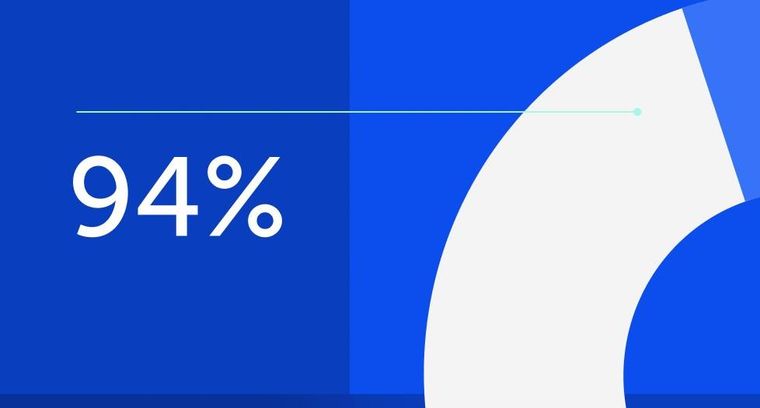
94% of researchers rate our articles as excellent or good
Learn more about the work of our research integrity team to safeguard the quality of each article we publish.
Find out more
REVIEW article
Front. Environ. Sci., 05 January 2023
Sec. Water and Wastewater Management
Volume 10 - 2022 | https://doi.org/10.3389/fenvs.2022.1080536
This article is part of the Research TopicDecentralized Wastewater Treatment TechnologiesView all 8 articles
Nutrient recovery and recycling are of great importance in sustainable development. Blackwater (BW) refers to wastewater from toilets, which contains feces, urine, water, and toilet paper from flush toilets. The highly concentrated nutrients of blackwater could be collected through source separation and treated adequately to recover nutrients efficiently and economically. The review intends to give an overview of the characteristics of BW and different techniques to recover nutrients and other valuable products. A number of these technologies are currently under development or being tested at laboratory or pilot scale. The perspective for blackwater nutrient recovery technologies is very positive due to their great potential. For application of source-oriented sanitation infrastructure and systems, there is still a long way to go for development of commercial technologies and valuable products.
Issues related to the decreasing quality and quantity of freshwater resources, which may be partly due to the currently applied sanitation systems, are becoming increasingly serious. In response to the limits of these sanitation systems, alternative solutions focusing on source separation and resource recovery, besides hygiene and environmental protection, are gradually emerging. Such solutions show great potential for promoting environmental and ecological sustainable development. Generally, wastewater streams (as shown in Figure 1) can be separated into greywater and blackwater (BW). Compared with mixed wastewater treatment, greywater recovery involves smaller hygienic concerns, has a reduced “yuck” factor, and demands less treatment effort. Furthermore, energy and nutrients are more easily recovered from BW (Larsen et al., 2016). Thibodeau et al. (2014) compared a BW source-separation sanitation system and a conventional system using life cycle assessment and environmental life cycle costing to show that the former is significantly superior to the latter in terms of climate change, resources, and human health, although their cost remains comparable.
BW refers to wastewater sourced exclusively from toilets; thus, it consists of urine, feces, toilet paper, and flushing water. It also contains large amounts of nutrients, around half of the domestic COD load, and the major proportion of pathogens excreted from the human body (Otterpohl, 2002; Vinnerås, 2007; Fidjeland et al., 2015). According to the calculated estimation in Table 1, the amounts of nutrients (N, P, and K) are considerable for resource recovery. Besides, according to the analysis of Mihelcic et al. (2011), the K available from urine was approximately 1.68 million metric tons (with a similar mass available in feces). If collected appropriately, the K available from urine and feces could account for 22% of the total global K demand. Researchers estimate that, in 2050, the available K from urine associated with the population increase only will increase to 2.16 million metric tons (with a similar mass available in feces). Because of its specific composition, BW requires separate collection, less dilution, adequate treatment and final recycling of the nutrients.
TABLE 1. Calculated estimation of the yearly nutrients in urine and feces (source: Larsen et al., 2016; Malila et al., 2019; Otterpohl, 2002; Stintzing and Salomon, 2004).
Applying source-separation systems in rural areas may enable the recovery of four times more K and over thirty times more N compared with those obtained in current wastewater treatment systems (Malila et al., 2019). Kjerstadius et al. (2017) used an attributional life cycle assessment to investigate the carbon footprint and potential for nutrient recovery of two sanitation systems for a hypothetical urban area in Southern Sweden. The carbon footprint and nutrient recovery (P and N) results obtained revealed that the source separation system could increase nutrient recovery while decreasing the carbon footprint to a greater extent compared with the conventional system. Therefore, if properly managed, BW may provide not only a source of different valuable resources, such as nutrients, energy, and reclaimed water, but also multiple benefits to society. Nutrient recovery technologies for BW have drawn great concerns but are far from well development.
In this article, a overview of the characteristics of BW and different techniques to recover nutrients and other valuable products are given. This work can also help researchers develop ideas concerning future research areas and make more informed decisions.
BW includes urine, feces, and flushing water. Faecal sludge is raw or partially digested, a slurry or semisolid, and results from the collection, storage or treatment of combinations of excreta and blackwater, with or without greywater. In specific cases, the characteristics of faecal sludge are similar to BW. The characteristics of urine and feces vary widely depending on person’s health and diet, as presented in Table 2. Flushing water is also an important factor which determines the concentrations of nutrients in BW. Generally, three toilet flushing system were commonly mentioned in literatures, i.e., conventional toilet (using 9 L water per flush), dual-flush toilet (using 3/6 L water per flush), and vacuum toilet (using .5/1.2 L water per flush) (de Graaff et al., 2010; Gao et al., 2019b). As shown in Table 3, the characteristics of BW varied widely in different source-separated systems. Compared with conventional toilet and dual-flush toilet, the sewage system employed vacuum toilet obtains more concentrated BW, which generally is beneficial for nutrient recovery, however, Gao et al. (2019a) figured out that BW from vacuum toilet exhibited lower bio-methane potential than that from conventional/dual-flush toilet because of ammonia inhibition. In domestic sewage, BW includes about 97% N, while greywater only includes about 3% N (Otterpohl, 2002). Considering the high concentration of N in BW, ammonia inhibition is one of the main challenges when employing biotechnology for nutrient recovery. However, as real BW has highly variable characteristics and involves pathogenic and individual feces, it is difficult to execute repeatable experiments. In literatures, synthetic feces were commonly used for providing consistently reproducible substrate and alleviating these challenges. Penn et al. (2018) provide a critical literature review of synthetic feces and faecal sludge used for human waste related research.
TABLE 2. Characteristics of feces found in the literature (source: Levitt and Duane, 1972; Otterpohl, 2002; Penn et al., 2018; Rose et al., 2015).
For safe and clean nutrient recovery from BW, multiple-step treatment processes are required. In this part, the individual core processes employed in research or practical applications are presented firstly. As urine and feces are collected separately in some specific systems, the raw materials for nutrient recovery technologies include urine, feces, and BW.
Processes for nutrient recovery by physicochemical means reported in the literature are summarized in Table 4, including evaporation, precipitation, pyrolysis, drying, and adsorption.
