- 1Key Laboratory of Forest Ecology and Environment of National Forestry and Grassland Administration, Institute of Forest Ecology, Environment and Nature Conservation, Chinese Academy of Forestry, Beijing, China
- 2Key Laboratory of Tree Breeding and Cultivation of the State Forestry Administration, Research Institute of Forestry Chinese Academy of Forestry, Beijing, China
- 3Northwest Institute of Plateau Biology, Chinese Academy of Science, Xining, China
- 4Monitoring and Evaluation Center of Qinghai National Park, Xining, China
- 5Forest Inventory and Planning Institute of Tibet Autonomous Region, Lhasa, China
Although soil total nitrogen (STN) and soil total phosphorus (STP) play significant roles in terrestrial ecosystem function, their storage and driving factors in the alpine wetlands of the Qinghai-Tibetan Plateau remain unclear. In this study, we estimated STN and STP storage and their controlling factors, including vegetation, soil, and climate characteristics, using data collected from 50 sites across the wetlands in the Three Rivers Source Region. STN and STP storage in the top 30 cm of soil were 62.12 ± 37.55 Tg N and 9.24 ± 2.90 Tg P, respectively. Although STN density did not differ significantly for different vegetation types (i.e., alpine meadow and alpine wetland), belowground biomass showed a positive relationship with STN density. Mean annual precipitation (MAP) showed a significant positive relationship with STN density, whereas the effects of mean annual temperature on STN density were minor. Compared with the effects of vegetation and climatic factors, soil characteristics were found to not only exert a significant effect on STN density, but also influence the effects of climate and vegetation on STN density. For STP density, soil characteristics were found to be a significant controlling factor, whereas the effects of biomass and climatic factors were minor. The studied climate, soil, and vegetation characteristics jointly explained ∼54% of STN variance, whereas soil characteristics explained only 20% of STP variation. MAP indirectly affected STN density via effects on vegetation and soil, and its direct effect on STN density was minor. This indicated a strong relationship between biotic and abiotic effects and STN density. Identification of the factors influencing STN and STP variance in alpine wetlands contributes to our understanding of the biogeochemical cycle in high-altitude regions.
Introduction
Nitrogen (N) and phosphorus (P) are prominent soil nutrients (Augusto et al., 2017). Although N was first to be demonstrated as a significant limiting nutrient (Vitousek and Howarth, 1991), research has since shown that P limitation or N and P co-limitation were also common (Harpole et al., 2011; Bracken et al., 2014). Soil N and P have significant effects on ecosystem functions through the regulation of soil properties such as physicochemical characteristics (Hati et al., 2008), soil microbial activity (Liu E. et al., 2010), and plant growth and competition in terrestrial ecosystems (Quilchano et al., 2008; Müller et al., 2015). Therefore, relative to other soil nutrients, N and P act as important biomacromolecule components in all organisms, and they are considered to be indispensable nutrients that have a substantial impact on soil productivity (Giesler et al., 2002; Vrede et al., 2004). As important references for soil quality and fertility, soil total nitrogen (STN) and soil total phosphorus (STP) can be used to determine the overall N and P contents in soil (Liu et al., 2013). Soil N and P have also been demonstrated to have a close relationship with organic carbon levels (Todd-Brown et al., 2014). As a result, soil N and P are linked to global climate change because of their dynamic influence on greenhouse gas emissions (Vitousek and Farrington, 1997; Lal, 2004). Therefore, understanding the characteristics of N and P in soil alongside their driving factors is of great importance. This not only applies to evaluating the dynamics of their contributions to nutrient cycles, but also to their potential effects on ecosystem functions, climate change, and related terrestrial ecosystem feedbacks (Jennings et al., 2009; Zhao et al., 2018).
Over the last decade, the mechanisms of N and P storage in soil and their controlling factors have received less attention compared with those of soil organic carbon (SOC) (Wang et al., 2009). As is the case for other soil components, such as SOC, the distributions of N and P in soil are heterogeneous, and the extent of their variation is dependent on the study scale (Wang et al., 2009). This spatial heterogeneity is caused by the influences of different factors, e.g., land use (Wang et al., 2009), climate (Patil et al., 2010), geography (Rezaei and Gilkes, 2005), or parent material (Lin et al., 2009). At a large scale, both mean annual precipitation (MAP) and mean annual temperature (MAT) have negative relationships with STN and STP in China forest (Chen et al., 2016b). However, at a regional scale, both temperature and precipitation showed positive relationships with STN and STP in the forest area of the Loess Plateau (Liu et al., 2013). This inconsistency may be attributed to different research scales (Fang et al., 2019), which highlights the need for furthermore exploring the relationships at a regional scale. In addition to climatic factors, vegetation characteristics (e.g., vegetation types and biomass) may also affect soil nutrient contents (Rodríguez et al., 2009; Zhao et al., 2018). For example, on the Qinghai-Tibetan Plateau, the STN in alpine meadow has been found to be 0.77 kg m−2, which is markedly higher than the values for alpine steppe (0.27 kg m−2) and alpine desert (0.12 kg m−2) (Zhao et al., 2018). The characteristics of soil, which control substrate qualities and microenvironments, also have a substantial effect on STN and STP (Lin et al., 2009; Nie et al., 2020). There is no doubt that the lack of studies of STN and STP characteristics and their controlling factors inevitably limits the prediction of biogeochemical cycles and policy formulation. Therefore, investigating STN and STP at a regional scale will not only contribute to soil management, but may also improve modeling accuracy for spatial data input (Lin et al., 2009).
Despite numerous studies being conducted, our understanding of the driving factors of STN and STP remain limited by the following two aspects. First, previous studies have focused mainly on forests, shrublands, and grasslands, concluding that vegetation types have substantial effects on soil nutrient contents (Liu et al., 2013; Chen et al., 2016b; Tashi et al., 2016; Nie et al., 2020). However, studies of STN and STP storage and their driving factors in alpine wetlands are rare. Little is yet known about STN and STP storage in alpine wetlands across the Three Rivers Source Region, which creates a major gap in the current understanding of the soil nutrient biogeochemical cycle in high-altitude regions. Second, although MAT and MAP are controlling factors, soil physical and chemical properties and vegetation also affect soil nutrient cycles (Yang et al., 2007; Chen et al., 2016a; Tashi et al., 2016); however, information on the total effects of climate, soil properties, and vegetation on STN and STP in alpine wetlands is lacking. Furthermore, it remains unclear as to which factor has the greatest effects on STN and STP.
Here, we aimed to evaluate STN and STP storage and the driving factors, including climate (MAP and MAT), soil characteristics (soil pH and SOC), and vegetation characteristics, i.e., aboveground biomass (AGB), belowground biomass (BGB), and vegetation types, in the Three Rivers Source Region wetland. We hypothesized that variations in STN and STP are related primarily to soil characteristics rather than climate and vegetation factors.
Materials and Methods
Study Area
Sampling sites were in the hinterland of the Qinghai-Tibetan Plateau (31.65°–37.02° N, 89.40°–102.25° E) (Supplementary Figure S1), which includes the headwaters of the Yangtze, Yellow, and Lancang rivers (Luo et al., 2014). The Three Rivers Source Region is characterized by low MAT and received ∼470 mm of MAP (Liang et al., 2013). Alpine wetland and meadow are the main vegetation types at the sampling sites. Alpine meadow areas are dominated by Kobresia. humilis and K. pygmaea, whereas K. tibetica occurs mainly in alpine wetland (Chang et al., 2014).
