- 1Department of Soil Science, Bangladesh Agricultural University, Myensingh, Bangladesh
- 2Bangladesh Agricultural Research Institute, Gazipur, Bangladesh
- 3Global Centre for Environmental Remediation, College of Engineering Science and Environment, The University of Newcastle, Callaghan, NSW, Australia
- 4Department of Biology, College of Science, Taif University, Taif, Saudi Arabia
- 5Genetics Department, Faculty of Agriculture, Cairo University, Oula, Egypt
Soil acidification and charland formation through alluvial sand deposition are emerging threats to food security in Bangladesh in that they endanger crop production in about 35% of its territory. The integrated plant nutrient system (IPNS) is a globally accepted nutrient management approach designed to revive the damaged soils’ fertility level. Total organic carbon (TOC) in soil is a composite index of soil quality that has consequences for agricultural productivity and natural soil ecosystems. This study assesses the impacts of using biochar, compost, poultry litter, and vermicompost-based IPNS approaches on labile and TOC pools, TOC stocks, lability and management indices, and microbial populations under different cropping patterns after 2 years in acidic and charland soils. The application of IPNS treatments increased microbial biomass carbon (MBC) by 9.1–50.0% in acidic soil and 8.8–41.2% in charland soil compared to the untreated soil, with the largest increase in poultry manure biochar (PMB). Microbial biomass nitrogen (MBN) rose from 20 to 180% in charland soil compared to the control, although no effect was observed in acidic soil. Basal respiration (BR) rose by 43–429% in acidic soil and 16–189% in charland soil compared to the control, exhibiting the highest value in PMB. IPNS treatments significantly improved SOC and POC but did not affect POXc and bulk density in both soils. The PMB and organic fertilizer (OF, compost)-based IPNS wielded the greatest influence on the lability index of MBC in acidic soils and the management index of MBC in both soils. This is despite the fact that IPNS did not affect the lability and management indices of active carbon (AC). IPNS treatments increased the stocks of SOC and MBC in both the soils and POC stock in acidic soil. IPNS treatments significantly boosted the bacterial and fungal populations in both soils, despite having no effect on phosphorus-solubilizing bacteria (PSB). Thus, PMB and OF (compost)-based IPNS may be a better nutrient management practice in degraded acidic and charland soils. This is especially the case in terms of soil quality improvement, soil carbon sequestration, and microbial enrichment.
Introduction
Acidic and charland soils currently constitute a major problem in soils comprising about 7.52 Mha of land and seriously affecting crop production in more than 36% of the territory in Bangladesh (Satter and Islam, 2010; FRG, 2018; Islam et al., 2021a; Uddin et al., 2021). Acidic soils have only small amounts of organic matter, low macronutrient availability, and micronutrient toxicity, thereby contributing to poor soil fertility (Kumar et al., 2019; Islam et al., 2021b). The poor fertility of charland soils is due to their coarse texture, low soil organic carbon (SOC) concentration, macronutrient content, and soil moisture retention capability (Ali et al., 2020; Rahman et al., 2021). Continuous and unchecked use of synthetic chemical fertilizers in these soils causes acute nutrient deficiencies and impairs soil physicochemical characteristics by increasing soil acidity, resulting in rapid degradation of soil health, productivity, stability, and sustainability (Selim and Al-Owied, 2017; Selim, 2018).
Conversely, the integrated plant nutrient system (IPNS), which combines chemical and organic fertilizers, is a widely accepted technology for sustaining soil health and its fertility, and supplying crops with their nutrient demands on a regular basis (Zhang et al., 2014a; Selim and Al-Owied, 2017; Selim, 2018; Jahangir et al., 2021a). Soil amendments such as poultry manure can significantly increase the SOC content, total exchangeable bases, pH, and microbial population (Kobierski et al., 2017; Islam et al., 2021a; Islam et al., 2021b). By contrast, biochar application in crop fields has attracted much research interest while its effects on soil quality and crop production are being debated. For example, applying biochar can improve plant growth by boosting soil microbial activity, soil moisture retention capacity, cation exchange capacity (CEC), and pH (Thomas and Gale, 2015; Gao et al., 2017; Sandhu et al., 2017; El-Naggar et al., 2018; Karimi et al., 2020). However, Deenik et al. (2010) and Dempster et al. (2012) observed diminished soil microbial biomass and activity when biochar was applied to soils. Likewise, Yu et al. (2018), Chen et al. (2018), and Zhou et al. (2020) reported that biochar has the potential to improve the population of bacteria, fungus, and phosphorus-solubilizing bacteria, as well as the total microbial diversity index. Very differently, Elzobair et al. (2016) discovered that biochar treatment (22 t ha−1) wielded no great influence on the composition and abundance of microbes when compared to manure amendment. Lack of experimental evidence on biochar application as an IPNS approach in tropical nutrient-poor soils is hindering our understanding of the potential of biochar application for better soil health and crop productivity in these soils.
The SOC is a major driver of key soil ecosystem functions and subsequently, thus, quantitative and qualitative changes in SOC levels can exert a large effect on the functional components of terrestrial ecosystems (Li et al., 2018). It emerges as a vital component that shapes soil quality and crop growth performance (Kundu et al., 2007; Liu et al., 2014). In contrast, the amount of SOC is simply a balanced outcome of input and decomposition rates, but it cannot express variations in SOC attributes. It should be noted that because soils have a high C baseline and a high degree of heterogenicity, small or rapid changes in SOC are difficult to detect (Yang et al., 2005; Smith et al., 2020). Evaluation of the IPNS effects on carbon sequestration requires knowing not only the total amount of carbon but also the quality of carbon with regard to its pools and dynamics, which is not yet well reported. For example, labile organic carbon refers to fractions with a high activity level (Cheng et al., 2010), which has proven to be a useful metric for estimating soil quality and productivity (Sun et al., 2014; Yang et al., 2017) and an early indicator of changes in SOC due to management interventions.
Active carbon (AC) or permanganate oxidizable C (POXc), total microbial biomass C (MBC), potentially mineralizable C (PMC) and particulate organic C (POC) are the SOC pools deemed to be labile C (Islam and Weil, 2000; Vieira et al., 2007; Banger et al., 2010; Ghimire et al., 2012; Li et al., 2012; Yang et al., 2018; Zhang et al., 2020a). Furthermore, indices such as CPI, CLi, and CMI, derived from the SOC, SMB, BR, and POXc, respectively, have been proposed as sensitive and early indicators of SOC lability (Anderson and Domsch, 1993; Islam and Weil, 2000; Yang et al., 2018; Zhang et al., 2020a). Examining the changes in soil microbial diversity and SOC pools and indices as a function of organic amendments, including biochar, will provide new information about the management impacts on the sustenance of soil fertility.
At the location where the research took place, our understanding of SOC pools and soil microbial diversity as impacted by IPNS methods is largely limited. This research is the first attempt to investigate this issue in such soils. Our hypothesis is that using organic amendments, particularly biochar, in combination with chemical fertilizer as a means of IPNS will increase the concentration, stocks, and lability of TOC pools, as well as the soil microbial population. This is with reference to acidic and charland agroecosystems with a diverse cropping pattern. The objectives of our research were to: 1) measure the effects of different IPNS approaches on labile and TOC pools; 2) evaluate their impacts on TOC stocks, lability, and management indices; and 3) determine the microbial population under different IPNS approaches.
Materials and Methods
Experimental Site and Soil Properties
The study focused on acidic soils in Madhupur, Tangail (24º59.82ʹ N, 90º03.99ʹ E) and charland soils in Islampur, Jamalpur (25º80.73ʹ N, 89º81.90ʹ E) in two farmers’ fields with major cropping patterns at both sites. The climate in the region is subtropical monsoon, with an average annual temperature of 26°C, average annual rainfall of 1800 mm, and an average relative humidity of 85% (Local weather station). The general soil type of acidic soil in Madhupur, Tangail with a deep red to brown terrace soils (FAO and UNDP, 1988) is located in the Agro-ecological zone 28 (AEZ-28; Madhupur Tract), while charland soil in Islampur, Jamalpur with a Non-Calcareous Dark Grey Floodplain Soils (FAO and UNDP, 1988) is situated in Agro-ecological zone 9 (AEZ-9; Old Brahmaputra Floodplain). The initial physicochemical properties of soils in Madhupur and Islampur site are presented in Table 1.
Experimental Design and Management of Crop
At both locations, the experiment began in mid-October of 2018 and was completed by the end of November in 2020. The experiment was set up in a randomized complete block design, with the experimental area divided into four blocks representing the replications, in order to minimize the heterogenic effects of soil. The single plot size was 4 m × 5 m at both locations, with 0.75 m of inter-plot space, 1 m of inter-block space, and a total of twenty-four plots.
On a farmer’s field at the Madhupur site, the treatments included poultry manure (PM), rice husk biochar (RHB), poultry manure biochar (PMB), and Dolomite whereas PM, PMB, compost (OF), and vermicompost (VC) served as treatments for a farmer’s field on the charland soils at the Islampur site. At both locations, organic materials were applied at a rate of 3 t ha−1, biochar at a rate of 2 t ha−1, while the remaining nutrients were applied from chemical fertilizers derived from the IPNS approach on the basis of AEZ number and following the fertilizer recommendation guide-2018 (FRG-2018). The other two treatments at both sites were recommended dose (RD following FRG-2018), and no organic and inorganic fertilizer (control). Each manure had a moisture level of 15% when it was applied and the chemical compositions of the organic amendments are presented in Table 2.
At the Madhupur site, the cropping pattern was Mustard (Brassica napus)—Boro rice (Winter rice-Oryza sativa L.)–Transplanted Aman rice (monsoon rice–Oryza sativa L.), while the cropping pattern at Islampur site was Maize (Zea mays)—Jute (Corchorus capsularis)–T. Aman rice (monsoon rice). At both locations, three rice seedlings were transplanted per hill, with 20 cm × 20 cm spacing. Maize seed was sown in line at a rate of 20–25 kg ha−1 with a spacing of 60 cm × 20 cm. The seed rate of mustard was 6–7 kg ha−1 and seeds were subjected to line sowing with a distance of 25 cm between lines. Similarly, jute seed rate was 5–6 kg ha−1 and seeds were sown by line sowing with a 25 cm × 5 cm spacing. The crop varieties were BARI Sarisa-14 for mustard, BRRI dhan71 for T. Aman rice, BRRI dhan28 for boro rice, Kaveri 50 for maize and Tosha paat for jute, respectively.
Seeds of boro rice were sown in the seedbed between 15 and 29 November 2018 and transplanted to the main field from the last week of January to the first week of February 2019. Similarly, seeds of T. Aman rice were sown in the seedbed between 5 and 15 July 2019 and transplanted in mid-August 2019. Mustard seed was planted between mid-October and mid-November 2019 whereas jute and maize were planted in April, and between October and November 2019, respectively. The recommended fertilizer doses at the Madhupur site were: 90 kg N, 18 kg P, 40 kg K, 5 kg S, 1 kg B per ha for mustard; 144 kg N, 9 kg P, 60 kg K, 4 kg S, 1.5 kg Zn per ha for boro rice; and 90 kg N, 7 kg P, 50 kg K, 4 kg S, 1 kg Zn per ha for T. Aman rice. Likewise, the recommended fertilizer doses at the Islampur site were: 225 kg N, 40 kg P, 80 kg K, 30 kg S, 5 kg Mg, 2 kg Zn per ha for maize; 90 kg N, 8 kg P, 50 kg K, 8 kg S per ha for jute; and 90 kg N, 8 kg P, 50 kg K, 4 kg S per ha for T. Aman rice. The sources of N, P, K, S, Zn, and B were Urea, Triple superphosphate (TSP), Muriate of Potash (MoP), Gypsum, Zinc sulphate, and Boric acid, respectively.
During the land preparation, organic amendments, Dolomite, and all synthetic fertilizers except urea were applied. In the case of rice, urea was applied in three equal portions: 7–10 days after transplanting (DAT), 25–30 days after transplanting (DAT), and 5–7 days before panicle initiation (DAT). During the field preparation for maize, one-third of the urea and other fertilizers were applied. The rest of the urea was divided into two equal amounts. The 1st installment was applied at 8 to 10 leaf stage (30–35 days after sowing, DAS), while the 2nd one was applied at 60–65 DAS. Organic amendment, half of the urea, and all other nutrients were supplied during the land preparation in jute and mustard. The other half of N was applied at 40–45 days after seed sowing in jute and during the flowering stage in mustard.
