- 1Key Laboratory of Ecological Safety and Sustainable Development in Arid Lands, Key Laboratory of Ecohydrology of Inland River Basin, Alax Desert Eco-hydrology Experimental Research Station, Qilian Mountains Eco-environment Research Center in Gansu Province, Northwest Institute of Eco-Environment and Resources, Chinese Academy of Sciences, Lanzhou, China
- 2Lanzhou Branch of the Chinese Academy of Sciences, Lanzhou, China
Introduction: Global watershed sustainable development has experienced world-wide threats from continuing anthropogenic stressors, and the need to deepen and broaden research encompassing the intersection in global environmental change as well as environmentally oriented watershed sustainable development (EOWSD) has been noticed. However, there is not yet a widely recognized cognition on the applicability and scope of various EOWSD issues, and the zoning of global EOWSD issues is remains uncertain despite it is crucial for achieving global watershed sustainable development.
Methods: This research was conducted to both clarify the zoning and evolution of various EOWSD issues around the world, and differentiate the relative impacts on EOWSD of climate change and human activities. The global EOWSD issues were summarized from 62 watersheds around the world as 6 categories associated with different aspects of global watershed sustainability. And the partition method, in which the spatial and temporal variations of global summer Normalized Difference Vegetation Index in summer were examined and the quantitative climate classification were conducted, indicates a clear and definite relationship between the zoning of EOWSD issues and 8 natural geographical zones. Meanwhile, we selected 34 watersheds either or both are the 100 most populous river basins and the 100 largest (by area) river basins in the world from the 62 watersheds to assess relative effects of human impact on watershed sustainability.
Results: Results from the numerical analyses of baseline water stress (BWS) values, which was used to provide a robust measure of human impact and evaluate the impact and relative importance of human-induced changes on watershed sustainability, indicate that the human activities do not affect the zoning of EOWSD issues at global scale while the environmental change induced by water engineering development should be certain to affect that on the long-term.
Discussion: Our findings present a new perspective to illustrate the relationship among global EOWSD, environmental change and human impacts, and will also provide a scientific basis on setting future emphasizes of global watershed sustainable development and furthering the related disciplines.
1 Introduction
Global watershed sustainable development, which plays important and unique roles in the provision of ecological and economic services and advancement of human civilization (Postal and Carpenter, 1997; Covich et al., 2004; Wang et al., 2022), has experienced worldwide threats from continuing anthropogenic stressors. Thus, it is necessary to deepen and broaden research encompassing the intersection of watershed sustainable development and the evolution of global environmental change and water engineering development (Brinson and Malvarez, 2002; Malmqvist and Rundle, 2002; Covich et al., 2004). According to the most frequently quoted definition of sustainable development from Our Common Future (WCED, 1987), the Brundtland Report recognizes the dependency of humans on the environment to meet present and future needs and regards the environmentally oriented development issues as one of the two key concepts in sustainable development definition. Given that interactions with global change dynamics (Walker et al., 2009) and the development of water engineering (Vormoor, 2010) are not addressed, sustainable development is no longer possible, and promoting global watershed sustainable development requires research on a wide range of environmentally oriented watershed sustainable development issues, such as the potential impact of environmental changes and water engineering development on regional water supplies, biodiversity, food security, and feedback from watershed itself. Environmentally oriented watershed sustainable development (EOWSD) is of importance in meeting both economic development goals and global environmental risks, so many studies have been conducted on one specific drainage basin or part of it (Covich et al., 2004; Palmer et al., 2007). However, there is not yet a widely recognized cognition on the applicability and scope of various EOWSD issues, and the zoning of global EOWSD issues remains uncertain, although it is crucial for achieving global watershed sustainable development.
Furthermore, in addition to global warming and relevant changes in the hydrological cycle, which are likely to increase the frequency and severity of extreme climate events, the increase in human activities by way of water engineering, including cultivation, afforestation, irrigation, deforestation, and urbanization, has also resulted in changes to flow regimes, especially large-scale changes in land cover or management in watersheds (Bates et al., 2008; Milliman et al., 2008; Déry et al., 2009; Jung et al., 2012; Thompson, 2012). Meanwhile, the close connection between watershed sustainable development and water engineering development and its impact on socio-economic conditions has been demonstrated (Wang and Gao, 2002; Vormoor, 2010). Thus, understanding the impact and relative importance of water engineering development on EOWSD has recently drawn considerable concerns. Assessing the relative impact of climate change and human activities is important, both for understanding the mechanism of global watershed sustainability and for local water resources management as well as drought and flood protection (Ye et al., 2013; Luan et al., 2021; Ahmad et al., 2023).
Given the importance of setting future emphasis on global EOWSD and furthering the related disciplines, there is a clear need to clarify the zoning and evolution of various EOWSD issues around the world and distinguish the relative effects of human activities and climate change on EOWSD. In this study, we integrated the EOWSD issues from 62 watersheds located in different parts of the world (Figure 1). To study the distribution characteristics of these EOWSD issues and to explore the underlying evolutionary mechanisms, the zoning of global EOWSD issues, which is based on the assessment of environmental variables, including climate change and vegetation condition, was proposed by producing a global map of climate on the basis of observational data and examining the spatial and temporal variations of global summer vegetation conditions. Meanwhile, baseline water stress and similar withdrawal-to-availability indicators were quantitatively analyzed to provide a robust measure of human impact on the hydrological context at the catchment scale and evaluate the effect and relative importance of human-induced change on watershed sustainability and distribution of EOWSD issues. In addition, simulated climate data were obtained to predict the evolution of global EOWSD and address the future emphasis on global watershed sustainable development.
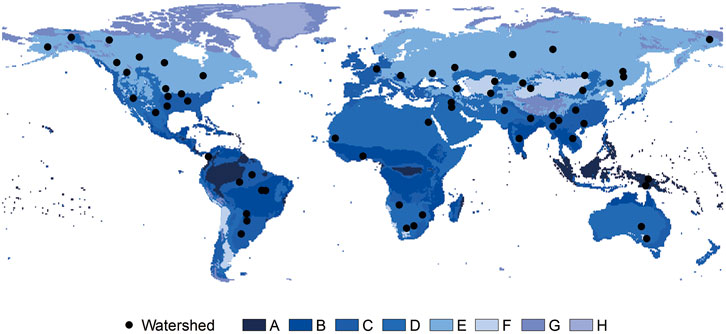
FIGURE 1. Eight natural geographical zones based on the Köppen–Geiger system and terrestrial vegetation, and the locations of the 62 watersheds. The letters in legends represent different natural geographical zones: A, humid tropical rainforest zone; B, tropical savanna climatic zone; C, humid evergreen broad-leaved forest zone; D, arid steppe-desert zone; E, humid and semihumid mixed broadleaf–conifer forest zone; F, arid and semiarid steppe zone; G, subpolar tundra zone; and H, polar ice sheet zone.
2 Materials and methods
2.1 Global data
As the existing research aiming to achieve EOWSD is highly fragmented and always focuses on one specific aspect, we have selected 62 watersheds for the unbiased collection and cataloging of global environmentally oriented watershed sustainability (Table 1). To investigate the interrelationship between environmental changes and watershed sustainable development at global scales, we categorized EOWSD issues according to the goals specified in the data source (Bernhardt et al., 2005) or based on key phrases in the title and description when the goals were not clearly articulated in the data source. We particularly avoided inferring the intent of a study or project from the data record.
2.2 Global climate and vegetation zoning
A zoning method, in which a global map of climate based on observational data was produced and spatial and temporal variations of the global normalized difference vegetation index (NDVI) in summer were examined, was used here to achieve a better understanding of the relationship between global climate and vegetation zoning and the distribution of EOWSD issues and to explore the underlying evolutionary mechanisms.
In order to assess the influence of climate change on global EOWSD issues, the Köppen–Geiger system was used to represent long-term mean climate conditions. There have been many modifications proposed to the Köppen system, but here we followed the criteria from Köppen’s last publication about his classification system in the Köppen–Geiger Handbook (Köppen, 1936). The NCEP Reanalysis data for the period of 2001–2014 were downloaded from the NOAA/OAR/ESRL PSD, Boulder, Colorado, USA (http://www.esrl.noaa.gov/psd/). Data from the Community Earth System Model version 1 with the Community Atmosphere Model version 5 [CESM1 (CAM5)] (Hurrell et al., 2013), which is a single climate model of the Coupled Model Intercomparison Project Phase 5 (CMIP5), during 2041–2050 and 2091–2100 were both processed by the Köppen–Geiger system to examine the current status and evolution of emphasis on EOWSD in different parts of the world. The description and definition criteria for the Köppen climate symbols are listed in Table 2.
One series of 16-day NDVI data from a Moderate Resolution Imaging Spectroradiometer (MODIS) sensor at spatial resolutions of 1,000 m, which were downloaded from the Distribution Active Archive Center (DAAC) at the NASA Goddard Space Flight Center (GSFC) during the summer (June to August in the Northern Hemisphere and December to February in the Southern Hemisphere) of 2000–2014, was quantitatively analyzed using the EOF method to evaluate the influence of vegetation change on EOWSD issues around the world.
