- 1Instituto de Genética “E. A. Favret”, Instituto Nacional de Tecnología Agropecuaria (INTA) – Grupo Vinculado al Instituto de Agrobiotecnología y Biología Molecular (IABIMO-CONICET), Hurlingham, Argentina
- 2Instituto de Recursos Biológicos, INTA, Hurlingham, Argentina
- 3Estación Experimental Agropecuaria Reconquista, INTA, Santa Fe, Argentina
- 4Estación Experimental Agropecuaria Rafaela, INTA, Santa Fe, Argentina
- 5Unidad Integrada INTA Balcarce – Universidad Nacional de Mar del Plata, Buenos Aires, Argentina
- 6Estación Experimental H. Ascasubi, INTA, Buenos Aires, Argentina
The Western honey bee, Apis mellifera, is an important species in providing honey and pollination services globally. The mite Varroa destructor is the major threat to A. mellifera, and it is associated with the severe colony winter mortality reported in recent decades. However, Varroa mite tolerant or resistant populations of A. mellifera have been detected around the world. A proposed mechanism responsible for limiting mite population growth in the colonies is grooming behavior, the physical removal and injury of mites from the adult bee bodies by individual workers or by their nest-mates. This behavioral strategy has been poorly studied in V. destructor-resistant colonies worldwide, especially in honey bee populations of European origin. In Argentina, honey bee stocks showing survival without mite treatment have been reported. In the present study, European-derived A. mellifera populations established in the Transition Chaco eco-region (Santa Fe province), with a subtropical climate, were characterized at the colony level. A honey bee stock showing natural Varroa-resistance (M) was compared to a Varroa-susceptible stock (C) for parameters of colony status (colony strength, percentage of Varroa infestation in adults and brood, hygienic behavior) and for indirect measures of grooming (percentage of fallen mites and damaged mites). M colonies showed lower phoretic and brood infestation and higher hygienic behavior in early autumn, and higher survival and population strength after wintering, in comparison with C colonies. The mean percentages of fallen mites and of damaged mites, and the injury to mites were higher in M than in C colonies. Our results suggest that, by modulating the parasitization dynamics in colonies, grooming behavior would be associated with the higher survival of Varroa-resistant stock. This study sheds light on how honey bee colonies can adaptively respond to mite pressure by modeling their behavior to resist Varroosis and provides evidence for grooming as an emerging factor evolving by natural selection. Percentage of damaged mites appears to be a reliable measure to enhance this behavior in honey bee colonies by selective breeding. Finally, the importance of improving and protecting locally adapted honey bee populations with natural Varroa resistance for regional apiculture is discussed.
Introduction
The Western honey bee, Apis mellifera (Linnaeus), is one of the most valuable pollinators worldwide (Aizen and Harder, 2009; Gallai et al., 2009; Hung et al., 2018), providing essential pollination services to agroecosystems as well as profitable hive products for the apicultural sector (Morse and Calderone, 2000; Klein et al., 2007). Over the last few decades, honey bee colony losses have increased dramatically, as reported mainly in the Northern Hemisphere (Neumann and Carreck, 2010; Potts et al., 2010b; vanEngelsdorp et al., 2011), but also in South Africa (Pirk et al., 2014), Oceania (Brown et al., 2018), and South America (Vandame and Palacio, 2010; Maggi et al., 2016; Antúnez et al., 2017; Requier et al., 2018). The possible driving factors of these losses include a growing number of interacting threats, such as environment and climate change, nutritional deficiencies, pesticides, parasites, and pathogens (reviewed by Le Conte and Navajas, 2008; Potts et al., 2010a; Goulson et al., 2015).
The mite Varroa destructor (Anderson and Trueman) is considered the main parasite threatening A. mellifera colony survival, mainly in honey bee populations of European origin (Rosenkranz et al., 2010). Although the mite does not directly kill the bees, it has strong effects by weakening brood and adults through feeding on them (Amdam et al., 2004; Zaobidna et al., 2017; Ramsey et al., 2019) and transmitting several honey bee viruses (Dainat and Neumann, 2013; Francis et al., 2013; Mondet et al., 2014; McMenamin and Genersch, 2015). Together, these effects can affected the wing development and shorten the life span of worker bees and generate an epidemic disease within the colony, eventually resulting in colony death (Boecking and Genersch, 2008; Neumann et al., 2012; Van Dooremalen et al., 2012).
Today, most managed A. mellifera colonies depend on mite control treatments to survive (Rosenkranz et al., 2010). However, several Varroa-surviving honey bee populations have been documented around the world as a result of selective breeding or natural selection (e.g., Locke, 2016; Le Conte and Mondet, 2017). Bees may survive Varroa through the expression of resistance or tolerance traits. Resistance involves a reduction in Varroa growth, while tolerance reduces parasitic burden despite similar levels of Varroa growth (Schneider and Ayres, 2008; Kurze et al., 2016). Resistance or tolerance to V. destructor mites is a typical characteristic of Africanized bees from South and Central America (e.g., Martin and Medina, 2004; Mondragón et al., 2005; Rivera-Marchand et al., 2012). There are also accounts of Varroa resistant and tolerant European-derived A. mellifera populations from North America, Europe, and other parts of the world (e.g., Fries et al., 2006; Le Conte et al., 2007; Seeley, 2007; Pritchard, 2016). Specific adaptive behaviors have evolved in these honey bee populations, mainly related to resistance mechanisms, such as hygienic behavior specifically targeting Varroa-infested capped brood cells (VSH), recapping, and grooming (reviewed by Mondet et al., 2020).
Grooming behavior involves the physical removal, and often injury, of parasitic mites from the body of adult bees by individual workers or by their nest-mates. Through this behavior, the parasitized bees can dislodge mites themselves using their legs and mandibles (autogrooming) or receiving help from other bees (allogrooming) (Boecking and Spivak, 1999). Grooming is one of the main mechanisms of resistance against mite in A. cerana (Peng et al., 1987), and it is also observed in A. mellifera but expressed at a lower frequency (Boecking and Ritter, 1993; Fries et al., 1996). Despite these observations, several studies have evidenced that grooming behavior confers some degree of resistance against the Varroa mite in populations of Africanized bees (Moretto et al., 1993; Guzman-Novoa et al., 1999, 2002; Arechavaleta-Velasco and Guzmán-Novoa, 2001; Martin and Medina, 2004; Mondragón et al., 2005, 2006; Invernizzi et al., 2015). In European races of honey bees, grooming effectiveness against varroa mites is still unclear, although phenotypic variation for this behavior has been documented (Moosbeckhofer, 1997; Currie and Tahmasbi, 2008; Andino and Hunt, 2011; Ba̧k and Wilde, 2015). Guzman-Novoa et al. (2012) compared mite-resistant and susceptible honey bee stocks of different origins (Africanized bees, Russian, and European races) and found that all resistant stocks showed comparatively higher proportions of injured mites falling from colonies and increased intensity of individual grooming actions in laboratory assays, which underscores the importance of this trait in Varroa resistance. In fact, higher proportions of mite injuries inflicted by grooming have been associated with decreases in mite infestation levels observed in A. mellifera colonies (Moosbeckhofer, 1992; Arechavaleta-Velasco and Guzmán-Novoa, 2001; Mondragón et al., 2005; Guzman-Novoa et al., 2012).
Over the last three decades, numerous breeding programs have been initiated to selectively enhance heritable resistance or tolerance to the mite on specific honey bee populations (reviewed by Guichard et al., 2020). Such developments relied on the identification of specific traits that characterize these populations. This is a critical point since some characteristics that strongly confer mite resistance to some bee populations may not have a great influence on others (Locke, 2016). In Argentina, efforts have been made to identify and select local stocks that survive without mite treatment and characterize the underlying mechanisms. One of the criteria used by local breeding programs is the selection of hygienic behavior. This behavior involves the workers’ detection, uncapping and removal of unhealthy or dead brood (Rothenbuhler, 1964). Based on the pin-killed brood method, Argentinian honey bee populations have been studied and selected (Palacio et al., 2000, 2010). These honey bee stocks were later evaluated in relation to Varroa resistance in regions of temperate climate, where the mite has become a serious problem (Merke, 2016; Visintini, 2018). However, the phenotypic variation of grooming behavior and its contribution to colony survival has not been previously addressed in Varroa-resistant stocks from the country.
The objectives of this study were to characterize a Varroa-surviving honey bee stock located in North-Central Argentina, a region with a subtropical climate, and to evaluate the contribution of grooming behavior to mite-resistance. The integral characterization of this naturally selected honey bee population and the associated varroa mite provides a better understanding of the adaptive ways in which honey bee colonies can respond to mite infestation. Our results contribute to enhancing the management and breeding strategies for regional apiculture.
Materials and Methods
Colonies for the Present Survey
A. mellifera colonies from two stocks were sampled: (1) a Varroa-surviving honey bee population (M, 22 colonies), and (2) a susceptible honey bee population (considered a control to our assays, C, 11 colonies) located at the apiary of Reconquista Agricultural Experimental Station (EEA Reconquista, 29°15′31.8″S 59°44′36.0″W) of the National Institute of Agricultural Technology (INTA). They were surveyed during the 2017–2018 season (Figure 1). This region is defined as Transition Chaco and characterized by a subtropical climate with a dry season. The control population was chosen for its geographical sympatry with the surviving population. Control colonies were headed by commercial queens of European origin and were known to require synthetic acaricide treatments against V. destructor twice a year (one in early autumn and one in early spring) or else suffer severe losses. A previous study (Russo et al., 2018) evidence 60% of overwinter colony mortality for this stock in absence of mite treatment. Colonies of both stocks received the same beekeeping practices and were not subjected to acaricide treatment during the survey.
