- 1Department of Environmental Science, School of Earth and Environmental Sciences, University of Kashmir, Srinagar, India
- 2Department of Environmental Science, Govt. Degree College Beerwah, Budgam, India
High altitude lakes (HALs) of Kashmir Himalaya are the important ecosystems in the mountain ecology of the broader Hindukush Himalayan region. This article provides a comprehensive information about the plankton (phytoplankton and periphyton) assemblages, water quality (WQ), bathymetry, morphometry, and land use land cover (LULC) of some select high altitude mountain lakes of Kashmir Himalaya. LULC analysis revealed that the catchment of the lakes spread over an area of about 16179 ha, is covered by different land cover types dominated by pastures (50.8%), followed by barren rocky (32.6%), snow and glaciers (11.9%), lakes (2.5%), forest (2%), and streams (0.2%). Bathymetric and morphometric analysis revealed that the Gangbal Lake is the deepest (84 m) and largest (162.4 ha) among the investigated lakes. The water quality index revealed that all the HALs have the excellent water quality category. Statistical analysis (Wilk’s λ) depicted that nitrate-nitrogen (NO3−-N), nitrite nitrogen (NO2−-N), ammoniacal nitrogen (NH3-N), total phosphorus (TP), and magnesium hardness (Mg-H) are responsible for major variability between all HALs sites. The cations followed the order of Ca2+ > Mg2+ > Na+ > K+ while as anions followed the order as HCO3− > Cl− > SO42−. Algal composition (phytoplankton and periphyton) assessment revealed the presence of 61 taxa belonging to Bacillariophyceae (45), Chlorophyceae (14), Cyanophyceae (1), and Xanthophyceae (1). The higher dominance of Bacillariophyceae indicates oligotrophic nature of the lakes. Canonical correspondence analysis (CCA) highlighted the role of various water quality parameters like pH, EC, and TDS on the composition of phytoplankton and periphyton species among the lakes. The present study therefore generated a baseline database for some of the HALs of Kashmir Himalaya that can act as a precursor for more research on future changes in the lake ecosystems of the region.
Introduction
High Altitude Lakes (HALs) are the most important, pristine, and ecologically sensitive ecosystems which lie usually above 3,000 m altitude (Chatterjee et al., 2010; Pastorino et al., 2020; Dar et al., 2021a). They are considered as one of the most significant natural resources for the survival of living beings (Walsh and Milon, 2016). HALs provide a variety of ecological services and functions, apart from being a major source of the transboundary river ecosystems, and play an important role in hydropower generation, irrigation facilities, besides they help in satisfying the drinking water demand to a noteworthy percentage of the world population (Chatterjee et al., 2010; UNCSD, 2012). HAL’s are known as “natural laboratories” wherein global biological diversity and water quality changes could be examined (Rogora et al., 2018; Pastorino and Prearo, 2020) to identify the important anthropogenic pressures (Battarbee et al., 2009). HALs are categorized by a distinctive water sources, species, habitats, and communities, and have particular spiritual, and cultural importance (WWF, 2009; Gurung et al., 2018). Although apparent to be in a pristine state, they are potentially at risk from overgrazing (Pastorino et al., 2018), climate change (Pastorino and Prearo, 2020), long-range transport of atmospheric pollutants (Schindler and Smol, 2006), water abstraction and exploitation (Tiberti et al., 2019), alien species introduction (Pastorino et al., 2020b), mountain tourism activities (Rupakheti et al., 2017) and mountain farming (Tiberti et al., 2014). Remote HALs are highly vulnerable to pollution from human dominated mid and low altitude lake systems due to combined effect of high ultra-violet radiation exposure and cold distillery phenomenon (Elser et al., 2020; Dar et al., 2021a). The concentration of precipitation borne pollutants increases in ice and snow because of sublimation and lower temperature slows down contaminant degradation (Daly and Wania 2005; Elser et al., 2020). HALs function as a natural storeroom for ecological evidence linked to changing climate and other environmental parameters (Raut et al., 2012; Kaphle et al., 2021). Lake/river water chemistry along with sediment chemistry plays a significant part in explaining the underlying forces of earth surface (Sheikh et al., 2014). As lakes are relatively less dynamic, they are prone to exogenic influences and deposit pollutants gradually (Saini et al., 2008). The hydro-chemical profile of these lake systems is mostly influenced by atmospheric deposition and climatic parameters; therefore, they act as indicators of climate change, atmospheric deposition, and air pollution (Rogora et al., 2008). Generally, the hydrochemistry of the lakes explains the temporal changes in the behavior of ions and watershed features (Anshumali and Ramanathan, 2007). LULC change is most effective factor responsible for changing landscapes (Dar et al., 2021c; 2021d). Land cover maps represent inclusive information about the status of specific landmarks under study. Bathymetric and morphometric attributes are important factors for better understanding of lacustrine system structure, physical topography, and functioning (Raut et al., 2012). GIS based quantitative morphometric, bathymetric measurements and LULC maps provides a pivotal information in support of concluding results to proper lake management and protection (Dar et al., 2021c; 2021d).
The interest of the scientific community towards the phytoplankton and periphytic assemblages increased massively after Hutchinson’s (1961) seminal publication on the paradox of the plankton. Hutchinson contrast Hardin’s competitive exclusion theory (Hardin, 1960) by providing a plausible explanation for the co-existence of the species in a homogeneous environment. Ever since the resurgence in phytoplankton research might be elucidated by development in the theoretical and methodological characteristics. Lakes at high altitudes are unique ecosystems that are often described by lower temperatures, oligotrophy, low productivity, simple food web structure, and strong ultra-violet radiation in surface waters (Xing et al., 2009; Kuefner et al., 2021). Biological assemblages at higher elevations are modified to severe environmental conditions of unfavorable boreo-alpine environment (Kuefner et al., 2021). Plankton display a significant role in the productivity and transmission of nutrients in the aquatic ecosystems and therefore acts as a vital bioindicator for monitoring and evaluating the degree of contamination in the aquatic systems (Gao et al., 2018). Increasing anthropogenic pressures and global warming induced latitudinal shifts in climate zones, lead to the modification in the hydrological regime with harmful inference for aquatic systems inclusive of planktons. This contemporary confronts may influence the near future advancements in phytoplankton related studies (Borics et al., 2020). The hydro-biological status of HALs acts as a reference system for complex biophysical and chemical processes occurring within their catchment areas (Steingruber and Colombo, 2006; Singh et al., 2008).
Previously, the studies on the phytoplankton community of the HALs were limited to large species like dinoflagellates and diatoms, as large mesh-size plankton nets were used (Tolotti et al., 2006). The findings guided to the intention that HALs are very low in productivity, and thus aren’t capable of supporting a well-established phytoplankton community (Naumann, 1924; Monti and Stella, 1934). This hypothesis was not annulled till the late 1950s when the autotrophic nature of HALs was identified (Rodhe, 1962). This realization stirred a chain of intensive studies which considerably enhanced the acquaintance of plankton distribution and their ecological status in HALs. In Kashmir Himalaya, the limnological investigation on HALs began with the works of Hutchinson, (1937), Löffler, (1969), and (Zutshi et al., 1972). The restricted research that exists is limited in diffuse and at times in unpublished sources. Although, several studies have been conducted to understand biological diversity and hydro-chemical profile of the valley lakes in Kashmir Himalaya (Romshoo and Muslim, 2011; Parvez and Bhat, 2014; Nissa and Bhat, 2016; Rashid et al., 2017a; Khanday et al., 2018; Bashir et al., 2020; Dar et al., 2020a, b; Dar et al., 2021b), very few studies have focused on hydrobiological assessments of the HALs in Kashmir Himalaya (Zutshi, 1980; Zutshi and Vass, 1982; Zutshi, 1991; Shah et al., 2014). Thus, to fulfill this research gap the five HALs in the Kashmir Himalaya were selected to provide a comprehensive information on the LULC, bathymetry, hydro-chemical profile including the plankton diversity. The study will contribute to the further understanding of the hydro-chemical and biological (Phytoplankton and Periphyton) spectrum and physical status of alpine lake ecosystems and will also serve as a reference for assessing future alterations in these alpine systems of the Kashmir Himalayas especially in the climate change impact scenario.
Materials and Methods
Study Area
In the Indian Himalayan region, a total of 4,703 HALs have been mapped, which are situated above 3,000 m, covering an area of 126,249 ha (SAC, 2011; Singh et al., 2016). In the Jammu and Kashmir state alone, there are about 2014 HALs. The present study is focused on the five HALs viz., Gangbal, Nundkul, Gadsar, Kishansar, and Vishansar (also known as the Great Lakes of Kashmir) and their associated streams in Kashmir Himalaya (Figure 1). Since these HALs are located in remote, tough mountain terrains, these are assessed only through marathon trekking. The scale of landscape makes the study area a moderately-difficult trek spanning over 10 days. The trek introduces many challenging situations and great skills are needed to overcome them. The trekking route passes through many picturesque flower-stern meadows, stupefied by the sight of Himalayan wildlife and striking flora. The study area is a conglomerate of wild landscapes, tough rugged mountains, and turquoise HALs. The lakes under investigation are fed by glacier melt, precipitation, and springs. The Gangbal and Nundkol Lakes are situated at the foothills of Mount Haramukh in the Sindh basin of the Ganderbal district. Both the lakes are alpine high altitude oligotrophic lakes and home to many species of fish including the brown trout. Gadsar Lake also known as Yemsar Lake is a picturesque, alpine high altitude oligotrophic lake, the floating icebergs could be easily seen on its surface in the month of July-August. The Gadsar Lake outflows through a stream, north-westwards, and joins Neelum River at Tulail which flows to Pakistan. The Kishansar and Vishansar Lake are situated 10 kms away from the Sonamarg hill station. Kishansar Lake outflows through a small channel to the Vishansar Lake. The Vishansar Lake outflows in a north-westward direction up to Badoab and then westwards through Gurez along the Line of Control to Pakistan. The watershed as dominated by exposed barren and rocky areas, the vegetation is comprised of grasses, herbs, and a patch of forest on one side. The catchment area of all the lakes is heavily used as a grazing land by the herders and the area is also used for the collection of medicinal plants. Threats of pollution from both mountain tourism and herders exist in the area. Animals such as bears, Snow leopard, Musk deer, and Yak have been reported in the area.
