- 1Key Laboratory of Mountain Surface Processes and Ecological Regulation, Institute of Mountain Hazards and Environment, Chinese Academy of Sciences, Chengdu, China
- 2Chinese Academy of Sciences, University of the Chinese Academy of Sciences, Beijing, China
This study presents the first detailed estimate of Rwanda’s nitrogen (N) flows and N footprint for food (NFfood) from 1961 to 2018. Low N fertilizer inputs, substandard production techniques, and inefficient agricultural management practices are focal causes of low crop yields, environmental pollution, and food insecurity. We therefore assessed the N budget, N use efficiency (NUE), virtual N factors (VNFs), soil N mining factors (SNMFs), and N footprint for the agro-food systems of Rwanda with consideration of scenarios of fertilized and unfertilized farms. The total N input to croplands increased from 14.6 kg N ha−1 yr−1 (1960s) to 34.1 kg N ha−1 yr−1 (2010–2018), while the total crop N uptake increased from 18 kg N ha−1yr−1 (1960s) to 28.2 kg N ha−1yr−1 (2010–2018), reflecting a decline of NUE from 124% (1960s) to 85% (2010–2018). Gaseous N losses of NH3, N2O, and NO increased from 0.45 (NH3), 0.03 (N2O), and 0.00 (NO) Gg N yr−1 (1960s) to 6.98 (NH3), 0.58 (N2O), and 0.10 (NO) Gg N yr−1 (2010–2018). Due to the low N inputs, SNMFs were in the range of 0.00 and 2.99 and the rice production, cash-crop production, and livestock production systems have greater SNMFs in Rwanda. The weighted NFfood per capita that presents the actual situation of fertilized and unfertilized croplands increased from 4.0 kg N cap−1 yr−1 (1960s) to 6.3 kg N cap−1 yr−1 (2010–2018). The NFfood per capita would increase from 3.5 kg N cap−1 yr−1 to 4.8 kg N cap−1 yr−1 under a scenario of all croplands without N fertilizer application and increase from 6.0 to 8.7 kg N cap−1 yr−1 under the situation of all croplands receiving N fertilizer. The per capita agro-food production accounted for approximately 58% of the national NFfood. The present study indicates that Rwanda is currently suffering from low N inputs, high soil N depletion, food insecurity, and environmental N losses. Therefore, suggesting that the implementation of N management policies of increasing agricultural N inputs and rehabilitating the degraded soils with organic amendments of human and animal waste needs to be carefully considered in Rwanda.
1 Introduction
By 2050, the world’s population is projected to reach 9.7 billion, with the populations of Sub-Saharan Africa (SSA) being doubled (United Nations, 2019). The rapid growth of the world population demands increasing food demand and production (Katz, 2020). These demands of agro-food products require a highly productive agroecosystems with high inputs of fertilizers, particularly nitrogen (N) fertilizers. Since the innovation of the Haber–Bosch process in the early 20th century, the N produced by the industrial Haber-Bosch process (220 × 109 kg N yr−1) was twice the natural N fixation (60 × 109 kg N yr−1) (Fowler et al., 2013). It should be noted that worldwide, synthetic N fertilizers (SNF) are not equally distributed and there are large differences in SNF use between the African continent and the other continents (Raza et al., 2018). Nevertheless, excess N applied to arable lands, particularly with low N use efficiency (NUE), has caused several issues, such as soil degradation, water pollution, and greenhouse gas emissions (Spiertz, 2009).
The rate of crop N uptake remains below 50% of total applied N, and over half of N inputs are released to the environment in gaseous and hydrological pathways (Lassaletta et al., 2014a). However, most SSA countries still use less than 7 kg ha−1 yr−1 (Hickman et al., 2015), resulting in low crop productivity and food insecurity. Improving crop NUE is critical to increasing crop productivity and enhancing environmental performance (Guo et al., 2017). The approach of N footprint (NF) is a useful tool to estimate the environmental impacts of N losses through the whole agro-food production chain (Leach et al., 2012; Einarsson and Cederberg, 2019). To date, the approach of NF estimation for the agro-food system has been applied worldwide, such as in China (Guo et al., 2017), Japan (Shibata et al., 2014), the United States (Leach et al., 2012), the United Kingdom (Stevens et al., 2014), Australia (Liang et al., 2016), Portugal (Cordovil et al., 2020), Egypt (Elrys et al., 2019b) and Tanzania (Hutton et al., 2017). These studies have indicated substantial variations in NF for agro-food systems across the world, and the NF per capita ranges from 7.0 kg N cap−1yr−1 in developing countries to over 100 kg N cap−1yr−1 in developed countries.
Rwanda, a landlocked country, has one of Africa’s densest populations, with 13 million people living on a surface area of 26,338 km2 (Worldometer, 2020). The agriculture sector employs more than 70% of the total population (FAO, 2015) and contributed 25% to the national gross domestic product (World Bank, 2018). Although the agriculture sector is growing, it is impossible to achieve enough crops and livestock products to feed the growing population (Abdulaziz et al., 2009; Taiz, 2013). Rwanda’s low N fertilizer input is insufficient to sustain crop productivity (Kelly et al., 2001). However, SNF application has increased by 187 folds in the last 5 decades, i.e., from 0.05 Gg N yr−1 to 4.30 Gg N yr−1. The daily diets of Rwandans are mainly based on cereals, starchy roots and tubers, beans, and cooking bananas (Custodio et al., 2019; Miklyaev et al., 2021), while rarely consuming livestock products (FAO, 2019). Although 19% of Rwandans are food insecure, 40% of the annual food produced is lost and/or wasted (World Bank, 2020).
In Rwanda, the main issues of agricultural N management include high N losses in fertilized arable lands and serious soil mining of N in unfertilized arable lands (Masso et al., 2017). Thus, it is a great challenge to manage agricultural N flows to promote food security, improve NUE while sustaining soil fertility, and minimize environmental pollution in Rwanda. Thus, to meet the challenges, it is urgent to improve our understanding of Rwanda’s N flow and budget of agro-food systems. Nevertheless, several studies have estimated the N budget and NF on national and global scales. There were few studies focused on developing countries, in particular the Sub-Saharan countries. No study has yet combined both estimates into a single study and counted for N mining issue. Therefore, we conducted the present study to estimate the national N budget and NF for the agro-food systems of Rwanda over the last 5 decades (1961–2018). The specific objectives of this study were 1) to estimate N flows and budget in the agro-food systems of Rwanda during 1961–2018, 2) to evaluate NF for Rwandan agro-food systems in comparison with other countries, and 3) to propose suitable agricultural N management practices for sustaining productivity while reducing environmental N pollution for the agro-food systems in Rwanda based on proposed future scenarios.
2 Materials and Methods
2.1 Data Collection
This study relies on secondary data from different publications and the Food and Agriculture Organization Corporate Statistical Database (FAOSTAT) (FAO, 2021) accessed in February 2021.
2.2 Description of Rwanda
Rwanda is located in Central Eastern Africa (1°56'25" S 29°52.433' E, GeoDatos, 2021). The mountains dominate the northwestern, while the central part landscape is characterized by continuing hills creating savanna, plains and swamps. It has a steep topography lying at an altitude ranging from 915 m to 4,486 m above sea level (Karamage et al., 2016). It shares borders with Uganda to the north, Burundi to the south, Tanzania to the east, and the Democratic Republic of Congo to the west (Worldatlas, 2019). The climate is temperate tropical, comprising two seasons of rain, the short rains (September to December) and the long rains (March to May); the dry seasons are a short dry season (January to February) and a prolonged dry season (June to August) (Karamage et al., 2016; Nyesheja et al., 2018). The annual mean national rainfall is 1,116 mm, and temperatures range from 16 to 20°C (Karamage et al., 2016).
In the past half-century, several changes have occurred in the food production (FP) system of Rwanda (Supplementary Table S1). The total population hugely increased (239%), and the rural areas decreased (13%). Agricultural area and arable land increased by (34%) and (128%), respectively. The yields increased for most of the crops, accounting as for maize:56%, wheat:104%, rice: 98%, and vegetables: 12%, whereas a decrease in the yields of fruits (42%) and sugar cane (41%) was recorded in the last 58 years (Supplementary Table S1). There was an exciting increase in livestock in the previous 5 decades, including cattle, goats, sheep, poultry, pigs, and rabbits (Supplementary Table S1). Protein consumption has tardily increased (9%), where animal protein proportion increased by 181% during the last 5 decades (Supplementary Table S1). The quantity of N fertilizers imported to Rwanda has generally increased, and nearly all have been used in agriculture over the previous 5 decades (Supplementary Table S2). Rwanda traded agricultural products with other nations; in the last 5 decades, both N imported and exported from Rwanda rose, with plant-derived food products dominating (Supplementary Table S2).
2.3 N Budget Calculation
This study estimated Rwanda’s overall N budget during the last 58 years (1961–2018). We quantified the total annual cultivated land by combining all cropland areas during the previous 58 years mentioned in the FAOSTAT. For cases where the sum of the cropland areas was higher than that stated in the FAOSTAT resources module, we retained the latest area to avoid overestimating the actual cropland area because two or more crops can be intercropped in the same year (Lassaletta et al., 2014a). We computed the total crop production per year by combining its annual harvest and N content (Lassaletta et al., 2014a). The total N input (TNI) applied to croplands was estimated by summing up the overall SNF, animal N manure (ANM), biological N fixation (BNF), and atmospheric N deposition (AND). Due to the lack of documentation related to N applied to plain, we did not account for N applied to grassland; we assumed that nearly all N fertilizers were added to croplands. The historical data on SNF and ANM consumptions were derived from the FAOSTAT. The quantity of BNF on croplands was calculated using a yield-based model by the following equation (Lassaletta et al., 2014a):
Where %Ndfa: the fraction of N up taken resulting from N fixation, Yield: the harvest produced (kg N ha−1yr−1), NHI: the N harvest index (a ratio of N collected in grain to the total N amassed in grain and straw), and BGN: a multiplicative factor that considers the share of underground fixation to total N2 fixation. We used a constant BNF rate ha−1for rice paddies and sugar cane suggested by Herridge et al. (2008).
We assessed the overall AND by multiplying the total cropland area for each year from the FAOSTAT with the regional AND estimated rate (Dentener et al., 2006). We further estimated the quantity of gaseous NH3 emissions after applying ANM and SNF during the study period following the regional NH3 volatilization emissions rate based on cropland types (wetland-rice and upland-crops) (Supplementary Table S3) described in (Bouwman et al., 2002a). Similarly, we estimated the NO volatilization rate based on developing countries’ emission factors (FAO, 2001). Data on N2O emissions from ANM and SNF was attained from the FAOSTAT. To estimate the total N trade, we considered the N amount of agro-products imported to or exported from Rwanda from 1961 to 2018, using data from the FAOSTAT. The total N import and export were computed using food product N concentrations from Lassaletta et al. (2014b).
2.4 NUE and N Surplus
We calculated the NUE and N surplus based on the equations below (Elrys et al., 2020).
2.5 NF of the Agro-Food System
We expressed NF for food (NFfood) as the whole quantity of reactive N (Nr) released to the environment from losses associated with FP and food consumption (FC). The entire losses from the FP to FC chain are called food production NF (FPNF), while the total amounts of N consumed by the citizens are called food consumption NF (FCNF). The estimation of the total NFfood in Rwanda was developed based on the modified N-calculator version (Leach et al., 2012), following the equation below:
Where i and n symbolize various food products and the food product numbers, respectively.
We obtained data on FC from the FAOSTAT. To compute NFfood, data for specific food products used were in food supply data per capita [protein supply quantity (g/capita/day)]. The following equation was used (Leach et al., 2012):
Where PSproteini refers to the protein supply per capita for a given food product, NCproteini refers to the protein’s N content, and FWFoodi is the food waste per capita for a specific food product consumed. We subtracted food waste data from the protein supply per capita data using specific ratios SSA available in the FAO bulletin (FAO, 2011) to compute per capita FCNF.
The estimation of FPNF began with computing the virtual N factors (VNFs) according to (Leach et al., 2012) for the major food products. The following equation was used to calculate the FPNF for a single year:
The VNF refers to the ratio of Nr freed to the environment all across the production to the N content of that food, and virtual N refers to the N used during the FP process but absent in the consumed food (Leach et al., 2012).
2.5.1 Development of Crop and Animal Food Products VNFs
To estimate NFfood, the amount of N released at each stage, starting from FP to FC, was quantified. Rwanda accounts for farms that receive inorganic fertilizers and others that do not receive fertilizers. A survey carried out by the National Institute of Statistics of Rwanda (NISR) in three consecutive growing seasons A, B, and C (2016–20,017) showed that only 24% of small-scale farmers used inorganic fertilizer (NISR, 2018). The remaining 76% represents farms that do not receive fertilizer where crops rely on soil reservoirs to obtain nutrients. In line with both situations, we proposed two scenarios for N fertilizer use. The first scenario considers farms that utilize N fertilizer (fertilized scenario), which accounts for a small percentage of farms in Rwanda. In this situation, the highest loss of N occurs in the initial phases of the FP process. The second scenario takes into account farms that do not utilize inorganic N fertilizer (unfertilized scenario). Crops take N nutrients from soils in this situation, and N losses are minimal since N from soil stock is not recharged, resulting in soil mining (Hutton et al., 2017).
The VNF calculation requires an analysis of each stage in the FP process. FP passes through a long pathway from field to final consumption, and a given amount of Nr gets lost to the environment. Leach et al., 2012 detailed five stages by which N flux passes through during the production and consumption process of crop-derived food: 1) input of new N, 2) crop production (uptake), 3) crop harvesting, 4) plant-derived food processing, and 5) food consumption. Livestock-derived food passes through seven stages: 1) input of new N, 2) feed production, 3) feed processing, 4) animal production, 5) animal slaughtering, 6) animal-derived food processing, and 7) food consumption. The VNF calculations considered six variables for both plant and animal-derived foods: 1) available N, 2) percentage of available N (product N), 3) N waste produced, 4) percentage of N recycled, 5) N recycled, and N losses at each stage (Leach et al., 2012). To estimate N losses, we quantified Nr losses at each production stage. We further assumed that the N recycled was 50% at each step after crop harvesting. This ratio is acceptable because waste recycling still faces several difficulties in Africa (Hutton et al., 2017). Food waste is measured only for human consumable products by accounting for food loss during retail, food service, and final consumption (FAO, 2011).
