- 1College of Resources and Environmental Engineering, Guizhou University, Guiyang, Guizhou, China
- 2Key Laboratory of Karst Geological Resources and Environment, Ministry of Education, Guizhou University, Guiyang, Guizhou, China
Heavy metal pollution derived from the oxidation of sulfides in Pb-Zn mine tailings has been a common public concern. Although the oxidative dissolution of sphalerite will release cadmium into the soil environment, it is still unclear whether other minerals contain a large amount of cadmium and how much these minerals contribute to the surrounding environmental media. In this paper, XRD, particle size analyzer, SEM-EDS and improved BCR sequential extraction method were used to analyze cadmium rich zinc mine tailing and adjacent farmland soil in Duyun, Guizhou Province. The results show that the average contents of Pb, Zn, and Cd in tailing are 214 ± 71 mg/kg, 2668 ± 773 mg/kg, and 37.5 ± 9.97 mg/kg respectively. While in the surrounding soil, they were 519 ± 280 mg/kg, 3,779 ± 2614 mg/kg, and 30.8 ± 14.4 mg/kg respectively. The mineralogical composition of tailing is mainly dolomite and less pyrite. Cadmium in tailing is mainly in weak acid extractable state, indicating that cadmium mainly exists in dolomite of tailing. Pyrite in tailing generates acid through oxidation, which accelerates the dissolution of dolomite, thus releasing Cd from minerals and migrating to surrounding farmland, resulting in soil cadmium pollution. This study reveals an important process that has been neglected. Carbonate minerals play an important role in the migration of cadmium in the tailing of the Mississippi Valley-type (MVT) lead-zinc mine and the soils affected by the tailing.
1 Introduction
Cadmium is a highly toxic and mobile element in the environment (Clemens and Ma, 2016). It can substitute zinc or calcium in living organisms because of its similar ionic radius and chemical behavior to zinc and calcium. It can also accumulate in several human organs (Wang et al., 2019a; Mamatchi et al., 2022) and cause kidney dysfunction and osteoporosis (Aoshima, 2016). Human exposure to cadmium is predominated by the food chain (Inaba et al., 2005; Luo et al., 2022). In recent years, Cd concentrations in rice grains exceeding the Chinese food limit have been reported in southern China (Chen et al., 2018; Wang et al., 2019a). The natural sources of cadmium include weathering of rocks, forest fires, and volcanic eruptions (Richardson et al., 2001), while anthropogenic sources consist of non-ferrous metal production, steel production, fossil fuel combustion, cement production, and waste incineration (Pacyna and Pacyna, 2001). The production of nonferrous metals from mines contributes the most cadmium to the environment (Nriagu, 1990). During mining and milling, Cd-bearing minerals at certain depths underground are exposed to the surface environment, causing sulfide oxidation and dissolution, which accelerates Cd release to the environment (Xu et al., 2019). Tailing produced by mineral processing have attracted much attention due to their large volume, high cadmium content and potential pollution risks to the surrounding environment (Sasmaz et al., 2019; Kan et al., 2020).
Cadmium is commonly recovered from Zn deposits because the independent Cd deposit is rarely formed in nature. Mississippi Valley type (MVT) lead-zinc deposit is one of the most important Zn ones. The deposit is named after the Mississippi Valley basin in the central United States in which it is produced. The MVT Zn deposits provide about 25% of the world’s Zn resources (Hedenquist et al., 2005) and are regarded as the most Cd-rich ones among the various Zn deposits (Schwartz, 2000; Frenzel et al., 2016; Zhu et al., 2020). MVT deposit is hosted by carbonate rocks and characterized by weak alteration and simple mineralogical assemblage with sphalerite (ZnS), galena (PbS), and pyrite being ore minerals and calcite and/or dolomite being major gangue minerals. Due to its geochemical affinity for Zn, Cd is mainly hosted by sphalerite in primary ores or smithsonite in oxidized ores. Limited by mineral processing technology, tailing often contain a large number of sulfide minerals, of which the content of heavy metals (Cd, Zn, and Pb) is high (Tao et al., 2019; Wei et al., 2021). These heavy metals in tailing pose a potential threat to human health when spreading into the surrounding environment and causing crops to exceed the national standard (Boussen et al., 2013; Wang et al., 2019a; Kan et al., 2020; Sun, et al., 2022). Therefore, the Zn tailing produced during the beneficiation of Zn ores have become one of the main sources of Cd in the environment.
The impact of the tailing heavy metals on the environment is closely linked to their speciation. In previous studies, it has been recognized that the total content of heavy metals in environmental media (e.g., soil, sediment, and tailing) usually does not reflect their hazard to the surrounding environment. A five step sequential extraction procedure based on single chemical reagent extraction (Tessier et al., 1979; Rosado et al., 2016; Lohmann et al., 2022) and the three-step protocol developed by Bureau Community of Reference (BCR) (Alan and Kara, 2019; Khoeurn et al., 2019; Qureshi et al., 2020) have been well-established to study the operationally-defined speciation of heavy metals in tailing and other materials, which have greatly improved our understanding of the impact of heavy metals. However, the variety of the mineral composition of tailing frequently leads to a lack of selectivity in extracting reagents, which makes the schemes been criticized (Förstner, 1993; Hsu et al., 2015; Uchimiya et al., 2020). As a result, the heavy metal chemical speciation of tailing should be more effectively assessed by combining the mineral composition through a sequential extraction procedure (Smieja-Król et al., 2022; Yaciuk et al., 2022).
Traditionally, Cd in Zn mine tailing is regarded to be hosted by sphalerite and its release is closely linked to sphalerite oxidation dissolution (O’Day et al., 1998; Mazeina and Bessonov, 1999; Rodríguez et al., 2009; Long et al., 2022). Nevertheless, high Cd contents in the mineralized carbonate rocks reported by some studies (Quezada-Hinojosa et al., 2009; Jacquat et al., 2011; Ye et al., 2011) raise the possibility that carbonate minerals could be a major host phase of Cd in MVT Zn tailing. In this paper, Niujiaotang Zn mine in Guizhou, Southwestern China, with an unusually high Cd content (4,081 mg/kg on average) (Ye et al., 2011), were selected as the study area. The particle size, mineralogy, pH, and content of Cd were analyzed to decipher Cd speciation in the tailing. Paddy soil samples impacted by the tailing were also collected to assess Cd environmental risk. It is suggested that carbonate mineral dolomite, not sphalerite, is the predominant Cd host mineral in Niujiaotang Cd-rich Zn tailing. This provides a new insight into the environmental impact of cadmium on MVT zinc deposit, because it is rich in cadmium and widely distributed around the world.
2 Materials and methods
2.1 Site description and sample collection
Niujiaotang Zn deposit in Guizhou Province of southwest China is the highest Cd grade deposit ever discovered (Ye et al., 2011). The deposit is located about 20 km east of Duyun City, Guizhou Province, and the elevation of the study area is in the range of 800–1251 m. The study area has a humid subtropical monsoon climate with an average annual temperature of 16.1°C, and an average annual rainfall of 1,431 mm. The karst landforms are well developed in the study area. The Niujiaotang Zn deposit is an MVT Zn deposit hosted within lower Cambrian Qingxudong Formation dolostone. The grade of the ore is ranged from 5.9% to 24.5% (average 19.8%) Zn and 3,000–9,800 mg/kgCd (average 4081 mg/kg) with the sulfide ore being the major mining target (Ye et al., 2011). The main ore minerals are sphalerite, pyrite, and minor galena, while gangue mineralogy is characterized by dolomite, subordinate calcite, and minor quartz (Ye et al., 2011). The Niujiaotang Zn deposit has the proven zinc reserves of 350*103t and cadmium reserves of 5299t (Ye et al., 2011). Zinc concentrate ore was obtained through flotation and the flotation waste was discharged to the tailing dumps.
