- 1Department of Biology and Ecology of Fishes, Leibniz-Institute of Freshwater Ecology and Inland Fisheries, Berlin, Germany
- 2Faculty of Life Sciences, Albrecht Daniel Thaer-Institute, Humboldt University of Berlin, Berlin, Germany
- 3Ichthyology Section, Zoological Research Museum Alexander Koenig, Bonn, Germany
- 4Cluster of Excellence “Science of Intelligence,” Technical University of Berlin, Berlin, Germany
- 5Dahlem Center of Machine Learning and Robotics, Institute of Computer Science, Free University of Berlin, Berlin, Germany
Understanding the linkage between behavioral types and dispersal tendency has become a pressing issue in light of global change and biological invasions. Here, we explore whether dispersing individuals exhibit behavioral types that differ from those remaining in the source population. We investigated a feral population of guppies (Poecilia reticulata) that undergoes a yearly range shift cycle. Guppies are among the most widespread invasive species in the world, but in temperate regions these tropical fish can only survive in winter-warm freshwaters. Established in a thermally-altered stream in Germany, guppies are confined to a warm-water influx in winter, but can spread to peripheral parts as these become thermally accessible. We sampled fish from the source population and a winter-abandoned site in March, June and August. Fish were tested for boldness, sociability and activity involving open-field tests including interactions with a robotic social partner. Guppies differed consistently among each other in all three traits within each sample. Average trait expression in the source population differed across seasons, however, we could not detect differences between source and downstream population. Instead, all populations exhibited a remarkably stable behavioral syndrome between boldness and activity despite strong seasonal changes in water temperature and associated environmental factors. We conclude that random drift (opposed to personality-biased dispersal) is a more likely dispersal mode for guppies, at least in the investigated stream. In the face of fluctuating environments, guppies seem to be extremely effective in keeping behavioral expressions constant, which could help explain their successful invasion and adaptation to new and disturbed habitats.
Introduction
Consistent behavioral differences among individuals (e.g., behavioral types or animal personality; Réale et al., 2007) seem to be a ubiquitous biological feature (Bell et al., 2009; Bierbach et al., 2017) that was proposed to have substantial ecological and evolutionary importance (Sih et al., 2012; Wolf and Weissing, 2012). For one, behavioral types are thought to be relevant in determining success (Chapple et al., 2012; Carere and Gherardi, 2013; Canestrelli et al., 2015) and impact of biological invasions (Cote et al., 2010a; Fogarty et al., 2011; Juette et al., 2014), which is becoming an ever more pressing issue in the light of global change and ongoing translocations of species among habitats (Wong and Candolin, 2015; Piria et al., 2017; Seebens et al., 2017, 2018).
It is assumed that individuals that invade novel habitats are a non-random subset of their source population (Shine et al., 2011; Sih et al., 2012; Juette et al., 2014; Canestrelli et al., 2015; Spiegel et al., 2017). These invaders may share certain phenotypic and life-history characteristics that are associated with their behavioral types (Juette et al., 2014). Behavioral types of invading individuals may determine whether they are transported (by humans) or move (by themselves) into new environments (first invasion stage; Lockwood et al., 2011), whether they can survive and establish a non-native population (second stage), and whether they may spread to new habitats thereafter (third stage). Behavioral types shown to be relevant in this regard are boldness (Cote et al., 2011; Myles-Gonzalez et al., 2015), aggression (Duckworth and Badyaev, 2007; Hudina et al., 2015), activity (Brown and Irving, 2014; Thorlacius et al., 2015) as well as sociability (Cote et al., 2010b, 2011; Rasmussen and Belk, 2012; Camacho-Cervantes et al., 2015). Further, it was proposed that individuals carrying correlated suites (i.e., behavioral syndromes, Sih et al., 2012) of bold, aggressive, active and asocial types may predominantly be found at the invasion front (Duckworth and Badyaev, 2007; Cote et al., 2010b, 2011; Juette et al., 2014). However, such spatially and/or temporally assorted compositions of behavioral types among populations have only rarely been investigated along naturally occurring invasion gradients.
The difficulty to study invasion phenomena in the wild is their random and unpredictable occurrence (Lockwood et al., 2011), which makes it nearly impossible to follow active invasion fronts. In the rare cases where range expansions could be tracked in real time (e.g., western bluebirds: Duckworth and Badyaev, 2007; African jewelfish: Lopez et al., 2012; signal crayfish: Hudina et al., 2015; round goby: Thorlacius et al., 2015; cane toad: Gruber et al., 2017), the invasion origin is often unknown or unclear due to multiple introduction events. Thus, we need to identify study systems that allow us to investigate dispersal across sensible temporal and spatial scales. Thermally altered freshwater systems in the temperate zones provide fruitful conditions to study this phenomenon. Here, natural and/or anthropogenic warm water influxes create unique temperature refuges that allow non-native species (especially those of tropical origin) to establish populations (Langford, 1990; Jourdan et al., 2014). While ambient water temperatures drop in winter (e.g., sometimes below 1°C in the river Rhine; Jourdan et al., 2014), areas close to constant warm water influx will remain suitable for tropical non-native species to survive. Once peripheral areas become thermally suitable again in warmer seasons, certain individuals from the sources may disperse into these areas. Such a scenario enables us to investigate the role of behavioral types during in situ dispersal of non-native species from a single, well-determined source into new (i.e., winter-abandoned) habitats.
Under a personality-biased dispersal hypothesis, where individuals with a certain set of personality traits are assumed to leave the source population to colonize new habitats, we predicted that (i) the source population's average personality traits should change over the course of the year due to the drain of the emigrating individuals, (ii) newly founded populations should differ in their average personality traits compared to their source populations, and (iii) behavioral variation should decrease both in the source as well as in the newly founded population due to the non-random migration patterns. In addition, (iv) population-wide correlations among personality traits (behavioral syndromes) may emerge or disappear because of assumed non-random migration patterns.
In Germany, tropical fishes have established populations in several artificially heated stream systems (Lukas et al., 2017b), the most investigated being the Gillbach near Cologne (see Figure 1A, Jourdan et al., 2014; Lukas et al., 2017a; Kempkes et al., 2018). To estimate seasonal fluctuations in temperature-associated abiotic and biotic conditions within the sampled range of the stream, we continuously recorded water temperatures with submerged data-loggers. We sampled feral guppies (Poecilia reticulata) from the Gillbach during different seasons (temporal scale: spring, early summer, late summer) and, if possible, at different sites (spatial scale: core of warm water influx vs. more peripheral downstream area; see Figure 1A) and repeatedly assessed behavioral types in terms of boldness, sociability and activity in a laboratory setting.