2.5.1.1 Crop Derived Food VNF
The first step of the model quantifies the amount of new N input, assuming that the plant uptakes all N at this level (100% N uptake). In the next step, the recovery rate for maize, rice, and wheat was 23, 24, and 18%, respectively (Krupnik et al., 2004). For vegetable-fruit, starchy roots and tubers were 40% (Asare et al., 2009). The next step quantifies N removed during crop harvesting; the minor quantity of N was left behind in leaves, roots, stems, and husks. 2/3 of N for maize plants accumulated in grain (Sanchez et al., 1997). After harvesting, N is recycled or lost to the environment from leaves and stacks left behind. In the next step, after harvesting, the fractions of N for maize, rice, and wheat were found to be 70, 50, and 80% (Desai and Bhatia, 1978; Hossain et al., 2005; Hutton et al., 2017). Each left 30, 50, and 20% of N respectively in the stove, then got lost to the environment, except for maize that got recycled. The ratios for vegetable-fruit (55%) of N were concentrated in the edible parts (Hutton et al., 2017); greens and roots, 25 and 75% of N were concentrated in plants and tubers, respectively (Hutton et al., 2017). Next step, the plant delivery process. Some amount of N is lost during harvesting, storing, or transportation. Approximately 5, 16, and 10% for cereals, vegetable-fruit, and tubers, respectively. This loss might occur due to infestations, decay, or other losses (FAO, 2011; Hutton et al., 2017). End step, food consumption. After purchasing delivered foods, some waste happens during the cooking and serving process before getting consumed 1, 2, and 5% of N for legumes, cereals, and vegetable-fruit, respectively (FAO, 2011). Since household livestock, such as small ruminants (sheep, goats, and pigs; hereafter small ruminants), and poultry, feed on food waste, about 8% of household food waste was deducted from total N waste during consumption, representing the amount consumed by the small animals at home (Hutton et al., 2017). In determining VNFs for unfertilized farms, no loss happened for the first two steps (input of new N and crop production). Legumes can fix N biologically, and they use their N, not from fertilizer (Hutton et al., 2017), and their soil N mining factor (SNMF) is equal to 0. Of 70% of N from legumes was accumulated in grains (Sanchez et al., 1997). Table 1 presents details of different parameters used to develop VNFs for different crop products.
2.5.1.2 Livestock Derived Food VNF
In this model, all cattle were agro-pastoral because non-agro pastoral cattle made a small proportion in Rwanda (FAO, 2011). An integrated crop-livestock production system is very productive, sustainable, and economical. The main feed for livestock, about 92%, came from natural pastural, such as Napier grass (Pennisetum purpureum), roadside grass, etc., and about 8% came from maize stover (Mutimura et al., 2013). Livestock is used for both milk and meat production (FAO, 2011). For quantifying the stover N portion, the initial production step is similar to that of grain production, where the crop recovered 23% of applied N fertilizer (Krupnik et al., 2004). In the next step, 33% of maize N is within the stover since the waste in the “waste product,” in this case, is actually a grain. About 20% of the N ingested is accumulated as the tissue in big ruminates. The remaining N is accumulated in manure (National Research Council, 2003). In the next step, some of the meat is lost to spoilage, hides, or other forms of wastage between the finished carcass and the butcher shop or market. In SSA, 12% of meat is wasted at this stage (FAO, 2011). Next step, in Rwanda, meat is costly; a small number of families can afford to buy it. A small amount is wasted at this stage, only 2% (FAO, 2011). The milk production pathway is similar to that of beef. Milk, manure, and meat account for 5.6, 74, and 20% of animals ingested N (Hutton et al., 2017). Of the non-milk “waste” (94% of the previous steps), 20/94 = 21% is removed from the waste category because it is correspondingly to meat. This step prevents overestimating N loss and double-counting meat and milk. Estimations for small ruminants are principally the same as for beef.
Concerning small animals, the meat yield per kg grain is higher than that of beef; about 25% of the carcass (Animal slaughtering) is discharged (Clottey, 1985). Poultry obtains food primarily from the household by eating insects and sometimes wild plants; 8% of the domestic grain waste is “discounted” in the above sections to account for poultry’s move. Of all the N moving through a flock of chickens, 50% is wasted as manure, and 45% moves to egg (Summers et al., 1964). 47% of poultry “waste” belongs to the eggs in the model, so it is deducted from the total waste. The model’s remaining computations are similar to those of beef (Hutton et al., 2017). Eggs are in a particular grouping; the step pathways are similar to those of poultry. 8% of the N that is not used in eggs accumulates as tissues in a growing poultry flock; the remaining 92% is excreted as manure. Fish are not agro-products, being wild-caught from ponds and lakes in Rwanda. Thus, the early steps in the model are disregarded, and the SNMF equals zero. The processing, distribution, and consumption steps are from FAO estimates (FAO, 2011). Table 2 presents details of different parameters used to develop VNFs for different livestock products.
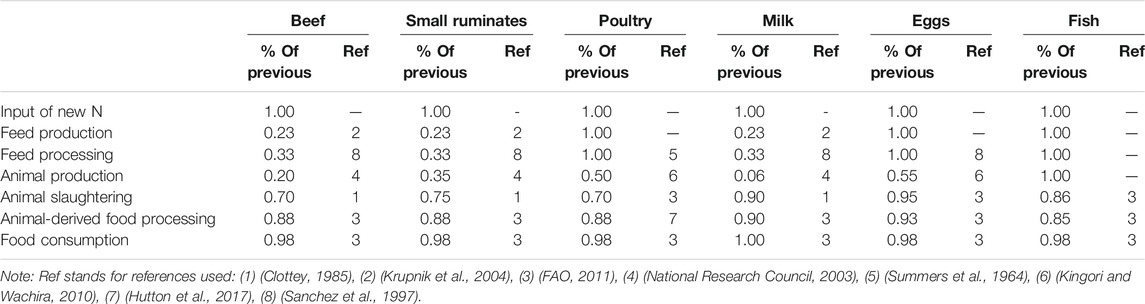
TABLE 2. Parameters and references used for the calculation of the VNFs of Rwanda: livestock products.
2.5.2 Calculation of a Combined VNF
We generated a combined VNF (combined scenario) as the average weighted unfertilized and fertilized scenarios for Rwanda to couple the unfertilized and fertilized scenarios. The combined scenario quantity was computed using the percentage of farms that receive N fertilizer and the percentage of N in food products as a result of soil depletion (PNSD). The combined VNFs and PNSD calculations are based on the following equations (Hutton et al., 2017):
Where VNFcombined is the combined VNF, VNFunferilized is the unfertilized VNF, VNFfertilized is the fertilized VNF, ƒunfertilized refers to the unfertilized farm’s percentage, ƒyield refers to the factor balancing unfertilized and fertilized yields. Existing data suggested that the ƒyield value is 0.5, as fertilized farms yield twofold of what the unfertilized farms produce (Carsky et al., 1999; Hutton et al., 2017). The value of ƒunfertilized is 76%, as 24% of farms are only fertilized (NISR, 2018). The assumption is that 24% of fertilized farms yield double what unfertilized farms yield. By replacing these values in (Eq. 8), the value of PNSD is 61%, reflecting the amount of N that came from soil reserves instead of N fertilization.
2.5.3 Development of SNMF
When TNI into agricultural land results in a negative N nutrient balance, soil N mining occurs. To compute the SNMFs for cereals, starchy roots, and vegetable-fruit, we assumed that the N released is 100% recovered during the soil mineralization course. For animal products, when grazing, some mining happens. Afterward, some amount of N in the livestock excretion (manure) returns to the grazing area. However, about 34% of this N gets lost in the volatilization process (Brouwer and Powell, 1998). To value N mined during grazing, the share of N derived from pasture area and excretion wasted by livestock is multiplied by a factor of 0.34 (Hutton et al., 2017). Fish has a SNMF of zero because it is not an agricultural product. To quantify soil mining caused by inadequate fertilizer application, we multiplied crop N use by 61%. We developed SNMF based on the following equation (Hutton et al., 2017) to quantify N coming from soil mining but not considered in the NFfood calculation:
Assuming that all livestock are fed food from unfertilized farms, we can estimate the amount of N in agro-food products supplied protein that originated from mining by the following equation:
Where NPmined refers to the quantity of N in protein supplied by food products mined from soil N stock.
Assuming that the N surplus reflects the amount of N that remains in the soil after crops use the TNI for production. Without accounting for the different N losses within the soil, we can calculate the quantity of N kept after TNI recharge and N taken during FP as follows:
Where SNR refers to soil N reserved.
2.6 Development of Future Scenarios
In this study, we focused on simple scenarios that could be realized by 2050. Five different scenarios were developed to depict potential future changes in N cycling within the Rwandan agro-food system. Two paths were created to achieve this projection: Business-as-usual path (BAU) and the Self-sufficiency (Diet equitable) path (SSD).
In BAU, we assumed that the trends in N inputs into cropland, FP, FC, and the unhealthy human diet would remain the same as they were in the last 58 years. The SSD path aimed to achieve a balanced diet of plant and animal-fish-derived food for Rwandan citizens for sustainable self-sufficiency agro-food systems (Lassaletta et al., 2016) by 2050. In all of them, we utilized the projected population of Rwandans by 2050 available from United Nations (2017). Based on these two pathways, we created five different scenarios that account for future possible N use, NFfood, and N mining situations by 2050 by proposing possible measures that we believe could be achieved by 2050:
1) BAU, 2) SNF users remained constant and would quadruple the current SNF rate by 2050 (scenario S1), and 3) all farmers used SNF and would double the current SNF rate by 2050 (scenario S2). Under scenarios (S1 and S2), we hypothesized that no future agro-food system management policies would be improved by 2050 and that Rwandans would achieve SSD of 4 kg cap−1yr−1 (60% plant, 24% animal, and 16% fish food) by that time. In addition, we have used the current combined VNFs for the BAU scenario, fertilized VNFs for S2, and SNMFs for BAU, S1, and S3 scenarios to predict future situations. 4) We assumed the same outcome in scenario S3 as we did in scenario S1, and 5) we assumed the same outcome in scenario S4 as we did in S2. However, alternative agro-food system management strategies were assumed to be realized by 2050 in both scenarios (S3 and S 4), such as:
We believe that by 2050, the final household wastage for all FC will decrease by 4%, as also shown by a recent estimate by Niyitanga and Naramabuye (2020). We also believe in meeting the Malabo Declaration’s goal of decreasing post-harvest losses by 50% (African Union, 2020). We agree that this ratio is reasonable to fulfill the food demands of a growing population. We also assume a 10% increase in nutrient usage efficiency; this rate is reasonable because it has been proven in several studies under varied conditions (Pasley et al., 2020).
We predicted that ANM application to cropland would continue to rise at the same rate as animal-derived food demand in the SSD scenarios (S1 and S2). It is reasonable since animal FC will rise with the amount of manure excretion as well as ANM. We hypothesized a 10% increase in SSD scenarios (S3 and S4) relative to S1 and S2 by 2050, assuming improved manure management practices. We determined the per capita food supply by 2050 under SSD scenarios by dividing the per capita equitable diet protein consumption of each food product predicted by 2050 by the protein content of that food product (Elrys et al., 2021a). We also developed new VNFs for future NFfood predictions to account for these expected changes in S3 and S4 scenarios by 2050.
3 Results
3.1 Sources of N Inputs
In Rwanda, the TNI to croplands increased from 9.0 Gg N yr−1 (14.6 kg N ha−1yr−1) during the 1960s to 47.8 Gg N yr−1 (34.2 kg N ha−1yr−1) during 2010–2018 (Figure 1). The arable lands increased from 507 × 103 ha to 1,154 × 103 ha (Supplementary Table S1). Compared with all N inputs, ANM presented the highest increase from 2.40 kg N ha−1yr−1 in the 1960s to 15.08 kg N ha−1yr−1 during 2010–2018. The N input from BNF increased from 7.53 kg N ha−1yr−1 in the 1960s to 10.93 kg N ha−1yr−1 during 2010–2018. During the 1960s, BNF was the dominant source of N input to croplands, providing 51.7% of the overall N input, followed by AND and ANM, with shares of 31.5 and 16.50%, respectively. The contribution of SNF of 0.30% remained minimal. During 2010–2018, an additional increase in ANM share of 44.13% and a coinciding decrease in BNF contribution of 31.98% were noticed.
3.2 Gaseous N Emissions
The N gaseous emissions increased as cropland N usage increased (Figure 2). The total N losses through gaseous emissions of NH3-N, N2O-N, and NO-N increased from 0.45, 0.03, and 0.00 Gg N yr−1 during the 1960s to 6.98, 0.58, and 0.10 Gg N yr−1 during 2010–2018, respectively (Figure 2A). The share of NH3-N was 91% of the overall N emitted. The contributions deriving from N2O-N and NO-N emitted were 7.5 and 1.3%, respectively. The tally of N gas emitted (NH3-N, N2O-N, and NO-N) was 5.4% of the TNI in the 1960s, increasing to 19.3% during 2010–2018.
