- 1School of Mathematics and Physics, University of Portsmouth, Portsmouth, United Kingdom
- 2Sustainable Use, Management and Reclamation of Soil and Water Research Group (GARSA), Department of Agricultural Engineering, Universidad Politécnica de Cartagena, Cartagena, Spain
- 3Consiglio per la Ricerca in Agricoltura e l’analisi Dell’economia Agraria (CREA), Centro di Ricerca Agricoltura e Ambiente—Research Centre for Agriculture and Environment (AA), Roma, Italy
- 4Natural Resources Institute Finland (LUKE)/Natural Resources, Jokioinen, Finland
- 5Departamento de Suelo y Agua, Estación Experimental de Aula Dei, Consejo Superior de Investigaciones Científicas (CSIC), Zaragoza, Spain
Sustainable agriculture has been identified as key to achieving the 2030 Agenda for the Sustainable Development Goals, which aims to end poverty and hunger and address climate change while maintaining natural resources. Soil organic carbon (SOC) sequestration is a key soil function for ecosystem services, and storing carbon (C) in soil by changing traditional management practices can represent an important step toward the development of more sustainable agricultural systems in Europe. Within the European project Diverfarming, the process-based ecosystem model ECOSSE was modified and evaluated in four long-term experiments (>8 years) to assess the impact of crop diversification and agricultural management in SOC dynamics. ECOSSE was able to simulate SOC under dry conditions in Mediterranean regions in Spain and Italy. In the site of Murcia, Spain, the addition of manure and cover crop in the diversified systems produced an increase of SOC in 9 years, when compared with the conventional management (16% measured increase, 32% simulated increase). The effect of tillage management on SOC stock in dry soil, in Foggia, Italy and Huesca, Spain, was also modeled, and a positive impact on SOC was predicted when no tillage was practiced. Finally, ECOSSE was used to understand the impact of diversifications in Boreal regions, Finland, where different proportions of legumes and grass were considered in a 4-year crop rotation compared with conventional cereal rotations. Experiments and modeling showed that the loss of SOC in conventional cereal was compensated when grass was introduced in the rotations. A good agreement (NRMSE <10%) and a nonsignificant bias were observed between model and experimental data for all sites. Mitigation scenarios considered in the modeling analysis for the test site Huesca showed that an integrated management of no tillage and manure is the best strategy to increase SOC, ∼51% over 20 years, compared with the baseline scenario (current farmers practice). This study demonstrated the ability of the modified version of ECOSSE to simulate SOC dynamics in diversified cropping systems, with various soil management practices and different climatic conditions.
Introduction
For many years intensive agricultural systems, e.g., large use of fertilizers, pesticides, intense mechanization, and monocultures, have been practised to increase crop productivity, and to meet the demand of a growing population and food security challenges. However, such simplified farming systems had detrimental effects not only on the cropping systems themselves, but also on the environment with respect to resilience, adaptation to climate change, groundwater pollution, biodiversity loss, reduction of ecosystem functions, soil erosion (Lichtenberg et al., 2017; Francaviglia et al., 2020), and economic loss (Pretty, 2018). Consequently, in the last few years greater attention has been paid to sustainability and to a use of resources that would minimize the costs and maximize the benefits, while enhancing resilience and increasing productivity. Sustainable agriculture has been identified as an important strategy to achieve the 2030 agenda for Sustainable Development Goals (SDGs). As addressed by Nhemachena et al. (2018), the sustainability challenge faced by the agriculture sector is to be able to provide enough food for the growing population without increasing the use of primary resources such as water and farmland, and without reducing biodiversity. The implementation of crop diversification strategies under low-input management in place of traditional farming systems is gaining attention in agriculture, not only to meet the demand of food for an ever-growing population, but also with respect to environmental resilience and sustainability.
Increasing depleting Soil Organic Carbon (SOC) stocks can represent an important step toward the development of more sustainable agricultural systems. The reservoir capacity of SOC in soil carbon (C) pool was estimated at 1,550 Gigaton (Gt), which is nearly twice as large as the atmospheric pool, and three times larger than the C present in the biotic pool (Lal, 2016). Soil organic carbon is considered one of the most important indicators of soil quality and agronomic sustainability because of its impact on other physical, chemical, and biological properties of the soil (Reeves, 1997; Lal, 2013; Yigini and Panagos 2016; Sanz-Cobena et al., 2017). Soil productivity and SOC storage are largely affected by the agricultural managements, e.g., crop cover, residue, and tillage management (Paustian et al., 2016). Approximately 90% of total greenhouse gas (GHG) mitigation potential could be derived from SOC sequestration (Smith et al., 2007; Begum et al., 2017), and the SOC sequestration potential is mainly derived from agricultural land management (Minasny et al., 2017). However, there are also studies suggesting that SOC sequestration potential is a limited tool to mitigate climate change (Schlesinger and Amundson, 2019). Using the “4 per mille” approach, the SOC stock from the global agricultural land is estimated to be 2 to 3 Gt C yr−1 from the top 1 m, which effectively offset 20–35% of global anthropogenic GHG emissions (Minasny et al., 2017). Improving agricultural management can contribute to prevent the global warming potential from exceeding between 1.5°C and 2°C, as mentioned in the Intergovernmental Panel on Climate Change (IPCC) sixth report. Since a small change in SOC can impact the global C cycle (Smith, 2004) and soil ecosystems, it is crucial to store SOC in the soil for longer periods (Smith, 2004; Rodeghiero et al., 2011).
In Europe with the exception of the Netherlands, other countries, e.g., Belgium, France, Finland, England, Wales, etc, found a decreasing trend in SOC stock in cropland (Heikkinen et al., 2013) and overall a decline of ∼28% has been observed in the last 30 years (FAOSTAT, 2021). A modeling analysis by Smith et al. (2005) predicted a loss of 4–6 Gt from the cropland SOC stock under different climate change scenarios by 2080, which is a 39–54% decrease compared with the 1990 level. Loss of SOC has been identified as one of the major soil threats in the European Union’s Thematic Strategy for Soil Protection (EC, 2006). Increasing SOC stock by agricultural means has also been emphasized in the Kyoto Protocol and Paris Climate Agreement (Begum et al., 2017). In the 21st conference of the parties to the United Nations (UN) Framework Convention on Climate Change (COP21), soil C and agriculture were first presented in the agenda, and a voluntary initiative called “4 per mille” was proposed, to increase the global SOC stocks annually by 0.4% at 40 cm depth (Lal, 2016; Begum et al., 2018a).
In this context, the H2020 Diverfarming project (www.diverfarming.eu) aims to increase SOC stock by crop diversification and reduction of external inputs across six European pedoclimatic regions. In this study we focus on the Mediterranean and Boreal regions, which occupy 11% and 14% of the EU 28 cropland area, respectively (EUROSTAT, 2015). Generally, in the Mediterranean climatic regions the SOC content is low (0.5–1%). Climate, e.g., high temperature, evapotranspiration, and low precipitation, crop and soil management, e.g., bare fallow practised for long periods of time (up to 1.5 years), crop residue removal, intensive tillage, and soil erosion favor the SOC decline (Hernanz et al., 2009; Alvaro-Fuentes and Paustian, 2011; Rodeghiero et al., 2011; Aguilera et al., 2018). Low crop growth rates, difficult land recovery after degradation, and potential high mineralization rates are also common phenomena in Mediterranean ecosystems (Almagro et al., 2017). Dry soils are significantly affected by agricultural management practices. However, there is potential to improve and increase SOC stock by adoption of innovative management practices, e.g., no tillage (NT), use of cover crops as green manure (GM) in the rotation, incorporation of residue, and C input by exogenous source (Mazzoncini et al., 2008; Álvaro Fuentes et al., 2009; Rodeghiero et al., 2011; Aguilera et al., 2018). A data-analysis carried out by Francaviglia et al. (2017) found that SOC in dry regions increases by 11% under diversified crop managements and by 25% under diversified fertilization managements in comparison with humid and subhumid regions. In European Boreal ecosystems the SOC content depends on the type of soil. A study on Finnish cropland (11% of total area covered with organic soils) found a decrease in SOC of 0.4% yr−1 and 0.2–0.3% yr−1 for mineral and organic soils, respectively, due to climate change, land use, and management practices. Moreover, a high decomposition rate due to peatland drainage increases CO2 emissions and reduces SOC gain (Heikkinen et al., 2013).
The analysis of experimental data is necessary to appreciate changes in SOC (if any) due to diversification. However, since changes in SOC are slow, long-term field experiments are of key importance to get insights into the SOC dynamics in soil-atmosphere-plant interactions. Furthermore, due to diverse climatic pattern, the detailed studies on SOC dynamics across Mediterranean ecosystems are limited (Rodeghiero et al., 2011). The aid of process-based models gives the opportunity to extend the information obtained experimentally to future scenarios. This adds a new level of relevance to the research, and provides key information to farmers and stakeholders when they consider diversified cropping systems as a potential improvement to their own agroecosystems. In the last few years, the process-based model ECOSSE (Model to Estimate Carbon in Organic Soils-Sequestration and Emissions; Smith et al., 2010) was extensively modified and used to assess GHG emissions from different soils and cropping systems in Europe (Dondini et al., 2015). New land uses such as those for the production of bioenergy-woody crops were introduced (Dondini et al., 2015, 2016). Soil respiration was simulated in the European peatland sites (Abdalla et al., 2014) and in Ireland arable soil (Flattery et al., 2018), and Bell et al. (2012) simulated soil nitrogen (N) and nitrous oxide (N2O) emissions in cropland sites in Europe. However, the use of ECOSSE to model SOC sequestration potential in arable cropland with diversified crops (e.g., multiple cropping, crop rotation) and agricultural managements (e.g., tillage, irrigation, fertilization) in the Mediterranean pedoclimatic regions is limited. SOC dynamics was modeled in barley cropping systems in association with different tillage practices, considering current (Álvaro Fuentes et al., 2009) and future climate scenarios (Alvaro-Fuentes and Paustian, 2011). Aguilera et al. (2018) analyzed SOC in the cropland under different managements. Both studies however were limited to Spain. Modeling work on SOC dynamics was also done in Mediterranean Italian sites (Farina et al., 2013, 2018) and at a regional level (Farina et al., 2017) by adapting the process-based model RothC (Jenkinson et al., 1999) to Mediterranean conditions.
The main aim of this study was to assess how SOC dynamics change in relation to diversified farming and soil management. We show how the model ECOSSE was modified, parameterized, and used to simulate C dynamics in the European Mediterranean and Boreal ecosystems. Through the numerical simulations of all considered management practices we could explore which factors in diversified managements have the largest impact on SOC in contrast to conventional agricultural systems, gaining insights into the effect of diversification and sustainable soil management on SOC. Simulations of long-term rotations in Mediterranean and Boreal croplands allowed us to compare the SOC sequestration potential in different European pedoclimatic regions and to attain a deeper understanding of SOC dynamics in contrasting regions. Finally, different mitigation scenarios were considered for the test site of Huesca (Spain) to estimate how different managements affect SOC dynamics in Mediterranean ecosystems.
Materials and Methods
Site Description
The SOC data used in this study are from four long-term (>8 years) experimental sites, three in the Mediterranean regions in Murcia, Spain, in Foggia, Italy, and in Huesca, Spain; and one in the boreal region in Toholampi, Finland. The top layer soil properties (0–30 cm depth) are presented in Table 1 for each of these sites. Soils in Mediterranean regions are generally basic (∼pH 8) whereas Finnish soil is acidic (∼pH 6). The average clay content is between 15% and 35% in Mediterranean regions and 6% circa in the Boreal region. In each region several tailored diversification systems and soil managements are considered as alternatives to conventional management. All sites and agricultural management practices are described below. Diversification codes with the main management features, including yearly amounts of C input, can be found in Supplementary Table S1.
