- Department of Chemistry, Life Sciences and Environmental Sustainability, University of Parma, Parma, Italy
Chemical analyses are inadequate for assessing soil biological quality. Instead, the soil living community can be used both for monitoring and restoring soil health. The aim of this research was to verify vermiremediation efficiency in PCDD/F and PCB contaminated soils from Brescia-Caffaro (Italy), using an ecotoxicity approach. To gauge whether Caffaro soil could sustain a living community, a characterization of the arthropod community was conducted. Earthworms’ suitability for soil bioremediation was assessed applying ecotoxicity tests. Five treatments were set up: 1) contaminated soil; 2) contaminated soil + Eisenia fetida; 3) contaminated soil + Lepidium sativum; 4) contaminated soil + E. fetida + L. sativum, 5) uncontaminated soil + E. fetida. The ecotoxicity tests were: L. sativum germination index and root elongation inhibition, and Folsomia candida survival and reproduction, applied on soil and elutriate on: starter soil (T0), after 56 and 112 days (T56 and T112), the last after water percolation. Soil arthropod community was dominated by Hypogastruridae, Oribatida and, to a lesser degree, Formicidae and Coleoptera larvae. Ecotoxicity tests showed that F. candida reproduction and L. sativum root elongation were more adversely affected by pollutants than survival and germination. The higher soil ecotoxicity at T112 than at T56, suggested higher contaminant bioavailability after water addition. F. candida showed more variability between soil and elutriate than L. sativum. Both bioassays suggested earthworm treatment as the most promising. The importance of selecting different organisms in soil ecotoxicity monitoring, and the role of elutriate like a solid phase complement, was highlighted.
Introduction
Polychlorobiphenyls (PCBs), polychlorodibenzo-p-dioxins and furans (PCDDs and PCDFs) are representative of a group called “Persistent Organic Pollutants (POPs)” that threaten human health and the environment (Campanella et al., 2002). They are regarded as ubiquitously persistent environmental pollutants in the ecosystem (Rathna et al., 2018). Once emitted, they tend to bind to the organic matter in the upper soil surface, where they can transfer to animals by ingestion and, through biomagnification along the trophic chain, to humans (Pereira, 2004).
Nowadays, numerous removal technologies are commercially available, but the effectiveness of these technologies depends on investment cost and operating expenses, which in turn depend on the national economy. Against this background, bioremediation has proved to be an inexpensive and effective tool, with the potential to respond to the remediation need of restoring a functioning ecosystem (Curry and Majer, 1990).
Soil fauna have been considered not only a reliable tool in the bioremediation process but also in monitoring action to assess toxicity and the risk of contaminated soil before and after bioremediation (Haimi, 2000). In fact, soil biota is very dynamic and susceptible to soil disturbance, unlike most chemical and physical properties, which take longer to change; edaphic organisms are thus considered good indicators of soil health (Cardoso et al., 2013).
Diversity and abundance of soil fauna has been used as an indicator of anthropogenic impacts on terrestrial ecosystems because they are strictly correlated with physical, chemical, and microbiological soil attributes (Decaëns et al., 2004; Eggleton et al., 2005). Among the most representative organisms generally used as soil health indicators, mesofauna, being involved in many processes, such as organic matter translocation, breaking and decomposition, nutrient cycling, soil structure formation and water regulation, plays a key role in monitoring plans (Lavelle and Spain, 2001; Menta and Remelli, 2020). In aged contaminated sites, the number of tolerant specimens to be increased and the replacement of pollution-sensitive species with less sensitive ones can lead to a different species assemblage inside the community (Salminen et al., 2001). These changes are not only indicators of potentially contaminated soils but also could be useful for assessing the rate of bioremediation, both natural and supported (Rusin and Gospodarek, 2016). Among bioremediation techniques, vermiremediation is found to be a reliable tool, enhancing microbial activity and assisting phytoremediation, which are often adversely affected by low PCDD/F and PCB bioavailability, and supporting plant growth (Aken et al., 2009; Campanella et al., 2002; Haimi, 2000; Rodriguez-Campos et al., 2014).
When assessing the validity of remediation techniques, chemical analyses characterize the contamination level of the medium (soil or water), but are inadequate in assessing its biological quality. Combining such testing with bioassays, which can reflect the effects of bioavailable contaminants, can help to highlight the link between actual contamination levels and adverse effects on biota (Leitgib et al., 2007). The bioavailability of contaminants depends on several factors, such as physico-chemical properties of the contaminant itself, the characteristics of the environment and the organisms used in the bioassay (Aqeel et al., 2014). Thus, the use of multiple target species, especially if representative of different trophic levels and having different degrees of sensitivity toward toxicants, is highly recommended, since it allows a more complete evaluation of potential contaminant toxicity by considering different exposure routes and endpoints (e.g. survival, reproduction, growth and development) (Burton et al., 2002; Picone et al., 2016).
More than 1,800,000 potentially contaminated sites have been identified in Europe and, among them, 39 are in Italy (“SIN - Siti di Interesse Nazionale”, or National Priority Sites) (EEA, 2007). The SIN Brescia-Caffaro, deriving from the activities of the former Caffaro S.p.A. chemical factory, is one of the most polluted sites in Italy. Caffaro produced PCBs and PCB mixtures, such as Fenclor and Apirolio, from 1930 to 1984. During those years contaminated surface water went into the soil (as irrigation water) of adjacent agricultural areas. This resulted in significant concentrations of persistent chlorinated organic pollutants, with a prevalence of PCBs and PCDDs/Fs exceeding residential (0.001 mg/kg) and industrial (5 mg/kg) legal limits respectively (di Guardo et al., 2017).
In the present study, we sought to: 1) characterize the soil arthropod community in order to assess whether highly PCB and PCDD/F contaminated soils, like those in Caffaro, can support a well-structured soil living community; 2) monitor the effectiveness of vermiremediation and/or phytoremediation treatments in PCB and PCDD/F contaminated soil by using an ecotoxicological test. Since the determination of contaminants using traditional chemical analyses cannot predict their impact on living organisms, to obtain an ecosystemic approach PCDD and PCB biodegradation in the soil was monitored, both for soil and soil-elutriate, using a multi-organism and multi-endpoint approach in which the bioindicators belonged to different taxonomic groups: Lepidium sativum for higher plants, and Folsomia candida for mesofauna.
