontal longitudinal section). (k,l) Connective tissue (pointed empty arrowheads) between the posterior trachea (pt) and the subgenual organ (SGO), located proximally to the DO. The section shown in (k) is located 27 μm dorsally to the section in panel (l). Note that in panel (l), the connective strand extends distally to the level of the DO but runs to the posterior cuticle and not the DO placed more anteriorly (empty arrowhead). Scales: (a,b,d,e,j–l) = 200 μm, (c) = 300 μm, (f–i) = 100 μm. at, anterior trachea; CS, campaniform sensilla; DO, distal organ; ft, fat tissue; lg, ligament between tracheae; pt, posterior trachea; SGO, subgenual organ.
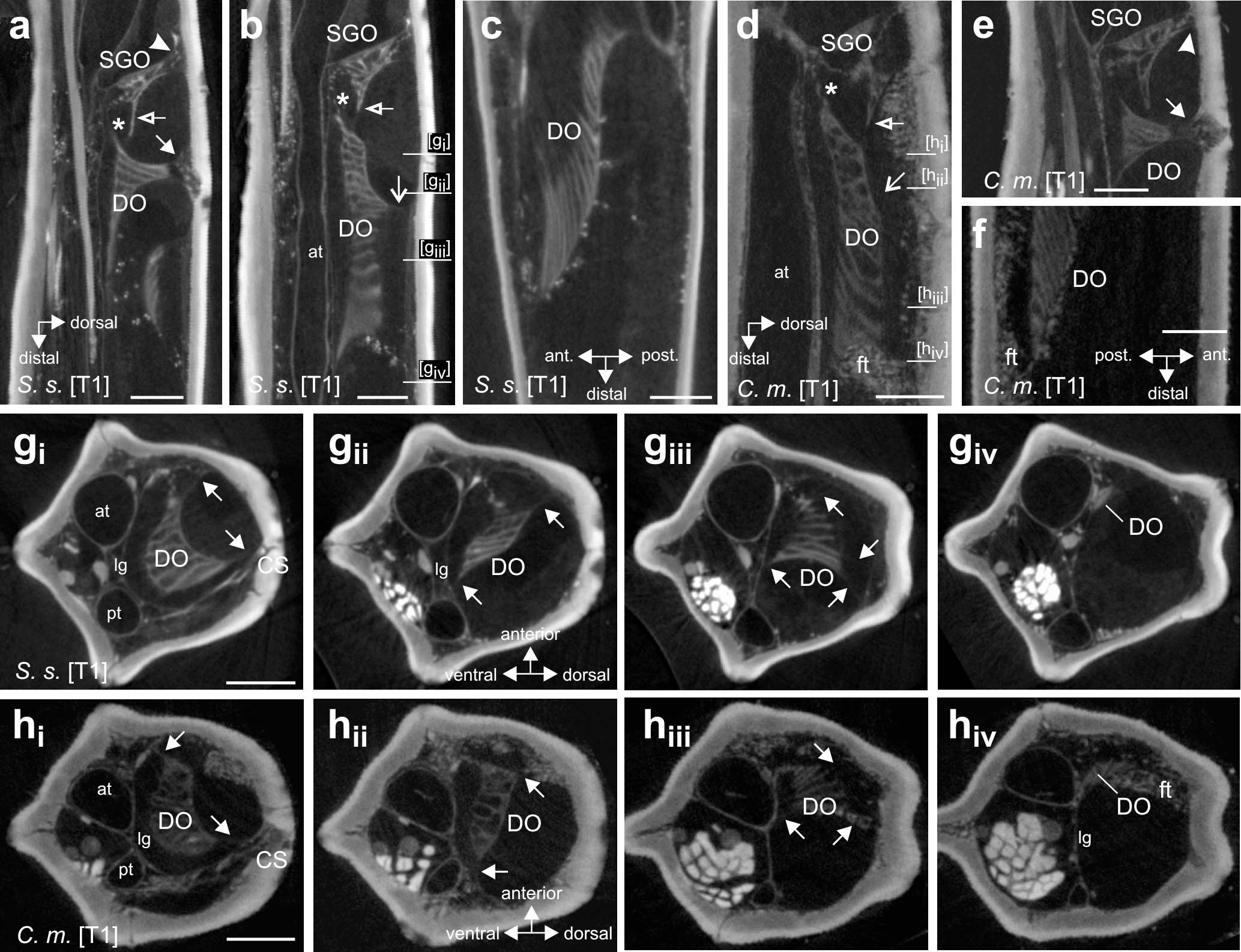
Figure 5. Morphology and attachment structures of the distal organ in S. sipylus and C. morosus forelegs. In (a) S. sipylus and (e) C. morosus, the distal organ (DO) shows a proximal strand of connective tissue to the dorsal cuticle (solid arrow) (vertical longitudinal section). From the DO extends a strand to the ventral SGO (asterisk). The subgenual organ (SGO) is linked to the dorsal cuticle by a separate tissue strand (solid arrowhead). A thin membrane (empty arrow) connects the SGO and DO in panel (b) S. sipylus and (d) C. morosus (vertical longitudinal sections). More distally, the main body of the DO shows a dorsal connection to the cuticle (open arrow) in panel (b) S. sipylus and (d) C. morosus. The distal DO is located near the anterior leg cuticle but is not directly inserted or linked to it in (c) S. sipylus and (f) C. morosus (horizontal longitudinal sections). Transversal sections of the foreleg tibia in (g) S. sipylus and (h) C. morosus show the DO and strands to the cuticle (arrows); the levels of the sections are indicated in (b,d). The most distal part of the DO is thinner in diameter and is located just dorsally to the anterior trachea. Scales: (a,b) = 200 μm, (c,f) = 100 μm, and (d,e,g,h) = 150 μm. at, anterior trachea; C. m., Carausius morosus; CS, campaniform sensilla; DO, distal organ; ft, fat tissue; lg, ligament; pt, posterior trachea; SGO, subgenual organ; S. s., Sipyloidea sipylus.