LT1 Diversified Horticulture in Spain
In Murcia, Spain, the long-term effect of multiple cropping and rotations on SOC dynamics with the addition of organic amendment was evaluated. The experimental period is 2010–2018 with melon (Cucumis melo), pepper (Capsicum annum), and pumpkin (Cucurbita moschata) as rotated summer crops and cabbage (Brassica oleracea var. sabellica), celery (Apium graveolens), lettuce (Lactuca sativa), broccoli (Brasica oleracea var. italica), and fennel (Foeniculum vulgare) as rotated winter crops. Three managements were practised on this site: 1) conventional, 2) organic, and 3) biodynamic, with the same multiple cropping and rotation pattern. All of them included conventional tillage (CT) to a depth of 30 cm and fertigation. The conventional management received yearly sheep manure applications with inorganic mineral N fertilizer under fertigation and application of pesticides (LT1DV0). The organic management included sheep manure, soluble organic fertilizers for fertigation, and biological control of pests/diseases (LT1DV1). The biodynamic management included sheep compost, soluble organic fertilizers and compost tea for fertigation, biological control of pests/diseases, and cover crops (LT1DV2). Manure and compost were applied before the winter cycle, around September. Inorganic/organic fertilizers and compost tea were applied by fertigation according to crop needs. Cover crops consisted of a mixture of oats (Avena sativa) and vetch (Vicia sativa), grown in between the two main crops and incorporated in the field in April/May before summer crop was established. The residues from all treatments were incorporated in the field with CT, up to 30 cm depth.
1) Conventional (LT1DV0): 12–15 t ha−1 sheep manure (% C 22.5, %N 0.98%) + inorganic fertilizer containing 6.18 kg N ha−1 in fertigation.
2) Organic (LT1DV1): 15 t ha−1 sheep manure (% C 22.5, %N 0.98%), organic fertilizer containing 1.23 kg N ha−1 in fertigation.
3) Biodynamic (LT1DV2): 10 t ha−1 sheep compost (% C 20, % N 1.3), organic fertilizer containing 1.23 kg N ha−1, compost tea (140 mgL−1 C and 21 mgL−1 N) + cover crop. Carbon and nitrogen input by compost tea are 140 mg C/L and 21 mg N/L. Fertigation varies on a yearly basis and the simulations showed that between 0.9 and 1.7 t ha−1 C are added through fertigation in LT1DV2.
LT2 Durum Wheat in Italy
The impact of tillage and crop rotation on the soil physicochemical properties of the Italian site in Foggia has been analyzed. Two field experiments were established in 1995 to observe the soil physico-chemical properties in monocropping wheat (Triticum aestivum) under CT, tillage depth 35–40 cm), and NT. From 2008 two other management practices were included, wheat in rotation with tick bean (Vicia faba) under CT and NT systems. No tillage was associated with direct seeding to the field. The tick bean grown in the rotation systems were incorporated in the field as GM. Inorganic fertilizer containing 36 kg ha−1 N was applied during the wheat growing season. In conclusion, the four management practices for this site were the following: Conventional (LT2DV0), that is Durum wheat monocrop with CT; Diversification 1 (LT2DV1), Durum wheat monocrop with NT; Diversification 2 (LT2DV2), rotation tick bean-durum wheat with CT; and Diversification 3 (LT2DV3), rotation tick bean-durum wheat with NT.
LT4 Tillage in Rainfed Systems in Spain
The experiment designed to study the long-term impact of tillage on SOC dynamics in the Mediterranean semiarid regions started in 2010 in Huesca (Spain) and run for 9 years. Two tillage managements, reduced tillage (RT, 15 cm depth with disk ploughing and cultivator) and NT, were practised in barley (Hordeum vulgare L.) rainfed cropping systems.
The site was mostly dominated by barley monocropping, except in 2014 and 2016 when pea (Pisum sativum) and wheat were introduced. About 75 kg N ha−1 yr−1 was applied as an inorganic fertilizer in both management practices. All crop residues were left in the field. In summary, the two management practices considered in this site were as follows: Conventional (LT4DV0), with Barley monocrop under RT, and Diversification 1 (LT4DV1), with Barley monocrop under NT. The impact of tillage on SOC was assessed on a 0–30 cm depth as this was the considered sampling depth, and it is the minimum depth recommended by IPCC to estimate carbon stock with a minimum error. Also, many studies analyze the effect of tillage on SOC at a 0–30 cm depth making possible a comparison between their and our results (Alvarez et al., 1995; Álvaro Fuentes et al., 2009; Meurer et al., 2018).
LT7 Cereals and Forage Crop Rotations in Finland
The common rotation in Finnish croplands includes cereal, leys, and legumes (Hakala et al., 2016; Palosuo et al., 2016). However, cereal monoculture was observed quite commonly on crop production farms in southern Finland. It was practised on 20.1% of field parcels in 2007–2011 (Peltonen-Sainio et al., 2017).
In a sandy soil experimental field in Toholampi Ostrobothnia, western Finland, four crop rotations were practised from 1997 to study erosion and nutrient leaching, crop yield, and soil properties in crop rotations of organic and conventional farming. First 4-year crop rotations (1997–2000) included one conventional and three organic forage crop rotations. Cultivated plants and tillage of crop rotations were kept as similar as possible, the main difference was in fertilization (different nitrogen sources and fertilization intensities). In 2001, the unfertilized organic crop rotation was changed from organic cereal crop rotation to conventional cereal rotation. Organic and conventional crop rotations, fertilized with composted or un-composted farmyard manure, continued similarly, but farmyard manure was changed to cow slurry. The idea and implementation of crop rotations in 2001–2018 is described more precisely in Peltoniemi et al. (2021).
To summarize, the managements for this site are the following:
1) Conventional (LT7DV0): Conventional cereal [barley-barley-rye (Secale cereale L.)-oats (Avena sativa L.)], synthetic fertilizers (2001–2018). In 1997–2000: [barley-timothy (Phleum pratense L.) + clover (Trifolium) ley-timothy + clover ley-mixture of oats and common vetch (Vicia sativa L.)], fertilization with composted fox manure.
2) Diversification 1 (LT7DV1): Organic cereal [barley-timothy + red clover (Trifolium pratense L.) ley -rye-oats] fertilized with cow slurry (2001–2018). In 1997–2000 (barley-timothy + clover ley-timothy + clover ley-mixture of oats and common vetch), no fertilization.
3) Diversification 2 (LT7DV2): Conventional forage [barley-timothy + meadow fescue (Festuca pratensis Huds.) ley-timothy + meadow fescue ley-barley) fertilized with cow slurry + synthetic fertilizers in 2001–2008. In 1997–2000 fertilized with cow farmyard manure + urine.
4) Diversification 3 (LT7DV3): Organic forage (barley-timothy + red clover ley-timothy + clover ley-mixture of oats and common vetch) fertilized with cow slurry in 2001–2018 and composted cow farmyard manure + urine in 1997–2000.
The conventional management (LT7DV0, 2001–2018) in the 4-year rotation included the following cereals: barley (twice), rye (Secale cereale L.), and oats. Sowing and harvesting times for spring cereals, oats, and common vetch were in the beginning of June and in late August or early September, respectively. Rye was sown at the end of August or beginning of September and harvested in August of the next year.
In the diversified systems LT7DV1, LT7DV3, barley was under sown with grass and clover seeds and in diversified system LT7DV2 with grass seed. After the establishment year, ley was grown for 2 years and cut twice per year. In diversified systems LT7DV2 and LT7DV3 ley yields of both cuts were removed. In diversified cereal rotation (LT7DV1) the establishment year was followed by one ley year. Ley was cut twice, the first cut was removed and the second cut was measured and left on the soil surface as GM, except in 2006 when both cuts were removed.
The straw was removed from all crop rotations in the first year of each 4-year crop rotation (1997, 2001, 2005, 2009, 2013, and 2017). Otherwise, straw was left on the field and incorporated into soil during ploughing. Legumes and leys in crop rotation, tillage, and different types of manure (fox manure, farmyard manure, cow slurry) were practised on this site. The amount of manure varied over the study period.
SOC, Soil Properties and Yield Data Used for Simulations
All information required by ECOSSE to run the simulations in the long-term experiments, e.g., SOC, soil properties, and yield data, were collected by the Diverfarming project partners. The supplied SOC concentration in the four arable cropland sites was expressed in g kg−1. To get the SOC stock in t ha−1 at 0–30 cm depth, as required for simulations with ECOSSE, the value was converted into %, and calculated based on the following equations as reported by Nayak et al. (2015):
n: number of soil layers; Cc: carbon concentration; BD: bulk density; D: sampling depth.
In 2018 data were measured separately at 0–10 cm and 10–30 cm depth. Data for previous years were available at 0–30 cm depth without layering (LT1, LT2, LT4, LT7). In 2010 SOC in LT4 was measured at 0–5, 5–10, and 10–25 cm depth. In the absence of further information on SOC depth gradients in that year, SOC concentration at 25–30 cm depth was assumed to be the same as at 15–25 cm depth. In 1997, 2001, and 2009 the SOC concentration in LT7 was measured at 0–23 and 23–35 depth. The SOC concentration of layer 23–35 cm was assumed to represent the concentration of the 23–30 cm soil layer. The available soil water at field capacity and at saturation, and the yield data were supplied by the project partners. Three or more replicated values were available for each of the studied sites.
Weather Characteristics
The weather of three of the test sites Murcia (Spain), Foggia (Italy), and Huesca (Spain), located in the Mediterranean regions, are characterized by warm, dry summers, and wet winters. The annual precipitation averages are between 280 and 529 mm, and occur mostly between October and December and from March to April. The annual temperature averages are between 13°C and 17°C. July and August are the driest months (∼30°C). The mean annual potential evapotranspiration (PET) at these sites reaches 730–1,200 mm maximum, which occurs in July and August. The test site in Finland has colder winters and summers than the other sites. The mean annual temperature in the Finland boreal regions Toholampi is 3°C with annual precipitation of 600 mm and PET of 480 mm. The temperature in July-August reaches between 12 and 20°C. The weather characteristics of the four sites are presented in Figure 1.
