- 1State Key Laboratory of Biobased Material and Green Papermaking, Shandong, China
- 2Key Laboratory of Pulp and Paper Science & Technology of Ministry of Education/Shandong Province, Shandong, China
- 3School of Bioengineering, Qilu University of Technology, Shandong Academy of Sciences, Shandong, China
Heavy metal immobilization using poly-γ-glutamic acid-producing bacteria is a novel and environmentally friendly technique for the remediation of heavy metal-contaminated soil. However, only a few studies have investigated the effects of γ-PGA-producing bacteria on the Cd uptake of wheat plants and the Cd distribution in soil aggregates in Cd-polluted soils. In this study, solution culture and pot experiments were used to investigate the Cd immobilization effect and mechanism of the γ-PGA-producing bacteria Bacillus subtilis W7 and Bacillus amyloliquefaciens W25. In the two bacteria-inoculated culture media, the concentration of Cd decreased, whereas the pH, cell growth, γ-PGA production and cell-immobilized Cd significantly increased over time. Strain W25 exhibited a higher ability to produce γ-PGA and immobilize Cd than strain W7. In the pot experiments, the grain Cd content of wheat was reduced by 24–35% and the DTPA-Cd content was decreased by 22–37% in the rhizosphere soils inoculated with both strains compared to the control. Furthermore, strain W25 had a greater ability to decrease the grain Cd uptake than strain W7. Inoculation with the two strains significantly increased the pH, organic matter content, and urease activity and promoted the migration of Cd from large fractions (>0.25 mm) to small fractions (<0.048 mm) and the transformation of available Cd to unavailable Cd in wheat rhizosphere soil. Our results highlight the potential of γ-PGA-producing bacteria in remediating Cd-polluted soils for safe wheat producing.
1 Introduction
Human activities such as mining and the use of fertilizers and pesticides can result in soil Cd contamination (Ma et al., 2020a). Excessive Cd threatens the ecosystem and human health (Pi et al., 2019; Ma et al., 2022). Wheat (Triticum aestivum L.) accounts for 21% of the global food crop (FAO, 2020) and is one of the most important and widely distributed crops (Klymiuk et al., 2018). In wheat production, Cd is readily taken up via the root system and translocated to the grain (Jafarnejadi et al., 2011). Therefore, dietary uptake of Cd through wheat consumption is one of the major sources of Cd in the human body (Rizwan et al., 2016). Thus, an efficient approach to reduce Cd uptake by wheat in Cd-contaminated soils is critically needed.
In-situ stabilization has received considerable attention among the techniques for the remediation of heavy metal-contaminated soils because it is environmentally friendly, cost-effective, and dose not land use management (Liu et al., 2018a; Xia et al., 2019). Recently, the application of microbes to immobilize heavy metals in soils has been extensively researched (Etesami, 2018; Cheng et al., 2020b). Metal-resistant bacteria can decrease the availability of metals in soils and metal uptake by wheat (Hassan et al., 2016; Han et al., 2020). For example, Pseudomonas aeruginosa CPSB1, isolated from metal-contaminated chili rhizosphere soils, decreased the Cd content in shoots and grains of wheat (Rizvi and Khan, 2017). In another study, Ralstonia eutropha Q2-8 and Exiguobacterium aurantiacum Q3-11 increased the abundance of Fe- and Mn-oxidizing Leptothrix species (which may involve the development of Fe and/or Mn oxides and the adsorption of Cd in the soil) and reduced the grain Cd content in wheat to meet the Cd threshold (Wang et al., 2018). Also, Serratia liquefaciens CL-1 increased the pH of wheat rhizosphere soil resulting in decreased available Cd in rhizosphere soil and, consequently, a lower Cd content of the wheat (Cheng et al., 2020a).
Poly-γ-glutamic acid (γ-PGA), produced by Bacillus species, is a biopolymer made up of D/L-glutamic acid units. Because of its many carboxy groups, it is used to adsorb heavy metals (Inbaraj et al., 2009; Luo et al., 2016). Studies have shown that γ-PGA can improve salt tolerance of wheat and significantly increase the plant yield (Xu et al., 2013; Guo et al., 2017). In a previous study, γ-PGA decreased Cd and Pb uptake by cucumber seedlings (Pang et al., 2018). Also, the γ-PGA-producing bacteria Bacillus subtilis W7 and Bacillus amyloliquefaciens W25 (strains W7 and W25) decreased Cd availability and accumulation in lettuce (Wang et al., 2020b). However, the effects of these two strains on wheat Cd uptake and Cd distribution in soil aggregates and the underlying mechanisms are still largely unclear. To develop effective and environmentally friendly bioremediation technologies, we need an in-depth understanding of the mechanisms of γ-PGA-producing bacteria involved in reducing Cd uptake by wheat.
Soil aggregates, the basic units of soil structure, are formed by organic matter, metals, and primarily minerals (Sithole et al., 2019). Heavy metal immobilization and mobility in soils are strongly associated with soil particle size and controlled by their interactions with the components of soil aggregates (Zhang and Zhang, 2020; Shentu et al., 2022). Studies have shown that γ-PGA has a great potential in promoting the formation and stability of soil aggregates, thus improving the soil structure (Chen et al., 2018; Liang and Shi, 2018). Fine soil particles have a higher ability to keep heavy metals because of their larger surface area and higher amounts of clay and organic matter (Li et al., 2020). Inoculation of plant growth-promoting bacteria can affect the structure of soil aggregates. For example, Neorhizobium huautlense T1-17 and Serratia liquefaciens CL-1 increased the ratio of small soil aggregates, reducing heavy metal availability in soil (Wang et al., 2016; Han et al., 2018). However, there is no report on the effects of γ-PGA-producing bacteria on the accumulation and distribution of Cd in soil aggregates.
The objectives of this study were: 1) to explore the impacts of the γ-PGA-producing bacteria Bacillus subtilis W7 and Bacillus amyloliquefaciens W25 on Cd immobilization in solution and Cd uptake by wheat in Cd-contaminated soil. 2) to investigate the effects of these two strains on soil available Cd, pH, organic matter content, and soil enzyme activities, as well as the Cd distribution in wheat rhizosphere soil aggregates. This study improves our understanding of the remediation mechanisms in Cd-polluted soil and the environmental impact of γ-PGA-producing bacteria.
2 Materials and methods
2.1 Bacteria and wheat
The isolates of Bacillus subtilis W7 and Bacillus amyloliquefaciens W25 (accession numbers MN894000 and MN894001, respectively) were obtained from the rhizosphere soil of Lactuca sativa L. grown in a Cd-contaminated environment. Strains W7 and W25 exhibited several traits, including the ability to tolerate high concentrations of Cd (1.5 and 2.5 mm, respectively) and produce IAA (31.7 and 50.4 mg L−1, respectively), siderophore (60.1% and 30.6%, respectively) and γ-PGA (6.4 and 8.5 g L−1, respectively). The strains also decreased lettuce Cd uptake (Wang et al., 2020b). Jimai 22 is a high-yielding winter wheat cultivar cultivated over large areas in China (Xia et al., 2018).
2.2 Determination of Cd immobilization by strains W7 and W25
The effect of strains W7 and W25 on Cd immobilization was analyzed as described previously (Han et al., 2021) with some modifications described in the Supporting Information (method 1). Both strains were cultured in sterile LB medium, harvested, washed, and resuspended in sterile deionized water to a final concentration of 108 cells mL−1. The bacterial suspensions of the two strains were inoculated in culture flasks (in triplicate) containing 150 ml sterile LB medium supplemented with 0 and 20 mg L−1 Cd (Cd cannot precipitate under these conditions). The cultures were then shaken at 150 rpm under 37°C. The culture solution was taken for the following measurements on days 0, 1, 3, 5, and 7. Bacterial growth was analyzed by measuring the optical density (OD600), and the pH of the culture medium was determined with a pH meter. The NH4+ concentration in the solution was analyzed using the salicylatehypochlorous acid method (Hu and Wu, 2005). The Cd concentration of the culture medium was determined by an inductively coupled-plasma optical emission spectrometer (ICP-OES) (Optima 2100DV, Perkin Elmer). Another 10 ml of supernatant was used to analyze the strain’s γ-PGA production according to previously described methods (Zeng et al., 2013).