The EOF analysis is similar to the principal component analysis (PCA) commonly used for decorrelating a set of variables. The dataset of the observed parameter can be treated as a function s(x, y, t) of spatial coordinates (x and y) and time t (Eq. 1). The EOF analysis basically decomposes s(x, y, t) into a series of orthogonal functions fi (x, y) of the spatial coordinates only. The temporal variation is captured in a series of temporal functions g(t) such that
where N is the total number of observations made in time t, which is taken for the 15-year period from 2000 to 2014 in this study. The orthogonal functions fi (x, y) of EOF and their respective coefficients g(t) can be determined by solving the eigenvalue equation constructed from the covariance matrix of s(x, y, t). The orthogonal functions fi (x, y) are arranged in decreasing order of the corresponding eigenvalues of the covariance matrix. Thus, the first few orthogonal functions usually account for most of the spatial variance that exists in the dataset.
2.3 The human impact: baseline water stress for 34 specific basin watersheds and numerical analyses
Based on the locations and ecosystem service value of watersheds, we have selected 62 watersheds to summarize their EOWSD issues, of which 34 watersheds, from either or both the 100 most populous river basins (Bernhard et al., 2008; CIESIN, 2010) and the 100 largest (by area) river basins (CIESIN, 2010) in the world, were chosen to assess the relative effect of human impact on watersheds. Baseline water stress (BWS), a commonly used indicator also known as relative water demand (Brown and Matlock, 2011), indicates the level of competition for available water and estimates the demand for freshwater (Gassert, 2013). The raw data of BWS we used here were obtained from the Aqueduct Water Risk Atlas (Aqueduct) (Reig et al., 2013), which is a publicly available, global database and interactive tool developed by the World Resources Institute (WRI). It evaluates, maps, and scores water risks globally based on 12 indicators, including baseline water stress. Baseline water stress measures the ratio of total annual water withdrawal (Ut) to average annual available blue water (Ba) (Eq. 2). It is important to note that most estimates of relative water demand do not account for upstream consumptive use as BWS here. A long time series of supply (1950–2010) were used to reduce the effect of multi-year climate cycles and to ignore complexities of short-term water storage (e.g., dams and floodplains) for which global operational data are nonexistent (Beek et al., 2011; Wada et al., 2011). Baseline water stress thus measures chronic stress rather than drought stress.
We masked catchments with less than 0.012 m/m2/year of withdrawal and 0.03 m/m2/year of available blue water as “arid and low water use” since catchments with low values were more prone to error in estimates of BWS.
The BWS values were then mapped to thresholds and normalized to a score between 0 and 5 such that scores 0–1 correspond to the lowest category and scores 4–5 correspond to the highest category. The general function for mapping indicators like BWS, whose thresholds are on a logarithmic scale (Eq. 3), is
where r is the raw value, t1 is the lowest category’s upper threshold, and base is the rate of increase between thresholds. Values greater than 5 or less than 0 are truncated to remain within the range of 0–5. For BWS, t1 = 0.1 and base = 2. The threshold method of indicator normalization has several advantages. Foremost is it creates clear categories and enables scores to be matched with guidelines. Relative to purely mathematical or statistical methods of normalization, thresholds are unaffected by extreme values. They allow for comparison even when using new sources of data.
The subprogram discriminant in SPSS 16.0 was used to perform the descriptive statistics, bivariate analysis, discriminant analysis, and hierarchical cluster analysis of BWS scores in order to examine whether human activities affect the distribution of environmentally oriented watershed sustainable development issues at a global scale.
3 Results
3.1 Global distribution of EOWSD issues
A great deal of research aiming to achieve global EOWSD has attracted significant attention all over the world. In the tropical region, EOWSD issues mainly focus on rainforest recovery and restoration and maintenance of natural ecosystem functions (Dudgeon and Smith, 2006; Coe et al., 2009; Laura et al., 2016; Richard et al., 2022). The impact of human activities, which has led to the introduction of chemical pollutants, altered flows, and system instability (Wang and Chang, 2006; Sarkar et al., 2007), is a crucial part of EOWSD in mid-latitude regions, which is the origin of many ancient civilizations and also has the world’s important economic belts. The primary issue of EOWSD in the polar regions is how to deal with the effects of global warming (Westmacott and Burn, 1997; Hay and Mccabe, 2010; Lin et al., 2019), while a rational allocation of water resources is an essential component in regions of water scarcity and flood (Richardson et al., 2005; Karimov et al., 2010). The main global EOWSD issues were summarized into six categories associated with different aspects of watershed sustainable development to explore the interrelations of environmental changes and watershed sustainable development at global scales (Supplementary Figures S1–S8). The six categories of EOWSD issues are as follows (Figure 2): A) water scarcity and allocation of water resources, which exists in Central Asia, the southern tip and northern tip of Africa, Central and southern Australia, and the mid-east of North America. B) Floods and water resource allocation could mainly be found in Central and Western Asia. In South America, northern North America, and South Asia, there exists the issue of C) interaction between the watershed itself and the potential impact of environmental changes and human behaviors on watershed sustainable development. D) Prevention and control of water quality deterioration particularly occurs in Europe and some plain areas of Asia. E) Prevention and control of damage to habitats and fishery resources mainly exists around 50° north latitude. F) Changes in biodiversity concentrate in Eastern and Western Asia.
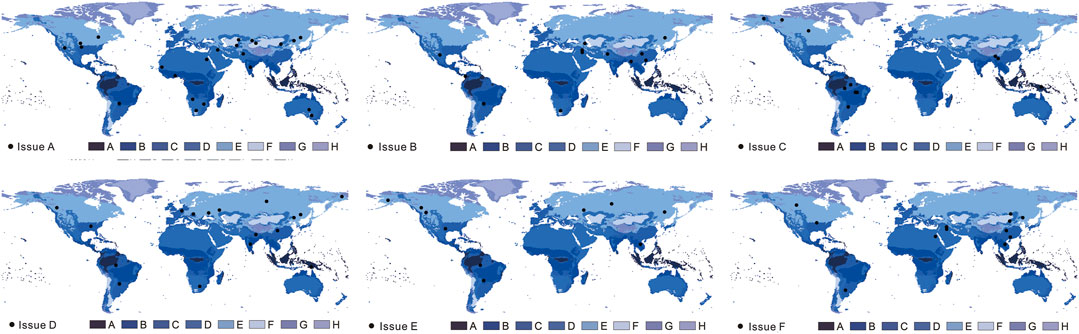
FIGURE 2. Global distribution of EOWSD issues. The six categories of the issues are as follows: (A) Water scarcity and allocation of water resources. (B) Floods and water resource allocation. (C) Interaction between the watershed itself and the potential impact of environmental changes and human behaviors on watershed sustainable development. (D) Prevention and control of water quality deterioration. (E) Prevention and control of damage to habitats and fishery resources. (F) Changes in biodiversity. The letters in legends represent different natural geographical zones: A, humid tropical rainforest zone; B, tropical savanna climatic zone; C, humid evergreen broad-leaved forest zone; D, arid steppe-desert zone; E, humid and semihumid mixed broadleaf–conifer forest zone; F, arid and semiarid steppe zone; G, subpolar tundra zone; and H, polar ice sheet zone.
3.2 The relationship between the zoning of global EOWSD issues and climate and vegetation zoning
In order to examine the influence of climate and vegetation changes on the distribution of global EOWSD issues, the global NDVI in the summer of 2000–2014 was quantitatively analyzed using the EOF method (Figure 3A; Supplementary Figure S9). The first three EOF modes account for 21.64%, 14.03%, and 11.17% of the total variance in the Northern Hemisphere and 20.49%, 12.60% and 10.33% of the total variance in the Southern Hemisphere, respectively. Since there is a distinct correspondence between EOF modes and distribution of the main sustainable development issues and the global vegetation growth is mainly dominated by climate change, a partition method based on the Köppen–Geiger system and terrestrial vegetation was used to achieve a better understanding of the distribution of global EOWSD issues and to verify their applicability and scope. The first three temporal EOFs show that the responses of vegetation to climate change in different parts of the world have consistency even though the responsiveness varies, as shown in the associated EOF modes (Figure 3B). Ultimately, a clear and definite relationship between the distribution of the main EOWSD issues and different natural geographical zones is shown (Figure 2). In the humid tropical rainforest zone (A), issue C exists in six of all eight rivers, issues B and E occur in one river each, and three rivers have issue D. All five rivers in the tropical savanna climatic zone (B) have issue A, while two rivers have issue B, and one river has issue E. Issue A–F all exist in the humid evergreen broad-leaved forest zone (C), whilst the numbers of theories are various. Issue A is the main watershed sustainable development issue in the arid steppe-desert zone (D), and the sustainable development practices implemented in the Nile also led to issue F. Issue D can be found in most rivers in the humid and semihumid mixed broadleaf–conifer forest zone (E), which is the origin of many ancient civilizations and also has many of the world’s important economic belts. Issues A and E are also obvious in this area. The main watershed sustainable development issues in the arid and semiarid steppe zone (F) concentrate on issues A, B, E, and F, while issues A, C, D, E, and F are found in the subpolar tundra zone (G). The main watershed sustainable development issues in the polar ice sheet zone (H) are issues C and D. It is worth noting that using the NDVI as a standard for global analyses has its limitations, especially in tropical regions, and should be improved in subsequent studies.