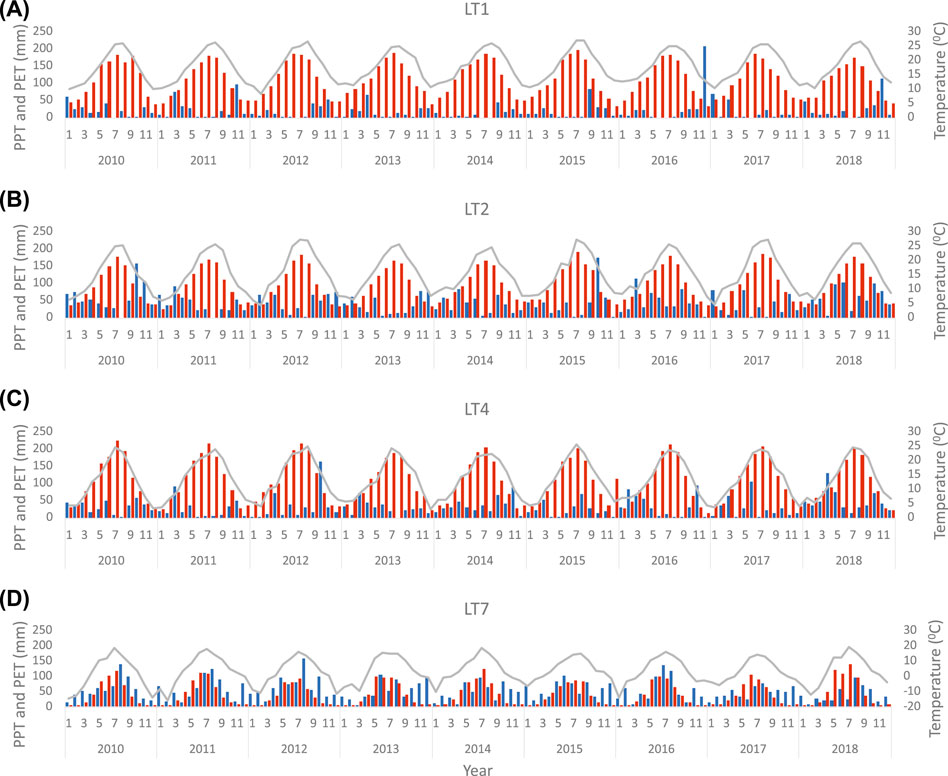
FIGURE 1. Monthly climatic characteristics of European cropland during the 2010–2018 period. (A) LT1 Murcia Spain, (B) LT2 Foggia Italy, (C) LT3 Huesca Spain and (D) LT7 Toholampi, Finland [PPT, Precipitation mm, (blue bars); PET, Potential evapotranspiration (mm, red bars), Temperature, Average temperature (°C, gray line)].
The ECOSSE Model
ECOSSE was developed in 2007 to examine the impact of changes in land-use and climate on thin organo-mineral soils with <50 cm surface organic horizon, which tend to undergo more land-use changes than the deeper peat soils and are more accessible for agriculture (Smith et al., 2010). In particular, Smith et al. (2010) aimed to simulate how land-use and climate change affect SOC and GHG emissions from organo-mineral, mineral, and peat soils. ECOSSE simulates the major below-ground C and N turnover in mineral and highly organic soils using concepts derived from two well-established models, RothC (Colman and Jenkinson, 1996) and SUNDIAL (Bradbury et al., 1993). In ECOSSE soil organic matter (SOM) is described as five pools: active pools of humus (HUM), biomass (BIO), resistant plant material (RPM) and decomposable plant material (DPM), and an inert organic matter (IOM) pool. The DPM/RPM ratio determines the decay of plant material added to the soil, this ratio being derived from standard values for each land use type or modified for new land-uses. Material in each pool decomposes at a specific rate depending on soil temperature, soil moisture, pH, and cover crop. The C in the IOM pool is not active in the decomposition process. ECOSSE works with different levels of input detail, namely in limited data and site-specific mode. In the limited data mode, the only inputs are the commonly available meteorological data, such as monthly air temperature, precipitation, and PET; soil data such as soil pH, soil clay content, initial total SOC content, and soil texture class; and land-use (or management data) such as vegetation type, cultivation/planting schedules, and amount and timing of nutrient amendments. With these drivers the model can simulate daily N-gas flux (N2O, N2, and NH3), C-gas flux (CH4 and CO2), dissolved organic C, dissolved organic N, and leached nitrate N, and therefore to predict how land-use and climate change impact C and N dynamics in organic and mineral soils. A complete and more detailed description of the structure and formulation of C and N stocks dynamics in ECOSSE is given Smith et al., 2010. The limited data mode is well suited for simulations at both national and field scales, thus allowing us to use results to directly inform policy decisions. In limited mode, the plant input is estimated from the provided SOC or estimated using the MIAMI model (Smith et al., 2010). In site-specific mode, along with the above-mentioned input, the user needs to provide detailed management data describing planting times, cultivation, fertilizer applications, manure, and crop type. Also, the user can decide to either provide detailed soil parameters, plant input and soil water parameters, or to have them calculated by the program as in limited mode. Simulations in site-specific mode allow us to get a better approximation of the contribution of factors that determine the activity of the SOM, and of the plant inputs needed to calculate the changes in SOC. The site-specific version has been usually applied to arable land use (Smith et al., 2010). Therefore, also in this study ECOSSE was run in site-specific mode, and all data used for the simulations were obtained from field measurements. ECOSSE was parameterized for 35 arable crops and one perennial crop Miscanthus. In the site version, C input from plant is obtained from the yield, using five empirical parameters as in the following equation (Smith et al., 2010):
Plant residues can be either incorporated or removed. Unlike other C models, e.g., RothC, ECOSSE considers the effect of tillage in the different C and N pools. Based on the depth, tillage is classified as CT, RT, minimum tillage (MT), and NT. In ECOSSE CT represents a cultivation depth of 20–30cm, RT refers to 15–20 cm, and MT to 5–10 cm depth (Smith et al., 2011). Less tillage results in lower or no soil disturbance down to the cultivation depth which enhances SOC stabilization processes (Bell et al., 2012). The model can run with daily/weekly or monthly time steps. In this study the model was run in site mode with a monthly time step.
Model Modifications
To adapt the ECOSSE model to all European arable land uses, the object of this study, the following modifications were made.
Moisture Modifier
The original version of ECOSSE does not consider the dynamics of SOC below the permanent wilting point. Under aerobic conditions, the decomposition of soil organic matter is modified in response to soil temperature, moisture, pH, and crop cover. The soil moisture rate modifier for aerobic decomposition follows the equation proposed by Bradbury et al. (1993) for the SUNDIAL model and varies between 0.2 and 1.0. The aerobic decomposition proceeds at a maximum rate of 1.0 as the soil dries from field capacity (
The above field capacity,
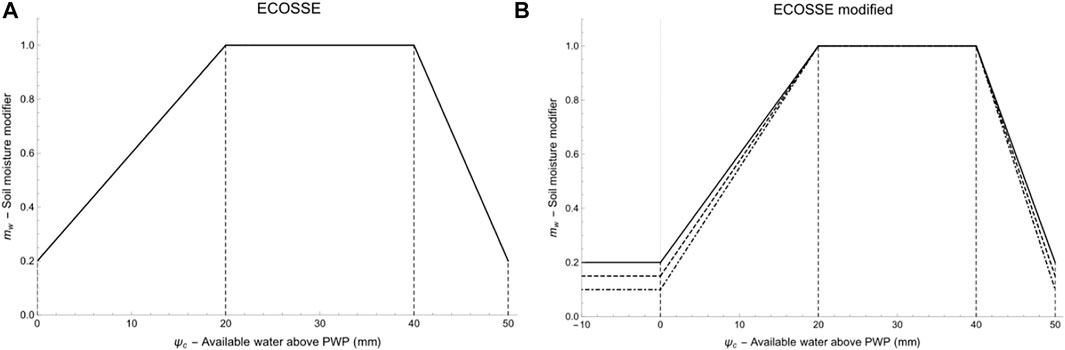
FIGURE 2. (A) Soil moisture rate modifier for aerobic decomposition as a function of the available water above permanent wilting point (PWP) in ECOSSE; (B) and in its modified version–the continuous line refers to normal soil, dashed line to dry soil, and dot-dashed line to semiarid soil.
Following the method used for RothC by Farina et al. (2013), the ECOSSE model was modified to account for dry and semiarid soil conditions, both in limited and site-specific mode. In the modified ECOSSE, when no crop is present (bare fallow) the soil can dry below wilting point, and the soil decomposition rate is assumed to be lower than 0.2 in dry and semiarid soils (Figure 2). The user can now choose between three types of dryness categories: normal (
Multiple Cropping, Manure application, and Irrigation
To be able to consider the managements practised in LT1 and LT2, ECOSSE was modified to allow simulations of multiple cropping systems, i.e., two crops growing one after the other in the same year. The crop melon was added to the arable crop list, and it was parameterized following the crop code for cauliflower as suggested by Bell et al. (2012). Although different types of manure were already included in ECOSSE, several other types have been used within Diverfarming and were added to the new version. These are sheep manure, sheep compost, compost tea, farmyard manure, cow slurry, and GM. ECOSSE computes C input from manure using C and N content in kg t−1 fresh manure. These values were provided for each manure type. Finally, the possibility of adding irrigation was implemented in the model.
Cover Crop
As previously mentioned, cover crops were sown in the LT1 and LT2 sites and all biomass was incorporated into the soil as GM, rather than harvested. In the simulations for LT1, the application of 2.8 t ha−1 yr−1 GM (41.9%C, 2.24%N, Almagro et al., 2021) was considered to simulate the effect of fully incorporated cover crops on soil properties. For LT2, plant C input from each crop was available for the entire period. The yield of tick bean necessary to obtain the equivalent (measured) amount of carbon in the soil (Farina et al., 2017) was calculated using the information provided, and simulations were run accordingly. An additional in silico experiment was run by adding 2.4 t ha−1 yr−1 GM instead of a growing crop after wheat, which reproduced a similar impact on SOC simulations.
Grass Ley
Like other process-based models (DayCent, DNDC), in site-specific mode ECOSSE is not able to simulate C dynamics in intercropping managements. Also, arable crops are assumed to have one sowing and one harvest date; therefore, grass with multiple mowing dates cannot be simulated. However, based on yield values it was possible to evaluate the C input for each crop and rotation in LT7. Carbon input was calculated according to Palosuo et al. (2016). A C input of 1 t ha−1 yr−1 was estimated from the grass incorporated in the soil as GM, which amounts to 2.5 t ha−1 yr−1 of GM in the field for the simulations. The already parameterized crop “oats” was used to model the combined oats + vetch crop (Smith et al., 2011). All other plots were run without further modifications.
Statistical Analysis
For each case study, simulated and measured SOC values were compared. The model performance was evaluated with MODEVAL (Smith and Smith, 2007; Smith et al., 1997a), which allows determining coincidence and association between measured and modeled SOC. MODEVAL calculates the normalized root mean square error (NRMSE), which indicates the total difference between the observed and predicted values (Eq. 5). The significance of NRMSE was assessed by comparing it to the value NRMSE95% obtained assuming a deviation corresponding to the 95% confidence interval of the measurements Eq. 6. An NRMSE value that is less than NRMSE95% indicates that the simulated values fall within the 95% confidence interval of the measurements. The degree of association between the modeled and measured values is determined using the correlation coefficient r. The value of r ranges from −1 to +1. Values close to −1 indicate a negative correlation between simulations and measured values, 0 indicates no correlation, and values close to +1 indicate a positive correlation (Smith et al., 1996). The significance of the association between simulations and measurements is assigned using a Student’s t-test as outlined in Smith and Smith (2007). The mean difference between observation and simulation (M, Eq. 8) is calculated to assess bias in the modeled values and is expressed in the same unit as the analyzed data (Dondini et al., 2015). The bias is expressed as a percentage of the normalized error, EN (Eq. 9). The significance of EN was determined by comparing it to the value EN95% obtained assuming a deviation corresponding to the 95% confidence interval of the measurements (eq. 10). An EN value greater than EN95% indicates that the bias in the simulation is greater than the 95% confidence interval of the measurements
where
Simulations With Mitigation Scenarios
To understand the most suitable mitigation options in the Mediterranean ecosystem, ECOSSE was used to model the mitigation potential of different agricultural management scenarios (SCN) for SOC in the test site LT4. The model was run in this site for the period 2010–2018 using current weather and then for 21 more years using future weather data. The future weather data (denoted as METO-HC-HadRM3Q0-HadCM3Q0) were obtained from the UK Met Office Hadley Centre for Climate Prediction and Research (Christensen et al., 2010). This selected simulated scenario represents the warmest forecasted temperature. Eight management scenarios were evaluated to observe the impact of alternative management practices in the SOC dynamics compared with the baseline management. Here, the baseline management refers to the conventional management of LT4, i.e., LT4DV0. The alternative management scenarios are presented in Table 2. The cropping systems, inorganic N fertilizer management, residue management, and cropping seasons of all scenarios were considered the same as in the baseline management. Yields under the management scenarios associated with RT were obtained as average yield values from LT4DV0 and the management scenarios associated with NT were obtained from LT4DV1. Since different types of diversified managements were already practised in the long-term case studies LT1, LT2, and LT7, no other practices were considered. However, long-term simulations were run for these sites using the same weather scenarios used for LT4 (Supplementary Figures S1–S3).
