- 1College of Natural Resources and Environment, South China Agricultural University, Guangzhou, China
- 2Department of Civil and Environmental Engineering, Shantou University, Shantou, China
- 3School of Resources and Planning, Guangzhou Xinhua University, Guangzhou, China
A comprehensive understanding of the influence of earthworms on the growth and Pb accumulation of leafy vegetables is significant for soil management and human health. This study was aimed to evaluate the different influences of two ecological earthworm species on the growth and Pb accumulation of Brassica campestris in a Pb-contaminated soil and their relationship with soil physico-chemical properties. In a 30-day microcosm experiment, the anecic and native earthworm species Amynthas aspergillum and the epigeic species Eisenia fetida were inoculated in soil artificially contaminated with Pb at different levels (i.e., 0, 100, 500, and 1,000 mg kg−1), and B. campestris was grown. With a survival rate of 81%–100%, A. aspergillum was more tolerant to Pb contamination than E. fetida with 46%–84%. At the same time, earthworm inoculation significantly increased soil Pb bioavailability (p < 0.05). At the 500 and 1,000 mg kg−1 Pb levels, the treatments with earthworm inoculation showed higher plant biomass, leaf area, and chlorophyll concentration than the treatments without earthworm. The principal component analysis (PCA) showed that earthworm inoculation exerted a stronger effect on soil properties than Pb contamination, but the latter had a stronger effect on plant growth and Pb accumulation. Compared with A. aspergillum, E. fetida had a greater effect on soil cation exchange capacity, available Pb, and plant growth and Pb accumulation. In contrast, A. aspergillum had a greater effect on soil C and N contents than E. fetida. The co-inertia analysis revealed that plant Pb accumulation was positively correlated with soil available Pb and CEC. The leaf chlorophyll concentration was closely related to soil Eh, pH, and Dissolved organic carbon. The findings of this study showed that in the Pb-contaminated soils, earthworm inoculation exerted a strong effect on soil physicochemical properties and the growth and Pb accumulation of the leafy vegetable B. campestris. Both the epigeic earthworm species E. fetida and the anecic species A. aspergillum were associated with higher Pb accumulation or concentration in B. campestris, which may bring a possible risk to food security.
1 Introduction
Soil Pb contamination has a detrimental impact on soil health and functionality, and it also poses a risk to humans and other living organisms due to biomagnification via the food chain (Zulfiqar et al., 2019; Kumar et al., 2020). Brassica campestris is one of the most widely consumed and grown leafy vegetables in Asia; meanwhile, it is a significant route for Pb to enter the food chain. When B. campestris is cultivated in Pb-contaminated soils, Pb is absorbed by the roots and translocated to the edible organs (Liu et al., 2020; Ali et al., 2022), which causes concern for vegetables by highlighting a potential food safety problem. Therefore, information regarding Pb accumulation and possible risks in leafy vegetables is urgently needed to raise public awareness.
When it comes to the bioavailability of Pb, the total concentration in the soil does not always indicate how Pb is readily accessible to plants. The bioavailable Pb is the fraction of Pb taken up by plants, useful in accessing potential metal bioaccumulation in plants. However, some studies have shown that Pb uptake by B. campestris is related to Pb bioavailability in soil (Yang et al., 2016; Liu et al., 2019). Many factors influence the transformation of Pb from unavailable to available forms. Soil attributes such as pH, redox potential (Eh), organic matter content, cation exchange capacity (CEC), and competing cations govern the bioavailability of Pb in soil (Romero-Freire et al., 2015; Xiao et al., 2017a; Xiao et al., 2017b). Xiao et al. (2020b) found that soil microbial properties (e.g., soil microbial biomass, soil respiration, soil enzyme activities) significantly affect the accumulation of Pb in brassica leaves. So far, few studies have focused on the effect of soil macrofauna on Pb accumulation in leafy vegetables, especially earthworms, which are keystone species in the soil ecosystem (Gluhar et al., 2021).
Pb bioavailable fraction in soil reflects Pb accumulation in plants. Earthworm activities such as feeding, digging, and metabolite excreting strongly influence soil properties and Pb availability (Porfido et al., 2022; Zhang et al., 2022), hence enhancing soil Pb participation in the food chain. The abilities of earthworms to regulate soil properties and Pb bioavailability are intimately connected to the diverse ecotypes of earthworms that differ in lifestyles, digestive systems, and feeding behaviors (Jeyanthi et al., 2016; Sinkakarimi et al., 2020). There are two distinct processes in which various ecological earthworms can mediate the fate of Pb. First, the intake of soil Pb incorporated in mineral particles or organic staff ingested by earthworms varies with the feeding habits of earthworms, resulting in varying Pb bioavailability and accumulation. Second, earthworm activities such as borrowing, casting, and composting influence soil physicochemical properties, leading to changes in Pb bioavailability (Wang et al., 2018; Richardson et al., 2020). Therefore, a new insight to be gained is how earthworm ecotypes affect the chemical behavior of Pb to involve the food chain of vegetable-soil systems. In previous studies, different ecological earthworm species have been investigated for their influences on metal accumulation in plant leaves, stems, and roots (Du et al., 2014; Wang et al., 2020; Guo et al., 2022). However, there is still a lack of knowledge on how earthworms of different ecotypes influence the soil physicochemical properties and Pb bioavailability in the vegetable-soil system.
Based upon the above argument, a microcosmic experiment was set up with two ecological earthworm species. The anecic species Amynthas aspergillum feeds on a mixture of soil and little-decomposed organic debris, showing an exceptional soil-ingesting ability. The epigeic species Eisenia fetida, which is a composting earthworm, lives on high quality organic matters (compost or manure heaps) (Zhang et al., 2020; Paul et al., 2022). Most previous studies relied on the impact of E. fetida on vermicompost. So far, few studies have focused on the effects of these two ecological earthworm species on Pb accumulation, the growth of leafy vegetables, and the underlying physico-chemical mechanism. In this study, firstly, we expected that Pb accumulation and the growth of B. campestris are sensitive to the presence of earthworms. Secondly, we hypothesized that the anecic species Amynthas aspergillum would be more efficient in Pb transformation and accumulation than E. fetida because Pb is bound to soil particles and has a high affinity to organic matter and different ecological earthworm species have different feeding habits. Last but not the least, we hypothesized that Pb transformation in the soil-plant system and the growth of B. campestris would be influenced by the changes in soil physico-chemical properties caused by earthworms and/or Pb input levels.
2 Materials and Methods
2.1 Soil, Earthworm Species, and Plant
The soil was collected from the 0–20 cm layer of a fallow vegetable field located at 23°54′ N and 113°27′ E in Qingyuan City, Guangdong Province, China. After plant residues and stones were removed, the soil was air-dried and sieved to <2 mm. The soil pH, organic C, total N, and C: N ratio were 6.06, 16.6 g·kg−1, 1.30 g·kg−1, and 12.7, respectively, and Pb content was 20 mg·kg−1.
Earthworms of A. aspergillum and E. fetida were purchased from a biofertilizer company in Qingyuan, Guangdong Province, China. Before the experiment, the earthworms were cultured in uncontaminated soil with a moisture content of 40%–60% of field water capacity at 25°C, and organic matter was added for better growth.
Seeds of B. campestris were provided by the Guangdong Academy of Agricultural Sciences.
2.2 Experimental Design
A total of 96 pots (18.5 cm × 12 cm × 16 cm) were used in this experiment. Each pot was filled with 1 kg soil, which had been spiked with PbCl2 to set up 4 Pb contamination levels of 0, 100, 500, and 1,000 mg∙kg−1 (OECD, 2000) and had been aged for 10 months. Six treatments were further set up in four replicates for each Pb level: 1) no plant or earthworm (S), 2) B. campestris (SP), 3) A. aspergillum (SA), 4) E. fetida (SE), 5) B. campestris + A. aspergillum (SPA), and 6) B. campestris + E. fetida (SPE). In each pot of the SP, SPA, and SPE treatments, three seedlings of B. campestris were transplanted, and to each pot of SA, SE, SPA, and SPE, 23 ± 1 g of healthy clitellate adult earthworms were introduced. The transplanted seedlings were healthy and uniform in size. Similarly, the introduced earthworms were uniform in weight, 5.73 ± 0.4 g individual−1 for A. aspergillum and 0.4 ± 0.1 g individual−1 for E. fetida.
During the experiment, the temperature was maintained at 25°C, and the light intensity was 400–800 lx. The earthworms were prevented from escaping according to Zhou et al. (2016) and Chen et al. (2017). Soil moisture in each pot was maintained at field capacity. The day before harvesting, leaf chlorophyll concentration was measured on the newest fully expanded leaf using a Konica Minolta SPAD-502 chlorophyll meter (Alordzinu et al., 2021). After 30 days, plant height, shoot diameter, and the number of branches were recorded. The plants were harvested, washed, and oven-dried. The earthworms in each pot were collected, counted, and weighed before being cultured for 7 days at 25°C to empty the guts. The soil in each pot was air-dried and passed through 2-mm and 0.149-mm sieves for chemical analysis.
2.3 Laboratory Analyses
Soil pH and Eh were measured at 1:2.5 soil: water ratio. Soil total N was quantified by Kjeldahl digestion, organic C was determined by the dichromate digestion method, and CEC was estimated by the ammonium acetate (1 mol L−1, pH 7.0) method (Sparks et al., 1996). Dissolved organic carbon (DOC) was quantified according to Dai et al. (2004). Available N was measured by the alkaline diffusion method (Cornfield, 1960).
Soil total Pb was determined by flame atomic absorption spectroscopy (FAAS) after soil samples were digested with HCl–HF–HNO3–HClO4 in an electrically heated block digester (Amacher, 1996). Soil bioavailable Pb was extracted with pH 7.3 diethylenetriaminepentaacetic acid (DTPA) extractant consisting of 0.005M DTPA, 0.1M triethanolamine (TEA), and 0.01M calcium chloride (CaCl2) at a soil: solution ratio of 1:2 for 2 h, centrifuged at 4,000 g for 20 min, then filtered. Finally, Pb was quantified by FAAS (Lindsay and Norvell, 1978).