Materials and Methods
The study site (45°31′24.75″N, 10°11′18.60″E), covering a surface area of about 460 m2, lies in a green area outside the National Priority Site (SIN) Brescia-Caffaro, about 5 km south of the Brescia-Caffaro factory (Northern Italy). The SIN is the result of activity performed at the Caffaro factory, and includes soils polluted by PCBs and PCDD/Fs that often exceed both residential (0.001 mg/kg) and industrial (5 mg/kg) legal limits (Vergani et al., 2019).
From Brescia municipality data, the stratigraphy of the study area is thus represented: 0—0.2 m silty topsoil with gravel, containing plant frustules and root systems, 0.2—0.8 m slightly silty to brown silty sand with gravel and root systems and heterometric, polygenic, from sub-angular to sub-rounded clasts, 0.8—1.7 fine and brown sandy silt with spread gravel and decimetre sandy gravel lens and heterometric, polygenic, from sub-angular to sub-rounded clasts, 1.7—2.0 slightly silty to brown silty sand with gravel and heterometric, polygenic, from sub-angular to sub-rounded clasts.
Soil Arthropod Characterization
To characterize the arthropod community at the study site, five soil samples (10 × 10 × 10 cm) about 5 m from each other were collected using a spade. Soil arthropods (200 µm–2 mm) were extracted from each soil sample using a Berlese–Tullgren funnel for 10 days. The extracted specimens were collected and preserved in a solution consisting of 75% ethyl alcohol and 25% glycerol by volume. Specimens were identified and counted using a stereomicroscope. Different taxonomic levels were considered: class for Myriapoda and, among them, order for Chilopoda, and order for Chelicerata, Crustacea and Hexapoda. We decided to maintain this different taxonomic level to obtain a wider vision of the edaphic fauna community living in such a contaminated site. It was decided to consider a lower taxonomic level for Coleoptera, Collembola and mites. In the case of Coleoptera, which indicates many kinds of alteration in the environment (e.g. pollution), different ecological requirements require that they be distinguished at least at family level (Menta and Remelli, 2020). Like Coleoptera, Collembola too have been seen to be useful in the assessment of bioremediation rates when considering at least family level (Rusin and Gospodarek, 2016). Finally, among mites, a distinction was made between Oribatida and other Acarina, considering the close relationship between Oribatida and soil organic matter. Within holometabolous insects, adults and larvae were separated.
Bioremediation Experimental Design
About 0.25 m3 of silty contaminated topsoil (C) was sampled (<20 cm deep), air-dried and mechanically homogenized following the “one-dimensional Japanese Slab-Cake” (JSC) technique (Low et al., 2010). As uncontaminated soil (control), biological potting soil was used.
For the microcosm setup, 15 polypropylene tanks, with a cut-off bottom, were overturned and filled with soil following this experimental design (3 replicates for each one): 1) uncontaminated soil + Eisenia fetida (control, NCEf), 2) contaminated soil (C), 3) contaminated soil + E. fetida (CEf), 4) contaminated soil + L. sativum (CLs), 5) contaminated soil + E. fetida + L. sativum (CEfLs).
E. fetida was supplied by a worm breeding company. For treatments with earthworms, 15 sexually mature individuals were placed in each microcosm, after being washed with deionized water, dried, and weighed. Food, consisting of 20 g of air-dried cattle manure, was supplied weekly. At the end of the experiment, the number of earthworms in each microcosm was reported, considering both adults and juveniles. For treatments with L. sativum, 0.5 g of seeds were added to each microcosm.
During the test period, microcosms were maintained at 20 ± 2°C with 80–85% RH, and deionized water was added when water loss >2% of the initial WHC.
The experiment lasted 112 ays (from February 23, 2021 to June 15, 2021). Three sampling times were considered: before microcosms setup (T0), after 56 days (T56), and after 112 days (T112). At each sampling time, soil needed for ecotoxicological tests was collected using a brass drilling machine (Ø = 1.90 cm, height = 9.00 cm). At T0 and T112, pH and soil organic matter (SOM) analyses were carried out, together with the measurement of PCB and PCDD/F total and congener concentrations (toxic equivalent - TEQ values have been calculated using TEFWHO98). At the beginning of the test analyses were carried out on three replicates of C. After, they were measured at T112 in each microcosm. The pH analysis was conducted on a soil distilled water liquid mixture (1:2.5 w/v) using, besides pH electrode, a temperature probe to achieve automatic temperature compensation (Società Italiana della Scienza del Suolo, 1986). SOM was determined by using LOI - Loss on Ignition, i.e. the ignition of 1 g of dried soil at 550°C for 4 h (Heiri et al., 2001). For each sample measured, 50 g of soil were collected in glass vials for PCB and PCDD/PCDF analyses, which were performed at Biochemie Lab S.r.l. following the EPA 1668C 2010 protocol for PCB and the EPA 1613B 1994 protocol for PCDD/PCDF (US EPA, 1994; US EPA, 2010).
At T112, soil was taken after having percolated 2 L of water (needed to simulate bioremediation impact on contaminants’ mobility into groundwater, unpublished data), in order to keep earthworm tunnels intact and be able to assess their effect on water percolation.
Ecotoxicological Tests
Before the microcosm setup, a T0 test on contaminated soil from the Caffaro factory was carried out for E. fetida to determine its suitability for use in the bioremediation test. The sexually mature E. fetida belonged to the same breeding subsequently used for earthworm treatments applied to microcosms. Survival and reproduction tests were carried out according to standard ISO 11268 (ISO 11268-2:2012, 2012). Test containers (three replicates) were filled with 500 g of Caffaro soil, to which deionised water was added to achieve a soil moisture of 40–60% of the WHC. Ten earthworms were cleaned with distilled water, dried and placed in the container and kept at 20 ± 2°C with 80–85% relative humidity (RH) for 28 days. During the test period, earthworms were fed weekly with cattle manure, and water was added when water loss >2% of the initial water holding capacity (WHC). After 28 days, surviving earthworms were counted; containers with (possible) cocoons were incubated for a further 28 days under the previous conditions, except that food was administered only once, after the removal of adults. At the end of the test (56 days), newborn juveniles were counted.
F. candida (Collembola: Isotomidae) for soil invertebrates and L. sativum (Brassicales: Brassicaceae) for plants were used to test soil and elutriate toxicity at T0, T56 and T112. Three replicates for each microcosm, for both test organisms and for both soil and elutriate, were carried out in the three trials. Before running the test procedure, these soil samples were dried at 50°C for 16 h, sieved at 2 mm, and homogenised. To test soil toxicity, Petri dishes were filled with 30 g of testing soil, wetted with deionized water to reach 40–60% of the total water holding capacity (WHC). To test elutriate toxicity, Petri dishes containing 5 ml of an elutriate solution on filter paper were used. The elutriate was the liquid phase, obtained by shaking a solution of testing soil:water (1:4 w/v) for 30 min, and then allowing it to sediment at room temperature for 24 h.
