- 1Department of Agronomy, University of Agriculture Faisalabad, Faisalabad, Pakistan
- 2College of Agriculture, Fujian Agriculture and Forestry University, Fuzhou, China
- 3Department of Agronomy, Northwest A&F University, Xianyang, China
- 4Department of Soil and Environmental Sciences, Ghazi University, Dera Ghazi Khan, Pakistan
- 5Department of Agronomy, Gomal University, Dera Ismail Khan, Pakistan
- 6Department of Soil Science and Plant Nutrition, Faculty of Agriculture, Tekirdag Namık Kemal University, Tekirdağ, Turkey
- 7Faculty of Process and Environmental Engineering, Lodz University of Technology, Lodz, Poland
- 8Department of Biology, College of Science, Taif University, Taif, Saudi Arabia
With the provoked environmental constraints under extreme climatic events, a better understanding of plant responses to these environmental stresses helps in obtaining sustainable productivity. Wheat is a significant cereal crop for the burgeoning population; its yield is significantly limited by too little water in the rhizosphere. The ramifications of water deficiency on the wheat crop can be reduced by the application of vermicompost. With the objective to cope with drought stress, a wire-house experiment was established where seedlings of two cultivars, viz., Faisalabad-08 and Galaxy-13 (drought-tolerant and -sensitive cultivar, respectively) were grown in pots and exposed to mild drought (D1, 45% field capacity) and severe drought stress (D2, 30% field capacity). A control with well-watered condition (70% field capacity) was kept for comparison. Various application rates of wheat straw vermicompost (control (VT0), 4 t ha−1 (VT1), 6 t ha−1 (VT2), and 8 t ha−1 (VT3)), were used in soil-filled pots under drought and control treatments. Our data depicted that compared with control, drought treatments recorded a significant reduction in morpho-physiological and biochemical attributes with maximum reduction under severe drought conditions. Nonetheless, it was observed that soil application of vermicompost, particularly at a high rate, ameliorated the negative effects of drought. Under severe drought conditions, a significant and positive influence on morphological and physiological traits was recorded for VT3 treatment, which increased root and shoot length by 27.55 and 27.85%, root and shoot fresh weight by 26.98 and 28.20%, root and shoot dry weight by 40 and 50.05%, and photosynthesis and transpiration rate by 27.65 and 49.25%, respectively, on average of two cultivars. Similarly, VT3 also significantly ameliorated the adverse effect of drought by enhancing the antioxidant enzyme activities as it increased superoxide dismutase activity by 14.28%, peroxidase by 27.28%, and catalase by 50% compared to the control treatment. Among cultivars, Faisalabad-2008 showed comparatively more resistance against drought stress. The findings of this work revealed that drought drastically reduced the growth and productivity of wheat; however, soil-applied vermicompost positively influenced the performance of wheat cultivars.
Introduction
Wheat (Triticum aestivum L.) is a significant crop that is cultivated throughout the world. The crop is cultivated on an area of 214.79 million hectares with a total production of 735.17 million tons on the globe (FAO, 2019). It is a dominant cereal crop that provides a food source for over 1.2 billion people and is of significant importance as a commercial product (Iqbal et al., 2018). Wheat is a C3 crop and is particularly vulnerable to harsh environments, including drought stress (Wang et al., 2014), which affects the growth and developmental processes, particularly when it occurs at early developmental stages. Drought stress also disrupts the physiological events in crop plants as it reduces chlorophyll content (Ahmad et al., 2022), photosynthetic assimilation of CO2 (Golldack et al., 2011; Wei et al., 2015), inhibits cell division and elongation (Avramova et al., 2015), and decreases photosynthesis-related parameters and relative water contents (Hussain et al., 2019; Ahmad et al., 2021a). Inevitably, prolonged drought also results in oxidative damage owing to increasing the production of reactive oxygen species (ROS) that markedly affected cell membranes and caused tissue injuries (Reddy et al., 2004; Liu et al., 2015; Farooq et al., 2020). Nonetheless, different enzymatic and non-enzymatic antioxidants, also called scavenging enzymes (including ascorbic acid, superoxide dismutase, peroxidase, and catalase), are activated in plants as a defense response to detoxify the ROS (Abid et al., 2016; Hussain et al., 2018). Prolonged drought episodes also caused a significant reduction in grain yield which accounted for a 20–40% reduction in wheat yield globally (Daryanto et al., 2016). In recent years, various approaches are being used to minimize the negative effects of drought; these include the application of osmoprotectants, cultivation of drought-tolerant cultivars, and the conservation of agricultural practices using conservation tillage, natural and synthetic mulches, and vermicompost application.
In today’s agriculture scenario, the main concern of researchers and farmers is to enhance crop productivity in a sustainable way to maintain soil fertility and protect the environment. Hence, vermicompost application has great potential to promote plant growth and grain yield while protecting the soil environment (Varghese and Prabha, 2014). Its application enhances the physiochemical and organic properties of the soil. Vermicompost, degraded solid organic residues formed under aerobic conditions, contains a bulk quantity of earthworms and other microfauna that enhance the availability of growth regulators/hormones, degrading enzymes (including chitinase, cellulase, lipase, amylase, and proteases), and vitamins. These earthworms also help in the modification and fragmentation of organic residues by lowering the C/N ratio and exposed area for microfauna to react with cellulolytic-degrading microfauna for complete degradation. Earthworm guts can release bacterial excrements which are the product called humus (Dominguez and Edwars, 2004). The epigeic earthworms, Eisenia fetida and E. andrei, are among the most commonly used worm types in vermiculture; however, some other species including Polipheretima elongate, Millsonia anomala, and Pontoscolex corethurus are also used in the preparation of vermicompost (Lattaud et al., 1997a, b; Urbasek and Pizl 1991; Zhang et al., 1993; Zhang et al., 2000).
Vermicompost application plays a pivotal role in regulating drought tolerance in crop plants. In this regard, Curtis and Classsen (2005) demonstrated that applying vermicompost significantly enhanced the availability of soil water, increased leaf water content, and enhanced plant biomass production. Working with maize and soybean crops, Bowden et al. (2010) confirmed that vermicompost application enhanced drought tolerance as it promoted the antioxidant enzyme activities and photosynthesis traits. According to Suthar (2009), the application of vermicompost improves the drought tolerance of garlic (Allium sativum L.) grown under pot conditions. The positive influence of vermicompost was also reported for other field-grown crops, such as Triticum aestivum L. (wheat), Capsicum annum L. (peppers), Brassica oleracea L. (cabbage), and Lycopersicum esculentom L. (tomatoes) (reviewed by Subler et al., 1998). Working with chickpea (Cicer arietinum L.) crop, Jat and Ahlawat (2006) reported that vermicompost improved the soil nutrient status, thereby promoting crop growth.