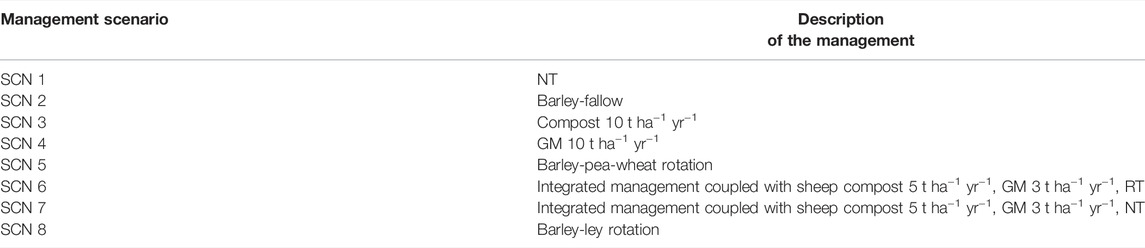
TABLE 2. Management scenarios considered for the Mediterranean region of Spain (Huesca) for the period 2010–2039.
Results
Modeled and Measured SOC
LT1 Diversified Horticulture in Spain
Each year the application of organic amendment under all managements led to a ∼60% increase in SOC over the experimental period 2010–2018 as shown in Figure 3. The highest increase in SOC stock at 0–30 cm depth, from 31 t ha−1 in 2010 to 51 t ha−1, was measured in 2018 under LT1DV2. This represents a 16% gain when compared with the conventional management (LT1DV0). The simulated SOC also showed a similar increasing trend over the 9-year period with the highest gain (32% as compared with LT1DV0) under LT1DV2. The measured changes in SOC under the three managements were 16.56, 16.26, and 19.23 t ha−1 respectively, whereas the predicted SOC changes were 12.83, 13.22, and 16.95 t ha−1 respectively. The simulations are highly significantly correlated (p < 0.001, NRMSE <10%) with the measured data under the three management practices without any bias (Table 3).
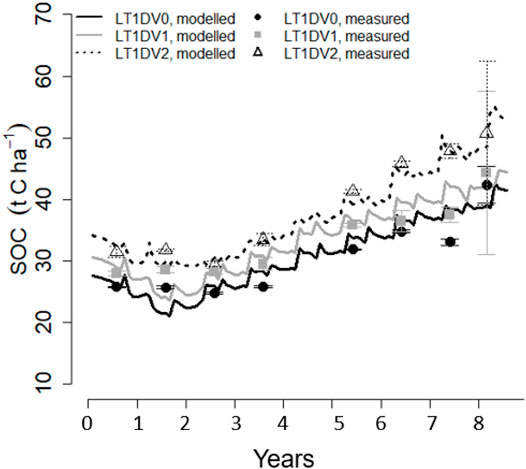
FIGURE 3. Measured (point) and modeled (line) SOC in Spain (Murcia) Horticulture site, LT1, under conventional (LT1DV0), organic (LT1DV1), and diversified management (LT1DV2) for the period 2010–2018 at 0–30 cm depth. The error bar indicates standard deviation of the measured SOC.

TABLE 3. Statistics for the evaluation of the performance of the ECOSSE model on SOC simulations in diversified cropping systems in the European agriculture at 0–30 cm soil depth.
LT2 Durum Wheat in Italy
Long-term impact of tillage in wheat monocropping was observed in the Italian dry soil. When the experiment started in 1995, the measured SOC was 49 t ha−1 and it increased to 62 t ha−1 in 2018 under CT (LT2DV0). Practising NT instead of CT led to a further 21% difference in the increase of SOC (LT2DV1) by the end of the experimental period (Figure 4). The impact of rotation was also observed under both tillage systems between 2010 and 2018. The introduction of tick bean in rotation with wheat led to a measured increase in SOC of 25% under CT (LT2DV2) and 21% under NT (LT2DV3). As in the observations, ECOSSE predicted that under monocropping wheat the long-term NT practice (LT2DV1) will lead to a larger SOC value, nearly 19%, than that simulated in the CT system (LT2DV0). Similarly, when wheat tick bean rotations were considered, the model demonstrated a ∼24% increase in both LT2DV2 and LT2DV3 sites. The increase in measured SOC under LT2DV0 and LT2DV1 for the period 1996–2018 was of 13 and 15 t ha−1 respectively, whereas ECOSSE predicted an increase of 19 and 23 t ha−1 respectively. The increase in SOC stock for the period 2010–2018 under LT2DV2 and LT2DV3 was 13 and 11 t ha−1 respectively, while the model predicted 13 t ha−1 for both managements. A good agreement between the observed and predicted values (NRMSE <10%) was found for all of these sites under the four different treatments. A significant positive association between measured and modeled SOC was attained under all managements (p < 0.05) except LT2DV3. However, a highly significant association between modeled and measured SOC was observed when all values were compared under the three managements. In addition, a nonsignificant bias was found between modeled and measured SOC (Table 3).
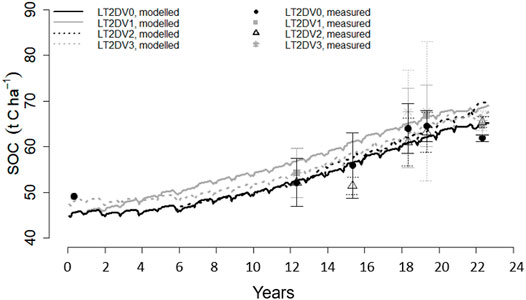
FIGURE 4. Measured (point) and modeled (line) SOC in Foggia (Italy) arable cropland site, LT2, under conventional (LT2DV0), diversification 1 (LT2DV1), diversification 2 (LT2DV2), and diversification 3 (LT2DV3) for the period 1996–2018 at 0–30 cm depth (The field experimental period of LT2DV2 and LT2DV3 is 2010–2018). The error bar indicates standard deviation of the measured SOC. Please note that the simulated and experimental values of LT2DV2 slightly overlap with those of LT2DV0.
LT4 Tillage in Rainfed Systems in Spain
Both modeled and measured SOC showed a similar increasing trend over the studied period under the conventional management associated with RT in cereal rotations (LT4DV0, Figure 5). SOC increased from 35 t ha−1 in 2010 to ∼38 t ha−1 in 2018 as revealed in both the simulations and observations with this management. The increasing trend was also observed when RT was replaced with NT (LT4DV1). The field data showed 6% SOC increase, while the model predicted 8% increase in SOC under NT compared to RT in 9 years. A highly significant positive association between modeled and measured SOC values without bias was observed in this site. Moreover, the NRMSE95% value was greater than the NRMSE values indicating that the simulated values fall within the 95% confidence interval of the measurements.
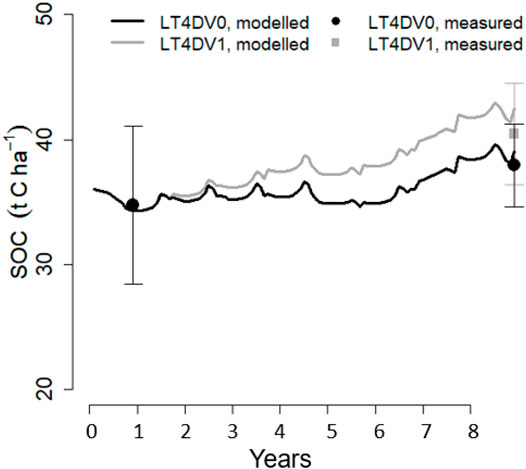
FIGURE 5. Measured (point) and modeled (line) SOC in Huesca (Spain) cereal cropland site, LT4, under conventional (LT4DV0), and diversified management (LT4DV1) for the period 2010–2018 at 0–30 cm depth. The error bar indicates standard deviation of the measured SOC.
LT7 Cereals and forage crop rotations in Finland.
In the Boreal site of Toholampi, Finland, the long-term impact of 4-year cereal and forage rotations under diverse tillage, residue, and manure management practices was observed over 20 years, from 1997 to 2018. In all considered managements, the highest SOC value was observed in 2001, after 4 years from the start of the experiment and then followed a declining trend until the end of the experiment (Figure 6). The maximum SOC loss (nearly 23 t ha−1) was observed under the conventional cereal rotation (LT7DV0) where the value decreased to 127 t ha−1 during 2018 from 150 t ha−1 in 1997. The SOC loss however was comparatively lower under the management with grass in the rotations. Besides, application of manure, tillage, and residue management led to a loss of 10 t ha−1 in LT7DV1 and 5 t ha−1 in LT7DV3, respectively. A SOC gain of nearly 4 t ha−1 was observed under the management of conventional grass (LT7DV2), where an increase in SOC stock was observed from 151 t ha−1 in 1997 to 155 t ha−1 in 2018. As in the measured data, the model also predicted highest SOC gain under the management LT7DV2 amounting to 10 t ha−1 SOC stock increase over the 23 years of simulations. An SOC gain was also observed by the model under the clover-grass management (LT7DV3), predicting an increase of 6 t ha−1. As in observations the maximum decline in SOC was predicted under LT7DV0, with a value of 36 t ha−1 at the end of the simulated period. A positive but nonsignificant association between the modeled and measured SOC values was found for this site, considering all measured data points. However, no bias between simulations and observations was found in the simulations for any management. Also, the NRMSE95% value was greater than the NRMSE values of 7%.
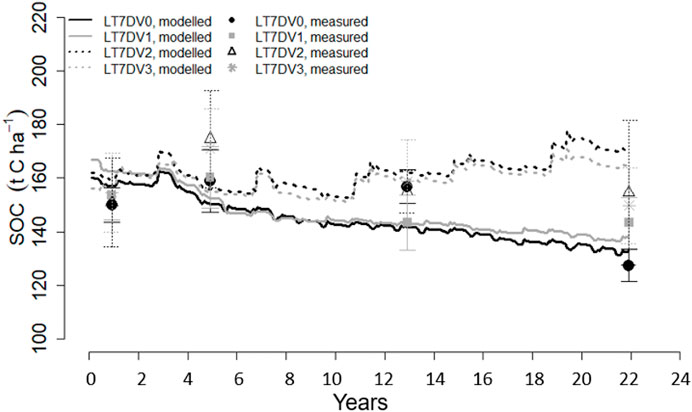
FIGURE 6. Measured (point) and modeled (line) SOC under conventional (LT7DV0), diversification 1 (LT7DV1), diversification 2 (LT7DV2), and diversification 3 (LT7DV3) management in Toholampi (Finland) fodder cropland site, LT7, for the period 2010–2018 at 0–30 cm depth. The error bar indicates standard deviation of the measured SOC.