For Pb concentration in earthworms, 0.20 g of dried and crushed (0.2 mm) earthworm sample was homogenized with 8 ml concentrated HNO3 and 2 ml concentrated HClO4 for 12 h and digested at 250°C for 2 h. After cooling, the solution was diluted to a final volume of 50 ml using deionized water, and Pb concentration was quantified by FAAS. Using a microwave digestion system, 0.20 g of plant sample was wet-digested with aqua regia solution (4 ml HNO3 and 1 ml HCl), and Pb concentration was quantified using FAAS (Moral et al., 1996).
2.4 Calculations
Leaf area was calculated according to the Montgomery equation (Shi et al., 2019; He et al., 2020):
Where LA is leaf area (cm2), LL is leaf length (cm), LW is leaf width (cm), and MP is Montgomery coefficient, which is 0.68.
The transfer factor (TF) of Pb was calculated as (Gupta et al., 2008):
The survival rate (SR) of earthworms (%) was calculated as (Wu et al., 2020a):
2.5 Statistical Analysis
Data were processed using the SPSS statistical software and analyzed using one-way ANOVA, followed by Duncan’s multiple range test and t-test. Data are presented as mean ± standard deviation, and the significance level was set at p < 0.05. Principal component analysis (PCA) and co-inertia analysis were performed using the Ade-4 package in R to explore the influences of earthworms on soil properties, Pb bioavailability in soil, and Pb accumulation in the plant (Thioulouse et al., 1997).
3 Results
3.1 Earthworm Biomass, Survival Rate, and Pb Accumulation
In all treatments, A. aspergillum tolerated Pb contamination better than E. fetida (Table 1). At the 0 and 100 mg kg−1 Pb contamination levels, the biomass of A. aspergillum was decreased by 16% and 5%, respectively, and at the 500 and 1,000 mg kg−1 Pb levels, it was increased by 11% and 2%, respectively, in SPA at the end of the experiment compared with at the beginning of the experiment (p > 0.05). At the 0 and 1,000 mg kg−1 Pb contamination levels, the biomass of A. aspergillum was reduced by 12% and 13%, respectively, and at the 100 and 500 mg kg−1 Pb levels, it was increased by 2% and 18% (p < 0.05), respectively, in SA at the end of the experiment compared with at the start of the experiment. The survival rate of A. aspergillum was high in all treatments, ranging from 81% to 100% (Table 1).
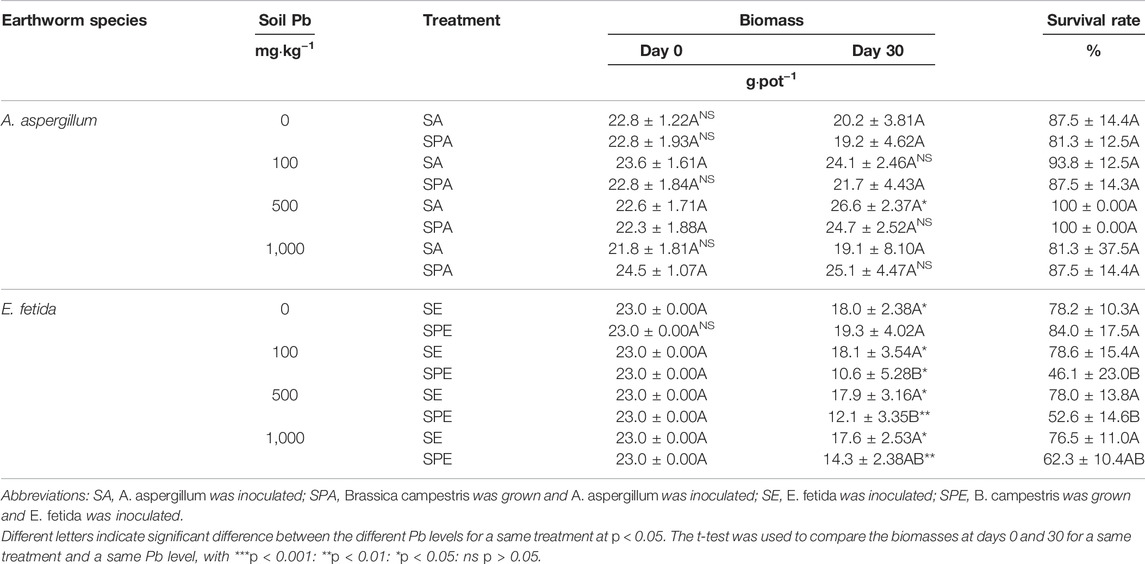
TABLE 1. Biomass and survival rate of the two earthworm species, Amynthas aspergillum and Eisenia fetida, in the treatments with different soil Pb concentrations.
The biomass of E. fetida was markedly decreased at the end of the experiment compared with at the beginning of the experiment. At the 100, 500, and 1,000 mg kg−1 Pb contamination levels, the biomass of E. fetida was significantly decreased by 54% (p < 0.05), 47% (p < 0.01), and 38% (p < 0.01), respectively, in SPE at the end of the experiment compared with at the start of the experiment. It decreased significantly by 22% (p < 0.05), 21% (p < 0.05), 22% (p < 0.05), and 24% (p < 0.05) in SE at the 0, 100, 500, and 1,000 mg kg−1 Pb levels, respectively. Generally, E. fetida survived better in the SE treatments, with the survival rates ranging from 77% to 79% across the Pb contamination levels. It displayed lower survival rates in the SPE treatments at the 100, 500, and 1,000 mg kg−1 Pb levels, which were 46%, 53%, and 62%, respectively (Table 1). At the higher Pb contamination levels, the earthworms accumulated more Pb in their bodies (p < 0.05, Figure 1). At the 500 mg kg−1 Pb level, E. fetida accumulated significantly more Pb in SE than A. aspergillum in SPA (p < 0.05).
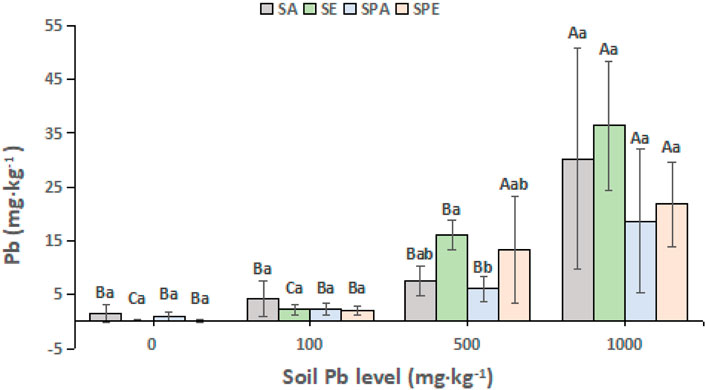
FIGURE 1. Pb accumulation in the two earthworm species, Amynthas aspergillum and Eisenia fetida, under different Pb contamination conditions. *SA: A. aspergillum was inoculated, SE: E. fetida was inoculated, SPA: Brassica campestris was grown and A. aspergillum was inoculated, SPE: B. campestris was grown and E. fetida was inoculated. Different uppercase letters indicate significant differences for a same treatment between different soil Pb levels at p < 0.05; different lowercase letters indicate significant differences between treatments for a same soil Pb level at p < 0.05.
3.2 Soil Properties and DTPA-Extractable Pb
3.2.1 Soil Properties
At the end of the experiment, soil pH ranged from 5.20 to 6.27, with the highest values in SP and the lowest in SE (p < 0.001, Table 2). At the 0, 100, 500, and 1,000 mg kg−1 Pb levels, the inoculation of A. aspergillum alone (i.e., the SA treatment) substantially decreased soil pH by 0.3, 0.2, 0.4, and 0.4 units, respectively, the inoculation of E. fetida alone (i.e., the SE treatment) significantly decreased soil pH by 0.5, 0.7, 0.8, and 0.7 units, respectively, while the growth of plant alone (i.e., the SP treatment) increased soil pH by 0.3, 0.3, 0.3, and 0.1 units, respectively, compared with the respective S treatments (p < 0.05).
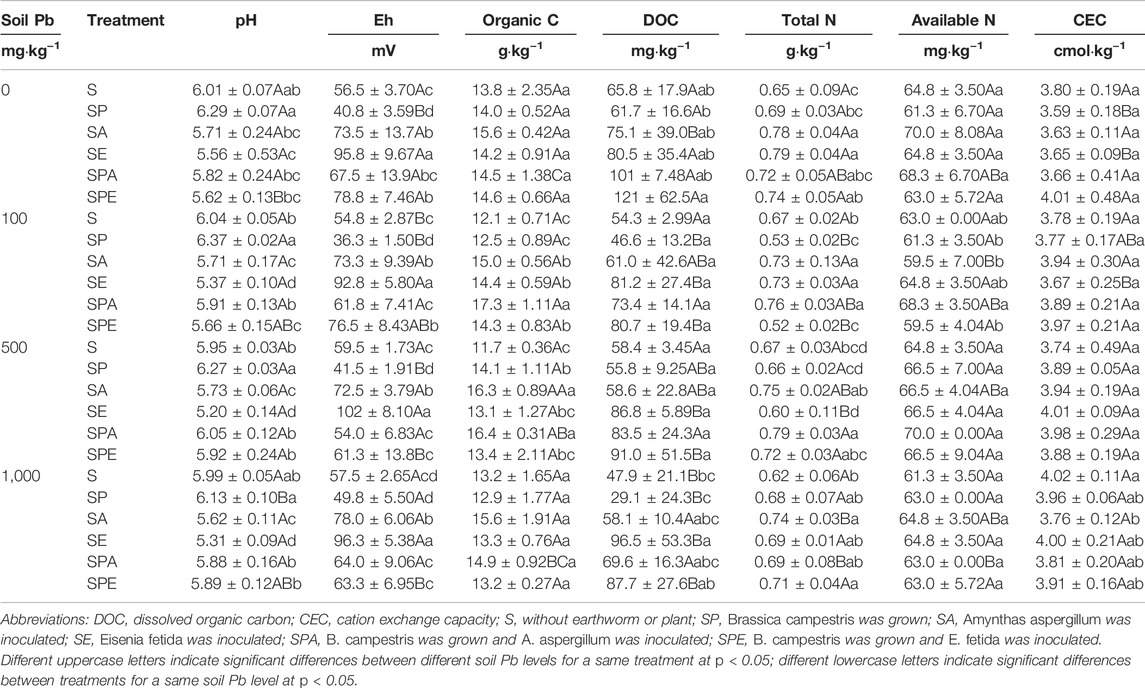
TABLE 2. Effects of earthworm inoculation and plant growth on soil chemical properties under different Pb contamination conditions.