In mustard, first irrigation was provided at 20–25 days after sowing (before flowering) and the second one within 55 days after seed sowing (during fruit setting). Depending on soil type and crop requirement, four to five irrigations were applied in maize and T. Aman whereas boro rice required eight irrigations during the cropping season. When the plants reached a height of 10–12 cm and had formed three to four leaves, jute was irrigated. Supplemental irrigation was applied to the crop as needed. The rice fields were irrigated a day prior to the land preparation and then as needed during the growing season to keep standing water at roughly 3 cm above the soil surface.
Three days before land preparation, a non-selective herbicide called Glyphosate (Round up®) was sprayed across the area at a rate of 1.85 kg ha−1. Moreover, 7 days after transplanting rice seedlings, Pretilachlor (Superhit®, post-emergence herbicide) was applied at a dosage of 450 g ha−1. To control rice insects, Brifer 5G and Cidial 5G (ACI Bangladesh Ltd.) were used as needed.
To control Alternaria leaf blight of mustard, seeds should be treated with Provax-200 before sowing and Ripcord 10 EC was sprayed to control cut worm. Diazinon 60 EC, Karate 2.5 EC and Dithane M-45 were sprayed to control major diseases and pests that can ruin jute. Contact insecticides like Ripcord 10 EC and DCC 100 EC were sprayed to control maize insects.
Soil Sampling and Analysis
Three soil samples were taken at 0–15 cm depth from geo-referenced sites in each replicated plot using a 10 cm internal diameter auger, composited, and kept in sealed plastic bags. At both the Madhupur and Islampur sites we collected a total of 24 soil samples. After sieving through a 2-mm mesh to remove visible organic residues and rock particles (if any), a portion of field-moist soil was treated and evaluated for MBC and associated biological characteristics. The remaining field-moist soil was air-dried for 2 weeks in the shade at room temperature (25°C) and then processed (2 mm sieved) for the purpose of physical and chemical analysis.
Soil Carbon Pools and Basal Respiration
The fumigation extraction method (Vance et al., 1987; Wang et al., 2020; Begum et al., 2021; Jahangir et al., 2021b) served to calculate microbial biomass carbon (MBC) from the following relationship:
Where, Fc is the difference between [(CO2-C developed from fumigated soil, 0–10 days)–(CO2-C evolved from non-fumigated soil)]. For 10-days incubations at 25°C, the proportion of microbial C evolved as CO2 = 0.45. (Jenkinson and Ladd, 1981).
Brookes et al. (1985) described a method for determining microbial biomass N (MBN). In brief, fresh soil samples (10 g oven dry equivalent) were placed in tubes (six samples at a time), which were kept in a vacuumed desiccator containing 3 ml chloroform in another tube and incubated at 25°C for 72 h. Then the soil samples were mixed with 40 ml 0.5 M K2SO4, shaken at 300 rpm for 30 min and filtered to extract the MBC and MBN from the fumigated soil. Simultaneously, soil extraction was carried out on soils that had been incubated under the same conditions but without fumigation, these were referred to as non-fumigated samples. The wet oxidation method (Walkley and Black, 1934) was employed to examine MBC, while the semi-micro Kjeldahl method helped to analyze MBN (Bremner and Mulvaney, 1982). The anticipated differences between the fumigated and non-fumigated samples provided the MBC and MBN, respectively. The results for MBC and MBN were expressed as mg kg−1.
Basal respiration (BR) was determined following the alkali absorption method for quantification of CO2 evolution (Cheng et al., 2013; Diniz et al., 2020; Meena and Rao, 2021). In brief, a 20 g air-dried soil sample was adjusted to 60% of its water-holding capacity by adding water and pre-incubated at room temperature (25 °C) for 7 days. Then the pre-incubated soils were spread on the bottom of a 1-L glass jar in which a glass vial with 10 ml of NaOH (0.1 M) solution was hung. After 24 h of incubation at 25°C, 2 ml of 0.5 M BaCl2 and two drops of phenolphthalein indicator were added to the glass vial, and then titrated with 0.1 M HCl. The controls were the jars that did not have any soil in them. The quantity of CO2 evolution from soil microbes was calculated using the difference in consumed volume of HCl between the treatment and the control in titration; 1 ml of 0.1 M NaOH was equivalent to 2.2 mg of CO2.
The SOC concentration was determined by following the standard wet oxidation method (Walkley and Black, 1934; Begum et al., 2021; Islam et al., 2021c). 1 g of ground, sieved, and oven-dried sample was placed into a 250 ml conical flask. Then 10 ml of 1 N K2Cr2O7 solution was added to the soil and the flask was swirled gently to disperse the soil in the solution. After that, 20 ml of concentrated H2SO4 was added to the conical flask, and the flask was swirled until the soil and the reagent were mixed well. The conical flask was then placed in a hot water bath, with the stream directed into the suspension and heated until the temperature reached 135°C (about 12 min). After heating, the content in the conical flask was cooled for 300 min, and then 200 ml of deionized water and 10 ml of ortho-phosphoric acid were added. Following this, 2 ml of o-phenanthroline indicator were added to the content and titrated the solution with 0.5 M (NH4)2 Fe (SO4)2.6H2O until a dark green colour appeared. Particulate organic carbon (POC) was determined according to the method utilized by Cambardella and Elliott, (1992) and Begum et al. (2021). In short, 20 g of soil was dispersed in 100 ml of 5 g L−1 sodium hexametaphosphate solution by shaking for 15 h on an end-over-end shaker. The dispersed soil suspension was passed through a 53 mm sieve, and the material retained in the sieve was dried at 105°C after being rinsed several times with distilled water.
Organic carbon was measured in dried samples that were ground and analyzed. After mild oxidation of air-dried soil with a neutral KMnO4 solution, the Permanganate oxidizable carbon (POXc) was quantified spectrophotometrically as a measure of chemically labile C pool (Weil et al., 2003; Begum et al., 2021). In brief, we used 20 ml of neutral KMnO4 in water to react 1.0 g oven dry equivalent (ODE) of air-dried soil at concentrations of 0.005, 0.01, 0.0125, 0.025, 0.05, and 0.1 M. In screw-cap polycarbonate centrifuge tubes, the soil KMnO4 suspensions were agitated at 200 rpm for 15 min at room temperature. To separate the dirt particles from the solution, the tubes were centrifuged at 3,000 rpm for 5 min after shaking. We then diluted 0.20 ml of the clear centrifuge with 10.0 ml of distilled water in a glass cuvette tube with a strong stream to ensure complete mixing. Using a Bosch and Lomb 2,500 spectrophotometer, we measured the absorbance of 565 nm light and compared the results to a standard curve made up of 0.20 ml of each unreacted KMnO4 solution and 10.0 ml pure water. The standard core method was used to determine the bulk density of the soil (McKenzie et al., 2004). The thermo-gravimetric method determined the antecedent soil moisture content (Black, 1965).
Soil Carbon Lability and Management Indices
Using the measured SOC, MBC, POC, and POXC data, the CMI was calculated (Islam et al., 2021d; Begum et al., 2021; Franzluebbers, (2002)) as follows:
where CPI is the C pool index and CLI is the C lability index, which were calculated as:
CPI = TOC in the treatment soil/TOC in the control.
CLI = CL in the treatment soil/CL in the control.where CL refers to the lability of C (CL = Labile C/Non-labile C).
The POXc pool consisted of the labile C pool, which was quantified as a percentage of SOC. By subtracting the POXc content from the TOC, the non-labile C pool was estimated. The calculated CMIs were normalized (CMI) by dividing the values with the highest CMI values in the database to a relative scale of >0 to ≤ 100. It was considered that higher CMI values are better indicators of SOC accumulation and lability in response to different treatments.
Soil Carbon Stocks
TOC, POC, POXc, and MBC stocks at 0–15 cm depth were estimated by multiplying their concentrations by 15 cm sample depth and measuring antecedent bulk density at the same time.
Microbial Population
The microbial population in soil was determined using the dilution spread plate technique as described in previous studies (Kakosová et al., 2017; Akande and Adekayode, 2019; Kumar et al., 2021). For bacteria and fungi, Nutrient Agar (NA) and Potato Dextrose agar (PDA) were the preferred growth media. To produce a dilution ratio of 10−6, a 1 ml aliquot of the material was pipetted into a sterile test tube and serially diluted in six more test tubes, each holding 9 ml of sterile distilled water. 20 ml of cold (45°C) sterile molten agar medium was pipette separated aseptically into various sterile Petri dishes, spun gently for equal distribution of the inocula, allowed to set, and incubated at 30–37°C for 24 h (for bacteria), and at 25–27°C for 72 h (for fungi). At the end of the incubation time (24 h), bacteria colonies were counted and documented accurately, while fungus colonies were tallied and reported correctly at the end of the incubation period (72 h).
PSB was isolated and counted using a dilution plate method using hydroxy apatite media (Katzenelson and Bose, 1959; Narsian et al., 1994; Baliah et al., 2016; Ameena et al., 2020). The soil samples were serially diluted up to a 10−6 dilution, plated on Petri dishes, and incubated at 35 ± 2°C for 7 days to isolate PSB. At the end of the incubation period, PSB colonies were visually identified by the clear zone surrounding the bacterial colony. PSB was counted after incubation lasting 3–5 days by checking for a halo/solubilization zone around bacterial colonies that were surrounded by a turbid white background.
Statistical Analysis
A one-way analysis of variance (ANOVA) was done using different treatments as a random variable. Before using ANOVA, the data distribution was tested for normality. With the help of Statistix 10 software, the data were statistically evaluated to determine the significant differences in treatment effects. Using Tukey’s multiple comparison test, a post-hoc test was used to find the differences between treatments. Unless otherwise stated, all statistical analyses were considered significant at p ≤ 0.05.
Results
The research work was conducted in two nutrient poor soil sites (i.e., acidic and charland soils) in Bangladesh to evaluate the impacts of different integrated plant nutrient systems (IPNS) on: firstly, soil labile and total organic carbon (TOC) pools; and secondly, indices and microbial populations under different cropping patterns. For this purpose, soil samples were collected after two consecutive years of field experiments to analyze soil chemical and biological properties following standard protocols. We hypothesized that integrated nutrient management would increase carbon sequestration and microbial populations in both the degraded soils.
Effects of Integrated Nutrient Management on MBC and MBN
At the Madhupur site, MBC was significantly influenced by different treatments (p < 0.05, Figure 1A) being higher in PMB than all other treatment combinations except the RHB and PM, where the latter two were also similar to each other. The MBC in the control was the lowest which was statistically identical to all the treatments except the PMB (ca. 275.0, 233.3, 225.0, 212.5, 200.0 and 183.3 mg kg−1 soil in PMB, PM, RHB, Dolomite + RD, RD and control, respectively) (Figure 1A). MBC increased by 9.1% in RD to 50.0% in PMB over the control. In contrast, the effect of incorporating different organic and inorganic treatments to soil on MBN was not statistically significant (Figure 1A).