The positive influence of vermicompost application combined with other organic fertilizers on morpho-physiological and yield traits under drought stress has been well-discussed in previous studies (Aboelsoud and Ahmed, 2020; Hafez et al., 2020). The vermicompost fertilizer and humic acid application have been reported to induce the activities of scavenging enzymes, including antioxidant enzyme catalase, superoxide dismutase, and peroxidase, to detoxify the ROS (Garcia et al., 2012; Kiran, 2019). A similar phenomenon has also been reported in chickpea (Gholipoor et al., 2011; Hosseinzadeh et al., 2018), tomato (Solanum lycopersicon L.) (Ahanger et al., 2021), mungbean (Mahmoudi et al., 2016), lentil (Ahmadpour and Hosseinzadeh, 2017), and the oilseed crop: canola (Rashtbari et al., 2012). Considering the aforementioned scenario, the present investigation was focused on studying the effect of wheat straw vermicompost application on wheat morphology and physiology under water stress and investigating the damaging effects of drought stress on wheat growth. In the current study, we hypothesized that wheat straw vermicompost will improve wheat growth and drought tolerance by modulating the physiological and biochemical characteristics of our crop.
Materials and Methods
Experimental Station and Plant Material
The concurrent study was executed as a pot experiment in the wirehouse at the Student Research Farm, Department of Agronomy, Faculty of Agriculture, University of Agriculture, Faisalabad (UAF) (latitude 31°-04′ N, longitude 73°-06′ E, and an elevation of 184.4 m above sea level) during the winter season of 2020–21. Clay loam soil with pH 8.31, Ec 1.46 dSm−1, available N 0.056%, accessible phosphorus 36.5 ppm, exchangeable potassium 325 ppm, and organic matter 1.08% was used in this experiment to fill the pots. The seeds of two contradicting wheat cultivars, drought-tolerant (cv. Faisalabad-2008) and drought-sensitive (cv. Galaxy-2013), were obtained from the Directorate of Farms, Department of Agronomy, UAF and Seed Department, Ayub Agricultural Research Institute, Faisalabad (AARI).
Analysis of Raw Materials and Vermicompost
The raw material used for the preparation of vermicompost was wheat straw obtained from the Department of Agronomy, UAF. The potential of hydrogen (pH), electrical conductivity (EC), ash content, available nitrogen (N) content, available phosphorus (P), available potassium (K), calcium (Ca), magnesium (Mg), iron (Fe), sulfur (S), and heavy metals such as, cadmium (Cd), nickel (Ni), lead (Pb), mercury (Hg), chromium (Cr), and stannum (Sn) of raw material and vermicompost were measured according to standard protocols and are shown in Tables 1, 2.
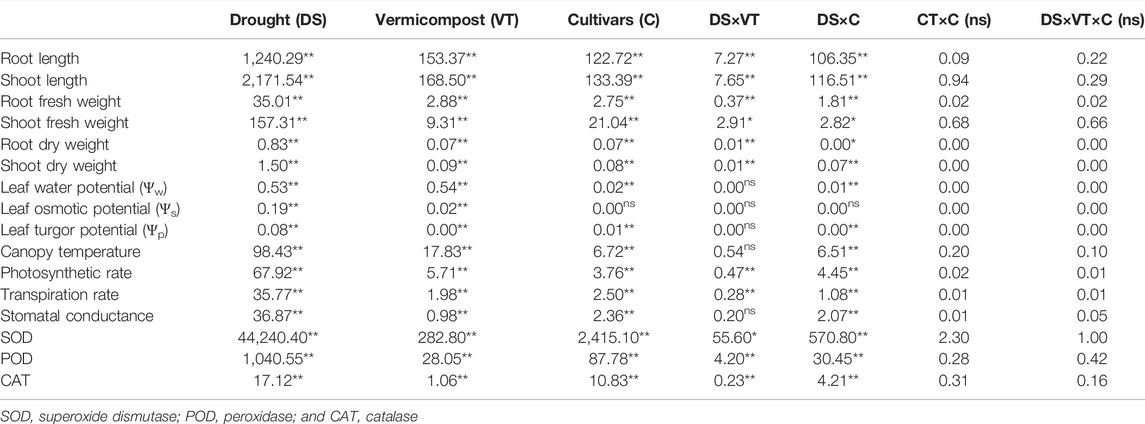
TABLE 2. Mean sum of squares regarding the effect of soil-applied wheat straw vermicompost on the growth and physio-biochemical attributes of wheat cultivars under drought stress.
Meteorological Observation
Weather data were gaged throughout the growing period of the wheat crop from the meteorological observatory at Agronomy Department, UAF, and are manifested in Figure 1.
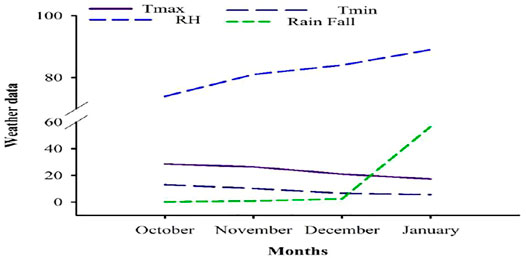
FIGURE 1. Meteorological data, mean monthly maximum and minimum temperatures (Tmax and Tmin, respectively), relative humidity (RH), and rainfall during the crop growing season.
Experimental Design and Treatments
This wirehouse experiment consisted of three factors, viz., (a) two contrasting wheat cultivars (Faisalabad-08 and Galaxy-13 as drought-tolerant and drought-sensitive, respectively), (b) three drought levels (control, 70% of field capacity (D0); mild drought, 45% of field capacity (D1); and severe drought, 30% of field capacity (D2)), and (c) different application rates of vermicompost [control (VT0), 4 t ha−1 (VT1), 6 t ha−1 (VT2) and 8 t ha−1 (VT3)]. The experimental pots were intended under a complete randomized design with factorial arrangements where each pot gets three replicates. Initially, vermicompost was applied before sowing and wheat seeds were sown in uniform soil moisture conditions. After that, water stress treatments were started after first irrigation until harvesting. In each replicate pot, water losses were compensated by applying a measured amount of water to prevail the required field capacity. For each treatment, soil moisture contents were measured on a daily basis by using a soil moisture meter (TZS-W). After 35 days of stress imposition, plant samples, from each replicate pot, were harvested to analyze the morpho-physio-biochemical traits.
Crop Husbandry
The seeds of wheat cultivars were sown in pots (with a measurement of 20 cm × 20 cm), filled with sieved soil 5 kg each, in the rabi season (sown on 06 October 2020). Urea and diammonium phosphate (60 and 57 mg kg−1 soil, respectively) were mixed and applied to each pot at the time of sowing. Initially, fifteen seeds were sown at identical distances; later, ten seedlings were maintained in each replicate pot. Except for treatments, all other practices, including chemical application, were kept uniform for all pots.
Data Recorded
Growth-Related Traits
A rod meter scale was used to calculate the seedling lengths (cm). In order to calculate the seedling weights, first, shoots and roots were separated, and an electrical balance was used to record the fresh weights, which were expressed in g. Then, seedling parts were transferred to an oven at 70 ± 02°C for 72 h and the aforementioned weighing balance was used to calculate the dry weights in g.
Physiological Attributes
Water Potential (Ψw)
At 60 days after sowing, a water potential apparatus (Chas W. Cook and Sons., Birmingham-B, Industrial manufacturer, England) was used to measure the Ψw, where a fully emerged flag leaf was used (Scholander et al., 1964). First, pressure-filled gas was exerted into the leaves until the xylem components are visible on the cut section. To ensure no evaporative loss, the samples were taken between 6.00 p.m. and 8.00 a.m., and plant leaves were placed as early as possible in the pressure chamber. From each replicate pot, three samples were taken from each plant, and the average value was measured.