In contrast, soil Eh (36.25–102.75 mV) showed its lowest values in SP and the highest in SE (Table 2). Earthworm inoculation alone (i.e., the SA and SE treatments) significantly increased soil Eh in comparison with the S and SP treatments. The growth of B. campestris alone (i.e., the SP treatment) substantially lowered soil Eh as compared with the S treatment except at the 1,000 mg kg−1 Pb level (Table 2). At the 0, 100, 500, and 1,000 mg kg−1 Pb levels, the inoculation of E. fetida alone (i.e., the SE treatment) significantly increased soil Eh by 39, 38, 43, and 39 mV, respectively, while the growth of B. campestris alone (i.e., the SP treatment) substantially decreased soil Eh by 16, 19, 18, and 8 mV, respectively, compared with the respective S treatments. Soil Eh was generally higher in the SPE treatment than in the SPA treatment except at the 1,000 mg kg−1 Pb level.
The treatments with earthworm inoculation generally increased soil organic C content compared with the earthworm-free treatments except at the 500 mg kg−1 Pb level, where soil organic C content in the SP treatment was higher than those in the E. fetida addition treatments (Table 2). The treatments with inoculation of A. aspergillum (i.e., SA and SPA) generally had higher contents of soil organic C than the treatments with inoculation of E. fetida (i.e., SE and SPE), with significant differences at the 100 and 500 mg kg−1 Pb levels (p < 0.05). At the 100 mg kg−1 Pb level, soil organic C was 24%, 19%, 43%, and 18% higher in SA, SE, SPA, and SPE, respectively, than in S. At the 500 mg kg−1 Pb level, soil organic C was 39%, 12%, 40%, and 15% higher in SA, SE, SPA, and SPE, respectively than in S (Table 2).
Soil DOC was higher in SA, SE, SPA, and SPE than in S and SP (p > 0.05) and had the lowest values in SP (Table 2). The SPE treatment had a higher DOC than the SPA treatment regardless of Pb contamination level (p > 0.05). At the 0 and 500 mg·kg−1 Pb levels, SPE exhibited the highest DOC among all treatments, which was 84% and 56%, respectively, higher than that in S, but a significant difference was only observed between SPE and SP at the 0 mg·kg−1 Pb level (p < 0.05). However, at the 100 and 1,000 mg·kg−1 Pb levels, the highest DOC was found in the SE treatment, which was 50% and 101%, respectively, higher than that in S, but significant differences were only observed between SE and S, SE and SP, and SPE and SP at the 1,000 mg kg−1 Pb level.
Earthworm activity generally increased soil total N content (Table 2). At the 0 mg kg−1 Pb level, soil total N was increased by 20% and 22% (p < 0.05) in SA and SE, respectively, compared with S. At the other Pb levels, soil total N was also increased in SA and SE except at the 500 mg kg−1 level where total N was considerably lower in the SE treatment than in the S treatment. The SPA and SPE treatments had close values of soil total N at all Pb contamination levels except at 100 mg kg−1 Pb levels where SPA had a much higher total N. At the 0, 100, 500, and 1,000 mg kg−1 Pb levels, soil total N in SPA was 11%, 13%, 18%, and 11%, respectively, higher than that in the respective S treatment. At the 0, 500, and 1,000 mg kg−1 Pb levels, soil total N in SPE was increased by 14%, 7%, and 15%, respectively, compared with the respective S treatments (Table 2).
At both the 100 and 500 mg kg−1 Pb levels, the highest soil available N was found in SPA (Table 2). For the SA treatments, soil available N was 70.0 mg kg−1 at the 0 mg kg−1 Pb level but decreased significantly to 59.9 mg kg−1 at the 100 mg kg−1 Pb level. For the SPA treatments, soil available N was 70.0 mg kg−1 at the 500 mg kg−1 Pb level but decreased significantly to 63.0 mg kg−1 at the 1,000 mg kg−1 Pb level.
For the same Pb contamination level, there were no significant differences in soil CEC between the treatments except at the 1,000 mg kg−1 Pb level, where the CEC in S was significantly higher than that in SA.
3.2.2 DTPA-Extractable Pb
For a same treatment, there were significant differences (p < 0.05) in soil DTPA-extractable Pb content between the 4 Pb contamination levels (Figure 2). At the end of the experiment, soil DTPA-extractable Pb was generally higher in the treatments with inoculation of earthworms than in S and SP, and the differences were significant at the 0 mg kg−1 Pb level (p < 0.05). At the 100 mg kg−1 Pb level, soil DTPA-extractable Pb was significantly higher (p < 0.05) in SPE than in S, SP, SE, and SPA. At the 500 mg kg−1 Pb level, soil DTPA-extractable Pb was significantly higher (p < 0.05) in SA and SPA than in SP and SE. At the 1,000 mg kg−1 Pb level, soil DTPA-extractable Pb was significantly higher (p < 0.05) in SA and SPA than in S and SPE. Compared with SP, soil DTPA-extractable Pb in SPE was increased by 22% (p < 0.05), 13% (p < 0.05), and 8% (p > 0.05) at the 0, 100, and 500 mg kg−1 Pb levels, respectively, but was decreased by 0.8% at the 1,000 mg kg−1 level. Similarly, compared with SP, soil DTPA-extractable Pb in SPA was raised by 26% (p < 0.05), 12% (p < 0.05), and 2% (p > 0.05) at the 0, 500, and 1,000 mg kg−1 Pb levels, respectively, but was decreased by 2% at the 100 mg kg−1 Pb level.
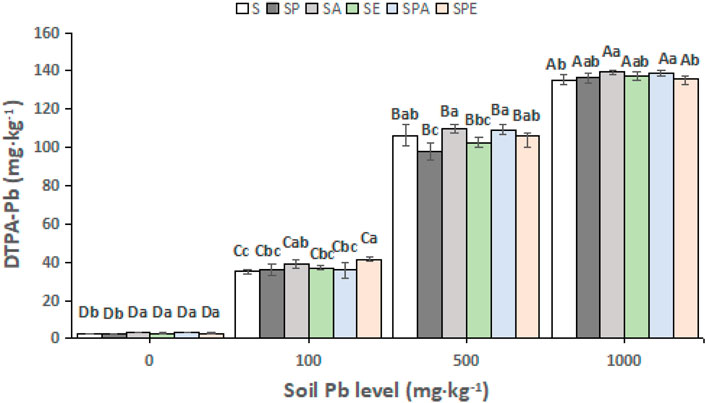
FIGURE 2. Diethylenetriaminepentaacetic acid (DTPA)-extractable Pb contents in different treatments at 0, 100, 500, and 1,000 mg kg−1 Pb contamination levels. *S: without plant or earthworm, SP: Brassica campestris was grown, SA: Amynthas aspergillum was inoculated, SE: Eisenia fetida was inoculated, SPA: B. campestris was grown and A. aspergillum was inoculated, SPE: B. campestris was grown and E. fetida was inoculated. Different uppercase letters indicate significant differences between different Pb levels for a same treatment at p < 0.05; different lowercase letters indicate significant differences between treatments for a same soil Pb level at p < 0.05.
3.2.3 PCA Analysis of the Soil Properties
PCA analysis revealed significant differences in soil properties between treatments at the same Pb pollution level (Figures 3A,B). The first factor extracted (36.3% of the variance explained) indicated the effects of earthworm inoculation and plant growth on soil pH, Eh, and C and N forms across all treatments (Figure 3A). The presence of earthworms and plants increased the contents of different soil C and N forms and soil Eh but decreased soil pH (Figure 3B, p < 0.05). The second factor extracted (23.3% of the variance explained) characterized the influence of Pb contamination level on soil CEC and available Pb content (Figure 3C). The high Pb contamination levels resulted in high soil CEC and available Pb content. Compared with A. aspergillum, the inoculation of E. fetida led to greater increases in soil CEC and DTPA-extractable Pb at the 1,000 mg kg−1 Pb level and smaller decreases in soil pH. The distance between SPA and SPE was longer than that between SA and SE, indicating greater interspecific differences in the plant + earthworm treatments than in the earthworm alone treatments.