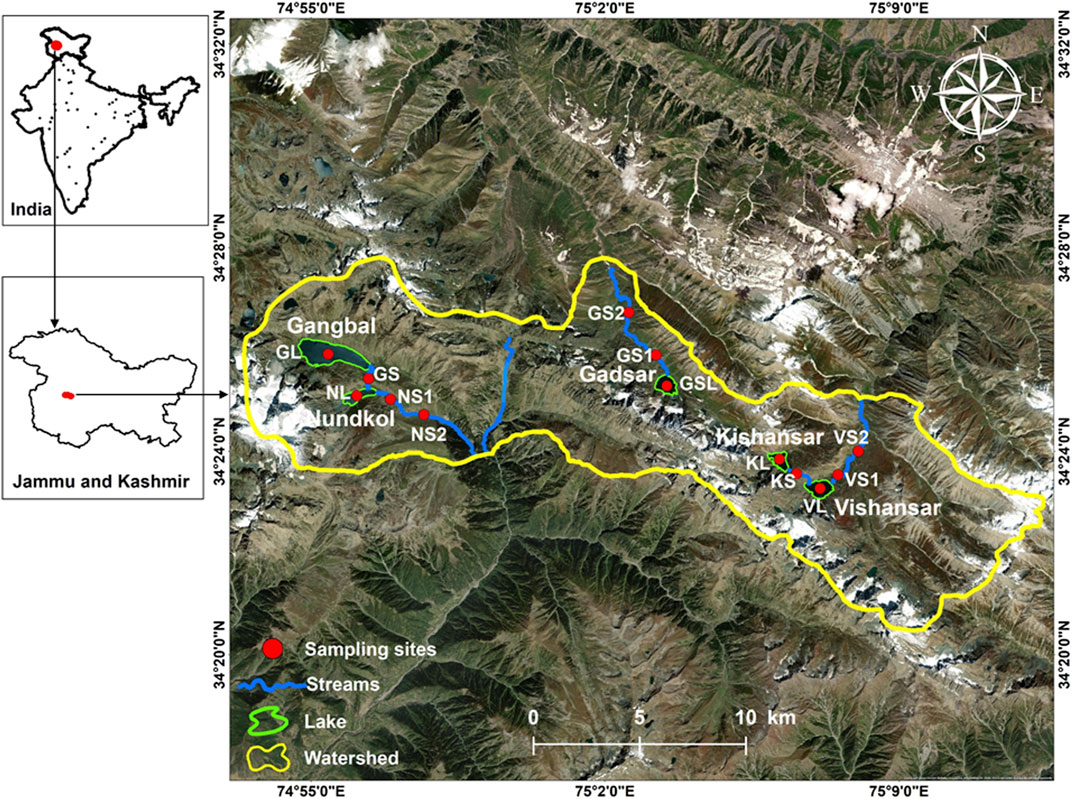
FIGURE 1. Study area map showing some important alpine high altitude lakes in Kashmir Himalaya. Background image ESRI Basemap.
Water Quality
The sampling for collection of water and biological samples was carried out in ice free period (July–August) during 2015 and 2017. A total of thirteen sites comprising five sampling sites from lakes and eight sampling sites from associated streams were selected for collection of water and biological samples (Figure 1). A detailed description of the selected sites is provided in Table 1. As the study sites are located in deep mountainous terrains, the sampling was carried out by trekking which extended for a period of 10 days each year. Collection, preservation, and transfer of samples to the laboratory was carried out as per the standard methods (APHA, 2017). A portable global positioning system (GPS) was employed to assess the geographical coordinates of the sampling stations. Water temperature (WT), electrical conductivity (EC), pH, and total dissolved solids (TDS) were measured with the assistance of the Digital multi-parameter probe (Eutech PCSTEST35-01x441506). Dissolved oxygen (DO) was determined by using Hannah’s digital DO meter (Hanna HI 9146). Nitrate nitrogen (NO3−-N), nitrite nitrogen (NO2−-N), ammoniacal nitrogen (NH3-N), orthophosphate phosphorus (PO4), total phosphorus (TP), sulphate (SO42-), and dissolved silica (DS) were estimated using the UV-VIS Spectrophotometer. Total hardness (TH), calcium hardness (Ca-H), magnesium hardness (Mg-H), total alkalinity (TA), and chloride ion (Cl−) were determined by titrimetric method, following the standard protocols (APHA, 2017).
Morphometry and Bathymetric Analysis
Bathymetric maps for most of the HALs worldwide are still lacking, except for a small number of lakes that were measured mainly by sonar (Khattab et al., 2017). Kashmir Himalaya has a rich heritage of HALs. These lakes have high ecological, economic, and cultural importance but most of these are yet to be studied due to remote location, tough terrain, and lack of accessibility. A bathymetric survey was performed in August 2017 in the lakes under investigation to generate baseline information. Depth measurements were obtained from different locations in a boat using a graduated rope. The lake bathymetry surfaces were created using natural neighbor interpolation (NNI)/Sibson’s interpolant in ArcMap 10.1 (Ledoux and Gold, 2005; Dar et al., 2020b). The NNI uses measured values of points and their associated areas to calculate weights of the neighboring unsampled points. If we consider that each data point in S (a set of n points) has an attribute ai (a scalar value), the NNI function is given as
where f(x) is the interpolated function value at the location x and wi is the value or weight of a sample.
For the morphometric characterization of the lakes, the following morphometric parameters were obtained viz: lake area, maximum length (Lmax), and maximum width (Bmax). Values for these parameters were obtained from measurements performed with the ArcGIS 10.1 software (ESRI Inc.). The boundary of the lakes was digitized manually using high-resolution Google base map satellite imagery as a data source in ArcGIS 10.1 (Dar et al., 2021c). Area of the lakes was calculated using Calculate Geometry tool in ArcGIS 10.1. Scale tool was used to measure the Lmax along North-South axis and Bmax along East-West axis.
LULC Analysis
Since, catchment characteristics determine the water quality of lakes, the main rationale behind the LULC analysis was to look over the percent land area available for grazing. This was pursued keeping in view the fact that the catchment area of the lakes under investigation undergoes heavy grazing and browsing and it transports all the nutrients and fecal matter of cattle and livestock into the lakes. High resolution Google base map imagery was used for generating a LULC map. The imagery was co-registered for removal of geometric incongruity. Owing to the high resolution of the satellite data, six LULC classes including forest, lakes, pastures, barren rocky, snow/glaciers and streams were delineated using digital interpretation method at 1:2000 scale in ArcMap 10.1 (Rashid et al., 2016). The methodological sequence consisted of the following steps: image selection, digital processing, interpretation, ground truthing, quantification of land use classes, and qualitative analysis of the results.
Phytoplankton and Periphyton Collection, Identification, and Enumeration
Phytoplankton samples for qualitative and quantitative analysis were collected from different lakes. Phytoplankton net with mesh size of 64 µm was employed for filtration and a volume of 50 L of water were filtered from each site. The Periphytic algal samples were collected by scraping the 3 cm2 surface area of stones, boulders etc. using blade, scale, and brushes from stream sites. The material collected was then stored in vials having capacity of 25 ml. Samples were preserved in 4% formalin and algal identification and enumeration was carried out with the help of standard taxonomic works (Prescott, 1939; Edmondson, 1992; Cox, 1996; Jumppanen, 1976; Biggs and Kilroy, 2000; APHA, 2017). 1 ml of preserved phytoplankton sample was placed in Sedgwick-Rafter (SR) cell, it was allowed to settle for about 15 min and counted. Counting and identification of phytoplankton community was carried out using Binocular microscope (Magnus MLX-DX Olympus (India) pvt. ltd.) at 10x and 40x magnification. Samples were discarded and replaced by diluted ones if it was too dense to count, 1 cm of filamentous organisms was taken as one individual (Ahmed et al., 2017). Phytoplankton diversity indices were estimated using Paleontological statistics software for education and data Analysis (PAST 3.21 software) (Hammer et al., 2001).
Water Quality Index
Water quality index (WQI) is appropriate mathematical approach for assessing the quality of water (Sanchez et al., 2007; Sener et al., 2017; Khanday et al., 2021). To each WQ parameter, weights were given ranging from 1 (least impact on WQ) to 5 (highest impact on WQ).
WQI is estimated by the following equations:
where, Rwi is relative weight, wi is WQ parameter weight and n is the total number of WQ parameters. After that to every WQ parameter quality rating is assigned as below:
where Qri is the quality rating, CI is WQ parameter concentration in each sample and SI is drinking water standard for every parameter as per the guidelines of Bureau of Indian Standards (BIS) and World Health Organization (WHO).
Then, first sub-index (SIi) is calculated as:
Where in, SI is the sub-index of ith parameter, relative weight and quality rating of ith parameter are given by Rwi and Qri respectively.
Then water quality index is calculated as
The calculated WQI values are classified into five classes as: <50 as excellent water quality category; 50–100 highlights good water quality category; 100–200 denotes poor water quality category; 200–300 symbolizes very poor water quality class and >300 specifies the water quality category which is unsafe for drinking (Sanchez et al., 2007; Khanday et al., 2021).
Statistical Treatment
Cluster analysis (CA) a multivariate statistical tool was applied to data sets to find the resemblance and dissimilarities among the investigated sampling sites on the basis of physico-chemical parameters using Euclidean distance (Dar et al., 2021e). In order to evaluate whether the dataset contains meaningful cluster as highlighted in the cluster plot. “Hopkins’s statistic” was used to assess the clustering tendency in the dataset using 0.5 as threshold. Cluster validation was carried out using Silhouette coefficient measures, which indicates how similar or different an object is in its own cluster or neighboring cluster. Wilk’s λ test was performed to check which physico-chemical parameters were mainly responsible for the clustering of the sites. Correlation plots were plotted for determining the relation between the physicochemical parameters (p < 0.05). Canonical correspondence analysis (CCA) is a linear depiction learning technique that seeks maximally correlated parameters in multi-view data and this method was employed to establish the association among algal assemblages and various environmental variables (Devi et al., 2016). CA, clustering tendency, cluster validation, Wilk’s λ and correlation, was performed using R statistical package with “NbClust”, “cluster”, clustertend”, “factoextra”. “fpc”, and “clValid” packages and SPSS-16. CCA was performed using package “lab dsv” (Roberts, 2016).