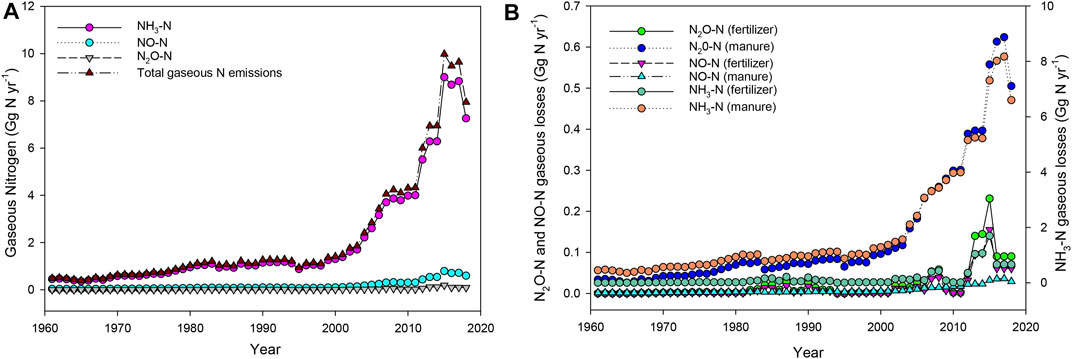
FIGURE 2. Historical changes in total NH3-N, NO2-N, and NO emissions (A), and total N gaseous emissions from animal N manure and synthetic N fertilizer (B) in Rwanda.
3.3 N Trade in Rwanda
Rwanda has imported nearly all the SNF used for crop production from abroad countries. The total SNF imported during the 1960s was 0.03 Gg N yr−1, increasing to 5.02 Gg N yr−1 from 2010–2018 (Supplementary Table S1). Rwanda has traded various plant and animal-derived products worldwide (Figure 3). The overall N trade increased from 0.2 Gg N yr−1 in the 1960s to 13 Gg N yr−1 during 2010–2018. In the 1960s, the contributions of N imports and exports to the total N trade were 36% (Figure 3A) and 64% (Figure 3B), respectively. Plant-derived products have dominated over animal-derived products by contributing 96.8% during the 1960s and 92.5% during 2010–2018 of the total N traded.
3.4 NUE, TCNP, and N Surplus
The NUE successively dropped in Rwanda, decreasing from 124% during the 1960s to 85% during 2010–2018 (Figure 4). The TCNP increased from 11.1 Gg N yr−1 (18 kg N ha−1yr−1) during the 1960s to 39.6 Gg N yr−1 (28.3 kg N ha−1yr−1) during 2010–2018 (Figure 4). The rate of N surplus to TCNP increased from −19% during the 1960s to 21% during 2010–2018.
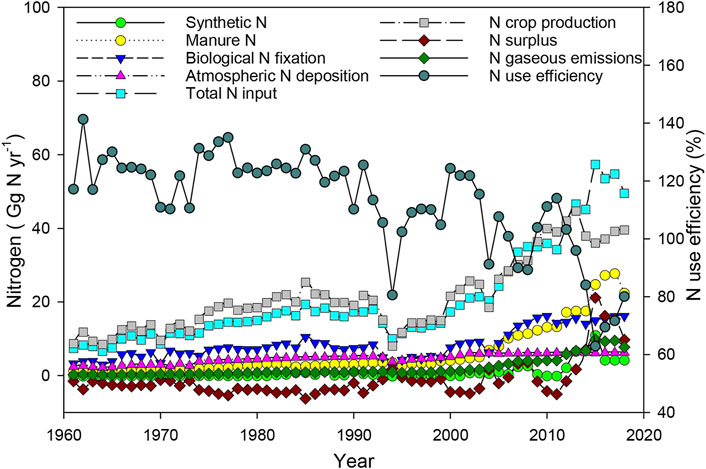
FIGURE 4. Historical changes in the total N input, NUE, crop N production, total N gas emission, and N surplus in Rwanda.
3.5 VNFs of Major Food Products
There are substantial differences between the VNFs of fertilized and unfertilized farms for various food products (Table 3). The VNF of legumes, poultry, eggs, and fish are equal under fertilized and unfertilized scenarios. Milk has a relatively higher VNF in the unfertilized scenario than in the fertilized scenario. Fertilized rice holds the highest VNF of crop products, while unfertilized vegetable-fruit has the tallest VNF. For animal products, milk and beef have the highest VNFs. Unfertilized scenarios for wheat, rice, maize, starchy roots, vegetable-fruit, beef, and small ruminants have lower VNFs than those of fertilized scenarios (Table 3).
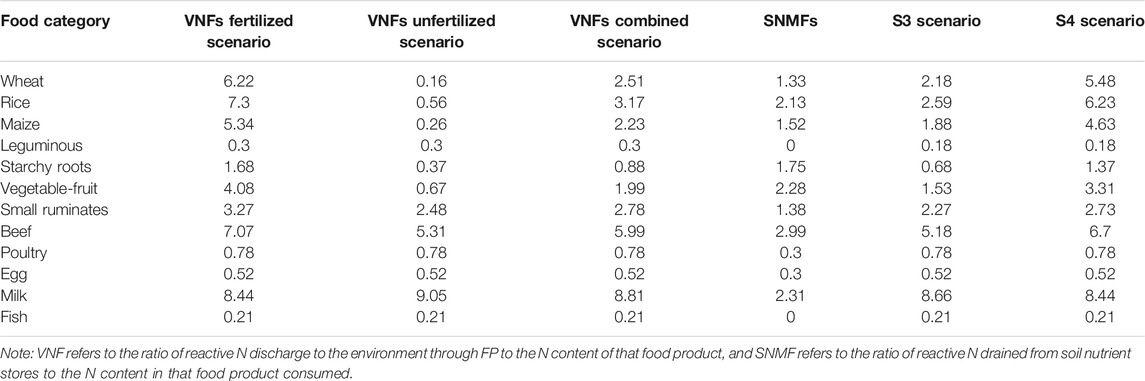
TABLE 3. Mean virtual N factors (VNFs) and soil nitrogen mining factors (SNMFs) of various food products under different scenarios in Rwanda.
3.6 N Mining Within the Agro-Food System
Unfertilized farms SNMFs basically pursued similar directions as those of VNFs, showing that crops and livestock products that lose lots of N are likely to pull in lots of N (Table 3). The plant products with the highest SNMFs in Rwanda are vegetable-fruit and rice. Beef holds the largest SNMF of livestock products and altogether, followed by milk and small ruminants, respectively (Table 3). Over the last 5 decades, the overall NPmined increased from 4.4 Gg N yr−1 in the 1960s to 19.5 Gg N yr−1 during 2010–2018. Simultaneously, SNR decreased from −6.6 Gg N yr−1 to −11.2 Gg N yr−1 (Figure 5A). Plant-derived products such as starchy roots and vegetable-fruit have the largest share of the total NPmined, while milk and beef have the highest NPmined of animal-derived food (Figure 5B).
3.7 Per Capita and National NFfood
A small change in dietetic food regime selection in Rwanda has been noticed in the last 58 years, with a gentle decrease in crop protein sources from 95% in the 1960s to 84% during 2010–2018. The animal protein share increased from 5% in the 1960s to 13% during 2010–2018, along with an increase of the fraction of fish protein from 0% in the 1960s to 3% during 2010–2018. The total average NFfood would increase from 6.0 kg N cap−1yr−1 during the 1960s to 8.7 kg N cap−1yr−1 during 2010–2018 if all farms used fertilizers (Figure 6A). However, the overall average NFfood would increase from 3.5 kg N cap−1yr−1 during the 1960s to 4.8 kg N cap−1yr−1 during 2010–2018 if no fertilizer was applied to crops (Figure 6B). The balanced total average NFfood representing the actual ratios of fertilized and unfertilized scenarios increased from 4.0 kg N cap−1yr−1 during the 1960s to 6.3 kg N cap−1yr−1 during 2010–2018 (Figure 6C).
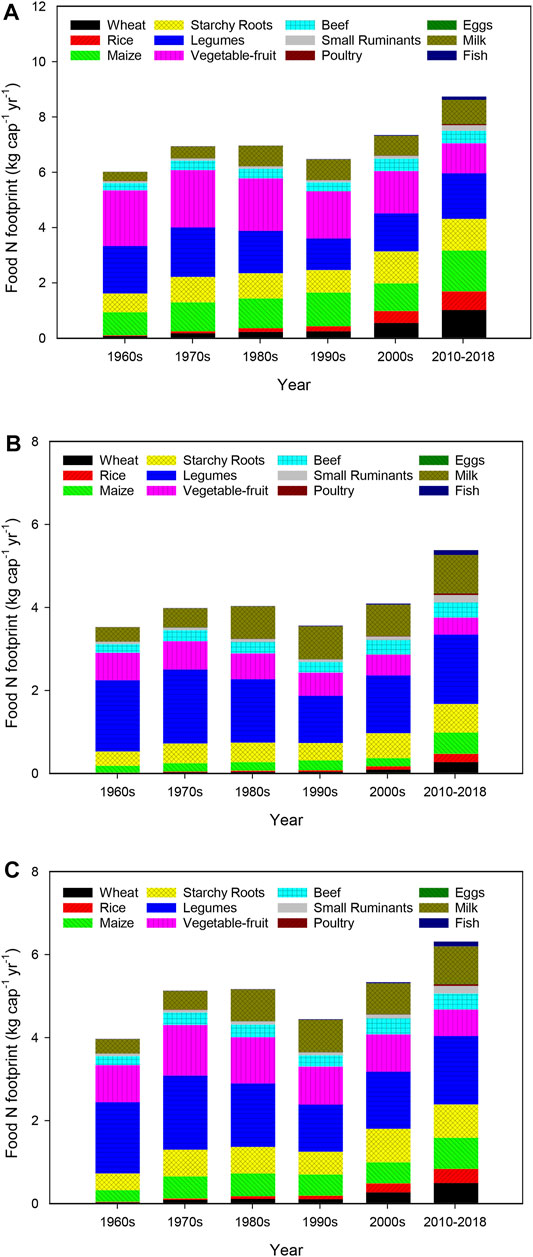
FIGURE 6. Crop and livestock-derived food per capita food N footprint under fertilized scenario (A), unfertilized scenario (B), and combined scenario (C).
The share coming from crop-derived products to the total FCNF decreased from 96 to 88%. Animal-derived products portion increased from 3.9 to 8%, at once the share derived from fish increased from 0.2 to 3.7% of the total FCNF. The FPNF would increase from 3.8 kg N cap−1yr−1 to 6.0 kg N cap−1yr−1 if all farms were fertilized, but it would increase from 1.3 kg N cap−1yr−1 to 2.1 kg N cap−1yr−1 if no fertilizer was applied (Figures 7A,B). In the fertilized farms, the shares derived from crops to the NFfood were dominated by vegetable-fruit, legumes, starchy roots, and maize, by sharing 33.4, 28.5, 11.2, and 13.9%, respectively, in the 1960s and 11.7, 17.9, 12.4, and 16%, respectively, during 2010–2018 (Figure 6A).
The national NFfood increased from 13.4 Gg N yr−1 in the 1960s to 71.1 Gg N yr−1 during 2010–2018 (Figure 8A). It should have increased from 20.2 Gg N yr−1 to 98.3 Gg N yr−1 if all farms were fertilized (Figure 8B) and from 11.8 Gg N yr−1 to 54 Gg N yr−1 if no fertilizer were used in all farms (Figure 8C). The national FCNF increased from 7.4 Gg N yr−1 in the 1960s to 30.2 Gg N yr−1 during 2010–2018 (Figure 8), which accounted for about 42% of the total national NFfood. If all farms were fertilizer or not used fertilizer, the national FPNF would have contributed 68 and 44%, respectively, of the total national NFfood (Figures 8B,C).
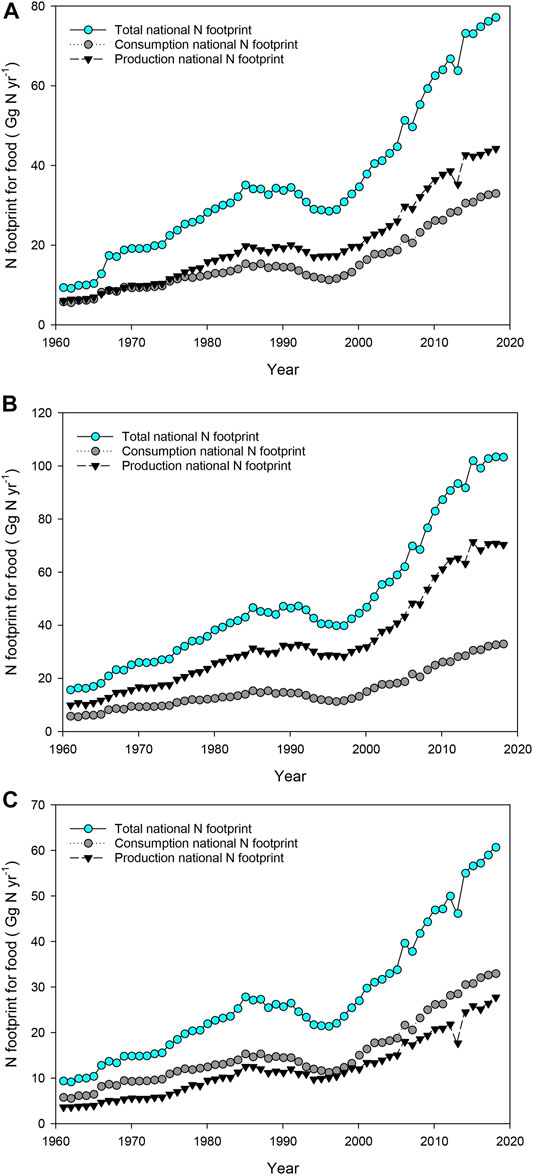
FIGURE 8. Mean national N footprint for food (combined scenario) (A), fertilized scenario (B) and unfertilized scenario (C) in Rwanda.