To determine Cd immobilization on the cell walls of the two strains, 2.5 ml of bacterial suspension (108 CFU ml−1) was added into a conical flask containing 250 ml of LB medium (0 and 20 mg L−1 Cd) and incubated the cultures at 150 rpm under 37°C for 3 days. Subsequently, 5 g samples of wet bacterial cells of strains W7 and W25 were collected, and Cd immobilization on the cell walls of the two strains was observed using a scanning electron microscope coupled with an energy dispersive spectrometer (SEM-EDS) analysis. To understand the contributions of strains W7 and W25 to the immobilization of Cd, we evaluated the Cd contents in the extracellular adsorption, intracellular accumulation, bioprecipitation, and supernatant fractions using the methods described previously (Wang et al., 2022), with some modifications as elaborated in the Supporting Information (method 2).
2.3 Pot experiment
Non-metal-contaminated yellow brown soil (Alfisols) in Jinan (China) was sampled at a depth of 0–15 cm and had the following properties: pH 7.14; organic matter (OM), 20.4 g kg−1; available P, 91.7 mg kg−1; available K, 236 mg kg−1, cation exchange capacity, 14.7 cmol kg−1. The pot experiment was performed based on the method described previously (Wang et al., 2018), with some modifications described in the Supporting Information (method 3). Each pot was 32 cm in diameter × 35 cm in height and contained 10.0 kg of soil supplemented with 0, 1.5, and 3 mg kg−1 Cd. Fifteen surface-sterilized wheat seeds (Shandong Academy of Agricultural Sciences) were sown in each pot in October 2019. Bacterial inoculation was performed as previously described (Wang et al., 2022) with some modifications. Briefly, bacterial suspensions (100 ml per pot) were poured into the ditches (1–2 cm deep) around the roots 2 weeks post seedling emergence; non-bacterial inoculation was considered the control. Each treatment consisted of three pots, which were placed under open-air conditions in a completely randomized design at the experimental station of Qilu University of Technology (China). The plants were harvested in June 2020.
2.4 Plant and soil sample analyses
Wheat plant roots, straws, and grains were separated, washed, dried at 80°C, ground, and digested to determine the Cd content by ICP-OES. The rhizosphere soils that firmly adhered to the roots were collected, and soil pH, organic matter content and available Cd (DTPA-Cd) were determined using previously described methods (Chen et al., 2016). Urease and invertase activities of rhizosphere soils were determined colorimetrically using sodium phenol sodium hypochlorite and 3, 5-dinitrosalicylic acid, respectively (Chen et al., 2022).
2.4.1 Cd distribution in wheat rhizosphere soils
The impacts of strains W7 and W25 on the Cd distribution in the soil were analyzed according to the sequential extraction procedures (He et al., 2019), including the exchangeable Cd (EX-Cd), the carbonate-bound (CB-Cd), the Fe-Mn oxides (OX-Cd) and the organic matter (OM-Cd). The Cd fractions in the extracting solutions were measured through ICP-OES.
2.4.2 Aggregate fractionation in wheat rhizosphere soils
Soil aggregates distribution was determined using the dry-sieving method (Blaud et al., 2017), with some modifications described in the Supporting Information (method 4). Briefly, the soil samples were placed in a sieve system containing a 2-mm sieve, a 0.25-mm sieve, a 0.075-mm sieve, and a 0.048-mm sieve, from top to bottom, and vibrated at 1,000 rpm for 10 min. Subsequently, the different aggregate sizes (>2 mm, 2–0.25 mm, 0.25–0.075 mm, 0.075–0.048 mm, and <0.048 mm) were collected and weighed. The >0.25 mm aggregates were defined as macro-aggregates and the 0–0.25 mm aggregates as the micro-aggregates. The available Cd content (DTPA-Cd) in the soil aggregates was measured by ICP-OES. Total Cd content in the different soil aggregate samples was digested (HCl: HNO3: HClO4, 3:1:1, v/v/v) and determined by ICP-OES.
2.4.3 Cd loading and accumulation in soil aggregates
The grain size fraction metals loading (GSF) and the accumulation factor (AFx) of Cd in each aggregate size fraction were calculated using the following equations:
where GSFloading is the grain size fraction metal loading, HMi is the heavy metal content of individual aggregate size class i (mg kg−1), and GSi is the percentage of the weight of the individual aggregate size class i (Sutherland, 2003).
where AFx is the accumulation factor, and Xfraction and Xbulk are the heavy metal contents in a given fraction and bulk sample (mg kg−1) (Acosta et al., 2009).
2.4.4 Soil relative abundance of γ-PGA-producing bacteria and colonization of strains W7 and W25
The screening of γ-PGA-producing bacteria was performed as described previously (Wang et al., 2020b). Briefly, CFUs of the suspensions of the soil samples were analyzed according to the dilution-plate method on LB agar, and colonies were collected. Colonies that formed a specific concentric zone (with a color change from red to yellow) on the isolation medium were considered γ-PGA-producing bacteria and selected for further analysis. The colonization of both strains was analyzed as described previously (He et al., 2009) with some modifications described in the Supporting Information (method 5).
2.5 Quality control/quality assurance
Quality assurance/quality control (QA/QC) was performed to test the accuracy and precision of the results. The Chinese standardized reference materials GBW07401a for soil samples and GBW10011 for wheat samples were used, and the measured values were 2.523 ± 0.254 mg kg−1 (certified value 2.500 ± 0.200 mg kg−1) and 0.049 ± 0.002 mg kg−1 (certified value 0.053 ± 0.007 mg kg−1), respectively, with recoveries of 90.5% and 110.2%, respectively. The detection limit of Cd was 0.025 mg kg−1.
2.6 Statistical analyses
One-way analysis of variance and Tukey’s test (p < 0.05) were used to compare the treatment means. All statistical analyses were performed using the SPSS 20.0 software (SPSS Inc, United States).
3 Results
3.1 Cd immobilization by strains W7 and W25
The γ-PGA production by strains W7 and W25 at different Cd2+ concentrations in LB medium is shown in Figure 1A. The γ-PGA concentration increased over time. Notably, the γ-PGA concentrations of both strains were significantly increased on days 3, 5, and 7 at 20 mg L−1 Cd2+ compared to the treatments without Cd2+. Particularly, the γ-PGA production of strain W25 was higher than that of strain W7, with or without Cd2+. In W7- and W25-inoculated solutions, the Cd concentration significantly decreased over time by 17–36% and 21–45%, respectively (Figure 1B). The water-soluble Cd concentration of strain W25 was significantly lower than that of strain W7 on days 3, 5, and 7. The total amount of Cd in LB solution was 5 mg. After 3 days of culture, inoculation with strains W7 and W25 decreased the Cd concentration in the supernatant by 30 and 42% through extracellular adsorption (11 and 16%), intracellular accumulation (3 and 4%), and bioprecipitation (16 and 23%), respectively (Figure 1C). Based on the SEM, both strains were long rods, and some precipitates which contained Cd, according to the EDS analysis, were found on the surface of the two strains (Figure 2). Besides, more Cd precipitates were found on the W25 cell surfaces than on the W7 cell surfaces. The OD600 values of both strains increased with time, and Cd2+ did not affect bacterial growth (Supplementary Figure S1A). The pH value and the NH4+ concentration of the solutions inoculated with these two strains also increased with time (Supplementary Figures S1B, C). These results suggest that both strains are resistant to Cd and could immobilize Cd in the Cd solution.