Results
Water Quality
The results of the different physico-chemical parameters of the five HALs measured from 13 sampling sites are presented in Table 2. The WT varied from 9–14˚C. The lakes and streams were having good concentration of dissolved oxygen ranging from 7.6–8.4 mg L−1. The pH of the lakes highlighted neutral to alkaline pH with values ranging from 7.3 to 8.1. The lakes showed the lowest concentration of dissolved ions with EC values ranging from 15–53 μS cm−1, and TDS values ranging from 10–37 mg L−1. Total alkalinity values ranging from 36–70 mg L−1 is indicative of the good buffering capacity of the lakes. The order of equivalency of cations followed the order of Ca2+ > Mg2+ > Na+ > K+, while as anions followed the order of HCO32− > Cl− > SO42−. The concentration of chloride content ranging from 8–20 mg L−1 in the lakes clearly shows the role of catchment characteristics being largely exposed to extensive grazing activities by cattle and livestock (Gurung et al., 2018). All the nutrients recorded low concentration in the lakes with NO2−-N values ranging from 82–30 μg L−1, NO3−-N between 64 to 208 μg L−1, and NH3-N ranging from 35 to 108 μg L−1. During the present study, concentration of PO4 ranged from 21–72 μg L−1 and that of total phosphorus ranged from 32–168 μg L−1. Measurements of the flow data revealed that Nundkul stream has the highest discharge (14.6 m3 S−1) and velocity (1.2 m S−1) among all the investigated streams. The measurements of the WQI revealed that all the 13 sites fall within the excellent category with the WQI values ranging from 23.3–39.9 (Supplementary Table S1).
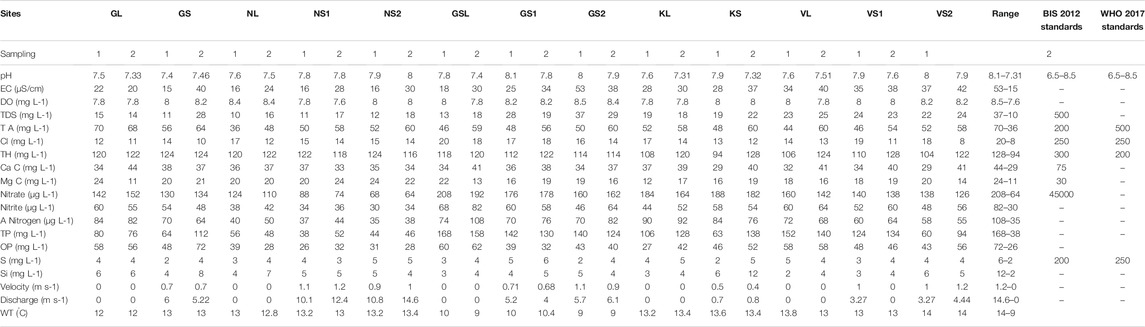
TABLE 2. Showing the variation in physicochemical parameters in the five HALs and associated streams.
It is evident from the Wilk’s λ quotient Mg-H, TDS, NO3-N, NO2-N, NH3-N, TP and ortho-P varied significantly (p < 0.05) throughout the study period (Supplementary Table S2). Highest values of TDS (37 mg L−1) and Mg+ (20 mg L−1), were recorded at VS2, GS2. While as highest values of NO3-N (208 μg L−1), NO2-N (68 μg L−1), NH3-N (108 μg L−1), TP (168 μg L−1) and Ortho-P (60 μg L−1) were recorded at GSL site.
Bathymetry and Morphometry
The Bathymetric analysis of the lakes revealed that the Gangbal lake is the deepest lake with a maximum depth of 84 m (Figure 2). This lake has a crevice as the deepest zone near the Mount Harmukh in the western part of the lake. The altitudinal variation of the lakes ranges from 3,505 m to 3,832 m. The morphometric features of the lakes are provided in Table 3. All the lakes are situated in an asymmetrical basin. Gangbal and Nundkol lakes are crescent shaped and are fed by the snow and glacial melt originating from the Harmukh glacier located on the western side of both the lakes. Besides snow and glacial melt, Nundkol lake is also getting its inflow through an outflow channel from the Gangbal lake. The outlet from the Nundkol lake forms Wangath nallah which after traversing a distance of 22 kms joins the Sindh River at Kangan. Gadsar Lake is more or less circular in shape and receives the snow melt water from the glaciers on the southern side. An outflow channel from the Gadsar Lake joins Neelum river at Tulail which flows through the Pakistan. Kishansar and Vishansar lakes are asymmetrical in shape. Kishansar Lake is fed by the melting of snow and glaciers and drains out through a small stream which falls into the Vishansar Lake. There is one outflow from the Vishansar Lake forming a tributary of Neelum river flowing through Pakistan.
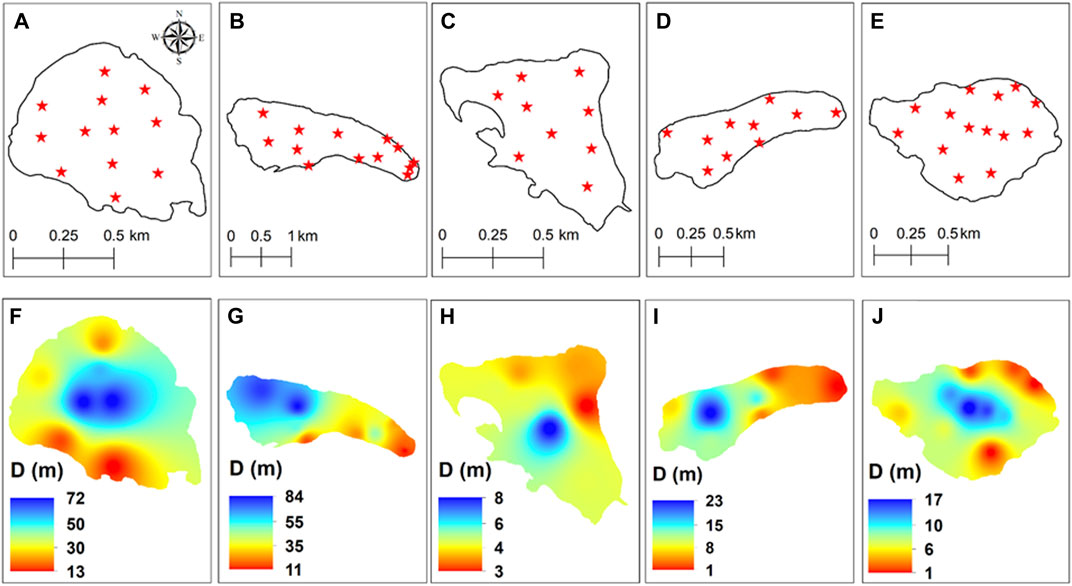
FIGURE 2. Bathymetry locations for measuring the depth of the lakes (A) Gadsar, (B) Gangbal, (C) Kishansar, (D) Nundkol, and (E) Vishansar and interpolated bathymetric maps of lakes (F) Gadsar, (G) Gangbal, (H) Kishansar, (I) Nundkol, and (J) Vishansar.
LULC
Using on-screen digitization of High resolution Basemap imagery, six LULC classes which include Forest, Lakes, Pasture, Barren rocky, Snow/Glacier, and Streams were delineated. The LULC map is shown in Figure 3, and details are provided in Table 4. From the analysis of the 2018 satellite data, the catchment of the lakes comprises of an area of 16179.5 ha. The predominant LULC feature in the catchment of the lakes is covered by pastures covering an area of 8,216.7 ha (50.8%). These pastures are mostly used for grazing by cattle and livestock. Second predominant LULC feature in the catchment comprises of barren rocks covering an area of 5,273.9 ha (32.6%). Snow and glaciers covering 1922.3 ha (11.9%) of the catchment area, are the main contributing sources to lakes and streams. Lakes cover an area of 401.7 ha (2.5%), followed by forest 324.9 ha (2%), and least area was covered by streams 40 ha (0.2%).
Algal Composition
The analysis of the data collected from the 13 sites revealed the presence of 63 genera of planktonic algae wherein the class Bacillariophyceae was dominant with 46 taxa followed by Chlorophyceae having 16 taxa, and 1 taxon of Xanthophyceae (Supplementary Table S3, S4). Floristically, Bacillariophyceae and Chlorophyceae were dominant in both the lake and the stream sites. Among Bacillariophyceae, Amphora sp., Cymbella sp., Diatoma sp., Didymosphenia sp., Pinnularia sp., Navicula sp., Navicularadiosa., Fragilaria sp., Fragilariforma sp., Frustulia sp. and Gomphonema sp., were dominant. In case of stream periphytic algae, Cymbella aspera, Cymbella kappi, Cymbella sp., Diatoma sp. Diatomella sp., Ghomphonema sp., Gomphonema minutum, Gomphonies sp., Navicula radiosa, Navicula sp., were found to dominate the overall species diversity. The highest number of phytoplankton individuals were found in Kishansar Lake (12987 ind/ L), followed by Vishansar Lake (11334 ind/ L), and Gangbal Lake (9,750 ind/ L), whereas in case of High-altitude streams, Gadsar stream reported highest number of periphytic algal density (1,020 Ind/ cm2), followed by Vishansar stream (954 ind/ cm2). The Shannon-Weiner index didn’t show much variation throughout the lakes and varied between 3.330 and 2.098 (Table 5). The Simpson and Evenness coefficients in both lakes and stream sites ranged between 0.9675 to 0.7754 and 0.7890 to 0.5178 respectively.

TABLE 5. Biodiversity indices of planktonic species collected at different sites during the course of study.