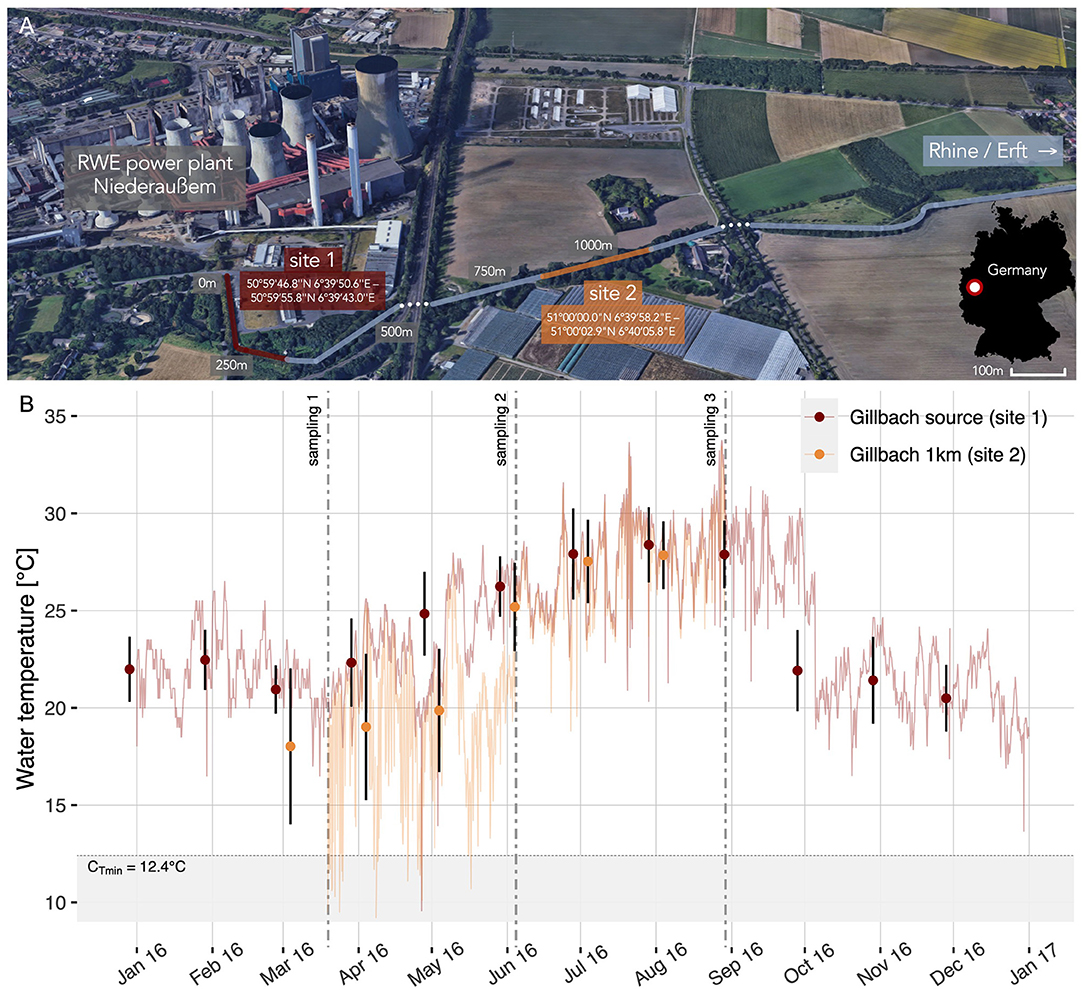
Figure 1. The Gillbach stream and its altered temperature regime. (A) Warm water discharge from a power plant feeds into the Gillbach (map created using ©Google Earth; Imagery ©2019 Google, Map data ©2009 GeoBasis-DE/BKG) and generates a thermal gradient above ambient water temperatures that is preserved for several kilometers (site 1–site 2). (B) 4-hourly water temperature with error bars indicating monthly means ± SD for each site (site 1 Gillbach source: red; site 2 one kilometer downstream: orange). Dotted lines correspond to the three sampling occasions. The shaded region indicates temperatures below the critical minimum temperature (CTmin) of Gillbach guppies. Note that due to a loss of loggers, continuous monitoring at site 2 was interrupted (no data from 01.01.2016 to 19.03.2016 or from 29.08.2016 to 31.12.2016).
Materials and Methods
Study Site
The Gillbach (Rhine catchment, Germany) has been under the influence of thermal pollution and anthropogenic modifications for over six decades. Its headwaters are exclusively fed by the warm water discharge of the brown coal power plant Niederaußem (Figure 1A). This creates a temperature regime that is highly dependent on the volume and temperature of the plant's water discharge. Generally exhibiting above ambient water temperatures (Figure 1B), the Gillbach harbors several established populations of non-native fish, invertebrate and plant species, many of which are of tropical or subtropical origin (see Supplementary Table 1 for a list of native and non-native fish species found in the Gillbach).
Here, feral guppies (Poecilia reticulata) are highly abundant and as successful global invaders (Deacon et al., 2011) they are well-suited to investigate the expression of behavioral traits across time and space. First records of guppies at the Gillbach date back to 1978 and the population has existed continuously for a conservative minimum of 10 years (Lukas et al., 2017b; Kempkes et al., 2018). The Gillbach population likely originated from a small number of released domesticated guppies (see Supplementary Figure 4, Jourdan et al., 2014; Lukas et al., 2017b), and thus would have undergone a demographic bottleneck (i.e., founders' effect) with potential consequences on genetic diversity. The Gillbach has since been subject to repeated introduction of guppies through intentional release (personal observation of JL, GK, FWM and DB). However, release points downstream of the source are unlikely due to limited access to the waterbody, thus all individuals captured below the source site (site 1, Figure 1A) will have dispersed from the source at some point. The population's maximum spatial range is estimated to be <2 km downstream, as no guppies have been found beyond this point during multiple samplings over multiple years (see Supplementary Tables 1, 2, Höfer and Staas, 1998; Jourdan et al., 2014; Lukas et al., 2017a).
Sampling
Sampling was conducted at two sites (~300 m transects, see Figure 1A) in March, June and August 2016. The Gillbach's source population (site 1) grew from a small core population surviving the winter to more than 4000 individuals by June, but decreased again in late August to a few hundred (Supplementary Table 2). This extreme population decline was likely predation-induced as densities of convict cichlids (Amatitlania nigrofasciata) and European chub (Squalius cephalus) also increased during this period (Supplementary Table 1). Sampling commenced until sufficient numbers for behavioral testing were reached (i.e., ~40 adult individuals). Captured fish were transferred into well-aerated coolers and kept separately by sampling site. To reduce a possible sampling bias of certain behavioral types, we collected specimens by seine and dip netting and actively drove fish into the net as recommended by Biro and Dingemanse (2009). While we acknowledge some size selectivity against juvenile guppies introduced by the 2 mm mesh size, our population sample was representative of adult body size variation (see Figure 3B and Supplementary Figure 3), thus avoiding indirect bias of behavioral traits that are correlated with size (Polverino et al., 2016).
Water temperatures at the source (site 1) and one kilometer downstream (site 2) were monitored using HOBO data loggers (Onset Computer Corporation, Bourne, MA, USA) for a period of 12 months (Figure 1B). For the period of sampling and behavioral testing, the Gillbach's thermal gradient resulted in a between-site temperature difference of 4°C in spring (March – May 2016, mean ± SD, site 1: 23.1 ± 2.6°C; site 2: 19.2 ± 3.6°C; Figure 1B) and ~1°C in summer (June – August, site 1: 27.5 ± 2.2°C; site 2: 26.8 ± 2.4°C; Figure 1B). However, we expect the temperature difference between site 1 and 2 to be even more pronounced in colder months [e.g., difference between site 1 and 1 km downstream of site 2: 7°C in November (Klotz et al., 2013); 8.4°C in February (Jourdan et al., 2014)]. With temperatures below the population's critical minimum temperature (~12.4°C after Jourdan et al., 2014, see Figure 1B), survival of guppies outside the core area during winter is highly unlikely.
Husbandry
Guppies collected for behavioral testing were transferred to the lab at Humboldt Universität zu Berlin, Germany. We determined sex and body size distribution of each population sample before introduction to the housing tank (pre-acclimation; see Supplementary Figure 3). Females and males obtained from the same site were housed communally in separate compartments of a 250-l fiberglass holding tank (LxWxH: 120 × 58 × 40 cm). Each compartment contained artificial java moss to serve as a shelter. The water was kept under continuous aeration and filtration, while the temperature was maintained at 26°C. Fish were held under diurnal lighting (12:12 h light:dark cycle). Offspring was removed twice a week. Adults were fed commercially available flake food (TetraMin® Tetra GmbH) twice a day. Feeding was halted 24 h before experiments and commenced afterwards. To acclimate the fish to the new laboratory conditions, no behavioral testing was conducted for at least 30 days (Figure 2A). Previous studies on poeciliids indicated that 4 weeks are sufficient for thermal up- or downward acclimation of the magnitude of 5–6°C (as experienced by spring-caught fish, see Chung, 2001; Seebacher et al., 2014). One week prior to testing, fish were marked using VIE tagging (see Jourdan et al., 2014 for procedure) to allow for individual recognition throughout the behavioral assessment.