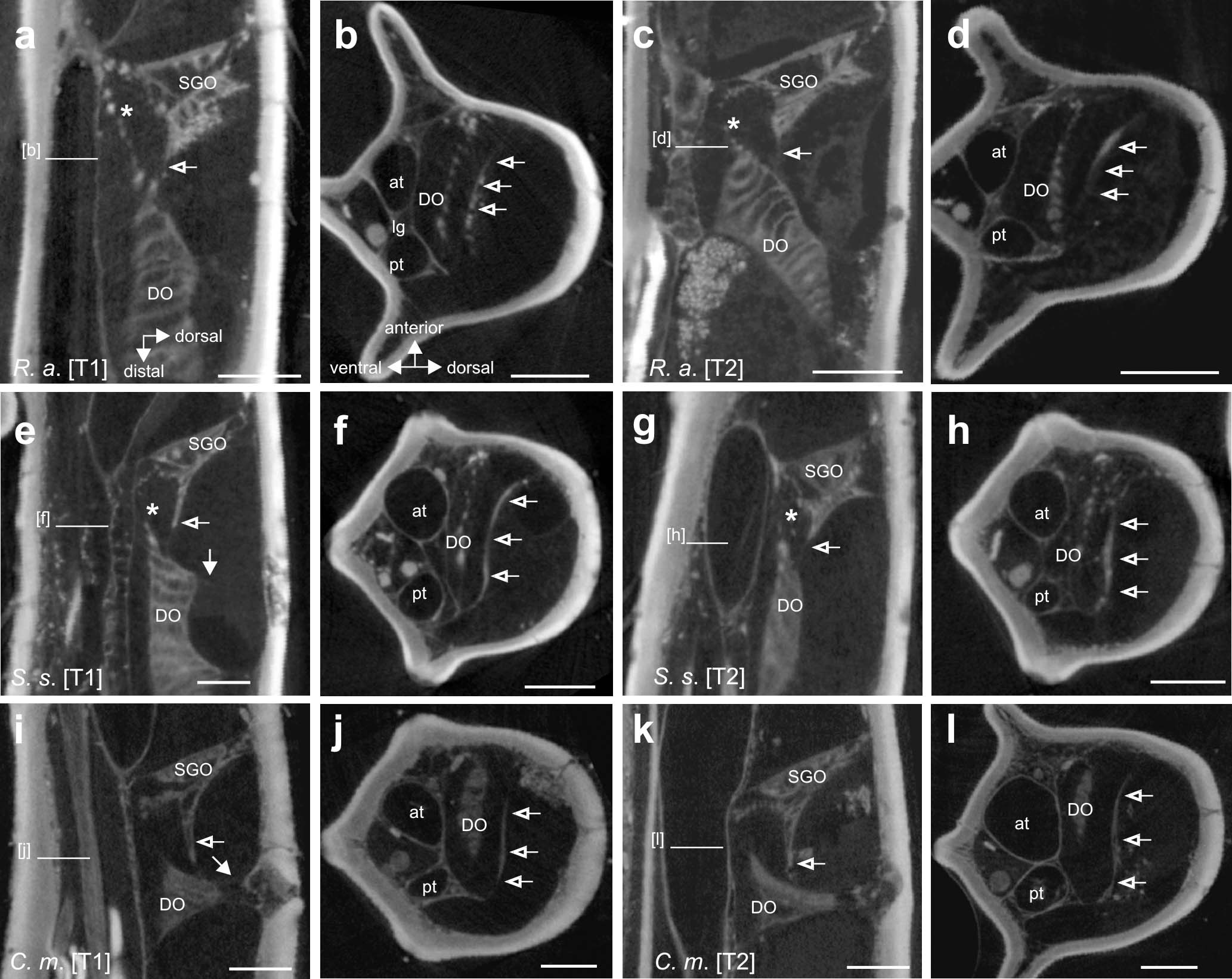
Figure 6. The membranous connection between the subgenual organ (SGO) and distal organ (DO) in (a–d) R. artemis, (e–h) S. sipylus, and (i–l) C. morosus, indicated by empty arrows. The tissue connection is present in forelegs (a,e,i) and midlegs (c,g,k) (vertical longitudinal sections). The tissue spans the tibia in transversal sections between the anterior and posterior cuticle in (b,f,j) forelegs and (d,h,l) midlegs (empty arrows). Ventral to this, a strand from the DO extends to the SGO. Scales: (a–d) = 200 μm, (e) = 100 μm, and (f,h-l) = 150 μm. at, anterior trachea; DO, distal organ; pt, posterior trachea; SGO, subgenual organ; [T1], foreleg; [T2], midleg.
The subgenual organ complex was located dorsally to the two tibial tracheae (Figures 3a–c). The SGO spanned the proximal tibia, and was suspended in the hemolymph channel with attachment to the dorsal and anterior cuticle (Figures 3, 4a,b, 5b,e). The SGO tissue attached most strongly at the posterior side of the tibia (Figures 3a–c). The dendrites of the SGO sensilla with their distal orientation inserted into the rounded SGO tissue located almost perpendicularly to the leg’s main axis (Figure 3).
The DO was located at the anterior side of the tibia (Figures 2, 4a,j, 5c). The dendrites, cap and accessory cells of the DO formed a triangular, continuous structure at the anterior side of the tibia that became narrower at the distal end (Figures 3, 4a,j, 5c). The accessory cells of the DO are long and give a lamellar structure (Figures 3d–f). The DO extended posteriorly in the proximal part (R. artemis; Figures 3d, 4a,di,ei; S. sipylus: Figures 3e,f; Supplementary Videos 1,2) but the sensilla did not directly contact the leg’s cuticle (Figures 4a,di and Supplementary Figure 2). The proximal DO was attached to the dorsal and anterior cuticle by separate tissue strands (Figures 3, 4b,di,ei, 5a,e). This organization was identical in all leg pairs (Figures 3a–c, 4d,e and Supplementary Figures 3A,B, 5, 6). The attachment to the dorsal cuticle also supplies the dorsal campaniform sensilla (dorsal group 6B) located at the level of the SGO (Figures 4b,di). In cleared tracing preparations of the tibia, the stained axons from the campaniform sensilla can be seen (not shown). These structures from the DO and campaniform sensilla can also be more separated (Figures 5gi,hi). During preparations, no attachment of the DO to a tendon or a receptor apodeme was noted.
In the central part, the DO extended toward the dorsal cuticle (Figures 4c,dii,iii, 5b,d,gii,iii,hii,iii). At the extension of the DO in dorsal direction toward the cuticle, the organ is suspended by fine strands (Figures 4c, 5b, 6a). Similar thin strands occurred more distally between the DO and the cuticle (Figures 4d–f,h,i, 5b,g,h). The space between the DO and the dorsal leg cuticle was filled with a diffuse tissue (Figures 4c,dii,iii,g, 5d,gii,iii,hii,iii). While this could represent hemolymph, especially at the distal DO it appeared more consistent than hemolymph seen in the remaining hemolymph channel (Figures 4div,v, 5giv,hiv). At the distal end, the DO was located in the hemolymph channel close to the anterior cuticle (Figures 4j, 5c,f and Supplementary Figures 3–7). The DO was placed dorsally to the anterior trachea (Figures 4dv,ev, 5giv,hiv). The elongated accessory cells run in parallel (Figures 4j, 5c and Supplementary Figures 3C, 4C, 5D). In some preparations, fat depositions occurred in the legs adjacent to the SGO and the DO, for the latter at the proximal and distal end (Figures 4a,b,j, 5d and Supplementary Figures 3C, 4C, 5D,E). At the distal end, fat cells were in some legs placed between the DO and the anterior cuticle (Figures 5c,f and Supplementary Figures 3C, 6C), while fat was largely absent in other legs (Figure 4j and Supplementary Figure 5D). At the distal end, no attachment of the DO to a ligament or an apodeme was noted.
The proximal DO extends a fine tissue strand to the ventral SGO (Figures 4c, 5a,b,d, 6a). Dorsally to this strand runs a fine membrane, which connects the SGO and the DO in the middle of the tibia (Figure 6). In transverse sections, this membrane is located dorsally to the DO in anterior-posterior direction in all legs from all species studied here (Figure 6). Due to the low contrast of the membrane compared to surrounding tissue in the μCT scans, the membrane is not visible in the 3D-rendering of R. artemis (Figure 3d) and only represented as a grainy structure in S. sipylus (open arrows, Figures 3e,f). Both the SGO and the DO were located dorsally of two tracheae, which run in the ventral tibia (Figures 3, 4, 5). The proximal and middle DO was placed in the hemolymph channel with a clear gap to the underlying anterior trachea (Figures 4di–iii,ei–iii, 5gi–iii,hi–iii). The middle DO formed a strand to the anterior trachea (Figures 4diii,eiii). At the distal section, the DO was placed at the dorsal side of the anterior trachea (Figures 4c,div,v,eiv,v, 5d,giv,hiv). Notably, the anterior trachea expanded slightly in diameter between the proximal and distal end of the DO (Figures 4c,d, 5b,d,g,h). This expansion did not affect the position of the DO, which was located more dorsally with a gap to the trachea (Figure 5d). The DO showed a coupling to the anterior trachea by a strand in the middle part of the DO, while the SGO was linked by a stronger tissue strand to the posterior trachea (Figures 4k,l). The leg tracheae did not show obvious tracheal vesicles at the level of the DO.
Discussion
The subgenual organ complex in stick insects consists of the subgenual organ (SGO) and the distal organ (DO), and the latter has a notable linear organization of sensilla (Strauß and Lakes-Harlan, 2013). Here, we show a unique functional morphology for the DO by different types of attachments. This structural complexity raises the question for its physiological function, and for the adaptations driving the evolutionary convergence of linear sets of sensilla which occur in the stick insect DO as well as the tympanal hearing organs in Orthoptera (Strauß and Lakes-Harlan, 2013).