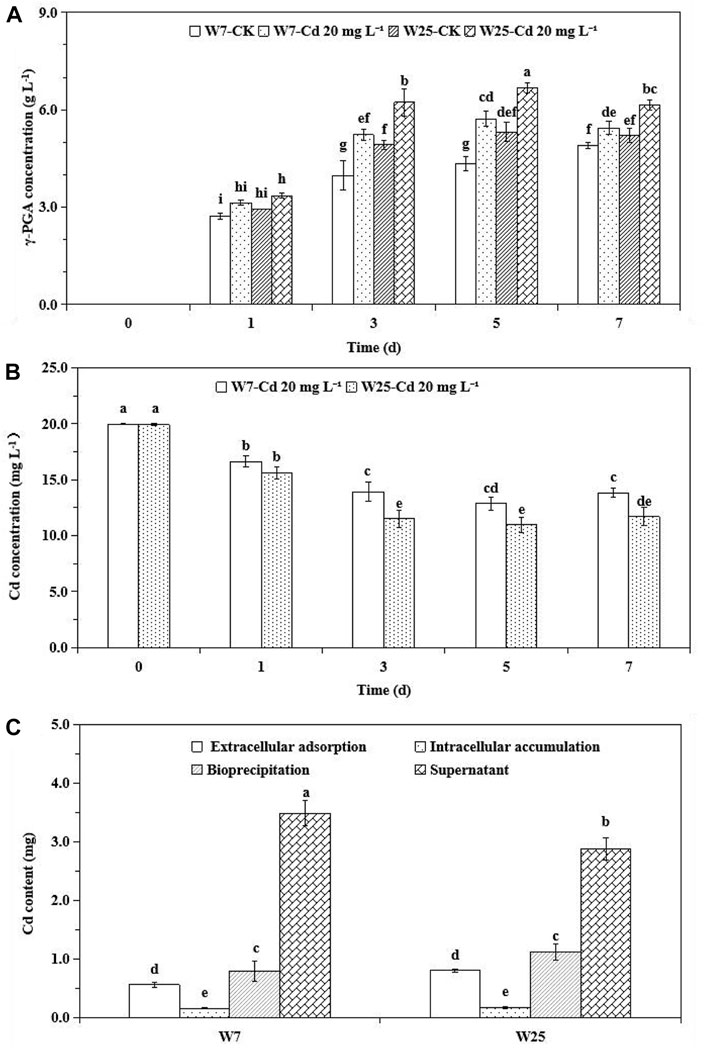
FIGURE 1. γ-PGA (A) and Cd concentration (B) in the culture solution inoculated with strains W7 and W25 and extracellular adsorption, intracellular accumulation, and bioprecipitation of Cd by strains W7 and W25 (C). Error bars are mean ± standard error (n = 3). Bars with the same letter are not significantly different (p > 0.05) according to Tukey’s test.
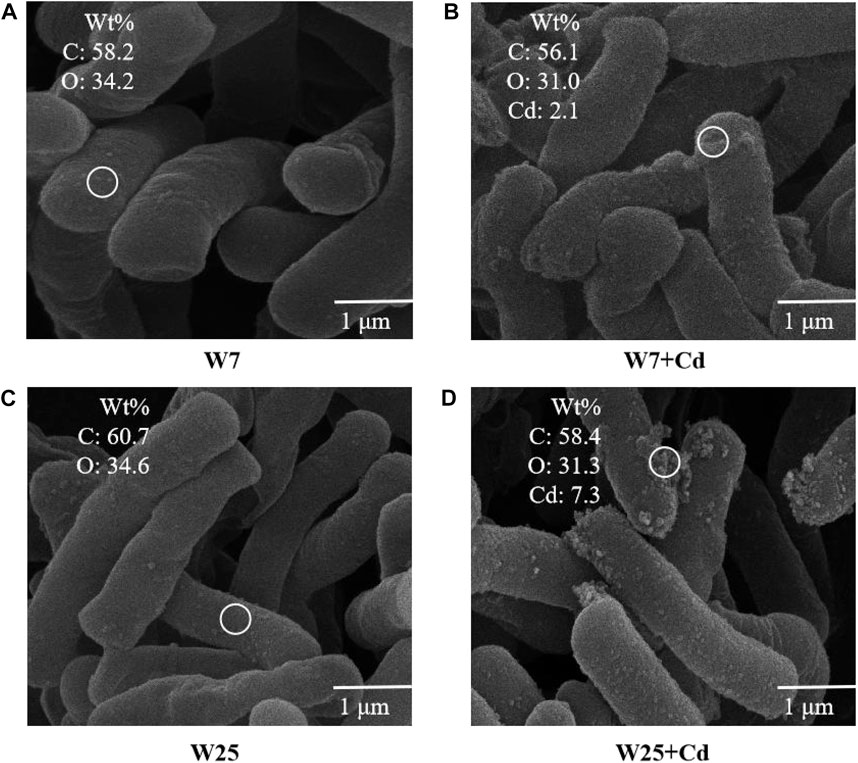
FIGURE 2. SEM-EDS images of strains W7 and W25 with or without Cd. (A) SEM-EDS image of strain W7 in the absence of Cd; (B) SEM-EDS image of strain W7 in the presence of 20 mg L−1 Cd; (C) SEM-EDS image of strain W25 in the absence of Cd; (D) SEM-EDS image of strain W25 in the presence of 20 mg L−1 Cd. The white circles indicate selected spots for EDS.
3.2 Effects of strains W7 and W25 on wheat biomass and Cd content
The application of Cd or bacteria had no significant effect on the biomass of wheat tissues (Supplementary Figure S2). In highly Cd-polluted soil, inoculation with strains W7 and W25 significantly reduced the Cd contents of wheat roots, straw, and grains by 15–34%, 17–33%, and 24–35%, respectively. Strain W25 had a higher ability to decrease wheat tissue Cd content than strain W7 (Figure 3). Furthermore, the Cd uptake by roots (28%), straw (27%), and grains (22%) were significantly decreased when the soil was inoculated with strain W25 at low Cd levels in comparison to the controls (Figure 3). Particularly, the grain Cd content (0.19 mg kg−1) inoculated with strain W25 met the maximum allowable concentration set by the FAO/WHO for Cd (0.2 mg kg−1) in wheat (FAO/WHO, 2011) in low Cd-polluted soil.
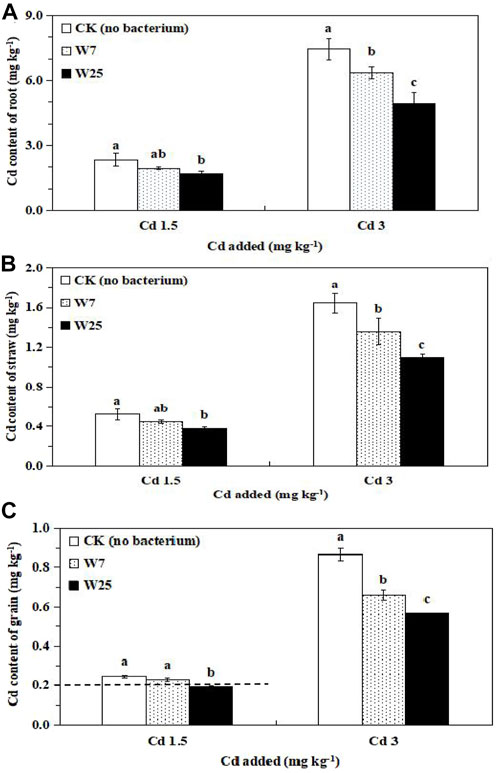
FIGURE 3. Effects of strains W7 and W25 on Cd contents of root (A), straw (B) and grain (C) of wheat plants grown in low and high Cd-contaminated soils. Error bars are ± standard error (n = 3). Bars with the same letter are not significantly different (p > 0.05) according to Tukey’s test. Dotted line corresponds to the maximum allowable concentration of Cd in wheat grain set by FAO/WHO (0.2 mg kg−1).
3.3 Effects of strains W7 and W25 on DTPA-Cd content and relative abundance of γ-PGA-producing bacteria in rhizosphere soils
Both strains significantly reduced the DTPA-Cd contents in high Cd-polluted rhizosphere soils by 22 and 37%, respectively, compared to the control (Table 1). In addition, strain W25 had a significantly higher ability to decrease the DTPA-Cd content than strain W7. Similarly, inoculation with strain W25 significantly decreased the DTPA-Cd content in low Cd-polluted soil (Table 1). Compared with the control, inoculation with both strains significantly increased the relative abundance of γ-PGA-producing bacteria (27–53%) in Cd-polluted rhizosphere soils (Supplementary Figure S3). Notably, strain W25 had a significantly higher ability to increase the relative abundance of γ-PGA-producing bacteria than strain W7 at high Cd levels (Supplementary Figure S3). Regarding the colonization by the two strains of the rhizosphere, the cell numbers for strains W7 and W25 were 3.3–5.7 × 104 and 4.2–6.4 × 104 cfu g−1 of fresh soil, respectively.