Biomass, Soil, and Climate Data
Field sampling was conducted in the Three Rivers Source Region of the central Qinghai-Tibetan Plateau during the growing season from 2019 to 2020. There were 50 sampling sites, including 32 alpine wetland sites and 18 alpine meadow sites. At each site, five quadrats of 1 m × 1 m were set up, and they were located at each corner and the center of a 10 m × 10 m plot. The AGB in the five quadrats was clipped at the ground level, and a total of 250 AGB samples (50 sites × 5 replications) were obtained, including 90 (18 sites × 5 replications) from alpine meadow and 160 (32 sites × 5 replications) from alpine wetland. Corresponding BGB samples were obtained by coring using a 7.5 cm auger at soil depths of 0–10, 10–20, and 20–30 cm in each of the five plots. Separating live roots from dead roots was difficult in the field, so both dead and live roots were sampled and washed using a sieve (Mu et al., 2018). AGB and BGB were dried at 65°C for 48 h and weighted to a constant value.
A soil pit of 30 m length × 30 m width × 30 m height was dug at each site, and three samples were collected from different soil profiles at depths of 0–10, 10–20, and 20–30 cm, respectively. For each site, three soil samples were blended into a single sample for each depth interval. Samples for estimating bulk density were collected in a standard 100 cm3 container with a height of 50 mm and a diameter of 50.46 mm, then dried in an oven at 105°C for 24 h. Bulk density was determined by dividing the soil dry mass by volume. Dried soil samples were passed through a 2-mm sieve before analyses of soil pH and SOC, STN, and STP contents. Visible stones and roots were removed, and samples were then ground in a ball mill. Soil pH was determined using a soil water suspension with a water:soil ratio of 2.5:1 The method of Walkley-Black wet oxidation was used for the determination of SOC. STN was determined using an element analyzer (2400 Ⅱ CHNS/O elemental analyzer, Perkin-Elmer, Waltham, MA), and STP was estimated using the molybdate/ascorbic acid method after H2SO4-H2O2 digestion.
To explore the effects of climatic factors on STN and STP, MAT and MAP data for 1950–2000 were calculated from the WorldClim database (http://www.worldclim.org/) using geographical coordinates and a spatial resolution was 1 km × 1 km (Hijmans et al., 2005).
Statistical Analyses
To determine the STN and STP densities and their storage at each site, the following formulae were used:
where STN densityi was soil total nitrogen density (kg m−2), Ti was soil thickness (cm), STN content was soil total nitrogen content (g kg−1), BDi was the bulk density (g cm−3), STP densityi was the soil total phosphorus density (kg m−2), STP contenti was the soil total phosphorus content (g kg−1), SOC densityi was the soil organic carbon density (kg m−2), SOC contenti was the soil organic carbon content (g kg−1), STN storagei was the soil total nitrogen storage (Tg N), STP storagei was the soil total phosphorus storage (Tg P), and area was the vegetation area.
The ordinary least squares method was used to explore the effects of specific controlling factors such as MAP, AGB, BGB, soil pH, and SOC on STN and STP densities in alpine wetland and alpine meadow, except for exploring the effects of BGB on STN and STP densities, and AGB on STP density in alpine meadow. Curve fitting of the power function was used for the latter relationship. Variance analysis was conducted to determine the difference of STN and STP at different soil depths (p < 0.05) using statistical software of SPSS 16.0 (SPSS Inc., Chicago, IL).
The controlling factors that had significant effects on the dependent variables were used in variation partitioning analysis. Three explained variables were used, including soil, climate, and plant factors. For STN density, explanatory variables included soil properties referring to soil pH, SOC density, climate factor referring to MAP, and vegetation factors referring to BGB in alpine wetland, and AGB in alpine meadow, their joints effects, and unexplained variation. In addition, for STP density, only SOC density and pH were used in the variation partitioning analysis. This method allows the estimation of the amount of variation in the responses of specific variables to certain controlling factors (Legendre, 2007). The “VEGAN” package was used to perform the method by R software (R Development Core Team, 2012).
The independent effects of soil, climate, and vegetation factors on STN and STP were estimated using partial correlation by controlling other factors. Partial correlation analysis allows the estimation of the effects of given factors on the relationship between predictor and response variable (Doetterl et al., 2015). These analyses were performed using the “GGM” package in R software (R Development Core Team, 2012).
Structural equation modeling was used to estimate the indirect and direct pathways for STN density from controlling factors of climate, soil, and vegetation. A hypothetical conceptual model was designed using the same factors as those used for the variation partitioning analysis (Supplementary Figure S2) before executing the structural equation model. STN density, MAP, soil pH, AGB, BGB, and SOC were scaled to mean = 0, and SD = 1. A p value >0.05 for Fisher’s C test is necessary for a good model fit (Leifeld, 2015). The Akaike information criterion and standardized path coefficients were shown in the model. The “PIECEWISE” package was used for structural equation modeling in the statistical R software (R Development Core Team, 2012).
Graphs were constructed using SigmaPlot 12.5 (SYSTAT Software, Inc., Point Richmond, United States).
Results
Storage and Density of Soil Total Nitrogen and Soil Total Phosphorus
STN density showed a decreasing trend with increasing soil depth in alpine wetland, with values of 0.39 ± 0.26 and 0.39 ± 0.17, 0.32 ± 0.23 and 0.27 ± 0.13, and 0.26 ± 0.20 and 0.22 ± 0.08 kg m−2 at soil depths of 0–10, 10–20, and 20–30 cm in alpine wetland and alpine meadow, respectively (Table 1). STN storage at a soil depth of 0–30 cm was 65.90 ± 41.47 and 59.44 ± 22.70 Tg N in alpine wetland and alpine meadow, respectively (Table 1). Although the calculated STN density for alpine wetlands (0.98 ± 0.62 kg m−2) was larger than that for alpine meadow (0.88 ± 0.34 kgm−2), and the difference was not significant (p > 0.05) (Table 1).
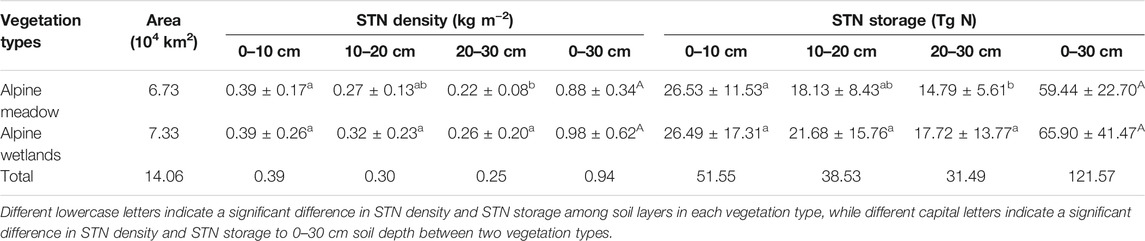
TABLE 1. Soil total nitrogen (STN) density and storage in alpine meadow and alpine wetlands in the Three Rivers Source Region. Different lowercase letters indicate a significant difference in STN density and STN storage among soil layers in each vegetation type, while different capital letters indicate a significant difference in STN density and STN storage to 0–30 cm soil depth between two vegetation types.