The Subgenual Organ Complex in Stick Insects
The overall neuroanatomy of the subgenual organ complex in R. artemis is identical to two other stick insect species investigated previously (S. sipylus, C. morosus; Strauß and Lakes-Harlan, 2013). Few minor differences occur, such as the relatively long nerve branches of T1 and T2 splitting off in the femur-tibia-joint in R. artemis, as in S. sipylus the nerve branches split off more distally in the proximal tibia (Strauß, 2020a). The number of SGO sensilla (averages between 36–39) is slightly lower than in the two other species studied previously which have on average 40–44 sensilla, while the number of DO sensilla is similar to C. morosus but slightly lower than in S. sipylus in the fore- and midleg (Strauß and Lakes-Harlan, 2013). The presence of the elaborate DO in three different groups of stick insects (Clitumninae, Lonchodinae, and Necrosciinae) supports the common physiological relevance of this mechanosensory organ. The three species investigated here belong to Oriophasmata, the Old World Phasmatodea (Simon et al., 2019). The DO likely also occurs in further groups of stick insects, but the distribution including the New World Phasmatodea (Occidophasmata) and the earliest branching Euphasmatodea requires a broader taxonomic study, also relating to differences in ecology, body size, and anatomy. The current data show that the elaborate DO anatomy occurs in species with cryptic body shape and elongated, slender legs, and appears to be independent of wings and flight capacity or secondary chemical defense [see Carlberg (1984, 1985) and Bradler and Buckley (2018)].
Functional Morphology of the Sensory Organs and Possible Sensory Adaptations
For chordotonal organs, the functional morphology and the attachment of the sensilla’s dendrites determine the detectable stimuli and their parameters like frequency and amplitude (eg., Shaw, 1994b; Barth, 2019), since both substrate-borne vibrations and airborne sound mechanically interact with parts of the insect body (Cocroft et al., 2000; Römer and Schmidt, 2016; Stritih Peljhan and Strauß, 2018). In several cases, chordotonal organs can respond to both airborne sound and substrate vibrations if they attach to soft membranes or trachea (Shaw, 1994a; Jeram et al., 1995; Stumpner, 1996; Pflüger and Field, 1999). Other chordotonal organs can respond to proprioceptive stimuli, cuticular strain, as well as low-frequency substrate vibrations. In such cases, the functional differentiation of distinct groups of sensilla depends on different mechanical couplings of these groups, which are connected to a receptor apodeme and the inside of the cuticle. Such differentiation is described for the femoral chordotonal organ in locusts, crickets, weta, and stick insects (Field and Pflüger, 1989; Matheson and Field, 1990; Kittmann and Schmitz, 1992; Stein and Sauer, 1999; Nishino, 2000, 2003).
The SGO neuroanatomy in stick insects is similar to that in other orthopteroid insects (Lakes-Harlan and Strauß, 2014). The DO in stick insects shows different types of attachments, but there is no connection with a tendon or a tissue strand toward muscles or joints to support a proprioceptive function, as it would be typical for a connective chordotonal organ. The linear organization of sensilla in the DO neuroanatomically resembles the auditory sensilla in tympanal organs in crickets and tettigoniids, where it forms the anatomical basis for frequency analysis (Michel, 1974; Imaizumi and Pollack, 1999; Stumpner and Nowotny, 2014; Montealegre-Z and Robert, 2015). In crickets and tettigoniids, the auditory sensilla are closely associated with the trachea in the leg (Figure 7; Michel, 1974; Schumacher, 1975; Oldfield et al., 1986; Lin et al., 1994; Hummel et al., 2017; Schneider et al., 2017; Nishino et al., 2019). In tettigoniids, the hemolymph channel in certain species is adapted as an acoustic vesicle which is filled with fluid and plays an important role for frequency analysis (Montealegre-Z and Robert, 2015). In the stick insect species studied here, the ventral side of the DO at the proximal end floats in the hemolymph, while the middle DO connects to the leg cuticle as well as the anterior trachea by fine strands, and the distal DO locates dorsally of the anterior trachea (Figures 4d,e,f, 5g,h). No auditory adaptations are evident from the morphological structures. In the hearing organs of Orthoptera, sound energy can reach the auditory sensilla via tympanal membranes or through enlarged auditory trachea and spiracles. In comparison to auditory systems in Orthoptera, the stick insect DO shows only a weak coupling of sensilla to the tracheal system (Figure 7). In the stick insects, the anterior tibial trachea is larger in diameter than the posterior trachea (Figures 4–6), but not notably enlarged either at the DO, or at the thoracic spiracle as a potential pathway for sound input (J. Strauß, in preparation). However, an auditory detection of far-field sound can also occur without the elaboration of tympanal membranes (Shaw, 1994a; van Staaden and Römer, 1998). Based on the functional anatomy, any possible physiological and behavioral roles of such sound stimuli require further experiments. With respect to acoustic behaviors, sound production is known in few stick insects in defense to predators (Bedford, 1978; Carlberg, 1989). Signaling behavior using substrate vibrations is so far not described for species of stick insects, while many insects use the detection of vibrations in predator avoidance (Cocroft and Rodriguez, 2005; Hill, 2008).
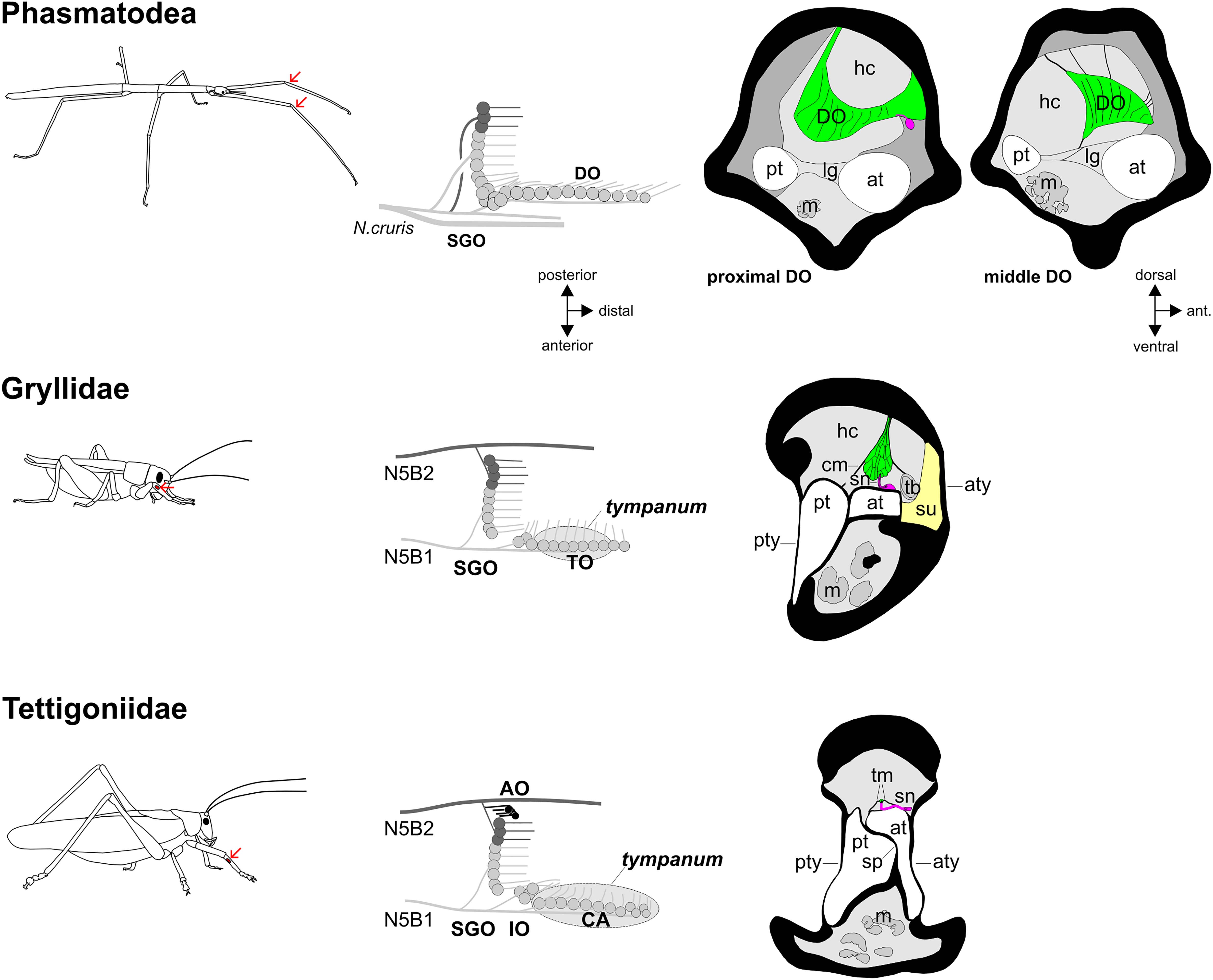
Figure 7. Functional morphology of the stick insect distal organ (DO) and of orthopteran hearing organs in the foreleg. The position of the subgenual organ complex in forelegs is indicated by arrows (the subgenual organ complex is present in all leg pairs). Schematics of the subgenual organ complex show the sensory organs and innervating nerves. In the schematics, only the anterior tympanal membrane is shown. Transversal sections of the distal organ and auditory organs show the leg tracheae, sensory neurons (magenta), accessory cells (green) and cuticle/tympanal membranes, with hemolymph channels (light grey) and tissue in the leg (dark grey). AO, accessory organ; at, anterior trachea; aty, anterior tympanum; CA, crista acustica; cm, covering membrane; DO, distal organ; hc, hemolymph channel; IO, intermediate organ; lg, ligament; m, muscle; pt, posterior trachea; pty, posterior tympanum; SGO, subgenual organ; sn, sensory neuron; sp, septum; su, suspensorium; tb, tracheal body; tm, tectorial membrane; TO, tympanal organ. Cross-section of cricket ear reprinted by permission from Springer Nature: Springer, Zeitschrift für Morphologie der Tiere, Das Tympanalorgan von Gryllus bimaculatus Degeer (Saltatoria, Gryllidae), K. Michel, copyright 1974, https://link.springer.com/article/10.1007/BF00298805). Cross-section of tettigoniid ear reprinted by permission from J Comp Neurol, J. Hummel, M. Kössl, and M. Nowotny, Morphological basis for a tonotopic design of an insect ear, pp. 2443–2455, copyright 2017, with permission from John Wiley and Sons.