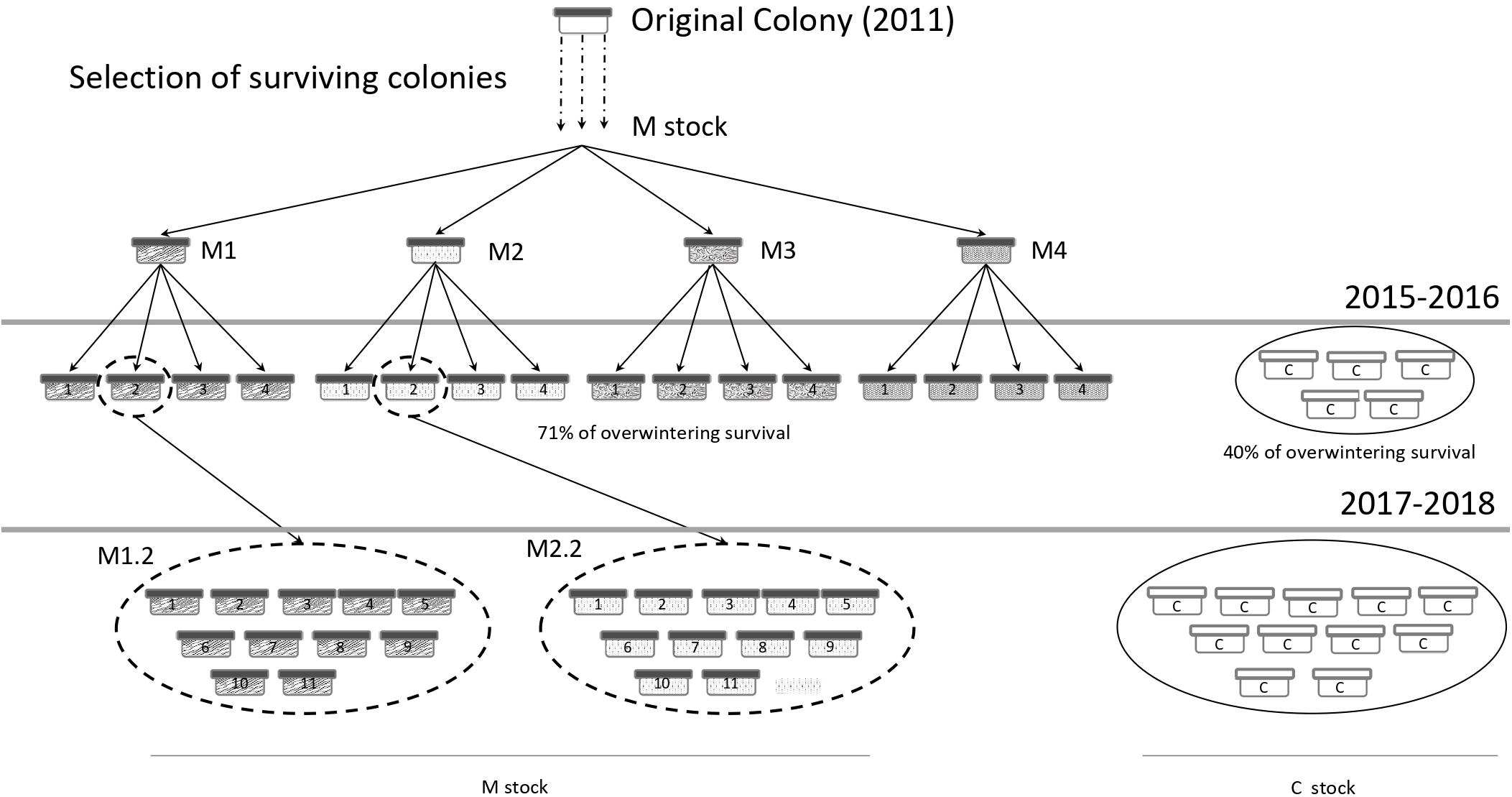
Figure 1. Selection scheme performed to obtain the Varroa-surviving honey bee stock (M) and experimental design of the present survey (2017–2018 season). C corresponds to control colonies.
Origin and Selection of the Varroa-Surviving Honey Bee Stock
The Varroa-surviving stock (M) is a honey bee population that had been kept without mite treatment for 6 years prior to the beginning of the present study (March 2017) (Figure 1). This stock was derived from a single colony from an abandoned commercial apiary at Reconquista locality (north of Santa Fe province, Argentina), where most of the colonies had died. The surviving colony was transported to the EEA Reconquista in 2011 and multiplied. Every spring, daughter colonies that survived winter without Varroa treatment and showed vitality in terms of colony growth were selected for the new generation. In the early spring of 2014, four colonies of M stock (named M1, M2, M3, and M4; Figure 1) were selected as mothers of the next generation and split into four new colonies each. The resulting sixteen daughter honey bee colonies were firstly monitored during the 2015–2016 season (Figure 1) and they showed higher overwintering survival and a higher proportion of fallen mites than the colonies from a commercial control stock (Russo et al., 2018). For the next generation, two colonies of M stock were selected as mothers and multiplied in 11 daughter colonies each to perform the present survey. During the selection process, queens of all colonies were naturally mated.
Genetic Characterization of Stocks
The mitochondrial (mt) haplotypes of all surveyed colonies were analyzed. Briefly, adult workers were collected from all colonies of M and C stocks during spring 2017. Total DNA was extracted from the thorax of one worker per colony following a high-salt protocol (Baruffi et al., 1995). DNA samples of honey bee workers were analyzed using a PCR-RFLP-based method. A fragment of 1,001 bp from the mitochondrial COI-COII region was amplified by PCR using the primers and conditions described by Hall and Smith (1991) and Lobo Segura (2000). PCR products were digested with HinfI (Promega, Madison, MN, United States) following the manufacturer’s recommendations. The restriction fragments were separated on 4% (wt/v) agarose gels, stained with GelRed, and photographed under UV light. The mt haplotypes detected in the restriction analysis using HinfI were assigned as previously described by Agra et al. (2018).
Parameters Measured During the Survey
During the 2017–2018 season, the experimental apiary at EEA Reconquista was visited once in March 2017 (early autumn) and monthly during the active season, from September 2017 (early spring) to February 2018. During the visits, the following measurements were registered in each colony from both stocks (M and C): populations of adult bees and brood, percentage of mite infestation of adult bees, number of naturally fallen mites, and number of damaged mites. Overwintering survival of each stock was also registered. In addition, hygienic behavior and percentage of mite infestation on brood were measured twice, in March 2017 and September 2017.
Overwintering Colony Survival and Bee Population
The number of colonies that survived the winter was registered in spring (October 2017) for both stocks. Adult and brood populations were assessed in each colony by estimating the total area of comb covered by adult bees and brood according to DeGrandi-Hoffman et al. (2008). Briefly, once each hive was opened, frames were sequentially removed, and the percentage of the comb surface covered by adults and brood on both sides were registered. Then, the number of total frames fully occupied by adults and brood was estimated for each colony. The total number of bees per colony (total worker population) was estimated according to Delaplane et al. (2013).
Phoretic and Brood Infestation
The percentage of phoretic Varroa was determined by collecting and examining samples of approximately 300 workers from each colony. The samples were taken from the three central frames of each hive, by collecting the bees in plastic flasks previously filled with 70% ethanol. The number of mites detected in each sample was divided by the number of bees in the sample and multiplied by 100 to obtain the percentage of phoretic Varroa (De Jong et al., 1982). The total phoretic mite population was estimated for each colony using the percentage of phoretic Varroa and the estimated total worker population.
In addition, the percentage of mite infestation on brood was assessed once in autumn (March) and once in spring (October) 2017. Briefly, in each colony from both stocks, a frame with recently sealed brood (pupae not older than the purple- to dark-purple-eye stage) was identified. Fifty sealed brood cells from each side of the frame (a total of 100 cells per colony) were randomly selected and examined for the presence of adult female mites (Branco et al., 2006). The percentage of mite infestation on brood was the number of mite-infested cells.
Grooming Behavior
Grooming behavior was estimated by registering the mite fall and the damaged mites (indirect measures of grooming) (Boecking and Spivak, 1999). To this end, the screen bottom board method described by Pettis and Shimanuki (1999) was used. The original bottom board of each colony was replaced with a screened bottom board, allowing only the mites to fall through it and onto the slide-out inspection board. Before each measurement, the slide board of each colony was removed, cleaned, and reintroduced. Forty-eight hours later, the sliding boards were pulled out and the fallen mites were collected from the debris using a fine hairbrush. All fallen mites from each colony were counted and examined under a stereoscopic magnifying glass. Each mite received a binary score of “undamaged” or “damaged” for the analysis. In these cases, damage to the dorsal shield, gnathosoma, and legs was identified according to Rosenkranz et al. (1997) and Corrêa-Marques et al. (2000). The proportion of damaged mites in each colony was obtained by dividing the number of damaged mites by the total number of fallen mites collected at the end of the collection period (48 h). The proportion of fallen mites was obtained by dividing the number of fallen mites by the estimated total Varroa population of each colony, which represents the fraction of the mite removed by honey bees off their bodies relative to the total mite population present in the colonies.
Hygienic Behavior
Hygienic behavior was measured using the pin-killed brood assay (Newton and Ostasiewski, 1986; Palacio et al., 2000). Briefly, one frame of each colony containing a uniform capped brood was selected. On each frame, capped brood cells contained in a 10 × 5 cm comb section were perforated using an entomological pin (No. 1) to kill the brood. The frames were reintroduced in the original colony and inspected 24 h later to count the number of cells that had been cleaned by the bees. The hygienic activity of the colony was determined using the following equation:
Statistical Analysis
Overwintering colony survival was compared between stocks using a contingency-table analysis. To investigate whether adult bee population (number of frames fully occupied by bees), brood population (number of frames fully occupied by brood), and the percentage of phoretic Varroa differed between stocks and months across the season, separate generalized linear models (GLM) were performed including stock (M, C) and months of the active season (March, September, October, December, January, February) as fixed factors, and colonies as random factors. Logit transformation (ln) was applied to phoretic Varroa data. Similarly, the percentage of hygienic behavior was compared between stocks (M, C) and seasons (early autumn, early spring) by using GLM. Multiple comparisons were performed using Fisher LSD (α = 0.05) in all cases.
Fallen mites and damaged mites were analyzed separately by using the general linear mixed model (GLMM) with a binomial distribution and logit link function (fallen vs. not fallen mites and damaged vs. undamaged mites, respectively) considering stocks and months, as fixed factors, and colonies, as a random factor. In the case of damaged mites, the comparisons between months were performed separately for each stock to obtain a better adjustment to the model. Multiple comparisons were performed using Fisher LSD (α = 0.05). In all cases (GLMs and GLMMs), the Shapiro-Wilks and Levene tests and the residue normality were analyzed. To obtain the most appropriate structure of variance, the Akaike information criterion was used.
In addition, to find relationships between the measurements of grooming behavior and phoretic infestation of adults, the percentages of fallen mites, damaged mites, and phoretic mites were subjected to Spearman Rank Correlation analysis for each stock.
Possible differences in the types of damage on fallen mites from C and M colonies were analyzed with contingency tables. Specifically, the frequency of different categories of damage described above (legs, dorsal shield, gnathosoma) and the frequencies of multiple (legs + body) vs. simple (legs or body) damage were considered.
The frequencies of mite infestation on brood were compared between stocks by using contingency-table analysis. All statistical analyses were performed using InfoStat (Di Rienzo and Montiglio, 2016).
Results
The genetic characterization of honey bee colonies used in the present study showed the presence of 100% of European haplotypes (C1) in both M and C colonies.