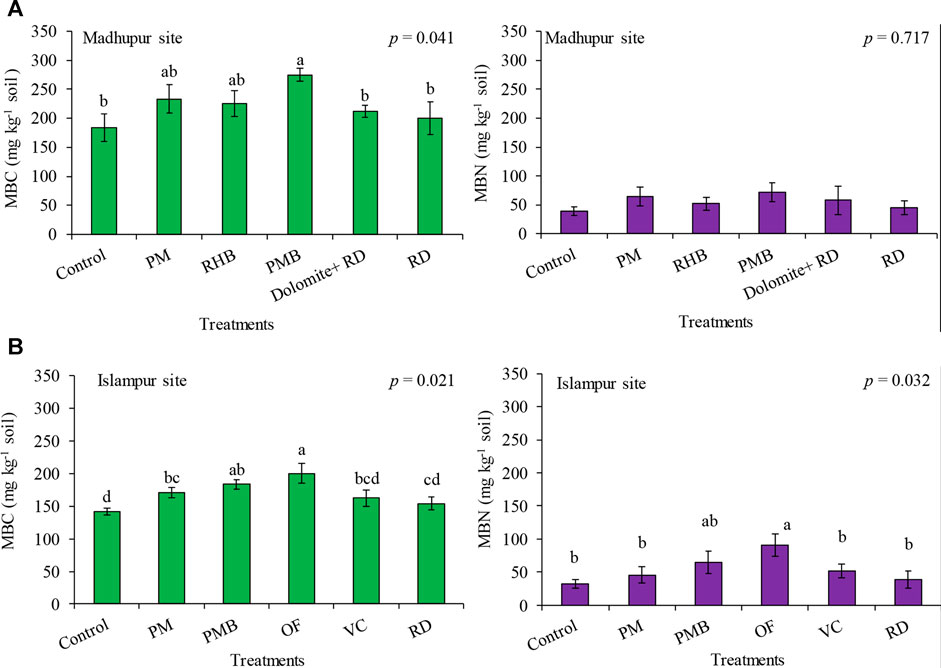
FIGURE 1. Effects of different organic and inorganic treatments on MBC and MBN at Madhupur site (A) and Islampur site (B). Data are average ± SE (n = 4); PM = poultry manure, RHB = rice husk biochar, PMB = poultry manure biochar, RD = recommended dose only from chemical fertilizer, OF = compost, VC = vermicompost.
Likewise, at the Islampur site, different organic and inorganic treatments significantly influenced MBC and MBN (p < 0.05, Figure 1B). MBC in different treatments ranged from 141.7 to 200.0 mg kg−1 soil showing the highest value in OF (200.0 mg kg−1 soil), which was statistically similar to PMB (183.3 mg kg−1 soil) and the lowest in the control (141.7 mg kg−1 soil), being identical to VC (162.5 mg kg−1 soil) and RD (154.2 mg kg−1 soil). The MBC content in the soil increased from approximately 8.8–41.2% over the control. Similarly, MBN in different treatments ranged from 32.4 to 90.8 μg g−1 soil, exhibiting the highest value in OF (90.8 mg kg−1 soil), which was statistically similar to PMB (64.9 mg kg−1 soil), and the lowest in the control (32.4 mg kg−1 soil), which was identical to all the treatments except OF (Figure 1B). The MBN content in the soil increased by about 20% in RD to 180% in OF over the control.
Effects of Integrated Nutrient Management on Basal Respiration
Different treatments significantly influenced BR at both the Madhupur and Islampur sites (p < 0.05; Figures 2A,B). At the Madhupur site, the highest BR was observed in PMB and it was statistically similar to all the treatments except the control, whereas the lowest value was observed in the control. This was statistically identical to all the treatments except PMB. The mean BR was 154.17, 135.42, 72.92, 54.17, 41.67, and 29.17 mg CO2-C h−1 kg−1 soil, in PMB, Dolomite + RD, RHB, RD, PM, and control, respectively (Figure 2A). The increase in BR due to the incorporation of different treatments varied from 43% in PM to 429% in PMB. Likewise, at the Islampur site, BR ranged from 39.58 to 114.58 mg CO2-C h−1 kg−1 soil. The highest mean BR was observed in PMB and it was statistically similar to OF, while the lowest value was observed in the control, which was statistically similar to VC, RD, and PM (Figure 2B). The BR rose from 16% in PM to 189% in PMB over the control (Figure 2B).
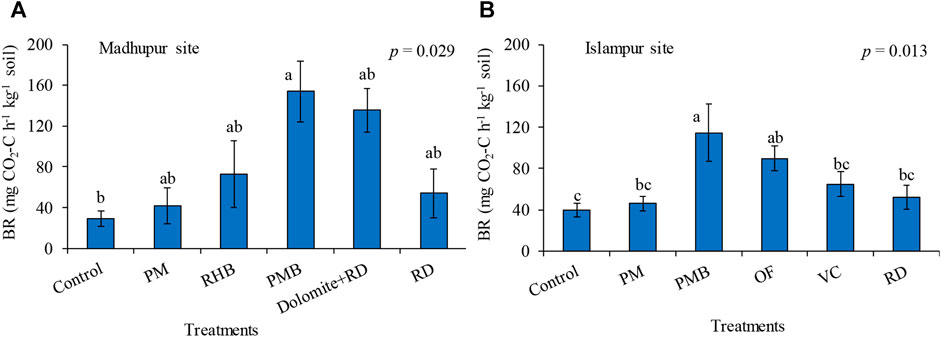
FIGURE 2. Effects of different organic and inorganic treatments on basal respiration at Madhupur site (A) and Islampur site (B). Data are average ± SE (n = 4); PM = poultry manure, RHB = rice husk biochar, PMB = poultry manure biochar, RD = recommended dose only from chemical fertilizer, OF = compost, VC = vermicompost.
Effects of Integrated Nutrient Management on Organic Carbon Pools
At the Madhupur site, application of biochar (PMB and RHB) and organic amendment (PM) based IPNS and inorganic fertilizer (Dolomite + RD and RD) had a significant impact on SOC content (p < 0.05, Table 3), being higher in Dolomite + RD than all other treatments except RHB, PMB, and RD, where the later three were also similar to each other. The SOC content in control was the lowest which was statistically similar to all the treatment combinations except Dolomite + RD. The increase in SOC content in soil over the control ranged from 1.1% in PM to 23.6% in Dolomite + RD. Similarly, the POC content in soil was also significantly influenced by the organic and inorganic treatments (p < 0.05, Table 3), being higher in PMB which was statistically identical to all the treatments except in the control. The POC content in the control was the smallest which was identical to all the treatments except PMB and RD (Table 3). The increase in POC content in soil over the control ranged from 88.0% in PM to 176.2% in PMB. Unlike this, the effects of application of biochar (PMB and RHB) and organic amendment (PM) based IPNS and inorganic fertilizer (Dolomite + RD and RD) on POXc and soil bulk density were not significant (Table 3).
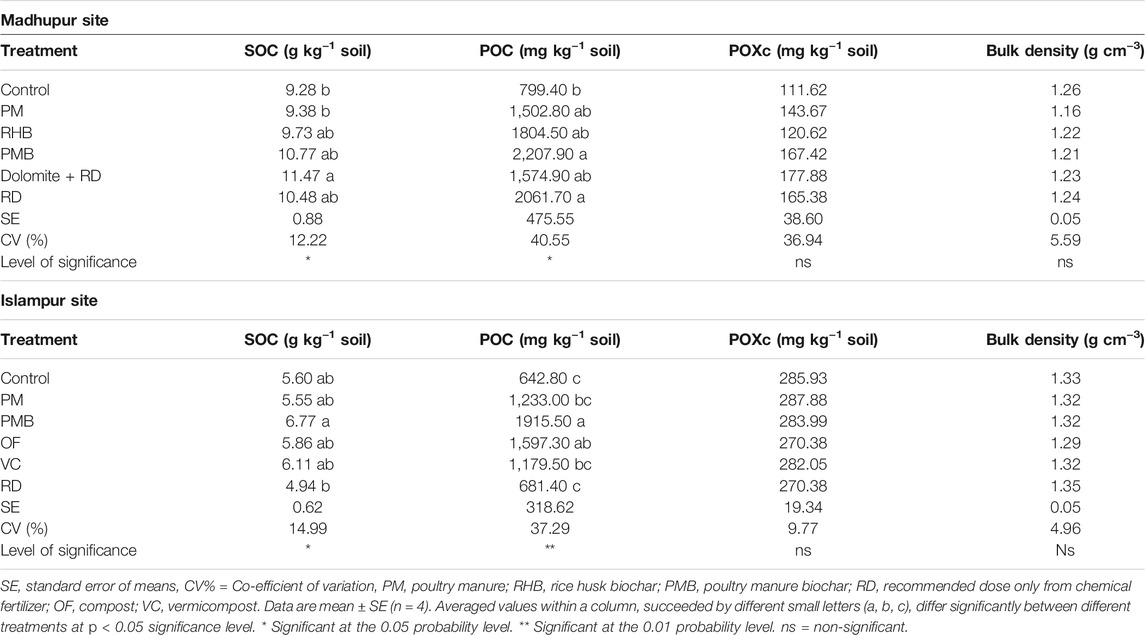
TABLE 3. Effects of different treatments on SOC, POC, POXc and bulk density at Madhupur and Islampur sites at a depth of 0–15 cm.
At the Islampur site, biochar (PMB and RHB) and organic amendment (PM) based IPNS and inorganic fertilizer (Dolomite + RD and RD) significantly influenced both SOC (p < 0.05) and POC (p < 0.01) content in soil (Table 3). The SOC content in soil varied from 4.9 to 6.8 g kg−1 soil in different treatments. The highest SOC content was determined in PMB which proved to be statistically similar to all the treatments except RD, whereas the lowest value was observed in RD. It was identical to all the treatments except PMB. SOC content in soil deminished over the control by about 0.9 and 11.8% when treated with PM and RD, respectively, whereas SOC content increased by 4.6, 9.1, and 20.9% in OF, VC, and PMB treated soil, respectively. Likewise, the POC content in soil varied from 642.8 to 1915.5 mg kg−1 soil in different treatments. The highest POC content in soil was observed in PMB, which was statistically similar to OF. Conversely, the lowest value was observed in the control, which was identical to all the treatments except PMB and OF. The increase in POC content in soil over the control ranged from 6.0% in RD to 198.0% in PMB. In contrast, application of biochar (PMB and RHB) and organic amendment (PM) based IPNS and inorganic fertilizer (Dolomite + RD and RD) did not affect POXc content and soil bulk density (Table 3).
Effects of Integrated Nutrient Management on Soil Carbon Lability and Management Indices
At the Madhupur site, the CLI and CMI of AC were not significantly influenced by different organic and inorganic treatments (Table 4). In contrast, the CLI and CMI of MBC significantly differed due to the application of different treatments (p < 0.05, Table 4). The CLI of MBC ranged from 1.0 to 1.5 in different treatments. The highest value was observed in PMB, which was statistically similar to all the treatments except the control, whereas the lowest value was observed in the control; it was identical to all the treatments except PMB. Likewise, the highest value of CMI (1.8) was observed in PMB and it was identical to PM (1.6), and RHB (1.5), whereas the lowest value was noted in the control (1.1), which was identical to RD (1.3), Dolomite + RD (1.4), and RHB (1.5).
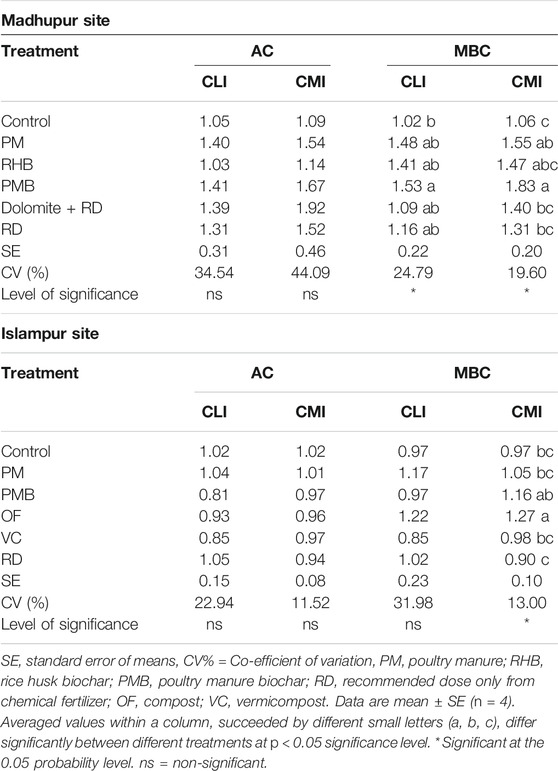
TABLE 4. Effects of different treatments on carbon lability and management indices of active and microbial biomass at Madhupur and Islampur sites at a depth of 0–15 cm.
For the Islampur site, the CLI and CMI of AC were not significantly influenced by different treatments (Table 2). Similarly, the CLI of MBC was not affected by different treatments (Table 4). In contrast, the CMI of MBC was significantly influenced by different treatments (p < 0.05, Table 4). The CMI of MBC ranged from 0.9 to 1.3 in different treatments, exhibiting the highest value in OF, which was statistically similar to PMB. In contrast, the lowest value was observed in RD, being identical to VC, control, and PM, respectively.
