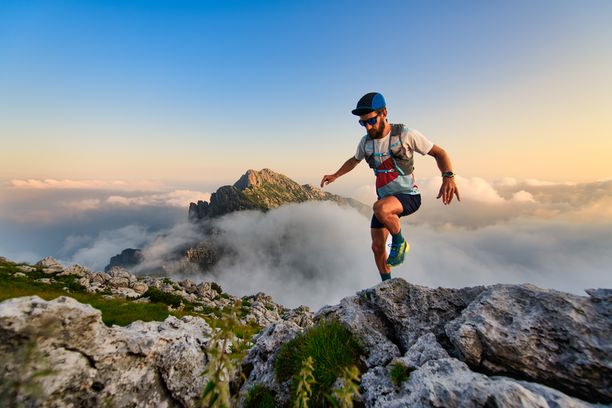
94% of researchers rate our articles as excellent or good
Learn more about the work of our research integrity team to safeguard the quality of each article we publish.
Find out more
ORIGINAL RESEARCH article
Front. Environ. Sci., 10 May 2022
Sec. Soil Processes
Volume 10 - 2022 | https://doi.org/10.3389/fenvs.2022.869194
This article is part of the Research TopicSoil-Plant-Microbe Interactions: An Innovative Approach Towards Improving Soil Health and Plant GrowthView all 26 articles
The northwestern Himalayas (NWH) in India have low rice productivity (∼2 t ha−1) and quality due to poor crop and nutrient management in predominantly Zn-deficient soils. Hence, a field experimentation in the NWH compared the conventionally transplanted rice (CTR) and the system of rice intensification (SRI) under three nutrient management practices (NMPs), viz., 1) farmers’ fertilization practice, FYM @ 5 t ha−1 + N:P2O5:K2O @ 50:40:20 kg ha−1 (FFP); 2) recommended dose of fertilization, FYM @ 10 t ha−1 + N:P2O5:K2O @ 90:40:40 kg ha−1 (RDF); and 3) RDF + Zn fertilization using ZnSO4 @ 25 kg ha−1 (RDF + Zn). The results revealed that SRI practice harnessed a significantly higher rice yield under different NMPs (6.59–8.69 t ha−1) with ∼1.3–1.4- and ∼3.3–4.3-fold enhancements over the CTR and average rice productivity in NWH, respectively. SRI had the greatest improvement in panicle number hill−1 by ∼2.4 folds over the CTR. RDF + Zn had a significantly higher grain (10.7; 7.9%) and straw yield (28.9; 19.7%) over FFP and RDF, respectively, with significant augmentation of Zn biofortification in grains (11.8%) and Zn uptake (23.9%) over the RDF. SRI also enhanced the Zn concentrations in rice grains and straws by ∼4.0 and 2.7% over CTR with respective increases of 36.9 and 25.9% in Zn uptake. The nutrient harvest index and partial factor productivity of applied nutrients (NPK) had a higher magnitude under SRI and RDF + Zn over their respective counterparts, i.e., CTR and RDF. In addition, SRI had higher AE-Zn, CRE-Zn, and PE-Zn to the tune of 119.6, 63.4, and 34%, respectively, over the CTR. Overall, SRI coupled with RDF + Zn in hybrid rice assumes greater significance in enhancing the rice productivity with better Zn-biofortified grains besides higher nutrient use efficiencies to combat widespread malnutrition and acute Zn deficiencies in humans and livestock in the northwestern Himalayas.
Rice (Oryza sativa L.) is an important food crop of Asia where it provides ∼35–80% of the total calorie intake (Pooniya et al., 2019). Again, in India, rice is the staple food to tackle the widespread malnutrition and hunger in the nation (Narayanan, 2017), where it provides ∼43% of the calorie requirement for more than 70% of the Indian population (Choudhary and Suri, 2018a; 2018b). Demographic projections reveal that India would require ∼130 million ton (mt) rice by 2025 (Rao, 2012), with ∼14 mt additional rice to the current levels with an annual hike of ∼3 mt year−1 to ensure national food and nutritional security vis-à-vis achieving the United Nations’ Sustainable Development Goals (SDGs). The Indo–Gangetic plain region (IGPR), the food bowl of India, has been the major contributor in the nation’s rice production (Biswakarma et al., 2021). However, the conventional rice–wheat cropping system followed for over 6 decades in IGPR is already facing acute decline in factor productivity and yield stagnation owing to acute nutrient deficiencies, nutrient mining, poor soil health, and degradation of natural resources. (Singh P. et al., 2021, Singh et al., 2021 U.; Biswakarma et al., 2021; Harish et al., 2022; Pooniya et al., 2022). The IGPR also faces severe water shortage due to poor and uneven rainfall patterns and depleting groundwater table (Heba et al., 2021; Rajpoot et al., 2021; Kumar et al., 2021, 2022), thus posing a threat to rice sustainability owing to its high water requirement ∼1,566–2,262 mm under conventionally transplanted rice (CTR) (Dass et al., 2017). These resource and production vulnerabilities in the IGPR have made India to focus on non-conventional rice areas such as the northwestern Himalayas (NWH) and northeastern India. However, the non-use of high-yielding cultivars/hybrids, poor crop and nutrient management, and inappropriate water management practices have again led to stagnant rice yield in the Indian sub-continent in general and in the NWH (∼2 t ha−1) in particular (Choudhary and Suri, 2018a, 2018b; Kakraliya et al., 2018; Singh P. et al., 2021). In order to boost the rice production in the NWH region (Himachal Pradesh, Uttarakhand, and Jammu and Kashmir provinces), we have to rely on high-yielding rice hybrids coupled with efficient crop and nutrient management practices (Adhikari et al., 2018; Choudhary and Suri 2018a, 2018b).
During the last 2 decades, Asian rice systems have undergone many technological breakthroughs to boost rice production (Barison and Uphoff 2011). One such technological innovation has been a shift from CTR to SRI for more rice with less water (Adhikari et al., 2018). The SRI technique utilizing ∼50% less seed and ∼25–50% less irrigation water than CTR (Choudhary et al., 2010) has greatly enhanced the rice productivity in various agro-ecologies across the globe (Stoop et al., 2002; Latif et al., 2005; Uphoff 2010; Barison and Uphoff 2011; Sharif 2011; Styger et al., 2011; Dass et al., 2016a; Thakur and Uphoff 2017; Uphoff 2017; Choudhary and Suri 2018a; and Adhikari et al., 2018). Under SRI, transplanting single young seedlings (8–15 days old, two to three leaf stage) in 25 × 25 cm wider square spacing immediately after uprooting minimizes the transplant shock and also reduces the initial inter-plant competition for light, space, nutrients, and water, later resulting in a prolific root–shoot system (Dass et al., 2016a) and improved soil microbiome and nutrient bio-availability (Thakur et al., 2010; Dass et al., 2017), leading to profuse tillering with greater yields (Kassam et al., 2011; Adhikari et al., 2018). Another game-changing technology has been the hybrid rice cultivation (Yamauchi 1994; Choudhary and Suri 2018a, 2018b). Rice hybrids possess vigorous root and shoot systems with higher yields compared to conventional varieties (Yamauchi 1994). Hybrid rice cultivation under SRI may further result in a more robust root and shoot system with better yield traits due to their genetic make-up and favorable soil microbiome and nutrient bio-availability under SRI as reported by various researchers (Thakur et al., 2010; Veeramani and Singh 2011; Wu and Uphoff 2015; Dass et al., 2016a, 2017; and Choudhary and Suri 2018a, 2018b). Rice grain yield is a quantitative trait characterized by low heritability and a high genotype × environment (G × E) interaction (Farooq et al., 2009). Hence, it is pertinent to use high-yielding genotypes and rice hybrids with efficient crop and nutrient management to harness the benefits of SRI innovation (Stoop et al., 2009; Choudhary and Rahi, 2018). As rice hybrids are nutrient-exhaustive, it inevitable to revisit their fertilizer management schedules both for CTR and SRI methods of rice farming (Styger et al., 2011; Dass et al., 2016a; Choudhary and Suri 2018a). Thus, the SRI technology coupled with rice hybrids under appropriate nutrient management practices primarily essential for rice hybrids may hold the key to harness their full benefits (Choudhary and Suri 2018b). Already, the conventional rice–wheat cropping system of north-west India including the NWH followed for over 6 decades is facing an acute decline in factor productivity, food quality, yield stagnation owing to acute nutrient deficiencies, nutrient mining, poor soil health, and degradation of natural resources. (Choudhary and Suri 2014; Paul et al., 2014, 2016; Sharma et al., 2020; Singh et al., 2020, Singh et al., 2021 U.; Harish et al., 2022; Pooniya et al., 2022).
Furthermore, most parts of the rice-dominated north-west India and NWH are facing a widespread zinc (Zn) deficiency causing numerous health risks to both humans and animals (Heba et al., 2021; Sharma et al., 2021). Ozkutlu et al. (2006) reported that Zn deficiency may cause yield losses by ∼40% in various field crops in Turkey. Likewise, Zn nutrition holds prime importance in rice farming as it induces drought tolerance and improves plant–water relations and photosynthesis due to better stomatal regulation and cell membrane stability (Hassan et al., 2020; Heba et al., 2021; Kumar et al., 2021). Zn nutrition also enhances the protein content due to its vital role in tryptophan amino acid and protein biosynthesis (Hanafy-Ahmed et al., 2012; Kumar et al., 2021). Hence, Zn fertilization may play a vital role in enhancing the crop productivity, quality, and Zn biofortification in the field crops (Hussain et al., 2010, 2012; Kumar et al., 2021, 2022; Bana RC. et al., 2022, Bana et al., 2022 RS.). As earlier stated, the NWH soils are Zn deficient (Sharma et al., 2021). Likewise from the viewpoint of curtailing the malnutrition and hunger in the NWH (Rasul et al., 2018; FAO 2019), it is again essential to devise Zn-imbedded nutrient management practices to harvest more rice with enhanced quality and better Zn-biofortified grains. In alluvial soils of north Indian plains, soil application of ZnSO4 @ 25 kg ha−1 have been proved to be highly beneficial in enhancing the rice productivity and Zn-biofortification in rice grains (Pooniya et al., 2012, 2019). Hence, the soil application of Zn may also prove equally effective in hybrid rice in the NWH. Likewise, the performance of the SRI technique is also reported to be favorably influenced by the organic manure additions (Stoop et al., 2009; Choudhary and Suri 2018a). As rice hybrids are nutrient-exhaustive (Dass et al., 2017), it inevitable to revisit their fertilizer management schedules both for CTR and SRI to ensure higher productivity and soil-health sustenance (Styger et al., 2011; Dass et al., 2016a; and Choudhary and Suri 2018a). However, pertinent information on the comparative performance of rice hybrids under CTR and SRI under such efficient nutrient management schedules is entirely lacking for the NWH region. Therefore, the current study assessed the influence of three nutrient management practices under CTR and SRI with respect to rice productivity, grain quality, Zn-biofortification, and nutrient-use efficiency to tackle widespread malnutrition and Zn-deficiency in the Himalayan region; besides abridging the yield gaps when we are outbidding to ensure the country’s food and nutritional security targets by 2025.