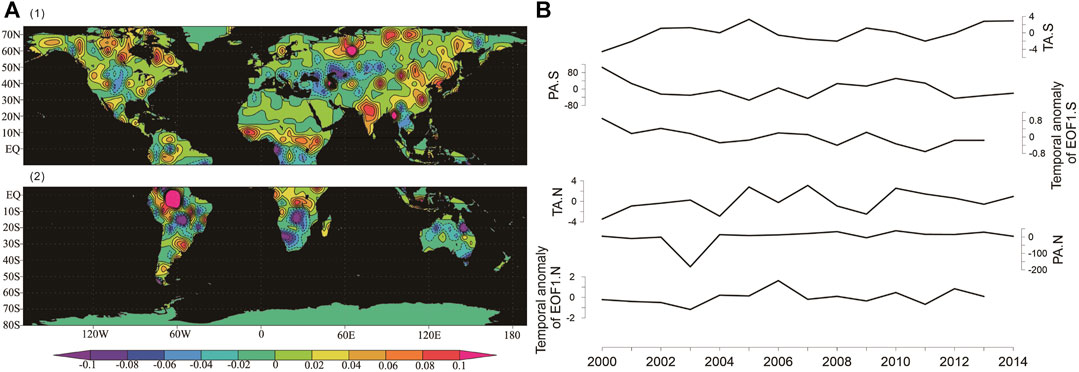
FIGURE 3. Spatial and temporal variations of the global NDVI in summer. (A) First EOF mode of the global NDVI in the summer (June to August in the Northern Hemisphere (1) and December to February in the Southern Hemisphere (2)) during 2000–2014. (B) Temporal anomaly of EOF1, temperature anomaly, and precipitation anomaly (Bernhard et al., 2008) in the Northern Hemisphere and Southern Hemisphere (Harris et al., 2014). TA.N, temperature anomaly in the Northern Hemisphere; TA.S, temperature anomaly in the Southern Hemisphere; PA.N, precipitation anomaly in the Northern Hemisphere; PA.S, precipitation anomaly in the Southern Hemisphere.
3.3 Evaluation of watershed human impacts on the zoning of global EOWSD issues
In the context of global climate change and anthropogenic pressures, intensified regional environmental change and human water engineering efforts have been identified as two factors influencing EOWSD. Here, we selected 34 watersheds from 62 watersheds, from the 100 most populated watersheds globally (Bernhard et al., 2008; CIESIN, 2010) and the 100 largest watersheds globally (in terms of area) (CIESIN, 2010), in order to assess the relative impacts of human activities on watersheds (Table 3). When evaluating the influence and relative importance of human impact on watersheds (Vörösmarty et al., 2000), it is particularly important to understand baseline water stress (BWS), which measures total annual water withdrawals expressed in percentage of total available flow. Two variables determine baseline water stress: water supply availability and demand for that water. Water supply estimates are obtained from a model that considers a wide variety of variables, including temperature, precipitation, wind speed, and soil moisture absorption. The demand for water is computed by adding the total annual withdrawals from municipal, industrial, and agricultural sources based on a series of reported and modeled global datasets (Gassert et al., 2013). Water withdrawals of the three sectors (agricultural, domestic, and industrial) are used as a weight to measure the exposure of each sector’s water users to BWS (Figure 4), which allows us to explore the internal characteristics in specific river basins. The baseline water stress of all 34 river basins was used in the numerical analyses.
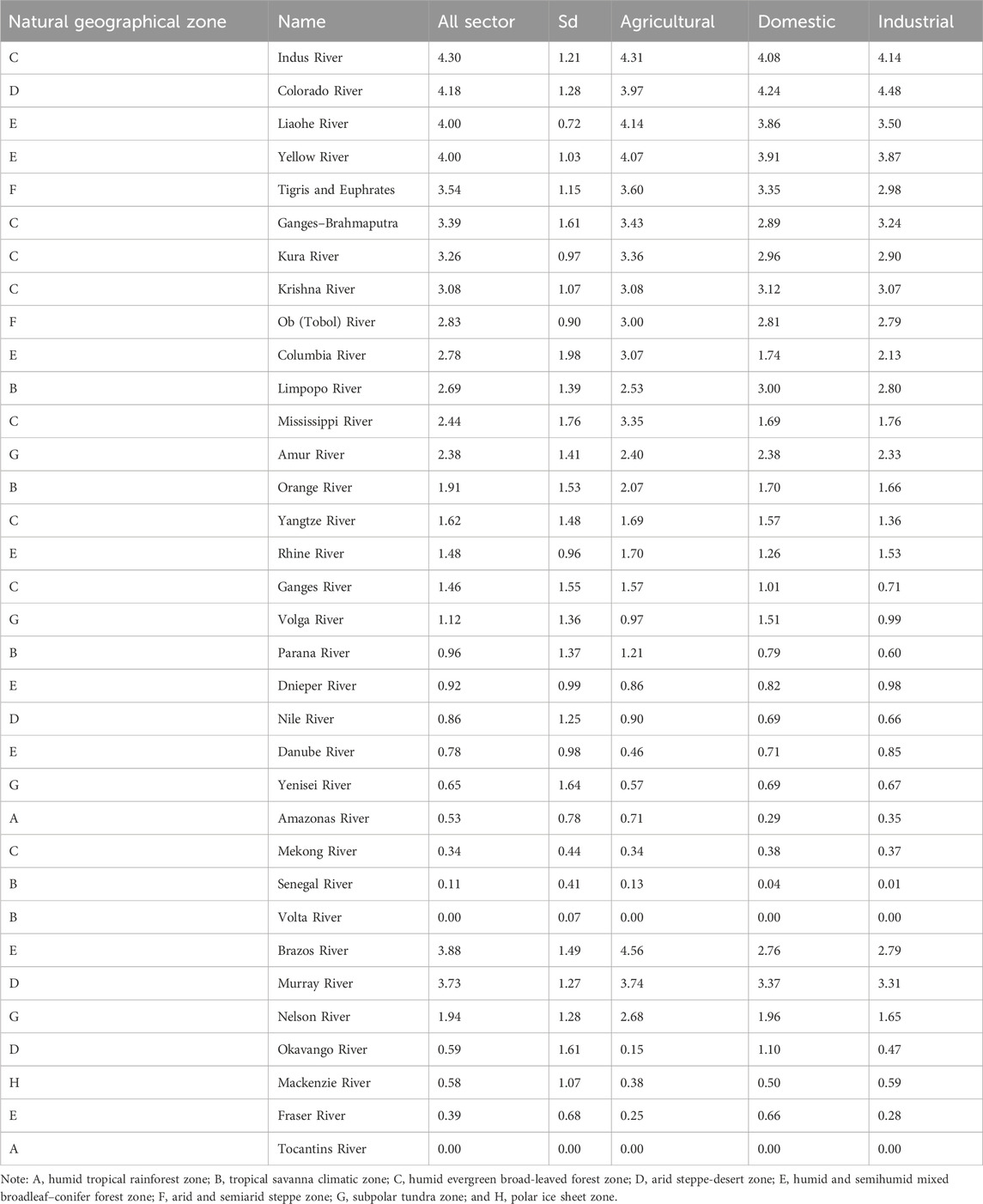
TABLE 3. BWS scores of 34 watersheds from either or both the 100 most populous river basins and the 100 largest (by area) river basins in the world from the 62 watersheds.
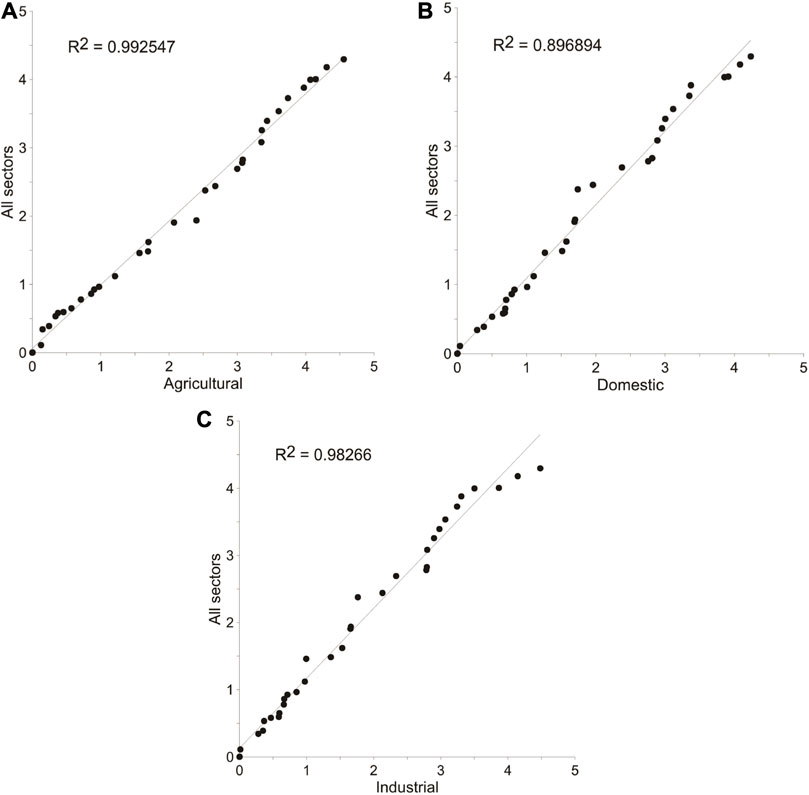
FIGURE 4. Quantile–quantile plots of the total baseline water stress vs (A) agricultural, (B) domestic, and (C) industrial (AID) scores in the 34 watersheds.