Tailing samples and agricultural soil samples were collected from Heli tailing dump and the surrounding paddy soil besides Fanjia River, respectively (Figure 1). Heli tailing dump was constructed in 2,000 and closed in 2021 covering an area of about 3.2 hm2 and accumulating about 350,000 m3 of tailing. We selected nine samples of tailing (0–20 cm) in Heli tailing dump with a depth of 0–20 cm using the random point distribution method corresponding to the possible ore variation and 10 samples of paddy soil (0–20 cm) along Fanjia River, each about 1 kg. All tailing and soil samples were collected with a plastic shovel and stored in polyethylene bags and transported to the laboratory.
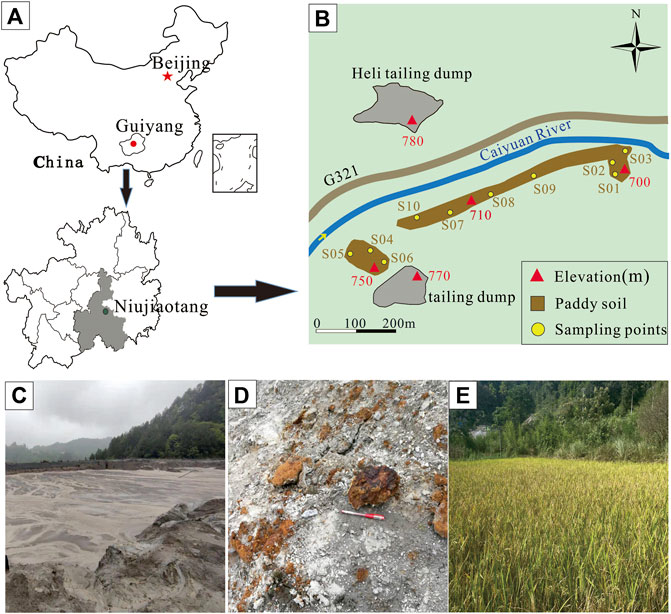
FIGURE 1. Niujiaotang Zn-rich mine (A), tailing and soil sample locations (B), tailing dump (C), iron (oxyhydr) oxides in tailing (D), and paddy soil (E).
2.2 Analysis of physical and chemical parameters
The collected samples were air-dried at room temperature. Then, the sample was ground and sieved to below 200 mesh. Sample particle size fraction of the soils and tailings were measured by a Laser particle size analyzer (Bettersize 3,000 Plus) and the pH was determined with a solid: water ratio of 1:2.5 (w/v) with a Mettler Toledo FE28 potentiometer. Mineralogy of soil and tailing was determined with a diffractometer PANalytical X’Pert PRO with CuKα (λ = 1.5406 Å) and Ni filter. Scans settings were from 5° < 2θ < 90° (0.02° step size). Microstructural morphology and element mapping of tailing particles were measured by scanning electron microscope coupled with energy dispersive spectroscopy (SEM-EDS) HITACHI SU8010 using an accelerating voltage of 20 kV. The SEM-EDS analyses were conducted at the State Key Laboratory of Public Big Data, Guizhou University, China.
2.3 Total concentration of Cd, Zn, and Pb
The total concentrations of Cd, Zn, and Pb were measured by inductively coupled plasma mass spectrometry (ICP- MS XII, Thermo Fisher, United States) in the Key Laboratory of Karst Geological Resources and Environment, Ministry of Education, Guizhou University, China. The aliquot (50 mg) of tailing or soil samples were dissolved with a concentrated HNO3- HF (1:4) mixture in a Teflon bomb maintained in an oven at 185°C for 72 h. The obtained solutions were dried on a hot plate to evaporate HF and then were re-dissolved in a final 3 ml 2 N HNO3 stock solution. Finally, the obtained solution was diluted 4,000 times with ∼2% HNO3. During analysis, Rh (10 μg/kg) was added as an internal spike to calibrate instrument drift. USGS standards W-2a and BHVO-2 were used for data quality control. The detailed ICP-MS analytical procedure follows the protocol of Liang et al. (2000). The analytical error for Cd, Zn, and Pb is less than 10%.
2.4 Modified bureau community of reference sequential extraction procedure
The tailing samples were selected for modified three-step sequential extractions (Rauret et al., 1999). Accordingly, 0.2 g of dry tailing was extracted in a 50 ml centrifuge tube with reagents. The first fraction (F1) was extracted with 20 ml 0.11 mol/L acetic acid at 50°Ceshaking for 16 h. The tube was centrifuged for 10 min at 4500r/min and the supernatant was collected in another tube. This step was repeated once with a shaking time of 12 h. The residue in the tube was extracted with 10 ml of de-ionized water and centrifuged again. The second fraction (F2) was obtained by 20 ml 0.5 mol/L NH2OHHCl extracting the residue from the first fraction. The subsequent centrifugation and deionized water extraction are the same as the first fraction. The third fraction (F3) was obtained by adding 5 ml 8.8 mol/L H2O2 at 85°C for 1 h and 1 mol/L ammonium acetate at 25°C for 16 h. The subsequent centrifugation and deionized water extraction are the same as the first fraction. The residual fraction (F4) was estimated as the difference between Cd concentration in the tailing samples and the sum of the above three fractions.
All the supernatants in each fraction were filtered with a 0.45 μm membrane filter and diluted by 2% HNO3. The Cd concentration in the tailing extracts obtained during each sequential extraction step was determined by ICP-MS (ICP- MS XII, Thermo Fisher, United States). The tailing extracts and blanks were analyzed in duplicates. The recovery of the sample is 107% through certified reference material for the chemical composition of the soil (GBW07980).
3 Results and discussion
3.1 Mineralogy and size distribution of the tailings and soils
The results of mineral phases identified by XRD in tailing and soil samples of the Niujiaotang Cd-rich Zn mine are presented in Table 1. The representative XRD patterns are presented in Figure 2. It is noticeable that dolomite [CaMg(CO3)2] is present in all tailing samples as an abundant phase. Pyrite is presented in minor quantities as revealed by the intensity of its peak and no independent Pb and Zn minerals are detected. Soil samples present more abundant quartz and kaolinite and slightly less dolomite compared to the tailing samples. SEM element mapping results confirm the dominance of dolomite and pyrite in the tailing samples (Figure 3). The consistent element mapping distribution of S, Fe, Zn, and Pb and dominance of S and Fe implies that pyrite is the mainly sulfide phase and independent Cd, Zn, and Pb minerals [e.g., greenockite (CdS), sphalerite (ZnS) and galena (PbS)] are not detected by SEM analyses. Particle size distribution of the tailings and paddy soils showed that both the tailings and soils were composed of primarily silt and clay (71% and 79%, respectively) with median particle size (D50) being 32.94 μm and 21.76 μm, respectively.
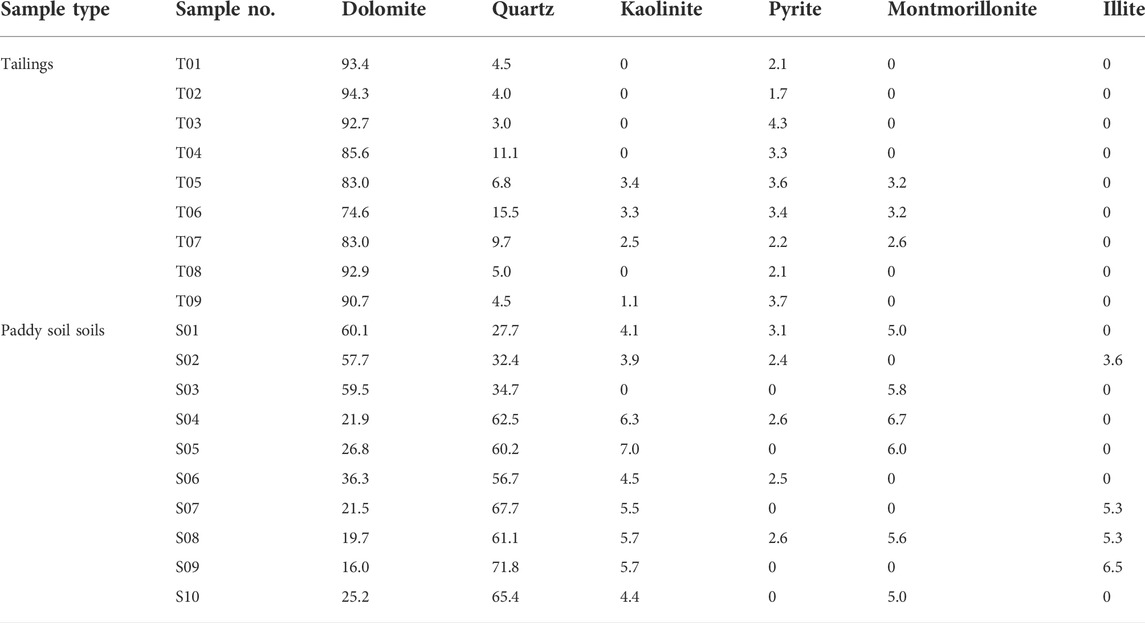
TABLE 1. Mineralogical composition (%) of the tailing and paddy soil samples based upon XRD patterns.