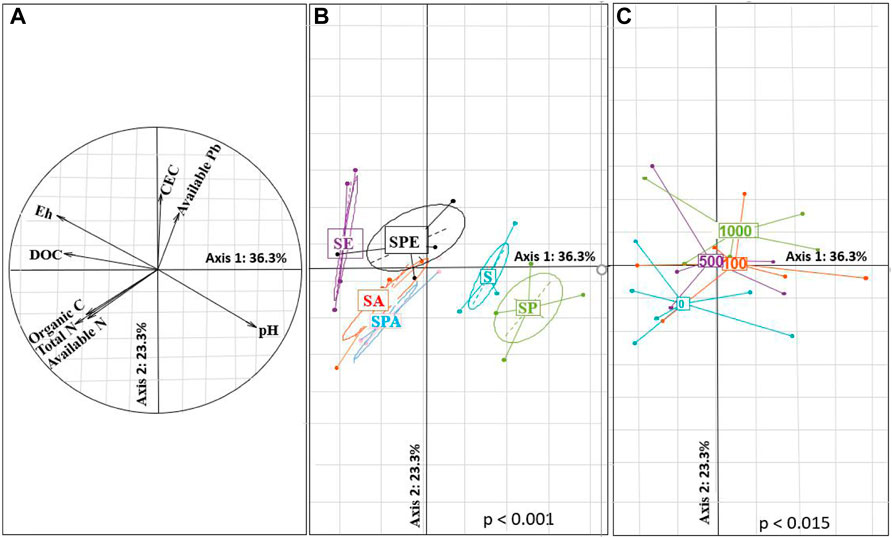
FIGURE 3. Principal component analysis of soil characteristics in the different treatments. (A): Correlation circle of the soil properties; projection of experimental points according to treatments; (B) Treatments with or without earthworms. (C) Treatments at different Pb contamination levels of 0, 100, 500, and 1,000 mg∙kg-1 Pb. DOC: dissolved organic carbon; CEC: cation exchange capacity. S: without plant or earthworm, SP: Brassica campestris was grown, SA: Amynthas aspergillum was inoculated, SE: Eisenia fetida was inoculated, SPA: B. campestris was grown and A. aspergillum was inoculated, SPE: B. campestris was grown and E. fetida was inoculated.
3.3 Plant Growth, Pb Accumulation, and TF
3.3.1 Plant Growth
Generally, the plant growth was not much affected by the Pb contamination levels. Only at the 500 and 1,000 mg kg−1 Pb levels, yellow leaves of B. campestris were observed in the treatment without earthworms (i.e., SP), indicating that plant growth was impaired (Table 3).
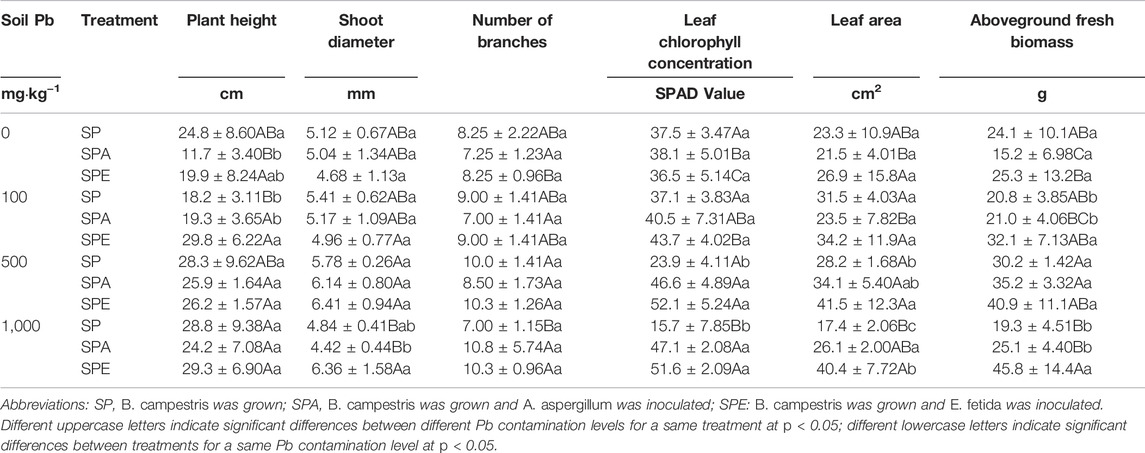
TABLE 3. Effects of the two earthworms species, Amynthas aspergillum and Eisenia fetida, on the growth of Brassica campestris.
The addition of earthworms mitigated Pb phytotoxicity and markedly improved plant growth, which was evident by the increases in plant biomass, leaf chlorophyll concentration, and leaf area at the end of the experiment across all Pb contamination levels (p < 0.05, Table 3). Compared with plants alone (i.e., SP), the addition of E. fetida in SPE increased plant biomass by 55% (p < 0.05), 36% (p > 0.05), and 138% (p < 0.05) at the 100, 500, and 1,000 mg kg−1 Pb levels, respectively, and the addition of A. aspergillum in SPA increased plant biomass by 17% and 30% at the 500 and 1,000 mg kg−1 Pb levels, respectively. At the 500 and 1,000 mg kg−1 Pb levels, the introduction of A. aspergillum significantly increased leaf chlorophyll concentration by 95% (p < 0.05) and 199% (p < 0.05), respectively, and the introduction of E. fetida significantly increased leaf chlorophyll concentration by 118% (p < 0.05) and 228% (p < 0.05), respectively. Also, at the 500 and 1,000 mg kg−1 Pb levels, the introduction of A. aspergillum increased leaf area by 21% (p > 0.05) and 51% (p < 0.05), respectively, and the introduction of E. fetida increased leaf area by 47% (p < 0.05) and 133% (p < 0.05), respectively (Table 3).
3.3.2 Pb Accumulation in the Plants
The plants treated with E. fetida addition (i.e., SPE) had higher Pb accumulation in both the belowground and aboveground parts than the plants in SP and SPA regardless of Pb contamination level (Table 4). Compared with SP, Pb accumulation in the aboveground plant parts increased by 257% (p < 0.05), 70%, and 13%, and that in the belowground plant parts increased by 118% (p < 0.05), 17%, and 0.5% in SPE at the 100, 500, and 1,000 mg kg−1 Pb levels, respectively. In contrast, at the 0 mg kg−1 Pb level, Pb accumulation in the aboveground and belowground plant parts was reduced by 22% and 48%, respectively, in SPE compared with SP. For the SPA treatments, Pb accumulation was decreased in the belowground plant part regardless of Pb contamination level but was decreased in the aboveground plant parts only at the 500 and 1,000 mg kg−1 Pb levels as compared with the SP treatments.
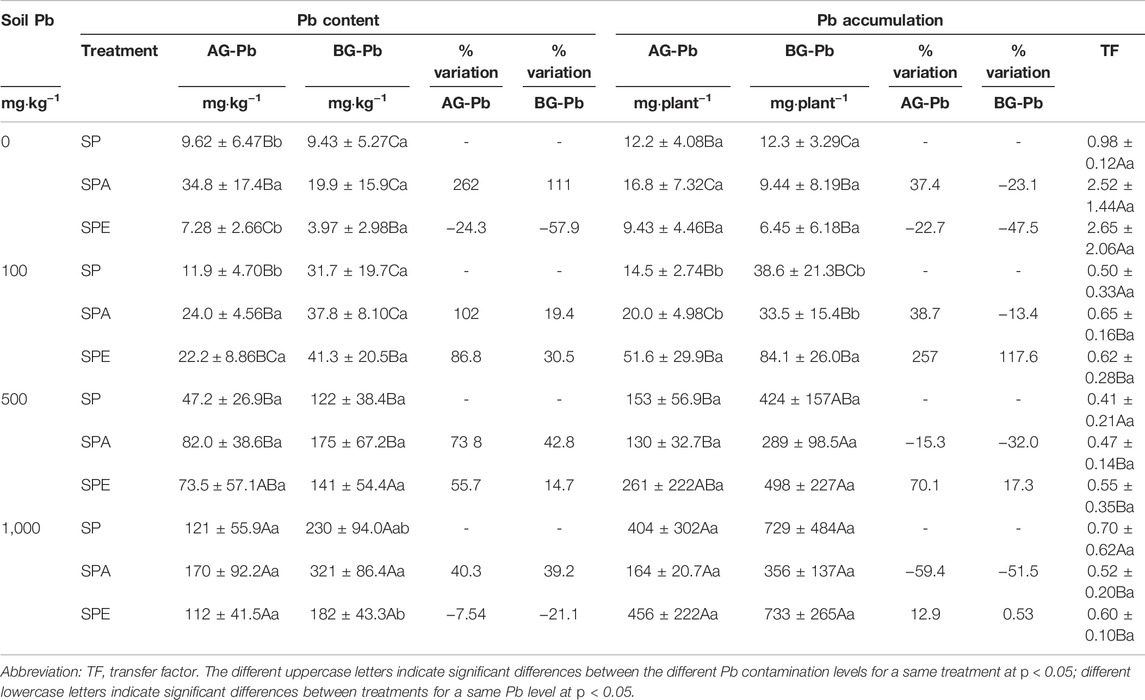
TABLE 4. Accumulations and contents of Pb in the aboveground (AG) and belowground (BG) parts of Brassica campestris.
The concentration of Pb in aboveground plant parts was higher in SPA than in S and SPE regardless of Pb contamination level (Table 4). Compared with SP, the Pb accumulation in SPA was increased by 262% (p < 0.05), 102% (p < 0.05), 74% (p > 0.05), and 40% (p > 0.05) in the aboveground plant parts and by 111% (p > 0.05), 19% (p > 0.05), 43% (p > 0.05), and 39% (p > 0.05) in the belowground plant part at the 0, 100, 500, and 1,000 mg kg−1 Pb levels, respectively.
3.3.3 TF of Pb
The TF index reflects the distribution of Pb between the aboveground and belowground plant parts (Table 4). The TF ranged from 2.65 to 0.41, with significantly higher values at the 0 mg kg−1 Pb level as compared with at the other Pb levels. At the 0 mg kg−1 Pb level, high TF values of 0.98, 2.52, and 2.65 were obtained for SP, SPA, and SPE, respectively. In contrast, the TF values at 100, 500, and 1,000 mg kg−1 Pb were notably low, indicating that a larger proportion of Pb was kept in the roots and prevented from being transferred to the aboveground edible parts when B. campestris was grown in the Pb-contaminated soils.