The F. candida specimens used in the test came from laboratory cultures at Parma University. Growth, survival and reproduction tests were carried out according to standard ISO 11267 (ISO 11267:1999, 1999). Individual specimens were kept at 20 ± 2°C (with 50–55% RH) and fed weekly on dry yeast. Specimens used for egg deposition (aimed to obtain age-synchronized juveniles to be used in the test) were collected from breeding containers and mixed to prevent them originating from a single breeding line. All springtails used for testing were 10 days old and age-synchronized by removing eggs from the deposition cultures and, once hatched, inserting juveniles into Petri dishes with a moistened breeding substrate of plaster of Paris:activated carbon powder (8:1 w/w).
Ten F. candida specimens aged 10 days were added to each Petri dish using an exhauster, ensuring that none of the exemplars died during the process. Springtails were kept at 20 ± 2°C with 70–80% relative humidity (RH) and fed with the same dry yeast used during the breeding phase. The Petri dishes were incubated for 28 days, aerated once a week and watered when water loss exceeded 2% of the initial WHC. At the end of this period, adults and juveniles (when present) were euthanized by freezing. Vessels were filled with water and gently stirred with a spatula, allowing the animals to float on the water surface (flotation technique). A small amount, approx. 0.5 ml, of black ink was added to the water to increase the contrast between the water and the white Collembola. Then a digital photograph was taken, and the number of surviving adults and new-born springtails were counted using image analysis software provided by ImageJ (version 1.53). The same procedure was applied to both contaminated and control soil.
The survival rate (SR%) was calculated as follows:
where Sc and Sn are the number of survivors in the control sample and in soil (or elutriate), respectively.
The reproduction rate (RR%) was calculated as follows:
where Rc and Rn are the number of juveniles in the control sample and in soil (or elutriate), respectively.
Non pesticide-treated L. sativum seeds were used to test germination and root elongation, in accordance with standard ISO 17126 (ISO 17126:2005, 2005). As for E. fetida, tests at T0 were conducted before the microcosm setup. To test soil toxicity, Petri dishes containing a filter paper onto which 5 g of soil saturated with deionised water were used; to test elutriate toxicity, Petri dishes with filter paper were prepared, as previously described for F. candida tests on elutriate. Ten seeds were added to each Petri dish; all dishes were wrapped in Parafilm™ to avoid cross contamination and incubated at 25 ± 1°C in a dark incubation chamber for 72 h. At the end of this period, germinated seeds were counted and their root elongation (the length from the tip of the root to the radicle) was measured. To assess the validity of the test, the same procedure was applied for the control soil.
The elongation inhibition rate (EI%) was calculated as follows:
where Lc and Ln are the mean values of root length in the control sample and in soil (or elutriate), respectively.
The germination index was calculated using the formula:
where Gn and Ln are the mean values of germinated seeds and root in soil (or elutriate), respectively, and Gc and Lc are the mean values of germinated seeds and root in the control sample, respectively.
Statistical Analysis
One-Way ANOVA, followed by the Tukey test, was used to evaluate differences in earthworm numbers (total abundance, adults and juveniles) between treatments and the control sample after 112 days. Since ANOVA assumptions were not met, Box Cox transformation was applied.
To understand relations between treatments, PCB and PCDD/F total concentrations in soil (at T112) and ecotoxicological results, considering the fact that the dataset contains both quantitative and qualitative variables, a Factor Analysis of Mixed Data (FAMD) was run (Pagès, 2004). Computation and visualization of FAMD data was carried out using “FactoMineR” and “factoextra” packages respectively (Kassambara and Mundt, 2016; Husson et al., 2020).
For ecotoxicological tests, F. candida survival and reproduction rates and L. sativum elongation inhibition rate and germination index were considered as dependent variables. ANOVA assumptions were not met, and the “lme4” package was used to estimate a mixed effects logistic regression model with treatments and time as predictors, a random intercept by microcosm, and family Gaussian (Bates et al., 2014). The “emmeans” package was used for pairwise post hoc multiple comparisons with Bonferroni adjustment (Lenth et al., 2017).
A p-value ≤ 0.05 was considered significant. All analyses were performed using R (version 4.0.5) (R Core Team, 2021).
Results
Data on pH and SOM, as well as PCB and PCDD/F total and congeners concentration in the starter soil and in microcosms after 112 days of bioremediation test are reported in the Supplementary Material (Supplementary Tables S1, S2). Briefly, pH and SOM content did not change significantly from T0 to T112, and no differences were observed between treatments. Indeed, PCB and PCDD/F concentrations fell significantly after 112 days in all treatments. Moreover, even if treatments did not differ in terms of total PCBs, CEfLs congeners were less environmentally threatening. Finally, among the treatments, CEf and CLs resulted in lower PCDD/F total concentrations, with a reduction in higher TEF congeners too.
Arthropod Community
Arthropods extracted in the sampling area totalled 7570.62 ± 1688.19 ind./m2, most were Collembola (46%) and Acarina (30%) (Acarina/Collembola ratio: 0.9 ± 0.5), followed by Hymenoptera Formicidae (12%), Coleoptera (5%), Diptera (larvae, 4%) and Hemiptera (2%) (Table 1). Isopoda, Araneae, Pauropoda, Protura and Thysanoptera accounted for the remaining 1%. Seven families of Collembola were identified, the most present was Hypogastruridae (80%), followed by Isotomidae (10%) and Sminthuridae (9%); the remaining 1% consisted of Entomobryidae, Bourletiellidae and Onychiuridae. Acarina consisted mainly of Oribatida (68%) and Coleptera in larvae (83%). Among Coleoptera adults three families were identified, the most abundant belonging to Carabidae (53%), followed by Staphilinidae (35%) and Curculionidae (12%).
Eisenia fetida
Preliminary tests on E. fetida resulted in a survival rate of 100% after two months in contaminated soil, with no reproduction in any replicate.
Inside the microcosms, earthworms differed in terms of total abundance, adults and juvenile presence after 112 days of the experiment (p < 0.001, all). CEf and CEfLs differed from NCEf for all the parameters evaluated (Figures 1A,B), while CEf and CEfLs differed from each other only in terms of the number of adults (Figure 1A).