The lack of obvious auditory specializations leaves the question for the DO adaptations still open. The strongest connection of the DO to other structures occurs at the proximal end by tissue strands to the dorsal and anterior cuticle in the three species studied (Figures 4d,e, 5g,h). The middle part of the DO is closer to the dorsal cuticle than the proximal part of the DO, and it is suspended by fine tissue strands to the dorsal and anterior cuticle, and also the anterior trachea (Figures 4d,e, 5g,h). The biomechanical properties of these strands or their biochemical components are not known. These would affect the possible movements of the DO, by determining if the organ is merely suspended from the cuticle, or if the DO could be displaced by hemolymph movements in the tibia. These strands are notably very thin, and can be assumed to have some flexibility. The DO in heelwalkers is also attached by stronger strands to the epidermis and posterior trachea (Eberhard et al., 2010). The ventral side of the DO is at the proximal end without contact sites to other tissues, and it is at the distal end placed dorsally of the anterior trachea. We did not note the connection of DO sensilla to a membrane covering the organ which was described previously for C. morosus (Friedrich, 1929). Given the elongated structure of the DO, this attachment would allow for more than one possible mechanical coupling. The strand connections to the cuticle could mediate the detection of vibrations transmitted over the leg cuticle. The relatively large diameter of the proximal DO, located in the dorsal hemolymph channel, could imply the detection of hemolymph vibrations caused by substrate-borne vibrations, similar to the tibial SGO [see Kilpinen and Storm (1997), on the bee SGO]. This is also supported by the direction of the DO dendrites in distal direction in the tibia, making them well suited to respond to forces like vibrations transferred in longitudinal direction of the tibia. While the bee SGO is best studied for the activation of this organ, the similarity may be restricted by the differences in the leg’s size, diameter, and also the attachment points of the SGO (Kilpinen and Storm, 1997). For bees, the role of mechanical signal detection in intraspecific communication and the leg mechanoreceptors are analyzed at different levels (Kilpinen and Storm, 1997; Rohrseitz and Kilpinen, 1997; Michelsen, 2014), while the behavioral roles of mechanical signal detection in stick insects is not documented in detail.
Other chordotonal organs located next to the SGO respond to substrate-borne vibrations with a tuning shifted to other frequencies than those detected by the SGO in tettigoniids and cave crickets (Ebendt et al., 1994; Kalmring et al., 1994; Čokl et al., 1995). This tuning shift has been shown for the intermediate organ (IO), a chordotonal organ located just distally to the SGO in some Ensifera. In these groups, the IO is located dorsally of the trachea, and is also attached to the inner cuticle which allows for multimodal stimulation (Lin et al., 1994; Jeram et al., 1995). The DO in stick insects could possibly complement the SGO by such a sensory specialization in vibrational frequency tuning. Contact to the leg cuticle is established by the fine tissue strands from the DO and the diffuse tissue in the space between DO and cuticle, though the latter seems to provide only a weak coupling to the cuticle. A mechanical interaction between the SGO and the proximal DO could be possible from the structural connections between both organs (Figure 6).
Another functional aspect of the DO in orthopteroid insects is that the organ could detect changes in the hemolymph pressure which occur during leg movements. This sensory function was discussed for the DO in cockroaches, which did not respond to substrate-borne vibrations (Schnorbus, 1971). So far, this remains a tentative function for chordotonal organs. In conclusion, two features may support the detection of substrate vibrations, apart from the attachment to the cuticle: with the relatively large diameter of the DO in the hemolymph channel and with DO dendrites oriented distally in the tibia, it is most likely that the sensilla respond to forces acting in the longitudinal axis of the leg. The position in the hemolymph channel would make it difficult to isolate the DO from hemolymph movements caused by external substrate vibrations, and restrict it to detecting only pressure changes occurring during locomotion. The enlarged size of the DO by the long, lamellar accessory cells (Figure 3) also supports the exposition to stimuli from the hemolymph.
Comparative Morphology and Evolutionary Convergence in Tibial Sensory Organs
The DO is homologous to a chordotonal organ identified distally of the SGO in some Orthoptera, the intermediate organ (IO) (Lin et al., 1995; Strauß and Lakes-Harlan, 2013). The IO in Ensifera is studied eg., in tettigoniids, cave crickets, or weta, where it contains 12–20 sensilla (Lin et al., 1994; Jeram et al., 1995; Nishino and Field, 2003; Strauß and Stritih, 2017). In tettigoniids and cave crickets, the IO responds to substrate vibration but also airborne sound (Kalmring et al., 1994; Čokl et al., 1995; Stölting and Stumpner, 1998). In orthopteroid insects, the IO and DO can occur in diverse neuroanatomical organizations, which are as a compact group of sensilla (cockroach: Schnorbus, 1971), to extending dorsally in the leg (raspy crickets: Strauß and Lakes-Harlan, 2008; weta: Strauß et al., 2017), and linear sensilla in part of the organ (cave cricket: Jeram et al., 1995) or the complete organ (stick insects: Strauß, 2020b). The organization of linear sensilla in Ensifera and stick insects, independent of tympanal membranes, is an evolutionary convergence in these two taxa (Strauß and Lakes-Harlan, 2013). The specific organization of the DO in the common ancestor of Orthoptera and Phasmatodea is not known, as species from more basal taxa of Polyneoptera (Wipfler et al., 2019) from Plecoptera (Wittig, 1955) and Dermaptera (Friedrich, 1929) lack a DO and have only the SGO. However, all other orthopteroid taxa studied so far have more compact sensilla in the DO/IO, which can differ in their attachment structures (Schnorbus, 1971; Lin et al., 1995; Eberhard et al., 2010). The DO in locusts (Lin et al., 1995) and especially the IO in cave crickets (Jeram et al., 1995) as early branching Ensifera (Song et al., 2020), are simpler in their neuroanatomy. This indicates that the elaboration in Ensifera was absent in the ground pattern of this group (Strauß and Lakes-Harlan, 2009).