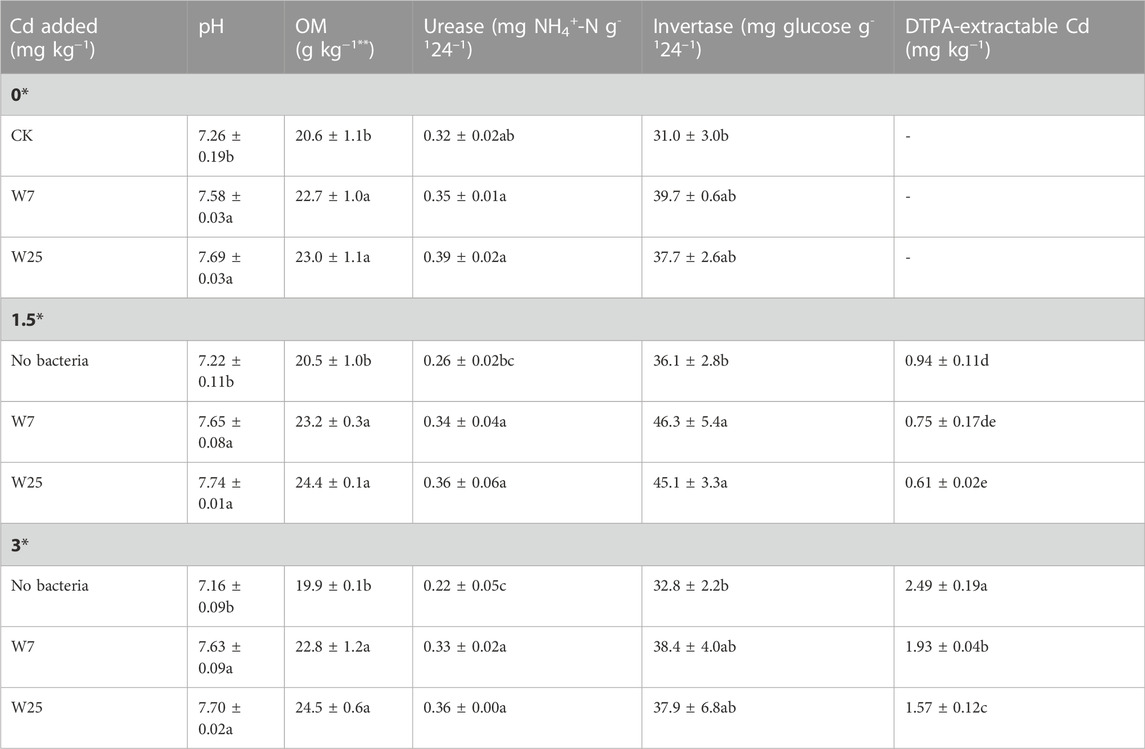
TABLE 1. Effects of strains W7 and W25 on the pH, organic matter content, enzyme activities and DTPA-extractable Cd content of the rhizosphere soils of wheat plants. The values are means ± standard error (n = 3). Mean followed by the same letters within the same column are not significantly different (p > 0.05) according to Tukey’s test. **OM: organic matter.
3.4 Effects of strains W7 and W25 on soil properties and Cd fractions in wheat rhizosphere soils
The impacts of both strains on pH value, organic matter content and enzyme activities of rhizosphere soils are shown in Table 1. Compared with the control, the two strains significantly increased the pH and organic matter content (10–23%) of the rhizosphere soils (Table 1). Also, the urease activity was significantly increased by 32–61% after inoculating the two strains into Cd-contaminated soils (Table 1). Moreover, strains W7 and W25 significantly increased the invertase activity of the rhizosphere soils at low Cd levels by 28 and 25%, respectively, compared to the control (Table 1). Table 2 shows the impacts of strains W7 and W25 on the Cd distribution in rhizosphere soils. Inoculation with both strains significantly decreased the available Cd content (EX-Cd) by 18–20% and increased the unavailable Cd content (OX-Cd) by 27–31% in high Cd-polluted rhizosphere soils compared to the control (Table 2). A similar decrease in EX-Cd content and an increase in OX-Cd content were also found in low Cd-polluted rhizosphere soil in the presence of strain W25 (Table 2). Furthermore, the content of Cd, in different chemical forms, was largest in EX-Cd (40–58%), followed by OX-Cd (18–31%).
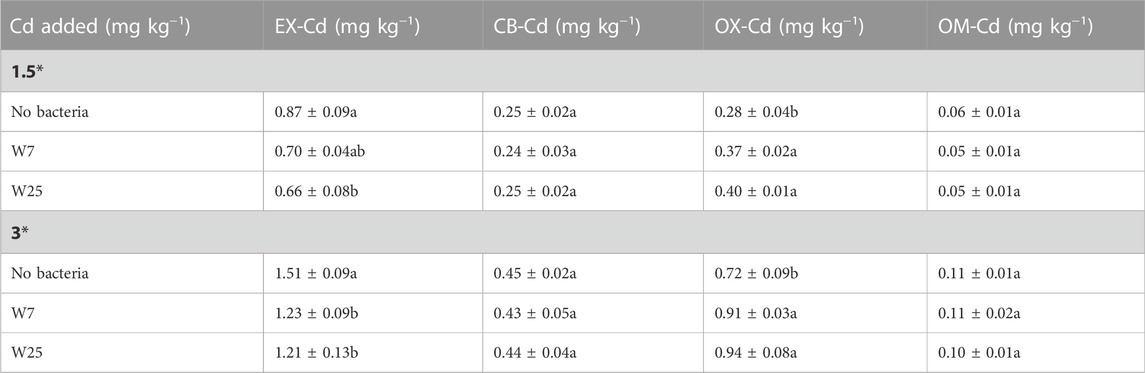
TABLE 2. Effects of strains W7 and W25 on Cd distributions of the rhizosphere soils of wheat plants grown in low and high Cdcontaminated soils. The values are means ± standard error (n = 3). Mean followed by the same letters within the same column are not significantly different (p > 0.05) according to Tukey’s test.
3.5 Effects of strains W7 and W25 on available and total Cd in soil aggregates
Supplementary Table S1 shows the impact of strains W7 and W25 on soil aggregate structure. The main soil particles were >2 mm, 2–0.25 mm, 0.25–0.075 mm, and <0.048 mm. The application of both strains did not affect soil aggregate distribution. In low Cd-polluted rhizosphere soils, the application of strains W7 and W25 significantly reduced the DTPA-Cd content in the 2–0.25 mm soil particles by 16 and 21%, respectively, compared with the controls (Figure 4A). The application of strain W25 significantly reduced the DTPA-Cd content in the >2 mm soil particles by 35%. In high Cd-polluted rhizosphere soils (Figure 4B), inoculation with both strains significantly reduced the DTPA-Cd content in >2 mm soil particles by 22 and 37%, respectively, and strain W25 significantly reduced the DTPA-Cd content in 2–0.25 mm soil particles by 26% compared to controls. In low Cd-contaminated rhizosphere soils, inoculation with both strains significantly increased the total Cd contents in the 0.075–0.048 mm and <0.048 mm soil particles by 29–33% and 30–46%, respectively (Figure 4C). The presence of both strains significantly decreased the total Cd concentrations in the >2 mm (16–18%) and 2–0.25 mm (18–21%) soil particles and increased the total Cd concentrations in the <0.048 mm (35–46%) soil particles in high Cd-polluted rhizosphere soils, compared to the controls (Figure 4D).