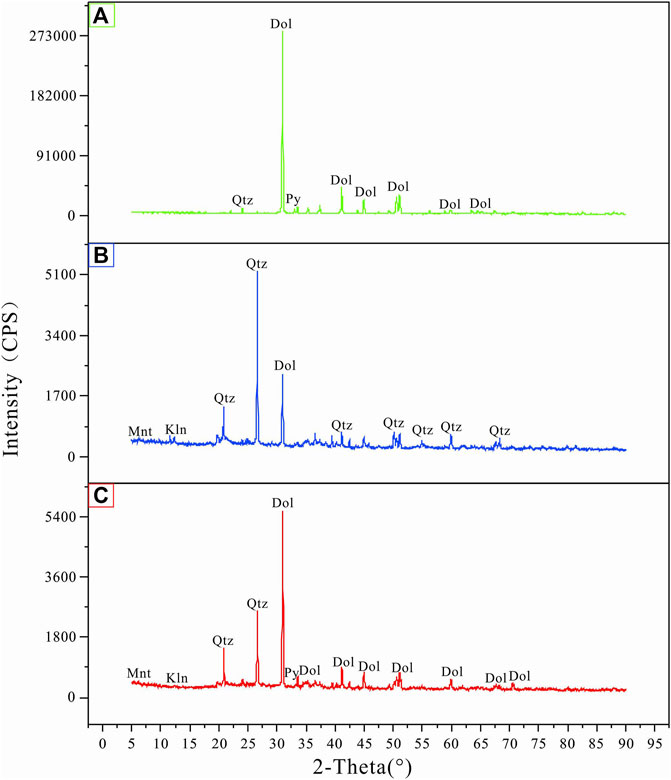
FIGURE 2. Representative XRD patterns of the tailing and soil samples. (A) T02 tailing, (B) S03 paddy soil, (C) S05 paddy soil. Dol: dolomite, Qtz: quartz, Mnt: Montmorillonite, Kln: Kaolinite, Py: pyrite.
3.2 pH of the tailings and soils
All tailing and soil samples present slightly alkaline with an average pH of 7.64 and 7.55, respectively (Table 2). The result that dolomite is dominant in tailing and soil samples is consistent with the karst background of the study area.
3.3 Total concentration of Cd, Pb, and Zn
The total contents of heavy metal elements Cd, Pb, and Zn in tailings and farmland soils are shown in Table 2. The maximum value of 55.1 mg/kg of Cd occurring in the Heli tailing dump and the minimum value of 10.6 mg/kg occurring in farmland soils. The Cd content in the Niujiaotang Pb-Zn tailing is comparable to the level of the Jalta tailing (55 mg/kg) in Northern Tunisia, a typical MVT Zn-Pb mining area (Boussen et al., 2013). The content of Cd in the paddy soil samples near Fanjia River (10.6–53.4 mg/kg, average 30.8 mg/kg) exceeded the risk screening value (0.8 mg/kg) and the risk control value (4.0 mg/kg) of the Chinese Soil Environmental (GB15618-2018). The maximum Cd level in the soil sample S04 (53.04 mg/kg) has reached more than 13 times of the risk control value of Cd, reflecting that the paddy soil has suffered from serious Cd contamination. The average values of Pb and Zn in the paddy soil samples were 214 and 2,667 mg/kg, respectively, which exceeded the risk screening values specified in the standard (240 mg/kg for Pb and 300 mg/kg for Zn) and were 6.7 and 28.2 times of the background values of soil in Guizhou Province (32.08 mg/kg and 94.57 mg/kg for Pb and Zn, respectively). It suggests that there is a greater risk of heavy metal Cd, Pb, and Zn contamination in the soil environment affected by the Niujiaotang Pb-Zn tailing dump. It is noteworthy that the contents of heavy metals (Cd, Zn, and Pb) in some paddy soils (S04-S06) were much higher than those from tailings. There are nine tailing dumps along the Fanjia River and four dumps are located in the upstream of the paddy soil sampling sites. Reported maximum average content of Cd, Zn, and Pb in the tailings is 174.85 mg/kg, 10,200 μg/g, and 900 μg/g respectively (Zhang et al., 2018). Therefore, we proposed other tailings with high heavy metal input to these paddy soils.
In the Zn-Cd elemental cross-plot (Figure 4A), eight tailing samples except tailing T09 showed a significant positive correlation with the paddy soils S01, S02, and S03 (n = 11, α < 0.001), while T09 was significantly positively correlated with S04, S05, S06, S07, S08, S09, and S10 (n = 8, α < 0.001). Correlation coefficient of dolomite content and Cd content in soil and sediment form Fanjiahe River r = 0.741 (Figure 4B). The tailing samples and soil samples present a better linear correlation for Zn and Pb (r = 0.877) than Zn and Cd (r = 0.659) (Figures 4C,D), which is odd with the affinity of Zn and Cd.
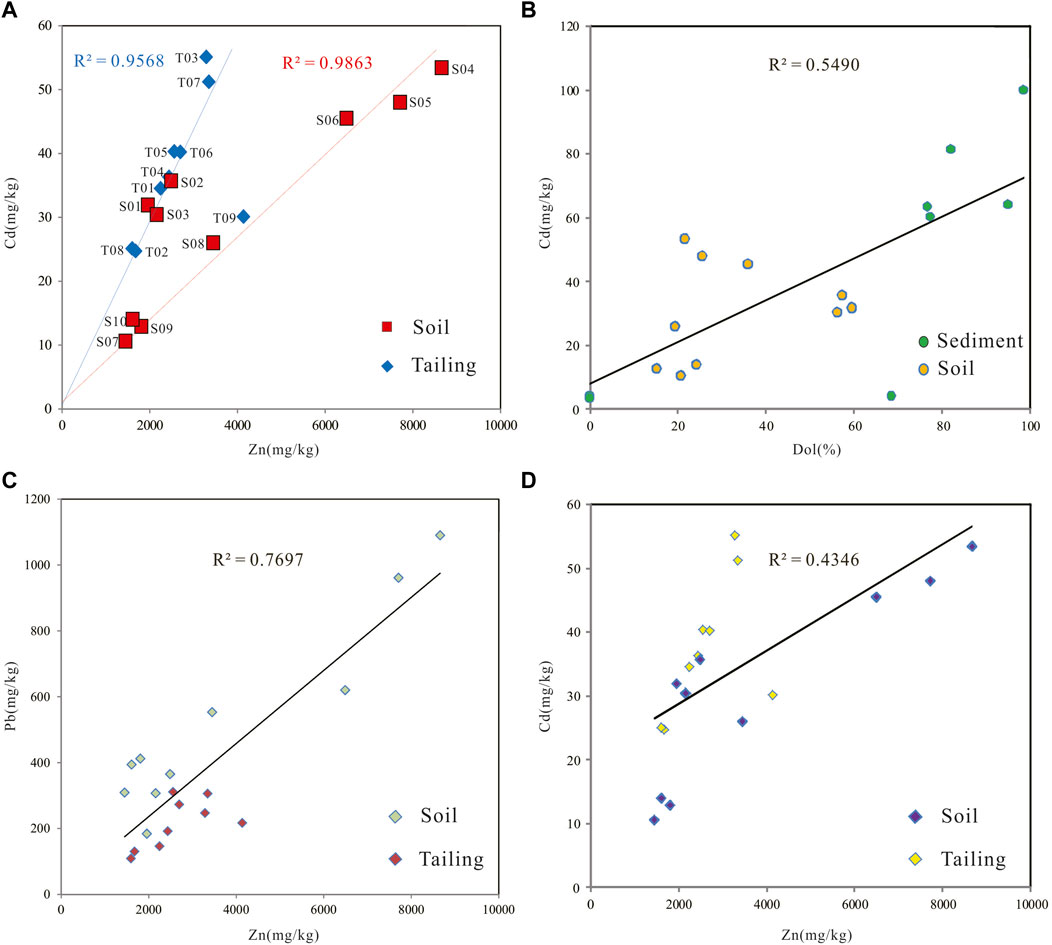
FIGURE 4. Cross-plot of Zn and Cd from tailing and soil samples (showing two different linear trends) (A) and correlation diagram between dolomite content and Cd content in soils and sediments from Fanjia River (B) (sediment samples from Liu et al. (2022a) (C) Cross-plot of Zn and Pb from tailing and soil samples (D) Linear correlation diagram between Zn and Cd contents from tailing and soil samples (showing total correlation).