3.3.4 PCA Analysis of the Plant Parameters
The results of PCA analysis indicated significant differences in the plant growth properties between treatments at the different Pb pollution levels (Figures 4A–C). The first factor extracted (44.4% of the variance explained) showed the influence of Pb addition on plant biomass, leaf area, shoot diameter, plant height, and Pb accumulation and translocation (Figure 4A). Higher Pb contamination levels showed higher plant biomass, leaf area, and Pb accumulation but lower TF values (p < 0.05, Figure 4C). The second factor extracted (20.8% of the variance explained) characterized the influence of earthworm introduction on leaf area, chlorophyll concentration, and Pb accumulation of plants (Figure 4B), showing that earthworm introduction increased leaf area and chlorophyll concentration compared with the respective earthworm-free treatments. The two earthworm species E. fetida, and A. aspergillum showed their interspecific difference in their influences on the growth and Pb accumulation of B. campestris.
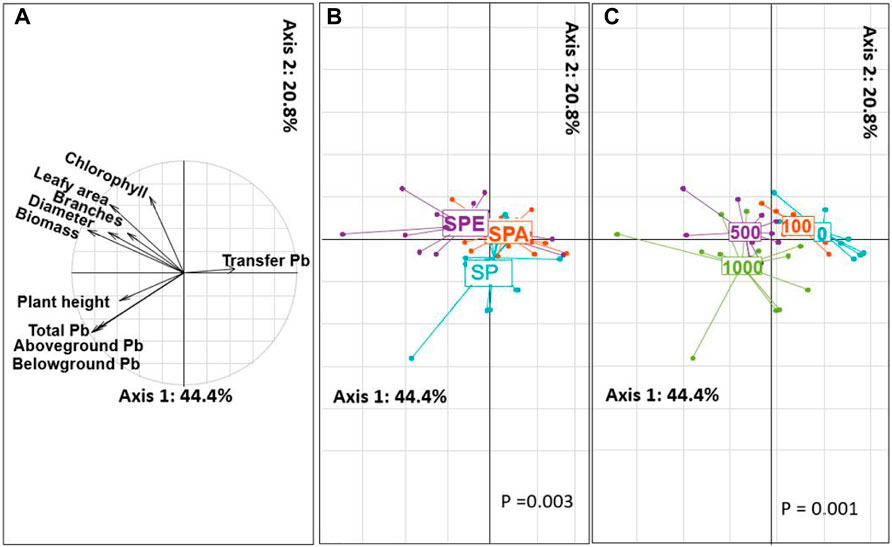
FIGURE 4. Principal component analysis of the plant growth properties in the different treatments. (A) PCA projection of plant growth properties and Pb uptake; (B) Treatments with or without earthworms; (C) Treatments at different Pb contamination levels of 0, 100, 500, and 1,000 mg∙kg-1 Pb; SP: Brassica campestris was grown, SPA: B. campestris was grown and Amynthas aspergillum was inoculated, SPE: B. campestris was grown and Eisenia fetida was inoculated.
3.4 Co-Inertia Analysis of the Soil and Plant Growth Properties
The co-inertia analysis showed that the first axis explained 34.2% of the total variation, and the second axis explained 18.6% of the total variation (Figure 5). Soil bioavailable Pb and CEC were significantly positively correlated with plant Pb accumulation, biomass, leaf area, height, and shoot diameter (Figures 5A,B), which clearly distinguished the treatments between high and low Pb contamination levels (Figure 5C). Compared with the treatments with low Pb contamination levels, those with high Pb contamination levels exhibited higher soil CEC, available Pb content, plant biomass, leaf area, and plant Pb accumulation. In addition, soil Eh was positively correlated with leaf chlorophyll concentration, while soil pH was negatively correlated with leaf chlorophyll concentration. The treatments with earthworm inoculation (SPA and SPE) were separated from those without earthworm inoculation (SP), with the former associated with higher projected values of soil Eh and leaf chlorophyll concentration but a lower projected value of soil pH.
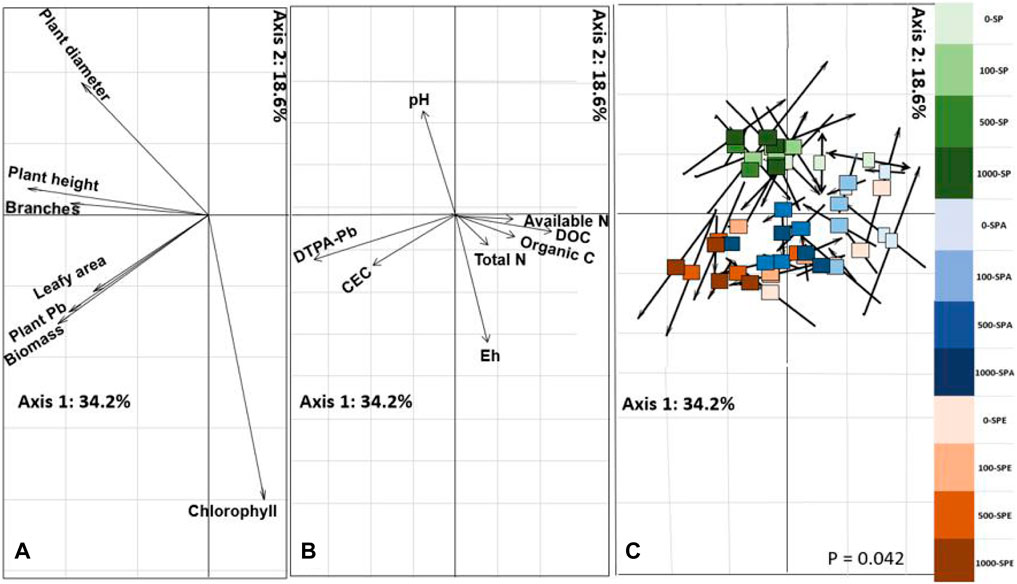
FIGURE 5. Co-inertia analysis between soil properties and plant characteristics in the different treatments. (A) Co-inertia projection of plant growth and Pb uptake properties; (B) Co-inertia projection of soil properties; (C) Score plot of treatments; The squares and arrows represent the projected coordinates of soil physio-chemical properties and plant growth parameters and Pb accumulation of each pot. DOC: dissolved organic carbon; CEC: cation exchange capacity. SP: Brassica campestris was grown, SPA: B. campestris was grown and A. aspergillum was inoculated, SPE: B. campestris was grown and E. fetida was inoculated. Pb contamination levels include 0, 100, 500, and 1,000 mg kg−1 Pb.
4 Discussion
4.1 Earthworm Survival and Pb Accumulation
Previous studies concern the effects of metals on earthworms in terms of loss of weight, mortality, and metal accumulation in tissue (Xiao et al., 2020a; Zhang et al., 2022). until yet, few studies have focused on the differential survival and Pb accumulation between the anecic species A. aspergillum and the epigeic species E. fetida in Pb-contaminated soils.
At all the tested Pb contamination levels in our study, the earthworm species A. aspergillum exhibited a higher survival rate than E. fetida. This earthworm species have been commonly found to survive well in stressful environments. According to Wu et al. (2020b), A. aspergillum survived against soil acidification and aluminum toxicity with a survival rate of 85.8% and an extremely low weight loss rate of 13.4%. In contrast, the biomass and survival rate of E. fetida were low at the tested Pb levels, especially the high Pb levels. Similar findings were reported by Nahmani et al. (2007a) and Sizmur et al. (2011) that the population, biomass, sexual development, and cocoon production of E. fetida were dramatically reduced when the concentrations of heavy metals (e.g., Zn, Cd, Cu, and Pb) in soil exceeded acceptable levels. However, E. fetida had a lower survival rate in treatments that contained plants than those without plants. Our findings could be explained by the possibility that soil Pb and certain compounds in plant root exudates interact to generate toxic molecules that impair E. fetida. For better survival of E. fetida in highly polluted soils, organic matter is often added as a nutrient and energy source (Nahmani et al., 2007b; Zhang et al., 2016; Wu et al., 2020a; Raiesi et al., 2020).
In this study, both earthworm species accumulated Pb in their bodies and accumulated more Pb in the more heavily polluted soils. Studies show that earthworm species differ in feeding preference, behavioral characteristics, and metal bioaccumulation (Hickman and Reid, 2008; Butt and Lowe, 2011; Zhang et al., 2022). Compared with A. aspergillum, E. fetida accumulated more Pb at the 500 and 1,000 mg kg−1 Pb levels. The reason may be because Pb is readily bound to organic matter, and E. fetida requires a large amount of organic matter for food. Therefore, more Pb was accumulated by E. fetida with the ingestion of organic matter. Other researchers reported similar results that E. Veneta, an epigeic species, accumulated significantly more Pb than Lumbricus Terrestris, an anecic species, and Allolobophora chlorotica in 112 days (Sizmur et al., 2011). Earthworm bioaccumulation of Pb can be a useful indicator of soil bioavailable Pb for Pb risk assessment and remediation strategy development (Xiao et al., 2020a; Oorts et al., 2021).