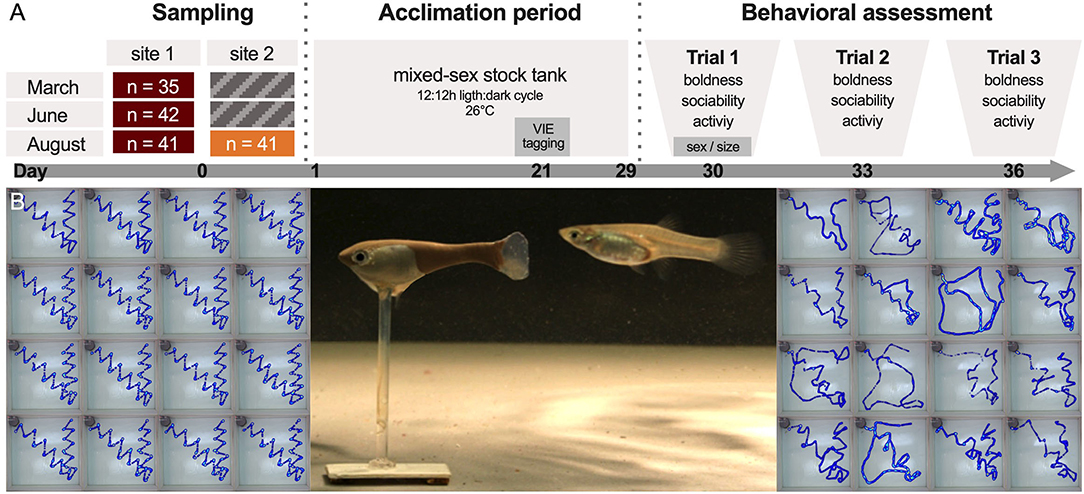
Figure 2. Behavioral testing with robotic fish. (A) Test fish were collected three times and, when possible, at two sites (site 1 and 2, see Figure 1A). After an initial 30-day acclimation period, fish were tested a total of three times (trial 1–3, sample sizes indicate tested individuals, for total catch numbers see Supplementary Table 2). (B) During trials, the fish replica was moved remotely through the test tank (center). Once the focal individual had emerged from its shelter, the biomimetic robot initiated its predefined path (left) and the fish's swimming trajectory was recorded (right).
Behavioral Assessment
We tested a total of 159 guppies (95 females, 64 males; for population-specific sample sizes see Figure 2A). Fish were included if their body size exceeded 15 mm standard length (SL) and if they showed external signs of maturity (females: gravid spot, males: fully developed gonopodium, see Supplementary Figure 4). Test fish were introduced to an open field test arena (88 × 88 cm, 75 mm water depth) filled with aged tap water kept at 26°C. To reduce disturbing stimuli, the arena was enclosed in black, opaque plastic and illuminated with artificial light reproducing the daylight spectrum. Behavioral observations were recorded via a camera (Ximea 4K USB 3.1 camera) mounted above the arena. Position information was extracted using EthoVision 10 XT software (Noldus Information Technology, Wageningen, Netherlands).
(1) Boldness: Latency to emerge from a shelter was used as a measure of boldness with a low latency time indicating high levels of boldness. Fish were introduced into a gray opaque shelter box (diameter: 100 mm; top left of each image in Figure 2B) and allowed to acclimate. After 1 min, a researcher removed the sponge from the entrance (40 × 25 mm), allowing the fish to emerge and explore the test arena. A fish was scored as having emerged when its full body was visible outside of the shelter. If fish did not emerge after 5 min (15 cases in 477 trials), the lid of the shelter box was removed. Fish that failed to emerge within 8 min (11 cases in 477 trials) were given the maximum score of 480 s and the box was removed entirely. As these interventions could have disturbed fish and influenced subsequent measures, we confirmed that the results were qualitatively similar if we fitted models excluding these data points (results not shown).
(2) Sociability: A three-dimensional fish replica was stationed 1 cm away from the shelter entrance. The replica, which resembled a large-sized guppy female (SL = 30 mm; left fish in Figure 2B), could be moved by a robot via a magnetic base (see Landgraf et al., 2016; Bierbach et al., 2018 for details). Upon the fish's emergence (or the removal of the shelter box), the biomimetic robot started to move through the arena in a standardized sequence (Figure 2B) imitating a conspecific. We calculated the mean distance the fish kept to the biomimetic robot as a proxy of sociability with lower values indicating higher levels of sociability. Consequently, fish that followed the robot closer were assumed to be more sociable (see Bierbach et al., 2018).
(3) Activity: After completion of the robotic fish's predefined path, the replica was removed from the arena by hand. Once the fish resumed swimming behavior (e.g., after a period of freezing), it was allowed to explore the arena. As a measure of activity, we calculated the mean velocity with which the fish explored the open arena during 3 min.
To minimize handling and exposure to stress (Animal Behaviour, 2020), all three traits were assessed consecutively (same test sequence) and within the same arena. We are aware that testing multiple traits within one behavioral assay and in the same order can introduce carryover effects and give rise to correlation artifacts (Carter et al., 2013; Seebacher et al., 2014), both of which are common caveats in personality research, but neither affected our interpretation of between-population differences. After the completion of the first behavioral assay, we sexed each individual and recorded its body length by photographing the fish in a water-filled petri dish with a reference grid. Fish were transferred back to their respective compartment and allowed 72 h resting before being retested following the same protocol. To reduce acclimatization effects, we only assessed short-term repeatability by testing fish a total of three times within the span of a week. The order in which fish were tested was randomized for each trial.
Statistical Analysis
To test for differences in average trait expression over a temporal and/or spatial scale, we used linear mixed models (LMM, GENLINMIXED procedure) with each behavioral trait (boldness, sociability, activity) as a separate response variable. Boldness and sociability were log10-transformed to fulfill normality assumptions. All models included sample “population” as the fixed effect of interest (four levels: March site 1, June site 1, August site 1, August site 2). The low sample size collected at site 2 during March and June (n = 8 and n = 2, respectively; see Supplementary Table 2) made these estimates uncertain. After verifying that there were no substantial differences found when running the analyses with and without the above population samples, these estimates were excluded from final analyses. Behavior in poeciliids is often size- and/or sex-dependent (Brown and Braithwaite, 2004; Harris et al., 2010), thus we included “standard length” (SL) as a covariate and “sex” as an additional fixed factor. To account for the repeated testing of individuals, we further included “trial” as a fixed factor and “fish ID” as a random factor. We allowed for trial effects to vary across populations (interaction “population x trial”), but removed the interaction term when it did not significantly improve the model (results not shown). Post-hoc LSD tests were employed in case fixed factors were found to have a significant effect. For the covariate “body size,” we also performed Pearson's correlations to better evaluate the relationship.
(Co)Variance structure was nested within “population,” which enabled us to calculate the total behavioral variance as well as within- and among-individual variance components in each population. Based on this, we calculated behavioral repeatability for each of the four populations as a measure of consistency. We assumed that repeatability and/or variance estimates differed among populations when 95% confidence intervals (CIs) were not overlapping (see Nakagawa and Schielzeth, 2010).
To evaluate the existence of behavioral syndromes (i.e., correlation among behavioral traits) at certain times and/or sites, we first estimated the raw phenotypic correlation (Pearson's correlation). To partition phenotypic correlation coefficients into a between-individual and within-individual correlation component (after Dingemanse and Dochtermann, 2013), we used multivariate mixed-effect models (MMM, MIXED procedure) separated by sample population. In order to minimize the effects of mean differences in behavioral traits on trait correlation, we mean-centered each behavioral trait within “trial” and “population” (z-transformation). Each mixed model included “sex” and “body length” as covariates and “fish ID” as a random factor. We assumed that trait correlations differed among populations when 95% confidence intervals (CIs) were not overlapping (see Nakagawa and Schielzeth, 2010).
All statistical analyses were performed with SPSS 25.0 software (IBM Corp., NY, USA) and visualization of the results was done with GraphPad Prism 8.01 (GraphPad Software Inc., CA, USA) or R (v. 4.0.2; R Core Team, 2020).