3.4 Cd geochemical speciation in the tailings
The Cd contents and their percentages of acid extractable/exchangeable fraction (F1), easily reducible fraction (F2), the oxidizable fraction (F3), and the residual fraction (F4) were obtained by the improved BCR sequential extraction procedure, as shown in Table 3; Figure 5. The Cd contents of various fractions varied among tailing samples with the average contents of Cd in the F1, F2, F3, and F4 19.56 mg/kg, 11.07 mg/kg, 2.53 mg/kg, and 3.94 mg/kg, accounting for 52.72%, 29.84%, 6.82%, and 10.62% of the total Cd content, respectively. The average Cd content of F2 much greater than that of F3 is unexpected given the sulfide ore being the main mining and processing object in the Niujiaotang Zn mine, which indicates that a great portion of pyrite and/or sphalerite have been weathered and oxidized in the tailings as evidenced from Figure 1D. It is noteworthy that sample WK09 has the highest percentage Cd in F1, reaching 93% of the total Cd level. This reflects that cadmium mainly exists in carbonate minerals.
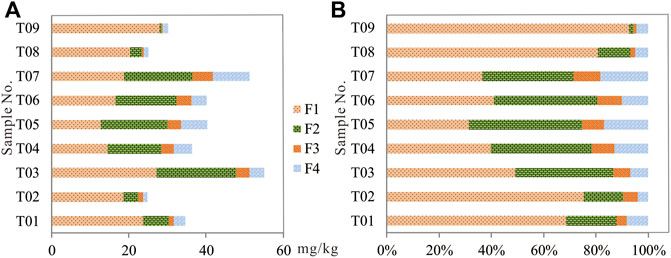
FIGURE 5. Tailing Cd concentration (A) and percentage (B) distribution in various fractions by modified BCR procedure.
3.5 Cd host phases in the tailings
The first step (F1) in the BCR procedure consists of exchangeable fraction and fraction bound to carbonates. The exchangeable fraction is commonly attributed to the metal that is loosely held by the clay minerals. As discussed in Section 3.1, kaolinite is the dominant clay mineral in the tailing samples. The reported Cd adsorption capacity of kaolinite is ranged from 3.0 mg/g to 14.11 mg/g dependent upon kaolinite purity and adsorption conditions (Ulmanu et al., 2003; Bhattacharyya and Gupta, 2008; Sari and Tuzen, 2014). Given the low cation exchange capacity of kaolinite and its low content in the tailing samples (Table 2; Figure 3), kaolinite is not regarded as the candidate phase to host Cd in the first step of the BCR procedure. Thus, the candidate phase in F1 fraction is a most likely carbonate mineral, namely, dolomite.
Cadmium has a similar ion radio to Ca, therefore, Cd can substitute Ca in the dolomite crystal lattice to form (Cd, Ca)Mg(CO3)2 (Goldsmith, 1972). Cadmium incorporated into dolomite is also through otavite (CdCO3) surface precipitate (Callagon et al., 2017; La Plante et al., 2018). As a result, dolomite is widely used to remove Cd in the environmental media (Mohammadi et al., 2015; Liu et al., 2021; Zhang and Liu, 2022). In Section 3.1, we suggested that dolomite is the predominant mineral in the tailing samples by XRD and SEM-EDS. Improved BCR sequential procedure demonstrates that Cd bound to carbonate is the main fraction of total Cd in the tailing samples. Dolomite in the tailing samples is derived from hydrothermal dolomite in ores and host rocks. Ye et al. (2011) reported that the average Cd content in the hydrothermal dolomite (n = 7) and host rock dolomite (n = 10) is 190 mg/kg and 30 mg/kg, respectively. The average Cd content in bulk samples (35 mg/kg) and F1 fraction (23 mg/kg) of the tailings indicate that dolomite in the host rock is the prevailing Cd host phase. Low average Zn grade (16.9%) of the ores and disseminated and brecciated texture of the ores (Ye et al., 2011) provide the evidence that dolomite from the wall rock predominated over hydrothermal dolomite.
The improved BCR sequential extraction result demonstrates that the easily reducible fraction and oxidizable fraction account for most of the rest of cadmium except for one bound to dolomite. As introduced in Section 3.4, these two fractions are ascribed to sulfide-bound cadmium in un-weathered tailings. While XRD and SEM-EDX analyses suggested that pyrite is the major sulfide mineral and no sphalerite and galena were detected, we propose that pyrite is the major Cd host phase of sulfide minerals in the fresh tailings. This argument is also evidenced by the consistent elemental mapping of Cd, Fe, and S from SEM-EDX analyses of the tailing samples. When pyrite was precipitated from Cd-bearing aqueous solution, Cd was incorporated into pyrite by interaction with the surface of the pyrite (Parkman et al., 1999; Borah and Senapati, 2006). Ye et al. (2011) reported that pyrite in the ore of the Niujiaotang has a Cd content of 183 mg/kg to 2500 mg/kg with an average of 1,345 mg/kg (n = 4). Therefore, pyrite is the important Cd-host phase following dolomite in the primary tailing of the Niujiaotang mine.
When the pyrite was exposed to ambient air and water, it was oxidized by microbial-mediated reactions (Singer and Stumm, 1970):
In the neutral or alkaline pH environment of the dolomite-dominated tailings, the ferrous ions (Fe2+) produced by the oxidation of pyrite (Eq. 1) were further oxidized by dissolved oxygen to form Fe3+ (Eq. 2), which was hydrolyzed to form iron (oxyhydr) oxides (Eq. 3; Figure 1D). Thus, iron (oxyhydr) oxides formation prevents ferric iron (Fe3+) from further oxidizing pyrite (Eq. 4). Since the primary ore mined at the Niujiaotang Zn mine is mainly sphalerite and pyrite, the iron (oxyhydr) oxides in the tailing dump are likely formed by the oxidation of pyrite within it. Meanwhile, the oxidative weathering process of pyrite released the Cd in it, which is consistent with the study of Ettler (2016) and others. Iron (oxyhydr)oxides of the tailings in Niujiaotang are amorphous material, rather than goethite (α-FeOOH), lepidocrocite (γ-FeOOH) and/or hematite (α-Fe2O3), as evidenced by the absence of the diffraction peaks of the goethite, lepidocrocite and hematite shown in the XRD patterns of the tailing samples. We hypothesize that iron (oxyhydr) oxide in the Niujiaotang tailings is ferrihydrite. Ferrihydrite has a high specific surface area and a significant number of reactive hydroxyl on the surface which interacts with cadmium in aqueous solution to form mono-nuclear or bi-nuclear complexes (Shi et al., 2020; Li et al., 2021). Therefore, the F2 fraction in the BCR procedure is Cd hosted by ferrihydrite which was formed by pyrite oxidation and scavenged Cd from pyrite oxidation. Khoeurn et al. (2019) reached a similar conclusion by leaching experiments for tailings.