3.8 Future Projection Scenarios
The TNI would continue to increase from 35.4 kg N ha−1yr−1 (2018) to 42.5, 58.1, 52, 61.3, and 53.6 kg Nha−1yr−1 by 2050 for BAU, S1, S2, S3, and S4 scenarios, respectively (Figure 9A). While the SNR would decrease from −9.5 Gg N yr−1 to -30.8 Gg N yr−1 for BAU, then increase to 4.5, and 9.0 Gg N yr−1, for S1, and S3 scenarios, respectively (Figure 9B). The per capita NFfood would continue to rise from 6.3 kg N cap−1yr−1 in 2018 to 7.0, 10.7, 12.6, 10.1, and 12.0 kg N cap−1yr−1 for BAU, S1, S2, S3, and S4 scenarios, respectively, by 2050 (Figure 9C). The share of FP to the total per capita NFfood would increase from 57% in 2018 to 60, 68, 73, 65, and 71% for BAU, S1, S2, S3, and S4 scenarios, respectively, by 2050. At that time, the national NFfood would increase from 77.2 Gg N yr−1 to 161.9, 246.2, 291.5, 232.4, and 275.9 Gg N yr−1 for BAU, S1, S2, S3, and S4 scenarios, respectively (Figure 9D).
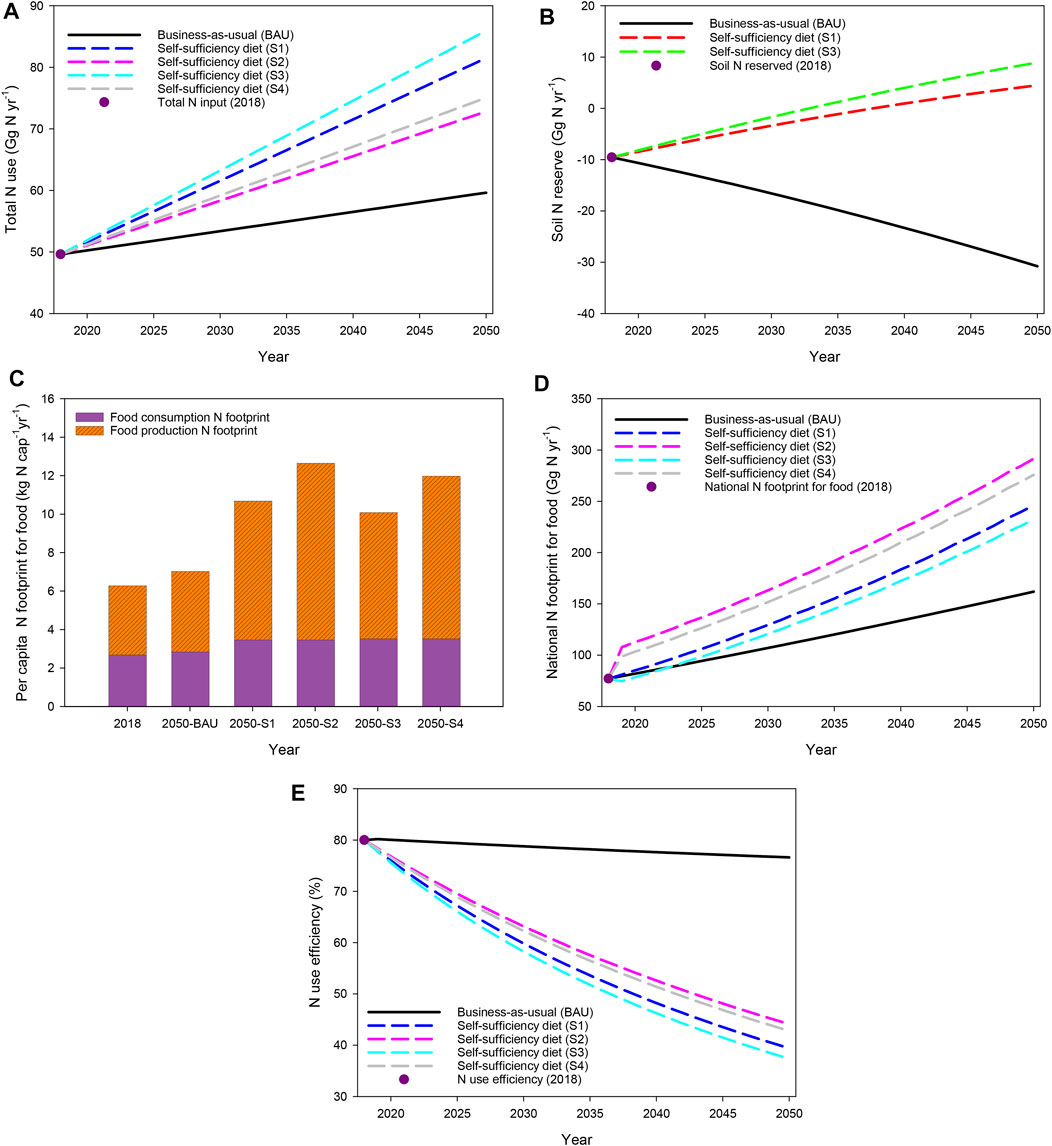
FIGURE 9. Future scenarios for total N use (A), total soil N reserve (B), per capita N footprint for food (C), national N footprint for food (D), and N use efficiency (E) by 2050.
4 Discussion
The present study introduces the first model that estimates the N budget and NFfood in Rwanda’s agroecosystem from 1961 to 2018. Significant changes have occurred in Rwanda’s N cycle over the last 58 years (Figures 10A,B). We present diverse regulators and factors that promote N distribution in Rwanda, pending environmental eyeing consequences for the agro-food system. Lastly, we identified possible strategies to win over soil N mining issues, which is a critical limiting factor in Rwanda’s agro-food production.
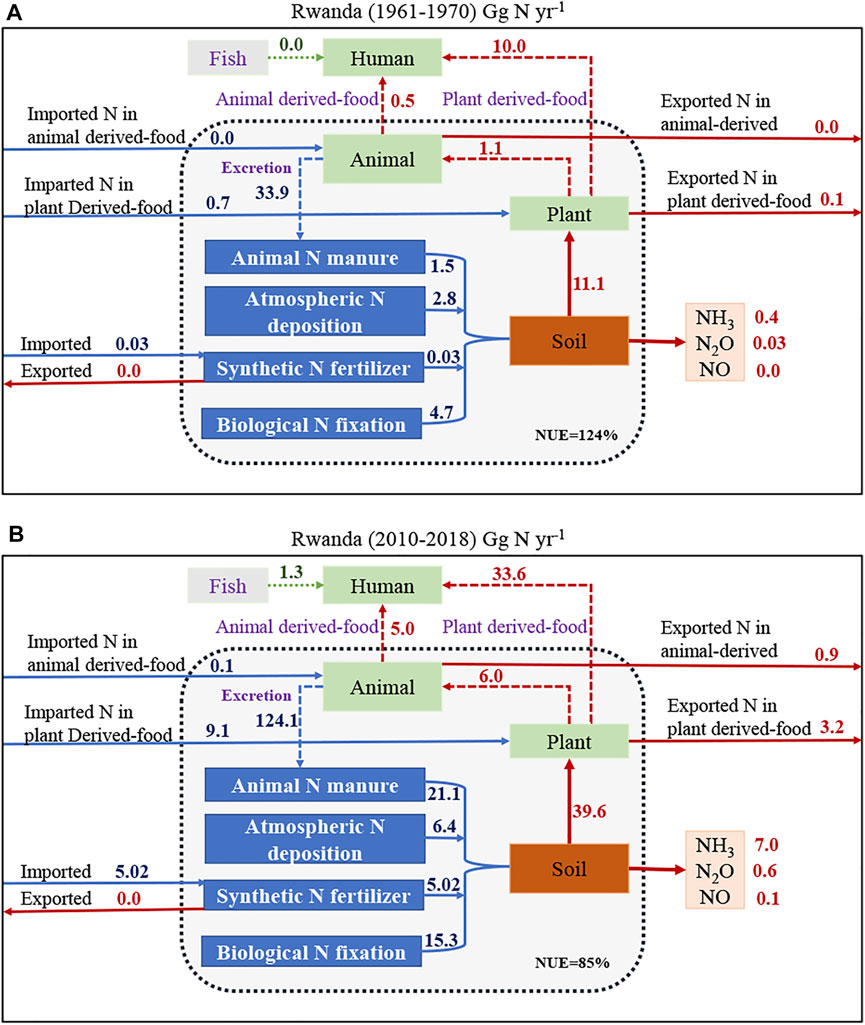
FIGURE 10. Notable changes in the N flux in Rwanda’s agro-food system from the 1960s (A) to 2010–2018 (B).
4.1 Agro-Food System N Flows and NUE in Rwanda
Our findings revealed inferior use of SNF in Rwandan croplands during the last 5 decades (Figure 1). The average SNF used for crop production increased by only about 3.5 kg N ha−1yr−1. Synthetic fertilizers play an essential role in boosting agricultural yields in Rwandan soil (Sabry, 2015), which is about 50% acidic with a pH < 5.2 and associated with high exchangeable aluminum (Nduwumuremyi et al., 2013; Nzeyimana et al., 2013). With an estimated mean soil erosion of 48.6 ton ha−1yr−1 (Nambajimana et al., 2019), nutrient (N, phosphorous (P), and potassium (K)) depletion rate of >100 kg ha−1yr−1 (Henao and Baanante, 1999), and low cation exchange capacity (Nabahungu et al., 2007), unfitted 24% of the national cropland unsuitable for cropping production (Karamage et al., 2016). A survey conducted in 2016, including 159 countries worldwide, on chemical fertilizer use per unit of arable land ranked Rwanda 140th (Miklyaev et al., 2021). Moreover, the proportions of farmers using inorganic fertilizers for cropping are low and inconsistent in Rwanda. For instance, households that used inorganic fertilizer were 10.5 and 8% in 1990 and 2005, respectively (Rwirahira, 2009), while during the 1995–1999 period, only 12% of farm households used inorganic fertilizer for once (Kelly et al., 2001). According to a recent survey, 24% of small-scale farmers used inorganic fertilizers (NISR, 2018). To attain SSD by 2050, Rwanda’s agro-food system would require more than 31.9, 23.2, 36.4, and 25.5 Gg N yr−1 for S1, S2, S3, and S4 scenarios, respectively, in addition to the current TNI to successfully feed a rising population (Figure 9A). This would assist in mitigating the problem of soil mining by enhancing SNR by 147 and 194%, respectively, by 2050 for S1 and S3 (Figure 9B). It will not be easy to achieve since the fertilizer sector in Rwanda currently faces several problems. Some key factors contributing to the low farming input in Rwanda are the sloppy topography of the country, inadequate inputs stocks, affordability, low incomes from sold yields, knowledge and skills of farmers, and lack of motivators (Kelly et al., 2001; Rwirahira, 2009; Mugabo et al., 2020). Achieving an SNF rate of 12.4 kg N ha−1yr−1 or 6.2 kg N ha−1yr−1 for (S1, S3) and (S2, S4) scenarios, respectively, by 2050 would be possible, but far less than the entire quantity of N fertilizer required to ensure food security for a growing population. Specifically, ANM and BNF will continue to be important sources of Nr in the future.
Inputs from ANM and BNF are primarily essential N inputs to the TNI, while they are still low compared to many African countries (Elrys et al., 2021b). Due to the overall increase in livestock numbers (Supplementary Table S1) and the fact of holding the highest per capita consumption of beans in the world of 164 g day−1 (Mulambu, 2017), the application rate of ANM and BNF is high in Rwanda >10 kg N ha−1yr−1 (Figure 1). Beneficially, BNF reduces energy costs and improves sustainability for agricultural production. A study also showed that climbing beans adoption decreased the likelihood of households being poor by 0.6% and raised 4,714 families out of poverty between 1985 and 2012 (CGIAR, 2019). Despite the numerous benefits of ANM to the soil, optimizing crop production appears to be unachievable unless various farm management strategies are combined with specific N inputs. Organic manure, such as urine and liquid manure, is not frequently treated or even put to cropland in Rwanda; instead, it is let to run and sometimes discharges into the soil, or even spills over into water bodies, with no action taken (Teenstra et al., 2014). Due to that improper manure management and poor use of animal feed quality (Diogo et al., 2013), only a few quantities of livestock excrements are applied to cropland as ANM. Generally, policymakers in Africa are uninterested in using organic manure for cropping (Ciceri and Allanore, 2019), which has caused poor purchasing power and lower accessibility of good quality fertilizers (Elrys et al., 2019a).
The TCNP presented a slight increase from 18 kg N ha−1yr−1 to 28.2 kg N ha−1yr−1 during the last 58 years owing to little N inputs (Figure 4). Our estimate of TCNP was comparable to the rate obtained by Lassaletta et al. (2014a). Low harvest yields in Rwanda are due to highly depleted soil nutrients that reduce soil fertility and productivity. The present study observed a decline in NUE from 124 to 85% (Figure 4), possibly because crop production no longer depends widely on soil mining and relies on external N sources. Moreover, incorrect agronomic practices, namely low N fertilizer and pesticides (Rwirahira, 2009), have led to poor crop yields and the downfall of NUE in recent years (Figure 4); as a consequence, the N surplus increased from −24 to 17% of the TNI (Figure 4). In Rwanda, about 60 kg ha−1yr−1 of N is depleted (Chianu et al., 2012; Masso et al., 2017); several N losses channels that surplus inputs of N nutrients can be attributed to the highest soil N depletion (Stoorvogel et al., 1993; Smaling and Braun, 1996; Lederer et al., 2015; Masso et al., 2017).