Leaf Osmotic Potential and Turgor Pressure (Ψs & Ψp)
For Ψs, leaves were frozen for seven successive days at −20°C and later, the extracted secretion was used to assess the osmotic potential/solute potential using a vapor pressure osmometer, Wescor-5500. Then, the difference in water and osmotic potential was measured as turgor pressure.
Canopy Temperature (°C), Photosynthetic Rate (An), and Transpiration Rate (E)
An infrared temperature sensor (IRIS) was used to measure the canopy temperature. The infrared gas analyzer (IRGA) was used to measure An and E values where five plant leaves in each replicate pot were used, and the average was measured according to the protocol of Singh et al. (2018) and Rosolem et al. (2019).
Stomatal Conductance (gs)
An open system LCA-4 ADC-portable infrared gas analyzer (developed by Analytical Development Company, Hoddeson-England) with the following accommodations: ambient CO2 concentration-Cref and leaf surface area of 371 μmol mol−1 and 6.25 cm2, respectively; the temperature of leaf chamber-Tch varying from 25–28°C; leaf chamber molar gas flow rate-U and leaf chamber volume gas flow rate-v of 400 μmol s−1 and 296 ml min−1, respectively; ambient pressure-P, 97.95 kPa; PAR-Qleaf at the leaf surface, up to 770 μmol m−2 s−1 was used to measure gs, and the values were recorded from the 3rd leaf from the top.
Enzymatic Antioxidants
Enzyme Extraction
First, fresh leaves of 0.5 g were extracted in 50 mM of phosphate buffer solution (with pH 7.8); after that, the extract was then centrifuged through filtration at 16,000 × g for 20 min at 4 ± 0.5°C. Later, the filtrate supernatant was utilized for enzyme assay.
Catalase
A UV–Visible spectrophotometer was used to determine the CAT content, through decomposition and change in absorption by adding hydrogen peroxide at every 30 s interval for 5 min, at a wavelength of 240 nm. The reaction mixture for the estimation of CAT was 900 μl hydrogen peroxide (H2O2) solution (5.9 mM) and 2 ml phosphate buffer solution (50 mM). For measuring the enzyme activity, the reaction was initiated by adding an extract of 100 μl of the enzyme to the reaction mixture, and the CAT activity was manifested as μmol of H2O2 min−1 mg protein−1, according to the procedure of Chance and Maehly (1955).
Superoxide Dismutase
A method described by Giannopolitis and Ries (1977) based on the use of nitroblue tetrazolium (NBT) was used to determine the SOD activity spectrophotometrically (by using UV–Visible spectrophotometer, IRMECO U2020) at 560 nm wavelength. First, 50 μl of the enzyme extract was added to the working solution containing 50 μM of ethanol-containing NBT solution, 1.3 μM solution of riboflavin, 13 mM of methionine, 75 nM EDTA solution, and 50 Mm phosphate buffer solution (pH 7.8). Then, the solutions were transferred in a chamber under 30-W fluorescent lamp illumination for 5 min, and then the values were measured spectrophotometrically at 560 nm wavelength. The working solution without leaf extract was kept as the control. One unit of SOD was specified as the enzyme amount needed to diminish the rate of reduction of NBT at 560 nm by 50% compared to the reaction solution without plant extract.
Peroxidase
The estimation of POD content was performed by oxidation of guaiacol and specified as a change in absorbance min−1 mg−1 protein. At first, a 100-μl filtrate enzyme extract solution was supplemented with a reaction mixture containing 400 μl of guaiacol solution (20 mM), 500 μL of hydrogen peroxide (H2O2) (40 mM), and 2 ml of phosphate (50 mM). Later, the change in absorbance was observed spectrometrically at 470 nm, according to Chance and Maehly (1955).
Statistical Analysis
All accumulated data on wheat growth and morpho-physio-biochemical attributes were computed using Microsoft Excel 2016 and subjected to the analysis of variance technique (an ANOVA). Tukey’s honest significance test (HSD) was used to test the comparative significance of treatments with help of Statistics version 8.1 (Analytical Software ©, 1985–2005) according to the protocol of Steel et al. (1997). The software SigmaPlot 10.0 was used for graphical presentation.
Results
Morphological Growth
The analyzed data regarding the morphological traits of wheat crop (including seedling length and fresh and dry weights) showed that drought treatments, 45 and 30% FC, significantly (p ≤ 0.05) affected these parameters of both cultivars as compared to well-watered conditions (70% FC) (Table 2; Figure 2). The extent of reduction for these traits was higher in Galaxy-13 than that in Faisalabad-08. Nonetheless, the application of vermicompost significantly promoted the morphological traits of both cultivars under control and drought stress conditions. Significantly higher values of these traits were recorded in plants treated with a high rate of vermicompost (VT3) than those of the lower ones (VT0, VT1, and VT2). Under severe drought, VT3 increased root length by 27.57 and 37.19%, shoot length by 16.09 and 31.34%, root fresh weight by 14.74 and 22.90%, shoot fresh weight by 20.69 and 24.39%, root dry weight by 19.44 and 30.76%, and shoot dry weight by 28.57 and 27.26% in Faisalabad-08 and Galaxy-13, respectively. For studied cultivars, under well-watered conditions, Galaxy-13 depicted more biomass than Faisalabad-08; however, both tested cultivars showed a similar trend under drought treatments.