The present investigation was conducted during Kharif 2010–2013 in Himachal Pradesh, a northwestern Himalayan state of India. The Mandi district [31°13′20″–32°04′30″N latitude; 76°37′20″-77°23′15″ E longitude; 700–4,000 m altitude] of Himachal Pradesh geographically located centrally in the state and representing the wet-temperate agro-climatic conditions of the whole NWH region of India was selected as the study area (Figure 1). This district also constitutes the major rice producing district of Himachal Pradesh in terms of acreage and production (Figure 2A), besides falling under the rice suitability zone of the state (Figure 2B); hence, qualified for selecting as the study area for the current experimentation in the NWH. For carrying out the study during Kharif 2010–2013 in the study area, 05 rice-dominated Community Developmental Blocks (CDB) of Mandi district (Sundernagar, Balh, Sadar, Gopalpur, and Karsog) in Himachal Pradesh were selected randomly. Thereafter, 10 representative villages/locations/farmers’ fields having irrigation facility were selected randomly in these 6 CDBs to continuously conduct the field experimentation during 2010–2013. For this purpose, farm soils having medium nutrient status with respect to available nitrogen (N), phosphorus (P2O5), and potassium (K2O) were selected after analytical scrutiny (Table 1). These soils were silty-clay loam in texture, acid Alfisol in nature with high soil organic carbon (SOC), while DTPA extractable-Zn ranged between 0.59–0.68 mg kg−1 soil (Table 1). The response of rice to Zn was expected in these soils as the critical level of DTPA-extractable Zn in north India varies from 0.38 to 0.90 mg kg−1 soil (Takkar and Walker 1993; Heba et al., 2021). Rainfall and temperature data was recorded at “Agro-Meteorological Observatory” of CSKHPKV, Farm Science Centre, Sundernagar, India (Supplementary Figure S1). The study area receives an average annual rainfall of 1,700 mm, ∼75% of which is received during July to September, and the rest is received during December to February. The hottest months are May to July with the mean daily maximum temperature ranging between 32 and 35°C, whereas December to February are the coldest months, with a mean daily minimum temperature ranging between 2.6 and 3°C.
FIGURE 1. Maps of India and the Himachal Pradesh province showing the location of Mandi district, Himachal Pradesh, and India (study area).
FIGURE 2. Map of the Himachal Pradesh province of India showing (A) major/moderate rice producing areas and (B) rice suitability areas (Graphics Source: GIS Centre, CSKHPKV, Palampur, India).
TABLE 1. Physico-chemical properties of experimental soils at the initiation of field experimentation in the wet-temperate northwestern Himalayas.
In the current study, two crop establishment methods (CEMs) of rice cultivation, viz., conventionally transplanted rice (CTR) and system of rice intensification (SRI), were considered as factor A, and three nutrient management practices (NMPs), viz., 1) farmers’ fertilization practice (FFP), i.e., FYM @ 5 t ha−1 + N:P2O5:K2O @ 50:40:20 kg ha−1 (FYM5+N50P40K20); 2) recommended dose of fertilization (RDF), i.e., FYM @ 10 t ha−1 + N:P2O5:K2O @ 90:40:40 kg ha−1 (FYM10 + N90P40K40); and 3) recommended dose of fertilization + Zn-fertilization (RDF + Zn), i.e., FYM @ 10 t ha−1 + N:P2O5:K2O @ 90:40:40 kg ha−1+ ZnSO4 @ 25 kg ha−1 (FYM10 + N90P40K40 + Zn25), constituted as factor B, making six treatment combinations with a gross plot size of 400 m2 under each treatment at 10 locations in the NWH. Field experimentation was conducted under factorial randomized block design (FRBD); where six treatments were randomized logically for an analysis of variance (ANOVA) while considering 10 locations as 10 replications (Choudhary and Suri 2018a; Choudhary et al., 2021). Since the temperature in NW Himalayas after rice flowering goes down, long duration rice hybrids do not perform well. The short and medium duration rice hybrids can thrive well in wet-temperate NWH; hence, a promising medium-duration hybrid (Arize-6129) was selected as the test cultivar/hybrid for current experimentation. This rice hybrid (Arize-6129) was sown in the third week of May in the case of CTR and in the first week of June in the case of SRI during 2010–2013 in nursery plots at respective locations following CTR (CSKHPKV 2011) and SRI principles (Choudhary and Suri 2018a) (Table 2). The 30- and 15-day 4) old seedlings were then transplanted on the same dates in the third week of June during 2010–2013 both in CTR and SRI in their respective plot locations−1 at 20 × 15 cm and 25 × 25 cm spacing using two to three seedlings hill−1 and single seedling hill−1 in CTR and SRI, respectively (Table 2; Supplementary Figure S2).
TABLE 2. Crop management followed under conventionally transplanted rice (CTR) and SRI in the northwestern Himalayas, India.
Nutrient management was done strictly as per the treatment plan. Well-rotten FYM was added in respective treatments on fresh weight basis (35% moisture on an av.) during land preparation, which contained N, P, K, and Zn to the tune of 0.81, 0.45, 0.65% and 42.1 mg kg−1 (on oven dry-weight basis), respectively. The 1/3rd N and entire P, K, and Zn doses were applied basally at puddling time in the rice through urea (46% N), single super phosphate (16% P2O5), muriate of potash (60% K2O), and Zn-sulfate heptahydrate (21% Zn), respectively; while the remaining 2/3rd N was applied through broadcasting in two equal splits at maximum tillering and flowering stages following the treatment plan. Weeds were controlled by using Pretilachlor @ 0.75 kg a.i. ha−1 both in CTR and SRI. Two hand-weeding (HW) was done in CTR at 15–20 DAT and 30–35 DAT. In the SRI method, two mechanical weeding operations (at 10–12 and 20–22 DAT) using a manually-operated country-made cono-weeder in both directions followed by one HW (30–35 DAT) (Table 2), were performed to control the weeds, to add weed biomass into soil, and to promote the rhizosphere aeration (Choudhary and Suri 2018a). In case of CTR, continuous water standing was kept during the vegetative phase through flood irrigation. Under SRI, keeping in view the monsoon rains, the off and on irrigation scheduling was done at 3-day after the disappearance of ponded water (DADPW) to maintain saturation up to the panicle initiation stage so as to promote the aerobic soil conditions by alternate wetting and drying (AWD) (Supplementary Figure S2). However, right from panicle emergence to 10-days before crop maturity, a shallow submergence (2 cm) was continuously maintained in all the plots. The plots were also drained before N top-dressing and 1 week before harvest if it rained. Both CTR and SRI plots received uniform plant protection practices throughout the cropping season.
For recording the number of tillers hill−1 and the number of panicles m−2; three observational units of 1 m row length each were selected randomly for counting from the net-plots and the mean value was converted into the number of panicles hill−1, and the number of panicles m−2. Plant height and the panicle-length measurements were done from 10 randomly selected tagged plants in the net-plot area at the time of harvest. Samples were drawn from the rice grains produced from the net-plot after weighing, and the 1,000-grain weight was determined at 14% moisture content. Rice crop was harvested from each farm plot, dried in the sun, threshed, and then weighed. The rice grain, straw, and biological yield were determined using standard procedures (Rana et al., 2014), and expressed as t ha−1. Grain yield was expressed at 14% moisture content.
Plant samples of rice grains and straw collected from all the net-plots just after the crop harvest from different locations were air-dried and then dried in an hot air oven at 60 ± 2°C for 6–8 h. These dried plant samples were ground in a Macro Willey-Mill fitted with stainless steel parts and passed through a 40 mesh sieve and then subjected to chemical analysis for NPK and Zn. Plant samples and FYM both were analyzed for total N using the Kjeldahl digestion unit, while total P and K were determined using di-acid digestion [4:1 ratio of HNO3 and HClO4 (v/v)] as per standard procedure (Rana et al., 2014). The protein content (%) in grains was determined by multiplying respective grain–N content (%) by a factor 6.25. The respective N, P, and K uptakes (kg ha−1) were determined by multiplying grain and straw yield (kg ha−1) with their respective grain and straw nutrient concentrations (%) as follows:
Zn content in both rice grains and straw [g kg−1 dry matter (DM)] were determined after di-acid digestion [4:1 ratio of HNO3 and HClO4 (v/v)] of the above Macro Willey-Mill ground samples and then estimated using an atomic absorption spectrophotometer (Rana et al., 2014). The Zn uptake (g ha−1) was determined by multiplying grain and straw yield (kg ha−1) with their respective grain and straw nutrient concentrations (g kg−1 DM) as follows:
Nutrient harvest index of applied nutrients (N, P, K, and Zn) were computed by the following equation as suggested by Fageria and Baligar (2003):
where NHI, PHI, KHI, and ZnHI refer to nitrogen harvest index, phosphorus harvest index, potassium harvest index, and zinc harvest index, respectively. GUN/P/K/Zn refers to respective N/P/K/Zn uptake (kg or g ha−1) in grains, while UN/P/K/Zn refers to the respective total N/P/K/Zn uptake (kg or g ha−1) both in rice grains and straw in respective N/P/K/Zn applied plots, both through chemical fertilizers and FYM.
Partial factor productivity (PFP) of applied nutrients (N, P and K) as PFPn/PFPP/PFPk (kg ha−1 kg−1 of applied N/P/K) were calculated by computing the total applied nutrients (N/P/K) both through chemical fertilizers and FYM as suggested by Fageria and Baligar (2003) hereunder:
Where PFPn, PFPP, and PFPk refer to the partial factor productivity (PFP) of the applied N, P, and K, respectively. Yt refers to grain yield (kg ha−1) of rice while Na, Pa, Ka refer to respective N, P, or K applied (kg ha−1) both through chemical fertilizers and FYM.