STP density remained relatively stable with increasing soil depth, with values of 0.04 ± 0.02 and 0.05 ± 0.01, 0.05 ± 0.02 and 0.06 ± 0.02, and 0.05 ± 0.01 and 0.06 ± 0.01 kg m−2 at soil depths of 0–10, 10–20, and 20–30 cm in alpine wetland and alpine meadow, respectively (Table 2). STP storage at soil depths of 0–30 cm was 9.24 ± 2.90 Tg P and 11.35 ± 2.45 Tg P for alpine wetland and alpine meadow, respectively (Table 2). The STP density for alpine meadow (0.17 ± 0.04 kg m−2) was greater than that for alpine wetland (0.14 ± 0.04 kg m−2) (p < 0.05) (Table 2).

TABLE 2. Soil total phosphorus (STP) density and storage in alpine meadow and alpine wetlands in the Three Rivers Source Region. Different lowercase letters indicate a significant difference in STP density and STP storage among soil layers in each vegetation type, while different capital letters indicate a significant difference in STP density and STP storage to 0–30 cm soil depth between two vegetation types.
Drivers of Soil Total Nitrogen and Soil Total Phosphorus
Soil properties, including pH and SOC density, had significant effects on STN and STP densities, respectively (p < 0.05) (Figure 1). In alpine wetland, STN and STP showed significant increasing trends with increasing SOC density (Figures 1A,C), whereas STN and STP density exhibited significant decreasing trends with increasing soil pH in alpine wetlands (Figures 1B,D). Trends for these edaphic factors were similar in alpine meadow (Figures 1E–H).
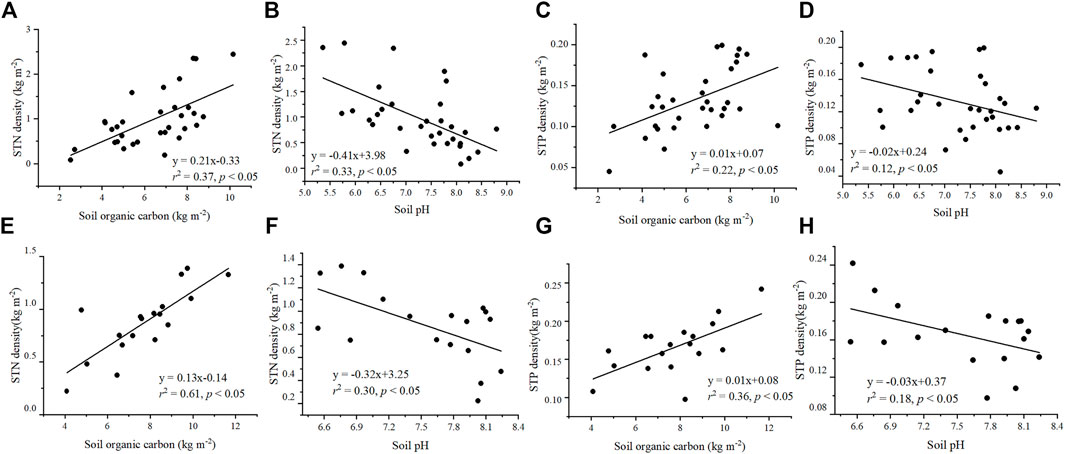
FIGURE 1. Relationships of STN density and STP density with soil variables, including soil organic carbon and soil pH. The relationships of STN density in alpine wetlands (A,B) and alpine meadow (E,F) with soil organic carbon (A,E) and soil pH (B,F). The relationships of STP density in alpine wetlands (C,D) and alpine meadow (G,H) with soil organic carbon density (C,G) and soil pH (D,H). STN density, soil total nitrogen density; STP density, soil total phosphorus density.
Biomass had a moderate effect on STN and STP densities in the Three Rivers Source Region. In general, biomass showed a significantly positive relationship with STN density, but its effects on STP density were minor (Figure 2). STN density significantly increased with increasing BGB in alpine wetland (Figure 2A) and alpine meadow (Figure 2E), and STN density also significantly increased with increasing AGB in alpine meadow (Figure 2F). However, the increasing trend was not significant (p > 0.05) in alpine wetlands (Figure 2B). The effects of biomass on STP density differed from those observed on STN. In alpine wetland, STP density exhibited a weak increasing trend with increasing AGB and BGB (p > 0.05) (Figures 2C,D). In alpine meadow, although the relationships were significant (p < 0.05), the effects of AGB and BGB were relatively weak, with parameters of r2 = 0.06, and r2 = 0.12 in the power functions (Figures 2G,H). The STN was no significant difference between the alpine meadow and alpine wetland (Table 1).
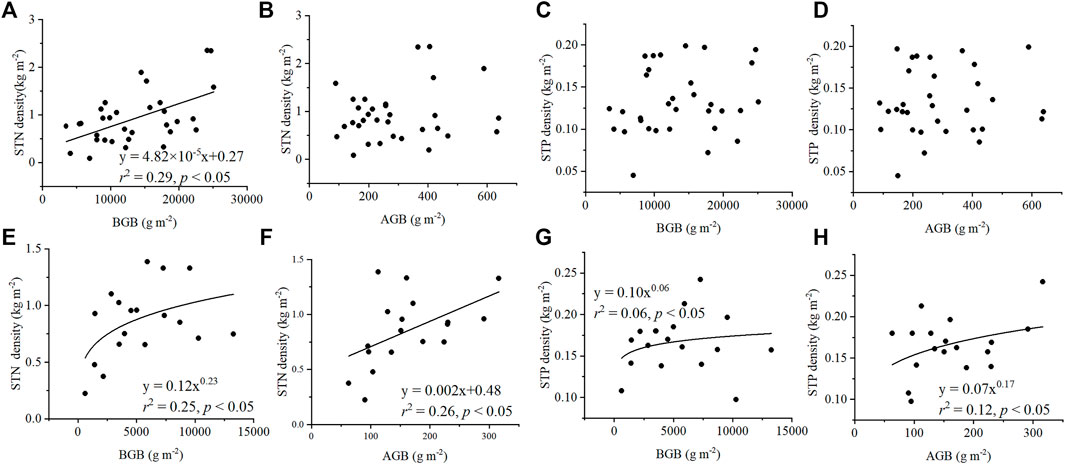
FIGURE 2. Relationships of STN density and STP density with plant variables, including AGB and BGB. The relationships of STN density in alpine wetlands (A,B) and alpine meadow (E,F) with BGB (A,E) and AGB (B,F). The relationships of STP density in alpine wetlands (C,D) and alpine meadow (G,H) with BGB (C,G) and AGB (D,H). STN density, soil total nitrogen density; STP density, soil total phosphorus density; AGB, aboveground biomass; and BGB, belowground biomass.
The effects of MAP on STN and STP densities differed for alpine wetlands and alpine meadow. In both alpine wetland and alpine meadow, STN density showed an increasing trend with increasing MAP, whereas the effects of MAP on STP density were minor (Figure 3). In contrast to the significant effects of MAP on STN density in alpine meadow and alpine wetland, the relationships of MAT with STN and STP densities were not significant (Supplementary Figure S3).