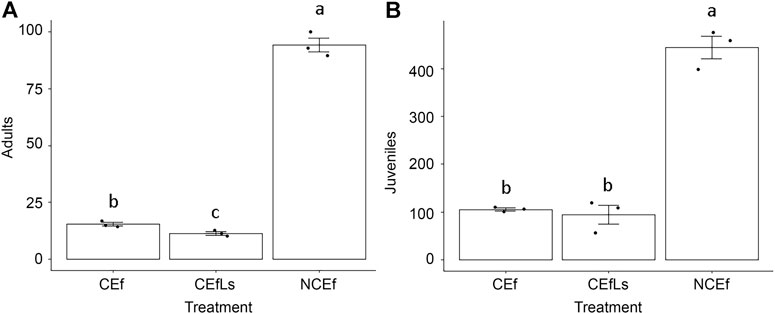
FIGURE 1. (A) Adults and (B) juveniles of E. fetida in contaminated soils (CEf and CEfLs) and control sample (NCEf) at the end of the experiment. Different letters correspond to significant differences (p ≤ 0.05).
Ecotoxicological Tests on Folsomia candida and Lepidium sativum
From the FAMD a weak susceptibility to soil PCB and PCDD/F concentrations (which were mainly linked to CEfLs treatment) could be observed on the survival and reproduction rates of F. candida in elutriate, unlike what happened in soil (Figure 2). on the other hand, L. sativum results showed a higher germination index related to CEf treatment and an increased elongation inhibition linked to C.
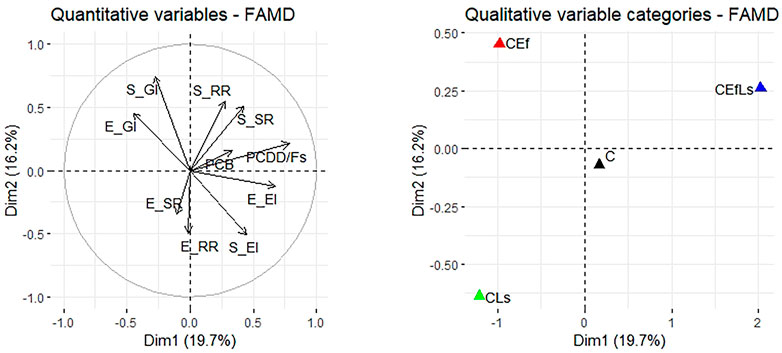
FIGURE 2. FAMD output for quantitative (ecotoxicological indexes) and qualitative (treatments) variables and their contribution to the dimensions 1 and 2 in soil. F. candida indicators were: S_SR and E_SR = survival rate in soil and elutriate respectively, S_RR and E_RR = reproduction rate in soil and elutriate respectively; L. sativum indicators were: S_GI and E_GI = germination index in soil and elutriate respectively, S_EI and E_EI = elongation inhibition in soil and elutriate respectively.
Folsomia candida
The F. candida survival rate in soil (Figure 3A) depended on time (p < 0.001) and on the interaction between time and treatment (p < 0.01).
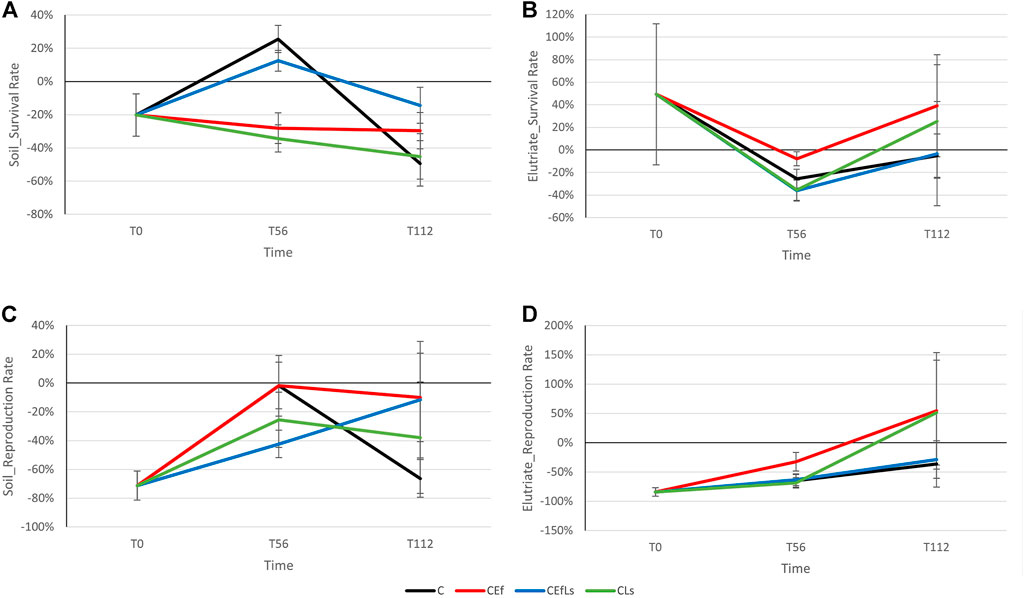
FIGURE 3. F. candida survival and reproduction rates in (A,C) soil and (B,D) elutriate respectively. The horizontal bar, corresponding to 0%, indicates results equal to the control sample, values above and below that bar indicate an increase and decrease, respectively, in treatment parameters compared to the control sample.
Differences were observed between T56 and T112 (p ≤ 0.001). Within C, T56 posted higher values than T0 and T112 (p ≤ 0.01 and p < 0.001, respectively). Within T56, differences were observed between C and CEf (p ≤ 0.01), C and CLs (p < 0.01), and CEfLs and CLs (p < 0.05), C and CEfLs having the higher survival rate. The reproduction rate (Figure 3C) was significantly lower than those of the control sample since T0 (intercept: p ≤ 0.001), with differences attributable to time, and lower values in T0 than T56 and T112 (p < 0.01 and p < 0.05).
For elutriate, neither survival nor reproduction rates showed patterns depending on treatment or time (Figures 3B–D), however at T0 contaminated soil resulted in higher survival than the control sample (p < 0.05).
Lepidium sativum
In soil, neither the germination index nor elongation inhibition showed a dependence on treatment or time (Figures 4A–C).