Results
Differences in Average Trait Expression Among Populations
Expression of average sociability and activity differed between populations, while boldness did not (“population” effect in Table 1; Figure 3A). On a temporal scale, fish sampled at site 1 in August showed higher levels of sociability (i.e., reduced distance to robotic fish) compared to March and June populations (post-hoc, August site 1 vs. March site 1: p = 0.003, vs. June site 1: p = 0.025). Similarly, fish sampled at site 1 in August were more active than populations sampled in March and June (post-hoc, August site 1 vs. March site 1: p = 0.002, vs. June site 1: p < 0.001). On a spatial scale, we found no evidence that fish sampled in August at site 1 and site 2 differed in boldness, sociability, nor activity (post-hoc, August site 1 vs. site 2 boldness: p = 0.76, sociability: p = 0.92, activity: p = 0.89; Figure 3A).
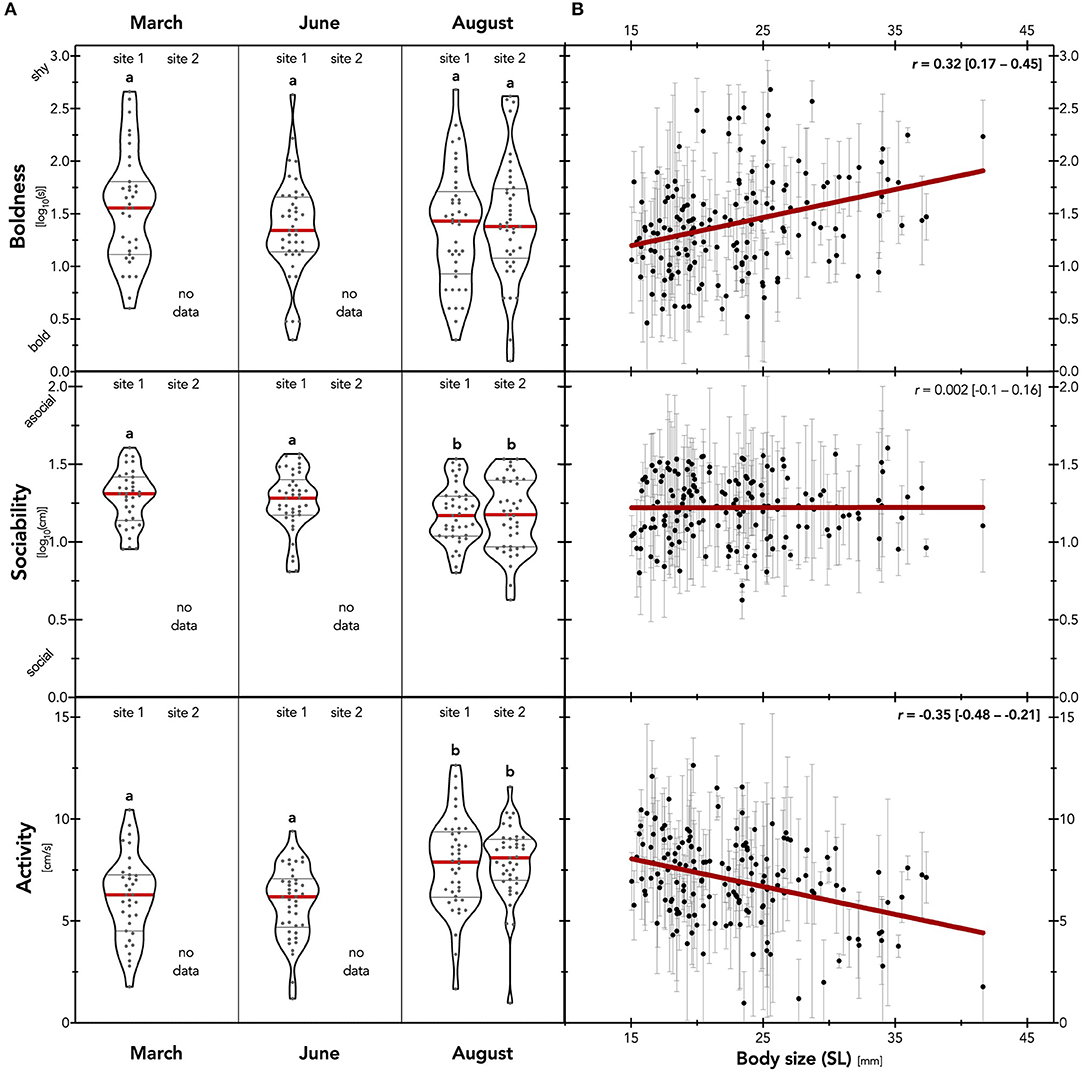
Figure 3. Average personality traits across temporal and spatial scale. (A) Mean trait scores (median ± IQR) for boldness (i.e., time to emerge from shelter), activity (i.e., velocity during open field exploration) and sociability (i.e., distance to biomimetic robot). Different letters depict significant differences based on post-hoc LSD tests after global linear mixed models (p < 0.05). (B) Mean trait scores (±SD) in relation to body size (standard length; n = 159). To visualize the relationships, we show linear regressions (red line) calculated from individual means (parameters significantly different from zero at the 95% confidence level are indicated in bold; see main text for detailed analysis). Note that low scores of boldness and sociability indicate high trait expression.
Boldness and activity showed a size-dependency (Figure 3B) with smaller individuals being bolder (i.e., reduced emergence time; Pearson's r = 0.32; p < 0.001) and more active (Pearson's r = 0.35; p < 0.001) than larger ones. We found no such relation between body size and sociability (Pearson's r = 0.002; p = 0.98). While sex is correlated with size in the sexually dimorphic guppy, sex did not affect any of the measured behavioral traits (non-sig. “sex” effect; Table 1). We further observed a habituation to the repeated testing during sociability and activity assays (sig. “trial” effect; Table 1), which was uniform across population samples (non-sig. interaction “population x trial” for all three traits; p never below 0.25). Fish were considerably less social and less active with each consecutive measurement. We note that the habituation effect observed for activity but not boldness serves as a validation that emergence into and activity within an open field measured two different traits.
Among- and Within-Individual Variation in Behavioral Traits
Fish within each sampled population differed consistently in their expression of boldness, sociability and activity across trials (sig. repeatability estimates; Table 2). Only fish sampled in August at site 2 had a significantly higher repeatability estimate for sociability than fish from the March sampling at site 1 (non-overlapping CIs; Table 2). There was no significant difference among populations in estimated overall behavioral variances in any of the examined traits (see “total variation” in Table 2; for within- and among-individual variance estimates see Supplementary Table 5).
Behavioral Syndrome Structure
Boldness and activity were correlated in all four population samples (significant phenotypic correlation, rraw in Figure 4). This was driven by high significant among-individual correlations (ramong in Figure 4) that did not differ among populations (overlapping CIs). This indicated that fish with high average activity levels also left the shelter quicker, confirming that activity and boldness are part of a larger behavioral syndrome (Figure 4).