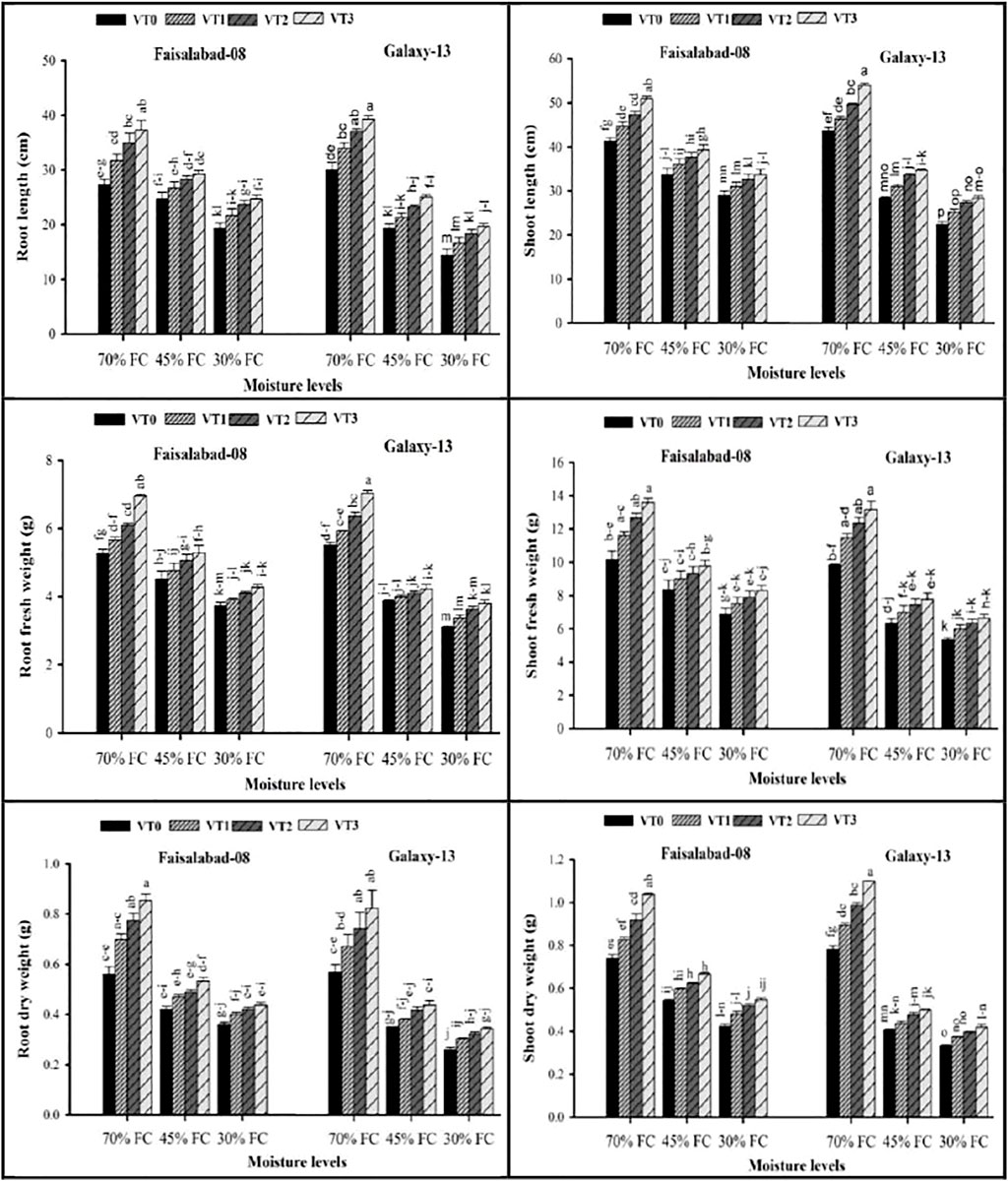
FIGURE 2. Effect of soil-applied wheat straw vermicompost (VT) on root length, shoot length, root fresh weight, shoot fresh weight, root dry weight, and shoot dry weight of two wheat cultivars under different drought levels [70% field capacity (FC), 45% FC, and 30% FC]. Bars with different alphabetical letters differ significantly at 5% probability, according to Tukey’s HSD test. VT0, control; VT1, 4 t ha−1; VT2, 6 t ha−1; and VT3 8 t ha−1.
Physiological Attributes
The physiological attributes (i.e., leaf water potential, osmotic potential, turgor potential, photosynthetic rate, transpiration rate, and stomatal conductance) were significantly reduced under drought stress treatments (45% FC and 30% FC) compared to well-watered condition (70% FC) in both cultivars. Severe drought (30% FC) caused more reduction in plant physiological attributes than moderate drought (45% FC). Among cultivars, the magnitude of reduction for these traits was greater in Galaxy-13 than in Faisalabad-08. However, the vermicompost application significantly affected these traits under control and drought stress in both cultivars where VT3 recorded significantly higher values followed by other treatments that were ordered as VT2>VT1>control (Figure 3). Under severe drought stress, VT3 treatment increased water potential by 9.43 and 12.17%, osmotic potential by 4.59 and 4.52%, turgor potential by 6.06 and 10.16%, photosynthetic rate by 15.20 and 23.44%, transpiration rate by 14.05 and 26.10%, and stomatal conductance by 14.15 and 13.10% in Faisalabad-08 and Galaxy-13, respectively, than control treatment without vermicompost application. For cultivars under well-watered conditions, the Galaxy-13 recorded significantly higher values than Faisalabad-08. However, under drought treatments, Faisalabad-08 depicted higher values than Galaxy-13, except for canopy temperature. We also noted that drought treatments increased the canopy temperature; however, vermicompost application decreased its values under normal and drought conditions in both cultivars.
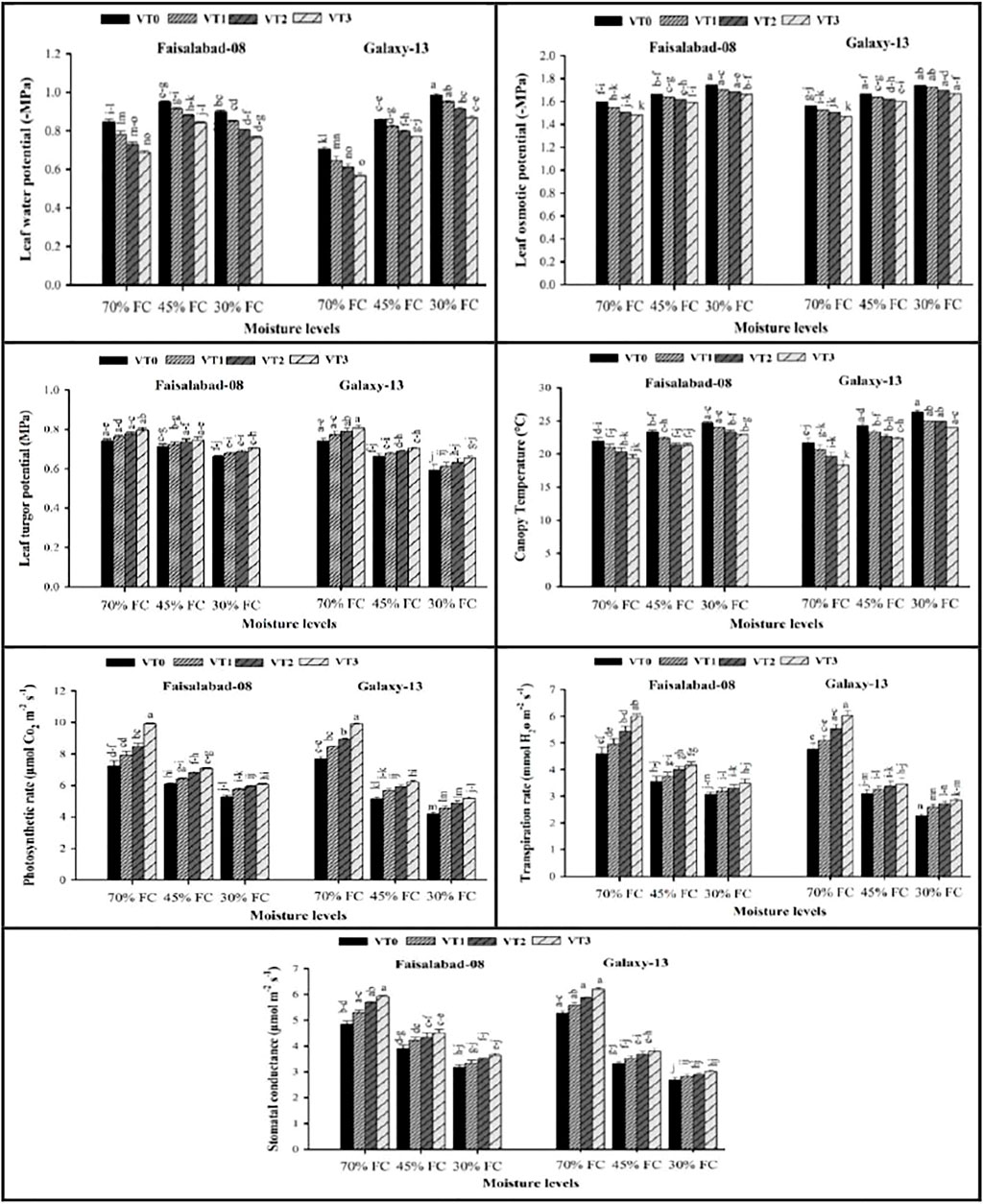
FIGURE 3. Effect of soil-applied wheat straw vermicompost (VT) on leaf water potential, leaf osmotic potential, leaf turgor potential, canopy temperature, photosynthetic rate, transpiration rate, and stomatal conductance of two wheat cultivars under different drought levels [70% field capacity (FC), 45% FC, and 30% FC]. Bars with different alphabetical letters differ significantly at 5% probability, according to Tukey’s HSD test. VT0, control; VT1, 4 t ha−1; VT2, 6 t ha−1; and VT3, 8 t ha−1.