The percentage of overwintering survival (March to September 2017) was higher for M (81.8%) than for C (45.4%) stock [ = 4.59, P = 0.032]. The adult bee population across the active season was similar between C and M stocks with a significant difference only in early spring [GLMM results: F(1,31) = 0.01, P = 0.92 for stock; F(5,105) = 24.45, P < 0.001 for month; F(5,105) = 2.59, P = 0.03 for interaction stock × month; post hoc comparisons in Figure 2A]. Specifically in September, the mean number of frames completely covered by bees was higher in M (6.76 ± 0.55) than in C (5.05 ± 0.98) (Figure 2A). Within C stock, the adult bee population was significantly lower in spring (September: 5.5 ± 0.9, and October: 5.8 ± 0.9) than in the other months evaluated (mean value: 8.6 ± 0.3), while no significant differences in this variable was detected across the season for M colonies (mean value: 7.4 ± 0.4) (Figure 2A).
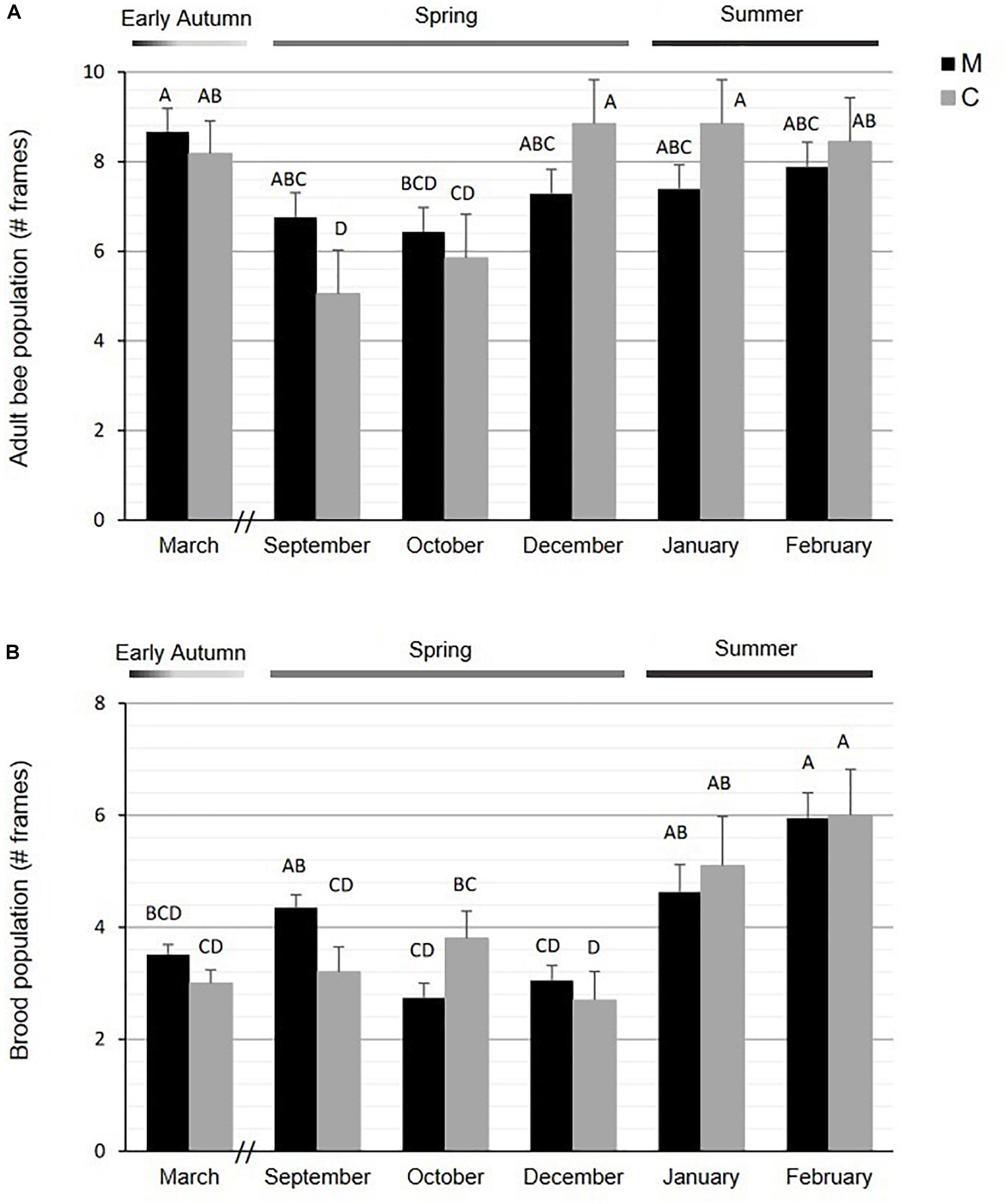
Figure 2. Mean number (± SE) of frames fully occupied by (A) adult bees and (B) brood in M and C stocks. Different letters indicate significant differences in post hoc comparisons by Fisher LSD (α = 005).
Regarding the estimated brood population, variation in the number of frames occupied by brood across the season was detected for both stocks [GLMM results: F(1,52) = 2.91, P = 0.09 for stock; F(5,76) = 20.01, P < 0.001 for month; F(5,76) = 4.47, P < 0.01 for interaction stock × month; Figure 2B]. Though similar brood patterns were observed between stocks in most monitored months, a significant difference was detected between M and C colonies for the mean number of frames with brood in early spring (September: M = 4.35 ± 0.23; C = 3.20 ± 0.45; Figure 2B).
According to the population dynamics of the colonies, the percentage of phoretic Varroa varied throughout the active season [GLMM results: F(1,31) = 2.66, P = 0.11 for stock; F(5,73) = 22.94, P < 0.001 for month; F(5,73) = 2.40, P = 0.049 for interaction stock × month, Figure 3A]. The dynamics of phoretic infestation was similar in M and C colonies, with growing levels from spring to summer, and a peak in early autumn (Figure 3A). The increase in the percentage of phoretic Varroa observed in March was significantly higher for C (5.2 ± 1.2) than for M colonies (11.5 ± 1.7) (Figure 3A).
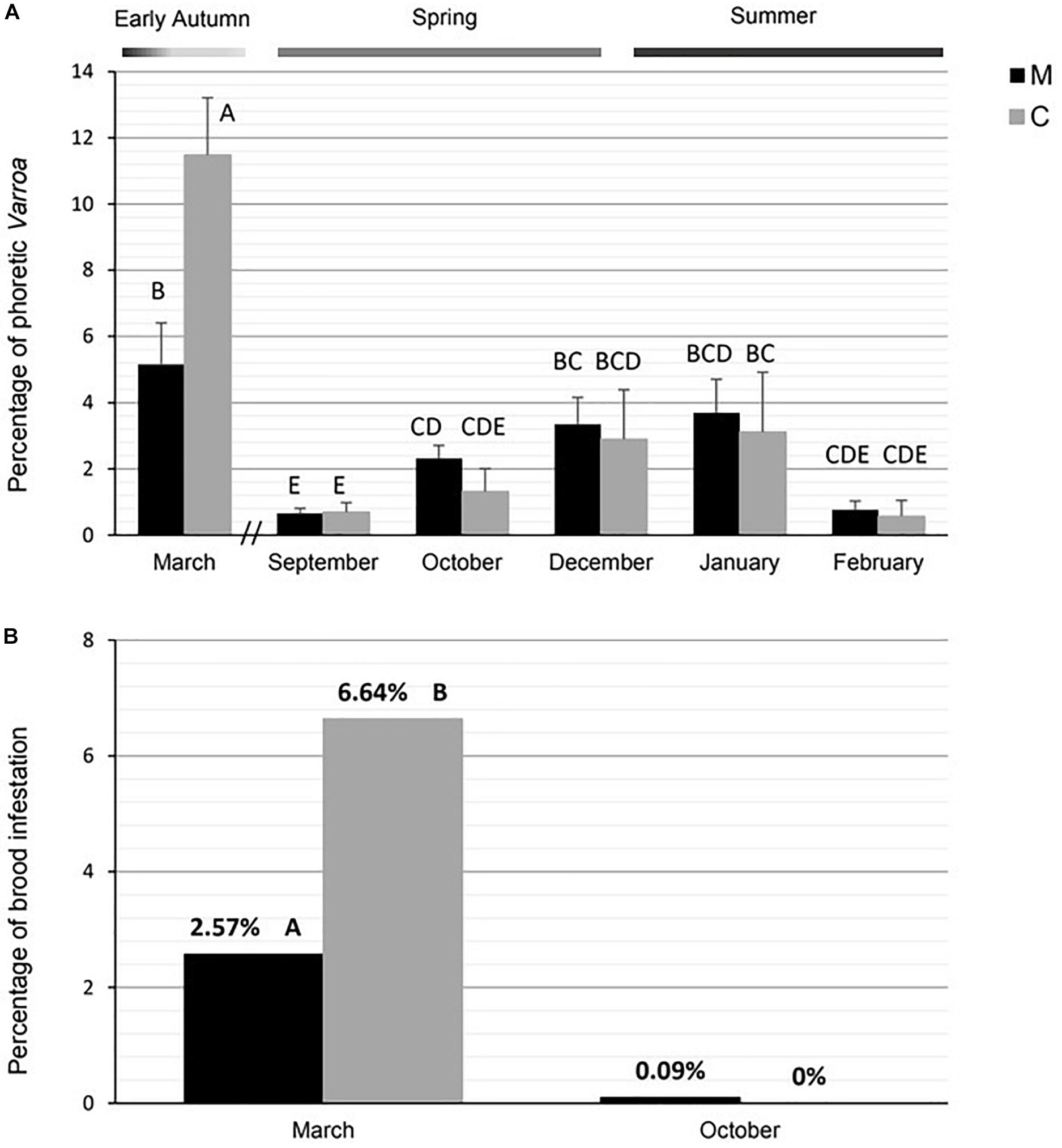
Figure 3. Mean percentage (± SE) of (A) phoretic Varroa and (B) brood infestation in M and C stocks. Different letters indicate significant differences between stocks or months in (A) post hoc comparisons by Fisher LSD or (B) contingency analysis.
Consistent with the results of phoretic Varroa, the percentage of mite infestation on brood was significantly higher in C (6.64%) than in M (2.57%) in early autumn χ2(1) = 31.28, P < 0.001; Figure 3B]. An extremely low percentage of infestation on brood was evident in both M and C stocks during spring [0.09% in M and 0% in C; χ2(1) = 1.05, P = 0.31; Figure 3B].