Effects of Integrated Nutrient Management on Soil Carbon Stocks
At the Madhupur site, TOC stock was significantly influenced by different organic and inorganic treatments (p < 0.05, Table 5), being higher in Dolomite + RD than all other treatments except PMB, RHB, and RD, where the latter three were statistically similar. The TOC stock in PM was the lowest, which was statistically similar to all the treatments except Dolomite + RD. The TOC stock fell by about 6.6% in PM compared to the control, while the highest increase (20.9%) in TOC stock was observed in Dolomite + RD (Table 5). Likewise, the POC stock was also significantly influenced by different treatments (p < 0.05, Table 5). The POC stock in soils treated with different treatments ranged from 1.5 to 3.9 Mg ha−1. The PMB had the highest POC stocks, which was identical to all the treatments except the control, which exhibited the lowest POC stock, being identical to all the treatments except PMB. The increase in POC stock in different treatments over the control ranged from 74% in PM to 162% in PMB. In contrast, the impact of different treatments on POXc stock was not significant. On the other hand, different treatments significantly influenced MBC stock (p < 0.05, Table 5). The highest MBC stock was observed in PMB, which was statistically similar to PM, RHB, and Dolomite + RD, whereas the lowest value was observed in the control, which was identical to all the treatments except PMB. The increase in MBC stock in different treatments over the control ranged from 23.3% in RD to 66.7% in PMB.
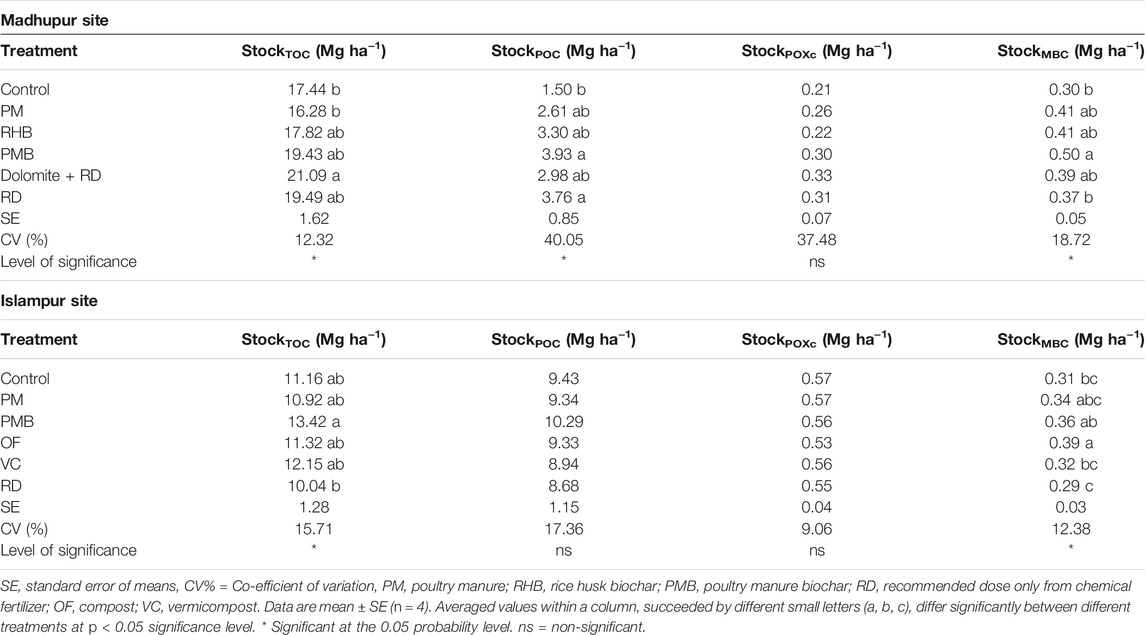
TABLE 5. Effects of different treatments on total organic carbon, particulate organic matter, particulate organic carbon, permanganate oxidizable carbon, microbial biomass carbon stocks at Madhupur and Islampur sites at a depth of 0–15 cm.
At the Islampur site, different organic and inorganic treatments significantly influenced TOC stock in the soil (p < 0.05, Table 5). The TOC stock in soils treated with different treatments ranged from 10.0 to 13.4 Mg ha−1. The highest TOC stock was observed in PMB, which was statistically similar to all the treatments except RD, whereas the lowest value was observed in RD, which was identical to all the treatments except PMB (Table 5). The TOC stock in soil declined by 2.2 and 10.0% in PM and RD, respectively, compared to the control. Meanwhile, OF, VC, and PMB increased TOC stock over the control by 1.4, 8.9, and 20.3%, respectively. In contrast, POC and POXc stocks were not influenced by different organic and inorganic treatments (Table 5). Unlike, different treatments significantly influenced MBC stock (p < 0.05, Table 5). The highest MBC stock was observed in OF, which was statistically similar to PM and PMB, whereas the lowest value was observed in RD, being identical to the control, PM, and VC. MBC stock declined by 6.5% in RD compared to the control, whereas MBC stock increased by about 3.2, 9.7, 16.1, and 25.8% in VC, PM, PMB, and OF, respectively, over the control (Table 5).
Effects of Integrated Nutrient Management on Microbial Population
At the Madhupur site, the soil bacterial population was significantly influenced by different organic and inorganic treatments (p < 0.05, Table 6), being higher in Dolomite + RD than in all the treatments except RHB and PMB, where the later two were statistically similar. The bacterial population in the control was the lowest, which was statistically similar to all the treatments except Dolomite + RD (Table 6). Likewise, the soil fungal population was also significantly enhanced due to the addition of various organic and inorganic treatments (p < 0.05, Table 6). The highest fungal population (3.54 × 105 CFU g−1 soil) was observed in RHB, and the smallest number was observed in the control (2.68 × 103 CFU g−1 soil), which was statistically similar to all the treatments except RHB. In contrast, the addition of different treatments did not significantly influence the PSB population, while different treatments did significantly influence the B:F ratio in soil. The highest B:F ratio was observed in PMB and it was identical to Dolomite + RD, while the lowest B:F ratio was observed in the control, which was statistically similar to PM, RHB, and RD (Table 6).
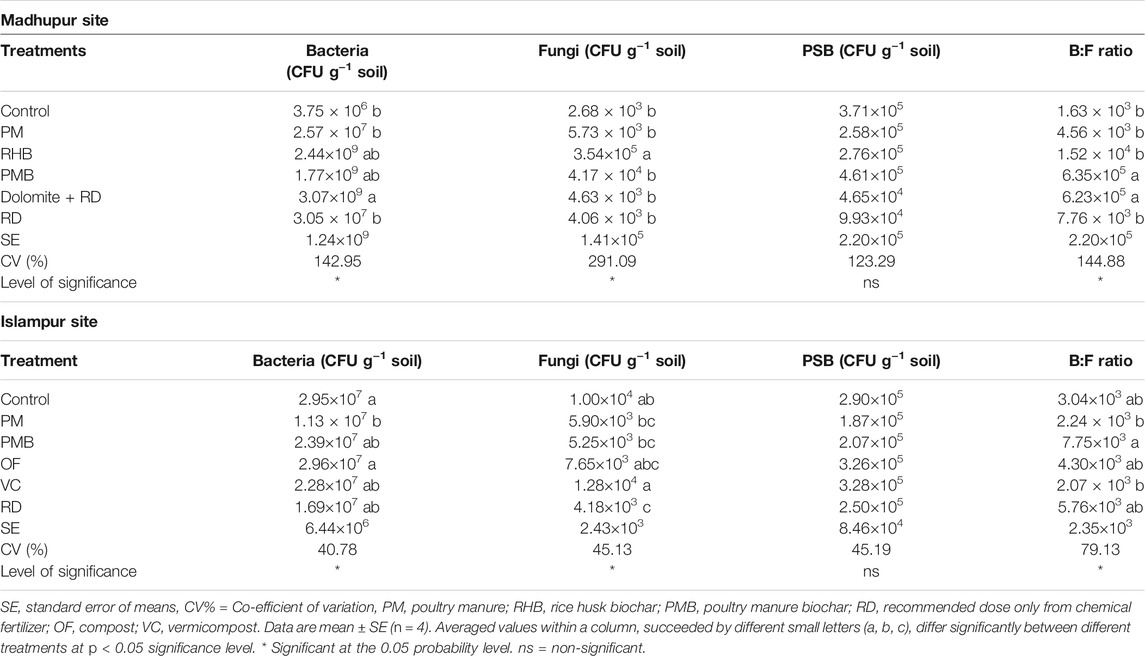
TABLE 6. Effects of different treatments on microbial population at Madhupur and Islampur sites at a depth of 0–15 cm.
Referring to the Islampur site, different organic and inorganic treatments significantly influenced the soil bacterial population (p < 0.05, Table 6). The bacterial population in soil ranged from 1.13×107 to 2.96 × 107 CFU g−1 soil. The largest number of bacteria was recorded in soils treated with OF, which was statistically identical to all the treatments except PM, whereas the lowest number was observed in PM, which was identical to VC and RD. Similarly, the fungal population in soil was also significantly influenced by different organic and inorganic treatments (p < 0.05, Table 6). The highest fungal population was observed in soil treated with VC, which was statistically similar to OF, and the lowest one in RD. It proved to be identical to all the treatments except the control and VC. In contrast, the PSB population was not significantly influenced by the treatments. However, the B:F ratio in soil was statistically significant due to the incorporation of different treatments (p < 0.05, Table 6). The highest B:F ratio (7.75×103) was observed in PMB, which was statistically similar to RD (5.76×103) and OF (4.30×103), whereas the lowest ratio was noted in VC (2.07×103), which was identical to PM (2.24×103), control (3.04×103), and RD (5.76×103).
Discussion
Effect of IPNS on Microbial Biomass Carbon and Nitrogen
Soil microbial biomass is an important biomarker for tracking rapid changes in soil quality due to human influences as well as agricultural management methods (Suman et al., 2006). MBC and MBN account for 3–7% of SOC and 1–5% of STN (Wu et al., 2007; Liddle et al., 2020). Our results demonstrated that IPNS treatments, especially biochar (PMB) and compost (OF)-based IPNS, significantly elevated MBC in both soils and MBN in charland soil. This is consistent with other research (Zhang et al., 2014b; Chaudhry et al., 2016; Liu et al., 2021). MBC and MBN increased by 8.8–50% and 20–180%, respectively, which was comparable with the recent work by Al-Suhaibani et al. (2020) who observed 20.0–47.5% and 32.2–65.2% increases in MBC and MBN, respectively, as a result of IPNS treatments. This is attributed to the decomposed organic manure and biochar itself being a carbon source, which provides an adequate energy supply in terms of C and N for various soil microbial biomass. The result is a heavy proliferation of soil microbial populations (Das et al., 2017; Li et al., 2017).
However, other studies discovered that: firstly, biochar application had no effect on soil MBC (Castaldi et al., 2011; Zavalloni et al., 2011); and secondly, MBC significantly diminished with biochar application while MBN remained undistorted in a coarse textured soil (Deenik et al., 2010; Castaldi et al., 2011; Zavalloni et al., 2011; Dempster et al., 2012). The build-up of organic compounds on the surface of the soil offers a substrate for increased microbial activity, which leads to greater MBC rates (Balota et al., 2004; Koković et al., 2021). The fastest mineralizable C and N in organic additions are the most important factors in biomass increase (Hao et al., 2008; Liu et al., 2010). A mixture of charcoal and inorganic fertilizer is more efficient for beneficial microbes in soil (Wardle et al., 2008; Brunn et al., 2012). The benefits flowing from IPNS treatments on MBN in charland soil are consistent with Kiem and Kandeler, (1997) findings that biological activity is greatest in coarse textured soils. This may be attributed to the more favorable habitat offered by the coarse textured soil for fungi in a microenvironment rich in bioavailable substrates formed by fresh unprotected organic manure. It results in the rapid mineralization of the substrate (Witzgall et al., 2021).