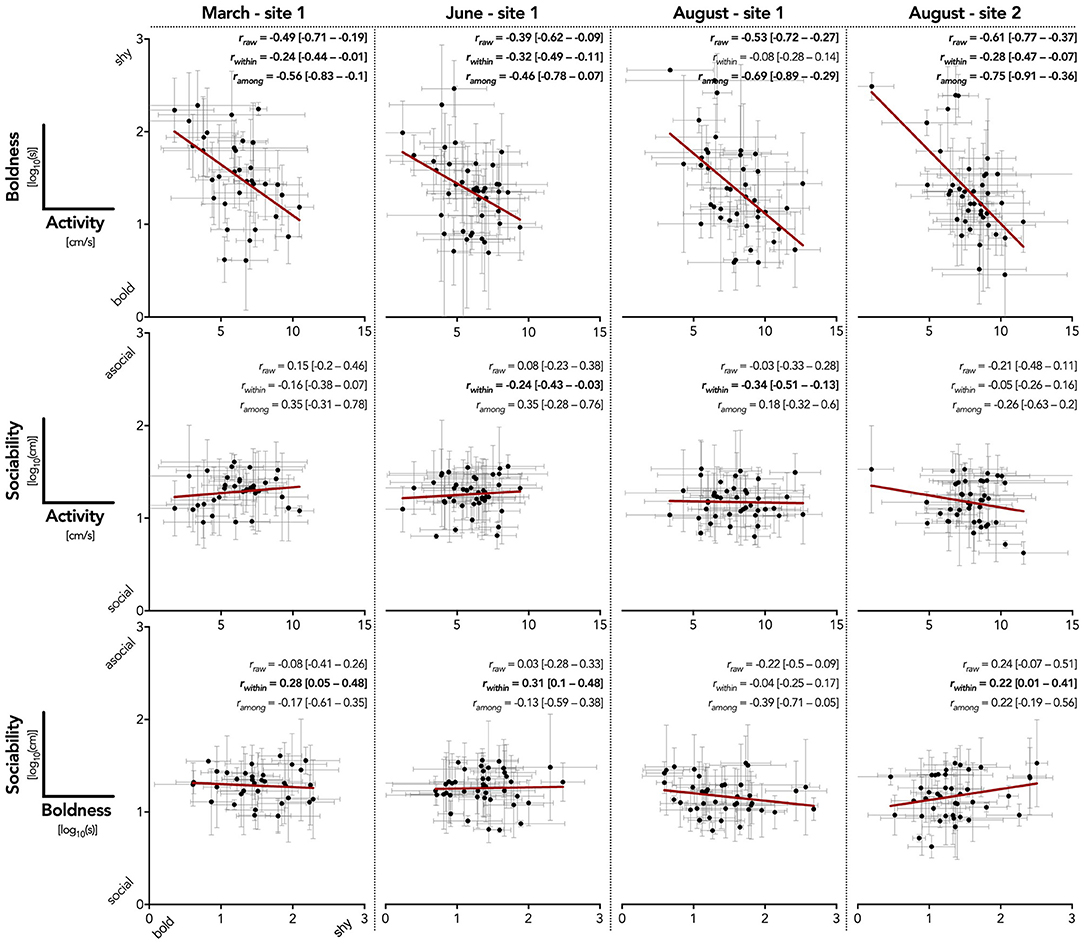
Figure 4. Correlations between behavioral traits across temporal and spatial scale. Mean trait scores (±SD) of individual test fish are shown for each population. Linear regressions (red line) and mixed model estimated correlation coefficients visualize the relationships between traits (parameters significantly different from zero at the 95% confidence level are indicated in bold; see main text for detailed analysis). Note that for boldness and sociability lower values indicate a high trait score.
There was no evidence for raw phenotypic or among-individual correlations between sociability and boldness, nor between sociability and activity among populations (non-significant rraw and ramong). Thus, sociability measured as distance kept to the moving robotic fish does not seem to be part of a behavioral syndrome with either boldness or activity. Nevertheless, we found several traits to show significant within-individual correlations (rwithin) which indicates that fish showing high variation in one trait also exhibited high variation in the other trait (for within- and among-individual variance estimates see Supplementary Table 6).
Discussion
Our study follows the annual range shifts of non-native fish in an artificially heated stream in temperate Germany. As guppies dispersed into winter-abandoned areas in warmer months, we expected this process to be driven by dispersal-enhancing behavioral traits. We found similar consistent among-individual variation (e.g., significant behavioral repeatability) in the behaviors “boldness,” “sociability,” and “activity” within all temporal and spatial samples (except a difference between sociability in March at site 1 and August at site 2). Contrary to our predictions, we did not detect a decrease in total behavioral variation of the sampled populations indicative of a “thinning out” of disperser's behavioral types. Further, we found no evidence that the source and the newly-founded population differed in terms of average behavioral trait expression, though a seasonal change in activity and sociability (but not boldness) was detectable in the source population: August-sampled individuals were more active and more sociable than those sampled earlier in the year. Instead, we found a behavioral syndrome among activity and boldness that was consistently detectable throughout the year and across sampled populations. To sum up, we found no strong evidence for a personality-biased dispersal in this feral guppy population and the detected seasonal differences in average trait expression (sociability, activity) are likely due to changed environmental factors such as temperature, predator abundance and/or resource availability.
Differences in behavioral traits across seasons but not between sites as found in our study could be explained by seasonal variation in environmental conditions rather than by a drain of individuals carrying certain behavioral types from source to downstream populations. The Gillbach's water temperatures show strongest differences between sites in the colder months (~4°C between site 1 and 2), but relatively small differences during the warmer months (<1°C between site 1 and 2; Figure 1B). Thus, when winter-abandoned peripheral sites are colonized again in summer, these habitats do not differ much in temperature from the source at this point in time. Nevertheless, the Gillbach still shows a strong seasonality in temperature which, in combination with a variation in day length, food quality/quantity and predation pressure, may likely cause population-wide changes in behaviors across seasons (e.g., Biro et al., 2010; Eccard and Herde, 2013; Spiegel et al., 2015; Uchida et al., 2016; Barbosa et al., 2018; Dhellemmes et al., 2020) as seen in the source population. For example, prior temperature acclimation during sensitive developmental phases can alter the phenotypes of subsequent adult phases (Beitinger et al., 2000; Seebacher et al., 2014). This might explain the increased activity exhibited by fish in August, as higher developmental temperatures lead to more active phenotypes (Seebacher et al., 2014). However, teasing apart the effects of abiotic (e.g., temperature) and biotic (e.g., predation pressure) fluctuations in environmental conditions will require additional experimentation.
Besides seasonal differences in average behavioral traits, we found stable behavioral syndromes among sampled populations that involved a strong positive correlation between boldness and activity. Such a boldness-activity syndrome has been documented in various taxa (van Oers et al., 2004; Pintor et al., 2008; Wilson and Godin, 2009; Cote et al., 2010b; Eccard and Herde, 2013; Muraco et al., 2014; Bierbach et al., 2015), including guppies (Brown and Irving, 2014). Natural selection through predation favors the evolution of behavioral correlations (Dingemanse et al., 2007; Harris et al., 2010; Dhellemmes et al., 2020). The Gillbach's guppy population underwent strong seasonal changes in size (Supplementary Appendix 2) and the observed population decline at the end of the summer, while primary production is highest in temperate regions, suggests strong predation pressure. A positive boldness-activity correlation might thus represent an optimal trait combination in the face of predation experienced by both populations (Smith and Blumstein, 2010). However, the lack of differences in the level of (individual) behavioral variation among populations might be a result of a random downstream drift and a low overall behavioral plasticity in this population. This can be a consequence of the assumed bottleneck origin of this feral population that most likely stems from an unknown number of released domesticated guppies (see Gertzen et al., 2008 for estimates of propagule pressure for popular aquarium species). Investigations involving other populations of the guppy and more distantly related species are highly recommended, especially as several environmental factors are predicted to impact behavioral variation, especially in fish (e.g., Harris et al., 2010; Laskowski et al., 2016; Barbosa et al., 2018). Alternatively, guppies and especially those from the Gillbach that experience highly fluctuating temperature regimes may have the ability to adjust their behavioral expressions within very short times, e.g., within the 30-days acclimatization period in the laboratory used here. It is known that guppies have a remarkable capacity for behavioral plasticity (Deacon and Magurran, 2016) and thermal adaptation (Chung, 2001; Jourdan et al., 2014), and thus have successfully established populations in environments with very different (thermal) regimes compared to their native habitats (Deacon et al., 2011). Testing of individuals after different acclimation periods could be a possibility to investigate this issue further.
Many large-scale field studies, especially on taxa of small-sized organisms, have the inherent problem that it is not (yet) feasible to track individuals continuously in real time. Without it, studies in the wild always carry an element of uncertainty concerning the dispersal process and our study is no exception. We assume that peripheral areas (site 2) are depopulated in winter and (re)colonized by dispersing individuals from the source (site 1) in warmer months based on the following: First, temperature data of site 2 (Figure 1B) suggests that several temperature drops occurred during spring (and likely many more in winter), thus inhibiting winter survival of guppies more than 1 kilometer downstream of the source. Second, the unique conditions of the Gillbach system render colonization from other areas than the source unlikely. Although our mark-recapture experiment (Supplementary Appendix 2) was not suited to find individuals dispersing between site 1 and 2, we argue that the Gillbach's source provides only one direction of dispersal simply because upstream dispersal to site 2 cannot take place as there are no guppy reports further downstream. Third, introductions by humans are similarly unlikely as this part of the stream is fenced and not well-accessible and the introduction of ornamental guppies to site 2 would have led to noticeable changes in fish's color patterns which was not the case. We are therefore confident that our assumptions on the dispersal route from source to downstream areas is justified and thus the reported results present an unbiased snapshot of the dispersing guppies' behavioral types during this journey.