Hygienic behavior (HB) differed between stocks and seasons [GLMM results: F(1,29) = 5.64, P = 0.02 for stock; F(1,16) = 11.21, P = 0.004 for season; F(1,16) = 3.79, P = 0.07 for interaction stock × season]. Specifically for M stock, HB was similar between spring and autumn, while for C stock, a lower level of HB was observed in autumn than in spring (Table 1). M showed higher HB than C only in autumn (Table 1).
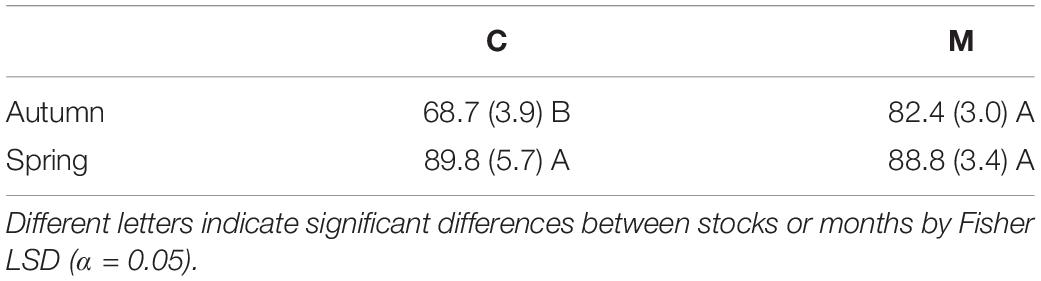
Table 1. Percentage of hygienic behavior (±SE) measured in early autumn (March) and spring (October) for M and C stocks.
The percentage of fallen mites on bottom boards differed between stocks and months, with a significant interaction between factors [GLMM results: F(1,127) = 23.67, P < 0.001 for stock; F(5,127) = 16.16, P < 0.001 for month; F(5,127) = 12.55, P < 0.001 for interaction stock × month; Figure 4A]. Significant variation in this variable was detected across the season for M colonies, with September and February being the months with the highest mite fall percentage (post hoc comparisons by Fisher LSD, Figure 4A). Conversely, C colonies evidenced a low percentage of fallen mites without significant differences across the season (Figure 4A).
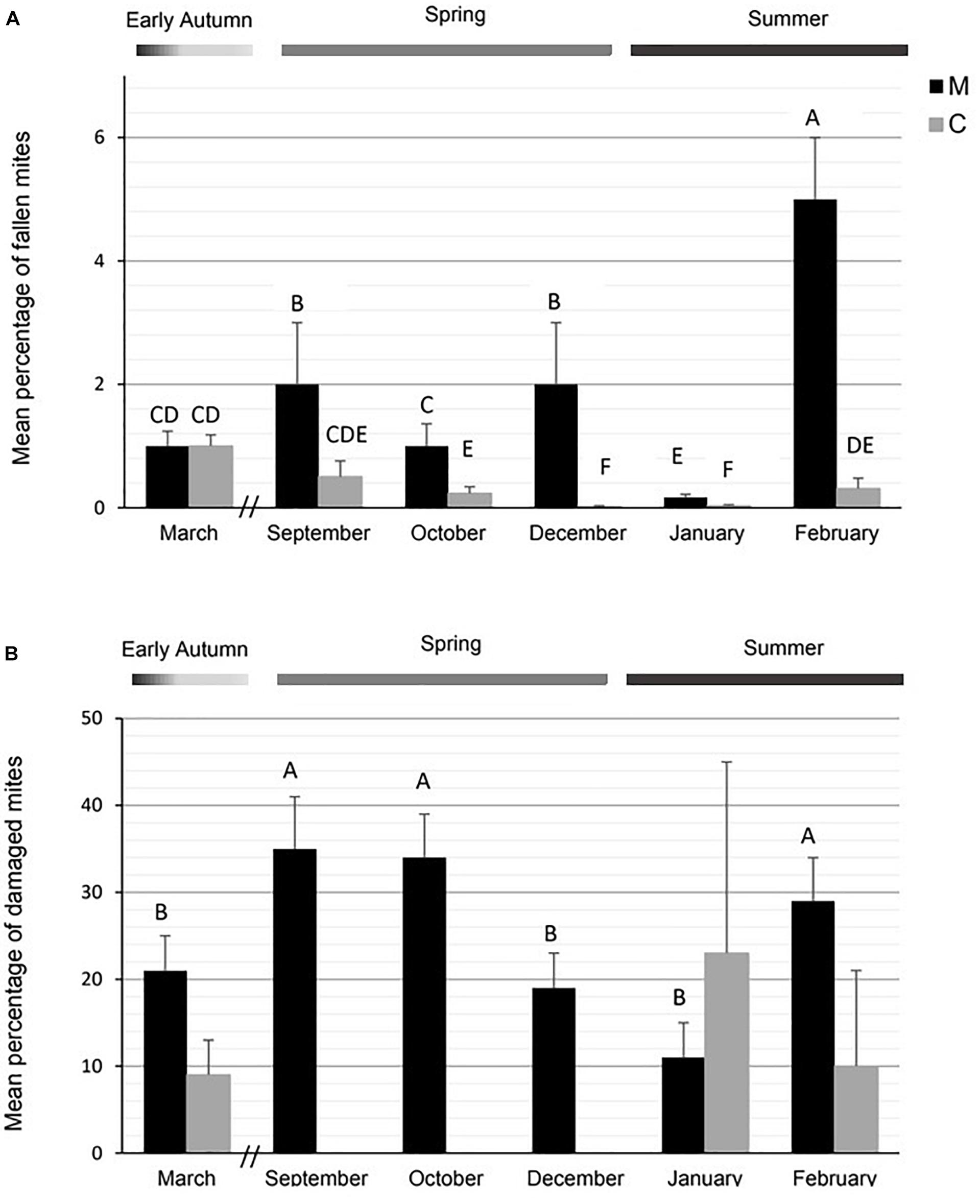
Figure 4. Mean percentage (± SE) of (A) fallen mites and (B) damaged mites in M and C stocks. Different letters indicate significant differences in GLMM between stocks or months by Fisher LSD (0.05). No significant differences were detected in the percentage of damaged mites between months for C stock.
The mean percentage of damaged mites over the season was higher in M (25%) than in C (9%) stock [F(1,97) = 8.51, P < 0.01]. C colonies exhibited a very low and similar number of damaged mites across the season [F(1,16) = 0.01, P > 0.05; Figure 4B]. Conversely, this parameter varied throughout the season for M line [F(1,71) = 5.18, P < 0.001; Figure 4B] with relatively greater damage during spring (September and October) and end of summer (February) (Figure 4B).
An association between the percentage of fallen mites and that of damaged mites was evident for M (Spearman’s rank correlation: r = 0.45, P < 0.001) but not for C colonies. No association was found between the percentage of phoretic Varroa and grooming parameters (fallen and damaged mites) in M stock. Conversely, a positive correlation was detected between the percentage of phoretic Varroa and that of fallen mites in C stock (Spearman’s rank correlation: r = 0.4, P < 0.05).
Different categories of damage to the mite were recorded in this study (Figures 5A–F). These categories were present in mites from colonies of both stocks, but at different relative frequencies depending on the colony origin (Table 2). Damaged leg (total or partial loss of one or more legs) was the predominant type of physical injury to the mite recorded in both M and C lines in similar percentages (Table 2), but with different intensity. In fact, significant differences were detected in the proportion of mites that presented more than 2 damaged legs in M (63.3%) than in C (10.5%) stock χ2(1) = 20.98, P < 0.001; Supplementary Table S1]. Moreover, 52.7% of the damaged mites from M colonies presented 4 or more damaged legs (Supplementary Table S1). Combined injury in body and legs (damaged legs + damaged gnathosoma or dorsal shield) was detected in 20.6 and 5% of the injured mites from M and C colonies, respectively. This difference was marginally significant (Table 2).
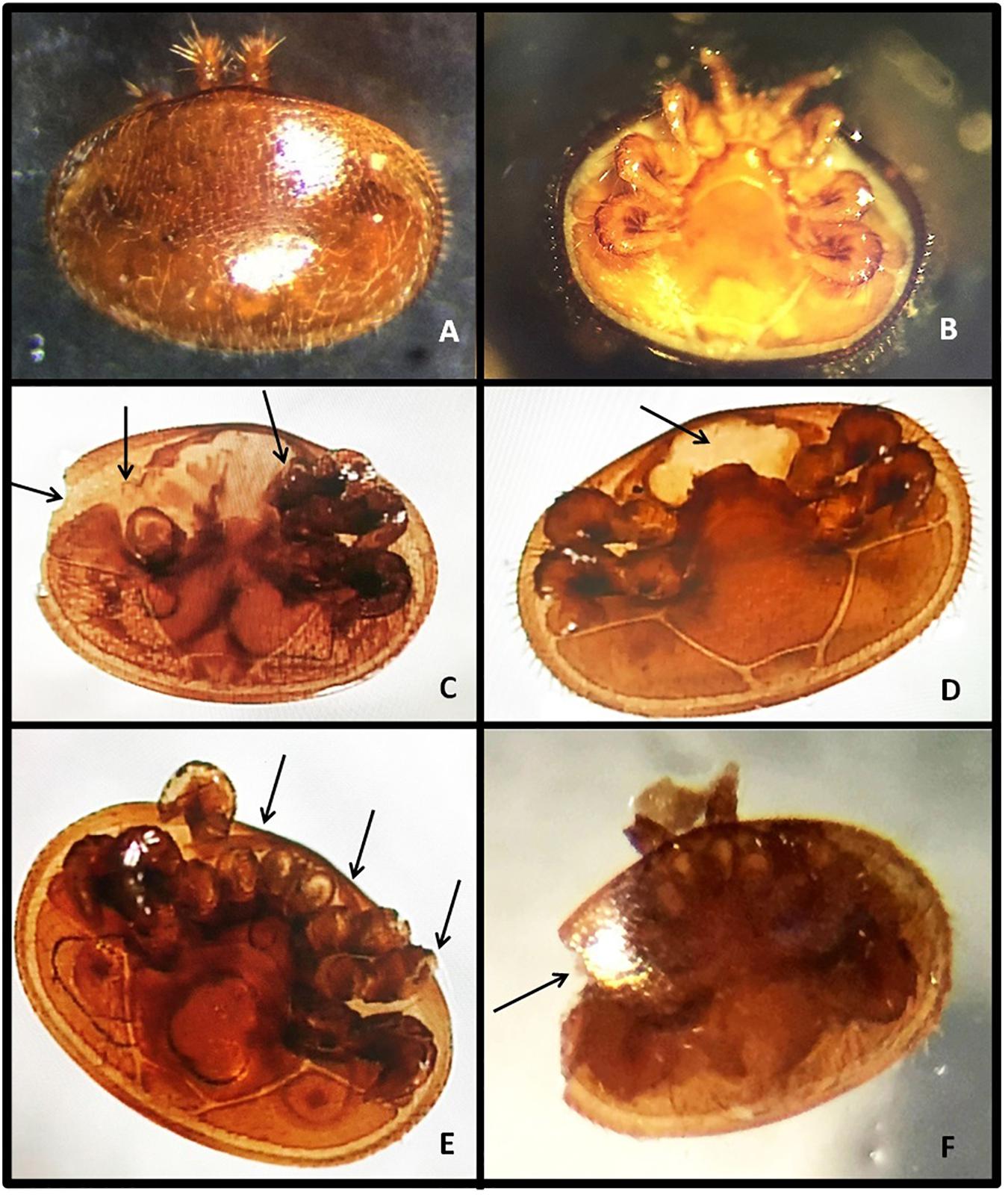
Figure 5. Photographs showing the different damage patterns in V. destructor mites. Arrows indicate the injuries on mite’s body, legs, and chelicerae. (A,B) Dorsal and ventral views of non-damaged mites, (C) damaged dorsal shield + damaged gnathosoma, and missing legs + chelicerae, (D,E) missing legs + chelicerae, (F) damaged dorsal shield + damaged gnathosoma. Classification of damage to the mites was previously reported by Corrêa-Marques et al. (2000). All the pictures were taken with an Olympus BX40 Microscope at 40x magnification.