Agronomic efficiency (AE–Zn), crop recovery efficiency (CRE–Zn,) and physiological efficiency (PE–Zn) of applied-Zn were computed by the following equations as suggested by Fageria and Baligar (2003):
where YZn and Y0 refer to grain yield (kg ha−1) in Zn-applied and non-Zn-applied plots/treatments, respectively. FZn refers to fertilizer-Zn applied (kg ha−1) which worked out to be 5.25 kg ha−1 in ZnSO4 supplied plots both under CTR and SRI. UZn and U0 refer to the total Zn uptake (kg ha−1) both in rice grains and straw in Zn-applied and non-Zn-applied plots/treatments, respectively. Here, the AE-Zn, CRE-Zn, and PE-Zn were worked out for Zn-applied treatment, i.e., recommended dose of fertilization + Zn fertilization (RDF + Zn: FYM10 + N90P40K40 + Zn25), and non-Zn-applied treatment, i.e., recommended dose of fertilization (RDF: FYM10 + N90P40K40), in the current study.
The experimental design was factorial randomized block design (FRBD) replicated 10 times (considering ten locations as the replications) and the statistical analysis was done by the standard procedure suggested by Gomez and Gomez (1984). Significance of differences among different treatments was tested using the standard F-test. Least significance difference (LSD) values at p = 0.05 were used to determine the significant differences between treatment means.
In general, the growing conditions at all the experimental locations were favorable for rice crop during all the 4 years (2010–2013) with an average annual rainfall of 1,503 mm across the four cropping seasons, except during the June months of 2010 and 2012, and the September month of 2013 which received a relatively scanty rainfall of 60, 90, and 72 mm, respectively (Supplementary Figure S1). About 80% of annual rainfall was received through the south-west monsoons during the fourth week of June to mid-September. During the cropping seasons of 2010–2013, the hottest month was June followed by July with the mean daily maximum temperature ranging between 28–37°C, whereas the mean daily minimum temperature ranged between 11–23°C. This set of production environments is highly congenial for yield expression of tested rice hybrid Arize-6129 in the NW Himalayas.
Plant height as well as the yield contributing characters of rice at harvest viz., number of panicles hill−1, panicles m−2, panicle length, and 1,000-grain weight remained significantly (p < 0.05) higher under SRI compared to the CTR crop establishment method (CEM) in the 4-year study with greatest improvement of ∼2.4 folds in the number of panicles hill−1 while other yield attributes were augmented by 11.5–23.9% under SRI (Table 3). Among different nutrient management practices (NMPs), the recommended dose of fertilization (RDF) + Zn-fertilization (RDF + Zn), i.e., FYM @ 10 t ha−1 + N:P2O5:K2O @ 90:40:40 kg ha−1 + ZnSO4 @ 25 kg ha−1 (FYM10 + N90P40K40 + Zn25) significantly (p < 0.05) enhanced the growth and yield attributes over the second best treatment supplied with the recommended dose of fertilization (RDF), i.e., FYM @ 10 t ha−1 + N:P2O5:K2O @ 90:40:40 kg ha−1 (FYM10 + N90P40K40), and the least performer, i.e., farmers fertilization practice (FFP) was supplied with 5 t FYM ha−1 + N:P2O5:K2O @ 50:40:20 kg ha−1 (FYM5+N50P40K20) in our current study. In general, RDF + Zn exhibited an increase of ∼4.4–18.3% enhancement in the yield attributes viz., panicles hill−1, panicles m−2, panicle length, and 1,000-grain weight compared to RDF; while RDF had an enhancement of 4.2–12.8% in these attributes over FFP (Table 3).
TABLE 3. Effect of different crop establishment methods (CEMs) and nutrient management practices (NMPs) on the growth and yield attributes of rice (4 years av.).
The CTR and SRI crop establishment methods (CEMs) of rice had a significant (p < 0.05) effect on the rice grain and straw yield. Across the years, rice grain yield ranged between 5.62–5.95 and 7.34–7.90 t ha−1 while straw yield ranged between 8.23–8.52 and 9.89–10.55 t ha−1 under CTR and SRI, respectively (Figures 3A,C). Likewise among NMPs, the Zn-imbedded treatment RDF + Zn consistently and significantly (p < 0.05) outperformed over RDF and FFP, with grain and straw yields ranging between 7.27–7.65 and 9.90–10.34 t ha−1, respectively (Figures 3B,D). Furthermore, the significant (p < 0.05) CTR vs. NMPs and SRI vs. NMPs interaction effects throughout the 4-year experimentation revealed that the CTR coupled with RDF + Zn could hardly produce higher grain and straw yield to the tune of 6.22–6.52 and 8.85–9.17 t ha−1, respectively (Figures 4A,C). However, compared to CTR, the SRI considerably enhanced the rice productivity under all NMPs with a significantly (p < 0.05) greater grain and straw yield under RDF + Zn to the tune of 8.32–8.88 and 10.94–11.58 t ha−1, respectively (Figures 4B,D). It was also noticed that the ill-distributed early monsoon rains and comparatively higher temperature during Kharif 2012 (Supplementary Figure S1), accounted for comparatively least rice grain and straw yields in the year 2012 over the normal rainfall rice seasons of Kharif 2010, 2011, and 2013 under all the CEMs and NMPs (Fig. 3, 4). The 4-year pooled data showed that the SRI had significantly (p < 0.05) higher grain (7.65 t ha−1), straw (10.29 t ha−1), and biological yield (17.95 t ha−1), and the harvest index (42.6%) over the CTR, with respective increases of 31.4, 22.5 and 26.2% in grain, straw, and biological yield over CTR (Table 4). The RDF + Zn consistently had the significant (p < 0.05) and greatest grain (7.54 t ha−1), straw (10.15 t ha−1) and biological yield (17.69 t ha−1), and harvest index (42.5%); which was followed by RDF and FFP, respectively. On an average, the RDF + Zn had 10.7, 7.87, and 9.1%; and 28.9, 19.7, and 23.4% higher grain, straw, and biological yield compared to FFP and RDF, respectively (Table 4).
FIGURE 3. Four years’ rice grain (A,B) and straw (C,D) yield trend of the rice under different CEMs and NMPs. The vertical bars indicate the LSD at p = 0.05.
FIGURE 4. Four years’ rice grain (A,B) and straw (C,D) yield trend under the CTR vs. NMPs, and SRI vs. NMPs. The vertical bars indicate the LSD at p = 0.05.
TABLE 4. Effect of different CEMs and NMPs on the grain, straw, and biological yield and harvest index of rice (4 years av.).
In general, the SRI proved superior to CTR with respect to (w.r.t.) NPK acquisition (pooled values) in rice grains and straw in the 4-year study (Figure 5). Between the two methods, SRI management for the same NMP level gave significantly (p < 0.05) larger concentrations of all nutrients (NPK) relative to the effects of CTR except for straw P content. Both in CTR and SRI, the NPK concentrations in rice grains and straw exhibited a consistent improvement under different NMPs following the trend of RDF + Zn > RDF > FFP, where RDF + Zn proved significantly (p < 0.05) superior to FFP but statistically at par to RDF, for NPK concentrations both in grains and straw. Among NMPs, the N, P, and K content in rice grains both under CTR and SRI varied between 1.261–1.345 and 1.319–1.373%; 0.319–0.331 and 0.322–0.342%; and 0.294–0.342 and 0.326–0.363%, respectively with highest grain NPK content under RDF + Zn under both CEMs (Figure 5).
FIGURE 5. NPK concentrations (%) in rice grains and straw as influenced by different (A) CEMs and (B) NMPs (4 years av.). The vertical bars indicate the LSD at p = 0.05. The “ns” represent the non-significant differences at p = 0.05.
Between the two CEMs, the N, P, and K uptakes both in rice grains and straw and the total NPK uptake (grains + straw) showed a significant (p < 0.05) variation with higher pooled values under SRI (Figures 6A–C). This was reflected in the higher concentrations of these elements in their respective plant parts, i.e., grains and straw. In general, SRI management had a higher grain-N and total-N uptake (103.2; 171.3 kg ha−1), P (25.45; 31.0 kg ha−1), and K uptake (26.4; 124.3 kg ha−1) with respective enhancements of 36.3 and 32.6%, 34.4 and 33.3%, and 42.7 and 28.7%, respectively, over CTR. Furthermore, the NMPs exhibited a consistent and significant (p < 0.05) increase in NPK uptake in rice grains and straw and a total uptake with the trend of RDF + Zn > RDF > FFP. Among NMPs, the RDF + Zn had an higher total N, P, and K uptake by 34.6, 35.1, and 12.5%; and 13.8, 13.7, and 6.43% over the FFP and RDF, respectively, in the 4-year study (Fig. 6a, 6b, 6c).
FIGURE 6. Influence of different CEMs and NMPs on (A) N uptake, (B) P uptake, (C) K uptake, and (D) Zn uptake in rice grains and straw and total nutrient uptake, and respective nutrient harvest indices (4 years av.). The vertical bars indicate the LSD at p = 0.05.
It was noticed that the SRI plots had a significantly (p < 0.05) higher protein content (8.41%) in rice grains and the protein yield (644.9 kg ha−1) compared to that of CTR (Table 5). Among NMPs, the RDF + Zn eventually resulted in protein-rich grains with a significantly (p < 0.05) higher protein content (8.49%) and protein yield (641.9 kg ha−1) over RDF and FFP. Averaged over 4 years, the SRI significantly raised the protein yield by 40.5% over CTR, while RDF + Zn raised it by 35.6 and 14.2% compared to FFP and RDF, respectively. Hence, the SRI coupled with RDF + Zn may prove as a boon to combat the protein malnutrition through this intervention in NWH.
TABLE 5. Effect of different CEMs and NMPs on the protein content in grains, protein yield, and Zn concentration in rice grains and straw (4 years av.).
Between the two methods, SRI gave significantly (p < 0.05) larger Zn concentrations (pooled values) both in rice grains (31.1 mg kg−1 DM) and straw (52.6 mg kg−1 DM) with respective enhancements of 4.0 and 2.7% over the CTR (Table 5). Among NMPs, the application of RDF + Zn proved highly beneficial for Zn biofortification of rice grains and straw both over RDF and FFP, respectively (Table 5). Both under CTR and SRI, the RDF + Zn nutrition expressed significantly (p < 0.05) higher Zn biofortification in rice grains (33.2 mg kg−1 DM) and straw (54.7 mg kg−1 DM), on an average, higher by 15.3 and 11.8% in grains; and 9.6 and 6.6% in straw over FFP and RDF, respectively (Table 5). RDF also had significantly (p < 0.05) higher Zn accumulation in grains and straw by 3.1 and 2.8%, respectively, over FFP irrespective of the CEMs. Furthermore, the Zn uptake in rice grains, straw, and the total Zn uptake was significantly influenced by the CEMs and NMPs (Figure 6D). SRI was perceptibly the best performer for augmenting the Zn biofortification with a significantly (p < 0.05) greater Zn uptake in grains (239.9 g ha−1), straw (543.4 g ha−1), and the total uptake (783.3 g ha−1) with respective increases of 36.9, 25.9, and 29.1% over the CTR. The RDF + Zn significantly recorded (p < 0.05) the greatest Zn uptake in grains (251.3 g ha−1), straw (555.9 g ha−1), and total uptake (807.2 g ha−1), on an average, higher by 23.9, 15.0, and 17.6%, respectively, over the RDF; while FFP had the least Zn uptake.