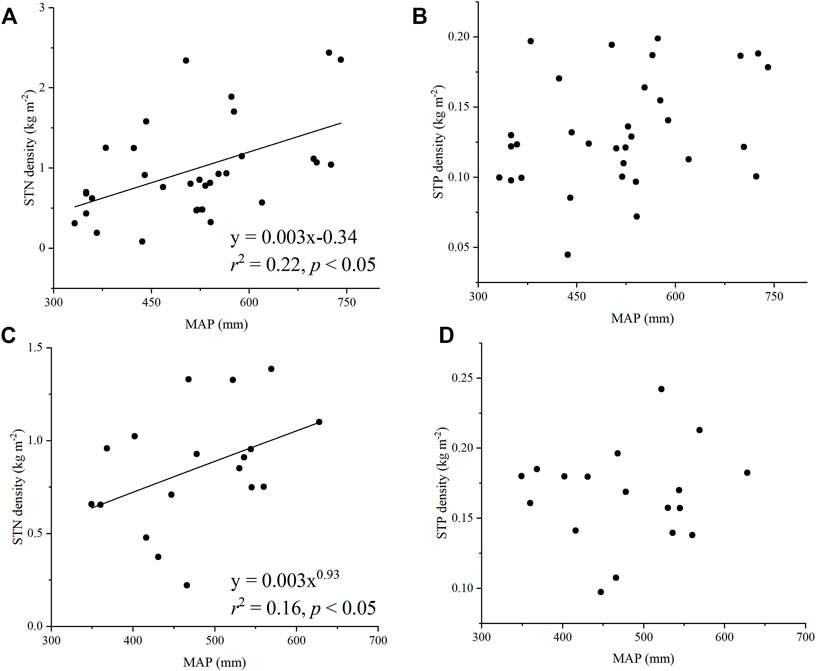
FIGURE 3. Relationships of STN density (A,C) and STP density (B,D) with MAP in alpine wetlands (A,B) and alpine meadow (C,D). STN density, soil total nitrogen density; STP density, soil total phosphorus density; MAP, mean annual precipitation.
More than half of the variations in STN density can be attributed to soil, climate, and vegetation properties. The variation partitioning analysis results showed that 54 and 56% of the variances in STN density were explained in alpine wetlands (Figure 4A) and alpine meadow (Figure 4B), respectively. Furthermore, soil played a significant role in STN density compared with climate and vegetation factors, when soil properties were used as a controlled factor, the effects of vegetation and climate factors on STN density were minor (p > 0.05). Furthermore, when vegetation or climate factors were used as controlled factors, soil properties still showed a significant effect on STN density (p < 0.05) (Figure 5). STP density was controlled mainly by soil properties, and 20 and 32% of the variation in STP density was explained by soil pH and SOC in alpine wetlands and alpine meadow, respectively (Figure 4).
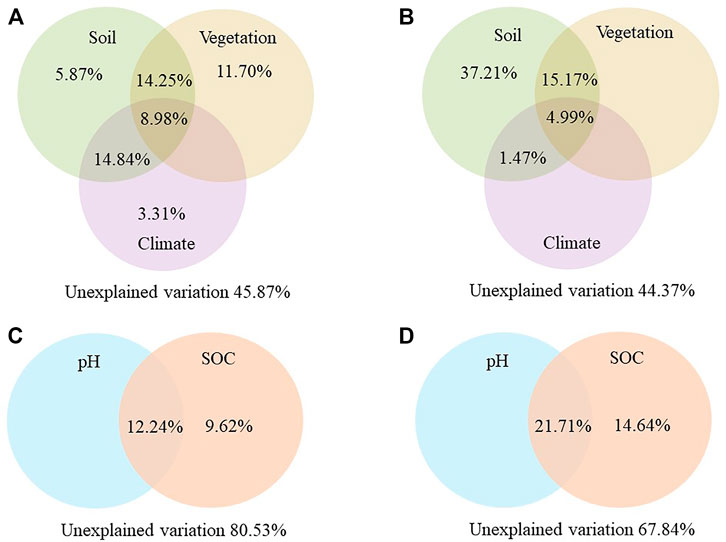
FIGURE 4. The results of variation partitioning analysis for soil total nitrogen density (A,B) and soil total phosphorus (C,D) in alpine wetlands (A,C) and alpine meadow (B,D). For soil total nitrogen density, variation partitioning analysis consisted of explained variation, including soil properties refer to soil pH, soil organic carbon density, climate factor refers to mean annual precipitation, and vegetation includes belowground biomass in alpine wetlands and aboveground biomass in alpine meadow, their joints effects, and unexplained variation. In addition, for soil total phosphorus, the variation partitioning analysis consisted of SOC and pH. SOC, soil organic carbon density; pH, soil pH.
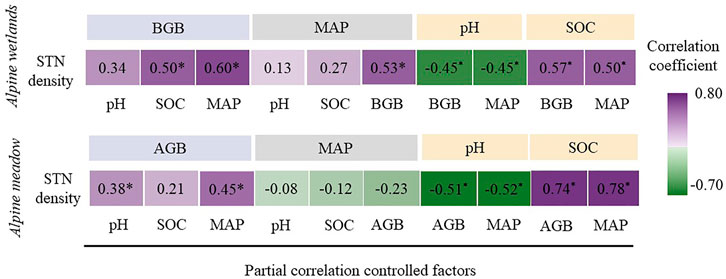
FIGURE 5. Partial correlations among soil factors, including pH and SOC, climatic and vegetation factors in alpine wetlands and alpine meadow. The numbers and color indicated the strength of correlation coefficient. Significant association was estimated at the level of 0.05 and was marked as *. MAP, mean annual precipitation; AGB, aboveground biomass; BGB, belowground biomass; SOC, soil organic carbon density; pH = soil pH.
The structural equation modeling results provided similar explanations for variances in STN density to those determined by variation partitioning analysis. In wetlands, MAP, BGB, soil pH, and SOC explained 60% of the total variance in STN density, which was similar to the variation partitioning analysis result (54%) (Figures 4A, 6A). Like alpine meadow, the explained variances in STN density were 66 and 56% in alpine wetlands with the method of structural equation mode and variation partitioning analysis, respectively (Figures 4B, 6B). For alpine wetlands, MAP indirectly affected STN density via BGB and soil pH/SOC, which indicated a tight relationship between biotic and abiotic effects on STN density (Figure 6A).
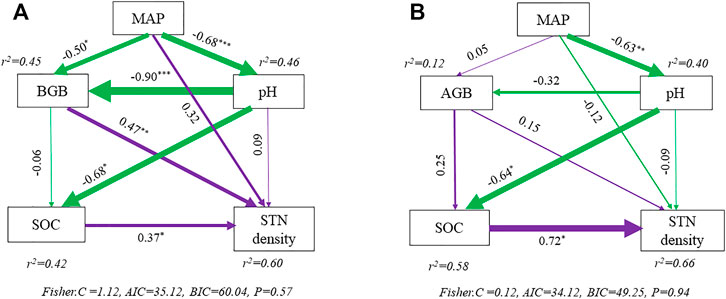
FIGURE 6. Structural equation models for depicting direct and indirect drivers to soil total nitrogen density in alpine wetlands (A) and alpine meadow (B). The numbers at the arrows indicate standardized path coefficients and arrow width indicates proportion of the relative strength for the relationship. The asterisks beside the numbers were used to indicate the significance of each path coefficient (*p < 0.05, **p < 0.01, ***p < 0.001). The colors purple and green inferred to positive effects and negative effects. STN density, soil total nitrogen density; MAP, mean annual precipitation; BGB, belowground biomass, AGB, aboveground biomass; pH, soil pH; SOC, soil organic carbon density.