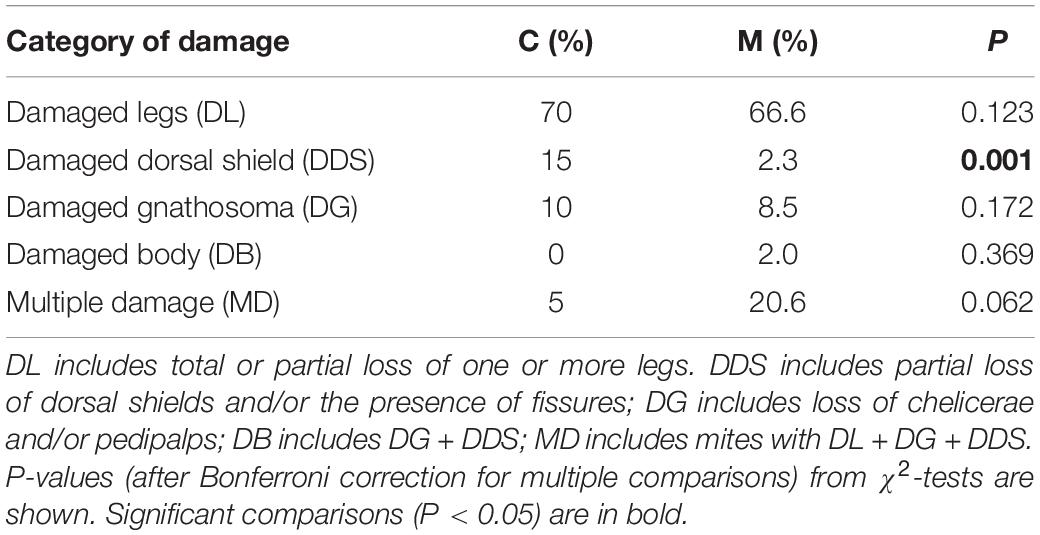
Table 2. Mean percentages for the different categories of damage to V. destructor recorded in the colony debris of M and C stocks.
Discussion
Here, we present a field survey of a naturally mite-surviving honey bee stock from north-east Argentina and explore the contribution of grooming behavior and colony dynamic to Varroa-resistance.
Our findings revealed that the Varroa-surviving honey bee stock (M) expressed a higher grooming behavior than that of the susceptible local control stock (C). This was evident in the higher mite damage recorded on the screened bottom boards of M compared to C colonies. The mean percentage of mite damage observed in our M population during spring (34.5%) appeared to be intermediate between those recorded in A. m. ligustica (26.4%) by Fries et al. (1996) and in Africanized A. mellifera (38.5%) by Moretto et al. (1991). We also detected phenotypic variation among stocks for the percentage of mite infestation in adults (phoretic Varroa) and in brood, particularly in early autumn. At this time of the season, C stock evidenced 2.2 and 2.6-fold more mites in adults and brood, respectively, compared to M stock. The difference in Varroa infestation between stocks in early autumn appeared to largely impact on the observed overwintering survival and the colony strength at the beginning of the season.
The high percentage of damaged mites observed during the active season and the more intense injuries inflicted on the mites by M bees, as discussed in more detail below, suggest that grooming behavior could increase mite mortality and hence modulate its population growth in the colonies. Our results are in line with a growing body of evidence (Morse et al., 1991; Moosbeckhofer, 1992; Ruttner and Hänel, 1992; Boecking and Ritter, 1993; Moretto et al., 1993; Bienefeld et al., 1999; Arechavaleta-Velasco and Guzmán-Novoa, 2001; Guzman-Novoa et al., 2012; Invernizzi et al., 2015; Nganso et al., 2017; Russo et al., 2018) suggesting that grooming behavior may be an important mechanism conferring resistance to honey bee colonies toward V. destructor, even in honey bee populations of European origin. Moreover, this trait may evolve by natural selection (as shown by the present results) and can be further developed or improved in ongoing selected stocks (e.g., Hunt et al., 2016).
The percentage of damaged mites showed seasonal variation, as previously suggested for grooming behavior (Büchler, 1994; Moosbeckhofer, 1997). Specifically, M colonies exhibited a high percentage of damaged mites during spring, where the phoretic infestation is low, in agreement with previous observations by Mondragón et al. (2005). In this sense, M (in contrast to C) colonies may strategically respond to mite phoretic infestation below a load threshold, slowing the population growth of the mite and ensuring fewer loads to deal with overwintering. Even though Kruitwagen et al. (2017) suggested that grooming would not be mite-density dependent and speculated that it would only be beneficial at high levels of mite infestation, this pattern was specifically observed in control colonies (like our C colonies) and at a small mite infestation range. On the one hand, our results can be used to recommend specific times of the season to measure and select the grooming behavior performance at the colony level. On the other hand, our results are in line with observations on colonies bred for hygienic behavior, which are more efficient at removing Varroa-infested brood only under low mite parasitism (Spivak and Reuter, 1998, 2001; Ibrahim and Spivak, 2006). This hypothesis must be further evaluated in controlled assays that test the response of groomer colonies against different parasite loads.
As the proportion of damaged mites can be a time-consuming measurement in field surveys (Rosenkranz et al., 1997; Bienefeld et al., 1999; Aumeier, 2001), it has been suggested that mite fall could be a simpler alternative to select the grooming behavior of a colony (e.g., Kruitwagen et al., 2017). The present results evidenced a positive correlation between the percentage of fallen and damaged mites in M stock, but not in C stock. Therefore, the validity of using only the percentage of mite fall as a measure to select colonies for increasing grooming abilities must be further evaluated on different stocks, specifically if the selection is initiated on a honey bee population or is used to increase this trait in already groomer stock (as in the present case). For unselected stocks, as our C colonies, the mite fall may reflect mainly the passive fall of the mite [consistent with its use as an estimator of mite infestation at colony level (Branco et al., 2006; Guzman-Novoa et al., 2012; Hunt et al., 2016)], and it may not strictly represent a measure of grooming activity by the adult bees.
To characterize the differences in grooming behavior intensity between the stocks, we analyzed the patterns of damage in mites using the known classification performed by Corrêa-Marques et al. (2000). In agreement with previous studies (Ruttner and Hänel, 1992; Lodesani et al., 1996; Rosenkranz et al., 1997; Corrêa-Marques et al., 2000; Stanimirovic et al., 2003), we found that leg damage was the most frequent damage in mites from colonies of both origins. While the percentage of this kind of damage did not differ between M and C colonies, the number of damaged legs was higher in mites of M colonies than C colonies. In fact, more than 50% of the mites from M colonies evidenced damage in 4 or more legs. This, together with an apparent higher frequency of multiple injuries (legs and gnathosoma or dorsal shield damage) to the mites from M stock, would reflect that more intense grooming, possibly collective behavior (allogrooming), was displayed by adult bees from this origin. Accordingly, Nganso et al. (2017) detected the same kind of combined injuries to mites from both African and European honey bee colonies, but at higher frequency in the former. Overall, the high rate of mite mutilations observed in our M stock reflects how robust is the mite damage as indirect measurement of grooming behavior at the colony level. Even this measurement is tedious and time-consuming in field surveys, it is the only reliable phenotypic trait to breed for increased grooming behavior so far known. Alternatively, the mite population growth, estimated by determining the difference between two measurements of mite fall assessments over time, could represent a simpler and less time-consuming method to predict Varroa-resistance in honey bee populations (Emsen et al., 2012) since it may estimate several mechanisms of mite resistance simultaneously (e.g., grooming behavior, VSH, etc.).
Despite the European mitochondrial lineage of our stocks, as the analyzed region represents a hybrid zone where Africanized and European honey bee populations coexist (Agra et al., 2018), our stock may be a local ecotype that carries genes from both origins. In fact, we observed differences in the temperament of the stocks during field monitoring, with more excitable behavior in M than in C workers. Consistently, previous studies revealed that subspecies of A. mellifera described as more excitable or even aggressive differed from other subspecies in their grooming behavior in laboratory assays (Aumeier, 2001; Wilde et al., 2003; Ba̧k and Wilde, 2015). Further laboratory assays on this stock will allow us to investigate the apparently greater intensity of the grooming reactions of M worker bees against V. destructor and to test the association between the proportion of damaged mites in field monitoring and the proportion of mites dislodged by the bees in lab grooming assays (as previously detected by Andino and Hunt, 2011; Guzman-Novoa et al., 2012; Invernizzi et al., 2015). Moreover, these experiments will enable us to elucidate the weight of individual (autogrooming) and social (allogrooming) responses in the behavioral resistance against V. destructor in this stock and the best parameters to quantify each response.
It is important to note that grooming behavior may not be the only sanitary trait involved in regulating Varroa parasitism in M colonies. In fact, this stock expressed a higher hygienic behavior toward dead brood than did the control stock during early autumn, when the percentage of brood infestation is high. This result suggests that the bees of the surviving stock display higher hygiene and can behaviorally respond to the infestation status of the colony. However, since the method used here to test hygienic behavior may overestimate the expression of this behavior (Espinosa-Montaño et al., 2008), these results have to be taken with caution and confirmed in future research using more reliable methods for testing this complex behavior. Additionally, although hygienic behavior against dead brood does not necessarily imply greater resistance to Varroa (e.g., Danka et al., 2013), it would be linked to other associated behaviors such as Varroa Sensitive Hygiene (VSH; Spivak, 1996; Visintini, 2018), which were not measured in this work. In this sense, the analysis of other host traits that can jointly determine the surviving phenotype of our M stock (as previously evidenced in other naturally surviving stocks: Fries et al., 2006; Harris et al., 2010; Locke and Fries, 2011; Panziera et al., 2017; Oddie et al., 2018) is needed.