The nitrogen harvest index (NHI), phosphorus harvest index (PHI), potassium harvest index (KHI), and Zn harvest index (ZnHI) had significantly (p < 0.05) higher magnitude (pooled data) under SRI compared to CTR (Figures 6A–D). Among NMPs, there was a consistent but non-significant increase in NHI (59.3–59.6%) while KHI (19–21.3%) had significant (p < 0.05) increase right from FFP to RDF and then to RDF + Zn application (Figures 6A,C). It was interesting, but not surprising that the PHI reported significantly (p < 0.05) higher values at RDF; thereafter, it showed a non-significant decline using RDF + Zn (Figure 6C), most probably a P×Zn antagonistic effect. Nonetheless, the ZnHI showed a consistent and significant (p < 0.05) improvement (28.4–31%) right from FFP to RDF + Zn (Figure 6D). Partial factor productivity (PFP) of applied N (PFPn), P (PFPp), and K (PFPk) showed a significant (p < 0.05) variation both under CEMs and NMPs (Figure 7). In general, the PFPn, PFPp, and PFPk were significantly higher under SRI cumulatively by 30.9–31.3% compared to CTR. Meanwhile, the FFP had significantly higher PFPn, PFPp, and PFPk, all of which then declined significantly under RDF, and again showed a slight improvement under RDF + Zn (Figure 7).
FIGURE 7. Influence of different (A) CEMs and (B) NMPs on the partial factor productivity (PFP) of applied nutrients viz. PFPn, PFPp, and PFPk in rice (4 year av.). The vertical bars indicate the LSD at p = 0.05.
Both under CTR and SRI, we assessed the agronomic efficiency (AE–Zn), crop recovery efficiency (CRE–Zn), and physiological efficiency (PE–Zn) of the applied Zn under RDF + Zn compared to RDF. It was again interesting to notice that the SRI had significantly higher AE-Zn (192.4 kg grain kg−1 Zn applied), CRE-Zn (28.6 kg Zn accumulated kg−1 Zn applied), and PE-Zn (6.7 kg grain kg−1 Zn uptake) to the tune of 119.6, 63.4, and 34%, respectively, over CTR (Figure 8). This was already reflected in the higher concentrations of Zn in rice grains and straw consequently to Zn-fertilization and its better bio-availability under RDF + Zn compared to RDF in the current study (Table 5).
FIGURE 8. Influence of Zn fertilization in rice on agronomic efficiency of applied Zn (AE-Zn) (kg grain kg−1 Zn applied), crop recovery efficiency of applied Zn (CRE-Zn) (kg Zn accumulated kg−1 Zn applied), and physiological efficiency of applied Zn (PE-Zn) (kg grain kg−1 Zn-uptake) under CTR and SRI (4 years av.). The vertical bars indicate the standard deviation.
Rice is a major food crop in the Indian Himalayas; however, non-adoption of high yielding cultivars, poor crop nutrition, and traditional rice farming practices (Choudhary and Suri 2018a; 2018b), coupled with low soil fertility, specifically Zn deficiency (Sharma et al., 2021), besides receding water resources and ill-distributed wet season rains are the major causes of concern which result in low rice productivity (∼2 t ha−1) and quality in this agro-ecology (Ceesav and Uphorr, 2003; Choudhary and Suri 2018a, 2018b). Hence, the widespread malnutrition and hunger among rural communities dependent on rice as a staple food in these remote hilly terrains (Sharma et al., 2021) has been a great cause of concern for the policy planners and the agricultural researchers in India. The current study validated the performance of conventionally transplanted rice (CTR) and the system of rice intensification (SRI) under three nutrient management practices (FFP, RDF, and RDF + Zn) to produce more rice with better Zn-biofortified and quality grains with enhanced nutrient-use efficiency to tackle the aforementioned issues in the NWH. The most important finding of this study was that the hybrid rice coupled with SRI produced higher yield attributes and the rice yield (6.59–8.69 t ha−1) under three nutrient management practices (NMPs); with perceptibly ∼3.3–4.3 fold higher yield compared to the average rice productivity (∼2 t ha−1) in the NWH (Figure 9). Under the conventionally transplanted rice (CTR), proper plant nutrition in hybrid rice also produced more rice (5.12–6.4 t ha−1) by ∼2.6–3.2 folds compared to the average rice productivity in the NWH. However, the SRI significantly out yielded over the CTR by ∼1.3–1.4 folds across the 4-year study in the wet-temperate environment spanning in India’s northwestern Himalayas.
FIGURE 9. Influence of different NMPs on grain yield enhancement both under CTR and SRI (4 years av.) over the average yield in the northwestern Himalayas. The vertical bars indicate the LSD at p = 0.05.
In NWH, comparisons were also made between CTR and SRI for growth and yield attributes where SRI produced taller plants with the greatest improvement by ∼2.4 folds in number of panicles hill−1 and 23.9% higher panicle number m−2 under wider spacing (25 × 25 cm) with ∼23% longer panicles and ∼12% heavier grain test-weight compared to CTR, which finally led to a 31.4% higher grain and 22.5% higher straw yield over the CTR. This was most evidently reflected in their respective grain and straw yield trends in the 4-year study despite insufficient early-season rainfall during 2012 compared to normal rainfall years (2010, 2011, and 2013). Under SRI management, planting of the healthy younger seedlings at two to three leaf stage (15 days old, or before the fourth phyllochron) in wider square spacing (25 × 25 cm) with minimal root damage and transplanting shock into a moist but not flooded seedbed (Stoop et al., 2002; Latif et al., 2005; McDonald et al., 2008; Styger et al., 2011; Dass et al., 2016a, 2017; and Choudhary and Suri 2018a, 2018b), are the key factors which played a pivotal role in producing healthier plants with better root and shoot growth, better photosynthetic rate, higher panicle count, and other yield attributes, which ultimately harnessed higher rice yield in hybrid rice under SRI compared to CTR in current study (Dass et al., 2016a, 2017; Choudhary and Suri, 2018a, 2018b).
The single young seedling plantings at wider spacing and less weed completion due to an efficient weed management through a cono-weeder, led to greater PAR interception and photosynthetic efficiency, resulting in higher growth and yield (Thakur et al., 2010; Dass et al., 2016a, 2016b). Moreover, the greater yield in a plant genotype is related to its ability to produce more biomass with better development of plant parts, the pre-requisites for effective utilization of environmental, soil, and water resources to develop and produce its economic sink (Choudhary and Suri, 2014; Dass et al., 2016a; Choudhary and Rahi, 2018; Bhupenchandra et al., 2022). Furthermore, the rice hybrids have a higher yield advantage over the conventional varieties (Choudhary and Suri, 2018a; 2018b); hence, tested hybrid “Arize-6129” in all the three NMPs had more rice yield both under CTR and SRI (Dass et al., 2016a). The optimum temperature for vegetative growth, anthesis, and ripening in rice ranges between 25 and 31, 30 and 33, and 20 and 25°C, respectively (Chandrasekaran et al., 2008). Hence, the planting of younger seedlings of short- and medium-duration rice hybrids under SRI in the wet-temperate climate of the NWH may skip the mild cool temperatures at anthesis, mainly responsible for impaired grain filling and low rice yield in the region (Dass et al., 2016a, 2017; Choudhary and Suri, 2018a, 2018b; Choudhary et al., 2020).
Furthermore, the rice hybrids have more vigorous growth, profuse tillering capacity, and higher yields over the conventional varieties, hence, require more plant nutrition to express their higher genetic potential (Yamauchi, 1994; Dass et al., 2017). It further becomes more essential to supply the balanced plant nutrition when we grow them under SRI management, that too under marginal fertility soils like acid Alfisol predominant in the NWH (Choudhary et al., 2010). In our study, we found that better plant nutrition under RDF and RDF + Zn proved highly rewarding over the FFP to produce better growth and yield attributes to harness a higher yield over the FFP following the trend of RDF + Zn > RDF > FFP both under CTR and SRI, although, SRI outperformed the CTR at all the fertilization levels with superior plant attributes and the grain and biomass yield under RDF + Zn, owing to balanced nutrient supply both through organic manures and chemical fertilizers especially the Zn-fertilization. Since, the DTPA extractable-Zn ranged between 0.59 and 0.68 mg kg−1 in the experimental soils; thus, we found a significant response under Zn-imbedded RDF + Zn treatment across the years (Takkar and Walker, 1993; Heba et al., 2021), making genotype × environment (G×E) interaction a reality for a better yield expression in our study (Farooq et al., 2009). Proper aeration and soil tilth and alternate wetting-drying (AWD) mechanism and efficient water-use under SRI, also resulted in better yields due to reduced leaching and deep percolation loses of N (Peng et al., 2010; Choudhary and Suri 2014, 2018a), enhanced nutrient bio-availability in the aerated rhizosphere (Santiago et al., 2011; Prasanna et al., 2012; Dass et al., 2016a, 2017; Singh U. et al., 2021, 2022), and better nutrient and water acquisition by the robust rooting system (Sharif, 2011; Styger et al., 2011; Adhikari et al., 2018); besides higher photosynthetic efficiency due to favorable stomatal regulation (Thakur et al., 2010; Dass et al., 2016a, 2017).