Discussion
Effects of Climate Factors on Soil Total Nitrogen and Soil Total Phosphorus
MAP was found to have a positive relationship with STN in alpine meadow and alpine wetland across the Three Rivers Source Region (Figure 3). This positive relationship was similar to that observed for desert shrublands on the Qinghai-Tibetan Plateau (Nie et al., 2017), forests and grasslands across the Loess Plateau (Liu et al., 2013), and the United States Great Plains (Epstein et al., 2002). However, our results were in contrast to those previously obtained for China forests, which showed a negative relationship between STN and MAP (Chen et al., 2016b). Both C and N are linked primarily to the biological processes, including photosynthesis, atmospheric N fixation, and subsequent microbial mineralization (Delgado-Baquerizo et al., 2013). On the Qinghai-Tibetan Plateau, increasing precipitation can lead to increased plant productivity (Yang et al., 2009), i.e., higher organic material input, which to some extent contribute to N accretion in topsoil (Li and Zhao, 2001; Liu et al., 2012). However, instead of having a positive effect on nutrient retention, increased precipitation can also cause greater nutrient leaching from soils, resulting in nutrient depletion and a negative relationship (Vitousek et al., 2010; Chen et al., 2016b). Considering that one of the most obvious characteristics on the Qinghai-Tibetan Plateau is arid (Yang, 2018), alongside increasing precipitation, accumulation of biomass and N input may also be more than leaching from soil, resulting in a positive relationship between STN and MAP observed in this study.
In contrast to the positive relationship between MAP and STN, the correlation between MAT and STN was not found to be significant in alpine wetland or alpine meadow. Our results were inconsistent with the significant negative relationship reported for forest (Chen et al., 2016b) and alpine shrubland on the Qinghai-Tibetan Plateau (Nie et al., 2017), and they were also incompatible with the results for Tibetan alpine grasslands, which STN showed a significant increasing trend with increasing MAT (Yang, 2008). On the one hand, increasing temperature contributes to more biomass and N inputs (Ding et al., 2017; Nie et al., 2018), thus stimulating STN accumulation. On the other hand, more intense decomposition tends to occur with increasing microbial activity as temperature increases (Yang et al., 2008), thus decreasing STN. In alpine wetland and alpine meadow, as MAT increases, N accumulation in soil may be roughly equivalent to N loss, resulting in a minor relationship between STN and MAT in the Three Rivers Source Region on the Qinghai-Tibetan Plateau.
Compared with the effects of MAP on STN, the effects of climatic factors, including MAT and MAP, on STP were found to be minor. The minor effects of climatic factors on STP in alpine wetlands was different from the trends reported for China forests, which STP showed significant decreasing trends with increasing MAT and MAP (Chen et al., 2016b). Our results also contrasted with the effects of MAP on STP in the Loess Plateau grasslands, which demonstrated that STP showed an increasing trend with MAP (Liu et al., 2013). However, the minor effects of MAT on STP in alpine wetland and alpine meadow were similar to those in the Loess Plateau grasslands (Liu et al., 2013). In general, P is weathered and released from its parent material more readily under high precipitation conditions (Lin et al., 2009). Furthermore, high temperatures accelerate the weathering process and microbial activity, which may result in a greater accumulation of P released from rocks (Lin et al., 2009). In this study, the effects of MAT and MAP on STP were minor in alpine wetland and alpine meadow areas of the Three Rivers Source Region, which may be due to the climatic characteristics of the Qinghai-Tibetan Plateau. Low temperatures are common across the Qinghai-Tibetan Plateau (Yang, 2018), especially at the sampling sites, as the mean altitude is 4078 m. At such high altitudes and in these cold conditions, increases in temperature may be minor to influence STP in both alpine wetland and alpine meadow areas of the Three Rivers Source Region. Increasing precipitation can contribute to P accumulation through increased weathering; however, it may also lead to P loss through leaching (Zhang et al., 2005; Tian et al., 2010; Chen et al., 2016b). P accumulation may offset P loss, resulting in the lack of a strong relation in alpine meadow and alpine wetland.
Effects of Vegetation Properties on Soil Total Nitrogen and Soil Total Phosphorus
STN was found to show a significant increasing trend with increasing biomass, whereas the relationship between STP and biomass was minor (Figure 2). In general, more biomass tends to cause a greater accumulation of forest litter (Nie et al., 2019), and this litter and plant residue contribute to increase in soil N input (Liu X. et al., 2010; Liang et al., 2019). As a result, increasing biomass triggered a positive relationship between STN and biomass in alpine wetland and alpine meadow in the Three Rivers Source Region on the Qinghai-Tibetan Plateau. BGB, rather than AGB, was found to have a positive relationship with STN density in alpine wetland, however, both AGB and BGB showed significant effects on STN in alpine meadow, highlighting the different roles of AGB in shaping STN in different ecosystems. Compared with AGB, BGB was more abundant in alpine wetland than in alpine meadow, which may be the cause of the significant relationship between BGB and STN in alpine wetland.
The correlation between biomass and STP was relatively weak (Figure 2). Soil properties (i.e., weathering stage and physicochemical characteristics) are significant pathways for STP accumulation (Chen et al., 2016b; Augusto et al., 2017), partly as a result of organic matter decomposition (Delgado-Baquerizo et al., 2013). Thus, increasing biomass only showed a relatively weak effect on STP in alpine meadow, even no linear relationship was observed between the two for alpine wetland.
Effects of Soil Properties on Soil Total Nitrogen and Soil Total Phosphorus
STN and STP had similar responses to increased soil pH and SOC, with both increasing in line with SOC and decreasing with the increase in soil pH in both alpine wetland and alpine meadow (Figure 1). Similar relationships between STN and soil pH and SOC have been observed in alpine shrubland on the Qinghai-Tibetan Plateau (Nie et al., 2020). Organic matter decomposition in soil is a significant pathway for soil N accumulation (Augusto et al., 2017; Zhou et al., 2018; Fang et al., 2019), which results in a positive correlation between STN and SOC. Meanwhile, soil P, to some extent, can also be affected by organic matter input and loss (Zhou et al., 2018), causing a positive relationship between STP and SOC in alpine wetland and alpine meadow of the Three Rivers Source Region.
Unlike their positive relationship with SOC, both STN and STP showed a significant negative relationship with soil pH. Moderately alkaline conditions are generally conducive to the decomposition of soil organic matter by stimulating microorganism activity (Whittinghill and Hobbie, 2011), whereas acidic conditions generally slow soil organic matter turnover (Kemmitt et al., 2006). Therefore, increasing soil pH contributed to a decrease in soil organic matter, which, to a certain extent, is the source of soil N and P. This explains the negative relationships among STN, STP, and soil pH in alpine wetland and alpine meadow of the Three Rivers Source Region on the Qinghai-Tibetan Plateau.
Limitations and Implications
Soil clay content has a significant effect on soil moisture and may therefore be a controlling factor for STN and STP. Vegetation biomass productivity and community compositions are all directly interrelated (Jorgenson et al., 2001; Wang et al., 2007; Liu et al., 2012), and the community compositions may also be potential indicators of STN and STP storage. However, these factors were not investigated in this study. Although the climate data extracted from WordClim has been used in numerous studies (Hijmans et al., 2005; Yang et al., 2014), measured MAT and MAP data from local climate stations may be more representative of the local areas, and thus may increase the accuracy of our study.