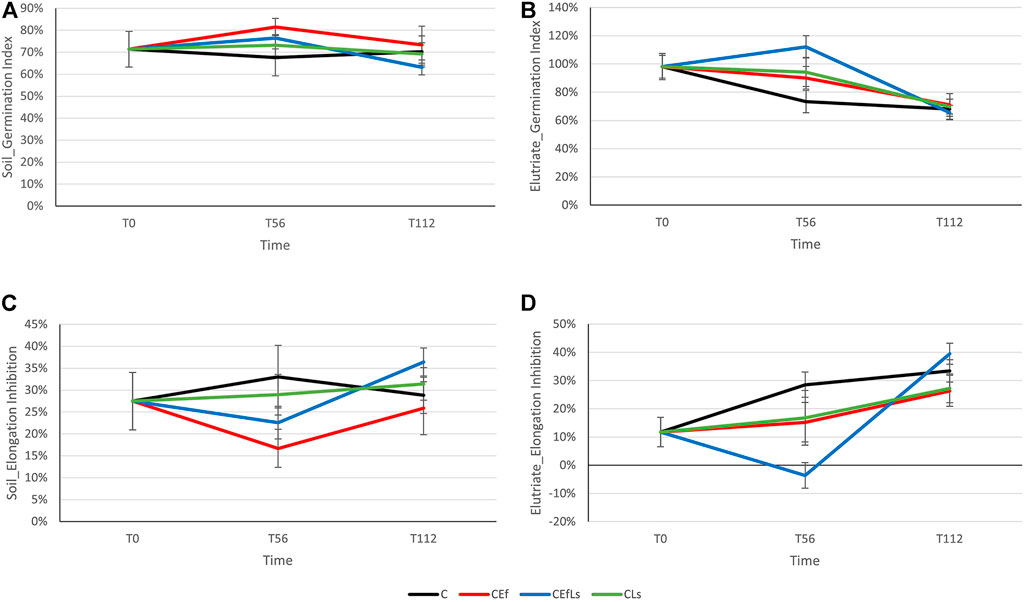
FIGURE 4. L. sativum germination index and elongation inhibition in (A,C) soil and (B,D) elutriate respectively. The horizontal bar, corresponding to 0%, indicates results equal to the control sample, values above and below that bar indicate an increase and decrease, respectively, in treatment parameters compared to the control sample.
For elutriate, a time effect was observed in the germination index (p < 0.05), which was lower at T112 than at T0 and T56 (p < 0.001, both; Figure 4B). Elongation inhibition not only increased with time (p ≤ 0.01), but was also affected by the interaction of time and treatment (p < 0.01; Figure 4D). Root elongation was more inhibited at T112 than at T0 and T56 (p < 0.001, both), with a treatment difference in C (with T112 inhibition higher than T0, p < 0.05) and CEfLs (with T112 inhibition higher than T0 and T56, p ≤ 0.001); moreover, a difference was observed between C and CEfLs in T56 (p < 0.01).
Discussion
Arthropod Community
Soils from Caffaro were collected in order to extract and characterize the arthropod community and assess whether highly PCB and PCDD/F contaminated soils could sustain a soil living community, and of what kind, before setting up vermiremediation tests.
Most arthropods extracted belonged to the Collembola and Acarina groups, with low values of the ratio Acarina/Collembola, typical of low-quality soils, confirming that in degraded soils Acarina numbers decrease (Visioli et al., 2013).
Within Collembola, Hypogastruridae was the dominant family, suggesting it was capable of withstanding some contaminants, as observed by García-Segura et al. (2018) in PAH contaminated sites. On the other hand, Rusin & Gospodarek (2016) found that Hypogastruridae and Isotomidae were the first families to recolonize soil contaminated by petroleum-derived substances after bioremediation, suggesting that in-field natural attenuation in Caffaro soils could be taking place, thus supporting results observed by the authors on soil contaminant concentration at the beginning and end of the experiment (Unpublished results).
With regard to Acarina, Oribatida were the most abundant mites, supporting the assumption of Iloba & Jarrett (2007) that adult Oribatid mites, having a stronger exoskeleton, could be consistently more tolerant or even active in extreme environments. Due to their lower permeable cuticle, the same applies to Coleoptera and Hymenoptera (Blakely et al., 2002). This is of particular interest since (Shen et al., 2021) observed that the use of beetle larvae in soil POP decontamination is feasible; while with ants being authentic ecosystem engineers that can affect the physical (soil porosity), chemical (nutrient content or pH), and biological (acceleration of decomposition rates) properties of soil (Frouz and Jilková, 2008), their influence on the redistribution of nutrients and even contaminants should be considered more carefully. Since invertebrate potential as bioreactors to treat environmental pollutants is considered promising, these results provide additional information about tolerant soil faunal groups that could be candidates for bioremediation studies (Remelli et al., 2020; Shen et al., 2021).
Bioremediation Ecotoxicological Effects
It is well established that over time a greater proportion of compound in soil becomes less extractable and less available for uptake or degradation than freshly added compound, reducing exposure and thus toxicity and risk (Alexander, 2000; Morrison et al., 2000). This has been demonstrated for plants, microorganisms and earthworms (Wilson and Naidu, 2008). This is the case for Caffaro soil, where the process of aging was supported by preliminary tests on E. fetida, with earthworm survival not being affected by the presence of PCB and PCDD/F. However, the absence of cocoons showed that E. fetida reproductive activity was damaged by contaminants, as already observed by (Remelli et al., 2020) at crude-oil contaminated sites. Nevertheless, even if in lower numbers than in the control soil, juvenile earthworms were observed after 4 months of bioremediation tests, supporting the hypothesis that reproductive activity was only slowed down. However, the difference in adult abundance observed between CEf and CEfLs suggested a negative impact of the presence of L. sativum on earthworm growth. Indeed, CEfLs was the treatment having overall most PCDD/F concentrations; moreover, this treatment was characterized by a higher presence of congeners frequently occurring in the environment and that are generally found in high concentrations in animal tissues (McFarland & Clarke, 1989; Unpublished results). CEfLs toxicity could in part be related to phytoremediation limits: even if CLs was shown to be the better bioremediation technique as regards dioxin content in our experiment, remediation by plants is often incomplete, since plants usually lack the biochemical pathways necessary to achieve the total mineralization of recalcitrant pollutants, leading therefore to undesirable effects, such as the accumulation of toxic metabolites that may be released into the soil and enter the food chain (Aken et al., 2009).
The toxicity effects of PCB and PCDD/F contaminated soils on F. candida and L. sativum has not been studied in depth, and there is little information in literature, especially for the effect of PCDDs on terrestrial invertebrates. In the present study, ecotoxicological tests with both bioassays confirmed that soil contaminants affected more negatively F. candida reproduction and L. sativum development than their survival and germination respectively. This is in accordance with (Domene et al., 2007), who observed that F. candida reproduction is generally affected at lower waste concentrations than survival, showing that this is a more sensitive parameter.