In addition, analyzing the performance of these colonies under different environments may help to clarify the influence of genotype x environment interactions (Büchler et al., 2014; Meixner et al., 2014) on grooming. It must be noted that this behavior can be influenced by environmental factors (Stanimirovic et al., 2003; Currie and Tahmasbi, 2008) and that Varroa damage thresholds can change under different environmental conditions (Meixner et al., 2014; Giacobino et al., 2017). In this sense, the development of regional breeding programs for mite-resistant honey bees that take advantage of the locally-adapted stocks deserves consideration, especially in Argentina where contrasting eco-regions coexist.
Given the complexity of measuring the mite damage at the colony level (the best way to phenotype grooming behavior according to our results) and the efforts involved in selecting it at a large scale, the genetic characterization of M stock would facilitate the identification of candidate genes associated with this trait. In turn, this identification would help to further develop marker-assisted selection tools for facilitating breeding efforts (Grozinger and Robinson, 2015; Guarna et al., 2017). Recent findings demonstrated a significant correlation between the expression of the gene neurexin and direct observations of grooming behavior (Hamiduzzaman et al., 2017). Furthermore, Morfin et al. (2019) found a correlation between this gene and mite mutilation, which reinforces the validity of analyzing mite damage as an indirect measurement of grooming behavior until the development of robust markers for marker-assisted selection. Finally, efforts are being made to characterize the productivity of the selected stock under standard beekeeping management. This information will greatly contribute to incorporating this genetic material into the breeding program conducted by INTA and to making it available for commercialization in the region.
Conclusion
Our data show that increased grooming behavior seems to be an important factor in reducing autumn Varroa infestation and enhancing overwintering survival of honey bee colonies of European origin, and suggest that mite damage would be the best proxy to evaluate and select this trait in the field. The characterization of this Argentinian stock, together with previously reported cases, clearly shows that honey bee populations can develop (different) traits and specific colony dynamics to overcome V. destructor infestations by means of natural selection. Taking advantage of these cases would be useful for a practical application in the apiculture and conservation of locally adapted honey bee populations.
Data Availability Statement
The raw data supporting the conclusions of this article will be made available by the authors, without undue reservation, to any qualified researcher.
Author Contributions
AS, JM, HP, SL, GR, and MP conceived and designed the research. RR, LL, HF, HP, JM, and IM conducted the experiments. ML contributed to statistical analyses. RR, SL, and AS analyzed the data and wrote the manuscript. All authors read and reviewed drafts of the manuscript and approved its final version.
Conflict of Interest
The authors declare that the research was conducted in the absence of any commercial or financial relationships that could be construed as a potential conflict of interest.
Funding
This study was funded by the Agencia Nacional de Promoción Científica y Tecnológica of Argentina through the project Foncyt-PICT 2016-0221 (AS), by Consejo Nacional de Investigaciones Científicas y Técnicas (CONICET) through the project PIP 2015-0517CO (AS), and by Instituto Nacional de Tecnología Agropecuaria (INTA) through the doctoral scholarship resolution N°1126/2013 (RR). We are grateful for the financial support of PNAPI through Grant 1112042 (GR, MP, SL) and FONTAGRO through the project FTG/RF-16112-RG.
Acknowledgments
We thank Rodrigo Muchiut for his assistance with honey bee colony management. On behalf of all authors, we would like to thank Dr. Giray for the invitation to this Special Edition and his encouragement to write this work.
Supplementary Material
The Supplementary Material for this article can be found online at: https://www.frontiersin.org/articles/10.3389/fevo.2020.590281/full#supplementary-material
References
Agra, M. N., Conte, C. A., Corva, P. M., Cladera, J. L., Lanzavecchia, S. B., and Palacio, M. A. (2018). Molecular characterization of Apis mellifera colonies from Argentina: genotypic admixture associated with ecoclimatic regions and apicultural activities. Entomol. Exp. Appl. 166, 724–738. doi: 10.1111/eea.12719
Aizen, M. A., and Harder, L. D. (2009). The global stock of domesticated honey bees is growing slower than agricultural demand for pollination. Curr. Biol. 19, 915–918. doi: 10.1016/j.cub.2009.03.071
Amdam, G. V., Hartfelder, K., Norberg, K., Hagen, A., and Omholt, S. W. (2004). Altered physiology in worker honey bees (Hymenoptera: Apidae) infested with the mite Varroa destructor (Acari: Varroidae): a factor in colony loss during overwintering? J. Econ. Entomol. 97, 741–747. doi: 10.1603/0022-0493(2004)097[0741:APIWHB]2.0.CO;2
Andino, G. K., and Hunt, G. J. (2011). A scientific note on a new assay to measure honeybee mite-grooming behavior. Apidologie 42, 481–484. doi: 10.1007/s13592-011-0004-1
Antúnez, K., Invernizzi, C., Mendoza, Y., and Zunino, P. (2017). Honeybee colony losses in Uruguay during 2013–2014. Apidologie 48, 364–370. doi: 10.1007/s13592-016-0482-2
Arechavaleta-Velasco, M. E., and Guzmán-Novoa, E. (2001). Relative effect of four characteristics that restrain the population growth of the mite Varroa destructor in honey bee (Apis mellifera) colonies. Apidologie 32, 157–174. doi: 10.1051/apido:2001121
Aumeier, P. (2001). Bioassay for grooming effectiveness towards Varroa destructor mites in Africanized and Carniolan honey bees. Apidologie 32, 81–90. doi: 10.1051/apido:2001113
Ba̧k, B., and Wilde, J. (2015). Grooming behavior by worker bees of various subspecies of honey bees to remove Varroa destructor mites. J. Apic. Res. 54, 207–215. doi: 10.1080/00218839.2016.1147791
Baruffi, L., Damiani, G., Guglielmino, C. R., Bandi, C., Malacrida, A. R., and Gasperi, G. (1995). Polymorphism within and between populations of Ceratitis capitata: comparison between RAPD and multilocus enzyme electrophoresis data. Heredity 74, 425–437. doi: 10.1038/hdy.1995.60
Bienefeld, K., Zautke, F., Pronin, D., and Mazeed, A. (1999). Recording the proportion of damaged Varroa jacobsoni Oud. in the debris of honey bee colonies (Apis mellifera). Apidologie 30, 249–256. doi: 10.1051/apido:19990401
Boecking, O., and Genersch, E. (2008). Varroosis–the ongoing crisis in bee keeping. J. Verbr. Lebensm. 3, 221–228. doi: 10.1007/s00003-008-0331-y
Boecking, O., and Ritter, W. (1993). Grooming and removal behaviour of Apis mellifera intermissa in Tunisia against Varroa jacobsoni. J. Apic. Res. 32, 127–134. doi: 10.1080/00218839.1993.11101297
Boecking, O., and Spivak, M. (1999). Behavioral defenses of honey bees against Varroa jacobsoni Oud. Apidologie 30, 141–158. doi: 10.1051/apido:19990205
Branco, M. R., Kidd, N. A., and Pickard, R. S. (2006). A comparative evaluation of sampling methods for Varroa destructor (Acari: Varroidae) population estimation. Apidologie 37, 452–461. doi: 10.1051/apido:2006010
Brown, P., Newstrom-Lloyd, L. E., Foster, B. J., Badger, P. H., and McLean, J. A. (2018). Winter 2016 honey bee colony losses in New Zealand. J. Apic. Res. 57, 278–291. doi: 10.1080/00218839.2018.1430980
Büchler, R. (1994). Varroa tolerance in honey bees-occurrence, characters and breeding. Bee World 75, 54–70. doi: 10.1080/0005772X.1994.11099201
Büchler, R., Costa, C., Hatjina, F., Andonov, S., Meixner, M. D., Conte, Y. L., et al. (2014). The influence of genetic origin and its interaction with environmental effects on the survival of Apis mellifera L. colonies in Europe. J. Apic. Res. 53, 230–232. doi: 10.3896/IBRA.1.53.2.03
Corrêa-Marques, M. H., Issa, M. R. C., and Jong, D. D. (2000). Classification and quantification of damaged Varroa jacobsoni found in the debris of honey bee colonies as criteria for selection? Am. Bee J. 140, 820–824.
Currie, R. W., and Tahmasbi, G. H. (2008). The ability of high-and low-grooming lines of honey bees to remove the parasitic mite Varroa destructor is affected by environmental conditions. Can. J. Zool. 86, 1059–1067. doi: 10.1139/Z08-083
Dainat, B., and Neumann, P. (2013). Clinical signs of deformed wing virus infection are predictive markers for honey bee colony losses. J. Invertebr. Pathol. 112, 278–280. doi: 10.1016/j.jip.2012.12.009
Danka, R. G., Harris, J. W., Villa, J. D., and Dodds, G. E. (2013). Varying congruence of hygienic responses to Varroa destructor and freeze-killed brood among different types of honeybees. Apidologie 44, 447–457. doi: 10.1007/s13592-013-0195-8
De Jong, D., Morse, R. A., and Eickwort, G. C. (1982). Mite pests of honey bees. Annu. Rev. Entomol. 27, 229–252. doi: 10.1146/annurev.en.27.010182.001305
DeGrandi-Hoffman, G., Wardell, G., Ahumada-Segura, F., Rinderer, T., Danka, R., and Pettis, J. (2008). Comparisons of pollen substitute diets for honey bees: consumption rates by colonies and effects on brood and adult populations. J. Apic. Res. 47, 265–270. doi: 10.1080/00218839.2008.11101473
Delaplane, K. S., Van Der Steen, J., and Guzman-Novoa, E. (2013). Standard methods for estimating strength parameters of Apis mellifera colonies. J. Apic. Res. 52, 1–12. doi: 10.3896/IBRA.1.52.4.12
Di Rienzo, N., and Montiglio, P. O. (2016). The contribution of developmental experience vs. condition to life history, trait variation and individual differences. J. Anim. Ecol. 85, 915–926. doi: 10.1111/1365-2656.12512
Emsen, B., Petukhova, T., and Guzman-Novoa, E. (2012). Factors limiting the growth of Varroa destructor populations in selected honey bee (Apis mellifera L.) colonies. J. Anim. Vet. Adv. 11, 4519–4525.
Espinosa-Montaño, L. G., Guzmán-Novoa, E., Sánchez-Albarrán, A., Montaldo, H. H., and Correa-Benítez, A. (2008). Comparative study of three assays to evaluate hygienic behavior in honey bee (Apis mellifera L.) colonies. Vet. Mexico 39, 39–54.