The ternary diagram shows that the distribution and variability of the agricultural/industrial/domestic (AID) withdrawals in the 34 watersheds (Figure 5A) do not suggest an evident relationship between the composition features of AID and the different natural geographical conditions since there is no obvious centrality of watersheds from each natural geographical zone. The raw BWS values are then mapped to thresholds (low (<10%); low to medium (10%–20%); medium to high (20%–40%); high (40%–80%); and extremely high (>80%)) using continuous functions and normalized to a score between 0 and 5 on account of thresholds that are unaffected by extreme values and allow for comparison even when using new sources of data relative to purely mathematical or statistical methods of normalization. Descriptive statistics and bivariate analysis were used to characterize scores of agricultural, industrial, domestic, and total baseline water stress and to examine whether the human impact could be well represented by the AID scores (Table 4). The results of the discriminant analysis confirm that the normalized scores of the 34 watersheds do not exhibit distinctive spatial signatures, as represented by the 8 natural geographical zones (Figure 5B). Meanwhile, two major groupings are indicated by hierarchical cluster analysis based on the BWS scores, and the clustering results are very different from the groups divided according to various natural geographical conditions of watersheds (Figure 5C). Therefore, human activities do not affect the distribution of EOWSD issues on a global scale, even though water engineering development may have significant influences on watershed sustainable development in each specific natural geographical zone.
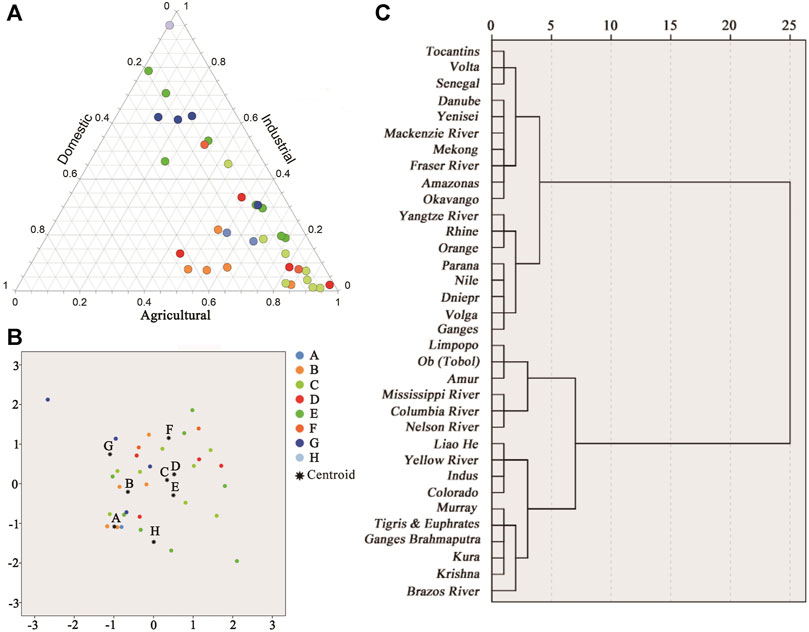
FIGURE 5. Numerical analyses of BWS values. (A) Ternary diagram of the agricultural/industrial/domestic withdrawals in the 34 watersheds. (B) Discriminant analysis of total baseline water stress scores. (C) Hierarchical cluster analysis of total baseline water stress scores. The solid circles in subgraph A and subgraph C represent different natural geographical zones as shown in the figure: A, humid tropical rainforest zone; B, tropical savanna climatic zone; C, humid evergreen broad-leaved forest zone; D, arid steppe-desert zone; E, humid and semihumid mixed broadleaf–conifer forest zone; F, arid and semiarid steppe zone; G, subpolar tundra zone; and H, polar ice sheet zone.

TABLE 4. Descriptive statistics and bivariate analysis of agricultural, industrial, domestic, and total baseline water stress.
4 Discussion
Environmental change and human activities are the two major factors that affect watershed sustainable development (Zhang et al., 2023). According to the zoning of global EOWSD issues in eight different natural geographical zones, it is quite evident that EOWSD issues vary under different natural geographical conditions and that the scientific basis of watershed sustainable development issues in identical natural geographical zones is always unified (Fu et al., 2019; Qi et al., 2020; Zhou et al., 2023). Meanwhile, under the condition that the number and magnitude of water engineering activities are growing rapidly (Postal and Carpenter, 1997; Malmqvist and Rundle, 2002), human activities were thought to be the main factor that threatens watershed sustainable development. However, our results based on BWS scores of 34 river basins and related numerical analyses suggest that water engineering development does not affect the distribution of EOWSD issues at a global scale directly, even though it may have significant influences on watershed sustainable development in each specific natural geographical zone. Nevertheless, environmental change induced by water engineering activities (Vörösmarty et al., 2010; Schewe et al., 2014) should be certain to affect the distribution of EOWSD issues in the long term. Therefore, as demonstrated in this paper, each natural geographical zone has a principal EOWSD issue, which provides the scientific basis for watershed sustainable development governance.
Research indicates that unabated climate change will exacerbate environmental risks and affect watershed sustainable development across the world (Brooks and Lake, 2007); hence, two maps of the eight natural geographical zones during 2041–2050 and 2091–2100 from simulated climate data were produced to predict the evolution of global watershed sustainability and address the future emphasis on sustainable development (Figure 6). There is an obvious change in the ranges of natural geographical zones, which presents various change trends in different parts of the world. The tropical savanna climatic zone (B) will increase by 9.11% (2.40 million km2) of its previous area, while the area of humid and semihumid mixed broadleaf–conifer forest zone (E) will decrease by approximately 1.07 million km2 from 2041–2050 to 2091–2100, as indicated by the simulated results. Therefore, water engineering aimed at water scarcity and water resource allocation will become even more important in the future, and the applicability and scope of the principal EOWSD issues of the areas in which the type of natural geographical zone will change should be appropriately adjusted.
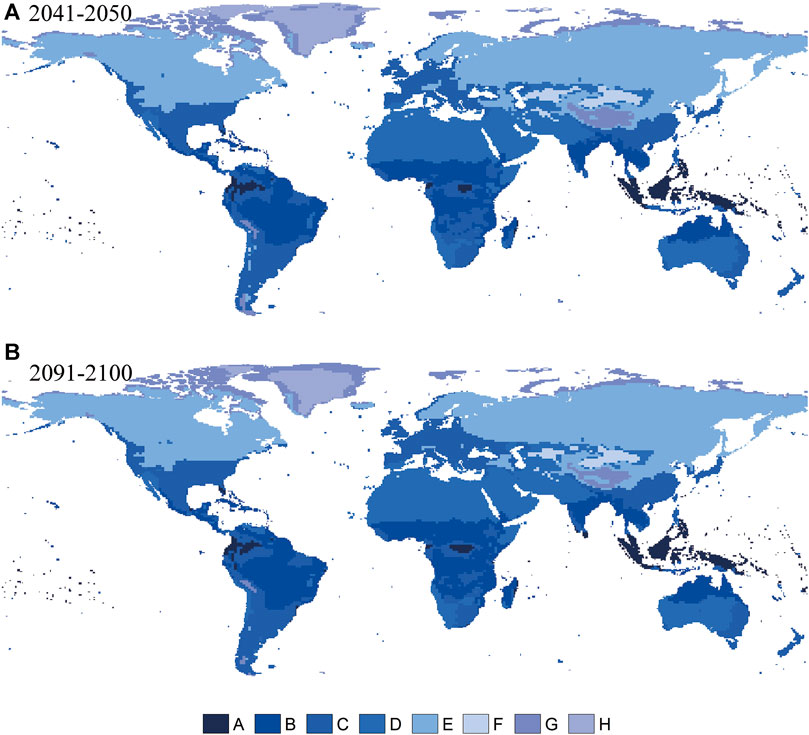
FIGURE 6. Evolution of the eight natural geographical zones during (A) 2041–2050 and (B) 2091–2100 from simulated climate data that follows the RCP 4.5 scenario. As indicated by the simulated results, the humid tropical rainforest zone (A), the tropical savanna climatic zone (B), and the arid steppe-desert zone (D) will increase by 9.55% (0.54 million km2), 9.11% (2.40 million km2), and 0.44% (0.13 million km2) of their previous area, while the area of the humid evergreen broad-leaved forest zone (C), the humid and semihumid mixed broadleaf–conifer forest zone (E), the arid and semiarid steppe zone (F), the subpolar tundra zone (G), and the polar ice sheet zone (H) will decrease by 0.12% (0.04 million km2), 2.57% (1.07 million km2), 25.97% (0.61 million km2), 14.90% (0.87 million km2), and 24.02% (0.48 million km2) from 2041–2050 to 2091–2100.