4.2 Effect of Earthworm Introduction on Plant Growth
Pb deactivates critical enzymes, inhibits enzyme activities, detrimentally affects various physiological and biochemical processes, impairs photosynthesis, causes cell death, and leads to leaf chlorosis and stunted growth of plants (Zulfiqar et al., 2019). Earthworm introduction has improved plant growth in Pb-contaminated soils (Wang et al., 2006; Yu et al., 2005a). In a Pb/Zn mine tailings-polluted soil with Pb at 1,202 mg·kg−1, the shoot biomass of Leucaena leucocephala was improved from 30.0 to 34 g after the addition of Pheretima guillelmi (Ma et al., 2006). Similarly, E. fetida was reported to considerably promote the growth of maize (Zea mays) and barley (Hordeum vulgare) in soil contaminated with Cu, Cd, Pb, and Zn (Ruiz et al., 2009). With the introduction of Pontoscolex corethrurus, an endogeic and geophagous earthworm species, to a Pb-contaminated soil (1,000 mg·kg−1 Pb), the shoot biomass of Lantana camara increased from 21.52 to 31.77 g, and its root biomass increased from 19.11 to 24.21 g (Jusselme et al., 2013; Jusselme et al., 2012). In this study, the introduction of earthworms significantly increased the biomass, leaf chlorophyll concentration, and leaf area of B. campestris at the high Pb contamination levels (Table 3; Figure 4). Chlorophyll, a primary pigment of green leafy vegetables, was most affected by earthworm inoculation (Figure 4). The significant relationships between leaf chlorophyll concentration and the soil properties of pH, Eh, and DOC (Figure 5) indicate that the earthworms influenced leaf chlorophyll concentration by increasing soil Eh and DOC content and decreasing soil pH. Soil Eh is an indicator of soil aeration status. Earthworm activity in soil improves soil aeration (higher Eh), which improves plant growth and quality (higher chlorophyll concentration) (Noh and Jeong, 2021). The digestion process of earthworms speeds up the decomposition of soil organic matter (higher DOC) (Zhang et al., 2016), which provides more nutrients for plant growth and results in better plant growth with a higher chlorophyll concentration. Soil microorganisms strongly influence the forming and decomposition of soil organic matter (Sokol et al., 2022). Further studies should be performed to elucidate the underlying mechanism of how earthworms affect plant growth at the molecular level (Jacquiod et al., 2020).
4.3 Effect of Earthworm Introduction on Plant Pb Uptake
The Pb uptake of B. campestris is affected by biotic and abiotic environmental factors (Kaur et al., 2018; Xu et al., 2018; Farahat et al., 2021).
In this study, Pb contamination was the deciding factor in Pb accumulation in the plants (Figure 4). When B. campestris was exposed to high levels of Pb, more Pb was accumulated in the roots, and less Pb was translocated to the aboveground organs (Table 4; Figure 4), consistent with the finding of Zhang Z. et al. (2020). The earthworms affected Pb bioavailability and plant accumulation as well by altering soil properties, increasing DOC, and reducing pH (Figure 5). Some studies indicated the association between the increased bioavailable Pb and the influences of earthworms on soil pH, organic carbon, and DOC (Lemtiri et al., 2016; Nannoni et al., 2014; Wen et al., 2004). The possible explanation is that earthworms may release soluble organic acids, which are responsible for soil pH decrease (Sizmur et al., 2011). Earthworm activities stimulate metal transformation. It was found that available Cd and Zn concentrations in A. morrisi casts were higher than those in the bulk soil, and E. fetida increased the organically bound Pb (Zhang C. et al., 2020). In this study, the inoculation of earthworms significantly affected soil CEC and available Pb content, resulting in higher Pb accumulation in B. campestris and higher biomass and leaf area (Figure 5). Wu et al. (2020b) also found more exchangeable base cations in earthworm casts than in the no-ingested soil. High soil CEC implies high concentrations of such exchangeable base cations as K, Ca, and Mg, which contribute to the increase of plant biomass (Cui et al., 2021). In addition, these cations compete with Pb for binding sites on soil organic matter and oxides, resulting in Pb release into the soil solution and absorption by the plants (Costa et al., 2017; Zhou et al., 2020).
E. fetida increased Pb accumulation in the aboveground and belowground parts of B. campestris by 12.9%–257% and 0.53%–118%, respectively (Table 4). This is mainly due to the increase in plant biomass (Table 3). Kaur et al. (2018) also showed that E. fetida addition mitigated the harmful effects of metals on plant growth, improved photosynthetic efficiency, and boosted metal uptake. Moreover, numerous studies have demonstrated that geophagous earthworms significantly increase Pb uptake of plants by improving Pb availability and plant growth (Ruiz et al., 2011; Ardestani et al., 2019; Zhang C. et al., 2020). In contrast, the introduction of A. aspergillum mainly increased the Pb concentration instead of its accumulation in plants (Table 4). This is due to the higher DTPA-extractable Pb concentration and lower plant biomass in SPA compared with SPE (Figure 2; Table 3). The higher DTPA-extractable Pb concentration in the soil led to higher Pb uptake by the plants, which resulted in the higher Pb concentration in the plants with lower plant biomass. The mechanisms of how different earthworm species influence metal accumulation in plants demand further studies.
5 Conclusion
In this study, the anecic and native earthworm species A. aspergillum presented a higher Pb tolerance than the epigeic species E. fetida, which is crucial for the operation of earthworm-assisted bioremediation techniques in the future. E. fetida had a greater effect on soil CEC and available Pb, while A. aspergillum had a greater effect on soil C and N contents. Inoculation of A. aspergillum mainly increased the Pb concentration rather than total accumulation in plants. These results revealed that different ecological earthworm species have different effects on the biogeochemical cycle of Pb and soil physico-chemical properties. Further studies are required to elucidate the mechanism of how different earthworm species influence soil properties and plant growth under heavy metal contamination conditions. Moreover, the high Pb concentration and accumulation in B. campestris in this short-term (30 days) experiment causes a concern. A hyperaccumulator plant may need to be introduced together with earthworms to heavy metal-contaminated leafy vegetable fields for safe production, which should focus on future studies.
Data Availability Statement
The original contributions presented in the study are included in the article/Supplementary Material, further inquiries can be directed to the corresponding author.
Author Contributions
CT: writing-original draft, methodology, data curation. MZ: methodology, writing-review and editing; HZ: writing–review and editing; LX: writing–review and editing. LW: data curation, investigation. JD: investigation. KL: methodology, formal analysis, data curation, writing review and editing. CZ: conceptualization, methodology, formal analysis, data curation, writing–original draft, writing–review and editing, funding acquisition, project administration.
Funding
Guangdong Natural Science Foundation (Grant No. 2021A1515011543), National Natural Science Foundation of China (Grant Nos. 41201305 and 41601575), National Science and Technology Fundamental Resources Investigation Program of China (2018FY100300) supported this work, in addition to support from the China Scholarship Council.
Conflict of Interest
The authors declare that the research was conducted in the absence of any commercial or financial relationships that could be construed as a potential conflict of interest.
Publisher’s Note
All claims expressed in this article are solely those of the authors and do not necessarily represent those of their affiliated organizations or those of the publisher, the editors, and the reviewers. Any product that may be evaluated in this article, or claim that may be made by its manufacturer, is not guaranteed or endorsed by the publisher.
References
Ali, I., Khan, M. J., Shah, A., Deeba, F., Hussain, H., Yazdan, F., et al. (2022). Screening of Various Brassica Species for Phytoremediation of Heavy Metals-Contaminated Soil of Lakki Marwat, Pakistan. Environ. Sci. Pollut. Res. doi:10.1007/s11356-021-18109-7
Alordzinu, K. E., Li, J., Lan, Y., Appiah, S. A., Al Aasmi, A., Wang, H., et al. (2021). Ground-Based Hyperspectral Remote Sensing for Estimating Water Stress in Tomato Growth in Sandy Loam and Silty Loam Soils. Sensors 21 (17), 5705. doi:10.3390/s21175705
Amacher, M. C. (1996). Nickel, Cadmium, and lead. Methods Soil Anal. Part 3 Chem. Methods 5, 739–768.