Mitigation Scenarios
Eight management scenarios were modeled in the Spanish dry soil for the period 2010–2039 to observe the mitigation potential for SOC dynamics at 0–30 cm depth in comparison with baseline scenario which is associated with barley-cereal rotations under RT (LT4DV0) (Figure 7). All scenarios considered in the modeling analysis tend to increase SOC, except SCN 2. Implementing barley-fallow rotations in this scenario leads to a 10% decrease in SOC. No changes, or a slight increase (∼4%), was observed in the barley-pea-wheat rotation (SCN 5), and barley-ley rotation (SCN 8) over 30 years, whereas NT in place of RT led to a 16% increase in SOC (SCN 1). The use of 10 t ha−1 yr−1 of sheep compost produced an increase in SOC of 36% (SCN 3), whereas the same amount of GM produced an increase of 106% (SCN 4), which is the highest gain among all scenarios. An integrated management coupling 5 t ha−1 yr−1 sheep compost and 3 t ha−1 yr−1 GM produced an increase of 59% under RT (SCN 6) and 71% under NT (SCN 7), respectively. The modeld SOC sequestration rate over the 30 years of simulations in this site suggested that an average increase of 1.28 t C ha−1 yr−1 SOC can be obtained by adopting an integrated management. Modifications of the tillage system from RT to NT generated an increase in SOC of 0.30 t C ha−1 yr−1. The SOC sequestration rate predicted by the model for sheep compost was 0.69 t C ha−1 yr−1, whereas with GM applications the simulated SOC sequestration rate was 2.02 t C ha−1 yr−1.
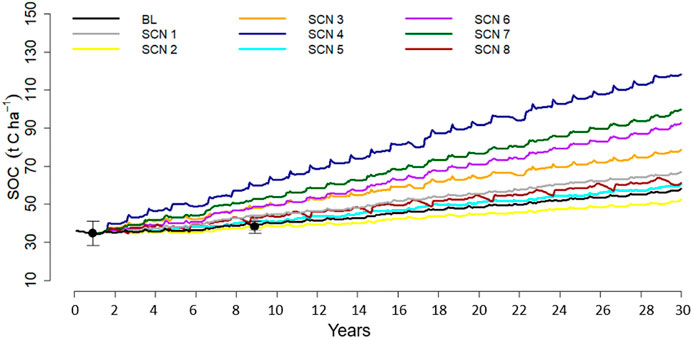
FIGURE 7. The mitigation potential for SOC under different scenarios in the long-term test sites Huesca (Spain) for the period of 2010–2039 at 0–30 cm depth using ECOSSE modeling. BL: Baseline associated with barley cereal rotations with RT. SCN 1: NT, SCN 2: Barley-fallow rotations, SCN 3: sheep compost 10 t ha−1 yr−1, SCN 4: GM 10 t ha−1 yr−1, SCN 5: Barley-pea-wheat crop cycle, SCN 6: Integrated management of sheep compost 5 t ha−1 yr−1, coupled with 3 t ha−1 yr-1 GM and RT, SCN 7: Integrated management of sheep compost 5 t ha−1 yr−1, coupled with 3 t ha−1 yr−1 GM and NT and SCN 8: barley-ley rotations.
Discussion
LT1
Organic amendments are an important exogenous source of C for the soil, and the use of manure/compost can improve soil quality and enhance ecosystem functioning. High SOC values under the organic amendment are often associated with the presence of microbial abundance. A study conducted in Ravenna, Italy, found that compost applications (municipal solid waste, ∼10 t C ha−1 yr−1) can produce an increase of SOC at 0–30 cm depth, which is twice as much as the SOC measured in plots that receive only mineral fertilizers (70–130 kg N C ha−1 yr−1). The microbial abundance did also increase by 50% compared to N fertilized plots (Baldi et al., 2018). An increase in SOC under organic amendment was also reported in the long-term wheat cropland in Broadbalk (Begum et al., 2017) and the barley cropland in Bavaria, Germany (Li et al., 2005). A similar pattern has been observed in all sites object of this work. As can be seen in Figure 3, over the 9-year field experiments in the semiarid Mediterranean region of Murcia, Spain (LT1), the addition of organic amendments led to a clear increase of SOC. An equivalent gain of SOC was observed in both LT1DV0 and LT1DV1, which might be due to the similar rate of sheep manure applied in both sites over the studied period. Among the three managements, the increase in SOC was relatively higher under LT1DV2, which received sheep compost and composted tea over the studied period. Compost is a source of stabilized organic matter, more prone to be incorporated in the soil under organic matter compared to direct applications of fresh manure, which rapidly mineralize (Farina et al., 2018). This site also received additional biomass from a cover crop which was then incorporated in the soil as GM and contributed to a further addition of C to the soil. The organic amendments used in the LT1DV2 produced an increase in SOC of 2.17 t C ha−1 yr−1.
A good agreement between modeled and measured SOC was observed in this experimental site. The measured increasing trend was well matched by the model. The SOC increased sharply in the LT1DV2 site compared to LT1DV0. The simulations in LT1DV2 were run replacing the cover crop with applications of GM equivalent to a plant input of 1 t C ha−1 yr−1. An initial underestimation of the SOC ∼3 t ha−1 suggested the need to change how ECOSSE represents the effect of soil moisture on the decomposition rate in the case of dry soil. ECOSSE was therefore modified following the adaptation to dry soils of the process-based model RothC proposed by Farina et al. (2013) to adjust the unrealistic high C input values for simulations in dry and bare fallow soils, when the water reaches values below wilting point.
Overall, observed and simulated results for this site demonstrated that in contrast to traditional manure, composted manure and application of GM contribute to a significant (p < 0.001) increase of SOC in dry ecosystems. This could depend on the ratio of applied amounts. In LT1DV2 composted manure and GM were combined in one treatment, while in LT1DV0 and LT1DV1 only sheep manure was applied. Reducing the minimum value of the moisture modifier from 0.2 to 0.15 slowed down the decomposition rate in the case of dry conditions. Such adaptation of the model has produced an increase of 0.4 t C ha−1 yr−1 that resulted into matching the measured SOC output by the end of the simulated period without any further addition of C input.
LT2
Adoption of NT practices proved to be an important diversified management in the semiarid region of Foggia, Italy. Compared to intense cultivations this practice promotes the accumulation of SOC in the topsoil. Previous studies showed that great benefits derive from practicing NT instead of CT in the Mediterranean cropland, including an increase in SOC (Aguilera et al., 2013; Sanz-Cobena et al., 2017), and that the reduced disturbance of soil, associated with NT, leads to a smaller decomposition rate therefore increasing SOC (Álvaro Fuentes et al., 2009; Begum et al., 2018a). A gain of about 13–20% was observed in NT compared to CT in different regions of Spain at 0–30 cm depth (Alvaro-Fuentes and Paustian, 2011) which agrees well with our findings of a 20% increase under NT with respect to CT in monocropping wheat. Over the 22 years in which the experiment ran, the measured gain of SOC under wheat monocropping was 0.58 and 0.70 t C ha−1 yr−1 for CT (LT2DV0) and NT (LT2DV1), respectively. The simulated SOC under these two managements was 0.84 and 1.00 t C ha−1 yr−1, respectively. Using the model Century to simulate monocropping barley under CT and NT in the semiarid Mediterranean region of Zaragoza, Spain, for 16 years, Álvaro Fuentes et al. (2009) found an increase in the rate of SOC of 0.21 (0.18, measured) and 0.37 (0.46, measured) t C ha−1 yr−1 under CT and NT, respectively. The results clearly demonstrated a greater increase in SOC under NT. The relatively higher SOC gain under monocropping wheat (compared to monocropping barley) could be due to a greater plant C input, as the measured annual average of above-ground plant C input from wheat monocropping is ∼2.50 t C ha−1 yr−1 which is nearly twice that estimated in Spain under barley monocropping systems (Álvaro Fuentes et al., 2009). A slight decrease in SOC was found under NT in the rotations systems compared to CT; however, the difference in C gain is not significant (p > 0.05). Overall in this site the rotation with tick bean showed a much higher impact on SOC compared to different tillage management practices. Since ECOSSE does not consider root growth we assume that higher increase in SOC is due to N fixation by the tick beans and the increased amount of C input.
The higher NRMSE95 and E95 values, in all sites, compared to NRMSE and EN respectively (Table 3) indicate that the total error in the simulations is less than the total error in the measurements at 95% confidence interval (Smith et al., 1997a; Bell et al., 2012). Also, no systematic bias was observed between simulations and observations with a nonsignificant t-statistics, and the observed NRMSE <6% is comparable to that in similar studies (Álvaro Fuentes et al., 2009) indicating an acceptable agreement between model output and experimental measurements.
LT4
The temporal dynamics of SOC was observed in the Mediterranean dry region Huesca (Spain) under two tillage regimes for the period 2010–2018. Both the simulations and observations demonstrated a higher SOC increase under NT compared to RT. In this long-term experiment, it was observed that in addition to the lower soil disturbance, the measured crop production, and therefore the C input returned to the soil, was significantly higher under NT than under RT (Plaza-Bonilla et al., 2017). In semiarid Mediterranean conditions, it has been demonstrated that conservation tillage systems, and particularly NT systems, promote soil water conservation and higher crop biomass production (Cantero-Martínez et al., 2007; Lampurlanés et al., 2016). Under NT, the crop residue layer in the soil surface reduces soil water losses by direct evaporation and also increases the infiltration to runoff ratio (Lampurlanés et al., 2016). These changes in soil water conservation result in a higher water use efficiency in NT compared to RT, and in the consequent increase in crop residue production.
Interestingly, even the RT system led to an increase in SOC levels. This is an opposite finding to what has been observed in other long-term tillage experiments located in NE Spain in which tillage systems reduced SOC stocks (Álvaro Fuentes et al., 2008). The increase in SOC stocks under RT can be explained by the change in tillage implements occurred in these areas during the last few decades. About 30 years ago, farmers adopted less intensive tillage systems, moving from moldboard ploughing to less intensive chisel or disk ploughing. This change in tillage systems resulted in an increase in SOC stocks which is still noticeable to date.
In simulations performed in a different long-term experiment located also in rainfed NE Spain, the RothC model predicted reasonably well the higher SOC accumulation rates observed under NT (Álvaro-Fuentes et al., 2012). In this last study, the higher C inputs measured under NT led to the increase in SOC accumulation rates. Similarly, in our study, the ECOSSE model was also able to capture the different SOC stock change rates observed between the two tillage systems and driven by the different C inputs in NT and RT.