In our study, the results obtained for F. candida in the soil matrix suggested a significant effect of time, with evidence of lower toxicity at T56. This reduction, compared to T0, might be explained by the higher aeration linked to the starter soil manipulation, which might have enhanced aerobic degradation by autochthonous microorganisms in the early stages of the experiment. On the other hand, lower toxicity in T56 compared to T112 suggests that toxicity was not related to contaminant concentrations, since they should have been reduced by water percolation in T112. Water percolation thus seems to enhance pollutants’ mobility and bioavailability, inducing a higher toxic effect on Collembola. Nevertheless, as time progressed, treatments involving earthworms were seen to be the better solution for lowering soil toxicity both for F. candida survival and reproduction, confirming that vermiremediation can improve soil quality and biological activity (Sinha et al., 2008). On the other hand, CLs probably caused a toxic effect relating to the presence of high hazardous congeners. Generally, the measurement of toxicity through solid phase tests is considered the most relevant way of estimating its ecotoxicological potential, as it is closer to real-life situations (Bakir et al., 2014). However, elutriate represents a useful tool in ecotoxicity assessment as it gives information on pollutants’ instantaneous bioavailability (McMillen et al., 2003). In this study, elutriate showed nonlinear results, suggesting that this parameter was more susceptible to PCB and PCDD/F total content, supporting the hypothesis that water enhanced contaminant mobility, disrupting their bindings with organic matter, and thus enhancing their bioavailability.
In this study a negative effect of time was observed on L. sativum, mostly in elutriate, with T112 soil more toxic than the starter soil. This result confirmed the suggestion for F. candida that the passage of water enhanced pollutants’ mobility and bioavailability, thus inducing a toxic effect on L. sativum. It has already been observed, by Vašíčková et al. (2016) for heavy metals, that composting and vermicomposting reduce contaminants’ mobility, regardless of changes in total concentration, thus reducing ecotoxicity on soil organisms which are mainly exposed to contaminants via pore water fraction. Indeed, at T56 less toxicity was observed in treatments with earthworms (probably because of the organic matter masking effect previously cited); while in T112, regardless of lower contaminant concentrations, water addition might have mobilized previously non-bioavailable contaminants bound to organic matter particles, especially humic substances, thus increasing ecotoxicity. This toxic effect was particularly evident in CEfLs, characterized by a high presence of dioxins. Moreover, while for F. candida at T112 CEfLs toxicity was noticeable only in elutriate, L. sativum sensibility was observable in soil too, confirming that plant uptake is not always necessarily directly related to that part of the compound available to soil fauna (Wilson and Naidu, 2008).
Conclusion
At the present time little is known about the ecotoxicity of PCB and PCDD/F contaminated soil on terrestrial organisms and on the consequences of bioremediation techniques adopted to treat the problem.
This study provides new insights on the soil fauna community in PCB and PCDD/F aged contaminated sites, which could be used to monitor Caffaro soil health in future. Moreover, results on tolerant soil faunal groups could be of interest for studies on invertebrate application in bioremediation systems.
A battery of bioassays was also conducted to monitor the ecotoxicity of PCB and PCDD/F contaminated soils during a bioremediation experiment lasting 112 days involving vermiremediation and/or phytoremediation treatments. Variable results observed for different experiment times and treatments, highlighting the necessity to give more attention to soil type in order to understand ecotoxicological dynamics and determine the bioavailability of contaminants for certain terrestrial organisms. Against this background, elutriate could help to complement soil testing, giving information about contaminant mobilization by means of water, responding to the several drawbacks associated with the organic matter matrix in solid-phase assays. However, it emerges clearly the importance of selecting different organisms when testing soil ecotoxicity. Finally, introducing ecotoxicological tests in risk assessment appears crucial to evaluate contamination impact, as some detrimental effects cannot be explained by contaminant chemistry data. In this study, bioassays confirmed earthworms as the most promising treatment among those considered, despite contaminant total concentration, thus supporting the need to consider bioassays as relevant tools for the assessment of remediation techniques.
Data Availability Statement
The raw data supporting the conclusion of this article could be made available by the authors.
Author Contributions
Conceptualization, SR and CM; methodology, SR and CM; validation, SR and CM; formal analysis, SR; investigation, SR; resources, FC and CM; data curation, SR; writing—original draft preparation, SR and CM; writing—review and editing, SR, FC, and CM; visualization, SR; supervision, CM; project administration, FC and CM; funding acquisition, FC. All authors have read and agreed to the published version of the manuscript.
Conflict of Interest
The authors declare that the research was conducted in the absence of any commercial or financial relationships that could be construed as a potential conflict of interest.
Publisher’s Note
All claims expressed in this article are solely those of the authors and do not necessarily represent those of their affiliated organizations, or those of the publisher, the editors and the reviewers. Any product that may be evaluated in this article, or claim that may be made by its manufacturer, is not guaranteed or endorsed by the publisher.
Supplementary Material
The Supplementary Material for this article can be found online at: https://www.frontiersin.org/articles/10.3389/fenvs.2022.907260/full#supplementary-material
References
Aken, B. V., Correa, P. A., and Schnoor, J. L. (2009). Phytoremediation of Polychlorinated Biphenyls: New Trends and Promises. Environ. Sci. Technol. 44, 2767–2776. doi:10.1021/ES902514D
Alexander, M. (2000). Aging, Bioavailability, and Overestimation of Risk from Environmental Pollutants. Environ. Sci. Technol. 34, 4259–4265. doi:10.1021/es001069+
Aqeel, M., Jamil, M., and Yusoff, I. (2014). “Soil Contamination, Risk Assessment and Remediation,” in Environmental Risk Assessment of Soil Contamination (London: IntechOpen). doi:10.5772/57287
Bakir, A., Rowland, S. J., and Thompson, R. C. (2014). Enhanced Desorption of Persistent Organic Pollutants from Microplastics under Simulated Physiological Conditions. Environ. Pollut. 185, 16–23. doi:10.1016/J.ENVPOL.2013.10.007
Bates, D., Mächler, M., Bolker, B., and Walker, S. (2014). Package Lme4: Linear Mixed-Effects Models Using Eigen and S4. R package version 67.