Evaporation is a simple technology for removing water from urine to recover water and concentrated hygienized liquid fertilizer or even solid fertilizer. It is often used to recycle water of the best possible quality from urine in space applications. Some pilot projects for nutrient recovery have been tested in Germany, Vietnam, and Canada (Antonini et al., 2012; Pahore et al., 2012; Bethune et al., 2015). The boiling point of the urine is 130°C, which is very high compared to the boiling point of water. This suggests that almost all water can be removed with minimal loss of the nutrient from urine with the loss of ammonia nitrogen (Patel et al., 2020). Urine evaporation is challenged by two major issues: ammonia loss and high energy consumption. Unless conducted in a sealed environment or reclaimed by ammonia stripping, up to 93% of the nitrogen bound in ammonia can be lost due to volatilization during urine evaporation (Chipako and Randall, 2020). Inhibition of urea hydrolysis, acidification, energy recovery, and solar energy utilization have been investigated and tested at the pilot scale to address these problems. Hydrochloric acid, acetic acid, sulfuric acid, and phosphoric acid have been reported succeeded in inhibiting urea hydrolysis by maintaining acidic conditions (Saetta et al., 2020; Moharramzadeh et al., 2022; Zuo et al., 2022). Ren et al. (2021) improved urea stabilization by nitrification at a 100 L pilot-scale aerobic MBR reactor, successfully converted 50% of the ammonia into nitrate and obtained vapour flux at 1.3 kg m−2 h−1 as well as high solar to vapour conversion efficiency of 87% by heat localized solar evaporation. The solar evaporation efficiency could be improved by developing photothermal conversion materials. Zhang L et al. (2023) recover 1.1 kg⋅m−2 of condensed freshwater (meeting WHO standards for drinkable water) from urine at 7.5 h under a low light intensity using cotton cloth photothermal conversion film-monolithic hydrophobic.
Struvite precipitation is a chemical reaction in which ammonia and phosphate are reacted with magnesium salt under alkaline conditions to form struvite [Mg(NH4)(PO4)•6H2O, MAP], which is an easily separable solid substance known to be a slow-release fertilizer. The pH of hydrolyzed urine (approximately 9) is suitable for struvite precipitation. Struvite precipitation generally has high removal efficiency and low initial operation cost (Pathy et al., 2021). Wei et al. (2018) recovered struvite and ammonium sulfate from urine at a pilot scale precipitation facility and achieved 94% struvite precipitation efficiency and 93% removal efficiencies for nitrogen. For forming struvite, human urine contains an excess of ammonium relative to phosphate but is deficient in Mg. Precipitation is triggered by addition of Mg, usually in the form of MgO, Mg(OH)2, MgCl2, or bittern (Maurer et al., 2006). Struvite precipitation has been extensively investigated to remove N and P from different water flows, such as digester supernatants, animal waste slurries, and landfill leachate, among others. Many laboratory studies and pilot scale experiments have also been conducted for N and P recovery from urine and BW (Ishii and Boyer, 2015; Tansel et al., 2018; Wang J et al., 2018; Wei et al., 2018; Aguado et al., 2019; Huang et al., 2019b). Li X et al. (2022) recovered phosphate as struvite from BW and obtained a high recovery rate of phosphorus (>98%) by recoverying product struvite with a median particle size of 32.96 μm, then conducting a pilot-scale test with a rural public toilet in Xiong’an New District, Hebei Province. Struvite precipitation has been used for N and P recovery from hydrolyzed urine in the Netherlands, Sweden, Germany, and Vietnam at the pilot scale (Winker et al., 2009; Wei et al., 2018).
However, the purity of the actual struvite production is generally only 30%–50% due to the simultaneous crystallization of Ca-P and CaCO3 during the recovery process (Zhang et al., 2022). Ongoing efforts are made to create new struvite productions. Recovery of calcium phosphate is attractive as calcium phosphate products (such as Ca(H2PO4)2⋅H2O and CaHPO4⋅2H2O) have comparable fertilization efficiency with struvite, and it has diverse applications in manufacturing (Tervahauta et al., 2014). Zhang B et al. (2023) harvest nutrients from hydrolyzed human urine via precisely regulating Ca/P dosing ratio, resulting in a P recovery efficiency of 100% and a purity for calcium phosphate of above 90%. K-struvite (i.e., magnesium potassium phosphate) can be harvested by potassium struvite precipitation (Wilsenach et al., 2007; Xu et al., 2015). In this case, the maximum P recovery efficiency could reach 100%, while the K recovery efficiency was relatively low. Tervahauta et al. (2014) harvasted calcium phosphate granulation without any addition of chemicals, proving that only 2% of the incoming K in the upflow anaerobic sludge blanket (UASB) reactor can be recovered with the calcium phosphate granules. Huang et al. (2019a) improved the K-struvite crystallization by using different additions, obtaining a K recovery efficiency of 25%–70%. Huang et al. (2019b) further observed the Na-struvite formation in the case that the P recovery efficiency was 96% and the Na content in the precipitates was 67 mg/g.
Pyrolysis (Liu et al., 2014; Yacob et al., 2018; Krueger et al., 2021), hydrothermal carbonization (Danso-Boateng et al., 2013; Danso-Boateng et al., 2015; Fakkaew et al., 2015; Fakkaew et al., 2018), and hydrothermal liquefaction (HTL) (Lu et al., 2017; Watson et al., 2017; Wang W et al., 2018; Hossain et al., 2022) are three thermochemical conversion processes conducted under different temperatures and pressures to hygienize and mineralize human feces from BW or brown water and produce valuable byproducts, such as biochar, hydro-char, and bio-crude oil. Given the high water content in BW, HTL is the most frequently used technology where water is directly used as a liquefacient (Xi et al., 2022). The HTL continuous reactor has been developed to provide efficient energy balance (Elliott et al., 2015). Some assistant methods have been conducted to impove the heating efficiency, such as microwave technology, electricity-driven method (Xi et al., 2022) and catalyst (Wang W et al., 2018). Afolabi and Sohail (2017) give an overview of treat and recover value from BW by microwave heating technology. Although such processes have only been tested at the laboratory scale, they also show great potential for practical applications in the near future.
Drying provokes the removal of moisture through natural drying, mechanical drying, or convective drying with hot air (Ling et al., 2022). It reduces the mass and volume, further destroys the pathogenic organisms due to the combined effect of heat and moisture removal during drying. The convective drying method is already widely studied in the wastewater sludge treatment, focusing on the direction of thin-layer drying, fluidized bed drying, spray drying and pneumatic drying. Solar energy can be used to reduce the cost of drying (Bennamoun, 2012). The thin-layer drying method could be improved by assisting with ultrasonic, microwave, and desiccant. Ling et al. (2022) systematically reviews the convective drying technology of sludge. However, the studies on convective drying of BW are still far from well developed. Feces and faecal sludge originates from onsite sanitation without greywater are suitable for drying. The dried product could be used as an organic fertilizer with a particularly high phosphorous content and a slow release of nitrogen and phosphorous (Septien et al., 2020). Besides, with high heating value, it could also be used as fuel.
Faecal sludge from ventilated improved pit latrines was dried in a convective drying thermobalance by varying the temperature from 40°C to 80°C, resulting in a drying rate ranging between 1 and 40 g/min/m2 and a moisture content of dried products ranging between 2.4 and 3.2 g/g db (Pocock et al., 2022). Seodigeng et al. (2022) dried human faeces using solar and wind energy under ambient conditions and further developed thin-layer drying semiempirical models. Venkata Sai and Reddy (2022) proposed a solar energy drying system of BW treatment with a BW capacity of 2.4 kg/day in which the moisture content of BW was reduced from 70% (w.b.) to 6.82% (w.b.). The drying technology could be combined with non-sewered sanitation system to provide safe local discharge and efficient nutrient/energy recovery.
Adsorbents such as biochar, zeolite, resins, activated carbon, alumina, and silica gel have been extensively studied for the nutrients recovery from urine (Patel et al., 2020). The adsorption efficiency of ammonium and potassium are not concerned with urine dilution, while the adsorption efficiency of orthophosphate is different for different diluted urine solutions. Adsorption of ammonium from urine on to the zeolites can be achieved up to 100% under appropriate conditions. In terms of the adsorption of ammonium, resins gave the highest efficiency for the ammonium compared to the zeolite and biochar (Tarpeh et al., 2017).