The DO/IO position in the hemolymph channel distal to the SGO could result in the extension in distal direction, when the number of sensilla is increased (Jeram et al., 1995; Strauß and Lakes-Harlan, 2013). In stick insects, the overall organization of tibial trachea and muscle/hemolymph channels and their proportions are similar to those seen in cave crickets, which also have long and very slender legs (Jeram et al., 1995; Stritih-Peljhan et al., 2019). The structure of the sensory organs seems not to be primarily affected by the leg elongation in stick insects, as their SGO morphology is similar to other orthopteroid insects (Lakes-Harlan and Strauß, 2014), and the distally located IO in cave crickets takes even more space in the anterior hemolymph channel (Jeram et al., 1995). Therefore, the stick insect DO would not be forced to extend only in the distal direction by forming a linear organ.
A linear organization is also found in atympanate sensory organs in some lineages of Orthoptera, suggested to contribute to the detection of vibrational signals from conspecifics (Strauß and Lakes-Harlan, 2008; Strauß et al., 2017). The organs distally of the SGO in Orthoptera are usually placed closely to the leg trachea (Jeram et al., 1995; Lin et al., 1995). This likely makes them sensitive to both vibrational stimuli and airborne sound (Kalmring et al., 1994; Čokl et al., 1995). In crickets, the auditory sensilla and accessory cells connect to the dorsal cuticle by a covering membrane (Michel, 1974; Oldfield et al., 1986; Nishino et al., 2019). In tettigoniids, the cap cells over the auditory sensilla’s dendrites are covered by the tectorial membrane and held by supporting bands (Schumacher, 1975; Lakes and Schikorski, 1990; Lin et al., 1994). The stick insects DO is broadest in the proximal part, where the organ attaches to the inner cuticle in the hemolymph channel, with a notable distance to the anterior trachea. Only the distal part, which is smaller in diameter, is close to the anterior trachea. Thus, the DO lacks the clear morphological adaptations seen in the elaborate tympanal hearing organs (Ball et al., 1989; Lakes and Schikorski, 1990; Lin et al., 1994; Hummel et al., 2017; Nishino et al., 2019). The stick insect DO therefore shows a unique organization among orthopteroid insects with the linearly arranged sensilla with distally oriented dendrites and long accessory cells. The neuronal convergence between the tympanal organs and the DO in this insect group is striking, while the functional anatomy of the stick insect DO indicates a sensory specialization other than detecting air-borne sound, and it likely detects vibrational stimuli.
Data Availability Statement
The cropped but otherwise unchanged μCT-scans presented in this study can be accessed at Zenodo: doi: 10.5281/zenodo.3856676.
Author Contributions
JS, LM, and PTR: carried out the experiments and edited the manuscript. JS: carried out the preparations, assembled figures, and drafted the manuscript. JS and PTR: analyzed the data. PTR: provided reconstructions and videos. All authors read the manuscript and agreed with the publication.
Funding
Funded by the Deutsche Forschungsgemeinschaft (DFG, German Research Foundation) – STR 1329/2-1.
Conflict of Interest
The authors declare that the research was conducted in the absence of any commercial or financial relationships that could be construed as a potential conflict of interest.
Acknowledgments
JS would like to thank Reinhard Lakes-Harlan (Gießen) for support of this study, as well as Mirjam Buß and Anja Schnecko for assisting with raising insects. PTR expresses his gratitude to Alexander Blanke (Bonn) for his support. LM thanks Thomas Wesener (ZFMK) for support and Benjamin Wipfler (ZFMK) for discussions. We thank Nataša Stritih-Peljhan for insightful comments on the manuscript. We thank two reviewers for their constructive criticism which improved the manuscript.
Supplementary Material
The Supplementary Material for this article can be found online at: https://www.frontiersin.org/articles/10.3389/fevo.2021.632493/full#supplementary-material
Supplementary Figure 1 | The tibia in the thoracic legs of R. artemis.
Supplementary Figure 2 | Posterior structures of the proximal distal organ in Ramulus artemis (foreleg).
Supplementary Figure 3 | The distal structure of the distal organ in Ramulus artemis (midleg).
Supplementary Figure 4 | The distal structure of the distal organ in Sipyloidea sipylus (foreleg).
Supplementary Figure 5 | The distal structure of the distal organ in Sipyloidea sipylus (midleg).
Supplementary Figure 6 | Attachment of the distal organ in S. sipylus (midleg).
Supplementary Figure 7 | The distal organ in Carausius morosus (midleg).
Supplementary Table 1 | Ramulus artemis SGOC sensilla.
Supplementary Video 1 | 3D-Reconstruction of the subgenual organ complex in Ramulus artemis.
Supplementary Video 2 | 3D-Reconstruction of the subgenual organ complex in Sipyloidea sipylus.
Footnotes
References
Ball, E. E., and Field, L. H. (1981). Structure of the auditory system of the weta Hemideina crassidens (Blanchard, 1851) (Orthoptera, Ensifera, Gryllacridoidea, Stenopelmatidae). 1. morphology and histology. Cell Tissue Res. 217, 321–344. doi: 10.1007/BF00233584
Ball, E. E., Oldfield, B. P., and Michel Rudolph, K. (1989). “Auditory organ structure, development, and function,” in Cricket Behavior and Neurobiology, eds F. Huber, T. Moore, and W. Loher (Ithaca, NY: Cornell University Press), 391–422. doi: 10.7591/9781501745904-015
Barth, F. G. (2019). Mechanics to pre-process information for the fine tuning of mechanoreceptors. J. Comp. Physiol. A 205, 661–686. doi: 10.1007/s00359-019-01355-z
Bässler, U. (1965). Proprioreceptoren am Subcoxal-und Femur-Tibia-Gelenk der Stabheuschrecke Carausius morosus und ihre Rolle bei der Wahrnehmung der Schwerkraftrichtung. Kybernetik 2, 168–193.
Bässler, U. (1977). Sense organs in the femur of the stick insect and their relevance to the control of position of the femur-tibia-joint. J. Comp. Physiol. 121, 99–113. doi: 10.1007/BF00614183
Bässler, U. (1983). Neural Basis of Elemantary Behavior in Stick Insects. Berlin: Springer. doi: 10.1007/978-3-642-68813-3
Bässler, U. (1993). The femur-tibia control system of stick insects – a model system for the study of the neural basis of joint control. Brain Res. Rev. 18, 207–226. doi: 10.1016/0165-0173(93)90002-H
Bässler, U., and Büschges, A. (1998). Pattern generation for stick insect walking movements – multisensory control of a locomotor program. Brain Res. Rev. 27, 65–88. doi: 10.1016/S0165-0173(98)00006-X
Bedford, G. O. (1978). Biology and ecology of the Phasmatodea. Annu. Rev. Entomol. 23, 125–149. doi: 10.1146/annurev.en.23.010178.001013
Bradler, S., and Buckley, T. R. (2018). “Biodiversity of Phasmatodea,” in Insect Biodiversity: Science and Society, Volume II, eds R. G. Foottit and P. H. Adler (Hoboken, NJ: Wiley-Blackwell), 281–313. doi: 10.1002/9781118945582.ch11
Carlberg, U. (1984). Flight in female Sipyloidea sipylus (Westwood) (Insecta: Phasmida). Zool. Jb. Physiol. 88, 9–14.