Francis, R. M., Nielsen, S. L., and Kryger, P. (2013). Varroa-virus interaction in collapsing honey bee colonies. PLoS One 8:e57540. doi: 10.1371/journal.pone.0057540
Fries, I., Huazhen, W., Wei, S., and Jin, C. S. (1996). Grooming behavior and damaged mites (Varroa jacobsoni) in Apis cerana cerana and Apis mellifera ligustica. Apidologie 27, 3–11. doi: 10.1051/apido:19960101
Fries, I., Imdorf, A., and Rosenkranz, P. (2006). Survival of mite infested (Varroa destructor) honey bee (Apis mellifera) colonies in a Nordic climate. Apidologie 37, 564–570. doi: 10.1051/apido:2006031
Gallai, N., Salles, J. M., Settele, J., and Vaissière, B. E. (2009). Economic valuation of the vulnerability of world agriculture confronted with pollinator decline. Ecol. Econ. 68, 810–821. doi: 10.1016/j.ecolecon.2008.06.014
Giacobino, A., Pacini, A., Molineri, A., Cagnolo, N. B., Merke, J., Orellano, E., et al. (2017). Environment or beekeeping management: What explains better the prevalence of honey bee colonies with high levels of Varroa destructor? Res. Vet. Sci. 112, 1–6. doi: 10.1016/j.rvsc.2017.01.001
Goulson, D., Nicholls, E., Botías, C., and Rotheray, E. L. (2015). Bee declines driven by combined stress from parasites, pesticides, and lack of flowers. Science 347:1255957. doi: 10.1126/science.1255957
Grozinger, C. M., and Robinson, G. E. (2015). The power and promise of applying genomics to honey bee health. Curr. Opin. Insect. Sci. 10, 124–132. doi: 10.1016/j.cois.2015.03.007
Guarna, M. M., Hoover, S. E., Huxter, E., Higo, H., Moon, K. M., Domanski, D., et al. (2017). Peptide biomarkers used for the selective breeding of a complex polygenic trait in honey bees. Sci. Rep. 7:8381. doi: 10.1038/s41598-017-08464-2
Guichard, M., Dietemann, V., Neuditschko, M., and Dainat, B. (2020). Three decades of selecting honey bees that survive infestations by the parasitic mite Varroa destructor: Outcomes, limitations and strategy. Preprints 2020, 1–83. doi: 10.20944/preprints202003.0044.v1
Guzman-Novoa, E., Emsen, B., Unger, P., Espinosa-Montaño, L. G., and Petukhova, T. (2012). Genotypic variability and relationships between mite infestation levels, mite damage, grooming intensity, and removal of Varroa destructor mites in selected strains of worker honey bees (Apis mellifera L.). J. Invertebr. Pathol. 110, 314–320. doi: 10.1016/j.jip.2012.03.020
Guzman-Novoa, E., Hunt, G. J., Page, R. E., and Fondrk, M. K. (2002). Genetic correlations among honey bee (Hymenoptera: Apidae) behavioral characteristics and wing length. Ann. Entomol. Soc. Am. 95, 402–406. doi: 10.1603/0013-8746(2002)095[0402:GCAHBH]2.0.CO;2
Guzman-Novoa, E., Vandame, R., and Arechavaleta, M. E. (1999). Susceptibility of European and Africanized honey bees (Apis mellifera L.) to Varroa jacobsoni Oud. in Mexico. Apidologie 30, 173–182. doi: 10.1051/apido:19990207
Hall, H. G., and Smith, D. R. (1991). Distinguishing African and European honeybee matrilines using amplified mitochondrial DNA. Proc. Natl. Acad. Sci. U.S.A. 88, 4548–4552. doi: 10.1073/pnas.88.10.4548
Hamiduzzaman, M. M., Emsen, B., Hunt, G. J., Subramanyam, S., Williams, C. E., Tsuruda, J. M., et al. (2017). Differential gene expression associated with honey bee grooming behavior in response to Varroa mites. Behav. Genet. 47, 335–344. doi: 10.1007/s10519-017-9834-6
Harris, J. W., Danka, R. G., and Villa, J. D. (2010). Honey bees (Hymenoptera: Apidae) with the trait of Varroa sensitive hygiene remove brood with all reproductive stages of Varroa mites (Mesostigmata: Varroidae). Ann. Entomol. Soc. Am. 103, 146–152. doi: 10.1603/AN09138
Hung, K. L. J., Kingston, J. M., Albrecht, M., Holway, D. A., and Kohn, J. R. (2018). The worldwide importance of honey bees as pollinators in natural habitats. Proc. R. Soc. B. 285:20172140. doi: 10.1098/rspb.2017.2140
Hunt, G., Given, J. K., Tsuruda, J. M., and Andino, G. K. (2016). Breeding mite-biting bees to control Varroa. Bee Cult. 8, 41–47.
Ibrahim, A., and Spivak, M. (2006). The relationship between hygienic behavior and suppression of mite reproduction as honey bee (Apis mellifera) mechanisms of resistance to Varroa destructor. Apidologie 37, 31–40. doi: 10.1051/apido:2005052
Invernizzi, C., Zefferino, I., Santos, E., Sánchez, L., and Mendoza, Y. (2015). Multilevel assessment of grooming behavior against Varroa destructor in Italian and Africanized honey bees. J. Apic. Res. 54, 321–327. doi: 10.1080/00218839.2016.1159055
Klein, A. M., Vaissiere, B. E., Cane, J. H., Steffan-Dewenter, I., Cunningham, S. A., Kremen, C., et al. (2007). Importance of pollinators in changing landscapes for world crops. Proc. R. Soc. B. 274, 303–313. doi: 10.1098/rspb.2006.3721
Kruitwagen, A., van Langevelde, F., van Dooremalen, C., and Blacquière, T. (2017). Naturally selected honey bee (Apis mellifera) colonies resistant to Varroa destructor do not groom more intensively. J. Apic. Res. 56, 354–365. doi: 10.1080/00218839.2017.1329797
Kurze, C., Routtu, J., and Moritz, R. F. (2016). Parasite resistance and tolerance in honeybees at the individual and social level. Zoology 119, 290–297. doi: 10.1016/j.zool.2016.03.007
Le Conte, Y., De Vaublanc, G., Crauser, D., Jeanne, F., Rousselle, J. C., and Bécard, J. M. (2007). Honey bee colonies that have survived Varroa destructor. Apidologie 38, 566–572. doi: 10.1051/apido:2007040
Le Conte, Y., and Mondet, F. (2017). “Natural selection of honey bees against Varroa destructor” in Beekeeping–From Science to Practice, eds R. H. Vreeland and D. Sammataro (Cham: Springer), 189–194. doi: 10.1007/978-3-319-60637-8_12
Le Conte, Y., and Navajas, M. (2008). Climate change: impact on honey bee populations and diseases. Rev. Sci. Tech. Off. Int. Épizoot. 27, 499–510. doi: 10.20506/rst.27.2.1819
Lobo Segura, J. A. (2000). Highly polymorphic DNA markers in an Africanized honey bee population in Costa Rica. Genet. Mol. Biol. 23, 317–322. doi: 10.1590/S1415-47572000000200013
Locke, B. (2016). Natural Varroa mite-surviving Apis mellifera honeybee populations. Apidologie 47, 467–482. doi: 10.1007/s13592-015-0412-8
Locke, B., and Fries, I. (2011). Characteristics of honey bee colonies (Apis mellifera) in Sweden surviving Varroa destructor infestation. Apidologie. 42, 533–542. doi: 10.1007/s13592-011-0029-5
Lodesani, M., Vecchi, M. A., Tommasini, S., and Bigliardi, M. (1996). A study on different kinds of damage to Varroa jacobsoni in Apis mellifera ligustica colonies. J. Apic. Res. 35, 49–56. doi: 10.1080/00218839.1996.11100912
Maggi, M., Antúnez, K., Invernizzi, C., Aldea, P., Vargas, M., Negri, P., et al. (2016). Honeybee health in South America. Apidologie 47, 835–854. doi: 10.1007/s13592-016-0445-7
Martin, S. J., and Medina, L. M. (2004). Africanized honeybees have unique tolerance to Varroa mites. Trends Parasitol. 20, 112–114. doi: 10.1016/j.pt.2004.01.001
McMenamin, A. J., and Genersch, E. (2015). Honey bee colony losses and associated viruses. Curr. Opin. Insect. Sci. 8, 121–129. doi: 10.1016/j.cois.2015.01.015
Meixner, M. D., Francis, R. M., Gajda, A., Kryger, P., Andonov, S., Uzunov, A., et al. (2014). Occurrence of parasites and pathogens in honey bee colonies used in a European genotype-environment interactions experiment. J. Apic. Res. 53, 215–229. doi: 10.3896/IBRA.1.53.2.04
Merke, J. (2016). Dinámica Poblacional de Varroa Destructor y Apis Mellifera L. Como Herramienta Para la Selección de Abejas Tolerantes. Doc. Thesis, Argentina: University of Mar del Plata..
Mondet, F., Beaurepaire, A., McAfee, A., Locke, B., Alaux, C., Blanchard, S., et al. (2020). Honey bee survival mechanisms against the parasite Varroa destructor: a systematic review of phenotypic and genomic research efforts. Int. J. Parasitol. 50, 433–447. doi: 10.1016/j.ijpara.2020.03.005
Mondet, F., de Miranda, J. R., Kretzschmar, A., Le Conte, Y., and Mercer, A. R. (2014). On the front line: quantitative virus dynamics in honeybee (Apis mellifera L.) colonies along a new expansion front of the parasite Varroa destructor. PLoS Pathog. 10:e1004323. doi: 10.1371/journal.ppat.1004323
Mondragón, L., Martin, S., and Vandame, R. (2006). Mortality of mite offspring: a major component of Varroa destructor resistance in a population of Africanized bees. Apidologie 37, 67–74. doi: 10.1051/apido:2005053
Mondragón, L., Spivak, M., and Vandame, R. (2005). A multifactorial study of the resistance of honeybees Apis mellifera to the mite Varroa destructor over one year in Mexico. Apidologie 36, 345–358. doi: 10.1051/apido:2005022
Moosbeckhofer, R. (1992). Observations on the occurrence of damaged Varroa mites in natural mite fall of Apis mellifera carnica. Apidologie 23, 523–531. doi: 10.1051/apido:19920604
Moosbeckhofer, R. (1997). Observations on reproduction rate of Varroa jacobsoni and the occurrence of mutilated mites in Apis mellifera carnica colonies. Apidologie 28, 193–195.