The residual fraction of the BCR procedure is Cd incorporated into the silicate crystal lattice and/or cannot be extracted by the reactants in three steps and accounts for a small percentage in the tailing samples. For its low bioavailability, we do not discuss it further.
3.6 Environmental risk of the Cd polluted paddy soil affected by Zn tailings
The mean values of Cd, Pb, and Zn in paddy soils were 30.8 mg/kg, 519 mg/kg, and 3,778 mg/kg, respectively, which were much higher than the background values of soil heavy metals in Guizhou Province (Cd, Pb, and Zn were 1.75 mg/kg, 32.08 mg/kg, and 94.57 mg/kg, respectively) (Zhu et al., 2014). Thus, it indicates that exogenous heavy metals were added to the paddy soils, most likely due to the migration of heavy metals from the Zn tailings to the Caiyuan River which is the most important irrigation water source for the paddy soils. Based on the migration mechanism of tailing and the chemical mechanism of dolomite dissolution, we establish the physical process of tailing migration to soil and the conceptual model of Cd environmental risk (Figure 6).
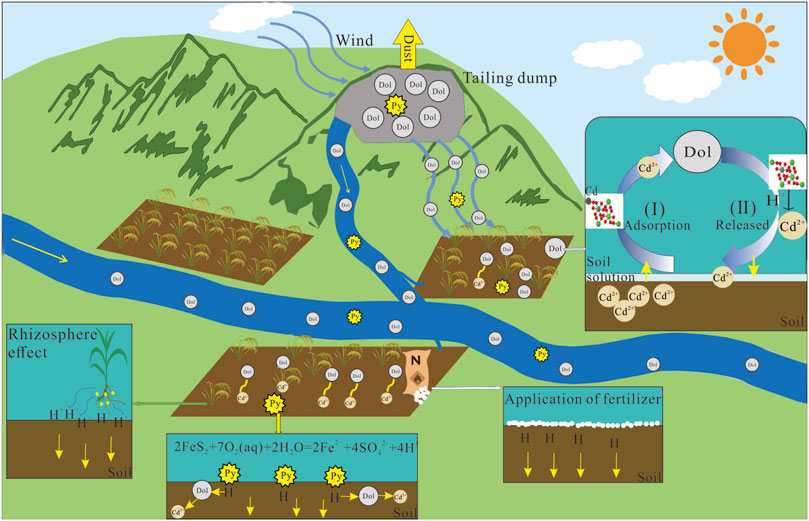
FIGURE 6. Conceptual model of soil environmental risk from Cd-rich dolomite derived from Zn mine tailing.
The XRD results found that high content of dolomite was present in both the tailings and the paddy soil (Figure 2). Mineralogy of the paddy soil in the background area not affected by Zn tailings is characterized by the dominance of quartz and illite and the absence of dolomite (Liu et al., 2022b). Combined with the similar size distribution of tailing and paddy soil, we speculate that the physical migration of Zn mine tailing is the major path of heavy metal Cd, Zn, and Pb enrichment in agricultural soils around the Caiyuan River. Grain size distribution, dolomite dominance, and its close relation to Cd content in the paddy soil and sediment samples (Figure 4), no dissolved Cd was detected in the Caiyuan River and Cd isotope composition (Liu et al., 2022b) of the paddy soils and sediments together point to dolomite is the predominant Cd host phase in the paddy soils. Thus, most Cd transport to the paddy soil from the tailing is physically through wind and surface runoff. In addition, significant correlations between Mg and Zn contents of sediments and paddy soils (Xia et al., 2020) also provide evidence that Zn, like Cd, is hosted predominantly by dolomite and was physically migrated to the surrounding soil and sediment.
Dolomite-dominated Cd enrichment in the paddy soils has two contrary effects on its environmental risk. On the one hand, elevated pH and dolomite’s high affinity for Cd immobilize Cd of the paddy soil through adsorption (Wang et al., 2001; Liu et al., 2022a). As a result, liming (e.g., addition of carbonate minerals) of acid paddy soil has become the economic and effective measure to decrease Cd accumulation in rice grain. On the other hand, the addition of Cd-rich dolomite from tailings to the paddy soil greatly increases environment risk given Cd levels in the paddy soil samples are 3.2–13.4 times than that of the risk control value (4.0 mg/kg) of the Chinese Soil Environmental (GB15618-2018).
It is widely agreed that Cd bioavailability in soils is highly pH dependent (Ma and Uren, 1998; Rajaie et al., 2006; Kubier et al., 2019; Feng et al., 2021). The decrease of soil pH enhances Cd entering rice grain and poses a potential environmental risk. Local decrease of soil pH could be caused by pyrite oxidation from tailings as indicated in Eqs 1–4 and evidenced from sample S05 in Figure 2. The agricultural soil acidification resulting from long-term nitrogen fertilizer addition is another possible reason for soil pH decrease (Li et al., 2019). Moreover, soil pH decrease is also caused by high CO2 levels after the flooding of the paddy soil (Kirk, 2004). Therefore, Cd solubility and bioavailability in paddy soil would increase about four folds with one unit pH decrease (Smolders and Mertens, 2012; Wang et al., 2019b). A large-scale survey demonstrates that 16 fold increase in Cd transfer ratio when soil pH decrease from 8.0 to 7.0 (Zhu et al., 2016). In the presence of dolomite, pH decrease from alkaline to neutral and an increase of sulfate generated by pyrite oxidation together facilitate dolomite dissolution (Pokrovsky and Schott, 2001). As a result, Cd released from the dolomite to the paddy soil solution. To evaluate Cd levels in rice grain compared to the national standard (0.2 mg/kg), we use the models developed by Zhu et al. (2016) to predict Cd contents in rice grain based upon total Cd contents and pH values of the paddy soils. Predicted average Cd contents of the rice grain is 0.98 ± 1.06 mg/kg, which points to the high Cd environmental risk posed by rice consumption. Accordingly, considering the high Cd levels in the paddy soil, the environmental risk of Cd from paddy soil to rice grain is of great concern.
As mentioned before, MVT zinc deposits are highly Cd enriched compared to other types of zinc deposits (Schwartz, 2000). Gangue mineral in MVT zinc deposit is dominated by calcite and/or dolomite. As the similar ion radius of Ca and Cd, Cd also is incorporated into calcite and/or dolomite by substituting Ca. However, although previous works paid great focus on Cd distribution in sphalerite, some studies conducted Cd speciation and found high Cd contents in carbonate minerals of the tailing samples (Carroll et al., 1998; O’Day et al., 1998; Boussen et al., 2013). Cd contents in oolitic carbonates in the Jura Mountains of Switzerland provide another line of evidence of Cd chiefly hosted by carbonate minerals associated with hydrothermal Zn mineralization (Quezada-Hinojosa et al., 2009; Jacquat et al., 2011). Given the dominance of calcite and/or dolomite in the tailings from MVT zinc mines, environmental risk derived from high Cd carbonate minerals in the tailings of MVT zinc deposits should be carefully assessed in future works.