Enzymatic Antioxidants
Drought stress and vermicompost application significantly (p ≤ 0.05) affected the activities of antioxidant enzymes including catalase, superoxide dismutase, and peroxidase in both cultivars (Figure 4). Under drought and well-watered conditions, antioxidant enzyme activities were increased by the application of vermicompost where VT3 recorded the highest increase than other rates in both cultivars. Under severe drought, VT3 increased superoxide dismutase activity by 8.53 and 11.96%, peroxidase activity by 20.01 and 18.66%, and catalase activity by 27.50 and 6.32% in Faisalabad-08 and Galaxy-13, respectively, than control treatment without vermicompost application. Between tested cultivars, Galaxy-13 depicted statistically similar values compared to those of Faisalabad-08, under sufficient water conditions; however, under drought stress, the Faisalabad-08 cultivar showed better performance than Galaxy-13.
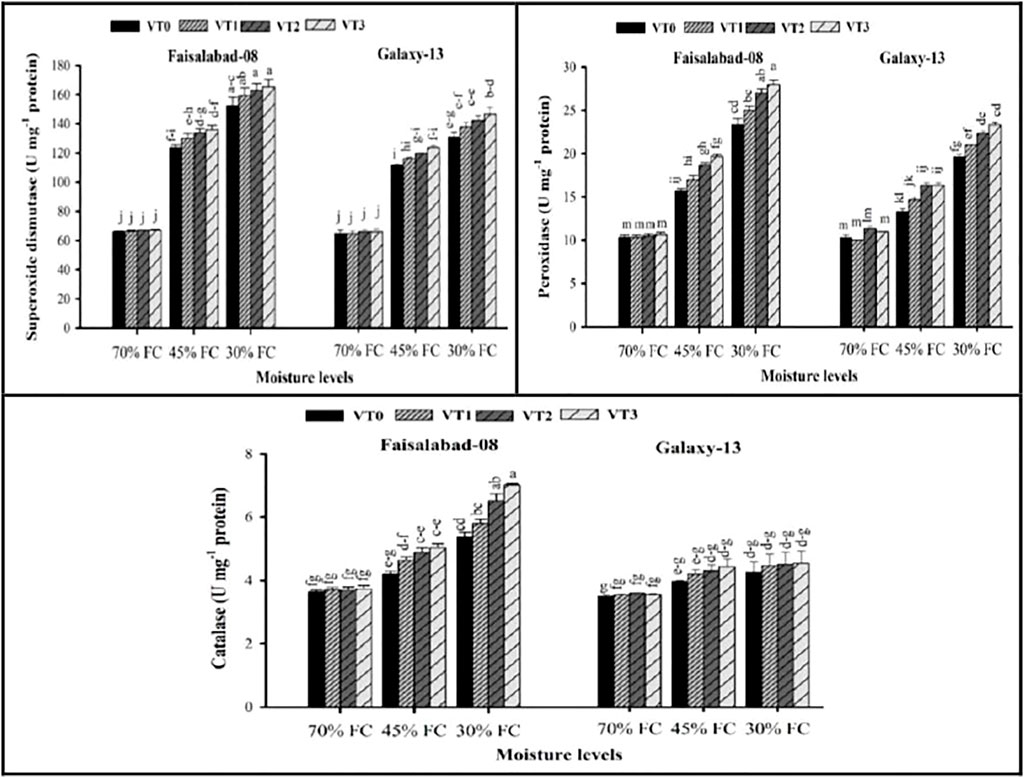
FIGURE 4. Effect of soil-applied wheat straw vermicompost on superoxide dismutase, peroxidase, and catalase activities of two wheat cultivars under different drought levels [70% field capacity (FC), 45% FC, and 30% FC]. Bars with different alphabetical letters differ significantly at 5% probability, according to Tukey’s HSD test. VT0, control; VT1, 4 t ha−1; VT2, 6 t ha−1; and VT3 8 t ha−1.
Discussion
The current study evaluated the effectiveness of wheat straw vermicompost application in improving plant performance under drought stress conditions. When compared to control, drought stress significantly affected the performance of wheat crop mainly through the production of reactive oxygen species (ROS), which redirected the leaf water potential, caused protein denaturation, and ultimately influenced crop productivity. Prolonged drought also retards plant growth and crop productivity due to severe loss in cell turgidity and dehydration of protoplasm. According to Chen et al. (2011), protoplasm dehydration is directly related to a decrease in cell division. Our results showed that vermicompost application significantly stimulated wheat morphological, physiological, and enzymatic antioxidant activities under drought stress.
It is well-reported that selecting suitable crop cultivars that have standard emergence; morphological, physiological, and antioxidant attributes; and the application of vermicompost are helpful in enhancing crop performance, in terms of better growth and yield under drought stress (Amiri et al., 2017; Ahmad et al., 2021). Similar to these reports, our results indicated that vermicompost application positively influenced the morpho-physiological traits and enzymatic antioxidant activities of both cultivars under drought stress, and the Faisalabad-08 was more productive and proved to be a drought-tolerant cultivar. Drought treatments caused significant reduction in seedling growth of studied wheat cultivars, and the reduction was more prominent in drought-susceptible wheat cultivars (Rahbarian et al., 2011). In this work, wheat straw vermicompost application significantly lowered the detrimental effects of drought. Similar findings were reported in a previous study where the authors have reported that vermicompost, when applied at a high rate, rendered more positive effects under control and drought conditions (Rahbarian et al., 2011; Kiran, 2019). Under drought stress, higher dehydration, denaturation of proteins, and excessive production of ROS caused significant reduction in seedling growth (Boyer and Westgate, 2004). Severe drought caused more reduction in seedling lengths and higher rates of vermicompost were more effective in reducing the negative effects of stress (Garcia et al., 2012). Similar findings were reported in our results.
Leaf water potential, osmotic and turgor potential, photosynthetic and transpiration rates, and stomatal conductance are the important indicators to determine the stress effects (Garcia et al., 2012; Kiran, 2019). In this study, moderate and severe drought resulted in significant decrease in the abovementioned physiological traits of both cultivars when compared to control, where drought-sensitive cultivars recorded a greater decrease than other studied varieties. Similar findings were reported in previous studies in which authors have reported that drought-resistant varieties had higher leaf water, osmotic and turgor potentials, photosynthetic and transpiration rates, and stomatal conductance (Gunes et al., 2007). In our work, the drought-resistant variety Faisalabad-08 exhibited better physiological traits under drought stress for all vermicompost treatments than the sensitive one Galaxy-13. Plants with higher water potential, turgor potential, stomatal conductance, and photosynthetic rates can store more assimilates for better growth and productivity. In stressed plants, reduced water losses help in stomatal closure for better tolerance to drought (Li-Ping et al., 2006; Sakuma et al., 2006).