Statistical Treatment
Cluster analysis on the basis of Hydro-chemical variables and algal assemblages categorized all the sites into three clusters 1, 2, and 3 (Figure 4). The cluster 1 comprises of sites Nundkul lake and Nundkul stream sites. Cluster two consists of Vishansar Lake and stream sites and Gangbal stream sites. Cluster three consists of Krishansar Lake and stream sites, Gadsar Lake and stream sites and Gangbal Lake site. The results of the Hopkins test are 0.60, which is greater than the threshold limit indicating that dataset is significantly clusterable. Three cluster solution gives us an average silhouette coefficient of 0.39 (Figure 5) Furthermore, no negative average silhouette coefficients were observed in three obtained clusters, indicating sites are present in the right cluster. Canonical discriminant functions depicted clear separation of sites into three clusters that were categorized by cluster analysis (Figure 5). Tests of Equality of Group Means (Wilk’s λ) depicted that nutrient concentration (TP, PO4, NH3-N, NO2−-N, NO3−-N) and Mg-H were major variables that are responsible for the variability among all selected sites (Supplementary Table S2). Boxplots depict that Mg-H was highest in cluster C1 comprising of Nundkul Lake and stream sites, while as nutrient concentration (TP, OP, NO2−-N, NO3−-N, and NH3-N) was highest at all remaining sites (Figure 6). Correlation plots depict that parameter like TDS and TP were positively correlated with EC (Figure 7). Variables such as NO2−N, NO3−N, and NH3-N were found negatively correlated with Mg-H whereas, Ca-H was negatively correlated with velocity and pH. Discharge was found to be in positive correlation with pH. CCA was conducted between density of the dominant genera belonging to algal assemblages and different physico-chemical parameters. This special set of scalars explained 61.55 and 27.86% of correlation along axis 1 and 2 between physico-chemical parameters of water and algal groups respectively (Figure 8). The variables such as pH, conductivity, and TDS showed positive correlation with the number of Taxa at the sampling stations VS2, NS1, NS2, KS, and GS whereas they were negatively correlated with Ca-H. During the study, it was observed that Xanthophyceae were positively correlated with nitrate, nitrite, and ammoniacal nitrogen at sampling sites GL and KL. However, Chlorophyceae and Bacillariophyceae reflected the negative relationships with DO, Mg-H, TH, and TA at sampling sites GS1, GS2, GGL, and VS.
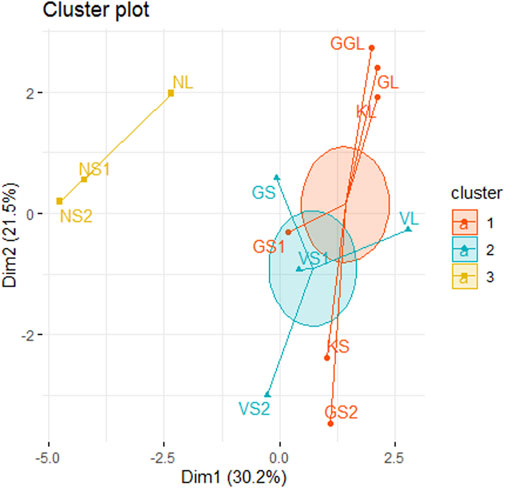
FIGURE 4. Hierarchical cluster analysis highlighting 3 clusters (1, 2, 3) of all sites on the basis of physicochemical parameters.
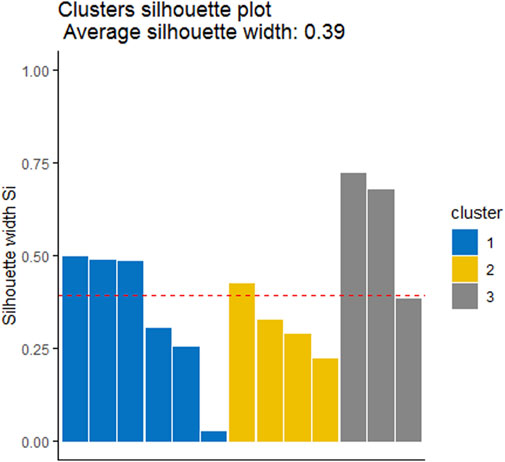
FIGURE 5. Cluster validation using Silhouette coefficient measures highlighting three cluster and placement of sites in their right cluster.
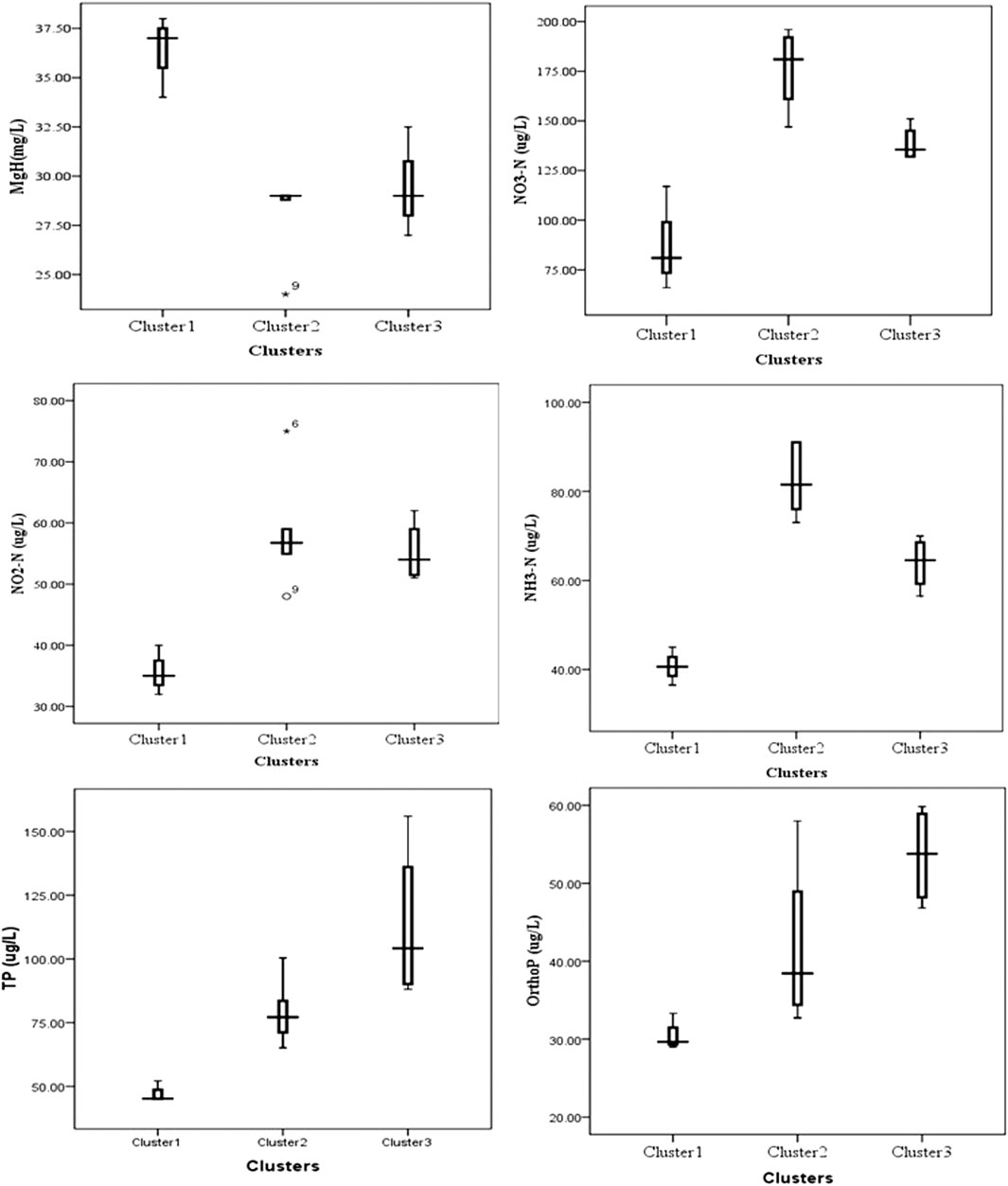
FIGURE 6. Boxplots of parameters (Mg-H, NO3-N, NO2-N, NH3-N, TP, and OP) responsible for major variability throughout the HAL sites.
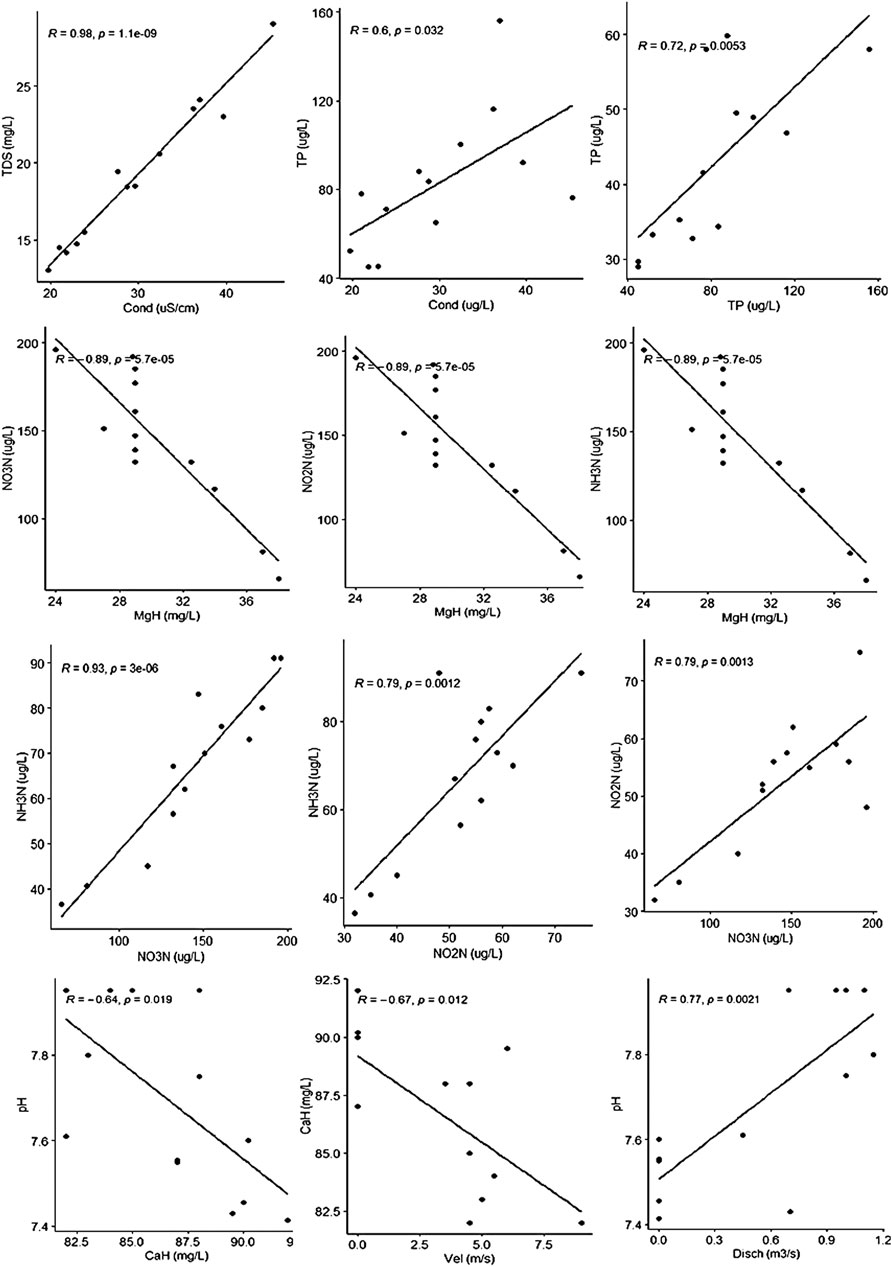
FIGURE 7. Correlation plots of various physicochemical parameters of water samples from the investigated sites (p ˂ 0.05).