4 Conclusion
We conducted a systematic work on the mineralogy of the Niujiaotang Cd-rich Zn tailings by XRD, SEM-EDS, grain size distribution, and BCR geochemical speciation of Cd. The results suggested that dolomite is the predominant Cd host phase in the Zn mine tailing. Cadmium was transported to the surrounding paddy soils from the tailing by runoff and aeolian processes. The paddy soils affected by Zn mine tailing had Cd contents greatly exceeding China’s national control standard and their high Cd contents also originated from Cd-rich dolomite accumulation derived from the tailing. The integrated evaluation demonstrates that the paddy soils affected by the tailing have a great environmental risk for the populations by rice consumption despite the slightly alkaline pH values. This study sheds new light on Cd speciation of tailings in MVT Zn deposits and its environmental and ecological risk for the tailing affecting soils .
Data availability statement
The original contributions presented in the study are included in the article/supplementary material, further inquiries can be directed to the corresponding author.
Author contributions
Conceptualization, SG, QL, and ZG; methodology, SG, QL, ZG, TZ, FZ, and XX; chemical analysis, ZG, TZ, FZ, and XX; investigation, SG, ZG, TZ, FZ, and XX; writing—original draft preparation, ZG, TZ, and XX; writing—review and editing, ZG, SG, and QL; supervision, SG; project administration, SG; funding acquisition, SG All authors have read and agreed to the published version of the manuscript.
Funding
This research was funded by the National Key R and D Program of China (No. 2018YFC1802601).
Acknowledgments
We would like to thank Jiangxun Huang at Guizhou University for his help in the laboratory experiment.
Conflict of interest
The authors declare that the research was conducted in the absence of any commercial or financial relationships that could be construed as a potential conflict of interest.
Publisher’s note
All claims expressed in this article are solely those of the authors and do not necessarily represent those of their affiliated organizations, or those of the publisher, the editors and the reviewers. Any product that may be evaluated in this article, or claim that may be made by its manufacturer, is not guaranteed or endorsed by the publisher.
References
Alan, M., and Kara, D. (2019). Comparison of a new sequential extraction method and the BCR sequential extraction method for mobility assessment of elements around boron mines in Turkey. Talanta 194, 189–198. doi:10.1016/j.talanta.2018.10.030
Aoshima, K. (2016). Itai-itai disease: Renal tubular osteomalacia induced by environmental exposure to cadmium—Historical review and perspectives. Soil Sci. Plant Nutr. 64 (4), 319–326. doi:10.1080/00380768.2016.1159116
Bhattacharyya, K. G., and Gupta, S. S. (2008). Adsorption of a few heavy metals on natural and modified kaolinite and montmorillonite: A review. Adv. Colloid Interface Sci. 140 (2), 114–131. doi:10.1016/j.cis.2007.12.008
Borah, D., and Senapati, K. (2006). Adsorption of Cd (II) from aqueous solution onto pyrite. Fuel 85 (12-13), 1929–1934. doi:10.1016/j.fuel.2006.01.012
Boussen, S., Soubrand, M., Bril, H., Ouerfelli, K., and Abdeljaouad, S. (2013). Transfer of lead, zinc and cadmium from mine tailings to wheat (Triticum aestivum) in carbonated Mediterranean (Northern Tunisia) soils. Geoderma 192, 227–236. doi:10.1016/j.geoderma.2012.08.029
Callagon, E. B. R., Lee, S. S., Eng, P. J., Laanait, N., Sturchio, N. C., Nagy, K. L., et al. (2017). Heteroepitaxial growth of cadmium carbonate at dolomite and calcite surfaces: Mechanisms and rates. Geochim. Cosmochim. Acta 205, 360–380. doi:10.1016/j.gca.2016.12.007
Carroll, S. A., O'day, P. A., and Piechowski, M. (1998). Rock-Water interactions controlling zinc, cadmium, and lead concentrations in surface waters and sediments, US Tri-state Mining District. 2. Geochemical interpretation. Environ. Sci. Technol. 32 (7), 956–965. doi:10.1021/es970452k
Chen, H. P., Yang, X. P., Wang, P., Wang, Z. X., Li, M., and Zhao, F. J. (2018). Dietary cadmium intake from rice and vegetables and potential health risk: A case study in xiangtan, southern China. Sci. Total Environ. 639, 271–277. doi:10.1016/j.scitotenv.2018.05.050
Clemens, S., and Ma, J. F. (2016). Toxic heavy metal and metalloid accumulation in crop plants and foods. Annu. Rev. Plant Biol. 67 (1), 489–512. doi:10.1146/annurev-arplant-043015-112301
Ettler, V. (2016). Soil contamination near non-ferrous metal smelters: A review. Appl. Geochem. 64, 56–74. doi:10.1016/j.apgeochem.2015.09.020
Feng, J., Shen, R. F., and Shao, J. F. (2021). Transport of cadmium from soil to grain in cereal crops: A review. Pedosphere 31 (1), 3–10. doi:10.1016/S1002-0160(20)60015-7
Förstner, U. (1993). Metal speciation-general concepts and applications. Int. J. Environ. Anal. Chem. 51, 5–23. doi:10.1080/03067319308027608
Frenzel, M., Hirsch, T., and Gutzmer, J. (2016). Gallium, germanium, indium, and other trace and minor elements in sphalerite as a function of deposit type—a meta-analysis. Ore Geol. Rev. 76, 52–78. doi:10.1016/j.oregeorev.2015.12.017
Goldsmith, J. R. (1972). Cadmium dolomite and the system CdCO3-MgCO3. J. Geol. 80, 617–626. doi:10.1086/627785
Hsu, L. C., Liu, Y. T., and Tzou, Y. M. (2015). Comparison of the spectroscopic speciation and chemical fractionation of chromium in contaminated paddy soils. J. Hazard. Mat. 296, 230–238. doi:10.1016/j.jhazmat.2015.03.044
Inaba, T., Kobayashi, E., Suwazono, Y., Uetani, M., Oishi, M., Nakagawa, H., et al. (2005). Estimation of cumulative cadmium intake causing Itai-itai disease. Toxicol. Lett. 159 (2), 192–201. doi:10.1016/j.toxlet.2005.05.011
Jacquat, O., Rambeau, C., Voegelin, A., Efimenko, N., Villard, A., Föllmi, K. B., et al. (2011). Origin of high Zn contents in jurassic limestone of the Jura mountain range and the burgundy: Evidence from Zn speciation and distribution. Swiss J. Geosci. 104 (3), 409–424. doi:10.1007/s00015-011-0086-9
Kan, X., Dong, Y., Feng, L., Zhou, M., and Hou, H. (2020). Contamination and health risk assessment of heavy metals in China’s lead-zinc mine tailings: A meta-analysis. Chemosphere 267, 128909. doi:10.1016/j.chemosphere.2020.128909
Khoeurn, K., Sakaguchi, A., Tomiyama, S., and Igarashi, T. (2019). Long-term acid generation and heavy metal leaching from the tailings of Shimokawa mine, Hokkaido, Japan: Column study under natural condition. J. Geochem. Explor. 201, 1–12. doi:10.1016/j.gexplo.2019.03.003
Kirk, G. (2004). “Reduction and oxidation,” in The biogeochemistry of submerged soils (Chichester: John Wiley and Sons), 93–131.