Carlberg, U. (1985). Secondary defence in Carausius morosus (de Sinety) (Insecta: Phasmida). Zool. Anz. 215, 373–384.
Carlberg, U. (1989). Defensive stridulation in Heteropteryx dilatata Parkinson (Insecta: Phasmida). Zool. Anz. 223, 165–173.
Cocroft, R. B., and Rodriguez, R. L. (2005). The behavioral ecology of insect vibrational communication. Bioscience 55, 323–334. doi: 10.1641/0006-3568(2005)055[0323:TBEOIV]2.0.CO;2
Cocroft, R. B., Tieu, T. D., Hoy, R. R., and Miles, R. N. (2000). Directionality in the mechanical response to substrate vibration in the treehopper (Hemiptera: Membracidae: Umbonia crassicornis). J. Comp. Physiol. A 186, 695–705. doi: 10.1007/s003590000123
Čokl, A., Kalmring, K., and Rössler, W. (1995). Physiology of atympanate tibial organs in forelegs and midlegs of the cave-living Ensifera, Troglophilus neglectus (Raphidophoridae, Gryllacridoidea). J. Exp. Zool. 273, 376–388. doi: 10.1002/jez.1402730503
Čokl, A., and Virant-Doberlet, M. (2009). ““Vibrational communication”,” in Encyclopedia of Insects, eds V. H. Resh and R. T. Cardé (Amsterdam: Academic Press), 1034–1038. doi: 10.1016/B978-0-12-374144-8.00271-X
Čokl, A., Blassioli-Moraes, M. C., Laumann, R. A., Žunič, A., and Borges, M. (2019). “Stinkbugs: multisensory communication with chemical and vibratory signals transmitted through different media,” in Biotremology: Studying Vibrational Behavior, eds P. S. M. Hill, R. Lakes-Harlan, V. Mazzoni, P. Narins, M. Virant-Doberlet, and A. Wessel (Cham: Springer), 91–122. doi: 10.1007/978-3-030-22293-2_7
Čokl, A., Virant-Doberlet, M., and Zorović, M. (2006). “Sense organs involved in the vibratory communication of bugs,” in Insect Sounds and Communication: Physiology, Behaviour, Ecology and Evolution, eds S. Drosopoulos and M. Claridge (Boca Raton FL: CRC Press), 71–80. doi: 10.1201/9781420039337.ch4
Devetak, D., and Amon, T. (1997). Substrate vibration sensitivity of the leg scolopidial organs in the green lacewing Chrysoperla carnea. J. Insect Physiol. 43, 433–437. doi: 10.1016/S0022-1910(96)00121-7
Ebendt, R., Friedel, J., and Kalmring, K. (1994). Central projection of auditory receptors in the prothoracic ganglion of the bushcricket Psorodonotus illyricus (Tettigoniidae): computer-aided analysis of the end branch pattern. J. Neurobiol. 25, 35–49. doi: 10.1002/neu.480250104
Eberhard, M. J. B., Lang, D., Metscher, B., Pass, G., Picker, M. D., and Wolf, H. (2010). Structure and sensory physiology of the leg scolopidial organs in Mantophasmatodea and their role in vibrational communication. Arthropod Struct. Dev. 39, 230–241. doi: 10.1016/j.asd.2010.02.002
Field, L. H., and Matheson, T. (1998). Chordotonal organs of insects. Adv. Insect Physiol. 27, 57–228. doi: 10.1016/S0065-2806(08)60013-2
Field, L. H., and Pflüger, H.-J. (1989). The femoral chordotonal organ: a bifunctional (Locusta migratoria) sense organ? Comp. Biochem. Physiol. A 93, 729–743. doi: 10.1016/0300-9629(89)90494-5
French, A. S. (1988). Transduction mechanisms of mechanosensilla. Annu. Rev. Entomol. 33, 39–58. doi: 10.1146/annurev.en.33.010188.000351
Friedrich, H. (1929). Vergleichende Untersuchungen über die tibialen scolopalorgane einiger orthopteren. Z. Wiss. Zool. 134, 84–148.
Godden, D. H. (1972). The motor innervation of the leg musculature and motor output duringthanatosis in the stick insect. Carausius morosus. Br. J. Comp. Physiol. 80, 201–225. doi: 10.1007/BF00696491
Hill, P. S. M. (2008). Vibrational Communication in Animals. Cambridge, MA: Harvard University Press.
Homberg, U., and Paech, U. (2002). Ultrastructure and orientation of ommatidia in the dorsal rim area of the locust compound eye. Arthropod Struct. Dev. 30, 271–280. doi: 10.1016/S1467-8039(02)00010-5
Howse, P. E. (1968). “The fine structure and functional organization of chordotonal organs”, in invertebrate receptors,” in Proceedings of the Symposium of the Zoological Society London, eds J. D. Carthy and G. E. Newell (London: Academic Press), 167–198.
Hummel, J., Kössl, M., and Nowotny, M. (2017). Morphological basis for a tonotopic design of an insect ear. J. Comp. Neurol. 525, 2443–2455. doi: 10.1002/cne.24218
Hustert, R. (1974). Morphologie und Atmungsbewegungen des 5. Abdominalsegments von Locusta migratoria migratorioides. Zool. Jb. Physiol. 78, 157–174.
Imaizumi, K., and Pollack, G. S. (1999). Neural coding of sound frequency by cricket auditory receptors. J. Neurosci. 19, 1508–1516. doi: 10.1523/JNEUROSCI.19-04-01508.1999
Jeram, S., Rössler, W., Čokl, A., and Kalmring, K. (1995). Structure of atympanate tibial organs in legs of the cave-living Ensifera, Troglophilus neglectus (Gryllacrididae, Raphidophoridae). J. Morphol. 223, 109–118. doi: 10.1002/jmor.1052230110
Kalmring, K., Rössler, W., and Unrast, C. (1994). Complex tibial organs in the forelegs, midlegs, and hindlegs of the bushcricket Gampsocleis gratiosa (Tettigoniidae): comparison of the physiology of the organs. J. Exp. Zool. 270, 155–161. doi: 10.1002/jez.1402700205
Kamikouchi, A., Inagaki, H. K., Effertz, T., Hendrich, O., Fiala, A., Göpfert, M. C., et al. (2009). The neural basis of drosophila gravity-sensing and hearing. Nature 458, 165–1671. doi: 10.1038/nature07810
Kavlie, R. G., and Albert, J. T. (2013). Chordotonal organs. Curr. Biol. 23, R334–R335. doi: 10.1016/j.cub.2013.03.048
Kilpinen, O., and Storm, J. (1997). Biophysics of the subgenual organ of the honeybee, Apis mellifera. J. Comp. Physiol. A 181, 309–318. doi: 10.1007/s003590050117
Kittmann, R., and Schmitz, J. (1992). Functional specialisation of the scoloparia of the femoral chordotonal organ in stick insects. J. Exp. Biol. 173, 91–108.