Our results showed that N emissions from applied ANM contributed 99% during the 1960s and 88% during 2010–2018 of the total N gaseous losses (Figure 2B). NH3-N was the highest emitted gas; consequently, it reduced crop N uptake and negatively affected air quality (Paramasivam et al., 2009). The overall increase in SNF and ANM use stimulates high N emissions in gaseous forms that deposit back onto agricultural land. In the SSA, the AND is comparable to the actual fertilizer use rate of 4–5 kg N ha−1yr−1 (Galy-Lacaux and Delon, 2014). N input from AND positively affects N balance by sustaining crop productivity, especially in a region affected by climate change (Masso et al., 2017), and by enhancing their responsiveness to changes in climate (Greaver et al., 2016; Li et al., 2016). However, the increased NH3 emissions generate a lot of environmental issues, including acid deposition and massive nutrient release into soil and water, resulting in eutrophication, toxicity, and a loss in water quality (Goulding et al., 1998; Bouwman et al., 2002b; Dentener et al., 2006; Liu et al., 2010; Elrys et al., 2021b). A recent study on two important lakes in Rwanda, namely Lake Burera and Ruhondo, showed that they are at risk of eutrophication due to increasing N and P accumulation in their water (Habimana and Nsabimana, 2020).
Despite a 5.3 fold increase in TNI, Rwanda has failed to reach the agreed target of 50 kg ha−1 (fertilizer application rate) by 2015 (Masso et al., 2017) and N inputs from ANM, BNF, and SNF still cannot overrate 30 kg N ha−1yr−1. A study on leading stable food grown in Rwanda such as maize, wheat, rice, beans, cassava, and Irish potatoes, conducted between 2000–2013, showed yield gaps of 60.7, 45.97, 36.28, 71.68, 63.99, and 76.40%, respectively, compared to their potential yields (Niyitanga et al., 2015). Inadequate agricultural inputs can directly be linked to declining soil fertility, low yields, and low incomes. Also, most farmers combine agricultural activities with auxiliary businesses, preventing them from concentrating on their farms superlatively.
Soil N depletion through different channels exceeds TNI (ANM, BNF, AND, and SNF) in Rwanda (Figure 10); the same issue was noticed by Elrys et al. (2019a). FP profits from soil mining even though soil fertility and environmental wellness are lost by mobilizing Nr (Hutton et al., 2017; Masso et al., 2017). Therefore, soil mining of N is still an issue to sweep away. Due to longtime companion with soil mining of N, it is necessary to raise the rate of SNF and the overall number of farmers applying fertilizer on their farms to prevent future crop failure. The low rate of N recovered from applied N fertilizer, ranging from 10 to 20% (Chianu et al., 2012), demoralizes small farmers from buying quality fertilizers in Africa (Woomer et al., 2008; Elrys et al., 2019a). Therefore, we should execute fair use of N input principles, such as using appropriate SNF and applying it at the proper rate, time, and place (Johnston and Bruulsema, 2014; Yuan and Peng, 2017).
A slight increase in SNF use from 0.04 kg N ha−1yr−1 to 3.58 kg N ha−1yr−1 caused an increase in N surplus from −3.46 kg N ha−1yr−1 to 5.9 kg N ha−1yr−1. In return, the total gaseous N emission rate increased from 0.79 kg N ha−1yr−1 to 5.47 kg N ha−1yr−1. In less sensitive soil, fertilizer leads to low NUE and cannot consent to harvest maximum yields unless suitable agronomic practices are accompanied by farming high-yielding crop varieties that fit local conditions and reuse accessible organic matter (Roobroeck et al., 2016). During 1991–1994, we noticed a rapid fall in NUE, followed by an increase (Figure 4). The rapid change in NUE is attributable to a broad fall in agricultural yields due to low SNF use, crop failures, and poor farm management practices, contributing to increased food insecurity. The main causes of the shortage are the weak economy and insecurity challenges, including civil war and genocide (1990–1994), which affected all national sectors (Akresh et al., 2011). Moreover, sporadic and occasional farm fertilization was prevalent during and before that period (Kelly et al., 2001). Still, NUE reduction remains a challenge for the future as the SNF rate increases in Rwanda. NUE is predicted to decline from 80% in 2018 to 77, 39, 44, 37, and 43% by 2050 for BAU, S1, S2, S3, and S4 scenarios, respectively (Figure 9E). It is therefore a big challenge to achieve SSD by increasing the rate of N fertilizer use. Educating food consumers about the idea of VNF and SNMF and encouraging Rwandans to alter their eating habits and adopt environmentally safe foods would be especially crucial by 2050 (Elrys et al., 2021a).
4.2 The NFfood, SNMFs, and Their Influences
For the past 58 years, agricultural productivity has remained low compared to Rwanda’s rapid population growth. We observed an inconsequential increase in the national NFfood resulting from the highest demographic growth (3.4 fold) with low consumption of high VNF food (Lassaletta et al., 2018). A slight increase in N inputs into FP and declining NUE induced the marginal increase in per capita NFfood (Zhang et al., 2015). Poor consumption of animal-derived foods, such as milk, beef, and small ruminants with the highest VNFs, resulted in a lowermost per capita NFfood of 6.3 kg N yr−1 than in other countries. Moreover, low NFfood is related to the consumption of low protein food, followed by low N releases in high protein FP (Hutton et al., 2017). The usage of very low N fertilizer has caused soil mining of N (Elrys et al., 2019b). Elevating SNF rate with adequate farmers’ knowledge would enhance plant nutrient absorption under fertilized farms, resulting in reduced VNFs of food products. Under SSD scenarios, the lowest VNFs would be seen under S3 and S4 scenarios (Table 3). This enhancement in nutrient absorption rate and reduction in FC waste and losses would result in lesser per capita NFfood under S3 and S4 scenarios by 2050 compared to S1 and S2 scenarios (Figure 9C). Under existing agricultural practices, the NFfood is expected to increase to 7.0, 10.7, and 12.6 kg N cap−1yr−1, respectively, by 2050, for the BAU, S1, and S2 scenarios (Figure 9C). With improved agricultural practices, the NFfood is expected to grow to 10.1 and 12.0 kg N cap−1yr−1, respectively, by 2050 for the S3 and S4 scenarios (Figure 9C). Therefore, practices that minimize food losses are now critical in reducing food insecurity in Rwanda. These practices would become much more essential in the future since if losses and waste continue to rise, they will generate plenty of environmental concerns by 2050.
Despite having the lowest NFfood (Figure 7), it still poses a significant challenge to food security because the majority of food products are produced on unfertilized farms that heavily mine soil N stock (Figure 6). In this study, a total of 61% of the yields produced came from unfertilized farms that mined soil N. There is a significant disparity between the amount of food produced today and the amount required to feed Rwandans in 2050. Beneficial, for a short period, soil N mining can sustain FP (Hutton et al., 2017), while it can lead to total crop failure in the long term (Masso et al., 2017). Longtime companion N mining resulted from years of low N fertilizer inputs, leaching, and typical erosion caused by abundant rainfall on hilly topography that harms Rwanda’s agro-food production sector. Therefore, estimation of NFfood for farms (fertilized and unfertilized) and the integration of SNMFs into the NFfood model for unfertilized plots in Rwanda is essential for N mining denigration by approximating possible FP process N losses and then determining the amount of N recycled from these losses (Hutton et al., 2017).
Beef, milk, and vegetable-fruit hold the highest SNMFs, while milk, beef, and small ruminants have the highest VNFs in unfertilized farms in Rwanda (Table 3). We should know that SNMFs and VNFs are complementary measurement tools but provide different pieces of information. A high VNF refers to a high ratio of Nr discharge to the environment through FP to the N content of that food product. In contrast, a high SNMF refers to a high ratio of Nr drained from soil nutrient stock to the N content in that food product consumed (Hutton et al., 2017). In this study, the quantity of Nr loss under fertilized and unfertilized plots was unequal (Table 3 and Figure 6). It is reasonable that food products would have higher VNFs on fertilized farms than on unfertilized farms, as no losses related to N fertilizer are linked to unfertilized plots (Elrys et al., 2021b).
Only 58% of the total NFfood resulted from FPNF. The estimated NFfood for the SSA is higher than that for Rwanda, reflecting how much daily human diets are deficient in proteins. However, there is little progress in Rwanda’s diet pattern; with a gentle increase in animal protein consumption, malnutrition is a severe problem in Rwanda (Weatherspoon et al., 2019). The food and nutrition security indicators report showed that about 48.7 and 22.1% of rural and urban populations in Rwanda were still food and nutrition insecure (FAO, 2018). It can be related to the lack of shift in low protein food products (Oita et al., 2018), insufficient N inputted during crop production, and declining NUE (Zhang et al., 2015).
Rwanda’s average protein consumption of only 3.5 kg N cap−1yr−1 (Figure 11) is still lower than the healthy protein consumption quantity recommended of 4.0 kg N cap−1yr−1 (Lassaletta et al., 2016). To achieve the daily protein mass advised, we suggest fortifying the consumption of high protein food products, such as animal-derived food products. Moreover, the development of rural food markets and rural nutrition education on reliable consumption of various foods should focus on growing specific nutritive crops rich in protein, micronutrients, and calories (Weatherspoon et al., 2019). Furthermore, farmers’ field schools in Rwanda could reinforce farmers’ awareness of ecological phenomena affecting their production of crops and animals. These can help farmers settle on successful N-efficiency decisions and help them save money on fertilizers and time (Hutton et al., 2017). Otherwise, valuable auxiliary to reduce N loss could lower the existing food insecurity and sustain environmental conservation (Elrys et al., 2021b; 2021a).
Commonly in Africa, the choice of food products based on those having lower VNFs and SNMFs is not a conscious concept because of poverty and ignorance (Elrys et al., 2021b). When deciding on dietary regimes, it is better to consider crops or livestock food products rich in protein and low VNFs, such as starch roots, legumes, vegetable-fruit, and maize; livestock products, small ruminants, poultry, and fish (Table 3). In reducing soil N mining, the best food could be legume crops because they hold SNMF of zero, are very rich in protein and can be a perfect choice over beef (Table 3). Moreover, livestock products such as small ruminants, poultry, and eggs can be prioritized since their SNMFs are lower than beef (Hutton et al., 2017; Elrys et al., 2019b). As most Rwandans are farmers, natural FP and FC choices can be based on foods with low VNFs and SNMFs to enhance soil fertility and limit Nr release, which can lead to environmental damage (Hutton et al., 2017; Elrys et al., 2021a). Food products with low SNMF promote food security because they do not intensify soil mining depletion (Hutton et al., 2017).
The major challenge facing the agro-food system in Africa is the lack of relevant knowledge concerning food losses and the statement of food security (Joshi and Visvanathan, 2019). According to the Food Smart Country Diagnostic report, while 19% of the population does not have enough food to eat, Rwanda loses 40% of total FP each year; crops with the highest loss rate are tomatoes, maize, and rice, at 49, 25, and 18%, respectively (World Bank, 2020). Most of those food losses happen before reaching markets or consumers, particularly in rural areas, due to poor infrastructure and physical topography. Decreasing food wastage during FC is a feasible way to reduce environmental N losses (Shibata et al., 2017). To overcome such wastage, consumers, food-service providers, and retailers could be liable for underrating wastage of food by adopting new technologies capable of converting Nr to atmospheric N2 (Zhang et al., 2018). Investment in food marketing infrastructures such as roads, market facilities, and electricity can motivate operations along agricultural supply chains (Sheahan and Barrett, 2017). For sustainable agriculture and alleviating depressing impacts on human health and the environment, appropriate management practice measures should focus on raising the TNI to cropland and promoting NUE (Zhang et al., 2015; Hutton et al., 2017; Yuan and Peng, 2017; Elrys et al., 2019a, 2019b). Therefore, it is essential to estimate NFfood to identify food products that induce high loss of N through soil mining/depletion and gaseous emission.
4.3 Global Comparisons
Rwanda’s weighted per capita NFfood was 5.3 kg N cap−1yr−1 in the 2000s (Figure 12), the smallest NFfood of all other countries. Australia has the topmost per capita NFfood of 32 kg N yr−1 which is about sixfold higher than that of Rwanda (Figure 12). Rwanda and Tanzania are the only countries with a per capita protein consumption rate lower than the World Health Organization’s recommended daily protein intake of 75 g day−1 adult −1 (Schönfeldt and Hall, 2012), due to low protein consumption and low rate of N fertilizer use (Elrys et al., 2021b). The per capita NFfood for Rwanda, Tanzania, and SSA were much lower than that of the remaining countries due to the lowest consumption of high VNF food products such as beef (Leach et al., 2012; Pierer et al., 2014; Shibata et al., 2014; Stevens et al., 2014; Liang et al., 2016; Oita et al., 2016; Guo et al., 2017; Elrys et al., 2019b, 2021b; Cordovil et al., 2020).
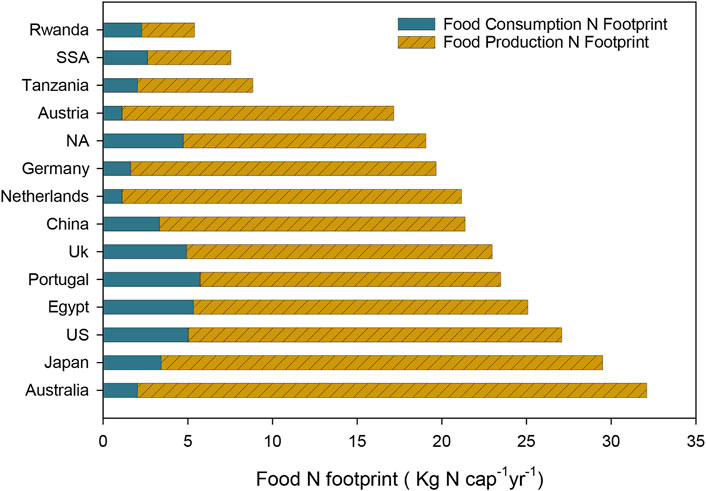
FIGURE 12. Comparison of the N footprint per capita of Rwanda with other countries and regions [Sub-Sahara Africa (SSA) and North Africa (NA)] in the 2000s. We utilized the weighted NFfood per capita for Rwanda, Tanzania, SSA, and NA.