Theoretical models (Cote et al., 2010a; Spiegel et al., 2017) as well as several studies across taxa (Duckworth and Badyaev, 2007; Pintor et al., 2009; Cote et al., 2010b; Myles-Gonzalez et al., 2015; Coates et al., 2019) provide evidence for the prominent role of individual differences in behavior during dispersal and invasion processes (Bowler and Benton, 2005; Chapple et al., 2012; Canestrelli et al., 2015). Nevertheless, the picture might be more complicated and context-dependent: spatial sorting of behavioral types did not emerge under predation pressure (Cote et al., 2013) and was lost as the population aged (Thorlacius et al., 2015). Moreover, even if there are significant (but small) effects of behavioral trait variation affecting relevant ecological phenomena, they might be non-detectable in benign laboratory environments (Schröder et al., 2016; Moiron et al., 2020). Our current study exemplifies that population-wide behavioral analysis can fail to provide evidence for behaviorally-mediated migration patterns and future analysis may focus on tracking individuals along their travel routes across seasons and locations. On top of this, several other feral guppy populations are known in Germany (Lukas et al., 2017b) and across Europe (Kempkes et al., 2018) for which similar investigations are highly advisable in order to test the generality of our herein presented results. Such analyses should strive to incorporate the invasion history, environmental factors as well as the population-specific genetic backgrounds which all can affect behavioral variation in wild populations (Sih and Bell, 2008; Bergmüller and Taborsky, 2010; Stamps and Groothuis, 2010).
Data Availability Statement
The raw data supporting the conclusions of this article is available at: https://doi.org/10.6084/m9.figshare.13292750.
Ethics Statement
The animal study was reviewed and approved by LaGeSo Berlin (Reg. 0117/16 to DB).
Author Contributions
JL and DB designed the study. JL, FM, GK, and DB jointly collected the fish. DB, TL, and JK developed the robotic fish system “Robofish.” JL and DB conducted the behavioral experiments, analyzed the data, and wrote the manuscript. All authors approved the final version of this manuscript.
Funding
The authors received financial support by the Elsa-Neumann-Scholarship from the state of Berlin [JL]; the German Ichthyological Society [DB, JL, and GK]; the German Research Foundation (BI 1828/2-1 [DB], LA 3534/1-1 [TL], KA 3029/2-1 [GK]) and through Germany's Excellence Strategy - EXC 2002/1 (project number 390523135 [JK]).
Conflict of Interest
The authors declare that the research was conducted in the absence of any commercial or financial relationships that could be construed as a potential conflict of interest.
Acknowledgments
The authors thank Dr. Udo Rose, biologist at the Erftverband and member of the Erftfischereigenossenschaft (Bergheim, Germany), for the permission to do research at the Gillbach and Dr. Sebastian Emde (LANUV NRW) for providing temperature profiles of the Gillbach prior to March 2016. Sampling efforts and monitoring was supported by several fish enthusiasts: Michael Kempkes, Bernd Neu, Geoffrey P.F. Mazué, Vivek Hari Sridhar, as well as Petr Zajicek and Marcus Ebert from IGB Berlin. Finally, we thank the editor as well as two reviewers for their valuable comments and effort to improve this manuscript. A previous version of this manuscript has been released as a pre-print (Lukas et al., 2020).
Supplementary Material
The Supplementary Material for this article can be found online at: https://www.frontiersin.org/articles/10.3389/fevo.2020.583670/full#supplementary-material
References
Animal Behaviour. (2020). Guidelines for the treatment of animals in behavioural research and teaching. Anim. Behav. 159, I–XI. doi: 10.1016/j.anbehav.2019.11.002
Barbosa, M., Deacon, A. E., Janeiro, M. J., Ramnarine, I., Morrissey, M. B., and Magurran, A. E. (2018). Individual variation in reproductive behaviour is linked to temporal heterogeneity in predation risk. Proc. R. Soc. B 285:20171499. doi: 10.1098/rspb.2017.1499
Beitinger, T. L., Bennett, W. A., and McCauley, R. W. (2000). Temperature tolerances of north american freshwater fishes exposed to dynamic changes in temperature. Environ. Biol. Fish 58:40. doi: 10.1023/A:1007676325825
Bell, A. M., Hankison, S. J., and Laskowski, K. L. (2009). The repeatability of behaviour: a meta-analysis. Anim. Behav. 77, 771–783. doi: 10.1016/j.anbehav.2008.12.022
Bergmüller, R., and Taborsky, M. (2010). Animal personality due to social niche specialisation. Trends Ecol. Evol. 25, 504–511. doi: 10.1016/j.tree.2010.06.012
Bierbach, D., Landgraf, T., Romanczuk, P., Lukas, J., Nguyen, H., Wolf, M., et al. (2018). Using a robotic fish to investigate individual differences in social responsiveness in the guppy. Roy. Soc. Open Sci. 5:181026. doi: 10.1098/rsos.181026
Bierbach, D., Laskowski, K. L., and Wolf, M. (2017). Behavioural individuality in clonal fish arises despite near-identical rearing conditions. Nat. Comm. 8:15361. doi: 10.1038/ncomms15361
Bierbach, D., Sommer-Trembo, C., Hanisch, J., Wolf, M., and Plath, M. (2015). Personality affects mate choice: bolder males show stronger audience effects under high competition. Behav. Ecol. 26, 1314–1325. doi: 10.1093/beheco/arv079
Biro, P. A., and Dingemanse, N. J. (2009). Sampling bias resulting from animal personality. Trends Ecol. Evol. 24, 66–67. doi: 10.1016/j.tree.2008.11.001
Biro, P. A., Beckmann, C., and Stamps, J. A. (2010). Small within-day increases in temperature affects boldness and alters personality in coral reef fish. Proc. R. Soc. B 277, 71–77. doi: 10.1098/rspb.2009.1346
Bowler, D. E., and Benton, T. G. (2005). Causes and consequences of animal dispersal strategies: relating individual behaviour to spatial dynamics. Biol. Rev. Camb. Philos. Soc. 80, 205–225. doi: 10.1017/S1464793104006645
Brown, C., and Braithwaite, V. A. (2004). Size matters: a test of boldness in eight populations of the poeciliid Brachyraphis episcopi. Anim. Behav. 68, 1325–1329. doi: 10.1016/j.anbehav.2004.04.004
Brown, C., and Irving, E. (2014). Individual personality traits influence group exploration in a feral guppy population. Behav. Ecol. 25, 95–101. doi: 10.1093/beheco/art090
Camacho-Cervantes, M., Ojanguren, A. F., and Magurran, A. E. (2015). Exploratory behaviour and transmission of information between the invasive guppy and native Mexican topminnows. Anim. Behav. 106, 115–120. doi: 10.1016/j.anbehav.2015.05.012
Canestrelli, D., Bisconti, R., and Carere, C. (2015). Bolder takes all? The behavioral dimension of biogeography. Trends Ecol. Evol. 31, 35–43. doi: 10.1016/j.tree.2015.11.004
Carere, C., and Gherardi, F. (2013). Animal personalities matter for biological invasions. Trends Ecol. Evol. 1, 5–6. doi: 10.1016/j.tree.2012.10.006
Carter, A. J., Feeney, W. E., Marshall, H. H., Cowlishaw, G., and Heinsohn, R. (2013). Animal personality: what are behavioural ecologists measuring? Biol. Rev. Camb. Philos. Soc. 88, 465–475. doi: 10.1111/brv.12007
Chapple, D. G., Simmonds, S. M., and Wong, B. B. M. (2012). Can behavioral and personality traits influence the success of unintentional species introductions? Trends Ecol. Evol. 27, 57–64. doi: 10.1016/j.tree.2011.09.010
Chung, K. S. (2001). Critical thermal maxima and acclimation rate of the tropical guppy Poecilla reticulata. Hydrobiologia 462, 253–257. doi: 10.1023/A:1013158904036
Coates, W. D., Hale, R., and Morrongiello, J. R. (2019). Dispersal decisions and personality in a freshwater fish. Anim. Behav. 157, 209–218. doi: 10.1016/j.anbehav.2019.07.012
Cote, J., Clobert, J., Brodin, T., Fogarty, S., and Sih, A. (2010a). Personality-dependent dispersal: characterization, ontogeny and consequences for spatially structured populations. Phil. Trans. R. Soc. B 365, 4065–4076. doi: 10.1098/rstb.2010.0176
Cote, J., Fogarty, S., Brodin, T., Weinersmith, K., and Sih, A. (2011). Personality-dependent dispersal in the invasive mosquitofish: group composition matters. Proc. R. Soc. B 278, 1670–1678. doi: 10.1098/rspb.2010.1892
Cote, J., Fogarty, S., Tymen, B., Sih, A., and Brodin, T. (2013). Personality-dependent dispersal cancelled under predation risk. Proc. R. Soc. B 280:20132349. doi: 10.1098/rspb.2013.2349
Cote, J., Fogarty, S., Weinersmith, K., Brodin, T., and Sih, A. (2010b). Personality traits and dispersal tendency in the invasive mosquitofish (Gambusia affinis). Proc. R. Soc. B 277, 1571–1579. doi: 10.1098/rspb.2009.2128
Deacon, A. E., and Magurran, A. E. (2016). “How behaviour contributes to the success of an invasive poeciliid fish: the trinidadian guppy (Poecilia reticulata) as a model species,” in Biological Invasions and Animal Behaviour, eds. J. S. Weis and D. Sol (Cambridge: Cambridge University Press), 266–290.