The recognition of the natural and social importance of watershed sustainability has resulted in a massive increase in efforts toward and research on watershed sustainable development. However, promoting sustainable development requires research on a wide range of social, economic, cultural, institutional, and environmental issues, and research dominated by the natural sciences must transition toward research involving the full range of sciences and humanities (Reid et al., 2010). Despite considerable consensus on the urgent need to improve watershed health and enhance ecological sustainability and the importance of understanding how to achieve watershed sustainability (Lake, 2005; Palmer et al., 2005), there is little evidence for a mechanism or a theory for guiding the practice of watershed sustainable development (Palmer et al., 1997; Lake, 2001; Bond and Lake, 2003; Brooks and Lake, 2007). The objectives and emphasis of global EOWSD efforts are multifarious since there are obvious differences in EOWSD issue types in various locations of the world, so it is essential for effective watershed sustainable development to ascertain the zoning of global EOWSD issues and identify the priorities of these issues as sustainable development emphasis could vary broadly. In this study, we made attempts, in which the distribution and evolution of various EOWSD issues were clarified and the relative impacts of environmental change and human activities on watershed sustainability were distinguished, to provide the scientific basis for setting future emphasis on global watershed sustainable development and furthering the related disciplines.
5 Conclusion
The zoning of global EOWSD issues suggested that EOWSD issues vary under different natural geographical conditions, while the scientific basis of EOWSD issues in identical natural geographical zones is always unified. Meanwhile, human activities do not affect the distribution of EOWSD issues at a global scale directly, even though they may have significant influences on watershed sustainability in each specific natural geographical zone, and environmental change induced by water engineering development should be certain to affect the distribution of EOWSD issues in the long term. Furthermore, water engineering aimed at water scarcity and the allocation of water resources will become even more important in the future according to simulated climate data, and the applicability and scope of the principal EOWSD issues of the areas in which the type of natural geographical zone will change should be appropriately adjusted. In light of these results, the zoning of global EOWSD issues presents a new perspective to understand the relationship between global EOWSD, human impacts, and environmental change, and our findings will also provide the scientific basis for setting future emphasis on global watershed sustainable development and furthering the related disciplines.
Data availability statement
The original contributions presented in the study are included in the article/Supplementary Material; further inquiries can be directed to the corresponding author.
Author contributions
ZM: conceptualization, formal analysis, funding acquisition, methodology, and writing–original draft. ZC-Q: conceptualization, formal analysis, methodology, and writing–original draft. FQ: conceptualization, methodology, project administration, supervision, and writing–review and editing. ZJ-T: methodology, validation, and writing–review and editing. LW: conceptualization, methodology, project administration, supervision, and writing–review and editing. WL-G: data curation, methodology, validation, and writing–review and editing. XY-Y: methodology, validation, and writing–review and editing. SY-Q: data curation and writing–review and editing.
Funding
The author(s) declare financial support was received for the research, authorship, and/or publication of this article. This research has been supported by the National Key R&D Program of China (No. 2022YFF1303301), the National Natural Science Fund of China (Grant Nos 42201133, 42101115, and 52179026), and the Science and Technology Program of Gansu Province (22JR5RA072).
Conflict of interest
The authors declare that the research was conducted in the absence of any commercial or financial relationships that could be construed as a potential conflict of interest.
Publisher’s note
All claims expressed in this article are solely those of the authors and do not necessarily represent those of their affiliated organizations, or those of the publisher, the editors, and the reviewers. Any product that may be evaluated in this article, or claim that may be made by its manufacturer, is not guaranteed or endorsed by the publisher.
Supplementary material
The Supplementary Material for this article can be found online at: https://www.frontiersin.org/articles/10.3389/fenvs.2023.1322308/full#supplementary-material
References
Abbasov, R. K., and Mahmudov, R. N. (2009). Analysis of non climatic origins of floods in the downstream part of the Kura River, Azerbaijan. Nat. Hazards 50 (2), 235–248. doi:10.1007/s11069-008-9335-2
Agostinho, A. A., Gomes, L. C., Veríssimo, S., and Okada, E. K. (2013). Flood regime, dam regulation and fish in the Upper Paraná River: effects on assemblage attributes, reproduction and recruitment. Rev. Fish. Biol. Fish. 108 (10), 11–19. doi:10.1007/s11160-004-3551-y
Ahmad, S., Jia, H. F., Ashraf, A., Yin, D. K., Chen, Z. X., Xu, C. Q., et al. (2023). Water resources and their management in Pakistan: a critical analysis on challenges and implications. Water-Energy Nexus 6, 137–150. doi:10.1016/j.wen.2023.10.001
Al-Ansari, N., and Knutsson, S. (2011). Toward prudent management of water resources in Iraq. J. Adv. Sci. Eng. Res. 1, 53–67. Avaliable at: https://api.semanticscholar.org/CorpusID:130096942.
Alexander, C. R., and Windom, H. L. (1999). Quantification of natural backgrounds and anthropogenic contaminants in a pristine arctic environment: the Anadyr river basin, Chukotka peninsula, Russia. Mar. Pollut. Bull. 38 (4), 276–284. doi:10.1016/S0025-326X(98)90146-1
Al-Yamani, F. Y., Bishop, J. M., Ismail, A. R. W., and Ismail, W. (2007). The effects of the river diversion, Mesopotamian marsh drainage and restoration, and river damming on the marine environment of the northwestern Arabian gulf. Aquat. Ecosyst. Health. 10 (10), 277–289. doi:10.1080/14634980701512384
Arthington, A. H., Balcombe, S. R., Wilson, G. A., Thoms, M. C., and Marshall, J. (2005). Spatial and temporal variation in fish-assemblage structure in isolated waterholes during the 2001 dry season of an arid-zone floodplain river, Cooper Creek, Australia. Mar. Freshw. Res. 56 (1), 25–35. doi:10.1071/MF04111
Barreteau, O., Bousquet, F., and Attonaty, J. M. (2001). Role-playing games for opening the black box of multi-agent systems: method and lessons of its application to Senegal River Valley irrigated systems. J. Artif. Soc. Simul. 4, 2. Avaliable at: https://api.semanticscholar.org/CorpusID:18719755.
Bastos, W. R., Almeida, R. D., Dórea, J. G., and Barbosa, A. C. (2007). Annual flooding and fish-mercury bioaccumulation in the environmentally impacted Rio Madeira (Amazon). Ecotoxicology 16 (3), 341–346. doi:10.1007/s10646-007-0138-0
Bates, B. C., Kundzewicz, Z. W., Wu, S., and Palutikof, J. P. (2008). “Climate change and water,” in Technical paper of the intergovernmental panel on climate change (Secretariat, Geneva: IPCC). Avaliable at: http://www.ipcc.ch/pdf/technical-papers/climate-change-water-en.pdf.
Beek, L. P. H. V., Wada, Y., and Bierkens, M. F. P. (2011). Global monthly water stress: 1. water balance and water availability. Water Resour. Res. 47 (7), 197–203. doi:10.1029/2010WR009791
Bernhard, L., Kristine, V., and Andy, J. (2008). New global hydrography derived from spaceborne elevation data. Eos, Trans. Am. Geophys. Union 89 (10), 93–94. doi:10.1029/2008EO100001
Bernhardt, E. S., Palmer, M. A., Allan, J. D., Alexander, G., Barnas, K., Brooks, S., et al. (2005). Synthesizing U.S. river restoration efforts. Science 308 (5722), 636–637. doi:10.1126/science.1109769
Bond, N. R., and Lake, P. S. (2003). Local habitat restoration in streams: constraints on the effectiveness of restoration for stream biota. Ecol. Manag. Restor. 4, 193–198. doi:10.1046/j.1442-8903.2003.00156.x
Braga, B., Varella, P., and Gonçalves, H. (2011). Transboundary water management of the Amazon basin. Int. J. Water. Resour. D. 27 (3), 477–496. doi:10.1080/07900627.2011.595382
Braune, E., and Rogers, K. H. (1987). The Vaal River catchment: problems and research needs. South African national scientific programmes report, NO. 143. Avaliable at: https://api.semanticscholar.org/CorpusID:129252903.
Brinson, M. M., and Malvarez, A. I. (2002). Temperate freshwater wetlands: types, status and threats. Environ. Conserv. 29, 115–133. doi:10.1017/S0376892902000085
Brooks, S. S., and Lake, P. S. (2007). River restoration in victoria, Australia: change is in the wind, and none too soon. Restor. Ecol. 15 (3), 584–591. doi:10.1111/j.1526-100X.2007.00253.x
Brown, A., and Matlock, M. D. (2011). A review of water scarcity indices and methodologies. Avaliable at: https://sustainabilityconsortium.org/download/a-review-of-water-scarcity-indices-and-methodologies/.