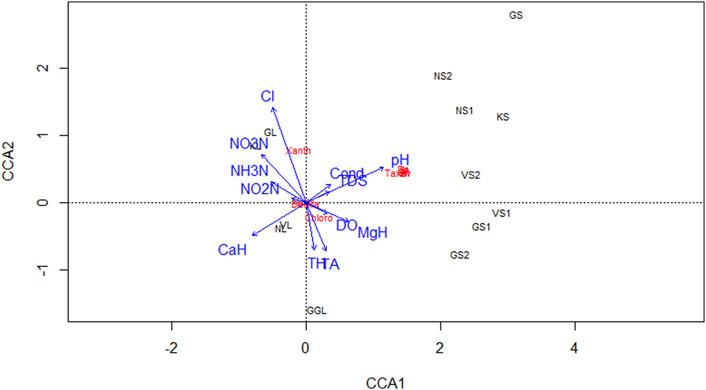
FIGURE 8. Canonical correspondence analysis (CCA) biplot, between physicochemical parameters and algal composition at HAL sites.
Discussion
Water quality of the lakes shows the variability in the behavior of ionic composition, nutrient dynamics, and catchment characteristics. pH values in all the HALs indicates the alkaline character, the results are in agreement with the work carried out on some other HALs of Kashmir valley Zutshi (1991) and Nepal region (Gurung et al., 2018). pH regulates the weathering processes and dissolved solids concentration in lake waters (Anshumali and Ramanathan, 2007). The variation in pH between different sites depends mainly on glacier melt water quality, and terrain (Deka et al., 2015), and biological processes such as photosynthesis and respiration (Hamid et el., 2020). EC is the assessment of the ionic potential and mainly depends on the magnitude and rate of movement of ionic species (Das and Kaur, 2001; Deka et al., 2015). Furthermore, EC indicates biotic and abiotic contamination in the water (Upadhyay et al., 2012; Sharma and Tiwari, 2018). EC varied from 15 to 53 μS/ cm, and was found to decrease with increase in the altitude, the results are in consonance with the findings of Zutshi et al. (1980), Sharma and Tiwari (2018) and Gurung et al. (2018). Lower values of EC of HALs could be credited to the lower values of all major ions. Ca2+ and Mg2+ ions were found to be the major dominant cations, while HCO32- was the major dominant anion in all the HALs. Ca2+ and Mg2+ ions contribute 88% of total cationic composition while as HCO32- contribute 70% of total anionic composition. The cations in decreasing order of concentration were Ca2+ > Mg2+ > Na+ > K+, while as anions in decreasing order of concentration were HCO32− > Cl− > SO42−. The higher concentration of Ca2+, Mg2+, and HCO32- suggests that weathering of carbonate rocks in the catchment areas is the major source of ions in these systems (Singh et al., 2016; Lone et al., 2019; Kaphle et al., 2021). Most of the Indian mountain lakes and glaciers also highlight that carbonate weathering is dominant process in these areas (Das and Kaur, 2001; Anshumali and Ramanathan, 2007; Singh et al., 2016). Ionic composition in freshwaters is mainly controlled by weathering process, atmospheric precipitation, and other existing atmospheric processes (Lacoul and Freedman, 2005; Anshumali and Ramanathan, 2007; Kaphle et al., 2021; Khadka and Ramanathan, 2021). Ca2+ is globally dominant cation in fresh water bodies (Wetzel, 2001; Gurung et al., 2018), but Mg2+ has also a significant role in defining water chemistry of lakes. The main source of these cations is the erosion of rocks (limestone and dolomite) and minerals (calcite and magnesite) (Hamid et al., 2020). The current study depicted that Mg-H is a dominant factor for clustering and categorizing the sites like Nundkul Lake and stream sites due to its higher values. Inorganic nitrogen forms (NO3−-N, NO2−-N, and NH3-N), TP and PO4 throughout the lakes were determining factors responsible for clustering and variability among all the sites. The values obtained during the study for NO3− and ammonium (NH4+) may be attributed to the hydrology of area which is snow-melt dominated and lead to seasonal spikes in the concentration (Campbell et al., 2000). Himalayan high altitude lake ecosystems are mostly phosphorus limited as the concentration of nitrogen is higher than that of phosphorus (Gurung et al., 2020). The concentrations of phosphorus in our study highlights that these lake ecosystems are phosphorus limited. The excellent WQI measured at all the sites may be attributed to their proximity to the water source, and less anthropogenic pressure (Yaseen et al., 2015). TDS is measurement of all the dissolved particles including organic as well as inorganic substances, TDS was positively correlated with EC and TP. This highlights that the concentration of TP increases with the concentration of TDS and EC. Concentration of TDS, EC, and TP mainly depends on surface runoff and sediment deposition in these lakes from the catchment area (Sharma and Tiwari 2018). All the inorganic forms of nitrogen (NO2−-N, NO3−-N, and NH3-N) were negatively correlated with the Mg-H. Ca-H was negatively correlated with stream velocity and pH at all the studied sites. Alpine stream outlets of all these lakes were having higher pH as evident from the correlation plot which shows discharge has a significant positive correlation with pH.
Land use changes directly influences the quality of freshwater ecosystems, particularly by enhancing the concentration of nutrient materials (Shi et al., 2017). Any alteration in the land cover of the watershed is considered as explanatory parameter behind the alterations of the hydrological unit, which ultimately changes runoff and water (Huang et al., 2013). The concentration of nutrients in the lake ecosystems are attributed to natural processes like heavy rainfall, soil erosion, and the runoff from the catchment areas which is laden with the fecal matter livestock (Kumar et al., 2019; Gurung et al., 2020). Same observations were recorded in the Sheshnag, a HAL in Kashmir Himalaya (Zutshi, 1991).
Bathymetric and morphometric analysis revealed that all the lakes are dammed glacier lakes, positioned in an asymmetrical basin. The lakes are deep because the lakes are formed in deep gorge like structures covered on all sides by the mountains and the water levels in these alpine systems are increasing presently, because of high melting of snow and glaciers in the catchment areas. The shoreline is gentle on the southern and eastern sides, but steep on the western and northern sides. Most of the shoreline portion in the lakes has no vegetation. A visual observation of the water markings in the riparian region of the lakes designates a seasonal water level fluctuation of 1–2 m as witnessed during sampling/field survey. The increase and changes in lake levels are in consonance with the results of Shekhar et al. (2010) and Srivastava et al. (2013) they attributed lake level fluctuations to seasonal snow and glacier melting due to increased air temperature in the northwestern Himalaya.
The land cover in the catchment of the lakes is dominated with meadows and pastures, glaciers, boulders/rocks, and eroding debris. Steep and eroding mountain slopes form the boundary of the lakes. Pastures are mainly covered by grasses and herbs are extensively used for grazing and browsing activities. There are bare land areas categorized as rocky in the catchment area. The Lakes are mainly fed by snow and glaciers which cover an area of 1922.31 ha. Forest area mainly comprises of Pinus, deodar, other herbs, and shrubby vegetation. There is also heavy grazing from July-September in the catchment area of the lakes.
Alpine lakes are remote and extremely sensitive ecosystems inhabited by scarce but well adapted species (Pastorino et al., 2020a). These lake ecosystems are characterized by small growing seasons, lower temperatures, longer ice periods, extended snow cover, higher elevations, and strong ultra-violet radiations. Small variations in these characteristics results in strong reactions of biodiversity, community structure, and productivity of alpine lake phototrophs, therefore forming planktonic biota sensitive senitals of minute environmental variations (Moser et al., 2019; Kuefner et al., 2021). Some studies on diversity and assemblage pattern of phytoplankton in HALs of Kashmir had been carried out by (Hutchinson, 1937); (Loffler, 1969); (Zutshi et al., 1972). As pollution is not the dominant factor in our study, altitude is one of the important parameters which structures the planktonic composition. The altitude determines its surface freezing duration (Pourriot et al., 1995), which may impact lake biota and thus diatom species composition. As only few diatomic species are able to survive in these extreme habitats, may explain to some extent the low diversity reported in this study (Lotter and Bigler, 2000). The low diversity is normally attributed to low productivity of the lakes and harsh environmental conditions Cartuche et al. (2019) but equally the number of samplings conducted may also have role to play in underestimation of the diversity. The overall phytoplankton composition and abundance suggest oligotrophic status of these lakes (Kumar et al., 2012). Low temperatures together with the low conversion efficiency of solar energy by phytoplankton have been observed to be responsible for low productivity elsewhere (Talling, 1965; Khan, 1986). One of the prominent characteristics of phytoplankton and periphyton assemblages of the HALs is the dominance of Bacillariophyceae (Shah et al., 2014; Bashir et al., 2020) in lakes and wetlands. This may be attributed to their ability to get adapted to lower temperatures than other algae (Lürling et al., 2013). Nutrient enrichment significantly alters the structure and functions of primary producers. Anthropogenic activities have been observed to influence on algal composition (diversity and density pattern) and biomass trends (Dubey and Dutta, 2020). The fallout of the grazing and tourist activity which currently is very low needs to be taken care of on long-term basis to protect and safeguard the environmental quality of these important lakes.