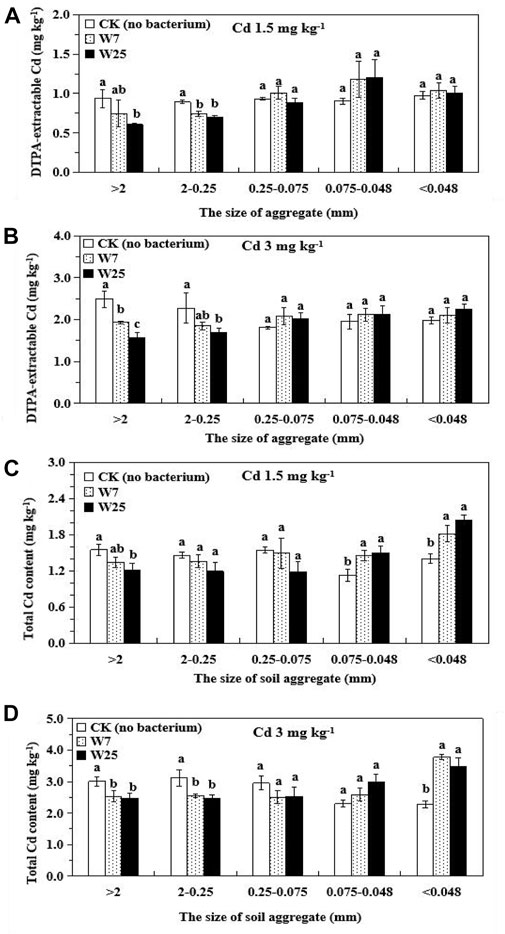
FIGURE 4. Effects of strains W7 and W25 on DTPA-extractable Cd contents (A,B) and total Cd contents (C,D) of soil aggregates in low and high Cd-contaminated rhizosphere soils of wheat. Error bars are ± standard error (n = 3). Bars with the same letter within each treatment are not significantly different (p > 0.05) according to Tukey’s test.
3.6 Effects of strains W7 and W25 on Cd loading and accumulation in soil aggregates
The loading of Cd in different soil particles was studied to evaluate the contribution of aggregate size fractions to total Cd accumulation. About 40% of the total metal loading was retained in soil particles of 2–0.25 mm (Figure 5A), indicating that Cd was preferentially accumulated in soil fractions of this size. Strains W7 and W25 had no significant effect on the GSF values in the first four aggregate fractions, but significantly increased the GSF values of Cd in the <0.048 mm soil particles (Figure 5A). Figure 5B shows the accumulation factors (AFx) of Cd. Inoculation with these two strains significantly decreased the AFx values in the >2 mm soil particles in Cd-contaminated soils, and similar decreases were found in the 2–0.25 mm aggregates in highly contaminated soils. Also, the presence of both strains significantly increased the AFx values in the <0.048 mm and 0.075–0.048 mm soil particles in low Cd-contaminated soils. These results indicate that inoculation with strains W7 and W25 reduced the AFx values of Cd in the macro-aggregates and increased those in the micro-aggregates.
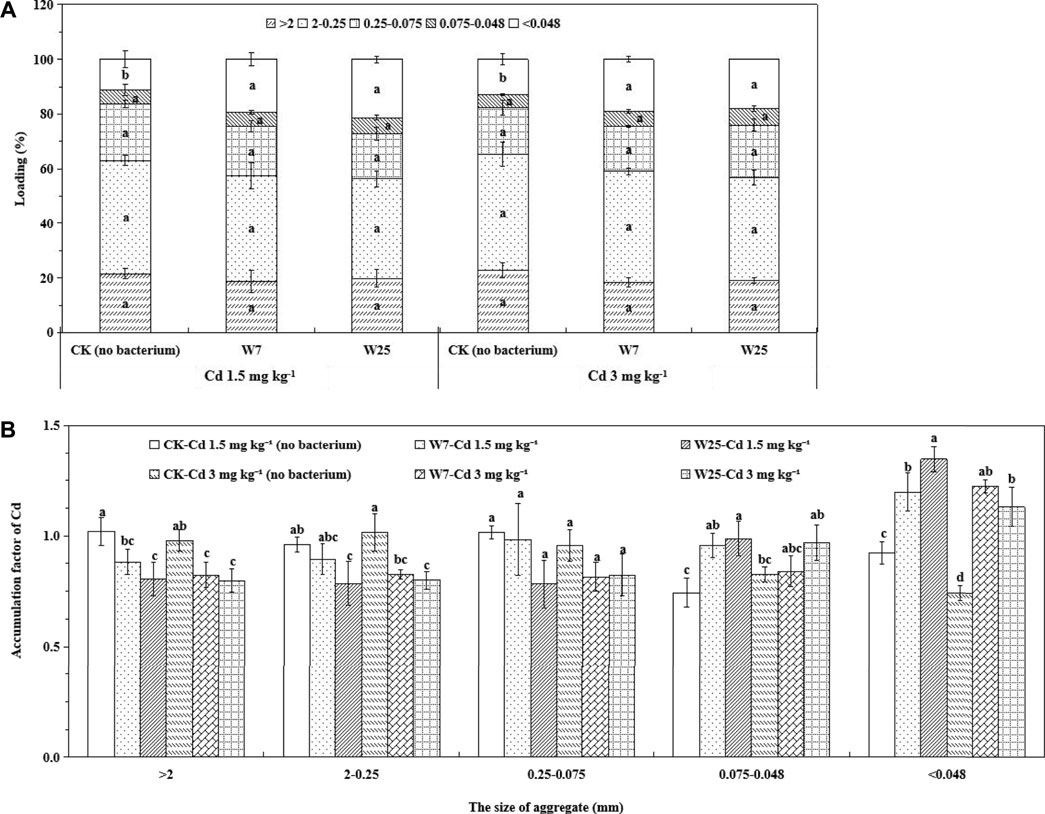
FIGURE 5. Effects of strains W7 and W25 on the GSFloading (A) and AFx (B) values of soil aggregates in low and high Cd-contaminated rhizosphere soils of wheat. Error bars are ± standard error (n = 3). Bars with the same letter within each treatment are not significantly different (p > 0.05) according to Tukey’s test.
4 Discussion
Bacterial immobilization of heavy metal is an effective, economical, and environmentally friendly strategy for remediating heavy metal-contaminated soil (Liu et al., 2018b; Shan et al., 2020). In the present study, the γ-PGA-producing bacteria W7 and W25 could significantly decrease the Cd contents in the wheat root, straw, and grain. In our pot experiments, the Cd content (0.19 mg kg−1 of dry weight) of wheat grains inoculated with strain W25 was lower than the maximum permitted Cd value established by the FAO/WHO (2011). In our previous study, we also found that these bacteria could significantly reduce Cd uptake by lettuce (Wang et al., 2020b). These results suggest that using of γ-PGA-producing bacteria to immobilize heavy metals and inhibit plant Cd uptake is a viable approach for soil remediation and the safe production of crops in Cd-polluted soils.
Resistance to heavy metals is essential for heavy metal-immobilizing bacteria to stabilize heavy metals. Bacteria perform Cd resistance by biosorption, extracellular binding, precipitation, intracellular accumulation and efflux of the metal (Ayangbenro and Babalola, 2017; Shan et al., 2019). In this study, strains W7 and W25 were confirmed to be Cd-resistant and can therefore be used for Cd stabilization in soils. In addition, they can produce IAA and siderophores, which may protect plants from Cd toxicity (El-Meihy et al., 2019), and γ-PGA, which contains numerous anionic functional groups that can bind metal ions (Inbaraj et al., 2009). These strains increased the solution pH value, cell growth and NH4+ production (Supplementary Figures S1B, C), which possibly led to Cd adsorption on the cell surface of the bacterial by competitive adsorption between H+ and Cd2+, thereby reducing the Cd availability in the solution. Similar changes in the cell numbers of both strains were found with and without Cd (Supplementary Figure S1A), suggesting that Cd had no distinct influence on bacterial growth. These findings indicated that the two strains survived and reduced the Cd2+ availability by increasing the solution pH value and producing γ-PGA to adsorb Cd2+. Furthermore, strains W7 and W25 immobilized Cd by precipitation (Figure 2). The cell wall anionic functional groups of both strains may be involved in the binding of Cd in solution (Xu et al., 2019), leading to increased Cd adsorption. In particular, strain W25 produced more γ-PGA and formed more Cd precipitate on its cell surface than strain W7, resulting in W25 having higher abilities to resist Cd and reduce the availability of Cd.