To the best of our knowledge, few studies have investigated STN and STP storage in alpine wetlands across the Three Rivers Source Region. Our study indicated that a tight relationship between biotic and abiotic factors and soil nutrient in alpine wetlands, is necessary to protect the wetlands ecosystem and reduce human disturbance, especially in global climate change scenario (Shang et al., 2013). This study not only provided an underlying database for soil nutrients but may also be applied to monitor changes in soil nutrient storage (e.g., at 10-year intervals) in the future. Soil nitrogen deposition has existed across the Qinghai-Tibetan Plateau (Lü and Tian, 2007), which potentially reduces soil pH (Yang et al., 2015). It had also been demonstrated that SOC storage has increased in alpine grasslands on the Qinghai-Tibetan Plateau owing to the warmer and wetter climate (Ding et al., 2017). Considering their significant relationships, both STN and STP may be potentially changed in the alpine wetlands and alpine meadow. As such, in global climate change and N deposition scenarios, further monitoring of dynamic changes in STN and STP is necessary to provide a more accurate assessment of soil nutrient conditions in alpine wetlands and alpine meadow in the Three Rivers Source Region on the Qinghai-Tibetan Plateau.
Conclusion
This study provides an estimation of the STN and STP storage and their controlling factors in the top 30 cm in the Three Rivers Source Region alpine wetland. The results revealed that the STN and STP were found to be 62.12 ± 37.55 Tg N and 9.24 ± 2.90 Tg P, respectively, across the study area. Compared with the effects of vegetation and climatic factors, soil characteristics performed a stronger effect on STN and STP in alpine wetlands. Soil pH was negatively correlated with both STN and STP, whereas SOC was positively correlated with them. This implies that monitoring soil organic matter and soil pH can provide a more accurate predication of STN and STP dynamic changes in alpine wetlands.
The MAP affected STN density indirectly via vegetation and soil characteristics and has a minor direct effect on STN density. This indicates a tight relationship between biotic and abiotic factors and STN density. This identification of the driving factors of STN and STP variation in alpine wetland contributes to our understanding of biogeochemical cycles in high-altitude regions.
Data Availability Statement
The raw data supporting the conclusion of this article will be made available by the authors, without undue reservation.
Author Contributions
DW designed the experiments; DW, GZ, FX, ZZ, YD, XN, YC, and KM performed the experiments and collected the data; XN, FX, ZZ, LR, and DW analyzed the data and wrote the paper.
Funding
This work was supported by National Natural Science Foundation of China (Grant No. 32001216), the National Non-Profit Institute Research Grant of Chinese Academy of Forestry (Grant No. CAFYBB2021MA015), Key Laboratory of Tree Breeding and Cultivation Special Fund Project (Grant No. ZDRIF201905), the Second Tibetan Plateau Scientific Expedition and Research Program (Grant No. 2019QZKK0302), the National Key Research and Development Program of China (Grant No. 2019YFC0507404), Qinghai Province International Exchange and Cooperation Project (Grant No. 2022-HZ-804, 2019-HZ-807), and the Natural Science Foundation of Qinghai (Grant No. 2019-ZJ-910).
Conflict of Interest
The authors declare that the research was conducted in the absence of any commercial or financial relationships that could be construed as a potential conflict of interest.
Publisher’s Note
All claims expressed in this article are solely those of the authors and do not necessarily represent those of their affiliated organizations, or those of the publisher, the editors and the reviewers. Any product that may be evaluated in this article, or claim that may be made by its manufacturer, is not guaranteed or endorsed by the publisher.
Supplementary Material
The Supplementary Material for this article can be found online at: https://www.frontiersin.org/articles/10.3389/fenvs.2022.806771/full#supplementary-material
Supplementary Figure S1 | Distribution of sampling sites in the Three Rivers Source Region.
Supplementary Figure S2 | Conceptual model of the direct and indirect effects from MAP, pH, BGB, and SOC on STN density in alpine wetlands (A), and MAP, pH, AGB, and SOC on STN density in alpine meadow (B). STN density, soil total nitrogen density; MAP, mean annual precipitation, BGB, belowground biomass, AGB, aboveground biomass, pH, soil pH, SOC, soil organic carbon density.
Supplementary Figure S3 | Relationships of STN density (A,C) and STP density (B,D) with MAT in alpine wetlands (A,B) and alpine meadow (C,D). STN density, soil total nitrogen density, STP density, soil total phosphorus density, MAT, mean annual temperature.
References
Augusto, L., Achat, D. L., Jonard, M., Vidal, D., and Ringeval, B. (2017). Soil Parent Material-A Major Driver of Plant Nutrient Limitations in Terrestrial Ecosystems. Glob. Change Biol. 23, 3808–3824. doi:10.1111/gcb.13691
Bracken, M. E. S., Hillebrand, H., Borer, E. T., Seabloom, E. W., Cebrian, J., Cleland, E. E., et al. (2014). Signatures of Nutrient Limitation and Co-limitation: Responses of Autotroph Internal Nutrient Concentrations to Nitrogen and Phosphorus Additions. Oikos 124, 113–121. doi:10.1111/oik.01215
Chang, X., Wang, S., Cui, S., Zhu, X., Luo, C., Zhang, Z., et al. (2014). Alpine Grassland Soil Organic Carbon Stock and its Uncertainty in the three rivers Source Region of the Tibetan Plateau. PLoS One 9, e97140. doi:10.1371/journal.pone.0097140
Chen, L. F., He, Z. B., and Du, J. (2016a). Patterns and Environmental Controls of Soil Organic Carbon and Total Nitrogen in alpine Ecosystems of Northwestern China. Catena 137, 37–43. doi:10.1016/j.catena.2015.08.017
Chen, L., Li, P., and Yang, Y. (2016b). Dynamic Patterns of Nitrogen: Phosphorus Ratios in forest Soils of China under Changing Environment. J. Geophys. Res. Biogeosciences 121, 2410–2421. doi:10.1002/2016jg003352
Delgado-Baquerizo, M., Maestre, F. T., Gallardo, A., Bowker, M. A., Wallenstein, M. D., Quero, J. L., et al. (2013). Decoupling of Soil Nutrient Cycles as a Function of Aridity in Global Drylands. Nature 502, 672–675. doi:10.1038/nature12670