Biochar, produced from the wastes through a process in which biomass wastes such as agricultural forest residue, food waste, solid waste, animal waste, and municipal sludge thermally decomposed in oxygen-less environmental conditions, is gaining the researcher’s attention in recent years (Li J et al., 2022). It is comparatively more economical compared with other conventional adsorbents due to adding value to the management of waste products at the same time (Pathy et al., 2021). The nutrient-loaded biochar can be directly applied in the agricultural field to act as slow realizing fertilizer and soil conditioner with no need for any other special treatment. The mechanisms of biochar adsorption include ion exchange; electrostatic interaction; formation of hydrogen bond; exchange of ligand; precipitation on the surface; complex formation between the target molecule and functional group present on the biochar surface and/or either through physical adsorption (Huang et al., 2020). Thus, researchers tried to increase the efficiency of nutrients recovery by improving the adsorbate properties of biochar, including increasing surface area, loading surface functional groups, modification with various metal oxides, adjusting environmental parameters (Pathy et al., 2021). Liu et al. (2021) recovered more than 70% of phosphate in the simulated urine using waste straw biochar modified with the Mg/Fe bimetallic oxide with a maximum adsorption capacity of 206.2 mg/g. Pathy et al. (2021) deliberated possible strategies of preparing engineered biochar aimed for recovering nutrients from urine with the potential challenges and opportunities in a review.
Biochar can also recovery nutrients and remove E. coli and faecal coliform bacteria from BW. Mamera et al. (2022) removed 89%–98% bacteria and recovered 68% N and 98% P from faecal sludge using pinewood biochar through different soil-bed biochar column. Besides the adsorbate properties of biochar, the solid content significantly impacts the efficiency of recovering nutrients from BW by biochar. However, the studies on recovering nutrients from BW by biochar are still insufficient. It generally act as additive for improving anaerobic digestion in terms of applying biochar in BW treatment.
Because of their low cost and easy operation and maintenance, biological treatment processes have been widely applied for BW, faecal sludge, or brown water treatment. Anaerobic digestion, composting, and partial nitrification processes are mainly used for nutrient recovery. Table 5 summerizes biological treatment processes.
Anaerobic digestion plays a key role as a well-known treatment technology for hygienizing concentrated wastewater, such as BW. Low water consumption helps achieve a low dilution of BW, which provides a good basis for efficient nutrient recovery. Thus, low-flush or vacuum toilets are preferred when collecting BW for anaerobic digestion (Mang and Li, 2010). Different types of digesters, such as anaerobic baffled reactors (ABRs), UASBs, continuous stirred tank reactors at the industrial level, as well as fixed domes, water jacked floating drums, and prefabricated plastic and glass fiber digesters at the household level (Luostarinen et al., 2007; Wendland et al., 2007; de Graaff et al., 2010), have been used for anaerobic digestion according to the characteristics of BW and hygienization demands.
Anaerobic digestion does not remove nutrients valuable as fertilizer and, therefore, presents an appropriate BW treatment for the agricultural cycle when combined with safe pasteurization. In practice, several factors drive the anaerobic digestion of BW within the scope of resource management sanitation:
• Safe sanitation: The hazardous compounds in excreta, pathogens, and medical residues, which present serious dangers to public health, are not spread in the water cycle.
• Production of biogas for cooking, lighting and electricity: The produced biogas is a reliable renewable energy source.
• Nutrients recovery for agriculture: The residues of anaerobic digestion are valuable fertilizers.
• Water saving: The application of pour or low-flush technology reduces the consumption of high-quality drinking water.
The efficiency of anaerobic processes depends on many influencing factors, such as temperature, the characteristics and concentration of organic matter, mass transfer, and fluctuations in composition and load, among others. Because of high N in BW, ammonia inhibition is a matter of concern which might inhibit microbial activities and reduce COD removal and methane production efficiencies. Fuchs et al. (2018) reviewed the state of technology as well as recent achievements and perspectives on tackling ammonia inhibition in anaerobic digetion.
BW can also be anaerobically co-digested with kitchen waste or other organic matter to improve the efficiency of biogas production (Gunnarsdóttir et al., 2014; Rajagopal et al., 2014; Hertel et al., 2015; Wang et al., 2020). The microbial community dynamics and inhibition effects of anaerobic digesters on BW have also been investigated (Gao et al., 2019a; Gao et al., 2019b).
Measures to enhance anaerobic digestion, such as granular activated carbon-assisted digestion, biochar addition, and zero-valent Fe addition, have been investigated (Florentino et al., 2019; Pan et al., 2019; Xu et al., 2019). Mamera et al. (2022) figured out that biochar contributed to remove N, P, E. coli and faecal coliform bacteria in BW.
Anaerobic digestion of BW or faecal sludge has been widely used at the household and residential levels and even in large-scale plants (Mang and Li, 2010). Post-treatment processes, such as solid/liquid separation, composting, and disinfection, should be integrated to achieve complete nutrient recycling (Mahmood et al., 2015; Thostenson et al., 2018). One large-scale co-digestion project can treat up to 400 tons of faecal sludge per day from septic tanks in Ouagadougou, Burkina Faso. This biogas system has a production capacity of approximately 5,000 m3 biogas daily. Arobic methods were also tried in some cases. Barrios-Hernández et al. (2020) co-treated synthetic faecal sludge with municipal synthetic wastewater in an aerobic granular sludge reactor, however, the addtion of faecal sludge decreased the sludge settle-ability and reduced the average granular size. More efforts are necessary to improve the efficiency and consistency for full-scal application.
Composting is an aerobic biological process that converts solid organic matter into stabilized organic compost, CO2, water, and other gases. Excessive heat energy generated in on-site composting due to microbes activity, peak temperatures during the thermophilic phase can reach up to 70°C (Zhou et al., 2022), thus the pathogens in BW could be killed by the high temperature. Proper composting of organic matter yields a nuisance-free, humus-like material that can be used as a soil conditioner in agriculture. Spreading BW compost on fields can improve the soil structure and raise the tolerance of plants to salts (Oarga Mulec et al., 2016).
A bulking agent, such as saw dust or wood chips, is usually added to reduce the moisture content of the raw material and improve its C:N ratio. Several studies on the composting of fecal matter and solid fraction of BW have been carried out (Vinnerås, 2007; Oarga Mulec et al., 2016). The effect of different bulking materials as a supporting matrix on the solid fraction of BW composting in terms of pathogen inactivation, nutrient loss, and CH4 emission have been studied (Oarga-Mulec et al., 2019). Zhu et al. (2022) composted faecal sludge with sawdust and rice straw in earthworm-based system and obtained significant promote in composting efficiency and global warming potential reduction.
Composting of human excreta from waterless toilets and the solid fraction or digestate of BW has been applied in practice. In cold regions, greenhouses could be used to keep a relatively constant ambient temperature for composting processes (Mahmood et al., 2015).
Nitrification refers to the biological oxidation of ammonia or ammonium to nitrite, followed by oxidation of nitrite to nitrate. Nitrification of urine can only oxidize half of the available ammonium until nitrification ceases due to low pH conditions and the absence of other relevant buffers at significant concentrations in urine (Maurer et al., 2006; Feng et al., 2008).
Experiments conducted by Udert and Wachter (2012), Udert et al. (2003) and other researchers reveal that the product of urine nitrification is either an ammonium-nitrate or ammonium-nitrite solution with an approximate composition of 1:1; urine nitrification could stabilize the free ammonia in the solution to prevent the nitrogen release loss from the urine. Fumasoli et al. (2016) employed a combined nitrification/distillation process to stabilize urine and concentrate the nutrient solution at the pilot scale to produce liquid fertilizer.
Urine storage seems to be a physical process, but, during storage, urea can be hydrolyzed by enzyme urease, which is a biological process. Urea is transformed into ammonia and ammonium, leading to a pH increase. Experimental results have revealed that temperature and elevated pH (to 9), in combination with ammonia, can affect the inactivation of microorganisms. The storage time also plays an important role in urine hygienization (Maurer et al., 2006). Inactivation of typical enteric pathogens and model organisms has been studied for hygienization by urine storage under different conditions of urine dilution and temperatures (Vinnerås et al., 2008). The results of this research showed that the recommended storage time for urine (i.e., 6 months at 20°C or higher) is safe for unrestricted use and could probably be shortened, especially for undiluted urine (Vinnerås et al., 2008).