Soil pH plays an important role in the distribution, transformation, and bioavailability of heavy metals (Lin et al., 2019). Heavy metal availability is influenced by soil pH; a higher pH increases the precipitation of insoluble complexes and lowers metal availability because of competition between H+ and metal ions (Li et al., 2017; Ma et al., 2020b). Previous studies have shown that inoculation with bacteria can increase the soil pH, thus decreasing the metal availability in the soil (Li et al., 2017; Wang et al., 2020a). Organic matter improves soil quality and function. It also significantly impacts the bioavailability of heavy metals in soil (Kwiatkowska-Malina, 2018). Organic matter can form insoluble complexes with metal ions and effectively affect the transport and transformation of metal speciation in soil becuase of its multitudinous composition and abundant functional groups, such as hydroxylic (-OH) (Li et al., 2019). Soil urease plays an important role in soil nitrogen transformation because it can hydrolyze urea into NH4+, NH3, and CO32− which increases soil pH and form carbonate precipitation of cations, thus reducing heavy metal bioavailability (Achal and Pan, 2011). In the present study, inoculation with the γ-PGA-producing strains W7 and W25 significantly increased the pH value, organic matter content, and urease activity of wheat rhizosphere soil (Table 1), leading to the decrease in DTPA-extractable Cd in wheat rhizosphere soils (Table 1), and consequently a decreased Cd uptake by wheat (Figure 3). Moreover, the presence of these two strains significantly decreased the content of EX-Cd and increased that of OX-Cd in wheat rhizosphere soils (Table 2), suggesting that these γ-PGA-producing bacteria could promote the transformation of Cd chemical forms from phytoavailable to invalid in wheat rhizosphere soil. This is consistent with the results observed previously (Wang et al., 2018).
Soil aggregates are the basic structural factors of soil and can affect the migration and accumulation of heavy metals in soil (Xiao et al., 2016). In this study, strains W7 and W25 did not influence the structure and composition of soil particles (Supplementary Table S1), most likely because the interaction time between these bacteria and soil was too short. The total content of Cd in soil aggregates was analyzed to study the impacts of γ-PGA-producing bacteria on Cd migration in soil aggregates. After inoculation with strains W7 and W25, the total Cd content in the macro-aggregates of wheat rhizosphere soil decreased, whereas that of micro-aggregates increased (Figure 4). Loading is strongly linked to the mass percentage and Cd content of each aggregate size fraction and is an important index for assessing the Cd distribution in soil aggregates (Sutherland, 2003). In this study, strains W7 and W25 increased the GSF values in micro-aggregates (Figure 5A). The accumulation factor (AFx) was used to estimate the enrichment of Cd in each particle size (Acosta et al., 2009). Both strains decreased the AFx values in macro-aggregates and increased those in micro-aggregates (Figure 5B). Currently, it is generally believed that micro-aggregates have a higher ability to retain Cd because of their large surface areas and numerous adsorption sites (Huang et al., 2020). The above results indicate that inoculation with the γ-PGA-producing bacteria W7 and W25 decreased the available Cd in macro-aggregates and promoted the migration of Cd from macro-to micro-aggregates, which may contribute to a decreased Cd availability in rhizosphere soils and, consequently, a reduced Cd content in wheat tissues.
5 Conclusion
Our results demonstrated that the γ-PGA-producing bacteria Bacillus subtilis W7 and Bacillus amyloliquefaciens W25 could immobilize Cd by increasing the pH value as well as extracellular adsorption, intracellular accumulation, and bioprecipitation in solution. Both strains reduced the Cd uptake of wheat tissues (grain, straw, and root) by decreasing soil Cd availability through increasing the pH value, OM content, and urease activity and by promoting the migration of total Cd from macro-to micro-aggregates, and transforming Cd from available into unavailable forms. The grain Cd content of W25 strain-inoculated wheat plants in Cd-polluted soil was below the threshold established by the FAO/WHO. Overall, these findings provide a new idea and basis for exploring the uptake of Cd by wheat plants inoculated with γ-PGA-producing bacteria. They also suggest an efficient, cost-effective, and environmentally friendly remediation technique for the safe production of wheat in Cd-polluted soils. However, further studies should be conducted to elucidate the mechanisms involved in Cd uptake of wheat inoculated with γ-PGA-producing bacteria and the possibility of using these bacteria for in situ remediations in metal-contaminated soils under field conditions.
Data availability statement
The original contributions presented in the study are included in the article/Supplementary Material, further inquiries can be directed to the corresponding authors.
Author contributions
XW and TX contributed to conception and design of the study. XL and HJ performed the statistical analysis. XW organized the database and wrote the first draft of the manuscript. All authors contributed to manuscript revision, read, and approved the submitted version.
Funding
The National Natural Science Foundation of China (Grant No. 42007140), the Natural Science Foundation of Shandong Province (ZR2020QD121), the Integration of Science and Education Program Foundation for the Talents by the Qilu University of Technology (No. 2018-81110268), the Foundation of Key Laboratory of Pulp and Paper Science and Technology of Ministry of Education (No. KF201817), the Foundation of State Key Laboratory of Biobased Material and Green Papermaking (No. ZZ20190309), and the Key Innovation Project of Qilu University of Technology (Shandong Academy of Sciences) (2022JBZ01-06).
Conflict of interest
The authors declare that the research was conducted in the absence of any commercial or financial relationships that could be construed as a potential conflict of interest.
Publisher’s note
All claims expressed in this article are solely those of the authors and do not necessarily represent those of their affiliated organizations, or those of the publisher, the editors and the reviewers. Any product that may be evaluated in this article, or claim that may be made by its manufacturer, is not guaranteed or endorsed by the publisher.