In this work, drought significantly lowered water and osmotic potentials. Similar findings were reported in a previous study by Xue and Loveridge (2004) who found that drought had decreased osmotic potential owing to decreased water availability than the untreated control. In addition, we found that vermicompost application increased the osmotic potential owing to re-established turgor pressure. Similarly, Kashem et al. (2015) reported that maintaining turgor pressures is crucial for proper cell growth, expansion, and stomatal conductivity.
The application of vermicompost significantly alleviated drought stress by increasing the concentrations of scavenging enzymes including SOD, POD, and CAT. Similar findings were reported by Hussain et al. (2010), where the authors have reported that higher antioxidant activities positively reduced oxidative damage caused by overproduction of activated oxygen species. In this work, a high rate of vermicompost significantly increased the plant tolerance to drought-induced oxidative stress by increasing the concentrations of antioxidant enzymes in both cultivars. The findings are consistent with those of Nazarideljou and Heidari (2014), who demonstrated that vermicompost application increased SOD, POD, and CAT activity.
Conclusion
Soil-applied vermicompost is an effective approach to alleviate drought damage in wheat. In response to drought stress, vermicompost application, particularly at a high rate, considerably promoted the growth and physiological attributes in Faisalabad-08 and Galaxy-13. Wheat genotypes, thereby, lead to better growth performance under drought stress. The upregulation of antioxidant enzymes such as catalase, peroxidase, and superoxide dismutase activities may also have contributed to better drought tolerance in wheat. Wheat straw vermicompost–induced improvement in physiological traits played a key role in improving maize growth under drought stress. This study advocates the possibility of soil-applied wheat straw vermicompost to improve drought tolerance by promoting the growth, physiology, and antioxidant defense system.
Data Availability Statement
The raw data supporting the conclusion of this article will be made available by the authors without undue reservation.
Author Contributions
All authors listed have made a substantial, direct, and intellectual contribution to the work and approved it for publication.
Funding
The current work was funded by the Taif University Researchers Supporting Project Number (TURSP-2020/94), Taif University, Taif, Saudi Arabia.
Conflict of Interest
The authors declare that the research was conducted in the absence of any commercial or financial relationships that could be construed as a potential conflict of interest.
Publisher’s Note
All claims expressed in this article are solely those of the authors and do not necessarily represent those of their affiliated organizations, or those of the publisher, the editors, and the reviewers. Any product that may be evaluated in this article, or claim that may be made by its manufacturer, is not guaranteed or endorsed by the publisher.
Acknowledgments
The results presented in this study are a part of PhD studies by AA. The authors gratefully acknowledge HEC for providing financial support to carry out this research work under HEC projects “NRPU-HEC project no. 7527/Punjab/NRPU/R&D/HEC/2017_Vermicomposting: A resourceful organic fertilizer to improve agriculture production and soil health and second project “Vermicomposting: An Agricultural Waste Management Technology,” Pak-Turk Researchers Mobility Grant Program Phase- II, vide letter No. (Ph-II-MG-9)/PAKTURK/R&D/HEC/2018.” The authors also extend their sincere appreciation to Taif University for funding the current work by Taif University Researchers Supporting Project number (TURSP-2020/94), Taif University, Taif, Saudi Arabia.
References
Abid, M., Tian, Z., Ata-Ul-Karim, S. T., Liu, Y., Cui, Y., Zahoor, R., et al. (2016). Improved Tolerance to post-anthesis Drought Stress by Pre-drought Priming at Vegetative Stages in Drought-Tolerant and -sensitive Wheat Cultivars. Plant Physiol. Biochem. 106, 218–227. doi:10.1016/j.plaphy.2016.05.003
Aboelsoud, H. M., and Ahmed, A. A. (2020). Effect of Biochar, Vermicompost and Polymer on Wheat and maize Productivity in sandy Soils under Drought Stress. Environ. Biodiverse. Soil Security 4, 85–102. doi:10.21608/jenvbs.2020.29442.1095
Ahanger, M. A., Qi, M., Huang, Z., Xu, X., Begum, N., Qin, C., et al. (2021). Improving Growth and Photosynthetic Performance of Drought Stressed Tomato by Application of Nano-Organic Fertilizer Involves Up-Regulation of Nitrogen, Antioxidant and Osmolyte Metabolism. Ecotoxicology Environ. Saf. 216, 112195. doi:10.1016/j.ecoenv.2021.112195
Ahmad, A., Aslam, Z., Bellitürk, K., Iqbal, N., Idrees, M., Nawaz, M., et al. (2021). Earth Worms and Vermicomposting: A Review on the story of Black Gold. J. Innov. Sci. 7 (1), 167–173. doi:10.17582/journal.jis/2021/7.1.167.173
Ahmad, A., Aslam, Z., Javed, T., Hussain, S., Raza, A., Shabbir, R., et al. (2022). Screening of Wheat (Triticum aestivum L.) Genotypes for Drought Tolerance through Agronomic and Physiological Response. Agronomy 12 (2), 287. doi:10.3390/agronomy12020287
Ahmad, A., Aslam, Z., Naz, M., Hussain, S., Javed, T., Aslam, S., et al. (2021a). Exogenous Salicylic Acid-Induced Drought Stress Tolerance in Wheat (Triticum aestivum L.) Grown under Hydroponic Culture. PloS one 16 (12), e0260556. doi:10.1371/journal.pone.0260556
Ahmadpour, R., and Hosseinzadeh, S. R. (2017). Effect of Vermicompost Fertilizer on Morphological Traits of Lentil under Water Stress. Conference: 3rd. Int. Conf. Agric. Eng. Nat. Resour. Iran.
Amiri, H., Ismaili, A., and Hosseinzadeh, S. R. (2017). Influence of Vermicompost Fertilizer and Water Deficit Stress on Morpho-Physiological Features of Chickpea (Cicer arietinumL.Cv. Karaj). Compost. Sci. Utilization 25, 152–165. doi:10.1080/1065657x.2016.1249313
Avramova, V., AbdElgawad, H., Zhang, Z., Fotschki, B., Casadevall, R., Vergauwen, L., et al. (2015). Drought Induces Distinct Growth Response, protection, and Recovery Mechanisms in the maize Leaf Growth Zone. Plant Physiol. 169 (2), 1382–1396. doi:10.1104/pp.15.00276
Bowden, C. L., Evanylo, G. K., Zhang, X., Ervin, E. H., and Seiler, J. R. (2010). Soil Carbon and Physiological Responses of Corn and Soybean to Organic Amendments. Compost. Sci. Utilization 18, 162–173. doi:10.1080/1065657x.2010.10736952
Boyer, J. S., and Westgate, M. (2004). Grain Yields with Limited Water. J. Exp. Bot. 55, 2385–2394. doi:10.1093/jxb/erh219
Chance, B., and Maehly, A. C. (1955). [136] Assay of Catalases and Peroxidases. Methods Enzymol. 2, 764–775. doi:10.1016/s0076-6879(55)02300-8
Chen, W., Yao, X., Cai, K., and Chen, J. (2011). Silicon Alleviates Drought Stress of rice Plants by Improving Plant Water Status, Photosynthesis and mineral Nutrient Absorption. Biol. Trace Elem. Res. 142, 67–76. doi:10.1007/s12011-010-8742-x
Curtis, M. J., and Claassen, V. P. (2005). Compost Incorporation Increases Plant Available Water in a Drastically Disturbed Serpentine Soil. Soil Sci. 170, 939–953. doi:10.1097/01.ss.0000187352.16740.8e
Daryanto, S., Wang, L., and Jacinthe, P. A. (2016). Global Synthesis of Drought Effects on maize and Wheat Production. PLoS ONE 11, e0156362–362. doi:10.1371/journal.pone.0156362
Dominguez, J., and Edwars, C. A. (2004). “Vermicomposting Organic Wastes: A Review,” in Soil Zoology for Sustainable Development in the 21st Century. Editors S. H. Shakir Hanna, and W. Z. A. Mikhatl Cairo, 369–395.