Lakes, R., and Schikorski, T. (1990). “Neuroanatomy of tettigoniids,” in The Tettigoniidae: Biology, Systematics and Evolution, eds W. J. Bailey and D. C. F. Rentz (Bathurst: Crawford House Press), 166–190. doi: 10.1007/978-3-662-02592-5_10
Lakes-Harlan, R., and Strauß, J. (2014). “Functional morphology and evolutionary diversity of vibration receptors in insects,” in Studying Vibrational Communication, eds R. Cocroft, M. Gogala, P. S. M. Hill, and A. Wessel (Berlin: Springer), 277–302. doi: 10.1007/978-3-662-43607-3_14
Land, M. F., and Nilsson, D.-E. (2012). Animal Eyes. Second edition. Oxford: Oxford University Press. doi: 10.1093/acprof:oso/9780199581139.001.0001
Limaye, A. (2012). “Drishti: a volume exploration and presentation tool,” in Proceedings of the. SPIE Developments in X-Ray Tomography VIII, 85060X, (San Diego, CA). doi: 10.1117/12.935640
Lin, Y., Klamring, K., Jatho, M., Sickmann, T., and Rössler, W. (1993). Auditory receptor organs in the forelegs of Gampsocleis gratiosa (Tettigoniidae): morphology and function of the organs in comparison to the frequency parameters of the conspecific song. J. Exp. Zool. 267, 377–388. doi: 10.1002/jez.1402670404
Lin, Y., Rössler, W., and Kalmring, K. (1994). Complex tibial organs in fore-, mid-, and hindlegs of the bushcricket Gampsocleis gratiosa (Tettigoniidae): comparison of morphology of the organs. J. Morphol. 221, 191–198. doi: 10.1002/jmor.1052210208
Lin, Y., Rössler, W., and Kalmring, K. (1995). Morphology of the tibial organs of Acrididae: comparison of subgenual and distal organs in fore-, mid-, and hindlegs of Schistocerca gregaria (Acrididae, Catantopidae) and Locusta migratoria (Acrididae, Oedipodinae). J. Morphol. 226, 351–360. doi: 10.1002/jmor.1052260310
Mantziaris, C., Bockemühl, T., and Büschges, A. (2020). Central pattern generating networks in insect locomotion. Dev. Neurobiol. 80, 16–30. doi: 10.1002/dneu.22738
Matheson, T., and Field, L. H. (1990). Innervation of the metathoracic femoral chordotonal organ of Locusta migratoria. Cell Tissue Res. 259, 551–560. doi: 10.1007/BF01740783
Matsuo, E., and Kamikouchi, A. (2013). Neuronal encoding of sound, gravity, and wind in the fruit fly. J. Comp. Physiol. A 199, 253–262. doi: 10.1007/s00359-013-0806-x
Metscher, B. D. (2009). MicroCT for comparative morphology: simple staining methodsallow high-contrast 3D imaging of diverse non-mineralized animal tissues. BMC Physiol. 9:11. doi: 10.1186/1472-6793-9-11
Michel, K. (1974). Das Tympanalorgan von Gryllus bimaculatus deGeer (Saltatoria. Gryllidae). Z. Morphol. Tiere 77, 285–315. doi: 10.1007/BF00298805
Michel, K., Amon, T., and Čokl, A. (1982). The morphology of the leg scolopidial organs in Nezara viridula (L.) (Heteroptera, Pentatomidae). Rev. Can. Biol. Exp. 42, 139–150.
Michelsen, A. (2014). “Mechanical signals in honeybee communication,” in Studying Vibrational Communication, eds R. Cocroft, M. Gogala, P. S. M. Hill, and A. Wessel (Berlin: Springer), 333–347. doi: 10.1007/978-3-662-43607-3_17
Montealegre-Z, F., and Robert, D. (2015). Biomechanics of hearing in katydids. J. Comp. Physiol. A 201, 5–18. doi: 10.1007/s00359-014-0976-1
Nishino, H. (2000). Topographic mapping of the axons of the femoral chordotonal organ neurons in the cricket Gryllus bimaculatus. Cell Tissue Res. 299, 145–157. doi: 10.1007/s004410050013
Nishino, H. (2003). Somatotopic mapping of chordotonal organ neurons in a primitive Ensiferan, the New Zealand tree weta Hemideina femorata: I. femoral chordotonal organ. J. Comp. Neurol. 464, 312–326. doi: 10.1002/cne.10779
Nishino, H., and Field, L. H. (2003). Somatotopic mapping of chordotonal organ neurons in a primitive Ensiferan, the New Zealand tree weta Hemideina femorata: II. complex tibial organ. J. Comp. Neurol. 464, 327–342. doi: 10.1002/cne.10780
Nishino, H., Domae, M., Takanashi, T., and Okajima, T. (2019). Cricket tympanal organ revisited: morphology, development and possible functions of the adult-specific chitin core beneath the anterior tympanal membrane. Cell Tissue Res. 377, 193–214. doi: 10.1007/s00441-019-03000-2
Nishino, H., Mukai, H., and Takanashi, T. (2016). Chordotonal organs in Hemipteran insects: unique peripheral structures but conserved central organization revealed by comparative neuroanatomy. Cell Tissue Res. 366, 549–572. doi: 10.1007/s00441-016-2480-0
Oldfield, B. P., Kleindienst, H. U., and Huber, F. (1986). Physiology and tonotopic organization of auditory receptors in the cricket Gryllus bimaculatus DeGeer. J. Comp. Physiol. A. 159, 457–464. doi: 10.1007/BF00604165
Pflüger, H.-J., and Field, L. H. (1999). A locust chordotonal organ coding for proprioceptive and acoustic stimuli. J. Comp. Physiol. A 184, 169–183. doi: 10.1007/s003590050316
Ridgel, A. L., Frazier, S. F., and Zill, S. N. (2001). Dynamic responses of tibial campaniform sensilla studied by substrate displacement in freely moving cockroaches. J. Comp. Physiol. A 187, 405–420. doi: 10.1007/s003590100213
Rohrseitz, K., and Kilpinen, O. (1997). Vibration transmission characteristics of the legs of freely standing honeybees. Zoology 100, 80–84.
Römer, H., and Schmidt, A. K. D. (2016). Directional hearing in insects with internally coupled ears. Biol. Cybern. 110, 247–254. doi: 10.1007/s00422-015-0672-4
Scherberich, J., Hummel, J., Schöneich, S., and Nowotny, M. (2017). Functional basis of the sexual dimorphism in the auditory fovea of the duetting bushcricket Ancylecha fenestrata. Proc. Royal Soc. B 284:20171426. doi: 10.1098/rspb.2017.1426
Schneider, E. S., Römer, H., Robillard, T., and Schmidt, A. K. D. (2017). Hearing with exceptionally thin tympana: ear morphology and tympanal membrane vibrations in eneopterine crickets. Sci. Rep. 7:15266. doi: 10.1038/s41598-017-15282-z
Schnorbus, H. (1971). Die subgenualen sinnesorgane von Periplaneta americana: histologie und vibrationsschwellen. Z. Vergl. Physiol. 71, 14–48.
Schumacher, R. (1975). Scanning-electron-microscope description of the tibial tympanal organ of the Tettigonioidea (Orthoptera, Ensifera). Z. Morph. Tiere 81, 209–219. doi: 10.1007/BF00278370
Shaw, S. R. (1994b). Re-evaluation of the absolute threshold and response mode of the most sensitive known “vibration” detector, the cockroach’s subgenual organ: a cochlea-like displacement threshold and a direct response to sound. J. Neurobiol. 25, 1167–1185. doi: 10.1002/neu.480250911
Shaw, S. R. (1994a). Detection of airborne sound by a cockroach ‘vibration detector’: a possible missing link in insect auditory evolution. J. Exp. Biol. 192, 13–47.
Simon, S., Letsch, H., Bank, S., Buckley, T. R., Donath, A., Liu, S., et al. (2019). Old world and New world Phasmatodea: phylogenomics resolve the evolutionary history of stick and leaf insects. Front. Ecol. Evol. 7:345. doi: 10.3389/fevo.2019.00345
Song, H., Béthoux, O., Shin, S., Donath, A., Letsch, H., and Liu, S. (2020). Phylogenomic analysis sheds light on the evolutionary pathways towards acoustic communication in Orthoptera. Nat. Commun. 11:4939. doi: 10.1038/s41467-020-18739-4
Stein, W., and Sauer, A. (1999). Physiology of vibration-sensitive afferents in the femoral chordotonal organ of the stick insect. J. Comp. Physiol. A 184, 253–263. doi: 10.1007/s003590050323
Steinbrecht, R. A. (1999). “Olfactory receptors,” in Atlas of Arthropod Sensory Receptors – Dynamic Morphology in Relation to Function, eds E. Eguchi and Y. Tominaga (Tokyo: Springer), 155–176.