Urine storage has been widely applied to hygenization and urine utilization as fertilizer at the household and residential levels in many countries, such as Sweden, Germany, Switzerland, China, and some African countries (Hanæus et al., 1997; Alemayehu et al., 2020).
Microalgae contains high oil content of 20%–50% on a dry weight basis and are efficient in removing nitrogen, phosphorus from BW and urine (Zubair et al., 2020; Nguyen et al., 2021). Different microalgae species have various efficiency on nutrients recovery. Chlorella sp. shows great tolerance to ammonia and can recovery 23%–100% N and 20%–100% P, respectively (Khan and Yoshida, 2008). Pathogens could be inhibited during the microalgae-based nutrient recovery process. Žitnik et al. (2019) removed 12%–51% E. coli from BW treated with microalgae Chlorella vulgaris.
As heterotrophic bacteria could decompose organic matter and promote plant growth by complex communication mechanisms and nutrient exchange, algal-bacterial systems were conducted to treat wastewater (Ramanan et al., 2016). In algal-bacterial systems, oxygen and organic carbon produced by microalgal photosynthesis could be used by heterotrophic bacteria while CO2 released by chemoorganotrophic bacterial respiration could be in turn fixed by algae (Li et al., 2018). Li et al. (2018) studied bacterium Vibrio. sp Y1-5 and microalgae S. platensis in their mutualistic system, obtaining a biomass yield of 4.28 g/L and recovering 86% of nitrogen and 91% of phosphorus. Algal-bacterial aerobic granular sludge is an emerging new method for wastewater treatment with more potentials for meeting the demands of sustainable bioeconomy due to its lower energy demand, higher nutrients removal, better biomass settleability, and CO2 capture capacity (Lee and Lei, 2019; Chen et al., 2022; Lee and Lei, 2022). However, further researches on recovering nutrients from BW by algal-bacterial aerobic granular sludge are required and meaningful.
This paper summarizes and reviews currently available processes for nutrient recovery of BW, urine, and feces. In most cases, nutrient recovery requires a treatment system consisting of multiple steps not only for nutrient recovery but also for discharge control and removal of harmful substances (Kakimoto et al., 2007).
A wide range of technological options are available for nutrient recovery from BW. Depending on the overall goal of the resource-oriented treatment process, a specific technical solution or combination of technologies may be determined to meet requirements. However, most of the techniques developed thus far present different weaknesses that limit their practical applications. Future BW treatment solutions will likely consist of a combination of treatment processes, for example, recovery of biogas energy by anaerobic digestion, followed by recovery of N and P by struvite precipitation, solid/liquid digestate separation, and then composting for solid digestates.
Source separation has shown to be advantageous for improving treatment capacity, food security, and efficiency; however, these systems are still immature and considered risky by professionals and, thus, scarcely implemented (McConville et al., 2017; Trimmer et al., 2019). Possible barriers to the practical application of these systems may include:
• Unproven technologies in practices
• Unproven cost benefit in comparison with conventional wastewater systems
• Complex operations and maintenance
• Lack of a source control collection system,
• Fluctuation of the target product value.
Therefore, additional research is needed to improve or enhance existing processes and find promising process combinations with great effectiveness.
However, non-sewered sanitation system, that is not connected to a networked sewer, and collects, conveys, and fully treats BW to allow for safe reuse or disposal, is a practical option for BW treatment and resource recovery. Non-sewered sanitation system was awarded as one of the top 10 breakthrough technologies in 2019 by MIT Technology Review (Winick, 2019), seems a feasible solution for decentralized BW management (Cheng et al., 2022). The developed technologies are useful for such systems.
Overall, the perspective for resource-oriented BW management technologies is very positive due to their great potential. More attention should be paid and more efforts should be spent to develop efficient technologies and improve the feasibility, reliability, economy, environment friendliness, and agronomic value of recovered products. It provides clear evidence that resource-oriented technologies are very important tools for sustainable development.
XW and ZL written the manuscript. JC, SC, H-PM, LZ, HH, and IJ discussed and edited the manuscript.
This study was supported by National Key Research and Development Plan (2019YFC0408700), MOST High-level Foreign Expert Project (G2022105016 and LG2022105013L), the USTB Research Center for International People-to-People Exchange in Science, Technology and Civilization (2022KFZD007, 2022KFYB020, and 2022KFYB024).
The authors would like to take this opportunity to express our sincere appreciation for the support of National Environmental and Energy Science and Technology International Cooperation Base.
The authors declare that the research was conducted in the absence of any commercial or financial relationships that could be construed as a potential conflict of interest.
All claims expressed in this article are solely those of the authors and do not necessarily represent those of their affiliated organizations, or those of the publisher, the editors and the reviewers. Any product that may be evaluated in this article, or claim that may be made by its manufacturer, is not guaranteed or endorsed by the publisher.
Afolabi, O. O. D., and Sohail, M. (2017). Microwaving human faecal sludge as a viable sanitation technology option for treatment and value recovery – a critical review. J. Environ. Manage. 187, 401–415. doi:10.1016/j.jenvman.2016.10.067
Aguado, D., Barat, R., Bouzas, A., Seco, A., and Ferrer, J. (2019). P-recovery in a pilot-scale struvite crystallisation reactor for source separated urine systems using seawater and magnesium chloride as magnesium sources. Sci. Total Environ. 672, 88–96. doi:10.1016/j.scitotenv.2019.03.485
Alemayehu, Y. A., Asfaw, S. L., and Terfie, T. A. (2020). Nutrient recovery options from human urine: A choice for large scale application. Sustain. Prod. Consum. 24, 219–231. doi:10.1016/j.spc.2020.06.016
Antonini, S., Nguyen, P. T., Arnold, U., Eichert, T., and Clemens, J. (2012). Solar thermal evaporation of human urine for nitrogen and phosphorus recovery in Vietnam. Sci. Total Environ. 414, 592–599. doi:10.1016/j.scitotenv.2011.11.055
Barrios-Hernández, M. L., Buenaño-Vargas, C., García, H., Brdjanovic, D., van Loosdrecht, M. C. M., and Hooijmans, C. M. (2020). Effect of the co-treatment of synthetic faecal sludge and wastewater in an aerobic granular sludge system. Sci. Total Environ. 741, 140480. doi:10.1016/j.scitotenv.2020.140480
Bennamoun, L. (2012). Solar drying of wastewater sludge: A review. Renew. Sustain. Energy Rev. 16, 1061–1073. doi:10.1016/j.rser.2011.10.005