Kubier, A., Wilkin, R. T., and Pichler, T. (2019). Cadmium in soils and groundwater: A review. Appl. Geochem. 108, 104388. doi:10.1016/j.apgeochem.2019.104388
La Plante, E. C., Lee, S. S., Eng, P. J., Stubbs, J. E., Fenter, P., Sturchio, N. C., et al. (2018). Dissolution kinetics of epitaxial cadmium carbonate overgrowths on dolomite. ACS Earth Space Chem. 3 (2), 212–220. doi:10.1021/acsearthspacechem.8b00115
Hedenquist, J. W., Thompson, J. F. H., Goldfarb, R. J., and Richards, J. P. (2005). “Sediment-hosted lead-zinc deposit: A global perspective,” in Economic Geology One Hundredth Anniversary Volume . Editors D. L. Leach (Littleton, Colorado: Society of Economic Geologists, inc.), 561–607. doi:10.5382/AV100.18
Li, Q., Li, S., Xiao, Y., Zhao, B., Wang, C., Li, B., et al. (2019). Soil acidification and its influencing factors in the purple hilly area of southwest China from 1981 to 2012. Catena 175, 278–285. doi:10.1016/j.catena.2018.12.025
Li, P., Luo, S., Zhang, L., Wang, Q., Huang, X., Zhang, Y., et al. (2021). Study on preparation and performance of iron tailings-based porous ceramsite filter materials for water treatment. Sep. Purif. Technol. 276, 119380. doi:10.1016/j.seppur.2021.119380
Liang, Q., jing, H., and Gregoire, D. C. (2000). Determination of trace elements in granites by inductively coupled plasma mass spectrometry. Talanta 51 (3), 507–513. doi:10.1016/S0039-9140(99)00318-5
Liu, B., He, Z., Liu, R., Montenegro, A. C., Ellis, M., Li, Q., et al. (2021). Comparative effectiveness of activated dolomite phosphate rock and biochar for immobilizing cadmium and lead in soils. Chemosphere 266, 129202. doi:10.1016/j.chemosphere.2020.129202
Liu, H., Zhang, T., Zhu, Q., Huang, D., and Zeng, X. (2022a). Effect of humic and calcareous substance amendments on the availability of cadmium in paddy soil and its accumulation in rice. Ecotoxicol. Environ. Saf. 231, 113186. doi:10.1016/j.ecoenv.2022.113186
Liu, Y. H., Xia, Y. F., Wang, Z. R., Gao, T., Zhu, J. M., Qi, M., et al. (2022b). Lithologic controls on the mobility of Cd in mining-impacted watersheds revealed by stable Cd isotopes. Water Res. 220, 118619. doi:10.1016/j.watres.2022.118619
Lohmann, M. S., Ramezany, S., Kästner, F., Raab, T., Heinrich, M., and Grimm, M. (2022). Using modified Tessier sequential extraction to specify potentially toxic metals at a former sewage farm. J. Environ. Manag. 304, 114229. doi:10.1016/j.jenvman.2021.114229
Long, Z., Zhu, H., Bing, H., Tian, X., Wang, X., Ma, Z., et al. (2022). Predicting soil cadmium uptake by plants in a tailings reservoir during 48-year vegetation restoration. Sci. Total Environ. 818, 151802. doi:10.1016/j.scitotenv.2021.151802
Luo, J., Xing, W. Q., Ippolito, J. A., Zhao, L. L., Han, K., Wang, Y. L., et al. (2022). Bioaccessibility, source and human health risk of Pb, Cd, Cu and Zn in windowsill dusts from an area affected by long-term Pb smelting. Sci. Total Environ. 842, 156707. doi:10.1016/j.scitotenv.2022.156707
Ma, Y. B., and Uren, N. C. (1998). Transformations of heavy metals added to soil — Application of a new sequential extraction procedure. Geoderma 84 (1-3), 157–168. doi:10.1016/S0016-7061(97)00126-2
Mamatchi, M., Rajaram, R., Ganeshkumar, A., Kpemissi, M., Pakoussi, T., Agbere, S., et al. (2022). Assessment of renal and hepatic dysfunction by co-exposure to toxic metals (Cd, Pb) and fluoride in people living nearby an industrial zone. J. Trace. Elem. Med. Bio. 69, 126890. doi:10.1016/j.jtemb.2021.126890
Mazeina, L. P., and Bessonov, D. U. (1999). Zinc and Cadmium behaviour in tailings. Geochemistry of the Earth's Surface: Proceedings of the 5th international symposium. Reykjavik, 16-20 August 1999. Rotterdam, Netherlands: CRC Press, 207.
Mohammadi, M., Ghaemi, A., Torab-Mostaedi, M., Asadollahzadeh, M., and Hemmati, A. (2015). Adsorption of cadmium (II) and nickel (II) on dolomite powder. Desalination Water Treat. 53 (1), 149–157. doi:10.1080/19443994.2013.836990
Nriagu, J. O. (1990). Global metal pollution: Poisoning the biosphere? Environ. Sci. Policy Sustain. Dev. 32 (7), 7–33. doi:10.1080/00139157.1990.9929037
O'Day, P. A., Carroll, S. A., and Waychunas, G. A. (1998). Rock-water interactions controlling zinc, cadmium, and lead concentrations in surface waters and sediments, US Tri-State mining district. 1. Molecular identification using X-ray absorption spectroscopy. Environ. Sci. Technol. 32 (7), 943–955. doi:10.1021/es970453c
Pacyna, J. M., and Pacyna, E. G. (2001). An assessment of global and regional emissions of trace metals to the atmosphere from anthropogenic sources worldwide. Environ. Rev. 9 (4), 269–298. doi:10.1139/a01-012
Parkman, R. H., Charnock, J. M., Bryan, N. D., Livens, F. R., and Vaughan, D. J. (1999). Reactions of copper and cadmium ions in aqueous solution with goethite, lepidocrocite, mackinawite, and pyrite. Am. Mineral. 84 (3), 407–419. doi:10.2138/am-1999-0326
Pokrovsky, O. S., and Schott, J. (2001). Kinetics and mechanisms of dolomite dissolution in neutral to alkaline solutions revisited. Am. J. Sci. 301 (7), 597–626. doi:10.2475/ajs.301.7.597
Quezada-Hinojosa, R. P., Matera, V., Adatte, T., Rambeau, C., and Föllmi, K. B. (2009). Cadmium distribution in soils covering Jurassic oolitic limestone with high Cd contents in the Swiss Jura. Geoderma 150 (3-4), 287–301. doi:10.1016/j.geoderma.2009.02.013
Qureshi, A. A., Kazi, T. G., Baig, J. A., Arain, M. B., and Afridi, A. H. (2020). Exposure of heavy metals in coal gangue soil, in and outside the mining area using BCR conventional and vortex assisted and single step extraction methods. Impact on orchard grass. Chemosphere 255, 126960. doi:10.1016/j.Chemosphere.2020.126960
Rajaie, M., Karimian, N., Maftoun, M., Yasrebi, J., and Assad, M. T. (2006). Chemical forms of cadmium in two calcareous soil textural classes as affected by application of cadmium-enriched compost and incubation time. Geoderma 136 (3-4), 533–541. doi:10.1016/j.geoderma.2006.04.007
Rauret, G., López-Sánchez, J. F., Sahuquillo, A., Rubio, R., Davidson, C., Ure, A., et al. (1999). Improvement of the BCR three step sequential extraction procedure prior to the certification of new sediment and soil reference materials. J. Environ. Monit. 1 (1), 57–61. doi:10.1039/a807854h
Richardson, M. G., Garrett, R., Mitchell, I. A., Mah-Paulson, M., and Hackbarth, T. (2001). “Critical review on natural global and regional emissions of six trace metals to the atmosphere,” in International lead zinc research organisation, the international copper association, and the nickel producers environmental research association, 52.