Effect of IPNS on Basal Respiration
Basal respiration is a measurement of fundamental recycling processes in soil that is assumed to represent the carbon available for microbial preservation (Dinesh et al., 2004; Vanhala et al., 2005; Li et al., 2019). Our findings strongly suggested that applying organic and inorganic fertilizers together boosted BR by 16–429% in acidic and charland soils, and this echoes what prior research has documented (Chang et al., 2008; Salehi et al., 2017; Li et al., 2018; Li et al., 2019). The increase in BR due to the addition of organic and inorganic fertilizers could be due to the increased amount of labile C in soils and microbial activities accelerated by inorganic phosphorus fertilizer addition, which will cause different impacts on soil microbial community and enzyme activities with N and P addition synchronously (Liu et al., 2019; Zhang et al., 2021). The positive effect of simultaneous application of inorganic N and P fertilizers on BR in forest ecosystems is also well documented in other recent studies (Zhang et al., 2020b; Xiao et al., 2020). According to Shrestha et al. (2013), cow manure had the highest BR, followed by compost, bare fallow, and cover crop treatments, yet Chang et al. (2008) detected no influence of soybean meal addition on BR. Besides, the addition of organic manures increased the quantity of organic matter available for soil respiration, resulting in increased BR. It also has the ability to greatly enhance both the number and activity of microorganisms, impacting both SOC transformation and CO2 release and subsequently raising the microbial respiration rate (Zang et al., 2015). As a result, the treatments with organic manure in our study considerably enhanced soil CO2 emissions and soil respiration rates.
Effect of IPNS on SOC, Carbon Pools and Bulk Density
The incorporation of manure amendment along with synthetic fertilizer enhanced SOC concentration by 0.9–23.6% in acidic and charland soils. Our finding is consistent with other analyses reporting an increase of about 2.9–46.7% in SOC content due to the application of both organic and inorganic nutrition sources (Libra et al., 2011; Roy and Kashem, 2014; Al-Suhaibani et al., 2020). Increased SOC supply from crop root exudation and high carbon content in readily biodegradable organic manures have resulted in an increase in SOC content (Yanardağ et al., 2014). The POC fraction is a labile SOC pool primarily composed of partially degraded crop residues not connected to soil minerals (Six et al., 2002). We observed a 6.0–198% increase in soil POC content and this is in line with previous research (Bongiorno et al., 2019; Zhang et al., 2020a). According to Liu et al. (2013) and Rudrappa et al. (2006), adding manure to the soil increased POC build-up. Increased POC is mostly due to increased subterranean biomass production, root exudation, and readily biodegradable organic input components (Purakayastha et al., 2008). (Zhang et al., 2021) reported that the POXc level of the soil had risen after a decade of wheat cultivation using synthetic fertilizer and residue application. Previous research has documented similar results (Rudrappa et al., 2006; Purakayastha et al., 2008; Cheng et al., 2010). Nevertheless, after 2 years of field trials, our findings revealed that IPNS had no effect on the build-up of POXc in soil. It may be due to the duration of the field experiment indicating that further long-term investigation is required.
Many studies have shown that including organic material decreases the bulk density of soil (Yazdanpanah et al., 2016; Ramos, 2017; Oladele et al., 2019). Surprisingly, after 2 years, we found no effect of IPNS on soil bulk density in either soil. This may be attributable to the balance in soil bulk density due to the combined application of organic and inorganic fertilizer.
Effect of IPNS on Carbon Lability and Management Indices and Carbon Stock
The carbon management index, which includes both the organic C pool and the C lability of the soil, may be used to assess the potential of management approaches to improve soil health. Incorporation of IPNS treatments into the soils had no impact on the lability and management indices of POXc (active carbon). After a short-term (2-years) trial, the IPNS treatments seemed to have no influence on POXc content in the soil, as expected. In previous studies, researchers reported an increased CLI and CMI after a long-term (11–32 years) combined fertilizer application (Benbi et al., 2015; Kumara et al., 2017; Tang et al., 2018). In our experiment, the CLI and CMI of MBC improved due to the use of IPNS treatments, which was comparable with other work (Benbi et al., 2015; Kumara et al., 2017; Tang et al., 2018). Such an outcome might be explained by soil microorganisms gaining access to organic carbon and other necessary nutrients (Kumara et al., 2017). Our results demonstrated that SOC, POC, and MBC stock increased significantly in both acidic and charland soils as a result of organic and inorganic inputs, which agrees with previous research (Maillard and Angers, 2014; Sun et al., 2014). This is due to the large C input through organic sources resulting in increased SOC, POC, and MBC content in the soil (Tang et al., 2018).
Effect of IPNS on Microbial Population
Soil microbes are crucial for OM decomposition, nutrient recycling, soil structure maintenance, insect and disease control, and the production of plant growth stimulants (Kirchman, 2018). Kumar et al. (2012) found that organic manures with or without chemical fertilizer had a beneficial impact on both the bacterial and fungal populations in soil. This could be due to the extra nutrients (N, P, K, and micronutrients) provided by the additives, which the bacteria break down for the plants to use. More exudates and plant compounds are released as a result of this breakdown product, which the rhizosphere bacteria can utilize. As a result, the bacterial biomass in the rhizosphere has increased (Richard and Ogunjobi, 2016).
Our results revealed that biochar-based IPNS increased the bacterial and fungal population in acidic soil, which was similar to what Narendrula-Kotha and Nkongolo (2017) and Alkharabsheh et al. (2021) reported. It might be due to its important properties such as pore space, surface area, porosity, minerals, surface volatile organic compounds, functional groups, free radicals, and high pH, which encourage bacterial development (Narendrula and Nkongolo, 2015; Gao et al., 2017; Zhu et al., 2017). Besides, biochar contains macronutrients like K+, Mg2+, Na+, N, P, and other nutrients that can benefit microbial growth in the long-term upon release into the soil solution (Rodríguez-Vila et al., 2016). Some bacteria and fungus with pore sizes smaller than the pore size of particular biochars can inhabit these pores to shield themselves from soil predators. Furthermore, soluble compounds contained in the mesopores and micropores of biochar, such as sugars, alcohols, acids, ketones, and water molecules, can enhance microbial activity and affect microbial abundance and composition in soil (Seleiman et al., 2019; Adnan et al., 2020).
It should be noted that the microbiome remained statistically similar or smaller compared to the control in charland soil, which is a mystery that requires further investigation. In both soils, our findings revealed that the bacterial community population was frequently larger than the fungal community population in all treatments, as previously mentioned by Kamaa et al. (2011). It has been demonstrated in previous research that organic and chemical fertilizers help PSB (Richard and Ogunjobi, 2016). In both acidic and charland soils, however, we found that IPNS or chemical fertilizer exerted on influence on PSB. This may be attributed to: firstly, the smaller amount of PSB population present in the organic amendments; and secondly, the inhibitory effect of inorganic phosphate applied via chemical fertilizer on the growth of PSB (Kaur et al., 2017).
Conclusion
This research applied different organic and inorganic fertilizers for two consecutive years in two contrasting soil sites in Bangladesh, and evaluated soil microbial diversity, C pools and indices. Our findings suggest that combining various soil additions with chemical fertilizer had varying impacts on soil microbial biomass, basal respiration, carbon pools, carbon stocks, lability index, management index, and microbial population. Treatments with IPNS, especially PMB and OF (compost)-based IPNS, significantly enhanced MBC (29.4–50.0% compared to the untreated soil) and MBN (100–180% compared to the untreated soil) in both the soils, except MBN in charland soil. Likewise, IPNS treatments improved SOC and POC but did not affect POXc. The PMB and OF (compost)-based IPNS had the highest impact on the lability index of MBC in acidic soil and the management index of MBC in both soils, indicating increased C pools and stocks in both soil sites. The IPNS treatments significantly boosted the bacterial and fungal populations in both soils, although no effect was observed in the PSB. Based on our findings, PMB and OF (compost)-based IPNS can be recommended as the best nutrient management practices in degraded acidic and charland soils, especially in terms of soil quality improvement or restoration.
Data Availability Statement
The original contributions presented in the study are included in the article/Supplementary Material, further inquiries can be directed to the corresponding author.
Author Contributions
MMR: Methodology, formal analysis, writing-original draft; MI: Conceptualization, methodology, funding acquisition, supervision, writing-review and editing; SU: Methodology, data analysis, writing-review and editing; MoR: Writing-review and editing. AG: Funding acquisition, writing-review and editing; AA: writing-reviewing and editing; MJ: Conceptualization, methodology, funding acquisition, supervision, writing-review and editing.
Funding
This research was funded by World Bank under National Agricultural Technology Program- Phase II Project (NATP-2) administered by Bangladesh Agricultural Research Council (BARC, 2005) (Project ID: 135). It was also partially supported by Taif University Researchers Supporting Project number (TURSP-2020/39), Taif University, Taif, Saudi Arabia.
Conflict of Interest
The authors declare that the research was conducted in the absence of any commercial or financial relationships that could be construed as a potential conflict of interest.
Publisher’s Note
All claims expressed in this article are solely those of the authors and do not necessarily represent those of their affiliated organizations, or those of the publisher, the editors, and the reviewers. Any product that may be evaluated in this article, or claim that may be made by its manufacturer, is not guaranteed or endorsed by the publisher.
Acknowledgments
The authors are grateful to the World Bank under National Agricultural Technology Program- Phase II Project (NATP-2) administered by Bangladesh Agricultural Research Council (BARC) (Project ID: 135) for its partial financial support. The authors also appreciate Taif University Researchers Supporting Project number (TURSP-2020/39), Taif University, Taif, Saudi Arabia.
Supplementary Material
The Supplementary Material for this article can be found online at: https://www.frontiersin.org/articles/10.3389/fenvs.2021.798729/full#supplementary-material
References
Adnan, M., Fahad, S., Zamin, M., Shah, S., Mian, I. A., Danish, S., et al. (2020). Coupling Phosphate-Solubilizing Bacteria with Phosphorus Supplements Improve maize Phosphorus Acquisition and Growth under Lime Induced Salinity Stress. Plants 9, 900. doi:10.3390/plants9070900
Akande, G. M., Adekayode, F. O., and Adekayode, F. O. (2019). Identification of Soil Microbial Population under Different Land Use. Trop. Plant Res. 6 (1), 90–100. doi:10.22271/tpr.2019.v6.i1.013
Al-Suhaibani, N., Selim, M., Alderfasi, A., and El-Hendawy, S. (2020). Comparative Performance of Integrated Nutrient Management between Composted Agricultural Wastes, Chemical Fertilizers, and Biofertilizers in Improving Soil Quantitative and Qualitative Properties and Crop Yields under Arid Conditions. Agronomy 10, 1503. doi:10.3390/agronomy10101503
Ali, M., Tarafder, M., Mohsin, N., and Haque, M. (2020). Soil Characterization and Fertility Assessment of Char Lands for Increased Cropping Intensity and Crop Productivity. Prog. Agric. 31 (1), 56–67. doi:10.3329/pa.v31i1.48313
Alkharabsheh, H. M., Seleiman, M. F., Battaglia, M. L., Shami, A., Jalal, R. S., Alhammad, B. A., et al. (2021). Biochar and its Broad Impacts in Soil Quality and Fertility, Nutrient Leaching and Crop Productivity: A Review. Agronomy 11, 993. doi:10.3390/agronomy11050993
Ameena, I., Aswaja, P., Anjana, S., Anumol, C. S., and Anila, M. (2020). Isolation, Characterization and Efficiency Study of Phosphate Solubilising Microorganisms from the Rock Samples of Western Ghats. Int. J. Sci. Res. Paper Multidiscipl. Stud. 6 (9), 22–28.
Anderson, T., and Domsch, K. (1993). The Metabolic Quotient for CO2 (qCO2) as a Specific Activity Parameter to Assess the Effects of Environmental Conditions, Such as Ph, on the Microbial Biomass of forest Soils. Soil Biol. Biochem. 25 (3), 393–395. doi:10.1016/0038-0717(93)90140-7
Baliah, N. T., Pandiarajan, A. G., and Kumar, B. M. (2016). Isolation, Identification and Characterization of Phosphate Solubilizing Bacteria from Different Crop Soils of Srivilliputtur Taluk, Virudhunagar District, Tamil Nadu. Trop. Ecol. 57 (3), 465–474.