Blakely, J. K., Neher, D. A., and Spongberg, A. L. (2002). Soil Invertebrate and Microbial Communities, and Decomposition as Indicators of Polycyclic Aromatic Hydrocarbon Contamination. Appl. Soil Ecol. 21, 71–88. doi:10.1016/S0929-1393(02)00023-9
Burton, G. A., Chapman, P. M., and Smith, E. P. (2002). Weight-of-evidence Approaches for Assessing Ecosystem Impairment. Hum. Ecol. Risk Assess. Int. J. 8, 1657–1673. doi:10.1080/20028091057547
Campanella, B. F., Bock, C., and Schröder, P. (20022002). Phytoremediation to Increase the Degradation of PCBs and PCDD/Fs. Environ. Sci. Pollut. Res 9, 73–85. doi:10.1007/BF02987318
Cardoso, E. J. B. N., Vasconcellos, R. L. F., Bini, D., Miyauchi, M. Y. H., Santos, C. A. d., Alves, P. R. L., et al. (2013). Soil Health: Looking for Suitable Indicators. What Should Be Considered to Assess the Effects of Use and Management on Soil Health? Sci. Agric. (Piracicaba, Braz.) 70, 274–289. doi:10.1590/S0103-90162013000400009
Curry, J., and Majer, J. D. (1990). Animals in Primary Succession. The Role of Fauna in Reclaimed Lands. J. Appl. Ecol. 27, 765. doi:10.2307/2404328
Decaëns, T., Jiménez, J. J., Barros, E., Chauvel, A., Blanchart, E., Fragoso, C., et al. (2004). Soil Macrofaunal Communities in Permanent Pastures Derived from Tropical Forest or Savanna. Agric. Ecosyst. Environ. 103, 301–312. doi:10.1016/J.AGEE.2003.12.005
di Guardo, A., Terzaghi, E., Raspa, G., Borin, S., Mapelli, F., Chouaia, B., et al. (2017). Differentiating Current and Past PCB and PCDD/F Sources: The Role of a Large Contaminated Soil Site in an Industrialized City Area. Environ. Pollut. 223, 367–375. doi:10.1016/j.envpol.2017.01.033
Domene, X., Alcañiz, J. M., and Andrés, P. (2007). Ecotoxicological Assessment of Organic Wastes Using the Soil Collembolan Folsomia candida. Appl. Soil Ecol. 35, 461–472. doi:10.1016/J.APSOIL.2006.10.004
EEA, European Environment Agency (2007). Progress in the Management of Contaminated Sites (CSI 015). Available at: https://www.eea.europa.eu/ (Accessed September 29, 2021).
Eggleton, P., Vanbergen, A. J., Jones, D. T., Lambert, M. C., Rockett, C., Hammond, P. M., et al. (2005). Assemblages of Soil Macrofauna across a Scottish Land-Use Intensification Gradient: Influences of Habitat Quality, Heterogeneity and Area. J. Appl. Ecol. 42, 1153–1164. doi:10.1111/J.1365-2664.2005.01090.X
Frouz, J., and Jilková, V. (2008). The Effect of Ants on Soil Properties and Processes (Hymenoptera: Formicidae). Myrmecol. News 11, 191–199.
García-Segura, D., Castillo-Murrieta, I. M., Martínez-Rabelo, F., Gomez-Anaya, A., Rodríguez-Campos, J., Hernández-Castellanos, B., et al. (2018). Macrofauna and Mesofauna from Soil Contaminated by Oil Extraction. Geoderma 332, 180–189. doi:10.1016/J.GEODERMA.2017.06.013
Haimi, J. (2000). Decomposer Animals and Bioremediation of Soils. Environ. Pollut. 107, 233–238. doi:10.1016/S0269-7491(99)00142-6
Heiri, O., Lotter, A. F., and Lemcke, G. (2001). Loss on Ignition as a Method for Estimating Organic and Carbonate Content in Sediments: Reproducibility and Comparability of Results. J. Paleolimnol. 25, 101–110. doi:10.1023/A:1008119611481
Husson, F., Josse, J., Le, S., and Maintainer, J. M. (2020). Package “FactoMineR” Title Multivariate Exploratory Data Analysis and Data Mining. Available at: http://factominer.free.fr (Accessed November 12, 2021).
Iloba, B. N., and Jarrett, I. E. (2006). Effect of Crude Oil Spills on the Abundance and Distribution of Soil Microartropods at Different Depths. Int. J. Zoological Res. 3, 24–32. doi:10.3923/IJZR.2007.24.32
ISO 11267:1999 (1999). Soil Quality — Inhibition of Reproduction of Collembola (Folsomia candida) by Soil Pollutants. Geneva, Switzerland: International Organization for Standardization. Available at: https://www.iso.org/standard/19245.html (Accessed January 12, 2022).
ISO 11268-2:2012 (2012). Soil Quality — Effects of Pollutants on Earthworms — Part 2: Determination of Effects on Reproduction of Eisenia fetida/Eisenia andrei. Geneva, Switzerland: International Organization for Standardization. Available at: https://www.iso.org/obp/ui/#iso:std:iso:11268:-2:ed-2:v1:en (Accessed November 12, 2021).
ISO 17126:2005 (2005). Soil Quality — Determination of the Effects of Pollutants on Soil Flora — Screening Test for Emergence of Lettuce Seedlings (Lactuca sativa L.). Geneva, Switzerland: International Organization for Standardization. Available at: https://www.iso.org/standard/31214.html (Accessed May 13, 2021).
Kassambara, A., and Mundt, F. (2016). Package “Factoextra” Type Package Title Extract and Visualize the Results of Multivariate Data Analyses. Available at: https://github.com/kassambara/factoextra/issues (Accessed November 12, 2021).
Leitgib, L., Kálmán, J., and Gruiz, K. (2007). Comparison of Bioassays by Testing Whole Soil and Their Water Extract from Contaminated Sites. Chemosphere 66, 428–434. doi:10.1016/J.CHEMOSPHERE.2006.06.024
Low, J. E., Whitfield Åslund, M. L., Rutter, A., and Zeeb, B. A. (2010). Effect of Plant Age on PCB Accumulation by Cucurbita Pepo Ssp. Pepo. J. Environ. Qual. 39, 245–250. doi:10.2134/JEQ2009.0169
McFarland, V. A., and Clarke, J. U. (1989). Environmental Occurrence, Abundance, and Potential Toxicity of Polychlorinated Biphenyl Congeners: Considerations for a Congener-specific Analysis. Environ. Health Perspect. 81, 225–239. doi:10.1289/EHP.8981225
McMillen, S. J., Gestel, C. A. M. van., Lanno, R. P., Linder, G. L., Pauwels, S. J., and Stephenson, G. L. (2003). “Biological Measures of Bioavailability,” in Proceedings from the Pellston Workshop on Assessing Contaminated Soils: From Soil-Contaminant Interactions to Ecosystem Management, Pellston, Michigan, USA, September 1998 (IEEE), 293–343.