FAO (2019). Crop Prospects and Food Situation.” in Global Information and Early Warning System. Food and Agriculture Organization of United Nations. Available online at https ://www.fao.org/giews/repor ts/crop-prosp ects/en/.
Farooq, A., Bukhari, S. A., Akram, N. A., Ashraf, M., Wijaya, L., Alyemeni, M. N., et al. (2020). Exogenously Applied Ascorbic Acid-Mediated Changes in Osmoprotection and Oxidative Defense System Enhanced Water Stress Tolerance in Different Cultivars of Safflower (Carthamus Tinctorious L.). Plants 9 (1), 104. doi:10.3390/plants9010104
García, A. C., Santos, L. A., Izquierdo, F. G., Sperandio, M. V. L., Castro, R. N., and Berbara, R. L. L. (2012). Vermicompost Humic Acids as an Ecological Pathway to Protect rice Plant against Oxidative Stress. Ecol. Eng. 47, 203–208. doi:10.1016/j.ecoleng.2012.06.011
Gholipoor, M., Karamzadeh, A., and Gholami, A. (2011). Vermicompost as a Soil Supplement to Relieve the Effects of Low-Intensity Drought Stress on Chickpea Yield. Int. Symp. Org. Matter Manage. Compost Use Hortic. 1018, 219. doi:10.17660/ActaHortic.2014.1018.22
Giannopolitis, C. N., and Ries, S. K. (1977). Superoxide Dismutases. Plant Physiol. 59, 309–314. doi:10.1104/pp.59.2.309
Golldack, D., Lüking, I., and Yang, O. (2011). Plant Tolerance to Drought and Salinity: Stress Regulating Transcription Factors and Their Functional Significance in the Cellular Transcriptional Network. Plant Cel Rep 30, 1383–1391. doi:10.1007/s00299-011-1068-0
Gunes, A., Inal, A., Alpaslan, M., Eraslan, F., Bagci, E. G., and Cicek, N. (2007). Salicylic Acid Induced Changes on Some Physiological Parameters Symptomatic for Oxidative Stress and mineral Nutrition in maize (Zea mays L.) Grown under Salinity. J. Plant Physiol. 164, 728–736. doi:10.1016/j.jplph.2005.12.009
Hafez, E. M., Omara, A. E. D., Alhumaydhi, F. A., and El‐Esawi, M. A. (2020). Minimizing hazard Impacts of Soil Salinity and Water Stress on Wheat Plants by Soil Application of Vermicompost and Biochar. Physiologia Plantarum 172 (2), 587–602. doi:10.1111/ppl.13261
Hosseinzadeh, S. R., Amiri, H., and Ismaili, A. (2018). Evaluation of Photosynthesis, Physiological, and Biochemical Responses of Chickpea (Cicer Arietinum L. Cv. Pirouz) under Water Deficit Stress and Use of Vermicompost Fertilizer. J. Integr. Agric. 17 (11), 2426–2437. doi:10.1016/s2095-3119(17)61874-4
Hussain, K., Nawaz, K., Majeed, A., Khan, A., Lin, F., Ghani, A., et al. (2010). Alleviation of Salinity Effects by Exogenous Applications of Salicylic Acid in Pearl Millet (Pennisetum Glaucum (L.) R. Br.) Seedlings. Afr. J. Biotechnol. 9, 8602. doi:10.5897/AJB10.850
Hussain, M., Farooq, S., Hasan, W., Ul-Allah, S., Tanveer, M., Farooq, M., et al. (2018). Drought Stress in sunflower: Physiological Effects and its Management through Breeding and Agronomic Alternatives. Agric. Water Manage. 201, 152–166. doi:10.1016/j.agwat.2018.01.028
Hussain, S., Hussain, S., Qadir, T., Khaliq, A., Ashraf, U., Parveen, A., et al. (2019). Drought Stress in Plants: An Overview on Implications, Tolerance Mechanisms and Agronomic Mitigation Strategies. Plant Sci. Today 6 (4), 389–402. doi:10.14719/pst.2019.6.4.578
Iqbal, M., Asif, S., Ilyas, N., Fayyaz-ul-Hassan, N. I., Raja, N. I., Ejaz, M. M., et al. (2018). Smoke Produced from Plants Waste Material Elicits Growth of Wheat ( Triticum aestivum L.) by Improving Morphological, Physiological and Biochemical Activity. Biotechnol. Rep. 17, 35–44. doi:10.1016/j.btre.2017.12.001
Jat, R. S., and Ahlawat, I. P. S. (2006). Direct and Residual Effect of Vermicompost, Biofertilizers and Phosphorus on Soil Nutrient Dynamics and Productivity of Chickpea-Fodder maize Sequence. J. Sust. Agric. 28, 41–54. doi:10.1300/j064v28n01_05
Kashem, M. A., Sarker, A., Hossain, I., and Islam, M. S. (2015). Comparison of the Effect of Vermicompost and Inorganic Fertilizers on Vegetative Growth and Fruit Production of Tomato (Solanum lycopersicum L.). Ojss 05, 53–58. doi:10.4236/ojss.2015.52006
Kiran, S. (2019). Effects of Vermicompost on Some Morphological, Physiological and Biochemical Parameters of Lettuce (Lactuca Sativa Var. Crispa) under Drought Stress. Not. Bot. Horti. Agrobot. 47 (2), 352. doi:10.15835/nbha47111260
Lattaud, C., Locati, S., Mora, P., and Rouland, C. (1997a). Origin and Activities of Glycolytic Enzymes in the Gut of the Tropical Geophagous Earthworm Millsoniaanomala from Lamto (Cote d’Ivoire). Pedobiologia 41, 242
Lattaud, C., Zhang, B. G., Locati, S., Rouland, C., and Lavelle, P. (1997b). Activities of the Digestive Enzymes in the Gut and in Tissue Culture of a Tropical Geophagous Earthworm, Polypheretima Elongata (Megascolecidae). Soil Biol. Biochem. 29, 335–339. doi:10.1016/s0038-0717(96)00021-1
Li-Ping, B., Fang-Gong, S., Ti-Da, G., Zhao-Hui, S., Yin-Yan, L., and Guang-Sheng, Z. (2006). Effect of Soil Drought Stress on Leaf Water Status, Membrane Permeability and Enzymatic Antioxidant System of maize. Pedosphere 16 (3), 326
Liu, H., Sultan, M. A. R. F., Liu, X. l., Zhang, J., Yu, F., and Zhao, H. x. (2015). Physiological and Comparative Proteomic Analysis Reveals Different Drought Responses in Roots and Leaves of Drought-Tolerant Wild Wheat (Triticum Boeoticum). PLoS ONE 10 (4), e0121852. doi:10.1016/S1002-0160(06)60059-3
Mahmoudi, S. V. R., Nasri, M., and Azizi, P. (2016). The Effect of Different Type of Vermicompost Organic Fertilizer Litter on Quantitative, Qualitative and Biochemical Characteristics of green Mung Bean (Vignaradiata L.) in Drought Stress Conditions in Varamin. Int. J. Adv. Biotechnol. Res. 7, 205