Ardestani, M. M., Giska, I., and van Gestel, C. A. M. (2019). The Effect of the Earthworm Lumbricus Rubellus on the Bioavailability of Cadmium and lead to the Springtail Folsomia candida in Metal-Polluted Field Soils. Environ. Sci. Pollut. Res. 26 (27), 27816–27822. doi:10.1007/s11356-019-05969-3
Butt, K. R., and Lowe, C. N. (2011). “Controlled Cultivation of Endogeic and Anecic Earthworms,” in Biology of Earthworms (Berlin, Heidelberg: Springer), 107–121. doi:10.11654/jaes.2016.07.00410.1007/978-3-642-14636-7_7
Chen, X., Wang, X., Gu, X., Jiang, Y., and Ji, R. (2017). Oxidative Stress Responses and Insights into the Sensitivity of the Earthworms Metaphire Guillelmi and Eisenia fetida to Soil Cadmium. Sci. Total Environ. 574, 300–306. doi:10.1016/j.scitotenv.2016.09.059
Cornfield, A. H. (1960). Ammonia Released on Treating Soils with N Sodium Hydroxide as a Possible Means of Predicting the Nitrogen-Supplying Power of Soils. Nature 187 (4733), 260–261. doi:10.1038/187260a0
Cui, X., Mao, P., Sun, S., Huang, R., Fan, Y., Li, Y., et al. (2021). Phytoremediation of Cadmium Contaminated Soils by Amaranthus Hypochondriacus L.: The Effects of Soil Properties Highlighting Cation Exchange Capacity. Chemosphere 283, 131067. doi:10.1016/j.chemosphere.2021.131067
Dai, J., Becquer, T., Henri Rouiller, J., Reversat, G., Bernhard-Reversat, F., Nahmani, J., et al. (2004). Heavy Metal Accumulation by Two Earthworm Species and its Relationship to Total and DTPA-Extractable Metals in Soils. Soil Biol. Biochem. 36 (1), 91–98. doi:10.1016/j.soilbio.2003.09.001
Du, Y.-L., He, M.-M., Xu, M., Yan, Z.-g., Zhou, Y.-Y., Guo, G.-l., et al. (2014). Interactive Effects between Earthworms and maize Plants on the Accumulation and Toxicity of Soil Cadmium. Soil Biol. Biochem. 72, 193–202. doi:10.1016/j.soilbio.2014.02.004
Farahat, E. A., Mahmoud, W. F., and Fahmy, G. M. (2021). Seasonal Variations of Heavy Metals in Water, Sediment, and Organs of Vossia Cuspidata (Roxb.) Griff. In River Nile Ecosystem: Implication for Phytoremediation. Environ. Sci. Pollut. Res. 28, 32626–32633. doi:10.1007/s11356-021-13033-2
Gluhar, S., Kaurin, A., Vodnik, D., Kastelec, D., Zupanc, V., and Lestan, D. (2021). Demonstration Gardens with EDTA-Washed Soil. Part III: Plant Growth, Soil Physical Properties and Production of Safe Vegetables. Sci. Total Environ. 792, 148521. doi:10.1016/j.scitotenv.2021.148521
González Costa, J. J., Reigosa, M. J., Matías, J. M., and Covelo, E. F. (2017). Soil Cd, Cr, Cu, Ni, Pb and Zn Sorption and Retention Models Using SVM: Variable Selection and Competitive Model. Sci. Total Environ. 593-594, 508–522. doi:10.1016/j.scitotenv.2017.03.195
Guo, F., Proctor, G., Larson, S. L., Ballard, J. H., Zan, S., Yang, R., et al. (2022). Earthworm Enhanced Phytoremediation of U in Army Test Range Soil with Indian Mustard and Sunflower. ACS Earth Space Chem. 6 (3), 746–754. doi:10.1021/acsearthspacechem.1c00403
Gupta, S., Nayek, S., Saha, R. N., and Satpati, S. (2008). Assessment of Heavy Metal Accumulation in Macrophyte, Agricultural Soil, and Crop Plants Adjacent to Discharge Zone of Sponge Iron Factory. Environ. Geol. 55 (4), 731–739. doi:10.1007/s00254-007-1025-y
He, J., Reddy, G. V. P., Liu, M., and Shi, P. (2020). A General Formula for Calculating Surface Area of the Similarly Shaped Leaves: Evidence from Six Magnoliaceae Species. Glob. Ecol. Conservation 23, e01129. doi:10.1016/j.gecco.2020.e01129
Hickman, Z. A., and Reid, B. J. (2008). Earthworm Assisted Bioremediation of Organic Contaminants. Environ. Int. 34 (7), 1072–1081. doi:10.1016/j.envint.2008.02.013
Jacquiod, S., Puga-Freitas, R., Spor, A., Mounier, A., Monard, C., Mougel, C., et al. (2020). A Core Microbiota of the Plant-Earthworm Interaction Conserved across Soils. Soil Biol. Biochem. 144, 107754. doi:10.1016/j.soilbio.2020.107754
Jeyanthi, V., Paul, J. A. J., Selvi, B. K., and Karmegam, N. (2016). Comparative Study of Biochemical Responses in Three Species of Earthworms Exposed to Pesticide and Metal Contaminated Soil. Environ. Process. 3 (1), 167–178. doi:10.1007/s40710-016-0131-9
Jusselme, M. D., Miambi, E., Mora, P., Diouf, M., and Rouland-Lefèvre, C. (2013). Increased lead Availability and Enzyme Activities in Root-Adhering Soil of Lantana Camara during Phytoextraction in the Presence of Earthworms. Sci. Total Environ. 445-446, 101–109. doi:10.1016/j.scitotenv.2012.12.054
Jusselme, M. D., Poly, F., Miambi, E., Mora, P., Blouin, M., Pando, A., et al. (2012). Effect of Earthworms on Plant Lantana Camara Pb-Uptake and on Bacterial Communities in Root-Adhering Soil. Sci. Total Environ. 416, 200–207. doi:10.1016/j.scitotenv.2011.10.070
Kaur, P., Bali, S., Sharma, A., Vig, A. P., and Bhardwaj, R. (2018). Role of Earthworms in Phytoremediation of Cadmium (Cd) by Modulating the Antioxidative Potential of Brassica Juncea L. Appl. Soil Ecol. 124, 306–316. doi:10.1016/j.apsoil.2017.11.017
Kumar, A., Kumar, A., M.M.S., C.-P., Chaturvedi, A. K., Shabnam, A. A., Subrahmanyam, G., et al. (2020). Lead Toxicity: Health Hazards, Influence on Food Chain, and Sustainable Remediation Approaches. Ijerph 17 (7), 2179. doi:10.3390/ijerph17072179
Lemtiri, A., Liénard, A., Alabi, T., Brostaux, Y., Cluzeau, D., Francis, F., et al. (2016). Earthworms Eisenia fetida Affect the Uptake of Heavy Metals by Plants Vicia faba and Zea mays in Metal-Contaminated soilsLindsay, W.L., and Norvell, W.A. (1978). Development of a DTPA Soil Test for Zinc, Iron, Manganese, and Copper. Appl. Soil EcologySoil Sci Soc Am J 10442 (3), 67421–78428. doi:10.1016/j.apsoil.2015.11.02110.2136/sssaj1978.03615995004200030009x
Liu, H.-L., Zhou, J., Li, M., Hu, Y.-m., Liu, X., and Zhou, J. (2019). Study of the Bioavailability of Heavy Metals from Atmospheric Deposition on the Soil-Pakchoi (Brassica Chinensis L.) System. J. Hazard. Mater. 362, 9–16. doi:10.1016/j.jhazmat.2018.09.032
Liu, Y., Sun, X., Li, S., Li, S., Zhou, W., Ma, Q., et al. (2020). Influence of green Waste Compost on Pb-Polluted Soil Remediation, Soil Quality Improvement, and Uptake by Pakchoi Cabbage (Brassica Campestris L. Ssp). Environ. Sci. Pollut. Res. 27 (7), 7693–7701. doi:10.1007/s11356-019-07505-9
Ma, Y., Dickinson, N. M., and Wong, M. H. (2006). Beneficial Effects of Earthworms and Arbuscular Mycorrhizal Fungi on Establishment of Leguminous Trees on Pb/Zn Mine Tailings. Soil Biol. Biochem. 38 (6), 1403–1412. doi:10.1016/j.soilbio.2005.10.016
Moral, R., Pedreño, J. N., Gómez, I., and Mataix, J. (1996). Quantitative Analysis of Organic Wastes: Effects of Sample Preparation in the Determination of Metals. Commun. Soil Sci. Plant Anal. 27 (3-4), 753–761. doi:10.1080/00103629609369592
Nahmani, J., Hodson, M. E., and Black, S. (2007b). A Review of Studies Performed to Assess Metal Uptake by Earthworms. Environ. Pollut. 145 (2), 402–424. doi:10.1016/j.envpol.2006.04.009
Nahmani, J., Hodson, M. E., and Black, S. (2007a). Effects of Metals on Life Cycle Parameters of the Earthworm Eisenia fetida Exposed to Field-Contaminated, Metal-Polluted Soils. Environ. Pollut. 149 (1), 44–58. doi:10.1016/j.envpol.2006.12.018
Nannoni, F., Rossi, S., and Protano, G. (2014). Soil Properties and Metal Accumulation by Earthworms in the Siena Urban Area (Italy). Appl. Soil Ecol. 77, 9–17. doi:10.1016/j.apsoil.2014.01.004
Noh, K., and Jeong, B. R. (2021). Increased Carbon Dioxide by Occupants Promotes Growth of Leafy Vegetables Grown in Indoor Cultivation System. Sustainability 13 (23), 13288. doi:10.3390/su132313288
OECD (2000). OECD Guideline for the Testing of Chemicals (Draft Document). Proposal for a New Guideline 227.
Oorts, K., Smolders, E., Lanno, R., and Chowdhury, M. J. (2021). Bioavailability and Ecotoxicity of Lead in Soil: Implications for Setting Ecological Soil Quality Standards. Environ. Toxicol. Chem. 40 (7), 1948–1961. doi:10.1002/etc.5051
Paul, S., Goswami, L., Pegu, R., Kumar Chatterjee, S., and Sundar Bhattacharya, S. (2022). Epigenetic Regulations Enhance Adaptability and Valorization Efficiency in Eisenia fetida and Eudrilus Eugeniae during Vermicomposting of Textile Sludge: Insights on Repair Mechanisms of Metal-Induced Genetic Damage and Oxidative Stress. Bioresour. Tech. 345, 126493. doi:10.1016/j.biortech.2021.126493
Porfido, C., Gattullo, C. E., Allegretta, I., Fiorentino, N., Terzano, R., Fagnano, M., et al. (2022). Investigating Lead Bioavailability in a Former Shooting Range by Soil Microanalyses and Earthworms Tests. Soil Syst. 6 (1), 25. doi:10.3390/soilsystems6010025
Raiesi, F., Motaghian, H. R., and Nazarizadeh, M. (2020). The Sublethal lead (Pb) Toxicity to the Earthworm Eisenia fetida (Annelida, Oligochaeta) as Affected by NaCl Salinity and Manure Addition in a Calcareous clay Loam Soil during an Indoor Mesocosm experiment. Ecotoxicology Environ. Saf. 190, 110083. doi:10.1016/j.ecoenv.2019.110083