Menta, C., and Remelli, S. (2020). Soil Health and Arthropods: From Complex System to Worthwhile Investigation. Insects 11, 54. doi:10.3390/insects11010054
Morrison, D. E., Robertson, B. K., and Alexander, M. (2000). Bioavailability to Earthworms of Aged DDT, DDE, DDD, and Dieldrin in Soil. Environ. Sci. Technol. 34, 709–713. doi:10.1021/es9909879
Pereira, M. D. S. (2004). Polychlorinated Dibenzo-P-Dioxins (PCDD), Dibenzofurans (PCDF) and Polychlorinated Biphenyls (PCB): Main Sources, Environmental Behaviour and Risk to Man and Biota. Quím. Nova 27, 934–943. doi:10.1590/S0100-40422004000600018
Picone, M., Bergamin, M., Losso, C., Delaney, E., Arizzi Novelli, A., and Ghirardini, A. V. (2016). Assessment of Sediment Toxicity in the Lagoon of Venice (Italy) Using a Multi-Species Set of Bioassays. Ecotoxicol. Environ. Saf. 123, 32–44. doi:10.1016/J.ECOENV.2015.09.002
R Core Team (2021). R: A Language and Environment for Statistical Computing. Available at: https://www.R-project.org/.
Rathna, R., Varjani, S., and Nakkeeran, E. (2018). Recent Developments and Prospects of Dioxins and Furans Remediation. J. Environ. Manag. 223, 797–806. doi:10.1016/J.JENVMAN.2018.06.095
Remelli, S., Rizzo, P., Celico, F., and Menta, C. (2020). Natural Surface Hydrocarbons and Soil Faunal Biodiversity: A Bioremediation Perspective. Water 12, 2358. doi:10.3390/W12092358
Rodriguez-Campos, J., Dendooven, L., Alvarez-Bernal, D., and Contreras-Ramos, S. M. (2014). Potential of Earthworms to Accelerate Removal of Organic Contaminants from Soil: A Review. Appl. Soil Ecol. 79, 10–25. doi:10.1016/j.apsoil.2014.02.010
Rusin, M., and Gospodarek, J. (2016). The Occurrence of Springtails (Collembola) and Spiders (Araneae) as anEffectiveness Indicator of Bioremediation of Soil Contaminated by Petroleum-Derived Substances. Int. J. Environ. Res. 10, 449–458. doi:10.22059/IJER.2016.58764
Salminen, J., Tan Anh, B., and van Gestel, C. A. M. (2001). Enchytraeids and Microbes in Zn Polluted Soil: No Link between Organism-Level Stress Responses and Ecosystem Functioning. Ecotoxicology 10, 351–361. doi:10.1023/A:1012266829555
Searle, S. R., Speed, F. M., and Milliken, G. A. (1980). Package “Emmeans” Type Package Title Estimated Marginal Means, Aka Least-Squares Means. doi:10.1080/00031305.1980.10483031
Shen, M.-W., Chen, H.-C., and Chen, S.-T. (20212021). A Pest or Otherwise? Encounter of Oryctes Rhinoceros (Coleoptera: Scarabaeidae) with Persistent Organic Pollutants. Insects 12, 818. doi:10.3390/INSECTS12090818
Sinha, R. K., Bharambe, G., and Ryan, D. (2008). Converting Wasteland into Wonderland by Earthworms-A Low-Cost Nature's Technology for Soil Remediation: a Case Study of Vermiremediation of PAHs Contaminated Soil. Environmentalist 28, 466–475. doi:10.1007/S10669-008-9171-7/FIGURES/3
Società Italiana della Scienza del Suolo (1986). Metodi normalizzati di analisi del suolo, ed. Edagricole.
US EPA (1994). Method 1613. Tetra- through Octa-Chlorinated Dioxins and Furans by Isotope Dilution HRGC/HRMS. SW-846. Washington, D.C. US EPA: United States Environmental Protection Agency, Office of Water.
US EPA (2010). Method 1668C: Chlorinated Biphenyl Congeners in Water, Soil, Sediment, Biosolids, and Tissue by HRGC/HRMS. Washington, D.C. US EPA: United States Environmental Protection Agency, Office of Water.
Vašíčková, J., Maňáková, B., Šudoma, M., and Hofman, J. (2016). Ecotoxicity of Arsenic Contaminated Sludge after Mixing with Soils and Addition into Composting and Vermicomposting Processes. J. Hazard. Mater. 317, 585–592. doi:10.1016/J.JHAZMAT.2016.06.029
Vergani, L., Mapelli, F., Suman, J., Cajthaml, T., Uhlik, O., and Borin, S. (2019). Novel PCB-Degrading Rhodococcus Strains Able to Promote Plant Growth for Assisted Rhizoremediation of Historically Polluted Soils. PLOS ONE 14, e0221253. doi:10.1371/JOURNAL.PONE.0221253
Visioli, G., Menta, C., Gardi, C., and Conti, F. D. (2013). Metal Toxicity and Biodiversity in Serpentine Soils: Application of Bioassay Tests and Microarthropod Index. Chemosphere 90, 1267–1273. doi:10.1016/J.CHEMOSPHERE.2012.09.081
Keywords: persistent organic pollutants, bioremediation assessment, earthworms, ecotoxicity tests, soil living community
Citation: Remelli S, Celico F and Menta C (2022) The Ecotoxicity Approach as a Tool for Assessing Vermiremediation Effectiveness in Polychlorobiphenyls, Polychlorodibenzo-p-Dioxins and Furans Contaminated Soils. Front. Environ. Sci. 10:907260. doi: 10.3389/fenvs.2022.907260
Received: 29 March 2022; Accepted: 26 April 2022;
Published: 11 May 2022.
Edited by:
Jaswinder Singh, Khalsa College, IndiaReviewed by:
C. S. K. Mishra, Orissa University of Agriculture and Technology, IndiaSalwinder Singh Dhaliwal, Punjab Agricultural University, India
Copyright © 2022 Remelli, Celico and Menta. This is an open-access article distributed under the terms of the Creative Commons Attribution License (CC BY). The use, distribution or reproduction in other forums is permitted, provided the original author(s) and the copyright owner(s) are credited and that the original publication in this journal is cited, in accordance with accepted academic practice. No use, distribution or reproduction is permitted which does not comply with these terms.
*Correspondence: Sara Remelli, c2FyYS5yZW1lbGxpQHVuaXByLml0