Supplementary material
The Supplementary Material for this article can be found online at: https://www.frontiersin.org/articles/10.3389/fenvs.2022.1097865/full#supplementary-material
References
Achal, V., and Pan, X. (2011). Characterization of urease and carbonic anhydrase producing bacteria and their role in calcite precipitation. Curr. Microbiol. 62, 894–902. doi:10.1007/s00284-010-9801-4
Acosta, J. A., Cano, A. F., Arocena, J. M., Debela, F., and Martínez-Martínez, S. (2009). Distribution of metals in soil particle size fractions and its implication to risk assessment of playgrounds in Murcia City (Spain). Geoderma 149, 101–109. doi:10.1016/j.geoderma.2008.11.034
Ayangbenro, A. S., and Babalola, O. O. (2017). A new strategy for heavy metal polluted environments: A review of microbial biosorbents. Int. J. Environ. Res. Public Health 14, 94–110. doi:10.3390/ijerph14010094
Blaud, A., Menon, M., Van Der Zaan, B., Lair, G. J., and Banwart, S. A. (2017). Effects of dry and wet sieving of soil on identification and interpretation of microbial community composition. Adv. Agron. 142, 119–142. doi:10.1016/bs.agron.2016.10.006
Chen, L., Fei, L., Mohamed, K., Liu, L., Wang, Z., Zhong, Y., et al. (2018). The effects of ploy (γ-glutamic acid) on spinach productivity and nitrogen use efficiency in North-West China. Plant, Soil Environ. 64, 517–522. doi:10.17221/371/2018-PSE
Chen, L., Han, L., Sun, K., Chen, G., Ma, C., Zhang, B., et al. (2022). Molecular transformation of dissolved organic carbon of rhizosphere soil induced by flooding and copper pollution. Geoderma 407, 115563. doi:10.1016/j.geoderma.2021.115563
Chen, L., He, L. Y., Wang, Q., and Sheng, X. F. (2016). Synergistic effects of plant growth-promoting Neorhizobium huautlense T1-17 and immobilizers on the growth and heavy metal accumulation of edible tissues of hot pepper. J. Hazard. Mater. 312, 123–131. doi:10.1016/j.jhazmat.2016.03.042
Cheng, C., Han, H., Wang, Y., Wang, R., He, L., and Sheng, X. (2020a). Biochar and metal-immobilizing Serratia liquefaciens CL-1 synergistically reduced metal accumulation in wheat grains in a metal-contaminated soil. Sci. Total Environ. 740, 139972. doi:10.1016/j.scitotenv.2020.139972
Cheng, C., Nie, Z. W., Wang, R., He, L. Y., and Sheng, X. F. (2020b). Metal(loid)-resistant Bacillus megaterium H3 reduces arsenic uptake in rice (Oryza sativa Nanjing 5055) at different growth stages in arsenic-contaminated soil. Geoderma 375, 114510. doi:10.1016/j.geoderma.2020.114510
Etesami, H. (2018). Bacterial mediated alleviation of heavy metal stress and decreased accumulation of metals in plant tissues: Mechanisms and future prospects. Ecotoxicol. Environ. Saf. 147, 175–191. doi:10.1016/j.ecoenv.2017.08.032
Guo, Z., Yang, N., Zhu, C., and Gan, L. (2017). Exogenously applied poly-γ-glutamic acid alleviates salt stress in wheat seedlings by modulating ion balance and the antioxidant system. Environ. Sci. Pollut. Res. 24, 6592–6598. doi:10.1007/s11356-016-8295-4
Han, H., Sheng, X., Hu, J., He, L., and Wang, Q. (2018). Metal-immobilizing Serratia liquefaciens CL-1 and Bacillus thuringiensis X30 increase biomass and reduce heavy metal accumulation of radish under field conditions. Ecotoxicol. Environ. Saf. 161, 526–533. doi:10.1016/j.ecoenv.2018.06.033
Han, H., Wu, X., Yao, L., and Chen, Z. (2020). Heavy metal-immobilizing bacteria combined with calcium polypeptides reduced the uptake of Cd in wheat and shifted the rhizosphere bacterial communities. Environ. Pollut. 267, 115432. doi:10.1016/j.envpol.2020.115432
Han, H., Zhang, H., Qin, S., Zhang, J., Yao, L., Chen, Z., et al. (2021). Mechanisms of Enterobacter bugandensis TJ6 immobilization of heavy metals and inhibition of Cd and Pb uptake by wheat based on metabolomics and proteomics. Chemosphere 276, 130157. doi:10.1016/j.chemosphere.2021.130157
Hassan, W., Bashir, S., Ali, F., Ijaz, M., Hussain, M., and David, J. (2016). Role of ACC-deaminase and/or nitrogen fixing rhizobacteria in growth promotion of wheat (Triticum aestivum L.) under cadmium pollution. Environ. Earth Sci. 75, 267. doi:10.1007/s12665-015-4902-9
He, D., Cui, J., Gao, M., Wang, W., Zhou, J., Yang, J., et al. (2019). Effects of soil amendments applied on cadmium availability, soil enzyme activity, and plant uptake in contaminated purple soil. Sci. Total Environ. 654, 1364–1371. doi:10.1016/j.scitotenv.2018.11.059
He, L. Y., Chen, Z. J., Ren, G. D., Zhang, Y. F., Qian, M., and Sheng, X. F. (2009). Increased cadmium and lead uptake of a cadmium hyperaccumulator tomato by cadmium-resistant bacteria. Ecotoxicol. Environ. Saf. 72, 1343–1348. doi:10.1016/j.ecoenv.2009.03.006
Hu, X., and Wu, P. (2005). Method of salicylate-hypochlorous acid ameliorated for determining ammonia nitrogen. Arid. Environ. Monit. 3, 184–185.
Huang, B., Yuan, Z., Li, D., Zheng, M., Nie, X., and Liao, Y. (2020). Effects of soil particle size on the adsorption, distribution, and migration behaviors of heavy metal(loid)s in soil: A review. Environ. Sci. Process. Impacts 22, 1596–1615. doi:10.1039/D0EM00189A
Inbaraj, B. S., Wang, J. S., Lu, J. F., Siao, F. Y., and Chen, B. H. (2009). Adsorption of toxic mercury(II) by an extracellular biopolymer poly(γ-glutamic acid). Bioresour. Technol. 100, 200–207. doi:10.1016/j.biortech.2008.05.014
Jafarnejadi, A. R., Homaee, M., Sayyad, G., and Bybordi, M. (2011). Large scale spatial variability of accumulated cadmium in the wheat farm grains. Soil Sediment Contam. Int. J. 20, 98–113. doi:10.1080/15320383.2011.528472
Klymiuk, V., Yaniv, E., Huang, L., Raats, D., Fatiukha, A., Chen, S., et al. (2018). Cloning of the wheat Yr15 resistance gene sheds light on the plant tandem kinase-pseudokinase family. Nat. Commun. 9, 3735. doi:10.1038/s41467-018-06138-9
Kwiatkowska-Malina, J. (2018). Functions of organic matter in polluted soils: The effect of organic amendments on phytoavailability of heavy metals. Appl. Soil Ecol. 123, 542–545. doi:10.1016/j.apsoil.2017.06.021
Li, R., Tan, W., Wang, G., Zhao, X., Dang, Q., Yu, H., et al. (2019). Nitrogen addition promotes the transformation of heavy metal speciation from bioavailable to organic bound by increasing the turnover time of organic matter: An analysis on soil aggregate level. Environ. Pollut. 255, 113170. doi:10.1016/j.envpol.2019.113170
Li, Y., Dong, S., Qiao, J., Liang, S., Wu, X., Wang, M., et al. (2020). Impact of nanominerals on the migration and distribution of cadmium on soil aggregates. J. Clean. Prod. 262, 121355. doi:10.1016/j.jclepro.2020.121355
Li, Y., Pang, H. D., He, L. Y., Wang, Q., and Sheng, X. F. (2017). Cd immobilization and reduced tissue Cd accumulation of rice (Oryza sativa wuyun-23) in the presence of heavy metal-resistant bacteria. Ecotoxicol. Environ. Saf. 138, 56–63. doi:10.1016/j.ecoenv.2016.12.024
Liang, J., and Shi, W. (2018). Poly-γ-glutamic acid improves water-stable aggregates, nitrogen and phosphorus uptake efficiency, water-fertilizer productivity, and economic benefit in barren desertified soils of Northwest China. Agric. Water Manag. 245, 106551. doi:10.1016/j.agwat.2020.106551
Lin, J., Sun, M., Su, B., Owens, G., and Chen, Z. (2019). Immobilization of cadmium in polluted soils by phytogenic iron oxide nanoparticles. Sci. Total Environ. 659, 491–498. doi:10.1016/j.scitotenv.2018.12.391
Liu, L., Li, W., Song, W., and Guo, M. (2018a). Remediation techniques for heavy metal-contaminated soils: Principles and applicability. Sci. Total Environ. 633, 206–219. doi:10.1016/j.scitotenv.2018.03.161