Bethune, D. N., Chu, A., and Ryan, M. C. (2015). Passive evaporation of source-separated urine from dry toilets: Prototype design and field testing using municipal water. J. Water Sanit. Hyg. Dev. 5, 392–401. doi:10.2166/washdev.2015.158
Chen, C., Dong, T., Han, M., Yao, J., and Han, L. (2020). Ammonium recovery from wastewater by donnan dialysis: A feasibility study. J. Clean. Prod. 265, 121838. doi:10.1016/j.jclepro.2020.121838
Chen, X., Wang, J., Wang, Q., Li, Z., Yuan, T., Lei, Z., et al. (2022). A comparative study on simultaneous recovery of phosphorus and alginate-like exopolymers from bacterial and algal-bacterial aerobic granular sludges: Effects of organic loading rate. Bioresour. Technol. 357, 127343. doi:10.1016/j.biortech.2022.127343
Cheng, S., Long, J., Evans, B., Zhan, Z., Li, T., Chen, C., et al. (2022). Non-negligible greenhouse gas emissions from non-sewered sanitation systems: A meta-analysis. Environ. Res. 212, 113468. doi:10.1016/j.envres.2022.113468
Chipako, T. L., and Randall, D. G. (2020). Urine treatment technologies and the importance of pH. J. Environ. Chem. Eng. 8, 103622. doi:10.1016/j.jece.2019.103622
Courtney, C., and Randall, D. G. (2022a). Concentrating stabilized urine with reverse osmosis: How does stabilization method and pre-treatment affect nutrient recovery, flux, and scaling? Water Res. 209, 117970. doi:10.1016/j.watres.2021.117970
Courtney, C., and Randall, D. G. (2022b). A hybrid nanofiltration and reverse osmosis process for urine treatment: Effect on urea recovery and purity. Water Res. 222, 118851. doi:10.1016/j.watres.2022.118851
Danso-Boateng, E., Holdich, R. G., Shama, G., Wheatley, A. D., Sohail, M., and Martin, S. J. (2013). Kinetics of faecal biomass hydrothermal carbonisation for hydrochar production. Appl. Energy 111, 351–357. doi:10.1016/j.apenergy.2013.04.090
Danso-Boateng, E., Holdich, R. G., Martin, S. J., Shama, G., and Wheatley, A. D. (2015). Process energetics for the hydrothermal carbonisation of human faecal wastes. Energy Convers. Manag. 105, 1115–1124. doi:10.1016/j.enconman.2015.08.064
de Graaff, M. S., Temmink, H., Zeeman, G., and Buisman, C. J. N. (2010). Anaerobic treatment of concentrated black water in a UASB reactor at a short HRT. WaterSwitzerl. 2, 101–119. doi:10.3390/w2010101
Elliott, D. C., Biller, P., Ross, A. B., Schmidt, A. J., and Jones, S. B. (2015). Hydrothermal liquefaction of biomass: Developments from batch to continuous process. Bioresour. Technol. 178, 147–156. doi:10.1016/j.biortech.2014.09.132
Fakkaew, K., Koottatep, T., and Polprasert, C. (2015). Effects of hydrolysis and carbonization reactions on hydrochar production. Bioresour. Technol. 192, 328–334. doi:10.1016/j.biortech.2015.05.091
Fakkaew, K., Koottatep, T., and Polprasert, C. (2018). Faecal sludge treatment and utilization by hydrothermal carbonization. J. Environ. Manage. 216, 421–426. doi:10.1016/j.jenvman.2017.09.031
Feng, D., Wu, Z., and Xu, S. (2008). Nitrification of human urine for its stabilization and nutrient recycling. Bioresour. Technol. 99, 6299–6304. doi:10.1016/j.biortech.2007.12.007
Fidjeland, J., Nordin, A., Pecson, B. M., Nelson, K. L., and Vinnerås, B. (2015). Modeling the inactivation of ascaris eggs as a function of ammonia concentration and temperature. Water Res. 83, 153–160. doi:10.1016/j.watres.2015.06.030
Florentino, A. P., Xu, R., Zhang, L., and Liu, Y. (2019). Anaerobic digestion of blackwater assisted by granular activated carbon: From digestion inhibition to methanogenesis enhancement. Chemosphere 233, 462–471. doi:10.1016/j.chemosphere.2019.05.255
Fuchs, W., Wang, X., Gabauer, W., Ortner, M., and Li, Z. (2018). Tackling ammonia inhibition for efficient biogas production from chicken manure: Status and technical trends in Europe and China. Renew. Sustain. Energy Rev. 97, 186–199. doi:10.1016/j.rser.2018.08.038
Fumasoli, A., Etter, B., Sterkele, B., Morgenroth, E., and Udert, K. M. (2016). Operating a pilot-scale nitrification/distillation plant for complete nutrient recovery from urine. Water Sci. Technol. 73, 215–222. doi:10.2166/wst.2015.485
Ganrot, Z., Dave, G., and Nilsson, E. (2007). Recovery of N and P from human urine by freezing, struvite precipitation and adsorption to zeolite and active carbon. Bioresour. Technol. 98, 3112–3121. doi:10.1016/j.biortech.2006.10.038
Gao, M., Guo, B., Zhang, L., Zhang, Y., and Liu, Y. (2019a). Microbial community dynamics in anaerobic digesters treating conventional and vacuum toilet flushed blackwater. Water Res. 160, 249–258. doi:10.1016/j.watres.2019.05.077
Gao, M., Zhang, L., Florentino, A. P., and Liu, Y. (2019b). Performance of anaerobic treatment of blackwater collected from different toilet flushing systems: Can we achieve both energy recovery and water conservation? J. Hazard. Mat. 365, 44–52. doi:10.1016/j.jhazmat.2018.10.055
Gunnarsdóttir, R., Heiske, S., Jensen, P. E., Schmidt, J. E., Villumsen, A., and Jenssen, P. D. (2014). Effect of anaerobiosis on indigenous microorganisms in blackwater with fish offal as co-substrate. Water Res. 63, 1–9. doi:10.1016/j.watres.2014.05.038
Hanæus, J., Hellström, D., and Johansson, E. (1997). A study of a urine separation system in an ecological village in northern Sweden. Water Sci. Technol. 35, 153–160. doi:10.2166/wst.1997.0339
Hellström, D., Johansson, E., and Grennberg, K. (1999). Storage of human urine: Acidification as a method to inhibit decomposition of urea. Ecol. Eng. 12, 253–269. doi:10.1016/S0925-8574(98)00074-3
Hertel, S., Navarro, P., Deegener, S., and Körner, I. (2015). Biogas and nutrients from blackwater, lawn cuttings and grease trap residues–experiments for Hamburg’s Jenfelder Au district. Energy. sustain. Soc. 5, 29. doi:10.1186/s13705-015-0057-5
Hossain, M. R., Khalekuzzaman, M., Kabir, S. B., Islam, M. B., and Bari, Q. H. (2022). Enhancing faecal sludge derived biocrude quality and productivity using peat biomass through co-hydrothermal liquefaction. J. Clean. Prod. 335, 130371. doi:10.1016/j.jclepro.2022.130371
Huang, H., Li, J., Li, B., Zhang, D., Zhao, N., and Tang, S. (2019a). Comparison of different K-struvite crystallization processes for simultaneous potassium and phosphate recovery from source-separated urine. Sci. Total Environ. 651, 787–795. doi:10.1016/j.scitotenv.2018.09.232
Huang, H., Zhang, D., Wang, W., Li, B., Zhao, N., Li, J., et al. (2019b). Alleviating Na+ effect on phosphate and potassium recovery from synthetic urine by K-struvite crystallization using different magnesium sources. Sci. Total Environ. 655, 211–219. doi:10.1016/j.scitotenv.2018.11.259
Huang, Y., Lee, X., Grattieri, M., Yuan, M., Cai, R., Macazo, F. C., et al. (2020). Modified biochar for phosphate adsorption in environmentally relevant conditions. Chem. Eng. J. 380, 122375. doi:10.1016/j.cej.2019.122375
Hug, A., and Udert, K. M. (2013). Struvite precipitation from urine with electrochemical magnesium dosage. Water Res. 47, 289–299. doi:10.1016/j.watres.2012.09.036
Ishii, S. K. L., and Boyer, T. H. (2015). Life cycle comparison of centralized wastewater treatment and urine source separation with struvite precipitation: Focus on urine nutrient management. Water Res. 79, 88–103. doi:10.1016/j.watres.2015.04.010