In all countries that estimated NF, FCNF holds the smallest share of the total per capita NFfood. Rwanda’s FCNF was 11% higher than that of Australia and Tanzania and was 51% higher than that of the Netherlands and Austria. It was also 29% higher than that of Germany. But then, it was 50, 121, 135, 152, 117, 46, 108, 15%, smaller relative to those of Japan, United States, Egypt, Portugal, United Kingdom, China, NA, SSA, respectively (Figure 12). Germany, Austria, the Netherlands, and Australia seem to have FCNF lower than that of Rwanda because they have developed sewage treatment plans that reduce environmental N losses during FC stages (Hutton et al., 2017; Elrys et al., 2019b). About 78, 79, 67% of the FCNF in the Netherlands, Austria, and Germany, respectively, are eliminated by advanced sewage treatment (Stevens et al., 2014). Only 2 and 5% of the FCNF in the United Kingdom and United States, respectively, are eliminated by modern sewage treatment (Stevens et al., 2014), and N losses during FC processes have always been higher in Portugal (Cordovil et al., 2020), this makes these countries have the highest FCNF compared to others.
The vital difference between the per capita NFfood of all countries was per capita FPNF (Figure 12). FPNF shared only 58% of the total per capita NFfood for Rwanda, which was smaller than that of Australia (878%), Japan (739%), the United States (610%), Egypt (535%), Portugal (471%), Netherlands (545%), Austria (416%), NA (361%), SSA (58%), Tanzania (119%), and was 481% smaller than that of China, United Kingdom, and Germany (Figure 12). The shares of the FPNF to the total per capita NFfood for Japan, Australia, Egypt, United States, Portugal, the Netherlands, Austria, NA, SSA, Tanzania, China, United Kingdom, and Germany were 88, 94, 79, 81, 76, 95, 94, 75, 65, 78, 86, 78, and 92%, respectively (Figure 12). The enormous losses of Nr during the FP process observed in other countries are narrowly occurring in Rwanda due to its resource flows and production patterns.
4.4 Implications and Perspectives
The overall increase in N input boosted crop yields to sustain the livelihood of the rapidly growing population in Rwanda. Increased crop yield demands SNF application in accordance with crop nutrient needs and the status of nutrients stocked in the soil (Elrys et al., 2019b). Moreover, by promoting NUE and reducing Nr environmental concerns, an emphasis on SNF use can be balanced with ANM and crop nutrient needs (Zhou et al., 2014; Raza et al., 2018). Since many farmers in Rwanda could not afford SNF, organic N inputs such as ANM and BNF have sustained crop productivity for many years. Combining crop and livestock farms with the primary purpose of recycling crop and livestock waste reduces N losses and promotes NUE (Yang et al., 2018). Moreover, strengthening the cultivation of highly protein legumes can supply much more N to the soil through BNF (Elrys et al., 2019b). These practices have a number of benefits for soil health and can help to reduce reliance on SNF.
We should note that soil fertility improvement by applying synthetic fertilizer can not only focus on N fertilizer, but also P and K fertilizers can boost soil fertility, since P and K are essential macronutrients for crop development, and P was found to be commonly deficient in Africa (Coetzee et al., 2016; Kok et al., 2018). Therefore, we should satisfy the “4Rs of nutrient stewardship”, namely, use the Right fertilizer type, apply it at the Right rate, Right time, and in the Right place (Johnston and Bruulsema, 2014). Since the amount of N fertilizer applied to cropland is the primary yield determining factor, followed by N sources, time, and the application technics (Verhulst et al., 2014; Masso et al., 2017; Yuan and Peng, 2017). These strategies are widely used in China to promote NUE in rice cultivation (Yuan and Peng, 2017). A soil test can be done prior to planting to determine the quantity of external fertilizers that will be needed to produce a potential yield. Moreover, cropping varieties of enhanced NUE can read to maximum yields for poor farmers that cannot afford SNF in high quality easily (Elrys et al., 2019a). A comprehensive and adequate FP system requires integrated farming management practices that minimize N fertilizer losses to water bodies and the air (Elrys et al., 2021b). Farm management practices, such as mulching combined with terracing, could enhance soil fertility and lessen soil losses by water erosion. Thus, Rwanda’s agricultural potential will necessitate substantial investment in N fertilizer acquisitions as well as in the farmers’ supply chain.
Our natural resources, namely land, water, and energy, are typically harassed by human waste. Reducing food wastage can contribute highly to the reduction of greenhouse gases emissions and natural resource conservation. Rwanda’s economy is now expanding across all sectors (Das and Bosco, 2020); sectors all the way up the food chain require economic growth that promotes food waste reduction. The tools proposed in this study can be employed to consider the core phases to regard on during the FP process to develop the greatest N sustainable management. The VNFs and SNMFs approaches serve to visualize the productiveness and environmental cost of food products linked to each other (Hutton et al., 2017). Distinguishing food products based on VNFs and SNMFs can help decision-makers, producers, and consumers decide which ones to consider for reducing Nr release into the environment (Elrys et al., 2021b). In Rwanda, the consumption market is developing faster each year. Packaging companies should include relevant information about NFfood of each component on product labels to assist customers in selecting low NFfood foods (Elrys et al., 2019b; Joshi and Visvanathan, 2019). More effective and efficient measures motivating food waste reduction along the food chain should be obvious (Goossens et al., 2019). Therefore, different approaches established in this research need stable incentives and continuing collaboration between soil scientists, agronomists, ecologists, agricultural economists, as well as politicians (Galloway et al., 2002). Finally, the tools developed in this study mainly target rural communities, which are particularly vulnerable to the crisis of poor N fertilizer usage and urban populations, which modestly promote N losses to the environment. Community leaders, decision-makers, environmental control agencies, and media should promote these tools to develop agricultural and environmental protection in Rwanda and other developing countries.
Agricultural and environmental research in Africa has a number of challenges due to a lack of consistent and comparable data (Hutton et al., 2017). Producing agricultural N budgets and determining the NFfood over an extended period involves several generalizations and extrapolations (Lassaletta et al., 2014a). Inevitable uncertainties and constraints accompany these extrapolations. We have used those we agreed on more meaningly. 1) To estimate gaseous emissions losses from soils, we have used regional emission factors suggested by Bouwman et al. (2002a) for NH3 and FAO, (2001) for NO emissions. These emissions seem to be outdated and might present transitional values for developing countries and could be the source of uncertainty. The N losses by leaching and N added by irrigation were not examined in this study due to the absence of related information. 2) To estimate the N budget, we calculated BNF based on the cropped area of legumes (Lassaletta et al., 2014a) and the constant rate for rice paddies and sugar cane from Herridge et al. (2008). We have used these values due to the absence of information concerning N fixation in Rwanda. Thus, the estimate of BNF based on the area could produce mistaken results. 3) To precisely calculate AND input in Rwanda, an AND monitoring station is needed. We have estimated AND inputs based on the estimated AND rate in the agricultural ecosystem available in Dentener et al. (2006) multiplied by the entire cropped area per year. However, N gaseous volatilization is inconstant even in the same field or can even depend on on-farm management practices, and weather may cause variation in AND within distant farmland. 4) To estimate Rwanda’s NFfood, we made different assumptions because Rwanda lacks specific systems to control N losses within the food chain. When calculating VNFs for various food products, maize and beef were reference points for all food products. The recent VNFs calculated were used to estimate NFfood from 1961 to 2018. It can be the source of uncertainty as N flowing through the food chain could change over time. 5) In this study, N recovery values used for rice and maize are explicit for farms in southern Africa (Krupnik et al., 2004), and for wheat, NUE used in the VNF were from studies in India (Cassman et al., 2002). Dobermann (2007) found NUE of around 50% for stable grains, whereas few studies were conducted, NUE was considerably less, ranging from 30 to 40% (Hutton et al., 2017). Agricultural practices, environmental conditions, and genetic diversity can result in different NUE within the country (Elrys et al., 2021b). In this study, we disregarded these variations due to the lack of data. 6) The current NFfood disregarded the influence of global trade. However, international trade is probably affecting the NFfood values in Rwanda because research done in Japan showed that global trade of food and feed affected NFfood for Japan (Shibata et al., 2014). Future studies should include the trade effect when modeling the NFfood for Rwanda or other countries. 7) To account for the amounts of food waste in this study, we used constant SSA values present in FAO, (2011). While food wastage may vary in SSA countries, these differences can affect the NFfood; due to limited data, these values were used. 8) When estimating the NFfood under the combined scenario during 1961–1966 and 1994–1995, all farm plots were assumed to be 100% unfertilized to avoid mistakes because no data was reported concerning SNF used during these periods in the FAOSTAT database. 9) The VNFs calculated in this study were for only 12 different crops and livestock-derived foods. Future studies should expand the NFfood methodology by calculating more VNFs for a wide range of various food products. 10) Finally, Rwanda lacks an inclusive database to quantify N losses throughout the FP and FC systems and related literature. These are serious issues that decision-makers and researchers should prioritize and support because they may help future calculations of NFfood to become much more precise.
5 Conclusion
We contribute the first national estimate of the N flows and NFfood for the agro-food systems of Rwanda during the 1961–2018 period. Inadequacy of SNF supply to cropland led to low crop yield and extended soil mining of N over the last 5 decades. The TNI and TCNP increased 5.3 and 3.6 folds, respectively, during this period. BNF was the primary source of N input to farmland in the 1960s, whereas ANM was the leading source of N input to cropland during 2010–2018. The NUE decreased from 124 to 85%, accompanying the increase in N surplus to 5.9 kgN ha−1yr−1 in 2010–2018. The emissions of gases NH3-N, N2O-N, and NO from croplands slightly increased while the per capita NFfood and national NFfood rapidly increased during this period. The FCNF of Rwanda is higher than that of some developed countries due to inadequate sewage treatment systems, while the FPNF is lower due to the low consumption of protein-rich foods. For overcoming the challenges of Rwanda’s agro-food system, it is necessary to adopt sustainable N management policies to promote NUE and minimize N loss during FP and FC processes in Rwanda.
Data Availability Statement
Publicly available datasets were analyzed in this study. This data can be found here: http://www.fao.org/faostat/en/#data.
Author Contributions
BH conducted the work, statistical analysis, figure drawing, and wrote the original draft. MZ performed conceptualization, supervision, review, and editing. MS and BZ performed the review and editing.
Funding
This study was supported by the National Natural Science Foundation of China (Grant No. U20A20107) and the National Key Research and Development Program (2019YFD1100503).
Conflict of Interest
The authors declare that the research was conducted in the absence of any commercial or financial relationships that could be construed as a potential conflict of interest.
Publisher’s Note
All claims expressed in this article are solely those of the authors and do not necessarily represent those of their affiliated organizations, or those of the publisher, the editors and the reviewers. Any product that may be evaluated in this article, or claim that may be made by its manufacturer, is not guaranteed or endorsed by the publisher.
Acknowledgments
The authors gratefully acknowledge the financial support provided by the Natural Science Foundation of China (Grant No. U20A20107) and the National Key Research and Development Program (2019YFD1100503).
Supplementary Material
The Supplementary Material for this article can be found online at: https://www.frontiersin.org/articles/10.3389/fenvs.2021.778699/full#supplementary-material
References
Abdulaziz, A. M., Hurtado, J., J. M., and Al‐Douri, R. (2009). Application of Multitemporal Landsat Data to Monitor Land Cover Changes in the Eastern Nile Delta Region, Egypt. Int. J. Remote Sensing 30, 2977–2996. doi:10.1080/01431160802558675
African Union (2020). Second Biennial Review Report of the African Union Commission on the Implementation of the Malabo Declaration on Accelerated Africa Agricultural Growth and Transformation for Shared prosperity and Improved Livelihoods. Assembly of the union Thirty-Third. Available at: https://au.int/sites/default/files/documents/38119-doc-2019_%0Abiennial_review-en.pdf.
Akresh, R., Verwimp, P., and Bundervoet, T. (2011). Civil War, Crop Failure, and Child Stunting in Rwanda. Econ. Dev. Cult. Change 59, 777–810. doi:10.1086/660003
Asare, D., Ayeh, E., and Amenorpe, G. (2009). Response of Rainfed Cassava to Methods of Application of Fertilizer-Nitrogen in a Coastal Savannah Environment of Ghana.
Bouwman, A. F., Boumans, L. J. M., and Batjes, N. H. (2002a). Emissions of N2O and NO from Fertilized fields: Summary of Available Measurement Data. Glob. Biogeochem. Cycles 16, 6–1. Cycles 16. doi:10.1029/2001gb001811
Bouwman, A. F., Boumans, L. J. M., and Batjes, N. H. (2002b). Estimation of Global NH3volatilization Loss from Synthetic Fertilizers and Animal Manure Applied to Arable Lands and Grasslands. Glob. Biogeochem. Cycles 16, 8–1. doi:10.1029/2000gb001389
Brouwer, J., and Powell, J. M. (1998). Increasing Nutrient Use Efficiency in West-African Agriculture: the Impact of Micro-topography on Nutrient Leaching from Cattle and Sheep Manure. Agric. Ecosyst. Environ. 71, 229–239. doi:10.1016/s0167-8809(98)00143-1
Carsky, R. J., Oyewole, B., and Tian, G. (1999). Integrated Soil Management for the savanna Zone of W . Africa : Legume Rotation and Fertilizer N, 55, 95–105. doi:10.1023/a:1009856032418
Cassman, K. G., Dobermann, A., and Walters, D. T. (2002). Agroecosystems, Nitrogen-Use Efficiency, and Nitrogen Management. AMBIO: A J. Hum. Environ. 31, 132–140. doi:10.1579/0044-7447-31.2.132
CGIAR (2019). Climbing Beans Reduce Poverty in Rwanda. Available at: https://www.cgiar.org/annual-report/performance-report-2019/(accessed February 02, 2021).