Balota, E. L., Colozzi Filho, A., Andrade, D. S., and Dick, R. P. (2004). Long-term Tillage and Crop Rotation Effects on Microbial Biomass and C and N Mineralization in a Brazilian Oxisol. Soil Tillage Res. 77, 137–145. doi:10.1016/j.still.2003.12.003
Banger, K., Toor, G. S., Biswas, A., Sidhu, S. S., and Sudhir, K. (2010). Soil Organic Carbon Fractions after 16-years of Applications of Fertilizers and Organic Manure in a Typic Rhodalfs in Semi-arid Tropics. Nutr. Cycl. Agroecosyst. 86 (3), 391–399. doi:10.1007/s10705-009-9301-8
BARC (2005). Fertilizer Recommendation Guide. Bangladesh Agricultural Research Council, BARC, Soils Pub. Farmgate, Dhaka: Bangladesh Agril. Res. Council.
Begum, R., Jahangir, M. M. R., Jahiruddin, M., Islam, M. R., Rahman, M. T., Rahman, M. L., et al. (2021). Nitrogen Fertilization Impact on Soil Carbon Pools and Their Stratification and Lability in Subtropical Wheat-Mungbean-rice Agroecosystems. PLoS One 16 (10), e0256397. doi:10.1371/journal.pone.0256397
Benbi, D. K., Brar, K., Toor, A. S., and Sharma, S. (2015). Sensitivity of Labile Soil Organic Carbon Pools to Long-Term Fertilizer, Straw and Manure Management in Rice-Wheat System. Pedosphere 25 (4), 534–545. doi:10.1016/s1002-0160(15)30034-5
Black, C. A. (1965). Methods of Soil Analysis, Part I, Agronomy Monographs 9. Wisconsin: ASA Madison.
Bongiorno, G., Bünemann, E. K., Oguejiofor, C. U., Meier, J., Gort, G., Comans, R., et al. (2019). Sensitivity of Labile Carbon Fractions to Tillage and Organic Matter Management and Their Potential as Comprehensive Soil Quality Indicators across Pedoclimatic Conditions in Europe. Ecol. Indicators 99, 38–50. doi:10.1016/j.ecolind.2018.12.008
Bremner, J. M., and Mulvaney, C. S. (1982). “Nitrogen- Total,” in Methods of Soil Analysis—Part. Editors A. L. Page, R. H. Miller, and D. R. Keeney (Madison, WI, USA: American Society of Agronomy, Inc. Publisher), 595–624.
Brookes, P. C., Landman, A., Pruden, G., and Jenkinson, D. S. (1985). Chloroform Fumigation and the Release of Soil Nitrogen: a Rapid Direct Extraction Method to Measure Microbial Biomass Nitrogen in Soil. Soil Biol. Biochem. 17 (6), 837–842. doi:10.1016/0038-0717(85)90144-0
Brunn, W. E., Ambus, P., Egsgaard, H., and Nielsen, H. H. (2012). Effects of Slow and Fast Pyrolysis Biochar on Soil C and N Turnover. Soil Biol. Biochem. 46, 73–79. doi:10.1016/j.soilbio.2011.11.019
Cambardella, C. A., and Elliott, E. T. (1992). Particulate Soil Organic‐Matter Changes across a Grassland Cultivation Sequence. Soil Sci. Soc. Am. J. 56 (3), 777–783. doi:10.2136/sssaj1992.03615995005600030017x
Castaldi, S., Riondino, M., Baronti, S., Esposito, F. R., Marzaioli, R., Rutigliano, F. A., et al. (2011). Impact of Biochar Application to a Mediterranean Wheat Crop on Soil Microbial Activity and Greenhouse Gas Fluxes. Chemosphere 85, 1464–1471. doi:10.1016/j.chemosphere.2011.08.031
Chang, E.-H., Chung, R.-S., and Wang, F.-N. (2008). Effect of Different Types of Organic Fertilizers on the Chemical Properties and Enzymatic Activities of an Oxisol under Intensive Cultivation of Vegetables for 4 Years. Soil Sci. Plant Nutr. 54 (4), 587–599. doi:10.1111/j.1747-0765.2008.00264.x
Chaudhry, U. K., Shahzad, S., Naqqash, M. N., Saboor, A., Abbas, M. S., Saeed, F., et al. (2016). Integration of Biochar and Chemical Fertilizer to Enhance Quality of Soil and Wheat Crop (Triticum aestivum L.). J. Biodivers. Environ. Sci. 9 (1), 348–358. doi:10.7287/peerj.preprints.1631v1
Chen, H.-L., Zhou, J.-M., and Xiao, B.-H. (2010). Characterization of Dissolved Organic Matter Derived from rice Straw at Different Stages of Decay. J. Soils Sediments 10, 915–922. doi:10.1007/s11368-010-0210-x
Chen, H., Ma, J., Wei, J., Gong, X., Yu, X., Guo, H., et al. (2018). Biochar Increases Plant Growth and Alters Microbial Communities via Regulating the Moisture and Temperature of green Roof Substrates. Sci. Total Environ. 635, 333–342. doi:10.1016/j.scitotenv.2018.04.127
Cheng, F., Peng, X., Zhao, P., Yuan, J., Zhong, C., Cheng, Y., et al. (2013). Soil Microbial Biomass, Basal Respiration and Enzyme Activity of Main Forest Types in the Qinling Mountains. PloS One 8 (6), e67353. doi:10.1371/journal.pone.0067353
Das, S., Jeong, S. T., Das, S., and Kim, P. J. (2017). Composted Cattle Manure Increases Microbial Activity and Soil Fertility More Than Composted Swine Manure in a Submerged rice Paddy. Front. Microbiol. 8, 1702. doi:10.3389/fmicb.2017.01702
Deenik, J. L., McClellan, T., Uehara, G., Antal, M. J., and Campbell, S. (2010). Charcoal Volatile Matter Content Influences Plant Growth and Soil Nitrogen Transformations. Soil Sci. Soc. Am. J. 74 (4), 1259–1270. doi:10.2136/sssaj2009.0115
Dempster, D. N., Gleeson, D. B., Solaiman, Z. I., Jones, D. L., and Murphy, D. V. (2012). Decreased Soil Microbial Biomass and Nitrogen Mineralisation with Eucalyptus Biochar Addition to a Coarse Textured Soil. Plant Soil 354 (1), 311–324. doi:10.1007/s11104-011-1067-5
Dinesh, R., Chaudhuri, S. G., Ganeshamurthy, A. N., and Pramanik, S. C. (2004). Biochemical Properties of Soils of Undisturbed and Disturbed Mangrove Forests of South Andaman (India). Wetlands Ecol. Manage. 12, 309–320. doi:10.1007/s11273-004-0777-3
Diniz, A. R., Silva, C. F. D., Pereira, M. G., Balieiro, F. C., Silva, E. V. D., and Santos, F. M. D. (2020). Microbial Biomass and Enzyme Activity of Soil under Clonal Rubber Tree Plantations. Floresta Ambient. 27 (3), e20171138. doi:10.1590/2179-8087.113817
El-Naggar, A., Lee, S. S., Awad, Y. M., Yang, X., Ryu, C., Rizwan, M., et al. (2018). Influence of Soil Properties and Feedstocks on Biochar Potential for Carbon Mineralization and Improvement of Infertile Soils. Geoderma 332, 100–108. doi:10.1016/j.geoderma.2018.06.017
Elzobair, K. A., Stromberger, M. E., Ippolito, J. A., and Lentz, R. D. (2016). Contrasting Effects of Biochar versus Manure on Soil Microbial Communities and Enzyme Activities in an Aridisol. Chemosphere 142, 145–152. doi:10.1016/j.chemosphere.2015.06.044
FAOUNDP (1988). Land Resources Appraisal of Bangladesh for Agricultural Development. Report 2. Agro- Ecological Regions of Bangladesh. Rome: Food and Agriculture Organization, 212–221.
Franzluebbers, A. J. (2002). Water Infiltration and Soil Structure Related to Organic Matter and its Stratification with Depth. Soil Tillage Res. 66, 197–205. doi:10.1016/s0167-1987(02)00027-2
FRG (2018). Fertilizer Recommendation Guide. Bangladesh Agricultural Research Council, BARC. Farmgate, Dhaka: Soils pub., Bangladesh Agril. Res. Council.
Gao, L., Wang, R., Shen, G., Zhang, J., Meng, G., and Zhang, J. (2017). Effects of Biochar on Nutrients and the Microbial Community Structure of Tobacco-Planting Soils. J. Soil Sci. Plant Nutr. 17, 884–896. doi:10.4067/s0718-95162017000400004
Ghimire, R., Adhikari, K. R., Chen, Z.-S., Shah, S. C., and Dahal, K. R. (2012). Soil Organic Carbon Sequestration as Affected by Tillage, Crop Residue, and Nitrogen Application in rice-wheat Rotation System. Paddy Water Environ. 10, 95–102. doi:10.1007/s10333-011-0268-0
Hao, X. H., Liu, S. L., Wu, J. S., Hu, R. G., Tong, C. L., and Su, Y. Y. (2008). Effect of Long-Term Application of Inorganic Fertilizer and Organic Amendments on Soil Organic Matter and Microbial Biomass in Three Subtropical Paddy Soils. Nutr. Cycl. Agroecosyst. 81, 17–24. doi:10.1007/s10705-007-9145-z
Islam, K. R., Roth, G., Rahman, M. A., Didenko, N. O., and Reeder, R. C. (2021a). Cover Crop Complements Flue Gas Desulfurized Gypsum to Improve No-Till Soil Quality. Commun. Soil Sci. Plant Anal. 52 (9), 926–947. doi:10.1080/00103624.2021.1872594
Islam, K. R., and Weil, R. R. (2000). Soil Quality Indicator Properties in Mid-Atlantic Soils as Influenced by Conservation Management. J. Soil Water Conserv. 55, 69–78. doi:10.2307/4003403
Islam, M. R., Akter, A., Hoque, M. A., Farzana, S., Uddin, S., Talukder, M. M. H., et al. (2021c). Lime and Organic Manure Amendment: A Potential Approach for Sustaining Crop Productivity of the T. Aman-Maize-Fallow Cropping Pattern in Acidic Piedmont Soils. Sustainability 13 (17), 9808. doi:10.3390/su13179808
Islam, M. R., Bilkis, S., Hoque, T. S., Uddin, S., Jahiruddin, M., Rahman, M. M., et al. (2021d). Mineralization of Farm Manures and Slurries for Successive Release of Carbon and Nitrogen in Incubated Soils Varying in Moisture Status under Controlled Laboratory Conditions. Agriculture 11 (9), 846. doi:10.3390/agriculture11090846
Islam, M. R., Jahan, R., Uddin, S., Harine, I. J., Hoque, M. A., Hassan, S., et al. (2021b). Lime and Organic Manure Amendment Enhances Crop Productivity of Wheat-Mungbean-T. Aman Cropping Pattern in Acidic Piedmont Soils. Agronomy 11 (8), 1595. doi:10.3390/agronomy11081595
Jahangir, M. M. R., Islam, S., Nitu, T. T., Uddin, S., Kabir, A. K. M. A., Meah, M. B., et al. (2021a). Bio-Compost-Based Integrated Soil Fertility Management Improves Post-Harvest Soil Structural and Elemental Quality in a Two-Year Conservation Agriculture Practice. Agronomy 11, 2101. doi:10.3390/agronomy11112101
Jahangir, M. M. R., Nitu, T. T., Uddin, S., Siddaka, A., Sarker, P., Khan, S., et al. (2021b). Carbon and Nitrogen Accumulation in Soils under Conservation Agriculture Practices Decreases with Nitrogen Application Rates. Appl. Soil Ecol. 168 (5), 104178. doi:10.1016/j.apsoil.2021.104178
Jenkinson, D. S., and Ladd, J. N. (1981). Microbial Biomass in Soil: Measurement and Turnover. Soil Biochem. 5 (1), 415–471. doi:10.1016/0038-0717(91)90183-K
Kakosová, E., Hrabák, P., Černík, M., Novotný, V., Czinnerová, M., TröglPopelk, J. J., et al. (2017). Effect of Various Chemical Oxidation Agents on Soil Microbial Communities. Chem. Engineer. J. 314, 257–265. doi:10.1016/j.cej.2016.12.065
Kamaa, M., Mburu, H., Blanchart, E., Chibole, L., Chotte, J.-L., Kibunja, C., et al. (2011). Effects of Organic and Inorganic Fertilization on Soil Bacterial and Fungal Microbial Diversity in the Kabete Long-Term Trial, Kenya. Biol. Fertil. Soils 47 (3), 315–321. doi:10.1007/s00374-011-0539-3