Nazarideljou, M. J., and Heidari, Z. (2014). Effects of Vermicompost on Growth Parameters, Water Use Efficiency and Quality of Zinnia Bedding Plants (Zinnia Elegance‘‘Dreamland Red’’) under Different Irrigation Regimes. Int. J. Hortic. Sci. Technol. 1 (2), 141. doi:10.22059/ijhst.2014.52786
Rahbarian, R., Khavari-Nejad, R., Ganjeali, A., Bagheri, A., and Najafi, F. (2011). Drought Stress Effects on Photosynthesis, Chlorophyll Fluorescence and Water Relations in Tolerant and Susceptible Chickpea (Cicer Arietinum L.) Genotypes. Acta Biol. Crac. Ser. Bot. 53, 47–56. doi:10.2478/v10182-011-0007-2
Rashtbari, M., Alikhani, H. A., and Ghorchiani, M. (2012). Effect of Vermicompost and Municipal Solid Waste Compost on Growth and Yield of Canola under Drought Stress Conditions. Int. J. Agric. Res. Rev. 2 (4), 395
Reddy, A. R., Chaitanya, K. V., and Vivekanandan, M. (2004). Drought-induced Responses of Photosynthesis and Antioxidant Metabolism in Higher Plants. J. Plant Physiol. 161, 1189–1202. doi:10.1016/j.jplph.2004.01.013
Rosolem, C. A., Sarto, M. V. M., Rocha, K. F., Martins, J. D. L., and Alves, M. S. (2019). Does the Introgression of Bt Gene Affect Physiological Cotton Response to Water Deficit? Planta Daninha 37, 1–7. doi:10.1590/s0100-83582019370100035
Sakuma, Y., Maruyama, K., Qin, F., Osakabe, Y., Shinozaki, K., and Yamaguchi-Shinozaki, K. (2006). Dual Function of an Arabidopsis Transcription Factor DREB2A in Water-Stress-Responsive and Heat-Stress-Responsive Gene Expression. Proc. Natl. Acad. Sci. U.S.A. 103, 18822–18827. doi:10.1073/pnas.0605639103
Scholander, P. F., Hammel, H. T., Bradstreet, E. A. E. D., and Hemminsoln, E. A. (1964). Hydrostatic Pressure and Osmotic Potential in Leaves of Mangroves and Some Other Plants. Proc. Natl. Acad. Sci. U.S.A. 52, 119–125. doi:10.1073/pnas.52.1.119
Singh, K., Wijewardana, C., Gajanayake, B., Lokhande, S., Wallace, T., Jones, D., et al. (2018). Genotypic Variability Among Cotton Cultivars for Heat and Drought Tolerance Using Reproductive and Physiological Traits. Euphytica 214, 1–22. doi:10.1007/s10681-018-2135-1
Steel, R. G. D., Torrie, J. H., and Dickey, D. A. (1997). Principles and Procedures of Statistics: A Biometrical Approach. 3rd ed. New York: McGraw Hill Book Co. Inc., 400–428.
Subler, S., Edwards, C., and Metzger, J. (1998). Comparing Vermicomposts and Composts. Biocycle 39, 63–66.
Suthar, S. (2009). Impact of Vermicompost and Composted Farmyard Manure on Growth and Yield of Garlic (Allium Stivum L.) Field Crop. Int. J. Plant Product. 3, 27
Urbasek, F., and Pizl, V. (1991). Activity of Digestive Enzymes in the Gut of Five Earthworm Species (Oligochaeta: Lumbricidae). Rev. Ecol. Biol. Sol. 28, 461
Varghese, S. M., and Prabha, M. L. (2014). Biochemical Characterization of Vermiwash and its Effect on Growth of Capsicum Frutescens. Malaya J. Biosci. 1, 86
Wang, X., Vignjevic, M., Jiang, D., Jacobsen, S., and Wollenweber, B. (2014). Improved Tolerance to Drought Stress after Anthesis Due to Priming before Anthesis in Wheat (Triticum aestivum L.) Var. Vinjett. J. Exp. Bot. 65, 6441–6456. doi:10.1093/jxb/eru362
Wei, W., Li, Q.-T., Chu, Y.-N., Reiter, R. J., Yu, X.-M., Zhu, D.-H., et al. (2015). Melatonin Enhances Plant Growth and Abiotic Stress Tolerance in Soybean Plants. J. Exp. Bot. 66, 695–707. doi:10.1093/jxb/eru392
Xue, G. P., and Loveridge, C. W. (2004). HvDRF1 Is Involved in Abscisic Acid‐mediated Gene Regulation in Barley and Produces Two Forms of AP2 Transcriptional Activators, Interacting Preferably with a CT‐rich Element. Plant J. 37, 326–339. doi:10.1046/j.1365-313x.2003.01963.x
Zhang, B.-G., Li, G.-T., Shen, T.-S., Wang, J.-K., and Sun, Z. (2000). Changes in Microbial Biomass C, N, and P and Enzyme Activities in Soil Incubated with the Earthworms Metaphire Guillelmi or Eisenia fetida. Soil Biol. Biochem. 32, 2055–2062. doi:10.1016/s0038-0717(00)00111-5
Keywords: antioxidant, cultivars, drought, straw, vermicompost, wheat
Citation: Ahmad A, Aslam Z, Hussain S, Javed T, Hussain S, Bashir S, Hussain I, Belliturk K, Adamski R, Siuta D, Dessoky ES and Hessini K (2022) Soil Application of Wheat Straw Vermicompost Enhances Morpho-Physiological Attributes and Antioxidant Defense in Wheat Under Drought Stress. Front. Environ. Sci. 10:894517. doi: 10.3389/fenvs.2022.894517
Received: 11 March 2022; Accepted: 23 March 2022;
Published: 26 April 2022.
Edited by:
Balasubramani Ravindran, Kyonggi University, South KoreaReviewed by:
Mursleen Yasin, Western Sydney University, AustraliaRajesh Singhal, Indian Grassland and Fodder Research Institute (ICAR), India
Copyright © 2022 Ahmad, Aslam, Hussain, Javed, Hussain, Bashir, Hussain, Belliturk, Adamski, Siuta, Dessoky and Hessini. This is an open-access article distributed under the terms of the Creative Commons Attribution License (CC BY). The use, distribution or reproduction in other forums is permitted, provided the original author(s) and the copyright owner(s) are credited and that the original publication in this journal is cited, in accordance with accepted academic practice. No use, distribution or reproduction is permitted which does not comply with these terms.
*Correspondence: Zubair Aslam, Zauaf@hotmail.com; Talha Javed, talhajaved54321@gmail.com; Sadam Hussain, ch.sadam423@gmail.com