The concentrations and uptakes of NPK and Zn were higher under SRI compared to CTR, which consistently and significantly improved with the increase in fertilization with greatest values under RDF + Zn. Hence, it indicates that efficient nutrient management is primarily essential for better root and shoot growth, higher nutrient acquisition by the plants and better yields, both resulting in higher nutrient uptake (Styger et al., 2011; Harish et al., 2021; Shrivas et al., 2021; 2022). In our study, Zn concentration enhancement in rice grains and straw under SRI was merely 4.0 and 2.7% over the CTR; however, these small increases in Zn concentration had pronounced an effect on the total Zn uptake, and hence, may prove beneficial biologically to eliminate the widespread Zn deficiency across South Asia in general and the NWH in particular with least farm investments (Paul et al., 2016; Heba et al., 2016, 2021; and Kumar et al., 2021, 2022). Furthermore, a distinct superiority of the RDF + Zn w.r.t. NPK and Zn concentrations and uptakes could be ascribed chiefly to higher NPK and Zn fertilization through soil application as compared to RDF and FFP (Pooniya et al., 2012, 2019). RDF + Zn increased the supply and bioavailability of the major (NPK) and Zn micronutrient in addition to improved soil organic matter (SOM) by the FYM addition making nutrients more bioavailable (Pooniya et al., 2019; Biswakarma et al., 2021). It further highlighted the vital role of adequate moisture and aeration, both for their nutrient bioavailability and their uptake (Santiago et al., 2011; Dass et al., 2017). Proper aeration and moisture regimes under AWD and mechanical cono-weeding under SRI, led to enhanced growth and activity of the soil microbes (Choudhary and Suri 2018a; 2018b), which in turn, mediated the nutrient transformations and dynamics, availability, and their uptake (Santiago et al., 2011; Prasanna et al., 2012; Singh U. et al., 2021, 2022). Zn availability is generally impaired by the frequent irrigations or continuous submergence (Sarwar and Khanif 2005; Xu et al., 2015). In contrast, the rhizospheric aeration under AWD is expected to make the plant nutrients more bio-available (Dass et al., 2017; Zulfiqar et al., 2020), specifically Zn in the Zn-efficient NWH (Sharma et al., 2021), besides least N-losses compared to continuous submergence (Peng et al., 2010; Choudhary and Suri 2018a); all of which led to the higher acquisition of NPK and Zn under SRI compared to CTR. The latter resulted in a higher concentration of these nutrients both in rice grains and straw, resulting in a higher nutrient uptake. As Bana RC. et al. (2022) had reported, the foliar application of 4.0% Zn coated urea (ZnCU) + 0.2% ZnSO4 (ZnSO4.7H2O) may prove effective in enhancing the rice yield and Zn concentrations in rice grains and straw. Thus, both under aerobic and submerged rice, the foliar application of Zn may prove equally effective. In our study, a higher FYM application under RDF and RDF + Zn enhanced the SOM, which is highly beneficial for higher nutrient holding and the Zn chelation (Lin et al., 2009; Pooniya et al., 2019; Biswakarma et al., 2021:; Faiz et al., 2022). It also releases organic acids which solubilize the fixed-P, bound in Al- and Fe-rich acid Alfisol of the NWH (Kumar et al., 2017; Bhupenchandra et al., 2022); thus, helped in more nutrient bio-availability, higher nutrient concentrations and the uptakes in rice grains and straw in the current study. Higher N concentrations in rice grains under SRI compared to CTR, as well as under RDF + Zn compared to RDF and FFP, proved to be rewarding in enhancing the grain protein content and the protein yield. Zn fertilization was directly helpful in Zn biofortification of rice grains and straw and Zn uptake in Zn-fertilized plots (Heba et al., 2016, 2021; Kumar et al., 2021, 2022), being beneficial both for humans and livestock facing acute Zn deficiency in the Himalayan region.
The nutrient harvest index w.r.t. NHI, PHI, KHI, and ZnHI, as well as partial factor productivity (PFP) of applied nutrients (NPK) showed a significant improvement cumulatively by 30.9–31.3% under SRI compared to CTR, owing to better nutrient acquisition and accumulation in rice grains and straw in SRI plots where favorable soil and physico-chemical and microbiological properties enhanced the nutrient bioavailability in SRI management (Thakur et al., 2010; Dass et al., 2017). Furthermore, there was a consistent and significant increase in the NHI, KHI, and ZnHI under different NMPs with greatest values under RDF + Zn, due to a better supply of plant nutrients and their accumulation in rice grains and straw vis-a-vis a higher rice yield (Choudhary and Suri 2018b; Kumar et al., 2022). In contrast, PHI reported significantly higher values at RDF; thereafter, it showed a slight decline using RDF + Zn owing to a P×Zn antagonistic effect. In a nutshell, the higher nutrient harvest indices and PFPs are the obvious outcomes of higher rice productivity owing to better nutrient acquisition (Kumar et al., 2017), and the genetic ability of the rice cultivar for better yield expression under SRI management and the RDF + Zn nutrition (Dass et al., 2016a; Kumar et al., 2017). The PFPn, PFPp, and PFPk were higher under FFP, all of which then declined under RDF, and again showed a significant improvement under RDF + Zn. This trend can be attributed to the fact that the lesser doses of NPK under FFP brought higher incremental gains over the RDF; while under RDF + Zn, the Zn being the limiting factor amply enhanced the grain yield resulting in better PFPn, PFPp, and PFPk. The Zn-use efficiency in terms of agronomic efficiency (AE-Zn), crop recovery efficiency (CRE-Zn), and physiological efficiency of applied Zn (PE-Zn) were considerably higher under SRI to the tune of 119.6, 63.4, and 34%, respectively, over the CTR owing to better Zn bioavailability, Zn uptake, and rice grain yield of the applied and native Zn under SRI as earlier stated (Pooniya et al., 2019; Heba et al., 2016, 2021). Overall, Zn fertilization under RDF + Zn had greater significance in improving the rice productivity, quality, and Zn biofortification in rice grains and straw which has great potential in curtailing Zn malnutrition both in humans and animals in the NWH, also a prime objective of the United Nations’ Sustainable Development Goals (SDGs). Likewise, the foliar application of Zn through Zn-coated urea (ZnCU) and ZnSO4 could be another viable option to enhance the rice yield and Zn concentrations in rice grains and straw in the NWH, as per a recent study (Bana RC. et al., 2022).
It is concluded that the system of rice intensification (SRI) proved highly beneficial over the CTR to harness higher rice yield (6.59–8.69tha−1) under different nutrient management practices (NMPs); with a yield enhancement of ∼1.3–1.4 folds over the CTR and ∼3.3–4.3 folds compared to the average rice productivity in the NWH. Among NMPs, the rice grain yield ranged between 5.85 and 7.54 t ha−1 where RDF + Zn (FYM @ 10 t ha−1 + NPK @ 90:40:40 kg ha−1 + ZnSO4 @ 25 kg ha−1) outperformed RDF and FFP. SRI also improved the respective Zn-uptake in rice grains and straw by 36.9 and 25.9% compared to CTR. The RDF + Zn enhanced the Zn-biofortification of rice grains and grain Zn-uptake by 11.8 and 23.9% over the RDF, respectively. Nutrient harvest index and partial factor productivity of applied nutrients (NPK) had a higher magnitude under SRI and the RDF + Zn over their respective counterparts, i.e., CTR and RDF. The SRI also had higher AE-Zn (192.4 kg grain kg−1 Zn applied), CRE-Zn (28.6 kg Zn accumulated kg−1 Zn applied), and PE-Zn (6.7 kg grain kg−1 Zn uptake) to the tune of 119.6, 63.4, and 34%, respectively, over the CTR. Overall, SRI management coupled with RDF + Zn nutrition in promising rice hybrids provides ample opportunities to enhance rice productivity with better Zn-biofortified quality grains with higher nutrient-use efficiencies in the NWH to combat widespread malnutrition and hunger besides curtailing acute Zn deficiencies in humans and livestock in the northwestern Himalayas and collateral agro-ecologies across the globe. As the hybrid rice has shown higher response to soil applied-Zn in the NWH, the foliar application of Zn through ZnCU and ZnSO4 may also exhibit ample future prospects to correct the mid-season Zn nutrition deficiencies to further boost the rice yield with better Zn-biofortified rice grains and straw in NWH.
The original contributions presented in the study are included in the article/Supplementary Material, further inquiries can be directed to the corresponding authors.
AC conducted the all experiments. PS, SR, DY, OT, and KS supported in experimental work. AC, AK, and MR contributed in conceptualization, design of experiment, and manuscript writing. KS, AD, YS, SV, IB, VD, RB, VP, and SS contributed in statistical analysis and graphical representation. SK, GR, MH, VT, and AnK contributed in language corrections. SD and ViT contributed in manuscript editing.
The authors declare that the research was conducted in the absence of any commercial or financial relationships that could be construed as a potential conflict of interest.
All claims expressed in this article are solely those of the authors and do not necessarily represent those of their affiliated organizations, or those of the publisher, the editors, and the reviewers. Any product that may be evaluated in this article, or claim that may be made by its manufacturer, is not guaranteed or endorsed by the publisher.
The authors are thankful to CSK Himachal Pradesh Agricultural University, Palampur, India, and Indian Council of Agricultural Research (ICAR), New Delhi, for providing the technical and financial support. The financial and technical support provided by the World Bank-funded project “Agricultural Technology Management Agency (ATMA)” through Government of Himachal Pradesh, India, is also highly acknowledged. They are grateful to the agricultural officials of State Department of Agriculture, Government of Himachal Pradesh, India, for their contributions to the study. They also acknowledge the scientists of ICAR-Indian Agricultural Research Institute, New Delhi, India, for providing the technical support.
The Supplementary Material for this article can be found online at: https://www.frontiersin.org/articles/10.3389/fenvs.2022.869194/full#supplementary-material
Supplementary Figure S1 | Rice cultivation under SRI in NW Himalayan state, Himachal Pradesh, India.
Supplementary Figure S2 | Monthly rainfall and mean temperature during rice crop growing seasons (2010–2013) [source: Agro-meteorological Observatory, CSKHPKV, Farm Science Centre, Sundernagar, India].
Adhikari, P., Araya, H., Aruna, G., Balamatti, A., Banerjee, S., Baskaran, P., et al. (2018). System of Crop Intensification for More Productive, Resource-Conserving, Climate-Resilient, and Sustainable Agriculture: Experience with Diverse Crops in Varying Agroecologies. Int. J. Agric. Sustainability 16 (1), 1–28. doi:10.1080/14735903.2017.1402504
Alloway, B. J. (2008). Zinc in Soils and Crop Nutrition. Brussels: A publication of International Zinc Association and International Fertilizer Industry Association.
Anonymous (2011). Complete Package and Practices for Cultivation of Kharif Crops in Himachal Pradesh. Palampur, India: Publication of CSK Himachal Pradesh Agricultural University, 179.
Bana, R. C., Gupta, A. K., Bana, R. S., Shivay, Y. S., Bamboriya, S. D., Thakur, N. P., et al. (2022a). Zinc-coated Urea for Enhanced Zinc Biofortification, Nitrogen-Use Efficiency and Yield of Basmati rice under Typic Fluvents. Sustainability 14 (1), 104.