Conclusion
The range of physico-chemical parameters and water quality index calculated after accumulating various hydro-biological parameters corroborate the excellent water quality of the investigated HALs. Bathymetric analysis revealed that all the lakes are deep with Gangbal Lake attaining a maximum depth of 84 m. The catchment of the lakes is dominated by pastures and the area undergoes grazing during summers which to some extent affects the nutrient concentration of the lakes. Phytoplankton being dominated by Bacilariophyceae would require more in-depth studies to reach some clear conclusion on diversity and productivity of such lakes. We anticipate the increasing grazing activity and the renewed tourist attraction towards these lakes can add to water quality degradation which needs attention of the concerned agencies at an early stage.
Data Availability Statement
The original contributions presented in the study are included in the article/Supplementary Materials, further inquiries can be directed to the corresponding author.
Author Contributions
SI, SD, MS, and SUB conceived, designed, and executed this research work. SI, SD, MS, SUB, AJ, AB carried out the field sampling while SI, SD, MS, IS, and AH carried out the analysis of the samples in the laboratory. SI, SD, and MS wrote the original manuscript draft reviewed and edited by SUB. All the authors at the end read the manuscript and approved the same for its submission for publication.
Conflict of Interest
The authors declare that the research was conducted in the absence of any commercial or financial relationships that could be construed as a potential conflict of interest.
Publisher’s Note
All claims expressed in this article are solely those of the authors and do not necessarily represent those of their affiliated organizations, or those of the publisher, the editors and the reviewers. Any product that may be evaluated in this article, or claim that may be made by its manufacturer, is not guaranteed or endorsed by the publisher.
Acknowledgments
The authors would like to thank Head, Department of Environmental Science, University of Kashmir, Hazratbal Srinagar for providing logistics and laboratory facilities for carrying out this study. We acknowledge the support and company of Suhail Ahmad Bhat and pony wallas without which the sampling could not have been possible.
Supplementary Material
The Supplementary Material for this article can be found online at: https://www.frontiersin.org/articles/10.3389/fenvs.2021.681965/full#supplementary-material
References
Ahmed, A., Qayoom, U., Sabha, I., Islam, S. T., and Bhat, S. U. (2017). An Assessment of Phytoplankton Communities of Various Freshwater Habitats of Gulmarg Area in Kashmir Valley. J. Himalayan Ecol. Sustain. Dev. 12, 89–99. Available at: http://envirsc.uok.edu.in/Main/JournalVolume.aspx?V=2017V12.
American Public Health Association (APHA) (2017). Standard Methods for the Examination of Water and Waste Water. 23rd ed. Washington, USA.
Anshumali, Ramanathan. A. L., and Ramanathan, A. L. (2007). Seasonal Variation in the Major Ion Chemistry of Pandoh Lake, Mandi District, Himachal Pradesh, India. Appl. Geochem. 22, 1736–1747. doi:10.1016/j.apgeochem.2007.03.045
Bashir, T., Islam, S. T., and Bhat, S. U. (2020). Phytoplankton Dynamics at Diverse Depths in the Nigeen Lake of Srinagar City, Kashmir Himalaya. J. Himalayan Ecol. Sustain. Dev. 15, 1–30. Available at: https://envirsc.uok.edu.in/Main/JournalVolume.aspx?V=2020V15\.
Battarbee, R. W., Kernan, M., and Rose, N. (2009). Threatened and Stressed mountain lakes of Europe: Assessment and Progress. Aquat. Ecosystem Health Manag. 12, 118–128. doi:10.1080/14634980902905742
Biggs, B. J. F., and Kilroy, C. (2000). Stream Periphyton Monitoring Manual. The New Zealand Ministry for the Environment. Christchurch: NIWA.
Borics, G., Abonyi, A., Salmaso, N., and Ptacnik, R. (2021). Freshwater Phytoplankton Diversity: Models, Drivers and Implications for Ecosystem Properties. Hydrobiologia 848, 53–75. doi:10.1007/s10750-020-04332-9
WWF (2009). “Sacred Waters – Cultural Values of Himalayan Wetlands,”. Editors B. S. Dongol, and K. Shrestha (Nepal: WWF). ISBN:987-9937-8154-1-3.
Campbell, D. H., Baron, J. S., Tonnessen, K. A., Brooks, P. D., and Schuster, P. F. (2000). Controls on Nitrogen Flux in alpine/subalpine Watersheds of Colorado. Water Resour. Res. 36 (1), 37–47. doi:10.1029/1999WR900283
Cartuche, A., Guan, Z., Ibelings, B. W., and Venail, P. (2019). Phytoplankton Diversity Relates Negatively with Productivity in Tropical High-Altitude Lakes from Southern Ecuador. Sustainability 11 (19), 5235. doi:10.3390/su1119523510.3390/su11195235
Chatterjee, A., Blom, E., Gujja, B., Jacimovic, R., Beevers, L., O'Keeffe, J., et al. (2010). WWF Initiatives to Study the Impact of Climate Change on Himalayan High-Altitude Wetlands (HAWs). Mountain Res. Dev. 30, 42–52. doi:10.1659/MRD-JOURNAL-D-09-00091.1
Cox, E. J. (1996). Identification of Freshwater Diatoms from Live Material. Ist Edition. London, U.K: Chapman & Hall.
Daly, G. L., and Wania, F. (2005). Organic Contaminants in Mountains. Environ. Sci. Technol. 39, 385–398. doi:10.1021/es048859u
Dar, S. A., Bhat, S. U., Rashid, I., and Dar, S. A. (2020a). Current Status of Wetlands in Srinagar City: Threats, Management Strategies, and Future Perspectives. Front. Environ. Sci. 7, 1–11. doi:10.3389/fenvs.2019.00199
Dar, S. A., Bhat, S. U., Aneaus, S., and Rashid, I. (2020b). A Geospatial Approach for Limnological Characterization of Nigeen Lake, Kashmir Himalaya. Environ. Monit. Assess. 192, 1–18. doi:10.1007/s10661-020-8091-y
Dar, S. A., Bhat, S. U., and Rashid, I. (2021a). “The Status of Current Knowledge, Distribution and Conservation Challenges of Wetland Ecosystems in Kashmir Himalaya, India,” in Wetlands Conservation. Editors S. Sharma, and P. Singh doi:10.1002/9781119692621.ch10
Dar, S. A., Rashid, I., and Bhat, S. U. (2021b). Land System Transformations Govern the Trophic Status of an Urban Wetland Ecosystem: Perspectives from Remote Sensing and Water Quality Analysis. Land Degrad. Dev. 32, 4087–4104. doi:10.1002/ldr.3924
Dar, S. A., Bhat, S. U., and Rashid, I. (2021c). Landscape Transformations, Morphometry and Water Quality of Anchar Wetland in Kashmir Himalaya, India: Implications for Urban Wetland Management. Water Air Soil Pollut. (In Press).
Dar, S. A., Rashid, I., and Bhat, S. U. (2021d). Linking Land System Changes (1980-2017) with the Trophic Status of an Urban Wetland Using Remote Sensing and Water Quality Analysis. Environ. Monit. Assess. (In Press).
Dar, S. A., Hamid, I., Rashid, I., and Bhat, S. U. (2021e). Identification of Anthropogenic Contribution to Wetland Degradation: Insights from the Environmetric Techniques. Stoch. Environ. Res. Risk Assess. (In Press).
Das, B., and Kaur, P. (2001). Major Ion Chemistry of Renuka Lake and Weathering Processes, Sirmaur District, Himachal Pradesh, India. Environ. Geology. 40, 908–917. doi:10.1007/s002540100268
Deka, J. P., Tayeng, G., Singh, S., Hoque, R. R., Prakash, A., and Kumar, M. (2015). Source and Seasonal Variation in the Major Ion Chemistry of Two Eastern Himalayan High Altitude Lakes, India. Arab. J. Geosci. 8, 10597–10610. doi:10.1007/s12517-015-1964-7
Devi, M. B., Gupta, S., and Das, T. (2016). Phytoplankton Community of Lake Baskandi Anua, Cachar District, Assam, North East India - an Ecological Study. Knowl. Manag. Aquat. Ecosyst. 417, 2–9. doi:10.1051/kmae/2015034
Dubey, D., and Dutta, V. (2020). “Nutrient Enrichment in Lake Ecosystem and its Effects on Algae and Macrophytes,” in Environmental Concerns and Sustainable Development. Editors V. Shukla, and N. Kumar (Singapore: Springer), 81–126. doi:10.1007/978-981-13-6358-0_5
Edmondson, W. T. (1992). Ward and Whipple’s Fresh Water Biology. 2nd ed. New Delhi: International Books and Periodicals Supply Service.
Elser, J. J., Wu, C., González, A. L., Shain, D. H., Smith, H. J., Sommaruga, R., et al. (2020). Key Rules of Life and the Fading Cryosphere: Impacts in alpine Lakes and Streams. Glob. Change Biol. 26 (12), 6644–6656. doi:10.1111/gcb.15362
Evelyn Hutchinson, G. (1937). Limnological Studies in Indian Tibet. Int. Revue Ges. Hydrobiol. Hydrogr.Gesamten Hydrobiol 35, 134–177. doi:10.1002/iroh.19370350110
Farooq, H., Farooq, J., Bhat, S. A., Sofi, M. S., Qayoom, U., and Islam, S. T. (2019). Hydrobiological Study of the Panner Water Reservoir. TralKashmir.J. Himalayan Ecol. Sustain. Dev. 14, 1–18. Available at: http://envirsc.uok.edu.in/Main/JournalVolume.aspx?V=2019V14.
Gao, H., Zhang, S., Zhao, R., and Zhu, L. (2018). Plankton Community Structure Analysis and Water Quality Bioassessment in Jiulong Lake. IOP Conf. Ser. Earth Environ. Sci. 199, 022031. doi:10.1088/1755-1315/199/2/022031
Gurung, S., Gurung, A., Sharma, C. M., Jüttner, I., Tripathee, L., Bajracharya, R. M., et al. (2018). Hydrochemistry of Lake Rara: A High mountain lake in Western Nepal. Lakes Reserv Res. Manage. 23 (2), 87–97. doi:10.1111/lre.12218
Gurung, S., Tripathee, L., Wang, X., Paudyal, R., Bhatta, R., and Sharma, C. M. (2020). “Nutrients and Organic Carbons in lake Waters of the Third Pole,” in Water Quality in the Third Pole (Elesvier), 261–285. doi:10.1016/B978-0-12-816489-1.00008-6
Hamid, A., Bhat, S. U., and Jehangir, A. (2020). Local Determinants Influencing Stream Water Quality. Appl. Water Sci. 10 (1), 1–16. doi:10.1007/s13201-019-1043-4
Hammer, O., Harper, D. A., and Ryan, P. D. (2001). PAST: Paleontological Statistics Software Package for Education and Data Analysis. Palaeontol. electronica 4 (1), 9. Available at: https://palaeo-electronica.org/2001_1/past/past.pdf.