Ding, J., Chen, L., Ji, C., Hugelius, G., Li, Y., Liu, L., et al. (2017). Decadal Soil Carbon Accumulation across Tibetan Permafrost Regions. Nat. Geosci. 10, 1–5. doi:10.1038/ngeo2945
Doetterl, S., Stevens, A., Six, J., Merckx, R., Van Oost, K., Casanova Pinto, M., et al. (2015). Soil Carbon Storage Controlled by Interactions between Geochemistry and Climate. Nat. Geosci. 8, 780–783. doi:10.1038/ngeo2516
Epstein, H. E., Burke, I. C., and Lauenroth, W. K. (2002). Regional Patterns of Decomposition and Primary Production Rates in the US Great Plains. Ecology 83, 320–327. doi:10.1890/0012-9658(2002)083[0320:rpodap]2.0.co;2
Fang, Z., Li, D. D., Jiao, F., Yao, J., and Du, H. T. (2019). The Latitudinal Patterns of Leaf and Soil C:N:P Stoichiometry in the Loess Plateau of China. Front. Plant Sci. 10, 1–10. doi:10.3389/fpls.2019.00085
Giesler, R., Peter, P., and Högberg, P. (2002). Phosphorus Limitation in Boreal Forests: Effects of Aluminum and Iron Accumulation in the Humus Layer. Ecosystems 5, 300–314. doi:10.1007/s10021-001-0073-5
Harpole, W. S., Ngai, J. T., Cleland, E. E., Seabloom, E. W., Borer, E. T., Bracken, M. E. S., et al. (2011). Nutrient Co-limitation of Primary Producer Communities. Ecol. Lett. 14, 852–862. doi:10.1111/j.1461-0248.2011.01651.x
Hati, K. M., Swarup, A., Mishra, B., Manna, M. C., Wanjari, R. H., Mandal, K. G., et al. (2008). Impact of Long-Term Application of Fertilizer, Manure and Lime under Intensive Cropping on Physical Properties and Organic Carbon Content of an Alfisol. Geoderma 148, 173–179. doi:10.1016/j.geoderma.2008.09.015
Hijmans, R. J., Cameron, S. E., Parra, J. L., Jones, P. G., and Jarvis, A. (2005). Very High Resolution Interpolated Climate Surfaces for Global Land Areas. Int. J. Climatology: A J. R. Meteorol. Soc. 25, 1965–1978. doi:10.1002/joc.1276
Jennings, E., Allott, N., Pierson, D. C., Schneiderman, E. M., Lenihan, D., Samuelsson, P., et al. (2009). Impacts of Climate Change on Phosphorus Loading from a Grassland Catchment: Implications for Future Management. Water Res. 43, 4316–4326. doi:10.1016/j.watres.2009.06.032
Jorgenson, M. T., Racine, C. H., Walters, J. C., and Osterkamp, T. E. (2001). Permafrost Degradation and Ecological Changes Associated with a Warming Climate in central Alaska. Climatic Change 48, 551–579. doi:10.1023/a:1005667424292
Kemmitt, S., Wright, D., Goulding, K., and Jones, D. (2006). pH Regulation of Carbon and Nitrogen Dynamics in Two Agricultural Soils. Soil Biol. Biochem. 38, 898–911. doi:10.1016/j.soilbio.2005.08.006
Lal, R. (2004). Soil Carbon Sequestration Impacts on Global Climate Change and Food Security. Science 304, 1623–1627. doi:10.1126/science.1097396
Legendre, P. (2007). Studying Beta Diversity: Ecological Variation Partitioning by Multiple Regression and Canonical Analysis. Chin. J. Plant Ecol. 31, 976–981. doi:10.17521/cjpe.2007.0124
Leifeld, J. S. (2015). PIECEWISESEM: Piecewise Structural Equation Modelling in R for Ecology, Evolution, and Systematics. Methods Ecol. Evol. 7, 573–579.
Li, Z., and Zhao, Q. (2001). Organic Carbon Content and Distribution in Soils under Different Land Uses in Tropical and Subtropical China. Plant and Soil 231, 175–185.
Liang, C., Yin, S. B., and Liu, J. P. (2019). Spatial Distribution and Ecological Stoichimometric Characteristics of Nitrogen and Phosphorus in Soils from Saucer-Shaped Depressions to Island Forests in the Sanjing Plain. Acta Ecologica Sinica 39, 7679–7685. doi:10.5846/stxb201808231793
Liang, L., Li, L., Liu, C., and Cuo, L. (2013). Climate Change in the Tibetan Plateau Three Rivers Source Region: 1960-2009. Int. J. Climatology 33, 2900–2916. doi:10.1002/joc.3642
Lin, J. S., Shi, X. Z., Lu, X. X., Yu, D. S., Wang, H., Zhao, Y., et al. (2009). Storage and Spatial Variation of Phosphorus in Paddy Soils of China. Pedosphere 19, 790–798. doi:10.1016/s1002-0160(09)60174-0
Liu, E., Yan, C., Mei, X., He, W., Bing, S. H., Ding, L., et al. (2010a). Long-term Effect of Chemical Fertilizer, Straw, and Manure on Soil Chemical and Biological Properties in Northwest China. Geoderma 158, 173–180. doi:10.1016/j.geoderma.2010.04.029
Liu, W., Chen, S., Qin, X., Baumann, F., Scholten, T., Zhou, Z., et al. (2012). Storage, Patterns, and Control of Soil Organic Carbon and Nitrogen in the Northeastern Margin of the Qinghai–Tibetan Plateau. Environ. Res. Lett. 7, 035401. doi:10.1088/1748-9326/7/3/035401
Liu, X., Zhou, G., Zhang, D., Liu, S., Chu, G., and Yan, J. (2010b). N and P Stoichiometry of Plant and Soil in Lower Subtropical forest Successional Series in Southern China. Chin. J. Plant Ecol. 34, 64–71.
Liu, Z. P., Shao, M. A., and Wang, Y. Q. (2013). Spatial Patterns of Soil Total Nitrogen and Soil Total Phosphorus across the Entire Loess Plateau Region of China. Geoderma 197, 67–78. doi:10.1016/j.geoderma.2012.12.011
Lü, C., and Tian, H. (2007). Spatial and Temporal Patterns of Nitrogen Deposition in China: Synthesis of Observational Data. J. Geophys. Res. 112, 1–10. doi:10.1029/2006jd007990
Luo, C., Wang, J., Liu, M., and Liu, Z. (2014). Analysis on the Change of Grassland Coverage in the Source Region of Three Rivers during 2000-2012. IOP Conf. Ser. Earth Environ. Sci. 17, 012062. doi:10.1088/1755-1315/17/1/012062
Mu, C., Li, L., Zhang, F., Li, Y., Xiao, X., Zhao, Q., et al. (2018). Impacts of Permafrost on above- and Belowground Biomass on the Northern Qinghai-Tibetan Plateau. Arctic, Antarctic, Alpine Res. 50, e1447192. doi:10.1080/15230430.2018.1447192
Müller, A. K., Matson, A. L., Corre, M. D., and Edzo, V. (2015). Soil N2O Fluxes along an Elevation Gradient of Tropical Montane Forests under Experimental Nitrogen and Phosphorus Addition. Front. Earth Sci. 3, 1–12. doi:10.3389/feart.2015.00066
Nie, X. Q., Yang, L. C., Xiong, F., Li, C. B., Fan, L., and Zhou, G. Y. (2018). Aboveground Biomass of the alpine Shrub Ecosystems in Three-River Source Region of the Tibetan Plateau. J. Mountain Sci. 15, 357–363. doi:10.1007/s11629-016-4337-0
Nie, X., Wang, D., Yang, L., and Zhou, G. (2020). Controlling Soil Total Nitrogen Factors across Shrublands in the Three Rivers Source Region of the Tibetan Plateau. Forest-Biogeosciences For. 12, 559–565. doi:10.3832/ifor3533-013
Nie, X., Wang, D., Yang, L., and Zhou, G. (2019). Storage and Climatic Controlling Factors of Litter Standing Crop Carbon in the Shrublands of the Tibetan Plateau. Forests 10, 1–12. doi:10.3390/f10110987
Nie, X., Xiong, F., Yang, L., Li, C., and Zhou, G. (2017). Soil Nitrogen Storage, Distribution, and Associated Controlling Factors in the Northeast Tibetan Plateau Shrublands. Forests 8, 1–13. doi:10.3390/f8110416