Kabir, S. B., and Khalekuzzaman, M. (2022). Co-liquefaction of organic solid waste with fecal sludge for producing petroleum-like biocrude for an integrated waste to energy approach. J. Clean. Prod. 354, 131718. doi:10.1016/j.jclepro.2022.131718
Kakimoto, T., Osawa, T., and Funamizu, N. (2007). Antibiotic effect of amoxicillin on the feces composting process and reactivation of bacteria by intermittent feeding of feces. Bioresour. Technol. 98, 3555–3560. doi:10.1016/j.biortech.2006.11.029
Khan, M., and Yoshida, N. (2008). Effect of l-glutamic acid on the growth and ammonium removal from ammonium solution and natural wastewater by Chlorella vulgaris NTM06. Bioresour. Technol. 99, 575–582. doi:10.1016/j.biortech.2006.12.031
Kjerstadius, H., Bernstad Saraiva, A., Spångberg, J., and Davidsson, A. (2017). Carbon footprint of urban source separation for nutrient recovery. J. Environ. Manage. 197, 250–257. doi:10.1016/j.jenvman.2017.03.094
Krueger, B. C., Fowler, G. D., Templeton, M. R., and Septien, S. (2021). Faecal sludge pyrolysis: Understanding the relationships between organic composition and thermal decomposition. J. Environ. Manage. 298, 113456. doi:10.1016/j.jenvman.2021.113456
Landry, K. A., Sun, P., Huang, C. H., and Boyer, T. H. (2015). Ion-exchange selectivity of diclofenac, ibuprofen, ketoprofen, and naproxen in ureolyzed human urine. Water Res. 68, 510–521. doi:10.1016/j.watres.2014.09.056
Larsen, T. A., Hoffmann, S., Lüthi, C., Truffer, B., and Maurer, M. (2016). Emerging solutions to the water challenges of an urbanizing world. Science 352, 928–933. doi:10.1126/science.aad8641
Lee, Y. J., and Lei, Z. (2019). Microalgal-bacterial aggregates for wastewater treatment: A mini-review. Bioresour. Technol. Rep. 8, 100199. doi:10.1016/j.biteb.2019.100199
Lee, Y. J., and Lei, Z. (2022). Wastewater treatment using microalgal-bacterial aggregate process at zero-aeration scenario: Most recent research focuses and perspectives. Bioresour. Technol. Rep. 17, 100943. doi:10.1016/j.biteb.2021.100943
Levitt, M. D., and Duane, W. C. (1972). Floating stools - flatus versus fat. N. Engl. J. Med. 286, 973–975. doi:10.1056/nejm197205042861804
Li, Y., Wang, Y., Gao, Y., Zhao, H., and Zhou, W. (2018). Seawater toilet flushing sewage treatment and nutrients recovery by marine bacterial-algal mutualistic system. Chemosphere 195, 70–79. doi:10.1016/j.chemosphere.2017.12.076
Li, J., Li, L., Suvarna, M., Pan, L., Tabatabaei, M., Ok, Y. S., et al. (2022). Wet wastes to bioenergy and biochar: A critical review with future perspectives. Sci. Total Environ. 817, 152921. doi:10.1016/j.scitotenv.2022.152921
Li, X., Zhao, X., Zhang, J., Hao, J., and Zhang, Q. (2022). Struvite crystallization by using active serpentine: An innovative application for the economical and efficient recovery of phosphorus from black water. Water Res. 221, 118678. doi:10.1016/j.watres.2022.118678
Ling, W., Xing, Y., Hong, C., Zhang, B., Hu, J., Zhao, C., et al. (2022). Methods, mechanisms, models and tail gas emissions of convective drying in sludge: A review. Sci. Total Environ. 845, 157376. doi:10.1016/j.scitotenv.2022.157376
Liu, X., Li, Z., Zhang, Y., Feng, R., and Mahmood, I. B. (2014). Characterization of human manure-derived biochar and energy-balance analysis of slow pyrolysis process. Waste Manag. 34, 1619–1626. doi:10.1016/j.wasman.2014.05.027
Liu, B., Giannis, A., Zhang, J., Chang, V. W. C., and Wang, J. Y. (2015). Air stripping process for ammonia recovery from source-separated urine: Modeling and optimization. J. Chem. Technol. Biotechnol. 90, 2208–2217. doi:10.1002/jctb.4535
Liu, H., Shan, J., Chen, Z., and Lichtfouse, E. (2021). Efficient recovery of phosphate from simulated urine by Mg/Fe bimetallic oxide modified biochar as a potential resource. Sci. Total Environ. 784, 147546. doi:10.1016/j.scitotenv.2021.147546
Lu, J., Zhang, J., Zhu, Z., Zhang, Y., Zhao, Y., Li, R., et al. (2017). Simultaneous production of biocrude oil and recovery of nutrients and metals from human feces via hydrothermal liquefaction. Energy Convers. Manag. 134, 340–346. doi:10.1016/j.enconman.2016.12.052
Luostarinen, S., Sanders, W., Kujawa-Roeleveld, K., and Zeeman, G. (2007). Effect of temperature on anaerobic treatment of black water in UASB-septic tank systems. Bioresour. Technol. 98, 980–986. doi:10.1016/j.biortech.2006.04.018
Mahmood, I. B., Li, Z., Uddin, S. M. N., Mang, H. P., and Germer, J. (2015). Short communication co-composting of fecal matter in Mongolia using two different technologies. J. Water Sanit. Hyg. Dev. 5, 165–171. doi:10.2166/washdev.2014.079
Malila, R., Lehtoranta, S., and Viskari, E. L. (2019). The role of source separation in nutrient recovery – comparison of alternative wastewater treatment systems. J. Clean. Prod. 219, 350–358. doi:10.1016/j.jclepro.2019.02.024
Mamera, M., van Tol, J. J., and Aghoghovwia, M. P. (2022). Treatment of faecal sludge and sewage effluent by pinewood biochar to reduce wastewater bacteria and inorganic contaminants leaching. Water Res. 221, 118775. doi:10.1016/j.watres.2022.118775
Mang, H. P., and Li, Z. (2010). Technology review of biogas sanitation - biogas sanitation for blackwater, brown water, or for excreta treatment and reuse in developing countries. Eschborn: Deutsche Gesellschaft für Internationale Zusammenarbeit GmbH, GIZ.
Maurer, M., Pronk, W., and Larsen, T. A. (2006). Treatment processes for source-separated urine. Water Res. 40, 3151–3166. doi:10.1016/j.watres.2006.07.012
McConville, J. R., Kvarnström, E., Jönsson, H., Kärrman, E., and Johansson, M. (2017). Source separation: Challenges & opportunities for transition in the Swedish wastewater sector. Resour. Conserv. Recycl. 120, 144–156. doi:10.1016/j.resconrec.2016.12.004
Mihelcic, J. R., Fry, L. M., and Shaw, R. (2011). Global potential of phosphorus recovery from human urine and feces. Chemosphere 84, 832–839. doi:10.1016/j.chemosphere.2011.02.046
Moharramzadeh, S., Ong, S. K., Alleman, J., and Cetin, K. S. (2022). Stabilization and concentration of nitrogen in synthetic urine with peracetic acid and progressive freeze concentration. J. Environ. Chem. Eng. 10, 107768. doi:10.1016/j.jece.2022.107768
Nguyen, T. T., Bui, X. T., Ngo, H. H., Nguyen, T. T. D., Nguyen, K. Q., Nguyen, H. H., et al. (2021). Nutrient recovery and microalgae biomass production from urine by membrane photobioreactor at low biomass retention times. Sci. Total Environ. 785, 147423. doi:10.1016/j.scitotenv.2021.147423
Oarga Mulec, A., Mihelič, R., Walochnik, J., and Griessler Bulc, T. (2016). Composting of the solid fraction of blackwater from a separation system with vacuum toilets - effects on the process and quality. J. Clean. Prod. 112, 4683–4690. doi:10.1016/j.jclepro.2015.07.080
Oarga-Mulec, A., Hanssen, J. F., Jenssen, P. D., and Bulc, T. G. (2019). A comparison of various bulking materials as a supporting matrix in composting blackwater solids from vacuum toilets. J. Environ. Manage. 243, 78–87. doi:10.1016/j.jenvman.2019.05.005
Otterpohl, R. (2002). Options for alternative types of sewerage and treatment systems directed to improvement of the overall performance. Water Sci. Technol. 45, 149–158. doi:10.2166/wst.2002.0074