Bana, R. S., Jat, G. S., Grover, M., Bamboriya, S. D., Singh, D., Bansal, R., et al. (2022b). Nutrient Supplementation with Micronutrient-Embedded Fertilizer Increases Biofortification in Eggplant Fruit and Soil Biological Activity while Enhancing Plant Productivity. Scientific Reports 12 (1), 5146. doi:10.1038/s41598-022-09247-0
Barison, J., and Uphoff, N. (2011). Rice Yield and its Relation to Root Growth and Nutrient-Use Efficiency under SRI and Conventional Cultivation: An Evaluation in Madagascar. Paddy Water Environ. 9, 65–78. doi:10.1007/s10333-010-0229-z
Bhupenchandra, I., Chongtham, S. K., Basumatary, A., Singh, A. H., Das, A., Choudhary, A. K., et al. (2022). Changes in Soil Properties, Productivity and Profitability as Influenced by the Adoption of Site-specific Integrated Crop Management Technology in Turmeric (Curcuma Longa L.) in Eastern Himalayan Acidic Inceptisol. Ind. Crops Prod. 180, 114745. doi:10.1016/j.indcrop.2022.114745
Biswakarma, N., Pooniya, V., Zhiipao, R. R., Kumar, D., Verma, A. K., Shivay, Y. S., et al. (2021). Five Years Integrated Crop Management in Direct Seeded rice-zero till Wheat Rotation of north-western India: Effects on Soil Carbon Dynamics, Crop Yields, Water Productivity and Economic Profitability. Agric. Ecosyst. Environ. 318, 107492. doi:10.1016/j.agee.2021.107492
Cakmak, I., Yilmaz, A., Kalayci, M., Ekiz, H., Torun, B., Ereno% MathType!Mtef!2!1!+-% feaafi, B., and Braun, H. J. (1996). Zinc Deficiency as a Critical Problem in Wheat Production in Central Anatolia. Plant Soil 180, 165–172. doi:10.1007/bf00015299
Cassman, K. G., Peng, S., Olk, D. C., Ladha, J. K., Reichardt, W., Dobermann, A., and Singh, U. (1998). Opportunities for Increased Nitrogen-Use Efficiency from Improved Resource Management in Irrigated rice Systems. Field Crops Res. 56, 7–39. doi:10.1016/s0378-4290(97)00140-8
Ceesay, M., and Uphoff, N. (2003). The Effects of Repeated Soil Wetting and Drying on lowland rice Yield with System of rice Intensification Methods. Available at: http/ciifad.cornell.edu.
Chandrasekaran, D., Khan, A. K., Behera, M. S., Anand, P. S. B., Ghosh, S., and Panda, D. K. (2008). Effect of Varying Irrigation Schedules and Fertility Levels on Water Saving and Yield of Hybrid rice. Indian J. Agric. Sci. 78 (2), 122.
Choudhary, A. K., Singh, A., Singh, A., Yadav, D. S., and Sood, P. (2010). “System of rice Intensification – A Boon to Enhance rice Productivity in NW Himalayas,” in Proc. Of 3rdInternational Rice Congress. Organized by IRRI, Philippines (Hanoi, Vietnam: & Government of Vietnam), 159.
Choudhary, A. K., Varatharajan, T., Rohullah, , Bana, R. S., Pooniya, V., Dass, A., Kumar, A., and Harish, M. N. (2020). Integrated Crop Management Technology for Enhanced Productivity, Resource-Use Efficiency and Soil Health in Legumes – A Review. Indian J. Agric. Sci. 90 (10), 1839–1849.
Choudhary, A. K., and Rahi, S. (2018). Organic Cultivation of High Yielding Turmeric (Curcuma Longa L.) Cultivars: a Viable Alternative to Enhance Rhizome Productivity, Profitability, Quality and Resource-Use Efficiency in Monkey-Menace Areas of north-western Himalayas. Ind. Crops Prod. 124, 495–504. doi:10.1016/j.indcrop.2018.07.069
Choudhary, A. K., and Suri, V. K. (2014). Integrated Nutrient-Management Technology for Direct-Seeded Upland Rice (Oryza Sativa) in Northwestern Himalayas. Commun. Soil Sci. Plant Anal. 45 (6), 777–784. doi:10.1080/00103624.2013.861914
Choudhary, A. K., and Suri, V. K. (2018b). System of rice Intensification in Promising rice Hybrids in north-western Himalayas: Crop and Water Productivity, Quality, and Economic Profitability. J. Plant Nutr. 41 (8), 1020–1034. doi:10.1080/01904167.2018.1431675
Choudhary, A. K., and Suri, V. K. (2018a). System of rice Intensification in Short Duration rice Hybrids under Varying Bio-Physical Regimes: New Opportunities to Enhance rice Productivity and Rural Livelihoods in North-Western Himalayas under a Participatory-Mode Technology Transfer Program. J. Plant Nutr. 41 (20), 2581–2605. doi:10.1080/01904167.2018.1510515
Choudhary, A. K., Yadav, D. S., Sood, P., Rahi, S., Arya, K., Thakur, S. K., Lal, R., Kumar, S., Sharma, J., Dass, A., Babu, S., Bana, R. S., Rana, D. S., Kumar, A., Rajpoot, S. K., Gupta, G., Kumar, A., M.N., H., Noorzai, A. U., Rajanna, G. A., Khan, M. H., Dua, V. K., and Singh, R. (2021). Post-emergence Herbicides for Effective Weed Management, Enhanced Wheat Productivity, Profitability and Quality in North-Western Himalayas: A 'Participatory-Mode' Technology Development and Dissemination. Sustainability 13 (10), 5425. doi:10.3390/su13105425
Dass, A., Shekhawat, K., Choudhary, A. K., Sepat, S., Rathore, S. S., Mahajan, G., and Chauhan, B. S. (2016b). Weed Management in rice Using Crop-Competition–A Review. Crop Prot. 95, 45–52.
Dass, A., Chandra, S., Choudhary, A. K., Singh, G., and Sudhishri, S. (2016a). Influence of Field Re-ponding Pattern and Plant Spacing on rice Root-Shoot Characteristics, Yield, and Water Productivity of Two Modern Cultivars under SRI Management in Indian Mollisols. Paddy Water Environ. 14 (1), 45–59. doi:10.1007/s10333-015-0477-z
Dass, A., Chandra, S., Uphoff, N., Choudhary, A. K., Bhattacharyya, R., and Rana, K. S. (2017). Agronomic Fortification of rice Grains with Secondary and Micronutrients under Differing Crop Management and Soil Moisture Regimes in the north Indian Plains. Paddy Water Environ. 15 (4), 745–760. doi:10.1007/s10333-017-0588-9
de Santiago, A., Quintero, J. M., Avilés, M., and Delgado, A. (2011). Effect of Trichoderma Asperellum Strain T34 on Iron, Copper, Manganese, and Zinc Uptake by Wheat Grown on a Calcareous Medium. Plant Soil 342, 97–104. doi:10.1007/s11104-010-0670-1
DOA (2009). District Agriculture Plan: Mandi District, Himachal Pradesh, VIII. Shimla, India: Government of Himachal Pradesh, 220.
Fageria, N. K., and Baligar, V. C. (2003). Methodology for Evaluation of Lowland Rice Genotypes for Nitrogen Use Efficiency. J. Plant Nutr. 26, 1315–1333. doi:10.1081/pln-120020373
Faiz, M. A., Bana, R. S., Choudhary, A. K., Laing, A. M., Bansal, R., Bhatia, A., Bana, R. C., Singh, Y. V., Kumar, V., Bamboriya, S. D., Padaria, R. N., Khaswan, S. L., and Dabas, J. P. S. (2022). Zero Tillage, Residue Retention and System-Intensification with Legumes for Enhanced Pearl Millet Productivity and mineral Biofortification. Sustainability 14 (1), 543. doi:10.3390/su14010543
FAO (2019). Mountain Agriculture: Opportunities for Harnessing Zero Hunger in Asia. Bangkok: FAO-Regional Office for Asia and Pacific Region, 278.
Farooq, M., Kobayashi, N., Wahid, A., Ito, O., and Basra, S. M. A. (2009). Strategies for Producing More rice with Less Water. Adv. Agron. 101, 351–388.
Gomez, K. A., and Gomez, A. A. (1984). Statistical Procedures for Agricultural Research. New York, USA: A Wiley-Interscience Publication, John Wiley and Sons Inc.
Hanafy-Ahmed, A. H., Khalil, M. K., Abd-Ei-Rahman, A. M., and Nadia, A. M. (2012). Effect of Zinc, Tryptophan and Indole Acetic Acid on Growth, Yield and Chemical Composition of Valencia orange Trees. J. Appl. Sci. Res. 8, 901–914.
Hanway, J. J., and Heidel, H. (1952). Soil Analysis Methods as Used in Iowa State College Soil Testing Laboratory, 57. BulletinUSA: Iowa State College of Agriculture, Iowa, 131.
Harish, M. N., Choudhary, A. K., Choudhary, A. K., Singh, Y. V., Pooniya, V., Das, A., Babu, S., Dass, A., and Varatharajan, T. (2021). Nutrient Management Practices for Improved Crop and Water Productivity, Grain Quality and Energy Productivity of Promising rice Cultivars in Eastern Himalayas. Jeb 42 (2), 309–318. doi:10.22438/jeb/42/2/mrn-1372
Harish, M. N., Choudhary, A. K., Kumar, S., Dass, A., Singh, V. K., and Sharma, V. K. (2022). Double Zero Tillage and Foliar Phosphorus Fertilization Coupled with Microbial Inoculants Enhance maize Productivity and Quality in a maize-wheat Rotation. Scientific Reports 12, 3161. doi:10.1038/s41598-022-07148-w
Heba, M. N., Rana, D. S., Choudhary, A. K., Dass, A., Rajanna, G. A., and Pande, P. (2021). Influence of sulphur and Zinc Nutrition on Productivity, Quality and Biofortification in Groundnut (Arachis Hypogea L.) in South-Asian Alluvial Soil. J. Plant Nutr. 44 (8), 1151–1174.
Heba, M. N., Rana, D. S., Choudhary, A. K., Rajpoot, S. K., and Paul, T. (2016). Sulphur and Zn Management in Groundnut (Arachis hypogaea)–wheat (Triticum aestivum) Cropping System: Direct Effects on System Productivity and Residual Effects on Yield, Energetics and Zn Biofortification in Wheat. Indian J. Agric. Sci. 86 (4), 441–447.