Hardin, G. (1960). The Competitive Exclusion Principle. Science 131, 1292–1297. doi:10.1126/science.131.3409.1292
Huang, J., Zhan, J., Yan, H., Wu, F., and Deng, X. (2013). Evaluation of the Impacts of Land Use on Water Quality: a Case Study in the Chaohu Lake basin. Scientific World J. 2013, 1–7. doi:10.1155/2013/329187
Hutchinson, G. E. (1961). The Paradox of the Plankton. The Am. Naturalist 95, 137–145. doi:10.1086/282171
Jumppanen, K. (1976). Effects of Waste Waters on a lake Ecosystem. AnnalesZoologiciFennici 13, 85–138.
Kaphle, B., Wang, J.-b., Kai, J.-l., Lyu, X.-m., Paudayal, K. N., and Adhikari, S. (2021). Hydrochemistry of Rara Lake: A Ramsar lake from the Southern Slope of the central Himalayas, Nepal. J. Mt. Sci. 18, 141–158. doi:10.1007/s11629-019-5910-0
Khadka, U. R., and Ramanathan, A. L. (2021). Hydrogeochemical Analysis of Phewa Lake: A Lesser Himalayan Lake in the Pokhara Valley, Nepal. Environ.Nat.Resour.j. 19, 68–83. doi:10.32526/ennrj/19/2020083
Khan, M. A. (1986). Hydrobiology and Organic Production in a Marl lake of Kashmir Himalayan Valley. Hydrobiologia 135, 233–242. doi:10.1007/bf00006535
Khanday, S. A., Bhat, S. U., Islam, S. T., and Sabha, I. (2021). Identifying Lithogenic and Anthropogenic Factors Responsible for Spatio-Seasonal Patterns and Quality Evaluation of Snow Melt Waters of the River Jhelum Basin in Kashmir Himalaya. Catena 196, 104853. doi:10.1016/j.catena.2020.104853
Khanday, S. A., Romshoo, S. A., Jehangir, A., Sahay, A., and Chauhan, P. (2018). Environmetric and GIS Techniques for Hydrochemical Characterization of the Dal Lake, Kashmir Himalaya, India. Stoch Environ. Res. Risk Assess. 32, 3151–3168. doi:10.1007/s00477-018-1581-6
Kuefner, W., Hofmann, A. M., Geist, J., Dubois, N., and Raeder, U. (2021). Algal Community Change in Mountain Lakes of the Alps Reveals Effects of Climate Warming and Shifting Treelines 1. J. Phycol. 57, 1266–1283. doi:10.1111/jpy.13163
Kumar, P., Wanganeo, A., Sonaullah, F., and Wanganeo, R. (2012). Limnological Study on Two High Altitude Himalayan Ponds, Badrinath, Uttarakhand. Ije 2, 103–111. doi:10.5923/j.ije.20120205.04
Kumar, R., Singh, S., and Sharma, R. C. (2019). Application of WQI for Assessment of Water Quality of High Altitude lake Dodi Tal, Garhwal Himalaya, India. Sustain. Water Resour. Manag. 5 (3), 1033–1042. doi:10.1007/s40899-018-0281-1
Kumari, R., and Sharma, R. C. (2019). Assessment of Water Quality index and Multivariate Analysis of High Altitude Sacred Lake Prashar, Himachal Pradesh, India. Int. J. Environ. Sci. Technol. 16, 6125–6134. doi:10.1007/s13762-018-2007-1
Lacoul, P., and Freedman, B. (2005). Physical and Chemical Limnology of 34 Lentic Waterbodies along a Tropical-To-Alpine Altitudinal Gradient in Nepal. Internat. Rev. Hydrobiol. 90, 254–276. doi:10.1002/iroh.200410766
Ledoux, H., and Gold, C. (2005). Developments in Spatial Data Handling. Berlin, Heidelberg: Springer, 97–108. doi:10.1007/3-540-26772-7_8An Efficient Natural Neighbour Interpolation Algorithm for Geoscientific Modelling
Löffler, H. (1969). High Altitude Lakes in Mt. Everest Region. SIL Proc. 1922-2010 17, 373–385. doi:10.1080/03680770.1968.11895862
Lone, A., Jeelani, G., Deshpande, R. D., Kang, S., and Huang, J. (2019). Hydrochemical Assessment (Major Ions and Hg) of Meltwater in High Altitude Glacierized Himalayan Catchment. Environ. Monit. Assess. 191 (4), 1–19. doi:10.1007/s10661-019-7338-y
Lotter, A. F., and Bigler, C. (2000). Do diatoms in the Swiss Alps Reflect the Length of Ice-Cover? Aquat. Sci. 62, 125–141. doi:10.1007/s000270050002
Lürling, M., Eshetu, F., Faassen, E. J., Kosten, S., and Huszar, V. L. M. (2013). Comparison of Cyanobacterial and green Algal Growth Rates at Different Temperatures. Freshw. Biol. 58, 552–559. doi:10.1111/j.1365-2427.2012.02866.x
Monti, R., and Stella, E. (1934). Illago di Molveno. La vita in unlagozootrofo. Mem. Mus. St. Nat. Ven. Trident. 2, 69–101.
Moser, K. A., Baron, J. S., Brahney, J., Oleksy, I. A., Saros, J. E., Hundey, E. J., et al. (2019). Mountain lakes: Eyes on Global Environmental Change. Glob. Planet. Change 178, 77–95. doi:10.1016/j.gloplacha.2019.04.001
Naumann, E. (1924). Einige allgemeine Gesichtspunkte betreffs des Studiums der regionalen Limnologie. SIL Proc. 1922-2010 2, 100–110. doi:10.1080/03680770.1924.11898298
Nissa, M., and Bhat, S. U. (2015). An Assessment of Phytoplankton in Nigeen Lake of Kashmir Himalaya. Asian J. Biol. Sci. 9, 27–40. doi:10.3923/ajbs.2016.27.40
O. Khattab, M. F., Abo, R. K., Al-Muqdadi, S. W., and Merkel, B. J. (2017). Generate Reservoir Depths Mapping by Using Digital Elevation Model: a Case Study of Mosul Dam lake, Northern Iraq. Ars 06, 161–174. doi:10.4236/ars.2017.63012
Parvez, S., and Bhat, S. U. (2014). Searching for Water Quality Improvement in Dal lake, Srinagar, Kashmir. J. Himalayan Ecol. Sustain. Dev. 9, 51–64. Available at: http://envirsc.uok.edu.in/Files/ab1ac1f1-07e3-42a2-85bc-83717ef39155/Custom/Paper%206.pdf.
Pastorino, P., Pizzul, E., Bertoli, M., Perilli, S., Brizio, P., Salvi, G., et al. (2020). Macrobenthic Invertebrates as Bioindicators of Trace Elements in High-mountain lakes. Environ. Sci. Pollut. Res. 27 (6), 5958–5970. doi:10.1007/s11356-019-07325-x
Pastorino, P., Polazzo, F., Bertoli, M., Santi, M., Righetti, M., Pizzul, E., et al. (2020). Consequences of Fish Introduction in Fishless Alpine Lakes: Preliminary Notes from a Sanitary point of View. Turk. J. Fish. Aquat. Sci. 20, 1–8. doi:10.4194/1303-2712-v20_1_01
Pastorino, P., Prearo, M., Bertoli, M., Menconi, V., Esposito, G., Righetti, M., et al. (2020). Assessment of Biological and Sanitary Condition of Alien Fish from a High-Mountain Lake (Cottian Alps). Water 12 (2), 559. doi:10.3390/w12020559
Pastorino, P., and Prearo, M. (2020). High-Mountain Lakes, Indicators of Global Change: Ecological Characterization and Environmental Pressures. Diversity 12, 260–265. doi:10.3390/d12060260
Ramanathan, A. L. (2007). Seasonal Variation in the Major Ion Chemistry of Pandoh Lake, Mandi District, Himachal Pradesh, India. Appl. Geochem. 22, 1736–1747. doi:10.1016/j.apgeochem.2007.03.045
Rashid, I., Romshoo, S. A., Amin, M., Khanday, S. A., and Chauhan, P. (2017a). Linking Human-Biophysical Interactions with the Trophic Status of Dal Lake, Kashmir Himalaya, India. Limnologica 62, 84–96. doi:10.1016/j.limno.2016.11.008
Rashid, I., Romshoo, S. A., Hajam, J. A., and Abdullah, T. (2016). A Semi-automated Approach for Mapping Geomorphology in Mountainous Terrain, Ferozpora Watershed (Kashmir Himalaya). J. Geol. Soc. India. 88, 206–212. doi:10.1007/s12594-016-0479-5
Raut, R., Sharma, S., Bajracharya, R. M., Sharma, C. M., and Gurung, S. (2012). Physico-chemical Characterization of Gosainkunda Lake. Nepal J. Sci. Tech. 13, 107–114. doi:10.3126/njst.v13i1.7449
Roberts, D. W. (2016). Labdsv: Ordination and Multivariate Analysis for Ecology. R Package Version 1.8-0. Available online at https://CRAN.R-project.org/package=labdsv.
Rodhe, W. (1962). Sulla produzione di fitoplancton in laghitrasparenti di altamontagna. Mem. Ist. Ital. Idrobiol 15, 21–28.
Rogora, M., Frate, L., Carranza, M. L., Freppaz, M., Stanisci, A., Bertani, I., et al. (2018). Assessment of Climate Change Effects on Mountain Ecosystems through a Cross-Site Analysis in the Alps and Apennines. Sci. Total Environ. 624, 1429–1442. doi:10.1016/j.scitotenv.2017.12.155
Rogora, M., Massaferro, J., Marchetto, A., Tartari, G., and Mosello, R. (2008). The Water Chemistry of Some Shallow Lakes in Northern Patagonia and Their Nitrogen Status in Comparison with Remote Lakes in Different Regions of the globe. J. Limnol. 67, 75–86. doi:10.4081/jlimnol.2008.75
Romshoo, S. A., and Muslim, M. (2011). Geospatial Modeling for Assessing the Nutrient Load of a Himalayan lake. Environ. Earth Sci. 64, 1269–1282. doi:10.1007/s12665-011-0944-9
R. Pourriot, M. Meybeck, P. Champ, and J. Arcady-Meyer (1995). in Limnologiegénérale (Paris: Masson), 965. ISBN 2-225-84687-1.