Liu, Y., Tie, B., Li, Y., Lei, M., Wei, X., Liu, X., et al. (2018b). Inoculation of soil with cadmium-resistant bacterium Delftia sp. B9 reduces cadmium accumulation in rice (Oryza sativa L.) grains. Ecotoxicol. Environ. Saf. 163, 223–229. doi:10.1016/j.ecoenv.2018.07.081
Luo, Z., Guo, Y., Liu, J., Qiu, H., Zhao, M., Zou, W., et al. (2016). Microbial synthesis of poly-γ-glutamic acid: Current progress, challenges, and future perspectives. Biotechnol. Biofuels 9, 134. doi:10.1186/s13068-016-0537-7
Ma, H., Wei, M., Wang, Z., Hou, S., Li, X., and Xu, H. (2020a). Bioremediation of cadmium polluted soil using a novel cadmium immobilizing plant growth promotion strain Bacillus sp. TZ5 loaded on biochar. J. Hazard. Mater. 388, 122065. doi:10.1016/j.jhazmat.2020.122065
Ma, Q. J., Sun, M. H., Lu, J., Hu, D. G., Kang, H., You, C. X., et al. (2020b). Phosphorylation of a malate transporter promotes malate excretion and reduces cadmium uptake in apple. J. Exp. Bot. 71, 3437–3449. doi:10.1093/jxb/eraa121
Ma, X., Hou, M., Liu, C., Li, J., Ba, Q., and Wang, H. (2022). Cadmium accelerates bacterial oleic acid production to promote fat accumulation in Caenorhabditis elegans. J. Hazard. Mater. 421, 126723. doi:10.1016/j.jhazmat.2021.126723
Pang, X., Lei, P., Feng, X., Xu, Z., Xu, H., and Liu, K. (2018). Poly-γ-glutamic acid, a bio-chelator, alleviates the toxicity of Cd and Pb in the soil and promotes the establishment of healthy Cucumis sativus L. seedling. Environ. Sci. Pollut. Res. 25, 19975–19988. doi:10.1007/s11356-018-1890-9
Pi, H., Li, M., Zou, L., Yang, M., Deng, P., Fan, T., et al. (2019). AKT inhibition-mediated dephosphorylation of TFE3 promotes overactive autophagy independent of MTORC1 in cadmium-exposed bone mesenchymal stem cells. Autophagy 15, 565–582. doi:10.1080/15548627.2018.1531198
Rizvi, A., and Khan, M. S. (2017). Biotoxic impact of heavy metals on growth, oxidative stress and morphological changes in root structure of wheat (Triticum aestivum L.) and stress alleviation by Pseudomonas aeruginosa strain CPSB1. Chemosphere 185, 942–952. doi:10.1016/j.chemosphere.2017.07.088
Rizwan, M., Ali, S., Abbas, T., Zia-Ur-Rehman, M., Hannan, F., Keller, C., et al. (2016). Cadmium minimization in wheat: A critical review. Ecotoxicol. Environ. Saf. 130, 43–53. doi:10.1016/j.ecoenv.2016.04.001
Shan, S., Guo, Z., Lei, P., Li, Y., Wang, Y., Zhang, M., et al. (2020). Increased biomass and reduced tissue cadmium accumulation in rice via indigenous Citrobacter sp. XT1-2-2 and its mechanisms. Sci. Total Environ. 708, 135224. doi:10.1016/j.scitotenv.2019.135224
Shan, S., Guo, Z., Lei, P., Wang, Y., Li, Y., Cheng, W., et al. (2019). Simultaneous mitigation of tissue cadmium and lead accumulation in rice via sulfate-reducing bacterium. Ecotoxicol. Environ. Saf. 169, 292–300. doi:10.1016/j.ecoenv.2018.11.030
Shentu, J., Li, X., Han, R., Chen, Q., Shen, D., and Qi, S. (2022). Effect of site hydrological conditions and soil aggregate sizes on the stabilization of heavy metals (Cu, Ni, Pb, Zn) by biochar. Sci. Total Environ. 802, 149949. doi:10.1016/j.scitotenv.2021.149949
Sithole, N. J., Magwaza, L. S., and Thibaud, G. R. (2019). Long-term impact of no-till conservation agriculture and N-fertilizer on soil aggregate stability, infiltration and distribution of C in different size fractions. Soil Tillage Res. 190, 147–156. doi:10.1016/j.still.2019.03.004
Sutherland, R. A. (2003). Lead in grain size fractions of road-deposited sediment. Environ. Pollut. 121, 229–237. doi:10.1016/S0269-7491(02)00219-1
Wang, Q., Chen, L., He, L. Y., and Sheng, X. F. (2016). Increased biomass and reduced heavy metal accumulation of edible tissues of vegetable crops in the presence of plant growth-promoting Neorhizobium huautlense T1-17 and biochar. Agric. Ecosyst. Environ. 228, 9–18. doi:10.1016/j.agee.2016.05.006
Wang, T., Wang, S., Tang, X., Fan, X., Yang, S., Yao, L., et al. (2020a). Isolation of urease-producing bacteria and their effects on reducing Cd and Pb accumulation in lettuce (Lactuca sativa L.). Environ. Sci. Pollut. Res. 27, 8707–8718. doi:10.1007/s11356-019-06957-3
Wang, X., Cai, D., Ji, M., Chen, Z., Yao, L., and Han, H. (2022). Isolation of heavy metal-immobilizing and plant growth-promoting bacteria and their potential in reducing Cd and Pb uptake in water spinach. Sci. Total Environ. 819, 153242. doi:10.1016/j.scitotenv.2022.153242
Wang, X., Dong, G., Liu, X., Zhang, S., Li, C., Lu, X., et al. (2020b). Poly-γ-glutamic acid-producing bacteria reduced Cd uptake and effected the rhizosphere microbial communities of lettuce. J. Hazard. Mater. 398, 123146. doi:10.1016/j.jhazmat.2020.123146
Wang, X. H., Luo, W. W., Wang, Q., He, L. Y., and Sheng, X. F. (2018). Metal(loid)-resistant bacteria reduce wheat Cd and as uptake in metal(loid)-contaminated soil. Environ. Pollut. 241, 529–539. doi:10.1016/j.envpol.2018.05.088
Xia, H., Xue, Y., Liu, D., Kong, W., Xue, Y., Tang, Y., et al. (2018). Rational application of fertilizer nitrogen to soil in combination with foliar Zn spraying improved Zn nutritional quality of wheat grains. Front. Plant Sci. 9, 677. doi:10.3389/fpls.2018.00677
Xia, W. Y., Du, Y. J., Li, F. S., Li, C. P., Yan, X. L., Arulrajah, A., et al. (2019). In-situ solidification/stabilization of heavy metals contaminated site soil using a dry jet mixing method and new hydroxyapatite based binder. J. Hazard. Mater. 369, 353–361. doi:10.1016/j.jhazmat.2019.02.031
Xiao, R., Zhang, M., Yao, X., Ma, Z., Yu, F., and Bai, J. (2016). Heavy metal distribution in different soil aggregate size classes from restored brackish marsh, oil exploitation zone, and tidal mud flat of the Yellow River Delta. J. Soils Sediments 16, 821–830. doi:10.1007/s11368-015-1274-4
Xu, S., Xing, Y., Liu, S., Huang, Q., and Chen, W. (2019). Role of novel bacterial Raoultella sp. strain X13 in plant growth promotion and cadmium bioremediation in soil. Appl. Microbiol. Biotechnol. 103, 3887–3897. doi:10.1007/s00253-019-09700-7
Xu, Z., Wan, C., Xu, X., Feng, X., and Xu, H. (2013). Effect of poly (γ-glutamic acid) on wheat productivity, nitrogen use efficiency and soil microbes. J. soil Sci. plant Nutr. 13, 0–755. doi:10.4067/S0718-95162013005000059
Zeng, W., Lin, Y., Qi, Z., He, Y., Wang, D., Chen, G., et al. (2013). An integrated high-throughput strategy for rapid screening of poly(γ-glutamic acid)-producing bacteria. Appl. Microbiol. Biotechnol. 97, 2163–2172. doi:10.1007/s00253-013-4717-0
Keywords: cadmium, Bacillus subtilis W7, Bacillus amyloliquefaciens W25, wheat, soil aggregates
Citation: Wang X, Liu X, Ji H and Xia T (2023) Poly-γ-glutamic acid-producing bacteria reduce wheat Cd uptake by promoting Cd transfer from macro-to micro-aggregates in Cd-contaminated soil. Front. Environ. Sci. 10:1097865. doi: 10.3389/fenvs.2022.1097865
Received: 14 November 2022; Accepted: 14 December 2022;
Published: 10 January 2023.
Edited by:
Junyuan Guo, Chengdu University of Information Technology, ChinaReviewed by:
Shuang Luo, Hunan Agricultural University, ChinaFuyong Wu, Northwest A&F University, China
Copyright © 2023 Wang, Liu, Ji and Xia. This is an open-access article distributed under the terms of the Creative Commons Attribution License (CC BY). The use, distribution or reproduction in other forums is permitted, provided the original author(s) and the copyright owner(s) are credited and that the original publication in this journal is cited, in accordance with accepted academic practice. No use, distribution or reproduction is permitted which does not comply with these terms.
*Correspondence: Xiaohan Wang, NTExODY3QHFsdS5lZHUuY24=; Tao Xia, dHhpYUBxbHUuZWR1LmNu