Richardson, J. B., Görres, J. H., and Sizmur, T. (2020). Synthesis of Earthworm Trace Metal Uptake and Bioaccumulation Data: Role of Soil Concentration, Earthworm Ecophysiology, and Experimental Design. Environ. Pollut. 262, 114126. doi:10.1016/j.envpol.2020.114126
Romero-Freire, A., Martin Peinado, F. J., and van Gestel, C. A. M. (2015). Effect of Soil Properties on the Toxicity of Pb: Assessment of the Appropriateness of Guideline Values. J. Hazard. Mater. 289, 46–53. doi:10.1016/j.jhazmat.2015.02.034
Ruiz, E., Alonso-Azcárate, J., and Rodríguez, L. (2011). Lumbricus Terrestris L. Activity Increases the Availability of Metals and Their Accumulation in maize and Barley. Environ. Pollut. 159 (3), 722–728. doi:10.1016/j.envpol.2010.11.032
Ruiz, E., Rodríguez, L., and Alonso-Azcárate, J. (2009). Effects of Earthworms on Metal Uptake of Heavy Metals from Polluted Mine Soils by Different Crop Plants. Chemosphere 75 (8), 1035–1041. doi:10.1016/j.chemosphere.2009.01.042
Shi, P., Liu, M., Ratkowsky, D. A., Gielis, J., Su, J., Yu, X., et al. (2019). Leaf Area-Length Allometry and its Implications in Leaf Shape Evolution. Trees 33 (4), 1073–1085. doi:10.1007/s00468-019-01843-4
Sinkakarimi, M. H., Solgi, E., and Hosseinzadeh Colagar, A. (2020). Interspecific Differences in Toxicological Response and Subcellular Partitioning of Cadmium and lead in Three Earthworm Species. Chemosphere 238, 124595. doi:10.1016/j.chemosphere.2019.124595
Sizmur, T., Tilston, E. L., Charnock, J., Palumbo-Roe, B., Watts, M. J., and Hodson, M. E. (2011). Impacts of Epigeic, Anecic and Endogeic Earthworms on Metal and Metalloid Mobility and Availability. J. Environ. Monit. 13 (2), 266–273. doi:10.1039/c0em00519c
Sokol, N. W., Slessarev, E., Marschmann, G. L., Nicolas., A., Blazewicz, S. J., Brodie, E. L., et al. (2022). Soil Microbiome Consortium., Pett-Ridge J., Life and Death in the Soil Microbiome: How Ecological Processes Influence Biogeochemistry. Nat. Rev. Microbiol. doi:10.1038/s41579-022-00695-z
Sparks, D. L., Page, A. L., Helmke, P. A., Loeppert, R. H., Soltanpour, P. N., Tabatabai, M. A., et al. (1996). Part 3-Chemical Methods.Methods of Soil Analysis
Tabelin, C. B., and Igarashi, T. (2009). Mechanisms of Arsenic and lead Release from Hydrothermally Altered Rock. J. Hazard. Mater. 169 (1-3), 980–990. doi:10.1016/j.jhazmat.2009.04.049
Thioulouse, J., Chessel, D., Dole´Dec, S., and Olivier, J.-M. (1997). ADE-4: a Multivariate Analysis and Graphical Display Software. Stat. Comput. 7, 75–83. doi:10.1023/A:1018513530268
Wang, D., Li, H., Wei, Z., Wang, X., and Hu, F. (2006). Effect of Earthworms on the Phytoremediation of Zinc-Polluted Soil by Ryegrass and Indian Mustard. Biol. Fertil. Soils. 43 (1), 120–123. doi:10.1007/s00374-006-0075-8
Wang, G., Wang, L., Ma, F., You, Y., Wang, Y., and Yang, D. (2020). Integration of Earthworms and Arbuscular Mycorrhizal Fungi into Phytoremediation of Cadmium-Contaminated Soil by Solanum nigrum L. J. Hazard. MaterialsJ. Hazard. Mater. 389, 121873. doi:10.1016/j.jhazmat.2019.121873
Wang, K., Qiao, Y., Zhang, H., Yue, S., Li, H., Ji, X., et al. (2018). Bioaccumulation of Heavy Metals in Earthworms from Field Contaminated Soil in a Subtropical Area of China. Ecotoxicology Environ. Saf. 148, 876–883. doi:10.1016/j.ecoenv.2017.11.058
Wen, B., Hu, X.-y., Liu, Y., Wang, W.-s., Feng, M.-h., and Shan, X.-q. (2004). The Role of Earthworms (Eisenia fetida) in Influencing Bioavailability of Heavy Metals in Soils. Biol. Fertil. Soils. 40 (3), 181–187. doi:10.1007/s00374-004-0761-3
Wu, J., Ren, Z., Zhang, C., Motelica-Heino, M., Deng, T., Wang, H., et al. (2020a). Effects of Soil Acid Stress on the Survival, Growth, Reproduction, Antioxidant Enzyme Activities, and Protein Contents in Earthworm (Eisenia fetida). Environ. Sci. Pollut. Res. 27 (27), 33419–33428. doi:10.1007/s11356-019-04643-y
Wu, J., Zhang, C., Xiao, L., Motelica-Heino, M., Ren, Z., Deng, T., et al. (2020b). Impacts of Earthworm Species on Soil Acidification, Al Fractions, and Base Cation Release in a Subtropical Soil from China. Environ. Sci. Pollut. Res. 27 (27), 33446–33457. doi:10.1007/s11356-019-05055-8
Xiao, L., Guan, D., Peart, M. R., Chen, Y., Li, Q., and Dai, J. (2017b). The Influence of Bioavailable Heavy Metals and Microbial Parameters of Soil on the Metal Accumulation in rice Grain. Chemosphere 185, 868–878. doi:10.1016/j.chemosphere.2017.07.096
Xiao, L., Guan, D., Peart, M. R., Chen, Y., and Li, Q. (2017a). The Respective Effects of Soil Heavy Metal Fractions by Sequential Extraction Procedure and Soil Properties on the Accumulation of Heavy Metals in rice Grains and Brassicas. Environ. Sci. Pollut. Res. 24 (3), 2558–2571. doi:10.1007/s11356-016-8028-8
Xiao, L., Li, M.-h., Dai, J., Motelica-Heino, M., Chen, X.-f., Wu, J.-L., et al. (2020a). Assessment of Earthworm Activity on Cu, Cd, Pb and Zn Bioavailability in Contaminated Soils Using Biota to Soil Accumulation Factor and DTPA Extraction. Ecotoxicology Environ. Saf. 195, 110513. doi:10.1016/j.ecoenv.2020.110513
Xiao, L., Zhang, C., Wang, G., Guan, D., Zhang, R., Chen, Y., et al. (2020b). Influencing pathways of soil microbial attributes on accumulation of heavy metals in Brassica (Brassica campestris L. ssp.chinensis var.utilis Tsen et Lee) leaves. Environ. Pollut. 262, 114215. doi:10.1016/j.envpol.2020.114215
Xu, Z.-M., Mei, X.-Q., Tan, L., Li, Q.-S., Wang, L.-L., He, B.-Y., et al. (2018). Low root/shoot (R/S) biomass ratio can be an indicator of low cadmium accumulation in the shoot of Chinese flowering cabbage (Brassica campestris L. ssp. chinensis var. utilis Tsen et Lee) cultivars. Environ. Sci. Pollut. Res. 25 (36), 36328–36340. doi:10.1007/s11356-018-3566-x
Yang, Y., Li, Y., and Zhang, J. (2016). Chemical Speciation of Cadmium and lead and Their Bioavailability to Cole (Brassica Campestris L.) from Multi-Metals Contaminated Soil in Northwestern China. Chem. Speciation Bioavailability 28 (1-4), 33–41. doi:10.1080/09542299.2016.1157005
Yu, X., Cheng, J., and Wong, M. H. (2005a). Earthworm-mycorrhiza Interaction on Cd Uptake and Growth of Ryegrass. Soil Biol. Biochem. 37, 195–201. doi:10.1016/j.soilbio.2004.07.029
Zhang, C., Dai, J., Chen, X., Li, H., and Lavelle, P. (2020a). Effects of a Native Earthworm Species (Amynthas Morrisi) and Eisenia fetida on Metal Fractions in a Multi-Metal Polluted Soil from South China. Acta Oecologica 102, 103503. doi:10.1016/j.actao.2019.103503
Zhang, C., Mora, P., Dai, J., Chen, X., Giusti-Miller, S., Ruiz-Camacho, N., et al. (2016). Earthworm and Organic Amendment Effects on Microbial Activities and Metal Availability in a Contaminated Soil from China. Appl. Soil Ecol. 104, 54–66. doi:10.1016/j.apsoil.2016.03.006
Zhang, M., Jouquet, P., Dai, J., Xiao, L., Du, Y., Liu, K., et al. (2022). Assessment of Bioremediation Potential of Metal Contaminated Soils (Cu, Cd, Pb and Zn) by Earthworms from Their Tolerance, Accumulation and Impact on Metal Activation and Soil Quality: A Case Study in South China. Sci. Total Environ. 820, 152834. doi:10.1016/j.scitotenv.2021.152834
Zhang, Z., Wu, X., Tu, C., Huang, X., Zhang, J., Fang, H., et al. (2020b). Relationships between Soil Properties and the Accumulation of Heavy Metals in Different Brassica Campestris L. Growth Stages in a Karst Mountainous Area. Ecotoxicology Environ. Saf. 206, 111150. doi:10.1016/j.ecoenv.2020.111150
Zhou, D., Ning, Y., Wang, B., Wang, G., Su, Y., Li, L., et al. (2016). Study on the Influential Factors of Cd2+ on the Earthworm Eisenia fetida in Oxidative Stress Based on Factor Analysis Approach. Chemosphere 157, 181–189. doi:10.1016/j.chemosphere.2016.05.045
Zhou, Y., Sherpa, S., and McBride, M. B. (2020). Pb and Cd Chemisorption by Acid mineral Soils with Variable Mn and Organic Matter Contents. Geoderma 368, 114274. doi:10.1016/j.geoderma.2020.114274
Keywords: earthworm, Pb-contaminated soil, leafy vegetable, Pb bioavailability, plant growth
Citation: Tibihenda C, Zhang M, Zhong H, Xiao L, Wu L, Dai J, Liu K and Zhang C (2022) Growth and Pb Uptake of Brassica campestris Enhanced by Two Ecological Earthworm Species in Relation to Soil Physicochemical Properties. Front. Environ. Sci. 10:884889. doi: 10.3389/fenvs.2022.884889
Received: 27 February 2022; Accepted: 04 April 2022;
Published: 27 April 2022.
Edited by:
Jaswinder Singh, Khalsa College, IndiaCopyright © 2022 Tibihenda, Zhang, Zhong, Xiao, Wu, Dai, Liu and Zhang. This is an open-access article distributed under the terms of the Creative Commons Attribution License (CC BY). The use, distribution or reproduction in otherforums is permitted, provided the original author(s) and the copyright owner(s)are credited and that the original publication in this journal is cited, in accordance with accepted academic practice. No use, distributionor;reproduction is permitted which does not comply with these terms.
*Correspondence: Chi Zhang, emhhbmdjaGkyMDEyQHNjYXUuZWR1LmNu; Kexue Liu, MjgyNTc0NDhAcXEuY29t