Karimi, A., Moezzi, A., Chorom, M., and Enayatizamir, N. (2020). Application of Biochar Changed the Status of Nutrients and Biological Activity in a Calcareous Soil. J. Soil Sci. Plant Nutr. 20, 450–459. doi:10.1007/s42729-019-00129-5
Katzenelson, H., and Bose, B. (1959). Metabolic Activity and Phosphate Dissolving Ability of Bacterial Isolates from Wheat Roots Rhizosphere and Non-rhizosphere Soil. Can. J. Microbiol. 5, 79–85. doi:10.1139/m59-010
Kaur, H., Gosal, S., and Walia, S. (2017). Synergistic Effect of Organic, Inorganic and Biofertilizers on Soil Microbial Activities in Rhizospheric Soil of Green Pea. Arrb 12 (4), 1–11. doi:10.9734/arrb/2017/32509
Kiem, R., and Kandeler, E. (1997). Stabilization of Aggregates by the Microbial Biomass as Affected by Soil Texture and Type. Appl. Soil Ecol. 5 (3), 221–230. doi:10.1016/s0929-1393(96)00132-1
Kobierski, M., Bartkowiak, A., Lemanowicz, J., and Piekarczyk, M. (2017). Impact of Poultry Manure Fertilization on Chemical and Biochemical Properties of Soils. Plant Soil Environ. 63, 558–563. doi:10.17221/668/2017-PSE
Koković, N., Saljnikov, E., Eulenstein, F., Cakmak, D., Buntić, A., ˇSikirić, B., et al. (2021). Changes in Soil Labile Organic Matter as Affected by 50 Years of Fertilization with Increasing Amounts of Nitrogen. Agronomy 11, 2026. doi:10.3390/agronomy11102026
Kumar, M., Baishaya, L. K., Ghosh, D. C., Gupta, V. K., Dubey, S. K., Das, A., et al. (2012). Productivity and Soil Health of Potato (Solanum tuberosum L.) Field as Influenced by Organic Manures, Inorganic Fertilizers and Biofertilizers under High Altitudes of Eastern Himalayas. J. Agric. Sci. 4 (5), 223–234. doi:10.5539/jas.v4n5p223
Kumar, S., Meena, R. S., Singh, R. K., Munir, T. M., Datta, R., Danish, S., et al. (2021). Soil Microbial and Nutrient Dynamics under Different Sowings Environment of Indian Mustard (Brassica Juncea L.) in rice Based Cropping System. Sci. Rep. 11, 5289. doi:10.1038/s41598-021-84742-4
Kumar, U., Rashid, H., Tithi, N. H., and Mia, M. Y. (2019). Status of Soil Properties in Relationship with Soil pH in Madhupur Tract of Tangail District in Bangladesh. Prog. Agric. 30 (3), 282–287. doi:10.3329/pa.v30i2.42488
Kumara, B. H., Antil, R. S., and Rao, C. S. (2017). Long-term Effect of Organic Manures and Fertilizers on Soil Fertility and Soil Carbon Management index after 16 Years Cycles of Pearl Millet–Wheat Cropping System in an Inceptisol of Subtropical India. Bull. Env. Pharmacol. Life Sci. 6 (1), 360–364. doi:10.1016/j.fcr.2012.07.002
Kundu, S., Bhattacharyya, R., Prakash, V., Ghosh, V., and Gupta, H. S. (2007). Carbon Sequestration and Relationship between Carbon Addition and Storage under Rainfed Soybean-Wheat Rotation in a sandy Loam Soil of Indian Himalayas. Soil Till. Res. 92 (1-2), 87–95. doi:10.1016/j.still.2006.01.009
Li, C.-F., Yue, L.-X., Kou, Z.-K., Zhang, Z.-S., Wang, J.-P., and Cao, C.-G. (2012). Short-term Effects of Conservation Management Practices on Soil Labile Organic Carbon Fractions under a Rape-rice Rotation in central China. Soil Tillage Res. 119, 31–37. doi:10.1016/j.still.2011.12.005
Li, C.-X., Ma, S.-C., Shao, Y., Ma, S.-T., and Zhang, L.-L. (2018). Effects of Long-Term Organic Fertilization on Soil Microbiologic Characteristics, Yield and Sustainable Production of winter Wheat. J. Integr. Agric. 17 (1), 210–219. doi:10.1016/s2095-3119(17)61740-4
Li, R., Tao, R., Ling, N., and Chu, G. (2017). Chemical, Organic and Bio-Fertilizer Management Practices Effect on Soil Physicochemical Property and Antagonistic Bacteria Abundance of a Cotton Field: Implications for Soil Biological Quality. Soil Tillage Res. 167, 30–38. doi:10.1016/j.still.2016.11.001
Li, W., Wang, J., Li, X., Wang, S., Liu, W., Shi, S., et al. (2019). Nitrogen Fertilizer Regulates Soil Respiration by Altering the Organic Carbon Storage in Root and Topsoil in alpine Meadow of the north-eastern Qinghai-Tibet Plateau. Sci. Rep. 9, 13735. doi:10.1038/s41598-019-50142-y
Libra, J. A., Ro, K. S., Kammann, C., Funke, A., Berge, N. D., Neubauer, Y., et al. (2011). Hydrothermal Carbonization of Biomass Residuals: a Comparative Review of the Chemistry, Processes and Applications of Wet and Dry Pyrolysis. Biofuels 2, 71–106. doi:10.4155/bfs.10.81
Liddle, K., McGonigle, T., and Koiter, A. (2020). Microbe Biomass in Relation to Organic Carbon and Clay in Soil. Soil Syst. 4, 41. doi:10.3390/soilsystems4030041
Liu, D., Ding, Z., Ali, E. F., Kheir, A. M. S., Eissa, M. A., and Ibrahim, O. H. M. (2021). Biochar and Compost Enhance Soil Quality and Growth of roselle (Hibiscus sabdariffa L.) under saline Conditions. Sci. Rep. 11, 8739. doi:10.1038/s41598-021-88293-6
Liu, E., Teclemariam, S. G., Yan, C., Yu, J., Gu, R., Liu, S., et al. (2014). Long-term Effects of No-Tillage Management Practice on Soil Organic Carbon and its Fractions in the Northern China. Geoderma 213, 379–384. doi:10.1016/j.geoderma.2013.08.021
Liu, E., Yan, C., Mei, X., He, W., Bing, S. H., Ding, L., et al. (2010). Long-term Effect of Chemical Fertilizer, Straw, and Manure on Soil Chemical and Biological Properties in Northwest China. Geoderma 158, 173–180. doi:10.1016/j.geoderma.2010.04.029
Liu, E., Yan, C., Mei, X., Zhang, Y., and Fan, T. (2013). Long-Term Effect of Manure and Fertilizer on Soil Organic Carbon Pools in Dryland Farming in Northwest China. PLoS One 8 (2), e56536. doi:10.1371/journal.pone.0056536
Liu, H., Zhou, G., Bai, S. H., Song, J., Shang, Y., He, M., et al. (2019). Differential Response of Soil Respiration to Nitrogen and Phosphorus Addition in a Highly Phosphorus-Limited Subtropical forest, China. For. Ecol. Management 448, 499–508. doi:10.1016/j.foreco.2019.06.020
Maillard, É., and Angers, D. A. (2014). Animal Manure Application and Soil Organic Carbon Stocks: a Meta-Analysis. Glob. Change Biol. 20 (2), 666–679. doi:10.1111/gcb.12438
McKenzie, N. J., Jacquier, D. J., Isbell, R. F., and Brown, K. (2004). Australian Soils and Landscapes – an Illustrated Compendium. Collingwood: Victoria: CSIRO Publishing.
Meena, A., and Rao, K. S. (2021). Assessment of Soil Microbial and Enzyme Activity in the Rhizosphere Zone under Different Land Use/cover of a Semiarid Region, India. Ecol. Process. 10, 16. doi:10.1186/s13717-021-00288-3
Narendrula, R., and Nkongolo, K. K. (2015). Fatty Acids Profile of Microbial Populations in a Mining Reclaimed Region Contaminated with Metals: Relation with Ecological Characteristics and Soil Respiration. J. Bioremedi. Biodegrad. 6, 1–9. doi:10.4172/2155-6199.1000274
Narendrula-Kotha, R., and Nkongolo, K. K. (2017). Microbial Response to Soil Liming of Damaged Ecosystems Revealed by Pyrosequencing and Phospholipid Fatty Acid Analyses. PLoS One 12 (1), e0168497. doi:10.1371/journal.pone.0168497
Narsian, V. J., Thakkar, H., and Patel, H. (1994). Isolation and Screening of Phosphate Solubilizing Fungi. Indian J. Microbiol. 34, 113–118.
Oladele, S., Adeyemo, A., Awodun, M., Ajayi, A., and Fasina, A. (2019). Effects of Biochar and Nitrogen Fertilizer on Soil Physicochemical Properties, Nitrogen Use Efficiency and upland rice (Oryza Sativa) Yield Grown on an Alfisol in Southwestern Nigeria. Int. J. Recycl. Org. Waste Agricult. 8, 295–308. doi:10.1007/s40093-019-0251-0
Purakayastha, T. J., Rudrappa, L., Singh, D., Swarup, A., and Bhadraray, S. (2008). Long-term Impact of Fertilizers on Soil Organic Carbon Pools and Sequestration Rates in maize-wheat-cowpea Cropping System. Geoderma 144, 370–378. doi:10.1016/j.geoderma.2007.12.006
Rahman, M. M., Uddin, S., Jahangir, M. M. R., Solaiman, Z. M., Alamri, S., Siddiqui, M. H., et al. (2021). Integrated Nutrient Management Enhances System Productivity and Nitrogen Use Efficiency of Crops with Subsequent Improvement in Physicochemical Properties of Acidic and Charland Soils. Plants 10, 2547. doi:10.3390/plants10112547
Ramos, M. C. (2017). Effects of Compost Amendment on the Available Soil Water and Grape Yield in Vineyards Planted after Land Levelling. Agric. Water Management 191, 67–76. doi:10.1016/j.agwat.2017.05.013
Richard, P. O., and Ogunjobi, A. A. (2016). Effect of Organic and Inorganic Fertilizer Applications on Phosphate Solubilizing Bacteria in the Rhizosphere of maize (Zea mays L.). Afr. J. Microbiol. Res. 10 (48), 2021–2028. doi:10.5897/AJMR2016.8318
Rodríguez-Vila, A., Forján, R., Guedes, R. S., and Covelo, E. F. (2016). Changes on the Phytoavailability of Nutrients in a Mine Soil Reclaimed with Compost and Biochar. Water Air Soil Pollut. 227, 453. doi:10.1007/s11270-016-3155-x
Roy, S., and Kashem, M. A. (2014). Effects of Organic Manures in Changes of Some Soil Properties at Different Incubation Periods. Ojss 04, 81–86. doi:10.4236/ojss.2014.43011
Rudrappa, L., Purakayastha, T. J., Singh, D., and Bhadraray, S. (2006). Long-term Manuring and Fertilization Effects on Soil Organic Carbon Pools in a Typic Haplustept of Semi-arid Sub-tropical India. Soil Tillage Res. 88, 180–192. doi:10.1016/j.still.2005.05.008
Salehi, A., Fallah, S., and Sourki, A. A. (2017). Organic and Inorganic Fertilizer Effect on Soil CO2 Flux, Microbial Biomass, and Growth of Nigella Sativa L. Int. Agrophys. 31, 103–116. doi:10.1515/intag-2016-0032
Sandhu, S. S., Ussiri, D. A. N., Kumar, S., Chintala, R., Papiernik, S. K., Malo, D. D., et al. (20172017). Analyzing the Impacts of Three Types of Biochar on Soil Carbon Fractions and Physiochemical Properties in a Corn-Soybean Rotation. Chemosphere 184, 473–481. doi:10.1016/j.chemosphere.2017.05.165
Satter, S. A., and Islam, M. N. (20102010). “Char Lands of Bangladesh: Their Extent, Management and Future Research Needs. Papers Presented in a Workshop on Soil Fertility,” in Fertilizer Management and Future Strategy held at Bangladesh Agricultural Research Council, Farmgate, Dhaka during 18-19 January, Farmgate, Dhaka, 18-19 January, 1–9.