Deacon, A. E., Ramnarine, I. W., and Magurran, A. E. (2011). How reproductive ecology contributes to the spread of a globally invasive fish. PLoS ONE 6:e24416. doi: 10.1371/journal.pone.0024416
Dhellemmes, F., Finger, J.-S., Laskowski, K. L., Guttridge, T. L., and Krause, J. (2020). Comparing behavioural syndromes across time and ecological conditions in a free-ranging predator. Anim. Behav. 162, 23–33. doi: 10.1016/j.anbehav.2020.01.009
Dingemanse, N. J., and Dochtermann, N. A. (2013). Quantifying individual variation in behaviour: mixed-effect modelling approaches. J. Anim. Ecol. 82, 39–54. doi: 10.1111/1365-2656.12013
Dingemanse, N. J., Wright, J., Kazem, A. J. N., Thomas, D. K., Hickling, R., and Dawnay, N. (2007). Behavioural syndromes differ predictably between 12 populations of three-spined stickleback. J. Anim. Ecol. 76, 1128–1138. doi: 10.1111/j.1365-2656.2007.01284.x
Duckworth, R. A., and Badyaev, A. V. (2007). Coupling of dispersal and aggression facilitates the rapid range expansion of a passerine bird. Proc. Natl. Acad. Sci. U.S.A. 104, 15017–15022. doi: 10.1073/pnas.0706174104
Eccard, J. A., and Herde, A. (2013). Seasonal variation in the behaviour of a short-lived rodent. BMC Ecol. 13:43. doi: 10.1186/1472-6785-13-43
Fogarty, S., Cote, J., and Sih, A. (2011). Social personality polymorphism and the spread of invasive species: a model. Am. Nat. 177, 273–287. doi: 10.1086/658174
Gertzen, E., Familiar, O., and Leung, B. (2008). Quantifying invasion pathways: fish introductions from the aquarium trade. Can. J. Fish. Aquat. Sci. 65, 1265–1273. doi: 10.1139/F08-056
Gruber, J., Brown, G., Whiting, M. J., and Shine, R. (2017). Geographic divergence in dispersal-related behaviour in cane toads from range-front versus range-core populations in Australia. Behav. Ecol. Sociobiol. 71:38. doi: 10.1007/s00265-017-2266-8
Harris, S., Ramnarine, I. W., Smith, H. G., and Pettersson, L. B. (2010). Picking personalities apart: estimating the influence of predation, sex and body size on boldness in the guppy Poecilia reticulata. Oikos 119, 1711–1718. doi: 10.1111/j.1600-0706.2010.18028.x
Höfer, S., and Staas, S. (1998). Bericht zur fischereibiologischen Untersuchung des Gillbaches im Bereich Bergheim-Auenheim. Zoologisches Institut der Universität zu Köln, Abt. Allgemeine Ökologie und Limnologie, Köln, Germany.
Hudina, S., Žganec, K., and Hock, K. (2015). Differences in aggressive behaviour along the expanding range of an invasive crayfish: an important component of invasion dynamics. Biol. Invasions 17, 3101–3112. doi: 10.1007/s10530-015-0936-x
Jourdan, J., Miesen, F. W., Zimmer, C., Gasch, K., Herder, F., Schleucher, E., et al. (2014). On the natural history of an introduced population of guppies (Poecilia reticulata Peters, 1859) in Germany. Bioinvasions Rec. 3, 175–184. doi: 10.3391/BIR.2014.3.3.07
Juette, T., Cucherousset, J., and Cote, J. (2014). Animal personality and the ecological impacts of freshwater non-native species. Curr. Zool. 60, 417–427. doi: 10.1093/czoolo/60.3.417
Kempkes, M., Lukas, J., and Bierbach, D. (2018). Tropische Neozoen in heimischen Fließgewässern. (Magdeburg: VerlagsKG Wolf).
Klotz, W., Miesen, F. W., Hüllen, S., and Herder, F. (2013). Two Asian fresh water shrimp species found in a thermally polluted stream system in North Rhine-Westphalia, Germany. Aquat. Invasions 8, 333–339. doi: 10.3391/ai.2013.8.3.09
Landgraf, T., Bierbach, D., Nguyen, H., Muggelberg, N., Romanczuk, P., and Krause, J. (2016). RoboFish: increased acceptance of interactive robotic fish with realistic eyes and natural motion patterns by live Trinidadian guppies. Bioinspir. Biomim. 11:15001. doi: 10.1088/1748-3190/11/1/015001
Langford, T. E. (1990). Ecological Effects of Thermal Discharges. Essex: Elsevier Applied Science Publishers Ltd.
Laskowski, K. L., Monk, C. T., Polverino, G., Alós, J., Nakayama, S., Staaks, G., et al. (2016). Behaviour in a standardized assay, but not metabolic or growth rate, predicts behavioural variation in an adult aquatic top predator Esox lucius in the wild. J. Fish Biol. 88, 1544–1563. doi: 10.1111/jfb.12933
Lockwood, J. L., Hoopes, M. F., and Marchetti, M. P. (2011). Non-natives: plusses of invasion ecology. Nature 475, 36–36. doi: 10.1038/475036c
Lopez, D. P., Jungman, A. A., and Rehage, J. S. (2012). Nonnative African jewelfish are more fit but not bolder at the invasion front: a trait comparison across an Everglades range expansion. Biol. Invasions 14, 2159–2174. doi: 10.1007/s10530-012-0221-1
Lukas, J., Kalinkat, G., Kempkes, M., Rose, U., and Bierbach, D. (2017b). Feral guppies in Germany – a critical evaluation of a citizen science approach as biomonitoring tool. Bull Fish Biol. 17, 13–27.