LT7
Perennial crops in rotation represent an important conservation farming system, which disturbs the soil less, reduces erosion, conserves soil moisture, and improves soil health overall (Smith et al., 2007; Di Bene et al., 2011; Sanz-Cobena et al., 2017). The three diversified managements in the Boreal region of Toholampi, Finland, included legume and/or perennial grass in rotation with cereals. Initial observations showed that after 4 years from the beginning of the experiment, in 2001, a nonsignificant increase (p > 0.05) in SOC was observed. This was the case in all four treated plots, including LT7DV1 where no organic amendments were applied. After 2001, it was possible to observe a slightly declining trend for LT7DV0 and LT7DV1, slightly increasing trend for LT7DV2 and a more stable one for LT7DV3. The possible reason for the difference in SOC dynamics between the first 4 years and the following ones might be due to the practice of having clover-grass ley in the rotation barley-grass-grass-oats + vetch, which was then changed with the cereal rotation barley-barley-rye-oats (LT7DV0), after 2001. Moreover, the LT7DV0 received 14 t ha−1 fox manure in 1997 whereas only chemical N fertilizers were applied for the rest of the study period. Numerical simulations suggest that intense cultivations without any organic amendments in the cereal rotations lead to increased respiration and consequently decreased SOC (not shown). Grass ley in LT7DV2 and clover-grass ley in LT7DV3 was included for two successive years. For the LT7DV2 plot, the modeled average annual C input through root rhizodeposition during the grass growing seasons was 1.8 t C ha−1 yr−1, which is nearly three times higher than that in the annual crops (LT7DV0). This supports the observation by Smith et al. (2007) that perennial crops accumulate more C in below ground biomass than annual crops. Despite the addition of 48 t ha−1 composted farmyard manure in 1997 and greater amount of cow slurry during most of the remaining period, nearly 28% more than that of the other manured plot, LT7DV3 had lower SOC stocks compared to LT7DV2 (p > 0.05). In the simulations, in agreement with the measurements, the model predicted a slight gain in SOC under LT7DV2 and LT7DV3. However, in both sites this increasing trend led to a negative correlation between modeled and measured values, with a nonsignificant r value of −0.55 and −0.77, respectively. The estimation of the uncertainty (NRMSE) for the four managements was found to be 7%, which falls within the values found by Falloon and Smith (2003) for the two process-based models Century and RothC applied to different management practices in European ecosystems (NRMSE between 1.8% and 16.4% for Century and 1.7%–29.2% for RothC). From this long-term study, it is clear that the factors with the largest impact on SOC are the type of crop, tillage, residue, and manure management/type. An additional benefit observed under LT7DV3 is associated with the fact that leguminous crops fix the atmospheric N so that the use of inorganic fertilizer can be reduced.
Modeling Mitigation Scenarios
Different mitigation scenarios have been modeled in Huesca to identify the most suitable managements to increase SOC sequestrations in Mediterranean ecosystems compared to a baseline scenario with RT and incorporation of crop residues (already considered in LT4). The simulation results, which could provide a guideline to policy makers on selecting best agricultural practices for this geographic region, showed that a few promising agricultural managements could be adopted to improve agricultural sustainability, soil resilience, SOC sequestration, and to mitigate the impact of climate change. The simulated changes in SOC were compared to the changes suggested by the IPCC after 20 years under different agricultural activities and categorized depending on climatic regions (Lasco et al., 2006). IPCC estimated an annualized stock change factor (SCF) of 1.10 for temperate dry climatic regions when changing from full tillage to NT, which indicates a 10% increase of SOC under such modification in arable cropland over 20 years. In our simulation a change of 13% (SCF, 1.13) over 20 years was obtained, which compares well with the IPCC value. Also, the 28%–74% range of predicted changes in SOC values under different management practices (SCN 3, SCN 4, SCN 6, and SCN 7) contains the value of 37% change in C stock reported by the IPCC under high nutrient inputs in temperate dry soil over the same period. According to the IPCC, low and medium input are classified as: low residue return, long time soil bare fallow, N fixing crop in rotations. The simulated annual variation in SOC values under these categories (SCN 2, SCN 5, and SCN 8) was between −0.19 and 0.12 t C ha−1 yr−1 compared to the reported SCF values of −0.05–−0.04 t C ha−1 yr−1. The difference of 0.30 C ha−1 yr−1 found in this analysis between RT (BL) and NT (SCN 1) is within the range reported by the previous literature, for changes from 0.25–0.5 t C ha−1 yr−1 under temperate dry climate (Lal., 2008) and of 0.09–0.71 t C ha−1 yr−1 in European continental Mediterranean semi-arid regions in Spain (Álvaro Fuentes et al., 2009; Gonzalez-Sanchez et al., 2012). Also, our results compare well to those reported in the meta-analysis by Aguilera et al. (2013) on SOC changes in Mediterranean cropland. The authors found an 11.3% increase under NT compared to CT and an increase in SOC of 1.31–5.29 t C ha−1 yr−1 under external organic inputs, which corresponds to 23.8%–98% increase, based on the type and amount of organic amendment. However, these estimations refer to the global Mediterranean climatic regions including arable land and woody cropland. An increase in SOC of 0.72 t C ha−1 yr−1 was also found under organic amendments by a meta-analysis carried out by Francaviglia et al. (2017) across European Mediterranean arable cropland, which compares well with the increase of 0.69–2.02 t C ha−1 yr−1 in simulated SOC found in our tested sites (SCN 3, SCN 4, SCN 6, and SCN 7).
The model results under SCN 3 suggest avoiding periods of bare fallow, as such practice leads to SOC loss. A long-term field experiment (16 years) in Mediterranean regions in Spain under RT management found an increase of SOC of 0.24 t C ha−1 yr−1 in continuous barley cropping systems, whereas the value reduced to 0.003 t C ha−1 yr−1 under barley-fallow cropping systems (Álvaro Fuentes et al., 2009). The maximum gain in SOC appeared to be under SCN 4 with GM applied at a rate of 10 t C ha−1 yr−1. However, this scenario might not be realistic as such high amount of GM is not practised in the European continent. Therefore, the best management scenarios considered in the modeling analysis appeared to be the integrated nutrient managements SCN 6 and SCN 7, followed by the NT scenario (SCN 1). The modeling analysis demonstrated that keeping the same soil management and cropping systems as in the baseline, there is scope to increase SOC by applying external organic C input, with compost being preferred to manure, as manure can lead to an increase in GHG emissions (Smith et al., 2007). In SCN 6 and SCN 7, in addition to the 5 t C ha−1 yr−1 compost, a 3 t C ha−1 yr−1 GM has been applied as a representative of cover crop. The adoption of the SCN 6 management could lead to a SOC increase of nearly 1.14 t C ha−1 yr−1 over 30 years.
When considering the 11.8 M ha of Spanish arable land (FAOSTAT, 2021), it is clear that the potential increase in SOC can be expected to be 3.4 Tg C yr−1 under NT and 13.3 Tg C yr−1 from diversified nutrient management practices, which correspond to a reduction in CO2 emission of 12.5 and 48.9 Tg CO2 yr−1 respectively. Applying the process-based model Century the mitigation potential for SOC in Spain was predicted to be 17.8 Tg CO2 yr−1 (Álvaro Fuentes et al., 2009). Our modeled results demonstrate that there is scope to increase SOC stock by adopting diversified management practices including no tillage, diverse cropping systems, and exogenous organic input. Practising an integrated management can contribute to mitigate nearly 14% of the total GHG emissions occurring in Spain (Álvaro Fuentes et al., 2009). Our study suggests that the 4 “per mille” change in SOC can be achieved within 10 years changing tillage practices and within 4 years using an integrated nutrient management.
It is clear that there is great uncertainty in estimating mitigation effects at national level based on one site only. However, this can be deemed as an interim set of estimates obtained by keeping soil, climate, management, and crop growth constant. The estimated figures compare well with other modeling studies in Spain (Álvaro Fuentes et al., 2009), and provide information on SOC sequestration potential under different tillage management, manure management, and cropping systems in Spain.
Conclusion
To achieve the SDGs, the current research on European agroecosystems is focusing on the adoption of diversified strategies that would promote soil resilience and agricultural sustainability. This study presents how the process-based ecosystem model ECOSSE was modified and used to simulate data from four European long-term experiments, to investigate changes in SOC under diversified soil and crop managements in comparison with traditional management practices. ECOSSE can now account for different levels of soil dryness, including semiarid regions and vertisols, and it is able to simulate multiple cropping systems and cover crops. ECOSSE was used to assess the mitigation potential for SOC of different management scenarios in semiarid regions in Spain.
The modified ECOSSE well simulated trend and significant associations (if enough data points were provided, >2) between the modeled and observed values of SOC over the study period in both the Mediterranean and Boreal regions. Both simulations and measurements revealed that with the considered diversifications the gain in SOC is higher in the test sites located in the Mediterranean regions than in the Boreal ecosystems. On the other hand, the initial SOC stock in the Boreal regions was much higher, 150 t C ha−1, than the one in the Mediterranean regions, 25 to 50 t C ha−1, therefore allowing for a lower SOC sequestration potential. The declining SOC stocks are also attributed to the fact that following relative recent land use changes, the SOC has not reached an equilibrium state yet (Kätterer et al., 2013). A study by Kätterer et al. (2013) on the Northern European agriculture found decreasing SOC stocks in ley arable rotations compared to cereal based rotations due to higher initial SOC stocks, which they associated with the previous land use history and management. In Finland decreasing trend in soil C stock has also been identified (0.4%/per year) by Heikkinen et al. (2013). Our results suggest that further loss of SOC could be avoided by introducing grass/legumes in the conventional cereal rotations and by adding manure. The higher SOC sequestration potential with lower initial SOC contents that was observed in the Mediterranean soils is consistent with previous findings (Smith et al., 1997a; Minasny et al., 2017; Begum et al., 2018b). The long-term simulations obtained with future weather scenarios for LT1, LT2, and LT7 (Supplementary Figures S1–S3) and the mitigation scenarios considered for LT4 confirm these findings suggesting that among all sites the highest SOC sequestration potential can be achieved in LT2 and LT4.
IPCC identified SOC sequestration as an important strategy for C mitigation (Smith et al., 1997b). The modeling exercise in the semiarid arable lands in Spain demonstrates the feasibility of different agricultural management options on sequestering SOC in Mediterranean ecosystems. Our modeled results suggested that an integrated approach considering manure application, cover crop, and NT in the barley cropping systems would be an effective management option. The simulations indicated that crop type, tillage management, and type of manure are the factors with the largest impact on SOC. As suggested by Smith et al. (1997b) other management options could be feasible in European cropland, e.g., afforestation of surplus land, which has greater C mitigation potential but was not covered in our analysis. The applicability of the modified ECOSSE model to European agro-ecosystems at site scale is a first step toward a more thorough analysis of SOC sequestration potential at regional scale, which is necessary to provide effective and clear guidelines for policy makers and stakeholders to mitigate the impact of climate change at the national and international levels.
Data Availability Statement
The raw data supporting the conclusion of this article will be made available by the authors, without undue reservation.
Author Contributions
KB: Writing-original draft, Model calibration and validation. RZ: Project Co-ordinator, Funding acquisition, Data collection, Writing-review and editing. RF, RL, and JÁ-F: Data collection, Writing-review and editing. MC: Study supervision, Model coding and parameterization, Writing-review and editing. All authors have read and agreed to the published version of the manuscript.
Funding
The work was funded by the Diverfarming project “Crop diversification and low-input farming across Europe: from practitioners’ engagement and ecosystems services to increased revenues and value chain organisation,” a European Union’s Horizon 2020 Programme for Research and Innovation, under grant agreement no. 728003.
Conflict of Interest
The authors declare that the research was conducted in the absence of any commercial or financial relationships that could be construed as a potential conflict of interest.
Publisher’s Note
All claims expressed in this article are solely those of the authors and do not necessarily represent those of their affiliated organizations, or those of the publisher, the editors and the reviewers. Any product that may be evaluated in this article, or claim that may be made by its manufacturer, is not guaranteed or endorsed by the publisher.