Seleiman, M. F., Refay, Y., Al-Suhaibani, N., Al-Ashkar, I., El-Hendawy, S., and Hafez, E. M. (2019). Integrative Effects of rice-straw Biochar and Silicon on Oil and Seed Quality, Yield and Physiological Traits of Helianthus Annuus L. Grown under Water Deficit Stress. Agronomy 9, 637. doi:10.3390/agronomy9100637
Selim, M. M., and Al-Owied, A. J. A. (2017). Genotypic Responses of Pearl Millet to Integrated Nutrient Management. Biosci. Res. 14 (2), 156–169.
Selim, M. (2018). Potential Role of Cropping System and Integrated Nutrient Management on Nutrients Uptake and Utilization by maize Grown in Calcareous Soil. Egypt. J. Agron. 40 (3), 297–312. doi:10.21608/agro.2018.6277.1134
Shrestha, R. K., Lal, R., and Rimal, B. (2013). Soil Carbon Fluxes and Balances and Soil Properties of Organically Amended No-Till Corn Production Systems. Geoderma 197-198, 177–185. doi:10.1016/j.geoderma.2013.01.005
Six, J., Conant, R. T., Paul, E. A., and Paustian, K. (2002). Stabilization Mechanisms of Soil Organic Matter: Implications for C-Saturation of Soils. Plant Soil 241, 155–176. doi:10.1023/a:1016125726789
Smith, P., Soussana, J. F., Angers, D., Schipper, L., Chenu, C., Rasse, D. P., et al. (2020). How to Measure, Report and Verify Soil Carbon Change to Realize the Potential of Soil Carbon Sequestration for Atmospheric Greenhouse Gas Removal. Glob. Change Biol. 26, 219–241. doi:10.1111/gcb.14815
Suman, A., Lal, M., Singh, A. K., and Gaur, A. (2006). Microbial Biomass Turnover in Indian Subtropical Soils under Different Sugarcane Intercropping Systems. Agron.j. 98 (3), 698–704. doi:10.2134/agronj2005.0173
Sun, B., Roberts, D. M., Dennis, P. G., Caul, S., Daniell, T. J., Hallett, P. D., et al. (2014). Microbial Properties and Nitrogen Contents of Arable Soils under Different Tillage Regimes. Soil Use Manage 30 (1), 152–159. doi:10.1111/sum.12089
Tang, H., Xiao, X., Tang, W., Li, C., Wang, K., Li, W., et al. (2018). Long-term Effects of NPK Fertilizers and Organic Manures on Soil Organic Carbon and Carbon Management index under a Double-Cropping rice System in Southern China. Commun. Soil Sci. Plant Anal. 49, 1976–1989. doi:10.1080/00103624.2018.1492600
Thomas, S. C., and Gale, N. (2015). Biochar and forest Restoration: a Review and Meta-Analysis of Tree Growth Responses. New Forests 46 (5), 931–946. doi:10.1007/s11056-015-9491-7
Uddin, S., Islam, M. R., Jahangir, M. M. R., Rahman, M. M., Hassan, S., Hassan, M. M., et al. (20212021). Nitrogen Release in Soils Amended with Different Organic and Inorganic Fertilizers under Contrasting Moisture Regimes: A Laboratory Incubation Study. Agronomy 11, 2163. doi:10.3390/agronomy11112163
Vance, E. D., Brookes, P. C., and Jenkinson, D. S. (1987). An Extraction Method for Measuring Soil Microbial Biomass C. Soil Biol. Biochem. 19, 703–707. doi:10.1016/0038-0717(87)90052-6
Vanhala, P., Tamminen, P., and Fritze, H. (2005). Relationship between Basal Soil Respiration Rate, Tree Stand and Soil Characteristics in Boreal Forests. Environ. Monit. Assess. 101, 85–92. doi:10.1007/s10661-005-9134-0
Vieira, F. C. B., Bayer, C., Zanatta, J. A., Dieckow, J., Mielniczuk, J., and He, Z. L. (2007). Carbon Management index Based on Physical Fractionation of Soil Organic Matter in an Acrisol under Long-Term No-Till Cropping Systems. Soil Tillage Res. 96, 195–204. doi:10.1016/j.still.2007.06.007
Walkley, A. J., and Black, A. I. (1934). Estimation of Organic Carbon by Chromic Acid Titration Method. J. Soil Sci. 25, 259–260.
Wang, Y., Liu, X., Chen, F., HuangDeng, R. X., Deng, X., and Jiang, Y. (2020). Seasonal Dynamics of Soil Microbial Biomass C and N of Keteleeria Fortunei Var. Cyclolepis Forests with Different Ages. J. For. Res. 31 (6), 2377–2384. doi:10.1007/s11676-019-01058-w
Wardle, D. A., Nilsson, M.-C., and Zackrisson, O. (2008). Fire-Derived Charcoal Causes Loss of Forest Humus. Science 320, 629. doi:10.1126/science.1154960
Weil, R. R., Islam, K. R., Stine, M. A., Gruver, J. B., and Samson-Liebig, S. E. (2003). Estimating Active Carbon for Soil Quality Assessment: a Simplified Method for Laboratory and Field Use. Am. J. Altern. Agric. 18, 3–17. doi:10.1079/AJAA200228
Witzgall, K., Vidal, A., Schubert, D. I., Höschen, C., Schweizer, S. A., Buegger, F., et al. (2021). Particulate Organic Matter as a Functional Soil Component for Persistent Soil Organic Carbon. Nat. Commun. 12, 4115. doi:10.1038/s41467-021-24192-8
Wu, J., Huang, M., Xiao, H. A., Su, Y. R., Tong, C. L., Huang, D. Y., et al. (2007). Dynamics in Microbial Immobilization and Transformations of Phosphorus in Highly Weathered Subtropical Soil Following Organic Amendments. Plant Soil 290 (1), 333–342. doi:10.1007/s11104-006-9165-5
Xiao, S., Wang, G. G., Tang, C., Fang, H., Duan, J., and Yu, X. (2020). Effects of One-Year Simulated Nitrogen and Acid Deposition on Soil Respiration in a Subtropical Plantation in China. Forests 11 (2), 235. doi:10.3390/f11020235
Yanardağ, I. H., Zornoza, R., Cano, A. F., Yanardağ, A. B., and Mermut, A. R. (2014). Evaluation of Carbon and Nitrogen Dynamics in Different Soil Types Amended with Pig Slurry, Pig Manure and its Biochar by Chemical and Thermogravimetric Analysis. Biol. Fertil. Soils. 51, 183–196. doi:10.1007/s00374-014-0962-3
Yang, C. M., Yang, L. Z., and Ouyang, Z. (2005). Organic Carbon and its Fractions in Paddy Soil as Affected by Different Nutrient and Water Regimes. Geoderma 124 (1-2), 133–142. doi:10.1016/j.geoderma.2004.04.008
Yang, X., Meng, J., Lan, Y., Chen, W., Yang, T., Yuan, J., et al. (2017). Effects of maize stover and its Biochar on Soil CO 2 Emissions and Labile Organic Carbon Fractions in Northeast China. Agric. Ecosyst. Environ. 240, 24–31. doi:10.1016/j.agee.2017.02.001
Yang, X., Wang, D., Lan, Y., Meng, J., Jiang, L., Sun, Q., et al. (2018). Labile Organic Carbon Fractions and Carbon Pool Management index in a 3-year Field Study with Biochar Amendment. J. Soils Sediments 18, 1569–1578. doi:10.1007/s11368-017-1874-2
Yazdanpanah, N., Mahmoodabadi, M., and Cerdà, A. (2016). The Impact of Organic Amendments on Soil Hydrology, Structure and Microbial Respiration in Semiarid Lands. Geoderma 266, 58–65. doi:10.1016/j.geoderma.2015.11.032
Yu, L., Yu, M., Lu, X., Tang, C., Liu, X., Brookes, P. C., et al. (2018). Combined Application of Biochar and Nitrogen Fertilizer Benefits Nitrogen Retention in the Rhizosphere of Soybean by Increasing Microbial Biomass but Not Altering Microbial Community Structure. Sci. Total Environ. 640-641, 1221–1230. doi:10.1016/j.scitotenv.2018.06.018
Zang, Y. F., Hao, M. D., Zhang, L. Q., and Zhang, H. Q. (2015). Effects of Wheat Cultivation and Fertilization on Soil Microbial Biomass Carbon, Soil Microbial Biomass Nitrogen and Soil Basal Respiration in 26 Years. Acta Ecologica. Sin. 35, 1445–1451. doi:10.5846/stxb201305070967
Zavalloni, C., Alberti, G., Biasiol, S., Vedove, G. D., Fornasier, F., Liu, J., et al. (2011). Microbial Mineralization of Biochar and Wheat Straw Mixture in Soil: A Short-Term Study. Appl. Soil Ecol. 50, 45–51. doi:10.1016/j.apsoil.2011.07.012
Zhang, H.-L., Lal, R., Zhao, X., Xue, J.-F., and Chen, F. (2014a). Opportunities and Challenges of Soil Carbon Sequestration by Conservation Agriculture in China. Adv. Agron. 124, 1–36. doi:10.1016/b978-0-12-800138-7.00001-2
Zhang, H., Qian, Z., and Zhuang, S. (2020a). Effects of Soil Temperature, Water Content, Species, and Fertilization on Soil Respiration in Bamboo forest in Subtropical China. Forests 11 (1), 99. doi:10.3390/f11010099
Zhang, J., Li, Y., Wang, J., Chen, W., Tian, D., Niu, S., et al. (2021). Different Responses of Soil Respiration and its Components to Nitrogen and Phosphorus Addition in a Subtropical Secondary forest. For. Ecosyst. 8, 37. doi:10.1186/s40663-021-00313-z
Zhang, L., Chen, X., Xu, Y., Jin, M., Ye, X., Gao, H., et al. (2020b). Soil Labile Organic Carbon Fractions and Soil Enzyme Activities after 10 Years of Continuous Fertilization and Wheat Residue Incorporation. Sci. Rep. 10, 11318. doi:10.1038/s41598-020-68163-3
Zhang, Q.-z., Dijkstra, F. A., Liu, X.-r., Wang, Y.-d., Huang, J., and Lu, N. (2014b). Effects of Biochar on Soil Microbial Biomass after Four Years of Consecutive Application in the north China plain. PLoS One 9 (7), e102062. doi:10.1371/journal.pone.0102062
Zhou, C., Heal, K., Tigabu, M., Xia, L., Hu, H., Yin, D., et al. (2020). Biochar Addition to forest Plantation Soil Enhances Phosphorus Availability and Soil Bacterial Community Diversity. For. Ecol. Management 455, 117635. doi:10.1016/j.foreco.2019.117635
Keywords: poultry manure biochar, integrated nutrient management, microbial biomass, carbon pools, lability index, management index, microbial population
Citation: Rahman MM, Islam MR, Uddin S, Rahman MM, Gaber A, Abdelhadi AA and Jahangir MMR (2022) Biochar and Compost-Based Integrated Nutrient Management: Potential for Carbon and Microbial Enrichment in Degraded Acidic and Charland Soils. Front. Environ. Sci. 9:798729. doi: 10.3389/fenvs.2021.798729
Received: 20 October 2021; Accepted: 23 November 2021;
Published: 07 January 2022.
Edited by:
Rosa Francaviglia, Council for Agricultural and Economics Research (CREA), ItalyReviewed by:
Mukesh Kumar Awasthi, Northwest A and F University, ChinaHongyu Chen, Freie Universität Berlin, Germany
Copyright © 2022 Rahman, Islam, Uddin, Rahman, Gaber, Abdelhadi and Jahangir. This is an open-access article distributed under the terms of the Creative Commons Attribution License (CC BY). The use, distribution or reproduction in other forums is permitted, provided the original author(s) and the copyright owner(s) are credited and that the original publication in this journal is cited, in accordance with accepted academic practice. No use, distribution or reproduction is permitted which does not comply with these terms.
*Correspondence: Md. Rafiqul Islam, rafiqss69@bau.edu.bd