Pahore, M. M., Ushijima, K., Ito, R., and Funamizu, N. (2012). Fate of nitrogen during volume reduction of human urine using an on-site volume reduction system. Environ. Technol. 33, 229–235. doi:10.1080/09593330.2011.560192
Palmquist, H., and Hanæus, J. (2005). Hazardous substances in separately collected grey- and blackwater from ordinary Swedish households. Sci. Total Environ. 348, 151–163. doi:10.1016/j.scitotenv.2004.12.052
Pan, J., Ma, J., Zhai, L., Luo, T., Mei, Z., and Liu, H. (2019). Achievements of biochar application for enhanced anaerobic digestion: A review. Bioresour. Technol. 292, 122058. doi:10.1016/j.biortech.2019.122058
Patel, A., Mungray, A. A., and Mungray, A. K. (2020). Technologies for the recovery of nutrients, water and energy from human urine: A review. Chemosphere 259, 127372. doi:10.1016/j.chemosphere.2020.127372
Pathy, A., Ray, J., and Paramasivan, B. (2021). Challenges and opportunities of nutrient recovery from human urine using biochar for fertilizer applications. J. Clean. Prod. 304, 127019. doi:10.1016/j.jclepro.2021.127019
Penn, R., Ward, B. J., Strande, L., and Maurer, M. (2018). Review of synthetic human faeces and faecal sludge for sanitation and wastewater research. Water Res. 132, 222–240. doi:10.1016/j.watres.2017.12.063
Pocock, J., Septien, S., Makununika, B. S. N., Velkushanova, K. V., and Buckley, C. A. (2022). Convective drying kinetics of faecal sludge from VIP latrines. Heliyon 8, e09221. doi:10.1016/j.heliyon.2022.e09221
Pronk, W., Biebow, M., and Boller, M. (2006). Treatment of source-separated urine by a combination of bipolar electrodialysis and a gas transfer membrane. Water Sci. Technol. 53, 139–146. doi:10.2166/wst.2006.086
Rajagopal, R., Ahamed, A., and Wang, J. Y. (2014). Hydrolytic and acidogenic fermentation potential of food waste with source segregated feces-without-urine as co-substrate. Bioresour. Technol. 167, 564–568. doi:10.1016/j.biortech.2014.06.024
Ramanan, R., Kim, B. H., Cho, D. H., Oh, H. M., and Kim, H. S. (2016). Algae-bacteria interactions: Evolution, ecology and emerging applications. Biotechnol. Adv. 34, 14–29. doi:10.1016/j.biotechadv.2015.12.003
Ren, X., Chen, H., Cheng, Y., Wu, L., and Jiang, H. (2018). Full-scale practice of domestic wastewater source separation and collection in a semicentralized treatment system: A case study. Water Sci. Technol. 78, 2193–2203. doi:10.2166/wst.2018.505
Ren, J., Hao, D., Jiang, J., Phuntsho, S., Freguia, S., Ni, B. J., et al. (2021). Fertiliser recovery from source-separated urine via membrane bioreactor and heat localized solar evaporation. Water Res. 207, 117810. doi:10.1016/j.watres.2021.117810
Rodrigues, M., Sleutels, T., Kuntke, P., Hoekstra, D., ter Heijne, A., Buisman, C. J. N., et al. (2020). Exploiting Donnan Dialysis to enhance ammonia recovery in an electrochemical system. Chem. Eng. J. 395, 125143. doi:10.1016/j.cej.2020.125143
Ronteltap, M., Maurer, M., Hausherr, R., and Gujer, W. (2010). Struvite precipitation from urine - influencing factors on particle size. Water Res. 44, 2038–2046. doi:10.1016/j.watres.2009.12.015
Rose, C., Parker, A., Jefferson, B., and Cartmell, E. (2015). The characterization of feces and urine: A review of the literature to inform advanced treatment technology. Crit. Rev. Environ. Sci. Technol. 45, 1827–1879. doi:10.1080/10643389.2014.1000761
Saetta, D., Zheng, C., Leyva, C., and Boyer, T. H. (2020). Impact of acetic acid addition on nitrogen speciation and bacterial communities during urine collection and storage. Sci. Total Environ. 745, 141010. doi:10.1016/j.scitotenv.2020.141010
Seodigeng, R., Kabuba, J., and Rutto, H. (2022). Modelling the drying characteristics of human faeces using thin-layer drying models and calculation of mass transfer properties at ambient conditions. Environ. Challenges 9, 100648. doi:10.1016/j.envc.2022.100648
Septien, S., Mirara, S. W., Makununika, B. S. N., Singh, A., Pocock, J., Velkushanova, K., et al. (2020). Effect of drying on the physical and chemical properties of faecal sludge for its reuse. J. Environ. Chem. Eng. 8, 103652. doi:10.1016/j.jece.2019.103652
Stintzing, A. R., and Salomon, E. (2004). Guidelines on the use of urine and faeces in crop production. Stockholm: The EcoSanRes Programme and the Stockholm Environment Institute.
Tansel, B., Lunn, G., and Monje, O. (2018). Struvite formation and decomposition characteristics for ammonia and phosphorus recovery: A review of magnesium-ammonia-phosphate interactions. Chemosphere 194, 504–514. doi:10.1016/j.chemosphere.2017.12.004
Tarpeh, W. A., Udert, K. M., and Nelson, K. L. (2017). Comparing ion exchange adsorbents for nitrogen recovery from source-separated urine. Environ. Sci. Technol. 51, 2373–2381. doi:10.1021/acs.est.6b05816
Tervahauta, T., van der Weijden, R. D., Flemming, R. L., Hernández Leal, L., Zeeman, G., and Buisman, C. J. N. (2014). Calcium phosphate granulation in anaerobic treatment of black water: A new approach to phosphorus recovery. Water Res. 48, 632–642. doi:10.1016/j.watres.2013.10.012
Thibodeau, C., Monette, F., and Glaus, M. (2014). Comparison of development scenarios of a black water source-separation sanitation system using life cycle assessment and environmental life cycle costing. Resour. Conserv. Recycl. 92, 38–54. doi:10.1016/j.resconrec.2014.08.004
Thostenson, J. O., Mourouvin, R., Hawkins, B. T., Ngaboyamahina, E., Sellgren, K. L., Parker, C. B., et al. (2018). Improved blackwater disinfection using potentiodynamic methods with oxidized boron-doped diamond electrodes. Water Res. 140, 191–199. doi:10.1016/j.watres.2018.04.022
Trimmer, J. T., Margenot, A. J., Cusick, R. D., and Guest, J. S. (2019). Aligning product chemistry and soil context for agronomic reuse of human-derived resources. Environ. Sci. Technol. 53, 6501–6510. doi:10.1021/acs.est.9b00504
Udert, K. M., Fux, C., Münster, M., Larsen, T. A., Siegrist, H., and Gujer, W. (2003). Nitrification and autotrophic denitrification of source-separated urine. Water Sci. Technol. 48, 119–130. doi:10.2166/wst.2003.0031
Udert, K. M., and Wächter, M. (2012). Complete nutrient recovery from source-separated urine by nitrification and distillation. Water Res. 46, 453–464. doi:10.1016/j.watres.2011.11.020
Venkata Sai, P., and Reddy, K. S. (2022). Techno-enviro-economic investigations on self-sustainable solar powered blackwater treatment system. Sol. Energy 231, 297–316. doi:10.1016/j.solener.2021.11.019
Vinnerås, B. (2007). Comparison of composting, storage and urea treatment for sanitising of faecal matter and manure. Bioresour. Technol. 98, 3317–3321. doi:10.1016/j.biortech.2006.07.011
Vinnerås, B., Nordin, A., Niwagaba, C., and Nyberg, K. (2008). Inactivation of bacteria and viruses in human urine depending on temperature and dilution rate. Water Res. 42, 4067–4074. doi:10.1016/j.watres.2008.06.014
Wang, H., Li, Z., Zhou, X., Wang, X., and Zuo, S. (2020). Anaerobic co-digestion of kitchen waste and blackwater for different practical application scenarios in decentralized scale : From wastes to energy recovery. Water 12, 2556. doi:10.3390/w12092556