Hussain, S., Maqsood, M. A., and Rahmatullah, (2010). Increasing Grain Zinc and Yield of Wheat for the Developing World: A Review. Emirates J. Food Agric. 22 (5), 326–339. doi:10.9755/ejfa.v22i5.4821
Hussain, S., Maqsood, M. A., Rengel, Z., and Aziz, T. (2012). Biofortification and Estimated Human Bioavailability of Zinc in Wheat Grains as Influenced by Methods of Zinc Application. Plant and Soil 361 (1&2), 279–290. doi:10.1007/s11104-012-1217-4
Jackson, M. L. (1967). Soil Chemical Analysis. New Delhi, India: Prentice Hall of India Pvt. Limited, 331–334.
Kakraliya, S. K., Jat, H. S., Singh, I., Sapkota, T. B., Singh, L. K., Sutaliya, J. M., Sharma, P. C., Jat, R. D., Choudhary, M., Lopez-Ridaura, S., and Jat, M. L. (2018). Performance of Portfolios of Climate Smart Agriculture Practices in a rice-wheat System of Western Indo-Gangetic plains. Agric. Water Manag. 202, 122–133. doi:10.1016/j.agwat.2018.02.020
Kassam, A., Stoop, W., and Uphoff, N. (2011). Review of SRI Modifications in rice Crop and Water Management and Research Issues for Making Further Improvements in Agricultural and Water Productivity. Paddy Water Environ. 9, 163–180. doi:10.1007/s10333-011-0259-1
Kediyal, V. K., and Dimri, S. (2009). Traditional Methods of rice Cultivation and SRI in Uttarakhand hills. Asian Agri-History 13 (4), 293–306.
Kumar, A., Choudhary, A. K., and Suri, V. K. (2017). Agronomic Bio-Fortification and Quality Enhancement in Okra-Pea Cropping System through Arbuscular Mycorrhizal Fungi at Varying Phosphorus and Irrigation Regimes in Himalayan Acid Alfisol. J. Plant Nutr. 40 (8), 1213–1229. doi:10.1080/01904167.2016.1267208
Kumar, A., Rana, K. S., Choudhary, A. K., Bana, R. S., Sharma, V. K., Gupta, G., Rajpoot, S. K., Bhupenchandra, I., Choudhary, M., Jakhar, P., Kumar, A., Kumar, A., Kishore, P., Pradhan, A., Tyagi, V., and Kumar, K. (2022). Sole- or Dual-Crop Basis Residue Mulching and Zn Fertilization Lead to Improved Productivity, Rhizo-Modulation and Soil Health in Zero-Tilled Pigeonpea-Wheat Cropping System. J. Soil Sci. Plant Nutr. doi:10.1007/s42729-021-00723-6
Kumar, A., Rana, K. S., Choudhary, A. K., Bana, R. S., Sharma, V. K., Prasad, S., Gupta, G., Choudhary, M., Pradhan, A., Rajpoot, S. K., Kumar, A., Kumar, A., and Tyagi, V. (2021). Energy Budgeting and Carbon Footprints of Zero-Tilled Pigeonpea-Wheat Cropping System under Sole or Dual Crop Basis Residue Mulching and Zn-Fertilization in a Semi-arid Agro-Ecology. Energy 231, 120862. doi:10.1016/j.energy.2021.120862
Latif, M. A., Islam, M. R., Ali, M. Y., and Saleque, M. A. (2005). Validation of the System of rice Intensification (SRI) in Bangladesh. Field Crops Res. 93, 281–292. doi:10.1016/j.fcr.2004.10.005
Lin, X. Q., Zhu, D. F., Chen, H. Z., Cheng, S. H., and Uphoff, N. (2009). Effect of Plant Density and Nitrogen Fertilizer Rates on Grain Yield and Nitrogen Uptake of Hybrid rice. J. Agric. Biotechnol. Sust. Dev. 1, 44–53.
Lindsay, W. L., and Norvell, W. A. (1978). Development of a DTPA Soil Test for Zinc, Iron, Manganese, and Copper. Soil Sci. Soc. America J. 42, 421–428. doi:10.2136/sssaj1978.03615995004200030009x
McDonald, A. J., Hobbs, P. R., and Riha, S. J. (2008). Stubborn Facts: Still No Evidence that the System of Rice Intensification Out-Yields Best Management Practices (BMPs) beyond Madagascar. Field Crops Res. 108, 188–191. doi:10.1016/j.fcr.2008.06.002
Narayanan, S. (2017). “Rice in the Public Distribution System,” in The Future rice Strategy for India. Editor S. Mohanty (London, UK: Academic Press), 15–37. doi:10.1016/b978-0-12-805374-4.00002-6
Olsen, S. R., Cole, C. V., Watanabe, F. S., and Dean, L. A. (1954). Estimation of Available Phosphorus in Soils by Extraction with Sodium Bicarbonate (USDA Circular No. 939). Washington DC: US Government Printing Office.
Ozkutlu, F., Torun, B., and Cakmak, I. (2006). Effect of Zinc Humate on Growth of Soybean and Wheat in Zinc‐Deficient Calcareous Soil. Commun. Soil Sci. Plant Anal. 37, 2769–2778. doi:10.1080/00103620600832167
Pandey, S., Mortimer, M., Wade, L., Tuong, T. P., Lopez, K., and Hardy, B. (2002). “Direct Seeding: Research Issues and Opportunities,” in Proc. Of International Workshop on Direct-Seeding in Asian rice Systems: Strategic Research Issues and Opportunities (Philippines: An International Rice Research Institute Publication), 383.
Paul, J., Choudhary, A. K., Suri, V. K., Sharma, A. K., Kumar, V., and Shobhna, (2014). Bioresource Nutrient Recycling and its Relationship with Biofertility Indicators of Soil Health and Nutrient Dynamics in Rice-Wheat Cropping System. Commun. Soil Sci. Plant Anal. 45 (7), 912–924. doi:10.1080/00103624.2013.867051
Paul, T., Rana, D. S., Choudhary, A. K., Das, T. K., and Rajpoot, S. K. (2016). Crop Establishment Methods and Zn Nutrition in Bt-Cotton: Direct Effects on System Productivity, Economic–Efficiency and Water–Productivity in Bt-Cotton–Wheat Cropping System and Their Residual Effects on Yield and Zn Biofortification in Wheat. Indian J. Agric. Sci. 86 (11), 1406–1412.
Peng, S., Buresh, R. J., Huang, J., Zhong, X., Zou, Y., Yang, J., Wang, G., Liu, Y., Hu, R., Tang, Q., Cui, K., Zhang, F., and Dobermann, A. (2010). Improving Nitrogen Fertilization in rice by Sitespecific N Management. A Review. Agron. Sustain. Dev. 30, 649–656. doi:10.1051/agro/2010002
Pooniya, V., Choudhary, A. K., Bana, R. S., and Pankaj, (2019). Zinc Bio-Fortification and Kernel Quality Enhancement in Elite Basmati rice Cultivars of South-Asia through Legume Residue-Recycling and Zinc Fertilization. Indian J. Agric. Sci. 89 (2), 279–287.
Pooniya, V., Shivay, Y. S., Rana, A., Nain, L., and Prasanna, R. (2012). Enhancing Soil Nutrient Dynamics and Productivity of Basmati rice through Residue Incorporation and Zinc Fertilization. Eur. J. Agron. 41, 28–37. doi:10.1016/j.eja.2012.03.004
Pooniya, V., Zhiipao, R. R., Biswakarma, N., Kumar, D., Shivay, Y. S., Babu, S., Das, K., Choudhary, A. K., Swarnalakshmi, K., Jat, R. D., Choudhary, R. L., Ram, H., Khokhar, M. K., Mukri, G., Lakhena, K. K., Puniya, M. M., Jat, R., Muralikrishnan, L., Singh, A. K., and Lama, A. (2022). Conservation Agriculture Based Integrated Crop Management Sustains Productivity and Economic Profitability along with Soil Properties of the maize-wheat Rotation. Sci. Rep. 12, 1962. doi:10.1038/s41598-022-05962-w
Prasanna, R., Joshi, M., Rana, A., Shivay, Y. S., and Nain, L. (2012). Influence of Co-inoculation of Bacteria-Cyanobacteria on Crop Yield and C-N Sequestration in Soil under rice Crop. World J. Microbiol. Biotechnol. 28, 1223–1235. doi:10.1007/s11274-011-0926-9
Qingquan, Y. (2002). “The SRI and its Use with Hybrid rice Varieties in China,” in Proc. International Conference on Assessments of SRI. Organized by CNHRRDC, Sanya (Ithaca, USA: China & CIIFAD), 109–111.
Rajpoot, S. K., Rana, D. S., and Choudhary, A. K. (2021). Crop and Water Productivity, Energy Auditing, Carbon Footprints and Soil Health Indicators of Bt-Cotton Transplanting Led System Intensification. J. Environ. Manage. 300, 113732. doi:10.1016/j.jenvman.2021.113732
Rana, K. S., Choudhary, A. K., Sepat, S., Bana, R. S., and Dass, A. (2014). Methodological and Analytical Agronomy. New Delhi, India: Post Graduate School, Indian Agricultural Research Institute, 276.
Rao, Y. Y. (2012). Rice Seed Production Scenario in India rice Knowledge Management portal. Hyderabad, India: Directorate of Rice Research.