Patil, R. H., Laegdsmand, M., Olesen, J. E., and Porter, J. R. (2010). Effect of Soil Warming and Rainfall Patterns on Soil N Cycling in Northern Europe. Agric. Ecosyst. Environ. 139, 195–205. doi:10.1016/j.agee.2010.08.002
Quilchano, C., Marañón, T., Pérez-Ramos, I., Noejovich, L., Valladares, F., and Zavala, M. (2008). Patterns and Ecological Consequences of Abiotic Heterogeneity in Managed Cork Oak Forests of Southern Spain. Ecol. Res. 23, 127–139. doi:10.1007/s11284-007-0343-6
Rezaei, S. A., and Gilkes, R. J. (2005). The Effects of Landscape Attributes and Plant Community on Soil Physical Properties in Rangelands. Geoderma 125, 145–154. doi:10.1016/j.geoderma.2004.07.011
Rodríguez, A., Durán, J., Fernández-Palacios, J. M., and Gallardo, A. (2009). Spatial Pattern and Scale of Soil N and P Fractions under the Influence of a Leguminous Shrub in a Pinus Canariensis forest. Geoderma 151, 303–310. doi:10.1016/j.geoderma.2009.04.019
Shang, Z. H. Q. S., Feng, G. L., WuRen, G. H. R. J., and Long, (2013). Grasslandification Has Significant Impacts on Soil Carbon, Nitrogen and Phosphorus of alpine Wetlands on the Tibetan Plateau. Ecolgocial Eng. 58, 170–179. doi:10.1016/j.ecoleng.2013.06.035
Tashi, S., Singh, B., Keitel, C., and Adams, M. (2016). Soil Carbon and Nitrogen Stocks in Forests along an Altitudinal Gradient in the Eastern Himalayas and a Meta-Analysis of Global Data. Glob. Change Biol. 22, 2255–2268. doi:10.1111/gcb.13234
Tian, H., Chen, G., Zhang, C., Melillo, J. M., and Hall, C. A. J. B. (2010). Pattern and Variation of C: N: P Ratios in China’s Soils: a Synthesis of Observational Data. Biogeochemistry 98, 139–151. doi:10.1007/s10533-009-9382-0
Todd-Brown, K. E. O., Randerson, J. T., Hopkins, F., Arora, V., Hajima, T., Jones, C., et al. (2014). Changes in Soil Organic Carbon Storage Predicted by Earth System Models during the 21st century. Biogeosciences 11, 2341–2356. doi:10.5194/bg-11-2341-2014
Vitousek, P. M., and Farrington, H. (1997). Nutrient Limitation and Soil Development: Experimental Test of a Biogeochemical Theory. Biogeochemistry 37, 63–75. doi:10.1023/a:1005757218475
Vitousek, P. M., and Howarth, R. W. (1991). Nitrogen Limitation on Land and in the Sea: How Can it Occur? Biogeochemistry 13, 87–115. doi:10.1007/bf00002772
Vitousek, P., Porder, M. S., Houlton, B., and Chadwick, O. (2010). Terrestrial Phosphorus Limitation: Mechanisms, Implications, and Nitrogen–Phosphorus Interactions. Ecol. Appl. 20, 5–15. doi:10.1890/08-0127.1
Vrede, T., Dobberfuhl, D. R., and Kooijman, S. (2004). Fundamental Connections Among Organism C:N:P Stoichiometry, Macromolecular Composition, and Growth. Ecology 85, 1217–1229. doi:10.1890/02-0249
Wang, G., Wang, Y., Li, Y., and Cheng, H. (2007). Influences of alpine Ecosystem Responses to Climatic Change on Soil Properties on the Qinghai–Tibet Plateau, China. Catena 70, 506–514. doi:10.1016/j.catena.2007.01.001
Wang, Y., Zhang, X., and Huang, C. (2009). Spatial Variability of Soil Total Nitrogen and Soil Total Phosphorus under Different Land Uses in a Small Watershed on the Loess Plateau, China. Geoderma 150, 141–149. doi:10.1016/j.geoderma.2009.01.021
Whittinghill, K. A., and Hobbie, S. E. (2011). Effects of pH and Calcium on Soil Organic Matter Dynamics in Alaskan Tundra. Biogeochemistry 111, 569–581. doi:10.1007/s10533-011-9688-6
Yang, X., Tang, Z., Ji, C., Liu, H., Ma, W., Mohhamot, A., et al. (2014). Scaling of Nitrogen and Phosphorus across Plant Organs in Shrubland Biomes across Northern China. Scientific Rep. 4, 1–7. doi:10.1038/srep05448
Yang, Y. (2008). Carbon and Nitrogen in alpine Grasslands on the Tibetan Plateau. Ph. D. Dissertation. Peking Universtiy.
Yang, Y. (2018). Ecological Processes in alpine Ecosystems under Changing Environment. Chin. J. Plant Ecol. 42, 1–5.
Yang, Y., Fang, J., Tang, Y., Ji, C., Zheng, C., He, J., et al. (2008). Storage, Patterns and Controls of Soil Organic Carbon in the Tibetan Grasslands. Glob. Change Biol. 14, 1592–1599. doi:10.1111/j.1365-2486.2008.01591.x
Yang, Y. H., Fang, J. Y., Pan, Y. D., and Ji, C. J. (2009). Aboveground Biomass in Tibetan Grasslands. J. Arid Environments 73, 91–95. doi:10.1016/j.jaridenv.2008.09.027
Yang, Y., Li, P., He, H., Zhao, X., Datta, A., Ma, W., et al. (2015). Long-term Changes in Soil pH across Major forest Ecosystems in China. Geophys. Res. Lett. 42, 933–940. doi:10.1002/2014gl062575
Yang, Y., Ma, W., and Fang, J. (2007). Storage, Patterns and Controls of Soil Nitrogen in China. Pedosphere 17, 776–785. doi:10.1016/s1002-0160(07)60093-9
Zhang, C., Tian, H., Liu, J., Wang, S., Liu, M., Pan, S., et al. (2005). Pools and Distributions of Soil Phosphorus in China. Glob. Biogeochem. Cycles 19, 1–8. doi:10.1029/2004gb002296
Zhao, L., Wu, X., Wang, Z., Sheng, Y., Fang, H., Zhao, Y., et al. (2018). Soil Organic Carbon and Total Nitrogen Pools in Permafrost Zones of the Qinghai-Tibetan Plateau. Scientific Rep. 8, 1–9. doi:10.1038/s41598-018-22024-2
Keywords: alpine wetlands, Tibetan plateau, soil total nitrogen, soil total phosphorus, controlling factors
Citation: Nie X, Wang D, Zhou G, Xiong F, Ren L, Chen Y, Ma K, Zhong Z and Du Y (2022) Drivers of Soil Total Nitrogen and Phosphorus Storage in Alpine Wetland Across the Three Rivers Source Region on the Qinghai-Tibetan Plateau. Front. Environ. Sci. 10:806771. doi: 10.3389/fenvs.2022.806771
Received: 01 November 2021; Accepted: 24 January 2022;
Published: 04 March 2022.
Edited by:
Jianjun Cao, Northwest Normal University, ChinaReviewed by:
Gang Fu, Institute of Geographic Sciences and Natural Resources Research (CAS), ChinaZhenchao Zhang, Northwest A&F University, China
Yanfu Bai, Lanzhou University, China
Yingwen Yu, Lanzhou University, China
Copyright © 2022 Nie, Wang, Zhou, Xiong, Ren, Chen, Ma, Zhong and Du. This is an open-access article distributed under the terms of the Creative Commons Attribution License (CC BY). The use, distribution or reproduction in other forums is permitted, provided the original author(s) and the copyright owner(s) are credited and that the original publication in this journal is cited, in accordance with accepted academic practice. No use, distribution or reproduction is permitted which does not comply with these terms.
*Correspondence: Dong Wang, ZHdhbmdAY2FmLmFjLmNu; Guoying Zhou, emhvdWd5QG53aXBiLmNhcy5jbg==