Chianu, J. N., Chianu, J. N., and Mairura, F. (2012). Mineral Fertilizers in the Farming Systems of Sub-saharan Africa. A Review. Agron. Sustain. Dev. 32, 545–566. doi:10.1007/s13593-011-0050-0
Ciceri, D., and Allanore, A. (2019). Local Fertilizers to Achieve Food Self-Sufficiency in Africa. Sci. Total Environ. 648, 669–680. doi:10.1016/j.scitotenv.2018.08.154
Clottey, S. J. A. (1985). Manual for the slaughter of Small Ruminants in Developing Countries. Available at: http://www.fao.org/3/X6552E/X6552E00.htm (Accessed January 25, 2021).
Coetzee, P.-E., Ceronio, G. M., and du Preez, C. C. (2016). 1862, Effect of Phosphorus and Nitrogen Sources on Essential Nutrient Concentration and Uptake by maize (Zea maysL.) during Early Growth and Development. South Afr. J. Plant Soil 34, 55–64. doi:10.1080/02571862.2016.1180714
Cordovil, C. M. S., Gonçalves, V., Varennes, A. De., Leach, A. M., and Galloway, J. N. (2020). A First Approach to the Calculation of Nitrogen Footprint in Lisbon. Portugal: Springer International Publishing. doi:10.1007/978-3-030-58065-0
Custodio, E., Herrador, Z., Nkunzimana, T., Węziak-Białowolska, D., Perez-Hoyos, A., and Kayitakire, F. (2019). Children's Dietary Diversity and Related Factors in Rwanda and Burundi: A Multilevel Analysis Using 2010 Demographic and Health Surveys. PLoS One 14, e0223237–17. doi:10.1371/journal.pone.0223237
Das, G. G., and Bosco, R., and Editors, (2020). Frontiers in African Business Research Rwandan Economy at the Crossroads of Development Key Macroeconomic and Microeconomic Perspectives. Available at: http://www.springer.com/series/13889.
Dentener, F., Drevet, J., Lamarque, J. F., Bey, I., Eickhout, B., Fiore, A. M., et al. (2006). Nitrogen and Sulfur Deposition on Regional and Global Scales: A Multimodel Evaluation. Glob. Biogeochem. Cycles 20, a–n. doi:10.1029/2005GB002672
Desai, R. M., and Bhatia, C. R. (1978). Nitrogen Uptake and Nitrogen Harvest index in Durum Wheat Cultivars Varying in Their Grain Protein Concentration. Euphytica 27, 561–566. doi:10.1007/BF00043182
Diogo, R. V. C., Schlecht, E., Buerkert, A., Rufino, M. C., and van Wijk, M. T. (2013). Increasing Nutrient Use Efficiency through Improved Feeding and Manure Management in Urban and Peri-Urban Livestock Units of a West African City: A Scenario Analysis. Agric. Syst. 114, 64–72. doi:10.1016/j.agsy.2012.09.001
Dobermann, A. (2007). IFA Int. Work. Fertil. Best Manag. Pract. Brussels, Belgium, 7–9. Available at: http://sustainablecropnutrition.net/ifacontent/download/7163/113016/version/1/file/2007_IFA_FBMP+Workshop_Brussels.pdf#page=8 March 28, 2007).Nutrient Use Efficiency–Measurement and Management
Einarsson, R., and Cederberg, C. (2019). Is the Nitrogen Footprint Fit for Purpose? an Assessment of Models and Proposed Uses. J. Environ. Manage. 240, 198–208. doi:10.1016/j.jenvman.2019.03.083
Elrys, A. S., Abdel-Fattah, M. K., Raza, S., Chen, Z., and Zhou, J. (2019a). Spatial Trends in the Nitrogen Budget of the African Agro-Food System over the Past Five Decades. Environ. Res. Lett. 14, 124091. doi:10.1088/1748-9326/ab5d9e
Elrys, A. S., Desoky, E.-S. M., Ali, A., Zhang, J.-b., Cai, Z.-c., and Cheng, Y. (2021a). Sub-Saharan Africa's Food Nitrogen and Phosphorus Footprints: A Scenario Analysis for 2050. Sci. Total Environ. 752, 141964. doi:10.1016/j.scitotenv.2020.141964
Elrys, A. S., Desoky, E.-S. M., Alnaimy, M. A., Zhang, H., Zhang, J.-b., Cai, Z.-c., et al. (2021b). The Food Nitrogen Footprint for African Countries under Fertilized and Unfertilized Farms. J. Environ. Manage. 279, 111599. doi:10.1016/j.jenvman.2020.111599
Elrys, A. S., Metwally, M. S., Raza, S., Alnaimy, M. A., Shaheen, S. M., Chen, Z., et al. (2020). How Much Nitrogen Does Africa Need to Feed Itself by 2050? J. Environ. Manage. 268, 110488. doi:10.1016/j.jenvman.2020.110488
Elrys, A. S., Raza, S., Abdo, A. I., Liu, Z., Chen, Z., and Zhou, J. (2019b). Budgeting Nitrogen Flows and the Food Nitrogen Footprint of Egypt during the Past Half century: Challenges and Opportunities. Environ. Int. 130, 104895. doi:10.1016/j.envint.2019.06.005
FAO (2021). FAOSTAT Statistical Database. Available from: http://www.fao.org/faostat/en/#data (Accessed January 26, 2021).
FAO (2001). Global Estimates of Gaseous Emissions of NH3, NO and N2O from Agricultural Land. Available at: http://www.fao.org/3/Y2780E/y2780e00.htm (Accessed January 27, 2021).
FAO (2011). Global Food Losses and Food Waste: Extent, Causes, and Prevention Study Conducted for the International Congress Save Food Interpack Dusseldorf Germany. Available online at: www.fao.org/3/a-i2697e.pdf.
FAO (2019). Modernizing Rwanda's Livestock to Attract Investment and Enhance Food. Available at: http://www.fao.org/rwanda/news/detail-events/en/c/1185157/ (Accessed February 22, 2021).
FAO (2015). Rwanda at a Glance. Available at: http://www.fao.org/rwanda/our-office-in-rwanda/rwanda-at-a-glance/en/(Accessed February 21, 2021).
FAO (2018). Rwanda:Hunger and Food Insecurity. Available at: http://www.fao.org/faostat/en/#country/184 (Accessed February 22, 2021).
Fowler, D., Coyle, M., Skiba, U., Sutton, M. A., Cape, J. N., Reis, S., et al. (2013). The Global Nitrogen Cycle in the Twenty-First century. Phil. Trans. R. Soc. B 368, 20130164. doi:10.1098/rstb.2013.0164
Galloway, J. N., Cowling, E. B., Seitzinger, S. P., and Socolow, R. H. (2002). Reactive Nitrogen: Too Much of a Good Thing? AMBIO: A J. Hum. Environ. 31, 60–63. doi:10.1579/0044-7447-31.2.60
Galy-Lacaux, C., and Delon, C. (2014). Nitrogen Emission and Deposition Budget in West and Central Africa. Environ. Res. Lett. 9, 125002. doi:10.1088/1748-9326/9/12/125002
GeoDatos, (2021). Geographic Information: Maps, Data and Statistics from All the Countries and Cities in the World. Available at: https://www.geodatos.net/en (Accessed February 3, 2021).
Goossens, Y., Wegner, A., and Schmidt, T. (2019). Sustainability Assessment of Food Waste Prevention Measures: Review of Existing Evaluation Practices. Front. Sustain. Food Syst. 3. doi:10.3389/fsufs.2019.00090
Goulding, K. W. T., Bailey, N. J., Bradbury, N. J., Hargreaves, P., Howe, M., Murphy, D. V., et al. (1998). Nitrogen Deposition and its Contribution to Nitrogen Cycling and Associated Soil Processes. New Phytol. 139, 49–58. doi:10.1046/j.1469-8137.1998.00182.x
Greaver, T. L., Clark, C. M., Compton, J. E., Vallano, D., Talhelm, A. F., Weaver, C. P., et al. (2016). Key Ecological Responses to Nitrogen Are Altered by Climate Change. Nat. Clim Change 6, 836–843. doi:10.1038/nclimate3088
Guo, M., Chen, X., Bai, Z., Jiang, R., Galloway, J. N., Leach, A. M., et al. (2017). How China's Nitrogen Footprint of Food Has Changed from 1961 to 2010. Environ. Res. Lett. 12, 104006. doi:10.1088/1748-9326/aa81d9
Habimana, V., and Nsabimana, A. (2020). Water Physico-Chemical Characteristics of the Lakes Burera and Ruhondo, Rwanda. Rjeste 3, 80–87. doi:10.4314/rjeste.v3i2.5
Henao, J., and Baanante, C. (1999). Estimating Rates of Nutrient Depletion in Soils of Agricultural Lands of Africa. Muscle Shoals, Alabama: Muscle Shoals: International Fertilizer Development Center.
Herridge, D. F., Peoples, M. B., and Boddey, R. M. (2008). Global Inputs of Biological Nitrogen Fixation in Agricultural Systems. Plant Soil 311, 1–18. doi:10.1007/s11104-008-9668-3
Hickman, J. E., Tully, K. L., Groffman, P. M., Diru, W., and Palm, C. A. (2015). A Potential Tipping point in Tropical Agriculture: Avoiding Rapid Increases in Nitrous Oxide Fluxes from Agricultural Intensification in Kenya. J. Geophys. Res. Biogeosci. 120, 938–951. doi:10.1002/2015JG002913
Hossain, M. F., White, S. K., Elahi, S. F., Sultana, N., Choudhury, M. H. K., Alam, Q. K., et al. (2005). The Efficiency of Nitrogen Fertiliser for rice in Bangladeshi Farmers' fields. Field Crops Res. 93, 94–107. doi:10.1016/j.fcr.2004.09.017
Hutton, M. O., Leach, A. M., Leip, A., Galloway, J. N., Bekunda, M., Sullivan, C., et al. (2017). Toward a Nitrogen Footprint Calculator for Tanzania. Environ. Res. Lett. 12, 034016. doi:10.1088/1748-9326/aa5c42
Johnston, A. M., and Bruulsema, T. W. (2014). 4R Nutrient Stewardship for Improved Nutrient Use Efficiency. Proced. Eng. 83, 365–370. doi:10.1016/j.proeng.2014.09.029
Joshi, P., and Visvanathan, C. (2019). Sustainable Management Practices of Food Waste in Asia: Technological and Policy Drivers. J. Environ. Manage. 247, 538–550. doi:10.1016/j.jenvman.2019.06.079
Karamage, F., Zhang, C., Ndayisaba, F., Shao, H., Kayiranga, A., Fang, X., et al. (2016). Extent of Cropland and Related Soil Erosion Risk in Rwanda. Sustainability 8, 609–619. doi:10.3390/su8070609
Katz, B. G. (2020). Nitrogen Overload: Environmental Degradation, Ramifications, and Economic Costs. John Wiley & Sons. doi:10.1002/9781119513933 Nitrogen Overload
Kelly, V., Mpyisi, E., Shingiro, E., and Nyarwaya, J. (2001). Agricultural Intensification in Rwanda: An Elusive Goal Fertilizer Use and Conservation Investments, 1092, 2016–87649.
Kingori, A. M., Wachira, A. M., and Tuitoek, J. K. (2010). Indigenous Chicken Production in Kenya: A Review. Int. J. Poult. Sci. 9, 309–316. doi:10.3923/ijps.2010.309.316
Kok, D.-J. D., Pande, S., Ortigara, A. R. C., Savenije, H., and Uhlenbrook, S. (2018). Socio-Hydrological Approach to the Evaluation of Global Fertilizer Substitution by Sustainable Struvite Precipitants from Wastewater. Proc. IAHS 376, 83–86. doi:10.5194/piahs-376-83-2018
Krupnik, T. J., Six, J., Ladha, J. K., and Kessel, C. Van. (2004). An Assessment of Fertilizer Nitrogen Recovery Efficiency by Grain Crops across an Assessment of Fertilizer Nitrogen Recovery Efficiency by Grain Crops across Scales Department of Agronomy and Range Science. University of California , One Shields Ave.