Rasul, G., Hussain, A., Mahapatra, B., and Dangol, N. (2018). Food and Nutrition Security in the Hindu Kush Himalayan Region. J. Sci. Food Agric. 98 (2), 429–438. doi:10.1002/jsfa.8530
Sarwar, M. J., and Khanif, Y. M. (2005). The Effect of Different Water Levels on rice Yield and Cu and Zn Concentration. J. Agron. 4, 116–121. doi:10.3923/ja.2005.142.146
Sharif, A. (2011). Technical Adaptations for Mechanized SRI Production to Achieve Water Saving and Increased Profitability in Punjab, Pakistan. Paddy Water Environ. 9, 111–119. doi:10.1007/s10333-010-0223-5
Sharma, M., Parmar, D. K., Sharma, S. K., Kumar, P., and Kumar, P. (2021). Influence of Zinc Nutrition on Zinc Availability, Uptake and Crop Yield of Rainfed maize-pea Sequence in Northwestern Himalaya. J. Plant Nutr. 44 (14), 2069–2080. doi:10.1080/01904167.2021.1889596
Sharma, S., Singh, P., and Kumar, S. (2020). Responses of Soil Carbon Pools, Enzymatic Activity, and Crop Yields to Nitrogen and Straw Incorporation in a Rice-Wheat Cropping System in North-Western India. Front. Sustain. Food Syst. 4, 532704. doi:10.3389/fsufs.2020.532704
Shrivas, V. L., Choudhary, A. K., Hariprasad, P., and Sharma, S. (2021). Nutrient Concentrations Affect the Antimicrobial Resistance Profiles of Cattle Manures. Environ. Sci. Pollut. Res. doi:10.1007/s11356-021-16700-6
Singh, P., Benbi, D. K., and Verma, G. (2021a). Nutrient Management Impacts on Nutrient Use Efficiency and Energy, Carbon, and Net Ecosystem Economic Budget of a Rice-Wheat Cropping System in Northwestern India. J. Soil Sci. Plant Nutr. 21 (1), 559–577. doi:10.1007/s42729-020-00383-y
Singh, U., Choudhary, A. K., and Sharma, S. (2022). A 3-year Field Study Reveals that Agri-Management Practices Drive the Dynamics of Dominant Bacterial Taxa in the Rhizosphere of Cajanus Cajan. Symbiosis. doi:10.1007/s13199-022-00834-3
Singh, U., Choudhary, A. K., and Sharma, S. (2021b). Agricultural Practices Modulate the Bacterial Communities, and Nitrogen Cycling Bacterial Guild in Rhizosphere: Field experiment with Soybean. J. Sci. Food Agric. 101, 2687–2695. doi:10.1002/jsfa.10893
Singh, U., Choudhary, A. K., and Sharma, S. (2020). Comparative Performance of Conservation Agriculture Vis-A-Vis Organic and Conventional Farming, in Enhancing Plant Attributes and Rhizospheric Bacterial Diversity in Cajanus Cajan: A Field Study. Eur. J. Soil Biol. 99, 103197. doi:10.1016/j.ejsobi.2020.103197
Stein, A. J., Nestel, P., Meenakshi, J., Qaim, M., Sachdev, H., and Bhutta, Z. A. (2007). Plant Breeding to Control Zinc Deficiency in India: How Cost-Effective Is Biofortification? Public Health Nutr. 10, 492–501. doi:10.1017/s1368980007223857
Stoop, W. A., Adam, A., and Kassam, A. (2009). Comparing rice Production Systems: A challenge for Agronomic Research and for the Dissemination of Knowledge-Intensive Farming Practices. Agric. Water Manag. 96, 1491–1501. doi:10.1016/j.agwat.2009.06.022
Stoop, W. A., Uphoff, N., and Kassam, A. (2002). A Review of Agricultural Research Issues Raised by the System of rice Intensification (SRI) from Madagascar: Opportunities for Improving Farming Systems for Resource-Poor Farmers. Agric. Syst. 71, 249–274. doi:10.1016/s0308-521x(01)00070-1
Styger, E., Aboubacrine, G., Attaher, M. A., and Uphoff, N. (2011). The System of rice Intensification as a Sustainable Agricultural Innovation: Introducing, Adapting and Scaling up a System of rice Intensification Practices in the Timbuktu Region of Mali. Int. J. Agric. Sustainability 9, 67–75. doi:10.3763/ijas.2010.0549
Subbiah, B. V., and Asija, G. L. (1956). A Rapid Procedure for Assessment of Available-N in rice Soils. Curr. Sci. 25, 259–260.
Swaminathan, M. S., and Kesavan, P. C. (2012). Agricultural Research in an Era of Climate Change. Agric. Res. 1 (1), 3–11. doi:10.1007/s40003-011-0009-z
Takkar, P. N., and Walker, C. D. (1993). “The Distribution and Correction of Zinc Deficiency,” in Zn in Soils and Plants. Developments in Plant and Soil Sciences Book Series. Editor A. D. Robson (Dordrecht: Springer), 55, 151–165. doi:10.1007/978-94-011-0878-2_11
Thakur, A. K., and Uphoff, N. (2017). How the System of rice Intensification Can Contribute to Climate-Smart Agriculture? Agron. J. 109, 1–20. doi:10.2134/agronj2016.03.0162
Thakur, A. K., Uphoff, N., and Antony, E. (2010). An Assessment of Physiological Effects of System of Rice Intensification (Sri) Practices Compared with Recommended Rice Cultivation Practices in India. Ex. Agric. 46, 77–98. doi:10.1017/s0014479709990548
Umair Hassan, M., Aamer, M., Umer Chattha, M., Haiying, T., Shahzad, B., Barbanti, L., Nawaz, M., Rasheed, A., Afzal, A., Liu, Y., and Guoqin, H. (2020). The Critical Role of Zinc in Plants Facing the Drought Stress. Agriculture 10 (9), 396. doi:10.3390/agriculture10090396
Uphoff, N. (2010). “Alternate Management Methods and Impacts with the SRI in Responding to Climate Change Effects,” in Proc. Of 3rd International Rice Congress. Organized by IRRI (Hanoi: Philippines & Government of Vietnam).
Uphoff, N., Kassam, A., and Harwood, R. (2011). SRI as a Methodology for Raising Crop and Water Productivity: Productive Adaptations in rice Agronomy and Irrigation Water Management. Paddy Water Environ. 9, 3–11. doi:10.1007/s10333-010-0224-4
Uphoff, N. (2017). SRI: An Agroecological Strategy to Meet Multiple Objectives with Reduced reliance on Inputs. Agroecology Sust. Food Syst. 41, 825–854. doi:10.1080/21683565.2017.1334738
Varatharajan, T., Choudhary, A. K., PooniyaV, , Dass, A., and Harish, M. N. (2019a). Integrated Crop Management Practices for Enhancing Productivity, Profitability, Production-Efficiency and Monetary-Efficiency of Pigeonpea in Indo-Gangetic plains Region. Indian J. Agric. Sci. 89 (3), 559–563.
Varatharajan, T., Choudhary, A. K., Choudhary, A. K., Pooniya, V., Dass, A., Meena, M. C., Gurung, B., and Harish, M. N. (2019b). Influence of Integrated Crop Management Practices on Yield, PAR Interception, Resource-Use-Efficiency and Energetics in Pigeonpea in north Indian plains. Jeb 40 (6), 1204–1210. doi:10.22438/jeb/40/6/mrn-1073
Veeramani, P., and Singh, R. D. (2011). System of rice Intensification in Hybrid rice-A Review. Agric. Rev. 32 (1), 32–35.
Walkley, A., and Black, I. A. (1934). An Examination of the Degtjareff Method for Determining Soil Organic Matter, and a Proposed Modification of the Chromic Acid Titration Method. Soil Sci. 37, 29–38. doi:10.1097/00010694-193401000-00003
Wu, W., Ma, B., and Uphoff, N. (2015). A Review of the System of rice Intensification in China. Plant Soil 393, 361–381. doi:10.1007/s11104-015-2440-6
Xu, J., Lv, Y., Yang, S., Wei, Q., and Qiao, Z. (2015). Water Saving Irrigation Improves the Solubility and Bioavailability of Zinc in Rice Paddy. Ijab 17, 1001–1006. doi:10.17957/ijab/14.0029
Yamauchi, M. (1994). Hybrid rice Technology: New Development, Future Prospects. Int. Rice Res. Notes 11 (2), 71–80.
Yang, X., and Zhang, J. (1999). Characteristics of Nitrogen Nutrition in Hybrid rice. Int. Rice Res. Notes 24 (1), 5–8.
Zhao, L., Wu, L., Li, Y., Lu, X., Zhu, D., and Uphoff, N. (2009). Influence of the System of Rice Intensification on Rice Yield and Nitrogen and Water Use Efficiency with Different N Application Rates. Ex. Agric. 45, 275–286. doi:10.1017/s0014479709007583
Zulfiqar, U., Hussain, S., Ishfaq, M., Matloob, A., Ali, N., Ahmad, M., Alyemeni, M. N., and Ahmad, P. (2020). Zinc-Induced Effects on Productivity, Zinc Use Efficiency, and Grain Biofortification of Bread Wheat under Different Tillage Permutations. Agronomy 10 (10), 1566. doi:10.3390/agronomy10101566
Keywords: conventionally transplanted rice, Zn-use efficiency, Zn biofortification, system of rice intensification, nutrient management, rice productivity, grain quality
Citation: Choudhary AK, Sood P, Rahi S, Yadav DS, Thakur OC, Siranta KR, Dass A, Singh YV, Kumar A, Vijayakumar S, Bhupenchandra I, Dua VK, Shivadhar , Bana RS, Pooniya V, Sepat S, Kumar S, Rajawat MVS, Rajanna GA, Harish MN, Varatharajan T, Kumar A and Tyagi V (2022) Rice Productivity, Zn Biofortification, and Nutrient-Use Efficiency as Influenced by Zn Fertilization Under Conventional Transplanted Rice and the System of Rice Intensification. Front. Environ. Sci. 10:869194. doi: 10.3389/fenvs.2022.869194
Received: 04 February 2022; Accepted: 25 March 2022;
Published: 10 May 2022.
Edited by:
Rahul Mahadev Shelake, Gyeongsang National University, South KoreaReviewed by:
Rajesh Ramdas Waghunde, Navsari Agricultural University, Gujarat, IndiaCopyright © 2022 Choudhary, Sood, Rahi, Yadav, Thakur, Siranta, Dass, Singh, Kumar, Vijayakumar, Bhupenchandra, Dua, Shivadhar, Bana, Pooniya, Sepat, Kumar, Rajawat, Rajanna, Harish, Varatharajan, Kumar and Tyagi. This is an open-access article distributed under the terms of the Creative Commons Attribution License (CC BY). The use, distribution or reproduction in other forums is permitted, provided the original author(s) and the copyright owner(s) are credited and that the original publication in this journal is cited, in accordance with accepted academic practice. No use, distribution or reproduction is permitted which does not comply with these terms.
*Correspondence: Anil K. Choudhary, YW5pbGhwYXUyMDEwQGdtYWlsLmNvbQ==; Adarsh Kumar, YWRhcnNoMjAxNDlAZ21haWwuY29t; Mahendra Vikram Singh Rajawat, cmFqYXdhdC5tdnNAZ21haWwuY29t
Disclaimer: All claims expressed in this article are solely those of the authors and do not necessarily represent those of their affiliated organizations, or those of the publisher, the editors and the reviewers. Any product that may be evaluated in this article or claim that may be made by its manufacturer is not guaranteed or endorsed by the publisher.
Research integrity at Frontiers
Learn more about the work of our research integrity team to safeguard the quality of each article we publish.