Acknowledgments
We are grateful to Dr Claudia Di Bene, Council for Agricultural Research and Economics (CREA), Diverfarming project partner, Italy, Professor Kristina Regina, MTT Agrifood Research, FI-31600 Jokioinen, Co-ordinator Finland, Diverfarming, Project partner, Finland for providing valuable information of their measured test sites. We also would like to thank Professor Jo Smith, Martin Michael, and the Environmental Modelling group at the University of Aberdeen, UK, for providing information about the ECOSSE model. Finally, we would like to thank all reviewers for their insightful comments.
Supplementary Material
The Supplementary Material for this article can be found online at: https://www.frontiersin.org/articles/10.3389/fenvs.2022.819162/full#supplementary-material
References
Abdalla, M., Hastings, A., Bell, M. J., Smith, J. U., Richards, M., Nilsson, M. B., et al. (2014). Simulation of CO2 and Attribution Analysis at Six European Peatland Sites Using the ECOSSE Model. Water Air Soil Pollut. 225. doi:10.1007/s11270-014-2182-8
Aguilera, E., Guzmán, G. I., Álvaro-Fuentes, J., Infante-Amate, J., García-Ruiz, R., Carranza-Gallego, G., et al. (2018). A Historical Perspective on Soil Organic Carbon in Mediterranean Cropland (Spain, 1900-2008). Sci. Total Environ. 621, 634–648. doi:10.1016/j.scitotenv.2017.11.243
Aguilera, E., Lassaletta, L., Gattinger, A., and Gimeno, B. S. (2013). Managing Soil Carbon for Climate Change Mitigation and Adaptation in Mediterranean Cropping Systems: A Meta-Analysis. Agric. Ecosyst. Environ. 168, 25–36. doi:10.1016/j.agee.2013.02.003
Almagro, M., Garcia-Franco, N., and Martínez-Mena, M. (2017). The Potential of Reducing Tillage Frequency and Incorporating Plant Residues as a Strategy for Climate Change Mitigation in Semiarid Mediterranean Agroecosystems. Agric. Ecosyst. Environ. 246, 210–220. doi:10.1016/j.agee.2017.05.016
Almagro, M., Ruiz-Navarro, A., Díaz-Pereira, E., Albaladejo, J., and Martínez-Mena, M. (2021). Plant Residue Chemical Quality Modulates the Soil Microbial Response Related to Decomposition and Soil Organic Carbon and Nitrogen Stabilization in a Rainfed Mediterranean Agroecosystem. Soil Biol. Biochem. 156, 108198. doi:10.1016/j.soilbio.2021.108198
Alvarez, R., Diaz, R. A., Barbero, N., Santanatoglia, O. J., and Blotta, L. (1995). Soil Organic Carbon, Microbial Biomass and CO2-C Production from Three Tillage Systems. Soil Tillage Res. 33 (1), 17–28. doi:10.1016/0167-1987(94)00432-E
Álvaro-Fuentes, J., López, M. v., Arrúe, J. L., Moret, D., and Paustian, K. (2009). Tillage and Cropping Effects on Soil Organic Carbon in Mediterranean Semiarid Agroecosystems: Testing the Century Model. Agric. Ecosyst. Environ. 134, 211–217. doi:10.1016/j.agee.2009.07.001
Álvaro-Fuentes, J., López, M. V., Cantero-Martinez, C., and Arrúe, J. L. (2008). Tillage Effects on Soil Organic Carbon Fractions in Mediterranean Dryland Agroecosystems. Soil Sci. Soc. Am. J. 72, 541–547. doi:10.2136/sssaj2007.0164
Álvaro-Fuentes, J., Morell, F. J., Plaza-Bonilla, D., Arrúe, J. L., and Cantero-Martínez, C. (2012). Modelling Tillage and Nitrogen Fertilization Effects on Soil Organic Carbon Dynamics. Soil Tillage Res. 120, 32–39. doi:10.1016/j.still.2012.01.009
Álvaro-Fuentes, J., and Paustian, K. (2011). Potential Soil Carbon Sequestration in a Semiarid Mediterranean Agroecosystem under Climate Change: Quantifying Management and Climate Effects. Plant Soil 338, 261–272. doi:10.1007/s11104-010-0304-7
Baldi, E., Cavani, L., Margon, A., Quartieri, M., Sorrenti, G., Marzadori, C., et al. (2018). Effect of Compost Application on the Dynamics of Carbon in a Nectarine Orchard Ecosystem. Sci. Total Environ. 637-638, 918–925. doi:10.1016/j.scitotenv.2018.05.093
Begum, K., Kuhnert, M., Yeluripati, J., Glendining, M., and Smith, P. (2017). Simulating Soil Carbon Sequestration from Long Term Fertilizer and Manure Additions under Continuous Wheat Using the DailyDayCent Model. Nutr. Cycl Agroecosyst 109, 291–302. doi:10.1007/s10705-017-9888-0
Begum, K., Kuhnert, M., Yeluripati, J., Ogle, S., Parton, W., Kader, M. A., et al. (2018b). Soil Organic Carbon Sequestration and Mitigation Potential in a rice Cropland in Bangladesh - a Modelling Approach. Field Crops Res. 226, 16–27. doi:10.1016/j.fcr.2018.07.001
Begum, K., Kuhnert, M., Yeluripati, J., Ogle, S., Parton, W., Kader, M., et al. (2018a). Model Based Regional Estimates of Soil Organic Carbon Sequestration and Greenhouse Gas Mitigation Potentials from rice Croplands in Bangladesh. Land 7, 82. doi:10.3390/land7030082
Bell, M. J., Jones, E., Smith, J., Smith, P., Yeluripati, J., Augustin, J., et al. (2012). Simulation of Soil Nitrogen, Nitrous Oxide Emissions and Mitigation Scenarios at 3 European Cropland Sites Using the ECOSSE Model. Nutr. Cycl Agroecosyst 92, 161–181. doi:10.1007/s10705-011-9479-4
Bradbury, N. J., Whitmore, A. P., Hart, P. B. S., and Jenkinson, D. S. (1993). Modelling the Fate of Nitrogen in Crop and Soil in the Years Following Application of 15N-Labelled Fertilizer to winter Wheat. J. Agric. Sci. 121, 363–379. doi:10.1017/S0021859600085567
Cantero-Martínez, C., Angás, P., Lampurlanés, J., and Castel, L (2007). Long-term Yield and Water Use Efficiency under Various Tillage Systems in Mediterranean Rainfed Conditions. Ann. Appl. Biol. 150 (3), 293–305. doi:10.1111/j.1744-7348.2007.00142.x
Christensen, J., Kjellström, E., Giorgi, F., Lenderink, G., and Rummukainen, M. (2010). Weight Assignment in Regional Climate Models. Clim. Res. 44, 179–194. doi:10.3354/cr00916
Colman, K., and Jenkinson, D. S. (1996). RothC-26.3 — a Model for the Turnover of Carbon in Soil. In: D. S. Powlson, P. Smith, and J. U. Smith (Eds.), Evaluation of Soil Organic Matter Models Using Existing Long-Term Datasets. NATO ASI Series I. Springer-Verlag, Heidelberg
Di Bene, C., Tavarini, S., Mazzoncini, M., and Angelini, L. G. (2011). Changes in Soil Chemical Parameters and Organic Matter Balance after 13 Years of Ramie [Boehmeria Nivea (L.) Gaud.] Cultivation in the Mediterranean Region. Eur. J. Agron. 35, 154–163. doi:10.1016/j.eja.2011.05.007
Dondini, M., Jones, E. O., Richards, M., Pogson, M., Rowe, R. L., Keith, A. M., et al. (2015). Evaluation of the ECOSSE Model for Simulating Soil Carbon under Short Rotation Forestry Energy Crops in Britain. GCB Bioenergy 7, 527–540. doi:10.1111/gcbb.12154
Dondini, M., Richards, M. I. A., Pogson, M., McCalmont, J., Drewer, J., Marshall, R., et al. (2016). Simulation of Greenhouse Gases Following Land-Use Change to Bioenergy Crops Using the ECOSSE Model: a Comparison between Site Measurements and Model Predictions. GCB Bioenergy 8, 925–940. doi:10.1111/gcbb.12298
Ec, (2006). European Commission, Thematic Strategy for Soil protection. Available at: https://ec.europa.eu/environment/soil/three_en.htm (Accessed on October 12, 2021).
Eurostat, (2015). Available at: http://ec.europa.eu/eurostat/.
Falloon, P., and Smith, P. (2003). Accounting for Changes in Soil Carbon under the Kyoto Protocol: Need for Improved Long-Term Data Sets to Reduce Uncertainty in Model Projections. Soil Use Manag. 19, 265–269. doi:10.1079/sum200320110.1111/j.1475-2743.2003.tb00313.x
Faostat, (2021). Available at: https://www.fao.org/faostat/en/#data/RL (Accessed October 12, 2021).
Farina, R., Coleman, K., and Whitmore, A. P. (2013). Modification of the RothC Model for Simulations of Soil Organic C Dynamics in Dryland Regions. Geoderma 200-201, 18–30. doi:10.1016/j.geoderma.2013.01.021
Farina, R., Marchetti, A., Francaviglia, R., Napoli, R., and Bene, C. D. (2017). Modeling Regional Soil C Stocks and CO2 Emissions under Mediterranean Cropping Systems and Soil Types. Agric. Ecosyst. Environ. 238, 128–141. doi:10.1016/j.agee.2016.08.015
Farina, R., Testani, E., Campanelli, G., Leteo, F., Napoli, R., Canali, S., et al. (2018). Potential Carbon Sequestration in a Mediterranean Organic Vegetable Cropping System. A Model Approach for Evaluating the Effects of Compost and Agro-Ecological Service Crops (ASCs). Agric. Syst. 162, 239–248. doi:10.1016/j.agsy.2018.02.002
Flattery, P., Fealy, R., Fealy, R. M., Lanigan, G., and Green, S. (2018). Simulation of Soil Carbon Efflux from an Arable Soil Using the ECOSSE Model: Need for an Improved Model Evaluation Framework? Sci. Total Environ. 622-623, 1241–1249. doi:10.1016/j.scitotenv.2017.12.077
Francaviglia, R., Álvaro-Fuentes, J., di Bene, C., Gai, L., Regina, K., and Turtola, E. (2020). Diversification and Management Practices in Selected European Regions. A Data Analysis of Arable Crops Production. Agronomy 10, 297. Agronomy 10. doi:10.3390/agronomy10020297
Francaviglia, R., di Bene, C., Farina, R., and Salvati, L. (2017). Soil Organic Carbon Sequestration and Tillage Systems in the Mediterranean Basin: a Data Mining Approach. Nutr. Cycl Agroecosyst 107, 125–137. doi:10.1007/s10705-016-9820-z
González-Sánchez, E. J., Ordóñez-Fernández, R., Carbonell-Bojollo, R., Veroz-González, O., and Gil-Ribes, J. A. (2012). Meta-analysis on Atmospheric Carbon Capture in Spain through the Use of Conservation Agriculture. Soil Tillage Res. 122, 52–60. doi:10.1016/j.still.2012.03.001
Hakala, K., Heikkinen, J., Sinkko, T., and Pahkala, K. (2016). Field Trial Results of Straw Yield with Different Harvesting Methods, and Modelled Effects on Soil Organic Carbon. A Case Study from Southern Finland. Biomass and Bioenergy 95, 8–18. doi:10.1016/j.biombioe.2016.08.021
Heikkinen, J., Ketoja, E., Nuutinen, V., and Regina, K. (2013). Declining Trend of Carbon in Finnish Cropland Soils in 1974-2009. Glob. Change Biol. 19, 1456–1469. doi:10.1111/gcb.12137
Hernanz, J. L., Sánchez-Girón, V., and Navarrete, L. (2009). Soil Carbon Sequestration and Stratification in a Cereal/leguminous Crop Rotation with Three Tillage Systems in Semiarid Conditions. Agric. Ecosyst. Environ. 133, 114–122. doi:10.1016/j.agee.2009.05.009
Jenkinson, D. S., Harris, H. C., Ryan, J., Mcneill, A. M., Pilbeam, C. J., and Coleman, K. (1999). Organic Matter Turnover in a Calcareous clay Soil from Syria under a Two-Course Cereal Rotation. doi:10.1016/S0038-0717(98)00157-6
Kätterer, T., Bolinder, M. A., Thorvaldsson, G., and Kirchmann, H.(2013). The Role of Grasslands in a Green Future Influence of Ley-Arable Systems on Soil Carbon Stocks in Northern Europe and Eastern Canada. http://www-markinventeringen.slu.se.