Rupakheti, D., Tripathee, L., Kang, S., Sharma, C. M., Paudyal, R., and Sillanpää, M. (2017). Assessment of Water Quality and Health Risks for Toxic Trace Elements in Urban Phewa and Remote Gosainkunda Lakes, Nepal. Hum. Ecol. Risk Assess. Int. J. 23, 959–973. doi:10.1080/10807039.2017.1292117
SAC (2011). High Altitude Himalayan Lakes. National Wetland Inventory and Assessment. Ahmedabad: Space Application Centre, Indian Space Research Organisation.
Saini, R. K., Swain, S., Patra, A., Khanday, G. J., Gupta, H., Purushothaman, P., et al. (2008). Water Chemistry of Three Himalayan Lakes: Dal (Jammu & Kashmir), Khajjiar (Himachal Pradesh) and Nainital (Uttarakhand). Himalayan Geology. 29 (1), 63–72.
Sánchez, E., Colmenarejo, M. F., Vicente, J., Rubio, A., García, M. G., Travieso, L., et al. (2007). Use of the Water Quality index and Dissolved Oxygen Deficit as Simple Indicators of Watersheds Pollutionficit as Simple Indicators of Basins Pollution. Ecol. Indicators 7, 315–328. doi:10.1016/j.ecolind.2006.02.005
Schindler, D. W., and Smol, J. P. (2006). Cumulative Effects of Climate Warming and Other Human Activities on Freshwaters of Arctic and Subarctic North America. Ambio 35, 160–168. doi:10.1579/0044-7447(2006)35[160:ceocwa]2.0.co;2
Sener, S., Sener, E., and Davraz, A. (2017). Evaluation of Water Quality Using Water Quality index (WQI) Method and GIS in Aksu River (SW-Turkey). Sci. Total Environ. 584-585, 131–144.
Shah, J. A., Wanganeo, R., Pandit, A. K., and Farooq, S. (2014). Periphytic Algal Community of Dal Lake in Kashmir Valley, India. Res. J. Environ. Sci. 8, 391–398. doi:10.3923/rjes.2014.391.398
Sharma, R. C., and Tiwari, V. (2018). Seasonal Physico-Chemical Characterization of Water of Sacred lake Nachiketa Tal, Garhwal Himalaya. Appl. Water Sci. 8, 1–9. doi:10.1007/s13201-018-0802-y
Sheikh, J. A., Jeelani, G., Gavali, R. S., and Shah, R. A. (2014). Weathering and Anthropogenic Influences on the Water and Sediment Chemistry of Wular Lake, Kashmir Himalaya. Environ. Earth Sci. 71 (6), 2837–2846. doi:10.1007/s12665-013-2661-z
Shekhar, M. S., Chand, H., Kumar, S., Srinivasan, K., and Ganju, A. (2010). Climate-change Studies in the Western Himalaya. Ann. Glaciol. 51, 105–112. doi:10.3189/172756410791386508
Shi, P., Zhang, Y., Li, Z., Li, P., and Xu, G. (2017). Influence of Land Use and Land Cover Patterns on Seasonal Water Quality at Multi-Spatial Scales. Catena 151, 182–190. doi:10.1016/J.CATENA.2016.12.017
Singh, O., Rai, S. P., Kumar, V., Sharma, M. K., and Choubey, V. K. (2008). “Water Quality and Eutrophication Status of Some Lakes of the Western Himalayan Region (India),” in Proceedings of Taal 2007: The 12th World Lake Conference (Jaipur, Rajasthan: World Lake Conference), 286–291.
Singh, V. B., Ramanathan, A., and Mandal, A. (2016). Hydrogeochemistry of High-Altitude lake: a Case Study of the Chandra Tal, Western Himalaya, India. Arab. J. Geosci. 9, 1–8. doi:10.1007/s12517-016-2358-1
Srivastava, P., Bhambri, R., Kawishwar, P., and Dobhal, D. P. (2013). Water Level Changes of High Altitude Lakes in Himalaya-Karakoram from ICESat Altimetry. J. Earth Syst. Sci. 122, 1533–1543. doi:10.1007/s12040-013-0364-1
Steingruber, S., and Colombo, L. (2006). Impacts of Air Pollution on Alpine Lakes and Rivers. Chemistry and Biology in Alpine Lakes and Rivers in Southern Switzerland Related to Acidification from Long-Range Transboundary Air Pollution: Monitoring Results from 1980–2004. Bern, Switzerland: Federal Office for the Environment. Environmental Studies No. UW-0619. (74).
Talling, J. F. (1965). The Photosynthetic Activity of Phytoplankton in East African Lakes. Int. Revue Ges. Hydrobiol. Hydrogr. 50, 1–32. doi:10.1002/iroh.19650500102
Tiberti, R., Buscaglia, F., Armodi, M., Callieri, C., Ribelli, F., Rogora, M., et al. (2019). Mountain lakes of Mont Avic Natural Park: Ecological Features and Conservation Issues. J. Limnol. 79, 43–58. doi:10.4081/jlimnol.2019.1923
Tiberti, R., von Hardenberg, A., and Bogliani, G. (2014). Ecological Impact of Introduced Fish in High Altitude Lakes: A Case of Study from the European Alps. Hydrobiologia 724, 1–19. doi:10.1007/s10750-013-1696-1
Tolotti, M., Manca, M., Angeli, N., Morabito, G., Thaler, B., Rott, E., et al. (2006). Phytoplankton and Zooplankton Associations in a Set of Alpine High Altitude Lakes: Geographic Distribution and Ecology. Hydrobiologia 562, 99–122. doi:10.1007/s10750-005-1807-8
UNCSD (2012). Report of the United Nations Conference on sustainable development, Rio de Janeiro, Brazil 20–- 22 June, 2012. New York: United Nations Digital Library, 41–42.
Upadhyay, R., Pandey, A. K., Upadhyay, S. K., Bassin, J. K., and Misra, S. M. (2012). Limnochemistry and Nutrient Dynamics in Upper Lake, Bhopal, India. Environ. Monit. Assess. 184, 7065–7077. doi:10.1007/s10661-011-2480-1
Walsh, P. J., and Milon, J. W. (2016). Nutrient Standards, Water Quality Indicators, and Economic Benefits from Water Quality Regulations. Environ. Resource Econ. 64, 643–661. doi:10.1007/s10640-015-9892-2
Wetzel, R. G. (2001). Limnology: Lake and River Ecosystems, 3rd Ed. (P. +xvi=1006). London, UK: Academic Press.
WWF (2005). An Overview of Glacial Retreat, and Subsequent Impacts in Nepal, India and China. Kathmandu, Nepal: World Wide Fund for Nature, 1–80.
Xing, P., Hahn, M. W., and Wu, Q. L. (2009). Low Taxon Richness of Bacterioplankton in High-Altitude Lakes of the Eastern Tibetan Plateau, with a Predominance of Bacteroidetes and Synechococcus Spp. Appl. Environ. Microbiol. 75, 7017–7025. doi:10.1128/AEM.01544-09
Yaseen, S., Pandit, A. K., and Shah, J. A. (2015). Water Quality index of Fresh Water Streams Feeding Wular Lake, in Kashmir Himalaya, India. Int. J. Water Res. Environ. Eng. 7 (4), 50–57. doi:10.5897/IJWREE2014.0557
Zutshi, D. P., Kaul, V., and Vass, K. K. (1972). Limnology of High Altitude Kashmir Lakes. SIL Proc. 1922-2010 18, 599–604. doi:10.1080/03680770.1972.11899514
Zutshi, D. P. (1991). Limnology of High Altitude Lakes of Himalayan Region. SIL Proc. 1922-2010 24, 1077–1080. doi:10.1080/03680770.1989.11898915
Zutshi, D. P., Subla, B. A., Khan, M. A., and Wanganeo, A. (1980). Comparative Limnology of Nine Lakes of Jammu and Kashmir Himalayas. Hydrobiologia 72, 101–112. doi:10.1007/BF00016238
Zutshi, D. P., and Vass, K. K. (1982). Limnological Studies on Dal Lake, Srinagar. III. Biological Features. Proc. Indian Natl. Sci. Acad. 48, 234–241. Available at: https://insa.nic.in/writereaddata/UpLoadedFiles/PINSA/Vol48B_1982_2_Art11.pdf.
Keywords: high altitude lakes, biodiversity, bathymetry, morphometry, Kashmir Himalaya
Citation: Islam ST, Dar SA, Sofi MS, Bhat SU, Sabha I, Hamid A, Jehangir A and Bhat AA (2021) Limnochemistry and Plankton Diversity in Some High Altitude Lakes of Kashmir Himalaya. Front. Environ. Sci. 9:681965. doi: 10.3389/fenvs.2021.681965
Received: 17 March 2021; Accepted: 25 August 2021;
Published: 04 October 2021.
Edited by:
Muhammad Hasan Ali Baig, Pir Mehr Ali Shah Arid Agriculture University Rawalpindi, PakistanReviewed by:
Reinaldo Luiz Bozelli, Federal University of Rio de Janeiro, BrazilGuoqing Zhang, Institute of Tibetan Plateau Research (CAS), China
Shanlong Lu, Aerospace Information Research Institute (CAS), China
Sher Muhammad, International Centre for Integrated Mountain Development, Nepal
Copyright © 2021 Islam, Dar, Sofi, Bhat, Sabha, Hamid, Jehangir and Bhat. This is an open-access article distributed under the terms of the Creative Commons Attribution License (CC BY). The use, distribution or reproduction in other forums is permitted, provided the original author(s) and the copyright owner(s) are credited and that the original publication in this journal is cited, in accordance with accepted academic practice. No use, distribution or reproduction is permitted which does not comply with these terms.
*Correspondence: Sami Ullah Bhat, samiullahbhat11@gmail.com