Cai, D. K. (2011). The study on the development modes of the Nujiang valley based on the balance between development rights and sustainability. Chengdu, China: Southwest. Univ. Fin. Econ. Avaliable at: https://www.dissertationtopic.net/doc/1755865.
Chen, X. Q., and Chen, J. (2005). Water resources allocation and integrated water resources management in the Yangtze River Basin. Res. Environ. Yangtze. 14 (2), 163–167. doi:10.1007/s11769-005-0030-x
Chen, Y. N., Cui, W. C., and Li, Y. M. (2003). Utilization of water resources and ecological protection in the Tarim River. J. Geoge. Sci. 58 (02), 215–222.
Christensen, N. S., Wood, A. W., Voisin, N., Lettenmaier, D. P., and Palmer, R. N. (2004). The effects of climate change on the hydrology and water resources of the Colorado River basin. Clim. Change 62 (1–3), 337–363. doi:10.1023/B:CLIM.0000013684.13621.1f
CIESIN (Center for International Earth Science Information Network) (2010). Columbia university, FAO (united nations food and agriculture organization), and CAIT (centro internacional de Agricultura tropical) gridded population of the world version 3 (GPWv3): population count grid, future estimates. Avaliable at: http://sedac.ciesin.columbia.edu/gpw.
Coe, M. T., Costa, M. H., and Soares-Filho, B. S. (2009). The influence of historical and potential future deforestation on the stream flow of the Amazon River-Land surface processes and atmospheric feedbacks. J. Hydrol. 369 (1–2), 165–174. doi:10.1016/j.jhydrol.2009.02.043
Coe, M. T., Latrubesse, E. M., Ferreira, M. E., and Amsler, M. L. (2011). The effects of deforestation and climate variability on the streamflow of the Araguaia River, Brazil. Biogeochemistry 105 (1–3), 119–131. doi:10.1007/s10533-011-9582-2
Conley, A. H., and Niekerk, P. H. V. (2000). Sustainable management of international waters: the Orange River case. Water Policy 2 (1–2), 131–149. doi:10.1016/S1366-7017(99)00026-4
Costa, M. H., Botta, A., and Cardille, J. A. (2003). Effects of large-scale changes in land cover on the discharge of the Tocantins River, Southeastern Amazonia. J. Hydrol. 283 (1–4), 206–217. doi:10.1016/S0022-1694(03)00267-1
Covich, A. P., Ewel, K. C., Hall, R. O., Giller, P. S., Goedkoop, W., and Merritt, D. M. (2004). “Ecosystem services provided by freshwater benthos,” in Sustaining biodiversity and ecosystem services in soils and sediments. Editor D. H. Wall (Washington, DC: Island Press), 45–72. doi:10.1007/s10750-021-04739-y
Cutlac, I. M., and Horbulyk, T. M. (2010). Optimal water allocation under short-run water scarcity in the South Saskatchewan River Basin. J. Water Res. 137 (1), 92–100. doi:10.1061/(ASCE)WR.1943-5452.0000092
Day, J. W., Boesch, D. F., Clairain, E. J., Kemp, G. P., Laska, S. B., Mitsch, W. J., et al. (2007). Restoration of the Mississippi delta: lessons from hurricanes Katrina and Rita. Science 315 (5819), 1679–1684. doi:10.1126/science.1137030
Deng, M. J., Long, A. H., Gong, Y., and Ma, J. L. (2011). Water resources utilization in the middle reach of Irtysh River and its impact. J. Hydraul. Eng. 42 (12), 1487–1495. doi:10.13243/j.cnki.slxb.2011.12.008
Déry, S. J., Hernández–Henríquez, M. A., Burford, J. E., and Wood, E. F. (2009). Observational evidence of an intensifying hydrological cycle in northern Canada. Geophys. Res. Lett. 36, L13402. doi:10.1029/2009GL038852
Dubnyak, S., and Timchenko, V. (2000). Ecological role of hydrodynamic processes in the Dnieper reservoirs. Ecol. Eng. 16 (1), 181–188. doi:10.1016/S0925-8574(00)00103-8
Dudgeon, D., and Smith, R. E. W. (2006). Exotic species, fisheries and conservation of freshwater biodiversity in tropical Asia: the case of the Sepik River, Papua New Guinea. Aquat. Conserv. 16 (2), 203–215. doi:10.1002/aqc.713
Ferguson, J. W., Healey, M., Dugan, P., and Barlow, C. (2010). Potential effects of dams on migratory fish in the Mekong River: lessons from salmon in the Fraser and Columbia rivers. Environ. Manage. 47 (47), 141–159. doi:10.1007/s00267-010-9563-6
Fu, Y. C., Cui, X. Y., Liu, L. S., Zhao, J. Y., Leng, J. W., and Zhang, S. P. (2019). Equilibrium cost of water environmental protection based on watershed sustainability. J. Hydrol. 579, 124216. doi:10.1016/j.jhydrol.2019.124216
Gassert, F. (2013). Aqueduct country and river basin rankings: a weighted aggregation of spatially distinct hydrological indicators. Avaliable at: http://wri.org/publication/aqueduct–country–river–basin–rankings.
Gassert, F., Landis, M., Luck, M., Reig, P., and Shiao, T. (2013). Aqueduct global maps 2.0. Avaliable at: http://www.wri.org/publication/aqueductmetadata–global.
Gisclair, B. R. (2009). Salmon bycatch management in the Bering Sea walleye pollock fishery: threats and opportunities for western Alaska. Avaliable at: http://innfiiini.fisheries.org/proofs/ayk/gisclair.pdf.
Glantz, M. H. (2005). Water, climate, and development issues in the amu darya basin. Mitig. Adapt. Strat. Gl. 10 (1), 23–50. doi:10.1007/S11027-005-7829-8
Gober, P., and Wheater, H. S. (2013). Socio-hydrology and the science-policy interface, a case study of the Saskatchewan River basin. Hydrol. Earth. Syst. Sc. 10 (5), 1413–1422. doi:10.5194/hess-18-1413-2014
Goel, N. K., Than, H. H., and Arya, D. S. (2005). “Flood hazard mapping in the lower part of Chindwin river basin, Myanmar,” in International conference on innovation advances and implementation of flood forecasting technology (Tromsø, Norway: UNECE). Avaliable at: https://api.semanticscholar.org/CorpusID:130002930.
Gosain, A. K., Raoand, S., and Basuray, D. (2006). Climate change impact assessment on hydrology of Indian river basins. Curr. Sci. India. 90 (3), 346–353. Avaliable at: https://www.jstor.org/stable/24091868.
Gottgens, J. F., Perry, J. E., Fortney, R. H., Meyer, J. E., Benedict, M., and Rood, B. E. (2001). The Paraguay-paraná hidrovía: protecting the Pantanal with lessons from the past. Bioscience 51 (4), 301–308. doi:10.1641/0006-3568(2001)051[0301:TPPHAP]2.0.CO;2
Guo, Y., Zhang, R. M., and Li, H. (2001). Degeneration causes of the aboriginal Fishes and some measures for protecting them in the Ergis River, Xinjiang, China. J. Arid. Land. 20 (02), 152–155. doi:10.13866/j.azr.2003.02.020
Hanjra, M. A., and Francis, G. (2008). Investments in agricultural water management for poverty reduction in Africa: case studies of Limpopo, Nile, and Volta river basins. Nat. Resour. Forum. 32, 185–202. doi:10.1111/j.1477-8947.2008.00191.x
Harris, I., Jones, P. D., Osborn, T. J., and Lister, D. H. (2014). Updated high-resolution grids of monthly climatic observations-the CRU TS3.10 Dataset. Int. J. Climatol. 34, 623–642. doi:10.1002/joc.3711
Hay, L. E., and Mccabe, G. J. (2010). Hydrologic effects of climate change in the yukon river basin. Clim. Change 100 (3), 509–523. doi:10.1007/s10584-010-9805-x
Hideyuki, K., Minjiao, L. U., Hironori, D., Dambaravjaa, O., and Gombo, D. (2004). “Spatial variation and long-term change of hydrological regime of Kerulen river basin, Mongolia,” in Proceedings of the 3rd international workshop on terrestrial change in Mongolia (Tsukuba, Japan: Wordpress), 50–53. Avaliable at: https://www.ied.tsukuba.ac.jp/wordpress/wp-content/uploads/pdf_papers/tercbull05s/t5supple_50.pdf.
Hurrell, J. W., Holland, M. M., Gent, P. R., Ghan, S., Kay, J. E., Kushner, P. J., et al. (2013). The community earth system model: a framework for collaborative research. B. Am. Meteorol. Soc. 94, 1339–1360. doi:10.1175/BAMS-D-12-00121.1
Jung, G., Wagner, S., and Kunstmann, H. (2012). Joint climate-hydrology modeling: an impact study for the data-sparse environment of the Volta Basin in West Africa. Hydrol. Res. 43 (3), 231–248. doi:10.2166/nh.2012.044
Karimov, A., Smakhtin, V., Mavlonov, A., and Gracheva, I. (2010). Water ‘banking’ in Fergana valley aquifers-A solution to water allocation in the Syrdarya river basin? Agr. Water. manage. 97 (10), 1461–1468. doi:10.1016/j.agwat.2010.04.011
Kassas, M. (1971). The river Nile ecological system: a study towards an international programme. Biol. Conserv. 4 (1), 19–25. doi:10.1016/0006-3207(71)90049-8
Kim, U., and Kaluarachchi, J. J. (2009). Climate change impacts on water resources in the upper blue Nile River Basin, Ethiopia. J. Am. Water Resour. As. 45 (6), 1361–1378. doi:10.1111/j.1752-1688.2009.00369.x
Köppen, W. (1936). Das geographische system der Klimate borntraeger. Avaliable at: https://api.semanticscholar.org/CorpusID:128639822.