Moretto, G., Gonçalves, L. S., and De Jong, D. (1993). Heritability of Africanized and European honey bee defensive behavior against the mite Varroa jacobsoni. Rev. Bras. Genét. 16, 71–71.
Moretto, G., Gonçalves, L. S., De Jong, D., and Bichuette, M. Z. (1991). The effects of climate and bee race on Varroa jacobsoni Oud infestations in Brazil. Apidologie 22, 197–203. doi: 10.1051/apido:19910303
Morfin, N., Given, K., Evans, M., Guzman-Novoa, E., and Hunt, G. J. (2019). Grooming behavior and gene expression of the Indiana “mite-biter” honey bee stock. Apidologie 51, 267–275. doi: 10.1007/s13592-019-00710-y
Morse, R. A., and Calderone, N. W. (2000). The value of honey bees as pollinators of US crops in 2000. Bee Cult. 128, 1–15.
Morse, R. A., Miksa, D., and Masenheimer, J. A. (1991). Varroa resistance in US honey bees. Am. Bee J. 131, 433–434.
Neumann, P., and Carreck, N. (2010). Honey bee colony losses. J. Apic. Res. 49, 1–6. doi: 10.3896/IBRA.1.49.1.01
Neumann, P., Yañez, O., Fries, I., and de Miranda, J. R. (2012). Varroa invasion and virus adaptation. Trends Parasitol. 28, 353–354. doi: 10.1016/j.pt.2012.06.004
Newton, D. C., and Ostasiewski, N. J. Jr. (1986). A simplified bioassay for behavioral resistance to American foulbrood in honey bees. Am. Bee J. 126, 278–281.
Nganso, B. T., Fombong, A. T., Yusuf, A. A., Pirk, C. W., Stuhl, C., and Torto, B. (2017). Hygienic and grooming behaviors in African and European honeybees-New damage categories in Varroa destructor. PLoS One 12:e0179329. doi: 10.1371/journal.pone.0179329
Oddie, M., Büchler, R., Dahle, B., Kovacic, M., Le Conte, Y., Locke, B., et al. (2018). Rapid parallel evolution overcomes global honey bee parasite. Sci. Rep. 8:7704. doi: 10.1038/s41598-018-26001-7
Palacio, M. A., Figini, E. E., Ruffinengo, S. R., Rodriguez, E. M., del Hoyo, M. L., and Bedascarrasbure, E. L. (2000). Changes in a population of Apis mellifera L. selected for hygienic behaviour and its relation to brood disease tolerance. Apidologie 31, 471–478. doi: 10.1051/apido:2000139
Palacio, M. A., Rodriguez, E., Goncalves, L., Bedascarrasbure, E., and Spivak, M. (2010). Hygienic behaviors of honey bees in response to brood experimentally pin-killed or infected with Ascosphaera Apis. Apidologie 41, 602–612. doi: 10.1051/apido/2010022
Panziera, D., van Langevelde, F., and Blacquière, T. (2017). Varroa sensitive hygiene contributes to naturally selected Varroa resistance in honey bees. J. Apic. Res. 56, 635–642. doi: 10.1080/00218839.2017.1351860
Peng, Y. S., Fang, Y., Xu, S., and Ge, L. (1987). The resistance mechanism of the Asian honey bee, Apis cerana Fabr., to an ectoparasitic mite, Varroa jacobsoni Oudemans. J. Invertebr, Pathol. 49, 54–60. doi: 10.1016/0022-2011(87)90125-X
Pettis, J. S., and Shimanuki, H. (1999). A hive modification to reduce Varroa populations. Am. Bee J. 139, 471–473.
Pirk, C. W., Human, H., Crewe, R. M., and vanEngelsdorp, D. (2014). A survey of managed honey bee colony losses in the Republic of South Africa 2009 to 2011. J. Apic. Res. 53, 35–42. doi: 10.3896/IBRA.1.53.1.03
Potts, S. G., Biesmeijer, J. C., Kremen, C., Neumann, P., Schweiger, O., and Kunin, W. E. (2010a). Global pollinator declines: trends, impacts and drivers. Trends Eco. Evol. 25, 345–353. doi: 10.1016/j.tree.2010.01.007
Potts, S. G., Roberts, S. P., Dean, R., Marris, G., Brown, M. A., Jones, R., et al. (2010b). Declines of managed honey bees and beekeepers in Europe. J. Apic. Res. 49, 15–22. doi: 10.3896/IBRA.1.49.1.02
Pritchard, D. J. (2016). Grooming by honey bees as a component of Varroa resistant behavior. J. Apic. Res. 55, 38–48. doi: 10.1080/00218839.2016.1196016
Ramsey, S. D., Ochoa, R., Bauchan, G., Gulbronson, C., Mowery, J. D., Cohen, A., et al. (2019). Varroa destructor feeds primarily on honey bee fat body tissue and not hemolymph. Proc. Natl. Acad. Sci. U.S.A. 116, 1792–1801. doi: 10.1073/pnas.1818371116
Requier, F., Antúnez, K., Morales, C. L., Aldea Sánchez, P., Castilhos, D., Garrido, P. M., et al. (2018). Trends in beekeeping and honey bee colony losses in Latin America. J. Apic. Res. 57, 657–662. doi: 10.1080/00218839.2018.1494919
Rivera-Marchand, B., Oskay, D., and Giray, T. (2012). Gentle Africanized bees on an oceanic island. Evol. Appl. 5, 746–756. doi: 10.1111/j.1752-4571.2012.00252.x
Rosenkranz, P., Aumeier, P., and Ziegelmann, B. (2010). Biology and control of Varroa destructor. J. Invertebr. Pathol. 103, S96–S119. doi: 10.1016/j.jip.2009.07.016
Rosenkranz, P., Fries, I., Boecking, O., and Stürmer, M. (1997). Damaged Varroa mites in the debris of honey bee (Apis mellifera L) colonies with and without hatching brood. Apidologie 28, 427–437. doi: 10.1051/apido:19970609
Rothenbuhler, W. C. (1964). Behaviour genetics of nest cleaning in honey bees. I. Responses of four inbred lines to disease-killed brood. Anim. Behav. 12, 578–583. doi: 10.1016/0003-3472(64)90082-X
Russo, R. M., Merke, J., Landi, L., Pietronave, H., Fain, H., Liendo, M. C., et al. (2018). The Grooming Behavior as a Varroa-Tolerance Mechanism in Apis Mellifera Colonies from Argentina. Guarujá: IUSSI.
Ruttner, F., and Hänel, H. (1992). Active defense against Varroa mites in a Carniolan strain of honeybee (Apis mellifera carnica Pollmann). Apidologie 23, 173–187. doi: 10.1051/apido:19920210
Schneider, D. S., and Ayres, J. S. (2008). Two ways to survive infection: what resistance and tolerance can teach us about treating infectious diseases. Nat. Rev. Immunol. 8, 889–895. doi: 10.1038/nri2432
Seeley, T. D. (2007). Honey bees of the Arnot Forest: a population of feral colonies persisting with Varroa destructor in the northeastern United States. Apidologie 38, 19–29. doi: 10.1051/apido:2006055
Spivak, M. (1996). Honey bee hygienic behavior and defense against Varroa jacobsoni. Apidologie 27, 245–260. doi: 10.1051/apido:19960407
Spivak, M., and Reuter, G. S. (1998). Performance of hygienic honey bee colonies in a commercial apiary. Apidologie 29, 291–302. doi: 10.1051/apido:19980308
Spivak, M., and Reuter, G. S. (2001). Varroa destructor infestation in untreated honey bee (Hymenoptera: Apidae) colonies selected for hygienic behavior. J. Econ. Entomol. 94, 326–331. doi: 10.1603/0022-0493-94.2.326
Stanimirovic, Z., Stevanovic, J., and Cirkovic, D. (2003). Investigations of reproductive, productive, hygienic and grooming features of Syenichko-Peshterski honey bee ecotype. Apidologie 34, 487–488.
Van Dooremalen, C., Gerritsen, L., Cornelissen, B., van der Steen, J. J., van Langevelde, F., and Blacquiere, T. (2012). Winter survival of individual honey bees and honey bee colonies depends on level of Varroa destructor infestation. PLoS One 7:e36285. doi: 10.1371/journal.pone.0036285
Vandame, R., and Palacio, M. A. (2010). Preserved honey bee health in Latin America: a fragile equilibrium due to low-intensity agriculture and beekeeping? Apidologie 41, 243–255. doi: 10.1051/apido/2010025
vanEngelsdorp, D., Hayes, J. Jr., Underwood, R. M., Caron, D., and Pettis, J. (2011). A survey of managed honey bee colony losses in the USA, fall 2009 to winter 2010. J. Apic. Res. 50, 1–10. doi: 10.3896/IBRA.1.50.1.01
Visintini, M. G. (2018). Factores Relacionados con la Tolerancia Frente a Varroa Destructor de Colmenas de Apis Mellifera en Climas Templados. Bach. Thesis degree, Universidad Nacional del Litoral: Argentina.
Wilde, J., Bratkowski, J., and Siuda, M. (2003). Defensive behavior of three breeds of Apis mellifera L. Ann. Univ. Mariae Curie Sklodowska Sect. EE Zootech. 21, 87–93.
Keywords: grooming behavior, honey bee health, Varroa-resistance, hygienic behavior, natural selection, breeding programs
Citation: Russo RM, Liendo MC, Landi L, Pietronave H, Merke J, Fain H, Muntaabski I, Palacio MA, Rodríguez GA, Lanzavecchia SB and Scannapieco AC (2020) Grooming Behavior in Naturally Varroa-Resistant Apis mellifera Colonies From North-Central Argentina. Front. Ecol. Evol. 8:590281. doi: 10.3389/fevo.2020.590281
Received: 31 July 2020; Accepted: 28 September 2020;
Published: 22 October 2020.
Edited by:
Tugrul Giray, University of Puerto Rico, Puerto RicoReviewed by:
Ernesto Guzman-Novoa, University of Guelph, CanadaHongmei Li-Byarlay, Central State University, United States
Copyright © 2020 Russo, Liendo, Landi, Pietronave, Merke, Fain, Muntaabski, Palacio, Rodríguez, Lanzavecchia and Scannapieco. This is an open-access article distributed under the terms of the Creative Commons Attribution License (CC BY). The use, distribution or reproduction in other forums is permitted, provided the original author(s) and the copyright owner(s) are credited and that the original publication in this journal is cited, in accordance with accepted academic practice. No use, distribution or reproduction is permitted which does not comply with these terms.
*Correspondence: Alejandra C. Scannapieco, c2Nhbm5hcGllY28uYUBpbnRhLmdvYi5hcg==; YWxlamFuZHJhc2Nhbm5hcGllY29AZ21haWwuY29t