Lal, R. (2016). Beyond COP 21: Potential and Challenges of the "4 Per Thousand" Initiative. J. Soil Water Conservation 71, 20A–25A. doi:10.2489/jswc.71.1.20A
Lal, R. (2013). Soil Carbon Management and Climate Change. Carbon Manag. 4, 439–462. doi:10.4155/cmt.13.31
Lal, R. (2008). Soil Carbon Stocks under Present and Future Climate with Specific Reference to European Ecoregions. Nutr. Cycl Agroecosyst 81 (2), 113–127. doi:10.1007/s10705-007-9147-x
Lampurlanés, J., Plaza-Bonilla, D., Álvaro-Fuentes, J., and Cantero-Martínez, C. (2016). Long-term Analysis of Soil Water Conservation and Crop Yield under Different Tillage Systems in Mediterranean Rainfed Conditions. Field Crops Res. 189, 59–67. doi:10.1016/j.fcr.2016.02.010
Lasco, R. D., Ogle, S., Raison, J., Verchot, L., Wassmann, R., Yagi, K., et al. (2006). "Chapter 5: Cropland. 2006 IPCC Guidelines for National Greenhouse Gas Inventories," in Intergovernmental Panel on Climate Change, Vol. 4. Japan: IGES.
Li, C., Frolking, S., and Butterbach-Bahl, K. (2005). Carbon Sequestration in Arable Soils Is Likely to Increase Nitrous Oxide Emissions, Offsetting Reductions in Climate Radiative Forcing. Climatic Change 72 (3), 321–338. doi:10.1007/s10584-005-6791-5
Lichtenberg, E. M., Kennedy, C. M., Kremen, C., Batáry, P., Berendse, F., Bommarco, R., et al. (2017). A Global Synthesis of the Effects of Diversified Farming Systems on Arthropod Diversity within fields and across Agricultural Landscapes. Glob. Change Biol. 23, 4946–4957. doi:10.1111/gcb.13714
Mazzoncini, M., di Bene, C., Coli, A., Antichi, D., Petri, M., and Bonari, E. (2008). Rainfed Wheat and Soybean Productivity in a Long‐Term Tillage Experiment in Central Italy. Agron.j. 100, 1418–1429. doi:10.2134/agronj2007.0173
Meurer, K. H. E., Haddaway, N. R., Bolinder, M. A., and Kätterer, T. (2018)., 177. Elsevier B.V, 613–622. doi:10.1016/j.earscirev.2017.12.015Tillage Intensity Affects Total SOC Stocks in Boreo-Temperate Regions Only in the Topsoil-A Systematic Review Using an ESM ApproachEarth-Science Rev.
Minasny, B., Malone, B. P., McBratney, A. B., Angers, D. A., Arrouays, D., Chambers, A., et al. (2017). Soil Carbon 4 Per Mille. Geoderma 292, 59–86. doi:10.1016/j.geoderma.2017.01.002
Nayak, D., Saetnan, E., Cheng, K., Wang, W., Koslowski, F., Cheng, Y.-F., et al. (2015). Management Opportunities to Mitigate Greenhouse Gas Emissions from Chinese Agriculture. Agric. Ecosyst. Environ. 209, 108–124. doi:10.1016/j.agee.2015.04.035
Nhemachena, C., Matchaya, G., Nhemachena, C., Karuaihe, S., Muchara, B., and Nhlengethwa, S. (2018). Measuring Baseline Agriculture-Related Sustainable Development Goals index for Southern Africa. Sustainability 10, 849. doi:10.3390/su10030849
Palosuo, T., Heikkinen, J., and Regina, K. (2016). Method for Estimating Soil Carbon Stock Changes in Finnish mineral Cropland and Grassland Soils. Carbon Manag. 6, 207–220. doi:10.1080/17583004.2015.1131383
Paustian, K., Lehmann, J., Ogle, S., Reay, D., Robertson, G. P., and Smith, P. (2016). Climate-smart Soils. Nature 532, 49–57. doi:10.1038/nature17174
Peltonen-Sainio, P., Jauhiainen, L., and Sorvali, J. (2017). Diversity of High-Latitude Agricultural Landscapes and Crop Rotations: Increased, Decreased or Back and Forth? Agric. Syst. 154, 25–33. doi:10.1016/j.agsy.2017.02.011
Peltoniemi, K., Velmala, S., Fritze, H., Lemola, R., and Pennanen, T. (2021). Long-term Impacts of Organic and Conventional Farming on the Soil Microbiome in Boreal Arable Soil. Eur. J. Soil Biol. 104, 103314. doi:10.1016/j.ejsobi.2021.103314
Plaza-Bonilla, D., Cantero-Martínez, C., Bareche, J., Arrúe, J. L., Lampurlanés, J., and Álvaro-Fuentes, J. (2017). Do no-till and Pig Slurry Application Improve Barley Yield and Water and Nitrogen Use Efficiencies in Rainfed Mediterranean Conditions? Field Crops Res. 203, 74–85. doi:10.1016/j.fcr.2016.12.008
Pretty, J. (2018). Intensification for Redesigned and Sustainable Agricultural Systems. Science 362. doi:10.1126/science.aav0294
Reeves, D. W. (1997). The Role of Soil Organic Matter in Maintaining Soil Quality in Continuous Cropping Systems. Soil Tillage Res. 43 (1-2), 131–167. doi:10.1016/S0167-1987(97)00038-X
Rodeghiero, M., Rubio, A., Díaz-Pinés, E., Romanyà, J., Marañón-Jiménez, S., Levy, G. J., et al. (2011). “Soil Carbon in Mediterranean Ecosystems and Related Management Problems,” in Soil Carbon in Sensitive European Ecosystems: From Science to Land Management (John Wiley & Sons), 175–218. doi:10.1002/9781119970255.ch8
Sanz-Cobena, A., Lassaletta, L., Aguilera, E., Prado, A. d., Garnier, J., Billen, G., et al. (2017). Strategies for Greenhouse Gas Emissions Mitigation in Mediterranean Agriculture: A Review. Agric. Ecosyst. Environ. 238, 5–24. doi:10.1016/j.agee.2016.09.038
Schlesinger, W. H., and Amundson, R. (2019). Managing for Soil Carbon Sequestration: Let's Get Realistic. Glob. Change Biol. 25 (2), 386–389. doi:10.1111/gcb.14478
Smith, J., Gottschalk, P., Bellarby, J., Chapman, S., Lilly, A., Towers, W., et al. (2010). Estimating Changes in Scottish Soil Carbon Stocks Using ECOSSE. I. Model Description and Uncertainties. Clim. Res. 45, 179–192. doi:10.3354/cr00899
Smith, J., Smith, P., and Addiscott, T. (1996). “Quantitative Methods to Evaluate and Compare Soil Organic Matter (SOM) Models,” in sEvaluation of Soil Organic Matter Models Using Existing Long-Term Datasets NATO ASI Series I: Global Environmental Change. Editors D.S. Powlson, P. Smith, and J.U. Smith (Berlin: Springer), Vol. 38, 181–199. doi:10.1007/978-3-642-61094-3_13
Smith, J., Smith, P., Wattenbach, M., Zaehle, S., Hiederer, R., Jones, R. J. A., et al. (2005). Projected Changes in mineral Soil Carbon of European Croplands and Grasslands, 1990-2080. Glob. Change Biol. 11, 2141–2152. doi:10.1111/j.1365-2486.2005.001075.x
Smith, P., Gottschalk, P., Bellarby, J., Richards, M., Nayak, D., Hillier, C. K., et al. (2011). Model to Estimate Carbon in Organic Soils-Sequestration and Emissions (ECOSSE). User-Manual.
Smith, P., Martino, D., Cai, Z., Gwary, D., Janzen, H., Kumar, P., et al. (2007). “Agriculture. Chapter 8 of Climate Change 2007: Mitigation,” in Contribution of Working Group III to the Fourth Assessment Report of the Intergovernmental Panel on Climate Change (Cambridge, United Kingdom and New York, NY, USA: Cambridge University Press).
Smith, P., Powlson, D., Glendining, M., and Smith, J. (1997b). Potential for Carbon Sequestration in European Soils: Preliminary Estimates for Five Scenarios Using Results from Long-Term Experiments. Glob. Change Biol. 3, 67–79. doi:10.1046/j.1365-2486.1997.00055.x
Smith, P., Smith, J. U., Powlson, D. S., Mcgill, W. B., Arah, J. R. M., Chertov, O. G., et al. (1997a). A Comparison of the Performance of Nine Soil Organic Matter Models Using Datasets from Seven Long-Term Experiments. Geoderma 81. doi:10.1016/s0016-7061(97)00087-6
Smith, P., and Smith, P. (2004). Monitoring and Verification of Soil Carbon Changes under Article 3.4 of the Kyoto Protocol. soil use manage 20, 264–270. doi:10.1079/sum2004239
Keywords: soil organic carbon, sustainable agriculture, modeling, ECOSSE, mediterranean, boreal
Citation: Begum K, Zornoza R, Farina R, Lemola R, Álvaro-Fuentes J and Cerasuolo M (2022) Modeling Soil Carbon Under Diverse Cropping Systems and Farming Management in Contrasting Climatic Regions in Europe. Front. Environ. Sci. 10:819162. doi: 10.3389/fenvs.2022.819162
Received: 20 November 2021; Accepted: 07 February 2022;
Published: 08 April 2022.
Edited by:
Lu-Jun Li, Northeast Institute of Geography and Agroecology (CAS), ChinaReviewed by:
Lucila González Molina, Instituto Nacional de Investigación Forestal, Agropecuaria (INIFAP), MexicoRene Dechow, Federal Ministry of Food and Agriculture, Germany
Tiago Morais, Universidade de Lisboa, Portugal
Julius Diel, Helmholtz Association of German Research Centres (HZ), Germany
Copyright © 2022 Begum, Zornoza, Farina, Lemola, Álvaro-Fuentes and Cerasuolo. This is an open-access article distributed under the terms of the Creative Commons Attribution License (CC BY). The use, distribution or reproduction in other forums is permitted, provided the original author(s) and the copyright owner(s) are credited and that the original publication in this journal is cited, in accordance with accepted academic practice. No use, distribution or reproduction is permitted which does not comply with these terms.
*Correspondence: Khadiza Begum, Yi5raGFkaXphMjRAZ21haWwuY29t; Marianna Cerasuolo, bWFyaWFubmEuY2VyYXN1b2xvQHBvcnQuYWMudWs=