Rodríguez, L., Ruiz, E., Alonso-Azcárate, J., and Rincón, J. (2009). Heavy metal distribution and chemical speciation in tailings and soils around a Pb-Zn mine in Spain. J. Environ. Manage. 90 (2), 1106–1116. doi:10.1016/j.jenvman.2008.04.007
Rosado, D., Usero, J., and Morillo, J. (2016). Ability of 3 extraction methods (BCR, Tessier and protease K) to estimate bioavailable metals in sediments from Huelva estuary (Southwestern Spain). Mar. Pollut. Bull. 102 (1), 65–71. doi:10.1016/j.marpolbul.2015.11.057
Sari, A., and Tuzen, M. (2014). Cd (II) adsorption from aqueous solution by raw and modified kaolinite. Appl. Clay Sci. 88, 63–72. doi:10.1016/j.clay.2013.12.021
Sasmaz, M., Öbek, E., and Sasmaz, A. (2019). Bioaccumulation of cadmium and thallium in Pb-Zn tailing waste water by Lemna minor and Lemna gibba. Appl. Geochem. 100, 287–292. doi:10.1016/j.apgeochem.2018.12.011
Schwartz, M. O. (2000). Cadmium in zinc deposits: Economic geology of a polluting element. Int. Geol. Rev. 42 (5), 445–469. doi:10.1080/00206810009465091
Shi, M. Q., Min, X. B., Ke, Y., Lin, Z., Yang, Z. H., Wang, S., et al. (2020). Recent progress in understanding the mechanism of heavy metals retention by iron (oxyhydr)oxides. Sci. Total Environ. 752, 141930. doi:10.1016/j.scitotenv.2020.141930
Singer, P. C., and Stumm, W. (1970). Acidic mine drainage: The rate-determining step. Science 167 (3921), 1121–1123. doi:10.1126/science.167.3921.1121
Smieja-Król, B., Pawlyta, M., and Gałka, M. (2022). Ultrafine multi-metal (Zn, Cd, Pb) sulfide aggregates formation in periodically water-logged organic soil. Sci. Total Environ. 820, 153308. doi:10.1016/j.scitotenv.2022.153308
Smolders, E., and Mertens, J. (2012). “Chapter 10. Cadmium,” in Heavy metals in soils: Trace metals and metalloids in soils and their bioavailability. Editor B. J. Alloway (Dordrecht: Springer Science + Business Media), 283–311.
Sun, R. G., Gao, Y., and Yang, Y. (2022). Leaching of heavy metals from lead-zinc mine tailings and the subsequent migration and transformation characteristics in paddy soil. Chemosphere 291, 132792. doi:10.1016/j.chemosphere.2021.132792
Tao, M., Zhang, X., Wang, S., Cao, W., and Jiang, Y. (2019). Life cycle assessment on lead-zinc ore mining and beneficiation in China. J. Clean. Prod. 237, 117833. doi:10.1016/j.jclepro.2019.117833
Tessier, A., Campbell, P. G. C., and Bisson, M. (1979). Sequential extraction procedure for the speciation of particulate trace metals. Anal. Chem. 51 (7), 844–851. doi:10.1021/ac50043a017
Uchimiya, M., Bannon, D., Nakanishi, H., McBride, M. B., Williams, M. A., and Yoshihara, T. (2020). Chemical speciation, plant uptake, and toxicity of heavy metals in agricultural soils. J. Agric. Food Chem. 68 (46), 12856–12869. doi:10.1021/acs.jafc.0c00183
Ulmanu, M., Marañón, E., Fernández, Y., Castrillón, L., Anger, I., and Dumitriu, D. (2003). Removal of copper and cadmium ions from diluted aqueous solutions by low cost and waste material adsorbents. Water Air Soil Pollut. 142 (1), 357–373. doi:10.1023/a:1022084721990
Wang, H., Zhou, W., and Lin, B. (2001). Effect of calcium carbonate on adsorptin and esorption of cadmium in soils. Acta Ecol. Sinica 21 (6), 932–937. doi:10.3321/j.issn:1000-0933.2001.06.012
Wang, J., Wang, P. M., Gu, Y., Kopittke, P. M., Zhao, F. J., and Wang, P. (2019a). Iron-manganese (oxyhydro)oxides, rather than oxidation of sulfides, determine mobilization of Cd during soil drainage in paddy soil systems. Environ. Sci. Technol. 53 (5), 2500–2508. doi:10.1021/acs.est.8b06863
Wang, P., Sun, Z., Hu, Y., and Cheng, H. (2019b). Leaching of heavy metals from abandoned mine tailings brought by precipitation and the associated environmental impact. Sci. Total Environ. 695, 133893. doi:10.1016/j.scitotenv.2019.133893
Wei, Q., Dong, L., Qin, W., Jiao, F., Qi, Z., Feng, C., et al. (2021). Efficient flotation recovery of lead and zinc from refractory lead-zinc ores under low alkaline conditions. Geochemistry 81 (4), 125769. doi:10.1016/j.chemer.2021.125769
Xia, Y., Gao, T., Liu, Y., Wang, Z., Liu, C., Wu, Q., et al. (2020). Zinc isotope revealing zinc's sources and transport processes in karst region. Sci. Total Environ. 724, 138191. doi:10.1016/j.scitotenv.2020.138191
Xu, D., Zhan, C., Liu, H., and Lin, H. (2019). A critical review on environmental implications, recycling strategies, and ecological remediation for mine tailings. Environ. Sci. Pollut. Res. 26 (35), 35657–35669. doi:10.1007/s11356-019-06555-3
Yaciuk, P. A., Colombo, F., Lecomte, K. F., Micco, G. D., and Bohé, A. E. (2022). Cadmium sources, mobility, and natural attenuation in contrasting environments (carbonate-rich and carbonate-poor) in the Capillitas polymetallic mineral deposit, NW Argentina. Appl. Geochem. 136, 105152. doi:10.1016/j.apgeochem.2021.105152
Ye, L., Cook, N. J., Liu, T., Ciobanu, C. L., Gao, W., and Yang, Y. (2011). The Niujiaotang Cd-rich zinc deposit, Duyun, Guizhou province, southwest China: Ore Genesis and mechanisms of cadmium concentration. Min. Depos. 47 (6), 683–700. doi:10.1007/s00126-011-0386-z
Zhang, D., and Liu, Z. (2022). Immobilization of chromium and cadmium in contaminated soil using layered double hydroxides prepared from dolomite. Appl. Clay Sci. 228, 106654. doi:10.1016/j.clay.2022.106654
Zhang, J., Wei, H., Yang, R., Gao, J., and Ou, Y. (2018). Study on distribution characteristics of heavy metals in tailings from NiujiaotangLead-zinc mine area, Duyun city, Guizhou province. Nonferrous Met. Eng. 8 (1), 122–127. (in Chinese). doi:10.3969/j.issn.2095-1744.2018.01.024
Zhu, H. L., Liu, H. Y., Long, J. H., and Yan, Z. Y. (2014). Contamination characteristics analysis of heavy metals in soils of typical contaminated areas in Guizhou Province. Erath Environ. 42, 505–511. doi:10.14050/j.cnki.1672-9250.2014.04.017
Zhu, H. H., Chen, C., Xu, C., Zhu, Q. H., and Huang, D. Y. (2016). Effects of soil acidification and liming on the phytoavailability of cadmium in paddy soils of central subtropical China. Environ. Pollut. 219, 99–106. doi:10.1016/j.envpol.2016.10.043
Keywords: cadmium, dolomite, environmental risk, MVT Zn-Pb mine, niujiaotang, tailings
Citation: Guo Z, Gu S, Li Q, Zhang T, Xie X and Zhao F (2022) Carbonate mineral controls the transport of Cd from tailings to surrounding soils: An example from Cd-rich Niujiaotang Zn mine in Guizhou Province, Southwest China. Front. Environ. Sci. 10:1045093. doi: 10.3389/fenvs.2022.1045093
Received: 15 September 2022; Accepted: 03 October 2022;
Published: 17 October 2022.
Edited by:
Hongbiao Cui, Anhui University of Science and Technology, ChinaReviewed by:
Tangfu Xiao, Guangzhou University, ChinaYanshan Cui, University of Chinese Academy of Sciences, China
Hailong Liu, Yangzhou University, China
Copyright © 2022 Guo, Gu, Li, Zhang, Xie and Zhao. This is an open-access article distributed under the terms of the Creative Commons Attribution License (CC BY). The use, distribution or reproduction in other forums is permitted, provided the original author(s) and the copyright owner(s) are credited and that the original publication in this journal is cited, in accordance with accepted academic practice. No use, distribution or reproduction is permitted which does not comply with these terms.
*Correspondence: Shangyi Gu, sygu@gzu.edu.cn