Lake, P. S. (2001). On the maturing of restoration: linking ecological research and restoration. Ecol. Manag. Restor. 2, 110–115. doi:10.1046/j.1442-8903.2001.00074.x
Lake, P. S. (2005). Perturbation, restoration and seeking ecological sustainability in Australian flowing waters. Hydrobiologia 552, 109–120. doi:10.1007/s10750-005-1509-2
Laura, M. A., Michiel, V. E., Zhang, G. P., Marta, P., Lucieta, G. M., Leila, S. L., et al. (2016). Impact of agricultural expansion on water footprint in the Amazon under climate change scenarios. Sci. Total Environ. 569–570, 1159–1173. doi:10.1016/j.scitotenv.2016.06.191
Liao, D., Barnes, R. W., Chambless, L. E., Simpson, R. J., Sorlie, P., and Heiss, G. (2003). A conceptual analysis of the relation between human activities and river quality deterioration in the Volga-Don basin (Russia). Eur. Water Manage. 76 (12), 906–1012. Avaliable at: https://api.semanticscholar.org/CorpusID:195741463.
Lin, H. X., Huang, J. C., Fang, C. L., Qi, X. X., and Chen, Y. Q. (2019). A preliminary study on the theory and method of comprehensive regionalization of cryospheric services. Adv. Clim. Chang. Res. 10 (2), 115–123. doi:10.1016/j.accre.2019.06.002
Luan, J. K., Zhang, Y. Q., Ma, N., Tian, J., Li, X. J., and Liu, D. F. (2021). Evaluating the uncertainty of eight approaches for separating the impacts of climate change and human activities on streamflow. J. Hydrol. 601, 126605. doi:10.1016/j.jhydrol.2021.126605
Malmqvist, B., and Rundle, S. (2002). Threats to the running water ecosystems of the world. Environ. Conserv. 29, 134–153. doi:10.1017/S0376892902000097
Martins, E. G., Hinch, S. G., Patterson, D. A., Hague, M. J., Cooke, S. J., Miller, K. M., et al. (2010). Effects of river temperature and climate warming on stock-specific survival of adult migrating Fraser River sockeye salmon (Oncorhynchus nerka). Glob. Change Biol. 17 (1), 99–114. doi:10.1111/j.1365-2486.2010.02241.x
Mbaiwa, J. E. (2004). Causes and possible solutions to water resource conflicts in the Okavango River Basin: the case of Angola, Namibia and Botswana. Phys. Chem. Earth. 29 (15–18), 1319–1326. doi:10.1016/j.pce.2004.09.015
Milliman, J. D., Farnsworth, K. L., Jones, P. D., Xu, K. H., and Smith, L. C. (2008). Climatic and anthropogenic factors affecting river discharge to the global ocean, 1951–2000. Glob. Planet Change 62, 187–194. doi:10.1016/j.gloplacha.2008.03.001
Morris, M., and de Loë, R. C. (2014). Exploring the prospects for collaborative transboundary water governance in the Mackenzie River Basin, Canada. Avaliable at: http://paperroom.ipsa.org/papers/paper_30369.pdf.
Mosquera–Machado, S., and Ahmad, S. (2007). Flood hazard assessment of atrato river in Colombia. Water Resour. Manag. 21 (3), 591–609. doi:10.1007/s11269-006-9032-4
Naiman, R. J., Lamberson, A., Wood, C. C., Bisson, P. A., Congleton, J., Henny, C. J., et al. (2012). Developing a broader scientific foundation for river restoration: columbia River food webs. P. Natl. Acad. Sci. Usa. 109 (52), 21201–21207. doi:10.1073/pnas.1213408109
Newton, K. L., Hart, D. D., Pizzuto, J. E., Thomson, J. R., Egan, J., Ashley, J. T., et al. (2007). An integrative approach towards understanding ecological responses to dam removal: the manatawny creek study 1. J. Am. Water Resour. As. 38 (6), 1581–1599. doi:10.1111/j.1752-1688.2002.tb04366.x
Palmer, M., Allan, J. D., Meyer, J., and Bernhardt, E. S. (2007). River restoration in the twenty-first century: data and experiential knowledge to inform future efforts. Restor. Ecol. 15 (3), 472–481. doi:10.1111/j.1526-100X.2007.00243.x
Palmer, M. A., Ambrose, R. F., and Poff, N. L. (1997). Ecological theory and community restoration ecology. Restor. Ecol. 5, 291–300. doi:10.1046/j.1526-100X.1997.00543.x
Palmer, M. A., Bernhardt, E., Allan, J. D., Lake, P., Alexander, G., Brooks, S., et al. (2005). Standards for ecologically successful river restoration. J. Appl. Ecol. 42, 208–217. doi:10.1111/j.1365-2664.2005.01004.x
Phukan, A., Goswami, R., Borah, D., Nath, A., and Mahanta, C. (2012). River bank erosion and restoration in the Brahmaputra River in India. Clarion 1 (1), 1–7. Avaliable at: https://api.semanticscholar.org/CorpusID:220754846.
Pinheiro, A. C. T., Arsenault, K., Houser, P., Toll, D., Kumar, S., Matthews, D., et al. (2004). “Improved evapotranspiration estimates to aid water management practices in the Rio Grande River Basin,” in IGARSS 2004. 2004 IEEE International Geoscience and Remote Sensing Symposium, Anchorage, AK, USA, 20-24 September 2004 (IEEE). doi:10.1109/IGARSS.2004.1370777
Postal, S., and Carpenter, S. (1997). “Freshwater ecosystem services,” in Nature’s services: societal dependence on natural ecosystems. Editor G. Daly (Washington DC: Island Press), 195–214. doi:10.1023/A:1023307309124
Qi, Y., Lian, X. H., Wang, H. W., Zhang, J. L., and Yang, R. (2020). Dynamic mechanism between human activities and ecosystem services: a case study of Qinghai lake watershed, China. Ecol. Indic. 117, 106528. doi:10.1016/j.ecolind.2020.106528
Qiao, M., Zhu, Z. A., Xu, H. L., Zhou, S. B., and Li, Y. L. (2007). Studies on the counter measures for recovering ecology of Yili river vally and harmonizing big agriculture development. J. Agric. Res. Reg. 28 (03), 45–48.
Reid, W. V., Chen, D., Goldfarb, L., Hackmann, H., Lee, Y. T., Mokhele, K., et al. (2010). Earth system science for global sustainability: grand challenges. Science 330 (6006), 916–917. doi:10.1126/science.1196263
Reig, P., Shiao, T., and Gassert, F. (2013). Aqueduct water risk framework. Avaliable at: http://www.wri.org/publication/aqueduct–water–riskframework.
Richard, V. D. H., Nathália, N., Ailton, F., Carolina, J., Geanderson, A., Julia, A., et al. (2022). Policy-oriented ecosystem services research on tropical forests in South America: a systematic literature review. Ecosyst. Serv. 56, 101437. doi:10.1016/j.ecoser.2022.101437
Richardson, C. J., Reiss, P., Hussain, N. A., Alwash, A. J., and Pool, D. J. (2005). The restoration potential of the Mesopotamian marshes of Iraq. Science 307 (5713), 1307–1311. doi:10.1126/science.1105750
Rob, J. H. M., Veeren, D. V., and Tol, R. S. J. (2003). Game theoretic analyses of nitrate emission reduction strategies in the Rhine river basin. Int. J. Glob. Environ. 3 (1), 74–103. doi:10.1504/IJGENVI.2003.002412
Sarkar, S. K., Saha, M., Takada, H., Bhattacharya, A., Mishra, P., and Bhattacharya, B. (2007). Water quality management in the lower stretch of the river Ganges, east coast of India: an approach through environmental education. J. Clean. Prod. 15 (16), 1559–1567. doi:10.1016/j.jclepro.2006.07.030
Schewe, J., Heinke, J., Gerten, D., Haddeland, I., Arnell, N. W., Clark, D. B., et al. (2014). Multimodel assessment of water scarcity under climate change. P. Natl. Acad. Sci. 111 (9), 3245–3250. doi:10.1073/pnas.1222460110
Schoenholtz, S. H., James, J. P., Kaminski, R. M., Leopold, B. D., and Ezell, A. W. (2001). Afforestation of bottomland hardwoods in the lower Mississippi alluvial valley: status and trends. Wetlands 21 (4), 602–613. doi:10.1672/0277-5212(2001)021[0602:AOBHIT]2.0.CO;2
Seitz, N. E., Westbrook, C. J., Dubé, M. G., and Squires, A. J. (2013). Assessing large spatial scale landscape change effects on water quality and quantity response in the lower athabasca river basin. Integr. Environ. Asses. 9 (3), 392–404. doi:10.1002/ieam.1336
Smolders, A. J. P., Guerrero, H. M. A., Van, d.V. G., and Roelofs, J. G. M. (2002). Dynamics of discharge, sediment transport, heavy metal pollution and sábalo (prochilodus lineatus) catches in the lower Pilcomayo river (Bolivia). River. Res. Appl. 18 (18), 415–427. doi:10.1002/rra.690
Sommerwerk, N., Baumgartner, C., Venohr, M., Schneider-Jacoby, M., Hein, T., Tockner, K., et al. (2010). Managing the world's most international river: the Danube river basin. Mar. Freshw. Res. 61 (7), 736–748. doi:10.1071/MF09229
Standring, W. J. F., Brown, J. E., Dowdall, M., Korobova, E. M., Linnik, V. G., and Volosov, A. G. (2009). Vertical distribution of anthropogenic radionuclides in cores from contaminated floodplains of the Yenisey River. J. Environ. Radioact. 100 (12), 1109–1120. doi:10.1016/j.jenvrad.2009.04.011
Supalla, R. J., Gopalakrishnan, C., and Huffaker, R. (2000). A game theoretic analysis of institutional arrangements for Platte River management. Int. J. Water Resour. D. 16 (2), 253–264. doi:10.1080/07900620050003152
Swales, S. (2002). United nations environment programme fish and fisheries of the fly river, Papua New Guinea: population changes associated with natural and anthropogenic factors and lessons to be learned. Avaliable at: http://www.unep.org/bpsp/Fisheries/Fisheries%20Case%20Studies/SWALES.pdf.