Stölting, H., and Stumpner, A. (1998). Tonotopic organization of auditory receptors of the bushcricket Pholidoptera griseoaptera (Tettigoniidae, Decticinae). Cell Tissue Res. 294, 377–386. doi: 10.1007/s004410051187
Strauß, J., Stritih, N., and Lakes-Harlan, R. (2014). The subgenual organ complex in the cave cricket Troglophilus neglectus (Orthoptera: Rhaphidophoridae): comparative innervation and sensory evolution. Royal Soc. Open. sci. 1:140240. doi: 10.1098/rsos.140240
Strauß, J. (2017). The scolopidial accessory organs and Nebenorgans in orthopteroid insects: comparative neuroanatomy, mechanosensory function and evolutionary origin. Arthropod Struct. Dev. 46, 765–776. doi: 10.1016/j.asd.2017.08.004
Strauß, J. (2020a). Neuronal innervation of the subgenual organ complex and the tibial campaniform sensilla in the stick insect midleg. Insects 11:40. doi: 10.3390/insects11010040
Strauß, J. (2020b). Early postembryogenic development of the subgenual organ complex in the stick insect Sipyloidea sipylus. Arthropod Struct. Dev. 56:100933. doi: 10.1016/j.asd.2020.100933
Strauß, J., and Lakes-Harlan, R. (2008). Neuroanatomy and physiology of the complex tibial organ of an atympanate Ensiferan, Ametrus tibialis (Brunner von Wattenwyl, 1888) (Gryllacrididae, Orthoptera) and evolutionary implications. Brain Behav. Evol. 71, 167–180. doi: 10.1159/000114405
Strauß, J., and Lakes-Harlan, R. (2009). The evolutionary origin of auditory receptors in Tettigonioidea: the complex tibial organ of Schizodactylidae. Naturwissenschaften 96, 143–146. doi: 10.1007/s00114-008-0450-4
Strauß, J., and Lakes-Harlan, R. (2013). Sensory neuroanatomy of stick insects highlights the evolutionary diversity of the orthopteroid subgenual organ complex. J. Comp. Neurol. 521, 3791–3803. doi: 10.1002/cne.23378
Strauß, J., and Lakes-Harlan, R. (2017). Vibrational sensitivity of the subgenual organ complex in female Sipyloidea sipylus stick insects in different experimental paradigms of stimulus direction, leg attachment, and ablation of a connective tibial sense organ. Comp. Biochem. Physiol. A 203, 100–108. doi: 10.1016/j.cbpa.2016.09.002
Strauß, J., and Stritih, N. (2017). Neuronal regression of internal leg vibroreceptor organs in a cave-dwelling insect (Orthoptera: Rhaphidophoridae: Dolichopoda araneiformis). Brain Behav. Evol. 89, 104–116. doi: 10.1159/000462957
Strauß, J., Lomas, K., and Field, L. H. (2017). The complex tibial organ of the New Zealand ground weta: sensory adaptations for vibrational signal detection. Sci. Rep. 7:2031. doi: 10.1038/s41598-017-02132-1
Stritih Peljhan, N., and Strauß, J. (2018). Mechanical leg response to vibration stimuli in cave crickets and implications for vibrosensory organ functions. J. Comp. Physiol. A 205, 687–702. doi: 10.1007/s00359-018-1271-3
Stritih-Peljhan, N., Rühr, P. T., Buh, B., and Strauß, J. (2019). Low-frequency vibration transmission and mechanosensory detection in the legs of cave crickets. Comp. Biochem. Physiol. A 233, 89–96. doi: 10.1016/j.cbpa.2019.04.003
Stumpner, A. (1996). Tonotopic organization of the hearing organ in a bushcricket. Naturwissenschaften 83, 81–84. doi: 10.1007/BF01141875
Stumpner, A., and Nowotny, M. (2014). “Neural processing in the bush-cricket auditory pathway,” in Insect Hearing and Acoustic Communication, ed. B. Hedwig (Berlin: Springer), 143–166. doi: 10.1007/978-3-642-40462-7_9
Takanashi, T., Fukaya, M., Nakamuta, K., Skals, N., and Nishino, H. (2016). Substrate vibrations mediate behavioral responses via femoral chordotonal organs in a cerambycid beetle. Zool. Lett. 2:18. doi: 10.1186/s40851-016-0053-4
Tuthill, J. C., and Azim, E. (2018). Proprioception. Curr. Biol. 28, R194–R203. doi: 10.1016/j.cub.2018.01.064
van Staaden, M. J., and Römer, H. (1998). Evolutionary transition from stretch to hearing in ancient grasshoppers. Nature 394, 773–776. doi: 10.1038/29517
Windmill, J. F. C., and Jackson, J. C. (2016). “Mechanical specializations of insect ears,” in Insect Hearing, eds G. S. Pollack, A. C. Mason, A. N. Popper, and R. R. Fay (Cham: Springer), 125–157. doi: 10.1007/978-3-319-28890-1_6
Wipfler, B., Letsch, H., Frandsen, P. B., Kapli, P., Mayer, C., Bartel, D., et al. (2019). Evolutionary history of Polyneoptera and its implications for our understanding of early winged insects. Proc. Nat. Acad. Sci. 116, 3024–3029. doi: 10.1073/pnas.1817794116
Wright, B. R. (1976). “Limb and wing receptors in insects, chelicerates and myriapods,” in Structure and Function of Proprioceptors in the Invertebrates, ed. P. J. Mill (London: Chapman and Hall), 323–386.
Wittig, G. (1955). Untersuchungen am Thorax von Perla abdominalis Burm. (Larve und Imago) unter besonderer Berücksichtigung des peripheren Nervensystems und der Sinnesorgane. Zool. JB Anat. Ontogen. Tiere. 74, 491–570.
Yack, J. E. (1993). Janus green B as a rapid, vital stain for peripheral nerves and chordotonal organs in insects. J. Neurosci. Methods 49, 17–22. doi: 10.1016/0165-0270(93)90105-Z
Yack, J. E. (2004). The structure and function of auditory chordotonal organs in insects. Microsc. Res. Tech. 63, 315–337. doi: 10.1002/jemt.20051
Keywords: mechanoreception, chordotonal organ, stick insect, neuroanatomy, vibration, hearing, sensory evolution
Citation: Strauß J, Moritz L and Rühr PT (2021) The Subgenual Organ Complex in Stick Insects: Functional Morphology and Mechanical Coupling of a Complex Mechanosensory Organ. Front. Ecol. Evol. 9:632493. doi: 10.3389/fevo.2021.632493
Received: 23 November 2020; Accepted: 21 January 2021;
Published: 23 February 2021.
Edited by:
Fernando Montealegre-Z, University of Lincoln, United KingdomReviewed by:
Volker Dürr, Bielefeld University, GermanyBerthold Gerhard Hedwig, University of Cambridge, United Kingdom
Copyright © 2021 Strauß, Moritz and Rühr. This is an open-access article distributed under the terms of the Creative Commons Attribution License (CC BY). The use, distribution or reproduction in other forums is permitted, provided the original author(s) and the copyright owner(s) are credited and that the original publication in this journal is cited, in accordance with accepted academic practice. No use, distribution or reproduction is permitted which does not comply with these terms.
*Correspondence: Johannes Strauß, am9oYW5uZXMuc3RyYXVzc0BwaHlzem9vbC5iaW8udW5pLWdpZXNzZW4uZGU=
†ORCID: Johannes Strauß, orcid.org/0000-0002-0494-2578; Leif Moritz, orcid.org/0000-0002-6028-5189; Peter T. Rühr, orcid.org/0000-0003-2776-6172