Lassaletta, L., Billen, G., Garnier, J., Bouwman, L., Velázquez, E., Mueller, N. D., et al. (2016). Nitrogen Use in the Global Food System: Past Trends and Future Trajectories of Agronomic Performance, Pollution, Trade, and Dietary Demand. Environ. Res. Lett. 11, 095007. doi:10.1088/1748-9326/11/9/095007
Lassaletta, L., Billen, G., Garnier, J., Oita, A., Shibata, H., Shindo, J., et al. (2019). Nitrogen Embedded in Global Food Trade, 105, 109. doi:10.1016/B978-0-08-100596-5.21975-6
Lassaletta, L., Billen, G., Grizzetti, B., Anglade, J., and Garnier, J. (2014a). 50 Year Trends in Nitrogen Use Efficiency of World Cropping Systems: The Relationship between Yield and Nitrogen Input to Cropland. Environ. Res. Lett. 9, 105011. doi:10.1088/1748-9326/9/10/105011
Lassaletta, L., Billen, G., Grizzetti, B., Garnier, J., Leach, A. M., and Galloway, J. N. (2014b). Food and Feed Trade as a Driver in the Global Nitrogen Cycle: 50-year Trends. Biogeochemistry 118, 225–241. doi:10.1007/s10533-013-9923-4
Leach, A. M., Galloway, J. N., Bleeker, A., Erisman, J. W., Kohn, R., and Kitzes, J. (2012). A Nitrogen Footprint Model to Help Consumers Understand Their Role in Nitrogen Losses to the Environment. Environ. Dev. 1, 40–66. doi:10.1016/j.envdev.2011.12.005
Lederer, J., Karungi, J., and Ogwang, F. (2015). The Potential of Wastes to Improve Nutrient Levels in Agricultural Soils: A Material Flow Analysis Case Study from Busia District, Uganda. Agric. Ecosyst. Environ. 207, 26–39. doi:10.1016/j.agee.2015.03.024
Li, Y., Schichtel, B. A., Walker, J. T., Schwede, D. B., Chen, X., Lehmann, C. M. B., et al. (2016). Increasing Importance of Deposition of Reduced Nitrogen in the United States. Proc. Natl. Acad. Sci. USA 113, 5874–5879. doi:10.1073/pnas.1525736113
Liang, X., Leach, A. M., Galloway, J. N., Gu, B., Lam, S. K., and Chen, D. (2016). Beef and Coal Are Key Drivers of Australia's High Nitrogen Footprint. Sci. Rep. 6, 4–11. doi:10.1038/srep39644
Liu, X., Song, L., He, C., and Zhang, F. (2010). Nitrogen Deposition as an Important Nutrient from the Environment and its Impact on Ecosystems in China. null 2, 137–143. doi:10.3724/SP.J.1227.2010.00137
Masso, C., Baijukya, F., Ebanyat, P., Bouaziz, S., Wendt, J., Bekunda, M., et al. (2017). Dilemma of Nitrogen Management for Future Food Security in Sub-saharan Africa - a Review. Soil Res. 55, 425. a review Dilemma of nitrogen management for future food security in sub-Saharan Africa – a review. doi:10.1071/SR16332
Miklyaev, M., Jenkins, G., and Shobowale, D. (2021). Sustainability of Agricultural Crop Policies in Rwanda: An Integrated Cost-Benefit Analysis. Sustainability 13, 48–22. doi:10.3390/su13010048
Montagnac, J. A., Davis, C. R., and Tanumihardjo, S. A. (2009). Nutritional Value of Cassava for Use as a Staple Food and Recent Advances for Improvement. Compr. Rev. Food Sci. Food Saf. 8, 181–194. doi:10.1111/j.1541-4337.2009.00077.x
Mugabo, J. R., Tollens, E., Chianu, J. N., and Vanlauwe, B. (2020). Mineral Fertilizer Use in Land-Scarce Conditions: Case of Rwanda. Open Agric. 5, 690–702. doi:10.1515/opag-2020-0066
Mulambu, J. (2017). Iron Beans in Rwanda: Crop Development and Delivery Experience. Ajfand 17, 12026–12050. doi:10.18697/ajfand.78.HarvestPlus10
Mutimura, M., Lussa, A. B., Mutabazi, J., Myambi, C. B., Cyamweshi, R. A., and Ebong, C. (2013). Status of Animal Feed Resources in Rwanda. Trop. Grass - Forr Trop. 1, 109–110. doi:10.17138/tgft(1)109-110
Nabahungu, N. L., Semoka, J. M. R., and Zaongo, C. (2007). Limestone, Minjingu Phosphate Rock and Green Manure Application on Improvement of Acid Soils in Rwanda. Adv. Integr. Soil Fertil. Manag. Sub-saharan Africa Challenges Oppor, 703–712. doi:10.1007/978-1-4020-5760-1_65
Nambajimana, J. d. D., He, X., Zhou, J., Justine, M. F., Li, J., Khurram, D., et al. (2019). Land Use Change Impacts on Water Erosion in Rwanda. Sustainability 12, 50. doi:10.3390/su12010050
National-Research-Council (2003). Air Emissions from Animal Feeding Operations. Washington, D.C: The National Academic Press.Air Emissions from Animal Feeding Operations
Nduwumuremyi, A., Ruganzu, V., Mugwe, J. N., and Cyamweshi Rusanganwa, A. (2013). Effects of Unburned Lime on Soil pH and Base Cations in Acidic Soil. ISRN Soil Sci. 2013, 1–7. doi:10.1155/2013/707569
NISR (2018). National Institute of Statistics of Rwanda: Seasonal Agricultural Survey, 2017; May 2018. Available at: https://www.statistics.gov.rw/publication/seasonal-agricultural-survey-report-2017. pdf.
Niyitanga, Fidèle., and Naramabuye, F. X. (2020). Pathways to Sustainable Land-Use and Food Systems.
Niyitanga, F., Kabayiza, A., and Niyonzima, J. P. (2015). Assessment of Yield Gaps in Main Staple Crops in Rwanda. Int. J. Agric. Innov. Res. 3, 1267–1271. http://www.ijair.org/administrator/components/com_jresearch/files/publications/IJAIR-1241_Final.pdf.
Nyesheja, E. M., Chen, X., El-Tantawi, A. M., Karamage, F., Mupenzi, C., and Nsengiyumva, J. B. (2018). Soil Erosion Assessment Using RUSLE Model in the Congo Nile Ridge Region of Rwanda. Phys. Geogr. 40, 339–360. doi:10.1080/02723646.2018.1541706
Nzeyimana, I., Hartemink, A. E., and de Graaff, J. (2013). Coffee Farming and Soil Management in Rwanda. Outlook Agric. 42, 47–52. doi:10.5367/oa.2013.0118
Oita, A., Malik, A., Kanemoto, K., Geschke, A., Nishijima, S., and Lenzen, M. (2016). Substantial Nitrogen Pollution Embedded in International Trade. Nat. Geosci 9, 111–115. doi:10.1038/ngeo2635
Oita, A., Nagano, I., and Matsuda, H. (2018). Food Nitrogen Footprint Reductions Related to a Balanced Japanese Diet. Ambio 47, 318–326. doi:10.1007/s13280-017-0944-4
Paramasivam, S., Jayaraman, K., Wilson, T. C., Alva, A. K., Kelson, L., and Jones, L. B. (2009). Ammonia Volatilization Loss from Surface Applied Livestock Manure. J. Environ. Sci. Health B 44, 317–324. doi:10.1080/1093452080265962010.1080/03601230902728484
Pasley, H. R., Camberato, J. J., Cairns, J. E., Zaman-Allah, M., Das, B., and Vyn, T. J. (2020). Nitrogen Rate Impacts on Tropical maize Nitrogen Use Efficiency and Soil Nitrogen Depletion in Eastern and Southern Africa. Nutr. Cycl Agroecosyst 116, 397–408. doi:10.1007/s10705-020-10049-x
Pierer, M., Winiwarter, W., Leach, A. M., and Galloway, J. N. (2014). The Nitrogen Footprint of Food Products and General Consumption Patterns in Austria. Food Policy 49, 128–136. doi:10.1016/j.foodpol.2014.07.004
Raza, S., Zhou, J., Aziz, T., Afzal, M. R., Ahmed, M., Javaid, S., et al. (2018). Piling up Reactive Nitrogen and Declining Nitrogen Use Efficiency in Pakistan: a challenge Not Challenged (1961-2013). Environ. Res. Lett. 13, 034012. doi:10.1088/1748-9326/aaa9c5
Roobroeck, D., Van Asten, P. J. A., Jama, B., Harawa, R., and Vanlauwe, B. (2016). Integrated Soil Fertil. Manag. Contrib. framework practices Climate-Smart Agric. doi:10.13140/RG.2.1.1695.2400
Sanchez, P. A., Shepherd, K. D., Soule, M. J., Place, F. M., Buresh, R. J., Izac, A.-M. N., et al. (1997, Soil Fertility Replenishment in Africa: An Investment in Natural Resource Capital). 1–46. doi:10.2136/sssaspecpub51.c1
Schönfeldt, H. C., and Gibson Hall, N. (2012). Dietary Protein Quality and Malnutrition in Africa. Br. J. Nutr. 108, S69–S76. doi:10.1017/S0007114512002553
Sheahan, M., and Barrett, C. B. (2017). Review: Food Loss and Waste in Sub-saharan Africa. Food Policy 70, 1–12. doi:10.1016/j.foodpol.2017.03.012
Shibata, H., Cattaneo, L. R., Leach, A. M., and Galloway, J. N. (2014). First Approach to the Japanese Nitrogen Footprint Model to Predict the Loss of Nitrogen to the Environment. Environ. Res. Lett. 9, 115013. doi:10.1088/1748-9326/9/11/115013
Shibata, H., Galloway, J. N., Leach, A. M., Cattaneo, L. R., Cattell Noll, L., Erisman, J. W., et al. (2017). Nitrogen Footprints: Regional Realities and Options to Reduce Nitrogen Loss to the Environment. Ambio 46, 129–142. doi:10.1007/s13280-016-0815-4
Smaling, E. M. A., and Braun, A. R. (1996). Soil Fertility Research in sub‐Saharan Africa: New Dimensions, New Challenges. Commun. Soil Sci. Plant Anal. 27, 365–386. doi:10.1080/00103629609369562
Spiertz, J. H. J. (2009). Nitrogen, Sustainable Agriculture and Food Security: a Review. Sust. Agric., 635–651. doi:10.1007/978-90-481-2666-8_39
Stevens, C. J., Leach, A. M., Dale, S., and Galloway, J. N. (2014). Personal Nitrogen Footprint Tool for the United Kingdom. Environ. Sci. Process. Impacts 16, 1563–1569. doi:10.1039/c3em00690e
Stoorvogel, J. J., Smaling, E. M. A., and Janssen, B. H. (1993). Calculating Soil Nutrient Balances in Africa at Different Scales. Fertilizer Res. 35, 227–235. doi:10.1007/BF00750641
Summers, J. D., Slinger, S. J., Sibbald, I. R., and Pepper, W. F. (1964). Influence of Protein and Energy and Growth and Protein Utilization in the Growing Chicken. J. Nutr. 82, 463–468. doi:10.1093/jn/82.4.463
Taiz, L. (2013). Agriculture, Plant Physiology, and Human Population Growth: Past, Present, and Future. Theor. Exp. Plant Physiol. 25, 167–181. doi:10.1590/s2197-00252013000300001
Teenstra, E., Vellinga, T., Aektasaeng, A., Amatayakul, W., Ndambi, O. A., Pelster, D. E., et al. (2014). Global Assessment of Manure Management Policies and Practices. Wageningen Livest. Res. Rep. 844. doi:10.6084/m9.figshare.8251232
Toomsan, B., McDonagh, J. F., Limpinuntana, V., and Giller, K. E. (1995). Nitrogen Fixation by Groundnut and Soyabean and Residual Nitrogen Benefits to rice in Farmers' fields in Northeast Thailand. Plant Soil 175, 45–56. doi:10.1007/BF02413009
United Nations (2019). Resources for Different Audiences. https://www.un.org/en/sections/resources-different-audiences/(Accessed February 20, 2021).
United Nations, (2017). World Population Prospects. Available at: https://population.un.org/wpp/ (Accessed October 9, 2021).
Verhulst, N., François, I., Grahmann, K., Cox, R., and Govaerts, B. (2014). Nitrogen Use Efficiency and Optimization of Nitrogen Fertilization in Conservation Agriculture.
Weatherspoon, D. D., Miller, S., Ngabitsinze, J. C., Weatherspoon, L. J., and Oehmke, J. F. (2019). Stunting , Food Security , Markets and Food Policy in Rwanda, 1–13.
Westermann, D. T., Porter, L. K., and O'Deen, W. A. (1985). Nitrogen Partitioning and Mobilization Patterns in Bean Plants1. Crop Sci. 25, 225. doi:10.2135/cropsci1985.0011183x002500020007x
Woomer, P. L., Bokanga, M., and Odhiambo, G. D. (2008). Striga Management and the African Farmer. Outlook Agric. 37, 277–282. doi:10.5367/000000008787167790
World Bank (2018). DataBank. https://databank.worldbank.org/home.aspx (Accessed February 21, 2021).
World Bank (2020). Rwanda Food Smart Country Diagnostic. Washington, DC: International Bank for Reconstruction and Development.
Worldatlas (2019). Geography Website that Aids Students, Teachers, Travellers and Parents with Their Geography, Map and Travel Questions 100 - 7405 Transcanadienne St. Laurent Quebec Canada H4T1Z2. Worldatlas. Available at: https://www.worldatlas.com/(Accessed February 28, 2021).
Worldometer (2020). Worldometer - Real Time World Statistics. Available at: https://www.worldometers.info/(Accessed February 21, 2021).
Yang, K., Zhu, J., Gu, J., Xu, S., Yu, L., and Wang, Z. (2018). Effects of Continuous Nitrogen Addition on Microbial Properties and Soil Organic Matter in a Larix Gmelinii Plantation in China. J. For. Res. 29, 85–92. doi:10.1007/s11676-017-0430-7
Yuan, S., and Peng, S. (2017). Exploring the Trends in Nitrogen Input and Nitrogen Use Efficiency for Agricultural Sustainability. Sustainability 9, 1905. doi:10.3390/su9101905
Zhang, X., Davidson, E. A., Mauzerall, D. L., Searchinger, T. D., Dumas, P., and Shen, Y. (2015). Managing Nitrogen for Sustainable Development. Nature 528, 51–59. doi:10.1038/nature15743
Zhang, Y., Liu, Y., Shibata, H., Gu, B., and Wang, Y. (2018). Virtual Nitrogen Factors and Nitrogen Footprints Associated with Nitrogen Loss and Food Wastage of China's Main Food Crops. Environ. Res. Lett. 13, 014017. doi:10.1088/1748-9326/aa98a6
Keywords: nitrogen loss, agro-food system, soil nitrogen mining, nitrogen footprint, virtual nitrogen factor, nitrogen use efficiency, environmental pollution, Rwanda
Citation: Harerimana B, Zhou M, Shaaban M and Zhu B (2021) Estimating Nitrogen Flows and Nitrogen Footprint for Agro-Food System of Rwanda Over the Last Five Decades: Challenges and Measures. Front. Environ. Sci. 9:778699. doi: 10.3389/fenvs.2021.778699
Received: 17 September 2021; Accepted: 26 October 2021;
Published: 26 November 2021.
Edited by:
Laodong Guo, University of Wisconsin–Milwaukee, United StatesCopyright © 2021 Harerimana, Zhou, Shaaban and Zhu. This is an open-access article distributed under the terms of the Creative Commons Attribution License (CC BY). The use, distribution or reproduction in other forums is permitted, provided the original author(s) and the copyright owner(s) are credited and that the original publication in this journal is cited, in accordance with accepted academic practice. No use, distribution or reproduction is permitted which does not comply with these terms.
*Correspondence: Minghua Zhou, bWh1YW56aG91QGltZGUuYWMuY24=