Thompson, J. R. (2012). Modelling the impacts of climate change on upland catchments in southwest Scotland using MIKE SHE and the UKCP09 probabilistic projections. Hydrol. Res. 43 (4), 507–530. doi:10.2166/nh.2012.105
Thoms, M. C., and Sheldon, F. (2000). Water resource development and hydrological change in a large dryland river: the Barwon–Darling River, Australia. J. Hydrol. 228 (1–2), 10–21. doi:10.1016/S0022-1694(99)00191-2
Tockner, K., Schiemer, F., and Ward, J. V. (1998). Conservation by restoration: the management concept for a river-floodplain system on the Danube River in Austria. Aquat. Conserv. 8 (1), 71–86. doi:10.1002/(sici)1099-0755(199801/02)8:1<71::aid-aqc265>3.0.co;2-d
Tu, X., Peng, J. F., Duan, L., Song, Y. H., and Gao, H. J. (2013). Study on measures of natural habitat restoration in the mainstream of Liaohe conservation area. J. Environ. Eng. Tech. 3 (6), 503–507. doi:10.3969/j.issn.1674-991X.2013.06.078
Venot, J. P., Sharma, B. R., and Rao, K. V. G. K. (2008). Krishna basin development interventions to limit downstream environmental degradation. J. Environ. Dev. 17 (3), 269–291. doi:10.1177/1070496508320532
Vogl, A. L., and Lopes, V. L. (2009). Impacts of water resources development on flow regimes in the Brazos River. Environ. Monit. Assess. 157 (1–4), 331–345. doi:10.1007/s10661-008-0538-5
Vormoor, K. (2010). Water engineering, agricultural development and socio–economic trends in the Mekong Delta, Vietnam. Bonn: University of Bonn, Center for Development Research ZEF.
Vörösmarty, C. J., Green, P., Salisbury, J., and Lammers, R. B. (2000). Global water resources: vulnerability from climate change and population growth. Science 289 (5477), 284–288. doi:10.1126/science.289.5477.284
Vörösmarty, C. J., McIntyre, P. B., Gessner, M. O., Dudgeon, D., Prusevich, A., Green, P., et al. (2010). Global threats to human water security and river biodiversity. Nature 467 (7315), 555–561. doi:10.1038/nature09440
Wada, Y., Beek, L. P. H. V., Viviroli, D., Dürr, H. H., Weingartner, R., and Bierkens, M. F. P. (2011). Global monthly water stress: 2. water demand and severity of water stress. Water Resour. Res. 47 (7), 197–203. doi:10.1029/2010WR009792
Walker, B., Barrett, S., Polasky, S., Galaz, V., Folke, C., Engström, G., et al. (2009). Looming global–scale failures and missing institutions. Science 325 (5946), 1345–1346. doi:10.1126/science.1175325
Wang, G., Jager, H. I., Baskaran, L. M., Baker, T. F., and Brandt, C. C. (2016). SWAT modeling of water quantity and quality in the Tennessee River basin: spatiotemporal calibration and validation. Hydrol. Earth. Syst. Sc. 1, 33. doi:10.5194/hess-2016-34
Wang, L. G., Zhu, R., Yin, Z. L., Chen, Z. X., Lu, R., and Fang, C. S. (2022). Quantifying the spatial–temporal patterns and influencing factors of agricultural carbon emissions based on the coupling effect of water–land resources in arid inland regions. Front. Environ. Sci. 10, 908987. doi:10.3389/fenvs.2022.908987
Wang, S. W., Zhang, J. P., and Ni, W. (2013). Summary of comprehensive planning of Songhuajiang river basin. Water Res. hydro. China 31 (7), 13–17. doi:10.14124/j.cnki.dbslsd22-1097.2013.07.012
Wang, X. Q., and Gao, Q. Z. (2002). Sustainable development and management of water resources in the hei river basin of North-west China. Int. J. Water Resour. D. 18 (2), 335–352. doi:10.1080/07900620220135139
Wang, Y., and Chang, J. (2006). Status and conservation of sturgeons in Amur River, China: a review based on surveys since the year 2000. J. Appl. Ichthyol. 22 (s1), 44–52. doi:10.1111/j.1439-0426.2007.00928.x
Wang, Z. (2007). Preliminary study on water resources development and utilization problem in yili river basin. J. Yili Norm. Univ. Soc. Sci. Ed.) 3, 48–51.
Westmacott, J. R., and Burn, D. H. (1997). Climate change effects on the hydrologic regime within the churchill-nelson river basin. J. Hydrol. 202 (1–4), 263–279. doi:10.1016/S0022-1694(97)00073-5
World Commission on Environment and Development (WCED) (1987). Our Common future. Oxford: Oxford University Press. Avaliable at: http://www.un-documents.net/ocf-ov.htm.
Xia, H. P. (1999). Flood disasters, soil erosion, and eco-restoration of vegetation in the Yangtze and the Pearl River valleys. Trop. Geogr. 19 (02), 124–129. doi:10.13284/j.cnki.rddl.000435
Ye, X., Zhang, Q., Liu, J., Li, X., and Xu, C. Y. (2013). Distinguishing the relative impacts of climate change and human activities on variation of streamflow in the poyang lake catchment, China. J. Hydrol. 494 (12), 83–95. doi:10.1016/j.jhydrol.2013.04.036
Zhang, H. B., Wang, Y. M., Jiang, X. J., and Xin, C. (2011). Ecological regulation of reservoirs on the Yellow River mainstream oriented to ecological flow restoration. J. Hydro. Eng. 30 (3), 15–21.
Zhang, J. F., and Sun, Q. X. (2005). Causes of wetland degradation and ecological restoration in the Yellow River delta region. For. Stud. China 7 (2), 15–18. doi:10.1007/s11632-005-0015-y
Zhang, X. Q., Zheng, Z. W., Sun, S. F., Wen, Y. H., and Chen, H. Y. (2023). Study on the driving factors of ecosystem service value under the dual influence of natural environment and human activities. J. Clean. Prod. 420, 138408. doi:10.1016/j.jclepro.2023.138408
Zhong, H. X., Liu, S. Z., and Fan, J. R. (2003). Restoration and reconstruction of degraded ecosystems in the upper reaches of the Changjiang River. Res. Environ. Yangtze. 12 (2), 157–162.
Keywords: environmental change, sustainable development, zoning, climate change, human activities, watershed, global scale
Citation: Meng Z, Cheng-Qi Z, Qi F, Ju-Tao Z, Wei L, Ling-Ge W, Yuan-Yuan X and Ying-Qing S (2024) Environmental change induced by water engineering development dominates the global watershed sustainable development issues. Front. Environ. Sci. 11:1322308. doi: 10.3389/fenvs.2023.1322308
Received: 17 October 2023; Accepted: 18 December 2023;
Published: 08 January 2024.
Edited by:
Zhihao Xu, Guangdong University of Technology, ChinaCopyright © 2024 Meng, Cheng-Qi, Qi, Ju-Tao, Wei, Ling-Ge, Yuan-Yuan and Ying-Qing. This is an open-access article distributed under the terms of the Creative Commons Attribution License (CC BY). The use, distribution or reproduction in other forums is permitted, provided the original author(s) and the copyright owner(s) are credited and that the original publication in this journal is cited, in accordance with accepted academic practice. No use, distribution or reproduction is permitted which does not comply with these terms.
*Correspondence: Feng Qi, cWlmZW5nQGx6Yi5hYy5jbg==