Lukas, J., Jourdan, J., Kalinkat, G., Emde, S., Miesen, F. W., Jüngling, H., et al. (2017a). On the occurrence of three non-native cichlid species including the first record of a feral population of Pelmatolapia (Tilapia) mariae (Boulenger, 1899) in Europe. Roy. Soc. Open Sci. 4:170160. doi: 10.1098/rsos.170160
Lukas, J., Kalinkat, G., Miesen, F. W., Landgraf, T., Krause, J., and Bierbach, D. (2020). Consistent behavioral syndrome across seasons in an invasive freshwater fish. bioRxiv [Preprint]. doi: 10.1101/2020.03.03.974998
Moiron, M., Laskowski, K. L., and Niemelä, P. T. (2020). Individual differences in behaviour explain variation in survival: a meta-analysis. Ecol. Lett. 23, 399–408. doi: 10.1111/ele.13438
Muraco, J. J., Aspbury, A. S., and Gabor, C. R. (2014). Does male behavioral type correlate with species recognition and stress? Behav. Ecol. 25, 200–205. doi: 10.1093/beheco/art106
Myles-Gonzalez, E., Burness, G., Yavno, S., Rooke, A., and Fox, M. G. (2015). To boldly go where no goby has gone before: Boldness, dispersal tendency, and metabolism at the invasion front. Behav. Ecol. 26, 1083–1090. doi: 10.1093/beheco/arv050
Nakagawa, S., and Schielzeth, H. (2010). Repeatability for Gaussian and non-Gaussian data: a practical guide for biologists. Biol. Rev. Camb. Philos. Soc. 85, 935–956. doi: 10.1111/j.1469-185X.2010.00141.x
Pintor, L. M., Sih, A., and Bauer, M. L. (2008). Differences in aggression, activity and boldness between native and introduced populations of an invasive crayfish. Oikos 117, 1629–1636. doi: 10.1111/j.1600-0706.2008.16578.x
Pintor, L. M., Sih, A., and Kerby, J. L. (2009). Behavioral correlations provide a mechanism for explaining high invader densities and increased impacts on native prey. Ecology 90, 581–587. doi: 10.1890/08-0552.1
Piria, M., Copp, G., Dick, J., Duplić, A., Groom, Q., Jelić, D., et al. (2017). Tackling invasive alien species in Europe II: threats and opportunities until 2020. Manag. Biol. Invasions 8, 273–286. doi: 10.3391/mbi.2017.8.3.02
Polverino, G., Bierbach, D., Killen, S. S., Uusi-Heikkilä, S., and Arlinghaus, R. (2016). Body length rather than routine metabolic rate and body condition correlates with activity and risk-taking in juvenile zebrafish Danio rerio. J. Fish Biol. 89, 2251–2267. doi: 10.1111/jfb.13100
R Core Team (2020). R: A Language and Environment for Statistical Computing. (Vienna: R Foundation for Statistical Computing). Available online at: http://www.R-project.org/
Rasmussen, J. E., and Belk, M. C. (2012). Dispersal behavior correlates with personality of a North American fish. Curr. Zool. 58, 260–270. doi: 10.1093/czoolo/58.2.260
Réale, D., Reader, S. M., Sol, D., McDougall, P. T., and Dingemanse, N. J. (2007). Integrating animal temperament within ecology and evolution. Biol. Rev. Camb. Philos. Soc. 82, 291–318. doi: 10.1111/j.1469-185X.2007.00010.x
Schröder, A., Kalinkat, G., and Arlinghaus, R. (2016). Individual variation in functional response parameters is explained by body size but not by behavioural types in a poeciliied fish. Oecologia 182, 1129–1140. doi: 10.1007/s00442-016-3701-7
Seebacher, F., Beaman, J., and Little, A. G. (2014). Regulation of thermal acclimation varies between generations of the short-lived mosquitofish that developed in different environmental conditions. Funct. Ecol. 28, 137–148. doi: 10.1111/1365-2435.12156
Seebens, H., Blackburn, T. M., Dyer, E., Genovesi, P., Hulme, P. E., Jeschke, J. M., et al. (2017). No saturation in the accumulation of alien species worldwide. Nat. Comm. 8:14435. doi: 10.1038/ncomms14435
Seebens, H., Blackburn, T. M., Dyer, E. E., Genovesi, P., Hulme, P. E., Jeschke, J. M., et al. (2018). Global rise in emerging alien species results from increased accessibility of new source pools. Proc. Natl. Acad. Sci. U.S.A. 115, E2264–E2273. doi: 10.1073/pnas.1719429115
Shine, R., Brown, G. P., and Phillips, B. L. (2011). An evolutionary process that assembles phenotypes through space rather than through time. Proc. Natl. Acad. Sci. U.S.A. 108, 5708–5711. doi: 10.1073/pnas.1018989108
Sih, A., and Bell, A. M. (2008). Insights for behavioral ecology from behavioral syndromes. Adv. Stud. Behav. 38, 227–281. doi: 10.1016/S0065-3454(08)00005-3
Sih, A., Cote, J., Evans, M., Fogarty, S., and Pruitt, J. (2012). Ecological implications of behavioural syndromes. Ecol. Lett. 15, 278–289. doi: 10.1111/j.1461-0248.2011.01731.x
Smith, B. R., and Blumstein, D. T. (2010). Behavioral types as predictors of survival in Trinidadian guppies (Poecilia reticulata). Behav. Ecol. 21, 919–926. doi: 10.1093/beheco/arq084
Spiegel, O., Leu, S. T., Bull, C. M., and Sih, A. (2017). What's your move? Movement as a link between personality and spatial dynamics in animal populations. Ecol Lett. 20, 3–18. doi: 10.1111/ele.12708
Spiegel, O., Leu, S. T., Sih, A., Godfrey, S. S., and Bull, C. M. (2015). When the going gets tough: behavioural type-dependent space use in the sleepy lizard changes as the season dries. Proc. R. Soc. B 282:20151768. doi: 10.1098/rspb.2015.1768
Stamps, J., and Groothuis, T. G. G. (2010). The development of animal personality: relevance, concepts and perspectives. Biol. Rev. Camb. Philos. Soc. 85, 301–325. doi: 10.1111/j.1469-185X.2009.00103.x
Thorlacius, M., Hellstrom, G., and Brodin, T. (2015). Behavioral dependent dispersal in the invasive round goby Neogobius melanostomus depends on population age. Curr. Zool. 61, 529–542. doi: 10.1093/czoolo/61.3.529
Uchida, K., Suzuki, K., Shimamoto, T., Yanagawa, H., and Koizumi, I. (2016). Seasonal variation of flight initiation distance in Eurasian red squirrels in urban versus rural habitat. J. Zool. 298, 225–231. doi: 10.1111/jzo.12306
van Oers, K., Drent, P. J., de Goede, P., and van Noordwijk, A. J. (2004). Realized heritability and repeatability of risk-taking behaviour in relation to avian personalities. Proc. R. Soc. B 271, 65–73. doi: 10.1098/rspb.2003.2518
Wilson, A. D. M., and Godin, J.-G. J. (2009). Boldness and behavioral syndromes in the bluegill sunfish, Lepomis macrochirus. Behav. Ecol. 20, 231–237. doi: 10.1093/beheco/arp018
Wolf, M., and Weissing, F. J. (2012). Animal personalities: consequences for ecology and evolution. Trends Ecol. Evol. 27, 452–461. doi: 10.1016/j.tree.2012.05.001
Keywords: animal personality, dispersal, range expansion, invasive species, thermally altered freshwaters, guppy
Citation: Lukas J, Kalinkat G, Miesen FW, Landgraf T, Krause J and Bierbach D (2021) Consistent Behavioral Syndrome Across Seasons in an Invasive Freshwater Fish. Front. Ecol. Evol. 8:583670. doi: 10.3389/fevo.2020.583670
Received: 15 July 2020; Accepted: 30 November 2020;
Published: 07 January 2021.
Edited by:
Jerald B. Johnson, Brigham Young University, United StatesReviewed by:
Spencer J. Ingley, Brigham Young University–Hawaii, United StatesJared B. Lee, Southern Virginia University, United States
Copyright © 2021 Lukas, Kalinkat, Miesen, Landgraf, Krause and Bierbach. This is an open-access article distributed under the terms of the Creative Commons Attribution License (CC BY). The use, distribution or reproduction in other forums is permitted, provided the original author(s) and the copyright owner(s) are credited and that the original publication in this journal is cited, in accordance with accepted academic practice. No use, distribution or reproduction is permitted which does not comply with these terms.
*Correspondence: Juliane Lukas, Y29udGFjdEBqdWxpYW5lbHVrYXMuY29t