Wang, J., Ye, X., Zhang, Z., Ye, Z. L., and Chen, S. (2018). Selection of cost-effective magnesium sources for fluidized struvite crystallization. J. Environ. Sci. 70, 144–153. doi:10.1016/j.jes.2017.11.029
Wang, W., Yang, L., Yin, Z., Kong, S., Han, W., and Zhang, J. (2018). Catalytic liquefaction of human feces over Ni-Tm/TiO2 catalyst and the influence of operating conditions on products. Energy Convers. Manag. 157, 239–245. doi:10.1016/j.enconman.2017.11.081
Watson, J., Si, B., Li, H., Liu, Z., and Zhang, Y. (2017). Influence of catalysts on hydrogen production from wastewater generated from the HTL of human feces via catalytic hydrothermal gasification. Int. J. Hydrogen Energy 42, 20503–20511. doi:10.1016/j.ijhydene.2017.05.083
Wei, S. P., van Rossum, F., van de Pol, G. J., and Winkler, M. K. H. (2018). Recovery of phosphorus and nitrogen from human urine by struvite precipitation, air stripping and acid scrubbing: A pilot study. Chemosphere 212, 1030–1037. doi:10.1016/j.chemosphere.2018.08.154
Wendland, C., Deegener, S., Behrendt, J., Toshev, P., and Otterpohl, R. (2007). Anaerobic digestion of blackwater from vacuum toilets and kitchen refuse in a continuous stirred tank reactor (CSTR). Water Sci. Technol. 55, 187–194. doi:10.2166/wst.2007.144
Wilsenach, J. A., Schuurbiers, C. A. H., and van Loosdrecht, M. C. M. (2007). Phosphate and potassium recovery from source separated urine through struvite precipitation. Water Res. 41, 458–466. doi:10.1016/j.watres.2006.10.014
Winker, M., Vinnerås, B., Muskolus, A., Arnold, U., and Clemens, J. (2009). Fertiliser products from new sanitation systems: Their potential values and risks. Bioresour. Technol. 100, 4090–4096. doi:10.1016/j.biortech.2009.03.024
Wu, H., Foster, X., Kazemian, H., and Vaneeckhaute, C. (2022). N, P, K recovery from hydrolysed urine by Na-Chabazite adsorption integrated with ammonia stripping and (K-)struvite precipitation. SSRN Electron. J. 857, 159277. doi:10.1016/j.scitotenv.2022.159277
Xi, D., Wen, S., Zhang, X., Xie, W., Fang, Z., Zhou, R., et al. (2022). Plasma-electrolytic liquefaction of human waste for biofuels production and recovery of ammonium, chlorine and metals. Chem. Eng. J. 433, 134581. doi:10.1016/j.cej.2022.134581
Xu, K., Li, J., Zheng, M., Zhang, C., Xie, T., and Wang, C. (2015). The precipitation of magnesium potassium phosphate hexahydrate for P and K recovery from synthetic urine. Water Res. 80, 71–79. doi:10.1016/j.watres.2015.05.026
Xu, K., Zhang, C., Li, J., Cheng, X., and Wang, C. (2017). Removal and recovery of N, P and K from urine via ammonia stripping and precipitations of struvite and struvite-K. Water Sci. Technol. 75, 155–164. doi:10.2166/wst.2016.494
Xu, R., Xu, S., Zhang, L., Florentino, A. P., Yang, Z., and Liu, Y. (2019). Impact of zero valent iron on blackwater anaerobic digestion. Bioresour. Technol. 285, 121351. doi:10.1016/j.biortech.2019.121351
Yacob, T. W., Fisher, R., Linden, K. G., and Weimer, A. W. (2018). Pyrolysis of human feces: Gas yield analysis and kinetic modeling. Waste Manag. 79, 214–222. doi:10.1016/j.wasman.2018.07.020
Yahav Spitzer, R., Mau, V., and Gross, A. (2018). Using hydrothermal carbonization for sustainable treatment and reuse of human excreta. J. Clean. Prod. 205, 955–963. doi:10.1016/j.jclepro.2018.09.126
Zhang, M., Wang, S., Ji, B., and Liu, Y. (2019). Towards mainstream deammonification of municipal wastewater: Partial nitrification-anammox versus partial denitrification-anammox. Sci. Total Environ. 692, 393–401. doi:10.1016/j.scitotenv.2019.07.293
Zhang, B., Tian, S., and Wu, D. (2022). Phosphorus harvesting from fresh human urine: A strategy of precisely recovering high-purity calcium phosphate and insights into the precipitation conversion mechanism. Water Res. 227, 119325. doi:10.1016/j.watres.2022.119325
Zhang, B., Tian, S., and Wu, D. (2023). An integrated strategy for nutrient harvesting from hydrolyzed human urine as high-purity products: Tracking of precipitation transformation and precise regulation. Sci. Total Environ. 854, 158721. doi:10.1016/j.scitotenv.2022.158721
Zhang, L., Liu, J., Yang, L., Yu, Z., Chen, J., Chu, H., et al. (2023). Interfacial solar evaporation toward efficient recovery of clean water and concentration of nutrients from urine with polypyrrole-based photothermal conversion films. Resour. Conserv. Recycl. 188, 106645. doi:10.1016/j.resconrec.2022.106645
Zhou, Y., Xiao, R., Klammsteiner, T., Kong, X., Yan, B., Mihai, F. C., et al. (2022). Recent trends and advances in composting and vermicomposting technologies: A review. Bioresour. Technol. 360, 127591. doi:10.1016/j.biortech.2022.127591
Zhu, S., Yang, Y., Yan, Y., Causone, F., Jin, X., Zhou, X., et al. (2022). Co-composting of faecal sludge and carbon-rich wastes in the earthworm’s synergistic cooperation system: Performance, global warming potential and key microbiome. Sci. Total Environ. 857, 159311. doi:10.1016/j.scitotenv.2022.159311
Žitnik, M., Šunta, U., Godič Torkar, K., Krivograd Klemenčič, A., Atanasova, N., and Griessler Bulc, T. (2019). The study of interactions and removal efficiency of Escherichia coli in raw blackwater treated by microalgae Chlorella vulgaris. J. Clean. Prod. 238, 117865. doi:10.1016/j.jclepro.2019.117865
Zubair, M., Wang, S., Zhang, P., Ye, J., Liang, J., Nabi, M., et al. (2020). Biological nutrient removal and recovery from solid and liquid livestock manure: Recent advance and perspective. Bioresour. Technol. 301, 122823. doi:10.1016/j.biortech.2020.122823
Keywords: blackwater, toilet, feces, urine, source separation, nutrient recovery
Citation: Wang X, Chen J, Li Z, Cheng S, Mang H-P, Zheng L, Jan I and Harada H (2023) Nutrient recovery technologies for management of blackwater: A review. Front. Environ. Sci. 10:1080536. doi: 10.3389/fenvs.2022.1080536
Received: 26 October 2022; Accepted: 19 December 2022;
Published: 05 January 2023.
Edited by:
Xinjiang Hu, Central South University Forestry and Technology, ChinaReviewed by:
Junting Pan, Institute of Agricultural Resources and Regional Planning (CAAS), ChinaCopyright © 2023 Wang, Chen, Li, Cheng, Mang, Zheng, Jan and Harada. This is an open-access article distributed under the terms of the Creative Commons Attribution License (CC BY). The use, distribution or reproduction in other forums is permitted, provided the original author(s) and the copyright owner(s) are credited and that the original publication in this journal is cited, in accordance with accepted academic practice. No use, distribution or reproduction is permitted which does not comply with these terms.
*Correspondence: Zifu Li, emlmdWxpQHVzdGIuZWR1LmNu; Shikun Cheng, Y2hlbmdzaGlrdW5AdXN0Yi5lZHUuY24=
Disclaimer: All claims expressed in this article are solely those of the authors and do not necessarily represent those of their affiliated organizations, or those of the publisher, the editors and the reviewers. Any product that may be evaluated in this article or claim that may be made by its manufacturer is not guaranteed or endorsed by the publisher.
Research integrity at Frontiers
Learn more about the work of our research integrity team to safeguard the quality of each article we publish.